- 1Biomedical Research Networking Centre on Respiratory Diseases (CIBERES), Madrid, Spain
- 2Respiratory Diseases Group, Respiratory Diseases Department, Hospital La Paz Institute for Health Research – IdiPAZ, Madrid, Spain
- 3Servicio de Neumología, Hospital Universitario Ramón y Cajal, Madrid, Spain
- 4The Innate Immune Response Group, Hospital La Paz Institute for Health Research – IdiPAZ, Madrid, Spain
- 5Faculty of Medicine, Autonomous University of Madrid, Madrid, Spain
Introduction: Although higher incidence of cancer represents a major burden for obstructive sleep apnea (OSA) patients, the molecular pathways driving this association are not completely understood. Recently, the adhesion receptor P-selectin glycoprotein-1 (PSGL 1) has been identified as a novel immune checkpoint, which are recognized major hallmarks in several types of cancer and have revolutionized cancer therapy.
Methods: The expression of PSGL-1 and its ligands VISTA and SIGLEC-5 was assessed in the leucocytes of OSA patients and control subjects exploring the role of intermittent hypoxia (IH) using in vitro models. In addition, PSGL-1 impact on T-cells function was evaluated by ex vivo models.
Results: Data showed PSGL-1 expression is upregulated in the T-lymphocytes from patients with severe OSA, indicating a relevant role of hypoxemia mediated by intermittent hypoxia. Besides, results suggest an inhibitory role of PSGL-1 on T-cell proliferation capacity. Finally, the expression of SIGLEC-5 but not VISTA was increased in monocytes from OSA patients, suggesting a regulatory role of intermittent hypoxia.
Discussion: In conclusion, PSGL-1 might constitute an additional immune checkpoint leading to T-cell dysfunction in OSA patients, contributing to the disruption of immune surveillance, which might provide biological plausibility to the higher incidence and aggressiveness of several tumors in these patients.
1 Introduction
Obstructive sleep apnea (OSA) is a very prevalent disorder characterized by recurrent episodes of total or partial obstruction of the upper airway during sleep. As consequence, OSA patients exhibit intermittent hypoxemia, increased inspiratory effort and sleep disruption (1–3). Besides, there is growing evidence that OSA is associated with a higher incidence of cancer, tumor aggressiveness and cancer mortality. In fact, the prevalence of cancers in OSA patients reached 1.53 (95%CI, 1.01–2.31) times higher than non-OSA individuals (4–12). In this line, several studies highlight hypoxia as main player in this context. Intermittent hypoxia is widely recognized as a primary contributor to OSA effects on tumor development and progression, enhancing cell proliferation, triggering the release of pro-angiogenic factors, and modifying the immune surveillance system (13–22). The overexpression of hypoxia-inducible factor (HIF-1α) due to intermittent hypoxia (IH) compromises the immune surveillance system by modulating various immune components, which supports the development of a tumor-promoting environment (16, 17). In fact, previous studies carried out in OSA patients demonstrate that HIF-1α triggers the production of transforming growth factor β (TGF-β). Interestingly, this factor plays a crucial role in establishing an immunosuppressive state in the monocytes and natural killer cells of OSA patients (16). Furthermore, the IH induces the expression of the immune checkpoint axis PD-1/PD-L1 (Programmed Cell Death Protein 1/Programmed Cell Death Ligand 1), impairing T-cell function (13, 22). In this line, the potential contribution of additional immune checkpoints to impaired T-cell function in OSA patients remains to be investigated.
In the recent years, immune checkpoints have emerged as major hallmarks of different types of tumors and its inhibition have revolutionized cancer treatment (23). Among the immune checkpoint family, the adhesion receptor P-selectin glycoprotein-1 (PSGL-1) has been recently identified as a key T cell-intrinsic inhibitory receptor (24). Particularly, growing evidence demonstrated that PSGL-1 signaling reduces T cell proliferation (25), activation and survival (26, 27), increases inhibitory receptor expression and dampens T cell receptor (TCR) signals and cytokine production to promote T-cell exhaustion (28). Conversely, PSGL-1 has been typically reported to facilitate adaptive responses by contributing to effector T cell recruitment through selectin binding (29). However, less is known regarding PSGL-1 ligands driving T-cell inhibitory effect (30). In fact, PSGL-1 binds additional molecules other than selectins, including chemokines (CCL19 and CCL21), the sialic acid-binding immunoglobulin-like lectin (SIGLEC)-5 and the V-domain immunoglobulin suppressor of T-cell activation (VISTA) (31–33). The role of selectins and chemokines on PSGL-1 T-cell inhibitory function has been discarded in viral infection models (24), thereby this study focuses on SIGLEC-5 and VISTA. In this context, SIGLEC-5 exerts immune inhibitory roles not only by directly inhibiting T-cell migration by blocking PSGL-1 – selectins binding, but also by reducing T cell receptor TCR-induced activation (34). Moreover, VISTA has been recently identified as a novel promising target in tumor immunotherapy and as a ligand to PSGL-1 under acidic pH-conditions, such as those occurring in the tumor microenvironment.
In this context, we have explored the PSGL-1 pathway activation in OSA patients without clinical evidence of cancer, exploring its contribution to the disruption of immune surveillance prior to tumor initiation. Here, we analyzed PSGL-1 expression in T-cells from patients with OSA and control subjects (CS), addressing its inhibitory roles on T-cell proliferation and activation by ex vivo models. Also, we explored the expression of SIGLEC-5 and VISTA as ligands in monocytes from patients with OSA and control subjects and investigated the regulatory role of intermittent hypoxia on the PSGL-1 axis using different in vitro models.
2 Methods
2.1 Study subjects
This study includes 120 OSA patients and 60 control subjects. A detailed description of selection criteria is provided in supplement online. Briefly, OSA diagnosis was determined conducting respiratory polygraphy (using Embletta GOLD, ResMed). This process included continuous monitoring of oronasal airflow, pressure, heart rate, chest and abdominal breathing patterns, and oxygen saturation (SaO2). Tests were repeated if patients reported sleeping less than 4 hours or if there was less than 5 hours of nocturnal recording. Patients were categorized as severe OSA when their Apnea-Hypopnea Index (AHI) exceeded 15. Additionally, control subjects (CS), matched for gender and age with OSA patients, were selected from the census register of the Madrid, Spain metropolitan area. Respiratory polygraphy confirmed the absence of OSA in healthy subjects. All participants provided written informed consent and the study was approved by the local ethics committees.
2.2 Human cell isolation
Peripheral blood mononuclear cells (PBMCs) were separated through centrifugation, employing a Ficoll-Paque Plus (Amersham Bioscience, Uppsala, Sweden) density gradient. Subsequently, 5×106 PBMCs were seeded into each well of 6-well plates. These cells were then cultured in Roswell Park Memorial Institute (RPMI) 1640 medium supplemented with 100 U/mL penicillin and 100μg/mL streptomycin, along with 10% fetal bovine serum (35). Finally, the cells were incubated for 16 hours at 37°C in a 5% CO2 environment.
2.3 Flow cytometry
Following a 16-hour incubation, the cells were collected and stained for 30 minutes at 4°C in darkness, using the anti-human antibodies listed in Table S1. Subsequently, they were washed with PBS (Phosphate Buffered Saline) containing 1% FBS. Lastly, the cells were acquired using a BD FACS-Celesta flow cytometer (BD-Biosciences, Eysins, Switzerland), and the data were processed with FlowJo vX.0.7 software (FlowJo, USA).
2.4 Intermittent hypoxia in vitro model
In order to generate the intermittent hypoxia (IH) conditions, we cultured healthy PBMCs in an incubation chamber linked to an external computer-controlled oxygen/nitrogen controller, using the BioSpherix OxyCycler C42 system (Redfield, NY, USA). This system produces cyclical alterations in oxygen levels while maintaining CO2 levels, regulating the air gas composition within each chamber (36, 37). In addition, to inhibit Hypoxia Inducible Factor (HIF-1α), two methods were employed: First, monocytes were treated with 30 μM PX-478 (MedKoo Biosciences, Morrisville, NC, USA) for 16 hours (38). Second, cells were transfected using a specific pre-designed silencer for HIF-1α siRNA (s6539, Ambion Inc, Austin, TX, USA) or a control plasmid following the standard protocol for Amaxa™ Human Monocyte Nucleofector Kit (Lonza, Basel, Switzerland). Briefly, cells were transferred to an electroporation cuvette and nucleofected, and cultured for 16h under routine culture conditions or IH conditions. Finally, for the DMOG assay, cells were treated with 100μM of Dimethyloxallyl Glycine (DMOG) for 16 hours in routine culture conditions.
2.5 Proliferation ex vivo assays
For the proliferation assays 5×105 PBMCs were labeled with Carboxy Fluorescein Succinimidyl Ester (CFSE, ThermoFisher, Darmstadt, Germany) and treated or not with anti-PSGL-1 antibody (Clone KPL-1, Merck Life Science ‘s, Bayswater, Victoria, Australia). The cells were cultured for 4 days and then stained with specific human antibodies for CD4 (APC) or CD8 (APC) (Inmunostep, Salamanca, Spain). Cells were acquired by flow cytometry with the FACS-Calibur flow cytometer (BD-Biosciences, Eysins, Switzerland) and data were analyzed using FlowJo vX.0.7 software (FlowJo, USA).
2.6 Statistical analysis
Comparisons between groups were conducted using Mann-Whitney U test, two-way ANOVA with post-hoc Tukey’s or Bonferroni tests, or the chi-squared test statistical methods, depending on the nature and distribution of the variables. Correlations were evaluated using Spearman’s rank correlation. To assess data distribution, the Anderson-Darling and D’Agostino-Pearson tests were employed. For all analyses, a significance level of p < 0.05 was applied. Statistical analyses were carried out using Prism 8.0 software (GraphPad, USA) or SPSS (IBM, USA).
3 Results
3.1 Characteristics of the participants
In this study, 120 patients with OSA were prospectively enrolled and 60 healthy individuals were included as the control group. The key characteristics of the study participants are presented in Table 1. Notably, there were no significant differences between the groups in relation to sex, age, BMI, or smoking habits, as detailed in Table 1.
3.2 PSGL-1 is overexpressed on T-cells from patients with OSA
As a first approach, we assessed PSGL-1 expression on T-cells. Our results show that percentage of cells that expressed high levels of PSGL-1 (PSGL-1hi, determined as shown in Figure S1A) was higher in CD4+ and CD8+ T-lymphocytes from OSA patients than in those from control subjects (Figures 1A, B). Moreover, the percentage of PSGL-1hi CD4+ T-cells was related to OSA severity parameters such as the Apnea Hypopnea Index (AHI), Oxygen Desaturation Index (ODI) and mean nocturnal oxygen saturation (mean SatO2) (Figures 1C, D, S1B). Besides, the percentage of PSGL-1hi CD8+ T-cells correlated with mean the mean SatO2 (Figure S1C), although no significant correlation was found with AHI or ODI (data not shown). Finally, PSGL-1 mRNA was overexpressed in PBMCs from OSA patients in comparison with controls subjects and negatively correlated with the mean SatO2 (Figures S1D–E). Collectively, these data suggested that PSGL-1 expression is elevated in OSA T-cells and related with hypoxia severity.
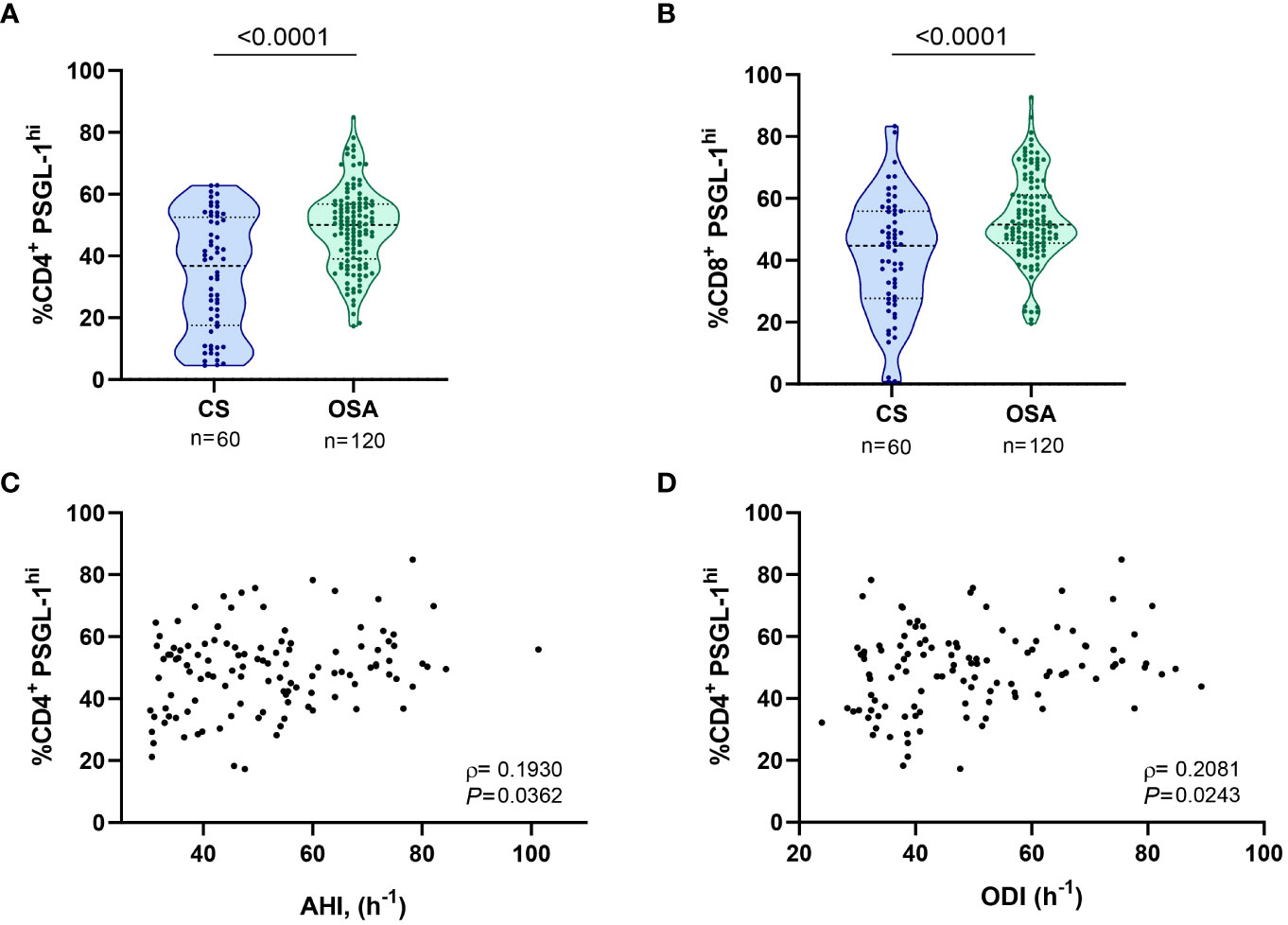
Figure 1 PSGL-1 expression in T-lymphocytes from patients with obstructive sleep apnea. (A) Percentage of CD4+ T-lymphocytes that expressed high levels of PSGL-1 (PSGL-1hi) determined by flow cytometry in control subjects (CS, n=60) and OSA patients (OSA, n=120). (B) Percentage of CD8+ T-lymphocytes that expressed high levels of PSGL-1 (PSGL-1hi) determined by flow cytometry in control subjects (CS, n=60) and OSA patients (OSA, n=120). Comparisons between groups were performed by Mann-Whitney U-test, p-values are shown. (C, D) Correlation between the percentage of PSGL-1hi CD4+ T-lymphocytes and (C) apnea-hypopnea index [AHI] (n=120) and (D) oxygen desaturation index [ODI] (n=120). Spearman’s correlation coefficients (ρ) and p-values are shown.
3.3 PSGL-1 expression on T-cells is induced by intermittent hypoxia through HIF-1α
Considering that the expression of PSGL-1 is associated to hypoxemia severity, we explore the role of intermittent hypoxia in the upregulation of PSGL-1. As a first approach, we observed that PSGL-1 expression was related to HIF-1α, the master regulator of the molecular response to hypoxia (Figure S2A), suggesting an implication of the hypoxia factor in upregulating PSGL-1. Then, we corroborate that OSA patients showed a higher mRNA expression of HIF-1α than control subjects (Figure S2B). To further assess this hypoxia role, PBMCs from healthy volunteers were cultured under normoxia or intermittent hypoxia conditions. Concomitantly, in order to clarify the effect of HIF-1α on PSGL-1 expression, we used different approaches targeting HIF-1α expression and/or activity. Firstly, we used the specific agent, PX-478 (S-2-amino-3-[4V-N,N,-bis (2-chloroethyl) amino]-Phenyl Propionic Acid N-oxide Dihydrochloride), which suppresses constitutive and hypoxia-induced levels of HIF-1α (Figure S2C). Secondly, HIF-1α expression was silenced using and specific RNA silencer for HIF-1α (siHIF) (Figure S2D). Finally, HIF-1α activity was enhanced using DMOG (dimethyloxallyl glycine), a cell permeable prolyl-4-hydroxylase inhibitor that increases endogenous HIF-1α levels as a complementary strategy (Figure S2E). Our results showed that exposure to intermittent hypoxia enhanced PSGL-1 expression in both CD4+ and CD8+ T-cells compared with the cells cultured under normoxic conditions (Figures 2A–D). Interestingly, the IH effect was suppressed either when cells were treated with PX-478 or upon HIF-1α silencing, indicating that HIF-1α mediates hypoxia-induced PSGL-1 overexpression in T-cells (Figures 2A–D). Indeed, PSGL-1 mRNA expression in healthy PBMCs was also enhanced by IH stimulation and this effect was suppressed when HIF-1α pathway was inhibited (Figures S2F–G). Also, PSGL-1 mRNA expression increases when cells are stimulated with DMOG, (Figure S2H). Taken together, these data suggest that intermittent hypoxia, through HIF-1α, mediates PSGL-1 overexpression.
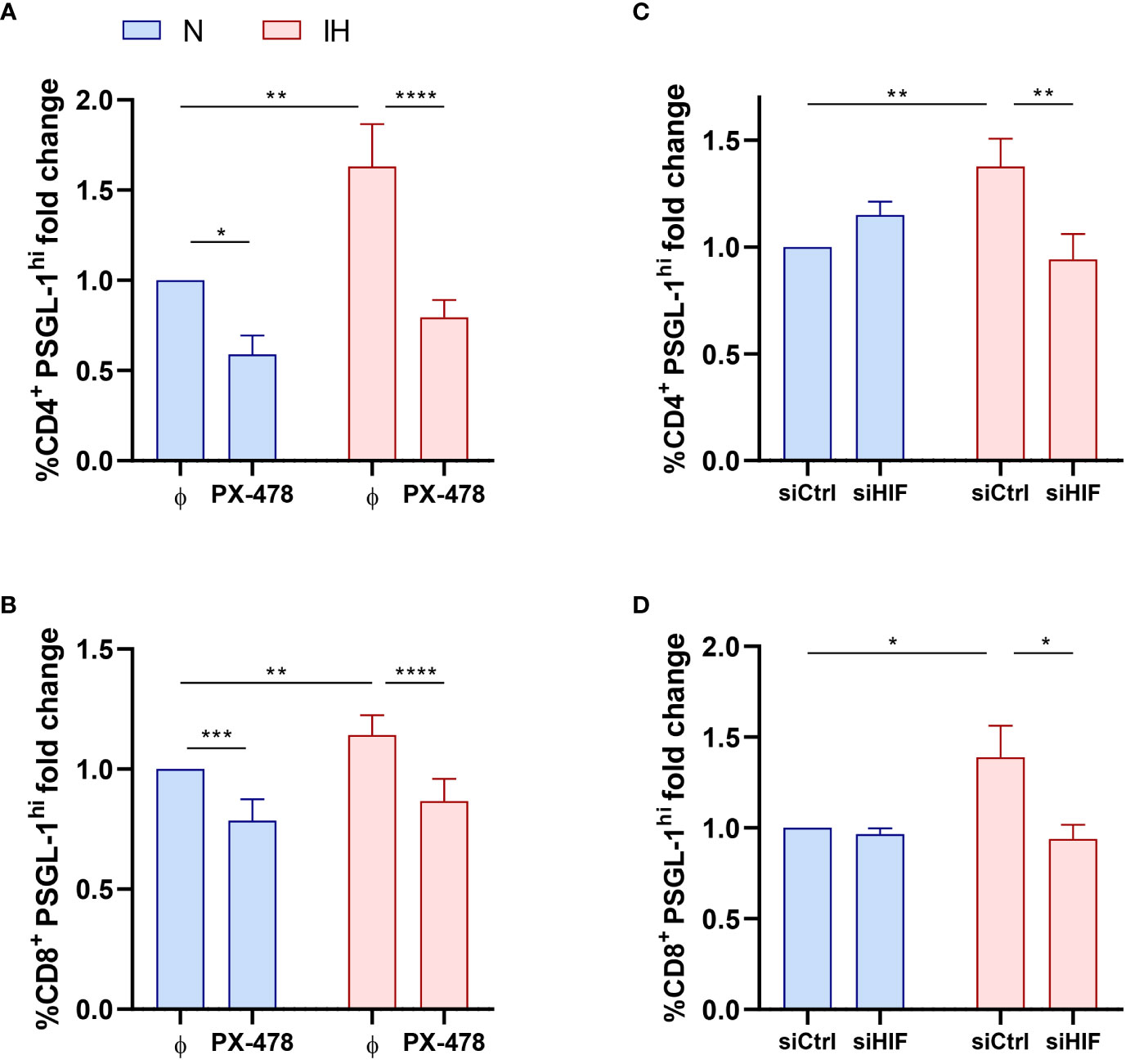
Figure 2 Intermittent hypoxia effect on PSGL-1 expression. (A, B) Fold change of the percentage of CD4+ (A) or CD8+ (B) T-lymphocytes that expressed high levels of PSGL-1 (PSGL-1hi, normalized to the control normoxia condition, CD4+: 35.64% ± 5.01%; CD8+: 48.40% ± 4.85%) determined by flow cytometry in healthy volunteer’s cells (n=9) treated or not with a specific inhibitor for HIF-1α (PX-478, 30μM) cultured under normoxia (N) or intermittent hypoxia (IH) conditions for 16 hours. (C, D) Fold change of the percentage of CD4+ (C) or CD8+ (D) T-lymphocytes that expressed high levels of PSGL-1 (PSGL-1hi, normalized to the control normoxia condition, CD4+: 23.30% ± 2.81%; CD8+: 32.51% ± 4.80%) determined by flow cytometry in healthy volunteers’ cells (n=9) transfected with a control silencing RNA (siCtrl) or with a silencing RNA targeting HIF-1α (siHIF), cultured under normoxia (N) or intermittent hypoxia (IH) conditions for 16 hours. Comparisons between groups were performed by Two-way ANOVA with Tukey’s correction for multiple comparison tests. *P<0.05, **P<0.01, ***P<0.001, ****P<0.0001.
3.4 Blocking PSGL-1 axis restores T cell proliferation in OSA patients
To assess the functional effect of PSGL-1 upregulation on T-cell function, we first evaluated the ex vivo proliferation capacity of T-cells from OSA patients and control subjects, blocking or not PSGL-1 axis with a neutralizing αPSGL-1 antibody. In this context, we observed that CD4+ T-cell proliferation was significantly impaired in OSA patients in comparison to control subjects (Figures 3A, S3A). Indeed, the percentage of proliferating T-cells negatively correlated with sleep parameters such as AHI and ODI, suggesting that the proliferation capacity of CD4+ T-cell decreases along OSA severity (Figures 3B, C). Interestingly, when OSA CD4+ T-cells were treated with αPSGL-1 antibody this effect was reverted (Figure 3A), indicating that T-cell proliferation impairment in OSA patients may be mediated by PSGL-1. Interestingly, we observed that the percentage of proliferating CD4+T-cells is inversely related to the percentage of CD4+ T-cells that expressed high levels of PSGL-1 (PSGL-1hi) in OSA patients (Figure 3D). Besides, CD8+ T-cell proliferation was also impaired in OSA patients in comparison to control subjects (Figures 3E, S3B) and the percentage of proliferating CD8+ T-cells negatively correlated with AHI and ODI (Figures 3F, G). In addition, CD8+ T-cell proliferation was restored after αPSGL-1 antibody treatment (Figure 3E) and proliferating CD8+T-cells negatively correlated with percentage of PSGL-1hi CD8+ T-cells (Figure 3H). Altogether, these data indicate that PSGL-1 dampens T-cell proliferation in OSA patients. Moreover, we also found that the percentage of T-cells that expressed high levels of PSGL-1 (PSGL-1hi) negatively correlated with plasma levels of immune mediators such as IFN-γ or TNF-α (Figures S4A–B). Finally, PSGL-1hi T-cells were related to the exhaustion marker PD-1 (Figure S4C), supporting the PSGL-1 role on T-cell function impairment in OSA patients. Altogether these data indicate that PSGL-1 could drive T-cell dysfunction in OSA patients.
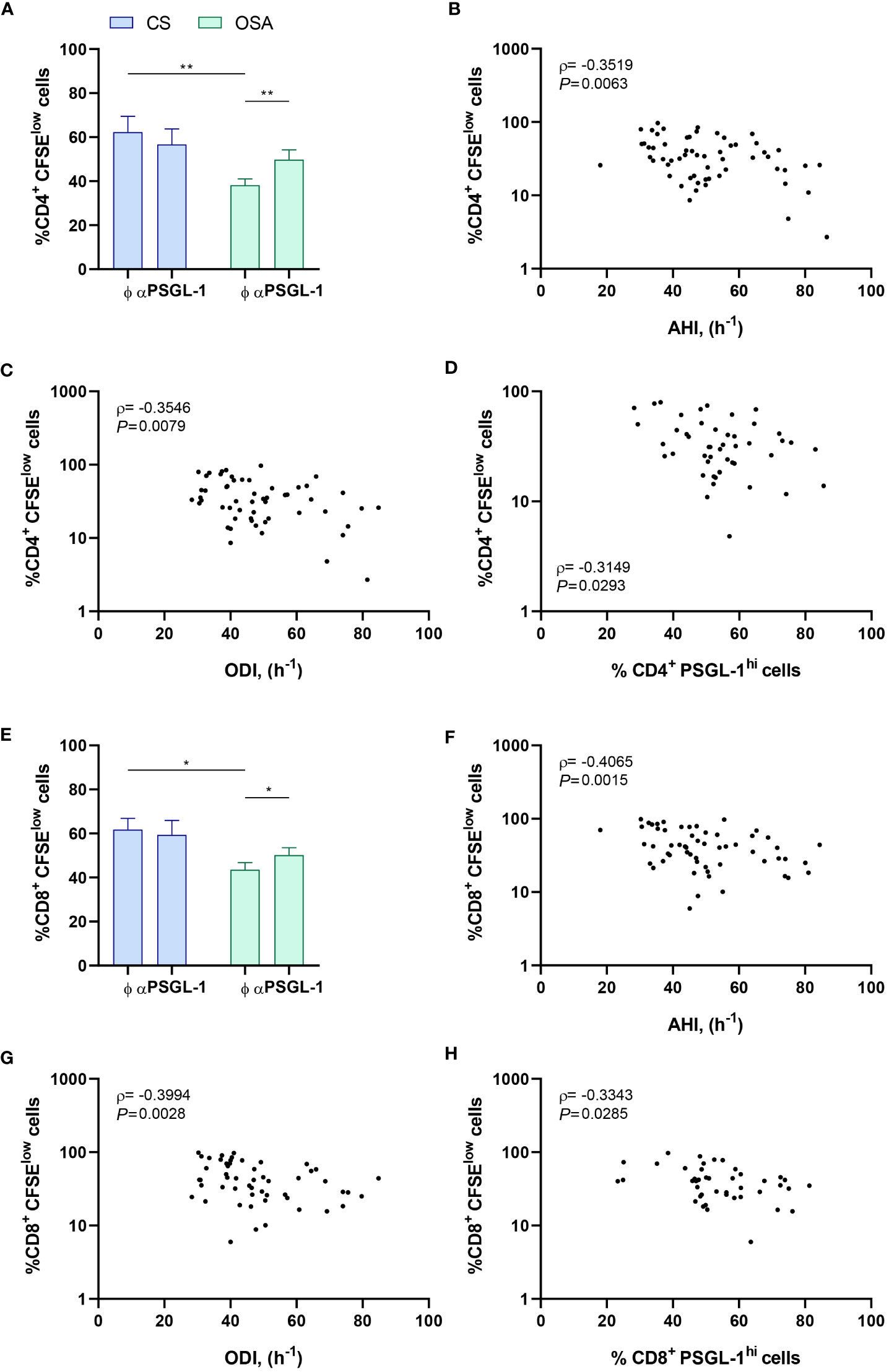
Figure 3 PSGL-1 effect on T-cell proliferation. (A) CD4+ T-lymphocytes proliferation capacity estimated by flow cytometry using carboxyfluorescein succinimidyl ester (CFSE) staining in cells from control subjects (CS, n=20) or patients with OSA (OSA, n=60) which were cultured for 4 days with or without a neutralizing α-PSGL-1 antibody. (B–D) Correlation between the percentage of proliferating (CFSElow) CD4+ T-lymphocytes and (B) apnea-hypopnea index [AHI] (n=60), (C) oxygen desaturation index [ODI] (n=60), and (D) percentage of CD4+ T-lymphocytes that expressed high levels of PSGL-1 (PSGL-1hi) determined by flow cytometry. (E) CD8+ T-lymphocytes proliferation capacity estimated by flow cytometry using CFSE staining in cells from control subjects (CS, n=20) or patients with OSA (OSA, n=60) which were cultured for 4 days with or without a neutralizing α-PSGL-1 antibody. (F–H) Correlation between the percentage of proliferating (CFSElow) CD8+ T-lymphocytes and (F) apnea-hypopnea index [AHI] (n=60), (G) oxygen desaturation index [ODI] (n=60), and (H) percentage of CD8+ T-lymphocytes that expressed high levels of PSGL-1 (PSGL-1hi) determined by flow cytometry. Comparisons between groups were performed by Two-way ANOVA with Bonferroni’s correction for multiple comparison tests. Spearman’s correlation coefficients (ρ) and p-values are shown. *P<0.05, **P<0.01.
3.5 Intermittent hypoxia induces T-cell proliferation impairment through PSGL-1 upregulation
To further check whether T-cell proliferation impairment was mediated by intermittent hypoxia and subsequent PSGL-1 overexpression; we performed an in vitro proliferation assay using T-cells from healthy volunteers. Strikingly, exposure to intermittent hypoxia dampens the proliferation of healthy CD4+ T-cells and PSGL-1 axis blockade suppressed this effect (Figure 4A). Moreover, IH also decreases CD8+ T-cell proliferation. However, although PSGL-1 axis blockade induce a tendency to proliferation recovery, it did not reach statistical significance (Figure 4B). Collectively, these results suggest that IH, through PSGL-1 overexpression contribute to dampen T-cell proliferation in OSA patients.
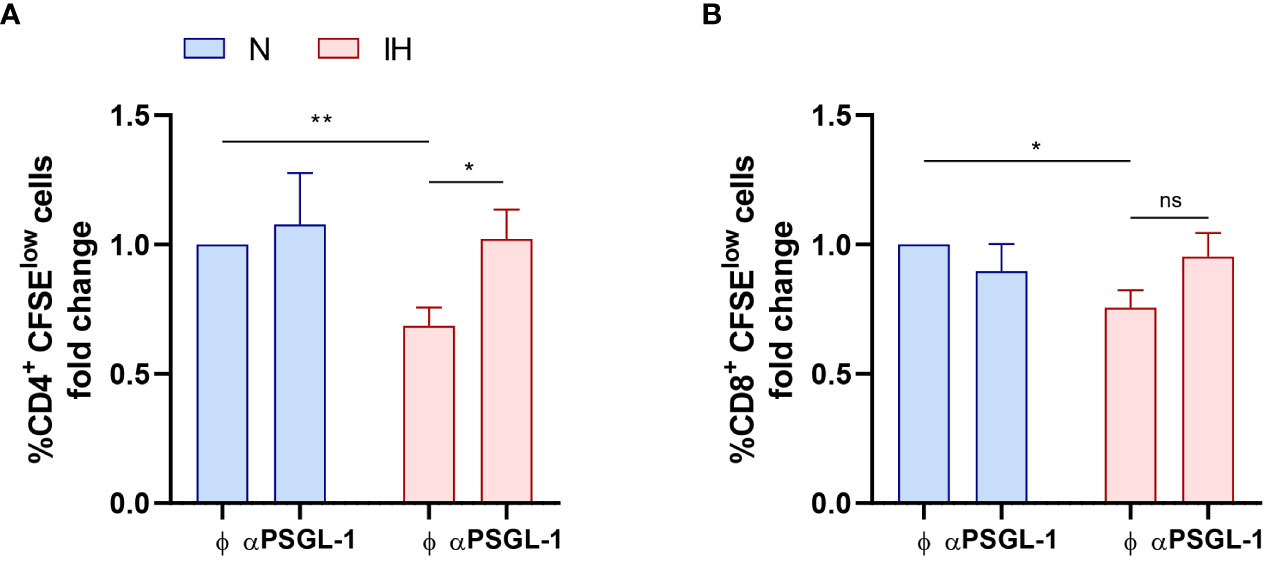
Figure 4 Intermittent hypoxia effect on T-cell proliferation. Fold change of (A) CD4+ and (B) CD8+ T-lymphocytes proliferation capacity (normalized to the control normoxia condition, CD4+: 47.13% ± 7.66%; CD8+: 64.46% ± 8.48%) estimated by flow cytometry using carboxyfluorescein succinimidyl ester (CFSE) staining in cells from healthy volunteers (n=9) which were cultured for 4 days with or without a neutralizing α-PSGL-1 antibody under normoxia (N) or intermittent hypoxia conditions (IH). Comparisons between groups were performed by Two-way ANOVA with Tukey’s correction for multiple comparison tests.*P<0.05, **P<0.01, ns, non-significant.
3.6 SIGLEC-5 is overexpressed on monocytes from patients with OSA
We then assessed the expression on monocytes of SIGLEC-5 and VISTA as potential PSGL-1 ligands driving T-cell impairment. Our data indicate an overexpression of SIGLEC-5 but not VISTA in monocytes from OSA patients in comparison to control subjects (Figures 5A, S5A, B). In this line, the expression of VISTA did not correlate with OSA severity parameters (data not shown), while the percentage of monocytes that expressed high levels of SIGLEC-5 (SIGLEC-5hi,determined as shown in Supplementary Figure 5A) positively correlated to AHI and ODI parameters, indicating that SIGLEC-5 increases along OSA severity (Figures 5B, C). Accordingly, SIGLEC-5 mRNA expression was higher in monocytes from OSA patients and negatively correlated with the mean SatO2 (Figures S5C–D). Collectively, these data suggested that SIGLEC-5 expression is elevated in OSA monocytes and related with hypoxia severity.
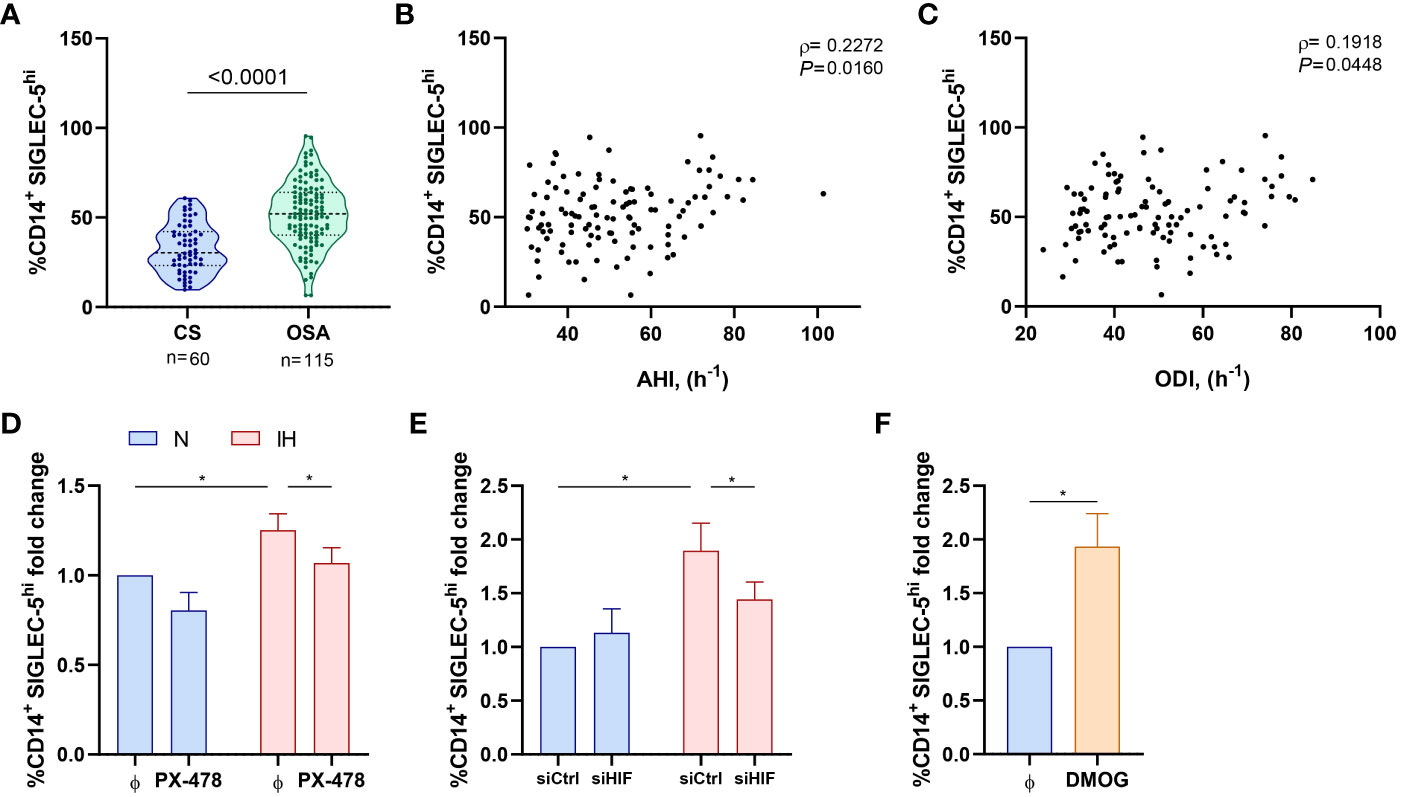
Figure 5 SIGLEC-5 is overexpressed in patients with obstructive sleep apnea. (A) Percentage of monocytes (CD14+) that expressed high levels of SIGLEC-5 (SIGLEC-5hi) determined by flow cytometry in control subjects (CS, n=60) and OSA patients (OSA, n=115). Comparison between groups was performed by Mann-Whitney U-test, p-value is shown. (B, C) Correlation between the percentage of SIGLEC-5hi monocytes and (B) apnea-hypopnea index [AHI] (n=115) and (C) oxygen desaturation index [ODI] (n=115). Spearman’s correlation coefficients (ρ) and p-values are shown. (D) Fold change of the percentage of monocytes that expressed high levels of SIGLEC-5 (SIGLEC-5hi, normalized to the control normoxia condition, 41.93% ± 7.92%) determined by flow cytometry in healthy volunteer’s cells (n=11) treated or not with a specific inhibitor for HIF-1α (PX-478, 30μM) cultured under normoxia (N) or intermittent hypoxia (IH) conditions for 16 hours. (E) Fold change of the percentage of monocytes that expressed high levels of SIGLEC-5 (SIGLEC-5hi, normalized to the control normoxia condition, 32.38% ± 4.35%) determined by flow cytometry in healthy volunteers’ cells (n=7) transfected with a control silencing RNA (siCtrl) or with a silencing RNA targeting HIF-1α (siHIF), cultured under normoxia (N) or intermittent hypoxia (IH) conditions for 16 hours. Comparisons between groups were performed by Two-way ANOVA with Tukey’s correction for multiple comparison tests. (F) Fold change of the percentage of monocytes that expressed high levels of SIGLEC-5 (SIGLEC-5hi, normalized to the control normoxia condition, 41.93% ± 7.92%) determined by flow cytometry in healthy volunteers’ cells treated or not with 100μM of dimethyloxallyl glycine (DMOG) for 16 hours in routine culture conditions (n=7) *P<0.05.
3.7 SIGLEC-5 overexpression is induced by intermittent hypoxia
Given that SIGLEC-5 is upregulated in OSA monocytes, we then explored intermittent hypoxia role in this context. Interestingly, we found that SIGLEC-5 expression was related to HIF-1α mRNA expression (Figure S5E). In this context, we explored the potential role of hypoxemia in the SIGLEC-5 upregulation, using the intermittent hypoxia in vitro model in combination with the HIF-1α inhibitor PX-478 or the specific RNA silencer (siRNA) for HIF-1α (siHIF). Our data indicate that monocytes cultured under intermittent hypoxia exhibit higher levels of SIGLEC-5 when compared to cells cultured under normoxia condition (Figures 5D, E). Interestingly, this effect was blocked when cells were treated with PX-478 or transfected with the HIF-1α siRNA (Figures 5D, E). Moreover, this effect was also observed at the mRNA level (Figures S5F–G). Finally, DMOG treatment also enhanced SIGLEC-5 expression both at the protein and mRNA level (Figures 5F, S5H). Collectively, these results suggest that intermittent hypoxia, through HIF-1α, could mediate SIGLEC-5 upregulation in OSA monocytes.
4 Discussion
The higher risk of cancer represents a major burden for sleep apnea patients. In this study, we have explored the PSGL-1 expression as an immune checkpoint and its capacity to reduce T-cell function. In addition, we analyzed the intermittent hypoxia effect on the PSGL-1 expression and function using hypoxemia clinical parameters and ex vivo and in vitro strategies. Ultimately, in order to have a complete picture of the PSGL-1 function, we analyzed known potential ligands in monocytes, however, only SIGLEC-5 was overexpressed in OSA monocytes and associated with hypoxemia conditions. Overall, this study suggests that OSA patients exhibited an impaired T cell response through PSGL-1, providing a reasonable explanation to understand the impairment of immune surveillance in these patients.
4.1 Impact of the intermittent hypoxia in PSGL-1 pathway
The hypoxemia is one of the main intermediate mechanisms of OSA. In this regard, a recent study reported that PSGL-1 expression increased susceptibility in patients with acute respiratory distress syndrome, demonstrating the PSGL-1 promoter activity was strongly regulated by HIF-1α and HIF-2α (39). Remarkably, hypoxia develops in most solid tumors because of the rapid growth of the tumor that outstrips the oxygen supply, and is one of the main hallmarks of tumor microenvironment (40). In this line, we speculate that the effect of hypoxia may directly or indirectly impact tumor growth in OSA patients. Nevertheless, additional studies are needed to understand the implications of PSGL-1 axis in the context of OSA-related tumor initiation. On the other side, Sun et al., suggest that additional inflammatory and epigenetic factors may regulate PSGL-1 expression (39). Moreover, previous studies indicate an upregulation of PSGL-1 in acute inflammation (41). In line, we previously demonstrated a systemic inflammation in OSA patients resulting from the inflammasome activation (42, 43), also, others authors reported the low-grade-chronic inflammatory state in these patients (44–49). On the other hand, there are two studies in OSA patients focusing on potential role of circulating PSGL-1, as a driver of leucocyte infiltration facilitating the development of atherosclerosis; however, assessing PSGL-1 circulating levels provided discrepant results (50, 51). Particularly, Horvath et al., reported no differences on circulating PSGL-1 levels, while Winiarska et al., concluded that circulating PSGL-1 levels were significantly increased in OSA patients and correlated with AHI. In this line, our study provides new insight by assessing PSGL-1 expression on leucocyte membrane, where it exerts its biological function (28). More importantly, the present study focused on recently described PSGL-1 immune checkpoint function, addressing its potential contribution as a negative regulator of T-cell function in OSA patients, possibly eliciting cancer development and progression.
4.2 Connections to T-cell dysfunction
Previous studies performed in animal models have shown that genetic deletion of PSGL-1 enhanced CD4+ and CD8+ T-cell responses by preventing development of exhausted T-cells, while increasing T-cell effector function and decreasing inhibitory receptor expression (24, 25, 52, 53). Moreover, many efforts have focused in unravelling the signaling pathway driving T-cell exhaustion upon PSGL-1 engagement. So far, PSGL-1 ligation on exhausted T-cells resulted in diminished ERK (extracellular signal-regulated kinases) and AKT (Protein kinase B) signaling (24), and constrains its metabolic activity, thereby, limiting anti-tumor response (54). In addition, PSGL-1 has been shown to suppress the expression of TCF1 (T cell factor 1), a transcription factor with a key role in the self-renewal, expansion, and development of effector function in T-cells. Simultaneously, PSGL-1 also enhances the expression of TOX (thymocyte selection-associated high mobility group box factor), a pivotal controller of T-cell irreversible exhaustion (54). Furthermore, PSGL-1 has been reported to act upstream of PD-1 (another immune checkpoint), requiring direct interaction with TCR to directly restrain its signaling, thus attenuating T-cell activation (54). Interestingly, PD-1/PD-L1 pathway has also been shown to be implicated in T-cell decreased proliferation in OSA subjects (13). In agreement, our results showed that although the blockage of PSGL-1 pathway significantly recovers T-cell function, is a partial recovery not reaching the control subject proliferation levels. This indicates that other pathways, such as PD-1/PD-L1 may be implicated in T-cell dysfunction in OSA patients. In agreement, recent study showed that PD-1 immune checkpoint blockade and PSGL-1 inhibition synergize to reinvigorate exhausted T cells (55). Indeed, in animal models PSGL-1 blockade reduced anti-PD-1 resistant melanoma tumor growth, one of the most common types of tumor among OSA patients (12, 54, 56). Altogether, this evidence suggests that the impairment of T- lymphocyte function in OSA patients involve the activity of both immune checkpoints, PSGL-1 and PD-1/PD-L1.
4.3 PSGL-1 ligands: VISTA
In this context, a key outstanding question is the ligand driving PSGL-1 mediated T-cell dysfunction. Therefore, we have focused on PSGL-1 ligand candidates SIGLEC-5 and VISTA, as previous studies have reported (32, 33). Interestingly, VISTA is a well-established immune regulatory receptor independently of PSGL-1 (57–59). Indeed, VISTA promotes the suppressive function of myeloid-derived suppressor cells in the tumor microenvironment dampening T-cell response (60). In this line, the role of VISTA on myeloid cells is complex and remains to be completely understood. For instance, while overexpression of VISTA on monocytes induced elevated levels of cytokine expression (61), VISTA deficient myeloid cells showed an enhanced inflammatory phenotype (62). Also, VISTA-deficient myeloid cells showed a marked dysregulation in the surface expression of chemokine receptors, and their responses to inflammatory chemokines is profoundly impaired. Altogether this evidence underscores a central function of VISTA controlling both innate and adaptive immunity (63). Besides, Deng et al., reported that VISTA is preferentially upregulated under hypoxic conditions, through direct binding of HIF-1α to VISTA gene promoter (60), which prompted us to speculate that this molecule could play a central role engaging PSGL-1 in OSA patients. Intriguingly, we did not find an upregulation of VISTA in OSA monocytes. In this line, a previous study reported a downregulation of VISTA in the microglia from patients with sepsis and in chronic multiple sclerosis lesions (64). Moreover, evidence showed that VISTA was downregulated under certain inflammatory conditions (e.g., stimulation by LPS, CFA, and poly-IC) (57, 64). Given that OSA patients exhibit a proinflammatory state, it is plausible that inflammation and hypoxia are counteracting each other, resulting in no differences in VISTA expression. Besides, current studies have shown that PSGL-1-VISTA interaction occurs in a pH-dependent manner (pH < 6.2) in vitro, so that binding in vivo has yet to be confirmed. Moreover, VISTA seems to utilize pH sensitivity to suppress T-cell function primarily in acidic and inflamed environments like tumors, rather than in lymphoid organs or the bloodstream (33). In this line, the OSA patients included in this study had no evidence of cancer at the time of recruitment, also, VISTA analysis was performed in circulating monocytes where the pH do not reach the acidic conditions as happen in tumor microenvironment (TME), so, we speculate that probably VISTA could not engage PSGL-1 under this conditions. Nevertheless, VISTA may probably function as an additional ligand for PSGL-1 under acidic TME conditions, enhancing PSGL-1 overexpression effect in OSA patients with stablished tumors. However, further evidence is needed to uncover the precise role of VISTA in this context.
4.4 PSGL-1 ligands: SIGLEC-5
Furthermore, SIGLEC-5 is upregulated in several types of tumors, including glioma and colorectal cancer and has been proposed as a prognosis marker to predict patient outcome (65, 66). Indeed, SIGLEC-5 has been reported to exert an anti-inflammatory role by directly blocking PSGL-1 interaction with selectins, hindering leukocyte infiltration (32). Additionally, SIGLEC-5 suppresses T cell activation by abrogating antigen receptor induced activation of NFAT (nuclear factor of activated T cells) and AP-1 (activating protein-1), independently of PSGL-1 (34). In turn, SIGLEC-5 also suppresses the inflammatory response of innate immune cells, such as monocytes (67–69). As a consequence, the interaction between PSGL-1 and SIGLEC-5 may not only impair T-cell function but also impact the innate subset, inducing a broad immunosuppressive state, thus facilitating tumor progression. Moreover, it has been shown that Siglec-expressing cells are specifically recruited to the TME (70), were they could fulfill specific functions in the tumor progression (71, 72). Herein, we showed that SIGLEC-5 is upregulated in OSA monocytes, also, its expression significantly increased along the hypoxemia severity. Indeed, we corroborated by in vitro assays the relevant role of the hypoxemia in this context. In agreement, previous evidence suggested that blocking SIGLEC-5 could serve as a new immune checkpoint blockade strategy to enhance anti-tumor T cell functions (34). Thus, we have performed a SIGLEC-5 ex vivo assay to explore the antigen presentation role; however, our data not show a significant effect on the lymphocyte proliferation using a functional blocking anti-SIGLEC-5 (data not shown). Furthermore, there is scarce literature assessing SIGLEC-5 interaction with PSGL-1 and its role as an immune checkpoint; probably because SIGLEC-5 is not present in mice, and much of what is known regarding PSGL-1 role in T-cell dysfunction is based on mouse models rather. Therefore, further investigations are needed either to understand SIGLEC-5 and VISTA PSGL-1 engaging; or to unravel other yet-to-be-identified ligands, which could potentially contribute to PSGL-1 mediated T-cell exhaustion.
4.5 Limitations
Our study has several limitations, which we acknowledge. First, the diagnosis of OSA in patients was based on validated respiratory polygraphy, and while we believe their clinical characterization is sufficient, this method does not enable us to evaluate the role of sleep fragmentation in the upregulation of PSGL-1. Second, while our data demonstrate that IH modulate PSGL-1 and SIGLEC-5 expression, it is predictable that other pathways contribute to modulate PSGL-1 expression. Third, although our study demonstrates an upregulation of SIGLEC-5 it does not provide information about its potential engaging PSGL-1 and/or modulating T-cell function. Fourth, on our in vitro model the intermittent hypoxia cycles are longer than those suffered by OSA patients. Fifth, our study includes patients without any evidence of cancer, so we cannot conclude PSGL-1 effect on tumor development or progression. Sixth, this study does not provide any information on the effect of OSA treatment on PSGL-1 expression or its effect on the development or progression of cancer.
4.6 Conclusions
In conclusion, this study demonstrates that PSGL-1 expression is upregulated in the lymphocytes from patients with severe OSA, indicating a relevant role of the hypoxemia by HIF-1α mediation. Furthermore, our data showed that PSGL-1 could constitute an additional immune checkpoint leading to T-cell dysfunction in OSA patients, thereby potentially contributing to the higher cancer incidence and aggressiveness. Finally, our data show an upregulation of SIGLEC-5 in OSA monocytes, suggesting it potential contribution to the PSGL-1 axis and highlighting the need of further studies assessing PSGL-1 engaging (Figure 6).
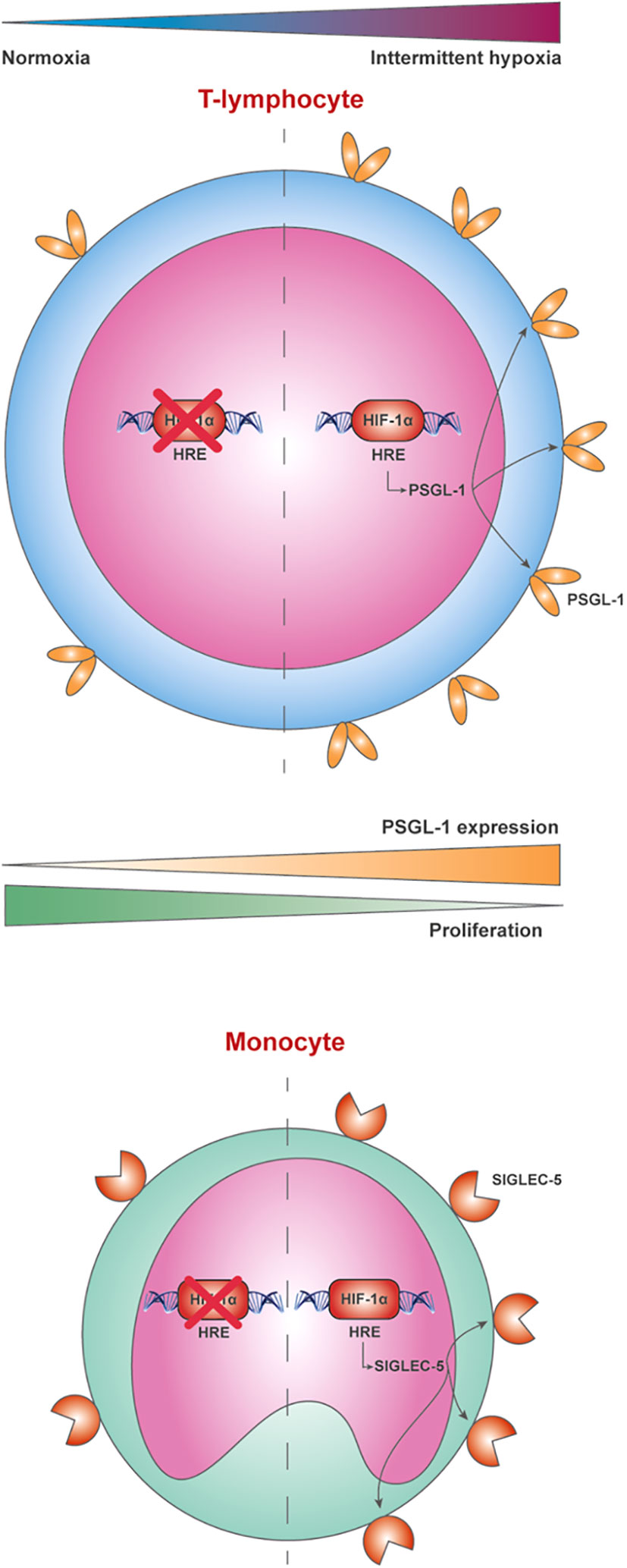
Figure 6 P-selectin glycoprotein-1 (PSGL-1) is overexpressed in lymphocytes from patients with severe OSA through a hypoxemia-dependent mechanism mediated by HIF-1α. The behavior of PSGL-1 as an immune checkpoint leading to T-cell dysfunction appears to be mediated through the expression of the sialic acid-binding immunoglobulin-like lectin (SIGLEC-5) in monocytes, which reduces T-cell proliferation, compromising the immunosurveillance system of these patients.
Data availability statement
The raw data supporting the conclusions of this article will be made available by the authors, without undue reservation.
Ethics statement
The studies involving humans were approved by CEIm Hospital Universitario La Paz (PI-3620). The studies were conducted in accordance with the local legislation and institutional requirements. The participants provided their written informed consent to participate in this study.
Author contributions
ED-G: Data curation, Formal Analysis, Investigation, Methodology, Supervision, Writing – original draft, Writing – review & editing. AG-S: Conceptualization, Data curation, Investigation, Methodology, Formal Analysis, Software, Writing – original draft, Writing – review & editing. EA: Data curation, Formal Analysis, Methodology, Writing – review & editing. CL-F: Methodology, Writing – review & editing. EM: Formal Analysis, Methodology, Writing – review & editing. IC-P: Conceptualization, Formal Analysis, Methodology, Writing – review & editing. EL-C: Conceptualization, Resources, Validation, Writing – review & editing. FG-R: Conceptualization, Formal Analysis, Funding acquisition, Investigation, Project administration, Resources, Supervision, Validation, Writing – original draft, Writing – review & editing. CC-Z: Conceptualization, Data curation, Formal Analysis, Funding acquisition, Investigation, Methodology, Project administration, Resources, Supervision, Validation, Writing – original draft, Writing – review & editing.
Funding
This study was supported by Instituto de Salud Carlos III (ISCIII) through the projects PI13/01512, PI16/00201 and PI19/01612, PI22/01262, P2022/BMD-7224 to F. García-Río and CP18/00028, PI19/01363 and PI22/01257 to C. Cubillos-Zapata; and co-funded by the European Union, Ayudas Luis Alvarez 2021 FIBHULP. CL-F was supported by Investigo technician fellowship CAM.
Acknowledgments
We thank the blood donor’s service of Hospital Universitario La Paz (Madrid, Spain) for their help in control subject recruitment.
Conflict of interest
The authors declare that the research was conducted in the absence of any commercial or financial relationships that could be construed as a potential conflict of interest.
Publisher’s note
All claims expressed in this article are solely those of the authors and do not necessarily represent those of their affiliated organizations, or those of the publisher, the editors and the reviewers. Any product that may be evaluated in this article, or claim that may be made by its manufacturer, is not guaranteed or endorsed by the publisher.
Supplementary material
The Supplementary Material for this article can be found online at: https://www.frontiersin.org/articles/10.3389/fimmu.2023.1277551/full#supplementary-material
Glossary
References
1. Veasey SC, Rosen IM. Obstructive sleep apnea in adults. N Engl J Med (2019) 380(15):1442–9. doi: 10.1056/NEJMcp1816152
2. Gottlieb DJ, Punjabi NM. Diagnosis and management of obstructive sleep apnea: A review. JAMA (2020) 323(14):1389–400. doi: 10.1001/jama.2020.3514
3. Senaratna CV, Perret JL, Lodge CJ, Lowe AJ, Campbell BE, Matheson MC, et al. Prevalence of obstructive sleep apnea in the general population: A systematic review. Sleep Med Rev (2017) 34:70–81. doi: 10.1016/j.smrv.2016.07.002
4. Nieto F, Peppard P, Young T, Finn L, Hla K, Farré R. Sleep-disordered breathing and cancer mortality: results from the Wisconsin Sleep Cohort Study. Am J Respir Crit Care Med (2012) 186(2):190–4. doi: 10.1164/rccm.201201-0130OC
5. Martinez-Garcia MA, Campos-Rodriguez F, Duran-Cantolla J, de la Pena M, Masdeu MJ, Gonzalez M, et al. Obstructive sleep apnea is associated with cancer mortality in younger patients. Sleep Med (2014) 15(7):742–8. doi: 10.1016/j.sleep.2014.01.020
6. Gozal D, Ham SA, Mokhlesi B. Sleep apnea and cancer: analysis of a nationwide population sample. Sleep (2016) 39(8):1493–500. doi: 10.5665/sleep.6004
7. Campos-Rodriguez F, Martinez-Garcia M, Martinez M, Duran-Cantolla J, Peña ML, Masdeu M, et al. Association between obstructive sleep apnea and cancer incidence in a large multicenter Spanish cohort. Am J Respir Crit Care Med (2013) 187(1):99–105. doi: 10.1164/rccm.201209-1671OC
8. Chen CY, Hu JM, Shen CJ, Chou YC, Tian YF, Chen YC, et al. Increased incidence of colorectal cancer with obstructive sleep apnea: a nationwide population-based cohort study. Sleep Med (2020) 66:15–20. doi: 10.1016/j.sleep.2019.02.016
9. Chang WP, Liu ME, Chang WC, Yang AC, Ku YC, Pai JT, et al. Sleep apnea and the subsequent risk of breast cancer in women: a nationwide population-based cohort study. Sleep Med (2014) 15(9):1016–20. doi: 10.1016/j.sleep.2014.05.026
10. Campos-Rodriguez F, Cruz-Medina A, Selma MJ, Rodriguez-de-la-Borbolla-Artacho M, Sanchez-Vega A, Ripoll-Orts F, et al. Association between sleep-disordered breathing and breast cancer aggressiveness. PloS One (2018) 13(11):e0207591. doi: 10.1371/journal.pone.0207591
11. Cabezas E, Perez-Warnisher MT, Troncoso MF, Gomez T, Melchor R, Pinillos EJ, et al. Sleep disordered breathing is highly prevalent in patients with lung cancer: results of the sleep apnea in lung cancer study. Respiration (2019) 97(2):119–24. doi: 10.1159/000492273
12. Martinez-Garcia MA, Campos-Rodriguez F, Nagore E, Martorell A, Rodriguez-Peralto JL, Riveiro-Falkenbach E, et al. Sleep-disordered breathing is independently associated with increased aggressiveness of cutaneous melanoma: A multicenter observational study in 443 patients. Chest (2018) 154(6):1348–58. doi: 10.1016/j.chest.2018.07.015
13. Cubillos-Zapata C, Avendano-Ortiz J, Hernandez-Jimenez E, Toledano V, Casas-Martin J, Varela-Serrano A, et al. Hypoxia-induced PD-L1/PD-1 crosstalk impairs T-cell function in sleep apnoea. Eur Respir J (2017) 50(4):1700833. doi: 10.1183/13993003.00833-2017
14. Martinez-Garcia MA, Campos-Rodriguez F, Farre R. Sleep apnoea and cancer: current insights and future perspectives. Eur Respir J (2012) 40(6):1315–7. doi: 10.1183/09031936.00127912
15. Cubillos-Zapata C, Díaz-García E, García-Río F. Cancer immunosurveillance in respiratory diseases. In: Martínez-García MÁ, Gaga M, Fong KM eds. Lung Diseases and Cancer (ERS Monograph). Sheffield, European Respiratory Society (2022) pp. 1––4. doi: 10.1183/2312508X.10018021
16. Hernandez-Jimenez E, Cubillos-Zapata C, Toledano V, Perez de Diego R, Fernandez-Navarro I, Casitas R, et al. Monocytes inhibit NK activity via TGF-beta in patients with obstructive sleep apnoea. Eur Respir J (2017) 49(6):1602456. doi: 10.1183/13993003.02456-2016
17. Cubillos-Zapata C, Hernandez-Jimenez E, Avendano-Ortiz J, Toledano V, Varela-Serrano A, Fernandez-Navarro I, et al. Obstructive sleep apnea monocytes exhibit high levels of vascular endothelial growth factor secretion, augmenting tumor progression. Mediators Inflamm (2018) 2018:7373921. doi: 10.1155/2018/7373921
18. Cubillos-Zapata C, Martinez-Garcia MA, Campos-Rodriguez F, Sanchez de la Torre M, Nagore E, Martorell-Calatayud A, et al. Soluble PD-L1 is a potential biomarker of cutaneous melanoma aggressiveness and metastasis in obstructive sleep apnoea patients. Eur Respir J (2019) 53(2):1801298. doi: 10.1183/13993003.01298-2018
19. Diaz-Garcia E, Garcia-Tovar S, Casitas R, Jaureguizar A, Zamarron E, Sanchez-Sanchez B, et al. Intermittent hypoxia mediates paraspeckle protein-1 upregulation in sleep apnea. Cancers (Basel) (2021) 13(15):3888. doi: 10.3390/cancers13153888
20. Cubillos-Zapata C, Martinez-Garcia MA, Diaz-Garcia E, Garcia-Tovar S, Campos-Rodriguez F, Sanchez-de-la-Torre M, et al. Obstructive sleep apnoea is related to melanoma aggressiveness through paraspeckle protein-1 upregulation. Eur Respir J (2023) 61(2):2200707. doi: 10.1183/13993003.00707-2022
21. Sanchez-de-la-Torre M, Cubillos C, Veatch OJ, Garcia-Rio F, Gozal D, Martinez-Garcia MA. Potential pathophysiological pathways in the complex relationships between OSA and cancer. Cancers (Basel) (2023) 15(4):1061. doi: 10.3390/cancers15041061
22. Cubillos-Zapata C, Balbas-Garcia C, Avendano-Ortiz J, Toledano V, Torres M, Almendros I, et al. Age-dependent hypoxia-induced PD-L1 upregulation in patients with obstructive sleep apnoea. Respirology (2019) 24(7):684–92. doi: 10.1111/resp.13470
23. Haslam A, Prasad V. Estimation of the percentage of US patients with cancer who are eligible for and respond to checkpoint inhibitor immunotherapy drugs. JAMA Netw Open (2019) 2(5):e192535. doi: 10.1001/jamanetworkopen.2019.2535
24. Tinoco R, Carrette F, Barraza ML, Otero DC, Magana J, Bosenberg MW, et al. PSGL-1 is an immune checkpoint regulator that promotes T cell exhaustion. Immunity (2016) 44(5):1190–203. doi: 10.1016/j.immuni.2016.04.015
25. Matsumoto M, Miyasaka M, Hirata T. P-selectin glycoprotein ligand-1 negatively regulates T-cell immune responses. J Immunol (2009) 183(11):7204–11. doi: 10.4049/jimmunol.0902173
26. Angiari S, Rossi B, Piccio L, Zinselmeyer BH, Budui S, Zenaro E, et al. Regulatory T cells suppress the late phase of the immune response in lymph nodes through P-selectin glycoprotein ligand-1. J Immunol (2013) 191(11):5489–500. doi: 10.4049/jimmunol.1301235
27. Perez-Frias A, Gonzalez-Tajuelo R, Nunez-Andrade N, Tejedor R, Garcia-Blanco MJ, Vicente-Rabaneda E, et al. Development of an autoimmune syndrome affecting the skin and internal organs in P-selectin glycoprotein ligand 1 leukocyte receptor-deficient mice. Arthritis Rheumatol (2014) 66(11):3178–89. doi: 10.1002/art.38808
28. Tinoco R, Otero DC, Takahashi AA, Bradley LM. PSGL-1: A new player in the immune checkpoint landscape. Trends Immunol (2017) 38(5):323–35. doi: 10.1016/j.it.2017.02.002
29. Hirata T, Merrill-Skoloff G, Aab M, Yang J, Furie BC, Furie B. P-Selectin glycoprotein ligand 1 (PSGL-1) is a physiological ligand for E-selectin in mediating T helper 1 lymphocyte migration. J Exp Med (2000) 192(11):1669–76. doi: 10.1084/jem.192.11.1669
30. Abadier M, Ley K. P-selectin glycoprotein ligand-1 in T cells. Curr Opin Hematol (2017) 24(3):265–73. doi: 10.1097/MOH.0000000000000331
31. Veerman KM, Williams MJ, Uchimura K, Singer MS, Merzaban JS, Naus S, et al. Interaction of the selectin ligand PSGL-1 with chemokines CCL21 and CCL19 facilitates efficient homing of T cells to secondary lymphoid organs. Nat Immunol (2007) 8(5):532–9. doi: 10.1038/ni1456
32. Pepin M, Mezouar S, Pegon J, Muczynski V, Adam F, Bianchini EP, et al. Soluble Siglec-5 associates to PSGL-1 and displays anti-inflammatory activity. Sci Rep (2016) 6:37953. doi: 10.1038/srep37953
33. Johnston RJ, Su LJ, Pinckney J, Critton D, Boyer E, Krishnakumar A, et al. VISTA is an acidic pH-selective ligand for PSGL-1. Nature (2019) 574(7779):565–70. doi: 10.1038/s41586-019-1674-5
34. Vuchkovska A, Glanville DG, Scurti GM, Nishimura MI, White P, Ulijasz AT, et al. Siglec-5 is an inhibitory immune checkpoint molecule for human T cells. Immunology (2022) 166(2):238–48. doi: 10.1111/imm.13470
35. Hernandez-Jimenez E, Gutierrez-Fernandez M, Cubillos-Zapata C, Otero-Ortega L, Rodriguez-Frutos B, Toledano V, et al. Circulating monocytes exhibit an endotoxin tolerance status after acute ischemic stroke: mitochondrial DNA as a putative explanation for poststroke infections. J Immunol (2017) 198(5):2038–46. doi: 10.4049/jimmunol.1601594
36. Cubillos-Zapata C, Almendros I, Diaz-Garcia E, Toledano V, Casitas R, Galera R, et al. Differential effect of intermittent hypoxia and sleep fragmentation on PD-1/PD-L1 upregulation. Sleep (2020) 43(5):zsz285. doi: 10.1093/sleep/zsz285
37. Diaz-Garcia E, Jaureguizar A, Casitas R, Garcia-Tovar S, Sanchez-Sanchez B, Zamarron E, et al. SMAD4 overexpression in patients with sleep apnoea may be associated with cardiometabolic comorbidities. J Clin Med (2020) 9(8):2378. doi: 10.3390/jcm9082378
38. Koh MY, Spivak-Kroizman T, Venturini S, Welsh S, Williams RR, Kirkpatrick DL, et al. Molecular mechanisms for the activity of PX-478, an antitumor inhibitor of the hypoxia-inducible factor-1alpha. Mol Cancer Ther (2008) 7(1):90–100. doi: 10.1158/1535-7163.MCT-07-0463
39. Sun X, Sammani S, Hufford M, Sun BL, Kempf CL, Camp SM, et al. Targeting SELPLG/P-selectin glycoprotein ligand 1 in preclinical ARDS: Genetic and epigenetic regulation of the SELPLG promoter. Pulm Circ (2023) 13(1):e12206. doi: 10.1002/pul2.12206
40. Petrova V, Annicchiarico-Petruzzelli M, Melino G, Amelio I. The hypoxic tumour microenvironment. Oncogenesis (2018) 7(1):10. doi: 10.1038/s41389-017-0011-9
41. Almulki L, Noda K, Amini R, Schering A, Garland RC, Nakao S, et al. Surprising up-regulation of P-selectin glycoprotein ligand-1 (PSGL-1) in endotoxin-induced uveitis. FASEB J (2009) 23(3):929–39. doi: 10.1096/fj.08-118760
42. Diaz-Garcia E, Garcia-Tovar S, Alfaro E, Jaureguizar A, Casitas R, Sanchez-Sanchez B, et al. Inflammasome activation: A keystone of proinflammatory response in obstructive sleep apnea. Am J Respir Crit Care Med (2022) 205(11):1337–48. doi: 10.1164/rccm.202106-1445OC
43. Diaz-Garcia E, Sanz-Rubio D, Garcia-Tovar S, Alfaro E, Cubero P, Gil AV, et al. Inflammasome activation mediated by oxidised low-density lipoprotein in patients with sleep apnoea and early subclinical atherosclerosis. Eur Respir J (2023) 61(3). doi: 10.1183/13993003.01401-2022
44. Kheirandish-Gozal L, Gozal D. Obstructive sleep apnea and inflammation: proof of concept based on two illustrative cytokines. Int J Mol Sci (2019) 20(3):459. doi: 10.3390/ijms20030459
45. Unnikrishnan D, Jun J, Polotsky V. Inflammation in sleep apnea: an update. Rev Endocr Metab Disord (2015) 16(1):25–34. doi: 10.1007/s11154-014-9304-x
46. Ryan S, Taylor CT, McNicholas WT. Predictors of elevated nuclear factor-kappaB-dependent genes in obstructive sleep apnea syndrome. Am J Respir Crit Care Med (2006) 174(7):824–30. doi: 10.1164/rccm.200601-066OC
47. Ryan S, Taylor CT, McNicholas WT. Selective activation of inflammatory pathways by intermittent hypoxia in obstructive sleep apnea syndrome. Circulation (2005) 112(17):2660–7. doi: 10.1161/CIRCULATIONAHA.105.556746
48. Garvey JF, Taylor CT, McNicholas WT. Cardiovascular disease in obstructive sleep apnoea syndrome: the role of intermittent hypoxia and inflammation. Eur Respir J (2009) 33(5):1195–205. doi: 10.1183/09031936.00111208
49. Nadeem R, Molnar J, Madbouly EM, Nida M, Aggarwal S, Sajid H, et al. Serum inflammatory markers in obstructive sleep apnea: a meta-analysis. J Clin Sleep Med (2013) 9(10):1003–12. doi: 10.5664/jcsm.3070
50. Horvath P, Lazar Z, Galffy G, Puskas R, Kunos L, Losonczy G, et al. Circulating P-selectin glycoprotein ligand 1 and P-selectin levels in obstructive sleep apnea patients. Lung (2020) 198(1):173–9. doi: 10.1007/s00408-019-00299-0
51. Winiarska HM, Cofta S, Bielawska L, Plociniczak A, Piorunek T, Wysocka E. Circulating P-selectin and its glycoprotein ligand in nondiabetic obstructive sleep apnea patients. Adv Exp Med Biol (2020) 1279:61–9. doi: 10.1007/5584_2020_501
52. Tinoco R, Neubert EN, Stairiker CJ, Henriquez ML, Bradley LM. PSGL-1 is a T cell intrinsic inhibitor that regulates effector and memory differentiation and responses during viral infection. Front Immunol (2021) 12:677824. doi: 10.3389/fimmu.2021.677824
53. Veerman KM, Carlow DA, Shanina I, Priatel JJ, Horwitz MS, Ziltener HJ. PSGL-1 regulates the migration and proliferation of CD8(+) T cells under homeostatic conditions. J Immunol (2012) 188(4):1638–46. doi: 10.4049/jimmunol.1103026
54. Hope JL, Otero DC, Bae EA, Stairiker CJ, Palete AB, Faso HA, et al. PSGL-1 attenuates early TCR signaling to suppress CD8(+) T cell progenitor differentiation and elicit terminal CD8(+) T cell exhaustion. Cell Rep (2023) 42(5):112436. doi: 10.1016/j.celrep.2023.112436
55. Viramontes KM, Neubert EN, DeRogatis JM, Tinoco R. PD-1 immune checkpoint blockade and PSGL-1 inhibition synergize to reinvigorate exhausted T cells. Front Immunol (2022) 13:869768. doi: 10.3389/fimmu.2022.869768
56. DeRogatis JM, Viramontes KM, Neubert EN, Henriquez ML, Guerrero-Juarez CF, Tinoco R. Targeting the PSGL-1 immune checkpoint promotes immunity to PD-1-resistant melanoma. Cancer Immunol Res (2022) 10(5):612–25. doi: 10.1158/2326-6066.CIR-21-0690
57. ElTanbouly MA, Zhao Y, Nowak E, Li J, Schaafsma E, Le Mercier I, et al. VISTA is a checkpoint regulator for naive T cell quiescence and peripheral tolerance. Science (2020) 367(6475):eaay0524. doi: 10.1126/science.aay0524
58. Huang X, Zhang X, Li E, Zhang G, Wang X, Tang T, et al. VISTA: an immune regulatory protein checking tumor and immune cells in cancer immunotherapy. J Hematol Oncol (2020) 13(1):83. doi: 10.1186/s13045-020-00917-y
59. Wang L, Rubinstein R, Lines JL, Wasiuk A, Ahonen C, Guo Y, et al. VISTA, a novel mouse Ig superfamily ligand that negatively regulates T cell responses. J Exp Med (2011) 208(3):577–92. doi: 10.1084/jem.20100619
60. Deng J, Li J, Sarde A, Lines JL, Lee YC, Qian DC, et al. Hypoxia-induced VISTA promotes the suppressive function of myeloid-derived suppressor cells in the tumor microenvironment. Cancer Immunol Res (2019) 7(7):1079–90. doi: 10.1158/2326-6066.CIR-18-0507
61. Bharaj P, Chahar HS, Alozie OK, Rodarte L, Bansal A, Goepfert PA, et al. Characterization of programmed death-1 homologue-1 (PD-1H) expression and function in normal and HIV infected individuals. PloS One (2014) 9(10):e109103. doi: 10.1371/journal.pone.0109103
62. Ceeraz S, Eszterhas SK, Sergent PA, Armstrong DA, Ashare A, Broughton T, et al. VISTA deficiency attenuates antibody-induced arthritis and alters macrophage gene expression in response to simulated immune complexes. Arthritis Res Ther (2017) 19(1):270. doi: 10.1186/s13075-017-1474-y
63. Broughton TWK, ElTanbouly MA, Schaafsma E, Deng J, Sarde A, Croteau W, et al. Defining the signature of VISTA on myeloid cell chemokine responsiveness. Front Immunol (2019) 10:2641. doi: 10.3389/fimmu.2019.02641
64. Borggrewe M, Grit C, Den Dunnen WFA, Burm SM, Bajramovic JJ, Noelle RJ, et al. VISTA expression by microglia decreases during inflammation and is differentially regulated in CNS diseases. Glia (2018) 66(12):2645–58. doi: 10.1002/glia.23517
65. Li GZ, Zhang KN, Wang Z, Hu HM, Wang ZL, Huang RY, et al. Siglecs, novel immunotherapy targets, potentially enhance the effectiveness of existing immune checkpoint inhibitors in glioma immunotherapy. Onco Targets Ther (2019) 12:10263–73. doi: 10.2147/OTT.S223406
66. Montalban-Hernandez K, Cantero-Cid R, Casalvilla-Duenas JC, Avendano-Ortiz J, Marin E, Lozano-Rodriguez R, et al. Colorectal cancer stem cells fuse with monocytes to form tumour hybrid cells with the ability to migrate and evade the immune system. Cancers (Basel) (2022) 14(14):3445. doi: 10.3390/cancers14143445
67. Cornish AL, Freeman S, Forbes G, Ni J, Zhang M, Cepeda M, et al. Characterization of siglec-5, a novel glycoprotein expressed on myeloid cells related to CD33. Blood (1998) 92(6):2123–32. doi: 10.1182/blood.V92.6.2123
68. Ali SR, Fong JJ, Carlin AF, Busch TD, Linden R, Angata T, et al. Siglec-5 and Siglec-14 are polymorphic paired receptors that modulate neutrophil and amnion signaling responses to group B Streptococcus. J Exp Med (2014) 211(6):1231–42. doi: 10.1084/jem.20131853
69. Nordstrom T, Movert E, Olin AI, Ali SR, Nizet V, Varki A, et al. Human Siglec-5 inhibitory receptor and immunoglobulin A (IgA) have separate binding sites in streptococcal beta protein. J Biol Chem (2011) 286(39):33981–91. doi: 10.1074/jbc.M111.251728
70. Santegoets KCM, Gielen PR, Bull C, Schulte BM, Kers-Rebel ED, Kusters B, et al. Expression profiling of immune inhibitory Siglecs and their ligands in patients with glioma. Cancer Immunol Immunother (2019) 68(6):937–49. doi: 10.1007/s00262-019-02332-w
71. Beatson R, Tajadura-Ortega V, Achkova D, Picco G, Tsourouktsoglou TD, Klausing S, et al. The mucin MUC1 modulates the tumor immunological microenvironment through engagement of the lectin Siglec-9. Nat Immunol (2016) 17(11):1273–81. doi: 10.1038/ni.3552
Keywords: PSGL-1, T-cells, immunecheckpoints, sleep apnea, intermittent hypoxia, immune surveillance, SIGLEC-5, VISTA
Citation: Díaz-García E, García-Sánchez A, Alfaro E, López-Fernández C, Mañas E, Cano-Pumarega I, López-Collazo E, García-Río F and Cubillos-Zapata C (2023) PSGL-1: a novel immune checkpoint driving T-cell dysfunction in obstructive sleep apnea. Front. Immunol. 14:1277551. doi: 10.3389/fimmu.2023.1277551
Received: 14 August 2023; Accepted: 12 September 2023;
Published: 03 October 2023.
Edited by:
Lewis Zhichang Shi, University of Alabama at Birmingham, United StatesReviewed by:
Enrique Hernández Jiménez, Institut d’Investigacio Biomedica de Bellvitge (IDIBELL), SpainHongxing Shen, University of Alabama at Birmingham, United States
Glenda Ernst, Hospital Británico de Buenos Aires, Argentina
Copyright © 2023 Díaz-García, García-Sánchez, Alfaro, López-Fernández, Mañas, Cano-Pumarega, López-Collazo, García-Río and Cubillos-Zapata. This is an open-access article distributed under the terms of the Creative Commons Attribution License (CC BY). The use, distribution or reproduction in other forums is permitted, provided the original author(s) and the copyright owner(s) are credited and that the original publication in this journal is cited, in accordance with accepted academic practice. No use, distribution or reproduction is permitted which does not comply with these terms.
*Correspondence: Carolina Cubillos-Zapata, cubilloszapata@gmail.com; Francisco García-Río, fgr01m@gmail.com