- 1School of Medical and Life Sciences, Chengdu University of Traditional Chinese Medicine, Chengdu, China
- 2Institute of Translational Pharmacology and Clinical Application, Sichuan Academy of Chinese Medical Science, Chengdu, Sichuan, China
Triple-negative breast cancer (TNBC) is the most aggressive subtype of breast cancer. Due to the lack of specific therapeutic targets, treatment options are limited, and the recurrence and metastasis rate is high, the overall survival of patients is poor. However, with the discovery of some new targets and the corresponding immune regulation after targeting these targets, TNBC has a new hope in treatment. The peptide has a simple structure, strong binding affinity, and high stability, and has great potential in targeted therapy and immune regulation against TNBC. This review will discuss how single peptides and peptide combinations target triple-negative breast cancer to exert immunomodulatory effects. Among them, single peptides target specific receptors on TNBC cells, act as decoys to target key ligands in the regulatory pathway, and target TME-related cells. The combinations of peptides work in the form of cancer vaccines, engineered exosomes, microRNAs and other immune-related molecular pathways, immune checkpoint inhibitors, chimeric antigen receptor T cells, and drug-peptide conjugates. This article is mainly dedicated to exploring new treatment methods for TNBC to improve the curative effect and prolong the survival time of patients.
1 Introduction
Breast cancer is one of the most frequently diagnosed cancers among all cancers and the most common cancer among women worldwide (1), posing a serious threat to the health of women. Breast cancer is a highly heterogeneous malignant tumor. Breast cancer cell lines are usually used as the original model to study it. However, because the cell line is cultured in vitro alone, it lacks the influence of the microenvironment and cannot fully simulate the heterogeneity of cancer cells at the real tumor level. It is also necessary to combine tumor samples or mouse models to verify the results (2). In addition, breast cancer can be divided into different subtypes, but often because of different classification criteria and seem to be somewhat confused, so after considering the genetic and epigenetic aspects of the tumor, it can be divided into lumen A, lumen B, HER2 positive, triple negative A and triple-negative B five subtypes(2). The luminal A and B cell lines are distinguished from each other, and the HER2-positive line is identified as a single subtype, while triple-negative breast cancer (TNBC) is due to the absence of estrogen receptor (ER), progesterone receptor (PR) and human epidermal growth factor receptor 2 (HER2)(3). In addition, TNBC contains at least four subcategories, namely, metaplastic breast cancer, core basal carcinoma, low-density protein, and interferon-rich breast cancer, but there are only two subgroups of TNBC cell lines currently available, namely triple-negative A and B cell lines. Among them, triple-negative A is mainly characterized by the expression of basal keratin (KRT4/5/6/13/14/15/16/17), similar to the core basal tumor, while triple-negative B is characterized by cancer stem cell patterns such as CD44+, CD24- and migration markers such as VIM(2). It is hoped that more TNBC cell lines, such as interferon-rich cell lines, can be established in the future to cover the current subclass of TNBC more comprehensively.
TNBC accounts for about 10% to 20% of all types of breast cancer and is more common in young women of African and Hispanic origin (4). However, the incidence of TNBC in China has also increased year by year since 2000. Therefore, TNBC also poses new challenges to the treatment of breast cancer. Due to the lack of iconic ER, PR, and HER2 receptors in TNBC, endocrine therapy and targeted therapy commonly used in breast cancer treatment cannot be used (5). At present, the treatment of TNBC includes surgery, adjuvant chemotherapy, and adjuvant radiotherapy (6). Usually, surgical resection of the tumor mass is combined with a variety of drug treatments such as chemotherapy, radiotherapy, and immunotherapy (7). Chemotherapy is the main treatment (8). The commonly used chemotherapeutic drugs include anthracyclines, taxanes, and cyclophosphamide (9). Although TNBC is sensitive to chemotherapy, chemotherapy drugs lack specificity and often damage healthy tissues. Toxic side effects often occur during treatment, such as neurotoxicity and nephrotoxicity (10). With the resistance of tumor cells to chemotherapeutic drugs, it is gradually unable to control the proliferation of tumor cells (11). And chemotherapy drugs have poor pharmacokinetics and rapid systemic clearance caused by poor water solubility (12). Due to these reasons, only 20% of TNBC patients showed pathological complete response (PCR) to neoadjuvant chemotherapy (13), and many patients experienced treatment failure. Although adjuvant radiotherapy using radiotherapy and imaging technology has a certain therapeutic effect on TNBC, systemic reactions caused by off-target toxicity and radiation resistance of tumor cells limit the effectiveness of clinical treatment(14). In addition, it has the characteristics of rapid progression and strong invasiveness (5). TNBC patients often have distant brain, liver, lung, and bone metastasis, and poor prognosis (15). Therefore, TNBC is still the most difficult subtype of breast cancer (16). Although the overall 5-year survival rate of breast cancer is 90%, the 5-year mortality rate of TNBC is 77% regardless of stage, and the median death time of patients is 4.2 years (17).
In the face of the lack of effective treatment for TNBC, it is particularly critical to find new treatment methods (18). One of the ideas is to explore new molecular targets for targeted therapy and immune regulation to control the growth and metastasis of tumor cells, reduce targeted toxicity and prevent recurrence (19). The reason why there is currently no targeted therapy for TNBC is closely related to the fact that TNBC is a heterogeneous tumor (20). Tumor heterogeneity refers to the changes in the genetic or epigenetic evolution of tumor cells during clonal evolution (19). Despite this, there are still overexpressed targets in TNBC that can be used for specific targeted therapy (14). Now there are targeted therapy methods under study such as immune checkpoint inhibitors, cell DNA key repair enzyme inhibitors (21), vascular endothelial growth factor inhibitors, and integrin inhibitors (22). These new molecular targets are up-regulated receptors such as folate receptors and integrin receptors in TNBC. The development of new therapies for new molecular targets in the treatment of TNBC tumors is becoming a research hotspot (23).
Another problem to be solved after finding new molecular targets is to develop active targeting ligands, and peptides are a good candidate (24). Peptides have a series of advantages such as simple structure, low synthesis cost, easy engineering (25), strong binding affinity, and high stability(26), which can efficiently reach the target and have great potential in the treatment of TNBC. Many targeted peptides have been found in phage display and in vivo biopanning techniques and can be further engineered (25). These peptides can achieve immune regulation and anti-tumor by targeting the corresponding molecular targets in TNBC, and these peptides can work alone or in combination with other substances such as adjuvants. Among them, a single peptide plays a role mainly by targeting specific receptors on TNBC tumor cells and inhibiting the growth, proliferation, and metastasis of tumor cells through certain molecular mechanisms; or as an inducing peptide targeting a key ligand in the regulatory pathway of triple-negative breast cancer to promote tumor cell death; there is also a peptide single peptide targeting triple-negative breast cancer-related cells to play an immunomodulatory role. The combinations of peptides work by forming cancer vaccines, engineered exosomes, microRNAs and other immune-related molecular pathways, immune checkpoint inhibitors (ICI), chimeric antigen receptor T cells, and drug-peptide conjugates to improve efficacy Figure 1. It can be seen that peptides have great hope in targeted therapy and immune regulation of TNBC.
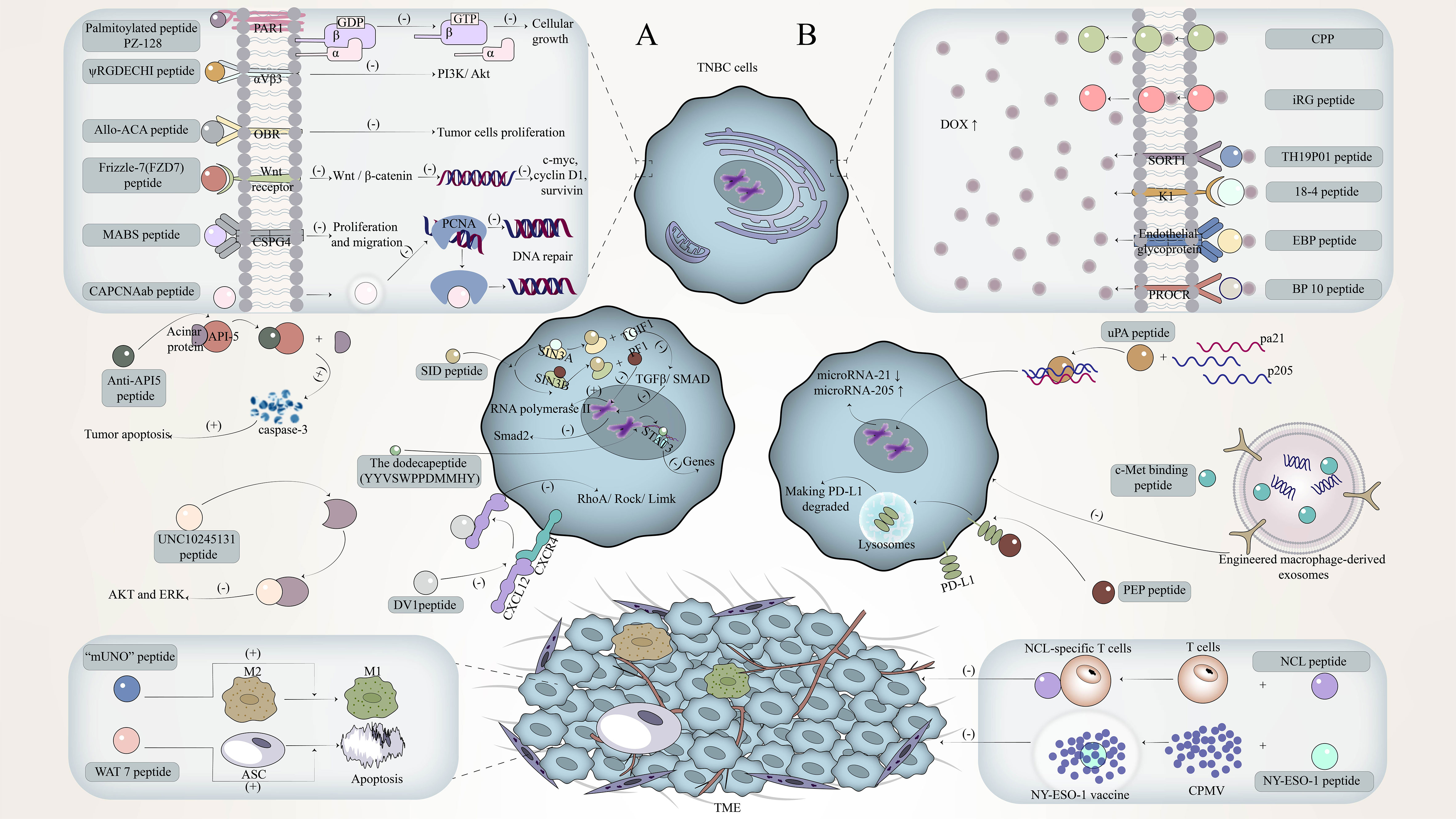
Figure 1 The mechanism of peptide in targeted therapy and immune regulation against triple-negative breast cancer (TNBC). (A) The mechanism by which single peptides target TNBC to exert its immunomodulatory effects. (B) The mechanism by which the combinations of peptides target TNBC to exert its immunomodulatory effects.
Although peptides are promising for the treatment of TNBC in the future, current research has focused on reporting the discovery of new therapeutic molecular targets or potential therapeutic molecular targets, and there are few reports summarizing peptides for targeted therapy and immune regulation of TNBC. Therefore, this article will focus on how the peptide is targeted therapy and immune regulation to achieve anti-TNBC, which will provide a reference for exploring new treatment methods for TNBC, enhancing the clinical treatment effect of TNBC, and improving the survival rate of TNBC patients.
2 Single peptides target TNBC to exert immunomodulatory effects
Single peptides are often widely studied because of their simple structure and ease to explore their independent mechanism of action (27). For how a single peptides target TNBC and play an immunomodulatory role, we will discuss it in three parts: targeting specific receptors on TNBC cells, as decoys targeting key ligands in the regulatory pathway, and targeting TME-related cells Table 1.
2.1 Single peptides target specific receptors on TNBC cells
TNBC cells were once unable to target therapy due to the lack of specific receptors, but recent studies have found some potential targets or become the key to targeting triple-negative breast cancer. Using a single peptide to target these receptors has a good anti-TNBC effect.
2.1.1 G protein-coupled receptors
G protein-coupled receptors (GPCRs) are cell surface proteins that are closely related to the occurrence and development of tumors (29). They are involved in tumor growth, angiogenesis, invasion, and metastasis (46), and the expression of GPR1 in TNBC tissues will increase (3). Therefore, GPCRs have also become a good therapeutic target.
Studies have found that an adipokine Chemerin secreted by white adipose tissue can activate GPR1 to release intracellular calcium, thereby inhibiting the accumulation of cyclic adenosine monophosphate, achieving phosphorylation of P42-P44 MAP kinase through G1 heterotrimeric G protein(47). The corresponding peptide antagonist LRH7-G5 can competitively bind to GPR1 with Chemerin, thereby blocking the signal transduction of Chemerin/GPR1 through the P13K/Akt signaling pathway and inhibiting the growth of TNBC cells (3). In addition, protease-activated receptor 1 (PAR1) is also a G protein-coupled receptor. A palmitoylated peptide PZ-128, which targets the intracellular loop of PAR1, can inhibit PAR1/G protein signaling by interacting with GPCRs to significantly inhibit the growth of MDA-MB-231 TNBC cells (5). In addition to the G protein-coupled receptors mentioned above, there are G protein-coupled adenosine receptors, such as the adenosine A2B receptor (ADORA2B)(48), which is a member of the G protein-coupled adenosine receptor superfamily. ADORA2B and adenosine signal transduction can affect the occurrence and invasion of TNBC, especially in mutant TP53, because the expression of ADORA2B is enhanced by the interaction between the CCAAT box and NF-γ protein (28). In addition, ADORA2B is selectively up-regulated under hypoxic conditions, and hypoxia activates hypoxia factor 1α (HIF-1α) through signal transduction pathways such as Akt and extracellular regulated kinase 1/2 (ERK1/2), and HIF-1α stimulates more adenosine production (49). The study found that cytotoxic cyclic peptide SA-1 can inhibit the expression of ADORA2B (28), to achieve the purpose of inhibiting the occurrence of TNBC. Although GPCRs are almost always located on the cell surface, some GPCRs retain atypical intracellular/nuclear locations and are involved in intracellular signal transduction. These intracellular/nuclear GPCRs, such as kallikrein B1/B2 receptors (B1R/B2R), affect tumor growth, invasion, and angiogenesis. (18), affect tumor growth, invasion, and angiogenesis. For kinin receptors, cell-penetrating kinin receptor antagonists, such as cell-penetrating B1R antagonists (CP-B1Ras) and a cell-penetrating B2R antagonist (CP-B2Ras) (18), are generally used to target B1R and B2R, respectively. For kinin receptors, cell penetrating kinin receptor antagonists, such as cell penetrating B1R antagonists (CP-B1Ras) and cell penetrating B2R antagonists (CP-B2Ras), are generally used to target B1R and B2R, respectively(18). Among them, CP-B1Ras such as SSR240612, NG67, N2000, etc. showed strong anti-cancer activity (29), which could inhibit the proliferation effect caused by the transactivation of EGF receptor and the phosphorylation of MAPK p42/p44 after the activation of B1R. CP-B2Ras can activate the p38kinase/p27kip1 pathway, which in turn arrests the growth of TNBC in the G1 phase and leads to apoptosis (18).
2.1.2 Integrin receptor
The expression of integrin receptors is up-regulated on the surface of tumor cells and is related to the promotion of angiogenesis, cancer cell adhesion, migration, and invasion (31). The common integrin is mainly αVβ3 (50). Studies have found that some peptides can specifically target αVβ3, thereby disrupting integrin-dependent signaling pathways to achieve anti-tumor purposes. A type IV collagen-derived peptide AXT050 specifically binds to αVβ3 to produce an anti-vascular effect unrelated to VEGF inhibits the formation of new blood vessels in TNBC(30), and makes it lack the supply of oxygen and nutrients(51). Another novel peptide ψRGDECHI inhibits the PI3K/Akt pathway involved in integrin-mediated EMT activation after targeting αVβ3 (52), thereby inhibiting the migration and invasion of TNBC cells.
2.1.3 Leptin receptor
Leptin (obesity hormone) is related to obesity-related stimuli, which can be produced by adipose tissue and breast cancer cells (53). Leptin and its receptor (OBR) are overexpressed in TNBC(54). Therefore, the leptin receptor is also a target for TNBC-targeted therapy. The leptin receptor antagonist peptide allo-ACA can specifically bind to OBR, inhibit the expression of downstream signal channels and cyclins induced by OBR activation, and inhibit leptin-stimulated tumor cell proliferation (55). Because leptin is also an angiogenic factor (56), the use of allo-ACA peptides can also achieve anti-angiogenic therapy (32).
2.1.4 Chondroitin sulfate receptor
Chondroitin sulfate proteoglycan 4 (CSPG4) is an antigen receptor with increased expression in tumors, including TNBC. CSPG4 can promote the survival and adhesion of cancer cells and promote tumor growth and metastasis (57). Correspondingly, the polypeptide targeting CSPG4 has an anti-CSPG4 monoclonal antibody (MABS), which can effectively inhibit the proliferation and migration of TNBC by targeting CSPG4 (33).
2.1.5 Wnt receptor
Studies have shown that Wnt receptors are overexpressed in TNBC and induce the proliferation and invasion of TNBC cells through the Wnt/β-catenin signaling pathway(58). RHFZD7 is a recombinant soluble peptide fragment, which can effectively target and antagonize a Wnt receptor Frizzle-7 (FZD7)(34), inhibit the activation of Wnt/β-catenin signaling pathway, and block the initiation of downstream target genes caused by β-catenin entering the nucleus, such as inhibition of c-myc, cyclin D1, survivin, and other target genes can inhibit tumor growth and drug resistance(59), while inhibition of VEGF target genes can inhibit tumor angiogenesis.
2.1.6 Proliferating cell nuclear antigen
Proliferating cell nuclear antigen (PCNA) is essential for DNA replication and repair. During DNA replication, PCNA forms a homotrimer around the DNA chain and loads related proteins and enzymes(60). There are some interdomain connector loop (IDCL) domains in the PCNA monomer, and IDCL contains the motif of the PCNA interacting protein box (PIP-box). The proteins interacting with PCNA regulate DNA replication and repair by binding to these motifs. Therefore, the use of polypeptides to target IDCL to inhibit the interaction between proteins required for DNA replication and repair and PCNA can hinder cell function and promote cell death. The study found a rabbit polyclonal antibody (CAPCNAab) that specifically recognizes the tumor-associated PCNA subtype. CAPCNAab can compete with CAPCNA binding auxiliary proteins and specifically target the CAPCNA in the IDCL of PCNA (35). The replication of DNA in TNBC tumor cells is blocked, and the damaged DNA cannot be repaired and then dies.
2.2 Single peptides as decoys target key ligands in the regulatory pathway
In addition to binding to certain receptors on triple-negative breast cancer cells, single peptides can also bind to ligands related to tumor regulation. These peptides can be called peptide decoys. Peptide decoys are molecular traps that bind to certain ligands and play a confusing role, which are the same as receptor proteins. They can be soluble proteins, that is, binding proteins, or inactive cell surface receptors. Soluble peptide decoys have different production mechanisms, including proteolytic cleavage of cell surface receptors, phospholipase C-mediated cleavage, selective splicing of receptor mRNA transcripts, and selective intron polyadenylation (61). These peptide decoys can target key ligands in the breast cancer regulatory pathway, including chemokines, SIN3, etc.
2.2.1 Chemotactic factor
Chemokines are small proteins with low molecular weight and play a key role in tumor immunity and communication between tumor cells and their surrounding environment(62), such as CCR9/CCL25, CXCR5/CXCL13, CXCR4/CXCL12 and CCR7/CCL19 (CCL21), which play a role in cancer growth and metastasis. The decoy peptide can inhibit tumor cells by targeting chemokines, such as the DV1 peptide targeting CXCL12. CXCL12 is a chemokine stromal cell-derived factor-1, and its specific receptor is the chemokine receptor CXCR4. In tumors, CXCR4-CXCL12 is involved in activating a variety of cancer-promoting regulatory mechanisms, thereby promoting tumor proliferation, inhibiting cancer cell apoptosis, and promoting metastasis. DV1 peptide mainly blocks CXCR4 signal transduction by binding to CXCL12 to silence the expression of CXCR4 (36), inhibits Rho-involved RhoA/Rock/Limk signaling pathway (63), and makes Rho protein unable to regulate the tissue, focal adhesion arrangement and intracellular transport of actin stress fibers, thereby inhibiting the migration of tumor cells (64). Of course, silencing the expression of CXCR4 can also inhibit some targets that induce tumor cell migration activity. These targets refer to Akt and its downstream targets activated when stimulating PI3K in CXCR4 signal transduction (36).
2.2.2 SIN3
SIN3 is a key adaptor protein in the co-repressor complex of histone deacetylase (HDAC1/2). As a scaffold connecting DNA-binding transcription factors and chromatin regulators (65), it has a regulatory effect on the proliferation and differentiation of TNBC tumor cells (66). Sin3 includes Sin3A and Sin3B (67), and its structure is composed of four pairs of amphipathic α-helix (PAH1-PAH4) motifs.
The Sin 3-interacting domain (SID) bait is a peptide designed to target the SIN3 complex (PAH2 domain) and induce epigenetic reprogramming in TNBC (68). SID peptide binding to SIN3A can inhibit TGIF1 and then bind to SIN3A, while TGIF1 is a transcription factor involved in the regulation of Wnt signal transduction. TGIF1 can not bind to the PAH2 domain of SIN3A, and then can not promote the expression of the Wnt gene, while the expression of nuclear β-catenin and its target is also inhibited, because the activity of Wnt/β-catenin is not increased, resulting in downstream media such as Axin2, Bcl9 can not be activated(69). It can not further promote the EMT and invasion and metastasis of TNBC tumor cells. The activity was not increased, resulting in downstream mediators such as Axin2 and Bcl9 could not be activated, and it could not further promote the EMT and invasion and metastasis of TNBC tumor cells(70). In addition, some other proteins that can bind to the PAH2 domain, such as the adaptor PF1 (PHF12) and TIEG1 (TGFβ-induced early gene), can not only interact with SIN3A but also interact with SIN3B. First of all, the SID peptide can down-regulate the transcription of genes related to epithelial-mesenchymal transition (EMT) after blocking the interaction between SIN3A and PF1(71). Blocking the interaction between PF1 and SIN3B can inhibit the modification of downstream chromatin of the transcription initiation site by PF1 and restore the effect of RNA polymerase II. SID peptide inhibits the combination of TIEG1 and PAH2, which is to inhibit the TGFβ/SMAD signal transduction pathway involved in TIEG1, so that the expression of Smad2 is down-regulated, and the EMT of tumor is inhibited to a certain extent (72). Of course, the interaction between MAD and SIN3-PAH2 is blocked, which enhances the expression of CDH1 and ESR1 and restores the sensitivity of tumor cells to chemotherapeutic drugs (37).
2.2.3 Transcription factor
Transcription factors are an important part of the signaling pathway. Most of them are proteins. Transcription factors are also related to the progression of tumors by binding to DNA to regulate gene transcription. Targeting transcription factors with inducing peptides can achieve the purpose of treating TNBC.
Among them, STAT3 is one of the many transcription factors, and its overexpression is related to the malignant transformation of tumors including breast cancer. STAT3 has six conserved domains, namely the N-terminal domain followed by the CCD domain, the C-terminal trans-activation domain, the DNA binding domain, the SH2 domain, and the linker domain (38). The activation of STAT3 depends on phosphorylation and dimerization(39). There are several peptides targeting STAT3. The first is a small regulatory peptide ASRPS of STAT3, which mainly regulates the STAT3 vascular endothelial growth factor (VEGF) signaling pathway (38). The combination of ASRPS and the CCD domain of STAT3 can inhibit the phosphorylation of STAT3, thereby reducing the expression of VEGF and inhibiting the formation of tumor blood vessels. The dodecapeptide of the YYVSWPPDMMHY sequence is another peptide targeting STAT3 (39), which mainly prevents the homodimerization of STAT3 and inhibits the stimulation of tumor genes by recognizing and binding to the promoter region of the target gene (73). Studies have shown that YYVSWPPDMMHY peptide down-regulated genes related to angiogenesis (VECGF-A), proliferation-related genes (BIRC5, CDK2, and MCL1) and invasion-related genes (MMP1 and MMP7)(39), which can effectively induce apoptosis and inhibit the growth of TNBC tumor cells.
2.2.4 Anti-apoptotic
Caspase-3 is a protease related to apoptosis and plays an important role in promoting apoptosis. However, in some tumor cells, the function of caspase-3 is inhibited, resulting in the inhibition of apoptosis. For example, after the anti-apoptotic protein-5 (API-5) binds to the acinar protein, the acinar protein cannot be cleaved by caspase-3, and the formation of the active p17 fragment is inhibited, resulting in DNA breakage(74). Therefore, the anti-API5 peptide targeting API-5 promotes the apoptosis of TNBC tumor cells by preventing the API-5/acinar interaction and reactivating the caspase-3 pathway (40).
2.2.5 CIB1
CIB1 is a small cytoplasmic protein, 22kDa (75). It is composed of 10 alpha helices, of which 8 alpha helices form 4 EF-hand domains, and the other 2 alpha helices are binding to the Ca2+ C-terminal domain (76). CIB1 can not only interact with the cytoplasmic tail of integrin αIIb through the hydrophobic pocket hidden by the C-terminal helix 10 to participate in the adhesion and migration of tumor cells(77). CIB1 can also interact with PAK1 to affect the downstream signaling pathways of related signaling pathways such as PI3K/Akt and Ras/RAF/MEK/ERK and regulate the growth and proliferation of TNBC tumor cells (78). Studies have found that CIB1 cyclic peptide inhibitors such as UNC10245131 (41) and UNC10245092 (1), these cyclic peptide inhibitors by targeting CIB1 to prevent its regulation of AKT and ERK carcinogenic pathways, achieve the purpose of intervention TNBC.
2.3 Single peptides target TME-related cells
Tumor cells and their environment are a functional whole. Tumor cells and tumor microenvironment (TME) affect each other and jointly promote the occurrence and development of tumors. TME-related cells include immune cells, fibroblasts, and adipocytes (17). These cells are also closely related to tumor growth and metastasis (79). Some single peptides can also play a good role in immune regulation and inhibition of tumor cells by targeting TME-related cells.
2.3.1 Tumor-infiltrating immune cell
Tumor-associated immune cells include tumor-infiltrating lymphocytes, macrophages, neutrophils, etc. Among them, tumor-associated macrophages (TAM) are classically divided into two major groups: M1 and M2. The M1 group is associated with anti-tumor activity, while the M2 group is associated with tumor growth, angiogenesis, migration and invasion, and metastasis, and promotes epithelial-mesenchymal transition (EMT) in TNBC(80). Generally, macrophages are in a balance between M1 and M2 in healthy tissues, while in cancer tissues, the phenotype shifts to M2. Therefore, targeting M2-like macrophages and restoring them to anti-tumor Ml-like macrophages is a direction for cancer treatment. Researchers have found several peptides that can effectively target M2-like macrophages. One is the melittin KLA8-26, which can distinguish M0/M1/M2. When the melittin is attached to the lipid membrane of M2-like macrophages, the membrane will be disturbed, resulting in asymmetry between the two lipid layers and temporary formation of pores, thereby inducing cell death (42). The other is a cyclic peptide with a structure of CSSTRESAC, which can specifically bind to the protein disulfide isomerase A3 (PDIA3) expressed on the cell surface of TAM, namely vitamin D receptor, to achieve the purpose of reducing M2-like macrophages. In addition, there is an “mUNO” peptide, which mainly targets CD206-positive M2-like TAM and converts it into M1-like TAM (43).
In addition to the commonly used method of targeting M2-like macrophages, it can also target other tumor-associated immune cells. C24D peptide is an immunoregulatory therapeutic peptide derived from placental immunoregulatory ferritin targeting CD45 molecule, which consists of 24 amino acids (81). The CD45 receptor is a transmembrane protein tyrosine phosphatase receptor type C, which is present in T cells and NK cells and can inhibit the activation of Src family tyrosine kinases and immune cells in TNBC patients (82). Therefore, after binding to the CD45 receptor on the inhibited leukocytes in TNBC, the C24D peptide can re-activate the Src family of tyrosine kinases, break the inhibition and trigger the intracellular signaling cascade, resulting in an increase in 69+ T cells and 69+ NK cells, inducing CD8+ and activating CD56+ tumor-infiltrating cells to achieve specific killing of TNBC cells (44).
2.3.2 Cancer-associated fibroblasts and adipose stromal cells
Cancer-associated fibroblasts (CAF), also known as tumor mesenchymal stromal cells (MSC), are associated with extracellular matrix (ECM) remodeling, leukocyte recruitment, and immunosuppression in cancer (83), and can induce epithelial-mesenchymal transition (EMT) of cancer cells. Related studies have shown that CAF is heterogeneous and can be derived from different lineages. Some of them are derived from white adipose tissue (WAT) around the tumor, and adipose stromal cells (ASC) are MSC in WAT (84). It is one of the most abundant cell components around the breast tissue, which can cause changes in tumor cell phenotype and promote epithelial-mesenchymal transition and invasiveness of TNBC cells by secreting tumor growth-related hormones and cytokines. Therefore, targeting ASC and depleting it is a new method for the treatment of TNBC. D-CAN peptide, which is made of cyclic peptide WAT7 (sequence CSWKYWFGEC), can induce the apoptosis of ASC and interfere with invasive TNBC (45).
3 The combinations of peptides target TNBC to exert immunomodulatory effects
In addition to targeting triple-negative breast cancer in a single form to exert immunomodulatory effects, there are many combinations of peptides, such as vaccines, exosomes, microRNAs, checkpoint inhibitors, etc. These combinations show good results in TNBC treatment (85) Table 2.
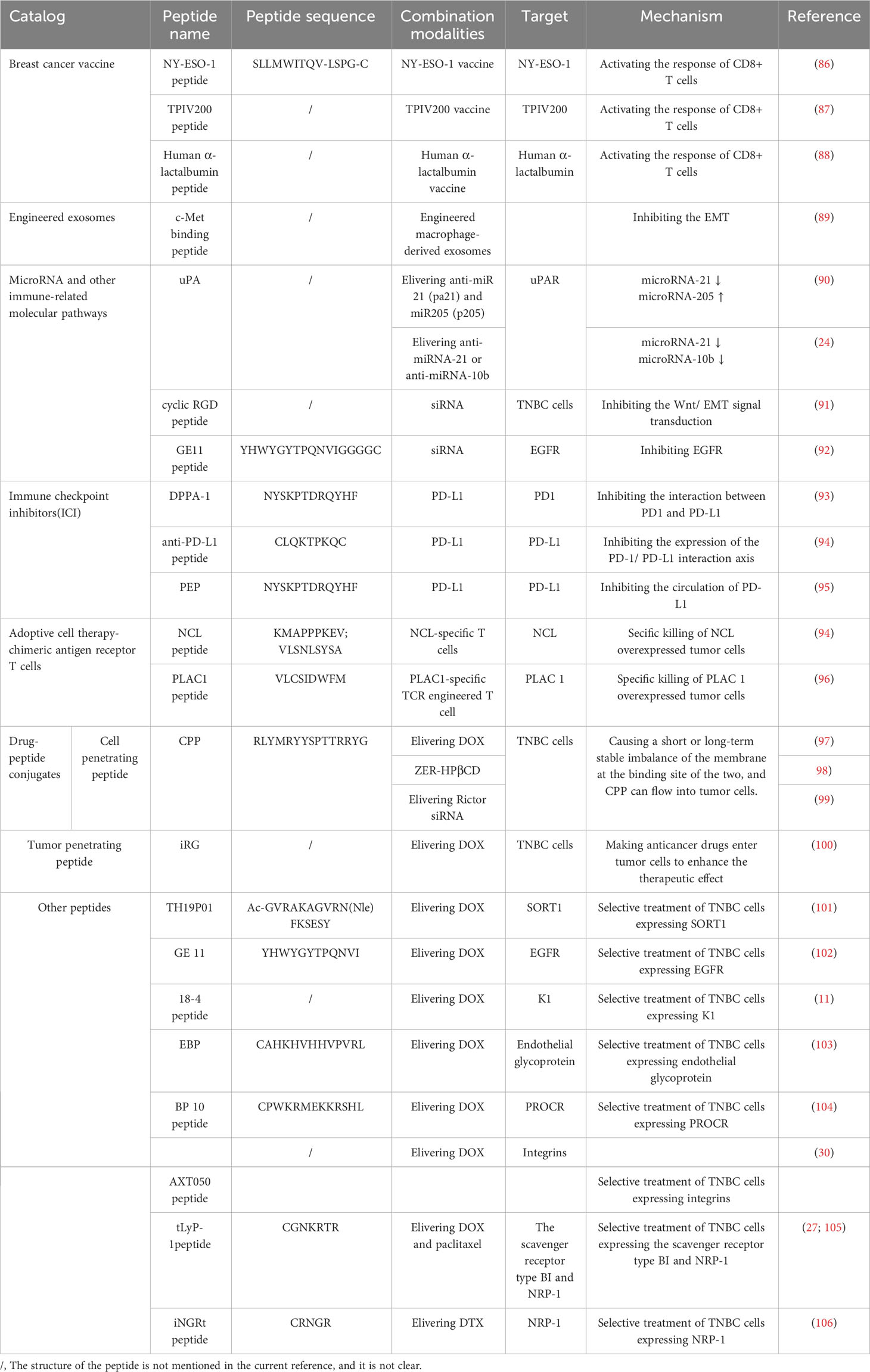
Table 2 The mechanism by which the combinations of peptides target TNBC to exert its immunomodulatory effects.
3.1 Breast cancer vaccine
Cancer vaccines mainly recognize tumor-associated antigens by stimulating the immune system to produce corresponding antibodies to achieve the effect of prevention and treatment. In TNBC, many new antigens have been identified, such as a cancer-testis antigen, folate receptor α (Frα), human α-lactalbumin, and so on (87). However, many of these antigens are short peptides with poor immunogenicity and cannot stimulate the cellular immune response well. Therefore, adjuvants are generally added to these antigenic peptides to enhance the immune response and improve the efficacy of the vaccine. Common adjuvants are MPL or MF (107). NY-ESO-1, the first cancer-testis antigen, is a highly immunogenic antigen target present in triple-negative breast cancer. Studies have developed the NY-ESO-1 vaccine using cowpea mosaic virus (CPMV) as an adjuvant (86). CPMV has strong immune stimulation due to its protein structure and capsized nucleic acid, which enhances the uptake of NY-ESO-1 peptide by antigen-presenting cells and the subsequent response of CD8+ T cells. Secondly, folate receptor α (Frα) TPIV200 is also a candidate vaccine and is being studied (87). Human α-lactalbumin is an antigen that is only expressed in the lactating mammary gland of normal tissues, but is also expressed in TNBC, and has the potential to be a candidate vaccine target (88). The combination of antigen peptides and adjuvants to make vaccines stimulate the body to strengthen the humoral immune response and a cellular immune response is believed to play a greater role in the prevention and treatment of triple-negative breast cancer in the future.
3.2 Engineered exosomes
Exosomes are extracellular vesicles that can be found in almost all cells and affect the growth and metastasis of tumor cells by participating in many cell signal transduction processes. They have now been used to deliver some important molecules (87). It has been reported that when the antigen peptide in cancer is carried by exosomes, the body will produce stronger immune stimulation on it, so exosomes are also tried to be used as immunogen carriers(108). Studies have produced engineered macrophage-derived exosomes for carrying c-Met binding peptides. C-Met is a mesenchymal-epithelial transforming factor, and its abnormal signals will lead to poor prognosis and increased metastasis of triple-negative breast cancer (89). Using c-Met binding peptides combined with exosomes can enhance the immune response and inhibit the expression of c-Met, thereby achieving therapeutic effects.
3.3 MicroRNA and other immune-related molecular pathways
The growth, metastasis, and recurrence of TNBC are also closely related to the imbalance of immune-related molecules such as microRNAs (miRNA) and short interfering RNA (siRNA) (87). Regulating the expression of these molecules by targeting the delivery of miRNA or siRNA can well inhibit breast cancer cells.
MiRNAs are generally small non-coding RNA molecules composed of about 20 nucleotides. MiRNAs can bind to the complementary sequence bases of endogenous mRNAs and regulate gene expression by interacting with the 3’ untranslated region of the target gene, thereby interfering with the transcription and translation of specific genes (109). In TNBC, the disordered expression of miRNAs can lead to the growth, metastasis, and recurrence of tumor cells. Among them, the overexpression of microRNA-21, miRNA-10b, and miRNA-21 and the down-regulation of microRNA-205 often occur (24, 90). In the corresponding study, plasmid anti-miR21 (pa21) and plasmid miR205 (p205) were coated in urokinase plasminogen activator peptide (uPA). UPA can target urokinase plasminogen activator receptor (uPAR) in the TNBC cell membrane, thereby delivering anti-miR 21 (pa21) and miR205 (p205) to tumor cells, playing a role in simultaneously down-regulating microRNA-21 levels and up-regulating microRNA-205 levels (90). At the same time, there are also studies using uPA to target the delivery of anti-miRNA-21 or anti-miRNA-10b, antagonizing the accumulation of multiple endogenous miRNAs to achieve the purpose of treatment (24).
Immune-related molecules In addition to the aforementioned miRNAs, there are short interfering RNAs (siRNAs). The siRNA molecule is a double-stranded short-chain oligonucleotide that can specifically match the sequence of the mRNA molecule, thereby causing specific gene silencing, which is of great significance for the treatment of cancer(110). The experimental cyclic RGD peptide delivery therapeutic siRNA can down-regulate PRC 2-mediated H3 K27 trimethylation and Wnt/EMT signal transduction, which changes the phosphorylation spectrum of several kinases in TNBC cells and affects the carcinogenic relationship (91). In addition to the use of cyclic RGD peptides to deliver siRNA, GE11 peptide is also used, which is an anti-EGFR peptide that can actively bind to TNBC cells overexpressing epidermal growth factor receptor (EGFR), which is beneficial for siRNA to better target these tumor cells (92).
3.4 Immune checkpoint inhibitors
Immune checkpoints determine the activation or inhibition of the host immune response in anti-tumor immunity. Common immune checkpoints are PD-L1 and PD-L2. Tumor cells evade immune surveillance of T cells by up-regulating programmed death ligand (PD-L1) on the cell surface (111). Moreover, studies have shown that the expression of PD-L1 is much higher in TNBC cells than in other subtypes of breast cancer(112). Therefore, the use of immune checkpoint inhibitors (ICI) can restore the host’s immune response and enhance the clearance of tumor cells. At the same time, some studies have found that some peptides can act as immune checkpoint inhibitors and have good anti-tumor effects. The general method used to block immune checkpoints is to block the interaction between programmed cell death protein 1 (PD1) and PD-L1, such as a D-peptide antagonist (DPPA-1), DPPA-1 is the hydrolysis release product LAG-DPPA-1 of the sequence MMP-2 on the CD peptide (93). In addition, the anti-PD-L1 peptide can be used to directly target PD-L1, activate autophagy by inhibiting PD-L1, and restore T cell-mediated killing in TNBC tumors (94). Although blocking PD-L1 does prevent the expression of the PD-1/PD-L1 interaction axis to a certain extent, it generally shows a good therapeutic effect. In some patients, PD-L1 is circulating in tumor cells, and then PD-L1 is re-expressed on the cell surface (113). Therefore, blocking the circulation of PD-L1 is the key. Some studies have proposed the use of PD-L1 targeting D peptide (NYSKPTDRQYHF, PEP). After PEP binds to PD-L1 on the surface of cancer cells, it will guide PD-L1 into lysosomes, making PD-L1 degraded and unable to circulate again, thereby down-regulating the expression of PD-L1 and restoring the killing effect of T cells on TNBC tumor cells (95).
3.5 Adoptive cell therapy-chimeric antigen receptor T cells
Adoptive cell therapy (ACT) is a highly effective and promising treatment method, which is generally activated by tumor-specific lymphocytes including TIL, CD8 + cells and CD4 + helper cells, or other cells of the immune system such as NK cells and DC cells, and then infused into patients to kill tumor cells. Chimeric antigen receptor T cell therapy is a kind of adoptive cell therapy. These T cells can specifically recognize tumor-specific antigen (TSA) or tumor-associated antigen (TAA), especially TSA, through specific T cell receptors (TCR) to achieve tumor-targeted therapy (87).
One of the chimeric antigen receptor T cells mentioned first is nucleolin (NCL) specific T cells. NCL is a protein that exists in the nucleolus, cytoplasm, and cell surface of eukaryotic cells, and has a variety of biological functions because NCL is overexpressed in TNBC and is associated with metastasis and poor prognosis of TNBC cells (114). Therefore, NCL has become a potential target for TNBC treatment. Researchers have engineered NCL-specific T cells and found that they have a good specific killing effect on NCL-overexpressing tumor cells (94). In addition, there is also a PLAC1-specific TCR-engineered T cell. Placenta-specific antigen 1 (PLAC1) is one of the cancer-testis antigens (CTA), which is expressed in malignant tumors such as breast cancer and is related to the proliferation and migration of tumor cells(115). Effective engineering of CD8 + T cells to express TCRs that recognize human leukocyte antigen (HLA) -restricted PLAC1 peptides can specifically kill tumor cells overexpressing PLAC1. Although PLAC1 is also expressed in normal tissues of the testis and placenta, it is not affected by TCR-engineered T cells because germ cells do not express MHC molecules and do not present antigens(116).
3.6 Drug-peptide conjugates
Drug-peptide conjugates (PDC) are a unique class of drug carriers that couple small molecule drugs with peptides. These peptides can modify and deliver the corresponding drugs to tumor cells, enhance the intracellular transport of drugs, and improve the lethality of the corresponding drugs (117). There are many kinds of peptides applied in PDC, among which cell-penetrating peptide (CPP) and tumor-penetrating peptide (TTP) are the most widely used. At the same time, some other peptides are being studied, which are mainly modified by small-sized drugs such as oligonucleotides and chemotherapeutic drugs.
3.6.1 Cell-penetrating peptide
CPP is composed of natural amino acids with high biocompatibility and effective tissue penetration. The positively charged amino acids on CPP and the negatively charged membrane phospholipid bilayer on the surface of tumor cells form an electrostatic interaction. At the same time, the hydrophobic amino acids of CPP interact with the hydrophobic core of the tumor cell membrane, which makes the lipid bilayer sparse, causing a short or long-term stable imbalance of the membrane at the binding site of the two, and CPP can flow into tumor cells (117).
Because the lack of targeting of chemotherapeutic drugs often damages normal tissue cells to produce toxic effects, the use of CPP-modified chemotherapeutic drugs has become a research hotspot. Some studies have modified the chemotherapeutic drug doxorubicin (DOX) with tumor-homing CPP (RLYMRYYSPTTRRYG) and loaded it into nanoparticles (NP) and found that it has good blood compatibility and biocompatibility. The effect of inhibiting the growth of TNBC cells is more significant than that of DOX alone (97). In addition to some commonly used chemotherapeutic drugs, some natural anticancer drugs have gradually attracted people’s attention. Here, the anticancer drug Zerumbone (ZER, M.218.34g/mol) derived from the rhizome of wild ginger Zingiber Zerumbet Smith is mentioned. It is a sesquiterpene compound that can increase the activation of Bax to induce apoptosis of breast cancer cells and inhibit the proliferation of tumor cells by inhibiting the expression of the Ki-67 protein (118). ZER achieves targeted therapy of ZER by using CPP-modified ZER (ZER-HPβCD) encapsulated in hydroxypropyl-β-cyclodextrin (98). In addition, oligonucleotides have always been a good choice for the treatment of cancer, such as small interfering RNA (siRNA), which can effectively target the table of silent genes (119). However, due to the relatively unstable physiological environment of naked siRNA in the blood system, digestive system, and intracellular lysosomes, it is easy to be removed when used alone, so the CPP delivery method shows great potential. In some experiments, CPP-modified GO nanoparticles loaded with Rictor siRNA were injected into nude mice, which significantly inhibited the growth of TNBC tumors. This may be because Rictor siRNA further inhibited the phosphorylation of Akt and p70s6k by inhibiting the expression of Rictor, as well as the subsequent PI3K/Akt/mTOR signal transduction (99).
3.6.2 Tumor penetrating peptide
TTP is mainly targeted at tumor cells, and drug delivery destroys cancer cells through different mechanisms. IRG is a commonly used cyclic tumor penetrating peptide, which is composed of a disulfide-linked RGD module and an overlapping C-terminal R module (120). The RGD module can bind to the highly expressed αvβ3 or αvβ5 integrin on TNBC tumor cells, making anticancer drugs enter tumor cells to enhance the therapeutic effect (121). Studies have confirmed that RGD-modified RBC membrane and then encapsulating DOX can ensure its good biological stability and low cytotoxicity in vivo (99).
3.6.3 Other peptides
PDC has a class of antibody-drug conjugates (ADC), which recognize TAA or TSA through corresponding antibodies. These peptides as antibodies do not have anti-cancer effects themselves, but after binding to tumor cells, they can deliver the corresponding drugs and kill the target cancer cells by internalizing the cells (87). There are many potential peptides in ADC. The following will list some peptides that are being studied in recent years.
The first thing to be mentioned is the newly developed peptide TH19P01 targeting neurotensin receptor 3 (SORT1). SORT1 belongs to the receptor of the VPS10P family and is abnormally expressed in human cancers such as breast cancer, ovarian cancer, and pancreatic cancer. TH19P01 can be combined with many anticancer drugs such as DOX, and then selectively treat TNBC cells containing SORT1 (101). Epidermal growth factor receptor (EGFR) has become a promising target because it is overexpressed in TNBC cancer cells. A twelve peptide named GE 11 (YHWYGYTPQNVI) can effectively bind EGFR and release DOX to kill tumor cells (102). In addition, keratin 1 (K1) is a novel receptor that is highly expressed on breast cancer cells and can also be used as a target for drug delivery. Peptide 18-4 was designed and then conjugated with a DOX-containing acid-sensitive hydrazone linker to achieve targeted killing of cancer cells(11). In addition, endothelial glycoprotein binding peptide (EBP) can also be used to modify DOX and target TNBC cells(103). Similarly, peptide BP 10 has a strong affinity for the new cancer stem cell marker protein C receptor (PROCR) in tumor tissues of TNBC patients. By targeting TNBC cells expressing PROCR, DOX is released and the treatment efficiency is improved (104). The AXT 050 peptide is a collagen-IV-derived peptide that can target tumor-associated integrins and increase the accumulation of drugs in tumor cells (30). Here we also mention a tumor-homing peptide tLyP-1 peptide that can load DOX and paclitaxel and target tumor cells by binding to the overexpressed scavenger receptor type B I and neuropilin receptor 1 (NRP-1) in TNBC cells(27, 91, 105). The iNGRt peptide can also target NRP-1, which enhances anticancer activity by attaching to the surface of NP loaded with the anticancer drug docetaxel (DTX) (106).
4 Conclusion
TNBC is a highly heterogeneous subtype of breast cancer, and its incidence is increasing year by year. Due to the lack of effective therapeutic targets, it has a higher recurrence and metastasis rate, and mortality rate than ordinary breast cancer. Surgery plus chemotherapy is the main method of clinical treatment of TNBC, but the curative effect is very little (17). So far, the treatment progress of TNBC has lagged far behind other breast cancer subtypes, and the treatment of TNBC is still a huge challenge. With the continuous improvement of the understanding of the immunogenicity of TNBC, TNBC immunotherapy has developed rapidly in recent years and has shown strong therapeutic potential (122). However, there are still some challenges in the immunotherapy of TNBC. For example, some patients have poor responses to immunotherapy, and highly accurate prediction methods are needed to screen out responders for treatment and further explore the reasons for poor responses. Many TNBC-associated antigens are not only highly expressed in tumor cells, but also expressed in normal cells to varying degrees. Immunotherapy targeting these antigens may damage normal tissues and cause adverse reactions(123). TNBC cells have strong migration ability and poor response to targeted drug treatment. Therefore, it is necessary to further find new TNBC-specific therapeutic targets and promote personalized treatment in the direction of future development.
In the future, the combination of targeted therapy and immunotherapy is also expected to improve the efficacy of anti-tumor therapy. The combination of the two has a synergistic therapeutic effect, which may be better than the simple superposition of the effects of a single component (124). Targeted therapy can accurately overcome various forms of immunosuppression in the tumor microenvironment and promote anti-tumor immune regulation. The combination of targeted therapy and immunotherapy includes a combination of various immunotherapy drugs and new treatments such as standard therapy and targeted therapy (125). Among these new treatments, peptides have become a new star in anti-tumor therapy because of their advantages such as small molecular weight, high biocompatibility, and easy synthesis, and numerous researchers have been competing to study them. Of course, peptides also have some shortcomings, such as being unstable and easily decomposed in vivo, having short half-life, and most cannot be taken orally(123). For various reasons, the research of many peptides as drugs has been stuck in the theoretical stage, failing to carry out clinical trials and further promotion, but it is believed that there will be a breakthrough shortly.
At the same time, with the development of sequencing technology and medical big data processing, it has become a hot spot in current medical development to adopt accurate medical strategies to deal with highly heterogeneous diseases such as tumors and to select reasonable combination therapy according to the tumor characteristics of each patient (126). Using whole exome sequencing, targeted sequencing, and transcriptome sequencing, we can analyze the tumor-specific cancer driver genes and the abnormal signaling pathways involved in each patient’s tumor, such as PI3K/AKT, RAS/MAPK, DNA damage repair, cell cycle regulation, and transcriptional regulation (127). Most of the drugs targeting these pathways are currently in clinical trials, and the treatment strategies combined with other therapies, including anti-PD-1/PDL1 antibodies, have attracted much attention. In the future, clinical trials with larger sample sizes will be carried out to explore their effects on survival and disease development (17). It is believed that through further research, the treatment of TNBC will achieve greater breakthroughs in the future.
Author contributions
YH: conceptualization, writing – original draft, writing – review & editing. LS: funding acquisition, writing – review & editing. AZ: conceptualization, visualization.
Funding
The work is supported by the Foundation of National Natural Science Foundation of China (82004176) and the Foundation of Science and Technology Department of Sichuan Province (2020YJ0147).
Conflict of interest
The authors declare that the research was conducted in the absence of any commercial or financial relationships that could be construed as a potential conflict of interest.
Publisher's note
All claims expressed in this article are solely those of the authors and do not necessarily represent those of their affiliated organizations, or those of the publisher, the editors and the reviewers. Any product that may be evaluated in this article, or claim that may be made by its manufacturer, is not guaranteed or endorsed by the publisher.
Glossary
References
1. Puhl AC, Bogart JW, Haberman VA, Larson JE, Godoy AS, Norris-Drouin JL, et al. Discovery and characterization of peptide inhibitors for calcium and integrin binding protein 1. ACS Chem Biol (2020) 15(6):1505–16. doi: 10.1021/acschembio.0c00144
2. Dai X, Cheng H, Bai Z, Li J. Breast cancer cell line classification and its relevance with breast tumor subtyping. J Cancer (2017) 8(16):3131–41. doi: 10.7150/jca.18457
3. Huang C, Dai XY, Cai JX, Chen J, Wang BB, Zhu W, et al. A screened GPR1 peptide exerts antitumor effects on triple-negative breast cancer. Mol Ther Oncolytics (2020) 18:602–12. doi: 10.1016/j.omto.2020.08.013
4. Rakha EA, Reis-Filho JS, Ellis IO. Basal-like breast cancer: a critical review. J Clin Oncol (2008) 26(15):2568–81. doi: 10.1200/JCO.2007.13.1748
5. Chen YH, Yu MM, Wang ZG. Inhibition of MDA-MB-231 cell proliferation by pHLIP(Var7)-P1AP and SPECT imaging of MDA-MB-231 breast cancer-bearing nude mice using 125I-pHLIP(Var7)-P1AP. Nuklearmedizin (2021) 60(3):240–8. doi: 10.1055/a-1307-1923
6. Staquicini FI, Hajitou A, Driessen WH, Proneth B, Cardo-Vila M, Staquicini DI, et al. Targeting a cell surface vitamin D receptor on tumor-associated macrophages in triple-negative breast cancer. Elife (2021) 10:e65145. doi: 10.7554/eLife.65145
7. Oner G, Altintas S, Canturk Z, Tjalma W, Verhoeven Y, Van Berckelaer C, et al. Triple-negative breast cancer-Role of immunology: A systemic review. Breast J (2020) 26(5):995–9. doi: 10.1111/tbj.13696
8. Gotwals P, Cameron S, Cipolletta D, Cremasco V, Crystal A, Hewes B, et al. Prospects for combining targeted and conventional cancer therapy with immunotherapy. Nat Rev Cancer (2017) 17(5):286–301. doi: 10.1038/nrc.2017.17
9. Huang Y, Peng H, Zeng A, Song L. The role of peptides in reversing chemoresistance of breast cancer: current facts and future prospects. Front Pharmacol (2023) 14:1188477. doi: 10.3389/fphar.2023.1188477
10. Liu L, Bi Y, Zhou M, Chen X, He X, Zhang Y, et al. Biomimetic human serum albumin nanoparticle for efficiently targeting therapy to metastatic breast cancers. ACS Appl Mater Interfaces (2017) 9(8):7424–35. doi: 10.1021/acsami.6b14390
11. Saghaeidehkordi A, Chen S, Yang S, Kaur K. Evaluation of a keratin 1 targeting peptide-doxorubicin conjugate in a mouse model of triple-negative breast cancer. Pharmaceutics (2021) 13(5):661. doi: 10.3390/pharmaceutics13050661
12. Mustacchi G, De Laurentiis M. The role of taxanes in triple-negative breast cancer: literature review. Drug Des Devel Ther (2015) 9:4303–18. doi: 10.2147/DDDT.S86105
13. Swain SM, Kim SB, Cortes J, Ro J, Semiglazov V, Campone M, et al. Pertuzumab, trastuzumab, and docetaxel for HER2-positive metastatic breast cancer (CLEOPATRA study): overall survival results from a randomised, double-blind, placebo-controlled, phase 3 study. Lancet Oncol (2013) 14(6):461–71. doi: 10.1016/S1470-2045(13)70130-X
14. Wu Z, Stangl S, Hernandez-Schnelzer A, Wang F, Hasanzadeh Kafshgari M, Bashiri Dezfouli A, et al. Functionalized hybrid iron oxide-gold nanoparticles targeting membrane Hsp70 radiosensitize triple-negative breast cancer cells by ROS-mediated apoptosis. Cancers (Basel) (2023) 15(4):1167. doi: 10.3390/cancers15041167
15. O'Reilly D, Sendi MA, Kelly CM. Overview of recent advances in metastatic triple negative breast cancer. World J Clin Oncol (2021) 12(3):164–82. doi: 10.5306/wjco.v12.i3.164
16. Lehmann BD, Bauer JA, Chen X, Sanders ME, Chakravarthy AB, Shyr Y, et al. Identification of human triple-negative breast cancer subtypes and preclinical models for selection of targeted therapies. J Clin Invest (2011) 121(7):2750–67. doi: 10.1172/JCI45014
17. Yin L, Duan JJ, Bian XW, Yu SC. Triple-negative breast cancer molecular subtyping and treatment progress. Breast Cancer Res (2020) 22(1):61. doi: 10.1186/s13058-020-01296-5
18. Dubuc C, Savard M, Bovenzi V, Lessard A, Fortier A, Cote J, et al. Targeting intracellular B2 receptors using novel cell-penetrating antagonists to arrest growth and induce apoptosis in human triple-negative breast cancer. Oncotarget (2018) 9(11):9885–906. doi: 10.18632/oncotarget.24009
19. Cho YS, Kim HR, Park SJ, Chung SW, Ko YG, Yeo JH, et al. Sustained potentiation of bystander killing via PTEN-loss driven macropinocytosis targeted peptide-drug conjugate therapy in metastatic triple-negative breast cancer. Biomaterials (2022) 289:121783. doi: 10.1016/j.biomaterials.2022.121783
20. Kang SP, Martel M, Harris LN. Triple negative breast cancer: current understanding of biology and treatment options. Curr Opin Obstet Gynecol (2008) 20(1):40–6. doi: 10.1097/GCO.0b013e3282f40de9
21. De Laurentiis M, Cianniello D, Caputo R, Stanzione B, Arpino G, Cinieri S, et al. Treatment of triple negative breast cancer (TNBC): current options and future perspectives. Cancer Treat Rev (2010) 36 Suppl 3:S80–6. doi: 10.1016/S0305-7372(10)70025-6
22. Diana A, Franzese E, Centonze S, Carlino F, Della Corte CM, Ventriglia J, et al. Triple-negative breast cancers: systematic review of the literature on molecular and clinical features with a focus on treatment with innovative drugs. Curr Oncol Rep (2018) 20(10):76. doi: 10.1007/s11912-018-0726-6
23. Danhier F, Le Breton A, Preat V. RGD-based strategies to target alpha(v) beta(3) integrin in cancer therapy and diagnosis. Mol Pharm (2012) 9(11):2961–73. doi: 10.1021/mp3002733
24. Bose RJ, Kumar US, Garcia-Marques F, Zeng Y, Habte F, McCarthy JR, et al. Engineered cell-derived vesicles displaying targeting peptide and functionalized with nanocarriers for therapeutic microRNA delivery to triple-negative breast cancer in mice. Adv Healthc Mater (2022) 11(5):e2101387. doi: 10.1002/adhm.202101387
25. Scodeller P, Asciutto EK. Targeting tumors using peptides. Molecules (2020) 25(4):808. doi: 10.3390/molecules25040808
26. Vlieghe P, Lisowski V, Martinez J, Khrestchatisky M. "Synthetic therapeutic peptides: science and market.". Drug Discovery Today (2010) 15(1-2):40–56. doi: 10.1016/j.drudis.2009.10.009
27. Jiang C, Wang X, Teng B, Wang Z, Li F, Zhao Y, et al. Peptide-targeted high-density lipoprotein nanoparticles for combinatorial treatment against metastatic breast cancer. ACS Appl Mater Interfaces (2021) 13(30):35248–65. doi: 10.1021/acsami.1c02074
28. Horigome E, Fujieda M, Handa T, Katayama A, Ito M, Ichihara A, et al. Mutant TP53 modulates metastasis of triple negative breast cancer through adenosine A2b receptor signaling. Oncotarget (2018) 9(77):34554–66. doi: 10.18632/oncotarget.26177
29. Dubuc C, Savard M, Bovenzi V, Lessard A, Cote J, Neugebauer W, et al. Antitumor activity of cell-penetrant kinin B1 receptor antagonists in human triple-negative breast cancer cells. J Cell Physiol (2019) 234(3):2851–65. doi: 10.1002/jcp.27103
30. Bressler EM, Kim J, Shmueli RB, MIrando AC, Bazzazi H, Lee E, et al. Biomimetic peptide display from a polymeric nanoparticle surface for targeting and antitumor activity to human triple-negative breast cancer cells. J BioMed Mater Res A (2018) 106(6):1753–64. doi: 10.1002/jbm.a.36360
31. Hill BS, Sarnella A, Capasso D, Comegna D, Del Gatto A, Gramanzini M, et al. Therapeutic potential of a novel alpha(v)beta(3) antagonist to hamper the aggressiveness of mesenchymal triple negative breast cancer sub-type. Cancers (Basel) (2019) 11(2):139. doi: 10.3390/cancers11020139
32. Otvos L Jr., Kovalszky I, Riolfi M, Ferla R, Olah J, Sztodola A, et al. Efficacy of a leptin receptor antagonist peptide in a mouse model of triple-negative breast cancer. Eur J Cancer (2011) 47(10):1578–84. doi: 10.1016/j.ejca.2011.01.018
33. Nounamo B, Jousheghany F, Siegel ER, Post SR, Kelly T, Ferrone S, et al. VT68.2: an antibody to chondroitin sulfate proteoglycan 4 (CSPG4) displays reactivity against a tumor-associated carbohydrate antigen. Int J Mol Sci (2023) 24(3):2506. doi: 10.3390/ijms24032506
34. Xie W, Zhang Y, He Y, Zhang K, Wan G, Huang Y, et al. A novel recombinant human Frizzled-7 protein exhibits anti-tumor activity against triple negative breast cancer via abating Wnt/beta-catenin pathway. Int J Biochem Cell Biol (2018) 103:45–55. doi: 10.1016/j.biocel.2018.08.004
35. Smith SJ, Gu L, Phipps EA, Dobrolecki LE, Mabrey KS, Gulley P, et al. A Peptide mimicking a region in proliferating cell nuclear antigen specific to key protein interactions is cytotoxic to breast cancer. Mol Pharmacol (2015) 87(2):263–76. doi: 10.1124/mol.114.093211
36. Liu D, Guo P, McCarthy C, Wang B, Tao Y, Auguste D. Peptide density targets and impedes triple negative breast cancer metastasis. Nat Commun (2018) 9(1):2612. doi: 10.1038/s41467-018-05035-5
37. Kwon YJ, Petrie K, Leibovitch BA, Zeng L, Mezei M, Howell L, et al. Selective inhibition of SIN3 corepressor with avermectins as a novel therapeutic strategy in triple-negative breast cancer. Mol Cancer Ther (2015) 14(8):1824–36. doi: 10.1158/1535-7163.MCT-14-0980-T
38. Wang Y, Wu S, Zhu X, Zhang L, Deng J, Li F, et al. LncRNA-encoded polypeptide ASRPS inhibits triple-negative breast cancer angiogenesis. J Exp Med (2020) 217(3):jem.20190950. doi: 10.1084/jem.20190950
39. Ambaye ND, Yu HE. Novel anti-cancer candidates from a combinatorial peptide library. Chem Biol Drug Des (2021) 97(1):87–96. doi: 10.1111/cbdd.13763
40. Bousquet G, Feugeas JP, Gu Y, Leboeuf C, Bouchtaoui ME, Lu H, et al. High expression of apoptosis protein (Api-5) in chemoresistant triple-negative breast cancers: an innovative target. Oncotarget (2019) 10(61):6577–88. doi: 10.18632/oncotarget.27312
41. Haberman VA, Fleming SR, Leisner TM, Puhl AC, Feng E, Xie L, et al. Discovery and development of cyclic peptide inhibitors of CIB1. ACS Med Chem Lett (2021) 12(11):1832–9. doi: 10.1021/acsmedchemlett.1c00438
42. Kim S, Choi I, Han IH, Bae H. Enhanced therapeutic effect of optimized melittin-dKLA, a peptide agent targeting M2-like tumor-associated macrophages in triple-negative breast cancer. Int J Mol Sci (2022) 23(24):15751. doi: 10.3390/ijms232415751
43. Figueiredo P, Lepland A, Scodeller P, Fontana F, Torrieri G, Tiboni M, et al. Peptide-guided resiquimod-loaded lignin nanoparticles convert tumor-associated macrophages from M2 to M1 phenotype for enhanced chemotherapy. Acta Biomater (2021) 133:231–43. doi: 10.1016/j.actbio.2020.09.038
44. Raiter A, Zlotnik O, Lipovetsky J, Mugami S, Dar S, Lubin I, et al. A novel role for an old target: CD45 for breast cancer immunotherapy. Oncoimmunology (2021) 10(1):1929725. doi: 10.1080/2162402X.2021.1929725
45. Su F, Wang X, Pearson T, Lee J, Krishnamurthy S, Ueno NT, et al. Ablation of stromal cells with a targeted proapoptotic peptide suppresses cancer chemotherapy resistance and metastasis. Mol Ther Oncolytics (2020) 18:579–86. doi: 10.1016/j.omto.2020.08.012
46. Dorsam RT, Gutkind JS. G-protein-coupled receptors and cancer. Nat Rev Cancer (2007) 7(2):79–94. doi: 10.1038/nrc2069
47. Wittamer V, Franssen JD, Vulcano M, Mirjolet JF, Le Poul E, Migeotte I, et al. Specific recruitment of antigen-presenting cells by chemerin, a novel processed ligand from human inflammatory fluids. J Exp Med (2003) 198(7):977–85. doi: 10.1084/jem.20030382
48. Gao ZG, Jacobson KA. Emerging adenosine receptor agonists. Expert Opin Emerg Drugs (2007) 12(3):479–92. doi: 10.1517/14728214.12.3.479
49. Merighi S, Benini A, MIrandola P, Gessi S, Varani K, Simioni C, et al. Caffeine inhibits adenosine-induced accumulation of hypoxia-inducible factor-1alpha, vascular endothelial growth factor, and interleukin-8 expression in hypoxic human colon cancer cells. Mol Pharmacol (2007) 72(2):395–406. doi: 10.1124/mol.106.032920
50. Graf N, Bielenberg DR, Kolishetti N, Muus C, Banyard J, Farokhzad OC, et al. alpha(V)beta(3) integrin-targeted PLGA-PEG nanoparticles for enhanced anti-tumor efficacy of a Pt(IV) prodrug. ACS Nano (2012) 6(5):4530–9. doi: 10.1021/nn301148e
51. Kim J, MIrando AC, Popel AS, Green JJ. Gene delivery nanoparticles to modulate angiogenesis. Adv Drug Delivery Rev (2017) 119:20–43. doi: 10.1016/j.addr.2016.11.003
52. Luo J, Yao JF, Deng XF, Zheng XD, Jia M, Wang YQ, et al. 14, 15-EET induces breast cancer cell EMT and cisplatin resistance by up-regulating integrin alphavbeta3 and activating FAK/PI3K/AKT signaling. J Exp Clin Cancer Res (2018) 37(1):23. doi: 10.1186/s13046-018-0694-6
53. Garofalo C, Koda M, Cascio S, Sulkowska M, Kanczuga-Koda L, Golaszewska J, et al. Increased expression of leptin and the leptin receptor as a marker of breast cancer progression: possible role of obesity-related stimuli. Clin Cancer Res (2006) 12(5):1447–53. doi: 10.1158/1078-0432.CCR-05-1913
54. Surmacz E. Obesity hormone leptin: a new target in breast cancer? Breast Cancer Res (2007) 9(1):301. doi: 10.1186/bcr1638
55. Ray A, Nkhata KJ, Cleary MP. Effects of leptin on human breast cancer cell lines in relationship to estrogen receptor and HER2 status. Int J Oncol (2007) 30(6):1499–509. doi: 10.3892/ijo.30.6.1499
56. Ishikawa M, Kitayama J, Nagawa H. Enhanced expression of leptin and leptin receptor (OB-R) in human breast cancer. Clin Cancer Res (2004) 10(13):4325–31. doi: 10.1158/1078-0432.CCR-03-0749
57. Wang X, Wang Y, Yu L, Sakakura K, Visus C, Schwab JH, et al. CSPG4 in cancer: multiple roles. Curr Mol Med (2010) 10(4):419–29. doi: 10.2174/156652410791316977
58. Clevers H, Nusse R. Wnt/beta-catenin signaling and disease. Cell (2012) 149(6):1192–205. doi: 10.1016/j.cell.2012.05.012
59. Flanagan DJ, Phesse TJ, Barker N, Schwab RH, Amin N, Malaterre J, et al. Frizzled7 functions as a Wnt receptor in intestinal epithelial Lgr5(+) stem cells. Stem Cell Rep (2015) 4(5):759–67. doi: 10.1016/j.stemcr.2015.03.003
60. Prosperi E. Multiple roles of the proliferating cell nuclear antigen: DNA replication, repair and cell cycle control. Prog Cell Cycle Res (1997) 3:193–210. doi: 10.1007/978-1-4615-5371-7_15
61. Rouzier R, Perou CM, Symmans WF, Ibrahim N, Cristofanilli M, Anderson K, et al. Breast cancer molecular subtypes respond differently to preoperative chemotherapy. Clin Cancer Res (2005) 11(16):5678–85. doi: 10.1158/1078-0432.CCR-04-2421
62. Mehraj U, Mushtaq U, Mir MA, Saleem A, Macha MA, Lone MN, et al. Chemokines in triple-negative breast cancer heterogeneity: New challenges for clinical implications. Semin Cancer Biol (2022) 86(Pt 2):769–83. doi: 10.1016/j.semcancer.2022.03.008
63. Tan W, Martin D, Gutkind JS. The Galpha13-Rho signaling axis is required for SDF-1-induced migration through CXCR4. J Biol Chem (2006) 281(51):39542–9. doi: 10.1074/jbc.M609062200
64. Ridley AJ, Hall A. The small GTP-binding protein rho regulates the assembly of focal adhesions and actin stress fibers in response to growth factors. Cell (1992) 70(3):389–99. doi: 10.1016/0092-8674(92)90163-7
65. Kadamb R, Mittal S, Bansal N, Batra H, Saluja D. Sin3: insight into its transcription regulatory functions. Eur J Cell Biol (2013) 92(8-9):237–46. doi: 10.1016/j.ejcb.2013.09.001
66. Ellison-Zelski SJ, Alarid ET. Maximum growth and survival of estrogen receptor-alpha positive breast cancer cells requires the Sin3A transcriptional repressor. Mol Cancer (2010) 9:263. doi: 10.1186/1476-4598-9-263
67. Wotton D, Knoepfler PS, Laherty CD, Eisenman RN, Massague J. The Smad transcriptional corepressor TGIF recruits mSin3. Cell Growth Differ (2001) 12(9):457–63. doi: 10.1038/sj.cdd.4400904
68. Mahjoubin-Tehran M, Rezaei S, Jalili A, Aghaee-Bakhtiari SH, Orafai HM, Jamialahmadi T, et al. Peptide decoys: a new technology offering therapeutic opportunities for breast cancer. Drug Discovery Today (2020) 25(3):593–8. doi: 10.1016/j.drudis.2020.01.010
69. Silverstein RA, Ekwall K. Sin3: a flexible regulator of global gene expression and genome stability. Curr Genet (2005) 47(1):1–17. doi: 10.1007/s00294-004-0541-5
70. Stewart TA, Pattengale PK, Leder P. Spontaneous mammary adenocarcinomas in transgenic mice that carry and express MTV/myc fusion genes. Cell (1984) 38(3):627–37. doi: 10.1016/0092-8674(84)90257-5
71. Bansal N, Petrie K, Christova R, Chung CY, Leibovitch BA, Howell L, et al. Targeting the SIN3A-PF1 interaction inhibits epithelial to mesenchymal transition and maintenance of a stem cell phenotype in triple negative breast cancer. Oncotarget (2015) 6(33):34087–105. doi: 10.18632/oncotarget.6048
72. Johnsen SA, Subramaniam M, Janknecht R, Spelsberg TC. TGFbeta inducible early gene enhances TGFbeta/Smad-dependent transcriptional responses. Oncogene (2002) 21(37):5783–90. doi: 10.1038/sj.onc.1205681
73. Song H, Wang R, Wang S, Lin J. A low-molecular-weight compound discovered through virtual database screening inhibits Stat3 function in breast cancer cells. Proc Natl Acad Sci U.S.A. (2005) 102(13):4700–5. doi: 10.1073/pnas.0409894102
74. Rigou P, Piddubnyak V, Faye A, Rain JC, Michel L, Calvo F, et al. The antiapoptotic protein AAC-11 interacts with and regulates Acinus-mediated DNA fragmentation. EMBO J (2009) 28(11):1576–88. doi: 10.1038/emboj.2009.106
75. Naik UP, Patel PM, Parise LV. Identification of a novel calcium-binding protein that interacts with the integrin alphaIIb cytoplasmic domain. J Biol Chem (1997) 272(8):4651–4. doi: 10.1074/jbc.272.8.4651
76. Gentry HR, Singer AU, Betts L, Yang C, Ferrara JD, Sondek J, et al. Structural and biochemical characterization of CIB1 delineates a new family of EF-hand-containing proteins. J Biol Chem (2005) 280(9):8407–15. doi: 10.1074/jbc.M411515200
77. Naik MU, Naik UP. Contra-regulation of calcium- and integrin-binding protein 1-induced cell migration on fibronectin by PAK1 and MAP kinase signaling. J Cell Biochem (2011) 112(11):3289–99. doi: 10.1002/jcb.23255
78. Leisner TM, Liu M, Jaffer ZM, Chernoff J, Parise LV. Essential role of CIB1 in regulating PAK1 activation and cell migration. J Cell Biol (2005) 170(3):465–76. doi: 10.1083/jcb.200502090
79. Zhang Y, Liu C, Wu C, Song L. Natural peptides for immunological regulation in cancer therapy: Mechanism, facts and perspectives. BioMed Pharmacother (2023) 159:114257. doi: 10.1016/j.biopha.2023.114257
80. Hayashi G, Okamoto A. Probe design for the effective fluorescence imaging of intracellular RNA. Chem Rec (2013) 13(2):209–17. doi: 10.1002/tcr.201200026
81. Solodeev I, Zahalka MA, Moroz C. The novel C24D synthetic polypeptide inhibits binding of placenta immunosuppressive ferritin to human T cells and elicits anti-breast cancer immunity in vitro and in vivo. Neoplasia (2014) 16(9):741–50. doi: 10.1016/j.neo.2014.08.005
82. Barreira M, Rodriguez-Fdez S, Bustelo XR. New insights into the Vav1 activation cycle in lymphocytes. Cell Signal (2018) 45:132–44. doi: 10.1016/j.cellsig.2018.01.026
83. Gascard P, Tlsty TD. Carcinoma-associated fibroblasts: orchestrating the composition of Malignancy. Genes Dev (2016) 30(9):1002–19. doi: 10.1101/gad.279737.116
84. Zhang T, Tseng C, Zhang Y, Sirin O, Corn PG, Li-Ning-Tapia EM, et al. CXCL1 mediates obesity-associated adipose stromal cell trafficking and function in the tumour microenvironment. Nat Commun (2016) 7:11674. doi: 10.1038/ncomms11674
85. So JY, Ohm J, Lipkowitz S, Yang L. Triple negative breast cancer (TNBC): Non-genetic tumor heterogeneity and immune microenvironment: Emerging treatment options. Pharmacol Ther (2022) 237:108253. doi: 10.1016/j.pharmthera.2022.108253
86. Patel BK, Wang C, Lorens B, Levine AD, Steinmetz NF, Shukla S. Cowpea mosaic virus (CPMV)-based cancer testis antigen NY-ESO-1 vaccine elicits an antigen-specific cytotoxic T cell response. ACS Appl Bio Mater (2020) 3(7):4179–87. doi: 10.1021/acsabm.0c00259
87. Razazan A, Behravan J. Single peptides and combination modalities for triple negative breast cancer. J Cell Physiol (2020) 235(5):4089–108. doi: 10.1002/jcp.29300
88. Tuohy VK, Jaini R, Johnson JM, Loya MG, Wilk D, Downs-Kelly E, et al. Targeted Vaccination against Human alpha-Lactalbumin for Immunotherapy and Primary Immunoprevention of Triple Negative Breast Cancer. Cancers (Basel) (2016) 8(6):56. doi: 10.3390/cancers8060056
89. Li S, Wu Y, Ding F, Yang J, Li J, Gao X, et al. Engineering macrophage-derived exosomes for targeted chemotherapy of triple-negative breast cancer. Nanoscale (2020) 12(19):10854–62. doi: 10.1039/d0nr00523a
90. Song L, Yang J, Qin Z, Ou C, Luo R, Yang W, et al. Multi-targeted and on-demand non-coding RNA regulation nanoplatform against metastasis and recurrence of triple-negative breast cancer. Small (2023) 19(23):e2207576. doi: 10.1002/smll.202207576
91. Vaidya AM, Sun Z, Ayat N, Schilb A, Liu X, Jiang H, et al. Systemic Delivery of Tumor-Targeting siRNA Nanoparticles against an Oncogenic LncRNA Facilitates Effective Triple-Negative Breast Cancer Therapy. Bioconjug Chem (2019) 30(3):907–19. doi: 10.1021/acs.bioconjchem.9b00028
92. Chen G, Wang Y, Xie R, Gong S. Tumor-targeted pH/redox dual-sensitive unimolecular nanoparticles for efficient siRNA delivery. J Control Release (2017) 259:105–14. doi: 10.1016/j.jconrel.2017.01.042
93. Wang X, Zhu X, Li B, Wei X, Chen Y, Zhang Y, et al. Intelligent biomimetic nanoplatform for systemic treatment of metastatic triple-negative breast cancer via enhanced EGFR-targeted therapy and immunotherapy. ACS Appl Mater Interfaces (2022). 14(20):23152–63.doi: 10.1021/acsami.2c02925
94. Thongchot S, Jirapongwattana N, Luangwattananun P, Chiraphapphaiboon W, Chuangchot N, Sa-Nguanraksa D, et al. Adoptive transfer of anti-nucleolin T cells combined with PD-L1 inhibition against triple-negative breast cancer. Mol Cancer Ther (2022) 21(5):727–39. doi: 10.1158/1535-7163.MCT-21-0823
95. Hu D, Zhang W, Xiang J, Li D, Chen Y, Yuan P, et al. A ROS-responsive synergistic delivery system for combined immunotherapy and chemotherapy. Mater Today Bio (2022) 14:100284. doi: 10.1016/j.mtbio.2022.100284
96. Li Q, Liu M, Wu M, Zhou X, Wang S, Hu Y, et al PLAC1-specific TCR-engineered T cells mediate antigen-specific antitumor effects in breast cancer. Oncol Lett (2018) 15(4):5924–32. doi: 10.3892/ol.2018.8075
97. Niu S, Williams GR, Wu J, Wu J, Zhang X, Chen X, et al. A chitosan-based cascade-responsive drug delivery system for triple-negative breast cancer therapy. J Nanobiotechnology (2019) 17(1):95. doi: 10.1186/s12951-019-0529-4
98. Eid E,EM, Alanazi A S, Koosha S, Alrasheedy A A, Azam F, Taban I M, et al. Zerumbone Induces Apoptosis in Breast Cancer Cells by Targeting alphavbeta3 Integrin upon Co-Administration with TP5-iRGD Peptide. Molecules (2019) 24(14)2554. doi: 10.3390/molecules24142554
99. Yang YY, Zhang W, Liu H, Jiang JJ, Wang WJ, Jia ZY. Cell-penetrating peptide-modified graphene oxide nanoparticles loaded with rictor siRNA for the treatment of triple-negative breast cancer. Drug Des Devel Ther (2021) 15:4961–72. doi: 10.2147/DDDT.S330059
100. Huang J, Lai W, Wang Q, Tang Q, Hu C, Zhou M, et al Effective triple-negative breast cancer targeted treatment using iRGD-modified RBC membrane-camouflaged nanoparticles. Int J Nanomed (2021) 16:7497–515. doi: 10.2147/IJN.S321071
101. Demeule M, Charfi C, Currie JC, Larocque A, Zgheib A, Kozelko S, et al. TH1902, a new docetaxel-peptide conjugate for the treatment of sortilin-positive triple-negative breast cancer. Cancer Sci (2021) 112(10):4317–34. doi: 10.1111/cas.15086
102. Li D, Ma S, Xu D, Meng X, Lei N, Liu C, et al. Peptide-functionalized therapeutic nanoplatform for treatment orthotopic triple negative breast cancer and bone metastasis. Nanomedicine (2023) 50:102669. doi: 10.1016/j.nano.2023.102669
103. Mu QG, Lin G, Jeon M, Wang H, Chang FC, Revia RA, et al. Iron oxide nanoparticle targeted chemo-immunotherapy for triple negative breast cancer. Mater Today (Kidlington) (2021) 50:149–69. doi: 10.1016/j.mattod.2021.08.002
104. Xiao C, Han J, Bai J, Xia Y, Wang S. Trojan-like peptide drug conjugate design and construction for application in treatment of triple-negative breast cancer. J BioMed Nanotechnol (2021) 17(8):1554–63. doi: 10.1166/jbn.2021.3104
105. Wang Y, Yin S, Mei L, Yang Y, Xu S, He X, et al. A dual receptors-targeting and size-switchable "cluster bomb" co-loading chemotherapeutic and transient receptor potential ankyrin 1 (TRPA-1) inhibitor for treatment of triple negative breast cancer. J Control Release (2020) 321:71–83. doi: 10.1016/j.jconrel.2020.02.010
106. Conte C, Longobardi G, Barbieri A, Palma G, Luciano A, Dal Poggetto G, et al. Non-covalent strategies to functionalize polymeric nanoparticles with NGR peptides for targeting breast cancer. Int J Pharm (2023) 633:122618. doi: 10.1016/j.ijpharm.2023.122618
107. Ko EJ, Kang SM. Immunology and efficacy of MF59-adjuvanted vaccines. Hum Vaccin Immunother (2018) 14(12):3041–5. doi: 10.1080/21645515.2018.1495301
108. Wein L, Savas P, Luen SJ, Virassamy B, Salgado R, Loi S. Clinical validity and utility of tumor-infiltrating lymphocytes in routine clinical practice for breast cancer patients: current and future directions. Front Oncol (2017) 7:156. doi: 10.3389/fonc.2017.00156
109. Xie XP, Laks DR, Sun D, Ganbold M, Wang Z, Pedraza AM, et al. Quiescent human glioblastoma cancer stem cells drive tumor initiation, expansion, and recurrence following chemotherapy. Dev Cell (2022) 57(1):32–46.e8. doi: 10.1016/j.devcel.2021.12.007
110. Kim DH, Rossi JJ. Strategies for silencing human disease using RNA interference. Nat Rev Genet (2007) 8(3):173–84. doi: 10.1038/nrg2006
111. Peng J, Hamanishi J, Matsumura N, Abiko K, Murat K, Baba T, et al. "Chemotherapy induces programmed cell death-ligand 1 overexpression via the nuclear factor-kappaB to foster an immunosuppressive tumor microenvironment in ovarian cancer.". Cancer Res (2015) 75(23):5034–45. doi: 10.1158/0008-5472.CAN-14-3098
112. Pardoll DM. The blockade of immune checkpoints in cancer immunotherapy. Nat Rev Cancer (2012) 12(4):252–64. doi: 10.1038/nrc3239
113. Ren Y, Qian Y, Ai L, Xie Y, Gao Y, Zhuang Z, et al. TRAPPC4 regulates the intracellular trafficking of PD-L1 and antitumor immunity. Nat Commun (2021) 12(1):5405. doi: 10.1038/s41467-021-25662-9
114. Qi J, Li H, Liu N, Xing Y, Zhou G, Wu Y, et al. The implications and mechanisms of the extra-nuclear nucleolin in the esophageal squamous cell carcinomas. Med Oncol (2015) 32(3):45. doi: 10.1007/s12032-015-0484-3
115. Old LJ. Cancer is a somatic cell pregnancy. Cancer Immun (2007) 7:19. doi: 10.1158/1424-9634.DCL-19.7.1
116. Leclerc D, Beauseigle D, Denis J, Morin H, Pare C, Lamarre A, et al. "Proteasome-independent major histocompatibility complex class I cross-presentation mediated by papaya mosaic virus-like particles leads to expansion of specific human T cells.". J Virol (2007) 81(3):1319–26. doi: 10.1128/JVI.01720-06
117. Fatima M, Abourehab MAS, Aggarwal G, Jain GK, Sahebkar A, Kesharwani P. Advancement of cell-penetrating peptides in combating triple-negative breast cancer. Drug Discovery Today (2022) 27(11):103353. doi: 10.1016/j.drudis.2022.103353
118. Sehrawat A, Arlotti JA, Murakami A, Singh SV. Zerumbone causes Bax- and Bak-mediated apoptosis in human breast cancer cells and inhibits orthotopic xenograft growth in vivo. Breast Cancer Res Treat (2012) 136(2):429–41. doi: 10.1007/s10549-012-2280-5
119. Husain SR, Han J, Au P, Shannon K, Puri RK. Gene therapy for cancer: regulatory considerations for approval. Cancer Gene Ther (2015) 22(12):554–63. doi: 10.1038/cgt.2015.58
120. Teesalu T, Sugahara KN, Kotamraju VR, Ruoslahti E. C-end rule peptides mediate neuropilin-1-dependent cell, vascular, and tissue penetration. Proc Natl Acad Sci U S A (2009) 106(38):16157–62. doi: 10.1073/pnas.0908201106
121. Li M, Tang Z, Zhang D, Sun H, Liu H, Zhang Y, et al. Doxorubicin-loaded polysaccharide nanoparticles suppress the growth of murine colorectal carcinoma and inhibit the metastasis of murine mammary carcinoma in rodent models. Biomaterials (2015) 51:161–72. doi: 10.1016/j.biomaterials.2015.02.002
122. Yang F, Xiao Y, Ding JH, Jin X, Ma D, Li DQ, et al. Ferroptosis heterogeneity in triple-negative breast cancer reveals an innovative immunotherapy combination strategy. Cell Metab (2023) 35(1):84–100.e8. doi: 10.1016/j.cmet.2022.09.021
123. Keenan TE, Tolaney SM. Role of immunotherapy in triple-negative breast cancer. J Natl Compr Canc Netw (2020) 18(4):479–89. doi: 10.6004/jnccn.2020.7554
124. Zhang R, Yang Y, Dong W, Lin M, He J, Zhang X, et al. D-mannose facilitates immunotherapy and radiotherapy of triple-negative breast cancer via degradation of PD-L1. Proc Natl Acad Sci U S A (2022) 119(8):e2114851119. doi: 10.1073/pnas.2114851119
125. Deepak KGK, Vempati R, Nagaraju GP, Dasari VR, N. S, Rao DN, et al. Tumor microenvironment: Challenges and opportunities in targeting metastasis of triple negative breast cancer. Pharmacol Res (2020) 153:104683. doi: 10.1016/j.phrs.2020.104683
126. Quereda V, Bayle S, Vena F, Frydman SM, Monastyrskyi A, Roush WR, et al. Therapeutic targeting of CDK12/CDK13 in triple-negative breast cancer. Cancer Cell (2019) 36(5):545–58.e7. doi: 10.1016/j.ccell.2019.09.004
Keywords: triple-negative breast cancer, peptide, immunity, target, combination
Citation: Huang Y, Zeng A and Song L (2023) Facts and prospects of peptide in targeted therapy and immune regulation against triple-negative breast cancer. Front. Immunol. 14:1255820. doi: 10.3389/fimmu.2023.1255820
Received: 09 July 2023; Accepted: 11 August 2023;
Published: 25 August 2023.
Edited by:
Yingfeng Wan, University of Michigan, United StatesReviewed by:
Yihang Qi, Chinese Academy of Medical Sciences and Peking Union Medical College, ChinaJia Li, University of North Carolina at Charlotte, United States
Copyright © 2023 Huang, Zeng and Song. This is an open-access article distributed under the terms of the Creative Commons Attribution License (CC BY). The use, distribution or reproduction in other forums is permitted, provided the original author(s) and the copyright owner(s) are credited and that the original publication in this journal is cited, in accordance with accepted academic practice. No use, distribution or reproduction is permitted which does not comply with these terms.
*Correspondence: Linjiang Song, c29uZ2xpbmppYW5nQGNkdXRjbS5lZHUuY24=; Anqi Zeng, emVuZzYwMDJhcUAxNjMuY29t