- 1Key Laboratory of Medical Science and Laboratory Medicine of Jiangsu Province, School of Medicine, Jiangsu University, Zhenjiang, China
- 2The People’s Hospital of Danyang, Affiliated Danyang Hospital of Nantong University, Zhenjiang, Jiangsu, China
- 3Changzhou Key Laboratory of Molecular Diagnostics and Precision Cancer Medicine, Wujin Hospital Affiliated with Jiangsu University, Changzhou, Jiangsu, China
Inflammation is a characteristic symptom of the occurrence and development of many diseases, which is mainly characterized by the infiltration of inflammatory cells such as macrophages and granulocytes, and the increased release of proinflammatory factors. Subsequently, macrophage differentiates and T cells and other regulated factors exhibit anti-inflammatory function, releasing pro- and anti-inflammatory factors to maintain homeostasis. Although reports define various degrees of metabolic disorders in both the inflamed and non-inflamed parts of inflammatory diseases, little is known about the changes in amino acid metabolism in such conditions. This review aims to summarize amino acid changes and mechanisms involved in the progression of inflammatory bowel disease (IBD) and other inflammatory diseases. Since mesenchymal stem cells (MSCs) and their derived exosomes (MSC-EXO) have been found to show promising effects in the treatment of IBD and other inflammatory diseases,their potential in the modulation of amino acid metabolism in the treatment of inflammation is also discussed.
1 Introduction
Inflammation is the activation of immune cells and other non-immune cells during the defense against harmful invasion of life, protecting the host from bacteria, viruses, toxins, and infections by eliminating pathogens and promoting tissue repair and recovery. The normal inflammatory response is timely. However, inflammation for a long time can lead to chronic inflammation and a range of autoimmune diseases. The occurrence and development of disease are mostly accompanied by inflammation whose main feature is significantly increased expression of pro-inflammatory cytokines such as interleukin (IL) - 1β, IL - 6, TNF -α, etc. (1), leading to the anti-inflammatory and pro-inflammatory cytokines imbalance, promoting the incidence of inflammatory and autoimmune diseases including IBD and rheumatoid arthritis (RA) (2). How to eliminate inflammation in the later stage of the disease is the focus of experimental research and clinical treatment. Inflammatory bowel disease (IBD) is a non-infectious, chronic, systemic, and recurrent inflammatory disease of the gastrointestinal tract. IBD is characterized by recurrent and prolonged episodes of diarrhea and abdominal pain, mainly including ulcerative colitis (UC) and Crohn’s disease (CD). From the aspects of immunity, IBD can be regarded as a kind of inappropriate immune response. IBD is characterized by recurrent and prolonged episodes of diarrhea and abdominal pain and can affect any location throughout the gastrointestinal tract. IBD not only affects the quality of life, work, and leisure but also greatly increases the risk of colorectal cancer (3). This disease is not only widespread in the West but also a problem for many people in Asian countries, including China. At present, there is no specific therapy or drug to completely cure IBD, and most treatments only target its clinical symptoms to relieve it. The recurrence of the disease brings economic and mental burdens to the patients and their families, so it is urgent to actively seek effective treatment. The pathogenesis of IBD is still unclear and involves complex factors such as genetics, environment, and immunity. More studies have reported that IBD is associated with intestinal mucosal integrity, permeability and barrier function, intestinal immune status, intestinal flora, gastrointestinal anti-inflammatory cytokine and pro-inflammatory cytokine balance, and intestinal oxidative stress.
Amino acids are an important class of substances, mainly composed of five elements, which are carbon, hydrogen, oxygen, nitrogen, and sulfur. There are 20 amino acids known to make up proteins in the human body. The biological functions of amino acids include basic units of protein, energy metabolizers, precursors of nitrogen compounds, etc. The essential amino acid/non-essential amino acid ratio in the blood is usually 3-3.5. Studies have shown that this ratio can change in disease, and some amino acid levels can also be affected (4, 5). Amino acid metabolism is an important process of normal life activities, including deamination, decarboxylation, transamination, ammonia metabolism, oxidation energy supply, and other general amino acid metabolism processes as well as individual amino acid metabolism, taking branched-chain amino acid metabolism as an example (4, 6). Exogenous amino acids (produced by digestion and degradation of protein in food) and endogenous amino acids (produced by the degradation of tissue protein in the body) are distributed throughout the body, known as the amino acid metabolic pool. As an important component of metabolism, amino acids are not only used for protein synthesis but also participate in important human life activities mainly through amino acid catabolism. For example, some amino acids can be decarboxylated under the action of a specific amino acid decarboxylase to produce corresponding amines. The amines produced after amino acid decarboxylation are low in content but have important physiological functions. For example, glutamic acid (Glu) decarboxylates and then generates gamma-aminobutyric acid (GABA), an inhibitory neurotransmitter which has a suppressive effect on the central nervous system (7); Histamine produced by histidine (His) catalyzed by histidine decarboxylase is widely distributed in the body, with strong vasodilation function, and histamine is released at the wound site and inflammation site (8). Another example is tryptophan (Trp) catalyzed by tryptophan hydroxylase (TPH) to produce 5-hydroxytryptamine (5-HT), which is widely present in the gastrointestinal tract and nervous tissue (9). 5-HT acts as a neurotransmitter in the brain and has the function of constricting blood vessels in peripheral tissues. It can be seen that amino acids and their metabolites are important participants in human life activities.
Exosomes are nano-scale vesicles that can be secreted by a variety of cells. They are about 30 to 150nm in diameter, and their composition varies according to their origin. Exosomes are spherical bilayer lipid particles containing various types of proteins, lipids, DNA, non-coding RNA, miRNA, and mRNA, which can cause the exchange and reprogramming of genetic information in recipient cells, that is, have regulatory effects on target cells (10). The typical representatives of exosome proteins are annexin and G protein, which is closely related to trans-membrane transport and is also the driving factor that determines the secretion of exosomes into cells through membrane fusion. Exosomes express biomarkers such as CD63, CD9, and CD81 on the surface (11). Since exosomes show therapeutic effects as transfer vectors and participate in immune system regulation, more and more studies have been conducted to explore the relationship between exosomes and diseases. For example, In one study, colonic epithelial-derived exosomes carrying transforming growth factor-β1 (TGF-β1) exhibited immuno-suppressive activity by inducing both Treg and immuno-suppressive dendritic cells (DC) to treat colitis (12). Exosomes also serve as good drug carriers to deliver proteins, mRNA, and other biological macro-molecules or active substances. Thus, exosomes are active natural nanocarriers that can be used to provide innate anti-inflammatory biological components for the treatment of IBD (13).
This review mainly summarizes the changes in amino acid metabolism in the pathogenesis of IBD and other inflammatory diseases. And the review additionally talks about what effects MSCs and their derived exosomes have on the treatment in inflammatory diseases and aims at finding some novel therapeutic targets of this method.
2 Amino acids and immune cells
Some amino acid catabolic pathways have become key checkpoints of immunity (14, 15).
2.1 Amino acids and the regulation of macrophages
Macrophages are key cells in the occurrence and development of inflammatory diseases (16). They maintain the internal environment by polarization into pro-inflammatory macrophages M1 and anti-inflammatory macrophages M2 and regulating the balance of pro-inflammatory/anti-inflammatory cytokines. At the site of inflammation, amino acids as vital substances contribute greatly to the nodes involved in the elimination of inflammation. Relevant studies and literature over the years indicate that the participation of amino acids in eliminating the inflammatory process can be achieved through mutual regulation with macrophages (Figure 1). Several major amino acids and their metabolites directly or indirectly inhibit the levels of inflammatory factors such as TNF- α, IFN- γ, and IL-6 by promoting M2 polarization, inhibiting NF- κ B, STST 1, and STAT 5, and simultaneously reducing the levels of ROS, iNOS, and COX 2 in macrophages (Figure 1). As shown in the figure, Trp acts on 5-hydroxytryptamine receptor (5-HTR) expressed in macrophages through catabolism leading to serotonin (5-HT), inhibiting the release of pro-inflammatory factors by macrophages (17, 18); Under inflammatory conditions, pro-inflammatory factors such as TNF-α can up-regulate indoleamine 2, 3-dioxygenase 1(IDO1) and then increase the activity of Trp catabolism leading to kynurenine (KYN) (19). Histidine inhibits NF-κB activity in a concentration-dependent manner and then reduces the release levels of TNF- α, and IL-6 in macrophages (20). Glutamine inhibits the production of ROS, NOS, iNOS, COX-2, and other pro-inflammatory mediators to protect tissue (21). Reducing the level of NF-κB activation in inflammation while simultaneously inhibiting STAT1, STAT5, and Akt phosphorylation, inhibiting the increase of TNF-α and IFN-γ (22). Glu also promotes the conversion of M1 to M2 in muscle, down-regulating IL-1β et al. (23). Glycine (Gly) blunted calcium influx in macrophages to inhibit the production of toxic free radicals by macrophages (24). Additionally, Macrophage M1 polarization promotes iNOS expression and stimulates arginine to generate NO while inhibiting ROS activity (25). Thus, amino acids can function by interacting with macrophages.
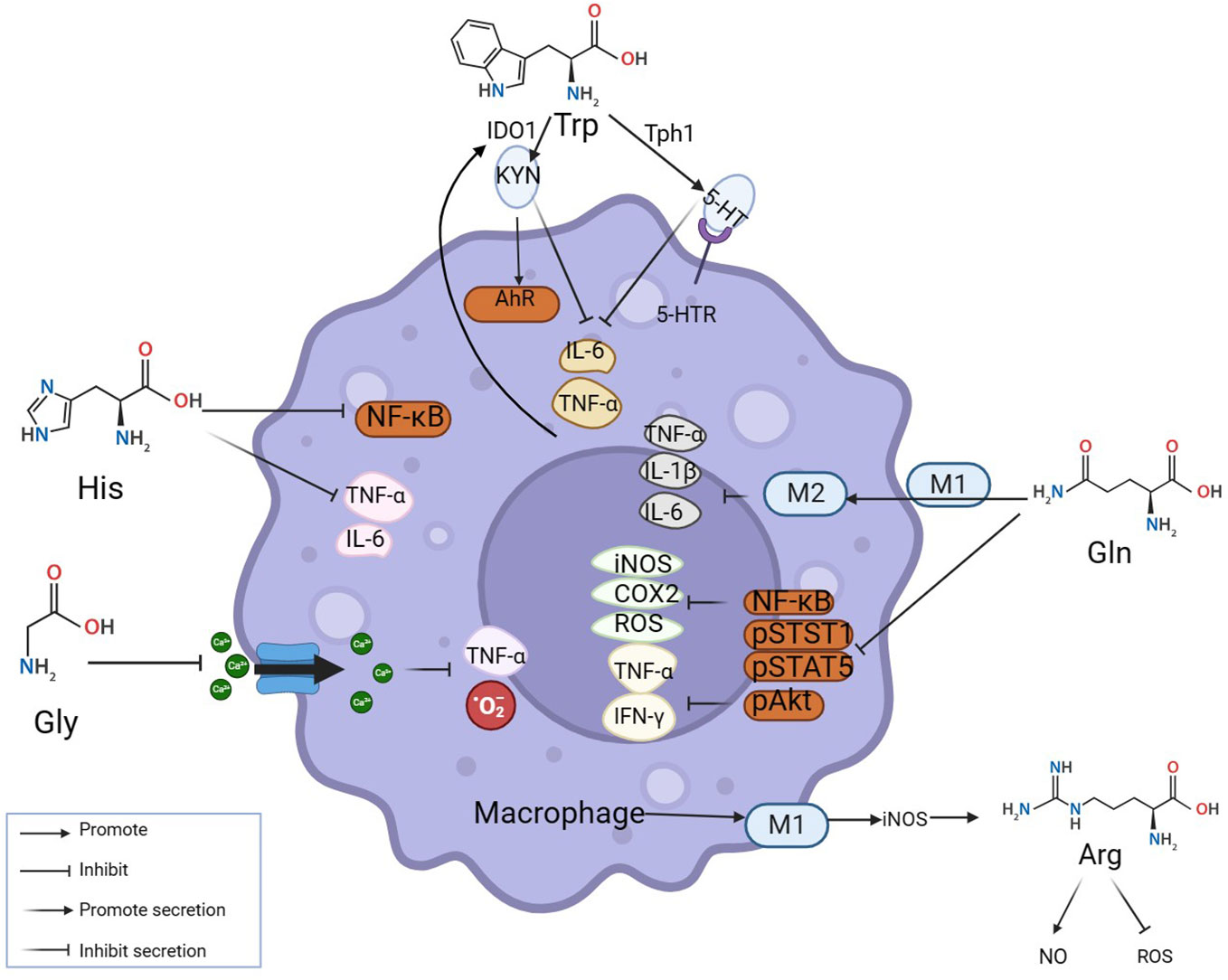
Figure 1 Amino acids modulate inflammation by reciprocal regulation of macrophages. The mechanisms at inflammatory sites by which amino acids exert anti-inflammatory effects by interacting with macrophages are illustrated above. Amino acids make an impact on macrophages by mediating their polarization and secretion. Many classic inflammatory pathways in macrophages, such as NF-κB, STAT1, and STAT5, are inhibited by amino acids.
2.2 Amino acids and the regulation of T cells
T lymphocytes are also important players and balancers at the sites of inflammation. Among them, CD8 + T cells receive antigen signals submitted by major histocompatibility complex I (MHCI) to directly eliminate pathogens or tumor cells through cytotoxic effects. Therefore, it is considered the key to the anti-tumor immune response (26). CD4 + T cells mainly receive the presenting signal of major histocompatibility complexII (MHCII) to eliminate the antigen by releasing various cytokines. There are many subsets of CD4 + T cells, among which the Th subset is important in the pathogenic process of inflammatory diseases, capable of mediating a variety of chronic inflammation and autoimmune diseases. For example, the Th 17, which has been discovered for more than a decade, is indispensable for the onset of IBD and is also found to be actively involved in experimental autoimmune encephalomyelitis (EAE) by releasing IL-17 (27). However, CD4+ T cells can differentiate into regulatory T cells (Tregs). As immunosuppressive T cells, Tregs can inhibit the activation of other T cells and become a mainstay in controlling the proper immunity of the body under normal physiological conditions (28). Tregs have been proven to be dysfunctional when the body experiences chronic inflammation or autoimmune diseases, so Tregs have become an important regulatory target to deal with excessive immune responses (29, 30). In recent years, studies on treating inflammatory diseases by regulating Th 17/Treg levels have emerged. Researchers use many methods to restore the balance of the Th 17/Treg axis to regulate the inflammatory response (31–33).
Amino acids and their metabolites play an important role in the growth and function of T cells. First, amino acids and their metabolites can participate in T cell internal nutrient metabolism through the intracellular entry of various amino acid transport proteins widely expressed on the T cell membrane to maintain T cell survival and proliferation. For example, glutamine (Gln) enters T cells through Recombinant Solute Carrier Family 1, Member 5 (SLC1A5)/Recombinant Solute Carrier Family 38, Member 1(SLC38A1) and is metabolized to α-ketoglutarate, and then participates in the intra-cellular TCA cycle to generate ATP to provide energy for T cell proliferation (34). Arginine (Arg) enters the cell through SLC7A1 and can generate polyamines to maintain the needs of T cell growth (35). Secondly, the mammalian target of rapamycin (mTOR) and general control nonderepressible 2 (GCN2) are important amino acid sensors. Various amino acids and their metabolites, such as leucine (Leu), arginine (Arg), and glutathione (GSH), regulate the proliferation and differentiation of T cells by activating the amino acid sensor mTORC1 (36). Trp and its metabolite kynurenine (Kyn) can enter T cells through Solute Carrier Family7, Member5 (SLC7A5) and activate intra-cellular GCN2 to inhibit Th 17 differentiation (37), and T cell proliferation is partly dependent on the activity of GCN2 (38). In addition, amino acid post-translational modifications, such as methylation, acetylation, etc., are important players in directly regulating T cell typing from the genetic level. The methionine (Met) metabolite S-adenosine methionine (SAM) is the main methyl donor (39), while Gln and branched-chain amino acids can be metabolically metabolized to CoA to provide acetyl groups (40) (Figure 2).
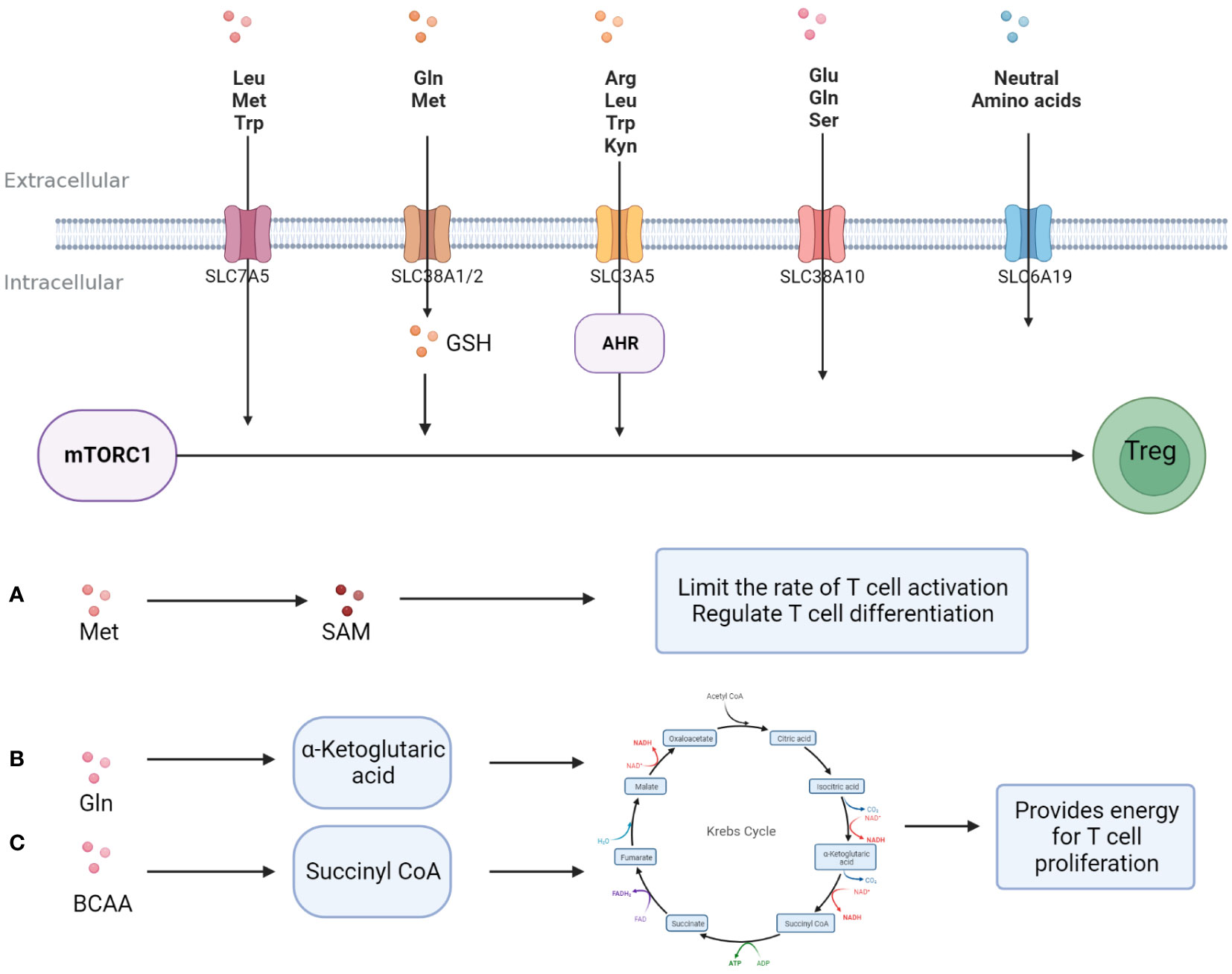
Figure 2 Amino acids promote T -cell proliferation and differentiation. Amino acids enter T cells and support T cell proliferation and differentiation in three major ways; (A) A variety of amino acids activates mTORC1 directly or indirectly after entering T cells, promoting Treg differentiation and activity. (B) Methionine is metabolized to S-adenosine methionine, provides a substrate for methylation, and regulates the typing and activity of T cells. (C) Some amino acid metabolites are involved in glucose metabolism and provide energy for T cell proliferation.
3 Amino acid metabolism and the progression and treatment of inflammatory diseases
3.1 Amino acids and the pathogenesis of IBD
The body’s metabolism is disturbed by a pathological state, and amino acid metabolism is no exception. In recent years, there has been constant new evidence that amino acid metabolism is closely related to inflammation (Table 1). A randomized controlled trial of the relationship between branched-chain amino acids and inflammation directly revealed this (52). A large number of studies have shown that compared with the normal state, in the course of inflammatory diseases, the serum levels of 20 amino acids are reduced to varying degrees (5, 53), with glutamic acid, arginine, glycine, etc. being the most significant. Metagenomic analysis showed major perturbations in amino acid metabolism in IBD: the abundance of metabolic and biosynthetic genes decreased for almost all amino acids (especially histidine and lysine), while the abundance of arginine, histidine, and lysine transporter genes increased (54). These findings indicate that amino acid metabolism is affected by IBD pathological status. Table 2 summarizes some amino acid changes during the IBD process (Table 2). Mingyan Jing et al. reviewed the real location of amino acids in the body under inflammation or disease, especially mentioning that threonine is closely related to intestinal metabolism and plays an important role in maintaining the non-specific immune barrier of intestinal mucosa (6).
A typical example is 5-HT. 5-HT is an important regulator in the human body, derived from the catabolism of tryptophan. More than 90% of 5-HT in the human body is produced in the gut, specifically in intestinal chromaffin cells (ECs), a specific subtype of intestinal epithelial cells (63). This process occurs through the action of the Trp hydroxylase 1 enzyme (TpH1), which produces 5-hydroxytryptophan (5-HTP), and is further metabolized into 5-HT (64). Under physiological conditions, peripheral 5-HT does not cross the blood-brain barrier. Peripheral 5-HT triggers many functions in the gastrointestinal tract and is associated with a wide range of human physiological functions through the activation of specific 5-HT receptors. Specifically, 5-HT is an important gastrointestinal signaling molecule that transmits signals from the gut to internal or external neurons and influences intestinal peristalsis and movement, secretion, vasodilation, and nutrient absorption (58).The intestinal microbiota is a major player in intestinal 5-HT production (65). Its effect has been demonstrated in germ-free mice that exhibit impaired 5-HT production in the colon (but not in the small intestine) and low 5-HT concentration in the blood. The mechanism by which intestinal microbiota regulates 5-HT production 3 is not fully understood, but the role of SCFAs in stimulating TpH1 expression has been proposed (66). In addition, some secondary bile acids, such as deoxycholate produced by microbial bioconversion of cholate, can also stimulate 5-HT biosynthesis. Most studies (67–70) report a decrease in intestinal microbiome diversity in patients with IBD, and this change is considered to be an important factor in the development of IBD (54, 71, 72). IBD patients have obvious intestinal flora imbalance, which is mainly manifested as a decreased abundance of intestinal flora, decreased stability of intestinal dominant flora, decreased protective flora, and increased pathogenic bacteria. Intestinal inflammation is usually associated with a significant decrease in Bacteroides (gram-negative microorganisms) and Firmicutes (gram-positive) populations (67, 68), and an increase in the ratio of gram-negative to gram-positive microbes in the gut leads to an increase in the release of inflammatory LPS (73).
3.2 Amino acids and the treatment of IBD
It has been reported that glutamine can reduce the damage caused by oxidative stress in DSS-induced colitis mice (74). Mechanistically, glutamine can up-regulate the expression of tight junction proteins and maintain intestinal barrier integrity (75); Meanwhile, glutamine can also inhibit the activity of NF-κB and STAT and increase the stability of IκB, and this may be related to the increased expression of HSP 70 and HSP 25 (76). There is also a conjecture that glutamine may influence the production of IL-8, thereby targeting intestinal inflammation (77). Arginine can stimulate the synthesis of collagen, reduce the expression of iNOS, and reduce the level of pro-inflammatory cytokine IL-17 (74) so that the improvement of the colitis mouse model can be observed. There have been several articles on dietary treatments for IBD that have demonstrated the involvement of exogenous amino acids in improving the intestinal environment. Amino acid-balanced diet-fed mice can effectively alleviate inflammation and this effect is associated with amino acid interaction with gut microbes (78). Thus, amino acids and their metabolites have strong links with intestinal health.
Some special amino acids play a key role in inflammation and immune response (6). Nikolaus et al. found that changes in Trp metabolism are closely related to the progression of IBD (79). Allison et al. also found that Trp metabolism is associated with host intestinal inflammation, epithelial barrier, and energy homeostasis in IBD (80), and is closely related to the intestinal flora. Intestinal microbes such as Bacteroides and Fusobacterium have been shown to act as modulators of intestinal tryptophan metabolism (81). Examples include Clostridium, which is involved in Trp decarboxylation and leads to the production of the neurotransmitter tryptamine (64). Escherichia coli and Lactobacillus, which directly convert Trp to indole and its derivatives, are key to enhancing intestinal AHR activity. AHR signaling is considered to be a key component of the barrier site immune response and is essential for intestinal homeostasis (82, 83).. The AHR also plays an extremely important role in controlling the inflammatory function of macrophages. AHR can be expressed in microglia. A study showed that loss of microglial AHR aggravates inflammation in the central nerve system in mice (84) and dietary amino acid deficiency further aggravates the symptoms of autoimmune encephalomyelitis. Previous studies have also demonstrated that damage to AHR ligand, equally tryptophan metabolite, is detected in patients with IBD (82, 85), resulting in decreased activation of AHR in intestinal epithelial cells and immune cells, thus affecting intestinal homeostasis. Studies have shown that CARD 9, a susceptibility gene for IBD, promotes the recovery of colitis by promoting the production of interleukin (IL) -22, and that CARD 9-/-mice are more likely to develop colitis. The microbiota from Card9-/-mice cannot metabolize tryptophan as a metabolite acting as a ligand for the aryl hydrocarbon receptor (AHR) (85). This may directly indicate that the Trp-AHR pathway is a key therapeutic target in colitis. In addition, other tryptophan metabolites also play an important role in the process of anti-inflammatory. For example, under the catalysis of tryptophan decarboxylase (TrpD), tryptophan oxygenase - 2 - sheet (TMO), and bacteria tryptophan gradually turns into indole - 3 - acetic acid (IAA) and indole - 3 - propionic acid (IPA) which even have direct anti-inflammatory effects (86).
It has been found that in the large intestine, Escherichia coli participates in the process of amino acid fermentation, mainly using lysine, arginine, glycine, and branched amino acids as substrates to produce a large number of complex metabolites including ammonia, acetic acid, butyric acid, and branched fatty acids. These products have been shown to affect the physiological function of intestinal epithelial cells by affecting their signaling pathways and regulating the mucosal immune system. Butyric acid (87), in particular, plays such major roles and is a major source of energy for intestinal lining cells. Butyric acid provides 90 percent of its total energy requirements. These cells need this SCFA to perform their important functions, especially maintaining the integrity of the intestinal wall (88). The intestinal wall is very important because it acts as a barrier between the intestinal environment and the rest of the body.
All the above examples illustrate that intestinal flora plays an important role in the metabolism of amino acids in the process of IBD. Intestinal bacteria can decompose proteins into their constituent amino acids, which are then absorbed by the human body and used in various metabolic processes. Under the condition of inflammation, intestinal flora may affect the bioavailability of amino acids (67). Therefore, it is necessary to attach importance to the regulation of the intestinal microbial environment in future research concerning the treatment of IBD.
Several studies (89, 90) report that there is a link between amino acid metabolisms and IBD process, which may be realized through various signaling pathways. There are many examples, such as glutamine and glutamate, which promote the proliferation of intestinal epithelial cells by regulating the activity of the mTOR pathway, activate the MAPK pathway to promote the proliferation and differentiation of intestinal epithelial cells, increase the expression of tight junction protein, and maintain the stability of intestinal mucosa to ensure its barrier function. Glutamine can regulate NF-κB signal transduction and STAT signaling pathway activation, to inhibit the release of IFN-γ, TNF-α, and other pro-inflammatory cytokines and reduce intestinal inflammatory response. Hsp70 has been reported to inhibit the production of LPs-stimulated inflammatory mediators such as TNF-α, IL-1, and inducible nitric oxide synthase (iNOS) (91). However, another study on the signal transduction pathway of extracellular heat shock protein HSP70 found that an exogenous (e.g., Exosome-derived) HSP70-induced signaling cascade uses TLR2 and TLR4 to activate NF-κB activity (92). However, no adverse effects caused by HSP70 have been found in the treatment of IBD. Although there are no reports, we may assume that exosomes HSP70 can be antagonized by amino acids such as glutamine in the gut to avoid the activation of NF-κB and the up-regulation of the release of TNF-α, IL-1β, IL-6, and other pro-inflammatory factors.
Amino acid transporters are essential in amino acid absorption in the gut. As early as 2006, some scholars discovered that the L-Arg transporter CAT-2 (SLC7A2) could regulate macrophage function by controlling the activities of NOS2 and arginase, thus regulating gastrointestinal inflammation (93). Recovery of the inflammatory-injured colonic epithelium also depends on the ability of CAT2 to transport L-Arg into the cells (94). Previously reported mutations in the neutral amino acid transporter B0AT1(SLC6A19) in Hartnup disease may result in an almost complete lack of tryptophan absorption in the gut (95). In addition to being regulated by the B0AT1 transporter, tryptophan absorption is also mainly regulated by the neutral amino acid transporter B0,+AT(SLC6A14) and the aromatic amino acid transporter TAT1(SLC16A10) (96), but the extent to which this is impaired remains to be determined. It has been reported that mRNA of neutral amino acid transporter SLC6A19/B0AT1 is significantly down-regulated in biopsies of patients with active CD and UC (79). It is easy to speculate that when inflammation occurs, the intestinal mucosa is damaged, permeability is damaged, and amino acid transporter expression is decreased, in particular the tryptophan transporters, which further increases the degree of intestinal inflammation. Tryptophan is also an upstream regulator of the recovery process of intestinal inflammation (97, 98). Voluntary ingestion of exogenous tryptophan can control the expression of antimicrobial peptides in the mouse small intestine and affect the regeneration and repair of the damaged intestinal epithelium.
There are many key enzymes and rate-limiting enzymes in amino acid metabolism, which may also be therapeutic targets for diseases. For instance, biopsies of the diseased colon in patients with IBD significantly express IDO1mRNA (99). Pro-inflammatory cytokines, including IFN-γ, TNF-α, IL1β, IL2, IL6, IL18, and IFN-α induce IDO1 expression in chronic inflammatory conditions. IDO1 plays an important role in immune regulation under pathophysiological conditions, and is one of the most overexpressed genes in CD, mediating potent anti-inflammatory effects through tryptophan metabolism along the Canine uric acid pathway (100).
Amino acid metabolism demonstrates an integral role in disease progression, and the importance of the gut microbiome in gut metabolism cannot be overemphasized. There are still some mechanisms that have not been clarified in the progression and treatment of IBD. For example, the microbial community involved in intestinal amino acid metabolism is regulated by the IBD susceptibility gene NOD2, which may indicate that intestinal amino acid metabolism is more regulated at the genetic level. However, due to fewer studies, this remain s to be fully understood.
3.3 Amino acids and other inflammatory diseases
An article on patients with rheumatoid arthritis, spinal joint disease, and osteoarthritis reported that glycine in dog urine (endogenous metabolites of tryptophan) in inflammatory state local defects (101). Sun etc. summarized the immunomodulatory effects of several specific amino acids, such as glutamine and glycine (102). These examples indicate that amino acids have great potential in the treatment of these inflammatory diseases.
Rheumatoid arthritis characterized by synovial inflammation is a kind of autoimmune disease. Although the etiology of RA is not fully evident, it is clear that amino acid metabolism disorders definitely engage in the chronic inflammation of later RA (103). Several amino acids and amines including citrulline, succinate, and glutamine were reported to be potential biomarkers for RA (103). According to a study, compared with healthy people, RA patients’ serum analysis shows the kynurenine pathway is active (104). Trp content decreased at the same time the dog urine glycine content increased significantly. One of the most obvious factors is the IDO1 increased significantly in RA (105). IDO1 plays a protective role in RA. Research has shown that IDO1 deficiency can exacerbate tissue damage (106), and the kynurenine pathway mediated by IDO1 can inhibit T cell immune response (107). However, the process of IDO1 in RA is not static from beginning to end. In the inflammatory synovial region, hypoxia conditions actually lead to a decrease in IDO1 levels (108), while IFN-γ expressed by extensive immune cells in the inflammatory environment can induce IDO activation. Therefore, the detection of IDO1 activation in RA patients may be a forward regulation of inflammation development.
Multiple sclerosis(MS) is a chronic inflammatory disease of the central nervous system. Similar to RA, the two most representative regulatory amino acids are Trp and Arg (109). Amino acids have long been found to be necessary in the activation of the mTOR pathway (110). In multiple sclerosis (MS), IDO1 and ARG1 defects cause a decline in the catabolism of Trp and Arg, increase mTOR activity, and decrease Treg and proinflammatory factor increases (44). Although amino acids have been recognized as key inputs in this pathway, the mechanism of action of the mTORC1 amino acid sensor is still not clear (111).
Systemic lupus erythematosus (SLE) is a chronic autoimmune disease characterized by abnormal responses to self-antigens (112). In the SLE process, the most significant immunol regulatory amino acid metabolism is Glutamine catabolism. Glu catabolism is essential to T cell immune response in SLE (113). A research pointed out that glutaminolysis enzymes are significantly elevated in CD4+T cells from lupus-prone TC mice (114). Therefore, inhibiting overwhelming glutaminolysis may be an important target for treating SLE (115).
4 Exosomes modulate inflammation partly through the regulation of amino acid metabolism
Currently, antibiotics, anti-inflammatory drugs (e.g., 5-aminosalicylic acid, corticosteroids), or immunosuppressants (e.g., azathioprine, 6-mercaptopurine) are used to treat IBD (116). Some of these drugs are effective at relieving early inflammatory symptoms, but their long-term effectiveness is compromised by toxic buildup. Nanoparticle-based (NP) drugs are widely studied for their potential to solve such problems (13). The exosomes derived from mesenchymal stem cells (MSC-EXO) have attracted much attention. Exosomes have been widely studied for the treatment of clinical diseases due to their low immunogenicity and biocompatibility. In recent years, people have begun to pay attention to the physiological and pathological functions of exosomes and their complex components. Recent studies have shown that exosome cross-talk mechanisms may influence major IBD-related pathways such as immune response, barrier function, and intestinal flora (117, 118). For example, previous studies have shown that canine adipose tissue-derived MSC extracellular vesicles increase the polarization of M2 macrophages and Tregs in vitro through TSG-6 (119–121). MSC- EXO has been shown to play immunosuppressive and intestinal barrier repair functions in IBD by secreting the protein TSG-6 (122, 123). Moreover, exosomes regulate macrophage differentiation to modulate the level of cytokines and protein expression (124). IL-7 is considered to be an important cytokine in the activation of mucosal inflammation in the progression of IBD and Tao Ji et al. found that in DSS-induced colitis mice, the expression of AHR is down-regulated, while IL-7 from epithelium is up-regulated, indicating that the AHR-driven signal may regulate IL-7 (125). In experimental colitis, the AHR agonist beta-naphthalene flavone attenuates the symptom of colitis and reduces the response of human epithelial colon cells induced by LPS treatment, suggesting that AHR activation in epithelial cells may be an important mechanism regulating intestinal inflammation (126–128). Mao et al. found that exosomes derived from human umbilical cord MSCs (hucMCSs) may restore enterocyte proliferation capacity (129) by reducing macrophage infiltration in colon tissue and down-regulating NOS as well as IL-7 expression in macrophages. It is therefore reasonable to speculate that human umbilical cord mesenchymal stem cell-derived exosomes can also act on aromatics receptors, probably through the AHR ligand tryptophan. Moreover, the ultraviolet irradiation product of tryptophan is also one of the ligands with a high affinity to AHR (128).
Aside from some of the assumptions about the effect of MSC-EXO in IBD, there is real evidence holding that in other diseases. A recent research shows that hucMSC-EXO can increase the level of GSH to improve non-alcoholic steatohepatitis (NASH) although the increase is not direct (130). Another research reveals that Bone marrow MSC-derived exosomes (BMSC-EXO) regulate the level of chondrocyte glutamine metabolism and then alleviate Osteoarthritis (OA) (131).
Non-coding RNA in exosomes plays an important role in anti-inflammatory. One study showed that MSC-derived exosome miR-326 reduced the neddylation of IκB, inhibiting NF-κB activity and effectively slowing the inflammation (132). In addition, an edible plant (i.e., ginger)-derived exosome-like nanoparticle (ELN) RNA has been shown to induce IL-22 by activating the AHR pathway, thereby improving intestinal inflammation (133). Human umbilical cord-derived mesenchymal stem cell exosome-derived miRNA-181c regulates systemic inflammation by reducing TLR4 (toll-like receptor 4, LPS receptor) expression, causing decreased expression of p65/NF-κB phosphorylation, inhibiting TNF-α and IL-1β secretion, and increasing IL-10 secretion (10, 129) (Figure 3). Zhao et. also found that miR-140-5p in adipose tissue macrophage-derived exosomes can target SLC7A11 to inhibit GSH synthesis and then induce cardiac injury, which provides a clear and novel therapeutic strategy (134).
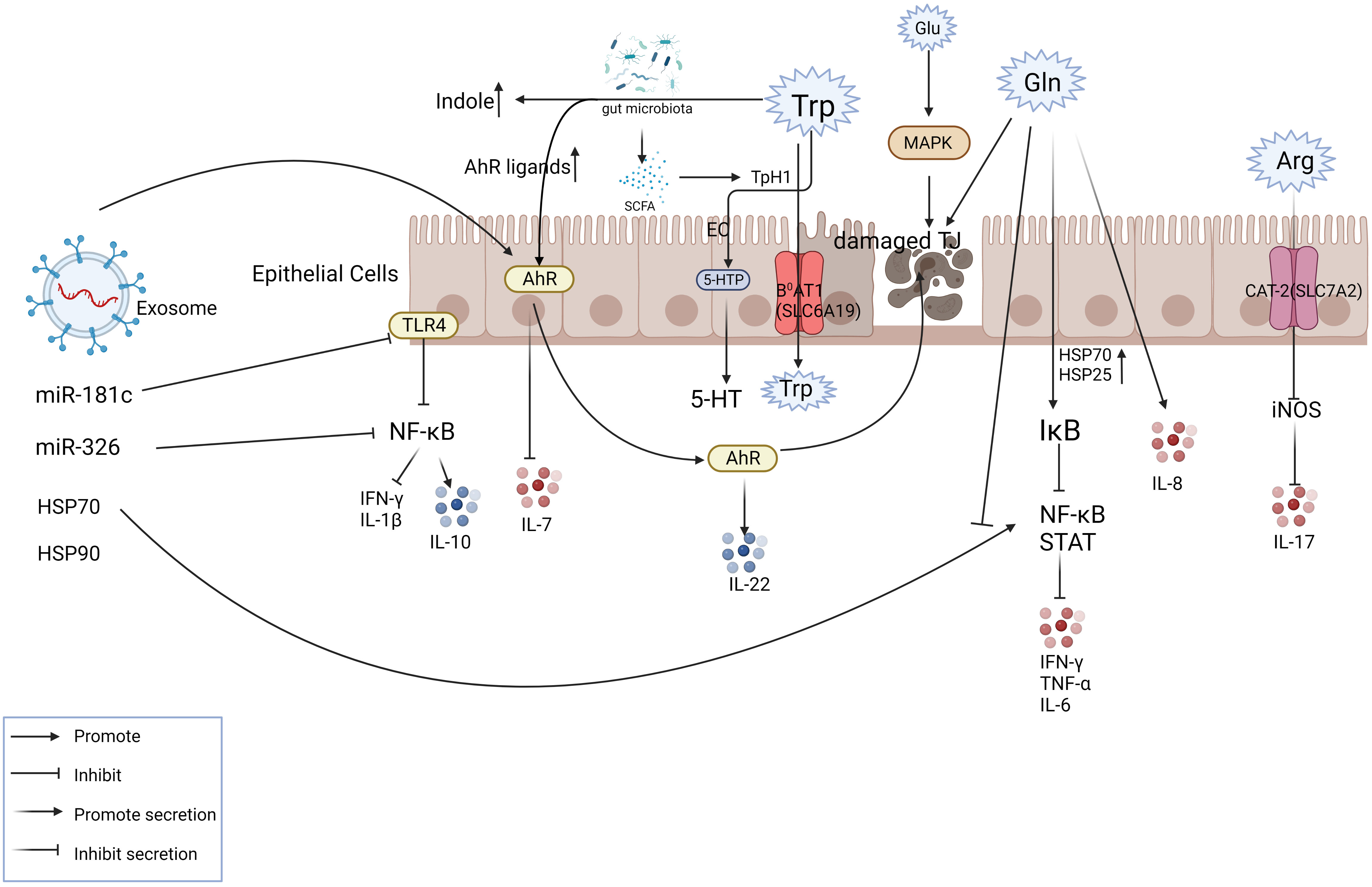
Figure 3 Exosomes play a role in mitigating IBD partly via amino acid metabolism. The figure shows the transport mode of amino acids inside and outside the intestinal epithelial cells with some of the key nodes involved in IBD. The involvement of exosome components in intestinal amino acid metabolism plays a therapeutic role at the site of inflammation. For example, exosomes can activate AHR, inhibit IL-7 levels, promote IL-22 release, and relieve inflammation.
5 Conclusion and prospect
In inflammatory diseases, amino acid metabolism is disturbed by the disease state or inflammation, mainly manifested in the reduction of absorption and utilization of most amino acids, decrease of related intestinal flora, and tissue functional damage. Both neutral amino acids or aromatic amino acids or aromatic amino acid transport proteins are destroyed to varying degrees. In addition to tryptophan, glutamine, and arginine also play an important role in the anti-inflammatory process. Most studies have observed the improvement of these exogenous amino acids by adding one or multiple dietary amino acids, most of which are animal experiments. An improvement in inflammation can indeed be observed, but the specific mechanisms need to be thoroughly studied. The link between inflammation and amino acid metabolism goes through key nodes, such as MAPK, NF-κB, and other signaling pathways, as well as key immune cells.
Amino acid metabolism is involved in the various anti-inflammatory mechanisms of exosomes. Mesenchymal stem cells and their derived exosomes directly or indirectly regulate key signaling pathways in inflammation, such as NF-κB and STAT. This is only the anti-inflammatory mechanism of the large framework, under which the amino acid metabolism constantly operates and functions in the regulation and being regulated. Exosomes can even directly regulate the activity of the AHR, acting on intestinal inflammation by controlling the Trp-AHR pathway. Some components of exosomes, such as non-coding RNA, are significant in anti-inflammatory processes and are easily linked to amino acid metabolism by regulating pathways such as NF-κB, which is well worth further exploration in later studies.
Although some assumptions have not been directly demonstrated for the time being, there are other emerging treatments for IBD, such as fecal flora transplantation, dietary fiber intake, and other methods that have been effectively employed in the treatment of IBD, from the perspective of amino acid metabolism. The functions of many components of exosomes exhibit good feedback in the treatment and anti-inflammation of IBD, but there are still many mechanisms and pathways that are not fully understood. We hope that there will be more practical evidence for the involvement of amino acid metabolism in the treatment of IBD in future studies.
Author contributions
XZ: Writing – original draft. YZ: Writing – original draft. ZZ: Writing – review & editing. YC: Funding acquisition, Writing – review & editing. WY: Conceptualization, Writing – original draft.
Funding
The author(s) declare financial support was received for the research, authorship, and/or publication of this article. This project is supported by the Grant of the Scientific Project of Jiangsu Health Commission (Grant no.Z2020038); the Grant of the Open Project of Jiangsu Key Laboratory of New Drug Research and Clinical Pharmacy (Grant no.XZSYSKF2020032); Jiangsu 333 High-Level Talents Training Project and Changzhou High-Level Medical Talents Training Project (Grant no.2022CZBJ111).
Conflict of interest
The authors declare that the research was conducted in the absence of any commercial or financial relationships that could be construed as a potential conflict of interest.
Publisher’s note
All claims expressed in this article are solely those of the authors and do not necessarily represent those of their affiliated organizations, or those of the publisher, the editors and the reviewers. Any product that may be evaluated in this article, or claim that may be made by its manufacturer, is not guaranteed or endorsed by the publisher.
References
1. Dinarello CA. Biologic basis for interleukin-1 in disease. Blood (1996) 87(6):2095–147. doi: 10.1182/blood.V87.6.2095.bloodjournal8762095
2. Pasparakis M. IKK/NF-kappaB signaling in intestinal epithelial cells controls immune homeostasis in the gut. Mucosal Immunol (2008) 1 Suppl 1:S54–7. doi: 10.1038/mi.2008.53
3. Duran NE, Hommes DW. Stem cell-based therapies in inflammatory bowel disease: promises and pitfalls. Therap Adv Gastroenterol (2016) 9(4):533–47. doi: 10.1177/1756283X16642190
4. Miao M. Amino acid metabolism and clinical use. Chin Gen Surg Literature (electronic version) (2008) 01):58–61. doi: 10.3969/j.issn.1674-0793.2008.01.02
5. Feng M. (2021) Construction of amino acid detection method and supplementary rule in inflammatory bowel disease. (Jilin University: China).
6. Jing M, Liu B, Sun J, Zhao S. Amino-acid metabolism and the immune response. Chin Anim Husbandry J (2007) 05):37–9. doi: 10.3969/j.issn.0258-7033.2007.05.01
7. Petroff OA. GABA and glutamate in the human brain. Neuroscientist (2002) 8(6):562–73. doi: 10.1177/1073858402238515
8. Deng X, Wu X, Yu Z, Arai I, Sasano T, Sugawara S, et al. Inductions of histidine decarboxylase in mouse tissues following systemic antigen challenge: contributions made by mast cells, non-mast cells, and IL-1. Int Arch Allergy Immunol (2007) 144(1):69–78. doi: 10.1159/000102617
9. McLean PG, Borman RA, Lee K. 5-HT in the enteric nervous system: gut function and neuropharmacology. Trends Neurosci (2007) 30(1):9–13. doi: 10.1016/j.tins.2006.11.002
10. Li X, Liu L, Yang J, Yu Y, Chai J, Wang L, et al. Exosome derived from human umbilical cord mesenchymal stem cell mediates miR-181c attenuating burn-induced excessive inflammation. EBioMedicine (2016) 8:72–82. doi: 10.1016/j.ebiom.2016.04.030
11. Ma F, Chen R, Ding S, Li X, Wu L. (2022) Progress in the anti-infective effects of exosomes. Chin J Comp Med. 32(12):115–119. doi: 10.3969/j.issn.1671-7856.2022.12.01
12. Cai Z, Zhang W, Yang F, Yu L, Yu Z, Pan J, et al. Immunosuppressive exosomes from TGF-β1 gene-modified dendritic cells attenuate Th17-mediated inflammatory autoimmune disease by inducing regulatory T cells. Cell Res (2012) 22(3):607–10. doi: 10.1038/cr.2011.196
13. Yang C, Merlin D. Nanoparticle-mediated drug delivery systems for the treatment of IBD: current perspectives. Int J Nanomedicine. (2019) 14:8875–89. doi: 10.2147/IJN.S210315
14. Grohmann U, Bronte V. Control of immune response by amino acid metabolism. Immunol Rev (2010) 236:243–64. doi: 10.1111/j.1600-065X.2010.00915.x
15. Murray PJ. Amino acid auxotrophy as a system of immunological control nodes. Nat Immunol (2016) 17(2):132–9. doi: 10.1038/ni.3323
16. Netea MG, Balkwill F, Chonchol M, Cominelli F, Donath MY, Giamarellos-Bourboulis EJ, et al. A guiding map for inflammation. Nat Immunol. 2017 Jul 19;18(8):826-831. doi: 10.1038/ni.3790. Erratum: Nat Immunol (2021) 22(2):254. doi: 10.1038/ni.3790
17. Haq S, Grondin JA, Khan WI. Tryptophan-derived serotonin-kynurenine balance in immune activation and intestinal inflammation. FASEB J (2021) 35(10):e21888. doi: 10.1096/fj.202100702R
18. de las Casas-Engel M, Domínguez-Soto A, Sierra-Filardi E, Bragado R, Nieto C, Puig-Kroger A, et al. Serotonin skews human macrophage polarization through HTR2B and HTR7. J Immunol (2013) 190(5):2301–10. doi: 10.4049/jimmunol.1201133
19. Munn DH, Mellor AL. Indoleamine 2,3 dioxygenase and metabolic control of immune responses. Trends Immunol (2013) 34(3):137–43. doi: 10.1016/j.it.2012.10.001
20. Andou A, Hisamatsu T, Okamoto S, Chinen H, Kamada N, Kobayashi T, et al. Dietary histidine ameliorates murine colitis by rinhibition of proinflammatory cytokine production from macrophages. Gastroenterology (2009) 136(2):564–74. doi: 10.1053/j.gastro.2008.09.062
21. Fillmann H, Kretzmann NA, San-Miguel B, Llesuy S, Marroni N, González-Gallego J, et al. Glutamine inhibits over-expression of pro-inflammatory genes and down-regulates the nuclear factor kappaB pathway in an experimental model of colitis in the rat. Toxicology (2007) 236(3):217–26. doi: 10.1016/j.tox.2007.04.012
22. Kretzmann NA, Fillmann H, Mauriz JL, Marroni CA, Marroni N, González-Gallego J, et al. Effects of glutamine on proinflammatory gene expression and activation of nuclear factor kappa B and signal transducers and activators of transcription in TNBS-induced colitis. Inflammation Bowel Dis (2008) 14(11):1504–13. doi: 10.1002/ibd.20543
23. Hou YC, Pai MH, Wu JM, Yang PJ, Lee PC, Chen KY, et al. Protective effects of glutamine and leucine supplementation on sepsis-induced skeletal muscle injuries. Int J Mol Sci (2021) 22(23):13003. doi: 10.3390/ijms222313003
24. Wheeler MD, Thurman RG. Production of superoxide and TNF-alpha from alveolar macrophages is blunted by glycine. Am J Physiol (1999) 277(5):L952–9. doi: 10.1152/ajplung.1999.277.5.L952
25. Korish AA. Multiple antioxidants and L-arginine modulate inflammation and dyslipidemia in chronic renal failure rats. Ren Fail (2010) 32(2):203–13. doi: 10.3109/08860221003592820
26. Zhang L, Romero P. Metabolic control of CD8+ T cell fate decisions and antitumor immunity. Trends Mol Med (2018) 24(1):30–48. doi: 10.1016/j.molmed.2017.11.005
27. Cua DJ, Sherlock J, Chen Y, Murphy CA, Joyce B, Seymour B, et al. Interleukin-23 rather than interleukin-12 is the critical cytokine for autoimmune inflammation of the brain. Nature (2003) 421(6924):744–8. doi: 10.1038/nature01355
28. Dikiy S, Rudensky AY. Principles of regulatory T cell function. Immunity (2023) 56(2):240–55. doi: 10.1016/j.immuni.2023.01.004
29. Josefowicz SZ, Lu LF, Rudensky AY. Regulatory T cells: mechanisms of differentiation and function. Annu Rev Immunol (2012) 30:531–64. doi: 10.1146/annurev.immunol.25.022106.141623
30. Hirahara K, Nakayama T. CD4+ T-cell subsets in inflammatory diseases: beyond the Th1/Th2 paradigm. Int Immunol (2016) 28(4):163–71. doi: 10.1093/intimm/dxw006
31. Xu YQ, Lv W, Wu HJ, Shi S. Ginsenoside regulates Treg/Th17 cell ratio and inhibits inflammation to treat COPD. Pharmazie (2020) 75(11):590–4. doi: 10.1691/ph.2020.0696
32. Sun JK, Zhang WH, Chen WX, Wang X, Mu XW. Effects of early enteral nutrition on Th17/Treg cells and IL-23/IL-17 in septic patients. World J Gastroenterol (2019) 25(22):2799–808. doi: 10.3748/wjg.v25.i22.2799
33. Higashiyama M, Hokari R, Hozumi H, Kurihara C, Ueda T, Watanabe C, et al. HIF-1 in T cells ameliorated dextran sodium sulfate-induced murine colitis. J Leukoc Biol (2012) 91(6):901–9. doi: 10.1189/jlb.1011518
34. Klysz D, Tai X, Robert PA, Craveiro M, Cretenet G, Oburoglu L, et al. Glutamine-dependent α-ketoglutarate production regulates the balance between T helper 1 cell and regulatory T cell generation. Sci Signal (2015) 8(396):ra97. doi: 10.1126/scisignal.aab2610
35. Wu R, Chen X, Kang S, Wang T, Gnanaprakasam JR, Yao Y, et al. De novo synthesis and salvage pathway coordinately regulate polyamine homeostasis and determine T cell proliferation and function. Sci Adv (2020) 6(51):eabc4275. doi: 10.1126/sciadv.abc4275
36. Shi H, Chapman NM, Wen J, Guy C, Long L, Dhungana Y, et al. Amino Acids License Kinase mTORC1 Activity and Treg Cell Function via Small G Proteins Rag and Rheb. Immunity (2019) 51(6):1012–27. doi: 10.1016/j.immuni.2019.10.001
37. Keil M, Sonner JK, Lanz TV, Oezen I, Bunse T, Bittner S, et al. General control non-derepressible 2 (GCN2) in T cells controls disease progression of autoimmune neuroinflammation. J Neuroimmunol. (2016) 297:117–26. doi: 10.1016/j.jneuroim.2016.05.014
38. Van de Velde LA, Guo XJ, Barbaric L, Smith AM, Oguin TH 3rd, Thomas PG, et al. Stress kinase GCN2 controls the proliferative fitness and trafficking of cytotoxic T cells independent of environmental amino acid sensing. Cell Rep (2016) 17(9):2247–58. doi: 10.1016/j.celrep.2016.10.079
39. Klein Geltink RI, Pearce EL. The importance of methionine metabolism. Elife (2019) 8:e47221. doi: 10.7554/eLife.47221
40. Yerinde C, Siegmund B, Glauben R, Weidinger C. Metabolic control of epigenetics and its role in CD8+ T cell differentiation and function. Front Immunol (2019) 10:2718. doi: 10.3389/fimmu.2019.02718
41. Lorin J, Zeller M, Guilland JC, Cottin Y, Vergely C, Rochette L. Arginine and nitric oxide synthase: regulatory mechanisms and cardiovascular aspects. Mol Nutr Food Res (2014) 58(1):101–16. doi: 10.1002/mnfr.201300033
42. Siasos G, Tousoulis D, Antoniades C, Stefanadi E, Stefanadis C. L-Arginine, the substrate for NO synthesis: an alternative treatment for premature atherosclerosis? Int J Cardiol (2007) 116(3):300–8. doi: 10.1016/j.ijcard.2006.04.062
43. Zhu Q, Wu Y, Mai J, Guo G, Meng J, Fang X, et al. Comprehensive metabolic profiling of inflammation indicated key roles of glycerophospholipid and arginine metabolism in coronary artery disease. Front Immunol (2022) 13:829425. doi: 10.3389/fimmu.2022.829425
44. Negrotto L, Correale J. Amino acid catabolism in multiple sclerosis affects immune homeostasis. J Immunol (2017) 198(5):1900–9. doi: 10.4049/jimmunol.1601139
45. Yoon BR, Oh YJ, Kang SW, Lee EB, Lee WW. Role of SLC7A5 in metabolic reprogramming of human monocyte/macrophage immune responses. Front Immunol (2018) 9:53. doi: 10.3389/fimmu.2018.00053
46. Suzuki T. Regulation of intestinal epithelial permeability by tight junctions. Cell Mol Life Sci (2013) 70(4):631–59. doi: 10.1007/s00018-012-1070-x
47. Seth A, Basuroy S, Sheth P, Rao RK. L-Glutamine ameliorates acetaldehyde-induced increase in paracellular permeability in Caco-2 cell monolayer. Am J Physiol Gastrointest Liver Physiol (2004) 287(3):G510–7. doi: 10.1152/ajpgi.00058.2004
48. Perl A, Hanczko R, Lai ZW, Oaks Z, Kelly R, Borsuk R, et al. Comprehensive metabolome analyses reveal N-acetylcysteine-responsive accumulation of kynurenine in systemic lupus erythematosus: implications for activation of the mechanistic target of rapamycin. Metabolomics (2015) 11(5):1157–74. doi: 10.1007/s11306-015-0772-0
49. Kato H, Perl A. Mechanistic target of rapamycin complex 1 expands Th17 and IL-4+ CD4-CD8- double-negative T cells and contracts regulatory T cells in systemic lupus erythematosus. J Immunol (2014) 192(9):4134–44. doi: 10.4049/jimmunol.1301859
50. Jian H, Xu Q, Wang X, Liu Y, Miao S, Li Y, et al. Amino acid and fatty acid metabolism disorders trigger oxidative stress and inflammatory response in excessive dietary valine-induced NAFLD of laying hens. Front Nutr (2022) 9:849767. doi: 10.3389/fnut.2022.849767
51. Zhou X, He L, Zuo S, Zhang Y, Wan D, Long C, et al. Serine prevented high-fat diet-induced oxidative stress by activating AMPK and epigenetically modulating the expression of glutathione synthesis-related genes. Biochim Biophys Acta Mol Basis Dis (2018) 1864(2):488–98. doi: 10.1016/j.bbadis.2017.11.009
52. Cosentino RG, Churilla JR, Josephson S, Molle-Rios Z, Hossain MJ, Prado WL, et al. Branched-chain amino acids and relationship with inflammation in youth with obesity: A randomized controlled intervention study. J Clin Endocrinol Metab (2021) 106(11):3129–39. doi: 10.1210/clinem/dgab538
53. Gao N, Dou X, Yang S’A. Role of amino acids in inflammatory bowel disease and their signaling pathways. J Anim Husbandry Veterinary Med (2020) 51(10):10. doi: 10.11843/j.issn.0366-6964.2020.10.0
54. Morgan XC, Tickle TL, Sokol H, Gevers D, Devaney KL, Ward DV, et al. Dysfunction of the intestinal microbiome in inflammatory bowel disease and treatment. Genome Biol (2012) 13(9):R79. doi: 10.1186/gb-2012-13-9-r79
55. Ooi M, Nishiumi S, Yoshie T, Shiomi Y, Kohashi M, Fukunaga K, et al. GC/MS-based profiling of amino acids and TCA cycle-related molecules in ulcerative colitis. Inflammation Res (2011) 60(9):831–40. doi: 10.1007/s00011-011-0340-7
56. Scoville EA, Allaman MM, Brown CT, Motley AK, Horst SN, Williams CS, et al. Alterations in lipid, amino acid, and energy metabolism distinguish Crohn’s disease from ulcerative colitis and control subjects by serum metabolomic profiling. Metabolomics (2018) 14(1):17. doi: 10.1007/s11306-017-1311-y
57. Danilczyk U, Sarao R, Remy C, Benabbas C, Stange G, Richter A, et al. Essential role for collectrin in renal amino acid transport. Nature (2006) 444(7122):1088–91. doi: 10.1038/nature05475
58. Mawe GM, Hoffman JM. Serotonin signaling in the gut–functions, dysfunctions and therapeutic targets. Nat Rev Gastroenterol Hepatol (2013) 10(8):473–86. doi: 10.1038/nrgastro.2013.105
59. Dawiskiba T, Deja S, Mulak A, Ząbek A, Jawień E, Pawełka D, et al. Serum and urine metabolomic fingerprinting in diagnostics of inflammatory bowel diseases. World J Gastroenterol (2014) 20(1):163–74. doi: 10.3748/wjg.v20.i1.163
60. Tsune I, Ikejima K, Hirose M, Yoshikawa M, Enomoto N, Takei Y, et al. Dietary glycine prevents chemical-induced experimental colitis in the rat. Gastroenterology (2003) 125(3):775–85. doi: 10.1016/s0016-5085(03)01067-9
61. Mao X, Sun R, Wang Q, Chen D, Yu B, He J, et al. l-isoleucine administration alleviates DSS-induced colitis by regulating TLR4/myD88/NF-κB pathway in rats. Front Immunol (2022) 12:817583. doi: 10.3389/fimmu.2021.817583
62. Linder M, Liko D, Kancherla V, Piscuoglio S, Hall MN. Colitis is associated with loss of the histidine phosphatase LHPP and upregulation of histidine phosphorylation in intestinal epithelial cells. Biomedicines (2023) 11(8):2158. doi: 10.3390/biomedicines11082158
63. Jonnakuty C, Gragnoli C. What do we know about serotonin? J Cell Physiol (2008) 217(2):301–6. doi: 10.1002/jcp.21533
64. Williams BB, Van Benschoten AH, Cimermancic P, Donia MS, Zimmermann M, Taketani M, et al. Discovery and characterization of gut microbiota decarboxylases that can produce the neurotransmitter tryptamine. Cell Host Microbe (2014) 16(4):495–503. doi: 10.1016/j.chom.2014.09.001
65. Yano JM, Yu K, Donaldson GP, Shastri GG, Ann P, Ma L, et al. Indigenous bacteria from the gut microbiota regulate host serotonin biosynthesis. Cell. 2015 Apr 9;161(2):264-76. doi: 10.1016/j.cell.2015.02.047. Erratum: Cell (2015) 163:258. doi: 10.1016/j.cell.2015.02.047
66. Reigstad CS, Salmonson CE, Rainey JF 3rd, Szurszewski JH, Linden DR, Sonnenburg JL, et al. Gut microbes promote colonic serotonin production through an effect of short-chain fatty acids on enterochromaffin cells. FASEB J (2015) 29(4):1395–403. doi: 10.1096/fj.14-259598
67. Zhang X. (2021) Studying amino acid metabolism in the human gut using an in vitro fermentation model. (Zhejiang Normal University: China).
68. Ott SJ, Musfeldt M, Wenderoth DF, Hampe J, Brant O, Fölsch UR, et al. Reduction in diversity of the colonic mucosa associated bacterial microflora in patients with active inflammatory bowel disease. Gut (2004) 53(5):685–93. doi: 10.1136/gut.2003.025403
69. Frank DN, St Amand AL, Feldman RA, Boedeker EC, Harpaz N, Pace NR. Molecular-phylogenetic characterization of microbial community imbalances in human inflammatory bowel diseases. Proc Natl Acad Sci U S A. (2007) 104(34):13780–5. doi: 10.1073/pnas.0706625104
70. Manichanh C, Rigottier-Gois L, Bonnaud E, Gloux K, Pelletier E, Frangeul L, et al. Reduced diversity of faecal microbiota in Crohn’s disease revealed by a metagenomic approach. Gut (2006) 55(2):205–11. doi: 10.1136/gut.2005.073817
71. Matsuoka K, Kanai T. The gut microbiota and inflammatory bowel disease. Semin Immunopathol (2015) 37(1):47–55. doi: 10.1007/s00281-014-0454-4
72. Chen L, Wang J. Gut microbiota and inflammatory bowel disease. WIREs Mech Dis (2022) 14(2):e1540. doi: 10.1002/wsbm.1540
73. Cani PD, Amar J, Iglesias MA, Poggi M, Knauf C, Bastelica D, et al. Metabolic endotoxemia initiates obesity and insulin resistance. Diabetes (2007) 56(7):1761–72. doi: 10.2337/db06-1491
74. Huldani H, Margiana R, Ahmad F, Opulencia MJC, Ansari MJ, Bokov DO, et al. Immunotherapy of inflammatory bowel disease (IBD) through mesenchymal stem cells. Int Immunopharmacol. (2022) 107:108698. doi: 10.1016/j.intimp.2022.108698
75. Beutheu S, Ghouzali I, Galas L, Déchelotte P, Coëffier M. Glutamine and arginine improve permeability and tight junction protein expression in methotrexate-treated Caco-2 cells. Clin Nutr (2013) 32(5):863–9. doi: 10.1016/j.clnu.2013.01.014
76. Malhotra V, Eaves-Pyles T, Odoms K, Quaid G, Shanley TP, Wong HR. Heat shock inhibits the activation of NF-kappaB in the absence of heat shock factor-1. Biochem Biophys Res Commun (2002) 291(3):453–7. doi: 10.1006/bbrc.2002.6470
77. Hubert-Buron A, Leblond J, Jacquot A, Ducrotté P, Déchelotte P, Coëffier M. Glutamine pretreatment reduces IL-8 production in human intestinal epithelial cells by limiting IkappaBalpha ubiquitination. J Nutr (2006) 136(6):1461–5. doi: 10.1093/jn/136.6.1461
78. Sugihara K, Morhardt TL, Kamada N. The role of dietary nutrients in inflammatory bowel disease. Front Immunol (2019) 9:3183. doi: 10.3389/fimmu.2018.03183
79. Nikolaus S, Schulte B, Al-Massad N, Thieme F, Schulte DM, Bethge J, et al. Increased tryptophan metabolism is associated with activity of inflammatory bowel diseases. Gastroenterology (2017) 153(6):1504–1516.e2. doi: 10.1053/j.gastro.2017.08.028
80. Agus A, Planchais J, Sokol H. Gut microbiota regulation of tryptophan metabolism in health and disease. Cell Host Microbe (2018) 23(6):716–24. doi: 10.1016/j.chom.2018.05.003
81. Paeslack N, Mimmler M, Becker S, Gao Z, Khuu MP, Mann A, et al. Microbiota-derived tryptophan metabolites in vascular inflammation and cardiovascular disease. Amino Acids (2022) 54(10):1339–56. doi: 10.1007/s00726-022-03161-5
82. Lamas B, Natividad JM, Sokol H. Aryl hydrocarbon receptor and intestinal immunity. Mucosal Immunol (2018) 11(4):1024–38. doi: 10.1038/s41385-018-0019-2
83. Gutiérrez-Vázquez C, Quintana FJ. Regulation of the immune response by the aryl hydrocarbon receptor. Immunity (2018) 48(1):19–33. doi: 10.1016/j.immuni.2017.12.012
84. Rothhammer V, Borucki DM, Tjon EC, Takenaka MC, Chao CC, Ardura-Fabregat A, et al. Microglial control of astrocytes in response to microbial metabolites. Nature (2018) 557(7707):724–8. doi: 10.1038/s41586-018-0119-x
85. Lamas B, Richard ML, Leducq V, Pham HP, Michel ML, Da Costa G, et al. CARD9 impacts colitis by altering gut microbiota metabolism of tryptophan into aryl hydrocarbon receptor ligands. Nat Med (2016) 22(6):598–605. doi: 10.1038/nm.4102
86. Wlodarska M, Luo C, Kolde R, d’Hennezel E, Annand JW, Heim CE, et al. Indoleacrylic acid produced by commensal peptostreptococcus species suppresses inflammation. Cell Host Microbe (2017) 22(1):25–37.e6. doi: 10.1016/j.chom.2017.06.007
87. Donohoe DR, Garge N, Zhang X, Sun W, O’Connell TM, Bunger MK, et al. The microbiome and butyrate regulate energy metabolism and autophagy in the mammalian colon. Cell Metab (2011) 13(5):517–26. doi: 10.1016/j.cmet.2011.02.018
88. Chen G, Ran X, Li B, Li Y, He D, Huang B, et al. Sodium butyrate inhibits inflammation and maintains epithelium barrier integrity in a TNBS-induced inflammatory bowel disease mice model. EBioMedicine (2018) 30:317–25. doi: 10.1016/j.ebiom.2018.03.030
89. Chen S, Xia Y, Zhu G, Yan J, Tan C, Deng B, et al. Glutamine supplementation improves intestinal cell proliferation and stem cell differentiation in weanling mice. Food Nutr Res (2018) 62(62):v62.1439. doi: 10.29219/fnr.v62.1439
90. Jiang J, Yin L, Li JY, Li Q, Shi D, Feng L, et al. Glutamate attenuates lipopolysaccharide-induced oxidative damage and mRNA expression changes of tight junction and defensin proteins, inflammatory and apoptosis response signaling molecules in the intestine of fish. Fish Shellfish Immunol (2017) 70:473–84. doi: 10.1016/j.fsi.2017.09.035
91. Ding XZ, Fernandez-Prada CM, Bhattacharjee AK, Hoover DL. Over-expression of hsp-70 inhibits bacterial lipopolysaccharide-induced production of cytokines in human monocyte-derived macrophages. Cytokine (2001) 16(6):210–9. doi: 10.1006/cyto.2001.0959
92. Asea A, Rehli M, Kabingu E, Boch JA, Bare O, Auron PE, et al. Novel signal transduction pathway utilized by extracellular HSP70: role of toll-like receptor (TLR) 2 and TLR4. J Biol Chem (2002) 277(17):15028–34. doi: 10.1074/jbc.M200497200
93. Yeramian A, Martin L, Serrat N, Arpa L, Soler C, Bertran J, et al. Arginine transport via cationic amino acid transporter 2 plays a critical regulatory role in classical or alternative activation of macrophages. J Immunol (2006) 176(10):5918–24. doi: 10.4049/jimmunol.176.10.5918
94. Singh K, Coburn LA, Barry DP, Boucher JL, Chaturvedi R, Wilson KT. L-arginine uptake by cationic amino acid transporter 2 is essential for colonic epithelial cell restitution. Am J Physiol Gastrointest Liver Physiol (2012) 302(9):G1061–73. doi: 10.1152/ajpgi.00544.2011
95. Bröer S. Amino acid transport across mammalian intestinal and renal epithelia. Physiol Rev (2008) 88(1):249–86. doi: 10.1152/physrev.00018.2006
96. Mariotta L, Ramadan T, Singer D, Guetg A, Herzog B, Stoeger C, et al. T-type amino acid transporter TAT1 (Slc16a10) is essential for extracellular aromatic amino acid homeostasis control. J Physiol (2012) 590(24):6413–24. doi: 10.1113/jphysiol.2012.239574
97. Kim CJ, Kovacs-Nolan JA, Yang C, Archbold T, Fan MZ, Mine Y. l-Tryptophan exhibits therapeutic function in a porcine model of dextran sodium sulfate (DSS)-induced colitis. J Nutr Biochem (2010) 21(6):468–75. doi: 10.1016/j.jnutbio.2009.01.019
98. Hashimoto T, Perlot T, Rehman A, Trichereau J, Ishiguro H, Paolino M, et al. ACE2 links amino acid malnutrition to microbial ecology and intestinal inflammation. Nature (2012) 487(7408):477–81. doi: 10.1038/nature11228
99. Wolf AM, Wolf D, Rumpold H, Moschen AR, Kaser A, Obrist P, et al. Overexpression of indoleamine 2,3-dioxygenase in human inflammatory bowel disease. Clin Immunol (2004) 113(1):47–55. doi: 10.1016/j.clim.2004.05.004
100. Lee A, Kanuri N, Zhang Y, Sayuk GS, Li E, Ciorba MA. IDO1 and IDO2 non-synonymous gene variants: correlation with Crohn’s disease risk and clinical phenotype. PLoS One. 2014 Dec 26;9(12):e115848. doi: 10.1371/journal.pone.0115848. Erratum: PloS One (2015) 10(7):e0133098. doi: 10.1371/journal.pone.0115848
101. Parada-Turska J, Zgrajka W, Majdan M. Kynurenic acid in synovial fluid and serum of patients with rheumatoid arthritis, spondyloarthropathy, and osteoarthritis. J Rheumatol (2013) 40(6):903–9. doi: 10.3899/jrheum.121035
102. Sun Q, Wu H, Li S, Sun Z. Regulation of amino acids in inflammatory bowel disease. J Bioengineering (2022) 38(6):11. doi: 10.13345/j.cjb.210697
103. Kim S, Hwang J, Xuan J, Jung YH, Cha HS, Kim KH. Global metabolite profiling of synovial fluid for the specific diagnosis of rheumatoid arthritis from other inflammatory arthritis. PloS One (2014) 9(6):e97501. doi: 10.1371/journal.pone.0097501
104. Forrest CM, Kennedy A, Stone TW, Stoy N, Darlington LG. Kynurenine and neopterin levels in patients with rheumatoid arthritis and osteoporosis during drug treatment. Adv Exp Med Biol (2003) 527:287–95. doi: 10.1007/978-1-4615-0135-0_32
105. Parada-Turska J, Rzeski W, Zgrajka W, Majdan M, Kandefer-Szerszeń M, Turski W. Kynurenic acid, an endogenous constituent of rheumatoid arthritis synovial fluid, inhibits proliferation of synoviocytes in vitro. Rheumatol Int (2006) 26(5):422–6. doi: 10.1007/s00296-005-0057-4
106. Chalise JP, Pallotta MT, Narendra SC, Carlsson B, Iacono A, Namale J, et al. IDO1 and TGF-β Mediate protective effects of IFN-α in antigen-induced arthritis. J Immunol (2016) 197(8):3142–51. doi: 10.4049/jimmunol.1502125
107. Tykocinski LO, Lauffer AM, Bohnen A, Kaul NC, Krienke S, Tretter T, et al. Synovial fibroblasts selectively suppress th1 cell responses through IDO1-mediated tryptophan catabolism. J Immunol (2017) 198(8):3109–17. doi: 10.4049/jimmunol.1600600
108. Kaul NC, Mohapatra SR, Adam I, Tucher C, Tretter T, Opitz CA, et al. Hypoxia decreases the T helper cell-suppressive capacity of synovial fibroblasts by downregulating IDO1-mediated tryptophan metabolism. Rheumatol (Oxford). (2020) 59(5):1148–58. doi: 10.1093/rheumatology/kez587
109. Correale J. Immunosuppressive amino-acid catabolizing enzymes in multiple sclerosis. Front Immunol (2021) 11:600428. doi: 10.3389/fimmu.2020.600428
110. Sabatini DM. Twenty-five years of mTOR: Uncovering the link from nutrients to growth. Proc Natl Acad Sci USA. (2017) 114(45):11818–25. doi: 10.1073/pnas.1716173114
111. Wolfson RL, Sabatini DM. The dawn of the age of amino acid sensors for the mTORC1 pathway. Cell Metab (2017) 26(2):301–9. doi: 10.1016/j.cmet.2017.07.001
112. Tsokos GC, Lo MS, Costa Reis P, Sullivan KE. New insights into the immunopathogenesis of systemic lupus erythematosus. Nat Rev Rheumatol (2016) 12(12):716–30. doi: 10.1038/nrrheum.2016.186
113. Cruzat V, Macedo Rogero M, Noel Keane K, Curi R, Newsholme P. Glutamine: metabolism and immune function, supplementation and clinical translation. Nutrients (2018) 10(11):1564. doi: 10.3390/nu10111564
114. Yin Y, Choi SC, Xu Z, Perry DJ, Seay H, Croker BP, et al. Normalization of CD4+ T cell metabolism reverses lupus. Sci Transl Med (2015) 7(274):274ra18. doi: 10.1126/scitranslmed.aaa0835
115. Zhang X, Wang G, Bi Y, Jiang Z, Wang X. Inhibition of glutaminolysis ameliorates lupus by regulating T and B cell subsets and downregulating the mTOR/P70S6K/4EBP1 and NLRP3/caspase-1/IL-1β pathways in MRL/lpr mice. Int Immunopharmacol. (2022) 112:109133. doi: 10.1016/j.intimp.2022.109133
116. Mu Y, Luo J, Miao Y. Progress in tryptophan metabolism in inflammatory bowel diseases based on the involvement of intestinal flora. Int J Digestive Dis (2022) 03):141–5. doi: 10.3969/j.issn.1673-534X.2022.03.00
117. Zhang H, Wang L, Li C, Yu Y, Yi Y, Wang J, et al. Exosome-induced regulation in inflammatory bowel disease. Front Immunol (2019) 10:1464. doi: 10.3389/fimmu.2019.01464
118. Yaghoubi Y, Movassaghpour A, Zamani M, Talebi M, Mehdizadeh A, Yousefi M. Human umbilical cord mesenchymal stem cells derived-exosomes in diseases treatment. Life Sci (2019) 233:116733. doi: 10.1016/j.lfs.2019.116733
119. Arabpour M, Saghazadeh A, Rezaei N. Anti-inflammatory and M2 macrophage polarization-promoting effect of mesenchymal stem cell-derived exosomes. Int Immunopharmacol. (2021) 97:107823. doi: 10.1016/j.intimp.2021.107823
120. Cao L, Xu H, Wang G, Liu M, Tian D, Yuan Z. Extracellular vesicles derived from bone marrow mesenchymal stem cells attenuate dextran sodium sulfate-induced ulcerative colitis by promoting M2 macrophage polarization. Int Immunopharmacol. (2019) 72:264–74. doi: 10.1016/j.intimp.2019.04.020
121. Heidari N, Abbasi-Kenarsari H, Namaki S, Baghaei K, Zali MR, Ghaffari Khaligh S, et al. Adipose-derived mesenchymal stem cell-secreted exosome alleviates dextran sulfate sodium-induced acute colitis by Treg cell induction and inflammatory cytokine reduction. J Cell Physiol (2021) 236(8):5906–20. doi: 10.1002/jcp.30275
122. Yang S, Liang X, Song J, Li C, Liu A, Luo Y, et al. A novel therapeutic approach for inflammatory bowel disease by exosomes derived from human umbilical cord mesenchymal stem cells to repair intestinal barrier via TSG-6. Stem Cell Res Ther (2021) 12(1):315. doi: 10.1186/s13287-021-02404-8
123. An JH, Li Q, Ryu MO, Nam AR, Bhang DH, Jung YC, et al. TSG-6 in extracellular vesicles from canine mesenchymal stem/stromal is a major factor in relieving DSS-induced colitis. PloS One (2020) 15(2):e0220756. doi: 10.1371/journal.pone.0220756
124. Liu H, Liang Z, Wang F, Zhou C, Zheng X, Hu T, et al. Exosomes from mesenchymal stromal cells reduce murine colonic inflammation via a macrophage-dependent mechanism. JCI Insight (2019) 4(24):e131273. doi: 10.1172/jci.insight.131273
125. Ji T, Xu C, Sun L, Yu M, Peng K, Qiu Y, et al. Aryl hydrocarbon receptor activation down-regulates IL-7 and reduces inflammation in a mouse model of DSS-induced colitis. Dig Dis Sci (2015) 60(7):1958–66. doi: 10.1007/s10620-015-3632-x
126. Pernomian L, Duarte-Silva M, de Barros Cardoso CR. The aryl hydrocarbon receptor (AHR) as a potential target for the control of intestinal inflammation: insights from an immune and bacteria sensor receptor. Clin Rev Allergy Immunol (2020) 59(3):382–90. doi: 10.1007/s12016-020-08789-3
127. Furumatsu K, Nishiumi S, Kawano Y, Ooi M, Yoshie T, Shiomi Y, et al. A role of the aryl hydrocarbon receptor in attenuation of colitis. Dig Dis Sci (2011) 56(9):2532–44. doi: 10.1007/s10620-011-1643-9
128. Nguyen LP, Bradfield CA. The search for endogenous activators of the aryl hydrocarbon receptor. Chem Res Toxicol (2008) 21(1):102–16. doi: 10.1021/tx7001965
129. Mao F, Wu Y, Tang X, Kang J, Zhang B, Yan Y, et al. Exosomes derived from human umbilical cord mesenchymal stem cells relieve inflammatory bowel disease in mice. BioMed Res Int (2017) 2017:5356760. doi: 10.1155/2017/5356760
130. Kang Y, Song Y, Luo Y, Song J, Li C, Yang S, et al. Exosomes derived from human umbilical cord mesenchymal stem cells ameliorate experimental non-alcoholic steatohepatitis via Nrf2/NQO-1 pathway. Free Radic Biol Med (2022) 192:25–36. doi: 10.1016/j.freeradbiomed.2022.08.037
131. Jiang K, Jiang T, Chen Y, Mao X. Mesenchymal stem cell-derived exosomes modulate chondrocyte glutamine metabolism to alleviate osteoarthritis progression. Mediators Inflamm (2021) 2021:2979124. doi: 10.1155/2021/2979124
132. Wang G, Yuan J, Cai X, Xu Z, Wang J, Ocansey DKW, et al. HucMSC-exosomes carrying miR-326 inhibit neddylation to relieve inflammatory bowel disease in mice. Clin Transl Med (2020) 10(2):e113. doi: 10.1002/ctm2.113
133. Teng Y, Ren Y, Sayed M, Hu X, Lei C, Kumar A, et al. Plant-derived exosomal microRNAs shape the gut microbiota. Cell Host Microbe (2018) 24(5):637–652.e8. doi: 10.1016/j.chom.2018.10.001
Keywords: inflammation, inflammatory bowel disease, amino acid metabolism, mesenchymal stem cell, exosome
Citation: Zheng X, Zhu Y, Zhao Z, Chu Y and Yang W (2023) The role of amino acid metabolism in inflammatory bowel disease and other inflammatory diseases. Front. Immunol. 14:1284133. doi: 10.3389/fimmu.2023.1284133
Received: 12 September 2023; Accepted: 10 October 2023;
Published: 23 October 2023.
Edited by:
Jiong Chen, Ningbo University, ChinaReviewed by:
Ning Liu, Chinese Academy of Medical Sciences, ChinaJosé Luis Fachi, Washington University in St. Louis, United States
Copyright © 2023 Zheng, Zhu, Zhao, Chu and Yang. This is an open-access article distributed under the terms of the Creative Commons Attribution License (CC BY). The use, distribution or reproduction in other forums is permitted, provided the original author(s) and the copyright owner(s) are credited and that the original publication in this journal is cited, in accordance with accepted academic practice. No use, distribution or reproduction is permitted which does not comply with these terms.
*Correspondence: Ying Chu, chuying@wjrmyy.cn
†These authors have contributed equally to this work