- 1Department of Pediatrics, Yantai Affiliated Hospital of Binzhou Medical University, Yantai, China
- 2Department of Gastrointestinal Surgery, Yantai Affiliated Hospital of Binzhou Medical University, Yantai, China
- 3Department of Biochemistry and Molecular Biology, Binzhou Medical University, Yantai, China
Leukemia cells prevent immune system from clearing tumor cells by inducing the immunosuppression of the bone marrow (BM) microenvironment. In recent years, further understanding of the BM microenvironment and immune landscape of leukemia has resulted in the introduction of several immunotherapies, including checkpoint inhibitors, T-cell engager, antibody drug conjugates, and cellular therapies in clinical trials. Among them, the programmed cell death protein 1 (PD-1)/programmed death-ligand 1 (PD-L1) axis is a significant checkpoint for controlling immune responses, the PD-1 receptor on tumor-infiltrating T cells is bound by PD-L1 on leukemia cells. Consequently, the activation of tumor reactive T cells is inhibited and their apoptosis is promoted, preventing the rejection of the tumor by immune system and thus resulting in the occurrence of immune tolerance. The PD-1/PD-L1 axis serves as a significant mechanism by which tumor cells evade immune surveillance, and PD-1/PD-L1 checkpoint inhibitors have been approved for the treatment of lymphomas and varieties of solid tumors. However, the development of drugs targeting PD-1/PD-L1 in leukemia remains in the clinical-trial stage. In this review, we tally up the basic research and clinical trials on PD-1/PD-L1 inhibitors in leukemia, as well as discuss the relevant toxicity and impacts of PD-1/PD-L1 on other immunotherapies such as hematopoietic stem cell transplantation, bi-specific T-cell engager, chimeric antigen receptor T-cell immunotherapy.
1 Introduction
The current standard clinical treatment for leukemia, as a non-solid malignant tumor, mainly includes chemotherapy and hematopoietic stem cell transplantation (HSCT). However, the treatment process faces a series of problems such as chemotherapy insensitivity, chemoresistance, post-transplant relapse, and intolerance in elderly patients (1–4), thereby greatly limiting the progress of treatment for patients with leukemia. Therefore, developing effective methods with low adverse reactions is currently imperative to ameliorate the prognoses of leukemia patients. The immune milieu of bone marrow (BM) is dramatically altered in patients with leukemia, where tumor cells prevent themselves from being cleared by immune system by affecting suppressive immune responses (5–8). Moreover, tumor cells in the blood, BM, and lymphoid tissue are also more accessible to immune cells than solid tumors. Furthermore, the efficacy of allogeneic HSCT (allo-HSCT) demonstrates that leukemia is a typical immune-responsive tumor type (9). Thus, immunotherapy is an obvious choice for treating hematological malignant tumors. In hematologic tumors, currently used immunotherapies include allo-HSCT, bi-specific T-cell engager (BiTE), chimeric antigen receptor (CAR) T-cell immunotherapy (CAR-T), immune-checkpoint inhibitors (ICIs), and other monoclonal antibodies (mAbs) targeting tumor-cell surface antigens (10–13). In recent years, the role of immune escape in leukemia progression and development of immunotherapy have been elucidated, employing ICIs to block suppressor molecules on the surface of T cells, thereby reversing the “exhausted” state of T cells to an “activated” one to kill tumor cells, has proved to be a promising option.
Immune checkpoint (IC) is a signal regulating T-cell receptor (TCR) antigen recognition during immune response. Programmed cell death protein 1 (PD-1)/programmed death-ligand 1 (PD-L1) as an important IC modulating immune response. PD-1 (CD279), a type I transmembrane protein inhibitory checkpoint molecule is expressed on various immune cells, such as naive and activated B cells, effector T cells, regulatory T cells (Tregs), dendritic cells (DCs), activated monocytes, macrophages, natural killer (NK), and immature Langerhans cells (14). PD-1 receptors bind two ligands of the B7 family, PD-L1 and programmed death-ligand 2 (PD-L2). PD-L1 (CD274) is expressed on the surface of hematopoietic cells, such as DCs, macrophages, T cells, and B cells (15, 16). PD-L2 (PDCD1LG2) is expressed on monocytes, myeloid DCs, and activated CD4+ or CD8+ T-cell subsets (17). PD-L1 and PD-L2 differ in expression patterns but have the same effect, and binding of PD-1 to either ligand leads to T-cell dysfunction or exhaustion, resulting in diminished intensity of antigen-specific T-cell response in tumor tissues (18–21). In hematological malignant tumors, the expression rate of PD-L1 in malignant cells is 37%–58% (22). Leukemia cells highly express checkpoint-inhibitor receptors for sharing an immune-cell lineage (9, 23), making them potential targets for this therapy. This review centers around PD-1 signaling, summarizes its molecular functions in hematological malignant tumors and the achievements of ICIs in preclinical development and clinical settings.
2 Mechanisms involved in tumor immune escape through PD-1/PD-L1
As a pair of co-stimulatory signals, PD-1 and PD-L1 jointly constitute PD-1/PD-L1 signaling pathway. Under physiological conditions, the binding of PD-L1 on cell surface to PD-1 on lymphocyte surface inhibits lymphocyte function and induces the apoptosis of activated lymphocytes. The activation of the PD-1/PD-L1 pathway reduces the damage of immunoreactions to surrounding tissues and prevents the progression of autoimmune diseases (24). However, the activation of this pathway causes the binding of PD-L1 expressed on tumor cells to PD-1 on tumor infiltrating lymphocytes, decreasing the immune effect of T cells in the local tumor microenvironment (TME), thereby mediating tumor immune escape and promoting cancer progression (25–27). Researches have shown that PD-L1 expression is upregulated in tumor cells, which activates PD-1/PD-L1 downstream pathways by specifically binding to PD-1 on the surface of cytotoxic T lymphocytes (CTLs) to deliver negative regulatory signals. In turn, it induces the exhaustion of activated T cells and the loss of immunoreactivity, leading to a diminished intensity of antigen-specific CTL responses in tumor tissues (18–21). Besides, Tregs as important suppressive immune cells in TME contribute to cancer initiation and progression. The PD-1/PD-L1 pathway promotes Tregs transformation and enhances their immunosuppressive capacity (28–30). In addition to T cells, other immune cells are implicated in the regulation of immune tolerance induced by the PD-1/PD-L1 pathway. Tumor-associated macrophages (TAMs) upregulate PD-L1 expression of tumor cells (31), whereas tumor cell-secreted versican and derived exosomes induce upregulation of PD-L1 expression in TAMs, which is associated with M2 polarization of TAMs. TAMs with high expression of PD-L1 more significantly inhibit effector T cells and promote tumor growth and metastasis (32–34). Tumor cells increase PD-1 expression on B cells (35, 36), and PD-1+ B cells significantly suppress the proliferation and reduce the viability of CD4+ and CD8+ T cells via the PD-1/PD-L1-dependent pathway (37). NK cells can obtain PD-1 from leukemia cells by endocytosis in tumor cells, and PD-L1 in tumor cells interacts with PD-1 of NK cells to reduce NK cell responses and produce more aggressive tumors (38–40) (Figure 1).
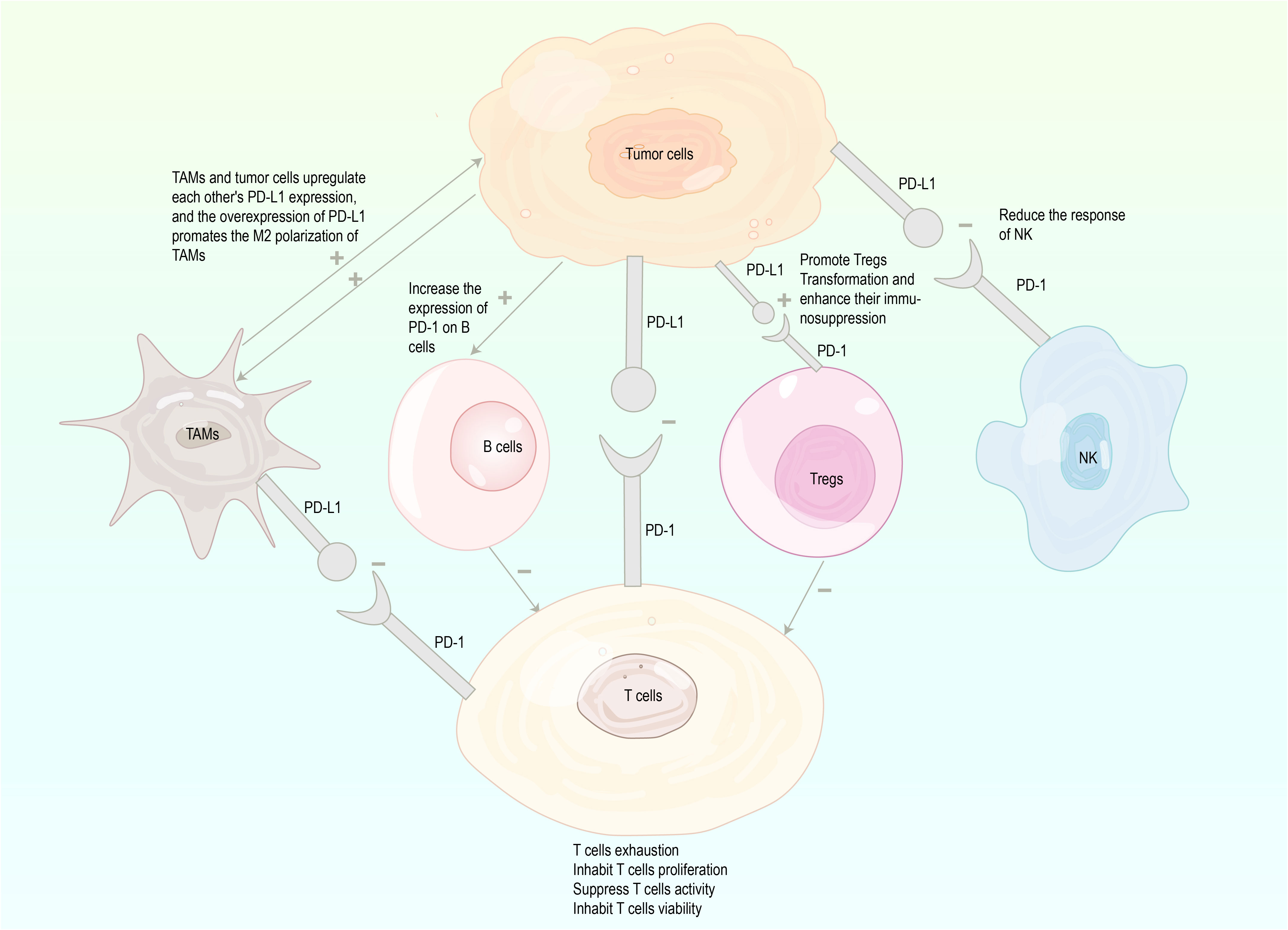
Figure 1 Mechanisms involved in tumor immune escape through the PD-1/PD-L1 (inhibition marked with -, enhancement marked with+), PD-1, programmed cell death protein 1; PD-L1, programmed death-ligand 1; TAMs, tumor associated macrophages; Tregs, regulatory T cells; NK, natural killer.
PD-1 signaling is a pivotal molecule mediating immune escape in TME. Blocking PD-1 signaling attenuates tumor cell suppression of immune cells and improves immune system recognition and cytotoxicity of tumor cells. The increasing understanding of immune function and immune escape mechanisms has led to exploitation of therapeutic mAbs targeting PD-1 signaling (25). Up to now, FDA has successively approved four mAbs (pembrolizumab, nivolumab, cemiplimab, and dostarlimba) targeting PD-1 and three mAbs (atezolizumab, avelumab, and durvalumab) targeting PD-L1 for the treatment of solid and hematological malignancies (15, 16, 41–46), as presented in Table 1.
3 Role of PD-1/PD-L1 in the development of leukemia
Leukemia can be divided four major clinical categories: acute myeloid leukemia (AML), chronic myeloid leukemia (CML), acute lymphoblastic leukemia (ALL), and chronic lymphocytic leukemia (CLL). This review provides a theoretical basis for drug discovery and clinical application of PD-1/PD-L1 pathway by summarizing and analyzing the role of PD-1 signaling in various types of leukemia.
3.1 AML
AML is a heterogeneous disease with various genetic and epigenetic alterations. Its pathogenesis is the accumulation and expansion of immature myeloid cells in the peripheral blood (PB) and BM, resulting in hematopoietic dysfunction. Historically, AML has been regarded as an immunoreactive malignancy and remains the most common indication to receive allo-HSCT (7). PD-1 expression is generally high on T cells in AML patients with de novo and relapsed/refractory (R/R) after chemotherapy, and partial recovery is achieved in patients with complete remission (47–49). Moreover, the level of PD-1 on NK cells and PD-L1 on regulatory B cells (Bregs) increases in AML patients (47, 48, 50, 51). High expression of PD-1 coincides with the T-cell exhaustion (52–54). The overexpression of PD-1 signaling is relevant to poor overall survival of AML patients (55). Above studies suggest PD-1 signaling may influence the development and poor prognosis of AML by increasing T-cell exhaustion. Contrary to this conclusion, Schnorfeil et al. (56) found the level of PD-1 expression on PB CD4+ and CD8+ T cells of AML patients at diagnosis was similar to that of healthy controls, but significantly increased in relapse after stem cell transplantation. T-cell function is not impaired during this process. They thought that this pattern is associated with a shift toward effector memory cells in patients with recurrent AML and T-cell exhaustion does not play a major role in AML. Besides, AML cells induce generation and expansion of Tregs by PD-1 signaling, and Tregs promote the proliferation of AML cells by secreting IL-10 and IL-35 (57, 58). In addition to regulating immune cells, PD-1/PD-L1 drives AML progression by regulating tumor-associated proteins, for example, the expression of PI3K and p-AKT decreases after PD-L1 knockdown, which induces G2/M cell cycle arrest and apoptosis, and the upregulation of PD-L1 increases the expression of PI3K/AKT and enhances the proliferation of tumor cells (59). PD-L1 is overexpressed in AML leukemia-initiating cells, where it increases cyclin D2 expression by enhancing JNK phosphorylation, ultimately promoting the entry of leukemia-initiating cells into cell cycle and proliferation (60). Epigenetic therapy (EGT), particularly with hypomethylating agents (HMAs) either alone or in combination, continues to be successfully used in treating elderly AML, although resistance is a frequent and ultimately near universal outcome (61). Liu et al. (62) found that EGT treatment induces the expression of PD-L1 mRNA and PD-L1 induces the occurrence of EGT resistance. To sum up, PD-1 signaling can promote AML progression by regulating immune cells, oncoproteins, and the occurrence of drug resistance, inhibition of PD-1 signaling can be a breakthrough for successful treatment of AML.
3.2 CML
CML is a myeloproliferative disorder characterized by BCR-ABL oncoprotein with high tyrosine kinase activity, which promotes the proliferation and inhibits the apoptosis of cancer cells (63). PD-1 signaling on specific T cells leads to T-cell exhaustion, and leukemia cells inhibit effector T-cell proliferation through PD-1/PD-L1 interactions, blocking PD-1 signaling contributes to improved CML control in pre-clinical mouse models by restoring the function of CML-specific CTLs (64). The quantity of bcr-abl fusion gene, as the initiation and core factor of CML pathogenesis, is positively correlated with the PD-1 expression level on CD8+ T cells. When CML is treated with tyrosine kinase inhibitors (TKIs), a target drug for bcr-abl, the PD-1 expression level of CD8+ T cells in the complete hematological response group is significantly lower than that in the control group, chronic phase, and blast phase (22). However, leukemia stem cells (LSCs) are resistant to specific TKIs and cause disease relapse after drug discontinuation in CML, besides, CTL transfer therapy leads to upregulation of PD-L1 on LSCs, which protects LSCs from CTL-mediated elimination. In contrast, PD-1 blockade during CTL transfer results in long-term survival of CML mice, suggesting that LSCs were either eliminated or effectively controlled by PD-1 blockade (65, 66). The Tregs are also increased in CML patients at diagnosis and in patients refractory to TKI treatment, and these Tregs have higher levels of PD-1 expression (67, 68). Which suggests PD-1-blocking antibodies given directly prior to and temporarily after TKI discontinuation may block the immune inhibitory effects of Tregs on CD4+/CD8+T-cells, blocking aberrant PD-1 signaling may result in greater success in TKI cessation studies.
3.3 ALL
ALL results from a clonal expansion of abnormal lymphoid progenitors of B cell (BCP-ALL) or T cell (T-ALL) origin that invades BM, PB, and extramedullary sites (69). Similar to other types of leukemia, PD-1 expression increases on T-cell subsets in B-ALL patients and is more prominent at relapse, PD-L1/L2 expression increases on LSCs (70). PD-1+ LSCs are used for T-ALL initiation and relapse, they can upregulate genes related to the MYC pathway, leukemic stemness, and early T-cell progenitor development, and downregulate genes related to apoptosis, cell cycle, and PI3K/AKT signal pathway (71). To determine whether PD-L1 expression on ALL cells inhibits T-cell responses, Blaeschke et al. (72) co-cultured second-generation anti-CD19 CAR-T cells with CD19+ and CD19+/PD-L1+ target cells. Result shows that CAR-T cells co-cultured with PD-L1+ target cells decrease the levels of Th1 cytokine secretion. Which indicates that PD-1 signaling mediates T-cell inhibition after/during T cells against BCP-ALL. In summary, above studies suggest that enhancing T-cell response by inhibiting PD-L1/L2 is a promising therapeutic option.
3.4 CLL
CLL is characterized by the accumulation and clonal proliferation of mature and typically CD5+CD23+ B-cells within PB, BM, lymph nodes, and spleen (73). Several studies have shown that PD-1 signaling is significantly upregulated in CLL patients, and the high level of PD-1/PD-L1 is closely related to disease grade and poor prognosis (74–78). Epstein–Barr virus (EBV) is one of the human tumor viruses, it can transform B-cells into tumor cells. In CLL patients, EBV load is positively correlated with the expression of PD-1 signaling on CD4+ and CD8+ T cells. In EBV (+) patients, the higher the level of PD-1 signaling on T cells, the higher the risk of lymphocyte doubling and treatment initiation (79). Gassner et al. (80) found that inhibiting the interaction of PD-1/PD-L1 can reactivate the cytotoxic effect of exhausted T cells in CLL mouse model. To study the mechanism of PD-1 signaling in CLL, Qorraj et al. (81) collected PB mononuclear cells from CLL patients. They found that triggering PD-1 on monocytes hampers phagocytosis, glycolysis, and Bruton’s tyrosine kinase (BTK)-signaling. Conversely, the immune metabolic dysfunctions and antitumor activity of monocytes can be reversed by disrupting PD-1 signaling. In conclusion, PD-1 signaling inhibits immune cell activity and interferes with immune metabolic processes. The blockade of PD-1 signaling may improve the prognosis of CLL.
4 Regulation of the PD-1/PD-L1 pathway in leukemia
In addition to PD-1 and PD-L1 antibodies directly acting on PD-1 signaling, other proteins, genes, and drugs affect the level of PD-1/PD-L1. When mAbs are insensitive or patients are intolerant to adverse reactions, we may consider indirectly inhibiting immune escape of tumor cells by regulating related proteins and genes or applying relevant drugs (Figure 2).
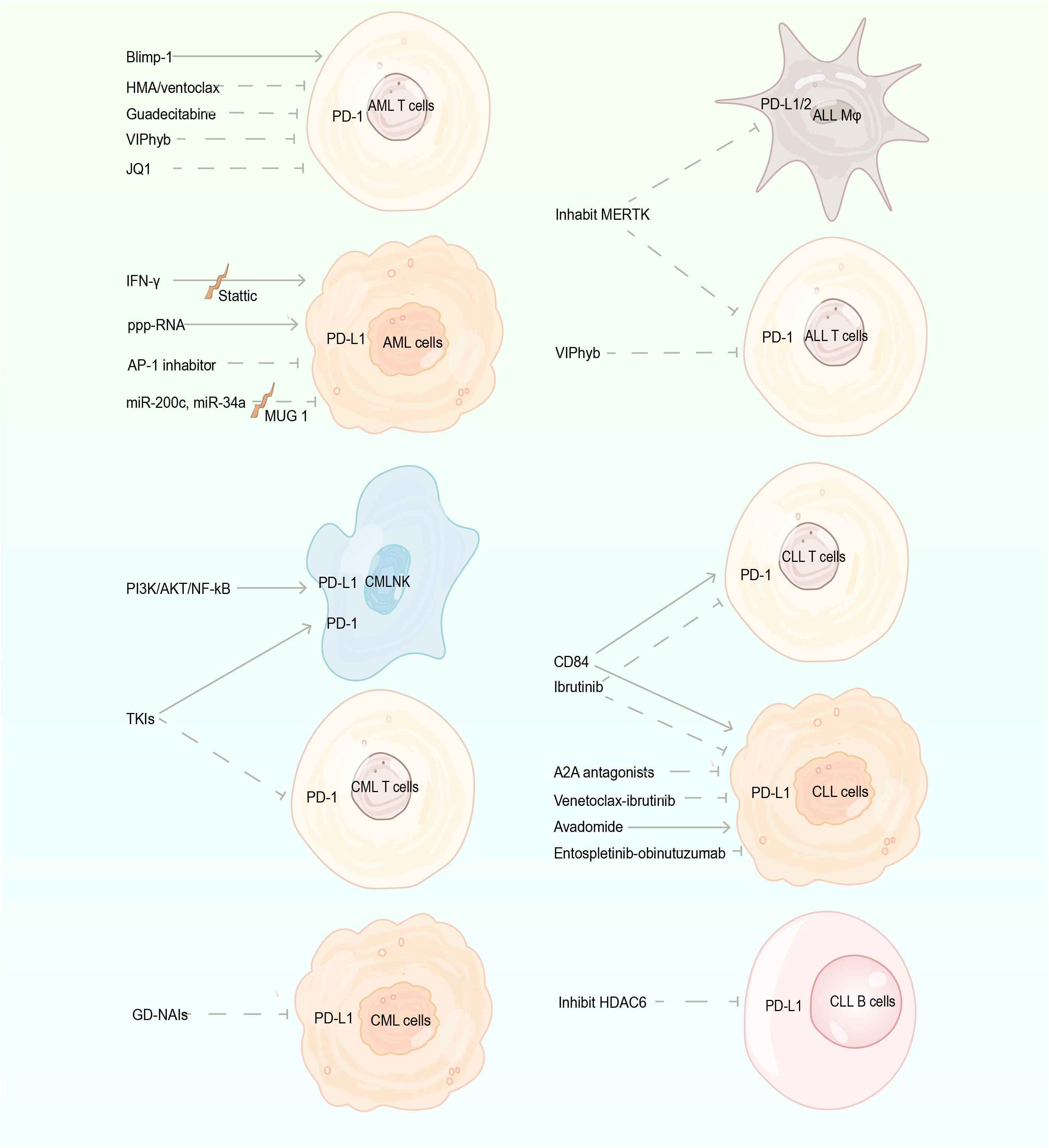
Figure 2 The regulation of the PD-1/PD-L1 pathway. PD-1, programmed cell death protein 1; PD-L1, programmed death-ligand 1; AML, acute myeloid leukemia; ALL, acute lymphoblastic leukemia; CML, chronic myeloid leukemia; CLL, chronic lymphocytic leukemia; Blimp-1, B lymphocyte-induced maturation protein 1; HMA, hypomethylating agents; TKIs, tyrosine kinase inhibitors; GD-NAIs, Gynura divaricate-non-alkaline ingredients; Mφ, monocytes/macrophages; A2A, Adenosine A2A receptor.
4.1 AML
In AML patients, B lymphocyte-induced maturation protein 1 (Blimp-1) directly binds to the promoter of PD-1 and impairs T-cell activity by upregulating PD-1. The knockdown of Blimp-1 can reverse the T-cell functional defect (82). IFN-γ induces PD-L1 expression in myeloid precursor cells and primary cells (57, 83). Stattic, a small molecule inhibitor of STAT3, interferes with IFN-γ-induced PD-L1 expression in AML (84). PD-1 level decreases after the initial HMA/ventoclax (Bcl-2 inhibitor) treatment on all CD4+ T-cell subpopulations except naïve in AML patients (48). In an immunocompetent murine leukemia model, guadecitabine (a second-generation HMA) negatively regulates inhibitory accessory cells in TME by reducing PD-1+ T cells and the AML-mediated expansion of myeloid-derived suppressor cells. Consequently, functionally active leukemia specific T cells increase (85). NA-AML (NrasG12D/-; Asxl-/–AML) cells overexpress PD-L1/PD-L2, and the level of PD-L1 is associated with the upregulation of AP-1 transcription factor (TF). AP-1 inhibitor or short-hairpin RNAs against AP-1 TF Jun decreases PD-L1 expression (86). The overexpression of miR-200c and miR-34a causes the significant downregulation of PD-L1 level. MUC1 attenuates the interference of miR-34a and miR-200c on PD-L1 translation by negatively regulating the expression of miR-34a and miR-200c, and silencing of MUC1 leads to increased miR-34a and miR-200c. In turn, PD-L1 expression is reduced (87).
4.2 CML
Myeloid leukemia cells induce PD-L1 expression on NK cells via PI3K/AKT/NF-kB pathway (88), thus, inhibiting this pathway may block PD-L1 expression. The level of PD-1 on CD8+ T cells is reduced in CML patients treated with TKIs dasatinib and imatinib (22). Gynura divaricata (L.) DC. is a widely used herbal medicine, whose non-alkaline ingredients regulate PD-1 signaling, significantly inducing apoptosis and inhibiting proliferation of CML cells (89).
4.3 ALL
A leukemic microenvironment supports the survival of ALL cells and their immune evasion through multiple interactions (69). In an ALL mouse model, inhibition of MERTK significantly decreases the expression PD-L1/L2 on CD11b+ monocytes/macrophages and PD-1 on CD4+ and CD8+ T cells in the leukemic microenvironment, reducing the incidence of splenic FOXP3+ Tregs at sites of leukemic infiltration. Consequently, T-cell activation increases, and immune-mediated ALL clearance is promoted (90). Murine models of AML and T-ALL reveal that VIPhyb, a peptide antagonist of VIP signaling, enhances IFN-γ secretion and suppresses PD-1 expression in CD4+ and CD8+ T cells (91).
4.4 CLL
CD84-mediated intercellular interactions upregulate the level of PD-1 on T cells and PD-L1 on CLL-cells via the Akt-mTOR pathway, resulting in T-cell exhaustion. Conversely, the downregulation of CD84 expression reverses these phenomena and reduces the expression level of other exhaustion markers (92). The activation of Adenosine A2A receptor (A2A) induces immune tolerance and is closely associated with immune escape of tumor cells (93). In CLL cells, hypoxia causes the emergence of a population of PD-1+ and IL-10–secreting T cells, and adding A2A antagonists attenuates Tregs generation, TGF-β induction, PD-1 expression, and IL-10 synthesis and secretion. Thus, leukemia cells become more susceptible to pharmacological agents while restoring immune competence and T-cell proliferation (94). Ibrutinib, a covalent inhibitor of BTK, is approved for treatment of patients with R/R or treatment-naive CLL (95). Cubillos et al. (96) found that ibrutinib can decrease PD-1 and PD-L1 expression by driving Th1-selective pressure in T cells. Kondo et al. (95) suggested that ibrutinib enhances antitumor immune responses by inhibiting STAT3-induced selective and persistent downregulation of PD-L1 on CLL cells and PD-1 in CD4+ and CD8+ T cells. In a venetoclax (VEN)–ibrutinib combination treatment, the number of PD-1+CD8+ T cells, Tregs, and follicular helper T cells decreases more than fivefold, thereby reducing the immunosuppressive characteristics of CLL (97). The SYK inhibitor entospletinib in combination with obinutuzumab downregulates the expression of PD-1 in CD4+ and CD8+ T-cell subsets of CLL patients, partially reversing the T-cell exhausted phenotype (98).
5 PD-1/PD-L1 and allo-HSCT
Allo-HSCT is a potentially curative therapy for various hematologic malignancies. It relies on the graft-versus-leukemia (GVL) effect mediated by donor-derived alloreactive T cells. However, graft-versus-host disease (GVHD) is also mediated by the same T cells and remains a major clinical problem related to considerable morbidity and mortality (99, 100). The occurrence of GVHD and T-cell suppression is positively correlated with the expression level of PD-L1 (101). The loss of GVL effect is relevant to PD-1 overexpression in allograft recipients, and blocking PD-L1 largely restores GVL efficacy without triggering GVHD (102). Besides, HSCT leads to differential upregulation of PD-1 ligands in tissues, which compartmentalizes CTL activity and thus creates niches for tumor escape. PD-1 blockage can restore CTL sensitivity to antigens and homogenize the effect of graft against tumor (103). These suggest that improving GVL and reducing GVHD by blocking PD-1 signaling can yield considerable results. Ni et al. (100) found that the exhaustion of CD4+ T cells leads to PD-L1 upregulation in donor CD8+ T cells and recipient tissues. which increased PD-L1/PD-1 interplay between donor CD8+ T cells and recipient tissues contributes to preventing GVHD by promoting the apoptosis and exhaustion of T-cell in GVHD target tissues, and enhanced PD-L1/CD80 interplay between CD8+ T cells contributes to retaining GVL responses by improving T-cell expansion and survival. Accordingly, the influence of the PD-L1-mediated effect on HSCT depends on the tissue microenvironment, the existence of CD4+ T cells, and the natural interacting partner expressed by CD8+ T cells. This suggests that we can enhance the PD-1 signaling-mediated GVL effect and reduce the PD-1 signaling-mediated GVHD by changing the above conditions. Besides, VIPhyb also increases the anti-leukemic effect after allogeneic BM transplantation by downregulating PD-1 and PD-L1 expression on donor immune cells (104). In clinical trial, Tschernia et al. (105) found that the use of pembrolizumab before allo-SCT reduced 100-day mortality in AML patients (17% vs 0%) and did not increase grade III-IV acute GVHD. The chronic GVHD is not found in patients who have received pembrolizumab before allo-SCT and cyclophosphamide after transplantation. This suggests that ICI treatment prior to allo-SCT is effective and safe, and post-transplant cyclophosphamide can eliminate the GVHD risk and severity. The above studies provide an empirical and theoretical basis for ICIs combined with HSCT in the treatment of leukemia.
In addition to utilizing the GVL effect of hematopoietic stem cells (HSCs), Hu et al. (106) enhanced the delivery of checkpoint inhibitors by using the in situ activation of platelets and the homing ability of HSCs. They constructed HSC-platelet-aPD-1 conjugates and then injected them into mice bearing AML cells, the therapeutic effect of checkpoint blocking is significantly enhanced. With regard to the drug-delivery mode of PD-1/PD-L1, Chen et al. (107) introduced a transdermal cold atmospheric plasma (CAP)-mediated IC blockade (ICB) therapy. The ICB delivered via microneedles enhanced the immune response mediated by T cells. Han et al. (108) used HEK293T-derived vesicles with PD-1 receptors on their surface to destroy PD-1 signaling, while the internal space of the vesicle allows for the packaging of an indoleamine 2,3-dioxygenase inhibitor, which further enhanced the antitumor effect. This suggests that in addition to drug development for ICIs, new technologies for applying ICIs are also of interest worthy of attention.
6 Efficacy of PD-1/PD-L1 mAbs treating leukemia alone or in combination
Studies on leukemia treatment with PD-1/PD-L1 mAbs are rapidly increasing in number. They are primarily divided into basic research and clinical stages. Herein, we provide guidance and rationale for subsequent clinical applications by analyzing their pooled data.
6.1 PD-1/PD-L1 mAbs for AML
Current clinical treatments for AML are primarily chemotherapy and allo-HSCT. However, due to the emergence of resistance to chemotherapy and GVHD, more effective and safer drugs to treat AML need to be developed (109, 110). Nivolumab, a PD-1 mAbs, is applied in an index case of recurrent myeloproliferative neoplasms after HSCT. Before infusion of nivolumab, AML blasts show high expression of chemokines, whereas T cells are characterized by the expression of interferon-responsive genes. This baseline inflammatory signature disappears after infusion of nivolumab, and the clinical responses are characterized by the temporary expansion of polyclonal CD4+ T-cell populations, the contraction of AML subsets exhibiting megakaryocytic characteristics, and elevated PD-L1 expression (111). Several studies show that the combination of PD-1/PD-L1 mAbs is promising research, for instance, the combined blockade of PD-1 signaling and Tim-3 have an additive effect on inhibiting tumor growth in advanced AML mouse models (112). The combination of IL-15 and PD-1 blockers activates AML-NK cells and enhances the killing ability of NK by increasing the release of perforin, granzyme, and IFN-γ (113). In addition, inhibiting the effects of other therapies on PD-1 expression can also yield considerable results, for instance, exogenous short 5′-triphosphate-modified RNA (ppp-RNA) can direct the immune response toward tumor cells. However, ppp-RNA treatment induces PD-L1 expression on AML cells and establishes therapeutic sensitivity to anti-PD-1 in vivo, the combination of anti-PD-1 and ppp-RNA is superior to either regimen alone in the survival rate of a mouse model (114). The DAC/VEN therapy (HMA decitabine combined with BCL‐2 inhibitor venetoclax) effectively targets leukemia cells while upregulating PD-1 expression in AML patients. Nivolumab combined with DAC/VEN can enhance antitumor effect and eliminate circulating blasts and LSCs/progenitor cells (115). The above studies indicate that considering PD-1/PD-L1 antibodies as an adjuvant treatment scheme for AML can effectively enhance the sensitivity of cell therapy and chemotherapeutic agents, which is a promising combination-chemotherapy option. A number of clinical studies have been conducted on PD-1 mAbs combined with other chemotherapeutic drugs in the treatment of AML, such as cytarabine (116), azacytidine (117), decitabine (118), and these treatment regimens are clinically feasible and have shown encouraging results.
Tumor progression leads to increased Tregs and elevated PD-1 expression on CD8+ CTLs in AML mouse model, which reduces the recognition and activation of tumor-specific CTLs (58). PD-L1 siRNA-mediated silencing augments the expression of T cell activation markers (CD69 and CD137) and improves CTL degranulation (CD107a) (119). CTL infusion combined with PD-1 blockade suppresses Tregs (120). PD-1 blockade in combination with Tregs exhaustion or CTL infusion induces significantly more AML tumor reduction than either treatment alone (58, 120). Additionally, combining DC-based immunotherapy with PD-1 blockade might be a promising approach to eliminating LSCs (121). In sum, PD-1/PD-L1 blockade combined with cell therapy represents a significant new approach that can be easily translated into clinical applications to enhance T cell-mediated cytotoxic responses.
6.2 PD-1/PD-L1 mAbs for CML
TKIs and HSCT are the mainstay of treatment for CML (122, 123), and immune mechanisms may help maintain treatment-free remission. The direct interplay between NK cells and K562 myeloid leukemia cells induces the PD-L1 expression of NK cells. Compared with PD-L1-NK cells, PD-L1+ NK cells are activated effector cells with strong killing activity against tumor cells in vitro. The binding of the PD-L1 mAbs atezolizumab to PD-L1 upregulates PD-L1 expression on the surface of NK cells and provides more binding sites for PD-L1 mAbs, resulting in continuous activation of p38. This phenomenon further propagates strong activation signals toward NK cells to maintain their cytotoxic and cytokine-secretion features. In vivo, the combination of PD-L1 mAbs and NK cell-activating cytokines significantly enhances the antitumor activity of NK cells against myeloid leukemia lacking PD-L1 expression (88). This finding suggests that PD-L1 mAbs have a unique therapeutic effect on PD-L1- tumors, this is independent of PD-1. Dasatinib, a second-generation TKI, upregulates PD-1 expression on CD56dimNK cells and increases dysfunctional CD56negNK cells that highly express PD-1. Nivolumab enhances the cytotoxic activity of both subsets but more efficiently in the CD56dim subset compared with the CD56neg subset (39). Which suggests the combination of TKIs and PD-1/PD-L1 mAbs may be an approach for the successful treatment of CML patients. Recent evidence shows PD-1 expression on CD4+ and CD8+ T-cells, including on CML-reactive PR1-CTL in TKI-naive but also TKI-treated remission CML patients (124–126), which suggests T-cell exhaustion also in deep molecular remission, this provides a rationale for the treatment with checkpoint blocking antibodies to PD-1/PD-L1. However, a clinical trial of the combination of dasatinib and nivolumab for the treatment of CML showed that this approach did not show meaningful clinical activity in patients with CML in chronic phase or accelerated phase who received ≥2 prior TKIs with progression, resistance, or suboptimal response to most recent therapy (127). A phase II trial of the effectiveness of pembrolizumab and dasatinib, imatinib mesylate, or nilotinib in treating patients with CML and consistently detecting minimal residual disease (defined as the level of a gene product called bcr-abl in the blood) is currently underway (www.clinicaltrials.gov as # NCT03516279).
6.3 PD-1/PD-L1 mAbs for ALL
ALL has genetic heterogeneity, and the incidence is much higher in children. The current therapies for ALL are primarily multidrug chemotherapy, which has a high response rate but also has a high recurrence rate, leaving much room for improvement (128, 129). The phenotypic exhaustion of CD4+ T-cells predicts recurrence and poor overall survival in B-ALL. In a Ph+ B-ALL mouse model, the application of PD-L1 antibody clonally expands leukemia-specific CD4+ T-cells with helper/cytotoxic phenotype and reduces the expression of exhaustion markers. The combination of PD-L1 mAbs and TKI nilotinib also significantly improves the efficacy of nilotinib against BCR-ABL+ B-ALL (130). Axl ablation in macrophages can elicit the susceptibility of PD-1 refractory treatment naive B-ALL to PD-1 checkpoint blockade and promote antileukemia immunity (131). A new peptide, nABPD1, is designed to specifically bind PD-1. It enhances cytokine-induced killer (ICIK) cell-mediated antitumor activity by protecting ICIK cells through blockade of PD-1 signaling (44). There is a lack of clinical studies on the use of PD-1/PD-L1 mAbs in ALL, especially in young people, and as far as the current studies are concerned, they show unsatisfactory results for MRD in adults(median age is 52.5y) with ALL (132). Two studies of PD-1 mAbs for the treatment of ALL in children, adolescents, and young adults are currently underway (www.clinicaltrials.gov as # NCT05310591, NCT04546399).
6.4 PD-1/PD-L1 mAbs for CLL
Chemotherapy and anti-CD20 mAbs therapy are the standard of care for patients with CLL (133–135). Currently, it is prominent to improve the complete remission rate and reduce chemotherapy-induced immunosuppression. Studies suggest that early blockade of PD-L1 effectively prevents the immune dysfunction induced by tumor cells and thus avoids CLL development in mice. This includes the prevention of exhaustion-like and aberrant T-cell phenotypes, and the restoration of MHC class II-expressing dendritic cells and mature macrophages (108, 136). Ioannou et al. (137) concluded that although PD-L1 mAbs are superior to PD-1 mAbs in inducing anti-CLL T-cell activity, PD-1 mAbs and PD-L1 mAbs monotherapies are largely ineffective in overcoming T-cell tolerance in CLL. Avadomide is a cereblon E3 ligase modulator drug that stimulates T-cell immune synapse while increasing PD-L1 expression, it triggers IFN-driven T-cell responses and converts noninflamed CLL tumors into CD8+ T cell-inflamed ones, making CLL sensitive to PD-1/PD-L1 immunotherapy. The combination of avadomide and PD-1/PD-L1 blockade effectively reinvigorates previously exhausted patient T cells and contributes to more T cell killing in CLL. HDAC6 gene silencing or inhibition decreases PD-L1 expression on B cells of Eµ-TCL1 mice model, and the combination of HDAC6 inhibitor ACY738 and anti-PD-1/anti-PD-L1 further enhances the cytotoxicity of T cells (138). Rivas et al. (139) found that the treatment of CLL with anti-PD-L1 in combination with IL-10 produces more IFN-γ+, memory CD8+ T-cells, and cytotoxic effector KLRG1+, and fewer exhausted T-cells than anti-PD-L1 alone. CLL animal experiments show that PD-1/PD-L1 antibody as a combination chemotherapy regimen definitely affects tumor inhibition. However, according to the results of the current clinical study, PD-1 mAbs have limited efficacy in CLL patients, but reassuringly they show a promising therapeutic option in patients with Richter’s transformation (140–142).
6.5 PD-1/PD-L1 mAbs and CAR-T
CAR-T cell therapy has contributed to a revolution in the therapy of patients with hematological malignancies (143). However, the activation of CAR-T cells can lead to persistently high levels of PD−1 and eventually cause the exhaustion of T cells (144). Several studies have shown that the integration of PD-1-mediated inhibitory signaling into CAR-T significantly improves the function of conventional CAR-T, and it even may have an almost equivalent or better anti-tumor effect and a lower side effect compared with the CAR-T plus PD-1 antibody (72, 145, 146). More studies on PD-1 signaling with CAR-T are shown in Table 2. These studies suggest that PD-1 signaling blockade combined with CAR-T can enhance the efficacy of CAR-T. To date, a variety of CAR-T with PD-1 inhibition have been designed, and they have achieved gratifying results in preclinical studies. PD-1 signal blocking combined with CAR-T may produce greater benefits compared with chemotherapeutic drugs. However, there is a lack of clinical studies in this area, and the clinical effects and adverse effects are unclear.
6.6 PD-1/PD-L1 mAbs and BiTE
Blinatumomab (BiTE antibody) is a novel immunotherapy that recruits the forces of T cells and guides them against lymphoblastic cells by binding CD3 expressed on the surface of T cells and CD19 expressed on the surface of B cell lines (151, 152). It was approved by FDA in 2014 for the treatment of Ph-negative R/R precursor B-ALL. However, approximately 50% of R/R B-ALL patients do not respond to blinatumomab. Non-responders consistently express higher levels of PD-1 during blinatumomab treatment, and the levels of PD-L1 and PD-L2 increase on residual tumor cells in BM after treatment. The T-cell responses of blinatumomab against leukemia are potentiated by blocking CTLA-4 and PD-L1 signaling pathways (153, 154). This finding illustrates that the response of blinatumomab is correlated with the molecular level of IC. Wunderlich et al. (155) reported that pembrolizumab combined with blinatumomab increases the clearance of B-ALL in mice and reverses T-cell lymphopenia induced by blinatumomab. PD-1 inhibition also enhances the efficacy of blinatumomab in a UCB/PDX model of recurrent pediatric B-ALL. Krupka et al. (156) constructed the CD33/CD3 BiTE antibody AMG 330. They found that PD-L1 on primary AML cells is strongly upregulated after adding AMG 330 in the ex vivo culture, and blocking PD-1/PD-L1 axis enhances the AMG 330-induced lysis of AML cells by reversing T-cell-induced immune escape. Herrmann et al. (157) fused the extracellular domain of PD-1 (PD-1ex), which naturally holds a low affinity to PD-L1, with an αCD3.αCD33 BiTE®-like scaffold to form a bifunctional checkpoint inhibitory T-cell-binding (CiTE) antibody. The CiTE antibody is more potent in binding to AML cells and T cells, thereby increasing the function of T-cell effectors, and minimizing iRAEs associated with the systemic application of ICB. From the above several ex vivo studies and animal experiments, we can conclude that BiTE and PD-1 signaling blockade have good synergy in leukemia treatment. Nevertheless, there are few completed clinical studies on the combination therapy of blinatumomab and PD-1/PD-L1 mAbs for leukemia. A large sample phase II trial comparing blinatumomab alone to blinatumomab with nivolumab in patients with relapsed B-ALL is currently underway (www.clinicaltrials. as # gov NCT04546399).
6.7 Clinical trials of PD-1/PD-L1 mAbs in leukemia
A number of clinical trials on leukemia treatment with PD-1/PD-L1 mAbs have been performed nowadays. The overall response rate (ORR) of pembrolizumab alone is 0% in eight patients with AML (158). When combined with cytarabine, the ORR is 46% (116); the former grade 3–4 iRAEs are 25% (158), and the latter grade ≥3 iRAEs are 14% and self-limiting (116). The median recurrence-free survival (RFS) of AML patients treated with nivolumab alone is 8.48 months (159). When combined with cytarabine–idarubicin, the RFS is 18.54 months (160); the former grade 3–4 iRAEs are 27% (159), and the latter are 13.6% (160). From the above data, we assume that the efficacy of pembrolizumab and nivolumab alone is significantly lower than that of the combination, and the incidence of iRAEs is also higher with the single agent than with the combination. The median overall survival (mOS) is 11.1 months for AML with 200 mg of pembrolizumab in combination with 1.5–2 g/m2 cytarabine (116), 21 months when combined with 1.5–2 mg/m2 cytarabine (105), and 10.8 months when combined with decitabine (118). Regarding the data from the current sample, the efficacy of pembrolizumab combined with low-dose cytarabine is superior to that of the high-dose one, and the efficacy of pembrolizumab combined with decitabine or high-dose cytarabine is similar. The iRAEs are 42% in AML patients treated with pembrolizumab alone, the grade 3–4 iRAEs are 25% (158), the iRAEs are 40% when treated with nivolumab alone, the grade 3–4 iRAEs are 27% (159), and the incidence of adverse events is similar for both drugs. The mOS for AML treated with avelumab–azacitidine combination is 4.8 months (161), and that with durvalumab–azacitidine combination is 13.0 months (162). The ORR for AML treated with avelumab–azacitidine combination is 10.5% (161), that with nivolumab–azacitidine combination is 33% (117), and that with durvalumab–azacitidine combination is 31.3% (162). However, the grade 3–4 iRAEs in the avelumab combination are less than 7.7% (163). Based on the current sample alone, avelumab is less effective than pembrolizumab, nivolumab, and durvalumab, but its incidence of iRAEs is much lower. Due to differences in sample size and patient disease status among studies, comparisons of efficacy and adverse effect assessments of PD-1/PD-L1 mAbs among studies are subject to large errors. Regarding the current sample, PD-1/PD-L1 mAbs are effective in the treatment of leukemia, but the effect of single drug therapy is weak, and the effect of combination is more considerable. The occurrence of iRAEs is also not negligible, and large sample data are required to clarify the curative effects and adverse effects of PD-1/PD-L1 mAbs. Studies on PD-1/PD-L1 mAbs in CML, ALL, and CLL are few, and more details about clinical trials on PD-1/PD-L1 mAbs in the treatment of leukemia are shown in Table 3.
6.8 Future clinical scenarios of PD-L1/PD-1 inhibitors in AML
In sum, setting of either consolidation or maintenance where, in the presence of PD-L1/PD-1 inhibitors at least partially restored immune system, they could promote measurable residual disease negativity. A very interesting therapeutic application, albeit of limited use, of checkpoint inhibitors in AML, could be in the post allo-HSCT setting, where, in the presence of AML relapse/progression, these agents might be useful in augmenting the immune reactivity of the graft, boosting the GVL effect, at the expense of also enhancing iRAEs, in combination with other chemotherapeutic drugs might improve drug sensitivity in patients with R/R AML, and in combination with other T-cell based immunotherapies such as CAR-T, BiTE, and Treg exhaustion might enhance cytotoxic responses.
7 Limitations of ICB in the treatment of leukemia
7.1 Limited efficacy of ICB treatment
Different from other preclinical studies, co-blockade of PD-1 with Tim-3 or PD-1 with TIGIT fails to restore the proliferation and degranulation of CD8+ T-cells from CLL patients (173, 174). There are many other suppressive checkpoint molecules in T cells such as A2A receptor, CD276, B7-H4, CD272, CTLA-4, LAG-3, etc. (16), and they may also play an important role in exhaustion of CD8+ T-cells. Besides, Radpour et al. (175) suggest that CD8+ T cells in AML are dysfunctional mainly due to epigenetic silencing of activating IC receptors rather than signaling by immune inhibitory IC receptors. Kalinin et al. (176) block PD-1/PD-L1 signaling in CD19 CAR-T cells by co-expression of CD19-CAR and PD-1-specific VHH domain of anti-PD-1 nanobody. Results show that although the activation of CAR-T cells with low PD-1 level increases, the survival and cytotoxicity of these cells are diminished. Functional impairment caused by disrupted PD-1 signaling is accompanied by faster maturation and upregulation of exhaustion marker TIGIT in CAR-T cells. This result proves that for prolonged CAR-T activity and successful target cell killing, the strength of activation signal provided by CAR should be balanced by negative signal from IC. It suggests simply eliminating/knocking out PD-1 is not enough if one wants to optimize CAR-T cells by disposing of negative co-stimulation. Moreover, AML is an aggressive, rapid progressive disease, which does not allow the immune system to develop a proper antileukemic response. A study shows robust antigen-specific T cell responses are generated against AML cells after localized implantation (subcutaneous), but not a systemic (intravenous) route, the latter generates a tolerant state towards the malignant cells. Which suggests the ideal scenario for promoting a leukemia-specific T cell response will likely be in the minimal residual disease setting (177). Furthermore, AML has a low mutational burden and the newly formed antigens are expressed in different other tissues of the host (16). In conclusion, there are some experiments that have not found the exact effect of PD-1 signal blocking, and the reasons for poor PD-1 efficacy are complex. This may explain why PD-1 mAbs have suboptimal clinical efficacy.
7.2 Adverse reactions of ICB treatment
Additionally, the application of PD-1/PD-L1 mAbs is greatly limited by adverse drug reactions during the clinical treatment of leukemia patients. Godfrey et al. (158) concluded from a prospective study that treatment with pembrolizumab after allo-SCT is feasible, but it may be associated with serious iRAEs. A case study has reported the combined use of azacitidine and tislelizumab (an PD-1 mAbs) to treat relapsed AML posttransplantation. AML patients achieve complete remission, but the patients successively develop serious iRAEs and GVHD, eventually dying from GVHD complications (178). Significant ICI-related toxicity can occur in multiple tissues and organs, such as pneumonia, glomerulonephritis, hepatitis, gastroenteritis, dermatitis, neurotoxicity, and others. Fortunately, these symptoms are usually alleviated with the prompt use of steroids (179). However, among the 75 R/R AML patients treated with nivolumab, 85% develop infections during the study period, and they are mostly severe. R/R AML patients treated with nivolumab are more likely to develop infections when treated with corticosteroids than those who are not (164). More adverse events during leukemia treatment with ICIs are shown in Table 4. Chemotherapy intolerance is an important cause of treatment discontinuation in leukemia patients, and reducing adverse effects during ICI therapy while aiming to improve their efficacy is equally important. Accordingly, the development of well-tolerated ICIs and the exploration of clinical protocols with few adverse effects of ICIs are the keys to solving the problem. However, given the insufficient data on the clinical application of ICIs for leukemia, further exploration is required to optimize ICI therapy.
8 Conclusion
Blocking PD-1/PD-L1 achieves encouraging outcomes as shown by ex vivo studies and animal models, but clinical trials on PD-1/PD-L1 mAbs as single-agent in leukemia treatment show suboptimal results and varying degrees of adverse drug reactions. Fortunately, combinations of PD-1/PD-L1mAbs with other immunotherapies have shown quite promising, including the enhancement of GVL effect and reduction of GVHD in HSCT, the improvement of T-cell response in BiTE or CAR-T, and the application to multidrug chemotherapy to enhance drug sensitivity. In conclusion, ICB therapy opens new horizons for tumor immunotherapy, and future research will focus on refining combination regimens of ICIs to modulate the immune environment so that leukemia patients can maximize the benefits of ICB therapy.
Author contributions
HC: Data curation, Investigation, Writing – original draft, Conceptualization. TW: Conceptualization, Investigation, Writing – original draft. XZ: Investigation, Writing – original draft, Data curation, Formal Analysis. SX: Conceptualization, Supervision, Writing – review & editing. HS: Supervision, Writing – review & editing, Investigation. YS: Conceptualization, Supervision, Writing – review & editing, Funding acquisition, Project administration, Resources. YL: Supervision, Writing – review & editing, Conceptualization, Funding acquisition, Project administration, Resources.
Funding
The authors declare financial support was received for the research, authorship, and/or publication of this article. The research was supported by The Shandong Science and Technology Committee (grant nos. ZR2023MH223, ZR2020QH221, ZR2022LSW002), The Support Plan for Youth Entrepreneurship and Technology of Colleges and Universities in Shandong (grant no. 2019KJK014), The National Natural Science Foundation of China (grant nos. 81800169, 82002604), The Foundation of Binzhou Medical University (grant no. BY2021LCX04).
Conflict of interest
The authors declare that the research was conducted in the absence of any commercial or financial relationships that could be construed as a potential conflict of interest.
Publisher’s note
All claims expressed in this article are solely those of the authors and do not necessarily represent those of their affiliated organizations, or those of the publisher, the editors and the reviewers. Any product that may be evaluated in this article, or claim that may be made by its manufacturer, is not guaranteed or endorsed by the publisher.
References
1. Tamiro F, Weng AP, Giambra V. Targeting leukemia-initiating cells in acute lymphoblastic leukemia. Cancer Res (2021) 81:4165–73. doi: 10.1158/0008-5472.CAN-20-2571
2. Thompson PA, Kantarjian HM, Cortes JE. Diagnosis and treatment of chronic myeloid leukemia in 2015. Mayo Clin Proc (2015) 90:1440–54. doi: 10.1016/j.mayocp.2015.08.010
3. Chiorazzi N, Chen S, Rai KR. Chronic lymphocytic leukemia. Csh Perspect Med (2021) 11:a35220. doi: 10.1101/cshperspect.a035220
4. Newell LF, Cook RJ. Advances in acute myeloid leukemia. BMJ (2021) 375:n2026. doi: 10.1136/bmj.n2026
5. Xu B, Hu R, Liang Z, Chen T, Chen J, Hu Y, et al. Metabolic regulation of the bone marrow microenvironment in leukemia. Blood Rev (2021) 48:100786. doi: 10.1016/j.blre.2020.100786
6. Toffalori C, Zito L, Gambacorta V, Riba M, Oliveira G, Bucci G, et al. Immune signature drives leukemia escape and relapse after hematopoietic cell transplantation. Nat Med (2019) 25:603–11. doi: 10.1038/s41591-019-0400-z
7. Vago L, Gojo I. Immune escape and immunotherapy of acute myeloid leukemia. J Clin Invest. (2020) 130:1552–64. doi: 10.1172/JCI129204
8. Griggio V, Perutelli F, Salvetti C, Boccellato E, Boccadoro M, Vitale C, et al. Immune dysfunctions and immune-based therapeutic interventions in chronic lymphocytic leukemia. Front Immunol (2020) 11:594556. doi: 10.3389/fimmu.2020.594556
9. Liao D, Wang M, Liao Y, Li J, Niu T. A review of efficacy and safety of checkpoint inhibitor for the treatment of acute myeloid leukemia. Front Pharmacol (2019) 10:609. doi: 10.3389/fphar.2019.00609
10. Lv M, Gorin NC, Huang X. A vision for the future of allogeneic hematopoietic stem cell transplantation in the next decade. Sci Bull (2022) 67:1921–4. doi: 10.1016/j.scib.2022.09.004
11. Brown PA, Ji L, Xu X, Devidas M, Hogan LE, Borowitz MJ, et al. Effect of postreinduction therapy consolidation with blinatumomab vs chemotherapy on disease-free survival in children, adolescents, and young adults with first relapse of b-cell acute lymphoblastic leukemia. JAMA (2021) 325:833. doi: 10.1001/jama.2021.0669
12. Melenhorst JJ, Chen GM, Wang M, Porter DL, Chen C, Collins MA, et al. Decade-long leukaemia remissions with persistence of cd4+ car t cells. Nature (2022) 602:503–9. doi: 10.1038/s41586-021-04390-6
13. Tabata R, Chi S, Yuda J, Minami Y. Emerging immunotherapy for acute myeloid leukemia. Int J Mol Sci (2021) 22:1944. doi: 10.3390/ijms22041944
14. Ok CY, Young KH. Checkpoint inhibitors in hematological Malignancies. J Hematol Oncol (2017) 10:103. doi: 10.1186/s13045-017-0474-3
15. Salmaninejad A, Valilou SF, Shabgah AG, Aslani S, Alimardani M, Pasdar A, et al. Pd-1/pd-l1 pathway: basic biology and role in cancer immunotherapy. J Cell Physiol (2019) 234:16824–37. doi: 10.1002/jcp.28358
16. Jimbu L, Mesaros O, Popescu C, Neaga A, Berceanu I, Dima D, et al. Is there a place for pd-1-pd-l blockade in acute myeloid leukemia? Pharmaceuticals (2021) 14:288. doi: 10.3390/ph14040288
17. Messal N, Serriari N, Pastor S, Nunès JA, Olive D. Pd-l2 is expressed on activated human t cells and regulates their function. Mol Immunol (2011) 48:2214–9. doi: 10.1016/j.molimm.2011.06.436
18. Mueller SN, Ahmed R. High antigen levels are the cause of t cell exhaustion during chronic viral infection. Proc Natl Acad Sci - PNAS. (2009) 106:8623–8. doi: 10.1073/pnas.0809818106
19. Schönrich G, Raftery MJ. The pd-1/pd-l1 axis and virus infections: a delicate balance. Front Cell Infect Mi. (2019) 9:207. doi: 10.3389/fcimb.2019.00207
20. Butte MJ, Keir ME, Phamduy TB, Sharpe AH, Freeman GJ. Programmed death-1 ligand 1 interacts specifically with the b7-1 costimulatory molecule to inhibit t cell responses. Immun (Cambridge Mass.). (2007) 27:111–22. doi: 10.1016/j.immuni.2007.05.016
21. Francisco LM, Salinas VH, Brown KE, Vanguri VK, Freeman GJ, Kuchroo VK, et al. Pd-l1 regulates the development, maintenance, and function of induced regulatory t cells. J Exp Med (2009) 206:3015–29. doi: 10.1084/jem.20090847
22. Lee MY, Park C, Cho Y, You E, Jang S, Seol CA, et al. Differences in pd-1 expression on cd8+ t-cells in chronic myeloid leukemia patients according to disease phase and tki medication. Cancer Immunol Immunother (2020) 69:2223–32. doi: 10.1007/s00262-020-02617-5
23. Norde WJ, Maas F, Hobo W, Korman A, Quigley M, Kester MGD, et al. Pd-1/pd-l1 interactions contribute to functional t-cell impairment in patients who relapse with cancer after allogeneic stem cell transplantation. Cancer Res (2011) 71:5111–22. doi: 10.1158/0008-5472.CAN-11-0108
24. Sun C, Mezzadra R, Schumacher TN. Regulation and function of the pd-l1 checkpoint. Immun (Cambridge Mass.). (2018) 48:434–52. doi: 10.1016/j.immuni.2018.03.014
25. Jelinek T, Mihalyova J, Kascak M, Duras J, Hajek R. Pd-1/pd-l1 inhibitors in haematological Malignancies: update 2017. Immunology (2017) 152:357–71. doi: 10.1111/imm.12788
26. Isogawa M, Furuichi Y, Chisari FV. Oscillating cd8+ t cell effector functions after antigen recognition in the liver. Immunity (2005) 23:53–63. doi: 10.1016/j.immuni.2005.05.005
27. Kataoka K, Shiraishi Y, Takeda Y, Sakata S, Matsumoto M, Nagano S, et al. Aberrant pd-l1 expression through 3′-utr disruption in multiple cancers. Nature (2016) 534:402–6. doi: 10.1038/nature18294
28. Kumagai S, Togashi Y, Kamada T, Sugiyama E, Nishinakamura H, Takeuchi Y, et al. The pd-1 expression balance between effector and regulatory t cells predicts the clinical efficacy of pd-1 blockade therapies. Nat Immunol (2020) 21:1346–58. doi: 10.1038/s41590-020-0769-3
29. Kamada T, Togashi Y, Tay C, Ha D, Sasaki A, Nakamura Y, et al. Pd-1+regulatory t cells amplified by pd-1 blockade promote hyperprogression of cancer. Proc Natl Acad Sci (2019) 116:9999–10008. doi: 10.1073/pnas.1822001116
30. Cai J, Wang D, Zhang G, Guo X. the role of pd-1/pd-l1 axis in treg development and function: implications for cancer immunotherapy. Onco Targets Ther (2019) 12:8437–45. doi: 10.2147/OTT.S221340
31. Xia Q, Jia J, Hu C, Lu J, Li J, Xu H, et al. Tumor-associated macrophages promote pd-l1 expression in tumor cells by regulating pkm2 nuclear translocation in pancreatic ductal adenocarcinoma. Oncogene (2022) 41:865–77. doi: 10.1038/s41388-021-02133-5
32. Li W, Wu F, Zhao S, Shi P, Wang S, Cui D. Correlation between pd-1/pd-l1 expression and polarization in tumor-associated macrophages: a key player in tumor immunotherapy. Cytokine Growth F R (2022) 67:49–57. doi: 10.1016/j.cytogfr.2022.07.004
33. Yang H, Zhang Q, Xu M, Wang L, Chen X, Feng Y, et al. Ccl2-ccr2 axis recruits tumor associated macrophages to induce immune evasion through pd-1 signaling in esophageal carcinogenesis. Mol Cancer. (2020) 19:41. doi: 10.1186/s12943-020-01165-x
34. Pu Y, Ji Q. Tumor-associated macrophages regulate pd-1/pd-l1 immunosuppression. Front Immunol (2022) 13:874589. doi: 10.3389/fimmu.2022.874589
35. Ren Z, Peng H, Fu Y. Pd-1 shapes b cells as evildoers in the tumor microenvironment. Cancer Discovery (2016) 6:477–8. doi: 10.1158/2159-8290.CD-16-0307
36. Sun X, Zhang T, Li M, Yin L, Xue J. Immunosuppressive B cells expressing pd-1/pd-l1 in solid tumors: a mini review. QJM (2019), hcz162. doi: 10.1093/qjmed/hcz162
37. Wang X, Wang G, Wang Z, Liu B, Han N, Li J, et al. Pd-1-expressing b cells suppress cd4+ and cd8+ t cells via pd-1/pd-l1-dependent pathway. Mol Immunol (2019) 109:20–6. doi: 10.1016/j.molimm.2019.02.009
38. Hasim MS, Marotel M, Hodgins JJ, Vulpis E, Makinson OJ, Asif S, et al. When killers become thieves: trogocytosed pd-1 inhibits nk cells in cancer. Sci Adv (2022) 8:j3286. doi: 10.1126/sciadv.abj3286
39. Ishiyama KI, Kitawaki T, Otsuka Y, Takaori Kondo A, Kadowaki N. Programmed cell death 1-expressing cd56-negative natural killer (nk) cell expansion is a hallmark of chronic nk cell activation during dasatinib treatment. Cancer Sci (2021) 112:523–36. doi: 10.1111/cas.14692
40. Hsu J, Hodgins JJ, Marathe M, Nicolai CJ, Bourgeois-Daigneault M, Trevino TN, et al. Contribution of nk cells to immunotherapy mediated by pd-1/pd-l1 blockade. J Clin Invest. (2018) 128:4654–68. doi: 10.1172/JCI99317
41. Akinleye A, Rasool Z. Immune checkpoint inhibitors of pd-l1 as cancer therapeutics. J Hematol Oncol (2019) 12:92. doi: 10.1186/s13045-019-0779-5
42. BaSudan AM. The role of immune checkpoint inhibitors in cancer therapy. Clinics Practice. (2023) 13:22–40. doi: 10.3390/clinpract13010003
43. Liu J, Chen Z, Li Y, Zhao W, Wu J, Zhang Z. Pd-1/pd-l1 checkpoint inhibitors in tumor immunotherapy. Front Pharmacol (2021) 12:731798. doi: 10.3389/fphar.2021.731798
44. Chen Y, Huang H, Liu Y, Wang Z, Wang L, Wang Q, et al. Engineering a high-affinity pd-1 peptide for optimized immune cell-mediated tumor therapy. Cancer Res Treat (2022) 54:362–74. doi: 10.4143/crt.2021.424
45. Migden MR, Rischin D, Schmults CD, Guminski A, Hauschild A, Lewis KD, et al. Pd-1 blockade with cemiplimab in advanced cutaneous squamous-cell carcinoma. New Engl J Med (2018) 379:341–51. doi: 10.1056/NEJMoa1805131
46. Saha A, Connor RS O, Thangavelu G, Lovitch SB, Dandamudi DB, Wilson CB, et al. Programmed death ligand-1 expression on donor t cells drives graft-versus-host disease lethality. J Clin Invest. (2016) 126:2642–60. doi: 10.1172/JCI85796
47. Liu G, Zhang Q, Yang J, Li X, Xian L, Li W, et al. Increased tigit expressing nk cells with dysfunctional phenotype in aml patients correlated with poor prognosis. Cancer Immunol Immunother (2022) 71:277–87. doi: 10.1007/s00262-021-02978-5
48. Zhigarev D, Varshavsky A, MacFarlane AW, Jayaguru P, Barreyro L, Khoreva M, et al. Lymphocyte exhaustion in aml patients and impacts of hma/venetoclax or intensive chemotherapy on their biology. Cancers (2022) 14:3352. doi: 10.3390/cancers14143352
49. Xu L, Liu L, Yao D, Zeng X, Zhang Y, Lai J, et al. Pd-1 and tigit are highly co-expressed on cd8+ t cells in aml patient bone marrow. Front Oncol (2021) 11:686156. doi: 10.3389/fonc.2021.686156
50. Shi Y, Liu Z, Wang H. Expression of pd-l1 on regulatory b cells in patients with acute myeloid leukaemia and its effect on prognosis. J Cell Mol Med (2022) 26:3506–12. doi: 10.1111/jcmm.17390
51. Jia B, Wang L, Claxton DF, Ehmann WC, Rybka WB, Mineishi S, et al. Bone marrow cd8 t cells express high frequency of pd-1 and exhibit reduced anti-leukemia response in newly diagnosed aml patients. Blood Cancer J (2018) 8:34. doi: 10.1038/s41408-018-0069-4
52. Tan J, Chen S, Lu Y, Yao D, Xu L, Zhang Y, et al. Higher pd-1 expression concurrent with exhausted cd8+ t cells in patients with de novo acute myeloid leukemia. Chin J Cancer Res (2017) 29:463–70. doi: 10.21147/j.issn.1000-9604.2017.05.11
53. Tan J, Yu Z, Huang J, Chen Y, Huang S, Yao D, et al. Increased pd-1+tim-3+ exhausted t cells in bone marrow may influence the clinical outcome of patients with aml. biomark Res (2020) 8:6. doi: 10.1186/s40364-020-0185-8
54. Wang M, Bu J, Zhou M, Sido J, Lin Y, Liu G, et al. Cd8+t cells expressing both pd-1 and tigit but not cd226 are dysfunctional in acute myeloid leukemia (aml) patients. Clin Immunol (2017) 190:64–73. doi: 10.1016/j.clim.2017.08.021
55. Chen C, Liang C, Wang S, Chio CL, Zhang Y, Zeng C, et al. Expression patterns of immune checkpoints in acute myeloid leukemia. J Hematol Oncol (2020) 13:28. doi: 10.1186/s13045-020-00853-x
56. Schnorfeil FM, Lichtenegger FS, Emmerig K, Schlueter M, Neitz JS, Draenert R, et al. T cells are functionally not impaired in aml: increased pd-1 expression is only seen at time of relapse and correlates with a shift towards the memory t cell compartment. J Hematol Oncol (2015) 8:93. doi: 10.1186/s13045-015-0189-2
57. Dong Y, Han Y, Huang Y, Jiang S, Huang Z, Chen R, et al. Pd-l1 is expressed and promotes the expansion of regulatory t cells in acute myeloid leukemia. Front Immunol (2020) 11:1710. doi: 10.3389/fimmu.2020.01710
58. Zhou Q, Munger ME, Highfill SL, Tolar J, Weigel BJ, Riddle M, et al. Program death-1 signaling and regulatory t cells collaborate to resist the function of adoptively transferred cytotoxic t lymphocytes in advanced acute myeloid leukemia. Blood (2010) 116:2484–93. doi: 10.1182/blood-2010-03-275446
59. Wang F, Yang L, Xiao M, Zhang Z, Shen J, Anuchapreeda S, et al. Pd-l1 regulates cell proliferation and apoptosis in acute myeloid leukemia by activating pi3k-akt signaling pathway. Sci Rep-Uk. (2022) 12:11444. doi: 10.1038/s41598-022-15020-0
60. Fang X, Chen C, Xia F, Yu Z, Zhang Y, Zhang F, et al. Cd274 promotes cell cycle entry of leukemia-initiating cells through jnk/cyclin d2 signaling. J Hematol Oncol (2016) 9:124. doi: 10.1186/s13045-016-0350-6
61. Yun S, Vincelette ND, Abraham I, Robertson KD, Fernandez-Zapico ME, Patnaik MM. Targeting epigenetic pathways in acute myeloid leukemia and myelodysplastic syndrome: a systematic review of hypomethylating agents trials. Clin Epigenetics. (2016) 8:68. doi: 10.1186/s13148-016-0233-2
62. Liu H, Hu Y, Rimoldi R, Von Hagt C, Khong E, Lee N, et al. Epigenetic treatment-mediated modulation of pd-l1 predicts potential therapy resistance over response markers in myeloid Malignancies: a molecular mechanism involving effectors of pd-l1 reverse signaling. Oncol Lett (2019) 17:2543–50. doi: 10.3892/ol.2018.9841
63. Cortes J, Pavlovsky C, Saußele S. Chronic myeloid leukaemia. Lancet (2021) 398:1914–26. doi: 10.1016/S0140-6736(21)01204-6
64. Mumprecht S, Schurch C, Schwaller J, Solenthaler M, Ochsenbein AF. Programmed death 1 signaling on chronic myeloid leukemia-specific t cells results in t-cell exhaustion and disease progression. Blood (2009) 114:1528–36. doi: 10.1182/blood-2008-09-179697
65. Riether C, Gschwend T, Huguenin A, Schürch CM, Ochsenbein AF. Blocking programmed cell death 1 in combination with adoptive cytotoxic t-cell transfer eradicates chronic myelogenous leukemia stem cells. Leukemia (2015) 29:1781–5. doi: 10.1038/leu.2015.26
66. Schürch C, Riether C, Amrein MA, Ochsenbein AF. Cytotoxic t cells induce proliferation of chronic myeloid leukemia stem cells by secreting interferon-γ. J Exp Med (2013) 210:605–21. doi: 10.1084/jem.20121229
67. Riether C. Regulation of hematopoietic and leukemia stem cells by regulatory t cells. Front Immunol (2022) 13:1049301. doi: 10.3389/fimmu.2022.1049301
68. Harrington P, Dillon R, Radia D, McLornan D, Woodley C, Asirvatham S, et al. Chronic myeloid leukaemia patients at diagnosis and resistant to tyrosine kinase inhibitor therapy display exhausted t-cell phenotype. Brit J Haematol (2022) 198:1011–5. doi: 10.1111/bjh.18302
69. Pastorczak A, Domka K, Fidyt K, Poprzeczko M, Firczuk M. Mechanisms of immune evasion in acute lymphoblastic leukemia. Cancers (2021) 13:1536. doi: 10.3390/cancers13071536
70. Park SH, You E, Park CJ, Cho YU, Jang S, Im HJ, et al. Increased expression of immune checkpoint programmed cell death protein-1 (pd-1) on t cell subsets of bone marrow aspirates in patients withb-lymphoblastic leukemia, especially in relapse and at diagnosis. Cytometry Part B: Clin Cytometry. (2020) 98:336–47. doi: 10.1002/cyto.b.21879
71. Xu X, Zhang W, Xuan L, Yu Y, Zheng W, Tao F, et al. Pd-1 signalling defines and protects leukaemic stem cells from t cell receptor-induced cell death in t cell acute lymphoblastic leukaemia. Nat Cell Biol (2023) 25:170–82. doi: 10.1038/s41556-022-01050-3
72. Blaeschke F, Stenger D, Apfelbeck A, Cadilha BL, Benmebarek M, Mahdawi J, et al. Augmenting anti-cd19 and anti-cd22 car t-cell function using pd-1-cd28 checkpoint fusion proteins. Blood Cancer J (2021) 11:108. doi: 10.1038/s41408-021-00499-z
73. Bosch F, Dalla-Favera R. Chronic lymphocytic leukaemia: from genetics to treatment. Nat Rev Clin Oncol (2019) 16:684–701. doi: 10.1038/s41571-019-0239-8
74. Gamaleldin MA, Ghallab OM, Nadwan EA, Abo Elwafa RA. Pd-1 and pd-l1 gene expressions and their association with epstein-barr virus infection in chronic lymphocytic leukemia. Clin Trans Oncol (2021) 23:2309–22. doi: 10.1007/s12094-021-02657-y
75. Brusa D, Serra S, Coscia M, Rossi D, D’Arena G, Laurenti L, et al. The pd-1/pd-l1 axis contributes to t-cell dysfunction in chronic lymphocytic leukemia. Haematologica (2013) 98:953–63. doi: 10.3324/haematol.2012.077537
76. Taghiloo S, Allahmoradi E, Ebadi R, Tehrani M, Hosseini-Khah Z, Janbabaei G, et al. Upregulation of galectin-9 and pd-l1 immune checkpoints molecules in patients with chronic lymphocytic leukemia. Asian Pac J Cancer Prev (2017) 18:2269–74. doi: 10.22034/APJCP.2017.18.8.2269
77. Grzywnowicz M, Karczmarczyk A, Skorka K, Zajac M, Zaleska J, Chocholska S, et al. Expression of programmed death 1 ligand in different compartments of chronic lymphocytic leukemia. Acta Haematol-Basel. (2015) 134:255–62. doi: 10.1159/000430980
78. Rusak M, Eljaszewicz A, Bolkun L, Luksza E, Lapuc I, Piszcz J, et al. Prognostic significance of pd-1 expression on peripheral blood cd4+ t cells in patients with newly diagnosed chronic lymphocytic leukemia. Pol Arch Med Wewn. (2015) 125:553–9. doi: 10.20452/pamw.2967
79. Grywalska E, Pasiarski M, Sosnowska-Pasiarska B, Macek P, Rolińska A, Samardakiewicz M, et al. Programmed cell death 1 expression and epstein-barr virus infection in chronic lymphocytic leukaemia: a prospective cohort study. Cancer Manag Res (2019) 11:7605–18. doi: 10.2147/CMAR.S212069
80. Gassner FJ, Zaborsky N, Catakovic K, Rebhandl S, Huemer M, Egle A, et al. Chronic lymphocytic leukaemia induces an exhausted t cell phenotype in the tcl1 transgenic mouse model. Brit J Haematol (2015) 170:515–22. doi: 10.1111/bjh.13467
81. Qorraj M, Bruns H, Böttcher M, Weigand L, Saul D, Mackensen A, et al. The pd-1/pd-l1 axis contributes to immune metabolic dysfunctions of monocytes in chronic lymphocytic leukemia. Leukemia (2017) 2:470–8. doi: 10.1038/leu.2016.214
82. Zhu L, Kong Y, Zhang J, Claxton DF, Ehmann WC, Rybka WB, et al. Blimp-1 impairs t cell function via upregulation of tigit and pd-1 in patients with acute myeloid leukemia. J Hematol Oncol (2017) 10:124. doi: 10.1186/s13045-017-0486-z
83. Kronig H, Kremmler L, Haller B, Englert C, Peschel C, Andreesen R, et al. Interferon-induced programmed death-ligand 1 (pd-l1/b7-h1) expression increases on human acute myeloid leukemia blast cells during treatment. Eur J Haematol (2014) 92:195–203. doi: 10.1111/ejh.12228
84. Yoyen-Ermis D, Tunali G, Tavukcuoglu E, Horzum U, Ozkazanc D, Sutlu T, et al. Myeloid maturation potentiates stat3-mediated atypical ifn-γ signaling and upregulation of pd-1 ligands in aml and mds. Sci Rep-Uk. (2019) 9:11697. doi: 10.1038/s41598-019-48256-4
85. Nahas MR, Stroopinsky D, Rosenblatt J, Cole L, Pyzer AR, Anastasiadou E, et al. Hypomethylating agent alters the immune microenvironment in acute myeloid leukaemia (aml) and enhances the immunogenicity of a dendritic cell/aml vaccine. Brit J Haematol (2018) 185:679–90. doi: 10.1111/bjh.15818
86. You X, Liu F, Binder M, Vedder A, Lasho T, Wen Z, et al. Asxl1 loss cooperates with oncogenic nras in mice to reprogram the immune microenvironment and drive leukemic transformation. Blood (2022) 139:1066–79. doi: 10.1182/blood.2021012519
87. Pyzer AR, Stroopinsky D, Rosenblatt J, Anastasiadou E, Rajabi H, Washington A. Muc1 inhibition leads to decrease in pd-l1 levels via upregulation of mirnas. Leukemia (2017) 12:2780–90. doi: 10.1038/leu.2017.163
88. Dong W, Wu X, Ma S, Wang Y, Nalin AP, Zhu Z, et al. The mechanism of anti–pd-l1 antibody efficacy against pd-l1–negative tumors identifies nk cells expressing pd-l1 as a cytolytic effector. Cancer Discovery (2019) 9:1422–37. doi: 10.1158/2159-8290.CD-18-1259
89. Ye X, Wang L, Yang X, Yang J, Zhou J, Lan C, et al. Integrated chemical characterization, network pharmacology and transcriptomics to explore the mechanism of sesquiterpenoids isolated from gynura divaricata (l.) Dc. Against chronic myelogenous leukemia. Pharmaceuticals (2022) 15:1435. doi: 10.3390/ph15111435
90. Lee-Sherick AB, Jacobsen KM, Henry CJ, Huey MG, Parker RE, Page LS, et al. Mertk inhibition alters the pd-1 axis and promotes anti-leukemia immunity. JCI Insight (2020) 5:e97941. doi: 10.1172/jci.insight.145847
91. Petersen CT, Li JM, Waller EK. Administration of a vasoactive intestinal peptide antagonist enhances the autologous anti-leukemia t cell response in murine models of acute leukemia. Oncoimmunology (2017) 6:e1304336. doi: 10.1080/2162402X.2017.1304336
92. Lewinsky H, Barak AF, Huber V, Kramer MP, Radomir L, Sever L, et al. Cd84 regulates pd-1/pd-l1 expression and function in chronic lymphocytic leukemia. J Clin Invest. (2018) 128:5465–78. doi: 10.1172/JCI96610
93. Yu F, Zhu C, Ze S, Wang H, Yang X, Liu M, et al. Design, synthesis, and bioevaluation of 2-aminopteridin-7(8h)-one derivatives as novel potent adenosine a(2a) receptor antagonists for cancer immunotherapy. J Med Chem (2022) 65:4367–86. doi: 10.1021/acs.jmedchem.1c02199
94. Serra S, Vaisitti T, Audrito V, Bologna C, Buonincontri R, Chen SS, et al. Adenosine signaling mediates hypoxic responses in the chronic lymphocytic leukemia microenvironment. Blood Adv (2016) 1:47–61. doi: 10.1182/bloodadvances.2016000984
95. Kondo K, Shaim H, Thompson PA, Burger JA, Keating M, Estrov Z, et al. Ibrutinib modulates the immunosuppressive cll microenvironment through stat3-mediated suppression of regulatory b-cell function and inhibition of the pd-1/pd-l1 pathway. Leukemia (2018) 4:960–70. doi: 10.1038/leu.2017.304
96. Cubillos-Zapata C, Avendaño-Ortiz J, Córdoba R, Hernández-Jiménez E, Toledano V, Pérez De Diego R, et al. Ibrutinib as an antitumor immunomodulator in patients with refractory chronic lymphocytic leukemia. Oncoimmunology (2016) 5:e1242544. doi: 10.1080/2162402X.2016.1242544
97. de Weerdt I, Hofland T, de Boer R, Dobber JA, Dubois J, van Nieuwenhuize D, et al. Distinct immune composition in lymph node and peripheral blood of cll patients is reshaped during venetoclax treatment. Blood Adv (2019) 3:2642–52. doi: 10.1182/bloodadvances.2019000360
98. Lam V, Best S, Kittai A, Orand K, Spurgeon SE, Liu T, et al. Proapoptotic and immunomodulatory effects of syk inhibitor entospletinib in combination with obinutuzumab in patients with chronic lymphocytic leukaemia. Brit J Clin Pharmaco. (2022) 88:836–41. doi: 10.1111/bcp.14962
99. Brennan TV, Yang Y. Pd-l1 serves as a double agent in separating gvl from gvhd. J Clin Invest. (2017) 127:1627–30. doi: 10.1172/JCI94196
100. Ni X, Song Q, Cassady K, Deng R, Jin H, Zhang M, et al. Pd-l1 interacts with cd80 to regulate graft-versus-leukemia activity of donor cd8+ t cells. J Clin Invest. (2017) 127:1960–77. doi: 10.1172/JCI91138
101. Baur R, Karl F, Böttcher-Loschinski R, Stoll A, Völkl S, Gießl A, et al. Accumulation of t-cell-suppressive pd-l1high extracellular vesicles is associated with gvhd and might impact gvl efficacy. J Immunother Cancer. (2023) 11:e6362. doi: 10.1136/jitc-2022-006362
102. Koestner W, Hapke M, Herbst J, Klein C, Welte K, Fruehauf J, et al. Pd-l1 blockade effectively restores strong graft-versus-leukemia effects without graft-versus-host disease after delayed adoptive transfer of t-cell receptor gene-engineered allogeneic cd8+t cells. Blood (2011) 3:1030–41. doi: 10.1182/blood-2010
103. Michonneau D, Sagoo P, Breart B, Garcia Z, Celli S, Bousso P. The pd-1 axis enforces an anatomical segregation of ctl activity that creates tumor niches after allogeneic hematopoietic stem cell transplantation. Immunity (2016) 44:143–54. doi: 10.1016/j.immuni.2015.12.008
104. Li J, Petersen CT, Li J, Panjwani R, Chandra DJ, Giver CR, et al. Modulation of immune checkpoints and graft-versus-leukemia in allogeneic transplants by antagonizing vasoactive intestinal peptide signaling. Cancer Res (2016) 76:6802–15. doi: 10.1158/0008-5472.CAN-16-0427
105. Tschernia NP, Kumar V, Moore DT, Vincent BG, Coombs CC, Van Deventer H, et al. Safety and efficacy of pembrolizumab prior to allogeneic stem cell transplantation for acute myelogenous leukemia. Transplant Cell Ther (2021) 27:1021. doi: 10.1016/j.jtct.2021.08.022
106. Hu Q, Sun W, Wang J, Ruan H, Zhang X, Ye Y, et al. Conjugation of haematopoietic stem cells and platelets decorated with anti-pd-1 antibodies augments anti-leukaemia efficacy. Nat BioMed Eng. (2018) 2:831–40. doi: 10.1038/s41551-018-0310-2
107. Chena G, Chen Z, Wen DI, Wang Z, Li H, Zeng Y. Transdermal cold atmospheric plasma-mediated immune checkpoint blockade therapy. P Natl Acad Sci USA (2020) 7:3687–92. doi: 10.1073/pnas.1917891117/-/DCSupplemental
108. Han X, Li H, Zhou D, Chen Z, Gu Z. Local and targeted delivery of immune checkpoint blockade therapeutics. Accounts Chem Res (2020) 53:2521–33. doi: 10.1021/acs.accounts.0c00339
109. Ogbue O, Unlu S, Ibodeng G, Singh A, Durmaz A, Visconte V, et al. Single-cell next-generation sequencing to monitor hematopoietic stem-cell transplantation: current applications and future perspectives. Cancers (2023) 15:2477. doi: 10.3390/cancers15092477
110. Pollyea DA, Altman JK, Assi R, Bixby D, Fathi AT, Foran JM, et al. Acute myeloid leukemia, version 3.2023. J Natl Compr Cancer Network: JNCCN. (2023) 5:503–13. doi: 10.6004/jnccn.2023.0025
111. Penter L, Gohil SH, Huang T, Thrash EM, Schmidt D, Li S, et al. Coevolving jak2v617f+relapsed aml and donor t cells with pd-1 blockade after stem cell transplantation: an index case. Blood Adv (2021) 5:4701–9. doi: 10.1182/bloodadvances.2021004335
112. Zhou Q, Munger ME, Veenstra RG, Weigel BJ, Hirashima M, Munn DH. Coexpression of tim-3 and pd-1 identifies a CD8+ t-cell exhaustion phenotype in mice with disseminated acute myelogenous leukemia. Blood (2011) 17:4501–10. doi: 10.1182/blood-2010
113. Firouzi J, Hajifathali A, Azimi M, Parvini N, Ghaemi F, Shayan Asl N, et al. Hsp70, in combination with il-15 and pd-1 blocker, interferes with the induction of cytotoxic nk cells in relapsed acute myeloid leukemia patients. Cell J (Yakhteh). (2023) 25:92–101. doi: 10.22074/CELLJ.2023.561054.1123
114. Ruzicka M, Koenig LM, Formisano S, Boehmer DFR, Vick B, Heuer E, et al. Rig-i-based immunotherapy enhances survival in preclinical aml models and sensitizes aml cells to checkpoint blockade. Leukemia (2020) 34:1017–26. doi: 10.1038/s41375-019-0639-x
115. Zeng Z, Maiti A, Herbrich S, Cai T, Cavazos A, Manzella T, et al. Triple combination targeting methyltransferase, bcl-2, and pd-1 facilitates antileukemia responses in acute myeloid leukemia. Cancer-Am Cancer Soc (2022) 129:531–40. doi: 10.1002/cncr.34566
116. Zeidner JF, Vincent BG, Ivanova A, Moore D, McKinnon KP, Wilkinson AD, et al. Phase ii trial of pembrolizumab after high-dose cytarabine in relapsed/refractory acute myeloid leukemia. Blood Cancer discovery. (2021) 2:616–29. doi: 10.1158/2643-3230.BCD-21-0070
117. Daver N, Garcia-Manero G, Basu S, Boddu PC, Alfayez M, Cortes JE, et al. Efficacy, safety, and biomarkers of response to azacitidine and nivolumab in relapsed/refractory acute myeloid leukemia: a nonrandomized, open-label, phase ii study. Cancer Discovery (2019) 9:370–83. doi: 10.1158/2159-8290.CD-18-0774
118. Goswami M, Gui G, Dillon LW, Lindblad KE, Thompson J, Valdez J, et al. Pembrolizumab and decitabine for refractory or relapsed acute myeloid leukemia. J Immunother Cancer. (2022) 10:e3392. doi: 10.1136/jitc-2021-003392
119. van Ens D, Mousset CM, Hutten TJA, van der Waart AB, Campillo-Davo D, van der Heijden S, et al. Pd-l1 sirna-mediated silencing in acute myeloid leukemia enhances anti-leukemic t cell reactivity. Bone Marrow Transpl. (2020) 55:2308–18. doi: 10.1038/s41409-020-0966-6
120. Deng R, Fan F, Yi H, Liu F, He G, Sun H, et al. Pd-1 blockade potentially enhances adoptive cytotoxic t cell potency in a human acute myeloid leukaemia animal model. Hematol (Luxembourg). (2018) 23:740–6. doi: 10.1080/10245332.2018.1486357
121. Schürch CM, Riether C, Ochsenbein AF. Dendritic cell-based immunotherapy for myeloid leukemias. Front Immunol (2013) 4:496. doi: 10.3389/fimmu.2013.00496
122. Niederwieser C, Kroger N. Transplantation in cml in the tki era: who, when, and how? Hematol Am Soc Hematol Educ Program. (2022) 2022:114–22. doi: 10.1182/hematology.2022000329
123. Senapati J, Sasaki K, Issa GC, Lipton JH, Radich JP, Jabbour E, et al. Management of chronic myeloid leukemia in 2023 - common ground and common sense. Blood Cancer J (2023) 13:58. doi: 10.1038/s41408-023-00823-9
124. Hsieh Y, Kirschner K, Copland M. Improving outcomes in chronic myeloid leukemia through harnessing the immunological landscape. Leukemia (2021) 35:1229–42. doi: 10.1038/s41375-021-01238-w
125. Brück O, Blom S, Dufva O, Turkki R, Chheda H, Ribeiro A, et al. Immune cell contexture in the bone marrow tumor microenvironment impacts therapy response in cml. Leukemia (2018) 32:1643–56. doi: 10.1038/s41375-018-0175-0
126. Molldrem JJ, Lee PP, Wang C, Felio K, Kantarjian HM, Champlin RE, et al. Evidence that specific t lymphocytes may participate in the elimination of chronic myelogenous leukemia. Nat Med (2000) 6:1018–23. doi: 10.1038/79526
127. Martínez-López J, Mustjoki S, Porkka K, Klisovic RB, Wolf D, Busque L, et al. The safety and efficacy of dasatinib plus nivolumab in patients with previously treated chronic myeloid leukemia: results from a phase 1b dose-escalation study. Leukemia Lymphoma. (2021) 62:2040–3. doi: 10.1080/10428194.2021.1889536
128. Gregory S. Adult acute lymphoblastic leukemia: treatment and management updates. Semin Oncol Nurs. (2019) 35:150951. doi: 10.1016/j.soncn.2019.150951
129. DeAngelo DJ, Jabbour E, Advani A. Recent advances in managing acute lymphoblastic leukemia. Am Soc Clin Oncol Educ book. (2020) 40:330. doi: 10.1200/EDBK_280175
130. Tracy SI, Venkatesh H, Hekim C, Heltemes-Harris LM, Knutson TP, Bachanova V, et al. Combining nilotinib and pd-l1 blockade reverses cd4+ t-cell dysfunction and prevents relapse in acute b-cell leukemia. Blood (2022) 140:335–48. doi: 10.1182/blood.2021015341
131. Tirado-Gonzalez I, Descot A, Soetopo D, Nevmerzhitskaya A, Schaffer A, Kur IM, et al. Axl inhibition in macrophages stimulates host-versus-leukemia immunity and eradicates naive and treatment-resistant leukemia. Cancer Discovery (2021) 11:2924–43. doi: 10.1158/2159-8290.CD-20-1378
132. Cassaday RD, Garcia KA, Fromm JR, Percival MM, Turtle CJ, Nghiem PT, et al. Phase 2 study of pembrolizumab for measurable residual disease in adults with acute lymphoblastic leukemia. Blood Advances. (2020) 4:3239–45. doi: 10.1182/bloodadvances.2020002403
133. Hallek M, Shanafelt TD, Eichhorst B. Chronic lymphocytic leukaemia. Lancet (2018) 391:1524–37. doi: 10.1016/S0140-6736(18)30422-7
134. Eichhorst B, Niemann CU, Kater AP, Fürstenau M, von Tresckow J, Zhang C, et al. First-line venetoclax combinations in chronic lymphocytic leukemia. New Engl J Med (2023) 19:1739–54. doi: 10.1056/NEJMoa2213093
135. Eyre TA, Riches JC. The evolution of therapies targeting bruton tyrosine kinase for the treatment of chronic lymphocytic leukaemia: future perspectives. Cancers (2023) 15:2596. doi: 10.3390/cancers15092596
136. McClanahan F, Hanna B, Miller S, Clear AJ, Lichter P, Gribben JG, et al. Pd-l1 checkpoint blockade prevents immune dysfunction and leukemia development in a mouse model of chronic lymphocytic leukemia. Blood (2015) 126:203–11. doi: 10.1182/blood-2015-01-622936
137. Ioannou N, Hagner PR, Stokes M, Gandhi AK, Apollonio B, Fanous M, et al. Triggering interferon signaling in t cells with avadomide sensitizes cll to anti-pd-l1/pd-1 immunotherapy. Blood (2021) 137:216–31. doi: 10.1182/blood.2020006073
138. Maharaj K, Powers JJ, Mediavilla-Varela M, Achille A, Gamal W, Quayle S, et al. Hdac6 inhibition alleviates cll-induced t-cell dysfunction and enhances immune checkpoint blockade efficacy in the eμ-tcl1 model. Front Immunol (2020) 11:590072. doi: 10.3389/fimmu.2020.590072
139. Rivas JR, Liu Y, Alhakeem SS, Eckenrode JM, Marti F, Collard JP, et al. Interleukin-10 suppression enhances t-cell antitumor immunity and responses to checkpoint blockade in chronic lymphocytic leukemia. Leukemia (2021) 35:3188–200. doi: 10.1038/s41375-021-01217-1
140. Gregory GP, Kumar S, Wang D, Mahadevan D, Walker P, Wagner-Johnston N, et al. Pembrolizumab plus dinaciclib in patients with hematologic Malignancies: the phase 1b keynote-155 study. Blood Adv (2022) 6:1232–42. doi: 10.1182/bloodadvances.2021005872
141. Younes A, Brody J, Carpio C, Lopez-Guillermo A, Ben-Yehuda D, Ferhanoglu B, et al. Safety and activity of ibrutinib in combination with nivolumab in patients with relapsed non-hodgkin lymphoma or chronic lymphocytic leukaemia: a phase 1/2a study. Lancet Haematol (2019) 6:e67–78. doi: 10.1016/S2352-3026(18)30217-5
142. Jain N, Senapati J, Thakral B, Ferrajoli A, Thompson P, Burger J, et al. A phase 2 study of nivolumab combined with ibrutinib in patients with diffuse large b-cell richter transformation of cll. Blood Advances. (2023) 7:1958–66. doi: 10.1182/bloodadvances.2022008790
143. Abbasi S, Totmaj MA, Abbasi M, Hajazimian S, Goleij P, Behroozi J, et al. Chimeric antigen receptor t (car-t) cells: novel cell therapy for hematological Malignancies. Cancer Med-Us. (2023) 12:7844–58. doi: 10.1002/cam4.5551
144. Lin G, Zhang Y, Yu L, Wu D. Cytotoxic effect of cll−1 car−t cell immunotherapy with pd−1 silencing on relapsed/refractory acute myeloid leukemia. Mol Med Rep (2021) 23:208. doi: 10.3892/MMR.2021.11847
145. Peng Q, Zhu X, Li C, Xin P, Zheng Y, Liu S. Apdl1-cart cells exhibit strong pd-l1-specific activity against leukemia cells. Aging (Albany NY). (2021) 13:7199–210. doi: 10.18632/aging.202578
146. Zhou J, Yu J, Wang Y, Wang H, Wang J, Wang Y, et al. Shrna-mediated silencing of pd-1 augments the efficacy of chimeric antigen receptor t cells on subcutaneous prostate and leukemia xenograft. BioMed Pharmacother. (2021) 137:111339. doi: 10.1016/j.biopha.2021.111339
147. Fraietta JA, Lacey SF, Orlando EJ, Pruteanu-Malinici I, Gohil M, Lundh S, et al. Determinants of response and resistance to cd19 chimeric antigen receptor (car) t cell therapy of chronic lymphocytic leukemia. Nat Med (2018) 24:563–71. doi: 10.1038/s41591-018-0010-1
148. Mengjun Zhong RGRZ. Bet bromodomain inhibition rescues pd-1-mediated t-cell exhaustion in acute myeloid leukemia. Cell Death disease. (2022) 13:671. doi: 10.1038/s41419-022-05123-x
149. Ma YJ, Dai HP, Cui QY, Cui W, Zhu WJ, Qu CJ, et al. Successful application of pd-1 knockdown cll-1 car-t therapy in two aml patients with post-transplant relapse and failure of anti-cd38 car-t cell treatment. Am J Cancer Res (2022) 12:615–21.
150. Lorenzini T, Cadilha BL, Obeck H, Benmebarek MR, Markl F, Michaelides S, et al. Rational design of pd-1-cd28 immunostimulatory fusion proteins for car t cell therapy. Br J Cancer. (2023) 129:696–705. doi: 10.1038/s41416-023-02332-9
151. Topp MSD, Gökbuget NM, Stein ASM, Zugmaier GM, O’Brien SM, Bargou RCP, et al. Safety and activity of blinatumomab for adult patients with relapsed or refractory b-precursor acute lymphoblastic leukaemia: a multicentre, single-arm, phase 2 study. Lancet Oncol (2015) 16:57–66. doi: 10.1016/S1470-2045(14)71170-2
152. Kantarjian H, Haddad FG, Jain N, Sasaki K, Short NJ, Loghavi S, et al. Results of salvage therapy with mini-hyper-cvd and inotuzumab ozogamicin with or without blinatumomab in pre-b acute lymphoblastic leukemia. J Hematol Oncol (2023) 16:44. doi: 10.1186/s13045-023-01444-2
153. Kobayashi T, Ubukawa K, Fujishima M, Takahashi N. Correlation between increased immune checkpoint molecule expression and refractoriness to blinatumomab evaluated by longitudinal t cell analysis. Int J Hematol (2021) 113:600–5. doi: 10.1007/s12185-020-03047-w
154. Feucht J, Kayser S, Gorodezki D, Hamieh M, Doring M, Blaeschke F, et al. T-cell responses against cd19+ pediatric acute lymphoblastic leukemia mediated by bispecific t-cell engager (bite) are regulated contrarily by pd-l1 and cd80/cd86 on leukemic blasts. Oncotarget (2016) 7:76902–19. doi: 10.18632/oncotarget.12357
155. Wunderlich M, Manning N, Sexton C, O Brien E, Byerly L, Stillwell C, et al. Pd-1 inhibition enhances blinatumomab response in a ucb/pdx model of relapsed pediatric b-cell acute lymphoblastic leukemia. Front Oncol (2021) 11:642466. doi: 10.3389/fonc.2021.642466
156. Krupka C, Kufer P, Kischel R, Zugmaier G, Lichtenegger FS, Lichtenegger TKBV. Blockade of the pd-1/pd-l1 axis augments lysis of aml cells by the cd33/cd3 bite antibody construct amg 330: reversing a t-cell-induced immune escape mechanism. Leukemia (2016) 2:484–91. doi: 10.1038/leu.2015.214
157. Herrmann M, Krupka C, Deiser K, Brauchle B, Marcinek A, Ogrinc Wagner A, et al. Bifunctional pd-1 × αcd3 × αcd33 fusion protein reverses adaptive immune escape in acute myeloid leukemia. Blood (2018) 132:2484–94. doi: 10.1182/blood-2018-05-849802
158. Godfrey J, Liu H, Yu J, Tallarico M, Curran E, Artz AS, et al. Pembrolizumab for the treatment of disease relapse following allogeneic hematopoietic stem cell transplantation. Blood Adv (2022) 7:963–70. doi: 10.1182/bloodadvances.2022008403
159. Reville PK, Kantarjian HM, Ravandi F, Jabbour E, DiNardo CD, Daver N, et al. Nivolumab maintenance in high-risk acute myeloid leukemia patients: a single-arm, open-label, phase ii study. Blood Cancer J (2021) 11:60. doi: 10.1038/s41408-021-00453-z
160. Ravandi F, Assi R, Daver N, Benton CB, Kadia T, Thompson PA, et al. Idarubicin, cytarabine, and nivolumab in patients with newly diagnosed acute myeloid leukaemia or high-risk myelodysplastic syndrome: a single-arm, phase 2 study. Lancet Haematol (2019) 6:e480–8. doi: 10.1016/S2352-3026(19)30114-0
161. Saxena K, Herbrich SM, Pemmaraju N, Kadia TM, DiNardo CD, Borthakur G, et al. A phase 1b/2 study of azacitidine with pd-l1 antibody avelumab in relapsed/refractory acute myeloid leukemia. Cancer-Am Cancer Soc (2021) 127:3761–71. doi: 10.1002/cncr.33690
162. Zeidan AM, Boss I, Beach C, Copeland WB, Thompson E, Fox BA, et al. Azacitidine and durvalumab in first-line treatment of elderly patients with acute myeloid leukemia. Blood advances. (2022) 7:2219–29. doi: 10.1182/bloodadvances.2021006138
163. Short NJ, Borthakur G, Pemmaraju N, Dinardo CD, Kadia TM, Jabbour E, et al. A multi-arm phase ib/ii study designed for rapid, parallel evaluation of novel immunotherapy combinations in relapsed/refractory acute myeloid leukemia. Leuk Lymphoma. (2022) 63:2161–70. doi: 10.1080/10428194.2022.2062345
164. Spallone A, Alotaibi AS, Jiang Y, Daver N, Kontoyiannis DP. Infectious complications among patients with aml treated with immune checkpoint inhibitors. Clin Lymphoma Myeloma Leukemia. (2022) 22:305–10. doi: 10.1016/j.clml.2021.10.012
165. Zheng H, Mineishi S, Claxton D, Zhu J, Zhao C, Jia B, et al. A phase i clinical trial of avelumab in combination with decitabine as first line treatment of unfit patients with acute myeloid leukemia. Am J Hematol (2021) 96:E46–E50. doi: 10.1002/ajh.26043
166. Prebet T, Goldberg AD, Jurcic JG, Khaled S, Dail M, Feng Y, et al. A phase 1b study of atezolizumab in combination with guadecitabine for the treatment of acute myeloid leukemia. Leuk Lymphoma. (2022) 63:2180–8. doi: 10.1080/10428194.2022.2057484
167. Daver N, Jonas BA, Medeiros BC, Patil U, Yan M. Phase 1b, open-label study evaluating the safety and pharmacokinetics of atezolizumab (anti-pd-l1 antibody) administered in combination with hu5f9-g4 to patients with relapsed and/or refractory acute myeloid leukemia. Leukemia Lymphoma. (2022) 63:2711–4. doi: 10.1080/10428194.2022.2092853
168. Gao X, Su Y, Li M, Jing Y, Wang J, Xu L, et al. Single-center phase 2 study of pd-1 inhibitor combined with dna hypomethylation agent + cag regimen in patients with relapsed/refractory acute myeloid leukemia. Cancer Immunol Immunother (2023) 72:2769–82. doi: 10.1007/s00262-023-03454-y
169. O’Connell CL, Baer MR, Ørskov AD, Saini SK, Duong VH, Kropf P, et al. Safety, outcomes, and t-cell characteristics in patients with relapsed or refractory mds or cmml treated with atezolizumab in combination with guadecitabine. Clin Cancer Res (2022) 28:5306–11. doi: 10.1158/1078-0432.CCR-22-1810
170. Tang Y, Zhou Z, Yan H, You Y. Case report: preemptive treatment with low-dose pd-1 blockade and azacitidine for molecular relapsed acute myeloid leukemia with runx1-runx1t1 after allogeneic hematopoietic stem cell transplantation. Front Immunol (2022) 13:810284. doi: 10.3389/fimmu.2022.810284
171. Albring JC, Inselmann S, Sauer T, Schliemann C, Altvater B, Kailayangiri S, et al. Pd-1 checkpoint blockade in patients with relapsed aml after allogeneic stem cell transplantation. Bone marrow Transplant (Basingstoke). (2017) 52:317–20. doi: 10.1038/bmt.2016.274
172. Chan TSY, Sim JPY, Kwong Y. Low-dose nivolumab-induced responses in acute lymphoblastic leukaemia relapse after allogeneic haematopoietic stem cell transplantation. Ann Hematol (2017) 9:1569–72. doi: 10.1007/s00277-017-3033-7
173. Hatefi F, Asgarian-Omran H, Hossein-Nataj H, Akbar A, Shekarriz R, Zaboli E, et al. Combined blockade of pd-1 and tigit is not sufficient to improve the function of cd8+ t-cells in chronic lymphocytic leukemia. Asian Pacific J Cancer Prev (2022) 23:2225–31. doi: 10.31557/APJCP.2022.23.7.2225
174. Rezazadeh H, Astaneh M, Tehrani M, Hossein-Nataj H, Zaboli E, Shekarriz R, et al. Blockade of pd-1 and tim-3 immune checkpoints fails to restore the function of exhausted cd8+ t cells in early clinical stages of chronic lymphocytic leukemia. Immunol Res (2020) 68:269–79. doi: 10.1007/s12026-020-09146-4
175. Radpour R, Stucki M, Riether C, Ochsenbein AF. Epigenetic silencing of immune-checkpoint receptors in bone marrow- infiltrating t cells in acute myeloid leukemia. Front Oncol (2021) 11:663406. doi: 10.3389/fonc.2021.663406
176. Kalinin RS, Ukrainskaya VM, Chumakov SP, Moysenovich AM, Tereshchuk VM, Volkov DV, et al. Engineered removal of pd-1 from the surface of cd19 car-t cells results in increased activation and diminished survival. Front Mol Biosciences. (2021) 8:745286. doi: 10.3389/fmolb.2021.745286
177. Zhang L, Chen X, Liu X, Kline DE, Teague RM, Gajewski TF, et al. Cd40 ligation reverses t cell tolerance in acute myeloid leukemia. J Clin Invest. (2013) 123:1999–2010. doi: 10.1172/JCI63980
178. Yao S, Jianlin C, Zhuoqing Q, Yuhang L, Jiangwei H, Guoliang H, et al. Case report: combination therapy with pd-1 blockade for acute myeloid leukemia after allogeneic hematopoietic stem cell transplantation resulted in fatal gvhd. Front Immunol (2021) 12:639217. doi: 10.3389/fimmu.2021.639217
179. Hagop M, Kantarjian M, Michael J. Keating MB, And Emil J Freireich M. Toward the potential cure of leukemias in the next decade. Cancer-Am Cancer Soc (2018) 22:4301–13. doi: 10.1002/cncr.31669
180. Yin ES, Totonchy MB, Leventhal JS. Nivolumab-associated vitiligo-like depigmentation in a patient with acute myeloid leukemia: a novel finding. JAAD Case Rep (2017) 3:90–2. doi: 10.1016/j.jdcr.2016.10.008
181. Hsiao C, Yao M, Liu J, Chen W. Pembrolizumab induced acute corneal toxicity after allogeneic stem cell transplantation. Clin Exp Ophthalmol (2018) 46:698–700. doi: 10.1111/ceo.13139
Keywords: leukemia, programmed cell death protein 1, programmed death-ligand 1, immunotherapy, PD-1/PD-L1 mAbs
Citation: Cao H, Wu T, Zhou X, Xie S, Sun H, Sun Y and Li Y (2023) Progress of research on PD-1/PD-L1 in leukemia. Front. Immunol. 14:1265299. doi: 10.3389/fimmu.2023.1265299
Received: 22 July 2023; Accepted: 13 September 2023;
Published: 26 September 2023.
Edited by:
Cory L. Brooks, California State University, Fresno, United StatesReviewed by:
Jifeng Yu, First Affiliated Hospital of Zhengzhou University, ChinaCarsten Riether, Bern University Hospital, Switzerland
Copyright © 2023 Cao, Wu, Zhou, Xie, Sun, Sun and Li. This is an open-access article distributed under the terms of the Creative Commons Attribution License (CC BY). The use, distribution or reproduction in other forums is permitted, provided the original author(s) and the copyright owner(s) are credited and that the original publication in this journal is cited, in accordance with accepted academic practice. No use, distribution or reproduction is permitted which does not comply with these terms.
*Correspondence: Yunxiao Sun, c3VueXVueGlhbzE5NzlAMTYzLmNvbQ==; Youjie Li, eW91amllMTk3OUAxNjMuY29t
†These authors share first authorship