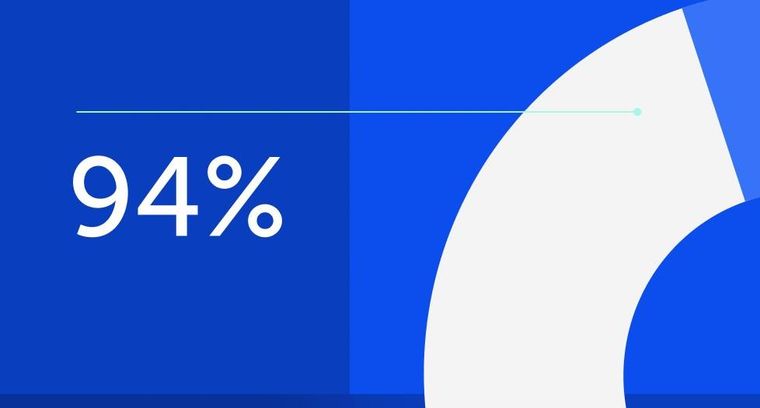
94% of researchers rate our articles as excellent or good
Learn more about the work of our research integrity team to safeguard the quality of each article we publish.
Find out more
ORIGINAL RESEARCH article
Front. Immunol., 27 September 2023
Sec. Microbial Immunology
Volume 14 - 2023 | https://doi.org/10.3389/fimmu.2023.1256425
This article is part of the Research TopicThe Role of Cytosolic Sensors in Host Defense to Intracellular PathogensView all 11 articles
Exosomes, organelles measuring 30-200nm, are secreted by various cell types. Leishmania exosomes consist of many proteins, including heat shock proteins, annexins, Glycoprotein 63, proteins exerting signaling activity and those containing mRNA and miRNA. Studies have demonstrated that Leishmania donovani exosomes downregulate IFN-γ and inhibit the expression of microbicidal molecules, such as TNF and nitric oxide, thus creating a microenvironment favoring parasite proliferation. Despite lacking immunological memory, data in the literature suggest that, following initial stimulation, mononuclear phagocytes may become “trained” to respond more effectively to subsequent stimuli. Here we characterized the effects of macrophage sensitization using L. braziliensis exosomes prior to infection by the same pathogen. Human macrophages were stimulated with L. braziliensis exosomes and then infected with L. braziliensis. Higher levels of IL-1β and IL-6 were detected in cultures sensitized prior to infection compared to unstimulated infected cells. Moreover, stimulation with L. braziliensis exosomes induced macrophage production of IL-1β, IL-6, IL-10 and TNF. Inhibition of exosome secretion by L. braziliensis prior to macrophage infection reduced cytokine production and produced lower infection rates than untreated infected cells. Exosome stimulation also induced the consumption/regulation of NLRP3 inflammasome components in macrophages, while the blockade of NLRP3 resulted in lower levels of IL-6 and IL-1β. Our results suggest that L. braziliensis exosomes stimulate macrophages, leading to an exacerbated inflammatory state that may be NLRP3-dependent.
Cutaneous leishmaniasis (CL), due to L. braziliensis infection, is characterized by the presence of few or rare parasites, a predominance of lymphocytes and mononuclear phagocytes in the inflammatory infiltrate (1). Host immunological factors are known to play an important role in the pathogenesis of this disease. Mononuclear cells from CL patients stimulated with soluble Leishmania antigen (SLA) produce exacerbated amounts of IFN- γ and TNF, as well as low levels of IL-10 in culture (2). While the production of IFN-γ is important for parasite killing, high levels of TNF have been associated with tissue damage and lesion development (2, 3). Studies on lesion tissue samples have confirmed the contribution of inflammation to ulcer development, as evidenced by the presence of Granzyme B produced by CD8+ and NK cells, in addition to metalloproteinases and inflammatory cytokines (4–11). Moreover, Leishmania braziliensis-infected mononuclear phagocytes have been shown to produce high levels of reactive oxygen species (ROS), molecules with leishmanicidal properties that have also been associated with tissue damage (12, 13).
An important role of IL-1β in CL pathogenesis has been documented in recent years, with the use of IL-1β inhibitors shown to improve the course of disease in murine models (8, 12). In humans, IL-1β concentrations were found to correlate with lesion size, and previous research by our group has documented elevated expression of the NLRP3 inflammasome in monocytes obtained from the peripheral blood of CL patients, in addition to high levels of IL-1β in cultured peripheral blood mononuclear cells (PBMC) stimulated with SLA (8, 14). Moreover, NLRP3-/- BALB/c mice infected with L. major demonstrated a greater ability to control Leishmania infection when compared to wild-type mice (15).
Leishmania, and its soluble products, can trigger immune responses in innate immune cells prior to the onset of mononuclear cellular infection (13, 16, 17). For instance, Leishmania lipophosphoglycan (LPG) and DNA can induce the production of inflammatory mediators by mononuclear phagocytes through the activation of toll-like receptors (TLR) 2, 4 and 9 (18–20). Pathogen molecules are often secreted via extracellular vesicles (EV), also known as exosomes.
Exosomes, organelles ranging in size from 30-200nm, are secreted by various cell types. The invagination of regions of the endosomal membrane results in the formation of multivesicular bodies (MVB); the fusion of MVBs with the plasm membrane leads to exosome secretion (21, 22). These vesicles play a major role in molecular trafficking, e.g., proteins and nucleic acids, which once delivered may modulate host macrophage function (23–29). For example, glycoprotein (gp) 63-containing Leishmania exosomes was shown to induce TNF production by macrophages and exacerbate pathology in a CL mouse model (28, 30–32).
The present study aimed to assess the effects of L. braziliensis exosomes on human macrophagic responses, revealing that these vesicles induce both IL-1β and IL-6 production. Moreover, the pre-exposure of macrophages to L. braziliensis exosomes was found to prime these cells to enhance the production of pro-inflammatory mediators in response to L. braziliensis infection, potentially contributing to the pathogenesis of disease.
An L. braziliensis isolate (MHOM/BR/LTCP11245) previously obtained from a CL patient’s skin lesion was initially cultivated in biphasic medium (NNN). After isolation, parasites were identified by multilocus enzyme electrophoresis and cryopreserved in liquid nitrogen. Following selection, parasites were expanded and cultivated in Schneider’s culture medium (SIGMA) supplemented with 20% inactive fetal bovine serum (FBS) (GIBCO), 1% L-glutamine and antibiotics.
L. braziliensis promastigotes were cultured at 37°C under 5% CO2 for 4 hours to optimize protein and vesicle secretion (31). Supernatants collected from in vitro cultures were centrifuged (2500rpm) and filtered (0.22 µm), after which exosomes were isolated through sequential ultracentrifugation (10 x 105g). The EV particle-size distribution was determined by diffraction analysis using a NS300 particle-size tracker and Nanosight NTA 3.0 software using light scatter mode (Malvern Instruments Ltd., Technologies, Malvern, UK) (Figure 1) (33–35).
Figure 1 (A) Image of L. Braziliensis exosomes taken by a NS300 particle-sike tracker and Nanosight NTA 3.0 software using light scatter mode. (B) Quantification and Characterization of extracellular vesicles extracted from isolates of L. braziliensis.
Peripheral blood mononuclear cells (PBMC) were obtained from heparinized venous blood from healthy subjects by Ficoll-paque density gradient centrifugation (GE Healthcare). Cells were washed twice in saline, and monocytes were isolated from PBMCs using magnetic beads in accordance with the manufacturer’s protocol (Dynabeads untouched human monocytes; Invitrogen Dynal AS, Oslo, Norway). This process was performed twice, after which cells were washed in phosphate-buffered saline (PBS), then adjusted to the desired concentration, and resuspended in RPMI 1640 (GIBCO BRL., Grand Island, NY USA) supplemented with 10% FBS (GIBCO BRL., Grand Island, NY USA) and antibiotics. Monocytes were then allowed to adhere to polystyrene culture plates and incubated for 6 days at 37°C under 5% CO2 until differentiation into macrophages, as previously described (36).
Monocyte-derived macrophages (3 x 105) were stimulated, using a transwell membrane, with soluble factors from L. braziliensis for 24 hours at 37 °C, 5% CO2 in RPMI (Figure 2A). Following stimulation, cells were washed twice in saline and infected or not with L. braziliensis (MOI 5:1) for 2 hours. Next, the remaining promastigotes were washed out, and cells were reincubated for another 24 hours at 37 °C, 5% CO2 in RPMI. The slides were subsequently submitted to panoptic staining for posterior quantification of infected macrophages and amastigotes per 100 cells via optical microscopy.
Figure 2 Macrophages from HS infected with L. braziliensis after sensitization with L. braziliensis through a transwell membrane (0,4µm). (A) Stimulation strategy. (B) Cytokine production by macrophages sensitized with soluble factors and infected with L. braziliensis. (C) Cytokine production by macrophages from HS sensitized with soluble factors from L. braziliensis. Levels of IL-1β, TNF, IL-10 and IL-6 were determined in culture supernatants by LUMINEX. Statistical analyses were performed using the Wilcoxon rank test *p<.05, **p<.01.
Monocyte-derived macrophages (3x105) were stimulated with exosomes from L. braziliensis (300 vesicles/macrophage) for 24 hours at 37°C under 5% CO2 in RPMI. After stimulation, cells were washed twice in saline and infected or not with L. braziliensis (MOI 5:1) for 2 hours. The remaining promastigotes were washed out, then cells were incubated for another 24 hours (37 °C, 5% CO2) in RPMI.
For some experiments involving the inhibition of exosome/vesicle generation, 1x107 Leishmania/mL were treated with GW4869 (20ng/ml), a vesicle generation inhibitor, (https://www.sigmaaldrich.com/BR/pt/product/sigma/d1692) for 30 minutes at room temperature, then washed three times to prevent contact between the inhibitor and human cells during stimulation/infection protocols. To assess GW4869 toxicity against Leishmania parasites, we evaluated L. braziliensis viability after treatment with GW4869 using propidium iodide as cell death marker, by flow cytometry.
For the blockade of the NLRP3 inflammasome, monocyte-derived macrophages (3x105) were first treated with the NLRP3 inhibitor glibenclamide (100µM) for 2 hours, then stimulated with L. braziliensis exosomes (300:1) for 24 hours. Cells were then washed twice and infected or not with L. braziliensis (MOI 5:1) for 2 hours, washed again to remove any remaining promastigotes, and finally incubated for 24 hours at 37 °C under 5% CO2in RPMI. Unstimulated macrophages and untreated stimulated cells were used as controls.
To evaluate infection rate, 3x105 macrophages/well were plated on Nunc® Labtek® plates and stimulated with exosomes from L. braziliensis (300 vesicles/macrophage), then incubated for 24 hours at 37° C with 5% CO2. Cells were then washed twice and infected or not with L. braziliensis (MOI 5:1) for 2 hours, washed again to remove any remaining promastigotes, and finally incubated for 24 hours at 37 °C under 5% CO2 in RPMI. The slides were submitted to panoptic staining for the later quantification of infected macrophages and the number of amastigotes per 100 cells, performed via optical microscopy.
Following stimulation and/or infection protocols, the supernatants from cultures were collected for cytokine (IL-1β, TNF, IL-6 and IL-10) quantification via Luminex (Bio-Plex Pro Human Cytokine 27-plex Assay) or ELISA.
Monocyte-derived macrophages from healthy subjects were stimulated with L. braziliensis exosomes for 24 hours and infected or not with L. braziliensis for another 24 hours, as described above. After the final incubation, cells were collected and placed in 5mL polystyrene FACS tubes (BD Biosciences Falcon™ 352052) for cell labeling with conjugated antibodies αCD14 (APC) and αHLA-DR (PerCP) to determine cell populations of interest, as well as αNLRP3 (PE). Events were acquired on a flow cytometer (BD LSRFortessa™ Cell analyzer) and data were analyzed using Flowjo® software.
To evaluate reactive oxygen species (ROS) production, macrophages were stimulated as described in “Exosome Stimulation and Macrophage infection with L. braziliensis” section, then treated with dihydrorhodamine-123 at 10ng/mL (Cayman Chemical Company) for 10 minutes. Cells were subsequently labeled with αHLA-DR and αCD14 to evaluate fluorescence intensity by flow cytometry, with data analysis performed via FlowJo® software.
Cells stimulated with exosomes and infected or not with L. braziliensis, followed by incubation at 37°C under 5% CO2 for 2 hours, were harvested in TRIzol Reagent (Invitrogen). RNA extraction was performed using TRIzol RNA isolation, according to manufacturer’s instructions. RNA concentration and integrity were determined by spectrophotometric optical density measurements (260 and 280 nm). Gene expression was analyzed performed as previously described (37).
C57BL/6 mice, both wild-type and others genetically deficient for NLRP3−/−, were obtained as previously described (38). All animals were maintained at the UFMG Animal Facility and used for experimentation at 6–8 weeks of age. Bone marrow-derived macrophages (BMDM) were prepared and infected as previously described (39, 40). Briefly, bone marrow cells were isolated from the femurs and tibias of the animals, cultured in RPMI 1640 supplemented with 30% L929 cell-conditioned medium and 20% FBS for 7 days. BMDM (0.5×106) were treated or not with lipopolysaccharide (LPS) for 6 hours (500 ng/ml) and stimulated or not with L. braziliensis exosomes (300:1), followed by infection with stationary phase Leishmania braziliensis (MOI 10:1) for 24 hours. After 48 hours, supernatants were harvested and IL-1β, TNF and IL-6 concentrations were detected by ELISA.
Statistical analysis was performed using the Wilcoxon test for paired variables and Mann-Whitney rank test for unpaired measurements (*p<0.05, **p<0.01, ***p<0.001, ****p<0.0001). All experimental data were analyzed using Prism GraphPad® 8.0.2, which was also used for graphical data representations.
Macrophages can be “trained” to enhance response to infection (41). For instance, macrophages exposed to Saccharomyces cerevisiae demonstrated an increased ability to produce TNF in response to TLR ligands, such as LPS (42). To investigate whether soluble factors from L. braziliensis would interfere in cytokine production, human macrophages were cultured with L. braziliensis separated by a membrane, which allowed only soluble factors/small molecules to cross the barrier. Macrophages exposed to L. braziliensis products through the membrane produced more IL-1β upon infection than those that were not previously exposed to parasite factors (Figure 2B). Moreover, exposure to L. braziliensis soluble factors was also shown to induce the production of inflammatory mediators TNF, IL-6 and IL-1β by uninfected macrophages (Figure 2C). This data provides evidence that exposure to L. braziliensis modulates immune response prior to the establishment of cellular infection.
We first investigated whether L. braziliensis exosomes induced an inflammatory response in macrophages, and then assessed the effects of stimulating macrophages with these vesicles prior to infection with L. braziliensis. Compared to unstimulated cells, macrophages stimulation with EVs were found to induce IL-1β, TNF, IL-10 and IL-6 production (Figure 3). Pre-sensitization of macrophages with exosomes prior to infection was shown to increase IL-1β and IL-6 levels (Figure 3). Similarly, pre-sensitization with exosomes produced no influence on infection rate, nor the number of parasites internalized (data not shown).
Figure 3 Cytokine production by macrophages from HS sensitized with L. braziliensis exosomes and infected with L. braziliensis. Macrophages from HS (n=8) were stimulated with exosomes isolated from L. braziliensis (300:1) for 24 hours and afterwards infected or not with L. braziliensis for another 24 hours. Levels of IL-1β, TNF, IL-10 and IL-6 were determined in culture supernatants by LUMNEX. Statistical analyses were performed using the Wilcoxon rank test *p<.05, **p<.01.
To further ascertain the role of exosomes in inducing inflammatory cytokine production, we infected human macrophages with L. braziliensis previously treated with GW4869, and then compared cytokine expression with other untreated Leishmania-infected cells. As in the experiments above, our data shows that cells infected with exosome-free L. braziliensis produced significantly less IL-1β, TNF, IL-10 and IL-6 upon infection (Figure 4A). Although no differences were observed in parasite internalization by macrophages pre-sensitized with exosomes compared to unstimulated cells (data not shown), the cells infected with exosome-free L. braziliensis presented less infectivity, as evidenced by fewer numbers of infected macrophages and lower numbers of amastigotes within the cells (Figure 4B). As treatment with GW4869 could have interfered with L. braziliensis survival, we performed a dose-response curve with different GW4869 concentrations. Our experiments show no toxicity of GW4869 over L. braziliensis (Figure 4C).
Figure 4 (A) Cytokine production and (B) Infection rate of macrophages from HS infected with L. braziliensis with exosome production inhibited. Axenic culture of L. braziliensis promastigotes was treated with GW4869 (20ng/ml), exosome production inhibitor, for 2 hours. Macrophages from HS (n=8) were infected with GW4869 treated L. braziliensis (5:1) for 24 hours. (C) L. braziliensis viability after GW4869 treatment was assessed using propidium iodide as cell death marker, by flow cytometry. (D) optical microscopy picture of panoptic stained macrophages infected with L. braziliensis treated (left) or not (right) with GW4869. Levels of IL-1β, TNF, IL-10 and IL-6 were determined in culture supernatants by LUMNEX. The percentage of infected cells as well as the number of intracellular parasites were determined by microscopic evaluation after panoptic staining. Statistical analyses were performed using the Wilcoxon rank test *p<.05, **p<.01.
Most of the data presented in this study demonstrate the important association between L. braziliensis exosomes and IL-1β production. Consequently, the participation of NLRP3 in IL-1β production was investigated by evaluating both the expression of NLRP3 protein by cultured macrophages (Figure 5A) and the production of cytokines by cells stimulated with exosomes after the blockade of NLRP3 through glibenclamide treatment (Figure 5B). Our results indicate lower NLRP3 protein expression in cells stimulated with exosomes for 24 hours compared to basal level cells, while exosome stimulation in macrophages prior to infection induced more consumption, and probably further expression regulation, of this inflammasome than unsensitized cells (Figure 5A), which corroborates our previous results. Moreover, glibenclamide-treated cells were found to secrete less IL-1β, TNF, IL-6 and IL-10 than untreated cells, regardless of L. braziliensis infection or exosome stimulation. (Figure 5B), which suggests that cytokine expression induced by exosome stimulation is dependent on NLRP3.
Figure 5 (A) Exosome stimulation enhance NLRP3 consumption by macrophages. Macrophages from healthy subjects (n=8) were sensitized with exosomes (300:1) for 24 hours and infected with L. braziliensis promastigotes for another 24 hours at a ratio of 5:1. Cells were stained with anti-CD14 and anti-NLRP3. Data were collected using flow cytometry and analyzed with FLOWJO® software. Representative gating strategy on CD14+ expression in macrophages from one healthy subject. NLRP3 MFI was taken from CD14+ population. The data represent the mean of fluorescence intensity (MFI) of NLRP3 expression by macrophages in the different stimulated groups. (B) Glyburide downmodulates exosome-induced cytokine production in macrophages from healthy subjects. Macrophages from 8 individuals were treated with glyburide (100µM) for 2 hours. Afterwards cells were stimulated for 24 hours with L. braziliensis exosomes and infected with the parasite for another 24 hours. Levels of IL-1β, TNF, IL-10, IL-10 and IL-6 were measured in culture supernatant by ELISA. Statistical analyses were performed using the Mann-Whitney test for unpaired groups and Wilcoxon rank test for paired measurements *p<.05 **p<.01.
The secretion of IL-1β may be dependent on inflammasome activation (8, 14, 39). NLRP3, the main inflammasome receptor responsible for inducing IL-1β production, forms a complex with ASC and Caspase-1 for the processing and secretion of IL-1β and IL-18 (43, 44). Since our results showed that L. braziliensis exosome stimulation followed by infection induced high levels of IL-1β secretion by human macrophages, we decided to investigate whether NLRP3 activation is required to drive IL-1β production upon L. braziliensis infection in a murine model.
Bone marrow-derived macrophages (BMDM) from C57BL/6 WT mice and mice deficient for NLRP3 were infected with L. braziliensis after previous stimulation with L. braziliensis exosomes. Levels of IL-1β, IL-6 and TNF were quantified by ELISA in cell supernatants. IL-1β production in mice was found to be dependent on the NLRP3 inflammasome, as IL-1β production was completely abrogated in cells from NLRP3-/- mice, regardless of stimulation. However, NLRP3 did not appear to be involved in the production of the other cytokines evaluated, as levels did not differ between WT and NLRP3-/- mouse cells (Figure 6).
Figure 6 NLRP is key for L. braziliensis induced IL-1β production in mouse macrophages. BMDMs from wild-type C57BL/6 mices and deficient for NLRP3 were prepared, pulsed with LPS (500 ng/ml), stimulated with exosomes (300:1) and infected with L. braziliensis (MOI 10:1) or not. After 48 hours of culture ELISA for IL-1β was performed on supernatants. Statistical analyses were performed using the Mann-Whitney test and the Wilcoxon rank test. *p<0.05, **p<0.01.
Our group previously showed that reactive oxygen species (ROS) constitute a major endogenous factor in Leishmania killing (13, 45). Furthermore, TNF production is directly associated with ROS production through the activation of the NF-κB signaling pathway. Therefore, to investigate the role of L. braziliensis exosomes on ROS production, ROS expression (Figure 7) was evaluated in macrophages stimulated with exosomes further infected with L. braziliensis. Our data show that while L. braziliensis exosomes induced ROS production by uninfected macrophages, pre-sensitization did not interfere in ROS production following in vitro macrophage infection (Figure 7).
Figure 7 Macrophagic stimulation with L. braziliensis exosomes enhance reactive oxygen species production. Macrophages from healthy subjects were evaluated after being stimulated with exosomes for 24 hours and infected with L. braziliensis for 24 hours (n=6) or not. The cells were treated with DHR (10ng/mL – 10 min) and stained with anti-CD14 and anti-HLA-DR. Data were collected using flow cytometry and analyzed by FLOWJO® software. (A) Representative gating strategy on CD14+ and HLA-DR+ expression in macrophages. DHR MFI were taken from CD14+ HLA-DR+ population. (B) The data represent the mean of fluorescence intensity (MFI) of oxidative burst production by macrophages stimulated with exosomes and infected with L. braziliensis. Statistical analyses were performed using the Wilcoxon rank test for paired measurements *p<.05.
Herein L. braziliensis exosomes were found to induce TNF and ROS production by human macrophages. It has been shown that NF-kB activation induces TNF production through the activation of TLRs 2-4 upon L. braziliensis infection (18). To determine the role of L. braziliensis exosomes in TLR2 and NFkB expression, human macrophages were stimulated with EVs for 2 hours and infected or not with L. braziliensis for another 2 hours. Uninfected macrophages stimulated with exosomes were found to induce higher expression of NF-κB and TLR2 (Figure 8), yet infected cells previously stimulated with EVs demonstrated increased TLR2 expression, yet no effect on NF-κB expression (Figure 8). No differences were observed among the groups with regard to TLR4 expression. These data corroborate with our findings on exosome-induced TNF and ROS production, as these molecules are formed as a result of the NF-κB pathway.
Figure 8 Macrophages stimulated with L. braziliensis exosomes express high levels of NF-κB and Toll-Like Receptor 2. (A) TLR2 and (B) NF-κ B gene expression, represented as 2−ΔΔCT, following RT-PCR of RNA from macrophages-derived monocytes of HS (N=7) stimulated with exosomes for 24 hours and infected with L. braziliensis for 24 hours or not. Statistical analyses were performed using the Mann-Whitney test and the Wilcoxon rank test. *p<0.05, **p<0.01. ***p<0.001.
The initial events occurring upon Leishmania infection orchestrate immune response, constituting determinants in parasite proliferation and disease development. Among the factors contributing to disease expression, vector molecules, including salivary gland proteins, parasite-derived molecules and host immune response are of great importance (46, 47). On the parasite side, attention has been paid to the role of exosomes due to the close relationship between molecules present in the exosomes and their ability to modulate host immune response (28, 31, 32). The present study documented that L. braziliensis exosomes indeed modulate immune response by priming macrophages to produce more inflammatory molecules.
It has recently been suggested that macrophages could become “trained” to enhance infection response (41). For instance, macrophages exposed to Saccharomyces cerevisiae demonstrated an increased ability to produce TNF in response to TLR ligands, such as LPS. Moreover, monocytes “trained” with S. cerevisiae demonstrated heightened microbial activity (42). In the same vein, our results additionally indicate that L. braziliensis exosomes effectively “train” macrophages to produce higher cytokine concentrations in response to posterior L. braziliensis infection, suggesting that epigenetics in macrophages may play a role in subsequent exposure to L. braziliensis components.
Clearly, Leishmania vesicle contents vary across species, and thereby exert variable effects on host immune response. For instance, studies have shown that exosomes from L. donovani modulate monocyte response to IFN-γ and inhibit TNF while inducing IL-10 production. In this manner, mice stimulated with L. donovani exosomes prior to infection with the same species were found to exhibit higher macrophage infection rates within the spleen (26, 27). Also, stimulation with L. major exosomes prior to L. major parasite challenge produced a shift towards a Th2-type response in mice, as evidenced by a high frequency of CD4+ T cells producing IL-4, which led to disease exacerbation (27). Moreover, it was demonstrated that L. major exosomes contribute to CL pathology through the induction of an overproduction of inflammatory cytokines IL-23 and IL-17 in the lymph nodes of BALB/c mice (29).
The pathogenesis of Leishmania infection varies widely depending on the Leishmania species involved, and its specific effect on host immune response. In all cases, parasite control is associated with the expansion of CD4+ Th1 cells producing IFN-γ, which promotes the killing of parasites within infected cells (48). In some cases, the parasite evades the immune system silently, without inducing an immunological response, as is the case in infection by L. donovani. In L. braziliensis infection, however, an exaggerated Th1 response is observed, together with high levels of proinflammatory cytokines and a predominance of lymphocytes and mononuclear phagocytes at the lesion site. Herein we demonstrate that stimulation with L. braziliensis exosomes induces high levels of proinflammatory cytokines (TNF and IL-1β) as well as ROS, molecules known to be involved in CL due to L. braziliensis pathogenesis. In our experiments where we treated L. braziliensis with GW4869, a vesicle secretion inhibitor, we observed a decrease in cytokines production in infected macrophages. However, since GW4869 also decreased L. braziliensis internalization, further studies need to be performed to understand the mechanism by which L. braziliensis exosomes interfere in Leishmania uptake.
Some Leishmania species are known to inhibit several macrophage functions, such as macrophage activation, cytokine release and antigen presentation. For instance, down-regulation of class II MHC expression and the inability to produce IL-12 has been observed in several studies (49–54). Also, TLR-induced up-regulation of co-stimulatory molecules, as well as TNF-alpha and IL-12 production, was notably impaired in L. major, L. chagasi, L. donovani and L. mexicana-infected macrophages, while in the case of L. mexicana, disruption of NF-κB signaling was observed (55, 56). In contrast, our results show that exosome sensitization increased the expression of NF-κB and TLR2, corroborating previously published data indicating that L. braziliensis infection promotes an inflammatory environment.
Inflammation is often associated with macrophage activation and intracellular parasite killing. Here we found that in spite of L. braziliensis exosomes enhancing IL-1b production by human macrophages, these vesicles have no effect on Leishmania parasite killing. These results are in accordance with our previous data showing no association between IL-1b production and L. brazileisns killing (8). Also, in our current results we found that exosomes do not increase the ability of macrophages to produce ROS in response to L. braziliensis infection, corroborating that these vesicles have no effect on L. braziliensis killing by macrophages. In the present study we found that the IL-1b production driven by L. braziliensis vesicles is NLPR3-dependent, corroborating our previous data involving L. braziliensis-infected macrophages (8). These findings favor the potential role of L. braziliensis EVs in the immunopathology observed in CL arising from L. braziliensis, in which ulcer development has been associated with an exaggerated inflammatory response that leads to tissue damage. Previous results have shown that IL-1β concentrations in CL due to L. braziliensis correlate positively with lesion size and healing time (8, 12). It has been documented that L. braziliensis infection activates the NLRP3 inflammasome, thereby inducing ROS (8, 39). Therefore, the blockade of NLRP3 may constitute a sound therapeutic approach.
Considering the physiology and dynamics of vesicle secretion, studies involving L. mexicana demonstrated a substantial increase in vesicle secretion at a temperature of 37˚C (31). Herein we observed a similar phenomenon in L. braziliensis. The up-regulation in vesicle release induced at infection-like temperatures suggests that parasites release vesicles into the extracellular environment prior to invading host cells. Similarly, it has been suggested that Leishmania exosomes interact with the host cell prior to the parasite itself, as evidenced by the presence of Leishmania molecules within uninfected macrophages (57). Moreover, as previous studies have shown that Leishmania secretes exosomes into the midgut of the sandfly vector, it has been hypothesized that these vesicles then become inoculated into the host alongside the parasite during sandfly blood-feeding, possibly enhancing the vesicle-induced effects of the parasite on immune response (29, 32). Although both L. major and L. braziliensis cause CL, the magnitude of inflammation caused by both parasite species is quite different, as infection with L. braziliensis induces higher inflammatory response in humans than L. major. Thus, further experiments need to be performed to determine differences in the contents of exosomes between both species.
In contrast to previously published data indicating the downregulatory effects of L. donovoni and L. major exosomes, L. braziliensis exosomes do not appear to contribute to a microenvironment favorable for parasite growth, but instead participate in an exacerbated pathologic inflammatory response, which may potentially exacerbate lesion development.
The original contributions presented in the study are included in the article/supplementary materials. Further inquiries can be directed to the corresponding authors.
The studies involving humans were approved by Institution Review Board of the Federal University of Bahia Medical School and the National Commission of Ethics in Research (CONEP) under the number 3.252.513. The studies were conducted in accordance with the local legislation and institutional requirements. The human samples used in this study were acquired from Peripheral blood was collected from healthy volunteers. Written informed consent for participation was not required from the participants or the participants’ legal guardians/next of kin in accordance with the national legislation and institutional requirements. The animal study was approved by Institutional Animal Care and Use Committee of the Federal University of Minas Gerais (CEUA no. 165/2019). The study was conducted in accordance with the local legislation and institutional requirements.
FP: Conceptualization, Data curation, Formal Analysis, Investigation, Methodology, Writing – original draft, Writing – review & editing. DZ: Investigation, Methodology, Formal Analysis, Writing – review & editing. TC: Formal Analysis, Methodology, Writing – review & editing. MN: Investigation, Methodology, Writing – review & editing. RS: Methodology, Resources, Writing – review & editing. MA: Investigation, Writing – review & editing. PS: Funding acquisition, Resources, Visualization, Writing – review & editing. SO: Resources, Writing – review & editing. EC: Formal Analysis, Funding acquisition, Project administration, Resources, Supervision, Visualization, Writing – review & editing. LC: Conceptualization, Data curation, Formal Analysis, Funding acquisition, Investigation, Project administration, Resources, Supervision, Visualization, Writing – original draft, Writing – review & editing.
This study was sponsored by the National Council for Scientific and Technological Development (CNPq), the National Institute of Health (NIH AI 136862) and AI149456a grant US-Brazil grant. The authors declare financial support was received for the research, authorship, and/or publication of this article.
We would like to thank Cristiano Sampaio Franco for secretarial assistance in the submission of this manuscript. The authors are also grateful to Andris K. Walter for English-language revision services and manuscript copyediting assistance and for the personal at Electronic Microscopy Platform- Fiocruz Bahia for assistance with acquiring the images. We thank Dr. Rachel DeRita from The Extracellular Vesicle Core Facility at The University of Pennsylvania.
The authors declare that the research was conducted in the absence of any commercial or financial relationships that could be construed as a potential conflict of interest. The authors declared that they were an editorial board member of Frontiers, at the time of submission. This had no impact on the peer review process and the final decision.
All claims expressed in this article are solely those of the authors and do not necessarily represent those of their affiliated organizations, or those of the publisher, the editors and the reviewers. Any product that may be evaluated in this article, or claim that may be made by its manufacturer, is not guaranteed or endorsed by the publisher.
1. Bittencourt AL, Barral A. Evaluation of the histopathological classifications of American cutaneous and mucocutaneous leishmaniasis. Mem Inst Oswaldo Cruz (1991) 86(1). doi: 10.1590/S0074-02761991000100009
2. Bacellar O, Lessa H, Schriefer A, MaChado P, De Jesus AR, Dutra WO, et al. Up-regulation of Th1-type responses in mucosal leishmaniasis patients. Infect Immun (2002) 70(12). doi: 10.1128/IAI.70.12.6734-6740.2002
3. Passos S, Carvalho LP, Costa RS, Campos TM, Novais FO, Magalhães A, et al. Intermediate monocytes contribute to pathologic immune response in leishmania Braziliensis infections. J Infect Dis (2015) 211(2). doi: 10.1093/infdis/jiu439
4. Amorim CF, Novais FO, Nguyen BT, Misic AM, Carvalho LP, Carvalho EM, et al. Variable gene expression and parasite load predict treatment outcome in cutaneous leishmaniasis. Sci Transl Med (2019) 11(519). doi: 10.1126/scitranslmed.aax4204
5. Saldanha MG, Pagliari C, Queiroz A, MaChado PRL, Carvalho L, Scott P, et al. Tissue damage in human cutaneous leishmaniasis: correlations between inflammatory cells and molecule expression. Front Cell Infect Microbiol (2020) 10. doi: 10.3389/fcimb.2020.00355
6. Campos TM, Passos ST, Novais FO, Beiting DP, Costa RS, Queiroz A, et al. Matrix metalloproteinase 9 production by monocytes is enhanced by TNF and participates in the pathology of human cutaneous leishmaniasis. PloS Negl Trop Dis (2014) 8(11). doi: 10.1371/journal.pntd.0003282
7. Campos TM, Novais FO, Saldanha M, Costa R, Lordelo M, Celestino D, et al. Granzyme B produced by natural killer cells enhances inflammatory response and contributes to the immunopathology of cutaneous leishmaniasis. J Infect Dis (2020) 221(6). doi: 10.1093/infdis/jiz538
8. Santos D, Campos TM, Saldanha M, Oliveira SC, Nascimento M, Zamboni DS, et al. IL-1β Production by intermediate monocytes is associated with immunopathology in cutaneous leishmaniasis. J Invest Dermatol (2018) 138(5). doi: 10.1016/j.jid.2017.11.029
9. Novais FO, Carvalho LP, Graff JW, Beiting DP, Ruthel G, Roos DS, et al. Cytotoxic T cells mediate pathology and metastasis in cutaneous leishmaniasis. PloS Pathog (2013) 9(7). doi: 10.1371/journal.ppat.1003504
10. Novais FO, Scott P. CD8+ T cells in cutaneous leishmaniasis: the good, the bad, and the ugly. Semin Immunopathology (2015) 37. doi: 10.1007/s00281-015-0475-7
11. Santos CDS, Boaventura V, Ribeiro Cardoso C, Tavares N, Lordelo MJ, Noronha A, et al. CD8+ granzyme B+ -mediated tissue injury vs. CD4 + IFNγ+ -mediated parasite killing in human cutaneous leishmaniasis. J Invest Dermatol (2013) 133(6). doi: 10.1038/jid.2013.4
12. Novais FO, Carvalho AM, Clark ML, Carvalho LP, Beiting DP, Brodsky IE, et al. CD8+T cell cytotoxicity mediates pathology in the skin by inflammasome activation and IL-1β production. PloS Pathog (2017) 13(2). doi: 10.1371/journal.ppat.1006196
13. Carneiro PP, Conceição J, Macedo M, Magalhães V, Carvalho EM, Bacellar O. The role of nitric oxide and reactive oxygen species in the killing of Leishmania Braziliensis by monocytes from patients with cutaneous leishmaniasis. PloS One (2016) 11(2). doi: 10.1371/journal.pone.0148084
14. Carvalho AM, Novais FO, Paixão CS, de Oliveira CI, MaChado PRL, Carvalho LP, et al. Glyburide, a NLRP3 inhibitor, decreases inflammatory response and is a candidate to reduce pathology in leishmania Braziliensis infection. J Invest Dermatol (2020) 140(1). doi: 10.1016/j.jid.2019.05.025
15. Gurung P, Karki R, Vogel P, Watanabe M, Bix M, Lamkanfi M, et al. An NLRP3 inflammasome’triggered Th2-biased adaptive immune response promotes leishmaniasis. J Clin Invest (2015) 125(3):1329–38. doi: 10.1172/JCI79526
16. Carvalho LP, Pearce EJ, Scott P. Functional dichotomy of dendritic cells following interaction with leishmania braziliensis: Infected cells produce high levels of TNF-α, whereas bystander dendritic cells are activated to promote T cell responses. J Immunol (2008) 181(9). doi: 10.4049/jimmunol.181.9.6473
17. Becker I, Salaiza N, Aguirre M, Delgado J, Carrillo-Carrasco N, Kobeh LG, et al. Leishmania lipophosphoglycan (LPG) activates NK cells through toll-like receptor-2. Mol Biochem Parasitol (2003) 130(2). doi: 10.1016/S0166-6851(03)00160-9
18. Carneiro PP, Dórea AS, Oliveira WN, Guimarães LH, Brodskyn C, Carvalho EM, et al. Blockade of TLR2 and TLR4 attenuates inflammatory response and parasite load in cutaneous leishmaniasis. Front Immunol (2021) 12. doi: 10.3389/fimmu.2021.706510
19. Carvalho LP, Petritus PM, Trochtenberg AL, Zaph C, Hill DA, Artis D, et al. Lymph Node Hypertrophy following Leishmania major Infection Is Dependent on TLR9. J Immunol (2012) 188(3). doi: 10.4049/jimmunol.1101018
20. Sacramento LA, da Costa JL, de Lima MHF, Sampaio PA, Almeida RP, Cunha FQ, et al. Toll-like receptor 2 is required for inflammatory process development during Leishmania infantum infection. Front Microbiol (2017) 8(FEB). doi: 10.3389/fmicb.2017.00262
21. Bobrie A, Colombo M, Raposo G, Théry C. Exosome secretion: molecular mechanisms and roles in immune responses. Traffic (2011) 12. doi: 10.1111/j.1600-0854.2011.01225.x
22. Théry C, Zitvogel L, Amigorena S. Exosomes: Composition, biogenesis and function. Nat Rev Immunol (2002) 2. doi: 10.1038/nri855
23. Théry C, Ostrowski M, Segura E. Membrane vesicles as conveyors of immune responses. Nat Rev Immunol (2009) 9. doi: 10.1038/nri2567
24. Raposo G, Stoorvogel W. Extracellular vesicles: Exosomes, microvesicles, and friends. J Cell Biol (2013) 200. doi: 10.1083/jcb.201211138
25. Tkach M, Théry C. Communication by extracellular vesicles: where we are and where we need to go. (2016) Cellpress 164:Cell. doi: 10.1016/j.cell.2016.01.043
26. Silverman JM, Clos J, De’Oliveira CC, Shirvani O, Fang Y, Wang C, et al. An exosome-based secretion pathway is responsible for protein export from Leishmania and communication with macrophages. J Cell Sci (2010) 123(6). doi: 10.1242/jcs.056465
27. Silverman JM, Clos J, Horakova E, Wang AY, Wiesgigl M, Kelly I, et al. Leishmania exosomes modulate innate and adaptive immune responses through effects on monocytes and dendritic cells. J Immunol (2010) 185(9). doi: 10.4049/jimmunol.1000541
28. Hassani K, Shio MT, Martel C, Faubert D, Olivier M. Absence of metalloprotease GP63 alters the protein content of leishmania exosomes. PloS One (2014) 9(4). doi: 10.1371/journal.pone.0095007
29. Atayde VD, Aslan H, Townsend S, Hassani K, Kamhawi S, Olivier M. Exosome secretion by the parasitic protozoan leishmania within the sand fly midgut. Cell Rep (2015) 13(5). doi: 10.1016/j.celrep.2015.09.058
30. Alcolea PJ, Alonso A, Degayón MA, Moreno-Paz M, Jiménez M, Molina R, et al. In vitro infectivity and differential gene expression of Leishmania infantum metacyclic promastigotes: Negative selection with peanut agglutinin in culture versus isolation from the stomodeal valve of Phlebotomus perniciosus. BMC Genomics (2016) 17(1). doi: 10.1186/s12864-016-2672-8
31. Hassani K, Antoniak E, Jardim A, Olivier M. Temperature-induced protein secretion by leishmania mexicana modulates macrophage signalling and function. PloS One (2011) 6(5). doi: 10.1371/journal.pone.0018724
32. Silverman JM, Reiner NE. Leishmania exosomes deliver preemptive strikes to create an environment permissive for early infection. Front Cell Infection Microbiol (2011) 1. doi: 10.3389/fcimb.2011.00026
33. Dragovic RA, Gardiner C, Brooks AS, Tannetta DS, Ferguson DJP, Hole P, et al. Sizing and phenotyping of cellular vesicles using Nanoparticle Tracking Analysis. Nanomedicine (2011) 7(6):780–8. doi: 10.1016/j.nano.2011.04.003
34. Lötvall J, Hill AF, Hochberg F, Buzás EI, Di Vizio D, Gardiner C, et al. Minimal experimental requirements for definition of extracellular vesicles and their functions: a position statement from the International Society for Extracellular Vesicles. J Extracell Vesicles (2014) 3(1):26913. doi: 10.3402/jev.v3.26913
35. Cruz FF, Borg ZD, Goodwin M, Sokocevic D, Wagner DE, Coffey A, et al. Systemic administration of human bone marrow-derived mesenchymal stromal cell extracellular vesicles ameliorates aspergillus hyphal extract-induced allergic airway inflammation in immunocompetent mice. Stem Cells Transl Med (2015) 4(11):1302–16. doi: 10.5966/sctm.2014-0280
36. Giudice A, Vendrame C, Bezerra C, Carvalho LP, Delavechia T, Carvalho EM, et al. Macrophages participate in host protection and the disease pathology associated with Leishmania Braziliensis infection. BMC Infect Dis (2012) 12. doi: 10.1186/1471-2334-12-75
37. Almeida L, Silva JA, Andrade VM, MaChado P, Jamieson SE, Carvalho EM, et al. Analysis of expression of FLI1 and MMP1 in American cutaneous leishmaniasis caused by Leishmania Braziliensis infection. Infection Genet Evol (2017) 49. doi: 10.1016/j.meegid.2017.01.018
38. Vandanmagsar B, Youm YH, Ravussin A, Galgani JE, Stadler K, Mynatt RL, et al. The NLRP3 inflammasome instigates obesity-induced inflammation and insulin resistance. Nat Med (2011) 17(2). doi: 10.1038/nm.2279
39. Lima-Junior DS, Costa DL, Carregaro V, Cunha LD, Silva ALN, Mineo TWP, et al. Inflammasome-derived IL-1β production induces nitric oxide-mediated resistance to Leishmania. Nat Med (2013) 19(7). doi: 10.1038/nm.3221
40. Marim FM, Silveira TN, Lima DS, Zamboni DS. A method for generation of bone marrow-derived macrophages from cryopreserved mouse bone marrow cells. PloS One (2010) 5(12). doi: 10.1371/journal.pone.0015263
41. Netea MG, Latz E, Mills KHG, O’Neill LAJ. Innate immune memory: A paradigm shift in understanding host defense. Nat Immunol (2015) 16:675–9. doi: 10.1038/ni.3178
42. Rizzetto L, Ifrim DC, Moretti S, Tocci N, Cheng SC, Quintin J, et al. Fungal chitin induces trained immunity in human monocytes during cross-talk of the host with Saccharomyces cerevisiae. J Biol Chem (2016) 291(15). doi: 10.1074/jbc.M115.699645
43. Connolly DJ, Bowie AG. The emerging role of human PYHIN proteins in innate immunity: Implications for health and disease. Biochem Pharmacol (2014) 92. doi: 10.1016/j.bcp.2014.08.031
44. Man SM, Karki R, Kanneganti TD. AIM2 inflammasome in infection, cancer, and autoimmunity: Role in DNA sensing, inflammation, and innate immunity. Eur J Immunol (2016) 46. doi: 10.1002/eji.201545839
45. Novais FO, Carvalho LP, Passos S, Roos DS, Carvalho EM, Scott P, et al. Genomic profiling of human leishmania Braziliensis lesions identifies transcriptional modules associated with cutaneous immunopathology. J Invest Dermatol (2015) 135(1). doi: 10.1038/jid.2014.305
46. Carvalho AM, Viana SM, Andrade BB, Oliveira F, Valenzuela JG, Carvalho EM, et al. Immune response to linB13, a lutzomyia intermedia salivary protein correlates with disease severity in tegumentary leishmaniasis. Clin Infect Dis (2022) 75(10). doi: 10.1093/cid/ciac258
47. Carvalho AM, Fukutani KF, Sharma R, Curvelo RP, MIranda JC, Barral A, et al. Seroconversion to Lutzomyia intermedia LinB-13 as a biomarker for developing cutaneous leishmaniasis. Sci Rep (2017) 7(1). doi: 10.1038/s41598-017-03345-0
48. Carvalho LP, Passos S, Schriefer A, Carvalho EM. Protective and pathologic immune responses in human tegumentary leishmaniasis. Front Immunol (2012) 3. doi: 10.3389/fimmu.2012.00301
49. Carrera L, Gazzinelli RT, Badolato R, Hieny S, Müller W, Kühn R, et al. Leishmania promastigotes selectively inhibit interleukin 12 induction in bone marrow-derived macrophages from susceptible and resistant mice. J Exp Med (1996) 183(2). doi: 10.1084/jem.183.2.515
50. Kane MM, Mosser DM. Leishmania parasites and their ploys to disrupt macrophage activation. Curr Opin Hematol (2000) 7. doi: 10.1097/00062752-200001000-00006
51. Forget G, Gregory DJ, Whitcombe LA, Olivier M. Role of host protein tyrosine phosphatase SHP-1 in Leishmania donovani-induced inhibition of nitric oxide production. Infect Immun (2006) 74(11). doi: 10.1128/IAI.00853-05
52. Shweash M, Adrienne McGachy H, Schroeder J, Neamatallah T, Bryant CE, Millington O, et al. Leishmania mexicana promastigotes inhibit macrophage IL-12 production via TLR-4 dependent COX-2, iNOS and arginase-1 expression. Mol Immunol (2011) 48(15–16). doi: 10.1016/j.molimm.2011.05.013
53. Gupta P, Srivastav S, Saha S, Das PK, Ukil A. Leishmania donovani inhibits macrophage apoptosis and pro-inflammatory response through AKT-mediated regulation of β-catenin and FOXO-1. Cell Death Differ (2016) 23(11). doi: 10.1038/cdd.2016.101
54. Weinheber N, Wolfram M, Harbecke D, Aebischer T. Phagocytosis of Leishmania mexicana amastigotes by macrophages leads to a sustained suppression of IL-12 production. Eur J Immunol (1998) 28(8). doi: 10.1002/(SICI)1521-4141(199808)28:08<2467::AID-IMMU2467>3.0.CO;2-1
55. Parmar N, Chandrakar P, Vishwakarma P, Singh K, Mitra K, Kar S. Leishmania donovani exploits tollip, a multitasking protein, to impair TLR/IL-1R signaling for its survival in the host. J Immunol (2018) 201(3). doi: 10.4049/jimmunol.1800062
56. Cameron P, McGachy A, Anderson M, Paul A, Coombs GH, Mottram JC, et al. Inhibition of lipopolysaccharide-induced macrophage IL-12 production by leishmania mexicana amastigotes: the role of cysteine peptidases and the NF-κB signaling pathway. J Immunol (2004) 173(5). doi: 10.4049/jimmunol.173.5.3297
Keywords: Leishmania braziliensis, exosome, macrophage, immune response, innate immunity
Citation: Peixoto FC, Zanette DL, Cardoso TM, Nascimento MT, Sanches RCO, Aoki M, Scott P, Oliveira SC, Carvalho EM and Carvalho LP (2023) Leishmania braziliensis exosomes activate human macrophages to produce proinflammatory mediators. Front. Immunol. 14:1256425. doi: 10.3389/fimmu.2023.1256425
Received: 10 July 2023; Accepted: 30 August 2023;
Published: 27 September 2023.
Edited by:
Ulisses Gazos Lopes, Federal University of Rio de Janeiro, BrazilReviewed by:
Peter Epeh Kima, University of Florida, United StatesCopyright © 2023 Peixoto, Zanette, Cardoso, Nascimento, Sanches, Aoki, Scott, Oliveira, Carvalho and Carvalho. This is an open-access article distributed under the terms of the Creative Commons Attribution License (CC BY). The use, distribution or reproduction in other forums is permitted, provided the original author(s) and the copyright owner(s) are credited and that the original publication in this journal is cited, in accordance with accepted academic practice. No use, distribution or reproduction is permitted which does not comply with these terms.
*Correspondence: Lucas P. Carvalho, Y2FydmFsaG9scDc2QGdtYWlsLmNvbQ==; Fabio C. Peixoto, ZmFiaW85OXBlaXhvdG9AZ21haWwuY29t
Disclaimer: All claims expressed in this article are solely those of the authors and do not necessarily represent those of their affiliated organizations, or those of the publisher, the editors and the reviewers. Any product that may be evaluated in this article or claim that may be made by its manufacturer is not guaranteed or endorsed by the publisher.
Research integrity at Frontiers
Learn more about the work of our research integrity team to safeguard the quality of each article we publish.