- 1Institute of Animal Husbandry and Veterinary Medicine, Zhejiang Academy of Agricultural Sciences, Hangzhou, Zhejiang, China
- 2College of Animal Science and Technology, Henan University of Science and Technology, Luoyang, Henan, China
- 3Department of Epidemic Surveillance, Lingcheng Center for Disease Control and Prevention, Dezhou, Shandong, China
- 4Department of Animal Parasitology, College of Animal and Veterinaty Sciences, Southwest Minzu University, Chengdu, Sichuan, China
Background: Rhoptry organelle proteins (ROPs) secreted by apicomplexan parasites play important roles during parasites invasion and survival in host cells, and are potential vaccine candidates against apicomplexan diseases. Eimeria tenella (E. tenella) is one of the most noteworthy apicomplexan species, which causes hemorrhagic pathologies. Although dozens of putative E. tenella ROP sequences are annotated, most ROP proteins are not well studied.
Methods: In this study, an E. tenella ROP21 gene was identified and the recombinant EtROP21 protein (rEtROP21) was expressed in Escherichia coli. The developmental expression levels, localization, and protective efficacy against E. tenella infection in chickens were studied.
Results: An EtROP21 gene fragment with an open reading frame (ORF) of 981 bp was obtained from the Beijing strain of E. tenella. The rEtROP21 has a molecular weight of approximately 50 kDa and was recognized by rEtROP21-immunized mouse serum. Two specific protein bands, about 43 KDa and 95 KDa in size, were detected in the whole sporozoite proteins using the rEtROP21-immunized chicken serum. RT-qPCR analysis of the E. tenella ROP21 gene (EtROP21) revealed that its mRNA levels were higher in merozoites and sporozoites than in sporulated and unsporulated oocysts. Immunofluorescence and immunoelectron analyses showed that the EtROP21 protein predominantly localizes in the bulb region of rhoptries distributed at anterior, posterior, and perinuclear regions of E. tenella sporozoites. Immunization and challenge experiments revealed that immunizing chickens with rEtROP21 significantly increased their average body weight gain while decreasing mean lesion score and oocyst output (P <0.05). When compared with the challenged control group, the rEtROP21-immunized group was associated with a significantly higher relative weight gain (90.2%) and a greater reduction in oocyst output (67%) (P <0.05). The anticoccidial index of the rEtROP21-immunized group was 163.2. Chicken serum ELISA revealed that the levels of the specific anti- rEtROP21 antibody, IFN-γ, and IL-4 were significantly higher in the rEtROP21-immunized group than in the challenged control group (P <0.05).
Conclusion: These results indicate that rEtROP21 can induce a high level of specific immune response and it is a potential candidate for the development of vaccines against E. tenella infection in chickens.
1 Introduction
Eimeria tenella is the causative agent of chicken coccidiosis, which causes an estimated annual loss of ~ £10.4 billion annually worldwide (1). Traditional measures against chicken coccidiosis include the use of anticoccidial drugs and live oocyst vaccines (2). However, the effectiveness of these measures has been limited by the emergence of drug-resistant strains, growing consumer concerns about food safety, virulence reversion, and production challenges. Therefore, novel and cost-effective anticoccidial control measures are needed (3, 4). The development of effective coccidiosis vaccines require the identification of highly protective antigens (5). However, because E. tenella expresses about 6,700 proteins across its life cycle (6), it is necessary to first screen the E. tenella proteins and identify the protective antigens among so many parasite proteins. Apicomplexan parasites, including Eimeria, Toxoplasma gondii, and Plasmodium, have unique apical secretory organelles called rhoptries, dense granules, and micronemes, which are necessary for cell invasion, survival, and parasite growth (7, 8). Rhoptries are an arsenal of secreted virulence factors involved in apicomplexan pathogenicity (9). Hence, rhoptry organelle proteins (ROPs) are considered promising vaccine candidates against apicomplexan diseases (10). Indeed, several T. gondii ROPs, including TgROP1, 5, 7, and 17, have been reported to effectively stimulate protective immunity and to significantly reduce brain cyst loads in immunized animals (11–14). Several E. tenella ROPs, including Etrop5405, Etrop5905 (EtROP35), Etrop27705 (EtROP30), and Etrop5190 (EtROP17) have been also reported to effectively stimulate protective immunity and reduce oocyst output in immunized chickens (15–18). In this study, we identified EtROP21, an E. tenella ROP protein, and investigated its localization in sporozoites, as well as its protective efficacy against E. tenella infection. Our results provide a foundation for the development of effective vaccines against chicken coccidiosis.
2 Materials and methods
2.1 Animals and ethics
Coccidia-free chickens (one-day-old) were obtained from a local poultry breeding center (Xiaoshan, Hangzhou, China). These chickens were then raised at the experimental animal center of Zhejiang Academy of Agricultural Sciences in coccidia-free conditions and were given free access to forage (without anticoccidial drugs) and cold boiled water. All animal studies and protocols were approved by the institutional animal care committee of the Zhejiang Academy of Agricultural Sciences (approval No. 2022ZAASLA001).
2.2 E. tenella propagation, isolation, and purification
Two-week-old specific pathogen free (SPF) chickens were used to propagate oocysts of the E. tenella Beijing strain, which was obtained from Professor Xun Suo’s laboratory at China Agricultural University. Briefly, 1×104 sporulated oocysts of the Beijing strain were orally inoculated to the chickens. Seven to nine days after the inoculation, unsporulated oocysts were collected and purified according to a modified method (19). The gained oocysts were sporulated in 2.5% potassium dichromate solution in a constant-temperature shaker (Shanghai Zhichu Instrument, China) at 28°C with a rotation speed of 200 rpm. In order to purify sporozoites from the oocysts, a tissue-preparation instrument (Gering, China) was used to release sporocysts. Then, sporozoites were excysted using a modified excystation buffer made of phosphate-buffered saline (1×PBS, pH 7.4), 0.75% trypsin, and 10% chicken bile extract, and purified using DE-52 anion-exchange chromatography (20). Merozoites were isolated and purified according to a modified method (21) as follows: ceca were taken from the chickens at 120 h post the inoculation of E. tenella sporulated oocysts and washed with the sterilized 1×PBS. And then mucosal tissues were scraped from the ceca and harvested with a digestion solution containing 1 mg/mL hyaluronidase, 120 mmol/L NaCl, 20 mmol/L Tris-HCl (pH7.4), 3 mmol/L K2HPO4, 1 mmol/L CaCl2, and 1 mg/mL bovine serum albumin (BSA) at 37°C for 60 min. The digestive mixture containing merozoites was filtrated with 200-mesh sieves and centrifuged to gain merozoite precipitation. The merozoites were further purified using erythrocyte lysis buffer (Solarbio, China), and Percoll density gradient centrifugation at 4,000 rpm for 5 min. The finally purified merozoites were stored at 4°C for use. All oocysts, sporocysts, sporozoites, and merozoites were enriched using horizontal centrifugation (Cence, China).
2.3 Amplification of the EtROP21 gene and bioinformatics analysis
E. tenella sporozoites (5×107) RNA was extracted using Trizol reagent (Invitrogen, USA), and cDNA was prepared using a TransScript® First-Strand cDNA Synthesis SuperMix kit (TransGen, China). The gene sequence of EtROP21 was amplified by polymerase chain reaction (PCR). Primers for PCR amplification of the ROP21 gene were designed according to the nucleotide sequence (NCBI accession No. XM_013377531) of E. tenella (Houghton strain); 12 specific primers (11 upstream and one downstream) were synthesized, and the actin gene was used as control. All of the primers are listed in Table 1. The PCR products were identified using 1% agarose gel and purified fragments were cloned into the pMD19-T vector. Then, the pMD19-T- EtROP21 plasmid was transformed into trans-t1 E. coli cells (TransGen, China). After cultivation on Luria broth (LB) agar plates at 37°C for 12 h, the clones were picked and identified by PCR, and the positive plasmid of pMD19-T- EtROP21 was further verified via sequencing. Finally, the obtained sequences were blasted in NCBI, and a phylogenetic tree was constructed using the MEGA 6 software (all of the reference sequences are listed in Table 2). In order to predict the molecular weight and isoelectric point of ROP21 protein gained in the study, the ExPASy ProtParam tool was applied. The SignalP 4.1 server was utilized for prediction of the signal peptide. In addition, ROPs are usually considered promising vaccine antigens; in order to analyze the antigenicity of the ROP21 protein, the DNAMAN (9.0) tool was utilized for prediction of the antigenic epitopes.
2.4 Analysis of EtROP21 expression at various E. tenella developmental stages
EtROP21 mRNA levels in the developmental stages of sporozoites, second-generation merozoites, sporulated oocysts, and unsporulated oocysts were determined using RT-qPCR as previously described (22). Briefly, E. tenella total RNA was extracted at these four stages and reverse transcribed as described in section 2.3. EtROP21 transcript levels were then determined on a 7500 real-time PCR system (ThermoFisher, USA) using the primers shown in Table 1 and actin as the reference gene. Relative gene expression was determined using the 2-ΔΔCt method (23). The data represent three independent experiments.
2.5 Expression and preparation of recombinant EtROP21 protein
The EtROP21 gene fragment was cloned in pMD19-T vector, and it was then double-digested by Kpn I/Xho I restriction enzymes. The purified EtROP21 fragment was ligated into the pET32a expression vector leading to a recombinant plasmid, pET32a-EtROP21. Then, the recombinant plasmid was transfected into competent E. coli BL21 (DE3) cells (TransGen, China), and the bacteria were grown in LB plates containing 100 μg/ml ampicillin. After an overnight incubation at 37°C, the clones were picked and confirmed with PCR, double digestion with restriction enzymes, and gene sequencing. The confirmed positive clone was inoculated into LB medium with ampicillin and cultured at 37°C with shaking at 200 rpm until it reached an optical density (OD600) of 0.6-0.8. The rEtROP21 protein was induced with 1 m M IPTG and shaking at 37°C with 200 rpm for 4 h, and the precipitate of the bacteria expressing rEtROP21 protein was collected after being centrifuged at 8,000 rpm for 5 min at 4°C. The precipitate was re-suspended in PBS and sonicated at 40 W for 30 min (Ningbo Scientz, China), and then the recombinant protein was identified by SDS-PAGE. The rEtROP21 protein was further purified using Ni2+-NTA (Thermo Scientific, USA). Finally, the concentration of the protein was measured using a BCA protein assay kit (Beyotime, China), and the obtained protein was stored at -80°C until use.
2.6 Western blot analysis
In order to prepare sporozoite proteins, 5×107 E. tenella sporozoites were treated with RIPA lysis buffer (Solarbio, China) containing 1 mM protease inhibitor phenylmethanesulfonyl fluoride (PMSF) and sonicated on ice for 10 min. The treated sporozoite mixture was centrifuged at 12,000 rpm for 10 min at 4°C to gain supernatant. The rEtROP21 recombinant protein and the sporozoite proteins were separated using 12% SDS-PAGE gels, and then were transferred onto nitrocellulose (NC) filter membrane at 200 mA for 15 min using a Bio-Rad transfer system (Bio-Rad, USA); bands of the transferred NC membrane were blocked with 5% skim milk at 4°C overnight. Sera (1:250 dilution with PBS) from rEtROP21-immunized or unimmunized mice or chicken were added and incubated at 37°C for 2 h. The bands were then incubated with horseradish peroxidase (HRP)-conjugated goat anti-mouse IgG (1:6000 dilution with PBS, Beyotime, China) for 2 h at 37°C after being washed with PBST three times. Finally, an enhanced chemiluminescence kit (Sangon Biotech, China) was used to visualize the bands. Six-week-old SPF mice were used to produce polyclonal antibodies for rEtROP21. Briefly, 100 μg of purified rEtROP21 formulated with Freund’s complete adjuvant (Thermo Fisher Scientific, USA) (1:1) were subcutaneously injected into mice. The second and third booster immunization were performed at 14-day intervals, with 50 ug of rEtROP21 protein plus Freund’s incomplete adjuvant. Seven days after the third immunization, mouse sera were separated and stored at -80°C for use.
2.7 Immunofluorescence assay and immunoelectron assay
EtROP21 localization was observed using indirect immunofluorescence assay (IFA) and immunoelectron assay (IEA). The IFA protocol was as follows: purified sporozoites (1×107) were fixed with 2% paraformaldehyde for 20 min and centrifuged at 4,000 rpm for 5 min. The sporozoites were permeabilized with 0.2% Triton-X-100 for 10 min at 37°C and then were blocked with 5% BSA after being washed three times with 0.01 M PBS. Primary anti-rEtROP21 antibody (mouse) diluted 1/100 with PBS was incubated for 1 h at room temperature. After washes with PBS, the sporozoites were incubated with secondary antibody Alexa Fluor® labeled goat anti-mouse 488 (Beyotime, China, 1/500 dilution). DAPI was used to stain the nuclei following further washes, and the sample was examined by fluorescent microscope (SOPTOP, China). For IEA, 1×108 freshly purified sporozoites were fixed in 3% paraformaldehyde (Shanghai Macklin Biochemical Ltd, China) + 2% glutaraldehyde (ThermoFisher Scientific Ltd, USA) in 0.2 M phosphate buffer (PB), pH 7.2, at 4°C overnight. They were then washed thrice using PB and centrifugation at 4,000 rpm for 5 min at 4°C. The sporozoites were then dehydrated with 30% ethanol for 30 min at 4°C, and then with 50%, 70%, 80%, 90%, 95%, and 100% ethanol for 60 min at −20°C. They were then infiltrated with 1:1 and 2:1 LR gold resin/ethanol at −20°C for 2 h, with 100% LR gold resin (Agar Scientific Ltd, UK) overnight, at −20°C, and then with 0.06% and 0.12% benzil (London Resin company, UK) in LR gold resin at −20°C for 6 h and overnight, respectively. The sporozoites were centrifuged at 4,000 rpm for 5 min at 4°C following each step of dehydration or infiltration. The supernatants were discarded. Samples were then embedded in 0.12% benzil in LR gold resin with ultraviolet radiation at −20°C for 96 h. They were then trimmed, frozen in liquid nitrogen, and sectioned using an ultra-thin microtome (Leica UC6, Germany). The nanometer sections were then blocked with 1% BSA and 0.02% PEG2000 in PBS (pH 7.0) for 30 min at room temperature and incubated with serum from rEtROP21-immunized mice for 1 h at room temperature, followed by incubation with rabbit-anti-mouse IgG secondary antibody conjugated with 15-nm gold particles (Abcam, UK) for 1 h at room temperature. After each reaction, the sections were washed thrice with PBS and imaged using a transmission electron microscope (Hitachi 7650, Japan).
2.8 Analysis of immuno-protection induced by rEtROP21
Sixty (one-week-old) coccidia-free chickens were randomly divided into three groups of 20 chickens with similar mean body weights per group (Table 3). Immunization procedures for the experimental chickens were as follows: the rEtROP21 protein with 100 μg/chicken was used for chickens of the EtROP21 group. PBS was used for chickens of the challenged and unchallenged control groups. The rEtROP21 protein and PBS were emulsified in complete Freund’s adjuvant before use and injected subcutaneously into the neck of the chickens. Two hundred µg of rEtROP21 and PBS emulsified in Freund’s incomplete adjuvant were used in the second and third immunizations with 14-day intervals. Blood samples were collected from all the experimental groups on the seventh day after the third immunization and sera were isolated for use in antibody and cytokine (IFN-γ, IL-2, IL-4, TGF-β) detection. Chickens in the rEtROP21-immunized and challenged control group were orally challenged with 2×104 sporulated E. tenella oocysts and chickens in the unchallenged control group were orally administered the same volumes of PBS. In order calculate E. tenella oocysts, chicken droppings from each experimental group were collected separately on the sixth to ninth day post the challenge. All the chickens were weighed and slaughtered on the ninth day after the challenge. The cecum and its content from chickens of each group was collected separately. The McMaster counting method was used to count E. tenella oocysts in the chicken droppings and the cecal contents. The oocyst decrease ratio was calculated using the formula: (total number of oocysts from the challenge control group-total number of oocysts from rEtROP21-immunized group)/total number of oocysts from the challenge control group × 100%. Body weight gain was calculated as the following: mean body weight per group on the slaughter day-mean body weight per group on the challenge day. Cecal lesion scores were determined on a graded scale from 0 (normal) to 4 (severe), as previously described by Johnson and Reid (24). The anticoccidial index (ACI) is a synthetic criterion for determining anticoccidial effects and was calculated using the formula: (relative rate of weight gain + survival rate) × (lesion value + oocyst value). ACIs of >180, 160 < ACI ≤ 179, 120 < ACI ≤ 159, and <120 were considered as an excellent anticoccidial effect, good anticoccidial effect, generally effective anticoccidial effect, and invalid, respectively (25).
2.9 Antibody and cytokines assays
The level of the specific anti-EtROP21 antibody was determined using enzyme-linked immunosorbent assay (ELISA). Briefly, rEtROP21 protein was diluted to 1 μg/mL with 50 mM carbonate buffer (PH 9.6) and the ELISA plate was coated with 100 μg/well of rEtROP21 protein at 4°C overnight. The ELISA plate was blocked for 2 h using 100 μL of 5% skim milk and washed with PBST three times. Diluted sera (a gradient of 1:100 to 1:12,800) were added in triplicate and incubated for 2 h at room temperature. HRP-conjugated goat anti-chicken IgG secondary antibody (Beyotime, China, 1/10,000 dilution) was added after three washes, and the plate was incubated for 1 h at 37°C. After the final washes, a tetramethylbenzidine (TMB) substrate solution was added into the wells followed by incubation at 37°C for 20 min and subsequently the reaction was stopped after adding 50 μL of stop solution (2M H2SO4). The absorbance was read at 450 nm. Cytokine levels, IFN-γ, IL-2, IL-4, and TGF-β in the chicken sera from each group were detected using ELISA kits (Cusabio, Wuhan Huamei Biotech, China). The assay was performed in three independent experiments.
2.10 Statistical analysis
Statistical analyses were performed using GraphPad Prism version 8.0 (GraphPad Prism, San Diego, CA). The differences in cytokines production, antibody level, mean body weight gain, oocyst output per chicken, and mean lesion scores were determined with a one-way analysis of variance (ANOVA). All of the experiments were done in triplicate and three independent experiments and the results are shown as the arithmetic mean ± standard deviation. P <0.05 indicates statistically significant differences.
3 Results
3.1 Determination of EtROP21 transcript levels and construction of a recombinant EtROP21 expression plasmid
Of the 12 specific primers used to amplify EtROP21 gene fragments PCR, only one primer pair (rop21-F11 and rop21-R) generated a single, 981-bp DNA band from the E. tenella Beijing strain (Figure S1A). Actin, which was used as the reference gene, was amplified correctly. Sequence analysis indicated that the EtROP21 gene fragment amplified from the Beijing strain was 100% identical to the partial sequence of a putative E. tenella ROP21 gene from the Houghton strain (Gene ID: 25252056). A phylogenetic tree constructed using four ROP21 gene sequences from other apicomplexan parasites (Table 2) indicated that they share a lower percent identity with the sequence from E. tenella (Figure 1). Bioinformatics analysis revealed that the 981-bp EtROP21 gene fragment encoded a protein with a predicted molecular weight of 36.89 kDa. The isoelectric point of EtROP21 protein was 8.69, and eight potential antigenic epitopes were predicted. There were no signal peptide regions in the EtROP21 protein (Table 4). A recombinant EtROP21 expression plasmid (pET32a (+)-EtROP21) was successfully constructed (Figure S1B). RT-qPCR analysis of RNA from sporozoites, second-generation merozoites, and sporulated and unsporulated oocysts revealed that the EtROP21 mRNA levels were markedly high in second-generation merozoites and sporozoites than in sporulated and unsporulated oocysts (Figure 2).
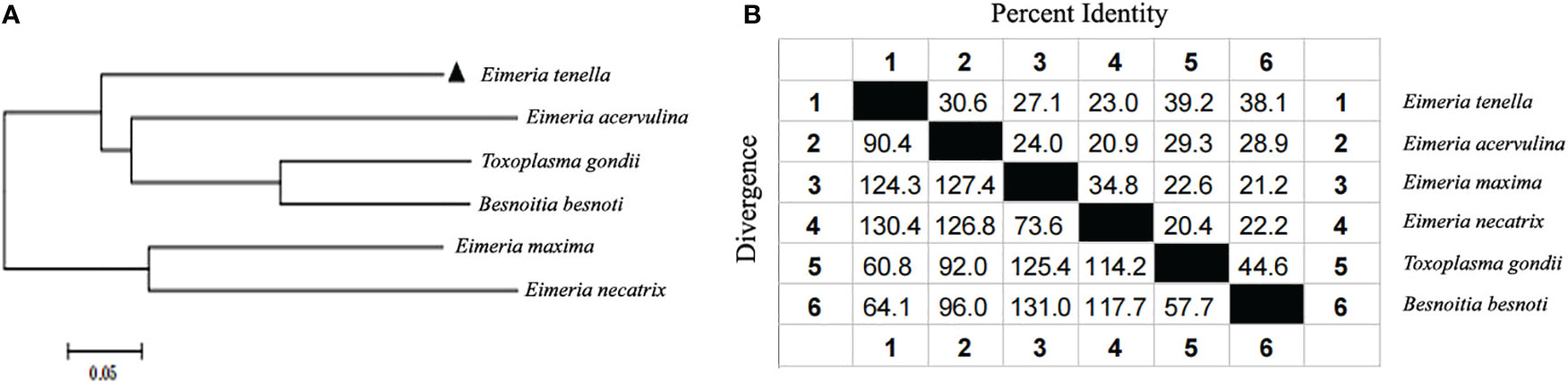
Figure 1 Phylogenetic tree construction and homology analysis based on ROP21 genes. (A) A phylogenetic tree based on ROP21 gene sequences from different apicomplexan species. (B) Similarity and differences between the ROP21 gene sequences of various apicomplexan species.
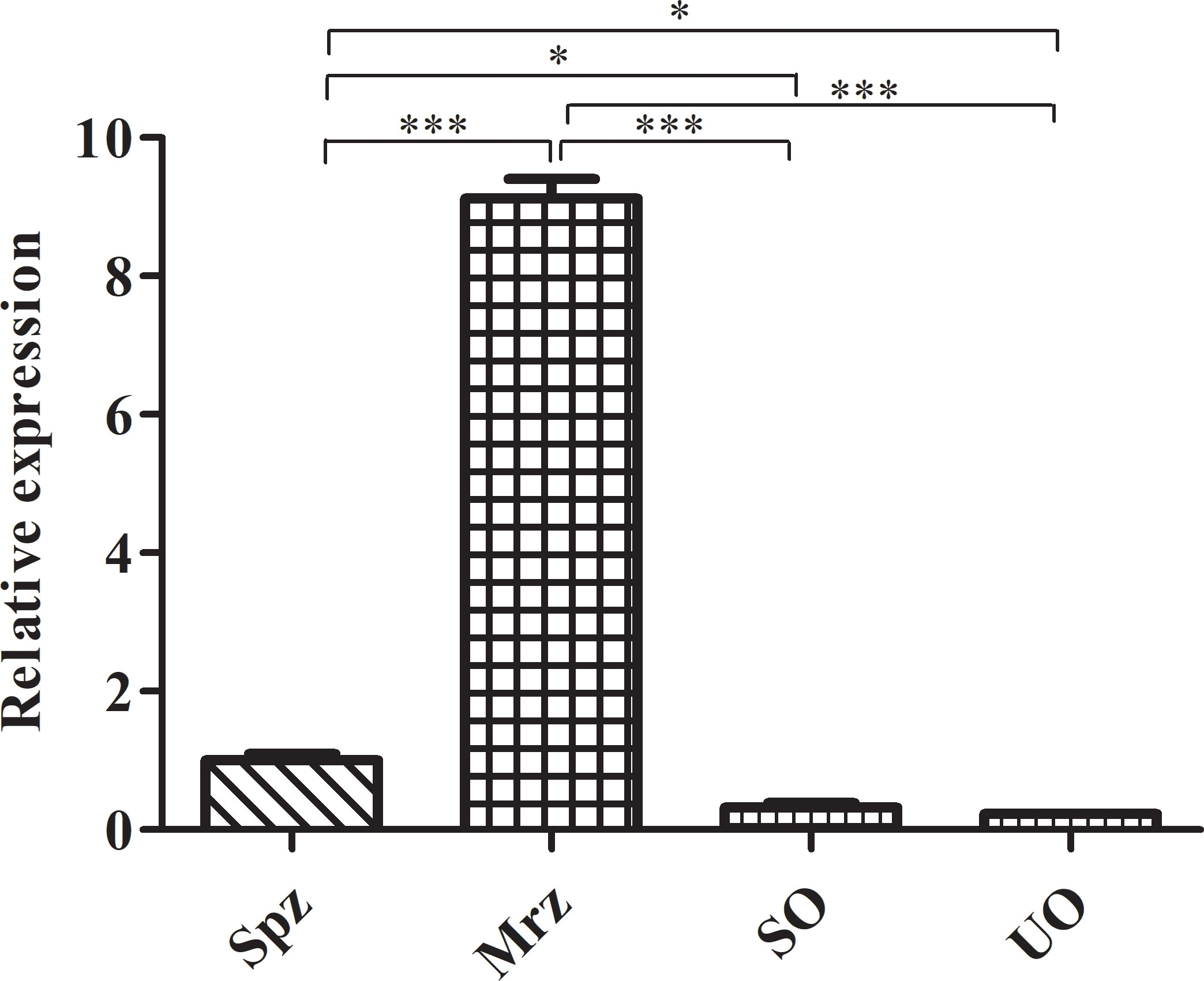
Figure 2 RT-qPCR analysis of ROP21 mRNA levels at different E. tenella developmental stages. UO, unsporulated oocysts; SO, sporulated oocysts; Spz, sporozoites; Mrz, merozoites. * and *** indicate P <0.05 and <0.001, respectively.
3.2 Expression and purification of recombinant EtROP21 protein
The recombinant target EtROP21 protein (rEtROP21) was induced in E. coli strain BL21 (DE3) by the addition of IPTG to a final concentration of 1 mM/L and incubation at 37°C and 200 rpm for 4 h. The rEtROP21 had a molecular weight of approximately 50 kDa, which included the Trx and His-tag protein expressed from the pET32a (+) vector. The recombinant rEtROP21 protein appeared as inclusion bodies. Therefore, the actual size of rEtROP21 (50 kDa) in the SDS-PAGE was in agreement with the theoretical size. Purified rEtROP21 was isolated using nickel ion affinity chromatography and recovery from SDS-PAGE (Figure 3A). Western blot analysis revealed the presence of a specific anti-EtROP21 antibody in sera from rEtROP21-immunized mice or chicken, and that the antibody reacted with both the recombinant protein and whole sporozoite proteins. The band size of the EtROP21 protein in the Western blot was consistent with its theoretical size and sera from unimmunized mice or chicken did not react with rEtROP21 or with whole sporozoite proteins (Figures 3B, C). In addition, there was another larger protein band, about 95 KDa in size, seen in the whole parasite protein. We speculate that the larger protein may be the dimer form of the EtROP21 protein.
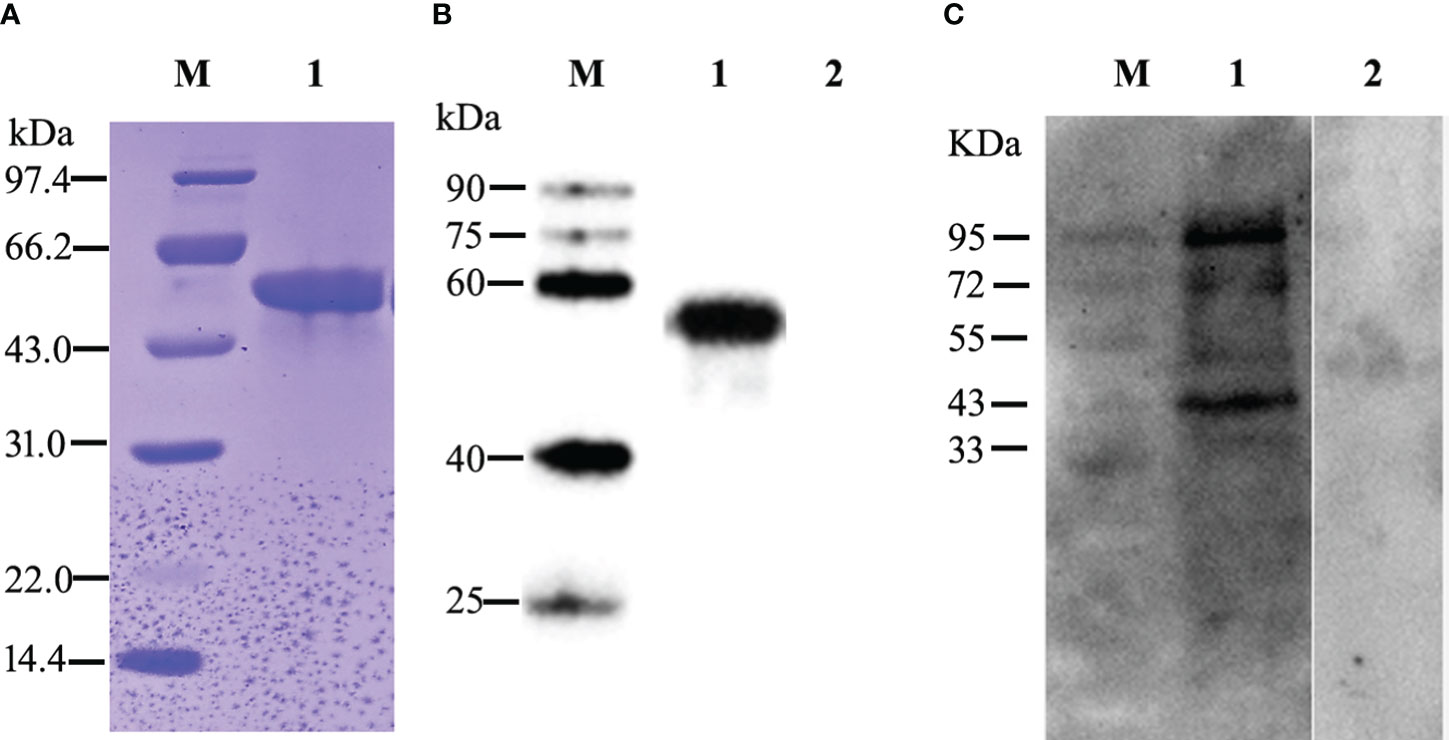
Figure 3 Expression, purification, and Western blot analysis of the rEtROP21 protein. M: protein marker. (A) SDS-PAGE analysis of the purified rEtROP21 protein. Lane 1: the rEtROP21 protein was purified using nickel ion affinity chromatography. (B) Western blot analysis of the recombinant EtROP21 protein. Lanes 1 and 2: recombinant EtROP21 reacted with immunized and unimmunized mouse sera, respectively. (C) Western blot analysis of the EtROP21 protein in E. tenella. Lanes 1 and 2: total proteins from E. tenella sporozoites reacted with immunized and unimmunized chicken sera, respectively.
3.3 EtROP21 localization in E. tenella sporozoites
IFA indicated that EtROP21 was mainly distributed at the anterior, posterior, and perinuclear regions of E. tenella sporozoites but not in the refractive bodies (Figure 4). IEA further confirmed that EtROP21 was mainly localized in the bulb region of the rhoptries (Figure 5).
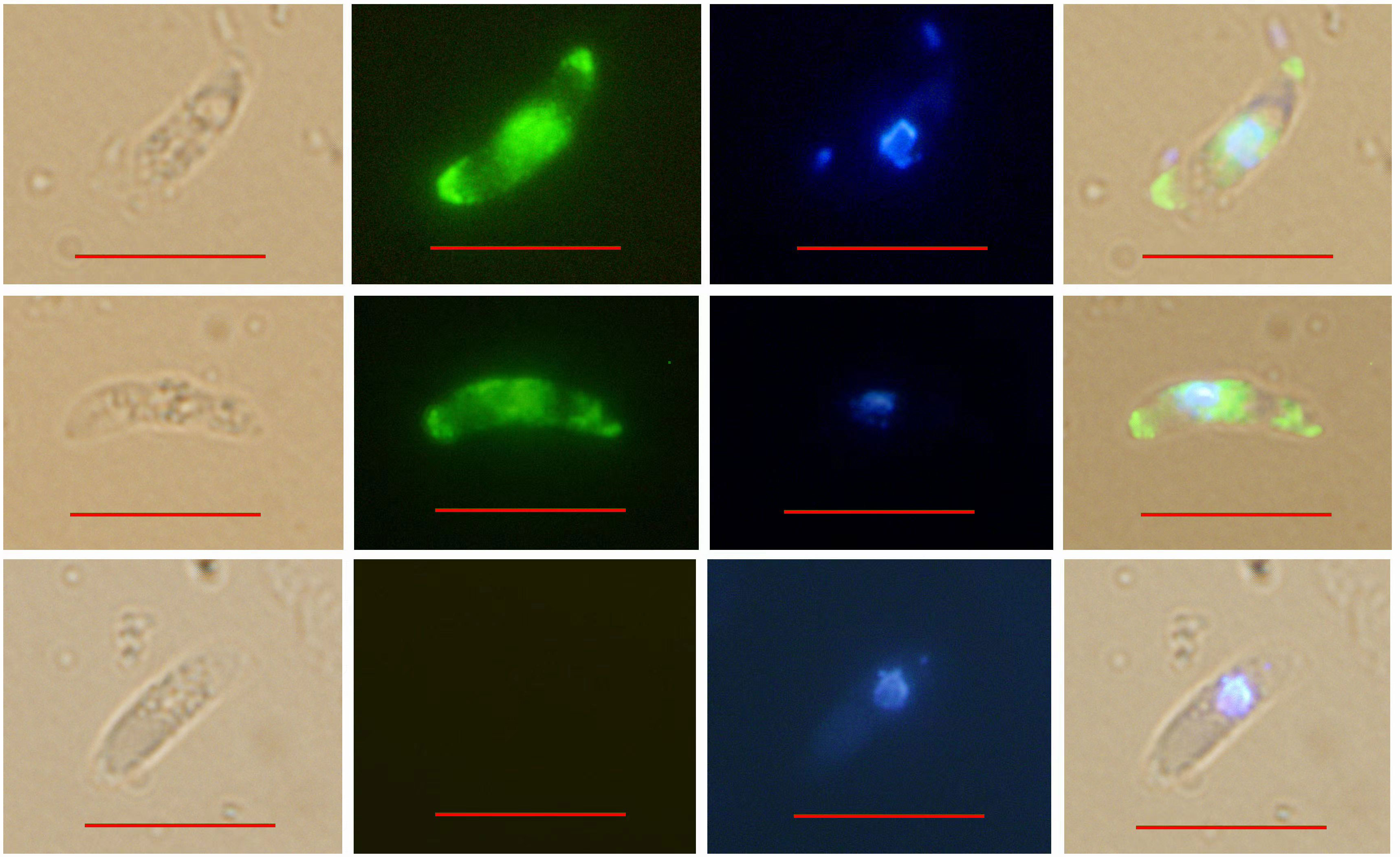
Figure 4 Determination of EtROP21 localization in E. tenella sporozoites using indirect immunofluoresence. BRI: sporozoites under bright field imaging. FLU: sporozoites stained with Alexa Fluor 488-labeled goat anti-mouse IgG under green fluorescence imaging. UV: sporozoites stained with DAPI under UV imaging. Mer: merged images. Scale bar: 10 μm.
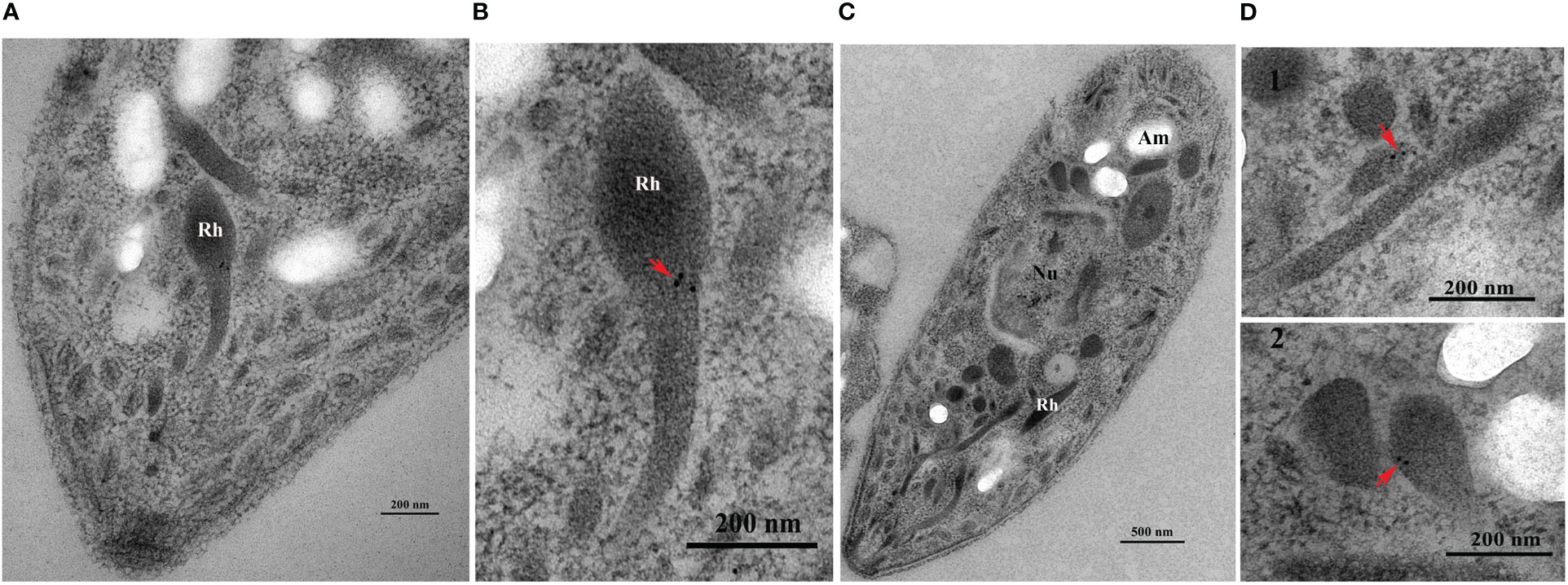
Figure 5 Determination of EtROP21 localization in E. tenella sporozoites’ rhoptries using immunoelectron analysis. (A, B) The anterior region (A) and rhoptry (B) of a sporozoite stained with gold particles. (C, D) A whole sporozoite (C) and its rhoptries (D). (D1–D2) Rhoptries localization at the anterior (D1) and posterior (D2) regions of a sporozoite stained with gold particles. “Rh”, “Nu”, and “Am” represents “Rhoptries”, “Nucleus”, and “Amylopectin granules”, respectively.
3.4 rEtROP21 exhibits immunoprotection against E. tenella infection
No deaths were observed in all experimental groups (the rEtROP21-immunized group and PBS- immunized group). Whereas the challenged control group exhibited severe clinical symptoms, including bloody droppings, decreased appetite, and depression, the rEtROP21-immunized group showed only mild symptoms on the fifth day after the challenge. The average weight gain was significantly higher in the rEtROP21-immunized group (443.3 ± 47.30 g) than in the challenged control group (P <0.05), which had a relative weight gain of 90.23%. The average oocyst output was 8.98×107 ± 9.21×106 per chicken in the rEtROP21-immunized group and 2.76×108 ± 3.58×107 in the challenged control group. No oocysts were observed in the unchallenged control group. Compared with the challenged control group, the rEtROP21-immunized group had significantly lower oocyst excretion (67.42% oocyst reduction, P <0.05). The cecal lesion score of the rEtROP21-immunized group was lower than that of the challenged control group. No lesions were observed in the unchallenged controls. The ACI value of the rEtROP21-immunized group was 163.23 (Table 5). According to the judgment criteria of ACI in the Materials and Methods section, rEtROP21 showed a good anticoccidial effect. The ELISA results revealed that levels of specific anti-EtROP21 antibody, IFN-γ, and IL-4 in chicken sera were significantly higher in the rEtROP21-immunized group than in the unimmunized control group (P <0.05, Figures 6A–E). Because IFN-γ and IL-4 are mainly associated with host humoral and cellular immune responses, this indicates that the rEtROP21 protein stimulated the chickens’ humoral and cellular immune responses.
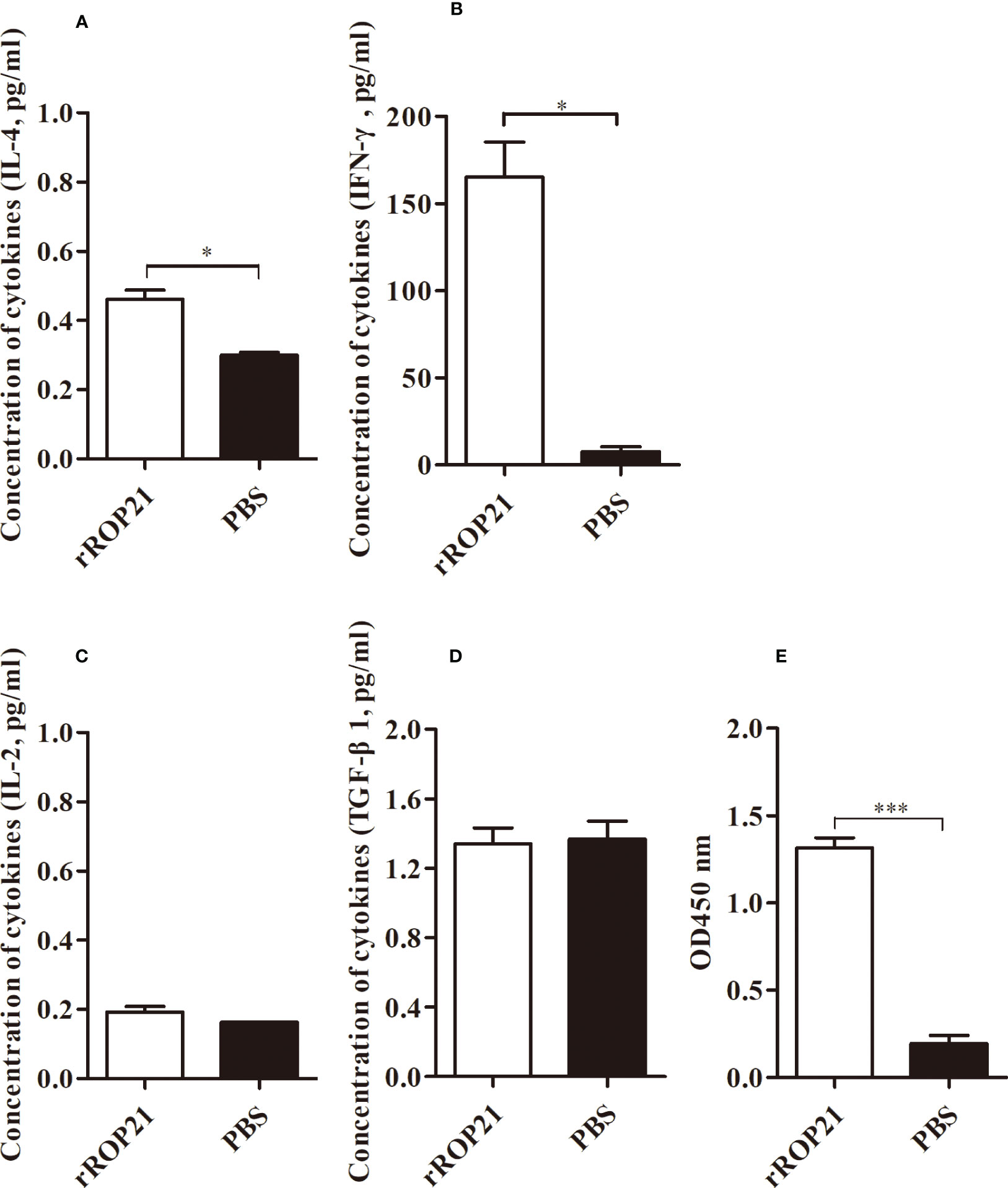
Figure 6 ELISA analyses of the levels of IL-4, IFN-γ, IL-2, TGF-β, and a specific anti-rEtROP21 antibody in chicken sera (n=4). (A–D) Levels of IL-4, IFN-γ, IL-2, and TGF-β in the rEtROP21 and PBS-immunized groups, respectively. (E) Levels of the anti-rEtROP21 specific antibody in the rEtROP21 and the PBS-immunized control group, respectively. * and *** indicate P <0.05 and P <0.001, respectively.
4 Discussion
This study identified a rhoptry protein, EtROP21, from the E. tenella Beijing strain. Based on RT-qPCR analysis, we estimated that the EtROP21 gene fragment transcribed in the E. tenella sporozoite stage was 981-bp long, which was markedly shorter than the putative whole EtROP21 gene from the E. tenella Houghton strain (Gene ID: 25252056), probably because only a partial sequence of the EtROP21 gene was expressed by E. tenella sporozoites. RT-qPCR analysis showed that the expression levels of EtROP21 were higher in the extracellular sporozoite and second-generation merozoite stages (26), which is consistent with our observation. Rhoptry organelle proteins, one type of specific secretory proteins of apicomplexa, are usually considered as having a signal peptide in the N-terminal region. However, no signal peptide was predicted in the EtROP21 protein identified in this study. The reasons could be that the EtROP21 protein identified in this study is a truncated version of a larger protein, lacking its signal peptide. In addition, considering the two specific protein bands detected in the whole sporozoite proteins with the Western bot, in which the smaller protein about 43 KDa was similar in molecular size with the theoretical molecular weight calculated from the 981-bp EtROP21 gene fragment amplified in the study, the other main possibility was that the EtROP21 protein could be another type of ROP without the signal peptide. Similar findings have been reported in T. gondii ROPs, in which T. gondii ROP30 was also predicted containing no signal peptide in the N-terminal region (27). We speculate that the larger protein, about 95 Kda, observed in the whole sporozoite protein may be the dimer form of the EtROP21 protein. This was in agreement with the study in T. gondii, in which two forms of TgROP21 also occurs, with the predicted molecular weights of 86 kDa and 51.4 kDa, and the smaller form was highly expressed both at tachyzoite and bradyzoite stages, but the larger form was poorly expressed at the bradyzoite stage (27).
Using IFA, we found that in E. tenella sporozoites, the EtROP21 protein localizes at two terminal and perinuclear regions. This is consistent with the findings by Burrell et al., that although rhoptries predominantly localize at the apical end of sporozoites, they are also present at the central and posterior parts of the cell (28). This EtROP21 immunolocalization in E. tenella sporozoites was consistent with previous reports on the immunolocalization of EtROP30 and EtROP35 (16, 18). Rhoptries are club-shaped organelles that contain a rounded bulb region and an elongated neck region. Our immunoelectron analysis showed that the EtROP21 protein mainly localized in the bulb region of rhoptries, which is different from the localization of the T. gondii ROP21 protein. TgROP21 is primarily secreted in constitutive secretory vesicles into parasitophorous vacuoles (PV) rather than localizing in rhoptries in storage form (27).
Both host humoral and cellular immunity defend against pathogen infection. In this study, we found that rEtROP21-immunized chickens have high levels of a specific anti-rEtROP21 IgG. Higher levels of IFN-γ and IL-2 (Th1-like cytokines) and IL-4 (Th2-like cytokines) were also detected in the rEtROP21-immunized group, which showed a mixed Th1/Th2- immune response. Because IFN-γ plays an important role in the immunoregulation of avian coccidia infection (29), its release has been used to screen for effective protective antigens against E. tenella infection (30). IL-4 also enhances IFN-γ production during the chronic stage of T. gondii infection (31) and is prominently involved in counterbalancing immune responses by downregulating IFN-γ secretion (32). In the current study, chickens vaccinated using the rEtROP21 protein exhibited high levels of IFN-γ and IL-4, indicating that rEtROP21 could protect chicken from E. tenella infection by inducing humoral and cellular immune responses.
In addition, animal protection experiments revealed that rEtROP21 robustly protects against E. tenella infection, suppressing oocyst levels by up to 67.42%. Such a high level of protection has also been reported using a DNA vaccine based on T. gondii’s ROP21, which significantly prolonged survival time and decreased the number of brain cysts (33), as well as other E. tenella rhoptry proteins, including Etrop5405, Etrop5905 (EtROP35), Etrop27705 (EtROP30), and Etrop5190 (EtROP17), which have been associated with oocyst reduction rates of 82.75%, 63.4%, 61.43%, and 64.29%, respectively (15–18). Thus, EtROP21 might be a good antigen candidate for the development of vaccines against chicken coccidiosis, and its functions in the invasion, survival, and growth of E. tenella need further investigation.
5 Conclusion
Our analysis revealed that the actual length of the EtROP21 gene fragment expressed by E. tenella sporozoite is shorter than the putative whole EtROP21 gene recorded in the database of National Center for Biotechnology Information (NCBI). The recombinant EtROP21 (rEtROP21) expressed in E. coli had a molecular weight of approximately 50 kDa. Two specific protein bands, about 43 KDa and 95 KDa in size, were detected in the whole sporozoite proteins using the rEtROP21-immunized chicken serum in the Western blot. The larger protein (95 KDa) was speculated to be the dimer form of the EtROP21 protein. Immunofluorescence and immunoelectron assays showed that in E. tenella sporozoites, the EtROP21 protein was localized at the bulb region of rhoptries and that it was predominantly distributed at two terminals and perinuclear regions. Furthermore, rEtROP21 could induce specific humoral and cellular immune responses, offer robust immunoprotection against E. tenella infection, and suppress oocyst levels by 67.42%. These findings highlight EtROP21 as a potential candidate for the development of vaccines against coccidiosis in chickens. However, its functions in the invasion, survival, and virulence of E. tenella require further study.
Data availability statement
The original contributions presented in the study are included in the article/Supplementary Material. Further inquiries can be directed to the corresponding authors.
Ethics statement
The animal study was approved by Institutional animal care committee of the Zhejiang Academy of Agricultural Sciences. The study was conducted in accordance with the local legislation and institutional requirements.
Author contributions
T-yS: Funding acquisition, Project administration, Conceptualization, Data curation, Writing – original draft. S-hZ: Writing – original draft, Methodology, Software. Y-rK: Formal Analysis, Investigation, Writing – review & editing. YF: Conceptualization, Methodology, Resources, Validation, Writing – review & editing. YL: Data curation, Resources, Software, Writing – review & editing. W-cY: Conceptualization, Methodology, Supervision, Validation, Writing – review & editing. Y-xZ: Conceptualization, Data curation, Formal Analysis, Writing – review & editing. LZ: Investigation, Methodology, Visualization, Writing – review & editing. L-lH: Formal Analysis, Methodology, Software, Writing – review & editing. H-cS: Funding acquisition, Methodology, Project administration, Writing – review & editing.
Funding
The author(s) declare financial support was received for the research, authorship, and/or publication of this article. This work was supported by the National Natural Science Foundation of China (32072883), Hangzhou Agricultural and Social Development Project (202203B19), Zhejiang Province “Sannongjiufang” Science and Technology Cooperation Project (No. 2023SNJF058), Hangzhou Science and Technology Commissioner Project (20221122I30), the China Agriculture Research System of MOF and MARA (No. CARS-43-C-2), International Cooperation Projects of Zhejiang Academy of Agricultural Sciences, and Natural Science Foundation of Zhejiang province (LQ20C170001).
Conflict of interest
The authors declare that the research was conducted in the absence of any commercial or financial relationships that could be construed as a potential conflict of interest.
Publisher’s note
All claims expressed in this article are solely those of the authors and do not necessarily represent those of their affiliated organizations, or those of the publisher, the editors and the reviewers. Any product that may be evaluated in this article, or claim that may be made by its manufacturer, is not guaranteed or endorsed by the publisher.
Supplementary material
The Supplementary Material for this article can be found online at: https://www.frontiersin.org/articles/10.3389/fimmu.2023.1277955/full#supplementary-material
Supplementary Figure 1 | Construction of the prokaryotic expression plasmid, pET32a-EtROP21. M1 & M2: DNA markers. (A) The EtROP21 fragment was amplified using PCR. Lane 1: the product of the PCR analysis of the actin gene in E. tenella. Lane 2: the product of the PCR using the primer pair, rop21-F11 and rop21-R. (B) The expression plasmid, pET32a-EtROP21, was identified via double digestion. Lane 1: the product of the recombinant plasmid, pET32a-EtROP21, after digestion with the restriction enzymes, KpnI and XhoI.
References
1. Blake DP, Knox J, Dehaeck B, Huntington B, Rathinam T, Ravipati V, et al. Re-calculating the cost of coccidiosis in chickens. Vet Res (2020) 51(1):115. doi: 10.1186/s13567-020-00837-2
2. Blake DP, Tomley FM. Securing poultry production from the ever-present Eimeria challenge. Trends Parasitol (2014) 30(1):12–9. doi: 10.1016/j.pt.2013.10.003
3. Peek HW, Landman WJ. Coccidiosis in poultry: anticoccidial products, vaccines and other prevention strategies. Vet Q (2011) 31(3):143–61. doi: 10.1080/01652176.2011.605247
4. Martins RR, Silva LJG, Pereira AMPT, Esteves A, Duarte SC, Pena A. Coccidiostats and poultry: a comprehensive review and current legislation. Foods (2022) 11(18):2738. doi: 10.3390/foods11182738
5. Gupta SK, Shukla P. Advanced technologies for improved expression of recombinant proteins in bacteria: perspectives and applications. Crit Rev Biotechnol (2016) 36(6):1089–98. doi: 10.3109/07388551.2015.1084264
6. Reid AJ, Blake DP, Ansari HR, Billington K, Browne HP, Bryant J, et al. Genomic analysis of the causative agents of coccidiosis in domestic chickens. Genome Res (2014) 24(10):1676–85. doi: 10.1101/gr.168955.113
7. Dubremetz JF, Garcia-Réguet N, Conseil V, Fourmaux MN. Apical organelles and host-cell invasion by Apicomplexa. Int J Parasitol (1998) 28(7):1007–13. doi: 10.1016/s0020-7519(98)00076-9
8. Gubbels MJ, Duraisingh MT. Evolution of apicomplexan secretory organelles. Int J Parasitol (2012) 42(12):1071–81. doi: 10.1016/j.ijpara.2012.09.009
9. Bradley PJ, Sibley LD. Rhoptries: an arsenal of secreted virulence factors. Curr Opin Microbiol (2007) 10(6):582–7. doi: 10.1016/j.mib.2007.09.013
10. Foroutan M, Ghaffarifar F, Sharifi Z, Dalimi A, Jorjani O. Rhoptry antigens as Toxoplasma gondii vaccine target. Clin Exp Vaccin Res (2019) 8(1):4–26. doi: 10.7774/cevr.2019.8.1.4
11. Zheng B, Lu S, Tong Q, Kong Q, Lou D. The virulence-related rhoptry protein 5 (ROP5) of Toxoplasma Gondii is a novel vaccine candidate against toxoplasmosis in mice. Vaccine (2013) 31(41):4578–84. doi: 10.1016/j.vaccine.2013.07.058
12. Wang HL, Zhang TE, Yin LT, Pang M, Guan L, Liu HL, et al. Partial protective effect of intranasal immunization with recombinant Toxoplasma gondii rhoptry protein 17 against toxoplasmosis in mice. PloS One (2014) 9(9):e108377. doi: 10.1371/journal.pone.0108377
13. Sonaimuthu P, Ching XT, Fong MY, Kalyanasundaram R, Lau YL. Induction of protective immunity against toxoplasmosis in BALB/c mice vaccinated with Toxoplasma gondii Rhoptry-1. Front Microbiol (2016) 7:808. doi: 10.3389/fmicb.2016.00808
14. Sun HC, Huang J, Fu Y, Hao LL, Liu X, Shi TY. Enhancing immune responses to a DNA vaccine encoding Toxoplasma gondii GRA7 using calcium phosphate nanoparticles as an adjuvant. Front Cell Infect Microbiol (2021) 11:787635. doi: 10.3389/fcimb.2021.787635
15. Song X, Yang X, Zhang T, Liu J, Liu Q. A novel rhoptry protein as candidate vaccine against Eimeria tenella infection. Vaccines (Basel). (2020) 8:452. doi: 10.3390/vaccines8030452
16. Bingxiang W, Ningning Z, Yakun W, Lingyu S, Hongmei L, Zhang X, et al. Characterization of the Eimeria tenella rhoptry protein with a nuclear localization sequence (EtROP30). Parasitol Res (2022) 121(5):1507–16. doi: 10.1007/s00436-022-07499-3
17. Liu X, Mu B, Zheng W, Meng Y, Yu L, Gao W, et al. Identification and Protective Efficacy of Eimeria tenella Rhoptry Kinase Family Protein 17. Anim (Basel). (2022) 12(5):556. doi: 10.3390/ani12050556
18. Wang B, Zhao N, Sun J, Sun L, Li H, Wu Z, et al. Identification and characterization of Eimeria tenella rhoptry Protein 35 (EtROP35). Vet Sci (2022) 9(9):465. doi: 10.3390/vetsci9090465
19. Long PL, Millard BJ, Joyner LP, Norton C. A guide to laboratory techniques used in the study and diagnosis of avian coccidiosis. Folia Vet Lat. (1976) 6(3):201–17.
20. Schmatz DM, Crane MS, Murray PK. Purification of Eimeria sporozoites by DE-52 anion exchange chromatography. J Protozool. (1984) 31(1):181–83. doi: 10.1111/j.1550-7408.1984.tb04314.x
21. Xie MQ, Gilbert JM, Fuller AL, McDougald LR. A new method for purification of Eimeria tenella merozoites. Parasitol Res (1990) 76(7):566–9. doi: 10.1007/BF00932562
22. Han HY, Zhu SH, Jiang LL, Li Y, Dong H, Zhao QP, et al. Molecular characterization and analysis of a novel calcium-dependent protein kinase from Eimeria tenella. Parasitology (2013) 140(6):746–55. doi: 10.1017/S0031182012002107
23. Michaelidou K, Tzovaras A, Missitzis I, Ardavanis A, Scorilas A. The expression of the CEACAM19 gene, a novel member of the CEA family, is associated with breast cancer progression. Int J Oncol (2013) 42(5):1770–7. doi: 10.3892/ijo.2013.1860
24. Johnson J, Reid WM. Anticoccidial drugs: Lesion scoring techniques in battery and floor-pen experiments with chickens. Exp Parasitol (1970) 28(1):30–6. doi: 10.1016/0014-4894(70)90063-9
25. McManus EC, Campbell WC, Cuckler AC. Development of resistance to quinoline coccidiostats under field and laboratory conditions. J Parasitol (1968) 54(6):1190–3. doi: 10.2307/3276989
26. Ribeiro E Silva A, Sausset A, Bussière FI, Laurent F, Lacroix-Lamandé S, Silvestre A. Genome-wide expression patterns of rhoptry kinases during the Eimeria tenella life-cycle. Microorganisms (2021) 9(8):1621. doi: 10.3390/microorganisms9081621
27. Jones NG, Wang Q, Sibley LD. Secreted protein kinases regulate cyst burden During chronic toxoplasmosis. Cell Microbiol (2017) 19(2):e12651. doi: 10.1111/cmi.12651
28. Burrell A, Marugan-Hernandez V, Wheeler R, Moreira-Leite F, Ferguson DJP, Tomley FM, et al. Cellular electron tomography of the apical complex in the apicomplexan parasite Eimeria tenella shows a highly organised gateway for regulated secretion. PLoS Pathog (2022) 18(7):e1010666. doi: 10.1371/journal.ppat.1010666
29. Dalloul RA, Lillehoj HS. Poultry coccidiosis: recent advancements in control measures and vaccine development. Expert Rev Vaccines (2006) 5(1):143–63. doi: 10.1586/14760584.5.1.143
30. Breed DG, Schetters TP, Verhoeven NA, Boot-Groenink A, Dorrestein J, Vermeulen AN. Vaccination against Eimeria tenella infection using a fraction of E. tenella sporozoites selected by the capacity to activate T cells. Int J Parasitol (1999) 29(8):1231–40. doi: 10.1016/s0020-7519(99)00110-1
31. Jin D, Takamoto M, Hu T, Taki S, Sugane K. STAT6 signalling is important in CD8 T-cell activation and defence against Toxoplasma gondii infection in the brain. Immunology (2009) 127(2):187–95. doi: 10.1111/j.1365-2567.2008.02935.x
32. Hunter CA, Sibley LD. Modulation of innate immunity by Toxoplasma gondii virulence effectors. Nat Rev Microbiol (2012) 10(11):766–78. doi: 10.1038/nrmicro2858
Keywords: Eimeria tenella, rhoptry protein, EtROP21, localization, protective efficacy
Citation: Shi T-y, Zhou S-h, Kong Y-r, Fu Y, Liu Y, Yan W-c, Zhou Y-x, Zhang L, Hao L-l and Sun H-c (2023) A rhoptry protein, localizing in the bulb region of rhoptries, could induce protective immunity against Eimeria tenella infection. Front. Immunol. 14:1277955. doi: 10.3389/fimmu.2023.1277955
Received: 15 August 2023; Accepted: 17 November 2023;
Published: 04 December 2023.
Edited by:
Kun Li, Nanjing Agricultural University, ChinaReviewed by:
Carlos Esteban Suarez, United States Department of Agriculture (USDA), United StatesNanshan Qi, Guangdong Academy Agricultural Sciences, China
Hongyu Han, Chinese Academy of Agricultural Sciences, China
Copyright © 2023 Shi, Zhou, Kong, Fu, Liu, Yan, Zhou, Zhang, Hao and Sun. This is an open-access article distributed under the terms of the Creative Commons Attribution License (CC BY). The use, distribution or reproduction in other forums is permitted, provided the original author(s) and the copyright owner(s) are credited and that the original publication in this journal is cited, in accordance with accepted academic practice. No use, distribution or reproduction is permitted which does not comply with these terms.
*Correspondence: Hong-chao Sun, sunhongchao1988@126.com; Li-li Hao, leelee-hao@126.com
†These authors have contributed equally to this work and share first authorship