- 1Faculty of Medicine, University of Hamburg, Hamburg, Germany
- 2Department of Rheumatology and Clinical Immunology, University Medical Center Schleswig-Holstein, Lübeck, Germany
- 3Faculty of Medicine, University of Lübeck, Lübeck, Germany
- 4Department of Oncology, Hematology and Bone Marrow Transplantation with Division of Pneumology, University Medical Center Eppendorf, Hamburg, Germany
Engineering immune cells to treat hematological malignancies has been a major focus of research since the first resounding successes of CAR-T-cell therapies in B-ALL. Several diseases can now be treated in highly therapy-refractory or relapsed conditions. Currently, a number of CD19- or BCMA-specific CAR-T-cell therapies are approved for acute lymphoblastic leukemia (ALL), diffuse large B-cell lymphoma (DLBCL), mantle cell lymphoma (MCL), multiple myeloma (MM), and follicular lymphoma (FL). The implementation of these therapies has significantly improved patient outcome and survival even in cases with previously very poor prognosis. In this comprehensive review, we present the current state of research, recent innovations, and the applications of CAR-T-cell therapy in a selected group of hematologic malignancies. We focus on B- and T-cell malignancies, including the entities of cutaneous and peripheral T-cell lymphoma (T-ALL, PTCL, CTCL), acute myeloid leukemia (AML), chronic myeloid leukemia (CML), chronic lymphocytic leukemia (CLL), classical Hodgkin-Lymphoma (HL), Burkitt-Lymphoma (BL), hairy cell leukemia (HCL), and Waldenström’s macroglobulinemia (WM). While these diseases are highly heterogenous, we highlight several similarly used approaches (combination with established therapeutics, target depletion on healthy cells), targets used in multiple diseases (CD30, CD38, TRBC1/2), and unique features that require individualized approaches. Furthermore, we focus on current limitations of CAR-T-cell therapy in individual diseases and entities such as immunocompromising tumor microenvironment (TME), risk of on-target-off-tumor effects, and differences in the occurrence of adverse events. Finally, we present an outlook into novel innovations in CAR-T-cell engineering like the use of artificial intelligence and the future role of CAR-T cells in therapy regimens in everyday clinical practice.
1 Introduction
The diverse cluster of mature hematologic malignancies can broadly be classified into five groups using genetic, molecular, and clinical parameters: mature B-cell neoplasms, classic Hodgkin-Lymphomas, mature T- and natural killer (NK)-cell-neoplasms, histiocytic and dendritic cell neoplasms, and immunodeficiency-associated lymphoproliferative disorders (1).
These groups feature a wide spectrum of pathophysiological mechanisms of disease, pathological features, and therapeutic options. The clinical characteristics of these diseases differ significantly: While some, like CLL, do not necessarily require therapy upon diagnosis (2), diseases such as aggressive T-cell malignancies still present a highly complex clinical challenge with a median overall survival of less than 12 months (3). Patient groups like people living with HIV (PLWH) are at increased risk of developing hematologic malignancies (4). Some diseases are more common in pediatric (acute leukemias) or geriatric (CLL) patients (5). The treatment and management of these malignancies have significantly benefited from the implementation of CAR-T-cell therapies and this technology remains the most successful of several cellular immunotherapies developed in the 21st century (6, 7).
Research currently focuses on expanding this technology towards treatment of solid tumors. Other possible areas of application are infectious diseases (e.g. HIV, HBV, other viral and fungal infections), and auto-immune disorders (such as rheumatoid arthritis, systemic lupus erythematosus). While these areas are prominently featured in the current CAR-T-cell research landscape, there are also approaches for its implementation in novel hematologic malignancies. Currently, many of these diseases are treated utilizing a combination of chemotherapy and immunotherapy, and in some cases, autologous or allogeneic hematopoietic stem cell transplantation (allo-HSCT), leading to high response rates and potential long-lasting complete remission. However, patients with relapsed or refractory disease often have a poor prognosis (8, 9). Furthermore, particular patient populations like heavily pretreated patients and those over the age of 60 years often suffer from reduced response to therapy or limited life expectancy (10).
To extend the remarkable achievements of CAR-T-cell therapy to more diseases and patient populations, researchers are currently employing an array of different technologies and approaches. These include CRISPR/Cas9- and TALEN-based (Transcription activator-like effector nucleases) gene-editing, OMICs methods (including transcriptomics, surfaceomics, and proteomics), nanotechnology, single-cell technologies, and advanced combination regimens with established therapies. Through these approaches, more and more malignant entities become possible targets of CAR-T-cell therapy (11–13).
However, severe adverse events including immune effector cell-associated neurotoxicity syndrome (ICANS) and cytokine release syndrome (CRS) (14) as well as T-cell dysfunction, resistance, and tumor escape mechanisms still pose difficulties (15, 16). Novel approaches for the management of these adverse events are currently under development, including subcutaneous injection of IL-6-adsorbing hydrogel (17) and the implementation of inducible “on-and-off” systems (18). Recent research has highlighted the possibility to employ sequential CAR-T-cell therapies to tackle post-CAR-T-cell relapse, a major cause of death in CAR-T-cell treated patients (19). Additionally, tools are being developed to aid in the management of CAR-T-cell patients and improve clinical care (20).
After initial hopes that CAR-T cells could present a potentially universal therapeutic approach for malignant diseases, researchers are currently understanding that CAR-T cells are most likely just one of several options in personalized therapies (21). Therefore, other innovative immunotherapeutic strategies are being investigated, including different immune cell groups, oncolytic viruses, technologies like T-cell engagers, T-cell receptor (TCR) engineering, and combinations of these technologies with CAR-T cells (22). The ultimate goal of these approaches and innovations is the improvement of clinical outcomes like overall survival (OS), progression-free survival (PFS), prevention of adverse events, improvements in patients’ quality of life, and above all the identification of curative therapeutic strategies.
While many of these techniques are still in their early development, several CAR-T-cell therapies have already been approved and are utilized in the clinical setting. Therefore, we highlight potential applications of CAR-T-cell technology in hematologic malignancies beyond the currently approved indications. We also provide an overview of the current research and possible innovations regarding different lymphomas and leukemias. We further focus on aspects such as safety, efficacy, and organizational issues.
2 T-cell malignancies
2.1 Current state of treatment
T-cell malignancies include lymphomas and leukemias originating from T cells and their precursor cells. Within the past decade, the prognosis of acute lymphatic T-cell leukemia (T-ALL) has improved, especially due to new chemotherapy protocols and monitoring of minimal residual disease (MRD) after therapy (23). However, these diseases still pose a difficult task for oncologists as the treatment and management of complications remain complex with poor outcome (24). Especially patients with cases of refractory or relapsing disease rarely respond well to established salvage protocols (25). Standard therapy regimens include chemotherapy, histone deacetylase inhibitors (HDACi) and monoclonal antibodies targeting antigens such as CD30 or CCR4 (26).
The lack of malignant T-cell-specific target antigens for CAR-T cells is one of the main difficulties as most of the targeted antigens in T-cell malignancies (such as CD3, CD5, CD7) are expressed by healthy T cells as well. A T-cell depleting therapy would lead to a complete eradication of T cells, resulting in detrimental infectious complications (27). The second fundamental challenge in employing CAR-T-cell therapy for T-cell malignancies is the apheresis of exclusively healthy T cells from the patient in order to generate CAR-T cells without contamination with circulating tumor cells (28).
2.2 CD7-directed CAR-T-cell therapy
To account for these limitations, a CAR-T-cell therapy needs to be able to distinguish between healthy and malignant T cells to a required extent. This poses a substantial challenge to researchers as the expressed proteins on healthy and malignant T cells differ only marginally (29). The most comprehensively studied T-cell-specific target is CD7 due to its abundant expression in T-cell malignancies compared to limited presence on healthy T cells (30).
An important issue in the use of anti-CD7 CAR-T cells against T-cell malignancies is the concept of “fratricide”, meaning the killing of therapeutic CAR-T cells by the CAR-T cells themselves due to shared expression of CD7 (Figure 1) (31). This protein is also expressed on NK cells, where it is related to activation and maturation (32). Fratricide results in reduced anti-tumoral activity, decreased survival of CAR-T cells and limited therapeutic success (33). Approaches to reduce fratricide include nanobody-based techniques (34, 35), natural selection of fratricide-resistant CAR-T cells (36, 37), and the use of antibodies (38) or protein expression blockers (39).
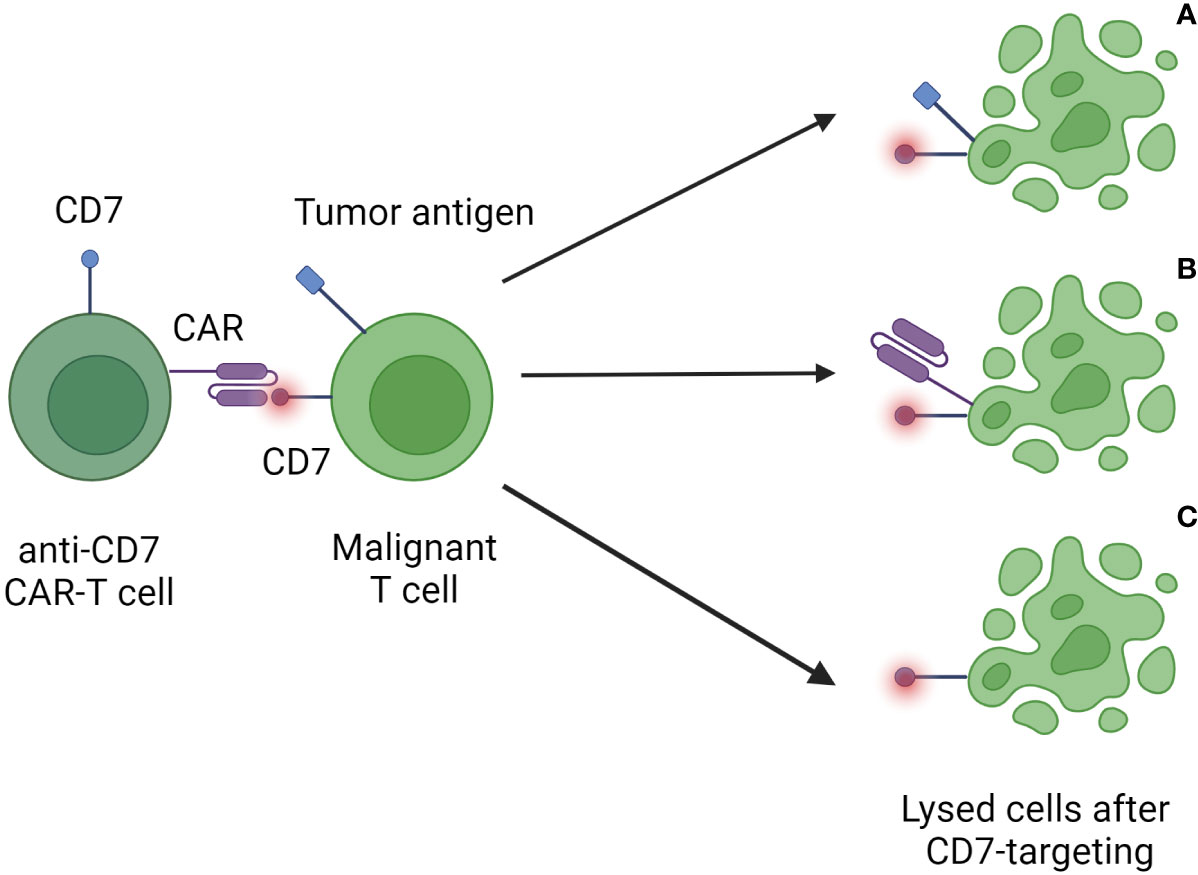
Figure 1 Fratricide elicited by anti-CD7 CAR-T-cell therapy. After infusion CAR-T cells recognize CD7 present on tumor cells (A), other CAR-T cells (B), and healthy T cells (C) in the recipient. These targeted cells are then destroyed by the immune system. The consequences are the depletion of the patient’s healthy T-cell reservoir as well as a reduced capacity and longevity of applied CAR-T cells.
Chiesa et al. have recently shown the benefits of using base-editing to inactivate the genes encoding for the CD52 and CD7 receptors, and the β chain of the αβ TCR in a phase I trial of CD7-targeting CAR-T cells (40).The implementation of targeted pharmacotherapy with the objective of diminishing fratricide has the potential to facilitate the utilization of unedited anti-CD7 CAR-T cells, thereby enhancing the targetability of CD7. Previous studies have investigated the combination of ibrutinib and dasatinib for this purpose (41).
Another approach for the circumvention of fratricide is to make use of the naturally occurring subset of CD7- T cells for the generation of CAR-T cells. These could be resistant to fratricide and provide a more sustained anti-tumor activity (42). Furthermore, to avoid fratricide, CD7 can be gene-edited with techniques like CRISPR/Cas9 (43, 44). If the expression of targeted antigens can be sufficiently reduced on the CAR-T cell itself, even bi-specific CAR-T cells are potential therapeutic tools without eliciting fratricide (45). These bi-specific CAR-T cells can be generated through the use of nanobodies as demonstrated by Xia et al. with the manufacturing of CAR-T cells targeting CD30 and CD5 (46).
Investigating another way to tackle fratricide, Jiang et al. have recently developed a “2-in-1 strategy” of knocking out the CD7 locus and inserting an EF1α-driven CD7-CAR in this locus to achieve improved tumor rejection in a mouse xenograft model (47). A similar technique was employed by Liao et al. for the generation of CD38-specific CAR-T cells (48). Similar to this is the editing of the T-cell receptor α constant (TRAC) locus (49). By this modification, allogeneic cell attack by T and NK cells can be reduced. There are multiple techniques to disrupt the TRAC expression, focusing on different parts of the receptor. The main technologies used for these editing approaches include CRISPR/Cas9 and TALENs (50). Xie et al. have recently demonstrated how CRISPR-based CD7- and TRAC-knockout CAR-T cells can efficiently proliferate and kill T-ALL cells in vitro and in vivo. The researchers showed increased frequency of CD8- T cells and a higher number of activated CD4+ memory T cells (51). The application of gene-edited CD7- hematopoietic stem cells could help sustain a sufficient number of healthy T cells after anti-CD7 CAR-T-cell therapy (52).
So far, clinical trials have utilized both autologous and allogeneic anti-CD7 CAR-T cells and have shown great promise regarding survival, tumor regression, and PFS (53–55). Recently, Chen et al. demonstrated the complete eradication of CD7+ T cells and the rapid expansion of the functional CD7- T-cell subset following anti-CD7 CAR-T-cell therapy (56). Li et al. observed a patient achieving >3 years of PFS in their cohort of 12 patients treated with “off-the-shelf” anti-CD7 CAR-T-cell therapy (57). However, these studies have also confirmed the risks of T-cell aplasia as the result of on-target-off-tumor effects with associated severe adverse events like viral reactivation and infection, and fungal pneumonia. This is described by a case report of a hepatosplenic γδ-T-cell lymphoma treated with HLA fully-mismatched allogeneic anti-CD7 CAR-T-cell therapy. Researchers observed CRS, cytopenia and infections to a manageable extent and rapid decrease of circulating CAR-T cells after infusion. However, the patient did receive allo-HSCT and achieved lasting CR (58).
2.3 Potential targets
To circumvent CD7-related fratricide, researchers are also focusing on finding more suitable biological markers to differentiate between malignant, healthy, and therapeutic T cells. Recent studies have investigated the T-cell receptor β-chain constant domains TRBC1 and TRBC2 (24, 59), CD1a (60), CD2 (61), CD4 (62), CD5 (63, 64), CD21 (65), CD26 (66), CD38 (67), CD99 (68, 69), CCR9 (70), the natural CD7 ligand SECTM-1 (71), and the dual targeting of CD38 and LMP1 (72). Shaw et al. have shown the efficacy and antigen-specificity of TCRvβ-targeting CAR-T cells in cell lines, patient samples, and mice (73). To enhance the functionality of CAR-T-cells in the treatment of T-cell malignancies, researchers are also investigating other parts of the CAR-T-construct. This includes the hinge region, which has recently been shown to influence the cytotoxicity of anti-CD5 CAR-T cells and can be enhanced through specific modifications (74).
There are particular target antigens for different patient populations like targets specifically investigated for pediatric T-cell malignancies (75). Today, many of these targets seem promising, but CD7 is still the most extensively tested CAR-T-cell target antigen in clinical trials.
2.4 Increasing CAR-T-cell therapy safety
To reduce CAR-T-cell toxicities and adverse events, CAR-T cells incorporating “safety switches” or suicide genes have been proposed. These mechanisms limit the CAR-T-cell life span and persistence due to depletion upon administration of a prodrug (metabolic switch) or using monoclonal antibodies (76). In an effort to control CRS after CAR-T-cell therapy, Li et al. have shown promising results utilizing the Januskinase inhibitor ruxolitinib after infusion of anti-CD7 CAR-T cells (77). This application has also illustrated potential in preventing severe cases of CRS by limiting cytokine release and proliferation of CAR-T cells and is used for the treatment of CRS in other diseases (78). These advances towards a safer CAR-T-cell therapy are accompanied by innovations reducing the cost and complexity. These include retroviral vector-based gene therapy approaches based on in vivo delivery of the CAR gene (79).
To account for the risks and complexities of acquiring autologous T cells from patients, researchers are also investigating the application of allogeneic CAR-T cells. Hu et al. have shown the potential of allogeneic anti-CD7 CAR-T cells derived from healthy donors to target T-ALL cells (80). In the phase I trial, adverse events like high-grade ICANS and CRS or Graft-versus-Host-Disease (GvHD) were not observed in the study cohort of 11 patients. In contrast, Pan et al. administered allogeneic anti-CD7 CAR-T cells in 20 patients with 2 of them experiencing CRS grade 3-4 and 60% of patients experiencing GvHD grade 1 or 2 (53).
2.5 Cutaneous T-cell lymphoma
A special entity within the group of hematologic T-cell malignancies is the cutaneous T-cell lymphoma (CTCL), derived from the CD4+ T-cell subset. The most common forms of CTCL are mycosis fungoides and Sézary syndrome. While both diseases can be managed through treatment, achieving a complete cure is only feasible with allo-HSCT (81–83).
Currently investigated targets to combat CTCL with CAR-T cells include CD30, CD38, CD56 (84), and CCR4 (85). The latter is of particular interest as CCR4 is needed for cell trafficking and homing, as well as recruiting of regulatory T cells to the TME and has also shown to be upregulated in advanced stages of CTCL (86). However, anti-CCR4 CAR-T cells have been shown to elicit fratricide and selectively attack TH2-, TH17- and Treg-cells (87). Recent findings have indicated CD37 and TRBC as potential targets (88, 89). Gluud et al. have highlighted the importance of the JAK/STAT signaling pathway in CTCL and its potential targeting in immunotherapy (90).
Relevant obstacles in CAR-T-cell therapy for CTCL include antigen overlap with healthy T cells, transduction of malignant cells, fratricide, and prolonged T-cell aplasia (91). Tumor heterogeneity plays an important role in CTCL immunotherapy and shifts researcher’s attention towards dual- or multi-targeting therapy approaches. These could target malignant cells comprehensively and specifically (92). Further approaches include in-depth genotyping of tumor cells and monitoring of intra-tumoral activity of T cells (93). Due to these advances, CAR-T-cell therapy is seen as an option to achieve long-term remission in CTCL patients (94) and could offer a promising chance for these currently incurable diseases (95).
2.6 Peripheral T-cell lymphoma
These peripheral T-cell lymphomas (PTCL) are difficult to treat as there are few established treatment methods and the prognosis is mostly poor (96). Thus, PTCL proves to be a potentially rewarding target of CAR-T-cell therapy.
As shown in other T-cell malignancies, major problems include fratricide, the consequences of therapy-induced T-cell aplasia and the contamination of the CAR-T-cell product with malignant T cells (97). Similar problems in the implementation of CAR-T-cell therapy have also been independently described for entities such as T-follicular helper cell lymphoma (98).
Wu et al. have shown the efficacy of anti-CD30 CAR-T cells against PTCL cells in vitro and in vivo mouse models (99). As TRBC1/2 is already being investigated as a CAR-T-cell target antigen (24), current research is aiming at increasing the specificity towards this target in T-cell malignancies (100). A key aspect of this approach is the finding that T-cell malignancies are restricted to either TRBC1 or TRBC2. These can be targeted differentially. While clinical trials currently investigate other targets like CD5 and CD7 for T-cell malignancies, many of these trials do not include patients with PTCL (101). Other groups are investigating CD37, CD70, and CD147 as potential targets for PTCL CAR-T-cell therapy (102). As CD4 is also frequently upregulated on subtypes of PTCL like angioimmunoblastic T-cell lymphoma, anti-CD4 CAR-T cells are another area of investigation (103). Fang et al. could recently demonstrate the effect of CD4-/CD8- anti-CD4 CAR-T cells against T-ALL and PTCL in vitro and in vivo without eliciting fratricide (104).
2.7 Outlook
CAR-T-cell therapy can offer a novel therapy option for patients with advanced, relapsed or therapy-refractory T-cell malignancies (105). While side effects could potentially be detrimental, the general risk-benefit evaluation could yield an overall positive outcome for a significant subgroup of patients. In a follow-up of 2 years after application of anti-CD7 CAR-T cells, Tan et al. observed durable efficacy but serious adverse events and potential disease relapse (106). Li et al. have recently highlighted the potential role of anti-CD7 CAR-T cells for bridging patients towards allo-HSCT (107).
In conclusion, anti-CD7 CAR-T-cell therapy remains the most promising approach to T-cell malignancies. So far, clinical trials with this target have shown potential regarding PFS and OS. However, other targets such as TRBC1/2, CD30, and specific targets for subgroups like CTCL and PTCL could provide potential benefits for patients.
3 Acute myeloid leukemia
3.1 Potential targets
Acute myeloid leukemia (AML) comprises a group of acute hematologic malignancies that arise from myeloid precursor cells and is often associated with a variety of genetic aberrations. As AML is the second most common type of leukemia in adults, there are numerous approaches to make this type of disease feasible to CAR-T-cell therapy (108, 109). Previous studies using CAR-T-cell therapy in AML patients have shown that the technique could prove a potentially valid strategy for patients with relapsed or refractory disease (110–114), a patient group that currently has only very limited therapy options.
Potential target antigens for CAR-T cells in AML include CD7 (110, 115), CD33/Siglec-3 (116, 117), CD38 (67, 111), CD41 (118), CD44 (119), CD64 (120), CD70 (121–123), CD117 (124), CD123 (125), CLL-1 (112, 114, 126, 127), B7-H3 (128), PR1 (129), FLT3 (130–132), IL1-RAP (133, 134), Siglec-6 (135), NKG2D (136), PRAME (137), and GRP78 (138). As it has recently been shown to be associated with the highly aggressive subcategory AML-MR, CD5 is another potential target of CAR-T cells (139). Similarly, CD36 is being investigated as a central driver of dissemination, disease progression, and relapse in AML patients and association with an unfavorable disease prognosis (140). In a high-precision approach, Giannakopoulou et al. have recently shown the possibility of targeting a single driver mutation (D835Y) in FLT3. This lead to the successful elimination of both CD34+ and CD34- AML cells in mice through a mutation-specific TCR (141).
Other studies investigate bi-specific or dual CAR-T cells targeting CD13/TIM3 (142), CD123/FR-β (143), IL3-zetakine/CD33 (144), CD123/NKG2DLs (145), and FLT3scFv/NKG2D (113). These multi-targeted CAR-T-cell therapies could help to increase the specificity of CAR-T cells towards leukemic cells, limiting on-target-off-tumor effects and thereby enhance safety and efficacy for patients (146). Targeting multiple targets, especially those with low surface expression, can control tumor growth even in genetically heterogenous AML cases (147). Alberti et al. have recently proposed the dual targeting of stromal and non-stromal targets by CAR-T cells, investigating CD33/CD146 cytokine-induced killer cells (CIKs) (148). However, stromal syntenin appears to be downregulated in AML, potentially enhancing AML cell survival and increasing translational activity (149).
Kim et al. have also shown that the genetic inactivation of potential CAR-T-cell targets like CD33 on healthy hematopoietic stem cells might reduce the risk of bone marrow suppression (150). Studies investigating the role of CD33 have found this protein to be a non-vital marker of myeloid cells and its depletion does not hamper development and function of cells (151). Further studies underlined the role of CD33 on malignant AML cells by correlating its presence to clinically unfavorable outcomes and parameters (152). The presence of AML fusion-genes in CD33- cells might be the reason for relapses after CD33-targeted AML therapy and necessitates the highly-precise engineering of CARs targeting this structure (153). This need is being addressed in current clinical trials through systematic preclinical structural evaluations (154). These evaluations also consider known modes of resistance to CD33-targeted therapy, including CD33-gene polymorphisms and upregulation of downstream pathways (155).
In another promising approach, Hino et al. have investigated the complex crosstalk between CAR-T cells and thymoid tissue in AML. The authors hypothesized a potential enhancement of patient’s endogenous anti-tumor capacities through elimination of tumor-antigen carrying APCs. Additionally, the thymus plays a central role in the development of the T-cell repertoire and could therefore be integral to the TME and success of immunotherapies for AML (156). Future research could investigate if the thymus is another possible focus of action to enhance the functionality of CAR-T-cell therapies.
The search for leukemia-specific target antigens remains a central challenge in the efforts to design CAR-T cells for AML therapy. While some targets are chosen because of their functional relevance to AML pathogenesis (CD5, CD33, CD36), others are of particular interest due to their relatively upregulated expression on AML cells or potency in pre-clinical trials (CD44, CD64, CD123).
3.2 Overcoming immunosuppression in AML
To transfer the success of currently available CAR-T-cell therapies, researchers are focusing on a diverse range of approaches to enhance the immune response towards AML cells. Major obstacles towards this goal are summarized in Figure 2.
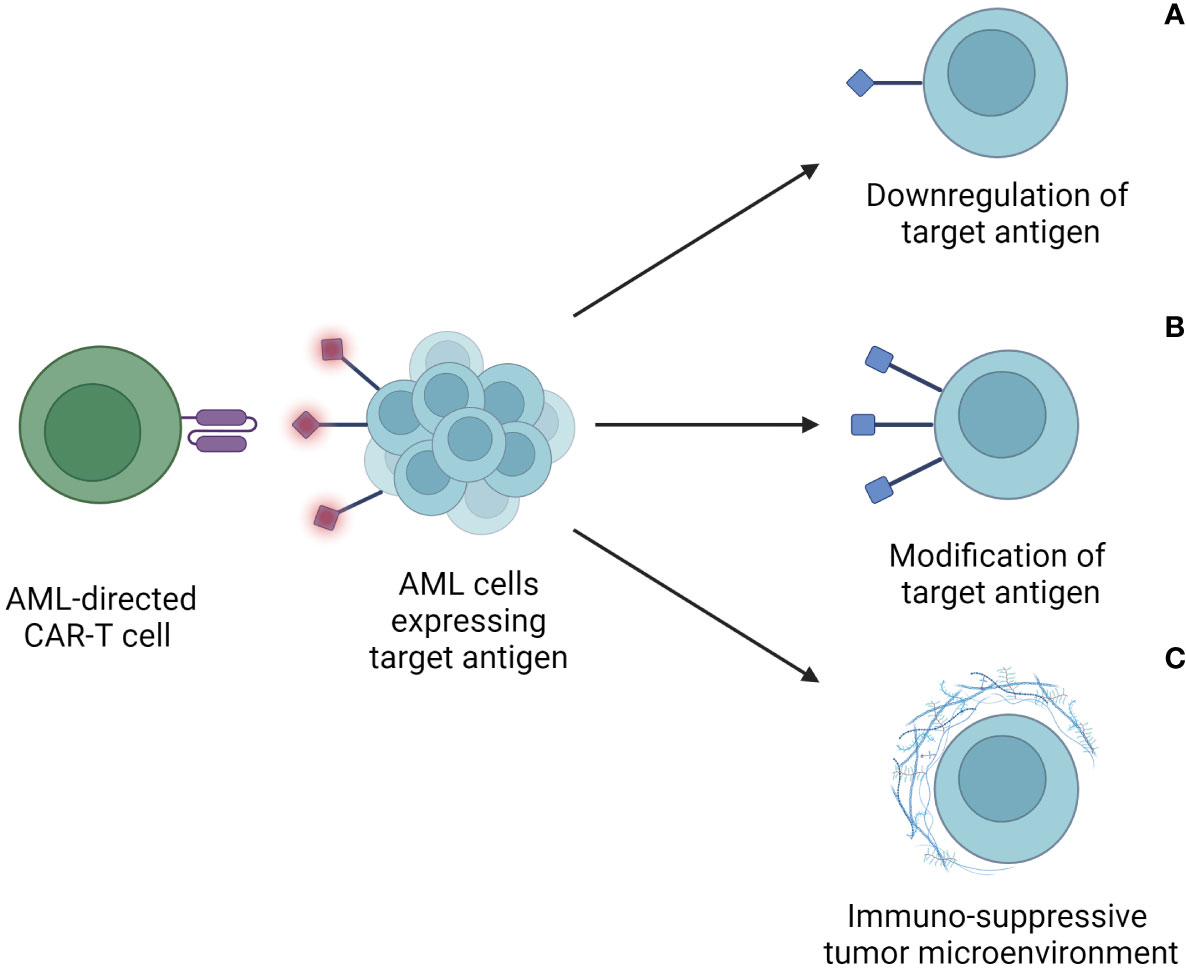
Figure 2 Escape mechanisms of AML cells after CAR-T-cell therapy. These include downregulation or loss of target antigen expression on tumor cells (A), modification of the target antigen to escape recognition and binding by the CAR-T cell (B), and an immunosuppressive tumor microenvironment (TME) (C). Through these mechanisms, AML cells avoid detection and lysis through CAR-T cells, thus limiting the therapeutic efficacy of CAR-T cells.
One of the most prominent challenges is the limited persistence of CAR-T cells in vivo and decreasing anti-tumoral effects over time with resulting disease relapse (157). Relapse can occur either with antigen-positive AML cells or accompanied by the phenomenon of antigen-loss. An important mechanism leading to antigen-loss is the selection of antigen-negative malignant clones through homozygous mutations of the B-cell receptor complex, splicing or target mutations. Newly developed, highly affine CAR-T cells have the ability to target even low antigen-expressing cells (158) and overcome epitope masking. The application of these T cells could help to target AML cells in patients after allo-HSCT with relapsed disease due to immune escape (159).
To further enhance CAR-T-cell efficacy in AML, An et al. and Leick et al. have shown positive effects of other fine-tuning approaches of CAR-T cells like the targeted down-regulation of PI3K-δ (160) and the design of a non-cleavable hinge region (161). Current studies also underline the potential use of unconventional T cells like invariant natural killer T cells and γδ-T cells, since they can function without HLA-signaling and possess natural anti-tumoral activity (162).
Naturally occurring mutations in target regions and molecular resistance mechanisms as well as an immuno-suppressive, leukemia-specific TME are further obstacles in effective CAR-T-cell therapy for AML (119, 163). As CAR-T-cell therapy is currently mostly investigated as a salvage therapy in relapsed or refractory disease, decreased T-cell fitness could play a role in the performance of CAR-T cells. In accordance with this, Vadakekolathu et al. have recently highlighted T-cell exhaustion and senescence as well as unique cellular signaling processes and chemokines as central components of this immunosuppressive microenvironment (164). This is supported by recent investigations into the role of ferroptosis signatures that highlighted their role in the TME and T-cell function (165). The effects of a functional T-cell immune response in AML patients on therapy efficacy (166), as well as clinical (167) and laboratory parameters (168, 169) are known.
3.3 Approaches to increase CAR-T specificity
Another obstacle of adoptive cell therapy in AML is the insufficient discrimination between healthy and malignant myeloid cells through specific antigens. This can lead to severe neutropenia and life-threatening infections due to myelosuppression (170). To overcome this, innovative techniques aim to increase the CAR-T-cell specificity in targeting malignant myeloid cells and therefore reduce on-target-off-tumor effects. Furthermore, researchers aim to increase the chance of long-term persistence of immunological tumor control.
To achieve these goals, researchers are employing multiple methods. These include suicide switches (anti-CD20 agents against CAR-T cells carrying CD20), logic gating (typically AND, NOT, and OR) to improve target recognition, and the genetic engineering of hematopoietic stem cells (HSCs) to reduce target antigen expression on their surface (171). Specific logic gating strategies have been proposed for anti-CD93 CAR-T cells (172) and an IF-BETTER gate for the targeting of ADGRE2 and CLEC12A (173).
So far, progress has been made in epitope-engineering with the altering of CD123 expressed on HSCs while maintaining their functional capabilities (174). Similarly, Casirati et al. have shown success in engineering epitopes of FLT3, CD123 and KIT and introducing these into CD34+ stem and progenitor cells resulting in the maintenance of functional hematopoiesis and the eradication of AML cells (175).. Another approach to sustain hematopoiesis is the targeting of leukemia-specific signaling pathways like the newly discovered IL33-ST2 interaction on myeloid leukemic stem cells (176). Nelde et al. have recently investigated non-mutated and neo-epitopes of common driver mutations of NPM1 and IDH2 shared between AML cells and leukemic progenitor and stem cells. This study further emphasizes the possible functional relevance of these epitopes and their association with clinical outcomes (177).
3.4 Novel CAR-T platforms
From a clinical perspective, the management of adverse events and therapy-associated risks is possibly more difficult due to the high number of older AML patients. This could intensify several adverse events including CRS, off-target-effects, and the risks associated with myelosuppression such as life-threatening infections (178).
To mitigate these risks, “suicide genes” have been implemented into CAR-T cells. This approach aims to control the infused suicide gene-modified CAR-T cells in vivo through its activation with a nontherapeutic agent like rapamycin (179). Constructs like this can lead to accelerated recovery of the patient’s immune system after CAR-T-cell therapy as demonstrated for anti-FLT3 CAR-T cells (180).
Wemke et al. have reported on first-in-human proof-of-concept for rapidly switchable anti-CD123 CAR-T cells based on the universal chimeric antigen receptor platform (UniCAR) that is currently further investigated in a phase Ia trial. This platform could allow for the sequential or parallel targeting of multiple target antigens. So far, all three patients who have gone through the treatment protocol in this study have experienced partial or complete remission without dose-limiting toxicities (125). CAR-constructs based on the UniCAR platform can be rapidly switched off upon the occurrence of adverse events by withholding the antigen targeting module. Ehninger et al. have shown the success of this approach for the handling of ICANS after administration of anti-CD123 CAR-T-cell therapy (181). These results are further supported by the reports of Peschke et al. who have developed an anti-FLT3 UniCAR-T-cell product. They observed killing of AML cells in vitro and in vivo in a murine xenograft model (182).
Nixdorf et al. have recently investigated the approach of using the AdCAR platform to specifically target AML cells using three adapter molecules targeting different antigens (CD33, CD123 and CLL-1). In these ex vivo experiments, AdCAR-T cells could be applied, targeted at one antigen, and later on re-targeted towards another, achieving high anti-tumor activity (183). This approach could address the problem of therapy-induced escape variants and target downregulation on AML cells. Using the UniCAR or AdCAR platforms, it could be possible to improve the management of CAR-T-cell induced adverse events like CRS and ICANS by limiting the exposure of CAR-T cells to their target antigen.
Overall, engineering CAR-T cells with mechanisms to reduce their activity after infusion appears as a central innovation towards making them a safer and more reliable therapeutic option. Novel CAR-T platforms provide the basis for innovative approaches, multi-targeting, and can help to address escape mechanisms and adverse clinical events.
3.5 Combinatorial regimens
Another field of investigation is the combination of CAR-T cells with established therapeutics like rapamycin (184), DNA hypomethylating agents like azacytidine (185) or decitabine (186, 187), and anti-PD-1 antibody therapy (166). Combinations of novel CAR-T-cell products with agents like chidamide and decitabine have proven superior to mono-therapy in vitro (122).
Using CAR-T cells to “bridge” the time towards allo-HSCT or in patients ineligible for allo-HSCT could help enhancing their usability in AML patients (188, 189). Anti-CD83 CAR-T cells even have the potential to be used in GvHD or AML relapse after allo-HSCT (190). Similarly, the combination with other pharmaceuticals might prove to be another therapeutic pathway (191, 192). However, major concerns against using CAR-T cells as a salvage therapy in AML relapse after HSCT include the occurrence of cytopenia and the risk of GvHD as well as limited efficacy (193). This may be due to increased T-cell exhaustion phenotype and a depletion in absolute T-cell numbers as hypothesized by Jia et al. using a newly developed Graft-versus-Leukemia (GvL) model in AML. The authors highlight the possibility of enhancing T-cell function in this model through the blockage of T-cell-inhibitory pathways (194).
As has been shown, valproic acid has the capacity to increase the cytotoxicity of anti-CD123 and anti-CLL-1 CAR-T cells against AML cells in mice (195). Cummins and Gill have recently highlighted the proposed advantages of either increasing antigen expression through combinatorial treatment or investigation of possible dose escalation schemes of the CAR-T-cell therapy (196).
3.6 Outlook
Despite disappointing progress in the field of immunotherapeutic approaches in AML (197), the implementation of proteomics (198) and transcriptomics (199) in designing a more personalized CAR-T-cell therapies holds promises. Single-cell sequencing offers the possibility to investigate mutational changes across the DNA, epigenetic and protein level (200). A recent review by Shahzad et al. has investigated 10 clinical trials and 3 case reports, overall calculating the response rate of patients with relapsed or refractory AML to CAR-T-cell therapy at 49.5% (201).
Finally, novel production pipelines of CAR-T cells like piggyBac-transposon-based technologies offer the possibility of cost reduction and shortening of production cycles (202). Approaches combining such transposon-based delivery systems with CRISPR technologies have the potential to facilitate allogeneic CAR-T-cell therapy through depletion of HLA-I and the TCR on donor cells (203). It should be noted that the induction of mutations and limited specificity restrict the utility of gene editing technology (204).
4 Chronic myeloid leukemia
4.1 Current state of treatment
Chronic myeloid leukemia (CML) is a disease with malignant transformation of myeloid precursor cells similar to AML. Unlike in AML, targeted therapies have significantly improved the treatment outcomes for CML using inhibitors of tyrosine kinases (TKI) – enzymes that are pathologically activated in most of the CML cases (205). Many patients can be treated safely and effectively with these oral inhibitors. Severe cases with progression into the acceleration phase or a potentially lethal blast crisis are relatively rare. Under optimal therapy and comprehensive management of comorbidities, patients can achieve a close to normal life expectancy (206).
However, there are patients that cannot be treated with TKIs, experience insufficient therapy success or excessive side effects (207). The current ultima ratio for CML patients is an allo-HSCT, which makes use of the highly advantageous GvL effect. Adoptive cell therapies could be employed as a salvage option for patients with relapsing disease even after allo-HSCT (208, 209) or long-time remission after TKI therapy (210).
4.2 Potential targets
In the past years, CD19 (211, 212), CD26 (213), CD38 (214), and IL-1-Receptor-associated Protein (IL-1-RAP) (215) have been investigated as potential target antigens for a CAR-T-cell therapy approach in CML. IL-1-RAP is of pronounced interest as its expression correlates with the formation of the Philadelphia chromosome in CML cells (216) and is often expressed in increased levels on malignant hematopoietic cells (217). CAR-T cells targeting IL-1-RAP can be produced semi-automatically and GMP-compliant since 2023 (218). In 2021, a digital droplet PCR (ddPCR)-based method was established to monitor both anti-CD19 and anti-IL-1-RAP CAR-T cells (219) to accurately assess their presence in vivo.
4.3 Outlook
Zhang et al. have shown that the combination of anti-CD19 CAR-T-cell therapy with the TKI dasatinib can potentially provide a curative therapy option even in the phenomenon of multilinear disease with several lines of mutated cells present (220). While several studies have shown substantial success in middle- to long-term follow-up of treated patients, the current research landscape on CAR-T cells focuses on other malignancies due to the success of TKI therapy (221).
Future studies have the potential to establish CAR-T-cell therapy as a valid and even potentially curative treatment option for CML through the targeting of promising antigens like IL-1-RAP. Especially patients refractory towards TKI therapy or after progression to acceleration phase/blast crisis can profit from this novel approach (222). Importantly, Jiang et al. have recently shown the preserved functional capacity of CD4+ T cells in CML patients, suggesting that this subgroup could be suitable for the generation of CAR-T cells (223). Additionally, success in implementing novel technologies like streamlined production processes and digital PCR allow for an easier implementation of CAR-T cells into clinical practice.
5 Chronic lymphocytic leukemia
5.1 Current state of treatment
Chronic lymphocytic leukemia (CLL) is a disease that primarily affects patients over the age of 65 years and is the most common type of Non-Hodgkin Lymphoma (NHL) in the western hemisphere (224). Today, there are numerous treatment options available for patients including monoclonal antibodies like rituximab and obinutuzumuab (anti-CD20), the BCL-2 inhibitor venetoclax, and BTK inhibitors (i.e. ibrutinib, acalabrutinib). Due to these available and well-established therapeutics with very high efficacy, research in CAR-T-cell therapy in CLL lacks behind that in other hematological malignancies of comparable clinical relevance (225).
Still, many patients develop a resistance towards established therapeutics (226). Disease progression into a highly malignant NHL (“Richter transformation”) remains a major issue (227) despite significant progress in the understanding of its genetics and mechanisms (228). Early results of phase I/II trials indicate a possible role for CAR-T cells in prevention or treatment of Richter transformation (229, 230), but highlight the importance of further research in this approach (231). Research has further identified IL-10, IL-6 and reduced levels of CD27+/CD45RO-/CD8+ T cells as potential biomarkers for refractoriness to immunotherapy (232). In contrast, IL-27 has been shown to boost CD8+ T-cell anti-tumor activity against CLL and is decreased in the peripheral blood of CLL patients (233). MALAT1 expression has recently been found to be associated with an aggressive course of disease in CLL and may hint towards previously not understood mechanisms of disease (234).
5.2 Overcoming resistance mechanisms
A challenging aspect of immunotherapy in CLL is a pathologically reduced immunocompetence. Main reasons for this include an immuno-suppressive microenvironment generated by malignant cells and systemic extracellular vesicles (235), changes in immune-synaptic signaling (236), and disruptions in T-cell metabolism (237). Additionally, Treg cells seem to disturb the expression of CD62L and IL-4R on neutrophils, reducing their natural immunological capacities in CLL models (238).
T cells of CLL patients frequently display an exhausted phenotype and show increased expression of PD-1, CTLA-4, TIGIT, CD160, and CD244 (239). Agarwal et al. have recently demonstrated how the selective deletion of CTLA4 can enable CD28 signaling in CAR-T cells targeting CD19 in CLL (240). Epigenetic studies have highlighted changes in the profile of cytokine secretion, reduced cytotoxic capacities, and exhaustion as factors leading to reduced T-cell function in CLL (241). Possible solutions include the application of allogeneic CAR-T cells that are not affected by these defects or the combination with modulators of epigenetic reading (242).
T-cell-focused studies have further indicated the CLL-associated depletion of polyfunctional CD26+ T cells, which represent another possible target for adoptive cell therapy (243) and unique subsets and transcriptional signatures of T cells in CLL patients (244). These pathological mechanisms can disrupt the efficacy of CAR-T cells as well as their long-term establishment in the recipient (Figure 3) (245).
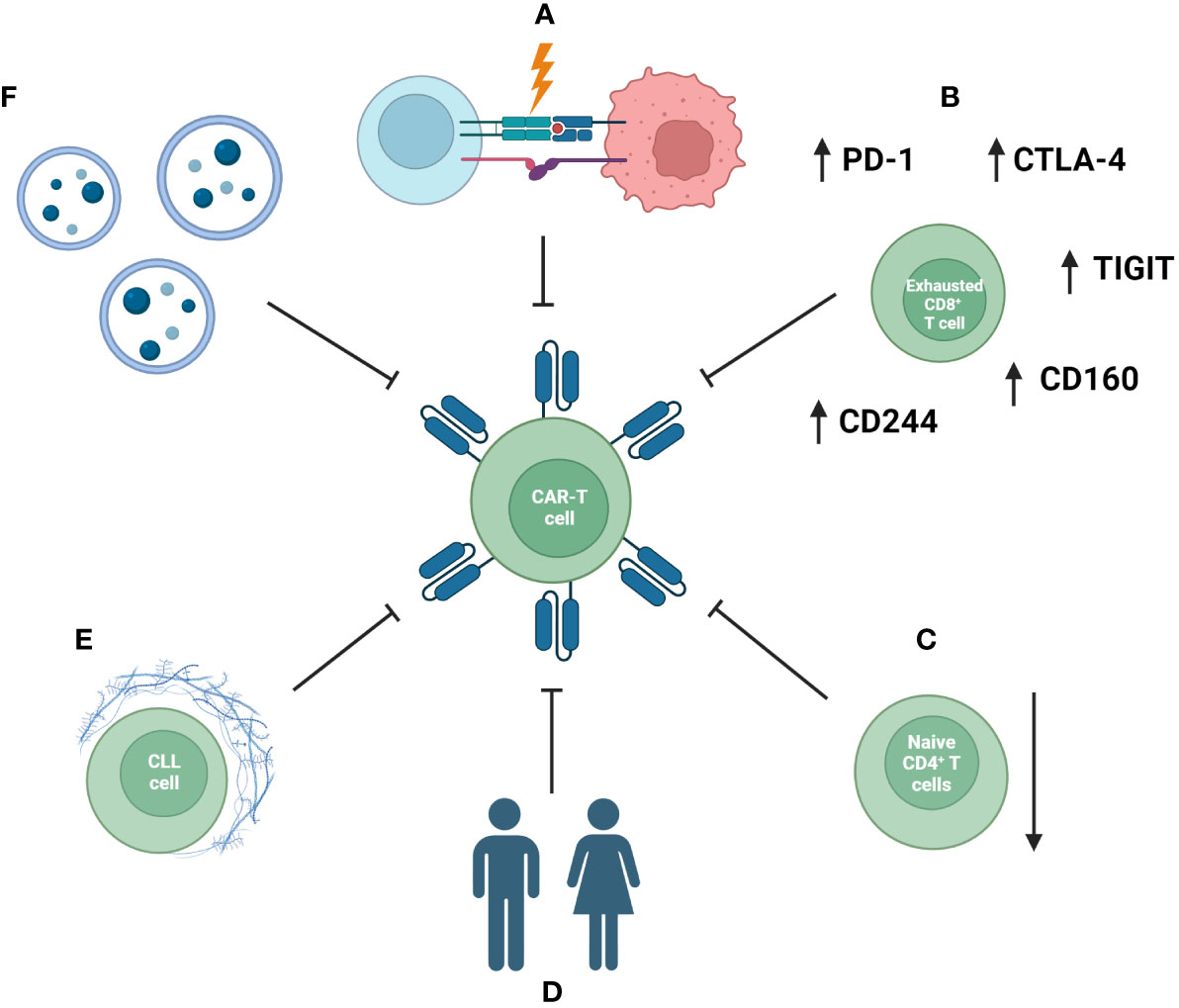
Figure 3 Mechanisms impacting the efficacy of CAR-T-cell therapy in CLL patients. These include disruption of formation of the immunological synapse and communication between an immune cell and a tumor cell (A), exhausted phenotype of CAR-T cells (B) and a reduced naïve compartment of T cells (C). Additionally, patient-specific factors and comorbidities (D), an immunosuppressive tumor microenvironment (E), as well as systemic extracellular vesicles (EVs) inducing exhaustion phenotype CAR-T cells with reduced anti-tumor efficacy (F) are important factors.
Specific T cells like Vγ9Vδ2 cells have been identified as a central player in the TME and γδ-T cells can be used as carriers of anti-CD19 CARs (246–248). Investigations into different ways of cultivating Vγ9Vδ2 cells and using them as cytotoxic agents are showing promising results (249). Engineered γδ-T cells have further been shown to impact the TME and potentially show lower rates of on-target-off-tumor effects (250). Donor-derived γδ-T cells could be another therapeutic option as they exhibit natural anti-tumor activity (251). Another aspect of fine-tuning γδ-T cells for antitumor use includes the specific expression of chemokines like IL-15 to increase longevity and tumor control (252).
Recently, receptors of the SLAM-family have been investigated as central players of disease progression. So far, SLAMF3 and SLAMF7 are in focus of CAR-T-cell research. However, these approaches have been limited by fratricide induced by SLAMF-targeting CAR-T cells (253).
5.3 Previous studies
CAR-T-cell therapy has been employed since 2011 for CLL patients (254). While the first CAR-T-cell treated CLL patients have shown both comprehensive as well as sustained responses (255, 256), this is not the case for all patients. Using a CD19-targeting CAR-T-cell therapy (lisocabtagene maraleucel, initially approved for DLBCL, PMBCL and FL), 45% of patients after multiple disease relapses achieved a complete remission (257). In 2020, Cappell et al. demonstrated a duration of response of over 3 years for 50% of CLL patients treated with anti-CD19 CAR-T-cell therapy with a limited prevalence of serious adverse events and a median event free survival of 40.5 months (258).
Recently, Siddiqi et al. have reported a phase I/II study investigating lisocabtagene maraleucel in 117 patients who had previously experienced BTK-inhibitor therapy failure. The investigators observed a complete response rate of 18% with 9% of patients experiencing grade 3 CRS and 18% experiencing grade 3 neurological events. Overall, within 90 days after CAR-T-cell infusion 5 treatment-emergent deaths were reported (259).
Detailed investigations into the kinetics of CAR-T-cell persistence have shown correlations between the numbers of CAR-T cells present in the peripheral blood and adverse events like the occurrence of CRS (260). In line with these findings, patients treated with anti-CD19 CAR-T-cell therapy for CLL have experienced side effects like bacterial, viral, and fungal infections due to myelosuppression similar to patients suffering from other hematological malignancies (261). Through the comprehensive analysis of 47 patients with CLL or Richter transformation receiving anti-CD19 CAR-T-cell therapy, Liang et al. have determined several factors associated with longer PFS. These include peak CD4+ and CD8+ CAR-T-cell expansion, MRD negativity and CAR-T-cell persistence (262).
Other studies underline the complex immune mechanisms influencing the efficacy, survival and functionality of CAR-T cells (263). Until today, CD19 is still the most comprehensively investigated target antigen for CAR-T-cell therapies directed against CLL (264). However, there have also been promising results with the use of CARs targeting malignancy-associated κ-light chains (265), CD32b (266), the Fc μ receptor (267), and Siglec-6 (268) in CLL patients. Luo, Qie et al. have recently reported the success of BAFF-R-targeted CAR-T cells even in CD19- cell lines (269).
5.4 Outlook
Currently, CAR-T-cells are being investigated as part of an integrated therapeutic algorithm including established lines of therapy to maximize the percentage of patients being treated optimally (270). Studies have shown favorable results for the implementation of CAR-T cells into the therapeutic repertoire for CLL through combination with PI3K-γ-δ inhibitors (idelalisib) (271, 272), ibrutinib (273, 274), and lenalidomide (275). The sequential application of BTK inhibitors and CAR-T cells seems to provide synergistic effects in CLL treatment (276).
The combination of fludarabine and cyclophosphamide prior to anti-CD19 CAR-T-cell infusion has been shown to improve CAR-T-cell functionality and clinical outcomes, a phenomenon also seen in other hematological malignancies (277). Studies suggest favorable effects of these combinations on severe CAR-T-cell associated side effects like CRS (278). Several advantages set CAR-T-cell therapy apart from options like allo-HSCT: possibly milder profile of adverse events, shorter duration of treatment, and the prospect of long-term CAR-T-cell persistence and therefore disease control (279).
However, there is still a multitude of factors keeping CAR-T-cell therapy from becoming a widely available and reliably applicable therapy for CLL (280, 281). CAR-T-cell therapy could prove especially viable for patients suffering from refractory or relapsed disease, as well as those developing secondary malignancies or suffering from intense symptoms (282). In a subset of patients, CAR-T-cell therapy can offer a potential way to disease eradication (283). Additionally, recent approaches like the killing of CLL cells through targeting of the Lck-IP3R protein-protein interaction hint towards other innovative methods of immunotherapy targeting in CLL (284).
6 Classical Hodgkin-Lymphoma
6.1 Current state of treatment
Classical Hodgkin-Lymphoma (HL) is considered a highly curable disease today. The majority of patients achieve deep and long-lasting complete remission with standard therapy (285). However, cases of refractory or relapsing disease still pose an important problem (286). Furthermore, treatment-related toxicities and side effects, especially in individuals over the age of 60 years, still prove life-threatening and therapy-limiting.
6.2 CD30 and the TME in HL
CAR-T-cell investigations in HL patients mainly focus on CD30 as a target antigen (287) due to the abundant expression on malignant Hodgkin Reed-Sternberg cells and malignant B cells (288, 289). Previously, this expression has successfully been exploited through the antibody-drug conjugate (ADC) brentuximab–vedotin (BV) targeting CD30 (290). CD30 plays an important pathophysiological role in cell-morphology and chromosomal instability (291). First phase I and II trials utilizing anti-CD30 CAR-T cells showed substantial success in heavily pre-treated patients with an overall response rate of 39-72% and mostly tolerable side effects (292, 293).
Since these initial clinical trials, the RELY-30 study has shown further improvements in the safety profile and efficacy of anti-CD30 CAR-T-cell therapy but also limited durability of responses with 36% 1-year PFS and additional relapses occurring after this point of time (294). Kim et al. have also reported a decrease or loss of CD30 expression in relapse after targeting through different modes of immunotherapy (295).
Since recent research has highlighted the importance of the TME in HL, it has become a target of CAR-T-cell therapy approaches (296, 297). Studies have investigated CAR-T-cell-mediated targeting of CD19 (298) and CD123 (299) to influence the TME of HL cells and induce a long-lasting immune response to lymphoma cells. These substances could potentially be combined with anti-CD30 CAR-T cells to achieve synergistic effects (300). Such an effect has been shown in vitro for the combined targeting of CD30 and CCR4 (301). The varying persistence of CD30 expression on malignant cells could mean that antigenic escape is unlikely to occur in CAR-T-cell therapy (302). Like CD30, the expression and signaling of PD-1 and PD-L1 is highly characteristic for Hodgkin Reed-Sternberg cells and could prove a valuable target in HL patients (303).
6.3 Outlook
Since CD30 has already been shown to be a potentially targetable structure, CAR-T-cell research for HL focuses on optimization of lymphodepletion regimens (304), improvement and high-precision engineering of CAR-T cells (305, 306), and the development of combinatorial regimens (307) with agents like PD-1 inhibitors (308) or allo-HSCT (309). Thereby, CAR-T-cell therapy can provide a path for refractory patients after BV and checkpoint-inhibitor therapy who lack clear and defined therapy options in current guidelines (310). Recent results from the CHARIOT trial have shown a generally safe side effect profile for CAR-T-cell therapy in a cohort of heavily pretreated HL patients (311).
More clinical trials are needed, but anti-CD30 CAR-T-cell therapy could prove to be a new tool in the treatment arsenal in HL. It can provide an option for patients insufficiently responding to conventional therapy (312). Reduction of toxicities, optimization of CAR-design and combination regimens with other therapeutic agents are further areas of promising research (313). The integration of technologies like mass cytometry, single-cell RNA sequencing, and monitoring of circulating tumor DNA promise more detailed insights into the pathophysiology of HL and potential new molecular targets (314).
7 Burkitt-Lymphoma
7.1 Current state of treatment
Burkitt-Lymphoma (BL) is a highly aggressive NHL and commonly found in children and adolescents. BL cases are categorized accordingly to their etiology into endemic, sporadic, and immunodeficiency-associated (315). Long-term remission through high-intensity chemotherapy is achieved in over 90% of pediatric but only 75-85% of adult patients (316).
The application of intense chemotherapy regimens in adult patients is especially limited by the occurrence of toxic side-effects. Treatment failure among adult patients is common and occurs in up to 35% of patients (3-year PFS of 64%) (317). Thus, new and comprehensive treatment options for refractory and relapsed disease as well as for special patient cohorts are needed.
7.2 Current research
Case reports have shown considerable success in the treatment of relapsed and refractory BL cases (318–327), mostly utilizing CAR-T-cell therapies targeting CD19. These reports include both adult and pediatric BL cases and show substantial therapeutic responses in both patient groups. Despite this success, a recent case series by Geerts et al. has also shown a case of BL disease progression and patient death despite objective CAR-T-cell expansion in vivo and adequate management of side effects (CRS grade 2) (328). A recent case report has highlighted the possibility of using unedited HLA-matched allogeneic CAR-T-cells, in this case directed against CD20 and CD22, for the treatment of BL. Due to persistent pancytopenia, the patient also received an allo-HSCT from the same donor 55 days after application of the CAR-T-cell therapy (329).
Hsieh and Rouce have comprehensively compiled three major targets of research to make CAR-T-cell therapy usable in Burkitt-Lymphoma and other pediatric hematologic malignancies: Tackling the immunosuppressive TME, antigen escape mechanisms, and optimizing CAR-T-cell efficacy and functionality (330). This was further refined by the ACCELERATE study group to resistance mechanisms, best tumor targets, possibilities of double-/triple-targeting, and the evaluation of CAR-T-cell therapy in the context of T-cell engagers, ADCs, and autologous HSCT (Figure 4) (331).
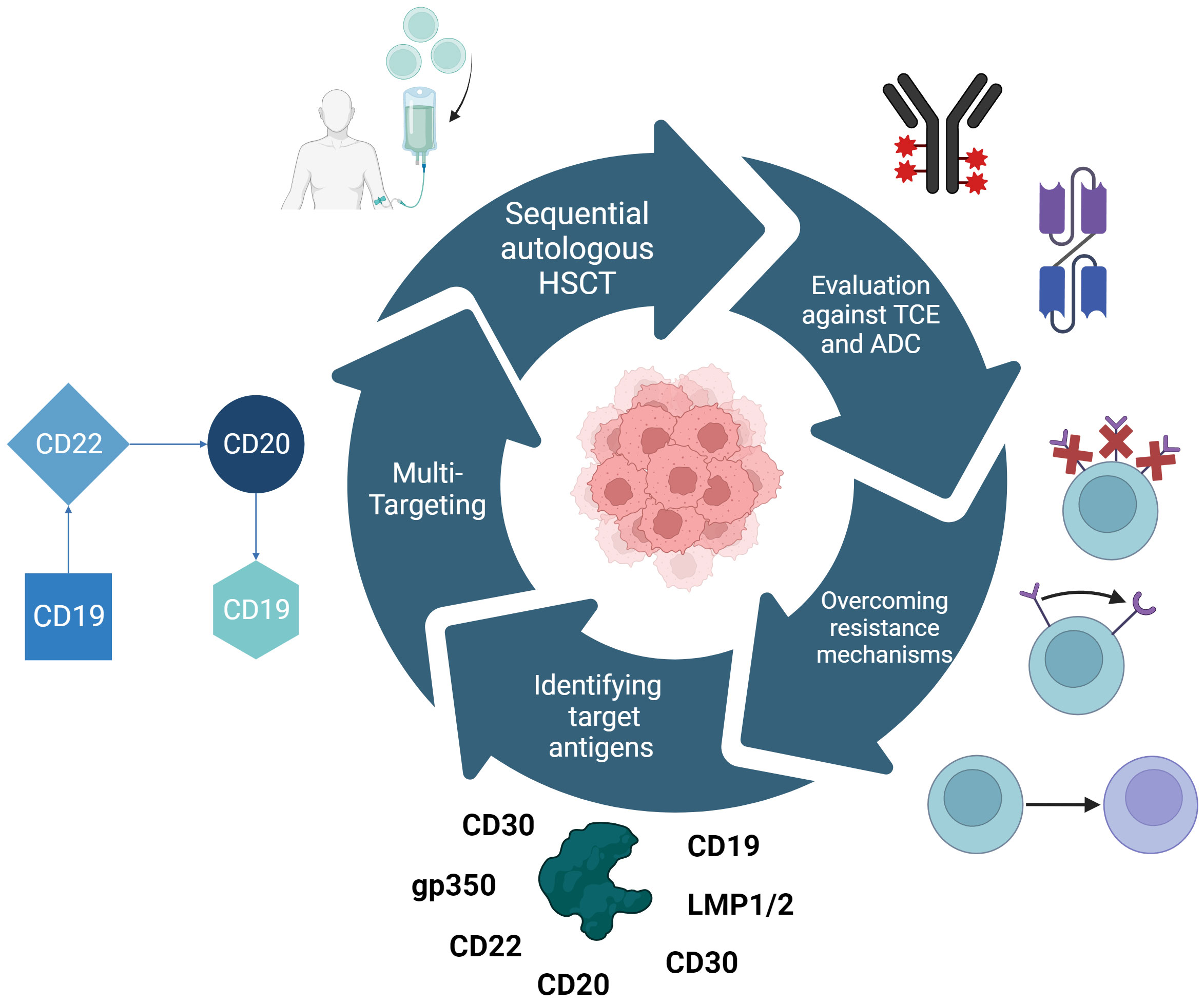
Figure 4 Research approaches for making CAR-T-cell therapy useable for Burkitt lymphoma as defined by the ACCELERATE study group. These are the identification of novel target antigens and the repurposing of those already in use for other hematologic malignancies, multi-targeting CAR therapy to prevent antigen escape as currently employed with CD19, CD20, and CD22. Furthermore, CAR-T-cell therapy needs to be evaluated in the context of combinational therapies e.g. sequential autologous HSCT, T-cell engagers (TCEs), and antibody-drug conjugates (ADCs). Challenging resistance mechanisms that need to be overcome include the downregulation of targeted antigens, structural changes in these antigens or the switch of myeloid linages.
To tackle these challenges, Laurent et al. have employed high-resolution investigations into the phenotype of post-CAR-T-cell therapy cancers and have shown a highly variable decrease of one or more B-cell markers in relapsed patients (332). These findings indicate extensive genetic changes, remodeling and acquired mutations in PI3K- and KRAS-pathways, leading to an impaired B-cell differentiation.
7.3 Combinatorial regimens
In line with these laboratory findings, Du et al. reported promising outcomes of a pediatric patient treated sequentially with anti-CD19, -CD22 and -CD20 CAR-T cells (319). This was expanded upon by Zhang et al. in a clinical trial with 5 pediatric patients each achieving complete remission through the sequential application of anti-CD19, anti-CD22 and anti-CD20 CAR-T cells, depending on histological tumor staining analyses (333). In the largest trial of its kind in 2022, Liu et al. treated 23 patients with up to 4 cycles of CAR-T-cell-therapy: 23 with anti-CD19, 13 of those with anti-CD22, 6 of those with anti-CD20, and 1 patient with a second anti-CD19 CAR-T-cell therapy (334). Over the course of the four therapy cycles, 18 of 23 patients achieved complete remission and 4 patients died due to rapid disease progression or CNS involvement with intracranial mass progression.
Further studies have investigated the combination of anti-CD19/CD22 CAR-T-cell therapy with allo-HSCT (335) and showed an overall survival rate of 55.6% in a cohort of heavily pretreated r/r BL patients. The investigators highlighted the beneficial effects of early initiation of CAR-T-cell therapy in combination with allo-HSCT. There is evidence that CAR-T-cell therapy could also play a valuable role in bridging BL patients towards allo-HSCT (336). Conversely, established therapeutic approaches like radiation therapy are also investigated as possible bridges towards CAR-T-cell therapy with the goal of reducing tumor load in high-risk patients (337).
Like in other hematological malignancies, the combination of established therapeutic agents with CAR-T-cell therapies is being investigated in BL patients. This includes the histone deacetylase inhibitor romidepsin that induced BL cell death in vitro and in vivo mouse models when combined with anti-CD20 CAR-T cells (338).
7.4 Outlook
Translational approaches in CAR-T-cell design also include targeting of EBV-associated structures like the abundantly found gp350, as EBV-infection is frequently associated with BL (339). In vivo studies in mouse models have so far shown limited success in reducing EBV DNA load, tumor development and growth, and inflammation parameters (340, 341). Further CAR-T-cell targets to combat EBV in lymphoma tissue include latent membrane proteins LMP1 and LMP2 (340), and Gb3 (342).
In conclusion, Burkitt-Lymphoma appears to be a promising target for CAR-T-cell therapy. Especially the availability of CD30 as a potential target, the possibility of employing sequential regimens of CAR-T cells targeting different B-cell markers and substantial response rates in initial clinical trials may provide hope for the successful treatment of patients with relapsed or refractory disease. Future research could offer the possibility of establishing CAR-T-cell therapy in the context of other established therapeutics, and larger and more diverse patient cohorts.
8 Hairy cell leukemia
8.1 Current state of treatment
Hairy cell leukemia (HCL) is an uncommon type of B-cell NHL. It rarely presents in a prolymphocytic, more aggressive variant known as HCL-v. Both diseases can be controlled through established therapy regimens including cladribine and pentostatine as first-line therapies. More than 95% of patients can be treated adequately with these therapies (343).
However, nearly 50% of all patients encounter disease relapse within 10 years, often being unable to undergo first-line treatment again due to novel mutations that induce resistance and the need for more intensive therapeutic approaches (344). Currently, proposed therapeutic options for r/r HCL include BRAF-inhibitors, BTK-inhibitors and immunotherapy targeting CD22 (345). Further, HCL-v patients are faced with inferior outcomes after standard therapy lines (346). HCL can also arise from Richter-transformation of CLL, a rare occurrence without defined therapeutic pathways (347). Therefore, these patient groups can potentially benefit from CAR-T-cell therapy (348).
8.2 Potential targets
A challenging aspect of CAR-T-cell therapy targeting HCL/-v is the diverse expression profile of surface antigens on malignant cells. While HCL cells usually express CD103, CD123, CD25, and CD11c abundantly, other markers that have been targeted in other CAR-T-cell trials like CD5, CD26 or CD38 are expressed very heterogeneously (349). This is an additional difficulty in differentiating HCL from other hematological malignancies and can be addressed by technologies like flow cytometry (CD5, CD200) (350, 351). Bhatti et al. have reported on 10 HCL cases with all of them expressing CD11c, CD19, CD20, CD22, and CD123 (352). HCL-v is often characterized by lower expression of CD25 and CD123 (353). Furthermore, there are reports of CD10+/- biclonality (354), CD103- cells (355), variable expression of CD123 on HCL-v cells (356), or cases with expression of both CD38 and CD10 (357).
Maitre et al. have recently approached this problem through a comprehensive gene expression analysis that could provide the basis for identification of novel therapeutic targets (358). Another potential target is ROR1 (359), already investigated for therapeutic use in patients with CLL, MCL, and ALL (360). Current research has also shown success in utilizing anti-CD22 immunotoxins, proving this could be another experimental target of HCL/HCL-v CAR-T-cell therapy (361).
8.3 Outlook
Overall, CD123 and more established CAR-T-cell targets like CD19/20/22 are the most promising targets for CAR-T-cell therapy in HCL (362, 363). The generally low and at best variable expression on the cells of the aggressive HCL-v is an important problem keeping CAR-T-cell therapy from being implemented for the most pressing cases of HCL. This can be addressed through comprehensive analysis of antigen expression and diagnostics, establishment of sequential therapy regimens and further research into novel HCL-specific target antigens. According to current knowledge, a full flow cytometric HCL/-v panel could include CD5, CD10, CD11c, CD19, CD20, CD22, CD25, CD26, CD38, CD103, CD123, and CD200 to comprehensively assess marker expression and individual therapy options.
9 Waldenström’s macroglobulinemia
9.1 Current state of treatment
Waldenström’s macroglobulinemia (WM), also called Lymphoplasmacytic Lymphoma, is an indolent NHL characterized by infiltration of multiple malignant lymphocytic cells (plasma cells, plasmacytoid lymphocytes, lymphocytes) into the bone marrow (364). These cells secrete monoclonal IgM and show constitutive B-cell activation signaling (365). WM is commonly treated with immune-chemotherapy or BTK-inhibitors. These therapy lines often lead to remission but either induce significant side effects (ICT) or necessitate constant therapy (BTK-inhibitors) (366).
Currently available therapies are not curative for WM and no consensus on the adequate treatment of relapsed or refractory disease exists. Main therapy regimens include toxicity- and progression-free-oriented application of differential therapy lines (367, 368). Ahmed et al. have shown how diverse factors like complex karyotype and refractoriness to multiple lines of therapy significantly impact prognosis after auto-HSCT. Patients receiving auto-HSCT still experience disease relapse in 46% of cases (369).
9.2 Potential targets
Palomba et al. have reported the treatment of three patients using anti-CD19 CAR-T-cell therapy and clinical remission in all patients but also disease recurrence 3 – 26 months after infusion (370). Another case report has shown more promising results in a patient suffering from histologically transformed disease after treatment with anti-CD19 CAR-T-cell therapy. The patient reached complete remission and remained in this state for 12 months up to the publication of the case (371).
Other investigated targets include NF-κB and MALT1. However, their usability for CAR-T-cell therapy has not yet been explored (372). CD40 is another promising target since it plays a central role in shaping the TME and has an impact on WM cell growth (373). Qiu et al. have recently proposed several distinct cell lines in WM patients with a rare entity of CD3+/CD19+ cells with “stemness” features (374). This cellular distinct and potentially crucial subgroup could be a central target to effectively combat WM since CD19 is an established target antigen of CAR-T-cell therapy in other diseases.
BCMA, the target antigen of currently approved CAR-T-cell therapies in Multiple Myeloma has also been shown to be elevated in the blood of WM patients (375). CD5 is expressed in up to 50% of WM cases and could be a CAR-T-cell target in these patients (376). Kaiser et al. have recently highlighted the role of CXCR4 in WM and its possible use as a therapeutic target (377). Additionally, CD138 has recently emerged as a potentially identifying feature of WM tumor cells, associated with IgM peaks and MYD88 mutations (378). Recent research has shown the role of nanoscale organization of CARs and TCRs for CAR-T cells targeting CD138 with yet unknown consequences (379). Future investigations can determine whether these structures are suitable targets for CAR-T-cell therapy in WM.
9.3 Outlook
Despite the limited research conducted so far in the field of CAR-T-cell therapy in WM, the success of treating CLL with anti-CD19 CAR-T cells sparked hope to translate these findings (380). This hope is supported by recent investigations, indicating that T-cell number, distribution, and functionality in WM patients are conserved, in contrast to CLL patients (381).
Still, other potentially WM-specific targets could prove to be even more suitable. Intra-tumor heterogeneity is of particular interest in WM as it increases the difficulty of defining targets sufficiently broadly expressed on tumor cells (364). Especially young patients, who might tolerate the potential side effects of a CAR-T-cell therapy better, could profit from the curative therapeutic approach (382).
10 Discussion
10.1 Common challenges and approaches
In this review, our objective was to outline the potential uses of CAR-T-cell therapy in emerging indications that extend far beyond the hematologic diseases currently approved for treatment. We have discussed the use of targets already established in different malignancies, the identification of novel targets, and innovative approaches of defining these.
In a recent review, Mishra et al. have highlighted antigen loss after CAR-T-cell therapy as a major driver of treatment failure and compiled several main reasons: Genetic alterations of antigens, epigenetic modifications (methylation), development of immunosuppressive escape mechanisms, clonal selection of antigen-negative subclones, and antigen shedding into the TME (383). Current research focuses on many of these mechanisms to enhance and sustain CAR-T-cell functionality in vivo (384).
While many of the diseases we have addressed are already approachable with currently available therapies, none of them provide comprehensive treatment options to refractory or relapsed disease status. CAR-T cells have the potential to fill this gap and provide a potential curative treatment option in many currently incurable hematologic malignancies (385).
10.2 Integration of CAR-T-cell therapy into clinical practice
While the potential side effects and costs of the technology still hinder its extensive implementation into clinical practice, these problems are being tackled and are expected to be resolved or at least substantially improved in the near future (386, 387). To make these advances available to patients and employ them for improvement of care, CAR-T-cell therapy needs to be economically feasible, inclusive, and access for all patient groups must be ensured (388–390). A recently proposed approach to these requirements is the “Cocoon Platform” by Lonza (Basel, Switzerland) (391). A distinct advantage of CAR-T-cell therapy over currently available treatment options such as tyrosine-kinase inhibitors is that patients may experience sustained long-term remissions after one single CAR-T-cell therapy and therefore are spared the burden of continuous treatment regimens (255).
Further research highlights the potential application of CAR-T-cell therapy in the context of combinatorial, bridging or sequential treatment modalities. These types of regimens have been implemented into clinical practice for a long time and promise a personalized and highly refined therapeutic approach for each individual patient. In comparison to this, several research approaches highlight the possibility of establishing multi- or “pan-leukemic” targets (392, 393). A promising candidate is CD45, which has recently been investigated in an epitope base editing approach. Through this modification, Wellhausen et al. were able to design cells with the ability to engraft, persist and differentiate in an in vivo model and were not attacked by anti-CD45 CAR-T cells. This effect could be shown for models of AML, B-cell lymphoma and T-ALL (394).
The recently developed YTB323 CAR-T-cell therapy, based on the anti-CD19 tisagenleucel, is another particularly promising approach. Through the novel T-Charge manufacturing platform, this therapy can be provided to patients within less than 10 days after leukapheresis (395). Potential benefits include an enhanced clinical safety profile, high response rates and preservation of a higher T-cell stemness. This final aspect is assumed to play a major role in CAR-T-cell functionality and survival (396).
Future research is urgently needed to expand upon our current knowledge of the applicability of CAR-T-cell therapy in other diseases and particular clinical courses. Clinical research needs to include currently underrepresented disease groups into CAR-T-cell trials to broadly establish them in the clinical practice (397).
10.3 The role of AI in CAR-T-cell research
Artificial intelligence (AI) and machine learning (ML) are two of the most fascinating topics in the currently evolving medical research landscape (398). Their effect on multiple disciplines cannot yet be reliably estimated but early reports hint towards their relevance in tackling biological and structural challenges (399). Because of an intense attention to the topic, hematologic research is also investigating potential uses of the technologies in the diagnosis and treatment of hematologic malignancies.
In fact, there are several areas of CAR-T-cell therapy in which AI and ML can benefit patients and clinicians. One key example might be the prediction and assessment of adverse events like CRS (400–402). Since early detection of an overbearing immune response is of critical importance to adequately support patients, this could prove beneficial to improve clinical outcome and therapy management. Similarly, AI models can assess clinical parameters for their prognostic value and predict long-term outcome (403). This includes the close investigation of the crosstalk between CAR-T cells and the human gut microbiome, a challenging task that requires the collection, curation and handling of large datasets (404).
Identification of possible targets for CAR-T-cell therapy represents another excellent challenge to be solved by AI. Through multi-OMIC approaches, researchers have a previously unknown amount of data at their disposal to investigate potential targets for CAR-T-cell therapy (405). ML algorithms can aid in filtering, clustering, and interpreting these data to assess neoantigens or design TCRs (406, 407). Through the implementation of multi-dimensional ML algorithms it is possible to investigate large datasets on CAR-T-cell phenotype (408) as well as correlations between cellular and clinical data (409).
As the production of CAR-T cells is highly elaborate and requires multiple complex steps as well as close monitoring and data collection for quality and process control, researchers are employing AI to determine optimal production conditions and workflows (410–412). Similar approaches have shown success in other biological production systems. Since the clinical performance of CAR-T-cell therapy is highly dependent on the quality of its production, previous promising results in improving cell culture and expansion can hopefully be translated to similar processes in CAR-T-cell technology (413).
Gil and Grajek have recently outlined potential applications of AI in CAR-T-cell therapy. These include: Improvement of lymphodepletion regimens, identification of novel target antigens, designing of new therapeutic molecules, and the construction of predictive clinical models based on biomarkers, antigen loss, TME and T-cell phenotype (414). Furthermore, AI can aid in the improvement of gene editing technologies employed for CAR-T-cell design, the combination of currently separately tested approaches (expression of cytokines or transcription factors), and the screening of large CAR-T-cell libraries (415).
In conclusion, AI can potentially address a number of the most pressing issues in the application of CAR-T-cell therapy for hematologic malignancies. Both clinical as well as experimental problems can potentially be approached through AI and ML, especially to harness large datasets, predict novel designs, and improve high-precision workflows. This decade of CAR-T-cell and AI research will show if the technology can live up to these expectations.
Author contributions
HK: Conceptualization, Investigation, Methodology, Visualization, Writing – original draft, Writing – review & editing. LM: Methodology, Visualization, Writing – review & editing. SC: Investigation, Validation, Visualization, Writing – review & editing. WF: Supervision, Visualization, Writing – review & editing, Writing – original draft. WA: Supervision, Visualization, Writing – review & editing. AB: Conceptualization, Funding acquisition, Methodology, Project administration, Resources, Supervision, Validation, Visualization, Writing – original draft, Writing – review & editing.
Funding
The author(s) declare financial support was received for the research, authorship, and/or publication of this article. This research was funded in part by the “Andreas Andresen-Stiftung für Krebsforschung”. SC was supported by the University Cancer Center Hamburg Research fellowship. The funders had no role in the design of the study; in the collection, analyses, or interpretation of data; in the writing of the manuscript; or in the decision to publish the results.
Acknowledgments
The authors would like to thank the staff of the II. Medical Clinic and Polyclinic of the University clinic center Hamburg-Eppendorf for their clinical guidance and help in the interpretation of compiled knowledge. We further want to thank Suzette M.T. Block, MD for proof reading and the staff of the clinical studies department for their support and input. All figures were created with BioRender.com.
Conflict of interest
WA has received honoraria from GSK and Janssen, payment of expenses by Janssen and Biontech and research funding received by institution for clinical research by Affimed and Biontech. WF participated in advisory boards for Morphosys, AbbVie, Pfizer, Amgen, Jazz Pharmaceuticals, and Clinigen, received support for meeting attendance from Amgen, Jazz Pharmaceuticals, Daiichi Sankyo Oncology, Bristol Myers Squibb, and Servier, received support for medical writing from Amgen, Boehringer Ingelheim, Pfizer, and AbbVie, and received research funding from Apis Technologies.
The remaining authors declare that the research was conducted in the absence of any commercial or financial relationships that could be construed as a potential conflict of interest.
Publisher’s note
All claims expressed in this article are solely those of the authors and do not necessarily represent those of their affiliated organizations, or those of the publisher, the editors and the reviewers. Any product that may be evaluated in this article, or claim that may be made by its manufacturer, is not guaranteed or endorsed by the publisher.
References
1. Campo E, Jaffe ES, Cook JR, Quintanilla-Martinez L, Swerdlow SH, Anderson KC, et al. The international consensus classification of mature lymphoid neoplasms: a report from the clinical advisory committee. Blood (2022) 140:1229–53. doi: 10.1182/blood.2022015851
2. Hallek M, Al-Sawaf O. Chronic lymphocytic leukemia: 2022 update on diagnostic and therapeutic procedures. Am J Hematol (2021) 96:1679–705. doi: 10.1002/ajh.26367
3. Kameda T, Kataoka K, Kamiunten A, Hidaka M, Miyoshi H, Nakano N, et al. Integrated genetic and clinical prognostic factors for aggressive adult T-cell leukemia/lymphoma. 1 (2023) 108:2178–91. doi: 10.3324/haematol.2022.281510
4. Carbone A, Vaccher E, Gloghini A. Hematologic cancers in individuals infected by HIV. Blood (2022) 139:995–1012. doi: 10.1182/blood.2020005469
5. Bispo JA, Pinheiro PS, Kobetz EK. Epidemiology and etiology of leukemia and lymphoma. Cold Spring Harb Perspect Med (2020) 10:a034819. doi: 10.1101/cshperspect.a034819
6. Rosenbaum L. Tragedy, perseverance, and chance - the story of CAR-T therapy. N Engl J Med (2017) 377:1313–5. doi: 10.1056/NEJMp1711886
7. Johnson PC, Abramson JS. Engineered T cells: CAR T cell therapy and beyond. Curr Oncol Rep (2022) 24:23–31. doi: 10.1007/s11912-021-01161-4
8. Thol F, Heuser M. Treatment for relapsed/refractory acute myeloid leukemia. Hemasphere (2021) 5:e572. doi: 10.1097/HS9.0000000000000572
9. Othman T, Herrera A, Mei M. Emerging therapies in relapsed and refractory hodgkin lymphoma: what comes next after brentuximab vedotin and PD-1 inhibition? Curr Hematol Malig Rep (2021) 16:1–7. doi: 10.1007/s11899-020-00603-3
10. de Leeuw DC, Ossenkoppele GJ, Janssen JJ. Older patients with acute myeloid leukemia deserve individualized treatment. Curr Oncol Rep (2022) 24:1387–400. doi: 10.1007/s11912-022-01299-9
11. Sun W, Liang A-B, Huang H, Huang X-J. Strategies to optimize CAR-T cell therapy in hematological Malignancies: Chinese experience. Haematologica (2023) 1:2011–28. doi: 10.3324/haematol.2022.282316
12. Hajifathali A, Lasemi MV, Mehravar M, Moshari MR, Alizadeh AM, Roshandel E. Novelty in improvement of CAR T cell-based immunotherapy with the aid of CRISPR system. Hematol Transfusion Cell Ther (2023). doi: 10.1016/j.htct.2023.05.009
13. Barboy O, Katzenelenbogen Y, Shalita R, Amit I. In synergy: optimizing CAR T development and personalizing patient care using single-cell technologies. Cancer Discovery (2023) 13:1546–55. doi: 10.1158/2159-8290.CD-23-0010
14. Sievers S, Watson G, Johncy S, Adkins S. Recognizing and grading CAR T-cell toxicities: an advanced practitioner perspective. Front Oncol (2020) 10:885. doi: 10.3389/fonc.2020.00885
15. Song M-K, Park B-B, Uhm J-E. Resistance mechanisms to CAR T-cell therapy and overcoming strategy in B-cell hematologic Malignancies. Int J Mol Sci (2019) 20:5010. doi: 10.3390/ijms20205010
16. Labanieh L, Mackall CL. CAR immune cells: design principles, resistance and the next generation. Nature (2023) 614:635–48. doi: 10.1038/s41586-023-05707-3
17. Li X, Gong N, Tian F, Zhang S, Zhang Y, Wang Y, et al. Suppression of cytokine release syndrome during CAR-T-cell therapy via a subcutaneously injected interleukin-6-adsorbing hydrogel. Nat BioMed Eng (2023) 7:1129–41. doi: 10.1038/s41551-023-01084-4
18. Cheng J, Liu M, Zhang J. Intelligent tunable CAR-T cell therapy leads the new trend. Synth Syst Biotechnol (2023) 8:606–9. doi: 10.1016/j.synbio.2023.09.001
19. Holland EM, Yates B, Steinberg SM, Yuan CM, Wang H-W, Annesley C, et al. Chimeric antigen receptor T cells as salvage therapy for post-chimeric antigen receptor T cell failure. Transplant Cell Ther (2023) 29:574:e1–574.e10. doi: 10.1016/j.jtct.2023.06.019
20. Kampouri E, Little JS, Rejeski K, Manuel O, Hammond SP, Hill JA. Infections after chimeric antigen receptor (CAR)-T-cell therapy for hematologic Malignancies. Transpl Infect Dis (2023):e14157. doi: 10.1111/tid.14157
21. Sharma R, Suravarjhula L, Banerjee M, Kumar G, Kumar N. Chimeric antigen receptor T-cell therapy in cancer: A critical review. Curr Drug Res Rev (2023) 15(3):241–61. doi: 10.2174/2589977515666230220092125
22. Gao X, Liu J, Sun R, Zhang J, Cao X, Zhang Y, et al. Alliance between titans: combination strategies of CAR-T cell therapy and oncolytic virus for the treatment of hematological Malignancies. . Ann Hematol (2023), 1–21. doi: 10.1007/s00277-023-05488-9
23. O’Dwyer KM. Optimal approach to T-cell ALL. Hematology. Am Soc Hematol Educ Program (2022) 2022:197–205. doi: 10.1182/hematology.2022000337C
24. Maciocia PM, Wawrzyniecka PA, Philip B, Ricciardelli I, Akarca AU, Onuoha SC, et al. Targeting the T cell receptor β-chain constant region for immunotherapy of T cell Malignancies. Nat Med (2017) 23:1416–23. doi: 10.1038/nm.4444
25. Cooper ML, DiPersio JF. Chimeric antigen receptor T cells (CAR-T) for the treatment of T-cell Malignancies. Best Pract Res Clin haematol (2019) 32:101097. doi: 10.1016/j.beha.2019.101097
26. Luo L, Zhou X, Zhou L, Liang Z, Yang J, Tu S, et al. Current state of CAR-T therapy for T-cell Malignancies. Ther Adv Hematol (2022) 13:20406207221143025. doi: 10.1177/20406207221143025
27. Chakraverty R, Robinson S, Peggs K, Kottaridis P, Watts M, Ings S, et al. Excessive T cell depletion of peripheral blood stem cells has an adverse effect upon outcome following allogeneic stem cell transplantation. Bone marrow Transplant (2001) 28:827–34. doi: 10.1038/sj.bmt.1703248
28. Braun T, Schrader A. Education and empowering special forces to eradicate secret defectors: immune system-based treatment approaches for mature T- and NK-cell Malignancies. Cancers (Basel) (2023) 15:2532. doi: 10.3390/cancers15092532
29. Bayón-Calderón F, Toribio ML, González-García S. Facts and challenges in immunotherapy for T-cell acute lymphoblastic leukemia. Int J Mol Sci (2020) 21:7685. doi: 10.3390/ijms21207685
30. Polgárová K, Otáhal P, Šálek C, Pytlík R. Chimeric antigen receptor based cellular therapy for treatment of T-cell Malignancies. Front Oncol (2022) 12:876758. doi: 10.3389/fonc.2022.876758
31. Gomes-Silva D, Srinivasan M, Sharma S, Lee CM, Wagner DL, Davis TH, et al. CD7-edited T cells expressing a CD7-specific CAR for the therapy of T-cell Malignancies. Blood (2017) 130:285–96. doi: 10.1182/blood-2017-01-761320
32. Andualem H, Lemma M, Keflie A, Workeneh M, Ayelign B, Tassachew Y, et al. Elevated KIR expression and diminished intensity of CD7 on NK cell subsets among treatment naïve HIV infected Ethiopians. Sci Rep (2022) 12:14747. doi: 10.1038/s41598-022-18413-3
33. Huang Q, Zhang X-H, Liu D. CAR T cell therapy for T cell leukemia and lymphoma: latest updates from 2022 ASH Annual Meeting. J Hematol Oncol (2023) 16:18. doi: 10.1186/s13045-023-01406-8
34. Chen D, You F, Xiang S, Wang Y, Li Y, Meng H, et al. Chimeric antigen receptor T cells derived from CD7 nanobody exhibit robust antitumor potential against CD7-positive Malignancies. Am J Cancer Res (2021) 11:5263–81.
35. Zhang M, Chen D, Fu X, Meng H, Nan F, Sun Z, et al. Autologous nanobody-derived fratricide-resistant CD7-CAR T-cell therapy for patients with relapsed and refractory T-cell acute lymphoblastic leukemia/lymphoma. Clin Cancer Res an Off J Am Assoc Cancer Res (2022) 28:2830–43. doi: 10.1158/1078-0432.CCR-21-4097
36. Lu P, Liu Y, Yang J, Zhang X, Yang X, Wang H, et al. Naturally selected CD7 CAR-T therapy without genetic manipulations for T-ALL/LBL: first-in-human phase 1 clinical trial. Blood (2022) 140:321–34. doi: 10.1182/blood.2021014498
37. Lu Y, Liu Y, Wen S, Kuang N, Zhang X, Li J, et al. Naturally selected CD7 CAR-T therapy without genetic editing demonstrates significant antitumour efficacy against relapsed and refractory acute myeloid leukaemia (R/R-AML). J Trans Med (2022) 20:600. doi: 10.1186/s12967-022-03797-7
38. Ye J, Jia Y, Tuhin IJ, Tan J, Monty MA, Xu N, et al. Feasibility study of a novel preparation strategy for anti-CD7 CAR-T cells with a recombinant anti-CD7 blocking antibody. Mol Ther oncol (2022) 24:719–28. doi: 10.1016/j.omto.2022.02.013
39. Png YT, Vinanica N, Kamiya T, Shimasaki N, Coustan-Smith E, Campana D. Blockade of CD7 expression in T cells for effective chimeric antigen receptor targeting of T-cell Malignancies. Blood Adv (2017) 1:2348–60. doi: 10.1182/bloodadvances.2017009928
40. Chiesa R, Georgiadis C, Syed F, Zhan H, Etuk A, Gkazi SA, et al. Base-edited CAR7 T cells for relapsed T-cell acute lymphoblastic leukemia. N Engl J Med (2023) 389:899–910. doi: 10.1056/NEJMoa2300709
41. Watanabe N, Mo F, Zheng R, Ma R, Bray VC, van Leeuwen DG, et al. Feasibility and preclinical efficacy of CD7-unedited CD7 CAR T cells for T cell Malignancies. Mol Ther J Am Soc Gene Ther (2023) 31:24–34. doi: 10.1016/j.ymthe.2022.09.003
42. Freiwan A, Zoine JT, Crawford JC, Vaidya A, Schattgen SA, Myers JA, et al. Engineering naturally occurring CD7- T cells for the immunotherapy of hematological Malignancies. Blood (2022) 140:2684–96. doi: 10.1182/blood.2021015020
43. Cooper ML, Choi J, Staser K, Ritchey JK, Devenport JM, Eckardt K, et al. An “off-the-shelf” fratricide-resistant CAR-T for the treatment of T cell hematologic Malignancies. Leukemia (2018) 32:1970–83. doi: 10.1038/s41375-018-0065-5
44. Georgiadis C, Rasaiyaah J, Gkazi SA, Preece R, Etuk A, Christi A, et al. Base-edited CAR T cells for combinational therapy against T cell Malignancies. Leukemia (2021) 35:3466–81. doi: 10.1038/s41375-021-01282-6
45. Dai Z, Mu W, Zhao Y, Cheng J, Lin H, Ouyang K, et al. T cells expressing CD5/CD7 bispecific chimeric antigen receptors with fully human heavy-chain-only domains mitigate tumor antigen escape. Signal transduct target Ther (2022) 7:85. doi: 10.1038/s41392-022-00898-z
46. Xia B, Lin K, Wang X, Chen F, Zhou M, Li Y, et al. Nanobody-derived bispecific CAR-T cell therapy enhances the anti-tumor efficacy of T cell lymphoma treatment. Mol Ther oncol (2023) 30:86–102. doi: 10.1016/j.omto.2023.07.007
47. Jiang J, Chen J, Liao C, Duan Y, Wang Y, Shang K, et al. Inserting EF1α-driven CD7-specific CAR at CD7 locus reduces fratricide and enhances tumor rejection. Leukemia (2023) 37:1660–70. doi: 10.1038/s41375-023-01948-3
48. Liao C, Wang Y, Huang Y, Duan Y, Liang Y, Chen J, et al. CD38-specific CAR integrated into CD38 locus driven by different promoters causes distinct antitumor activities of T and NK cells. Adv Sci (2023) 10(27):e2207394. doi: 10.1002/advs.202207394
49. Fleischer LC, Spencer HT, Raikar SS. Targeting T cell Malignancies using CAR-based immunotherapy: challenges and potential solutions. J Hematol Oncol (2019) 12:141. doi: 10.1186/s13045-019-0801-y
50. Poirot L, Philip B, Schiffer-Mannioui C, Le Clerre D, Chion-Sotinel I, Derniame S, et al. Multiplex genome-edited T-cell manufacturing platform for “Off-the-shelf” Adoptive T-cell immunotherapies. Cancer Res (2015) 75:3853–64. doi: 10.1158/0008-5472.CAN-14-3321
51. Xie L, Gu R, Yang X, Qiu S, Xu Y, Mou J, et al. Universal anti-CD7 CAR-T cells targeting T-ALL and functional analysis of CD7 antigen on T/CAR-T cells. Hum Gene Ther (2023). doi: 10.1089/hum.2023.029
52. Kim MY, Cooper ML, Jacobs MT, Ritchey JK, Hollaway J, Fehniger TA, et al. CD7-deleted hematopoietic stem cells can restore immunity after CAR T cell therapy. JCI Insight (2021) 6. doi: 10.1172/jci.insight.149819
53. Pan J, Tan Y, Wang G, Deng B, Ling Z, Song W, et al. Donor-derived CD7 chimeric antigen receptor T cells for T-cell acute lymphoblastic leukemia: first-in-human, phase I trial. J Clin Oncol (2021) 39:3340–51. doi: 10.1200/JCO.21.00389
54. Zhang Y, Li C, Du M, Jiang H, Luo W, Tang L, et al. Allogenic and autologous anti-CD7 CAR-T cell therapies in relapsed or refractory T-cell Malignancies. Blood Cancer J (2023) 13:61. doi: 10.1038/s41408-023-00822-w
55. Zhang X, Yang J, Li J, Qiu L, Zhang J, Lu Y, et al. Analysis of 60 patients with relapsed or refractory T-cell acute lymphoblastic leukemia and T-cell lymphoblastic lymphoma treated with CD7-targeted chimeric antigen receptor-T cell therapy. Am J Hematol (2023) 98(12):1898–1908. doi: 10.1002/ajh.27094
56. Chen W, Shi H, Liu Z, Yang F, Liu J, Zhang L, et al. Single-cell transcriptomics reveals immune reconstitution in patients with R/R T-ALL/LBL treated with donor-derived CD7 CAR-T therapy. Clin Cancer Res an Off J Am Assoc Cancer Res (2023) 29:1484–95. doi: 10.1158/1078-0432.CCR-22-2924
57. Li S, Wang X, Liu L, Liu J, Rao J, Yuan Z, et al. CD7 targeted “off-the-shelf” CAR-T demonstrates robust in vivo expansion and high efficacy in the treatment of patients with relapsed and refractory T cell Malignancies. Leukemia (2023) 37:2176–86. doi: 10.1038/s41375-023-02018-4
58. Xu X, Zu C, Zhang M, Xiao P, Hong R, Feng J, et al. HLA fully-mismatched sibling-derived CD7 CAR-T therapy bridging to haploidentical hematopoietic stem cell transplantation for hepatosplenic γδ T-cell lymphoma. Cell Transplant (2023) 32:9636897231194265. doi: 10.1177/09636897231194265
59. Horna P, Shi M, Olteanu H, Johansson U. Emerging role of T-cell receptor constant β Chain-1 (TRBC1) expression in the flow cytometric diagnosis of T-cell Malignancies. Int J Mol Sci (2021) 22:1817. doi: 10.3390/ijms22041817
60. Sánchez-Martínez D, Baroni ML, Gutierrez-Agüera F, Roca-Ho H, Blanch-Lombarte O, González-García S, et al. Fratricide-resistant CD1a-specific CAR T cells for the treatment of cortical T-cell acute lymphoblastic leukemia. Blood (2019) 133:2291–304. doi: 10.1182/blood-2018-10-882944
61. Xiang J, Devenport JM, Carter AJ, Staser KW, Kim MY, O’ Neal J, et al. An “off-the-shelf” CD2 universal CAR-T therapy for T-cell Malignancies. Leukemia (2023), 1–9. doi: 10.1038/s41375-023-02039-z
62. Feng J, Xu H, Cinquina A, Wu Z, Zhang W, Sun L, et al. Treatment of aggressive T-cell lymphoma/leukemia with anti-CD4 CAR T cells. Front Immunol (2022) 13:997482. doi: 10.3389/fimmu.2022.997482
63. Feng J, Xu H, Cinquina A, Wu Z, Chen Q, Zhang P, et al. Treatment of aggressive T cell lymphoblastic lymphoma/leukemia using anti-CD5 CAR T cells. Stem Cell Rev Rep (2021) 17:652–61. doi: 10.1007/s12015-020-10092-9
64. Lato MW, Przysucha A, Grosman S, Zawitkowska J, Lejman M. The new therapeutic strategies in pediatric T-cell acute lymphoblastic leukemia. Int J Mol Sci (2021) 22:4502. doi: 10.3390/ijms22094502
65. Patel J, Gao X, Wang H. An update on clinical trials and potential therapeutic strategies in T-cell acute lymphoblastic leukemia. Int J Mol Sci (2023) 24:7201. doi: 10.3390/ijms24087201
66. Kobayashi E, Kamihara Y, Arai M, Wada A, Kikuchi S, Hatano R, et al. Development of a novel CD26-targeted chimeric antigen receptor T-cell therapy for CD26-expressing T-cell Malignancies. Cells (2023) 12. doi: 10.3390/cells12162059
67. Glisovic-Aplenc T, Diorio C, Chukinas JA, Veliz K, Shestova O, Shen F, et al. CD38 as a pan-hematologic target for chimeric antigen receptor T cells. Blood Adv (2023) 7(16):4418–30. doi: 10.1182/bloodadvances.2022007059
68. Shi J, Zhang Z, Cen H, Wu H, Zhang S, Liu J, et al. CAR T cells targeting CD99 as an approach to eradicate T-cell acute lymphoblastic leukemia without normal blood cells toxicity. J Hematol Oncol (2021) 14:162. doi: 10.1186/s13045-021-01178-z
69. Ren A, Zhao Y, Zhu H. T-ALL cells as tool cells for CAR T therapy. Vaccines (2023) 11:854. doi: 10.3390/vaccines11040854
70. Maciocia PM, Wawrzyniecka PA, Maciocia NC, Burley A, Karpanasamy T, Devereaux S, et al. Anti-CCR9 chimeric antigen receptor T cells for T-cell acute lymphoblastic leukemia. Blood (2022) 140:25–37. doi: 10.1182/blood.2021013648
71. Wei W, Ma H, Yang D, Sun B, Tang J, Zhu Y, et al. SECTM1-based CAR-T cells enriched with CD7low/negative subsets exhibit efficacy in CD7-positive Malignancies. Blood Adv (2023) 7(13):2941–51. doi: 10.1182/bloodadvances.2022008402
72. Li H, Song W, Wu J, Shi Z, Gao Y, Li J, et al. CAR-T cells targeting CD38 and LMP1 exhibit robust antitumour activity against NK/T cell lymphoma. BMC Med (2023) 21:330. doi: 10.1186/s12916-023-03040-0
73. Shaw LC, Poussin M, Rodriguez-Garcia A, Eggold J, Minutolo NG, Wang J, et al. TCRvβ-CART therapy mediates high-precision targeting of Malignant T-cell clones. Blood Adv (2023) 7:1885–98. doi: 10.1182/bloodadvances.2022008798
74. Wu H, Yu Y, Zhao Y, Liu W, Liu Z, Zhang G, et al. A CH2CH3 hinge region enhances the cytotoxicity of anti-CD5 CAR-T cells targeting T cell acute lymphoblastic leukemia. Int Immunopharmacol (2023) 124:110904. doi: 10.1016/j.intimp.2023.110904
75. Newman H, Teachey DT. A bright horizon: immunotherapy for pediatric T-cell Malignancies. Int J Mol Sci (2022) 23:8600. doi: 10.3390/ijms23158600
76. Assi R, Salman H. Harnessing the potential of chimeric antigen receptor T-cell therapy for the treatment of T-cell Malignancies: A dare or double dare? Cells (2022) 11:3971. doi: 10.3390/cells11243971
77. Li S, Wang X, Yuan Z, Liu L, Luo L, Li Y, et al. Eradication of T-ALL cells by CD7-targeted universal CAR-T cells and initial test of ruxolitinib-based CRS management. Clin Cancer Res an Off J Am Assoc Cancer Res (2021) 27:1242–6. doi: 10.1158/1078-0432.CCR-20-1271
78. Xu N, Yang X-F, Xue S-L, Tan J-W, Li M-H, Ye J, et al. Ruxolitinib reduces severe CRS response by suspending CAR-T cell function instead of damaging CAR-T cells. Biochem Biophys Res Commun (2022) 595:54–61. doi: 10.1016/j.bbrc.2022.01.070
79. Nawaz W, Huang B, Xu S, Li Y, Zhu L, Yiqiao H, et al. AAV-mediated in vivo CAR gene therapy for targeting human T-cell leukemia. Blood Cancer J (2021) 11:119. doi: 10.1038/s41408-021-00508-1
80. Hu Y, Zhou Y, Zhang M, Zhao H, Wei G, Ge W, et al. Genetically modified CD7-targeting allogeneic CAR-T cell therapy with enhanced efficacy for relapsed/refractory CD7-positive hematological Malignancies: a phase I clinical study. Cell Res (2022) 32:995–1007. doi: 10.1038/s41422-022-00721-y
81. Atilla PA, Atilla E. Are we there yet? cellular therapies for cutaneous T cell lymphoma. Curr Res Trans Med (2023) 71:103390. doi: 10.1016/j.retram.2023.103390
82. Brazel D, Pinter-Brown L. SOHO state-of-the-art updates and next questions: A modern approach to the systemic treatment of advanced CTCL. Clin lymphoma myeloma leukemia (2023) 23:401–9. doi: 10.1016/j.clml.2023.03.003
83. Dai J, Duvic M. Cutaneous T-cell lymphoma: current and emerging therapies. MJH Life Sci (2023) 37(2):55–62. doi: 10.46883/2023.25920984
84. Dummer R, Vermeer MH, Scarisbrick JJ, Kim YH, Stonesifer C, Tensen CP, et al. Cutaneous T cell lymphoma. Nat Rev Dis Primers (2021) 7:61. doi: 10.1038/s41572-021-00296-9
85. Perera LP, Zhang M, Nakagawa M, Petrus MN, Maeda M, Kadin ME, et al. Chimeric antigen receptor modified T cells that target chemokine receptor CCR4 as a therapeutic modality for T-cell Malignancies. Am J Hematol (2017) 92:892–901. doi: 10.1002/ajh.24794
86. Nicolay JP, Albrecht JD, Alberti-Violetti S, Berti E. CCR4 in cutaneous T-cell lymphoma: Therapeutic targeting of a pathogenic driver. Eur J Immunol (2021) 51:1660–71. doi: 10.1002/eji.202049043
87. Watanabe K, Aznar MA, Kuramitsu S, Siurala M, Da T, Agarwal S, et al. Identifying highly active anti-CCR4-CAR T cells for the treatment of T-cell lymphoma. Blood Adv (2023) 7(14):3416–30. doi: 10.1182/bloodadvances.2022008327
88. Scarfò I, Ormhøj M, Frigault MJ, Castano AP, Lorrey S, Bouffard AA, et al. Anti-CD37 chimeric antigen receptor T cells are active against B- and T-cell lymphomas. Blood (2018) 132:1495–506. doi: 10.1182/blood-2018-04-842708
89. Xu S, Foss F. New nonchemotherapy treatment options for cutaneous T-cell lymphomas. Expert Rev Anticancer Ther (2021) 21:1017–28. doi: 10.1080/14737140.2021.1882859
90. Gluud M, Pallesen EM, Buus TB, Gjerdrum LM, Lindahl LM, Kamstrup MR, et al. Malignant T cells induce skin barrier defects through cytokine-mediated JAK/STAT signaling in cutaneous T-cell lymphoma. Blood (2023) 141:180–93. doi: 10.1182/blood.2022016690
91. Pelcovits A, Ollila TA, Olszewski AJ. Advances in immunotherapy for the treatment of cutaneous T-cell lymphoma. CMAR (2023) 15:989–98. doi: 10.2147/CMAR.S330908
92. To V, Evtimov VJ, Jenkin G, Pupovac A, Trounson AO, Boyd RL. CAR-T cell development for Cutaneous T cell Lymphoma: current limitations and potential treatment strategies. Front Immunol (2022) 13:968395. doi: 10.3389/fimmu.2022.968395
93. Sivanand A, Surmanowicz P, Alhusayen R, Hull P, Litvinov IV, Zhou Y, et al. Immunotherapy for cutaneous T-cell lymphoma: current landscape and future developments. J Cutan Med Surg (2019) 23:537–44. doi: 10.1177/1203475419867610
94. Weiner DM, Durgin JS, Wysocka M, Rook AH. The immunopathogenesis and immunotherapy of cutaneous T cell lymphoma: Current and future approaches. J Am Acad Dermatol (2021) 84:597–604. doi: 10.1016/j.jaad.2020.12.026
95. Scarfò I, Frigault MJ, Maus MV. CAR-based approaches to cutaneous T-cell lymphoma. Front Oncol (2019) 9:259. doi: 10.3389/fonc.2019.00259
96. Nakahata S, Morishita K. Peripheral T-cell lymphoma: from biological research to new therapies. Cancers (Basel) (2023) 15:4192. doi: 10.3390/cancers15164192
97. Angelos MG, Ballard HJ, Barta SK. Advances and personalized approaches in the frontline treatment of T-cell lymphomas. J Personal Med (2022) 12:267. doi: 10.3390/jpm12020267
98. Du J, Jin S, Zhang M, Fu X, Yang J, Zhang L, et al. Precise diagnosis and targeted therapy of nodal T-follicular helper cell lymphoma (T-FHCL). Front Oncol (2023) 13:1163190. doi: 10.3389/fonc.2023.1163190
99. Wu Y, Chen D, Lu Y, Dong S-C, Ma R, Tang W, et al. A new immunotherapy strategy targeted CD30 in peripheral T-cell lymphomas: CAR-modified T-cell therapy based on CD30 mAb. Cancer Gene Ther (2022) 29:167–77. doi: 10.1038/s41417-021-00295-8
100. Saetang J, Sangkhathat S, Jangphattananont N, Khopanlert W, Julamanee J, Tipmanee V. Computational discovery of binding mode of anti-TRBC1 antibody and predicted key amino acids of TRBC1. Sci Rep (2022) 12:1760. doi: 10.1038/s41598-022-05742-6
101. Rogers AM, Brammer JE. Hematopoietic cell transplantation and adoptive cell therapy in peripheral T cell lymphoma. Curr Hematol Malig Rep (2020) 15:316–32. doi: 10.1007/s11899-020-00590-5
102. Couto SC, Kowes A, Aurabi CS, Oliveira TG, Klinger P, Rocha V. Autologous, allogeneic hematopoietic cell transplantation and CAR-T/NK therapy: what is their real importance in PTCL? Front. Oncol (2023) 13:1195759. doi: 10.3389/fonc.2023.1195759
103. Krug A, Tari G, Saidane A, Gaulard P, Ricci J-E, Lemonnier F, et al. Novel T follicular helper-like T-cell lymphoma therapies: from preclinical evaluation to clinical reality. Cancers (Basel) (2022) 14:2392. doi: 10.3390/cancers14102392
104. Fang KK-L, Lee J, Khatri I, Na Y, Zhang L. Targeting T-cell Malignancies using allogeneic double-negative CD4-CAR-T cells. J immunother Cancer (2023) 11:e007277. doi: 10.1136/jitc-2023-007277
105. Dai H-P, Cui W, Cui Q-Y, Zhu W-J, Meng H-M, Zhu M-Q, et al. Haploidentical CD7 CAR T-cells induced remission in a patient with TP53 mutated relapsed and refractory early T-cell precursor lymphoblastic leukemia/lymphoma. biomark Res (2022) 10:6. doi: 10.1186/s40364-022-00352-w
106. Tan Y, Shan L, Zhao L, Deng B, Ling Z, Zhang Y, et al. Long-term follow-up of donor-derived CD7 CAR T-cell therapy in patients with T-cell acute lymphoblastic leukemia. J Hematol Oncol (2023) 16:34. doi: 10.1186/s13045-023-01427-3
107. Li Z, An N, Yang K, Meng F, Xu T, Peng X, et al. Donor CD7 chimeric antigen receptor T cell bridging to allogeneic hematopoietic stem cell transplantation for T cell hematologic Malignancy. Transplant Cell Ther (2023) 29:167–73. doi: 10.1016/j.jtct.2022.11.013
108. Vishwasrao P, Li G, Boucher JC, Smith DL, Hui SK. Emerging CAR T cell strategies for the treatment of AML. Cancers (Basel) (2022) 14:1241. doi: 10.3390/cancers14051241
109. Fetsch V, Zeiser R. Chimeric antigen receptor T cells for acute myeloid leukemia. Eur J Haematol (2023) 1–8. doi: 10.1111/ejh.14047
110. Cao X, Dai H, Cui Q, Li Z, Shen W, Pan J, et al. CD7-directed CAR T-cell therapy: a potential immunotherapy strategy for relapsed/refractory acute myeloid leukemia. Exp Hematol Oncol (2022) 11:67. doi: 10.1186/s40164-022-00318-6
111. Cui Q, Qian C, Xu N, Kang L, Dai H, Cui W, et al. CD38-directed CAR-T cell therapy: a novel immunotherapy strategy for relapsed acute myeloid leukemia after allogeneic hematopoietic stem cell transplantation. J Hematol Oncol (2021) 14:82. doi: 10.1186/s13045-021-01092-4
112. Jin X, Zhang M, Sun R, Lyu H, Xiao X, Zhang X, et al. First-in-human phase I study of CLL-1 CAR-T cells in adults with relapsed/refractory acute myeloid leukemia. J Hematol Oncol (2022) 15:88. doi: 10.1186/s13045-022-01308-1
113. Li K, Wu H, Pan W, Guo M, Qiu D, He Y, et al. A novel approach for relapsed/refractory FLT3mut+ acute myeloid leukaemia: synergistic effect of the combination of bispecific FLT3scFv/NKG2D-CAR T cells and gilteritinib. Mol Cancer (2022) 21:66. doi: 10.1186/s12943-022-01541-9
114. Zhang H, Wang P, Li Z, He Y, Gan W, Jiang H. Anti-CLL1 chimeric antigen receptor T-cell therapy in children with relapsed/refractory acute myeloid leukemia. Clin Cancer Res (2021) 27:3549–55. doi: 10.1158/1078-0432.CCR-20-4543
115. Gomes-Silva D, Atilla E, Atilla PA, Mo F, Tashiro H, Srinivasan M, et al. CD7 CAR T cells for the therapy of acute myeloid leukemia. Mol Ther J Am Soc Gene Ther (2019) 27:272–80. doi: 10.1016/j.ymthe.2018.10.001
116. Albinger N, Pfeifer R, Nitsche M, Mertlitz S, Campe J, Stein K, et al. Primary CD33-targeting CAR-NK cells for the treatment of acute myeloid leukemia. Blood Cancer J (2022) 12:61. doi: 10.1038/s41408-022-00660-2
117. Liu Y, Wang S, Schubert M-L, Lauk A, Yao H, Blank MF, et al. CD33-directed immunotherapy with third-generation chimeric antigen receptor T cells and gemtuzumab ozogamicin in intact and CD33-edited acute myeloid leukemia and hematopoietic stem and progenitor cells. Int J Cancer (2022) 150:1141–55. doi: 10.1002/ijc.33865
118. Tigu AB, Constantinescu CS, Teodorescu P, Kegyes D, Munteanu R, Feder R, et al. Design and preclinical testing of an anti-CD41 CAR T cell for the treatment of acute megakaryoblastic leukaemia. J Cell Mol Med (2023) 27:2864–75. doi: 10.1111/jcmm.17810
119. Marvin-Peek J, Savani BN, Olalekan OO, Dholaria B. Challenges and advances in chimeric antigen receptor therapy for acute myeloid leukemia. Cancers (Basel) (2022) 14:497. doi: 10.3390/cancers14030497
120. Sun X, Wang G, Zuo S, Niu Q, Chen X, Feng X. Preclinical evaluation of CD64 as a potential target for CAR-T-cell therapy for acute myeloid leukemia. J Immunother (2022) 45:67–77. doi: 10.1097/CJI.0000000000000406
121. Sauer T, Parikh K, Sharma S, Omer B, Sedloev D, Chen Q, et al. CD70-specific CAR T cells have potent activity against acute myeloid leukemia without HSC toxicity. Blood (2021) 138:318–30. doi: 10.1182/blood.2020008221
122. Cheng J, Ge T, Zhu X, Wang J, Zeng Y, Mu W, et al. Preclinical development and evaluation of nanobody-based CD70-specific CAR T cells for the treatment of acute myeloid leukemia. Cancer immunol immunother CII (2023) 72:2331–46. doi: 10.1007/s00262-023-03422-6
123. Wu G, Guo S, Luo Q, Wang X, Deng W, Ouyang G, et al. Preclinical evaluation of CD70-specific CAR T cells targeting acute myeloid leukemia. Front Immunol (2023) 14:1093750. doi: 10.3389/fimmu.2023.1093750
124. Myburgh R, Kiefer JD, Russkamp NF, Magnani CF, Nuñez N, Simonis A, et al. Anti-human CD117 CAR T-cells efficiently eliminate healthy and Malignant CD117-expressing hematopoietic cells. Leukemia (2020) 34:2688–703. doi: 10.1038/s41375-020-0818-9
125. Wermke M, Kraus S, Ehninger A, Bargou RC, Goebeler M-E, Middeke JM, et al. Proof of concept for a rapidly switchable universal CAR-T platform with UniCAR-T-CD123 in relapsed/refractory AML. Blood (2021) 137:3145–8. doi: 10.1182/blood.2020009759
126. Pei K, Xu H, Wang P, Gan W, Hu Z, Su X, et al. Anti-CLL1-based CAR T-cells with 4-1-BB or CD28/CD27 stimulatory domains in treating childhood refractory/relapsed acute myeloid leukemia. Cancer Med (2023) 12:9655–61. doi: 10.1002/cam4.5916
127. Zhang H, Bu C, Peng Z, Li G, Zhou Z, Ding W, et al. Characteristics of anti-CLL1 based CAR-T therapy for children with relapsed or refractory acute myeloid leukemia: the multi-center efficacy and safety interim analysis. Leukemia (2022) 36:2596–604. doi: 10.1038/s41375-022-01703-0
128. Fan S, Wang T, You F, Zhang T, Li Y, Ji C, et al. B7-H3 chimeric antigen receptor-modified T cell shows potential for targeted treatment of acute myeloid leukaemia. Eur J Med Res (2023) 28:129. doi: 10.1186/s40001-023-01049-y
129. Ma Q, Garber HR, Lu S, He H, Tallis E, Ding X, et al. A novel TCR-like CAR with specificity for PR1/HLA-A2 effectively targets myeloid leukemia in vitro when expressed in human adult peripheral blood and cord blood T cells. Cytotherapy (2016) 18:985–94. doi: 10.1016/j.jcyt.2016.05.001
130. Wang Y, Xu Y, Li S, Liu J, Xing Y, Xing H, et al. Targeting FLT3 in acute myeloid leukemia using ligand-based chimeric antigen receptor-engineered T cells. J Hematol Oncol (2018) 11:60. doi: 10.1186/s13045-018-0603-7
131. Pedersen MG, Møller BK, Bak RO. Recent advances in the development of anti-FLT3 CAR T-cell therapies for treatment of AML. Biomedicines (2022) 10:2441. doi: 10.3390/biomedicines10102441
132. Tecik M, Adan A. Therapeutic targeting of FLT3 in acute myeloid leukemia: current status and novel approaches. OTT (2022) 15:1449–78. doi: 10.2147/OTT.S384293
133. Da Rocha MN, Guiot M, Nicod C, Trad R, Bouquet L, Haderbache R, et al. Coated recombinant target protein helps explore IL-1RAP CAR T-cell functionality. vitro. Immunol Res (2023) 71:276–82. doi: 10.1007/s12026-022-09348-y
134. Trad R, Warda W, Alcazer V, Da Neto Rocha M, Berceanu A, Nicod C, et al. Chimeric antigen receptor T-cells targeting IL-1RAP: a promising new cellular immunotherapy to treat acute myeloid leukemia. J immunother Cancer (2022) 10. doi: 10.1136/jitc-2021-004222
135. Jetani H, Navarro-Bailón A, Maucher M, Frenz S, Verbruggen C, Yeguas A, et al. Siglec-6 is a novel target for CAR T-cell therapy in acute myeloid leukemia. Blood (2021) 138:1830–42. doi: 10.1182/blood.2020009192
136. Baumeister SH, Murad J, Werner L, Daley H, Trebeden-Negre H, Gicobi JK, et al. Phase I trial of autologous CAR T cells targeting NKG2D ligands in patients with AML/MDS and multiple myeloma. Cancer Immunol Res (2019) 7:100–12. doi: 10.1158/2326-6066.CIR-18-0307
137. Kirkey DC, Loeb AM, Castro S, McKay CN, Perkins L, Pardo L, et al. Therapeutic targeting of PRAME with mTCRCAR T cells in acute myeloid leukemia. Blood Adv (2023) 7:1178–89. doi: 10.1182/bloodadvances.2022008304
138. Hebbar N, Epperly R, Vaidya A, Thanekar U, Moore SE, Umeda M, et al. CAR T cells redirected to cell surface GRP78 display robust anti-acute myeloid leukemia activity and do not target hematopoietic progenitor cells. Nat Commun (2022) 13:587. doi: 10.1038/s41467-022-28243-6
139. Zhang Y, Ding Y, Zhang R, Zhang L, Yan X, Wang P. Aberrant expression of CD5 in adult acute myeloid leukemia belonged to AML-MR, may represent a uniquely aggressive subcategory. Leuk Lymphoma (2023) 64(10):1711–14. doi: 10.1080/10428194.2023.2230332
140. Farge T, Nakhle J, Lagarde D, Cognet G, Polley N, Castellano R, et al. CD36 drives metastasis and relapse in acute myeloid leukemia. Cancer Res (2023) 83(17):2824–38. doi: 10.1158/0008-5472.CAN-22-3682
141. Giannakopoulou E, Lehander M, Virding Culleton S, Yang W, Li Y, Karpanen T, et al. A T cell receptor targeting a recurrent driver mutation in FLT3 mediates elimination of primary human acute myeloid leukemia in vivo. Nat Cancer (2023) 4:1474–90. doi: 10.1038/s43018-023-00642-8
142. He X, Feng Z, Ma J, Ling S, Cao Y, Gurung B, et al. Bispecific and split CAR T cells targeting CD13 and TIM3 eradicate acute myeloid leukemia. Blood (2020) 135:713–23. doi: 10.1182/blood.2019002779
143. Ghamari A, Pakzad P, Majd A, Ebrahimi M, Hamidieh AA. Design and Production An Effective Bispecific Tandem Chimeric Antigen Receptor on T Cells against CD123 and Folate Receptor ß towards B-Acute Myeloid Leukaemia Blasts. Cell J (2021) 23:650–7. doi: 10.22074/cellj.2021.7314
144. Perriello VM, Rotiroti MC, Pisani I, Galimberti S, Alberti G, Pianigiani G, et al. IL3-zetakine combined with a CD33 costimulatory receptor as a Dual CAR approach for safer and selective targeting of AML. Blood Adv (2022) 7(12):2855–71. doi: 10.1182/bloodadvances.2022008762
145. Jin X, Xie D, Sun R, Lu W, Xiao X, Yu Y, et al. CAR-T cells dual-target CD123 and NKG2DLs to eradicate AML cells and selectively target immunosuppressive cells. Oncoimmunology (2023) 12:2248826. doi: 10.1080/2162402X.2023.2248826
146. Marofi F, Rahman HS, Al-Obaidi ZM, Jalil AT, Abdelbasset WK, Suksatan W, et al. Novel CAR T therapy is a ray of hope in the treatment of seriously ill AML patients. Stem Cell Res Ther (2021) 12:465. doi: 10.1186/s13287-021-02420-8
147. Atilla PA, McKenna MK, Watanabe N, Mamonkin M, Brenner MK, Atilla E. Combinatorial antigen targeting strategies for acute leukemia: application in myeloid Malignancy. Cytotherapy (2022) 24:282–90. doi: 10.1016/j.jcyt.2021.10.007
148. Alberti G, Arsuffi C, Pievani A, Salerno D, Mantegazza F, Dazzi F, et al. Engineering tandem CD33xCD146 CAR CIK (cytokine-induced killer) cells to target the acute myeloid leukemia niche. Front Immunol (2023) 14:1192333. doi: 10.3389/fimmu.2023.1192333
149. Leblanc R, Ghossoub R, Goubard A, Castellano R, Fares J, Camoin L, et al. Downregulation of stromal syntenin sustains AML development. EMBO Mol Med (2023) 15(11):e17570. doi: 10.15252/emmm.202317570
150. Kim MY, Yu K-R, Kenderian SS, Ruella M, Chen S, Shin T-H, et al. Genetic inactivation of CD33 in hematopoietic stem cells to enable CAR T cell immunotherapy for acute myeloid leukemia. Cell (2018) 173:1439–1453.e19. doi: 10.1016/j.cell.2018.05.013
151. Borot F, Wang H, Ma Y, Jafarov T, Raza A, Ali AM, et al. Gene-edited stem cells enable CD33-directed immune therapy for myeloid Malignancies. Proc Natl Acad Sci U.S.A. (2019) 116:11978–87. doi: 10.1073/pnas.1819992116
152. Liu J, Tong J, Yang H. Targeting CD33 for acute myeloid leukemia therapy. BMC Cancer (2022) 22:24. doi: 10.1186/s12885-021-09116-5
153. Chen Y, Liu Q, Xing H, Rao Q, Wang M, Mi Y, et al. Acute myeloid leukemia fusion genes can be found in CD33-negative cells. Int J Lab Hematol (2022) 44:1111–4. doi: 10.1111/ijlh.13942
154. Qin H, Yang L, Chukinas JA, Shah N, Tarun S, Pouzolles M, et al. Systematic preclinical evaluation of CD33-directed chimeric antigen receptor T cell immunotherapy for acute myeloid leukemia defines optimized construct design. J Immunother Cancer (2021) 9:e003149. doi: 10.1136/jitc-2021-003149
155. Mecklenbrauck R, Heuser M. Resistance to targeted therapies in acute myeloid leukemia. Clin Exp Metastasis (2023) 40:33–44. doi: 10.1007/s10585-022-10189-0
156. Hino C, Xu Y, Xiao J, Baylink DJ, Reeves ME, Cao H. The potential role of the thymus in immunotherapies for acute myeloid leukemia. Front Immunol (2023) 14:1102517. doi: 10.3389/fimmu.2023.1102517
157. Schuster SJ, Svoboda J, Chong EA, Nasta SD, Mato AR, Anak Ö, et al. Chimeric antigen receptor T cells in refractory B-cell lymphomas. N Engl J Med (2017) 377:2545–54. doi: 10.1056/NEJMoa1708566
158. Warda W, Da Rocha MN, Trad R, Haderbache R, Salma Y, Bouquet L, et al. Overcoming target epitope masking resistance that can occur on low-antigen-expresser AML blasts after IL-1RAP chimeric antigen receptor T cell therapy using the inducible caspase 9 suicide gene safety switch. Cancer Gene Ther (2021) 28:1365–75. doi: 10.1038/s41417-020-00284-3
159. Pagliuca S, Gurnari C, Hercus C, Hergalant S, Hong S, Dhuyser A, et al. Leukemia relapse via genetic immune escape after allogeneic hematopoietic cell transplantation. Nat Commun (2023) 14:3153. doi: 10.1038/s41467-023-38113-4
160. An N, Pan Y, Yang L, Zhang Q, Deng S, Zhang Q, et al. Anti-acute myeloid leukemia activity of CD38-CAR-T cells with PI3Kδ Downregulation. Mol pharma (2023) 20:2426–35. doi: 10.1021/acs.molpharmaceut.2c00913
161. Leick MB, Silva H, Scarfò I, Larson R, Choi BD, Bouffard AA, et al. Non-cleavable hinge enhances avidity and expansion of CAR-T cells for acute myeloid leukemia. Cancer Cell (2022) 40:494–508.e5. doi: 10.1016/j.ccell.2022.04.001
162. Kent A, Crump LS, Davila E. Beyond αβ T cells: NK, iNKT, and γδT cell biology in leukemic patients and potential for off-the-shelf adoptive cell therapies for AML. Front Immunol (2023) 14:1202950. doi: 10.3389/fimmu.2023.1202950
163. Vanhooren J, Dobbelaere R, Derpoorter C, Deneweth L, van Camp L, Uyttebroeck A, et al. CAR-T in the treatment of acute myeloid leukemia: barriers and how to overcome them. Hemasphere (2023) 7:e937. doi: 10.1097/HS9.0000000000000937
164. Vadakekolathu J, Rutella S. Escape from T-cell targeting immunotherapies in acute myeloid leukemia. . Blood (2023). doi: 10.1182/blood.2023019961
165. Fu D, Zhang B, Wu S, Feng J, Jiang H. Molecular subtyping of acute myeloid leukemia through ferroptosis signatures predicts prognosis and deciphers the immune microenvironment. Front Cell Dev Biol (2023) 11:1207642. doi: 10.3389/fcell.2023.1207642
166. Abbas HA, Hao D, Tomczak K, Barrodia P, Im JS, Reville PK, et al. Single cell T cell landscape and T cell receptor repertoire profiling of AML in context of PD-1 blockade therapy. Nat Commun (2021) 12:6071. doi: 10.1038/s41467-021-26282-z
167. Bansal AK, Sharawat SK, Gupta R, Vishnubhatla S, Dhawan D, Bakhshi S. Regulatory T cells in pediatric AML are associated with disease load and their serial assessment suggests role in leukemogenesis. Am J Blood Res (2020) 10:90–6.
168. Williams P, Basu S, Garcia-Manero G, Hourigan CS, Oetjen KA, Cortes JE, et al. The distribution of T-cell subsets and the expression of immune checkpoint receptors and ligands in patients with newly diagnosed and relapsed acute myeloid leukemia. Cancer (2019) 125:1470–81. doi: 10.1002/cncr.31896
169. Drumheller B, Gebre K, Lockhart B, Margolskee E, Obstfeld A, Paessler M, et al. Haematology laboratory parameters to assess efficacy of CD19-, CD22-, CD33-, and CD123-directed chimeric antigen receptor T-cell therapy in haematological Malignancies. Int J Lab Hematol (2022) 44:750–8. doi: 10.1111/ijlh.13850
170. Riva C, Vernarecci C, Minetto P, Goda R, Greppi M, Pesce S, et al. Harnessing immune response in acute myeloid leukemia. J Clin Med (2023) 12:5824. doi: 10.3390/jcm12185824
171. Chen EC, Garcia JS. Immunotherapy for acute myeloid leukemia: current trends, challenges, and strategies. Acta Haematol (2023), 1–21. doi: 10.1159/000533990
172. Richards RM, Zhao F, Freitas KA, Parker KR, Xu P, Fan A, et al. NOT-gated CD93 CAR T cells effectively target AML with minimized endothelial cross-reactivity. Blood Cancer Discovery (2021) 2:648–65. doi: 10.1158/2643-3230.BCD-20-0208
173. Haubner S, Mansilla-Soto J, Nataraj S, Kogel F, Chang Q, de StanChina E, et al. Cooperative CAR targeting to selectively eliminate AML and minimize escape. Cancer Cell (2023) 41(11):1871–91. doi: 10.1016/j.ccell.2023.09.010
174. Marone R, Landmann E, Devaux A, Lepore R, Seyres D, Zuin J, et al. Epitope-engineered human hematopoietic stem cells are shielded from CD123-targeted immunotherapy. J Exp Med (2023) 220. doi: 10.1084/jem.20231235
175. Casirati G, Cosentino A, Mucci A, Salah Mahmoud M, Ugarte Zabala I, Zeng J, et al. Epitope editing enables targeted immunotherapy of acute myeloid leukaemia. Nature (2023) 621:404–14. doi: 10.1038/s41586-023-06496-5
176. Naef P, Radpour R, Jaeger-Ruckstuhl CA, Bodmer N, Baerlocher GM, Doehner H, et al. IL-33-ST2 signaling promotes stemness in subtypes of myeloid leukemia cells through the Wnt and Notch pathways. Sci Signal (2023) 16:eadd7705. doi: 10.1126/scisignal.add7705
177. Nelde A, Schuster H, Heitmann JS, Bauer J, Maringer Y, Zwick M, et al. Immune surveillance of acute myeloid leukemia is mediated by HLA-presented antigens on leukemia progenitor cells. Blood Cancer Discovery (2023) 4(6):468–89. doi: 10.1158/2643-3230.BCD-23-0020
178. Pasvolsky O, Daher M, Alatrash G, Marin D, Daver N, Ravandi F, et al. CARving the path to allogeneic CAR T cell therapy in acute myeloid leukemia. Front Oncol (2021) 11:800110. doi: 10.3389/fonc.2021.800110
179. Bouquet L, Bôle-Richard E, Warda W, Da Neto Rocha M, Trad R, Nicod C, et al. RapaCaspase-9-based suicide gene applied to the safety of IL-1RAP CAR-T cells. Gene Ther (2023) 30:706–13. doi: 10.1038/s41434-023-00404-2
180. Sommer C, Cheng H-Y, Nguyen D, Dettling D, Yeung YA, Sutton J, et al. Allogeneic FLT3 CAR T cells with an off-switch exhibit potent activity against AML and can be depleted to expedite bone marrow recovery. Mol Ther J Am Soc Gene Ther (2020) 28:2237–51. doi: 10.1016/j.ymthe.2020.06.022
181. Ehninger G, Kraus S, Sala E, Metzelder SK, Vucinic V, Fiedler W, et al. Phase 1 dose escalation study of the rapidly switchable universal CAR-T therapy unicar-T-CD123 in relapsed/refractory AML. Blood (2022) 140:2367–8. doi: 10.1182/blood-2022-168877
182. Peschke JC, Bergmann R, Mehnert M, Gonzalez Soto KE, Loureiro LR, Mitwasi N, et al. FLT3-directed UniCAR T-cell therapy of acute myeloid leukaemia. Br J haematol (2023) 202:1137–50. doi: 10.1111/bjh.18971
183. Nixdorf D, Sponheimer M, Berghammer D, Engert F, Bader U, Philipp N, et al. Adapter CAR T cells to counteract T-cell exhaustion and enable flexible targeting in AML. Leukemia (2023) 37:1298–310. doi: 10.1038/s41375-023-01905-0
184. Nian Z, Zheng X, Dou Y, Du X, Zhou L, Fu B, et al. Rapamycin pretreatment rescues the bone marrow AML cell elimination capacity of CAR-T cells. Clin Cancer Res (2021) 27:6026–38. doi: 10.1158/1078-0432.CCR-21-0452
185. El Khawanky N, Hughes A, Yu W, Myburgh R, Matschulla T, Taromi S, et al. Demethylating therapy increases anti-CD123 CAR T cell cytotoxicity against acute myeloid leukemia. Nat Commun (2021) 12:6436. doi: 10.1038/s41467-021-26683-0
186. You L, Han Q, Zhu L, Zhu Y, Bao C, Yang C, et al. Decitabine-mediated epigenetic reprograming enhances anti-leukemia efficacy of CD123-targeted chimeric antigen receptor T-cells. Front Immunol (2020) 11:1787. doi: 10.3389/fimmu.2020.01787
187. Wang Y, Tong C, Dai H, Wu Z, Han X, Guo Y, et al. Low-dose decitabine priming endows CAR T cells with enhanced and persistent antitumour potential via epigenetic reprogramming. Nat Commun (2021) 12:409. doi: 10.1038/s41467-020-20696-x
188. Badar T, Manna A, Gadd ME, Kharfan-Dabaja MA, Qin H. Prospect of CAR T-cell therapy in acute myeloid leukemia. Expert Opin Investig Drugs (2022) 31:211–20. doi: 10.1080/13543784.2022.2032642
189. Daver N, Alotaibi AS, Bücklein V, Subklewe M. T-cell-based immunotherapy of acute myeloid leukemia: current concepts and future developments. Leukemia (2021) 35:1843–63. doi: 10.1038/s41375-021-01253-x
190. Holtan SG, Savid-Frontera C, Walton K, Eaton AA, Demorest C, Hoeschen A, et al. Human effectors of acute and chronic GVHD overexpress CD83 and predict mortality. Clin Cancer Res an Off J Am Assoc Cancer Res (2023) 29:1114–24. doi: 10.1158/1078-0432.CCR-22-2837
191. Voorhees TJ, Grover NS, Beaven A, Park SI, Morrison JK, Cheng CJ, et al. Retrospective cohort study analyzing the safety and efficacy of anti-PD-1 therapy following CD30 CAR-T cell therapy in relapsed/refractory hodgkin lymphoma. Blood (2019) 134:3233. doi: 10.1182/blood-2019-122846
192. Dhakal P, Bates M, Tomasson MH, Sutamtewagul G, Dupuy A, Bhatt VR. Acute myeloid leukemia resistant to venetoclax-based therapy: What does the future hold? Blood Rev (2023) 59:101036. doi: 10.1016/j.blre.2022.101036
193. Liberatore C, Di Ianni M. Novel approaches to treatment of acute myeloid leukemia relapse post allogeneic stem cell transplantation. Int J Mol Sci (2023) 24:15019. doi: 10.3390/ijms241915019
194. Jia B, Zhao C, Minagawa K, Shike H, Claxton DF, Ehmann WC, et al. Acute myeloid leukemia causes T cell exhaustion and depletion in a humanized graft-versus-leukemia model. J Immunol (2023) 211:1426–37. doi: 10.4049/jimmunol.2300111
195. Wen J, Chen Y, Yang J, Dai C, Yu S, Zhong W, et al. Valproic acid increases CAR T cell cytotoxicity against acute myeloid leukemia. J Immunother Cancer (2023) 11:e006857. doi: 10.1136/jitc-2023-006857
196. Cummins K, Gill S. Chimeric antigen receptor T cells in acute myeloid leukemia. Hematol Oncol Clin North Am (2023) 37(6):1125–47. doi: 10.1016/j.hoc.2023.06.004
197. Gale RP. Can immune therapy cure acute myeloid leukemia? Curr. Treat Options Oncol (2023) 24:381–6. doi: 10.1007/s11864-023-01066-3
198. Perna F, Berman SH, Soni RK, Mansilla-Soto J, Eyquem J, Hamieh M, et al. Integrating proteomics and transcriptomics for systematic combinatorial chimeric antigen receptor therapy of AML. Cancer Cell (2017) 32:506–519.e5. doi: 10.1016/j.ccell.2017.09.004
199. Gottschlich A, Thomas M, Grünmeier R, Lesch S, Rohrbacher L, Igl V, et al. Single-cell transcriptomic atlas-guided development of CAR-T cells for the treatment of acute myeloid leukemia. Nat Biotechnol (2023) 41:1618–32. doi: 10.1038/s41587-023-01684-0
200. Atilla E, Benabdellah K. The black hole: CAR T cell therapy in AML. Cancers (Basel) (2023) 15:2713. doi: 10.3390/cancers15102713
201. Shahzad M, Nguyen A, Hussain A, Ammad-Ud-Din M, Faisal MS, Tariq E, et al. Outcomes with chimeric antigen receptor t-cell therapy in relapsed or refractory acute myeloid leukemia: a systematic review and meta-analysis. Front Immunol (2023) 14:1152457. doi: 10.3389/fimmu.2023.1152457
202. Yagyu S, Nakazawa Y. piggyBac-transposon-mediated CAR-T cells for the treatment of hematological and solid Malignancies. Int J Clin Oncol (2023) 28:736–47. doi: 10.1007/s10147-023-02319-9
203. Calviño C, Ceballos C, Alfonso A, Jauregui P, Calleja-Cervantes ME, San Martin-Uriz P, et al. Optimization of universal allogeneic CAR-T cells combining CRISPR and transposon-based technologies for treatment of acute myeloid leukemia. Front Immunol (2023) 14:1270843. doi: 10.3389/fimmu.2023.1270843
204. Peroni E, Randi ML, Rosato A, Cagnin S. Acute myeloid leukemia: from NGS, through scRNA-seq, to CAR-T. dissect cancer heterogeneity and tailor the treatment. J Exp Clin Cancer Res CR (2023) 42:259. doi: 10.1186/s13046-023-02841-8
205. Kantarjian H, O’Brien S, Jabbour E, Garcia-Manero G, Quintas-Cardama A, Shan J, et al. Improved survival in chronic myeloid leukemia since the introduction of imatinib therapy: a single-institution historical experience. Blood (2012) 119:1981–7. doi: 10.1182/blood-2011-08-358135
206. Bower H, Björkholm M, Dickman PW, Höglund M, Lambert PC, Andersson TM-L. Life expectancy of patients with chronic myeloid leukemia approaches the life expectancy of the general population. J Clin Oncol (2016) 34:2851–7. doi: 10.1200/JCO.2015.66.2866
207. Mahon F-X, Réa D, Guilhot J, Guilhot F, Huguet F, Nicolini F, et al. Discontinuation of imatinib in patients with chronic myeloid leukaemia who have maintained complete molecular remission for at least 2 years: the prospective, multicentre Stop Imatinib (STIM) trial. Lancet Oncol (2010) 11:1029–35. doi: 10.1016/S1470-2045(10)70233-3
208. Sun K, Zhang X, Wang Z, Chen Y, Zhang L, Cheng W, et al. Allogeneic CAR-T cell therapy for treatment of relapse after allo-HSCT in patients with refractory CML lymphoid blast crisis: significance of HLA matched donor/patient pair in the safety/efficacy of CAR-T cell therapy. Blood (2018) 132:4275. doi: 10.1182/blood-2018-99-115768
209. Mardiana S, Gill S. CAR T cells for acute myeloid leukemia: state of the art and future directions. Front Oncol (2020) 10:697. doi: 10.3389/fonc.2020.00697
210. Miao Y-R, Liu W, Zhong Z, You Y, Tang Y, Li W, et al. Case report: multi-omics analysis and CAR-T treatment of a chronic myeloid leukemia blast crisis case 5 years after the discontinuation of TKI. Front Oncol (2021) 11:739871. doi: 10.3389/fonc.2021.739871
211. Liu F, Sha S, Ma G, Su Y, Xiong Y, He G, et al. Treatment of CML-transformed B cell acute lymphoblastic leukemia (B-ALL) in adults with anti-CD19 chimeric antigen receptor T cell (CAR T) therapy. Stem Cell Rev Rep (2020) 16:1356–8. doi: 10.1007/s12015-020-10008-7
212. Venkataraman V, Casey KS, Onozato M, Cin PD, Nardi V, Amrein PC, et al. Long: molecular tracking of CML with bilineal inv(16) myeloid and del(9) lymphoid blast crisis and durable response to CD19-directed CAR-T therapy. Leukemia (2020) 34:3050–4. doi: 10.1038/s41375-020-0983-x
213. Zhou S, Li W, Xiao Y, Zhu X, Zhong Z, Li Q, et al. A novel chimeric antigen receptor redirecting T-cell specificity towards CD26+ cancer cells. Leukemia (2021) 35:119–29. doi: 10.1038/s41375-020-0824-y
214. Cui Q, Liang P, Dai H, Cui W, Cai M, Ding Z, et al. Case report: CD38-directed CAR-T cell therapy: A novel immunotherapy targeting CD38- positive blasts overcomes TKI and chemotherapy resistance of myeloid chronic myeloid leukemia in blastic phase. Front Immunol (2022) 13:1012981. doi: 10.3389/fimmu.2022.1012981
215. Warda W, Larosa F, Da Neto Rocha M, Trad R, Deconinck E, Fajloun Z, et al. CML hematopoietic stem cells expressing IL1RAP can be targeted by chimeric antigen receptor-engineered T cells. Cancer Res (2019) 79:663–75. doi: 10.1158/0008-5472.CAN-18-1078
216. Järås M, Johnels P, Hansen N, Agerstam H, Tsapogas P, Rissler M, et al. Isolation and killing of candidate chronic myeloid leukemia stem cells by antibody targeting of IL-1 receptor accessory protein. Proc Natl Acad Sci U.S.A. (2010) 107:16280–5. doi: 10.1073/pnas.1004408107
217. Frenay J, Bellaye P-S, Oudot A, Helbling A, Petitot C, Ferrand C, et al. IL-1RAP, a key therapeutic target in cancer. Int J Mol Sci (2022) 23:14918. doi: 10.3390/ijms232314918
218. Nicod C, Da Rocha MN, Warda W, Roussel X, Haderbache R, Seffar E, et al. CAR-T cells targeting IL-1RAP produced in a closed semiautomatic system are ready for the first phase I clinical investigation in humans. Curr Res Trans Med (2023) 71:103385. doi: 10.1016/j.retram.2023.103385
219. Haderbache R, Warda W, Hervouet E, Da Rocha MN, Trad R, Allain V, et al. Droplet digital PCR allows vector copy number assessment and monitoring of experimental CAR T cells in murine xenograft models or approved CD19 CAR T cell-treated patients. J Transl Med (2021) 19:265. doi: 10.1186/s12967-021-02925-z
220. Zhang H, Hu Y, Chang AH, Huang H. Successful treatment of T315I BCR-ABL mutated lymphoid blast phase chronic myeloid leukemia with chimeric antigen receptor T cell therapy followed by dasatinib. Regenerative Ther (2020) 14:40–2. doi: 10.1016/j.reth.2019.11.003
221. Pratap S, Zhao ZJ. Finding new lanes: Chimeric antigen receptor (CAR) T-cells for myeloid leukemia. Cancer Rep (2020) 3:e1222. doi: 10.1002/cnr2.1222
222. Matsushita M. Novel treatment strategies utilizing immune reactions against chronic myelogenous leukemia stem cells. Cancers (Basel) (2021) 13:5435. doi: 10.3390/cancers13215435
223. Jiang Y, Yao J, Yao Z, Huang Y, Li Z, Fan Z, et al. CD4 T cells in chronic myeloid leukemia present MHC class II-dependent and IFN-γ-dependent cytotoxic capacity. Med Oncol (2023) 40:253. doi: 10.1007/s12032-023-02123-5
224. Sharma S, Rai KR. Chronic lymphocytic leukemia (CLL) treatment: So many choices, such great options. Cancer (2019) 125:1432–40. doi: 10.1002/cncr.31931
225. Zinzi A, Gaio M, Liguori V, Cagnotta C, Paolino D, Paolisso G, et al. Late relapse after CAR-T cell therapy for adult patients with hematologic Malignancies: A definite evidence from systematic review and meta-analysis on individual data. Pharmacol Res (2023) 190:106742. doi: 10.1016/j.phrs.2023.106742
226. Peters FS, Kater AP. Increasing CART cell engine performance in CLL. Blood (2022) 139:473–4. doi: 10.1182/blood.2021013895
227. Petrackova A, Turcsanyi P, Papajik T, Kriegova E. Revisiting Richter transformation in the era of novel CLL agents. Blood Rev (2021) 49:100824. doi: 10.1016/j.blre.2021.100824
228. Abrisqueta P, Nadeu F, Bosch-Schips J, Iacoboni G, Serna A, Cabirta A, et al. From genetics to therapy: Unraveling the complexities of Richter transformation in chronic lymphocytic leukemia. Cancer Treat Rev (2023) 120:102619. doi: 10.1016/j.ctrv.2023.102619
229. Bajwa A, Voorhees TJ, Kittai AS. Cellular therapy advances in chronic lymphocytic leukemia and richter’s syndrome. Curr Probl Cancer (2022) 46:100827. doi: 10.1016/j.currproblcancer.2021.100827
230. Barbanti MC, Appleby N, Kesavan M, Eyre TA. Cellular therapy in high-risk relapsed/refractory chronic lymphocytic leukemia and richter syndrome. Front Oncol (2022) 12:888109. doi: 10.3389/fonc.2022.888109
231. Coombs CC, Easaw S, Grover NS, O’Brien SM. Cellular therapies in chronic lymphocytic leukemia and richter’s transformation: recent developments in chimeric antigen receptor T-cells, natural killer cells, and allogeneic stem cell transplant. Cancers (Basel) (2023) 15:1838. doi: 10.3390/cancers15061838
232. Maher N, Mouhssine S, Matti BF, Alwan AF, Gaidano G. Treatment refractoriness in chronic lymphocytic leukemia: old and new molecular biomarkers. Int J Mol Sci (2023) 24:10374. doi: 10.3390/ijms241210374
233. Pagano G, Botana IF, Wierz M, Roessner PM, Ioannou N, Zhou X, et al. Interleukin-27 potentiates CD8+ T-cell-mediated anti-tumor immunity in chronic lymphocytic leukemia. 1 (2023) 108(11):3011–24. doi: 10.3324/haematol.2022.282474
234. Fernández-Garnacho EM, Nadeu F, Martín S, Mozas P, Rivero A, Delgado J, et al. MALAT1 expression is associated with aggressive behavior in indolent B-cell neoplasms. Sci Rep (2023) 13:16839. doi: 10.1038/s41598-023-44174-8
235. Cox MJ, Lucien F, Sakemura R, Boysen JC, Kim Y, Horvei P, et al. Leukemic extracellular vesicles induce chimeric antigen receptor T cell dysfunction in chronic lymphocytic leukemia. Mol Ther J Am Soc Gene Ther (2021) 29:1529–40. doi: 10.1016/j.ymthe.2020.12.033
236. Can I, Cox MJ, Siegler EL, Sakemura R, Kenderian SS. Challenges of chimeric antigen receptor T-cell therapy in chronic lymphocytic leukemia: lessons learned. Exp Hematol (2022) 108:1–7. doi: 10.1016/j.exphem.2022.02.001
237. van Bruggen JA, Martens AW, Fraietta JA, Hofland T, Tonino SH, Eldering E, et al. Chronic lymphocytic leukemia cells impair mitochondrial fitness in CD8+ T cells and impede CAR T-cell efficacy. Blood (2019) 134:44–58. doi: 10.1182/blood.2018885863
238. Goral A, Sledz M, Manda-Handzlik A, Cieloch A, Wojciechowska A, Lachota M, et al. Regulatory T cells contribute to the immunosuppressive phenotype of neutrophils in a mouse model of chronic lymphocytic leukemia. Exp Hematol Oncol (2023) 12:89. doi: 10.1186/s40164-023-00452-9
239. van Bruggen JA, Martens AW, Tonino SH, Kater AP. Overcoming the hurdles of autologous T-cell-based therapies in B-cell non-hodgkin lymphoma. Cancers (Basel) (2020) 12:3837. doi: 10.3390/cancers12123837
240. Agarwal S, Aznar MA, Rech AJ, Good CR, Kuramitsu S, Da T, et al. Deletion of the inhibitory co-receptor CTLA-4 enhances and invigorates chimeric antigen receptor T cells. Immunity (2023) 56:2388–2407.e9. doi: 10.1016/j.immuni.2023.09.001
241. Peters FS, Strefford JC, Eldering E, Kater AP. T-cell dysfunction in chronic lymphocytic leukemia from an epigenetic perspective. Haematologica (2021) 106:1234–43. doi: 10.3324/haematol.2020.267914
242. Kong W, Dimitri A, Wang W, Jung I-Y, Ott CJ, Fasolino M, et al. BET bromodomain protein inhibition reverses chimeric antigen receptor extinction and reinvigorates exhausted T cells in chronic lymphocytic leukemia. J Clin Invest (2021) 131:1–16. doi: 10.1172/JCI145459
243. Bozorgmehr N, Hnatiuk M, Peters AC, Elahi S. Depletion of polyfunctional CD26highCD8+ T cells repertoire in chronic lymphocytic leukemia. Exp Hematol Oncol (2023) 12:13. doi: 10.1186/s40164-023-00375-5
244. Roessner PM, Llaó Cid L, Lupar E, Roider T, Bordas M, Schifflers C, et al. EOMES and IL-10 regulate antitumor activity of T regulatory type 1 CD4+ T cells in chronic lymphocytic leukemia. Leukemia (2021) 35:2311–24. doi: 10.1038/s41375-021-01136-1
245. Heyman BM, Tzachanis D, Kipps TJ. Recent advances in CAR T-cell therapy for patients with chronic lymphocytic leukemia. Cancers (Basel) (2022) 14:1715. doi: 10.3390/cancers14071715
246. Giannotta C, Autino F, Massaia M. Vγ9Vδ2 T-cell immunotherapy in blood cancers: ready for prime time? Front. Immunol (2023) 14:1167443. doi: 10.3389/fimmu.2023.1167443
247. Rozenbaum M, Meir A, Aharony Y, Itzhaki O, Schachter J, Bank I, et al. Gamma-delta CAR-T cells show CAR-directed and independent activity against leukemia. Front Immunol (2020) 11:1347. doi: 10.3389/fimmu.2020.01347
248. Rafia C, Loizeau C, Renoult O, Harly C, Pecqueur C, Joalland N, et al. The antitumor activity of human Vγ9Vδ2 T cells is impaired by TGF-β through significant phenotype, transcriptomic and metabolic changes. Front Immunol (2022) 13:1066336. doi: 10.3389/fimmu.2022.1066336
249. Du S-H, Li Z, Chen C, Tan W-K, Chi Z, Kwang TW, et al. Co-expansion of cytokine-induced killer cells and Vγ9Vδ2 T cells for CAR T-cell therapy. PloS One (2016) 11:e0161820. doi: 10.1371/journal.pone.0161820
250. Gao Z, Bai Y, Lin A, Jiang A, Zhou C, Cheng Q, et al. Gamma delta T-cell-based immune checkpoint therapy: attractive candidate for antitumor treatment. Mol Cancer (2023) 22:31. doi: 10.1186/s12943-023-01722-0
251. Katz OB, Yehudai-Ofir D, Zuckerman T. Cellular therapy in chronic lymphocytic leukemia: have we advanced in the last decade? Acta Haematol (2023) 1. doi: 10.1159/000534341
252. Mensurado S, Blanco-Domínguez R, Silva-Santos B. The emerging roles of γδ T cells in cancer immunotherapy. Nat Rev Clin Oncol (2023) 20:178–91. doi: 10.1038/s41571-022-00722-1
253. Farhangnia P, Ghomi SM, Mollazadehghomi S, Nickho H, Akbarpour M, Delbandi A-A. SLAM-family receptors come of age as a potential molecular target in cancer immunotherapy. Front Immunol (2023) 14:1174138. doi: 10.3389/fimmu.2023.1174138
254. Porter DL, Levine BL, Kalos M, Bagg A, June CH. Chimeric antigen receptor-modified T cells in chronic lymphoid leukemia. N Engl J Med (2011) 365:725–33. doi: 10.1056/NEJMoa1103849
255. Melenhorst JJ, Chen GM, Wang M, Porter DL, Chen C, Collins MA, et al. Decade-long leukaemia remissions with persistence of CD4+ CAR T cells. Nature (2022) 602:503–9. doi: 10.1038/s41586-021-04390-6
256. Hiramatsu H. Current status of CAR-T cell therapy for pediatric hematologic Malignancies. Int J Clin Oncol (2023) 28:729–35. doi: 10.1007/s10147-023-02346-6
257. Shadman M. Diagnosis and treatment of chronic lymphocytic leukemia: A review. JAMA (2023) 329:918–32. doi: 10.1001/jama.2023.1946
258. Cappell KM, Sherry RM, Yang JC, Goff SL, Vanasse DA, McIntyre L, et al. Long-term follow-up of anti-CD19 chimeric antigen receptor T-cell therapy. J Clin Oncol (2020) 38:3805–15. doi: 10.1200/JCO.20.01467
259. Siddiqi T, Maloney DG, Kenderian SS, Brander DM, Dorritie K, Soumerai J, et al. Lisocabtagene maraleucel in chronic lymphocytic leukaemia and small lymphocytic lymphoma (TRANSCEND CLL 004): a multicentre, open-label, single-arm, phase 1-2 study. Lancet (2023) 402:641–54. doi: 10.1016/S0140-6736(23)01052-8
260. Mueller KT, Maude SL, Porter DL, Frey N, Wood P, Han X, et al. Cellular kinetics of CTL019 in relapsed/refractory B-cell acute lymphoblastic leukemia and chronic lymphocytic leukemia. Blood (2017) 130:2317–25. doi: 10.1182/blood-2017-06-786129
261. Gao Z, Lian Y, Ti J, Ren R, Ma L. Therapeutic efficacy and infectious complications of CD19-targeted chimeric antigen receptor-modified T cell immunotherapy. Anti-Cancer Drugs (2023) 34:551–7. doi: 10.1097/CAD.0000000000001485
262. Liang EC, Albittar A, Huang JJ, Hirayama AV, Kimble EL, Portuguese AJ, et al. Factors associated with long-term outcomes of CD19 CAR T-cell therapy for relapsed/refractory CLL. Blood Adv (2023). doi: 10.1182/bloodadvances.2023011399
263. Fraietta JA, Lacey SF, Orlando EJ, Pruteanu-Malinici I, Gohil M, Lundh S, et al. Determinants of response and resistance to CD19 chimeric antigen receptor (CAR) T cell therapy of chronic lymphocytic leukemia. Nat Med (2018) 24:563–71. doi: 10.1038/s41591-018-0010-1
264. Kharfan-Dabaja MA, Yassine F, Gadd ME, Qin H. Driving out chronic lymphocytic leukemia with CAR T cells. Transplant Cell Ther (2022) 28:5–17. doi: 10.1016/j.jtct.2021.10.005
265. Ramos CA, Savoldo B, Torrano V, Ballard B, Zhang H, Dakhova O, et al. Clinical responses with T lymphocytes targeting Malignancy-associated κ light chains. J Clin Invest (2016) 126:2588–96. doi: 10.1172/JCI86000
266. Wang G, Sun X, Zuo S, Li C, Niu Q, Xia Y, et al. Homogeneously high expression of CD32b makes it a potential target for CAR-T therapy for chronic lymphocytic leukemia. J Hematol Oncol (2021) 14:149. doi: 10.1186/s13045-021-01160-9
267. Faitschuk E, Hombach AA, Frenzel LP, Wendtner C-M, Abken H. Chimeric antigen receptor T cells targeting Fc μ receptor selectively eliminate CLL cells while sparing healthy B cells. Blood (2016) 128:1711–22. doi: 10.1182/blood-2016-01-692046
268. Kovalovsky D, Yoon JH, Cyr MG, Simon S, Voynova E, Rader C, et al. Siglec-6 is a target for chimeric antigen receptor T-cell treatment of chronic lymphocytic leukemia. Leukemia (2021) 35:2581–91. doi: 10.1038/s41375-021-01188-3
269. Luo Y, Qie Y, Gadd ME, Manna A, Rivera-Valentin R, To T, et al. Translational development of a novel BAFF-R CAR-T therapy targeting B-cell lymphoid Malignancies. Cancer immunol immunother CII (2023), 1–17. doi: 10.1007/s00262-023-03537-w
270. Shadman M, Maloney DG. Immune therapy for chronic lymphocytic leukemia: allogeneic transplant, chimeric antigen receptor T-cell therapy, and beyond. Hematol Oncol Clin North Am (2021) 35:847–62. doi: 10.1016/j.hoc.2021.03.011
271. Funk CR, Wang S, Chen KZ, Waller A, Sharma A, Edgar CL, et al. PI3Kδ/γ inhibition promotes human CART cell epigenetic and metabolic reprogramming to enhance antitumor cytotoxicity. Blood (2022) 139:523–37. doi: 10.1182/blood.2021011597
272. Stock S, Übelhart R, Schubert M-L, Fan F, He B, Hoffmann J-M, et al. Idelalisib for optimized CD19-specific chimeric antigen receptor T cells in chronic lymphocytic leukemia patients. Int J Cancer (2019) 145:1312–24. doi: 10.1002/ijc.32201
273. Gauthier J, Hirayama AV, Purushe J, Hay KA, Lymp J, Li DH, et al. Feasibility and efficacy of CD19-targeted CAR T cells with concurrent ibrutinib for CLL after ibrutinib failure. Blood (2020) 135:1650–60. doi: 10.1182/blood.2019002936
274. Fan F, Yoo HJ, Stock S, Wang L, Liu Y, Schubert M-L, et al. Ibrutinib for improved chimeric antigen receptor T-cell production for chronic lymphocytic leukemia patients. Int J Cancer (2021) 148:419–28. doi: 10.1002/ijc.33212
275. Tettamanti S, Rotiroti MC, Giordano Attianese GM, Arcangeli S, Zhang R, Banerjee P, et al. Lenalidomide enhances CD23.CAR T cell therapy in chronic lymphocytic leukemia. Leuk Lymphoma (2022) 63:1566–79. doi: 10.1080/10428194.2022.2043299
276. Shah PS, Jacobson CA. Chimeric antigen receptor T-cells in indolent lymphoma, mantle cell lymphoma, chronic lymphocytic leukemia. Hematol Oncol Clin North Am (2023) 37:1077–88. doi: 10.1016/j.hoc.2023.07.002
277. Turtle CJ, Berger C, Sommermeyer D, Hanafi L-A, Pender B, Robinson EM, et al. Anti-CD19 chimeric antigen receptor-modified T cell therapy for B cell non-hodgkin lymphoma and chronic lymphocytic leukemia: fludarabine and cyclophosphamide lymphodepletion improves in vivo expansion and persistence of CAR-T cells and clinical outcomes. Blood (2015) 126:184. doi: 10.1182/blood.V126.23.184.184
278. Leclercq G, Steinhoff N, Haegel H, de Marco D, Bacac M, Klein C. Novel strategies for the mitigation of cytokine release syndrome induced by T cell engaging therapies with a focus on the use of kinase inhibitors. Oncoimmunology (2022) 11:2083479. doi: 10.1080/2162402X.2022.2083479
279. Iovino L, Shadman M. CAR T-cell therapy for CLL: a new addition to our treatment toolbox? Clin Adv Hematol Oncol H&O (2023) 21:134–41.
280. Zhao Z, Grégoire C, Oliveira B, Chung K, Melenhorst JJ. Challenges and opportunities of CAR T-cell therapies for CLL. Semin Hematol (2023) 60:25–33. doi: 10.1053/j.seminhematol.2023.01.002
281. Vitale C, Strati P. CAR T-cell therapy for B-cell non-hodgkin lymphoma and chronic lymphocytic leukemia: clinical trials and real-world experiences. Front Oncol (2020) 10:849. doi: 10.3389/fonc.2020.00849
282. Todorovic Z, Todorovic D, Markovic V, Ladjevac N, Zdravkovic N, Djurdjevic P, et al. CAR T cell therapy for chronic lymphocytic leukemia: successes and shortcomings. Curr Oncol (2022) 29:3647–57. doi: 10.3390/curroncol29050293
283. Porter DL, Hwang W-T, Frey NV, Lacey SF, Shaw PA, Loren AW, et al. Chimeric antigen receptor T cells persist and induce sustained remissions in relapsed refractory chronic lymphocytic leukemia. Sci Trans Med (2015) 7:303ra139. doi: 10.1126/scitranslmed.aac5415
284. Harr MW, Lavik A, McColl K, Zhong F, Haberer B, Aldabbagh K, et al. A novel peptide that disrupts the lck-IP3R protein-protein interaction induces widespread cell death in leukemia and lymphoma. Arch Microbiol Immunol (2023) 7:165–77. doi: 10.26502/ami.936500114
285. Mohty R, Dulery R, Bazarbachi AH, Savani M, Hamed RA, Bazarbachi A, et al. Latest advances in the management of classical Hodgkin lymphoma: the era of novel therapies. Blood Cancer J (2021) 11:126. doi: 10.1038/s41408-021-00518-z
286. Maaroufi M. Immunotherapy for Hodgkin lymphoma: From monoclonal antibodies to chimeric antigen receptor T-cell therapy. Crit Rev Oncology/Hematol (2023) 182:103923. doi: 10.1016/j.critrevonc.2023.103923
287. Andrade-Gonzalez X, Ansell SM. Novel therapies in the treatment of hodgkin lymphoma. Curr Treat Options Oncol (2021) 22:42. doi: 10.1007/s11864-021-00840-5
288. Savoldo B, Rooney CM, Di Stasi A, Abken H, Hombach A, Foster AE, et al. Epstein Barr virus specific cytotoxic T lymphocytes expressing the anti-CD30zeta artificial chimeric T-cell receptor for immunotherapy of Hodgkin disease. Blood (2007) 110:2620–30. doi: 10.1182/blood-2006-11-059139
289. Abbasi S, Totmaj MA, Abbasi M, Hajazimian S, Goleij P, Behroozi J, et al. Chimeric antigen receptor T (CAR-T) cells: Novel cell therapy for hematological Malignancies. Cancer Med (2023) 12:7844–58. doi: 10.1002/cam4.5551
290. Meier JA, Savoldo B, Grover NS. The emerging role of CAR T cell therapy in relapsed/refractory hodgkin lymphoma. J Personal Med (2022) 12:197. doi: 10.3390/jpm12020197
291. Watanabe M, Hatsuse H, Nagao K, Nakashima M, Uchimaru K, Otsu M, et al. CD30 induces Reed-Sternberg cell-like morphology and chromosomal instability in classic Hodgkin lymphoma cell lines. Cancer Sci (2023) 114:3433–45. doi: 10.1111/cas.15874
292. Wang C-M, Wu Z-Q, Wang Y, Guo Y-L, Dai H-R, Wang X-H, et al. Autologous T cells expressing CD30 chimeric antigen receptors for relapsed or refractory hodgkin lymphoma: an open-label phase I trial. Clin Cancer Res (2017) 23:1156–66. doi: 10.1158/1078-0432.CCR-16-1365
293. Ramos CA, Ballard B, Zhang H, Dakhova O, Gee AP, Mei Z, et al. Clinical and immunological responses after CD30-specific chimeric antigen receptor-redirected lymphocytes. J Clin Invest (2017) 127:3462–71. doi: 10.1172/JCI94306
294. Ramos CA, Grover NS, Beaven AW, Lulla PD, Wu M-F, Ivanova A, et al. Anti-CD30 CAR-T cell therapy in relapsed and refractory hodgkin lymphoma. J Clin Oncol (2020) 38:3794–804. doi: 10.1200/JCO.20.01342
295. Kim DH, Vega F. Relapsed classic Hodgkin lymphoma with decreased CD30 expression after brentuximab and anti-CD30 CAR-T therapies. Blood (2022) 139:951. doi: 10.1182/blood.2021013881
296. Aoki T, Chong LC, Takata K, Milne K, Hav M, Colombo A, et al. Single-cell transcriptome analysis reveals disease-defining T-cell subsets in the tumor microenvironment of classic hodgkin lymphoma. Cancer Discovery (2020) 10:406–21. doi: 10.1158/2159-8290.CD-19-0680
297. Georgoulis V, Papoudou-Bai A, Makis A, Kanavaros P, Hatzimichael E. Unraveling the immune microenvironment in classic hodgkin lymphoma: prognostic and therapeutic implications. Biology (2023) 12:862. doi: 10.3390/biology12060862
298. Svoboda J, Rheingold SR, Gill SI, Grupp SA, Lacey SF, Kulikovskaya I, et al. Nonviral RNA chimeric antigen receptor-modified T cells in patients with Hodgkin lymphoma. Blood (2018) 132:1022–6. doi: 10.1182/blood-2018-03-837609
299. Ruella M, Klichinsky M, Kenderian SS, Shestova O, Ziober A, Kraft DO, et al. Overcoming the immunosuppressive tumor microenvironment of hodgkin lymphoma using chimeric antigen receptor T cells. Cancer Discovery (2017) 7:1154–67. doi: 10.1158/2159-8290.CD-16-0850
300. Ho C, Ruella M, Levine BL, Svoboda J. Adoptive T-cell therapy for Hodgkin lymphoma. Blood Adv (2021) 5:4291–302. doi: 10.1182/bloodadvances.2021005304
301. Di Stasi A, de Angelis B, Rooney CM, Zhang L, Mahendravada A, Foster AE, et al. T lymphocytes coexpressing CCR4 and a chimeric antigen receptor targeting CD30 have improved homing and antitumor activity in a Hodgkin tumor model. Blood (2009) 113:6392–402. doi: 10.1182/blood-2009-03-209650
302. Marques-Piubelli ML, Kim DH, Medeiros LJ, Lu W, Khan K, Gomez-Bolanos LI, et al. CD30 expression is frequently decreased in relapsed classic Hodgkin lymphoma after anti-CD30 CAR T-cell therapy. Histopathology (2023) 83(1):143–8. doi: 10.1111/his.14910
303. Sadaf H, Ambroziak M, Binkowski R, Kluebsoongnoen J, Paszkiewicz-Kozik E, Steciuk J, et al. New molecular targets in Hodgkin and Reed-Sternberg cells. Front Immunol (2023) 14:1155468. doi: 10.3389/fimmu.2023.1155468
304. Voorhees TJ, Zhao B, Oldan J, Hucks G, Khandani A, Dittus C, et al. Pretherapy metabolic tumor volume is associated with response to CD30 CAR T cells in Hodgkin lymphoma. Blood Adv (2022) 6:1255–63. doi: 10.1182/bloodadvances.2021005385
305. Alvarez-Fernández C, Escribà-Garcia L, Caballero AC, Escudero-López E, Ujaldón-Miró C, Montserrat-Torres R, et al. Memory stem T cells modified with a redesigned CD30-chimeric antigen receptor show an enhanced antitumor effect in Hodgkin lymphoma. Clin Trans Immunol (2021) 10:e1268. doi: 10.1002/cti2.1268
306. Hazane Leroyer E, Ziegler C, Moulin C, Campidelli A, Jacquet C, Rubio MT, et al. Filling the gap: the immune therapeutic armamentarium for relapsed/refractory hodgkin lymphoma. J Clin Med (2022) 11. doi: 10.3390/jcm11216574
307. Grover NS, Savoldo B. Challenges of driving CD30-directed CAR-T cells to the clinic. BMC Cancer (2019) 19:203. doi: 10.1186/s12885-019-5415-9
308. Sang W, Wang X, Geng H, Li T, Li D, Zhang B, et al. Anti-PD-1 therapy enhances the efficacy of CD30-directed chimeric antigen receptor T cell therapy in patients with relapsed/refractory CD30+ Lymphoma. Front Immunol (2022) 13:858021. doi: 10.3389/fimmu.2022.858021
309. Zhang P, Yang X, Cao Y, Wang J, Zhou M, Chen L, et al. Autologous stem cell transplantation in tandem with Anti-CD30 CAR T-cell infusion in relapsed/refractory CD30+ lymphoma. Exp Hematol Oncol (2022) 11:72. doi: 10.1186/s40164-022-00323-9
310. Maranzano M, Mead M. The role of transplantation in Hodgkin lymphoma. Front Oncol (2022) 12:1054314. doi: 10.3389/fonc.2022.1054314
311. Ahmed S, Flinn IW, Mei M, Riedell PA, Armand P, Grover NS, et al. Updated results and correlative analysis: autologous CD30.CAR-T-cell therapy in patients with relapsed or refractory classical hodgkin lymphoma (CHARIOT trial). Blood (2022) 140:7496–7. doi: 10.1182/blood-2022-158869
312. Ullah F, Dima D, Omar N, Ogbue O, Ahmed S. Advances in the treatment of Hodgkin lymphoma: Current and future approaches. Front Oncol (2023) 13:1067289. doi: 10.3389/fonc.2023.1067289
313. Che Y, Sun X. Recent advances in CAR T-cell therapy for lymphoma in China. Clin Trans Oncol Off Publ Fed Spanish Oncol Societies Natl Cancer Instit Mexico (2023) 25:2793–2800. doi: 10.1007/s12094-023-03153-1
314. Randall MP, Spinner MA. Optimizing treatment for relapsed/refractory classic hodgkin lymphoma in the era of immunotherapy. Cancers (Basel) (2023) 15:4509. doi: 10.3390/cancers15184509
315. Crombie J, LaCasce A. The treatment of Burkitt lymphoma in adults. Blood (2021) 137:743–50. doi: 10.1182/blood.2019004099
316. Roschewski M, Staudt LM, Wilson WH. Burkitt’s lymphoma. N Engl J Med (2022) 387:1111–22. doi: 10.1056/NEJMra2025746
317. Evens AM, Danilov A, Jagadeesh D, Sperling A, Kim S-H, Vaca R, et al. Burkitt lymphoma in the modern era: real-world outcomes and prognostication across 30 US cancer centers. Blood (2021) 137:374–86. doi: 10.1182/blood.2020006926
318. Avigdor A, Shouval R, Jacoby E, Davidson T, Shimoni A, Besser M, et al. CAR T cells induce a complete response in refractory Burkitt Lymphoma. Bone marrow Transplant (2018) 53:1583–5. doi: 10.1038/s41409-018-0235-0
319. Du J, Zhang Y. Sequential anti-CD19, 22, and 20 autologous chimeric antigen receptor T-cell (CAR-T) treatments of a child with relapsed refractory Burkitt lymphoma: a case report and literature review. J Cancer Res Clin Oncol (2020) 146:1575–82. doi: 10.1007/s00432-020-03198-7
320. Nattes T, Camus V, François A, Dallet G, Ferrand C, Guerrot D, et al. Kidney transplant T cell-mediated rejection occurring after anti-CD19 CAR T-cell therapy for refractory aggressive burkitt-like lymphoma with 11q aberration: A case report. Am J Kidney Dis (2022) 79:760–4. doi: 10.1053/j.ajkd.2021.07.012
321. Portuguese AJ, Gauthier J, Tykodi SS, Hall ET, Hirayama AV, Yeung CC, et al. CD19 CAR-T therapy in solid organ transplant recipients: case report and systematic review. Bone marrow Transplant (2023) 58:353–9. doi: 10.1038/s41409-022-01907-z
322. Seitter SJ, McClelland PH, Ahlman MA, Goff SL, Yang JC, McIntyre L, et al. Durable remissions in two adult patients with Burkitt lymphoma following anti-CD19 CAR T-cell therapy: a single center experience. Leuk Lymphoma (2022) 63:2469–73. doi: 10.1080/10428194.2022.2076853
323. Wang T, Feng M, Luo C, Wan X, Pan C, Tang J, et al. Successful Treatment of Pediatric Refractory Burkitt Lymphoma PTLD after Liver Transplantation using Anti-CD19 Chimeric Antigen Receptor T-Cell Therapy. Cell Transplant (2021) 30:963689721996649. doi: 10.1177/0963689721996649
324. Ye M, Gao L, Wang T, Yu J, Gui J, Yang J. CD19 chimeric antigen receptor T-cell therapy following autologous stem cell transplantation against relapsed or refractory Burkitt lymphoma/leukemia: A case report and literature review. Front Oncol (2022) 12:932254. doi: 10.3389/fonc.2022.932254
325. Zhang Q, Zhu X, Liu B, Zhang Y, Xiao Y. Case report: Sandwich therapy of CAR-T combined with ASCT: Sequential CAR-T cell therapy with ASCT after remission with CAR-T therapy caused long-term survival in a patient with relapsed/refractory Burkitt’s lymphoma with TP53 mutations. Front Immunol (2023) 14:1127868. doi: 10.3389/fimmu.2023.1127868
326. Zhou W, Chen W, Wan X, Luo C, Du X, Li X, et al. Benefits of chimeric antigen receptor T-cell therapy for B-cell lymphoma. Front Genet (2021) 12:815679. doi: 10.3389/fgene.2021.815679
327. Zhou X, Ge T, Li T, Huang L, Cao Y, Xiao Y, et al. CAR19/22 T cell therapy in adult refractory Burkitt’s lymphoma. Cancer immunol immunother CII (2021) 70:2379–84. doi: 10.1007/s00262-021-02850-6
328. Geerts PA, ‘t Hart N, Visser O, Ortiz-Maldonado V, Chamuleau ME. Chemotherapy resistant Burkitt lymphoma: Possible novel therapies including CAR-T cell infusion. Clin Case Rep (2023) 11:e7361. doi: 10.1002/ccr3.7361
329. Yang X, Luo C, Qian J, Huang X, Zhang J, Wang J, et al. Case Report: Unedited allogeneic chimeric antigen receptor T cell bridging to conditioning-free hematopoietic stem cell transplantation for a child with refractory Burkitt lymphoma. Front Immunol (2023) 14:1219872. doi: 10.3389/fimmu.2023.1219872
330. Hsieh EM, Rouce RH. Chimeric antigen receptor T cells for mature B-cell lymphoma and Burkitt lymphoma. Hematol Am Soc Hematol Educ Program (2020) 2020:487–93. doi: 10.1182/hematology.2020000133
331. Pearson AD, Rossig C, Mackall C, Shah NN, Baruchel A, Reaman G, et al. Paediatric Strategy Forum for medicinal product development of chimeric antigen receptor T-cells in children and adolescents with cancer: ACCELERATE in collaboration with the European Medicines Agency with participation of the Food and Drug Administration. Eur J Cancer (2022) 160:112–33. doi: 10.1016/j.ejca.2021.10.016
332. Laurent C, Syrykh C, Hamon M, Adélaïde J, Guille A, Escudié F, et al. Resistance of B-cell lymphomas to CAR T-cell therapy is associated with genomic tumor changes which can result in transdifferentiation. Am J Surg Pathol (2022) 46:742–53. doi: 10.1097/PAS.0000000000001834
333. Zhang W, Yang J, Zhou C, Hu B, Jin L, Deng B, et al. Early response observed in pediatric patients with relapsed/refractory Burkitt lymphoma treated with chimeric antigen receptor T cells. Blood (2020) 135:2425–7. doi: 10.1182/blood.2019002008
334. Liu Y, Deng B, Hu B, Zhang W, Zhu Q, Liu Y, et al. Sequential different B-cell antigen-targeted CAR T-cell therapy for pediatric refractory/relapsed Burkitt lymphoma. Blood Adv (2022) 6:717–30. doi: 10.1182/bloodadvances.2021004557
335. Wu J, Cao Y, Zhang Q, Liu W, Zhou X, Ming X, et al. Chimeric antigen receptor-modified T cell immunotherapy for relapsed and refractory adult burkitt lymphoma. Front Immunol (2022) 13:879983. doi: 10.3389/fimmu.2022.879983
336. Cheng L, Huang M, Cao Y, Wang J, Xu H, Jiang L. Allogeneic CAR-T bridging to allo-HSCT as a treatment strategy for refractory adult Burkitt’s lymphoma: a case report. Trans Cancer Res (2022) 11:2996–3002. doi: 10.21037/tcr-22-174
337. Hubbeling H, Silverman EA, Michaud L, Tomas AA, Shouval R, Flynn J, et al. Bridging radiation rapidly and effectively cytoreduces high-risk relapsed/refractory aggressive B cell lymphomas prior to chimeric antigen receptor T cell therapy. Transplant Cell Ther (2023) 29:259.e1–259.e10. doi: 10.1016/j.jtct.2022.12.021
338. Chu Y, Yahr A, Huang B, Ayello J, Barth M, Cairo MS. Romidepsin alone or in combination with anti-CD20 chimeric antigen receptor expanded natural killer cells targeting Burkitt lymphoma in vitro and in immunodeficient mice. Oncoimmunology (2017) 6:e1341031. doi: 10.1080/2162402X.2017.1341031
339. Braun T, Pruene A, Darguzyte M, vom Stein AF, Nguyen P-H, Wagner DL, et al. Non-viral TRAC-knocked-in CD19KICAR-T and gp350KICAR-T cells tested against Burkitt lymphomas with type 1 or 2 EBV infection: In vivo cellular dynamics and potency. Front Immunol (2023) 14:1086433. doi: 10.3389/fimmu.2023.1086433
340. Slabik C, Kalbarczyk M, Danisch S, Zeidler R, Klawonn F, Volk V, et al. CAR-T cells targeting epstein-barr virus gp350 validated in a humanized mouse model of EBV infection and lymphoproliferative disease. Mol Ther oncol (2020) 18:504–24. doi: 10.1016/j.omto.2020.08.005
341. Zhang Y, Lyu H, Guo R, Cao X, Feng J, Jin X, et al. Epstein-Barr virus-associated cellular immunotherapy. Cytotherapy (2023) 25(9):903–12. doi: 10.1016/j.jcyt.2023.04.003
342. Meléndez AV, Velasco Cárdenas RM-H, Lagies S, Strietz J, Siukstaite L, Thomas OS, et al. Novel lectin-based chimeric antigen receptors target Gb3-positive tumour cells. Cell Mol Life Sci (2022) 79:513. doi: 10.1007/s00018-022-04524-7
343. Mendez-Hernandez A, Moturi K, Hanson V, Andritsos LA. Hairy cell leukemia: where are we in 2023? Curr Oncol Rep (2023) 25:833–40. doi: 10.1007/s11912-023-01419-z
344. Paillassa J, Troussard X. Patients with relapsed/refractory hairy-cell leukemia. Cancer Rep (2022) 5:e1495. doi: 10.1002/cnr2.1495
345. Troussard X, Maître E, Cornet E. Hairy cell leukemia 2022: Update on diagnosis, risk-stratification, and treatment. Am J Hematol (2022) 97:226–36. doi: 10.1002/ajh.26390
346. Liu Q, Harris N, Epperla N, Andritsos LA. Current and emerging therapeutic options for hairy cell leukemia variant. OncoTargets Ther (2021) 14:1797–805. doi: 10.2147/OTT.S242247
347. D’Addona M, Giudice V, Pezzullo L, Ciancia G, Baldi C, Gorrese M, et al. Hodgkin lymphoma and hairy cell leukemia arising from chronic lymphocytic leukemia: case reports and literature review. J Clin Med (2022) 11. doi: 10.3390/jcm11164674
348. Gargiulo E, Giordano M, Niemann CU, Moussay E, Paggetti J, Morande PE. The protective role of the microenvironment in hairy cell leukemia treatment: Facts and perspectives. Front Oncol (2023) 13:1122699. doi: 10.3389/fonc.2023.1122699
349. Maitre E, Cornet E, Salaün V, Kerneves P, Chèze S, Repesse Y, et al. Immunophenotypic analysis of hairy cell leukemia (HCL) and hairy cell leukemia-like (HCL-like) disorders. Cancers (Basel) (2022) 14. doi: 10.3390/cancers14041050
350. Cenariu D, Rus I, Bergthorsson JT, Grewal R, Cenariu M, Greiff V, et al. Flow cytometry of CD5-positive hairy cell leukemia. Mol Diagn Ther (2023) 27:593–9. doi: 10.1007/s40291-023-00658-x
351. Al-Zubaidi HK, Hughes SF. The use of CD200 in the differential diagnosis of B-cell lymphoproliferative disorders. Br J BioMed Sci (2023) 80:11573. doi: 10.3389/bjbs.2023.11573
352. Bhatti V, Kaur G, Ahuja A, Isaacs R. Hairy cell leukemia: hematological and immunophenotypic profile of 13 patients. Cureus (2023) 15:e44876. doi: 10.7759/cureus.44876
353. Shao H, Calvo KR, Grönborg M, Tembhare PR, Kreitman RJ, Stetler-Stevenson M, et al. Distinguishing hairy cell leukemia variant from hairy cell leukemia: development and validation of diagnostic criteria. Leukemia Res (2013) 37:401–9. doi: 10.1016/j.leukres.2012.11.021
354. Gorrese M, Guariglia R, Campana A, Bertolini A, Fresolone L, Martorelli MC, et al. Biclonality in hairy cell leukemia: Co-occurrence of CD10+ and CD10- clones with different surface membrane immunoglobulin expression. Cytometry Part B Clin cytometry (2022) 102:458–61. doi: 10.1002/cyto.b.22096
355. de Propris MS, Musiu P, Intoppa S, Nardacci MG, Pucciarini A, Santi A, et al. Hairy cell leukaemia with low CD103 expression: A rare but important diagnostic pitfall. Br J haematol (2022) 198:e28–31. doi: 10.1111/bjh.18224
356. Venkataraman G, Aguhar C, Kreitman RJ, Yuan CM, Stetler-Stevenson M. Characteristic CD103 and CD123 expression pattern defines hairy cell leukemia: usefulness of CD123 and CD103 in the diagnosis of mature B-cell lymphoproliferative disorders. Am J Clin Pathol (2011) 136:625–30. doi: 10.1309/AJCPKUM9J4IXCWEU
357. Nguyen TT, Do ATV, Phan Thi P, Anh LL, Vu MT. A case of atypical hairy cell leukemia with CD10+ and CD38+: diagnosis and treatment. Cureus (2022) 14:e3188. doi: 10.7759/cureus.31882
358. Maitre E, Cornet E, Debliquis A, Drenou B, Gravey F, Chollet D, et al. Hairy cell leukemia: a specific 17-gene expression signature points to new targets for therapy. J Cancer Res Clin Oncol (2022) 148:2013–22. doi: 10.1007/s00432-022-04010-4
359. Maitre E, Paillassa J, Troussard X. Novel targeted treatments in hairy cell leukemia and other hairy cell-like disorders. Front Oncol (2022) 12:1068981. doi: 10.3389/fonc.2022.1068981
360. Kipps TJ. ROR1: an orphan becomes apparent. Blood (2022) 140:1583–91. doi: 10.1182/blood.2021014760
361. Paillassa J, Safa F, Troussard X. Updates in hairy cell leukemia (HCL) and variant-type HCL (HCL-V): rationale for targeted treatments with a focus on ibrutinib. Ther Adv Hematol (2022) 13:20406207221090886. doi: 10.1177/20406207221090886
362. Testa U, Pelosi E, Castelli G. CD123 as a therapeutic target in the treatment of hematological Malignancies. Cancers (Basel) (2019) 11:1358. doi: 10.3390/cancers11091358
363. Sugita M, Guzman ML. CD123 as a therapeutic target against malignant stem cells. Hematol Oncol Clin North Am (2020) 34:553–64. doi: 10.1016/j.hoc.2020.01.004
364. Boutilier AJ, Huang L, Elsawa SF. Waldenström macroglobulinemia: mechanisms of disease progression and current therapies. Int J Mol Sci (2022) 23:11145. doi: 10.3390/ijms231911145
365. Smyth E, Cheah CY, Seymour JF. Management of indolent B-cell Lymphomas: A review of approved and emerging targeted therapies. Cancer Treat Rev (2023) 113:102510. doi: 10.1016/j.ctrv.2023.102510
366. Buske C, Jurczak W, Salem J-E, Dimopoulos MA. Managing Waldenström’s macroglobulinemia with BTK inhibitors. Leukemia (2023) 37:35–46. doi: 10.1038/s41375-022-01732-9
367. Amaador K, Kersten MJ, Minnema MC, Vos JM. Treatment of relapsed and refractory Waldenstrom Macroglobulinemia. Leuk Lymphoma (2023) 64:30–41. doi: 10.1080/10428194.2022.2131423
368. García-Sanz R, Tedeschi A. The management of relapsed or refractory waldenström’s macroglobulinemia. Hematol Oncol Clin North Am (2023) 37(4):727–49. doi: 10.1016/j.hoc.2023.04.006
369. Ahmed S, Zhao Q, Hanel W, Qazilbash MH, Patel K, Narra R, et al. Post-relapse survival in Waldenstrom macroglobulinemia patients experiencing therapy failure following autologous transplantation. Hematol Oncol (2022) 40:48–56. doi: 10.1002/hon.2946
370. Palomba ML, Qualls D, Monette S, Sethi S, Dogan A, Roshal M, et al. CD19-directed chimeric antigen receptor T cell therapy in Waldenström macroglobulinemia: a preclinical model and initial clinical experience. J Immunother Cancer (2022) 10. doi: 10.1136/jitc-2021-004128
371. Bansal R, Jurcic JG, Sawas A, Mapara MY, Reshef R. Chimeric antigen receptor T cells for treatment of transformed Waldenström macroglobulinemia. Leuk Lymphoma (2020) 61:465–8. doi: 10.1080/10428194.2019.1665668
372. Sarosiek S, Castillo JJ. Novel agents in waldenström macroglobulinemia. Hematol Oncol Clin North Am (2023) 37(4):751–60. doi: 10.1016/j.hoc.2023.04.001
373. Sacco A, Desantis V, Celay J, Giustini V, Rigali F, Savino FD, et al. Targeting the immune microenvironment in Waldenström Macroglobulinemia via halting the CD40/CD40-ligand axis. Blood (2023) 141(21):2615–28. doi: 10.1182/blood.2022019240
374. Qiu Y, Wang X, Yao Y, Si Y, Wang X, Jia M, et al. Single-cell transcriptome analysis reveals stem cell-like subsets in the progression of Waldenström’s macroglobulinemia. Exp Hematol Oncol (2023) 12:18. doi: 10.1186/s40164-023-00382-6
375. Sanchez E, Tanenbaum EJ, Patil S, Li M, Soof CM, Vidisheva A, et al. The clinical significance of B-cell maturation antigen as a therapeutic target and biomarker. Expert Rev Mol diagn (2018) 18:319–29. doi: 10.1080/14737159.2018.1448269
376. Marchand A, Swalchick WG, Wang H-Y. A fatal case of a CD5-positive lymphoplasmacytic lymphoma with associated AL-type amyloidosis. Int J Lab Hematol (2022) 44:995–6. doi: 10.1111/ijlh.13879
377. Kaiser LM, Hunter ZR, Treon SP, Buske C. CXCR4 in Waldenström’s Macroglobulinema: chances and challenges. Leukemia (2021) 35:333–45. doi: 10.1038/s41375-020-01102-3
378. Gayet M, Leymarie V, Derouault P, Guérin E, Vaidié J, Pascal V, et al. Flow cytometry detection of CD138 expression continuum between monotypic B and plasma cells is associated with both high IgM peak levels and MYD88 mutation and contributes to diagnosis of Waldenström macroglobulinemia. . Cytometry Part B Clin cytometry (2022) 102:62–9. doi: 10.1002/cyto.b.21995
379. Sajman J, Yakovian O, Unger Deshet N, Almog S, Horn G, Waks T, et al. Nanoscale CAR organization at the immune synapse correlates with CAR-T effector functions. Cells (2023) 12:2261. doi: 10.3390/cells12182261
380. Stephens DM, Byrd JC. Resistance to Bruton tyrosine kinase inhibitors: the Achilles heel of their success story in lymphoid Malignancies. Blood (2021) 138:1099–109. doi: 10.1182/blood.2020006783
381. Amaador K, Martens A, de Boer R, Rietveld J, Heemskerk M, Rutten CE, et al. T-cell subset composition and functionality in patients with Waldenström’s macroglobulinemia. Leuk Lymphoma (2022) 63:1469–73. doi: 10.1080/10428194.2022.2030478
382. Sermer D, Sarosiek S, Branagan AR, Treon SP, Castillo JJ. SOHO State of the Art Updates and Next Questions: Targeted therapies and emerging novel treatment approaches for Waldenström Macroglobulinemia. Clin lymphoma myeloma leukemia (2022) 22:547–56. doi: 10.1016/j.clml.2022.02.005
383. Mishra A, Maiti R, Mohan P, Gupta P. Antigen loss following CAR-T cell therapy: Mechanisms, implications, and potential solutions. Eur J Haematol (2023). doi: 10.1111/ejh.14101
384. Xu J, Luo W, Li C, Mei H. Targeting CD22 for B-cell hematologic Malignancies. Exp Hematol Oncol (2023) 12:90. doi: 10.1186/s40164-023-00454-7
385. Dagar G, Gupta A, Masoodi T, Nisar S, Merhi M, Hashem S, et al. Harnessing the potential of CAR-T cell therapy: progress, challenges, and future directions in hematological and solid tumor treatments. J Trans Med (2023) 21:449. doi: 10.1186/s12967-023-04292-3
386. Wang H, Tang L, Kong Y, Liu W, Zhu X, You Y. Strategies for reducing toxicity and enhancing efficacy of chimeric antigen receptor T cell therapy in hematological Malignancies. Int J Mol Sci (2023) 24:9115. doi: 10.3390/ijms24119115
387. McGann M, Velayati A, Roubal K, Granger K, Davis JA, Gaffney KJ, et al. Early cytopenias and infections following chimeric antigen receptor T-Cell therapy for hematologic Malignancies. Leuk Lymphoma (2023) 64(9):1592–5. doi: 10.1080/10428194.2023.2220455
388. Ahmed N, Mahmoudjafari Z, Hamadani M, Hashmi H. Bridging the gap: early transition and hybrid models of care to improve access to chimeric antigen receptor T cell therapy. Transplant Cell Ther (2023) 29:399–402. doi: 10.1016/j.jtct.2023.03.021
389. Hall AG, Winestone LE, Sullivan EM, Wu Q, Lamble AJ, Walters MC, et al. Access to chimeric antigen receptor T cell clinical trials in underrepresented populations: A multicenter cohort study of pediatric and young adult acute lymphobastic leukemia patients. Transplant Cell Ther (2023) 29:356.e1–7. doi: 10.1016/j.jtct.2023.03.022
390. Petrou P. Is it a chimera? A systematic review of the economic evaluations of CAR-T cell therapy - an update. Expert Rev Pharmacoecon Outcomes Res (2023) 23:625–50. doi: 10.1080/14737167.2023.2214731
391. Trainor N, Purpura KA, Middleton K, Fargo K, Hails L, Vicentini-Hogan M, et al. Automated production of gene-modified chimeric antigen receptor T cells using the Cocoon Platform. Cytotherapy (2023) doi: 10.1016/j.jcyt.2023.07.012
392. Backert L, Kowalewski DJ, Walz S, Schuster H, Berlin C, Neidert MC, et al. A meta-analysis of HLA peptidome composition in different hematological entities: entity-specific dividing lines and “pan-leukemia” antigens. Oncotarget (2017) 8:43915–24. doi: 10.18632/oncotarget.14918
393. Peng Y, Tao Y, Zhang Y, Wang J, Yang J, Wang Y. CD25: A potential tumor therapeutic target. Int J Cancer (2023) 152:1290–303. doi: 10.1002/ijc.34281
394. Wellhausen N, O’Connell RP, Lesch S, Engel NW, Rennels AK, Gonzales D, et al. Epitope base editing CD45 in hematopoietic cells enables universal blood cancer immune therapy. Sci Trans Med (2023) 15:eadi1145. doi: 10.1126/scitranslmed.adi1145
395. Dickinson MJ, Barba P, Jäger U, Shah NN, Blaise D, Briones J, et al. A novel autologous CAR-T therapy, YTB323, with preserved T-cell stemness shows enhanced CAR T-cell efficacy in preclinical and early clinical development. Cancer Discovery (2023) 13:1982–97. doi: 10.1158/2159-8290.CD-22-1276
396. Noraldeen SA, Rasulova I, Lalitha R, Hussin F, Alsaab HO, Alawadi AH, et al. Involving stemness factors to improve CAR T-cell-based cancer immunotherapy. Med Oncol (2023) 40:313. doi: 10.1007/s12032-023-02191-7
397. Mills GS, Chadwick V, Tang C, Perram J, Anderson MA, Anazodo A, et al. Immunochemotherapy for life-threatening haematological Malignancies in pregnancy: a systematic review of the literature and cross-sectional analysis of clinical trial eligibility. Lancet Haematol (2023) 10:e458–67. doi: 10.1016/S2352-3026(23)00059-5
398. Rajpurkar P, Chen E, Banerjee O, Topol EJ. AI in health and medicine. Nat Med (2022) 28:31–8. doi: 10.1038/s41591-021-01614-0
399. Beck JT, Rammage M, Jackson GP, Preininger AM, Dankwa-Mullan I, Roebuck MC, et al. Artificial intelligence tool for optimizing eligibility screening for clinical trials in a large community cancer center. JCO Clin Cancer Inform (2020) 4:50–9. doi: 10.1200/CCI.19.00079
400. Wei Z, Xu J, Zhao C, Zhang M, Xu N, Kang L, et al. Prediction of severe CRS and determination of biomarkers in B cell-acute lymphoblastic leukemia treated with CAR-T cells. Front Immunol (2023) 14:1273507. doi: 10.3389/fimmu.2023.1273507
401. Bogatu A, Wysocka M, Wysocki O, Butterworth H, Pillai M, Allison J, et al. Meta-analysis informed machine learning: Supporting cytokine storm detection during CAR-T cell Therapy. J Biomed Inf (2023) 142:104367. doi: 10.1016/j.jbi.2023.104367
402. Banerjee R, Shah N, Dicker AP. Next-generation implementation of chimeric antigen receptor T-cell therapy using digital health. JCO Clin Cancer Inform (2021) 5:668–78. doi: 10.1200/CCI.21.00023
403. Le Goff E, Blanc-Durand P, Roulin L, Lafont C, Loyaux R, MBoumbae D-L, et al. Baseline circulating tumour DNA and total metabolic tumour volume as early outcome predictors in aggressive large B-cell lymphoma. A real-world 112-patient cohort. Br J haematol (2023) 202:54–64. doi: 10.1111/bjh.18809
404. Asokan S, Cullin N, Stein-Thoeringer CK, Elinav E. CAR-T cell therapy and the gut microbiota. Cancers (Basel) (2023) 15:794. doi: 10.3390/cancers15030794
405. Yang J, Chen Y, Jing Y, Green MR, Han L. Advancing CAR T cell therapy through the use of multidimensional omics data. Nat Rev Clin Oncol (2023) 20:211–28. doi: 10.1038/s41571-023-00729-2
406. Bujak J, Kłęk S, Balawejder M, Kociniak A, Wilkus K, Szatanek R, et al. Creating an innovative artificial intelligence-based technology (TCRact) for designing and optimizing T cell receptors for use in cancer immunotherapies: protocol for an observational trial. JMIR Res Protoc (2023) 12:e45872. doi: 10.2196/45872
407. Gartner JJ, Parkhurst MR, Gros A, Tran E, Jafferji MS, Copeland A, et al. A machine learning model for ranking candidate HLA class I neoantigens based on known neoepitopes from multiple human tumor types. Nat Cancer (2021) 2:563–74. doi: 10.1038/s43018-021-00197-6
408. Daniels KG, Wang S, Simic MS, Bhargava HK, Capponi S, Tonai Y, et al. Decoding CAR T cell phenotype using combinatorial signaling motif libraries and machine learning. Science (2022) 378:1194–200. doi: 10.1126/science.abq0225
409. Naghizadeh A, Tsao W-C, Hyun Cho J, Xu H, Mohamed M, Li D, et al. In vitro machine learning-based CAR T immunological synapse quality measurements correlate with patient clinical outcomes. PloS Comput Biol (2022) 18:e1009883. doi: 10.1371/journal.pcbi.1009883
410. Hort S, Herbst L, Bäckel N, Erkens F, Niessing B, Frye M, et al. Toward rapid, widely available autologous CAR-T cell therapy - artificial intelligence and automation enabling the smart manufacturing hospital. Front Med (Lausanne) (2022) 9:913287. doi: 10.3389/fmed.2022.913287
411. Bäckel N, Hort S, Kis T, Nettleton DF, Egan JR, Jacobs JJ, et al. Elaborating the potential of Artificial Intelligence in automated CAR-T cell manufacturing. Front Mol Med (2023) 3:1250508. doi: 10.3389/fmmed.2023.1250508
412. Goldrick S, Sandner V, Cheeks M, Turner R, Farid SS, McCreath G, et al. Multivariate data analysis methodology to solve data challenges related to scale-up model validation and missing data on a micro-bioreactor system. Biotechnol J (2020) 15:e1800684. doi: 10.1002/biot.201800684
413. Mehrian M, Lambrechts T, Marechal M, Luyten FP, Papantoniou I, Geris L. Predicting in vitro human mesenchymal stromal cell expansion based on individual donor characteristics using machine learning. Cytotherapy (2020) 22:82–90. doi: 10.1016/j.jcyt.2019.12.006
414. Gil L, Grajek M. Artificial intelligence and chimeric antigen receptor T-cell therapy. Acta Haematol Pol (2022) 53:176–9. doi: 10.5603/AHP.a2022.0019
Keywords: CAR-T-cell therapy, T-cell malignancies, AML, CML, CLL, lymphoma, hairy cell leukemia, Waldenström’s macroglobulinemia
Citation: Karsten H, Matrisch L, Cichutek S, Fiedler W, Alsdorf W and Block A (2023) Broadening the horizon: potential applications of CAR-T cells beyond current indications. Front. Immunol. 14:1285406. doi: 10.3389/fimmu.2023.1285406
Received: 29 August 2023; Accepted: 10 November 2023;
Published: 27 November 2023.
Edited by:
Stephen Goldrick, University College London, United KingdomCopyright © 2023 Karsten, Matrisch, Cichutek, Fiedler, Alsdorf and Block. This is an open-access article distributed under the terms of the Creative Commons Attribution License (CC BY). The use, distribution or reproduction in other forums is permitted, provided the original author(s) and the copyright owner(s) are credited and that the original publication in this journal is cited, in accordance with accepted academic practice. No use, distribution or reproduction is permitted which does not comply with these terms.
*Correspondence: Andreas Block, block@uke.de