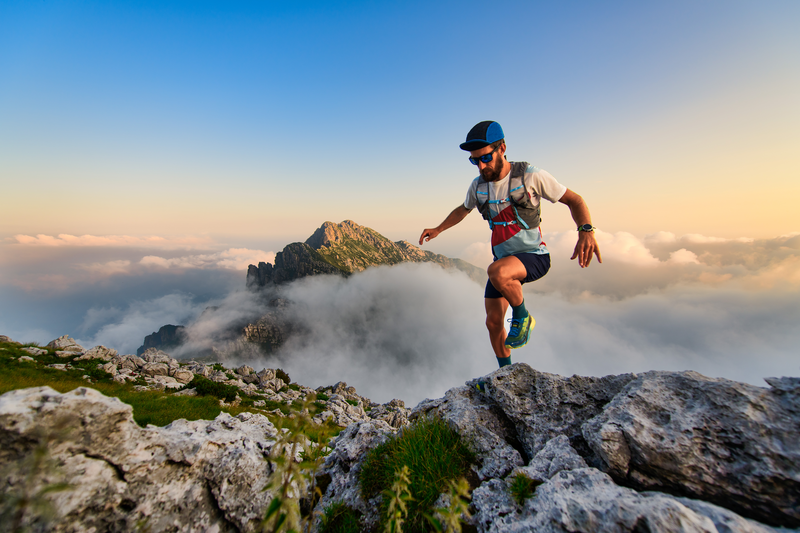
95% of researchers rate our articles as excellent or good
Learn more about the work of our research integrity team to safeguard the quality of each article we publish.
Find out more
REVIEW article
Front. Immunol. , 22 September 2023
Sec. Inflammation
Volume 14 - 2023 | https://doi.org/10.3389/fimmu.2023.1259515
This article is part of the Research Topic Epithelial Immune Microenvironment and Inflammatory Skin Diseases View all 8 articles
The skin is exposed to environmental challenges and contains heterogeneous cell populations such as epithelial cells, stromal cells, and skin-resident immune cells. As the most abundant type of stromal cells, fibroblasts have been historically considered silent observers in the immune responses of the cutaneous epithelial immune microenvironment (EIME), with little research conducted on their heterogeneity and immune-related functions. Single-cell RNA sequencing (scRNA-seq) and spatial transcriptomics (ST) have overcome the limitations of bulk RNA sequencing and help recognize the functional and spatial heterogeneity of fibroblasts, as well as their crosstalk with other types of cells in the cutaneous EIME. Recently, emerging single-cell sequencing data have demonstrated that fibroblasts notably participate in the immune responses of the EIME and impact the initiation and progression of inflammatory skin diseases. Here, we summarize the latest advances in the role of fibroblasts in the cutaneous EIME of inflammatory skin diseases and discuss the distinct functions and molecular mechanisms of activated fibroblasts in fibrotic skin diseases and non-fibrotic inflammatory skin diseases. This review help unveil the multiple roles of fibroblasts in the cutaneous EIME and offer new promising therapeutic strategies for the management of inflammatory skin diseases by targeting fibroblasts or the fibroblast-centered EIME.
The skin contains heterogeneous cell populations, such as epithelial cells, skin-resident immune cells, and stromal cells, and acts as both a physical barrier and an immune organ that can defend against external damage and adverse factors (1, 2). Based on the current knowledge of skin structure, immune responses predominantly develop in the epithelial immune microenvironment (EIME), situated in the epidermis and papillary dermis of the skin (3, 4). Facing ever-changing internal and external stimuli, the cutaneous EIME develops an intricate system for immune responses, including the proliferation and differentiation of multiple cell types and the local interactions among activated immune cells, keratinocytes, and stromal cells in the EIME (4). Disruptions in the cutaneous EIME lead to dysregulated immune responses, inducing various inflammatory skin disorders.
Fibroblasts, characterized by collagen alpha-1 chain and decorin, are the major type of stromal cells in the cutaneous EIME and can be spatially categorized into papillary and reticular fibroblasts (5–7). Fibroblasts are the fundamental cellular component supporting the cutaneous framework because they can produce collagens to form the extracellular matrix (ECM) (8, 9). Historically, fibroblasts have been regarded as silent observers occupying a secondary role within the cutaneous EIME. Studies on the heterogeneity and immune-related functions of fibroblasts are limited (10, 11). Recently, the newly developed single-cell technologies have significantly facilitated our exploration of the morphological and functional heterogeneity of dermal fibroblasts, including single-cell RNA sequencing (scRNA-seq) and spatial transcriptomics (ST), which can recognize unique alterations in gene expression for each cell and provide quantitative visualization of the distribution of gene expression within tissue sections (12–15). Emerging evidence has revealed the dynamic changes and specific functions of fibroblasts in the cutaneous EIME during inflammation progression (16–18).
Under different pathological conditions, such as wound healing, malignancy, or other inflammatory disorders, normal fibroblasts can be activated and secrete cytokines to interact with other cells. Fibroblasts and other types of cells in the cutaneous EIME, such as epithelial cells, endothelial cells, pericytes, and adipocytes, can develop into myofibroblasts, or cancer-associated fibroblasts (CAFs) which are a heterogenous population of activated fibroblasts playing key roles in tumor microenvironment and affecting tumor proliferation, metastasis, and chemotherapy resistance (Figure 1) (19, 20). The activation states of fibroblasts are closely involved in the pathogenesis of diverse inflammatory skin diseases. Based on the distinct roles of fibroblasts in the cutaneous EIME, we divided inflammatory skin diseases into two major categories: (I) fibrotic skin diseases like keloid and systemic sclerosis (SSc), in which fibroblasts act as hallmark cells (21–23); and (II) non-fibrotic inflammatory skin diseases like psoriasis, atopic dermatitis (AD), vitiligo, and systemic lupus erythematosus (SLE), in which fibroblasts act as fundamental and active participants in the immune response by interacting with other types of cells in the cutaneous EIME (24–27).
Figure 1 The activation states of fibroblasts and their precursors in the cutaneous epithelial immune microenvironment (EIME). Under different pathological conditions, such as wound healing, malignancy, or other inflammatory disorders, normal fibroblasts and other types of cells in the cutaneous EIME, including epithelial cells, endothelial cells, pericytes, and adipocytes, can be activated to develop into myofibroblasts or cancer-associated fibroblasts (CAFs). These activated fibroblasts can result in the abnormal accumulation of extracellular matrix (ECM), or secrete cytokines to interact with other types of cells. Created with Figdraw.
In this review, we summarize the latest advances in the role of fibroblasts in the cutaneous EIME of inflammatory skin disorders and discuss the distinct functions and potential molecular mechanisms of activated fibroblasts in fibrotic skin diseases and non-fibrotic inflammatory skin diseases. We aim to unveil the multiple roles of fibroblasts in the cutaneous EIME and explore new promising therapeutic strategies targeting fibroblasts or fibroblast-centered EIME in inflammatory skin diseases.
Fibrotic skin diseases, including local and systematic fibrotic diseases, are characterized by the excessive accumulation of fibroblasts and the abnormal buildup of the ECM and exert a major healthcare burden worldwide (28, 29). Fibroblasts act as the hallmark cells in fibrotic skin diseases. Under pathological conditions, normal fibroblasts and other types of cells can be stimulated to develop into fibroblastic activation states, resulting in excessive collagen accumulation and abnormal tissue fibrosis (19, 30). This section summarizes recent studies on the functions and underlying molecular mechanisms of fibroblasts in various fibrotic skin diseases to provide potential targets for treating refractory fibrotic skin diseases.
Keloid is a typical local fibrotic skin disorder characterized by hypervascularity and excessive accumulation of the ECM (31, 32). Associated with progressive tissue fibrosis and a high recurrence rate, keloid leads to heavy burden and psychological issues for patients. Abnormal activation and excessive proliferation of fibroblasts are the core pathological manifestations in keloid. However, the underlying mechanisms and molecular changes remain unclear.
In a recent study, Yang et al. conducted a scRNA-seq analysis on dermis tissues from keloid and normal scar samples. They obtained transcriptomes of 40,655 cells (keloid: 21,488; normal scar: 19,167) and identified 13 fibroblast subclusters with further heterogeneity (17). Among the identified fibroblast subsets, a notable increase in the proportion of mesenchymal fibroblasts was observed in keloids compared to regular scars, along with a consistent upregulation of genes related to ECM formation, such as collagen type I alpha 1/2 (COL1A1/2), periostin (POSTN) and fibronectin 1 (FN1). Although prior research has documented the increased expression of certain proteins, including POSTN and FN1, the precise cellular origins of these proteins were uncertain. The use of scRNA-seq offers a valuable approach to unveil the cell-specific molecular alterations and mechanisms involved in keloid pathogenesis, a task that conventional bulk RNA sequencing and microarray techniques have struggled to achieve. In that study, keloid exhibited marked increases in the interaction between transforming growth factor-β (TGFβ) and its receptor as well as the interaction between POSTN and integrin alpha-V (ITGAV)/ITGB5 compared to normal scars, suggesting that the TGFβ pathway and POSTN may actively participate in the initiation and progression of keloid. These results were further validated through immunofluorescence staining assay. Consequently, inhibiting mesenchymal fibroblasts by blocking the TGFβ pathway or targeting POSTN might potentially serve as viable therapeutic approaches for treating keloid. The notable upregulation of mesenchymal fibroblasts and ECM-related genes, namely COL1A1, POSTN, COL1A2, FN1 and ASPN, in keloid was similarly documented in a distinct scRNA-seq study conducted by Huang et al. (33). Additionally, the researchers observed that mesenchymal fibroblasts can promote the differentiation and proliferation of macrophages, significantly contributing to the macrophage-centered regulatory network within the EIME of keloid. These findings substantially advanced our comprehension of the immunological characteristics of keloid.
As the key contributor to keloid, mesenchymal fibroblasts can originate from several types of cells, such as normal fibroblasts, adipocytes and endothelial cells. Endothelial-to-mesenchymal transition (EndoMT) is an intrinsic transdifferentiated process that remarkably participates in the occurrence and development of tissue fibrosis under different pathological conditions (34, 35). The EndoMT process involves the reduction of specific endothelial markers, such as CD31 and vascular endothelial (VE)-cadherin, and the acquisition of mesenchymal markers, such as fibroblast-specific protein (FSP1) and alpha-smooth muscle actin (α-SMA) (Figure 2). Endothelial cells (ECs) can be stimulated by TGFβ, secreted by activated immune cells, and transdifferentiate into myofibroblasts or other overactive fibroblasts, leading to collagen accumulation and tissue fibrosis. For a long time, EndoMT has been commonly observed in cardiac fibrosis and kidney fibrosis, yet few studies have been conducted on its occurrence in skin fibrosis. Lee et al. recently performed a scRNA-seq analysis on 35,424 cells from two keloid samples and five normal skin samples. They use the single-cell data from a previously published cohort as normal skin control (36). Remarkably, mesenchymal activation, marked by dysregulated TGFβ/Smad signaling, was widely observed in keloid ECs, which is a characteristic of EndoMT. Moreover, the integration of ST allowed them to observe that the disease-related mesenchymal fibroblasts predominantly clustered around the endothelial regions and colocalized with the keloid ECs. These findings further suggested the potential involvement of fibroblast-EC communication and the EndoMT process in the pathogenesis of keloid. However, given the relatively limited sample size in this study and the lack of extensive prior research on the EndoMT process in keloid, further investigation is necessary to fully understand the impact of EndoMT on the occurrence and progression of keloid.
Figure 2 Sketch map of the endothelial to mesenchymal transition (EndoMT) and epithelial to mesenchymal transition (EMT) processes. In response to diverse inflammatory stimuli, endothelial cells or epithelial cells can be activated, transdifferentiating into mesenchymal cells, known as EndoMT or EMT respectively. These intrinsic processes entail the gradual loss of endothelial/epithelial markers, including CD31, vascular endothelial (VE)-cadherin or epithelial (E)-cadherin, and β-catenin, and the acquisition of mesenchymal markers, including fibroblast-specific protein (FSP1) and alpha-smooth muscle actin (α-SMA).
Endothelin-1 (ET-1), a powerful vasoactive peptide responsible for regulating vascular tone and produced by ECs, was observed to be highly expressed in keloid (37). Notably, the RhoA/Rho-kinase (ROCK) pathway can be activated by ET-1 to promote myofibroblast differentiation and ECM accumulation in the dermis, thereby markedly participating in the pathophysiology of abnormal scar/keloid formation. Therefore, targeting the expression of ET-1 or blocking the RhoA/ROCK pathway may provide powerful approaches for inhibiting ET-1-induced keloid (38).
CAF-like fibroblasts, with the increased expression of CAF-related genes, such as COL11A1, POSTN, WNT5A, and ASPN, is another key subset enriched in fibrotic diseases (33, 39). This fibroblast subset can originate from epithelial cells though the epithelial-to-mesenchymal transition (EMT) process (Figure 2) or the activation of normal fibroblasts under various inflammatory stimuli (30, 40, 41). Several recent studies have confirmed that CAF-like fibroblasts can remodel and boost the accumulation of the ECM or interact with other types of cells, resulting in the progression and expansion of fibrotic lesions in the EIME (42–44). These findings help us understand the mechanisms of tissue fibrosis and provide potential new targets for preventing and treating keloid and other skin diseases characterized by fibrosis.
Systemic sclerosis is a complicated disorder characterized by excessive fibrosis of the skin and multisystem organs. Continuous activation of fibroblasts into myofibroblasts is the core process in the pathogenesis of SSc (45, 46). However, the specific origins of myofibroblasts and their molecular changes in the skin of SSc patients remain uncertain. Recently, Lafyatis et al. performed a scRNA-seq analysis on mid-forearm skin biopsies from 12 patients with SSc and 10 healthy controls (47). They identified a new fibroblast subcluster mainly in SSc skin, named SFRP2hi (expressing high levels of SFRP2) PRSS23+ fibroblasts, which might be associated with the ECM and extracellular structure organization. Moreover, the researchers used pseudotime analysis to ascertain the differentiation trajectory of cells and demonstrated a linear progression from SFRP2hi WIF+ to SFRP2hi PRSS23+ WIF1− and to SFRP2hi PRSS23+ SFRP4+ fibroblasts during the progression of SSc. Additionally, a series of transcription factors (TFs) implicated in myofibroblast differentiation was elucidated, including fos-like antigen 2 (FOSL2), runt-related transcription factor 1 (RUNX1), signal transducer and activator of transcription (STAT1), forkhead box prote1 (FOXP1), and interferon regulatory factor 7 (IRF7). These findings not only revealed a significant shift in fibroblast phenotypes but also unveiled the underlying alterations in gene expression in SSc skin, which can broaden our comprehension of the pathogenesis of SSc and help explore the origin and differentiation of myofibroblast in other fibrotic diseases.
The involvement of EndoMT in dermal fibrosis of SSc was determined through in vitro and in vivo experiments (48). CD31/α-SMA and VE-cadherin/α-SMA colocalization was markedly higher in SSc lesional dermis than in healthy skin dermis. Additionally, the ECs in SSc lesions showed a functional phenotype similar to that of myofibroblasts, indicating the potential participation of EndoMT in the progression of SSc. Furthermore, in vitro experimental results indicated that healthy dermal microvascular ECs (H-dMVECs) exposed to SSc sera developed a myofibroblast-like appearance, marked by a decrease in endothelial indicators and the activation of mesenchymal indicators. This phenotypic transition of H-dMVECs treated with SSc sera was consistent with that of H-dMVECs treated with TGFβ, a universally acknowledged stimulus for EndoMT, further suggesting the key role played by EndoMT in the progression of SSc. These studies revealed the close link between EC dysfunction and the progression of fibrosis, and thus, inhibiting EndoMT may be an effective approach for managing skin fibrosis in patients with SSc.
In addition to conventional fibrotic skin disorders, certain inflammatory skin conditions exhibit focal fibrosis, such as prurigo nodularis (PN). As a chronic inflammatory skin disorder, PN is characterized by stubborn pruritic firm nodules on the extremities and trunk and has recently been associated specifically with the follicular epithelium in the cutaneous EIME (49–51). In the epidermis, it presents as orthohyperkeratosis, hypergranulosis, and acanthosis, occasionally reaching the extent of pseudoepitheliomatous hyperplasia (52). However, recent studies have provided new evidence indicating the unique fibroproliferative characteristics of PN compared with other inflammatory skin diseases, such as psoriasis and atopic dermatitis (23, 53). That reminds us the activated fibroblasts might potentially play a key role in the formation of PN lesions, which is consistent with its clinical features of firm fibrotic nodules.
Gudjonsson et al. conducted a scRNA-seq analysis on 72,782 cells from the skin of 15 healthy donors and the nonlesional and lesional skin of 6 patients with PN (54). They observed the presence of a specific fibroblast subset in the papillary dermis of PN skin, characterized by elevated expression levels of COL11A1, POSTN, and serine protease 23 (PRSS23). This subset, identified as COL11A1+ fibroblasts, demonstrated distinct profibrotic properties in the cutaneous EIME of PN. Additionally, the researchers also found that the receptor of interleukin-31 (IL-31), which is a favorable immune mediator in PN, was expressed in diseased fibroblasts, and therapy targeting IL-31R (Nemolizumab) could revert the changes in PN lesions. This finding provides convincing evidence of the critical role of immune-fibroblast crosstalk in the pathogenesis of PN. Remarkably, that study also used ST to ascertain the spatial heterogeneity and cell-cell interaction of fibroblasts in the cutaneous EIME. The incorporation of suitable controls and the integration of ST and scRNA-seq enhance the reliability and importance of these findings.
A separate single-cell analysis conducted by Kwatra et al. revealed a significant increase in CAF-like (WNT5A+ POSTN+) fibroblasts within PN lesions compared with nonlesional skin of PN patients (55). Moreover, ligand-receptor analyses demonstrated that the WNT5A and POSTN expressed by fibroblasts could frequently interact with the neuronal receptors MCAM and ITGAV in PN lesions, further suggesting the potential role of the fibroblast-neuronal axis in the initiation and progression of PN. However, this study solely focused on patients diagnosed with PN, without incorporating healthy individuals as a control group. Therefore, further extensive research is necessary to acquire a more comprehensive and persuasive understanding of the specific roles of fibroblasts in the development of PN.
The above studies at single-cell level enhanced our understanding of the roles of morphologically and functionally heterogeneous fibroblasts in the pathogenesis of PN. For a long time, most studies on prurigo nodularis focused on “itch”, and limited studies were conducted on firm “nodules”. Hence, exploring fibroblast-related molecular mechanisms of firm nodules may provide optional and promising strategies for treating refractory PN.
In addition to playing a central role in fibrotic skin diseases, fibroblasts also actively participate in the cutaneous EIME of non-fibrotic inflammatory skin diseases, such as psoriasis, AD, SLE, and vitiligo, mostly by frequent crosstalk with immune cells, epithelial cells, and others. Single-cell data provides a unique opportunity to analyze cell-cell communication mediated by ligand-receptor interactions. This section discusses the new insight into the role of crosstalk between fibroblasts and multiple types of cells in non-fibrotic inflammatory skin diseases to discover novel and powerful targets for managing diverse inflammatory skin diseases.
Psoriasis is one of the most common immune-regulated skin diseases, exerting a substantial medical burden on patients and society (56, 57). Previous studies have confirmed that psoriasis is closely related to T-cell immune dysregulation, and IL-17 and IL-23 are regarded as the core initiators of the pathogenesis of psoriasis (58). Recently, researchers have combined scRNA-seq and ST to identify several novel fibroblast subsets in the cutaneous EIME of psoriasis that may drive and participate in the progression of psoriasis. Meanwhile, the crosstalk between fibroblasts and immune cells has also been demonstrated as the key contributor to psoriatic inflammation.
Hu et al. conducted a scRNA-seq analysis on full-thickness skins obtained from the psoriatic lesions of 3 patients and the corresponding regions of 3 healthy individuals, and a total of 24,234 cells were further clustered into 35 cellular subsets (59). They analyzed cell-specific gene expression and observed that the expression of MHC molecules, including HLA-A/B/C and HLA-DRA, was greatly increased on the surface of fibroblasts under psoriatic-inflammation conditions, which can stimulate the activation of T and NK cells by interactions with CD4, CD8, and CD94 (60, 61). Additionally, fibroblasts in psoriatic lesions showed a significant increase in the release of key chemokines and cytokines, including C-C motif chemokine ligand 26 (CCL26), IL-6, leukemia inhibitory factor (LIF), IL-17B, CCL19, and stromal cell-derived factor 1 (SDF-1), which greatly enhanced the accumulation of a variety of immune cells around fibroblasts. The release of a series of cytokines further confirmed the proinflammatory roles exerted by fibroblasts. Notably, these key molecules, such as CCL26, IL-6, IL-17B, and CCL19, have been previously considered active participants in the inflammatory process and are solely secreted by immune cells. In contrast, single-cell data help to reveal that fibroblasts can also secrete inflammatory cytokines and contribute to the immune responses of psoriasis.
Perivascular fibroblasts, an important subtype of fibroblasts, are widely distributed in human tissues and involved in various inflammatory diseases, such as myocardial infarction and fibrotic diseases. In contrast, their roles in psoriasis are rarely studied. By combining scRNA-seq and ST, a novel subset of PDGFRβ+ perivascular fibroblasts was identified in psoriatic lesions. This subset exhibited a remarkable increase in the release of IL-17B and activation of dendritic cells (DCs). The presence of PDGFRβ+ fibroblasts might be relevant to the surface expression of CD80/86 proteins in DCs, thereby promoting the progression of psoriatic inflammation (62). Nevertheless, the underlying mechanisms by which PDGFRβ+ fibroblasts secrete cytokines and affect the expression of CD80/86 proteins in DCs remain to be elucidated. These findings confirmed that fibroblasts could interact with immune cells by secreting a series of inflammatory factors and significantly regulate the immune responses in the EIME of psoriasis, providing promising targets and pathways for treating psoriasis.
Atopic dermatitis is a refractory inflammatory skin disease with clinical features of dry skin, intense itching, and eczema-like rashes (63–66). Although the etiology and underlying mechanisms have not yet been fully elucidated, AD has been consistently characterized by a robust stimulation of TH2 immune dysfunction in skin lesions and normal skin (67, 68). Compared to conventional bulk transcriptomics, single-cell analysis offer a more precise, comprehensive, and reliable characterization of immune subsets in AD. Researchers have identified novel fibroblast subpopulations in the cutaneous EIME that might contribute to the progression of inflammation and pathological disruption of AD.
Recently, Guttman et al. conducted a comprehensive scRNA-seq analysis on 39,042 cells obtained from lesional and nonlesional skin samples from 5 patients with moderate-to-severe AD and from healthy individuals. The inclusion criteria for the patients were strictly defined. Using cell lineage markers, the researchers identified a novel COL6A5/18A1+ fibroblast subset uniquely distributed in the AD lesions, associated with a higher release of the CCL2, CCL19, and IL-32 (25). COL6A5, also present in other locations of individuals with allergic conditions, has been identified as a gene involved in the development of AD (69). The potential contribution of COL6A5 in AD may be attributed to the creation of unstable heterotrimers, resulting in abnormal fibroblast adhesion and barrier dysfunction (70). COL18A1 can strongly bind to the ECM component and potentially disrupt and destabilize the ECM in AD lesions. Interestingly, they also observed that CD3+ T cells accumulated mostly around COL6A5/18A1+ fibroblasts, indicating that these fibroblasts may be involved in the recruitment and organization of T cells by secreting a series of cytokines. Additionally, as the best indicator of TH2 immune responses (71, 72), CCL26 upregulated the expression in COL6A5/18A1+ and MFAP5+ FBN1+ fibroblasts, further suggesting the critical roles of these fibroblast subsets in the TH2 immune responses of AD (25). As an important chemokine involved in several inflammatory processes, CCL26 has been widely studied and might be a favorable target for addressing inflammatory skin disorders (73, 74). Nevertheless, the cell-cell interactions and underlying pathways by which CCL26 contributes to the pathogenesis of AD remain unclear and need further investigation. In addition to COL6A5/18A1+ fibroblasts, AD lesions also exhibited higher infiltration of CCL2+ fibroblasts, with consistent patterns observed for CCL19 and POSTN. The CCL2+ fibroblasts are mostly distributed next to CD3+ T cells and can interact with macrophages and DCs via the receptors CCR1 and CCR2, further suggesting the critical roles of CCL2+ fibroblasts in the recruitment and organization of T cells, macrophages, and DCs. Despite the relatively small sample size, this study represents the initial comprehensive scRNA-seq analysis covering all cell types and expression conditions within the skin tissues of AD patients compared to healthy individuals. Therefore, the findings in this research are reliable, representative, and of substantial value.
Combining scRNA-seq with a series of in vivo experiments, Ko et al. first identified a unique paired related homeobox-1 (Prx1+) fibroblast subpopulation in AD lesions in a classical experimental mouse model, in which the IKKB-NF-kB pathway was disrupted under homeostatic conditions (75). These Prx1+ fibroblasts can overexpress CCL11 and lead to skin inflammation of AD, which is characterized by the infiltration of eosinophils and followed by TH2 immune responses. The effectiveness of monoclonal antibody therapy against CCL11 in reducing eosinophilia and TH2 inflammation confirmed the proinflammatory effect of CCL11 (75). Additionally, the researchers also examined human AD samples and confirmed that the perturbation of IKKB-NF-kB could upregulate CCL11 in human AD fibroblasts, further suggesting the critical roles of Prx1+ fibroblasts and CCL11 in the pathogenesis of AD. However, as a key chemokine and medium in the crosstalk between fibroblasts and immune cells, CCL11 has never been studied in inflammatory skin diseases other than AD (76). Hence, the underlying pathway by which CCL11 stimulates eosinophil infiltration and the TH2 immune response in the human dermis deserves further investigation (77).
In previous studies, the C-X-C motif receptor (CXCR4)/C-X-C motif ligand 12 (CXCL12) axis was reported to participate in psoriasis-like inflammation both by promoting keratinocyte proliferation and recruiting T cells (78). Likewise, the upregulation of CXCL12 was also identified in AD lesions. Sun et al. reported that thymic stromal lymphopoietin (TSLP) could induce fibroblasts to produce CXCL12 and stimulate the trafficking and migration of natural killer T cells (NKT) via CXCR4, resulting in inflammation progression in AD skin lesions (79). Thus, blocking CXCR4/CXCL12 could also be a feasible treatment option for individuals with AD.
SLE is a severe inflammatory disease characterized by diverse clinical and immunopathological manifestations (80, 81). Dysregulated activation of immune cells and aberrant secretion of autoantibodies and proinflammatory cytokines participate in the pathogenesis of SLE (82, 83). As a critical type of lupus erythematosus (LE), cutaneous lupus erythematosus (CLE) has been commonly studied, and the cellular heterogeneity and underlying mechanisms in cutaneous lesions have been revealed at single-cell resolution (84–86). Recently, Zhao et al. reported the scRNA-seq data of 23 skin biopsy samples from 8 discoid lupus erythematosus (DLE), 10 SLE patients, and 5 healthy controls (85). The researchers observed that CXCL1+ fibroblasts and HLA+ fibroblasts were the most abundant fibroblast subtypes in cutaneous lesions, and CXCL1, known for its ability to facilitate the infiltration of immune cells, was highly expressed in these activated fibroblasts. Additionally, the receptors of fibroblast-secreted CCL19, CXCL12, and tumor necrosis factor superfamily member 13b (TNFSF13B), which participate in the immune responses of the cutaneous EIME, exhibited higher expression in the immune cells of LE cutaneous lesions compared with healthy skin. These findings were also validated by immunofluorescence staining assay, indicating that the crosstalk between fibroblasts and immune cells contributes to the pathological processes of lupus cutaneous lesions.
In addition to releasing cytokines and chemokines, researchers found that activated fibroblasts might interact with immune cells by presenting antigens. HLA-DRB1 and HLA-DRA1, typically marked in antigen-presenting cells, were found to be highly expressed in HLA+ fibroblasts, suggesting the potential role of HLA+ fibroblasts as nonclassical antigen-presenting cells in immune responses (59). Nevertheless, the underlying pathways by which HLA+ fibroblasts present antigens and the specific types of involved immune cells remain to be elucidated.
Keratocytes are the most abundant cell type in the epidermis, chiefly involved in the immune responses in the cutaneous EIME (87, 88). Consistent with immune cells, abundant crosstalk between fibroblasts and keratinocytes has also been confirmed in the pathogenesis of psoriasis. Using a skin equivalent model containing both psoriatic and healthy cells, researchers observed that psoriatic fibroblasts could stimulate the excessive proliferation of healthy keratinocytes, which is the main pathological manifestation of psoriasis (89). Moreover, the epidermis isolated from psoriatic skin continued to be hyperproliferative for at least 15 days, with no inhibition by normal fibroblasts, further demonstrating the key roles played by psoriatic fibroblasts in keratocyte hyperproliferation (90). However, revealing the underlying molecular mechanisms and signaling pathways by which fibroblasts interact with keratinocytes is critical for studies on psoriasis.
More recently, Miossec et al. observed that fibroblasts from individuals with psoriasis exhibit a significant increase in proliferation and enhance the growth of keratinocytes through the expression of SDF-1, also named CXCL12 (90, 91). SDF-1, a protein specific to fibroblasts, possesses the ability to trigger the ERK pathway and acts as a growth factor to induce the proliferation of epidermal keratinocytes. In-depth studies also confirmed that the overexpression of SDF-1 could significantly increase the quantity of keratinocyte layers and the thickness of the epidermis (92). SDF-1 has been widely studied as a stable chemokine that induces the migration and activation of various cell types, and SDF-1/CXCR4 targeted therapies have been successfully developed and applied in diverse diseases (93, 94). Thus, although SDF-1 is less studied in psoriasis than in tumors, and the underlying molecular mechanisms remain unclear, SDF-1 is still a promising target in the cutaneous EIME to inhibit keratinocyte proliferation and subsequently treat or prevent the occurrence of psoriasis.
Fibroblasts can alter the proliferation and differentiation of keratinocytes in a series of inflammatory diseases, but their role in AD has not been fully elucidated (90, 95). Recent studies confirmed the contribution of crosstalk between fibroblasts and keratinocytes to the pathogenesis of AD in the cutaneous EIME. Through atopic-like organotypic culture (OTC) skin models, Gitta et al. first revealed that atopic fibroblasts could impact the characteristics of the epidermis by promoting cell proliferation and hindering the layering of keratinocytes (18). Furthermore, atopic fibroblasts significantly downregulate the expression of LIF, a key regulator of the cytokine cascade (96), and release several cytokines, including IL-4, IL-3, and IL-31. These cytokines can diminish filaggrin expression in keratinocytes, leading to dysfunctions of the epidermal barrier in AD skin (97, 98). Notably, filaggrin is a major protein in maintaining the normal function of the epidermal barrier, and the lack or dysfunction of filaggrin can result in a range of skin disorders (99, 100). Hence, the phenomenon that filaggrin expression in keratinocytes can be downregulated by atopic fibroblasts and restored by healthy fibroblasts offers promising strategies for treating AD.
Melanocytes are an unremarkable cell type situated at the basal layer of the epidermis, and they can secrete melanin to maintain the appearance of the skin and protect it from ultraviolet damage (101, 102). Vitiligo is a unique inflammatory skin disease in which the immune system targets melanocytes, leading to the formation of depigmentation (103, 104). However, whether fibroblasts participate in the immune responses in vitiligo remains unclear.
Recently, Chen et al. conducted an extensive investigation into the distinct functions of fibroblast subsets in the pathogenesis of vitiligo (105). Using scRNA-seq, cell-type-specific knockouts, and engraftment experiments, the researchers demonstrated the importance of interferon γ (IFNγ) responsive fibroblasts in the recruitment and activation of CD8+ T cells. The CD8+ T cells subsequently attacked melanocytes, leading to the loss of melanocytes and skin depigmentation (105, 106). This subset of IFNγ-responsive fibroblasts and its pivotal role in the cutaneous EIME of vitiligo lesions have never been elucidated in prior research. Additionally, the researchers revealed that the activated IFNγ-responsive fibroblasts can recruit CXCR3+ T cells and interact with melanocytes by secreting chemokines CXCL9/CXCL10 in vitiligo lesions (105, 107). The CXCL9/CXCL10-CXCR3 axis is closely involved in the progression of vitiligo and deserves further attention. The highlight of this study lies in its comprehensive and logical experimental design. In addition to conducting scRNA-seq analysis on skin specimens obtained from vitiligo patients, the researchers employed a diverse range of in vitro and in vivo experiments, such as gene knockouts, engraftment experiments, and transwell migration assays, to investigate the specific functions of different fibroblast subsets in vitiligo. These comprehensive approaches enhance the credibility and validity of the research findings. Consequently, the triangulated crosstalk between fibroblasts, T cells, and melanocytes in the EIME of vitiligo provide promising methods and targeted strategies for preventing and treating vitiligo.
(Note: The identified fibroblast subsets and their crosstalk with other types of cells in the cutaneous EIME of diverse inflammatory skin disorders are illustrated in Figure 3)
Figure 3 Crosstalk between fibroblasts and other types of cells in the cutaneous epithelial immune microenvironment (EIME) of inflammatory skin diseases. Blue, red, green, purple, black and golden words represent psoriasis-specific, atopic dermatitis (AD)-specific, prurigo nodularis (PN)-specific, keloid-specific, vitiligo-specific and systemic lupus erythematosus (SLE)-specific inflammation, respectively. SDF1, stromal-derived factor 1; TGF-β, transforming growth factor-β; CXCL, C-X-C motif ligand; CCL, C-C motif chemokine ligand; LIF, leukemia inhibitory factor; CAF, cancer-associated fibroblast; COL6A5, collagen type VI alpha 5; POSTN, periostin; TNFSF13B, tumor necrosis factor superfamily member 13b. IFNγ, interferon-γ; EMT, epithelial-mesenchymal transition; EndoMT, endothelial to mesenchymal transition.
Thanks to the newly comprehensive analysis of the morphological and functional heterogeneity of fibroblasts by combining scRNA-seq and spatial transcriptomics, new insights can be obtained into the multiple roles of fibroblasts in the cutaneous EIME of inflammatory skin disorders. The present review discussed the distinct roles of fibroblasts in two major categories of inflammatory skin diseases.
In fibrotic skin diseases, the fibrous hyperproliferation and overaccumulation of the ECM caused by overactivated fibroblasts, as a hallmark, are crucial in the progression of these diseases. Therefore, strategies directly targeting fibroblasts or blocking the activation of fibroblasts are valuable for treating fibrotic skin diseases. In fact, new drugs or therapies targeting fibroblasts have emerged in recent years, especially in cancer research.
In the past few years, there has been a significant increase in the investigation of cancer-associated fibroblasts (108, 109). CAFs are commonly considered nonmutant cells within the tumor microenvironment that can significantly regulate cancer progression and metastasis (110, 111). Here, we summarize the current research on the roles of CAF-like fibroblasts in inflammatory skin disorders beyond the conventional understanding of CAFs in cancer research. We think CAFs are not a unique phenotype of tumors but a special phenotype or state of activated fibroblasts during wound healing or other inflammatory processes. Therefore, strategies targeting CAF-like fibroblasts may provide promising approaches for managing inflammatory skin diseases. Currently, although the phenotypes, origin, and functions of CAFs remain controversial, a series of novel drugs or biologics have been developed and prepared for anticancer clinical practice, such as galunisertib, JNJ-42756493, AMD3100, and PT630 (Table 1). These drugs can inhibit CAF activation or action by targeting a series of key molecules, such as fibroblast growth factor receptor (FGFR), TGFβ, CXCR4, ROCK, and fibroblast activation protein-α (FAP) (110, 123, 124). Given the crucial roles of CAFs/CAF-like fibroblasts in the cutaneous EIME of inflammatory skin diseases, these novel drugs targeting CAFs may also provide promising therapies for various inflammatory skin diseases with CAF-like fibroblasts, such as keloid, PN, and other diseases characterized by excessive fibrosis: this deserves further research.
Activated fibroblasts can also release chemokines or other proinflammatory substances to interact with other types of cells in the cutaneous EIME (Figure 3). The crosstalk between fibroblasts and multiple types of cells significantly participates in the pathogenesis of fibrotic and non-fibrotic inflammatory diseases. It would be a valuable strategy to disrupt the crosstalk between fibroblasts and other cells for managing non-fibrotic inflammatory diseases. These findings at the single-cell level broaden our understanding of the roles of fibroblasts in the cutaneous EIME under inflammatory conditions and provide new targets and pathways to prevent and treat diverse inflammatory skin diseases (Table 2).
Table 2 Summary of potential targets of fibroblasts in the cutaneous EIME for the management of diverse inflammatory skin diseases.
Although single-cell technologies are available with perfect accuracy, we still need wide dynamic observation of the identified cell “subtypes”. The cell “subtypes” may indicate varying reactions to internal and external stimuli or damages instead of consistent functional specializations in a stable condition. This concept is similar to that established for a group of functionally unique subcategories of T cells, including Tregs, CD8+ T cells, CD4+ T cells, and double-negative T cells. Moreover, single-cell analyses via scRNA-seq and spatial transcriptomics cannot completely reflect the cellular protein levels or posttranslational protein modifications of molecular complexity and heterogeneity (127–129). Hence, the single-cell data should be analyzed more objectively and comprehensively, and other approaches should be integrated to reach a relatively precise conclusion.
LW: Investigation, Resources, Writing – original draft. BW: Investigation, Resources, Writing – original draft. EK: Methodology, Writing – original draft. LD: Supervision, Visualization, Writing – review & editing. YZ: Conceptualization, Funding acquisition, Supervision, Writing – review & editing.
The authors declare financial support was received for the research, authorship, and/or publication of this article. This study was funded by the Shanghai Collaborative Innovation Project (XTCX-KJ-2023-44), the National Natural Science Foundation of China (32201251), the Basic scientific research project of Naval Medical University (2022MS009) and the Clinical research project of Shanghai Changning District Science and Technology Commission (CNKW2022Y60).
The authors declare that the research was conducted in the absence of any commercial or financial relationships that could be construed as a potential conflict of interest.
All claims expressed in this article are solely those of the authors and do not necessarily represent those of their affiliated organizations, or those of the publisher, the editors and the reviewers. Any product that may be evaluated in this article, or claim that may be made by its manufacturer, is not guaranteed or endorsed by the publisher.
1. Chambers ES, Vukmanovic-Stejic M. Skin barrier immunity and ageing. Immunology (2020) 160(2):116–25. doi: 10.1111/imm.13152
2. Orsmond A, Bereza-Malcolm L, Lynch T, March L, Xue M. Skin barrier dysregulation in psoriasis. Int J Mol Sci (2021) 22(19):10841. doi: 10.3390/ijms221910841
3. Zhou Y, Xu F, Chen XY, Yan BX, Wang ZY, Chen SQ, et al. The epidermal immune microenvironment plays a dominant role in psoriasis development, as revealed by mass cytometry. Cell Mol Immunol (2022) 19(12):1400–13. doi: 10.1038/s41423-022-00940-8
4. Dainichi T, Kitoh A, Otsuka A, Nakajima S, Nomura T, Kaplan DH, et al. The epithelial immune microenvironment (Eime) in atopic dermatitis and psoriasis. Nat Immunol (2018) 19(12):1286–98. doi: 10.1038/s41590-018-0256-2
5. Schuster R, Rockel JS, Kapoor M, Hinz B. The inflammatory speech of fibroblasts. Immunol Rev (2021) 302(1):126–46. doi: 10.1111/imr.12971
6. Liu B, Li A, Xu J, Cui Y. Single-cell transcriptional analysis deciphers the inflammatory response of skin-resident stromal cells. Front Surg (2022) 9:935107. doi: 10.3389/fsurg.2022.935107
7. Ahuja AK, Pontiggia L, Moehrlen U, Biedermann T. The dynamic nature of human dermal fibroblasts is defined by marked variation in the gene expression of specific cytoskeletal markers. Life (Basel) (2022) 12(7):935. doi: 10.3390/life12070935
8. Woodley DT. Distinct fibroblasts in the papillary and reticular dermis: implications for wound healing. Dermatol Clin (2017) 35(1):95–100. doi: 10.1016/j.det.2016.07.004
9. Lynch MD, Watt FM. Fibroblast heterogeneity: implications for human disease. J Clin Invest (2018) 128(1):26–35. doi: 10.1172/jci93555
10. Suárez-Fariñas M, Ungar B, Correa da Rosa J, Ewald DA, Rozenblit M, Gonzalez J, et al. Rna sequencing atopic dermatitis transcriptome profiling provides insights into novel disease mechanisms with potential therapeutic implications. J Allergy Clin Immunol (2015) 135(5):1218–27. doi: 10.1016/j.jaci.2015.03.003
11. Nomura I, Gao B, Boguniewicz M, Darst MA, Travers JB, Leung DY. Distinct patterns of gene expression in the skin lesions of atopic dermatitis and psoriasis: a gene microarray analysis. J Allergy Clin Immunol (2003) 112(6):1195–202. doi: 10.1016/j.jaci.2003.08.049
12. Xia D, Wang Y, Xiao Y, Li W. Applications of single-cell rna sequencing in atopic dermatitis and psoriasis. Front Immunol (2022) 13:1038744. doi: 10.3389/fimmu.2022.1038744
13. Kim D, Chung KB, Kim TG. Application of single-cell rna sequencing on human skin: technical evolution and challenges. J Dermatol Sci (2020) 99(2):74–81. doi: 10.1016/j.jdermsci.2020.06.002
14. Du J, Yang YC, An ZJ, Zhang MH, Fu XH, Huang ZF, et al. Advances in spatial transcriptomics and related data analysis strategies. J Transl Med (2023) 21(1):330. doi: 10.1186/s12967-023-04150-2
15. Liao J, Lu X, Shao X, Zhu L, Fan X. Uncovering an organ's molecular architecture at single-cell resolution by spatially resolved transcriptomics. Trends Biotechnol (2021) 39(1):43–58. doi: 10.1016/j.tibtech.2020.05.006
16. Soliman H, Tung LW, Rossi FMV. Fibroblast and myofibroblast subtypes: single cell sequencing. Methods Mol Biol (2021) 2299:49–84. doi: 10.1007/978-1-0716-1382-5_4
17. Deng CC, Hu YF, Zhu DH, Cheng Q, Gu JJ, Feng QL, et al. Single-cell RNA-seq reveals fibroblast heterogeneity and increased mesenchymal fibroblasts in human fibrotic skin diseases. Nat Commun (2021) 12(1):3709. doi: 10.1038/s41467-021-24110-y
18. Berroth A, Kühnl J, Kurschat N, Schwarz A, Stäb F, Schwarz T, et al. Role of fibroblasts in the pathogenesis of atopic dermatitis. J Allergy Clin Immunol (2013) 131(6):1547–54. doi: 10.1016/j.jaci.2013.02.029
19. Gauthier V, Kyriazi M, Nefla M, Pucino V, Raza K, Buckley CD, et al. Fibroblast heterogeneity: keystone of tissue homeostasis and pathology in inflammation and ageing. Front Immunol (2023) 14:1137659. doi: 10.3389/fimmu.2023.1137659
20. Plikus MV, Wang X, Sinha S, Forte E, Thompson SM, Herzog EL, et al. Fibroblasts: origins, definitions, and functions in health and disease. Cell (2021) 184(15):3852–72. doi: 10.1016/j.cell.2021.06.024
21. Valenzi E, Bulik M, Tabib T, Morse C, Sembrat J, Trejo Bittar H, et al. Single-cell analysis reveals fibroblast heterogeneity and myofibroblasts in systemic sclerosis-associated interstitial lung disease. Ann Rheum Dis (2019) 78(10):1379–87. doi: 10.1136/annrheumdis-2018-214865
22. Shook BA, Wasko RR, Rivera-Gonzalez GC, Salazar-Gatzimas E, López-Giráldez F, Dash BC, et al. Myofibroblast proliferation and heterogeneity are supported by macrophages during skin repair. Science (2018) 362(6417):eaar2971. doi: 10.1126/science.aar2971
23. Sutaria N, Alphonse MP, Roh YS, Choi J, Parthasarathy V, Deng J, et al. Cutaneous transcriptomics identifies fibroproliferative and neurovascular gene dysregulation in prurigo nodularis compared with psoriasis and atopic dermatitis. J Invest Dermatol (2022) 142(9):2537–40. doi: 10.1016/j.jid.2022.02.010
24. Qie C, Jiang J, Liu W, Hu X, Chen W, Xie X, et al. Single-cell rna-seq reveals the transcriptional landscape and heterogeneity of skin macrophages in vsir(-/-) murine psoriasis. Theranostics (2020) 10(23):10483–97. doi: 10.7150/thno.45614
25. He H, Suryawanshi H, Morozov P, Gay-Mimbrera J, Del Duca E, Kim HJ, et al. Single-cell transcriptome analysis of human skin identifies novel fibroblast subpopulation and enrichment of immune subsets in atopic dermatitis. J Allergy Clin Immunol (2020) 145(6):1615–28. doi: 10.1016/j.jaci.2020.01.042
26. Leyva-Castillo JM, Sun L, Wu SY, Rockowitz S, Sliz P, Geha RS. Single-cell transcriptome profile of mouse skin undergoing antigen-driven allergic inflammation recapitulates findings in atopic dermatitis skin lesions. J Allergy Clin Immunol (2022) 150(2):373–84. doi: 10.1016/j.jaci.2022.03.002
27. Seneschal J, Boniface K, D'Arino A, Picardo M. An update on vitiligo pathogenesis. Pigment Cell Melanoma Res (2021) 34(2):236–43. doi: 10.1111/pcmr.12949
28. Kramann R, DiRocco DP, Humphreys BD. Understanding the origin, activation and regulation of matrix-producing myofibroblasts for treatment of fibrotic disease. J Pathol (2013) 231(3):273–89. doi: 10.1002/path.4253
29. Allanore Y, Simms R, Distler O, Trojanowska M, Pope J, Denton CP, et al. Systemic sclerosis. Nat Rev Dis Primers (2015) 1:15002. doi: 10.1038/nrdp.2015.2
30. Biffi G, Tuveson DA. Diversity and biology of cancer-associated fibroblasts. Physiol Rev (2021) 101(1):147–76. doi: 10.1152/physrev.00048.2019
31. Xia Y, Wang Y, Shan M, Hao Y, Liang Z. Decoding the molecular landscape of keloids: new insights from single-cell transcriptomics. Burns Trauma (2023) 11:tkad017. doi: 10.1093/burnst/tkad017
32. Liu X, Chen W, Zeng Q, Ma B, Li Z, Meng T, et al. Single-cell Rna-sequencing reveals lineage-specific regulatory changes of fibroblasts and vascular endothelial cells in keloids. J Invest Dermatol (2022) 142(1):124–35.e11. doi: 10.1016/j.jid.2021.06.010
33. Feng C, Shan M, Xia Y, Zheng Z, He K, Wei Y, et al. Single-cell Rna sequencing reveals distinct immunology profiles in human keloid. Front Immunol (2022) 13:940645. doi: 10.3389/fimmu.2022.940645
34. Lamouille S, Xu J, Derynck R. Molecular mechanisms of epithelial-mesenchymal transition. Nat Rev Mol Cell Biol (2014) 15(3):178–96. doi: 10.1038/nrm3758
35. Bischoff J. Endothelial-to-mesenchymal transition. Circ Res (2019) 124(8):1163–5. doi: 10.1161/circresaha.119.314813
36. Shim J, Oh SJ, Yeo E, Park JH, Bae JH, Kim SH, et al. Integrated analysis of single-cell and spatial transcriptomics in keloids: highlights on fibrovascular interactions in keloid pathogenesis. J Invest Dermatol (2022) 142(8):2128–39.e11. doi: 10.1016/j.jid.2022.01.017
37. Kiya K, Kubo T, Kawai K, Matsuzaki S, Maeda D, Fujiwara T, et al. Endothelial cell-derived endothelin-1 is involved in abnormal scar formation by dermal fibroblasts through rhoa/Rho-kinase pathway. Exp Dermatol (2017) 26(8):705–12. doi: 10.1111/exd.13264
38. Piera-Velazquez S, Li Z, Jimenez SA. Role of endothelial-mesenchymal transition (Endomt) in the pathogenesis of fibrotic disorders. Am J Pathol (2011) 179(3):1074–80. doi: 10.1016/j.ajpath.2011.06.001
39. Peng D, Fu M, Wang M, Wei Y, Wei X. Targeting Tgf-B Signal transduction for fibrosis and cancer therapy. Mol Cancer (2022) 21(1):104. doi: 10.1186/s12943-022-01569-x
40. Asif PJ, Longobardi C, Hahne M, Medema JP. The role of cancer-associated fibroblasts in cancer invasion and metastasis. Cancers (Basel) (2021) 13(18):4720. doi: 10.3390/cancers13184720
41. Yang J, Antin P, Berx G, Blanpain C, Brabletz T, Bronner M, et al. Guidelines and definitions for research on epithelial-mesenchymal transition. Nat Rev Mol Cell Biol (2020) 21(6):341–52. doi: 10.1038/s41580-020-0237-9
42. Zeltz C, Primac I, Erusappan P, Alam J, Noel A, Gullberg D. Cancer-associated fibroblasts in desmoplastic tumors: emerging role of integrins. Semin Cancer Biol (2020) 62:166–81. doi: 10.1016/j.semcancer.2019.08.004
43. Caja L, Dituri F, Mancarella S, Caballero-Diaz D, Moustakas A, Giannelli G, et al. Tgf-B and the tissue microenvironment: relevance in fibrosis and cancer. Int J Mol Sci (2018) 19(5):1294. doi: 10.3390/ijms19051294
44. Szabo PM, Vajdi A, Kumar N, Tolstorukov MY, Chen BJ, Edwards R, et al. Cancer-associated fibroblasts are the main contributors to epithelial-to-mesenchymal signatures in the tumor microenvironment. Sci Rep (2023) 13(1):3051. doi: 10.1038/s41598-023-28480-9
45. Corallo C, Cutolo M, Kahaleh B, Pecetti G, Montella A, Chirico C, et al. Bosentan and macitentan prevent the endothelial-to-mesenchymal transition (Endomt) in systemic sclerosis: in vitro study. Arthritis Res Ther (2016) 18(1):228. doi: 10.1186/s13075-016-1122-y
46. Denton CP, Khanna D. Systemic sclerosis. Lancet (2017) 390(10103):1685–99. doi: 10.1016/s0140-6736(17)30933-9
47. Tabib T, Huang M, Morse N, Papazoglou A, Behera R, Jia M, et al. Myofibroblast transcriptome indicates SFRP2hi fibroblast progenitors in systemic sclerosis skin. Nat Commun (2021) 12(1):4384. doi: 10.1038/s41467-021-24607-6
48. Manetti M, Romano E, Rosa I, Guiducci S, Bellando-Randone S, De Paulis A, et al. Endothelial-to-mesenchymal transition contributes to endothelial dysfunction and dermal fibrosis in systemic sclerosis. Ann Rheum Dis (2017) 76(5):924–34. doi: 10.1136/annrheumdis-2016-210229
49. Kwatra SG. Breaking the itch-scratch cycle in prurigo nodularis. N Engl J Med (2020) 382(8):757–8. doi: 10.1056/NEJMe1916733
50. Tsoi LC, Hacini-Rachinel F, Fogel P, Rousseau F, Xing X, Patrick MT, et al. Transcriptomic characterization of prurigo nodularis and the therapeutic response to nemolizumab. J Allergy Clin Immunol (2022) 149(4):1329–39. doi: 10.1016/j.jaci.2021.10.004
51. Williams KA, Huang AH, Belzberg M, Kwatra SG. Prurigo nodularis: pathogenesis and management. J Am Acad Dermatol (2020) 83(6):1567–75. doi: 10.1016/j.jaad.2020.04.182
52. Weigelt N, Metze D, Ständer S. Prurigo nodularis: systematic analysis of 58 histological criteria in 136 patients. J Cutan Pathol (2010) 37(5):578–86. doi: 10.1111/j.1600-0560.2009.01484.x
53. Shao Y, Zhu Y, Xiao Z, Shen Y, Dai B, Tang H, et al. Rna sequencing reveals the transcriptome profile of the atopic prurigo nodularis with severe itching. Exp Dermatol (2023) 32(1):30–40. doi: 10.1111/exd.14678
54. Ma F, Gharaee-Kermani M, Tsoi LC, Plazyo O, Chaskar P, Harms P, et al. Single-cell profiling of prurigo nodularis demonstrates immune-stromal crosstalk driving profibrotic responses and reversal with nemolizumab. J Allergy Clin Immunol (2023) S0091-6749(23):00925–9. doi: 10.1016/j.jaci.2023.07.005
55. Patel JR, Joel MZ, Lee KK, Kambala A, Cornman H, Oladipo O, et al. Single-cell rna sequencing reveals dysregulated fibroblast subclusters in prurigo nodularis. bioRxiv (2023). doi: 10.1101/2023.01.29.526050
56. Griffiths CE, Barker JN. Pathogenesis and clinical features of psoriasis. Lancet (2007) 370(9583):263–71. doi: 10.1016/s0140-6736(07)61128-3
57. Boehncke WH, Schön MP. Psoriasis. Lancet (2015) 386(9997):983–94. doi: 10.1016/s0140-6736(14)61909-7
58. Griffiths CEM, Armstrong AW, Gudjonsson JE, Barker J. Psoriasis. Lancet (2021) 397(10281):1301–15. doi: 10.1016/s0140-6736(20)32549-6
59. Gao Y, Yao X, Zhai Y, Li L, Li H, Sun X, et al. Single cell transcriptional zonation of human psoriasis skin identifies an alternative immunoregulatory axis conducted by skin resident cells. Cell Death Dis (2021) 12(5):450. doi: 10.1038/s41419-021-03724-6
60. Fleury S, Thibodeau J, Croteau G, Labrecque N, Aronson HE, Cantin C, et al. Hla-Dr polymorphism affects the interaction with Cd4. J Exp Med (1995) 182(3):733–41. doi: 10.1084/jem.182.3.733
61. Sun J, Leahy DJ, Kavathas PB. Interaction between Cd8 and major histocompatibility complex (Mhc) class I mediated by multiple contact surfaces that include the alpha 2 and alpha 3 domains of Mhc class I. J Exp Med (1995) 182(5):1275–80. doi: 10.1084/jem.182.5.1275
62. Shan B, Shao M, Zhang Q, Hepler C, Paschoal VA, Barnes SD, et al. Perivascular mesenchymal cells control adipose-tissue macrophage accrual in obesity. Nat Metab (2020) 2(11):1332–49. doi: 10.1038/s42255-020-00301-7
63. Agrawal R, Woodfolk JA. Skin barrier defects in atopic dermatitis. Curr Allergy Asthma Rep (2014) 14(5):433. doi: 10.1007/s11882-014-0433-9
64. Schuler C, Billi AC, Maverakis E, Tsoi LC, Gudjonsson JE. Novel insights into atopic dermatitis. J Allergy Clin Immunol (2023) 151(5):1145–54. doi: 10.1016/j.jaci.2022.10.023
65. Weidinger S, Beck LA, Bieber T, Kabashima K, Irvine AD. Atopic dermatitis. Nat Rev Dis Primers (2018) 4(1):1. doi: 10.1038/s41572-018-0001-z
67. Brandt EB, Sivaprasad U. Th2 cytokines and atopic dermatitis. J Clin Cell Immunol (2011) 2(3):110. doi: 10.4172/2155-9899.1000110
68. Sugaya M. The role of th17-related cytokines in atopic dermatitis. Int J Mol Sci (2020) 21(4):1314. doi: 10.3390/ijms21041314
69. Sabatelli P, Gara SK, Grumati P, Urciuolo A, Gualandi F, Curci R, et al. Expression of the collagen vi A5 and A6 chains in normal human skin and in skin of patients with collagen vi-related myopathies. J Invest Dermatol (2011) 131(1):99–107. doi: 10.1038/jid.2010.284
70. Szalus K, Zysk W, Gleń J, Zabłotna M, Nowicki RJ, Trzeciak M. The associations of single nucleotide polymorphisms of the Col3a1, Col6a5, and Col8a1 genes with atopic dermatitis. J Pers Med (2023) 13(4):661. doi: 10.3390/jpm13040661
71. Esaki H, Ewald DA, Ungar B, Rozenblit M, Zheng X, Xu H, et al. Identification of novel immune and barrier genes in atopic dermatitis by means of laser capture microdissection. J Allergy Clin Immunol (2015) 135(1):153–63. doi: 10.1016/j.jaci.2014.10.037
72. Renert-Yuval Y, Thyssen JP, Bissonnette R, Bieber T, Kabashima K, Hijnen D, et al. Biomarkers in atopic dermatitis-a review on behalf of the international eczema council. J Allergy Clin Immunol (2021) 147(4):1174–90.e1. doi: 10.1016/j.jaci.2021.01.013
73. He H, Del Duca E, Diaz A, Kim HJ, Gay-Mimbrera J, Zhang N, et al. Mild atopic dermatitis lacks systemic inflammation and shows reduced nonlesional skin abnormalities. J Allergy Clin Immunol (2021) 147(4):1369–80. doi: 10.1016/j.jaci.2020.08.041
74. Sugaya M. Chemokines and skin diseases. Arch Immunol Ther Exp (Warsz) (2015) 63(2):109–15. doi: 10.1007/s00005-014-0313-y
75. Ko KI, Merlet JJ, DerGarabedian BP, Zhen H, Suzuki-Horiuchi Y, Hedberg ML, et al. Nf-Kb perturbation reveals unique immunomodulatory functions in Prx1(+) fibroblasts that promote development of atopic dermatitis. Sci Transl Med (2022) 14(630):eabj0324. doi: 10.1126/scitranslmed.abj0324
76. Pavel AB, Del Duca E, Cheng J, Wu J, Ungar B, Estrada YD, et al. Delayed type hypersensitivity reactions to various allergens may differently model inflammatory skin diseases. Allergy (2023) 78(1):178–91. doi: 10.1111/all.15538
77. Roth N, Städler S, Lemann M, Hösli S, Simon HU, Simon D. Distinct eosinophil cytokine expression patterns in skin diseases - the possible existence of functionally different eosinophil subpopulations. Allergy (2011) 66(11):1477–86. doi: 10.1111/j.1398-9995.2011.02694.x
78. Zgraggen S, Huggenberger R, Kerl K, Detmar M. An important role of the Sdf-1/Cxcr4 axis in chronic skin inflammation. PloS One (2014) 9(4):e93665. doi: 10.1371/journal.pone.0093665
79. Sun Z, Kim JH, Kim SH, Kim HR, Zhang K, Pan Y, et al. Skin-resident natural killer T cells participate in cutaneous allergic inflammation in atopic dermatitis. J Allergy Clin Immunol (2021) 147(5):1764–77. doi: 10.1016/j.jaci.2020.11.049
80. Ribero S, Sciascia S, Borradori L, Lipsker D. The cutaneous spectrum of lupus erythematosus. Clin Rev Allergy Immunol (2017) 53(3):291–305. doi: 10.1007/s12016-017-8627-2
81. Fava A, Petri M. Systemic lupus erythematosus: diagnosis and clinical management. J Autoimmun (2019) 96:1–13. doi: 10.1016/j.jaut.2018.11.001
82. Aringer M. Inflammatory markers in systemic lupus erythematosus. J Autoimmun (2020) 110:102374. doi: 10.1016/j.jaut.2019.102374
83. Durcan L, O'Dwyer T, Petri M. Management strategies and future directions for systemic lupus erythematosus in adults. Lancet (2019) 393(10188):2332–43. doi: 10.1016/s0140-6736(19)30237-5
84. Zhao Z, Zhu H, Li Q, Liao W, Chen K, Yang M, et al. Skin Cd4(+) Trm cells distinguish acute cutaneous lupus erythematosus from localized discoid lupus erythematosus/subacute cutaneous lupus erythematosus and other skin diseases. J Autoimmun (2022) 128:102811. doi: 10.1016/j.jaut.2022.102811
85. Zheng M, Hu Z, Mei X, Ouyang L, Song Y, Zhou W, et al. Single-cell sequencing shows cellular heterogeneity of cutaneous lesions in lupus erythematosus. Nat Commun (2022) 13(1):7489. doi: 10.1038/s41467-022-35209-1
86. Li Q, Wu H, Liao W, Zhao M, Chan V, Li L, et al. A comprehensive review of immune-mediated dermatopathology in systemic lupus erythematosus. J Autoimmun (2018) 93:1–15. doi: 10.1016/j.jaut.2018.07.007
87. Zhou X, Chen Y, Cui L, Shi Y, Guo C. Advances in the pathogenesis of psoriasis: from keratinocyte perspective. Cell Death Dis (2022) 13(1):81. doi: 10.1038/s41419-022-04523-3
88. Deng Y, Chang C, Lu Q. The inflammatory response in psoriasis: A comprehensive review. Clin Rev Allergy Immunol (2016) 50(3):377–89. doi: 10.1007/s12016-016-8535-x
89. Jevtić M, Löwa A, Nováčková A, Kováčik A, Kaessmeyer S, Erdmann G, et al. Impact of intercellular crosstalk between epidermal keratinocytes and dermal fibroblasts on skin homeostasis. Biochim Biophys Acta Mol Cell Res (2020) 1867(8):118722. doi: 10.1016/j.bbamcr.2020.118722
90. Saiag P, Coulomb B, Lebreton C, Bell E, Dubertret L. Psoriatic fibroblasts induce hyperproliferation of normal keratinocytes in a skin equivalent model in vitro. Science (1985) 230(4726):669–72. doi: 10.1126/science.2413549
91. Priestley GC, Adams LW. Hyperactivity of fibroblasts cultured from psoriatic skin: I. Faster proliferation and effect of serum withdrawal. Br J Dermatol (1983) 109(2):149–56. doi: 10.1111/j.1365-2133.1983.tb07075.x
92. Quan C, Cho MK, Shao Y, Mianecki LE, Liao E, Perry D, et al. Dermal fibroblast expression of stromal cell-derived factor-1 (Sdf-1) promotes epidermal keratinocyte proliferation in normal and diseased skin. Protein Cell (2015) 6(12):890–903. doi: 10.1007/s13238-015-0198-5
93. Cambier S, Gouwy M, Proost P. The chemokines cxcl8 and Cxcl12: molecular and functional properties, role in disease and efforts towards pharmacological intervention. Cell Mol Immunol (2023) 20(3):217–51. doi: 10.1038/s41423-023-00974-6
94. Mousavi A. Cxcl12/cxcr4 signal transduction in diseases and its molecular approaches in targeted-therapy. Immunol Lett (2020) 217:91–115. doi: 10.1016/j.imlet.2019.11.007
95. Marchese C, Felici A, Visco V, Lucania G, Igarashi M, Picardo M, et al. Fibroblast growth factor 10 induces proliferation and differentiation of human primary cultured keratinocytes. J Invest Dermatol (2001) 116(4):623–8. doi: 10.1046/j.0022-202x.2001.01280.x
96. Zhu M, Oishi K, Lee SC, Patterson PH. Studies using leukemia inhibitory factor (Lif) knockout mice and a lif adenoviral vector demonstrate a key anti-inflammatory role for this cytokine in cutaneous inflammation. J Immunol (2001) 166(3):2049–54. doi: 10.4049/jimmunol.166.3.2049
97. Howell MD, Kim BE, Gao P, Grant AV, Boguniewicz M, Debenedetto A, et al. Cytokine modulation of atopic dermatitis filaggrin skin expression. J Allergy Clin Immunol (2007) 120(1):150–5. doi: 10.1016/j.jaci.2007.04.031
98. Cornelissen C, Marquardt Y, Czaja K, Wenzel J, Frank J, Lüscher-Firzlaff J, et al. Il-31 regulates differentiation and filaggrin expression in human organotypic skin models. J Allergy Clin Immunol (2012) 129(2):426–33. doi: 10.1016/j.jaci.2011.10.042
99. Palmer CN, Irvine AD, Terron-Kwiatkowski A, Zhao Y, Liao H, Lee SP, et al. Common loss-of-function variants of the epidermal barrier protein filaggrin are a major predisposing factor for atopic dermatitis. Nat Genet (2006) 38(4):441–6. doi: 10.1038/ng1767
100. Kim Y, Lim KM. Skin barrier dysfunction and filaggrin. Arch Pharm Res (2021) 44(1):36–48. doi: 10.1007/s12272-021-01305-x
101. Lin JY, Fisher DE. Melanocyte biology and skin pigmentation. Nature (2007) 445(7130):843–50. doi: 10.1038/nature05660
102. Chen J, Li S, Li C. Mechanisms of melanocyte death in vitiligo. Med Res Rev (2021) 41(2):1138–66. doi: 10.1002/med.21754
103. Frisoli ML, Essien K, Harris JE. Vitiligo: mechanisms of pathogenesis and treatment. Annu Rev Immunol (2020) 38:621–48. doi: 10.1146/annurev-immunol-100919-023531
104. Ezzedine K, Eleftheriadou V, Whitton M, van Geel N. Vitiligo. Lancet (2015) 386(9988):74–84. doi: 10.1016/s0140-6736(14)60763-7
105. Xu Z, Chen D, Hu Y, Jiang K, Huang H, Du Y, et al. Anatomically distinct fibroblast subsets determine skin autoimmune patterns. Nature (2022) 601(7891):118–24. doi: 10.1038/s41586-021-04221-8
106. Riding RL, Harris JE. The role of memory Cd8(+) T cells in vitiligo. J Immunol (2019) 203(1):11–9. doi: 10.4049/jimmunol.1900027
107. Gellatly KJ, Strassner JP, Essien K, Refat MA, Murphy RL, Coffin-Schmitt A, et al. Scrna-Seq of human vitiligo reveals complex networks of subclinical immune activation and a role for Ccr5 in T(Reg) function. Sci Transl Med (2021) 13(610):eabd8995. doi: 10.1126/scitranslmed.abd8995
108. Sahai E, Astsaturov I, Cukierman E, DeNardo DG, Egeblad M, Evans RM, et al. A framework for advancing our understanding of cancer-associated fibroblasts. Nat Rev Cancer (2020) 20(3):174–86. doi: 10.1038/s41568-019-0238-1
109. Lavie D, Ben-Shmuel A, Erez N, Scherz-Shouval R. Cancer-associated fibroblasts in the single-cell era. Nat Cancer (2022) 3(7):793–807. doi: 10.1038/s43018-022-00411-z
110. Chen X, Song E. Turning foes to friends: targeting cancer-associated fibroblasts. Nat Rev Drug Discovery (2019) 18(2):99–115. doi: 10.1038/s41573-018-0004-1
111. Chen Y, McAndrews KM, Kalluri R. Clinical and therapeutic relevance of cancer-associated fibroblasts. Nat Rev Clin Oncol (2021) 18(12):792–804. doi: 10.1038/s41571-021-00546-5
112. US National Library of Medicine. ClinicalTrials.gov (2018). Available at: https://clinicaltrials.gov/ct2/show/NCT01373164.
113. Hurwitz HI, Uppal N, Wagner SA, Bendell JC, Beck JT, Wade SM 3rd, et al. Randomized, double-blind, phase ii study of ruxolitinib or placebo in combination with capecitabine in patients with metastatic pancreatic cancer for whom therapy with gemcitabine has failed. J Clin Oncol (2015) 33(34):4039–47. doi: 10.1200/jco.2015.61.4578
114. US National Library of Medicine. ClinicalTrials.gov (2020). Available at: https://clinicaltrials.gov/ct2/show/NCT02699606.
115. Olive KP, Jacobetz MA, Davidson CJ, Gopinathan A, McIntyre D, Honess D, et al. Inhibition of hedgehog signaling enhances delivery of chemotherapy in a mouse model of pancreatic cancer. Science (2009) 324(5933):1457–61. doi: 10.1126/science.1171362
116. US National Library of Medicine. ClinicalTrials.gov (2018). Available at: https://clinicaltrials.gov/ct2/show/NCT01585701.
117. Feig C, Jones JO, Kraman M, Wells RJ, Deonarine A, Chan DS, et al. Targeting cxcl12 from fap-expressing carcinoma-associated fibroblasts synergizes with anti-pd-L1 immunotherapy in pancreatic cancer. Proc Natl Acad Sci U.S.A. (2013) 110(50):20212–7. doi: 10.1073/pnas.1320318110
118. US National Library of Medicine. ClinicalTrials.gov (2017). Available at: https://clinicaltrials.gov/ct2/show/NCT01951690.
119. US National Library of Medicine. ClinicalTrials.gov (2015). Available at: https://clinicaltrials.gov/ct2/show/NCT01472198.
120. Neesse A, Frese KK, Bapiro TE, Nakagawa T, Sternlicht MD, Seeley TW, et al. Ctgf antagonism with mab fg-3019 enhances chemotherapy response without increasing drug delivery in murine ductal pancreas cancer. Proc Natl Acad Sci U.S.A. (2013) 110(30):12325–30. doi: 10.1073/pnas.1300415110
121. Hingorani SR, Zheng L, Bullock AJ, Seery TE, Harris WP, Sigal DS, et al. Halo 202: randomized phase ii study of pegph20 plus Nab-paclitaxel/gemcitabine versus Nab-paclitaxel/gemcitabine in patients with untreated, metastatic pancreatic ductal adenocarcinoma. J Clin Oncol (2018) 36(4):359–66. doi: 10.1200/jco.2017.74.9564
122. Santos AM, Jung J, Aziz N, Kissil JL, Puré E. Targeting fibroblast activation protein inhibits tumor stromagenesis and growth in mice. J Clin Invest (2009) 119(12):3613–25. doi: 10.1172/jci38988
123. Valkenburg KC, de Groot AE, Pienta KJ. Targeting the tumour stroma to improve cancer therapy. Nat Rev Clin Oncol (2018) 15(6):366–81. doi: 10.1038/s41571-018-0007-1
124. Hah N, Sherman MH, Yu RT, Downes M, Evans RM. Targeting transcriptional and epigenetic reprogramming in stromal cells in fibrosis and cancer. Cold Spring Harb Symp Quant Biol (2015) 80:249–55. doi: 10.1101/sqb.2015.80.027185
125. Kim Y, Park JY, Kim H, Chung DK. Differential role of lipoteichoic acids isolated from staphylococcus aureus and lactobacillus plantarum on the aggravation and alleviation of atopic dermatitis. Microb Pathog (2020) 147:104360. doi: 10.1016/j.micpath.2020.104360
126. Nguyen HN, Noss EH, Mizoguchi F, Huppertz C, Wei KS, Watts GFM, et al. Autocrine loop involving Il-6 family member Lif, Lif receptor, and stat4 drives sustained fibroblast production of inflammatory mediators. Immunity (2017) 46(2):220–32. doi: 10.1016/j.immuni.2017.01.004
127. Longo SK, Guo MG, Ji AL, Khavari PA. Integrating single-cell and spatial transcriptomics to elucidate intercellular tissue dynamics. Nat Rev Genet (2021) 22(10):627–44. doi: 10.1038/s41576-021-00370-8
128. Bridges K, Miller-Jensen K. Mapping and validation of Scrna-Seq-derived cell-cell communication networks in the tumor microenvironment. Front Immunol (2022) 13:885267. doi: 10.3389/fimmu.2022.885267
Keywords: fibroblasts, epithelial immune microenvironment, inflammatory skin diseases, single-cell RNA sequencing, spatial transcriptomics, cell crosstalk, prurigo nodularis
Citation: Wang L, Wang B, Kou E, Du L and Zhu Y (2023) New insight into the role of fibroblasts in the epithelial immune microenvironment in the single-cell era. Front. Immunol. 14:1259515. doi: 10.3389/fimmu.2023.1259515
Received: 16 July 2023; Accepted: 11 September 2023;
Published: 22 September 2023.
Edited by:
Zhongjian Chen, Tongji University, ChinaReviewed by:
Xilin Zhang, Shanghai Dermatology Hospital, ChinaCopyright © 2023 Wang, Wang, Kou, Du and Zhu. This is an open-access article distributed under the terms of the Creative Commons Attribution License (CC BY). The use, distribution or reproduction in other forums is permitted, provided the original author(s) and the copyright owner(s) are credited and that the original publication in this journal is cited, in accordance with accepted academic practice. No use, distribution or reproduction is permitted which does not comply with these terms.
*Correspondence: Yuanjie Zhu, MTM5MTgzMzg5NjBAMTI2LmNvbQ==; Lin Du, bHlubmllX2R1QDEyNi5jb20=
†These authors have contributed equally to this work and share first authorship
Disclaimer: All claims expressed in this article are solely those of the authors and do not necessarily represent those of their affiliated organizations, or those of the publisher, the editors and the reviewers. Any product that may be evaluated in this article or claim that may be made by its manufacturer is not guaranteed or endorsed by the publisher.
Research integrity at Frontiers
Learn more about the work of our research integrity team to safeguard the quality of each article we publish.