- 1Transfusion Medicine, Faculty of Medicine Carl Gustav Carus, Dresden University of Technology, Dresden, Germany
- 2Institute for Transfusion Medicine Dresden, German Red Cross Blood Donation Service North-East, Dresden, Germany
- 3CRTD - Center for Regenerative Therapies TU Dresden, Dresden University of Technology, Dresden, Germany
- 4Center for Healthy Aging, Faculty of Medicine Carl Gustav Carus, Dresden University of Technology, Dresden, Germany
- 5Georg-Speyer-Haus, Institute for Tumor Biology and Experimental Therapy, Frankfurt am Main, Germany
- 6Frankfurt Cancer Institute, Goethe University, Frankfurt am Main, Germany
- 7German Cancer Consortium (DKTK), Partner Site Frankfurt/Mainz, Frankfurt am Main, Germany
- 8German Cancer Consortium (DKTK), Partner Site Dresden, Dresden, Germany
Natural killer (NK) cells are attractive effectors for adoptive immunotherapy of cancer. Results from first-in-human studies using chimeric antigen receptor (CAR)-engineered primary NK cells and NK-92 cells are encouraging in terms of efficacy and safety. In order to further improve treatment strategies and to test the efficacy of CAR-NK cells in a personalized manner, preclinical screening assays using patient-derived tumor samples are needed. Zebrafish (Danio rerio) embryos and larvae represent an attractive xenograft model to study growth and dissemination of patient-derived tumor cells because of their superb live cell imaging properties. Injection into the organism’s circulation allows investigation of metastasis, cancer cell-to-immune cell-interactions and studies of the tumor cell response to anti-cancer drugs. Here, we established a zebrafish larval xenograft model to test the efficacy of CAR-NK cells against metastatic breast cancer in vivo by injecting metastatic breast cancer cells followed by CAR-NK cell injection into the Duct of Cuvier (DoC). We validated the functionality of the system with two different CAR-NK cell lines specific for PD-L1 and ErbB2 (PD-L1.CAR NK-92 and ErbB2.CAR NK-92 cells) against the PD-L1-expressing MDA-MB-231 and ErbB2-expressing MDA-MB-453 breast cancer cell lines. Injected cancer cells were viable and populated peripheral regions of the larvae, including the caudal hematopoietic tissue (CHT), simulating homing of cancer cells to blood forming sites. CAR-NK cells injected 2.5 hours later migrated to the CHT and rapidly eliminated individual cancer cells throughout the organism. Unmodified NK-92 also demonstrated minor in vivo cytotoxicity. Confocal live-cell imaging demonstrated intravascular migration and real-time interaction of CAR-NK cells with MDA-MB-231 cells, explaining the rapid and effective in vivo cytotoxicity. Thus, our data suggest that zebrafish larvae can be used for rapid and cost-effective in vivo assessment of CAR-NK cell potency and to predict patient response to therapy.
Introduction
Retargeting of T cells with CAR against a tumor marker has shown remarkable clinical efficacy in relapsed or refractory B-cell malignancies and multiple myeloma (1). This success has led to the approval of several CAR T cell products targeting hematologic malignancies by the U.S. Food and Drug Administration (FDA) and the European Medicines Agency (EMA). Despite significant clinical efficacy in some cancers, CAR T cell therapy is often associated with several side effects, such as a cytokine storm or neurological toxicity. In addition, CAR T cell products must be produced autologously due to T cell product incompatibility between patients, resulting in high therapy costs. As an alternative, NK cells have recently been recognized as a promising cytotoxic cell type for CAR-based cancer therapy, overcoming many of the obstacles of T cell-based systems. Primary NK cells and the NK cell line NK-92 have been shown to be safe with no adverse effects in clinical trials, and early clinical data in hematologic malignancies are encouraging in terms of efficacy (2–4). NK cells do not express the T cell receptor and therefore an NK cell product can be infused into multiple recipients in an allogeneic scenario without the risk of graft versus host disease (GvHD). In contrast, NK cells express an inherited set of activating or inhibitory receptors that allow them to recognize tumor or infected cells, making them unique cytotoxic cells. Although NK cells can recognize and kill many cancer cells, some cancers can evade NK cell-mediated killing. To overcome this resistance, NK cells can also be genetically engineered with CAR, similar to T cells (5–7). Indeed, several sources of NK cells are in preclinical or clinical development, including primary blood-derived NK cells, NK cells differentiated from hematopoietic or induced pluripotent stem cells (3, 8, 9). In addition, stable NK cell lines such as NK-92 have been established for clinical use (2, 10).
Despite the success of CAR-based therapies in hematological malignancies, solid tumors such as breast cancer remain a challenge. CAR T cells have not shown clinical efficacy and CAR-NK cells are currently in clinical investigation. The difficulties in targeting solid tumors are determined by inter-tumor heterogeneity, heterogeneity in antigen expression, resistance mechanisms to CAR T or NK cells and often generate metastatic diaspores that are difficult to reach by immune cells. Therefore, a personalized approach is required to select the most appropriate CAR system for each patient. Novel CAR-based strategies are rapidly developing and good preclinical models are needed to apply them in a personalized manner. Currently, novel CAR-NK cell therapies are tested in immunodeficient mouse models. However, these models are very expensive, laborious, inflexible and slow to yield results. Furthermore, the lack of transparency of the host tissues hampers in vivo observations of homing processes and the antitumor response. Therefore, in order to bridge the gap from in vitro studies to clinical trials, medium to high-throughput preclinical models with optimal live-cell imaging properties and fast experimental readout are needed to test the efficacy of CAR-NK cells against patient cancer cells.
Zebrafish (Danio rerio) embryos and larvae are prime candidates for the development of such a xenograft screening system. Their small size and transparency allow for medium-throughput injections with the prospect of automated high-throughput upscaling in the near future. Likewise, imaging of cancer and immune cells in transplanted embryos and larvae can be performed at high throughput at low cost. Notably, the lack of a fully functional adaptive immune system during early larval stages prevents rejection of transplanted cells in zebrafish (11). Zebrafish xenografts have been developed for the investigation of human tumor cell proliferation, apoptosis, invasion, extravasation and small molecule anti-cancer drug screening (12, 13). Recent work has shown that the zebrafish model can be used to assess the in vivo efficacy of CAR T cells (14–16). This provides proof of principle that embryonic zebrafish xenografts can be used for preclinical testing of CAR T cell efficacy; however, NK cells are biologically different from T cells in terms of their proliferation, migration, activation, metabolic properties and cytokine requirements (17). Whether zebrafish are suitable to evaluate the antitumor action of CAR-NK cells, especially the relatively large NK-92 cells (18), is currently unclear.
Here, we established zebrafish embryos and larvae as a preclinical xenograft model to assess the efficacy of CAR-NK cells against metastatic cancer cells of solid tumor origin. We validated the CAR-NK cell system with two different specificities and two different breast cancer models. Furthermore, we investigated the live interaction of CAR-NK and cancer cells and the migration of CAR-NK cells through the microvasculature to the site of metastatic breast cancer cells. This demonstrates the functionality and usefulness of CAR-NK cells in cancer therapy and reaffirms the power of zebrafish xenografts in preclinical cancer research.
Materials and methods
Cells and cell culture
The established human NK cell line NK-92 was provided by H.G. Klingemann (Chicago, IL, USA) (19). ErbB2.CAR NK-92 (NK-92/5.28.z) cells were generated previously (6). NK cell lines were cultured in X-vivo 10 medium (Lonza) containing 5% heat-inactivated human AB plasma (German Red Cross Blood Donation Service North-East, Dresden, Germany), 500 IU/mL IL-2 (Proleukin; Novartis Pharma), 100 IU/mL penicillin, and 100 µg/mL streptomycin (Merck/Biochrom). MDA-MB-453, MDA-MB-231 cells were purchased from the American Type Culture Collection (ATCC, Manassas, VA, USA) and cultured in DMEM medium (Merck/Biochrom) supplemented with 10% HI-FBS (Merck/Biochrom), 2 mM L-glutamine (Merck/Biochrom), 100 IU/mL penicillin, and 100 μg/mL streptomycin. All cells were cultured at 37°C in a humidified atmosphere with 5% CO2 and routinely checked for Mycoplasma contamination.
Generation of transgenic cells
PD-L1.CAR NK-92 were generated by lentiviral transduction with a second-generation CAR consisting of PD-L1-specific scFv, CD8 hinge, CD28 transmembrane/costimulatory, and CD3ζ intracellular signaling domains cloned into the pSIEW backbone as described previously (6). PD-L1.CAR NK-92 cells were further immuno-magnetically enriched according to the manufacturer’s protocol (Miltenyi Biotec) using human recombinant PD-L1-Fc combined with biotinylated anti-human Fc. Enriched cells resulted in >95% purity of PD-L1.CAR-expressing NK-92 cells at the time of analysis. MDA-MB-231 and MDA-MB-453 cells were transduced with pSIEW-GFP/Puro vector at MOI<1 by 30 min spinoculation at 1000xg in the presence of 8 µg/ml Polybrene (Sigma-Aldrich) to produce MDA-MB-231 GFP and MDA-MB-453 GFP. Cells were purified by puromycin selection (Sigma-Aldrich).
Lentivirus production
Lentivirus particles were produced in the packaging cell line HEK293T using packaging vectors psPAX2 and pMD2.G (both from Addgene). Plasmids were transfected with polyethyleneimine (PEI) and supernatants were harvested after 48 hours. Virus was concentrated with PEG-it solution (System Biosciences) according to the manufacturer’s instructions.
Flow cytometry
Cells were stained with antibodies specific for PD-L1 (Miltenyi Biotec) and ErbB2 (R&D Systems) for 30 min on ice. For CAR expression, CAR-NK cells were loaded with human recombinant PD-L1-Fc (Biolegend) or ErBb2-Fc fusion proteins (R&D Systems) for 30 min on ice, washed, and stained with anti-human Fc secondary antibody (Jackson Immunoresearch) for 30 min on ice. Live cells were discriminated using 7-AAD (BD Biosciences) or DAPI (Sigma Aldrich). Samples were acquired using a BD FACSCanto II flow cytometer and data were analyzed using FlowJo software version 9 (BD Biosciences).
Europium-TDA cytotoxicity assay
The specific cytotoxicity of the NK-92 cell lines against target cells was determined using the Europium (EuTDA) cytotoxicity assay (DELFIA, PerkinElmer #C135-100) according to the manufacturer’s protocol and as previously described (20). Briefly, target cells were loaded with an acetoxymethyl ester of the fluorescence-enhancing ligand (BATDA; Perkin Elmer #C136-100) and then co-cultured at 10,000 cells/well in triplicate with effector cells at the indicated E:T ratios. After 2 hours of co-culture, supernatants were collected for measurement of the fluorescent signal reflecting target cell lysis using a VICTOR X4 fluorometer (PerkinElmer). Specific lysis was calculated according to the standard formula in the manufacturer’s instructions.
Zebrafish husbandry
Zebrafish were maintained in the fish facility of the Center for Regenerative Therapies, Dresden (CRTD) in accordance with the guidelines of the Landesdirektion Dresden (DD25-5131/450/4 and 25-5131/564/2). The experiments were performed with zebrafish larvae up to a maximum age of 5 dpf (days post fertilization). Adult fish were mated in mating tanks and the eggs were collected 2-3 hours after mating. Fertilized eggs were raised in E3 medium (5 mM NaCl, 0.17 mM KCL, 0.33 mM CaCl2, 0.33 mM MgSO4) at 28°C.
Cell preparation and labelling
At 2 dpf, MDA-MB-231 GFP or MDA-MB-453 GFP cells were detached using Accutase (Biolegend) and concentrated to 1x106 cells/ml in DMEM. NK-92 or CAR NK-92 cells were labeled with PKH26 (Sigma Aldrich) according to the manufacturer’s instructions. Briefly, 2x106 NK cells were washed with serum-free X-vivo medium followed by resuspension in 200 µl diluent C (DC), mixed with PKH26 staining solution (1 µl PKH26 in 250 µl DC) and incubated for 5 min at RT. The reaction was stopped by adding FBS for 1 min. The labeled cells were washed twice with pure X-vivo medium and finally concentrated to 1x106 cells/ml in X-vivo medium. The cells were validated for staining by flow cytometry. Shortly before injection, cancer and NK cells were washed with 1X PBS, followed by Tx buffer (1% Pen/Strep, 1.5 mM EDTA, 1X PBS) and finally resuspended in 10 µl of Tx buffer (1x106 cells/10 µl, 100 cells/nl) and kept on ice.
Microinjection
Wild type (WT) AB or transgenic Tg(flk1:GFP) zebrafish embryos were dechorionated at 2 dpf using forceps (Dumostar Biology, Dumont 55), anesthetized in 0.02% tricaine methanesulfonate (MESAB; Sigma Aldrich #A5040) in E3, aligned on 1.5% agarose plates with grooves, and placed under a dissecting microscope (Olympus SZX10) equipped with a microinjector (Marzhauser, MM3301R) and a vacuum pump (WPI, Pneumatic PicoPump PV 820). Filamented glass needles (TW100F-3, World Precision Instruments) pulled with a P-97 Flaming/Brown micropipette puller (Sutter Instrument) were used to inject 1 nl (in average 100 cells) of cancer cells into the Duct of Cuvier (DoC), followed by fresh addition of E3 to the injected larvae and incubation at 33 °C to allow recovery. After 2.5 hours, larvae were sorted by fluorescence microscopy to discard improperly injected larvae. The second microinjection was performed to inject 1 nl of labeled NK cells followed by E3 addition and incubation at 33 °C.
Zebrafish imaging
For imaging, larvae were anesthetized in 0.02% MESAB in E3, embedded in 2.5% methylcellulose in a glass cavity slide and imaged using the SteREO Discovery V12 microscope equipped with an AxioCam MRm (Zeiss) and an AxioVision 4.7 software (Zeiss). Fluorescence imaging was performed on both sides of the larvae in brightfield, GFP and RFP channels, wherein unsaturated images were obtained.
Confocal microscopy
Zebrafish larvae [WT AB and Tg(flk1:GFP)] were injected with MDA-MB-231 GFP followed by PKH26-labeled PD-L1.CAR NK-92 cells. For confocal imaging, larvae were anesthetized with 0.02% MESAB in E3 and embedded in 1% low melt agarose (LMA) filled with 0.01% MESAB in E3. High-resolution live imaging of larvae was performed using a Dragonfly spinning disk confocal microscope (Andor) equipped with a sCMOS camera, and Fusion software (Andor, version 2.3.0.44). Time-lapse imaging combined with z-stacks was performed every minute.
Image analysis and quantification
Image analysis and quantification were performed using Fiji ImageJ 1.52p (NIH) with the cell counter plugin for manual counting of GFP+ cells in zebrafish larvae from both sides.
Statistical analysis
Statistical analysis was performed with GraphPad Prism 7 (Graphpad Software). A P value <0.05 was considered statistically significant. ****P<0.0001; ***P<0.001; **P<0.01; *P<0.05; ns (not significant) P ≥ 0.05.
Results
Cytotoxicity of PD-L1.CAR.NK-92 cells against breast cancer cells in vitro
We first constructed a second-generation CAR containing the CD28 costimulatory and the CD3ζ activation domain with specificity for PD-L1 (PD-L1.CAR; Figure 1A), a checkpoint molecule frequently expressed in many solid tumors. The PD-L1.CAR was lentivirally transduced into the clinically applicable NK cell line NK-92 (PD-L1.CAR NK-92; Figure 1B), which has many similarities to primary NK cells and is a common model for CAR-NK cell studies (19, 21). As a cancer model, we used the PD-L1-expressing metastatic breast cancer cell line MDA-MB-231 (Figure 1C). The PD-L1.CAR NK-92 cells showed strong CAR expression on the cell surface and exhibited efficient in vitro cytotoxicity against MDA-MB-231 cells (Figures 1D, E).
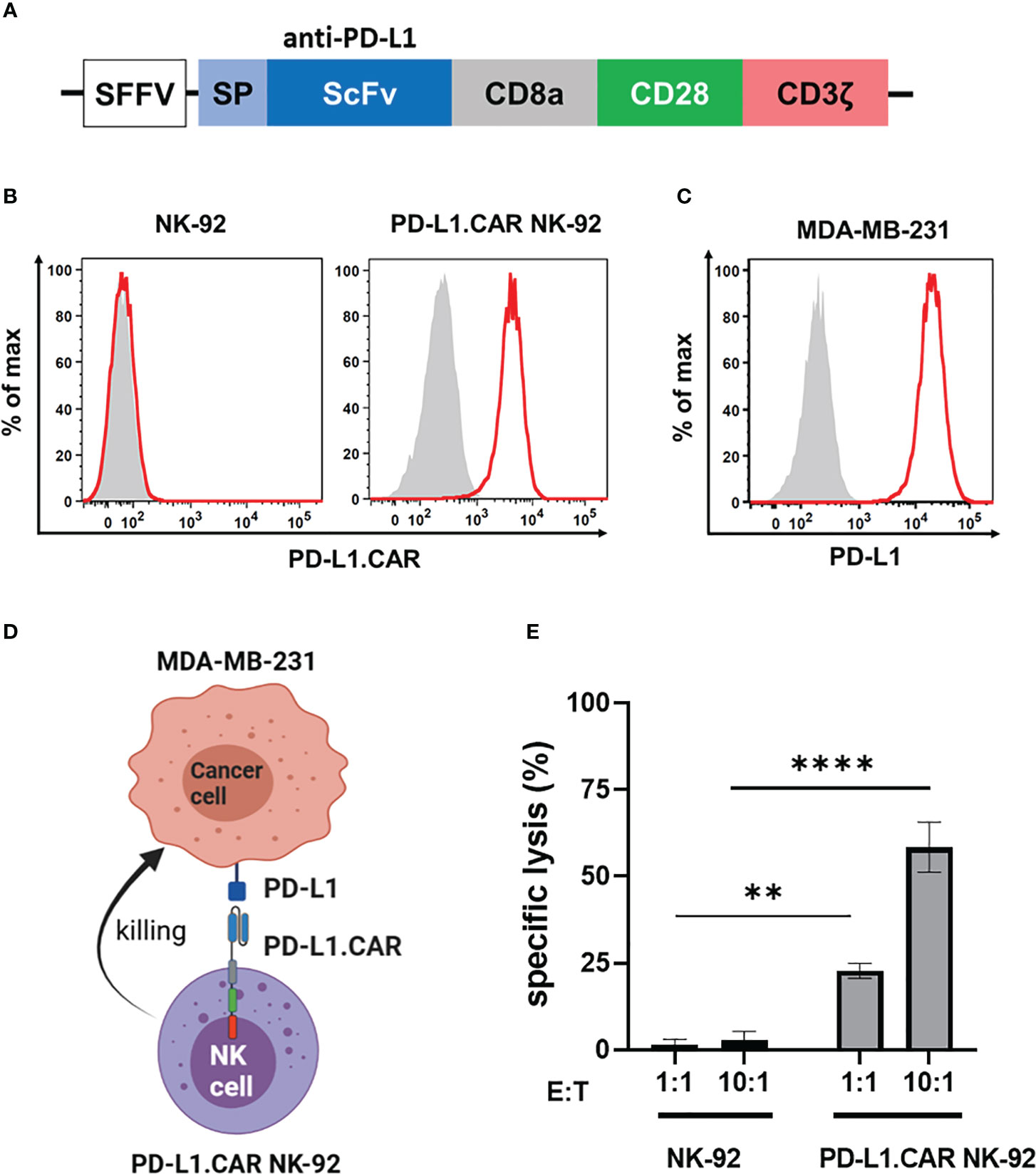
Figure 1 PD-L1.CAR NK-92 cells are highly cytotoxic against PD-L1+ targets in vitro. (A) Schematic representation of the PD-L1.CAR construct. A second-generation CAR under the spleen focus-forming virus (SFFV) promoter consists of PD-L1-specific scFv, CD8 hinge, CD28 transmembrane/costimulatory, and CD3ζ intracellular signaling domains. (B) Flow cytometric analysis of PD-L1.CAR expression on immunomagnetically enriched PD-L1.CAR NK-92 cells and parental NK-92 cells. PD-L1.CAR was detected using human recombinant PD-L1-Fc protein combined with anti-Fc secondary antibody. Filled gray areas indicate negative controls stained with secondary antibody only. (C) Flow cytometric analysis of PD-L1+ expression on the cell surface of MDA-MB-231 cells. Representative data from at least 3 independent experiments are shown. (D) Illustration of the NK cell and cancer cell model. NK-92 cells were lentivirally transduced with PD-L1.CAR, and the PD-L1+ metastatic breast adenocarcinoma cell line MDA-MB-231 was used as a target. Created with BioRender. (E) PD-L1.CAR NK-92 or parental NK-92 cells were co-cultured with MDA-MB-231 cells at E:T ratios of 1:1 and 10:1 for 2 hours as indicated and specific in vitro cytotoxicity was measured by Europium-based cytotoxicity assay. One-way ANOVA with Tukey’s multiple comparisons was used to calculate statistics. Data were pooled from 3 independent experiments, and means ± SEM are shown. **P<0.01; ****P<0.0001.
Injection of labeled cancer and CAR-NK cells into zebrafish embryos
To visualize the CAR-NK and cancer cells in the zebrafish xenograft model, we differentially labeled the effector and target cells. The MDA-MB-231 cells were stably transduced with GFP (MDA-MB-231 GFP) without affecting PD-L1 expression (Figure 2A). The PD-L1.CAR NK-92 cells were labeled with the red membrane dye PKH26 prior to injection to distinguish them from the cancer cells (Figure 2B). We further confirmed that MDA-MB-231 GFP cells were efficiently killed by PD-L1.CAR NK-92 cells in vitro, with comparable efficacy compared to wild-type (WT) MDA-MB-231 target cells (Figure 2C). The MDA-MB-231 GFP cells were then injected into the circulation of zebrafish embryos via the DoC at 2 days post fertilization (dpf) using a micromanipulation device. Correctly injected embryos were sorted under the microscope, and 2.5 hours later red-labeled PD-L1.CAR NK-92 cells were injected into the DoC. Zebrafish embryos were then imaged 1 hour later. Both cancer and CAR-NK cells were clearly visible in the body of the zebrafish embryos. Cancer cells successfully migrated within the organism, especially to the tail region (including the CHT), head and ventral region of the zebrafish embryo (Figure 2D). Circulating CAR-NK cells were detected in the blood stream which is consistent with their subsequent injection, thus representing an optimal CAR-NK cell homing model to sites of diffuse metastatic cancer cell accumulation. With this approach, we established a novel assay for the visualization of CAR-NK cells and metastatic cancer cells in zebrafish embryos in vivo.
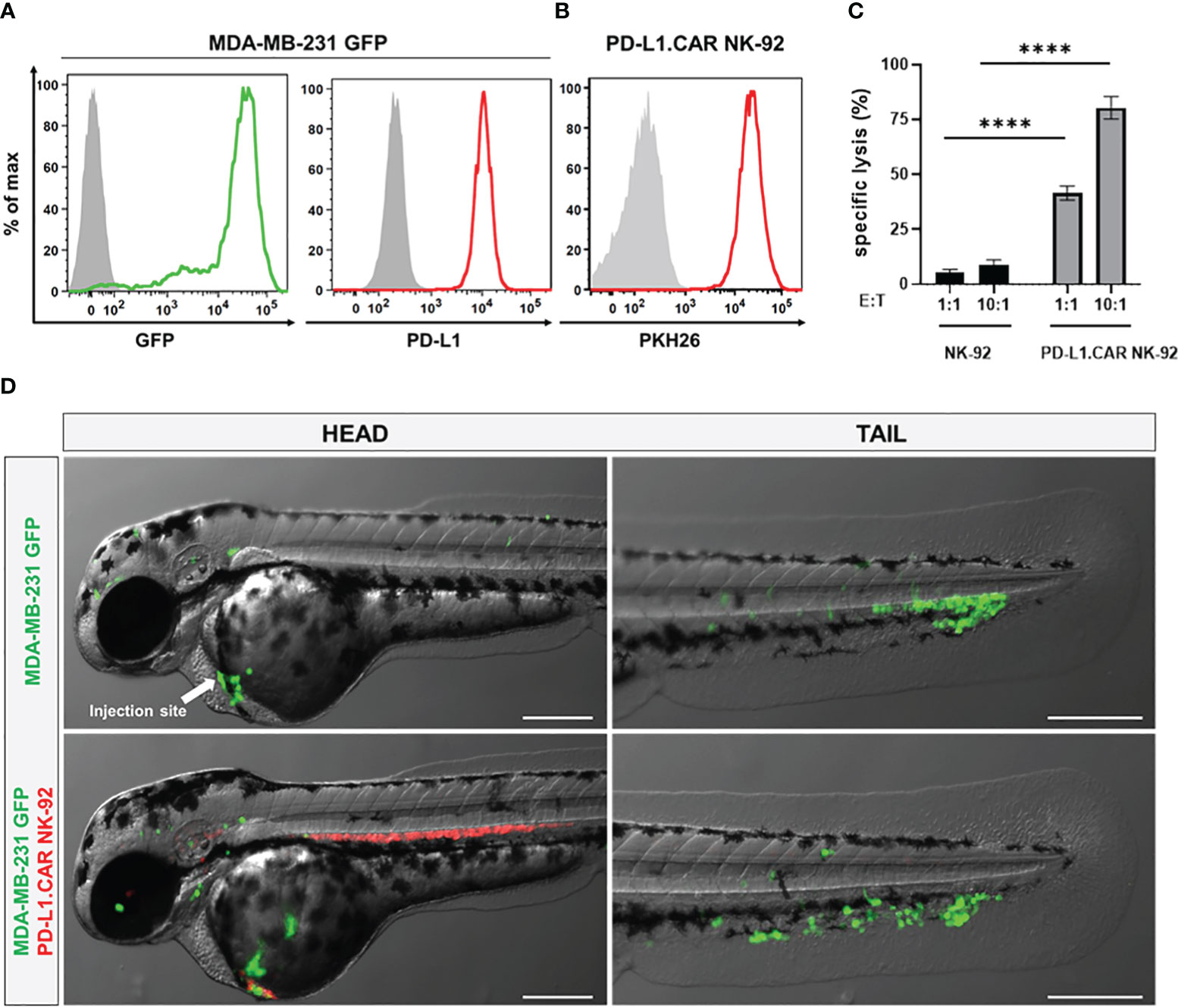
Figure 2 Labeled cancer and NK cells retain in vitro cytotoxicity and circulate in zebrafish larvae after injection. (A) MDA-MB-231 cells were transduced with GFP (MDA-MB-231 GFP). Expression of GFP and PD-L1 was analyzed by flow cytometry. Non-transduced and unstained cells shown in gray as negative controls for GFP and PD-L1, respectively. (B) PD-L1.CAR NK-92 cells were labeled with PKH26 and confirmed by flow cytometry. The filled gray histogram represents the unstained control. (C) PD-L1.CAR NK-92 or parental NK-92 cells were co-cultured with MDA-MB-231 GFP cells at E:T ratios of 1:1 and 10:1 for 2 hours as indicated, and specific in vitro cytotoxicity was measured by Europium-based cytotoxicity assay. Data were pooled from at least 3 independent experiments, and means ± SEM are shown. One-way ANOVA with Tukey’s multiple comparisons was used to calculate statistics. (D) MDA-MB-231 GFP cells were injected into the DoC alone (top) or injected at the same site 2.5 hours later with PD-L1.CAR NK-92 PKH26-labeled cells (bottom). Images of the head or tail region of zebrafish larvae were captured by fluorescence microscopy. Scale bar = 250 μm. Representative images are shown. ****P<0.0001. ns, not significant.
Kinetics of in vivo cytotoxicity of PD-L1.CAR NK-92 cells in zebrafish
Next, we tested the in vivo cytotoxicity of the PD-L1.CAR NK-92 cells against the MDA-MB-231 GFP cells to identify the optimal time point for the final analysis. Therefore, we injected MDA-MB-231 GFP cells into the DoC of a small cohort of zebrafish embryos followed by injection of red-labeled PD-L1.CAR NK-92 cells, unmodified parental NK-92 cells 2.5 hours later or without any follow-up cell injection (control). Individual zebrafish embryos were imaged immediately after NK cell injection and again at 24, 48 and 72 hours post-injection (hpi, Figure 3A). Cancer cells in the control group were distributed throughout the body of the zebrafish larvae, while in the NK-92 treated group, NK cells were found in close contact with the cancer cells. However, surviving cancer cells were detectable also at later time points (Figure 3B). In contrast, larvae treated with PD-L1.CAR NK-92 cells were almost devoid of cancer cells at 48 and 72 hpi, while NK cells remained at the sites of interest. We quantified surviving cancer cells in the zebrafish across time points and confirmed significant in vivo cytotoxicity by PD-L1.CAR NK-92 cells compared to NK-92 and untreated controls as early as 24 hpi (Figure 3C). The cytotoxic effect was further enhanced at 48 and 72 hpi (10 ± 5 and 5 ± 3 remaining cells in PD-L1.CAR NK-92 compared to 39 ± 6 and 36 ± 5 remaining cells in NK-92, respectively). These data demonstrate that PD-L1.CAR NK-92 cells rapidly detect cancer cells in vivo, kill the majority of them within 24 hpi, and eliminated nearly all residual cancer cells within the following two days.
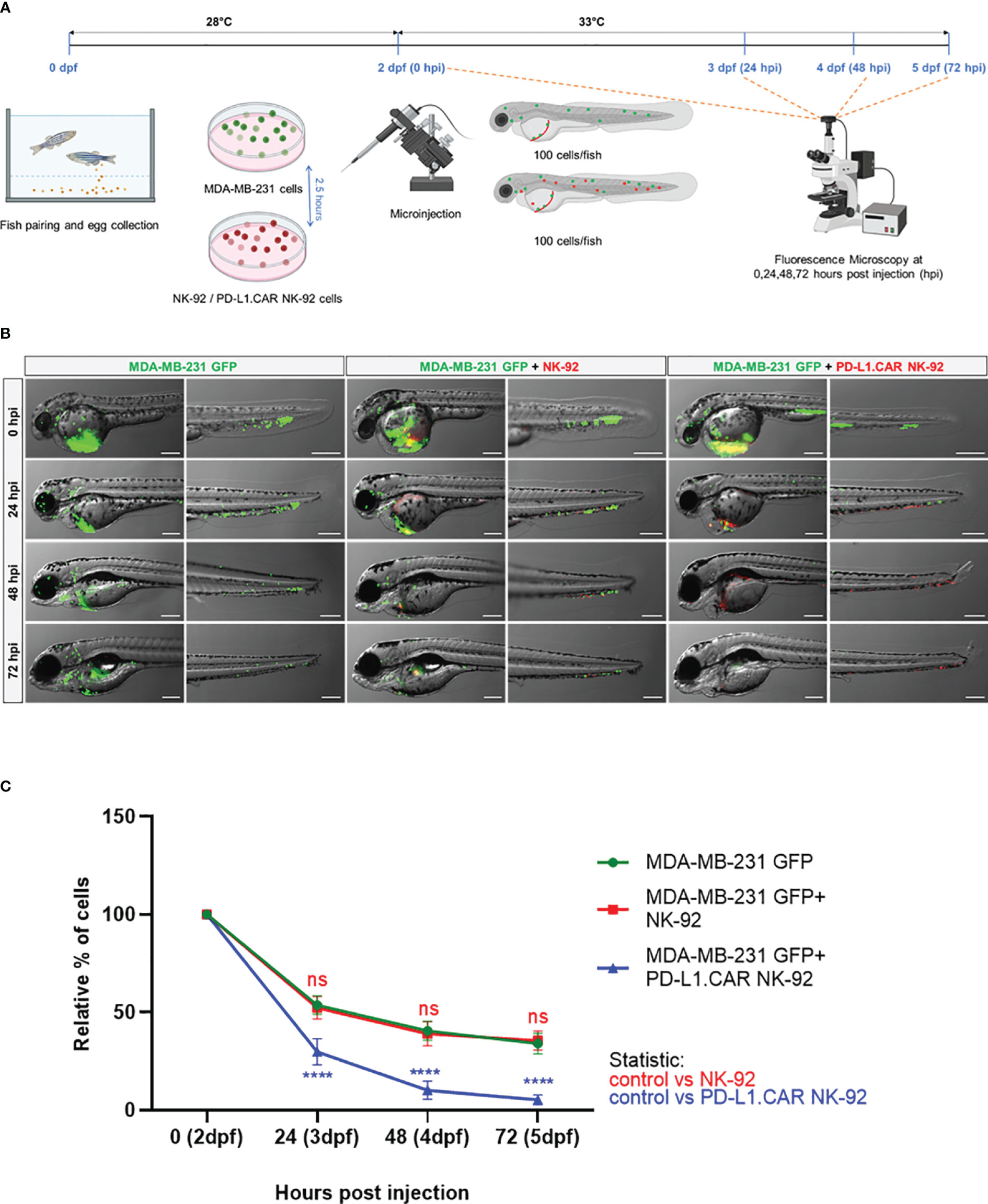
Figure 3 Kinetics of PD-L1.CAR NK-92 cytotoxicity in zebrafish in vivo. (A) Schematic time course of the experimental setup. Zebrafish larvae were injected with MDA-MB-231 GFP cells at 2 days post fertilization (dpf) and 2.5 hours later with PKH26-labeled PD-L1.CAR NK-92 or parental NK-92 cells. For each cell type, 100 cells were injected on average. Images were captured by fluorescence microscopy at 24, 48, and 72 hours post injection (hpi). (B) Fluorescence micrographs showing zebrafish larvae injected with MDA-MB-231 GFP cells or combinations with NK-92 or PD-L1.CAR NK-92 at the indicated time points. MDA-MB-231 GFP cells are shown in green and PKH26-labeled NK cells are shown in red. Representative images are shown. Scale bar = 250 μm. (C) Quantification of MDA-MB-231 GFP cells throughout the zebrafish at the indicated time points and plotted as relative percentage of cells at 0 hpi. Data are pooled from 2 independent experiments and shown as mean ± SEM. n=3 (MDA-MB-231 GFP, MDA-MB-231 GFP+NK-92), n=4 (MDA-MB-231 GFP+PD-L1.CAR NK-92). Two-way ANOVA analysis with Tukey’s multiple comparison was used for statistical analysis. ****P<0.0001; ns (not significant).
Evaluation of in vivo killing efficacy of PD-L1.CAR-NK and ErbB2.CAR-NK cells
To further validate the in vivo killing efficacy of PD-L1.CAR NK-92 cells in a pooled setup, we injected a larger cohort of zebrafish larvae with MDA-MB-231 GFP cells. Positively injected larvae were selected, divided into three groups and injected 2.5 hours later with PD-L1.CAR NK-92 cells, NK-92 control or left without any follow-up cell injection (control). Based on previous results, larvae were imaged at 48 hpi (Figure 4A). The quantification revealed that parental NK-92 cells exhibited low cytotoxicity with less than 50% cancer cell reduction (23 ± 16 remaining cells) compared to the control group (38 ± 18 remaining cells). In contrast, PD-L1.CAR NK-92 cells almost completely eliminated cancer cells (7 ± 7 remaining cells) in the majority of zebrafish larvae (Figure 4B). To test whether this system is applicable to other CAR-NK cell models, we used NK-92 cells with CAR targeting the ErbB2 (HER2) molecule (NK-92/5.28.z, here ErbB2.CAR NK-92) (6). ErbB2 is overexpressed in many solid tumor cancer cells. To mimic this, we used the ErbB2-expressing breast cancer cell line MDA-MB-453 transduced with GFP (Figure 4C). Using this model we observed rapid killing of MDA-MB-453 GFP cells by ErbB2.CAR NK-92 cells as early as 8 hpi (18 ± 22 remaining cells), whereas NK-92 cells mediated only moderate killing (47 ± 28) compared to the control (96 ± 36) (Figure 4D). Taken together, this demonstrates that CAR-NK cells with different lysis specificities can eliminate metastatic breast cancer cells in zebrafish larval xenografts.
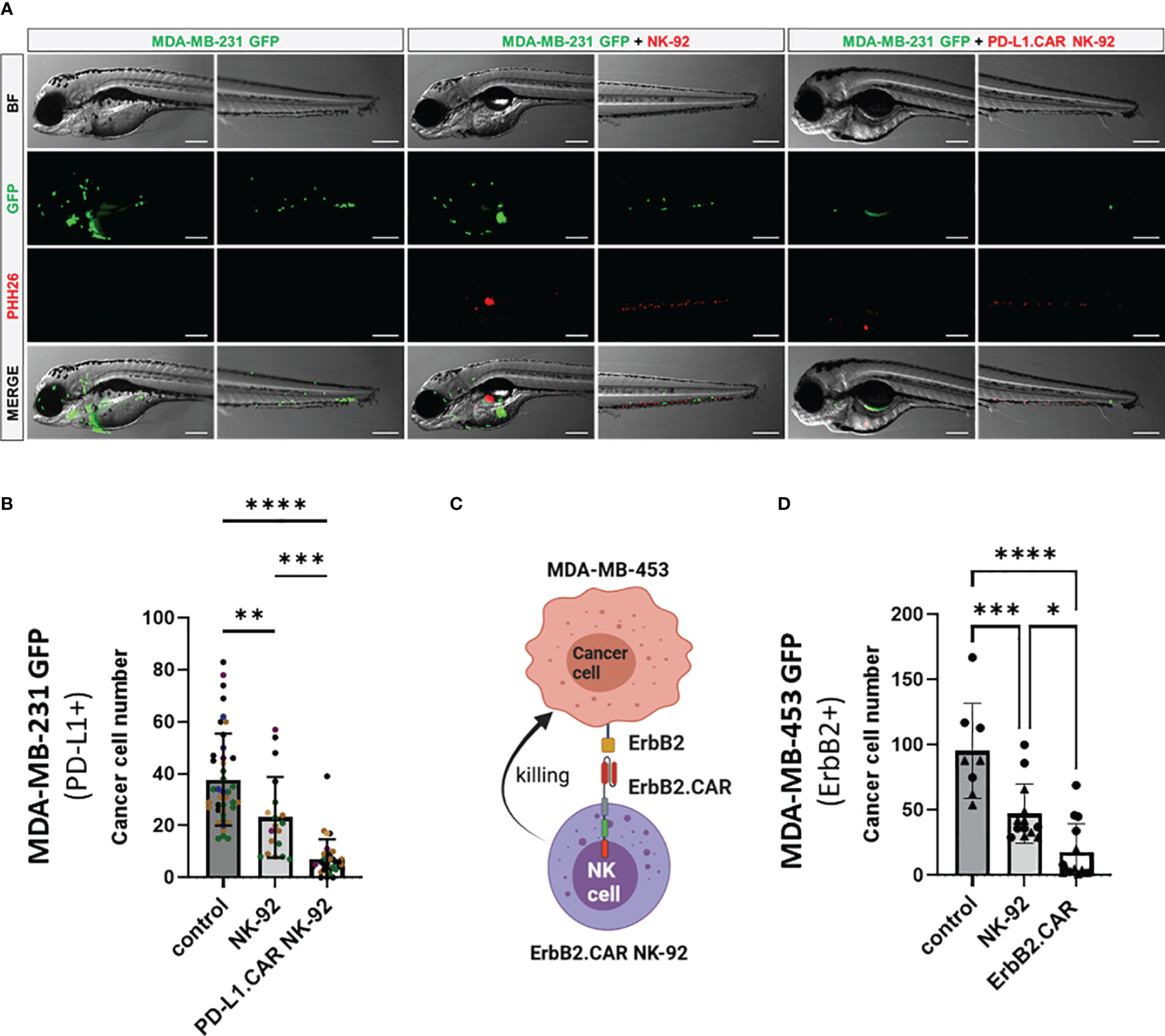
Figure 4 CAR NK-92 cells specific for PD-L1 or ErbB2 show high efficacy against resistant breast cancer cells in a zebrafish xenograft model. (A) Zebrafish larvae were injected at 2 dpf with MDA-MB-231 GFP cells and 2.5 hours later with PKH26-labeled PD-L1.CAR NK-92 or parental NK-92 cells. For each cell type an average 100 cells was injected. Images were captured by fluorescence microscopy at time points of 48 hpi. Scale bar = 250 μm. (B) The number of MDA-MB-231 GFP cells was quantified at 48 hpi. Data are pooled from 6 independent experiments and shown as mean ± SD. n=41 (MDA-MB-231 GFP), n=19 (MDA-MB-231 GFP+NK-92), n=31 (MDA-MB-231 GFP+PD-L1.CAR NK-92). (C) Schematic representation of ErbB2-specific CAR NK-92 (ErbB2.CAR NK-92) targeting ErbB2+ MDA-MB-453 metastatic breast cancer cell line. Illustration created with BioRender. (D) Zebrafish larvae were injected at 2 dpf with MDA-MB-453 GFP cells and 2.5 hours later with PKH26-labeled ErbB2.CAR NK-92 or parental NK-92 cells. For each cell type, 100 cells were injected on average. Images were captured by fluorescence microscopy at 8 hpi and the number of cancer cells was quantified. Data were pooled from 2 independent experiments and means ± SD are shown. n=8 (MDA-MB-453 GFP) n=13 (MDA-MB-453 GFP+NK-92), n=14 (MDA-MB-453 GFP+ErbB2.CAR NK-92). One-way ANOVA analysis with Tukey’s multiple comparison was used for statistical analysis. *p=0.0154. **P<0.01; ***P<0.001; ****P<0.0001.
Live observation of CAR-NK cells killing tumor cells in the zebrafish xenograft
To further investigate the interaction of CAR-NK and cancer cells within the zebrafish host, we injected MDA-MB-231 GFP cells, followed by PD-L1.CAR NK-92 cell injection as described above, and performed confocal in vivo imaging of the zebrafish tail region 1 hour later (Figure 5A). The PD-L1.CAR NK-92 cells migrated to the cancer cells, conjugated with them, and subsequently killed the cancer cells (Figure 5B, Supplementary Video 1). An important ability of CAR-NK cells is to migrate through the vasculature to reach metastatic cancer cells. To study this, we used the transgenic Tg(flk1:GFP) zebrafish strain with labeled endothelial cells (22). We observed that PD-L1.CAR NK-92 cells migrated to the disseminated metastatic cancer cells, demonstrating that CAR-NK cells can migrate through the zebrafish vasculature and identify individual metastatic cancer cells (Figure 6, Supplementary Video 2). Taken together, our data demonstrate that zebrafish larvae are a suitable host to study the migration properties and efficacy of CAR-NK cells to eliminate metastatic cancer cells of solid tumor origin in vivo.
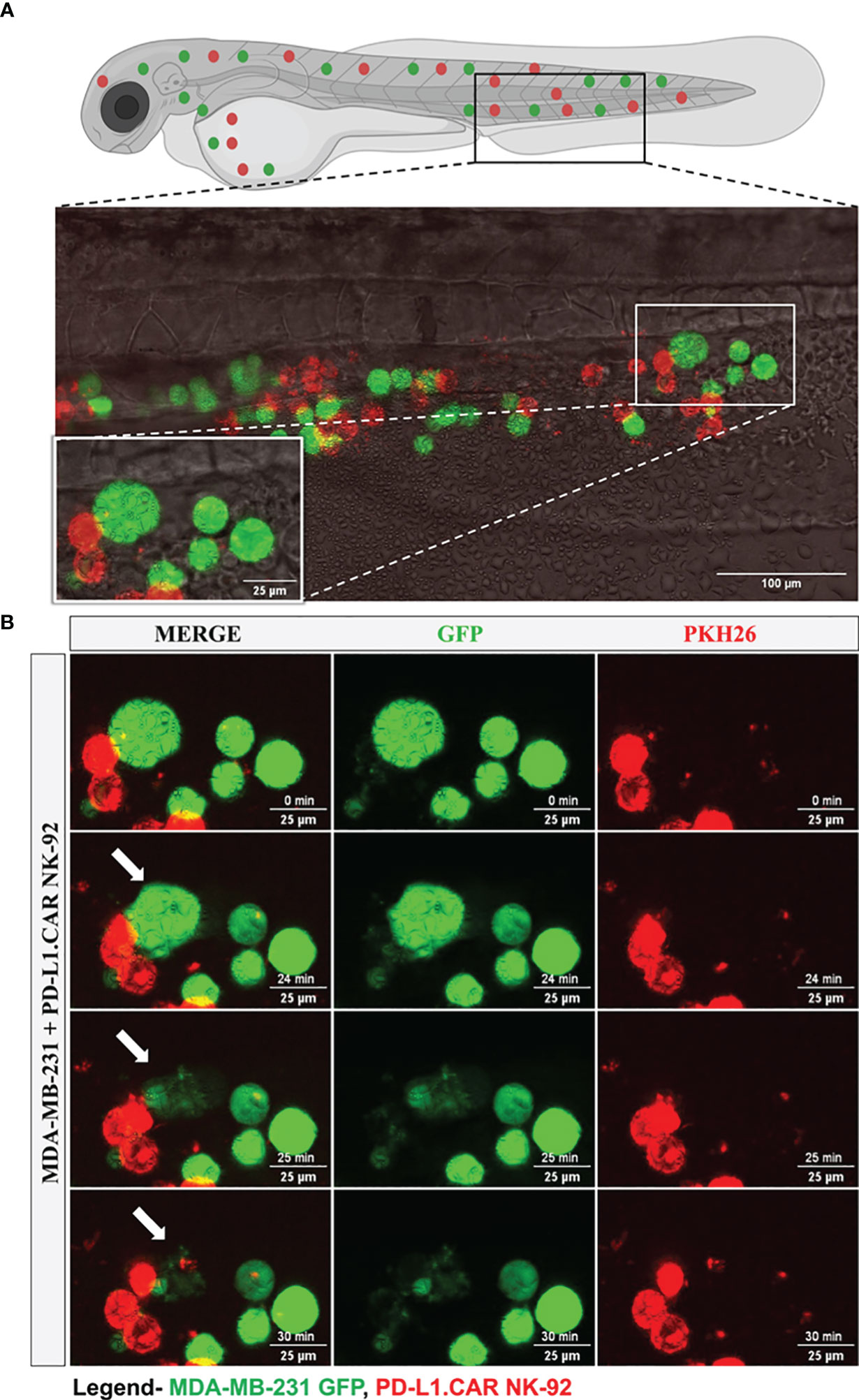
Figure 5 Time-lapse imaging of CAR-NK cell interaction with cancer cells and cytotoxicity in the zebrafish tail. MDA-MB-231 GFP cells and 2 hours later PD-L1.CAR NK-92 cells (PKH26-labeled) were injected into the DoC. One hour after injection, confocal microcopy images were taken in the tail region. (A) Representative picture showing the location of imaging. (B) Time-lapse images showing the interaction between NK cells (red) and cancer cells (green). The arrow indicates cancer cell killing. Scale bar = 25 μm.
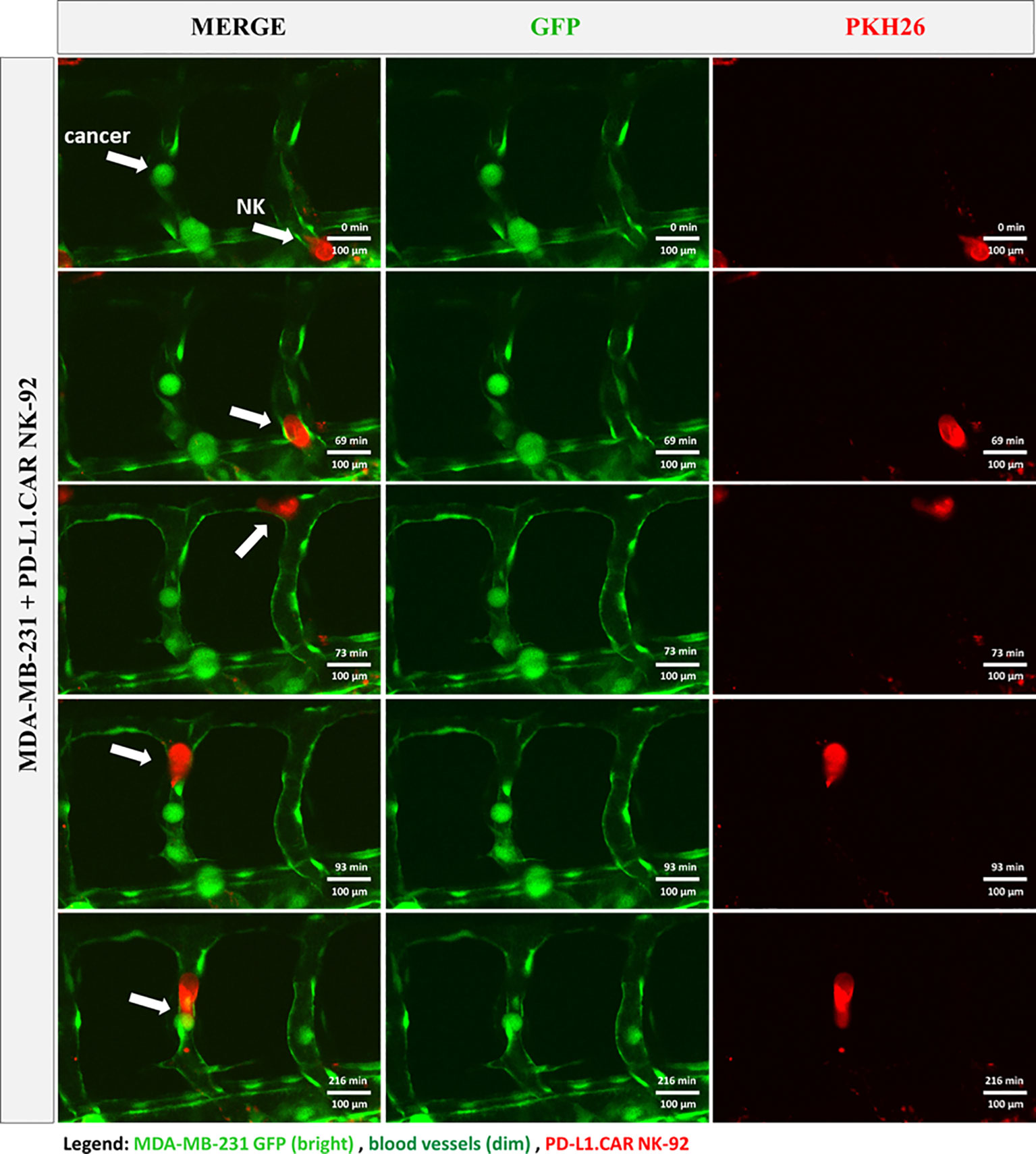
Figure 6 CAR-NK cells migrate to cancer cells within the vasculature. MDA-MB-231 GFP cells and PKH26-labeled PD-L1.CAR NK-92 cells were injected into transgenic Tg(flk1:GFP) zebrafish with endothelial cells expressing low levels of GFP. Confocal time-lapse microscopy shows NK cell migration to the metastatic breast cancer cell (indicated by arrows). Representative images are shown. Scale bar = 100 μm.
Discussion
CAR-NK cell therapies have recently emerged as a promising platform to treat otherwise incurable cancers without severe side effects. Despite promising clinical results in hematological malignancies, solid tumors remain challenging mainly due to their heterogeneity, resistance mechanisms, suppressive microenvironment, immune exhaustion and metastatic properties. Novel CAR or other targeted NK cell systems are rapidly emerging and therefore quick preclinical models to test their in vivo efficacy are required to facilitate the development of NK cell therapy. Currently, immunodeficient mouse xenografts are used, but they are limited in their use by high costs and long read out times.
Here, we describe a preclinical zebrafish xenograft model as an alternative and reliable system to test the efficacy of CAR-NK cells against metastatic breast cancer. Using two different CAR NK-92 systems targeting PD-L1 or ErbB2 molecules, we demonstrate that human NK cells survive in the zebrafish environment and retain specific cytotoxicity against metastatic breast cancer cells. Due to the absence of adaptive immune cells in zebrafish larvae, neither CAR-NK cells nor cancer cells appear to be rejected by the host´s endogenous immune system, although we cannot exclude that innate immune cells such as macrophages diminish CAR-NK and breast cancer cell numbers. In our metastatic model, we observed rapid killing of cancer cells in the periphery within 8 and 24 hours, respectively, depending on the target. We also observed partial elimination of cancer cells by unmodified NK-92 cells in our bulk embryo analysis setup (a time course setup would likely require similar numbers of embryos to demonstrate such activity), suggesting that this model can also provide information on the natural killing of NK cells against certain tumors of interest. While natural cytotoxicity may be difficult to test in short-term in vitro cytotoxicity assays, the zebrafish may certainly provide clinically relevant information on cytotoxicity in vivo. Although unmodified NK cells are unable to eliminate the resistant cancer cells and CAR targeting is required, additional natural cytotoxicity may support CAR-based cancer cell elimination of cells in which the target antigen is less expressed, blocked or shed.
The small size and transparency of the system allowed microscopic tracking of CAR-NK cells as well as of breast cancer cells in individual animals over time to obtain kinetic data on killing efficacy. The small size of the animals and high progeny number allowed us to inject larger cohorts of zebrafish, resulting in reliable data also in a pooled analysis setting. Our system will allow for medium- to high-throughput testing of novel CAR-NK cell products in early developmental stages of zebrafish before initiating time consuming mouse models, which in addition require the injection of a much higher cancer cell number. In this regard, the zebrafish system will be a promising tool to test the reaction of primary patient cancer cells to CAR-NK cell therapy. In fact, most cancer patients do not benefit from immunotherapy, and distinguishing responders from non-responders prior to treatment will allow for a reliable prediction of the treatment outcome. Because of the large number of available zebrafish embryos, low haltering costs and the small number of required patient cancer cells for injection (100-200 cells per embryo) the system is ideally suited for personalized clinical testing. Such a testing model allows fast recapitulation of the patient’s disease and testing of multiple CAR-NK cell products alone or in combination with other cell-based therapeutics such as CAR-T cells, whose action has recently been demonstrated in zebrafish embryos as a basis for T-cell based therapy (14, 15). Of note, zebrafish xenografts also offer the possibility of testing the CAR-NK cells in combination with small drugs for synergistic effects, in analogy to previous studies using zebrafish for screening small drug libraries (23).
The zebrafish embryos’ small size and transparency allowed us to track CAR-NK cells and cancer cells at the single cell level, and to study CAR-NK/cancer cell interaction and cytotoxicity in real-time. In the future, confocal microscopy of cancer cell plus CAR-NK injected embryonic zebrafish could be used to study lytic granule polarization toward the immunological synapse, which is an important parameter associated with cancer cell resistance to NK cell cytotoxicity (20). Furthermore, using transgenic zebrafish with a fluorescently labeled vasculature, we observe homing of metastatic cancer cells to the zebrafish periphery and intravascular migration of CAR-NK cells to these individual tumor cells, suggesting that CAR-NK cells can effectively find and eradicate circulating tumor cells and potentially micrometastases that are difficult to eliminate with standard therapies. In a clinical setting, these capacities can be exploited. For instance, the homing and persistence of novel improved CAR-NK cells including CIS (cytokine-inducible SH2-containing protein) knock out, transgenic IL-15 production and others can be tested in the zebrafish xenograft model (24, 25), as recently done to assess the efficacy of CAR-T cells expressing IL-15 and CCL19 (16). Although active homing of NK cells has previously been shown for solid tumors in a mouse model (26), further studies are required to determine whether CAR-NK cells in the zebrafish xenograft model randomly encounter cancer cells within the bloodstream or whether this is an active migration process involving specific mechanisms. If accurate quantification of NK cells is required, a brighter and photobleach-resistant cell dye or engineered cells expressing a fluorescent protein would be beneficial.
Although the used zebrafish xenograft model has many advantages, it is important to be aware of its limitations, one of which is its brevity itself. The model is very short-term and does not allow for the development of secondary (acquired) resistance mechanisms within the transplanted animal, as ultimately transplants might be rejected after development of innate immunity. However, recently an adult zebrafish model has been introduced that allows a minimum of 14 days of cancer-immune cell interaction albeit it is not clear whether this time is sufficient to study secondary resistance (27). Importantly, patient treatment needs to be started promptly in most cases, which is why short-term assays are generally desirable. Furthermore, the very first response to CAR-NK cell therapy is the first important parameter for healthcare professionals to apply the treatment. In line with this, we have shown that the CAR-NK cell efficacy can be measured as early as 24 hpi, but assessment can be extended to 72 hours if necessary. Similar to other xenograft animal models, zebrafish do not contain human immune and stromal cells, limiting the ability to study complex immune cell interactions. This drawback can be overcome in part by co-injecting human stromal cells along with the cancer cells, as recently shown for CAR-T cells (14) or even by co-injecting immune cells from the patient. In order to enhance the survival of human cells in zebrafish, genetic engineering approaches introducing the production of human cytokines and growth factors can be taken, such as shown for human GM-CSF expression in zebrafish (28). It is also important to note that although zebrafish have a wide temperature range due to temperature variation in their natural habitat, xenotransplanted individuals are usually maintained at a comparatively low temperature of 33 to 34°C, opposite to the standard 37°C of the mouse and human body. This could affect cancer and NK cell growth, proliferation and metabolism. However, we did not observe any effect of the lower temperature on CAR-NK cell killing efficacy, suggesting that this may be a minor issue. In addition, protocols for maintaining adult zebrafish at 37°C were recently established, suggesting that zebrafish xenografts can be further adopted for preclinical cell therapy models in the future (23).
In this work, we successfully assessed the efficacy of the CAR-engineered NK-92 cell line to eliminate breast cancer cells within xenotransplanted zebrafish larvae. NK-92 cells have many similarities with primary NK cells. Several other NK sources are currently being investigated as CAR effectors (3, 9) and further studies are required to test whether zebrafish xenografts can also be used for these cell types. In addition, NK cells have recently been shown to be successfully retargeted by various engagers such as monoclonal antibodies or bispecific antibodies, and the zebrafish model provides an attractive system for high-throughput in vivo testing of these drugs (29–33). Therefore, we propose that the combination of novel engineered CAR-NK cells along with the versatility of zebrafish xenografts may facilitate the future advancement of CAR NK-based therapeutic approaches to the benefit of patients suffering from progressive disease.
Conclusion
Here, we demonstrate that zebrafish embryos and larvae are a suitable in vivo system for testing the killing efficacy of CAR-NK cells against metastatic solid tumor cells. This system is flexible and will allow mid-throughput screening of new drugs at low cost, leading to faster development of highly effective CAR-NK cell products. It can also be refined to personalize testing of CAR-NK cell efficacy against patient-derived cancer cells and as a predictive tool to select the best-possible therapy for the patient.
Data availability statement
The original contributions presented in the study are included in the article/Supplementary Material. Further inquiries can be directed to the corresponding author.
Ethics statement
Ethical approval was not required for the studies on humans in accordance with the local legislation and institutional requirements because only commercially available established cell lines were used. The animal study was approved by Landesdirektion Dresden (DD25-5131/450/4 and 25-5131/564/2 for breeding). The study was conducted in accordance with the local legislation and institutional requirements.
Author contributions
NM: Investigation, Writing – original draft, Writing – review and editing. PM: Writing – review and editing. AK: Writing – review and editing. WR: Writing – review and editing. SK: Writing – review and editing. WW: Writing – review and editing. TT: Conceptualization, Supervision, Writing – review and editing. FK: Conceptualization, Supervision, Writing – original draft, Writing – review and editing. JE: Conceptualization, Supervision, Writing – original draft, Writing – review and editing.
Funding
This research was supported in part by the German Federal Ministry of Education (Clusters4Future SaxoCell, 03ZU1111DA) and German Red Cross Blood Donation Service internal grants to TT, the German Research Foundation (DFG) through EI1223/2-1 to JE, the DFG TRR67 (project 387653785), SPP2084 µBone (project KN 1102/2–1) and Transcampus (project tC2020_02_MED) to FK. The work at the TU Dresden is co-financed with tax revenues based on the budget agreed by the Saxonian Landtag. This work was supported by the Light Microscopy Facility of the TU Dresden CMCB Technology Platform.
Acknowledgments
The authors would like to thank Erik Zenker and Madeleine Teichert for outstanding technical assistance, Hella Hartmann and Michael Gerlach for excellent assistance within the light microscopy facility, Akash Emmanuel Aravindan for help with confocal data analysis. We are grateful to Katrin Lambert for teaching the microinjection procedure, to Alejandra Cristina López-Delgado for general support and to Marika Fischer, Daniela Mögel and Silvio Kunadt for excellent fish care. BioRender software was used for the graphical illustrations in the figures.
Conflict of interest
TT and WW are named as inventors on patents in the field of cancer immunotherapy owned by their respective institutions.
The remaining authors declare that the research was conducted in the absence of any commercial or financial relationships that could be construed as a potential conflict of interest.
Publisher’s note
All claims expressed in this article are solely those of the authors and do not necessarily represent those of their affiliated organizations, or those of the publisher, the editors and the reviewers. Any product that may be evaluated in this article, or claim that may be made by its manufacturer, is not guaranteed or endorsed by the publisher.
Supplementary material
The Supplementary Material for this article can be found online at: https://www.frontiersin.org/articles/10.3389/fimmu.2023.1254821/full#supplementary-material
Supplementary Video 1 | Video showing in vivo interaction and killing of MDA-MB-231 GFP cells by PD-L1.CAR NK-92 cells in the zebrafish larval xenograft model. Refers to Figure 5.
Supplementary Video 2 | Video showing migration of PD-L1.CAR NK-92 cells (red) in the transgenic zebrafish larval xenograft model with dim GFP vessels. The NK cell migrates within the circulation to the metastatic breast cancer cell (bright green). Refers to Figure 6.
References
1. Finck AV, Blanchard T, Roselle CP, Golinelli G, June CH. Engineered cellular immunotherapies in cancer and beyond. Nat Med (2022) 28(4):678–89. doi: 10.1038/s41591-022-01765-8
2. Tonn T, Schwabe D, Klingemann HG, Becker S, Esser R, Koehl U, et al. Treatment of patients with advanced cancer with the natural killer cell line NK-92. Cytotherapy (2013) 15(12):1563–70. doi: 10.1016/j.jcyt.2013.06.017
3. Liu E, Marin D, Banerjee P, Macapinlac HA, Thompson P, Basar R, et al. Use of CAR-transduced natural killer cells in CD19-positive lymphoid tumors. N Engl J Med (2020) 382(6):545–53. doi: 10.1056/NEJMoa1910607
4. Burger MC, Forster MT, Romanski A, Strassheimer F, Macas J, Zeiner PS, et al. Intracranial injection of NK cells engineered with a HER2-targeted chimeric antigen receptor in patients with recurrent glioblastoma. Neuro Oncol (2023) 6:noad087. doi: 10.1093/neuonc/noad087
5. Uherek C, Tonn T, Uherek B, Becker S, Schnierle B, Klingemann HG, et al. Retargeting of natural killer-cell cytolytic activity to ErbB2-expressing cancer cells results in efficient and selective tumor cell destruction. Blood (2002) 100(4):1265–73. doi: 10.1182/blood.V100.4.1265.h81602001265_1265_1273
6. Schonfeld K, Sahm C, Zhang C, Naundorf S, Brendel C, Odendahl M, et al. Selective inhibition of tumor growth by clonal NK cells expressing an ErbB2/HER2-specific chimeric antigen receptor. Mol Ther (2015) 23(2):330–8. doi: 10.1038/mt.2014.219
7. Romanski A, Uherek C, Bug G, Seifried E, Klingemann H, Wels WS, et al. CD19-CAR engineered NK-92 cells are sufficient to overcome NK cell resistance in B-cell Malignancies. J Cell Mol Med (2016) 20(7):1287–94. doi: 10.1111/jcmm.12810
8. Miller JS, Soignier Y, Panoskaltsis-Mortari A, McNearney SA, Yun GH, Fautsch SK, et al. Successful adoptive transfer and in vivo expansion of human haploidentical NK cells in patients with cancer. Blood (2005) 105(8):3051–7. doi: 10.1182/blood-2004-07-2974
9. Zhu H, Lai YS, Li Y, Blum RH, Kaufman DS. Concise review: human pluripotent stem cells to produce cell-based cancer immunotherapy. Stem Cells (2018) 36(2):134–45. doi: 10.1002/stem.2754
10. Nowakowska P, Romanski A, Miller N, Odendahl M, Bonig H, Zhang C, et al. Clinical grade manufacturing of genetically modified, CAR-expressing NK-92 cells for the treatment of ErbB2-positive Malignancies. Cancer Immunol Immunother (2018) 67(1):25–38. doi: 10.1007/s00262-017-2055-2
11. Lam SH, Chua HL, Gong Z, Lam TJ, Sin YM. Development and maturation of the immune system in zebrafish, Danio rerio: a gene expression profiling, in situ hybridization and immunological study. Dev Comp Immunol (2004) 28(1):9–28. doi: 10.1016/s0145-305x(03)00103-4
12. Dietrich K, Fiedler IA, Kurzyukova A, Lopez-Delgado AC, McGowan LM, Geurtzen K, et al. Skeletal biology and disease modeling in zebrafish. J Bone Miner Res (2021) 36(3):436–58. doi: 10.1002/jbmr.4256
13. Miao KZ, Kim GY, Meara GK, Qin X, Feng H. Tipping the scales with zebrafish to understand adaptive tumor immunity. Front Cell Dev Biol (2021) 9:660969. doi: 10.3389/fcell.2021.660969
14. He X, Yin X, Wu J, Wickström SL, Duo Y, Du Q, et al. Visualization of human T lymphocyte-mediated eradication of cancer cells in vivo. Proc Natl Acad Sci U.S.A. (2020) 117(37):22910–9. doi: 10.1073/pnas.2009092117
15. Pascoal S, Salzer B, Scheuringer E, Wenninger-Weinzierl A, Sturtzel C, Holter W, et al. A preclinical embryonic zebrafish xenograft model to investigate CAR T cells in vivo. Cancers (Basel) (2020) 12(3):567. doi: 10.3390/cancers12030567
16. Zhou Z, Li J, Hong J, Chen S, Chen M, Wang L, et al. Interleukin-15 and chemokine ligand 19 enhance cytotoxic effects of chimeric antigen receptor T cells using zebrafish xenograft model of gastric cancer. Front Immunol (2022) 13:1002361. doi: 10.3389/fimmu.2022.1002361
17. Shimasaki N, Jain A, Campana D. NK cells for cancer immunotherapy. Nat Rev Drug Discovery (2020) 19(3):200–18. doi: 10.1038/s41573-019-0052-1
18. Gong JH, Maki G, Klingemann HG. Characterization of a human cell line (NK-92) with phenotypical and functional characteristics of activated natural killer cells. Leukemia (1994) 8(4):652–8.
19. Maki G, Klingemann HG, Martinson JA, Tam YK. Factors regulating the cytotoxic activity of the human natural killer cell line, NK-92. J Hematother Stem Cell Res (2001) 10(3):369–83. doi: 10.1089/152581601750288975
20. Eitler J, Wotschel N, Miller N, Boissel L, Klingemann HG, Wels W, et al. Inability of granule polarization by NK cells defines tumor resistance and can be overcome by CAR or ADCC mediated targeting. J Immunother Cancer (2021) 9(1):e001334. doi: 10.1136/jitc-2020-001334
21. Klingemann H. The NK-92 cell line-30 years later: its impact on natural killer cell research and treatment of cancer. Cytotherapy (2023) 25(5):451–7. doi: 10.1016/j.jcyt.2022.12.003
22. Beis D, Bartman T, Jin SW, Scott IC, D’Amico LA, Ober EA, et al. Genetic and cellular analyses of zebrafish atrioventricular cushion and valve development. Development (2005) 132(18):4193–204. doi: 10.1242/dev.01970
23. Yan C, Brunson DC, Tang Q, Do D, Iftimia NA, Moore JC, et al. Visualizing engrafted human cancer and therapy responses in immunodeficient zebrafish. Cell (2019) 177(7):1903–1914 e1914. doi: 10.1016/j.cell.2019.04.004
24. Delconte RB, Kolesnik TB, Dagley LF, Rautela J, Shi W, Putz EM, et al. CIS is a potent checkpoint in NK cell-mediated tumor immunity. Nat Immunol (2016) 17(7):816–24. doi: 10.1038/ni.3470
25. Liu E, Tong Y, Dotti G, Shaim H, Savoldo B, Mukherjee M, et al. Cord blood NK cells engineered to express IL-15 and a CD19-targeted CAR show long-term persistence and potent antitumor activity. Leukemia (2018) 32(2):520–31. doi: 10.1038/leu.2017.226
26. Daldrup-Link HE, Meier R, Rudelius M, Piontek G, Piert M, Metz S, et al. In vivo tracking of genetically engineered, anti-HER2/neu directed natural killer cells to HER2/neu positive mammary tumors with magnetic resonance imaging. Eur Radiol (2005) 15(1):4–13. doi: 10.1007/s00330-004-2526-7
27. Yan C, Yang Q, Zhang S, Millar DG, Alpert EJ, Do D, et al. Single-cell imaging of T cell immunotherapy responses in vivo. J Exp Med (2021) 218(10):e20210314. doi: 10.1084/jem.20210314
28. Rajan V, Melong N, Hing Wong W, King B, Tong SR, Mahajan N, et al. Humanized zebrafish enhance human hematopoietic stem cell survival and promote acute myeloid leukemia clonal diversity. Haematologica (2020) 105(10):2391–9. doi: 10.3324/haematol.2019.223040
29. Snyder KM, Hullsiek R, Mishra HK, Mendez DC, Li Y, Rogich A, et al. Expression of a recombinant high affinity igG fc receptor by engineered NK cells as a docking platform for therapeutic mAbs to target cancer cells. Front Immunol (2018) 9:2873. doi: 10.3389/fimmu.2018.02873
30. Vallera DA, Oh F, Kodal B, Hinderlie P, Geller MA, Miller JS, et al. A HER2 tri-specific NK cell engager mediates efficient targeting of human ovarian cancer. Cancers (Basel) (2021) 13(16):3994. doi: 10.3390/cancers13163994
31. Wingert S, Reusch U, Knackmuss S, Kluge M, Damrat M, Pahl J, et al. Preclinical evaluation of AFM24, a novel CD16A-specific innate immune cell engager targeting EGFR-positive tumors. MAbs (2021) 13(1):1950264. doi: 10.1080/19420862.2021.1950264
32. Demaria O, Gauthier L, Vetizou M, Blanchard Alvarez A, Vagne C, Habif G, et al. Antitumor immunity induced by antibody-based natural killer cell engager therapeutics armed with not-alpha IL-2 variant. Cell Rep Med (2022) 3(10):100783. doi: 10.1016/j.xcrm.2022.100783
Keywords: CAR-NK cells, zebrafish xenograft, cancer immunotherapy, NK-92, PD-L1, ErbB2 (HER2), breast cancer, in vivo imaging
Citation: Murali Shankar N, Ortiz-Montero P, Kurzyukova A, Rackwitz W, Künzel SR, Wels WS, Tonn T, Knopf F and Eitler J (2023) Preclinical assessment of CAR-NK cell-mediated killing efficacy and pharmacokinetics in a rapid zebrafish xenograft model of metastatic breast cancer. Front. Immunol. 14:1254821. doi: 10.3389/fimmu.2023.1254821
Received: 07 July 2023; Accepted: 12 September 2023;
Published: 11 October 2023.
Edited by:
David Wald, Case Western Reserve University, United StatesReviewed by:
Kamalakannan Rajasekaran, Genentech Inc., United StatesFrank Momburg, Antigen Presentation and T/NK Cell Activation Group, Clinical Cooperation Unit Applied Tumor Immunity, German Cancer Research Centre, Germany
Copyright © 2023 Murali Shankar, Ortiz-Montero, Kurzyukova, Rackwitz, Künzel, Wels, Tonn, Knopf and Eitler. This is an open-access article distributed under the terms of the Creative Commons Attribution License (CC BY). The use, distribution or reproduction in other forums is permitted, provided the original author(s) and the copyright owner(s) are credited and that the original publication in this journal is cited, in accordance with accepted academic practice. No use, distribution or reproduction is permitted which does not comply with these terms.
*Correspondence: Torsten Tonn, dC50b25uQGJsdXRzcGVuZGUuZGU=; Franziska Knopf, ZnJhbnppc2thLmtub3BmQHR1LWRyZXNkZW4uZGU=
†These authors have contributed equally to this work and share senior authorship