- 1Department of Gastroenterology, The Third Xiangya Hospital of Central South University, Changsha, China
- 2Hunan Key Laboratory of Non-Resolving Inflammation and Cancer, Cancer Research Institute, Central South University, Changsha, China
Inflammatory Bowel Disease (IBD) is a chronic, relapsing inflammatory disorder of the gastrointestinal tract. Though the pathogenesis of IBD remains unclear, diet is increasingly recognized as a pivotal factor influencing its onset and progression. Fatty acids, essential components of dietary lipids, play diverse roles in IBD, ranging from anti-inflammatory and immune-regulatory functions to gut-microbiota modulation and barrier maintenance. Short-chain fatty acids (SCFAs), products of indigestible dietary fiber fermentation by gut microbiota, have strong anti-inflammatory properties and are seen as key protective factors against IBD. Among long-chain fatty acids, saturated fatty acids, trans fatty acids, and ω-6 polyunsaturated fatty acids exhibit pro-inflammatory effects, while oleic acid and ω-3 polyunsaturated fatty acids display anti-inflammatory actions. Lipid mediators derived from polyunsaturated fatty acids serve as bioactive molecules, influencing immune cell functions and offering both pro-inflammatory and anti-inflammatory benefits. Recent research has also highlighted the potential of medium- and very long-chain fatty acids in modulating inflammation, mucosal barriers, and gut microbiota in IBD. Given these insights, dietary intervention and supplementation with short-chain fatty acids are emerging as potential therapeutic strategies for IBD. This review elucidates the impact of various fatty acids and lipid mediators on IBD and delves into potential therapeutic avenues stemming from these compounds.
Introduction
Inflammatory Bowel Disease (IBD) is a gastrointestinal chronic inflammatory disorder characterized by mucosal barrier disruption, dysbiosis, and immune dysregulation, which mainly comprises ulcerative colitis (UC) and Crohn’s disease (CD). Although the etiology of IBD remains unclear, genetic susceptibility and multiple environmental factors play significant roles (1). As one of the modifiable risk factors, diet has gained increasing attention from researchers (2, 3), where fatty acids derive mostly from diet. Growing evidence demonstrated that “western diet” rich in high fat is closely associated with the risk and progression of IBD (3, 4), with supplementation exacerbating colitis severity in both IBD patients and dextran sulfate sodium (DSS) induced colitis mice (5, 6). This may be attributed to the effects of fatty acids on the regulation of inflammatory pathways, the mucosal barrier, and the intestinal microbiota, as fatty acids are essential components of dietary lipids (7).
Fatty acid is a diverse class of molecules consisting of hydrocarbon chains of different lengths and degrees of desaturation. Classification based on carbon chain length divides them into short-chain fatty acids (SCFAs), medium-chain fatty acids (MCFAs), long-chain fatty acids (LCFAs), and very long-chain fatty acids (VLCFAs) (8). LCFAs and VLCFAs are mainly derived from dietary intake, whereas SCFAs are formed by the conversion of indigestible dietary fibers by specific gut bacteria. In LCFAs, the anti-inflammatory effect of ω-3 fatty acids has been widely confirmed, while ω-6 is connected with impacts of pro-inflammation (9). ω-3 and ω-6 fatty acids can also produce lipid mediators that regulate inflammation resolution (10). SCFAs, which interact with the gut microbiota, are considered important factors in regulating physiological processes such as metabolism and inflammation in the human body (11, 12). Briefly, fatty acids vary in length and type, playing crucial roles in physiological regulation within the body.
Intriguingly, emerging research highlights the varying roles that different types of fatty acids play in the progression of IBD. SCFAs are closely connected with gut microbiota and have been reported to alleviate intestinal inflammation, maintain the mucosal barrier, and regulate immunity. On the contrary, LCFAs promote inflammation and contribute to IBD induction and aggravation. In addition, an imbalance in ω-6 and ω-3 intake is among the factors contributing to IBD onset. ω-3 polyunsaturated fatty acids are thought to be anti-inflammatory and ameliorate IBD (13), while ω-6 fatty acids are considered to contribute to IBD pathogenesis (14). Lipid mediators, derived from PUFA through at least one oxidation step, possess inflammation-regulating capabilities (10). Fatty acids and lipid mediators wield critical roles in IBD, particularly LCFAs and SCFAs, holding promise as prospective therapeutic targets for IBD. However, there is limited knowledge of what forms and amounts of fatty acids and lipid mediators should be consumed to benefit patients with IBD, and evidence-based dietary guidance is relatively scarce. Thus, this review categorizes fatty acids and lipid mediators, clarifies their roles in IBD pathogenesis, and introduces fatty acid-based therapeutic approaches (Figure 1).
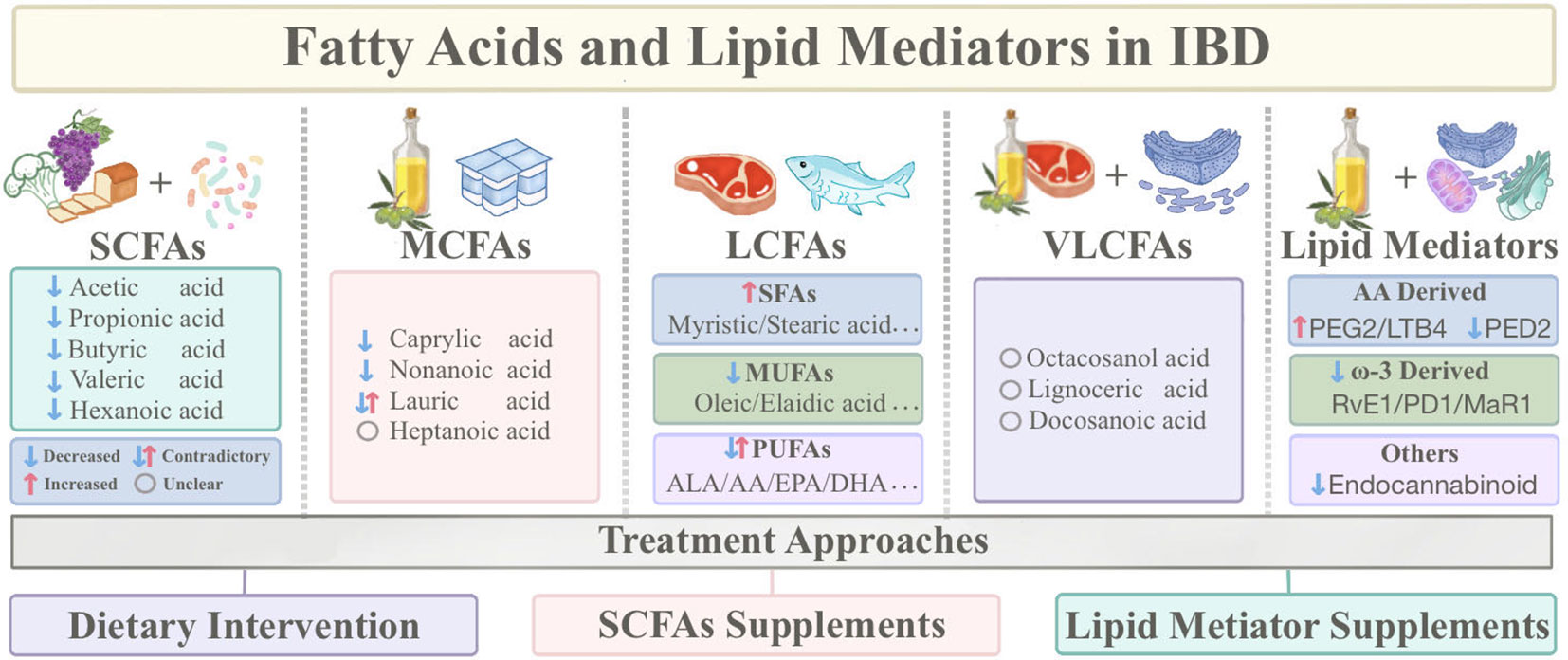
Figure 1 Sources of fatty acids and lipid mediators and classification based on carbon chain length, as well as therapeutic approaches based on fatty acids. (IBD, Inflammatory Bowel Disease; SCFAs, Short-chain fatty acids; MCFAs, Medium-chain fatty acids; LCFAs, Long-chain fatty acids; VLCFAs, Very long-chain fatty acids; SFAs, Saturated fatty acids; MUFAs, Monounsaturated fatty acids; PUFAs, Polyunsaturated fatty acids; DHA, Docosahexaenoic acid; EPA, Eicosapentaenoic acid; ALA, Alpha-linolenic acid; AA, Arachidonic acid).
Short-chain fatty acids
SCFAs mainly include butyric acid, propionic acid, and acetic acid, which not only provide nourishment to the intestinal mucosal epithelium but also exhibit potent anti-inflammatory effects, and regulate immune functions (15). They also collaborate with the gut microbiota to maintain intestinal homeostasis (16). Dietary fiber serves as a substrate for SCFAs production. Gut microbiota capable of breaking down indigestible dietary fibers has the capacity to produce SCFAs (17). The inflammatory response in the gut is modulated by the intestinal microbial ecosystem, with an imbalanced microbiota being a hallmark of IBD (18). At a molecular level, SCFAs act as ligands for G-protein-coupled receptors, exerting their physiological effects (19).
Epidemiological studies have illustrated the role of SCFAs in IBD. Evidence from population-based research implies lower levels of isobutyric, butyric, propionic, and acetic acids in patients with active IBD, accompanied by diverse alterations in SCFAs patterns between CD and UC (20). The dysbiosis of the intestinal microbiota is a significant manifestation of IBD. In CD, the composition of the gut microbiota undergoes distinct changes, including the loss of SCFA-producing bacteria such as Roseburia, Eubacterium, Subdoligranumum, and Ruminococcus, alongside an increase in pro-inflammatory microbial groups (21). As a result, the diminished ability to synthesize butyrate in CD was more pronounced than in UC, potentially linked to reduced dietary fiber intake (22) and decreased butyrate-producing bacteria (23). During mucosal healing in pediatric CD, Dialister species and levels of butyrate salts notably increased (24). Furthermore, compared to first-time surgical patients, recurrent patients exhibited significant downregulation in levels of cyclohexanoic acid, 2-methylbutyric acid, and isobutyric acid (25), and CD patients with a history of abdominal surgery showed lower levels of butyrate-producing bacteria (26). However, Kiasat A et al. suggested that plasma SCFAs changes are unrelated to CD and UC after adjusting for gender, age, and diet (27).
The bulk of SCFAs research has predominantly centered around butyrate. Thus, we will detail the implications of SCFAs concerning IBD, encompassing facets of modulation of inflammation and immunity, regulation of intestinal microbiota, and maintenance of the intestinal barrier (Table 1, Figure 2).
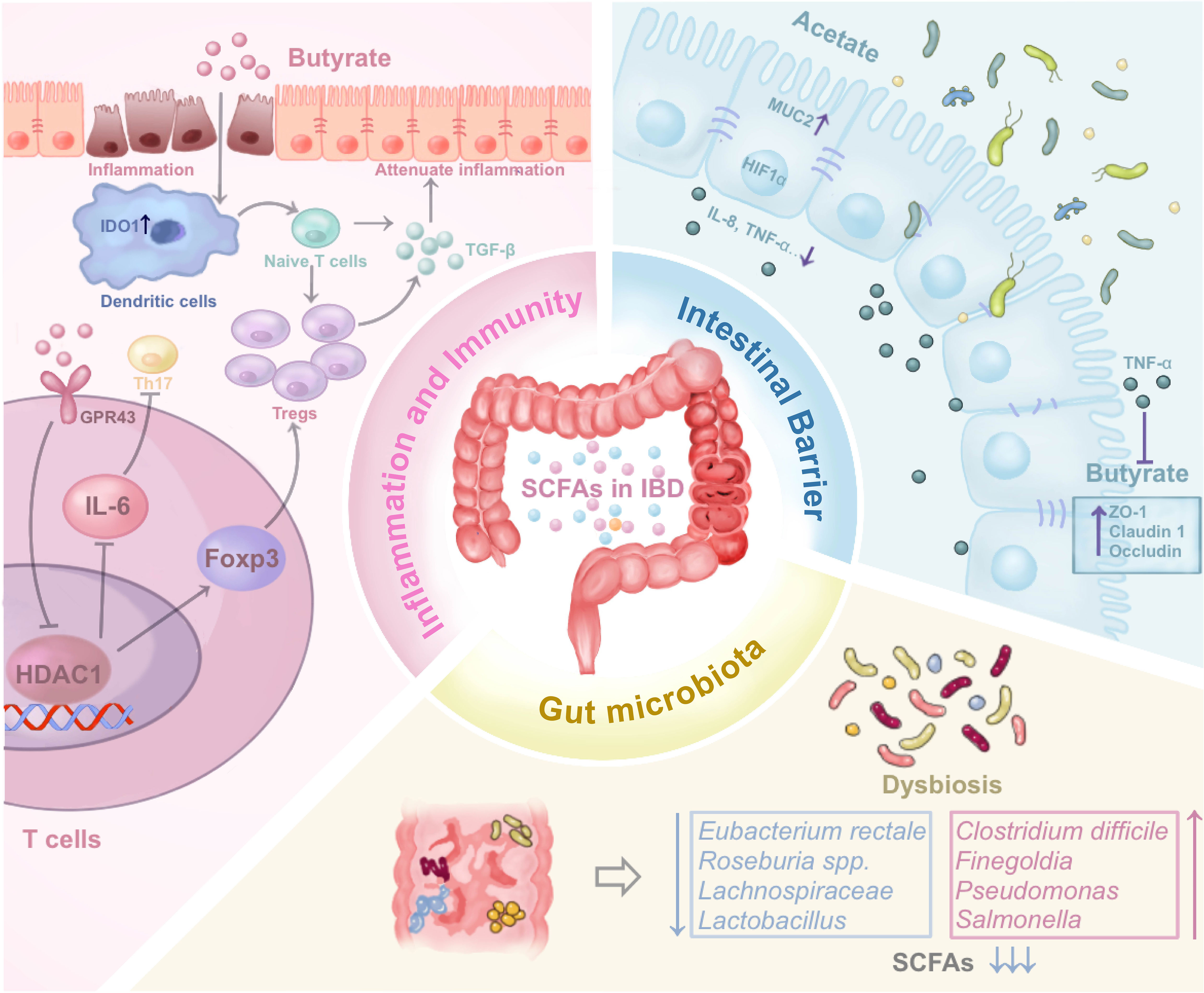
Figure 2 The impact of SCFAs on IBD by regulating inflammation and immune, regulating gut microbiota, and maintaining intestinal barrier through various mechanisms. The figure illustrates the three potential mechanism of SCFAs mentioned in the review. (SCFAs, Short-chain fatty acids; IBD, Inflammatory Bowel Disease; HDAC1, Histone Deacetylase 1; MUC2, Mucin 2; ZO-1, Zonula occludens-1).
Inflammation and immune regulation
SCFAs, particularly butyrate, exhibit anti-inflammatory properties. Among SCFAs, butyrate is most extensively studied related to inflammation and immune regulation in IBD. Acting on receptors GPR43 and GPR109a, butyrate effectively suppresses the development of IBD (28, 29), and its supplementation reduces the expression of inflammation-related genes in UC (30). Nonetheless, the activation of the inflammasome sensor Nlrp1 triggers IL-18, which paradoxically inhibits the benefits of butyrate-producing bacteria, exacerbating inflammation in UC (31). EHLJ7, a quaternary coptisine derivative, not only stimulates butyrate production but also collaborates with butyrate to inhibit the JAK2/STAT3/SOCS1 signalling pathway, ultimately alleviating UC symptoms (32). Prophylactic administration of butyrate can reverse the reduced activity of cytochrome P450 2A5 induced by UC, a phenomenon associated with the gut microbiota (33). In addition to butyrate, acetate supplementation decreases levels of pro-inflammatory cytokines such as IL-8 and TNF-α in UC-derived epithelial cells while simultaneously upregulating hypoxia-inducible factor (HIF1α) and mucin 2 (34). Valproic Acid, functioning as a histone deacetylase (HDAC) inhibitor, elevates H3K27ac levels in UC mice, effectively suppressing the production of inflammatory cytokines (35).
Maintaining the balance of T cells has always been a hot spot for the exploration of the pathogenesis of IBD. Dendritic cells exposed to butyrate can induce the differentiation of naïve T cells into Foxp3-expressing Treg cells (36). Moreover, SCFAs can directly enhance Foxp3 expression by activating GPR43 on the surface of T cells (37). A butyrate producer, Faecalibacterium prausnitzii, fosters forkhead box protein P3 (Foxp3) expression by inhibiting HDAC1, thereby disrupting the IL-6/STAT3/IL-17 pathway and altering the Treg/Th17 equilibrium (38, 39). The administration of a probiotic combination comprising Saccharomyces boulardii, Lactobacillus rhamnosus, Lactobacillus acidophilus, and Bifidobacterium breve to SAMP1/YitFc mice resulted in increased SCFAs levels and gene expression changes involved in memory B cell development and T cell infiltration (40). Employing poly-D-3-hydroxybutyric acid as a controlled-release agent for 3-hydroxybutyrate, sustained butyrate release enhances regulatory T cell presence (41).
While considerable research has delved into the anti-inflammatory effects of butyrate, challenges persist in its application, with some IBD patients exhibiting suboptimal responses to butyrate treatment (42). Investigations have revealed that the reduction of butyrate-producing bacteria in the feces of IBD patients is unrelated to the decrease in butyrate concentration, and the sensitivity of IBD patients to butyrate remains unchanged, but the elevation of TNF-α diminishes the response of intestinal epithelial cells to butyrate (43). This “disabling” effect of butyrate during active UC might be linked to altered gene expression patterns in UC (44) or possibly associated with compromised regulation of the CTLA-4 receptor on T cell surfaces induced by butyrate (45). These studies could potentially offer novel insights for enhancing the application of SCFAs.
Regulation of gut microbiota
Dysbiosis of the gut ecosystem stands as a significant hallmark in IBD, with beneficial probiotics producing SCFAs being recognized for their favorable impact on IBD. Conversely, the proliferation of certain pathogenic bacteria contributes to intestinal inflammation associated with disease onset and relapse (54). Among these probiotics, butyrate-producing bacteria have garnered attention for their capacity to maintain mucosal barriers, regulate immune functions, and alleviate inflammation (55). A reduction in butyrate-producing bacteria, including Clostridium coccoides/Eubacterium rectale, Clostridium leptum, and Faecalibacterium prausnitzii in the intestinal lumen, as well as Roseburia spp. in the mucosa of UC patients (56). The abundance of Lachnospiraceaeis significantly diminished regardless of active or remission phase of UC (57). Apigenin mitigates DSS-induced colitis by modulating Akkermansia, Turicibacter, Klebsiella, Romboutsia (58). Conversely, Paraclostridium bifermentans exacerbates UC symptoms, potentially linked to SCFAs reduction (59). The expansion of gut microbiota in UC patients can stimulate the occurrence of cancer through the activation of T helper cell types 1 and 17 cytokines (60). Acetate suppresses uvrY-dependent type 1 pilus expression and hinders the adherence of invasive Escherichia coli (61).
Dietary fiber serves as a source of SCFAs, and diets rich in dietary fiber are advantageous for the amelioration of inflammatory conditions (62). A Cohort study discovered that dietary fiber intake can reduce the occurrence of CD exacerbations (63). Conversely, the lack of dietary fiber prompts a shift in the gut microbiota’s nutritional preference to mucin glycans, detrimentally impacting mucosal epithelium (64). Soluble dietary fiber from quinoa bran increases tight junction protein expression, enhances gut microbial diversity, boosts SCFAs production, and alleviates DSS-induced colitis (65). Similarly, highly purified insoluble dietary fiber extracted from soybean bran exhibits similar effects (66). However, A case-control study found that dietary fiber had a limited impact on microbial and SCFAs profiles (67).
Maintenance of intestinal barrier
SCFAs play a pivotal role in maintaining intestinal barrier integrity. Prior research has indicated that HIF coordinates intestinal protection (46). Butyrate reduces damage to the intestinal barrier inflicted by Clostridium difficile (48), regulates intestinal epithelial cell autophagy (51), modulates tight junction (74) through a HIF-dependent mechanism, and thereby alleviates DSS-induced colitis. The stabilization of HIF by butyrate is hypothesized to occur through the inhibition of HIF prolyl hydroxylase (75).
The maintenance of tight junction components is a critical factor in protecting the intestinal barrier. Among these, butyrate takes center stage in SCFAs (47). Reduced tight junction protein 1 (TJP1) expression and elevated claudin-1 expression characterize the intestinal epithelium of IBD patients. Butyricicoccus can upregulate claudin-1 expression, and maintain the integrity of intestinal epithelial tight junctions (76). Butyrate might also enhance tight junction protein abundance by activating the AKT/mTOR pathway (77). Heat shock transcription factor 2 can inhibit mTOR and promote butyrate-induced autophagy, thereby protecting intestinal epithelial cells (50). Isobutyric acid upregulates claudin-23 protein through the SP1 and AMPK pathways (53). Butyrate-producing bacteria also activate epidermal growth factor receptors (EGFR), contributing to tight junction protection (52). However, research also pointed out that co-culturing butyrate with TNF-α and IFN-γ can lead to epithelial damage, suggesting potential contrasting effects of butyrate in IBD (49).
Besides, exploration of acetate and propionate has enriched the spectrum of SCFAs effects on IBD. Acetate, exhibiting lower epithelial toxicity, maintained the epithelial barrier and reduced levels of pro-inflammatory factors such as IL-8 and TNF-α (34). Propionate enhanced the expression of tight junction proteins zonula occludens-1 (ZO-1) and occludin, which exerted anti-inflammatory effects by inhibiting macrophages (78). Furthermore, propionate upregulated endothelial cell-selective adhesion molecules, reinforcing intestinal epithelial tight junctions (79).
Supplementing bacteria that produce butyrate for CD patients (80), or administering butyrate derivatives orally (81), can achieve protection of the intestinal barrier. The intricate relationship between gut microbiota and intestinal barrier function is pivotal, where probiotics can mitigate epithelial damage and regulate inflammatory responses. The combination of Faecalibacterium prausnitzii, a butyrate-producing bacterium, with chitosan oligosaccharides, can restore tight junction levels and enhance intestinal epithelial barrier function (82). Supplementation with prebiotics, probiotics, IgG, and amino acids collectively improves IBD intestinal barrier function (83). Soluble dietary fiber from quinoa bran (65) and Panax quinquefolius polysaccharides both elevate tight junction protein expression, subsequently augmenting SCFAs production.
SCFAs as biomarkers and potential therapeutic targets
A notable feature of IBD patients is the reduced levels of SCFAs, evident in feces, urine, plasma, intestinal fluid, and exhaled gas, highlighting the potential of SCFAs as diagnostic and predictive biomarkers for IBD remission and relapse. Total SCFAs in rectal fluid are reduced in recurrent IBD patients (106). Butyrate levels in feces decrease during active CD and UC phases, implying its potential as a marker for active IBD (107). The diminished capacity for butyrate synthesis is more pronounced in CD than in UC (22). Regardless of relapse status, IBD patients have lower isobutyrate levels than healthy individuals (106). Furthermore, isobutyrate and Verrucomicrobiota could serve as markers for diagnosing UC remission (108). Additionally, hexanoate might serve as a predictive indicator for CD patient relapse risk (25). Targeted delivery of SCFAs to the colon alleviated inflammation in DSS-induced colitis mice, enhancing SCFAs application for IBD (109).
Medium-chain fatty acids
MCFAs are usually defined as fatty acids with carbon chain lengths ranging from 7 to 12 carbon atoms. As directly absorbable fatty acids, MCFAs facilitate quick energy supply, promote intestinal mucosal repair, and regulate inflammation (132). The colon plays a pivotal role in the digestion and absorption of MCFAs. Moreover, MCFAs exhibit inherent antibacterial effects, and maintain gut microbiota balance by reducing pathogenic bacteria and promoting beneficial bacteria (132).
The role of MCFAs in patients suffering from UC and CD has been elucidated by various epidemiological investigations. Observations from a case-control study have demonstrated significantly reduced levels of lauric acid (C12:0) in the serum of patients with autoimmune diseases (133). MCFAs such as heptanoate, octanoate and nonanoate, exhibit markedly decreased levels in IBD patients (134). In pediatric patients, there were variations of lauric acid between CD and UC, and higher levels of lauric acid were observed during UC active phases compared to remission (135). In summary, reduced levels of MCFAs are common among IBD patients, and proportions of MCFAs differ between CD and UC.
MCFAs alleviates IBD symptoms by regulating inflammation and intestinal epithelial barrier (Table 2). Jun Wang et al. investigated the impact of octanoic acid and nonanoic acid on intestinal mucosal barrier. They found that treatment with these acids enhanced intestinal barrier function and reduced bacterial translocation by reducing activity of histone deacetylases, resulting in increased secretion of porcine β-defensin 1 and porcine β-defensin 2 (69). Octanoic acid can also serve as a molecular conjugate to enhance the activity of other drugs. The novel drug CLX-103, composed of mesalazine, eicosapentaenoic acid, and octanoic acid, shows prolonged intestinal retention and greater efficacy compared to mesalazine alone (68). Decanoic acid can induce increased paracellular permeability, influencing tight junctions in intestinal epithelial cells (70). Lauric acid has been found to have anti-inflammatory effects in other diseases (136), though its role in IBD remains unclear. Black soldier fly larvae (BSFL) oil, rich in lauric acid, is proposed to modulate mTOR signalling and enhance PPAR target gene expression related to fatty acid oxidation. This demonstrates anti-inflammatory activity and amelioration of colitis symptoms in mice (72). However, several studies have demonstrated the pro-inflammatory activity of MCFAs. MCFAs regulate inflammation in human fetal intestinal epithelial cells by promoting IL-8 secretion induced by IL-1β and TNF-α (137). Additionally, lauric acid could induce NOD2 signalling and increase NF-kB activation and IL-8 expression (71).
MCFAs have been employed for the prediction and treatment of IBD. Serum lauric acid serves as a significant biomarker for autoimmune diseases, while the relationship between MCFAs and IBD cannot be firmly established due to the limited sample size (133). Medium-chain triglycerides (MCTs) hold potential therapeutic value. However, due to the risk of formulations with MCTs lacking essential PUFAs and fat-soluble vitamins, the use as additives is not recommended for healthy children (138). Additionally, a novel amphiphilic C10 and C18 cyclobutene and cyclobutane fatty acid has demonstrated inhibition of Mycobacterium avium subsp. paratuberculosis associated with CD (73, 139).
Long-chain fatty acids
LCFAs generally encompass fatty acids with carbon atoms ranging from 13 to 22 (8) and are categorized into saturated fatty acids (SFAs), monounsaturated fatty acids (MUFAs), and polyunsaturated fatty acids (PUFAs) based on unsaturation degrees. Dietary intake is a common means of obtaining LCFAs, such as ω-3 and ω-6 fatty acids found in fish oil (140). The significant variations among various LCFAs yield distinct implications for IBD development (Table 3).
Saturated fatty acids and trans fatty acid
SFAs have been identified to exhibit pro-inflammatory effects. Interestingly, trans fatty acids (TFAs), a distinct subset of unsaturated fatty acids, seem to induce similar responses to SFAs (141). Both SFAs and TFAs potentially regulate inflammatory responses by impacting the PPAR-γ and retinoid X receptor (RXR) pathways (142). Despite the fact that the precise roles of SFAs and TFAs in IBD remains enigmatic, extensive studies have shown their contribution to IBD onset and progression. A genetic study focusing on fatty acid profiles found that C18 TFAs, total TFAs, and palmitic acid may positively correlate with the onset of IBD (143). Several epidemiological studies also demonstrated that higher intake of SFAs and TFAs is associated with elevated UC risk (4, 5), whereas a meta-analysis did not observe a significant correlation between SFA intake and IBD (144). Furthermore, a diet rich in TFAs could exacerbate pathological inflammation in the intestinal epithelium of individuals with IBD (94). In addition, a multicenter prospective study found that consuming high intake of myristic acid increased the risk of UC recurrence in remission (145). Another cohort study indicated a significant difference in urinary TFAs among patients with UC recurrence (146).
Palmitic acid is a key component of SFAs, which is associated with pro-inflammation. Plasma metabolomics measurements suggested that palmitic acid was a potential diagnostic marker for IBD (147). Palmitic acid contributes to inflammation by enhancing intestinal epithelial permeability (85), activating NF-κB pathway and cytokines (86), and inducing endoplasmic reticulum stress leading to intestinal epithelial cell lipotoxicity (84).
Monounsaturated fatty acids
MUFAs, characterized by a single double bond, have been observed to possess anti-inflammatory properties in animal experiments (148, 149). However, investigations on the effects of MUFAs on IBD are limited and contradictory. S. Bühner et al. identified a reduction in MUFAs (18:1, ω-9) levels by analyzing fatty acid profiles of biopsies from CD patients (150), while another study reported increased oleic acid levels in IBD patients (14). Mounting case-control studies have associated increased MUFA intake with elevated UC incidence (4, 151). In the case of palmitoleic acid, a study revealed elevated serum levels in CD patients, correlating with increased surgical intervention risk (152). Conversely, a cross-sectional analysis found lower MUFA intake in CD patients (153), and a meta-analysis incorporating four case-control and five prospective studies did not establish a significant association between MUFAs and UC incidence (144). In conclusion, further epidemiological and clinical research is required to elucidate the role of MUFAs in IBD.
As one of the prominent MUFAs, oleic acid has garnered considerable attention from researchers. Early experiments indicated decreased levels of oleic and palmitoleic acids in the inflamed intestinal mucosa (154). Oleic acid exerts a protective effect on CD patient intestinal epithelial cells without upregulating interleukin-8 (IL-8) levels (87). Furthermore, oleic acid-containing Javanese Bruise Oil Emulsion and ellagic acid-containing thymol polyphenols can inhibit the NF-κB pathway in DSS-induced colitis mice (90, 155). In addition, 9- or 10-nitrooctadec-9-enoic acid activates the PPAR-γ pathway, displaying superior anti-inflammatory efficacy in IBD compared to natural oleic acid (91). Mice consuming oleic acid-rich acorn-fed ham exhibited enhanced anti-inflammatory microbial abundance and cecal SCFAs concentration after DSS induction, suggesting potential preventive effects against UC (88). On the contrary, excessive consumption of oleic acid-rich extra virgin olive oil failed to ameliorate symptoms in DSS-induced colitis mice and even elevated TNF-α levels (89). Therefore, MUFAs potentially serve as an anti-inflammatory fatty acid with preventative and therapeutic roles in IBD. Nonetheless, contradictions across studies necessitate further experimental exploration into the specific mechanisms underlying MUFAs’ actions in IBD.
Polyunsaturated fatty acids
PUFAs comprise a category of fatty acids characterized by multiple double bonds. Among the various PUFAs, ω-6 fatty acids promote IBD progression (14), whereas ω-3 fatty acids possess anti-inflammatory properties (13). High ω-6/ω-3 fatty acid ratio in Western diets is a pivotal feature of IBD.
ω-6 fatty acids include arachidonic acid (AA) and linoleic acid, which are often associated with promoting UC pathogenesis (14). Accumulating evidence from population-based revealed an increased level of AA in the colonic mucosa of UC patients (154) associated with IBD-related colorectal cancer progression (156, 157). Metabolomic analysis indicated perturbed AA metabolism in UC mucosa (158), and an increased likelihood of ω-6 fatty acid depletion in pediatric IBD patients (159). Observations from a cohort inquiry highlight a positive correlation between dietary AA intake and UC risk (160). A Mendelian analysis proposed ω-6 fatty acids as causative for CD (161).
ω-3 fatty acids exert anti-inflammatory effects and are often considered protective against IBD. Diminished levels of ω-3 fatty acids were observed in both CD and UC patients (162). A Japanese case-control study suggested that PUFA intake serves as a protective factor for UC, while excessive docosahexaenoic acid (DHA), eicosapentaenoic acid (EPA), and docosapentaenoic acid (DPA) intake might increase UC risk (163). Conversely, two Mendelian analyses independently associated ω-3 fatty acids with protection against IBD (164, 165). A nurse cohort study found a connection between ω-3 fatty acid intake and reduced UC risk (5). Meta-analytic investigations revealed protective effects of fish oil and dietary ω-3 fatty acids against UC (13). Conversely, a systematic review highlighted that supplementing PUFAs has a negligible impact on IBD prevention and treatment, with limited long-term inflammation improvement (166). Another scoping review revealed inconclusive effects of ω-3 fatty acids on IBD inflammation and symptom alleviation (167).
PUFAs can impact IBD through influencing intestinal inflammation. Elevated AA levels in UC patient’s colonic mucosa are linked to inflammation and colonic mucosal phospholipid AA composition (154, 168). PUFAs can modulate anti-inflammatory signalling pathways and maintain the integrity of the intestinal barrier by binding to GPR120 (169, 170). The anti-inflammatory effects of ω-3 fatty acids were linked to decreased levels of prostaglandin E2 (PGE2), alkaline phosphatase (AP) (92), and the downregulation of NF-κB, TNF-α, inducible nitric oxide synthase, and IL-1β activity (93), along with upregulation of PPAR-γ (94). Alpha-linolenic acid (ALA) could reduce ionized calcium-binding adaptor molecule 1-positive macrophages (96) and downregulate the expression of pro-inflammatory genes IL-8, cyclooxygenase-2 (COX2), and inducible nitric oxide synthase to reduce intestinal inflammation (95). EPA moderates TGF-β/P-EGFR and NF-κB inflammatory pathways, modulates the redox balance, and mitigates rat UC progression (97). Recent research revealed the antioxidative activity of glutathione peroxidase 4 against PUFA-induced oxidative stress. Excessive dietary PUFAs could activate toll-like receptor 2 (TLR2) through inositol-requiring enzyme 1α (IRE1α)-induced endoplasmic reticulum stress (98), and AA might trigger cytokine production akin to ferroptosis in intestinal epithelial cells, leading to epithelial injury (99).
Microbial dysbiosis and impaired mucosal barrier are pivotal features in IBD pathogenesis, and PUFAs might influence IBD by altering microbial balance and maintaining mucosal barrier. Flaxseed supplementation and ω-6-rich fatty acids have been associated with promoting microbial dysbiosis in CD and altering mucosal barrier functions (100, 171). Supplementation of DPA increases gut microbial diversity and changes microbial composition in DSS-induced colitis mice (102). Furthermore, DHA and EPA alleviate endoplasmic reticulum stress in goblet cells, reducing the synthesis and secretion of Muc2 protective mucin barriers (84). Limited evidence suggested DPA possesses stronger anti-inflammatory and protective effects against IBD than EPA and DHA (101), offering new directions for PUFAs research.
Eicosatetraynoic acid (ETYA) (20:4) in a patient-specific human intestinal organoid (HIO) model developed by Ingrid Jurickova et al. demonstrated reduced extracellular matrix gene expression and alleviated CD ileal stricture (103). Icosapent ethyl can mitigate CD ileitis through the silent information regulator 1 (SIRT1) pathway, decreasing the expression of various pro-inflammatory cytokines and exerting anti-inflammatory, antioxidant, and anti-apoptotic effects (104). Lifang Wang et al. synthesized 7S, 15R-dihydroxy-16S, 17S-epoxy-22-carbon-5-docosenoic acid and demonstrated its anti-inflammatory effects by reducing TNF-α, IL-6, and IL-1β levels (105). Notably, traditional Chinese herbal formulas may modify IBD progression by regulating PUFAs (172–174).
Researches have extensively been exploring the regulation of inflammation and intestinal barrier by PUFAs. Recent studies elucidate PUFAs mechanisms impacting IBD progression via ferroptosis-related pathways and the influence of PUFAs on microbial ecology, thereby expanding perspectives in IBD research.
Very long-chain fatty acids
The role of VLCFAs, defined as fatty acids with a carbon chain length exceeding 23, is gradually being elucidated in inflammation. VLCFAs can originate from dietary sources and can also be endogenously synthesized from long-chain fatty acids and Elongated by the elongase of very long fatty acid (ELOVL) family (175). Saturated VLCFAs can mediate necroptosis (176) and activate macrophages to generate an inflammatory response (177). However, research on VLCFAs has primarily focused on X-linked adrenoleukodystrophy, a disorder characterized by VLCFAs accumulation. There is a gap in understanding of VLCFAs’ role in IBD.
Research on VLCFAs in IBD is limited. Only some gene sequencing revealed differences in the ELOVL gene between IBD patients and healthy individuals. Genetic sequencing of two CD patients in Japan revealed variations in ELOVL6 (178), and gene analysis of immune cells from UC patients demonstrated significant upregulation of ELOVL5 (179). Furthermore, ELOVL7 gene regions were identified as possible novel loci associated with adalimumab response in CD patients (180). Additionally, octacosanol was demonstrated to alleviate DSS-induced colitis by protecting intestinal barrier and modulating gut microbiota and SCFAs levels (181).
Lipid derive mediators
Lipid mediator (or oxylipins) is a group of bioactive molecules that derive from fatty acid involving at least one step of dioxygen-dependent oxidation (10). PUFAs serve as major precursors for lipid mediators. Inflammatory responses and resolution are perceived as a controlled, ongoing process involving sequential release of diverse lipid mediators. Prostaglandins (PGs) and leukotrienes (LTs) derived from AA are pro-inflammatory mediators at inflammation onset, while lipoxin A4 (LXA4) and LXB4 from AA and ω-3 fatty acids derived lipid mediators are recognized as pro-resolving mediators during resolution. Furthermore, the interaction of a novel lipid mediator endocannabinoid system with exogenous cannabinoids is considered to be a potential therapeutic avenue for IBD (182). Lipid mediators are increasingly recognized for their significance in the pathophysiology and treatment of IBD (Table 4).
Arachidonic acid derived lipid mediators
Lipid mediators derived from AA primarily include PGs, LTs, and LXA4. A case-control study has identified a series of pro-inflammatory lipid mediators, such as PGE2, PGD2, and thromboxane (TXB2) in the intestinal mucosa of UC patients, correlated with the severity of inflammation (183). Interestingly, PGD2 also exhibited a protective effect, and its upregulation was associated with prolonged remission in UC patients (183). Elevated LXA4 expression promotes mucosal homeostasis and can be found only in the intestinal mucosa during remission (184). EPA, an AA metabolite generated through P450 metabolism, has been observed at elevated levels in UC tissues in comparison to the surrounding tissues, suggesting a potential protective role against UC (185). Evidence from observational study points to higher levels of AA-derived oxylipins in CD patients (186).
PGs serve as key regulators of vascular responses, modulating inflammatory cell infiltration (187). PGE2 and PGD2 play a crucial role in inflammation modulation, originating from the common precursor PGH2 through enzymatic catalysis. In IL-10 knockout mice, PGD2, PGE2, and other lipid mediators are upregulated. This may result from local inflammatory cell induced breakdown of PUFA to maintain inflammatory homeostasis (188). PGE2 exerts pro-inflammatory effects in IBD, possibly through the IL-23/IL-17 axis regulation (110). PGD2 may have both pro-inflammatory and anti-inflammatory effects under different circumstances. For instance, the increased presence of lipocalin-type prostaglandin D synthase (L-PGDS) in UC may exacerbate inflammation (111). Conversely, PGD2 also has protective effects in IBD. Nicotinic acid regulates suppressed macrophage pro-inflammatory gene expression through PGD2 release (114). The effects of PGD2 depend on its receptors; activation of CRTH2 may mediate inflammation, while stimulation of another PGD2 receptor DP is inversely correlated with neutrophil activity to mitigate inflammation (112, 113).
LTs promote leukocyte activation and cytokine production. In trinitrobenzene sulfonic acid (TNBS)-induced colitis mice, LTB4 inhibition accelerates colitis healing. Furthermore, LTB4 synthesis in colonic epithelial cells is enhanced in TNBS-induced colitis mice, which modulates Th1 and Th17 cell differentiation (115). LXA4 and LXB4, anti-inflammatory pro-resolving lipid mediators derived from AA, inhibit vascular inflammation (189). Knocking out COX2 reduces colonic LXA4 levels, exacerbating inflammation (116).
AA derived lipid mediators can also serve as biomarkers and potential therapeutic targets. Due to its significant role in inflammation, the major urinary metabolite of PGE is considered to be a predictive biomarker of mucosal healing and relapse (190). Furthermore, in a lipidomics study of UC patients, PGE1 and PGD2 are critical factors influencing mucosal healing (191). Inhibition of LTB4 might hold potential therapeutic implications for UC (192).
ω-3 fatty acids derived lipid mediators
ALA can be metabolized into DHA and EPA, which are subsequently transformed by LOX and CYP enzymes into various ω-3 fatty acid-derived lipid mediators (193). ω-3 fatty acids derived lipid mediators include resolvins, protectins, and maresins, are considered potential tools for controlling IBD due to their remarkable pro-resolving capabilities, which are also named specialized pro-resolving mediators (SPMs) (194). In an observational study, RvE1 was found to be positively correlated with disease activity in UC patients (195). Resolvins and protectins from ω-3 fatty acids have a significant up-regulation in IBD colon biopsies (122). A case-control study has revealed lower levels of ω-3 fatty acid-derived lipid mediators in CD patients (186).
Resolvins are interactive products generated during the inflammation resolution phase, contributing to alleviating inflammatory stress (10). Protectin, a lipid mediator produced by DHA, exhibits the ability to inhibit neutrophil migration and promote neutrophil apoptosis (196, 197). Maresins, macrophage mediators derived from DHA, demonstrate pronounced anti-inflammatory effects (198). Resolvin E1 (RvE1) derived from EPA induces the expression and activity of alkaline phosphatase in intestinal epithelial cells to detoxify lipopolysaccharide (LPS) (117). RvE1 suppresses pro-inflammatory cytokine TNF-α production, reducing leukocyte-mediated tissue damage and gene expression (119), ultimately improving histological scores in colitis mice and preventing colitis onset (118, 119). BLT1 can serve as a receptor for RvE1, accelerating the intestinal epithelial barrier repair (199). RvD1, sourced from DHA, confers protection against IBD by strengthening tight junctions, maintaining intestinal homeostasis, and attenuating inflammation (120). RvD1 also reduces mesenchymal transition of intestinal epithelium and prevents IBD-associated fibrosis (121). Both RvD1 and RvD5 diminish leukocyte adhesion, migration, and endothelial adhesion activated by TNF-α, which exhibit protective effects against colitis (122). Maresin 1 diminishes IL-1β, TNF-α, IL-6, and IFN-γ levels and suppresses NF-κB pathway (123). Besides, maresin 1 regulates NRF2 and TLR4/NF-kB pathways to control colonic inflammation, concurrently improves tight junction protein expression, and maintains mucosal barrier (124). Targeted transport of maresin 2 via nanoparticle packaging promotes mucosal repair (200). Besides, DHA-derived epoxides can restore the ability of the endothelium to resolve intestinal inflammation through major facilitator superfamily domain containing 2A (MFSD2A), and overexpression of MFSD2A reduces colitis in mice (201).
Certain lipid mediators derived from ω-3 fatty acids have the potential to serve as biomarkers reflecting the progression of IBD. Compared to CD, RvE1 levels are significantly elevated in active UC, while during CD remission, protectin DX concentrations are higher than UC (202). However, another study suggests that RvE1 may not be a biomarker for UC (195). Enteral nutrition can stimulate the release of RvD1-RvD5 through innate lymphoid cell activation to alleviate CD symptoms (203).
Other lipid mediators
Hydroxyeicosatetraenoic acid (HETE) and cannabinoids are important lipid mediators influencing IBD. Accumulating case-control research suggests elevated levels of 5-HETE, 11-HETE, 12-HETE, 15-HETE, and endocannabinoids in inflamed mucosa of IBD (186, 203, 204). Pro-inflammatory AA derivative 15S-HETE elevated in CD and 12S-HETE higher during CD remission (204). Functional variants of cannabinoid receptor 2 increase the risk of pediatric CD (205). Distinct differences exist in the endocannabinoid systems between individuals with IBD and colorectal cancer (206).
HETEs derived from various PUFAs could regulate inflammation. HETE is upregulated in IL-10 knockout mice, for local inflammatory cell induce PUFA breakdown (188). 5-HETE enhances intestinal epithelial cell permeability through the cAMP-independent protein kinase A pathway and phospholipase C/Ca (2+)/protein kinase C pathway (125). Exogenous supplementation of 12-HETE reduces the expression of tight junction protein ZO-1, impairing the intestinal barrier function in UC (126). Conversely, ω-3 supplementation exerts a protective effect on UC by increasing 15-HETE (127, 207). Mechanistic study on 15-HETE reveals its role in reducing intestinal epithelial permeability by inhibiting AMPK and increasing the activity of ZO-1 (128).
Endocannabinoid system has recently been identified to offer protective effects in IBD. Under chronic IBD conditions, the endocannabinoid system undergoes downregulation, while the activation of cannabinoid receptor 2 mitigates inflammation by enhancing regulatory T cell (Treg) function and IL-10 secretion (129). DSS-induced colitis mice exhibit an increase in colonic mucosal endocannabinoids (130). Disruption of the endocannabinoid system in mice by a high ω-6 fatty acid diet heightens susceptibility to UC (208). Recent findings suggest that inflammation induced in germ-free mice by DNBS is attenuated, attributing to the role of the endocannabinoid system in the endocannabinoidome (eCBome) (131).
These lipid mediators also serve as biomarkers and potential therapeutic targets. The 20- HETE-d6 could serve as a diagnostic biomarker for IBD (147). Patients with IBD might self-administer cannabis to alleviate abdominal pain symptoms (209). Phycocyanin has been found to activate cannabinoid receptors and promote UC healing (210).
Therapeutic approaches based on fatty acids
A myriad of therapeutic strategies is available for IBD, encompassing surgical interventions, dietary interventions, corticosteroid hormone therapy, biologics, fecal microbiota transplantation, probiotic therapy, and enteral nutrition. In this context, we will specifically highlight three treatment approaches closely associated with fatty acids and lipid mediators, including dietary interventions, SCFAs and lipid mediator supplementation.
Biological agents, 5-aminosalicylic acid (5-ASA), glucocorticoids, and immunosuppressive drugs are widely used for induction and maintenance treatment in IBD patients. The therapeutic mechanisms of these drugs are partly related to fatty acids. Butyrate salts might synergistically enhance the therapeutic effects of anti-TNF treatments (211). An increase in SCFA-producing bacterial levels is one of the mechanisms by which Infliximab therapy exerts its therapeutic effect (212), and serves as a biomarker for patient response to Infliximab therapy (213). Levels of butyrate and substrates are correlated with the remission outcomes of Infliximab treatment (214). Similarly, butyric acid and isobutyric acid can serve as biomarkers for patient responses to Vedolizumab (108). Furthermore, glucocorticoids, such as prednisolone, can directly reduce luminal lipid mediators like PGE2 and LTB4, improving IBD symptoms (215, 216). The effects of 5-ASA on UC intestinal mucosa SCFA have been elaborated on in other reviews (217). A recent clinical study found that UC patients treated with a combination of 5-ASA and FEEDColon involving Butyrate achieved better subjective symptom improvement than those treated with 5-ASA alone (218). Additionally, animal studies have found that 1000mg/kg EPA in alleviating colitis-related oxidative stress, serum LDH, colonic GLP-1 expression, and NF-κB capacity is comparable to Sulfasalazine (97). These studies illuminate the role of fatty acids in IBD drug therapy.
Dietary intervention: particularly the therapeutic role of fatty acids in IBD
Fatty acids play a pivotal role in the pathological and physiological processes of IBD by regulating intestinal mucosal barrier, modulating pro-inflammatory/anti-inflammatory balance, influencing immune function, and regulating gut microbiota homeostasis. Therefore, a rational intake of fatty acids is crucial for both IBD prevention and treatment.
Increasing the intake of ω-3 fatty acids is generally deemed advantageous for IBD. Consumption of anti-inflammatory diets rich in ω-3 fatty acids has been linked to alterations in the gut microbiota of UC patients during remission, resulting in a reduction of subclinical inflammation (219). Incorporating a Japanese diet, which is high in fish oil and has high ω-3 fatty acids, is also beneficial for clinical remission in UC patients (220). ω-3 fatty acids exhibit anti-inflammatory effects in postoperative patients without influencing the risk of postoperative complications (221). The use of EPA has shown promise in lowering calprotectin levels and decreasing UC relapse (222), as well as improving gut microbiota composition and inflammation (223). Supplementing with cis-palmitoleic acid can downregulate the expression of hepatocyte nuclear factor (HNF4α) and HNF4γ, potentially mitigating inflammation in UC patients (224). Moreover, the combination of amino-salicylic acid and ω-3 fatty acids effectively maintains remission in pediatric CD (225).
However, ω-3 fatty acids exhibit contradictory effects. Combined supplementation of ω-3 fatty acids and vitamin D does not significantly improve CD inflammation and clinical remission (226). Multicenter randomized controlled trials have demonstrated the ineffectiveness of using free ω-3 fatty acids for preventing CD relapse (227). Hence, the European Society of Parenteral and Enteral Nutrition suggests a dietary approach characterized by lower ω-6 and higher ω-3 content to prevent IBD, while advising against ω-3 supplementation for maintaining IBD remission (228).
The Mediterranean diet, rich in fish oil and PUFAs and low in SFAs, was considered a relatively healthful dietary pattern (229). It has been recommended as a part of auxiliary treatment for patients with IBD (230). Embracing a healthful lifestyle, such as the Mediterranean diet, has demonstrated potential in ameliorating IBD disease activity and inflammation status (229). Mediterranean diet can elevate the levels of SCFA-producing bacteria, maintaining clinical remission in patients with quiescent UC (230), and reducing the mortality associated with IBD (231). The Mediterranean diet might exhibit superior therapeutic efficacy for mild-to-moderate CD compared to specific carbohydrate-based diets (232).
The composition of fatty acids can also play a guiding role in the proportion of enteral nutrition (EN). Early EN stands as a primary therapeutic choice for CD patients (233), offering a relatively low-cost option. EN formulations contain fewer SFAs than the National Diet and Nutrition Survey (234). Prior research has highlighted the varied impacts of EN with different lipid compositions on CD patients, with higher proportions of oleic acid showing a stronger relief impact compared to higher proportions of stearic acid (235). The ratio of ω-6 to ω-3 fatty acids exhibits a significant positive correlation with CD remission, while total MCFAs and LCFAs show an inverse correlation with remission (236). This insight contributes to a better understanding of optimal EN fatty acid ratios.
SCFAs supplements
The anti-inflammatory potential of SCFAs has been well established in animal experiments, with clinical trials showcasing their promise in treating IBD. Butyrate enema therapy was previously deemed as an efficacious treatment for distal colitis (237). However, its effectiveness has not been substantiated in large-scale RCTs, and the inconvenience associated with the long-term administration of enemas has led to its gradual phasing out (238). Oral microencapsulated sodium butyrate has been found to lower calprotectin levels, sustain remission, enhance quality of life and promote SCFA-producing bacteria in IBD patients (239). Nevertheless, issues of potential poor tolerance to butyrate salts have been raised (240). The use of an herbal mixture containing chamomile flowers and charcoal has demonstrated an ability to elevate butyrate concentrations in UC patients, resulting in reduced relapses (241). Butyrate salts derived from dietary fiber fermentation have shown promise in raising butyrate levels and improving symptoms like abdominal pain and reflux (242). However, Sunil Thomas et al. suggest caution in supplementing with dietary fiber, as high-fiber diets might amplify immunotherapy-associated toxicity in UC (243). Moreover, the efficacy of sodium butyrate in pediatric IBD appears limited (244). Further extensive research is warranted to further develop therapeutic protocols centered around butyrate interventions.
Probiotic supplementation contributes to rectifying gut microbiota balance and alleviates IBD symptoms through elevated SCFAs. Probiotic supplements also reduced inflammation (244). Intake of fermented vegetable beverages containing Pediococcus pentosaceus led to improved loose stool symptoms associated with higher levels of acetic, propionic, and butyric acids (245). A composite formulation of calcium butyrate, Bifidobacterium bifidum, Bifidobacterium lactis, and fructooligosaccharides improved the quality of life and alleviated abdominal pain and diarrhea (218). Prebiotics can promote the growth and reproduction of beneficial microbacteria. Oral fructooligosaccharide prebiotics rich in inulin augmented butyrate production, lowering UC severity (246).
Lipid mediator supplements
The endocannabinoid system is a significant lipid mediator, the homeostasis of which can be modulated by exogenous cannabinoids. In UC patients, supplementation with cannabinoids led to significant downregulation of AA, palmitoylethanolamine, and anandamide levels, reshaping the tone of endocannabinoid system and ameliorating UC symptoms (247). Cannabinoid supplementation exhibited improvement in active CD and reduced corticosteroid dependency (248). The exploration of lipid mediator-derived therapies remains underdeveloped, pointing toward a potential avenue for future research.
Conclusion
In summary, this review encompasses the mechanisms of fatty acid impact on IBD, the current epidemiological landscape, and therapeutic strategies. Fatty acids, as essential dietary components, have been identified to exert anti-inflammatory, immune-regulatory, gut-microbiota-modulating, and barrier-maintaining effects within the pathological processes of IBD. However, the mechanisms of specific fatty acids like MCFAs and VLCFAs in IBD necessitate further elucidation, potentially directing future research endeavors. We have conducted an overview of epidemiological research investigating the impact of fatty acids on IBD. It reveals inconsistencies among certain epidemiological studies and disparities between findings from animal experiments and epidemiological investigations. Furthermore, the availability of robust evidence from large-scale cohorts and randomized controlled trials is limited, and translating results from animal models to human populations presents challenges.
Fatty acids can serve as adjunctive therapeutic tools in managing IBD. We compile existing dietary recommendations and supplementary strategies utilizing fatty acids for therapeutic intents. However, pure fatty acid supplementation often falls short of meeting diverse patient needs. As the effects of each fatty acid and lipid mediator are unveiled, demands for refined dietary strategies or supplement regimens for IBD treatment or relapse prevention intensify. More precise fatty acid ratios and composite formulations may pave the way for future investigations. Additionally, as a disease influenced by genetics and environment, IBD manifests varied sensitivity to different fatty acids or lipid mediators among different genotypes. Leveraging personalized fatty acid supplements based on individual genetic phenotypes through artificial intelligence and extensive data systems could illuminate the path toward tailored interventions.
Author contributions
DY: Conceptualization, Investigation, Methodology, Writing – original draft, Writing – review & editing. SY: Conceptualization, Data curation, Methodology, Validation, Writing – original draft. YH: Validation, Writing – review & editing. SW: Writing – original draft, Writing – review & editing. YX: Resources, Writing – review & editing. XX: Validation, Writing – review & editing. MD: Resources, Supervision, Writing – review & editing. WL: Data curation, Resources, Writing – review & editing. XC: Conceptualization, Project administration, Supervision, Writing – original draft, Writing – review & editing. XW: Conceptualization, Funding acquisition, Project administration, Supervision, Writing – review & editing.
Funding
This work was supported by the National Natural Science Foundation of China (81970494) and Key Project of Research and Development Plan of Hunan Province (2019SK2041).
Conflict of interest
The authors declare that the research was conducted in the absence of any commercial or financial relationships that could be construed as a potential conflict of interest.
Publisher’s note
All claims expressed in this article are solely those of the authors and do not necessarily represent those of their affiliated organizations, or those of the publisher, the editors and the reviewers. Any product that may be evaluated in this article, or claim that may be made by its manufacturer, is not guaranteed or endorsed by the publisher.
References
1. Ananthakrishnan AN. Epidemiology and risk factors for ibd. Nat Rev Gastroenterol Hepatol (2015) 12(4):205–17. doi: 10.1038/nrgastro.2015.34
2. Khalili H, Chan SSM, Lochhead P, Ananthakrishnan AN, Hart AR, Chan AT. The role of diet in the aetiopathogenesis of inflammatory bowel disease. Nat Rev Gastroenterol Hepatol (2018) 15(9):525–35. doi: 10.1038/s41575-018-0022-9
3. Lewis JD, Abreu MT. Diet as a trigger or therapy for inflammatory bowel diseases. Gastroenterology (2017) 152(2):398–414.e6. doi: 10.1053/j.gastro.2016.10.019
4. Rashvand S, Somi MH, Rashidkhani B, Hekmatdoost A. Dietary fatty acid intakes are related to the risk of ulcerative colitis: A case-control study. Int J Colorectal Dis (2015) 30(9):1255–60. doi: 10.1007/s00384-015-2232-8
5. Ananthakrishnan AN, Khalili H, Konijeti GG, Higuchi LM, de Silva P, Fuchs CS, et al. Long-term intake of dietary fat and risk of ulcerative colitis and Crohn’s disease. Gut (2014) 63(5):776–84. doi: 10.1136/gutjnl-2013-305304
6. Gruber L, Kisling S, Lichti P, Martin FP, May S, Klingenspor M, et al. High fat diet accelerates pathogenesis of murine Crohn’s disease-like ileitis independently of obesity. PloS One (2013) 8(8):e71661. doi: 10.1371/journal.pone.0071661
7. Piotrowska M, Binienda A, Fichna J. The role of fatty acids in Crohn’s disease pathophysiology - an overview. Mol Cell Endocrinol (2021) 538:111448. doi: 10.1016/j.mce.2021.111448
8. Milligan G, Shimpukade B, Ulven T, Hudson BD. Complex pharmacology of free fatty acid receptors. Chem Rev (2017) 117(1):67–110. doi: 10.1021/acs.chemrev.6b00056
9. D’Angelo S, Motti ML, Meccariello R. Ω-3 and Ω-6 polyunsaturated fatty acids, obesity and cancer. Nutrients (2020) 12(9):2751. doi: 10.3390/nu12092751
10. Dyall SC, Balas L, Bazan NG, Brenna JT, Chiang N, da Costa Souza F, et al. Polyunsaturated fatty acids and fatty acid-derived lipid mediators: recent advances in the understanding of their biosynthesis, structures, and functions. Prog Lipid Res (2022) 86:101165. doi: 10.1016/j.plipres.2022.101165
11. Morrison DJ, Preston T. Formation of short chain fatty acids by the gut microbiota and their impact on human metabolism. Gut Microbes (2016) 7(3):189–200. doi: 10.1080/19490976.2015.1134082
12. He J, Zhang P, Shen L, Niu L, Tan Y, Chen L, et al. Short-chain fatty acids and their association with signalling pathways in inflammation, glucose and lipid metabolism. Int J Mol Sci (2020) 21(17):6356. doi: 10.3390/ijms21176356
13. Mozaffari H, Daneshzad E, Larijani B, Bellissimo N, Azadbakht L. Dietary intake of fish, N-3 polyunsaturated fatty acids, and risk of inflammatory bowel disease: A systematic review and meta-analysis of observational studies. Eur J Nutr (2020) 59(1):1–17. doi: 10.1007/s00394-019-01901-0
14. Wiese DM, Horst SN, Brown CT, Allaman MM, Hodges ME, Slaughter JC, et al. Serum fatty acids are correlated with inflammatory cytokines in ulcerative colitis. PloS One (2016) 11(5):e0156387. doi: 10.1371/journal.pone.0156387
15. Parada Venegas D, de la Fuente MK, Landskron G, González MJ, Quera R, Dijkstra G, et al. Short chain fatty acids (Scfas)-mediated gut epithelial and immune regulation and its relevance for inflammatory bowel diseases. Front Immunol (2019) 10:277. doi: 10.3389/fimmu.2019.00277
16. Deleu S, Machiels K, Raes J, Verbeke K, Vermeire S. Short chain fatty acids and its producing organisms: an overlooked therapy for ibd? EBioMedicine (2021) 66:103293. doi: 10.1016/j.ebiom.2021.103293
17. Zhang Z, Zhang H, Chen T, Shi L, Wang D, Tang D. Regulatory role of short-chain fatty acids in inflammatory bowel disease. Cell Commun Signal (2022) 20(1):64. doi: 10.1186/s12964-022-00869-5
18. Chen Y, Cui W, Li X, Yang H. Interaction between commensal bacteria, immune response and the intestinal barrier in inflammatory bowel disease. Front Immunol (2021) 12:761981. doi: 10.3389/fimmu.2021.761981
19. Carretta MD, Quiroga J, López R, Hidalgo MA. Burgos RA. Participation of short-chain fatty acids and their receptors in gut inflammation and colon cancer. Front Physiol (2021) 12:662739. doi: 10.3389/fphys.2021.662739
20. Zhuang X, Li T, Li M, Huang S, Qiu Y, Feng R, et al. Systematic review and meta-analysis: short-chain fatty acid characterization in patients with inflammatory bowel disease. Inflammation Bowel Dis (2019) 25(11):1751–63. doi: 10.1093/ibd/izz188
21. Buffet-Bataillon S, Bouguen G, Fleury F, Cattoir V, Le Cunff Y. Gut microbiota analysis for prediction of clinical relapse in crohn’s disease. Sci Rep (2022) 12(1):19929. doi: 10.1038/s41598-022-23757-x
22. Laserna-Mendieta EJ, Clooney AG, Carretero-Gomez JF, Moran C, Sheehan D, Nolan JA, et al. Determinants of reduced genetic capacity for butyrate synthesis by the gut microbiome in Crohn’s disease and ulcerative colitis. J Crohns Colitis (2018) 12(2):204–16. doi: 10.1093/ecco-jcc/jjx137
23. Earley H, Lennon G, Coffey JC, Winter DC, O’Connell PR. Colonisation of the colonic mucus gel layer with butyrogenic and hydrogenotropic bacteria in health and ulcerative colitis. Sci Rep (2021) 11(1):7262. doi: 10.1038/s41598-021-86166-6
24. Taylor H, Serrano-Contreras JI, McDonald JAK, Epstein J, Fell JM, Seoane RC, et al. Multiomic features associated with mucosal healing and inflammation in paediatric Crohn’s disease. Aliment Pharmacol Ther (2020) 52(9):1491–502. doi: 10.1111/apt.16086
25. Russo E, Cinci L, Di Gloria L, Baldi S, D’Ambrosio M, Nannini G, et al. Crohn’s disease recurrence updates: first surgery vs. Surgical relapse patients display different profiles of ileal microbiota and systemic microbial-associated inflammatory factors. Front Immunol (2022) 13:886468. doi: 10.3389/fimmu.2022.886468
26. Handa O, Miura H, Gu T, Osawa M, Matsumoto H, Umegaki E, et al. Reduction of butyric acid-producing bacteria in the ileal mucosa-associated microbiota is associated with the history of abdominal surgery in patients with Crohn’s disease. Redox Rep (2023) 28(1):2241615. doi: 10.1080/13510002.2023.2241615
27. Kiasat A, Rautiainen S, Prast-Nielsen S, Engstrand L, Schuppe-Koistinen I, Gustafsson UO, et al. Evaluation of plasma short chain fatty acid levels as markers for inflammatory bowel disease. Scand J Gastroenterol (2023), 1–7. doi: 10.1080/00365521.2023.2219357
28. Masui R, Sasaki M, Funaki Y, Ogasawara N, Mizuno M, Iida A, et al. G protein-coupled receptor 43 moderates gut inflammation through cytokine regulation from mononuclear cells. Inflammation Bowel Dis (2013) 19(13):2848–56. doi: 10.1097/01.MIB.0000435444.14860.ea
29. Singh N, Gurav A, Sivaprakasam S, Brady E, Padia R, Shi H, et al. Activation of gpr109a, receptor for niacin and the commensal metabolite butyrate, suppresses colonic inflammation and carcinogenesis. Immunity (2014) 40(1):128–39. doi: 10.1016/j.immuni.2013.12.007
30. Ikeda Y, Matsuda S. Gut protective effect from D-methionine or butyric acid against dss and carrageenan-induced ulcerative colitis. Molecules (2023) 28(11):4392. doi: 10.3390/molecules28114392
31. Tye H, Yu CH, Simms LA, de Zoete MR, Kim ML, Zakrzewski M, et al. Nlrp1 restricts butyrate producing commensals to exacerbate inflammatory bowel disease. Nat Commun (2018) 9(1):3728. doi: 10.1038/s41467-018-06125-0
32. Tang X, Li X, Wang Y, Zhang Z, Deng A, Wang W, et al. Butyric acid increases the therapeutic effect of ehlj7 on ulcerative colitis by inhibiting jak2/stat3/socs1 signaling pathway. Front Pharmacol (2019) 10:1553. doi: 10.3389/fphar.2019.01553
33. Satka S, Frybortova V, Zapletalova I, Anzenbacher P, Anzenbacherova E, Kozakova H, et al. Effect of dss-induced ulcerative colitis and butyrate on the cytochrome P450 2a5: contribution of the microbiome. Int J Mol Sci (2022) 23(19):11627. doi: 10.3390/ijms231911627
34. Deleu S, Arnauts K, Deprez L, Machiels K, Ferrante M, Huys GRB, et al. High acetate concentration protects intestinal barrier and exerts anti-inflammatory effects in organoid-derived epithelial monolayer cultures from patients with ulcerative colitis. Int J Mol Sci (2023) 24(1):768. doi: 10.3390/ijms24010768
35. Felice C, Lewis A, Iqbal S, Gordon H, Rigoni A, Colombo MP, et al. Intestinal inflammation is linked to hypoacetylation of histone 3 lysine 27 and can be reversed by valproic acid treatment in inflammatory bowel disease patients. Cell Mol Gastroenterol Hepatol (2021) 11(3):889–91.e6. doi: 10.1016/j.jcmgh.2020.11.009
36. Gurav A, Sivaprakasam S, Bhutia YD, Boettger T, Singh N, Ganapathy V. Slc5a8, a Na+-Coupled High-Affinity Transporter for Short-Chain Fatty Acids, Is a Conditional Tumour Suppressor in Colon That Protects against Colitis and Colon Cancer under Low-Fibre Dietary Conditions. Biochem J (2015) 469(2):267–78. doi: 10.1042/bj20150242
37. Smith PM, Howitt MR, Panikov N, Michaud M, Gallini CA, Bohlooly YM, et al. The microbial metabolites, short-chain fatty acids, regulate colonic treg cell homeostasis. Science (2013) 341(6145):569–73. doi: 10.1126/science.1241165
38. Zhou L, Zhang M, Wang Y, Dorfman RG, Liu H, Yu T, et al. Faecalibacterium prausnitzii produces butyrate to maintain th17/treg balance and to ameliorate colorectal colitis by inhibiting histone deacetylase 1. Inflammation Bowel Dis (2018) 24(9):1926–40. doi: 10.1093/ibd/izy182
39. Sitkin S, Pokrotnieks J. Clinical potential of anti-inflammatory effects of faecalibacterium prausnitzii and butyrate in inflammatory bowel disease. Inflammation Bowel Dis (2019) 25(4):e40–e1. doi: 10.1093/ibd/izy258
40. Di Martino L, Osme A, Ghannoum M, Cominelli F. A novel probiotic combination ameliorates crohn’s disease-like ileitis by increasing short-chain fatty acid production and modulating essential adaptive immune pathways. Inflammation Bowel Dis (2023) 29(7):1105–17. doi: 10.1093/ibd/izac284
41. Suzuki R, Mishima M, Nagane M, Mizugaki H, Suzuki T, Komuro M, et al. The novel sustained 3-hydroxybutyrate donor poly-D-3-hydroxybutyric acid prevents inflammatory bowel disease through upregulation of regulatory T-cells. FASEB J (2023) 37(1):e22708. doi: 10.1096/fj.202200919R
42. Hamer HM, Jonkers D, Venema K, Vanhoutvin S, Troost FJ, Brummer RJ. Review article: the role of butyrate on colonic function. Aliment Pharmacol Ther (2008) 27(2):104–19. doi: 10.1111/j.1365-2036.2007.03562.x
43. Ferrer-Picón E, Dotti I, Corraliza AM, Mayorgas A, Esteller M, Perales JC, et al. Intestinal inflammation modulates the epithelial response to butyrate in patients with inflammatory bowel disease. Inflammation Bowel Dis (2020) 26(1):43–55. doi: 10.1093/ibd/izz119
44. Magnusson MK, Isaksson S, Öhman L. The anti-inflammatory immune regulation induced by butyrate is impaired in inflamed intestinal mucosa from patients with ulcerative colitis. Inflammation (2020) 43(2):507–17. doi: 10.1007/s10753-019-01133-8
45. Magnusson MK, Vidal A, Maasfeh L, Isaksson S, Malhotra R, Olsson HK, et al. Impaired butyrate induced regulation of T cell surface expression of ctla-4 in patients with ulcerative colitis. Int J Mol Sci (2021) 22(6):3084. doi: 10.3390/ijms22063084
46. Kelly CJ, Zheng L, Campbell EL, Saeedi B, Scholz CC, Bayless AJ, et al. Crosstalk between microbiota-derived short-chain fatty acids and intestinal epithelial hif augments tissue barrier function. Cell Host Microbe (2015) 17(5):662–71. doi: 10.1016/j.chom.2015.03.005
47. Chen G, Ran X, Li B, Li Y, He D, Huang B, et al. Sodium butyrate inhibits inflammation and maintains epithelium barrier integrity in a tnbs-induced inflammatory bowel disease mice model. EBioMedicine (2018) 30:317–25. doi: 10.1016/j.ebiom.2018.03.030
48. Fachi JL, Felipe JS, Pral LP, da Silva BK, Corrêa RO, de Andrade MCP, et al. Butyrate protects mice from clostridium difficile-induced colitis through an hif-1-dependent mechanism. Cell Rep (2019) 27(3):750–61.e7. doi: 10.1016/j.celrep.2019.03.054
49. Vancamelbeke M, Laeremans T, Vanhove W, Arnauts K, Ramalho AS, Farré R, et al. Butyrate does not protect against inflammation-induced loss of epithelial barrier function and cytokine production in primary cell monolayers from patients with ulcerative colitis. J Crohns Colitis (2019) 13(10):1351–61. doi: 10.1093/ecco-jcc/jjz064
50. Zhang F, Wang W, Niu J, Yang G, Luo J, Lan D, et al. Heat-shock transcription factor 2 promotes sodium butyrate-induced autophagy by inhibiting mtor in ulcerative colitis. Exp Cell Res (2020) 388(1):111820. doi: 10.1016/j.yexcr.2020.111820
51. Zhou C, Li L, Li T, Sun L, Yin J, Guan H, et al. Scfas induce autophagy in intestinal epithelial cells and relieve colitis by stabilizing hif-1α. J Mol Med (Berl) (2020) 98(8):1189–202. doi: 10.1007/s00109-020-01947-2
52. Wu J, Zhou B, Pang X, Song X, Gu Y, Xie R, et al. Clostridium butyricum, a butyrate-producing potential probiotic, alleviates experimental colitis through epidermal growth factor receptor activation. Food Funct (2022) 13(13):7046–61. doi: 10.1039/d2fo00478j
53. Xu W, Ishii Y, Rini DM, Yamamoto Y, Suzuki T. Microbial metabolite N-butyrate upregulates intestinal claudin-23 expression through sp1 and ampk pathways in mouse colon and human intestinal caco-2 cells. Life Sci (2023) 329:121952. doi: 10.1016/j.lfs.2023.121952
54. Cantó E, Zamora C, Garcia-Planella E, Gordillo J, Ortiz MA, Perea L, et al. Bacteria-related events and the immunological response of onset and relapse adult Crohn’s disease patients. J Crohns Colitis (2019) 13(1):92–9. doi: 10.1093/ecco-jcc/jjy138
55. Stoeva MK, Garcia-So J, Justice N, Myers J, Tyagi S, Nemchek M, et al. Butyrate-producing human gut symbiont, clostridium butyricum, and its role in health and disease. Gut Microbes (2021) 13(1):1–28. doi: 10.1080/19490976.2021.1907272
56. Vermeiren J, Van den Abbeele P, Laukens D, Vigsnaes LK, De Vos M, Boon N, et al. Decreased colonization of fecal clostridium coccoides/eubacterium rectale species from ulcerative colitis patients in an in vitro dynamic gut model with mucin environment. FEMS Microbiol Ecol (2012) 79(3):685–96. doi: 10.1111/j.1574-6941.2011.01252.x
57. Sasaki K, Inoue J, Sasaki D, Hoshi N, Shirai T, Fukuda I, et al. Construction of a model culture system of human colonic microbiota to detect decreased lachnospiraceae abundance and butyrogenesis in the feces of ulcerative colitis patients. Biotechnol J (2019) 14(5):e1800555. doi: 10.1002/biot.201800555
58. Fu R, Wang L, Meng Y, Xue W, Liang J, Peng Z, et al. Apigenin remodels the gut microbiota to ameliorate ulcerative colitis. Front Nutr (2022) 9:1062961. doi: 10.3389/fnut.2022.1062961
59. Kutsuna R, Tomida J, Morita Y, Kawamura Y. Paraclostridium bifermentans exacerbates pathosis in a mouse model of ulcerative colitis. PloS One (2018) 13(5):e0197668. doi: 10.1371/journal.pone.0197668
60. Tian Y, Zuo L, Guan B, Wu H, He Y, Xu Z, et al. Microbiota from patients with ulcerative colitis promote colorectal carcinogenesis in mice. Nutrition (2022) 102:111712. doi: 10.1016/j.nut.2022.111712
61. Miki T, Hoshino Y, Sudo N, Ito M, Haneda T, Okada N. Uvry deletion and acetate reduce gut colonization of crohn’s disease-associated adherent-invasive escherichia coli by decreasing expression of type 1 fimbriae. Infect Immun (2022) 90(3):e0066221. doi: 10.1128/iai.00662-21
62. Bhatt S, Gupta M. Dietary fiber from fruit waste as a potential source of metabolites in maintenance of gut milieu during ulcerative colitis: A comprehensive review. Food Res Int (2023) 164:112329. doi: 10.1016/j.foodres.2022.112329
63. Brotherton CS, Martin CA, Long MD, Kappelman MD, Sandler RS. Avoidance of fiber is associated with greater risk of Crohn’s disease flare in a 6-month period. Clin Gastroenterol Hepatol (2016) 14(8):1130–6. doi: 10.1016/j.cgh.2015.12.029
64. Desai MS, Seekatz AM, Koropatkin NM, Kamada N, Hickey CA, Wolter M, et al. A dietary fiber-deprived gut microbiota degrades the colonic mucus barrier and enhances pathogen susceptibility. Cell (2016) 167(5):1339–53.e21. doi: 10.1016/j.cell.2016.10.043
65. Liu J, Wang Z, Mai P, Hao Y, Wang Z, Wang J. Quinoa bran soluble dietary fiber ameliorates dextran sodium sulfate induced ulcerative colitis in balb/C mice by maintaining intestinal barrier function and modulating gut microbiota. Int J Biol Macromol (2022) 216:75–85. doi: 10.1016/j.ijbiomac.2022.06.194
66. Lyu B, Wang Y, Fu H, Li J, Yang X, Shen Y, et al. Intake of high-purity insoluble dietary fiber from okara for the amelioration of colonic environment disturbance caused by acute ulcerative colitis. Food Funct (2022) 13(1):213–26. doi: 10.1039/d1fo02264d
67. Gerasimidis K, Nichols B, McGowan M, Svolos V, Papadopoulou R, Kokkorou M, et al. The effects of commonly consumed dietary fibres on the gut microbiome and its fibre fermentative capacity in adults with inflammatory bowel disease in remission. Nutrients (2022) 14(5):1053. doi: 10.3390/nu14051053
68. Kandula M, Sunil Kumar KB, Palanichamy S, Rampal A. Discovery and preclinical development of a novel prodrug conjugate of mesalamine with eicosapentaenoic acid and caprylic acid for the treatment of inflammatory bowel diseases. Int Immunopharmacol (2016) 40:443–51. doi: 10.1016/j.intimp.2016.09.013
69. Wang J, Huang N, Xiong J, Wei H, Jiang S, Peng J. Caprylic acid and nonanoic acid upregulate endogenous host defense peptides to enhance intestinal epithelial immunological barrier function via histone deacetylase inhibition. Int Immunopharmacol (2018) 65:303–11. doi: 10.1016/j.intimp.2018.10.022
70. Söderholm JD, Olaison G, Peterson KH, Franzén LE, Lindmark T, Wirén M, et al. Augmented increase in tight junction permeability by luminal stimuli in the non-inflamed ileum of crohn’s disease. Gut (2002) 50(3):307–13. doi: 10.1136/gut.50.3.307
71. Huang S, Zhao L, Kim K, Lee DS, Hwang DH. Inhibition of nod2 signaling and target gene expression by curcumin. Mol Pharmacol (2008) 74(1):274–81. doi: 10.1124/mol.108.046169
72. Richter H, Gover O, Schwartz B. Anti-inflammatory activity of black soldier fly oil associated with modulation of tlr signaling: A metabolomic approach. Int J Mol Sci (2023) 24(13):10634. doi: 10.3390/ijms241310634
73. Zinniel DK, Sittiwong W, Marshall DD, Rathnaiah G, Sakallioglu IT, Powers R, et al. Novel amphiphilic cyclobutene and cyclobutane cis-C(18) fatty acid derivatives inhibit mycobacterium avium subsp. Paratuberculosis growth. Vet Sci (2019) 6(2):46. doi: 10.3390/vetsci6020046
74. Yin J, Zhou C, Yang K, Ren Y, Qiu Y, Xu P, et al. Mutual regulation between butyrate and hypoxia-inducible factor-1α in epithelial cell promotes expression of tight junction proteins. Cell Biol Int (2020) 44(6):1405–14. doi: 10.1002/cbin.11336
75. Wang RX, Henen MA, Lee JS, Vögeli B, Colgan SP. Microbiota-derived butyrate is an endogenous hif prolyl hydroxylase inhibitor. Gut Microbes (2021) 13(1):1938380. doi: 10.1080/19490976.2021.1938380
76. Devriese S, Eeckhaut V, Geirnaert A, Van den Bossche L, Hindryckx P, Van de Wiele T, et al. Reduced mucosa-associated butyricicoccus activity in patients with ulcerative colitis correlates with aberrant claudin-1 expression. J Crohns Colitis (2017) 11(2):229–36. doi: 10.1093/ecco-jcc/jjw142
77. Yan H, Ajuwon KM. Butyrate modifies intestinal barrier function in ipec-J2 cells through a selective upregulation of tight junction proteins and activation of the akt signaling pathway. PloS One (2017) 12(6):e0179586. doi: 10.1371/journal.pone.0179586
78. Tong LC, Wang Y, Wang ZB, Liu WY, Sun S, Li L, et al. Propionate ameliorates dextran sodium sulfate-induced colitis by improving intestinal barrier function and reducing inflammation and oxidative stress. Front Pharmacol (2016) 7:253. doi: 10.3389/fphar.2016.00253
79. Isayama K, Rini DM, Yamamoto Y, Suzuki T. Propionate regulates tight junction barrier by increasing endothelial-cell selective adhesion molecule in human intestinal caco-2 cells. Exp Cell Res (2023) 425(2):113528. doi: 10.1016/j.yexcr.2023.113528
80. Geirnaert A, Calatayud M, Grootaert C, Laukens D, Devriese S, Smagghe G, et al. Butyrate-producing bacteria supplemented in vitro to Crohn’s disease patient microbiota increased butyrate production and enhanced intestinal epithelial barrier integrity. Sci Rep (2017) 7(1):11450. doi: 10.1038/s41598-017-11734-8
81. Simeoli R, Mattace Raso G, Pirozzi C, Lama A, Santoro A, Russo R, et al. An orally administered butyrate-releasing derivative reduces neutrophil recruitment and inflammation in dextran sulphate sodium-induced murine colitis. Br J Pharmacol (2017) 174(11):1484–96. doi: 10.1111/bph.13637
82. Huang X, Hu J, Zhang H, Li J, Zhu X, Liu Y, et al. Clostridium butyricum and chitooligosaccharides in synbiotic combination ameliorate symptoms in a dss-induced ulcerative colitis mouse model by modulating gut microbiota and enhancing intestinal barrier function. Microbiol Spectr (2023) 11(2):e0437022. doi: 10.1128/spectrum.04370-22
83. Marzorati M, Bubeck S, Bayne T, Krishnan K, Giusto M. Effects of combined prebiotic, probiotic, igg and amino acid supplementation on the gut microbiome of patients with inflammatory bowel disease. Future Microbiol (2022) 17:1307–24. doi: 10.2217/fmb-2022-0066
84. Escoula Q, Bellenger S, Narce M, Bellenger J. Docosahexaenoic and eicosapentaenoic acids prevent altered-muc2 secretion induced by palmitic acid by alleviating endoplasmic reticulum stress in ls174t goblet cells. Nutrients (2019) 11(9):2179. doi: 10.3390/nu11092179
85. Gori M, Altomare A, Cocca S, Solida E, Ribolsi M, Carotti S, et al. Palmitic acid affects intestinal epithelial barrier integrity and permeability in vitro. Antioxidants (Basel) (2020) 9(5):417. doi: 10.3390/antiox9050417
86. Bashllari R, Molonia MS, Muscarà C, Speciale A, Wilde PJ, Saija A, et al. Cyanidin-3-O-glucoside protects intestinal epithelial cells from palmitate-induced lipotoxicity. Arch Physiol Biochem (2023) 129(2):379–86. doi: 10.1080/13813455.2020.1828480
87. Alzoghaibi MA, Walsh SW, Willey A, Fowler AA 3rd, Graham MF. Linoleic acid, but not oleic acid, upregulates the production of interleukin-8 by human intestinal smooth muscle cells isolated from patients with Crohn’s disease. Clin Nutr (2003) 22(6):529–35. doi: 10.1016/s0261-5614(03)00083-9
88. Fernández J, de la Fuente VG, García MTF, Sánchez JG, Redondo BI, Villar CJ, et al. A diet based on cured acorn-fed ham with oleic acid content promotes anti-inflammatory gut microbiota and prevents ulcerative colitis in an animal model. Lipids Health Dis (2020) 19(1):28. doi: 10.1186/s12944-020-01205-x
89. de Paula do Nascimento R, Lima AV, Oyama LM, Paiotti APR, Cardili L, Martinez CAR, et al. Extra virgin olive oil and flaxseed oil have no preventive effects on dss-induced acute ulcerative colitis. Nutrition (2020) 74:110731. doi: 10.1016/j.nut.2020.110731
90. Huang YF, Zhou JT, Qu C, Dou YX, Huang QH, Lin ZX, et al. Anti-inflammatory effects of brucea javanica oil emulsion by suppressing nf-Kb activation on dextran sulfate sodium-induced ulcerative colitis in mice. J Ethnopharmacol (2017) 198:389–98. doi: 10.1016/j.jep.2017.01.042
91. Borniquel S, Jansson EA, Cole MP, Freeman BA, Lundberg JO. Nitrated oleic acid up-regulates ppargamma and attenuates experimental inflammatory bowel disease. Free Radic Biol Med (2010) 48(4):499–505. doi: 10.1016/j.freeradbiomed.2009.11.014
92. Nieto N, Torres MI, Ríos A, Gil A. Dietary polyunsaturated fatty acids improve histological and biochemical alterations in rats with experimental ulcerative colitis. J Nutr (2002) 132(1):11–9. doi: 10.1093/jn/132.1.11
93. Hudert CA, Weylandt KH, Lu Y, Wang J, Hong S, Dignass A, et al. Transgenic mice rich in endogenous omega-3 fatty acids are protected from colitis. Proc Natl Acad Sci U.S.A. (2006) 103(30):11276–81. doi: 10.1073/pnas.0601280103
94. Yao J, Lu Y, Zhi M, Hu P, Wu W, Gao X. Dietary N−3 polyunsaturated fatty acids ameliorate Crohn’s disease in rats by modulating the expression of ppar−Γ/nfat. Mol Med Rep (2017) 16(6):8315–22. doi: 10.3892/mmr.2017.7673
95. Reifen R, Karlinsky A, Stark AH, Berkovich Z, Nyska A. A-linolenic acid (Ala) is an anti-inflammatory agent in inflammatory bowel disease. J Nutr Biochem (2015) 26(12):1632–40. doi: 10.1016/j.jnutbio.2015.08.006
96. Kim J, Ahn M, Choi Y, Kang T, Kim J, Lee NH, et al. Alpha-linolenic acid alleviates dextran sulfate sodium-induced ulcerative colitis in mice. Inflammation (2020) 43(5):1876–83. doi: 10.1007/s10753-020-01260-7
97. El Mahdy RN, Nader MA, Helal MG, Abu-Risha SE, Abdelmageed ME. Eicosapentaenoic acid mitigates ulcerative colitis-induced by acetic acid through modulation of nf-Kb and tgf-B/egfr signaling pathways. Life Sci (2023) 327:121820. doi: 10.1016/j.lfs.2023.121820
98. Schwärzler J, Mayr L, Vich Vila A, Grabherr F, Niederreiter L, Philipp M, et al. Pufa-induced metabolic enteritis as a fuel for crohn’s disease. Gastroenterology (2022) 162(6):1690–704. doi: 10.1053/j.gastro.2022.01.004
99. Mayr L, Grabherr F, Schwärzler J, Reitmeier I, Sommer F, Gehmacher T, et al. Dietary lipids fuel gpx4-restricted enteritis resembling crohn’s disease. Nat Commun (2020) 11(1):1775. doi: 10.1038/s41467-020-15646-6
100. Plissonneau C, Sivignon A, Chassaing B, Capel F, Martin V, Etienne M, et al. Beneficial effects of linseed supplementation on gut mucosa-associated microbiota in a physically active mouse model of Crohn’s disease. Int J Mol Sci (2022) 23(11):5891. doi: 10.3390/ijms23115891
101. Zheng Z, Dai Z, Cao Y, Shen Q, Zhang Y. Docosapentaenoic acid (Dpa, 22:5n-3) ameliorates inflammation in an ulcerative colitis model. Food Funct (2019) 10(7):4199–209. doi: 10.1039/c8fo02338g
102. Dong Y, Huang C, Yang J, Zheng Z, Dai Z. Docosapentaenoic acid (Dpa, 22:5n-3) alleviates ulcerative colitis via modification of gut microbiota and their metabolism. Nutrients (2022) 14(19):4204. doi: 10.3390/nu14194204
103. Jurickova I, Bonkowski E, Angerman E, Novak E, Huron A, Akers G, et al. Eicosatetraynoic acid and butyrate regulate human intestinal organoid mitochondrial and extracellular matrix pathways implicated in Crohn’s disease strictures. Inflammation Bowel Dis (2022) 28(7):988–1003. doi: 10.1093/ibd/izac037
104. Abdelsameea AA, Alsemeh AE, Alabassery N, Samy W, Fawzy A, Abbas NAT. Icosapent ethyl alleviates acetic acid-induced ulcerative colitis via modulation of sirt1 signaling pathway in rats. Int Immunopharmacol (2023) 115:109621. doi: 10.1016/j.intimp.2022.109621
105. Wang L, Choi HS, Su Y, Lee B, Choi JH, Jang SH, et al. Protective effect of 17s−Epoxy−Docosapentaenoic acid against dextran sulfate sodium induced ulcerative colitis in balb/C mice. Mol Med Rep (2022) 26(3):278. doi: 10.3892/mmr.2022.12794
106. Vertzoni M, Goumas K, Söderlind E, Abrahamsson B, Dressman JB, Poulou A, et al. Characterization of the ascending colon fluids in ulcerative colitis. Pharm Res (2010) 27(8):1620–6. doi: 10.1007/s11095-010-0158-y
107. Kaczmarczyk O, Dąbek-Drobny A, Woźniakiewicz M, Paśko P, Piątek-Guziewicz A, Zwolińska-Wcisło M. Altered fecal short−Chain fatty acid profile as a potential marker of disease activity in patients with ulcerative colitis and Crohn’s disease: A pilot study. Pol Arch Intern Med (2022) 132(6):16254. doi: 10.20452/pamw.16254
108. Liu J, Fang H, Hong N, Lv C, Zhu Q, Feng Y, et al. Gut microbiome and metabonomic profile predict early remission to anti-integrin therapy in patients with moderate to severe ulcerative colitis. Microbiol Spectr (2023) 11(3):e0145723. doi: 10.1128/spectrum.01457-23
109. Dianzani C, Foglietta F, Ferrara B, Rosa AC, Muntoni E, Gasco P, et al. Solid lipid nanoparticles delivering anti-inflammatory drugs to treat inflammatory bowel disease: effects in an in vivo model. World J Gastroenterol (2017) 23(23):4200–10. doi: 10.3748/wjg.v23.i23.4200
110. Sheibanie AF, Yen JH, Khayrullina T, Emig F, Zhang M, Tuma R, et al. The proinflammatory effect of prostaglandin E2 in experimental inflammatory bowel disease is mediated through the il-23–>Il-17 axis. J Immunol (2007) 178(12):8138–47. doi: 10.4049/jimmunol.178.12.8138
111. Hokari R, Kurihara C, Nagata N, Aritake K, Okada Y, Watanabe C, et al. Increased expression of lipocalin-type-prostaglandin D synthase in ulcerative colitis and exacerbating role in murine colitis. Am J Physiol Gastrointest Liver Physiol (2011) 300(3):G401–8. doi: 10.1152/ajpgi.00351.2010
112. Sturm EM, Radnai B, Jandl K, Stančić A, Parzmair GP, Högenauer C, et al. Opposing roles of prostaglandin D2 receptors in ulcerative colitis. J Immunol (2014) 193(2):827–39. doi: 10.4049/jimmunol.1303484
113. Radnai B, Sturm EM, Stančić A, Jandl K, Labocha S, Ferreirós N, et al. Eosinophils contribute to intestinal inflammation via chemoattractant receptor-homologous molecule expressed on th2 cells, crth2, in experimental Crohn’s disease. J Crohns Colitis (2016) 10(9):1087–95. doi: 10.1093/ecco-jcc/jjw061
114. Li J, Kong D, Wang Q, Wu W, Tang Y, Bai T, et al. Niacin ameliorates ulcerative colitis via prostaglandin D(2)-mediated D prostanoid receptor 1 activation. EMBO Mol Med (2017) 9(5):571–88. doi: 10.15252/emmm.201606987
115. Zhou J, Lai W, Yang W, Pan J, Shen H, Cai Y, et al. Blt1 in dendritic cells promotes th1/th17 differentiation and its deficiency ameliorates tnbs-induced colitis. Cell Mol Immunol (2018) 15(12):1047–56. doi: 10.1038/s41423-018-0030-2
116. Meriwether D, Sulaiman D, Volpe C, Dorfman A, Grijalva V, Dorreh N, et al. Apolipoprotein a-I mimetics mitigate intestinal inflammation in cox2-dependent inflammatory bowel disease model. J Clin Invest (2019) 129(9):3670–85. doi: 10.1172/jci123700
117. Campbell EL, MacManus CF, Kominsky DJ, Keely S, Glover LE, Bowers BE, et al. Resolvin E1-induced intestinal alkaline phosphatase promotes resolution of inflammation through lps detoxification. Proc Natl Acad Sci U.S.A. (2010) 107(32):14298–303. doi: 10.1073/pnas.0914730107
118. Arita M, Yoshida M, Hong S, Tjonahen E, Glickman JN, Petasis NA, et al. Resolvin E1, an endogenous lipid mediator derived from omega-3 eicosapentaenoic acid, protects against 2,4,6-trinitrobenzene sulfonic acid-induced colitis. Proc Natl Acad Sci U.S.A. (2005) 102(21):7671–6. doi: 10.1073/pnas.0409271102
119. Ishida T, Yoshida M, Arita M, Nishitani Y, Nishiumi S, Masuda A, et al. Resolvin E1, an endogenous lipid mediator derived from eicosapentaenoic acid, prevents dextran sulfate sodium-induced colitis. Inflammation Bowel Dis (2010) 16(1):87–95. doi: 10.1002/ibd.21029
120. Zeng C, Liu X, Zhu S, Xiong D, Zhu L, Hou X, et al. Resolvin D1 ameliorates hepatic steatosis by remodeling the gut microbiota and restoring the intestinal barrier integrity in dss-induced chronic colitis. Int Immunopharmacol (2022) 103:108500. doi: 10.1016/j.intimp.2021.108500
121. Zeng C, Liu X, Xiong D, Zou K, Bai T. Resolvin D1 prevents epithelial-to-mesenchymal transition and reduces collagen deposition by stimulating autophagy in intestinal fibrosis. Dig Dis Sci (2022) 67(10):4749–59. doi: 10.1007/s10620-021-07356-w
122. Gobbetti T, Dalli J, Colas RA, Federici Canova D, Aursnes M, Bonnet D, et al. Protectin D1(N-3 dpa) and resolvin D5(N-3 dpa) are effectors of intestinal protection. Proc Natl Acad Sci U.S.A. (2017) 114(15):3963–8. doi: 10.1073/pnas.1617290114
123. Marcon R, Bento AF, Dutra RC, Bicca MA, Leite DF, Calixto JB. Maresin 1, a proresolving lipid mediator derived from omega-3 polyunsaturated fatty acids, exerts protective actions in murine models of colitis. J Immunol (2013) 191(8):4288–98. doi: 10.4049/jimmunol.1202743
124. Qiu S, Li P, Zhao H, Li X. Maresin 1 alleviates dextran sulfate sodium-induced ulcerative colitis by regulating nrf2 and tlr4/nf-kb signaling pathway. Int Immunopharmacol (2020) 78:106018. doi: 10.1016/j.intimp.2019.106018
125. Rodríguez-Lagunas MJ, Storniolo CE, Ferrer R, Moreno JJ. 5-hydroxyeicosatetraenoic acid and leukotriene D4 increase intestinal epithelial paracellular permeability. Int J Biochem Cell Biol (2013) 45(7):1318–26. doi: 10.1016/j.biocel.2013.04.005
126. Kroschwald S, Chiu CY, Heydeck D, Rohwer N, Gehring T, Seifert U, et al. Female mice carrying a defective alox15 gene are protected from experimental colitis via sustained maintenance of the intestinal epithelial barrier function. Biochim Biophys Acta Mol Cell Biol Lipids (2018) 1863(8):866–80. doi: 10.1016/j.bbalip.2018.04.019
127. Rohwer N, Chiu CY, Huang D, Smyl C, Rothe M, Rund KM, et al. Omega-3 fatty acids protect from colitis via an alox15-derived eicosanoid. FASEB J (2021) 35(4):e21491. doi: 10.1096/fj.202002340RR
128. Pochard C, Coquenlorge S, Jaulin J, Cenac N, Vergnolle N, Meurette G, et al. Defects in 15-hete production and control of epithelial permeability by human enteric glial cells from patients with Crohn’s disease. Gastroenterology (2016) 150(1):168–80. doi: 10.1053/j.gastro.2015.09.038
129. Leinwand KL, Jones AA, Huang RH, Jedlicka P, Kao DJ, de Zoeten EF, et al. Cannabinoid receptor-2 ameliorates inflammation in murine model of Crohn’s disease. J Crohns Colitis (2017) 11(11):1369–80. doi: 10.1093/ecco-jcc/jjx096
130. Calzadilla N, Qazi A, Sharma A, Mongan K, Comiskey S, Manne J, et al. Mucosal metabolomic signatures in chronic colitis: novel insights into the pathophysiology of inflammatory bowel disease. Metabolites (2023) 13(7):873. doi: 10.3390/metabo13070873
131. Venneri T, Giorgini G, Leblanc N, Flamand N, Borrelli F, Silvestri C, et al. Altered endocannabinoidome bioactive lipid levels accompany reduced dnbs-induced colonic inflammation in germ-free mice. Lipids Health Dis (2023) 22(1):63. doi: 10.1186/s12944-023-01823-1
132. Jia M, Zhang Y, Gao Y, Ma X. Effects of medium chain fatty acids on intestinal health of monogastric animals. Curr Protein Pept Sci (2020) 21(8):777–84. doi: 10.2174/1389203721666191231145901
133. Tsoukalas D, Fragoulakis V, Sarandi E, Docea AO, Papakonstaninou E, Tsilimidos G, et al. Targeted metabolomic analysis of serum fatty acids for the prediction of autoimmune diseases. Front Mol Biosci (2019) 6:120. doi: 10.3389/fmolb.2019.00120
134. De Preter V, Machiels K, Joossens M, Arijs I, Matthys C, Vermeire S, et al. Faecal metabolite profiling identifies medium-chain fatty acids as discriminating compounds in ibd. Gut (2015) 64(3):447–58. doi: 10.1136/gutjnl-2013-306423
135. Kikut J, Drozd A, Mokrzycka M, Grzybowska-Chlebowczyk U, Ziętek M, Szczuko M. There is a differential pattern in the fatty acid profile in children with cd compared to children with uc. J Clin Med (2022) 11(9):2365. doi: 10.3390/jcm11092365
136. Khan HU, Aamir K, Jusuf PR, Sethi G, Sisinthy SP, Ghildyal R, et al. Lauric acid ameliorates lipopolysaccharide (Lps)-induced liver inflammation by mediating tlr4/myd88 pathway in sprague dawley (Sd) rats. Life Sci (2021) 265:118750. doi: 10.1016/j.lfs.2020.118750
137. Andoh A, Takaya H, Araki Y, Tsujikawa T, Fujiyama Y, Bamba T. Medium- and long-chain fatty acids differentially modulate interleukin-8 secretion in human fetal intestinal epithelial cells. J Nutr (2000) 130(11):2636–40. doi: 10.1093/jn/130.11.2636
138. Łoś-Rycharska E, Kieraszewicz Z, Czerwionka-Szaflarska M. Medium chain triglycerides (Mct) formulas in paediatric and allergological practice. Prz Gastroenterol (2016) 11(4):226–31. doi: 10.5114/pg.2016.61374
139. Kuenstner JT, Naser S, Chamberlin W, Borody T, Graham DY, McNees A, et al. The consensus from the mycobacterium avium ssp. Paratuberculosis (Map) conference 2017. Front Public Health (2017) 5:208. doi: 10.3389/fpubh.2017.00208
140. Erez İ, Serbester U. Fish oil supplementation as an omega-3 fatty acid source during gestation: effects on the performance of weaned male goat kids. Trop Anim Health Prod (2023) 55(4):268. doi: 10.1007/s11250-023-03681-6
141. Valenzuela CA, Baker EJ, Miles EA, Calder PC. Eighteen−Carbon trans fatty acids and inflammation in the context of atherosclerosis. Prog Lipid Res (2019) 76:101009. doi: 10.1016/j.plipres.2019.101009
142. Mozaffarian D. Trans fatty acids - effects on systemic inflammation and endothelial function. Atheroscler Suppl (2006) 7(2):29–32. doi: 10.1016/j.atherosclerosissup.2006.04.007
143. Jezernik G, Potočnik U. Comprehensive genetic study of fatty acids helps explain the role of noncoding inflammatory bowel disease associated snps and fatty acid metabolism in disease pathogenesis. Prostaglandins Leukot Essent Fatty Acids (2018) 130:1–10. doi: 10.1016/j.plefa.2018.02.002
144. Wang F, Lin X, Zhao Q, Li J. Fat intake and risk of ulcerative colitis: systematic review and dose-response meta-analysis of epidemiological studies. J Gastroenterol Hepatol (2017) 32(1):19–27. doi: 10.1111/jgh.13416
145. Barnes EL, Nestor M, Onyewadume L, de Silva PS, Korzenik JR. High dietary intake of specific fatty acids increases risk of flares in patients with ulcerative colitis in remission during treatment with aminosalicylates. Clin Gastroenterol Hepatol (2017) 15(9):1390–6.e1. doi: 10.1016/j.cgh.2016.12.036
146. Keshteli AH, van den Brand FF, Madsen KL, Mandal R, Valcheva R, Kroeker KI, et al. Dietary and metabolomic determinants of relapse in ulcerative colitis patients: A pilot prospective cohort study. World J Gastroenterol (2017) 23(21):3890–9. doi: 10.3748/wjg.v23.i21.3890
147. Guan S, Jia B, Chao K, Zhu X, Tang J, Li M, et al. Uplc-qtof-ms-based plasma lipidomic profiling reveals biomarkers for inflammatory bowel disease diagnosis. J Proteome Res (2020) 19(2):600–9. doi: 10.1021/acs.jproteome.9b00440
148. Tamer F, Ulug E, Akyol A, Nergiz-Unal R. The potential efficacy of dietary fatty acids and fructose induced inflammation and oxidative stress on the insulin signaling and fat accumulation in mice. Food Chem Toxicol (2020) 135:110914. doi: 10.1016/j.fct.2019.110914
149. Howe AM, Burke S, O’Reilly ME, McGillicuddy FC, Costello DA. Palmitic acid and oleic acid differently modulate tlr2-mediated inflammatory responses in microglia and macrophages. Mol Neurobiol (2022) 59(4):2348–62. doi: 10.1007/s12035-022-02756-z
150. Bühner S, Nagel E, Körber J, Vogelsang H, Linn T, Pichlmayr R. Ileal and colonic fatty acid profiles in patients with active Crohn’s disease. Gut (1994) 35(10):1424–8. doi: 10.1136/gut.35.10.1424
151. Rahmani J, Kord-Varkaneh H, Ryan PM, Rashvand S, Clark C, Day AS, et al. Dietary total antioxidant capacity and risk of ulcerative colitis: A case-control study. J Dig Dis (2019) 20(12):636–41. doi: 10.1111/1751-2980.12823
152. Akazawa Y, Morisaki T, Fukuda H, Norimatsu K, Shiota J, Hashiguchi K, et al. Significance of serum palmitoleic acid levels in inflammatory bowel disease. Sci Rep (2021) 11(1):16260. doi: 10.1038/s41598-021-95923-6
153. Taylor L, Almutairdi A, Shommu N, Fedorak R, Ghosh S, Reimer RA, et al. Cross-sectional analysis of overall dietary intake and mediterranean dietary pattern in patients with Crohn’s disease. Nutrients (2018) 10(11):1761. doi: 10.3390/nu10111761
154. Nishida T, Miwa H, Shigematsu A, Yamamoto M, Iida M, Fujishima M. Increased arachidonic acid composition of phospholipids in colonic mucosa from patients with active ulcerative colitis. Gut (1987) 28(8):1002–7. doi: 10.1136/gut.28.8.1002
155. Zhou Z, He W, Tian H, Zhan P, Liu J. Thyme (Thymus vulgaris L.) polyphenols ameliorate dss-induced ulcerative colitis of mice by mitigating intestinal barrier damage, regulating gut microbiota, and suppressing tlr4/nf-Kb-nlrp3 inflammasome pathways. Food Funct (2023) 14(2):1113–32. doi: 10.1039/d2fo02523j
156. Tang Q, Liu R, Chu G, Wang Y, Cui H, Zhang T, et al. A comprehensive analysis of microflora and metabolites in the development of ulcerative colitis into colorectal cancer based on the lung-gut correlation theory. Molecules (2022) 27(18):5838. doi: 10.3390/molecules27185838
157. Tang Q, Cang S, Jiao J, Rong W, Xu H, Bi K, et al. Integrated study of metabolomics and gut metabolic activity from ulcerative colitis to colorectal cancer: the combined action of disordered gut microbiota and linoleic acid metabolic pathway might fuel cancer. J Chromatogr A (2020) 1629:461503. doi: 10.1016/j.chroma.2020.461503
158. Diab J, Hansen T, Goll R, Stenlund H, Jensen E, Moritz T, et al. Mucosal metabolomic profiling and pathway analysis reveal the metabolic signature of ulcerative colitis. Metabolites (2019) 9(12):291. doi: 10.3390/metabo9120291
159. Socha P, Ryzko J, Koletzko B, Celinska-Cedro D, Woynarowski M, Czubkowski P, et al. Essential fatty acid depletion in children with inflammatory bowel disease. Scand J Gastroenterol (2005) 40(5):573–7. doi: 10.1080/00365520510012136
160. de Silva PS, Luben R, Shrestha SS, Khaw KT, Hart AR. Dietary arachidonic and oleic acid intake in ulcerative colitis etiology: A prospective cohort study using 7-day food diaries. Eur J Gastroenterol Hepatol (2014) 26(1):11–8. doi: 10.1097/MEG.0b013e328365c372
161. Di’Narzo AF, Houten SM, Kosoy R, Huang R, Vaz FM, Hou R, et al. Integrative analysis of the inflammatory bowel disease serum metabolome improves our understanding of genetic etiology and points to novel putative therapeutic targets. Gastroenterology (2022) 162(3):828–43.e11. doi: 10.1053/j.gastro.2021.11.015
162. Jiang J, Chen L, Sun R, Yu T, Jiang S, Chen H. Characterization of serum polyunsaturated fatty acid profile in patients with inflammatory bowel disease. Ther Adv Chronic Dis (2023) 14:20406223231156826. doi: 10.1177/20406223231156826
163. Kobayashi Y, Ohfuji S, Kondo K, Fukushima W, Sasaki S, Kamata N, et al. Association of dietary fatty acid intake with the development of ulcerative colitis: A multicenter case-control study in Japan. Inflammation Bowel Dis (2021) 27(5):617–28. doi: 10.1093/ibd/izaa140
164. He J, Luo X, Xin H, Lai Q, Zhou Y, Bai Y. The effects of fatty acids on inflammatory bowel disease: A two-sample mendelian randomization study. Nutrients (2022) 14(14):2883. doi: 10.3390/nu14142883
165. Astore C, Nagpal S, Gibson G. Mendelian randomization indicates a causal role for omega-3 fatty acids in inflammatory bowel disease. Int J Mol Sci (2022) 23(22):14380. doi: 10.3390/ijms232214380
166. Ajabnoor SM, Thorpe G, Abdelhamid A, Hooper L. Long-term effects of increasing omega-3, omega-6 and total polyunsaturated fats on inflammatory bowel disease and markers of inflammation: A systematic review and meta-analysis of randomized controlled trials. Eur J Nutr (2021) 60(5):2293–316. doi: 10.1007/s00394-020-02413-y
167. Sabo CM, Simiras C, Ismaiel A, Dumitrascu DL. Diet and gut inflammation: the effect of diet on inflammatory markers in inflammatory bowel disease - a scoping review. J Gastrointest Liver Dis (2023). doi: 10.15403/jgld-5090
168. Pacheco S, Hillier K, Smith C. Increased arachidonic acid levels in phospholipids of human colonic mucosa in inflammatory bowel disease. Clin Sci (Lond) (1987) 73(4):361–4. doi: 10.1042/cs0730361
169. Rubbino F, Garlatti V, Garzarelli V, Massimino L, Spanò S, Iadarola P, et al. Gpr120 prevents colorectal adenocarcinoma progression by sustaining the mucosal barrier integrity. Sci Rep (2022) 12(1):381. doi: 10.1038/s41598-021-03787-7
170. Mobraten K, Haug TM, Kleiveland CR, Lea T. Omega-3 and omega-6 pufas induce the same gpr120-mediated signalling events, but with different kinetics and intensity in caco-2 cells. Lipids Health Dis (2013) 12:101. doi: 10.1186/1476-511x-12-101
171. Martinez-Medina M, Denizot J, Dreux N, Robin F, Billard E, Bonnet R, et al. Western diet induces dysbiosis with increased E coli in ceabac10 mice, alters host barrier function favouring aiec colonisation. Gut (2014) 63(1):116–24. doi: 10.1136/gutjnl-2012-304119
172. Yuan Z, Yang L, Zhang X, Ji P, Hua Y, Wei Y. Mechanism of huang-lian-jie-du decoction and its effective fraction in alleviating acute ulcerative colitis in mice: regulating arachidonic acid metabolism and glycerophospholipid metabolism. J Ethnopharmacol (2020) 259:112872. doi: 10.1016/j.jep.2020.112872
173. Wu J, Luo Y, Shen Y, Hu Y, Zhu F, Wu J, et al. Integrated metabonomics and network pharmacology to reveal the action mechanism effect of shaoyao decoction on ulcerative colitis. Drug Des Devel Ther (2022) 16:3739–76. doi: 10.2147/dddt.S375281
174. Wang L, Tao JH, Chen YF, Shen YM, Jiang S. Lizhong decoction ameliorates ulcerative colitis in mice via regulation of plasma and urine metabolic profiling. Chin J Integr Med (2022) 28(11):1015–22. doi: 10.1007/s11655-021-3299-4
175. Lemaitre RN, King IB. Very long-chain saturated fatty acids and diabetes and cardiovascular disease. Curr Opin Lipidol (2022) 33(1):76–82. doi: 10.1097/mol.0000000000000806
176. Pradhan AJ, Lu D, Parisi LR, Shen S, Berhane IA, Galster SL, et al. Protein acylation by saturated very long chain fatty acids and endocytosis are involved in necroptosis. Cell Chem Biol (2021) 28(9):1298–309.e7. doi: 10.1016/j.chembiol.2021.03.012
177. Zierfuss B, Buda A, Villoria-González A, Logist M, Fabjan J, Parzer P, et al. Saturated very long-chain fatty acids regulate macrophage plasticity and invasiveness. J Neuroinflamm (2022) 19(1):305. doi: 10.1186/s12974-022-02664-y
178. Motoi Y, Ito Z, Suzuki S, Takami S, Matsuo K, Sato M, et al. Fads2 and elovl6 mutation frequencies in Japanese Crohn’s disease patients. Drug Discovery Ther (2019) 13(6):354–9. doi: 10.5582/ddt.2019.01081
179. Tan M, Ye J, Zhou Z, Ke X, Yu X, Huang K. Fatty acid metabolism in immune cells: A bioinformatics analysis of genes involved in ulcerative colitis. DNA Cell Biol (2020) 39(9):1573–82. doi: 10.1089/dna.2020.5582
180. Gorenjak M, Zupin M, Jezernik G, Skok P, Potočnik U. Omics Data Integration Identifies elovl7 and mmd gene Regions as Novel Loci for Adalimumab Response in Patients with Crohn’s Disease. Sci Rep (2021) 11(1):5449. doi: 10.1038/s41598-021-84909-z
181. Miao ST, Lu QS, Zhou YJ, Chang YN, Xu T, Zhu MY. Oral administration of octacosanol modulates the gut bacteria and protects the intestinal barrier in ulcerative colitis mice. J Food Biochem (2022) 46(10):e14284. doi: 10.1111/jfbc.14284
182. Ambrose T, Simmons A. Cannabis, cannabinoids, and the endocannabinoid system-is there therapeutic potential for inflammatory bowel disease? J Crohns Colitis (2019) 13(4):525–35. doi: 10.1093/ecco-jcc/jjy185
183. Masoodi M, Pearl DS, Eiden M, Shute JK, Brown JF, Calder PC, et al. Altered colonic mucosal polyunsaturated fatty acid (Pufa) derived lipid mediators in ulcerative colitis: new insight into relationship with disease activity and pathophysiology. PloS One (2013) 8(10):e76532. doi: 10.1371/journal.pone.0076532
184. Vong L, Ferraz JG, Dufton N, Panaccione R, Beck PL, Sherman PM, et al. Up-regulation of annexin-A1 and lipoxin a(4) in individuals with ulcerative colitis may promote mucosal homeostasis. PloS One (2012) 7(6):e39244. doi: 10.1371/journal.pone.0039244
185. Qiu YE, Qin J, Luo Y, Qin SL, Mu YF, Cun R, et al. Increased epoxyeicosatrienoic acids may be part of a protective mechanism in human ulcerative colitis, with increased cyp2j2 and reduced soluble epoxide hydrolase expression. Prostaglandins Other Lipid Mediat (2018) 136:9–14. doi: 10.1016/j.prostaglandins.2018.03.004
186. Ben-Mustapha Y, Ben-Fradj MK, Hadj-, Serghini M, Ben Ahmed M, Boubaker J, et al. Altered mucosal and plasma polyunsaturated fatty acids, oxylipins, and endocannabinoids profiles in Crohn’s disease. Prostaglandins Other Lipid Mediat (2023) 168:106741. doi: 10.1016/j.prostaglandins.2023.106741
187. Levy BD, Clish CB, Schmidt B, Gronert K, Serhan CN. Lipid mediator class switching during acute inflammation: signals in resolution. Nat Immunol (2001) 2(7):612–9. doi: 10.1038/89759
188. Nishiumi S, Izumi Y, Yoshida M. Alterations in docosahexaenoic acid-related lipid cascades in inflammatory bowel disease model mice. Dig Dis Sci (2018) 63(6):1485–96. doi: 10.1007/s10620-018-5025-4
189. Brancaleone V, Gobbetti T, Cenac N, le Faouder P, Colom B, Flower RJ, et al. A vasculo-protective circuit centered on lipoxin A4 and aspirin-triggered 15-epi-lipoxin A4 operative in murine microcirculation. Blood (2013) 122(4):608–17. doi: 10.1182/blood-2013-04-496661
190. Sakurai T, Akita Y, Miyashita H, Miyazaki R, Maruyama Y, Saito T, et al. Prostaglandin E-major urinary metabolite diagnoses mucosal healing in patients with ulcerative colitis in remission phase. J Gastroenterol Hepatol (2022) 37(5):847–54. doi: 10.1111/jgh.15782
191. Bjerrum JT, Wang Y, Zhang J, Riis LB, Nielsen OH, Seidelin JB. Lipidomic trajectories characterize delayed mucosal wound healing in quiescent ulcerative colitis and identify potential novel therapeutic targets. Int J Biol Sci (2022) 18(5):1813–28. doi: 10.7150/ijbs.67112
192. Junek R, Kverka M, Jandera A, Panajotová V, Satinský D, Machácek M, et al. Antileukotrienic phenethylamido derivatives of arylalkanoic acids in the treatment of ulcerative colitis. Eur J Med Chem (2009) 44(1):332–44. doi: 10.1016/j.ejmech.2008.02.030
193. Ungaro F, Rubbino F, Danese S, D’Alessio S. Actors and factors in the resolution of intestinal inflammation: lipid mediators as a new approach to therapy in inflammatory bowel diseases. Front Immunol (2017) 8:1331. doi: 10.3389/fimmu.2017.01331
194. Lawrence T, Willoughby DA, Gilroy DW. Anti-inflammatory lipid mediators and insights into the resolution of inflammation. Nat Rev Immunol (2002) 2(10):787–95. doi: 10.1038/nri915
195. Günay S, Taşova F, Yılmaz HE, Paköz ZB, Çekiç C. Serum resolvin E1 levels and its relationship with disease activity in ulcerative colitis. Gastroenterol Res Pract (2019) 2019:6258327. doi: 10.1155/2019/6258327
196. Serhan CN, Gotlinger K, Hong S, Lu Y, Siegelman J, Baer T, et al. Anti-inflammatory actions of neuroprotectin D1/protectin D1 and its natural stereoisomers: assignments of dihydroxy-containing docosatrienes. J Immunol (2006) 176(3):1848–59. doi: 10.4049/jimmunol.176.3.1848
197. Li X, Li C, Liang W, Bi Y, Chen M, Dong S. Protectin D1 promotes resolution of inflammation in a murine model of lipopolysaccharide-induced acute lung injury via enhancing neutrophil apoptosis. Chin Med J (Engl) (2014) 127(5):810–4. doi: 10.3760/cma.j.issn.0366-6999.20131104
198. Saito-Sasaki N, Sawada Y, Nakamura M. Maresin-1 and inflammatory disease. Int J Mol Sci (2022) 23(3):1367. doi: 10.3390/ijms23031367
199. Hayashi S, Muraleedharan CK, Oku M, Tomar S, Hogan SP, Quiros M, et al. Intestinal epithelial blt1 promotes mucosal repair. JCI Insight (2022) 7(23):e162392. doi: 10.1172/jci.insight.162392
200. Miranda J, Brazil JC, Morris AH, Parkos CA, Quiros M, Nusrat A. Maresin-2 promotes mucosal repair and has therapeutic potential when encapsulated in thermostable nanoparticles. Proc Natl Acad Sci U.S.A. (2023) 120(4):e2218162120. doi: 10.1073/pnas.2218162120
201. Ungaro F, Tacconi C, Massimino L, Corsetto PA, Correale C, Fonteyne P, et al. Mfsd2a promotes endothelial generation of inflammation-resolving lipid mediators and reduces colitis in mice. Gastroenterology (2017) 153(5):1363–77.e6. doi: 10.1053/j.gastro.2017.07.048
202. Kikut J, Drozd A, Mokrzycka M, Grzybowska-Chlebowczyk U, Ziętek M, Szczuko M. Are epa and dha derivatives involved in ibd remission? J Clin Med (2022) 11(9):2388. doi: 10.3390/jcm11092388
203. Zhao D, Yang B, Ye C, Zhang S, Lv X, Chen Q. Enteral nutrition ameliorates the symptoms of Crohn’s disease in mice via activating special pro-resolving mediators through innate lymphoid cells. Innate Immun (2021) 27(7-8):533–42. doi: 10.1177/17534259211057038
204. Kikut J, Mokrzycka M, Drozd A, Grzybowska-Chlebowczyk U, Ziętek M, Szczuko M. Involvement of proinflammatory arachidonic acid (Ara) derivatives in Crohn’s disease (Cd) and ulcerative colitis (Uc). J Clin Med (2022) 11(7):1861. doi: 10.3390/jcm11071861
205. Strisciuglio C, Bellini G, Miele E, Martinelli M, Cenni S, Tortora C, et al. Cannabinoid receptor 2 functional variant contributes to the risk for pediatric inflammatory bowel disease. J Clin Gastroenterol (2018) 52(5):e37–43. doi: 10.1097/mcg.0000000000000755
206. Grill M, Högenauer C, Blesl A, Haybaeck J, Golob-Schwarzl N, Ferreirós N, et al. Members of the endocannabinoid system are distinctly regulated in inflammatory bowel disease and colorectal cancer. Sci Rep (2019) 9(1):2358. doi: 10.1038/s41598-019-38865-4
207. Schäfer M, Reisch F, Labuz D, Machelska H, Stehling S, Püschel GP, et al. Humanization of the reaction specificity of mouse alox15b inversely modified the susceptibility of corresponding knock-in mice in two different animal inflammation models. Int J Mol Sci (2023) 24(13):11034. doi: 10.3390/ijms241311034
208. Deol P, Ruegger P, Logan GD, Shawki A, Li J, Mitchell JD, et al. Diet high in linoleic acid dysregulates the intestinal endocannabinoid system and increases susceptibility to colitis in mice. Gut Microbes (2023) 15(1):2229945. doi: 10.1080/19490976.2023.2229945
209. Phatak UP, Rojas-Velasquez D, Porto A, Pashankar DS. Prevalence and patterns of marijuana use in young adults with inflammatory bowel disease. J Pediatr Gastroenterol Nutr (2017) 64(2):261–4. doi: 10.1097/mpg.0000000000001474
210. El-Maadawy WH, Hafiz E, Okasha H, Osman NA, Ali GH, Hussein RA. Phycocyanin stimulates ulcerative colitis healing via selective activation of cannabinoid receptor-2, intestinal mucosal healing, treg accumulation, and P38mapk/mk2 signaling inhibition. Life Sci (2022) 305:120741. doi: 10.1016/j.lfs.2022.120741
211. Chen J, Vitetta L. Butyrate in inflammatory bowel disease therapy. Gastroenterology (2020) 158(5):1511. doi: 10.1053/j.gastro.2019.08.064
212. Wang Y, Gao X, Zhang X, Xiao F, Hu H, Li X, et al. Microbial and metabolic features associated with outcome of infliximab therapy in pediatric Crohn’s disease. Gut Microbes (2021) 13(1):1–18. doi: 10.1080/19490976.2020.1865708
213. Ventin-Holmberg R, Eberl A, Saqib S, Korpela K, Virtanen S, Sipponen T, et al. Bacterial and fungal profiles as markers of infliximab drug response in inflammatory bowel disease. J Crohns Colitis (2021) 15(6):1019–31. doi: 10.1093/ecco-jcc/jjaa252
214. Aden K, Rehman A, WasChina S, Pan WH, Walker A, Lucio M, et al. Metabolic functions of gut microbes associate with efficacy of tumor necrosis factor antagonists in patients with inflammatory bowel diseases. Gastroenterology (2019) 157(5):1279–92.e11. doi: 10.1053/j.gastro.2019.07.025
215. Lauritsen K, Laursen LS, Bukhave K, Rask-Madsen J. Effects of topical 5-aminosalicylic acid and prednisolone on prostaglandin E2 and leukotriene B4 levels determined by equilibrium in vivo dialysis of rectum in relapsing ulcerative colitis. Gastroenterology (1986) 91(4):837–44. doi: 10.1016/0016-5085(86)90684-0
216. Lauritsen K, Laursen LS, Bukhave K, Rask-Madsen J. In vivo effects of orally administered prednisolone on prostaglandin and leucotriene production in ulcerative colitis. Gut (1987) 28(9):1095–9. doi: 10.1136/gut.28.9.1095
217. Roediger W, Schapel G, Lawson M, Radcliffe B, Nance S. Effect of 5-aminosalicylic acid (5-asa) and other salicylates on short-chain fat metabolism in the colonic mucosa. Pharmacological implications for ulcerative colitis. Biochem Pharmacol (1986) 35(2):221–5. doi: 10.1016/0006-2952(86)90517-4
218. Caviglia GP, De Blasio F, Vernero M, Armandi A, Rosso C, Saracco GM, et al. Efficacy of a preparation based on calcium butyrate, bifidobacterium bifidum, bifidobacterium lactis, and fructooligosaccharides in the prevention of relapse in ulcerative colitis: A prospective observational study. J Clin Med (2021) 10(21):4961. doi: 10.3390/jcm10214961
219. Keshteli AH, Valcheva R, Nickurak C, Park H, Mandal R, van Diepen K, et al. Anti-inflammatory diet prevents subclinical colonic inflammation and alters metabolomic profile of ulcerative colitis patients in clinical remission. Nutrients (2022) 14(16):3294. doi: 10.3390/nu14163294
220. Miyaguchi K, Tsuzuki Y, Ichikawa Y, Shiomi R, Ohgo H, Nakamoto H, et al. Positive zinc intake and a Japanese diet rich in N-3 fatty acids induces clinical remission in patients with mild active ulcerative colitis: A randomized interventional pilot study. J Clin Biochem Nutr (2023) 72(1):82–8. doi: 10.3164/jcbn.22-72
221. Sorensen LS, Thorlacius-Ussing O, Rasmussen HH, Lundbye-Christensen S, Calder PC, Lindorff-Larsen K, et al. Effects of perioperative supplementation with omega-3 fatty acids on leukotriene B4 and leukotriene B5; Production by stimulated neutrophils in patients with colorectal cancer: A randomized, placebo-controlled intervention trial. Nutrients (2014) 6(10):4043–57. doi: 10.3390/nu6104043
222. Scaioli E, Sartini A, Bellanova M, Campieri M, Festi D, Bazzoli F, et al. Eicosapentaenoic acid reduces fecal levels of calprotectin and prevents relapse in patients with ulcerative colitis. Clin Gastroenterol Hepatol (2018) 16(8):1268–75.e2. doi: 10.1016/j.cgh.2018.01.036
223. Prossomariti A, Scaioli E, Piazzi G, Fazio C, Bellanova M, Biagi E, et al. Short-term treatment with eicosapentaenoic acid improves inflammation and affects colonic differentiation markers and microbiota in patients with ulcerative colitis. Sci Rep (2017) 7(1):7458. doi: 10.1038/s41598-017-07992-1
224. Bueno-Hernández N, Sixtos-Alonso MS, Milke García MDP, Yamamoto-Furusho JK. Effect of cis-palmitoleic acid supplementation on inflammation and expression of hnf4γ, hnf4α and il6 in patients with ulcerative colitis. Minerva Gastroenterol Dietol (2017) 63(3):257–63. doi: 10.23736/s1121-421x.17.02367-4
225. Romano C, Cucchiara S, Barabino A, Annese V, Sferlazzas C. Usefulness of omega-3 fatty acid supplementation in addition to mesalazine in maintaining remission in pediatric Crohn’s disease: A double-blind, randomized, placebo-controlled study. World J Gastroenterol (2005) 11(45):7118–21. doi: 10.3748/wjg.v11.i45.7118
226. Brennan Laing B, Cavadino A, Ellett S, Ferguson LR. Effects of an omega-3 and vitamin D supplement on fatty acids and vitamin D serum levels in double-blinded, randomized, controlled trials in healthy and crohn’s disease populations. Nutrients (2020) 12(4):1139. doi: 10.3390/nu12041139
227. Feagan BG, Sandborn WJ, Mittmann U, Bar-Meir S, D’Haens G, Bradette M, et al. Omega-3 free fatty acids for the maintenance of remission in Crohn disease: the epic randomized controlled trials. Jama (2008) 299(14):1690–7. doi: 10.1001/jama.299.14.1690
228. Bischoff SC, Escher J, Hébuterne X, Kłęk S, Krznaric Z, Schneider S, et al. Espen practical guideline: clinical nutrition in inflammatory bowel disease. Clin Nutr (2020) 39(3):632–53. doi: 10.1016/j.clnu.2019.11.002
229. Chicco F, Magrì S, Cingolani A, Paduano D, Pesenti M, Zara F, et al. Multidimensional impact of mediterranean diet on ibd patients. Inflammation Bowel Dis (2021) 27(1):1–9. doi: 10.1093/ibd/izaa097
230. Haskey N, Estaki M, Ye J, Shim RK, Singh S, Dieleman LA, et al. A mediterranean diet pattern improves intestinal inflammation concomitant with reshaping of the bacteriome in ulcerative colitis: A randomized controlled trial. J Crohns Colitis (2023). doi: 10.1093/ecco-jcc/jjad073
231. Lo CH, Khalili H, Song M, Lochhead P, Burke KE, Richter JM, et al. Healthy lifestyle is associated with reduced mortality in patients with inflammatory bowel diseases. Clin Gastroenterol Hepatol (2021) 19(1):87–95.e4. doi: 10.1016/j.cgh.2020.02.047
232. Lewis JD, Sandler RS, Brotherton C, Brensinger C, Li H, Kappelman MD, et al. A randomized trial comparing the specific carbohydrate diet to a mediterranean diet in adults with Crohn’s disease. Gastroenterology (2021) 161(3):837–52.e9. doi: 10.1053/j.gastro.2021.05.047
233. Ruemmele FM, Veres G, Kolho KL, Griffiths A, Levine A, Escher JC, et al. Consensus guidelines of ecco/espghan on the medical management of pediatric Crohn’s disease. J Crohns Colitis (2014) 8(10):1179–207. doi: 10.1016/j.crohns.2014.04.005
234. Logan M, Gkikas K, Svolos V, Nichols B, Milling S, Gaya DR, et al. Analysis of 61 Exclusive Enteral Nutrition Formulas Used in The management of Active Crohn’s Disease-New Insights into Dietary Disease Triggers. Aliment Pharmacol Ther (2020) 51(10):935–47. doi: 10.1111/apt.15695
235. Gassull MA, Fernández-Bañares F, Cabré E, Papo M, Giaffer MH, Sánchez-Lombraña JL, et al. Fat composition may be a clue to explain the primary therapeutic effect of enteral nutrition in crohn’s disease: results of a double blind randomised multicentre european trial. Gut (2002) 51(2):164–8. doi: 10.1136/gut.51.2.164
236. Ajabnoor SM, Forbes A. Effect of fat composition in enteral nutrition for crohn’s disease in adults: A systematic review. Clin Nutr (2019) 38(1):90–9. doi: 10.1016/j.clnu.2017.12.018
237. Vernia P, Marcheggiano A, Caprilli R, Frieri G, Corrao G, Valpiani D, et al. Short-chain fatty acid topical treatment in distal ulcerative colitis. Aliment Pharmacol Ther (1995) 9(3):309–13. doi: 10.1111/j.1365-2036.1995.tb00386.x
238. Breuer RI, Soergel KH, Lashner BA, Christ ML, Hanauer SB, Vanagunas A, et al. Short chain fatty acid rectal irrigation for left-sided ulcerative colitis: A randomised, placebo controlled trial. Gut (1997) 40(4):485–91. doi: 10.1136/gut.40.4.485
239. Vernero M, De Blasio F, Ribaldone DG, Bugianesi E, Pellicano R, Saracco GM, et al. The usefulness of microencapsulated sodium butyrate add-on therapy in maintaining remission in patients with ulcerative colitis: A prospective observational study. J Clin Med (2020) 9(12):3941. doi: 10.3390/jcm9123941
240. Wedenoja S, Saarikivi A, Mälkönen J, Leskinen S, Lehto M, Adeshara K, et al. Fecal microbiota in congenital chloride diarrhea and inflammatory bowel disease. PloS One (2022) 17(6):e0269561. doi: 10.1371/journal.pone.0269561
241. Langhorst J, Koch AK, Voiss P, Dobos GJ, Rueffer A. Distinct patterns of short-chain fatty acids during flare in patients with ulcerative colitis under treatment with mesalamine or a herbal combination of myrrh, chamomile flowers, and coffee charcoal: secondary analysis of a randomized controlled trial. Eur J Gastroenterol Hepatol (2020) 32(2):175–80. doi: 10.1097/meg.0000000000001582
242. Hallert C, Björck I, Nyman M, Pousette A, Grännö C, Svensson H. Increasing fecal butyrate in ulcerative colitis patients by diet: controlled pilot study. Inflammation Bowel Dis (2003) 9(2):116–21. doi: 10.1097/00054725-200303000-00005
243. Thomas S, Dilbarov N, Kelly J, Mercogliano G, Prendergast GC. Diet effects on colonic health influence the efficacy of bin1 mab immunotherapy for ulcerative colitis. Sci Rep (2023) 13(1):11802. doi: 10.1038/s41598-023-38830-2
244. Pietrzak A, Banasiuk M, Szczepanik M, Borys-Iwanicka A, Pytrus T, Walkowiak J, et al. Sodium butyrate effectiveness in children and adolescents with newly diagnosed inflammatory bowel diseases-randomized placebo-controlled multicenter trial. Nutrients (2022) 14(16):3283. doi: 10.3390/nu14163283
245. Bamba S, Takahashi K, Imaeda H, Nishida A, Kawahara M, Inatomi O, et al. Effect of fermented vegetable beverage containing pediococcus pentosaceus in patients with mild to moderate ulcerative colitis. BioMed Rep (2018) 9(1):74–80. doi: 10.3892/br.2018.1099
246. Valcheva R, Koleva P, Martínez I, Walter J, Gänzle MG, Dieleman LA. Inulin-type fructans improve active ulcerative colitis associated with microbiota changes and increased short-chain fatty acids levels. Gut Microbes (2019) 10(3):334–57. doi: 10.1080/19490976.2018.1526583
247. Tartakover Matalon S, Azar S, Meiri D, Hadar R, Nemirovski A, Abu Jabal N, et al. Endocannabinoid levels in ulcerative colitis patients correlate with clinical parameters and are affected by cannabis consumption. Front Endocrinol (Lausanne) (2021) 12:685289. doi: 10.3389/fendo.2021.685289
Keywords: inflammatory bowel disease, fatty acid, lipid mediators, pathogenesis, therapeutic approaches
Citation: Yan D, Ye S, He Y, Wang S, Xiao Y, Xiang X, Deng M, Luo W, Chen X and Wang X (2023) Fatty acids and lipid mediators in inflammatory bowel disease: from mechanism to treatment. Front. Immunol. 14:1286667. doi: 10.3389/fimmu.2023.1286667
Received: 31 August 2023; Accepted: 25 September 2023;
Published: 05 October 2023.
Edited by:
Charalampia Amerikanou, Harokopio University, GreeceReviewed by:
Federica Rubbino, Humanitas Research Hospital, ItalyKang Xu, Chinese Academy of Sciences (CAS), China
Copyright © 2023 Yan, Ye, He, Wang, Xiao, Xiang, Deng, Luo, Chen and Wang. This is an open-access article distributed under the terms of the Creative Commons Attribution License (CC BY). The use, distribution or reproduction in other forums is permitted, provided the original author(s) and the copyright owner(s) are credited and that the original publication in this journal is cited, in accordance with accepted academic practice. No use, distribution or reproduction is permitted which does not comply with these terms.
*Correspondence: Weiwei Luo, bHVvd2Vpd2VpQGNzdS5lZHUuY24=; Xuejie Chen, eHVlamllY2hlbkBjc3UuZWR1LmNu; Xiaoyan Wang, d2FuZ3hpYW95YW5AY3N1LmVkdS5jbg==
†These authors have contributed equally to this work and share first authorship