- 1Center for Energy Metabolism and Reproduction, Shenzhen Institutes of Advanced Technology, Chinese Academy of Sciences, Shenzhen, China
- 2Department of Research and Development, Shenzhen Innovation Immunotechnology Co., Ltd, Shenzhen, China
- 3Department of Research and Development, Shenzhen Institute for Innovation and Translational Medicine, Shenzhen, China
New York-esophageal cancer 1 (NY-ESO-1) belongs to the cancer testis antigen (CTA) family, and has been identified as one of the most immunogenic tumor-associated antigens (TAAs) among the family members. Given its ability to trigger spontaneous humoral and cellular immune response and restricted expression, NY-ESO-1 has emerged as one of the most promising targets for cancer immunotherapy. Cancer vaccines, an important element of cancer immunotherapy, function by presenting an exogenous source of TAA proteins, peptides, and antigenic epitopes to CD4+ T cells via major histocompatibility complex class II (MHC-II) and to CD8+ T cells via major histocompatibility complex class I (MHC-I). These mechanisms further enhance the immune response against TAAs mediated by cytotoxic T lymphocytes (CTLs) and helper T cells. NY-ESO-1-based cancer vaccines have a history of nearly two decades, starting from the first clinical trial conducted in 2003. The current cancer vaccines targeting NY-ESO-1 have various types, including Dendritic cells (DC)-based vaccines, peptide vaccines, protein vaccines, viral vaccines, bacterial vaccines, therapeutic whole-tumor cell vaccines, DNA vaccines and mRNA vaccines, which exhibit their respective benefits and obstacles in the development and application. Here, we summarized the current advances in cancer vaccines targeting NY-ESO-1 for solid cancer treatment, aiming to provide perspectives for future research.
1 Introduction
The NY-ESO-1 gene is located in chromosome Xq28 with a total length of 747 bp and encodes an 18-KDa protein (1). NY-ESO-1 is a 180-amino acid protein with a glycine-rich N-terminal region and a strongly hydrophobic C-terminal region that contains a conserved Pcc-1 domain (2). The expression of this gene is predominantly restricted to a variety of solid tumors, germ cells and placental cells, exhibiting little or no expression in normal adult somatic tissues (3). The positive expression rate of NY-ESO-1 varies among different solid tumors. For instance, myxoid and round cell liposarcoma shows the highest expression rate (89-100%) of NY-ESO-1 by immunohistochemistry with the monoclonal antibodies ES121 and E978 (4), followed by neuroblastoma (82%), synovial sarcoma (80%), melanoma (46%), and epithelial ovarian cancer (43%) (5–9). The highly restricted expression of NY-ESO-1 antigen in normal tissues (male germ cells) and its widespread expression in different tumor types make it a promising candidate target for tumor immunotherapy. However, the heterogeneous expression pattern of NY-ESO-1 in tumor tissues may affect the treatment effect of immunotherapy. The most homogeneous expression of NY-ESO-1 has been reported in synovial sarcomas (70%) (6), making these tumors a promising candidate for immunotherapy targeting the NY-ESO-1 antigen. Multiple clinical trials are currently in progress to explore the potential of NY-ESO-1 as a target for cancer immunotherapy. One strategy involves the use of genetically modified T cells that are engineered to specifically recognize and eliminate cancer cells expressing NY-ESO-1. Another strategy is to use cancer vaccines or other treatments to induce and activate the endogenous immune system to recognize and eliminate NY-ESO-1-positive cancer cells (3).
Cancer vaccines are a type of vaccine that is designed to activate the immune system to recognize and attack cancer cells (10). Unlike traditional vaccines, which are designed to prevent infectious diseases, cancer vaccines are used to treat cancer or to prevent cancer from recurring after treatment (10). Although cancer vaccines have shown promising results in preclinical and clinical trials, they are not yet widely available or approved for use as a standard cancer treatment (11, 12). Sipuleucel-T (Provenge, or APC8015) is currently only one FDA-approved therapeutic cancer vaccine, which is a DC-based vaccine to treat prostate cancer that has metastasized (11, 13). Cancer cells may evade immune attack induced by cancer vaccines through various mechanisms, including antigen depletion, alterations in antigen processing and the decreased surface expression of human leukocyte antigen class I (HLA-I) molecules (11). In addition, since the successful treatment with cancer vaccines depends on the activation of T cells, an effective response may not occur if the patient’s cancer cells have an insufficient capacity for tumor antigen processing and presentation (14, 15). Therefore, future studies may focus on combining appropriate cancer vaccines with innovative immunomodulatory strategies and standard-of-care treatment for overcoming impaired antitumor responses and therapy resistance (11). Research in this area is ongoing, and it is hoped that cancer vaccines will one day become an important tool in fighting against cancer. Here, we summarized the current advances in cancer vaccines targeting NY-ESO-1 for solid cancer treatment, aiming to provide perspectives for future research.
2 Cancer vaccines targeting NY-ESO-1
The efficacy of cancer vaccines targeting NY-ESO-1 antigen has been extensively investigated using various formulations, including DC vaccines, peptide vaccines, protein vaccines, viral vaccines, bacterial vaccines, therapeutic whole-tumor cell vaccines, DNA vaccines and mRNA vaccines. These studies have validated the safety of the NY-ESO-1 antigen-targeted vaccines and demonstrated their immunogenicity. (Figure 1)
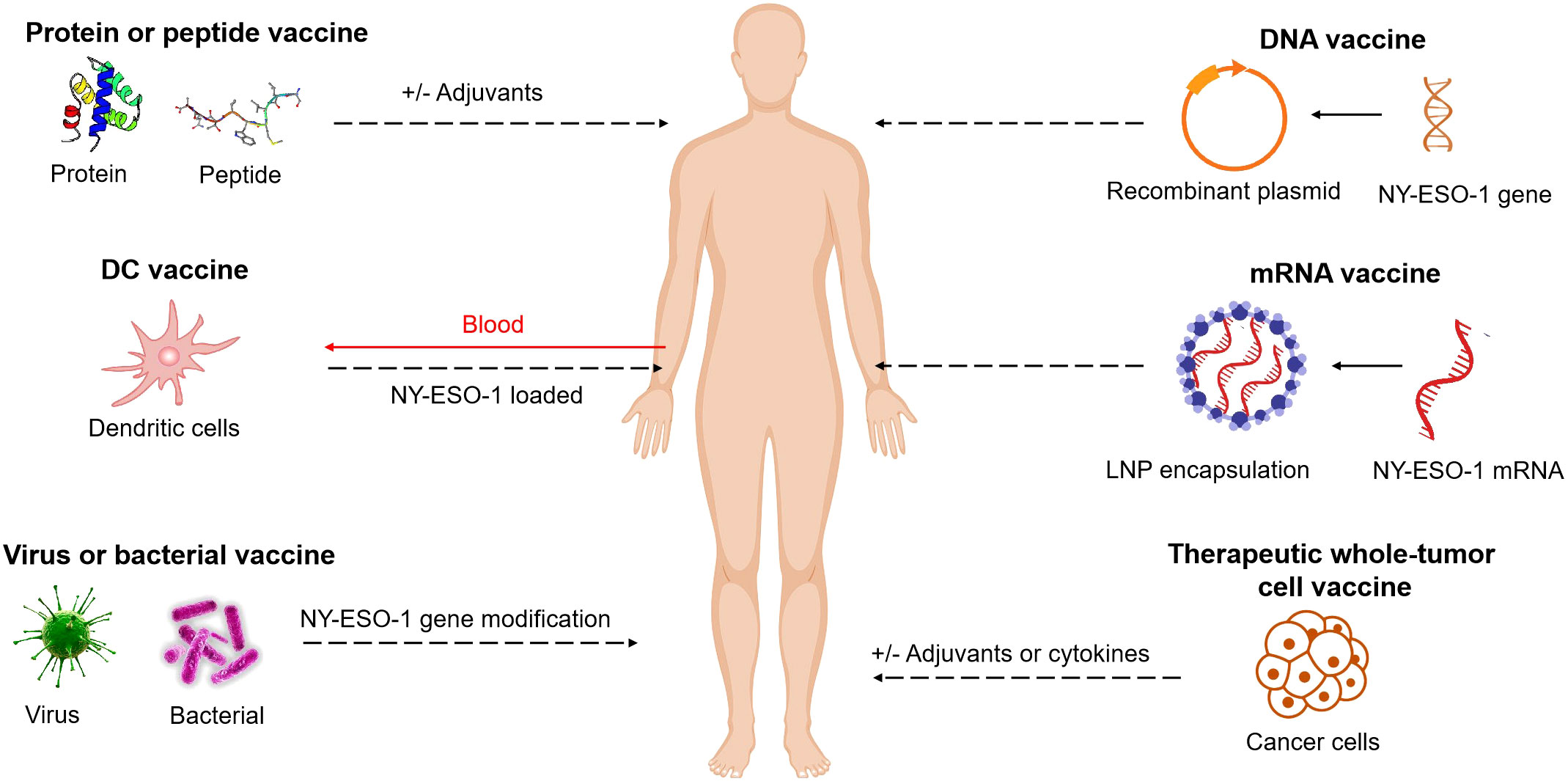
Figure 1 NY-ESO-1-based cancer vaccine approaches. Vaccines include short peptides, full length proteins (with and without adjuvants), DC vaccines, viruses or bacterial vaccines with NY-ESO-1 gene modification, DNA or mRNA vaccines (with NY-ESO-1 in sequences), therapeutic whole-tumor cell vaccines. These elements can be modified, added to adjuvants, or combined together.
2.1 Bacterial vaccines
Bacteria possess pathogen-associated molecular patterns (PAMPs) which can be recognized by pattern recognition receptors (PRRs) like Toll-like receptors (TLRs)on immune cells and trigger an innate immune response against the bacteria. This innate immune response can also influence the adaptive immune system, making attenuated or avirulent recombinant bacterial vectors highly effective in stimulating targeted and durable immune responses to the antigens carried in the bacterial vectors (16, 17). These bacterial vectors not only produce and deliver antigens, but also act as immune-stimulating adjuvants. Several preclinical and clinical trials had investigated the effectiveness of bacterial vaccines targeting NY-ESO-1. The Salmonella typhimurium vaccine was engineered to deliver NY-ESO-1 antigen through a type III protein secretion system, and was proved to be effective in mice and could elicit NY-ESO-1-specific CD8+ and CD4+ T cells responses in vitro (18). Interestingly, the antigen-specific T cell responses induced by S. typhimurium-NY-ESO-1 vaccine were resistant to suppression by CD4+CD25+ Treg cells (19). In addition, a phase I clinical trial (NCT01967758) on a live-attenuated listeria monocytogenes vaccine (ADU-623), which was engineered to express NY-ESO-1 and EGFRvIII for the treatment of glioma, was performed, but clinical results for safety and efficacy have not been reported yet.
In contrast to viral vaccine vectors, bacterial vectors under development for human use are susceptible to antibiotics, enabling treatment in case of adverse reactions during extensive vaccination trials (20). This characteristic has led to increased interest in bacterial vectors for the development of cancer vaccines.
2.2 DC vaccines
DCs are the dominant antigen-presenting cells in the immune system and play a crucial role in initiating adaptive immune responses (21). Extensive clinical trials have been conducted, particularly in patients with advanced melanoma, to investigate the potential of DCs in immunotherapy (22–24). In these trials, patients were treated with autologous DCs, which were loaded with antigens in order to induce specific T cell responses against cancer cells. DCs can be loaded with the antigens in various forms, including peptides, whole protein, tumor lysate, apoptotic debris or complexed with antibody (25). Moreover, antigens encoded by mRNA or cDNA is particularly appealing as it offers a more straightforward approach compared to the use of externally provided peptides and proteins. This approach simplifies the isolation and utilization of antigens in clinical settings (25–27). However, the transfection of DCs with cDNA encoding antigens has not been demonstrated effectiveness (28). In contrast, the transfection of DCs with mRNA encoding antigens has been found to be a highly efficient method for antigen loading (26, 28).
Several ongoing clinical trials are exploring the safety and efficacy of NY-ESO-1 peptide-pulsed DCs, either alone or in combination with NY-ESO-1 protein vaccine and TLR agonists. The information of these trials are included in Table 1. As an illustrative example, in a phase IIa trial (NCT02692976), DCs were activated with protamine/mRNA and loaded with TAAs including NY-ESO-1, MAGE-C2 and MUC-1. After vaccination, antigen-specific T cells were detectable in peripheral blood of 12 out of 21 patients (57%). Specifically, NY-ESO-1, MAGE-C2 and MUC-1 specific T cells were identified in the blood of 10 out of 21 patients (48%), 4 out of 21 patients (19%), and 2 out of 21 patients (10%), respectively. Importantly, all patients tolerated the DC vaccines well, with only grade 1-2 toxicity reported (29). Additional trials have been performed to evaluate the safety and feasibility of combining NY-ESO-1 peptide-pulsed DC vaccine with NY-ESO-1 transduced T cell therapy and Nivolumab in advanced solid cancers (NCT02775292, Table 1). This combination therapy demonstrated temporary anti-tumor activity. Reconstitution of NY-ESO-1-specific T cells in the peripheral blood reached its peak within two weeks, indicating rapid in vivo expansion. The transferred lymphocytes exhibited predominantly an effector memory phenotype, without progressing to exhaustion or terminal differentiation. On-treatment biopsy revealed complete loss of NY-ESO-1 expression, accompanied by extensive methylation of the promoter sequence (30). The combination of NY-ESO-1 pulsed DC vaccination named CMB305 with Atezolizumab has been investigated in patients with sarcoma (NCT02609984, Table 1). While the combination of CMB305 and atezolizumab did not show significant improvements in progression-free survival (PFS) or overall survival (OS) compared to atezolizumab alone, some patients exhibited indications of an anti-NY-ESO-1 immune response and showed better outcomes in imaging assessments (31).
Another approach to target cancer vaccines toward DCs is through TLRs. A notable example is the utilization of a NY-ESO-1 encoding LV305 lentivirus, which specifically targets DCs through TLR3 and TLR7. This approach induced a robust cellular immune response and resulted in significant disease regression in one patient with metastatic, treatment-refractory synovial sarcoma (32). The mentioned case report is part of a completed phase I clinical trial (NCT02122861, Table 1) investigating the intradermal administration of NY-ESO-1-specific lentiviral DC-targeting in various cancer types, including melanoma, non-small cell lung cancer (NSCLC), ovarian cancer, and sarcoma.
DC vaccines have the capability to incorporate tumor proteins, mRNAs, and DNAs, enabling the rapid activation of T cells independent of the patients’ HLA type. Moreover, DC-based vaccines have demonstrated a favorable safety profile. However, the clinical effectiveness of NY-ESO-1 peptide-pulsed DC vaccines has not consistently met expectations, which may be due to a low proportion of patients achieving specific anti-NY-ESO-1 immune responses. Additionally, the complexity of the procedure poses challenges in ensuring the reliability of DC vaccines.
2.3 DNA vaccines
DNA vaccines are a type of vaccine that contain a DNA fragment encoding a specific protein antigen. These vaccines work by activating the body’s immune responses through the expression of the antigen, which helps to fight against the tumor development and progression. In particular, DNA vaccines targeting NY-ESO-1 have been studied in both preclinical and clinical trials as a potential immunotherapy.
A phase I clinical trials (NCT00199849) on NY-ESO-1 DNA vaccine (pPJV7611, plasmid) has been completed for tumor vaccination (33). The vaccine was administered safely through particle-mediated epidermal delivery (PMED) and evaluated for safety and immunogenicity in patients with confirmed NY-ESO-1 antigen expression. Among 15 patients who had no antigen-specific immune responses prior to vaccination, 14 (93%) developed antigen-specific CD4+ T cell responses, and 5 patients (33%) developed CD8+ T cell responses. However, the durability of T cell responses was not strong, possibly due to the suppressive effects of regulatory T cells. Additionally, the study found that T cells had different specificity for various regions of the NY-ESO-1 protein at different time points, which may be due to the effect of regulatory T cells on different subpopulations of effector T cells, resulting in changes in T cell responses to specific peptides (33).
SCIB2 is an antibody DNA vaccine that encodes the NY-ESO-1 antigen and covers over 80% of HLA phenotypes by encoding 16 NY-ESO-1 epitopes (34). Compared to peptide vaccines, it generates an increased frequency and enhanced affinity of T cell responses, which exhibit potent cytotoxic activity against tumor cells expressing NY-ESO-1. In mice, SCIB2 demonstrated the ability to effectively suppress the growth of B16-NY-ESO-1-expressing tumor cells, leading to a long-term survival rate of 35%. When administered in conjunction with Treg depletion, CTLA-4 blockade, or PD-1 blockade, the long-term survival rates of mice exhibited substantial improvements, reaching 56%, 67%, and 100%, respectively (34).However, clinical results of SCIB2 have not been reported yet.
DNA vaccine is simple, stable and cost effective. However, one of the initial worries regarding the use of DNA vaccines was the possibility of integrating into the human genome. According to FDA guidelines, the rate of plasmid integration in DNA vaccines should be significantly lower than the rate of spontaneous mutation. Moreover, based on the existing clinical trial results, the NY-ESO-1 DNA vaccine cannot elicit satisfactory immune responses. Consequently, due to the non-genomic integration advantages and the remarkable achievements of COVID-19 vaccines, there is a growing interest among researchers in exploring the potential of NY-ESO-1 mRNA cancer vaccines as a promising approach.
2.4 mRNA vaccines
The COVID-19 pandemic has brought widespread attention to mRNA vaccine technology (35, 36). In fact, the rapid development of COVID-19 mRNA vaccines may be attributed to extensive prior research on mRNA vaccines as a potential therapeutic approach for cancer in both preclinical and clinical trials (36). mRNA vaccines offer several advantages in the vaccination process: firstly, mRNA vaccines are readily degradable and exhibit a favorable safety profile, and secondly, mRNA vaccines are a non-infectious, non-integrating platform technology, thereby eliminating the potential risks of infection or insertional mutations. Thirdly, mRNA vaccines have the potential to stimulate both humoral and cellular responses; finally, mRNA vaccines can be produced quickly at low cost (36). mRNA cancer vaccines can be custom-designed to target specific tumor antigens expressed by cancer cells, stimulating robust in vivo immune responses mediated by T cells or B cells against the tumors (37, 38). Since the inception and validation of the first mRNA cancer vaccine (39), a multitude of clinical studies have provided evidence for the safety and efficacy of mRNA vaccines in cancer therapy.
Up to now, there are three mRNA cancer vaccines targeting NY-ESO-1 that have entered clinical stages, named BNT111, CV9201 and CV9202. BNT111 is a nano-liposomal mRNA vaccine developed by BioNTech. It was granted orphan drug designation by the US FDA in September 2021. BNT111 encodes 4 TAAs: NY-ESO-1, MAGE-A3, tyrosinase, and TPTE. These 4 TAAs are expressed in over 90% of skin melanomas and have high immunogenicity. A Phase I clinical trial (NCT02410733) evaluating the use of BNT111 alone or in combination with PD-1 inhibitors in patients with unresectable melanoma demonstrated favorable safety profiles and initial signs of anti-tumor efficacy. Among 50 patients, over 39 patients (75%) were found to have immune responses to one or more TAAs, and BNT111 was able to induce CD4+ and CD8+ T cell responses. 17 patients received combination therapy with BNT111 and anti-PD-1, of whom 6 (35%) had a partial response and 2 (12%) had stable disease. 25 patients received BNT111 monotherapy, of whom 3 (12%) had a partial response and 7 (28%) had stable disease (40). Based on the success of NCT02410733 trial, the BNT111 vaccine received fast track qualification in November 2021. A randomized phase II clinical trial (NCT04526899) is currently evaluating FixVac BNT111, either alone or in combination with the PD-1 antibody cemiplimab, for the treatment of unresectable stage III and IV melanoma patients.
In another Phase I/IIa clinical trial (NCT00923312), 7 patients with locally advanced disease and 39 patients with metastatic NSCLC were treated with 5 doses of intradermal injection of CV9201, an active vaccine encoding 5 antigens including NY-ESO-1, MAGE-C1/2, survivin, trophoblast glycoprotein and 5T4 (41). The results showed that 63% of patients exhibited specific immune responses to one or more TAAs, and 60% of patients showed increased activation of IgD+CD38high B cells. Disease stabilization (SD) was observed in 31% of patients, while the remaining patients exhibited disease progression (41). In a similar Phase I/IIa clinical trial (NCT03164772), CV9202 was administered in combination with local radiotherapy in patients with advanced NSCLC (42, 43). CV9202 is an RNA-based active vaccine encoding 6 TAAs including NY-ESO-1, MAGE-C1, MAGE-C2, 5T4, survivin, and MUC-1. The results showed that compared to baseline, 80% of patients experienced an increase in antigen-specific antibody levels, 40% of patients showed an increase in functional T cells, and 52% of patients exhibited evident involvement of multiple antigen-specific responses. One patient achieved PR with combined therapy of pembrolizumab, and 46.2% of patients achieved SD (42).
The development of NY-ESO-1 mRNA cancer vaccines has progressed rapidly, and future improvements may focus on achieving higher expression levels and prolonged expression time specifically within the target tissue.
2.5 protein and peptide vaccines
Protein- and peptide-based cancer vaccines are a form of immunotherapy that stimulate an immune response against TAAs or tumor-specific antigens (TSAs) by utilizing purified, recombinant, or synthetically engineered epitopes and proteins to trigger host immune responses (44, 45).
The NY-ESO-1 protein and peptide cancer vaccine trials have undergone significant advancements since their initial clinical trials over a decade ago. The discovery of proteins and the formulation of vaccines have been improved greatly, resulting in a range of synthetic peptides, recombinant proteins (both individual and complexed), and adjuvant formulations. Numerous clinical trials evaluating NY-ESO-1 recombinant protein vaccines have been conducted (Table 1). However, nearly none of these trials have advanced to phase III. The use of recombinant proteins can result in suboptimal or non-specific immune responses due to protein misfolding and inadequate epitope presentation (46–48). Thus, some researchers have redirected their attention towards NY-ESO-1 peptide vaccines (Table 1), Peptides derived from the NY-ESO-1 antigen bypass the requirement for protein antigen expression and processing, allowing for direct loading of epitopes onto MHC-I/MHC-II molecules. Currently, researchers have identified 21 unique epitopes that are restricted to at least 5 different HLA-class II alleles in the NY-ESO-1 antigen (3). Among these epitopes, NY-ESO-180–109 and NY-ESO-1157–165 peptides demonstrated the highest immunogenicity, eliciting both CD4+ and CD8+ T cell responses (49). However, short peptides are poorly immunogenic and require adjuvants for enhanced and prolonged immune responses (50, 51). Various adjuvants have been extensively evaluated in conjunction with the NY-ESO-1 peptides and protein vaccines in clinical trials (48, 49, 52–66). These adjuvants include TLR agonists, such as OK-432, CpG, poly-ICLC, or MIS416, saponin-based adjuvant (ISCOMATRIX), incomplete Freund’s adjuvant (IFA) Montanide ISA-51. In a phase I clinical trial (NCT00616941) (55, 67), researchers investigated the impact of poly-ICLC and Montanide adjuvant on pre- and post-vaccine NY-ESO-1-specific CD4+ T cells. Vaccination with overlapping long peptides (OLP) from NY-ESO-1 alone did not induce CD4+ T cell responses. However, emulsifying OLP in Montanide was necessary for expanding high-avidity NY-ESO-1-specific CD4+ T cell precursors. Additionally, poly-ICLC significantly enhanced CD4+ Th1 responses while suppressing the generation of interleukin (IL)-4-producing Th2 and IL-9-producing Th9 cells (55). Another clinical trial was conducted to evaluate the safety and efficacy of CHP-NY-ESO-1 with MIS416 adjuvant in patients with refractory solid tumors expressing NY-ESO-1 (57). A total of 26 patients were enrolled in the study, and 7 patients (38%) continued receiving vaccinations during the maintenance phase. Throughout the trial, 6 patients (23%) experienced grade 3 drug-related adverse events, with one patient exhibiting anorexia and 5 patients experiencing hypertension. No grade 4-5 drug-related adverse events were reported. Among the patients, 8 (31%) demonstrated SD.
Additionally, NY-ESO-1 vaccination is being combined with other treatments, such as the mTOR inhibitor Sirolimus (NCT01522820), Decitabine (NCT01673217, NCT03358719), Azacitidine (NCT02750995), Cyclophosphamide (NCT00819806, NCT00518206 (68)), IDO1 inhibitor Epacadostat (NCT02166905) and PD-1 antibody Nivolumab (NCT05479045, NCT01176461 (69), NCT02737787, NCT01176474, NCT03358719) etc. And further investigation is needed to explore the potential of NY-ESO-1 protein and peptide vaccine in combination with other treatments to enhance the efficacy of the therapy.
The protein and peptide vaccines are simple, cheap to manufacture and stable when transported, which makes large-scale manufacture and transportation possible. Preclinical and clinical results have demonstrated the potency of NY-ESO-1 protein and peptide vaccines. However, the efficacy of individual peptide vaccines is often limited to specific HLA subtypes. Consequently, patients who do not express the commonly targeted HLA types may not be eligible for treatment with this vaccine. Besides, the immune response induced by synthetic peptide-based cancer vaccines may not accurately reflects or complements the natural immune response to endogenous antigen expression. Studies comparing the TCR features of naturally and vaccine-elicited NY-ESO-1 specific CTLs have indicated that these cells exhibited a highly conserved structure but distinct TCR features (70). These results indicate that the synthetic peptides used for vaccination may fail to accurately reflect the naturally processed antigen and antitumor immune response (3, 70). Since the vaccine peptides might not be the ones naturally processed. Thus, the actual presence on the tumor cells of the peptides used in vaccines should better be validated. Further research is needed to determine the optimal approach for developing effective NY-ESO-1 cancer vaccines that accurately reflect the natural immune response.
2.6 Therapeutic whole-tumor cell vaccines
The whole-tumor cell vaccine consists of genetically modified human tumor cell lines, which can be either autologous or allogeneic tumor cells. This vaccine approach exposes the immune system to a diverse range of tumor antigens, many of which are often unknown. To date, no therapeutic whole-tumor cell vaccine targeting NY-ESO-1 has advanced to the clinical stage. In a preclinical study, Xu et al. genetically engineered Renca cancer cells to express NY-ESO-1 and injected them into the renal cancer tumor (lacking NY-ESO-1) mice model (71). After treatment, the tumor size was significantly reduced in comparison to the control group. This reduction was attributed to the increased interaction between DCs and T cells with the NY-ESO-1 expressed Renca cancer cells. This suggests that NY-ESO-1 may be effective in training T cells to recognize and target the tumor-specific epitopes with high immunogenicity, even in tumors lacking NY-ESO-1, if coupled with the appropriate antigens.
2.7 Viral vaccines
Recombinant virus can act as vectors to express antigen gene, which represent a promising platform for vaccines. As the immune system has evolved to efficiently control viral infections, viral gene products may activate APCs, such as DCs, by triggering PRRs. At present, several viruses have been exploited as cancer vaccine platforms for encoding NY-ESO-1 antigens. NY-ESO-1 encoding recombinant fowlpox and recombinant vaccinia have been evaluated in clinical trials (72). Considering host-neutralizing immunity of subsequent vaccinations, the most viral vaccines, such as adenoviruses, vaccinia, MVA and other mammalian poxviruses, can only be given once. On the contrary, multiple injections of recombinant avipox (i.e., fowlpox) have been shown to induce non-neutralizing antibodies. Therefore, the recombinant fowlpox can be used for multiple booster vaccinations. In a clinical trial conducted under the Cancer Vaccine Collaborative (NCT00112957) (72), researchers used recombinant vaccinia-NY-ESO-1 (rV-NY-ESO-1) as prime and recombinant fowlpox-NY-ESO-1 (rF-NY-ESO-1) as booster in patients with various advanced solid tumors, and this diversified prime-boost regimen was certified to be safe and successfully induced both humoral and cellular immune responses specific to NY-ESO-1 in the majority of patients. Moreover, two parallel phase II clinical trials were conducted in 25 melanoma and 22 epithelial ovarian cancer (EOC) patients with advanced disease who were at high risk for recurrence/progression to test the clinical efficacy of rV-NY-SO-1 and rF-NY-ESO-1 prime-boost regimen (73). The results showed that a significant proportion of melanoma and EOC patients exhibited induction of NY-ESO-1-specific antibody, as well as CD4+ and CD8+ T cells. In the melanoma patients, CR rate was 9.5% (2/21), PR rate was 4.8% (1/21), mixed response rate (MR) was 4.8% (1/21), and SD rate was 52.4% (11/21), resulting in a clinical benefit rate (CBR) of 71.5%. In the melanoma patients, the median PFS and the median OS was 9 months and 48 months, respectively. In the 22 EOC patients, the median PFS and the median OS was 21 months and 48 months, respectively (73).
vCP2292 [ALVAC (2)-NY-ESO-1(M)-TRICOM] is a recombinant ALVAC (2) poxviruse containing transgenes NY-ESO-1 and co-stimulatory molecules (TRICOM: B7-1, ICAM-1, and LFA-3). TRICOM genes have been shown to have the ability to induce higher numbers of antigen-specific T cells, enhance the avidity of these T cells, and improve tumor activity (74). In preclinical trials, it was observed that vCP2292 could elicit NY-ESO-1 specific T cell responses in mice. Unexpectedly, vectors lacking TRICOM generated higher NY-ESO-1-specific responses than those containing TRICOM. This could be due to difference between human TRICOM with its murine counterpart, and the human TRICOM inserted in the vectors was recognized as foreign antigen in the mice, the immune response against the foreign human TRICOM was further competed with the anti-TAA responses, leading to a reduction in the responses elicited by vectors containing TRICOM. However, this effect is not considered worrisome in a human context, as no immune responses against TRICOM were observed in clinical trials involving other vectors carrying human TRICOM (74).
As cancer advances, malignant cells have the ability to evade detection by the immune system. Boehmer et al (75) found the existence of immunoediting and immune escape in a melanoma patient, whose primary tumor displayed expression of NY-ESO-1, MAGE-C1, and Melan-A. The patient was immunized with a recombinant NY-ESO-1 fowlpox vaccine followed by immunization with NY-ESO-1 protein + CpG. Spontaneous humoral and cellular responses against NY-ESO-1 were observed, which were further enhanced by subsequent immunizations. However, despite induction of the immune responses, in the following years, all developing lesions were found to be negative for NY-ESO-1, while being positive for MAGE-C1, Melan-A, and MHC-I (75). Thus, a multivalent NY-ESO-1 vaccine (including two or more cancer antigens) might be more effective at preventing the outgrowth of NY-ESO-1-negative tumors.
The virus-based NY-ESO-1 vaccines demonstrate natural immunogenicity, and the oncolytic virus vaccines exhibit the capacity to directly eradicate tumor cells. However, the emergence of neutralizing antibodies in patients and safety concerns may impose limitations on its clinical application.
3 Conclusion and perspectives
NY-ESO-1 is a potential prognostic factor and a promising target for immunotherapy. With an enhanced comprehension of antitumor immune mechanisms, various approaches, including cancer vaccines, adoptive T cell therapy (ACT) and combination therapies targeting NY-ESO-1 against solid cancers have made significant progress in recent years. Cancer vaccines targeting NY-ESO-1 have come a long way, different types have shown promising results in preclinical trials, leading to the initiation of new clinical trials for the treatment of solid cancers. Currently, there are more than 70 clinical trials registered using NY-ESO-1-based cancer vaccines, and they have gained significant attention in clinical prevention and treatment. While there have not yet been any successful clinical applications of NY-ESO-1-based cancer vaccines, it is crucial to note that they hold significant potential for antitumor applications following additional refinement and assessment in clinical trials. Safety and effectiveness are crucial factors that must be considered in all preclinical and clinical studies. To ensure the successful development of cancer vaccines targeting NY-ESO-1 in clinical trials, several critical questions must be addressed. Firstly, it is essential to activate and balance both the innate and adaptive immune responses through various mechanisms and pathways. Secondly, the enhanced immune monitoring function after vaccination is essential to accurately assess the vaccine’s efficacy. Finally, the issue of tumor immune evasion must be addressed, as tumors can downregulate antigen presentation or induce immune suppression, which can limit the effectiveness of tumor vaccines.
As the self-antigens are expressed in the thymus, the T cell repertoire of CD8+ or/and CD4+ T cell against self-antigen will be blunted by central tolerance (76), the immunogenicity of cancer vaccines targeting NY-ESO-1 in patients with advanced cancer may be affected by the immuno-editing and tumor evasion. To address this issue, future research efforts should focus on optimizing the immune microenvironment to enhance the activation of immune cells and promote tumor recognition and rejection. Additionally, the protective immune effect of cancer vaccines targeting NY-ESO-1 can be influenced by the immunization route. Therefore, further investigation into the optimization of immune processes, improvement of vaccine design and the potential advantages of cancer vaccines targeting NY-ESO-1 is warranted.
As a monotherapy, cancer vaccines have not yet been demonstrated to show outstanding clinical results in malignant tumor treatment. Recently, there has been a growing interest in the integration of cancer vaccines with ACTs to combat tumor evasion of immune surveillance and resistance (77–79). Ma et al (77, 78) identified that cancer vaccination induces metabolic changes in CAR-T cells, leading to an increase in the production of interferon gamma (IFN-γ), which may potentially contribute to overcoming the immunosuppressive tumor microenvironment. Furthermore, the enhanced efficacy of CAR-T cells induced by tumor vaccination may be associated with DCs, including factors such as DCs recruitment, uptake of tumor antigens, and activation (77, 78). In another study, researchers discovered that the combination of tumor vaccines with TCR-T cell therapy significantly enhanced the anti-tumor response of TCR-T cells and induced epitope spreading among the endogenous T cell population, leading to durable eradication of established solid tumors in syngeneic tumor models (79). The improved anti-tumor efficacy was associated with pro-inflammatory transcriptional reprogramming in lymph nodes and enhanced maturation of antigen presenting cells. This led to the expansion and functional enhancement of TCR-T cells in both lymph nodes and the solid tumor tissue, without the need for lymphodepletion (79). It is important to note that the aforementioned conclusions are based on experimental results obtained from mouse models, further research and validation are required to determine the feasibility and effectiveness of these findings in clinical applications. Additionally, it is crucial to delve deeper into the mechanisms underlying the interaction between vaccines and ACTs in order to optimize immune therapeutic strategies.
Author contributions
MW: Writing – review & editing. HZ: Writing – original draft. YM: Writing – review & editing. FL: Writing – review & editing. BL: Writing – review & editing. DQ: Writing – review & editing. PR: Writing – review & editing.
Funding
This work was supported by grants from the Shenzhen Science and Technology Research Program (grant number CJGJZD20200617102403009), Guangdong Basic and Applied Basic Research Foundation (grant number 2020B1515120018).
Conflict of interest
Authors HZ, YM, FL, BL, DQ and MW are employed by the company Shenzhen Innovation Immunotechnology Co., Ltd.
The remaining author declares that the research was conducted in the absence of any commercial or financial relationships that could be construed as a potential conflict of interest.
Publisher’s note
All claims expressed in this article are solely those of the authors and do not necessarily represent those of their affiliated organizations, or those of the publisher, the editors and the reviewers. Any product that may be evaluated in this article, or claim that may be made by its manufacturer, is not guaranteed or endorsed by the publisher.
References
1. Chen YT, Boyer AD, Viars CS, Tsang S, Old LJ, Arden KC. Genomic cloning and localization of ctag, a gene encoding an autoimmunogenic cancer-testis antigen ny-eso-1, to human chromosome xq28. Cytogenet Cell Genet (1997) 79(3-4):237–40. doi: 10.1159/000134734
2. Gnjatic S, Nishikawa H, Jungbluth AA, Gure AO, Ritter G, Jager E, et al. Ny-eso-1: review of an immunogenic tumor antigen. Adv Cancer Res (2006) 95:1–30. doi: 10.1016/S0065-230X(06)95001-5
3. Thomas R, Al-Khadairi G, Roelands J, Hendrickx W, Dermime S, Bedognetti D, et al. Ny-eso-1 based immunotherapy of cancer: current perspectives. Front Immunol (2018) 9:947. doi: 10.3389/fimmu.2018.00947
4. Hemminger JA, Ewart Toland A, Scharschmidt TJ, Mayerson JL, Kraybill WG, Guttridge DC, et al. The cancer-testis antigen ny-eso-1 is highly expressed in myxoid and round cell subset of liposarcomas. Mod Pathol (2013) 26(2):282–8. doi: 10.1038/modpathol.2012.133
5. Pollack SM, Jungbluth AA, Hoch BL, Farrar EA, Bleakley M, Schneider DJ, et al. Ny-eso-1 is a ubiquitous immunotherapeutic target antigen for patients with myxoid/round cell liposarcoma. Cancer (2012) 118(18):4564–70. doi: 10.1002/cncr.27446
6. Jungbluth AA, Antonescu CR, Busam KJ, Iversen K, Kolb D, Coplan K, et al. Monophasic and biphasic synovial sarcomas abundantly express cancer/testis antigen ny-eso-1 but not mage-A1 or ct7. Int J Cancer (2001) 94(2):252–6. doi: 10.1002/ijc.1451
7. Odunsi K, Jungbluth AA, Stockert E, Qian F, Gnjatic S, Tammela J, et al. Ny-eso-1 and lage-1 cancer-testis antigens are potential targets for immunotherapy in epithelial ovarian cancer. Cancer Res (2003) 63(18):6076–83.
8. Rodolfo M, Luksch R, Stockert E, Chen YT, Collini P, Ranzani T, et al. Antigen-specific immunity in neuroblastoma patients: antibody and T-cell recognition of ny-eso-1 tumor antigen. Cancer Res (2003) 63(20):6948–55.
9. Barrow C, Browning J, MacGregor D, Davis ID, Sturrock S, Jungbluth AA, et al. Tumor antigen expression in melanoma varies according to antigen and stage. Clin Cancer Res (2006) 12(3 Pt 1):764–71. doi: 10.1158/1078-0432.CCR-05-1544
10. Chehelgerdi M, Chehelgerdi M. The use of rna-based treatments in the field of cancer immunotherapy. Mol Cancer (2023) 22(1):106. doi: 10.1186/s12943-023-01807-w
11. Saxena M, van der Burg SH, Melief CJM, Bhardwaj N. Therapeutic cancer vaccines. Nat Rev Cancer (2021) 21(6):360–78. doi: 10.1038/s41568-021-00346-0
12. Lin MJ, Svensson-Arvelund J, Lubitz GS, Marabelle A, Melero I, Brown BD, et al. Cancer vaccines: the next immunotherapy frontier. Nat Cancer (2022) 3(8):911–26. doi: 10.1038/s43018-022-00418-6
13. Kantoff PW, Higano CS, Shore ND, Berger ER, Small EJ, Penson DF, et al. Sipuleucel-T immunotherapy for castration-resistant prostate cancer. N Engl J Med (2010) 363(5):411–22. doi: 10.1056/NEJMoa1001294
14. Sharma P, Hu-Lieskovan S, Wargo JA, Ribas A. Primary, adaptive, and acquired resistance to cancer immunotherapy. Cell (2017) 168(4):707–23. doi: 10.1016/j.cell.2017.01.017
15. Wang S, He Z, Wang X, Li H, Liu XS. Antigen presentation and tumor immunogenicity in cancer immunotherapy response prediction. Elife (2019) 8:e49020. doi: 10.7554/eLife.49020
16. da Silva AJ, Zangirolami TC, Novo-Mansur MT, Giordano Rde C, Martins EA. Live bacterial vaccine vectors: an overview. Braz J Microbiol (2014) 45(4):1117–29. doi: 10.1590/s1517-83822014000400001
17. Janeway CA Jr., Medzhitov R. Innate immune recognition. Annu Rev Immunol (2002) 20:197–216. doi: 10.1146/annurev.immunol.20.083001.084359
18. Nishikawa H, Sato E, Briones G, Chen LM, Matsuo M, Nagata Y, et al. In vivo antigen delivery by a salmonella typhimurium type iii secretion system for therapeutic cancer vaccines. J Clin Invest (2006) 116(7):1946–54. doi: 10.1172/JCI28045
19. Nishikawa H, Tsuji T, Jager E, Briones G, Ritter G, Old LJ, et al. Induction of regulatory T cell-resistant helper cd4+ T cells by bacterial vector. Blood (2008) 111(3):1404–12. doi: 10.1182/blood-2007-09-113761
20. Shata MT, Stevceva L, Agwale S, Lewis GK, Hone DM. Recent advances with recombinant bacterial vaccine vectors. Mol Med Today (2000) 6(2):66–71. doi: 10.1016/s1357-4310(99)01633-0
21. Banchereau J, Steinman RM. Dendritic cells and the control of immunity. Nature (1998) 392(6673):245–52. doi: 10.1038/32588
22. Figdor CG, de Vries IJ, Lesterhuis WJ, Melief CJ. Dendritic cell immunotherapy: mapping the way. Nat Med (2004) 10(5):475–80. doi: 10.1038/nm1039
23. Palucka K, Banchereau J. Dendritic-cell-based therapeutic cancer vaccines. Immunity (2013) 39(1):38–48. doi: 10.1016/j.immuni.2013.07.004
24. Bol KF, Figdor CG, Aarntzen EH, Welzen ME, van Rossum MM, Blokx WA, et al. Intranodal vaccination with mrna-optimized dendritic cells in metastatic melanoma patients. Oncoimmunology (2015) 4(8):e1019197. doi: 10.1080/2162402X.2015.1019197
26. Gilboa E, Vieweg J. Cancer immunotherapy with mrna-transfected dendritic cells. Immunol Rev (2004) 199:251–63. doi: 10.1111/j.0105-2896.2004.00139.x
27. Schuler G, Schuler-Thurner B, Steinman RM. The use of dendritic cells in cancer immunotherapy. Curr Opin Immunol (2003) 15(2):138–47. doi: 10.1016/s0952-7915(03)00015-3
28. Van Tendeloo VF, Ponsaerts P, Lardon F, Nijs G, Lenjou M, Van Broeckhoven C, et al. Highly efficient gene delivery by mrna electroporation in human hematopoietic cells: superiority to lipofection and passive pulsing of mrna and to electroporation of plasmid cdna for tumor antigen loading of dendritic cells. Blood (2001) 98(1):49–56. doi: 10.1182/blood.v98.1.49
29. Westdorp H, Creemers JHA, van Oort IM, Schreibelt G, Gorris MAJ, Mehra N, et al. Blood-derived dendritic cell vaccinations induce immune responses that correlate with clinical outcome in patients with chemo-naive castration-resistant prostate cancer. J Immunother Cancer (2019) 7(1):302. doi: 10.1186/s40425-019-0787-6
30. Frankiw L, Singh A, Peters C, Comin-Anduix B, Berent-Maoz B, Macabali M, et al. Immunotherapy resistance driven by loss of ny-eso-1 expression in response to transgenic adoptive cellular therapy with pd-1 blockade. J Immunother Cancer (2023) 11(5):e006930. doi: 10.1136/jitc-2023-006930
31. Chawla SP, Van Tine BA, Pollack SM, Ganjoo KN, Elias AD, Riedel RF, et al. Phase ii randomized study of cmb305 and atezolizumab compared with atezolizumab alone in soft-tissue sarcomas expressing ny-eso-1. J Clin Oncol (2022) 40(12):1291–300. doi: 10.1200/JCO.20.03452
32. Pollack SM, Lu H, Gnjatic S, Somaiah N, O’Malley RB, Jones RL, et al. First-in-human treatment with a dendritic cell-targeting lentiviral vector-expressing ny-eso-1, lv305, induces deep, durable response in refractory metastatic synovial sarcoma patient. J Immunother (2017) 40(8):302–6. doi: 10.1097/CJI.0000000000000183
33. Gnjatic S, Altorki NK, Tang DN, Tu SM, Kundra V, Ritter G, et al. Ny-eso-1 DNA vaccine induces T-cell responses that are suppressed by regulatory T cells. Clin Cancer Res (2009) 15(6):2130–9. doi: 10.1158/1078-0432.CCR-08-2632
34. Xue W, Metheringham RL, Brentville VA, Gunn B, Symonds P, Yagita H, et al. Scib2, an antibody DNA vaccine encoding ny-eso-1 epitopes, induces potent antitumor immunity which is further enhanced by checkpoint blockade. Oncoimmunology (2016) 5(6):e1169353. doi: 10.1080/2162402X.2016.1169353
35. Wei J, Hui AM. The paradigm shift in treatment from covid-19 to oncology with mrna vaccines. Cancer Treat Rev (2022) 107:102405. doi: 10.1016/j.ctrv.2022.102405
36. Lorentzen CL, Haanen JB, Met O, Svane IM. Clinical advances and ongoing trials on mrna vaccines for cancer treatment. Lancet Oncol (2022) 23(10):e450–e8. doi: 10.1016/S1470-2045(22)00372-2
37. Lang F, Schrors B, Lower M, Tureci O, Sahin U. Identification of neoantigens for individualized therapeutic cancer vaccines. Nat Rev Drug Discovery (2022) 21(4):261–82. doi: 10.1038/s41573-021-00387-y
38. Pastor F, Berraondo P, Etxeberria I, Frederick J, Sahin U, Gilboa E, et al. An rna toolbox for cancer immunotherapy. Nat Rev Drug Discovery (2018) 17(10):751–67. doi: 10.1038/nrd.2018.132
39. Conry RM, LoBuglio AF, Wright M, Sumerel L, Pike MJ, Johanning F, et al. Characterization of a messenger rna polynucleotide vaccine vector. Cancer Res (1995) 55(7):1397–400. doi: 10.1007/BF01519900
40. Sahin U, Oehm P, Derhovanessian E, Jabulowsky RA, Vormehr M, Gold M, et al. An rna vaccine drives immunity in checkpoint-inhibitor-treated melanoma. Nature (2020) 585(7823):107–12. doi: 10.1038/s41586-020-2537-9
41. Sebastian M, Schroder A, Scheel B, Hong HS, Muth A, von Boehmer L, et al. A phase I/iia study of the mrna-based cancer immunotherapy cv9201 in patients with stage iiib/iv non-small cell lung cancer. Cancer Immunol Immunother (2019) 68(5):799–812. doi: 10.1007/s00262-019-02315-x
42. Papachristofilou A, Hipp MM, Klinkhardt U, Fruh M, Sebastian M, Weiss C, et al. Phase ib evaluation of a self-adjuvanted protamine formulated mrna-based active cancer immunotherapy, bi1361849 (Cv9202), combined with local radiation treatment in patients with stage iv non-small cell lung cancer. J Immunother Cancer (2019) 7(1):38. doi: 10.1186/s40425-019-0520-5
43. Sebastian M, Papachristofilou A, Weiss C, Fruh M, Cathomas R, Hilbe W, et al. Phase ib study evaluating a self-adjuvanted mrna cancer vaccine (Rnactive(R)) combined with local radiation as consolidation and maintenance treatment for patients with stage iv non-small cell lung cancer. BMC Cancer (2014) 14:748. doi: 10.1186/1471-2407-14-748
44. Bezu L, Kepp O, Cerrato G, Pol J, Fucikova J, Spisek R, et al. Trial watch: peptide-based vaccines in anticancer therapy. Oncoimmunology (2018) 7(12):e1511506. doi: 10.1080/2162402X.2018.1511506
45. Tang M, Cai JH, Diao HY, Guo WM, Yang X, Xing S. The progress of peptide vaccine clinical trials in gynecologic oncology. Hum Vaccin Immunother (2022) 18(5):2062982. doi: 10.1080/21645515.2022.2062982
46. Ordaz ML, Larmonier N, Lybarger L. Dc-Expressed Mhc Class I Single-Chain Trimer-Based Vaccines Prime Cytotoxic T Lymphocytes against Exogenous but Not Endogenous Antigens. Cell Immunol (2010) 262(2):141–9. doi: 10.1016/j.cellimm.2010.02.006
47. Ebert LM, MacRaild SE, Zanker D, Davis ID, Cebon J, Chen W. A cancer vaccine induces expansion of ny-eso-1-specific regulatory T cells in patients with advanced melanoma. PloS One (2012) 7(10):e48424. doi: 10.1371/journal.pone.0048424
48. Nicholaou T, Ebert LM, Davis ID, McArthur GA, Jackson H, Dimopoulos N, et al. Regulatory T-cell-mediated attenuation of T-cell responses to the ny-eso-1 iscomatrix vaccine in patients with advanced Malignant melanoma. Clin Cancer Res (2009) 15(6):2166–73. doi: 10.1158/1078-0432.CCR-08-2484
49. Davis ID, Chen W, Jackson H, Parente P, Shackleton M, Hopkins W, et al. Recombinant ny-eso-1 protein with iscomatrix adjuvant induces broad integrated antibody and cd4(+) and cd8(+) T cell responses in humans. Proc Natl Acad Sci U.S.A. (2004) 101(29):10697–702. doi: 10.1073/pnas.0403572101
50. Patel BK, Wang C, Lorens B, Levine AD, Steinmetz NF, Shukla S. Cowpea mosaic virus (Cpmv)-based cancer testis antigen ny-eso-1 vaccine elicits an antigen-specific cytotoxic T cell response. ACS Appl Bio Mater (2020) 3(7):4179–87. doi: 10.1021/acsabm.0c00259
51. Apostolico Jde S, Lunardelli VA, Coirada FC, Boscardin SB, Rosa DS. Adjuvants: classification, modus operandi, and licensing. J Immunol Res (2016) 2016:1459394. doi: 10.1155/2016/1459394
52. Ishikawa T, Kageyama S, Miyahara Y, Okayama T, Kokura S, Wang L, et al. Safety and antibody immune response of chp-ny-eso-1 vaccine combined with poly-iclc in advanced or recurrent esophageal cancer patients. Cancer Immunol Immunother (2021) 70(11):3081–91. doi: 10.1007/s00262-021-02892-w
53. Pavlick A, Blazquez AB, Meseck M, Lattanzi M, Ott PA, Marron TU, et al. Combined vaccination with ny-eso-1 protein, poly-iclc, and montanide improves humoral and cellular immune responses in patients with high-risk melanoma. Cancer Immunol Res (2020) 8(1):70–80. doi: 10.1158/2326-6066.CIR-19-0545
54. Takeoka T, Nagase H, Kurose K, Ohue Y, Yamasaki M, Takiguchi S, et al. Ny-eso-1 protein cancer vaccine with poly-iclc and ok-432: rapid and strong induction of ny-eso-1-specific immune responses by poly-iclc. J Immunother (2017) 40(4):140–7. doi: 10.1097/CJI.0000000000000162
55. Tsuji T, Sabbatini P, Jungbluth AA, Ritter E, Pan L, Ritter G, et al. Effect of montanide and poly-iclc adjuvant on human self/tumor antigen-specific cd4+ T cells in phase I overlapping long peptide vaccine trial. Cancer Immunol Res (2013) 1(5):340–50. doi: 10.1158/2326-6066.CIR-13-0089
56. Adams S, O’Neill DW, Nonaka D, Hardin E, Chiriboga L, Siu K, et al. Immunization of Malignant melanoma patients with full-length ny-eso-1 protein using tlr7 agonist imiquimod as vaccine adjuvant. J Immunol (2008) 181(1):776–84. doi: 10.4049/jimmunol.181.1.776
57. Ishihara M, Tono Y, Miyahara Y, Muraoka D, Harada N, Kageyama S, et al. First-in-human phase I clinical trial of the ny-eso-1 protein cancer vaccine with nod2 and tlr9 stimulants in patients with ny-eso-1-expressing refractory solid tumors. Cancer Immunol Immunother (2020) 69(4):663–75. doi: 10.1007/s00262-020-02483-1
58. Kakimi K, Isobe M, Uenaka A, Wada H, Sato E, Doki Y, et al. A phase I study of vaccination with ny-eso-1f peptide mixed with picibanil ok-432 and montanide isa-51 in patients with cancers expressing the ny-eso-1 antigen. Int J Cancer (2011) 129(12):2836–46. doi: 10.1002/ijc.25955
59. Valmori D, Souleimanian NE, Tosello V, Bhardwaj N, Adams S, O’Neill D, et al. Vaccination with ny-eso-1 protein and cpg in montanide induces integrated antibody/th1 responses and cd8 T cells through cross-priming. Proc Natl Acad Sci U.S.A. (2007) 104(21):8947–52. doi: 10.1073/pnas.0703395104
60. Karbach J, Gnjatic S, Bender A, Neumann A, Weidmann E, Yuan J, et al. Tumor-reactive cd8+ T-cell responses after vaccination with ny-eso-1 peptide, cpg 7909 and montanide isa-51: association with survival. Int J Cancer (2010) 126(4):909–18. doi: 10.1002/ijc.24850
61. Diefenbach CS, Gnjatic S, Sabbatini P, Aghajanian C, Hensley ML, Spriggs DR, et al. Safety and immunogenicity study of ny-eso-1b peptide and montanide isa-51 vaccination of patients with epithelial ovarian cancer in high-risk first remission. Clin Cancer Res (2008) 14(9):2740–8. doi: 10.1158/1078-0432.CCR-07-4619
62. Karbach J, Neumann A, Atmaca A, Wahle C, Brand K, von Boehmer L, et al. Efficient in vivo priming by vaccination with recombinant ny-eso-1 protein and cpg in antigen naive prostate cancer patients. Clin Cancer Res (2011) 17(4):861–70. doi: 10.1158/1078-0432.CCR-10-1811
63. Patel SP, Petroni GR, Roszik J, Olson WC, Wages NA, Chianese-Bullock KA, et al. Phase I/ii trial of a long peptide vaccine (Lpv7) plus toll-like receptor (Tlr) agonists with or without incomplete freund’s adjuvant (Ifa) for resected high-risk melanoma. J Immunother Cancer (2021) 9(8):e003220. doi: 10.1136/jitc-2021-003220
64. Legat A, Maby-El Hajjami H, Baumgaertner P, Cagnon L, Abed Maillard S, Geldhof C, et al. Vaccination with lag-3ig (Imp321) and peptides induces specific cd4 and cd8 T-cell responses in metastatic melanoma patients–report of a phase I/iia clinical trial. Clin Cancer Res (2016) 22(6):1330–40. doi: 10.1158/1078-0432.CCR-15-1212
65. Baumgaertner P, Costa Nunes C, Cachot A, Maby-El Hajjami H, Cagnon L, Braun M, et al. Vaccination of stage iii/iv melanoma patients with long ny-eso-1 peptide and cpg-B elicits robust cd8(+) and cd4(+) T-cell responses with multiple specificities including a novel dr7-restricted epitope. Oncoimmunology (2016) 5(10):e1216290. doi: 10.1080/2162402X.2016.1216290
66. Kageyama S, Wada H, Muro K, Niwa Y, Ueda S, Miyata H, et al. Dose-dependent effects of ny-eso-1 protein vaccine complexed with cholesteryl pullulan (Chp-ny-eso-1) on immune responses and survival benefits of esophageal cancer patients. J Transl Med (2013) 11:246. doi: 10.1186/1479-5876-11-246
67. Sabbatini P, Tsuji T, Ferran L, Ritter E, Sedrak C, Tuballes K, et al. Phase I trial of overlapping long peptides from a tumor self-antigen and poly-iclc shows rapid induction of integrated immune response in ovarian cancer patients. Clin Cancer Res (2012) 18(23):6497–508. doi: 10.1158/1078-0432.CCR-12-2189
68. Klein O, Davis ID, McArthur GA, Chen L, Haydon A, Parente P, et al. Low-dose cyclophosphamide enhances antigen-specific cd4(+) T cell responses to ny-eso-1/iscomatrix vaccine in patients with advanced melanoma. Cancer Immunol Immunother (2015) 64(4):507–18. doi: 10.1007/s00262-015-1656-x
69. Weber JS, KudChadkar RR, Yu B, Gallenstein D, Horak CE, Inzunza HD, et al. Safety, efficacy, and biomarkers of nivolumab with vaccine in ipilimumab-refractory or -naive melanoma. J Clin Oncol (2013) 31(34):4311–8. doi: 10.1200/JCO.2013.51.4802
70. Le Gal FA, Ayyoub M, Dutoit V, Widmer V, Jager E, Cerottini JC, et al. Distinct structural tcr repertoires in naturally occurring versus vaccine-induced cd8+ T-cell responses to the tumor-specific antigen ny-eso-1. J Immunother (2005) 28(3):252–7. doi: 10.1097/01.cji.0000161398.34701.26
71. Xu L, Zheng J, Nguyen DH, Luong QT, Zeng G. Enhancing whole-tumor cell vaccination by engaging innate immune system through ny-eso-1/dendritic cell interactions. J Immunother (2013) 36(8):412–22. doi: 10.1097/CJI.0b013e3182a80263
72. Jager E, Karbach J, Gnjatic S, Neumann A, Bender A, Valmori D, et al. Recombinant vaccinia/fowlpox ny-eso-1 vaccines induce both humoral and cellular ny-eso-1-specific immune responses in cancer patients. Proc Natl Acad Sci U.S.A. (2006) 103(39):14453–8. doi: 10.1073/pnas.0606512103
73. Odunsi K, Matsuzaki J, Karbach J, Neumann A, Mhawech-Fauceglia P, Miller A, et al. Efficacy of vaccination with recombinant vaccinia and fowlpox vectors expressing ny-eso-1 antigen in ovarian cancer and melanoma patients. Proc Natl Acad Sci U.S.A. (2012) 109(15):5797–802. doi: 10.1073/pnas.1117208109
74. Vogel TU, Visan L, Ljutic B, Gajewska B, Caterini J, Salha D, et al. Preclinical qualification of a new multi-antigen candidate vaccine for metastatic melanoma. J Immunother (2010) 33(8):743–58. doi: 10.1097/CJI.0b013e3181eccc87
75. von Boehmer L, Mattle M, Bode P, Landshammer A, Schafer C, Nuber N, et al. Ny-eso-1-specific immunological pressure and escape in a patient with metastatic melanoma. Cancer Immun (2013) 13:12.
76. van der Burg SH, Arens R, Ossendorp F, van Hall T, Melief CJ. Vaccines for established cancer: overcoming the challenges posed by immune evasion. Nat Rev Cancer (2016) 16(4):219–33. doi: 10.1038/nrc.2016.16
77. Ma L, Dichwalkar T, Chang JYH, Cossette B, Garafola D, Zhang AQ, et al. Enhanced Car-T Cell Activity against Solid Tumors by Vaccine Boosting through the Chimeric Receptor. Science (2019) 365(6449):162–8. doi: 10.1126/science.aav8692
78. Ma L, Hostetler A, Morgan DM, Maiorino L, Sulkaj I, Whittaker CA, et al. Vaccine-boosted car T crosstalk with host immunity to reject tumors with antigen heterogeneity. Cell (2023) 186(15):3148–65. doi: 10.1016/j.cell.2023.06.002
Keywords: CTA, NY-ESO-1, cancer vaccine, immunotherapy, solid cancer
Citation: Zhou H, Ma Y, Liu F, Li B, Qiao D, Ren P and Wang M (2023) Current advances in cancer vaccines targeting NY-ESO-1 for solid cancer treatment. Front. Immunol. 14:1255799. doi: 10.3389/fimmu.2023.1255799
Received: 09 July 2023; Accepted: 22 August 2023;
Published: 05 September 2023.
Edited by:
Yunlang She, Tongji University, ChinaReviewed by:
Hans-Georg Rammensee, University of Tübingen, GermanyXingbing Wang, The First Affiliated Hospital of University of Science and Technology of China Anhui Provincial Hospital, China
Yanhui Yin, Peking University, China
Copyright © 2023 Zhou, Ma, Liu, Li, Qiao, Ren and Wang. This is an open-access article distributed under the terms of the Creative Commons Attribution License (CC BY). The use, distribution or reproduction in other forums is permitted, provided the original author(s) and the copyright owner(s) are credited and that the original publication in this journal is cited, in accordance with accepted academic practice. No use, distribution or reproduction is permitted which does not comply with these terms.
*Correspondence: Mingjun Wang, bWluZ2p1bndAc3ppbm5vLmNvbQ==; Peigen Ren, cGcucmVuQHNpYXQuYWMuY24=