- 1Department of Clinical Haematology, Alfred Hospital, Melbourne, VI, Australia
- 2Australian Centre for Blood Diseases, Monash University, Melbourne, VI, Australia
- 3Atherothrombosis and Vascular Biology Laboratory, Baker Heart and Diabetes Institute, Melbourne, VI, Australia
- 4Department of Plastic and Hand Surgery, Medical Center – University of Freiburg, Medical Faculty of the University of Freiburg, Freiburg, Germany
- 5Department of Cardiology, Alfred Hospital, Melbourne, VI, Australia
- 6Department of Cardiometabolic Health, The University of Melbourne, Parkville, VI, Australia
- 7Department of Medicine, Monash University, Melbourne, VI, Australia
The canonical role of platelets as central players in cardiovascular disease by way of their fundamental role in mediating thrombosis and haemostasis is well appreciated. However, there is now a large body of experimental evidence demonstrating that platelets are also pivotal in various physiological and pathophysiological processes other than maintaining haemostasis. Foremost amongst these is the emerging data highlighting the key role of platelets in driving cancer growth, metastasis and modulating the tumour microenvironment. As such, there is significant interest in targeting platelets therapeutically for the treatment of cancer. Therefore, the purpose of this review is to provide an overview of how platelets contribute to the cancer landscape and why platelets present as valuable targets for the development of novel cancer diagnosis tools and therapeutics.
Introduction
The relationship between platelets, thrombosis, and cancer has long been acknowledged. Already in the nineteenth century, Armand Trousseau reported the association between cancer and thrombosis, describing migratory thrombophlebitis as a presenting feature of visceral cancer (1). Thrombocytosis is a frequent finding in cancer patients, and the link between thrombocytosis and malignancy was first noted by Reiss et al. in 1872 (2). Thrombocytosis remains widely recognised as an adverse prognostic marker correlating with shortened survival in a wide range of common malignancies, including lung, breast, colorectal, gastric, renal, and ovarian cancers (3–11).
There is now a large and constantly growing body of evidence illustrating the bidirectional relationship between platelets and cancer. In 1968, Gasic et al. first described the correlation between platelets and metastatic potential, demonstrating that neuraminidase-induced thrombocytopenia was able to inhibit tumour metastasis in mice and that this antimetastatic effect could be reversed by the transfusion of platelet-rich plasma (12). Further experimental evidence identified that thrombopoietic cytokine production by tumour cells, specifically interleukin (IL)-6, induces platelet production, thereby creating a feedback loop that further enhances tumour growth (10). Here, platelet-derived factors not only offer stability to the tumour vasculature but have the capacity to increase the metastatic potential of tumour cells (13, 14).
Notably, platelets have been shown to influence the efficacy of cancer therapies. Large cohort studies have revealed that in comparison to the general population, the risk of developing thrombosis is much higher in cancer patients (15). Elevated platelet count in ovarian cancer patients is associated with higher relapse rates and lower response rates to taxane chemotherapy, indicating that platelets may offer cancer cells protection against apoptosis (16). Experimental models of adenocarcinoma have also demonstrated that the presence of platelets conferred increased tumour cell survival rates against treatment with standard anticancer drugs, 5-fluorouracil and paclitaxel (17). These findings emphasise the intimate and bidirectional crosstalk that exists between platelets and tumours. This review will discuss the mechanisms by which platelets and tumours interact and explore the consequences of platelet-tumour interactions. Finally, we will provide a critical appraisal of how these new insights may be exploited for the optimisation of cancer treatments.
Platelet-tumour crosstalk
There has been a shift in the understanding of cancerous tumours in recent years, where tumours are no longer seen as mere insular masses of proliferating cancer cells. Indeed, there is now a widely accepted appreciation that recruited immune cells in the surrounding tumour environment play fundamental roles in mediating tumour growth and behaviour (18, 19). This dynamic crosstalk between cancer cells and non-malignant cells creates the tumour microenvironment (TME), and our growing awareness of the importance of the TME in all steps of tumorigenesis has led to an increased emphasis on therapies that may target elements of the TME (19, 20).
Beyond their canonical role in haemostasis and thrombosis, platelets are also critical immune modulators of the innate and adaptive immune responses, by way of their surface expression of adhesion receptors and capacity to release an array of cytokines and growth factors from intracellular granules (21–23). Several platelet adhesion receptors relevant to haemostasis have been directly implicated in malignancy, in this regard, platelets and tumour cells maintain a complex, bidirectional interaction directly linked to the survival of tumour cells (Figure 1) (24, 25).
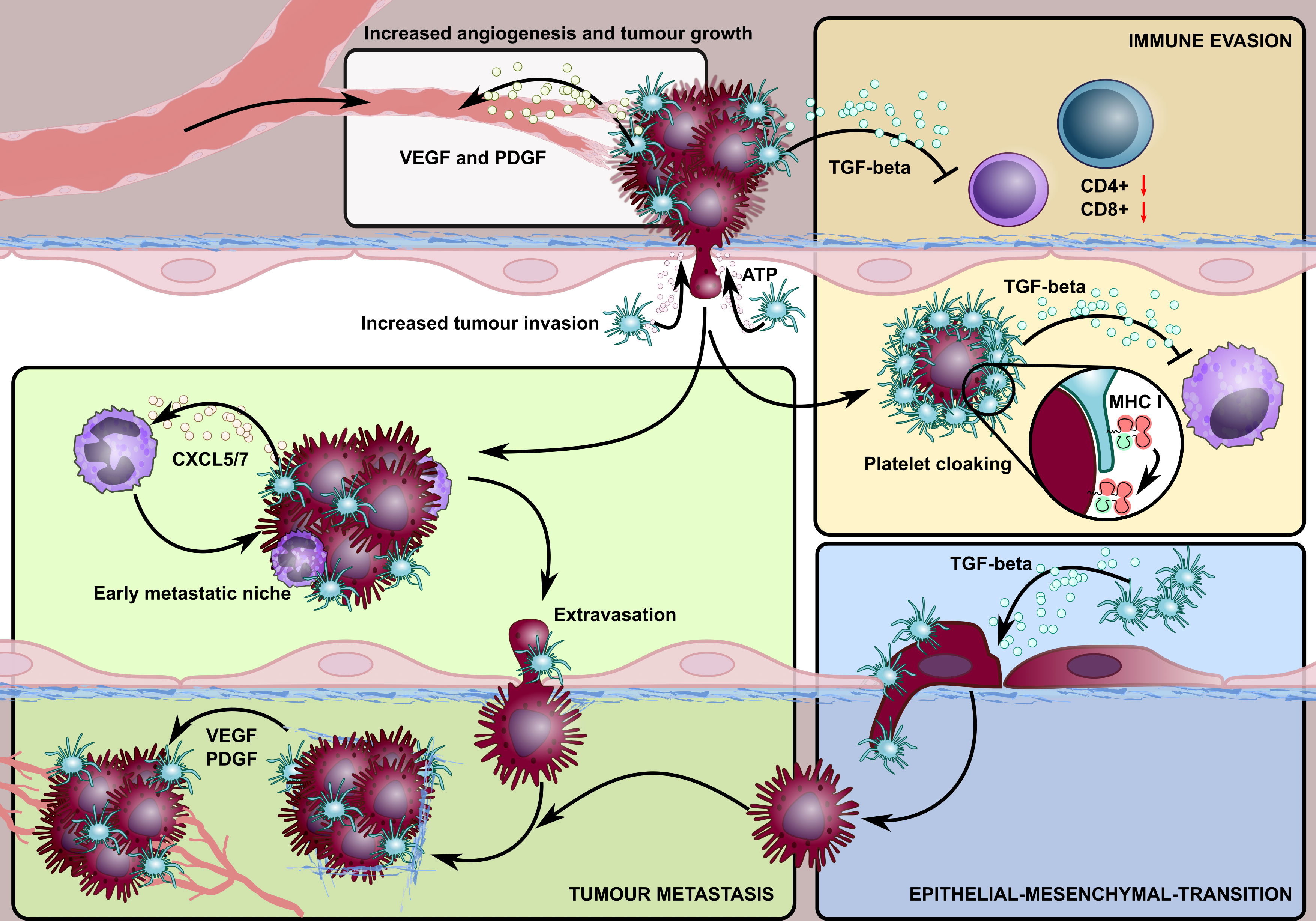
Figure 1 A schematic overview highlighting the involvement of platelets in different stages of the cancer lifecycle. Platelet granules contain growth factors, such as VEGF and PDGF that promote tumour angiogenesis. Platelets also stabilise neovessels and maintain tumour vascular integrity, in a process mediated by platelet focal adhesion kinase, where platelets within intra-tumoural blood vessels extravasate to the tumour microenvironment and enhance the survival rate of detached cancer cells. Activated platelets enable tumour cells to escape detection by the immune system (immune evasion) by 1) adhering to and ‘cloaking’ disseminating tumour cells, offering protection against NK cell detection by transferring their MHC class I molecules to tumour cells, and 2) releasing TGF-β which suppresses T cell immune responses in the tumour microenvironment. The release of platelet-derived TGF-β is also critical in promoting tumour epithelial-mesenchymal transformation which enhances their metastatic potential. Platelet-derived ATP activates endothelial cells, triggering an opening that allows for the endothelial transmigration of tumour cells. Lastly, the release of platelet-derived chemokines, CXCR5/7, recruits granulocytes to form platelet-tumour aggregates, allowing the formation of a specialised microenvironment, the so-called early metastatic niche, which promotes efficient metastatic seeding.
Various studies have now demonstrated that tumour cells induce platelet activation in a process known as tumour cell-induced platelet aggregation (TCIPA). Interestingly, this facultative ability of tumour cells also correlates with their metastatic potential (26, 27). TCIPA confers several survival advantages to the platelet-coated tumour cells – namely, the evasion of immune detection, platelet release of growth factors supporting tumour growth and protection against the potentially detrimental effects of high shear forces of the flowing blood (26, 28–30). Moreover, TCIPA facilitates the embolization of large tumour-platelet clusters within the microvasculature at the new extravasation site (26, 28). Although detailed mechanisms of these interactions remain to be elucidated, tumour cells have demonstrated the ability to activate and aggregate platelets through thrombin generation, the release of ADP, thromboxane A2 (TXA2) and matrix metalloproteinase 2 (MM2) (31–33).
Major platelet receptors have been described to mediate platelet-tumour cell interactions, including P-selectin, glycoproteins (GP) IIb/IIIa, GPIb, GPVI, and C-type lectin-like receptor 2 (CLEC-2) (25, 34–37). As will be discussed below, the role of these platelet receptors in governing platelet-tumour interactions has led to significant interest in the therapeutic targeting of platelets for the treatment of cancer (Figure 2).
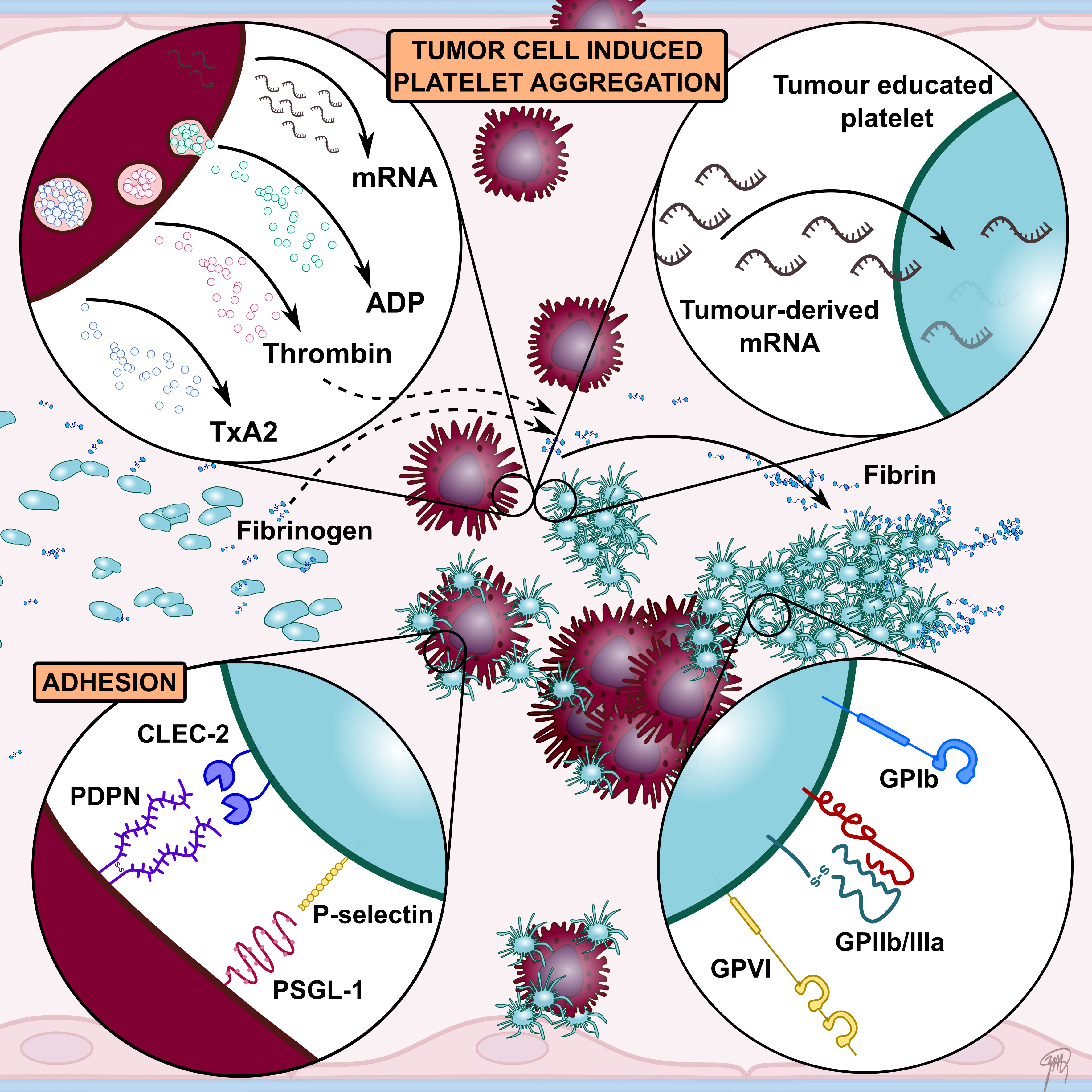
Figure 2 A schematic overview highlighting key interactions between platelets and tumour cells facilitating tumour cell-induced platelet aggregation (TCIPA). Tumour cells can activate and aggregate platelets directly through the release of platelet agonists such as ADP and thrombin. A number of platelet adhesion receptors have been implicated to contribute to TCIPA, including GPIIb/IIIa, GPIb, GPVI, P-selectin and CLEC-2. Their involvement in promoting tumour growth and metastasis has led to numerous preclinical studies investigating platelet receptors as therapeutic targets to enhance cancer therapy. Tumour cells have also been shown to alter platelet mRNA content. These so called tumour-educated platelets (TEPs) have been investigated as novel liquid biopsy tool for cancer diagnosis.
Platelets support tumour growth
Angiogenesis, – the formation of new blood vessels – is fundamental for tumour growth and spread (38). Platelet α-granules are a major reservoir for pro-angiogenic factors including vascular endothelial growth factor (VEGF), platelet-derived growth factor (PDGF) and basic fibroblast growth factor (bFGF) (39). Therefore, as a consequence of the ability of tumour cells to induce platelet activation, platelets serve as transporters that release angiogenic regulators, thus enhancing tumour angiogenesis (40). In murine models, platelet depletion reduced the formation of bFGF-induced neoangiogenesis in the cornea (41). Moreover, in thrombocytopenic mice bearing the Lewis lung carcinoma and B16F10 melanoma, the occurrence of haemorrhage was markedly increased, implicating that platelets serve to stabilize newly formed vessels and maintain tumour vascular integrity (13, 41). The latter study also found that tumoral bleeding occurred immediately following antibody-induced platelet depletion, resulting in intra-tumour haemorrhage, reduced tumour cell proliferation and increased tumour necrosis (13). Although the transfusion of platelets was able to arrest such bleeding, the transfusion of thrombin-activated degranulated platelets could not, highlighting the notion that granular contents in platelets are crucial for the regulation and formation of tumour vasculature (13). Moreover, preclinical studies have demonstrated that platelet inhibition with aspirin is associated with a reduction of platelet-mediated cancer cell proliferation and metastasis (42). These effects were due to the inhibition of platelet COX-1 and the subsequent suppression of thromboxane A2 and prostaglandin E2 generation, thereby limiting metastasis in a colon cancer model (42). Additionally, platelet inhibition with aspirin diminishes platelet VEGF release, which leads to a suppression of angiogenesis in breast cancer cell lines (43).
Importantly, platelets in cancer patients are functionally altered. Compared to healthy controls, platelets from early breast cancer patients were found to release an increased amount of VEGF upon activation with thrombin and tissue factor (44). Similar clinical observations were also made where platelet lysates from breast cancer patients and tumour aspirates from sarcoma patients contained higher concentrations of VEGF compared to healthy controls (45, 46).
More recently, it has emerged that platelets within intra-tumour blood vessels can extravasate into the extracellular matrix of the TME in a process mediated by platelet focal adhesion kinase (FAK) (47). In a mouse model of platelet-specific FAK deficiency, platelet infiltration into the TME was reduced (47). Additionally, the co-incubation of platelets with human ovarian cancer cells reduced the rate of anoikis (detachment-induced apoptosis), leading to the increased survival of detached cancer cells and enhanced metastatic potential (48). Following platelet co-incubation, signalling pathway analysis revealed that yes-associated protein 1 (YAP1), a transcription co-regulator, was differentially regulated (48). The phosphorylation of YAP1 was observed to be associated with platelet-mediated protection from anoikis, implicating that the activation of YAP1 in the signalling pathway results in the expression of a pro-survival gene signature, promoting tumour survival and metastasis (48).
Platelets support tumour invasion and metastasis
Cancer metastasis is a cascade of events involving the detachment of metastatic cells from the primary tumour, extravasation into the bloodstream, evasion of immune detection, and finally the settlement and growth at distant organs (49, 50). Sequalae related to metastasis remains the leading cause of cancer mortality, accounting for 90% of all cancer-related deaths (49). Recent data have highlighted several critical roles platelets play in regulating these key steps required for tumour metastasis, which is underscored by the observation that platelet inhibition or depletion affords protection from metastasis in a range of experimental tumour models (12, 51, 52).
During the haematogenous phase of metastasis, tumour cells are in a hostile environment interacting directly with effectors of the host immune system, a stage where the majority of circulating tumour cells do not survive (53). Natural killer (NK) cells play an important role in the immunosurveillance of tumours by eliminating malignant cells through direct cytolysis or interferon (IFN)-γ secretion to induce an efficient T-cell-mediated response, preventing both local tumour progression and metastasis (54). However, platelets activated by metastatic tumours in the bloodstream assist with tumour dissemination by adhering to and ‘cloaking’ circulating tumour cells, masking them from NK cell recognition and subsequent destruction (14). Here, platelets transfer their major histocompatibility complex (MHC) class I molecules to metastasising tumour cells, imparting a platelet-derived “pseudo-self” phenotype to tumour cells thereby hiding malignant cells from NK cell recognition (55). Additionally, activated platelets can inhibit NK cell effector functions through the release of humoral factors such as TGF-β, which downregulates NKG2D – an activation receptor of NK cells to sense stress-associated molecules, in which its depletion results in the inhibition of NK cytotoxicity and IFN-γ transcription (56, 57). In doing so, platelets undermine the second mainstay of NK cells to recognise stress-induced ligands, further impairing NK cell immunosurveillance (57).
Platelet adhesion receptors mediating tumour growth and metastasis
Glycoprotein IIb/IIIa
GPIIb/IIIa is the most abundant platelet surface receptor and has been demonstrated to play an important role in cancer growth and metastasis. Indeed, pharmacological inhibition of GPIIb/IIIa with eptifibatide reduced cell migration and invasion of breast cancer carcinoma cells in vitro (34). Furthermore, the targeted deletion of β3 integrin has been demonstrated to afford protection against osteolytic bone metastasis in a mouse model of melanoma (52). Accordingly, conformation-specific antibodies, using a single-chain variable fragment (scFv) antibody platform, targeting the activated conformation of GPIIb/IIIa on platelets has been used as a molecular targeting epitope for imaging tumours in several types of cancers in mice (58). Subsequent studies from the same group also explored the potential of an scFv antibody-drug conjugate that targeted the activated GPIIb/IIIa receptor whilst carrying a potent chemotherapeutic microtubule inhibitor (59). This antibody-drug conjugate demonstrated efficacy in a murine xenograft model of triple-negative breast cancer, highlighting its application to target platelets in the setting where tumour cell-specific epitopes are absent (59).
Glycoprotein Ibα
Platelet GPIbα is a subunit of the GPIb-IX-V receptor complex constitutively and exclusively expressed on the plasma membrane of circulating platelets and megakaryocytes (60). However, the precise role of GPIbα in mediating tumour metastasis remains controversial. Indeed, previous studies have been conflicting with pulmonary metastasis being reduced in GPIbα-deficient mice yet antibody-mediated functional inhibition of GPIbα led to a significant increase in pulmonary metastasis (35, 61). Similarly, the deletion of von Willebrand factor (vWF), the major ligand of GPIbα, markedly increased pulmonary metastasis following the injection of tumour cells (62). It is hypothesized that the adverse metastatic effects observed with GPIbα blockage are a result of abolishing interactions between GPIbα and vWF, which occurs physiologically in high shear stress conditions (35). Instead, tumour cells are arrested in mechanically less stressful environments, favouring their survival and growth (35, 60). Furthermore, some anti-GPIbα monoclonal antibodies have been shown to induce platelet activation, suggesting the possibility of enhancing TCIPA through platelet aggregation, resulting in the observed increase in metastasis in experimental models (21, 63).
Glycoprotein VI
GPVI is a platelet-specific receptor with a critical role in mediating collagen-induced platelet activation. GPVI deficiency led to a 50% decrease in metastasis in B16F10.1 melanoma and Lewis lung carcinoma cells (36). Moreover, GPVI has been shown to support platelet adhesion to breast and colon cancer cells through binding to galectin-3, which resulted in the promotion of tumour extravasation (64). Recently, the inhibition of GPVI was shown to increase intra-tumour haemorrhage, which was associated with a decrease in tumour growth and enhanced efficacy of chemotherapy (65). Further supporting this finding, the repurposing of revacept, a soluble dimeric GPVI-Fc fusion protein developed for the treatment of atherothrombotic diseases, has been demonstrated in a model of colon carcinoma to inhibit aberrant COX-2 expression and tumour EMT (66). Whilst these data support the important role of GPVI in cancer growth and metastasis, further investigation of GPVI inhibition is required. This is particularly relevant since the reported intra-tumoral haemorrhage associated with GPVI inhibition may in turn limit the feasibility of clinical translation given the potential to develop deleterious sequelae of bleeding in metastatic lesions, particularly intracerebral lesions.
P-selectin
P-selectin belongs to the selectin family of cell adhesion molecules. In platelets, P-selectin is stored in the α-granules and is rapidly translocated to the platelet surface via exocytosis upon cell activation (67). P-selectin has been demonstrated to bind to a variety of human cancers and cancer-derived cell lines in vitro, including colon cancer, small cell lung cancer, breast cancer, melanoma, neuroblastoma and adenoid cancer, where P-selectin has been shown to contribute to tumour cell extravasation (68–73). Accordingly, several studies have shown that P-selectin-deficient mice demonstrate attenuation of metastasis (50, 74, 75). Furthermore, the use of the anticoagulant heparin – a highly sulphated glycosaminoglycan that binds and inhibits P-selectin – was able to attenuate metastasis in mouse models (50, 75, 76). However, the benefit of low molecular weight heparin on the survival of cancer patients in large, randomised clinical trials has not been replicated (77, 78).
CLEC-2
CLEC-2 is a platelet activation receptor expressed almost exclusively in platelets and megakaryocytes and at very low levels in liver Kupffer and sinusoidal cells (79). Originally identified as a platelet-activating receptor to snake venom rhodocytin, CLEC-2 induces platelet activation via similar signalling pathways to that of GPVI, resulting in platelet aggregation (80). The only known endogenous ligand of CLEC-2, podoplanin, has been identified on the surface cells from osteosarcoma, squamous cell carcinoma and glioblastoma tumours (81–84). The surface expression of podoplanin on tumour cells induces platelet binding, and a study examining 26 cell lines of the mouse colon adenocarcinoma observed that metastatic clones were associated with higher podoplanin expression and a greater platelet aggregation capacity (85). Additionally, in experimental models of lung metastasis, the use of an anti-podoplanin antibody was able to inhibit platelet aggregation and markedly reduced the number of sites of metastatic foci (37).
Platelets promote tumour cell epithelial-mesenchymal transition
Recent data have highlighted a key step governing the metastatic potential of tumour cells is the ability of primary tumour cells to undergo epithelial-mesenchymal transition (EMT)-like transformation, which promotes tumour cell motility, invasion, and dissemination (86). This process is enhanced by TGF-β, as evidenced in metastatic breast cancer models, where an induced increase of TGF-β levels is associated with increased circulating tumour cells and metastasis in the lung (87). Such findings were also reflected in the clinical setting where increased plasma levels of TGF-β correlated with colorectal cancer progression, and an increase in immunostaining of TGF-β has been implicated with metastasis in prostate, breast and colon cancer patients (88–91). Importantly, platelets are a major source of bioavailable TGF-β, and seminal work from the Hynes group demonstrated that the metastatic capacity of tumour cells can be further increased by active signals from platelets in which they come into transient contact during their transit in the blood (86, 92). This notion is supported by the finding that pre-treating tumour cells with platelets lacking TGF-β were not able to enhance metastasis formation (86). Further investigations revealed that platelet-derived TGF-β appears to be critical in inducing a pro-metastatic gene profile in tumour cells, strongly upregulating EMT-related genes through activation of the Smad signalling pathway (86). Interestingly, platelet-derived TGF-β alone is insufficient to support metastasis in vivo, as tumours treated with platelets but not releasates from activated platelets were able to undergo EMT (86). Rather, tumour cell NF-κB signalling through direct platelet-tumour cell contact is required in synergy with platelet-derived TGF-β-Smad activation to prime tumour cells for metastasis (86).
Critically, platelets facilitate the early phases of metastatic seeding. Activated platelets release ATP, activating endothelial cells via the P2Y purinoreceptor 2 (P2Y2) receptor, which triggers an opening in the endothelial barrier for endothelial transmigration of tumour cells to occur (93). Importantly, mice deficient in P2Y2 or lacking platelet-derived ATP demonstrated a marked reduction in metastasis (93). Moreover, platelets interact with tumour cells to help guide the formation of the early metastatic niche, a specialised microenvironment to promote metastatic progression (51). Upon entry into the circulation, tumour cells will quickly encounter platelets and granulocytes, and the release of platelet-derived chemokines CXCR5/7 from tumour-bound platelets actively recruits granulocytes to platelet-tumour aggregates (51). This process appears essential for efficient metastatic seeding since platelet depletion or the inhibition of platelet chemokine signalling affords protection from metastasis (51).
These findings have been emphasised in ovarian cancer models, where the P2Y12 inhibitor, ticagrelor, as well as P2Y12 deficiency, were both associated with a reduction in tumour growth (94). Furthermore, ticlopidine, an irreversible P2Y12 receptor inhibitor, demonstrated inhibitory effects in the metastasis of rodent melanoma, Lewis lung carcinoma and hepatoma (95). Likewise, pulmonary metastasis was also inhibited through P2Y12 deficiency, decreasing the secretion of TGF-β from activated platelets, and diminished EMT in a Lewis lung carcinoma model (96). Moreover, the use of P2Y12 inhibitors as adjunct therapies has been explored with data demonstrating that ticagrelor enhances the chemotherapeutic efficiency in pancreatic ductal adenocarcinoma, displaying synergism when combined with gemcitabine in vitro (97). The synergistic effect of P2Y12 receptor inhibitors has also been shown with cisplatin in 4T1 breast cancer mouse models (98).
Platelets as modulators of the immune response in the TME
Recent clinical advancement in cancer therapy has been the adoption of immunotherapy as a means to harness the immune system for cancer treatment. However, not all patients respond to immunotherapy, which at least in part, likely reflects the differences in the immune constitution of the TME between individuals (99, 100). Recent findings have demonstrated that platelets play a key role in regulating T cell responses within the TME, potentially limiting the efficacy of immunotherapy (101). Platelets within the TME are capable of suppressing functional responses of CD4+ and CD8+ T cells through the release of TGF-β (101). Whilst platelets contribute significantly to the circulating pool of TGF-β, they also constitutively express the glycoprotein-A repetitions predominant (GARP) receptor – a cell surface docking receptor crucial for regulating the activation of latent TGF-β (102). It appears in this context, the ability of platelet GARP to capture and activate latent TGF-β systemically is the major contributor to the platelet immunosuppressive effects within the TME (101). More recent studies have demonstrated that thrombin is responsible for the cleavage of GARP on the surface of platelets to liberate biologically active TGF-β, presenting as a potential mechanism of cancer immune evasion (103). In support of this hypothesis, platelet depletion, anti-platelet therapy and direct thrombin inhibitors demonstrate efficacy in enhancing the anti-tumour effects of adoptive T-cell transfer therapy (ACT) and immunotherapy in preclinical cancer models (103).
Exploiting the platelet cancer interplay for therapeutic benefit
Tumour-educated platelets for cancer diagnosis
Platelets can in turn be altered or ‘educated’ by interactions with tumour cells, leading to platelets with a modified protein and RNA profile or a “tumour-educated platelet” (TEP) (104, 105). Tumour-mediated platelet education, either via direct or indirect mechanisms, has the potential to be exploited as a biomarker for liquid biopsy diagnostics.
Despite being anucleate cells, protein synthesis by platelets was described over half a century ago (106, 107). Since then, studies have revealed that platelets contain different types of RNA including protein-coding messenger RNAs (mRNAs) as well as a functional megakaryocyte-derived spliceosome for the processing of pre-mRNAs (108, 109). Activated platelets synthesise various functional proteins under signal-dependent controls, including the oncogene B-cell lymphoma-3, interleukin-1β (IL-1β), and tissue factor (110–112). The platelet transcriptome can be altered in response to external stimuli, including tumour cells via direct or indirect stimulation (105).
Platelets can be educated directly through the sequestration of tumour-derived microvesicles. Nilsson et al. established that cancer cells transfer mutant RNA into platelets in both in vitro and in vivo studies and showed that platelets from glioma and prostate cancer patients contained tumour-derived mRNA biomarkers EGFRvIII and PCA3, respectively (113). Furthermore, the fusion oncogene EML4-ALK – an essential growth driver in human cancer – was detected in TEPs from patients with non-small-cell lung cancer (NSCLC) and was proven relevant for prognosis and monitoring of therapy, whilst KLK3, FOLH1, and NPY genes were sequestered in platelets from patients with prostate cancer and enabled prediction of treatment outcomes (114, 115).
Platelets can also be indirectly altered through alternative RNA splicing, with various studies revealing that cancer patients display distinct platelet mRNA profiles. Calverley et al. reported that mRNA platelet profiles of metastatic NSCLC patients differ from control, where 197 out of the 200 platelet genes with the most altered expression were downregulated (116). Likewise, Nilsson et al. demonstrated a distinct RNA signature in TEPs from glioma patients, and Best et al. further characterised TEP RNA profiles and explored their diagnostic potential (104, 113). RNA sequencing combined with self-learning algorithms distinguished patients with localised and metastatic cancer from healthy controls with 96% accuracy, located the primary tumour for six different tumour types (NSCLC, colorectal cancer, glioblastoma, pancreatic cancer, hepatobiliary cancer, and breast cancer) with 71% accuracy, and allowed discrimination of tumour molecular subtypes (104). Later, Best et al. accurately used TEP RNA profiles in the detection of early- and late-stage NSCLC, further demonstrating that differential splicing was independent of age and various inflammatory conditions (117). The role of TEPs in haematological malignancies is less well defined but has been supported by a report of upregulated IL-1β detected by RNA sequencing of platelets in smouldering and plasma cell myeloma (118).
However, the exact mechanism of tumour cell-induced alternative RNA splicing in TEPs remains elusive. It is hypothesized that signal-dependent splicing of platelet RNA, differential binding of RNA-binding protein to the untranslated region of platelet RNA, differential transfer of RNA molecules into platelets by tumour-educated megakaryocytes, and a shift in the evolution of different platelet subpopulations towards RNA-rich reticulated platelets contribute to this process (109, 112, 117, 119–121). Whether the tumour-derived RNA or spliced RNA in TEPs is subsequently translated into functional proteins remains to be determined.
Liquid biopsies offer a simple, non-invasive, and repeatable alternative to tissue biopsies, allowing diagnosis, prognostication, individualised treatment, and treatment monitoring of cancer. Circulating tumour DNA or RNA and circulating tumour cells have been well studied, and given their abundance in peripheral blood, TEP-derived RNA and proteins have been proposed as novel cancer biomarkers in several cancers such as glioblastoma and lung, breast, colorectal, prostate, and ovarian cancers (104, 114, 115, 117, 122, 123). Several analysis methods have been reported, including next-generation sequencing with machine learning algorithms, quantitative reverse-transcriptase polymerase chain reaction, and proteomic analysis (104, 113, 117, 122, 124). However, technical standardisation and clinical validation are required before TEPs can be deployed in clinical practice as novel liquid biopsy biomarkers in cancer.
Targeting platelets for cancer therapy
With an expanding body of evidence demonstrating the fundamental importance of platelets for tumour growth and survival, the prospect of targeting platelets for the treatment of cancer has gained significant interest. To date, as discussed above, a number of preclinical studies have focused on established anti-platelet drugs and antagonists that target platelet receptors and signalling pathways important for platelet-tumour interactions. However, data from clinical studies, regarding the effects on cancer remains more limited.
Aspirin
Several clinical studies have investigated the use of aspirin as a chemopreventive agent. An early case-control study published in 1988 found aspirin use to be associated with a lower incidence of colorectal cancer (125). Various case-control and cohort studies followed, including a prospective mortality study of 662,424 patients that reported reduced death rates from colon cancer with regular aspirin use (126). Furthermore, the first two published randomised controlled trials in patients with previous colorectal cancer found a decreased risk of recurrence at one year with aspirin use (127, 128). The randomised controlled APACC trial similarly found daily aspirin decreased adenoma recurrence at one year, but this chemopreventive effect was not maintained at the final four-year colonoscopy although issues with methodology may have influenced the latter result (129, 130).
However, primary prevention trials have provided conflicting results. Trials initially designed to study cardiovascular disease prevention found reductions in colorectal cancer incidence with various doses of aspirin, although benefits were only observed after ten years (131, 132). Meta-analyses have demonstrated that aspirin modestly decreased the twenty-year risk of colon cancer incidence and mortality (133, 134). Despite these findings, the large randomised controlled ASPREE trial of healthy older adults found an increase in the secondary endpoint of overall mortality in patients randomised to low-dose aspirin, attributed predominantly to cancer-related deaths including from colorectal cancer (135). In a further analysis, aspirin use was associated with an increased risk of incident cancers that had metastasised or stage 4 cancers at diagnosis (136). These unanticipated findings may be due to the contrasting biological effects of aspirin in older adults and the lack of sufficient follow-up, findings from the observational trial ASPREE-XT evaluating the long-lasting effects of aspirin treatment in such demographics are awaited.
P2Y12 inhibitors
Whilst preclinical studies have been encouraging, results from clinical studies for the use of P2Y12 inhibitors are mixed. Data from large randomised controlled trials in patients with cardiovascular disease have previously shown potential associations between P2Y12 receptor inhibitors and cancer. The TRITON-TIMI 38 trial found a higher incidence of cancer and cancer-related mortality in patients treated with prasugrel compared to clopidogrel as part of dual antiplatelet therapy, whilst the DAPT study and PEGASUS-TIMI trial reported higher incidences of cancer-related mortality with dual antiplatelet therapy using clopidogrel and ticagrelor, respectively (137–139). In contrast, a meta-analysis including 282,084 patients from six randomised controlled trials and three retrospective cohort studies found no increased risk of cancer incidence or mortality with P2Y12 receptor inhibitors (140).
Perspectives and future directions
Our deepening understanding of platelets as important mediators of immunity and inflammation has afforded significant insights into their role in tumour growth and metastasis. However, a critical outstanding issue is how these findings can be translated to ultimately improve the therapeutic landscape and prognosis of patients with cancer. In this regard, the ongoing quest to develop novel anti-platelet approaches that do not cause bleeding may provide the ultimate hope to uncouple the potential benefit of antiplatelet therapy in cancer patients without the potentially significant bleeding risk. Whether specific aspects of platelet function that have been demonstrated to be important in cancer biology, such as platelet-derived ATP or TGF-β, can be inhibited without unwanted off-target effects remains to be tested. In the interim, further investigations regarding the potential of liquid biopsies for providing prognostic information and the role of anti-platelet therapies in modulating the tumour immune response are needed given the growing importance of immunotherapy in cancer therapy.
Author contributions
JZh: Writing – original draft, Writing – review & editing. AH: Writing – original draft, Writing – review & editing. JZe: Writing – original draft, Writing – review & editing. KP: Writing – original draft, Writing – review & editing, Conceptualization. JM: Conceptualization, Writing – original draft, Writing – review & editing.
Funding
JZ is supported by a RTP PhD Scholarship, AH is supported by a Wheaton Fellowship, Monash University, Gordan Wheaton Fellowship and the RTP PhD Scholarship. KP is supported by a NHMRC Investigator Grant. JM is supported by a Heart Foundation Future Leader Fellowship.
Conflict of interest
The authors declare that the research was conducted in the absence of any commercial or financial relationships that could be construed as a potential conflict of interest.
Publisher’s note
All claims expressed in this article are solely those of the authors and do not necessarily represent those of their affiliated organizations, or those of the publisher, the editors and the reviewers. Any product that may be evaluated in this article, or claim that may be made by its manufacturer, is not guaranteed or endorsed by the publisher.
Glossary
References
1. Metharom P, Falasca M, Berndt MC. The history of armand trousseau and cancer-associated thrombosis. Cancers (2019) 11(2):158. doi: 10.3390/cancers11020158
2. Riess L. Zur pathologischen anatomie des blutes. Arch Anat Physiol Wissensch Med (1872) 39:237–40.
3. Pedersen LM, Milman N. Prognostic significance of thrombocytosis in patients with primary lung cancer. Eur Respir J (1996) 9(9):1826–30. doi: 10.1183/09031936.96.09091826
4. Aoe K, Hiraki A, Ueoka H, Kiura K, Tabata M, Tanaka M, et al. Thrombocytosis as a useful prognostic indicator in patients with lung cancer. Respiration (2004) 71(2):170–3. doi: 10.1159/000076679
5. Taucher S, Salat A, Gnant M, Kwasny W, Mlineritsch B, Menzel R-C, et al. Impact of pretreatment thrombocytosis on survival in primary breast cancer. Thromb Haemost. (2003) 89(6):1098–106. doi: 10.1055/s-0037-1613413
6. Sasaki K, Kawai K, Tsuno NH, Sunami E, Kitayama J. Impact of preoperative thrombocytosis on the survival of patients with primary colorectal cancer. World J Surg (2012) 36(1):192–200. doi: 10.1007/s00268-011-1329-7
7. Ikeda M, Furukawa H, Imamura H, Shimizu J, Ishida H, Masutani S, et al. Poor prognosis associated with thrombocytosis in patients with gastric cancer. Ann Surg Oncol (2002) 9(3):287–91. doi: 10.1007/BF02573067
8. Bensalah K, Leray E, Fergelot P, Rioux-Leclercq N, Tostain J, Guillé F, et al. Prognostic value of thrombocytosis in renal cell carcinoma. J Urol (2006) 175(3 Pt 1):859–63. doi: 10.1016/S0022-5347(05)00526-4
9. Allensworth SK, Langstraat CL, Martin JR, Lemens MA, McGree ME, Weaver AL, et al. Evaluating the prognostic significance of preoperative thrombocytosis in epithelial ovarian cancer. Gynecol Oncol (2013) 130(3):499–504. doi: 10.1016/j.ygyno.2013.05.038
10. Stone RL, Nick AM, McNeish IA, Balkwill F, Han HD, Bottsford-Miller J, et al. Paraneoplastic thrombocytosis in ovarian cancer. N Engl J Med (2012) 366(7):610–8. doi: 10.1056/NEJMoa1110352
11. Sierko E, Wojtukiewicz MZ. Platelets and angiogenesis in Malignancy. Seminars in thrombosis and hemostasis. 333 Seventh Avenue, New: Thieme Medical Publishers, Inc. (2004) p. 95–108.
12. Gasic GJ, Gasic TB, Stewart CC. Antimetastatic effects associated with platelet reduction. Proc Natl Acad Sci U S A. (1968) 61(1):46–52. doi: 10.1073/pnas.61.1.46
13. Ho-Tin-Noé B, Goerge T, Cifuni SM, Duerschmied D, Wagner DD. Platelet granule secretion continuously prevents intratumor hemorrhage. Cancer Res (2008) 68(16):6851–8. doi: 10.1158/0008-5472.CAN-08-0718
14. Amo L, Tamayo-Orbegozo E, Maruri N, Eguizabal C, Zenarruzabeitia O, Riñón M, et al. Involvement of platelet-tumor cell interaction in immune evasion. Potential role of podocalyxin-like protein 1. Front Oncol (2014) 4:245–5. doi: 10.3389/fonc.2014.00245
15. Blom JW, Vanderschoot JPM, OostindiËR MJ, Osanto S, van der Meer FJM, Rosendaal FR. Incidence of venous thrombosis in a large cohort of 66°329 cancer patients: results of a record linkage study. J Thromb Haemostasis. (2006) 4(3):529–35. doi: 10.1111/j.1538-7836.2006.01804.x
16. Bottsford-Miller J, Choi H-J, Dalton HJ, Stone RL, Cho MS, Haemmerle M, et al. Differential platelet levels affect response to taxane-based therapy in ovarian cancer. Clin Cancer Res (2015) 21(3):602–10. doi: 10.1158/1078-0432.CCR-14-0870
17. Radziwon-Balicka A, Medina C, O’Driscoll L, Treumann A, Bazou D, Inkielewicz-Stepniak I, et al. Platelets increase survival of adenocarcinoma cells challenged with anticancer drugs: mechanisms and implications for chemoresistance. Br J Pharmacol (2012) 167(4):787–804. doi: 10.1111/j.1476-5381.2012.01991.x
18. Hanahan D, Weinberg Robert A. Hallmarks of cancer: the next generation. Cell (2011) 144(5):646–74. doi: 10.1016/j.cell.2011.02.013
19. Scott DW, Gascoyne RD. The tumour microenvironment in B cell lymphomas. Nat Rev Cancer. (2014) 14(8):517–34. doi: 10.1038/nrc3774
20. Balkwill FR, Capasso M, Hagemann T. The tumor microenvironment at a glance. J Cell Science. (2012) 125(23):5591–6. doi: 10.1242/jcs.116392
21. Xu XR, Yousef GM, Ni H. Cancer and platelet crosstalk: opportunities and challenges for aspirin and other antiplatelet agents. Blood (2018) 131(16):1777–89. doi: 10.1182/blood-2017-05-743187
22. Li C, Li J, Li Y, Lang S, Yougbare I, Zhu G, et al. Crosstalk between platelets and the immune system: old systems with new discoveries. Adv Hematol (2012) 2012:384685. doi: 10.1155/2012/384685
23. Semple JW, Italiano JE, Freedman J. Platelets and the immune continuum. Nat Rev Immunol (2011) 11(4):264–74. doi: 10.1038/nri2956
24. Bambace N, Holmes C. The platelet contribution to cancer progression. J Thromb haemostasis. (2011) 9(2):237–49. doi: 10.1111/j.1538-7836.2010.04131.x
25. Chen M, Geng J-G. P-selectin mediates adhesion of leukocytes, platelets, and cancer cells in inflammation, thrombosis, and cancer growth and metastasis. Archivum Immunologiae Therapiae Experimentalis. (2006) 54(2):75–84. doi: 10.1007/s00005-006-0010-6
26. Jurasz P, Alonso-Escolano D, Radomski MW. Platelet–cancer interactions: mechanisms and pharmacology of tumour cell-induced platelet aggregation. Br J Pharmacol (2004) 143(7):819–26. doi: 10.1038/sj.bjp.0706013
27. Pearlstein E, Salk PL, Yogeeswaran G, Karpatkin S. Correlation between spontaneous metastatic potential, platelet-aggregating activity of cell surface extracts, and cell surface sialylation in 10 metastatic-variant derivatives of a rat renal sarcoma cell line. Proc Natl Acad Sci (1980) 77(7):4336. doi: 10.1073/pnas.77.7.4336
28. Malik AB. Pulmonary microembolism. Physiol Rev (1983) 63(3):1114–207. doi: 10.1152/physrev.1983.63.3.1114
29. Philippe C, Philippe B, Fouqueray B, Perez J, Lebret M, Baud L. Protection from tumor necrosis factor-mediated cytolysis by platelets. Am J Pathol (1993) 143(6):1713–23.
30. Honn KV, Tang D. Hemostasis and Malignancy: an overview. Cancer Metastasis Rev (1992) 11(3):223–6. doi: 10.1007/BF01307178
31. Nierodzik ML, Klepfish A, Karpatkin S. Role of platelets, thrombin, integrin llb-llla, fibronectin and von willebrand factor on tumor adhesion in vitro and metastasis in vivo. Thromb haemostasis. (1995) 74(07):282–90. doi: 10.1055/s-0038-1642691
32. Ugen KK, Mahalingam M, Klein PA, Kao K-J. Inhibition of tumor cell-induced platelet aggregation and experimental tumor metastasis by the synthetic Gly-Arg-Gly-Asp-Ser peptide2. JNCI: J Natl Cancer Institute. (1988) 80(18):1461–6. doi: 10.1093/jnci/80.18.1461
33. Jurasz P, Sawicki G, Duszyk M, Sawicka J, Miranda C, Mayers I, et al. Matrix metalloproteinase 2 in tumor cell-induced platelet aggregation: regulation by nitric oxide. Cancer Res (2001) 61(1):376.
34. Zhao F, Li L, Guan L, Yang H, Wu C, Liu Y. Roles for GP IIb/IIIa and αvβ3 integrins in MDA-MB-231 cell invasion and shear flow-induced cancer cell mechanotransduction. Cancer Lett (2014) 344(1):62–73. doi: 10.1016/j.canlet.2013.10.019
35. Erpenbeck L, Nieswandt B, Schön M, Pozgajova M, Schön MP. Inhibition of platelet GPIbα and promotion of melanoma metastasis. J Invest Dermatol (2010) 130(2):576–86. doi: 10.1038/jid.2009.278
36. Jain S, Russell S, Ware J. Platelet glycoprotein VI facilitates experimental lung metastasis in syngenic mouse models. J Thromb Haemost. (2009) 7(10):1713–7. doi: 10.1111/j.1538-7836.2009.03559.x
37. Kato Y, Kaneko MK, Kunita A, Ito H, Kameyama A, Ogasawara S, et al. Molecular analysis of the pathophysiological binding of the platelet aggregation-inducing factor podoplanin to the C-type lectin-like receptor CLEC-2. Cancer Science. (2008) 99(1):54–61. doi: 10.1111/j.1349-7006.2007.00634.x
38. Nishida N, Yano H, Nishida T, Kamura T, Kojiro M. Angiogenesis in cancer. Vasc Health Risk Management. (2006) 2(3):213–9. doi: 10.2147/vhrm.2006.2.3.213
39. Braun A, Anders H-J, Gudermann T, Mammadova-Bach E. Platelet-cancer interplay: molecular mechanisms and new therapeutic avenues. Front Oncol (2021) 11(2576). doi: 10.3389/fonc.2021.665534
40. Verheul HM, Hoekman K, Luykx-de Bakker S, Eekman CA, Folman CC, Broxterman HJ, et al. Platelet: transporter of vascular endothelial growth factor. Clin Cancer Res (1997) 3(12):2187–90.
41. Kisucka J, Butterfield CE, Duda DG, Eichenberger SC, Saffaripour S, Ware J, et al. Platelets and platelet adhesion support angiogenesis while preventing excessive hemorrhage. Proc Natl Acad Sci U S A. (2006) 103(4):855–60. doi: 10.1073/pnas.0510412103
42. Guillem-Llobat P, Dovizio M, Bruno A, Ricciotti E, Cufino V, Sacco A, et al. Aspirin prevents colorectal cancer metastasis in mice by splitting the crosstalk between platelets and tumor cells. Oncotarget (2016) 7(22):32462–77. doi: 10.18632/oncotarget.8655
43. Battinelli EM, Markens BA, Italiano JE Jr. Release of angiogenesis regulatory proteins from platelet alpha granules: modulation of physiologic and pathologic angiogenesis. Blood (2011) 118(5):1359–69. doi: 10.1182/blood-2011-02-334524
44. McDowell G, Temple I, Li C, Kirwan CC, Bundred NJ, McCollum CN, et al. Alteration in platelet function in patients with early breast cancer. Anticancer Res (2005) 25(6b):3963–6.
45. Caine G, Lip G, Blann A. Platelet-derived VEGF, Flt-1, Angiopoietin-1 and P-selectin in breast and prostate cancer: further evidence for a role of platelets in tumour angiogenesis. Ann Med (2004) 36(4):273–7. doi: 10.1080/07853890410026098
46. Verheul HMW, Hoekman K, Lupu F, Broxterman HJ, van der Valk P, Kakkar AK, et al. Platelet and coagulation activation with vascular endothelial growth factor generation in soft tissue sarcomas. Clin Cancer Res (2000) 6(1):166–71. doi: 10.1038/s41467-017-00411-z
47. Haemmerle M, Bottsford-Miller J, Pradeep S, Taylor ML, Choi H-J, Hansen JM, et al. FAK regulates platelet extravasation and tumor growth after antiangiogenic therapy withdrawal. J Clin Invest (2016) 126(5):1885–96. doi: 10.1172/JCI85086
48. Haemmerle M, Taylor ML, Gutschner T, Pradeep S, Cho MS, Sheng J, et al. Platelets reduce anoikis and promote metastasis by activating YAP1 signaling. Nat Commun (2017) 8(1):310. doi: 10.1038/s41467-017-00411-z
49. Guan X. Cancer metastases: challenges and opportunities. Acta Pharm Sin B (2015) 5(5):402–18. doi: 10.1016/j.apsb.2015.07.005
50. Borsig L, Wong R, Feramisco J, Nadeau DR, Varki NM, Varki A. Heparin and cancer revisited: Mechanistic connections involving platelets, P-selectin, carcinoma mucins, and tumor metastasis. Proc Natl Acad Sci (2001) 98(6):3352. doi: 10.1073/pnas.061615598
51. Labelle M, Begum S, Hynes RO. Platelets guide the formation of early metastatic niches. Proc Natl Acad Sci (2014) 111(30):E3053–61. doi: 10.1073/pnas.1411082111
52. Bakewell SJ, Nestor P, Prasad S, Tomasson MH, Dowland N, Mehrotra M, et al. Platelet and osteoclast beta3 integrins are critical for bone metastasis. Proc Natl Acad Sci U S A. (2003) 100(24):14205–10. doi: 10.1073/pnas.2234372100
53. Nieswandt B, Hafner M, Echtenacher B, Männel DN. Lysis of tumor cells by natural killer cells in mice is impeded by platelets. Cancer Res (1999) 59(6):1295–300.
54. Waldhauer I, Steinle A. NK cells and cancer immunosurveillance. Oncogene (2008) 27(45):5932–43. doi: 10.1038/onc.2008.267
55. Placke T, Örgel M, Schaller M, Jung G, Rammensee H-G, Kopp H-G, et al. Platelet-derived MHC class I confers a pseudonormal phenotype to cancer cells that subverts the antitumor reactivity of natural killer immune cells. Cancer Res (2012) 72(2):440. doi: 10.1158/0008-5472.CAN-11-1872
56. Schmied L, Höglund P, Meinke S. Platelet-mediated protection of cancer cells from immune surveillance - possible implications for cancer immunotherapy. Front Immunol (2021) 12:640578. doi: 10.3389/fimmu.2021.640578
57. Kopp H-G, Placke T, Salih HR. Platelet-derived transforming growth factor-β Down-regulates NKG2D thereby inhibiting natural killer cell antitumor reactivity. Cancer Res (2009) 69(19):7775. doi: 10.1158/0008-5472.CAN-09-2123
58. Yap ML, McFadyen JD, Wang X, Zia NA, Hohmann JD, Ziegler M, et al. Targeting activated platelets: A unique and potentially universal approach for cancer imaging. Theranostics (2017) 7(10):2565–74. doi: 10.7150/thno.19900
59. Yap ML, McFadyen JD, Wang X, Ziegler M, Chen YC, Willcox A, et al. Activated platelets in the tumor microenvironment for targeting of antibody-drug conjugates to tumors and metastases. Theranostics (2019) 9(4):1154–69. doi: 10.7150/thno.29146
60. Erpenbeck L, Schön MP. Deadly allies: the fatal interplay between platelets and metastasizing cancer cells. Blood (2010) 115(17):3427–36. doi: 10.1182/blood-2009-10-247296
61. Jain S, Zuka M, Liu J, Russell S, Dent J, Guerrero JA, et al. Platelet glycoprotein Ibα supports experimental lung metastasis. Proc Natl Acad Sci (2007) 104(21):9024. doi: 10.1073/pnas.0700625104
62. Terraube V, Marx I, Denis CV. Role of von Willebrand factor in tumor metastasis. Thromb Res (2007) 120:S64–70. doi: 10.1016/S0049-3848(07)70132-9
63. Li J, van der Wal DE, Zhu G, Xu M, Yougbare I, Ma L, et al. Desialylation is a mechanism of Fc-independent platelet clearance and a therapeutic target in immune thrombocytopenia. Nat Commun (2015) 6(1):7737. doi: 10.1038/ncomms8737
64. Mammadova-Bach E, Gil-Pulido J, Sarukhanyan E, Burkard P, Shityakov S, Schonhart C, et al. Platelet glycoprotein VI promotes metastasis through interaction with cancer cell-derived galectin-3. Blood (2020) 135(14):1146–60. doi: 10.1182/blood.2019002649
65. Volz J, Mammadova-Bach E, Gil-Pulido J, Nandigama R, Remer K, Sorokin L, et al. Inhibition of platelet GPVI induces intratumor hemorrhage and increases efficacy of chemotherapy in mice. Blood J Am Soc Hematology. (2019) 133(25):2696–706. doi: 10.1182/blood.2018877043
66. Dovizio M, Maier TJ, Alberti S, Luigia Di F, Emanuela M, Götz M, et al. Pharmacological inhibition of platelet-tumor cell cross-talk prevents platelet-induced overexpression of cyclooxygenase-2 in HT29 human colon carcinoma cells. Mol Pharmacol (2013) 84(1):25–40. doi: 10.1124/mol.113.084988
67. Mantovani A, Dejana E. Endothelium. In: Delves PJ, editor. Encyclopedia of immunology, 2nd ed. Oxford: Elsevier (1998). p. 802–6.
68. Goetz DJ, Ding H, Atkinson WJ, Vachino G, Camphausen RT, Cumming DA, et al. A human colon carcinoma cell line exhibits adhesive interactions with P-selectin under fluid flow via a PSGL-1-independent mechanism. Am J Pathol (1996) 149(5):1661–73.
69. Stone JP, Wagner DD. P-selectin mediates adhesion of platelets to neuroblastoma and small cell lung cancer. J Clin Invest (1993) 92(2):804–13. doi: 10.1172/JCI116654
70. Li L, Short HJ, Qian K-X, Elhammer ÅP, Geng J-G. Characterization of glycoprotein ligands for P-selectin on a human small cell lung cancer cell line NCI-H345. Biochem Biophys Res Commun (2001) 288(3):637–44. doi: 10.1006/bbrc.2001.5806
71. Aigner S, Ramos CL, Hafezi-Moghadam A, Lawrence MB, Friederichs J, Altevogt P, et al. CD24 mediates rolling of breast carcinoma cells on P-selectin. FASEB J (1998) 12(12):1241–51. doi: 10.1096/fasebj.12.12.1241
72. Kaytes PS, Geng J-G. P-selectin mediates adhesion of the human melanoma cell line NKI-4: identification of glycoprotein ligands. Biochemistry (1998) 37(29):10514–21. doi: 10.1021/bi9730846
73. Ma Y-Q, Geng J-G. Obligatory requirement of sulfation for P-selectin binding to human salivary gland carcinoma acc-M cells and breast carcinoma ZR-75-30 cells. J Immunol (2002) 168(4):1690. doi: 10.4049/jimmunol.168.4.1690
74. Kim YJ, Borsig L, Varki NM, Varki A. P-selectin deficiency attenuates tumor growth and metastasis. Proc Natl Acad Sci (1998) 95(16):9325. doi: 10.1073/pnas.95.16.9325
75. Ludwig RJ, Boehme B, Podda M, Henschler R, Jager E, Tandi C, et al. Endothelial P-selectin as a target of heparin action in experimental melanoma lung metastasis. Cancer Res (2004) 64(8):2743. doi: 10.1158/0008-5472.CAN-03-1054
76. Borsig L. The role of platelet activation in tumor metastasis. Expert Rev Anticancer Ther (2008) 8(8):1247–55. doi: 10.1586/14737140.8.8.1247
77. Nelson RM, Cecconi O, Roberts WG, Aruffo A, Linhardt RJ, Bevilacqua MP. Heparin oligosaccharides bind L- and P-selectin and inhibit acute inflammation. Blood (1993) 82(11):3253–8. doi: 10.1182/blood.V82.11.3253.3253
78. Kozlowski EO, Pavao MS, Borsig L. Ascidian dermatan sulfates attenuate metastasis, inflammation and thrombosis by inhibition of P-selectin. J Thromb Haemost. (2011) 9(9):1807–15. doi: 10.1111/j.1538-7836.2011.04401.x
79. Suzuki-Inoue K, Osada M, Ozaki Y. Physiologic and pathophysiologic roles of interaction between C-type lectin-like receptor 2 and podoplanin: partners from in utero to adulthood. J Thromb Haemostasis. (2017) 15(2):219–29. doi: 10.1111/jth.13590
80. Suzuki-Inoue K, Inoue O, Ozaki Y. Novel platelet activation receptor CLEC-2: from discovery to prospects. J Thromb haemostasis (2011) 9:44–55. doi: 10.1111/j.1538-7836.2011.04335.x
81. Kunita A, Kashima TG, Ohazama A, Grigoriadis AE, Fukayama M. Podoplanin is regulated by AP-1 and promotes platelet aggregation and cell migration in osteosarcoma. Am J Pathol (2011) 179(2):1041–9. doi: 10.1016/j.ajpath.2011.04.027
82. Schacht V, Dadras SS, Johnson LA, Jackson DG, Hong YK, Detmar M. Up-regulation of the lymphatic marker podoplanin, a mucin-type transmembrane glycoprotein, in human squamous cell carcinomas and germ cell tumors. Am J Pathol (2005) 166(3):913–21. doi: 10.1016/S0002-9440(10)62311-5
83. Peterziel H, Müller J, Danner A, Barbus S, Liu H-K, Radlwimmer B, et al. Expression of podoplanin in human astrocytic brain tumors is controlled by the PI3K-AKT-AP-1 signaling pathway and promoter methylation. Neuro Oncol (2012) 14(4):426–39. doi: 10.1093/neuonc/nos055
84. Breiteneder-Geleff S, Soleiman A, Kowalski H, Horvat R, Amann G, Kriehuber E, et al. Angiosarcomas express mixed endothelial phenotypes of blood and lymphatic capillaries: podoplanin as a specific marker for lymphatic endothelium. Am J Pathol (1999) 154(2):385–94. doi: 10.1016/S0002-9440(10)65285-6
85. Tsuruo T, Yamori T, Naganuma K, Tsukagoshi S, Sakurai Y. Characterization of metastatic clones derived from a metastatic variant of mouse colon adenocarcinoma 26. Cancer Res (1983) 43(11):5437–42.
86. Labelle M, Begum S, Hynes Richard O. Direct signaling between platelets and cancer cells induces an epithelial-mesenchymal-like transition and promotes metastasis. Cancer Cell (2011) 20(5):576–90. doi: 10.1016/j.ccr.2011.09.009
87. Biswas S, Guix M, Rinehart C, Dugger TC, Chytil A, Moses HL, et al. Inhibition of TGF-β with neutralizing antibodies prevents radiation-induced acceleration of metastatic cancer progression. J Clin Invest (2007) 117(5):1305–13. doi: 10.1172/JCI30740
88. Wikström P, Stattin P, Franck-Lissbrant I, Damber J-E, Bergh A. Transforming growth factor β1 is associated with angiogenesis, metastasis, and poor clinical outcome in prostate cancer. Prostate. (1998) 37(1):19–29. doi: 10.1002/(SICI)1097-0045(19980915)37:1<19::AID-PROS4>3.0.CO;2-3
89. Tsushima H, Kawata S, Tamura S, Ito N, Shirai Y, Kiso S, et al. High levels of transforming growth factor beta 1 in patients with colorectal cancer: association with disease progression. Gastroenterology (1996) 110(2):375–82. doi: 10.1053/gast.1996.v110.pm8566583
90. Walker RA, Dearing SJ. Transforming growth factor beta1 in ductal carcinoma in situ and invasive carcinomas of the breast. Eur J Cancer. (1992) 28(2):641–4. doi: 10.1016/S0959-8049(05)80116-9
91. Friedman E, Gold LI, Klimstra D, Zeng ZS, Winawer S, Cohen A. High levels of transforming growth factor beta 1 correlate with disease progression in human colon cancer. Cancer Epidemiol Biomarkers & Prev (1995) 4(5):549.
92. Assoian RK, Komoriya A, Meyers CA, Miller DM, Sporn MB. Transforming growth factor-beta in human platelets. Identification of a major storage site, purification, and characterization. J Biol Chem (1983) 258(11):7155–60. doi: 10.1016/S0021-9258(18)32345-7
93. Schumacher D, Strilic B, Sivaraj KK, Wettschureck N, Offermanns S. Platelet-derived nucleotides promote tumor-cell transendothelial migration and metastasis via P2Y2 receptor. Cancer Cell (2013) 24(1):130–7. doi: 10.1016/j.ccr.2013.05.008
94. Cho MS, Noh K, Haemmerle M, Li D, Park H, Hu Q, et al. Role of ADP receptors on platelets in the growth of ovarian cancer. Blood (2017) 130(10):1235–42. doi: 10.1182/blood-2017-02-769893
95. Kohga S, Kinjo M, Tanaka K, Ogawa H, Ishihara M, Tanaka N. Effects of 5-(2-chlorobenzyl)-4,5,6,7-tetrahydrothieno[3,2-c]pyridine hydrochloride (Ticlopidine), a platelet aggregation inhibitor, on blood-borne metastasis. Cancer Res (1981) 41(11 Pt 1):4710–4.
96. Wang Y, Sun Y, Li D, et al. Platelet P2Y12 is involved in murine pulmonary metastasis. PloS One (2013) 8(11):e80780. doi: 10.1371/journal.pone.0080780
97. Elaskalani O, Domenichini A, Abdol Razak NB, ED D, Falasca M, Metharom P. Antiplatelet drug ticagrelor enhances chemotherapeutic efficacy by targeting the novel P2Y12-AKT pathway in pancreatic cancer cells. Cancers (Basel) (2020) 12(1). doi: 10.3390/cancers12010250
98. Sarangi S, Pandey A, Papa AL, Sengupta P, Kopparam J, Dadwal U, et al. P2Y12 receptor inhibition augments cytotoxic effects of cisplatin in breast cancer. Med Oncol (2013) 30(2):567. doi: 10.1007/s12032-013-0567-y
99. Sharma P, Hu-Lieskovan S, Wargo JA, Ribas A. Primary, adaptive, and acquired resistance to cancer immunotherapy. Cell (2017) 168(4):707–23. doi: 10.1016/j.cell.2017.01.017
100. Shah NN, Fry TJ. Mechanisms of resistance to CAR T cell therapy. Nat Rev Clin Oncol (2019) 16(6):372–85. doi: 10.1038/s41571-019-0184-6
101. Rachidi S, Metelli A, Riesenberg B, Wu BX, Li A, Li X, et al. Platelets subvert T cell immunity against cancer via GARP-TGFβ axis. Sci Immunol (2017) 2(11):eaai7911. doi: 10.1126/sciimmunol.aai7911
102. Metelli A, Salem M, Wallace CH, Wu BX, Li A, Li X, et al. Immunoregulatory functions and the therapeutic implications of GARP-TGF-β in inflammation and cancer. J Hematol Oncol (2018) 11(1):24. doi: 10.1186/s13045-018-0570-z
103. Metelli A, Wu BX, Riesenberg B, Guglietta S, Huck JD, Mills C, et al. Thrombin contributes to cancer immune evasion via proteolysis of platelet-bound GARP to activate LTGF-β. Sci Transl Med (2020) 12(525):eaay4860. doi: 10.1126/scitranslmed.aay4860
104. Best MG, Sol N, Kooi I, Tannous J, Westerman Bart A, Rustenburg F, et al. RNA-Seq of tumor-educated platelets enables blood-based pan-cancer, multiclass, and molecular pathway cancer diagnostics. Cancer Cell (2015) 28(5):666–76. doi: 10.1016/j.ccell.2015.09.018
105. In ‘t Veld SGJG, Wurdinger T. Tumor-educated platelets. Blood (2019) 133(22):2359–64. doi: 10.1182/blood-2018-12-852830
106. Warshaw AL, Laster L, Shulman NR. Protein synthesis by human platelets. J Biol Chem (1967) 242(9):2094–7. doi: 10.1016/S0021-9258(18)96021-7
107. Booyse FM, Rafelson ME Jr. Stable messenger RNA in the synthesis of contractile protein in human platelets. Biochim Biophys Acta (1967) 145(1):188–90. doi: 10.1016/0005-2787(67)90673-9
108. Newman PJ, Gorski J, White GC 2nd, Gidwitz S, Cretney CJ, Aster RH. Enzymatic amplification of platelet-specific messenger RNA using the polymerase chain reaction. J Clin Invest. (1988) 82(2):739–43. doi: 10.1172/JCI113656
109. Denis MM, Tolley ND, Bunting M, Schwertz H, Jiang H, Lindemann S, et al. Escaping the nuclear confines: signal-dependent pre-mRNA splicing in anucleate platelets. Cell (2005) 122(3):379–91. doi: 10.1016/j.cell.2005.06.015
110. Weyrich AS, Dixon DA, Pabla R, Denis MM, Risenmay BW, Buerke M, et al. Signal-dependent translation of a regulatory protein, Bcl-3, in activated human platelets. Proc Natl Acad Sci U S A. (1998) 95(10):5556–61. doi: 10.1073/pnas.95.10.5556
111. Lindemann S, Tolley ND, Dixon DA, McIntyre TM, Prescott SM, Zimmerman GA, et al. Activated platelets mediate inflammatory signaling by regulated interleukin 1beta synthesis. J Cell Biol (2001) 154(3):485–90. doi: 10.1083/jcb.200105058
112. Schwertz H, Tolley ND, Foulks JM, Denis MM, Risenmay BW, Buerke M, et al. Signal-dependent splicing of tissue factor pre-mRNA modulates the thrombogenicity of human platelets. J Exp Med (2006) 203(11):2433–40. doi: 10.1084/jem.20061302
113. Nilsson RJ, Balaj L, Hulleman E, van Rijn S, Pegtel DM, Walraven M, et al. Blood platelets contain tumor-derived RNA biomarkers. Blood (2011) 118(13):3680–3. doi: 10.1182/blood-2011-03-344408
114. Nilsson RJA, Karachaliou N, Berenguer J, Gimenez-Capitan A, Schellen P, Teixido C, et al. Rearranged EML4-ALK fusion transcripts sequester in circulating blood platelets and enable blood-based crizotinib response monitoring in non-small-cell lung cancer. Oncotarget (2016) 7(1):1066–75. doi: 10.18632/oncotarget.6279
115. Tjon-Kon-Fat LA, Lundholm M, Schröder M, Wurdinger T, Thellenberg-Karlsson C, Widmark A, et al. Platelets harbor prostate cancer biomarkers and the ability to predict therapeutic response to abiraterone in castration resistant patients. Prostate (2018) 78(1):48–53. doi: 10.1002/pros.23443
116. Calverley DC, Phang TL, Choudhury QG, Gao B, Oton AB, Weyant MJ, et al. Significant downregulation of platelet gene expression in metastatic lung cancer. Clin Transl Sci (2010) 3(5):227–32. doi: 10.1111/j.1752-8062.2010.00226.x
117. Best MG, Sol N, In ‘t Veld SGJG, Vancura A, Muller M, Niemeijer A-LN, et al. Swarm intelligence-enhanced detection of non-small-cell lung cancer using tumor-educated platelets. Cancer Cell (2017) 32(2):238–252.e239. doi: 10.1016/j.ccell.2017.07.004
118. Takagi S, Tsukamoto S, Park J, Johnson KE, Kawano Y, Moschetta M, et al. Platelets enhance multiple myeloma progression via IL-1β upregulation. Clin Cancer Res (2018) 24(10):2430–9. doi: 10.1158/1078-0432.CCR-17-2003
119. Zaslavsky A, Baek K-H, Lynch RC, Short S, Grillo J, Folkman J, et al. Platelet-derived thrombospondin-1 is a critical negative regulator and potential biomarker of angiogenesis. Blood J Am Soc Hematology. (2010) 115(22):4605–13. doi: 10.1182/blood-2009-09-242065
120. Cecchetti L, Tolley ND, Michetti N, Bury L, Weyrich AS, Gresele P. Megakaryocytes differentially sort mRNAs for matrix metalloproteinases and their inhibitors into platelets: a mechanism for regulating synthetic events. Blood (2011) 118(7):1903–11. doi: 10.1182/blood-2010-12-324517
121. Best MG, Wesseling P, Wurdinger T. Tumor-educated platelets as a noninvasive biomarker source for cancer detection and progression monitoring. Cancer Res (2018) 78(13):3407–12. doi: 10.1158/0008-5472.CAN-18-0887
122. Lomnytska M, Pinto R, Becker S, Engström U, Gustafsson S, Björklund C, et al. Platelet protein biomarker panel for ovarian cancer diagnosis. biomark Res (2018) 6:2. doi: 10.1186/s40364-018-0118-y
123. Sol N, ‘t Veld SGJG, Vancura A, Tjerkstra M, Leurs C, Rustenburg F, et al. Tumor-educated platelet RNA for the detection and (Pseudo)progression monitoring of glioblastoma. Cell Rep Med (2020) 1(7). doi: 10.1016/j.xcrm.2020.100101
124. Park C-K, Kim J-E, Kim M-S, Kho B-G, Park H-Y, Kim T-O, et al. Feasibility of liquid biopsy using plasma and platelets for detection of anaplastic lymphoma kinase rearrangements in non-small cell lung cancer. J Cancer Res Clin Oncol (2019) 145(8):2071–82. doi: 10.1007/s00432-019-02944-w
125. Kune GA, Kune S, Watson LF. Colorectal cancer risk, chronic illnesses, operations, and medications: case control results from the Melbourne Colorectal Cancer Study. Cancer Res (1988) 48(15):4399–404.
126. Thun MJ, Namboodiri MM, Heath CW Jr. Aspirin use and reduced risk of fatal colon cancer. N Engl J Med (1991) 325(23):1593–6. doi: 10.1056/NEJM199112053252301
127. Baron JA, Cole BF, Sandler RS, Haile RW, Ahnen D, Bresalier R, et al. A randomized trial of aspirin to prevent colorectal adenomas. N Engl J Med (2003) 348(10):891–9. doi: 10.1056/NEJMoa021735
128. Sandler RS, Halabi S, Baron JA, Budinger S, Paskett E, Keresztes R, et al. A randomized trial of aspirin to prevent colorectal adenomas in patients with previous colorectal cancer. N Engl J Med (2003) 348(10):883–90. doi: 10.1056/NEJMoa021633
129. Benamouzig R, Deyra J, Martin A, Girard B, Jullian E, Piednoir B, et al. Daily soluble aspirin and prevention of colorectal adenoma recurrence: one-year results of the APACC trial. Gastroenterology (2003) 125(2):328–36. doi: 10.1016/S0016-5085(03)00887-4
130. Benamouzig R, Uzzan B, Deyra J, Martin A, Girard B, Little J, et al. Prevention by daily soluble aspirin of colorectal adenoma recurrence: 4-year results of the APACC randomised trial. Gut (2012) 61(2):255–61. doi: 10.1136/gutjnl-2011-300113
131. Cook NR, Lee IM, Gaziano JM, Gordon D, Ridker PM, Manson JE, et al. Low-dose aspirin in the primary prevention of cancer: the Women’s Health Study: a randomized controlled trial. Jama (2005) 294(1):47–55. doi: 10.1001/jama.294.1.47
132. Flossmann E, Rothwell PM. Effect of aspirin on long-term risk of colorectal cancer: consistent evidence from randomised and observational studies. Lancet (2007) 369(9573):1603–13. doi: 10.1016/S0140-6736(07)60747-8
133. Rothwell PM, Wilson M, Elwin C-E, Norrving B, Algra A, Warlow CP, et al. Long-term effect of aspirin on colorectal cancer incidence and mortality: 20-year follow-up of five randomised trials. Lancet (2010) 376(9754):1741–50. doi: 10.1016/S0140-6736(10)61543-7
134. Chubak J, Whitlock EP, Williams SB, Kamineni A, Burda BU, Buist DSM, et al. Aspirin for the prevention of cancer incidence and mortality: systematic evidence reviews for the U.S. preventive services task force. Ann Intern Med (2016) 164(12):814–25. doi: 10.7326/M15-2117
135. McNeil JJ, Nelson MR, Woods RL, Lockery JE, Wolfe R, Reid CM, et al. Effect of aspirin on all-cause mortality in the healthy elderly. N Engl J Med (2018) 379(16):1519–28. doi: 10.1056/NEJMoa1803955
136. McNeil JJ, Gibbs P, Orchard SG, Lockery JE, Bernstein WB, Cao Y, et al. Effect of aspirin on cancer incidence and mortality in older adults. J Natl Cancer Inst (2021) 113(3):258–65. doi: 10.1093/jnci/djaa114
137. Wiviott SD, Braunwald E, McCabe CH, Montalescot G, Ruzyllo W, Gottlieb S, et al. Prasugrel versus clopidogrel in patients with acute coronary syndromes. New Engl J Med (2007) 357(20):2001–15. doi: 10.1056/NEJMoa0706482
138. Mauri L, Kereiakes DJ, Yeh RW, Driscoll-Shempp P, Cutlip DE, Steg PG, et al. Twelve or 30 months of dual antiplatelet therapy after drug-eluting stents. New Engl J Med (2014) 371(23):2155–66. doi: 10.1056/NEJMoa1409312
139. Bonaca MP, Bhatt DL, Cohen M, Steg PG, Storey RF, Jensen EC, et al. Long-term use of ticagrelor in patients with prior myocardial infarction. New Engl J Med (2015) 372(19):1791–800. doi: 10.1056/NEJMoa1500857
Keywords: cancer, platelet, tumour microenvirnonment, anti platelet therapy, metastasis (cancer metastasis)
Citation: Zhao J, Huang A, Zeller J, Peter K and McFadyen JD (2023) Decoding the role of platelets in tumour metastasis: enigmatic accomplices and intricate targets for anticancer treatments. Front. Immunol. 14:1256129. doi: 10.3389/fimmu.2023.1256129
Received: 11 July 2023; Accepted: 15 November 2023;
Published: 01 December 2023.
Edited by:
David George Menter, University of Texas MD Anderson Cancer Center, United StatesReviewed by:
Meinrad Paul Gawaz, Universität Tübingen, GermanyOwen McCarty, Oregon Health and Science University, United States
Copyright © 2023 Zhao, Huang, Zeller, Peter and McFadyen. This is an open-access article distributed under the terms of the Creative Commons Attribution License (CC BY). The use, distribution or reproduction in other forums is permitted, provided the original author(s) and the copyright owner(s) are credited and that the original publication in this journal is cited, in accordance with accepted academic practice. No use, distribution or reproduction is permitted which does not comply with these terms.
*Correspondence: James D. McFadyen, amFtZXMubWNmYWR5ZW5AbW9uYWguZWR1
†These authors share first authorship
‡These authors share senior authorship