- 1Departamento de Ciencias de la Salud, Universidad Autónoma Metropolitana-Lerma (UAM-L), Lerma, Mexico
- 2Facultad de Medicina, Universidad Autónoma del Estado de México (UAEMéx), Toluca de Lerdo, Mexico
- 3Laboratorio de Psicoinmunología, Dirección de Investigaciones en Neurociencias, Instituto Nacional de Psiquiatría Ramón de la Fuente Muñiz, Mexico City, Mexico
- 4Unidad de Fomento a la Investigación, Dirección de Servicios Clínicos, Instituto Nacional de Psiquiatría Ramón de la Fuente Muñiz, Mexico City, Mexico
- 5Departamento de Inmunología, Escuela Nacional de Ciencias Biológicas, Instituto Politécnico Nacional, Mexico City, Mexico
Maternal Immune Activation (MIA) has been linked to the pathogenesis of pre-eclampsia and adverse neurodevelopmental outcomes in the offspring, such as cognitive deficits, behavioral abnormalities, and mental disorders. Pre-eclampsia is associated with an activation of the immune system characterized by persistently elevated levels of proinflammatory cytokines, as well as a decrease in immunoregulatory factors. The Cholinergic Anti-inflammatory Pathway (CAP) may play a relevant role in regulating the maternal inflammatory response during pre-eclampsia and protecting the developing fetus from inflammation-induced damage. Dysregulation in the CAP has been associated with the clinical evolution of pre-eclampsia. Some studies suggest that therapeutic stimulation of this pathway may improve maternal and fetal outcomes in preclinical models of pre-eclampsia. Modulation of vagal activity influences the CAP, improving maternal hemodynamics, limiting the inflammatory response, and promoting the growth of new neurons, which enhances synaptic plasticity and improves fetal neurodevelopment. Therefore, we postulate that modulation of vagal activity may improve maternal and fetal outcomes in pre-eclampsia by targeting underlying immune dysregulation and promoting better fetal neurodevelopment. In this perspective, we explore the clinical and experimental evidence of electrical, pharmacological, physical, and biological stimulation mechanisms capable of inducing therapeutical CAP, which may be applied in pre-eclampsia to improve the mother’s and offspring’s quality of life.
1 Introduction
Pre-eclampsia is a condition characterized by hypertension, inflammation, and organ damage during pregnancy, and it has been associated with cognitive deficits, behavioral abnormalities, and neurodevelopmental issues in the offspring (1). Evidence indicates that the Cholinergic Anti-inflammatory Pathway (CAP) could significantly impact the development of the fetus and the newborn exposed to pre-eclampsia by functioning as a neuroimmunological network that facilitates internal monitoring. This pathway connects the central nervous system (CNS) through the vagus nerve, regulating inflammation in the body (2). Previous research indicates that the downregulation of α7 nicotinic acetylcholine receptor (α7nAChR) has a significant impact on the CAP to regulate systemic inflammation, particularly in cases of pre-eclampsia (3). However, this downregulation and its potential link to the onset of pre-eclampsia are areas still under investigation. Similarly, while there is some evidence to suggest that dysregulation of the CAP may contribute to the development of pre-eclampsia, further studies are needed to substantiate this claim (4).
This perspective article explores some vagal stimulation techniques that modulate the CAP and improve outcomes in preclinical pre-eclampsia models. Particularly, we explored the potential of modulating vagal activity, including the use of electrical Vagus Nerve Stimulation (VNS), to enhance maternal and fetal outcomes by targeting immune dysregulation and promoting fetal neurodevelopmental alterations caused by inflammation. This perspective article discusses the role of inflammatory response in triggering pre-eclampsia and its impact on neurodevelopment, emphasizing the increased risk of neurodevelopmental problems and mental disorders in the offspring. We position the modulation of vagal activity, including but not limited to VNS, as a promising, safe, and efficient therapeutic intervention, primarily backed by preclinical findings, warranting further exploration. This perspective proposes that modulating vagal activity using electrical, pharmacological, magnetic, and physical techniques, such as breathing and respiratory stimulation, might be potential approaches to address inflammatory dysregulation. It is hypothesized that such techniques could potentially improve outcomes in pre-eclampsia, minimize neurodevelopmental damage, and enhance the quality of life for affected mothers and their offspring (a visual abstract is available as Supplementary Material).
2 The maternal immune system in pregnancy: implications for pre-eclampsia
During pregnancy, the maternal immune system undergoes essential adaptations to protect both the mother and fetus from antigenic challenges while maintaining tolerance to the fetal allograft (5). Evidence has described an association between the balance of pro- and anti-inflammatory cytokines and placental development and function (6). Some research suggests that an inflammatory response during pregnancy is associated with complications like pre-eclampsia (7, 8). In animal models of pre-eclampsia, it has been found an increase in serum concentration of IFN-γ, IL-6, TNF-α, and IL-17, in addition to a decrease in IL-4 and IL-10, accompanied by placental damage (9–12). Meanwhile, in women with pre-eclampsia, it has been found an increase in pro-inflammatory cytokine serum levels such as IL-2, IL-4, IL-6, IL-15, IL-16, IL-17, IL-22, IL-35, IFN-γ, and TNF-α, as well as a decrease in IL-10 concentration (13–19). Therefore, the IL-2/IL-10 and TNF-α/IL-10 ratios have been proposed to assess the risk of developing pre-eclampsia (20). IL-22/CCL22 and IL-2/IL-4 ratios have also been considered (19). Conversely, some other authors (18, 21) have reported no changes in pro-inflammatory cytokine levels in pre-eclampsia. Consequently, it has been proposed that differences in the inflammatory profile reported in the studies could be explained by the sample size, the polymorphisms found in the population, and the sample collection conditions (22). Present findings predominantly point towards an association between inflammation and pre-eclampsia, rather than a conclusive cause-and-effect dynamic. This accentuates the need for more in-depth investigations to elucidate the relationship between cytokines and pre-eclampsia.
Furthermore, growth restriction has been observed in animal models of pre-eclampsia (23, 24). Similarly, pre-eclampsia induces fetal growth restriction in humans, which has been considered a predictor of maternal and neonatal prognoses (25, 26). Additionally, prenatal exposure to pre-eclampsia has been identified as a risk factor for developing type 2 diabetes and cardiovascular disease (27). Also, in recent years the study of neurodevelopmental repercussions following prenatal exposure to pre-eclampsia has gained interest and has been associated with inflammatory alterations (12, 28).
It has been described that the presence of pro-inflammatory cytokines during pregnancy due to Maternal Immune Activation (MIA) affects neurodevelopmental processes in the fetus (29). IL-6 and IL-17 have been highly implicated in causing the priming of microglia and leukocytes, which increases the risk of developing neuropsychiatric diseases characterized by inflammatory cytokines (30–33).
IL-6 has been implicated in altered synaptogenesis in animal models and impaired functional connectivity in human frontoparietal networks (8, 34, 35). Conversely, IL-17 and TNF-α have been implicated in increased Blood-Brain Barrier (BBB) permeability and damage to the cerebral vasculature, as well as in neural tube defects (36–38). Finally, increased IFN-γ has been associated with white matter damage in the central nervous system in preterm neonates (39).
3 Maternal immune activation and the cholinergic anti-inflammatory pathway in pre-eclampsia
MIA is a term that refers to an increase in proinflammatory markers during pregnancy, it is characterized by the activation of the maternal immune system in response to infectious or infectious-like stimuli (40). This activation leads to a series of changes in cytokine levels and immune responses that can affect the developing fetus, particularly the central nervous system, and give rise to adverse phenotypes (41). MIA typically occurs during the middle to late stages of gestation which are considered a critical period for brain development. During this time, adverse environmental conditions can impact neurogenesis (42). Thus, MIA can impact fetal development and have long-term effects on the offspring, leading to various neurological disorders. According to relevant findings, these disorders share genes and molecular mechanisms and are potentially associated with the abnormal structure and dysregulation of the amygdala (43). Furthermore, evidence has shown a link between MIA and increased emotional and behavioral problems in offspring throughout their childhood and adolescence. The findings support the idea that MIA can pose a risk to children’s mental and neurodevelopmental health during prenatal programming (44).
Several pregnancy complications, such as preterm birth, fetal growth restriction, and pre-eclampsia, may involve MIA. Pre-eclampsia is mainly linked to neurodevelopmental problems such as Autism Spectrum Disorder (ASD) and neurodevelopmental delay (45). However, it is possible that the inflammatory immune response seen in preeclamptic women has similar consequences on microglia, as observed in MIA, and may affect fetal microglia stability (45). A recent preclinical study revealed that fetal brain inflammation might be linked to the pathological mechanism connecting maternal pre-eclampsia and brain dysfunction in offspring. These findings are intriguing, as they suggest that pre-eclampsia in mothers might lead to altered inflammatory conditions in the fetal brain (46).
CAP inhibits the release of pro-inflammatory cytokines. This process requires the activation of the vagus nerve through α7 receptors (47). Tracey’s research has highlighted the vagally mediated CAP as an effective means of rapidly reducing inflammation (48). Relevant preclinical findings suggest that the activation of the α7nAChR attenuates pre-eclampsia-like symptoms, and this protective effect is likely the result of inhibiting inflammation through the NF-κB p65 pathway (49). According to a pertinent review by Wedn et al., the involvement of both peripheral and central cholinergic pathways plays a crucial role in the development and progression of pre-eclampsia. Interestingly, increasing the CAP could be a potential strategy for effectively managing pre-eclampsia and protecting against its associated maternal and fetal complications (50).
4 The impact of pre-eclampsia on neurodevelopment: insights into cognitive, behavioral, and mental disorders in offspring
Neurodevelopmental disorders are an issue that, according to research, may be related to insults during maternal pregnancy (51). Therefore, maternal health care during this period is crucial, as it is vital for both the mother's and the offspring's health (52). The inflammatory process and MIA caused by pre-eclampsia in the mother can affect both the mother's well-being and potentially lead to short, medium, and long-term problems in the neurodevelopment of the offspring (1, 53, 54). This association can generate an impact on the neurological basis of the offspring in the acquisition, retention, or application of specific skills or sets of information, as well as in memory, perception, language, problem-solving, executive function control, or social interaction; which produces a deficit in personal, social, academic, or occupational development (55). Several reports have found a significant association between pre-eclampsia and an increased risk of neurodevelopmental problems in offspring and mental disorders (56). In a recent study, researchers found that children born to mothers with pre-eclampsia were at higher risk of developmental delays, including cognitive, motor, and language difficulties (57), an increased risk of loss of cognitive functioning (58–60), Attention Deficit-Hyperactivity Disorder or ADHD (1, 28, 60–62), (1, 60, 61, 63), schizophrenia (28, 61), and epilepsy (1, 60, 61).
The aforementioned clinical conditions may be associated with functional and neurochemical changes described in the offspring of mothers with pre-eclampsia, such as altered dopamine levels, a neurotransmitter involved in learning and reward in specific brain regions (58, 64, 65), and the regulation of oxidative stress in the developing brain (61). The underlying mechanisms of this association are not yet fully understood. However, it is thought to involve disrupting normal fetal brain development due to impaired blood flow and oxygen supply. Preclinical studies have shown that pre-eclampsia can cause changes in the fetal brain’s structure and function, leading to altered behavior and cognitive deficits (4).
On the other hand, cardiovascular and metabolic diseases have been associated with the late-life outcomes of preeclamptic offspring (66). Furthermore, some neurodevelopmental and psychiatric disorders, such as ADHD and disruptive behavioral disorders, were significantly observed as early as six years after birth in the offspring of mothers with pre-eclampsia and perinatal complications in Finland (67). These findings have been reported in Taiwan, where not only ADHD was significantly increased, but also ASD and intellectual disability (60). Also, these psychiatric disorders cause significant distress in parents and children, resulting in disproportionate expenses during the assessment and treatment of these conditions. Further, the economic impact of these situations can be seen in the financial dependence of young adults with childhood ADHD (68).
5 Modulation of vagal nerve activity and potential applications in pregnancy
Electrical Vagus Nerve Stimulation (VNS) is a contemporary method that applies electrical pulses to the vagus nerve. This affects both upward- and downward-projecting nerve fibers, influencing the brainstem and internal organs. It plays a role in modulating autonomic functions as well as neuroendocrine and neuroimmunological systems (69). Vagal efferent fibers, which are prevalent in internal organs, facilitate communication between the nervous and immune systems, primarily via the CAP. The VNS has been used for over 20 years, but there is limited information on its safety during pregnancy. Voinescuo and Meador analyzed data from the International Registry of Antiepileptic Drugs and Pregnancy (EURAP) to assess pregnancy outcomes in 26 pregnancies among 25 women with epilepsy who used implanted VNS device during pregnancy. The sample size was too small to draw firm conclusions on the safety of implanted vagus nerve stimulators in pregnancy, but the study adds to the literature and encourages further research to improve the evidence for managing women with epilepsy during pregnancy (70). Preclinical relevant evidence has studied the effect of VNS during pregnancy and its underlying mechanisms in pre-eclampsia. Pregnant rats were used as a model, and implanted VNS therapy was found to decrease systolic blood pressure and urinary protein levels while mitigating abnormal pregnancy outcomes. VNS also reduced the inflammatory response by decreasing cytokine levels in the maternal serum, increasing the expression of placental α7nAChR and effectively inhibited placental NF-κB p65 activation. The study suggests that maternal VNS treatment is safe and protective in a pregnant rat model (71).
Transcutaneous auricular vagus nerve stimulation (taVNS), a non-invasive alternative to implanted VNS, has garnered substantial research interest due to its comparable benefits (72). Recognized as a potentially safe and feasible treatment, it is imperative for upcoming research on taVNS to meticulously evaluate any adverse events (73). Moreover, there is evidence indicating that taVNS can amplify cardiac vagal activity, reflected by heart rate variability (HRV) (74). Consequently, we speculate that, unlike the invasive implanted VNS, taVNS might serve as a pivotal tool in future studies on preeclamptic women to potentially alleviate their symptoms and possibly mitigate neurodevelopmental damage in their offspring.
In addition to the electrical VNS, other molecules may stimulate the CAP, such as extracellular monomeric ubiquitin (mUB) or pyridostigmine, a carbamate inhibitor of acetylcholinesterase whose administration can increase the concentration of circulating acetylcholine, presents an extensive range of safety in recommended doses (30-60 mg every 4-8 h) since the drug does not cross the placenta in significant amounts (75). However, its consumption may induce mainly intestinal side effects in patients (76). The clinical relevance of increasing the circulating cholinergic tone in an individual is that a high vagal tone favors the decreased serum levels of inflammatory molecules such as TNF-α, as well as cortisol and epinephrine (76), a very significant positive fact of this therapeutic strategy was observed when pyridostigmine was administered in patients affected by COVID-19 in which its administration increases the survival levels in patients (77). An even more novel option is the use of mUB, which is a small (8.6 kDa) and heat-stable protein higly-conserved in all eukaryotic cells (78). A large body of evidence shows in vitro and in vivo that pure mUB administration induces immunomodulatory effects, including decreasing macrophage and lymphocyte cellular functions, such as chemotaxis, proliferation, and cytokine secretion (78, 79). It is suggested that these immunomodulatory effects induced by mUB result from partial blockade of the CXCR4/CXCL12 axis. CXCR4 is constitutively expressed in the membrane of leukocytes in monomeric or dimeric form; it is associated with G proteins α and βγ subunits, β-arrestin, and JAK/STAT, and its activation favors chemotaxis, cell proliferation, and other cell activation phenomena (78).
mUB has been described as the main component of a Dialyzable Leukocyte Extract (DLE) (71). Interestingly, this complex drug induces a decrease in circulating levels of proinflammatory cytokines (TNF-α, IL-6), cortisol, and catecholamines when orally administered in major depression patients (72) as well as in murine (73) and canine infectious models (74, 75). Interestingly, the DLE and mUB alone increase the percent of survival of HSV-1-infected mice (80). This body of evidence suggests that the biological effects of the DLE in infectious models could be partially attributed to mUB, its main component.
Finally, the utilization of relaxation methods involves various mind-body techniques, including breathing training, progressive relaxation, and guided imagery, as supported by Geranmayeh et al. (81). Deep breathing-based yoga, a commonly practiced approach (82), has shown evidence of stimulating the vagus nerve, activating the parasympathetic nervous system and enhancing HRV. Moreover, the Food and Drug Administration (FDA) has approved magnetic stimulation of the vagus nerve to treat epilepsy and depression (83), demonstrating the potential of modulating vagal nerve activity through safe and established practices.
6 Perspective
Based on insights garnered from various studies, we propose that the modulation of vagal activity might offer a beneficial avenue for improving maternal and fetal outcomes in cases of pre-eclampsia. Pre-eclampsia, known for its links to unfavorable neurodevelopmental results in offspring, stems from a potential dysregulation within the Cholinergic Anti-inflammatory Pathway (CAP) and Maternal Immune Activation (MIA). There have been encouraging observations of reduced inflammation through electrical (VNS), physical, magnetic, and pharmacological methodologies that modulate the CAP. Such interventions may potentially improve maternal hemodynamics and bolster fetal neurodevelopment (as seen in Table 1 and Figure 1). Furthermore, exploring other molecular agents, such as extracellular monomeric ubiquitin (mUB) or pyridostigmine, to modulate the CAP seems promising. These strategies might address immune system imbalances, thus enhancing the quality of life for mothers dealing with pre-eclampsia. As a result, these experimental insights pave the way for the proposition that stimulating the CAP in pre-eclampsia offers a novel therapeutic tactic to mitigate the neurodevelopmental challenges posed by the condition. However, it is imperative to emphasize the necessity for additional research, particularly those studies backed by robust scientific evidence. This is crucial to ascertain the efficacy and safety of techniques to modulate vagal activity, especially when considering its application in pregnant women. As with any potential therapeutic approach, the weight of the evidence will guide its widespread acceptance and application in the clinical realm.
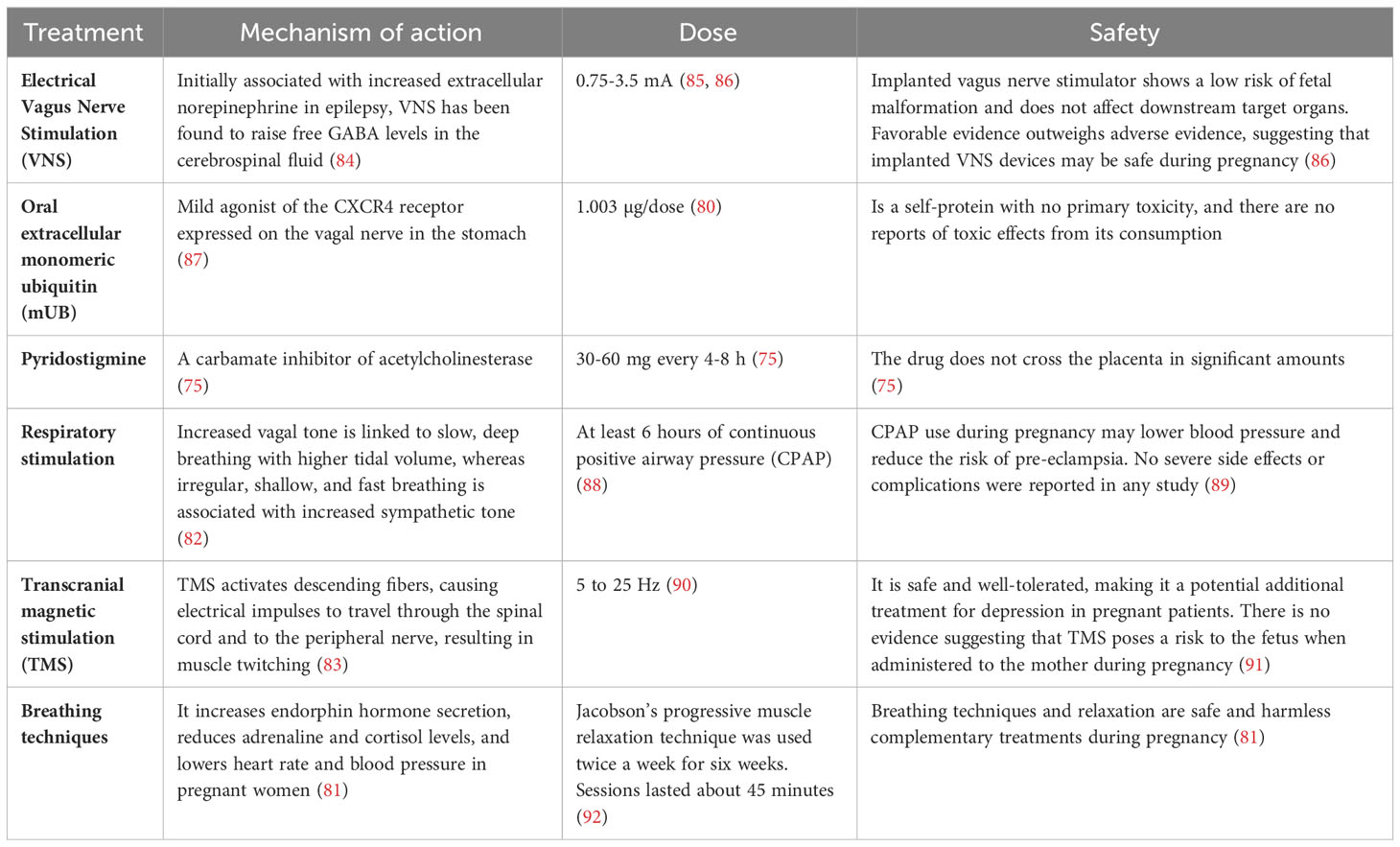
Table 1 Modulation of vagal nerve activity through electrical, pharmacological, physical, and magnetic stimulation.
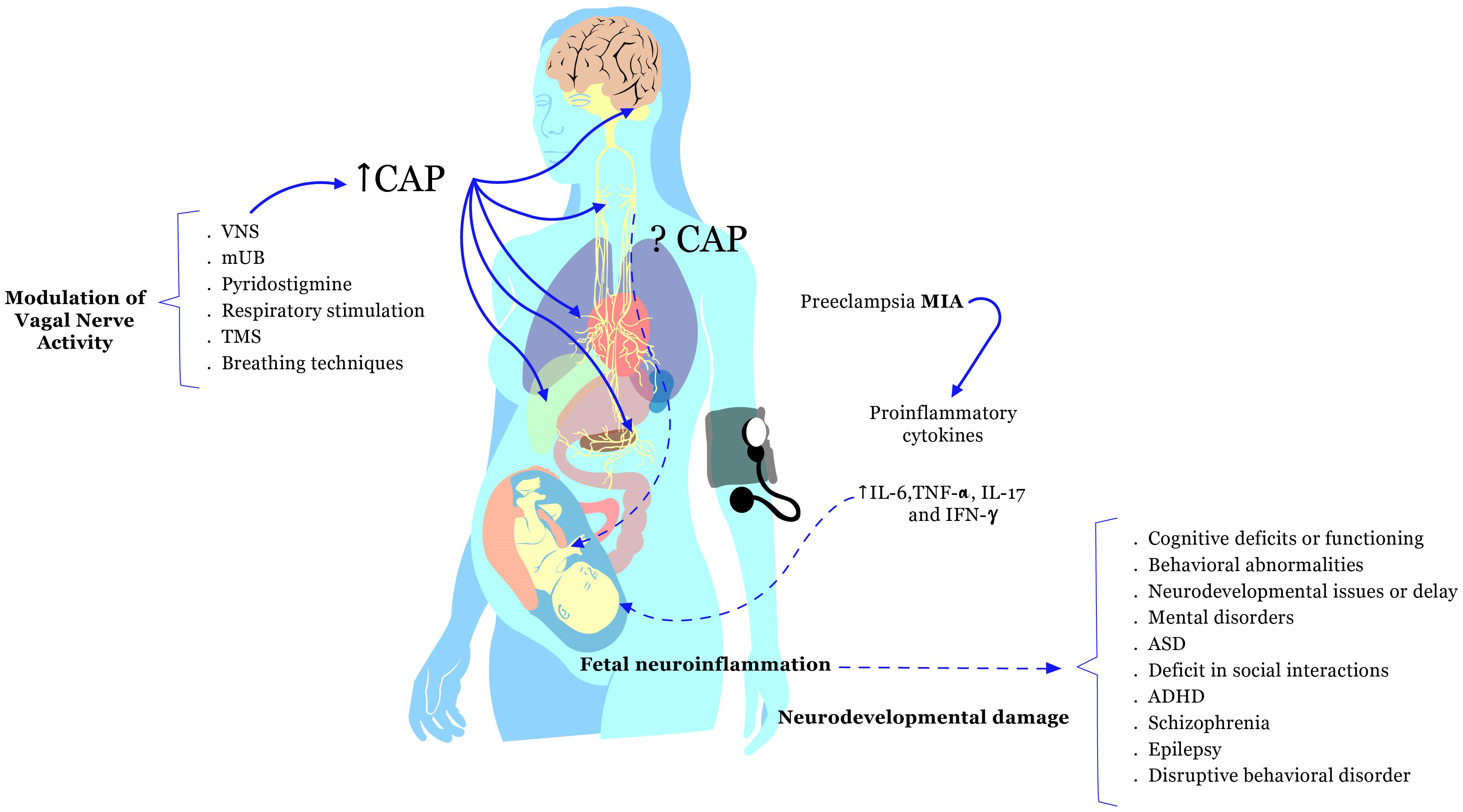
Figure 1 The inflammatory response during pregnancy can initiate complications, such as pre-eclampsia. Elevated pro-inflammatory cytokines, including interleukin-6 (IL-6), IL-17, tumor necrosis factor-alpha (TNF-α), and interferon-gamma (IFN-γ), are linked to this phenomenon. These pro-inflammatory cytokines may primarily mediate Maternal Immune Activation (MIA) during pregnancy and could impact fetal neurodevelopmental processes. The pathogenesis of pre-eclampsia might involve the disruption of the Cholinergic Anti-inflammatory Pathway (CAP) and fetal neuroinflammation. Pre-eclampsia has been associated with adverse neurodevelopmental outcomes in the offspring, such as cognitive deficits, behavioral abnormalities, mental disorders, neurodevelopmental issues, Autism Spectrum Disorder (ASD), Attention Deficit-Hyperactivity Disorder (ADHD), and others. Potential strategies to enhance maternal and fetal outcomes in pre-eclampsia involve modulating vagal nerve activity. This modulation can be achieved through techniques like Electrical Vagus Nerve Stimulation (VNS), pharmacological interventions (oral extracellular monomeric ubiquitin or mUB, and pyridostigmine), physical approaches (respiratory stimulation and breathing techniques), and Transcranial Magnetic Stimulation (TMS) in preeclamptic pregnant women.
Author contributions
EA-C: Conceptualization, Investigation, Resources, Writing – original draft, Writing – review & editing. AT-P: Conceptualization, Investigation, Resources, Writing – original draft, Writing – review & editing. JR-L: Resources, Writing – original draft, Writing – review & editing. EB-V: Resources, Writing – original draft, Writing – review & editing. GP-S: Resources, Writing – original draft, Writing – review & editing. FD-O: Resources, Writing – original draft, Writing – review & editing. JM-G: Resources, Writing – original draft, Writing – review & editing. LP: Conceptualization, Investigation, Resources, Writing – original draft, Writing – review & editing.
Funding
The authors declare that this study received funding partially by the Instituto Nacional de Psiquiatría Ramón de la Fuente Muñiz (grant numbers: NC23189.0) and providing partially financial support the Secretaría de Investigación y Estudios Avanzados (SIEA) for in research project 6725/2022CIB. The funders was not involved in the study design, collection, analysis, interpretation of data, the writing of this article or the decision to submit it for publication.
Conflict of interest
The authors declare that the research was conducted in the absence of any commercial or financial relationships that could be construed as a potential conflict of interest.
Publisher’s note
All claims expressed in this article are solely those of the authors and do not necessarily represent those of their affiliated organizations, or those of the publisher, the editors and the reviewers. Any product that may be evaluated in this article, or claim that may be made by its manufacturer, is not guaranteed or endorsed by the publisher.
Supplementary material
The Supplementary Material for this article can be found online at: https://www.frontiersin.org/articles/10.3389/fimmu.2023.1280334/full#supplementary-material
References
1. Sun BZ, Moster D, Harmon QE, Wilcox AJ. Association of preeclampsia in term births with neurodevelopmental disorders in offspring. JAMA Psychiatry (2020) 77:823–9. doi: 10.1001/jamapsychiatry.2020.0306
2. Garzoni L, Faure C, Frasch MG. Fetal cholinergic anti-inflammatory pathway and necrotizing enterocolitis: the brain-gut connection begins in utero. Front Integr Neurosci (2013) 7:57. doi: 10.3389/fnint.2013.00057
3. Xu H, Shi Q, Mo Y, Wu L, Gu J, Xu Y. Downregulation of α7 nicotinic acetylcholine receptors in peripheral blood monocytes is associated with enhanced inflammation in preeclampsia. BMC Pregnancy Childbirth (2019) 19:1–9. doi: 10.1186/S12884-019-2340-5
4. Reyes-Lagos JJ, Abarca-Castro EA. Nonlinear analysis of heart rhythm in preeclampsia: a route for translational clinical applications in neuroinflammation. Clin Hypertens (2021) 27:1–8. doi: 10.1186/s40885-021-00182-2
5. Abu-Raya B, Michalski C, Sadarangani M, Lavoie PM. Maternal immunological adaptation during normal pregnancy. Front Immunol (2020) 11:575197. doi: 10.3389/fimmu.2020.575197
6. Raghupathy R. Cytokines as key players in the pathophysiology of preeclampsia. Med Principles Pract (2013) 22:8–19. doi: 10.1159/000354200
7. Raghupathy R, Kalinka J. Cytokine imbalance in pregnancy complications and its modulation. Front Biosci (2008) 13. doi: 10.2741/2737
8. Yockey LJ, Iwasaki A. Interferons and proinflammatory cytokines in pregnancy and fetal development. Immunity (2018) 49(3):397–412. doi: 10.1016/j.immuni.2018.07.017
9. Sandgren JA, Deng G, Linggonegoro DW, Scroggins SM, Perschbacher KJ, Nair AR, et al. Arginine vasopressin infusion is sufficient to model clinical features of preeclampsia in mice. JCI Insight (2018) 3:e99403. doi: 10.1172/jci.insight.99403
10. Yockey LJ, Iwasaki A. Role of interferons and cytokines in pregnancy and fetal development. Immunity (2018) 49:397–412. doi: 10.1016/j.immuni.2018.07.017.Role
11. Cornelius DC, Amaral LM, Wallace K, Campbell N, Thomas AJ, Scott J, et al. Reduced uterine perfusion pressure T-helper 17 cells cause pathophysiology associated with preeclampsia during pregnancy. Am J Physiol Regul Integr Comp Physiol (2016) 311:R1192–9. doi: 10.1152/ajpregu.00117.2016
12. Choi GB, Yim YS, Wong H, Kim S, Kim H, Kim SV, et al. The maternal interleukin-17a pathway in mice promotes autism-like phenotypes in offspring. Sci (1979) (2016) 351:933–9. doi: 10.1126/science.aad0314
13. Udenze I, Amadi C, Awolola N, Makwe CC. The role of cytokines as inflammatory mediators in preeclampsia. Pan Afr Med J (2015) 20:219. doi: 10.11604/pamj.2015.20.219.5317
14. Aggarwal R, Jain AK, Mittal P, Kohli M, Jawanjal P, Rath G. Association of pro- and anti-inflammatory cytokines in preeclampsia. J Clin Lab Anal (2019) 33:1–10. doi: 10.1002/jcla.22834
15. Spence T, Allsopp PJ, Yeates AJ, Mulhern MS, Strain JJ, McSorley EM. Maternal serum cytokine concentrations in healthy pregnancy and preeclampsia. J Pregnancy (2021) 2021:6649608. doi: 10.1155/2021/6649608
16. Barrera D, Díaz L, Noyola-Martínez N, Halhali A. Vitamin D and inflammatory cytokines in healthy and preeclamptic pregnancies. Nutrients (2015) 7:6465–90. doi: 10.3390/nu7085293
17. El Shahaway AA, Elhady RRA, Abdelrhman AA, Yahia S. Role of maternal serum interleukin 17 in preeclampsia: Diagnosis and prognosis. J Inflamm Res (2019) 12:175–80. doi: 10.2147/JIR.S206800
18. Batebi A, Namavar-Jahromi B, Ali-Hassanzadeh M, Ahmadi M, Hosseini MS, Gharesi-Fard B. Evaluation of IL-17 and IL-35 serum levels in patients with preeclampsia. J Reprod Infertil (2019) 20:237–43.
19. Stefańska K, Zieliński M, Jankowiak M, Zamkowska D, Sakowska J, Adamski P, et al. Cytokine imprint in preeclampsia. Front Immunol (2021) 12:667841. doi: 10.3389/fimmu.2021.667841
20. Dong M, Wang Z, He J. Serum T helper 1- and 2-type cytokines in preeclampsia. Int J Gynecol Obstetrics (2005) 89:288–90. doi: 10.1016/j.ijgo.2004.11.039
21. Mosimann B, Wagner M, Poon LCY, Bansal AS, Nicolaides KH. Maternal serum cytokines at 30-33weeks in the prediction of preeclampsia. Prenat Diagn (2013) 33:823–30. doi: 10.1002/pd.4129
22. Mora-Palazuelos C, Bermúdez M, Aguilar-Medina M, Ramos-Payan R, Ayala-Ham A, Romero-Quintana JG. Cytokine-polymorphisms associated with Preeclampsia: A review. Med (United States) (2022) 101:e30870. doi: 10.1097/MD.0000000000030870
23. Mallette JH, Crudup BF, Alexander BT. Growth restriction in preeclampsia: lessons from animal models. Curr Opin Physiol (2023) 32:100647. doi: 10.1016/j.cophys.2023.100647
24. Chau K, Welsh M, Makris A, Hennessy A. Progress in preeclampsia: the contribution of animal models. J Hum Hypertens (2022) 36:705–10. doi: 10.1038/s41371-021-00637-x
25. Takahashi M, Makino S, Oguma K, Imai H, Takamizu A, Koizumi A, et al. Fetal growth restriction as the initial finding of preeclampsia is a clinical predictor of maternal and neonatal prognoses: a single-center retrospective study. BMC Pregnancy Childbirth (2021) 21:678. doi: 10.1186/s12884-021-04152-2
26. Zhu YC, Lin L, Li BY, Li XT, Chen DJ, Zhao XL, et al. Incidence and clinical features of fetal growth restriction in 4 451 women with hypertensive disorders of pregnancy. Matern-Fetal Med (2020) 2:207–10. doi: 10.1097/FM9.0000000000000062
27. Sławek-Szmyt S, Kawka-Paciorkowska K, Ciepłucha A, Lesiak M, Ropacka-Lesiak M. Preeclampsia and fetal growth restriction as risk factors of future maternal cardiovascular disease—A review. J Clin Med (2022) 11:6048. doi: 10.3390/jcm11206048
28. Gumusoglu SB, Chilukuri ASS, Santillan DA, Santillan MK, Stevens HE. Neurodevelopmental outcomes of prenatal preeclampsia exposure. Trends Neurosci (2020) 43:253–68. doi: 10.1016/j.tins.2020.02.003
29. Kreitz S, Zambon A, Ronovsky M, Budinsky L, Helbich TH, Sideromenos S, et al. Maternal immune activation during pregnancy impacts on brain structure and function in the adult offspring. Brain Behav Immun (2020) 83:56–67. doi: 10.1016/j.bbi.2019.09.011
30. Han VX, Patel S, Jones HF, Nielsen TC, Mohammad SS, Hofer MJ, et al. Maternal acute and chronic inflammation in pregnancy is associated with common neurodevelopmental disorders: a systematic review. Transl Psychiatry (2021) 11:71. doi: 10.1038/s41398-021-01198-w
31. Goeden N, Velasquez J, Arnold KA, Chan Y, Lund BT, Anderson GM, et al. Maternal inflammation disrupts fetal neurodevelopment via increased placental output of serotonin to the fetal brain. J Neurosci (2016) 36(22):6041–9. doi: 10.1523/JNEUROSCI.2534-15.2016
32. Kwon HK, Choi GB, Huh JR. Maternal inflammation and its ramifications on fetal neurodevelopment. Trends Immunol (2022) 43:230–44. doi: 10.1016/j.it.2022.01.007
33. Han VX, Patel S, Jones HF, Dale RC. Maternal immune activation and neuroinflammation in human neurodevelopmental disorders. Nat Rev Neurol (2021) 17:564–79. doi: 10.1038/s41582-021-00530-8
34. La Marca B, Brewer J, Wallace K. IL-6-induced pathophysiology during pre-eclampsia: Potential therapeutic role for magnesium sulfate? Int J Interferon Cytokine Mediat Res (2011) 3:59–64. doi: 10.2147/IJICMR.S16320
35. Mirabella F, Desiato G, Mancinelli S, Fossati G, Rasile M, Morini R, et al. Prenatal interleukin 6 elevation increases glutamatergic synapse density and disrupts hippocampal connectivity in offspring. Immunity (2021) 54:2611–31.e8. doi: 10.1016/j.immuni.2021.10.006
36. Cipollini V, Anrather J, Orzi F, Iadecola C. Th17 and cognitive impairment: possible mechanisms of action. Front Neuroanat (2019) 13:95. doi: 10.3389/fnana.2019.00095
37. Duncan JW, Younes ST, Hildebrandt E, Ryan MJ, Granger JP, Drummond HA. Tumor necrosis factor-α impairs cerebral blood flow in pregnant rats: Role of vascular β-epithelial Na+ channel. Am J Physiol Heart Circ Physiol (2020) 318:H1018–27. doi: 10.1152/ajpheart.00744.2019
38. Warrington JP, Drummond HA, Granger JP, Ryan MJ. Placental ischemia-induced increases in brain water content and cerebrovascular permeability: Role of TNF-α. Am J Physiol Regul Integr Comp Physiol (2015) 309:R1425–31. doi: 10.1152/ajpregu.00372.2015
39. Hansen-Pupp I, Harling S, Berg AC, Cilio C, Hellström-Westas L, Ley D. Circulating interferon-gamma and white matter brain damage in preterm infants. Pediatr Res (2005) 58:946–52. doi: 10.1203/01.PDR.0000182592.76702.E8
40. Hall MB, Willis DE, Rodriguez EL, Schwarz JM. Maternal immune activation as an epidemiological risk factor for neurodevelopmental disorders: Considerations of timing, severity, individual differences, and sex in human and rodent studies. Front Neurosci (2023) 17:1135559. doi: 10.3389/fnins.2023.1135559
41. Minakova E, Warner BB. Maternal immune activation, central nervous system development and behavioral phenotypes. Birth Defects Res (2018) 110:1539–50. doi: 10.1002/bdr2.1416
42. McEwan F, Glazier JD, Hager R. The impact of maternal immune activation on embryonic brain development. Front Neurosci (2023) 17:1146710. doi: 10.3389/fnins.2023.1146710
43. Keever MR, Zhang P, Bolt CR, Antonson AM, Rymut HE, Caputo MP, et al. Lasting and sex-dependent impact of maternal immune activation on molecular pathways of the amygdala. Front Neurosci (2020) 14:774. doi: 10.3389/fnins.2020.00774
44. Patel S, Cooper MN, Jones H, Whitehouse AJO, Dale RC, Guastella AJ. Maternal immune-related conditions during pregnancy may be a risk factor for neuropsychiatric problems in offspring throughout childhood and adolescence. Psychol Med (2021) 51:2904–14. doi: 10.1017/S0033291720001580
45. Prins JR, Eskandar S, Eggen BJL, Scherjon SA. Microglia, the missing link in maternal immune activation and fetal neurodevelopment; and a possible link in preeclampsia and disturbed neurodevelopment? J Reprod Immunol (2018) 126:18–22. doi: 10.1016/j.jri.2018.01.004
46. Katoh Y, Iriyama T, Yano E, Sayama S, Seyama T, Kotajima-Murakami H, et al. Increased production of inflammatory cytokines and activation of microglia in the fetal brain of preeclamptic mice induced by angiotensin II. J Reprod Immunol (2022) 154:103752. doi: 10.1016/j.jri.2022.103752
47. Czura CJ, Rosas-Ballina M, Tracey KJ. CHAPTER 3 - Cholinergic Regulation of Inflammation. In Ader R. Psychoneuroimmunology (Fourth Edition), Academic Press. (2006) 85–96. doi: 10.1016/B978-012088576-3/50007-1
49. Liu Y, Yang J, Bao J, Li X, Ye A, Zhang G, et al. Activation of the cholinergic anti-inflammatory pathway by nicotine ameliorates lipopolysaccharide-induced preeclampsia-like symptoms in pregnant rats. Placenta (2017) 49:23–32. doi: 10.1016/j.placenta.2016.11.003
50. Wedn AM, El-Bassossy HM, Eid AH, El-Mas MM. Modulation of preeclampsia by the cholinergic anti-inflammatory pathway: Therapeutic perspectives. Biochem Pharmacol (2021) 192:114703. doi: 10.1016/j.bcp.2021.114703
51. Bergdolt L, Dunaevsky A. Brain changes in a maternal immune activation model of neurodevelopmental brain disorders. Prog Neurobiol (2019) 175:1–19. doi: 10.1016/j.pneurobio.2018.12.002
52. Fine R, Zhang J, Stevens HE. Prenatal stress and inhibitory neuron systems: Implications for neuropsychiatric disorders. Mol Psychiatry (2014) 19:641–51. doi: 10.1038/mp.2014.35
53. Koulouraki S, Paschos V, Pervanidou P, Christopoulos P, Gerede A, Eleftheriades M. Short- and long-term outcomes of preeclampsia in offspring: review of the literature. Children (2023) 10:826. doi: 10.3390/children10050826
54. Katsuki S, Ushida T, Kidokoro H, Nakamura N, Iitani Y, Fuma K, et al. Hypertensive disorders of pregnancy and alterations in brain metabolites in preterm infants: A multi-voxel proton MR spectroscopy study. Early Hum Dev (2021) 163:105479. doi: 10.1016/j.earlhumdev.2021.105479
55. Asociación Americana de Psiquiatrı́a. Manual diagnóstico y estadístico de los trastornos mentales (DSM-5®), 5a. Ed. Asociación Americana de Psiquiatría (2014).
56. Tuovinen S, Räikkönen K, Pesonen AK, Lahti M, Heinonen K, Wahlbeck K, et al. Hypertensive disorders in pregnancy and risk of severe mental disorders in the offspring in adulthood: The Helsinki Birth Cohort Study. J Psychiatr Res (2012) 46:303–10. doi: 10.1016/j.jpsychires.2011.11.015
57. Koparkar S, Srivastava L, Randhir K, Dangat K, Pisal H, Kadam V, et al. Cognitive function and behavioral problems in children born to mothers with preeclampsia: an Indian study. Child Neuropsychol (2022) 28:337–54. doi: 10.1080/09297049.2021.1978418
58. Rätsep MT, Paolozza A, Hickman AF, Maser B, Kay VR, Mohammad S, et al. Brain structural and vascular anatomy is altered in offspring of pre-eclamptic pregnancies: A pilot study. Am J Neuroradiol (2016) 37:939–45. doi: 10.3174/ajnr.A4640
59. Tuovinen S, Eriksson JG, Kajantie E, Räikkönen K. Maternal hypertensive pregnancy disorders and cognitive functioning of the offspring: A systematic review. J Am Soc Hypertension (2014) 8:832–47.e1. doi: 10.1016/j.jash.2014.09.005
60. Chen KR, Yu T, Kang L, Lien YJ, Kuo PL. Childhood neurodevelopmental disorders and maternal hypertensive disorder of pregnancy. Dev Med Child Neurol (2021) 63:1107–13. doi: 10.1111/dmcn.14893
61. Barron A, McCarthy CM, O’Keeffe GW. Preeclampsia and neurodevelopmental outcomes: potential pathogenic roles for inflammation and oxidative stress? Mol Neurobiol (2021) 58:2734–56. doi: 10.1007/s12035-021-02290-4
62. Maher GM, Dalman C, O’Keeffe GW, Kearney PM, McCarthy FP, Kenny LC, et al. Association between preeclampsia and attention-deficit hyperactivity disorder: a population-based and sibling-matched cohort study. Acta Psychiatr Scand (2020) 142:275–83. doi: 10.1111/acps.13162
63. Jenabi E, Karami M, Khazaei S, Bashirian S. The association between preeclampsia and autism spectrum disorders among children: A meta-analysis. Korean J Pediatr (2019) 62:126–30. doi: 10.3345/kjp.2018.07010
64. Mak LE, Croy BA, Kay V, Reynolds JN, Rätsep MT, Forkert ND, et al. Resting-state functional connectivity in children born from gestations complicated by preeclampsia: A pilot study cohort. Pregnancy Hypertens (2018) 12:23–8. doi: 10.1016/J.PREGHY.2018.02.004
65. Kay VR, Rätsep MT, Figueiró-Filho EA, Croy BA. Preeclampsia may influence offspring neuroanatomy and cognitive function: A role for placental growth factor†. Biol Reprod (2019) 101:271–83. doi: 10.1093/biolre/ioz095
66. Stojanovska V, Scherjonm SA, Plösch T. Preeclampsia as modulator of offspring health. Biol Reprod (2016) 94:53. doi: 10.1095/biolreprod.115.135780
67. Kong L, Chen X, Liang Y, Forsell Y, Gissler M, Lavebratt C. Association of preeclampsia and perinatal complications with offspring neurodevelopmental and psychiatric disorders. JAMA Netw Open (2022) 5:e2145719. doi: 10.1001/jamanetworkopen.2021.45719
68. Altszuler AR, Page TF, Gnagy EM, Coxe S, Arrieta A, Molina BSG, et al. Financial dependence of young adults with childhood ADHD. J Abnorm Child Psychol (2016) 44:1217–29. doi: 10.1007/s10802-015-0093-9
69. Fang YT, Lin YT, Tseng WL, Tseng P, Hua GL, Chao YJ, et al. Neuroimmunomodulation of vagus nerve stimulation and the therapeutic implications. Front Aging Neurosci (2023) 15:1173987. doi: 10.3389/fnagi.2023.1173987
70. Voinescu PE, Meador KJ. Is neurostimulation through the vagal nerve safe during pregnancy? Epilepsy Res (2017) 137:163–4. doi: 10.1016/j.eplepsyres.2017.07.008
71. Zheng L, Tang R, Shi L, Zhong M, Zhou Z. Vagus nerve stimulation ameliorates L-NAME-induced preeclampsia-like symptoms in rats through inhibition of the inflammatory response. BMC Pregnancy Childbirth (2021) 21:177. doi: 10.1186/s12884-021-03650-7
72. Wang Y, Li SY, Wang D, Wu MZ, He JK, Zhang JL, et al. Transcutaneous auricular vagus nerve stimulation: from concept to application. Neurosci Bull (2021) 37:853–62. doi: 10.1007/s12264-020-00619-y
73. Kim AY, Marduy A, de Melo PS, Gianlorenco AC, Kim CK, Choi H, et al. Safety of transcutaneous auricular vagus nerve stimulation (taVNS): a systematic review and meta-analysis. Sci Rep (2022) 12:22055. doi: 10.1038/s41598-022-25864-1
74. Forte G, Favieri F, Leemhuis E, De Martino ML, Giannini AM, De Gennaro L, et al. Ear your heart: transcutaneous auricular vagus nerve stimulation on heart rate variability in healthy young participants. PeerJ (2022) 10:e14447. doi: 10.7717/peerj.14447
75. Gilhus NE. Myasthenia gravis can have consequences for pregnancy and the developing child. Front Neurol (2020) 11:554. doi: 10.3389/fneur.2020.00554
76. Pellissier S, Dantzer C, Mondillon L, Trocme C, Gauchez AS, Ducros V, et al. Relationship between Vagal Tone, Cortisol, TNF-alpha, epinephrine and negative affects in Crohn’s disease and irritable bowel syndrome. PloS One (2014) 9:e105328. doi: 10.1371/journal.pone.0105328
77. Fragoso-Saavedra S, Núñez I, Audelo-Cruz BM, Arias-Martínez S, Manzur-Sandoval D, Quintero-Villegas A, et al. Pyridostigmine reduces mortality of patients with severe SARS-CoV-2 infection: A phase 2/3 randomized controlled trial. Mol Med (2022) 28:131. doi: 10.1186/s10020-022-00553-x
78. Majetschak M. Extracellular ubiquitin: immune modulator and endogenous opponent of damage-associated molecular pattern molecules. J Leukoc Biol (2010) 89:205–19. doi: 10.1189/jlb.0510316
79. Sujashvili R. Advantages of extracellular ubiquitin in modulation of immune responses. Mediators Inflammation (2016) 2016:4190390. doi: 10.1155/2016/4190390
80. Vallejo-Castillo L, Favari L, Vázquez-Leyva S, Mellado-Sánchez G, Macías-Palacios Z, López-Juárez LE, et al. Sequencing analysis and identification of the primary peptide component of the dialyzable leukocyte extract “Transferon oral”: the starting point to understand its mechanism of action. Front Pharmacol (2020) 11:569039. doi: 10.3389/fphar.2020.569039
81. Geranmayeh M, Bikdeloo S, Azizi F, Mehran A. Effect of relaxation exercise on fasting blood glucose and blood pressure in gestational diabetes. Br J Midwifery (2019) 27:572–7. doi: 10.12968/bjom.2019.27.9.572
82. Jerath R, Barnes VA, Fadel HE. Mechanism of development of pre-eclampsia linking breathing disorders to endothelial dysfunction. Med Hypotheses (2009) 73:163–6. doi: 10.1016/j.mehy.2009.03.007
83. George MS, Aston-Jones G. Noninvasive techniques for probing neurocircuitry and treating illness: Vagus nerve stimulation (VNS), transcranial magnetic stimulation (TMS) and transcranial direct current stimulation (tDCS). Neuropsychopharmacology (2010) 35:301–16. doi: 10.1038/npp.2009.87
84. Ekmekçi H, Kaptan H. Vagus nerve stimulation. Open Access Maced J Med Sci (2017) 5:391–4. doi: 10.3889/oamjms.2017.056
85. Suller Marti A, Mirsattari SM, Steven DA, Parrent AG, MacDougall KW, McLachlan RS, et al. Experience on the use of Vagus Nerve Stimulation during pregnancy. Epilepsy Res (2019) 156:106186. doi: 10.1016/j.eplepsyres.2019.106186
86. Ding J, Wang L, Wang C, Gao C, Wang F, Sun T. Is vagal-nerve stimulation safe during pregnancy? A mini review. Epilepsy Res (2021) 174:106671. doi: 10.1016/j.eplepsyres.2021.106671
87. Hermann GE, Van Meter MJ, Rogers RC. CXCR4 receptors in the dorsal medulla: Implications for autonomic dysfunction. Eur J Neurosci (2008) 27:855–64. doi: 10.1111/j.1460-9568.2008.06058.x
88. Skovorodina TV, Vishnyakova PA, Tsvirkun DV, Shmakov RG, Vysokikh MY, Kalachin KA, et al. Impact of noninvasive respiratory therapy in pregnant women with early severe preeclampsia on the levels of preeclampsia markers and mitochondrial damps. Akusherstvo i Ginekologiya (Russian Federation) (2018) 2018. doi: 10.18565/aig.2018.10.52-58
89. Nugent R, Wee A, Kearney L, de Costa C. The effectiveness of continuous positive airway pressure for treating obstructive sleep apnoea in pregnancy: A systematic review. Aust New Z J Obstetrics Gynaecol (2023) 63(3):290–300. doi: 10.1111/ajo.13654
90. Hizli Sayar G, Ozten E, Tufan E, Cerit C, Kağan G, Dilbaz N, et al. Transcranial magnetic stimulation during pregnancy. Arch Womens Ment Health (2014) 17:311–5. doi: 10.1007/s00737-013-0397-0
91. Pridmore S, Turnier-Shea Y, Rybak M, Pridmore W. Transcranial Magnetic Stimulation (TMS) during pregnancy: a fetal risk factor. Australas Psychiatry (2021) 29:226–9. doi: 10.1177/1039856221992636
92. Ghorbannejad S, MehdizadehTourzani Z, Kabir K, Yazdkhasti M. The effectiveness of Jacobson’s progressive muscle relaxation technique on maternal, fetal and neonatal outcomes in women with non-severe preeclampsia: a randomized clinical trial. Heliyon (2022) 8:e09709. doi: 10.1016/j.heliyon.2022.e09709
Keywords: maternal immune activation, pre-eclampsia, cholinergic anti-inflammatory pathway, neurodevelopmental outcomes, vagus nerve stimulation, quality of life
Citation: Abarca-Castro EA, Talavera-Peña AK, Reyes-Lagos JJ, Becerril-Villanueva E, Pérez-Sanchez G, de la Peña FR, Maldonado-García JL and Pavón L (2023) Modulation of vagal activity may help reduce neurodevelopmental damage in the offspring of mothers with pre-eclampsia. Front. Immunol. 14:1280334. doi: 10.3389/fimmu.2023.1280334
Received: 20 August 2023; Accepted: 16 October 2023;
Published: 02 November 2023.
Edited by:
Martin Gerbert Frasch, University of Washington, United StatesReviewed by:
Cristina Wide Pissetti, Federal University of Paraíba, BrazilCopyright © 2023 Abarca-Castro, Talavera-Peña, Reyes-Lagos, Becerril-Villanueva, Pérez-Sanchez, de la Peña, Maldonado-García and Pavón. This is an open-access article distributed under the terms of the Creative Commons Attribution License (CC BY). The use, distribution or reproduction in other forums is permitted, provided the original author(s) and the copyright owner(s) are credited and that the original publication in this journal is cited, in accordance with accepted academic practice. No use, distribution or reproduction is permitted which does not comply with these terms.
*Correspondence: Ana Karen Talavera-Peña, YS50YWxhdmVyYUBjb3JyZW8ubGVyLnVhbS5teA==; Lenin Pavón, bGt1cmlha2lAaW5wcmYuZ29iLm14