- 1Department of Medicine IV, LMU University Hospital, LMU Munich, Munich, Germany
- 2Division of Clinical Pharmacology, University Hospital, LMU Munich, Munich, Germany
- 3Department of Medicine III, LMU University Hospital, LMU Munich, Munich, Germany
- 4German Cancer Consortium (DKTK), Partner Site Munich, Munich, Germany
- 5Einheit für Klinische Pharmakologie (EKLiP), Helmholtz Munich, Research Center for Environmental Health (HMGU), Neuherberg, Germany
- 6Klinik für Endokrinologie, Diabetologie und Klinische Ernährung, Universitätsspital Zürich (USZ) und Universität Zürich (UZH), Zurich, Switzerland
Introduction: Patients with primary adrenal insufficiency (PAI) suffer from increased risk of infection, adrenal crises and have a higher mortality rate. Such dismal outcomes have been inferred to immune cell dysregulation because of unphysiological cortisol replacement. As the immune landscape of patients with different types of PAI has not been systematically explored, we set out to immunophenotype PAI patients with different causes of glucocorticoid (GC) deficiency.
Methods: This cross-sectional single center study includes 28 patients with congenital adrenal hyperplasia (CAH), 27 after bilateral adrenalectomy due to Cushing’s syndrome (BADx), 21 with Addison’s disease (AD) and 52 healthy controls. All patients with PAI were on a stable GC replacement regimen with a median dose of 25 mg hydrocortisone per day. Peripheral blood mononuclear cells were isolated from heparinized blood samples. Immune cell subsets were analyzed using multicolor flow cytometry after four-hour stimulation with phorbol myristate acetate and ionomycin. Natural killer (NK-) cell cytotoxicity and clock gene expression were investigated.
Results: The percentage of T helper cell subsets was downregulated in AD patients (Th1 p = 0.0024, Th2 p = 0.0157, Th17 p < 0.0001) compared to controls. Cytotoxic T cell subsets were reduced in AD (Tc1 p = 0.0075, Tc2 p = 0.0154) and CAH patients (Tc1 p = 0.0055, Tc2 p = 0.0012) compared to controls. NKCC was reduced in all subsets of PAI patients, with smallest changes in CAH. Degranulation marker CD107a expression was upregulated in BADx and AD, not in CAH patients compared to controls (BADx p < 0.0001; AD p = 0.0002). In contrast to NK cell activating receptors, NK cell inhibiting receptor CD94 was upregulated in BADx and AD, but not in CAH patients (p < 0.0001). Although modulation in clock gene expression could be confirmed in our patient subgroups, major interindividual-intergroup dissimilarities were not detected.
Discussion: In patients with different etiologies of PAI, distinct differences in T and NK cell-phenotypes became apparent despite the use of same GC preparation and dose. Our results highlight unsuspected differences in immune cell composition and function in PAI patients of different causes and suggest disease-specific alterations that might necessitate disease-specific treatment.
Introduction
This study focuses on three different subtypes of primary adrenal insufficiency (PAI) with either an inborn lack of cortisol but maintained adrenal androgen synthesis in congenital adrenal hyperplasia (CAH) versus complete loss of adrenal function over time due to an autoimmune disorder in Addison’s disease (AD) or abruptly due to bilateral adrenalectomy (1, 2). BADx is recommended as a second-line therapy for occult or metastatic ectopic adrenocorticotropic hormone (ACTH) secretion, as a life-preserving emergency treatment or ultima ratio in patients with severe treatment-refractory ACTH-dependent Cushing’s syndrome (CS) and in the rare event of endogenous adrenal CS due to bilateral disease (3–5).
Adrenal insufficiency (AI) requires life-long glucocorticoid (GC) substitution therapy as well as stress adaptation in order to prevent life-threatening events of adrenal crises. This is usually accomplished by replacing hydrocortisone (HC) with a total daily dose of 15-25 mg divided into two to three doses with the highest in the morning to mimic the circadian rhythmicity of cortisol secretion (6–9).
The physiologic rise in serum cortisol levels as a reaction to stressful events such as systemic infection with fever can only be achieved by patients with AI by temporarily elevating their required GC dose (10). Acute deficiency of cortisol results in adrenal crisis, triggering a rise in cytokine levels and altered immune cell populations due to the missing physiological suppressive function of cortisol. This contributes to a systemic response including hypotension, hyponatremia, hyperkalemia and severe fatigue and weakness (11, 12).
Episodes of adrenal crises are common in patients with PAI ranging from 4.9-5.8/100 patient years (py) (13–15) in CAH to 8.3 and 9.3 events per 100 py in patients with AD or following BADx, respectively. This goes in hand with a higher mortality rate in PAI patients compared to healthy controls in population-based studies with increased risk ratios of 2-3 in AD (16) as well as increased hazard ratios of 2-3 in BADx (17) and CAH (18).
To unravel underlying immunological causes of the increased risk of adrenal crisis in these patients, Bancos et al. already investigated natural killer cell (NK cell) function in a cross-sectional study of 42 PAI patients. They could demonstrate an inhibition of NK cell cytotoxicity (NKCC) by means of decreased expression of NK cell activating receptors, which was linked to increased incidence of respiratory infections in this patient cohort (19). Further prospective trials demonstrated a pro-inflammatory state in PAI patients with dysregulations in immune cell profiles, such as increased numbers of classical monocytes and decreased numbers of CD16+ NK cells (20). As immune cell profiles could be restored after switch from standard GC substitution therapy to modified-release HC preparation – which more accurately mimics physiologic circadian rhythmicity of cortisol secretion – it was proposed that modulations of clock gene expression would explain these changes, which could ultimately be shown in an ancillary study (20, 21).
However, to the best of our knowledge there are no studies explicitly analyzing the specific immunophenotype in PAI patients with different causes of GC deficiency compared to healthy controls. We hypothesized that due to the nature and pathophysiology of these different causes of PAI, they might ultimately have different impact on immunophenotype beyond the sole effect of GC replacement therapy.
Methods
Subjects
In total 128 patients were recruited from the endocrine outpatient clinic of the University Hospital Munich, Germany, including 28 patients with classic CAH, 27 patients after BADx due to CS (Cushing’s disease (n=17), adrenal (n=7) and ectopic CS (n=3)), 21 patients with isolated AD (AD (n=4), autoimmune polyglandular syndrome type 2 (n=15)) and 52 age-, sex- and BMI-matched healthy controls. Of those patients with autoimmune polyglandular syndrome type 2, two patients suffered from diabetes mellitus type I and one patient from autoimmune gastritis. Therefore, the majority of patients with AD had Hashimoto’s thyroiditis (15 patients with APS2) and also some of the patients in the other cohorts suffered from Hashimoto’s thyroiditis with euthyreosis on L-thyroxin replacement (3 BADx, 4 CAH, 1 control). As exclusion of these patients in the subgroup of BADx, CAH and controls did not alter the results, they were not excluded from the analysis. All patients and controls provided written informed consent to participate in the German Cushing’s Registry (NeoExNET, ethical approval no. 152-10) or our registry and biobank for adrenal insufficiency and differences of sex development (Bio AI/DSD, ethical approval no. 19-558). Hypercortisolism was excluded in all control patients by use of recommended standard biochemical testing including low dose dexamethasone suppression test, late-night salivary cortisol and urinary free cortisol in a 24-h collection using validated assays. Adrenal insufficiency was excluded upon clinical suspicion. Exclusion criteria for healthy controls were intake of GCs in the last 12 months, current/past malignancy, autoimmune diseases other than Hashimoto’s thyroiditis or immunosuppressive/immunomodulatory medication. Patient samples were recruited during a regularly conducted follow-up visit between 8-12 am after intake of morning medication.
Isolation of immune cells
Blood samples were collected over all four seasons in all of the analyzed patient subgroups. Heparinized blood samples were processed for the isolation of peripheral blood mononuclear cells (PBMC) on the same day (withing 12 hours) to minimize apoptotic effects. We collected three tubes (each 7.5 ml) of heparinized blood from each patient to isolate PBMCs using a standardized protocol for density centrifugation. All isolated cells were cryopreserved using 10% dimethyl sulfoxide (DMSO, Sigma-Aldrich®) in fetal calf serum (FCS, Thermo Fisher Scientific®). Cells were cryopreserved at -80°C using an isopropanol freezing chamber (Mr. Frosty®, Sigma-Aldrich®) before further analysis by multicolor flow cytometry.
Thawing and staining of PBMCs
Cryopreserved PBMCs were first thawed in a 37°C water bath and washed in complete medium (450 x g, RT, 10 minutes) before incubating at 37°C and 5% CO2 for overnight rest. Before staining, samples were stimulated using working concentrations of phorbol myristate acetate (PMA)/ionomycin (eBioscienceTM Cell Stimulation Cocktail (500X), diluted 1/500 with 2µl/ml final concentration, InvitrogenTM). PMA mimics the function of diacylglycerol by diffusing into the cytoplasm and activating Protein Kinase C (PKC). In combinatory use with ionomycin, it results in a moderate level of cytokine release (22). After blocking of unspecific binding sites with Human TruStain FcX®, BioLegend®, cells were stained with appropriate anti-human antibodies or their correspondent isotype control (panels shown below) for 30 minutes at 4°C with a concentration of 0.7/100 µl.
For further intracellular staining required for the T cell panel we used the eBioscienceTM Foxp3/Transcription Factor Staining Buffer Set (Invitrogen®) according to the manufacturer’s protocol again using PMA/ionomycin as stimulating agents and Brefeldin A (Invitrogen®) as a protein transport inhibitor.
Samples were fixed using 4% paraformaldehyde (PFA), before transfer to Dulbecco’s Balanced Salt Solution (DPBS) for analysis by multicolor flow cytometry.
Assessment of T cell and NK cell subsets and surface phenotypes
We performed analysis of immune cell subsets via multicolor flow cytometry for T cell subsets under unstimulated and stimulated conditions, respectively. The T cell panel consisted of CD3-PECy5 (Clone HIT3a), CD4-BV786 (Clone OKT4), CD8-PECy7 (Clone RPA-T8), CD25-BV510 (Clone M-A251), FOXP3-BV421 (Clone 206D), IFN-γ-BV605 (Clone 4S.B3), IL-4-AF488 (MP4-25D2), IL-9-PE (Clone MH9A4), IL-17A-BV711 (Clone BL168) and IL-22-APC (Clone 2G12A41). The NK cell panel included the following antibodies: CD45-BV605 (Clone HI30), CD3-PECy5 (Clone HIT3a), CD56-FITC (Clone HCD56), CD16-BV785 (Clone 3G8), NKG2D-BV510 (Clone 1D11), NKp30-PE (Clone P30-15), NKp46-BV711 (Clone 9E2), CD107a-BV421 (Clone H4A3), NKG2A-PECy7 (Clone S19004C), CD94-PerCPCy5.5 (Clone DX22) and KIR-APC (Clone Clone HP-MA4).
All antibodies and their respective isotype controls were purchased from BioLegend®. All cytometric measurements were performed using LSFortessa II® (BD Biosciences®) and FlowJo® 10.8.1 software.
In the following, data is presented in terms of percentage of parent population.
Assessment of Natural Killer cell cytotoxicity
NKCC was evaluated in vitro by co-cultivating isolated PBMCs (effector-cells, E) with K562 cells (targeT cells, T) for 4 hours at 37°C and 5% CO2 in three replicates. Two E:T-ratios of 50:1 and 200:1 were used. K562 cells were purchased from ATCC (USA). Cells were lentivirally transduced with a pCDH-EF1a-eFly-eGFP plasmid (23) and enhanced green-fluorescent protein (eGFP) positive cells were sorted with a BD FACSAria™ III Cell Sorter. STR profiling was carried out at the Institute for forensic medicine of the LMU Munich to ensure correct origin of all cell lines. Mycoplasma contamination was excluded by polymerase chain reaction (PCR). Cells were cultured in RPMI containing 20% FBS, 2 mM L-Glutamine, 100 U/ml penicillin and 100 µg/ml streptomycin. All cells were grown at 37°C in a humidified incubator with 5% CO2. Cells were stored at -80°C or in the liquid nitrogen after addition of freezing medium (90% FCS and 10% DMSO).
The assay was performed using the Bio-GloTM Luciferase assay system (Promega®) according to the manufacturer’s protocol. TargeT cell killing is presented as specific lysis in 1 - (Luminescence of sample/negative control) x 100%.
Analysis of clock gene expression
After ribonucleic acid (RNA)-isolation using the Quick-RNA™ Miniprep Kit (Zymo Research®) and complementary deoxyribonucleic acid (cDNA) transcription, qPCR analysis was used to quantify mRNA expression of the following clock genes: circadian locomotor output cycles kaput (CLOCK), aryl hydrocarbon receptor nuclear translocator-like protein 1 (ARNTL), nuclear receptor subfamily 1 group D member 1 (NR1D1), Cryptochrome Circadian Regulator 1 (Cry1) and Cry2, WEE1 G2 Checkpoint Kinase (WEE1), Timeless Circadian Regulator (TIMELESS), CAMP Responsive Element Binding Protein 1 (CREB1) and Period Circadian Regulator 3 (PER3). Data was obtained as cycle threshold (Ct), and ΔCt = (Ct of target gene) - (Ct of β-Actin). Relative mRNA expression was expressed according to the delta delta CT method calculated by the formula 2-ΔΔCt. ΔΔCt = (ΔCt of patients) - (ΔCt of mean of controls). Some samples could not be analyzed due to missing sample volume [controls (n=32), CAH (n=24), AD (n=13), BADx (n=19)].
Statistical analysis
Based on a post-hoc analysis of previously analyzed data, with an effect size of d = 0.858 and a power of (1-β) = 0.947, we calculated that a total sample size of 30 patients is required at an α-error of 0.10 (adjusted α-error for rare diseases). For statistical analysis data was tested for normality using the Shapiro-Wilk test. Column statistics were calculated using GraphPad Prism (Mean, SEM, Quartiles). Non-normally distributed data were analyzed using Kruskal-Wallis test and Dunn’s multiple comparisons test. The confidence interval was defined as 95% and a p-value of < 0.05 was considered statistically significant P ≤ 0.05 (*), ≤ 0.01 (**), ≤ 0.001 (***), < 0.0001 (****)). Statistical analysis and graphical presentation were carried out using GraphPad Prism 7.03 and Adobe Illustrator 2020.
Results
Characteristics of study participants
As summarized in Table 1, 128 patients were recruited in our outpatient clinic including 28 patients with classic CAH due to 21-hydroxylase deficiency (71.4% females, 28.6% males), 27 after BADx (77.8% females, 22.2% males) and 21 patients with AD (66.7% females, 33.3% males) as well as 52 healthy controls (76.9% females, 23.1% males). Patients with AD [median 58 years (interquartile range (IQR) 17)] or after BADx [median 55 years (IQR 28)] did not significantly differ from controls [median 51 (IQR 29.5) years] regarding age, however patients with CAH [median 29 years (IQR 24.8)] were younger. Therefore, the CAH subgroup was split into patients above and below the age of 30 resulting in a subgroup of patients with median age of 50 years comparable to the other patient groups. As there were no differences between younger and older CAH patients regarding the results on immunophenotype data is not shown in the manuscript.
BMI did not significantly differ between the patient subgroups (Table 1). All patients were of the same ethnicity. All patients with PAI were on a stable GC replacement regimen using twice or thrice daily administration of HC. A median dose of 25 mg was used in all subtypes of PAI with no significant difference in HC/BMI or body surface area (BSA) between the subgroups.
Mineralocorticoid replacement was used in 17 (60.7%) of patients with CAH, 26 (96.3%) of BADx patients and 16 (76.2%) of patients with AD. Besides use of mineralocorticoid treatment, doses used also varied between a median of 0.05 – 0.1 mg/d as seen in Table 1.
Dysregulation of T cell subsets in different types of PAI
Analysis of T cell subsets using multicolor flow cytometry revealed a lower percentage of CD3+ T cells in BADx patients [median 53.7% (range 47.5 – 61.0%)] compared to those with CAH [median 61.5% (range 58.0 – 69.1%), p = 0.003] (compare Table 2). The percentage of CD4+ T cells was generally lower in patients with CAH [median 68.2% (range 60.7 – 75.9%), p = 0.0035] or after BADx [median 63.9% (range 49.0 – 82.7%)] compared to controls. Cytotoxic CD3+CD8+ T cells (Tc) were upregulated in patients with BADx [median 22.5% (range 13.2 – 41.7%)] compared to healthy controls [median 13.1% (range 7.8 – 16.5%), p = 0.0011].
Interferon-gamma (IFNγ) producing Th1 cells were reduced in AD patients (median 8.1% (range 4.8 – 16.4%) compared to controls (median 18.0% (range 10.4 – 28.0, p = 0.0024) as shown in Table 2 and Figure 1A. The same effect was seen in Tc1 cells (AD median 39.0% (range 26.3 – 46.5) vs. controls median 56.2% (range 36.7 – 69.2%), p = 0.0075), with however similar effects in CAH patients [median 39.2 (range 25.7 – 49.9), p = 0.0055].
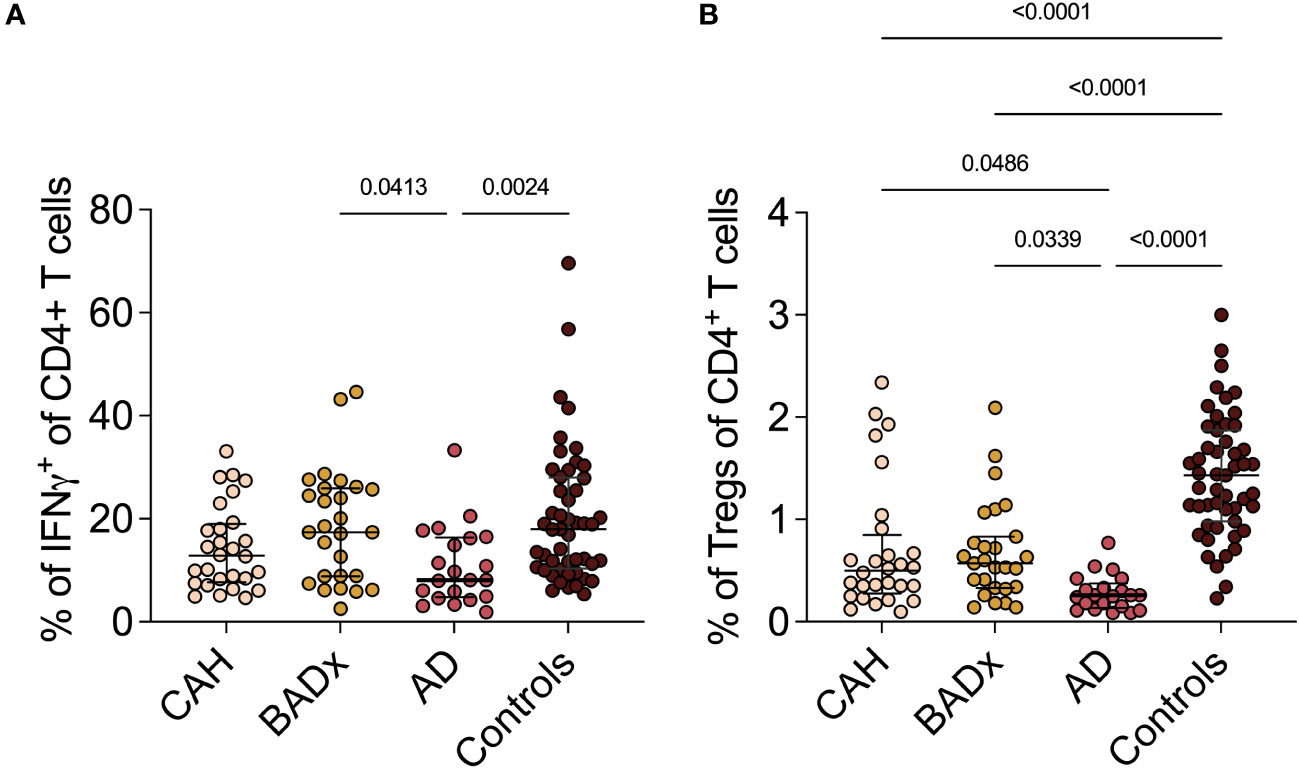
Figure 1 Dysregulation of Th1 and Treg subsets in PAI patients. Whole PBMCs were analyzed by multicolor flow cytometry for expression of different T cell subset specific cytokines. Percentage of (A) IFNγ+ Th1 cells and (B) CD3+CD4+CD25+FoxP3+ Tregs is presented in terms of percentage of parent population. Samples were analyzed after 4 hour stimulation with PMA/ionomycin. Kruskal–Wallis test and Dunn’s multiple comparisons test were used for statistical analysis. P-values are indicated in the pairwise comparison. A p-value of < 0.05 was considered statistically significant.
In the same way, the strongest reduction in Interleukin-4 (IL-4) secreting Th2 or Tc2 cells was observed in AD patients (Th1 median 2.4% (range 1.6 – 3.9%), p = 0.0157; Tc1 median 2.5% (range 1.2 – 5.9%), p = 0.0075) compared to controls (median 4.4% (range 3.0 – 6.2%); Tc1 median 6.8% (range 3.9 – 10.8%)).
The same effect could be observed regarding Interleukin-17 (IL-17) secreting Th17 and Tc17 cells. The percentage of Th17 and Tc17 cells was most reduced in the subgroup of AD patients [Th17 median 1.7% (range 1.2 – 2.4%), p < 0.0001; Tc17 median 1.9% (1.1 – 3.6%), p < 0.0001] compared to controls [Th17 median 8.7% (11.1 – 13.6%); Tc17 median 16.6% (range 13.5 – 18.9%)].
Interleukin-9 (IL-9) secreting Th9 cells were upregulated in both CAH [median 2.1% (range 0.9 – 3.8%), p < 0.0001] and BADx patients [median 3.3% (range 1.2 – 6.5%), p < 0.0001] compared to controls [median 0.2% (range 0.2 – 0.6%)] with no major difference in AD patients [median 0.5% (range 0.2 – 0.7%), p > 0.9999].
Similarly, percentage of Interleukin-22 (IL-22) positive Th22 and Tc22 cells was higher in BADx patients compared to a healthy control cohort [Th22 BADx median 2.9% (range 0.9 – 5.4%) vs. controls median 0.8% (range 0.5 – 1.2%), p = 0.0009; Tc22 BADx median 1.4% (range 0.6 – 5.3%) vs. controls median 0.5% (range 0.3 – 0.8%), p = 0.0149].
Regarding the subset of CD4+CD25+FoxP3+ regulatory T cells (Tregs), the percentage of Tregs (% of CD25+Foxp3+ cells of all CD4 + T cells) was reduced in CAH (median 0.50% (range 0.28 – 0.85%), p < 0.0001), BADx [median 0.57% (range 0.33 – 0.83), p < 0.0001] and AD patients [median 0.26% (range 0.14 – 0.38), p < 0.0001] compared to controls (median 1.43% (range 0.98- 1.87%), with the lowest percentage of Tregs present in the subgroup of AD patients (CAH vs. AD p = 0.0486 and BADx vs. AD p = 0.0339, Figure 1B).
As use of mineralocorticoid treatment and dose of fludrocortisone replacement differed between the different PAI subtypes, we also analyzed the effect on T cell subsets by correlation analysis. The presence of mineralocorticoid treatment correlated with the percentage of stimulated Th22 cells (r = 0.3239, p = 0.0043) and the dose of fludrocortisone with the percentage of stimulated Th1 cells (r = 0.2929, p = 0.0043). There was however no statistically significant correlation between HC dose and levels of T cell subsets nor between daily HC and mineralocorticoid dose used.
Dysregulation of NK cell receptor expression and impact on NK cell cytotoxicity in different types of PAI
In addition to the above illustrated effects on T cell subsets, we could show that NK cell cytotoxicity is reduced in all subsets of PAI patients using a luciferase-based killing assay (Figure 2A). While at an effector to target cell ratio of 200:1 median specific lysis was 57.5% (range 18.6 – 71.3%) in the control cohort, a median specific lysis of 21.7% (14.7 – 31.6%) was detected in the subgroup of CAH patients and even lower rates of specific lysis was observed in AD (-0.5% (range -10.8 – 4.7%)) and for patients after BADx (median -28.7% (range -37.9 – (-14.8)%). Negative values indicate insufficient killing capacity resulting in target cell replication.
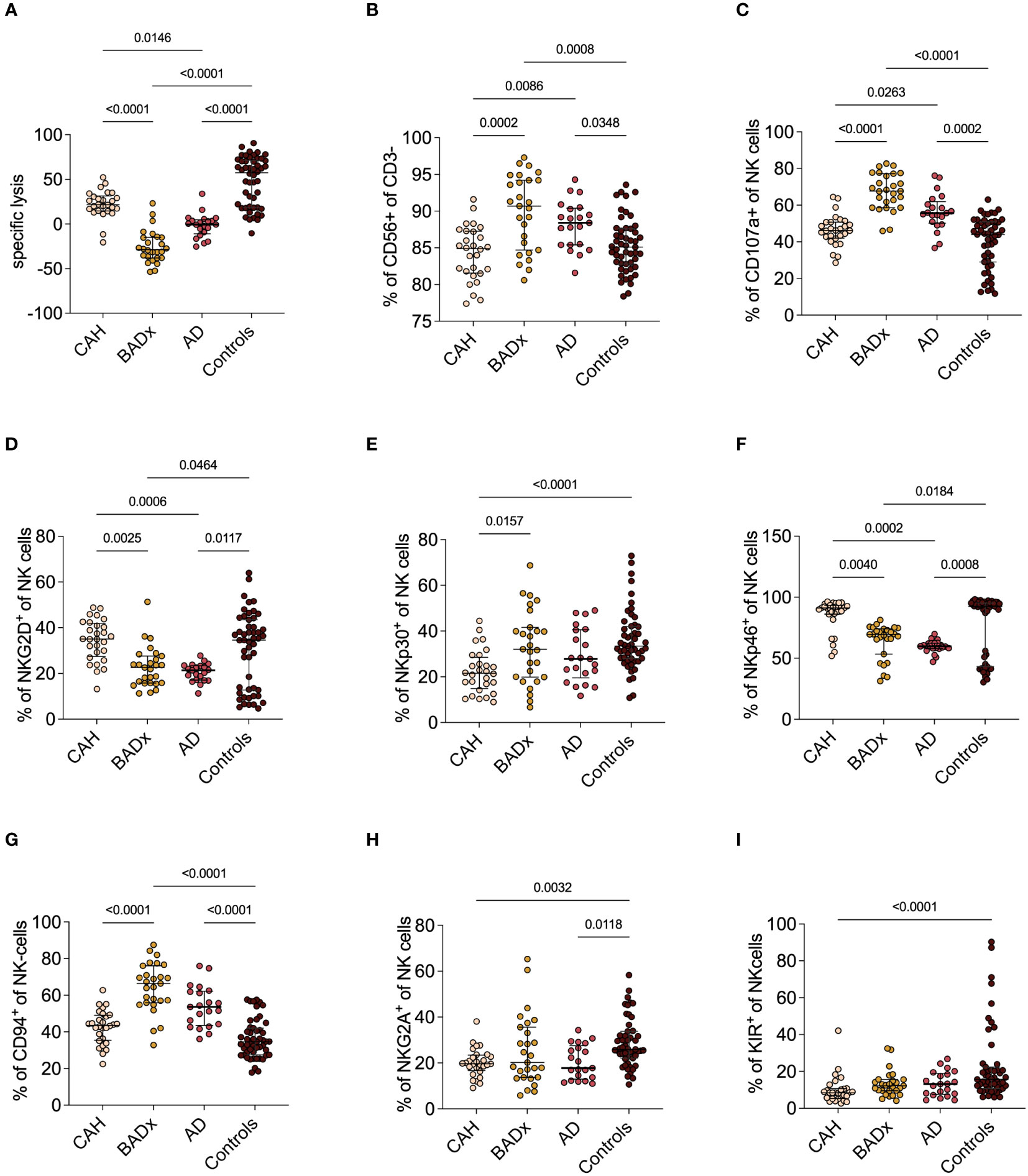
Figure 2 NK cell phenotype and function in different etiologies of PAI. (A) NKCC was analyzed using a luciferase-based killing assay including whole PBMCs as effector cells and luciferase and GFP-transduced K562 cells at an E:T of 200:1. Data presented in terms of specific lysis (luminescence of sample/positive control). (B-I) Whole PBMC samples were analyzed by multicolor flow cytometry for expression of different NK cell markers, including analysis of whole percentage of (B) CD3-CD56+ NK cells, (C) percentage expression of degranulation marker CD107a (C-F) percentage expression of activating receptors NKG2D, NKp30 and NKp46 and (G-I) percentage expression of inhibiting receptors CD94, NKG2A and KIR. Data is presented in terms of percentage of parent population. Samples were analyzed after 4 hour stimulation with PMA/ionomycin. Kruskal–Wallis test and Dunn’s multiple comparisons test were used for statistical analysis. P-values are indicated in the pairwise comparison. A p-value of < 0.05 was considered statistically significant.
At the same time, we could observe an upregulation of the percentage of NK cells compared to controls (BADx median 90.7% (range 84.7 – 94.2%), p = 0.0008; AD median 88.4% (range 85.4 – 90.4%), p = 0.0348; controls median 85.1% (range 82.8 – 87.6%) also expressed as an increase in CD107a expression as a marker of NK cell degranulation (BADx median 67.7% (range 58.6 – 77.2%), p < 0.0001; AD median 55.7% (range 50.2 – 61.9%), p = 0.0002; controls median 44.0% (range 29.0 – 50.9%)) (Figures 2B, C). The percentage of NK cell activating receptor expressing NK cells, such as natural killer group 2D (NKG2D), NK cell-protein with 30/46 kilodalton (NKp30 and NKp46) did either not differ (NKp30) or was even slightly lower compared to levels of controls (NKG2D: BADx median 22.6% (range 15.8 – 27.6%), p = 0.0464; AD median 21.4% (range 17.1 – 23.4%) p = 0.0117; controls median 34.6% (range 12.8 – 43.5%); NKp46: BADx median 69.6% (range 53.4 – 74.1%), p = 0.0184; AD median 59.8% (range 57.9 – 62.3%), p = 0.0008; controls median 92.5% (range 43.3 – 95.2%)) (compare Figures 2D-F). Percentage expression of NK cell inhibiting receptor CD94, however, was upregulated in both BADx and AD patients [BADx median 66.4% (range 55.9 – 76.1%), p < 0.0001; AD median 53.6% (range 43.6 – 62.2%), p < 0.0001; controls median 34.6% (range 27.5 – 42.0%)] (Figures 2G-I).
The use of mineralocorticoid replacement therapy correlated with CD94 expression (r = 0.2289, p = 0.0467), as well as use of mineralocorticoids and fludrocortisone dose with NKp30 expression (r = 0.2562, p = 0.0255; dose: r = 0.2316, p = 0.0441).
CLOCK Gene expression in patients with different causes of PAI
Analysis of relative mRNA expression of different clock genes in corresponding PBMC samples of the same patient subgroups revealed significant differences in CLOCK, CRY1, CRY2, WEE1 (no change in AD patients) and TIMELESS expression in all subgroups with PAI compared to controls, despite a difference regarding CLOCK expression in CAH and BADx patients (Figure 3). ARNTL expression was downregulated in BADx patients compared to controls (Figure 3A, p=0.0035). CREB1 expression was upregulated in AD patients (Figure 3H, p = 0.0178), while PER3 expression was downregulated in BADx patients compared to all other patient subgroups (Figure 3I, p<0.0001). No differences were observed regarding relative NR1D1 expression (Figure 3E, p=0.1257).
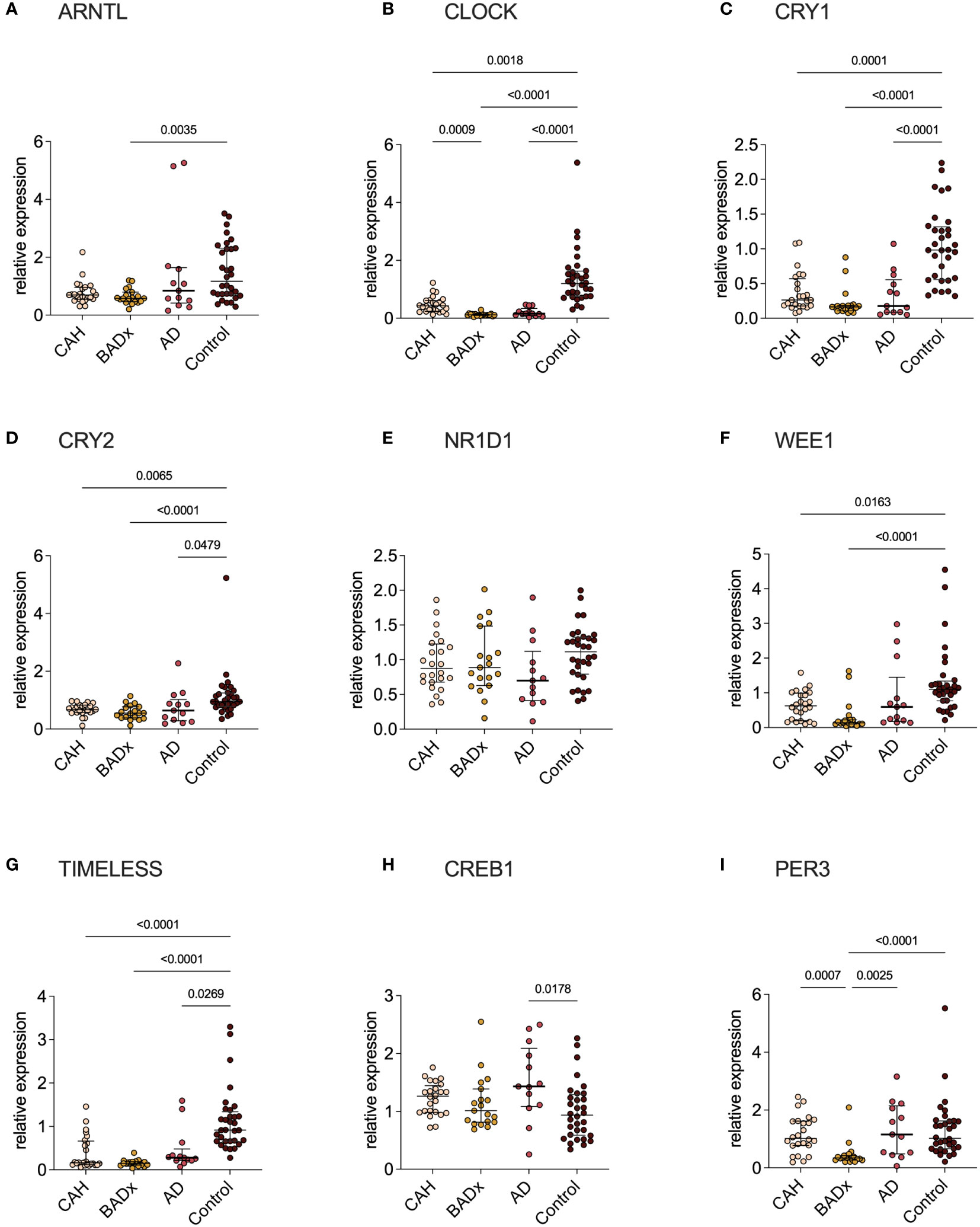
Figure 3 Clock gene expression is equally impaired in all three subtypes of PAI. mRNA expression of different clock genes [(A) – ARNTL, (B) – CLOCK, (C) – CRY1, (D) – CRY2, (E) – NR1D1, (F) – WEE1, (G) – TIMELESS, (H) – CREB1, (I) – PER3] was analyzed using qPCR analysis after RNA isolation of whole PBMCs and cDNA transcription. Data is presented as relative mRNA expression. Kruskal–Wallis test and Dunn’s multiple comparisons test were used for statistical analysis. P-values are indicated in the pairwise comparison. A p-value of < 0.05 was considered statistically significant.
Discussion
The main findings of our study in a cohort of PAI patients due to three different etiologies – inborn defect of steroidogenesis in CAH, autoimmune destruction of adrenocortical cells in AD and surgical removal of the adrenal glands due to CS – are distinct differences in immunophenotype that became apparent despite the use of the same GC preparation and dose in all three groups. This includes relevant changes in levels of Th/Tc1, Th/Tc2, Th/Tc17, Th9, Th/Tc22 and NKCC which might account for higher rates of intercurrent infections and higher incidences of related autoimmune disorders (19, 24–28). Previous publications demonstrated a dysregulation of immune-cell profiles and an impairment of immune function in PAI patients under conventional GC therapy, which was proposed to be the effect of modulations of clock gene expression, as a switch to modified-release preparations restored immunophenotype (19–21). Although modulation in clock gene expression could be confirmed in all our patient subgroups (Figure 3), major interindividual-intergroup dissimilarities were not detected.
While pleiotropic effects of both endogenous and exogenous GCs on the immune system have long been described, it appears illusive to summarize these immunosuppressive and immunoregulatory cellular and molecular mechanisms in order to create a clear picture (29). Endogenous GCs for example can both promote and suppress T cell immunity. GCs are involved in selection of T cell receptor (TCR) self-affinities in the thymus, T cell trafficking, suppression of Th1 and Th17 responses, while promoting Th2 and Treg activity and memory T cell differentiation by means of target gene (e.g. cytokine) expression and transcription factor activation via the intracellular GC receptor (30–32). GCs also have the potential to inhibit T cell activation by interference with TCR signaling (29, 33, 34).
The most impressive changes in levels of T cell subpopulation in our study were decreased percentages of Th1 in AD patients and Tc1 cells in AD and CAH patients compared to those of controls. Moreover, we observed a downregulation of the percentage of IL-4+ Th2 and Tc2 cells as well as Th17 and Tc17 cells in patients with AD. Previously published analysis of chemokine profiles in small cohorts of up to 15 patients with autoimmune AD indicated a Th1 dominant chemokine profile, which we however could not confirm in our study (35, 36). Downregulation of Th1, Th2 and Th17 subsets however fits PAI patients’ susceptibility to infections as Th1 and Th2 cells play important roles in identification and eradication of intracellular pathogens such as parasites, viruses and bacteria and also the defensive mechanisms of Th17 cells particularly at the mucosal and epithelial barrier are not to be neglected (14, 37–39). Especially the lower expression of IFNγ, which is a key cytokine involved in several cellular programs, signaling mechanisms and surveillance of immune function, could have detrimental effects on immune protective mechanisms (40).
Our data additionally revealed a lower percentage of Tregs, especially in patients after BADx and those with AD. Defective suppressor function of Tregs has previously been shown in a cohort of patients with polyendocrine autoimmune syndrome and the critical role of Tregs in maintenance of self-tolerance and immune cell homeostasis is widely known (41, 42). Differences from percentage expression in healthy controls can therefore be at least partially explained by the pathophysiology of the disease in AD patients, however similar disparities in patients after BADx indicate other accountable factors. Previous studies demonstrated development of autoimmune disease in patients successfully treated for CS, which the authors hypothesized might have been masked in patients with glucocorticoid excess (25, 26). In this study, we could now observe a reduction in the percentage of Tregs in all PAI subgroups, which could provide an additional explanation for a higher prevalence of autoimmune diseases in these patient subgroups.
Further effects on immunophenotype discovered are an upregulation of the percentage of Th9 cells in the subgroup of patients with CAH and those after BADx compared to patients with AD and healthy controls, which might be relevant regarding patients’ T cell-dependent allergic and antiparasitic immune response (43). We could also observe an upregulation of the percentage of Th22 and Tc22 cells in patients after BADx compared to the other two subsets of PAI patients and healthy controls.
Moreover, we could demonstrate an inhibition of NK cell cytotoxicity to varying degrees in AD and after BADx but with only minimal changes in the subset of CAH patients (Figure 2A). NK cells comprise a crucial barrier of the innate immune system by their early production of cytokines and chemokines and cell lysis potential irrespective of prior sensitization (44). Previous publications have demonstrated that GCs influence NK cell function through epigenetic modifications resulting in dysregulation of effector protein expression and hence inhibition of NK cell cytotoxicity, at least partially explaining the effect of chronic stress on incidence of infections and cancer (44–46). GCs however can also – dependent on the cytokine profile present – support NK cell proliferation and reactivity (35). Our data supports this hypothesis. On the one hand, GC treatment in PAI patients seems to result in dysregulation of NK cell receptor (NKCR) expression, meaning an upregulation of inhibitory receptors such as CD94, while the percentage of activating receptor (e.g. NKG2D, NKp30, Nkp46) positive NK cells was reduced (Figures 2D-I). On the other hand, patients with PAI show an upregulation of CD3-CD56+ NK cells and an upregulation of the degranulation marker CD107a (Figure 2C). This data suggests a generally activated phenotype of NK cells in PAI patients with however a lack of actual cytotoxic function in in vitro testing. This might be interpreted as a failure to compensate the dysregulation of NKCR expression. Comparing the different subtypes of PAI, it becomes apparent, that most changes in NK cell levels, NKCR and degranulation marker expression as well as NKCC are present in the subgroup of BADx and AD patients, while CAH patients consistently present with only slight changes in NK cell phenotype.
It has previously been shown that in patients with CAH, hormone precursors accumulating upstream of the genetic defect in steroidogenesis are able to potently bind and transactivate the human GC receptor, explaining cortisol functions in patients even with null mutations (47). This could possibly explain why NKCC is less affected in this patient subgroup. In contrast to patients with AD and after BADx, CAH patients do not lack adrenal androgen production, but rather have elevated adrenal androgen concentrations potentially influencing their immunophenotype.
It is known that sex hormones are variables influencing the immune system as well as the susceptibility to all types of diseases and malignancies (48). Interestingly, however, we did not observe any gender differences in none of the PAI subtypes (data not shown). We did however previously demonstrate that adrenal-derived 11-oxygenated 19-carbon (11oxC19) steroids, which are known to be the dominant androgens in classic 21-hydroxylase deficiency, are also elevated in CAH patients with good therapeutic control (49, 50). As AKR1C3 is expressed on human PBMCs and 11oxC19 androgens the primary substrate of AKR1C3 expressed on human NK cells, differences in immunophenotype of CAH patients in comparison to those with AD or after BADx might also be conveyed by this subset of steroids, despite the sole effect of GC substitution therapy (51).
The pathophysiological differences and disparities in etiology of these different types of PAI promote the idea of further factors influencing the distinct differences in immunophenotype in the different PAI groups. In the subgroup of patients after BADx a long-term imprinting effect of the previous GC excess on the immune system might play a role. Patients with CS in remission show significant improvements in comorbidities in follow-ups, depending on the extent and possibly also the total duration of hypercortisolism (52). Nonetheless, despite long-term remission, comorbidities, risk of infectious complications and mortality remain higher compared to the general population (53, 54). Further influencing factors might also be the use and dose of mineralocorticoid replacement via action at the mineralocorticoid receptor (55, 56) or direct effects of ACTH as melanocortin receptors are also known to be expressed on most immune cell lineages (57).
Limitations of this study include variability in the timeframe of sampling including both the exact time of day (time frame from 8-12 am) as well as seasons (collected over the whole year), however this time-dependent bias is present in all groups and was shown to be able to be neglected in whole group comparisons. Handling, isolation of PBMCs and sample storage was standardized as well as lay and transport times and duration of sample processing.
To conclude, this manuscript presents novel data on immunophenotypic differences in subsets of PAI of differential etiology. Our data highlights the importance of further fundamental analysis of potential influencing factors of immune regulation, such as imprinting effects of long-term GC excess and effects of elevated GC and androgen precursors or 11oxC19 concentrations.
Data availability statement
The raw data supporting the conclusions of this article will be made available by the authors, without undue reservation.
Ethics statement
The studies involving humans were approved by Ethikkommission der Medizinischen Fakultät der LMU München. The studies were conducted in accordance with the local legislation and institutional requirements. The participants provided their written informed consent to participate in this study.
Author contributions
HN: Conceptualization, Data curation, Formal Analysis, Funding acquisition, Investigation, Methodology, Visualization, Writing – original draft, Writing – review & editing. TMS: Conceptualization, Data curation, Formal Analysis, Investigation, Methodology, Visualization, Writing – original draft. JJ: Formal Analysis, Investigation, Writing – review & editing. AG: Methodology, Writing – review & editing. HS: Methodology, Writing – review & editing. SZ: Data curation, Writing – review & editing. FV: Methodology, Writing – review & editing. LT: Methodology, Writing – review & editing. MA: Methodology, Writing – review & editing. CL: Methodology, Writing – review & editing. SK: Methodology, Writing – review & editing. SR: Methodology, Writing – review & editing. FB: Methodology, Writing – review & editing. MR: Funding acquisition, Methodology, Project administration, Resources, Supervision, Writing – review & editing. LB: Funding acquisition, Methodology, Project administration, Resources, Supervision, Writing – original draft, Writing – review & editing. NR: Funding acquisition, Methodology, Project administration, Resources, Supervision, Writing – original draft, Writing – review & editing.
Funding
The author(s) declare financial support was received for the research, authorship, and/or publication of this article. This work was supported by the Deutsche Forschungsgemeinschaft (DFG, Heisenberg Professorship 325768017 to NR and Projektnummer: 314061271-TRR205 to HS, FB, MR and NR), Eva Luise und Horst Köhler Stiftung & Else Kröner-Fresenius-Stiftung (2019_KollegSE.03 to HN and LB and 2012_A103 and 2015_A228 to MR), by the Förderprogramm für Forschung und Lehre (FöFoLe) Reg.-Nr. 37/2019 to TMS and LB, by the FöFoLe grant number 1138 to AG, the Bavarian Cancer Research Center (BZKF) (AG), the DFG (grant number GO 3823/1-1 to AG; grant number: KO5055/3-1 to SK), the international doctoral program ‘i-Target: immunotargeting of cancer’ (funded by the Elite Network of Bavaria; to SK), the Melanoma Research Alliance (grant number 409510 to SK), Marie Sklodowska-Curie Training Network for the Immunotherapy of Cancer (IMMUTRAIN) (funded by the Horizon 2020 programme of the European Union; to SK), Marie Sklodowska-Curie Training Network for Optimizing Adoptive T Cell Therapy of Cancer (funded by the Horizon 2020 programme of the European Union; grant 955575 to SK), Else Kröner-Fresenius-Stiftung (to SK), German Cancer Aid (to SK), the Wilhelm-Sander-Stiftung (to SK), Ernst Jung Stiftung (to SK), Institutional Strategy LMUexcellent of LMU Munich (within the framework of the German Excellence Initiative; to SK), the Go-Bio-Initiative (to SK), the m4-Award of the Bavarian Ministry for Economical Affairs (to SK), Bundesministerium für Bildung und Forschung (to SK), European Research Council (Starting Grant 756017 and PoC Grant 101100460 to SK), Deutsche Forschungsgemeinschaft (DFG; to SK), by the SFB-TRR 338/1 2021–452881907 (to SK), Fritz-Bender Foundation (to SK), Deutsche José Carreras Leukämie Stiftung (to SK), the Bayerische Forschungsstiftung (BAYCELLator to SK) and Hector Foundation (to SK).
Acknowledgments
Cytometry data were obtained in the Core Facility Flow Cytometry of the University Hospital, LMU Munich using a BD LSRFortessa™ II. We also thank all participants of the study.
Conflict of interest
SK has received honoraria from TCR2 Inc, Novartis, BMS and GSK. SK is inventor of several patents in the field of immuno-oncology. SK received license fees from TCR2 Inc and Carina Biotech. A.G. received research support from Tabby Therapeutics for work unrelated to the manuscript. SK received research support from TCR2 Inc., Plectonic GmbH and Arcus Bioscience for work unrelated to the manuscript.
The remaining authors declare that the research was conducted in the absence of any commercial or financial relationships that could be construed as a potential conflict of interest.
The author(s) declared that they were an editorial board member of Frontiers, at the time of submission. This had no impact on the peer review process and the final decision.
Publisher’s note
All claims expressed in this article are solely those of the authors and do not necessarily represent those of their affiliated organizations, or those of the publisher, the editors and the reviewers. Any product that may be evaluated in this article, or claim that may be made by its manufacturer, is not guaranteed or endorsed by the publisher.
Supplementary material
The Supplementary Material for this article can be found online at: https://www.frontiersin.org/articles/10.3389/fimmu.2023.1275828/full#supplementary-material
Supplementary Figure 1 | Gating strategy for flow cytometric analysis of NK- and T cells. Gating strategy for both NK- and T cell subsets and NK cell surface receptors.
Glossary
References
1. Chabre O, Goichot B, Zenaty D, Bertherat J. Group 1. Epidemiology of primary and secondary adrenal insufficiency: Prevalence and incidence, acute adrenal insufficiency, long-term morbidity and mortality. Ann Endocrinol (Paris) (2017) 78(6):490–4. doi: 10.1016/j.ando.2017.10.010
2. Rubinstein G, Osswald A, Hoster E, Losa M, Elenkova A, Zacharieva S, et al. Time to diagnosis in cushing’s syndrome: A meta-analysis based on 5367 patients. J Clin Endocrinol Metab (2020) 105(3):dgz136. doi: 10.1210/clinem/dgz136
3. Nieman LK, Biller BM, Findling JW, Murad MH, Newell-Price J, Savage MO, et al. Treatment of cushing’s syndrome: an endocrine society clinical practice guideline. J Clin Endocrinol Metab (2015) 100(8):2807–31. doi: 10.1210/jc.2015-1818
4. Biller BM, Grossman AB, Stewart PM, Melmed S, Bertagna X, Bertherat J, et al. Treatment of adrenocorticotropin-dependent Cushing’s syndrome: a consensus statement. J Clin Endocrinol Metab (2008) 93(7):2454–62. doi: 10.1210/jc.2007-2734
5. Chevalier B, Vantyghem MC, Espiard S. Bilateral adrenal hyperplasia: pathogenesis and treatment. Biomedicines (2021) 9(10):1397. doi: 10.3390/biomedicines9101397
6. Pazderska A, Pearce SH. Adrenal insufficiency - recognition and management. Clin Med (Lond) (2017) 17(3):258–62. doi: 10.7861/clinmedicine.17-3-258
7. Bornstein SR, Allolio B, Arlt W, Barthel A, Don-Wauchope A, Hammer GD, et al. Diagnosis and treatment of primary adrenal insufficiency: an endocrine society clinical practice guideline. J Clin Endocrinol Metab (2016) 101(2):364–89. doi: 10.1210/jc.2015-1710
8. Nowotny H, Ahmed SF, Bensing S, Beun JG, Brosamle M, Chifu I, et al. Therapy options for adrenal insufficiency and recommendations for the management of adrenal crisis. Endocrine (2021) 71(3):586–94. doi: 10.1007/s12020-021-02649-6
9. Barbetta L, Dall’Asta C, Re T, Libe R, Costa E, Ambrosi B. Comparison of different regimens of glucocorticoid replacement therapy in patients with hypoadrenalism. J Endocrinol Invest (2005) 28(7):632–7. doi: 10.1007/BF03347262
10. Allolio B. Extensive expertise in endocrinology. Adrenal crisis. Eur J Endocrinol (2015) 172(3):R115–24. doi: 10.1530/EJE-14-0824
11. Papanicolaou DA, Tsigos C, Oldfield EH, Chrousos GP. Acute glucocorticoid deficiency is associated with plasma elevations of interleukin-6: does the latter participate in the symptomatology of the steroid withdrawal syndrome and adrenal insufficiency? J Clin Endocrinol Metab (1996) 81(6):2303–6. doi: 10.1210/jcem.81.6.8964868
12. Rushworth RL, Torpy DJ, Falhammar H. Adrenal crisis. N Engl J Med (2019) 381(9):852–61. doi: 10.1056/NEJMra1807486
13. Reisch N, Willige M, Kohn D, Schwarz HP, Allolio B, Reincke M, et al. Frequency and causes of adrenal crises over lifetime in patients with 21-hydroxylase deficiency. Eur J Endocrinol (2012) 167(1):35–42. doi: 10.1530/EJE-12-0161
14. Hahner S, Loeffler M, Bleicken B, Drechsler C, Milovanovic D, Fassnacht M, et al. Epidemiology of adrenal crisis in chronic adrenal insufficiency: the need for new prevention strategies. Eur J Endocrinol (2010) 162(3):597–602. doi: 10.1530/EJE-09-0884
15. Ritzel K, Beuschlein F, Mickisch A, Osswald A, Schneider HJ, Schopohl J, et al. Clinical review: Outcome of bilateral adrenalectomy in Cushing’s syndrome: a systematic review. J Clin Endocrinol Metab (2013) 98(10):3939–48. doi: 10.1210/jc.2013-1470
16. Bergthorsdottir R, Leonsson-Zachrisson M, Oden A, Johannsson G. Premature mortality in patients with Addison’s disease: a population-based study. J Clin Endocrinol Metab (2006) 91(12):4849–53. doi: 10.1210/jc.2006-0076
17. Ragnarsson O, Olsson DS, Papakokkinou E, Chantzichristos D, Dahlqvist P, Segerstedt E, et al. Overall and disease-specific mortality in patients with cushing disease: A swedish nationwide study. J Clin Endocrinol Metab (2019) 104(6):2375–84. doi: 10.1210/jc.2018-02524
18. Falhammar H, Frisen L, Norrby C, Hirschberg AL, Almqvist C, Nordenskjold A, et al. Increased mortality in patients with congenital adrenal hyperplasia due to 21-hydroxylase deficiency. J Clin Endocrinol Metab (2014) 99(12):E2715–21. doi: 10.1210/jc.2014-2957
19. Bancos I, Hazeldine J, Chortis V, Hampson P, Taylor AE, Lord JM, et al. Primary adrenal insufficiency is associated with impaired natural killer cell function: a potential link to increased mortality. Eur J Endocrinol (2017) 176(4):471–80. doi: 10.1530/EJE-16-0969
20. Isidori AM, Venneri MA, Graziadio C, Simeoli C, Fiore D, Hasenmajer V, et al. Effect of once-daily, modified-release hydrocortisone versus standard glucocorticoid therapy on metabolism and innate immunity in patients with adrenal insufficiency (DREAM): a single-blind, randomised controlled trial. Lancet Diabetes Endocrinol (2018) 6(3):173–85. doi: 10.1016/S2213-8587(17)30398-4
21. Venneri MA, Hasenmajer V, Fiore D, Sbardella E, Pofi R, Graziadio C, et al. Circadian rhythm of glucocorticoid administration entrains clock genes in immune cells: A DREAM trial ancillary study. J Clin Endocrinol Metab (2018) 103(8):2998–3009. doi: 10.1210/jc.2018-00346
22. Manger B, Hardy KJ, Weiss A, Stobo JD. Differential effect of cyclosporin A on activation signaling in human T cell lines. J Clin Invest (1986) 77(5):1501–6. doi: 10.1172/JCI112464
23. Sandhofer N, Metzeler KH, Rothenberg M, Herold T, Tiedt S, Groiss V, et al. Dual PI3K/mTOR inhibition shows antileukemic activity in MLL-rearranged acute myeloid leukemia. Leukemia (2015) 29(4):828–38. doi: 10.1038/leu.2014.305
24. Tresoldi AS, Sumilo D, Perrins M, Toulis KA, Prete A, Reddy N, et al. Increased infection risk in addison’s disease and congenital adrenal hyperplasia. J Clin Endocrinol Metab (2020) 105(2):418–29. doi: 10.1210/clinem/dgz006
25. Tatsi C, Keil M, Lyssikatos C, Belyavskaya E, Stratakis CA, Lodish MB. Incidence of autoimmune and related disorders after resolution of endogenous cushing syndrome in children. Horm Metab Res (2018) 50(4):290–5. doi: 10.1055/s-0044-101144
26. Petramala L, Olmati F, Conforti MG, Concistre A, Bisogni V, Alfieri N, et al. Autoimmune diseases in patients with cushing’s syndrome after resolution of hypercortisolism: case reports and literature review. Int J Endocrinol (2018) 2018:1464967. doi: 10.1155/2018/1464967
27. Pivonello R, Isidori AM, De Martino MC, Newell-Price J, Biller BM, Colao A. Complications of Cushing’s syndrome: state of the art. Lancet Diabetes Endocrinol (2016) 4(7):611–29. doi: 10.1016/S2213-8587(16)00086-3
28. Braun LT, Vogel F, Reincke M. Long-term morbidity and mortality in patients with Cushing’s syndrome. J Neuroendocrinol (2022) 34(8):e13113. doi: 10.1111/jne.13113
29. Cain DW, Cidlowski JA. Immune regulation by glucocorticoids. Nat Rev Immunol (2017) 17(4):233–47. doi: 10.1038/nri.2017.1
30. Taves MD, Ashwell JD. Glucocorticoids in T cell development, differentiation and function. Nat Rev Immunol (2021) 21(4):233–43. doi: 10.1038/s41577-020-00464-0
31. Marcenaro E, Carlomagno S, Pesce S, Della Chiesa M, Parolini S, Moretta A, et al. NK cells and their receptors during viral infections. Immunotherapy (2011) 3(9):1075–86. doi: 10.2217/imt.11.99
32. Chen L, Jondal M, Yakimchuk K. Regulatory effects of dexamethasone on NK and T cell immunity. Inflammopharmacology (2018) 26(5):1331–8. doi: 10.1007/s10787-017-0418-0
33. Elenkov IJ. Glucocorticoids and the th1/th2 balance. Ann N Y Acad Sci (2004) 1024:138–46. doi: 10.1196/annals.1321.010
34. Liberman AC, Refojo D, Druker J, Toscano M, Rein T, Holsboer F, et al. The activated glucocorticoid receptor inhibits the transcription factor T-bet by direct protein-protein interaction. FASEB J (2007) 21(4):1177–88. doi: 10.1096/fj.06-7452com
35. Bellastella G, Rotondi M, Pane E, Costantini S, Colella C, Calemma R, et al. Simultaneous evaluation of the circulating levels of both Th1 and Th2 chemokines in patients with autoimmune Addison’s disease. J Endocrinol Invest (2011) 34(11):831–4. doi: 10.3275/7414
36. Ekman B, Alstrand N, Bachrach-Lindstrom M, Jenmalm MC, Wahlberg J. Altered chemokine Th1/Th2 balance in Addison’s disease: relationship with hydrocortisone dosing and quality of life. Horm Metab Res (2014) 46(1):48–53. doi: 10.1055/s-0033-1351291
37. Minnetti M, Hasenmajer V, Sbardella E, Angelini F, Simeoli C, Di Paola N, et al. Susceptibility and characteristics of infections in patients with glucocorticoid excess or insufficiency: the ICARO tool. Eur J Endocrinol (2022) 187(5):719–31. doi: 10.1530/EJE-22-0454
38. Smans LC, Souverein PC, Leufkens HG, Hoepelman AI, Zelissen PM. Increased use of antimicrobial agents and hospital admission for infections in patients with primary adrenal insufficiency: a cohort study. Eur J Endocrinol (2013) 168(4):609–14. doi: 10.1530/EJE-12-0879
39. Guglani L, Khader SA. Th17 cytokines in mucosal immunity and inflammation. Curr Opin HIV AIDS (2010) 5(2):120–7. doi: 10.1097/COH.0b013e328335c2f6
40. Thakur A, Mikkelsen H, Jungersen G. Intracellular pathogens: host immunity and microbial persistence strategies. J Immunol Res (2019) 2019:1356540. doi: 10.1155/2019/1356540
41. Lan RY, Ansari AA, Lian ZX, Gershwin ME. Regulatory T cells: development, function and role in autoimmunity. Autoimmun Rev (2005) 4(6):351–63. doi: 10.1016/j.autrev.2005.01.007
42. Kriegel MA, Lohmann T, Gabler C, Blank N, Kalden JR, Lorenz HM. Defective suppressor function of human CD4+ CD25+ regulatory T cells in autoimmune polyglandular syndrome type II. J Exp Med (2004) 199(9):1285–91. doi: 10.1084/jem.20032158
43. Kaplan MH, Hufford MM, Olson MR. The development and in vivo function of T helper 9 cells. Nat Rev Immunol (2015) 15(5):295–307. doi: 10.1038/nri3824
44. Capellino S, Claus M, Watzl C. Regulation of natural killer cell activity by glucocorticoids, serotonin, dopamine, and epinephrine. Cell Mol Immunol (2020) 17(7):705–11. doi: 10.1038/s41423-020-0477-9
45. Krukowski K, Eddy J, Kosik KL, Konley T, Janusek LW, Mathews HL. Glucocorticoid dysregulation of natural killer cell function through epigenetic modification. Brain Behav Immun (2011) 25(2):239–49. doi: 10.1016/j.bbi.2010.07.244
46. Vitale C, Chiossone L, Cantoni C, Morreale G, Cottalasso F, Moretti S, et al. The corticosteroid-induced inhibitory effect on NK cell function reflects down-regulation and/or dysfunction of triggering receptors involved in natural cytotoxicity. Eur J Immunol (2004) 34(11):3028–38. doi: 10.1002/eji.200425418
47. Pijnenburg-Kleizen KJ, Engels M, Mooij CF, Griffin A, Krone N, Span PN, et al. Adrenal steroid metabolites accumulating in congenital adrenal hyperplasia lead to transactivation of the glucocorticoid receptor. Endocrinology (2015) 156(10):3504–10. doi: 10.1210/en.2015-1087
48. Klein SL, Flanagan KL. Sex differences in immune responses. Nat Rev Immunol (2016) 16(10):626–38. doi: 10.1038/nri.2016.90
49. Turcu AF, Nanba AT, Chomic R, Upadhyay SK, Giordano TJ, Shields JJ, et al. Adrenal-derived 11-oxygenated 19-carbon steroids are the dominant androgens in classic 21-hydroxylase deficiency. Eur J Endocrinol (2016) 174(5):601–9. doi: 10.1530/EJE-15-1181
50. Nowotny HF, Auer MK, Lottspeich C, Schmidt H, Dubinski I, Bidlingmaier M, et al. Salivary profiles of 11-oxygenated androgens follow a diurnal rhythm in patients with congenital adrenal hyperplasia. J Clin Endocrinol Metab (2021) 106(11):e4509–e19. doi: 10.1210/clinem/dgab446
51. Schiffer L, Bossey A, Kempegowda P, Taylor AE, Akerman I, Scheel-Toellner D, et al. Peripheral blood mononuclear cells preferentially activate 11-oxygenated androgens. Eur J Endocrinol (2021) 184(3):353–63. doi: 10.1101/2020.09.08.288316
52. Schernthaner-Reiter MH, Siess C, Gessl A, Scheuba C, Wolfsberger S, Riss P, et al. Factors predicting long-term comorbidities in patients with Cushing’s syndrome in remission. Endocrine (2019) 64(1):157–68. doi: 10.1007/s12020-018-1819-6
53. Papakokkinou E, Olsson DS, Chantzichristos D, Dahlqvist P, Segerstedt E, Olsson T, et al. Excess morbidity persists in patients with cushing’s disease during long-term remission: A swedish nationwide study. J Clin Endocrinol Metab (2020) 105(8):dgaa291. doi: 10.1210/clinem/dgaa291
54. van Haalen FM, Broersen LH, Jorgensen JO, Pereira AM, Dekkers OM. Management of endocrine disease: Mortality remains increased in Cushing’s disease despite biochemical remission: a systematic review and meta-analysis. Eur J Endocrinol (2015) 172(4):R143–9. doi: 10.1530/EJE-14-0556
55. Bene NC, Alcaide P, Wortis HH, Jaffe IZ. Mineralocorticoid receptors in immune cells: emerging role in cardiovascular disease. Steroids (2014) 91:38–45. doi: 10.1016/j.steroids.2014.04.005
56. van der Heijden C, Bode M, Riksen NP, Wenzel UO. The role of the mineralocorticoid receptor in immune cells in cardiovascular disease. Br J Pharmacol (2022) 179(13):3135–51. doi: 10.1111/bph.15782
Keywords: primary adrenal insufficiency, congenital adrenal hyperplasia, bilateral adrenalectomy, Addison’s disease, immunophenotype, ACTH, cortisol
Citation: Nowotny HF, Marchant Seiter T, Ju J, Gottschlich A, Schneider H, Zopp S, Vogel F, Tschaidse L, Auer MK, Lottspeich C, Kobold S, Rothenfusser S, Beuschlein F, Reincke M, Braun L and Reisch N (2023) Major immunophenotypic abnormalities in patients with primary adrenal insufficiency of different etiology. Front. Immunol. 14:1275828. doi: 10.3389/fimmu.2023.1275828
Received: 10 August 2023; Accepted: 27 October 2023;
Published: 15 November 2023.
Edited by:
Pier Paolo Sainaghi, University of Eastern Piedmont, ItalyReviewed by:
Mona Mashayekhi, Vanderbilt University Medical Center, United StatesXiaofan Jia, University of Colorado Anschutz Medical Campus, United States
Copyright © 2023 Nowotny, Marchant Seiter, Ju, Gottschlich, Schneider, Zopp, Vogel, Tschaidse, Auer, Lottspeich, Kobold, Rothenfusser, Beuschlein, Reincke, Braun and Reisch. This is an open-access article distributed under the terms of the Creative Commons Attribution License (CC BY). The use, distribution or reproduction in other forums is permitted, provided the original author(s) and the copyright owner(s) are credited and that the original publication in this journal is cited, in accordance with accepted academic practice. No use, distribution or reproduction is permitted which does not comply with these terms.
*Correspondence: Nicole Reisch, Tmljb2xlLnJlaXNjaEBtZWQudW5pLW11ZW5jaGVuLmRl
†These authors share first authorship