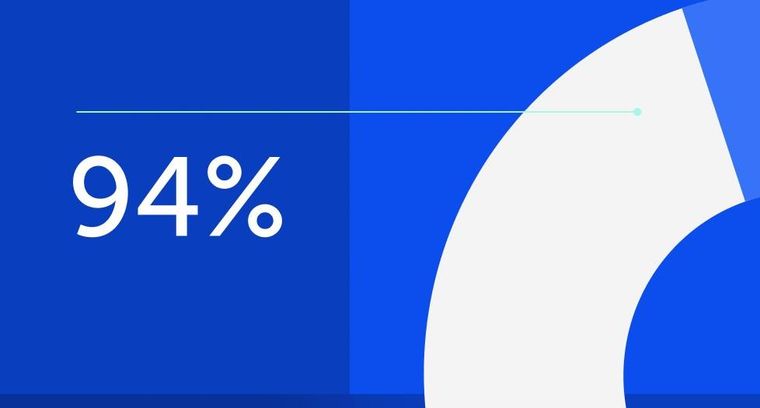
94% of researchers rate our articles as excellent or good
Learn more about the work of our research integrity team to safeguard the quality of each article we publish.
Find out more
MINI REVIEW article
Front. Immunol., 21 August 2023
Sec. Viral Immunology
Volume 14 - 2023 | https://doi.org/10.3389/fimmu.2023.1253395
This article is part of the Research TopicChimeric Antigen Receptor (CAR) Engineered Immune Cells for Infectious Viral DiseasesView all 4 articles
Chimeric Antigen Receptor (CAR) T cell therapies are tremendously successful in hematological malignancies and show great promise as treatment and curative strategy for HIV. A major determinant for effective CAR T cell therapy is the persistence of CAR T cells. Particularly, antigen density and target cell abundance are crucial for the engagement, engraftment, and persistence of CAR T cells. The success of HIV-specific CAR T cells is challenged by limited antigen due to low cell surface expression of viral proteins and the scarcity of chronically infected cells during antiretroviral therapy. Several strategies have been explored to increase the efficacy of CAR T cells by enhancing expansion and persistence of the engineered cells. This review highlights the challenges of designing CAR T cells against HIV and other chronic viral infections. We also discuss potential strategies to enhance CAR T cell expansion and persistence in the setting of low antigen exposure.
While Chimeric antigen receptor (CAR) T cell therapy has emerged as a potent treatment against several hematological malignancies (1–5), it was initially developed as a strategy for durable control of HIV. Although the concept was proved safe and engineered cells could be detected 10 years after infusion, results from early clinical trials did not show any impact of HIV-specific CAR T cell therapy on the latent viral reservoir (6–12). Subsequent improvements to the design of the CAR, specifically inclusion of an intracellular co-stimulatory signaling domain along with the CD3ζ domain, have proved vital to increase the efficacy of CAR T cells (13, 14). After the clinical success of CAR T cells targeting CD19 or BCMA in B cell malignancies, the field has expanded substantially and interest in CAR T cells as treatment for other diseases has been renewed. CAR T cells are now being explored against a range of diverse conditions, including solid tumors, rheumatological disease and chronic viral infections (15–19). However, the immunological landscape varies in these conditions giving rise to new challenges for effective CAR T cell therapy. Following the initial trials with HIV-specific CAR T cells and the considerable advances to the CAR design achieved in hematological indications, there is a renewed interest in using CAR T cells as a curative approach for HIV. Several HIV-specific CAR T cells are now being evaluated in published (20) and ongoing clinical trials (NCT04648046 and NCT03617198). HIV-specific CAR constructs are typically based on the CD4 ectodomain or single-chain variable fragments (scFv) derived from broadly neutralizing anti-Envelope (Env) antibodies. Several studies have further engineered the CAR T cells to become resistant to HIV infection through HIV co-receptor disruption or expression of entry inhibitors (20–28). However, one key obstacle to effective anti-HIV CAR T cell therapy is the persistence of the engineered cells in vivo.
Persistence of CAR T cells has been identified as a major determinant for treatment response (3, 4, 29). Several studies have demonstrated that the antigen density and subsequent CAR engagement with the antigen are crucial for CAR efficacy and engraftment (30–32). During ART-suppressed chronic HIV infection, infected cells typically have low levels of HIV antigen on their surface and in addition, latently infected cells are rare (33–36). These properties are in stark contrast to the high tumor burden and high antigen density observed in B cell malignancies. This gives rise to a barrier in ensuring persistence of CAR T cells directed towards HIV. In this mini-review, we address strategies to enhance the expansion and persistence of CAR T cells that are required for effective HIV-specific CAR T cell therapies in chronic HIV infection (Figure 1).
Figure 1 Strategies for enhancing CAR T cell persistence. (A) CAR design. The design of the CAR with inclusion and choice of co-stimulatory domain has great impact on persistence. (B) T cell phenotype. A less differentiated memory like phenotype of infused CAR T cells is favorable. (C) Preconditioning. Effective lymphodepleting preconditioning prior to CAR T cell infusion can increase CAR T cell persistence. (D) Receptor engagement. Target antigen encounter is crucial for the CAR T cells to persist after infusion. In the setting of low antigen exposure CAR engagement can be ensured by either vaccination (yellow box) or antigen supplementation (red box). Another approach to accommodate low level target antigen is engagement of the native TCR (blue box). Figure created with BioRender.com.
A major determinant for treatment response to CAR T cell therapies in B cell malignancies is the expansion and persistence of the CAR T cells (3, 4, 29). Several trials have demonstrated that the presence of CAR T cells in circulation months after infusion is associated with long-term treatment response (3, 29, 37). Both the cumulative CAR T cell expansion and peak numbers of CAR T cells after the initial expansion phase have been found to be significantly higher for patients responding to anti-CD19 CAR T cell therapy compared to non-responders (29, 38, 39). Additionally, patients with long-term persistence of CAR T cells have a greater initial peak of CAR T cell levels than patients without persistence of the CAR T cells (29, 37). Due to the essential role of target antigen density, patients with higher pre-treatment tumor burden and higher target antigen density have been shown to have a greater peak and accompanying expansion of the CAR T cells (38, 40). However, high pre-treatment tumor burden is generally associated with worse outcome. These studies demonstrate that the ability of the infused CAR T cells to expand and persist are crucial for the treatment success.
In order for CAR T cells to expand, the CAR or T cell receptor (TCR) has to engage with the specific antigen. A native TCR is 100-fold more sensitive to antigen than a CAR where it is estimated that a threshold of 200 target antigen molecules per cell is required for lytic activity of the CAR T cells and 10-fold higher for cytokine production (31, 41). The requirement for high antigen exposure poses a challenge for effective CAR T cell therapy in diseases where the antigen density is low, such as HIV. The sole surface antigen available for immune recognition of HIV-infected cells is the Env composed of the glycoprotein subunits gp120 and gp41. However, Env is expressed at very low levels both on virions and on the surface of infected cells (33, 35). Additionally, during ART suppression the Env expression decrease and latently infected cells become exceedingly rare (36). Combined, the low antigen density of HIV Env and the scarcity of latently infected cells challenge the expansion and persistence of anti-HIV CAR T cells due to insufficient CAR engagement.
In the first generation of CARs, signaling through CD3ζ alone did not induce an adequate treatment response. In subsequent generations of CARs two or more co-stimulatory signaling domains have been incorporated with greatly increased efficacy (11, 42) (Figure 1A). The most common design is the second generation CARs composed of an extracellular scFv fused to the intracellular signaling domains of either CD28 or 4-1BB along with CD3ζ (43). Several studies have compared the impact on proliferative capacity and persistence of second generation CARs using either CD28 or 4-1BB co-stimulatory signaling. Pre-clinical studies typically favor 4-1BB CARs although differences in efficacy are not as evident in clinical trials where other variations in CAR design and choice of delivery vector might also affect the differences observed in CAR T cell function (43–46).
Engagement of CD28-based CARs rapidly activates T cell effector functions relying on glycolytic metabolism inducing an effector phenotype. Engagement of 4-1BB-based CARs promotes a less differentiated memory phenotype of the T cell relying on oxidative metabolism and mitochondrial biogenesis. This leads to an enhanced persistence of the 4-1BB-based CAR T cells (45, 47–50). Additionally, the increased persistence of CAR T cells incorporating 4-1BB is further hypothesized to be due to a slower initial tumor clearance compared to CD28 CAR T cells. The slower tumor clearance results in longer antigenic stimulus of the 4-1BB-incorporating CAR T cells (51).
Furthermore, CD28-based CARs usually comes with an increased incidence of cytokine release syndrome, neurotoxicity and with higher rates of severe adverse events (13). For HIV-specific CAR T cells, Maldini et al. explored the choice of co-stimulatory domain in the CAR design in a murine study where 4-1BB-based CARs showed a proliferative advantage (23). However, T cells transduced with both a CD28 and a 4-1BB-based CAR, showed superior proliferation and mitigation of CD4+ T cell loss which was not observed for third generation CARs composed of both CD28 and 4-1BB in the same construct (23). Similarly, Leibman et al. showed in mice that 4-1BB-based CAR T cells reach greater numbers in both HIV-infected and uninfected humanized mice (22).
Further modifications of the CAR architecture could potentiate the expansion and persistence of CAR T cells in the setting of low-level antigen exposure (Figure 1A). The scFv linker, extracellular hinge/spacer and transmembrane domain all have profound impact on the functionality of the CAR T cells (52–56). Similarly, mutations in the intracellular signaling domains to avert tonic signaling have led to increased persistence and antitumor efficacy (57, 58). Several other co-stimulatory signaling domains and combinations thereof have been evaluated along with co-expression of cytokines or gene circuits with promising results. These additional CAR modifications have been reviewed extensively elsewhere (59–61).
The phenotype of engineered CAR T cells greatly influence the potency and proliferative capacity of the cells (Figure 1B). CAR T cells produced from less differentiated memory phenotypes, e.g., naïve and stem cell memory T cells, compared to either bulk or more differentiated phenotypes have higher antileukemic potency and greater proliferation in pre-clinical trials (62–67). Analyses of responders to anti-CD19 CAR T cell therapy in clinical trials further reveal that a less differentiated memory phenotype positively impact the efficacy and persistence of the CAR T cells (68–71). CAR T cells with a specific phenotype can be attained by either enrichment of cells with the desired phenotype or by supplementing the cell culture medium with specific cytokines known to modulate the phenotype of T cells (72–76). By expanding the CAR T cells in the presence of a combination of IL-7, IL-12, IL-15 and IL-21, cells exhibiting a less differentiated phenotype are retained resulting in a more durable antitumor response and greater persistence (72–74). Alternatively, anti-HIV CAR T cells derived from hematopoietic stem and progenitor cells (HSPC) have shown long-term persistence in NHPs (77–80). The regenerative nature of HSPCs makes these a promising choice for producing CAR T cells with long-term persistence although obtaining sufficient HSPCs from adult patients will be limiting with current technologies.
Several clinical trials have shown enhanced persistence of CAR T cells when patients are preconditioned with effective lymphodepleting most often using cyclophosphamide and fludarabine (40, 46, 81–83) (Figure 1C). Lymphodepleting preconditioning could have an additional beneficial effect when HIV-specific CAR T cells are used as a curative strategy as a means of lowering the size of the latent viral reservoir. Published cases of HIV-1 cure after CCR5Δ32/Δ32 HSPC transplantation have all received lymphodepleting preconditioning which is known to drastically reduce the size of the viral reservoir by eliminating host CD4+ T cells (84–91). However, careful consideration and optimization of the pre-CAR T cell lymphodepletion regimens to lessen adverse effects are required before use in otherwise healthy people with HIV.
Due to the crucial role of target antigen density for CAR T cell persistence, multiple strategies for ensuring receptor engagement have been employed. CAR engagement can be facilitated by increasing the level of available antigen. Increased HIV antigen can be achieved through the use of latency reversing agents (LRAs) which is a thoroughly studied concept in HIV cure trials. Several LRAs have been evaluated in clinical trials where they are safe and can induce viral reactivation in ART-suppressed participants (92–96). Target antigen density and subsequent CAR engagement can also be increased through vaccination or antigen supplementation (Figure 1D). An alternative approach is to engage the native T cell receptor (Figure 1D). In this section, these concepts will be discussed.
In indications with low CAR-specific antigen densities, sufficient antigen levels can be achieved through vaccination with the CAR-specific antigen (Figure 1D). CAR engagement through vaccination has the advantage of having the CAR-specific antigen presented by antigen presenting cells (APC) in lymphoid tissues and thus providing additional immunostimulatory signaling. Ma and colleagues (97) evaluated an amphiphile CAR T cell ligand that traffics to draining lymph nodes upon injection where it decorates the surface of APCs. Mice receiving a low dose of 5 x 104 CAR T cells followed by vaccination with the amphiphile CAR T cell ligand had significantly more CAR T cells in the peripheral blood 14 days after vaccination than mice receiving a 20-fold higher dose of 10 x 106 CAR T cells (97). Likewise, Reinhard and colleagues (98) developed a lipid nanoparticle (LNP) RNA vaccine encoding the CAR-specific antigen. Similar to Ma et al, mice receiving a low dose of 1 x 103 CAR T cells followed by LNP-RNA vaccination achieved greater CAR T cell levels 11 days after CAR T cell dosing than mice receiving a 1000-fold higher dose of 1 x 106 CAR T cells but not the vaccine. Finally, the authors hypothesize that administration of a lower initial dose of CAR T cells followed by serial vaccination will lead to CAR T cell levels in the optimal therapeutic range while avoiding dose limiting toxicities observed by high dose administration and insufficient therapeutic activity observed when CAR T cells fail to persist (98).
Another approach to increase CAR engagement is to engineer cells to express the CAR-specific antigen and thus provide antigen supplementation (Figure 1D). Two studies have explored antigen supplementation to enhance HIV-specific CAR T cell persistence in the absence of HIV viremia (23, 99). Uninfected BLT humanized mice or ART-suppressed non-human primates (NHP), respectively, were administered a cell line, K562, engineered to express HIV Env. This led to proliferation of the HIV-specific CAR T cells in both models and prolonged time to viral rebound in the NHPs (23, 99). K562 cells have been explored extensively as artificial APC given their lack of HLA surface expression, ease of engineering, and good safety profile (100–102). Antigen supplementation by artificial APCs therefore also represents a potential “vaccination-like” strategy for expanding CAR T cells in vivo in the absence of high antigen levels.
Most CAR T cells retain their native TCR which has been exploited in an effort to enhance the persistence of the CAR T cells (Figure 1D). Adoptive transfer of T cells with TCR-specificity toward chronic viral infections can persist when administered to recipients of allogeneic bone marrow transplant and reconstitute the cellular immunity toward these infections (103–105). By enriching virus-specific T cells these can be engineering to express a CAR directed against a different antigen while retaining the TCR specificity. The CAR T cells can then expand through engagement with the TCR-specific antigen in the absence of the CAR-specific antigen (106–111). Several pre-clinical studies have demonstrated improved expansion and persistence in vivo of CAR T cells engineered from virus-specific T cells when vaccination directed to the TCR is administered (112–114) Importantly, virus-specific CAR T cells does not expand when a vaccine directed to a different TCR-specificity is administered (112).
This indirect CAR T cell expansion approach has been explored in clinical trials using Epstein-Barr virus (EBV)-specific T cells engineered to express a CAR against a tumor antigen (115–118). In a clinical trial from 2008, Pule and colleagues (115) evaluated EBV-specific GD-2-CAR T cells in 11 participants. The EBV-specific GD-2-CAR T cells were detectable twice as long as GD-2-CAR T cells without EBV-TCR specificity. Additionally, CAR T cells sampled at different time points up to 6 months after infusion were cultured with autologous EBV-expressing B cells and the CAR transgene was quantified by qPCR. Only EBV-specific GD-2-CAR T cells expanded in response to EBV-TCR stimulation (115). In a 2019 clinical trial, Lapteva and colleagues (116) assessed EBV-specific CD19-CAR T cells. They found that the EBV-specific CD19-CAR T cells expanded and persisted in participants with reactivation of EBV leading to engagement of the native EBV-specific TCR (116). Lastly, Cruz and colleagues (117) performed a clinical trial assessing the safety of infusing virus-specific allogeneic CD19-CAR T cells to recipients of allogeneic HSPC transplantation. No graft-versus-host reaction was observed, which the authors hypothesized was due to the virus-specificity of the TCR. Two study participants had viral reactivation of EBV which coincided with an increase in the EBV-specific CD19-CAR T cells suggesting that EBV-TCR engagement induced an expansion of the EBV-specific CAR T cells (117). Lastly, Guan and colleagues (114) evaluated HIV-specific CAR T cells made from Cytomegalovirus (CMV)-specific T cells with CMV vaccination during HIV-suppressive ART. They observed that for the same dose of HIV CAR T cells, only the CAR T cells with CMV-specific TCR expanded after administration of a CMV vaccine (114).
Although TCR-engagement can lead to substantial CAR T cell expansion, TCR and CAR cross-talk have important implications for the functionality of CAR T cells (119). Yang and colleagues (120) demonstrated that CAR T cells produced from T cells with TCR specificity to the ubiquitously expressed male minor histocompatibility antigen HY have diverse in vivo activity in the presence of TCR- or CAR antigens (120). In the presence of TCR antigen, CD8+ CAR T cells did not expand and had diminished efficacy while CD4+ CAR T cells expanded and retained their effector functions (120). Furthermore, the indirect CAR T cell expansion strategy require the presence of the native TCR. Recent efforts in allogeneic CAR T cell development have targeted CAR integration to the TCR alpha constant locus (TRAC) (121). This results in disruption of the native TCR while driving the CAR from the endogenous TRAC promoter leading to greater antitumor activity (121). Disruption of the native TCR would thus preclude the indirect CAR T cell expansion method but may become an “off-the-shelf” CAR T cell product by bypassing the need for individual cell engineering.
Ensuring long-term persistence of HIV-specific CAR T cells is key to achieving durable control of the infection in the absence of ART. Here, we have described strategies to enhance the expansion and persistence of CAR T cells. We argue that successful CAR T cell trials in HIV will likely require combinations of the described approaches to ensure persistence of the CAR T cells and ultimately achieve a cure for HIV.
FR: Conceptualization, Visualization, Writing – original draft, Writing – review & editing. NL: Visualization, Writing – review & editing. OS: Writing – review & editing. MT: Conceptualization, Funding acquisition, Supervision, Writing – review & editing.
This work was supported by from amfAR (Target Grant # 110533-74-RPRL to MT) and the Novo Nordisk Foundation (NNF19OC0054577 to MT).
The authors declare that the research was conducted in the absence of any commercial or financial relationships that could be construed as a potential conflict of interest.
All claims expressed in this article are solely those of the authors and do not necessarily represent those of their affiliated organizations, or those of the publisher, the editors and the reviewers. Any product that may be evaluated in this article, or claim that may be made by its manufacturer, is not guaranteed or endorsed by the publisher.
1. Grupp SA, Kalos M, Barrett D, Aplenc R, Porter DL, Rheingold SR, et al. Chimeric antigen receptor-modified T cells for acute lymphoid leukemia. N Engl J Med (2013) 368(16):1509–18. doi: 10.1056/NEJMoa1215134
2. Schuster SJ, Svoboda J, Chong EA, Nasta SD, Mato AR, Anak O, et al. Chimeric antigen receptor T cells in refractory B-cell lymphomas. N Engl J Med (2017) 377(26):2545–54. doi: 10.1056/NEJMoa1708566
3. Park JH, Riviere I, Gonen M, Wang X, Senechal B, Curran KJ, et al. Long-term follow-up of CD19 CAR therapy in acute lymphoblastic leukemia. N Engl J Med (2018) 378(5):449–59. doi: 10.1056/NEJMoa1709919
4. Maude SL, Frey N, Shaw PA, Aplenc R, Barrett DM, Bunin NJ, et al. Chimeric antigen receptor T cells for sustained remissions in leukemia. N Engl J Med (2014) 371(16):1507–17. doi: 10.1056/NEJMoa1407222
5. Maude SL, Laetsch TW, Buechner J, Rives S, Boyer M, Bittencourt H, et al. Tisagenlecleucel in children and young adults with B-cell lymphoblastic leukemia. N Engl J Med (2018) 378(5):439–48. doi: 10.1056/NEJMoa1709866
6. Romeo C, Seed B. Cellular immunity to HIV activated by CD4 fused to T cell or Fc receptor polypeptides. Cell (1991) 64(5):1037–46. doi: 10.1016/0092-8674(91)90327-U
7. Roberts MR, Qin L, Zhang D, Smith DH, Tran AC, Dull TJ, et al. Targeting of human immunodeficiency virus-infected cells by CD8+ T lymphocytes armed with universal T-cell receptors. Blood (1994) 84(9):2878–89. doi: 10.1182/blood.V84.9.2878.2878
8. Yang OO, Tran A-C, Kalams SA, Johnson RP, Roberts MR, Walker BD, et al. Lysis of HIV-1-infected cells and inhibition of viral replication by universal receptor T cells. Proc Natl Acad Sci (1997) 94(21):11478–83. doi: 10.1073/pnas.94.21.11478
9. Mitsuyasu RT, Anton PA, Deeks SG, Scadden DT, Connick E, Downs MT, et al. Prolonged survival and tissue trafficking following adoptive transfer of CD4ζ gene-modified autologous CD4+ and CD8+ T cells in human immunodeficiency virus–infected subjects. Blood (2000) 96(3):785–93. doi: 10.1182/blood.V96.3.785.015k10_785_793
10. Walker RE, Bechtel CM, Natarajan V, Baseler M, Hege KM, Metcalf JA, et al. Long-term in vivo survival of receptor-modified syngeneic T cells in patients with human immunodeficiency virus infection. Blood (2000) 96(2):467–74. doi: 10.1182/blood.V96.2.467
11. Deeks SG, Wagner B, Anton PA, Mitsuyasu RT, Scadden DT, Huang C, et al. A phase II randomized study of HIV-specific T-cell gene therapy in subjects with undetectable plasma viremia on combination antiretroviral therapy. Mol Ther (2002) 5(6):788–97. doi: 10.1006/mthe.2002.0611
12. Scholler J, Brady TL, Binder-Scholl G, Hwang W-T, Plesa G, Hege KM, et al. Decade-long safety and function of retroviral-modified chimeric antigen receptor T cells. Sci Trans Med (2012) 4(132):132ra53–132ra53. doi: 10.1126/scitranslmed.3003761
13. Cappell KM, Kochenderfer JN. A comparison of chimeric antigen receptors containing CD28 versus 4-1BB costimulatory domains. Nat Rev Clin Oncol (2021) 18(11):715–27. doi: 10.1038/s41571-021-00530-z
14. Guedan S, Calderon H, Posey AD, Maus MV. Engineering and design of chimeric antigen receptors. Mol Ther Methods Clin Dev (2019) 12:145–56. doi: 10.1016/j.omtm.2018.12.009
15. Mackensen A, Muller F, Mougiakakos D, Boltz S, Wilhelm A, Aigner M, et al. Anti-CD19 CAR T cell therapy for refractory systemic lupus erythematosus. Nat Med (2022) 28(10):2124–32. doi: 10.1038/s41591-022-02017-5
16. Rurik JG, Tombácz I, Yadegari A, Méndez Fernández PO, Shewale SV, Li L, et al. CAR T cells produced in vivo to treat cardiac injury. Science (2022) 375(6576):91–6. doi: 10.1126/science.abm0594
17. Bhatia V, Kamat NV, Pariva TE, Wu LT, Tsao A, Sasaki K, et al. Targeting advanced prostate cancer with STEAP1 chimeric antigen receptor T cell and tumor-localized IL-12 immunotherapy. Nat Commun (2023) 14(1):2041. doi: 10.1038/s41467-023-37874-2
18. O’Rourke DM, Nasrallah MP, Desai A, Melenhorst JJ, Mansfield K, Morrissette JJD, et al. A single dose of peripherally infused EGFRvIII-directed CAR T cells mediates antigen loss and induces adaptive resistance in patients with recurrent glioblastoma. Sci Trans Med (2017) 9(399):eaaa0984. doi: 10.1126/scitranslmed.aaa0984
19. Zhang X, Wang T, Zhu X, Lu Y, Li M, Huang Z, et al. GMP development and preclinical validation of CAR-T cells targeting a lytic EBV antigen for therapy of EBV-associated Malignancies. Front Immunol (2023) 14:1103695. doi: 10.3389/fimmu.2023.1103695
20. Liu B, Zhang W, Xia B, Jing S, Du Y, Zou F, et al. Broadly neutralizing antibody-derived CAR T cells reduce viral reservoir in individuals infected with HIV-1. J Clin Invest (2021) 131(19):e150211. doi: 10.1172/JCI150211
21. Hale M, Mesojednik T, Romano Ibarra GS, Sahni J, Bernard A, Sommer K, et al. Engineering HIV-resistant, anti-HIV chimeric antigen receptor T cells. Mol Ther (2017) 25(3):570–9. doi: 10.1016/j.ymthe.2016.12.023
22. Leibman RS, Richardson MW, Ellebrecht CT, Maldini CR, Glover JA, Secreto AJ, et al. Supraphysiologic control over HIV-1 replication mediated by CD8 T cells expressing a re-engineered CD4-based chimeric antigen receptor. PloS Pathog (2017) 13(10):e1006613. doi: 10.1371/journal.ppat.1006613
23. Maldini CR, Claiborne DT, Okawa K, Chen T, Dopkin DL, Shan X, et al. Dual CD4-based CAR T cells with distinct costimulatory domains mitigate HIV pathogenesis in vivo. Nat Med (2020) 26(11):1776–87. doi: 10.1038/s41591-020-1039-5
24. Maldini CR, Gayout K, Leibman RS, Dopkin DL, Mills JP, Shan X, et al. HIV-resistant and HIV-specific CAR-modified CD4(+) T cells mitigate HIV disease progression and confer CD4(+) T cell help in vivo. Mol Ther (2020) 28(7):1585–99. doi: 10.1016/j.ymthe.2020.05.012
25. Ali A, Kitchen SG, Chen ISY, Ng HL, Zack JA, Yang OO, et al. HIV-1-specific chimeric antigen receptors based on broadly neutralizing antibodies. J Virol (2016) 90(15):6999–7006. doi: 10.1128/JVI.00805-16
26. Rothemejer FH, Lauritsen NP, Juhl AK, Schleimann MH, König S, Søgaard OS, et al. Development of HIV-resistant CAR T cells by CRISPR/cas-mediated CAR integration into the CCR5 locus. Viruses (2023) 15(1):202. doi: 10.3390/v15010202
27. Liu B, Zou F, Lu L, Chen C, He D, Zhang X, et al. Chimeric antigen receptor T cells guided by the single-chain fv of a broadly neutralizing antibody specifically and effectively eradicate virus reactivated from latency in CD4+ T lymphocytes isolated from HIV-1-infected individuals receiving suppressive combined antiretroviral therapy. J Virol (2016) 90(21):9712–24. doi: 10.1128/JVI.00852-16
28. Liu L, Patel B, Ghanem MH, Bundoc V, Zheng Z, Morgan RA, et al. Novel CD4-based bispecific chimeric antigen receptor designed for enhanced anti-HIV potency and absence of HIV entry receptor activity. J Virol (2015) 89(13):6685–94. doi: 10.1128/JVI.00474-15
29. Porter DL, Hwang W-T, Frey NV, Lacey SF, Shaw PA, Loren AW, et al. Chimeric antigen receptor T cells persist and induce sustained remissions in relapsed refractory chronic lymphocytic leukemia. Sci Trans Med (2015) 7(303):303ra139. doi: 10.1126/scitranslmed.aac5415
30. Ramakrishna S, Highfill SL, Walsh Z, Nguyen SM, Lei H, Shern JF, et al. Modulation of target antigen density improves CAR T-cell functionality and persistence. Clin Cancer Res (2019) 25(17):5329–41. doi: 10.1158/1078-0432.CCR-18-3784
31. Watanabe K, Terakura S, Martens AC, van Meerten T, Uchiyama S, Imai, et al. Target antigen density governs the efficacy of anti-CD20-CD28-CD3 zeta chimeric antigen receptor-modified effector CD8+ T cells. J Immunol (2015) 194(3):911–20. doi: 10.4049/jimmunol.1402346
32. Walker AJ, Majzner RG, Zhang L, Wanhainen K, Long AH, Nguyen SM, et al. Tumor antigen and receptor densities regulate efficacy of a chimeric antigen receptor targeting anaplastic lymphoma kinase. Mol Ther (2017) 25(9):2189–201. doi: 10.1016/j.ymthe.2017.06.008
33. Klein JS, Bjorkman PJ. Few and far between: how HIV may be evading antibody avidity. PloS Pathog (2010) 6(5):e1000908. doi: 10.1371/journal.ppat.1000908
34. Ho YC, Shan L, Hosmane NN, Wang J, Laskey SB, Rosenbloom DI, et al. Replication-competent noninduced proviruses in the latent reservoir increase barrier to HIV-1 cure. Cell (2013) 155(3):540–51. doi: 10.1016/j.cell.2013.09.020
35. Zhu P, Liu J, Bess J Jr., Chertova E, Lifson JD, Grise H, et al, et al. Distribution and three-dimensional structure of AIDS virus envelope spikes. Nature (2006) 441(7095):847–52. doi: 10.1038/nature04817
36. Ruelas DS, Greene WC. An integrated overview of HIV-1 latency. Cell (2013) 155(3):519–29. doi: 10.1016/j.cell.2013.09.044
37. Wittibschlager V, Bacher U, Seipel K, Porret N, Wiedemann G, Haslebacher C, et al. CAR T-cell persistence correlates with improved outcome in patients with B-cell lymphoma. Int J Mol Sci (2023) 24(6):5688. doi: 10.3390/ijms24065688
38. Mueller KT, Maude SL, Porter DL, Frey N, Wood P, Han X, et al. Cellular kinetics of CTL019 in relapsed/refractory B-cell acute lymphoblastic leukemia and chronic lymphocytic leukemia. Blood (2017) 130(21):2317–25. doi: 10.1182/blood-2017-06-786129
39. Hay KA, Gauthier J, Hirayama AV, Voutsinas JM, Wu Q, Li D, et al. Factors associated with durable EFS in adult B-cell ALL patients achieving MRD-negative CR after CD19 CAR T-cell therapy. Blood (2019) 133(15):1652–63. doi: 10.1182/blood-2018-11-883710
40. Turtle CJ, Hanafi LA, Berger C, Gooley TA, Cherian S, Hudecek M, et al. CD19 CAR-T cells of defined CD4+:CD8+ composition in adult B cell ALL patients. J Clin Invest (2016) 126(6):2123–38. doi: 10.1172/JCI85309
41. Salter AI, Rajan A, Kennedy JJ, Ivey RG, Shelby SA, Leung I, et al. Comparative analysis of TCR and CAR signaling informs CAR designs with superior antigen sensitivity and in vivo function. Sci Signaling (2021) 14(697):eabe2606. doi: 10.1126/scisignal.abe2606
42. Savoldo B, Ramos CA, Liu E, Mims MP, Keating MJ, Carrum G, et al. CD28 costimulation improves expansion and persistence of chimeric antigen receptor–modified T cells in lymphoma patients. J Clin Invest (2011) 121(5):1822–6. doi: 10.1172/JCI46110
43. Cappell KM, Kochenderfer JN. Long-term outcomes following CAR T cell therapy: what we know so far. Nat Rev Clin Oncol (2023) p:1–13. doi: 10.1038/s41571-023-00754-1
44. Cheng Z, Wei R, Ma Q, Shi L, He F, Shi Z, et al. In vivo expansion and antitumor activity of coinfused CD28- and 4-1BB-engineered CAR-T cells in patients with B cell leukemia. Mol Ther (2018) 26(4):976–85. doi: 10.1016/j.ymthe.2018.01.022
45. van der Stegen SJ, Hamieh M, Sadelain M. The pharmacology of second-generation chimeric antigen receptors. Nat Rev Drug Discov (2015) 14(7):499–509. doi: 10.1038/nrd4597
46. Elsallab M, Ellithi M, Hempel S, Abdel-Azim H, Abou-El-Enein M. Long-term response to autologous anti-CD19 chimeric antigen receptor T cells in relapsed or refractory B cell acute lymphoblastic leukemia: a systematic review and meta-analysis. Cancer Gene Ther (2023) 30:845–54. doi: 10.1038/s41417-023-00593-3
47. Salter AI, Ivey RG, Kennedy JJ, Voillet V, Rajan A, Alderman EJ, et al. Phosphoproteomic analysis of chimeric antigen receptor signaling reveals kinetic and quantitative differences that affect cell function. Sci Signaling (2018) 11(544):eaat6753. doi: 10.1126/scisignal.aat6753
48. Kawalekar OU, O'Connor RS, Fraietta JA, Guo L, McGettigan SE, Posey AD Jr., et al. Distinct signaling of coreceptors regulates specific metabolism pathways and impacts memory development in CAR T cells. Immunity (2016) 44(2):380–90. doi: 10.1016/j.immuni.2016.01.021
49. Milone MC, Fish JD, Carpenito C, Carroll RG, Binder GK, Teachey D, et al. Chimeric receptors containing CD137 signal transduction domains mediate enhanced survival of T cells and increased antileukemic efficacy in vivo. Mol Ther (2009) 17(8):1453–64. doi: 10.1038/mt.2009.83
50. Long AH, Haso WM, Shern JF, Wanhainen KM, Murgai M, Ingaramo M, et al. 4-1BB costimulation ameliorates T cell exhaustion induced by tonic signaling of chimeric antigen receptors. Nat Med (2015) 21(6):581–90. doi: 10.1038/nm.3838
51. Zhao Z, Condomines M, van der Stegen SJC, Perna F, Kloss CC, Gunset G, et al. Structural design of engineered costimulation determines tumor rejection kinetics and persistence of CAR T cells. Cancer Cell (2015) 28(4):415–28. doi: 10.1016/j.ccell.2015.09.004
52. Singh N, Frey NV, Engels B, Barrett DM, Shestova O, Ravikumar P, et al. Antigen-independent activation enhances the efficacy of 4-1BB-costimulated CD22 CAR T cells. Nat Med (2021) 27(5):842–50. doi: 10.1038/s41591-021-01326-5
53. Hudecek M, Sommermeyer D, Kosasih PL, Silva-Benedict A, Liu L, Rader C, et al. The nonsignaling extracellular spacer domain of chimeric antigen receptors is decisive for in vivo antitumor activity. Cancer Immunol Res (2015) 3(2):125–35. doi: 10.1158/2326-6066.CIR-14-0127
54. Alabanza L, Pegues M, Geldres C, Shi V, Wiltzius JJW, Sievers SA, et al. Function of novel anti-CD19 chimeric antigen receptors with human variable regions is affected by hinge and transmembrane domains. Mol Ther (2017) 25(11):2452–65. doi: 10.1016/j.ymthe.2017.07.013
55. Jonnalagadda M, Mardiros A, Urak R, Wang X, Hoffman LJ, Bernanke A, et al. Chimeric antigen receptors with mutated IgG4 Fc spacer avoid fc receptor binding and improve T cell persistence and antitumor efficacy. Mol Ther (2015) 23(4):757–68. doi: 10.1038/mt.2014.208
56. Li N, Quan A, Li D, Pan J, Ren H, Hoeltzel G, et al. The IgG4 hinge with CD28 transmembrane domain improves V(H)H-based CAR T cells targeting a membrane-distal epitope of GPC1 in pancreatic cancer. Nat Commun (2023) 14(1):1986. doi: 10.1038/s41467-023-37616-4
57. Feucht J, Sun J, Eyquem J, Ho YJ, Zhao Z, Leibold J, et al. Calibration of CAR activation potential directs alternative T cell fates and therapeutic potency. Nat Med (2019) 25(1):82–8. doi: 10.1038/s41591-018-0290-5
58. Guedan S, Madar A, Casado-Medrano V, Shaw C, Wing A, Liu F, et al. Single residue in CD28-costimulated CAR-T cells limits long-term persistence and antitumor durability. J Clin Invest (2020) 130(6):3087–97. doi: 10.1172/JCI133215
59. Fesnak AD, June CH, Levine BL. Engineered T cells: the promise and challenges of cancer immunotherapy. Nat Rev Cancer (2016) 16(9):566–81. doi: 10.1038/nrc.2016.97
60. Rafiq S, Hackett CS, Brentjens RJ. Engineering strategies to overcome the current roadblocks in CAR T cell therapy. Nat Rev Clin Oncol (2020) 17(3):147–67. doi: 10.1038/s41571-019-0297-y
61. Allen GM, Frankel NW, Reddy NR, Bhargava HK, Yoshida MA, Stark SR, et al. Synthetic cytokine circuits that drive T cells into immune-excluded tumors. Science (2022) 378(6625):eaba1624. doi: 10.1126/science.aba1624
62. Arcangeli S, Bove C, Mezzanotte C, Camisa B, Falcone L, Manfredi F, et al. CAR T cell manufacturing from naive/stem memory T lymphocytes enhances antitumor responses while curtailing cytokine release syndrome. J Clin Invest (2022) 132(12):e150807. doi: 10.1172/JCI150807
63. Sabatino M. Generation of clinical-grade CD19-specific CAR-modified CD8 memory stem cells for the treatment of human B-cell Malignancies. Blood (2016) 128(4):519–28. doi: 10.1182/blood-2015-11-683847
64. Meyran D, Zhu JJ, Butler J, Tantalo D, MacDonald S, Nguyen TN, et al. TSTEM-like CAR-T cells exhibit improved persistence and tumor control compared with conventional CAR-T cells in preclinical models. Sci Trans Med (2023) 15(690):eabk1900. doi: 10.1126/scitranslmed.abk1900
65. Gattinoni L. T memory stem cells in health and disease. Nat Med (2017) 23(1):18–27. doi: 10.1038/nm.4241
66. Sommermeyer D, Hudecek M, Kosasih PL, Gogishvili T, Maloney DG, Turtle CJ, et al. Chimeric antigen receptor-modified T cells derived from defined CD8+ and CD4+ subsets confer superior antitumor reactivity in vivo. Leukemia (2016) 30(2):492–500. doi: 10.1038/leu.2015.247
67. Gattinoni L, Lugli E, Ji Y, Pos Z, Paulos CM, Quigley MF, et al. A human memory T cell subset with stem cell-like properties. Nat Med (2011) 17(10):1290–7. doi: 10.1038/nm.2446
68. Fraietta JA, Lacey SF, Orlando EJ, Pruteanu-Malinici I, Gohil M, Lundh S, et al. Determinants of response and resistance to CD19 chimeric antigen receptor (CAR) T cell therapy of chronic lymphocytic leukemia. Nat Med (2018) 24(5):563–71. doi: 10.1038/s41591-018-0010-1
69. Deng Q, Han G, Puebla-Osorio N, Ma MCJ, Strati P, Chasen B, et al. Characteristics of anti-CD19 CAR T cell infusion products associated with efficacy and toxicity in patients with large B cell lymphomas. Nat Med (2020) 26(12):1878–87. doi: 10.1038/s41591-020-1061-7
70. Biasco L, Izotova N, Rivat C, Ghorashian S, Richardson R, Guvenel A, et al. Clonal expansion of T memory stem cells determines early anti-leukemic responses and long-term CAR T cell persistence in patients. Nat Cancer (2021) 2(6):629–42. doi: 10.1038/s43018-021-00207-7
71. Bai Z, Woodhouse S, Zhao Z, Arya R, Govek K, Kim D, et al. Single-cell antigen-specific landscape of CAR T infusion product identifies determinants of CD19-positive relapse in patients with ALL. Sci Adv (2022) 8(23):eabj2820. doi: 10.1126/sciadv.abj2820
72. Cieri N, Camisa B, Cocchiarella F, Forcato M, Oliveira G, Provasi E, et al. IL-7 and IL-15 instruct the generation of human memory stem T cells from naive precursors. Blood (2013) 121(4):573–84. doi: 10.1182/blood-2012-05-431718
73. Xu Y, Zhang M, Ramos CA, Durett A, Liu E, Dakhova O, et al. Closely related T-memory stem cells correlate with in vivo expansion of CAR.CD19-T cells and are preserved by IL-7 and IL-15. Blood (2014) 123(24):3750–9. doi: 10.1182/blood-2014-01-552174
74. Yang S, Ji Y, Gattinoni L, Zhang L, Yu Z, Restifo NP, et al. Modulating the differentiation status of ex vivo-cultured anti-tumor T cells using cytokine cocktails. Cancer Immunol Immunother (2013) 62(4):727–36. doi: 10.1007/s00262-012-1378-2
75. Joedicke JJ, Grosskinsky U, Gerlach K, Kunkele A, Hopken UE, Rehm A, et al. Accelerating clinical-scale production of BCMA CAR T cells with defined maturation stages. Mol Ther Methods Clin Dev (2022) 24:181–98. doi: 10.1016/j.omtm.2021.12.005
76. Arcangeli S, Falcone L, Camisa B, De Girardi F, Biondi M, Giglio F, et al. Next-generation manufacturing protocols enriching T(SCM) CAR T cells can overcome disease-specific T cell defects in cancer patients. Front Immunol (2020) 11:1217. doi: 10.3389/fimmu.2020.01217
77. Zhen A, Carrillo MA, Mu W, Rezek V, Martin H, Hamid P, et al. Robust CAR-T memory formation and function via hematopoietic stem cell delivery. PloS Pathog (2021) 17(4):e1009404. doi: 10.1371/journal.ppat.1009404
78. Zhen A, Kamata M, Rezek V, Rick J, Levin B, Kasparian S, et al. HIV-specific immunity derived from chimeric antigen receptor-engineered stem cells. Mol Ther (2015) 23(8):1358–67. doi: 10.1038/mt.2015.102
79. Zhen A, Peterson CW, Carrillo MA, Reddy SS, Youn CS, Lam BB, et al. Long-term persistence and function of hematopoietic stem cell-derived chimeric antigen receptor T cells in a nonhuman primate model of HIV/AIDS. PloS Pathog (2017) 13(12):e1006753. doi: 10.1371/journal.ppat.1006753
80. Barber-Axthelm IM, Barber-Axthelm V, Sze KY, Zhen A, Suryawanshi GW, Chen IS, et al. Stem cell-derived CAR T cells traffic to HIV reservoirs in macaques. JCI Insight (2021) 6(1):e141502. doi: 10.1172/jci.insight.141502
81. Amini L, Silbert SK, Maude SL, Nastoupil LJ, Ramos CA, Brentjens RJ, et al. Preparing for CAR T cell therapy: patient selection, bridging therapies and lymphodepletion. Nat Rev Clin Oncol (2022) 19(5):342–55. doi: 10.1038/s41571-022-00607-3
82. Turtle CJ, Hanafi L-A, Berger C, Hudecek M, Pender B, Robinson E, et al. Immunotherapy of non-Hodgkin’s lymphoma with a defined ratio of CD8+ and CD4+ CD19-specific chimeric antigen receptor–modified T cells. Sci Trans Med (2016) 8(355):355ra116–355ra116. doi: 10.1126/scitranslmed.aaf8621
83. Brentjens RJ, Rivière I, Park JH, Davila ML, Wang X, Stefanski J, et al. Safety and persistence of adoptively transferred autologous CD19-targeted T cells in patients with relapsed or chemotherapy refractory B-cell leukemias. Blood (2011) 118(18):4817–28. doi: 10.1182/blood-2011-04-348540
84. Allers K, Hutter G, Hofmann J, Loddenkemper C, Rieger K, Thiel E, et al. Evidence for the cure of HIV infection by CCR5Delta32/Delta32 stem cell transplantation. Blood (2011) 117(10):2791–9. doi: 10.1182/blood-2010-09-309591
85. Hütter G, Nowak D, Mossner M, Ganepola S, Müßig A, Allers K, et al. Long-term control of HIV by CCR5 delta32/delta32 stem-cell transplantation. New Engl J Med (2009) 360(7):692–8. doi: 10.1056/NEJMoa0802905
86. Gupta RK, Abdul-Jawad S, McCoy LE, Mok HP, Peppa D, Salgado M, et al. HIV-1 remission following CCR5Delta32/Delta32 haematopoietic stem-cell transplantation. Nature (2019) 568(7751):244–8. doi: 10.1038/s41586-019-1027-4
87. Gupta RK, Peppa D, Hill AL, Gálvez C, Salgado M, Pace M, et al. Evidence for HIV-1 cure after CCR5Δ32/Δ32 allogeneic haemopoietic stem-cell transplantation 30 months post analytical treatment interruption: a case report. Lancet HIV (2020) 7(5):e340–7. doi: 10.1016/S2352-3018(20)30069-2
88. Hsu J, Van Besien K, Glesby MJ, Pahwa S, Coletti A, Warshaw MG, et al. HIV-1 remission and possible cure in a wOman after haplo-cord blood transplant. Cell (2023) 186(6):1115–1126 e8. doi: 10.1016/j.cell.2023.02.030
89. Peterson CW, Benne C, Polacino P, Kaur J, McAllister CE, Filali-Mouhim A, et al. Loss of immune homeostasis dictates SHIV rebound after stem-cell transplantation. JCI Insight (2017) 2(4):e91230. doi: 10.1172/jci.insight.91230
90. Peterson CW, Kiem HP. Lessons from london and berlin: designing A scalable gene therapy approach for HIV cure. Cell Stem Cell (2019) 24(5):685–7. doi: 10.1016/j.stem.2019.04.010
91. Peterson CW, Wang J, Deleage C, Reddy S, Kaur J, Polacino P, et al. Differential impact of transplantation on peripheral and tissue-associated viral reservoirs: Implications for HIV gene therapy. PloS Pathog (2018) 14(4):e1006956. doi: 10.1371/journal.ppat.1006956
92. Rasmussen TA, Lewin SR. Shocking HIV out of hiding: where are we with clinical trials of latency reversing agents? Curr Opin HIV AIDS (2016) 11(4):394–401. doi: 10.1097/COH.0000000000000279
93. Rasmussen TA, Tolstrup M, Brinkmann CR, Olesen R, Erikstrup C, Solomon A, et al. Panobinostat, a histone deacetylase inhibitor, for latent-virus reactivation in HIV-infected patients on suppressive antiretroviral therapy: a phase 1/2, single group, clinical trial. Lancet HIV (2014) 1(1):e13–21. doi: 10.1016/S2352-3018(14)70014-1
94. Vibholm L, Schleimann MH, Højen JF, Benfield T, Offersen R, Rasmussen K, et al. Short-course toll-like receptor 9 agonist treatment impacts innate immunity and plasma viremia in individuals with human immunodeficiency virus infection. Clin Infect Dis (2017) 64(12):1686–95. doi: 10.1093/cid/cix201
95. Søgaard OSOS. The depsipeptide romidepsin reverses HIV-1 latency in vivo. PloS Pathog (2015) 11(9):e1005142. doi: 10.1371/journal.ppat.1005142
96. Gunst JD, Tolstrup M, Rasmussen TA, Sogaard OS. The potential role for romidepsin as a component in early HIV-1 curative efforts. Expert Rev Anti Infect Ther (2016) 14(5):447–50. doi: 10.1586/14787210.2016.1164031
97. Ma L, Dichwalkar T, Chang JYH, Cossette B, Garafola D, Zhang AQ, et al. Enhanced CAR–T cell activity against solid tumors by vaccine boosting through the chimeric receptor. Science (2019) 365(6449):162–8. doi: 10.1126/science.aav8692
98. Reinhard K, Rengstl B, Oehm P, Michel K, Billmeier A, Hayduk N, et al. An RNA vaccine drives expansion and efficacy of claudin-CAR-T cells against solid tumors. Science (2020) 367(6476):446–53. doi: 10.1126/science.aay5967
99. Rust BJ, Kean LS, Colonna L, Brandenstein KE, Poole NH, Obenza W, et al. Robust expansion of HIV CAR T cells following antigen boosting in ART-suppressed nonhuman primates. Blood (2020) 136(15):1722–34. doi: 10.1182/blood.2020006372
100. Maus MV, Thomas AK, Leonard DGB, Allman D, Addya K, Schlienger K, et al. Ex vivo expansion of polyclonal and antigen-specific cytotoxic T lymphocytes by artificial APCs expressing ligands for the T-cell receptor, CD28 and 4-1BB. Nat Biotechnol (2002) 20(2):143–8. doi: 10.1038/nbt0202-143
101. Kim JV, Latouche JB, Riviere I, Sadelain M. The ABCs of artificial antigen presentation. Nat Biotechnol (2004) 22(4):403–10. doi: 10.1038/nbt955
102. Butler MO, HIrano N. Human cell-based artificial antigen-presenting cells for cancer immunotherapy. Immunol Rev (2014) 257(1):191–209. doi: 10.1111/imr.12129
103. Walter EA, Greenberg PD, Gilbert MJ, Finch RJ, Watanabe KS, Thomas ED, et al. Reconstitution of cellular immunity against cytomegalovirus in recipients of allogeneic bone marrow by transfer of T-cell clones from the donor. New Engl J Med (1995) 333(16):1038–44. doi: 10.1056/NEJM199510193331603
104. Leen AM, Myers GD, Sili U, Huls MH, Weiss H, Leung KS, et al. Monoculture-derived T lymphocytes specific for multiple viruses expand and produce clinically relevant effects in immunocompromised individuals. Nat Med (2006) 12(10):1160–6. doi: 10.1038/nm1475
105. Heslop HE, Slobod KS, Pule MA, Hale GA, Rousseau A, Smith CA, et al. Long-term outcome of EBV-specific T-cell infusions to prevent or treat EBV-related lymphoproliferative disease in transplant recipients. Blood (2010) 115(5):925–35. doi: 10.1182/blood-2009-08-239186
106. Kershaw MH, Westwood JA, Hwu P. Dual-specific T cells combine proliferation and antitumor activity. Nat Biotechnol (2002) 20(12):1221–7. doi: 10.1038/nbt756
107. Cooper LJ, Al-Kadhimi Z, Serrano LM, Pfeiffer T, Olivares S, Castro A, et al. Enhanced antilymphoma efficacy of CD19-redirected influenza MP1-specific CTLs by cotransfer of T cells modified to present influenza MP1. Blood (2005) 105(4):1622–31. doi: 10.1182/blood-2004-03-1208
108. Murphy A, Westwood JA, Brown LE, Teng MW, Moeller M, Xu Y, et al. Antitumor activity of dual-specific T cells and influenza virus. Cancer Gene Ther (2007) 14(5):499–508. doi: 10.1038/sj.cgt.7701034
109. Savoldo B, Rooney CM, Di Stasi A, Abken H, Hombach A, Foster AE, et al. Epstein Barr virus–specific cytotoxic T lymphocytes expressing the anti-CD30ζ artificial chimeric T-cell receptor for immunotherapy of Hodgkin disease. Blood (2007) 110(7):2620–30. doi: 10.1182/blood-2006-11-059139
110. Terakura S, Yamamoto TN, Gardner RA, Turtle CJ, Jensen MC, Riddell SR, et al. Generation of CD19-chimeric antigen receptor modified CD8+ T cells derived from virus-specific central memory T cells. Blood (2012) 119(1):72–82. doi: 10.1182/blood-2011-07-366419
111. Tanaka M, Tashiro H, Omer B, Lapteva N, Ando J, Ngo M, et al. Vaccination targeting native receptors to enhance the function and proliferation of chimeric antigen receptor (CAR)-modified T cells. Clin Cancer Res (2017) 23(14):3499–509. doi: 10.1158/1078-0432.CCR-16-2138
112. Slaney CY, von Scheidt B, Davenport AJ, Beavis PA, Westwood JA, Mardiana S, et al. Dual-specific chimeric antigen receptor T cells and an indirect vaccine eradicate a variety of large solid tumors in an immunocompetent, self-antigen setting. Clin Cancer Res (2017) 23(10):2478–90. doi: 10.1158/1078-0432.CCR-16-1860
113. Wang X, Wong CW, Urak R, Mardiros A, Budde LE, Chang WC, et al. CMVpp65 vaccine enhances the antitumor efficacy of adoptively transferred CD19-redirected CMV-specific T cells. Clin Cancer Res (2015) 21(13):2993–3002. doi: 10.1158/1078-0432.CCR-14-2920
114. Guan M, Lim L, Holguin L, Han T, Vyas V, Urak R, et al. Pre-clinical data supporting immunotherapy for HIV using CMV-HIV-specific CAR T cells with CMV vaccine. Mol Ther Methods Clin Dev (2022) 25:344–59. doi: 10.1016/j.omtm.2022.04.007
115. Pule MA, Savoldo B, Myers GD, Rossig C, Russell HV, Dotti G, et al. Virus-specific T cells engineered to coexpress tumor-specific receptors: persistence and antitumor activity in individuals with neuroblastoma. Nat Med (2008) 14(11):1264–70. doi: 10.1038/nm.1882
116. Lapteva N, Gilbert M, Diaconu I, Rollins LA, Al-Sabbagh M, Naik S, et al. T-cell receptor stimulation enhances the expansion and function of CD19 chimeric antigen receptor-expressing T cells. Clin Cancer Res (2019) 25(24):7340–50. doi: 10.1158/1078-0432.CCR-18-3199
117. Cruz CRY, Micklethwaite KP, Savoldo B, Ramos CA, Lam S, Ku S, et al. Infusion of donor-derived CD19-redirected virus-specific T cells for B-cell Malignancies relapsed after allogeneic stem cell transplant: a phase 1 study. Blood (2013) 122(17):2965–73. doi: 10.1182/blood-2013-06-506741
118. Rossig C, Pule M, Altvater B, Saiagh S, Wright G, Ghorashian S, et al. Vaccination to improve the persistence of CD19CAR gene-modified T cells in relapsed pediatric acute lymphoblastic leukemia. Leukemia (2017) 31(5):1087–95. doi: 10.1038/leu.2017.39
119. Bridgeman JS, Hawkins RE, Bagley S, Blaylock M, Holland M, Gilham DE, et al. The optimal antigen response of chimeric antigen receptors harboring the CD3zeta transmembrane domain is dependent upon incorporation of the receptor into the endogenous TCR/CD3 complex. J Immunol (2010) 184(12):6938–49. doi: 10.4049/jimmunol.0901766
120. Yang Y, Kohler ME, Chien CD, Sauter CT, Jacoby E, Yan C, et al. TCR engagement negatively affects CD8 but not CD4 CAR T cell expansion and leukemic clearance. Sci Trans Med (2017) 9(417):eaag1209. doi: 10.1126/scitranslmed.aag1209
Keywords: chimeric antigen receptor, HIV, persistence, expansion, TCR-engagement, CAR T cell persistence
Citation: Rothemejer FH, Lauritsen NP, Søgaard OS and Tolstrup M (2023) Strategies for enhancing CAR T cell expansion and persistence in HIV infection. Front. Immunol. 14:1253395. doi: 10.3389/fimmu.2023.1253395
Received: 05 July 2023; Accepted: 04 August 2023;
Published: 21 August 2023.
Edited by:
Laura Margaret Snell, Indiana University School of Medicine, United StatesReviewed by:
Wei Li, Indiana University, United StatesCopyright © 2023 Rothemejer, Lauritsen, Søgaard and Tolstrup. This is an open-access article distributed under the terms of the Creative Commons Attribution License (CC BY). The use, distribution or reproduction in other forums is permitted, provided the original author(s) and the copyright owner(s) are credited and that the original publication in this journal is cited, in accordance with accepted academic practice. No use, distribution or reproduction is permitted which does not comply with these terms.
*Correspondence: Frederik Holm Rothemejer, ZnJlcm9AY2xpbi5hdS5kaw==; Martin Tolstrup, bXRvbEBjbGluLmF1LmRr
Disclaimer: All claims expressed in this article are solely those of the authors and do not necessarily represent those of their affiliated organizations, or those of the publisher, the editors and the reviewers. Any product that may be evaluated in this article or claim that may be made by its manufacturer is not guaranteed or endorsed by the publisher.
Research integrity at Frontiers
Learn more about the work of our research integrity team to safeguard the quality of each article we publish.