- 1Department of Translational Medical Sciences, University of Naples Federico II, Naples, Italy
- 2World Allergy Organization (WAO), Center of Excellence (CoE), Naples, Italy
- 3Unità Operativa (UO) Medicina Trasfusionale, Azienda Sanitaria Territoriale, Ascoli Piceno, Italy
- 4Center for Basic and Clinical Immunology Research (CISI), University of Naples Federico II, Naples, Italy
- 5Institute of Experimental Endocrinology and Oncology, National Research Council (CNR), Naples, Italy
Introduction: Common variable immunodeficiency (CVID) is the most prevalent symptomatic primary immunodeficiency. CVID is a heterogeneous disorder with a presumed multifactorial etiology. Intravenous or subcutaneous immunoglobulin replacement therapy (IgRT) can prevent severe infections but not underlying immune dysregulation.
Methods: In this study, we evaluated the serum concentrations of proinflammatory (TNF-α, IL-1β, IL-6) and immunoregulatory cytokines (IL-10), as well as lipopolysaccharide (LPS) and soluble CD14 (sCD14) in CVID individuals with infectious only (INF-CVID), and those with additional systemic autoimmune and inflammatory disorders (NIC-CVID), and healthy donors (HD).
Results: Our results showed increased serum concentrations of TNF-α, IL-1β, IL-6, and IL-10 in both INF-CVID and NIC-CVID subjects compared to HD. However, elevations of TNF-α, IL-1β, IL-6, and IL-10 were significantly more marked in NIC-CVID than INF-CVID. Additionally, LPS concentrations were increased only in NIC-CVID but not in INF-CVID compared to HD. Circulating levels of sCD14 were significantly increased in NIC-CVID compared to both INF-CVID and HD.
Discussion: These findings indicate persistent cytokine dysregulation despite IgRT in individuals with CVID. Moreover, the circulating cytokine profile reveals the heterogeneity of immune dysregulation in different subgroups of CVID subjects.
Introduction
Common variable immunodeficiency (CVID) is the most prevalent symptomatic primary immunodeficiency characterized by low immunoglobulin (Ig) levels (IgG and/or IgM) and impaired production of specific antibodies in response to vaccinations (1, 2). CVID is a heterogeneous disorder (3, 4) with a presumed multifactorial etiology and an estimated heritability of approximately 20% (2, 5–7).
Intravenous or subcutaneous immunoglobulin replacement therapy (IgRT) is effective in preventing severe infections and improving survival in CVID subjects (1, 3, 8). However, despite optimal IgRT, a significant percentage (30% to 50%) of these patients still experience complications related to immune dysregulation (3, 9–11). These complications include lymphoproliferative and autoimmune diseases, cytopenias, enteropathy, chronic lung diseases, and cancer (9, 12–16). The mechanisms underlying these heterogeneous manifestations of immune dysregulation remain unclear (17–19). Recent evidence suggests that gut microbiome alterations may be a driver of immune dysregulation in CVID subjects (20, 21).
Early identification of predictive biomarkers and risk factors for the development of immune dysregulation in CVID is crucial to improve the clinical care of these patients. Previous studies have suggested several potential risk factors, including low CD4+ T cells (22), increased CD21low B cells (23, 24), IgA deficiency (22, 24) and gut dysbiosis (21). More recently, the measurement of circulating cytokines and chemokines is gaining ground in identifying CVID immunophenotypes with different clinical manifestations (12, 13, 25–27). However, previous studies have reported conflicting results about serum cytokines in CVID subjects compared to healthy donors. For instance, serum concentrations of IL-10 have been found to be both increased (28, 29) or decreased (30) in CVID subjects compared to controls. These apparent discrepancies could be attributed, at least in part, to the selection of CVID individuals with different immunophenotypes.
In this study, we have evaluated the cytokine profile and biomarkers of systemic inflammation in CVID individuals with a clinical history of either infections only (INF-CVID) or autoimmune disease, GLID, lymphoproliferation, splenomegaly, bronchiectasis, and/or enteropathy (NIC-CVID) undergoing IgRT compared to healthy donors.
Materials and methods
Subjects
Forty-six Caucasian individuals with CVID were recruited from the outpatient clinic of the Division of Allergy and Clinical Immunology, University of Naples Federico II (Naples, Italy). Twenty-three healthy volunteers (HD) were enrolled as age and gender-matched controls. Patients were eligible for enrollment in the study if they had a CVID diagnosis based on the 2019 ESID criteria (31). Exclusion criteria for both healthy donors and CVID subjects were active bacterial or viral infections at the time of blood collection. There were no familiar cases of CVID. All patients were receiving chronic IgRT and were not undergoing immunosuppressive therapy. From the medical files of CVID subjects, we recorded serum Ig levels at diagnosis, T cell subsets, monocytes, B cells, clinical history of recurrent infections, chronic diarrhea, bronchiectasis, autoimmune diseases, polyclonal lymphoproliferation (splenomegaly, lymphadenopathy, and granulomatous disease), and malignancies. This study was approved by the institutional Ethics Committee of the University of Naples Federico II (Prot. 198/18), and informed consent was obtained from all participants prior to blood collection according to recommendations from the Declaration of Helsinki.
IgG, IgA, and IgM measurements
Serum samples obtained from venous blood were aliquoted and stored at − 80°C until testing. IgG, IgA, and IgM were measured by nephelometry employing the Behring BN™ II System (Siemens Healthcare Diagnostics Ltd, Erlangen, Germany) (32). To evaluate the precision of immunoglobulin measurement, the coefficient of variation (CV) was determined according to the Clinical and Laboratory Standards Institute (CLSI) EP05-A3 guidelines (33). The intra-assay CVs ranged from 1.8% to 3.6%. The linearity of the IgG measurement (coefficient of determination: R2) was assessed according to the CLSI EP06-A guidelines (34). The R2 values ranged from 0.97 to 0.99.
Cytokine, soluble CD14, and LPS measurements
Serum concentrations of TNF-α (Quantikine HS ELISA HSTA00E), IL-1β (Quantikine HS ELISA HSLB00D), IL-6 (Quantikine HS ELISA HS600C), and IL-10 (Quantikine HS HS100C) were measured in duplicate using ELISA assays from R&D Systems (Bio-Techne). The concentration of soluble CD14 (sCD14) was measured using the Quantikine ELISA DC140 kit from R&D Systems (Bio-Techne). The concentration of lipopolysaccharide (LPS) was also measured using an ELISA kit from Abbexa (Cambridge, UK). All reagents and chemicals used in these experiments were molecular biology grade to reduce endotoxin contamination. Disposable pipets, tubes, and plates were used in all experiments.
Statistical analysis
Statistical analysis was performed using GraphPad Prism 9 software (GraphPad Software, La Jolla, CA, USA). Data are presented as mean ± standard error of the mean (SEM). Group comparisons were made using Student’s t-test or Mann–Whitney U test, based on the parametric or nonparametric distribution of the continuous variables. Multiple comparisons were assessed using one-way analysis of variance (ANOVA) followed by Tukey’s post hoc test. Correlation analyses were conducted using Pearson’s correlation method. A p value less than 0.05 was considered statistically significant.
Results
Demographic and clinical characteristics of CVID subjects and healthy donors
CVID subjects were categorized as INF-CVID if they had a clinical history of recurrent infections only. NIC-CVID included patients with recurrent infections as well as one or more of the following non-infectious complications: enteropathy, lymphoproliferation, autoimmune disease, cytopenia, splenomegaly, granulomatous lymphocytic interstitial lung disease (GILD) or bronchiectasis. All CVID subjects were on IgRT at the time of sample collection.
Table 1 presents the demographic and clinical characteristics of healthy donors (HD), while Tables 2, 3 provide the characteristics of 23 subjects with INF-CVID and 23 subjects with NIC-CVID, respectively. The median age was 44.5 ± 2.5 years for INF-CVID, 41.3 ± 2.5 years for NIC-CVID, and 39.6 ± 2.2 years for HD. Among the INF-CVID patients, eleven (47.8%) were female and among NIC-CVID ten (43.4%) were female.
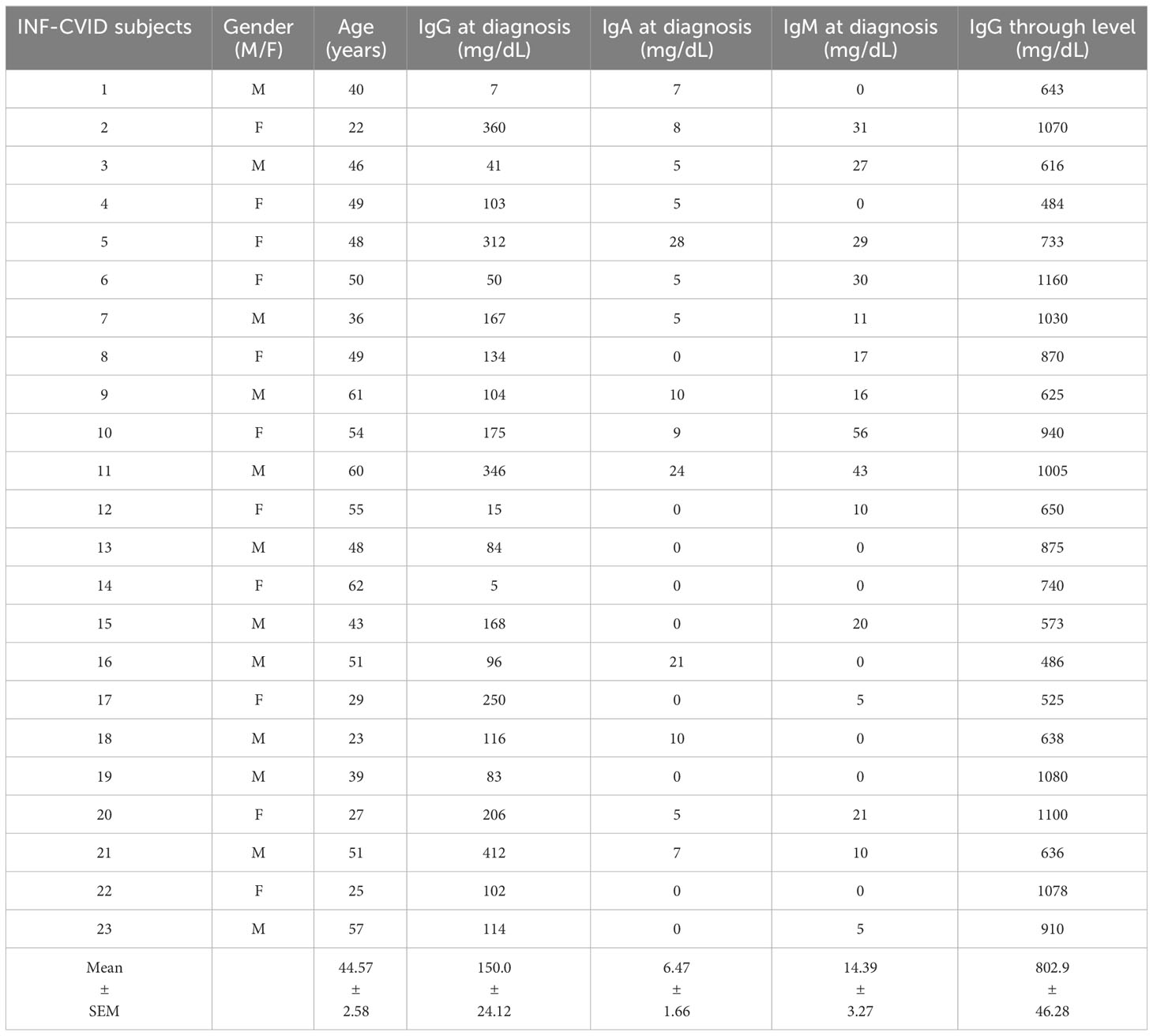
Table 2 Demographic and clinical characteristics of CVID subjects with recurrent infections (INF-CVID).
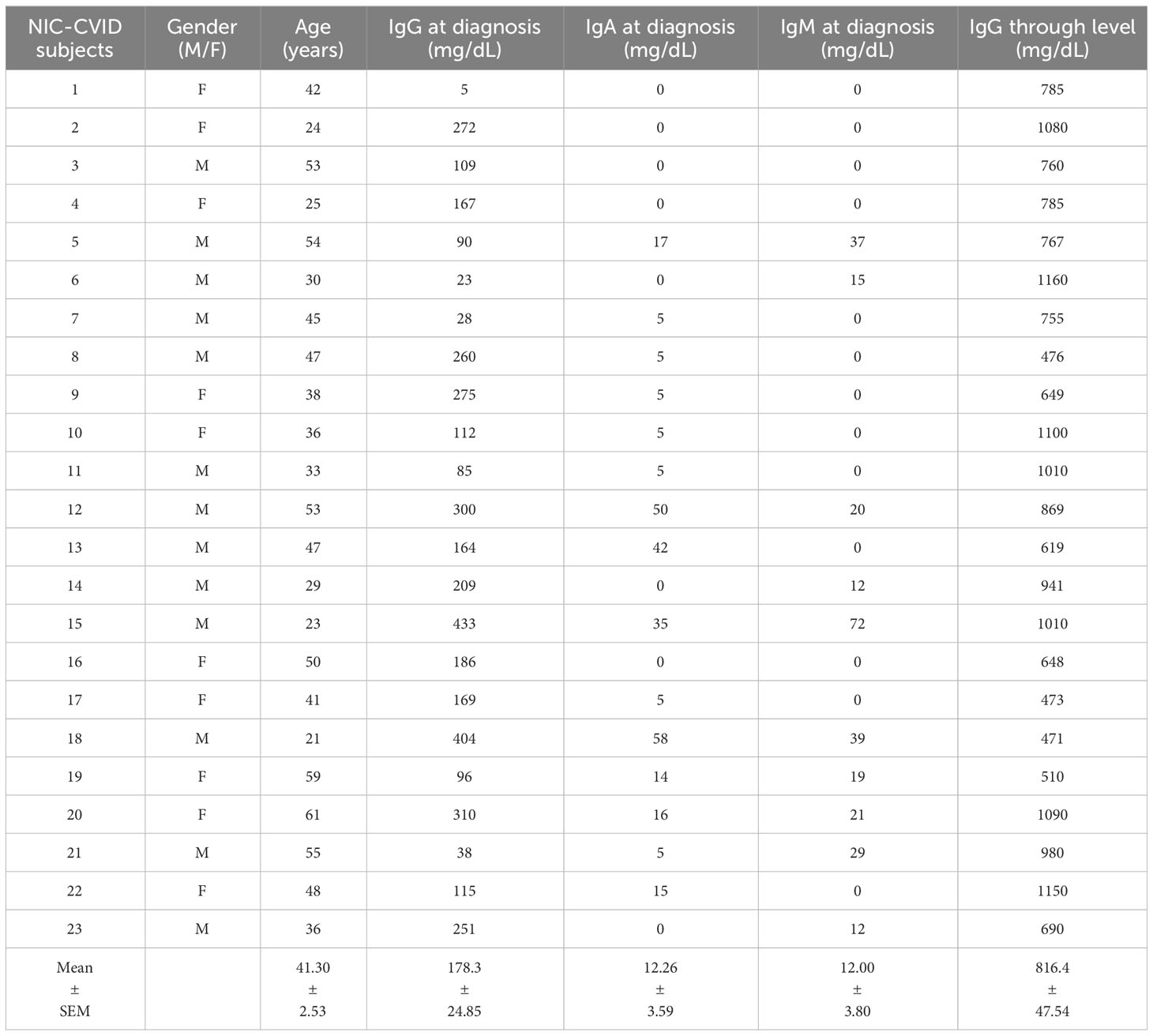
Table 3 Demographic and clinical characteristics of CVID subjects with addition of a variety of immune disorders (NIC-CVID).
Serum concentrations of IgG, IgA, and IgM in INF-CVID, NIC-CVID, and healthy donors
We evaluated the serum concentrations of IgG, IgA, and IgM in subjects with INF-CVID or NIC-CVID compared to HD. Figure 1A shows that both INF-CVID and NIC-CVID had significantly lower IgG serum concentrations than HD (p < 0.0001). N difference was found between the two groups of CVID. Similarly, serum concentrations of both IgA (Figure 1B) and IgM (Figure 1C) were markedly lower in both CVID groups compared to HD (p < 0.0001).
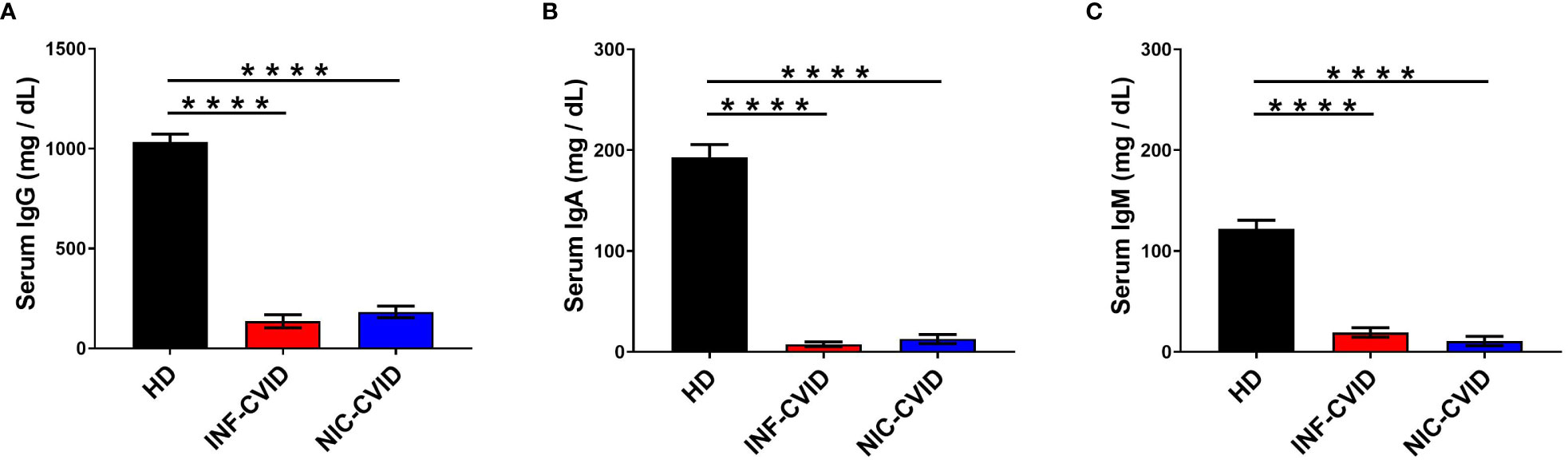
Figure 1 Serum concentrations of IgG (A), IgA (B), and IgM (C) in healthy donors (HD), CVID subjects with recurrent infections only (INF-CVID) or with addition of a variety of immune disorders (NIC-CVID). **** p < 0.0001.
Serum concentrations of cytokines in INF-CVID, NIC-CVID, and healthy donors
To investigate the mechanisms underlying chronic immune dysregulation in CVID, we measured serum levels of different cytokines in subjects with INF-CVID and NIC-CVID compared to HD. We found a significant elevation of TNF-α in both INF-CVID and NIC-CVID compared to HD (Figure 2A). Elevation of serum TNF-α was more marked in NIC-CVID compared to INF-CVID. Similarly, serum concentration of IL-1β were increased in both INF-CVID and NIC-CVID compared to HD (Figure 2B). The proinflammatory cytokine IL-6 was significantly increased in both INF-CVID and NIC-CVID, compared to HD (Figure 2C). The immunomodulatory cytokine IL-10 was significantly increased in both INF-CVID and NIC-CVID compared to HD (Figure 2D). Serum concentrations of IL-10 were more markedly increased in NIC-CVID compared to NF-CVID.
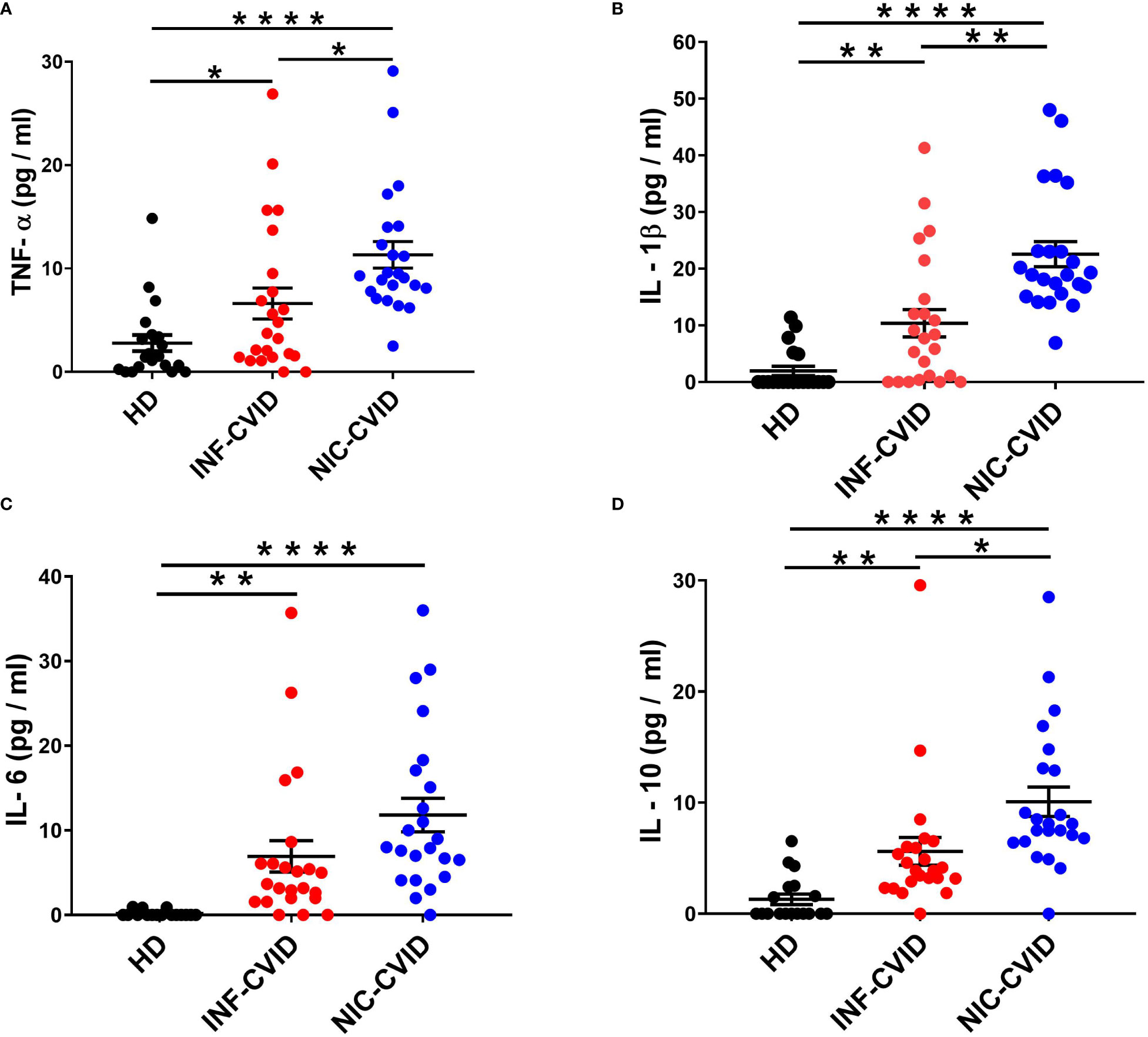
Figure 2 Serum concentrations of TNF-α (A), IL-1β (B), IL-6 (C), and IL-10 (D) in healthy donors (HD), CVID subjects with recurrent infections only (INF-CVID) or with addition of a variety of immune disorders (NIC-CVID). * p < 0.05; ** p < 0.01; **** p < 0.0001.
Serum concentrations of LPS and sCD14 in INF-CVID, NIC-CVID, and healthy donors
LPS is the major component of the outer membrane of Gram-negative bacteria (35, 36). LPS activates the multimeric receptor composed of Toll-like receptor-4 (TLR4), CD14, and MD2 on the membrane of several immune cells (e.g., macrophages, monocytes, neutrophils) (35–38). Circulating concentration of LPS is considered a biomarker of gut microbial translocation, a process facilitated by immune deficiency that may drive inflammation via endotoxemia (20, 39). Serum concentrations of LPS were not elevated in INF-CVID compared to HD (Figure 3A). By contrast, NIC-CVID subjects showed increased levels of LPS compared to both INF-CVID and HD (Figure 3A).
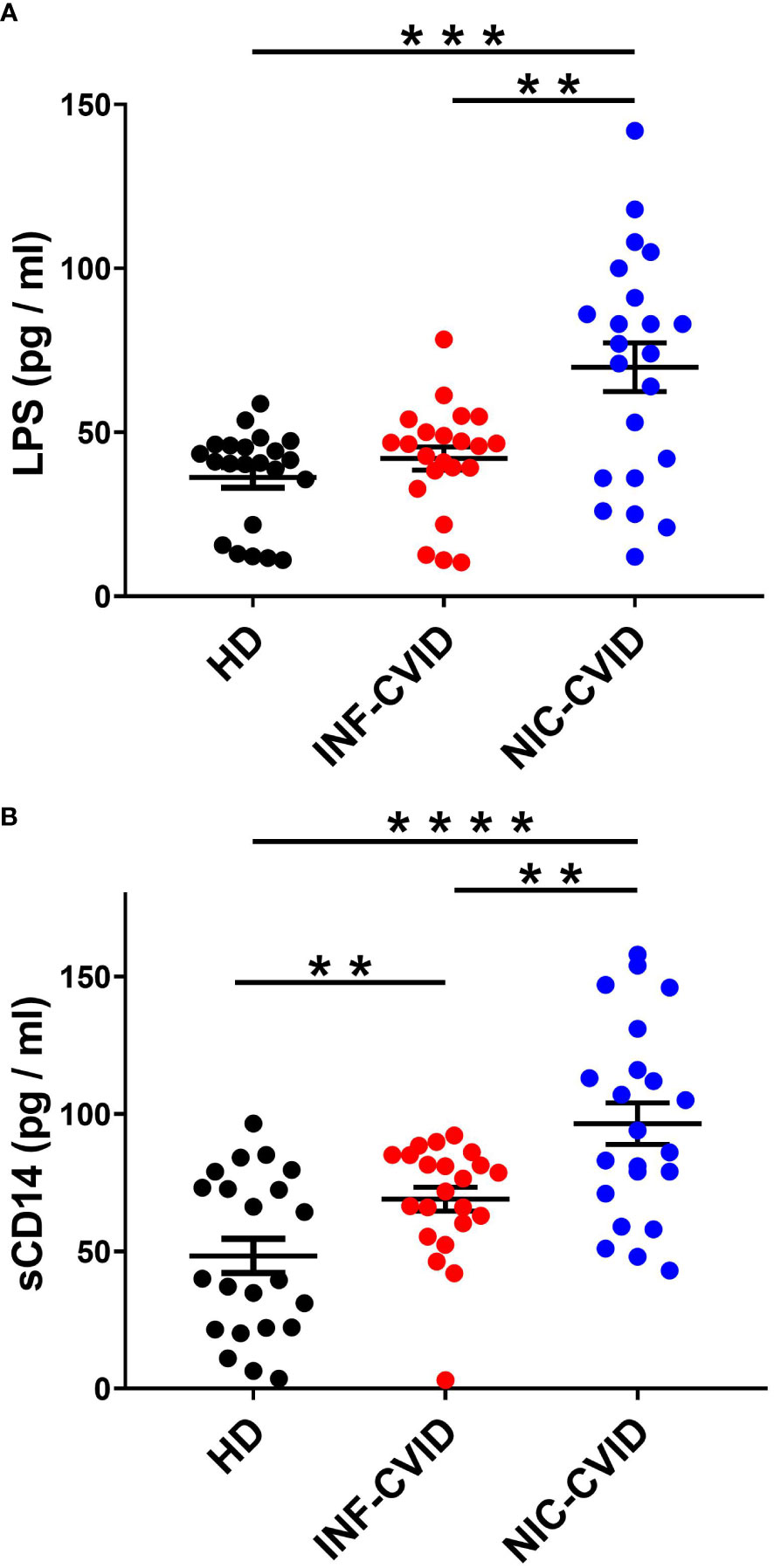
Figure 3 Serum concentrations of LPS (A) and sCD14 (B) in healthy donors (HD), CVID with recurrent infections only (INF-CVID) or with addition of a variety of immune disorders (NIC-CVID). ** p < 0.01; *** p < 0.001; **** p < 0.0001.
CD14 is a glycosylphosphatidylinositol (GPI)-anchored receptor which serves as a co-receptor for Toll-like receptor (TLR)-4 at the cell surface or can be secreted in a soluble form (sCD14) (40, 41). To assess the degree of systemic immune activation in CVID, we analyzed the serum levels of sCD14, a recognized marker of monocyte activation (42). Serum concentrations of sCD14 were increased in INF-CVID compared to HD. Moreover, sCD14 levels were significantly increased in NIC-CVID compared to both INF-CVID and HD (Figure 3B).
Correlations between cytokines, LPS, sCD14, and IgG concentrations
In our study, we examined the correlations between cytokine (TNF-α, IL-6, IL-1β, IL-10) levels and IgG concentrations after IgRT in patients with INF-CVID (Supplementary Figure 1: sFigure1) and NIC-CVID (Supplementary Figure 2: sFigure 2). No significant correlations were observed in both groups of CVID patients. Previous studies have reported an inverse correlation between endotoxemia and serum IgG levels in CVID patients (43). However, in our study, we did not find any correlation between LPS and sCD14 levels with IgG concentrations (Supplementary Figures 3, 4: sFigures 3, 4). No correlations were also found between cytokine levels and sCD14 concentrations in patients with INF-CVID (Supplementary Figure 5: sFigure 5) and NIC-CVID (Supplementary Figure 6: sFigure 6). Similarly, no correlations were found between cytokines and LPS in patients with INF-CVID (Supplementary Figure 7: sFigure 7) and NIC-CVID (Supplementary Figure 8: sFigure 8). No correlations were also found between sCD14 and LPS concentrations in both patients with INF-CVID and NIC-CVID (Supplementary Figure 9: sFigure 9).
Correlations between the different cytokines in the single subjects with INF-CVID and NIC-CVID
We have also examined the correlations between the different cytokines in the single subjects with both INF-CVID (Supplementary Figure 10: sFigure 10) and NIC-CVID (Supplementary Figure 11: sFigure 11). No correlations were found between the different cytokines with the exception of the significant correlation between IL-10 and IL-1β in INF-CVID subjects (p < 0.001).
Discussion
The results of this observational study demonstrate that individuals with different CVID phenotypes exhibit varying degrees of systemic cytokine dysregulation in peripheral blood. In particular, low-grade inflammation is more evident in subjects with NIC-CVID compared to INF-CVID. This condition of systemic inflammation, characterized by elevated levels of proinflammatory (i.e., TNF-α, IL-1β, IL-6) and immunoregulatory cytokines (i.e., IL-10) persists in CVID subjects despite receiving adequate IgG replacement therapy. Interestingly, we did not find significant correlations between serum concentrations of IgG and several inflammatory markers.
CVID is the most prevalent symptomatic primary immunodeficiency (44, 45). Although genetic etiologies have been identified in approximately 20% of CVID cases (2, 5–7), the pathobiological mechanisms underlying the inflammatory manifestations remain largely unknown (17, 18). Moreover, CVID is a highly heterogeneous condition (3, 4) with different immunological phenotypes characterized by various complications (12, 21, 26, 27, 46). For instance, serum and sputum cytokine levels are markedly increased in CVID subjects with chronic respiratory symptoms and airway disease compared to those with normal airways (26). Similarly, CVID subjects with autoimmune cytopenias (AIC) and lymphadenopathy have elevated levels of LPS and exhibit hyperplastic but inefficient germinal center responses compared to CVID individuals without AIC (46). Previous studies by Jorgensen and collaborators demonstrated that plasma levels of LPS, IL-6, and TNF-α were increased in CVID subjects with dysimmune complications (e.g., splenomegaly, lymphadenopathy, autoimmunity, enteropathy, granulomatous-lymphocytic interstitial lung disease [GLILD]) compared to CVID without dysimmune complications (27). Our findings confirm and extend the previous observations, showing that serum levels of inflammatory markers (LPS, sCD14) and cytokines (TNF-α, IL-1β, IL-6) are increased in NIC-CVID subjects compared to INF-CVID. Moreover, we found that circulating levels of the immunoregulatory cytokine IL-10 were also increased in both NIC-CVID and INF-CVID subjects.
LPS is a glycoprotein released by Gram-negative bacteria, which represent the vast majority of gut microbiota (47). Circulating LPS is considered a parameter of increased intestinal permeability and/or microbial translocation of Gram-negative bacteria (48). LPS activates the innate immune system by interacting with Toll-like receptor 4, an important mediator of innate immunity (35, 49). Previous studies investigating the circulating levels of LPS in individuals with CVID have yielded conflicting results, likely due to the inherent heterogeneity among CVID subjects (12, 27, 43, 46, 50, 51). Furthermore, the assays employed in these studies are known to exhibit high variability, making it challenging to compare findings obtained using different experimental methods (e.g., serum vs. plasma). Finally, it is known that sCD14 can directly bind to LPS (52, 53) and therefore can interfere with the measurement of circulating endotoxin (54). Initial studies reported that circulating levels of LPS were not increased in unselected CVID subjects (50, 51). By contrast, Perreau and coworkers found that plasma levels of LPS were markedly increased in CVID subjects before IVIG therapy (43). Also recent studies measuring circulating LPS in different CVID subgroups compared to HD have been controversial (12, 27, 46). Two studies highlighted significant differences between CVID subgroups showing that circulating levels of LPS are increased in CVID with inflammatory complications (27) and with autoimmunity (46) compared to uncomplicated CVID. By contrast, another recent study reported no differences in plasma LPS in patients with uncomplicated and complicated CVID and controls (12). In our study, we found that LPS levels are more markedly increased in NIC-CVID compared to INF-CVID.
Jorgensen and collaborators elegantly demonstrated that increased plasma levels of LPS in CVID subjects were associated with systemic inflammation and enhanced macrophage and T-cell activation (27). Interestingly, LPS concentration correlated with gut microbial dysbiosis in stool samples in CVID. More recently, it has been shown that gut mucosa of CVID subjects has altered response to LPS and viruses (55). Further studies are required to investigate the true extent of endotoxemia and its association with systemic and/or local (i.e., gastrointestinal) immune dysregulation in different immunophenotypes of CVID.
It should be pointed out that different commercial preparations of IVIG contain a broad spectrum of anti-carbohydrate antibodies, including bacterial glycans (56). In particular, the bacterial constituents containing these glycans include the cell wall component LPS. These findings suggest that administration of IVIG might interfere with the measurement of circulating LPS in CVID subjects. Indeed, a study examining the presence of endotoxin in CVID subjects before and after IVIG therapy reported a decrease in endotoxin levels following immunoglobulin treatment (43). This decrease was attributed to the reduction of bacterial translocation caused by IVIG in CVID subjects.
Soluble CD14 (sCD14) is a circulating marker of monocyte activation (40). Previous reports on circulating levels of sCD14 in CVID have shown conflicting results (12, 27, 50, 51, 57, 58). Initial studies reported increased serum concentrations of sCD14 in unselected CVID subjects (50, 51), while others found no significant difference compared to controls (58). Recently, two studies reported that plasma levels of sCD14 were increased in CVID, particularly in those with complications (12, 27). Our results are consistent with the latter findings, showing that sCD14 concentrations are more markedly increased in NIC-CVID compared to INF-CVID.
LPS activates the TLR4 on human macrophages inducing the release of several proinflammatory cytokines, including TNF-α, IL-1β, and IL-6 (36, 59). Circulating levels of TNF-α were found to be increased in CVID compared to controls by several investigators (12, 13, 26, 27, 29). Our results showing that circulating levels of TNF-α are more markedly increased in NIC-CVID compared to INF-CVID, are consistent with more recent findings (12, 26). IL-1β is a classical proinflammatory cytokine mainly produced by human macrophages and neutrophils (60, 61). To the best of our knowledge, there is only one published study that has investigated the serum concentration of IL-1β in CVID subjects (26). Schnell and collaborators reported that serum concentrations of IL-1β were increased in CVID compared to controls. Moreover, circulating and sputum levels of IL-1β were more markedly increased in CVID with abnormal airways compared to normal airways (26). Our results extend the previous findings showing that serum concentrations of IL-1β are more markedly increased in NIC-CVID compared to INF-CVID.
IL-6 is a proinflammatory cytokine mainly produced by human macrophages (62, 63). Several groups of investigators reported increased circulating levels of IL-6 in CVID (12, 13, 26, 27). In particular, elevated levels of IL-6 were found in CVID with abnormal airways (26) or with complications (12) compared to controls. Our study extends the latter observations showing that serum levels of IL-6 are particularly increased in NIC-CVID compared to INF-CVID.
IL-10 is a pleiotropic cytokine that stimulates several immune cells and exerts immunosuppressive effects on myeloid cells (64). IL-10 is a powerful immune mediator with complicated, even opposite, biological effects. Several investigators reported increased circulating IL-10 levels in CVID subjects (12, 13, 25, 28, 29). In particular, in a study performed in two large cohorts, serum concentrations of IL-10 were increased in CVID with immune dysregulation compared to CVID with infections only (13). In another study, circulating levels of IL-10 were found to be increased in CVID with non-infectious complications compared to CVID with infections only (12). Our results extend the previous observations showing more pronounced elevation of IL-10 in NIC-CVID compared to INF-CVID. These results are apparently surprising. IL-10 is constitutively expressed by regulatory T cells (Treg cells) (65, 66). In CVID subjects, a low number of Treg cells has been associated with autoimmune manifestations (67–69). Moreover, reduced IL-10 production by Treg cells has been found in CVID subjects (70). However, it is possible that increased circulating levels of IL-10 in CVID are influenced by IVIG therapy, which selectively activates Treg cells (71). This hypothesis is supported by the observation that IVIG administration in mice increased IL-10 levels compared to untreated mice (72). Moreover, polyclonal immunoglobulins induced IL-10 from mouse macrophages in vitro (73).
The alteration of circulating cytokines in CVID subjects, as reported by us and other investigators (12, 13, 25–27, 29), may be attributed to intrinsic immunologic changes or induced by exogenous immunoglobulin administration. The first hypothesis is indirectly supported by a wide spectrum of inflammatory and immunologic alterations in different phenotypes of CVID patients (25). The second hypothesis finds support in experimental mouse models. For instance, it has been demonstrated that IVIG administration in mice increased the circulating levels of several cytokines (e.g., IL-10, IL-33) (72, 74). Interestingly, IVIG potentiated LPS-induced IL-10 release from mouse bone marrow-derived macrophages (73). This observation raises the possibility that increased levels of circulating LPS in CVID subjects could modulate the serum concentrations of certain cytokines. Similarly, there is the possibility that IVIG might induce significant alterations of innate and adaptive compartments in peripheral blood of CVID subjects. For instance, it has been proposed that IVIG could decrease the number of circulating inflammatory monocytes (75).
CVID patients with autoimmune and/or inflammatory complications have a higher mortality rate compared to patients with infections only (3, 9, 76, 77). In addition, there is compelling evidence that CVID subjects are associated with increased prevalence of lymphoproliferative disorders and other malignancies (9, 10, 14, 15, 78–81). In particular, an increased prevalence of malignant lymphoma (82) and gastric cancer (15) has been reported in CVID compared to the general population. In the past, this phenomenon was simplistically attributed to defects in B and/or T cell levels or functions (14, 22, 83, 84). More recently, it has been recognized that several risk factors can contribute to malignant transformation in primary immunodeficiencies (78). Among these factors, low-grade inflammation is emerging as one of the hallmarks of cancer (78, 85–87). The present and previous studies have demonstrated the presence of increased concentrations of at least two biomarkers (e.g., cytokines, LPS) of low-grade inflammation in peripheral blood of CVID subjects (12, 13, 25–27, 29, 43, 46). The first biomarker is represented by an increase of circulating proinflammatory cytokines (e.g., IL-1β, TNF-α, IL-6) demonstrated in previous (12, 13, 26, 27, 29) and in our study. These cytokines are involved in tumor initiation and growth (61, 62, 88). Another relevant biomarker is represented by an increase in LPS concentrations, particularly in subjects with complicated CVID (17, 27, 43). Recent evidence has demonstrated that LPS can favor the onset of cancer (89, 90) and the formation of metastasis (91, 92). These observations suggest that persistent elevation of proinflammatory cytokines and LPS concentrations, despite IgRT, could contribute to chronic inflammation, favoring the onset of solid and hematologic cancers in CVID subjects.
Our study has several limitations that should be pointed out. First, our study is limited by the small sample size of subgroups of CVID subjects and healthy donors. Multicenter studies examining CVID patients with different complications (e.g., lymphoproliferative, autoimmune and gastrointestinal diseases, cancer) will be necessary to identify specific circulating biomarkers of immune dysregulation (e.g., cytokines, chemokines). In addition, the small sample sizes of INF-CVID and NIC-CVID subjects from a single center do not allow for meaningful statistical correlations with clinical features and phases of clinical activity. Moreover, in this study we measured a limited number of circulating proinflammatory and immunomodulatory cytokines. Additional investigations are required to evaluate a wider spectrum of cytokines, including epithelium-derived alarmins (e.g., TSLP, IL-33, IL-25) (93–96), and chemokines (25, 97), presumably involved in immune dysregulation in patients with different CVID phenotypes. Moreover, the enrolled individuals with different phenotypes of CVID lacked a molecular diagnosis. Finally, multiple LPS-binding proteins have been found in human serum. Among these, LPS-binding protein (LBP) and bactericidial/permeability increasing protein (BPI) both bind the Lipid A component of LPS (98, 99). In this study, we did not evaluate the concentrations of LBP, BPI, plasma phospholipid transfer protein (PLTP) (100, 101) and LPS-neutralizing capacity (LPS-NC) (102), which could interfere with LPS measurement.
Despite these limitations, our findings expand previous results (25, 28, 29), indicating that several biomarkers of systemic inflammation persist in CVID patients, particularly in those with inflammatory and autoimmune complications (12, 13, 17, 26, 27), despite receiving IgRT. Further efforts appear necessary to understand the possible pathophysiological implications, particularly in relation to cancer development, of cytokine dysregulation in CVID subjects with different phenotypes.
Data availability statement
The raw data supporting the conclusions of this article will be made available by the authors, without undue reservation.
Ethics statement
The studies involving humans were approved by Ethics Committee of the University of Naples Federico II (Prot. 198/18). The studies were conducted in accordance with the local legislation and institutional requirements. The participants provided their written informed consent to participate in this study.
Author contributions
RP: Conceptualization, Data curation, Formal Analysis, Investigation, Methodology, Validation, Writing – original draft, Writing – review & editing. AnP: Conceptualization, Data curation, Formal Analysis, Investigation, Writing – original draft, Writing – review & editing. AF: Data curation, Formal Analysis, Software, Writing – review & editing. AlP: Data curation, Formal Analysis, Software, Writing – review & editing. GL: Data curation, Formal Analysis, Software, Writing – review & editing. CM: Data curation, Formal Analysis, Software, Writing – review & editing. SL: Conceptualization, Data curation, Formal Analysis, Funding acquisition, Investigation, Methodology, Software, Writing – original draft, Writing – review & editing. GS: Conceptualization, Investigation, Supervision, Writing – original draft, Writing – review & editing. GV: Conceptualization, Formal Analysis, Funding acquisition, Investigation, Supervision, Writing – original draft, Writing – review & editing.
Funding
The authors declare financial support was received for the research, authorship, and/or publication of this article. This work was supported in part by grants from the CISI-Lab Project (University of Naples Federico II), TIMING Project and Campania Bioscience (Regione Campania).
Acknowledgments
The authors thank Dr. Gjada Criscuolo for her excellent managerial assistance in preparing this manuscript, and the administrative staff (Dr. Roberto Bifulco, Dr. Anna Ferraro, and Dr. Maria Cristina Fucci) without whom it would not be possible to work as a team.
Conflict of interest
The authors declare that the research was conducted in the absence of any commercial or financial relationships that could be construed as a potential conflict of interest.
The reviewer CP declared a shared affiliation with the authors to the handling editor at the time of review.
The author(s) declared that they were an editorial board member of Frontiers, at the time of submission. This had no impact on the peer review process and the final decision.
Publisher’s note
All claims expressed in this article are solely those of the authors and do not necessarily represent those of their affiliated organizations, or those of the publisher, the editors and the reviewers. Any product that may be evaluated in this article, or claim that may be made by its manufacturer, is not guaranteed or endorsed by the publisher.
Supplementary material
The Supplementary Material for this article can be found online at: https://www.frontiersin.org/articles/10.3389/fimmu.2023.1257398/full#supplementary-material
References
1. Bonilla FA, Barlan I, Chapel H, Costa-Carvalho BT, Cunningham-Rundles C, de la Morena MT, et al. International consensus document (ICON): common variable immunodeficiency disorders. J Allergy Clin Immunol In Pract (2016) 4(1):38–59. doi: 10.1016/j.jaip.2015.07.025
2. Peng XP, Caballero-Oteyza A, Grimbacher B. Common variable immunodeficiency: more pathways than roads to rome. Annu Rev Pathol (2023) 18:283–310. doi: 10.1146/annurev-pathmechdis-031521-024229
3. Chapel H, Lucas M, Lee M, Bjorkander J, Webster D, Grimbacher B, et al. Common variable immunodeficiency disorders: division into distinct clinical phenotypes. Blood (2008) 112(2):277–86. doi: 10.1182/blood-2007-11-124545
4. Ho HE, Cunningham-Rundles C. Non-infectious complications of common variable immunodeficiency: updated clinical spectrum, sequelae, and insights to pathogenesis. Front Immunol (2020) 11:149. doi: 10.3389/fimmu.2020.00149
5. Yazdani R, Abolhassani H, Rezaei N, Azizi G, Hammarstrom L, Aghamohammadi A. Evaluation of known defective signaling-associated molecules in patients who primarily diagnosed as common variable immunodeficiency. Int Rev Immunol (2016) 35(1):7–24. doi: 10.3109/08830185.2015.1136306
6. Salzer U, Warnatz K, Peter HH. Common variable immunodeficiency: an update. Arthritis Res Ther (2012) 14(5):223. doi: 10.1186/ar4032
7. Li J, Jorgensen SF, Maggadottir SM, Bakay M, Warnatz K, Glessner J, et al. Association of CLEC16A with human common variable immunodeficiency disorder and role in murine B cells. Nat Commun (2015) 6:6804. doi: 10.1038/ncomms7804
8. Jolles S, Chapel H, Litzman J. When to initiate immunoglobulin replacement therapy (IGRT) in antibody deficiency: a practical approach. Clin Exp Immunol (2017) 188(3):333–41. doi: 10.1111/cei.12915
9. Resnick ES, Moshier EL, Godbold JH, Cunningham-Rundles C. Morbidity and mortality in common variable immune deficiency over 4 decades. Blood (2012) 119(7):1650–7. doi: 10.1182/blood-2011-09-377945
10. Gathmann B, Mahlaoui N, Ceredih, Gerard L, Oksenhendler E, Warnatz K, et al. Clinical picture and treatment of 2212 patients with common variable immunodeficiency. J Allergy Clin Immunol (2014) 134(1):116–26. doi: 10.1016/j.jaci.2013.12.1077
11. Cunningham-Rundles C. The many faces of common variable immunodeficiency. Hematol Am Soc Hematol Educ Program (2012) 2012:301–5. doi: 10.1182/asheducation-2012.1.301
12. Abyazi ML, Bell KA, Gyimesi G, Baker TS, Byun M, Ko HM, et al. Convergence of cytokine dysregulation and antibody deficiency in common variable immunodeficiency with inflammatory complications. J Allergy Clin Immunol (2022) 149(1):315–26 e9. doi: 10.1016/j.jaci.2021.06.008
13. Berbers RM, Drylewicz J, Ellerbroek PM, van Montfrans JM, Dalm V, van Hagen PM, et al. Targeted proteomics reveals inflammatory pathways that classify immune dysregulation in common variable immunodeficiency. J Clin Immunol (2021) 41(2):362–73. doi: 10.1007/s10875-020-00908-1
14. Shavit R, Maoz-Segal R, Frizinsky S, Haj-Yahia S, Offengenden I, Machnas-Mayan D, et al. Combined immunodeficiency (CVID and CD4 lymphopenia) is associated with a high risk of Malignancy among adults with primary immune deficiency. Clin Exp Immunol (2021) 204(2):251–7. doi: 10.1111/cei.13579
15. Pulvirenti F, Pecoraro A, Cinetto F, Milito C, Valente M, Santangeli E, et al. Gastric cancer is the leading cause of death in italian adult patients with common variable immunodeficiency. Front Immunol (2018) 9:2546. doi: 10.3389/fimmu.2018.02546
16. Crescenzi L, Pecoraro A, Fiorentino A, Poto R, Varricchi G, Rispo A, et al. Liver stiffness assessment by transient elastography suggests high prevalence of liver involvement in common variable immunodeficiency. Dig Liver Dis (2019) 51(11):1599–603. doi: 10.1016/j.dld.2019.05.016
17. Romberg N, Lawrence MG. Birds of a feather: Common variable immune deficiencies. Ann allergy Asthma Immunol (2019) 123(5):461–7. doi: 10.1016/j.anai.2019.07.027
18. Maglione PJ. Autoimmune and lymphoproliferative complications of common variable immunodeficiency. Curr Allergy Asthma Rep (2016) 16(3):19. doi: 10.1007/s11882-016-0597-6
19. Cunningham-Rundles C. Common variable immune deficiency: Dissection of the variable. Immunol Rev (2019) 287(1):145–61. doi: 10.1111/imr.12728
20. Varricchi G, Poto R, Ianiro G, Punziano A, Marone G, Gasbarrini A, et al. Gut microbiome and common variable immunodeficiency: few certainties and many outstanding questions. Front Immunol (2021) 12:712915. doi: 10.3389/fimmu.2021.712915
21. Hajjar J, Voigt A, Conner M, Swennes A, Fowler S, Calarge C, et al. Common variable immunodeficiency patient fecal microbiota transplant recapitulates gut dysbiosis. Res square (2023). doi: 10.21203/rs.3.rs-2640584/v1
22. Giovannetti A, Pierdominici M, Mazzetta F, Marziali M, Renzi C, Mileo AM, et al. Unravelling the complexity of T cell abnormalities in common variable immunodeficiency. J Immunol (2007) 178(6):3932–43. doi: 10.4049/jimmunol.178.6.3932
23. Warnatz K, Denz A, Drager R, Braun M, Groth C, Wolff-Vorbeck G, et al. Severe deficiency of switched memory B cells (CD27(+)IgM(-)IgD(-)) in subgroups of patients with common variable immunodeficiency: a new approach to classify a heterogeneous disease. Blood (2002) 99(5):1544–51. doi: 10.1182/blood.v99.5.1544
24. Hartono S, Motosue MS, Khan S, Rodriguez V, Iyer VN, Divekar R, et al. Predictors of granulomatous lymphocytic interstitial lung disease in common variable immunodeficiency. Ann allergy Asthma Immunol (2017) 118(5):614–20. doi: 10.1016/j.anai.2017.01.004
25. Hultberg J, Ernerudh J, Larsson M, Nilsdotter-Augustinsson A, Nystrom S. Plasma protein profiling reflects T(H)1-driven immune dysregulation in common variable immunodeficiency. J Allergy Clin Immunol (2020) 146(2):417–28. doi: 10.1016/j.jaci.2020.01.046
26. Schnell A, Davrandi M, Saxenhofer M, Leboreiro C, Graeter S, Moreira F, et al. Airway inflammation and dysbiosis in antibody deficiency despite the presence of IgG. J Allergy Clin Immunol (2022) 149(6):2105–15 e10. doi: 10.1016/j.jaci.2021.12.778
27. Jorgensen SF, Troseid M, Kummen M, Anmarkrud JA, Michelsen AE, Osnes LT, et al. Altered gut microbiota profile in common variable immunodeficiency associates with levels of lipopolysaccharide and markers of systemic immune activation. Mucosal Immunol (2016) 9(6):1455–65. doi: 10.1038/mi.2016.18
28. Rezaei N, Aghamohammadi A, Kardar GA, Nourizadeh M, Pourpak Z. T- helper 1 and 2 cytokine assay in patients with common variable immunodeficiency. J investigational allergol Clin Immunol (2008) 18(6):449–53.
29. Hel Z, Huijbregts RP, Xu J, Nechvatalova J, Vlkova M, Litzman J. Altered serum cytokine signature in common variable immunodeficiency. J Clin Immunol (2014) 34(8):971–8. doi: 10.1007/s10875-014-0099-z
30. Polito R, Nigro E, Pecoraro A, Monaco ML, Perna F, Sanduzzi A, et al. Adiponectin receptors and pro-inflammatory cytokines are modulated in common variable immunodeficiency patients: correlation with Ig replacement therapy. Front Immunol (2019) 10:2812. doi: 10.3389/fimmu.2019.02812
31. Immunodeficiencies ES for Diagnostic Criteria . Available at: https://esid.org/Education/DiagnosticCriteria-PID.
32. Klein F, Skvaril F, Vermeeren R, Vlug A, Duimel WJ. The quantification of human IgG subclasses in reference preparations. Clin Chim Acta (1985) 150(2):119–27. doi: 10.1016/0009-8981(85)90262-1
33. CLS I. Evaluation of precision of quantitative measurement procedures: approved guideline. 3rd ed. Wayne, PA, United States: Clinical and Laboratory Standards Institute (2014). CLSI Document EP05-A3.
34. CLS I. Evaluation of the linearity of quantitative measurement procedures: a statistical approach; Approved guideline. Wayne, PA, United States: Clinical and Laboratory Standards Institute (2003). CLSI Document EP06-A.
35. Braile M, Cristinziano L, Marcella S, Varricchi G, Marone G, Modestino L, et al. LPS-mediated neutrophil VEGF-A release is modulated by cannabinoid receptor activation. J Leukoc Biol (2021) 109(3):621–31. doi: 10.1002/JLB.3A0520-187R
36. Staiano RI, Loffredo S, Borriello F, Iannotti FA, Piscitelli F, Orlando P, et al. Human lung-resident macrophages express CB1 and CB2 receptors whose activation inhibits the release of angiogenic and lymphangiogenic factors. J Leukoc Biol (2016) 99(4):531–40. doi: 10.1189/jlb.3HI1214-584R
37. Poto R, Shamji M, Marone G, Durham SR, Scadding GW, Varricchi G. Neutrophil extracellular traps in asthma: friends or foes? Cells (2022) 11(21):3521. doi: 10.3390/cells11213521
38. Poto R, Cristinziano L, Modestino L, de Paulis A, Marone G, Loffredo S, et al. Neutrophil extracellular traps, angiogenesis and cancer. Biomedicines (2022) 10(2):431. doi: 10.3390/biomedicines10020431
39. Marchetti G, Tincati C, Silvestri G. Microbial translocation in the pathogenesis of HIV infection and AIDS. Clin Microbiol Rev (2013) 26(1):2–18. doi: 10.1128/CMR.00050-12
40. Zanoni I, Granucci F. Role of CD14 in host protection against infections and in metabolism regulation. Front Cell Infect Microbiol (2013) 3:32. doi: 10.3389/fcimb.2013.00032
41. Ulevitch RJ, Tobias PS. Receptor-dependent mechanisms of cell stimulation by bacterial endotoxin. Annu Rev Immunol (1995) 13:437–57. doi: 10.1146/annurev.iy.13.040195.002253
42. Berbers RM, Nierkens S, van Laar JM, Bogaert D, Leavis HL. Microbial dysbiosis in common variable immune deficiencies: evidence, causes, and consequences. Trends Immunol (2017) 38(3):206–16. doi: 10.1016/j.it.2016.11.008
43. Perreau M, Vigano S, Bellanger F, Pellaton C, Buss G, Comte D, et al. Exhaustion of bacteria-specific CD4 T cells and microbial translocation in common variable immunodeficiency disorders. J Exp Med (2014) 211(10):2033–45. doi: 10.1084/jem.20140039
44. Lee TK, Gereige JD, Maglione PJ. State-of-the-art diagnostic evaluation of common variable immunodeficiency. Ann allergy Asthma Immunol (2021) 127(1):19–27. doi: 10.1016/j.anai.2021.03.005
45. Cunningham-Rundles C, Maglione PJ. Common variable immunodeficiency. J Allergy Clin Immunol (2012) 129(5):1425–6 e3. doi: 10.1016/j.jaci.2012.03.025
46. Romberg N, Le Coz C, Glauzy S, Schickel JN, Trofa M, Nolan BE, et al. Patients with common variable immunodeficiency with autoimmune cytopenias exhibit hyperplastic yet inefficient germinal center responses. J Allergy Clin Immunol (2019) 143(1):258–65. doi: 10.1016/j.jaci.2018.06.012
47. Poto R, Ianiro G, de Paulis A, Spadaro G, Marone G, Gasbarrini A, et al. Correction to: Is there a role for microbiome−based approach in common variable immunodeficiency? Clin Exp Med (2023). doi: 10.1007/s10238-023-01032-1
48. Kitchens RL, Thompson PA. Modulatory effects of sCD14 and LBP on LPS-host cell interactions. J Endotoxin Res (2005) 11(4):225–9. doi: 10.1179/096805105X46565
49. Kawai T, Akira S. The role of pattern-recognition receptors in innate immunity: update on Toll-like receptors. Nat Immunol (2010) 11(5):373–84. doi: 10.1038/ni.1863
50. Barbosa RR, Silva SP, Silva SL, Tendeiro R, Melo AC, Pedro E, et al. Monocyte activation is a feature of common variable immunodeficiency irrespective of plasma lipopolysaccharide levels. Clin Exp Immunol (2012) 169(3):263–72. doi: 10.1111/j.1365-2249.2012.04620.x
51. Litzman J, Nechvatalova J, Xu J, Ticha O, Vlkova M, Hel Z. Chronic immune activation in common variable immunodeficiency (CVID) is associated with elevated serum levels of soluble CD14 and CD25 but not endotoxaemia. Clin Exp Immunol (2012) 170(3):321–32. doi: 10.1111/j.1365-2249.2012.04655.x
52. Sandler NG, Wand H, Roque A, Law M, Nason MC, Nixon DE, et al. Plasma levels of soluble CD14 independently predict mortality in HIV infection. J Infect Dis (2011) 203(6):780–90. doi: 10.1093/infdis/jiq118
53. Tsukamoto H, Takeuchi S, Kubota K, Kobayashi Y, Kozakai S, Ukai I, et al. Lipopolysaccharide (LPS)-binding protein stimulates CD14-dependent Toll-like receptor 4 internalization and LPS-induced TBK1-IKKϵ-IRF3 axis activation. J Biol Chem (2018) 293(26):10186–201. doi: 10.1074/jbc.M117.796631
54. Lee CC, Avalos AM, Ploegh HL. Accessory molecules for Toll-like receptors and their function. Nat Rev Immunol (2012) 12(3):168–79. doi: 10.1038/nri3151
55. Kaarbo M, Yang M, Hov JR, Holm K, de Sousa MML, Macpherson ME, et al. Duodenal inflammation in common variable immunodeficiency has altered transcriptional response to viruses. J Allergy Clin Immunol (2023) 151(3):767–77. doi: 10.1016/j.jaci.2022.09.029
56. von Gunten S, Smith DF, Cummings RD, Riedel S, Miescher S, Schaub A, et al. Intravenous immunoglobulin contains a broad repertoire of anticarbohydrate antibodies that is not restricted to the IgG2 subclass. J Allergy Clin Immunol (2009) 123(6):1268–76 e15. doi: 10.1016/j.jaci.2009.03.013
57. dos Santos-Valente EC, da Silva R, de Moraes-Pinto MI, Sarni RO, Costa-Carvalho BT. Assessment of nutritional status: vitamin A and zinc in patients with common variable immunodeficiency. J investigational allergol Clin Immunol (2012) 22(6):427–31.
58. Paquin-Proulx D, Santos BAN, Silveira Barsotti N, Marinho AKBB, Kokron CM, Carvalho KI, et al. Loss of circulating mucosal-associated invariant T cells in common variable immunodeficiency is associated with immune activation and loss of eomes and PLZF. ImmunoHorizons (2017) 1(7):142–55. doi: 10.4049/immunohorizons.1700039
59. Palestra F, Poto R, Ciardi R, Opromolla G, Secondo A, Tedeschi V, et al. SARS-CoV-2 spike protein activates human lung macrophages. Int J Mol Sci (2023) 24(3):3036. doi: 10.3390/ijms24033036
60. Matarazzo L, Hernandez Santana YE, Walsh PT, Fallon PG. The IL-1 cytokine family as custodians of barrier immunity. Cytokine (2022) 154:155890. doi: 10.1016/j.cyto.2022.155890
61. Teufel LU, Arts RJW, Netea MG, Dinarello CA, Joosten LAB. IL-1 family cytokines as drivers and inhibitors of trained immunity. Cytokine (2022) 150:155773. doi: 10.1016/j.cyto.2021.155773
62. Atri C, Guerfali FZ, Laouini D. Role of human macrophage polarization in inflammation during infectious diseases. Int J Mol Sci (2018) 19(6):1801. doi: 10.3390/ijms19061801
63. Balestrieri B, Granata F, Loffredo S, Petraroli A, Scalia G, Morabito P, et al. Phenotypic and functional heterogeneity of low-density and high-density human lung macrophages. Biomedicines (2021) 9(5):505. doi: 10.3390/biomedicines9050505
64. Ouyang W, O'Garra A. IL-10 family cytokines IL-10 and IL-22: from basic science to clinical translation. Immunity (2019) 50(4):871–91. doi: 10.1016/j.immuni.2019.03.020
65. Maynard CL, Harrington LE, Janowski KM, Oliver JR, Zindl CL, Rudensky AY, et al. Regulatory T cells expressing interleukin 10 develop from Foxp3+ and Foxp3- precursor cells in the absence of interleukin 10. Nat Immunol (2007) 8(9):931–41. doi: 10.1038/ni1504
66. Kamanaka M, Kim ST, Wan YY, Sutterwala FS, Lara-Tejero M, Galan JE, et al. Expression of interleukin-10 in intestinal lymphocytes detected by an interleukin-10 reporter knockin tiger mouse. Immunity (2006) 25(6):941–52. doi: 10.1016/j.immuni.2006.09.013
67. Arumugakani G, Wood PM, Carter CR. Frequency of Treg cells is reduced in CVID patients with autoimmunity and splenomegaly and is associated with expanded CD21lo B lymphocytes. J Clin Immunol (2010) 30(2):292–300. doi: 10.1007/s10875-009-9351-3
68. Yu GP, Chiang D, Song SJ, Hoyte EG, Huang J, Vanishsarn C, et al. Regulatory T cell dysfunction in subjects with common variable immunodeficiency complicated by autoimmune disease. Clin Immunol (2009) 131(2):240–53. doi: 10.1016/j.clim.2008.12.006
69. Horn J, Manguiat A, Berglund LJ, Knerr V, Tahami F, Grimbacher B, et al. Decrease in phenotypic regulatory T cells in subsets of patients with common variable immunodeficiency. Clin Exp Immunol (2009) 156(3):446–54. doi: 10.1111/j.1365-2249.2009.03913.x
70. Arandi N, Mirshafiey A, Jeddi-Tehrani M, Abolhassani H, Sadeghi B, Mirminachi B, et al. Evaluation of CD4+CD25+FOXP3+ regulatory T cells function in patients with common variable immunodeficiency. Cell Immunol (2013) 281(2):129–33. doi: 10.1016/j.cellimm.2013.03.003
71. Tjon AS, Tha-In T, Metselaar HJ, van Gent R, van der Laan LJ, Groothuismink ZM, et al. Patients treated with high-dose intravenous immunoglobulin show selective activation of regulatory T cells. Clin Exp Immunol (2013) 173(2):259–67. doi: 10.1111/cei.12102
72. Lee SY, Jung YO, Ryu JG, Kang CM, Kim EK, Son HJ, et al. Intravenous immunoglobulin attenuates experimental autoimmune arthritis by inducing reciprocal regulation of Th17 and Treg cells in an interleukin-10-dependent manner. Arthritis Rheumatol (2014) 66(7):1768–78. doi: 10.1002/art.38627
73. Kozicky LK, Zhao ZY, Menzies SC, Fidanza M, Reid GS, Wilhelmsen K, et al. Intravenous immunoglobulin skews macrophages to an anti-inflammatory, IL-10-producing activation state. J Leukoc Biol (2015) 98(6):983–94. doi: 10.1189/jlb.3VMA0315-078R
74. Leontyev D, NesChadim A, Branch DR. Cytokine profiles in mouse models of experimental immune thrombocytopenia reveal a lack of inflammation and differences in response to intravenous immunoglobulin depending on the mouse strain. Transfusion (2014) 54(11):2871–9. doi: 10.1111/trf.12680
75. Siedlar M, Strach M, Bukowska-Strakova K, Lenart M, Szaflarska A, Weglarczyk K, et al. Preparations of intravenous immunoglobulins diminish the number and proinflammatory response of CD14+CD16++ monocytes in common variable immunodeficiency (CVID) patients. Clin Immunol (2011) 139(2):122–32. doi: 10.1016/j.clim.2011.01.002
76. Tabolli S, Giannantoni P, Pulvirenti F, La Marra F, Granata G, Milito C, et al. Longitudinal study on health-related quality of life in a cohort of 96 patients with common variable immune deficiencies. Front Immunol (2014) 5:605. doi: 10.3389/fimmu.2014.00605
77. Farmer JR, Ong MS, Barmettler S, Yonker LM, Fuleihan R, Sullivan KE, et al. Common variable immunodeficiency non-infectious disease endotypes redefined using unbiased network clustering in large electronic datasets. Front Immunol (2017) 8:1740. doi: 10.3389/fimmu.2017.01740
78. Hauck F, Voss R, Urban C, Seidel MG. Intrinsic and extrinsic causes of Malignancies in patients with primary immunodeficiency disorders. J Allergy Clin Immunol (2018) 141(1):59–68 e4. doi: 10.1016/j.jaci.2017.06.009
79. Quinti I, Agostini C, Tabolli S, Brunetti G, Cinetto F, Pecoraro A, et al. Malignancies are the major cause of death in patients with adult onset common variable immunodeficiency. Blood (2012) 120(9):1953–4. doi: 10.1182/blood-2012-05-431064
80. Mayor PC, Eng KH, Singel KL, Abrams SI, Odunsi K, Moysich KB, et al. Cancer in primary immunodeficiency diseases: Cancer incidence in the United States Immune Deficiency Network Registry. J Allergy Clin Immunol (2018) 141(3):1028–35. doi: 10.1016/j.jaci.2017.05.024
81. Rezaei N, Hedayat M, Aghamohammadi A, Nichols KE. Primary immunodeficiency diseases associated with increased susceptibility to viral infections and Malignancies. J Allergy Clin Immunol (2011) 127(6):1329–41 e2. doi: 10.1016/j.jaci.2011.02.047
82. Quinti I, Soresina A, Spadaro G, Martino S, Donnanno S, Agostini C, et al. Long-term follow-up and outcome of a large cohort of patients with common variable immunodeficiency. J Clin Immunol (2007) 27(3):308–16. doi: 10.1007/s10875-007-9075-1
83. Bateman EA, Ayers L, Sadler R, Lucas M, Roberts C, Woods A, et al. T cell phenotypes in patients with common variable immunodeficiency disorders: associations with clinical phenotypes in comparison with other groups with recurrent infections. Clin Exp Immunol (2012) 170(2):202–11. doi: 10.1111/j.1365-2249.2012.04643.x
84. Kutukculer N, Azarsiz E, Aksu G, Karaca NE. CD4+CD25+Foxp3+ T regulatory cells, Th1 (CCR5, IL-2, IFN-gamma) and Th2 (CCR4, IL-4, Il-13) type chemokine receptors and intracellular cytokines in children with common variable immunodeficiency. Int J Immunopathol Pharmacol (2016) 29(2):241–51. doi: 10.1177/0394632015617064
85. Hanahan D. Hallmarks of cancer: new dimensions. Cancer Discovery (2022) 12(1):31–46. doi: 10.1158/2159-8290.CD-21-1059
86. Cristinziano L, Modestino L, Antonelli A, Marone G, Simon HU, Varricchi G, et al. Neutrophil extracellular traps in cancer. Semin Cancer Biol (2022) 79:91–104. doi: 10.1016/j.semcancer.2021.07.011
87. Elia G, Ferrari SM, Galdiero MR, Ragusa F, Paparo SR, Ruffilli I, et al. New insight in endocrine-related adverse events associated to immune checkpoint blockade. Best Pract Res Clin Endocrinol Metab (2020) 34(1):101370. doi: 10.1016/j.beem.2019.101370
88. van Loo G, Bertrand MJM. Death by TNF: a road to inflammation. Nat Rev Immunol (2023) 23(5):289–303. doi: 10.1038/s41577-022-00792-3
89. Albrengues J, Shields MA, Ng D, Park CG, Ambrico A, Poindexter ME, et al. Neutrophil extracellular traps produced during inflammation awaken dormant cancer cells in mice. Science (2018) 361(6409):eaao4227. doi: 10.1126/science.aao4227
90. Demers M, Krause DS, Schatzberg D, Martinod K, Voorhees JR, Fuchs TA, et al. Cancers predispose neutrophils to release extracellular DNA traps that contribute to cancer-associated thrombosis. Proc Natl Acad Sci U.S.A. (2012) 109(32):13076–81. doi: 10.1073/pnas.1200419109
91. Heymann CJF, Bobin-Dubigeon C, Munoz-Garcia J, Cochonneau D, Ollivier E, Heymann MF, et al. Lipopolysaccharide-binding protein expression is associated to the metastatic status of osteosarcoma patients. J Bone Oncol (2022) 36:100451. doi: 10.1016/j.jbo.2022.100451
92. Wu X, Qian S, Zhang J, Feng J, Luo K, Sun L, et al. Lipopolysaccharide promotes metastasis via acceleration of glycolysis by the nuclear factor-kappaB/snail/hexokinase3 signaling axis in colorectal cancer. Cancer Metab (2021) 9(1):23. doi: 10.1186/s40170-021-00260-x
93. Varricchi G, Pecoraro A, Marone G, Criscuolo G, Spadaro G, Genovese A, et al. Thymic stromal lymphopoietin isoforms, inflammatory disorders, and cancer. Front Immunol (2018) 9:1595. doi: 10.3389/fimmu.2018.01595
94. Afferni C, Buccione C, Andreone S, Galdiero MR, Varricchi G, Marone G, et al. The pleiotropic immunomodulatory functions of IL-33 and its implications in tumor immunity. Front Immunol (2018) 9:2601. doi: 10.3389/fimmu.2018.02601
95. Gambardella AR, Poto R, Tirelli V, Schroeder JT, Marone G, Mattei F, et al. Differential effects of alarmins on human and mouse basophils. Front Immunol (2022) 13:894163. doi: 10.3389/fimmu.2022.894163
96. Cristinziano L, Poto R, Criscuolo G, Ferrara AL, Galdiero MR, Modestino L, et al. IL-33 and superantigenic activation of human lung mast cells induce the release of angiogenic and lymphangiogenic factors. Cells (2021) 10(1):145. doi: 10.3390/cells10010145
97. Tocchetti CG, Galdiero MR, Varricchi G. Cardiac toxicity in patients treated with immune checkpoint inhibitors: it is now time for cardio-immuno-oncology. J Am Coll Cardiol (2018) 71(16):1765–7. doi: 10.1016/j.jacc.2018.02.038
98. Yu Y, Song G. Lipopolysaccharide-binding protein and bactericidal/permeability-increasing protein in lipid metabolism and cardiovascular diseases. Adv Exp Med Biol (2020) 1276:27–35. doi: 10.1007/978-981-15-6082-8_3
99. Vreugdenhil AC, Snoek AM, van 't Veer C, Greve JW, Buurman WA. LPS-binding protein circulates in association with apoB-containing lipoproteins and enhances endotoxin-LDL/VLDL interaction. J Clin Invest (2001) 107(2):225–34. doi: 10.1172/JCI10832
100. Gautier T, Deckert V, Nguyen M, Desrumaux C, Masson D, Lagrost L. New therapeutic horizons for plasma phospholipid transfer protein (PLTP): Targeting endotoxemia, infection and sepsis. Pharmacol Ther (2022) 236:108105. doi: 10.1016/j.pharmthera.2021.108105
101. Deckert V, Lemaire S, Ripoll PJ, de Barros JP, Labbe J, Borgne CC, et al. Recombinant human plasma phospholipid transfer protein (PLTP) to prevent bacterial growth and to treat sepsis. Sci Rep (2017) 7(1):3053. doi: 10.1038/s41598-017-03285-9
Keywords: common variable immunodeficiency, CVID, lipopolysaccharide, IL-1, TNF-α, IL-6, IL-10
Citation: Poto R, Pecoraro A, Ferrara AL, Punziano A, Lagnese G, Messuri C, Loffredo S, Spadaro G and Varricchi G (2023) Cytokine dysregulation despite immunoglobulin replacement therapy in common variable immunodeficiency (CVID). Front. Immunol. 14:1257398. doi: 10.3389/fimmu.2023.1257398
Received: 12 July 2023; Accepted: 07 September 2023;
Published: 28 September 2023.
Edited by:
Laura Maggi, University of Florence, ItalyReviewed by:
Claudio Pignata, University of Naples Federico II, ItalyRiccardo Capecchi, Azienda USL Toscana nord ovest Livorno, Italy
Antonio Condino-Neto, University of São Paulo, Brazil
Copyright © 2023 Poto, Pecoraro, Ferrara, Punziano, Lagnese, Messuri, Loffredo, Spadaro and Varricchi. This is an open-access article distributed under the terms of the Creative Commons Attribution License (CC BY). The use, distribution or reproduction in other forums is permitted, provided the original author(s) and the copyright owner(s) are credited and that the original publication in this journal is cited, in accordance with accepted academic practice. No use, distribution or reproduction is permitted which does not comply with these terms.
*Correspondence: Gilda Varricchi, Z2lsZGFuZXRAZ21haWwuY29t
†ORCID: Remo Poto, orcid.org/0000-0002-4723-0167
Antonio Pecoraro, orcid.org/0000-0003-3617-2819
Anne Lise Ferrara, orcid.org/0000-0003-1839-8873
Alessandra Punziano, orcid.org/0000-0003-0151-9237
Gianluca Lagnese, orcid.org/0000-0001-9504-429X
Carla Messuri, orcid.org/0009-0000-8310-8081
Stefania Loffredo, orcid.org/0000-0002-5871-1898
Giuseppe Spadaro, orcid.org/0000-0001-7889-425X
Gilda Varricchi, orcid.org/0000-0002-9285-4657