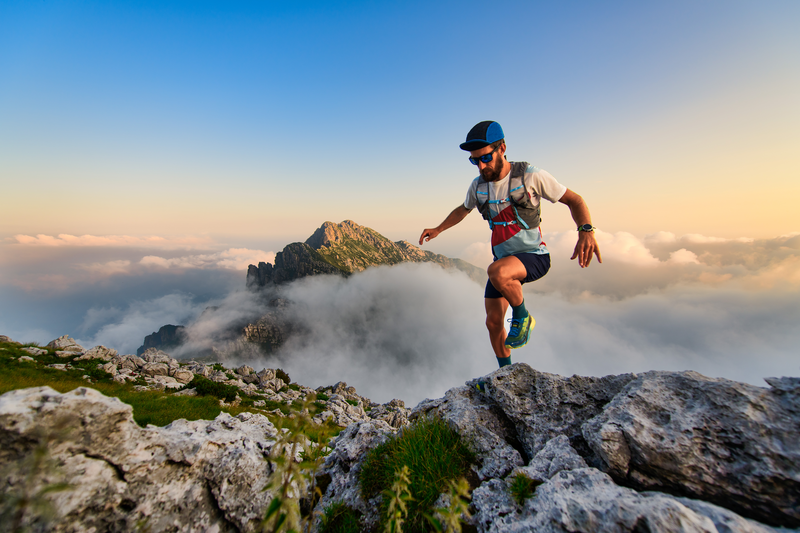
94% of researchers rate our articles as excellent or good
Learn more about the work of our research integrity team to safeguard the quality of each article we publish.
Find out more
ORIGINAL RESEARCH article
Front. Immunol. , 05 December 2023
Sec. Inflammation
Volume 14 - 2023 | https://doi.org/10.3389/fimmu.2023.1280884
Introduction: Heterotopic ossification (HO) is a complex pathology often observed in combat injured casualties who have sustained severe, high energy polytraumatic extremity injuries. Once HO has developed, prophylactic therapies are limited outside of surgical excision. Tourniquet-induced ischemia injury (IR) exacerbates trauma-mediated musculoskeletal tissue injury, inflammation, osteogenic progenitor cell development and HO formation. Others have shown that focal adhesion kinase-2 (FAK2) plays a key role in regulating early inflammatory signaling events. Therefore, we hypothesized that targeting FAK2 prophylactically would mitigate extremity trauma induced IR inflammation and HO formation.
Methods: We tested whether the continuous infusion of a FAK2 inhibitor (Defactinib, PF-573228; 6.94 µg/kg/min for 14 days) can mitigate ectopic bone formation (HO) using an established blast-related extremity injury model involving femoral fracture, quadriceps crush injury, three hours of tourniquet-induced limb ischemia, and hindlimb amputation through the fracture site. Tissue inflammation, infiltrating cells, osteogenic progenitor cell content were assessed at POD-7. Micro-computed tomography imaging was used to quantify mature HO at POD-56.
Results: In comparison to vehicle control-treated rats, FAK2 administration resulted in no marked wound healing complications or weight loss. FAK2 treatment decreased HO by 43%. At POD-7, marked reductions in tissue proinflammatory gene expression and assayable osteogenic progenitor cells were measured, albeit no significant changes in expression patterns of angiogenic, chondrogenic and osteogenic genes. At the same timepoint, injured tissue from FAK-treated rats had fewer infiltrating cells. Additionally, gene expression analyses of tissue infiltrating cells resulted in a more measurable shift from an M1 inflammatory to an M2 anti-inflammatory macrophage phenotype in the FAK2 inhibitor-treated group.
Discussion: Our findings suggest that FAK2 inhibition may be a novel strategy to dampen trauma-induced inflammation and attenuate HO in patients at high risk as a consequence of severe musculoskeletal polytrauma.
High energy blast-related trauma from improvised explosive devices is the leading cause of complicated multiple organ system injuries and death for servicemembers on the battlefield in recent military conflicts (1). Combat casualties with severe extremity wounds sustained in conflict (and once considered lethal) now survive due to advances in modern personal protective equipment technologies, far-forward positioning of critical care surgical teams, decreased medical evacuation times, and cutting-edge reconstructive surgery (2). While being fortunate to survive, these patients frequently experience complications in the convalescent period. A result of acute multi-mechanistic systemic responses to blast injury not commonly experienced in the civilian community, a delayed musculoskeletal pathologic condition that occurs with great frequency following wartime injuries is the formation of ectopic bone within soft-tissue, referred to as heterotopic ossification (HO) (3). Heterotopic ossification imposes morbidity resulting from impaired function and deceased use of affected limb secondary to joint contractures, tissue replacement and chronic pain (4).
Increasing evidence suggests hyperinflammation and immune dysregulation play a critical role in the onset and progression of the pathophysiology of complications induced by blast trauma (5). Nevertheless, the basis for this connection is not fully understood. The relationship between the immune system and trauma-induced HO formation is complex (6–11). However, an excessive proinflammatory response and hypoxia-mediated oxidative stress (iNOS, ROS) are considered to be the main mechanisms (10, 12, 13). Cytokine inflammatory pathways, mediated by activated monocytes-macrophages, are likely critical components of the microenvironmental cytokine milieu and play key roles in mediating HO formation following musculoskeletal trauma. Macrophage polarity plays a crucial role in the early innate immune response to trauma. During this process, accumulated macrophages transition from a polarized proinflammatory (M1) phenotype to an alternatively activated anti-inflammatory state (M2), a key in vivo cellular mechanism involved in mitigating inflammation, and promoting tissue repair and wound healing (14, 15)Pathogen-associated molecular patterns (PAMPs), damage-associated molecular patterns (DAMPs), and Th1 cell cytokines (IL-6, TNFα) activate resident macrophages to extend and augment inflammation, while Th2 cell cytokines (IL-4 and IL-13) polarize monocytes/macrophages to M2 macrophages releasing anti-inflammatory cytokines/chemokines (16, 17). M1/M2 polarization ratios in tissues have been suggested as potential prognostic factors to predict clinical outcome (18, 19).
The integrin-associated cytoplasmic protein tyrosine kinase, focal adhesion kinase-2 (FAK2), has been shown to be a key player of early signaling that orchestrates the initial development of focal adhesions. FAK2 has been shown to play important roles in the adherence of cells to the extracellular matrix (ECM) and cellular processes such cell adhesion, migration, proliferation, cytoskeletal organization, differentiation, and survival (20–23). FAK activity is regulated through extracellular integrin-ECM interactions and transmembrane receptors (G-protein-coupled, cytokine and growth factor receptors) at the plasma membrane), leading to downstream activation of phosphatidylinositol 3-kinase (PI3K) and AKT1, MAPK1/ERK2, MAPK3/ERK1 and MAP kinase signaling cascades (24). Together, these complex FAK-propagating signaling complexes are known to act as mechanosensors, responding to extracellular spatiotemporal environmental molecular and mechanical cues (25–28). Previously, focal adhesion kinase (FAK) and downstream YAP/TAZ have been identified as a link between mechanotransduction, hypoxic stress, inflammation, normal stem cell fate, and wound healing (29–32). A growing body of evidence suggest FAK overexpression is correlated with chronic inflammation which often leads to serious secondary complications and poor healing outcomes. The main immune cell implicated in in these FAK activation-mediated pathologies are macrophages. Chronic FAK-mediated proinflammatory molecule expression is promoted in endothelial cells by IL-1β and TNFα stimulation (29, 33, 34). Recent studies report that FAK signaling mediates LPS-induced inflammatory lung injury through increased transforming growth factor-β-activated kinase-1 (TAK1) and NFκB pathway activity (35) in macrophages. Moreover, FAK activation promotes lung fibrosis and myofibroblast formation following bleomycin lung injury (34). Others have reported that FAK signaling plays a critical role involved in the proliferation, migration, and proinflammatory activation of macrophages leading to chronic cardiac tissue inflammation in Chagas disease, diabetic cardiomyopathy, and other vascular diseases (36–38). Additionally, accumulating evidence demonstrates FAK signaling is overexpressed and activated in many tumor cells where it is critically involved with many diverse cell types (immune, stromal and tumor) and cellular/humoral processes (cytokines, chemokines, growth factors, ECM) within the tumor microenvironment that promote cell survival, proliferation, and metastasis resulting in poor prognosis (39, 40).
Increased FAK expression has been reported to increase angiogenesis and vascular permeability (41), two coupled processes essential for both normal and ectopic endochondral bone growth-osteogenesis (42–49). Intracellular FAK2 signaling regulates critical genes involved in the migration, proliferation, differentiation, survival, and osteogenesis of multipotent mesenchymal stromal cells (MSCs) and osteoblasts (23, 25, 46–48, 50, 51). Moreover, our initial studies have shown that FAK deletion in cells responsible for HO mitigates traumatic HO formation (52). Given that the resolution of inflammation is essential for proper wound healing along with bone and tissue regeneration, we hypothesized that the prophylactic inhibition of FAK2 with a pharmacologic inhibitor may attenuate inflammation in addition to ectopic bone formation in a rat poly-systemic trauma model (9, 10).
Adult male (11-12-week old; 350 - 450 g) pathogen free Sprague Dawley rats (Rattus norvegicus) were obtained from Taconic Biosciences (Germantown, New York, USA). Animals were housed for a minimum of 7 days for acclimatization and quarantine purposes. All rats were pair-housed in individually ventilated cages and exposed to a 12-hour light/dark cycle, with free access to food (standard rodent chow) and water, under veterinary care and supervision. All experiments and animal care procedures for this research were approved by the Uniformed Services University Institutional Animal and Care and Use Committees (IACUC; Protocol # SUR-21-069). All activities were conducted in accordance with all applicable regulations, best practices pertaining to the use of animals in research, and the ARRIVE guidelines (53).
Rats (n=20) were subjected to the well-established blast-associated complex lower limb injury model, as previously described (Figure 1) (9, 54, 55). In brief, rats received sequentially a head-on whole-body blast overpressure exposure (120 kPa), a femoral fracture, soft tissue crush injury of the quadriceps, three hours of prolonged limb ischemia, and hindlimb amputation through the zone of injury (ZOI). A small incision and a subcutaneous pocket were formed, by blunt dissection, between the scapulae for implantation of an Alzet osmotic mini-pump (model 2ML1, DURECT Corporation, Cupertino, California, USA) for 7 days of continuous subcutaneous infusion of either vehicle control (n =10) or FAK2 inhibitor (Defactinib; PF-573228; 10 mg/kg/day (6.94 µg/kg/min; n =10)). The skin incision was closed with 5-0 nylon sutures. Pumps were surgically exchanged under isoflurane sedation at post-operative day (POD)-7 for a total of 14 continuous days of subcutaneous infusion. In some of these studies, a separate cohort of rats (n=6) were used to measure the concentration of PF-573228 in the serum using a liquid chromatography/tandem mass spectrometric (LC-MS/MS) system (Agilent 1200 series, Agilent Technologies, Inc., Santa Clara, California, USA). Prior to pump implantation, PF-573228 was dissolved in 50% dimethyl sulfoxide (DMSO) and 50% polyethylene glycol 300 (PEG 300) (56). Per manufacturer instructions, loaded pumps were placed in 0.9% NaCl at 37°C overnight to equilibrate before implantation. Animal health status was observed daily using IACUC approved pain score chart and body weights were recorded twice per week. Animals were euthanized on either POD-7 (n=10) or POD-56 (n=10) for follow-on morphometric or molecular analyses. Serum and muscle tissue obtained from age matched naive rats (n=5) served as the uninjured, healthy control.
Figure 1 Illustration of the research study design and experimental approach. Adult male Sprague Dawley rats (350-450 g) underwent a sequential polysystem injury involving whole-body blast overpressure (120 kPa), a complex orthopaedic injury involving a mid-shaft femur fracture and medial quadriceps crush injury, followed by 180 min of tourniquet induced ischemia, and immediate hindlimb amputation. During the same anesthetic event, subcutaneous Alzet osmotic drug delivery pumps were implanted in the dorsum behind the scapulae containing either vehicle solution (1:1 DMSO to PEG300) or Defactinib (PF573228; mean pumping rate: 10 mg/kg/day; 6.94 µg/kg/min for 14 days). Cohorts of rats (n=5 per group for each timepoint) were euthanized on post-operative day (POD) 7 to assess inflammatory and early endochondral ossification signaling, or on POD-56 for morphometric analyses of ectopic bone formation. Schematic created with biorender.com web interface.
Formalin fixed limbs collected at POD-56 were shipped to collaborators at the Center for Organogenesis Research and Trauma. Limbs were radiographically imaged using a MedisoUSA nanoScan PET/CT system (Arlington, Virginia, USA). The scanning parameters were max zoom with 720 helical projections with an x-ray power of 70 kV at 980 μA and an exposure time of 300 ms. Digitized images were reconstructed using the manufacturer’s program, Nucline nanoScan, with a resulting voxel size of 40 × 40 × 40 μm. Reconstructions of rat hindlimbs were created and quantified using Dragonfly ORS (Montreal, Quebec, Canada) software on representative means at 800 Hounsfield units. Semi-quantification analyses of ectopic bone formation were performed at the amputation injury site of the rat hindlimb by a blinded, skilled operator.
Following established protocols (45, 57, 58), skeletal muscle tissue located within the medial ZOI was aseptically collected and placed in sterile D-PBS with 100 U/mL penicillin (Invitrogen, Gaithersburg, MD). In brief, we removed fascia and fat from the tissue, minced it finely, and digested it in a solution of DMEM F-12 (Gibco, Billings, Montana, USA), Collagenase Types I and II, and Neutral Protease (Worthington, Lakewood, New Jersey, USA), for two hours. Cell suspensions were passed through a series of cell strainers (100, 70, 40 µm) to remove debris. Next, residual red blood cells were removed using ACK lysis buffer (Sigma-Aldrich, St. Louis, MO), washed with D-PBS, and centrifuged. Cell pellets were resuspended in osteogenic medium consisting of DMEM-F12, supplemented with 10% FBS, 100 nM of dexamethasone, 200 μM of ascorbic acid, 10 mM of glycerol 2-phosphate, and 100 U/mL penicillin (MilliporeSigma, Burlington, Massachusetts, USA). Cell yields and viability were determined using trypan blue dye exclusion. Cells (1-4 x 104 per well; 6-well culture plate) were then plated in osteogenic medium for 10 days, with medium changes every 2 days. Culture wells were gently washed twice with D-PBS to remove non-adherent cells, air dried, fixed using 100% methanol and then stained with crystal violet. Entire plates were imaged using a fully-motorized Leica DMI6000B microscope (Leica, Wetzlar, Germany). Adjacent brightfield images from each well were stitched together using LAS X software (Leica, Wetzlar, Germany). Distinct CTP-Os (aggregates of > 50 cells) were manually counted as previously described (45, 59).
Skeletal muscle (100 μg) and remaining infiltrating cells (1-4×106) isolated within the ZOI surrounding the amputation site collected on POD-7 were flash frozen using liquid nitrogen, and stored at -80°C prior to RNA extraction. As previously optimized, a two-step RT-qPCR protocol was utilized to isolate RNA and transcribe cDNA (9, 55, 60) using the RNeasy Mini kit (Qiagen, Germantown, Maryland, USA) and the iScript Advanced cDNA synthesis kits (Bio-Rad, Hercules, California, USA). qPCR products were amplified using the SsoAdvanced ™ Universal SYBR Green Supermix, (Bio-Rad, Hercules, California, USA) according to the manufacturer’s instruction. The amplification was performed in 384-well plates using a QuantStudio real-time PCR system (QuantStudio 7 Pro, Applied Biosystems, Waltham, Massachusetts, USA). The data were acquired through ThermoFisher Connect software; the obtained mean Ct values were exported for statistical analysis. Muscle tissue collected from the medial quadriceps surrounding the ZOI was profiled for 120 gene targets consisting of chondrogenic, angiogenic, osteogenic, inflammatory, immune cell signaling, and early transcriptional activators for the assessment of early HO in injured muscle (Supplementary Table 1). Infiltrating cells harvested from muscle tissue were profiled for a panel of 40 gene for cell surface markers expressed on immune cells (Supplementary Table 2). Each gene array contained optimal housekeeping genes and assay controls (Bio-Rad, Hercules, California, USA).
The systemic concentration of Th1 interleukin-6 (IL-6), and Th2 interleukin-13 (IL-13) in serum specimens collected from POD-7 animals were analyzed using commercial protein arrays (Meso Scale Diagnostics, Rockville, Maryland, USA) according to the manufacturer’s instructions. Data acquisition was performed using a Meso Sector S600 (Meso Scale Diagnostics, Rockville, Maryland, USA) and quantitative results were generated using Methodical Mind software (version MMPR 1.0.27; Meso Scale Diagnostics, Rockville, Maryland, USA).
All data was curated using GraphPad Prism (version 9.5.1, San Diego, California, USA). Data analysis was performed using either SPSS (version 28.0.1.0 (142); SPSS Inc., Chicago, Illinois, USA) or Graph Pad Prism. Outliers, as necessary, were removed using the Rout method (Q=1%). Data are presented as mean ± SEM. Statistically significant comparisons were denoted as follows: * for p<0.05, ** for p<0.01, *** for p<0.001, or **** for p<0.0001, with brackets identifying the source of significance.
A two-tailed unpaired t-test with a 95% confidence level was conducted using GraphPad Prism to compute the differences in total ectopic bone volume at the ZOI of the injured limb between the vehicle and PF-573228-treated animals.
Each assessment, including the number of isolated cells, frequency of CTP-Os, and number of CTP-Os per gram of muscle, was evaluated with GraphPad Prism using a two-tailed unpaired t-test with a 95% confidence level. The t-test was conducted to determine statistical differences between cells or colonies derived from muscle tissue surrounding the ZOI in either vehicle or PF-573228-treated animals. Treatment effect size was calculated using Glass’s delta (GΔ) formula when variances between groups were significantly different (61–63). Ranking of effect size: small effect = 0.2 medium effect = 0.5, large effect > 0.8. A GΔ lower cutoff threshold of 0.75 was chosen to indicate significant effect size.
Relative expression (2-ΔCt) was calculated for naïve and injured samples using the optimal normalization strategy (55). A cycle threshold (Ct) level tagged as “undetermined” by the Cloud Connect software (Thermo Fisher Scientific) for any particular gene was considered not expressed. However, to explore changes in genes not expressed in naïve conditions but expressed following injury or vice versa, a Ct value of 40 was imputed for calculation purposes (64). All statistical analyses were conducted on ΔCt values. To assess for significant differences in relative gene expression between muscle tissue samples obtained from naïve or injured (vehicle- and PF-573228-treated) animals, we compared the ΔCt values using a one-way ANOVA on SPSS software (version 28.0.1.0 (142); SPSS Inc., Chicago, IL, USA), with α=0.05. A Tukey-Kramer post-hoc analysis test was utilized to determine the source of the significance.
A one-way ANOVA with Welch statistic, on GraphPad Prism, was used to assess significant differences between serum levels of individual analytes collected from naïve controls or injured animals on post-operative day 7. Dunnett’s T3 multiple comparisons test was used to determine the source of significance with α=0.05.
A continuous subcutaneous infusion of the FAK2 inhibitor Defactinib (PF-573228) at 10 mg/kg/day (6.94 µg/kg/min) resulted in a marked reduction (43%) in the amount of new bone formation at 8-weeks post trauma (Figure 2) when compared to the vehicle control group (22.03 ± 16.95 mm3 vs 38.88 ± 7.85 mm3 ectopic bone; p=0.0785). The independent samples t-test analysis revealed no significant difference in HO volume between vehicle-treated (M = 38.88, SD = 16.95, CI = 29.13-48.63) and FAK2-treated (M = 22.03, SD = 7.85, 95% CI 0.9-43.08)), t = 2.017, p = 0.0785. The effect size, as measured by GΔ = 1.05, indicating a large effect.
Figure 2 FAK2 inhibition mitigates trauma-induced heterotopic ossification (HO) formation. Micro-computed tomography scans were performed on vehicle- and PF573228-treated rats following sequential polysystem injury at week 8. (A) Total new bone was determined using Dragonfly software to identify the difference between new bone (green) and naïve bone (grey). (B) Quantitative measure of ectopic bone volume. Unpaired t-test analyses revealed a trend (p=0.0785) towards a reduced formation of ectopic bone at the amputation site in the PF573228-treated injured rats (n=5; 10 mg/kg/day; 6.94 µg/kg/min for 14 days) compared to the vehicle-treated injured rats (n=5). Data presented as mean values ± SEM. # indicates a large effect size (GΔ = 1.05).
Reconstructions from µCT analysis showed a clear development of new bone tissue proximal to the amputation site and within the surrounding soft-tissue. Histological evaluation confirmed the findings from the radiographic analysis (data not shown). LC-MS/MS determinations measurements showed a continuous intravenous dose of PF-573228 at 10 mg/kg/day (6.94 µg/kg/min) resulted in a serum concentration of 0.25 ± 0.17 ug/mL on day-3 (n=3). To investigate the effectiveness of short-term FAK2 inhibitor treatment without major adverse consequences, we monitored health status and body weight during the first 4 weeks of treatment. Compared to the vehicle-treated group, the PF-573228-treated group had slightly decreased weight gain, but there were no apparent differences in pain scores. Similar serum clinical chemistry measurements were detected between the two groups (Supplementary Figure 1) on POD-7. However, a significantly marked decrease of AST (p=0.028) was detected in the PF-573228-treated group compared to the vehicle. Compared to historical findings using this trauma model, no evidence of surgical site infections and/or adverse gross wound healing complications-wound breakdown resulting in delayed wound healing were noted in either group.
Inflammation is a major driver of heterotopic endochondral ossification process following tissue trauma. We therefore hypothesized, FAK2 inhibition may be a useful therapeutic to dampen the local early immune response induced by acute musculoskeletal trauma. To this end we investigated, at the peak of the tissue inflammatory response (POD-7), the level of inflammatory cell infiltration, presence of assayable connective tissue osteogenic progenitor cells (CTP-Os), and expression of levels of inflammatory, chondrogenic, angiogenic and osteogenic genes within muscle tissue obtained from the site of injury. The frequency and total number of isolated nucleated cells per gram of injured muscle tissue (Figures 3A, B) in the PF-573228-treated group (8.27×106 ± 2.76×106, n=5) was modestly reduced (p = 0.614) in comparison to the vehicle control group (11.21×106 ± 4.7×106; n =4). FAK2 inhibition treatment reduced the number assayable CTP-O colony forming progenitor cells (Figure 3C) by 86% when compared to vehicle control treatment The independent samples t-test analysis revealed a significant difference in number of tissue resident CTP-Os per gram of tissue between vehicle-treated (M = 1194, SD = 985, 95% CI = 636-4867) and FAK2-treated (M = 166, SD = 238, 95% CI = 24-850)), t = 2.290, p = .05). The effect size GΔ = 1.04, indicating a large effect.
Figure 3 FAK2 inhibition attenuates injured muscle tissue cellular infiltration and osteogenic connective tissue progenitor colony-forming cell activity (CTP-O). Following blast-related polytraumatic extremity injury and 6 days of vehicle or FAK2 inhibitor treatment (PF573228, 10 mg/kg/day, 6.94 µg/kg/min), muscle tissue surrounding the amputation site was harvested on POD-7. Viable nucleated cells were isolated using collagenase digestion, counted and plated in osteogenic culture media. (A) Number of nucleated cells isolated per gram of muscle. (B) Number of assayable CTP-Os per 1 x 106 plated cells. (C) Quantification of number of CTP-Os per gram of tissue by crystal violet staining after 10 days of in vitro culture in osteogenic media. Visible macroscopic colonies containing > 50 cells were enumerated. Data represents mean values ± SEM. * indicates p <0.05.
To further characterize the impact of FAK2 inhibition in our HO trauma model, we assessed transcript expression level of genes that regulate the early development of endochondral bone formation. As shown in Figures 4A-C, blast/extremity injury resulted in significant increases in expression levels of cell surface markers specific for immune cells (Cd4, Cd14, Faslg, Foxp3, Slc11a1, and Trem1), inflammatory signaling molecules and cytokines (Il1b, Il1r1, Il6, and Lcn2), and several chemokines (Cxcl5, Cxcl10, Cxcl2, and Ccl12). This significant reduction in inflammatory transcripts following PF573228 treatment corresponds with the measured reduction in the number of muscle infiltrating cells isolated at POD-7. Additionally, we found that FAK2 inhibition had a modest suppressive effect on a number of osteogenic genes (Atf3, Jun, Socs3, and Traf6) while upregulating the expression of a few chondrogenic genes including Acan, Comp, and Sox9 (Supplementary Figures 2A, B). Overall, short-term FAK2 inhibition had no impact on the expression of a number of angiogenic, toll-like receptors, adhesion/matrix proteins, or apoptotic-related gene transcripts (Supplementary Figures 2C-H).
Figure 4 Levels of inflammatory mediators in muscle tissue collected from the amputation site at POD-7 from rats treated with FAK2 inhibitor (PF573228;10 mg/kg/day; 10 mg/kg/day, 6.94 µg/kg/min)), vehicle control, or healthy muscle in age-matched controls. Panel (A) cell surface markers specific to immune cells; Panel (B) inflammatory signaling molecules and cytokines; Panel (C) chemokines. Relative expression (2-ΔCt) was calculated using an optimal normalization strategy. One-way ANOVAs for each gene were conducted on ΔCt values to determine treatment effects. Tukey-Kramer post-hoc analyses were utilized to determine the source of the significance. Data represents mean relative expression values ± SEM. * indicates p < 0.05, **indicates p < 0.01 and *** indicates p < 0.001.
To test whether FAK2 signaling also regulates macrophage transition in vivo, we explored the association of M1 and M2 macrophage cell composition by transcriptomic markers in cellular infiltrates collected from injured-regenerative muscle tissue from vehicle control-treated rats (Figure 5). Infiltrating cells collected from FAK2 inhibitor and vehicle-treated rats on POD-7 had very similar expression levels of pan-macrophage markers (Csf1r, Cd1, Cd47, CD68, Itgam, Itgax). Cells from vehicle control-treated rats had relatively higher expression levels of proinflammatory M1 molecular signature markers (Nos2, Mpo, Cxcl2, Cxcl5, Csf2, Csf3, Il1a, Clec7a, Cd80, Cd64) of traditional-classic activated macrophage phenotype. However, muscle infiltrating cells obtained from FAK2 inhibitor-treated rats showed relatively similar or higher expression levels of anti-inflammatory M2 markers (Arg1, CD163, Mrc1, Ccl2, Tgfb1) consistent with alternatively activated macrophage functional phenotype. The gene expression levels of three activation markers (Cd40, Elane, Il10) in both cell populations was similar and have been depicted as transitional repolarization M1-M2 signatures. Taken together, these results based on mRNA expression analysis, indicate that infiltrating cells differentiate and polarize more towards an M2 phenotype, with decreased M1 and increased M2 macrophage phenotype cytokine expression, in response to FAK2 inhibition. Notably, serum IL-6 and IL-13 levels (Figure 6), which are involved with M1 programming (IL-6) and M2 polarization (IL-13), were decreased and increased, respectively, in the FAK2 inhibitor treatment group when compared to levels in the vehicle control group.
Figure 5 Level of pan-macrophage, classic proinflammatory M1 and alternative activated anti-inflammatory M2 gene markers expressed in cellular infiltrates collected from injured muscle tissue at POD-7 from rats treated with the FAK2 inhibitor (PF-573228,10 mg/kg/day, 6.94 µg/kg/min), vehicle control and in healthy muscle from age-matched controls. Relative expression (2-ΔCt) was calculated using an optimal normalization strategy. One-way ANOVAs for each gene were conducted on ΔCt values to determine treatment effects. Tukey-Kramer post-hoc analyses were utilized to determine the source of the significance. Data represents mean relative expression values ± SEM. * indicates p < 0.05, **indicates p < 0.01 ands *** indicates p < 0.001.
Figure 6 The effect of FAK2 inhibitor (PF-573228; 10 mg/kg/day, 6.94 µg/kg/min) on systemic proinflammatory IL-6 and anti-inflammatory IL-13 secretion levels at POD-7. Results from non-injured naïve rats, vehicle control-treated rats, and FAK2 inhibitor-treated rats are expressed as mean value ± SEM, n = 25. * indicates p<0.05 and ** indicates p<0.01.
Combat-related wounds are normally associated with a persistent local and systemic inflammatory response that often result in delayed wound closure and healing (65, 66). Previous research has indicated that prolonged, elevated inflammation at the site of musculoskeletal trauma is one of the key contributors to impaired wound healing and development of heterotopic ossification (HO) (7, 8, 44, 45, 57, 59, 67–70). As an important regulator of cytokine and integrin-mediated signaling, FAK2 is involved in various cellular processes including cell adhesion, motility, proliferation, osteogenesis and bone homeostasis, through its kinase activity and scaffolding functions under steady and pathologic states (22, 23, 71–74). Tourniquet-induced ischemia-related injury (IR) exacerbates trauma-mediated musculoskeletal tissue injury, inflammation, osteogenic progenitor cell development and HO formation. Others have shown that FAK2 plays a key role in regulating early inflammatory signaling events. Therefore, we hypothesized that targeting FAK2 prophylactically may mitigate extremity trauma induced inflammation and HO formation using a validated extremity trauma model.
In this study, we found that early administration of PF-573228, an ATP analog that acts to inhibit FAK kinase activity, following polysystem extremity trauma involving blast blunted inflammation, osteogenic gene signaling, and the recruitment and growth of osteogenic progenitor cells at the site of injury resulting in reduced HO formation. We also provide gene signature evidence that macrophages with the M1 phenotype accumulate in the in the injured skeletal muscle. In vivo FAK2 signaling inhibition, promotes the differentiation and polarization switch of infiltrating macrophages into a M2 phenotype program, possibly contributing to earlier inflammation resolution, tissue repair, and wound healing (75). These results emphasize the interaction FAK2 signaling plays in regulating distinct cellular and molecular mechanisms post-traumatic injury. Therefore, inhibiting FAK2 signaling in vivo systemically or with topical application of pharmacological inhibitors might offer a new target attractive strategy for limiting inflammation and inhibiting HO.
Earlier studies demonstrate FAK adhesion-dependent signaling is critical for new bone formation, as it promotes osteoblast progenitor proliferation, differentiation, and mechanotransduction through enhancement of Akt, mTOR and WNT signaling (22, 23, 74, 76, 77). MSCs engineered to over-express FAK exhibit a marked increase in their osteogenic potential in vitro. FAK promotes the expression of osteoblast phenotypic markers as well as in vitro osteogenesis in human mesenchymal stromal cells (hMSCs). Interestingly, Kim et al. (73) showed in transgenic FAK−/− mice, osteogenic progenitors were able to migrate to the site of skeletal trauma, however a significant delay in osteoblast differentiation and matrix formation was measured. Further, Qi et al. (23) report that FAK-deficient osteoprogenitor cells have lessened proliferation and significantly reduced mammalian/mechanistic target of rapamycin complex 1 (mTORC1) signaling. Moreover, we previously demonstrated the robust efficacy of rapamycin in inhibiting blast trauma-induced HO (45). In this study, we show that inhibition of FAK2 during skeletal muscle regeneration results in reduced inflammation (IL-6), inflammatory cell accumulation, and expression of Runx2 and Osterix which are considered master transcription factors in regulating osteogenic differentiation of MSCs and regulation of bone matrix genes (72, 78). Mitogen-activated protein kinase (MAPK) and/or extracellular signal-regulated kinase (ERK) pathway activation of Runx2 triggers a cascade of the downstream osteoblast-related genes to promote the differentiation of osteoblasts for bone matrix synthesis and mineralization (79). Taken together, our findings are consistent with studies that report the loss or inhibition of FAK2 impairs osteoprogenitor cell differentiation (46, 73). More specifically, revealing that attenuated inflammation mediated FAK2 signaling may suppress the activation, proliferation, and/or in vivo migration of endogenous MSCs and osteogenic progenitor cells resulting in reduced ectopic bone formation following trauma. With regard to the muscle gene expression, blast/extremity injury resulted in significant increases in expression levels of cell surface markers specific for immune cells and inflammatory signaling molecules (interleukins, chemokines) as compared to naive muscle. In contrast, in the FAK2-treated muscle, there was a modest suppressive effect (reduction) in transcript levels of the same markers; even though these levels were elevated above naive levels these elevations were non- significant. The loss of significant elevations in gene expression of inflammatory mediators and cell surface markers is an important finding.
Macrophages play key roles in the inflammation, proliferation, and remodeling phases of wound healing. Throughout the wound healing process, converging inflammatory stimuli in the cellular microenvironment have been shown to facilitate the transition of local macrophages from a pro-inflammatory (M1-like phenotype) to anti-inflammatory (M2-like phenotype) state (14, 15). Robust inflammatory cell infiltration, macrophage activation as well as inflammatory and osteogenic cytokine/chemokine release contribute the microenvironmental niche that contributes to HO formation (80). Macrophages present in virtually all tissues are functionally regulated by their surrounding micro-environment stimuli (81).
Recently, evidence has indicated that the proinflammatory (M1) macrophage contributes to the hypoxia microenvironment and an excessive inflammatory, angio-chondro-osteogenic cytokine milieu which drives the differentiation of multipotent progenitors (MPPs) found in injured skeletal muscle into bone (80, 82). On POD-7, we detected elevated levels of pro-inflammatory cytokines along with cellular dysregulation consistent with increased reactive oxygen species (ROS) levels. Our findings demonstrate the proinflammatory M1 macrophage is the prominent inflammatory cell in traumatized muscle at the point when early angio-chondro-osteogenic signaling and progenitor cell activity is being established. In comparison, FAK2 inhibitor treatment (PF-573228) resulted in reduced cellular infiltration. Comparative gene expression studies and osteogenic progenitor cell assays of collected infiltrating cell populations, revealed infiltrating cells collected after FAK2 inhibition expressed less proinflammatory and angio-chondro-osteogenic genes with lesser prevalence of osteogenic colony-cells, respectively.
The signaling events underlying the crosstalk between M1-M2 macrophage phenotypic states, other immune cells, and MPPs within the osteogenic niche in the absence of FAK2 inhibition or before and after systemic macrophage depletion are unclear. M2 macrophages express IL-10, arginase I, and chemokines (TGFβ, IL-13), which play crucial roles in the resolution of inflammation, wound healing, and tissue remodeling (14, 15). M2, but not M1, macrophages have been reported to have anti-calcifying activity in vascular smooth muscle cell (VSMC) calcification (83),whereas others have reported in models of healing and sepsis the importance of wound macrophage transition from the predominantly pro-inflammatory phenotype present early post-injury to the anti-inflammatory phenotype to accelerate wound healing (84). On the other hand, Abshire et al. (85) report that loss of FAK expression results in reduced polarization, chemotaxis to CSF-1 (G-CSF) and recruitment to sites of inflammation (85). Likewise, Owen and colleagues (86) show bone marrow-derived macrophages from FAK−/− mice exhibit reduced chemotaxis toward CSF-1. A wide array of cell types produce CSF-1, a cytokine/growth factor, which possesses both autocrine and paracrine roles. It promotes the migration, proliferation, functionality, and survival of macrophages, while fostering M2 macrophage polarization (87). Our results bring new indications for the role of macrophages and their activation status in the initiation and development of HO. Future studies evaluating impact of FAK2 silence using knockout rats in this model may provide additional important information and strengthen our hypotheses.
Previous studies assessing wound healing and various pathologies demonstrated the mechanisms regulating macrophage polarization are diverse and complex. The mechanisms also involve the concurrent regulation of various cytokines, chemokines, and signaling pathways, to include PI3K/Akt, TLR4/NF-κB, mTOR, JAK/STAT, TGF-β/Smads, JNK/c-My, PPARγ and others (88) Zhang et al. (89) demonstrated that the promotion of macrophage polarization from M1 to M2 is facilitated by MCP-induced protein 1 through the suppression of the JNK/c-Myc signaling pathway. While our studies suggest a possible involvement of FAK2 in macrophage polarization regulation. Nonetheless, the function of FAK2 expression in regulating critical transcription pathways that control macrophage function and activity, has not yet been established in our wound healing model of acute musculoskeletal trauma. In summary, our transcriptomic studies have highlighted the necessity to further characterize the full spectrum of phenotypic changes of recruited-resident macrophage populations as these activated macrophages most likely fall into various states of polarization between M1 and M2.
This study has a number of limitations. First, PF-573228 inhibits FAK activity as well as the activity of other related kinases. Second, PYK2, a close paralogue to FAK has been shown to functionally compensate for loss of FAK (90, 91), albeit 50- to 250-fold less selective for FAK than PYKA (92). In oncological and chronic disease applications dual FAK/PYKA inhibition has been shown to be more efficacious (93). Hence, future therapeutic studies targeting both kinases are warranted. Third, while many of our results reached statistical significance, due to the increased biological variability encountered in a complex injury model, variance in results was often high, affecting the ability to detect statistical significance. Larger sample sizes for future studies should therefore be considered. Alternatively, we extended the HO analysis to calculation of mean effect size which was large, albeit the power of the experiment and sample size (number of animals per group) using a two-sample unpaired t-test was not able to detect this effect. The 95% Confidence Intervals support our conclusions that the mean sizes are large. While the measured differences between treatment groups may not be statistically significant (strong enough to reject the Null hypothesis), we suggest the strength of these results are highly clinically relevant for clinical patient care applications. Fourth, further work is required to characterize the impact of FAK2 inhibition in promoting the M1-M2 transition and identifying the biological variation of endogenous and tissue infiltrating cells. A comprehensive assessment using immunohistochemistry, single-cell RNA sequencing and multiparameter flow cytometry may provide additional critical insight. This approach could reveal the presence and activation status of regulatory/reparative cell subsets, detection of rare cell types in complex tissues, and highlight opportunities for further treatment refinement. Fifth, we did not investigate potential sex differences in this study; all the animals utilized were males. Notably, acute inflammatory responses differ between male and female rats, as well as in humans. Prior reports have shown that males are more susceptible to traumatic injury (94, 95). However, the utilization of only male rats in this study is justified, considering that fewer than 3% of severely combat-injured service members in recent conflicts were female (96). Last but not least, it is critical to transition and expand this knowledge to large animal model investigations.
Prolonged inflammation is a known critical driver of trauma-induced ectopic bone formation. In this study, we show continuous administration of the FAK2 inhibitor PF-573228 for 14-days post injury dampened tissue inflammation, suppressed osteogenic signaling and development, and promoted M1-M2 macrophage polarization thought to relieve inflammation and promote accelerated tissue repair resulting in reduced progenitor cell activity and subsequent HO formation.
The datasets used and/or analyzed during the current study are available from the corresponding author on reasonable request.
The animal study was approved by Uniformed Services University Institutional Animal and Care and Use Committees (IACUC; Protocol # SUR-21-069). The study was conducted in accordance with the local legislation and institutional requirements.
CR: Data curation, Formal analysis, Investigation, Methodology, Project administration, Validation, Writing – review & editing, Visualization. UN: Data curation, Investigation, Methodology, Writing – review & editing. DS: Writing – review & editing, Data curation, Investigation, Methodology. JH: Investigation, Writing – review & editing, Methodology. JN: Investigation, Writing – review & editing. JL: Investigation, Writing – review & editing. CM: Investigation, Writing – review & editing. BP: Writing – review & editing, Conceptualization, Funding acquisition. BL: Investigation, Methodology, Writing – review & editing, Conceptualization, Funding acquisition. TD: Investigation, Methodology, Writing – review & editing, Conceptualization, Data curation, Formal analysis, Funding acquisition, Project administration, Supervision, Validation, Writing – original draft.
The author(s) declare financial support was received for the research, authorship, and/or publication of this article. This work was partially supported by the Department of Defense Congressionally Directed Medical Research Programs (CDMRP) and Peer-Reviewed Orthopaedic Research Program (PRORP; grant award W81XWH-20-2-0057).
We gratefully acknowledge the institutional and technical support from the Pre-clinical Behavior and Modeling Core (PBMC) for their expertise with the ABS system (Amanda Fu and Yeohno Kim), the Department of Animal Laboratory Medicine for animal husbandry and veterinarian support (MAJ Kaufman and MAJ Hunter), and the Biomedical Instrumentation Center Genomics core for their assistance with mass spectroscopy (Dr. Sean Moran) and Microscopy core for their assistance with image acquisition (Dr. Dennis McDaniel).
Authors CR, UN and DS was employed by the company Henry M. Jackson Foundation for the Advancement of Military Medicine, Inc.
The remaining authors declare that the research was conducted in the absence of any commercial or financial relationships that could be construed as a potential conflict of interest.
All claims expressed in this article are solely those of the authors and do not necessarily represent those of their affiliated organizations, or those of the publisher, the editors and the reviewers. Any product that may be evaluated in this article, or claim that may be made by its manufacturer, is not guaranteed or endorsed by the publisher.
The Supplementary Material for this article can be found online at: https://www.frontiersin.org/articles/10.3389/fimmu.2023.1280884/full#supplementary-material
Supplementary Figure 1 | Treatment with PF-573228 results in no change in serum chemistry analytes at POD-7. Quantification of rat serum chemistry of naïve, or blast-extremity injury animals treated with vehicle solution or continuous infusion of PF573228 FAK inhibitor for 7 days. Statistical significance was calculated using a one-way ANOVA with Turkey post-hoc was used to test for statistical significance. Data are represented as mean values ± SEM. * indicates p < 0.05, ** indicates p < 0.01, *** indicates p < 0.001, **** indicates p < 0.0001).
Supplementary Figure 2 | Relative expression levels of early signaling genes involved in the development of heterotopic ossification including (A) osteogenesis (B) chondrogenesis (C) angiogenesis (D) adhesion and integrins (E) matrix metalloproteinases and tissue inhibitors of metalloproteinases (F) toll-like receptors (G) transcriptional activators and cytoplasmic protein kinases and (H) apoptosis. For this experiment, muscle tissue was collected from the amputation site at POD-7 from rats treated with FAK2 inhibitor (PF-573228;10 mg/kg/day; 10 mg/kg/day, 6.94 µg/kg/min)), vehicle controls, or in healthy muscle from age-matched controls. Relative expression (2-ΔCt) was calculated using an optimal normalization strategy. One-way ANOVAs for each gene were conducted on ΔCt values to determine treatment effects. Tukey-Kramer post-hoc analyses were utilized to determine the source of the significance. Data represents mean relative expression values ± SEM. * indicates p < 0.05, **indicates p < 0.01, *** indicates p < 0.001 and **** indicates p < 0.0001.
1. National Academies Press. Committee on gulf war and health: long-term effects of blast exposures. In: Gulf War and Health, vol. 9. Washington (DC): Board on the Health of Select Populations; Institute of Medicine (2014).
2. Bradley M, Nealiegh M, Oh JS, Rothberg P, Elster EA, Rich NM. Combat casualty care and lessons learned from the past 100 years of war. Curr Probl Surg (2017) 54(6):315–51. doi: 10.1067/j.cpsurg.2017.02.004
3. Daniels CM, Pavey GJ, Arthur J, Noller M, Forsberg JA, Potter BK. Has the proportion of combat-related amputations that develop heterotopic ossification increased? J Orthop Trauma (2018) 32(6):283–7. doi: 10.1097/BOT.0000000000001158
4. Alfieri KA, Forsberg JA, Potter BK. Blast injuries and heterotopic ossification. Bone Joint Res (2012) 1(8):192–7. doi: 10.1302/2046-3758.18.2000102
5. Cernak I. The importance of systemic response in the pathobiology of blast-induced neurotrauma. Front Neurol (2010) 1(1). doi: 10.3389/fneur.2010.00151
6. Forsberg JA, Potter BK, Wagner MB, Vickers A, Dente CJ, Kirk AD, et al. Lessons of war: turning data into decisions. EBioMedicine (2015) 2(9):1235–42. doi: 10.1016/j.ebiom.2015.07.022
7. Evans KN, Forsberg JA, Potter BK, Hawksworth JS, Brown TS, Andersen R, et al. Inflammatory cytokine and chemokine expression is associated with heterotopic ossification in high-energy penetrating war injuries. J Orthop Trauma (2012) 26(11):e204–13. doi: 10.1097/BOT.0b013e31825d60a5
8. Forsberg JA, Potter BK, Polfer EM, Safford SD, Elster EA. Do inflammatory markers portend heterotopic ossification and wound failure in combat wounds? Clin Orthop Relat Res (2014) 472(9):2845–54. doi: 10.1007/s11999-014-3694-7
9. Rowe CJ, Walsh SA, Dragon AH, Rhodes AM, Pak OL, Ronzier E, et al. Tourniquet-induced ischemia creates increased risk of organ dysfunction and mortality following delayed limb amputation. Injury (2023) 54(6):1792–803. doi: 10.1016/j.injury.2023.02.047
10. Spreadborough PJ, Strong AL, Mares J, Levi B, Davis TA. Tourniquet use following blast-associated complex lower limb injury and traumatic amputation promotes end organ dysfunction and amplified heterotopic ossification formation. J Orthop Surg Res (2021) 17:422. doi: 10.1186/s13018-022-03321-z
11. Fry DE. Article commentary: sepsis, systemic inflammatory response, and multiple organ dysfunction: the mystery continues. Am Surg (2012) 78(1):1–8. doi: 10.1177/000313481207800102
12. Zheng G, Peng X, Zhang Y, Wang P, Xie Z, Li J, et al. A novel Anti-ROS osteoblast-specific delivery system for ankylosing spondylitis treatment via suppression of both inflammation and pathological new bone formation. J Nanobiotechnology (2023) 21(1). doi: 10.1186/s12951-023-01906-2
13. Aini H, Ochi H, Iwata M, Okawa A, Koga D, Okazaki M, et al. Procyanidin B3 prevents articular cartilage degeneration and heterotopic cartilage formation in a mouse surgical osteoarthritis model. PloS One (2012) 7(5). doi: 10.1371/journal.pone.0037728
14. Wynn TA, Chawla A, Pollard JW. Macrophage biology in development, homeostasis and disease. Nature (2013) 496(7446):445–55. doi: 10.1038/nature12034
15. Chen S, Saeed AFUH, Liu Q, Jiang Q, Xu H, Xiao GG, et al. Macrophages in immunoregulation and therapeutics. Signal Transduction Targeted Ther (2023) 8(1):1–35. doi: 10.1038/s41392-023-01452-1
16. Lee H, Fessler MB, Qu P, Heymann J, Kopp JB. Macrophage polarization in innate immune responses contributing to pathogenesis of chronic kidney disease. BMC Nephrol (2020) 21(1):1–13. doi: 10.1186/s12882-020-01921-7
17. Arora S, Dev K, Agarwal B, Das P, Syed MA. Macrophages: Their role, activation and polarization in pulmonary diseases. Immunobiology (2018) 223(4–5):383–96. doi: 10.1016/j.imbio.2017.11.001
18. Orecchioni M, Ghosheh Y, Pramod AB, Ley K. Macrophage polarization: Different gene signatures in M1(Lps+) vs. Classically and M2(LPS-) vs. Alternatively activated macrophages. Front Immunol (2019) 10(MAY):451543. doi: 10.3389/fimmu.2019.01084
19. Wang N, Liang H, Zen K. Molecular mechanisms that influence the macrophage m1-m2 polarization balance. Front Immunol (2014) 5(NOV). doi: 10.3389/fimmu.2014.00614
20. Mitra SK, Hanson DA, Schlaepfer DD. Focal adhesion kinase: in command and control of cell motility. Nat Rev Mol Cell Biol (2005) 6(1):56–68. doi: 10.1038/nrm1549
21. Zhao X, Guan JL. Focal adhesion kinase and its signaling pathways in cell migration and angiogenesis. Adv Drug Delivery Rev (2011) 63(8):610–5. doi: 10.1016/j.addr.2010.11.001
22. Gunn SA, Kreps LM, Zhao H, Landon K, Ilacqua JS, Addison CL. Focal adhesion kinase inhibitors prevent osteoblast mineralization in part due to suppression of Akt-mediated stabilization of osterix. J Bone Oncol (2022) 34. doi: 10.1016/j.jbo.2022.100432
23. Qi S, Sun X, Choi HK, Yao J, Wang L, Wu G, et al. FAK promotes early osteoprogenitor cell proliferation by enhancing mTORC1 signaling. J Bone Miner Res (2020) 35(9):1798–811. doi: 10.1002/jbmr.4029
24. Takada Y, Ye X, Simon S. The integrins. Genome Biol (2007) 8(5):1–9. doi: 10.1186/gb-2007-8-5-215
25. El-Rashidy AA, El Moshy S, Radwan IA, Rady D, Abbass MMS, Dörfer CE, et al. Effect of polymeric matrix stiffness on osteogenic differentiation of mesenchymal stem/progenitor cells: concise review. Polymers (Basel) (2021) 13(17). doi: 10.3390/polym13172950
26. Zhou J, Aponte-Santamaría C, Sturm S, Bullerjahn JT, Bronowska A, Gräter F. Mechanism of focal adhesion kinase mechanosensing. PloS Comput Biol (2015) 11(11):e1004593. doi: 10.1371/journal.pcbi.1004593
27. Wang HB, Dembo M, Hanks SK, Wang YL. Focal adhesion kinase is involved in mechanosensing during fibroblast migration. Proc Natl Acad Sci U.S.A. (2001) 98(20):11295–300. doi: 10.1073/pnas.201201198
28. Bershadsky AD, Ballestrem C, Carramusa L, Zilberman Y, Gilquin B, Khochbin S, et al. Assembly and mechanosensory function of focal adhesions: experiments and models. Eur J Cell Biol (2006) 85(3–4):165–73. doi: 10.1016/j.ejcb.2005.11.001
29. Murphy JM, Jeong K, Rodriguez YAR, Kim JH, Ahn EYE, Lim STS. FAK and Pyk2 activity promote TNF-α and IL-1β-mediated pro-inflammatory gene expression and vascular inflammation. Sci Rep (2019) 9(1). doi: 10.1038/s41598-019-44098-2
30. Nag K, Chaudhary A. Mediators of tyrosine phosphorylation in innate immunity: from host defense to inflammation onto oncogenesis. Curr Signal Transduct Ther (2009) 4(2):76. doi: 10.2174/157436209788167501
31. Dayang EZ, Luxen M, Kuiper T, Yan R, Rangarajan S, van Meurs M, et al. Pharmacological inhibition of focal adhesion kinase 1 (FAK1) and anaplastic lymphoma kinase (ALK) identified via kinome profile analysis attenuates lipopolysaccharide-induced endothelial inflammatory activation. BioMed Pharmacother (2021) 133:133. doi: 10.1016/j.biopha.2020.111073
32. Nowell CS, Odermatt PD, Azzolin L, Hohnel S, Wagner EF, Fantner GE, et al. Chronic inflammation imposes aberrant cell fate in regenerating epithelia through mechanotransduction. Nat Cell Biol (2016) 18(2):168–80. doi: 10.1038/ncb3290
33. Murphy JM, Jeong K, Cioffi DL, Campbell PM, Jo H, Ahn EYE, et al. Focal adhesion kinase activity and localization is critical for TNF-α-induced nuclear factor-κB activation. Inflammation (2021) 44(3):1130–44. doi: 10.1007/s10753-020-01408-5
34. Lagares D, Busnadiego O, García-Fernández RA, Kapoor M, Liu S, Carter DE, et al. Inhibition of focal adhesion kinase prevents experimental lung fibrosis and myofibroblast formation. Arthritis Rheum (2012) 64(5):1653–64. doi: 10.1002/art.33482
35. Chen X, Zhao Y, Wang X, Lin Y, Zhao W, Wu D, et al. FAK mediates LPS-induced inflammatory lung injury through interacting TAK1 and activating TAK1-NFκB pathway. Cell Death Dis (2022) 13(7):1–12. doi: 10.1038/s41419-022-05046-7
36. Wan X, Chowdhury IH, Jie Z, Choudhuri S, Garg NJ. Origin of monocytes/Macrophages contributing to chronic inflammation in chagas disease: SIRT1 inhibition of FAK-NFκB-Dependent proliferation and proinflammatory activation of macrophages. Cells (2019) 9(1). doi: 10.3390/cells9010080
37. Jin B, Wang J, Chen Y, Zuo W, Hong B, Li J, et al. Focal adhesion kinase induces cardiac remodeling through NF-κB-mediated inflammatory responses in diabetic cardiomyopathy. Int Immunopharmacol (2023) 120. doi: 10.1016/j.intimp.2023
38. Murphy JM, Jeong K, Steve Lim ST. FAK family kinases in vascular diseases. Int J Mol Sci (2020) 21(10). doi: 10.3390/ijms21103630
39. Chuang HH, Zhen YY, Tsai YC, Chuang CH, Hsiao M, Huang MS, et al. FAK in cancer: from mechanisms to therapeutic strategies. Int J Mol Sci (2022) 23(3). doi: 10.3390/ijms23031726
40. Murphy JM, Rodriguez YAR, Jeong K, Ahn EYE, Lim STS. Targeting focal adhesion kinase in cancer cells and the tumor microenvironment. Exp Mol Med (2020) 52(6):877–86. doi: 10.1038/s12276-020-0447-4
41. Chen XL, Nam JO, Jean C, Lawson C, Walsh CT, Goka E, et al. VEGF-induced vascular permeability is mediated by FAK. Dev Cell (2012) 22(1):146–57. doi: 10.1016/j.devcel.2011.11.002
42. Grosso A, Burger MG, Lunger A, Schaefer DJ, Banfi A, Di Maggio N. It takes two to tango: coupling of angiogenesis and osteogenesis for bone regeneration. Front Bioeng Biotechnol (2017) 5(NOV). doi: 10.3389/fbioe.2017.00068
43. Dey D, Wheatley BM, Cholok D, Agarwal S, Yu PB, Levi B, et al. The traumatic bone: trauma-induced heterotopic ossification. Trans Res (2017) 186:95–111. doi: 10.1016/j.trsl.2017.06.004
44. Pavey GJ, Qureshi AT, Tomasino AM, Honnold CL, Bishop DK, Agarwal S, et al. Targeted stimulation of retinoic acid receptor-γ mitigates the formation of heterotopic ossification in an established blast-related traumatic injury model. Bone (2016) 90:159–67. doi: 10.1016/j.bone.2016.06.014
45. Qureshi AT, Dey D, Sanders EM, Seavey JG, Tomasino AM, Moss K, et al. Inhibition of mammalian target of rapamycin signaling with rapamycin prevents trauma-induced heterotopic ossification. Am J Pathol (2017) 187(11):2536–45. doi: 10.1016/j.ajpath.2017.07.010
46. Salasznyk RM, Klees RF, Williams WA, Boskey A, Plopper GE. Focal adhesion kinase signaling pathways regulate the osteogenic differentiation of human mesenchymal stem cells. Exp Cell Res (2007) 313(1):22. doi: 10.1016/j.yexcr.2006.09.013
47. Schreiber C, Saraswati S, Harkins S, Gruber A, Cremers N, Thiele W, et al. Loss of ASAP1 in mice impairs adipogenic and osteogenic differentiation of mesenchymal progenitor cells through dysregulation of FAK/Src and AKT signaling. PloS Genet (2019) 15(6):e1008216. doi: 10.1371/journal.pgen.1008216
48. Ma XY, Feng YF, Wang TS, Lei W, Li X, Zhou DP, et al. Involvement of FAK-mediated BMP-2/Smad pathway in mediating osteoblast adhesion and differentiation on nano-HA/chitosan composite coated titanium implant under diabetic conditions. Biomater Sci (2017) 6(1):225–38. doi: 10.1039/C7BM00652G
49. Tsai SY, Huang YL, Yang WH, Tang CH. Hepatocyte growth factor-induced BMP-2 expression is mediated by c-Met receptor, FAK, JNK, Runx2, and p300 pathways in human osteoblasts. Int Immunopharmacol (2012) 13(2):156–62. doi: 10.1016/j.intimp.2012.03.026
50. Yuh DY, Maekawa T, Li X, Kajikawa T, Bdeir K, Chavakis T, et al. The secreted protein DEL-1 activates a β3 integrin–FAK–ERK1/2–RUNX2 pathway and promotes osteogenic differentiation and bone regeneration. J Biol Chem (2020) 295(21):7261. doi: 10.1074/jbc.RA120.013024
51. Dejaeger M, Böhm AM, Dirckx N, Devriese J, Nefyodova E, Cardoen R, et al. Integrin-linked kinase regulates bone formation by controlling cytoskeletal organization and modulating BMP and Wnt signaling in osteoprogenitors. J Bone Miner Res (2017) 32(10):2087–102. doi: 10.1002/jbmr.3190
52. Pagani CA, Bancroft AC, Tower RJ, Livingston N, Sun Y, Hong JY, et al. Discoidin domain receptor 2 regulates aberrant mesenchymal lineage cell fate and matrix organization. Sci Adv (2022) 8(51). doi: 10.1126/sciadv.abq6152
53. Percie du Sert N, Hurst V, Ahluwalia A, Alam S, Avey MT, Baker M, et al. The ARRIVE guidelines 2.0: Updated guidelines for reporting animal research. PloS Biol (2020) 18(7):e3000410. doi: 10.1371/journal.pbio.3000410
54. Walsh SA, Davis TA. Key early proinflammatory signaling molecules encapsulated within circulating exosomes following traumatic injury. J Inflammation (2022) 19(1):6. doi: 10.1186/s12950-022-00303-0
55. Dragon AH, Rowe CJ, Rhodes AM, Pak OL, Davis TA, Ronzier E. Systematic identification of the optimal housekeeping genes for accurate transcriptomic and proteomic profiling of tissues following complex traumatic injury. Methods Protoc (2023) 6(2):22. doi: 10.3390/mps6020022
56. Zhang J, Fan G, Zhao H, Wang Z, Li F, Zhang P, et al. Targeted inhibition of focal adhesion kinase attenuates cardiac fibrosis and preserves heart function in adverse cardiac remodeling. Sci Rep (2017) 7. doi: 10.1038/srep43146
57. Wheatley BM, Cilwa KE, Dey D, Qureshi AT, Seavey JG, Tomasino AM, et al. Palovarotene inhibits connective tissue progenitor cell proliferation in a rat model of combat-related heterotopic ossification. J Orthopaedic Res (2018) 36(4):1135–44. doi: 10.1002/jor.23747
58. Strong AL, Spreadborough PJ, Pagani CA, Haskins RM, Dey D, Grimm PD, et al. Small molecule inhibition of non-canonical (TAK1-mediated) BMP signaling results in reduced chondrogenic ossification and heterotopic ossification in a rat model of blast-associated combat-related lower limb trauma. Bone (2020) 139:115517. doi: 10.1016/j.bone.2020.115517
59. Seavey JG, Wheatley BM, Pavey GJ, Tomasino AM, Hanson MA, Sanders EM, et al. Early local delivery of vancomycin suppresses ectopic bone formation in a rat model of trauma-induced heterotopic ossification. J Orthop Res (2017) 35:2397–406. doi: 10.1002/jor.23544
60. Rowe CJ, Mang J, Huang B, Dommaraju K, Potter BK, Schobel SA, et al. Systemic inflammation induced from remote extremity trauma is a critical driver of secondary brain injury. Mol Cell Neurosci (2023) 126:103878. doi: 10.1016/j.mcn.2023.103878
61. Durlak JA. How to select, calculate, and interpret effect sizes. J Pediatr Psychol (2009) 34(9):917–28. doi: 10.1093/jpepsy/jsp004
62. Maher JM, Markey JC, Ebert-May D. The other half of the story: effect size analysis in quantitative research. CBE Life Sci Educ (2013) 12(3):345–51. doi: 10.1187/cbe.13-04-0082
63. Glass GV. Primary, secondary, and meta-analysis of research. Educ Researcher (1976) 5(10):3. doi: 10.2307/1174772
64. Corman VM, Landt O, Kaiser M, Molenkamp R, Meijer A, Chu DKW, et al. Detection of 2019 novel coronavirus (2019-nCoV) by real-time RT-PCR. Euro Surveill (2020) 25(3). doi: 10.2807/1560-7917.ES.2020.25.3.2000045
65. Lisboa FA, Dente CJ, Schobel SA, Khatri V, Potter BK, Kirk AD, et al. Utilizing precision medicine to estimate timing for surgical closure of traumatic extremity wounds. Ann Surg (2019) 270(3):535–43. doi: 10.1097/SLA.0000000000003470
66. Thompson KB, Krispinsky LT, Stark RJ. Late immune consequences of combat trauma: a review of trauma-related immune dysfunction and potential therapies. Mil Med Res (2019) 6(1):11. doi: 10.1186/s40779-019-0202-0
67. Lisboa FA, Forsberg JA, Brown TS, Gage FA, Potter BK, Elster EA. Bilateral lower-extremity amputation wounds are associated with distinct local and systemic cytokine response. Surgery (2013) 154(2):282–90. doi: 10.1016/j.surg.2013.04.024
68. Cholok D, Chung MT, Ranganathan K, Ucer S, Day D, Davis TA, et al. Heterotopic ossification and the elucidation of pathologic differentiation. Bone (2018) 109:12–21. doi: 10.1016/j.bone.2017.09.019
69. Loder SJ, Agarwal S, Chung MT, Cholok D, Hwang C, Visser N, et al. Characterizing the circulating cell populations in traumatic heterotopic ossification. Am J Pathol (2018) 188(11):2464. doi: 10.1016/j.ajpath.2018.07.014
70. Kraft CT, Agarwal S, Ranganathan K, Wong VW, Loder S, Li J, et al. Trauma induced heterotopic bone formation and the role of the immune system: A Review. J Trauma Acute Care Surg (2016) 80(1):156. doi: 10.1097/TA.0000000000000883
71. Hanks SK, Ryzhova L, Shin NY, Brábek J. Focal adhesion kinase signaling activities and their implications in the control of cell survival and motility. Front Biosci (2003) 8. doi: 10.2741/1114
72. Rajshankar D, Wang Y, Mcculloch CA. Osteogenesis requires FAK-dependent collagen synthesis by fibroblasts and osteoblasts. FASEB J (2017) 31(3):937–53. doi: 10.1096/fj.201600645R
73. Kim JB, Leucht P, Luppen CA, Park YJ, Beggs HE, Damsky CH, et al. Reconciling the roles of FAK in osteoblast differentiation, osteoclast remodeling, and bone regeneration. Bone (2007) 41(1):39. doi: 10.1016/j.bone.2007.01.024
74. Sun C, Yuan H, Wang L, Wei X, Williams L, Krebsbach PH, et al. FAK promotes osteoblast progenitor cell proliferation and differentiation by enhancing Wnt signaling. J Bone Miner Res (2016) 31(12):2227. doi: 10.1002/jbmr.2908
75. Krzyszczyk P, Schloss R, Palmer A, Berthiaume F. The role of macrophages in acute and chronic wound healing and interventions to promote pro-wound healing phenotypes. Front Physiol (2018) 9(MAY). doi: 10.3389/fphys.2018.00419
76. Leucht P, Kim JB, Currey JA, Brunski J, Helms JA. FAK-mediated mechanotransduction in skeletal regeneration. PloS One (2007) 2(4):e390. doi: 10.1371/journal.pone.0000390
77. Castillo AB, Blundo JT, Chen JC, Lee KL, Yereddi NR, Jang E, et al. Focal adhesion kinase plays a role in osteoblast mechanotransduction in vitro but does not affect load-induced bone formation in vivo. PloS One (2012) 7(9). doi: 10.1371/journal.pone.0043291
78. Komori T. Regulation of bone development and extracellular matrix protein genes by RUNX2. Cell Tissue Res (2010) 339(1):189–95. doi: 10.1007/s00441-009-0832-8
79. Franceschi RT, Xiao G, Jiang D, Gopalakrishnan R, Yang S, Reith E. Multiple signaling pathways converge on the Cbfa1/Runx2 transcription factor to regulate osteoblast differentiation. Connect Tissue Res (2003) 44(Suppl 1):109. doi: 10.1080/03008200390152188
80. Huang Y, Wang X, Zhou D, Zhou W, Dai F, Lin H. Macrophages in heterotopic ossification: from mechanisms to therapy. NPJ Regenerative Med (2021) 6(1):1–12. doi: 10.1038/s41536-021-00178-4
81. Shapouri-Moghaddam A, Mohammadian S, Vazini H, Taghadosi M, Esmaeili SA, Mardani F, et al. Macrophage plasticity, polarization, and function in health and disease. J Cell Physiol (2018) 233(9):6425–40. doi: 10.1002/jcp.26429
82. Wosczyna MN, Biswas AA, Cogswell CA, Goldhamer DJ. Multipotent progenitors resident in the skeletal muscle interstitium exhibit robust BMP-dependent osteogenic activity and mediate heterotopic ossification. J Bone Miner Res (2012) 27(5):1004–17. doi: 10.1002/jbmr.1562
83. Villa-Bellosta R, Hamczyk MR, Andrés V. Alternatively activated macrophages exhibit an anticalcifying activity dependent on extracellular ATP/pyrophosphate metabolism. Am J Physiol Cell Physiol (2016) 310(10):C788–99. doi: 10.1152/ajpcell.00370.2015
84. Hassanshahi A, Moradzad M, Ghalamkari S, Fadaei M, Cowin AJ, Hassanshahi M. Macrophage-mediated inflammation in skin wound healing. Cells (2022) 11(19). doi: 10.3390/cells11192953
85. Abshire MY, Thomas KS, Owen KA, Bouton AH. Macrophage motility requires distinct α5β1/FAK and α4β1/paxillin signaling events. J Leukoc Biol (2011) 89(2):251. doi: 10.1189/jlb.0710395
86. Owen KA, Pixley FJ, Thomas KS, Vicente-Manzanares M, Ray BJ, Horwitz AF, et al. Regulation of lamellipodial persistence, adhesion turnover, and motility in macrophages by focal adhesion kinase. J Cell Biol (2007) 179(6):1275–87. doi: 10.1083/jcb.200708093
87. Jones CV, Ricardo SD. Macrophages and CSF-1: implications for development and beyond. Organogenesis (2013) 9(4):249–60. doi: 10.4161/org.25676
88. Wang C, Ma C, Gong L, Guo Y, Fu K, Zhang Y, et al. Macrophage polarization and its role in liver disease. Front Immunol (2021) 12:1. doi: 10.3389/fimmu.2021.803037
89. Zhang Y, Huang T, Jiang L, Gao J, Yu D, Ge Y, et al. MCP-induced protein 1 attenuates sepsis-induced acute lung injury by modulating macrophage polarization via the JNK/c-Myc pathway. Int Immunopharmacol (2019) 75. doi: 10.1016/j.intimp.2019.105741
90. Murphy JM, Park H, Lim STS. FAK and Pyk2 in disease. Front Biol (Beijing) (2016) 11(1):1–9. doi: 10.1007/s11515-016-1384-4
91. Corsi JM, Rouer E, Girault JA, Enslen H. Organization and post-transcriptional processing of focal adhesion kinase gene. BMC Genomics (2006) 7. doi: 10.1186/1471-2164-7-198
92. Selleckchem.com - bioactive compounds expert (Bioactive compounds,Compound libraries), cited 2023 Jul 24.
93. Wu X, Wang J, Liang Q, Tong R, Huang J, Yang X, et al. Recent progress on FAK inhibitors with dual targeting capabilities for cancer treatment. Biomedicine Pharmacother (2022) 151:113116. doi: 10.1016/j.biopha.2022.113116
94. Hart DA. Sex differences in musculoskeletal injury and disease risks across the lifespan: Are there unique subsets of females at higher risk than males for these conditions at distinct stages of the life cycle? Front Physiol (2023) 14:1127689. doi: 10.3389/fphys.2023.1127689
95. Ashcroft GS. Sex differences in wound healing. Elsevier BV (2004). Available at: https://research.manchester.ac.uk/en/publications/sex-differences-in-wound-healing.
Keywords: heterotopic ossification, FAK2 inhibition, musculoskeletal trauma, tourniquet induced ischemia, amputation, inflammation, M1-M2 macrophage transition
Citation: Rowe CJ, Nwaolu U, Salinas D, Hong J, Nunez J, Lansford JL, McCarthy CF, Potter BK, Levi BH and Davis TA (2023) Inhibition of focal adhesion kinase 2 results in a macrophage polarization shift to M2 which attenuates local and systemic inflammation and reduces heterotopic ossification after polysystem extremity trauma. Front. Immunol. 14:1280884. doi: 10.3389/fimmu.2023.1280884
Received: 21 August 2023; Accepted: 16 November 2023;
Published: 05 December 2023.
Edited by:
Gan Zhao, University of Pennsylvania, United StatesReviewed by:
Marialucia Gallorini, University of Studies G. d’Annunzio Chieti and Pescara, ItalyCopyright © 2023 Rowe, Nwaolu, Salinas, Hong, Nunez, Lansford, McCarthy, Potter, Levi and Davis. This is an open-access article distributed under the terms of the Creative Commons Attribution License (CC BY). The use, distribution or reproduction in other forums is permitted, provided the original author(s) and the copyright owner(s) are credited and that the original publication in this journal is cited, in accordance with accepted academic practice. No use, distribution or reproduction is permitted which does not comply with these terms.
*Correspondence: Thomas A. Davis, VGhvbWFzLmRhdmlzQHVzdWhzLmVkdQ==
Disclaimer: All claims expressed in this article are solely those of the authors and do not necessarily represent those of their affiliated organizations, or those of the publisher, the editors and the reviewers. Any product that may be evaluated in this article or claim that may be made by its manufacturer is not guaranteed or endorsed by the publisher.
Research integrity at Frontiers
Learn more about the work of our research integrity team to safeguard the quality of each article we publish.