- 1Light Chain Bioscience - Novimmune SA, Plan-les-Ouates, Switzerland
- 2Department of Internal Medicine and Medical Specialties, University of Genoa, Genoa, Italy
- 3Ospedale Policlinico San Martino IRCCS, Genoa, Italy
Nicotinamide phosphoribosyltransferase (NAMPT) plays a central role in mammalian cell metabolism by contributing to nicotinamide adenine dinucleotide biosynthesis. However, NAMPT activity is not limited to the intracellular compartment, as once secreted, the protein accomplishes diverse functions in the extracellular space. Extracellular NAMPT (eNAMPT, also called visfatin or pre-B-cell colony enhancing factor) has been shown to possess adipocytokine, pro-inflammatory, and pro-angiogenic activities. Numerous studies have reported the association between elevated levels of circulating eNAMPT and various inflammatory and metabolic disorders such as obesity, diabetes, atherosclerosis, arthritis, inflammatory bowel disease, lung injury and cancer. In this review, we summarize the current state of knowledge on eNAMPT biology, proposed roles in disease pathogenesis, and its potential as a disease biomarker. We also briefly discuss the emerging therapeutic approaches for eNAMPT inhibition.
1 Introduction
Nicotinamide phosphoribosyltransferase (NAMPT) is a homodimeric class II phosphoribosyltransferase (EC 2.4.2.12) ubiquitously expressed in mammalian tissues and playing a crucial role in nicotinamide adenine dinucleotide (NAD) metabolism (1, 2). NAMPT is the rate-limiting enzyme of the NAD salvage pathway, which catalyzes the production of nicotinamide mononucleotide (NMN) from nicotinamide (NAM) and 5-phosphoribosyl-1-pyrophosphate (PRPP) (3). NMN is converted to oxidized NAD (4), which then fuels cellular redox reactions and the activity of NAD-degrading enzymes, such as the cluster of differentiation (CD) 38 (CD38), poly (ADP-ribose) polymerases (PARPs), and sirtuins (SIRTs 1–7) (1, 5). These NAD-dependent enzymes mediate fundamental intracellular processes, including cell signaling, DNA repair, apoptosis and adaptive responses to cell stressors (1, 3, 5). Thus, by modulating NAD levels, NAMPT plays a pivotal role in cell metabolism and survival (3). The NAMPT gene is highly conserved (6–8), and orthologs of the mammalian NAMPT are found not only in other vertebrates [e.g., birds (8) and fish (9)], but even in sponges (9) and bacteria (10). The NAMPT enzyme is found both in the nucleus and in the cytosol, at varying levels, depending on the cell cycle phase (11, 12). Some studies have suggested that NAMPT may also be localized in mitochondria (13–15). In addition, Yoshida et al. demonstrated that NAMPT is transported through the systemic circulation via extracellular vesicles (EVs), before being reinternalized to participate in NAD production in recipient cells (16).
As mentioned above, NAMPT is not only found inside cells and EVs, but can also be directly secreted into the extracellular space. Historically, diverse names were attributed to extracellular NAMPT (eNAMPT), as its diverse functions were being characterized. eNAMPT was first identified as a cytokine secreted by pre-B cells and named “pre-B-cell colony enhancing factor” (PBEF) due to its ability to synergize with interleukin (IL)-7 and stem cell factor (SCF) in promoting pre-B-cell colony formation (17). eNAMPT was also baptized “visfatin”, reflecting its adipokine functions and its secretion from visceral adipose tissue (7, 18). Eventually, NAMPT was found to be released by all cellular types (2), and upregulated in various types of metabolic and inflammatory disorders (19–21). The present publication reviews relevant papers focusing on the biology of eNAMPT, its role as a biomarker and its contribution to disease pathogenesis. The emerging eNAMPT-targeting therapies are also examined. The references gathered in this review were found in PubMed/MEDLINE using “eNAMPT”, “visfatin” or “PBEF” as keywords. We included original and review articles published before July 2023.
2 NAMPT is released into extracellular space
As mentioned above, NAMPT is released by a wide range of cell types, including adipocytes, β-cells, immune cells, neurons, endothelial cells, cardiomyocytes and cancer cells (reviewed in Grolla et al. (2)). NAMPT secretion is an active phenomenon and is not a consequence of cell lysis or cell death (18, 22, 23). In healthy individuals, NAMPT secretion follows a diurnal rhythm, peaking in the afternoon (24). eNAMPT was found in serum (3), but also in synovial fluid (25), follicular fluid (26), bronchoalveolar lavage (BAL) fluid (27), saliva (28), cerebrospinal fluid (29) and stools (30).
As reviewed in Carbone et al. (3), the different stimuli promoting NAMPT secretion can be grouped into three main categories: 1) cellular stress [e.g., ischemia, oxygen-glucose deprivation, oxidative and endoplasmic reticulum [ER] stress, hypoxia (31)]; 2) nutritional cues (e.g., glucose or insulin); and 3) inflammatory signals [e.g., lipopolysaccharide [LPS], tumor necrosis factor-α [TNF-α], IL-1β, interferon-γ [IFN-γ] (32)]. However, the exact mechanism of NAMPT release into the extracellular space remains unclear, as eNAMPT lacks the peptide signal for secretion through the classical ER-Golgi secretory pathway (17). Furthermore, no canonical caspase-1 cleavage site is present in the NAMPT gene sequence (9), which suggests that NAMPT is not released via the conventional secretory pathway. In general, NAMPT secretion was found not to be affected by inhibitors of the ER-Golgi pathway such as brefeldin A or monensin (18, 32–35). Further studies are needed to clarify the mechanisms of NAMPT release into the extracellular space.
Under non-stimulating conditions, eNAMPT only accounts for 1% of total NAMPT protein (31). As compared to intracellular NAMPT, eNAMPT bears some distinctive post-translational modifications, such as different acetylation levels. Namely, SIRT-1-mediated deacetylation of NAMPT on lysine 53 was shown to facilitate its release (36). On the contrary, deacetylation of NAMPT by SIRT-6 reduced NAMPT secretion (37).
3 eNAMPT, a factor with multiple functions
Numerous and diverse functions have been reported for eNAMPT and they were associated with its enzymatic, pro-inflammatory, pro-angiogenic and adipocytokine activities. These are described in the paragraphs below and summarized in Figure 1.
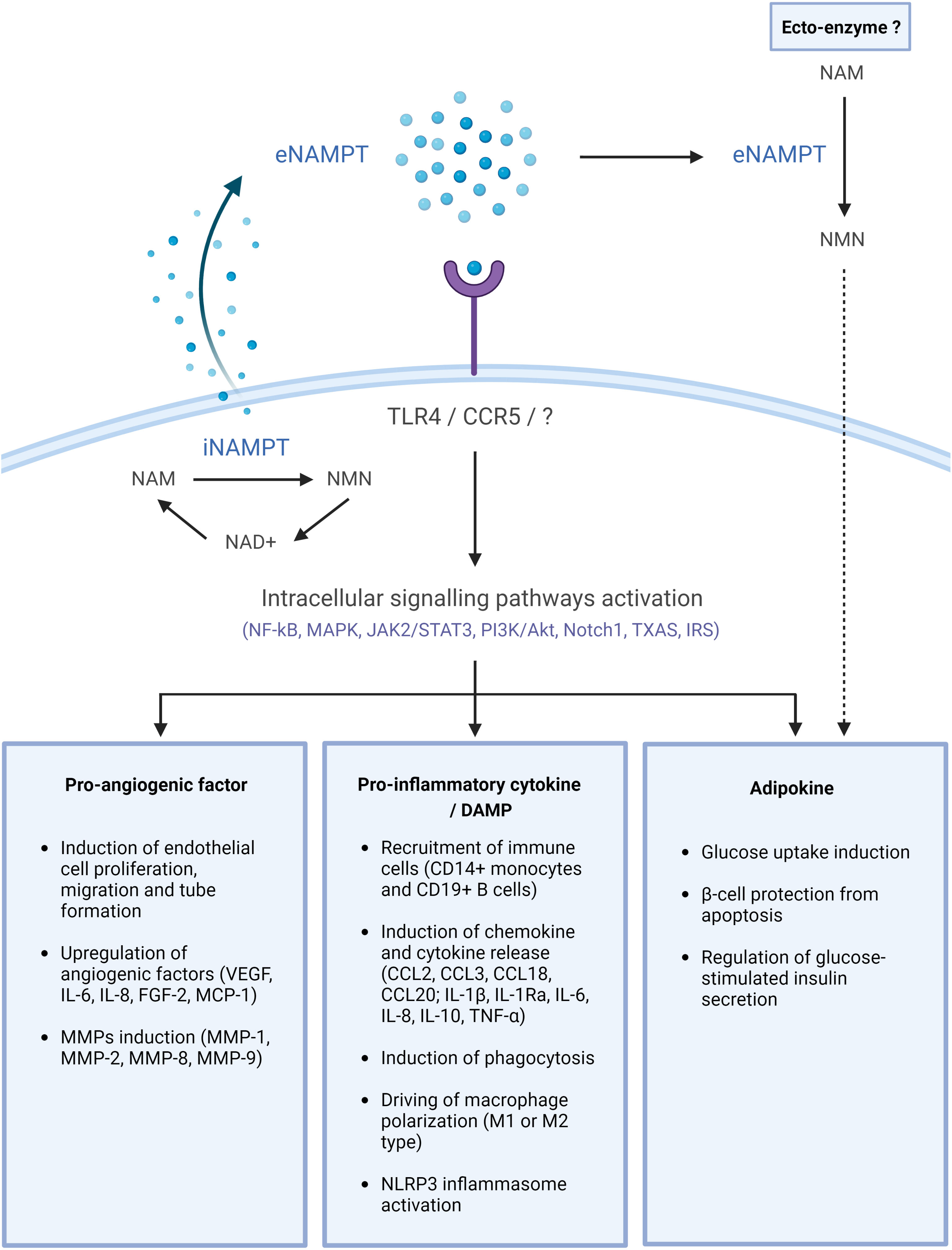
Figure 1 eNAMPT, a DAMP/adipocytokine with multiple functions. NAMPT is released into the extracellular space through an unknown mechanism. There, it may bind cell surface receptors, including TLR4 or CCR5, and activate intracellular signaling pathways, eventually leading to the initiation of diverse physiological and/or pathological processes. eNAMPT may possibly also act as an ectoenzyme in the extracellular space, generating NMN, which can subsequently be internalized and participate in intracellular NAD biosynthesis. This image was created with BioRender.com. Akt, protein kinase B; CD, cluster of differentiation; CCL, chemokine (C-C motif) ligand; eNAMPT, extracellular nicotinamide phosphoribosyltransferase; FGF, fibroblast growth factor; IL, interleukin; IR, insulin receptor; IRS, insulin receptor substrate; JAK, Janus kinase; MAPK, mitogen-activated protein kinases; MCP-1, monocyte chemoattractant protein-1; MMP, matrix metalloproteinase; NAD, nicotinamide adenine dinucleotide; NAM, nicotinamide; NF-κB, nuclear factor kappa-light-chain-enhancer of activated B cells; NMN, nicotinamide mononucleotide; NLRP3, NLR family pyrin domain containing 3; Nocht1, neurogenic locus notch homolog protein 1; PI3K, phosphatidylinositol-3-kinase; STAT3, signal transducer and activator of transcription 3; TXAS, thromboxane synthase; TNF, tumor necrosis factor; VEGF, vascular endothelial growth factor.
3.1 eNAMPT as ectoenzyme
It is not clear yet whether eNAMPT shows biologically relevant phosphoribosyltransferase activity in the extracellular milieu. NAMPT dimerization is essential for enzymatic activity, as shown by the reduced activity of non-dimerizing S199D and S200D NAMPT mutants (18, 38). Various studies have reported that eNAMPT, like its intracellular form, is also a homodimer (18, 34, 39, 40), suggesting that it may be enzymatically active in the extracellular space. In support of this, Revollo et al. demonstrated that adipocyte-derived eNAMPT exerts a strong NAD biosynthetic activity, which is even higher than that of intracellular NAMPT; the authors also found high levels of NMN in mouse plasma (18). eNAMPT has also been shown to increase extracellular NMN and NAD levels in vascular smooth muscle cell cultures and MCF7 cell cultured medium (41, 42). Furthermore, studies show that the extracellular NMN may enter cells and contribute to intracellular NAD biosynthesis (42–44).
On the other hand, the NAMPT substrates, NAM and PRPP, are present at low concentrations or are virtually absent from the extracellular milieu under physiological conditions (45). The same for ATP, which is required for the next enzymatic step, the conversion of NMN to NAD (1, 45). This implies that eNAMPT probably lacks sufficient substrates required for a sustained and functionally relevant enzymatic activity outside the cells. Nonetheless, certain pathological conditions could present a more favorable milieu for NAMPT enzymatic activity. For instance, the tumor microenvironment is characterized by hypoxic areas with elevated rates of necrosis and an acidic pH, and could thus bear increased levels of eNAMPT substrates (41).
3.2 eNAMPT as adipocytokine
Adipocytokines are molecules released from adipose tissues, which are involved in the regulation of glucose homeostasis, body weight, inflammation, blood pressure and tumorigenesis (46). The first description of eNAMPT as an adipocytokine was made in 2005 by Fukuhara et al. (47), demonstrating that eNAMPT is secreted by mouse adipocytes in vitro. This was subsequently confirmed in rats, where eNAMPT production was reported in perivascular adipose tissues (42). In addition, eNAMPT was shown to be expressed in human visceral, subcutaneous, and epicardial fat tissues (47–49). Fukuhara et al. (47) also showed that, similarly to insulin, eNAMPT lowers glucose levels in cultured adipocyte cells and in vivo by directly activating the insulin receptor (IR), but the publication was eventually retracted due to a lack of reproducibility of eNAMPT to IR binding experiments (50). Some subsequent studies have confirmed the insulin-mimetic effects of eNAMPT in human osteoblasts (51) and glomerular mesangial cells (52). Consistent with this, eNAMPT-mediated phosphorylation of IR (51, 53) and of the insulin receptor substrates (IRS)-1 and IRS-2 (51) was demonstrated in human osteoblasts and in mouse pancreatic β-cells. Yet another study reported that, to the contrary, eNAMPT-mediated increase in glucose transport did not involve IRS-1 phosphorylation in skeletal muscle (54). Thus, the interaction of eNAMPT with the IR signaling pathway remains unclear and highly controversial.
eNAMPT may also play a vital role in maintaining the viability and the function of pancreatic β-cells, since it was shown to prevent apoptosis and free-fatty-acid-induced metabolic dysfunction in the MIN6 pancreatic cell line (55). In line with that, Revollo et al. demonstrated that eNAMPT mediated the regulation of glucose-induced insulin release by β-cells (18). Of note, they showed that the enzymatic activity, rather than insulin-mimetic activity of eNAMPT is key for this process, supporting the notion that eNAMPT biosynthetic activity and its product, NMN, directly or indirectly maintain β-cell function (56, 57). Another study revealed that exogenous administration of NMN to mice on a high-fat diet (HFD) improved their impaired glucose tolerance and lipid profile by restoring normal NAD levels in white adipose tissue (WAT) and the liver (58).
3.3 eNAMPT as a mediator of inflammation
The pro-inflammatory activity of eNAMPT is well documented. Numerous studies have demonstrated that eNAMPT activates inflammatory signaling pathways, including the nuclear factor kappa-light-chain-enhancer of activated B cells (NF-κB) (59–61), the mitogen-activated protein kinase (MAPK) (61, 62), and the signal transducer and activator of transcription-3 (STAT3) (63, 64), leading to the production of pro-inflammatory cytokines (e.g., IL-1β, IL-1Ra, IL-6, CXCL8, IL-10, and TNF-α) and chemokines (e.g., C-C motif chemokine ligand [CCL]2, CCL3, CCL18, and CCL20) (59, 60, 62–67). As such, eNAMPT can be viewed either as a pro-inflammatory cytokine or as an alarmin, in other words, a Damage-Associated Molecular Pattern (DAMP). In addition, eNAMPT can function directly as a chemokine to induce the recruitment of monocytes and B cells in vitro, as well as that of neutrophils and monocytes in vivo (32, 66). eNAMPT was able not only to induce immune cell chemotaxis but also to promote their proliferation and differentiation (63, 68). Studies have shown eNAMPT capable of inducing context-dependent monocyte differentiation into either classically activated or alternatively activated macrophages (69) (i.e., M1-type (31, 32, 70), or M2-type (63, 71), respectively). For example, M2 polarization was observed with monocytes from leukemic patients (63), whereas M1 polarization was reported with monocytes from healthy donors (31).
In general, eNAMPT appears as a modulator of innate immune pro-inflammatory programs, particularly in monocytes and macrophages. For instance, eNAMPT supported the survival of mouse macrophages by suppressing the ER-stress-induced apoptosis of these cells through the activation of STAT3 (64). eNAMPT also induced the expression of co-stimulatory molecules in human monocytes, such as CD40, CD54, and CD80 (66). As a result, eNAMPT was able to promote monocyte effector functions, in particular, phagocytosis (66). The pro-inflammatory functions of eNAMPT are not limited to immune cells. eNAMPT is also involved in vascular inflammation by increasing the expression of endothelial cell adhesion molecules (intercellular adhesion molecule 1 [ICAM-1] and vascular cell adhesion molecule 1 [VCAM-1]) in endothelial cells, which boost the adhesion of monocytes (72, 73). Another study reported that eNAMPT promotes NLR family pyrin domain containing 3 (NLRP3) inflammasome complex activation and the subsequent release of pro-inflammatory IL-1β from endothelial cells (74). Neither the enzymatic activity (31, 32, 63, 64, 75) nor dimerization (64) were required for these cytokine/DAMP activities of eNAMPT.
3.4 eNAMPT as a pro-angiogenic factor
In addition to its pro-inflammatory effects, eNAMPT was shown to play a substantial role in angiogenesis. For instance, eNAMPT induced the proliferation, migration, and capillary-tube-forming activity of human umbilical vein endothelial cells in vitro (76–78). The pro-angiogenic function of eNAMPT was also confirmed in vivo, in angiogenesis models (76). In vitro, eNAMPT was able to induce the production and secretion of vascular endothelial growth factor (VEGF), as well as VEGF receptor 2 expression in endothelial and amniotic epithelial cells via the activation of MAPK and phosphatidylinositol-3 kinase/protein kinase B/mammalian target of rapamycin (PI3K/AKT/mTOR) signaling pathways (77–81). Yet other studies have suggested that eNAMPT mediates angiogenesis by eliciting IL-6, CXCL8, fibroblast growth factor 2, and monocyte chemoattractant protein-1 release from endothelial cells (82–86). Finally, eNAMPT was also shown to participate indirectly in extracellular matrix remodeling, a process creating a microenvironment favoring angiogenesis, via the upregulation of proteases involved in vascular basal membrane degradation (MMPs; gelatinases: MMP-2 and -9 and collagenases: MMP-1 and -8) (61, 77, 87–89).
4 eNAMPT cell surface receptors
The rapid activation of specific intracellular pathways, which occurs within minutes of exposure to eNAMPT, suggests that this protein exerts its functions via binding to one or more cell surface receptors (2, 63). Over the last few years, several putative eNAMPT cell surface receptors have been identified. Two of these, in particular, are supported by solid experimental evidence: The first one is the C-C chemokine receptor type 5 (CCR5). In 2012, Van den Bergh et al. demonstrated the direct binding of eNAMPT to CCR5, with an affinity (Kd) in the nM range (90). Later, Torretta et al. found that eNAMPT has a similar structural conformation to CCL7, a known CCR5 ligand and proposed that eNAMPT, like CCL7, acts as an antagonist of CCR5 (91). Eventually, Ratnayake and colleagues confirmed the interaction between eNAMPT and CCR5 in an ELISA binding assay (92, 93).
More recently, studies have focused on the interaction between eNAMPT and Toll-like receptor 4 (TLR4). In 2015, Camp et al. revealed that eNAMPT activates the NF-κB pathway by interacting with TLR4 (94). Consistent with this, another study demonstrated that TLR4 gene silencing in macrophages resulted in a significant reduction in eNAMPT-mediated NF-κB activation (59). Interestingly, eNAMPT has also been suggested to promote vascular dysfunction in mice through a TLR4-mediated pathway. In particular, the authors showed that a specific TLR4 inhibitor, CLI-095, prevented eNAMPT-mediated impairment of the endothelial cell responses to acetylcholine (74). Gasparrini et al. characterized the direct binding of eNAMPT to TLR4, unveiling a relatively high affinity value (KD of 18 nM (65)). Using site-directed mutagenesis, the authors identified two regions in the N-terminal part of eNAMPT that are involved in TLR4 binding (β1-β2 loop: 41–52 aa; and α1-α2 loop: 68–77 aa). The latter findings were recently confirmed by Kim et al. (95), who showed that the 57–65 aa region of eNAMPT interacts with the leucine-rich repeats (LRR) domain of TLR4. A putative TLR4-binding site in the C-terminal region of eNAMPT (445–457 aa) has also been identified (96).
Nonetheless, the question of biologically relevant eNAMPT receptors remains open-ended. For instance, Colombo et al. recently demonstrated that eNAMPT promoted the expression of inflammatory M1-related genes in macrophages (e.g., Il6, Il1b, Cox2, and Tnf), independently of TLR4 (32). They also reported that a competitive molecular antagonist of CCR5 (maraviroc) did not alter eNAMPT-induced activation of M1 macrophages (32). Furthermore, another group used the Retrogenix cell microarray platform to screen for potential eNAMPT protein-protein interactions with over 2,500 known human receptors (97). Using this approach, they identified multiple candidates, distinct from CCR5 and TLR4, suggesting that eNAMPT might interact rather pleiotropically with diverse receptors exposed at the cell surface (97).
5 eNAMPT in disease
Elevated eNAMPT levels are characteristic of numerous human metabolic and inflammatory disorders. Accumulated clinical and preclinical research data suggests eNAMPT could be involved in the pathogenesis of these conditions (represented in Figure 2) and/or serve as a disease biomarker.
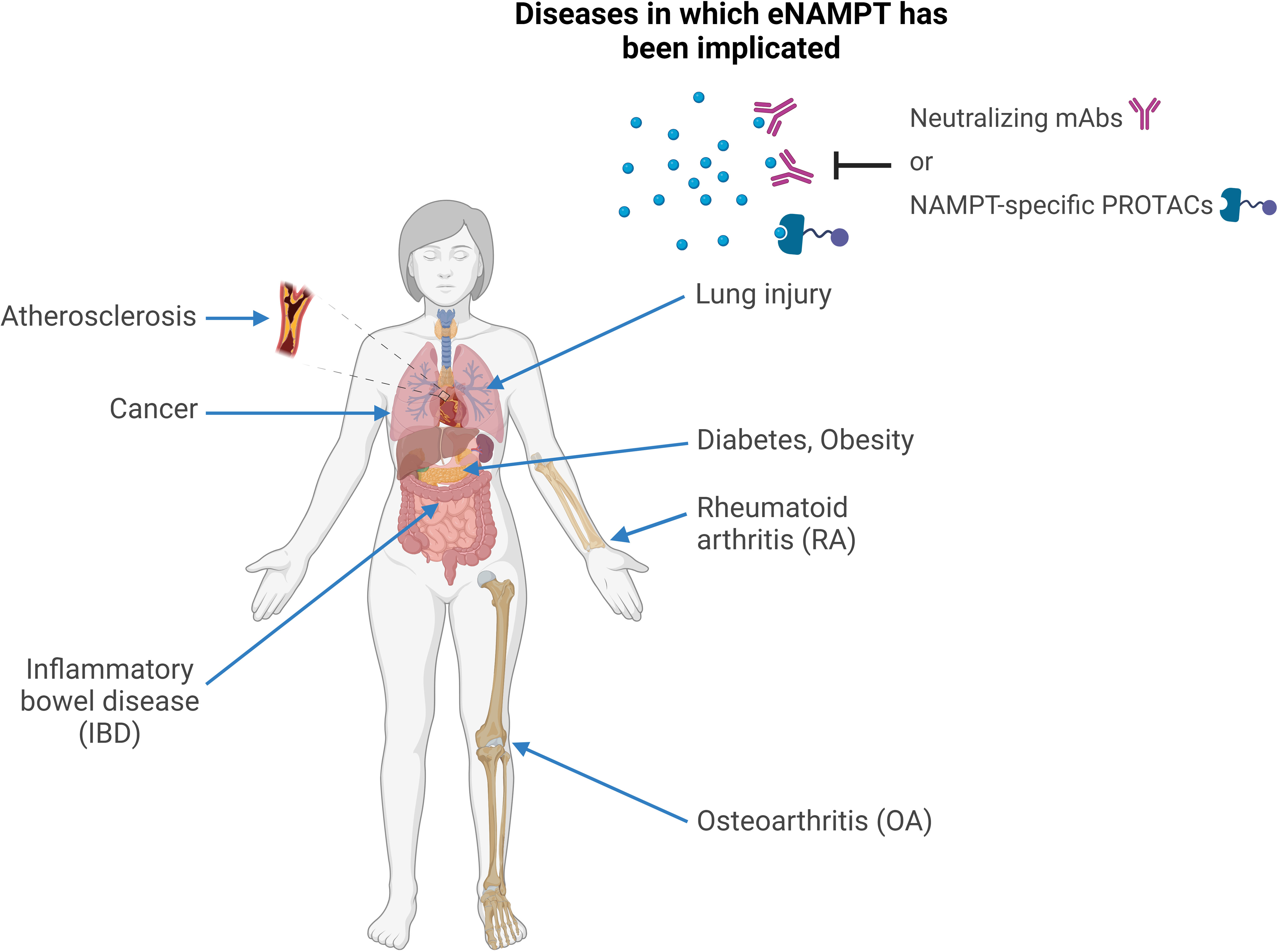
Figure 2 eNAMPT and human disease. Schematic representation of the human conditions in which eNAMPT was proposed to play a role. In all of these conditions, elevated eNAMPT levels were observed in patients’ sera and other bodily fluids, such as synovial fluid in rheumatoid arthritis or bronchoalveolar lavage in lung injury. In addition, preclinical studies pinpointed the mechanisms through which eNAMPT is likely to contribute to the pathophysiology of these conditions, mostly via pleiotropic effects on metabolism, inflammation, and immunity as a DAMP/adipocytokine/pro-angiogenic factor. For at least some of these diseases, neutralizing or reducing eNAMPT levels by monoclonal antibodies or by “molecular glues” (e.g., PROTACs), respectively, was shown to have therapeutic activity in preclinical models.
5.1 Metabolic and cardiovascular diseases
Over the past few years, multiple clinical studies have reported increased circulating eNAMPT concentrations in patients diagnosed with obesity, diabetes, or atherosclerosis, suggesting a role for eNAMPT as an universal biomarker for metabolic and cardiovascular diseases (19). Notably, numerous in vitro and in vivo studies suggest elevated eNAMPT levels might be a causative factor in these conditions, directly contributing to their pathophysiology.
5.1.1 Obesity
5.1.1.1 Preclinical studies
Similar to many other adipocytokines, eNAMPT secretion from adipose tissues is altered in obesity. One experimental study reported that plasma eNAMPT levels are significantly higher in obese, HFD-fed, mice than in controls (98). Whether eNAMPT contributes directly to the mechanisms underlying obesity remains still unclear. In any case, several studies have demonstrated that eNAMPT plays a role in the development of obesity-associated pathologies, including glomerular damage, endothelial dysfunction, and adipose tissue fibrosis (99). In particular, eNAMPT was shown to induce NLRP3 inflammasome, which is involved in the chronic, low-grade inflammation which is characteristic of obesity (74, 98, 100–102). Activation of the NLRP3 complex by eNAMPT was shown to promote glomerular injury, as evidenced by the increased expression of the injury factor desmin in podocytes (101). Moreover, Chen et al. (100) reported that eNAMPT-mediated NLRP3 inflammasome activation provoked the disassembly of junction proteins (zonula occludens [ZO]-1, ZO-2, occludin, and VE-cadherin) in mouse vascular endothelial cells, suggesting that eNAMPT promotes endothelial dysfunction. Finally, eNAMPT has been shown to enhance the expression of ECM proteins (e.g., collagen type C and osteopontin) and MMPs (MMP-2 and -9) in 3T3-L1 pre-adipocytes, leading to ECM accumulation and remodeling, and consequently, to adipose tissue fibrosis (87).
5.1.1.2 Clinical studies
Mounting evidence suggests that eNAMPT could be an attractive complementary biomarker in obesity, in particular of the chronic low-grade inflammation that is associated with this condition. Numerous clinical studies have reported significantly higher circulating eNAMPT levels in obese patients than in lean controls [reviewed by Carbone et al. (3) and summarized in Table 1 (87, 103–106, 117–120)]. Correlations between eNAMPT levels and unfavorable metabolic profiles, including high waist circumference and waist-to-hip ratio, as well as elevated levels of triglycerides, have also been observed (103, 118, 120). eNAMPT levels were also often positively correlated with body mass index (BMI) (87, 103, 104), although not in all studies (119, 121). These discrepancies could be explained by differences in the type of study, the type of population recruited, the number of cases per cohort, and the methods of sample collection and measurement (122). Consistent with the association between elevated eNAMPT levels and adiposity, several studies have reported a reduction in circulating eNAMPT levels after weight loss, and exercise has been shown to decrease eNAMPT levels in overweight/obese patients (123–125). Namely, Friebe et al. observed that serum eNAMPT concentrations became significantly reduced in obese subjects after bariatric surgery, and that this reduction correlated with a decline in white blood cell numbers (117). Another group demonstrated that in human visceral WAT, eNAMPT is mainly released by macrophages which accumulate with BMI increase (126, 127). Increased levels of circulating eNAMPT in obese patients are correlated with an inflammatory signature (e.g., IL-6, TNF-α, and C-reactive protein [CRP]) and endothelial markers (e.g., VCAM-1, ICAM-1, angiotensin-2 [ang-2], and E-selectin) (104, 120, 128). In addition, eNAMPT levels were also positively associated with endotrophin (r = 0.619, P < 0.001), a marker of increased fibrosis and metabolic abnormalities, in obese patients (87). Last but not least, plasma eNAMPT concentrations were shown to predict visceral adipose tissue accumulation in obese children (119). All this suggests a great potential for eNAMPT as a biomarker of obesity and chronic, low-grade inflammation. However, is it important to emphasise here that eNAMPT levels do not always correlate with metabolic and inflammatory disease. For example, no association was found between circulating eNAMPT levels and the severity of non-alcoholic fatty liver disease (manifested as fibrosis and non-alcoholic steatohepatitis) (129).
5.1.2 Diabetes
5.1.2.1 Preclinical studies
The role of eNAMPT in the pathophysiology of diabetes is controversial. Multiple studies have reported a beneficial effect of eNAMPT as an ectoenzyme on pancreatic function. For instance, eNAMPT and its product, NMN, were shown to maintain β-cell homeostasis by modulating cellular NAD levels (18). What is more, impaired islet function of mice fed a fructose-rich diet was associated with reduced plasma eNAMPT levels, which could be reversed by NMN administration (57). In line with this, injection of NMN into aged β-cell-specific SIRT-1-overexpressing transgenic mice restored the beneficial effect of SIRT-1 on glucose tolerance, which was lost with mice ageing (56). Conversely, one study reported that prolonged eNAMPT administration induced a diabetic phenotype in mice (130); suggesting that abnormally elevated eNAMPT levels may exert a detrimental effect on β-cell activity. Thus, the bimodal effect of eNAMPT on diabetes may be concentration-dependent: physiological concentrations of eNAMPT may help maintain pancreatic function, whereas higher concentrations of eNAMPT may drive pathological mechanisms (109). The authors demonstrated that low, physiological levels of eNAMPT increased static and dynamic glucose-stimulated insulin secretion (GSIS) and intracellular cytosolic calcium levels in mouse islets, whereas higher levels of eNAMPT resulted in islet inflammation and β-cell failure (109). These findings suggest that circulating blood levels of eNAMPT and its enzymatic activity may differentially affect diabetes pathophysiology.
5.1.2.2 Clinical studies
Multiple clinical studies have investigated the correlation between eNAMPT levels and the different types of diabetes. Increased eNAMPT levels are detected in patients with type 1 diabetes mellitus (T1DM) (131, 132), type 2 DM (T2DM) (107–109, 111, 132–135), and gestational diabetes (110, 136), compared with non-diabetic individuals (detailed in Table 1). The increase in serum eNAMPT levels coincided with the severity of diabetes, and particularly with the progression of β-cell deterioration, a major feature of T2DM. This was further attested by the positive correlation between eNAMPT and the levels of hemoglobin A1c, an important marker of glycemic control, whose levels directly correlate with the concentration of sugar in the blood during the previous three months (109, 132). The clinical association between eNAMPT and diabetes was independent of BMI (133, 134). No significant differences in circulating eNAMPT concentrations were found between pre-diabetic subjects and healthy controls (135). In addition, the levels of eNAMPT in newly diagnosed T2DM patients were similar to those of non-diabetic individuals, whereas eNAMPT levels of patients with chronic T2DM were higher (132). This suggests that elevated eNAMPT may play a role in the later stages of the disease, probably contributing to the maintenance of lingering inflammation. The link between eNAMPT and insulin resistance, another major characteristic of T2DM pathophysiology, was also extensively explored. Some studies (19, 108, 111, 137), but not others (138, 139), showed an association between eNAMPT levels and homeostasis model assessment of insulin resistance (HOMA-IR – in other words, a correlation between elevated eNAMPT and insulin resistance). Higher serum eNAMPT levels were also found in insulin-resistant children compared to insulin-sensitive children (117). Likewise, elevated eNAMPT levels were shown to predict gestational diabetes with high sensitivity and specificity (110). In conclusion, eNAMPT levels could still be a promising biomarker for monitoring diabetes progression, at least in specific medical conditions.
5.1.3 Atherosclerosis
5.1.3.1 Preclinical studies
Along with serum lipid levels, plasma eNAMPT levels are significantly elevated in ApoE knockout atherosclerotic mice (140). eNAMPT is also highly expressed in human symptomatic atherosclerotic plaques, localized to areas where foam cells are abundant (67, 141). Studies have shown that pro-atherogenic stimuli (e.g., oxidized low-density lipoprotein, hypoxia, and TNF-α) increase eNAMPT expression in THP-1 monocytes (67, 142) and promote eNAMPT release from RAW264.7 macrophage-like cells (140). In turn, elevated eNAMPT triggers cholesterol uptake and accumulation in macrophages (140) and induces the secretion of pro-inflammatory cytokines in peripheral blood mononuclear cells from patients with unstable angina (67). Thus, through its pro-inflammatory actions, eNAMPT may participate in foam cell formation and fatty streak development during atherogenesis. eNAMPT could also contribute to atherosclerosis development by promoting endothelial dysfunction. In this context, eNAMPT has been shown to alter the migration and adhesion of endothelial progenitor cells (EPCs, key cells in the regeneration of impaired blood vessels), and to induce their apoptosis (143, 144). What is more, eNAMPT may also directly impair microvascular endothelium-dependent vasorelaxation by reducing the endothelial response to vasodilators such as acetylcholine (74, 145), negatively affect vessel wall integrity and compound plaque stability by promoting the expression of MMPs in monocytes, macrophages (67, 88) and endothelial cells (77). Finally, eNAMPT could induce human vascular smooth muscle cell proliferation (42) and upregulate their nitric oxide synthase levels (146), thereby promoting vascular inflammation.
5.1.3.2 Clinical studies
Consistent with the experimental findings, elevated circulating eNAMPT levels were observed in patients with atherosclerotic plaques [see Table 1 (112–116, 147)]. Increased plasma eNAMPT concentrations were also measured after mechanical induction of plaque rupture (67) or in patients diagnosed with acute myocardial infarction (148), suggesting a relationship between eNAMPT levels and plaque instability. In line with that, a negative correlation between eNAMPT concentration and the gray-scale median score, which quantifies plaque vulnerability, in atherosclerotic patients, was found (115). Circulating eNAMPT concentrations are correlated with the extent of atherosclerosis, as measured by intima media thickness (116, 147) and with endothelial dysfunction, based on flow-mediated vasodilatation (149). Elevated circulating eNAMPT levels were proposed to be a risk factor for the development of atherosclerotic plaques (112, 114).
5.2 Osteoarthritis
5.2.1 Preclinical studies
Several studies have suggested that eNAMPT promotes tissue degradation in OA. For instance, Cheleschi et al. reported that eNAMPT contributes to cartilage turnover by promoting the apoptosis of OA chondrocytes and OA synovial fibroblasts (OASFs) (150, 151). eNAMPT can also contribute to joint degeneration during OA by affecting oxidative stress, in particular mitochondrial superoxide anion production as well as the expression of antioxidant enzymes (e.g., superoxide dismutase, catalase [CAT], and nuclear factor erythroid 2-related factor 2) (150–153). In addition, eNAMPT may exacerbate OA by promoting the expression of a variety of proteases: Studies show that eNAMPT stimulates the release of sulphated glycosaminoglycans from cartilage and meniscus explants, which is suggestive of elevated aggrecanase activity and proteoglycan loss (97, 154, 155). Gosset et al. reported that eNAMPT reduces aggrecan mRNA and the levels of high molecular weight aggregated proteoglycan in immature mouse articular chondrocytes and induces the expression of ADAMTS-4 and ADAMTS-5 aggrecanases (156). eNAMPT was also shown to upregulate the expression of MMPs (collagenases: MMP-1, -8, -13; gelatinases: MMP-2, -9; stromelysins: MMP-3, -10; matrilysin: MMP-7) in OA chondrocytes and OASFs (97, 150, 151, 156). In human and mouse OA chondrocytes, eNAMPT promoted the synthesis of prostaglandin E2, a well-known cartilage catabolic factor (156, 157). Indeed, eNAMPT-induced cartilage degradation seems to result, in large part, from its DAMP/pro-inflammatory activities, as eNAMPT was shown to activate NF-κB and MAPK signaling pathways in OASFs, leading to the downstream expression of pro-inflammatory cytokines and the activation of inflammatory processes (40, 97, 151, 158). On the other hand, eNAMPT may not only stimulate the activity of catabolic processes but could also directly alter the expression of cartilage structural proteins, thereby impairing cartilage production. For instance, eNAMPT was shown to reduce the expression of collagen type II and type X in OA chondrocytes and OASFs (151, 159). By the same token, another study demonstrated that eNAMPT prevents the insulin-like-growth-factor-1-mediated production of collagen type II and proteoglycan (160). Finally, another effect of eNAMPT was to increase VEGF production by human OASFs, which in turn induces EPC angiogenesis, a process involved in structural damage and pain during OA (161, 162). Also, Pecchi et al. revealed that eNAMPT induces nerve growth factor expression and release by chondrocytes, supporting the idea of a possible involvement of this DAMP/cytokine in OA-associated pain (163).
5.2.2 Clinical studies
Several studies have reported significantly elevated eNAMPT levels in the serum or synovial fluid of OA patients (see Table 2 (161, 164–167),). eNAMPT found in OA joints was shown to be released from many tissue components, including the synovium, the subchondral bone and cartilage, and the infrapatellar fat pad (40, 167). In line with preclinical evidence, synovial fluid eNAMPT levels positively correlated with OA severity and with biomarkers of cartilage degradation (166). eNAMPT was also positively associated with VEGF levels and with pain score (161, 179). As a corollary, experimental and clinical studies strongly suggest eNAMPT may serve as a complementary biomarker and/or a potential drug target in OA (180).
5.3 Rheumatoid arthritis (RA)
5.3.1 Preclinical studies
As in OA, elevated eNAMPT levels may contribute to the pathogenesis of RA, an autoimmune disease which is also characterized by joint damage. However, as compared to OA, human RA tissues exhibit higher serum and synovial fluid concentrations of eNAMPT, probably reflecting a higher-grade inflammatory state of the latter (25, 181). Accordingly, eNAMPT levels were particularly high in the serum and in the paw tissues of collagen-induced arthritis mice, an experimental model of RA (182). In human RA synovial fibroblasts (RASFs), eNAMPT secretion is induced by pro-inflammatory cytokines and TLR ligands such as poly(I:C) and LPS, typically found in RA joints (25, 181). In turn, elevated eNAMPT levels induce the expression of pro-inflammatory cytokines (e.g., IL-6 and CXCL8), chemotactic signals (e.g., chemokines of the CXC and CC family) and matrix-degrading enzymes (e.g., MMP-1 and MMP-3) promoting a vicious circle of self-propagating inflammation and destruction of RA joints (25, 183–185). In addition, eNAMPT was also proposed to promote cartilage invasion by RASFs by increasing their adhesion to endothelial cells (186) and enhancing their motility and migration (184).
5.3.2 Clinical studies
A growing body of research suggests that eNAMPT may be a useful biomarker for various autoimmune diseases characterized by chronic inflammation, in particular rheumatoid arthritis (RA) (187), inflammatory bowel disease (IBD) (188), and possibly also for psoriasis (189) and systemic lupus erythematosus (190).
As pointed out above, eNAMPT levels are high in RA (reviewed by Franco-Trepat et al. (187) and shown in Table 2 [(168–172, 191–194)]. In RA patients, eNAMPT was strongly expressed in the synovial lining layer and at sites of RASF invasion in the cartilage (25, 184). The protein was also detected in lymphoid aggregates and in perivascular areas (25, 181, 184). eNAMPT levels positively correlate with inflammatory mediators like TNF-α, IL-6, and CRP and with immune cells counts, namely neutrophils and B cells, both in the serum and in synovial fluid of RA patients (25, 170, 172, 191, 192). Overall, eNAMPT levels increase with RA severity and duration (170, 171, 191, 193) and are particularly high in patients showing radiographic joint damage (191, 193). In turn, a significant decrease in circulating eNAMPT levels could be observed in some RA studies following treatment with conventional synthetic disease modifying antirheumatic drugs (csDMARDs) (170), TNF-α blockers (195), anti-CD20 antibody (172), or with a combination of methotrexate and anti-IL-6 therapy (196). What is more, a reduction in serum eNAMPT levels after three months of csDMARD treatment could predict an improvement of disease activity score, suggesting that eNAMPT may serve as a complementary prognostic biomarker in RA (170). That said, other RA studies do not support this concept, as no significant changes in circulating eNAMPT levels have been observed there, either following treatment with DMARDs, TNF-α blockers, or a combination of the two (197, 198).
5.4 Inflammatory bowel disease (IBD)
5.4.1 Preclinical studies
While experimental studies of NAMPT role in IBD have mainly focused on the intracellular enzyme (199–201), Colombo et al. (188) recently reported that the administration of recombinant eNAMPT to mice with mild colitis exacerbated mucosal inflammation. They also observed an increased expression of TNF-α and IL-1β, and degradation of IκB-α in colonic tissues of eNAMPT-treated mice. Interestingly, an anti-NAMPT neutralizing monoclonal antibody was able to reduce the recruitment of pro-inflammatory monocytes and neutrophils and the activation of pathogenic Th1 and cytotoxic effector T cells in the colon in the dinitrobenzene sulfonic acid (DNBS)-induced model of colitis (188). The authors proposed that eNAMPT may fuel colonic inflammation by activating pro-inflammatory functions of myeloid cells and by triggering pathological Th1 and Th17-responses. Further studies are probably required to establish the role that eNAMPT plays in the pathogenesis of IBD, and particularly, in mucosal inflammation, a hallmark of this group of diseases.
5.4.2 Clinical studies
A bulk of clinical studies in IBD reported elevated circulating eNAMPT levels in patients with ulcerative colitis (UC) and Crohn’s disease (CD) [Table 2 (30, 173–176, 202–204)]. Interestingly, eNAMPT levels were found to be differently associated with disease activity in UC and CD patients. In CD patients, serum eNAMPT was elevated regardless of disease activity, whereas in UC patients, eNAMPT levels were significantly higher in patients with active disease as compared to inactive disease (66, 174, 175). Similar to the pattern observed in other inflammatory diseases, a positive correlation between the levels of eNAMPT and inflammation markers (e.g., IL-6, platelet count, erythrocyte sedimentation rate, or CRP) is apparent in IBD (173, 174). Intriguingly, Colombo et al. reported that after 14 weeks of therapy, IBD patients responding to anti-TNF-α treatment exhibited a strong decrease in circulating eNAMPT levels, while non-responders maintained elevated eNAMPT serum concentrations (188), suggesting that eNAMPT might be used as a predictive biomarker in IBD. Likewise, serum eNAMPT levels were shown to predict recurrence of active UC with a high sensitivity and specificity, underpinning the potential of eNAMPT as a diagnostic tool (173).
5.5 Lung injury
5.5.1 Preclinical studies
Growing evidence implicates eNAMPT in the lung injury-associated inflammatory responses that are associated with various pulmonary conditions, such as acute lung injury (ALI), acute respiratory distress syndrome (ARDS; a severe form of ALI), and ventilator-induced lung injury (VILI). Neutralization of eNAMPT with an antibody was shown to attenuate inflammatory lung injury in preclinical mouse, rat, and porcine ARDS/VILI models (205–207) and serum eNAMPT was found to be increased in different ALI mouse models (178). As with most, if not all, diseases in which eNAMPT is thought to be implicated, eNAMPT’s effects appear to result from its pro-inflammatory DAMP activities. In vivo studies show that eNAMPT acts as a leucocyte chemoattractant increasing polymorphonuclear leucocyte (PMN) counts and chemokine levels in bronchoalveolar lavage (BAL) fluid (94, 208). Consistent with this, tracheal administration of eNAMPT was shown to augment BAL pro-inflammatory cytokine levels in mice (208). eNAMPT also exacerbated mechanical VILI features, as evidenced by alveolar wall thickening and neutrophil infiltration observed in a murine model (208). In addition, eNAMPT may also elicit lung injury by promoting endothelial cell barrier disruption: For example, Quijada et al. observed a decrease in transendothelial electrical resistance in human lung endothelial cells treated with eNAMPT, reflecting a loss of endothelial barrier integrity (206). In line with these findings, silencing of NAMPT in human pulmonary artery endothelial cells, attenuated thrombin-induced endothelial barrier dysfunction (209). Finally, eNAMPT was shown to further contribute to pulmonary permeability by dysregulating NF-κB, MAPK, and AKT-mTOR-Rictor signaling pathways in human lung endothelial cells (94, 205, 206).
5.5.2 Clinical studies
Circulating levels of eNAMPT are increased in patients with ALI, ARDS [see Table 2 (27, 177, 178)], and ARDS-predisposing conditions like sepsis or acute pancreatitis (177, 210), and seem to be correlated with survival in patients presenting with these conditions. For instance, higher eNAMPT levels were found in non-survivors of sepsis-induced ARDS than in survivors (211), and the survival rate of ALI patients was also shown to negatively correlate with serum eNAMPT (178). Understandably, eNAMPT now became one of a panel of six prognostic biomarkers for 28-day ARDS mortality (212). Supporting a link between eNAMPT and pulmonary inflammation, a positive correlation was found between elevated serum eNAMPT levels and IL-6, CXCL8, IL-10, and MCP-1 in ALI patients (178, 211). Importantly, circulating eNAMPT levels can discriminate between healthy individuals and patients with ARDS (177) or ARDS-predisposing pathologies (177, 210). Taken together, these findings indicate that eNAMPT may serve as a novel diagnostic/prognostic biomarker for pulmonary inflammatory conditions, and, possibly, as a tool for patient stratification in clinical trials.
5.6 Cancer
5.6.1 Preclinical studies
Among all the pathologies that were investigated, elevated eNAMPT levels are probably the best documented in cancer. High levels of circulating eNAMPT are associated with various cancer types, ranging from solid tumors to hematological malignancies (213). eNAMPT, secreted both by the tumor (31, 34, 75) and by tumor-associated cells, is proposed to have a role in different hallmarks of cancer, promoting inflammation and cancer progression. To start with, eNAMPT could directly stimulate cancer cell proliferation in vitro, e.g., of breast cancer (41, 214–216), melanoma (217), hepatocellular carcinoma (218, 219), endometrial carcinoma (220), and prostate adenocarcinoma (221). eNAMPT is believed to exert its proliferative effects by activating various signaling pathways (c-Abl/STAT3, PI3K/AKT, MAPK/ERK, and Notch1/NF-κB) (214–216, 220) or by inducing the expression of cyclin D1 and cyclin-dependent kinase 2 (222). Moreover, eNAMPT was also shown to protect cancer cells from apoptosis by inhibiting survivin degradation (215) and from hydrogen-peroxide-induced DNA damage, presumably by increasing the activity of antioxidant enzymes (217). Some of these pro-tumorigenic effects of eNAMPT were confirmed in vivo (214, 220, 223).
Elevated eNAMPT levels may play an important role in promoting tumor cell migration and invasion. For example eNAMPT was shown to stimulate the migration of breast cancer, osteosarcoma, ovarian cancer, chondrosarcoma, and prostate cancer cells in vitro (214, 224–227). In colorectal cancer (CRC) cells, eNAMPT upregulated the expression of stromal-derived factor 1, a chemokine known to stimulate CRC cell migration (228). Importantly, a neutralizing anti-NAMPT antibody was able to successfully inhibit prostate cancer cell invasiveness in a mouse orthotopic xenograft model (229). As demonstrated by Soncini et al. (75), one of the mechanisms by which eNAMPT could promote cancer cell migration and metastasis is by inducing epithelial-to-mesenchymal transition (EMT) via transforming growth factor β signaling. eNAMPT could also trigger EMT via NF-κB/Snail signaling, as shown by others (230, 231). Additional mechanisms proposed to explain the pro-migratory and pro-invasive effects of eNAMPT are 1) the induction of matrix-degrading enzymes in cancer cells, such as MMP-2 and -9 gelatinases (214, 218, 221, 222, 226, 227, 232) and 2) the stimulation of angiogenesis by inducing pro-angiogenic factors in endothelial cells (81) and cancer cells (222).
Importantly, eNAMPT may contribute to cancer progression by fostering immunosuppression in the tumor micro-environment. For instance, studies reported that eNAMPT induced the polarization of macrophages towards the pro-tumorigenic M2 phenotype (63, 71). eNAMPT was also shown to boost the secretion of immunosuppressive, tumor-promoting factors such as indoleamine 2,3-dioxygenase, CCL18, IL-10, IL-1β, IL-6, and CXCL8 (63, 66). Audrito et al. reported that eNAMPT may contribute to immunosuppression in chronic lymphocytic leukemia by inducing the differentiation of monocytes into a specialized class of leukemia-associated macrophages called nurse-like cells that create an immunosuppressive niche to promote cancer cell survival and inhibit T cell proliferation (63).
Last but not least, in vitro studies suggest that eNAMPT could mediate resistance to cancer therapies. A recent study revealed that eNAMPT, by boosting thymidylate synthase expression in cancer cells, reduced the sensitivity of CRC to capecitabine (233). Likewise, eNAMPT was shown to induce the phosphorylation of estrogen receptor α, contributing to breast cancer resistance to tamoxifen (234, 235). Finally, the fact that eNAMPT enhances the antioxidant capacity of tumor cells may also contribute to their resilience and drug resistance (217, 236).
5.6.2 Clinical studies
Numerous studies of samples obtained from cancer patients assessed the association between circulating eNAMPT levels on the one hand, and cancer size/stage, patient survival or specific biomarkers, on the other. As summarized in Table 3 and as reviewed by Dalamaga et al. (213), elevated levels of eNAMPT are present in cancer patients, positively correlating with tumor size and cancer stage (23, 214, 219, 227, 232, 239, 241–246). eNAMPT levels in cancer patients could also be (negatively) correlated with disease-free and overall survival and (positively) with lymph node invasion and/or metastasis (214, 232, 245, 247). As an example, elevated eNAMPT levels were established as an independent risk factor for myometrial invasion in uterine cancer (248). Circulating eNAMPT levels also correlated with cancer biomarkers such as CA 15-3 in breast cancer (247), alpha-fetoprotein in hepatocellular carcinoma (243), and lactate dehydrogenase in metastatic melanoma (23). On a separate note, melanoma patients with Programmed death-ligand 1-positive (PD-L1+) lesions had significantly higher plasma eNAMPT concentrations than patients with PD-L1− lesions, hinting at a link between eNAMPT and the tumor-associated inflammatory response (23).
As a corollary, eNAMPT may represent an appealing novel therapeutic target in oncology, given its proposed implication in cancer pathogenesis and progression. In any case, eNAMPT prospects as a prognostic/predictive biomarker look particularly encouraging. Several studies demonstrated that eNAMPT could rather accurately predict cancer progression (227, 237, 239, 247) and a recent meta-analysis revealed a correlation between eNAMPT levels and cancer risk (21).
6 eNAMPT as a potential drug target
Historically, therapeutic treatment approaches have focused on NAMPT enzymatic activity and several low molecular weight NAMPT enzyme inhibitors (NAMPTi) are available (5, 249, 250). Among these, FK866 (also named APO866, (E)-Daporinad, and WK1) and GMX1778 (also named CHS-828) were among the first to be synthetized and tested. These “early” NAMPTi usually show potent anti-cancer activity in preclinical models, but could not progress beyond early clinical trials due to poor efficacy associated with dose-limiting toxicities such as thrombocytopenia and gastrointestinal toxicity (5, 249). In an attempt to broaden the therapeutic index, a second generation of NAMPTi (such as OT-82), as well as dual-function enzymatic inhibitors (i.e., inhibiting NAMPT enzymatic activity plus another target overexpressed in tumors, e.g., KPT-9274, which also blocks P21-activated kinase 4), were generated and are currently being evaluated in phase I clinical trials in cancer (250).
Concerning eNAMPT, its proposed pathogenic functions are typically not contingent on enzymatic activity, and thus cannot be inhibited by NAMPTi. Antibodies, in contrast, are well suited for the neutralization of extracellular factors like eNAMPT, and several in vitro and in vivo studies already explored this therapeutic strategy (summarized in Tables 4, 5). For example, Kieswich et al. successfully used a commercial anti-NAMPT polyclonal antibody in vivo to improve the diabetic phenotype of HFD-fed mice, pancreatic islet function, glycemic control, and insulin resistance (130). Another example is provided by Colombo et al., who developed a NAMPT-neutralizing mAb capable of limiting acute and chronic colitis in experimental mouse models (188). Unquestionably, the most advanced eNAMPT inhibitor is currently ALT-100, a humanized mAb developed by Joe G.N Garcia and coworkers. Since ALT-100 is cross-reactive with NAMPT from multiple mammalian species, it could be tested in mice but also in several non-murine in vivo models. Hence, ALT-100 demonstrated to reduce the severity of murine and porcine inflammatory lung injury (205–207), pulmonary hypertension in rats (255), radiation-induced lung fibrosis in mice (257), and prostate cancer cell proliferation, invasion, and metastasis (229). More recently, ALT-100 has also shown therapeutic efficacy in mouse models of lung vasculitis/hemorrhage, nonalcoholic fatty liver disease and intra-amniotic inflammation (190, 258, 259). ALT-100 is currently completing a first-in-human study in healthy volunteers (NCT05426746).
Recently, a novel therapeutic approach for targeted degradation of intracellular NAMPT was made possible thanks to the development of the “molecular glue” technology (PROTAC) (260). A mechanistic consequence of lowering intracellular NAMPT protein levels is the concomitant reduction of eNAMPT in the extracellular space. Thus, PROTAC is expected to limit both the enzymatic (principally intracellular) and non-enzymatic (extracellular) activities of NAMPT. Indeed, some promising preliminary results have been obtained with this class of molecules in vitro and in vivo (261, 262). One study reported that NAMPT-specific PROTACs outperformed FK866 in terms of tumor-killing activity (261). Strikingly, the authors showed that, unlike FK866, PROTACs were able to inhibit the NF-κB and MAPK/ERK1/2 pathways, implying that they also prevent eNAMPT pro-inflammatory DAMP activities (261). Notwithstanding these encouraging results, further studies are needed to determine whether NAMPT-targeting PROTACs could be developed into safe and efficacious drugs for patients.
7 Conclusions and perspectives
Numerous clinical studies, mostly published in the past decade, have reported a correlation between elevated levels of circulating eNAMPT and diverse metabolic and inflammatory disorders, presenting physicians with an opportunity of using eNAMPT levels as a disease activity indicator, prognostic biomarker, or even as a patient stratification tool. There is also mounting, well-documented, preclinical evidence suggesting that elevated eNAMPT levels may contribute to disease pathophysiology by exerting pleiotropic and systemic effects on metabolism and immunity via DAMP/adipocytokine/pro-angiogenic factor activities. Consequently, therapeutic approaches for eNAMPT inhibition, principally with mAbs, are now being developed and tested in various disease models. The leading experimental drug, mAb ALT-100, is in early clinical development, so we may soon learn more about the potential of eNAMPT as therapeutic target.
All that said, while eNAMPT may be a pleiotropic factor implicated in disease pathophysiology, it is also ubiquitously secreted and present at low physiological levels in healthy individuals, and the benefits of physiological eNAMPT release are not fully appreciated, aside from specific examples, such as maintaining pancreatic beta cell function. Likewise, little is known about how eNAMPT physiological levels in tissues and in the circulation are affected by diverse environmental and physiological cues, by age, activity, etc. Other aspects of eNAMPT biology remain unclear as well, in particular the exact modalities of eNAMPT interaction with target cells and its purported cell surface receptors. We hope that future research will help shed light on the currently unknown aspects of this fascinating protein.
Author contributions
ES: Conceptualization, Investigation, Writing – original draft, Writing – review & editing, Visualization. AN: Writing – review & editing, Visualization. KM: Conceptualization, Project administration, Supervision, Visualization, Writing - review & editing.
Funding
The author(s) declare financial support was received for the research, authorship, and/or publication of this article. This work received funding from the European Union’s Horizon 2020 research and innovation program under the Marie Skłodowska-Curie grant agreement no. 813284.
Acknowledgments
AN acknowledges the support of the Associazione Italiana per la Ricerca sul Cancro (IG#22098). The figures were created using BioRender software (https://www.biorender.com/).
Conflict of interest
ES and KM are employees of Light Chain Bioscience - Novimmune SA.
The remaining authors declare that the research was conducted in the absence of any commercial or financial relationships that could be constructed as a potential conflict of interest.
Publisher’s note
All claims expressed in this article are solely those of the authors and do not necessarily represent those of their affiliated organizations, or those of the publisher, the editors and the reviewers. Any product that may be evaluated in this article, or claim that may be made by its manufacturer, is not guaranteed or endorsed by the publisher.
References
1. Garten A, Schuster S, Penke M, Gorski T, de Giorgis T, Kiess W. Physiological and pathophysiological roles of NAMPT and NAD metabolism. Nat Rev Endocrinol (2015) 11(9):535–46. doi: 10.1038/nrendo.2015.117
2. Grolla AA, Travelli C, Genazzani AA, Sethi JK. Extracellular nicotinamide phosphoribosyltransferase, a new cancer metabokine. Br J Pharmacol (2016) 173(14):2182–94. doi: 10.1111/bph.13505
3. Carbone F, Liberale L, Bonaventura A, Vecchiè A, Casula M, Cea M, et al. Regulation and function of extracellular nicotinamide phosphoribosyltransferase/visfatin. Compr Physiol (2017) 7(2):603–21. doi: 10.1002/cphy.c160029
4. Chiarugi A, Dölle C, Felici R, Ziegler M. The NAD metabolome — a key determinant of cancer cell biology. Nat Rev Cancer. (2012) 12(11):741–52. doi: 10.1038/nrc3340
5. Ghanem MS, Monacelli F, Nencioni A. Advances in NAD-lowering agents for cancer treatment. Nutrients (2021) 13(5):1665. doi: 10.3390/nu13051665
6. Chen H, Xia T, Zhou L, Chen X, Gan L, Yao W, et al. Gene organization, alternate splicing and expression pattern of porcine visfatin gene. Domest Anim Endocrinol (2007) 32(3):235–45. doi: 10.1016/j.domaniend.2006.03.004
7. Revollo JR, Grimm AA, Imai S. The regulation of nicotinamide adenine dinucleotide biosynthesis by Nampt/PBEF/visfatin in mammals. Curr Opin Gastroenterol (2007) 23(2):164–70. doi: 10.1097/MOG.0b013e32801b3c8f
8. Li J, Meng F, Song C, Wang Y, Leung FC. Characterization of chicken visfatin gene: cDNA cloning, tissue distribution, and promoter analysis. Poult Sci (2012) 91(11):2885–94. doi: 10.3382/ps.2012-02315
9. Rongvaux A, Shea RJ, Mulks MH, Gigot D, Urbain J, Leo O, et al. Pre-B-cell colony-enhancing factor, whose expression is up-regulated in activated lymphocytes, is a nicotinamide phosphoribosyltransferase, a cytosolic enzyme involved in NAD biosynthesis. Eur J Immunol (2002) 32(11):3225–34. doi: 10.1002/1521-4141(200211)32:11<3225::AID-IMMU3225>3.0.CO;2-L
10. Martin PR, Shea RJ, Mulks MH. Identification of a plasmid-encoded gene from Haemophilus ducreyi which confers NAD independence. J Bacteriol (2001) 183(4):1168–74. doi: 10.1128/JB.183.4.1168-1174.2001
11. Kitani T, Okuno S, Fujisawa H. Growth phase-dependent changes in the subcellular localization of pre-B-cell colony-enhancing factor 1. FEBS Lett (2003) 544(1–3):74–8. doi: 10.1016/S0014-5793(03)00476-9
12. Svoboda P, Krizova E, Sestakova S, Vapenkova K, Knejzlik Z, Rimpelova S, et al. Nuclear transport of nicotinamide phosphoribosyltransferase is cell cycle–dependent in mammalian cells, and its inhibition slows cell growth. J Biol Chem (2019) 294(22):8676–89. doi: 10.1074/jbc.RA118.003505
13. Wang X, Zhang Z, Zhang N, Li H, Zhang L, Baines CP, et al. Subcellular NAMPT-mediated NAD + salvage pathways and their roles in bioenergetics and neuronal protection after ischemic injury. J Neurochem (2019) 151(6):732–48. doi: 10.1111/jnc.14878
14. Pittelli M, Formentini L, Faraco G, Lapucci A, Rapizzi E, Cialdai F, et al. Inhibition of nicotinamide phosphoribosyltransferase. J Biol Chem (2010) 285(44):34106–14. doi: 10.1074/jbc.M110.136739
15. Yang H, Yang T, Baur JA, Perez E, Matsui T, Carmona JJ, et al. Nutrient-sensitive mitochondrial NAD+ Levels dictate cell survival. Cell (2007) 130(6):1095–107. doi: 10.1016/j.cell.2007.07.035
16. Yoshida M, Satoh A, Lin JB, Mills KF, Sasaki Y, Rensing N, et al. Extracellular vesicle-contained eNAMPT delays aging and extends lifespan in mice. Cell Metab (2019) 30(2):329–42. doi: 10.1016/j.cmet.2019.05.015
17. Samal B, Sun Y, Stearns G, Xie C, Suggs S, McNiece I. Cloning and characterization of the cDNA encoding a novel human pre-B-cell colony-enhancing factor. Mol Cell Biol (1994) 14(2):1431–7. doi: 10.1128/mcb.14.2.1431-1437.1994
18. Revollo JR, Körner A, Mills KF, Satoh A, Wang T, Garten A, et al. Nampt/PBEF/visfatin regulates insulin secretion in β Cells as a systemic NAD biosynthetic enzyme. Cell Metab (2007) 6(5):363–75. doi: 10.1016/j.cmet.2007.09.003
19. Chang YH, Chang DM, Lin KC, Shin SJ, Lee YJ. Visfatin in overweight/obesity, type 2 diabetes mellitus, insulin resistance, metabolic syndrome and cardiovascular diseases: a meta-analysis and systemic review. Diabetes Metab Res Rev (2011) 27(6):515–27. doi: 10.1002/dmrr.1201
20. Yu PL, Wang C, Li W, Zhang FX. Visfatin level and the risk of hypertension and cerebrovascular accident: A systematic review and meta-analysis. Horm Metab Res Horm Stoffwechselforschung Horm Metab (2019) 51(4):220–9. doi: 10.1055/a-0867-1333
21. Mohammadi M, Mianabadi F, Mehrad-Majd H. Circulating visfatin levels and cancers risk: A systematic review and meta-analysis. J Cell Physiol (2019) 234(4):5011–22. doi: 10.1002/jcp.27302
22. Lin YC, Wu HC, Liao CC, Chou YC, Pan SF, Chiu CM. Secretion of one adipokine nampt/visfatin suppresses the inflammatory stress-induced NF- κ B activity and affects nampt-dependent cell viability in huh-7 cells. Mediators Inflamm (2015) 2015:1–9. doi: 10.1155/2015/392471
23. Audrito V, Managò A, Zamporlini F, Rulli E, Gaudino F, Madonna G, et al. Extracellular nicotinamide phosphoribosyltransferase (eNAMPT) is a novel marker for patients with BRAF-mutated metastatic melanoma. Oncotarget (2018) 9(27):18997–9005. doi: 10.18632/oncotarget.24871
24. Benedict C, Shostak A, Lange T, Brooks SJ, Schiöth HB, Schultes B, et al. Diurnal rhythm of circulating nicotinamide phosphoribosyltransferase (Nampt/visfatin/PBEF): impact of sleep loss and relation to glucose metabolism. J Clin Endocrinol Metab (2012) 97(2):E218–22. doi: 10.1210/jc.2011-2241
25. Brentano F, Schorr O, Ospelt C, Stanczyk J, Gay RE, Gay S, et al. Pre–B cell colony-enhancing factor/visfatin, a new marker of inflammation in rheumatoid arthritis with proinflammatory and matrix-degrading activities. Arthritis Rheumatol (2007) 56(9):2829–39. doi: 10.1002/art.22833
26. Tsouma I, Kouskouni E, Demeridou S, Boutsikou M, Hassiakos D, Chasiakou A, et al. Correlation of visfatin levels and lipoprotein lipid profiles in women with polycystic ovary syndrome undergoing ovarian stimulation. Gynecol Endocrinol (2014) 30(7):516–9. doi: 10.3109/09513590.2014.896896
27. Ye SQ, Simon BA, Maloney JP, Zambelli-Weiner A, Gao L, Grant A, et al. Pre–B-cell colony-enhancing factor as a potential novel biomarker in acute lung injury. Am J Respir Crit Care Med (2005) 171(4):361–70. doi: 10.1164/rccm.200404-563OC
28. Mamali I, Roupas ND, Armeni AK, Theodoropoulou A, Markou KB, Georgopoulos NA. Measurement of salivary resistin, visfatin and adiponectin levels. Peptides (2012) 33(1):120–4. doi: 10.1016/j.peptides.2011.11.007
29. Hallschmid M, Randeva H, Tan BK, Kern W, Lehnert H. Relationship between cerebrospinal fluid visfatin (PBEF/nampt) levels and adiposity in humans. Diabetes (2009) 58(3):637–40. doi: 10.2337/db08-1176
30. Colombo G, Caviglia GP, Ravera A, Tribocco E, Frara S, Rosso C, et al. NAMPT and NAPRT serum levels predict response to anti-TNF therapy in inflammatory bowel disease. Front Med (2023) 10:1116862. doi: 10.3389/fmed.2023.1116862
31. Grolla AA, Torretta S, Gnemmi I, Amoruso A, Orsomando G, Gatti M, et al. Nicotinamide phosphoribosyltransferase (NAMPT/PBEF/visfatin) is a tumoural cytokine released from melanoma. Pigment Cell Melanoma Res (2015) 28(6):718–29. doi: 10.1111/pcmr.12420
32. Colombo G, Travelli C, Porta C, Genazzani AA. Extracellular nicotinamide phosphoribosyltransferase boosts IFNγ-induced macrophage polarization independently of TLR4. iScience (2022) 25(4):104147. doi: 10.1016/j.isci.2022.104147
33. Lu Y, Chen C, Huang J, Tian Y, Xie X, Yang P, et al. Nicotinamide phosphoribosyltransferase secreted from microglia via exosome during ischemic injury. J Neurochem (2019) 150(6):723–37. doi: 10.1111/jnc.14811
34. Garten A, Petzold S, Barnikol-Oettler A, Körner A, Thasler WE, Kratzsch J, et al. Nicotinamide phosphoribosyltransferase (NAMPT/PBEF/visfatin) is constitutively released from human hepatocytes. Biochem Biophys Res Commun (2010) 391(1):376–81. doi: 10.1016/j.bbrc.2009.11.066
35. Tanaka M, Nozaki M, Fukuhara A, Segawa K, Aoki N, Matsuda M, et al. Visfatin is released from 3T3-L1 adipocytes via a non-classical pathway. Biochem Biophys Res Commun (2007) 359(2):194–201. doi: 10.1016/j.bbrc.2007.05.096
36. Yoon MJ, Yoshida M, Johnson S, Takikawa A, Usui I, Tobe K, et al. SIRT1-mediated eNAMPT secretion from adipose tissue regulates hypothalamic NAD+ and function in mice. Cell Metab (2015) 21(5):706–17. doi: 10.1016/j.cmet.2015.04.002
37. Sociali G, Grozio A, Caffa I, Schuster S, Becherini P, Damonte P, et al. SIRT6 deacetylase activity regulates NAMPT activity and NAD(P)(H) pools in cancer cells. FASEB J (2019) 33(3):3704–17. doi: 10.1096/fj.201800321R
38. Kim MK, Lee JH, Kim H, Park SJ, Kim SH, Kang GB, et al. Crystal structure of visfatin/pre-B cell colony-enhancing factor 1/nicotinamide phosphoribosyltransferase, free and in complex with the anti-cancer agent FK-866. J Mol Biol (2006) 362(1):66–77. doi: 10.1016/j.jmb.2006.06.082
39. Kuehnemann C, Hu KQ, Butera K, Patel SK, Bons J, Schilling B, et al. Extracellular nicotinamide phosphoribosyltransferase is a component of the senescence-associated secretory phenotype. Front Endocrinol (2022) 13:935106. doi: 10.3389/fendo.2022.935106
40. Laiguillon MC, Houard X, Bougault C, Gosset M, Nourissat G, Sautet A, et al. Expression and function of visfatin (Nampt), an adipokine-enzyme involved in inflammatory pathways of osteoarthritis. Arthritis Res Ther (2014) 16(1):R38. doi: 10.1186/ar4467
41. Behrouzfar K, Alaee M, Nourbakhsh M, Gholinejad Z, Golestani A. Extracellular NAMPT/visfatin causes p53 deacetylation via NAD production and SIRT1 activation in breast cancer cells. Cell Biochem Funct (2017) 35(6):327–33. doi: 10.1002/cbf.3279
42. Wang P, Xu TY, Guan YF, Su DF, Fan GR, Miao CY. Perivascular adipose tissue-derived visfatin is a vascular smooth muscle cell growth factor: role of nicotinamide mononucleotide. Cardiovasc Res (2009) 81(2):370–80. doi: 10.1093/cvr/cvn288
43. Formentini L, Moroni F, Chiarugi A. Detection and pharmacological modulation of nicotinamide mononucleotide (NMN) in vitro and in vivo. Biochem Pharmacol (2009) 77(10):1612–20. doi: 10.1016/j.bcp.2009.02.017
44. Grozio A, Mills KF, Yoshino J, Bruzzone S, Sociali G, Tokizane K, et al. Slc12a8 is a nicotinamide mononucleotide transporter. Nat Metab (2019) 1(1):47–57. doi: 10.1038/s42255-018-0009-4
45. Hara N, Yamada K, Shibata T, Osago H, Tsuchiya M. Nicotinamide phosphoribosyltransferase/visfatin does not catalyze nicotinamide mononucleotide formation in blood plasma. PloS One (2011) 6(8):e22781. doi: 10.1371/journal.pone.0022781
46. Recinella L, Orlando G, Ferrante C, Chiavaroli A, Brunetti L, Leone S. Adipokines: new potential therapeutic target for obesity and metabolic, rheumatic, and cardiovascular diseases. Front Physiol (2020) 11:578966. doi: 10.3389/fphys.2020.578966
47. Fukuhara A, Matsuda M, Nishizawa M, Segawa K, Tanaka M, Kishimoto K, et al. Visfatin: a protein secreted by visceral fat that mimics the effects of insulin. Science (2005) 307(5708):426–30. doi: 10.1126/science.1097243
48. Tan BK, Chen J, Digby JE, Keay SD, Kennedy CR, Randeva HS. Increased visfatin messenger ribonucleic acid and protein levels in adipose tissue and adipocytes in women with polycystic ovary syndrome: parallel increase in plasma visfatin. J Clin Endocrinol Metab (2006) 91(12):5022–8. doi: 10.1210/jc.2006-0936
49. Cheng KH, Chu CS, Lee KT, Lin TH, Hsieh CC, Chiu CC, et al. Adipocytokines and proinflammatory mediators from abdominal and epicardial adipose tissue in patients with coronary artery disease. Int J Obes (2008) 32(2):268–74. doi: 10.1038/sj.ijo.0803726
50. Fukuhara A, Matsuda M, Nishizawa M, Segawa K, Tanaka M, Kishimoto K, et al. Retraction. Science (2007) 318(5850):565. doi: 10.1126/science.318.5850.565b
51. Xie H, Tang SY, Luo XH, Huang J, Cui RR, Yuan LQ, et al. Insulin-like effects of visfatin on human osteoblasts. Calcif Tissue Int (2007) 80(3):201–10. doi: 10.1007/s00223-006-0155-7
52. Song HK, Lee MH, Kim BK, Park YG, Ko GJ, Kang YS, et al. Visfatin: a new player in mesangial cell physiology and diabetic nephropathy. Am J Physiol-Ren Physiol (2008) 295(5):F1485–94. doi: 10.1152/ajprenal.90231.2008
53. Brown JEP, Onyango DJ, Ramanjaneya M, Conner AC, Patel ST, Dunmore SJ, et al. Visfatin regulates insulin secretion, insulin receptor signalling and mRNA expression of diabetes-related genes in mouse pancreatic b-cells. J Mol Endocrinol (2010) 44(3):171–8. doi: 10.1677/JME-09-0071
54. Harasim E, Chabowski A, Górski J. Lack of downstream insulin-mimetic effects of visfatin/eNAMPT on glucose and fatty acid metabolism in skeletal muscles. Acta Physiol Oxf Engl (2011) 202(1):21–8. doi: 10.1111/j.1748-1716.2011.02254.x
55. Cheng Q, Dong W, Qian L, Wu J, Peng Y. Visfatin inhibits apoptosis of pancreatic β-cell line, MIN6, via the mitogen-activated protein kinase/phosphoinositide 3-kinase pathway. J Mol Endocrinol (2011) 47(1):13–21. doi: 10.1530/JME-10-0106
56. Ramsey KM, Mills KF, Satoh A, Imai S. Age-associated loss of Sirt1-mediated enhancement of glucose-stimulated insulin secretion in beta cell-specific Sirt1-overexpressing (BESTO) mice. Aging Cell (2008) 7(1):78–88. doi: 10.1111/j.1474-9726.2007.00355.x
57. Caton PW, Kieswich J, Yaqoob MM, Holness MJ, Sugden MC. Nicotinamide mononucleotide protects against pro-inflammatory cytokine-mediated impairment of mouse islet function. Diabetologia (2011) 54(12):3083–92. doi: 10.1007/s00125-011-2288-0
58. Yoshino J, Mills KF, Yoon MJ, Imai S. Nicotinamide mononucleotide, a key NAD+ Intermediate, treats the pathophysiology of diet- and age-induced diabetes in mice. Cell Metab (2011) 14(4):528–36. doi: 10.1016/j.cmet.2011.08.014
59. Managò A, Audrito V, Mazzola F, Sorci L, Gaudino F, Gizzi K, et al. Extracellular nicotinate phosphoribosyltransferase binds Toll like receptor 4 and mediates inflammation. Nat Commun (2019) 10(1):1–14. doi: 10.1038/s41467-019-12055-2
60. Yun MR, Seo JM, Park HY. Visfatin contributes to the differentiation of monocytes into macrophages through the differential regulation of inflammatory cytokines in THP-1 cells. Cell Signal (2014) 26(4):705–15. doi: 10.1016/j.cellsig.2013.12.010
61. Fan Y, Meng S, Wang Y, Cao J, Wang C. Visfatin/PBEF/Nampt induces EMMPRIN and MMP-9 production in macrophages via the NAMPT-MAPK (p38, ERK1/2)-NF-κB signaling pathway. Int J Mol Med (2011) 27(4):607–15. doi: 10.3892/ijmm.2011.621
62. Heo YJ, Choi SE, Lee N, Jeon JY, Han SJ, Kim DJ, et al. CCL20 induced by visfatin in macrophages via the NF-κB and MKK3/6-p38 signaling pathways contributes to hepatic stellate cell activation. Mol Biol Rep (2020) 47(6):4285–93. doi: 10.1007/s11033-020-05510-7
63. Audrito V, Serra S, Brusa D, Mazzola F, Arruga F, Vaisitti T, et al. Extracellular nicotinamide phosphoribosyltransferase (NAMPT) promotes M2 macrophage polarization in chronic lymphocytic leukemia. Blood (2015) 125(1):111–23. doi: 10.1182/blood-2014-07-589069
64. Li Y, Zhang Y, Dorweiler B, Cui D, Wang T, Woo CW, et al. Extracellular nampt promotes macrophage survival via a nonenzymatic interleukin-6/STAT3 signaling mechanism. J Biol Chem (2008) 283(50):34833–43. doi: 10.1074/jbc.M805866200
65. Gasparrini M, Mazzola F, Cuccioloni M, Sorci L, Audrito V, Zamporlini F, et al. Molecular insights into the interaction between human nicotinamide phosphoribosyltransferase and Toll-like receptor 4. J Biol Chem (2022) 298(3):101669. doi: 10.1016/j.jbc.2022.101669
66. Moschen AR, Kaser A, Enrich B, Mosheimer B, Theurl M, Niederegger H, et al. Visfatin, an adipocytokine with proinflammatory and immunomodulating properties. J Immunol Baltim Md (1950) 178(3):1748–58. doi: 10.4049/jimmunol.178.3.1748
67. Dahl TB, Yndestad A, Skjelland M, Øie E, Dahl A, Michelsen A, et al. Increased expression of visfatin in macrophages of human unstable carotid and coronary atherosclerosis: possible role in inflammation and plaque destabilization. Circulation (2007) 115(8):972–80. doi: 10.1161/CIRCULATIONAHA.106.665893
68. Skokowa J, Lan D, Thakur BK, Wang F, Gupta K, Cario G, et al. NAMPT is essential for the G-CSF–induced myeloid differentiation via a NAD+–sirtuin-1–dependent pathway. Nat Med (2009) 15(2):151–8. doi: 10.1038/nm.1913
69. Travelli C, Colombo G, Mola S, Genazzani AA, Porta C. NAMPT: A pleiotropic modulator of monocytes and macrophages. Pharmacol Res (2018) 135:25–36. doi: 10.1016/j.phrs.2018.06.022
70. Bermudez B, Dahl TB, Medina I, Groeneweg M, Holm S, Montserrat-de la Paz S, et al. Leukocyte overexpression of intracellular NAMPT attenuates atherosclerosis by regulating PPARγ-dependent monocyte differentiation and function. Arterioscler Thromb Vasc Biol (2017) 37(6):1157–67. doi: 10.1161/ATVBAHA.116.308187
71. Wang YY, Chen HD, Lo S, Chen YK, Huang YC, Hu SCS, et al. Visfatin enhances breast cancer progression through CXCL1 induction in tumor-associated macrophages. Cancers (2020) 12(12):3526. doi: 10.3390/cancers12123526
72. Kim SR, Bae YH, Bae SK, Choi KS, Yoon KH, Koo TH, et al. Visfatin enhances ICAM-1 and VCAM-1 expression through ROS-dependent NF-κB activation in endothelial cells. Biochim Biophys Acta BBA - Mol Cell Res (2008) 1783(5):886–95. doi: 10.1016/j.bbamcr.2008.01.004
73. Lin YT, Chen LK, Jian DY, Hsu TC, Huang WC, Kuan TT, et al. Visfatin Promotes Monocyte Adhesion by Upregulating ICAM-1 and VCAM-1 Expression in Endothelial Cells via Activation of p38-PI3K-Akt Signaling and Subsequent ROS Production and IKK/NF-κB Activation. Cell Physiol Biochem Int J Exp Cell Physiol Biochem Pharmacol (2019) 52(6):1398–411. doi: 10.33594/000000098
74. Romacho T, Valencia I, Ramos-González M, Vallejo S, López-Esteban M, Lorenzo O, et al. Visfatin/eNampt induces endothelial dysfunction in vivo: a role for Toll-Like Receptor 4 and NLRP3 inflammasome. Sci Rep (2020) 10(1):5386. doi: 10.1038/s41598-020-62190-w
75. Soncini D, Caffa I, Zoppoli G, Cea M, Cagnetta A, Passalacqua M, et al. Nicotinamide phosphoribosyltransferase promotes epithelial-to-mesenchymal transition as a soluble factor independent of its enzymatic activity. J Biol Chem (2014) 289(49):34189–204. doi: 10.1074/jbc.M114.594721
76. Kim SR, Bae SK, Choi KS, Park SY, Jun HO, Lee JY, et al. Visfatin promotes angiogenesis by activation of extracellular signal-regulated kinase 1/2. Biochem Biophys Res Commun (2007) 357(1):150–6. doi: 10.1016/j.bbrc.2007.03.105
77. Adya R, Tan BK, Punn A, Chen J, Randeva HS. Visfatin induces human endothelial VEGF and MMP-2/9 production via MAPK and PI3K/Akt signalling pathways: novel insights into visfatin-induced angiogenesis. Cardiovasc Res (2008) 78(2):356–65. doi: 10.1093/cvr/cvm111
78. Xiao J, Xiao ZJ, Liu ZG, Gong HY, Yuan Q, Wang S, et al. Involvement of dimethylarginine dimethylaminohydrolase-2 in visfatin-enhanced angiogenic function of endothelial cells. Diabetes Metab Res Rev (2009) 25(3):242–9. doi: 10.1002/dmrr.939
79. Park JW, Kim WH, Shin SH, Kim JY, Yun MR, Park KJ, et al. Visfatin exerts angiogenic effects on human umbilical vein endothelial cells through the mTOR signaling pathway. Biochim Biophys Acta BBA - Mol Cell Res (2011) 1813(5):763–71. doi: 10.1016/j.bbamcr.2011.02.009
80. Astern JM, Collier AC, Kendal-Wright CE. Pre-B cell colony enhancing factor (PBEF/NAMPT/Visfatin) and vascular endothelial growth factor (VEGF) cooperate to increase the permeability of the human placental amnion. Placenta (2013) 34(1):42–9. doi: 10.1016/j.placenta.2012.10.008
81. Dakroub A, Nasser SA, Kobeissy F, Yassine HM, Orekhov A, Sharifi-Rad J, et al. Visfatin: An emerging adipocytokine bridging the gap in the evolution of cardiovascular diseases. J Cell Physiol (2021) 236(9):6282–96. doi: 10.1002/jcp.30345
82. Kim JY, Bae YH, Bae MK, Kim SR, Park HJ, Wee HJ, et al. Visfatin through STAT3 activation enhances IL-6 expression that promotes endothelial angiogenesis. Biochim Biophys Acta BBA - Mol Cell Res (2009) 1793(11):1759–67. doi: 10.1016/j.bbamcr.2009.09.006
83. Kim SR, Jung YH, Park HJ, Kim MK, Jeong JW, Jang HO, et al. Upregulation of thromboxane synthase mediates visfatin-induced interleukin-8 expression and angiogenic activity in endothelial cells. Biochem Biophys Res Commun (2012) 418(4):662–8. doi: 10.1016/j.bbrc.2012.01.072
84. Bae YH, Park HJ, Kim SR, Kim JY, Kang Y, Kim JA, et al. Notch1 mediates visfatin-induced FGF-2 up-regulation and endothelial angiogenesis. Cardiovasc Res (2011) 89(2):436–45. doi: 10.1093/cvr/cvq276
85. Bae YH, Bae MK, Kim SR, Lee JH, Wee HJ, Bae SK. Upregulation of fibroblast growth factor-2 by visfatin that promotes endothelial angiogenesis. Biochem Biophys Res Commun (2009) 379(2):206–11. doi: 10.1016/j.bbrc.2008.12.042
86. Adya R, Tan BK, Chen J, Randeva HS. Pre-B cell colony enhancing factor (PBEF)/visfatin induces secretion of MCP-1 in human endothelial cells: Role in visfatin-induced angiogenesis. Atherosclerosis (2009) 205(1):113–9. doi: 10.1016/j.atherosclerosis.2008.11.024
87. Ezzati-Mobaser S, Malekpour-Dehkordi Z, Nourbakhsh M, Tavakoli-Yaraki M, Ahmadpour F, Golpour P, et al. The up-regulation of markers of adipose tissue fibrosis by visfatin in pre-adipocytes as well as obese children and adolescents. Cytokine (2020) 134:155193. doi: 10.1016/j.cyto.2020.155193
88. Li B, Zhao Y, Liu H, Meng B, Wang J, Qi T, et al. Visfatin destabilizes atherosclerotic plaques in apolipoprotein E–deficient mice. Xiao Q editor. PloS One (2016) 11(2):e0148273. doi: 10.1371/journal.pone.0148273
89. Adya R, Tan BK, Chen J, Randeva HS. Nuclear factor-κB induction by visfatin in human vascular endothelial cells. Diabetes Care (2008) 31(4):758–60. doi: 10.2337/dc07-1544
90. Van den Bergh R, Morin S, Sass HJ, Grzesiek S, Vekemans M, Florence E, et al. Monocytes contribute to differential immune pressure on R5 versus X4 HIV through the adipocytokine visfatin/NAMPT. PloS One (2012) 7(4):e35074. doi: 10.1371/journal.pone.0035074
91. Torretta S, Colombo G, Travelli C, Boumya S, Lim D, Genazzani AA, et al. The cytokine nicotinamide phosphoribosyltransferase (eNAMPT; PBEF; visfatin) acts as a natural antagonist of C-C chemokine receptor type 5 (CCR5). Cells (2020) 9(2):496. doi: 10.3390/cells9020496
92. Ratnayake D, Nguyen PD, Rossello FJ, Wimmer VC, Tan JL, Galvis LA, et al. Macrophages provide a transient muscle stem cell niche via NAMPT secretion. Nature (2021) 591(7849):281–7. doi: 10.1038/s41586-021-03199-7
93. Ratnayake D, Currie P, Martino M. inventor; Monash university, assignee. Methods and compositions. Australia patent AU 2021261045A1 (2019).
94. Camp SM, Ceco E, Evenoski CL, Danilov SM, Zhou T, Chiang ET, et al. Unique toll-like receptor 4 activation by NAMPT/PBEF induces NFκB signaling and inflammatory lung injury. Sci Rep (2015) 5:13135. doi: 10.1038/srep13135
95. Kim JS, Kim HK, Kim M, Jang S, Cho E, Mun SJ, et al. Colon-targeted eNAMPT-specific peptide systems for treatment of DSS-induced acute and chronic colitis in mouse. Antioxidants (2022) 11(12):2376. doi: 10.3390/antiox11122376
96. Garcia JGN. inventor; Arizona Board of Regents of University of Arizona, assignee. Compositions and methods for treating pulmonary arterial hypertension. United States patent US 10975167B2 (2018).
97. Philp AM, Butterworth S, Davis ET, Jones SW. eNAMPT is localised to areas of cartilage damage in patients with hip osteoarthritis and promotes cartilage catabolism and inflammation. Int J Mol Sci (2021) 22(13):6719. doi: 10.3390/ijms22136719
98. Xia M, Boini KM, Abais JM, Xu M, Zhang Y, Li PL. Endothelial NLRP3 inflammasome activation and enhanced neointima formation in mice by adipokine visfatin. Am J Pathol (2014) 184(5):1617–28. doi: 10.1016/j.ajpath.2014.01.032
99. Dakroub A A, Nasser S, Younis N, Bhagani H, Al-Dhaheri Y, Pintus G, et al. Visfatin: A possible role in cardiovasculo-metabolic disorders. Cells (2020) 9(11):2444. doi: 10.3390/cells9112444
100. Chen Y, Pitzer AL, Li X, Li PL, Wang L, Zhang Y. Instigation of endothelial Nlrp3 inflammasome by adipokine visfatin promotes inter-endothelial junction disruption: role of HMGB1. J Cell Mol Med (2015) 19(12):2715–27. doi: 10.1111/jcmm.12657
101. Koka S, Xia M, Zhang C, Zhang Y, Li PL, Boini KM. Podocyte NLRP3 inflammasome activation and formation by adipokine visfatin. Cell Physiol Biochem Int J Exp Cell Physiol Biochem Pharmacol (2019) 53(2):355–65. doi: 10.33594/000000143
102. Wani K, AlHarthi H, Alghamdi A, Sabico S, Al-Daghri NM. Role of NLRP3 inflammasome activation in obesity-mediated metabolic disorders. Int J Environ Res Public Health (2021) 18(2):511. doi: 10.3390/ijerph18020511
103. Alnowihi SM, Doghaither HAA, Osman NN. Serum visfatin concentration and its relationship with sex hormones in obese Saudi women. Int J Health Sci (2020) 14(3):5.
104. Yin C, Hu W, Wang M, Xiao Y. The role of the adipocytokines vaspin and visfatin in vascular endothelial function and insulin resistance in obese children. BMC Endocr Disord (2019) 19(1):127. doi: 10.1186/s12902-019-0452-6
105. Ahmadpour F. The association of plasma levels of mir-34A and mir-149 with obesity and insulin resistance in obese children and adolescents. Acta Endocrinol Buchar. (2018) 14(2):149–54. doi: 10.4183/aeb.2018.149
106. Nourbakhsh M, Nourbakhsh M, Gholinejad Z, Razzaghy-Azar M. Visfatin in obese children and adolescents and its association with insulin resistance and metabolic syndrome. Scand J Clin Lab Invest. (2015) 75(2):183–8. doi: 10.3109/00365513.2014.1003594
107. Mir MM, Mir R, Alghamdi MAA, Wani JI, Sabah ZU, Jeelani M, et al. Differential association of selected adipocytokines, adiponectin, leptin, resistin, visfatin and chemerin, with the pathogenesis and progression of type 2 diabetes mellitus (T2DM) in the asir region of Saudi Arabia: A case control study. J Pers Med (2022) 12(5):735. doi: 10.3390/jpm12050735
108. Mostafa TM, El-Gharbawy NM, Werida RH. Circulating IRAPe, irisin, and IL-34 in relation to insulin resistance in patients with type 2 diabetes. Clin Ther (2021) 43(7):e230–40. doi: 10.1016/j.clinthera.2021.05.003
109. Sayers SR, Beavil RL, Fine NHF, Huang GC, Choudhary P, Pacholarz KJ, et al. Structure-functional changes in eNAMPT at high concentrations mediate mouse and human beta cell dysfunction in type 2 diabetes. Diabetologia (2020) 63(2):313–23. doi: 10.1007/s00125-019-05029-y
110. Bawah AT, Seini MM, Abaka-Yawason A, Alidu H, Nanga S. Leptin, resistin and visfatin as useful predictors of gestational diabetes mellitus. Lipids Health Dis (2019) 18(1):221. doi: 10.1186/s12944-019-1169-2
111. Hetta HF, Ez-Eldeen ME, Mohamed GA, Gaber MA, ElBadre HM, Ahmed EA, et al. Visfatin serum levels in obese type 2 diabetic patients: relation to proinflammatory cytokines and insulin resistance. Egypt J Immunol (2018) 25(2):141–51.
112. Zheng LY, Xu X, Wan RH, Xia S, Lu J, Huang Q. Association between serum visfatin levels and atherosclerotic plaque in patients with type 2 diabetes. Diabetol Metab Syndr (2019) 11(1):60. doi: 10.1186/s13098-019-0455-5
113. Auguet T, Aragonès G, Guiu-Jurado E, Berlanga A, Curriu M, Martinez S, et al. Adipo/cytokines in atherosclerotic secretomes: increased visfatin levels in unstable carotid plaque. BMC Cardiovasc Disord (2016) 16(1):149. doi: 10.1186/s12872-016-0320-5
114. Kong Q, Xia M, Liang R, Li L, Cu X, Sun Z, et al. Increased serum visfatin as a risk factor for atherosclerosis in patients with ischaemic cerebrovascular disease. Singapore Med J (2014) 55(7):383–7. doi: 10.11622/smedj.2014091
115. Kadoglou NPE, Sailer N, Moumtzouoglou A, Kapelouzou A, Gerasimidis T, Kostakis A, et al. Adipokines: a novel link between adiposity and carotid plaque vulnerability: APELIN AND VISFATIN IN CAROTID PLAQUE VULNERABILITY. Eur J Clin Invest. (2012) 42(12):1278–86. doi: 10.1111/j.1365-2362.2012.02728.x
116. Zhong M, Tan HW, Gong HP, Wang SF, Zhang Y, Zhang W. Increased serum visfatin in patients with metabolic syndrome and carotid atherosclerosis. Clin Endocrinol (Oxf). (2008) 69(6):878–84. doi: 10.1111/j.1365-2265.2008.03248.x
117. Friebe D, Neef M, Kratzsch J, Erbs S, Dittrich K, Garten A, et al. Leucocytes are a major source of circulating nicotinamide phosphoribosyltransferase (NAMPT)/pre-B cell colony (PBEF)/visfatin linking obesity and inflammation in humans. Diabetologia (2011) 54(5):1200–11. doi: 10.1007/s00125-010-2042-z
118. Taşkesen D, Kirel B, Us T. Serum visfatin levels, adiposity and glucose metabolism in obese adolescents. J Clin Res Pediatr Endocrinol (2012) 4(2):76–81. doi: 10.4274/Jcrpe.547
119. Araki S, Dobashi K, Kubo K, Kawagoe R, Yamamoto Y, Kawada Y, et al. Plasma visfatin concentration as a surrogate marker for visceral fat accumulation in obese children. Obesity (2008) 16(2):384–8. doi: 10.1038/oby.2007.54
120. Li RZ, Ma XY, Hu XF, Kang SX, Chen SK, Cianflone K, et al. Elevated visfatin levels in obese children are related to proinflammatory factors. J Pediatr Endocrinol Metab (2012) 0(0):1–8. doi: 10.1515/jpem-2012-0237
121. Jin H, Jiang B, Tang J, Lu W, Wang W, Zhou L, et al. Serum visfatin concentrations in obese adolescents and its correlation with age and high-density lipoprotein cholesterol. Diabetes Res Clin Pract (2008) 79(3):412–8. doi: 10.1016/j.diabres.2007.09.019
122. Körner A, Garten A, Blüher M, Tauscher R, Kratzsch J, Kiess W. Molecular characteristics of serum visfatin and differential detection by immunoassays. J Clin Endocrinol Metab (2007) 92(12):4783–91. doi: 10.1210/jc.2007-1304
123. Makarewicz A, Jamka M, Geltz J, Śmidowicz A, Kokot M, Kaczmarek N, et al. Comparison of the effect of endurance, strength, and endurance-strength training on inflammatory markers and adipokines levels in overweight and obese adults: systematic review and meta-analysis of randomised trials. Healthcare (2022) 10(6):1098. doi: 10.3390/healthcare10061098
124. Saeidi A, Haghighi MM, Kolahdouzi S, Daraei A, Abderrahmane AB, Essop MF, et al. The effects of physical activity on adipokines in individuals with overweight/obesity across the lifespan: A narrative review. Obes Rev Off J Int Assoc Study Obes (2021) 22(1):e13090. doi: 10.1111/obr.13090
125. Kozłowska-Flis M, Rodziewicz-Flis E, Micielska K, Kortas J, Jaworska J, Borkowska A, et al. Short and long-term effects of high-intensity interval training applied alone or with whole-body cryostimulation on glucose homeostasis and myokine levels in overweight to obese subjects. Front Biosci Landmark Ed (2021) 26(11):1132–46. doi: 10.52586/5015
126. Curat CA, Wegner V, Sengenès C, Miranville A, Tonus C, Busse R, et al. Macrophages in human visceral adipose tissue: increased accumulation in obesity and a source of resistin and visfatin. Diabetologia (2006) 49(4):744–7. doi: 10.1007/s00125-006-0173-z
127. Weisberg SP, McCann D, Desai M, Rosenbaum M, Leibel RL, Ferrante AW. Obesity is associated with macrophage accumulation in adipose tissue. J Clin Invest. (2003) 112(12):1796–808. doi: 10.1172/JCI200319246
128. Jurdana M, Petelin A, Černelič Bizjak M, Bizjak M, Biolo G, Jenko-Pražnikar Z. Increased serum visfatin levels in obesity and its association with anthropometric/biochemical parameters, physical inactivity and nutrition. E-SPEN J (2013) 8(2):e59–67. doi: 10.1016/j.clnme.2013.02.001
129. Armandi A, Colombo G, Rosso C, Caviglia GP, Olivero A, Abate ML, et al. The predictive role of extracellular NAPRT for the detection of advanced fibrosis in biopsy-proven non-alcoholic fatty liver disease. Int J Mol Sci (2023) 24(2):1172. doi: 10.3390/ijms24021172
130. Kieswich J, Sayers SR, Silvestre MF, Harwood SM, Yaqoob MM, Caton PW. Monomeric eNAMPT in the development of experimental diabetes in mice: a potential target for type 2 diabetes treatment. Diabetologia (2016) 59(11):2477–86. doi: 10.1007/s00125-016-4076-3
131. Haider DG, Pleiner J, Francesconi M, Wiesinger GF, Müller M, Wolzt M. Exercise training lowers plasma visfatin concentrations in patients with type 1 diabetes. J Clin Endocrinol Metab (2006) 91(11):4702–4. doi: 10.1210/jc.2006-1013
132. López-Bermejo A, Chico-Julià B, Fernàndez-Balsells M, Recasens M, Esteve E, Casamitjana R, et al. Serum visfatin increases with progressive beta-cell deterioration. Diabetes (2006) 55(10):2871–5. doi: 10.2337/db06-0259
133. Esteghamati A, Alamdari A, Zandieh A, Elahi S, Khalilzadeh O, Nakhjavani M, et al. Serum visfatin is associated with type 2 diabetes mellitus independent of insulin resistance and obesity. Diabetes Res Clin Pract (2011) 91(2):154–8. doi: 10.1016/j.diabres.2010.11.003
134. Chen MP, Chung FM, Chang DM, Tsai JCR, Huang HF, Shin SJ, et al. Elevated plasma level of visfatin/pre-B cell colony-enhancing factor in patients with type 2 diabetes mellitus. J Clin Endocrinol Metab (2006) 91(1):295–9. doi: 10.1210/jc.2005-1475
135. Dogru T, Sonmez A, Tasci I, Bozoglu E, Yilmaz MI, Genc H, et al. Plasma visfatin levels in patients with newly diagnosed and untreated type 2 diabetes mellitus and impaired glucose tolerance. Diabetes Res Clin Pract (2007) 76(1):24–9. doi: 10.1016/j.diabres.2006.07.031
136. Krzyzanowska K, Krugluger W, Mittermayer F, Rahman R, Haider D, Shnawa N, et al. Increased visfatin concentrations in women with gestational diabetes mellitus. Clin Sci (2006) 110(5):605–9. doi: 10.1042/CS20050363
137. Shaker O, El-Shehaby A, Zakaria A, Mostafa N, Talaat S, Katsiki N, et al. Plasma visfatin and retinol binding protein-4 levels in patients with type 2 diabetes mellitus and their relationship to adiposity and fatty liver. Clin Biochem (2011) 44(17–18):1457–63. doi: 10.1016/j.clinbiochem.2011.08.1148
138. Retnakaran R, Youn BS, Liu Y, Hanley AJ, Lee NS, Park JW, et al. Correlation of circulating full-length visfatin (PBEF/NAMPT) with metabolic parameters in subjects with and without diabetes: a cross-sectional study. Clin Endocrinol (Oxf). (2008) 69(6):885–93.
139. Sandeep S, Velmurugan K, Deepa R, Mohan V. Serum visfatin in relation to visceral fat, obesity, and type 2 diabetes mellitus in Asian Indians. Metabolism (2007) 56(4):565–70. doi: 10.1016/j.metabol.2006.12.005
140. Zhou F, Pan Y, Huang Z, Jia Y, Zhao X, Chen Y, et al. Visfatin induces cholesterol accumulation in macrophages through up-regulation of scavenger receptor-A and CD36. Cell Stress Chaperones. (2013) 18(5):643–52. doi: 10.1007/s12192-013-0417-z
141. Chiu CA, Yu TH, Hung WC, Lu LF, Chung FM, Tsai IT, et al. Increased expression of visfatin in monocytes and macrophages in male acute myocardial infarction patients. Mediators Inflamm (2012) 2012:1–7. doi: 10.1155/2012/469852
142. Dahl T, Ranheim T, Holm S, Berge R, Aukrust P, Halvorsen B. Nicotinamide phosphoribosyltransferase and lipid accumulation in macrophages: NAMPT AND LIPID ACCUMULATION. Eur J Clin Invest. (2011) 41(10):1098–104. doi: 10.1111/j.1365-2362.2011.02515.x
143. Sun L, Chen S, Gao H, Ren L, Song G. Visfatin induces the apoptosis of endothelial progenitor cells via the induction of pro-inflammatory mediators through the NF-κB pathway. Int J Mol Med (2017) 40(3):637–46. doi: 10.3892/ijmm.2017.3048
144. Sun Y, Chen S, Song G, Ren L, Wei L, Liu N, et al. Effect of visfatin on the function of endothelial progenitor cells in high-fat-fed obese rats and investigation of its mechanism of action. Int J Mol Med (2012) 30(3):622–8. doi: 10.3892/ijmm.2012.1032
145. Vallejo S, Romacho T, Angulo J, Villalobos LA, Cercas E, Leivas A, et al. Visfatin impairs endothelium-dependent relaxation in rat and human mesenteric microvessels through nicotinamide phosphoribosyltransferase activity. PloS One (2011) 6(11):e27299. doi: 10.1371/journal.pone.0027299
146. Romacho T, Azcutia V, Vázquez-Bella M, Matesanz N, Cercas E, Nevado J, et al. Extracellular PBEF/NAMPT/visfatin activates pro-inflammatory signalling in human vascular smooth muscle cells through nicotinamide phosphoribosyltransferase activity. Diabetologia (2009) 52(11):2455–63. doi: 10.1007/s00125-009-1509-2
147. Kadoglou NPE, Sailer N, Moumtzouoglou A, Kapelouzou A, Tsanikidis H, Vitta I, et al. Visfatin (Nampt) and ghrelin as novel markers of carotid atherosclerosis in patients with type 2 diabetes. Exp Clin Endocrinol Diabetes. (2009) 118(02):75–80. doi: 10.1055/s-0029-1237360
148. Zheng M, Lu N, Ren M, Chen H. Visfatin associated with major adverse cardiovascular events in patients with acute myocardial infarction. BMC Cardiovasc Disord (2020) 20(1):271. doi: 10.1186/s12872-020-01549-3
149. Takebayashi K, Suetsugu M, Wakabayashi S, Aso Y, Inukai T. Association between plasma visfatin and vascular endothelial function in patients with type 2 diabetes mellitus. Metabolism (2007) 56(4):451–8. doi: 10.1016/j.metabol.2006.12.001
150. Cheleschi S, Tenti S, Barbarino M, Giannotti S, Bellisai F, Frati E, et al. Exploring the crosstalk between hydrostatic pressure and adipokines: an in vitro study on human osteoarthritic chondrocytes. Int J Mol Sci (2021) 22(5):2745. doi: 10.3390/ijms22052745
151. Cheleschi S, Gallo I, Barbarino M, Giannotti S, Mondanelli N, Giordano A, et al. MicroRNA mediate visfatin and resistin induction of oxidative stress in human osteoarthritic synovial fibroblasts via NF-κB pathway. Int J Mol Sci (2019) 20(20):E5200. doi: 10.3390/ijms20205200
152. Cheleschi S, Tenti S, Mondanelli N, Corallo C, Barbarino M, Giannotti S, et al. MicroRNA-34a and microRNA-181a mediate visfatin-induced apoptosis and oxidative stress via NF-κB pathway in human osteoarthritic chondrocytes. Cells (2019) 8(8):E874. doi: 10.3390/cells8080874
153. Zahan OM, Serban O, Gherman C, Fodor D. The evaluation of oxidative stress in osteoarthritis. Med Pharm Rep (2020) 93(1):12–22. doi: 10.15386/mpr-1422
154. McNulty AL, Miller MR, O’Connor SK, Guilak F. The effects of adipokines on cartilage and meniscus catabolism. Connect Tissue Res (2011) 52(6):523–33. doi: 10.3109/03008207.2011.597902
155. Nishimuta JF, Levenston ME. Meniscus is more susceptible than cartilage to catabolic and anti-anabolic effects of adipokines. Osteoarthritis Cartilage. (2015) 23(9):1551–62. doi: 10.1016/j.joca.2015.04.014
156. Gosset M, Berenbaum F, Salvat C, Sautet A, Pigenet A, Tahiri K, et al. Crucial role of visfatin/pre–B cell colony-enhancing factor in matrix degradation and prostaglandin E2 synthesis in chondrocytes: Possible influence on osteoarthritis. Arthritis Rheumatol (2008) 58(5):1399–409. doi: 10.1002/art.23431
157. Jacques C, Holzenberger M, Mladenovic Z, Salvat C, Pecchi E, Berenbaum F, et al. Proinflammatory actions of visfatin/nicotinamide phosphoribosyltransferase (Nampt) involve regulation of insulin signaling pathway and nampt enzymatic activity. J Biol Chem (2012) 287(18):15100–8. doi: 10.1074/jbc.M112.350215
158. Wu MH, Tsai CH, Huang YL, Fong YC, Tang CH. Visfatin Promotes IL-6 and TNF-α Production in Human Synovial Fibroblasts by Repressing miR-199a-5p through ERK, p38 and JNK Signaling Pathways. Int J Mol Sci (2018) 19(1):E190. doi: 10.3390/ijms19010190
159. Junker S, Frommer KW, Krumbholz G, Tsiklauri L, Gerstberger R, Rehart S, et al. Expression of adipokines in osteoarthritis osteophytes and their effect on osteoblasts. Matrix Biol (2017) 62:75–91. doi: 10.1016/j.matbio.2016.11.005
160. Yammani RR, Loeser RF. Extracellular nicotinamide phosphoribosyltransferase (NAMPT/visfatin) inhibits insulin-like growth factor-1 signaling and proteoglycan synthesis in human articular chondrocytes. Arthritis Res Ther (2012) 14(1):R23. doi: 10.1186/ar3705
161. Tsai CH, Liu SC, Chung WH, Wang SW, Wu MH, Tang CH. Visfatin increases VEGF-dependent angiogenesis of endothelial progenitor cells during osteoarthritis progression. Cells (2020) 9(5):1315. doi: 10.3390/cells9051315
162. MacDonald IJ, Liu SC, Su CM, Wang YH, Tsai CH, Tang CH. Implications of angiogenesis involvement in arthritis. Int J Mol Sci (2018) 19(7):2012. doi: 10.3390/ijms19072012
163. Pecchi E, Priam S, Gosset M, Pigenet A, Sudre L, Laiguillon MC, et al. Induction of nerve growth factor expression and release by mechanical and inflammatory stimuli in chondrocytes: possible involvement in osteoarthritis pain. Arthritis Res Ther (2014) 16(1):R16. doi: 10.1186/ar4443
164. Askari A, Arasteh P, Homayounfar R, Naghizadeh MM, Ehrampoush E, Mousavi SM, et al. The role of adipose tissue secretion in the creation and pain level in osteoarthritis. Endocr Regul (2020) 54(1):6–13. doi: 10.2478/enr-2020-0002
165. Fioravanti A, Cheleschi S, De Palma A, Addimanda O, Mancarella L, Pignotti E, et al. Can adipokines serum levels be used as biomarkers of hand osteoarthritis? Biomarkers (2018) 23(3):265–70. doi: 10.1080/1354750X.2017.1401665
166. Duan Y, Hao D, Li M, Wu Z, Li D, Yang X, et al. Increased synovial fluid visfatin is positively linked to cartilage degradation biomarkers in osteoarthritis. Rheumatol Int (2012) 32(4):985–90. doi: 10.1007/s00296-010-1731-8
167. Chen WP, Bao JP, Feng J, Hu PF, Shi ZL, Wu LD. Increased serum concentrations of visfatin and its production by different joint tissues in patients with osteoarthritis. Clin Chem Lab Med (2010) 48(8):1141–5. doi: 10.1515/CCLM.2010.230
168. Cheleschi S, Tenti S, Bedogni G, Fioravanti A. Circulating Mir-140 and leptin improve the accuracy of the differential diagnosis between psoriatic arthritis and rheumatoid arthritis: a case-control study. Transl Res (2022) 239:18–34. doi: 10.1016/j.trsl.2021.08.001
169. Ali D, Al-Fadhel S, Al-Ghuraibawi N, Al-Hakeim H. Serum chemerin and visfatin levels and their ratio as possible diagnostic parameters of rheumatoid arthritis. Reumatologia/Rheumatology (2020) 58(2):67–75. doi: 10.5114/reum.2020.95359
170. Sglunda O, Mann H, Hulejová H, Kuklová M, Pecha O, Pleštilová L, et al. Decreased circulating visfatin is associated with improved disease activity in early rheumatoid arthritis: data from the PERAC cohort. PloS One (2014) 9(7):e103495. doi: 10.1371/journal.pone.0103495
171. El-Hini SH, Mohamed FI, Hassan AA, Ali F, Mahmoud A, Ibraheem HM. Visfatin and adiponectin as novel markers for evaluation of metabolic disturbance in recently diagnosed rheumatoid arthritis patients. Rheumatol Int (2013) 33(9):2283–9. doi: 10.1007/s00296-013-2714-3
172. Šenolt L, Kryštůfková O, Hulejová H, Kuklová M, Filková M, Cerezo LA, et al. The level of serum visfatin (PBEF) is associated with total number of B cells in patients with rheumatoid arthritis and decreases following B cell depletion therapy. Cytokine (2011) 55(1):116–21. doi: 10.1016/j.cyto.2011.04.004
173. Saadoun MM, Nosair NAE, Abdel-Azeez HAH, Sharaf SM, Ahmed MH. Serum visfatin as a diagnostic marker of active inflammatory bowel disease. J Gastrointestin Liver Dis (2021) 30(3):339–45. doi: 10.15403/jgld-3504
174. Neubauer K, Bednarz-Misa I, Walecka-Zacharska E, Wierzbicki J, Agrawal A, Gamian A, et al. Oversecretion and overexpression of nicotinamide phosphoribosyltransferase/pre-B colony-enhancing factor/visfatin in inflammatory bowel disease reflects the disease activity, severity of inflammatory response and hypoxia. Int J Mol Sci (2019) 20(1):166. doi: 10.3390/ijms20010166
175. Dogan S, Guven K, Celikbilek M, Deniz K, Saraymen B, Gursoy S. Serum visfatin levels in ulcerative colitis: visfatin and ulcerative colitis. J Clin Lab Anal (2016) 30(5):552–6. doi: 10.1002/jcla.21901
176. Waluga M. Serum adipokines in inflammatory bowel disease. World J Gastroenterol (2014) 20(22):6912. doi: 10.3748/wjg.v20.i22.6912
177. Bime C, Casanova NG, Camp SM, Oita RC, Ndukum J, Hernon VR, et al. Circulating eNAMPT as a biomarker in the critically ill: acute pancreatitis, sepsis, trauma, and acute respiratory distress syndrome. BMC Anesthesiol. (2022) 22(1):182. doi: 10.1186/s12871-022-01718-1
178. Lee YC, Lin CY, Chen YH, Chiu WC, Wang YY, Hsu C, et al. Essential role of visfatin in lipopolysaccharide and colon ascendens stent peritonitis-induced acute lung injury. Int J Mol Sci (2019) 20(7):1678. doi: 10.3390/ijms20071678
179. Bas S, Finckh A, Puskas GJ, Suva D, Hoffmeyer P, Gabay C, et al. Adipokines correlate with pain in lower limb osteoarthritis: different associations in hip and knee. Int Orthop (2014) 38(12):2577–83. doi: 10.1007/s00264-014-2416-9
180. Franco-Trepat E, Guillán-Fresco M, Alonso-Pérez A, Jorge-Mora A, Francisco V, Gualillo O, et al. Visfatin connection: present and future in osteoarthritis and osteoporosis. J Clin Med (2019) 8(8):1178. doi: 10.3390/jcm8081178
181. Nowell MA, Richards PJ, Fielding CA, Ognjanovic S, Topley N, Williams AS, et al. Regulation of pre–B cell colony-enhancing factor by STAT-3–dependent interleukin-6trans-signaling: Implications in the pathogenesis of rheumatoid arthritis. Arthritis Rheumatol (2006) 54(7):2084–95. doi: 10.1002/art.21942
182. Busso N, Karababa M, Nobile M, Rolaz A, Leo O, So A, et al. Pharmacological inhibition of nicotinamide phosphoribosyltransferase/visfatin enzymatic activity identifies a new inflammatory pathway linked to NAD. PloS One (2008) 3(5):10. doi: 10.1371/journal.pone.0002267
183. Huber LC, Distler O, Tarner I, Gay RE, Gay S, Pap T. Synovial fibroblasts: key players in rheumatoid arthritis. Rheumatology (2006) 45(6):669–75. doi: 10.1093/rheumatology/kel065
184. Meier FMP, Frommer KW, Peters MA, Brentano F, Lefèvre S, Schröder D, et al. Visfatin/pre-B-cell colony-enhancing factor (PBEF), a proinflammatory and cell motility-changing factor in rheumatoid arthritis. J Biol Chem (2012) 287(34):28378–85. doi: 10.1074/jbc.M111.312884
185. Evans L, Williams AS, Hayes AJ, Jones SA, Nowell M. Suppression of leukocyte infiltration and cartilage degradation by selective inhibition of pre-B cell colony-enhancing factor/visfatin/nicotinamide phosphoribosyltransferase: Apo866-mediated therapy in human fibroblasts and murine collagen-induced arthritis. Arthritis Rheumatol (2011) 63(7):1866–77. doi: 10.1002/art.30338
186. Hasseli R, Frommer KW, Schwarz M, Hülser ML, Schreiyäck C, Arnold M, et al. Adipokines and inflammation alter the interaction between rheumatoid arthritis synovial fibroblasts and endothelial cells. Front Immunol (2020) 11:925. doi: 10.3389/fimmu.2020.00925
187. Franco-Trepat E, Alonso-Pérez A, Guillán-Fresco M, Jorge-Mora A, Gualillo O, Gómez-Reino JJ, et al. Visfatin as a therapeutic target for rheumatoid arthritis. Expert Opin Ther Targets. (2019) 23(7):607–18. doi: 10.1080/14728222.2019.1617274
188. Colombo G, Clemente N, Zito A, Bracci C, Colombo FS, Sangaletti S, et al. Neutralization of extracellular NAMPT (nicotinamide phosphoribosyltransferase) ameliorates experimental murine colitis. J Mol Med (2020) 98(4):595–612. doi: 10.1007/s00109-020-01892-0
189. Kiełbowski K, Bakinowska E, Ostrowski P, Pala B, Gromowska E, Gurazda K, et al. The role of adipokines in the pathogenesis of psoriasis. Int J Mol Sci (2023) 24(7):6390. doi: 10.3390/ijms24076390
190. Tumurkhuu G, Casanova NG, Kempf CL, Ercan Laguna D, Camp SM, Dagvadorj J, et al. eNAMPT/TLR4 inflammatory cascade activation is a key contributor to SLE Lung vasculitis and alveolar hemorrhage. J Transl Autoimmun (2023) 6:100181. doi: 10.1016/j.jtauto.2022.100181
191. Rho YH, Solus J, Sokka T, Oeser A, Chung CP, Gebretsadik T, et al. Adipocytokines are associated with radiographic joint damage in rheumatoid arthritis. Arthritis Rheumatol (2009) 60(7):1906–14. doi: 10.1002/art.24626
192. Otero M, Lago R, Gomez R, Lago F, Dieguez C, Gómez-Reino JJ, et al. Changes in plasma levels of fat-derived hormones adiponectin, leptin, resistin and visfatin in patients with rheumatoid arthritis. Ann Rheum Dis (2006) 65(9):1198–201. doi: 10.1136/ard.2005.046540
193. Mirfeizi Z, Noubakht Z, Rezaie AE, Jokar MH, Sarabi S. Plasma levels of leptin and visfatin in rheumatoid arthritis patients; is there any relationship with joint damage? Iran J Basic Med Sci (2014) 17(9):5.
194. Lee YH, Bae SC. Circulating adiponectin and visfatin levels in rheumatoid arthritis and their correlation with disease activity: A meta-analysis. Int J Rheum Dis (2018) 21(3):664–72. doi: 10.1111/1756-185X.13038
195. Klaasen R, Herenius MMJ, Wijbrandts CA, de Jager W, van Tuyl LH, Nurmohamed MT, et al. Treatment-specific changes in circulating adipocytokines: a comparison between tumour necrosis factor blockade and glucocorticoid treatment for rheumatoid arthritis. Ann Rheum Dis (2012) 71(9):1510–6. doi: 10.1136/annrheumdis-2011-200646
196. Fioravanti A, Tenti S, Bacarelli MR, Damiani A, Gobbi FL, Bandinelli F, et al. Tocilizumab modulates serum levels of adiponectin and chemerin in patients with rheumatoid arthritis: potential cardiovascular protective role of IL-6 inhibition. Clin Exp Rheumatol (2019) 37(2):293–300.
197. Kim KS, Choi HM, Ji HI, Song R, Yang HI, Lee SK, et al. Serum adipokine levels in rheumatoid arthritis patients and their contributions to the resistance to treatment. Mol Med Rep (2014) 9(1):255–60. doi: 10.3892/mmr.2013.1764
198. Gonzalez-Gay MA, Vazquez-Rodriguez TR, Garcia-Unzueta MT, Berja A, Miranda-Filloy JA, de Matias JM, et al. Visfatin is not associated with inflammation or metabolic syndrome in patients with severe rheumatoid arthritis undergoing anti-TNF-alpha therapy. Clin Exp Rheumatol (2010) 28(1):56–62.
199. Gerner RR, Klepsch V, Macheiner S, Arnhard K, Adolph TE, Grander C, et al. NAD metabolism fuels human and mouse intestinal inflammation. Gut (2018) 67(10):1813–23. doi: 10.1136/gutjnl-2017-314241
200. Travelli C, Aprile S, Rahimian R, Grolla AA, Rogati F, Bertolotti M, et al. Identification of novel triazole-based nicotinamide phosphoribosyltransferase (NAMPT) inhibitors endowed with antiproliferative and antiinflammatory activity. J Med Chem (2017) 60(5):1768–92. doi: 10.1021/acs.jmedchem.6b01392
201. Wnorowski A, Wnorowska S, Kurzepa J, Parada-Turska J. Alterations in kynurenine and NAD+ Salvage pathways during the successful treatment of inflammatory bowel disease suggest HCAR3 and NNMT as potential drug targets. Int J Mol Sci (2021) 22(24):13497. doi: 10.3390/ijms222413497
202. Valentini L, Wirth EK, Schweizer U, Hengstermann S, Schaper L, Koernicke T, et al. Circulating adipokines and the protective effects of hyperinsulinemia in inflammatory bowel disease. Nutrition (2009) 25(2):172–81. doi: 10.1016/j.nut.2008.07.020
203. Trejo-Vazquez F, Garza-Veloz I, Villela-Ramirez GA, Ortiz-Castro Y, Mauricio-Saucedo P, Cardenas-Vargas E, et al. Positive association between leptin serum levels and disease activity on endoscopy in inflammatory bowel disease: A case-control study. Exp Ther Med (2018) 15(4):3336–44. doi: 10.3892/etm.2018.5835
204. Terzoudis S, Malliaraki N, Damilakis J, Dimitriadou DA, Zavos C, Koutroubakis IE. Chemerin, visfatin, and vaspin serum levels in relation to bone mineral density in patients with inflammatory bowel disease. Eur J Gastroenterol Hepatol (2016) 28(7):814–9. doi: 10.1097/MEG.0000000000000617
205. Bermudez T, Sammani S, Song JH, Hernon VR, Kempf CL, Garcia AN, et al. eNAMPT neutralization reduces preclinical ARDS severity via rectified NFkB and Akt/mTORC2 signaling. Sci Rep (2022) 12(1):696. doi: 10.1038/s41598-021-04444-9
206. Quijada H, Bermudez T, Kempf CL, Valera DG, Garcia AN, Camp SM, et al. Endothelial eNAMPT amplifies pre-clinical acute lung injury: efficacy of an eNAMPT-neutralising monoclonal antibody. Eur Respir J (2021) 57(5):2002536. doi: 10.1183/13993003.02536-2020
207. Sammani S, Bermudez T, Kempf CL, Song JH, Fleming JC, Reyes Hernon V, et al. eNAMPT neutralization preserves lung fluid balance and reduces acute renal injury in porcine sepsis/VILI-induced inflammatory lung injury. Front Physiol (2022) 13:916159. doi: 10.3389/fphys.2022.916159
208. Hong SB, Huang Y, Moreno-Vinasco L, Sammani S, Moitra J, Barnard JW, et al. Essential role of pre-B-cell colony enhancing factor in ventilator-induced lung injury. Am J Respir Crit Care Med (2008) 178(6):605–17. doi: 10.1164/rccm.200712-1822OC
209. Ye SQ, Zhang LQ, Adyshev D, Usatyuk PV, Garcia AN, Lavoie TL, et al. Pre-B-cell-colony-enhancing factor is critically involved in thrombin-induced lung endothelial cell barrier dysregulation. Microvasc Res (2005) 70(3):142–51. doi: 10.1016/j.mvr.2005.08.003
210. Karampela I, Christodoulatos GS, Kandri E, Antonakos G, Vogiatzakis E, Dimopoulos G, et al. Circulating eNampt and resistin as a proinflammatory duet predicting independently mortality in critically ill patients with sepsis: A prospective observational study. Cytokine (2019) 119:62–70. doi: 10.1016/j.cyto.2019.03.002
211. Lee K, Huh JW, Lim CM, Koh Y, Hong SB. Clinical role of serum pre-B cell colony-enhancing factor in ventilated patients with sepsis and acute respiratory distress syndrome. Scand J Infect Dis (2013) 45(10):760–5. doi: 10.3109/00365548.2013.797600
212. Bime C, Casanova N, Oita RC, Ndukum J, Lynn H, Camp SM, et al. Development of a biomarker mortality risk model in acute respiratory distress syndrome. Crit Care (2019) 23(1):410. doi: 10.1186/s13054-019-2697-x
213. Dalamaga M, Christodoulatos GS, Mantzoros CS. The role of extracellular and intracellular Nicotinamide phosphoribosyl-transferase in cancer: Diagnostic and therapeutic perspectives and challenges. Metabolism (2018) 82:72–87. doi: 10.1016/j.metabol.2018.01.001
214. Hung AC, Lo S, Hou MF, Lee YC, Tsai CH, Chen YY, et al. Extracellular visfatin-promoted Malignant behavior in breast cancer is mediated through c-abl and STAT3 activation. Clin Cancer Res (2016) 22(17):4478–90. doi: 10.1158/1078-0432.CCR-15-2704
215. Gholinejad Z, Kheiripour N, Nourbakhsh M, Ilbeigi D, Behroozfar K, Hesari Z, et al. Extracellular NAMPT/Visfatin induces proliferation through ERK1/2 and AKT and inhibits apoptosis in breast cancer cells. Peptides (2017) 92:9–15. doi: 10.1016/j.peptides.2017.04.007
216. Park HJ, Kim SR, Kim SS, Wee HJ, Bae MK, Ryu MH, et al. Visfatin promotes cell and tumor growth by upregulating Notch1 in breast cancer. Oncotarget (2014) 5(13):5087–99. doi: 10.18632/oncotarget.2086
217. Bułdak RJ, Bułdak Ł, Polaniak R, Kukla M, Birkner E, Kubina R, et al. Visfatin affects redox adaptative responses and proliferation in Me45 human Malignant melanoma cells: An in vitro study. Oncol Rep (2013) 29(2):771–8. doi: 10.3892/or.2012.2175
218. Miethe C, Zamora M, Torres L, Raign KG, Groll CJ, Price RS. Characterizing the differential physiological effects of adipocytokines visfatin and resistin in liver cancer cells. Horm Mol Biol Clin Investig (2019) 38(2). doi: 10.1515/hmbci-2018-0068
219. Ninomiya S, Shimizu M, Imai K, Takai K, Shiraki M, Hara T, et al. Possible role of visfatin in hepatoma progression and the effects of branched-chain amino acids on visfatin-induced proliferation in human hepatoma cells. Cancer Prev Res Phila Pa. (2011) 4(12):2092–100. doi: 10.1158/1940-6207.CAPR-11-0340
220. Wang Y, Gao C, Zhang Y, Gao J, Teng F, Tian W, et al. Visfatin stimulates endometrial cancer cell proliferation via activation of PI3K/Akt and MAPK/ERK1/2 signalling pathways. Gynecol Oncol (2016) 143(1):168–78. doi: 10.1016/j.ygyno.2016.07.109
221. Patel ST, Mistry T, Brown JEP, Digby JE, Adya R, Desai KM, et al. A novel role for the adipokine visfatin/pre-B cell colony-enhancing factor 1 in prostate carcinogenesis. Peptides (2010) 31(1):51–7. doi: 10.1016/j.peptides.2009.10.001
222. Kim JG, Kim EO, Jeong BR, Min YJ, Park JW, Kim ES, et al. Visfatin stimulates proliferation of MCF-7 human breast cancer cells. Mol Cells (2010) 30(4):341–5. doi: 10.1007/s10059-010-0124-x
223. Chiang YF, Chen HY, Huang KC, Lin PH, Hsia SM. Dietary antioxidant trans-cinnamaldehyde reduced visfatin-induced breast cancer progression: in vivo and in vitro study. Antioxidants (2019) 8(12):625. doi: 10.3390/antiox8120625
224. Wang GJ, Shen NJ, Cheng L, Fang Y, Huang H, Li KH. Visfatin triggers the in vitro migration of osteosarcoma cells via activation of NF-κB/IL-6 signals. Eur J Pharmacol (2016) 791:322–30. doi: 10.1016/j.ejphar.2016.08.029
225. Li Y, Li X, Liu KR, Zhang JN, Liu Y, Zhu Y. Visfatin derived from ascites promotes ovarian cancer cell migration through Rho/ROCK signaling-mediated actin polymerization. Eur J Cancer Prev (2015) 24(3):231–9. doi: 10.1097/CEJ.0000000000000064
226. Hung SY, Lin CY, Yu CC, Chen HT, Lien MY, Huang YW, et al. Visfatin promotes the metastatic potential of chondrosarcoma cells by stimulating AP-1-dependent MMP-2 production in the MAPK pathway. Int J Mol Sci (2021) 22(16):8642. doi: 10.3390/ijms22168642
227. Sun BL, Sun X, Casanova N, Garcia AN, Oita R, Algotar AM, et al. Role of secreted extracellular nicotinamide phosphoribosyltransferase (eNAMPT) in prostate cancer progression: Novel biomarker and therapeutic target. EBioMedicine (2020) 61:103059. doi: 10.1016/j.ebiom.2020.103059
228. Huang WS, Chen CN, Sze CI, Teng CC. Visfatin induces stromal cell-derived factor-1 expression by β1 integrin signaling in colorectal cancer cells. J Cell Physiol (2013) 228(5):1017–24. doi: 10.1002/jcp.24248
229. Sun BL, Tang L, Sun X, Garcia AN, Camp SM, Posadas E, et al. A humanized monoclonal antibody targeting extracellular nicotinamide phosphoribosyltransferase prevents aggressive prostate cancer progression. Pharmaceuticals (2021) 14(12):1322. doi: 10.3390/ph14121322
230. Cao D, Chu L, Xu Z, Gong J, Deng R, Wang B, et al. Visfatin facilitates gastric cancer Malignancy by targeting snai1 via the NF-κB signaling. Hum Exp Toxicol (2021) 40(10):1646–55. doi: 10.1177/09603271211006168
231. Cheng G, Liu C, Sun X, Zhang L, Liu L, Ouyang J, et al. Visfatin promotes osteosarcoma cell migration and invasion via induction of epithelial-mesenchymal transition. Oncol Rep (2015) 34(2):987–94. doi: 10.3892/or.2015.4053
232. Wang G, Tian W, Liu Y, Ju Y, Shen Y, Zhao S, et al. Visfatin triggers the cell motility of non-small cell lung cancer via up-regulation of matrix metalloproteinases. Basic Clin Pharmacol Toxicol (2016) 119(6):548–54. doi: 10.1111/bcpt.12623
233. Wu KL, Lee KC, Yen CK, Chen CN, Chang SF, Huang WS. Visfatin and resveratrol differentially regulate the expression of thymidylate synthase to control the sensitivity of human colorectal cancer cells to capecitabine cytotoxicity. Life (2021) 11(12):1371. doi: 10.3390/life11121371
234. Zangooei M, Nourbakhsh M, Ghahremani MH, Meshkani R, Khedri A, Shadboorestan A, et al. Investigating the effect of visfatin on ERalpha phosphorylation (Ser118 and Ser167) and ERE-dependent transcriptional activity. EXCLI J (2018) 17:516–25. doi: 10.17179/excli2018-1299
235. de Leeuw R, Neefjes J, Michalides R. A role for estrogen receptor phosphorylation in the resistance to tamoxifen. Int J Breast Cancer. (2011) 2011:1–10. doi: 10.4061/2011/232435
236. Liu Y, Li Q, Zhou L, Xie N, Nice EC, Zhang H, et al. Cancer drug resistance: redox resetting renders a way. Oncotarget (2016) 7(27):42740–61. doi: 10.18632/oncotarget.8600
237. Sawicka-Gutaj N, Komarowska H, Gruszczyński D, Derwich A, Klimont A, Ruchała M. Serum visfatin/NAMPT as a potential risk predictor for Malignancy of adrenal tumors. J Clin Med (2022) 11(19):5563. doi: 10.3390/jcm11195563
238. Pazgan-Simon M, Kukla M, Zuwała-Jagiełło J, Derra A, Bator M, Menżyk T, et al. Serum visfatin and vaspin levels in hepatocellular carcinoma (HCC). PloS One (2020) 15(1):e0227459. doi: 10.1371/journal.pone.0227459
239. Cymbaluk-Płoska A, Chudecka-Głaz A, Pius-Sadowska E, Sompolska-Rzechuła A, Machaliński B, Menkiszak J. Circulating serum level of visfatin in patients with endometrial cancer. BioMed Res Int (2018) 2018:1–9. doi: 10.1155/2018/8576179
240. Sowa P, Misiolek M, Orecka B, Czecior E, Adamczyk-Sowa M. Serum levels of selected adipocytokines in benign and Malignant parotid gland tumor patients. Cytokine (2018) 106:40–4. doi: 10.1016/j.cyto.2018.03.004
241. Motawi T, Zakhary N, Darwish H, Abdullah H, Tadros S. Significance of some non-invasive biomarkers in the early diagnosis and staging of Egyptian breast cancer patients. Asian Pac J Cancer Prev (2020) 21(11):3279–84. doi: 10.31557/APJCP.2020.21.11.3279
242. Zhang LJ, Li XQ, Wang CD, Zhuang L, Gong Q, Li SJ, et al. The correlation of visfatin and its gene polymorphism with non-small cell lung cancer. Cancer Biother Radiopharm. (2018) 33(10):460–5. doi: 10.1089/cbr.2018.2526
243. Sun Y, Zhu S, Wu Z, Huang Y, Liu C, Tang S, et al. Elevated serum visfatin levels are associated with poor prognosis of hepatocellular carcinoma. Oncotarget (2017) 8(14):23427–35. doi: 10.18632/oncotarget.15080
244. Yang J, Zhang K, Song H, Wu M, Li J, Yong Z, et al. Visfatin is involved in promotion of colorectal carcinoma Malignancy through an inducing EMT mechanism. Oncotarget (2016) 7(22):32306–17. doi: 10.18632/oncotarget.8615
245. Lu GW, Wang QJ, Xia MM, Qian J. Elevated plasma visfatin levels correlate with poor prognosis of gastric cancer patients. Peptides (2014) 58:60–4. doi: 10.1016/j.peptides.2014.05.016
246. Nakajima TE, Yamada Y, Hamano T, Furuta K, Gotoda T, Katai H, et al. Adipocytokine levels in gastric cancer patients: resistin and visfatin as biomarkers of gastric cancer. J Gastroenterol (2009) 44(7):685–90. doi: 10.1007/s00535-009-0063-5
247. Dalamaga M, Archondakis S, Sotiropoulos G, Karmaniolas K, Pelekanos N, Papadavid E, et al. Could serum visfatin be a potential biomarker for postmenopausal breast cancer? Maturitas (2012) 71(3):301–8. doi: 10.1016/j.maturitas.2011.12.013
248. Ilhan TT, Kebapcilar A, Yilmaz SA, Ilhan T, Kerimoglu OS, Pekin AT, et al. Relations of serum visfatin and resistin levels with endometrial cancer and factors associated with its prognosis. Asian Pac J Cancer Prev (2015) 16(11):4503–8. doi: 10.7314/APJCP.2015.16.11.4503
249. Galli U, Colombo G, Travelli C, Tron GC, Genazzani AA, Grolla AA. Recent advances in NAMPT inhibitors: A novel immunotherapic strategy. Front Pharmacol (2020) 11:656. doi: 10.3389/fphar.2020.00656
250. Wei Y, Xiang H, Zhang W. Review of various NAMPT inhibitors for the treatment of cancer. Front Pharmacol (2022) 13:970553. doi: 10.3389/fphar.2022.970553
251. Pillai VB, Sundaresan NR, Kim G, Samant S, Moreno-Vinasco L, Garcia JGN, et al. Nampt secreted from cardiomyocytes promotes development of cardiac hypertrophy and adverse ventricular remodeling. Am J Physiol-Heart Circ Physiol (2013) 304(3):H415–26. doi: 10.1152/ajpheart.00468.2012
252. Liu T, Miao Z, Jiang J, Yuan S, Fang W, Li B, et al. Visfatin Mediates SCLC Cells Migration across Brain Endothelial Cells through Upregulation of CCL2. Int J Mol Sci (2015) 16(12):11439–51. doi: 10.3390/ijms160511439
253. Zhang C, Zhu R, Wang H, Tao Q, Lin X, Ge S, et al. Nicotinamide phosphate transferase (NAMPT) increases in plasma in patients with acute coronary syndromes, and promotes macrophages to M2 polarization. Int Heart J (2018) 59(5):1116–22. doi: 10.1536/ihj.17-363
254. Xiao L, Mao Y, Tong Z, Zhao Y, Hong H, Wang F. Radiation exposure triggers the Malignancy of non−small cell lung cancer cells through the activation of visfatin/Snail signaling. Oncol Rep (2021) 45(3):1153–61. doi: 10.3892/or.2021.7929
255. Ahmed M, Zaghloul N, Zimmerman P, Casanova NG, Sun X, Song JH, et al. Endothelial eNAMPT drives EndMT and preclinical PH: rescue by an eNAMPT-neutralizing mAb. Pulm Circ (2021) 11(4):204589402110597. doi: 10.1177/20458940211059712
256. Garcia AN, Casanova NG, Valera DG, Sun X, Song JH, Kempf CL, et al. Involvement of eNAMPT/TLR4 signaling in murine radiation pneumonitis: protection by eNAMPT neutralization. Transl Res (2022) 239:44–57. doi: 10.1016/j.trsl.2021.06.002
257. Garcia AN, Casanova NG, Kempf CL, Bermudez T, Valera DG, Song JH, et al. eNAMPT is a novel damage-associated molecular pattern protein that contributes to the severity of radiation-induced lung fibrosis. Am J Respir Cell Mol Biol (2022) 66(5):497–509. doi: 10.1165/rcmb.2021-0357OC
258. Sun BL, Sun X, Kempf CL, Song JH, Casanova NG, Camp SM, et al. Involvement of eNAMPT/TLR4 inflammatory signaling in progression of non-alcoholic fatty liver disease, steatohepatitis, and fibrosis. FASEB J Off Publ Fed Am Soc Exp Biol (2023) 37(3):e22825. doi: 10.1096/fj.202201972RR
259. Ahmed M, Casanova NG, Zaghloul N, Gupta A, Rodriguez M, Robbins IR, et al. The eNAMPT/TLR4 inflammatory cascade drives the severity of intra-amniotic inflammation in pregnancy and predicts infant outcomes. Front Physiol (2023) 14:1129413. doi: 10.3389/fphys.2023.1129413
260. Békés M, Langley DR, Crews CM. PROTAC targeted protein degraders: the past is prologue. Nat Rev Drug Discovery (2022) 21(3):181–200. doi: 10.1038/s41573-021-00371-6
261. Zhu X, Liu H, Chen L, Wu C, Liu X, Cang Y, et al. Addressing the Enzyme-independent tumor-promoting function of NAMPT via PROTAC-mediated degradation. Cell Chem Biol (2022) 29(11):1616–1629.e12. doi: 10.1016/j.chembiol.2022.10.007
Keywords: eNAMPT, adipocytokine, inflammation, metabolic diseases, cancer
Citation: Semerena E, Nencioni A and Masternak K (2023) Extracellular nicotinamide phosphoribosyltransferase: role in disease pathophysiology and as a biomarker. Front. Immunol. 14:1268756. doi: 10.3389/fimmu.2023.1268756
Received: 28 July 2023; Accepted: 03 October 2023;
Published: 17 October 2023.
Edited by:
Zhihao Jia, Purdue University, United StatesReviewed by:
Giorgia Colombo, University of Eastern Piedmont, ItalyValentina Audrito, Università del Piemonte Orientale, Italy
Copyright © 2023 Semerena, Nencioni and Masternak. This is an open-access article distributed under the terms of the Creative Commons Attribution License (CC BY). The use, distribution or reproduction in other forums is permitted, provided the original author(s) and the copyright owner(s) are credited and that the original publication in this journal is cited, in accordance with accepted academic practice. No use, distribution or reproduction is permitted which does not comply with these terms.
*Correspondence: Krzysztof Masternak, a3J6eXN6dG9mLm1hc3Rlcm5ha0BsaWdodGNoYWluYmlvLmNvbQ==