- 1Department of Oncology, The Affiliated Wuxi People’s Hospital of Nanjing Medical University, Wuxi, China
- 2Wuxi School of Medicine, Jiangnan University, Wuxi, China
- 3Suzhou Cancer Center Core Laboratory, The Affiliated Suzhou Hospital of Nanjing Medical University, Suzhou, China
- 4Department of Radiation Oncology, Shandong Cancer Hospital and Institute, Shandong First Medical University and Shandong Academy of Medical Sciences, Jinan, China
The emergence of immunotherapy, particularly programmed cell death 1 (PD-1) and programmed cell death ligand-1 (PD-L1) produced profound transformations for treating non-small cell lung cancer (NSCLC). Nevertheless, not all NSCLC patients can benefit from immunotherapy in clinical practice. In addition to limited response rates, exorbitant treatment costs, and the substantial threats involved with immune-related adverse events, the intricate interplay between long-term survival outcomes and early disease progression, including early immune hyperprogression, remains unclear. Consequently, there is an urgent imperative to identify robust predictive and prognostic biological markers, which not only possess the potential to accurately forecast the therapeutic efficacy of immunotherapy in NSCLC but also facilitate the identification of patient subgroups amenable to personalized treatment approaches. Furthermore, this advancement in patient stratification based on certain biological markers can also provide invaluable support for the management of immunotherapy in NSCLC patients. Hence, in this review, we comprehensively examine the current landscape of individual biological markers, including PD-L1 expression, tumor mutational burden, hematological biological markers, and gene mutations, while also exploring the potential of combined biological markers encompassing radiological and radiomic markers, as well as prediction models that have the potential to better predict responders to immunotherapy in NSCLC with an emphasis on some directions that warrant further investigation which can also deepen the understanding of clinicians and provide a reference for clinical practice.
1 Introduction
Lung carcinoma is widely acknowledged as being the foremost reason for mortality connected to neoplasms in both the U.S. and China, which is with around 85% of pulmonary neoplasms classified as non-small cell lung carcinomas (NSCLCs) (1, 2). And the advent of immunotherapeutic agents targeting programmed cell death 1 (PD-1) and programmed cell death ligand-1 (PD-L1), also known as one of immunoregulatory checkpoint inhibitors (ICIs), altered thoroughly the management of patients with progressive or metastatic NSCLC. Furthermore, the utilization of PD-1 inhibitors (Nivolumab, Pembrolizumab) and PD-L1 inhibitors (Atezolizumab) has been endorsed by the U.S. Food and Drug Administration (FDA) in terms of patients’ treatments with advanced NSCLC experiencing disease progression in or post initial-line therapy due to their superior disease progression-free survival (PFS) and overall survival (OS) outcomes in comparison to the conventional chemotherapy comparator (3–6). Nevertheless, the favorable response to PD-1/PD-L1 inhibitors is not universal, as merely 20 - 40% of patients exhibit a response, fewer achieve long-term disease remission, and even some patients could experience immune hyperprogression at the early stage of immunotherapy (7–9). Given the exorbitant costs and potential for severe adverse effects associated with ICIs, it becomes imperative to find patients with the most likely to derive therapeutic advantage from such treatments and enhance the efficacy of ICIs for precise therapeutic interventions. Consequently, the pursuit of reliable and effective biological markers for assessing the reaction to anti-PD-1/PD-L1 immunotherapy has become the focal point of oncoimmunology investigations in NSCLC patients. Although biological markers including PD-L1 expression levels, tumor mutational burden (TMB), hematological biological markers, and composite biological markers are presently under scrutiny as potential indicators for assessing the reaction to ICIs in NSCLC patients, none have gained widespread and accurate clinical utilization. Thus, in this review, we comprehensively examine the current landscape of biological markers for anti-PD-1/PD-L1 immunotherapy in NSCLC with an emphasis on some directions that warrant further investigation which can also deepen the understanding of clinicians and provide a reference for clinical practice.
2 Single biological marker
2.1 PD-L1 expression
Currently, PD-L1 expression serves as one of the highest extensively utilized biological markers to predict the ICIs efficiency in NSCLC. As per the guidelines by the National Comprehensive Cancer Network (NCCN), anti-PD-L1 immunotherapy (Atezolizumab) is suggested as first-line treatment for NSCLC patients exhibiting PD-L1 expression ≥ 50% or as second-line treatment for those with PD-L1 expression ≥ 1% (3). PD-1, an immunoregulatory checkpoint receptor expressed on activated T cells, including tumor-infiltrating lymphocytes, plays a pivotal role in immune evasion when it interacts with its ligands, PD-L1 and PD-L2, resulting in the suppression of cytotoxic T cell responses (10–13). Upregulation of PD-L1 is frequently observed on tumor cell surfaces, with PD-L1 expression reported in approximately 20% to 40% of NSCLC cases (14). Extensive investigations have delved into the connection between PD-L1 expression levels and immunotherapy efficacy, although the predictive value of PD-L1 remains controversial (Table 1) (15–20, 22–30, 34, 35). The KEYNOTE-024 trial showed that Pembrolizumab exhibited better OS in comparison to chemotherapy in patients displaying high PD-L1 expression (≥ 50%) (21). For PACIFIC trials, unresectable stage III NSCLC patients who completed radiotherapy and chemotherapy were administered Durvalumab for up to one year (36). It was observed that Durvalumab improved survival in patients with PD-L1 expression ≥ 25%. However, patients with low PD-L1 expression (< 1%) did not derive significant benefits from immune consolidation therapy. A meta-analysis involving 12 randomized clinical trials encompassing 6,932 patients with locally advanced NSCLC who received PD-1/PD-L1 inhibitors indicated that individuals with high PD-L1 expression levels exhibited longer PFS, OS, and overall response rate (ORR) in comparison to those with low PD-L1 levels (37). Of note, patients with PD-L1 expression ≥ 1% demonstrated a significant improvement in ORR, whilst little statistically significant disparity had been noted among patients with PD-L1 expression < 1% (P = 0.12).
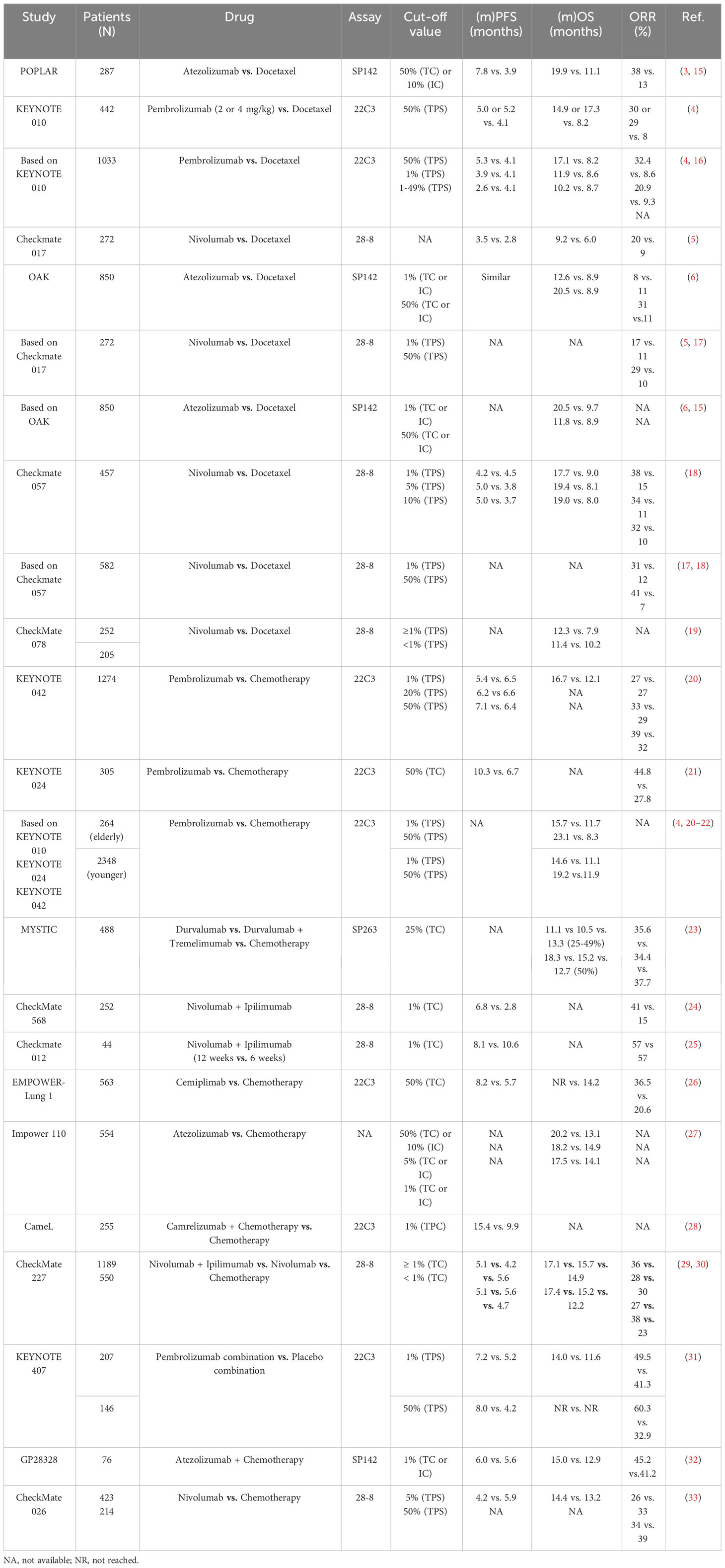
Table 1 Clinical outcomes of NSCLC patients treated with anti-PD-1/anti-PD-L1 agents according to the expression of PD-L1.
Even though individuals exhibiting elevated PD-L1 expression exhibited a higher likelihood of responding ICIs, around 10% of PD-L1-negative patients also displayed a reaction to ICIs. Conversely, there were instances where patients with substantial PD-L1 expression exhibited an unresponsive state (4, 5, 31, 32). Within the framework of the KEYNOTE-024 trial, the ORR for PD-L1 ≥ 50% NSCLC patients receiving Pembrolizumab was a mere 44.8%, thereby indicating a considerable proportion of individuals with heightened PD-L1 expression did not manifest a response to this treatment (28). Moreover, the CheckMate-026 trial revealed that a PD-L1 expression level of ≥ 50% failed to serve as a reliable predictor of the effectiveness of Nivolumab in first-line NSCLC treatment (ORR, 34% vs. 39%) (33). And CheckMate 227 also found that Nivolumab plus Ipilimumab increased 5-year survivorship verse chemotherapy regardless of PD-L1 expression (29). One of the most obstacles impeding the clinical implementation of PD-L1 as a biological marker for predicting response to anti-PD-1/PD-L1 could be the absence of standardization in PD-L1 testing. Presently, the FDA has sanctioned the utilization of three PD-L1 immunohistochemistry (IHC) assays as companion diagnostics: Dako 22C3 (22C3) for Pembrolizumab in patients with diverse solid tumors, including NSCLC; Ventana SP142 (SP142) for Atezolizumab in NSCLC patients; and Dako 28-8 (28-8) for the combination of Ipilimumab and Nivolumab in NSCLC patients (38). Then, discrepancies in the findings of various studies could potentially arise from disparities in antibodies, detection methodologies, environmental conditions at the time of analysis, and the threshold values employed to determine PD-L1 expression (39, 40). For instance, the KEYNOTE-028 phase Ib study necessitated PD-L1 expression exceeding 1% on tumor or stromal cells, per measurement by the 22C3 assay, for advanced-stage solid tumors patients who received Pembrolizumab (41). Furthermore, variations in previous treatments administered to patients across different studies could have also contributed to inconsistencies in the research outcomes (42, 43). Last, the expression of PD-L1 is dynamic, influenced by treatments such as chemotherapy, radiotherapy, or chemoradiotherapy, which can influence PD-L1 expression within the tumor (44–46). Consequently, studies encompassing larger cohorts are imperative to validate the significance of PD-L1 as a predictive biological marker for ICI response in NSCLC patients.
2.2 TMB
Apart from PD-L1 expression, TMB stands as another extensively studied biological marker for predicting the efficacy of immunotherapy in NSCLC (10, 47). Somatic mutations can induce substantial modifications in protein sequences, resulting in the generation of abnormal proteins. These anomalous proteins can act as novel antigenic peptides, exhibiting a strong affinity for major histocompatibility complexes (MHC) I or MHC II. Thereafter, these neoepitopes are presented on the cell surface, where they are identified as non-self by the immune system, thereby instigating the activation of T lymphocytes that are specific to these neoantigens. Consequently, the immunogenicity of a tumor is intimately associated with the accumulation of somatic mutations within tumor cells (48). Elevated tumor mutational burden (referred to as TMB-H) leads to an augmented production and release of neoantigens, thereby enhancing the recognition and elimination of tumor cells by activated cytotoxic T cells after treatment with ICIs (48). Notably, NSCLC demonstrates a comparatively higher TMB-H than other solid tumor types (49). Consequently, the potential value of TMB as a biological marker for predicting the efficacy of immunotherapy has been extensively investigated in NSCLC patients (Table 2) (50–52).
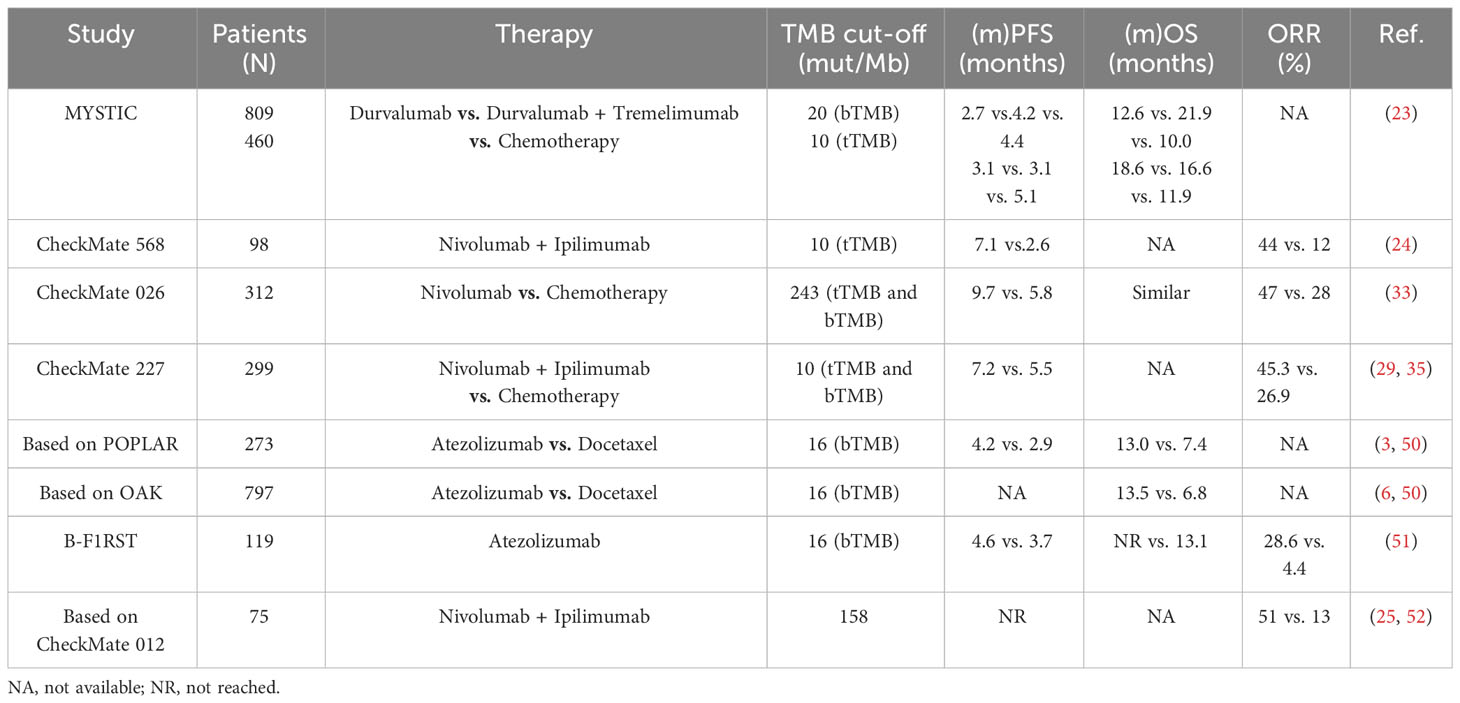
Table 2 Clinical outcomes of NSCLC patients treated with anti-PD-1/anti-PD-L1 agents according to the expression of TMB.
During the phase III clinical trial also known as CheckMate-026, researchers conducted a retrospective assessment of the TMB in 312 patients diagnosed with NSCLC who received either Nivolumab or chemotherapy through whole-exome sequencing (33). Among the patients with high TMB (≥ 243 mut/Mb), Nivolumab exhibited a superior ORR of 47% in comparison to 28% in the chemotherapy group, along with a longer PFS of 9.7 vs. 5.8 months. Gandara et al. conducted a retrospective analysis of blood-based tumor mutational burden (bTMB) in patients participating in the OAK and POPLAR studies. They confirmed that a bTMB of ≥ 16 mut/Mb could serve as a predictive factor for OS and PFS in NSCLC patients treated with second-line Atezolizumab (3, 6, 50). Subsequently, a prospective study called B-F1RST categorized NSCLC patients receiving Atezolizumab into two groups based on their bTMB levels: a high bTMB group (bTMB-H) with ≥ 16 mut/Mb, and a low bTMB group (bTMB-L) with < 16 mut/Mb (51). The bTMB-H group exhibited a significantly higher ORR of 28.6% in comparison to 4.4% in the bTMB-L group (P = 0.0002). Additionally, patients in the bTMB-H group experienced a longer PFS of 4.6 months in comparison to 3.7 months in the bTMB-L group (P = 0.12). Similarly, a positive correlation was observed between bTMB-H and improved OS (P = 0.48), despite that the correlations between OS and PFS yielded little statistical significance. Furthermore, the MYSTIC study, a phase III clinical trial involving NSCLC patients, demonstrated that Durvalumab, when in comparison to chemotherapy, did not provide a substantial overall survival benefit in patients with high bTMB (23). Another trial, CheckMate-227, compared the effectiveness of Nivolumab plus Ipilimumab to that of dual-drug chemotherapy containing platinum in patients with TMB ≥ 10 mut/Mb (29). In the TMB-H group, patients treated with the combination of Ipilimumab and Nivolumab showed higher ORR (45.3% vs. 26.9%) and a lower hazard ratio for PFS (0.58, P < 0.001) in comparison to those treated with chemotherapy. Descriptive analyses of overall survival in the CheckMate-227 trial revealed an improvement in patients with high TMB and low TMB (< 10 mut/Mb) who were treated with Nivolumab plus Ipilimumab. A meta-analysis encompassing 14,395 NSCLC patients demonstrated that the combination of programmed cell death ligand 1 (PD-L1) expression levels and TMB served as a reliable biological marker for predicting response to immunotherapy. The area under the curve (AUC) for 1-year and 3-year PFS exceeded 0.8 (53). Notably, the findings from CheckMate-026 indicated a lack of significant association between TMB and PD-L1 expression level (r = 0.059) (33).
However, it is important to note that TMB testing is a costly endeavor. At present, TMB determination primarily relies on two methods: tissue-based next-generation sequencing (FoundationOne) and whole exome sequencing (WES). WES necessitates samples with high DNA quality and a substantial tumor cell fraction, making high-quality samples a prerequisite for TMB testing. In the CheckMate-227 study, only 57.7% of the samples were available for TMB analysis, and a mere 10.3% of those patients tested positive for TMB (30, 35). Additionally, the variability in gene sequences and cutoff values employed by different studies to detect TMB using various panels contributes to inconsistencies in the results (54, 55). For instance, in the CheckMate-568 study, the ability of TMB = 10 mut/Mb to predict the effectiveness of Nivolumab plus Ipilimumab in NSCLC patients was ascertained via receiver operating characteristic (ROC) curve assessment (24). It is worth highlighting that the sample used for TMB analysis is obtained from a specific part of the tumor. Consequently, the molecular detection method based on biopsy is inevitably influenced by sampling deviation owing to intratumoral heterogeneity (56). In light of these factors, the combination of PD-L1 expression and TMB appears to hold more promise in clinical practice. Nonetheless, further prospective, randomized, controlled trials are warranted to ascertain the reliability of TMB as a biological marker for predicting the response to ICIs.
2.3 Hematological biological markers
2.3.1 CD8+ T cells
The key to eliciting the host’s immune response against malignant cells lies in the recognition of novel antigens by T cell receptors (TCR) (57). Consequently, biological markers founded on TCR may hold the potential to forecast the response to immunotherapy (Table 3). The sequencing of TCR lineages can be readily performed utilizing peripheral blood samples, thereby affording a non-intrusive approach for prognosticating the response to ICIs. Han and colleagues performed a sequencing analysis of PD-1+CD8+ TCRs in the peripheral blood of 25 patients diagnosed with NSCLC undergoing ICI treatment. The study revealed that patients exhibiting heightened diversity of PD-1+CD8+ TCRs before immunotherapy demonstrated superior treatment response and PFS in comparison to those with diminished TCR diversity (6.4 vs. 2.5 months, P = 0.021) (58). These findings were subsequently validated in a cohort comprising 15 patients. Importantly, the sensitivity and specificity of pre-immunotherapy PD-1+CD8+ TCR diversity in predicting PFS were determined to be 0.87 and 0.94, respectively. Patients with elevated clonality of PD-1+CD8+ TCRs following immunotherapy displayed prolonged PFS in contrast to those with diminished clonality (7.3 vs. 2.6 months, P = 0.002). Similarly, the proliferative response of peripheral blood PD-1+CD8+ T cells (quantified as the fold change in the percentage of Ki-67+ cells seven days post-treatment) proved to be a valuable surrogate biological marker for predicting the response to ICIs in NSCLC patients (59). Additionally, Kamphorst et al. ascertained that within the cohort of patients with advanced NSCLC, PD-1+CD8+ T cell responses were either delayed or absent in 70% of those experiencing disease progression, while 80% of the patients who positively responded to immunotherapy exhibited PD-1+CD8+ T cell responses within four weeks of treatment initiation (78). Given the paucity of clinical evidence, the amalgamation of TCR diversity and clonality in PD-1+CD8+ T cells derived from peripheral blood might serve as a non-invasive biological marker in conjunction with PD-L1 levels and TMB for predicting the response to immunotherapy and prognosticating outcomes in NSCLC patients.
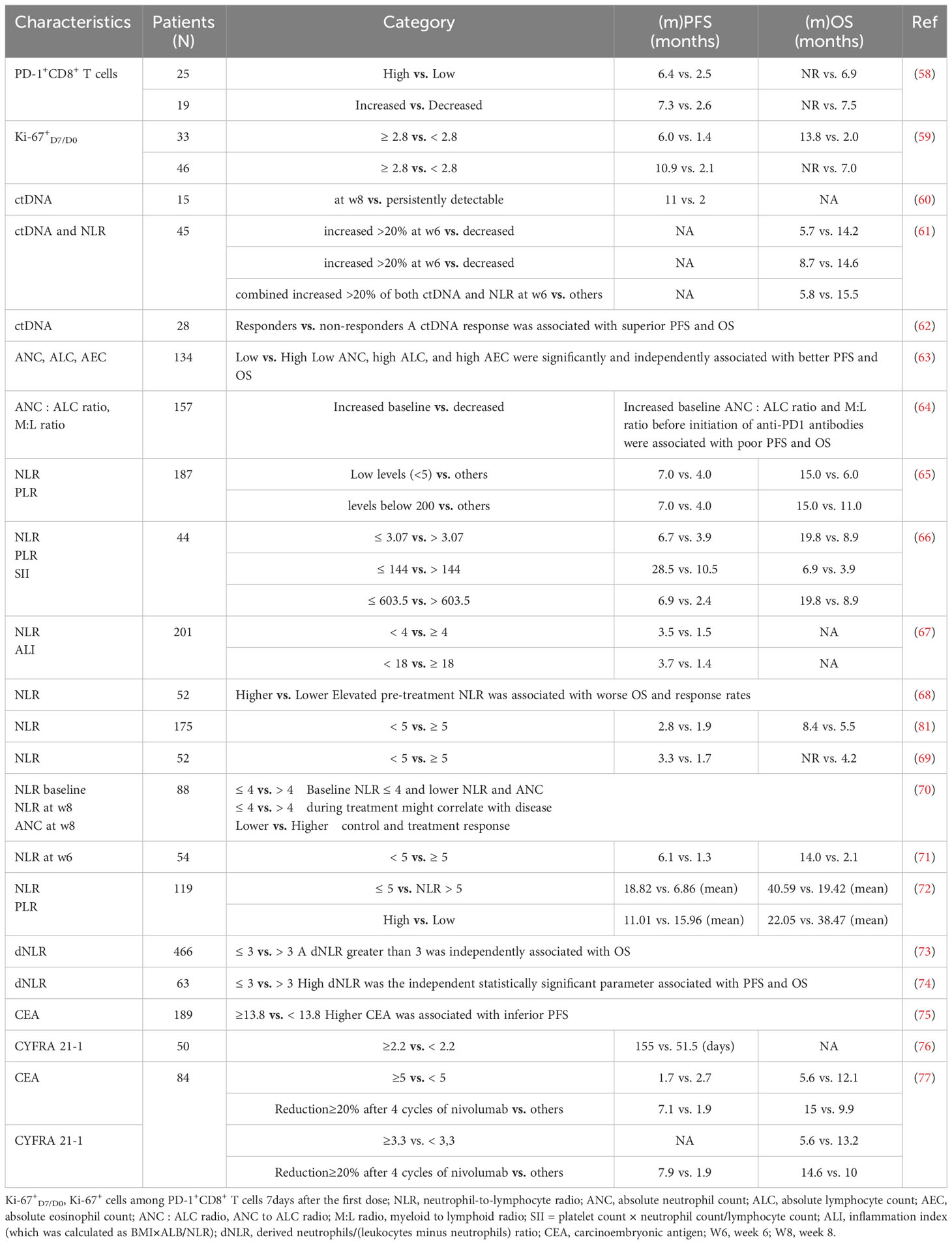
Table 3 Clinical outcomes of NSCLC patients treated with anti-PD-1/anti-PD-L1 agents according to hematological biological markers.
2.3.2 Circulating tumor DNA
Circulating tumor DNA (ctDNA) is a potent prognostic biological marker due to its resemblance to DNA obtained from solid tumor biopsies, often referred to as a “liquid tissue biopsy” (79–81). Many studies have demonstrated that alterations in ctDNA levels during chemotherapy are correlated with treatment response across various tumor types, including NSCLC (Table 3) (60–62, 82–86). In a study encompassing a cohort of five patients afflicted with metastatic melanoma who underwent treatment with Ipilimumab, alterations in ctDNA levels during therapy exhibited concurrence with the outcomes derived from imaging-based evaluation of treatment response (86). Hence, modifications in ctDNA levels during immunotherapy hold the potential to serve as a biological marker for monitoring immunotherapy response and prognosticating patient outcomes. Moreover, there exists evidence supporting the utility of ctDNA levels as a biological marker in foretelling the response to immunotherapy among NSCLC patients. Cabel et al. executed a study involving 15 patients diagnosed with advanced cancer, ten of whom had NSCLC (60). Pre-treatment blood specimens were procured and subsequently, eight weeks following the initiation of immunotherapy, ctDNA levels were evaluated. The findings demonstrated a positive correlation between tumor regression 8 weeks post-treatment and a decline in ctDNA levels (r = 0.86, P = 0.002). Furthermore, ctDNA levels assessed eight weeks after treatment exhibited a significant association with both PFS (P < 0.001) and OS (P = 0.004). In another study involving 45 NSCLC patients who underwent Nivolumab immunotherapy, Passiglia et al. demonstrated that a 20% increase in ctDNA levels observed 6 weeks after treatment was indicative of inferior OS (median OS, 5.7 vs. 14.2 months, P < 0.001) and time to progression (TTP) (3.3 vs. 10.2 months, P < 0.001) (61). Subsequent investigations further validated the capacity of ctDNA level alterations in predicting response to immunotherapy, emphasizing that changes in ctDNA levels could predict response earlier than assessments based on imaging (24.5 vs. 72.5 days) (62). Nevertheless, it is noteworthy that the sample sizes of the aforementioned studies were relatively small, and there exists a lack of uniformity in the threshold for ctDNA change and the timing of evaluating molecular response across each study. Consequently, it is imperative to establish a standardized framework for monitoring dynamic changes in ctDNA. The potential to proactively modify patients’ survival outcomes based on dynamic changes in ctDNA before observable imaging progression, as well as the prospect of employing liquid biopsy as a replacement for traditional imaging in the evaluation of early treatment response to immunotherapy, necessitate further deliberation.
2.3.3 Blood cell count
The changes of lymphocytes and neutrophils in peripheral blood can reflect the immune state of the body and can predict the curative effect to some certain extent. And tumor microenvironment with high neutrophils and low lymphocyte infiltration can promote blood tube formation, inhibit fine cell apoptosis, and promote tumor occurrence, resulting in poor prognosis. Tanizaki et al. substantiated that among individuals afflicted with locally advanced NSCLC subjected to Nivolumab treatment, PFS and OS experienced substantial elongation among those possessing elevated absolute neutrophil count and lymphocyte count before immunotherapy (63). Multiple investigations and meta-analyses have elucidated that a heightened neutrophil/lymphocyte ratio (NLR) correlates with an unfavorable prognosis in lung cancer patients (Table 3) (64–67, 87–89). Consequently, the association between NLR and immunotherapy response has been explored within the NSCLC patient cohort. In a study comprising 52 NSCLC patients, an elevated NLR exhibited an association with diminished OS (P < 0.001) and objective response rate (P = 0.013), albeit lacking correlation with PFS (P = 0.114). NLR demonstrated an AUC value of 0.738 for prognosticating the 10-month survival rate and 0.776 for predicting the efficacy of Nivolumab (66). Furthermore, the integration of NLR, among other variables, into a multivariate model substantially enhanced the prognostic capability of the model for predicting OS (68). Bagley et al. and Fukui et al. have corroborated the predictive worth of NLR in patients subjected to ICIs (69, 90). However, the optimal NLR threshold necessitates further establishment (70).
In addition to studies examining the potential utility of baseline NLR as a biological marker for immunotherapy response, various investigations have assessed the predictive and prognostic value of NLR during or post-treatment. Suh et al. made noteworthy observations regarding the median PFS and OS among patients with elevated NLR (≥ 5) six weeks after PD-1 blockade, demonstrating significantly shorter durations in comparison to patients with low NLR (median PFS: 1.3 vs. 6.1 months, P < 0.001; median OS: 2.1 vs. 14.0 months, P < 0.001) (71). Multivariate analysis confirmed that heightened NLR following treatment served as an independent prognostic indicator for OS (P = 0.003), indicating the potential of NLR six weeks after initiation of anti-PD-1 immunotherapy as a promising prognostic factor for patients with advanced NSCLC. Similarly, Passiglia et al. observed that a 20% or greater increase in NLR six weeks after Nivolumab treatment was associated with inferior OS (median OS: 8.7 vs. 14.6 months, P = 0.035) and TTP (5.2 vs. 10.3 months, P = 0.039) (61). The derived NLR (dNLR) may possess greater relevance than NLR, as it incorporates monocytes and other granulocyte subpopulations. Elevated dNLR has been linked to reduced survival in patients with multiple tumor types, including melanoma, pancreas, bladder, and renal cancer (72, 91–94). Mezquita et al. determined that a dNLR exceeding 3 represents the most suitable cutoff value for PFS and OS (73). Intriguingly, an inflammatory state (dNLR > 3) exhibited associations with shorter OS and durable clinical benefit among patients with advanced NSCLC receiving ICIs (95). Similarly, elevated dNLR (>3) demonstrated significant correlations with inferior PFS and OS (both P < 0.05) in patients with advanced NSCLC expressing at least 50% PD-L1 on tumor cells and treated with first-line Pembrolizumab (74). Although numerous studies have proposed the potential of peripheral blood cell count and its derivative indices as informative predictors of immunotherapy efficacy and prognosis, their clinical application remains limited due to considerations of peripheral blood cell count volatility and the absence of standardized research criteria.
2.3.4 Serum neoplasm biological markers
Serum neoplasm biological markers assume a pivotal function in monitoring the efficacy of interventions in individuals afflicted with NSCLC, specifically those undergoing chemotherapeutic or targeted interventions. Several frequently evaluated serum neoplasm biological markers encompass carcinoembryonic antigen (CEA), neuron-specific enolase (NSE), cytokeratin fragment 21-1 (Cyfra21-1), and carbohydrate antigen (CA19-9) (96, 97). Under the simplicity associated with acquiring serum samples, extensive scrutiny has been devoted to these markers as prognosticators of the response to immunotherapy (Table 3). In a multicenter investigation encompassing 189 subjects with advanced NSCLC subjected to Nivolumab as a second- or later-line treatment, Kataoka et al. uncovered an association linking pre-immunotherapy CEA concentrations to PFS; a crucial threshold of 13.8 ng/ml for CEA was established (75). The cohort surpassing 13.8 ng/ml of CEA exhibited a significantly abbreviated PFS (P = 0.002). Conversely, no such correlation manifested between Cyfra21-1 serum levels and the aforementioned outcome. In contrast, Shirasu et al. identified Cyfra21-1 as a prognostic indicator for individuals with lung adenocarcinoma undergoing Nivolumab (76). Discrepancies in the outcomes can be ascribed to the limited sample sizes and variations in subgroup categorizations based on pathological classifications. Intriguingly, an additional study demonstrated that a minimal 20% reduction in CEA and Cyfra21-1 concentrations after immunotherapy correlated with enhanced durable clinical rate (DCR; P = 0.021 for CEA; P < 0.001 for Cyfra21-1), PFS (P = 0.028 for CEA; P < 0.001 for Cyfra21-1), and OS (P = 0.026 for CEA; P = 0.019 for Cyfra21-1) (98). Moreover, Lang et al. substantiated that individuals experiencing diminished levels of serum neoplasm markers (CEA, Cyfra21-1, CA19-9, and NSE) after immunotherapy demonstrated significantly protracted PFS and OS (77).
2.4 Gene mutations
Multiple investigations have documented the correlation between the expression of programmed death-ligand 1 (PD-L1) and driver mutations in NSCLC; nevertheless, the findings exhibit contradictoriness (10, 99–102). The recent meta-analysis hath manifested a considerable level of heterogeneity amidst the expression of PD-L1 and driver mutations (10, 103). In accordance with the NCCN guidelines, immunotherapy is recommended for patients with NSCLC who test negative for EGFR mutations and ALK rearrangements (3). Ergo, data about the clinical efficacy of immunotherapy in patients with NSCLC harboring driver mutations art constrained. Another meta-analysis hath disclosed that, in comparison to patients with EGFR mutations, those afflicted with EGFR wild-type NSCLC evince significantly protracted progression-free survival (P < 0.00001) and OS (P < 0.05) after immunotherapy with programmed cell death protein 1 (PD-1)/PD-L1 inhibitors (37). Through the employment of second-generation sequencing and exon sequencing methodologies, it hath also been ascertained that the median progression-free survival of patients with EGFR mutations is significantly briefer than that of patients sans EGFR mutations (51.0 versus 70.5 days, P = 0.0037) (104). Cinausero et al. hath assessed the impact of KRAS mutations on the effectiveness of anti-PD-1 immunotherapy (105). The neoplastic deoxyribonucleic acid (DNA) of 47 patients was sequenced, and 43% of these individuals did present with KRAS mutations. An observable disparity in the frequency of KRAS mutations hath been observed betwixt responders and non-responders. The PFS and OS of patients with KRAS mutations hath been superior to that of patients with wild-type KRAS (P = 0.032, 0.010 separately). Notwithstanding, a comprehensive retrospective study hath debunked any association between KRAS mutations and the effectiveness of immunotherapy in a cohort of 328 patients with advanced NSCLC (106).
The effectiveness of immunotherapy in NSCLC has been examined concerning TP53 mutations. Non-synonymous TP53 mutations were observed in 57% (41 out of 65) of NSCLC individuals. Following a median follow-up period of 15.2 mos, individuals with TP53 mutations demonstrated a median OS of 18.1 months (95% CI, 6.6 – not reached), as opposed to 8.1 months for individuals with wild-type TP53 (95% CI: 2.2 – 14.5; P = 0.04). Patients with TP53 mutations also exhibited significantly longer median PFS (4.5 vs. 1.4 months, P = 0.03) and a higher ORR (51.2% vs. 20.7%; P = 0.01) in comparison to patients without TP53 mutations. Multivariate analysis disclosed an independent correlation between TP53 mutations and extended OS (P = 0.009) (107). Interestingly, Shi et al. have also found that patients with TP53/KMT2C co-mutation would get longer PFS and greater DCB (P = 0.033) when undergoing ICIs (108). A study involving 78 Chinese NSCLC individuals revealed that those with FAT1 mutations exhibited higher rates of clinical benefit and objective response rates in comparison to individuals without FAT1 mutations (71.4% vs. 22.7%, P = 0.01; 57.1% vs. 15.2%, P = 0.02) after undergoing ICIs. Furthermore, the loss of copy numbers in specific chromosome 3p fragments containing the tumor suppressor gene TIGA9 and several chemokine receptor genes were strongly associated with unfavorable clinical outcomes (6-month survival rate: 0% vs. 31%; P = 0.012) (104). Elevated PD-L1 expression has been linked to deficiencies in DNA repair through homologous recombination (109). Consequently, a study explored the methylation status of the Rad51B promoter (RAD51Bme), a crucial mediator of homologous recombination, PD-L1 expression, and the effectiveness of immunotherapy. RAD51Bme levels were markedly higher in PD-L1-expressing individuals in comparison to PD-L1-negative individuals (P < 0.05). Furthermore, RAD51Bme levels were significantly associated with the response to ICIs, suggesting its potential as a predictive indicator for the efficacy of immunotherapy in NSCLC (110). Although ICIs have demonstrated efficacy in NSCLC, the benefits of ICIs for individuals with positive driver gene mutations remain inconclusive. Targeted therapy is expected to continue playing a role in the treatment of NSCLC individuals with positive driver gene mutations in the future.
3 Combined biological markers
3.1 Radiology and radiomics
Radiomics encompasses the systematic assessment of tumor phenotype through the utilization of radiological images and bioinformatics tools. Its primary objective is to construct clinical models that enable the evaluation of tumor heterogeneity and the microenvironment in a high-throughput manner. Notably, multiple investigations have provided evidence that the radiological attributes of the peritumoral region hold potential as indicators of survival outcomes in individuals afflicted with lung cancer (111–113).
Artificial intelligence (AI)-assisted enhanced computed tomography (CT) was employed for the analysis of 1055 primary and metastatic lesions derived from patients diagnosed with advanced melanoma and NSCLC who underwent anti-PD-1 therapy. The objective of this study was to ascertain non-invasive biomarkers that could predict the response to treatment (114). In the case of NSCLC, the field of imageology revealed a highly significant marker (the highest AUC value was 0.83, P < 0.001), and a similar trend was observed in melanoma lymph nodes (AUC value = 0.64; P = 0.001). The pooled analysis yielded a maximum AUC value of 0.76 (P < 0.001) for the prediction of immunotherapy response, and it also highlighted a 24% disparity in the 1-year survival rate (P = 0.02). These findings suggest that radiological characteristics observed in CT imaging have the potential to serve as non-invasive biomarkers for the prediction of immunotherapy response. In a separate investigation conducted by Khorrami et al., machine learning techniques were employed to examine alterations in radiation texture parameters obtained from internal and external CT scans of tumor lesions, both before and after 2-3 cycles of ICIs therapy (DelRADx) (115). The retrospective analysis encompassed 139 NSCLC patients recruited from two research centers, with a division into a discovery group (D1 = 50) and two independent verification cohorts (D2 = 62 and D3 = 27). Through the implementation of machine learning methodologies, texture parameters within and surrounding the tumor were extracted, facilitating the computation of relative differences between pre-ICIs and post-ICIs treatment conditions. DelRADx proved capable of predicting both ICI response and overall survival (OS) among NSCLC patients. The linear discriminant analysis (LDA) classifier achieved AUC values of 0.88 ± 0.08 (D1), 0.85 (D2), and 0.81 (D3), effectively discriminating responders from non-responders. Moreover, the delta-radiomic risk score (DRS) demonstrated a significant correlation with OS (P = 0.0011; C-index = 0.72), thereby suggesting the potential utility of DelRADx as a tool for identifying NSCLC patients likely to exhibit a positive response to immunotherapy.
An analysis was undertaken encompassing 32 patients who had been diagnosed with NSCLC before undergoing treatment with Nivolumab. The utilization of 18F-FDG PET/CT scans facilitated this examination. The findings reveal a substantial disparity in the maximum standardized uptake value (SUVmax) between individuals who responded positively (N = 21) and those who did not (N = 11) (48.97 vs. 20.85, P = 0.002) (116). Furthermore, non-responders exhibited a tendency towards elevated tumor metabolic volume (MTVwb) and total lesion glycolysis (TLGwb) in comparison to responders. Likewise, a study involving one hundred and ninety-four patients with histologically confirmed stage IIIB-IV NSCLC investigated the potential of 18F-FDG PET/CT images acquired before treatment to identify a radiomics signature capable of predicting the response to immunotherapy (117). The resulting multiparametric radiomics signature (mpRS) demonstrated promising prognostic capabilities for durable clinical benefit (DCB), with respective AUC values of 0.86, 0.83, and 0.81 in the training, retrospective, and prospective test cohorts. Moreover, the nomogram models attained C-indexes of 0.74, 0.74, and 0.77 for prognosticating PFS, along with C-indexes of 0.83, 0.83, and 0.80 for predicting OS across the three cohorts. Furthermore, Seban et al. discovered through the analysis of FDG PET/CT that a total metabolic tumor volume (TMTV) surpassing 75 cm3 was linked to diminished overall survival and the absence of DCB in patients with advanced NSCLC who received treatment with ICIs (95). The study also established a significant correlation between high TMTV and unfavorable PFS and OS (both P < 0.05) in patients with advanced NSCLC who underwent first-line Pembrolizumab treatment (74). These findings suggest the potential usefulness of 18F-FDG PET/CT imaging parameters as predictive indicators for the efficacy of immunotherapy in patients with NSCLC. However, the clinical implementation of these parameters has been impeded by the redundancy and lack of replicability of several image features (118). Several ongoing prospective studies (NCT03304639, NCT03387761, and NCT03237780) are presently assessing the value of radiology and radiomics in predicting the response to ICIs.
3.2 Prediction models
3.2.1 Metabolic score
A composite prognostic biological marker employing dNLR and TMTV exhibits promising prospects in the domain of NSCLC. The metabolic scoring system classifies patients into three distinct categories: favorable prognosis (TMTV ≤ 75 cm3, dNLR ≤ 3), intermediate prognosis (TMTV > 75 cm3 or dNLR > 3), and unfavorable prognosis (TMTV > 75 cm3 and dNLR > 3). In a retrospective analysis encompassing 109 patients with advanced NSCLC who underwent ICIs, the metabolic score showcased noteworthy variances in terms of median OS and median PFS across the aforementioned categories (P < 0.001) (92). The favorable prognosis group exhibited the lengthiest median OS of 35.0 months, followed by the intermediate prognosis group (12.5 months), and finally, the unfavorable prognosis group (2.4 months). Median PFS values were recorded as 9.8, 2.7, and 1.4 months, respectively (P < 0.001). Notably, the metabolic score exhibited a correlation with ICI response, particularly in terms of durable clinical (P = 0.003) (Table 4). Within a multicenter study comprising 63 NSCLC patients possessing a PD-L1 tumor proportion score (TPS) ≥ 50, who were administered first-line Pembrolizumab and fluorodeoxyglucose positron emission tomography/computed tomography (FDG PET/CT), the favorable prognosis group exhibited a median OS of 17.9 months, in contrast to 13.8 and 6.6 months for the intermediate and unfavorable prognosis groups, respectively (74). Median PFS values were reported as 15.1, 5.2, and 1.9 months. The unfavorable prognosis group demonstrated associations with the DCR and ORR (P < 0.05). These findings lend support to the potential utilization of the metabolic score as a prognostic factor for NSCLC patients undergoing ICI treatment. Nonetheless, it is imperative to conduct prospective studies to validate the prognostic worthiness of this scoring system, given the retrospective nature of the existing evidence.
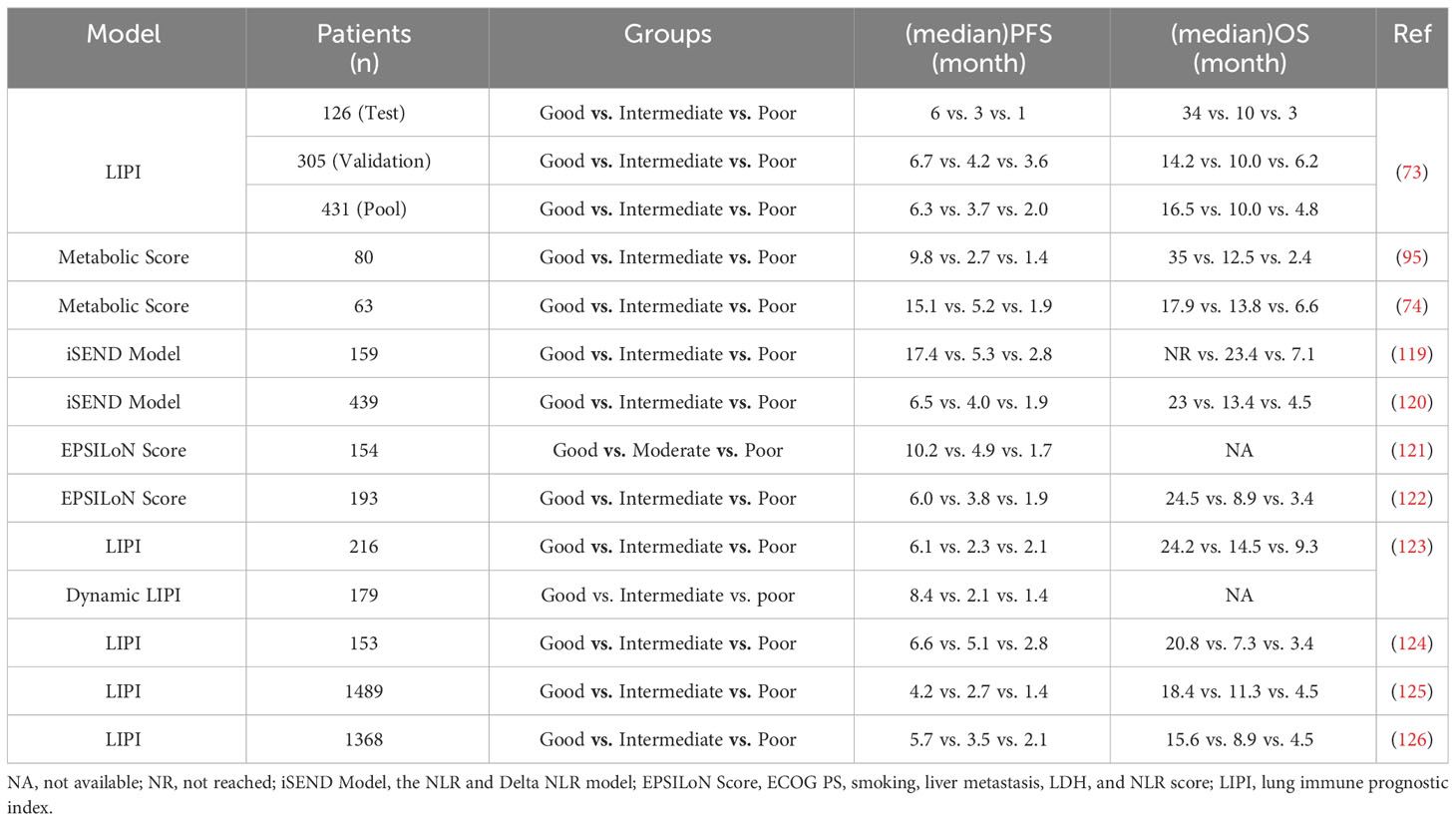
Table 4 Clinical outcomes of NSCLC patients received anti-PD-1/anti-PD-L1 agents according to different prediction models.
3.2.2 iSEND model
The NLR and Delta NLR (iSEND) model was initially introduced within the context of predicting the clinical efficacy of Nivolumab among patients diagnosed with NSCLC (119). An analysis conducted retrospectively on a cohort of 139 patients with locally advanced NSCLC, who had received second-line Nivolumab treatment, yielded correlations between sex, ECOG score, NLR, as well as pre-treatment and post-treatment changes in NLR, and PFS. The aforementioned variables were subsequently integrated into the iSEND model. By the iSEND model, patients were stratified into low-, medium-, and high-risk groups. The median follow-up period extended to 11.5 months. Within the low-risk group, the rates of 3, 6, 9, and 12-month PFS were observed at 78.4%, 63.7%, 55.3%, and 52.2%, respectively. Correspondingly, the medium-risk group experienced rates of 79.4%, 44.3%, 25.9%, and 19.2%, while the high-risk group exhibited rates of 65%, 25.9%, 22.8%, and 17.8%. As for the iSEND model’s ability to predict 3, 6, 9, and 12-month PFS, the respective AUC values amounted to 0.718, 0.74, 0.746, and 0.774. Significantly, disease progression within the high-risk group at 12 ± 2 weeks exhibited a strong correlation (P < 0.0001). These findings convey that the iSEND model can serve as a valuable tool in forecasting the prognosis of locally advanced NSCLC patients post Nivolumab treatment. In a subsequent investigation featuring a median follow-up duration of 18.2 months, patients classified as low-risk demonstrated notably superior OS rates in comparison to those deemed high-risk (P < 0.0001) (120). Furthermore, the prognostic capability of the iSEND model was assessed relative to PD-L1 expression levels. The time-dependent mortality rates in the iSEND low-risk group (N = 119) and the PD-L1 TPS = 0% group (N = 47) were found to be 0.75 vs. 0.53 at 12 months (P = 0.01) and 0.85 vs. 0.46 at 18 months (P = 0.03), respectively. Nevertheless, no significant distinction was observed in terms of the prognostic value between the iSEND model and PD-L1 TPS ≥ 50%. As such, the iSEND model holds potential as a prognostic factor applicable to patients with locally advanced NSCLC following Nivolumab therapy (Table 4).
3.2.3 EPSILoN score
The EPSILoN scoring system, which encompasses the ECOG PS, smoking history, liver metastasis, lactate dehydrogenase (LDH), and NLR, has been proposed as a prognostic tool for predicting the response to immunotherapy in individuals diagnosed with NSCLC (Table 4). Within a cohort comprising 154 patients with locally advanced NSCLC, who were administered second-line or later-line anti-PD-1 therapy, the ECOG score, smoking history, liver metastasis, LDH, and NLR demonstrated significant associations with both PFS and OS. Consequently, these aforementioned factors were incorporated into the EPSILoN scoring system. The patients were subsequently stratified into categories denoting favorable, moderate, and unfavorable prognoses based on their EPSILoN scores. The resulting median PFS values for each category were observed to be 10.2, 4.9, and 1.7 months, respectively (P < 0.001) (121). During the validation study of the EPSILoN scoring system among patients with locally advanced NSCLC who underwent anti-PD-1 immunotherapy, participants were divided into high, moderate, and low-risk groups (122). The corresponding PFS durations were 6.0, 3.8, and 1.9 months, respectively (P < 0.001), while the OS values were 24.5, 8.9, and 3.4 months (P < 0.001). These results affirm the prognostic significance of the EPSILoN scoring system for individuals with NSCLC who are receiving ICIs.
3.2.4 LIPI score
The lung immune prognostic index (LIPI) has been postulated as a novel categorical hematological biological marker to select individuals diagnosed with NSCLC who are suitable candidates for PD-1/PD-L1 therapy. LIPI integrates the dNLR and LDH to categorize patients into three distinct prognostic subsets. These subsets are demarcated by the subsequent thresholds: dNLR ≤ 3 and LDH ≤ upper limit of normal (ULN) to identify the low-risk cohort, dNLR ≥ 3 or LDH ≥ ULN to classify the moderate-risk group, and dNLR ≥ 3 and LDH ≥ ULN to allocate patients to the high-risk category (Table 4) (123–126). The work carried out by Mezquita et al. entailed a seminal investigation that established a noteworthy association between LIPI and treatment outcomes in patients with advanced NSCLC undergoing ICIs, namely Nivolumab, Pembrolizumab, Atezolizumab, Durvalumab, or Durvalumab plus Ipilimumab (73). The study demonstrated that dNLR values exceeding 3 and LDH levels surpassing the ULN were independently linked to OS. The high-risk, intermediate-risk, and low-risk groups exhibited median OS durations of 3, 10, and 34 months, respectively (P < 0.001). Median PFS values were measured at 2.0, 3.7, and 6.3 mos (P = 0.001), respectively. Moreover, the disease control rate displayed a positive correlation with dNLR values surpassing 3 and LDH levels exceeding the ULN (P = 0.004). Nevertheless, the prognostic value of the LIPI score failed to attain statistical significance within the chemotherapy cohort. The investigators concluded that pretreatment LIPI, encompassing dNLR values greater than 3 and LDH levels surpassing the ULN, was indicative of unfavorable outcomes in patients receiving ICIs, thereby suggesting its potential utility in identifying individuals unlikely to derive benefit from ICIs.
In a subsequent investigation conducted across multiple centers, the prognostic and predictive value of the LIPI score was examined among patients diagnosed with advanced NSCLC who were undergoing Nivolumab monotherapy (124). An insufficient LIPI score demonstrated a significant correlation with unfavorable OS according to both univariate analysis (P < 0.0001) and multivariate analysis (P < 0.0001). Although a noticeable association with diminished PFS was observed in the univariate analysis (P = 0.03), this correlation failed to reach statistical significance in the multivariate analysis (P = 0.09). Moreover, a low LIPI score displayed a statistically significant relationship with a reduced DCR based on both univariate analysis (P = 0.001) and multivariate analysis (P = 0.005). Sorich et al. conducted an extensive aggregated examination, assimilating data from the BIRCH, FIR, OAK, and POPLAR clinical trials, encompassing a total of 1489 patients who received Atezolizumab. The analysis unveiled a noteworthy correlation between the Lymphocyte Monocyte Ratio (LMR) score and the OS, PFS, and response rates, all possessing a level of significance below P < 0.001. Within the low-risk, intermediate-risk, and high-risk cohorts, the durations of median OS were found to be 18.4, 11.3, and 4.5 months, respectively (125). Importantly, the LMR score also exhibited correlations with survival (P < 0.001) and response rates (P = 0.005) in patients subjected to docetaxel treatment. In an additional pooled analysis comprising 11 clinical trials involving patients with metastatic NSCLC, a high LMR score displayed a favorable association with overall survival. Specifically, patients with a high LMR score demonstrated an estimated median survival of 15.6 months, whereas those with a low score had a median survival of 4.5 months (P < 0.001) (12). Analogous associations between elevated LMR scores and enhanced survival outcomes were observed among patients who underwent chemotherapy. In this context, patients with a high LMR score experienced a protracted period of survival in comparison to those with a low score, with an estimated median survival of 10.4 mos versus 5.3 mos (P < 0.001). Thus, the pretreatment LMR score exhibits promise as a valuable instrument for identifying patients who are likely to derive benefits from ICIs and chemotherapy.
4 Conclusion and future perspectives
The clinical application of PD-1/PD-L1 inhibitors in patients diagnosed with NSCLC has achieved unprecedented success in terms of enhancing long-term outcomes. However, the relatively low response rates, elevated treatment costs, and notable likelihood of immune-related adverse reactions necessitate an urgent quest for effective predictive and prognostic biological markers. At present, PD-L1 expression and TMB appear to hold the most promising potential as biological markers for predicting the response to immunotherapy. Nonetheless, these single biological markers require comprehensive exploration and optimization in various aspects due to certain limitations identified in specific cases (Table 5). Furthermore, emerging and promising biological markers encompass hematological biological markers, driver mutations, radiology, and radiomics. Going forward, it is imperative to standardize the diverse range of biological markers, leverage omics technologies to expedite the identification of robust biological markers, examine the feasibility of employing combination biological markers, and harness the capabilities of computer algorithms and AI technologies to establish innovative prognostic models. In the context of this review, it appears that prediction models incorporating multiple factors hold greater promise as tools for prognosticating the effectiveness of immunotherapy in NSCLC. During clinical trials, stratified analyses can be conducted based on the factors included in these models to identify subgroups that are more likely to benefit from immunotherapy.
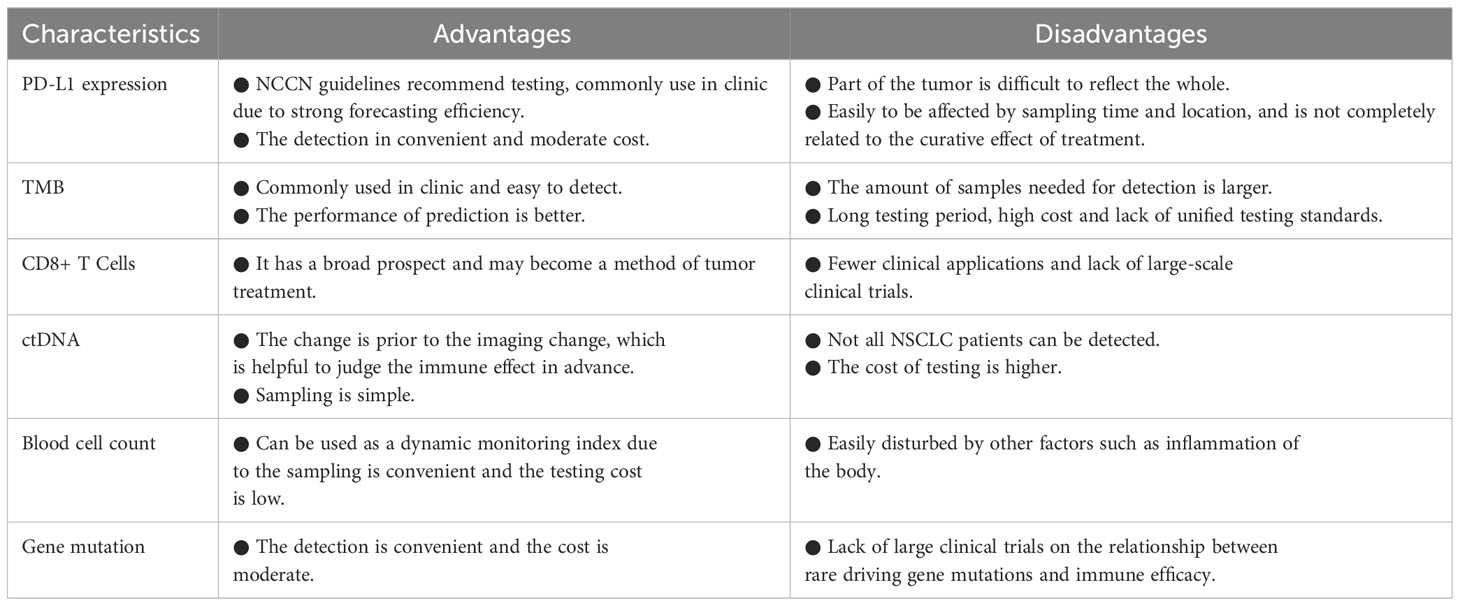
Table 5 Some advantages and disadvantages of single biological markers for predicting immunotherapy in NSCLC.
Author contributions
DH and CL conceived of the review and edited the manuscript. SL, HW, and HT analyzed the data and drafted the manuscript. ZX, and MW contributed to the data collection. All authors read and approved the final manuscript. All authors contributed to the article and approved the submitted version.
Funding
This work was supported by the Major Science and Technology Demonstration of Wuxi Science and Technology Bureau (No. WX18IVJN017), Top Medical Expert Team of 2021 Taihu Talent Plan Project, Medical Innovation Team of Wuxi (CXTD2021001).
Acknowledgments
The authors wish to thank editors as well as reviewers for reading the manuscript.
Conflict of interest
The authors declare that the research was conducted in the absence of any commercial or financial relationships that could be construed as a potential conflict of interest.
Publisher’s note
All claims expressed in this article are solely those of the authors and do not necessarily represent those of their affiliated organizations, or those of the publisher, the editors and the reviewers. Any product that may be evaluated in this article, or claim that may be made by its manufacturer, is not guaranteed or endorsed by the publisher.
Abbreviations
AUC, area under curve; bTMB, blood TMB; CA19-9, carbohydrate antigen; CEA, carcinoembryonic antigen; CT, computed tomography; ctDNA, circulating tumor DNA; Cyfra21-1, cytokeratin fragment 21-1; FDA, food and drug administration; DCB, disease clinical benefit; DCR, durable clinical rate; dNLR, derived NLR; HR, hazard ratio; ICIs, immune checkpoint inhibitors; LDA, linear discriminant analysis; LDH, lactate dehydrogenase; LIPI, lung immune prognostic index; MHC, major histocompatibility complexes; mOS, median OS; mPFS, median PFS; mpRS, multiparametric radiomics signature; MTVwb, tumor metabolic volume; NCCN, national comprehensive cancer network; NLR, neutrophil/lymphocyte ratio; NSCLC, non-small cell lung cancer; NSE, neuron specific enolase; ORR, overall response rate; OS, overall survival; PD-1, programmed cell death protein 1; PD-L1, programmed cell death ligand 1; PFS, progression-free survival; ROC, receiver operating characteristic curve; SUVmax, maximum standardized uptake value; TCR, T cell receptors; TLGwb, total lesion glycolysis; TMB, tumor mutation burden; TMB-H, high TMB; TMB-L, low TMB; TMTV, total metabolic tumor volume; TTP, time to progression; ULN, upper limit of normal.
References
1. Siegel RL, Miller KD, Wagle NS, Jemal A. Cancer statistics, 2023. CA Cancer J Clin (2023) 73(1):17–48. doi: 10.3322/caac.21763
2. Xia C, Dong X, Li H, Cao M, Sun D, He S, et al. Cancer statistics in China and United States, 2022: profiles, trends, and determinants. Chin Med J (Engl). (2022) 135(5):584–90. doi: 10.1097/CM9.0000000000002108
3. Fehrenbacher L, Spira A, Ballinger M, Kowanetz M, Vansteenkiste J, Mazieres J, et al. Atezolizumab versus docetaxel for patients with previously treated non-small-cell lung cancer (POPLAR): a multicentre, open-label, phase 2 randomised controlled trial. Lancet (2016) 387(10030):1837–46. doi: 10.1016/S0140-6736(16)00587-0
4. Herbst RS, Baas P, Kim DW, Felip E, Pérez-Gracia JL, Han JY, et al. Pembrolizumab versus docetaxel for previously treated, PD-L1-positive, advanced non-small-cell lung cancer (KEYNOTE-010): a randomised controlled trial. Lancet (2016) 387(10027):1540–50. doi: 10.1016/S0140-6736(15)01281-7
5. Brahmer J, Reckamp KL, Baas P, Crinò L, Eberhardt WE, Poddubskaya E, et al. Nivolumab versus docetaxel in advanced squamous-cell non-small-cell lung cancer. N Engl J Med (2015) 373(2):123–35. doi: 10.1056/NEJMoa1504627
6. Rittmeyer A, Barlesi F, Waterkamp D, Park K, Ciardiello F, von Pawel J, et al. Atezolizumab versus docetaxel in patients with previously treated non-small-cell lung cancer (OAK): a phase 3, open-label, multicentre randomised controlled trial. Lancet (2017) 389(10066):255–65. doi: 10.1016/S0140-6736(16)32517-X
7. Garon EB, Hellmann MD, Rizvi NA, Carcereny E, Leighl NB, Ahn MJ, et al. Five-year overall survival for patients with advanced non–small-cell lung cancer treated with pembrolizumab: results from the phase I KEYNOTE-001 study. J Clin Oncol (2019) 37:2518–27. doi: 10.1200/JCO.19.00934
8. Brahmer JR, Govindan R, Anders RA, Antonia SJ, Sagorsky S, Davies MJ, et al. The society for immunotherapy of cancer consensus statement on immunotherapy for the treatment of non-small cell lung cancer (NSCLC). J Immunother. Cancer (2018) 6:75. doi: 10.1186/s40425-018-0382-2
9. Hamid O, Robert C, Daud A, Hodi FS, Hwu WJ, Kefford R, et al. Five-year survival outcomes for patients with advanced melanoma treated with pembrolizumab in KEYNOTE-001. Ann Oncol (2019) 30:582–8. doi: 10.1093/annonc/mdz011
10. Tumeh PC, Harview CL, Yearley JH, Shintaku IP, Taylor EJ, Robert L, et al. PD-1 blockade induces responses by inhibiting adaptive immune resistance. Nature (2014) 515(7528):568–71. doi: 10.1038/nature13954
11. Hanahan D, Weinberg RA. Hallmarks of cancer: the next generation. Cell (2011) 144(5):646–74. doi: 10.1016/j.cell.2011.02.013
12. Petrelli F, Maltese M, Tomasello G, Conti B, Borgonovo K, Cabiddu M, et al. Clinical and molecular predictors of PD-L1 expression in non-small-cell lung cancer: systematic review and meta-analysis. Clin Lung Cancer. (2018) 19(4):315–22. doi: 10.1016/j.cllc.2018.02.006
13. Syn NL, Teng MWL, Mok TSK, Soo RA. De-novo and acquired resistance to immune checkpoint targeting. Lancet Oncol (2017) 18(12):e731–41. doi: 10.1016/S1470-2045(17)30607-1
14. Shi Y. Regulatory mechanisms of PD-L1 expression in cancer cells. Cancer Immunol Immunother. (2018) 67(10):1481–9. doi: 10.1007/s00262-018-2226-9
15. Mazieres J, Rittmeyer A, Gadgeel S, Hida T, Gandara DR, Cortinovis DL, et al. Atezolizumab versus docetaxel in pretreated patients with NSCLC: final results from the randomized phase 2 POPLAR and phase 3 OAK clinical trials. J Thorac Oncol (2021) 16(1):140–50. doi: 10.1016/j.jtho.2020.09.022
16. Herbst RS, Baas P, Perez-gracia JL, Felip E, Kim DW, Han J, et al. Use of archival versus newly collected tumor samples for assessing PD-L1 expression and overall survival: an updated analysis of KEYNOTE-010 trial. Ann Oncol (2019) 30(2):281–9. doi: 10.1093/annonc/mdy545
17. Horn L, Spigel DR, Vokes EE, Holgado E, Ready N, Steins M, et al. Nivolumab versus docetaxel in previously treated patients with advanced non-small-cell lung cancer: two-year outcomes from two randomized, open-label, phase III trials (CheckMate 017 and CheckMate 057). J Clin Oncol (2017) 35(35):3924–33. doi: 10.1200/JCO.2017.74.3062
18. Borghaei H, Paz-Ares L, Horn L, Spigel DR, Steins M, Ready NE, et al. Nivolumab versus docetaxel in advanced nonsquamous non-small-cell lung cancer. N Engl J Med (2015) 373(17):1627–39. doi: 10.1056/NEJMoa1507643
19. Wu YL, Lu S, Cheng Y, Zhou C, Wang J, Mok T, et al. Nivolumab versus docetaxel in a predominantly Chinese patient population with previously treated advanced NSCLC: CheckMate 078 randomized phase III clinical trial. J Thorac Oncol (2019) 14(5):867–75. doi: 10.1016/j.jtho.2019.01.006
20. Mok TSK, Wu YL, Kudaba I, Kowalski DM, Cho BC, Turna HZ, et al. Pembrolizumab versus chemotherapy for previously untreated, PD-L1-expressing, locally advanced or metastatic non-small-cell lung cancer (KEYNOTE-042): a randomised, open-label, controlled, phase 3 trial. Lancet (2019) 393(10183):1819–30. doi: 10.1016/S0140-6736(18)32409-7
21. Reck M, Rodriguez-Abreu D, Robinson AG, Hui R, Csőszi T, Fülöp A, et al. Pembrolizumab versus chemotherapy for PD-L1-positive non–small-cell lung cancer. N Engl J Med (2016) 375(19):1823–33. doi: 10.1056/NEJMoa1606774
22. Nosaki K, Saka H, Hosomi Y, Baas P, de Castro G Jr, Reck M, et al. Safety and efficacy of pembrolizumab monotherapy in elderly patients with PD-L1–positive advanced non–small-cell lung cancer: pooled analysis from the KEYNOTE-010, KEYNOTE-024, and KEYNOTE-042 studies. Lung Cancer. (2019) 135:188–95. doi: 10.1016/j.lungcan.2019.07.004
23. Peters S, Cho BC, Reinmuth N, Lee KH, Luft A, Ahn Mj , et al. Tumor mutational burden (TMB) as a biomarker of survival in metastatic non-small cell lung cancer (mNSCLC): Blood and tissue TMB analysis from MYSTIC, a Phase III study of first-line durvalumab ± tremelimumab vs chemotherapy. Cancer Res (2019). doi: 10.1158/1538-7445.AM2019-CT074
24. Ready N, Hellmann MD, Awad MM, Otterson GA, Gutierrez M, Gainor JF, et al. First-line nivolumab plus ipilimumab in advanced non-small-cell lung cancer (CheckMate 568): outcomes by programmed death ligand 1 and tumor mutational burden as biomarkers. J Clin Oncol (2019) 37(12):992–1000. doi: 10.1200/JCO.18.01042
25. Hellmann MD, Rizvi NA, Goldman JW, Gettinger SN, Borghaei H, Brahmer JR, et al. Nivolumab plus ipilimumab as first-line treatment for advanced non-small-cell lung cancer (CheckMate 012): results of an open-label, phase 1, multicohort study. Lancet Oncol (2017) 18(1):31–41. doi: 10.1016/S1470-2045(16)30624-6
26. Sezer A, Kilickap S, Gümüs M, Bondarenko I, Ozguroglu M, Gogishvili M, et al. EMPOWER-Lung 1: Phase III first-line (1L) cemiplimab monotherapy vs platinum-doublet chemotherapy (chemo) in advanced non-small cell lung cancer (NSCLC) with programmed cell death-ligand 1 (PD-L1) ≥50%. Ann Oncol (2020). doi: 10.1016/j.annonc.2020.10.372
27. Spigel D, Marinis FD, Giaccone G, Reinmuth N, Vergnenegre A, Barrios CH, et al. IMpower110: Interim overall survival (OS) analysis of a phase III study of atezolizumab (atezo) vs platinum-based chemotherapy (chemo) as first-line (1L) treatment (tx) in PD-L1–selected NSCLC. Ann Oncol (2019). doi: 10.1093/annonc/mdz394
28. Zhou CC, Chen GY, Huang YC, Zhou J, Lin L, Feng J, et al. Camrelizumab plus carboplatin and pemetrexed versus chemotherapy alone in chemotherapy-naive patients with advanced non-squamous non-small-cell lung cancer (CameL): a randomised, open-label, multicentre, phase 3 trial. Lancet Respir Med (2020) 9(3):305–14. doi: 10.1016/S2213-2600(20)30365-9
29. Brahmer JR, Lee JS, Ciuleanu TE, Bernabe Caro R, Nishio M, Urban L, et al. Five-year survival outcomes with nivolumab plus ipilimumab versus chemotherapy as first-line treatment for metastatic non-small-cell lung cancer in CheckMate 227. J Clin Oncol (2023) 41(6):1200–12. doi: 10.1200/JCO.22.01503.
30. Reck M, Ciuleanu TE, Lee JS, Schenker M, Audigier-Valette C, Zurawski , et al. First-line nivolumab plus ipilimumab vs chemotherapy in advanced non-small cell lung cancer with tumor PD-L1 ≥ 1%: patient-reported outcomes from CheckMate 227 part 1. J Thorac Oncol (2021) 16(4):665–76. doi: 10.1016/j.jtho.2020.12.019
31. Paz-Ares L, Luft A, Vicente D, Tafreshi A, Gümüş M, Mazières J, et al. Pembrolizumab plus chemotherapy for squamous non–small-cell lung cancer. N Engl J Med (2018) 379(21):2040–51. doi: 10.1056/NEJMoa1810865
32. Zinner RG, Obasaju CK, Spigel DR, Giaccone G, Heist RS, Hodi FS, et al. Long-term survival follow-up of atezolizumab in combination with platinum-based doublet chemotherapy in patients with advanced non-small-cell lung cancer. Eur J Cancer. (2018) 101:114–22. doi:10.1016/j.ejca.2018.06.033
33. Carbone DP, Reck M, Paz-Ares L, Creelan B, Horn L, Steins M, et al. First-line Nivolumab in stage IV or recurrent non-small-cell lung cancer. N Engl J Med (2017) 376(25):2415–26. doi: 10.1056/NEJMoa1613493
34. Gray JE, Villegas A, Daniel D, Vicente D, Murakami S, Hui R, et al. Three-year overall survival with durvalumab after chemoradiotherapy in stage III NSCLC-update from PACIFIC. J Thorac Oncol (2020) 15(2):288–93. doi: 10.1016/j.jtho.2019.10.002
35. Hellmann MD, Ciuleanu TE, Pluzanski A, Lee JS, Otterson GA, Audigier-Valette C, et al. Nivolumab plus ipilimumab in lung cancer with a high tumor mutational burden. N Engl J Med (2018) 378(22):2093–104. doi: 10.1056/NEJMoa1801946
36. Antonia SJ, Villegas A, Daniel D, Murakami S, Hui R, Kurata T, et al. Overall survival with durvalumab after chemoradiotherapy in stage III NSCLC. N Engl J Med (2018) 379(24):2342–50. doi: 10.1056/NEJMoa1809697
37. Li J, Gu J. PD-L1 expression and EGFR status in advanced non-small-cell lung cancer patients receiving PD-1/PD-L1 inhibitors: a meta-analysis. Future Oncol (2019) 15(14):1667–78. doi: 10.2217/fon-2018-0639
38. US Food and Drug Administration. List of cleared or approved companion diagnostic devices (in vitro and imaging tools). FDA (2020). Available at: https://www.fda.gov/medical-devices/vitro-diagnostics/list-cleared-or-approved-companion-diagnostic-devices-vitro-and-imaging-tools.
39. Fusi A, Festino L, Botti G, Masucci G, Melero I, Lorigan P, et al. PD-L1 expression as a potential predictive biomarker. Lancet Oncol (2015) 16(13):1285–7. doi: 10.1016/S1470-2045(15)00307-1
40. Meng X, Huang Z, Teng F, Xing L, Yu J. Predictive biomarkers in PD-1/PD-L1 checkpoint blockade immunotherapy. Cancer Treat Rev (2015) 41(10):868–76. doi: 10.1016/j.ctrv.2015.11.001
41. Ott PA, Bang YJ, Berton-Rigaud D, Elez E, Pishvaian MJ, Rugo HS, et al. Safety and antitumor activity of pembrolizumab in advanced programmed death ligand 1-positive endometrial cancer: results from the KEYNOTE-028 study. J Clin Oncol (2017) 35(22):2535–41. doi: 10.1200/JCO.2017.72.5952
42. Mclaughlin J, Han G, Schalper KA, Carvajal-Hausdorf D, Pelekanou V, Rehman J, et al. Quantitative assessment of the heterogeneity of PD-L1 expression in non-small-cell lung cancer. JAMA Oncol (2016) 2(1):46–54. doi: 10.1001/jamaoncol.2015.3638
43. Herbst RS, Soria JC, Kowanetz M, Fine GD, Hamid O, Gordon MS, et al. Predictive correlates of response to the anti-PD-L1 antibody MPDL3280A in cancer patients. Nature (2014) 515(7528):563–7. doi: 10.1038/nature14011
44. Formenti SC, Demaria S. Combining radiotherapy and cancer immunotherapy: a paradigm shift. J Natl Cancer Inst (2013) 105(4):256–65. doi: 10.1093/jnci/djs629
45. Ramakrishnan R, AsSudani D, Nagaraj S, Hunter T, Cho HI, Antonia S, et al. Chemotherapy enhances tumor cell susceptibility to CTL-mediated killing during cancer immunotherapy in mice. J Clin Invest. (2010) 120(4):1111–24. doi: 10.1172/JCI40269
46. Twymansaint VC, Rech AJ, Maity A, Rengan R, Pauken KE, Stelekati E, et al. Radiation and dual checkpoint blockade activate non-redundant immune mechanisms in cancer. Nature (2015) 520(7547):373–7. doi: 10.1038/nature14292
47. Rizvi NA, Hellmann MD, Snyder A, Kvistborg P, Makarov V, Havel JJ, et al. Cancer immunology. Mutational landscape determines sensitivity to PD-1 blockade in non-small cell lung cancer. Science (2015) 348:124–8. doi: 10.1126/science.aaa1348
48. Schumacher TN, Schreiber RD. Neoantigens in cancer immunotherapy. Science (2015) 348(6230):69–74. doi: 10.1126/science.aaa4971
49. Alexandrov LB, Nik-Zainal S, Wedge DC, Aparicio SA, Behjati S, Biankin AV, et al. Signatures of mutational processes in human cancer. Nature (2013) 500(7463):415–21. doi: 10.1038/nature12477
50. Gandara DR, Paul SM, Kowanetz M, Schleifman E, Zou W, Li Y, et al. Blood-based tumor mutational burden as a predictor of clinical benefit in non-small-cell lung cancer patients treated with atezolizumab. Nat Med (2018) 24(9):1441–8. doi: 10.1038/s41591-018-0134-3
51. Kim ES, Velcheti V, Mekhail T, et al. LBA55: Primary efficacy results from B-FIRST, a prospective phase II trial evaluating blood-based tumour mutational burden (bTMB) as a predictive biomarker for atezolizumab (atezo) in 1L non-small cell lung cancer (NSCLC). Annal Oncol (2018). doi: 10.1093/annonc/mdy424.067
52. Hellmann MD, Nathanson T, Rizvi H, Creelan BC, Sanchez-Vega F, Ahuja A, et al. Genomic features of response to combination immunotherapy in patients with advanced non-small-cell lung cancer. Cancer Cell (2018) 33(5):843–52.e4. doi: 10.1016/j.ccell.2018.03.018
53. Yu Y, Zeng D, Ou Q, Liu S, Li A, Chen Y, et al. Association of survival and immune-related biomarkers with immunotherapy in patients with non-small cell lung cancer: A meta-analysis and individual patient-level analysis. JAMA Netw Open (2019) 2(7):e196879. doi: 10.1001/jamanetworkopen.2019.6879
54. Camidge DR, Doebele RC, Kerr KM. Comparing and contrasting predictive biomarkers for immunotherapy and targeted therapy of NSCLC. Nat Rev Clin Oncol (2019) 16(6):341–55. doi: 10.1038/s41571-019-0173-9
55. Costantini A, Kamga PT, Dumenil C, Chinet T, Emile JF, Leprieur EG. Plasma biomarkers and immune checkpoint inhibitors in non-small cell lung cancer: new tools for better patient selection? Cancers (Basel) (2019) 11(9):1269. doi: 10.3390/cancers11091269
56. Ilie M, Long-Mira E, Bence C, Butori C, Lassalle S, Bouhlel L, et al. Comparative study of the PD-L1 status between surgically resected specimens and matched biopsies of NSCLC patients reveal major discordances: a potential issue for anti-PD-L1 therapeutic strategies. Ann Oncol (2016) 27(1):147–53. doi: 10.1093/annonc/mdv489
57. Riaz N, Havel JJ, Makarov V, Desrichard A, Urba WJ, Sims JS, et al. Tumor and microenvironment evolution during immunotherapy with nivolumab. Cell (2017) 171(4):934–49.e16. doi: 10.1016/j.cell.2017.09.028
58. Han J, Duan J, Bai H, Wang Y, Wan R, Wang X, et al. TCR repertoire diversity of peripheral PD-1+CD8+ T cells predicts clinical outcomes after immunotherapy in patients with non-small cell lung cancer. Cancer Immunol Res (2020) 8(1):146–54. doi: 10.1158/2326-6066.CIR-19-0398
59. Kim KH, Cho J, Ku BM, Koh J, Sun JM, Lee SH, et al. The first-week proliferative response of peripheral blood PD-1 + CD8 + T cells predicts the response to anti-PD-1 therapy in solid tumors. Clin Cancer Res (2019) 25(7):2144–54. doi: 10.1158/1078-0432.CCR-18-1449
60. Cabel L, Riva F, Servois V, Livartowski A, Daniel C, Rampanou A, et al. Circulating tumor DNA changes for early monitoring of anti-PD1 immunotherapy: a proof-of-concept study. Ann Oncol (2017) 28(8):1996–2001. doi: 10.1093/annonc/mdx212
61. Passiglia F, Galvano A, Castiglia M, Incorvaia L, Calò V, Listì A, et al. Monitoring blood biomarkers to predict nivolumab effectiveness in NSCLC patients. Ther Adv Med Oncol (2019) 11:1758835919839928. doi: 10.1177/1758835919839928
62. Goldberg SB, Narayan A, Kole AJ, Decker RH, Teysir J, Carriero NJ, et al. Early assessment of lung cancer immunotherapy response via circulating tumor DNA. Clin Cancer Res (2018) 24(8):1872–80. doi: 10.1158/1078-0432.CCR-17-1341
63. Tanizaki J, Haratani K, Hayashi H, Chiba Y, Nakamura Y, Yonesaka K, et al. Peripheral blood biomarkers associated with clinical outcome in non-small cell lung cancer patients treated with nivolumab. J Thorac Oncol (2018) 13(1):97–105. doi: 10.1016/j.jtho.2017.10.030
64. Soyano AE, Dholaria B, Marin-Acevedo JA, Diehl N, Hodge D, Luo Y, et al. Peripheral blood biomarkers correlate with outcomes in advanced non-small cell lung Cancer patients treated with anti-PD-1 antibodies. J Immunother Cancer. (2018) 6(1):129. doi: 10.1186/s40425-018-0447-2
65. Diem S, Schmid S, Krapf M, Migliorino MR, Aprile G, Mansueto G, et al. Neutrophil-to-lymphocyte ratio (NLR), platelet-to-lymphocyte ratio (PLR), and outcomes with nivolumab in pretreated non-small cell lung cancer (NSCLC): A large retrospective multicenter study. Adv Ther (2020) 37(3):1145–55. doi: 10.1007/s12325-020-01229-w
66. Liu J, Li S, Zhang S, Liu Y, Ma L, Zhu J, et al. Systemic immune-inflammation index, neutrophil-to-lymphocyte ratio, platelet-to-lymphocyte ratio can predict clinical outcomes in patients with metastatic non-small-cell lung cancer treated with nivolumab. J Clin Lab Anal (2019) 33(8):e22964. doi: 10.1002/jcla.22964
67. Bagley SJ, Kotharis S, Aggarwal C, Tamiya A, Tanaka A, Okamoto N, et al. Pretreatment advanced lung cancer inflammation index (ALI) for predicting early progression in nivolumab-treated patients with advanced non-small cell lung cancer. Cancer Med (2018) 7(1):13–20. doi: 10.1002/cam4.1234
68. Diem S, Schmid S, Krapf M, Flatz L, Born D, Jochum W, et al. Neutrophil- to-Lymphocyte Ratio (NLR) and Platelet-to- Lymphocyte ratio (PLR) as prognostic markers in patients with non-small cell lung cancer (NSCLC) treated with nivolumab. Lung Cancer. (2017) 111:176–81. doi: 10.1016/j.lungcan.2017.07.024
69. Fukui T, Okuma Y, Nakahara Y, Otani S, Igawa S, Katagiri M, et al. Activity of nivolumab and utility of neutrophil-to-lymphocyte ratio as a predictive biomarker for advanced non-small-cell lung cancer: a prospective observational study. Clin Lung Cancer. (2019) 20(3):208–14. doi: 10.1016/j.cllc.2018.04.021
70. Zer A, Sung MR, Walia P, Khoja L, Maganti M, Labbe C, et al. Correlation of Neutrophil to lymphocyte ratio and absolute neutrophil count with outcomes with PD-1 axis inhibitors in patients with advanced non-small- cell lung cancer. Clin Lung Cancer. (2018) 19(5):426–34. doi: 10.1016/j.cllc.2018.04.008
71. Suh KJ, Kim SH, Kim YJ, Kim M, Keam B, Kim TM, et al. Post-treatment neutrophil-to-lymphocyte ratio at week 6 is prognostic in patients with advanced non-small cell lung cancers treated with anti-PD-1 antibody. Cancer Immunol Immunother. (2018) 67(3):459–70. doi: 10.1007/s00262-017-2092-x
72. Petrova MP, Eneva MI, Arabadjiev JI, Conev NV, Dimitrova EG, Koynov KD, et al. Neutrophil to lymphocyte ratio as a potential predictive marker for treatment with pembrolizumab as a second line treatment in patients with non-small cell lung cancer. Biosci Trends. (2020) 14(1):48–55. doi: 10.5582/bst.2019.01279
73. Mezquita L, Auclin E, Ferrara R, Charrier M, Remon J, Planchard D, et al. Association of the lung immune prognostic index with immune checkpoint inhibitor outcomes in patients with advanced non-small cell lung cancer. JAMA Oncol (2018) 4(3):351–7. doi: 10.1001/jamaoncol.2017.4771
74. Seban RD, Assié JB, Giroux-Leprieur E, Massiani MA, Soussan M, Bonardel G, et al. Association of the metabolic score using baseline FDG-PET/CT and dNLR with immunotherapy outcomes in advanced NSCLC patients treated with first-line pembrolizumab. Cancers (Basel). (2020) 12(8):2234. doi: 10.3390/cancers12082234
75. Kataoka Y, HIrano K, Narabayashi T, Hara S, Fujimoto D, Tanaka T, et al. Carcinoembryonic antigen as a predictive biomarker of response to nivolumab in non-small cell lung cancer. Anticancer Res (2018) 38(1):559–63. doi: 10.21873/anticanres.12259
76. Shirasu H, Ono A, Omae K, Nakashima K, Omori S, Wakuda K, et al. CYFRA 21-1 predicts the efficacy of nivolumab in patients with advanced lung adenocarcinoma. Tumour Biol (2018) 40(2):1010428318760420. doi: 10.1177/1010428318760420
77. Lang D, Horner A, Brehm E, Akbari K, Hergan B, Langer K, et al. Early serum tumor marker dynamics predict progression-free and overall survival in single PD-1/PD-L1 inhibitor treated advanced NSCLC - A retrospective cohort study. Lung Cancer. (2019) 134:59–65. doi: 10.1016/j.lungcan.2019.05.033
78. Kamphorst AO, Pillai RN, Yang S, Nasti TH, Akondy RS, Wieland A, et al. Proliferation of PD-1+ CD8 T cells in peripheral blood after PD-1-targeted therapy in lung cancer patients. Proc Natl Acad Sci USA (2017) 114(19):4993–8. doi: 10.1073/pnas.1705327114
79. Bidard F-C, Weigelt B, Reis-Filho JS. Going with the flow: from circulating tumor cells to DNA. Sci Transl Med (2013). doi: 10.1126/scitranslmed.3006305
80. Diaz LA, Bardelli A. Liquid biopsies: genotyping circulating tumor DNA. J Clin Oncol (2014) 32(6):579–86. doi: 10.1200/JCO.2012.45.2011
81. Alix-Panabières C, Pantel K. Clinical applications of circulating tumor cells and circulating tumor DNA as liquid biopsy. Cancer Discovery (2016) 6(5):479–91. doi: 10.1158/2159-8290.CD-15-1483
82. Diehl F, Schmidt K, Choti MA, Romans K, Goodman S, Li M, et al. Circulating mutant DNA to assess tumor dynamics. Nat Med (2008) 14(9):985–90. doi: 10.1038/nm.1789
83. Siravegna G, Mussolin B, Buscarino M, Corti G, Cassingena A, Crisafulli G, et al (2015). Clonal evolution and resistance to EGFR blockade in the blood of colorectal cancer patients. Nat Med 21(7):795–801. doi: 10.1038/nm.3870
84. Riva F, Bidard F-C, Houy A, Saliou A, Madic J, Rampanou A, et al. Patient-specific circulating tumor DNA detection during neoadjuvant chemotherapy in triple-negative breast cancer. Clin Chem (2017) 63(3):691–9. doi: 10.1373/clinchem.2016.262337
85. Olsson E, Winter C, George A, Chen Y, Howlin J, Tang MH, et al. Serial monitoring of circulating tumor DNA in patients with primary breast cancer for detection of occult metastatic disease. EMBO Mol Med (2015) 7(8):1034–47. doi: 10.15252/emmm.201404913
86. Lipson EJ, Velculescu VE, Pritchard TS, Sausen M, Pardoll DM, Topalian SL, et al. Circulating tumor DNA analysis as a real-time method for monitoring tumor burden in melanoma patients undergoing treatment with immune checkpoint blockade. J Immunother Cancer. (2014) 2(1):42. doi: 10.1186/s40425-014-0042-0
87. Mei Z, Shi L, Wang B, Yang J, Xiao Z, Du P, et al. Prognostic role of pretreatment blood neutrophil-to-lymphocyte ratio in advanced cancer survivors: a systematic review and meta-analysis of 66 cohort studies. Cancer Treat Rev (2017) 58:1–13. doi: 10.1016/j.ctrv.2017.05.005
88. Yu Y, Qian L, Cui J. Value of neutrophil- to-lymphocyte ratio for predicting lung cancer prognosis: a meta-analysis of 7219 patients. Mol Clin Oncol (2017) 7(3):498–506. doi: 10.3892/mco.2017.1342
89. Derman BA, Macklis JN, Azeem MS, Sayidine S, Basu S, Batus M, et al. Relationships between longitudinal neutrophil to lymphocyte ratios, body weight changes, and overall survival in patients with non-small cell lung cancer. BMC Cancer. (2017) 17(1):141. doi: 10.1186/s12885-017-3122-y
90. Bagley SJ, Kothari S, Aggarwal C, Bauml JM, Alley EW, Evans TL, et al. Pretreatment neutrophil-to-lymphocyte ratio as a marker of outcomes in nivolumab-treated patients with advanced non-small-cell lung cancer. Lung Cancer. (2017) 106:1–7. doi: 10.1016/j.lungcan.2017.01.013
91. Ferrucci PF, Ascierto PA, Pigozzo J, Del Vecchio M, Maio M, Antonini Cappellini GC, et al. Baseline neutrophils and derived neutrophil-to-lymphocyte ratio: prognostic relevance in metastatic melanoma patients receiving ipilimumab. Ann Oncol (2016) 27(4):732–8. doi: 10.1093/annonc/mdw016
92. Suzuki R, Takagi T, Hikichi T, Konno N, Sugimoto M, Watanabe KO, et al. Derived neutrophil/lymphocyte ratio predicts gemcitabine therapy outcome in unresectable pancreatic cancer. Oncol Lett (2016) 11(5):3441–5. doi: 10.3892/ol.2016.4381
93. van Kessel KEM, de Haan LM, Fransen van de Putte EE, van Rhijn BW, de Wit R, van der Heijden MS, et al. Elevated derived neutrophil-to- lymphocyte ratio corresponds with poor outcome in patients undergoing pre-operative chemotherapy in muscle-invasive bladder cancer. Bladder Cancer. (2016) 2(3):351–60. doi: 10.3233/BLC-160055
94. Amato RJ, Flaherty A, Zhang Y, Ouyang F, Mohlere V. Clinical prognostic factors associated with outcome in patients with renal cell cancer with prior tyrosine kinase inhibitors or immunotherapy treated with everolimus. Urol Oncol (2014) 32(3):345–54. doi: 10.1016/j.urolonc.2013.09.008
95. Seban RD, Mezquita L, Berenbaum A, Dercle L, Botticella A, Le Pechoux C, et al. Baseline metabolic tumor burden on FDG PET/CT scans predicts outcome in advanced NSCLC patients treated with immune checkpoint inhibitors. Eur J Nucl Med Mol Imaging. (2020) 47(5):1147–57. doi: 10.1007/s00259-019-04615-x
96. Noonan SA, Patil T, Gao D, King GG, Thibault JR, Lu X, et al. Baseline and on-treatment characteristics of serum tumor markers in stage IV oncogene-addicted adenocarcinoma of the lung. J Thorac Oncol (2018) 13(1):134–8. doi: 10.1016/j.jtho.2017.08.005
97. Holdenrieder S, Wehnl B, Hettwer K, Simon K, Uhlig S, Dayyani F, et al. Carcinoembryonic antigen and cytokeratin-19 fragments for assessment of therapy response in non-small cell lung cancer: a systematic review and meta-analysis. Br J Cancer. (2017) 116(8):1037–45. doi: 10.1038/bjc.2017.45
98. Dal Bello MG, Filiberti RA, Alama A, Orengo AM, Mussap M, Coco S, et al. The role of CEA, CYFRA21-1 and NSE in monitoring tumor response to Nivolumab in advanced non-small cell lung cancer (NSCLC) patients. J Transl Med (2019) 17(1):74. doi: 10.1186/s12967-019-1828-0
99. Koh J, Go H, Keam B, Kim MY, Nam SJ, Kim TM, et al. Clinicopathologic analysis of programmed cell death-1 and programmed cell death-ligand 1 and 2 expressions in pulmonary adenocarcinoma: comparison with histology and driver oncogenic alteration status. Mod Pathol (2015) 28(9):1154–66. doi: 10.1038/modpathol.2015.63
100. Ota K, Azuma K, Kawahara A, Hattori S, Iwama E, Tanizaki J, et al. Induction of PD-L1 expression by the EML4-ALK oncoprotein and downstream signaling pathways in non-small cell lung cancer. Clin Cancer Res (2015) 21(17):4014–21. doi: 10.1158/1078-0432.CCR-15-0016
101. Rangachari D, VanderLaan PA, Shea M, Le X, Huberman MS, Kobayashi SS, et al. Correlation between classic driver oncogene mutations in EGFR, ALK, or ROS1 and 22C3-PD-L1 ≥50% Expression in lung adenocarcinoma. J Thorac Oncol (2017) 12(5):878–83. doi: 10.1016/j.jtho.2016.12.026
102. Song Z, Yu X, Cheng G, Zhang Y. Programmed death-ligand 1 expression associated with molecular characteristics in surgically resected lung adenocarcinoma. J Transl Med (2016) 14(1):188. doi: 10.1186/s12967-016-0943-4
103. Lan B, Ma C, Zhang C, Chai S, Wang P, Ding L, et al. Association between PD-L1 expression and driver gene status in non-small-cell lung cancer: a meta-analysis. Oncotarget (2018) 9(7):7684–99. doi: 10.18632/oncotarget.23969
104. Fang W, Ma Y, Yin JC, Hong S, Zhou H, Wang A, et al. Comprehensive genomic profiling identifies novel genetic predictors of response to anti-PD-(L)1 therapies in non-small cell lung cancer. Clin Cancer Res (2019) 25(16):5015–26. doi: 10.1158/1078-0432.CCR-19-0585
105. Cinausero M, Laprovitera N, De Maglio G, Gerratana L, Riefolo M, Macerelli M, et al. KRAS and ERBB-family genetic alterations affect response to PD-1 inhibitors in metastatic nonsquamous NSCLC. Ther Adv Med Oncol (2019) 11:1758835919885540. doi: 10.1177/1758835919885540
106. Gianoncelli L, Spitaleri G, Passaro A, Radice D, Fumagalli C, Del Signore E, et al. Efficacy of anti-PD1/PD-L1 therapy (IO) in KRAS mutant non-small cell lung cancer patients: A retrospective analysis. Anticancer Res (2020) 40(1):427–33. doi: 10.21873/anticanres.13970
107. Assoun S, Theou-Anton N, Nguenang M, Cazes A, Danel C, Abbar B, et al. Association of TP53 mutations with response and longer survival under immune checkpoint inhibitors in advanced non-small-cell lung cancer. Lung Cancer. (2019) 132:65–71. doi: 10.1016/j.lungcan.2019.04.005
108. Shi Y, Lei Y, Liu L, Zhang S, Wang W, Zhao J, et al. Integration of comprehensive genomic profiling, tumor mutational burden, and PD-L1 expression to identify novel biomarkers of immunotherapy in non-small cell lung cancer. Cancer Med (2021) 10(7):2216–31. doi: 10.1002/cam4.3649
109. Rodrigue A, LaFrance M, Gauthier MC, McDonald D, Hendzel M, West S, et al. Interplay between human DNA repair proteins at a unique double-strand break in vivo. EMBO J (2006) 25(1):222–31. doi: 10.1038/sj.emboj.7600914
110. Guerreiro IM, Barros-Silva D, Lopes P, Cantante M, Cunha AL, Lobo J, et al. RAD51Bme levels as a potential predictive biomarker for PD-1 blockade response in non-small cell lung cancer. J Clin Med (2020) 9(4):1000. doi: 10.3390/jcm9041000
111. Zeng H, Tohidinezhad F, De Ruysscher DKM, Willems YCP, Degens JHRJ, van Kampen-van den Boogaart VEM, et al. The association of gross tumor volume and its radiomics features with brain metastases development in patients with radically treated stage III non-small cell lung cancer. Cancers (Basel). (2023) 15(11):3010. doi: 10.3390/cancers15113010
112. Khorrami M, Khunger M, Zagouras A, Patil P, Thawani R, Bera K, et al. Combination of peri- and intratumoral radiomic features on baseline CT scans predicts response to chemotherapy in lung adenocarcinoma. Radiol Artif Intell (2019) 1(2):e180012. doi: 10.1148/ryai.2019180012
113. Khorrami M, Jain P, Bera K, Alilou M, Thawani R, Patil P, et al. Predicting pathologic response to neoadjuvant chemoradiation in resectable stage III non-small cell lung cancer patients using computed tomography radiomic features. Lung Cancer. (2019) 135:1–9. doi: 10.1016/j.lungcan.2019.06.020
114. Trebeschi S, Drago SG, Birkbak NJ, Cǎlin AM, Delli Pizzi A, et al. Predicting response to cancer immunotherapy using noninvasive radiomic biomarkers. Ann Oncol (2019) 30(6):998–1004. doi: 10.1093/annonc/mdz108
115. Khorrami M, Prasanna P, Gupta A, Patil P, Velu PD, Thawani R, et al. Changes in CT radiomic features associated with lymphocyte distribution predict overall survival and response to immunotherapy in non-small cell lung cancer. Cancer Immunol Res (2020) 8(1):108–19. doi: 10.1158/2326-6066.CIR-19-0476
116. Evangelista L, Cuppari L, Menis J, Bonanno L, Reccia P, Frega S, et al. 18F-FDG PET/CT in non-small-cell lung cancer patients: a potential predictive biomarker of response to immunotherapy. Nucl Med Commun (2019) 40(8):802–7. doi: 10.1097/MNM.0000000000001025
117. Mu W, Tunali I, Gray JE, Qi J, Schabath MB, Gillies RJ, et al. Radiomics of 18F-FDG PET/CT images predicts clinical benefit of advanced NSCLC patients to checkpoint blockade immunotherapy. Eur J Nucl Med Mol Imaging. (2020) 47(5):1168–82. doi: 10.1007/s00259-019-04625-9
118. Berengure R, Pastor-Juan MDR, Canals-Vazquez J, Castro-García M, Villas MV, Mansilla Legorburo F, et al. Radiomics of CT features may be nonreproducible and redundant: influence of CT acquisition parameters. Radiology (2018) 288(2):407–15. doi: 10.1148/radiol.2018172361
119. Park W, Kwon D, Saravia D, Desai A, Vargas F, El Dinali M, et al. Developing a predictive model for clinical outcomes of advanced non-small cell lung cancer patients treated with nivolumab. Clin Lung Cancer. (2018) 19(3):280–288.e4. doi: 10.1016/j.cllc.2017.12.007
120. Park W, Mezquita L, Okabe N, Chae YK, Kwon D, Saravia D, et al. Association of the prognostic model iSEND with PD-1/L1 monotherapy outcome in non-small-cell lung cancer. Br J Cancer. (2020) 122(3):340–7. doi: 10.1038/s41416-019-0643-y
121. Prelaj A, Rebuzzi SE, Pizzutilo P, Bilancia M, Montrone M, Pesola F, et al. EPSILoN: A prognostic score using clinical and blood biomarkers in advanced non-small-cell lung cancer treated with immunotherapy. Clin Lung Cancer. (2020) 21(4):365–77. doi: 10.1016/j.cllc.2019.11.017
122. Prelaj A, Ferrara R, Rebuzzi SE, Proto C, Signorelli D, Galli G, et al. EPSILoN: A prognostic score for immunotherapy in advanced non-small-cell lung cancer: A validation cohort. Cancers (Basel). (2019) 11(12):1954. doi: 10.3390/cancers11121954
123. Wang W, Huang Z, Yu Z, Zhuang W, Zheng W, Cai Z, et al. Prognostic value of the lung immune prognostic index may differ in patients treated with immune checkpoint inhibitor monotherapy or combined with chemotherapy for non-small cell lung cancer. Front Oncol (2020) 10:572853. doi: 10.3389/fonc.2020.572853
124. Ruiz-Bañobre J, Areses-Manrique MC, Mosquera-Martínez J, Cortegoso A, Afonso-Afonso FJ, de Dios-Álvarez N, et al. Evaluation of the lung immune prognostic index in advanced non-small cell lung cancer patients under nivolumab monotherapy. Transl Lung Cancer Res (2019) 8(6):1078–85. doi: 10.21037/tlcr.2019.11.07
125. Sorich MJ, Rowland A, Karapetis CS, Hopkins AM. Evaluation of the Lung Immune Prognostic Index for prediction of survival and response in patients treated with Atezolizumab for Non-Small Cell Lung Cancer: Pooled analysis of clinical trials. J Thorac Oncol (2019) 14(8):1440–6. doi: 10.1016/j.jtho.2019.04.006
Keywords: non-small cell lung cancer (NSCLC), immunotherapy, prognostic biological markers, anti-PD-(L)1, review
Citation: Liang S, Wang H, Tian H, Xu Z, Wu M, Hua D and Li C (2023) The prognostic biological markers of immunotherapy for non-small cell lung cancer: current landscape and future perspective. Front. Immunol. 14:1249980. doi: 10.3389/fimmu.2023.1249980
Received: 29 June 2023; Accepted: 24 August 2023;
Published: 11 September 2023.
Edited by:
Qun Xue, Affiliated Hospital of Nantong University, ChinaReviewed by:
Chuan Lan, the Affiliated Hospital of North Sichuan Medical College, ChinaHaiyan Zeng, West China Hospital, Sichuan University, China
Copyright © 2023 Liang, Wang, Tian, Xu, Wu, Hua and Li. This is an open-access article distributed under the terms of the Creative Commons Attribution License (CC BY). The use, distribution or reproduction in other forums is permitted, provided the original author(s) and the copyright owner(s) are credited and that the original publication in this journal is cited, in accordance with accepted academic practice. No use, distribution or reproduction is permitted which does not comply with these terms.
*Correspondence: Dong Hua, wx89211@163.com; Chengming Li, chengming0401@163.com
†These authors have contributed equally to this work