- Department of Gastroenterology and Digestive Endoscopy Center, The Second Hospital of Jilin University, Chang Chun, Jilin, China
Immune reconstitution inflammatory syndrome (IRIS) is characterized by exaggerated and dysregulated inflammatory responses that occur as a result of reconstitution of adaptive or innate immunity. A wide range of microorganisms have been found to be associated with IRIS, such as human immunodeficiency virus (HIV), Mycobacterium and actinobacteria. Whipple disease (WD) is an infectious disorder caused by the Gram-positive bacterium Tropheryma whipplei (T. whipplei) and IRIS also serves as a complication during its treament. Although many of these pathological mechanisms are shared with related inflammatory disorders, IRIS in WD exhibits distinct features and is poorly described in the medical literature. Novel investigations of the intestinal mucosal immune system have provided new insights into the pathogenesis of IRIS, elucidating the interplay between systemic and local immune responses. These insights may be used to identify monitoring tools for disease prevention and to develop treatment strategies. Therefore, this review synthesizes these new concepts in WD IRIS to approach the feasibility of manipulating host immunity and immune reconstitution of inflammatory syndromes from a newer, more comprehensive perspective and study hypothetical options for the management of WD IRIS.
1 Introduction
Whipple disease (WD) is a rare chronic disorder, which is caused by systemic infection with Tropheryma whipplei (T. whipplei) (1, 2). Studies have revealed that both the innate and adaptive mechanisms of the immune response are affected during WD, and immunosuppression, resulting in low CD4+ levels, has been identified as a risk factor for immune reconstitution inflammatory syndrome (IRIS), a phenomenon of acute immune-mediated pathology associated with the rapid reversal of immunosuppression, which has been described in association with HIV infection (3). In WD IRIS, non-specific T-helper 1(Th1) reconstitution and the outbreak of its response cytokines have been clearly delineated, and a consensus regarding the pathological mechanisms has been reached (4). Recent research demonstrated that the increased and synergistic action of tumor necrosis factor-a (TNF-a) and interferon-γ (IFN-γ) causes changes in epithelial apoptosis and tight junction (TJ) proteins which maintain intestinal mucosal epithelial barrier function, and the above changes can be measured using serum markers of microbial translocation (MT) (5). Clinical symptoms range from fever to death (4). Corticosteroids may be effective for some symptoms and currently recommended as a first-line treatment, thalidomide is more effective than corticosteroids in managing the early exaggerated immune response in IRIS (6, 7). It is important to identify the mechanisms underlying the progression of WD IRIS in order to provide a theoretical basis for the timely detection and limitation or avoidance of immune reconstitution. This review examines the pathogenesis and clinical presentation of WD IRIS and discusses potential strategies for disease monitoring and management.
2 Prevalence and case definitions of WD
WD is a rare and multisystemic chronic infectious disease with an estimated prevalence ranging 3/1000000 in Italy to 9.8/1000000 in the United States (8, 9). Previous literature also describes an incidence rate of 1 in a million and a susceptibility of middle-aged white males (10). A large sample size in the United States study showed that: it affects males and females at similar rates and is more common in Caucasians, non-Hispanics, and people > 65 of age (9). This difference is likely due to sample size and diversity in case series design. HLA-DRB1*13 and DQB1*06 class II alleles were significantly more common in patients with WD. In particular, HLA-DRB1*13, DQB1*06, and DRB1*15 are associated with classic WD (CWD) (11). CWD is the most commonly diagnosed form of WD, with an incidence of less than 1 in a million. CWD primarily affects the gastrointestinal tract, causing primary symptoms of diarrhea in approximately 70-80% of cases (12–14). Some in-depth studies on the intestinal barrier of WD complicated with IRIS are based on this type, which will be introduced in detail in the following. Localized WD (LWD) is one or more extraintestinal organs in the absence of the gastrointestinal tract, predominantly endocarditis and encephalitis. Furthermore, the extraenteric WD variants with cardiac and neurologic spread of infection are characterized by HLA-B*51 and B*44 class I alleles (11). The acute infection is considered to be the first contact with the bacterium. It has been observed in gastroenteritis, fever, or pneumonia mainly in children. Because of its self-limiting or transient nature, it is also called “transient WD” (TWD). Asymptomatic WD (AWD) has been described to occur in healthy carriers. The prevalence of AWD varies with geographic location, ranging from 2% to 4% in Europe and up to 75% in Senegal (15–17). Human leukocyte antigen-G (HLA-G), a non-classical HLA molecule with immunotolerogenic activity, has been found to have increased transcripts in the whole blood of patients with T. whipplei infection compared to controls and asymptomatic carriers. It may play a role in the pathogenesis of T. whipplei infection by mediating immunotolerance (18).
3 Definition of IRIS
Immune reconstitution inflammatory syndrome (IRIS) was proposed by Shelburne et al. in 2002 as “a paradoxical deterioration in clinical status attributable to the recovery of the immune system during highly active antiretroviral therapy.” (19) WD can present with a broad range of signs and symptoms, often leading to misdiagnosis (20). The diagnostic criteria for WD require two out of the following three tests to yield positive results: periodic acid-Schiff (PAS) staining, detection of T. whipplei by polymerase chain reaction (PCR), or immunohistochemistry. The gold standard diagnostic test is small bowel biopsy, and the classic histological presentation is PAS-positive foamy macrophages within the lamina propria (21). In WD IRIS, because the PCR shows negative results for T. whipplei and antibiotics are usually ineffective, re-inflammation is generally a complication of WD rather than a relapse of WD itself (22, 23). A 1.4% mortality rate has been reported (19). Furthermore, when IRIS is a common complication in patients starting antiretroviral therapy, underdiagnosis in resource-limited settings would contribute to high early mortality (24). Because of the wide variation in the clinical presentation, establishing a consensus on the definition of IRIS remains challenging (25).
4 Epidemiology and clinical progression of IRIS
A cohort study published in 2010 revealed that IRIS was detected in approximately 10% of individuals with WD while the prevalence of WD IRIS was twice as high in a study conducted at a tertiary referral center for WD in northern Italy (4, 22). However, there was no evidence that the difference in the prevalence of IRIS in patients with WD was associated with ethnicity (4). Risk factors identified for the development of WD IRIS include age between 40 and 60 years, Caucasian ethnicity, and a history of treatment with immunosuppressive therapy for joint pain, which is considered an inflammatory rheumatoid disorder (26). Other significant predictors included a lower baseline CD4+ T-cell percentage (19). WD is often misdiagnosed, with up to 15% of patients with WD presenting with non-specific symptoms; thus, the diagnosis is often missed or significantly delayed (27). WD is often diagnosed at a late stage once obvious signs have appeared (26). For example, weight loss and diarrhea, which typically occur up to 6 years before a definitive diagnosis of WD is made, although this duration is shorter in cases with immunosuppression (28). Determining the onset of IRIS and establishing the course of the disease is complicated by the fact that the symptoms of IRIS may develop gradually as the clinical symptoms of WD slowly disappear during the first weeks of successful antimicrobial treatment. Therefore, there may be an interval when the symptoms of IRIS and WD overlap (4, 29). Not all case reports clearly delineate WD and IRIS, and IRIS is sometimes incorrectly interpreted as an undertreatment of the primary disease or as a relapse (30). The diagnosis of IRIS relies on clinical judgment and requires careful scrutiny of the signs and symptoms over time and a high index of suspicion for this rare complication of WD.
5 Pathogenesis of WD and IRIS
5.1 WD displays an anergic and hyporesponsive state
Infection with T. whipplei results in atypical activation of bone marrow-derived macrophages (BMDMs) with M2 polarization which associated with Th2 response and type I interferon (IFN) response (31). The impaired T. whipplei-specific Th1 reactivity also plays an important pathogenetic role (32, 33). The combined effect of the above cause persistent immunological unresponsiveness and cytokine-induced activation of regulatory cells (Tregs) which may be attributed to a peculiar genetic polymorphism in cytokine genes (34).
Macrophage polarization is a dynamic process through which macrophages acquire specific features, including M1 and M2 polarization (35). T. whipplei-stimulated macrophages and duodenal tissues acquire phenotypes of M2/alternatively activated macrophages (36) which are linked to the persistence of bacterial pathogens in tissues and the chronic evolution of infectious diseases (37). Live T. whipplei also induces a strong type I IFN response, which is essential for bacterial pathogenicity, and induces c-Jun N-terminal kinase (JNK) phosphorylation to promote macrophage apoptosis. The JNK-independent intracellular replication of T. whipplei (compatible with M2) is also associated with type I IFN response (31). The crosstalk between type I and type II IFNs affects the host susceptibility to bacterial infection, and α/β IFNs downregulate the expression of IFN-γ receptors (38). As the production of IFN-γ and interleukin-12 (IL-12) is closely related (3), the reduction in IFN-γ may also result from reduced IL-12 production from antigen-presenting cells, which reveal an immature myeloid dendritic cell (M-DC) phenotype, supporting the systemic distribution of T. whipplei and perpetuating chronic infection (39) in all relevant tissues. As a result, marked reduction in the local inflammatory response has been reported (40).
The reduced T. whipplei-specific Th1 response in patients with WD (32) also leads to reduced production of IFN-γ in both peripheral blood and the lamina propria of the duodenum (36). Imperceptive IFN-γ production is thought to be critical in the pathophysiology of WD (33). In addition to inducing T. whipplei clearance by upregulating the small GTPase activity required for phagosome conversion and inducing the formation of phagosomes into phagolysosomes, IFN-γ also suppresses the production of IL-16, which inhibits the fusion of T. whipplei phagosomes with lysosomes. This is achieved by regulating the protein level of cathepsin D in macrophages and upregulating the expression of auto- and pro-apoptotic genes and immunomodulatory genes, such as IL-10 and transforming growth factor (TGF-β) (41). In the presence of TGF-β, precursor CD4+ T-cells differentiate towards regulatory cells (Tregs) characterized by the expression of foxhead box P3+ (FOXP3+) which express specific anti-inflammatory cytokines such as IL-10 and TGF-β (42). This is consistent with the immunopathological observations in WD. Patients with CWD have higher peripheral blood Tregs activation and increased duodenal IL-10 and TGF-β secretion (43). IL-16 also induces the recruitment and differentiation of CD4-expressing cells into tolerogenic cells (3). This contribution to local immune tolerance is also enhanced by a membrane or soluble HLA-G by inhibiting TNF expression (18).
5.2 IRIS is a rapid reversal of immunosuppression
The more frequently discussed questions about the pathogenesis of IRIS focus on the degree of immunosuppression resulting from the combined effects of the underlying antigenic burden and patient drug history. IRIS is ascribed to the activation of a non-specific Th1 response underlying the lower CD4+ T cell baseline coupled with reconstituted Treg inadequacy (44). The above characteristics can lead to barrier dysfunction, which can be the cause of prolonged microbial translocation (MT). The resulting entry of endotoxin into the bloodstream can lead to immune reconstitution disorders with systemic immune activation in IRIS CWD (5).
In summary, WD induces a combined effect in which the immune system is suppressed, leading to a high antigen load in affected local tissues. Furthermore, up to 50% patients with classic Whipple’s disease are initially misdiagnosed and treated with immunosuppressive drugs, such as disease-modifying antirheumatic drugs (DMARDs), anti-necrosis factor alpha, glucocorticoids, or drugs, with potentially fatal consequences (16). When treatment with these drugs is discontinued, inflammatory signs may rebound (4), and the degree of CD4+ T cells in the peripheral blood reduction increases with the time of immunosuppressant application (44).
Tumor necrosis factor inhibitors (TNFIs) may reduce phagolysosome fusion, resulting in increased intracellular replication and macrophage apoptosis (45, 46). Susceptibility to IRIS depends primarily on the absence of TNF-signaling (47). Moreover, antimicrobials per se can act as proinflammatory stimulants or upregulate Th1 responses (48). It has been shown that IFN-γ production by CD4+ T cells in response to staphylococcal enterotoxin B (SEB) increases significantly in patients with WD developing IRIS during antibiotic treatment but remains low in treated patients without IRIS (44). Antibiotics not only induce dysfunction of the intestinal epithelial tight junction (TJ) barrier but are also associated with dysbiosis of the intestinal microbiota (49).
Crossing of the defective mucosal barrier by gut microbiota was also demonstrated in a study evaluating pathological damage to the small intestinal mucosa in patients with CWD. The study revealed that elevated levels of lipopolysaccharide-binding protein (LBP), lipopolysaccharide (LPS), and sCD14, definitive alternative markers of increased intestinal permeability and MT, provide direct and indirect evidence of this invasion (50). The link between barrier defects in the intestinal mucosa and systemic immune activation in patients with WD developing IRIS has been further investigated and characterized as a storm in TNF-a expression (25), with TNF-a triggering the NF-κB signaling pathway. This pathway is also an index of inflammation and is synergized by the expression of MLCK activated by IFN-γ, an event that is necessary to promote redistribution of tight junction proteins and paracellular permeability (51). However, the disruption of TJs often leads to increased intestinal permeability, a pathological state termed “leaky gut syndrome” (LGS) (52). The leaky intestinal barrier enhances microbial translocation, which can be predicted by markers as described above.
Moreover, very recent studies have shown that endotoxaemic episode could be a potential mediator of dysbalanced T cell reconstitution (5). The diverse reservoir of LPS is the gut microbiota, on which LPS depends as a stimulator of the host immune response and as a promoter of pro-inflammatory cytokine secretion (53). The pathologic mechanism leads to an imbalance in T-cell recruitment and inflammatory activation in CWD IRIS, with a positive correlation with sCD14 levels and a negative correlation with the antibody (EndoCAb) titre (5). Therefore, monitoring of inflammatory and microbial translocation markers in patients with WD may be helpful to identify patients who are at risk of developing IRIS and preventing misdiagnosis of treatment failure due to the recurrence of inflammation. However, this intervention needs to be investigated in future studies. Figure 1 compares the brief process of CWD with CWD IRIS.
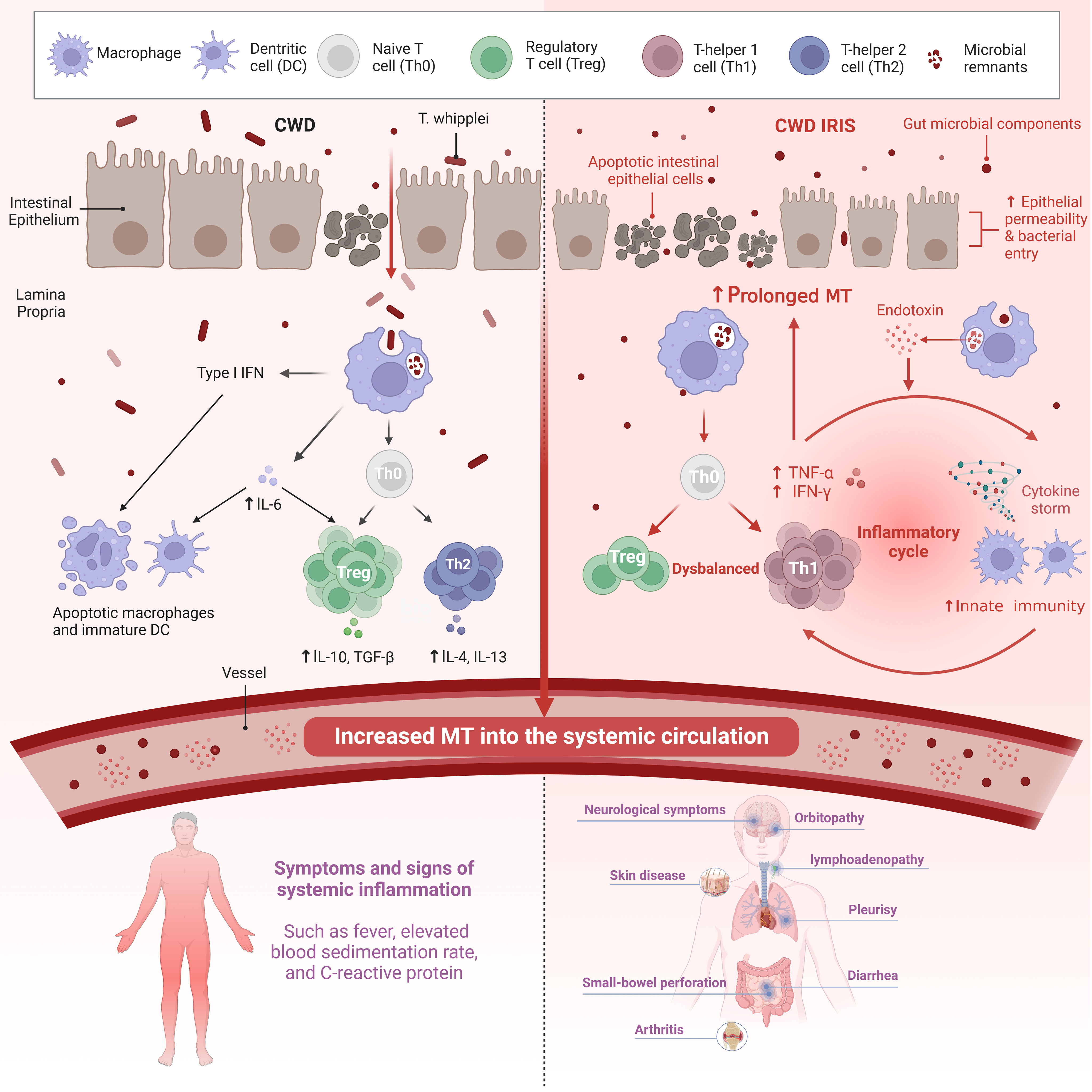
Figure 1 Both the innate and adaptive arms of the immune response are affected in CWD. There is also intestinal epithelial permeability caused by the Th2 cytokine IL-13 and macrophage-derived TNF-a. Increased translocation of gut microbial components across a defective mucosal barrier is an important stimulator of systemic inflammation in CWD. Systemic LPS levels indicate persistent abnormalities in CWD patients independent of active T. whipplei infection. In contrast, patients with WD IRIS had more severe damage to the intestinal mucosa, resulting in prolonged MT. Microbial products (in addition to T. whipplei) and other bacteria that enter the LP also due to impaired barrier function facilitate intestinal pathology and systemic immune response. However, the mechanism of non-specific T cell reconstitution remains unclear. Although IRIS is an immune overactivation that occurs in the context of a high bacterial load due to immunosuppression, it is driven by non-pathogens. A high systemic antigenic load may trigger inflammation in IRIS-related T cell repopulation, of which an endotoxemic episode may be a potential mediator. Recently, innate immunity has been implicated in IRIS, which may be mediated by endotoxin co-stimulation with non-specific T cell reconstitution. The coupling of innate immunity with adaptive immunity may be responsible for the reconstitution of activated T cells in patients with IRIS. CWD, Classic Whipple disease; IRIS, Immune reconstitution inflammatory syndrome; MT, microbial translocation; IFN, Interferon; TNF-a, Tumor necrosis factor-a; IL, Interleukin; TGF-β, Transforming growth factor. Created with BioRender.com.
6 Clinical and pathological manifestations
IRIS in WD usually presents as fever and arthralgia and may progress for several months. The primary pathogenic load area is the duodenal mucosa, with the organs outside of the intestines also posing a concern (44). Besides ocular and skin tissue. IRIS can also affect the lymph nodes, lungs, and central nervous system, as it gives rise to a heterogeneous range of clinical manifestations (57). A common feature when biopsies are obtained from lesions is that local CD4+ T-cell infiltration is typically observed, resulting in varying degrees of tissue destruction. A PCR for T. whipplei is usually negative, even if there might be a positive signal from remnants of dead bacteria (12). The reason is that the exaggerated immune response of WD IRIS is driven by pathogen-independent mechanisms (44). There were two articles that counted the clinical manifestations of WD IRIS. The prevalence of IRIS was not statistically different from each other and the overall mortality rate was 10.5% (4, 22).
6.1 Fever
Fever is described as a sudden inflammatory response in IRIS in non-HIV patients and can also be caused by an infectious agent (54). Fever is also a typical symptom of WD. Fever that resolves after 48 hours and does not recur is not considered to be IRIS in 80%-86.7% of cases (4, 22). The administration of oral corticosteroids for fever generally shows a response within 24 hours (4).
6.2 Arthritis
The incidence of arthritis is high, ranging from almost 93.3% to 100% (4, 22). The investigation of WD-associated arthropathy has been the focus of considerable research. In 1907, Whipple, the pathologist who first described the disease now bearing his name, noted the characteristics of joint involvement which was a part of the clinical manifestations of the first patient with the condition. In 2013, Krol and de Meijer entitled their letter to the editor “Palindromic rheumatism: consider Whipple’s disease.” (27) In 2021, logistic regression analysis was conducted to distinguish WD disease from rheumatoid arthritis, axial spondyloarthritis, psoriatic arthritis, and palindromic arthritis (55). Despite the fact that joint involvement is a general manifestation of WD IRIS, there has been limited research on this subject with some reports in the literature describing the condition as “recurrent arthritis.” (4).
6.3 Orbitopathy
Orbitopathy refers to an inflammatory orbital disease with symptoms, including orbital pain, pseudotumors of the eye socket, proptosis, diplopia, and vision loss after hormonal treatment, accounting for 26.7% (4). Unilateral and bilateral orbitopathy has also been reported but is very rare, and biopsy of the superior and lateral rectus extraocular muscles showed CD68-positive macrophages and occasional CD3-positive T cells between the muscle fibers (30). The observed ocular impairment in WD highlights the fact that antibiotics and steroids therapy should not be administered alone but in combination with other measures, such as ocular decompression to prevent ocular damage (56).
6.4 Skin disease
Skin disease occurred after WD treatment in 13.3% of cases (4). Subcutaneous erythematous nodules are the main cutaneous manifestations of IRIS in the treatment of WD; these are rare and are most commonly found on the lower limbs, but can also be found on the trunk and wrists (26, 57, 58). Inflammatory reactive dermatoses, which can occur at all stages of WD, including erythema nodosum (EN)-like lesions after antibiotic treatment, are distinct from dystrophic dermatoses, which can be improved with antibiotic treatment but occur only in the late stages of WD. EN-like lesions are a manifestation of high T. whipplei burden and have more common clinical, pathological, and immunological features than erythema nodosum leprosum (ENL) (57). IRIS in WD manifests as an ENL. The development of EN-like lesions after antibiotic therapy is also probably caused by lymphangiectasias and immune reconstitution secondary to considerable decrease in the number of viable replicating T. whipplei (59).
6.5 Small-bowel perforation
Patients with WD primarily affecting the muscular layer of the small-bowel wall may be at a risk of small-bowel perforation once IRIS develops. This incidence is the same as for skin diseases (4). This may be related to increased intestinal permeability caused by increased apoptosis of small intestinal mucosal epithelial cells and shorter villi length (5). This risk cannot be excluded with the use of oral steroid hormones. Evidence indicates that T. whipplei mainly infiltrates the submucosa or myenteric layer of the small intestine as PAS-positive material in macrophages containing T. whipplei remnants, and PCR for T. whipplei performed on biopsies shows negative results; this results in disruption to the integrity of the myenteric layer, and the infiltrate consists mainly of CD4+ T cells with a small number of CD8+ T cells (44).
6.6 Diarrhea and associated microscopic manifestations and laboratory tests
Diarrhea was not previously considered a symptom of WD IRIS (4), although some studies list diarrhea as a clinical presentation in patients with WD IRIS (4, 22, 44). Mounting evidence suggests that the reduced duodenal villus length and microbial translocation observed in patients with IRIS affect barrier dysfunction (5, 44) which may contribute to intestinal barrier leakage and gut microbiota and is another vital causative element of autoimmune disorders (60).
6.6.1 Altered epithelial barrier function
Although one hypothesis is that enteric T. whipplei does not directly cause diarrhea; rather, it might result from different sanitary and climatic conditions (61), and restrictive structural alignments of the intestinal mucosal barrier were observed. Diarrhea in patients with CWD is associated with the dysfunction and integrity of the intestinal mucosal epithelium (50). Duodenal villus length was shorter in patients with IRIS than in those with non-IRIS CWD both before and after treatment and displayed a lower regenerative potential of the small intestinal mucosa during the course of the disease, as exemplified by an increase in the number of apoptotic epithelial cells, reinforced by a persistently low proliferation rate in the crypt. This situation did not improve even after targeting with T. whipplei antimicrobials, whereas villus length was restored in patients with CWD, which seemed to be sufficient to achieve clinical remission (5).
6.6.2 The barrier dysfunction of the intestinal mucosa and systemic immune activation
The role of the inflammatory cytokines that can disrupt barrier function in IRIS was further investigated. TNF-α reduced the percentage of G2/M phase cells and IFN-γ treatment increased the apoptotic rate. Studies in cattle revealed their common role in directly disrupting the intestinal epithelial barrier (62). The intestinal mucosa of patients with CWD who later develop IRIS has a lower number of Tregs, which might involved in local inflammations. The local inflammations disrupts the integrity of the intestinal mucosal barrier and allows the translocation of luminal antigens displacement compared with patients with non-IRIS CWD (44). In a CWD research subject, gut mucosal barrier dysfunction has been shown to contribute to a systemic immune response, as reflected by an increased erythrocyte sedimentation rate, elevated C-reactive protein, and leukocytosis (50). However, the inflammatory activation observed in patients with colitis, which is accompanied by increase in IFN-γ, leads to colonic permeability, suggesting that it is only an early event that contributes to barrier dysfunction but is not sufficient to cause clinical symptoms (51). This may be one of the reasons why extraintestinal disease is prevalent and can present without any gastrointestinal signs (63).
The rates of diarrhea and weight loss differed significantly between different studies. According to the German article, the incidence of both was 33.3%, whereas the incidence in the Italian study was 100% and 80%, respectively. All participants in the Italian study had received immunosuppressive treatment, compared with 80% in the German article. Previous studies have shown that immunosuppressive therapy is associated with the emergence of diarrhea (64). Nevertheless, diarrhea in WD IRIS requires further investigation. Other clinical manifestations include pleurisy, which responds well to oral prednisone, with an incidence of approximately 13.3%. The incidence of lymphoadenopathy disease in the German and Italian studies was 46.7% and 80%, respectively. Neurological symptoms account for nearly 70% to 80% (4, 22).
7 Diagnosis of IRIS
The following criteria apply to IRIS in WD: 1) an initial clinical response of symptoms to antimicrobial treatment (cessation of diarrhea and fever, relief of arthritis, and normalization of the C-reactive protein level) within 3 weeks of treatment; 2) recurrence of systemic or local inflammation, with or without fever, lasting more than 1 week, after exclusion of hospital-related conditions; and 3) exclusion of WD recurrence based on histological examination and a negative PCR result for T. whipplei despite IRIS manifestation. A diagnosis of IRIS requires that all three criteria are met (4, 22).
PCR does not always differentiate between refractory WD and IRIS at the early stage of treatment, with a short interval between the initiation of antibiotic administration and the emergence of IRIS symptoms (26). There are no published data on the exact time interval for PCR conversion to negative findings following the initiation of antimicrobial therapy, as positive PCR outcomes may also be ascribed to the persistence of inactive pathogens (71) including dead and dying organisms and their residual antigens (12). This highlights the importance of carefully considering the clinical symptoms and signs in order to avoid delays in the management of IRIS.
8 When to suspect IRIS in WD
8.1 Previous medication regimen and clinical manifestation
As there is no established laboratory test for WD IRIS, the diagnosis is made by clinical observation (65). The immune response to WD IRIS most frequently occurs in patients previously treated with immunosuppressive drugs, such as analgesics, nonsteroidal anti-inflammatory drugs, steroid hormones, and TNFI, for misdiagnosed inflammatory rheumatism (66). Therefore, it is important to be aware of the possibility of IRIS in patients with WD and a history of any of the above medications. It is also important to consider that steroids suppress inflammatory reactions, and for all patients who present with fever or other inflammatory symptoms after the initiation of treatment for WD of no apparent origin, IRIS should be considered, and appropriate management should be instituted (67). In addition, in HIV patients treated with antiretroviral therapy (ART), ENL skin and eye socket pseudotumors that appeared during the first year of antibiotic therapy were all indications of IRIS. Despite treatment, patients with WD may still develop IRIS as the similar underlying pathology is still present. ENL skin is symbolic of a shift to Th1-mediated inflammation (57).
8.2 The levels of biomarkers or other laboratory tests
Compromised intestinal immunity with increased microbial translocation into the systemic circulation has been discussed as a mechanism of immune stimulation in IRIS. Intestinal epithelial dysfunction suggests the need for future research to investigate the length and regenerative potential of the small intestinal mucosa and to monitor inflammation and the MT markers discussed above in patients with CWD (5, 50). In CWD IRIS, reduced duodenal villus length and cytokines produced by non-specific Th1-responses lead to mucosal barrier dysfunction and leaky gut syndrome, which can be reflected by serum markers such as LBP, LPS and sCD14. Local immune activation is reflected by elevated levels of IL-6, CCL2, CCL5, CX3CL1 in the duodenal mucosa. In addition to cytokines, the combination of cerebrospinal fluid IFN-γ and TNF-α concentrations provided a predictive model for tuberculous meningitis (TBM)-IRIS, it has guiding significance for diagnosis and treatment (68). This is what WD IRIS is missing. Only when the auxiliary test is more perfect, the diagnostic prediction model is more likely to be established. In summary, further prospective studies are needed to generate robust evidence on WD IRIS.
9 Treatment
No trials have provided conclusive evidence on the optimal treatment of WD IRIS. Current management involves the empirical use of glucocorticoids, although these have been reported to be associated with a number of resistant events, and thalidomide, which is currently recommended as the first-line agent (25, 45).
9.1 Corticosteroids
The use of corticosteroids as immunosuppressants to treat seronegative arthritis in patients with no clear diagnosis of WD can exacerbate the disease and trigger progression to IRIS (69, 70). When antibiotic therapy fails or the diagnosis of IRIS is clear, oral corticosteroids are generally effective, and several case reports have indicated that oral corticosteroids can alleviate severe symptoms such as fever and subcutaneous nodules and reduce the levels of inflammatory markers such as C-reactive protein (26, 57, 71). However, with the exception of the use of corticosteroids in TB-IRIS (71), current treatment for IRIS is not evidence-based. Moreover, studies on TB-associated IRIS and IRIS associated with HIV infection have generated conflicting evidence regarding therapeutic benefits and appropriate doses of corticosteroids in terms of prevention and mortality rates (72–75). Effective treatment has been reported following the empirical administration of corticosteroids without distinguishing between refractory WD and WD IRIS (30, 56). Corticosteroid treatment for IRIS involves risks and benefits, and it is important to develop more effective and safer drugs (72).
9.2 Thalidomide and infliximab
Thalidomide has been successfully used in a case series and has been proposed as the first-line management for IRIS (76, 77) particularly in the presence of ENL reactions (25, 26). Experiments to investigate the mechanisms underlying the effectiveness of thalidomide have not been conducted, and it is speculated to achieve its effects by downregulating TNF-a expression (70). Successful cases of infliximab treatment for IRIS caused by mycobacteria have also been reported (78). However, in all cases, infliximab was used for the treatment of WD before progression to IRIS. This resulted in disease exacerbation with a negative PCR assay result for T. whipplei, which does not exclude the fact that infliximab may contribute to progression to IRIS (79, 80).
10 Discussion
WD or CWD is characterized by two stages. The first or prodromal stage is marked by protean symptoms, but notably arthralgias/arthritis and fatigue. The second or classic systemic/gastrointestinal stage is marked by diarrhea, abdominal pain, weight loss, and may include other systemic manifestations, such as fever, lymphadenopathy, anemia, skin pigmentation, and bone involvement (14). The two stages may be close, especially in cases on immunosuppressive therapy, in which aggravation occurs on an average of 26 months after immunosuppressive treatment initiation (1, 76).The clinical manifestations of unresolved WD and new-onset IRIS can overlap, and some signs and symptoms are caused by host immune responses rather than by infectious agents (81).
Although the underlying pathological mechanisms of exacerbated T-cell activation in WD IRIS remain unclear, cytokine storm and symptoms of diarrhea have been reported. WD IRIS is characterized by the overproduction of cytokines, and innate and adaptive immune reinforcement of this process enhances the persistence of LPS, sCD14, and LBP. LBP catalyzes the transfer of LPS to the membrane or soluble CD14 (sCD14), leading to NF-κB activation and cytokine release, which, in turn, promote abnormal immune activation. The pathology of MT in WD IRIS has been clearly demonstrated to be similar to IRIS in HIV. Thus, further research is warranted to determine the extent and mechanisms of MT involvement in IRIS, the probability of causing diarrhea in the course of leaky intestine, and the specific process responsible for the massive production of non-specific CD4+ T cells (82).
Regulatory T lymphocytes are essential for maintaining the homeostasis of the immune system, limiting the magnitude of effector responses and allowing the establishment of immunological tolerance (83). Therapeutic strategies aimed at reconstituting the mucosal barrier and controlling exacerbated inflammation may assist in the prevention of IRIS (5). In addition to thymic-derived Treg cells, the intestine is a preferential site for dependent induction of FOXP3+ Treg cells from naive CD4+ T-cell precursors (84). Recently, considerable efficacy has been achieved with immune tolerance therapies in animal and human models, and partial application to TB-IRIS has been reported; however, targeted experiments are required to determine whether these approaches can be replicated in non-specific T-cell-reconstituted WD IRIS (85). Promising results have been obtained in human Treg adoptive transfer therapy and in animal models of autoimmune diseases using biologicals that increase Treg numbers in vitro, including IL-2/anti-IL-2 complexes and rapamycin (86). However, the purification and expansion of Tregs remain problematic because of the lack of specific molecular markers, and Tregs employ several independent mechanisms to prevent different pathological immune responses, presenting both opportunities and challenges in the development of new therapies (87).
The body of knowledge regarding WD IRIS remains to be established, and the complete mechanism remains to be elucidated. Local intestinal responses are becoming increasingly important in systemic immune activation. We can focus on the mucosal immune system. For example, the role of gut-associated lymphoid tissue (GALT) and Peyer’s patch in the adaptive immune response; the mechanism by which T. whipplei is taken up from the intestinal lumen and whether it involves microfold cells; and the immunomodulatory role of the gut epithelium as an intestinal effector site in addition to the lamina propria. These need to be further investigated in WD IRIS. With respect to diagnosis and monitoring, a detailed analysis measuring the levels of immune response parameters in conjunction with markers of effector cell activation should be performed to more accurately predict the prevalence, severity, and prognosis of WD IRIS.
Author contributions
LW: Funding acquisition, Writing – review & editing. XS: Visualization, Writing – original draft. RD: Supervision, Writing – review & editing. LD: Writing – review & editing.
Funding
The authors declare financial support was received for the research, authorship, and/or publication of this article. This work was supported by Jilin Provincial Natural Science Foundation (YDZJ202301ZYTS477).
Acknowledgments
The successful completion of this article is especially thanks to the support of the corresponding author, L-JW. In addition, Dr. R-FD’s revision and review of the article were also decisive for the article.
Conflict of interest
The authors declare that the research was conducted in the absence of any commercial or financial relationships that could be construed as a potential conflict of interest.
Publisher’s note
All claims expressed in this article are solely those of the authors and do not necessarily represent those of their affiliated organizations, or those of the publisher, the editors and the reviewers. Any product that may be evaluated in this article, or claim that may be made by its manufacturer, is not guaranteed or endorsed by the publisher.
References
1. Puéchal X. Whipple's disease. Ann Rheumatic Diseases (2013) 72:797–803. doi: 10.1136/annrheumdis-2012-202684
2. Ferrieres L, Laroche M, Bonnet D, Alric L. Whipple’s disease: diagnosis and predictive factors of relapse. Eur J Gastroenterol Hepatol (2020) 32:325–8. doi: 10.1097/MEG.0000000000001611
3. Boumaza A, Ben Azzouz E, Arrindell J, Lepidi H, Mezouar S, Desnues B. Whipple's disease and Tropheryma whipplei infections: from bench to bedside. Lancet Infect Diseases (2022) 22:e280–e91. doi: 10.1016/S1473-3099(22)00128-1
4. Feurle GE, Moos V, Schinnerling K, Geelhaar A, Allers K, Biagi F, et al. The immune reconstitution inflammatory syndrome in whipple disease: A cohort study. Ann Internal Med (2010) 153:710. doi: 10.7326/0003-4819-153-11-201012070-00004
5. Friebel J, Schinnerling K, Geelhaar-Karsch A, Allers K, Schneider T, Moos V. Intestinal barrier dysfunction mediates Whipple's disease immune reconstitution inflammatory syndrome (IRIS). Immunity Inflammation Disease (2022) 10:e622. doi: 10.1002/iid3.622
6. Lagier J-C, Fenollar F, Raoult D. Current opinion in infectious diseases. La Rev Médecine Interne (2014) 35:801–7. doi: 10.1016/j.revmed.2014.04.016
7. Lagier J-C, Fenollar F, Lepidi H, Giorgi R, Million M, Raoult D. Treatment of classic Whipple's disease: from in vitro results to clinical outcome. J Antimicrobial Chemother (2014) 69:219–27. doi: 10.1093/jac/dkt310
8. Biagi F, Balduzzi D, Delvino P, Schiepatti A, Klersy C, Corazza GR. Prevalence of Whipple's disease in north-western Italy. Eur J Clin Microbiol Infect Diseases (2015) 34:1347–8. doi: 10.1007/s10096-015-2357-2
9. Elchert JA, Mansoor E, Abou-Saleh M, Cooper GS. Epidemiology of whipple’s disease in the USA between 2012 and 2017: A population-based national study. Digestive Dis Sci (2019) 64:1305–11. doi: 10.1007/s10620-018-5393-9
10. Schneider T, Moos V, Loddenkemper C, Marth T, Fenollar F, Raoult D. Whipple's disease: new aspects of pathogenesis and treatment. Lancet Infect Diseases (2008) 8:179–90. doi: 10.1016/s1473-3099(08)70042-2
11. Martinetti M, Biagi F, Badulli C, Feurle GE, Müller C, Moos V, et al. The HLA alleles DRB1*13 and DQB1*06 are associated to whipple's disease. Gastroenterol (2009) 136:2289–94. doi: 10.1053/j.gastro.2009.01.051
12. Moos V, Schneider T. Changing paradigms in Whipple’s disease and infection with Tropheryma whipplei. Eur J Clin Microbiol Infect Diseases (2011) 30:1151–8. doi: 10.1007/s10096-011-1209-y
13. Lagier J-C, Lepidi H, Raoult D, Fenollar F. Systemic tropheryma whipplei. Medicine (2010) 89:337–45. doi: 10.1097/MD.0b013e3181f204a8
14. El-Abassi R, Soliman MY, Williams F, England JD. Whipple's disease. J Neurological Sci (2017) 377:197–206. doi: 10.1016/j.jns.2017.01.048
15. Marth T. Whipple’s disease. Acta Clinica Belgica (2016) 71:373–8. doi: 10.1080/17843286.2016.1256586
16. Dolmans RAV, Boel CHE, Lacle MM, Kusters JG. Clinical manifestations, treatment, and diagnosis of tropheryma whipplei infections. Clin Microbiol Rev (2017) 30:529–55. doi: 10.1128/CMR.00033-16
17. Edouard S, Luciani L, Lagier J-C, Raoult D. Current knowledge for the microbiological diagnosis of Tropheryma whipplei infection. Expert Opin Orphan Drugs (2020) 8:237–44. doi: 10.1080/21678707.2020.1791700
18. Ben Azzouz E, Boumaza A, Mezouar S, Bardou M, Carlini F, Picard C, et al. Tropheryma whipplei increases expression of human leukocyte antigen-G on monocytes to reduce tumor necrosis factor and promote bacterial replication. Gastroenterol (2018) 155:1553–63. doi: 10.1053/j.gastro.2018.07.034
19. Nelson AM, Manabe YC, Lucas SB. Immune Reconstitution Inflammatory Syndrome (IRIS): What pathologists should know. Semin Diagn Pathol (2017) 34:340–51. doi: 10.1053/j.semdp.2017.04.010
20. Manini A, Querzola G, Lovati C, Pantoni L. Rapidly progressive dementia and intractable diarrhea: a teaching case report and a systematic review of cognitive impairment in Whipple’s disease. Neurological Sci (2022) 43:907–26. doi: 10.1007/s10072-021-05844-5
21. Thornton CS, Wang Y, Köebel M, Bernard K, Burdz T, Maitland A, et al. Another Whipple’s triad? Pericardial, myocardial and valvular disease in an unusual case presentation from a Canadian perspective. BMC Cardiovasc Disord (2019) 19:312. doi: 10.1186/s12872-019-1257-2
22. Biagi F, Trotta L, Di Stefano M, Balduzzi D, Marchese A, Vattiato C, et al. Previous immunosuppressive therapy is a risk factor for immune reconstitution inflammatory syndrome in Whipple's disease. Digestive Liver Disease (2012) 44:880–2. doi: 10.1016/j.dld.2012.05.008
23. Kapoor S. “Immune reconstitution inflammatory syndrome”—a rare complication of Whipple’s disease. Eur J Clin Microbiol Infect Dis (2016) 35:1045–6. doi: 10.1007/s10096-016-2626-8
24. Müller M, Wandel S, Colebunders R, Attia S, Furrer H, Egger M. Immune reconstitution inflammatory syndrome in patients starting antiretroviral therapy for HIV infection: a systematic review and meta-analysis. Lancet Infect Diseases (2010) 10:251–61. doi: 10.1016/S1473-3099(10)70026-8
25. Lagier J-C, Raoult D. Immune reconstitution inflammatory syndrome associated with bacterial infections. Expert Opin Drug Safety (2014) 13:341–50. doi: 10.1517/14740338.2014.887677
26. Sanchez A, Del Giudice P, Mantion C, Mazellier S, Boukari F, Roger P-M, et al. Erythematous skin nodules during treatment of Whipple's disease. Infect Dis Now (2021) 51:397–9. doi: 10.1016/j.medmal.2020.10.006
27. Krol CG, de Meijer PHEM. Palindromic rheumatism: consider Whipple's disease. Int J Rheumatic Diseases (2013) 16:475–6. doi: 10.1111/1756-185X.12084
28. Fenollar F, Lagier J-C, Raoult D. Tropheryma whipplei and Whipple's disease. J Infect (2014) 69:103–12. doi: 10.1016/j.jinf.2014.05.008
29. Feurle GE, Junga NS, Marth T. Efficacy of ceftriaxone or meropenem as initial therapies in whipple's disease. Gastroenterol (2010) 138:478–86. doi: 10.1053/j.gastro.2009.10.041
30. Parkash V, Mudhar HS, Wagner BE, Raoult D, Batty R, Lepidi H, et al. Bilateral ocular myositis associated with whipple's disease. Ocular Oncol Pathol (2017) 3:17–21. doi: 10.1159/000448622
31. Al Moussawi K, Ghigo E, Kalinke U, Alexopoulou L, Mege J-L, Desnues B. Type I Interferon Induction Is Detrimental during Infection with the Whipple's Disease Bacterium, Tropheryma whipplei. PloS Pathogens (2010) 6:e1000722. doi: 10.1371/journal.ppat.1000722
32. Moos V, Kunkel D, Marth T, Feurle GE, LaScola B, Ignatius R, et al. Reduced peripheral and mucosal tropheryma whipplei -specific th1 response in patients with whipple’s disease. J Immunol (2006) 177:2015–22. doi: 10.4049/jimmunol.177.3.2015
33. Marth T, Kleen N, Stallmach A, Ring S, Aziz S, Schmidt C, et al. Dysregulated peripheral and mucosal Th1/Th2 response in Whipple's disease. Gastroenterol (2002) 123:1468–77. doi: 10.1053/gast.2002.36583
34. Biagi F, Badulli C, Feurle GE, Müller C, Moos V, Schneider T, et al. Cytokine genetic profile in Whipple’s disease. Eur J Clin Microbiol Infect Dis (2012) 31:3145–50. doi: 10.1007/s10096-012-1677-8
35. Di Vincenzo S, Ferraro M, Taverna S, Malizia V, Buscetta M, Cipollina C, et al. Tyndallized bacteria preferentially induce human macrophage M1 polarization: an effect useful to balance allergic immune responses and to control infections. Antibiotics (2023) 12:571. doi: 10.3390/antibiotics12030571
36. Desnues B, Lepidi H, Raoult D, Mege JL. Whipple disease: intestinal infiltrating cells exhibit a transcriptional pattern of M2/alternatively activated macrophages. J Infect Diseases (2005) 192:1642–6. doi: 10.1086/491745
37. Benoit M, Desnues B, Mege J-L. Macrophage polarization in bacterial infections. J Immunol (2008) 181:3733–9. doi: 10.4049/jimmunol.181.6.3733
38. Mège J-L, Mehraj V, Capo C. Macrophage polarization and bacterial infections. Curr Opin Infect Diseases (2011) 24:230–4. doi: 10.1097/QCO.0b013e328344b73e
39. Schinnerling K, Geelhaar-Karsch A, Allers K, Friebel J, Conrad K, Loddenkemper C, et al. Role of dendritic cells in the pathogenesis of whipple's disease. Infect Immun (2015) 83:482–91. doi: 10.1128/IAI.02463-14
40. Lopes A, Santos AF, Alvarenga MJ, Mello e Silva A. Whipple’s disease: a rare case of malabsorption. BMJ Case Rep (2018), bcr–2017-222955. doi: 10.1136/bcr-2017-222955
41. Ghigo E, Barry AO, Pretat L, Al Moussawi K, Desnues B, Capo C, et al. IL-16 promotes T. whipplei replication by inhibiting phagosome conversion and modulating macrophage activation. PloS One (2010) 5:e13561. doi: 10.1371/journal.pone.0013561
42. Figueiredo AS, Schumacher A. The T helper type 17/regulatory T cell paradigm in pregnancy. Immunol (2016) 148:13–21. doi: 10.1111/imm.12595
43. Schinnerling K, Moos V, Geelhaar A, Allers K, Loddenkemper C, Friebel J, et al. Regulatory T cells in patients with whipple’s disease. J Immunol (2011) 187:4061–7. doi: 10.4049/jimmunol.1101349
44. Moos V, Feurle GE, Schinnerling K, Geelhaar A, Friebel J, Allers K, et al. Immunopathology of immune reconstitution inflammatory syndrome in whipple’s disease. J Immunol (2013) 190:2354–61. doi: 10.4049/jimmunol.1202171
45. Hannachi N, Arregle F, Lepidi H, Baudoin J-P, Gouriet F, Martel H, et al. A massive number of extracellular tropheryma whipplei in infective endocarditis: A case report and literature review. Front Immunol (2022) 13:900589. doi: 10.3389/fimmu.2022.900589
46. Boumaza A, Mezouar S, Bardou M, Raoult D, Mège J-L, Desnues B. Tumor necrosis factor inhibitors exacerbate whipple’s disease by reprogramming macrophage and inducing apoptosis. Front Immunol (2021) 12:667357. doi: 10.3389/fimmu.2021.667357
47. Barber DL, Andrade BB, Sereti I, Sher A. Immune reconstitution inflammatory syndrome: the trouble with immunity when you had none. Nat Rev Microbiol (2012) 10:150–6. doi: 10.1038/nrmicro2712
48. Sun H-Y, Singh N. Immune reconstitution inflammatory syndrome in non-HIV immunocompromised patients. Curr Opin Infect Diseases (2009) 22:394–402. doi: 10.1097/QCO.0b013e32832d7aff
49. Feng Y, Huang Y, Wang Y, Wang P, Song H, Wang F. Antibiotics induced intestinal tight junction barrier dysfunction is associated with microbiota dysbiosis, activated NLRP3 inflammasome and autophagy. PloS One (2019) 14:e0218384. doi: 10.1371/journal.pone.0218384
50. Epple H-J, Friebel J, Moos V, Troeger H, Krug SM, Allers K, et al. Architectural and functional alterations of the small intestinal mucosa in classical Whipple’s disease. Mucosal Immunol (2017) 10:1542–52. doi: 10.1038/mi.2017.6
51. Catalioto R-M A, Maggi C, Giuliani S. Intestinal epithelial barrier dysfunction in disease and possible therapeutical interventions. Curr Medicinal Chem (2011) 18:398–426. doi: 10.2174/092986711794839179
52. Kinashi Y, Hase K. Partners in leaky gut syndrome: intestinal dysbiosis and autoimmunity. Front Immunol (2021) 12:673708. doi: 10.3389/fimmu.2021.673708
53. Mohr AE, Crawford MS, Jasbi P, Fessler S, Sweazea KL. Lipopolysaccharide and the gut microbiota: considering structural variation. FEBS Letters (2022) 596:849–75. doi: 10.1002/1873-3468.14328
54. Kwiecień I, Rutkowska E, Sokołowski R, Bednarek J, Raniszewska A, Jahnz-Różyk K, et al. Effector memory T cells and CD45RO+ Regulatory T cells in metastatic vs. Non-metastatic lymph nodes in lung cancer patients. Front Immunol (2022) 13:864497. doi: 10.3389/fimmu.2022.864497
55. Feurle GE, Moos V, Stroux A, Gehrmann-Sommer N, Poddubnyy D, Fiehn C, et al. Differential diagnostic value of rheumatic symptoms in patients with Whipple’s disease. Sci Rep (2021) 11:5980. doi: 10.1038/s41598-021-85217-2
56. Lieger O, Otto S, Clemetson IA, Arnold M, Iizuka T. Orbital manifestation of whipple's disease: An atypical case. J Cranio-Maxillofacial Surg (2007) 35:393–6. doi: 10.1016/j.jcms.2007.03.005
57. Schaller J, Carlson JA. Erythema nodosum–like lesions in treated Whipple's disease: Signs of immune reconstitution inflammatory syndrome. J Am Acad Dermatol (2009) 60:277–88. doi: 10.1016/j.jaad.2008.09.024
58. Paul J, Schaller J, Rohwedder A, Carlson JA. Treated whipple disease with erythema nodosum leprosum–like lesions: cutaneous PAS-positive macrophages slowly decrease with time and are associated with lymphangiectases: A case report. Am J Dermatopathol (2012) 34:6. doi: 10.1097/DAD.0b013e318221ba55
59. Liersch J, Carlotti A, Theunis A, Leonard A, Barrett M, Carlson JA, et al. Erythema nodosum leprosum–like lesions are a histopathologic pattern in whipple's disease and a sign of the immune reconstitution inflammatory syndrome: A case series and review of the literature. Am J Dermatopathol (2017) 39:259–66. doi: 10.1097/DAD.0000000000000641
60. Luo XM. Leaky gut as a danger signal for autoimmune diseases. Front Immunol (2017) 8. doi: 10.3389/fimmu.2017.00598
61. Feurle GE, Moos V, Landt O, Corcoran C, Reischl U, Maiwald M. Tropheryma whipplei in feces of patients with diarrhea in 3 locations on different continents. Emerging Infect Diseases (2021) 27:932–5. doi: 10.3201/eid2703.200182
62. Crawford CK, Lopez Cervantes V, Quilici ML, Armién AG, Questa M, Matloob MS, et al. Inflammatory cytokines directly disrupt the bovine intestinal epithelial barrier. Sci Rep (2022) 12:14578. doi: 10.1038/s41598-022-18771-y
63. Hujoel IA, Johnson DH, Lebwohl B, Leffler D, Kupfer S, Wu T-T, et al. Tropheryma whipplei infection (Whipple disease) in the USA. Digestive Dis Sci (2019) 64:213–23. doi: 10.1007/s10620-018-5033-4
64. Mahnel R, Kalt A, Ring S, Stallmach A, Strober W, Marth T. Immunosuppressive therapy in whipple's disease patients is associated with the appearance of gastrointestinal manifestations. Am J Gastroenterol (2005) 100:1167–73. doi: 10.1111/j.1572-0241.2005.40128.x
65. Veld D, Sun H-Y, Hung C-C, Colebunders R. The immune reconstitution inflammatory syndrome related to HIV co-infections: a review. Eur J Clin Microbiol Infect Diseases (2012) 31:919–27. doi: 10.1007/s10096-011-1413-9. Huis in ‘t.
66. Glaser C, Rieg S, Wiech T, Scholz C, Endres D, Stich O, et al. Whipple’s disease mimicking rheumatoid arthritis can cause misdiagnosis and treatment failure. Orphanet J Rare Diseases (2017) 12:99. doi: 10.1186/s13023-017-0630-4
67. Keim VJ, Zachmann G, Faller G, Zöpf T. Seit 3 Wochen bestehende intestinale Beschwerden bei einem 67-jährigen Patienten mit chronischen Arthralgien. Der Internist (2021) 62:433–40. doi: 10.1007/s00108-020-00916-8
68. Marais S, Meintjes G, Pepper DJ, Dodd LE, Schutz C, Ismail Z, et al. Frequency, severity, and prediction of tuberculous meningitis immune reconstitution inflammatory syndrome. Clin Infect Diseases (2013) 56:450–60. doi: 10.1093/cid/cis899
69. Mori H, Yakabi C, Yonahara K, Hamahiga K, Yoshimura M, Sakihara M, et al. Whipple’s disease with normal duodenal histology diagnosed by ileal biopsy using balloon endoscopy. Clin J Gastroenterol (2022) 15:702–7. doi: 10.1007/s12328-022-01638-0
70. Lagier J-C, Raoult D. Whipple's disease and Tropheryma whipplei infections: when to suspect them and how to diagnose and treat them. Curr Opin Infect Diseases (2018) 31:463–70. doi: 10.1097/QCO.0000000000000489
71. Vayssade M, Tournadre A, D’Incan M, Soubrier M, Dubost J-J. Immune reconstitution inflammatory syndrome during treatment of Whipple's disease. Joint Bone Spine (2015) 82:122–4. doi: 10.1016/j.jbspin.2014.09.002
72. Bowen LN, Smith B, Reich D, Quezado M, Nath A. HIV-associated opportunistic CNS infections: pathophysiology, diagnosis and treatment. Nat Rev Neurol (2016) 12:662–74. doi: 10.1038/nrneurol.2016.149
73. Meintjes G, Stek C, Blumenthal L, Thienemann F, Schutz C, Buyze J, et al. Prednisone for the prevention of paradoxical tuberculosis-associated IRIS. New Engl J Med (2018) 379:1915–25. doi: 10.1056/NEJMoa1800762
74. Balasko A, Keynan Y. Shedding light on IRIS: from pathophysiology to treatment of cryptococcal meningitis and immune reconstitution inflammatory syndrome in HIV-infected individuals. HIV Med (2019) 20:1–10. doi: 10.1111/hiv.12676
75. Fernández-Sánchez M, Iglesias MC, Ablanedo-Terrazas Y, Ormsby CE, Alvarado-de La Barrera C, Reyes-Terán G. Steroids are a risk factor for Kaposi's sarcoma-immune reconstitution inflammatory syndrome and mortality in HIV infection. AIDS (2016) 30:909–14. doi: 10.1097/QAD.0000000000000993
76. Le Blay P, Rakotonirainy H, Lagier J-C, Raoult D, Puechal X, Pers Y-M. A severe Whipple disease with an immune reconstitution inflammatory syndrome: An additional case of thalidomide efficiency. Joint Bone Spine (2014) 81:260–2. doi: 10.1016/j.jbspin.2013.10.007
77. Lagier J-C, Fenollar F, Lepidi H, Liozon E, Raoult D. Successful treatment of immune reconstitution inflammatory syndrome in Whipple's disease using thalidomide. J Infect (2010) 60:79–82. doi: 10.1016/j.jinf.2009.09.017
78. Hsu DC, Faldetta KF, Pei L, Sheikh V, Utay NS, Roby G, et al. A paradoxical treatment for a paradoxical condition: infliximab use in three cases of mycobacterial IRIS. Clin Infect Diseases (2016) 62:258–61. doi: 10.1093/cid/civ841
79. Kneitz C, Suerbaum S, Beer M, Müller J, Jahns R, Tony HP. Exacerbation of Whipple's disease associated with infliximab treatment. Scandinavian J Rheumatol (2005) 34:148–51. doi: 10.1080/03009740510015230
80. Ramos JM, Pasquau F, Galipienso N, Valero B, Navarro A, Martinez A, et al. Whipple’s disease diagnosed during anti-tumor necrosis factor alpha treatment: two case reports and review of the literature. J Med Case Rep (2015) 9:165. doi: 10.1186/s13256-015-0632-6
81. Fujita J. Immune reconstitution inflammatory syndrome in the lung in non-human immunodeficiency virus patients. Respir Invest (2020) 58:36–44. doi: 10.1016/j.resinv.2019.11.001
82. Marchetti G, Tincati C, Silvestri G. Microbial translocation in the pathogenesis of HIV infection and AIDS. Clin Microbiol Rev (2013) 26:2–18. doi: 10.1128/CMR.00050-12
83. Bilate AM, Lafaille JJ. Induced CD4 + Foxp3 + Regulatory T cells in immune tolerance. Annu Rev Immunol (2012) 30:733–58. doi: 10.1146/annurev-immunol-020711-075043
84. Maloy KJ, Powrie F. Intestinal homeostasis and its breakdown in inflammatory bowel disease. Nature (2011) 474:298–306. doi: 10.1038/nature10208
85. Cevaal PM, Bekker L-G, Hermans S. TB-IRIS pathogenesis and new strategies for intervention: Insights from related inflammatory disorders. Tuberculosis (2019) 118:101863. doi: 10.1016/j.tube.2019.101863
86. Ulivieri C, Baldari CT. T-cell-based immunotherapy of autoimmune diseases. Expert Rev Vaccines (2013) 12:297–310. doi: 10.1586/erv.12.146
Keywords: immune reconstitution inflammatory syndrome, Whipple disease, intestinal barrier, microbial translocation, regulatory cells
Citation: Song X, Duan R, Duan L and Wei L (2023) Current knowledge of the immune reconstitution inflammatory syndrome in Whipple disease: a review. Front. Immunol. 14:1265414. doi: 10.3389/fimmu.2023.1265414
Received: 22 July 2023; Accepted: 25 September 2023;
Published: 13 October 2023.
Edited by:
Julien Pottecher, Hôpitaux Universitaires de Strasbourg, FranceReviewed by:
Katina Schinnerling, University of Chile, ChileJean-louis Mege, Aix-Marseille Université, France
Soraya Mezouar, Aix-Marseille University, France
Copyright © 2023 Song, Duan, Duan and Wei. This is an open-access article distributed under the terms of the Creative Commons Attribution License (CC BY). The use, distribution or reproduction in other forums is permitted, provided the original author(s) and the copyright owner(s) are credited and that the original publication in this journal is cited, in accordance with accepted academic practice. No use, distribution or reproduction is permitted which does not comply with these terms.
*Correspondence: Lijuan Wei, bTE1NTI2ODUyMzYyQDE2My5jb20=
†These authors share first authorship