- 1Department of Medical Biochemistry and Molecular Biology, School of Medicine, Virgen Macarena University Hospital, University of Seville, Seville, Spain
- 2Department of Laboratory Medicine, Virgen Macarena University Hospital, Seville, Spain
- 3Infectious Diseases and, Microbiology and Preventive Medicine Unit, Virgen Macarena University Hospital/Departments of Medicine and Microbiology, University of Seville/Biomedicine Institute of Seville (IBiS), Seville, Spain
- 4CIBERINFEC, Instituto de Salud Carlos III, Madrid, Spain
- 5Clinical Oncology Service, Virgen Macarena University Hospital, University of Seville, Seville, Spain
- 6Intensive Care Unit, Virgen Macarena University Hospital, Seville, Spain
SARS-CoV-2 infection is the cause of the disease named COVID-19, a major public health challenge worldwide. Differences in the severity, complications and outcomes of the COVID-19 are intriguing and, patients with similar baseline clinical conditions may have very different evolution. Myeloid-derived suppressor cells (MDSCs) have been previously found to be recruited by the SARS-CoV-2 infection and may be a marker of clinical evolution in these patients. We have studied 90 consecutive patients admitted in the hospital before the vaccination program started in the general population, to measure MDSCs and lymphocyte subpopulations at admission and one week after to assess the possible association with unfavorable outcomes (dead or Intensive Care Unit admission). We analyzed MDSCs and lymphocyte subpopulations by flow cytometry. In the 72 patients discharged from the hospital, there were significant decreases in the monocytic and total MDSC populations measured in peripheral blood after one week but, most importantly, the number of MDSCs (total and both monocytic and granulocytic subsets) were much higher in the 18 patients with unfavorable outcome. In conclusion, the number of circulating MDSCs may be a good marker of evolution in the follow-up of unvaccinated patients admitted in the hospital with the diagnosis of COVID-19.
Introduction
SARS-CoV-2 infection can lead to a wide range of symptoms and clinical manifestations, ranging from mild cold-like symptoms to fatal outcomes. Patients admitted to the hospital with COVID-19 may experience a spectrum of disease severity, with some individuals being discharged within a week due to mild disease, while others may develop severe bilateral pneumonia requiring oxygen and respiratory support in the Intensive Care Unit (ICU), and sadly, some may succumb to the infection. The mortality rate of COVID-19 has been estimated to be between 1% and 2% of infected patients, depending on factors such as age and co-morbidities (1, 2).
Myeloid-derived suppressor cells (MDSCs) constitute a heterogeneous group of immature immune cells originating from the myeloid lineage. This cell population is recruited and expanded in the inflammation sites through C-C motif chemokine ligand 2 (CCL2)/C-C motif chemokine receptor 2 (CCR2), CCL3/CCR5 or CXC motif chemokine ligand 13 (CXCL13)/CXC motif receptor 5 (CXCR5) pathways, and granulocyte-macrophage colony-stimulating factor (GM-CSF), interleukin (IL-6), or prostaglandin E2 (PGE2). MDSCs not only exhibit immunosuppressive functions on T-cell responses, but also participate in the suppression of dendritic cell (DC)-, B- and NK-mediated immune responses and have a role in the development and expansion of other suppressor cells such as regulatory T cells (Tregs) or M2-macrophages (3, 4).
Under normal conditions, these cells differentiate into mature macrophages, DCs, or granulocytes. However, under pathological conditions like cancer or viral and bacterial infections, this process may shift towards a pathological and immunosuppressive phenotype (5–9). Mouse MDSCs are characterized as Gr1+CD11b+ cells with expression of Ly6ChighLy6G- and Ly6ClowLy6G+, which are called monocytic (M-) and granulocytic (G-)MDSCs, respectively. In contrast, human MDSCs remain less defined. They are CD11b+HLA-DRlow/- cells that also express CD33+ and CD14+CD15- (M-MDSCs) or CD14-CD15+ (G-MDSCs). Of note, G-MDSCs can also express high levels of CD66b (10, 11).
In previous research, we observed that patients with mild COVID-19 exhibited increased levels of blood M-MDSCs, along with lymphopenia, an increased number of exhausted T cells, and a lower number of activated T cells, compared to healthy donors. Furthermore, blood M-MDSCs showed a negative correlation with activated T cells (12). In a recent study, we also found that peripheral blood G-MDSCs could serve as a marker for mortality in ICU-admitted patients with COVID-19 (13). Other studies have also suggested that MDSCs might influence both disease severity and mortality (14, 15). However, there is ongoing debate about which MDSC subtype (M-MDSCs or G-MDSCs) may predict COVID-19 severity (15, 16). Additionally, MDSCs have been proposed not only as potential biomarkers but also as therapeutic targets in COVID-19 (17).
MDSCs are known to inhibit T lymphocytes, leading to their inactivation, exhaustion, and apoptosis (18). In the context of COVID-19, T lymphocytes from patients have been found to exhibit increased expression of inhibitory markers, such as PD-1 or CTLA-4, which contribute to an ineffective immune response (19, 20). Moreover, exhausted CD4+ T cells have been associated with poor outcomes in COVID-19 (21). Consistent with these findings, we have observed a positive correlation between the numbers of MDSCs and exhausted T cells in severe COVID-19 patients admitted to the ICU (13). Conversely, we have found a lower number of activated T lymphocytes (CD3+ expressing OX-40) in COVID-19 patients (12, 13). Similar results have been reported for CD3+ expressing HLA-DR (22). Furthermore, we noted a negative correlation between MDSCs and activated T lymphocytes in both mild and severe COVID-19 patients (12, 13), suggesting that the increase in MDSCs might contribute to the prevention of T-cell activation.
Given these observations, we now seek to investigate whether MDSCs could serve as a valuable marker for the follow-up of COVID-19 patients, predicting their disease evolution from admission to discharge in cases of mild disease or ICU admission or exitus in cases of severe disease. To achieve this, we conducted a study on 90 consecutive patients admitted to the hospital during the second and third waves of COVID-19 before the initiation of the vaccination program in the general population.
Materials and methods
Study design
This is a prospective, observational cohort study that included adult patients (age ≥ 18 years) with COVID-19 admitted to the Virgen Macarena University Hospital in Seville, Spain, from January 2021 to March 2021. We excluded patients with previous immunosuppression, such as those with solid organ or hematologic transplantations, hematologic malignancies, or those taking immunosuppressants before hospital admission, as well as pregnant women.
The clinicopathological characteristics of the enrolled patients are presented in Table 1.
Patients
We studied the immunological characteristics of peripheral blood cells from 90 COVID-19 patients admitted at the Virgen Macarena University Hospital (Seville, Spain) after being tested positive as previously described (12, 13). Blood was obtained at admission and one week later, using samples sent to the hospital laboratory for routinary tests. Patients were divided into two groups, those with worst outcome (6 patients who died after one week of hospitalization and 12 patients admitted at ICU) and 72 patients who had a good evolution and were discharged from the hospital after the same follow-up time. The study was approved by the Institutional Review Board (12, 13).
Flow cytometry analysis in whole blood samples
Cell populations were assessed using the FACSCanto II flow cytometry system (Becton Dickinson, Franklin Lakes, NJ, USA) from EDTA-K3 tubes. MDSCs and lymphoid populations, including T, B, NK, CD4+, and CD8+ T cells, as well as Tregs, PD-1+OX40− T, and OX40+PD-1− T cells, were gated as previously described (12, 13). HLA-DR-expressing T cells were gated as illustrated in Supplementary Figure 1: CD3+CD8+HLA-DR+ and CD3+CD8-HLA-DR+. The latter population was considered as part of the CD4 compartment; therefore, we referred to it as CD4+HLA-DR+ T cells. The absolute count of these T-cell subsets was calculated by multiplying the percentage of CD4+HLA-DR+ and CD8+HLA-DR+ by the absolute count of CD4+ and CD8+ T cells, respectively. The absolute count of total HLA-DR+ T cells was calculated by summing up the CD3+CD4+HLA-DR+ cell counts and CD3+CD8+HLA-DR+ cell counts. Additionally, total lymphocyte, monocyte, and granulocyte counts were obtained from hematologic counts (Sysmex CS-1000). Table 2 displays the cell counts of granulocytes, lymphocytes, T cells, CD4+ T cells, CD8+ T cells, B cells, and NK cells.
Monoclonal antibodies
All antibodies were acquired from Becton Dickinson Immunocytometry Systems (San Jose, CA, USA) and employed at the concentrations recommended by the manufacturer (12, 13).
MDSCs: PerCP-Cy5.5 Mouse anti-human CD 45 (ref no. 564105), APC-Cy7 rat anti-CD11b (ref no. 557657), PE mouse anti-Human CD 33 (ref no. 555450), PE-Cy7 mouse anti-human HLA-DR (ref no. 560651), FITC mouse anti-human CD 14 (ref no. 555397) and APC mouse anti-human CD 15 (ref no. 551376).
Tregs: Human Treg cocktail (ref no. 560249), which includes PerCP Mouse Anti-Human CD4, PE mouse anti-Human CD127, and FITC anti-human CD25.
Activated OX40+PD-1- T cells: FITC Mouse anti-human OX-40 (CD134) (ref no. 555837), PerCP-Cy5.5 mouse anti-human CD8 (ref no. 565310), PE-Cy7 mouse anti-human CD4 (ref no. 557852), APC mouse anti-human PD-1 (CD279) (ref no. 558694), APC-H7 mouse anti-human CD3 (ref no. 560176).
Activated HLA-DR+ T cells: PE-Cy7 mouse anti-human HLA-DR (ref no. 560651), PerCP-Cy5.5 mouse anti-human CD8 (ref no. 565310), APC-H7 mouse anti-human CD3 (ref no. 560176).
Data analysis
Statistical analysis was conducted using GraphPad Prism 8.0.2 (GraphPad Software, San Diego, CA, USA), and graphs were generated accordingly. Continuous variables were presented as the median and 95% confidence intervals, while qualitative variables were expressed as absolute numbers and percentages. The normal distribution of analyzed variables was assessed through a histogram, box plot, the Q-Q plot, and the Kolmogorov-Smirnov normality test. As the data did not follow a normal distribution, non-parametric tests were employed. The comparison of cell distributions between discharged, deceased, or ICU-admitted COVID-19 patients was performed using the Mann-Whitney U test. Additionally, the Wilcoxon test was utilized to compare cell distributions within each group of patients. Bivariate correlations among cell populations were determined using Spearman’s coefficient. Statistically significant differences were considered for p-values ≤ 0.05.
Results
Clinico-pathological characteristics of COVID-19 patients
Ninety patients diagnosed with COVID-19 and hospitalized at the Virgen Macarena University Hospital (Seville, Spain) were included in the study. Table 1 displays their clinicopathological baseline characteristics.
The median age of the patients was 61 years old (95% CI, 56-65). Among them, 61.96% were male. Among the patients, 18 individuals experienced an unfavorable outcome, with 8 deaths and 10 ICU admissions due to either in-hospital mortality or post-hospitalization ICU care. The remaining 72 patients were successfully discharged without requiring ICU admission.
Table 2 presents the cell counts of granulocytes, lymphocytes, T cells, CD4+ T cells, CD8+ T cells, B cells, and NK cells from the patients.
Blood MDSC levels determined outcomes of hospitalized COVID-19 patients after the one-week follow-up
The follow-up of circulating MDSCs from COVID-19 patients after hospitalization is shown in Figures 1A–C. MDSC levels (both M-MDSCs and G-MDSCs, as well as total MDSCs) were similar in all patients at admission in the Hospital. However, individuals with a unfavorable outcome experienced a significant increment of MDSC levels (M-MDSCs, p=0.0034; G-MDSCs, p=0.0024; and total MDSCs, p=0.0017) one week later. COVID-19 patients who were discharged from the hospital experienced a significant decrease of M-MDSCs (p=0.0076) and total MDSCs (p=0.0493), whereas G-MDSC levels remained constant. Interestingly, after the follow-up time, both M-MDSC and total MDSC levels were remarkably higher in the group of patients with worst outcome compared to those who were discharged from the hospital (p=0.0004 and p=0.0001, respectively).
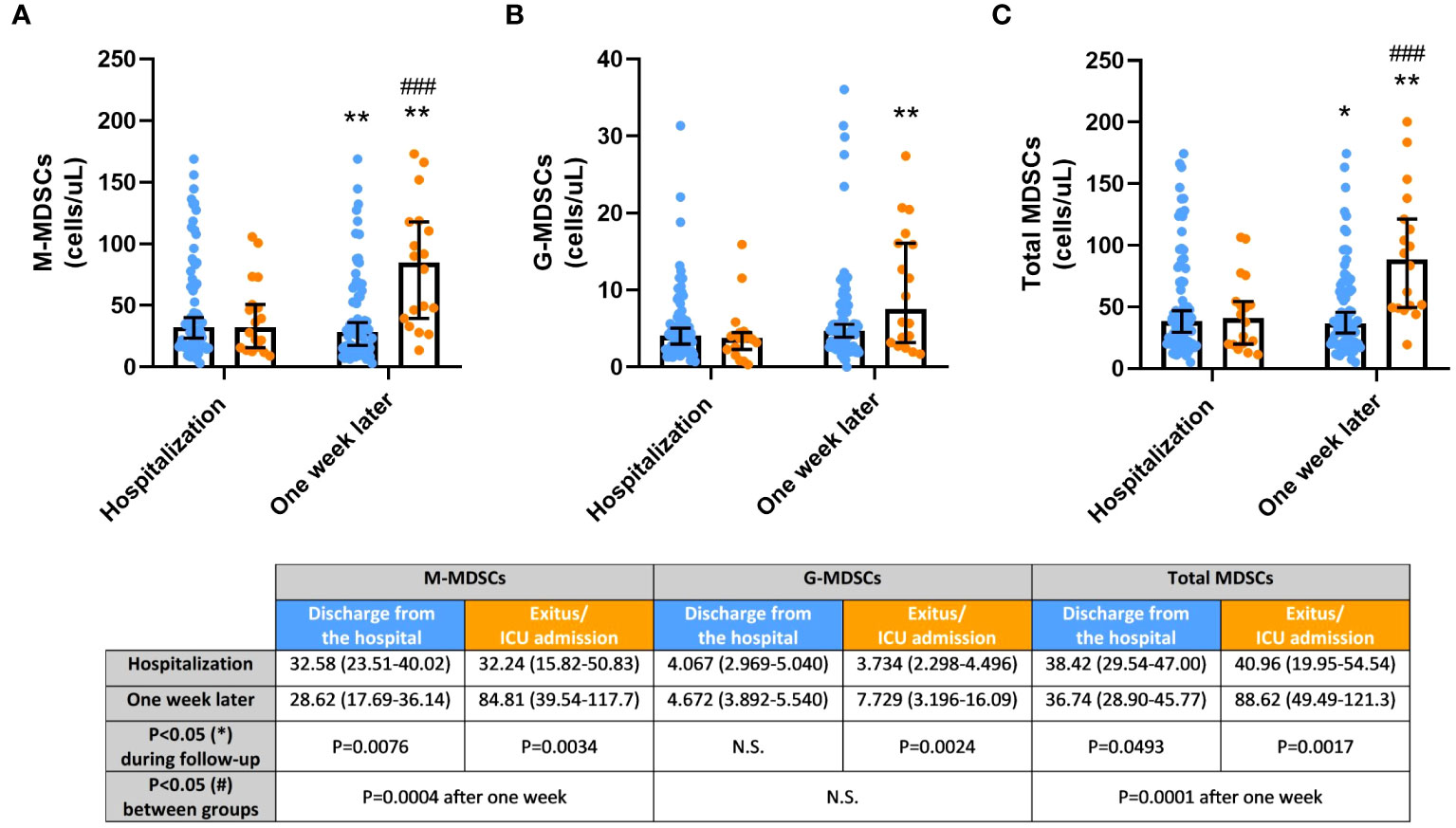
Figure 1 (A) Monocytic myeloid-derived suppressor cells (MDSCs); (B) Granulocytic MDSCs; (C) Total MDSCs, in COVID-19 patients discharged from the hospital (blue) and died after hospitalization or admitted at Intensive Care Unit (ICU; orange) during the one-week follow-up. Cell concentrations are represented as median and 95% confidence intervals (CI) of cells per microliter. *p ≤ 0.05 and **p ≤ 0.01, comparing determinations at hospitalization vs. one week later in every group of patients; ###p ≤ 0.001 comparing opposite groups in every determination. N.S., No significant.
Blood Treg levels after hospitalization determined clinical evolution of COVID-19 patients during the one-week follow-up
The proportion of circulating Tregs displayed a remarkable difference between both groups of patients after one week of hospitalization, as depicted in Supplementary Figure 2. Patients with an unfavorable outcome exhibited constant, yet overall low levels of Tregs, whereas patients with a better outcome experienced a significant increase in Tregs one week after hospitalization (p<0.0001). When comparing the two groups, blood Treg levels were significantly lower in the group with an unfavorable outcome both at hospitalization (p=0.0289) and one week later (p=0.0002) compared to the discharged patients.
OX40+PD-1- expression may activate cytotoxic T cells of COVID-19 patients discharged from the hospital
Figures 2A–C displays the evaluation of activated (OX40+PD-1-) T cells. The levels of activated CD4+ T cells were slightly higher in patients with a better outcome after hospitalization and one week later. Conversely, activated CD8+ T cells showed a significant increase during the follow-up in this patient cohort (p=0.0027), suggesting an intriguing OX40-mediated cytotoxic T-cell activation to facilitate immune responses against the SARS-COV-2 virus. Consequently, differences in the levels of total activated T cells between the groups were evident after one week of hospital stay (p=0.0496).
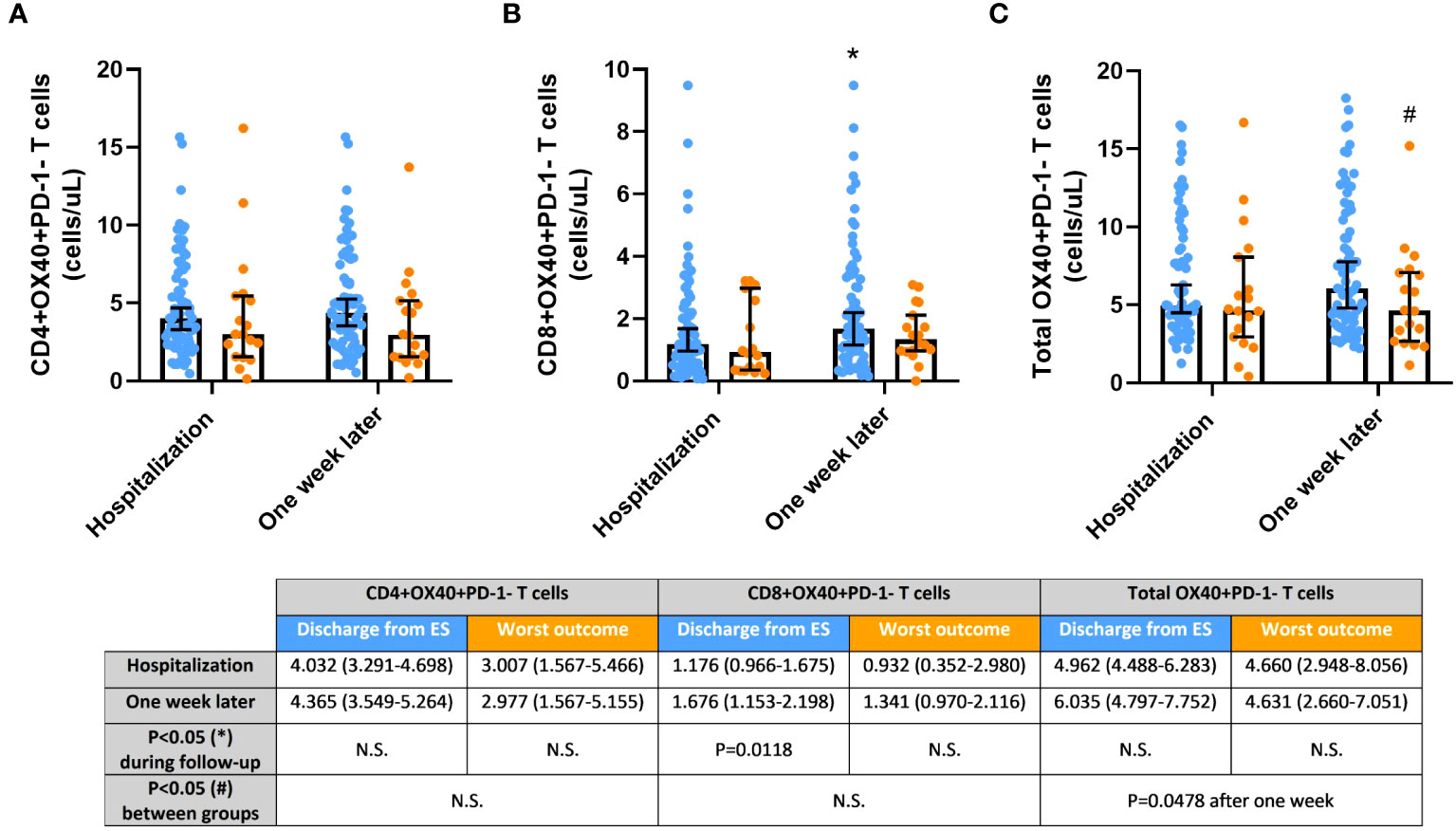
Figure 2 Activated OX40+PD-1- T cells, (A) CD4+; (B) CD8+; and (C) Total, in COVID-19 patients discharged from the hospital (blue) and passed away after hospitalization or admitted at Intensive Care Unit (ICU; orange) during the one-week follow-up. Cell concentrations are represented as median and 95% confidence intervals of cells per microliter. *p≤0.05, comparing determinations at hospitalization vs. one week later in every group of patients; #p ≤ 0.05, comparing opposite groups in every determination. N.S., No significant.
Activated HLA-DR-expressing T cells were significantly increased in blood of COVID-19 patients discharged after the one-week hospitalization
HLA-DR expression on CD4+, CD8+, and total T cells was also analyzed in COVID-19 patients (Figures 3A–C). The concentration of these cell populations in the blood was similar in both groups after hospitalization but remained constant in the group with an unfavorable outcome. However, HLA-DR-expressing T cells significantly expanded in the blood of patients with a better outcome after the follow-up period (CD4+HLA-DR+ T cells, p=0.0002; CD8+HLA-DR+ T cells, p=0.0150; and total HLA-DR+ T cells, p=0.0001), leading to significant differences between groups after one week of hospitalization (CD4+HLA-DR+ T cells, p=0.0068; CD8+HLA-DR+ T cells, p=0.0414; and total HLA-DR+ T cells, p=0.0083).
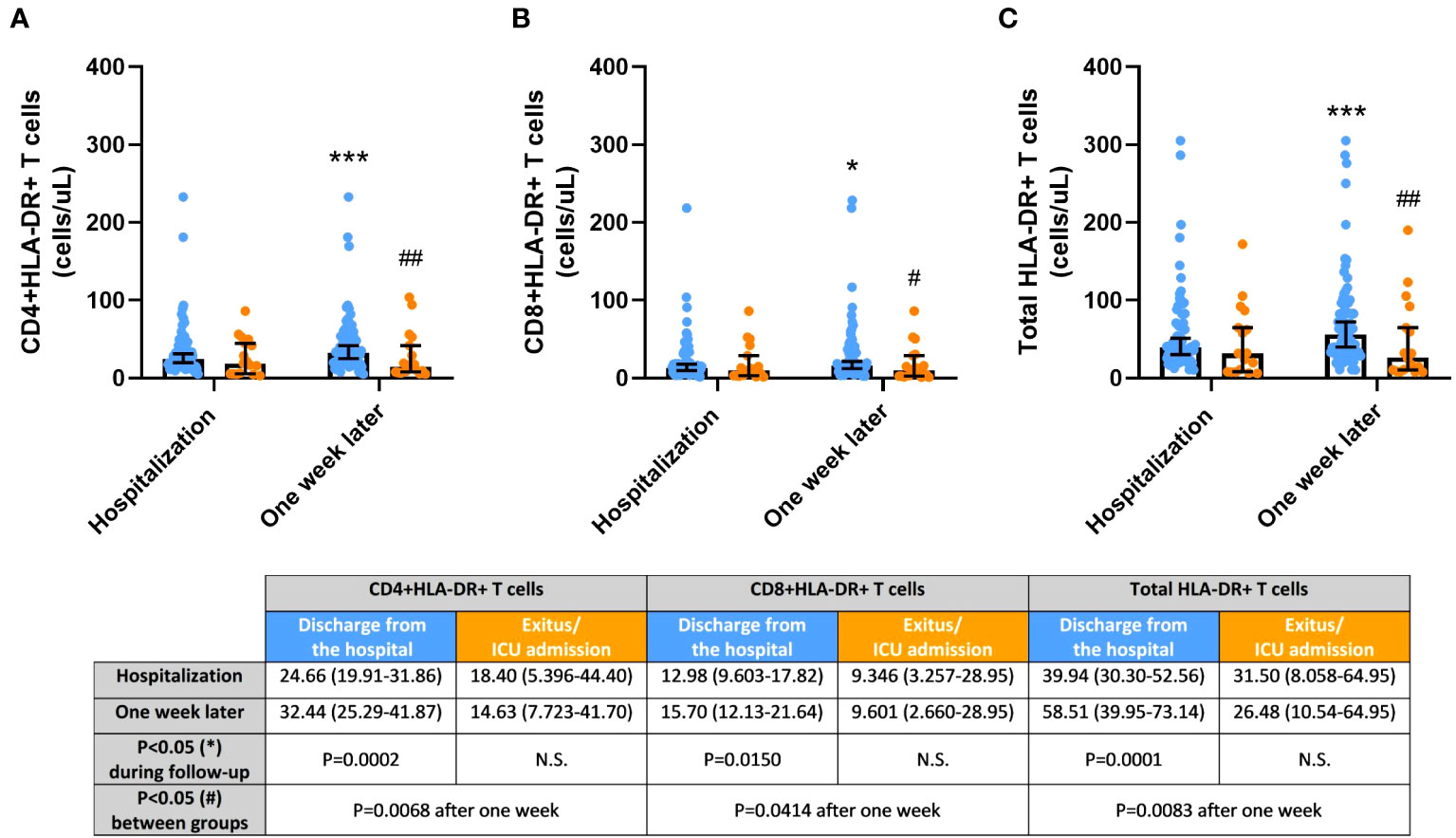
Figure 3 Activated HLA-DR+ T cells, (A) CD4+; (B) CD8+; and (C) Total, in COVID-19 patients discharged from the hospital (blue) and passed away after hospitalization or admitted at Intensive Care Unit (ICU; orange) during the one-week follow-up. Cell concentrations are represented as median and 95% confidence intervals of cells per microliter. *p ≤ 0.05 and ***p ≤ 0.001, comparing determinations at hospitalization vs. one week later in every group of patients; #p ≤ 0.05 and ##p ≤ 0.01, comparing opposite groups in every determination. N.S., No significant.
Activated OX40+PD-1- and HLA-DR+ T cells positively correlated each other and also with Tregs in COVID-19 patients
Table 3 illustrates strong correlations observed between different cell populations. Tregs showed positive correlations with every population (CD4+, CD8+, and total) of both activated OX40+PD-1- and HLA-DR+ T cells (p<0.0001 in all cases). Additionally, all cell populations of activated T cells exhibited positive correlations with each other (p<0.0001 in all cases, except p=0.0003 for CD4+OX40+PD-1- T cells vs. CD8+HLA-DR+ T cells).
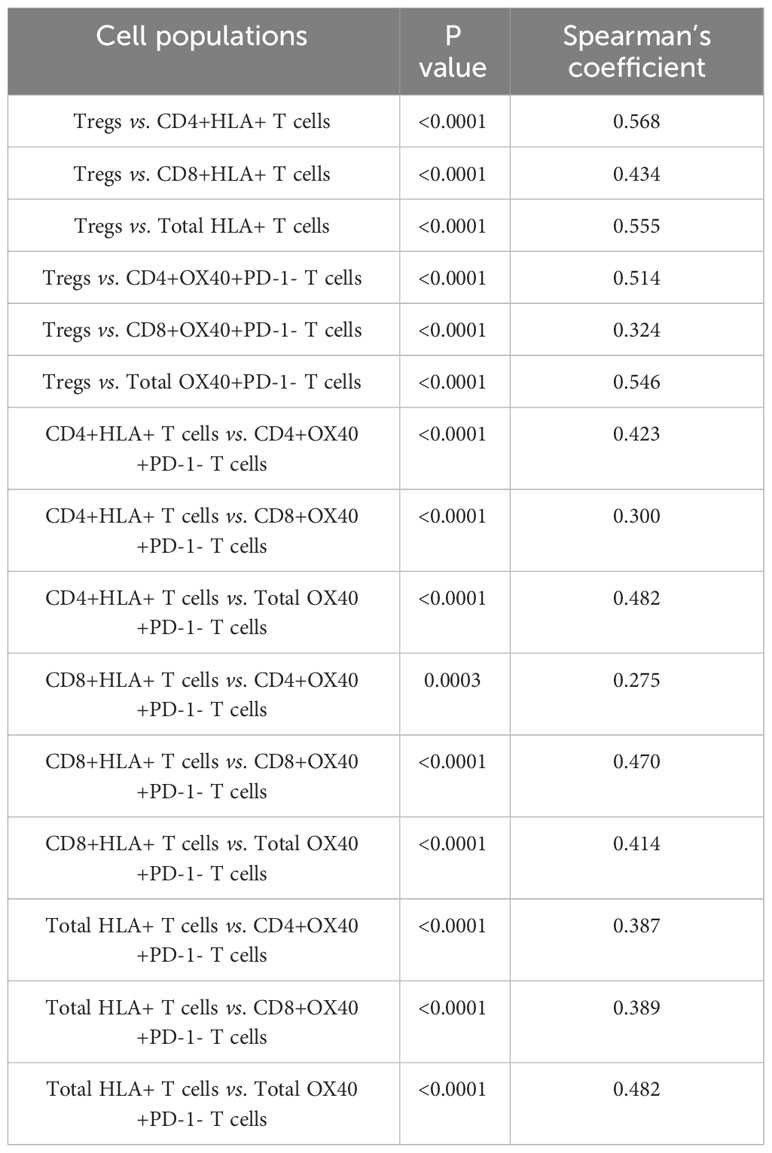
Table 3 Statistically significant (p<0.05) Spearman’s bivariate correlations between cell populations.
Discussion
COVID-19 exhibits a highly heterogeneous clinical evolution and outcomes, ranging from a mild cold-like illness to severe disease, influenced by various factors such as viral load, co-morbidities, and host immune response (23). However, the diverse responses of the innate immune system to the infection are not yet fully understood (2, 24). It is known that SARS-CoV-2 infection can lead to immune response suppression (25), partly due to the evasion of the innate immune system (26), resulting in significant lymphopenia (27, 28), which we previously detected in both mild and severe COVID-19 cases (12, 13). In this study, we found that patients with better outcomes (discharged from the hospital) had a higher proportion of lymphocytes at admission compared to those with an unfavorable outcome (deceased or admitted to the ICU). This suggests that low lymphocyte levels may predict severity or death in COVID-19 patients, as previously proposed (29, 30).
Suppression of T cell responses, as well as innate immune responses, is partly driven by the expansion of MDSCs, one of the major immunosuppressive mediators in the immune system (3). Consistent with this, we previously observed an increase in both circulating M-MDSCs in patients with mild COVID-19 (12) and G-MDSCs in patients with severe COVID-19 (13). Here, we demonstrate that MDSCs may serve as a useful biomarker for monitoring COVID-19 patients during one week of follow-up. Although all patients had similar clinical severity and levels of circulating MDSCs at admission, MDSC cell subsets significantly increased in the group of patients with worse outcomes after the follow-up period. Other studies have reported high levels of M-MDSCs in patients with acute COVID-19 (31), while others suggested G-MDSCs as the increased MDSC population in severe COVID-19 (14, 32).
Alternatively, MDSCs may also be a useful marker of immune suppression in COVID-19 patients with poor outcomes since Treg levels were low in peripheral blood, and even lower in those with a worse outcome after one week, whereas Tregs were increased in patients who were discharged from the hospital. The trend observed in the Tregs from peripheral blood of deceased/ICU-admitted patients suggests that Tregs may also be affected by the lymphopenic effects occurred in the SARS-COV-2 infection and may not mediate the immunosuppression driven by this viral disease (13). Also, Tregs has been previously found in a low percentage due to the proinflammatory cascade driven by IL-6, which could be diminished in recovered COVID-19 patients and may boost the levels of Tregs, as observed in the discharged COVID-19 patients after one week of follow-up. For these reasons, the application of Tregs has been proposed as a possible therapeutic approach to treat COVID-19 patients (33).
In addition, we analyzed T-cell activation by OX40 expression, as we did in previous studies with COVID-19 patients (12, 13). In this case, cytotoxic OX40+PD-1- T cells appear to be a promising biomarker for one-week follow-up since this cell population was significantly increased in discharged patients compared to those with poorer outcomes. To the best of our knowledge, previous reports regarding T-cell activation by OX40 have not been published by other research groups. However, we previously demonstrated the activation of both CD4+OX40+PD-1- and CD8+OX40+PD-1- T cells in patients discharged from the hospital, irrespective of the follow-up time (13). Here, we specifically checked the variations in the immune cell populations after one week of follow-up, and it appears that the activation of CD4+OX40+PD-1- T cells may take more time since no significant differences were found. The reason why only CD8+OX40+PD-1- T cells were increased in discharged patients after one week may be explained by the short period of stimulation that naïve CD8 T cells need to proliferate after activation (34). Also, the increase of CD8+OX40+PD-1- T cells may be sufficient for boosting T-cell responses in COVID-19 patients, as CD8 T cells play a critical role in mediating immune responses to acute viral infections in the lung (35).
Next, we analyzed activated HLA-DR-expressing T-cells. In previous studies involving COVID-19 patients, an overactivation of the immune system by HLA-DR+CD38+ T cells was found, even 12 months after infection (36), probably due to both the initial lymphopenia and the role these cells play in providing help to B lymphocytes (37–39). However, higher or lower expression of CD38 in HLA-DR+ T cells may promote different functions in co-activated T cells from severe COVID-19 patients (40). In this study, we analyzed the proportion of both circulating CD4+HLA-DR+ T and CD8+HLA-DR+ T cells (which may include variable CD38 expression). We observed that patients who were discharged from the hospital after one week of stay experienced a significant increase in every HLA-DR T cell population, suggesting that the activation of CD4 and CD8 T cells carried out by HLA-DR expression may be a promising biomarker for monitoring COVID-19 patients, at least during a short hospital stay. Importantly, we observed significant, positive correlations between every HLA-DR T cell population (CD4, CD8, and total) and every OX40+PD-1- T cell population (CD4, CD8, and total), highlighting the importance of T-cell activation biomarkers in monitoring COVID-19 patients. The evolution of this cell population (HLA-DR T cells without considering the expression of CD38) during the follow-up of COVID-19 patients has not been studied previously. However, HLA-DR T cells have been found increased in COVID-19 patients compared to healthy donors, especially the cytotoxic subset (41).
The inhibition of T cell activation may be mediated by both M-MDSC and G-MDSC (11). In fact, we have found lymphopenia and increased M-MDSC levels in mild COVID-19 (12), whereas higher G.MDSC levels were found in peripheral blood from severe COVI-19 patients (13). This expansion of G-MDSC could mediate at least in part the neutrophilia observed in severe COVID-19 patients, as previously suggested (42).
We have analyzed the immune profile of 90 unvaccinated, hospitalized COVID-19 patients, and we compared 72 individuals with good outcomes versus 18 individuals with worse outcomes. This imbalance in the sample size between groups makes us consider a limitation in the number of COVID-19 patients studied. Therefore, further studies in unvaccinated patients may be necessary to confirm the hypothesis that MDSCs may play a role in the evolution of COVID-19 patients admitted to the hospital. Additionally, considering that the vaccination program is already established and that some people have been reinfected, it could be interesting to study the immune profile of reinfected COVID-19 patients to consider MDSCs as a biomarker in the disease. Nevertheless, we have demonstrated that not only MDSCs may be considered as a biomarker in the follow-up of unvaccinated, hospitalized patients, and could be a plausible target for treatment in the infection with the SARS-CoV-2 virus, but also HLA-DR T cells may be a promising biomarker in the follow-up of these individuals.
Data availability statement
The raw data supporting the conclusions of this article will be made available by the authors, without undue reservation.
Ethics statement
The studies involving humans were approved by Virgen Macarena and Virgen del Rocio IRB. The studies were conducted in accordance with the local legislation and institutional requirements. The ethics committee/institutional review board waived the requirement of written informed consent for participation from the participants or the participants’ legal guardians/next of kin because of SARS-CoV-2 infection and to avoid contact.
Author contributions
CJ: Investigation, Data curation, Formal Analysis, Methodology, Writing – original draft. ES: Investigation, Methodology, Writing – review & editing. NP: Investigation, Writing – review & editing. FS: Investigation, Writing – review & editing. AP: Investigation, Writing – review & editing. TV: Investigation, Writing – review & editing. SF: Investigation, Writing – review & editing. SM: Investigation, Writing – review & editing. MJ: Investigation, Writing – review & editing. RG: Investigation, Writing – review & editing. CR: Investigation, Writing – review & editing. CS: Investigation, Writing – review & editing. EM: Investigation, Writing – review & editing. BG: Investigation, Writing – review & editing. NÁ: Investigation, Writing – review & editing. AS: Investigation, Writing – review & editing. JG: Writing – review & editing, Conceptualization. LD: Conceptualization, Writing – review & editing. JR: Conceptualization, Writing – review & editing. VS: Conceptualization, Writing – review & editing, Funding acquisition, Investigation, Supervision.
Funding
The author(s) declare financial support was received for the research, authorship, and/or publication of this article. CTS-151 Junta de Andalucía.
Acknowledgments
We acknowledge the technical work of the laboratory staff as well as the clinical support of the professionals involved in COVID-19 care.
Conflict of interest
The authors declare that the research was conducted in the absence of any commercial or financial relationships that could be construed as a potential conflict of interest.
Publisher’s note
All claims expressed in this article are solely those of the authors and do not necessarily represent those of their affiliated organizations, or those of the publisher, the editors and the reviewers. Any product that may be evaluated in this article, or claim that may be made by its manufacturer, is not guaranteed or endorsed by the publisher.
Supplementary material
The Supplementary Material for this article can be found online at: https://www.frontiersin.org/articles/10.3389/fimmu.2023.1266659/full#supplementary-material
Supplementary Figure 1 | Gating of activated CD8-HLA-DR+ T cells and CD8+HLA-DR+ T cells. Since CD8-HLD-DR+ cells were considered as a population within the CD4 compartment, this subset may be named as CD4+HLA-DR+ T cells.
Supplementary Figure 2 | Regulatory T cells in COVID-19 patients discharged from the hospital (blue) and passed away after hospitalization or admitted at Intensive Care Unit (ICU; orange) during the one-week follow-up. Cell concentrations are represented as median and 95% confidence intervals (CI) of cells per microliter. ****p ≤ 0.0001, comparing determinations at hospitalization vs. one week later in every group of patients. #p ≤ 0.05, and ###p ≤ 0.001 comparing opposite groups in every determination.
References
1. Zhu N, Zhang D, Wang W, Li X, Yang B, Song J, et al. A novel coronavirus from patients with pneumonia in China, 2019. N Engl J Med (2020) 382:727–33. doi: 10.1056/NEJMoa2001017
2. Chang FY, Chen HC, Chen PJ, Ho MS, Hsieh SL, Lin JC, et al. Immunologic aspects of characteristics, diagnosis, and treatment of coronavirus disease 2019 (COVID-19). J BioMed Sci (2020) 27:72. doi: 10.1186/s12929-020-00663-w
3. Gabrilovich DI, Nagaraj S. Myeloid-derived suppressor cells as regulators of the immune system. Nat Rev Immunol (2009) 9:162–74. doi: 10.1038/nri2506
4. Sinha P, Clements VK, Bunt SK, Albelda SM, Ostrand-Rosenberg S. Cross-talk between myeloid-derived suppressor cells and macrophages subverts tumor immunity toward a type 2 response. J Immunol (2007) 179:977–83. doi: 10.4049/jimmunol.179.2.977
5. Gabrilovich DI, Ostrand-Rosenberg S, Bronte V. Coordinated regulation of myeloid cells by tumours. Nat Rev Immunol (2012) 12:253–68. doi: 10.1038/nri3175
6. de la Cruz-Merino L, Chiesa M, Caballero R, Rojo F, Palazon N, Carrasco FH, et al. Breast cancer immunology and immunotherapy: current status and future perspectives. Int Rev Cell Mol Biol (2017) 331:1–53. doi: 10.1016/bs.ircmb.2016.09.008
7. O'Connor MA, Rastad JL, Green WR. The role of myeloid-derived suppressor cells in viral infection. Viral Immunol (2017) 30:82–97. doi: 10.1089/vim.2016.0125
8. Ost M, Singh A, Peschel A, Mehling R, Rieber N, Hartl D. Myeloid-derived suppressor cells in bacterial infections. Front Cell Infect Microbiol (2016) 6:37. doi: 10.3389/fcimb.2016.00037
9. Medina E, Hartl D. Myeloid-derived suppressor cells in infection: A general overview. J Innate Immun (2018) 10:407–13. doi: 10.1159/000489830
10. Jimenez-Cortegana C, Palazon-Carrion N, Martin Garcia-Sancho A, Nogales-Fernandez E, Carnicero-Gonzalez F, Rios-Herranz E, et al. Circulating myeloid-derived suppressor cells and regulatory T cells as immunological biomarkers in refractory/relapsed diffuse large B-cell lymphoma: translational results from the R2-GDP-GOTEL trial. J Immunother Cancer (2021) 9:e002323. doi: 10.1136/jitc-2020-002323
11. Veglia F, Sanseviero E, Gabrilovich DI. Myeloid-derived suppressor cells in the era of increasing myeloid cell diversity. Nat Rev Immunol (2021) 21:485–98. doi: 10.1038/s41577-020-00490-y
12. Jimenez-Cortegana C, Liro J, Palazon-Carrion N, Salamanca E, Sojo-Dorado J, de la Cruz-Merino L, et al. Increased blood monocytic myeloid derived suppressor cells but low regulatory T lymphocytes in patients with mild COVID-19. Viral Immunol (2021) 34:639–45. doi: 10.1089/vim.2021.0044
13. Jimenez-Cortegana C, Sanchez-Jimenez F, Perez-Perez A, Alvarez N, Sousa A, Canton-Bulnes L, et al. Low levels of granulocytic myeloid-derived suppressor cells may be a good marker of survival in the follow-up of patients with severe COVID-19. Front Immunol (2021) 12:801410. doi: 10.3389/fimmu.2021.801410
14. Sacchi A, Grassi G, Bordoni V, Lorenzini P, Cimini E, Casetti R, et al. Early expansion of myeloid-derived suppressor cells inhibits SARS-CoV-2 specific T-cell response and may predict fatal COVID-19 outcome. Cell Death Dis (2020) 11:921. doi: 10.1038/s41419-020-03125-1
15. Agrati C, Sacchi A, Bordoni V, Cimini E, Notari S, Grassi G, et al. Expansion of myeloid-derived suppressor cells in patients with severe coronavirus disease (COVID-19). Cell Death Differ (2020) 27:3196–207. doi: 10.1038/s41418-020-0572-6
16. Falck-Jones S, Vangeti S, Yu M, Falck-Jones R, Cagigi A, Badolati I, et al. Functional monocytic myeloid-derived suppressor cells increase in blood but not airways and predict COVID-19 severity. J Clin Invest (2021) 131:e144734. doi: 10.1172/JCI144734
17. Rowlands M, Segal F, Hartl D. Myeloid-derived suppressor cells as a potential biomarker and therapeutic target in COVID-19. Front Immunol (2021) 12:697405. doi: 10.3389/fimmu.2021.697405
18. Chen J, Ye Y, Liu P, Yu W, Wei F, Li H, et al. Suppression of T cells by myeloid-derived suppressor cells in cancer. Hum Immunol (2017) 78:113–9. doi: 10.1016/j.humimm.2016.12.001
19. Zheng HY, Zhang M, Yang CX, Zhang N, Wang XC, Yang XP, et al. Elevated exhaustion levels and reduced functional diversity of T cells in peripheral blood may predict severe progression in COVID-19 patients. Cell Mol Immunol (2020) 17:541–3. doi: 10.1038/s41423-020-0401-3
20. Zheng M, Gao Y, Wang G, Song G, Liu S, Sun D, et al. Functional exhaustion of antiviral lymphocytes in COVID-19 patients. Cell Mol Immunol (2020) 17:533–5. doi: 10.1038/s41423-020-0402-2
21. Rebillard RM, Charabati M, Grasmuck C, Filali-Mouhim A, Tastet O, Brassard N, et al. Identification of SARS-CoV-2-specific immune alterations in acutely ill patients. J Clin Invest (2021) 131:e145853. doi: 10.1172/JCI145853
22. Kazancioglu S, Yilmaz FM, Bastug A, Sakalli A, Ozbay BO, Buyuktarakci C, et al. Lymphocyte subset alteration and monocyte CD4 expression reduction in patients with severe COVID-19. Viral Immunol (2021) 34:342–51. doi: 10.1089/vim.2020.0166
23. Wu Z, McGoogan JM. Characteristics of and important lessons from the coronavirus disease 2019 (COVID-19) outbreak in China: summary of a report of 72314 cases from the Chinese center for disease control and prevention. JAMA (2020) 323:1239–42. doi: 10.1001/jama.2020.2648
24. Wang Y, Wu M, Li Y, Yuen HH, He ML. The effects of SARS-CoV-2 infection on modulating innate immunity and strategies of combating inflammatory response for COVID-19 therapy. J BioMed Sci (2022) 29:27. doi: 10.1186/s12929-022-00811-4
25. Tian W, Zhang N, Jin R, Feng Y, Wang S, Gao S, et al. Immune suppression in the early stage of COVID-19 disease. Nat Commun (2020) 11:5859. doi: 10.1038/s41467-020-19706-9
26. Taefehshokr N, Taefehshokr S, Hemmat N, Heit B. Covid-19: perspectives on innate immune evasion. Front Immunol (2020) 11:580641. doi: 10.3389/fimmu.2020.580641
27. Tavakolpour S, Rakhshandehroo T, Wei EX, Rashidian M. Lymphopenia during the COVID-19 infection: What it shows and what can be learned. Immunol Lett (2020) 225:31–2. doi: 10.1016/j.imlet.2020.06.013
28. Gutierrez-Gutierrez B, Del Toro MD, Borobia AM, Carcas A, Jarrin I, Yllescas M, et al. Identification and validation of clinical phenotypes with prognostic implications in patients admitted to hospital with COVID-19: a multicentre cohort study. Lancet Infect Dis (2021) 21:783–92. doi: 10.1016/S1473-3099(21)00019-0
29. Tan L, Wang Q, Zhang D, Ding J, Huang Q, Tang YQ, et al. Lymphopenia predicts disease severity of COVID-19: a descriptive and predictive study. Signal Transduct Target Ther (2020) 5:33. doi: 10.1038/s41392-020-0148-4
30. Xu B, Fan CY, Wang AL, Zou YL, Yu YH, He C, et al. Suppressed T cell-mediated immunity in patients with COVID-19: A clinical retrospective study in Wuhan, China. J Infect (2020) 81:e51–60. doi: 10.1016/j.jinf.2020.04.012
31. Thompson EA, Cascino K, Ordonez AA, Zhou W, Vaghasia A, Hamacher-Brady A, et al. Metabolic programs define dysfunctional immune responses in severe COVID-19 patients. Cell Rep (2021) 34:108863. doi: 10.1016/j.celrep.2021.108863
32. Takano T, Matsumura T, Adachi Y, Terahara K, Moriyama S, Onodera T, et al. Myeloid cell dynamics correlating with clinical outcomes of severe COVID-19 in Japan. Int Immunol (2021) 33:241–7. doi: 10.1093/intimm/dxab005
33. Liu Y, Qi G, Bellanti JA, Moser R, Ryffel B, Zheng SG. Regulatory T cells: A potential weapon to combat COVID-19? MedComm (2020) . 2020(1):157–64. doi: 10.1002/mco2.12
34. Bansal-Pakala P, Halteman BS, Cheng MH, Croft M. Costimulation of CD8 T cell responses by OX40. J Immunol (2004) 172:4821–5. doi: 10.4049/jimmunol.172.8.4821
35. Schmidt ME, Varga SM. The CD8 T cell response to respiratory virus infections. Front Immunol (2018) 9:678. doi: 10.3389/fimmu.2018.00678
36. Taeschler P, Adamo S, Deng Y, Cervia C, Zurbuchen Y, Chevrier S, et al. T-cell recovery and evidence of persistent immune activation 12 months after severe COVID-19. Allergy (2022) 77:2468–81. doi: 10.1111/all.15372
37. Shahbaz S, Xu L, Sligl W, Osman M, Bozorgmehr N, Mashhouri S, et al. The quality of SARS-CoV-2-specific T cell functions differs in patients with mild/moderate versus severe disease, and T cells expressing coinhibitory receptors are highly activated. J Immunol (2021) 207:1099–111. doi: 10.4049/jimmunol.2100446
38. Kuri-Cervantes L, Pampena MB, Meng W, Rosenfeld AM, Ittner CAG, Weisman AR, et al. Comprehensive mapping of immune perturbations associated with severe COVID-19. Sci Immunol (2020) 5:eabd7114. doi: 10.1126/sciimmunol.abd7114
39. Mathew D, Giles JR, Baxter AE, Oldridge DA, Greenplate AR, Wu JE, et al. Deep immune profiling of COVID-19 patients reveals distinct immunotypes with therapeutic implications. Science (2020) 369:eabc8511. doi: 10.1126/science.abc8511
40. Du J, Wei L, Li G, Hua M, Sun Y, Wang D, et al. Persistent high percentage of HLA-DR(+)CD38(high) CD8(+) T cells associated with immune disorder and disease severity of COVID-19. Front Immunol (2021) 12:735125. doi: 10.3389/fimmu.2021.735125
41. Song JW, Zhang C, Fan X, Meng FP, Xu Z, Xia P, et al. Immunological and inflammatory profiles in mild and severe cases of COVID-19. Nat Commun (2020) 11:3410. doi: 10.1038/s41467-020-17240-2
Keywords: SARS-CoV-2, COVID-19, MDSCs, T lymphocytes, biomarker
Citation: Jiménez-Cortegana C, Salamanca E, Palazón-Carrión N, Sánchez-Jiménez F, Pérez-Pérez A, Vilariño-García T, Fuentes S, Martín S, Jiménez M, Galván R, Rodríguez-Chacón C, Sánchez-Mora C, Moreno-Mellado E, Gutiérrez-Gutiérrez B, Álvarez N, Sosa A, Garnacho-Montero J, de la Cruz-Merino L, Rodríguez-Baño J and Sánchez-Margalet V (2023) Circulating myeloid-derived suppressor cells may be a useful biomarker in the follow-up of unvaccinated COVID-19 patients after hospitalization. Front. Immunol. 14:1266659. doi: 10.3389/fimmu.2023.1266659
Received: 25 July 2023; Accepted: 30 October 2023;
Published: 14 November 2023.
Edited by:
Getahun Abate, Saint Louis University, United StatesReviewed by:
Namrata Gautam, Moffitt Cancer Center, United StatesReza Elahi, Zanjan University of Medical Sciences, Iran
Copyright © 2023 Jiménez-Cortegana, Salamanca, Palazón-Carrión, Sánchez-Jiménez, Pérez-Pérez, Vilariño-García, Fuentes, Martín, Jiménez, Galván, Rodríguez-Chacón, Sánchez-Mora, Moreno-Mellado, Gutiérrez-Gutiérrez, Álvarez, Sosa, Garnacho-Montero, de la Cruz-Merino, Rodríguez-Baño and Sánchez-Margalet. This is an open-access article distributed under the terms of the Creative Commons Attribution License (CC BY). The use, distribution or reproduction in other forums is permitted, provided the original author(s) and the copyright owner(s) are credited and that the original publication in this journal is cited, in accordance with accepted academic practice. No use, distribution or reproduction is permitted which does not comply with these terms.
*Correspondence: Víctor Sánchez-Margalet, bWFyZ2FsZXRAdXMuZXM=; Jesús Rodríguez-Baño, amVzdXNyYkB1cy5lcw==