- 1Department of Laboratory Medicine, Division of Pathology, Karolinska Institutet, Karolinska University Hospital, Stockholm, Sweden
- 2Discovery & Front-End Innovation, Lesaffre Institute of Science & Technology, Lesaffre International, Marcq-en-Baroeul, France
- 3Global Health Institute, Antwerp University, Antwerp, Belgium
- 4Centre for Translational Microbiome Research (CTMR), Department of Microbiology, Tumor and Cell Biology, Karolinska Institute, Karolinska Hospital, Stockholm, Sweden
- 5Department of Head and Skin, Ghent University, Ghent, Belgium
PD-1 and PD-L1 are crucial regulators of immunity expressed on the surface of T cells and tumour cells, respectively. Cancer cells frequently use PD-1/PD-L1 to evade immune detection; hence, blocking them exposes tumours to be attacked by activated T cells. The synergy of PD-1/PD-L1 blockade with type I interferon (IFN) can improve cancer treatment efficacy. Type I IFN activates immune cells boosts antigen presentation and controls proliferation. In addition, type I IFN increases tumour cell sensitivity to the blockade. Combining the two therapies increases tumoral T cell infiltration and activation within tumours, and stimulate the generation of memory T cells, leading to prolonged patient survival. However, limitations include heterogeneous responses, the need for biomarkers to predict and monitor outcomes, and adverse effects and toxicity. Although treatment resistance remains an obstacle, the combined therapeutic efficacy of IFNα/β and PD-1/PD-L1 blockade demonstrated considerable benefits across a spectrum of cancer types, notably in melanoma. Overall, the phases I and II clinical trials have demonstrated safety and efficiency. In future, further investigations in clinical trials phases III and IV are essential to compare this combinatorial treatment with standard treatment and assess long-term side effects in patients.
Introduction
Cancer cells frequently exploit the Programmed Cell Death 1 (PD-1) signalling pathway to evade immune surveillance. Antibodies (Abs) that target PD-1 and its ligand PD-L1 can rescue exhausted T cells and revive immune responses against cancer cells. To date, anti-PD-1 Abs (camrelizumab cemiplimab, dostarlimab, nivolumab, pembrolizumab, prolgolimab, sintilimab, tislelizumab, toripalimab and zimberelimab) and anti-PD-L1 Abs (atezolizumab, avelumab, and durvalumab) have been approved for treating Hodgkin lymphoma, urothelial, hepatocellular, oesophagal, renal cell carcinomas, malignant pleural mesothelioma, head and neck, colorectal, skin, non-small cell lung, gastric, gastroesophageal junction, bladder, cervical and endometrial cancers (1).
Effective control of tumours by PD-1/PD-L1 therapy is associated with a higher level of tumour-infiltrating lymphocytes (TILs) (2). However, anti-PD1/PDL1 therapy benefits only a subset of patients. (3), i.e. PD-1/PD-L1 blockade therapy may not be sufficient to (re-) activate tumour-specific T lymphocytes (even in the presence of TILs) leading to intrinsic resistance (4). Furthermore, after initial responses, a large group of responders may develop acquired resistance. Major histocompatibility complex (MHC) dysfunction has been identified as one of the main resistance mechanisms to PD-1/PD-L1 therapy because antigen presentation in the tumour microenvironment (TME) is primarily accomplished via the MHC class I pathway. Consequently, tumours can evade T cell killing through inactivating the MHC class I complex. In other words, the presence of putative tumour rejection antigens provided by dendritic cells (DCs) to cross-priming CD8+ T lymphocytes results in less anticancer activity and inefficacy of PD-1/PD-L1 blockade (3).
IFN type I (IFNα, IFNβ, IFNω, IFNε, and IFNк) has multiple anti-tumour activities, such as direct tumour cell killing and the stimulation of immune cells including DCs and CD8+ T cells (5–7). To date, IFNα/β have been approved for the clinical treatment of multiple malignancies (e.g., Kaposi’s sarcoma, melanoma and renal cell carcinoma). However, monotherapy of recombinant IFNα/β is not well tolerated when administered systemically (causing a range of side effects in humans including fatigue, fever, muscle aches, depression, and liver damage) (8). To overcome monotherapy challenges with either IFNα/β or PD-1/PD-L1 blockade therapies; a combination of these two immunotherapies has been proposed to circumvent the resistance to PD-1/PD-L1 therapies and improve patient outcomes. For example, in patients with immune cell-poor melanomas, stimulating type I IFN puts forwards a rational approach to boost the therapeutic benefits of PD-1/PD-L1 inhibition (9).
This review gathered and systematically analyses the latest developments in combining PD-1/PD-L1 blockade with type I IFN application in preclinical and clinical stages to shed light on the current status and future research.
Type I IFN reinvigorates immune cells: mechanism of action
Inducing efficient tumour-specific cytotoxic T-cell responses is one of the objectives of anticancer therapies. DC can stimulate cross-priming with CD8+ T cells, known as cross-presentation by which antigen-presenting cells present tumour-associated antigens on their MHC class-I molecule (5). Type I IFN stands among the most potent activators for DC-induced cross-priming. Preclinical evidence suggests that type I IFN-stimulated cross-priming of DC against tumour-associated antigen is crucial for cancer immunosurveillance and can be used to effectively increase anti-tumour CD8+ T-cell responses (5). Mechanistically, type I IFNs promote the production of IP-10/CXCL10, a chemokine for attracting effector T cells to the TME. Type I IFNs also induces overexpression of MHC class I on tumour cells enhancing the effector response of anti-tumour CD8+ T cell in the TME (10). However, Type I IFN expression is limited or repressed within the TME. For instance, reduced cGAS-STING pathway signalling in certain tumour cells and the enhanced degradation of DNA and antigen within the TME can inhibit innate sensing and type I IFN production (11). Thereby, the intravenous application of IFNα/β might be an option to overcome limited expression in the TME. For example, in melanoma patients, peritumoral injection of IFNβ is known for recruiting CD8+ cytotoxic T cells, into the TME. This finding may elucidate the therapeutic advantages of IFNβ in melanoma treatment (12). Furthermore, IFNβ favours a shift in the phenotypes of tumour-associated macrophages from M2 to M1 phenotype, thereby reducing the proportion of Tregs among TILs within the TME (13). In murine melanoma, IFNβ increases the effects of anti-PD-1 Abs against melanoma by preferentially drawing effector cells, rather than Tregs, to tumour sites (13).
Current status
Combining PD-1/PD-L1 blockade with type I IFN presents potential benefits, including the activation of innate and adaptive immune cells, enhancement of antigen presentation on DCs, induction of PD-L1 expression on tumour cells, and sensitising cancer cells to PD-1/PD-L1 blockade (14) (Figure 1). Several preclinical and clinical studies have demonstrated that combining PD-1/PD-L1 blockade with IFN shows a therapeutically synergistic effect by increasing intratumoral T cell infiltration and activation, generating memory T cells, and prolonging the survival of both animals and patients (14, 15) (Tables 1, 2).
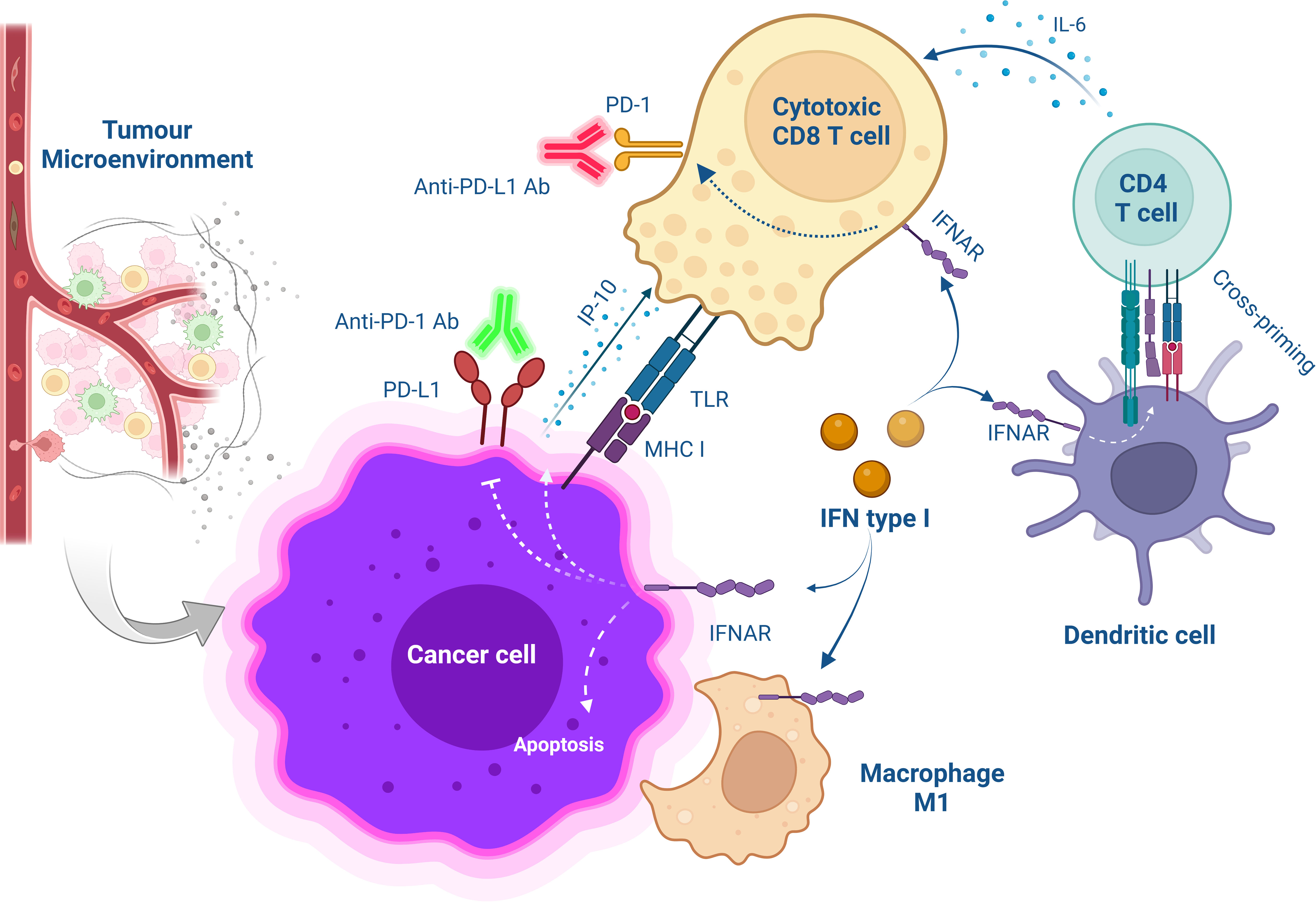
Figure 1 Schematic overview of cellular interaction after combinational treatment with type I IFN and PD-1/PD-L1 blockade. The treatment with anti-PD-1/PD-L1 Abs saves CD8+ T cells from exhaustion while targeting IFNα to the tumour microenvironment promotes the release of IP-10 from antigen-positive tumour cells increases T cell infiltration and improves CD4+ T cell function for anti-tumour immunity. Furthermore, MHC class I are upregulated on cancer cells increasing antitumor CD8+ T cell response. Also, IFNα activates DC-induced cross-priming by releasing IL-6.
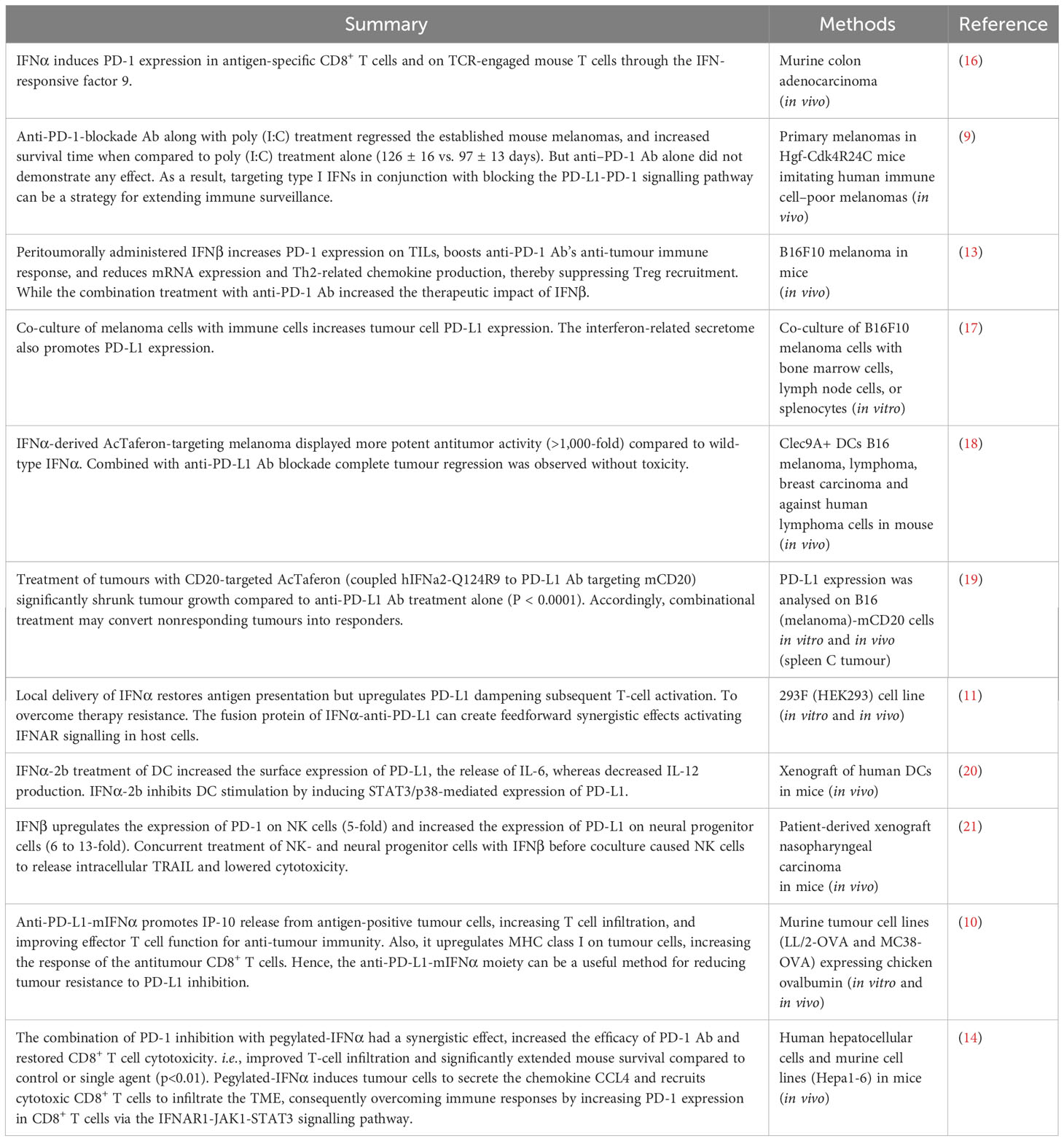
Table 1 Preclinical studies utilizing a combination of PD-1/PD-L1 blockade with type I IFN in cancer therapy.
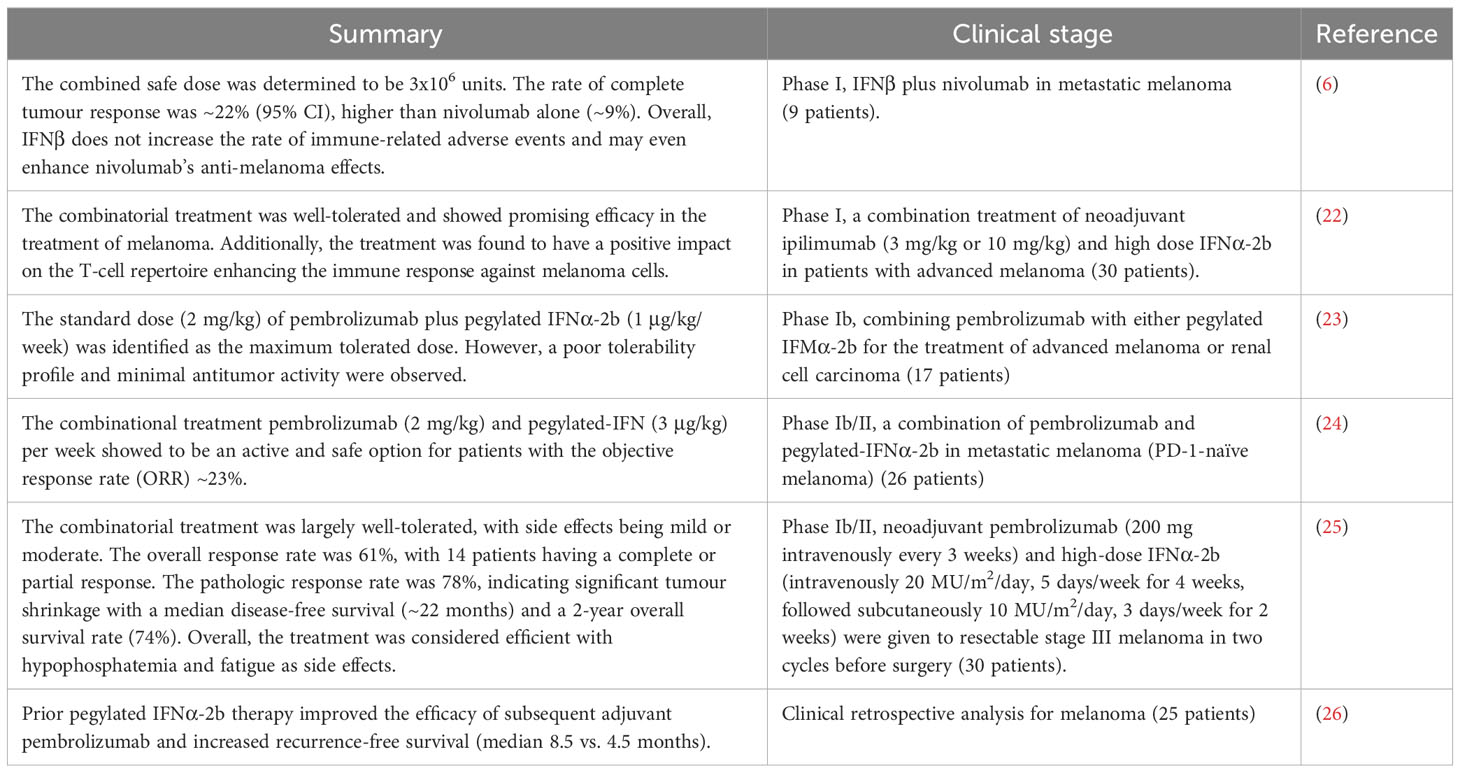
Table 2 Clinical trials of a combination of PD-1/PD-L1 blockade with type I interferon in cancer therapy.
To date, the phases I and II clinical trials (~130 patients in total) determined the optimal dose and showed that the combinatorial treatment of PD-1/PD-L1 blockade with IFNα/β is safe and efficient for particularly melanoma and to some extent for renal carcinoma patients (Table 2). Yet, further clinical phases III and IV trials are required to compare this combinatorial treatment with standard treatment and also to assess the side effects in the long term in patients. Later, other cancer types can also be evaluated in Phase III clinical trials for the effectiveness of this combinational treatment in larger populations.
Limitations and future prospects
Despite the determination of optimal dose and adverse effects of the combined type I IFN and PD-1/PD-L1 blockade therapy in phase I and II clinical trials (Table 2), challenges and limitations still include the need for biomarkers to predict and monitor treatment outcomes, understanding the tumour response across different cancer types and patients and more investigation on the optimal dose, timing and the potential toxicity and adverse events in later stages clinical trials (14). To address these limitations, the following suggestions might be considered.
Since the 1990s, clinical cancer research using DC-based vaccination has demonstrated validated safety and feasibility. However, DC vaccinations are still a new strategy for acute myeloid leukaemia (5-year overall survival rate >30%). Limitations include weak immune responses, time-consuming processes and high costs (27). Therefore, DC vaccination combined with immune checkpoint blockades such as PD-1 Abs may enhance DC-mediated activation of NK and T cells and prevent Treg stimulation (27, 28). In 2018, a phase II clinical trial indicated that activated autologous DC paired with PD-1 blockade (pembrolizumab) has a positive effect in patients with advanced solid tumours (ORR ~22%). This approach enabled the release of the brake on active T cells by inhibiting the PD-1/PD-L1 checkpoint leading to increased immune responsiveness and tumour elimination (29). In addition, both preclinical and clinical studies have indicated that combining DC vaccination and IFNα can reinvigorate the immune response against cancer cells, leading to improved outcomes (30). Thus, a combination of PD-1/PD-L1 checkpoint inhibitors with DC vaccines (reinvigorated by IFNα) might be a viable strategy to stimulate the patient’s immune system against the tumour in advance (30).
In contrast to general positive perception towards combined type I IFN and PD-1/PD-L1 blockade, one study debated that sustained type I IFN signalling may contribute to resistance to PD-1/PD-L1 blockade, by inducing nitric oxide synthase 2 (NOS2) expression in tumour and DCs, leading to T cell dysfunction and Treg accumulation (31). Also, resistance to anti-PD-1 monotherapy in melanoma patients was correlated with the induction of a type I IFN signature (31). Hence, this controversy may require more investigation in future.
Overall, the prospective effectiveness of a combined PD-1/PD-L1 blockade with type I IFN in cancer treatment depends on numerous factors including the type and dose of IFNα/β, the timing and duration of treatment, the tumour type and stage of cancer, the genetic and epigenetic alterations of tumour cells, the immune status of patients, and the interactions with other therapeutic interventions. Additional preclinical and clinical studies are crucial to elucidate the optimal conditions and underlying mechanisms of this combination strategy.
Conclusion
PD-1 serves as a receptor on T cells whereas PD-L1 is a ligand present in cancer cells or antigen-presenting cells, the latter of which exposes antigens to T cells. The binding of PD-1 and PD-L1 sends a signal that reduces the activity and survival of T cells. By blocking PD-1/PD-L1 interaction, the immune response against cancer cells can be enhanced.
Type I IFN represents a family of cytokines that exhibit dual roles in cancer immunity, manifesting both beneficial and detrimental effects. On one hand, type I IFN can increase the expression of antigens and co-stimulatory molecules on cancer cells and antigen-presenting cells, thereby increasing their visibility to T cells. Type I IFN can also stimulate the production and function of T cells and other immune cells, such as NK cells and DCs. On the other hand, type I IFN can induce the expression of PD-L1 inhibiting T cell function. Type I IFN also activates the NOS2 enzyme, which through the production of nitric oxide, can suppress T cell activity and promote the accumulation of Treg, subsequently followed by suppression of other immune cells.
Overall, the phases I and II clinical trials have demonstrated safety and efficiency, particularly for melanoma patients and to some extent for renal carcinoma patients. Further investigations in clinical trials phases III and IV are still needed to compare this combinatorial treatment with standard treatment and assess long-term side effects in patients. Later, other cancer types can also be evaluated in Phase III clinical trials for the effectiveness of this combinational treatment in larger populations.
Author contributions
AR: collecting information, writing and editing the manuscript and visualization. MD-D and NB: reviewing and editing the manuscript. MB: editing and funding. All authors contributed to the article and approved the submitted version.
Funding
This study was supported by grants from Cancerfonden, Cancer- och Allergifonden, Radiumhemmets forskningsfonder and CIMED to MB.
Conflict of interest
The authors have no conflict of interest. The authors declare that the research was conducted in the absence of any commercial or financial relationships that could be construed as a potential conflict of interest.
Publisher’s note
All claims expressed in this article are solely those of the authors and do not necessarily represent those of their affiliated organizations, or those of the publisher, the editors and the reviewers. Any product that may be evaluated in this article, or claim that may be made by its manufacturer, is not guaranteed or endorsed by the publisher.
Abbreviations
Ab, antibody; DC, dendritic cell; IFN, interferon; IFNAR, interferon α/β receptor; MHC, major histocompatibility complex; NK cell, natural killer cell; NOS2, nitric oxide synthase 2; ORR, objective response rate; PD-1, programmed cell death 1; PD-L1, programmed cell death ligand 1; TIL, tumour infiltrating lymphocyte; TME, tumour microenvironment; Treg, regulatory T cell.
References
1. Yi M, Zheng X, Niu M, Zhu S, Ge H, Wu K. Combination strategies with PD-1/PD-L1 blockade: current advances and future directions. Mol Cancer (2022) 21:28. doi: 10.1186/s12943-021-01489-2
2. Tumeh PC, Harview CL, Yearley JH, Shintaku IP, Taylor EJ, Robert L, et al. PD-1 blockade induces responses by inhibiting adaptive immune resistance. Nature (2014) 515:568–71. doi: 10.1038/nature13954
3. Lei Q, Wang D, Sun K, Wang L, Zhang Y. Resistance mechanisms of anti-PD1/PDL1 therapy in solid tumors. Front Cell Dev Biol (2020) 8:672. doi: 10.3389/fcell.2020.00672
4. Huang AC, Postow MA, Orlowski RJ, Mick R, Bengsch B, Manne S, et al. T-cell invigoration to tumour burden ratio associated with anti-PD-1 response. Nature (2017) 545:60–5. doi: 10.1038/nature22079
5. Schiavoni G, Mattei F, Gabriele L. Type I interferons as stimulators of DC-mediated cross-priming: impact on anti-tumor response. Front Immunol (2013) 4:483. doi: 10.3389/fimmu.2013.00483
6. Fujimura T, Hidaka T, Kambayashi Y, Furudate S, Kakizaki A, Tono H, et al. Phase I study of nivolumab combined with IFN-beta for patients with advanced melanoma. Oncotarget (2017) 8:71181–7. doi: 10.18632/oncotarget.17090
7. Shi W, Yao X, Fu Y, Wang Y. Interferon-alpha and its effects on cancer cell apoptosis. Oncol Lett (2022) 24:235. doi: 10.3892/ol.2022.13355
8. Razaghi A, Brusselaers N, Bjornstedt M, Durand-Dubief M. Copy number alteration of the interferon gene cluster in cancer: Individual patient data meta-analysis prospects to personalized immunotherapy. Neoplasia (2021) 23:1059–68. doi: 10.1016/j.neo.2021.08.004
9. Bald T, Landsberg J, Lopez-Ramos D, Renn M, Glodde N, Jansen P, et al. Immune cell-poor melanomas benefit from PD-1 blockade after targeted type I IFN activation. Cancer Discovery (2014) 4:674–87. doi: 10.1158/2159-8290.CD-13-0458
10. Guo J, Xiao Y, Iyer R, Lu X, Lake M, Ladror U, et al. Empowering therapeutic antibodies with IFN-alpha for cancer immunotherapy. PloS One (2019) 14:e0219829. doi: 10.1371/journal.pone.0219829
11. Liang Y, Tang H, Guo J, Qiu X, Yang Z, Ren Z, et al. Targeting IFNalpha to tumor by anti-PD-L1 creates feedforward antitumor responses to overcome checkpoint blockade resistance. Nat Commun (2018) 9:4586. doi: 10.1038/s41467-018-06890-y
12. Fujimura T, Okuyama R, Ohtani T, Ito Y, Haga T, Hashimoto A, et al. Perilesional treatment of metastatic melanoma with interferon-beta. Clin Exp Dermatol (2009) 34:793–9. doi: 10.1111/j.1365-2230.2009.03207.x
13. Kakizaki A, Fujimura T, Furudate S, Kambayashi Y, Yamauchi T, Yagita H, et al. Immunomodulatory effect of peritumorally administered interferon-beta on melanoma through tumor-associated macrophages. Oncoimmunology (2015) 4:e1047584. doi: 10.1080/2162402X.2015.1047584
14. Zhu Y, Chen M, Xu D, Li TE, Zhang Z, Li JH, et al. The combination of PD-1 blockade with interferon-alpha has a synergistic effect on hepatocellular carcinoma. Cell Mol Immunol (2022) 19:726–37. doi: 10.1038/s41423-022-00848-3
15. Burrack AL, Spartz EJ, Raynor JF, Wang I, Olson M, Stromnes IM. Combination PD-1 and PD-L1 blockade promotes durable neoantigen-specific T cell-mediated immunity in pancreatic ductal adenocarcinoma. Cell Rep (2019) 28:2140–2155 e2146. doi: 10.1016/j.celrep.2019.07.059
16. Terawaki S, Chikuma S, Shibayama S, Hayashi T, Yoshida T, Okazaki T, et al. IFN-alpha directly promotes programmed cell death-1 transcription and limits the duration of T cell-mediated immunity. J Immunol (2011) 186:2772–9. doi: 10.4049/jimmunol.1003208
17. Yang YQ, Dong WJ, Yin XF, Xu YN, Yang Y, Wang JJ, et al. Interferon-related secretome from direct interaction between immune cells and tumor cells is required for upregulation of PD-L1 in tumor cells. Protein Cell (2016) 7:538–43. doi: 10.1007/s13238-016-0281-6
18. Cauwels A, Van Lint S, Paul F, Garcin G, De Koker S, Van Parys A, et al. Delivering type I interferon to dendritic cells empowers tumor eradication and immune combination treatments. Cancer Res (2018) 78:463–74. doi: 10.1158/0008-5472.CAN-17-1980
19. Cauwels A, Van Lint S, Garcin G, Bultinck J, Paul F, Gerlo S, et al. A safe and highly efficient tumor-targeted type I interferon immunotherapy depends on the tumor microenvironment. Oncoimmunology (2018) 7:e1398876. doi: 10.1080/2162402X.2017.1398876
20. Bazhin AV, Von Ahn K, Fritz J, Werner J, Karakhanova S. Interferon-alpha up-regulates the expression of PD-L1 molecules on immune cells through STAT3 and p38 signaling. Front Immunol (2018) 9:2129. doi: 10.3389/fimmu.2018.02129
21. Makowska A, Braunschweig T, Denecke B, Shen L, Baloche V, Busson P, et al. Interferon beta and anti-PD-1/PD-L1 checkpoint blockade cooperate in NK cell-mediated killing of nasopharyngeal carcinoma cells. Transl Oncol (2019) 12:1237–56. doi: 10.1016/j.tranon.2019.04.017
22. Tarhini A, Lin Y, Lin H, Rahman Z, Vallabhaneni P, Mendiratta P, et al. Neoadjuvant ipilimumab (3 mg/kg or 10 mg/kg) and high dose IFN-α2b in locally/regionally advanced melanoma: safety, efficacy and impact on T-cell repertoire. J Immunother Cancer (2018) 6:112. doi: 10.1186/s40425-018-0428-5
23. Atkins MB, Hodi FS, Thompson JA, Mcdermott DF, Hwu WJ, Lawrence DP, et al. Pembrolizumab plus pegylated interferon alfa-2b or ipilimumab for advanced melanoma or renal cell carcinoma: dose-finding results from the phase Ib KEYNOTE-029 study. Clin Cancer Res (2018) 24:1805–15. doi: 10.1158/1078-0432.CCR-17-3436
24. Davar D, Wang H, Chauvin JM, Pagliano O, Fourcade JJ, Ka M, et al. Phase Ib/II study of pembrolizumab and pegylated-interferon Alfa-2b in advanced melanoma. J Clin Oncol (2018) 36:JCO1800632. doi: 10.1200/JCO.18.00632
25. Najjar YG, Mccurry D, Lin H, Lin Y, Zang Y, Davar D, et al. Neoadjuvant pembrolizumab and high-dose IFNalpha-2b in resectable regionally advanced melanoma. Clin Cancer Res (2021) 27:4195–204. doi: 10.1158/1078-0432.CCR-20-4301
26. Jia DD, Niu Y, Zhu H, Wang S, Ma T, Li T. Prior therapy with pegylated-interferon Alfa-2b improves the efficacy of adjuvant pembrolizumab in resectable advanced melanoma. Front Oncol (2021) 11:675873. doi: 10.3389/fonc.2021.675873
27. Yu J, Sun H, Cao W, Song Y, Jiang Z. Research progress on dendritic cell vaccines in cancer immunotherapy. Exp Hematol Oncol (2022) 11:3. doi: 10.1186/s40164-022-00257-2
28. Versteven M, Van Den Bergh JMJ, Marcq E, Smits ELJ, Van Tendeloo VFI, Hobo W, et al. Dendritic cells and programmed death-1 blockade: A joint venture to combat cancer. Front Immunol (2018) 9:. doi: 10.3389/fimmu.2018.00394
29. Chen CL, Pan QZ, Weng DS, Xie CM, Zhao JJ, Chen MS, et al. Safety and activity of PD-1 blockade-activated DC-CIK cells in patients with advanced solid tumors. Oncoimmunology (2018) 7:e1417721. doi: 10.1080/2162402X.2017.1417721
30. Lapenta C, Gabriele L, Santini SM. IFN-alpha-mediated differentiation of dendritic cells for cancer immunotherapy: advances and perspectives. Vaccines (2020) 8(4):617. doi: 10.3390/vaccines8040617
Keywords: cancer, interferon, immunotherapy, PD-1, PD-L1, interferon a (IFNa)
Citation: Razaghi A, Durand-Dubief M, Brusselaers N and Björnstedt M (2023) Combining PD-1/PD-L1 blockade with type I interferon in cancer therapy. Front. Immunol. 14:1249330. doi: 10.3389/fimmu.2023.1249330
Received: 28 June 2023; Accepted: 07 August 2023;
Published: 24 August 2023.
Edited by:
Nabiha Yusuf, University of Alabama at Birmingham, United StatesReviewed by:
Martina Musella, Catholic University of the Sacred Heart, Rome, ItalyMengwei Wu, Peking Union Medical College Hospital (CAMS), China
Jiashu Han, Peking Union Medical College Hospital (CAMS), China, in collaboration with reviewer MW
Copyright © 2023 Razaghi, Durand-Dubief, Brusselaers and Björnstedt. This is an open-access article distributed under the terms of the Creative Commons Attribution License (CC BY). The use, distribution or reproduction in other forums is permitted, provided the original author(s) and the copyright owner(s) are credited and that the original publication in this journal is cited, in accordance with accepted academic practice. No use, distribution or reproduction is permitted which does not comply with these terms.
*Correspondence: Ali Razaghi, YWxpLnJhemFnaGlAa2kuc2U=