- 1Department of Urology, Peking University First Hospital, Beijing, China
- 2The Institution of Urology, Peking University, Beijing, China
- 3Beijing Key Laboratory of Urogenital Diseases (Male) Molecular Diagnosis and Treatment Center, Beijing, China
- 4National Urological Cancer Center, Beijing, China
Renal cell carcinoma (RCC) is one of the most malignant urological tumors. Currently, there is a lack of molecular markers for early diagnosis of RCC. The 5-year survival rate for early-stage RCC is generally favorable; however, the prognosis takes a significant downturn when the tumor progresses to distant metastasis. Therefore, the identification of molecular markers for RCC is crucial in enhancing early diagnosis rates. Exosomes are a type of extracellular vesicle (EV) typically ranging in size from 30 nm to 150 nm, which contain RNA, DNA, proteins, lipids, etc. They can impact neighboring receptor cells through the autocrine or paracrine pathway, influence cellular communication, and regulate the local immune cells, consequently shaping the tumor immune microenvironment and closely associating with tumor development. The clinical application of exosomes as tumor markers and therapeutic targets has ignited significant interest within the research community. This review aims to provide a comprehensive summary of the advancements in exosome research within the context of RCC.
Introduction
Exosomes, a crucial subset of extracellular vesicles (EVs), typically range between 30 nm and 150 nm in diameter and transport a variety of biomolecules such as lipids, non-coding RNA, and proteins. Exosomes were first observed during maturation of sheep reticular cells (1). Exosomes primarily originate from cellular endocytosis. They are formed through the invagination of the limiting membrane of endosomes, leading to the creation of intraluminal vesicles (ILVs) within endosomes. These ILVs are eventually released from the cell as exosomes when multivesicular bodies fuse with the plasma membrane (2). The role of exosomes was originally thought to be the secretion of unneeded components into the extracellular space. As our understanding of exosomes grows, their high heterogeneity becomes evident because of their diverse cellular origins. Zhang et al. (3) identified two subpopulations of exosomes: large exosomal vesicles, 90 nm to 120 nm, Exo-L; and small exosomal vesicles, 60 nm to 80 nm, Exo-S. Exosomes facilitate intercellular communication and impact the homeostasis of the internal environment, playing pivotal roles in various diseases and tumors. Notably, exosomes exhibit significant heterogeneity, even among the same cell types, depending on environmental factors like oxygen tension, cell morphology (4), or cellular stimuli from apoptotic or autophagic signaling (5). Furthermore, the protein composition of specific EV subgroups varies in response to different agonists (6).
As exosome research advances, its role in tumor development has garnered significant attention. Exosome-derived RNA, mRNA, DNA, proteins, and metabolites can alter the fate of receptor cells via autocrine or paracrine pathways. For example, exosomes derived from chronic myeloid leukemia (CML) cells carry transforming growth factor-β (TGFβ1), which, in an autocrine manner, binds to the TGFβ1 receptor on CML cells, promoting tumor growth through activation of extracellular regulated MAP kinase (ERK), Akt kinase (AKT), and anti-apoptotic pathways (7). In breast cancer, miR-9 derived from breast cancer cells exerts paracrine effects on mammary fibroblasts, affecting their intrinsic properties and regulating transition of human mammary fibroblasts into cancer-associated fibroblasts (CAFs), ultimately fostering tumor growth (8).
Renal cell carcinoma (RCC) arises from the epithelial cells of renal tubules and stands as the third most common tumor within the urinary system, constituting approximately 90% of all malignant kidney tumors (9). Kidney cancer has an insidious onset, and reducing cancer mortality remains a major goal of the scientific community. Currently, the morbidity and mortality of clear cell RCC (ccRCC) continue to increase worldwide. Despite notable advancements in recent years in our understanding of ccRCC, the prognosis for this condition continues to be disheartening. Whereas the majority of tumors are considered benign, approximately one-third of them can become malignant, carrying a risk of metastasis as high as 70% (10). More than 60% of ccRCC cases are incidentally discovered. Despite improvements in imaging techniques, more than 30% of patients still present with tumor metastases, particularly in the bones and lungs, accounting for approximately 15% of all cases at the time of diagnosis (11). Therefore, the clinical challenge lies in identifying biomarkers and developing prognostic models for metastatic and advanced RCC to enhance treatment options and realize the goal of personalized oncology (12). Diagnostic biomarkers aid in disease detection, whereas prognostic biomarkers offer insights into disease progression and recurrence. Although abdominal ultrasound is a commonly used clinical screening method for renal cancer, its effectiveness in early diagnosis, particularly for small tumors, is limited. Valuable molecular markers for early screening or diagnosis of renal cancer have not yet been reported. The identification of reliable molecular markers could significantly reduce the number of cases detected at an advanced stage with a poor prognosis. Moreover, in cases where early detection is not feasible, biomarkers predicting treatment response can facilitate the timely initiation of second-line therapies. In addition, the scarcity of validated prognostic biomarkers poses a challenge in guiding the selection of optimal treatment approaches. Biomarkers encompass various categories, including nucleic acids, proteins, metabolites, isoenzymes, and hormones, and are typically classified as diagnostic, prognostic, or predictive (13).
Exosomes and the development of RCC
Exosomes play a pathologic role in renal carcinogenesis and metastasis. Exosome-derived non-coding RNAs in RCC can modulate the epithelial–mesenchymal transition (EMT) pathway in RCC cells, thereby promoting the development and metastasis of RCC (14). In addition, exosomes modulate the tumor immune microenvironment, facilitating immune escape (15). Furthermore, they also play a role in regulating various physiological processes, including tumor growth, angiogenesis, metastasis, drug resistance, and stem cell maintenance (16, 17). Wang et al (14). reported that cancer stem cell–derived miR-19b-3p promotes ccRCC metastasis by regulating the expression of EMT-related genes E-Cadherin, N-Cadherin, Vimentin, and twist in ccRCC cells. Hypoxia could induce the expression of induce the expression of hypoxia-induced long non-coding RNA associated with RCC (lncHILAR), which functions as a competing endogenous RNA (ceRNA) for miR-613/206/1-1-1-3p and competitively activates the Jagged1/Notch/CXCR4 pathway, thereby promoting invasion and migration of RCC in vitro and metastasis in vivo. In addition, hypoxic RCC can simultaneously secrete exosomes containing lncHILAR, which are internalized by normoxic RCC, enhancing their invasion phenotype through the acquisition of exogenous lncHILAR (18). Tsuruda et al. (19) reported the oncogenic effects of RAB27B, an exosome-secreted protein, in renal cancer cell lines, although the specific role of RAB27B in exocytosis has not yet been determined. Circular protein kinase C iota (Circ-PRKCI) is a microRNA (miRNA) sponge, and it has been shown that Circ-PRKCI can participate in tumor progression by stimulating migration and proliferation of esophageal squamous cell carcinoma cells through sponging miR-3680-3p (20). Qian et al. (21) demonstrated that the RCC cell line Caki-1–derived exosome circ-PRKCI promotes RCC proliferation by regulating the miR-545-3p/CCND1 axis. The expression level of miR-30c-5p in urinary exosomes of patients with ccRCC was lower than that of healthy controls. Overexpression of miR-30c-5p could inhibit the growth of renal clear cell carcinoma cells, suggesting that miR-30c-5p plays a role in the development of RCC (22). ApoC1 expression was upregulated in RCC samples and increased with ccRCC progression. ccRCC cells secrete ApoC1-containing exosomes that metastasize to vascular endothelial cells and promote ccRCC cell metastasis through activation of STAT3 (23).
Exosomes and drug resistance of RCC
Tyrosine kinase inhibitors (TKIs) were initially shown to be effective treatments for RCC. However, after a median of 14 months of treatment, the vast majority of patients develop drug resistance. Resistance to TKI therapy in RCC is also one of the major causes of post-treatment relapse (24). Exosomes are also important carriers for the propagation of cancer drug resistance (CDR), transferring drug-resistant mediators from donor cells to recipient cells, resulting in the acquisition of the CDR phenotype by previously drug-sensitive cells. Several mediators associated with drug resistance in cancer cells have been found to be transferred via exosomes, including drug efflux pumps (e.g., P-glycoprotein, ATP-binding cassette transporter G2, and ABCG2), miRNAs, lncRNAs, and other regulators such as transient receptor potential cation channel subfamily C member 5 (25).
Qu et al. (26) identified an uncharacterized lncRNA, activated in RCC with sunitinib resistance (lncARSR), that is highly expressed in sunitinib-resistant RCC and is functionally required for the RCC resistance phenotype. lncARSR could be secreted from drug-resistant cells via exosomes that convert sunitinib-sensitive cells into drug-resistant cells, thereby propagating drug resistance. Moreover, lncARSR promotes sunitinib resistance by competitively binding to miR-34 and miR-449, resulting in upregulation of AXL receptor tyrosine kinase-receptor tyrosine kinase mesenchymal-epithelial transition factor (AXL/c-MET) expression and activation of signal transducer and activator of transcription 3 (STAT3), AKT, and ERK signaling pathways. In contrast, activated AKT promotes lncARSR expression by inhibiting the transcription factors forkhead box, subgroup O 1 (FOXO1) and FOXO3a, creating a positive feedback loop in sunitinib-resistant renal cancer cells (26). Tipifarnib blocks exosome production and secretion in sunitinib-sensitive and sunitinib-resistant cells and downregulates PD-L1 expression by inhibiting endosomal sorting complex required for transport (ESCRT)-dependent and non–ESCRT-dependent exosome–endosome sorting complexes (27). Cancer cells frequently decrease DNA repair pathways and increase endogenous sources of mutation, which drive mutations, and therefore tend to be more dependent on a subset of DNA repair pathways to survive DNA damage. Targeting key DNA repair members such as poly(ADP-ribose) polymerases (PARPs) is a therapeutic strategy for cancer (28). Inhibition of PARPs by small-molecule compounds disrupts the ability of cancer cells to survive sustained DNA damage and leads to cell cycle arrest and/or cell death; however, PARP inhibitors (PARPis) have failed to significantly benefit patients, suggesting the need to develop new strategies. Liu et al. (29) reported that exosome component 1 (EXOSC1) sensitizes RCC cells to PARPis, suggesting that PARP inhibition may be an effective strategy for the treatment of Kidney renal clear cell carcinoma (KIRC) patients with high EXOSC1. EXOSC1 is associated with poorer poorer progression-free survival (PFS) and overall survival (OS) in patients with RCC, supporting its potential pathological significance.
Exosomes and the TME of RCC
In addition to simply affecting tumor cells, exosomes also affect the immune microenvironment of the tumor (Figure 1). Tumor-infiltrating macrophages exhibit two distinct polarization states, termed M1 and M2. RCC-derived exosomes carry a large amount of lncARSR, which interacts with local macrophages and activates the STAT3 pathway in macrophages, leading to the conversion of macrophages from M0/1 to M2, and this polarized state results in the secretion of large quantities of anti-inflammatory factors [e.g., interleukin-10 (IL-10) and TGFβ1] by macrophages to create a local microenvironment more suited for tumor metastasis and the formation of pre-metastatic lesions (30). In addition to tumor cell–derived exosomes regulating immune cells in the tumor microenvironment, immune cells also secrete exosomes to act on tumor cells. Liu et al. (31) reported that tumor-associated macrophage-derived exosome-loaded miR-193a-5p promotes ccRCC vasculogenic mimicry and immune invasion by inhibiting TIMP metallopeptidase inhibitor 2 (TIMP2) transcription through specific sponging the 3' untranslated regions (3′UTR) of TIMP2. Moreover, the RCC-derived exosome circSAFB2 mediates M2-macrophage polarization through the miR-620/JAK1/STAT3 axis, thereby promoting ccRCC immune escape and metastasis (32). lncRNA AP000439.2 was highly enriched in ccRCC-exo. ccRCC-exo significantly activated macrophage conversion to M2-type phenotype, which led to increased expression levels of TGFβ and IL-10 in macrophages, which, in turn, promoted the migration of ccRCC cells, suggesting that exosome AP000439.2 may be a new therapeutic target for ccRCC (33). In TME, in addition to tumor cell–derived exosomes that can act on and regulate immune cells, other cellular components in TME can also secrete exosomes to modulate tumor cells. CAF-derived exosomes can smoothly enter and be internalized by cancer cells. After co-culture of cancer cells with CAF-derived exosomes, cell proliferation, migration, and invasion were significantly enhanced, and apoptosis was reduced. In addition, the expression of fibronectin, matrix metalloproteinase 9 (MMP9), MMP2, and N-calmodulin and waveform protein in the EMT pathway was elevated in cancer cells, whereas the expression of E-calmodulin was decreased and the proportion of S-phase cells was increased, which proved that CAF-derived exosomes could promote the progression of RCC (34). Moreover, miR-224-5p in the exosomes secreted by CAFs can enter ccRCC cells and regulate the proliferation, migration, invasion, and apoptosis of ccRCC cells, which, in turn, promotes the malignant behaviors of ccRCC cells, suggesting that miR-224-5p has the potential to serve as a therapeutic target for ccRCC (35). Furthermore, enrichment of CAFs was positively correlated with tumor stemness and RCC progression, and exosomal miR-181d-5p delivered by CAFs directly targeted ring finger protein 43 (RNF43) and activated the Wnt/β-catenin signaling pathway, thereby enhancing tumor stemness and mediating RCC progression (36).
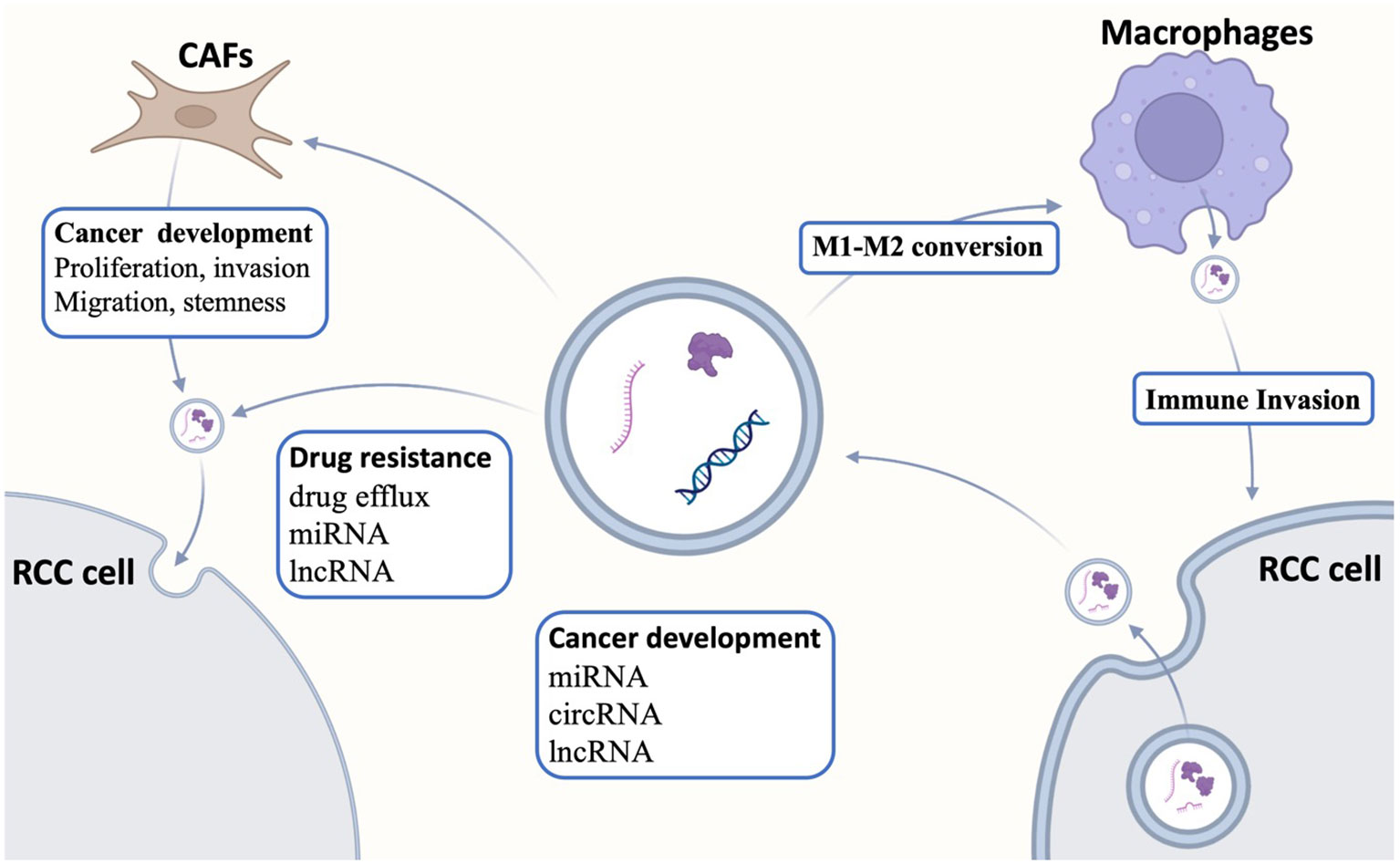
Figure 1 Interactions of exosomes with the tumor microenvironment. Exosomes regulate tumor differentiation, proliferation, apoptosis, and drug resistance by transmitting intercellular information, whereas exosomes are also related to the tumor microenvironment. Exosomes secreted by tumor cells can act on tumor-associated macrophages to regulate macrophage polarization, whereas exosomes secreted by tumor-associated fibroblasts regulate tumor development. CAFs, cancer-associated fibroblasts; RCC, renal cell carcinoma.
Exosomes as biomarkers of RCC
RCC has the highest mortality rate among genitourinary malignancies. Unfortunately, around 30% of patients are already in the metastatic stage at the time of diagnosis. Although localized RCC can often be cured with surgery, the prognosis dramatically worsens once metastasis occurs. Currently, aside from invasive biopsy, which often yields limited results, there is a notable absence of diagnostic tools for confirming renal masses. As a result, there is an urgent demand for non-invasive molecular testing of urine or serum as a viable alternative to biopsy (37). Although tissue biopsy remains the gold standard for tumor diagnosis, it is limited in that it reflects a single point in time at a specific tumor site. The emergence of next-generation sequencing technology has paved the way for the discovery of novel, more comprehensive, and less invasive biomarkers, bringing us closer to the realization of precision cancer medicine. Minimally invasive testing, commonly known as “liquid biopsy” (Figure 2), typically involves the analysis of circulating tumor cells, circulating tumor DNA, and tumor-derived exosomes to access tumor genomic signatures (38).
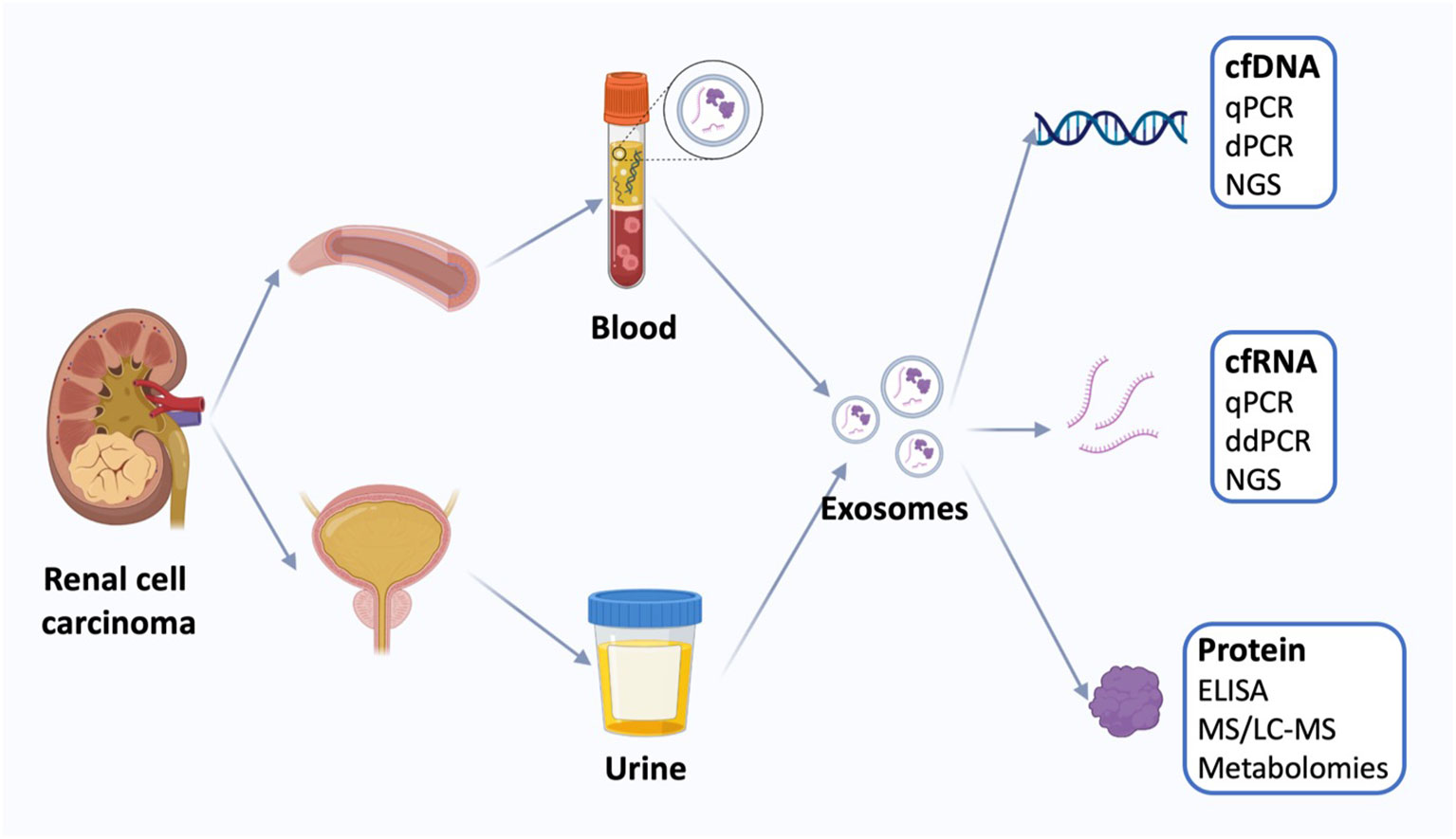
Figure 2 Liquid biopsy of renal cell carcinoma. Liquid biopsies for kidney cancer use mainly blood and urine samples, including exosomes and the cell-free DNA (cfDNA), cell-free RNA (cfRNA), and proteins. Quantification of circulating tumor markers at a single time point may be useful for disease staging and prognosis using a variety of techniques. cfDNA, cell-free DNA; qPCR, quantitative polymerase chain reaction; dPCR, digital polymerase chain reaction, NGS, next-generation sequencing; cfRNA, cell-free RNA; ddPCR, droplet digital polymerase chain reaction; ELISA, enzyme-linked immunosorbent assay; MS, Mass spectrometry; LS-MS, liquid chromatography-MS.
Recent studies have shown that EV RNA may be potential biomarkers for prostate cancer (39), hepatocellular carcinoma (40), and pancreatic ductal adenocarcinoma (41). In a single-center retrospective study, researchers collected serum from 106 patients with renal clear cell carcinoma and 73 patients with benign renal tumors and constructed a diagnostic tag for renal clear cell carcinoma by screening three candidate genes through multivariate stepwise logistic regression analysis. The diagnostic tag can differentiate between renal masses of indeterminate nature, such as cystic renal carcinomas, angiomyolipomas of lack of fat, and renal eosinophilic cell tumors, especially when the mass is small and imaging does not allow for identification of the tumor substance (42). In a case study containing 16 patients with ccRCC and healthy subjects, miR-30c-5p in urinary exosomes was the only one that was not differentially expressed in other urologic tumors, which demonstrated the specificity of its expression in ccRCC. Urinary exosomes of patients with ccRCC showed lower expression levels of miR-30c-5p than normal subjects, and overexpression of miR-30c-5p could inhibit the growth of ccRCC cells and tumorigenicity in nude mice, suggesting that miR-30c-5p is associated with the development of renal clear cell carcinoma (43). Bao et al. (44) identified six key miRNAs and developed a programmed necrosis–related miRNA signature to predict the prognosis of ccRCC. Exosome-derived nucleic acids can be used as therapeutic markers for RCC prognosis in addition to biomarkers for RCC diagnosis. Wang et al. (45) demonstrated that miR-210 is a good prognostic biomarker, and patients with renal cancer overexpressing miR-210 have higher chances of disease recurrence and shorter survival time. In a prospective study of 35 patients treated with immunotherapy, blood was drawn before and after treatment with immune checkpoint inhibitors. miRNA-146a levels were increased after treatment, and miRNA-126 levels were decreased compared with those before treatment, suggesting that miRNA-146a and miRNA-126 may serve as a predictor of the efficacy of ICI treatment (46).
At present, many mechanism of exosomes in RCC have been reported, and a variety of ways to isolate exosomes have been developed, suggesting that liquid biopsy holds promising prospects for the diagnosis of RCC. The mechanisms of exosome-promoted RCC progression and the ways of exosome isolation have been summarized in Table 1.
Discussion
In recent years, an increasing number of research studies have elucidated the physiological functions of exosomes that are critical to understand how they influence cancer development. Exosomes could activate signaling pathways through intercellular communication to promote cancer cell proliferation. In addition, exosomes are involved in many processes of tumor metastasis, including epithelial–mesenchymal transition, promotion of cancer cell proliferation, promotion of tumor angiogenesis, and extracellular matrix remodeling. Exosomes could also promote tumor drug resistance through a variety of mechanisms. Moreover, exosomes could help cancer cells evade immune surveillance and develop immune tolerance to evade attack by the immune system. The role of exosomes in tumorigenesis, metastasis, and drug resistance suggests that some new approaches could be developed for cancer diagnosis and treatment based on the nature of exosomes. Exosome-derived miRNAs can be obtained from a variety of bodily fluids, including urine, serum, plasma, saliva, and semen, making them valuable for large-scale cancer screening. Unlike tissue aspiration biopsy, which offers limited insight into a single tumor region at a specific time, exosome-derived miRNAs provide a solution to this limitation. On the basis of existing research, exosome-derived miRNAs not only complement current methods for grading and staging urinary system cancers but also hold promise in predicting tumor recurrence, metastasis, and evaluating treatment efficacy. This information aids patients in choosing the most suitable treatment approach (47). Exosome-derived cell-free DNA (cfDNA) and miRNA play crucial roles in tumor progression, drug resistance, and the regulation of the tumor microenvironment, establishing them as promising candidate biomarkers. Different from tissue aspiration biopsy, it is easy to obtain liquid biopsies and isolate exosomes from patients with cancer. In the future, more and more studies will focus on the role of exosomes in the early diagnosis of cancer. In clinical therapy, the ability of exosomes to regulate the tumor microenvironment allows them to be used as natural drug carriers with targeted properties that can be used to kill cancer cells without affecting healthy cells.
However, there remain notable limitations in current exosome research. Primarily, technical challenges persist in the separation and isolation of exosomes. Low-speed centrifugation, often employed for EV separation, lacks specificity and can introduce contamination from other membranous organelles. Conversely, high-speed centrifugation, while yielding higher quantities of exosomal miRNAs and proteins, produces different miRNA expression profiles compared to isolation kits with similarly high yields (48). This underscores the need for the development of more definitive exosome separation method. Furthermore, the mechanisms underlying exosome actions warrant further investigation. In tumor microenvironments, tumor cells release exosomes to modulate immune cell function, aiding in immune evasion. Tumor cells also utilize exosomes to transmit biological information among themselves, facilitating metastasis and drug resistance. Moreover, in highly vascularized cancers like renal cancer, the involvement of exosomes remains unclear. These findings highlight the promise of exosomes as potential targets for tumor therapy, suggesting that their biological properties could be further leveraged for drug development.
Author contributions
RR: Writing – original draft. LZ: Writing – review & editing. SH: Writing – review & editing.
Funding
The author(s) declare financial support was received for the research, authorship, and/or publication of this article. This work was supported by the National Natural Science Foundation of China (81972379, 82173216, 81872083, and 81972380), the Capital Health Research and Development of Special Fund (2022-1-4072), the Clinical Features Research of Capital (Z211100002921070), Clinical Medicine Plus X—Young Scholars Project, Peking University, the Fundamental Research Funds for the Central Universities (PKU2021LCXQ026), and Wuxi “Taihu Talents Program” Medical and Health High-level Talents Project.
Conflict of interest
The authors declare that the research was conducted in the absence of any commercial or financial relationships that could be construed as a potential conflict of interest.
Publisher’s note
All claims expressed in this article are solely those of the authors and do not necessarily represent those of their affiliated organizations, or those of the publisher, the editors and the reviewers. Any product that may be evaluated in this article, or claim that may be made by its manufacturer, is not guaranteed or endorsed by the publisher.
References
1. Johnstone RM, Adam M, Hammond JR, Orr L, Turbide C. Vesicle formation during reticulocyte maturation. Association of plasma membrane activities with released vesicles (exosomes). J Biol Chem (1987) 262:9412–20. doi: 10.1016/S0021-9258(18)48095-7
2. Colombo M, Raposo G, Théry C. Biogenesis, secretion, and intercellular interactions of exosomes and other extracellular vesicles. Annu Rev Cell Dev Biol (2014) 30:255–89. doi: 10.1146/annurev-cellbio-101512-122326
3. Zhang H, Freitas D, Kim HS, Fabijanic K, Li Z, Chen H, et al. Identification of distinct nanoparticles and subsets of extracellular vesicles by asymmetric flow field-flow fractionation. Nat Cell Biol (2018) 20:332–43. doi: 10.1038/s41556-018-0040-4
4. Tauro BJ, Greening DW, Mathias RA, Mathivanan S, Ji H, Simpson RJ. Two distinct populations of exosomes are released from LIM1863 colon carcinoma cell-derived organoids. Mol Cell Proteomics (2013) 12:587–98. doi: 10.1074/mcp.M112.021303
5. Pallet N, Sirois I, Bell C, Hanafi LA, Hamelin K, Dieudé M, et al. A comprehensive characterization of membrane vesicles released by autophagic human endothelial cells. Proteomics (2013) 13:1108–20. doi: 10.1002/pmic.201200531
6. Aatonen MT, Ohman T, Nyman TA, Laitinen S, Grönholm M, Siljander PR. Isolation and characterization of platelet-derived extracellular vesicles. J Extracell Vesicles (2014) 3. doi: 10.3402/jev.v3.24692
7. Raimondo S, Saieva L, Corrado C, Fontana S, Flugy A, Rizzo A, et al. Chronic myeloid leukemia-derived exosomes promote tumor growth through an autocrine mechanism. Cell Commun Signal (2015) 13:8. doi: 10.1186/s12964-015-0086-x
8. Baroni S, Romero-Cordoba S, Plantamura I, Dugo M, D’Ippolito E, Cataldo A, et al. Exosome-mediated delivery of miR-9 induces cancer-associated fibroblast-like properties in human breast fibroblasts. Cell Death Disease (2016) 7:e2312–2. doi: 10.1038/cddis.2016.224
9. Hsieh JJ, Purdue MP, Signoretti S, Swanton C, Albiges L, Schmidinger M, et al. Renal cell carcinoma. Nat Rev Dis Primers (2017) 3:17009. doi: 10.1038/nrdp.2017.9
10. Bukavina L, Bensalah K, Bray F, Carlo M, Challacombe B, Karam JA, et al. Epidemiology of renal cell carcinoma: 2022 update. Eur Urol (2022) 82:529–42. doi: 10.1016/j.eururo.2022.08.019
11. Barata PC, Rini BI. Treatment of renal cell carcinoma: Current status and future directions. CA: A Cancer J Clin (2017) 67:507–24. doi: 10.3322/caac.21411
12. Rosellini M, Marchetti A, Mollica V, Rizzo A, Santoni M, Massari F. Prognostic and predictive biomarkers for immunotherapy in advanced renal cell carcinoma. Nat Rev Urol (2023) 20:133–57. doi: 10.1038/s41585-022-00676-0
13. Jayanthi V, Das AB, Saxena U. Recent advances in biosensor development for the detection of cancer biomarkers. Biosens Bioelectron (2017) 91:15–23. doi: 10.1016/j.bios.2016.12.014
14. Wang L, Yang G, Zhao D, Wang J, Bai Y, Peng Q, et al. CD103-positive CSC exosome promotes EMT of clear cell renal cell carcinoma: role of remote MiR-19b-3p. Mol Cancer (2019) 18:86. doi: 10.1186/s12943-019-0997-z
15. Liu J, Ren L, Li S, Li W, Zheng X, Yang Y, et al. The biology, function, and applications of exosomes in cancer. Acta Pharm Sin B (2021) 11:2783–97. doi: 10.1016/j.apsb.2021.01.001
16. Ståhl AL, Johansson K, Mossberg M, Kahn R, Karpman D. Exosomes and microvesicles in normal physiology, pathophysiology, and renal diseases. Pediatr Nephrol (2019) 34:11–30. doi: 10.1007/s00467-017-3816-z
17. Li X, Corbett AL, Taatizadeh E, Tasnim N, Little JP, Garnis C, et al. Challenges and opportunities in exosome research-Perspectives from biology, engineering, and cancer therapy. APL Bioeng (2019) 3:011503. doi: 10.1063/1.5087122
18. Hu G, Ma J, Zhang J, Chen Y, Liu H, Huang Y, et al. Hypoxia-induced lncHILAR promotes renal cancer metastasis via ceRNA for the miR-613/206/ 1-1-3p/Jagged-1/Notch/CXCR4 signaling pathway. Mol Ther (2021) 29:2979–94. doi: 10.1016/j.ymthe.2021.05.020
19. Tsuruda M, Yoshino H, Okamura S, Kuroshima K, Osako Y, Sakaguchi T, et al. Oncogenic effects of RAB27B through exosome independent function in renal cell carcinoma including sunitinib-resistant. PloS One (2020) 15:e0232545. doi: 10.1371/journal.pone.0232545
20. Shi N, Shan B, Gu B, Song Y, Chu H, Qian L. Circular RNA circ-PRKCI functions as a competitive endogenous RNA to regulate AKT3 expression by sponging miR-3680-3p in esophageal squamous cell carcinoma. J Cell Biochem (2019) 120:10021–30. doi: 10.1002/jcb.28285
21. Qian Y, Li Y, Xu L, Chen K, Liu N, Yang X, et al. Tumor Cell-Derived Exosomal circ-PRKCI Promotes Proliferation of Renal Cell Carcinoma via Regulating miR-545-3p/CCND1 Axis. Cancers (Basel) (2022) 15. doi: 10.3390/cancers15010123
22. Song S, Long M, Yu G, Cheng Y, Yang Q, Liu J, et al. Urinary exosome miR-30c-5p as a biomarker of clear cell renal cell carcinoma that inhibits progression by targeting HSPA5. J Cell Mol Med (2019) 23:6755–65. doi: 10.1111/jcmm.14553
23. Li YL, Wu LW, Zeng LH, Zhang ZY, Wang W, Zhang C, et al. ApoC1 promotes the metastasis of clear cell renal cell carcinoma via activation of STAT3. Oncogene (2020) 39:6203–17. doi: 10.1038/s41388-020-01428-3
24. Sharma R, Kadife E, Myers M, Kannourakis G, Prithviraj P, Ahmed N. Determinants of resistance to VEGF-TKI and immune checkpoint inhibitors in metastatic renal cell carcinoma. J Exp Clin Cancer Res (2021) 40:186. doi: 10.1186/s13046-021-01961-3
25. Sousa D, Lima RT, Vasconcelos MH. Intercellular transfer of cancer drug resistance traits by extracellular vesicles. Trends Mol Med (2015) 21:595–608. doi: 10.1016/j.molmed.2015.08.002
26. Qu L, Ding J, Chen C, Wu ZJ, Liu B, Gao Y, et al. Exosome-transmitted lncARSR promotes sunitinib resistance in renal cancer by acting as a competing endogenous RNA. Cancer Cell (2016) 29:653–68. doi: 10.1016/j.ccell.2016.03.004
27. Greenberg JW, Kim H, Ahn M, Moustafa AA, Zhou H, Barata PC, et al. Combination of tipifarnib and sunitinib overcomes renal cell carcinoma resistance to tyrosine kinase inhibitors via tumor-derived exosome and T cell modulation. Cancers (Basel) (2022) 14. doi: 10.3390/cancers14040903
28. Tubbs A, Nussenzweig A. Endogenous DNA damage as a source of genomic instability in cancer. Cell (2017) 168:644–56. doi: 10.1016/j.cell.2017.01.002
29. Liu Q, Xiao Q, Sun Z, Wang B, Wang L, Wang N, et al. Exosome component 1 cleaves single-stranded DNA and sensitizes human kidney renal clear cell carcinoma cells to poly(ADP-ribose) polymerase inhibitor. Elife (2021) 10. doi: 10.7554/eLife.69454
30. Zhang W, Zheng X, Yu Y, Zheng L, Lan J, Wu Y, et al. Renal cell carcinoma-derived exosomes deliver lncARSR to induce macrophage polarization and promote tumor progression via STAT3 pathway. Int J Biol Sci (2022) 18:3209–22. doi: 10.7150/ijbs.70289
31. Liu Q, Zhao E, Geng B, Gao S, Yu H, He X, et al. Tumor-associated macrophage-derived exosomes transmitting miR-193a-5p promote the progression of renal cell carcinoma via TIMP2-dependent vasculogenic mimicry. Cell Death Dis (2022) 13:382. doi: 10.1038/s41419-022-04814-9
32. Huang X, Wang J, Guan J, Zheng Z, Hao J, Sheng Z, et al. Exosomal circsafb2 reshaping tumor environment to promote renal cell carcinoma progression by mediating M2 macrophage polarization. Front Oncol (2022) 12:808888. doi: 10.3389/fonc.2022.808888
33. Shen T, Miao S, Zhou Y, Yi X, Xue S, Du B, et al. Exosomal AP000439.2 from clear cell renal cell carcinoma induces M2 macrophage polarization to promote tumor progression through activation of STAT3. Cell Commun Signal (2022) 20:152. doi: 10.1186/s12964-022-00957-6
34. Fu W, Zhao H, Liu Y, Nie H, Gao B, Yin F, et al. Exosomes derived from cancer-associated fibroblasts regulate cell progression in clear-cell renal-cell carcinoma. Nephron (2022) 146:383–92. doi: 10.1159/000520304
35. Liu Y, Fu W, Cao X, Li S, Xiong T, Zhang X, et al. Delivery of miR-224-5p by exosomes from cancer-associated fibroblasts potentiates progression of clear cell renal cell carcinoma. Comput Math Methods Med (2021) 2021:5517747. doi: 10.1155/2021/5517747
36. Ding M, Zhao X, Chen X, Diao W, Kan Y, Cao W, et al. Cancer-associated fibroblasts promote the stemness and progression of renal cell carcinoma via exosomal miR-181d-5p. Cell Death Discovery (2022) 8:439. doi: 10.1038/s41420-022-01219-7
37. Butz H, Nofech-Mozes R, Ding Q, Khella HWZ, Szabó PM, Jewett M, et al. Exosomal microRNAs are diagnostic biomarkers and can mediate cell-cell communication in renal cell carcinoma. Eur Urol Focus (2016) 2:210–8. doi: 10.1016/j.euf.2015.11.006
38. Perakis S, Speicher MR. Emerging concepts in liquid biopsies. BMC Med (2017) 15:75. doi: 10.1186/s12916-017-0840-6
39. Ji J, Chen R, Zhao L, Xu Y, Cao Z, Xu H, et al. Circulating exosomal mRNA profiling identifies novel signatures for the detection of prostate cancer. Mol Cancer (2021) 20:58. doi: 10.1186/s12943-021-01349-z
40. Li Y, Zhao J, Yu S, Wang Z, He X, Su Y, et al. Extracellular Vesicles Long RNA Sequencing Reveals Abundant mRNA, circRNA, and lncRNA in Human Blood as Potential Biomarkers for Cancer Diagnosis. Clin Chem (2019) 65:798–808. doi: 10.1373/clinchem.2018.301291
41. Yu S, Li Y, Liao Z, Wang Z, Wang Z, Li Y, et al. Plasma extracellular vesicle long RNA profiling identifies a diagnostic signature for the detection of pancreatic ductal adenocarcinoma. Gut (2020) 69:540–50. doi: 10.1136/gutjnl-2019-318860
42. He X, Tian F, Guo F, Zhang F, Zhang H, Ji J, et al. Circulating exosomal mRNA signatures for the early diagnosis of clear cell renal cell carcinoma. BMC Med (2022) 20:270. doi: 10.1186/s12916-022-02467-1
43. Zhang W, Ni M, Su Y, Wang H, Zhu S, Zhao A, et al. MicroRNAs in serum exosomes as potential biomarkers in clear-cell renal cell carcinoma. Eur Urol Focus (2018) 4:412–9. doi: 10.1016/j.euf.2016.09.007
44. Bao JH, Li JB, Lin HS, Zhang WJ, Guo BY, Li JJ, et al. Deciphering a novel necroptosis-related miRNA signature for predicting the prognosis of clear cell renal carcinoma. Anal Cell Pathol (Amst) (2022) 2022:2721005. doi: 10.1155/2022/2721005
45. Wang X, Wang T, Chen C, Wu Z, Bai P, Li S, et al. Serum exosomal miR-210 as a potential biomarker for clear cell renal cell carcinoma. J Cell Biochem (2019) 120:1492–502. doi: 10.1002/jcb.27347
46. Ivanova E, Asadullina D, Gilyazova G, Rakhimov R, Izmailov A, Pavlov V, et al. Exosomal microRNA levels associated with immune checkpoint inhibitor therapy in clear cell renal cell carcinoma. Biomedicines (2023) 11. doi: 10.3390/biomedicines11030801
47. Diaz LA Jr., Bardelli A. Liquid biopsies: genotyping circulating tumor DNA. J Clin Oncol (2014) 32:579–86. doi: 10.1200/jco.2012.45.2011
Keywords: tumor-derived exosomes, extracellular vesicles, renal cell carcinoma, tumor biomarkers, lipid biopsy
Citation: Rui R, Zhou L and He S (2023) Advances in the research of exosomes in renal cell carcinoma: from mechanisms to applications. Front. Immunol. 14:1271669. doi: 10.3389/fimmu.2023.1271669
Received: 02 August 2023; Accepted: 09 October 2023;
Published: 24 October 2023.
Edited by:
Zhirui Zeng, Guizhou Medical University, ChinaReviewed by:
Liang-min Fu, The First Affiliated Hospital of Sun Yat-sen University, ChinaPeipei Wu, The First Affiliated Hospital of University of Science and Technology of China Anhui Provincial Hospital, China
Copyright © 2023 Rui, Zhou and He. This is an open-access article distributed under the terms of the Creative Commons Attribution License (CC BY). The use, distribution or reproduction in other forums is permitted, provided the original author(s) and the copyright owner(s) are credited and that the original publication in this journal is cited, in accordance with accepted academic practice. No use, distribution or reproduction is permitted which does not comply with these terms.
*Correspondence: Liqun Zhou, emhvdWxxbWFpbEBzaW5hLmNvbQ==; Shiming He, c2hpbWluZ2hlQGJqbXUuZWR1LmNu