- 1Division of Radiotherapy and Imaging, The Institute of Cancer Research, London, United Kingdom
- 2Department of Cardiovascular and Thoracic Surgery, Albert Einstein College of Medicine, Montefiore Health System, Bronx, NY, United States
While P2X7 receptor expression on tumour cells has been characterized as a promotor of cancer growth and metastasis, its expression by the host immune system is central for orchestration of both innate and adaptive immune responses against cancer. The role of P2X7R in anti-tumour immunity is complex and preclinical studies have described opposing roles of the P2X7R in regulating immune responses against tumours. Therefore, few P2X7R modulators have reached clinical testing in cancer patients. Here, we review the prognostic value of P2X7R in cancer, how P2X7R have been targeted to date in tumour models, and we discuss four aspects of how tumours skew immune responses to promote immune escape via the P2X7R; non-pore functional P2X7Rs, mono-ADP-ribosyltransferases, ectonucleotidases, and immunoregulatory cells. Lastly, we discuss alternative approaches to offset tumour immune escape via P2X7R to enhance immunotherapeutic strategies in cancer patients.
Introduction
P2X receptors (P2XR) belong to the ligand-gated ion channel family of receptors (1) and are characteristically gated by extracellular adenosine triphosphate (ATP). In mammals, seven subunits are expressed (P2X1-7), and form homo-trimeric or hetero-trimeric structures with diverse pharmacological characteristics (2). Agonist binding sites are found between subunit interfaces consisting of mostly positively charged amino acids, where activation of the receptor induces the opening of a cation permeable channel (2). Among the P2X receptors, the P2X7R is the least sensitive to ATP with EC50 values in the mM range and is also the most slowly desensitizing (3). Here, binding of two ATP molecules causes the opening of the channel, with the subsequent permeation of Ca2+, Na+, and K+ ions, whereas the binding of the third ATP generates a dilation of the channel which is known also as the “macropore”, resulting in ATP-induced cell death (AICD) (3–6). Although previously well described in various physiological and pathophysiological states (7), recent interest in the P2X7R has risen because of its involvement in diverse inflammatory conditions, including cancer (8).
The P2X7R is widely expressed by cells of the immune system. Because of the high threshold of ATP needed to activate the receptor, which can be found in conditions of cellular damage, it is considered a sensor for immunogenic danger signalling or a damage-associated molecular pattern (DAMP) which can trigger recruitment of various activated immune cells to the site of tissue damage, or alternatively AICD depending on ATP concentration (9–13). However, ATP can also be released through regulated mechanisms and in this manner control diverse immune-cell functions in an autocrine/paracrine fashion. In fact, activation of T-cells induces ATP release through pannexin-1 (Panx1) channels stimulating in an autocrine manner P2X7R, prompting cytokine release and proliferation (14). Furthermore, P2X7R plays a role in T cell migration and egress from lymph nodes following activation and differentiation by promoting shedding of L-selectin and by paracrine regulation of T cell motility via the P2X7R (15–17).
Dendritic cells (DCs) respond to ATP stimuli via P2X7R by activating Panx1 and causing an autocrine signalling loop that results in maturation of DCs as well as promoting cellular migration to lymph nodes facilitated by upregulation of the chemokine receptors CCR7 and CXCR4 (18, 19). P2X7R-induced upregulation of co-stimulatory molecules through a nuclear factor kappa B (NF-kB)-dependent mechanism promotes helper T (Th) cell differentiation (20, 21). Other innate immune populations show a similar effect, including monocytes (22), macrophages (23), eosinophils and neutrophils, facilitating actin polymerisation for transendothelial migration (24, 25). Further, P2X7R activation of DCs and other myeloid cells can trigger NLRP3 inflammasome complex induction, associated with efflux of cellular potassium, influx of calcium, reactive oxygen species (ROS) generation, and mitochondria depolarization. Altogether, it leads to the release of the pro-immunogenic cytokines interleukin (IL)-1β and IL-18, eventually inducing pyroptosis, a highly immunogenic form of cell death which further propagates the inflammatory signal (26, 27).
The P2X7R influences cell plasticity, differentiation, and metabolic fitness of immune populations. Genetic ablation of the P2X7R in the LCMV Armstrong infection model showed a normal expansion of effector T cells. However, the central memory T cell (TCM) and tissue-resident memory T cell (TRM) subsets were affected and lacked long-lasting protection against future reinfections. This was suggested to be due in part to metabolic impairment, specifically, a lower mitochondrial mass and a compromised spare respiratory capacity (28, 29). On the other hand, reduced mitochondrial mass makes T cells from P2X7R-deficient mice less susceptible to cell senescence derived from P2X7 activation (30).
P2X7R in tumour immune escape
P2X7R is expressed in most solid and haematological cancers, and there is ample evidence that tumour cell expression of P2X7R promotes proliferation, metabolism and facilitates tumour invasion and metastatic dissemination (31–35). While P2X7R expression has also been proven essential for mounting effective anti-tumour responses, preclinical studies evaluating how P2X7R governs anti-tumour immune responses have shown widely different and sometimes contradicting outcomes (36, 37). Ghiringelli and colleagues showed that P2X7R expression is mandatory for DC-mediated sensing of immunogenic cell death following anti-cancer therapy (36). Adinolfi and colleagues confirmed the derogatory immune effect of P2X7R-deletion in tumour-bearing mice, in particular the reduced activity of DCs (37). Further, a study by De Marchi and colleagues revealed that genetic deletion of P2X7R had adverse effects on tumour-infiltrating T cells and promoted tumour growth while systemic P2X7R antagonism in the same tumour model benefited T effector cells and reduced tumour burden (38).
In the context of adoptive T cell therapy, two studies assessing how P2X7R-expression affects the performance and anti-tumour effectiveness of transferred CD8 T cells reached opposite conclusions. Romagnani et al. described that P2X7R-expressing tumour-infiltrating lymphocytes (TILs) display signs of cellular senescence while Wanhainen et al. showed that they have superior mitochondrial fitness, proliferation and apoptosis-resistance compared to P2RX7-deficient TILs (30, 39). A potential explanation for the discrepancy between the two studies was the use of different T cell culture conditions prior to infusion. Work from Koch-Nolte’s lab suggest that discrepancies in immune readouts from pre-clinical P2X7R studies may be explained by selection bias following NICD- and AICD-mediated elimination of P2X7R-expressing immune cells exposed to high concentrations of NAD+ and ATP respectively during tissue dissociation and cell preparation (40, 41).
While the picture of how P2X7R regulates anti-tumour immunity is complex, there are key emerging areas where tumours have been shown to skew P2X7R signalling to promote immune escape. These are summarized below and illustrated in Figure 1.
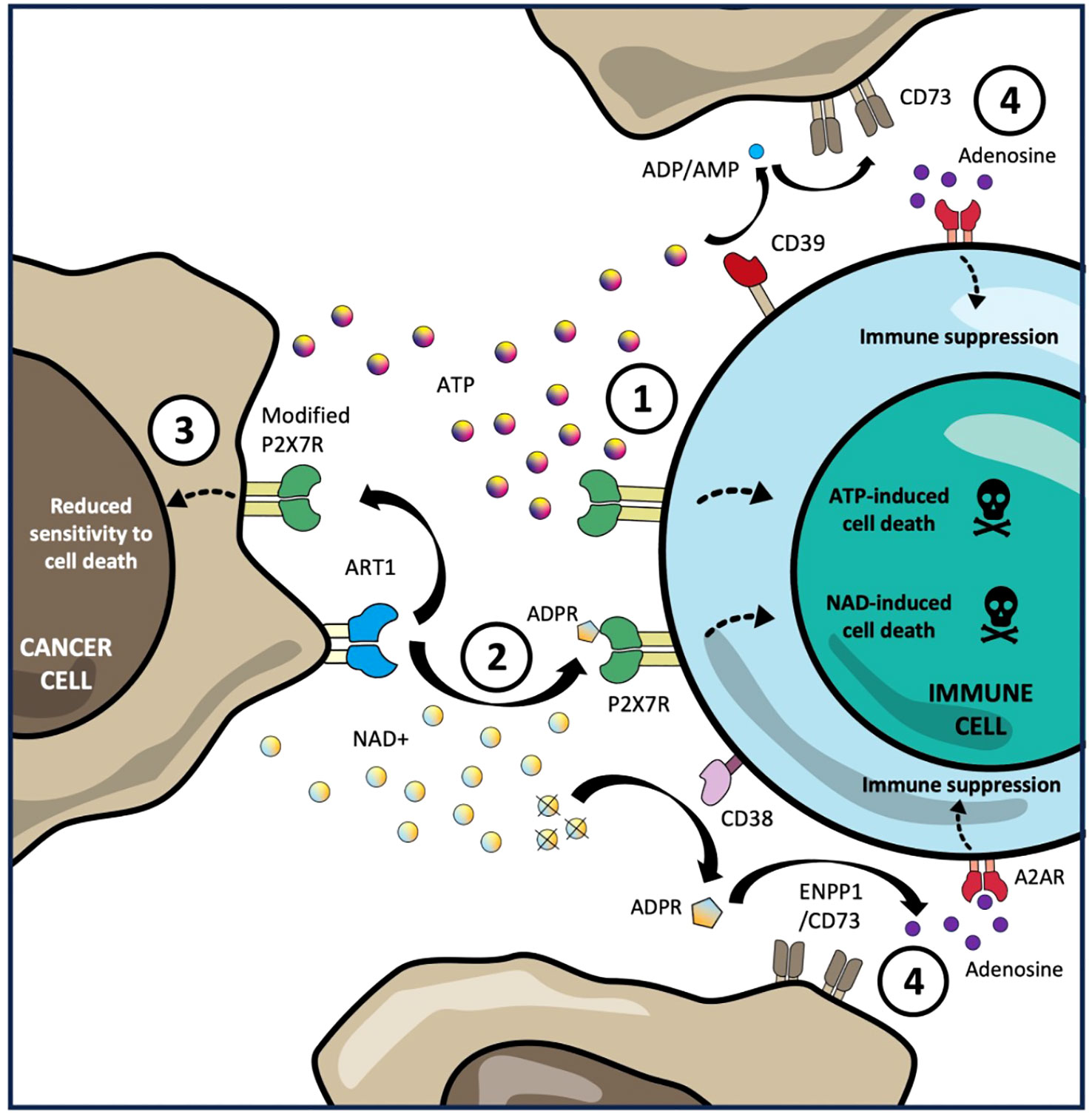
Figure 1 Tumour immune escape mechanisms through P2X7R signalling. 1. Sustained exposure of high concentrations of extracellular ATP, produced in the tumour microenvironment (TME), is sensed by the P2X7R on tumour infiltrating lymphocytes triggering macropore formation ATP-induced cell death (AICD). 2. Expression of ART1 by tumour cells can utilize extracellular NAD+ in TME mono-ADP-ribosylate the P2X7R on TILs in trans resulting in NAD-induced cell death (NICD). 3. Expression by tumour cells of non-pore functional variants of the P2X7R with low affinity for AICD and NICD allows them to avoid cell death while still exploiting P2X7R-mediated proliferation and growth signalling. 4. CD39-mediated catabolism of extracellular ATP and CD38-mediated catabolism of extracellular NAD into AMP and ADPR respectively, is converted into immunosuppressive adenosine by CD73, which is frequently overexpressed by tumour cells, resulting in polarization of intratumoural immune cells towards a regulatory phenotype (including Tregs and macrophages) as well as reduced proliferation, cytokine production and cytotoxic function of effector lymphocytes.
Non-pore functional P2X7R (nfP2X7R) variants
The high concentration of extracellular ATP found in tumours is proposed to drive the expression of modified P2X7Rs in cancers to favour tumorigenesis and progression (42, 43). These include single nucleotide polymorphisms and splice variants, leading to loss of the P2X7R macropore function, as well as reduced sensitivity to activation by MARylation which has further been associated with tumour growth and immune escape (Figure 1) (44, 45). In 2019, Gilbert et al, showed that the nfP2X7R E200, which does not induce large pore formation and cell death upon ATP-binding was expressed broadly across different human cancers. The authors showed that exposure of tumour cells to high concentrations of ATP expression of E200 nfP2X7R which was essential for survival of the cancer cells (43).
Antibodies targeting E200 nfP2X7R, was tested in an open-label phase I non-randomized clinical trial as a topical agent (BIL010t) for the treatment of basal cell carcinoma of the skin. The trial demonstrated that after being applied to primary lesions twice a day for 28 days (n=21), 65% of patients underwent reduction of lesion size, while 20% and 15% showed no changes, and increase in size, respectively. Although complete pathologic response was only observed in 3 of the patients after excision, treatment compliance was high, and the treatment was well tolerated (46). Further, A recent paper has shown that chimeric antigen receptor (CAR)-T cells targeting nfP2X7Rs have potent cytotoxic potential against human cancer cells. When adoptively transferred to NOD-scid-IL2Rγnull (NSG) mice, CAR-T cells were able to traffic to and infiltrate orthotopic breast and prostate tumours (47).
Mono-ADP-ribosyltransferases (ARTs)
In the presence of ARTs, the P2X7R can be activated by nicotinamide adenine dinucleotide (NAD+), a nucleotide that can be released extracellularly after cellular damage or from activated T cells (48, 49). In mice, expression of ART2 by peripheral T cells, allows them to mono-ADP-ribosylate (MARylate) the P2X7R in cis (50). This ART2-mediated MARylation results in Ca2+ increase, exposure of the phospholipid phosphatidylserine (PS), formation of a macropore, and ultimately NAD-induced cell death (NICD) (49–52). Importantly, the NAD+ concentration threshold for NICD via MARylation of the P2X7R is significantly lower than for P2X7R-mediated AICD and this process is irreversible (52). Stark and colleagues showed, in mouse models of tissue damage and infection, that ART2-mediated NICD of T cells serves as a mechanism for enrichment of antigen-specific T cells in inflamed tissues.
ART2 is a pseudogene in humans and no T cell-expressed ART with similar immune homeostatic function has been identified to date. However, ART1, which is expressed in various human tissues including lung epithelium and skeletal muscle as well as on a subset of CD39+ CD4 T cells, has been shown to MARylate immune cells in trans (53, 54). In non-small cell lung cancer (NSCLC), ART1 expressed by tumour cells has been characterized as a novel pathway of immune escape. Wennerberg and Mukherjee et al. showed that ART1 expression was associated with reduced tumour infiltration of P2X7+ CD8 T cells in non-small cell lung cancer (NSCLC) patients, while in murine immune competent lung tumour models, ART1 knockdown decreased tumour growth (55). Correspondingly, ART1 blockade with a therapeutic monoclonal antibody (22C12) reduced the growth and dissemination of ART1 expressing tumours in mice and promoted tumour infiltration of activated P2X7R+ CD8 T cells (Figure 1) (55).
Ectonucleotidases
Through its catabolism of eATP, CD39 produces precursors for CD73, which is overexpressed in several cancers, to generate immunosuppressive adenosine in the tumour microenvironment (TME). This has prompted testing of CD39 inhibitors in preclinical mouse models, which have shown impressive T cell and NK cell-mediated anti-tumour effects in immunogenic mouse tumour models (56–58). While CD39 expression on T cells has traditionally been described a marker of exhaustion and dysfunctionality, emerging patient reports show that CD39-expressing T cells are enriched in solid tumours, where they are shown to be preferentially tumour antigen-specific (59–61). Exposure of T cells and NK cells to high concentrations of ATP result in AICD and reduced cytotoxicity respectively in P2X7R-dependent manners (62, 63). Presumably, modulation of CD39 expression following ATP exposure, serves as a cytoprotective function for lymphocytes to maintain crucial effector functions in the TME (Figure 1) (38).
CD38 is primarily expressed on activated T cells and through its generation of cADPR, CD38 can function as a second messenger for Ca+ mobilization regulating T cell activation (64, 65). Further, CD38 expression clusters in the immune synapse upon T cell receptor (TCR) interaction with antigen-presenting cells, suggesting that CD38 plays a role in regulating T cell function (66). While CD38 expressed on tumour cells can mediate immune resistance by providing precursors for adenosine generation via the non-canonical pathway mediated by ENPP1 (CD203a) and CD73 (67, 68), its NADase function in immune cells is important for protection against ART-mediated NICD under NAD-rich conditions (Figure 1). Indeed, Krebs et al. showed that ART2-mediated mono-ADP-ribosylation following eNAD exposure was elevated in T cells lacking CD38 expression while Adriouch et al. showed that CD38-deficient mice experienced significantly more depletion of P2X7R+ T cells following NAD+ injection compared to wild-type mice (69, 70). In the tumour context, we have demonstrated that NICD of P2X7R+ CD8 T cells following exposure to recombinant ART1 is exacerbated in the presence of CD38-blocking antibodies. Consistent with these findings, analysis of P2X7R+ TILs from ART1-expressing human lung tumours showed enriched expression of CD38 (55).
Immunoregulatory cells
Regulatory T cells (Tregs) are recruited and polarized by tumours to blunt anti-tumour immune responses (71). Tregs are not affected by physiological concentrations of ATP, whereas concentrations approaching 1 mM triggers Treg-mediated immunosuppression (72). In lymph nodes, the expression of P2X7R in CD4 naïve cells or in Treg cells, can induce a posterior polarization towards the Th1/Th17 phenotype (73). Also, Tregs show cell plasticity based on the context. For example, in the presence of IL-6 and ATP, they become Th17 cells (74). If Th17 cells co-express CD39, they can differentiate into the IL-10-producing Tr1 phenotype (75). In mouse tumour models, P2X7R-deficient mice have elevated intratumoural Tregs compared to wild type mice (38, 74, 76). In these mice, the cytokine profile is shifted from pro-inflammatory to immunosuppressive mediators including TGF-β (38). In leukaemia patients, treatment with the chemotherapy agent daunorubicin promoted Tregs through P2X7R-dependent polarization of tolerogenic DCs (77).
Further, P2X7R is expressed on macrophages and on myeloid-derived suppressor cells (MDSCs). In a Lewis Lung carcinoma model, P2X7R expression on tumour-associated macrophages (TAMs) favoured immunosuppressive M2 polarization and anti-programmed cell death protein-1 (PD-1) resistance was overcome by administration of P2X7R inhibitors (78). In a murine neuroblastoma model, P2X7R signalling by MDSCs was associated with increased suppressive function including production of TGF-β, Arginase-1, and reactive oxygen species (Figure 1) (79). Recent work suggests that Toll-like receptor (TLR) mediated activation of diverse types of immune cell subsets can be used as a strategy to activate an immune response in the TME (80). In this regard, as the P2X7R is known to enhance the release of pro-inflammatory cytokines in macrophages and DCs after TLR2 and TLR4 activation, a dual therapeutic strategy could be conceived to obtain an increased anti-tumour immune effect (13, 81, 82).
As in other immune cells, microglial activation of the P2X7R causes a polarization of these cells towards a pro-inflammatory state, and as such the receptor has a clear role in diverse neuroinflammatory diseases such as Parkinsons and Multiple Sclerosis (83, 84). Mice microglia is known to express the P2X7a variant, that although it shows no variation of ATP sensitivity, it renders it insensitive to ART2-mediated NICD (85). Meanwhile, through diverse mutational studies, it is known that the retention of pore formation capabilities seems to be important for P2X7R driven microglial activation, proliferation, and cytokine release (86).
Prognostic value of P2X7R expression in cancer
In Acute Myeloid Leukemia (AML), P2X7R splice variants A and B have shown promising prognostic potential to identify patients with relapsing disease. P2X7RB has also been identified as a poor-prognosis marker in osteosarcoma, neuroblastoma, and lung adenocarcinoma (87, 88). In colorectal cancer, P2X7R expression is higher in undifferentiated tumours and has been associated with adverse oncologic features including invasiveness, advanced stages, metastatic disease, and worse overall survival (89). In addition, P2X7R-high tumours may correlate with increased carcinoembryonic antigen (CEA) expression, a tumour marker used for monitoring metastatic disease (90). In gastric cancer, P2X7R has also shown promise as a prognostic marker. In a study of gastric cancer specimens, P2X7R was overexpressed in specimens from patients with lymph-node metastases, vascular invasion, and advanced stages. Additionally, an inverse correlation was noted between P2X7R and CD8+ TILs (89).
In contrast, in hepatocellular carcinoma, intratumoral P2X7R expression did not correlate with oncologic outcomes. However, peri-tumoral expression of the receptor was inversely associated with overall survival in both an experimental and a validation cohort (91). Similarly, P2X7R expression has been correlated with decreased overall survival, and metastatic disease in metastatic melanoma. In this setting, splicing variants A and B have been associated with malignant transformation (88). High P2X7R expression was correlated with decreased overall survival in a cohort of patients with lung adenocarcinoma from The Cancer Genome Atlas (TCGA) dataset (92). In muscle-invasive bladder cancer, P2X7R has been reported as a negative predictor of overall survival (93). These findings have been validated through TCGA analysis (94). In the setting of renal-cell carcinoma, a study showed that P2X7R expression is an adverse prognostic indicator for postoperative cancer-specific survival (95).
Contrary to the above, a study showed that in non-small cell lung cancer, overexpression of P2X7R was associated with improved overall survival (96). However other studies have shown results that conflict with these findings (97). Similarly, a study showed that decreased P2X7R expression associated with development with cervical cancer in patients with epithelial precancerous lesions (98). Overall, most studies with clinical correlation associate high P2X7R expression with adverse prognosis and decreased survival in cancer. It is critical to note that most of the cited studies did not distinguish between tumour cell expression and immune cell expression of P2X7R or distinguish between distinct P2X7R isotypes, analyses that could potentially better stratify patients in terms of prognosis.
P2X7R antagonists and agonists in preclinical models
In the preclinical setting, a variety of antagonists of P2X7R have been described in recent literature, including Brilliant Blue G (BBG), oxidized ATP (oxATP), KN-04, KN-62, A740003, and A438079, although all are not specific for P2X7 and may block other purinergic receptors (99, 100). In AML, P2X7R blockade with AZ10606120 resulted in reduced leukemic growth when co-administered with daunorubicin, a process mediated through the blockade of P2X7 splice variant B (87). In neuro-oncological malignancies, AZ10606120 was shown to inhibit growth of human glioblastoma cells (101) and systemic administration of AZ10606120 in nude/nude mice reduced ACN-derived tumor growth of neuroblastoma. This was associated with downregulation of the Akt/hypoxia-inducable factor 1-alpha (HIF-1a) axis, and reduced VEGF and vessel formation as well as reduced the expression of MYCN, a crucial oncogene in neuroblastoma (32). In pancreatic cancer, AZ10606120 a non-selective inhibitor of P2X7R, inhibited the growth of stellate cells, a promoter of pancreatic adenocarcinoma progression (102). In contrast, a study by Mohammed et al, the inhibitors A438079 and AZ10606120 showed no chemopreventive effect, but instead promoted progression of intraepithelial lesions to cancer (103). In murine colorectal cancer models, P2X7R blockade with A438079 and AZD9056, inhibited tumour cell invasion, migration and TGF-B1 induced metastases (104). In addition, in murine models (CT26-mP2X7R), P2X7R blockade with intratumoral oxATP injections lead to reduction in tumour size and growth (31). Lastly, bilirubin has been found to interact with P2X7R, and decrease phosphorylation of mammalian target of rapamycin (mTOR), signal transducer and activator of transcription 3 (STAT3), and glycogen synthase kinase-3 beta (GSK-3beta), thus reducing oncogenicity (105).
In pancreatic cancer in-vivo murine models, the anti-P2X7 agent KN-62 abrogated tumor proliferation promoted by ATP (106). Alternatively, in a breast cancer in-vitro model, KN-62 inhibited ion currents, ethidium uptake, and calcium uptake, suggesting appropriate anti-P2X7 function (34). A similar isoquinoline derivative, KN-04 (an inactive analog), was found to inhibit ion fluxes in the nanomolar range. Subsequently, it was shown that both KN-62 and KN-04 only partially block pore formation (107). In vitro, A740003 a P2X7R blocker, has shown to reduce primary melanoma growth and to activate anti-tumor immune responses (38). In addition, A740003 has demonstrated a reduction in melanoma spread and tumour dissemination in-vivo (88). P2X7R antagonists have also been useful in establishing the mechanistic effects of P2X7R in lung models. For example, a specific P2X7R inhibitor GSK1370319A, was used to demonstrate that the macropore function of P2X7R may be impaired in immune cells of lung adenocarcinoma (92). Adamantane-1-carbonyl thiourea derivatives have also been shown to inhibit P2X7R activity, especially P2X7RB (108). Several natural compounds have been shown to antagonize P2X7 and have therapeutic potential. Teniposide, a podophyllotoxin derivative, acts as a topoisomerase inhibitor and is used in several types of cancer (109). Emodin, an anthraquinone derivative, specifically inhibited P2X7R-mediated currents and was shown to block cancer invasiveness in vitro and in an in-vivo zebrafish model of micrometastases (110). The agent GSK-1482160, a P2X7 blocker designed to target inflammatory conditions, was tested in a first-in-human blinded placebo-controlled study that was completed in 2009. The results of this study supported that the compound reduces the efficacy of ATP at the P2X7 receptor without affecting its affinity. With 29 subjects included, no safety or tolerability concerns were identified except for one case of asymptomatic accelerated idioventricular rhythm at the top dose (111).
Extracellular ATP has also been shown potential as anticancer therapy. In a study using a human prostate xenografts intraperitoneal injection of extracellular ATP resulted in significant tumour regression (112). However, this strategy failed to show clinical efficacy in a phase II study (113). In osteosarcoma, the P2X7R agonist benzoyl ATP (BzATP) promoted tumour proliferation and spread of osteosarcoma throughout the bone matrix. BzATP has also shown to have anti-tumour effect in glioblastoma stem cells (114). Non-nucleotide P2X7R agonists have also shown anti-tumour activity in pre-clinical models. A positive allosteric modulator against P2X7R, HEI3090, induced immune-mediated tumour regression in combination with anti-PD-1 antibodies in the immunotherapy-resistant Lewis Lung Carcinoma model (115),
Future strategies
It has become clear that P2X7R is essential for innate immune cell sensing of immunogenic cell death, which plays an important role for efficient priming of tumour-specific T cells. In these T cells, P2X7R signalling is involved in orchestrating their migration, metabolic fitness, memory cell differentiation, and survival. Altogether, the demonstrated crucial role of P2X7R in initiation and maintenance of adaptive immune responses sends a clear message to approach P2X7R-inhibition in cancer with caution. While there is a strong rationale for inhibiting P2X7R in multiple cancers which exploit it to promote proliferation, invasion and metastasis, the conflicting outcomes of preclinical testing of P2X7R inhibitors in immunocompetent mouse models, and the fact that few P2X7R antagonists have made it to clinical testing in cancer patients, are further indications that more refined ways of targeting this pathway need to be devised.
Nevertheless, the selective expression of nfP2X7R on tumour constitutes an attractive target that could be exploited for cancer therapy. Expression of nfP2X7R variants by tumour cells provides an explanation for how they benefit from the proliferative advantages of P2X7R activation while simultaneously avoiding NICD and AICD. Indeed, recent pre-clinical and clinical studies of nfP2X7R- targeting antibodies and CAR-T cells have generated encouraging results (46, 47).
Emerging findings describing immune regulation by ARTs in inflammation and cancer are rewriting the script for how P2X7R-expression shapes the TME (116, 117). ARTs are potent triggers of NICD via P2X7R, particularly in NAD+-rich conditions such as the TME. Cytotoxic therapy directed at tumours may increase local levels of NAD+ and prime ART-mediated NICD in treated tumours. The recent discovery of how tumour-expressed ART1 allows tumours to co-opt the immune homeostatic mechanism of NICD suggests that targeted ART1-inhibition would counter this immune escape mechanism and maintain the viability of critical anti-tumour immune cells (55).
It is plausible that tumour-engaged T cells rely on the ATPase activity of CD39 and NADase activity of CD38 not only to temper their activation by generating adenosine precursors but also to avoid AICD and NICD induced by sustained exposure to high concentrations of ATP and NAD+ in solid tumours (118). In fact, Tregs and TRMs upregulate CD39 and CD38 in response to activation and TCR activation (116, 119). This cytoprotective role of ectonucleotidase expression may be especially crucial in the context of immunotherapy, where increased susceptibility to activation-induced apoptosis has been reported (120). Considering these findings, re-evaluation of clinical trial designs where ectonucleotidase inhibitors in combination with immunotherapy are warranted. Indeed, clinical testing of anti-CD38 antibodies daratumumab and isatuximab in combination with PD-1/PD-L1 inhibitors in patients with solid malignancies have generated negative survival outcomes, including early termination of one of the studies due to increased mortality in the combined treatment arm (121–123).
In summary, new insights have provided both cautionary tales and important clues for how the dysregulated P2X7R-signalling that occurs in tumours could be alternatively targeted to optimize immunotherapeutic treatments of cancer patients.
Author contributions
RS: Writing – original draft, Writing – review & editing, Visualization. JR-Q: Writing – original draft, Writing – review & editing. MM: Writing – original draft, Writing – review & editing. BS: Writing – original draft, Writing – review & editing. EW: Writing – original draft, Writing – review & editing, Visualization.
Funding
The author(s) declare financial support was received for the research, authorship, and/or publication of this article. This work was supported by the ICR/RM CRUK RadNet Centre of Excellence.
Conflict of interest
BMS receives consulting fees and research support from Medtronic, AstraZeneca, Genentech, Pfizer, Merck, Regeneron, Arcus Biosciences, Bristol Myers Squibb, BMS Foundation, Gala Therapeutics, and the Lung Cancer Research Foundation.
The remaining authors declare that the research was conducted in the absence of any commercial or financial relationships that could be construed as a potential conflict of interest.
Publisher’s note
All claims expressed in this article are solely those of the authors and do not necessarily represent those of their affiliated organizations, or those of the publisher, the editors and the reviewers. Any product that may be evaluated in this article, or claim that may be made by its manufacturer, is not guaranteed or endorsed by the publisher.
References
1. North RA, Jarvis MF. P2X receptors as drug targets. Mol Pharmacol (2013) 83(4):759–69. doi: 10.1124/mol.112.083758
2. Illes P, Muller CE, Jacobson KA, Grutter T, Nicke A, Fountain SJ, et al. Update of P2X receptor properties and their pharmacology: IUPHAR Review 30. Br J Pharmacol (2021) 178(3):489–514. doi: 10.1111/bph.15299
3. Saul A, Hausmann R, Kless A, Nicke A. Heteromeric assembly of P2X subunits. Front Cell Neurosci (2013) 7:250. doi: 10.3389/fncel.2013.00250
4. Karoly R, Mike A, Illes P, Gerevich Z. The unusual state-dependent affinity of P2X3 receptors can be explained by an allosteric two-open-state model. Mol Pharmacol (2008) 73(1):224–34. doi: 10.1124/mol.107.038901
5. Sheng D, Hattori M. Recent progress in the structural biology of P2X receptors. Proteins (2022) 90(10):1779–85. doi: 10.1002/prot.26302
6. Rump A, Smolander OP, Ruutel Boudinot S, Kanellopoulos JM, Boudinot P. Evolutionary origin of the P2X7 C-ter region: capture of an ancient ballast domain by a P2X4-like gene in ancient jawed vertebrates. Front Immunol (2020) 11:113. doi: 10.3389/fimmu.2020.00113
7. Alves LA, de Melo Reis RA, de Souza CA, de Freitas MS, Teixeira PC, Neto Moreira Ferreira D, et al. The P2X7 receptor: shifting from a low- to a high-conductance channel - an enigmatic phenomenon? Biochim Biophys Acta (2014) 1838(10):2578–87. doi: 10.1016/j.bbamem.2014.05.015
8. Savio LEB, de Andrade Mello P, da Silva CG, Coutinho-Silva R. The P2X7 receptor in inflammatory diseases: angel or demon? Front Pharmacol (2018) 9:52. doi: 10.3389/fphar.2018.00052
9. Di Virgilio F, Vuerich M. Purinergic signaling in the immune system. Auton Neurosci (2015) 191:117–23. doi: 10.1016/j.autneu.2015.04.011
10. Khakh BS, North RA. P2X receptors as cell-surface ATP sensors in health and disease. Nature (2006) 442(7102):527–32. doi: 10.1038/nature04886
11. Martinez-Cuesta MA, Blanch-Ruiz MA, Ortega-Luna R, Sanchez-Lopez A, Alvarez A. Structural and functional basis for understanding the biological significance of P2X7 receptor. Int J Mol Sci (2020) 21(22):8454. doi: 10.3390/ijms21228454
12. Eltzschig HK, Sitkovsky MV, Robson SC. Purinergic signaling during inflammation. N Engl J Med (2012) 367(24):2322–33. doi: 10.1056/NEJMra1205750
13. Di Virgilio F, Dal Ben D, Sarti AC, Giuliani AL, Falzoni S. The P2X7 receptor in infection and inflammation. Immunity (2017) 47(1):15–31. doi: 10.1016/j.immuni.2017.06.020
14. Schenk U, Westendorf AM, Radaelli E, Casati A, Ferro M, Fumagalli M, et al. Purinergic control of T cell activation by ATP released through pannexin-1 hemichannels. Sci Signal (2008) 1(39):ra6. doi: 10.1126/scisignal.1160583
15. Le Gall SM, Bobe P, Reiss K, Horiuchi K, Niu XD, Lundell D, et al. ADAMs 10 and 17 represent differentially regulated components of a general shedding machinery for membrane proteins such as transforming growth factor alpha, L-selectin, and tumor necrosis factor alpha. Mol Biol Cell (2009) 20(6):1785–94. doi: 10.1091/mbc.e08-11-1135
16. Safya H, Mellouk A, Legrand J, Le Gall SM, Benbijja M, Kanellopoulos-Langevin C, et al. Variations in cellular responses of mouse T cells to adenosine-5’-triphosphate stimulation do not depend on P2X7 receptor expression levels but on their activation and differentiation stage. Front Immunol (2018) 9:360. doi: 10.3389/fimmu.2018.00360
17. Wang CM, Ploia C, Anselmi F, Sarukhan A, Viola A. Adenosine triphosphate acts as a paracrine signaling molecule to reduce the motility of T cells. EMBO J (2014) 33(12):1354–64. doi: 10.15252/embj.201386666
18. Saez PJ, Vargas P, Shoji KF, Harcha PA, Lennon-Dumenil AM, Saez JC. ATP promotes the fast migration of dendritic cells through the activity of pannexin 1 channels and P2X(7) receptors. Sci Signal (2017) 10(506):eaah7107. doi: 10.1126/scisignal.aah7107
19. Myrtek D, Idzko M. Chemotactic activity of extracellular nucleotideson human immune cells. Purinergic Signal (2007) 3(1-2):5–11. doi: 10.1007/s11302-006-9032-0
20. Killeen ME, Ferris L, Kupetsky EA, Falo L Jr., Mathers AR. Signaling through purinergic receptors for ATP induces human cutaneous innate and adaptive Th17 responses: implications in the pathogenesis of psoriasis. J Immunol (2013) 190(8):4324–36. doi: 10.4049/jimmunol.1202045
21. Yu Y, Feng S, Wei S, Zhong Y, Yi G, Chen H, et al. Extracellular ATP activates P2X7R-NF-kappaB (p65) pathway to promote the maturation of bone marrow-derived dendritic cells of mice. Cytokine (2019) 119:175–81. doi: 10.1016/j.cyto.2019.03.019
22. Parzych K, Zetterqvist AV, Wright WR, Kirkby NS, Mitchell JA, Paul-Clark MJ. Differential role of pannexin-1/ATP/P2X(7) axis in IL-1beta release by human monocytes. FASEB J (2017) 31(6):2439–45. doi: 10.1096/fj.201600256
23. Pelegrin P, Surprenant A. Pannexin-1 mediates large pore formation and interleukin-1beta release by the ATP-gated P2X7 receptor. EMBO J (2006) 25(21):5071–82. doi: 10.1038/sj.emboj.7601378
24. Dichmann S, Idzko M, Zimpfer U, Hofmann C, Ferrari D, Luttmann W, et al. Adenosine triphosphate-induced oxygen radical production and CD11b up-regulation: Ca(++) mobilization and actin reorganization in human eosinophils. Blood (2000) 95(3):973–8. doi: 10.1182/blood.V95.3.973.003k47_973_978
25. Idzko M, Dichmann S, Panther E, Ferrari D, Herouy Y, Virchow C Jr., et al. Functional characterization of P2Y and P2X receptors in human eosinophils. J Cell Physiol (2001) 188(3):329–36. doi: 10.1002/jcp.1129
26. Jin C, Flavell RA. Molecular mechanism of NLRP3 inflammasome activation. J Clin Immunol (2010) 30(5):628–31. doi: 10.1007/s10875-010-9440-3
27. Kepp O, Galluzzi L, Zitvogel L, Kroemer G. Pyroptosis - a cell death modality of its kind? Eur J Immunol (2010) 40(3):627–30. doi: 10.1002/eji.200940160
28. Borges da Silva H, Beura LK, Wang H, Hanse EA, Gore R, Scott MC, et al. The purinergic receptor P2RX7 directs metabolic fitness of long-lived memory CD8(+) T cells. Nature (2018) 559(7713):264–8. doi: 10.1038/s41586-018-0282-0
29. Mellouk A, Bobe P. CD8(+), but not CD4(+) effector/memory T cells, express the CD44(high)CD45RB(high) phenotype with aging, which displays reduced expression levels of P2X(7) receptor and ATP-induced cellular responses. FASEB J (2019) 33(3):3225–36. doi: 10.1096/fj.201800867R
30. Romagnani A, Rottoli E, Mazza EMC, Rezzonico-Jost T, De Ponte Conti B, Proietti M, et al. P2X7 receptor activity limits accumulation of T cells within tumors. Cancer Res (2020) 80(18):3906–19. doi: 10.1158/0008-5472.CAN-19-3807
31. Adinolfi E, Raffaghello L, Giuliani AL, Cavazzini L, Capece M, Chiozzi P, et al. Expression of P2X7 receptor increases in vivo tumor growth. Cancer Res (2012) 72(12):2957–69. doi: 10.1158/0008-5472.CAN-11-1947
32. Amoroso F, Capece M, Rotondo A, Cangelosi D, Ferracin M, Franceschini A, et al. The P2X7 receptor is a key modulator of the PI3K/GSK3beta/VEGF signaling network: evidence in experimental neuroblastoma. Oncogene (2015) 34(41):5240–51. doi: 10.1038/onc.2014.444
33. Xia J, Yu X, Tang L, Li G, He T. P2X7 receptor stimulates breast cancer cell invasion and migration via the AKT pathway. Oncol Rep (2015) 34(1):103–10. doi: 10.3892/or.2015.3979
34. Jelassi B, Chantome A, Alcaraz-Perez F, Baroja-Mazo A, Cayuela ML, Pelegrin P, et al. P2X(7) receptor activation enhances SK3 channels- and cystein cathepsin-dependent cancer cells invasiveness. Oncogene (2011) 30(18):2108–22. doi: 10.1038/onc.2010.593
35. Qiu Y, Li WH, Zhang HQ, Liu Y, Tian XX, Fang WG. P2X7 mediates ATP-driven invasiveness in prostate cancer cells. PloS One (2014) 9(12):e114371. doi: 10.1371/journal.pone.0114371
36. Ghiringhelli F, Apetoh L, Tesniere A, Aymeric L, Ma Y, Ortiz C, et al. Activation of the NLRP3 inflammasome in dendritic cells induces IL-1beta-dependent adaptive immunity against tumors. Nat Med (2009) 15(10):1170–8. doi: 10.1038/nm.2028
37. Adinolfi E, Capece M, Franceschini A, Falzoni S, Giuliani AL, Rotondo A, et al. Accelerated tumor progression in mice lacking the ATP receptor P2X7. Cancer Res (2015) 75(4):635–44. doi: 10.1158/0008-5472.CAN-14-1259
38. De Marchi E, Orioli E, Pegoraro A, Sangaletti S, Portararo P, Curti A, et al. The P2X7 receptor modulates immune cells infiltration, ectonucleotidases expression and extracellular ATP levels in the tumor microenvironment. Oncogene (2019) 38(19):3636–50. doi: 10.1038/s41388-019-0684-y
39. Wanhainen KM, Peng C, Zhou MH, Macedo BG, O'Flanagan S, Yang T, et al. P2RX7 enhances tumor control by CD8+ T cells in adoptive cell therapy. Cancer Immunol Res (2022) 10(7):871–84. doi: 10.1158/2326-6066.CIR-21-0691
40. Georgiev H, Ravens I, Papadogianni G, Malissen B, Forster R, Bernhardt G. Blocking the ART2.2/P2X7-system is essential to avoid a detrimental bias in functional CD4 T cell studies. Eur J Immunol (2018) 48(6):1078–81. doi: 10.1002/eji.201747420
41. Borges da Silva H, Wang H, Qian LJ, Hogquist KA, Jameson SC. ARTC2.2/P2RX7 signaling during cell isolation distorts function and quantification of tissue-resident CD8(+) T cell and invariant NKT subsets. J Immunol (2019) 202(7):2153–63. doi: 10.4049/jimmunol.1801613
42. Benzaquen J, Heeke S, Janho Dit Hreich S, Douguet L, Marquette CH, Hofman P, et al. Alternative splicing of P2RX7 pre-messenger RNA in health and diseases: Myth or reality? BioMed J (2019) 42(3):141–54. doi: 10.1016/j.bj.2019.05.007
43. Gilbert SM, Oliphant CJ, Hassan S, Peille AL, Bronsert P, Falzoni S, et al. ATP in the tumour microenvironment drives expression of nfP2X(7), a key mediator of cancer cell survival. Oncogene (2019) 38(2):194–208. doi: 10.1038/s41388-018-0426-6
44. Lara R, Adinolfi E, Harwood CA, Philpott M, Barden JA, Di Virgilio F, et al. P2X7 in cancer: from molecular mechanisms to therapeutics. Front Pharmacol (2020) 11:793. doi: 10.3389/fphar.2020.00793
45. Schwarz N, Drouot L, Nicke A, Fliegert R, Boyer O, Guse AH, et al. Alternative splicing of the N-terminal cytosolic and transmembrane domains of P2X7 controls gating of the ion channel by ADP-ribosylation. PloS One (2012) 7(7):e41269. doi: 10.1371/journal.pone.0041269
46. Gilbert SM, Gidley Baird A, Glazer S, Barden JA, Glazer A, Teh LC, et al. A phase I clinical trial demonstrates that nfP2X(7) -targeted antibodies provide a novel, safe and tolerable topical therapy for basal cell carcinoma. Br J Dermatol (2017) 177(1):117–24. doi: 10.1111/bjd.15364
47. Bandara V, Foeng J, Gundsambuu B, Norton TS, Napoli S, McPeake DJ, et al. Pre-clinical validation of a pan-cancer CAR-T cell immunotherapy targeting nfP2X7. Nat Commun (2023) 14(1):5546. doi: 10.1038/s41467-023-41338-y
48. Haag F, Adriouch S, Brass A, Jung C, Moller S, Scheuplein F, et al. Extracellular NAD and ATP: Partners in immune cell modulation. Purinergic Signal (2007) 3(1-2):71–81. doi: 10.1007/s11302-006-9038-7
49. Scheuplein F, Rissiek B, Driver JP, Chen YG, Koch-Nolte F, Serreze DV. A recombinant heavy chain antibody approach blocks ART2 mediated deletion of an iNKT cell population that upon activation inhibits autoimmune diabetes. J Autoimmun (2010) 34(2):145–54. doi: 10.1016/j.jaut.2009.08.012
50. Adriouch S, Bannas P, Schwarz N, Fliegert R, Guse AH, Seman M, et al. ADP-ribosylation at R125 gates the P2X7 ion channel by presenting a covalent ligand to its nucleotide binding site. FASEB J (2008) 22(3):861–9. doi: 10.1096/fj.07-9294com
51. Kawamura H, Aswad F, Minagawa M, Malone K, Kaslow H, Koch-Nolte F, et al. P2X7 receptor-dependent and -independent T cell death is induced by nicotinamide adenine dinucleotide. J Immunol (2005) 174(4):1971–9. doi: 10.4049/jimmunol.174.4.1971
52. Seman M, Adriouch S, Scheuplein F, Krebs C, Freese D, Glowacki G, et al. NAD-induced T cell death: ADP-ribosylation of cell surface proteins by ART2 activates the cytolytic P2X7 purinoceptor. Immunity (2003) 19(4):571–82. doi: 10.1016/S1074-7613(03)00266-8
53. Paone G, Stevens LA, Levine RL, Bourgeois C, Steagall WK, Gochuico BR, et al. ADP-ribosyltransferase-specific modification of human neutrophil peptide-1. J Biol Chem (2006) 281(25):17054–60. doi: 10.1074/jbc.M603042200
54. Cortes-Garcia JD, Lopez-Lopez C, Cortez-Espinosa N, Garcia-Hernandez MH, Guzman-Flores JM, Layseca-Espinosa E, et al. Evaluation of the expression and function of the P2X7 receptor and ART1 in human regulatory T-cell subsets. Immunobiology (2016) 221(1):84–93. doi: 10.1016/j.imbio.2015.07.018
55. Wennerberg E, Mukherjee S, Spada S, Hung C, Agrusa CJ, Chen C, et al. Expression of the mono-ADP-ribosyltransferase ART1 by tumor cells mediates immune resistance in non-small cell lung cancer. Sci Transl Med (2022) 14(636):eabe8195. doi: 10.1126/scitranslmed.abe8195
56. Moesta AK, Li XY, Smyth MJ. Targeting CD39 in cancer. Nat Rev Immunol (2020) 20(12):739–55. doi: 10.1038/s41577-020-0376-4
57. Warren MC, Matissek S, Rausch M, Panduro M, Hall RJ, Dulak A, et al. SRF617 is a potent inhibitor of CD39 with immunomodulatory and antitumor properties. Immunohorizons (2023) 7(5):366–79. doi: 10.4049/immunohorizons.2200089
58. Yan J, Li XY, Roman Aguilera A, Xiao C, Jacoberger-Foissac C, Nowlan B, et al. Control of metastases via myeloid CD39 and NK cell effector function. Cancer Immunol Res (2020) 8(3):356–67. doi: 10.1158/2326-6066.CIR-19-0749
59. Simoni Y, Becht E, Fehlings M, Loh CY, Koo SL, Teng KWW, et al. Bystander CD8(+) T cells are abundant and phenotypically distinct in human tumour infiltrates. Nature (2018) 557(7706):575–9. doi: 10.1038/s41586-018-0130-2
60. Chow A, Perica K, Klebanoff CA, Wolchok JD. Clinical implications of T cell exhaustion for cancer immunotherapy. Nat Rev Clin Oncol (2022) 19(12):775–90. doi: 10.1038/s41571-022-00689-z
61. Duhen T, Duhen R, Montler R, Moses J, Moudgil T, de Miranda NF, et al. Co-expression of CD39 and CD103 identifies tumor-reactive CD8 T cells in human solid tumors. Nat Commun (2018) 9(1):2724. doi: 10.1038/s41467-018-05072-0
62. Winzer R, Serracant-Prat A, Brock VJ, Pinto-Espinoza C, Rissiek B, Amadi M, et al. P2X7 is expressed on human innate-like T lymphocytes and mediates susceptibility to ATP-induced cell death. Eur J Immunol (2022) 52(11):1805–18. doi: 10.1002/eji.202249932
63. Baroja-Mazo A, Penin-Franch A, Lucas-Ruiz F, de Torre-Minguela C, Alarcon-Vila C, Hernandez-Caselles T, et al. P2X7 receptor activation impairs antitumour activity of natural killer cells. Br J Pharmacol (2023) 180(1):111–28. doi: 10.1111/bph.15951
64. Guse AH, da Silva CP, Berg I, Skapenko AL, Weber K, Heyer P, et al. Regulation of calcium signalling in T lymphocytes by the second messenger cyclic ADP-ribose. Nature (1999) 398(6722):70–3. doi: 10.1038/18024
65. Sandoval-Montes C, Santos-Argumedo L. CD38 is expressed selectively during the activation of a subset of mature T cells with reduced proliferation but improved potential to produce cytokines. J Leukoc Biol (2005) 77(4):513–21. doi: 10.1189/jlb.0404262
66. Munoz P, Mittelbrunn M, de la Fuente H, Perez-Martinez M, Garcia-Perez A, Ariza-Veguillas A, et al. Antigen-induced clustering of surface CD38 and recruitment of intracellular CD38 to the immunologic synapse. Blood (2008) 111(7):3653–64. doi: 10.1182/blood-2007-07-101600
67. Wennerberg E, Spada S, Rudqvist NP, Lhuillier C, Gruber S, Chen Q, et al. CD73 blockade promotes dendritic cell infiltration of irradiated tumors and tumor rejection. Cancer Immunol Res (2020) 8(4):465–78. doi: 10.1158/2326-6066.CIR-19-0449
68. Chen L, Diao L, Yang Y, Yi X, Rodriguez BL, Li Y, et al. CD38-mediated immunosuppression as a mechanism of tumor cell escape from PD-1/PD-L1 blockade. Cancer Discovery (2018) 8(9):1156–75. doi: 10.1158/2159-8290.CD-17-1033
69. Krebs C, Adriouch S, Braasch F, Koestner W, Leiter EH, Seman M, et al. CD38 controls ADP-ribosyltransferase-2-catalyzed ADP-ribosylation of T cell surface proteins. J Immunol (2005) 174(6):3298–305. doi: 10.4049/jimmunol.174.6.3298
70. Adriouch S, Hubert S, Pechberty S, Koch-Nolte F, Haag F, Seman M. NAD+ released during inflammation participates in T cell homeostasis by inducing ART2-mediated death of naive T cells in vivo. J Immunol (2007) 179(1):186–94. doi: 10.4049/jimmunol.179.1.186
71. Togashi Y, Shitara K, Nishikawa H. Regulatory T cells in cancer immunosuppression - implications for anticancer therapy. Nat Rev Clin Oncol (2019) 16(6):356–71. doi: 10.1038/s41571-019-0175-7
72. Trabanelli S, Ocadlikova D, Gulinelli S, Curti A, Salvestrini V, Vieira RP, et al. Extracellular ATP exerts opposite effects on activated and regulatory CD4+ T cells via purinergic P2 receptor activation. J Immunol (2012) 189(3):1303–10. doi: 10.4049/jimmunol.1103800
73. Grassi F. The P2X7 receptor as regulator of T cell development and function. Front Immunol (2020) 11:1179. doi: 10.3389/fimmu.2020.01179
74. Schenk U, Frascoli M, Proietti M, Geffers R, Traggiai E, Buer J, et al. ATP inhibits the generation and function of regulatory T cells through the activation of purinergic P2X receptors. Sci Signal (2011) 4(162):ra12. doi: 10.1126/scisignal.2001270
75. Fernandez D, Flores-Santibanez F, Neira J, Osorio-Barrios F, Tejon G, Nunez S, et al. Purinergic signaling as a regulator of th17 cell plasticity. PloS One (2016) 11(6):e0157889. doi: 10.1371/journal.pone.0157889
76. Hubert S, Rissiek B, Klages K, Huehn J, Sparwasser T, Haag F, et al. Extracellular NAD+ shapes the Foxp3+ regulatory T cell compartment through the ART2-P2X7 pathway. J Exp Med (2010) 207(12):2561–8. doi: 10.1084/jem.20091154
77. Lecciso M, Ocadlikova D, Sangaletti S, Trabanelli S, De Marchi E, Orioli E, et al. ATP release from chemotherapy-treated dying leukemia cells elicits an immune suppressive effect by increasing regulatory T cells and tolerogenic dendritic cells. Front Immunol (2017) 8:1918. doi: 10.3389/fimmu.2017.01918
78. Qin J, Zhang X, Tan B, Zhang S, Yin C, Xue Q, et al. Blocking P2X7-mediated macrophage polarization overcomes treatment resistance in lung cancer. Cancer Immunol Res (2020) 8(11):1426–39. doi: 10.1158/2326-6066.CIR-20-0123
79. Bianchi G, Vuerich M, Pellegatti P, Marimpietri D, Emionite L, Marigo I, et al. ATP/P2X7 axis modulates myeloid-derived suppressor cell functions in neuroblastoma microenvironment. Cell Death Dis (2014) 5(3):e1135. doi: 10.1038/cddis.2014.109
80. Cen X, Liu S, Cheng K. The role of toll-like receptor in inflammation and tumor immunity. Front Pharmacol (2018) 9:878. doi: 10.3389/fphar.2018.00878
81. de Torre-Minguela C, Barbera-Cremades M, Gomez AI, Martin-Sanchez F, Pelegrin P. Macrophage activation and polarization modify P2X7 receptor secretome influencing the inflammatory process. Sci Rep (2016) 6:22586. doi: 10.1038/srep22586
82. Babelova A, Moreth K, Tsalastra-Greul W, Zeng-Brouwers J, Eickelberg O, Young MF, et al. Biglycan, a danger signal that activates the NLRP3 inflammasome via toll-like and P2X receptors. J Biol Chem (2009) 284(36):24035–48. doi: 10.1074/jbc.M109.014266
83. Campagno KE, Mitchell CH. The P2X(7) receptor in microglial cells modulates the endolysosomal axis, autophagy, and phagocytosis. Front Cell Neurosci (2021) 15:645244. doi: 10.3389/fncel.2021.645244
84. Territo PR, Zarrinmayeh H. P2X(7) receptors in neurodegeneration: potential therapeutic applications from basic to clinical approaches. Front Cell Neurosci (2021) 15:617036. doi: 10.3389/fncel.2021.617036
85. Rissiek B, Stabernack J, Cordes M, Duan Y, Behr S, Menzel S, et al. Astrocytes and microglia are resistant to NAD(+)-mediated cell death along the ARTC2/P2X7 axis. Front Mol Neurosci (2019) 12:330. doi: 10.3389/fnmol.2019.00330
86. Monif M, Reid CA, Powell KL, Smart ML, Williams DA. The P2X7 receptor drives microglial activation and proliferation: a trophic role for P2X7R pore. J Neurosci (2009) 29(12):3781–91. doi: 10.1523/JNEUROSCI.5512-08.2009
87. Pegoraro A, De Marchi E, Adinolfi E. P2X7 variants in oncogenesis. Cells (2021) 10(1):189. doi: 10.3390/cells10010189
88. Pegoraro A, De Marchi E, Ferracin M, Orioli E, Zanoni M, Bassi C, et al. P2X7 promotes metastatic spreading and triggers release of miRNA-containing exosomes and microvesicles from melanoma cells. Cell Death Dis (2021) 12(12):1088. doi: 10.1038/s41419-021-04378-0
89. Calik I, Calik M, Sarikaya B, Ozercan IH, Arslan R, Artas G, et al. P2X7 receptor as an independent prognostic indicator in gastric cancer. Bosn J Basic Med Sci (2020) 20(2):188–96. doi: 10.17305/bjbms.2020.4620
90. Zhang Y, Ding J, Wang L. The role of P2X7 receptor in prognosis and metastasis of colorectal cancer. Adv Med Sci (2019) 64(2):388–94. doi: 10.1016/j.advms.2019.05.002
91. Liu H, Liu W, Liu Z, Liu Y, Zhang W, Xu L, et al. Prognostic value of purinergic P2X7 receptor expression in patients with hepatocellular carcinoma after curative resection. Tumour Biol (2015) 36(7):5039–49. doi: 10.1007/s13277-015-3155-2
92. Benzaquen J, Dit Hreich SJ, Heeke S, Juhel T, Lalvee S, Bauwens S, et al. P2RX7B is a new theranostic marker for lung adenocarcinoma patients. Theranostics (2020) 10(24):10849–60. doi: 10.7150/thno.48229
93. Ledderose S, Rodler S, Eismann L, Ledderose G, Rudelius M, Junger WG, et al. P2X1 and P2X7 receptor overexpression is a negative predictor of survival in muscle-invasive bladder cancer. Cancers (Basel) (2023) 15(8):2321. doi: 10.3390/cancers15082321
94. Dietrich F, Cappellari AR, Filippi-Chiela EC, de Paula PB, de Souza JB, Agatti SW, et al. High P2X6 receptor expression in human bladder cancer predicts good survival prognosis. Mol Cell Biochem (2022) 477(8):2047–57. doi: 10.1007/s11010-022-04425-0
95. Liu Z, Liu Y, Xu L, An H, Chang Y, Yang Y, et al. P2X7 receptor predicts postoperative cancer-specific survival of patients with clear-cell renal cell carcinoma. Cancer Sci (2015) 106(9):1224–31. doi: 10.1111/cas.12736
96. Boldrini L, Giordano M, Ali G, Melfi F, Romano G, Lucchi M, et al. P2X7 mRNA expression in non-small cell lung cancer: MicroRNA regulation and prognostic value. Oncol Lett (2015) 9(1):449–53. doi: 10.3892/ol.2014.2620
97. Li Q, Zhu X, Song W, Peng X, Zhao R. The P2X7 purinergic receptor: a potential therapeutic target for lung cancer. J Cancer Res Clin Oncol (2020) 146(11):2731–41. doi: 10.1007/s00432-020-03379-4
98. Gorodeski GI. P2X7-mediated chemoprevention of epithelial cancers. Expert Opin Ther Targets (2009) 13(11):1313–32. doi: 10.1517/14728220903277249
99. Drill M, Jones NC, Hunn M, O'Brien TJ, Monif M. Antagonism of the ATP-gated P2X7 receptor: a potential therapeutic strategy for cancer. Purinergic Signal (2021) 17(2):215–27. doi: 10.1007/s11302-021-09776-9
100. Khakh BS, Burnstock G, Kennedy C, King BF, North RA, Seguela P, et al. International union of pharmacology. XXIV. Current status of the nomenclature and properties of P2X receptors and their subunits. Pharmacol Rev (2001) 53(1):107–18.
101. Kan LK, Drill M, Jayakrishnan PC, Sequeira RP, Galea E, Todaro M, et al. P2X7 receptor antagonism by AZ10606120 significantly reduced in vitro tumour growth in human glioblastoma. Sci Rep (2023) 13(1):8435. doi: 10.1038/s41598-023-35712-5
102. Haanes KA, Schwab A, Novak I. The P2X7 receptor supports both life and death in fibrogenic pancreatic stellate cells. PloS One (2012) 7(12):e51164. doi: 10.1371/journal.pone.0051164
103. Mohammed A, Janakiram NB, Madka V, Pathuri G, Li Q, Ritchie R, et al. Lack of chemopreventive effects of P2X7R inhibitors against pancreatic cancer. Oncotarget (2017) 8(58):97822–34. doi: 10.18632/oncotarget.22085
104. Zhang WJ, Luo C, Huang C, Pu FQ, Zhu JF, Zhu ZM, et al. PI3K/Akt/GSK-3beta signal pathway is involved in P2X7 receptor-induced proliferation and EMT of colorectal cancer cells. Eur J Pharmacol (2021) 899:174041. doi: 10.1016/j.ejphar.2021.174041
105. Zhao Y, Chen X, He C, Gao G, Chen Z, Du J. Discovery of bilirubin as novel P2X7R antagonist with anti-tumor activity. Bioorg Med Chem Lett (2021) 51:128361. doi: 10.1016/j.bmcl.2021.128361
106. Choi JH, Ji YG, Ko JJ, Cho HJ, Lee DH. Activating P2X7 receptors increases proliferation of human pancreatic cancer cells via ERK1/2 and JNK. Pancreas (2018) 47(5):643–51. doi: 10.1097/MPA.0000000000001055
107. Watano T, Matsuoka I, Kimura J. Characteristics of ATP-induced current through P2X7 receptor in NG108-15 cells: unique antagonist sensitivity and lack of pore formation. Jpn J Pharmacol (2002) 88(4):428–35. doi: 10.1254/jjp.88.428
108. Mahmood A, Ali Shah SJ, Iqbal J. Design and synthesis of adamantane-1-carbonyl thiourea derivatives as potent and selective inhibitors of h-P2X4 and h-P2X7 receptors: An Emerging therapeutic tool for treatment of inflammation and neurological disorders. Eur J Med Chem (2022) 231:114162. doi: 10.1016/j.ejmech.2022.114162
109. Fischer W, Urban N, Immig K, Franke H, Schaefer M. Natural compounds with P2X7 receptor-modulating properties. Purinergic Signal (2014) 10(2):313–26. doi: 10.1007/s11302-013-9392-1
110. Jelassi B, Anchelin M, Chamouton J, Cayuela ML, Clarysse L, Li J, et al. Anthraquinone emodin inhibits human cancer cell invasiveness by antagonizing P2X7 receptors. Carcinogenesis (2013) 34(7):1487–96. doi: 10.1093/carcin/bgt099
111. Ali Z, Laurijssens B, Ostenfeld T, McHugh S, Stylianou A, Scott-Stevens P, et al. Pharmacokinetic and pharmacodynamic profiling of a P2X7 receptor allosteric modulator GSK1482160 in healthy human subjects. Br J Clin Pharmacol (2013) 75(1):197–207. doi: 10.1111/j.1365-2125.2012.04320.x
112. Shabbir M, Thompson C, Jarmulowiczc M, Mikhailidis D, Burnstock G. Effect of extracellular ATP on the growth of hormone-refractory prostate cancer in vivo. BJU Int (2008) 102(1):108–12. doi: 10.1111/j.1464-410X.2008.07578.x
113. Haskell CM, Mendoza E, Pisters KMW, Fossella FV, Figlin RA. Phase II study of intravenous adenosine 5’-triphosphate in patients with previously untreated stage IIIB and stage IV non-small cell lung cancer. Invest New Drugs (1998) 16(1):81–5. doi: 10.1023/A:1006018610986
114. D’Alimonte I, Nargi E, Zuccarini M, Lanuti P, Di Iorio P, Giuliani P, et al. Potentiation of temozolomide antitumor effect by purine receptor ligands able to restrain the in vitro growth of human glioblastoma stem cells. Purinergic Signal (2015) 11(3):331–46. doi: 10.1007/s11302-015-9454-7
115. Douguet L, Janho dit Hreich S, Benzaquen J, Seguin L, Juhel T, Dezitter X, et al. A small-molecule P2RX7 activator promotes anti-tumor immune responses and sensitizes lung tumor to immunotherapy. Nat Commun (2021) 12(1):653. doi: 10.1038/s41467-021-20912-2
116. Stark R, Wesselink TH, Behr FM, Kragten NAM, Arens R, Koch-Nolte F, et al. T (RM) maintenance is regulated by tissue damage via P2RX7. Sci Immunol (2018) 3(30):eaau1022. doi: 10.1126/sciimmunol.aau1022
117. Wennerberg E, Mukherjee S, Sainz RM, Stiles B. The ART of tumor immune escape. Oncoimmunology (2022) 11(1):2076310. doi: 10.1080/2162402X.2022.2076310
118. Rivas-Yanez E, Barrera-Avalos C, Parra-Tello B, Briceño P, Rosemblatt MV, Saavedra-Almarza J, et al. P2X7 receptor at the crossroads of T cell fate. Int J Mol Sci (2020) 21(14):4937. doi: 10.3390/ijms21144937
119. Read S, Mauze S, Asseman C, Bean A, Coffman R, Powrie F. CD38+ CD45RB(low) CD4+ T cells: a population of T cells with immune regulatory activities in vitro. Eur J Immunol (1998) 28(11):3435–47. doi: 10.1002/(SICI)1521-4141(199811)28:11<3435::AID-IMMU3435>3.0.CO;2-P
120. Shrimali RK, Ahmad S, Verma V, Zeng P, Ananth S, Gaur P, et al. Concurrent PD-1 blockade negates the effects of OX40 agonist antibody in combination immunotherapy through inducing T-cell apoptosis. Cancer Immunol Res (2017) 5(9):755–66. doi: 10.1158/2326-6066.CIR-17-0292
121. Pillai RN, Ramalingam SS, Thayu M, Lorenzini P, Alvarez Arias DA, Moy C, et al. Daratumumab plus atezolizumab in previously treated advanced or metastatic NSCLC: brief report on a randomized, open-label, phase 1b/2 study (LUC2001 JNJ-54767414). JTO Clin Res Rep (2021) 2(2):100104. doi: 10.1016/j.jtocrr.2020.100104
122. Zucali PA, Lin C-C, Carthon BC, Bauer TM, Tucci M, Italiano A, et al. Targeting CD38 and PD-1 with isatuximab plus cemiplimab in patients with advanced solid Malignancies: results from a phase I/II open-label, multicenter study. J Immunother Cancer (2022) 10(1):e003697. doi: 10.1136/jitc-2021-003697
Keywords: P2X7 receptor, ART1, CD38, CD39, tumour microenvironment, tumour immune escape, cancer immunotherapy
Citation: Sainz RM, Rodriguez-Quintero JH, Maldifassi MC, Stiles BM and Wennerberg E (2023) Tumour immune escape via P2X7 receptor signalling. Front. Immunol. 14:1287310. doi: 10.3389/fimmu.2023.1287310
Received: 01 September 2023; Accepted: 12 October 2023;
Published: 30 October 2023.
Edited by:
Dr Anirban Ganguly, All India Institute of Medical Sciences Deoghar, IndiaReviewed by:
Kaushik Banerjee, University of Michigan, United StatesCopyright © 2023 Sainz, Rodriguez-Quintero, Maldifassi, Stiles and Wennerberg. This is an open-access article distributed under the terms of the Creative Commons Attribution License (CC BY). The use, distribution or reproduction in other forums is permitted, provided the original author(s) and the copyright owner(s) are credited and that the original publication in this journal is cited, in accordance with accepted academic practice. No use, distribution or reproduction is permitted which does not comply with these terms.
*Correspondence: Erik Wennerberg, ZXJpay53ZW5uZXJiZXJnQGljci5hYy51aw==