- 1Department of Urology, The Quzhou Affiliated Hospital of Wenzhou Medical University, Quzhou People’s Hospital, Quzhou, Zhejiang, China
- 2Department of Hepatology, The Quzhou Affiliated Hospital of Wenzhou Medical University, Quzhou People’s Hospital, Quzhou, Zhejiang, China
- 3Department of Urology, The First Affiliated Hospital of Wenzhou Medical University, Wenzhou, Zhejiang, China
- 4Department of Psychiatry, Shulan Quzhou Hospital, Quzhou, Zhejiang, China
Background: Telomere length (TL) has been regarded as a biomarker of aging, and TL shortening is associated with numerous chronic illnesses. The mounting evidence has shown that inflammatory cytokines are involved in maintaining or shortening TL, the causality of cytokines with TL remains unknown. Therefore, we performed a two-sample Mendelian randomization (MR) analysis to estimate the underlying correlations of circulating inflammatory cytokines with TL.
Methods: Genetic instrumental variables for inflammatory cytokines were identified through a genome-wide association study (GWAS) involving 8,293 European individuals. Summary statistics of TL were derived from a UK Bio-bank cohort comprising 472,174 samples of individuals with European descent. We employed the inverse-variance weighted (IVW) approach as our main analysis, and to ensure the reliability of our findings, we also conducted additional analyses including the weighted median, MR-Egger, MR pleiotropy residual sum and outlier test, and weighted model. Lastly, the reverse MR analyses were performed to estimate the likelihood of inverse causality between TL and the cytokines identified in the forward MR analysis. Cochran’s Q test were employed to quantify the degree of heterogeneity.
Results: After applying Bonferroni correction, a higher circulating level of Interleukin-7 (IL-7) was suggestively associated with TL maintaining (OR:1.01, 95%CI:1.00-1.02, P=0.032 by IVW method). The study also revealed suggestive evidence indicating the involvement of Interleukin-2 receptor, alpha subunit (IL-2Rα) level was negatively associated with TL maintaining (OR:0.98, 95%CI:0.96-1.00, P=0.045 by IVW method), and the weighted median approach was consistent (OR:0.99, 95%CI:0.97-1.00, P=0.035). According to the findings of reverse MR analysis, no significant causal relationship between TL and cytokines was explored. Our analysis did not reveal any substantial heterogeneity in the Single nucleotide polymorphisms or horizontal pleiotropy.
Conclusions: Our MR analysis yielded suggestive evidence supporting the causality between circulating IL-7 and IL-2Rα and telomere length, necessitating further investigations to elucidate the mechanisms by which these inflammatory cytokines may impact the progression of telomeres.
1 Introduction
Telomeres are specific nucleoprotein structures composed of repetitive TTAGGG sequences at the end of linear chromosomes (1). Due to their critical biological character in maintaining chromosomal stability and integrity, preventing coalescence of chromosomal ends, and assessing cell proliferation (2). Telomeres have been widely recognized as a reliable biomarker for assessing survival, pressure, and senility (3–5). Telomere shortening is associated with an elevated risk of mellitus (6), malignant tumor (7), cardiovascular diseases (8), and obesity (9).
Previous studies have suggested that the acceleration of telomere shortening is primarily attributed to oxidative stress and inflammation, which facilitate cell renewal and promote the duplication of senescence cells (10–12). Moreover, the process of inflammation stimulates the generation of reactive oxygen species (ROS), resulting in telomere DNA damage, which subsequently leads to a loss of replication ability and accelerates cellular aging and apoptosis. Additionally, the consequence of this is the impairment of stem and progenitor cell function, resulting in functional recession and tissue atrophy (13, 14). Recently, accumulating evidence has shown that circulating inflammatory cytokines might be correlated with shorter telomeres. M. Groer et al. reported a negative association between telomere length shortening and IL-6 levels, which serves as a representative marker for inflammatory response (15). They reported that senescent cells not only shortened telomeres, but also caused overexpression of the transcription factor nuclear factor-kB during cell senescence, and these leaded to overproduction of circulating cytokines including IL-6, TNF-α, and macrophage interferon (16). Interestingly, an association has been also observed between hypoadiponectinemia and telomere shortening in obesity (17). What’s more, the verisimilitude of telomere DNA damage being related to the ascent of CRP levels has been supported by several epidemiological data (18). Another research conducted by T. Wang et al. revealed a natural correlation between telomere shortening-induced aging and the elevation of proinflammatory cytokines levels, which in turn stimulate the development of COPD through an inflammatory response (19). Considering the susceptibility of observational studies to potential control bias and reversed causality (20), further exploration into the underlying causal correlation between circulating levels of cytokines and telomere was still required.
Mendelian randomization (MR) study is a robust approach that utilizes single nucleotide polymorphisms as instrumental variables (IVs) to assess the causal link between exposures and outcomes (21). The evidence of causality provided by the MR research was reported to lie at the interface between conventional epidemiological studies and randomized controlled trials (RCTs) (22). Recently, a meta-analysis of genome-wide association study (GWAS) was conducted to assess the genetic basis for 41 circulating cytokines, providing an opportunity to explore their potential association with telomere length. Hence, by performing a two-sample MR analysis, we systematically evaluated the underlying causal associations between circulating levels of cytokines and telomeres.
2 Materials and methods
2.1 Study design
The workflow of our study is displayed in Figure 1. The study was on a basis of publicly available data from GWAS on inflammatory cytokines and telomere length, with explicit characteristics listed in Supplementary Table S1. To enhance the reliability of the MR analysis findings, this study endeavored to fulfill the following three assumptions. Firstly, the IVs are strongly associated with circulating levels of cytokines. Secondly, no confounders are related to the IVs. Thirdly, the IVs influence the outcome only via exposure and there are no other causal pathways for the IVs to influence the outcome (23). We extracted genetic IVs for each circulating cytokine to explore the causal link from each inflammatory cytokine to telomeres. The utilization of publicly available GWAS summary datasets obviated the need for ethical approval.
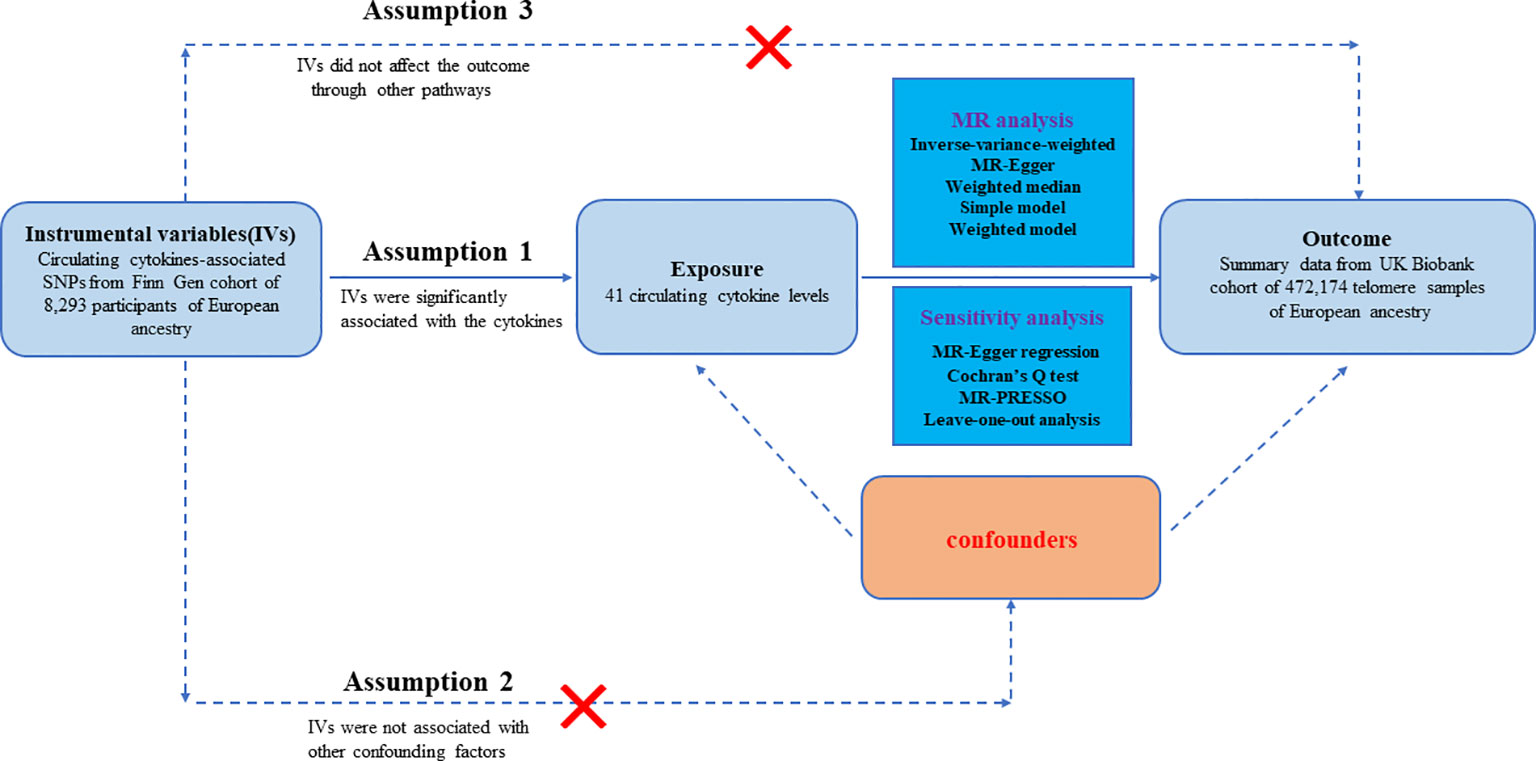
Figure 1 Datasets, assumptions, and study design of two-sample Mendelian randomization for circulating levels of 41 cytokines and telomere length.
2.2 Data sources and instruments
2.2.1 Cytokines
We collected genome-wide association summary datasets for 41 circulating inflammatory cytokines from the most proximate GWAS reported by Ahola-olli et al. (24), including 8,293 Finns from three independent cohorts: FINRISK1997, FINRISK2002, and the Young Finns Cardiovascular Risk Study (YFS). These 41 circulating cytokine distributions were normalized by converse transformation. An additive genetic model, adjusted for sex, age, body mass index (BMI), and the first ten genetic principal components, was employed to examine univariable correlations between 10.7 million genetic polymorphisms and 41 circulating cytokine concentrations.
Firstly, the genome-wide significant threshold of p<5e-08 was applied to each of the 41 circulating cytokines in order to identify robust IVs associated with their levels. Due to no or few (<3) IVs were extracted for a majority of the cytokines at the p-value<5e-08 level, we widen the threshold to p-value<5e-06 to select eligible IVs. Lastly, all 41 circulating cytokines were identified under this standard. Secondly, the impact of robust linkage disequilibrium (LD) between SNPs was mitigated by utilizing a LD threshold for the selected SNPs(r²<0.001,10000kb), ensuring independence among instrumental variables for each exposure. Thirdly, to avoid weak instrument bias, the average of SNPs F-statistics was computed (25, 26), and the F-statistics greater than 10 were regarded as robust IVs for our study. The F-statistic is a statistic approach that captures the magnitude and accuracy of the genetic effect on the trait. It can be calculated as F=R2(N-2)/(1-R2), where R2 represents the proportion of variance in the trait illuminated by the SNP, and N denotes the sample size of GWAS involving SNPs with the trait (27). Fourthly, intermediate allele frequency palindromic SNPs were excluded because allele frequencies were not provided in the GWAS of circulating cytokines, so we could not determine whether these SNPs were consistent with the direction of exposure and outcome. Additionally, to satisfy the independence assumption of MR, SNPs associated with confounders, including blood pressure, body mass index (BMI), and smoking, were excluded by applying the PhenoScannerV2 online platform. Furthermore, we performed a phenome-wide association study (including alcohol consumption, smoking, exercise, instant coffee intake, physical activity, sedentary, hypertension, atrial fibrillation, diabetes mellitus, and serum urate level, which can affect telomere length) of the instrumental variables. Finally, altogether 450 SNPs related to 41 cytokines were extracted as IVs in our study. The figure and F-statistics of the SNPs which are utilized in this study are displayed in Supplementary Table S2.
2.2.2 Telomere length
The genetically-related datasets for telomeres were selected from the publicly attainable GWAS (28), which was implemented using 488400 DNA samples from UK Biobank (UKB) participants. Mean telomere length was calculated utilizing an unequivocal quantitative PCR assay and underwent comprehensive quality inspection and technical change adjustments. Ultimately, 472,174 telomere length measurements were retained for our MR analysis. The individuals in the UK Biobank study were exclusively participants aged between 40 and 69 years, with a parallel percentage of men (45.8%) and women (54.2%). For reverse MR analyses, in all 117 genome-wide significant (p<5e-08) SNPs were authenticated for TL. Then, these IVs were fetched to assess the impact of genetic predisposition to TL on circulating cytokines.
2.3 Statistical analyses
To detect the causal effects of circulating levels of cytokines on TL by conjoining diverse SNPs, we performed a two-sample Mendelian randomization analysis employing five commonly employed analytical means, including MR-Egger, Weighted median, Inverse variance weighted (IVW), Simple mode, and Weighted mode (29, 30). The random-effects IVW approach is a dominant statistic method for aggregating Wald ratio estimations of various SNPs, exhibiting the highest statistic power among various MR methods. This method was recognized as the primary approach evaluating the underlying causal links between circulating cytokines levels and TL. Our analysis necessitated data on SNPs, alleles, effect sizes, P-values, and allele frequencies (EAF) (31). The weighted median estimator can yield reliable estimates even when incorporating 50% of the noneffective genetic instruments. Due to its widened threshold, the MR-Egger method yields valid estimates even in the presence of horizontal pleiotropy among SNPs, where all instrumental variables are invalid. Additionally, simple mode and weighted mode were also applied as auxiliary analysis methods.
2.4 Sensitivity analyses
The sensitivity analyses were executed by MR pleiotropy residual sum and outlier (MR-PRESSO), MR-Egger regression, Cochran’s Q Test, and leave-one-out analysis. Firstly, we utilized the simple-median method and weighted-median method to evaluate the underlying causal effects in situations where conventional assumptions were challenged (32). Secondly, The MR-Egger regression was conducted to assess the presence of horizontal pleiotropy, with statistical significance defined as p-values for the intercept being less than 0.05 (33). Thirdly, the MR-PRESSO global test was applied to investigate potential outliers as plausible pleiotropic biases and mitigate the impact of pleiotropy by excluding the specific SNPs that deviated from normality. Finally, the Cochran’s Q statistic was utilized for IVW and MR-Egger to estimate heterogeneity among SNPs. A p-value greater than 0.05 in the Cochran’s Q test indicated the absence of heterogeneity among the IVs (32).In addition, we further examined whether some SNPs could affect the results independently and evaluated the stability of effect sizes via leave-one-out analysis. Furthermore, the Bonferroni correction was utilized to resolve the issue of multiple comparisons, and a significance level of p<0.00024 (0.05/(41*5)) was adopted (Bonferroni correction with 41 tests* 5 models).
All the MR analysis and sensitivity analysis were performed with R (version 4.2.3). R packages “Two Sample MR” and “MR-PRESSO” packages were utilized.
3 Results
Firstly, the MR analysis was conducted by expanding the threshold to p-value<5e-06, taking account of the limited genetic variance, as well as the restricted number of SNPs and low statistical powers. By utilizing this standard (r²<0.001, p<5e-06), PhenoScannerV2 database screening, and removing the intermediate allele frequency palindromic SNPs, altogether 450 SNPs associated with 41 circulating cytokines were extracted. The F-statistics of each SNP employed in this study ranged from 20.01 to 345, indicating the robustness and strength of the IVs. In addition, we conducted a phenome-wide association study of the instrumental variables. We found no SNPs showing an association p-value<5e-06 with those relevant phenotypes affecting telomere length (including alcohol consumption, smoking, exercise, instant coffee intake, physical activity, sedentary, hypertension, atrial fibrillation, diabetes mellitus, and serum urate level), indicating that no potential confounding effect on results. The results of phenome-wide association study were shown in Figure 2.
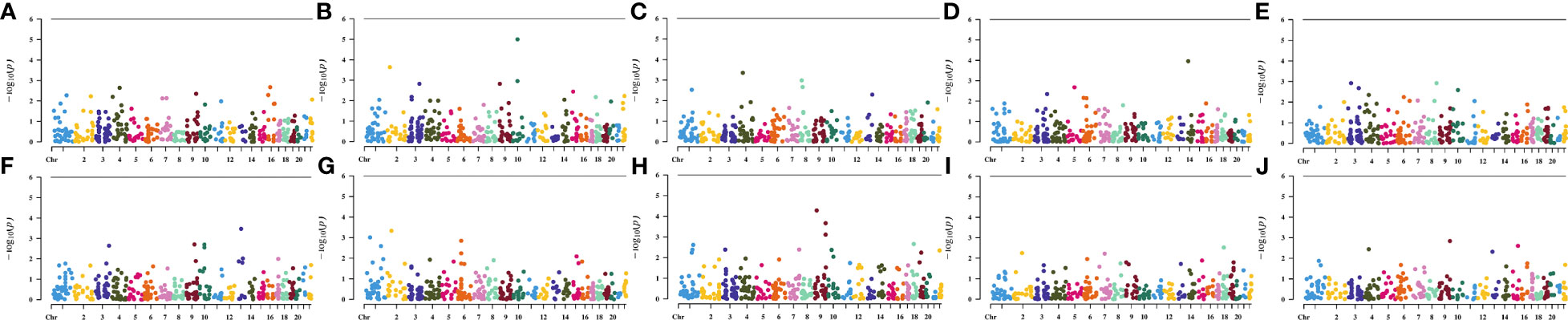
Figure 2 Manhattan plot of genetically predicted circulating levels of cytokines on relevant phenotypes affecting telomere length, (A) alcohol consumption, (B) smoking, (C) exercise, (D) instant coffee intake, (E) physical activity, (F) sedentary, (G) hypertension, (H) atrial fibrillation, (I) diabetes mellitus, and (J) serum urate.
The results from the MR analysis of the associations between 41 cytokines and TL are shown in Figure 3 and Supplementary Table S3. After applying the Bonferroni correction, only two cytokines (IL-7 and IL-2Rα) manifested suggestive associations with TL. The explicit characteristics for the associated SNPs were summarized in Supplementary Table S4. Genetically predicted elevation in circulating IL-7 levels exhibited a suggestively positive association with TL (OR:1.01, 95%CI:1.00-1.02, P=0.032 by IVW method). The findings of the alternative approach demonstrated comparable trends, although they did not reach statistical significance (OR:1.01, 95%CI:1.00-1.02, P=0.227 by Weighted median method; OR:1.00, 95%CI:0.98-1.02, P=0.783 by MR Egger method). Scatter plots of associations of genetically predicted IL-7 levels with TL were presented in Figure 4A. The leave-one-out approach was employed for sensitivity analysis and exhibited no impact in Figure 4B. The MR-Egger regression analysis did not investigate underlying directional pleiotropic effects among the SNPs (intercept p-value=0.139).
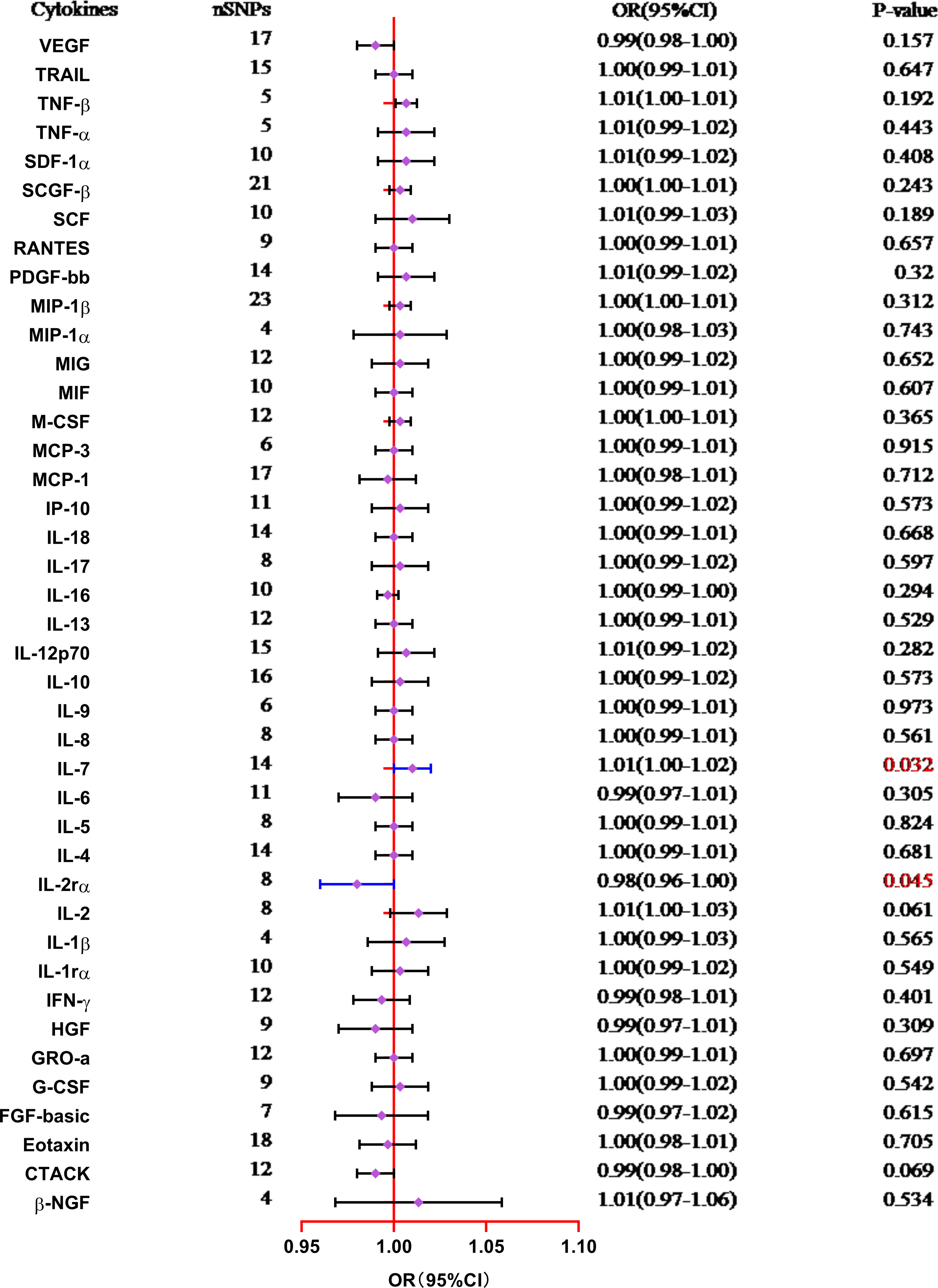
Figure 3 Forest plot of the Mendelian randomization analyses for the associations between circulating levels of cytokines and telomere length (IVW method). CI, confidence interval; OR, odds ratio; SNP, single nucleotide polymorphism.
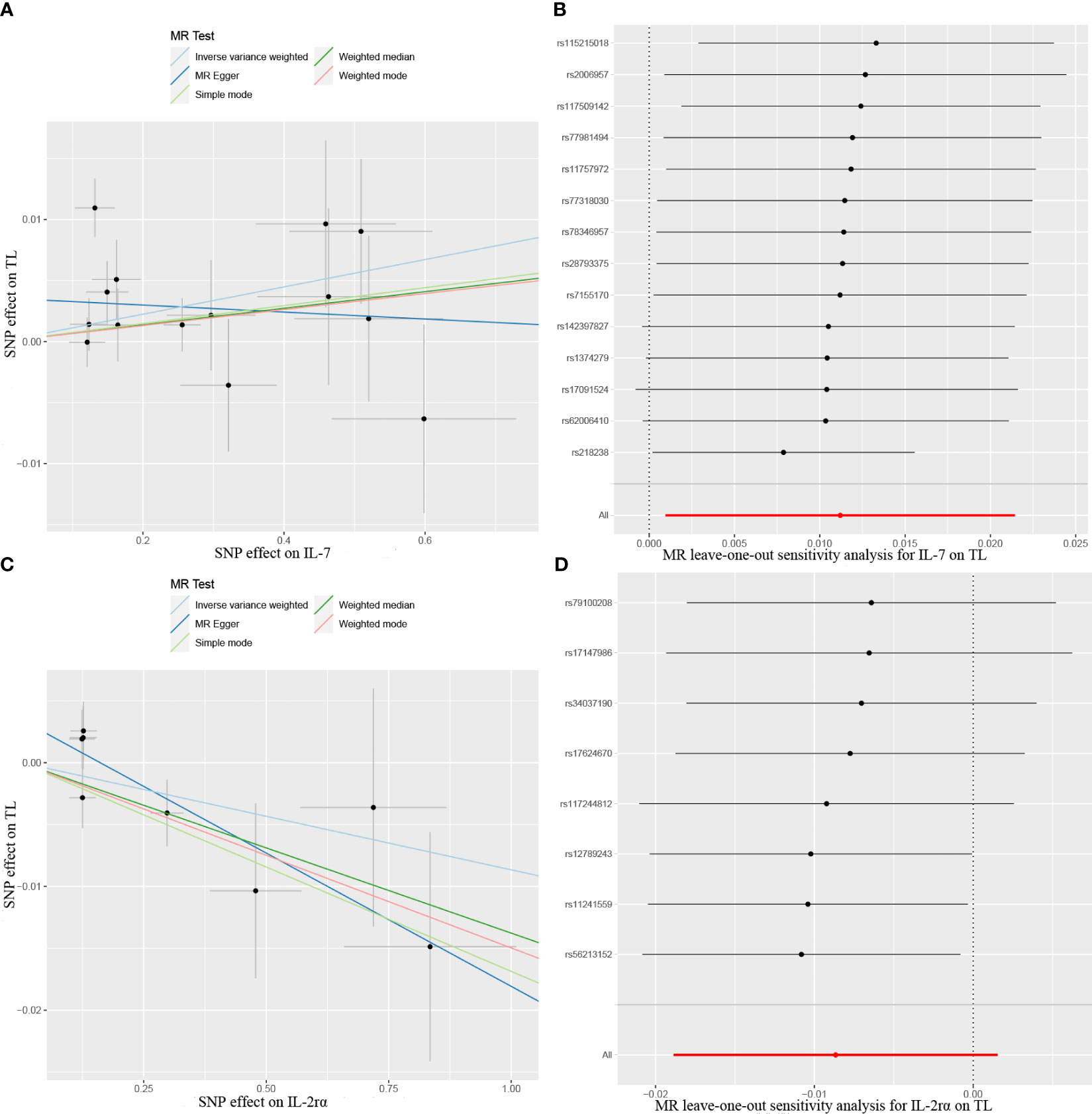
Figure 4 Scatter plots of Mendelian randomization (MR) analyses between circulating levels of cytokines and telomere length (TL), (A) IL-7; (C) IL-2rα. MR leave-one-out sensitivity analysis to assess whether every single SNP drove the causal association of cytokines on TL, (B) IL-7; (D) IL-2rα. SNP, single nucleotide polymorphism.
The findings of our study additionally manifested a suggestive inverse association between the circulating levels of IL-2Rα and TL (OR:0.98, 95%CI:0.96-1.00, P=0.045 by IVW method), and the weighted median approach was in accord with IVW method (OR:0.99, 95%CI:0.97-1.00, P=0.035), but the MR-Egger approach was non-significant (OR:0.99, 95%CI:0.98-1.00, P=0.096). Scatter plots for five methods highlighted the effect of IL-2Rα on TL in Figure 4C. Furthermore, the absence of pleiotropy was supported by the non-significant intercept observed in MR-Egger regression analysis (intercept p-value=0.113). The results of the leave-one-out method similarly indicated that none of the individual SNPs exerted significant influence on the analysis outcomes (Figure 4D).
The absence of evidence supported the lack of causal associations between other cytokines and TL (Supplementary Table S3). The MR-PRESSO global test showed no pleiotropy between the IVs and outcomes (Supplementary Table S5). Furthermore, MR-Egger and IVW Cochran’s Q tests indicated no significant heterogeneity of IVs (all p-values>0.05). The reverse MR analysis utilizing the IVW method did not identify any statistically significant associations of TL on IL-7 (OR:0.95, 95%CI:0.78-1.16, P=0.647) and IL-2Rα (OR:0.85, 95%CI:0.70-1.03, P=0.104) levels. The IVs we utilized and the outcomes of alternative approaches are documented in Supplementary Table S6.
4 Discussion
In this study, we conducted a two-sample Mendelian randomization approach to explore the underlying causal associations between circulating levels of 41 cytokines and TL. We have discovered suggestive evidence indicating a causal association between genetically predicted levels of circulating IL-7 and IL-2rα and TL. Nevertheless, we did not find any evidence indicating a causal relationship between the levels of other circulating cytokines and TL.
The cytokine IL-7 belongs to the cytokine family and possesses four anti-parallel α helixes that interact with Type I cytokine receptors. The production of this substance which plays a pivotal role in stimulating growth and maintaining homeostatic of lymphoid cells is attributed to stromal cells (34). Reports that have observed an association between IL-7 levels and maintaining telomere length are few. Previous experimental studies manifested that the cells stimulated by IL-7 or IL-15 maintain their naïve phenotype and preserve telomere length through the increased telomerase activity (35). These observational research results indicated that IL-7 was a potential protective factor for telomere length maintaining. Coincidentally, we observed a causal association between higher genetically predicted circulating IL-7 level and telomere maintenance, which aligns with previous study findings. The findings of our study were substantiated by the evidence obtained from experimental research through MR analysis. It has been reported that IL-17 exerts concentration, time, and subset-dependent effects by accelerating cell proliferation and protecting cells from apoptosis. Furthermore, long-drawn exposure of naïve CD8+ T cells to IL-7 facilitates proliferation without inducing differentiation or shortening of telomeres (35). These results suggested that IL-7 may serve as an underlying therapeutic target in maintaining telomere length, but further investigation to verify the potential biological mechanism were required.
The transmembrane protein IL-2Rα, also called CD25, is expressed on the surface of activated B cells, T cells, oligodendrocytes, myeloid precursors, and thymocytes (36). Additionally, human resting memory T cells constitutively expressed CD25 (36). The signaling pathways mediated by IL-2/IL-2Rα (CD25) are crucial in the regulation of adaptive immune reaction and the control of T cell proliferation and survival (37). Nevertheless, the epidemiological evidence for the association between IL-2Rα and telomere length was few, limited by employing a case-control study design and restricted by diminutive sample sizes. Previous research conducted by F. Albarran-Tamayo et al. (38) showed that the analysis did not reveal any significant correlation between IL-2Rα expression levels in circulation and telomere length shortening or reduced T cell proliferation. However, our results indicated that genetically predicted circulating IL-2Rα level was negatively associated with telomere length, indicating that the findings of observational studies may require validation through additional research.
In our study, the links between multiple circulating cytokines and telomere length were assessed utilizing MR analysis. Previously, S. Li et al. confirmed that the length of telomere exhibited a positive correlation with the expression levels of IL-6 and MIP-1α in bone marrow mesenchymal stem cells (MSCs) derived from patients with multiple myeloma (MM) (39). They found that the increase in telomerase activity induced by IL-6 was demonstrated to occur through AKT-mediated phosphorylation of hTERT in MM cell lines, without any variation observed in the expression of hTERT at the mRNA or protein level (40), suggesting that the biological mechanism employed by MSCs to maintain their telomere length in an inflammatory cytokine-rich microenvironment, such as IL-6, may also be utilized (39). In addition, they also reported that the contribution of MIP-1α to the progression of bone disease in MM is manifested through its promotion of tumor survival (41), inhibition of osteoblast function (42), and modulation of osteoblast differentiation (43). Moreover, the expression of MIP-1α in MM-MSCs was found to be significantly higher compared to MSCs from the control group, suggesting that MM-MSCs may play a vital role in the initiation and progression of MM (39). These results indicate that IL-6 and MIP-1α are protective factors for maintaining telomere length. However, in this study, we did not find any evidence indicating a correlation between higher genetically predicted levels of circulating IL-6 or MIP-1α and the maintenance of telomere length. Moreover, another study reported by R. M. Corbo et al. (44) showed that a significant negative linear correlation was observed between serum IL-1β levels and telomere length, after adjusting for age. Nevertheless, our study did not find a link between serum IL-1β levels and telomere length. The variations could be attributed to the diverse choice of IVs and GWAS summary data. Given the inconsistency of the results, more studies are needed to detect the accurate potential biological mechanisms of these cytokines in maintaining or shortening telomere length. For as we know, this is the first comprehensive and systematic MR analysis of the associations between circulating levels of cytokines and telomere length. Lastly, the reverse MR analysis we conducted yielded robust evidence to substantiate our primary research.
However, our study was subject to several limitations. First, the selection of IVs was conducted using a relaxed significance threshold of p<5e-06, and this might lead to consequence bias and false-positive variants. Nonetheless, the F-statistics of IVs all exceeded 10, indicating minimal presence of weak instrument bias. Similarly, several previous studies have also utilized the identical threshold (p<5e-06) when assessing the associations between inflammatory cytokines and systemic lupus erythematosus (45). Second, the participants of GWAS included in our study were limited to European descent, and it remains highly questionable whether the same results can be extrapolated to other races. Third, although pleiotropy cannot be completely ruled out, we have taken measures to exclude SNPs associated with underlying confounding factors and performed multiple sensitivity analyses using different assumptions, such as MR-Egger regression. Fourth, after applying the Bonferroni correction, no cytokines demonstrated a statistically significant correlation with telomere length, and the associations of only two cytokines (IL-7 and IL-2Rα) were found to be suggestive. Finally, MR analyses are not equal to a randomized controlled trial (RCT), the involvement of cytokines in maintaining or shortening of telomere length may not be causally established. Thus, these potential associations need to be validated in larger cohorts and the underlying involvement of cytokines in the regulation of telomere length maintenance or shortening should be further explored in future studies.
5 Conclusions
Our study provided support for the underlying causal associations between two circulating cytokines (IL-7 and IL-2Rα) and telomere length. Further investigations are warranted to verify these findings, elucidate potential biological mechanisms, and evaluate their utility as biomarkers and underlying therapeutic targets for telomere length development.
Data availability statement
The original contributions presented in the study are included in the article/Supplementary Material. Further inquiries can be directed to the corresponding authors.
Author contributions
RP: Conceptualization, Methodology, Writing – original draft. MX: Software, Writing – original draft, Funding acquisition. ZW: Visualization, Writing – review & editing. JL: Supervision, Writing – original draft. LW: Funding acquisition, Writing – review & editing.
Funding
The author(s) declare financial support was received for the research, authorship, and/or publication of this article. RP received grants from the Science and Technology Plan Project of Quzhou (ZD2020121). MX received grants from Science and Technology of Key Project of QuZhou (2023K124).
Acknowledgments
We appreciated all the genetics consortiums for making the GWAS summary data publicly available.
Conflict of interest
The authors declare that the research was conducted in the absence of any commercial or financial relationships that could be construed as a potential conflict of interest.
Publisher’s note
All claims expressed in this article are solely those of the authors and do not necessarily represent those of their affiliated organizations, or those of the publisher, the editors and the reviewers. Any product that may be evaluated in this article, or claim that may be made by its manufacturer, is not guaranteed or endorsed by the publisher.
Supplementary material
The Supplementary Material for this article can be found online at: https://www.frontiersin.org/articles/10.3389/fimmu.2023.1276257/full#supplementary-material
Glossary
References
1. Blackburn EH, Greider CW, Szostak JW. Telomeres and telomerase: the path from maize, Tetrahymena and yeast to human cancer and aging. Nat Med (2006) 12:1133–8. doi: 10.1038/nm1006-1133
2. Aksenova AY, Mirkin SM. At the beginning of the end and in the middle of the beginning: structure and maintenance of telomeric DNA repeats and interstitial telomeric sequences. Genes (Basel) (2019) 10, 1–38. doi: 10.3390/genes10020118
3. Turner KJ, Vasu V, Griffin DK. Telomere biology and human phenotype. Cells (2019) 8, 1–19. doi: 10.3390/cells8010073
4. Lin J, Epel E. Stress and telomere shortening: Insights from cellular mechanisms. Ageing Res Rev (2022) 73:101507. doi: 10.1016/j.arr.2021.101507
5. Liu J, Wang L, Wang Z, Liu JP. Roles of telomere biology in cell senescence, replicative and chronological ageing. Cells (2019) 8, 1–10. doi: 10.3390/cells8010054
6. Zee RY, Castonguay AJ, Barton NS, Germer S, Martin M. Mean leukocyte telomere length shortening and type 2 diabetes mellitus: a case-control study. Transl Res (2010) 155:166–9. doi: 10.1016/j.trsl.2009.09.012
7. Rode L, Nordestgaard BG, Bojesen SE. Long telomeres and cancer risk among 95 568 individuals from the general population. Int J Epidemiol (2016) 45:1634–43. doi: 10.1093/ije/dyw179
8. Deng Y, Li Q, Zhou F, Li G, Liu J, Lv J, et al. Telomere length and the risk of cardiovascular diseases: A Mendelian randomization study. Front Cardiovasc Med (2022) 9:1012615. doi: 10.3389/fcvm.2022.1012615
9. Kim S, Parks CG, DeRoo LA, Chen H, Taylor JA, Cawthon RM, et al. Obesity and weight gain in adulthood and telomere length. Cancer Epidemiol Biomarkers Prev (2009) 18:816–20. doi: 10.1158/1055-9965.EPI-08-0935
10. Halvorsen TL, Beattie GM, Lopez AD, Hayek A, Levine F. Accelerated telomere shortening and senescence in human pancreatic islet cells stimulated to divide in vitro. J Endocrinol (2000) 166:103–9. doi: 10.1677/joe.0.1660103
11. Kurz DJ, Decary S, Hong Y, Trivier E, Akhmedov A, Erusalimsky JD. Chronic oxidative stress compromises telomere integrity and accelerates the onset of senescence in human endothelial cells. J Cell Sci (2004) 117:2417–26. doi: 10.1242/jcs.01097
12. von Zglinicki T. Oxidative stress shortens telomeres. Trends Biochem Sci (2002) 27:339–44. doi: 10.1016/S0968-0004(02)02110-2
13. Jaskelioff M, Muller FL, Paik JH, Thomas E, Jiang S, Adams AC, et al. Telomerase reactivation reverses tissue degeneration in aged telomerase-deficient mice. Nature (2011) 469:102–6. doi: 10.1038/nature09603
14. Sahin E, Depinho RA. Linking functional decline of telomeres, mitochondria and stem cells during ageing. Nature (2010) 464:520–8. doi: 10.1038/nature08982
15. Groer M, Louis-Jacques A, Szalacha L, Redwine L, Dracxler R, Keefe D. Relationship of anxiety, inflammation, and telomere length in postpartum women: A pilot study. Biol Res Nurs (2020) 22:256–62. doi: 10.1177/1099800419890424
16. Zhang J, Rane G, Dai X, Shanmugam MK, Arfuso F, Samy RP, et al. Ageing and the telomere connection: An intimate relationship with inflammation. Ageing Res Rev (2016) 25:55–69. doi: 10.1016/j.arr.2015.11.006
17. Monickaraj F, Gokulakrishnan K, Prabu P, Sathishkumar C, Anjana RM, Rajkumar JS, et al. Convergence of adipocyte hypertrophy, telomere shortening and hypoadiponectinemia in obese subjects and in patients with type 2 diabetes. Clin Biochem (2012) 45:1432–8. doi: 10.1016/j.clinbiochem.2012.07.097
18. Harris SE, Martin-Ruiz C, von Zglinicki T, Starr JM, Deary IJ. Telomere length and aging biomarkers in 70-year-olds: the Lothian Birth Cohort 1936. Neurobiol Aging (2012) 33:1486 e3–8. doi: 10.1016/j.neurobiolaging.2010.11.013
19. Wang T, Jia Z, Li S, Li Y, Yu T, Lu T, et al. The association between leukocyte telomere length and chronic obstructive pulmonary disease is partially mediated by inflammation: a meta-analysis and population-based mediation study. BMC Pulm Med (2022) 22:320. doi: 10.1186/s12890-022-02114-8
20. Wang Q, Shi Q, Lu J, Wang Z, Hou J. Causal relationships between inflammatory factors and multiple myeloma: A bidirectional Mendelian randomization study. Int J Cancer (2022) 151:1750–9. doi: 10.1002/ijc.34214
21. Liu B, Lyu L, Zhou W, Song J, Ye D, Mao Y, et al. Associations of the circulating levels of cytokines with risk of amyotrophic lateral sclerosis: a Mendelian randomization study. BMC Med (2023) 21:39. doi: 10.1186/s12916-023-02736-7
22. Smith GD. Mendelian randomization for strengthening causal inference in observational studies: application to gene x environment interactions. Perspect Psychol Sci (2010) 5:527–45. doi: 10.1177/1745691610383505
23. Davies NM, Holmes MV, Davey Smith G. Reading Mendelian randomisation studies: a guide, glossary, and checklist for clinicians. BMJ (2018) 362:k601. doi: 10.1136/bmj.k601
24. Ahola-Olli AV, Wurtz P, Havulinna AS, Aalto K, Pitkanen N, Lehtimaki T, et al. Genome-wide association study identifies 27 loci influencing concentrations of circulating cytokines and growth factors. Am J Hum Genet (2017) 100:40–50. doi: 10.1016/j.ajhg.2016.11.007
25. Burgess S, Thompson SG, Collaboration CCG. Avoiding bias from weak instruments in Mendelian randomization studies. Int J Epidemiol (2011) 40:755–64. doi: 10.1093/ije/dyr036
26. Bowden J, Del Greco MF, Minelli C, Davey Smith G, Sheehan NA, Thompson JR. Assessing the suitability of summary data for two-sample Mendelian randomization analyses using MR-Egger regression: the role of the I2 statistic. Int J Epidemiol (2016) 45:1961–74. doi: 10.1093/ije/dyw220
27. Palmer TM, Lawlor DA, Harbord RM, Sheehan NA, Tobias JH, Timpson NJ, et al. Using multiple genetic variants as instrumental variables for modifiable risk factors. Stat Methods Med Res (2012) 21:223–42. doi: 10.1177/0962280210394459
28. Codd V, Wang Q, Allara E, Musicha C, Kaptoge S, Stoma S, et al. Polygenic basis and biomedical consequences of telomere length variation. Nat Genet (2021) 53:1425–33. doi: 10.1038/s41588-021-00944-6
29. Burgess S, Thompson SG. Interpreting findings from Mendelian randomization using the MR-Egger method. Eur J Epidemiol (2017) 32:377–89. doi: 10.1007/s10654-017-0255-x
30. Burgess S, Scott RA, Timpson NJ, Davey Smith G, Thompson SG, Consortium E-I. Using published data in Mendelian randomization: a blueprint for efficient identification of causal risk factors. Eur J Epidemiol (2015) 30:543–52. doi: 10.1007/s10654-015-0011-z
31. Choi KW, Chen CY, Stein MB, Klimentidis YC, Wang MJ, Koenen KC, et al. Major depressive disorder working group of the psychiatric genomics, assessment of bidirectional relationships between physical activity and depression among adults: A 2-sample mendelian randomization study. JAMA Psychiatry (2019) 76:399–408. doi: 10.1001/jamapsychiatry.2018.4175
32. Burgess S, Bowden J, Fall T, Ingelsson E, Thompson SG. Sensitivity analyses for robust causal inference from mendelian randomization analyses with multiple genetic variants. Epidemiology (2017) 28:30–42. doi: 10.1097/EDE.0000000000000559
33. Chang MJ, Liu MT, Chen MR, Li N, Zhao YH, Zhang SX, et al. Mendelian randomization analysis suggests no associations of herpes simplex virus infections with systemic lupus erythematosus. J Med Virol (2023) 95:e28649. doi: 10.1002/jmv.28649
34. Zhao Y, Wei K, Chi H, Xia Z, Li X. IL-7: A promising adjuvant ensuring effective T cell responses and memory in combination with cancer vaccines? Front Immunol (2022) 13:1022808. doi: 10.3389/fimmu.2022.1022808
35. Wallace DL, Berard M, Soares MV, Oldham J, Cook JE, Akbar AN, et al. Prolonged exposure of naive CD8+ T cells to interleukin-7 or interleukin-15 stimulates proliferation without differentiation or loss of telomere length. Immunology (2006) 119:243–53. doi: 10.1111/j.1365-2567.2006.02429.x
36. Chendi BH, Tveiten H, Snyders CI, Tonby K, Jenum S, Nielsen SD, et al. CCL1 and IL-2Ra differentiate Tuberculosis disease from latent infection Irrespective of HIV infection in low TB burden countries. J Infect (2021) 83:433–43. doi: 10.1016/j.jinf.2021.07.036
37. Malek TR, Bayer AL. Tolerance, not immunity, crucially depends on IL-2. Nat Rev Immunol (2004) 4:665–74. doi: 10.1038/nri1435
38. Albarran-Tamayo F, Murillo-Ortiz B, Gonzalez Amaro R, Lopez Briones S. Both in vitro T cell proliferation and telomere length are decreased, but CD25 expression and IL-2 production are not affected in aged men. Arch Med Sci (2021) 17:775–84. doi: 10.5114/aoms.2019.87593
39. Li S, Jiang Y, Li A, Liu X, Xing X, Guo Y, et al. Telomere length is positively associated with the expression of IL−6 and MIP−1alpha in bone marrow mesenchymal stem cells of multiple myeloma. Mol Med Rep (2017) 16:2497–504. doi: 10.3892/mmr.2017.6885
40. Akiyama M, Hideshima T, Hayashi T, Tai YT, Mitsiades CS, Mitsiades N, et al. Cytokines modulate telomerase activity in a human multiple myeloma cell line. Cancer Res (2002) 62:3876–82.
41. Lentzsch S, Gries M, Janz M, Bargou R, Dorken B, Mapara MY. Macrophage inflammatory protein 1-alpha (MIP-1 alpha) triggers migration and signaling cascades mediating survival and proliferation in multiple myeloma (MM) cells. Blood (2003) 101:3568–73. doi: 10.1182/blood-2002-08-2383
42. Vallet S, Pozzi S, Patel K, Vaghela N, Fulciniti MT, Veiby P, et al. A novel role for CCL3 (MIP-1alpha) in myeloma-induced bone disease via osteocalcin downregulation and inhibition of osteoblast function. Leukemia (2011) 25:1174–81. doi: 10.1038/leu.2011.43
43. Abe M, Hiura K, Wilde J, Moriyama K, Hashimoto T, Ozaki S, et al. Role for macrophage inflammatory protein (MIP)-1alpha and MIP-1beta in the development of osteolytic lesions in multiple myeloma. Blood (2002) 100:2195–202. doi: 10.1182/blood.V100.6.2195
44. Corbo RM, Businaro R, Scarabino D. Leukocyte telomere length and plasma interleukin-1beta and interleukin-18 levels in mild cognitive impairment and Alzheimer’s disease: new biomarkers for diagnosis and disease progression? Neural Regener Res (2021) 16:1397–8. doi: 10.4103/1673-5374.300986
Keywords: telomere length, cytokine, genome-wide association study, Mendelian randomization, single nucleotide polymorphisms
Citation: Pan R, Xiao M, Wu Z, Liu J and Wan L (2023) Associations of genetically predicted circulating levels of cytokines with telomere length: a Mendelian randomization study. Front. Immunol. 14:1276257. doi: 10.3389/fimmu.2023.1276257
Received: 11 August 2023; Accepted: 11 October 2023;
Published: 24 October 2023.
Edited by:
Annalisa Marcuzzi, University of Ferrara, ItalyReviewed by:
Edoardo Fiorillo, National Research Council (CNR), ItalyMaristella Steri, National Research Council (CNR), Italy
Copyright © 2023 Pan, Xiao, Wu, Liu and Wan. This is an open-access article distributed under the terms of the Creative Commons Attribution License (CC BY). The use, distribution or reproduction in other forums is permitted, provided the original author(s) and the copyright owner(s) are credited and that the original publication in this journal is cited, in accordance with accepted academic practice. No use, distribution or reproduction is permitted which does not comply with these terms.
*Correspondence: Lijun Wan, cGVyZmVjdHdsajIwMjJAMTYzLmNvbQ==; Jingwen Liu, cHN5MjAyM2xpdUAxNjMuY29t
†These authors have contributed equally to this work