- Department of Clinical Laboratory, Kunshan First People’s Hospital, Affiliated to Jiangsu University, Kunshan, China
The heterogeneity of the tumor microenvironment (TME) is a major obstacle in cancer treatment, making most therapeutic interventions palliative rather than curative. Previous studies have suggested that the reason for the low efficacy of immunotherapy and the relapse of the original responders over time may be due to the complex network of mesenchymal stem/stromal cells (MSCs), a population of multipotent progenitor cells existing in a variety of tissues. Cancer-associated MSCs (CA-MSCs) have already been isolated from various types of tumors and are characterized by their vigorous pro-tumorigenic functions. Although the roles of CA-MSCs from different sources vary widely, their origins are still poorly understood. Current evidence suggests that when local resident or distally recruited MSCs interact with tumor cells and other components in the TME, “naïve” MSCs undergo genetic and functional changes to form CA-MSCs. In this review, we mainly focus on the multiple roles of CA-MSCs derived from different sources, which may help in elucidating the formation and function of the entire TME, as well as discover innovative targets for anti-cancer therapies.
Introduction
The International Society for Cellular Therapy issued a set of minimal standards for the recognition of mesenchymal stem/stromal cells (MSCs) in 2006 based on three principles: Firstly, in typical culture settings, MSCs must adhere to the plastic. Secondly, it is essential that MSCs express CD105, CD90 and CD73, while they must be negative for CD 45, CD 34, CD 14 or 11b, CD 79α or 19, and HLA-DR. Lastly, MSCs are capable of differentiating into adipocytes, chondrocytes, and osteoblasts under certain circumstances (1–3). In healthy tissues, MSCs play a key role in wound healing and immune regulation. In the bone marrow niche, MSCs play an important role in hematopoietic stem cell maintenance and lineage determination by secreting factors, and contribute to the construction of the niche (4). Although MSCs account for a relatively small proportion of the tumor microenvironment (TME), with studies reporting that they make up 0.01–5% of the total cell population, they can amplify their effects by directly altering the matrix composition of the TME and influencing the behavior of their offspring given the capacity for multi-directional differentiation (4–7).
It has been found that MSCs that reside within tumor tissue have high tumorigenicity compared to MSCs from healthy tissue (8). In-depth knowledge of the origin, heterogeneity, and functions of MSCs is critical to understanding disease progression and elucidating potential targets for novel therapeutic interventions.
Origins of CA-MSCS
MSCs are a heterogeneous group of progenitor cells with both pro-tumor and anti-tumor effects (9–11). MSCs are recruited from adjacent tissues or circulating bone marrow to the tumor sites and reprogrammed into cancer-associated MSCs (CA-MSCs). Current evidence suggests that both recruited and local resident MSCs are major sources of CA-MSCs (12).
The recruitment and reprogramming of MSCS
Chemokines and cytokines secreted by tumor cells and the surrounding stroma have been shown to be involved in the mechanisms of migration or homing of the circulating bone marrow MSCs (BMMSCs) to the TME. It is well known that tumor-produced CCL2, CCL5, CXCL12 and CXCL16 play crucial roles in the chemotaxis of BMMSCs (13). In addition, cytokines such as PDGF, TGF-β, VEGF and TNF-α were also found to play important roles in BMMSCs homing (14, 15). For example, a study in prostate cancer showed that TGF-β1 is a key molecule that regulates the distant recruitment of BMMSCs into tumor sites (16). MSCs present in adjacent normal tissues express the homing spectrum of inflammatory signals from tumor cells, and are thus anchored in the tumor mass (17). However, the signaling pathways and underlying mechanisms by which MSCs migrate from adjacent normal tissues to tumor sites are still unknown.
Based on evidence, tumor cells can effectively reprogram the phenotype and function of “naïve” MSCs. Le et al. have demonstrated that ovarian cancer cells cause “naïve” MSCs to express CD73, CD90 and CD105, while CD14, CD20, CD34 and CD45 are absent. Moreover, the levels of CXCL1, CXCL2 and IL-8 secreted by MSCs increase through PI3K/Akt, MAPK and NF-κB signaling pathways, which in turn make tumor cells resistant to chemotherapy and the differentiation of monocytes into M2 type (18). Wang et al. have shown that gastric cancer cells induce “naïve” mesenchymal stem cells have pro-metastatic behavior by activating YAP signaling through the exosomal Wnt5a (19). Exosomes derived from gastric cancer cell cause abnormal activation of the NF-kB signaling pathway in MSCs, thus promoting the secretion of pro-inflammatory factors by macrophages and the activation of CD69 and CD25 on the surface of T cells (20). In addition to the tumor cells, the surrounding microenvironment also plays a critical role in the malignant transformation of “naïve” MSCs into CA-MSCs. For example, mononuclear/macrophage-derived TNF-a makes “naïve” MSCs similar to CA-MSCs in their chemokine profile and ability to promote tumorigenicity in lymphomas (21). Moreover, neutrophils treated with tumor cells activate the AKT and p38 pathways in “naïve” MSCs by secreting inflammatory factors such as IL-17, IL-23 and TNF-α, and transform them into CA-MSCs, thus significantly promoting the growth and metastasis of gastric cancer (22).
MSCS naturally residing in the TME
As one of the key stromal cell types in the tumor niche, CA-MSCs have been isolated from various tumors and have been found to play an important role in tumor progression (Table 1). MSCs isolated from gastric cancer tissues (GCMSCs) promote cancer progression and contribute to tumor immunotherapy tolerance through the CXCR2–HK2–PD-L1 pathway (23). IL-8 derived from GCMSCs induces the expression of PD-L1 in GC cells through c-Myc regulated by the STAT3 and mTOR signaling pathways and inhibits the anti-tumor immune response (24). GCMSCs also disrupt the Treg/Th17 balance in peripheral blood mononuclear cells (PBMCs) by inhibiting Th17 cell proliferation and inducing Tregs differentiation, thereby impairing the anti-tumor immune response (25). GCMSCs reduce the frequency of infiltrating NK cells and inhibit their effector function to promote tumor growth through the mTOR signaling pathway (26). Lung cancer-associated MSCs exert strong metastasis-promoting effects by producing complement C3, which is induced and maintained by Th2 cytokines in a STAT6-dependent manner and also promote tumor metastasis and tumorigenesis by induction of EMT and stem-like reprogram (27, 28). Single-cell transcriptional analysis of MSCs in invasive breast cancer TME revealed increased expression of the COL10A1 and COL8A1 genes, as well as pro-tumor effects driven by TGF-β-related signals (29). Breast cancer-associated MSCs also promote cancer proliferation and enhance mammosphere formation partially via EGF–EGFR–AKT pathway (30). MSCs residing in other solid tumors also reported to contribute to tumor progression, such as pancreatic cancer, cervical cancer, colon cancer, head and neck squamous cell cancer and neuroblastoma (31–35). In general, MSCs residing in TME not only up-regulate the proliferation, migration and invasion ability of tumor cells and their PD-L1 expression, but also disrupt the balance between immune cells, thus inhibiting the anti-tumor immune response (Figure 1).
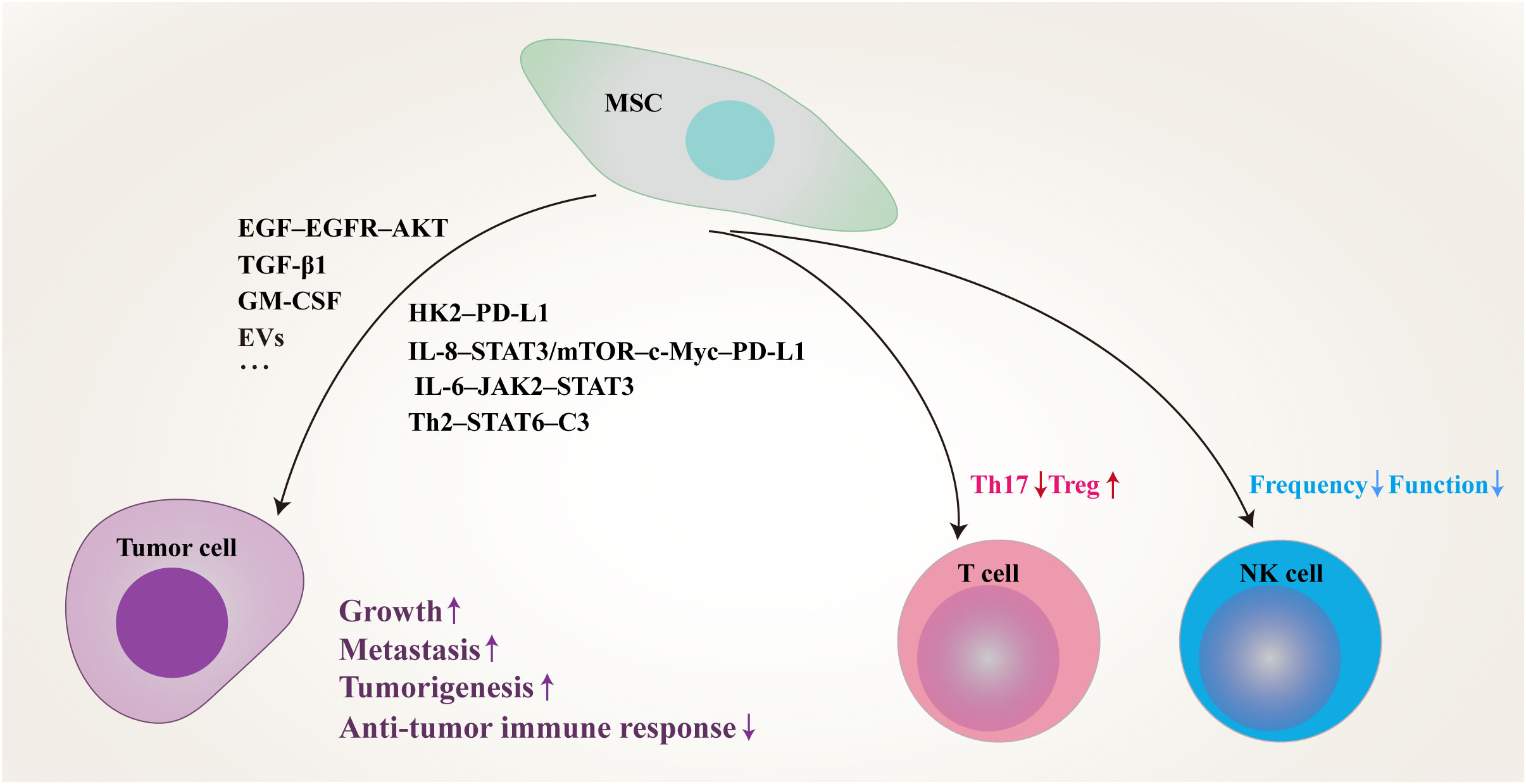
Figure 1 Schematic of proposed network of MSCs residing in TME inhibit anti-tumor immune responses and promote tumor progression by modulating tumor cells and immune cells through multiple signaling pathways. MSC, mesenchymal stem/stromal cell; PD-L1, programmed death-1 ligand; EVs, extracellular vesicles; IL, interleukin; STAT, signal transducer and activator of transcription; EGF, epidermal growth factor; EGFR, epidermal growth factor receptor; TGF-β, transforming growth factor-β; GM-CSF, granulocyte-macrophage colony-stimulating factor; HK2, hexokinase 2; mTOR, mammalian target of rapamycin; JAK2, janus kinase 2; Th, T helper; C3, complement 3; Treg, regulatory T cell; NK, natural killer.
Besides solid tumors, hematological malignancies also rely heavily on support of host cells, specifically MSCs (36). The development of multiple myeloma MSCs (MM-MSCs) may be the result of multiple factors, and changes may vary depending on the individual, lesion location, co-culture myeloma cell type, and cell subsets within the MSC population. MM-MSCs differ from non-diseased donors (ND-MSCs) in a number of ways, including cytokine production. MM-MSCs secrete higher levels of growth factors such as SCF, VEGF, and IL-6, than ND-MSCs. Recent studies have shown that, compared with ND-MSCs, MM-MSCs respond to myeloma cells with increased expression of IL-10, the TNF family member B-cell activating factor, and HGF (37, 38). MM-MSCs, but not ND-MSCs, can also induce bortezomib resistance via enhanced NF-κB activity in MM cells (39). MM-MSCs are spatially co-located with tumor cells and immune cells which express genes involved in tumor survival and immune regulation. Inflammatory MSCs (iMSCs) are specific for MM bone marrow. The development of iMSCs is the result of NF-κB signaling activated by inflammatory mediators (40).
Different properties between CA-MSCS and “naïve” MSCS
Interactions with tumor cells and other components in the TME lead to genetic and functional changes in “naïve” MSCs (18, 41). CA-MSCs show stronger proliferation and migration abilities and have stronger tumor-promoting effects than “naïve” MSCs. In addition, the number of G0/G1 phase cells is increased in CA-MSCs compared to their counterparts in neuroblastoma, suggesting that CA-MSCs play an important role in regulating cancer dormancy. Compared with “naïve” MSCs, CA-MSCs also exhibit different adipogenic differentiation and immunomodulatory abilities (12).
Regulatory effects of MSCS from different sources on tumor cells
Evidence indicates that the specific effects of MSCs on tumors depend on the origin and tumor type (Table 2).
Bone marrow MSCs
BMMSCs promote metastasis by activating the ABL–MMP9 signaling axis in lung cancer cells (42). BMMSCs secreted IL-6 contribute significantly to pancreatic cancer growth (43). However, BMMSCs-derived IL-28 trigger prostate cancer cell apoptosis via IL-28Rα–STAT1 signaling pathway (44). The plasticity of cancer stem-like cells (CSCs) is one of the primary elements responsible for the heterogeneity of the TME (63). Estrogen receptor-positive breast cancer cells make direct contact with BMMSCs and acquire CSCs phenotype with increased resistance to standard antiestrogenic drugs (45). Extracellular vesicles released by BMMSCs instruct breast cancer cells to become dormant CSCs with chemoresistance capacity (46). Cancer cell-stimulated BMMSCs also create a CSCs niche via the release of prostaglandin E2 (64). IL-6 secreted by tumor-stimulated BMMSCs, enhances the stemness and migration capacity of osteosarcoma cells (47). BMMSCs-derived exosomes promote colon CSCs traits via miR-142-3p (48).
Umbilical cord MSCs
Umbilical cord MSCs (UCMSCs) are known to facilitate the proliferation and movement of lung cancer cells through the ERK–phospho-c-Fos-S374 pathway and to induce malignant characteristics of cancer cells through the AMPK signaling pathway (49, 50). UCMSCs inhibit the growth and metastasis and promote the apoptosis of lung cancer cells by regulating the PI3K–Akt and NF-κB pathways (51). UCMSCs inhibit the proliferation, migration and invasion of glioblastoma cells by regulating the IL-6–JAK2–STAT3 signaling pathway, and promote the apoptosis of glioblastoma cells (52). Exosomes derived from UCMSCs have anti-proliferative, pro-apoptotic, and anti-angiogenic effects on hepatocellular carcinoma cells (53). Exosomes secreted by UCMSCs also inhibit macrophage M2 polarization and prevent liver metastasis of colorectal cancer by inducing miR-1827 to target SUCNR1 (54). Regulation of ITGA2/ITGA6 has been demonstrated to be the means by which UCMSCs suppress the proliferation, migration and invasion of colon cancer cells (55).
Adipose tissue-derived MSCs
Adipose tissue-derived MSCs (ADMSCs) can be used in the treatment of lung metastatic melanoma therapy by producing the anti-angiogenic factor TSP-1 (56). ADMSCs can increase the efficacy of chemotherapy in hepatocellular carcinoma treatment (57). ADMSCs also accelerate the progression of colon cancer by inducing an MSC-transformed cancer-associated fibroblast phenotype through the ICAM1–STAT3–AKT axis (58). ADMSCs promote the proliferation and migration of head and neck cancer cells (59). In addition, preclinical treatment of breast cancer is aided by ADMSCs, which exhibit anti-tumor activity (60). It has been demonstrated that CCL5 secreted by ADMSCs can induce PD-L1 expression in breast cancer cells (61). Exosomes derived from ADMSCs reduce glioblastoma proliferation and significantly down-regulate the invasion-related genes ITGα5 and ITGβ3, as well as the angiogenesis induction gene KDR (62).
Naturally residing MSCs
IL-6 derived from ameloblastoma MSCs (AMMSCs) induces epithelial–mesenchymal transition (EMT) and promotes the formation of CSCs through the STAT3 and ERK1/2 signaling pathways (65). Endometrial cancer-derived MSCs exhibit high expression of PD-L1 and PD-L2, which can be augmented by TNF-α and IFN-γ (66). Additionally, our previous studies have revealed that IL-8 derived from GCMSCs could promote immune escape by inducing PD-L1 expression in GC cells via c-Myc, which is regulated by the STAT3 and mTOR signaling pathways (24). Moreover, our findings have shown that GCMSCs upregulate PD-L1 expression not only in the cell membrane of GC cells, but also in the cytoplasm and nucleus. Checkpoint blocking antibodies only block immune checkpoints on cell membranes. Thus, their effects may be counteracted by checkpoints from cytoplasmic translocation (67). The accumulation of nuclear PD-L1, which is promoted by deacetylation, has been reported to activate genes involved in the immune response (68). Evidence has suggested that PD-L1 expression is linked to tumor cells’ intrinsic properties (69). GCMSCs can also induce changes in PD-L1 expression by regulating the intrinsic metabolism of tumor cells (23). We have shown that GCMSCs can augment the CSCs properties of GC cells through PD-L1, thus leading to GC cells’ resistance to chemotherapy (67).
Regulatory effects of MSCS from different sources on immune cells
MSCs not only regulate tumor cells to boost the growth and progression, but also have effects on a variety of immune cells to modify the complex immune microenvironment (Figure 2).
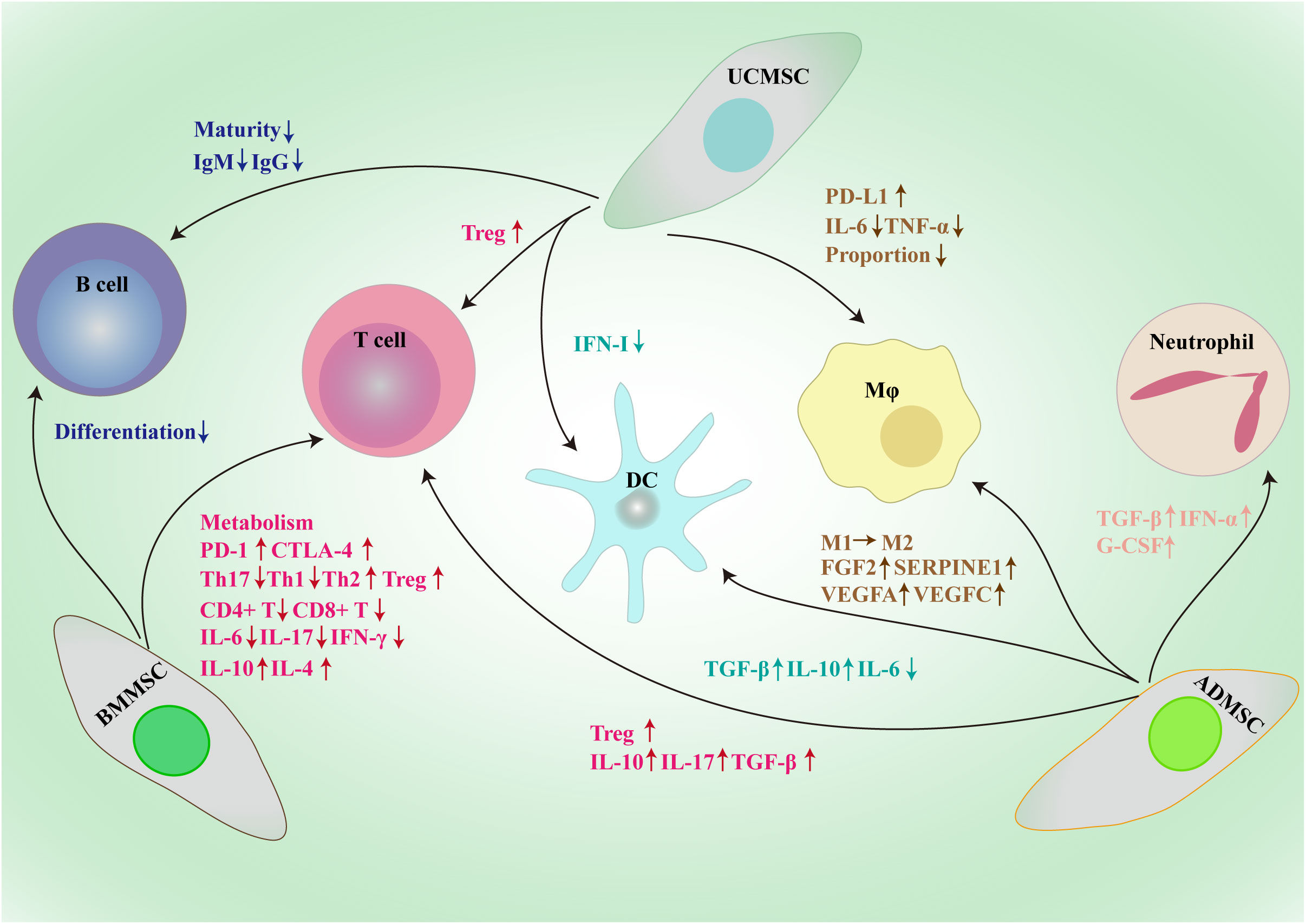
Figure 2 Framework diagram of MSCs derived from different sources exerting broad immunosuppressive effects through the interactions with immune cells, such as macrophages, dendritic cells, T cells, B cells and neutrophil. BMMSC, bone marrow mesenchymal stem/stromal cell; UCMSC, umbilical cord mesenchymal stem/stromal cell; ADMSC, adipose tissue-derived mesenchymal stem/stromal cell; PD-L1, programmed death-1 ligand; MΦ, macrophage; DC, dendritic cell; CTLA-1, cytotoxic T-lymphocyte antigen 4; Treg, regulatory T cell; Th, T helper; IL, interleukin; TGF-β, transforming growth factor-β; IFN, interferon; Ig, immunoglobulin; G-CSF, granulocyte colony-stimulating factor; FGF2, fibroblast growth factor 2; SERPINE1, serpin family E member 1; VEGF, vascular endothelial growth factor.
Bone marrow MSCs
The quantity and quality of tumor-killing T cells in the TME are essential for the success of immune therapeutics; however, due to the expression of immune checkpoints, the majority of infiltrating T cells are unable to identify and eliminate neighboring tumor cells (70, 71). The PD-1–PD-L1 axis is involved in the BMMSCs-mediated inhibition of T cell glycolysis by negatively regulating HK2 activity (72). IL-17 can also significantly increase the level of PD-L1 produced by BMMSCs through inducing iNOS expression to shape the immunosuppressive TME (73). Moreover, BMMSCs raise PD-1 expression on activated CD4+CD25− T cells and induce apoptosis of these cells (74). Endothelial cells were safeguarded from cytotoxic T lymphocyte-induced lysis by co-culturing with BMMSCs (75). Besides, BMMSCs help create an immunosuppressive microenvironment in tumors by reducing the proportion of Th17 cells and increasing the proportion of Tregs in PBMCs (76). Studies have demonstrated that BMMSCs can drastically impede the growth of CD4+ and CD8+ T cells through cell-contact and paracrine-dependent means (77). Moreover, MSCs not only have an effect on T cell viability and proliferation, but also impede the production and operation of Th1 and Th17 cells, while encouraging the production of Th2 cells and Tregs (78, 79). Dendritic cells educated by BMMSCs initiate a transition from Th1 to Th2, stimulating Tregs and leading to a decrease in pro-inflammatory cytokines such as IL-6, IL-17, and IFN-γ, as well as an upsurge in IL-4 and IL-10 production (80). Another important soluble factor derived from BMMSCs is IL-1RA; BMMSCs from IL-1RA-deficient mice do not inhibit B cell differentiation (81).
Umbilical cord MSCs
UCMSCs attenuate acute lung injury via PGE2-dependent reprogramming of macrophages to promote their PD-L1 expression (82). Besides, UCMSCs shift the cytokine profile of dendritic cells from proinflammatory to immunoregulatory (83). A study has reported that human UCMSCs disrupt the maturation of B cells by secreting TGF-β (84). Che et al. also have reported that UCMSCs inhibit the production of IgG and IgM in B cells (85). TGF-β secreted by UCMSCs enhances the induction of Tregs from “naïve” T cells to suppress colitis-associated colorectal cancer (86). UCMSCs inhibit the tumorigenesis of colon cancer by reducing the proportion of macrophages and inhibiting TNF-α and IL-6 secretion (87).
Adipose tissue-derived MSCs
ADMSCs promote the activity of neutrophils by inhibiting apoptosis mediated by the increased expression of TGF-β, IFN-α, and G-CSF (88). Moreover, ADMSCs-derived exosomes enhance the secretion of TGF-β and IL-10 and impede the secretion of IL-6 by dendritic cells (89). ADMSCs upregulate FGF2, SERPINE1, VEGFA and VEGFC in macrophages and supporting the proliferation of breast cancer cells (90). Exosomes secreted by ADMSCs enable macrophages to switch from the M1 to the M2 phenotype, thus possessing potent anti-inflammatory properties (91). In the presence of ADMSCs, the proportion of Tregs increases and the production of IL-10, IL-17, and TGF-β by T cells is enhanced (92).
Naturally residing MSCs
Endometrial cancer-derived MSCs inhibit the proliferation of PBMCs, which can be partially rescued by treatment with anti-PD-L1 antibodies (66). Glioma-associated MSCs enhance the immunosuppressive activity of MDSCs through a positive feedback loop of miR-21–SP1–DNMT1 (93). GCMSCs efficiently promote macrophage polarization toward the pro-tumor M2 subtype through several soluble molecules, including IL-6, IL-8, TGF-β, HGF, PGE2, IL-1RA, TSG6 and IDO (94). MSCs from head and neck squamous cell cancer inhibit the proliferation of CD4+ and CD8+ T cells through indoleamine 2,3 dioxygenase activity (34). GCMSCs inhibit the degranulation ability, perforin production, and cytotoxicity of NK cells by up-regulating the expression of fructose-bisphosphatase 1 (95). Moreover, Lung cancer-associated MSCs reduce NK cells cytotoxicity by expressing IL-6 and prostaglandin E2 (96).
Conclusions
MSCs from different sources play various biological roles, and different tumors react in different ways to MSCs. MSCs not only manipulate the stemness of tumor cells, but also change the expression of immune checkpoint molecules on tumor cells and have huge effects on immune cells. Compared with “naïve” MSCs, CA-MSCs exhibit several unique characteristics, including stronger pro-tumor effects; they are considered to be key regulators of tumor fate. Given the multifaceted role that MSCs play in the TME, they represent an untapped but promising target for improving cancer therapies. It is necessary to formulate targeted therapy plans according to individual conditions in the treatment of cancer patients.
Author contributions
LS: Funding acquisition, Writing – original draft. YY: Writing – review & editing.
Funding
The authors declare financial support was received for the research, authorship, and/or publication of this article. This work was supported by Natural Science Foundation of Jiangsu Province (BK20210136), National Natural Science Foundation of China (82203547) and China Postdoctoral Science Foundation (2022M721433).
Conflict of interest
The authors declare that the research was conducted in the absence of any commercial or financial relationships that could be construed as a potential conflict of interest.
Publisher’s note
All claims expressed in this article are solely those of the authors and do not necessarily represent those of their affiliated organizations, or those of the publisher, the editors and the reviewers. Any product that may be evaluated in this article, or claim that may be made by its manufacturer, is not guaranteed or endorsed by the publisher.
References
1. Olmedo-Moreno L, Aguilera Y, Baliña-Sánchez C, Martín-Montalvo A, Capilla-González V. Heterogeneity of in vitro expanded mesenchymal stromal cells and strategies to improve their therapeutic actions. Pharmaceutics (2022) 14(5):1112. doi: 10.3390/pharmaceutics14051112
2. Ishige I, Nagamura-Inoue T, Honda MJ, Harnprasopwat R, Kido M, Sugimoto M, et al. Comparison of mesenchymal stem cells derived from arterial, venous, and Wharton's jelly explants of human umbilical cord. Int J Hematol (2009) 90(2):261–9. doi: 10.1007/s12185-009-0377-3
3. Hass R, Kasper C, Böhm S, Jacobs R. Different populations and sources of human mesenchymal stem cells (MSC): A comparison of adult and neonatal tissue-derived MSC. Cell Commun Signal (2011) 9:12. doi: 10.1186/1478-811X-9-12
4. Frisbie L, Buckanovich RJ, Coffman L. Carcinoma-associated mesenchymal stem/stromal cells: architects of the pro-tumorigenic tumor microenvironment. Stem Cells (2022) 40(8):705–15. doi: 10.1093/stmcls/sxac036
5. Han X, Wang L, Li T, Zhang J, Zhang D, Li J, et al. Beyond blocking: engineering RNAi-mediated targeted immune checkpoint nanoblocker enables T-cell-independent cancer treatment. ACS Nano (2020) 14(12):17524–34. doi: 10.1021/acsnano.0c08022
6. Heinrich S, Craig AJ, Ma L, Heinrich B, Greten TF, Wang XW. Understanding tumour cell heterogeneity and its implication for immunotherapy in liver cancer using single-cell analysis. J Hepatol (2021) 74(3):700–15. doi: 10.1016/j.jhep.2020.11.036
7. Restifo NP, Smyth MJ, Snyder A. Acquired resistance to immunotherapy and future challenges. Nat Rev Cancer (2016) 16(2):121–6. doi: 10.1038/nrc.2016.2
8. Coffman LG, Pearson AT, Frisbie LG, Freeman Z, Christie E, Bowtell DD, et al. Ovarian carcinoma-associated mesenchymal stem cells arise from tissue-specific normal stroma. Stem Cells (2019) 37(2):257–69. doi: 10.1002/stem.2932
9. Chen Z, Xu P, Wang X, Li Y, Yang J, Xia Y, et al. MSC-NPRA loop drives fatty acid oxidation to promote stemness and chemoresistance of gastric cancer. Cancer Lett (2023) 565:216235. doi: 10.1016/j.canlet.2023.216235
10. Goldstein RH, Reagan MR, Anderson K, Kaplan DL, Rosenblatt M. Human bone marrow-derived MSCs can home to orthotopic breast cancer tumors and promote bone metastasis. Cancer Res (2010) 70(24):10044–50. doi: 10.1158/0008-5472
11. Zhu Y, Sun Z, Han Q, Liao L, Wang J, Bian C, et al. Human mesenchymal stem cells inhibit cancer cell proliferation by secreting DKK-1. Leukemia (2009) 23(5):925–33. doi: 10.1038/leu.2008.384
12. Li W, Yang J, Zheng P, Li H, Zhao S. The origins and generation of cancer-associated mesenchymal stromal cells: an innovative therapeutic target for solid tumors. Front Oncol (2021) 11:723707. doi: 10.3389/fonc.2021.723707
13. Pavon LF, Sibov TT, de Souza AV, da Cruz EF, Malheiros SMF, Cabral FR, et al. Tropism of mesenchymal stem cell toward CD133+ stem cell of glioblastoma in vitro and promote tumor proliferation in vivo. Stem Cell Res Ther (2018) 9(1):310. doi: 10.1186/s13287-018-1049-0
14. Belotti D, Capelli C, Resovi A, Introna M, Taraboletti G. Thrombospondin-1 promotes mesenchymal stromal cell functions via TGFβ and in cooperation with PDGF. Matrix Biol (2016) 55:106–16. doi: 10.1016/j.matbio.2016.03.003
15. Ishii M, Takahashi M, Murakami J, Yanagisawa T, Nishimura M. Vascular endothelial growth factor-C promotes human mesenchymal stem cell migration via an ERK-and FAK-dependent mechanism. Mol Cell Biochem (2019) 455(1-2):185–93. doi: 10.1007/s11010-018-3481-y
16. Barcellos-de-Souza P, Comito G, Pons-Segura C, Taddei ML, Gori V, Becherucci V, et al. Mesenchymal stem cells are recruited and activated into carcinoma-associated fibroblasts by prostate cancer microenvironment-derived TGF-β1. Stem Cells (2016) 34(10):2536–47. doi: 10.1002/stem.2412
17. Berger L, Shamai Y, Skorecki KL, Tzukerman M. Tumor specific recruitment and reprogramming of mesenchymal stem cells in tumorigenesis. Stem Cells (2016) 34(4):1011–26. doi: 10.1002/stem.2269
18. Le Naour A, Prat M, Thibault B, Mével R, Lemaitre L, Leray H, et al. Tumor cells educate mesenchymal stromal cells to release chemoprotective and immunomodulatory factors. J Mol Cell Biol (2020) 12(3):202–15. doi: 10.1093/jmcb/mjz090
19. Wang M, Zhao X, Qiu R, Gong Z, Huang F, Yu W, et al. Lymph node metastasis-derived gastric cancer cells educate bone marrow-derived mesenchymal stem cells via YAP signaling activation by exosomal Wnt5a. Oncogene (2021) 40(12):2296–208. doi: 10.1038/s41388-021-01722-8
20. Shen Y, Xue C, Li X, Ba L, Gu J, Sun Z, et al. Effects of gastric cancer cell-derived exosomes on the immune regulation of mesenchymal stem cells by the NF-kB signaling pathway. Stem Cells Dev (2019) 28(7):464–76. doi: 10.1089/scd.2018.0125
21. Ren G, Zhao X, Wang Y, Zhang X, Chen X, Xu C, et al. CCR2-dependent recruitment of macrophages by tumor-educated mesenchymal stromal cells promotes tumor development and is mimicked by TNFα. Cell Stem Cell (2012) 11(6):812–24. doi: 10.1016/j.stem.2012.08.013
22. Zhang J, Ji C, Li W, Mao Z, Shi Y, Shi H, et al. Tumor-educated neutrophils activate mesenchymal stem cells to promote gastric cancer growth and metastasis. Front Cell Dev Biol (2020) 8:788. doi: 10.3389/fcell.2020.00788
23. Huang C, Chen B, Wang X, Xu J, Sun L, Wang D, et al. Gastric cancer mesenchymal stem cells via the CXCR2/HK2/PD-L1 pathway mediate immunosuppression. Gastric Cancer (2023) 26(5):691–707. doi: 10.1007/s10120-023-01405-1
24. Sun L, Wang Q, Chen B, Zhao Y, Shen B, Wang H, et al. Gastric cancer mesenchymal stem cells derived IL-8 induces PD-L1 expression in gastric cancer cells via STAT3/mTOR-c-Myc signal axis. Cell Death Dis (2018) 9(9):928. doi: 10.1038/s41419-018-0988-9
25. Wang M, Chen B, Sun XX, Zhao XD, Zhao YY, Sun L, et al. Gastric cancer tissue-derived mesenchymal stem cells impact peripheral blood mononuclear cells via disruption of Treg/Th17 balance to promote gastric cancer progression. Exp Cell Res (2017) 361(1):19–29. doi: 10.1016/j.yexcr.2017.09.036
26. Guo S, Huang C, Han F, Chen B, Ding Y, Zhao Y, et al. Gastric Cancer Mesenchymal Stem Cells Inhibit NK Cell Function through mTOR Signalling to Promote Tumour Growth. Stem Cells Int (2021) 2021:9989790. doi: 10.1155/2021/9989790
27. Zheng Z, Li YN, Jia S, Zhu M, Cao L, Tao M, et al. Lung mesenchymal stromal cells influenced by Th2 cytokines mobilize neutrophils and facilitate metastasis by producing complement C3. Nat Commun (2021) 12(1):6202. doi: 10.1038/s41467-021-26460-z
28. Yan C, Chang J, Song X, Qi Y, Ji Z, Liu T, et al. Lung cancer-associated mesenchymal stem cells promote tumor metastasis and tumorigenesis by induction of epithelial-mesenchymal transition and stem-like reprogram. Aging (Albany NY) (2021) 13(7):9780–800. doi: 10.18632/aging.202732
29. Gordon JAR, Evans MF, Ghule PN, Lee K, Vacek P, Sprague BL, et al. Identification of molecularly unique tumor-associated mesenchymal stromal cells in breast cancer patients. PLoS One (2023) 18(3):e0282473. doi: 10.1371/journal.pone.0282473
30. Yan XL, Fu CJ, Chen L, Qin JH, Zeng Q, Yuan HF, et al. Mesenchymal stem cells from primary breast cancer tissue promote cancer proliferation and enhance mammosphere formation partially via EGF/EGFR/Akt pathway. Breast Cancer Res Treat (2012) 132(1):153–64. doi: 10.1007/s10549-011-1577-0
31. Waghray M, Yalamanchili M, Dziubinski M, Zeinali M, Erkkinen M, Yang H, et al. GM-CSF mediates mesenchymal-epithelial cross-talk in pancreatic cancer. Cancer Discovery (2016) 6(8):886–99. doi: 10.1158/2159-8290.CD-15-0947
32. Ávila-Ibarra LR, Mora-García ML, García-Rocha R, Hernández-Montes J, Weiss-Steider B, Montesinos JJ, et al. Mesenchymal stromal cells derived from normal cervix and cervical cancer tumors increase CD73 expression in cervical cancer cells through TGF-β1 production. Stem Cells Dev (2019) 28(7):477–88. doi: 10.1089/scd.2018.0183
33. Zhang X, Hu F, Li G, Li G, Yang X, Liu L, et al. Human colorectal cancer-derived mesenchymal stem cells promote colorectal cancer progression through IL-6/JAK2/STAT3 signaling. Cell Death Dis (2018) 9(2):25. doi: 10.1038/s41419-017-0176-3
34. Liotta F, Querci V, Mannelli G, Santarlasci V, Maggi L, Capone M, et al. Mesenchymal stem cells are enriched in head neck squamous cell carcinoma, correlates with tumour size and inhibit T-cell proliferation. Br J Cancer (2015) 112(4):745–54. doi: 10.1038/bjc.2015.15
35. Di Matteo S, Avanzini MA, Pelizzo G, Calcaterra V, Croce S, Spaggiari GM, et al. Neuroblastoma tumor-associated mesenchymal stromal cells regulate the cytolytic functions of NK cells. Cancers (Basel) (2022) 15(1):19. doi: 10.3390/cancers15010019
36. Reagan MR, Ghobrial IM. Multiple myeloma mesenchymal stem cells: characterization, origin, and tumor-promoting effects. Clin Cancer Res (2012) 18(2):342–9. doi: 10.1158/1078-0432.CCR-11-2212
37. Corre J, Mahtouk K, Attal M, Gadelorge M, Huynh A, Fleury-Cappellesso S, et al. Bone marrow mesenchymal stem cells are abnormal in multiple myeloma. Leukemia (2007) 21(5):1079–88. doi: 10.1038/sj.leu.2404621
38. Arnulf B, Lecourt S, Soulier J, Ternaux B, Lacassagne MN, Crinquette A, et al. Phenotypic and functional characterization of bone marrow mesenchymal stem cells derived from patients with multiple myeloma. Leukemia (2007) 21(1):158–63. doi: 10.1038/sj.leu.2404466
39. Markovina S, Callander NS, O'Connor SL, Xu G, Shi Y, Leith CP, et al. Bone marrow stromal cells from multiple myeloma patients uniquely induce bortezomib resistant NF-kappaB activity in myeloma cells. Mol Cancer (2010) 9:176. doi: 10.1186/1476-4598-9-176
40. de Jong MME, Kellermayer Z, Papazian N, Tahri S, Hofste Op Bruinink D, Hoogenboezem R, et al. The multiple myeloma microenvironment is defined by an inflammatory stromal cell landscape. Nat Immunol (2021) 22(6):769–80. doi: 10.1038/s41590-021-00931-3
41. Atiya H, Frisbie L, Pressimone C, Coffman L. Mesenchymal stem cells in the tumor microenvironment. Adv Exp Med Biol (2020) 1234:31–42. doi: 10.1007/978-3-030-37184-5_3
42. Gu JJ, Hoj J, Rouse C, Pendergast AM. Mesenchymal stem cells promote metastasis through activation of an ABL-MMP9 signaling axis in lung cancer cells. PLoS One (2020) 15(10):e0241423. doi: 10.1371/journal.pone.0241423
43. Antoon R, Wang XH, Saleh AH, Warrington J, Hedley DW, Keating A. Pancreatic cancer growth promoted by bone marrow mesenchymal stromal cell-derived IL-6 is reversed predominantly by IL-6 blockade. Cytotherapy (2022) 24(7):699–710. doi: 10.1016/j.jcyt.2021.12.005
44. McGuire JJ, Frieling JS, Lo CH, Li T, Muhammad A, Lawrence HR, et al. Mesenchymal stem cell-derived interleukin-28 drives the selection of apoptosis resistant bone metastatic prostate cancer. Nat Commun (2021) 12(1):723. doi: 10.1038/s41467-021-20962-6
45. Buschhaus JM, Rajendran S, Humphries BA, Cutter AC, Muñiz AJ, Ciavattone NG, et al. Effects of iron modulation on mesenchymal stem cell-induced drug resistance in estrogen receptor-positive breast cancer. Oncogene (2022) 41(29):3705–18. doi: 10.1038/s41388-022-02385-9
46. Sandiford OA, Donnelly RJ, El-Far MH, Burgmeyer LM, Sinha G, Pamarthi SH, et al. Mesenchymal Stem Cell-Secreted Extracellular Vesicles Instruct Stepwise Dedifferentiation of Breast Cancer Cells into Dormancy at the Bone Marrow Perivascular Region. Cancer Res (2021) 81(6):1567–82. doi: 10.1158/0008-5472.CAN-20-2434
47. Cortini M, Massa A, Avnet S, Bonuccelli G, Baldini N. Tumor-activated mesenchymal stromal cells promote osteosarcoma stemness and migratory potential via IL-6 secretion. PLoS One (2016) 11(11):e0166500. doi: 10.1371/journal.pone.0166500
48. Li H, Li F. Exosomes from BM-MSCs increase the population of CSCs via transfer of miR-142-3p. Br J Cancer (2018) 119(6):744–55. doi: 10.1038/s41416-018-0254-z
49. Chang YW, Wang CC, Yin CF, Wu CH, Huang HC, Juan HF. Quantitative phosphoproteomics reveals ectopic ATP synthase on mesenchymal stem cells to promote tumor progression via ERK/c-Fos pathway activation. Mol Cell Proteomics (2022) 21(6):100237. doi: 10.1016/j.mcpro.2022.100237
50. Wang L, Ouyang M, Xing S, Zhao S, Liu S, Sun L, et al. Mesenchymal Stem Cells and their Derived Exosomes Promote Malignant Phenotype of Polyploid Non-Small-Cell Lung Cancer Cells through AMPK Signaling Pathway. Anal Cell Pathol (Amst) (2022) 2022:8708202. doi: 10.1155/2022/8708202
51. Ye H, Pan J, Gong E, Cai X, Xu C, Li Y, et al. Inhibitory effect of immunologically activated mesenchymal stem cells on lung cancer cell growth and metastasis. Cancer Biother Radiopharm (2023) 38(5):322–35. doi: 10.1089/cbr.2020.3855
52. Wang M, Zhang Y, Liu M, Jia Y, He J, Xu X, et al. Inhibition of STAT3 signaling as critical molecular event in HUC-MSCs suppressed Glioblastoma Cells. J Cancer (2023) 14(4):611–27. doi: 10.7150/jca.77905
53. ElBadre HM, El-Deek SEM, Ramadan HK, Elbadr MM, Sabry D, Ahmed NM, et al. Potential role of human umbilical cord stem cells-derived exosomes as novel molecular inhibitors of hepatocellular carcinoma growth. Apoptosis (2023) 28(9-10):1346–56. doi: 10.1007/s10495-023-01863-z
54. Chen J, Li Z, Yue C, Ma J, Cao L, Lin J, et al. Human umbilical cord mesenchymal stem cell-derived exosomes carrying miR-1827 downregulate SUCNR1 to inhibit macrophage M2 polarization and prevent colorectal liver metastasis. Apoptosis (2023) 28(3-4):549–65. doi: 10.1007/s10495-022-01798-x
55. Guo G, Tan Z, Liu Y, Shi F, She J. The therapeutic potential of stem cell-derived exosomes in the ulcerative colitis and colorectal cancer. Stem Cell Res Ther (2022) 13(1):138. doi: 10.1186/s13287-022-02811-5
56. Bagheri-Mohammadi S, Moradian-Tehrani R, Noureddini M, Alani B. Novel application of adipose-derived mesenchymal stem cells via producing antiangiogenic factor TSP-1 in lung metastatic melanoma animal model. Biologicals (2020) 68:9–18. doi: 10.1016/j.biologicals.2020.09.004
57. Lou G, Chen L, Xia C, Wang W, Qi J, Li A, et al. MiR-199a-modified exosomes from adipose tissue-derived mesenchymal stem cells improve hepatocellular carcinoma chemosensitivity through mTOR pathway. J Exp Clin Cancer Res (2020) 39(1):4. doi: 10.1186/s13046-019-1512-5
58. Xue C, Gao Y, Sun Z, Li X, Zhang M, Yang Y, et al. Mesenchymal stem cells derived from adipose tissue accelerate the progression of colon cancer by inducing a MTCAF phenotype via ICAM1/STAT3/AKT axis. Front Oncol (2022) 12:837781. doi: 10.3389/fonc.2022.837781
59. Sharaf K, Eggersmann TK, Haider SP, Schwenk-Zieger S, Zhou J, Gires O, et al. Human adipose-derived stem/stromal cells promote proliferation and migration in head and neck cancer cells. Cancers (Basel) (2021) 13(11):2751. doi: 10.3390/cancers13112751
60. Li T, Zhou X, Wang J, Liu Z, Han S, Wan L, et al. Adipose-derived mesenchymal stem cells and extracellular vesicles confer antitumor activity in preclinical treatment of breast cancer. Pharmacol Res (2020) 157:104843. doi: 10.1016/j.phrs.2020.104843
61. Aboulkheyr Es H, Bigdeli B, Zhand S, Aref AR, Thiery JP, Warkiani ME. Mesenchymal stem cells induce PD-L1 expression through the secretion of CCL5 in breast cancer cells. J Cell Physiol (2021) 236(5):3918–28. doi: 10.1002/jcp.30135
62. Gečys D, Skredėnienė R, Gečytė E, Kazlauskas A, Balnytė I, Jekabsone A. Adipose tissue-derived stem cell extracellular vesicles suppress glioblastoma proliferation, invasiveness and angiogenesis. Cells (2023) 12(9):1247. doi: 10.3390/cells12091247
63. Kapoor-Narula U, Lenka N. Cancer stem cells and tumor heterogeneity: Deciphering the role in tumor progression and metastasis. Cytokine (2022) 157:155968. doi: 10.1016/j.cyto.2022.155968
64. Li HJ, Reinhardt F, Herschman HR, Weinberg RA. Cancer-stimulated mesenchymal stem cells create a carcinoma stem cell niche via prostaglandin E2 signaling. Cancer Discovery (2012) 2(9):840–55. doi: 10.1158/2159-8290.CD-12-0101
65. Jiang C, Zhang Q, Shanti RM, Shi S, Chang TH, Carrasco L, et al. Mesenchymal stromal cell-derived interleukin-6 promotes epithelial-mesenchymal transition and acquisition of epithelial stem-like cell properties in ameloblastoma epithelial cells. Stem Cells (2017) 35(9):2083–94. doi: 10.1002/stem.2666
66. Wang KH, Chang YH, Harnod T, Ding DC. Endometrial cancer-infiltrating mesenchymal stem cells exhibit immunosuppressive effects. Cell Transplant (2022) 31:9636897221104452. doi: 10.1177/09636897221104452
67. Sun L, Huang C, Zhu M, Guo S, Gao Q, Wang Q, et al. Gastric cancer mesenchymal stem cells regulate PD-L1-CTCF enhancing cancer stem cell-like properties and tumorigenesis. Theranostics (2020) 10(26):11950–62. doi: 10.7150/thno.49717
68. Gao Y, Nihira NT, Bu X, Chu C, Zhang J, Kolodziejczyk A, et al. Acetylation-dependent regulation of PD-L1 nuclear translocation dictates the efficacy of anti-PD-1 immunotherapy. Nat Cell Biol (2020) 22(9):1064–75. doi: 10.1038/s41556-020-0562-4
69. Hsu JM, Xia W, Hsu YH, Chan LC, Yu WH, Cha JH, et al. STT3-dependent PD-L1 accumulation on cancer stem cells promotes immune evasion. Nat Commun (2018) 9(1):1908. doi: 10.1038/s41467-018-04313-6
70. Marigo I, Zilio S, Desantis G, Mlecnik B, Agnellini AHR, Ugel S, et al. T cell cancer therapy requires CD40-CD40L activation of tumor necrosis factor and inducible nitric-oxide-synthase-producing dendritic cells. Cancer Cell (2016) 30(3):377–90. doi: 10.1016/j.ccell.2016.08.004
71. Hadrup S, Donia M, Thor Straten P. Effector CD4 and CD8 T cells and their role in the tumor microenvironment. Cancer Microenviron (2013) 6(2):123–33. doi: 10.1007/s12307-012-0127-6
72. Kawasaki Y, Sato K, Mashima K, Nakano H, Ikeda T, Umino K, et al. Mesenchymal stromal cells inhibit aerobic glycolysis in activated T cells by negatively regulating hexokinase II activity through PD-1/PD-L1 interaction. Transplant Cell Ther (2021) 27(3):231. doi: 10.1016/j.jtct.2020.11.012
73. Wang S, Wang G, Zhang L, Li F, Liu K, Wang Y, et al. Interleukin-17 promotes nitric oxide-dependent expression of PD-L1 in mesenchymal stem cells. Cell Biosci (2020) 10:73. doi: 10.1186/s13578-020-00431-1
74. Yan Z, Zhuansun Y, Liu G, Chen R, Li J, Ran P. Mesenchymal stem cells suppress T cells by inducing apoptosis and through PD-1/B7-H1 interactions. Immunol Lett (2014) 162(1 Pt A):248–55. doi: 10.1016/j.imlet.2014.09.013
75. Cahill EF, Sax T, Hartmann I, Haffner S, Holler E, Holler B, et al. Mesenchymal stromal cells protect endothelial cells from cytotoxic T lymphocyte-induced lysis. Scand J Immunol (2016) 84(3):158–64. doi: 10.1111/sji.12459
76. Yu Y, Valderrama AV, Han Z, Uzan G, Naserian S, Oberlin E. Human fetal liver MSCs are more effective than adult bone marrow MSCs for their immunosuppressive, immunomodulatory, and Foxp3 + T reg induction capacity. Stem Cell Res Ther (2021) 12(1):138. doi: 10.1186/s13287-021-02176-1
77. Krampera M, Glennie S, Dyson J, Scott D, Laylor R, Simpson E, et al. Bone marrow mesenchymal stem cells inhibit the response of naive and memory antigen-specific T cells to their cognate peptide. Blood (2003) 101(9):3722–9. doi: 10.1182/blood-2002-07-2104
78. Wu R, Fan X, Wang Y, Shen M, Zheng Y, Zhao S, et al. Mesenchymal stem cell-derived extracellular vesicles in liver immunity and therapy. Front Immunol (2022) 13:833878. doi: 10.3389/fimmu.2022.833878
79. Tang Y, Wu P, Li L, Xu W, Jiang J. Mesenchymal stem cells and their small extracellular vesicles as crucial immunological efficacy for hepatic diseases. Front Immunol (2022) 13:880523. doi: 10.3389/fimmu.2022.880523
80. Ge W, Jiang J, Arp J, Liu W, Garcia B, Wang H. Regulatory T-cell generation and kidney allograft tolerance induced by mesenchymal stem cells associated with indoleamine 2,3-dioxygenase expression. Transplantation (2010) 90(12):1312–20. doi: 10.1097/TP.0b013e3181fed001
81. Luz-Crawford P, Djouad F, Toupet K, Bony C, Franquesa M, Hoogduijn MJ, et al. Mesenchymal stem cell-derived interleukin 1 receptor antagonist promotes macrophage polarization and inhibits B cell differentiation. Stem Cells (2016) 34(2):483–92. doi: 10.1002/stem.2254
82. Tu C, Wang Z, Xiang E, Zhang Q, Zhang Y, Wu P, et al. Human umbilical cord mesenchymal stem cells promote macrophage PD-L1 expression and attenuate acute lung injury in mice. Curr Stem Cell Res Ther (2022) 17(6):564–75. doi: 10.2174/1574888X17666220127110332
83. Chen M, Peng J, Xie Q, Xiao N, Su X, Mei H, et al. Mesenchymal stem cells alleviate moderate-to-severe psoriasis by reducing the production of type I interferon (IFN-I) by plasmacytoid dendritic cells (pDCs). Stem Cells Int (2019) 2019:6961052. doi: 10.1155/2019/6961052
84. Park HH, Lee S, Yu Y, Yoo SM, Baek SY, Jung N, et al. TGF-β secreted by human umbilical cord blood-derived mesenchymal stem cells ameliorates atopic dermatitis by inhibiting secretion of TNF-α and IgE. Stem Cells (2020) 38(7):904–16. doi: 10.1002/stem.3183
85. Che N, Li X, Zhou S, Liu R, Shi D, Lu L, et al. Umbilical cord mesenchymal stem cells suppress B-cell proliferation and differentiation. Cell Immunol (2012) 274(1-2):46–53. doi: 10.1016/j.cellimm.2012.02.004
86. Tang RJ, Shen SN, Zhao XY, Nie YZ, Xu YJ, Ren J, et al. Mesenchymal stem cells-regulated Treg cells suppress colitis-associated colorectal cancer. Stem Cell Res Ther (2015) 6(1):71. doi: 10.1186/s13287-015-0055-8
87. Fu Y, Li J, Li M, Xu J, Rong Z, Ren F, et al. Umbilical cord mesenchymal stem cells ameliorate inflammation-related tumorigenesis via modulating macrophages. Stem Cells Int (2022) 2022:1617229. doi: 10.1155/2022/1617229
88. Park YS, Lim GW, Cho KA, Woo SY, Shin M, Yoo ES, et al. Improved viability and activity of neutrophils differentiated from HL-60 cells by co-culture with adipose tissue-derived mesenchymal stem cells. Biochem Biophys Res Commun (2012) 423(1):19–25. doi: 10.1016/j.bbrc.2012.05.049
89. Shahir M, Mahmoud Hashemi S, Asadirad A, Varahram M, Kazempour-Dizaji M, Folkerts G, et al. Effect of mesenchymal stem cell-derived exosomes on the induction of mouse tolerogenic dendritic cells. J Cell Physiol (2020) 235(10):7043–55. doi: 10.1002/jcp.29601
90. Kim J, Escalante LE, Dollar BA, Hanson SE, Hematti P. Comparison of breast and abdominal adipose tissue mesenchymal stromal/stem cells in support of proliferation of breast cancer cells. Cancer Invest (2013) 31(8):550–4. doi: 10.3109/07357907.2013.830737
91. Lo Sicco C, Reverberi D, Balbi C, Ulivi V, Principi E, Pascucci L, et al. Mesenchymal stem cell-derived extracellular vesicles as mediators of anti-inflammatory effects: endorsement of macrophage polarization. Stem Cells Transl Med (2017) 6(3):1018–28. doi: 10.1002/sctm.16-0363
92. Fakhimi M, Talei AR, Ghaderi A, Habibagahi M, Razmkhah M. Helios, CD73 and CD39 induction in regulatory T cells exposed to adipose derived mesenchymal stem cells. Cell J (2020) 22(2):236–44. doi: 10.22074/cellj.2020.6313
93. Qiu W, Guo Q, Guo X, Wang C, Li B, Qi Y, et al. Mesenchymal stem cells, as glioma exosomal immunosuppressive signal multipliers, enhance MDSCs immunosuppressive activity through the miR-21/SP1/DNMT1 positive feedback loop. J Nanobiotechnology (2023) 21(1):233. doi: 10.1186/s12951-023-01997-x
94. Li W, Zhao SL, Zheng P, Shi PQ, Zhou Y, Zhang T, et al. [Gastric cancer-derived mesenchymal stem cells regulate the M2 polarization of macrophages within gastric cancer microenvironment via JAK2/STAT3 signaling pathway]. Zhonghua Zhong Liu Za Zhi (2022) 44(7):728–36. doi: 10.3760/cma.j.cn112152-20200106-00008
95. Han F, Guo S, Huang C, Cui L, Zhao Y, Ma J, et al. Gastric cancer mesenchymal stem cells inhibit natural killer cell function by up-regulating FBP1. Cent Eur J Immunol (2021) 46(4):427–37. doi: 10.5114/ceji.2021.111753
Keywords: tumor microenvironment, heterogeneity, cancer-associated mesenchymal stem/stromal cells, stemness, immune cells
Citation: Sun L and Yao Y (2023) Mesenchymal stem/stromal cells- a principal element for tumour microenvironment heterogeneity. Front. Immunol. 14:1274379. doi: 10.3389/fimmu.2023.1274379
Received: 09 August 2023; Accepted: 28 September 2023;
Published: 09 October 2023.
Edited by:
Juan Francisco Santibanez, University of Belgrade, SerbiaReviewed by:
Abderrahim Naji, Kōchi University, JapanCopyright © 2023 Sun and Yao. This is an open-access article distributed under the terms of the Creative Commons Attribution License (CC BY). The use, distribution or reproduction in other forums is permitted, provided the original author(s) and the copyright owner(s) are credited and that the original publication in this journal is cited, in accordance with accepted academic practice. No use, distribution or reproduction is permitted which does not comply with these terms.
*Correspondence: Yongliang Yao, YYLGD313@126.com