- 1Department of Biomedical Sciences, Humanitas University, Pieve Emanuele, Italy
- 2Laboratory of Cellular and Humoral Innate Immunity, IRCCS Humanitas Research Hospital, Rozzano, Italy
Soluble pattern recognition molecules (PRMs) are a heterogenous group of proteins that recognize pathogen- and danger-associated molecular patterns (PAMPs and DAMPs, respectively), and cooperate with cell-borne receptors in the orchestration of innate and adaptive immune responses to pathogenic insults and tissue damage. Amongst soluble PRMs, pentraxins are a family of highly conserved proteins with distinctive structural features. Originally identified in the early 1990s as an early inflammatory gene, PTX3 is the prototype of long pentraxins. Unlike the short pentraxin C reactive protein (CRP), whose expression is mostly confined to the liver, PTX3 is made by several immune and non-immune cells at sites of infection and inflammation, where it intercepts fundamental aspects of infection immunity, inflammation, and tissue remodeling. Of note, PTX3 cross talks to components of the complement system to control cancer-related inflammation and disposal of pathogens. Also, it is an essential component of inflammatory extracellular matrices (ECMs) through crosslinking of hyaluronic acid and turn-over of provisional fibrin networks that assemble at sites of tissue injury. This functional diversity is mediated by unique structural characteristics whose fine details have been unveiled only recently. Here, we revisit the structure/function relationships of this long pentraxin in light of the most recent advances in its structural biology, with a focus on the interplay with complement and the emerging roles as a component of the ECM. Differences to and similarities with the short pentraxins are highlighted and discussed.
1 Introduction
The innate immune system is traditionally regarded as the first line of defense against invading pathogens (1). Cellular and molecular effector mechanisms of innate immunity are typically induced upon recognition of PAMPs (pathogen-associated molecular patterns, i.e. motifs shared by evolutionarily close microbial families that are often localized on the cell surface and are essential for fitness) by PRMs (cell-borne or soluble pattern recognition molecules that are expressed both by immune and non-immune cells and act as transducers of activation and modulation signals) (2). Fluid-phase PRMs are regarded as evolutionary ancestors of antibodies in that they exert immunoprotective and immunomodulatory effects by means of opsonic and neutralizing properties, promotion of phagocytosis and complement activation. In addition to their roles in pathogen recognition and disposal, PRMs are increasingly acknowledged as key players in tissue remodeling, whereby they recognize DAMPs (damage/danger-associated molecular patterns) and convey biochemical messages for removal of cellular debris and tissue regeneration (3).
Pentraxins are an evolutionarily conserved family of soluble PRMs that share a common sequence motif (i.e., the pentraxin signature His-x-Cys-x-Ser/Thr-Trp-x-Ser) and typical quaternary structures (4). This comprises short and long pentraxins, each with distinctive structural and functional characteristics. First identified in the ‘30s as an opsonin that recognizes the C-type polysaccharide of S. pneumoniae (5), C-reactive protein (CRP) is the prototypical short pentraxin with five identical protomer subunits assembled into symmetric disc-like pentamers stabilized by non-covalent interactions [Figure 1A and (15)]. A similar structural organization is found in serum amyloid P component (SAP) (16), another short pentraxin identified in the ‘70s that shares with CRP calcium-dependent recognition of several ligands (17). High levels of sequence and structural homology across short pentraxins from evolutionary distant species suggest that this PRMs play essential roles in innate immunity, even though their functions as opsonizing and complement-fixing molecules have probably become redundant, being overshadowed by other players of the immune system like immunoglobulins (18). Experimental evidence from gene-modified animals indicates that CRP exerts host protective functions in bacterial, especially pneumococcal, infections (19). Importantly, this protein is extensively used in the clinical practice as a non-specific systemic marker of inflammation (20). Serendipitously discovered in studies designed to evaluate the effects of CRP on lymphocytes (18), SAP has been recently acknowledged as an important player in the innate immune reaction to the opportunistic fungal pathogen A. fumigatus (21). Also, SAP is long-known as a an essential component of amyloid deposits (22). This property has been clinically exploited to develop SAP-based scintigraphy tracers for in vivo imaging of amyloid deposits (23, 24), and combination therapies to target these pathological fibrils in amyloidosis and Alzheimer’s disease (25, 26).
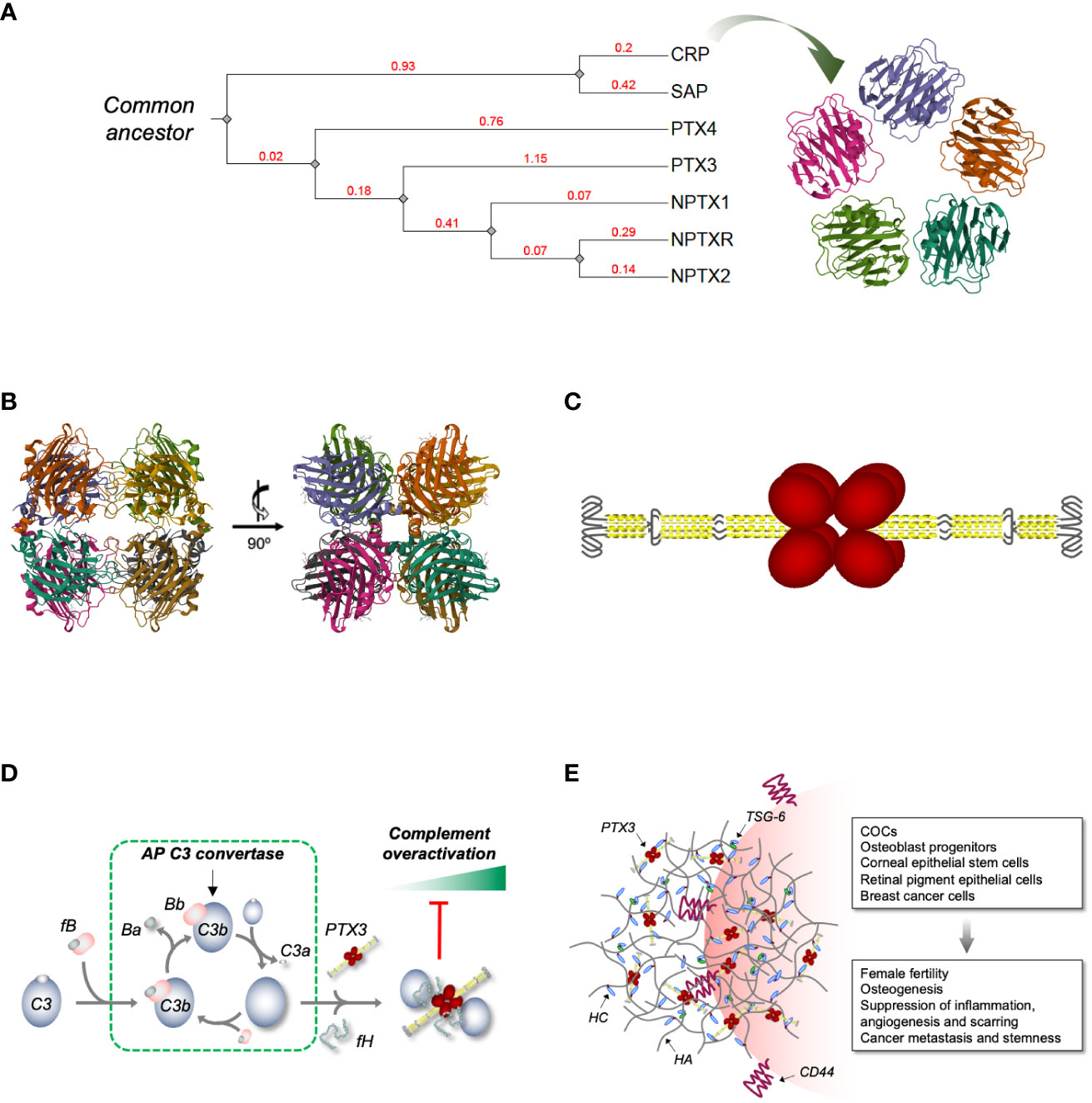
Figure 1 Phylogenetic relationships across human pentraxins, 3D model of PTX3 and its interplay with complement and HA ECM. (A) Phylogenetic tree of the human PTX family of proteins. The tree was constructed using the Phylogeny.fr web server [http://www.phylogeny.fr/ and (6)], edited and annotated with the iTOL (interactive Tree Of Life) online tool [https://itol.embl.de/ and (7)]. The extent of genetic variation across members of the family (i.e., the average number of amino acid substitutions per site) is indicated by the branch lengths. These are drawn to scale and expressed as arbitrary unit (in red) with tree nodes represented by grey diamonds. The accession numbers used for the analysis are NP_000558.2 (CRP), NP_001630.1 (SAP), NP_002843.2 (PTX3), NP_001013680.1 (PTX4), NP_002513.2 (NPTX1), NP_002514.1 (NPTX2) and NP_055108.2 (NPTXR). Also, a 3D model of human CRP is shown [PDB-ID: 1GNH and (8)] that highlights the symmetric pentameric quaternary structure of this short pentraxin. (B) Orthogonal views of a high-resolution model of the C-terminal pentraxin domains of the human PTX3 based on Cryo-EM that show these domains to fold into octamers with D4 symmetry [PDB-ID: 7ZL1 and (9)]. (C) Schematic drawing of the PTX3 protein with the N-terminal regions (in yellow) forming two parallel tetrameric coiled coils at the opposite sides of the C-terminal core (in red) [based on (9)]. Hinge and intrinsically disordered regions (represented by black lines) bring flexibility to the structure. (D) We have reported that surface bound PTX3 forms a ternary complex with fH and C3b that acts as a “hot spot” for AP inhibition (10). Indeed, when bound to PTX3 and fH, C3b is no longer accessible to factor B (fB, which during AP activation is proteolytically processed to Ba and Bb, the latter being a component of the AP C3 convertase) and loses the ability to amplify the complement cascade (with further cleavage of C3 to C3a and C3b) and the associated inflammatory response (including production of the anaphylatoxin C3a). (E) PTX3 is an essential component of the HA-rich ECMs that transiently form in inflammatory and inflammatory-like conditions. Incorporation of PTX3 in these matrices requires synthesis of covalent adducts between HA and heavy chains (HCs) of the proteoglycan IαI (HC•HA), a reaction that is catalyzed by the hyaladherin TSG-6. PTX3 makes multiple, non-covalent interactions with the HC components of the HC•HA complex, and in this way cross-links HA. This mechanism has major implications in female fertility (11) and has been associated with the anti-inflammatory, -angiogenic and -scarring properties of the PTX3/HC•HA complex isolated from the human amniotic membrane (12). Also, through the HA receptor CD44, HA-embedded PTX3 has been recently reported to promote osteogenesis (13) and breast cancer growth, stemness and metastasis (14).
Cloned in the early ‘90s, PTX3 is considered the paradigm of long pentraxins (27). Additional members of this subfamily are pentraxin 4 [PTX4; (28)], neuronal pentraxins 1 (NPTX1 or NP1 (29);) and 2 [NPTX2, also called Narp or NP2; (30)], and the transmembrane protein neuronal pentraxin receptor [NPTXR (31)]; (see Figure 1A for an overview of the phylogenetic relationships across human pentraxins). Like all other long pentraxins, the PTX3 protomer contains a compact C-terminal domain with sequence homology to the short pentraxins and an elongated N-terminal region (32–34). Initial functional evidence on PTX3 dates to the early 2000s, when a non-redundant immunoprotective role against A. fumigatus was documented by Garlanda et al. (35). Since this original discovery, other functions have emerged for this long pentraxin, including a crucial role in female fertility (11) and extrinsic oncosuppressive effects (36). Here, we reconsider the functional landscape of PTX3 in relation to the protein’s structure, a high-resolution model of which have been recently published. Within this frame, we will discuss emerging vistas on the interplay between PTX3, complement and ECM.
2 Structural biology of PTX3
PTX3 is made at sites of infection and inflammation by several immune and non-immune cell types upon stimulation with inflammatory cytokines (i.e., interleukin-1β, IL-1β, and tumor necrosis factor-α, TNF-α), microbial moieties (i.e., lipopolysaccharide, LPS) and intact microorganisms (i.e., A. fumigatus) (27). This marks a profound difference to CRP, whose synthesis is mostly induced in the liver by interleukin 6 (IL-6) and acts as a systemic (rather than local) marker of inflammation (37). Cellular sources and gene regulation of PTX3 are extensively reviewed in (27, 38). In this chapter, we will focus on the protein’s structure that, as anticipated by our own work, and refined in recent biophysical studies, marks an additional deviation from short pentraxins.
In 2022 Noone et al. reported the first high-resolution 3D model of PTX3 (first of this kind for a long pentraxin, actually) based on a hybrid cryoelectron microscopy (Cryo-EM)/AlphaFold strategy (9). In this study a Cryo-EM 2.5-Å map of the C-terminal pentraxin domains was generated where these regions folded into a rather unique (compared to the short pentraxins) D4 symmetrical octamer. More precisely, the C-domains were found to form a dimer of tetramers, with each tetramer arranged into a planar ring stabilized by noncovalent interactions, and the two tetramers held together by disulfide bonds (Figure 1B). Despite a low level of sequence identity between the C-domain of PTX3 and CRP or SAP (∼28%), these proteins all share a high degree of structural similarity (9). Nonetheless, the short pentraxins fold into pentamers (rather than tetramers; see Figure 1A) owing to poor conservation of the inter-subunit interfaces. Also, the metal-binding site present in CRP, SAP (17) and the other long pentraxin NPTX1 (9) is replaced by a disulfide bridge (C317/C318) (33) and an N-glycosylation site (39), which perhaps explains why PTX3 recognizes most of its ligand in a Ca2+-independent fashion (27). In the Cryo-EM map two water molecules were found proximal to the His residue of the pentraxin motif, raising the possibility that this residue might establish H-bonds with bulk water and thus act as a sensor of the microenvironment’s pH (9) (an intriguing mechanism, given that acidic pH values have a fundamental impact on the function of PTX3 in the ECM (40); see below).
The cryo-EM map revealed α-helical motifs protruding out of the pentraxin core that were partially resolved. AlphaFold was then used to generate in silico predictions of the remaining N-terminal regions and a 3D model was generated that showed the N-domains to form two long tetrameric coiled coils at opposite sides of the C-terminal complex (Figure 1C). Each tetramer contains two hinge regions (encompassing inter-chain disulfide bonds), which brings to the protein a high degree of flexibility (9). The two N-domains have a symmetric arrangement [as opposed to the asymmetric model we proposed based on mid-/low-resolution data (34)] and terminate with an intrinsically disordered region (initial 28-30 amino acids), which collectively provides an extended range of motion and possibly the ability to adapt to diverse interaction interfaces.
3 Structure/function relationships
The structural complexity of the PTX3 protein perhaps explains the rather vast spectrum of interactions and functions of this pentraxin, which ranges from infection immunity to regulation of inflammation, tissue remodeling and cancer (summarized in Table 1).
PTX3 acts as host protective soluble PRM towards a number of fungal, bacterial, and viral pathogens, including A. fumigatus (35, 46), S. pneumoniae (69), uropathogenic E. coli (49), influenza virus (44) and cytomegalovirus (45). Locally induced at sites of infection, PTX3 opsonizes cognate microorganisms upon engagement of selected PAMPs [e.g., outer membrane protein A, OmpA, of K. pneumoniae (47), outer membrane vesicles, OMV, and meningococcal antigens of N. meningitidis (48), nucleocapsid protein of SARS_COV_2 (41)] and promotes viral neutralization as well as fungal and bacterial phagocytosis and killing (by professional phagocytes, mostly neutrophils). The latter processes imply a tight interaction with complement components (discussed below) and Fcγ-receptors [reviewed in (70)].
Several studies point to PTX3 as a modulator of inflammation in sterile (in addition to infectious) settings. In this regard, PTX3 is known to interact with P-selectin in a glycosylation-dependent manner and dampen, via a negative feedback mechanism, the P-selectin-mediated extravasation of neutrophils to sites of tissue damage (50). Also, PTX3 participates in the clearance of apoptotic cells and debris (including histones) by modulating their uptake by dendritic cells (71) and acting as an “eat me” tag for late apoptotic neutrophils (72), in addition to regulating complement activation on dying cells (73). Moreover, it tames complement-dependent, tumor-promoting inflammation (36).
PTX3 is acknowledged as an important player in tissue remodeling and cancer. As for this, it is known to sequester FGF2 and FGF8 through its N-terminal region and inhibit their angiogenic and inflammatory activities in models of neovascularization and FGF-dependent tumorigenesis (51–55). Also, an interaction has been suggested for PTX3 with DC-SIGN (dendritic cell-specific intercellular adhesion molecule-3-grabbing nonintegrin) that might be relevant to leukocyte activation and differentiation to fibrocyte (74), which however requires experimental validation. Other than FGFs, other ligands have been proposed for PTX3 that are relevant to cancer biology, including components of the complement system and CD44 (see below) with however conflicting outcomes (see (75) for further discussion). Finally, PTX3 is known to intercept major mechanisms of fibrin- and HA-remodeling which will be discussed in a separate paragraph.
3.1 Crosstalk with the complement system
As “ante-antibodies”, pentraxins functionally cooperate with complement, de facto extending and modulating both recognition and effector phases of this system (76). The first complement ligand of CRP and SAP to be identified was C1q, whose interaction with these short pentraxins results into activation of the classical pathway (CP) (77, 78). Follow-up studies revealed that components of the lectin pathway (LP) too, with major regard to ficolins, form complexes with CRP and SAP that favor complement-mediated disposal of apoptotic cells and microbial pathogens (75). Importantly, both CP and LP cooperate with pentraxins through additive and synergistic effects that broaden the repertoire of PAMPs/DAMPs recognition and effector functions of the humoral innate immunity [reviewed in (79)]. Despite fundamental immunoprotective functions, over-activation of the complement system can be pathogenic. In this regard, short pentraxins have been proposed to play a dual role. For example, in myocardial infarction and ischemic stroke CRP binds DAMPs on injured cells and exacerbates complement-dependent tissue damage (80). On the other hand, short pentraxins have been involved in suppressive pathways that limit this process by recruiting complement inhibitors, like C4b-binding protein [C4BP, major soluble inhibitor of the CP and LP that is recognized by SAP (81)] and factor H [fH, primary fluid phase inhibitor of the alternative pathway, AP, that is bound by CRP (82)].
Like the short pentraxins, PTX3 tightly cross talks to the three pathways of complement with varying and context-dependent outcomes (83). For example, recognition units of the LP (i.e., mannose-binding-lectin, MBL, and ficolins) form with PTX3 hetero-complexes with LP amplifying activity towards fungal pathogens, like C. albicans (63) and A. fumigatus (64). However, the interaction of PTX3 with C1q (56) results into either activation (on surfaces) or inhibition (in solution) of the CP (57). Also, this long pentraxin is known to interact with complement inhibitors and restrain overactivation of this system. In this regard, PTX3 recruits C4BP to sites of tissue remodeling, including ECM and apoptotic cells, and inhibit activation of the CP/LP (62). More importantly, PTX3 is a ligand of members of the factor H family of proteins (i.e., fH, factor H-like protein 1, FHL-1, and factor H-related proteins 1 and 5, FHR-1 and -5) that collectively control the AP (58–61). This has implications in complement-driven cancer-related inflammation (36), opsonophagocytosis of A. fumigatus (46) and P. aeruginosa (84), atypical hemolytic uremic syndrome (aHUS) (60), and age-related macular degeneration (AMD) (85).
The retina is emerging as an important stage for the complement-modulating properties of PTX3. Indeed, this protein has been localized within and around the ECM of the outer blood-retinal barrier (oBRB), including the Bruch’s membrane (BrM), retinal pigment epithelium (RPE), and choriocapillaris, major sites of complement dysregulation in AMD (86). We recently reported that PTX3 forms a ternary complex with C3b (component of the AP C3 convertase) and fH on acellular surfaces (that mimic the BrM) and acts as a “molecular trap” for AP activation (10) (Figure 1D). Also, we documented that PTX3 interacts with FHL-1 (a truncated form of fH that comprises complement control proteins, CCPs, 1-7 and retains the ability to inhibit the AP), and this interaction is affected by the Y402H polymorphism (a major AMD-associated allele that maps in CCP7 and is thus present both in fH and FHL-1) (61). Given that FHL-1 is a primary inhibitor of the AP in the oBRB and displays Y402H-dependent binding to the BrM (87), we postulate that PTX3 exerts BrM (i.e., ECM) anchoring properties towards FHL-1 (in addition to fH) and these might compensate the pathological effects of the 402H allele (88). It is therefore conceived that this long pentraxin participates in the mechanisms of complement homeostasis in the eye whereby its multimeric and flexible structure allows at a time incorporation in the oBRB ECM and retention of complement inhibition. In this regard, it is worth reiterating here that ECM-embedded PTX3 exerts inhibitory (rather than activating) functions towards the CP/LP via a specific interaction with C4BP (62).
3.2 Roles in the ECM
In a pivotal study by Doni et al, primary components of hemostasis were identified as high affinity ligands of PTX3, i.e. fibrinogen/fibrin (FG) and plasminogen (PG) (40). These recognize non-overlapping sites in the PTX3 N-terminal domain in a calcium- and glycosylation-independent manner, which allows formation of a tripartite PTX3/FG/PG complex with fibrinolytic activity (in the presence of plasminogen activators). In an analogy with the AP components C3b and fH (10), PTX3 acted as a molecular scaffold to favor the interaction of PG with FG and ensure timely degradation of the fibrin clot (40). Consistent with this view, this long pentraxin had remodeling activity in several models of tissue injury, including skin wound, liver and lung damage, and arterial thrombosis. This activity is distinctive of PTX3 and its N-terminal domain (the short pentraxins lacks recognition of FG and PG) and, more importantly, is pH-dependent, whereby an acidic environment sets the PRM PTX3 in a tissue repair mode (40).
Back in 2002, Varani et al. reported that PTX3 deficiency is associated with severe subfertility in female mice (89). In a follow up study, Salustri et al. demonstrated that this is due to instability of the hyaluronic acid (HA) matrix that forms around the oocyte and the surrounding cumulus cells (a multicellular assembly known as cumulus oophorous complex, COC) prior to ovulation and is necessary for fertilization in vivo (11). More importantly, these preclinical findings were corroborated by epidemiological data, whereby PTX3 polymorphisms have been associated with frequency of offspring (90) and dizygotic twinning (91) in sub-Saharan females. The mechanisms underlying the role of PTX3 in fertility are paradigmatic examples of the structure/function complexity of this protein. In fact, in the preovulatory period the COC-associated HA undergoes physical and chemical remodeling due to the action of inter-α-trypsin inhibitor (IαI), a serum proteoglycan composed of two heavy chains (HCs) that enters the follicle due to permeabilization of the blood/follicle barrier (92), and the HA-binding protein TSG-6 (93), which is locally expressed by follicular cells and catalyzes the covalent transfer of HCs onto HA to form HC•HA complexes (94). These HC•HA adducts act as scaffolds for incorporation of the PTX3 protein that is secreted by cumulus cells upon stimulation with oocytic factors and second messengers (89). Owing to its multimeric structure, PTX3 has multiple Mg2+-dependent binding sites (in the N-domain) for the HC components of HC•HA, and thus acts as a node in crosslinking HA, providing stability to the HA ECM (33, 65–67) (Figure 1E). Beside ovulation, these mechanisms might be relevant to pathology, including inflammatory and infectious diseases of the bone (95), joint (96) and lung (97), and are distinctive of PTX3, again due to lack of the N-terminal domain in CRP and SAP. Also, HA-embedded PTX3 has been recently proposed as a promoter of synaptogenesis in the developing central nervous system, where it forms a rheostat with astrocyte-derived thrombospondin 1 (TSP1) (68).
Not only incorporation of PTX3 into HA-rich ECMs has scaffolding implications, but it also appears to convey intracellular signals. In this regard, PTX3 is present in the HA-dependent pericellular matrix of MC3T3-E1 osteoblasts, where it promotes a self-sustained osteogenic program in inflammatory conditions through a functional axis comprising HA, CD44 (major cellular receptor for HA) and the activated focal adhesion kinase (FAK)/protein kinase B (AKT) signaling cascade (13). On the same line, HA/HCs/PTX3 complexes isolated from the human amniotic membrane suppresses inflammation, angiogenesis and scarring in preclinical models of corneal and retinal pathology (12). Also, in a recent report by Hsiao et al, a direct interaction has been documented between CD44 and PTX3 that activates ERK1/2, AKT and NF‐κB pathways and contributes to metastasis/invasion and stemness of a triple‐negative breast cancer cell line (14) (see Figure 1D). It is not clear whether embedding into the HA pericellular matrix is required for PTX3 to recognize CD44, however, given that the CD44-binding interface is in the C-terminal domain (14), it is conceivable that, when incorporated into HA-ECMs [through its N-terminal region (66)], PTX3 retains binding to CD44 while modulating (e.g., via HA-crosslinking) its interaction with HA. This might have implications in leukocyte adhesion and activation (98), in addition to cancer metastasis and stemness (99).
4 Conclusion and perspectives
PTX3 has been implicated in various pathological conditions, including infections (100), cardiovascular diseases (101), bone disorders (95) and cancer (36, 102), where it has potential as a diagnostic and/or prognostic marker and therapeutic target. Importantly, the plasmatic levels of this pentraxin have been consistently associated with disease’s severity and outcome in sepsis and septic shock, tuberculosis, dengue and meningitis [reviewed in (100)], and, more recently, COVID-19 (42, 43). Also, polymorphisms in the PTX3 gene have been associated with the protein’s levels in the plasma and the risk of developing selected opportunistic fungal and bacterial infections [reviewed in (27)]. In this respect, the rs2305619, rs3816527 and rs1840680 single nucleotide polymorphisms (SNPs) form a common haplotypic block where the second SNP causes an amino acid substitution (Asp to Ala) at position 48 in the N-terminal domain. This polymorphism does not alter the protein structure substantially (56), neither does it affect the interaction of PTX3 with C1q (56) and A. fumigatus (103), however it is at present unknown whether it has any impact on the recognition of other ligands, with major regard to the complement proteins fH and C3b, and the incorporation of PTX3 in HA- and/or fibrin-rich ECMs.
CRP is widely used as a clinical biomarker to assess inflammation and predict the risk of cardiovascular diseases (104). Comparative analysis of the structure/function relationships of short and long pentraxins offers unprecedented opportunities to understand the roles of these PRMs in the immune response and their implications in the pathogenesis of several diseases. Recent insights into the structure of PTX3 (the first long pentraxin to be unraveled in such detail) have shed light on its unique architecture and pinpointed the molecular determinants of its immune-modulatory functions (9). In this regard, multi-domain composition of the protomer subunits, glycosylation and quaternary organization collectively contribute to the diverse ligand recognition and interaction capabilities of this long pentraxin. Here we highlighted emerging aspects of the PTX3 biology that intercept fundamental processes of inflammation and tissue remodeling and have translational value in clinical settings that are characterized by dysregulation of complement activation and ECM turnover.
Author contributions
AM: Data curation, Writing – original draft. FC: Data curation, Writing – original draft. BB: Writing – review & editing. AI: Conceptualization, Data curation, Funding acquisition, Project administration, Supervision, Validation, Visualization, Writing – original draft, Writing – review & editing.
Funding
The authors declare financial support was received for the research, authorship, and/or publication of this article. The article’s publication fees are funded by Fondazione Beppe e Nuccy Angiolini.
Acknowledgments
The authors gratefully acknowledge Fondazione Beppe e Nuccy Angiolini for funding a research project on the structure/function relationships of PTX3 in the bone ECM that inspired the writing of this manuscript.
Conflict of interest
The authors declare that the research was conducted in the absence of any commercial or financial relationships that could be construed as a potential conflict of interest.
Publisher’s note
All claims expressed in this article are solely those of the authors and do not necessarily represent those of their affiliated organizations, or those of the publisher, the editors and the reviewers. Any product that may be evaluated in this article, or claim that may be made by its manufacturer, is not guaranteed or endorsed by the publisher.
Glossary
References
1. Medzhitov R. Origin and physiological roles of inflammation. Nature (2008) 454(7203):428–35. doi: 10.1038/nature07201
2. Li D, Wu M. Pattern recognition receptors in health and diseases. Signal Transduct Target Ther (2021) 6(1):291. doi: 10.1038/s41392-021-00687-0
3. Zindel J, Kubes P. DAMPs, PAMPs, and LAMPs in immunity and sterile inflammation. Annu Rev Pathol (2020) 15:493–518. doi: 10.1146/annurev-pathmechdis-012419-032847
4. Bottazzi B, Doni A, Garlanda C, Mantovani A. An integrated view of humoral innate immunity: pentraxins as a paradigm. Annu Rev Immunol (2010) 28:157–83. doi: 10.1146/annurev-immunol-030409-101305
5. Abernethy TJ, Avery OT. The occurrence during acute infections of a protein not normally present in the blood : I. Distribution of the reactive protein in patients' Sera and the effect of calcium on the flocculation reaction with C polysaccharide of pneumococcus. J Exp Med (1941) 73(2):173–82. doi: 10.1084/jem.73.2.173
6. Dereeper A, Guignon V, Blanc G, Audic S, Buffet S, Chevenet F, et al. Phylogeny.fr: robust phylogenetic analysis for the non-specialist. Nucleic Acids Res (2008) 36(Web Server issue):W465–9. doi: 10.1093/nar/gkn180
7. Letunic I, Bork P. Interactive Tree Of Life (iTOL) v5: an online tool for phylogenetic tree display and annotation. Nucleic Acids Res (2021) 49(W1):W293–6. doi: 10.1093/nar/gkab301
8. Shrive AK, Cheetham GM, Holden D, Myles DA, Turnell WG, Volanakis JE, et al. Three dimensional structure of human C-reactive protein. Nat Struct Biol (1996) 3(4):346–54. doi: 10.1038/nsb0496-346
9. Noone DP, Dijkstra DJ, van der Klugt TT, van Veelen PA, de Ru AH, Hensbergen PJ, et al. PTX3 structure determination using a hybrid cryoelectron microscopy and AlphaFold approach offers insights into ligand binding and complement activation. Proc Natl Acad Sci U.S.A. (2022) 119(33):e2208144119. doi: 10.1073/pnas.2208144119
10. Stravalaci M, Davi F, Parente R, Gobbi M, Bottazzi B, Mantovani A, et al. Control of complement activation by the long pentraxin PTX3: implications in age-related macular degeneration. Front Pharmacol (2020) 11:591908. doi: 10.3389/fphar.2020.591908
11. Salustri A, Garlanda C, Hirsch E, De Acetis M, Maccagno A, Bottazzi B, et al. PTX3 plays a key role in the organization of the cumulus oophorus extracellular matrix and in in vivo fertilization. Development (2004) 131:1577–86. doi: 10.1242/dev.01056
12. Tseng SCG. HC-HA/PTX3 purified from amniotic membrane as novel regenerative matrix: insight into relationship between inflammation and regeneration. Invest Ophthalmol Visual Sci (2016) 57(5):ORSFh1-8. doi: 10.1167/iovs.15-17637
13. Dong W, Xu X, Luo Y, Yang C, He Y, Dong X, et al. PTX3 promotes osteogenic differentiation by triggering HA/CD44/FAK/AKT positive feedback loop in an inflammatory environment. Bone (2022) 154:116231. doi: 10.1016/j.bone.2021.116231
14. Hsiao YW, Chi JY, Li CF, Chen LY, Chen YT, Liang HY, et al. Disruption of the pentraxin 3/CD44 interaction as an efficient therapy for triple-negative breast cancers. Clin Trans Med (2022) 12(1):e724. doi: 10.1002/ctm2.724
15. Thompson D, Pepys MB, Wood SP. The physiological structure of human C-reactive protein and its complex with phosphocholine. Structure (1999) 7(2):169–77. doi: 10.1016/S0969-2126(99)80023-9
16. Emsley J, White HE, O'Hara BP, Oliva G, Srinivasan N, Tickle IJ, et al. Structure of pentameric human serum amyloid P component. Nature (1994) 367(6461):338–45. doi: 10.1038/367338a0
17. Kinoshita CM, Gewurz AT, Siegel JN, Ying SC, Hugli TE, Coe JE, et al. A protease-sensitive site in the proposed Ca(2+)-binding region of human serum amyloid P component and other pentraxins. Protein Sci (1992) 1(6):700–9. doi: 10.1002/pro.5560010602
18. Pepys MB. The pentraxins 1975-2018: serendipity, diagnostics and drugs. Front Immunol (2018) 9:2382. doi: 10.3389/fimmu.2018.02382
19. Simons JP, Loeffler JM, Al-Shawi R, Ellmerich S, Hutchinson WL, Tennent GA, et al. C-reactive protein is essential for innate resistance to pneumococcal infection. Immunology (2014) 142(3):414–20. doi: 10.1111/imm.12266
20. Pepys MB. C-reactive protein and the acute phase response. Nature (1982) 296(5852):12. doi: 10.1038/296012a0
21. Doni A, Parente R, Laface I, Magrini E, Cunha C, Colombo FS, et al. Serum amyloid P component is an essential element of resistance against Aspergillus fumigatus. Nat Commun (2021) 12(1):3739. doi: 10.1038/s41467-021-24021-y
22. Cathcart ES, Skinner MS, Cohen AS, Lawless OJ, Benson MD. Antigenic determinants in amyloid deposits. Nature (1970) 228(5276):1090–1. doi: 10.1038/2281090b0
23. Hawkins PN, Myers MJ, Lavender JP, Pepys MB. Diagnostic radionuclide imaging of amyloid: biological targeting by circulating human serum amyloid P component. Lancet (1988) 1(8600):1413–8. doi: 10.1016/S0140-6736(88)92235-0
24. Hawkins PN, Lavender JP, Pepys MB. Evaluation of systemic amyloidosis by scintigraphy with 123I-labeled serum amyloid P component. N Engl J Med (1990) 323(8):508–13. doi: 10.1056/NEJM199008233230803
25. Richards DB, Cookson LM, Berges AC, Barton SV, Lane T, Ritter JM, et al. Therapeutic clearance of amyloid by antibodies to serum amyloid P component. N Engl J Med (2015) 373(12):1106–14. doi: 10.1056/NEJMoa1504942
26. Al-Shawi R, Tennent GA, Millar DJ, Richard-Londt A, Brandner S, Werring DJ, et al. Pharmacological removal of serum amyloid P component from intracerebral plaques and cerebrovascular Abeta amyloid deposits in vivo. Open Biol (2016) 6(2):150202. doi: 10.1098/rsob.150202
27. Garlanda C, Bottazzi B, Magrini E, Inforzato A, Mantovani A. Ptx3, a humoral pattern recognition molecule, in innate immunity, tissue repair, and cancer. Physiol Rev (2018) 98(2):623–39. doi: 10.1152/physrev.00016.2017
28. Martinez de la Torre Y, Fabbri M, Jaillon S, Bastone A, Nebuloni M, Vecchi A, et al. Evolution of the pentraxin family: the new entry PTX4. J Immunol (2010) 184(9):5055–64. doi: 10.4049/jimmunol.0901672
29. Omeis IA, Hsu YC, Perin MS. Mouse and human neuronal pentraxin 1 (NPTX1): conservation, genomic structure, and chromosomal localization. Genomics (1996) 36(3):543–5. doi: 10.1006/geno.1996.0503
30. Hsu YC, Perin MS. Human neuronal pentraxin II (NPTX2): conservation, genomic structure, and chromosomal localization. Genomics (1995) 28(2):220–7. doi: 10.1006/geno.1995.1134
31. Dodds DC, Omeis IA, Cushman SJ, Helms JA, Perin MS. Neuronal pentraxin receptor, a novel putative integral membrane pentraxin that interacts with neuronal pentraxin 1 and 2 and taipoxin-associated calcium-binding protein 49. J Biol Chem (1997) 272(34):21488–94. doi: 10.1074/jbc.272.34.21488
32. Bottazzi B, Vouret-Craviari V, Bastone A, De Gioia L, Matteucci C, Peri G, et al. Multimer formation and ligand recognition by the long pentraxin PTX3. Similarities and differences with the short pentraxins C-reactive protein and serum amyloid P component. J Biol Chem (1997) 272(52):32817–23. doi: 10.1074/jbc.272.52.32817
33. Inforzato A, Rivieccio V, Morreale AP, Bastone A, Salustri A, Scarchilli L, et al. Structural characterization of PTX3 disulfide bond network and its multimeric status in cumulus matrix organization. J Biol Chem (2008) 283(15):10147–61. doi: 10.1074/jbc.M708535200
34. Inforzato A, Baldock C, Jowitt TA, Holmes DF, Lindstedt R, Marcellini M, et al. The angiogenic inhibitor long pentraxin PTX3 forms an asymmetric octamer with two binding sites for FGF2. J Biol Chem (2010) 285(23):17681–92. doi: 10.1074/jbc.M109.085639
35. Garlanda C, Hirsch E, Bozza S, Salustri A, De Acetis M, Nota R, et al. Non-redundant role of the long pentraxin PTX3 in anti-fungal innate immune response. Nature (2002) 420(6912):182–6. doi: 10.1038/nature01195
36. Bonavita E, Gentile S, Rubino M, Maina V, Papait R, Kunderfranco P, et al. PTX3 is an extrinsic oncosuppressor regulating complement-dependent inflammation in cancer. Cell (2015) 160(4):700–14. doi: 10.1016/j.cell.2015.01.004
37. Bottazzi B, Inforzato A, Messa M, Barbagallo M, Magrini E, Garlanda C, et al. The pentraxins PTX3 and SAP in innate immunity, regulation of inflammation and tissue remodelling. J Hepatol (2016) 64(6):1416–27. doi: 10.1016/j.jhep.2016.02.029
38. Doni A, Stravalaci M, Inforzato A, Magrini E, Mantovani A, Garlanda C, et al. The long pentraxin PTX3 as a link between innate immunity, tissue remodeling, and cancer. Front Immunol (2019) 10:712. doi: 10.3389/fimmu.2019.00712
39. Inforzato A, Peri G, Doni A, Garlanda C, Mantovani A, Bastone A, et al. Structure and function of the long pentraxin PTX3 glycosidic moiety: fine-tuning of the interaction with C1q and complement activation. Biochemistry (2006) 45(38):11540–51. doi: 10.1021/bi0607453
40. Doni A, Musso T, Morone D, Bastone A, Zambelli V, Sironi M, et al. An acidic microenvironment sets the humoral pattern recognition molecule PTX3 in a tissue repair mode. J Exp Med (2015) 212(6):905–25. doi: 10.1084/jem.20141268
41. Stravalaci M, Pagani I, Paraboschi EM, Pedotti M, Doni A, Scavello F, et al. Recognition and inhibition of SARS-CoV-2 by humoral innate immunity pattern recognition molecules. Nat Immunol (2022) 23(2):275–86. doi: 10.1038/s41590-021-01114-w
42. Capra AP, Ardizzone A, Panto G, Paterniti I, Campolo M, Crupi L, et al. The prognostic value of pentraxin-3 in COVID-19 patients: A systematic review and meta-analysis of mortality incidence. Int J Mol Sci (2023) 24(4):3537. doi: 10.3390/ijms24043537
43. Brunetta E, Folci M, Bottazzi B, De Santis M, Gritti G, Protti A, et al. Macrophage expression and prognostic significance of the long pentraxin PTX3 in COVID-19. Nat Immunol (2021) 22(1):19–24. doi: 10.1038/s41590-020-00832-x
44. Reading PC, Bozza S, Gilbertson B, Tate M, Moretti S, Job ER, et al. Antiviral activity of the long chain pentraxin PTX3 against influenza viruses. J Immunol (2008) 180(5):3391–8. doi: 10.4049/jimmunol.180.5.3391
45. Bozza S, Bistoni F, Gaziano R, Pitzurra L, Zelante T, Bonifazi P, et al. Pentraxin 3 protects from MCMV infection and reactivation through TLR sensing pathways leading to IRF3 activation. Blood (2006) 108(10):3387–96. doi: 10.1182/blood-2006-03-009266
46. Moalli F, Doni A, Deban L, Zelante T, Zagarella S, Bottazzi B, et al. Role of complement and Fc{gamma} receptors in the protective activity of the long pentraxin PTX3 against Aspergillus fumigatus. Blood (2010) 116(24):5170–80. doi: 10.1182/blood-2009-12-258376
47. Jeannin P, Bottazzi B, Sironi M, Doni A, Rusnati M, Presta M, et al. Complexity and complementarity of outer membrane protein A recognition by cellular and humoral innate immunity receptors. Immunity (2005) 22(5):551–60. doi: 10.1016/j.immuni.2005.03.008
48. Bottazzi B, Santini L, Savino S, Giuliani MM, Duenas Diez AI, Mancuso G, et al. Recognition of Neisseria meningitidis by the long pentraxin PTX3 and its role as an endogenous adjuvant. PloS One (2015) 10(3):e0120807. doi: 10.1371/journal.pone.0120807
49. Jaillon S, Moalli F, Ragnarsdottir B, Bonavita E, Puthia M, Riva F, et al. The humoral pattern recognition molecule PTX3 is a key component of innate immunity against urinary tract infection. Immunity (2014) 40(4):621–32. doi: 10.1016/j.immuni.2014.02.015
50. Deban L, Castro Russo R, Sironi M, Moalli F, Scanziani M, Zambelli V, et al. Regulation of leukocyte recruitment by the long pentraxin PTX3. Nat Immunol (2010) 11(4):328–34. doi: 10.1038/ni.1854
51. Presta M, Camozzi M, Salvatori G, Rusnati M. Role of the soluble pattern recognition receptor PTX3 in vascular biology. J Cell Mol Med (2007) 11(4):723–38. doi: 10.1111/j.1582-4934.2007.00061.x
52. Ronca R, Alessi P, Coltrini D, Salle ED, Giacomini A, Leali D, et al. Long pentraxin-3 as an epithelial-stromal fibroblast growth factor-targeting inhibitor in prostate cancer. J Pathol (2013). 230(2):228–38. doi: 10.1002/path.4181
53. Rodrigues PF, Matarazzo S, Maccarinelli F, Foglio E, Giacomini A, Silva Nunes JP, et al. Long pentraxin 3-mediated fibroblast growth factor trapping impairs fibrosarcoma growth. Front Oncol (2018) 8:472. doi: 10.3389/fonc.2018.00472
54. Turati M, Giacomini A, Rezzola S, Maccarinelli F, Gazzaroli G, Valentino S, et al. The natural FGF-trap long pentraxin 3 inhibits lymphangiogenesis and lymphatic dissemination. Exp Hematol Oncol (2022) 11(1):84. doi: 10.1186/s40164-022-00330-w
55. Leali D, Inforzato A, Ronca R, Bianchi R, Belleri M, Coltrini D, et al. Long pentraxin 3/tumor necrosis factor-stimulated gene-6 interaction: a biological rheostat for fibroblast growth factor 2-mediated angiogenesis. Arteriosclerosis Thrombosis Vasc Biol (2012) 32(3):696–703. doi: 10.1161/ATVBAHA.111.243998
56. Bally I, Inforzato A, Dalonneau F, Stravalaci M, Bottazzi B, Gaboriaud C, et al. Interaction of C1q with pentraxin 3 and igM revisited: mutational studies with recombinant C1q variants. Front Immunol (2019) 10:461. doi: 10.3389/fimmu.2019.00461
57. Nauta AJ, Bottazzi B, Mantovani A, Salvatori G, Kishore U, Schwaeble WJ, et al. Biochemical and functional characterization of the interaction between pentraxin 3 and C1q. Eur J Immunol (2003) 33(2):465–73. doi: 10.1002/immu.200310022
58. Deban L, Jarva H, Lehtinen MJ, Bottazzi B, Bastone A, Doni A, et al. Binding of the long pentraxin PTX3 to Factor H: Interacting domains and function in the regulation of complement activation. J Immunol (2008) 181(12):8433–40. doi: 10.4049/jimmunol.181.12.8433
59. Csincsi AI, Kopp A, Zoldi M, Banlaki Z, Uzonyi B, Hebecker M, et al. Factor H-related protein 5 interacts with pentraxin 3 and the extracellular matrix and modulates complement activation. J Immunol (2015) 194(10):4963–73. doi: 10.4049/jimmunol.1403121
60. Kopp A, Strobel S, Tortajada A, Rodriguez de Cordoba S, Sanchez-Corral P, Prohaszka Z, et al. Atypical hemolytic uremic syndrome-associated variants and autoantibodies impair binding of factor h and factor h-related protein 1 to pentraxin 3. J Immunol (2012) 189(4):1858–67. doi: 10.4049/jimmunol.1200357
61. Swinkels M, Zhang JH, Tilakaratna V, Black G, Perveen R, McHarg S, et al. C-reactive protein and pentraxin-3 binding of factor H-like protein 1 differs from complement factor H: implications for retinal inflammation. Sci Rep (2018) 8(1):1643. doi: 10.1038/s41598-017-18395-7
62. Braunschweig A, Jozsi M. Human pentraxin 3 binds to the complement regulator c4b-binding protein. PloS One (2011) 6(8):e23991. doi: 10.1371/journal.pone.0023991
63. Ma YJ, Doni A, Skjoedt MO, Honore C, Arendrup M, Mantovani A, et al. Heterocomplexes of mannose-binding lectin and the pentraxins PTX3 or serum amyloid P component trigger cross-activation of the complement system. J Biol Chem (2011) 286(5):3405–17. doi: 10.1074/jbc.M110.190637
64. Ma YJ, Doni A, Hummelshoj T, Honore C, Bastone A, Mantovani A, et al. Synergy between ficolin-2 and pentraxin 3 boosts innate immune recognition and complement deposition. J Biol Chem (2009) 284(41):28263–75. doi: 10.1074/jbc.M109.009225
65. Scarchilli L, Camaioni A, Bottazzi B, Negri V, Doni A, Deban L, et al. PTX3 interacts with inter-alpha-trypsin inhibitor: implications for hyaluronan organization and cumulus oophorus expansion. J Biol Chem (2007) 282(41):30161–70. doi: 10.1074/jbc.M703738200
66. Ievoli E, Lindstedt R, Inforzato A, Camaioni A, Palone F, Day AJ, et al. Implication of the oligomeric state of the N-terminal PTX3 domain in cumulus matrix assembly. Matrix Biol (2011) 30(5-6):330–7. doi: 10.1016/j.matbio.2011.05.002
67. Baranova NS, Inforzato A, Briggs DC, Tilakaratna V, Enghild JJ, Thakar D, et al. Incorporation of pentraxin 3 into hyaluronan matrices is tightly regulated and promotes matrix cross-linking. J Biol Chem (2014) 289(44):30481–98. doi: 10.1074/jbc.M114.568154
68. Fossati G, Pozzi D, Canzi A, Mirabella F, Valentino S, Morini R, et al. Pentraxin 3 regulates synaptic function by inducing AMPA receptor clustering via ECM remodeling and beta1-integrin. EMBO J (2019) 38(1):e99529. doi: 10.15252/embj.201899529
69. Porte R, Silva-Gomes R, Theroude C, Parente R, Asgari F, Sironi M, et al. Regulation of inflammation and protection against invasive pneumococcal infection by the long pentraxin PTX3. Elife (2023) 12:e78601. doi: 10.7554/eLife.78601
70. Parente R, Possetti V, Erreni M, D'Autilia F, Bottazzi B, Garlanda C, et al. Complementary roles of short and long pentraxins in the complement-mediated immune response to aspergillus fumigatus infections. Front Immunol (2021) 12:785883. doi: 10.3389/fimmu.2021.785883
71. Rovere P, Peri G, Fazzini F, Bottazzi B, Doni A, Bondanza A, et al. The long pentraxin PTX3 binds to apoptotic cells and regulates their clearance by antigen-presenting dendritic cells. Blood (2000) 96(13):4300–6. doi: 10.1182/blood.V96.13.4300
72. Jaillon S, Jeannin P, Hamon Y, Fremaux I, Doni A, Bottazzi B, et al. Endogenous PTX3 translocates at the membrane of late apoptotic human neutrophils and is involved in their engulfment by macrophages. Cell Death Differ (2009) 16(3):465–74. doi: 10.1038/cdd.2008.173
73. Baruah P, Dumitriu IE, Peri G, Russo V, Mantovani A, Manfredi AA, et al. The tissue pentraxin PTX3 limits C1q-mediated complement activation and phagocytosis of apoptotic cells by dendritic cells. J Leukocyte Biol (2006) 80(1):87–95. doi: 10.1189/jlb.0805445
74. Cox N, Pilling D, Gomer RH. DC-SIGN activation mediates the differential effects of SAP and CRP on the innate immune system and inhibits fibrosis in mice. Proc Natl Acad Sci U.S.A. (2015) 112(27):8385–90. doi: 10.1073/pnas.1500956112
75. Giacomini A, Ghedini GC, Presta M, Ronca R. Long pentraxin 3: A novel multifaceted player in cancer. Biochim Biophys Acta Rev Cancer (2018) 1869(1):53–63. doi: 10.1016/j.bbcan.2017.11.004
76. Haapasalo K, Meri S. Regulation of the complement system by pentraxins. Front Immunol (2019) 10:1750. doi: 10.3389/fimmu.2019.01750
77. Agrawal A, Shrive AK, Greenhough TJ, Volanakis JE. Topology and structure of the C1q-binding site on C-reactive protein. J Immunol (2001) 166(6):3998–4004. doi: 10.4049/jimmunol.166.6.3998
78. Sorensen IJ, Nielsen EH, Andersen O, Danielsen B, Svehag SE. Binding of complement proteins C1q and C4bp to serum amyloid P component (SAP) in solid contra liquid phase. Scand J Immunol (1996) 44(4):401–7. doi: 10.1046/j.1365-3083.1996.d01-326.x
79. Ma YJ, Garred P. Pentraxins in complement activation and regulation. Front Immunol (2018) 9:3046. doi: 10.3389/fimmu.2018.03046
80. Gill R, Kemp JA, Sabin C, Pepys MB. Human C-reactive protein increases cerebral infarct size after middle cerebral artery occlusion in adult rats. J Cereb Blood Flow Metab (2004) 24(11):1214–8. doi: 10.1097/01.WCB.0000136517.61642.99
81. Garcia de Frutos P, Dahlback B. Interaction between serum amyloid P component and C4b-binding protein associated with inhibition of factor I-mediated C4b degradation. J Immunol (1994) 152(5):2430–7. doi: 10.4049/jimmunol.152.5.2430
82. Okemefuna AI, Nan R, Miller A, Gor J, Perkins SJ. Complement factor H binds at two independent sites to C-reactive protein in acute phase concentrations. J Biol Chem (2010) 285(2):1053–65. doi: 10.1074/jbc.M109.044529
83. Inforzato A, Doni A, Barajon I, Leone R, Garlanda C, Bottazzi B, et al. PTX3 as a paradigm for the interaction of pentraxins with the Complement system. Semin Immunol (2013) 25(1):79–85. doi: 10.1016/j.smim.2013.05.002
84. Moalli F, Paroni M, Veliz Rodriguez T, Riva F, Polentarutti N, Bottazzi B, et al. The therapeutic potential of the humoral pattern recognition molecule PTX3 in chronic lung infection caused by Pseudomonas aeruginosa. J Immunol (2011) 186(9):5425–34. doi: 10.4049/jimmunol.1002035
85. Stravalaci M, Ferrara M, Pathak V, Davi F, Bottazzi B, Mantovani A, et al. The long pentraxin PTX3 as a new biomarker and pharmacological target in age-related macular degeneration and diabetic retinopathy. Front Pharmacol (2022) 12. doi: 10.3389/fphar.2021.811344
86. Wong JHC, Ma JYW, Jobling AI, Brandli A, Greferath U, Fletcher EL, et al. Exploring the pathogenesis of age-related macular degeneration: A review of the interplay between retinal pigment epithelium dysfunction and the innate immune system. Front Neurosci (2022) 16:1009599. doi: 10.3389/fnins.2022.1009599
87. Clark SJ, Schmidt CQ, White AM, Hakobyan S, Morgan BP, Bishop PN. Identification of factor H-like protein 1 as the predominant complement regulator in bruch's membrane: implications for age-related macular degeneration. J Immunol (2014) 193(10):4962–70. doi: 10.4049/jimmunol.1401613
88. Landowski M, Kelly U, Klingeborn M, Groelle M, Ding JD, Grigsby D, et al. Human complement factor H Y402H polymorphism causes an age-related macular degeneration phenotype and lipoprotein dysregulation in mice. Proc Natl Acad Sci United States America (2019) 116(9):3703–11. doi: 10.1073/pnas.1814014116
89. Varani S, Elvin JA, Yan C, DeMayo J, DeMayo FJ, Horton HF, et al. Knockout of pentraxin 3, a downstream target of growth differentiation factor-9, causes female subfertility. Mol Endocrinol (2002) 16:1154–67. doi: 10.1210/mend.16.6.0859
90. May L, Kuningas M, van Bodegom D, Meij HJ, Frolich M, Slagboom PE, et al. Genetic variation in pentraxin (PTX) 3 gene associates with PTX3 production and fertility in women. Biol Reprod (2010) 82(2):299–304. doi: 10.1095/biolreprod.109.079111
91. Sirugo G, Edwards DR, Ryckman KK, Bisseye C, White MJ, Kebbeh B, et al. PTX3 genetic variation and dizygotic twinning in the Gambia: could pleiotropy with innate immunity explain common dizygotic twinning in Africa? Ann Hum Genet (2012) 76(6):454–63. doi: 10.1111/j.1469-1809.2012.00723.x
92. Lord MS, Melrose J, Day AJ, Whitelock JM. The inter-alpha-trypsin inhibitor family: versatile molecules in biology and pathology. J Histochem Cytochem (2020) 68(12):907–27. doi: 10.1369/0022155420940067
93. Day AJ, Milner CM. TSG-6: A multifunctional protein with anti-inflammatory and tissue-protective properties. Matrix Biol (2019) 78-79:60–83. doi: 10.1016/j.matbio.2018.01.011
94. Baranova NS, Nileback E, Haller FM, Briggs DC, Svedhem S, Day AJ, et al. The inflammation-associated protein TSG-6 cross-links hyaluronan via hyaluronan-induced TSG-6 oligomers. J Biol Chem (2011) 286(29):25675–86. doi: 10.1074/jbc.M111.247395
95. Parente R, Sobacchi C, Bottazzi B, Mantovani A, Grcevic D, Inforzato A. The long pentraxin PTX3 in bone homeostasis and pathology. Front Immunol (2019) 10:2628. doi: 10.3389/fimmu.2019.02628
96. Boutet MA, Nerviani A, Lliso-Ribera G, Leone R, Sironi M, Hands R, et al. Circulating and synovial pentraxin-3 (PTX3) expression levels correlate with rheumatoid arthritis severity and tissue infiltration independently of conventional treatments response. Front Immunol (2021) 12:686795. doi: 10.3389/fimmu.2021.686795
97. Tighe RM, Garantziotis S. Hyaluronan interactions with innate immunity in lung biology. Matrix Biol (2019) 78-79:84–99. doi: 10.1016/j.matbio.2018.01.027
98. McDonald B, Kubes P. Interactions between CD44 and hyaluronan in leukocyte trafficking. Front Immunol (2015) 6. doi: 10.3389/fimmu.2015.00068
99. Senbanjo LT, Chellaiah MA. CD44: A multifunctional cell surface adhesion receptor is a regulator of progression and metastasis of cancer cells. Front Cell Dev Biol (2017) 5. doi: 10.3389/fcell.2017.00018
100. Porte R, Davoudian S, Asgari F, Parente R, Mantovani A, Garlanda C, et al. The long pentraxin PTX3 as a humoral innate immunity functional player and biomarker of infections and sepsis. Front Immunol (2019) 10:794. doi: 10.3389/fimmu.2019.00794
101. Ristagno G, Fumagalli F, Bottazzi B, Mantovani A, Olivari D, Novelli D, et al. Pentraxin 3 in cardiovascular disease. Front Immunol (2019) 10:823. doi: 10.3389/fimmu.2019.00823
102. Watt J, Siddique I, Dowe T, Crnogorac-Jurcevic T, Allavena P, Kocher H. Role of PTX3 in pancreatic cancer. Lancet (2014) 383:106–6. doi: 10.1016/S0140-6736(14)60369-X
103. Cunha C, Aversa F, Lacerda JF, Busca A, Kurzai O, Grube M, et al. Genetic PTX3 deficiency and aspergillosis in stem-cell transplantation. New Engl J Med (2014) 370(5):421–32. doi: 10.1056/NEJMoa1211161
Keywords: pattern recognition molecules (PRM), pentraxins, PTX3, structure, complement, extracellular matrix
Citation: Massimino AM, Colella FE, Bottazzi B and Inforzato A (2023) Structural insights into the biological functions of the long pentraxin PTX3. Front. Immunol. 14:1274634. doi: 10.3389/fimmu.2023.1274634
Received: 08 August 2023; Accepted: 27 September 2023;
Published: 09 October 2023.
Edited by:
Yi Wu, Xi’an Jiaotong University, ChinaReviewed by:
Chiara Agostinis, Institute for Maternal and Child Health Burlo Garofolo (IRCCS), ItalyJózsef Dobó, Hungarian Academy of Sciences (MTA), Hungary
Copyright © 2023 Massimino, Colella, Bottazzi and Inforzato. This is an open-access article distributed under the terms of the Creative Commons Attribution License (CC BY). The use, distribution or reproduction in other forums is permitted, provided the original author(s) and the copyright owner(s) are credited and that the original publication in this journal is cited, in accordance with accepted academic practice. No use, distribution or reproduction is permitted which does not comply with these terms.
*Correspondence: Antonio Inforzato, antonio.inforzato@humanitasresearch.it
†These authors have contributed equally to this work and share first authorship