- Institute of Regenerative and Translational Medicine, Tianyou Hospital, Wuhan University of Science and Technology, Wuhan, China
Dental pulp stem cells (DPSCs) have received a lot of attention as a regenerative medicine tool with strong immunomodulatory capabilities. The excessive inflammatory response involves a variety of immune cells, cytokines, and has a considerable impact on tissue regeneration. The use of DPSCs for controlling inflammation for the purpose of treating inflammation-related diseases and autoimmune disorders such as supraspinal nerve inflammation, inflammation of the pulmonary airways, systemic lupus erythematosus, and diabetes mellitus is likely to be safer and more regenerative than traditional medicines. The mechanism of the anti-inflammatory and immunomodulatory effects of DPSCs is relatively complex, and it may be that they themselves or some of the substances they secrete regulate a variety of immune cells through inflammatory immune-related signaling pathways. Most of the current studies are still at the laboratory cellular level and animal model level, and it is believed that through the efforts of more researchers, DPSCs/SHED are expected to be transformed into excellent drugs for the clinical treatment of related diseases.
1 Introduction
Adult dental pulp stem cells (DPSCs) and stem cells from human exfoliated deciduous teeth (SHED) are self-renewing mesenchymal stem cells (MSCs) present in the perivascular area of the dental pulp (1, 2). DPSCs can typically be obtained from pulp tissue of blocked wisdom teeth, orthodontic decimated teeth (3). SHED can be extracted from pulp tissue of deciduous milk teeth of children aged 6-12 years (2). These cells are generally believed to originate from the cranial neural crest and can express early markers of bone marrow mesenchymal stem cells and neural ectodermal stem cells (4). These cells have grown in significance in the field of regenerative medicine due to their capacity for self-renewal, flexibility, high proliferation, and other potentials (5). Similar to bone marrow mesenchymal stem cells, they are able to differentiate into dentin-forming cells, osteoblasts, chondrocytes, adipocytes, endothelial cells, and neurons in vitro under specific conditions (6). (Figure 1) More importantly, they can modulate a variety of immune cells such as T lymphocytes and B lymphocytes, dendritic cells (DCs) and natural killer (NK) cells, thus becoming effective immunomodulators that inhibit pro-inflammatory processes and stimulate anti-inflammatory mechanisms (7, 8).
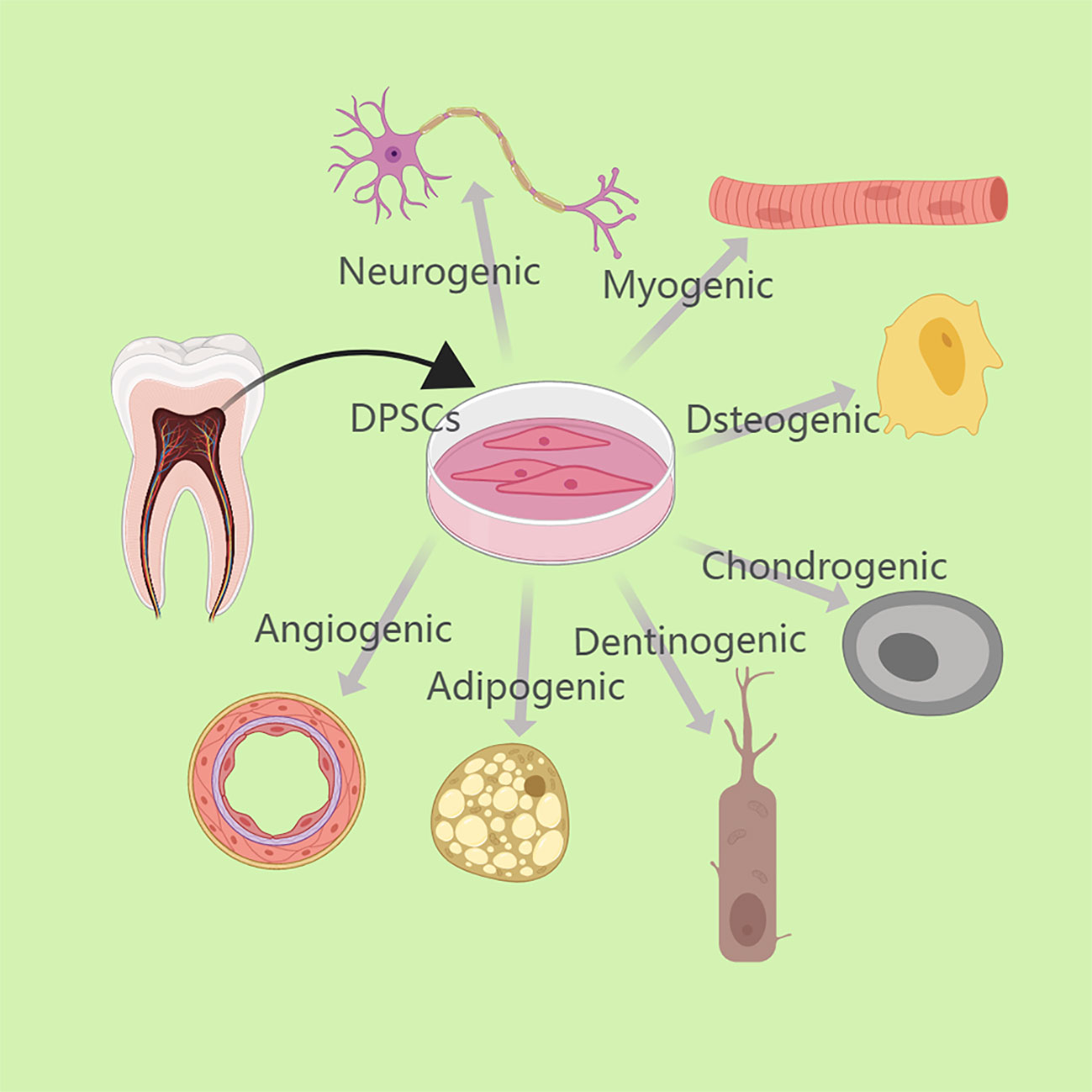
Figure 1 DPSCs can typically be obtained from pulp tissue of blocked wisdom teeth or orthodontic decimated teeth. Under particular circumstances, DPSCs can differentiate in vitro into dentinogenic cells, osteoblasts, chondrocytes, adipocytes, endothelial cells, neurons, etc.
Inflammation is the first response that occurs after an injury or infection and is an immune defense process of the body (9). If the inflammatory process continues for too long, an excessive number of activated cells are drawn to the site of the damage and release an excessive amount of enzymes, chemokines, etc., which is harmful to tissue repair and promotes further deterioration (7). Early inflammatory response management may be essential for regeneration.
The innate immune system is associated with the resolution of inflammation, and the adaptive immune system also contributes significantly to this process. The expression of several proteins and cytokines, the recruitment of numerous immune cells at various times and in varying numbers, and various signaling pathways all play major roles in the regulation of the regenerative process (10, 11). Excessive inflammation can develop in a variety of conditions, including supraspinal nerve inflammation, neonatal hypoxia-ischemia, pulmonary airway inflammation, systemic lupus erythematosus, and diabetes (8, 12). Controlling and eliminating inflammation and modulating immunity will help regeneration and contribute to the treatment of these diseases (Figure 2).
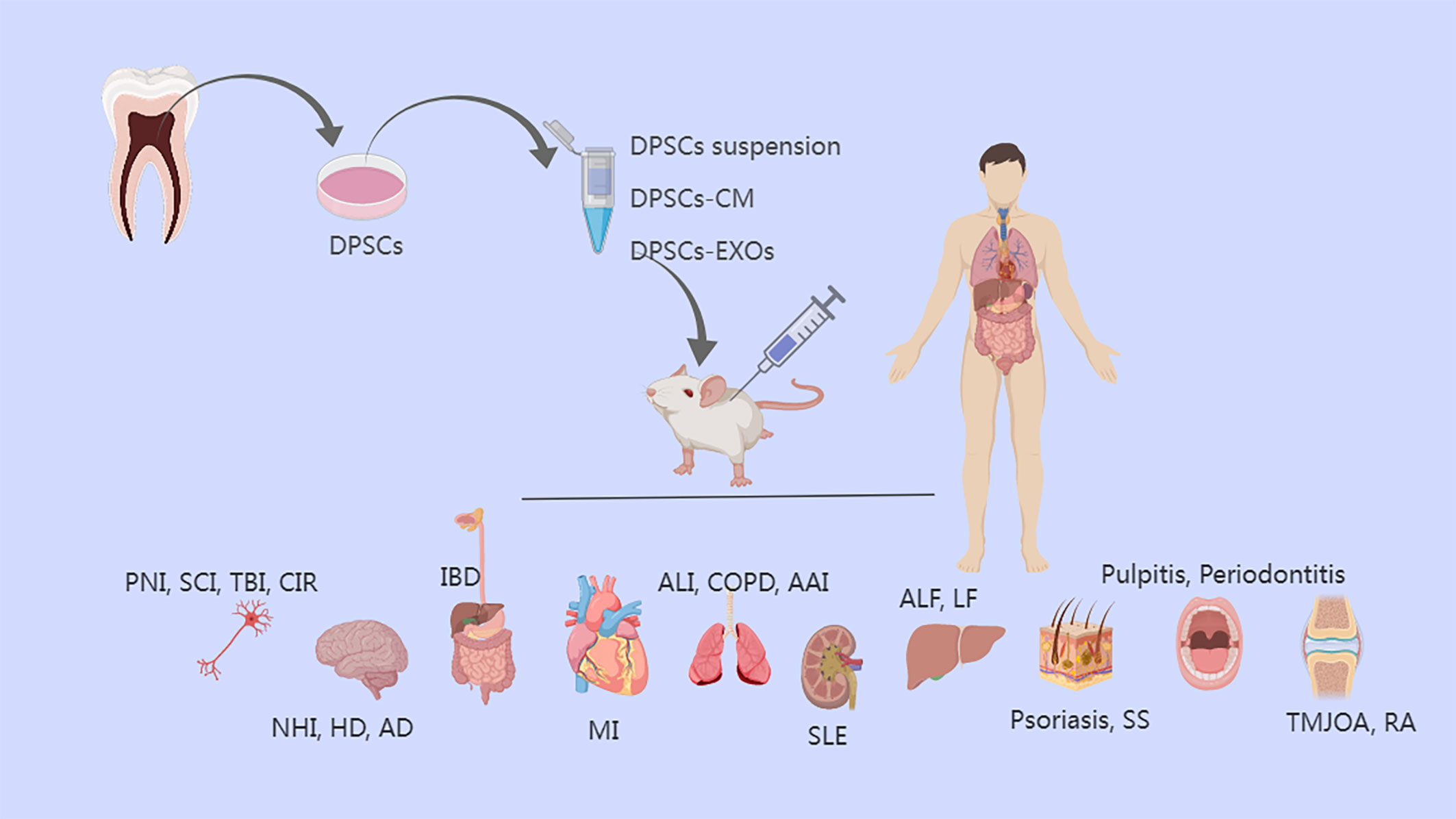
Figure 2 The treatment of diseases of various tissues/organs by anti-inflammatory effects of DPSCs and DPSCs-EXOs and DPSCs-CM. DPSCs, dental pulp stem cells; CM, conditioned medium; EXOs, exosomes; PNI, peripheral nerve injury; SCI, Spinal cord injury; TBI, traumatic brain injury; CIR, cerebral ischemia-reperfusion; NHI, neonatal hypoxia-ischemia; HD, Huntington’s disease; AD, Alzheimer’s disease, IBD, inflammatory bowel disease; MI, myocardial infarction; ALI, acute lung injury; COPD, Chronic Obstructive Pulmonary Disease; AAI, allergic airway inflammation; SLE, systemic lupus erythematosus; ALF, acute liver failure; LF, liver fibrosis; SS, Sjogren’s syndrome; TMJOA, temporomandibular joint osteoarthritis; RA, rheumatoid arthritis.
Clinical practice today favors oral or local injections of medications like nonsteroidal anti-inflammatory medicines, corticosteroids, etc. to treat disorders associated to inflammation (13). To achieve anti-inflammatory effects in a short period of time and to be applied to most types of inflammation, glucocorticoids are used in clinical practice (14). Glucocorticoids have a rapid, powerful, non-specific anti-inflammatory effect and are effective in a wide range of inflammatory conditions. However, all kinds of drugs have their limitations, and the side effects of drugs may make the benefits outweigh the disadvantages (9, 15). For example, long-term use of glucocorticoids can reduce the body’s resistance to pathogenic microorganisms, induce or aggravate infections, induce ulcers, aggravate mental illness, and cause osteoporosis, muscle atrophy, and delayed wound healing (15, 16).
In comparison to traditional MSCs, DPSCs are more proliferative, anti-inflammatory, and anti-fibrotic (17, 18). Proteomics was employed by Bousnaki et al. to examine the anti-inflammatory potential of the secretome of DPSCs under various settings, and they came to the conclusion that DPSCs are a promising therapeutic tool for the treatment of inflammatory illnesses (19). DPSCs may be as effective as glucocorticoids for a variety of inflammatory conditions, but are safer and more favorable to regeneration (20, 21).
Currently, more studies are focusing on the anti-inflammatory and immunomodulatory roles of DPSCs (22). In this review, we will discuss the current research progress on the anti-inflammatory effects of DPSCs in various organ- and tissue-related diseases, as well as the understanding of the related anti-inflammatory mechanisms and immunomodulatory functions, which will help the future clinical application of DPSCs.
2 Effects of DPSCs on nerve-associated inflammatory diseases
Peripheral nerve injury (PNI) can cause acute or chronic neuropathic pain. Neuropathic pain, characterized by sensory and emotional disturbances, is also known as a neuroimmune disorder and is the result of the interaction between neurons and inflammatory mediators of immune cell/immune cell origin, particularly chemokines and cytokines, as well as histamine, adenosine triphosphate and prostaglandins (23, 24). PNI induces the release of certain inflammatory mediators at the site of injury, in the dorsal root ganglion, and in spinal cord receptor segments, and also induces the release of inflammatory mediators in the brain, causing supraspinal nerve inflammation (25). Chronic inflammation also activates microglia, with elevated reactive oxygen species and Nuclear Factor-κ-gene Binding (NF-κB) activity and dysregulated homeostasis, accompanied by the release of pro-inflammatory cytokines, leading to neurodegeneration and altered synaptic transmission (26). In a study, researchers evaluated the alleviating effects of DPSCs and neuronal differentiated DPSCs on PNI-induced neuro-inflammation in a rat model of sciatic nerve damage (12). Microglia that had been activated by sciatic nerve injury produced more reactive oxygen species, which increased downstream NF-κB activation and started the transcription of pro-inflammatory cytokines by nuclear translocation. The expression of pAMPK and SIRT1 was decreased, and the pro-inflammatory responses were amplified while anti-inflammatory components were downregulated. By migrating to the site of injury and starting immune and anti-inflammatory effects, decreasing microglia activation, down-regulate pro-inflammatory markers, and up-regulate pAMPK/SIRT1, DPSCs may effectively balance the inflammation of the supraspinal nerve caused by PNI as well as alleviate pain and promote rapid regeneration (27–29).
Besides PNI, the central nervous system injury is also worthy of attention. Spinal cord injury (SCI) is a traumatic disease of the central nervous system in which a series of cellular responses, such as inflammation and apoptosis, occur near the site of injury due to increased release of biomolecules such as interleukin (IL)-6 and tumor necrosis factor (TNF)-α, as well as some other cytokines (30–32). Albashari et al. focused their attention on DPSCs when investigating treatments for SCI. Their work, in which Pluronic F-127, a biosynthetic hydrogel, was mixed with DPSCs and basic fibroblast growth factor and applied at the site of SCI, showed that it not only promoted tissue regeneration at the site of injury, but also contributed to the recovery of sensory and motor functions, which is inextricably linked to the low inflammatory microenvironment provided by DPSCs (33). DPSCs express IL-6, IL-8, and TGFβ via the Toll-like receptor (TLR) during the neural inflammation phase in treating central nerve diseases (34). Expression of IL-8 is increased in DPSCs in spinal cord injury, and IL-8 is associated with maintenance of neuronal cell integrity and reduction of injury (35). DPSCs modulate the immune response after nerve injury by increasing the release rate of anti-inflammatory factors IL-10 and transforming growth factor (TGF)-β and decreasing the release rate of pro-inflammatory factors IL-6 and TNF-α (36). The NF-κB pathway plays a key role in pro-inflammatory factor-induced neuronal cell inflammation and a central role in pro-inflammatory factor-induced apoptosis (37). At acute SCI stage, pro-inflammatory factors and NF-κB signaling pathways are activated, causing severe neuro-inflammation, and microglia are active and act as immune cells in direct contact with nerve cells (38, 39). DPSCs attenuate the inflammation of SCI by decreasing NF-κB expression. It is possible to treat SCI with tropical use of DPSCs by reducing inflammation.
Similar to SCI, traumatic brain injury is a trauma-induced disease of the central nervous system. It is caused by an external mechanical force striking the skull and causing intracranial damage to neurons, axons, glial cells, and blood vessels. Next, the blood-brain barrier is disrupted, which causes neuro-inflammation, oxidative stress, and excitotoxicity, which causes additional neuronal damage, primarily at the site of the injury and nearby tissues (40). And in the long term this secondary damage may even cause lesions in areas of the brain far from the site of injury or may be referred to as a neuro-degeneration (41). When traumatic brain injury occurs, to protect the site of injury from pathogens, an endogenous inflammatory response is activated and proliferating immune cells secrete various chemokines, pro-inflammatory cytokines such as TNF-α, IL-1β, IL-6, and IL-12, and other inflammatory mediators (42). Due to the immunomodulatory properties of MSCs, which can effectively suppress neuro-inflammation, it has been shown that stereotactic or intravenous administration of MSCs can reduce the levels of various pro-inflammatory cytokines, such as IL-1β, IL-6, IL-17, interferon (IFN)-γ or TNF-α, in the serum and brain after brain injury (43, 44). Its powerful anti-inflammatory effect inhibits the NF-κB signaling pathway, which facilitates the treatment of secondary brain injury (43). Importantly, investigators found that MSCs significantly altered blood-brain barrier function by decreasing endothelial permeability, increasing VE-cadherin expression and VE-cadherin/β-catenin interaction, which may be a doorway to break through multiple neurological diseases (45). DPSCs are derived from neural crest cells, which can induce apoptosis of activated T cells in vitro and modulate immune responses, and the application of DPSCs to resist neuro-inflammation is promising for the treatment of traumatic brain injury (43).
In addition to traumatic brain injury and SCI, many diseases of the central nervous system: Huntington’s disease (HD), Alzheimer’s disease, Parkinson’s disease, neuro-inflammatory multiple sclerosis, etc., are characterized by varying degrees of inappropriate inflammatory/immune responses and tissue destruction (8, 46). Among them, HD is a progressive neurodegenerative disease that can be fatal with autosomal dominant inheritance. The current consensus is that striatal degeneration due to chromosomal abnormalities leads to lateral ventricular effusion, and the main case is characterized by neuronal loss and neuro-inflammation within the striatum and cortex, resulting in a range of motor and cognitive symptoms (47). In the clinic, HD can be diagnosed, but there is no very clear treatment for this disease. Some scholars have then focused on the possibility of MSCs treatment for it. In a prior study, Eskandari et al. used transplanted DPSCs to treat a 3-nitropropionic acid rat model of HD. These cells were placed in the mid-posterior region of the rat striatum and were able to survive and treat motor dysfunction (48). DPSCs treatment inhibited gliosis and microgliosis in the striatum and reduced the expression of inflammatory cytokines at the mRNA level. This action of DPSCs is caused in part by their capacity to release neurotrophic substances, to reduce apoptosis, and to suppress the expression of inflammatory cytokines (4, 49). Treatment based on DPSCs seems to provide effective treatment for HD and other neurodegenerative diseases, and although this treatment has not yet been translated clinically, it is well worth looking forward to (50).
There is growing evidence that inflammatory processes are involved in the pathogenesis of diabetic neuropathy, which can occur in various parts of the peripheral and central nervous system (51, 52). Diabetes is considered to be a chronic low-grade inflammatory disease, manifested primarily by the transduction of various inflammatory signals and the release of molecular products that generate and maintain neurological inflammation through the activation of mononuclear phagocytes, including resident microglia and blood-derived macrophages (53, 54). Omi et al. discovered that injection of skeletal muscle DPSCs significantly decreased the number of sciatic nerve macrophages in diabetic rats while increasing the gene expression of M2 macrophages and decreasing that of M1 macrophages. This resulted in the treatment of diabetes-induced sciatic nerve inflammation, an improvement in the conduction velocity and blood flow of the sciatic nerve, and an increase in intraepidermal nerve fiber density (55). In conclusion, the anti-inflammatory effect of DPSCs by modulating the ratio of M1/M2 macrophages may be an effective treatment for diabetic polyneuropathy.
Based on the anti-inflammatory effects of DPSCs in neuro-inflammation above through multiple pathways, these chronic diseases causing neuro-inflammation may consider the application of DPSCs, not necessarily as the only treatment, but as an adjuvant or collaborative way to combat such diseases by counteracting inflammation, achieving relief of disease symptoms and relieving chronic pain.
Neonatal hypoxia-ischemia (NHI), also known as neonatal hypoxic-ischemic encephalopathy, can lead directly to neonatal death (56). Even if they live, some neonates may have one or more neuropsychiatric conditions, such as cerebral palsy, mental retardation, or learning difficulties (57). When the fetal blood-brain barrier is not fully developed, if intrauterine infection occurs, inflammatory cells and toxins will cross the barrier and enter the brain tissue, which will not only cause inflammation in the endothelial tissue of the brain, but also stimulate the production of inflammatory cytokines such as IL-1β, IL-6 and TNF-α, thus aggravating the condition of hypoxic-ischemic encephalopathy (58). Existing treatment modalities can partially improve the neurological deficits of NHI, but there is no effective clinical treatment for this devastating disease. Chiu et al. used human DPSCs to treat a hypoxia-ischemia model in rats and found that DPSCs in NHI decreased the expression of pro-inflammatory factors (IL-1β, IL-6, TNF-α, INF-γ) and increased the expression of anti-inflammatory factors (IL-10, TGF-β) (57). This suggests that the anti-inflammatory effects of DPSCs synergize with effects such as neuro-regeneration to promote neuroplasticity and improve neurological outcome for a desired improved prognosis (59, 60). DPSCs may provide a viable strategy for restoring NHI-induced disability.
3 Effects of DPSCs on intestinal-associated inflammatory diseases
Inflammatory bowel disease (IBD) is an umbrella term for Crohn’s disease and ulcerative colitis, and is closely related to genetics, flora, and immunity (61, 62). Pathogenic and benign commensal bacteria coexist in the gastrointestinal environment, and the human immune system regulates intestinal inflammation and tolerance. When this balance is upset, chronic inflammation and the development of unfavorable innate and adaptive immune mechanisms occur, which then cause IBD (63). Barrier disruption leads to altered intestinal flora, which leads to abnormal activation of the mucosal immune system and is an important factor in the development of IBD (64). Furthermore, misregulated Th cell responses play a central role in the progression of chronic inflammatory processes in the gut (65). It has been shown that in the intestinal mucosa of healthy mice, CX3CR1+ macrophages inhibit IL-17 production, express anti-inflammatory molecules such as IL-10, and induce differentiation of Foxp3+ regulatory T (Treg) cells (66). CD103+ DCs effectively induce Treg cells to suppress inflammation by producing IL-10, TGF-β and retinoic acid in intestinal inflammation (67). Elevated number of Th17 cells and upregulation of IL-17 in mucosa of IBD patients compared to normal mucosa (68).
Multiple researchers have used systemic infusion of DPSCs to treat DSS-induced colitis in mice. DPSCs significantly decreased inflammatory cell infiltration and downregulated inflammatory cytokines in the colon, ameliorating colonic transmural inflammation, which in turn resulted in a reduction in wall thickness, an inhibition of epithelial ulceration, and the restoration of normal intestinal architecture (69). In their study, they also found that DPSCs’ ability to inhibit T-cell viability in vitro was decreased when the transmembrane protein Fas ligand (FasL) was knocked down. This could have prevented DPSCs from inducing T-cell apoptosis, which would have reduced their ability to improve the colitis phenotype. This finding suggests that FasL may be necessary for DPSC-mediated immune regulation (69, 70). The study by Földes et al. also focused on a DSS-induced mouse model of colitis and used intravenous injections of human DPSCs to study the effects on IBD (8). Their results showed that a single intravenous injection of human DPSCs can beneficially modify the development of acute colitis in vivo, confirming the protective role of human DPSCs in experimental colitis. Different species sources of DPSCs and different culture methods may lead to different biological activities; exogenous and endogenous cytokine levels and the timing of administration may also affect the efficacy of DPSCs (71). For example, pre-stimulation with INF-γ and TNF-α significantly enhanced the protective effect of DPSCs against colitis in mice (72, 73). In conclusion, DPSCs’ contribution to intestinal inflammation should not be understated based on their immunomodulatory and anti-inflammatory actions. Although the mechanism needs to be clarified further, the outlook is generally favorable.
4 Effects of DPSCs on lung-associated inflammatory diseases
Acute lung injury (ALI) progresses to a severe stage called acute respiratory distress syndrome (ARDS), characterized by a variety of changes caused by lung injury, including increased pulmonary capillary permeability, enhanced inflammatory cell infiltration, and diffuse alveolar and interstitial edema (74). When paraquat enters the human body, it causes ALI, which develops into ARDS. As the disease progresses, pulmonary edema and hemorrhage occur along with the infiltration of inflammatory cells into the interstitium and alveoli of the lungs, fibrosis, and eventually respiratory failure, which results in death (75, 76).
Regulating and reducing inflammation is an extremely important therapeutic strategy for ALI and ARDs due to paraquat toxicity. Geng et al. focused on this problem with the idea of using stem cells to act on this ALI-induced inflammation and subsequent lung fibrosis, and their work concluded that DPSCs have more potential as a treatment for ALI by comparing the anti-inflammatory effects of umbilical cord mesenchymal stem cells and DPSCs (77). Using hepatocyte growth factor (HGF), a multifunctional cytokine that affects multiple pathophysiological processes involved in inflammatory and immune responses, modified DPSCs more significantly inhibit inflammatory mediators, maintain alveolar structural integrity, and attenuate interstitial hemorrhage and inflammatory cell infiltration in the lung (77, 78). Supporting the repair of ALI by this means of relieving inflammation also can achieve the goal of reducing mortality due to paraquat toxicity.
Additionally, exposure to some allergens, such as dust, can cause allergic airway inflammation, and eosinophil infiltration into the lungs can result in lung inflammation. The work of Laing et al. used egg yolk OVA to induce allergic lung inflammation in a mouse model. OVA sensitization and subsequent aerosol stimulation resulted in intense allergic airway inflammation with eosinophil infiltration into the lungs. By injecting live/apoptotic DPSCs, the number of inflammatory cells in the lung lavage fluid of mice decreased, demonstrating that apoptotic DPSCs are effective in controlling lung inflammation (79). Indeed, apoptotic cells and apoptotic cell fragments are known to contribute to the adoption of an immunosuppressive phenotype by antigen-presenting cells (80, 81). Injection of pre-killed DPSCs may be a more effective, clinically simpler and safer alternative.
Chronic Obstructive Pulmonary Disease (COPD) encompasses a wide range of diseases with common functional characteristics, such as chronic bronchitis, chronic respiratory failure and emphysema (82). Gao et al. used intratracheal injection to transplant DPSCs into a mouse model of emphysema for a therapeutic investigation on chronic obstructive pulmonary disease. They discovered that DPSCs improved lung function and emphysema-like changes in COPD by activating the Nrf2 signaling pathway and decreased IL-1β, TNF-α, and IL-6 levels in lung tissue and bronchoalveolar lavage fluid (83).
People will inevitably suffer from inflammation of the lungs and airways due to environmental pollution, work factors, personal habits and other factors. The long lasting chronic inflammatory response leads to destruction of the alveolar wall and thinning of the alveolar sac, which in turn causes breathing difficulties and reduced lung function, such as COPD, bronchopulmonary dysplasia, ARDS, asthma, pulmonary fibrosis and pulmonary hypertension (84, 85). Exploring a safe and effective therapeutic strategy to reduce inflammatory damage in the lung is a major topic to be addressed in the field of lung disease research. MSCs suppress deleterious immune responses and differentiate into alveolar epithelial type II cells in vitro to suppress inflammation (86). As a type of MSCs, DPSCs modulating lung inflammation and related immune functions are being noticed by more and more researchers, and there is reason to believe that there will be more and more evidence of the importance of the anti-inflammatory effects of DPSCs.
5 Effects of DPSCs on kidney-associated inflammatory diseases
As a typically fatal autoimmune disease, nearly half of patients with systemic lupus erythematosus develop complications of lupus nephritis (87). Kidney injury occurs, immune complexes are deposited in glomeruli, tubules, and microvessels, and clinically occult nephritis, nephritis syndrome, and nephrotic syndrome occur. The existing treatment methods mainly include immunosuppression, glucocorticoids, dialysis treatment and kidney transplantation. Some patients with lupus nephritis have no effect on both glucocorticoids and immunosuppressants, and long-term use of immunosuppressants has obvious toxic side effects, such as ovarian failure, secondary infection and secondary malignancy (88, 89). The immunomodulatory effect of MSCs can intervene and block the pathogenesis of this autoimmune disease, and the therapeutic effect and prospect are more advantageous than other diseases (90). In their study, Tang et al. treated mice with lupus nephritis using DPSCs; the mice showed a reduction in IgG and IgM deposition in the glomeruli, a significant decrease in the number of CD4+ T cells that produce IFN-γ, and a reduction in perivascular inflammatory infiltrates (91). It has been shown that MSCs transplantation in lupus nephritis alters the ratio of T cell subsets, upregulates the proportion of Treg cells, downregulates IL-17 and TGF-β, and increases Foxp3, thereby suppressing renal inflammation and improving disease conditions (92). MSCs also exhibit inhibition of secretion of multiple pro-inflammatory factors by B cells, as well as suppression of dendritic cell development through secretion of IL-6 and prostaglandin E2 maturation, inhibit the proliferation and activation of CD8+ T cells, and stimulate CD4+ T cells to produce Th2 responses, upregulate Treg cells, and attenuate the inflammatory response associated with lupus nephritis (93). It is believed that DPSCs can play a role in the treatment of systemic lupus and lupus nephritis.
6 Effects of DPSCs on skin-associated inflammatory diseases
Psoriasis is a chronic relapsing inflammatory skin disease that can be complicated by metabolic syndrome, cardiovascular diseases (e.g. myocardial infarction, stroke, hypertension) and other multisystem diseases. Available studies suggest that it is a result of excessive proliferation and abnormal differentiation of epidermal cells caused by excessive activation of immune cells (94). Its causes and may be related to genetic and environmental factors. Existing treatments are mainly aimed at relieving symptoms and have mixed results, and are not curative. A number of researchers have focused on the possibility of stem cell therapy for psoriasis (95). Meng et al. investigated the anti-inflammatory effects of DPSCs in a psoriasis-like mouse model established by imiquimod cream application. They found that intravenous infusion of DPSCs significantly decreased psoriasis-like pathological changes such inflammatory infiltration, epidermal thickening, hyperkeratosis, and hyperkeratosis in the skin of mice, and effectively improved the symptoms of psoriasis-like erythema, scaling, and thickening in the mice’s dorsal skin (96). The therapeutic effect of DPSCs on psoriasis was mainly achieved by reducing the inflammatory response. DPSCs treatment significantly downregulated T-bet (Th1 transcription factor) DPSCs treatment significantly downregulated the expression levels of T-bet (Th1-associated cytokine), IFN-γ (Th1-associated cytokine), RORγt (Th17 transcription factor), IL-17A, IL-17F and IL-23 (Th17-associated cytokine), and upregulated the expression levels of GATA3 (Th2 transcription factor), Foxp3 (Treg transcription factor) and IL-10. The mechanisms associated with DPSCs for the treatment of psoriasis lie in the down-regulation of Th1 and Th17 cell activity, as well as the promotion of Treg cell differentiation and the attenuation of the inflammatory response. HGF is mainly secreted by mesenchymal cells and helps to reduce the inflammatory response (97). The role of HGF was also verified by the work of Meng et al. Overexpression of HGF enhanced the inhibitory effect of DPSCs on Th1 and Th17 cell activity and the promotive effect of DPSCs on Treg cell activity (96).
7 Anti-inflammatory role of DPSCs in oral and other diseases
With their ease of access from teeth and better tissue repair/regeneration potential, DPSCs have gained a unique position in the field of regenerative dentistry (98). In the pulp tissue, the inflammatory and regenerative reactions are closely linked. Trauma or caries can trigger inflammation as well as regenerative effects at the molecular, cellular or tissue level in the dentin-pulp complex (99). As caries progresses and pulp structures become involved, the organism begins by fighting infection through an immune inflammatory response, followed by the recruitment of DPSCs that form the dentin-pulp complex for regeneration of lost portions of pulp structures including soft pulp tissue structures with vascular and neural components (100). Smaller and relatively slow injuries lead to dentin formation that compartmentalizes the injury from the pulp, whereas larger and rapidly progressive injuries lead to a more intense immune response in the pulp tissue, with clinical manifestations of pulpitis that can progress to pulp necrosis up to periapical periodontitis and alveolar bone inflammation (99).
Some current investigators have proposed that there are two main types of DPSCs to combat oral-associated inflammation, one relying on resident DPSCs in the pulp itself being stimulated, i.e., occurring during the process described above; the other can be transplanted into the root canal by ex vivo cultured DPSCs alone or in combination with appropriate scaffold material (101). When inflammation occurs, resident DPSCs are thought to exhibit opposing immunomodulatory and immunosuppressive effects at multiple cellular levels, particularly on DCs, macrophages and lymphocytes, reducing the amount of pro-inflammatory cytokines while locally increasing the amount of anti-inflammatory biomolecules (102). Meanwhile, inflammation-associated cytokines and growth factors significantly enhanced the proliferation, recruitment, and differentiation of DPSCs, which provided favorable conditions for further DPSCs to resist inflammation (103). Controlled activation of the intracellular p38MAPK and NF-KB signaling pathways in DPSCs during low-grade or short-term inflammation stimulates pulp repair or regenerative responses (104, 105). In the late stage of pulpal immunopathology, DPSCs control the inflammatory process together with endothelial cells, pulp fibroblasts, inflammatory cells from the peripheral circulation, and chemoattractive inflammatory cells (106). In conclusion, DPSCs directly or indirectly modulate the immune response to the anti-inflammatory phase (107).
Neves et al. found an increase in DPSCs when overlayed with Wnt agonist small molecules and an increase in macrophages near Wnt-receiving cells, indirectly regulating the anti-inflammatory state of macrophages from M1 to M2, with a possible molecular interaction between these two cell types (108). This suggests that Wnt/β-catenin signaling has a dual role in promoting restorative dentin formation by activating DPSCs and promoting anti-inflammatory macrophage responses, with a dual role in promoting restorative dentin formation (109). Fyn is a member of the protein tyrosine kinase family, whose overexpression is associated with various types of inflammation. Fyn forms a complex with Neuropilin-1, which inhibits adult dentin cell differentiation and amplifies inflammatory responses through the NF-κB signaling pathway. Fyn is involved in inflammatory and autoimmune disease imbalance, Fyn expression is reduced during adult dentin cell differentiation in DPSCs, where probably miR-125a-3p plays an important role in regulating its signaling pathway (110).
Periodontitis is a chronic inflammatory disease that leads to the destruction of alveolar bone and eventually to tooth loss (111). In genetically or environmentally susceptible individuals, periodontal pathogens trigger an inflammatory immune response in which activated macrophages secrete inflammatory cytokines and Th17 cells produce IL-17, NF-κB receptor activator ligand and TNF-α. The use of DPSCs offers the possibility to simultaneously target the inflammatory response to slow or stop its progression and to promote the regeneration of periodontal structures (63). Severe inflammation, progressive cartilage and bone destruction are typical pathological changes in temporomandibular joint arthritis, which makes treatment very difficult. Activation of immune cells and elevated expression of multiple inflammatory factors are closely associated with the development of inflammatory osteoarthritis (112, 113). Cui et al. used intra-articular drug injections to mimic the synovial inflammation and cartilage degradation of the temporomandibular joint. They then injected DPSCs locally and systemically, and discovered that local administration of the drug was superior to systemic administration in terms of reducing the progression of temporomandibular joint arthritis in rats by modifying the local immune-inflammatory response and cartilage matrix metabolism (114). Inhibition of the JAK-STAT1 pathway effectively downregulates immune cell activation and provides a target for DPSCs-based therapies (115). It was elaborated in their study that DPSCs effectively inhibit STAT1 pathway activation, leading to the downregulation of MMP3 and MMP13 (114).
8 Therapeutic effects of exosomes from DPSCs on inflammation associated with various diseases
The therapeutic potential of adult stem cells is highly dependent on the release of molecules and factors in the extracellular environment (116). DPSCs secrete soluble factors (proteins, lipids and nucleic acids) and extracellular vesicles through paracrine activity (117). Exosomes (EXOs) are a type of extracellular vesicles and are the main form of paracellular secretion. EXOs are small, double-layered, membranous lipid vesicles containing a variety of contents such as proteins and nucleic acids, with diameters ranging from 30 to 150 nm, involved in intercellular communication, promoting tissue repair and regeneration (118, 119). The morphology and structure of the EXOs that DPSCs secrete are not substantially different from those of other cell sources, and they maintain the same biological properties and functions as DPSCs. However, DPSCs-EXOs have the advantage of being more stable and easier to preserve than DPSCs (120).
Current studies suggest that the therapeutic effects of DPSCs are mainly attributed to their release of paracrine factors (121). As one of the most important paracrine mediators, DPSCs-derived EXOs show therapeutic effects through immunomodulation (122). Pivoraitė et al. injected SHED-EXOs intravenously into a mouse model of carrageenan-induced inflammation and found that SHED inhibited the activity of tissue proteinase B and matrix metalloproteinases at the site of acute inflammation, demonstrating for the first time that DPSCs can inhibit the carrageenan-induced acute inflammatory response in mice (123). In studying the protective effects of DPSC- EXOs against cerebral ischemia-reperfusion injury, Li et al. found that DPSC- EXOs inhibited neuro-inflammatory responses in brain cerebral ischemia-reperfusion mice by reducing the protein expression of IL-6, IL-1β and TNF-α through the HMGB1/TLR4/MyD88/NF-κB pathway (124). In another study, researchers established a periodontitis mouse model followed by local injection of DPSC- EXOs. They demonstrated that in mice with periodontitis, DPSC-EXOs conjugated chitosan hydrogel promoted the transformation of periodontal macrophages from a pro-inflammatory to an anti-inflammatory phenotype. The treatment also reduced periodontal damage by immune response modulation and suppression of periodontal inflammation, which sped up the healing of alveolar bone and periodontal epithelium in periodontitis mice through a mechanism that may be connected to miR-1246 in DPSC-EXOs (125).
The application of DPSC-EXOs does not require the direct use to cells, thus avoiding the limitations and risks associated with cell transplantation. Although DPSC-EXOs may face some limitations (heterogeneity, shortcomings of purification methods and tentative insurmountable large-scale production capacity), they are not only promising drugs, but may become effective vectors by engineering treatments that will provide particular ideas for the treatment of some diseases (15).
9 Therapeutic effects of conditioned media of DPSCs on inflammation associated with various diseases
As mentioned above, the paracrine role of DPSCs can express their therapeutic capacity to some extent. DPSCs secrete a large number of nutritional and immunomodulatory factors (126). Conditioned medium of DPSCs (DPSCs-CM) contain growth factors, cytokines and other active substances that mimic the regulatory effects of DPSCs on immunologically active cells. Conditioned media from various stem cell types have been shown to have considerable potential in the treatment of a variety of refractory diseases (127).
Temporomandibular joint osteoarthritis is a degenerative joint disease characterized by progressive cartilage degeneration, abnormal bone remodeling, and chronic pain (128). When Ogasawara et al. administered SHED-CM intravenously to mice in a model of temporomandibular joint osteoarthritis brought on by mechanical stress, they saw a decline in the number of chondrocytes expressing IL-1β, iNOS, and MMP-13, a significant reduction in temporal muscle inflammation, and a contribution to the regeneration and repair of the mice’s osteoarthritis (129). Rheumatoid arthritis is an autoimmune disease characterized by synovial hyperplasia and chronic inflammation leading to progressive destruction of articular cartilage and bone (130). The work of Ishikawa et al. by a single intravenous injection of SHED-CM into a mouse model of anti-collagen-type antibody-induced arthritis in rheumatoid arthritis-like mice yielded results that SHED-CM has therapeutic anti-inflammatory effects, ameliorating arthritic symptoms and inhibiting tissue damage by shifting the pro-inflammatory synovial environment to an anti-inflammatory synovial environment through induction of M2 macrophage polarization (131). As mentioned earlier, Alzheimer’s disease is a progressive neurodegenerative disease characterized by cognitive decline and the presence of β-amyloid plaques in the brain. The disease occurs when activated microglia release various neurotoxic factors, including pro-inflammatory cytokines (132). In a study conducted on a mouse model of Alzheimer’s disease, Mita et al. used SHED-CM and discovered that it reduced the pro-inflammatory response brought on by β-amyloid plaques and changed the pro-inflammatory M1-type microglia microenvironment into an M2-type anti-inflammatory/neuro-protective microenvironment (133). As was previously mentioned, anti-inflammation may be a crucial component of PNI treatment. The study by Fumiya Kano et al. demonstrated that SHED-CM is a crucial component of PNI treatment through monocyte chemoattractant protein-1 and the secreted ectodomain of sialic acid-binding Ig-like lectin-9. This allowed for the induction of tissue-repairing M2 macrophages and the promotion of facial nerve function recovery in rats following injury, confirming that the anti-inflammatory effect of SHEDs can have a therapeutic effect on severe PNI (134). Similarly, severe inflammation impedes functional recovery after SCI. Matsubara et al. injected SHED-CM intrathecally into the injured spinal cord of a rat model during the acute period of SCI and found that SHED-CM induced immunomodulatory activity of anti-inflammatory M2-like macrophages, thereby promoting axonal growth, peripheral neural tissue angiogenesis, schwann migration, proliferation and activation, and neuronal survival, leading to significant functional recovery, similar to the effect of direct SHEDs transplantation (135). Acute liver failure occurs with massive hepatocellular destruction and an intense inflammatory response (136). In a study by Matsushita et al., SHEDS or SHED-CM were intravenously injected into ALF rat models. The results showed attenuation of the pro-inflammatory response and induction of anti-inflammatory M2-like hepatic macrophages, proving that the anti-inflammatory effect of SHED helps create a favorable environment for tissue regeneration after ALF (137). Hepatocyte necrosis and apoptosis, which arise from chronic liver injury, then activate pro-inflammatory mediators and encourage the transdifferentiation of hepatic stellate cells into myofibroblasts, which leads to the development of liver fibrosis. Severe irreversible liver fibrosis is followed by liver failure (138). The work of Hirata et al. in which SHED-CM was injected into mouse model of liver fibrosis, inhibited gene expression of pro-inflammatory mediators, protected parenchymal hepatocytes from apoptosis, induced apoptosis in activated hepatic stellate cells, and induced apoptosis by inducing expression of MMP13 polarization of macrophages to promote fibrinolysis, ultimately leading to the regression of fibrous scarring (139). Ischemic heart diseases such as myocardial infarction can cause irreversible damage to the heart. Yamaguchi et al. showed that SHED-CM significantly reduced endotoxin-induced expression of pro-inflammatory genes, decreased the area of myocardial infarction after ischemia/reperfusion, decreased cardiomyocyte apoptosis under ischemic and hypoxic conditions, and decreased the levels of inflammatory cytokines in a mouse ischemia/reperfusion (I/R) model (140). Sjogren’s syndrome (SS) is a chronic systemic autoimmune disease with a complex pathogenesis involving multiple inflammatory cells and inflammatory factors. Compared to other inflammatory autoimmune diseases, including Rheumatoid arthritis, blocking TNF-α has little effect on SS patients (141). Kawashima et al. used DPSC-CM in a mouse SS model to promote the differentiation of Treg cells and inhibit the differentiation of Th17 cells in the spleen of mice, which reduced the inflammation of submandibular gland and improved the symptoms of SS (142). Ogata et al. also used DPSC-CM in a mouse SS model and obtained the same conclusion (143). As mentioned earlier, ARDS is a severe inflammatory disease characterized by acute respiratory failure caused by severe destructive pulmonary inflammation and irreversible pulmonary fibrosis (74). Wakayama et al. Subjected to ALI model mice, a single intravenous injection of SHED-CM attenuated the pro-inflammatory response and produced an anti-inflammatory/tissue regenerative environment, while inducing anti-inflammatory M2-like lung macrophages, which facilitated the treatment of lung injury (144).
The application of DPSC-CM/SHED-CM does not require direct use to the cells, similar to EXOs, avoiding risks such as potential tumorigenicity associated with direct use of the cells themselves, while not requiring the tedious and labor-intensive processing required to extract EXOs. This provides another exceptional idea for the treatment of multiple refractory diseases.
10 Mechanisms related to the anti-inflammatory and immunomodulatory effects of DPSCs
Like other MSCs, DPSCs can elude immune identification and inhibit immunological responses and are considered less immunogenic, probably because of the low expression levels of major histocompatibility complex class I molecules and the negativity of major histocompatibility complex class II cells (145, 146). Perhaps there are opportunities to use DPSCs’ immunosuppressive characteristics as a therapeutic immunomodulatory tool for the management of inflammatory and other inflammation-related autoimmune diseases, and even more so for the adjuvant management of allograft rejection and graft-versus-host disease (147).
As previously mentioned, several existing studies have addressed the anti-inflammatory effects and immunomodulatory capacity of DPSCs. DPSCs have a strong immunomodulatory capacity, either through intercellular contacts or through some of their secreted components, which can inhibit proliferation, reduce cytokine and antibody secretion, control immune cell maturation, and interfere with antigen presentation by T cells, B cells, NK cells, and DCs (148, 149). The main mechanism associated with the therapeutic effects of DPSCs is the indirect effect of the release of various cytokines, growth factors and chemokines through paracrine action (150). Available studies have pointed out that they secrete soluble factors mainly including HGF, nitric oxide, indoleamine 2,3-dioxygenase (IDO), prostaglandin E2 and TGF-β1, which help to mediate immunosuppression (151–153) (Figure 3).
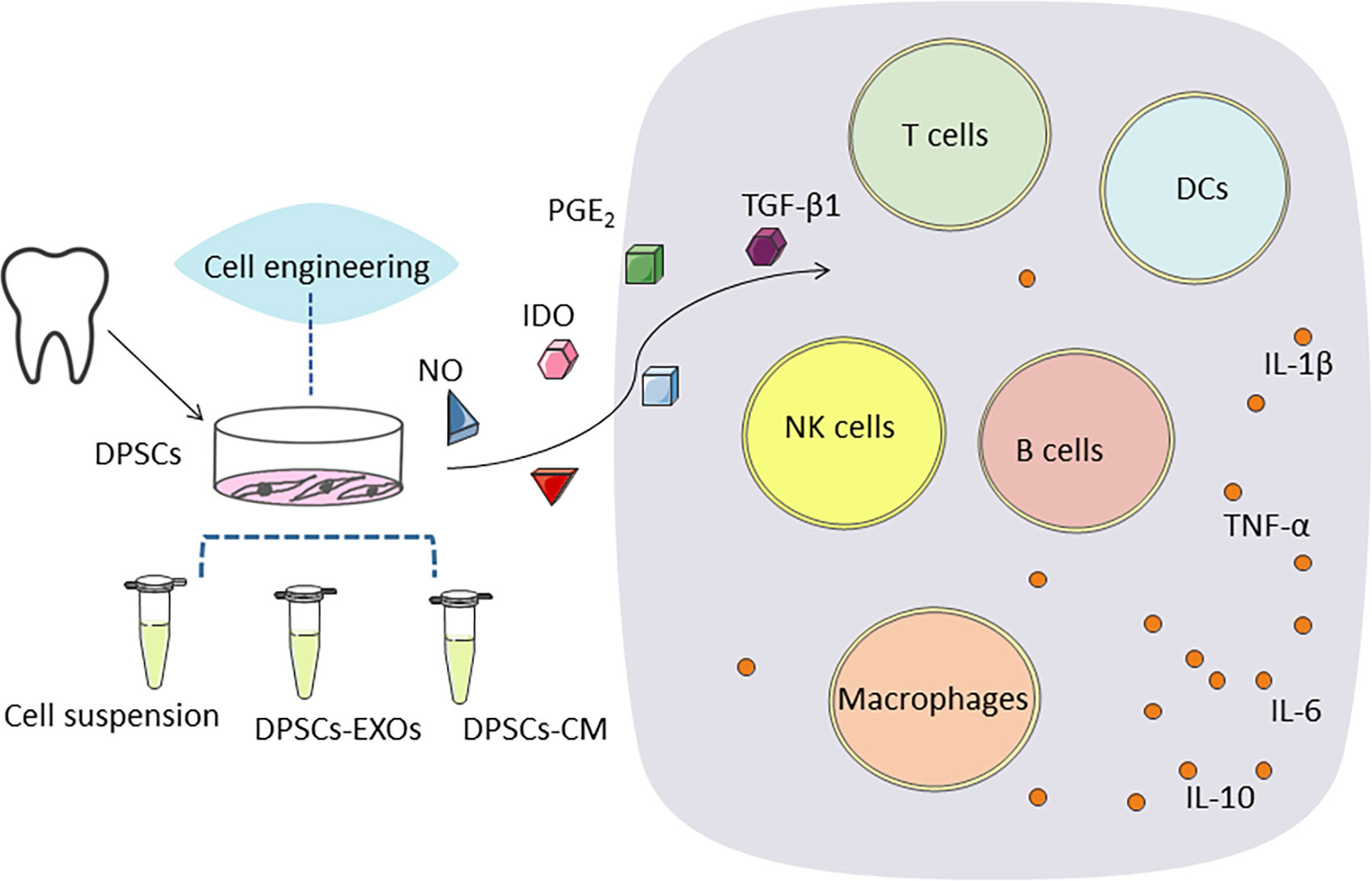
Figure 3 DPSCs secrete soluble factors mainly including, nitric oxide (NO), indoleamine 2,3-dioxygenase (IDO), prostaglandin E2 (PGE2) and transforming growth factor β1 (TGF-β1), which help to mediate immunosuppression. DPSCs stimulate regulatory T cell (Treg) function and inhibit all other cell types of the immune system, such as B and T cells, monocytes, dendritic cells. Gene transfer of an immunomodulatory or other factor may further enhance the efficacy of DPSCs therapy. DPSCs promote regeneration and healing by up-regulating anti-inflammatory immune cells and associated cytokines and down-regulating pro-inflammatory immune cells and associated cytokines to inhibit excessive inflammatory responses.
Lymphocytes are an important component of the immune system and consist mainly of T cells, B cells, NK cells and DCs. Among them, T cells and B cells are capable of producing specific immune responses to pathogens and become a major component of the adaptive immune system in addition to innate immunity (154). T-cell responses occur when antigen-presenting cells induce and control the differentiation of T cells. Initial CD4+ T helper cells may differentiate and transform into helper T cells1 (Th1), Th2, Th17 and Treg cells (155, 156). IL-17 induces fibroblasts, endothelial cells, macrophages and epithelial cells to produce pro-inflammatory cytokines, such as IL-1, IL-6 and TNF-α and certain chemokines, which generate and maintain an inflammatory environment (157, 158). Treg induces the secretion of IL-10 and TGF-β, which are used to regulate the immune response and suppress inflammation (159). B cells produce antibodies and interact closely with T cells, resulting in a variety of autoimmune diseases. Among soluble factors, TGF-β, HGF, prostaglandin E2 and IDO are thought to play an important role in MSCs-mediated B-cell immunosuppressive activity (160). The two most significant specialized antigen-presenting cells, DCs and macrophages, are essential for T-cells activation and the polarization of the adaptive immune response. Typically, macrophages are categorized as pro-inflammatory M1-type cells or anti-inflammatory M2-type cells (161, 162). M1 cells initiate inflammation by releasing high levels of pro-inflammatory cytokines, glutamate, reactive oxygen species, and nitric oxide. Correspondingly, M2 cells counteract inflammation and promote tissue repair and regeneration by secreting anti-inflammatory cytokines, phagocytic debris, and promoting tissue repair and regeneration (163). According to a study by Albashari et al., DPSCs significantly modulated the expression of NF-κB and its inhibitor IκB-α, attenuated the effect of lipopolysaccharide-induced polarization of inflammatory macrophages RAW 264.7 toward the M2 type, and decreased the expression of IL-6, which is achieved through the NF-κB signaling pathway (33). This has been the most likely effect of DPSCs in inflammatory diseases that many researchers have focused on, i.e., controlling the polarization of macrophages, inhibiting pro-inflammation and enhancing anti-inflammation.
Like Bone marrow mesenchymal stem cells, DPSC may inhibit T cell proliferation by secreting IDO induced by IFN-g (164). Recruitment of activated t cells by secreting fas (a death receptor known as tumor necrosis factor receptor superfamily member 6)-mediated monocyte chemoattractant protein-1 ensures intercellular contact, which in turn induces t cell apoptosis. Apoptotic t cells subsequently trigger the production of high levels of TGF-β by macrophages, which in turn leads to upregulation of CD4+CD25+Foxp3+ Tregs, thereby inducing the final immune effect (70). It has been shown that DPSCs can induce apoptosis of already activated T cells in vitro (69). DPSCs can inhibit the proliferation of stimulated T cells, and this inhibition is actually stronger than that of bone marrow mesenchymal stem cells (165). NK cells are important effector cells of innate immunity and play a key role in antitumor and antiviral effects through their cytotoxic and proinflammatory cytokines, including TNF-α and IFN-γ secretion. NK cells are also closely associated with inflammatory diseases, such as inflammatory bowel disease and periodontitis, among others (166). Regulation of B cells and NK cells by MSCs blocks pathogenic inflammatory and immune responses in vivo (167). The potential mechanism of DPSCs anti-inflammation is also closely related to the inhibition of NK cell proliferation, reduction of cytotoxicity, and promotion of apoptosis (168). DCs are the most potent antigen-presenting cells and play a key role in the effects of immunity and tolerance depending on the activation and maturation phases, as well as on the cytokine milieu at the site of inflammation (169). Mechanisms of DPSC anti-inflammation and immunomodulation may be related to interfering with the differentiation, maturation and function of DCs (170, 171). FasL is a transmembrane protein whose expression plays an important role in the induction of the Fas/FasL apoptotic pathway. In in vitro experiments, knockdown of FasL expression in DPSCs by siRNA led to a reduction in T cell apoptosis, making the anti-inflammatory effect of DPSCs greatly reduced which implies that FasL regulates the immunomodulatory properties of DPSCs in the context of inducing T cells apoptosis (69). DPSCs may be an effective treatment not only in oral inflammation, but in a variety of other acute and chronic inflammatory conditions.
As mentioned above, because DPSCs originate from the dental pulp, the pulp itself may be attacked by inflammation, complicating the relationship between inflammation and DPSCs. Inducing cell proliferation in DPSCs through chronic inflammation mediated by inflammatory biomolecules like TNF-α and C3a has been demonstrated; these proliferation-inducing effects are linked to NF-κB intracellular signaling stimulation, which may be related to the activation of the typical Wnt/β-Catenin pathway, while the atypical Wnt/Ca2+ pathway is inhibited (172, 173). When this stimulatory inflammatory challenge exceeds the capacity of the pulp tissue, the positive effect is diminished or even overridden. Stimulation by IFN-γ significantly upregulates the migratory capacity of DPSCs (173). Enhanced research in this area may provide more possibilities for DPSCs treatment of inflammatory diseases.
During inflammation caused by some bacterial injuries, NF-κB and p38MAPK intracellular signaling pathways are activated, releasing a large number of inflammation-associated biomolecules including IL-1α, IL -1β, IL-4, IL-6, IL-8, IL-10, TNF-α and a series of inflammatory molecular mediators (174). Neutrophils, macrophages, antigen-presenting DCs, T cells and later B cells are recruited and activated, producing a very complex effect. Multiple immune cells, in turn, produce additional inflammatory cytokines and antibodies to fight bacterial attack (151). Through their immunomodulatory effects, DPSCs play a decisive role in anti-inflammatory control through cell-cell contact and secreted substance mediation, especially IDO and TGF-β1 (33, 175). Through their action, DPSCs inhibit proliferation, maturation, antigen presentation and antibody/cytokine production of inflammatory cells (176).
11 Summary
In addition to their well-established self-renewal and pluripotent differentiation properties, MSCs also possess powerful anti-inflammatory and immunomodulatory functions in vitro and in vivo, making them candidates for the treatment of a variety of inflammation-related and autoimmune diseases (176). As a member of MSCs, DPSCs are favored by many researchers because of their easier availability and superior immunomodulatory ability than other MSCs (22, 176). The potentiality of DPSCs in the treatment of neurological, pulmonary, hepatic, renal, intestinal, skin-related inflammatory and autoimmune diseases should not be underestimated, probably because they themselves or some substances they secrete inhibit T cells proliferation and function through inflammatory immune-related signaling pathways, stimulate Treg cells, inhibit DCs differentiation, and convert macrophages to an anti-inflammatory phenotype role (103, 177) (Table 1).
Many countries have set up institutions specializing in the collection of DPSCs, and they have well-established processes for accessing DPSCs, including collection, transportation, stem cell isolation and culture, and cryogenic storage (178). Stockpiled DPSCs can be used in the future for both their personal use as well as for family members as necessary because of the low immunogenicity of DPSCs. Nagpal et al. administered appropriate concentrations of autologous DPSCs from elderly people into the infarct foci and surrounding areas of stroke survivors with moderate to severe disabilities using intracranial injections (179). After conducting a number of preoperative and postoperative observations and analyses, they concluded that autologous DPSC transplantation for the treatment of chronic symptoms following stroke was safe and practicable (179). Allogeneic DPSCs were used in a clinical trial by Ye et al. to treat severe COVID-19. They confirmed the safety and effectiveness of allogeneic DPSC transplantation by contrasting experimental and control groups in a limited sample size (180). Li et al. infused SHED in type 2 diabetes patients receiving insulin, and the outcomes demonstrated that SHED infusion is a secure and reliable treatment that enhances islet function and glucose metabolism in type 2 diabetes patients (181). The therapeutic potential and storage value of DPSCs are anticipated to get an increasing amount of attention as their safety and efficacy have been widely established.
However, as mentioned in this paper, DPSCs are derived from pulp tissue, which itself may experience inflammatory stimuli while in the oral cavity or during the process of being acquired, and furthermore, the means of culturing DPSCs, the environment, and the manner and method of delivering DPSCs or its products to the organism may affect the functional effects of DPSCs (3, 17). IFN-γ, TNF-α and IL-1β infusion into DPSCs may enhance their immunosuppressive capacity and can be considered as a feedback mechanism to suppress the exacerbated immune response (20). The role of the interface between DPSCs and immune cells is complex, and the roles of leader and leaderee may be interchanged under specific conditions (151). To really explain it in depth this is still a problem that needs to be overcome. Perhaps we can use such relationships to purposefully engineer DPSCs in order to obtain the drugs we are targeting to treat various diseases. For example, stimulating DPSCs to release engineered extracellular vesicles loaded with specific molecular cargoes for targeted delivery to cells and tissues treated with the ligand of interest (182).
We expect more research to be conducted on DPSCs, not only to achieve results in the laboratory and animal models, but also to serve clinical needs and transform them into practical tools that can truly contribute to the treatment of diseases and human health.
Author contributions
FL: Writing – original draft, Writing – review & editing. XW: Writing – review & editing. JS: Writing – review & editing. SW: Writing – review & editing. WX: Writing – review & editing. YH: Writing – review & editing.
Funding
The author(s) declare financial support was received for the research, authorship, and/or publication of this article. Wuhan University of Science and Technology startup fund (Chu Tian Scholars Program), the open laboratory fund of Hubei Province Key Laboratory of Oral and Maxillofacial Development and Regeneration, and the Hubei Provincial Health and Health Commission Research Project (No. WJ2023M121).
Conflict of interest
The authors declare that the research was conducted in the absence of any commercial or financial relationships that could be construed as a potential conflict of interest.
Publisher’s note
All claims expressed in this article are solely those of the authors and do not necessarily represent those of their affiliated organizations, or those of the publisher, the editors and the reviewers. Any product that may be evaluated in this article, or claim that may be made by its manufacturer, is not guaranteed or endorsed by the publisher.
References
1. Mattei V, Delle Monache S. Dental pulp stem cells (DPSCs) and tissue regeneration: mechanisms mediated by direct, paracrine, or autocrine effects. Biomedicines (2023) 11(2):386. doi: 10.3390/biomedicines11020386
2. Zheng Y, Lu H, Mu Q, Yi P, Lin L, Li P, et al. Effects of sEV derived from SHED and DPSC on the proliferation, migration and osteogenesis of PDLSC. Regener Ther (2023) 24:489–98. doi: 10.1016/j.reth.2023.09.009
3. Li Y, Zhang M, Yang C, Zou D, Zhang Z. Advances in studies on biological properties and pre-clinicalapplication of dental pulp stem cells. Stomatology (2022) 42(01):1–7. doi: 10.13591/j.cnki.kqyx.2022.01.001
4. Sakai K, Yamamoto A, Matsubara K, Nakamura S, Naruse M, Yamagata M, et al. Human dental pulp-derived stem cells promote locomotor recovery after complete transection of the rat spinal cord by multiple neuro-regenerative mechanisms. J Clin Invest (2012) 122(1):80–90. doi: 10.1172/jci59251
5. Altanerova U, Benejova K, Altanerova V, Tyciakova S, Rychly B, Szomolanyi P, et al. Dental pulp mesenchymal stem/stromal cells labeled with iron sucrose release exosomes and cells applied intra-nasally migrate to intracerebral glioblastoma. Neoplasma (2016) 63(6):925–33. doi: 10.4149/neo_2016_611
6. Ferro F, Spelat R, Baheney CS. Dental pulp stem cell (DPSC) isolation, characterization, and differentiation. Methods Mol Biol (Clifton N.J.) (2014) 1210:91–115. doi: 10.1007/978-1-4939-1435-7_8
7. Wang XD, Zhang JN, Gan YH, Zhou YH. Current understanding of pathogenesis and treatment of TMJ osteoarthritis. J Dental Res (2015) 94(5):666–73. doi: 10.1177/0022034515574770
8. Foldes A, Kadar K, Keremi B, Zsembery A, Gyires K, Zadori ZS, et al. Mesenchymal stem cells of dental origin-their potential for anti-inflammatory and regenerative actions in brain and gut damage. Curr Neuropharmacology (2016) 14(8):914–34. doi: 10.2174/1570159x14666160121115210
9. Su Y, Huang T, Sun H, Lin R, Zheng X, Bian Q, et al. High targeting specificity toward pulmonary inflammation using mesenchymal stem cell-hybrid nanovehicle for an efficient inflammation intervention. Adv Healthc Mater (2023) 12(23):e2300376. doi: 10.1002/adhm.202300376
10. Wiafe B, Adesida A, Churchill T, Metcalfe P. Mesenchymal stem cells inhibit hypoxia-induced inflammatory and fibrotic pathways in bladder smooth muscle cells. World J Urol (2018) 36(7):1157–65. doi: 10.1007/s00345-018-2247-1
11. Li D, Wang C, Chi C, Wang Y, Zhao J, Fang J, et al. Bone marrow mesenchymal stem cells inhibit lipopolysaccharide-induced inflammatory reactions in macrophages and endothelial cells. Mediators Inflamm (2016) 2016:2631439. doi: 10.1155/2016/2631439
12. Ullah I, Choe Y-h, Khan M, Bharti D, Shivakumar SB, Lee H-J, et al. Dental pulp-derived stem cells can counterbalance peripheral nerve injury-induced oxidative stress and supraspinal neuro-inflammation in rat brain. Sci Rep (2018) 8(1):15795. doi: 10.1038/s41598-018-34151-x
13. Liu Y, Wu J, Fei W, Cen X, Xiong Y, Wang S, et al. Is there a difference in intra-articular injections of corticosteroids, hyaluronate, or placebo for temporomandibular osteoarthritis? J Oral Maxillofac Surg (2018) 76(3):504–14. doi: 10.1016/j.joms.2017.10.028
14. Vandewalle J, Luypaert A, De Bosscher K, Libert C. Therapeutic mechanisms of glucocorticoids. Trends Endocrinol Metab (2018) 29(1):42–54. doi: 10.1016/j.tem.2017.10.010
15. Hongzhi HE, Dandan MA. Research progress on exosomes derived from dental pulp stem cells. J Prev Treat Stomatological Dis (2019) 27(10):652–7.
16. Oray M, Abu Samra K, Ebrahimiadib N, Meese H, Foster CS. Long-term side effects of glucocorticoids. Expert Opin Drug Saf (2016) 15(4):457–65. doi: 10.1517/14740338.2016.1140743
17. Sui B, Wu D, Xiang L, Fu Y, Kou X, Shi S. Dental pulp stem cells: from discovery to clinical application. J Endodontics (2020) 46(9):S46–55. doi: 10.1016/j.joen.2020.06.027
18. Ren H, Sang Y, Zhang F, Liu Z, Qi N, Chen Y. Comparative analysis of human mesenchymal stem cells from umbilical cord, dental pulp, and menstrual blood as sources for cell therapy. Stem Cells Int (2016) 2016:3516574. doi: 10.1155/2016/3516574
19. Bousnaki M, Bakopoulou A, Pich A, Papachristou E, Kritis A, Koidis P. Mapping the secretome of dental pulp stem cells under variable microenvironmental conditions. Stem Cell Rev Rep (2022) 18(4):1372–407. doi: 10.1007/s12015-021-10255-2
20. Andrukhov O, Behm C, Blufstein A, Rausch-Fan X. Immunomodulatory properties of dental tissue-derived mesenchymal stem cells: Implication in disease and tissue regeneration. World J Stem Cells (2019) 11(9):604–17. doi: 10.4252/wjsc.v11.i9.604
21. Buzoglu HD, Burus A, Bayazit Y, Goldberg M. Stem cell and oxidative stress-inflammation cycle. Curr Stem Cell Res Ther (2023) 18(5):641–52. doi: 10.2174/1574888x17666221012151425
22. Li P, Ou Q, Shi S, Shao C. Immunomodulatory properties of mesenchymal stem cells/dental stem cells and their therapeutic applications. Cell Mol Immunol (2023) 20(6):558–69. doi: 10.1038/s41423-023-00998-y
23. Austin PJ, Moalem-Taylor G. The neuro-immune balance in neuropathic pain: Involvement of inflammatory immune cells, immune-like glial cells and cytokines. J Neuroimmunology (2010) 229(1-2):26–50. doi: 10.1016/j.jneuroim.2010.08.013
24. Martini R, Willison H. Neuroinflammation in the peripheral nerve: cause, modulator, or bystander in peripheral neuropathies? Glia (2016) 64(4):475–86. doi: 10.1002/glia.22899
25. Fiore NT, Austin PJ. Are the emergence of affective disturbances in neuropathic pain states contingent on supraspinal neuroinflammation? Brain Behav Immun (2016) 56:397–411. doi: 10.1016/j.bbi.2016.04.012
26. Centonze D, Muzio L, Rossi S, Cavasinni F, De Chiara V, Bergami A, et al. Inflammation triggers synaptic alteration and degeneration in experimental autoimmune encephalomyelitis. J Neurosci (2009) 29(11):3442–52. doi: 10.1523/jneurosci.5804-08.2009
27. Ullah I, Subbarao RB, Rho GJ. Human mesenchymal stem cells - current trends and future prospective. Bioscience Rep (2015) 35(2):e00191. doi: 10.1042/bsr20150025
28. Strioga M, Viswanathan S, Darinskas A, Slaby O, Michalek J. Same or not the same? Comparison of adipose tissue-derived versus bone marrow-derived mesenchymal stem and stromal cells. Stem Cells Dev (2012) 21(14):2724–52. doi: 10.1089/scd.2011.0722
29. Grace PM, Hutchinson MR, Maier SF, Watkins LR. Pathological pain and the neuroimmune interface. Nat Rev Immunol (2014) 14(4):217–31. doi: 10.1038/nri3621
30. Saunders LL, Clarke A, Tate DG, Forchheimer M, Krause JS. Lifetime prevalence of chronic health conditions among persons with spinal cord injury. Arch Phys Med Rehabil (2015) 96(4):673–9. doi: 10.1016/j.apmr.2014.11.019
31. Witcher KG, Eiferman DS, Godbout JP. Priming the inflammatory puma of the CNS ater traumatic brain injury. Trends Neurosci (2015) 38(10):609–20. doi: 10.1016/j.tins.2015.08.002
32. Rouanet C, Reges D, Rocha E, Gagliardi V, Silva GS. Traumatic spinal cord injury: current concepts and treatment update. Arquivos Neuro-Psiquiatria (2017) 75(6):387–93. doi: 10.1590/0004-282x20170048
33. Albashari A, He Y, Zhang Y, Ali J, Lin F, Zheng Z, et al. Thermosensitive bFGF-modified hydrogel with dental pulp stem cells on neuroinflammation of spinal cord injury. ACS Omega (2020) 5(26):16064–75. doi: 10.1021/acsomega.0c01379
34. Bianco J, De Berdt P, Deumens R, des Rieux A. Taking a bite out of spinal cord injury: do dental stem cells have the teeth for it? Cell Mol Life Sci (2016) 73(7):1413–37. doi: 10.1007/s00018-015-2126-5
35. Luo L, He Y, Wang X, Key B, Lee BH, Li H, et al. Potential roles of dental pulp stem cells in neural regeneration and repair. Stem Cells Int (2018) 2018:1731289. doi: 10.1155/2018/1731289
36. Kuhla A, Ludwig SC, Kuhla B, Muench G, Vollmar B. Advanced glycation end products are mitogenic signals and trigger cell cycle reentry of neurons in Alzheimer's disease brain. Neurobiol Aging (2015) 36(2):753–61. doi: 10.1016/j.neurobiolaging.2014.09.025
37. Teeling JL, Perry VH. SYSTEMIC INFECTION AND INFLAMMATION IN ACUTE CNS INJURY AND CHRONIC NEURODEGENERATION: UNDERLYING MECHANISMS. Neuroscience (2009) 158(3):1062–73. doi: 10.1016/j.neuroscience.2008.07.031
38. Francos-Quijorna I, Amo-Aparicio J, Martinez-Muriana A, Lopez-Vales R. IL-4 drives microglia and macrophages toward a phenotype conducive for tissue repair and functional recovery after spinal cord injury. Glia (2016) 64(12):2079–92. doi: 10.1002/glia.23041
39. Papa S, Caron I, Erba E, Panini N, De Paola M, Mariani A, et al. Early modulation of pro-inflammatory microglia by minocycline loaded nanoparticles confers long lasting protection after spinal cord injury. Biomaterials (2016) 75:13–24. doi: 10.1016/j.biomaterials.2015.10.015
40. Gennai S, Monsel A, Hao Q, Liu J, Gudapati V, Barbier EL, et al. Cell-Based therapy for traumatic brain injury. Br J Anaesthesia (2015) 115(2):203–12. doi: 10.1093/bja/aev229
41. Acosta SA, Tajiri N, Shinozuka K, Ishikawa H, Sanberg PR, Sanchez-Ramos J, et al. Combination therapy of human umbilical cord blood cells and granulocyte colony stimulating factor reduces histopathological and motor impairments in an experimental model of chronic traumatic brain injury. PloS One (2014) 9(3):e90953. doi: 10.1371/journal.pone.0090953
42. Algattas H, Huang JH. Traumatic brain injury pathophysiology and treatments: early, intermediate, and late phases post-injury. Int J Mol Sci (2014) 15(1):309–41. doi: 10.3390/ijms15010309
43. Zhang R, Liu Y, Yan K, Chen L, Chen X-R, Li P, et al. Anti-inflammatory and immunomodulatory mechanisms of mesenchymal stem cell transplantation in experimental traumatic brain injury. J Neuroinflamm (2013) 10:106. doi: 10.1186/1742-2094-10-106
44. Galindo LT, Filippo TRM, Semedo P, Ariza CB, Moreira CM, Camara NOS, et al. Mesenchymal stem cell therapy modulates the inflammatory response in experimental traumatic brain injury. Neurol Res Int (2011) 2011:564089–9. doi: 10.1155/2011/564089
45. Pati S, Khakoo AY, Zhao J, Jimenez F, Gerber MH, Harting M, et al. Human mesenchymal stem cells inhibit vascular permeability by modulating vascular endothelial Cadherin/beta-catenin signaling. Stem Cells Dev (2011) 20(1):89–101. doi: 10.1089/scd.2010.0013
46. Tanna T, Sachan V. Mesenchymal stem cells: potential in treatment of neurodegenerative diseases. Curr Stem Cell Res Ther (2014) 9(6):513–21. doi: 10.2174/1574888x09666140923101110
47. Mu S, Wang J, Zhou G, Peng W, He Z, Zhao Z, et al. Transplantation of induced pluripotent stem cells improves functional recovery in Huntington's disease rat model. PLoS One (2014) 9(7):e101185. doi: 10.1371/journal.pone.0101185
48. Eskandari N, Boroujeni ME, Abdollahifar MA, Piryaei A, Khodagholi F, Mirbehbahani SH, et al. Transplantation of human dental pulp stem cells compensates for striatal atrophy and modulates neuro-inflammation in 3-nitropropionic acid rat model of Huntington's disease. Neurosci Res (2021) 170:133–44. doi: 10.1016/j.neures.2020.12.002
49. de Almeida FM, Marques SA, Ramalho B, Rodrigues RF, Cadilhe DV, Furtado D, et al. Human dental pulp cells: A new source of cell therapy in a mouse model of compressive spinal cord injury. J Neurotrauma (2011) 28(9):1939–49. doi: 10.1089/neu.2010.1317
50. Meffert MK, Baltimore D. Physiological functions for brain NF-kappaB. Trends Neurosci (2005) 28(1):37–43. doi: 10.1016/j.tins.2004.11.002
51. Schaper NC, Huijberts M, Pickwell K. Neurovascular control and neurogenic inflammation in diabetes. Diabetes-Metabolism Res Rev (2008) 24:S40–4. doi: 10.1002/dmrr.862
52. Vincent AM, Callaghan BC, Smith AL, Feldman EL. Diabetic neuropathy: cellular mechanisms as therapeutic targets. Nat Rev Neurol (2011) 7(10):573–83. doi: 10.1038/nrneurol.2011.137
53. Devaraj S, Dasu MR, Jialal I. Diabetes is a proinflammatory state: a translational perspective. Expert Rev Endocrinol Metab (2010) 5(1):19–28. doi: 10.1586/eem.09.44
54. Jing W, Peng-wei Z, Yan-jun Z. Research progress on role of neuroinflammation in pathogenesis of type 2 diabetic encephalopathy. Drugs Clinic (2015) 30(04):470–4. doi: 10.7501/j.issn.1674-5515.2015.04.027
55. Hata M, Omi M, Kobayashi Y, Nakamura N, Tosaki T, Miyabe M, et al. Transplantation of cultured dental pulp stem cells into the skeletal muscles ameliorated diabetic polyneuropathy: therapeutic plausibility of freshly isolated and cryopreserved dental pulp stem cells. Stem Cell Res Ther (2015) 6(1):162. doi: 10.1186/s13287-015-0156-4
56. Song L, Guo J, Song F, Peng Z. Neuroprotective effect of remote ischemic post-conditioning on neonatal hypoxic-ischemic encephalopathy. Chin J Child Health Care (2020) 28(11):1219–22. doi: 10.11852/zgetbjzz2019-1662
57. Chiu H-Y, Lin C-H, Hsu CY, Yu J, Hsieh C-H, Shyu W-C. IGF1R(+) dental pulp stem cells enhanced neuroplasticity in hypoxia-ischemia model. Mol Neurobiol (2017) 54(10):8225–41. doi: 10.1007/s12035-016-0210-y
58. Wang J, He P, Ze'en X, Qiao H, Wenjing W. Evaluation value of serum BDNF,IL-8,TNF -α and SOD levels for severity and prognosis of hypoxic-ischemic encephalopathy in neonates. Shaanxi Med J (2020) 49(11):1465–1468+1522. doi: 10.3969/j.issn.1000-7377.2020.11.024
59. Yuan J, Yang C, Chen J. Neuroprotective effect of mesenchymal stem cells on neonatal hypoxic-ischemic brain damage. Chin J Obstetrics Gynecology Ped (2020) 16(04):386–91. doi: 10.3877/cma.j.issn.1673-5250.2020.04.003
60. Yan N,WY, Tang X, Zhang X, Wang H, Yang T, Zhou M, et al. Bone marrow mesenchymal stem cells may alleviate brain damage caused by the microglial overactivation in the cortex around ischemic site of stroke. Chin J Tissue Eng Res (2022) 26(24):3790–5.
61. Murphy SF, Kwon JH, Boone DL. Novel players in inflammatory bowel disease pathogenesis. Curr Gastroenterol Rep (2012) 14(2):146–52. doi: 10.1007/s11894-012-0250-z
62. Loftus EV Jr. Clinical epidemiology of inflammatory bowel disease: Incidence, prevalence, and environmental influences. Gastroenterology (2004) 126(6):1504–17. doi: 10.1053/j.gastro.2004.01.063
63. Racz GZ, Kadar K, Foldes A, Kallo K, Perczel-Kovach K, Keremi B, et al. Immunomodulatory and potential therapeutic role of mesenchymal stem cells in periodontitis. J Physiol Pharmacol (2014) 65(3):327–39.
64. Hardenberg G, Steiner TS, Levings MK. Environmental influences on T regulatory cells in inflammatory bowel disease. Semin Immunol (2011) 23(2):130–8. doi: 10.1016/j.smim.2011.01.012
65. Himmel ME, Yao Y, Orban PC, Steiner TS, Levings MK. Regulatory T-cell therapy for inflammatory bowel disease: more questions than answers. Immunology (2012) 136(2):115–22. doi: 10.1111/j.1365-2567.2012.03572.x
66. Kamada N, Hisamatsu T, Okamoto S, Sato T, Matsuoka K, Arai K, et al. Abnormally differentiated subsets of intestinal macrophage play a key role in Th1-dominant chronic colitis through excess production of IL-12 and IL-23 in response to bacteria. J Immunol (Baltimore Md. 1950) (2005) 175(10):6900–8. doi: 10.4049/jimmunol.175.10.6900
67. Coombes JL, Siddiqui KRR, Arancibia-Carcamo CV, Hall J, Sun C-M, Belkaid Y, et al. A functionally specialized population of mucosal CD103(+) DCs induces Foxp3(+) regulatory T cells via a TGF-beta- and retinoic acid-dependent mechanism. J Exp Med (2007) 204(8):1757–64. doi: 10.1084/jem.20070590
68. Rovedatti L, Kudo T, Biancheri P, Sarra M, Knowles CH, Rampton DS, et al. Differential regulation of interleukin 17 and interferon gamma production in inflammatory bowel disease. Gut (2009) 58(12):1629–36. doi: 10.1136/gut.2009.182170
69. Zhao Y, Wang L, Jin Y, Shi S. Fas ligand regulates the immunomodulatory properties of dental pulp stem cells. J Dental Res (2012) 91(10):948–54. doi: 10.1177/0022034512458690
70. Akiyama K, Chen C, Wang D, Xu X, Qu C, Yamaza T, et al. Mesenchymal-stem-cell-induced immunoregulation involves FAS-ligand-/FAS-mediated T cell apoptosis. Cell Stem Cell (2012) 10(5):544–55. doi: 10.1016/j.stem.2012.03.007
71. Krampera M. Mesenchymal stromal cell 'licensing': a multistep process. Leukemia (2011) 25(9):1408–14. doi: 10.1038/leu.2011.108
72. Goncalves F, Schneider N, Pinto FO, Meyer FS, Visioli F, Pfaffenseller B, et al. Intravenous vs intraperitoneal mesenchymal stem cells administration: What is the best route for treating experimental colitis? World J Gastroenterol (2014) 20(48):18228–39. doi: 10.3748/wjg.v20.i48.18228
73. Cuerquis J, Romieu-Mourez R, Francois M, Routy J-P, Young YK, Zhao J, et al. Human mesenchymal stromal cells transiently increase cytokine production by activated T cells before suppressing T-cell proliferation: effect of interferon-gamma and tumor necrosis factor-alpha stimulation. Cytotherapy (2014) 16(2):191–202. doi: 10.1016/j.jcyt.2013.11.008
74. Matthay MA, Ware LB, Zimmerman GA. The acute respiratory distress syndrome. J Clin Invest (2012) 122(8):2731–40. doi: 10.1172/jci60331
75. Dinis-Oliveira RJ, Duarte JA, Sanchez-Navarro A, Remiao F, Bastos ML, Carvalho F. Paraquat poisonings: Mechanisms of lung toxicity, clinical features, and treatment. Crit Rev Toxicol (2008) 38(1):13–71. doi: 10.1080/10408440701669959
76. Gawarammana IB, Buckley NA. Medical management of paraquat ingestion. Br J Clin Pharmacol (2011) 72(5):745–57. doi: 10.1111/j.1365-2125.2011.04026.x
77. Geng P, Zhang Y, Zhang H, Dong X, Yang Y, Zhu X, et al. HGF-modified dental pulp stem cells mitigate the inflammatory and fibrotic responses in paraquat-induced acute respiratory distress syndrome. Stem Cells Int (2021) 2021:6662831. doi: 10.1155/2021/6662831
78. Molnarfi N, Benkhoucha M, Funakoshi H, Nakamura T, Lalive PH. Hepatocyte growth factor: A regulator of inflammation and autoimmunity. Autoimmun Rev (2015) 14(4):293–303. doi: 10.1016/j.autrev.2014.11.013
79. Laing AG, Riffo-Vasquez Y, Sharif-Paghaleh E, Lombardi G, Sharpe PT. Immune modulation by apoptotic dental pulp stem cells in vivo. Immunotherapy (2018) 10(3):201–11. doi: 10.2217/imt-2017-0117
80. Lee Y-J, Moon C, Lee SH, Park H-J, Seoh J-Y, Cho M-S, et al. Apoptotic cell instillation after bleomycin attenuates lung injury through hepatocyte growth factor induction. Eur Respir J (2012) 40(2):424–35. doi: 10.1183/09031936.00096711
81. Ren Y, Xie Y, Jiang G, Fan J, Yeung J, Li W, et al. Apoptotic cells protect mice against lipopolysaccharide-induced shock. J Immunol (2008) 180(7):4978–85. doi: 10.4049/jimmunol.180.7.4978
82. Raherison C, Girodet PO. Epidemiology of COPD. Eur Respir Rev (2009) 18(114):213–21. doi: 10.1183/09059180.00003609
83. Gao X, Liu Z, Wang Z. Dental pulp stem cells ameliorate elastase-induced pulmonary emphysema by regulating inflammation and oxidative stress. J Inflammation Res (2023) 16:1497–508. doi: 10.2147/jir.S402794
84. Nguyen JMK, Robinson DN, Sidhaye VK. Why new biology must be uncovered to advance therapeutic strategies for chronic obstructive pulmonary disease. Am J Physiology-Lung Cell Mol Physiol (2021) 320(1):L1–L11. doi: 10.1152/ajplung.00367.2020
85. Sundar IK, Rashid K, Sellix MT, Rahman I. The nuclear receptor and clock gene REV-ERB alpha regulates cigarette smoke-induced lung inflammation. Biochem Biophys Res Commun (2017) 493(4):1390–5. doi: 10.1016/j.bbrc.2017.09.157
86. Gazdic M, Volarevic V, Arsenijevic N, Stojkovic M. Mesenchymal stem cells: A friend or foe in immune-mediated diseases. Stem Cell Rev Rep (2015) 11(2):280–7. doi: 10.1007/s12015-014-9583-3
87. Flores-Mendoza G, Sanson SP, Rodriguez-Castro S, Crispin JC, Rosetti F. Mechanisms of tissue injury in lupus nephritis. Trends Mol Med (2018) 24(4):364–78. doi: 10.1016/j.molmed.2018.02.003
88. Mok CC. Therapeutic options for resistant lupus nephritis. Semin Arthritis Rheumatism (2006) 36(2):71–81. doi: 10.1016/j.semarthrit.2006.04.008
89. Rovin BH, Parikh SV. Lupus nephritis: the evolving role of novel therapeutics. Am J Kidney Dis (2014) 63(4):677–90. doi: 10.1053/j.ajkd.2013.11.023
90. Gao F, Chiu SM, Motan DAL, Zhang Z, Chen L, Ji HL, et al. Mesenchymal stem cells and immunomodulation: current status and future prospects. Cell Death Dis (2016) 7(1):e2062. doi: 10.1038/cddis.2015.327
91. Tang X, Li W, Wen X, Zhang Z, Chen W, Yao G, et al. Transplantation of dental tissue-derived mesenchymal stem cells ameliorates nephritis in lupus mice. Ann Trans Med (2019) 7(7):132. doi: 10.21037/atm.2019.02.41
92. Miyake K, Akahoshi M, Nakashima H. Th subset balance in lupus nephritis. J Biomedicine Biotechnol (2011) 2011:980286. doi: 10.1155/2011/980286
93. De Miguel MP, Fuentes-Julián S, Blázquez-Martínez A, Pascual CY, Aller MA, Arias J, et al. Immunosuppressive properties of mesenchymal stem cells: advances and applications. Curr Mol Med (2012) 12(5):574–91. doi: 10.2174/156652412800619950
94. Wu JJ, Kavanaugh A, Lebwohl MG, Gniadecki R, Merola JA-O. Psoriasis and metabolic syndrome: implications for the management and treatment of psoriasis. J Eur Acad Dermatol Venereol (2022) 36(6):797–806. doi: 10.1111/jdv.18044
95. Belinchon I, Rivera R, Blanch C, Comellas M, Lizan L. Adherence, satisfaction and preferences for treatment in patients with psoriasis in the European Union: a systematic review of the literature. Patient Preference Adherence (2016) 10:2357–67. doi: 10.2147/ppa.S117006
96. Meng H, Wei F, Zhou Y, Hu L, Ge Z, Jin J, et al. Overexpression of hepatocyte growth factor in dental pulp stem cells ameliorates the severity of psoriasis by reducing inflammatory responses. Stem Cells Dev (2021) 30(17):876–89. doi: 10.1089/scd.2021.0129
97. Nakamura T, Mizuno S. The discovery of Hepatocyte Growth Factor (HGF) and its significance for cell biology, life sciences and clinical medicine. Proc Japan Acad Ser B-Physical Biol Sci (2010) 86(6):588–610. doi: 10.2183/pjab.86.588
98. El-Sayed KMF, Elsalawy R, Ibrahim N, Gadalla M, Albargasy H, Zahra N, et al. The dental pulp stem/progenitor cells-mediated inflammatory-regenerative axis. Tissue Eng Part B-Reviews (2019) 25(5):445–60. doi: 10.1089/ten.teb.2019.0106
99. Cooper PR, Chicca IJ, Holden MJ, Milward MR. Inflammation and regeneration in the dentin-pulp complex: net gain or net loss? J Endodontics (2017) 43(9):S87–94. doi: 10.1016/j.joen.2017.06.011
100. Farges J-C, Alliot-Licht B, Renard E, Ducret M, Gaudin A, Smith AJ, et al. Dental pulp defence and repair mechanisms in dental caries. Mediators Inflammation (2015) 2015:230251. doi: 10.1155/2015/230251
101. Suzuki T, Lee CH, Chen M, Zhao W, Fu SY, Qi JJ, et al. Induced migration of dental pulp stem cells for in vivo pulp regeneration. J Dental Res (2011) 90(8):1013–8. doi: 10.1177/0022034511408426
102. Abd-Elmeguid A, Abdeldayem M, Kline LW, Moqbel R, Vliagoftis H, Yu DC. Osteocalcin expression in pulp inflammation. J Endodontics (2013) 39(7):865–72. doi: 10.1016/j.joen.2012.12.035
103. Leprince JG, Zeitlin BD, Tolar M, Peters OA. Interactions between immune system and mesenchymal stem cells in dental pulp and periapical tissues. Int Endodontic J (2012) 45(8):689–701. doi: 10.1111/j.1365-2591.2012.02028.x
104. Wang Z, Ma F, Wang J, Zhou Z, Liu B, He X, et al. Extracellular signal-regulated kinase mitogen-activated protein kinase and phosphatidylinositol 3-kinase/Akt signaling are required for lipopolysaccharide-mediated mineralization in murine odontoblast-like cells. J Endodontics (2015) 41(6):871–6. doi: 10.1016/j.joen.2015.01.006
105. Feng X, Feng G, Xing J, Shen B, Li L, Tan W, et al. TNF-alpha triggers osteogenic differentiation of human dental pulp stem cells via the NF-kappa B signalling pathway. Cell Biol Int (2013) 37(12):1267–75. doi: 10.1002/cbin.10141
106. Cooper PR, Takahashi Y, Graham LW, Simon S, Imazato S, Smith AJ. Inflammation-regeneration interplay in the dentine-pulp complex. J Dentistry (2010) 38(9):687–97. doi: 10.1016/j.jdent.2010.05.016
107. Wang Y, Chen X, Cao W, Shi Y. Plasticity of mesenchymal stem cells in immunomodulation: pathological and therapeutic implications. Nat Immunol (2014) 15(11):1009–16. doi: 10.1038/ni.3002
108. Neves VCM, Yianni V, Sharpe PT. Macrophage modulation of dental pulp stem cell activity during tertiary dentinogenesis. Sci Rep (2020) 10(1):20216. doi: 10.1038/s41598-020-77161-4
109. Sehgal A, Donaldson DS, Pridans C, Sauter KA, Hume DA, Mabbott NA. The role of CSF1R-dependent macrophages in control of the intestinal stem-cell niche. Nat Commun (2018) 9(1):1272. doi: 10.1038/s41467-018-03638-6
110. Wang J, Zheng Y, Bai B, Song Y, Zheng K, Xiao J, et al. MicroRNA-125a-3p participates in odontoblastic differentiation of dental pulp stem cells by targeting Fyn. Cytotechnology (2020) 72(1):69–79. doi: 10.1007/s10616-019-00358-7
111. Ray RR. Periodontitis: an oral disease with severe consequences. Appl Biochem Biotechnol (2023) 195(1):17–32. doi: 10.1007/s12010-022-04127-9
112. Penatti A, Facciotti F, De Matteis R, Larghi P, Paroni M, Murgo A, et al. Differences in serum and synovial CD4+T cells and cytokine profiles to stratify patients with inflammatory osteoarthritis and rheumatoid arthritis. Arthritis Res Ther (2017) 19(1):103. doi: 10.1186/s13075-017-1305-1
113. Monasterio G, Castillo F, Rojas L, Cafferata EA, Alvarez C, Carvajal P, et al. Th1/Th17/Th22 immune response and their association with joint pain, imagenological bone loss, RANKL expression and osteoclast activity in temporomandibular joint osteoarthritis: A preliminary report. J Oral Rehabil (2018) 45(8):589–97. doi: 10.1111/joor.12649
114. Cui SJ, Zhang T, Fu Y, Liu Y, Gan YH, Zhou YH, et al. DPSCs attenuate experimental progressive TMJ arthritis by inhibiting the STAT1 pathway. J Dental Res (2020) 99(4):446–55. doi: 10.1177/0022034520901710
115. Dong L, Chen X, Shao H, Bai L, Li X, Zhang X. Mesenchymal stem cells inhibited dendritic cells via the regulation of STAT1 and STAT6 phosphorylation in experimental autoimmune uveitis. Curr Mol Med (2017) 17(7):478–87. doi: 10.2174/1566524018666180207155614
116. Stanko P, Altanerova U, Jakubechova J, Repiska V, Altaner C. Dental mesenchymal stem/stromal cells and their exosomes. Stem Cells Int (2018) 2018:8973613. doi: 10.1155/2018/8973613
117. El Moshy S, Radwan IA, Rady D, Abbass MMS, El-Rashidy AA, Sadek KM, et al. Dental stem cell-derived secretome/conditioned medium: the future for regenerative therapeutic applications. Stem Cells Int (2020) 2020:7593402. doi: 10.1155/2020/7593402
118. Gao M, Gao W, Papadimitriou JM, Zhang C, Gao J, Zheng M. Exosomes-the enigmatic regulators of bone homeostasis (vol 6, 36, 2018). Bone Res (2019) 7(6):36. doi: 10.1038/s41413-018-0043-6
119. Kalluri R, LeBleu VS. The biology, function, and biomedical applications of exosomes. Science (2020) 367(6478):640–+. doi: 10.1126/science.aau6977
120. Yeo RWY, Lai RC, Zhang B, Tan SS, Yin Y, Teh BJ, et al. Mesenchymal stem cell: An efficient mass producer of exosomes for drug delivery. Advanced Drug Delivery Rev (2013) 65(3):336–41. doi: 10.1016/j.addr.2012.07.001
121. Galipeau J, Sensebe L. Mesenchymal stromal cells: clinical challenges and therapeutic opportunities. Cell Stem Cell (2018) 22(6):824–33. doi: 10.1016/j.stem.2018.05.004
122. Riazifar M, Mohammadi MA-O, Pone EJ, Yeri A, Lässer C, Segaliny AA-O, et al. Stem Cell-Derived Exosomes as Nanotherapeutics for Autoimmune and Neurodegenerative Disorders. ACS Nano (2019) 3(6):6670–88. doi: 10.1021/acsnano.9b01004
123. Pivoraite U, Jarmalaviciute A, Tunaitis V, Ramanauskaite G, Vaitkuviene A, Kaseta V, et al. Exosomes from human dental pulp stem cells suppress carrageenan-induced acute inflammation in mice. Inflammation (2015) 38(5):1933–41. doi: 10.1007/s10753-015-0173-6
124. Li S, Luo L, He Y, Li R, Xiang Y, Xing Z, et al. Dental pulp stem cell-derived exosomes alleviate cerebral ischaemia-reperfusion injury through suppressing inflammatory response. Cell Proliferation (2021) 54(8):e13093. doi: 10.1111/cpr.13093
125. Shen Z, Kuang S, Zhang Y, Yang M, Qin W, Shi X, et al. Chitosan hydrogel incorporated with dental pulp stem cell-derived exosomes alleviates periodontitis in mice via a macrophage-dependent mechanism. Bioactive Materials (2020) 5(4):1113–26. doi: 10.1016/j.bioactmat.2020.07.002
126. Konala VBR, Mamidi MK, Bhonde R, Das AK, Pochampally R, Pal R. The current landscape of the mesenchymal stromal cell secretome: A new paradigm for cell-free regeneration. Cytotherapy (2016) 18(1):13–24. doi: 10.1016/j.jcyt.2015.10.008
127. Vizoso FJ, Eiro N, Cid S, Schneider J, Perez-Fernandez R. Mesenchymal stem cell secretome: toward cell-free therapeutic strategies in regenerative medicine. Int J Mol Sci (2017) 18(9):1852. doi: 10.3390/ijms18091852
128. Tanaka E, Detamore MS, Mercuri LG. Degenerative disorders of the temporomandibular joint: Etiology, diagnosis, and treatment. J Dental Res (2008) 87(4):296–307. doi: 10.1177/154405910808700406
129. Ogasawara N, Kano F, Hashimoto N, Mori H, Liu Y, Xia L, et al. Factors secreted from dental pulp stem cells show multifaceted benefits for treating experimental temporomandibular joint osteoarthritis. Osteoarthritis Cartilage (2020) 28(6):831–41. doi: 10.1016/j.joca.2020.03.010
130. Firestein GS. Evolving concepts of rheumatoid arthritis. Nature (2003) 423(6937):356–61. doi: 10.1038/nature01661
131. Ishikawa J, Takahashi N, Matsumoto T, Yoshioka Y, Yamamoto N, Nishikawa M, et al. Factors secreted from dental pulp stem cells show multifaceted benefits for treating experimental rheumatoid arthritis. Bone (2016) 83:210–9. doi: 10.1016/j.bone.2015.11.012
132. Huang Y, Mucke L. Alzheimer mechanisms and therapeutic strategies. Cell (2012) 148(6):1204–22. doi: 10.1016/j.cell.2012.02.040
133. Mita T, Furukawa-Hibi Y, Takeuchi H, Hattori H, Yamada K, Hibi H, et al. Conditioned medium from the stem cells of human dental pulp improves cognitive function in a mouse model of Alzheimer's disease. Behav Brain Res (2015) 293:189–97. doi: 10.1016/j.bbr.2015.07.043
134. Kano F, Matsubara K, Ueda M, Hibi H, Yamamoto A. Secreted Ectodomain of Sialic Acid-Binding Ig-Like Lectin-9 and Monocyte Chemoattractant Protein-1 Synergistically Regenerate Transected Rat Peripheral Nerves by Altering Macrophage Polarity. Stem Cells (2017) 35(3):641–53. doi: 10.1002/stem.2534
135. Matsubara K, Matsushita YA-OX, Sakai K, Kano F, Kondo M, Noda MA-O, et al. Secreted ectodomain of sialic acid-binding Ig-like lectin-9 and monocyte chemoattractant protein-1 promote recovery after rat spinal cord injury by altering macrophage polarity. J Neurosci (2015) 35(6):2452–64.
136. Riordan SM, Williams R. Mechanisms of hepatocyte injury, multiorgan failure, and prognostic criteria in acute liver failure. Semin liver Dis (2003) 23(3):203–15. doi: 10.1055/s-2003-42639
137. Matsushita Y, Ishigami M, Matsubara K, Kondo M, Wakayama H, Goto H, et al. Multifaceted therapeutic benefits of factors derived from stem cells from human exfoliated deciduous teeth for acute liver failure in rats. J Tissue Eng Regenerative Med (2017) 11(6):1888–96. doi: 10.1002/term.2086
138. Kisseleva T, Brenner DA. Mechanisms of fibrogenesis. Exp Biol Med (2008) 233(2):109–22. doi: 10.3181/0707-mr-190
139. Hirata M, Ishigami M, Matsushita Y, Ito T, Hattori H, Hibi H, et al. Multifaceted therapeutic benefits of factors derived from dental pulp stem cells for mouse liver fibrosis. Stem Cells Trans Med (2016) 5(10):1416–24. doi: 10.5966/sctm.2015-0353
140. Yamaguchi S, Shibata R, Yamamoto N, Nishikawa M, Hibi H, Tanigawa T, et al. Dental pulp-derived stem cell conditioned medium reduces cardiac injury following ischemia-reperfusion. Sci Rep (2015) 5:16295. doi: 10.1038/srep16295
141. Kallenberg CGM, Vissink A, Kroese FGM, Abdulahad WH, Bootsma H. What have we learned from clinical trials in primary Sjogren's syndrome about pathogenesis? Arthritis Res Ther (2011) 13(1):205. doi: 10.1186/ar3234
142. Matsumura-Kawashima M, Ogata K, Moriyama M, Murakami Y, Kawado T, Nakamura S. Secreted factors from dental pulp stem cells improve Sjogren's syndrome via regulatory T cell-mediated immunosuppression. Stem Cell Res Ther (2021) 12(1):182. doi: 10.1186/s13287-021-02236-6
143. Ogata K, Matsumura-Kawashima M, Moriyama M, Kawado T, Nakamura S. Dental pulp-derived stem cell-conditioned media attenuates secondary Sjogren's syndrome via suppression of inflammatory cytokines in the submandibular glands. Regenerative Ther (2021) 16:73–80. doi: 10.1016/j.reth.2021.01.006
144. Wakayama H, Hashimoto N, Matsushita Y, Matsubara K, Yamamoto N, Hasegawa Y, et al. Factors secreted from dental pulp stem cells show multifaceted benefits for treating acute lung injury in mice. Cytotherapy (2015) 17(8):1119–29. doi: 10.1016/j.jcyt.2015.04.009
145. Morandi F, Raffaghello L, Bianchi G, Meloni F, Salis A, Millo E, et al. Immunogenicity of human mesenchymal stem cells in HLA-class I-restricted T-Cell responses against viral or tumor-associated antigens. Stem Cells (2008) 26(5):1275–87. doi: 10.1634/stemcells.2007-0878
146. Shi M, Liu ZW, Wang FS. Immunomodulatory properties and therapeutic application of mesenchymal stem cells. Clin Exp Immunol (2011) 164(1):1–8. doi: 10.1111/j.1365-2249.2011.04327.x
147. Taddio A, Biondi A, Piscianz E, Valencic E, Biagi E, Badolato R. From bone marrow transplantation to cellular therapies: possible therapeutic strategies in managing autoimmune disorders. Curr Pharm Design (2012) 18(35):5776–81. doi: 10.2174/138161212803530754
148. Tomic S, Djokic J, Vasilijic S, Vucevic D, Todorovic V, Supic G, et al. Immunomodulatory properties of mesenchymal stem cells derived from dental pulp and dental follicle are susceptible to activation by toll-like receptor agonists. Stem Cells Dev (2011) 20(4):695–708. doi: 10.1089/scd.2010.0145
149. Leprince JG, Zeitlin Bd, Tolar M, Peters OA. Interactions between immune system and mesenchymal stem cells in dental pulp and periapical tissues. Int Endod J (2012) 45(8):689–701. doi: 10.1111/j.1365-2591.2012.02028.x
150. Yamaguchi S, Shibata R, Yamamoto N, Nishikawa M, Hibi H, Tanigawa T, et al. Dental pulp-derived stem cell conditioned medium reduces cardiac injury following ischemia-reperfusion. Sci Rep (2015) 5:16295. doi: 10.1038/srep16295
151. Caplan AI, Correa D. The MSC: an injury drugstore. Cell Stem Cell (2011) 9(1):11–5. doi: 10.1016/j.stem.2011.06.008
152. Jiang W, Xu J. Immune modulation by mesenchymal stem cells. Cell Proliferation (2020) 53(1):e12712. doi: 10.1111/cpr.12712
153. Ren G, Zhang L, Zhao X, Xu G, Zhang Y, Roberts AI, et al. Mesenchymal stem cell-mediated immunosuppression occurs via concerted action of chemokines and nitric oxide. Cell Stem Cell (2008) 2(2):141–50. doi: 10.1016/j.stem.2007.11.014
154. Wang L, Zhao Y, Shi S. Interplay between mesenchymal stem cells and lymphocytes: implications for immunotherapy and tissue regeneration. J Dental Res (2012) 91(11):1003–10. doi: 10.1177/0022034512460404
155. Langrish CL, Chen Y, Blumenschein WM, Mattson J, Basham B, Sedgwick JD, et al. IL-23 drives a pathogenic T cell population that induces autoimmune inflammation. J Exp Med (2005) 201(2):233–40. doi: 10.1084/jem.20041257
156. Kumar V, Sercarz EE. The involvement of T cell receptor peptide-specific regulatory CD4+ T cells in recovery from antigen-induced autoimmune disease. J Exp Med (1993) 178(3):909–16. doi: 10.1084/jem.178.3.909
157. Hundorfean G, Neurath MF, Mudter J. Functional relevance of T helper 17 (Th17) cells and the IL-17 cytokine family in inflammatory bowel disease. Inflammatory Bowel Dis (2012) 18(1):180–6. doi: 10.1002/ibd.21677
158. Szkaradkiewicz AK, Karpinski TM, Zeidler A, Wyganowska-Swiatkowska M, Szkaradkiewicz A. Protective effect of oral Lactobacilli in pathogenesis of chronic periodontitis. J Physiol Pharmacol (2011) 62(6):685–9.
159. Suri-Payer E, Cantor H. Differential cytokine requirements for regulation of autoimmune gastritis and colitis by CD4(+)CD25(+) T cells. J Autoimmun (2001) 16(2):115–23. doi: 10.1006/jaut.2000.0473
160. Corcione A, Benvenuto F, Ferretti E, Giunti D, Cappiello V, Cazzanti F, et al. Human mesenchymal stem cells modulate B-cell functions. Blood (2006) 107(1):367–72. doi: 10.1182/blood-2005-07-2657
161. Mantovani A, Biswas SK, Galdiero MR, Sica A, Locati M. Macrophage plasticity and polarization in tissue repair and remodelling. J Pathol (2013) 229(2):176–85. doi: 10.1002/path.4133
162. Murray PJ, Allen JE, Biswas SK, Fisher EA, Gilroy DW, Goerdt S, et al. Macrophage activation and polarization: nomenclature and experimental guidelines. Immunity (2014) 41(1):14–20. doi: 10.1016/j.immuni.2014.06.008
163. Edwards JP, Zhang X, Frauwirth KA, Mosser DM. Biochemical and functional characterization of three activated macrophage populations. J Leukocyte Biol (2006) 80(6):1298–307. doi: 10.1189/jlb.0406249
164. Meisel R, Zibert A, Laryea M, Gobel U, Daubener W, Dilloo D. Human bone marrow stromal cells inhibit allogeneic T-cell responses by indoleamine 2,3-dioxygenase-mediated tryptophan degradation. Blood (2004) 103(12):4619–21. doi: 10.1182/blood-2003-11-3909
165. Sonoyama W, Liu Y, Yamaza T, Tuan RS, Wang S, Shi S, et al. Characterization of the apical papilla and its residing stem cells from human immature permanent teeth: A pilot study. J Endodontics (2008) 34(2):166–71. doi: 10.1016/j.joen.2007.11.021
166. Yadav PK, Chen C, Liu Z. Potential role of NK cells in the pathogenesis of inflammatory bowel disease. J Biomedicine Biotechnol (2011) p:1–6. doi: 10.1155/2011/348530
167. Gerdoni E, Gallo B, Casazza S, Musio S, Bonanni I, Pedemonte E, et al. Mesenchymal stem cells effectively modulate pathogenic immune response in experimental autoimmune encephalomyelitis. Ann Neurol (2007) 61(3):219–27. doi: 10.1002/ana.21076
168. Sotiropoulou PA, Perez SA, Gritzapis AD, Baxevanis CN, Papamichail M. Interactions between human mesenchymal stem cells and natural killer cells. Stem Cells (2006) 24(1):74–85. doi: 10.1634/stemcells.2004-0359
169. Pierdomenico L, Bonsi L, Calvitti M, Rondelli D, Arpinati M, Chirumbolo G, et al. Multipotent mesenchymal stem cells with immunosuppressive activity can be easily isolated from dental pulp. Transplantation (2005) 80(6):836–42. doi: 10.1097/01.tp.0000173794.72151.88
170. Rutella S, Danese S, Leone G. Tolerogenic dendritic cells: cytokine modulation comes of age. Blood (2006) 108(5):1435–40. doi: 10.1182/blood-2006-03-006403
171. Yang Y, Wang. J, Zhang R, Li C, Fan A, Cui D, et al. Effects of conditioned media of different sources on the proliferation of human dental pulp stem cells. Chin J Tissue Eng Res (2023) 27(01):49–53.
172. Rufas P, Jeanneau C, Rombouts C, Laurent P, About I. Complement C3a mobilizes dental pulp stem cells and specifically guides pulp fibroblast recruitment. J Endodontics (2016) 42(9):1377–84. doi: 10.1016/j.joen.2016.06.011
173. Strojny C, Boyle M, Bartholomew A, Sundivakkam P, Alapati S. Interferon gamma-treated dental pulp stem cells promote human mesenchymal stem cell migration in vitro. J Endodontics (2015) 41(8):1259–64. doi: 10.1016/j.joen.2015.02.018
174. Alkharobi H, Beattie J, Meade J, Devine D, El-Gendy R. Dental pulp cells isolated from teeth with superficial caries retain an inflammatory phenotype and display an enhanced matrix mineralization potential. Front Physiol (2017) 8:244. doi: 10.3389/fphys.2017.00244
175. Krampera M, Cosmi L, Angeli R, Pasini A, Liotta F, Andreini A, et al. Role for interferon-γ in the immunomodulatory activity of human bone marrow mesenchymal stem cells. Stem Cells (2006) 24(2):386–98. doi: 10.1634/stemcells.2005-0008
176. Cooper PR, Holder MJ, Smith AJ. Inflammation and regeneration in the dentin-pulp complex: A double-edged sword. J Endodontics (2014) 40(4):S46–51. doi: 10.1016/j.joen.2014.01.021
177. De Miguel MP, Fuentes-Julian S, Blazquez-Martinez A, Pascual CY, Aller MA, Arias J, et al. Immunosuppressive properties of mesenchymal stem cells: advances and applications. Curr Mol Med (2012) 12(5):574–91. doi: 10.2174/156652412800619950
178. Zeitlin BD. Banking on teeth - Stem cells and the dental office. Biomed J (2020) 43(2):124–33. doi: 10.1016/j.bj.2020.02.003
179. Nagpal A, Kremer KL, Hamilton-Bruce MA, Kaidonis X, Milton AG, Levi C, et al. TOOTH (The Open study Of dental pulp stem cell Therapy in Humans): Study protocol for evaluating safety and feasibility of autologous human adult dental pulp stem cell therapy in patients with chronic disability after stroke. Int J Stroke (2016) 11(5):575–85. doi: 10.1177/1747493016641111
180. Ye Q, Wang H, Xia X, Zhou C, Liu Z, Xia Z-e, et al. Safety and efficacy assessment of allogeneic human dental pulp stem cells to treat patients with severe COVID-19: structured summary of a study protocol for a randomized controlled trial (Phase I/II). Trials (2020) 21(1):520. doi: 10.1186/s13063-020-04380-5
181. Li W, Jiao X, Song J, Sui B, Guo Z, Zhao Y, et al. Therapeutic potential of stem cells from human exfoliated deciduous teeth infusion into patients with type 2 diabetes depends on basal lipid levels and islet function. Stem Cells Transl Med (2021) 10(7):956–67. doi: 10.1002/sctm.20-0303
182. Salazar-Puerta AA-O, Rincon-Benavides MA-OX, Cuellar-Gaviria TA-O, Aldana JA-O, Vasquez Martinez GA-O, Ortega-Pineda LA-O, et al. Engineered Extracellular Vesicles Derived from Dermal Fibroblasts Attenuate Inflammation in a Murine Model of Acute Lung Injury. Adv Mater (2023) 35(28):e2210579. doi: 10.1002/adma.202210579
Keywords: dental pulp stem cells, inflammation, anti-inflammatory, immune regulation, regeneration
Citation: Li F, Wang X, Shi J, Wu S, Xing W and He Y (2023) Anti-inflammatory effect of dental pulp stem cells. Front. Immunol. 14:1284868. doi: 10.3389/fimmu.2023.1284868
Received: 29 August 2023; Accepted: 06 November 2023;
Published: 23 November 2023.
Edited by:
Rosa M. Sainz, University of Oviedo, SpainReviewed by:
Aranka Ilea, University of Medicine and Pharmacy Iuliu Hatieganu, RomaniaIsabel Quiros Gonzalez, Universidad de Oviedo, Spain
Copyright © 2023 Li, Wang, Shi, Wu, Xing and He. This is an open-access article distributed under the terms of the Creative Commons Attribution License (CC BY). The use, distribution or reproduction in other forums is permitted, provided the original author(s) and the copyright owner(s) are credited and that the original publication in this journal is cited, in accordance with accepted academic practice. No use, distribution or reproduction is permitted which does not comply with these terms.
*Correspondence: Yan He, aGVsZW4tMTEwMUBob3RtYWlsLmNvbQ==
†These authors share first authorship