- 1Jenner Institute, Nuffield Department of Medicine, University of Oxford, Oxford, United Kingdom
- 2School of Biosciences, University of Surrey, Guilford, United Kingdom
- 3The Oxford Vaccine Group, Department of Paediatrics, University of Oxford, Oxford, United Kingdom
- 4Sexual Health and HIV, University Hospitals Birmingham NHS Trust, Birmingham, United Kingdom
Neisseria gonorrheoae is the causative agent of gonorrhea, a sexually transmitted infection responsible for a major burden of disease with a high global prevalence. Protective immunity to infection is often not observed in humans, possible due to high variability of key antigens, induction of blocking antibodies, or a large number of infections being relatively superficial and not inducing a strong immune response. N. gonorrhoeae is a strictly human pathogen, however, studies using mouse models provide useful insights into the immune response to gonorrhea. In mice, N. gonorrhoea appears to avoid a protective Th1 response by inducing a less protective Th17 response. In mouse models, candidate vaccines which provoke a Th1 response can accelerate the clearance of gonococcus from the mouse female genital tract. Human studies indicate that natural infection often induces a limited immune response, with modest antibody responses, which may correlate with the clinical severity of gonococcal disease. Studies of cytokine responses to gonococcal infection in humans provide conflicting evidence as to whether infection induces an IL-17 response. However, there is evidence for limited induction of protective immunity from a study of female sex workers in Kenya. A controlled human infection model (CHIM) has been used to examine the immune response to gonococcal infection in male volunteers, but has not to date demonstrated protection against re-infection. Correlates of protection for gonorrhea are lacking, which has hampered the progress towards developing a successful vaccine. However, the finding that the Neisseria meningitidis serogroup B vaccines, elicit cross-protection against gonorrhea has invigorated the gonococcal vaccine field. More studies of infection in humans, either natural infection or CHIM studies, are needed to understand better gonococcal protective immunity.
1 Introduction
Neisseria gonorrhoeae is a human-restricted Gram-negative bacterium responsible for infections of the genital tract as well as the rectum, the pharynx, and the eyes. Collectively these infections are known as gonorrhea and are primarily spread through sexual contact. Infection of the lower genital tract can range from asymptomatic to more invasive ascending infection. In women, this can be particularly severe and can result in pelvic inflammatory disease (PID), ectopic pregnancy and infertility (1). Severe outcomes of gonorrhea are particularly problematic in low- and middle-income countries (LMICs), where N. gonorrhoeae is a major cause of infertility (2). In addition, co-infections with human immunodeficiency virus (HIV) are common, and infection with N. gonorrhoeae increases the risk of transmission and acquisition of HIV infection (3).
There is an increasing prevalence of antimicrobial resistant (AMR) among N. gonorrhoeae. Strains that are resistant to the current treatment options have been characterized, including strains resistant to both ceftriaxone and azithromycin (4, 5), making research into alternate therapies as well as vaccines a priority. Moreover, reinfection following natural infection is common in humans, and broad protective immunity against multiple strains of gonococcus is not induced (6–8). A study from the UK of patients with gonorrhea showed that prior infection with N. gonorrhoeae was the strongest predictor of current infection (9). The association between previous infection and current infection was only true for prior gonococcal infection and not other STIs, suggesting that this is not just a consequence of high risk behavior. Furthermore, up to one third of gonorrhea patients in England have had some prior gonococcal infection (9). Since repeat infections are common and cannot completely be accounted for by high risk behavior, it appears that infection does not often lead to protective immunity.
What is not clear is whether the lack of protective immunity is due to immune evasion by the bacteria, the failure to mount the correct type of immune response that results in protection, the antigenic variation displayed by gonococcus, or a combination of factors. Recently, outer membrane vesicle (OMV) vaccines against capsular group B Neisseria meningitidis (MenB) was shown to cross-protect against N. gonorrhoeae. This finding suggests that it is possible to induce protection against gonococcus, reigniting efforts to develop a specific vaccine for gonorrhea (10–12). Further investigation is needed as both the antigens involved and the immune mechanisms of protection are unclear. There is a need to understand more about the natural immune response to gonococcus, why protective immunity is often not induced and why previous vaccine candidates have failed. Further knowledge is also required about the natural human immune response to N. gonorrhoeae infection, including whether there are individuals who do mount a protective immune response, either to the infecting strain or to heterologous strains. Identifying correlates of protection would help to clarify what immune response should be elicited by a candidate vaccine.
This article summarizes current knowledge regarding immunity to N. gonorrhoeae, from in vitro studies, the murine female genital tract model of gonococcal infection, human studies of natural infection and the controlled human infection model (Figure 1). Furthermore, we discuss current knowledge gaps that need to be addressed to facilitate vaccine design.
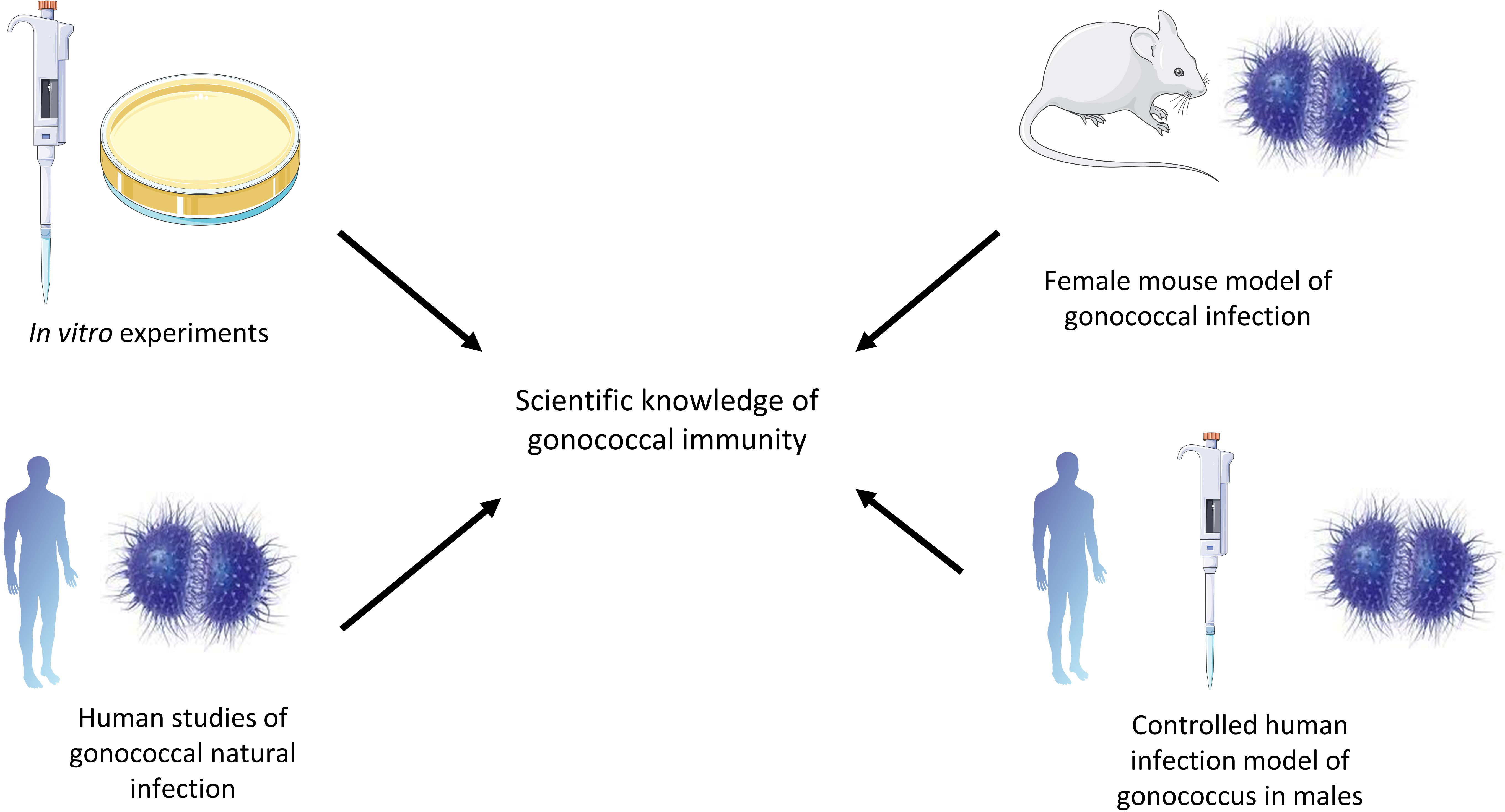
Figure 1 Different methods that have contributed to the field of gonococcal immunity. In vitro studies have been valuable in describing the interactions between gonococci and complement, for example. The murine female genital tract model is a transient infection model but is useful in describing the immune responses to gonococci and evasion mechanisms. Human studies of natural infection are valuable in studying gonococci in their natural host, while the controlled human infection model of gonococci can be used to describe the immune response to gonococci in the natural host in a controlled way, but only in males in a transient way.
2 Gonococcal biology and immunity
2.1 Variability of key antigens
N. gonorrhoeae has evolved many different routes to evade the human immune response. N. gonorrhoeae is a highly antigenically variable pathogen. It is naturally competent at taking up DNA from the environment, which may then be integrated into the genome by homologous recombination (13). This means variation between strains is a relatively common occurrence, and gives rise to populations which are not clonal (13–15). Gonococci are also subject to phase variation, and expression of specific genes is reversibly switched on or off leading to an additional source of variation between and within strains. Phase variation regulates the expression of opa genes, for example, which code for outer membrane proteins in N. gonorrhoeae. A single gonococcus can possess up to 11 different opa genes, all under phase variation due to differences in the number of CTCTT repeats in each gene caused by slipped-strand mispairing (13, 16, 17). In addition, a number of genes which encode glycosyltransferases (lgt) are subject to phase variation (18, 19). Expression of these genes results in differentially glycosylated lipooligosaccharide (LOS) on the surface of the bacteria. Gonococcal LOS has been shown to be immunogenic, and is the basis for a peptide mimetope candidate vaccine modelled on the 2C7 LOS epitope (20). It is possible that the expression of different glycosylation patterns of LOS are an immune evasion strategy, since mutant strains in which the expression of lgt genes is fixed are differentially bound and killed by a monoclonal antibody targeting 2C7 (21).
The genome of N. gonorrhoeae also contains several genes encoding pili, an adhesin mostly made up of PilE. Other copies of these genes, called pilS, are transcriptionally silent since they have no promoter (22). Each of these genes is variable in sequence, which results in different amino acid sequences in surface-exposed regions of the protein. Variation in pilE takes place by gene conversion (23, 24), which allows for the transfer of the DNA sequence from one donor pilS to target pilE, changing the sequence of the latter without changing the former. An efficacy study of a candidate vaccine based on purified pili failed possibly due to the variable nature of gonococcal pili (25).
PorB is an outer-membrane porin which can be used as a basis for gonococcal typing with one strain expressing one porB type. However, there is extensive variation in porB sequences between strains (26, 27). As gonococcus is a highly variable pathogen, especially within immunogenic antigens, a successful vaccine needs to consider worldwide strain coverage as well as account for continued evolution over time. The search for protective antigens that provide universal coverage in the face of a highly variable pathogen continues. It may be that there is a need for a reverse vaccinology approach to developing a successful gonococcal vaccine, as was the case for group B meningococcus (28). The specific antigens responsible for the cross-protective effect mediated by the Men B vaccine formulations are still to be identified (10, 11).
2.2 Evasion of complement
N. gonorrhoeae has developed many mechanisms to resist complement, suggesting a strong evolutionary pressure to do so. Firstly, gonococcus can recruit complement inhibitors. Gonococcal PorB, particularly the PorB1a allele, can bind the complement inhibitor C4BP, which correlates with the ability to evade complement-dependent killing (29, 30). Another complement inhibitor, factor H, can also be bound by PorB (31, 32). C4BP- and factor H-binding by gonococcus are human-specific. The gonococcal adhesin OpaA can bind the complement inhibitor vitronectin, which has been proposed to increase resistance to complement (33), and Opa proteins have been linked to serum resistance (34). In addition, N. gonorrhoeae has the ability to resist killing by normal human serum by scavenging CMP-Neu5Ac in order to sialylate lipo-oligosaccharide (LOS) (35, 36), which leads to a reduction in binding of anti-gonococcal antibodies and deposition of C4b (37, 38).
The importance of complement in immunity to N. gonorrhoeae can be inferred from studies indicating that individuals with primary immunodeficiencies of terminal complement components, who are unable to form the C5b-9 membrane attack complex, are at increased risk of disseminated gonococcal infection (39, 40). Though correlates of protection against gonorrhea are not known, this finding implies that antibody-dependent complement-mediated killing is an important mechanism of protection, at least in preventing invasive disease. Whether complement is involved in preventing more localized infection is not known. The finding that protection against meningococcal disease induced by meningococcal vaccines correlates with bactericidal antibody levels also supports the concept that complement is generally an important component of the immune response to genus Neisseria (41).
N. gonorrhoeae is able to evade immunity through the production of antibodies which block the action of protective antibodies targeting other antigens. An IgG antibody present in convalescent serum following gonococcal infection was found to block serum bactericidal activity against N. gonorrhoeae (42). This inhibitory activity is not the result of preventing the binding of complement since adding blocking antibody increases deposition of C3 and C5b-9, but due to deposition of complement at sites that does not lead to killing (42). These blocking antibodies were shown to bind bacterial target protein III (PIII), now called reduction modifiable protein (Rmp), which is present in all gonococcal strains and is highly conserved (42, 43). Antibodies to purified Rmp block killing in bactericidal assays, while depleting serum of anti-Rmp antibodies increases killing of N. gonorrhoeae by immune serum (43).
This finding was used to explain why bactericidal activity in convalescent serum from patients following disseminated gonococcal infection is usually very low despite high antibody levels (43). In a longitudinal study of 243 female sex workers in Nairobi, Kenya, those with anti-Rmp antibody were at increased risk of infection compared with those who lacked anti-Rmp antibody (44). Whether there is a need to remove Rmp from candidate whole cell N. gonorrhoeae vaccine strains is not known, but it is clear that induction of anti-Rmp antibodies can block the function of antibodies to other antigens. Serum from a mouse immunized with a mutant strain of N. gonorrhoeae lacking Rmp was more bactericidal than serum taken from a mouse immunized with the corresponding wild-type strain (45). Mice given anti-Rmp antibody by passive transfer or immunized intraperitoneally with recombinant Rmp, show delayed clearance of gonococcus following administration of anti-LOS bactericidal monoclonal antibody 2C7, as well as increased bacterial loads (46). In this study, anti-Rmp antibodies led to a reduction in the number of C3 molecules deposited on gonococci, in contrast to a previous study, which showed that anti-Rmp antibody increased deposition of C3 on cells but reduced killing (42). Together, these findings suggest that anti-Rmp antibodies can block killing of gonococci.
2.3 Evasion of bacterial killing by phagocytosis
N. gonorrhoeae infection induces a local immune response characterized by the infiltration of neutrophils to the site of infection, which is insufficient for clearance due to the ability of gonococci to evade phagocytosis by neutrophils and neutrophil extracellular traps (NETs) (47, 48). Mechanisms for this include failure to be taken up by neutrophils due to expression of pili (49) and resistance to intracellular killing (50, 51) which can be overcome by opsonophagocytosis following the addition of complement and serum (52). Gonococcus entering neutrophils via Opa binding to CEACAM1 or CEACAM6 causes inhibition of a pro-inflammatory response and neutrophil effectors (53, 54). Alternatively, the bacteria can adhere to the uropod of neutrophils to hide from their phagocytic activity (55).
Gonococcus can evade killing by neutrophils through modifying its peptidoglycan or LOS (56, 57), as well as resist killing by antimicrobial peptides through expression of the MtrCDE efflux pump (58, 59) and inhibit lysozyme killing (60). Gonococcus is also is able to degrade NETs through secretion of a nuclease (61) as well as inhibiting the maturation of the neutrophil phagosome, involving the pilus and PorB (48, 62). Further evasion of phagocytic killing by neutrophils takes place via binding to C4BP or by entering the neutrophil through CR3 inducing so-called ‘silent phagocytosis’ and reduced killing (63, 64).
N. gonorrhoeae can also evade killing by macrophages. Gonococci are phagocytosed by macrophages and while many of the bacteria are killed, some are able to survive and even replicate inside macrophages (65). It has been suggested that this is because N. gonorrhoeae is able to prevent the maturation of the phagosome, possibly mediated by PorB (65, 66). Furthermore, gonococci can prevent apoptosis in macrophages, suggesting preservation of a reservoir of bacteria for future replication (65). Additionally, N. gonorrhoeae can stimulate macrophages to take on an M2 phenotype associated with immunosuppressive functions (67). Gonococcal infection of M0 macrophages in vitro induces expression of M2 markers CD163 and CD206, but is not associated with the M1 markers CD86, MHCII, TLR-4 or CD64. IL-10 secretion occurs from macrophages stimulated with gonococci (67). Taken together, these findings suggest that gonococci induce a suppressive phenotype in macrophages, that increases the capacity for N. gonorrhoeae to survive after phagocytosis, but may also contribute to wider immune suppression through reduced capacity for antigen presentation and secretion of IL-10. For a detailed review of the interactions between N. gonorrhoeae and macrophages see Escobar et al. (68).
3 Mouse models
Despite the human restriction of N. gonorrhoeae, female BALB/c mice can be infected vaginally with gonococcus after pretreatment with 17-β-estradiol and the use of antibiotics to prevent the overgrowth of natural flora. Inoculation with 106 colony forming units (CFU) has resulted in a mean duration of infection of 12.8 days (69). The mouse model of gonococcal infection is therefore imperfect, since duration of infection is limited. The use of hormones and antibiotics to make the mouse permissible to gonococcal infection also sets this model apart from human infection. Nevertheless, this approach allows colonization and bacterial replication to take place since higher numbers of gonococci are recovered from mice than are administered (69). Following infection of mice in the 17-β-estradiol model, gonococci localize in the vaginal and cervical tissue where a local inflammatory response is induced, characterized by infiltration of macrophages and neutrophils (70), similar to human infection (48). Murine gonococcal infection induces a specific antibody response both in the genital mucosa and plasma, but this is transient and not sufficient to prevent reinfection with the same strain (70). The mouse model is of value for studying the immune response to N. gonorrhoeae with the caveats that gonococcus does not cause ascending or disseminated infection in mice, infection is quite transient, and human-specific immune evasion mechanisms exist for N. gonorrhoeae which cannot be studied in wild-type mice.
The 17-β-estradiol mouse model has permitted the discovery of mechanisms by which N. gonorrhoeae directly evades induction of a protective immune response. The immune response to N. gonorrhoeae in the model is Th17 polarized, driven by the induction of IL-17 and characterized by an influx of neutrophils into the genital tract (71). In early studies, blocking IL-17 led to prolonged infection, suggesting that IL-17 and the Th17 response play a role in the clearance of gonococcus. Subsequently, the Th17 response was found to be partially dependent on expression of TGF-β, which in turn suppressed Th1 and Th2 responses (72). Blocking TGF-β accelerated the clearance of N. gonorrhoeae, allowing for the induction of Th1 and Th2 responses, leading to the development of anti-gonococcal antibodies and enhanced resistance to reinfection. The use of mice with targeted immune gene deletions of IL-12 (Th1-deficient) or IL-4 (Th2-deficient) showed that accelerated clearance of infection was Th1-dependent while resistance to reinfection required both Th1 and Th2 responses. Therefore, the current understanding is that N gonorrhoeae actively promotes a Th17 response which suppresses Th1 and Th2 responses needed for protective immunity in mice. The Th17 response to N. gonorrhoeae was shown to be induced by gonococcal LOS signaling through TLR4, while induction of TGF-β at least partially depended on expression of gonococcal Opa, since incubation of spleen cells with an Opa-deficient mutant resulted in a lower production of TGF-β (73). In summary, N. gonorrhoeae induces a Th17 response in mice, which can result in bacterial clearance, but which is suboptimal for protection compared to Th1 responses and does not result in protective immunity. Through the induction of TGF-β, the Th17 response suppresses the Th1 and Th2 responses needed for accelerated clearance and protection from reinfection.
Gonococcal infection in the 17-β-estradiol mouse model also induces expression of IL-10 leading to the expansion of type 1 regulatory T cells (Tr1 cells) (74). Blocking IL-10 and Tr1 cells leads to an increased Th1 and Th2 response, accelerated clearance and protection from reinfection, with increased levels of anti-gonococcal antibodies. Therefore, it appears that N. gonorrhoeae has evolved two distinct mechanisms: induction of IL-10 and TGF-β, in order to suppress a protective Th1 and Th2 immune response. This may explain why the immune response to natural infection does not result in protection to reinfection, although it is unclear whether these findings apply to human infection. A summary of the cytokine responses to gonococcal infection in the female mouse model is shown in Figure 2.
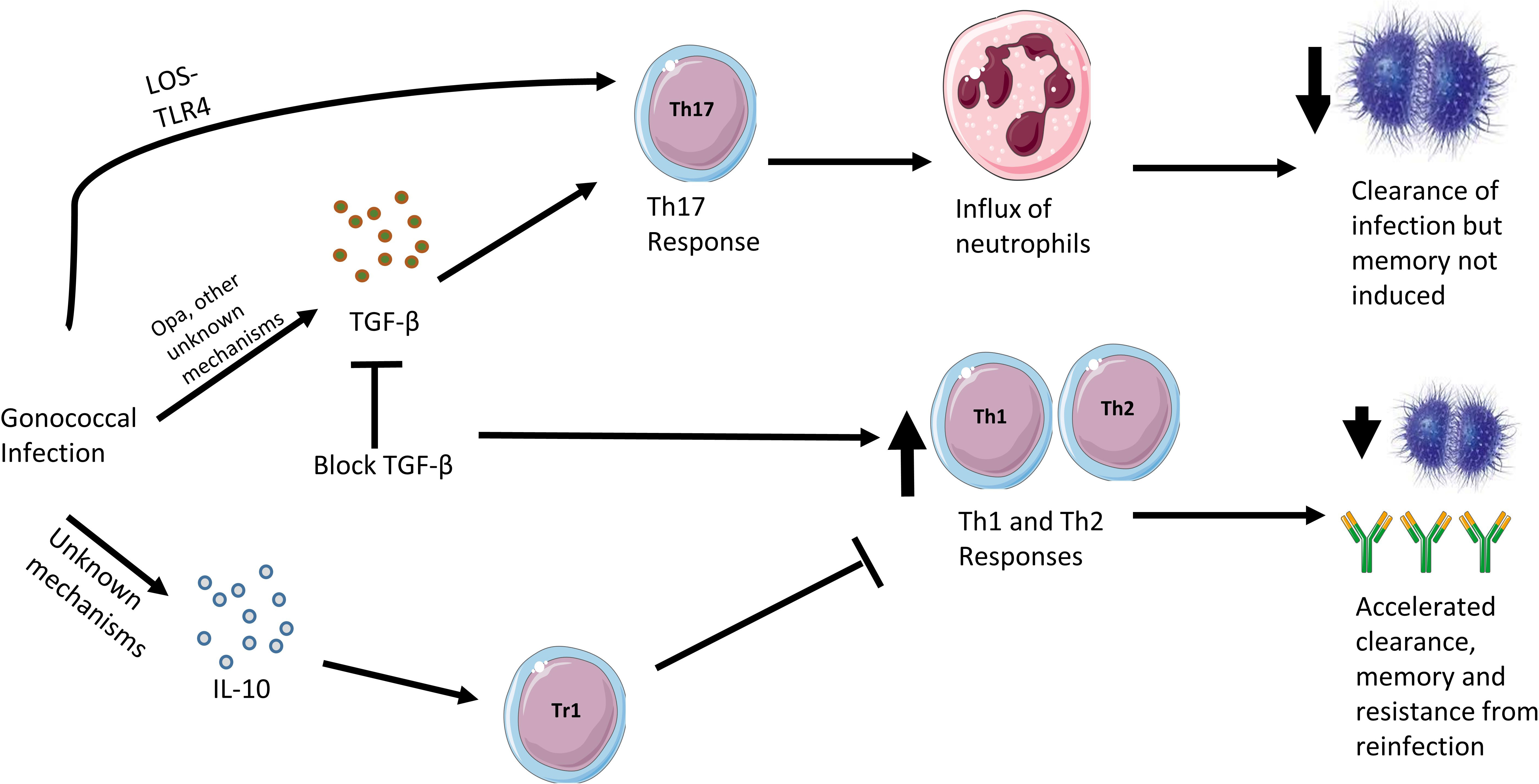
Figure 2 Cytokine responses in the murine female genital tract model of gonococcal infection. Gonococcal infection in the female mouse leads to a mostly Th17 response, driven by interactions between gonococcal LOS and murine TLR4 as well as stimulation of TGF-β, which is partly driven by gonococcal Opa surface proteins. The consequences of a Th17 response is an influx of neutrophils in the murine genital tract and clearance of the gonococci. However, if TGF-β action is blocked, Th1 and Th2 responses are observed, which leads to accelerated clearance, memory and resistance to reinfection. On infection with gonococci, the female mouse also responds by secreting IL-10 through an as yet undiscovered mechanism. This stimulates the differentiation of Tr1 cells and the inhibition of protective Th1 and Th2 responses.
Co-administration of N. gonorrhoeae and encapsulated IL-12 directly in the vagina (simulating a Th1 response) leads to accelerated clearance and protection from reinfection with increased anti-gonococcal antibodies (75). Protection against secondary reinfection extends to heterologous strains and persists for at least 6 months (76). The protective immunity induced by IL-12 co-administration is dependent on IFN-γ and B cells, suggesting that these are important for protection against N. gonorrhoeae. A further study found that co-administration of microencapsulated IL-12 and outer membrane vesicles from N. gonorrhoeae via the intranasal route accelerates clearance in a murine gonococcal challenge model (77). This co-administration leads to an increase in levels of serum IgG, salivary IgA and vaginal IgG and IgA anti-gonococcal antibodies as well as IFN-γ production from CD4+ T cells isolated from the iliac lymph nodes. Importantly, protection against heterologous strains of gonococcus was observed. MAP1, a vaccine candidate based on a peptide mimic of a conserved gonococcal LOS epitope (2C7), induces a Th1 polarized immune response in BALB/c mice and bactericidal anti-LOS antibodies (78). Immunization with MAP1 shortens carriage of N. gonorrhoeae and lowers bacterial burden, effects that can be achieved with passive administration of a monoclonal antibody to 2C7 (mAb2C7) (78). The protective mechanisms which reduce pathogen burden and length of infection in response to mAb2C7 administration are dependent on complement (79). While mAb2C7 is functional in neutrophil-depleted mice and mice with blocked C5a receptors, the protective effects are lost in C1q null mice, C9 null mice or in mice in which C5 function has been blocked. Furthermore, a version of mAb2C7 with amino acid substitutions resulting in reduced binding to C1q was ineffective at killing gonococci in vitro. Therefore, complement appears to be essential for protective immunity to gonococcus in mice, through antibody-directed complement-mediated killing. A vaccine candidate based on a tetrapeptide mimic of the 2C7 epitope was effective in accelerating clearance and reducing colonization in BALB/c mice, and stimulated production of bactericidal specific IgG (20).
4 Human immunology
4.1 Natural infection
In contrast to immunological studies in mice, which can be performed in a highly controlled way, human studies have largely been restricted to measuring the immune response to natural infection. Different studies on the human immune response often include cohorts of varying sizes and backgrounds, while measuring different types of samples often taken at different time-points post-infection. This makes comparisons of individual studies difficult. While there is much still to be understood about the immune response to natural gonococcal infection, a lot of valuable insight has already been gained by such studies. A summary of the studies of natural infection of gonococcus in humans is shown in Table 1.
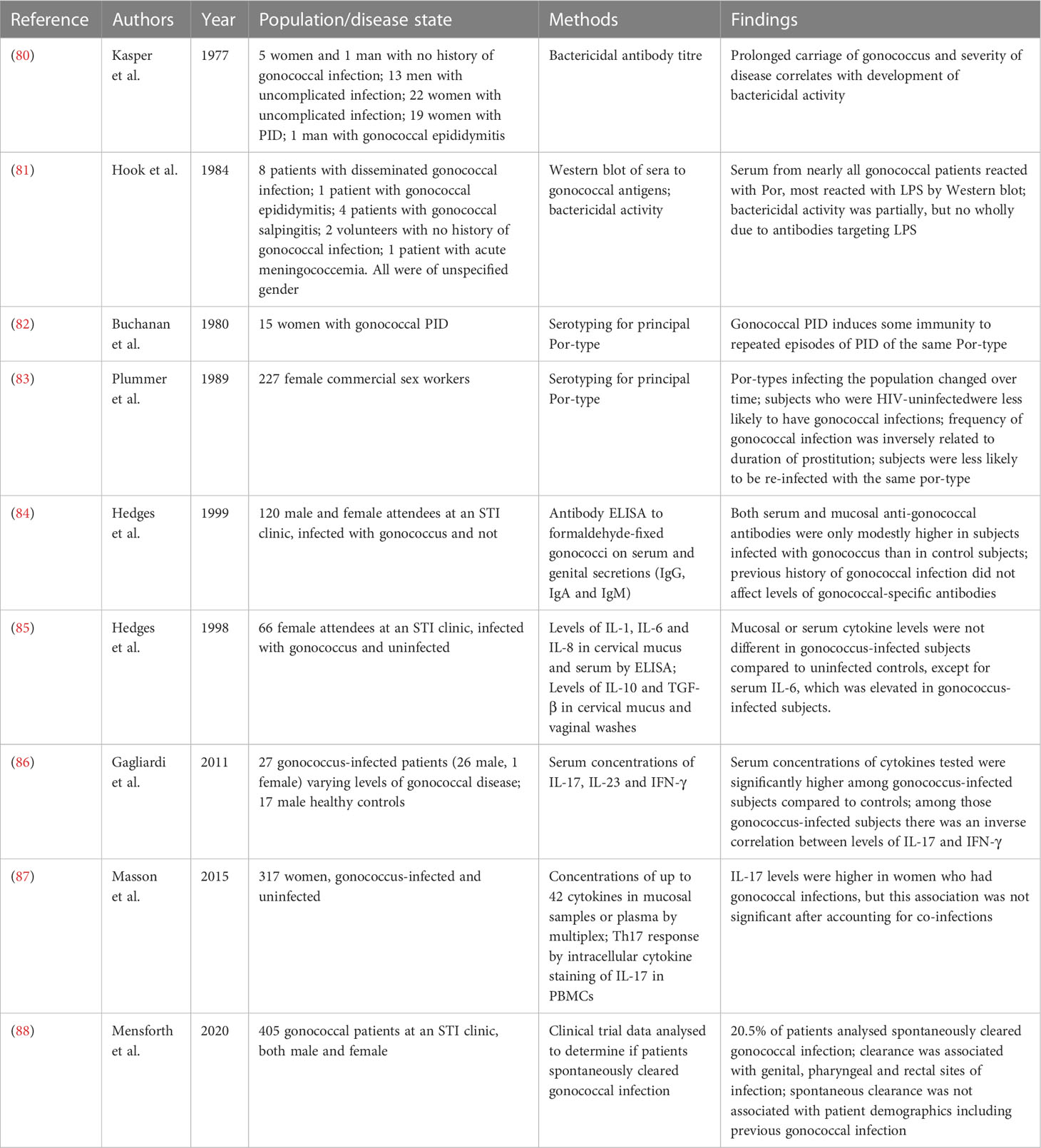
Table 1 Studies of natural infection of humans with Neisseria gonorrheoae detailing subjects, methods and key findings.
Sera from female laboratory workers who volunteered serum, and had no history of gonococcal infection had no detectable antibodies with bactericidal activity against gonococcal strains isolated from patients with pelvic inflammatory disease (PID). In the same study, only 4 out of 13 men with uncomplicated gonococcal urethritis had bactericidal antibodies to the infecting gonococcal strain in their pretreatment serum. Uncomplicated infection of male subjects was described as men who presented with a urethral discharge from which N. gonorrhoeae was isolated. Of 9 women found to have gonococcal infection during a routine pelvic examination and recalled for treatment within 33 days, one had bactericidal antibodies in pre-treatment sera to the infecting isolate. 4 out of the 5 of these women for whom the interval between isolation of the bacteria and treatment was more than 33 days had bactericidal antibodies suggesting a correlation between prolonged asymptomatic carriage of gonococcus and the development of bactericidal antibodies. Furthermore, in patients with PID, the severity of illness correlated with the development of bactericidal antibodies (80). Complications arise in comparing studies, which often study the immune response to gonococcus in different states of gonococcal infection in both male and female subjects, which could lead to different conclusions. The mechanisms of different outcomes of gonococcal infection, from asymptomatic infection to ascending infection of the upper reproductive tract in women, has been extensively investigated (89).
In a separate study (81), 12 out of 13 patients with gonococcal infection had serum antibodies to Por (protein I), a gonococcal outer membrane antigen, detectable by Western blotting. 9 out of the 13 patients had serum antibodies to LOS, and 8 out of 13 patients had serum antibodies to both Por and LOS. All patients regardless of disease status had antibodies against at least one gonococcal antigen tested. Therefore, it is important to note the variability in human antibody response to gonococcus in terms of breadth of response to different antigens (80, 81). Functional antibody response is often variable and difficult to measure, since non-immune sera contain endogenous antibodies which can have a high background level of activity against gonococcus (90). The development of blocking antibodies which inhibit the function of protective antibodies in immune sera further complicates the picture (43). Correlations between serum bactericidal antibody levels and severe disease or length of infection (80) need to be confirmed in a larger study. A key question is the reason for lack of protective immunity following infection. This could either be an inherent failure to develop protective immunity or the result of lack of breadth of protection against other gonococcal isolates due to their high antigenic variation. As discussed, animal studies appear to show that N. gonorrhoeae inhibits the generation of adaptive immunity (72–74). However, it is not known if this work translates to humans, where variation of infecting strains complicates the situation.
Recurrence of gonococcal salpingitis (inflammation of the fallopian tubes) is less likely with strains of the same Por (protein I) type, suggesting that Por-specific protective immunity can be induced, at least in the context of more disseminated infection (82). Por-type immunity was subsequently demonstrated in a larger study of 227 female sex workers in Nairobi, Kenya over 16 months, based on the testing of four hypotheses: 1. the prevalence of por-types making up the gonococcal population changed during the course of the study, which could have been driven by the development of protective immunity, 2. HIV-positive subjects (who were presumed to be immunodeficient) experienced more frequent gonococcal infections and were more at risk of reinfection, 3. the duration of prostitution correlated negatively with the frequency of infection (suggesting acquisition of immunity over time) and 4. for the majority of the serovars tested there was a reduced risk of reinfection with the same serovar (83). Therefore, there is epidemiological evidence for immunity to homologous gonococcal infection. A summary of the findings from this study is shown in Figure 3. Any immunity is apparently incomplete, since protection is observed only in the context of severe disease (82) or is Por-type specific (83). Further work is needed to establish the mechanistic basis for this incomplete protection.
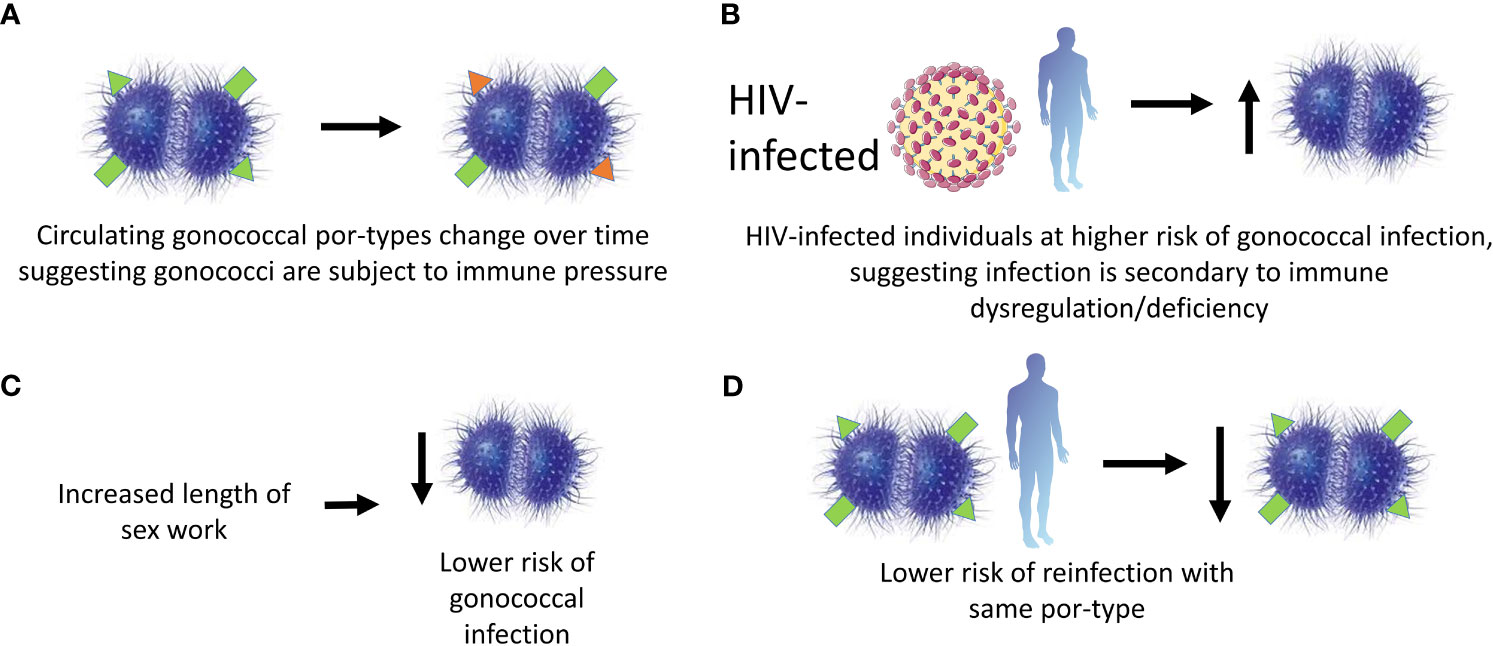
Figure 3 Evidence for protective immunity following gonococcal infection in a cohort of female commercial sex workers in Kenya. A cohort of 227 female sex workers in Kenya were followed in a longitudinal study over 16 months. Protective immunity in the cohort was said to be observed due to the findings supporting 4 hypotheses. These were: (A) the por-types of the infecting gonococci changed with time suggesting selective immune pressure; (B) HIV positive subjects were more likely to have gonococcal infections suggesting infection was associated with immune defects; (C) the likelihood of gonococcal infection decreased with the length of sex work suggesting a building-up of immunity with each exposure; and (D) subjects were unlikely to be re-infected with the same por-type suggesting at least por-type immunity is observed.
Gonococcal antibody levels, both serum and mucosal, from male and female patients with gonococcal infection attending an STI clinic in Birmingham, Alabama, to formaldehyde-fixed gonococcal bacteria were shown to be only slightly higher than in uninfected individuals (84). However, if formaldehyde affects key gonococcal epitopes then this could underestimate the antibody response. In these patients, previous history of gonococcal infection did not affect levels of specific antibodies suggesting that immunological memory was not induced. Since these were uncomplicated gonococcal infections, it is possible that the lack of antibody response was due to the absence of inductive tissues (organized lymphoid tissue) at the site of relatively superficial infection. In female patients attending an STI clinic in Birmingham, Alabama, aged between 17 and 57, cytokine levels in genital secretions were not higher in individuals who had gonococcal infection compared with those that did not, while the level of serum IL-6 was elevated in patients with gonorrhea (85). Levels of IL-10 and TGF-β were also not higher in those with gonococcal infection, suggesting that a suppressive cytokine response may not be responsible for a lack of detectable pro-inflammatory cytokine response. This is further evidence for a relatively mild immune response to uncomplicated genital gonococcal infection in humans.
Therefore, for uncomplicated genital infection, the immune response is relatively weak (84, 85), which may explain why reinfection occurs frequently. As previously explained, it is possible to correlate immune response to more severe disease (82), suggesting that lack of protection resulting from uncomplicated infection could in part be due to a lack of immune induction. Moreover, there is evidence that protective immunity could be Por-type-specific (83) which would suggest a large role for antigenic variation as a reason for lack of protective immunity. The reality is possibly a combination of the two, since incomplete protective immunity has been demonstrated (83). Additionally, active immune evasion resulting from induction of anti-inflammatory cytokines by N. gonorrhoeae has been shown in the mouse model (72–74), but not yet in human studies. There is scope for more in-depth studies of cytokine levels in human patients, to expand the range of cytokines previously studied, in order to understand if, and to what extent, an immunosuppressive environment is induced.
In a study from 2011, the serum concentrations of IL-17A, IL-23 and IFN-γ were analyzed in 27 patients (consisting of 16 men and 1 woman, recruited from Rome and Turin, Italy, with median age of 37.5 years) with gonorrhea and compared to 17 healthy controls (86). The concentrations of all three cytokines were significantly higher in gonorrhea patients compared to controls. Furthermore, there was an inverse correlation between IFN-γ and IL-17A, suggesting an antagonistic relationship between Th1 and Th17 responses. Another study from 2015 examined women with STIs from Cape Town and Durban, South Africa, and found that those who had gonorrhea had higher levels of IL-17 at the genital site than controls who had no STIs, although the association between gonococcal infection and increased levels of IL-17 was not significant after accounting for co-infection with other STIs detected included Chlamydia trachomatis, Trichomonas vaginalis, HSV-2, bacterial vaginosis and candida infection (87). The cellular source of IL-17 during human gonococcal infection is still unknown.
A recent report studied 405 gonorrhea patients enrolled in a clinical trial to test the efficacy of gentamicin as a treatment for gonorrhea in the UK and included both women and men, heterosexuals and men-who-have-sex-with-men. The results demonstrated that spontaneous clearance of N. gonorrhoeae occurred in 20.5% of patients within a median of 10 days (88). Ethical concerns prevent these kinds of studies from being conducted for a prolonged time period, since treatment must commence following diagnosis. However, clearance was observed at different anatomical sites (genital, pharyngeal and rectal), and was not associated with the specific demographics of participants (88). This implies that in some cases an immune response is generated that clears infection. There was no association with previous history of gonococcal infection, so it is unlikely that clearance in those specific patients was due to previously-acquired immunity. Those who spontaneously cleared were less likely to have had dysuria, which may suggest a lower bacterial load during the infection. Further work is needed to understand the specific immune mechanisms behind spontaneous clearance.
4.2 Controlled human infection models
Controlled human infection models (CHIMs) involve the inoculation of gonococcus into the urethra of male volunteers (91). This allows for the characterization of infection dynamics, immune response and pathogenesis within the natural host. However, there are limitations. Only studies with male volunteers are permitted since the risk of serious disease from local spread of infection is much lower than in women. Furthermore, ethically the infection must be treated within a limited period of time or when clinical symptoms appear, restricting study length to around five days. Therefore, studies on the pathogenesis and immune response in more severe disease or chronic infection are not possible in the human challenge model.
Despite the limitations, CHIM studies have furthered understanding of the pathogenesis of N. gonorrhoeae. For example, antigenic variation of the pilus and phase variation of Opa have both been demonstrated during infection (92, 93). LOS phase variation also occurs (94) and sialylation of LOS decreases infectivity in volunteers (95). A mutant strain of gonococcus lacking lptA, which, when functional, adds phosphoethanolamine onto lipid A of LOS, showed reduced survival compared to the wild-type strain in a competition challenge experiment (96). Since N. gonorrhoeae is a human restricted pathogen, some facets of the ability of the bacteria to grow in vivo can only be studied in man. For example, a human challenge model was used to demonstrate that N. gonorrhoeae does not require the expression of IgA1 protease to infect male humans since the course of infection with a gonococcal strain deficient for this protease was indistinguishable from that of the wild-type strain (97).
CHIM studies have been used to assess the immune response to gonococcal infection. In an early study from 1969, 10 male volunteers recruited from Atlanta, Georgia, were infected with gonococcal strain F62 and the antibody response measured (98). 9 out of the 10 volunteers showed a 4-fold increase in IgG titer to unspecified heat-labile formalin-fixed gonococcal antigens. 7 of those 9 with an increase in IgG reached peak titers within 10 days of infection. There was a 4-fold increase in IgM and IgA to the same antigens in only 4 and 3 patients respectively. The number of subjects, was small, and the inoculum used [a full 2 mm bacteriological loop (99)] was higher than is likely during natural transmission, highlighting the general limitations of human challenge models.
Cytokine responses were measured in the urine of volunteers exposed to experimental infection with N. gonorrhoeae (100). 17 volunteers were infected and treated with antibiotics at the onset of symptoms or 7 days after challenge. 10 subjects went on to show symptoms, while 7 remained asymptomatic. IL-8 was present in the urine of all symptomatic subjects, and rapidly declined upon treatment. Similarly, IL-6 levels were elevated in all symptomatic volunteers, declined on treatment, and correlated with the number of viable gonococci detected and number of leukocytes recovered from the urine. An increase was also observed in IL-8 and IL-6 levels in 4 of the 7 volunteers who were asymptomatic, but these levels rapidly returned to baseline. All symptomatic subjects had elevated levels of TNF-α and IL-1β in the urine, while IL-1α and GM-CSF were consistently undetectable (100). The number of subjects with a 4-fold elevation in IL-8, IL-6, TNF-α or IL-1β in plasma compared with pre-challenge was more variable. Levels were already high pre-challenge. The majority of symptomatic subjects had elevated levels of IL-8 in plasma post-challenge, but only around half had elevated levels of TNF-α or IL-1β, and only 3 subjects had increased IL-6 levels. PBMC mRNA levels from the same study indicate that expression of TNF-α, IL-6 and IL-8 is not significantly altered during experimental infection, although the data were quite limited. The rapid increase of IL-8, IL-6 and TNF-α levels measured in the urine suggests that they are produced at the mucosal site, while a relative delay in IL-1β production suggests that this cytokine is derived from the infiltrating leukocytes. A more detailed study of the origins of these cytokines during human infection is needed as well as a study of a wider range of cytokines.
In a reinfection study in the US in 2001, 14 volunteers out of 15 were initially successfully infected with N. gonorrhoeae strain MS11mkC at a dose of 104 CFU. Two weeks after treatment for the infection, the volunteers were re-challenged with a lower dose of 2.3 x 103 CFU of the same strain. 6 of the 14 previously successfully infected volunteers were successfully reinfected and showed symptoms, as did 5 of 10 of a control naïve group (101). Therefore, no protection to reinfection was detected. A limitation with the CHIM studies to date is that only low numbers of volunteers have been included in each study. Therefore, these studies do not have sufficient power to detect low levels of protection. Only 2 of the 10 volunteers who were able to complete 7 days of infection without early treatment became re-infected at phase 2, suggesting a correlation between protection and length of infection, although there was not enough power in the study to observe a significant difference. However, induction of serum antibodies targeting LOS was associated with resistance to reinfection. It is still unclear how serum antibodies protect against N. gonorrhoeae. Protection could occur through translocation to the mucosa, or serum antibodies could be a surrogate marker for mucosal antibody responses. It may be that protection is cumulative with each gonococcal infection, boosting immunity with each encounter.
5 Prospects for a protective vaccine
There is no licensed vaccine for gonorrhea, and all vaccine candidates to date tested in efficacy studies in humans have failed (25, 102, 103). Field trials with a lysed whole cell vaccine candidate showed no efficacy compared to a placebo group (102). Similarly, volunteers vaccinated with purified gonococcal pilus were not protected against infection compared to a placebo arm (25).
A retrospective cohort study of individuals who had received an OMV vaccine against N. meningitidis serogroup B (MeNZB) in New Zealand found vaccine effectiveness of 31% (95% CI 21-39) in preventing a diagnosis of gonorrhea (10). Furthermore, MeNZB vaccination significantly protected against hospitalization from gonorrhea (104). Similarly, the incidence of gonorrhea in Cuba declined at the end of the 1990s following the introduction of an OMV vaccine against N. meningitidis (105). At the same time incidences of other STIs such as genital warts and syphilis were unaffected suggesting this was a specific effect on gonorrhea. Cases of gonorrhea also decreased in Quebec, Canada following mass vaccination with the 4CMenB, another licensed vaccine for N. meningitidis group B, which contains MeNZB OMVs as one of its components (12). A further matched cohort study in Southern California found that gonorrhea rates were 46% lower among recipients of 4CMenB than recipients of MenACWY (a polysaccharide conjugate vaccine against N. meningitidis serotypes A, C, W and Y, which does not contains OMVs) (106). 4CMenB vaccination has also been associated with a lower risk of gonorrhea in a case-control study of men-who-have-sex-with-men living with HIV (107). Even a relatively low efficacy vaccine against gonococcus could none-the-less have a significant impact on the population disease prevalence (108–111) and the above findings have brought renewed hope to developing a bespoke vaccine against gonococcus.
While the mechanism of cross-protection of 4CMenB against gonococcus is not clear, sera from individuals vaccinated with 4CMenB were shown to react with gonococcal proteins in ELISA and Western blots (112). Immunization with 4CMenB in the female mouse model of gonococcal infection has shown that vaccination significantly accelerated clearance and reduced bacterial burden (113). Serum IgG and vaginal IgA from the mice cross-reacting with gonococcal OMVs recognized several gonococcal proteins and had serum bactericidal activity against both the challenge strain F62 and strain FA1090. However, no correlates of protection have yet been identified. There was no correlation between bactericidal activity of mouse serum and clearance, and sera from humans immunized with 4CMenB did not show increased bactericidal activity compared with unvaccinated controls. At least one randomized controlled clinical trial is due to investigate the efficacy of 4CMenB against N. gonorrhoeae (114). The primary endpoint will be the number of gonococcal infections, while secondary endpoints include vaccine-induced immune responses. More work is needed to uncover the mechanisms of protection of 4CMenB against N. gonorrhoeae in humans, which could help understand correlates of protection and inform the development of gonococcal-based vaccines for N. gonorrhoeae infection. A summary of current knowledge about mechanisms of protection to gonococcal infection is presented in Table 2.
Meningococcal OMVs accelerate clearance in the female mouse model of gonococcal infection, with OMVs made from a strain lacking PorA, PorB and RmpM stimulating the highest clearance rate (115). Evidence from this study suggests antibodies produced by these OMVs bind a wider range of targets than OMVs produced by wild-type strains. This is possibly because PorB is immunodominant but highly variable in gonococcus and so antibodies to PorB are likely not protective. Immune sera from mice immunized with these OMVs were used to identify target gonococcal antigens including PilQ, MtrE, NlpD and GuaB. Further work is required to discover the target antigens which lead to protective immunity to gonococcus. Proteomic methods have led to the discovery of a number of potential gonococcal antigens, with analyses mostly focusing on cell envelope proteins. One study identified five novel candidate antigens; BamA, LptD, TamA and the as yet uncharacterized NGO2054 and NGO2139 (116). These were ubiquitously expressed in different growth conditions, surface exposed and shown to elicit bactericidal antibodies that cross-reacted with a number of different gonococcal isolates. A further proteomic analysis of fifteen gonococcal strains reported nine novel vaccine candidates, based on conservation across most strains tested and localized to the cell envelope or outer membrane (117). While new vaccine candidates are being discovered, attention must be given to the heterogeneity of N. gonorrhoeae, and potential variation of antigens. A recent study compared the sequence variation of 34 gonorrhea antigens across more than 5000 clinical isolates. A single allele for eight antigens was represented in more than 80% of the isolates, while PorB and TbpB were highly variable. Thirteen candidate antigens were identified, based on high frequency distribution of a single allele, or low frequency sequence polymorphisms in surface loops (118). Potential vaccine antigens have also been discovered from in vivo studies. 36 targets were identified as expressed during human mucosal infection and predicted to be immunogenic, membrane-associated and conserved (119). Further studies will need to show that these proteins are immunogenic and drivers of functional immunity in either an animal model or in humans. A summary of gonococcal candidate antigens is shown in Table 3.
Knowledge of correlates of protection to gonococcal infection and disease is still lacking. This is largely due to the fact that natural infection doesn’t usually protect against reinfection. If this is due to a lack of inductive sites in the genital mucosa, to which gonococcal infection is often limited, it may be that a parenteral vaccine will induce protective immunity where natural infection cannot. Such a vaccine needs to improve on immunity stimulated by natural infection. The finding that N. gonorrhoeae induces the production of suppressive cytokines in mice (72–74), which can be overcome by vaginal administration of IL-12 (76) suggests that a vaccine should induce a Th1 polarized response in order to protect. However, it is not clear whether this holds true for a protective response in humans, although 4CMenB is formulated with alum as an adjuvant, which is Th2-promoting. Any vaccine candidate tested in mouse or human challenge studies should be used to understand better correlates of protection. It could be that repeat infections often occur because gonococcus is highly antigenically variable and so new infections could often occur with strains that are different from previously infecting strains. Another possibility is that any protective immunity is not long-lasting and wanes quickly. Clearly any successful vaccine would have to provide broad cross-protection against a number of distinct gonococcal strains, whilst having relatively long-lasting efficacy since boosters would not be desirable in LMICs, where the highest burden of gonococcal disease exists (2).
6 Summary
One of the main barriers to the development of a vaccine to N. gonorrhoeae is the lack of established correlates of protection. Reasons why previous vaccine efforts have failed are not well understood beyond the antigenic variation of the organism presenting an obstacle for broad protection. The establishment of a mouse model to investigate the immune response to gonococcus has provided information about the mechanisms by which N. gonorrhoeae evades immunity and prevents the generation of protective adaptive immunity. Studies in humans are critical due to the human-restricted nature of N. gonorrhoeae and have demonstrated the lack of protective immunity to natural infection with gonococcus, although reasons for this are not known to the same level of detail as in mice models.
Large cohort studies of naturally infected individuals have demonstrated varying degrees of protection gained from such infection. More work is needed to decipher the relevant functional antibody and T-cell responses to natural gonococcal infection in man. Human challenge studies come with drawbacks such as restriction to male volunteers and the short duration of study but have provided valuable information on the pathogenesis of the N. gonorrhoeae, as well as on the basic immune response to gonococcal infection. These models will be valuable going forward in providing a controlled environment for further investigations, such as whether protection can be achieved against a homologous second challenge, and to test responses to candidate vaccines. Such vaccines have to do better than nature in stimulating a protective response and have to provide protection against a broad-range of heterologous strains. Clinical trials investigating meningococcal serotype B vaccines for use against gonorrhea are beginning, summarised in Table 4. Nevertheless, a relatively low efficacy vaccine could have a significant impact on gonococcal infection and disease at a population level (108–111).
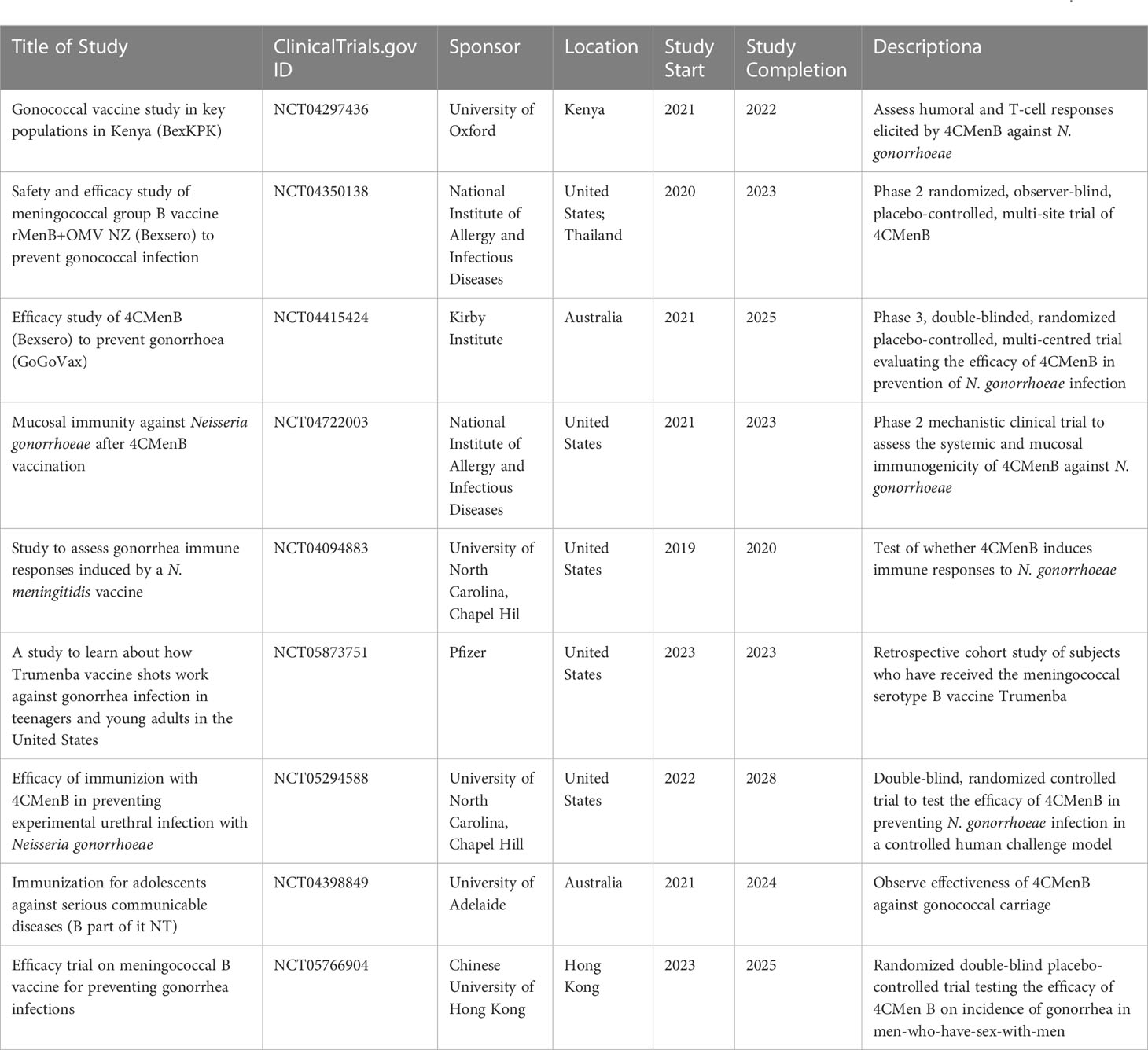
Table 4 Registered clinical studies assessing response to meningococcal serotype B vaccines against gonorrhea, found at ClinicalTrials.gov.
Author contributions
TB wrote the first draft of the manuscript. All authors contributed to the article and approved the submitted version.
Funding
Research reported in this publication is supported by CARB-X. CARB-X’s funding for this project is provided in part with federal funds from the U.S. Department of Health and Human Services; Administration for Strategic Preparedness and Response; Biomedical Advanced Research and Development Authority; under agreement number: 75A50122C00028, and by awards from Wellcome (WT224842), Germany’s Federal Ministry of Education and Research (BMBF), and the UK Global Antimicrobial Resistance Innovation Fund (GAMRIF) funded by the UK Government Department of Health and Social Care (DHSC). The content of this publication is solely the responsibility of the authors and does not necessarily represent the official views of CARB-X or any of its funders.
Acknowledgments
Figures constructed using icons from Bioicons (Servier and Marcel Tisch). Licence available at - https://creativecommons.org/licenses/by/3.0/legalcode.
Conflict of interest
Author JR reports personal fees from GSK Pharma; ownership of shares in GSK Pharma and AstraZeneca Pharma; lead author of the UK and European Guidelines on Pelvic Inflammatory Disease; Member of the European Sexually Transmitted Infections Guidelines Editorial Board. He is a UK National Institute for Health Research Journals Editor and associate editor of Sexually Transmitted Infections journal. He is treasurer for the International Union against Sexually Transmitted Infections and chair of charity trustees for the Sexually Transmitted Infections Research Foundation.
The remaining authors declare that the research was conducted in the absence of any commercial or financial relationships that could be construed as a potential conflict of interest.
Publisher’s note
All claims expressed in this article are solely those of the authors and do not necessarily represent those of their affiliated organizations, or those of the publisher, the editors and the reviewers. Any product that may be evaluated in this article, or claim that may be made by its manufacturer, is not guaranteed or endorsed by the publisher.
References
1. Unemo M, Seifert HS, Hook EW, Hawkes S, Ndowa F, Dillon JR. Gonorrhoea. Nat Rev Dis Primers (2019) 5(1):79. doi: 10.1038/s41572-019-0128-6
2. Joseph Davey DL, Shull HI, Billings JD, Wang D, Adachi K, Klausner JD. Prevalence of curable sexually transmitted infections in pregnant women in low- and middle-income countries from 2010 to 2015: A systematic review. Sex Transm Dis (2016) 43(7):450–8. doi: 10.1097/OLQ.0000000000000460
3. Fleming DT, Wasserheit JN. From epidemiological synergy to public health policy and practice: the contribution of other sexually transmitted diseases to sexual transmission of HIV infection. Sex Transm Infect (1999) 75(1):3–17. doi: 10.1136/sti.75.1.3
4. Zhang L, Zhang C, Zeng Y, Li Y, Huang S, Wang F, et al. Emergence and characterization of a ceftriaxone-resistant neisseria gonorrhoeae FC428 clone evolving moderate-level resistance to azithromycin in Shenzhen, China. Infect Drug Resist (2021) 14:4271–6. doi: 10.2147/IDR.S336212
5. Kueakulpattana N, Wannigama DL, Luk-In S, Hongsing P, Hurst C, Badavath VN, et al. Multidrug-resistant Neisseria gonorrhoeae infection in heterosexual men with reduced susceptibility to ceftriaxone, first report in Thailand. Sci Rep (2021) 11(1):21659. doi: 10.1038/s41598-021-00675-y
6. Platt R, Rice PA, McCormack WM. Risk of acquiring gonorrhea and prevalence of abnormal adnexal findings among women recently exposed to gonorrhea. JAMA (1983) 250(23):3205–9. doi: 10.1001/jama.1983.03340230057031
7. Fox KK, Thomas JC, Weiner DH, Davis RH, Sparling PF, Cohen MS. Longitudinal evaluation of serovar-specific immunity to Neisseria gonorrhoeae. Am J Epidemiol (1999) 149(4):353–8. doi: 10.1093/oxfordjournals.aje.a009820
8. Stupiansky NW, van der Pol B, Williams JA, Weaver B, Taylor SE, Fortenberry JD. The natural history of incident gonococcal infection in adolescent women. Sex Transm Dis (2011) 38(8):750–4. doi: 10.1097/OLQ.0b013e31820ff9a4
9. Fowler T, Caley M, Johal R, Brown R, Ross JD. Previous history of gonococcal infection as a risk factor in patients presenting with gonorrhoea. Int J STD AIDS (2010) 21(4):277–8. doi: 10.1258/ijsa.2009.009235
10. Petousis-Harris H, Paynter J, Morgan J, Saxton P, McArdle B, Goodyear-Smith F, et al. Effectiveness of a group B outer membrane vesicle meningococcal vaccine against gonorrhoea in New Zealand: a retrospective case-control study. Lancet (2017) 390(10102):1603–10. doi: 10.1016/S0140-6736(17)31449-6
11. Ochoa-Azze RF. Cross-protection induced by VA-MENGOC-BC® vaccine. Hum Vaccin Immunother (2018) 14(5):1064–8. doi: 10.1080/21645515.2018.1438028
12. Longtin J, Dion R, Simard M, Betala Belinga J-F, Longtin Y, Lefebvre B, et al. Possible impact of wide-scale vaccination against serogroup B neisseria meningitidis on gonorrhea incidence rates in one region of Quebec, Canada. Open Forum Infect Dis (2017) 4(Suppl 1):S734–S5. doi: 10.1093/ofid/ofx180.002
13. Rotman E, Seifert HS. The genetics of Neisseria species. Annu Rev Genet (2014) 48:405–31. doi: 10.1146/annurev-genet-120213-092007
14. O'Rourke M, Spratt BG. Further evidence for the non-clonal population structure of Neisseria gonorrhoeae: extensive genetic diversity within isolates of the same electrophoretic type. Microbiol (Reading) (1994) 140(Pt 6):1285–90. doi: 10.1099/00221287-140-6-1285
15. Unemo M, Dillon JA. Review and international recommendation of methods for typing neisseria gonorrhoeae isolates and their implications for improved knowledge of gonococcal epidemiology, treatment, and biology. Clin Microbiol Rev (2011) 24(3):447–58. doi: 10.1128/CMR.00040-10
16. Stern A, Brown M, Nickel P, Meyer TF. Opacity genes in Neisseria gonorrhoeae: control of phase and antigenic variation. Cell (1986) 47(1):61–71. doi: 10.1016/0092-8674(86)90366-1
17. Murphy GL, Connell TD, Barritt DS, Koomey M, Cannon JG. Phase variation of gonococcal protein II: regulation of gene expression by slipped-strand mispairing of a repetitive DNA sequence. Cell (1989) 56(4):539–47. doi: 10.1016/0092-8674(89)90577-1
18. Yang QL, Gotschlich EC. Variation of gonococcal lipooligosaccharide structure is due to alterations in poly-G tracts in lgt genes encoding glycosyl transferases. J Exp Med (1996) 183(1):323–7. doi: 10.1084/jem.183.1.323
19. Banerjee A, Wang R, Uljon SN, Rice PA, Gotschlich EC, Stein DC. Identification of the gene (lgtG) encoding the lipooligosaccharide beta chain synthesizing glucosyl transferase from Neisseria gonorrhoeae. Proc Natl Acad Sci USA (1998) 95(18):10872–7. doi: 10.1073/pnas.95.18.10872
20. Gulati S, Pennington MW, Czerwinski A, Carter D, Zheng B, Nowak NA, et al. Preclinical efficacy of a lipooligosaccharide peptide mimic candidate gonococcal vaccine. mBio (2019) 10(6):e02552-19. doi: 10.1128/mBio.02552-19
21. Chakraborti S, Lewis LA, Cox AD, St Michael F, Li J, Rice PA, et al. Phase-Variable Heptose I Glycan Extensions Modulate Efficacy of 2C7 Vaccine Antibody Directed against Neisseria gonorrhoeae Lipooligosaccharide. J Immunol (2016) 196(11):4576–86. doi: 10.4049/jimmunol.1600374
22. Haas R, Veit S, Meyer TF. Silent pilin genes of Neisseria gonorrhoeae MS11 and the occurrence of related hypervariant sequences among other gonococcal isolates. Mol Microbiol (1992) 6(2):197–208. doi: 10.1111/j.1365-2958.1992.tb02001.x
23. Haas R, Meyer TF. The repertoire of silent pilus genes in Neisseria gonorrhoeae: evidence for gene conversion. Cell (1986) 44(1):107–15. doi: 10.1016/0092-8674(86)90489-7
24. Segal E, Hagblom P, Seifert HS, So M. Antigenic variation of gonococcal pilus involves assembly of separated silent gene segments. Proc Natl Acad Sci USA (1986) 83(7):2177–81. doi: 10.1073/pnas.83.7.2177
25. Boslego JW, Tramont EC, Chung RC, McChesney DG, Ciak J, Sadoff JC, et al. Efficacy trial of a parenteral gonococcal pilus vaccine in men. Vaccine (1991) 9(3):154–62. doi: 10.1016/0264-410X(91)90147-X
26. Knapp JS, Tam MR, Nowinski RC, Holmes KK, Sandström EG. Serological classification of Neisseria gonorrhoeae with use of monoclonal antibodies to gonococcal outer membrane protein I. J Infect Dis (1984) 150(1):44–8. doi: 10.1093/infdis/150.1.44
27. Posada D, Crandall KA, Nguyen M, Demma JC, Viscidi RP. Population genetics of the porB gene of Neisseria gonorrhoeae: different dynamics in different homology groups. Mol Biol Evol (2000) 17(3):423–36. doi: 10.1093/oxfordjournals.molbev.a026322
28. Masignani V, Pizza M, Moxon ER. The development of a vaccine against meningococcus B using reverse vaccinology. Front Immunol (2019) 10:751. doi: 10.3389/fimmu.2019.00751
29. Bettoni S, Shaughnessy J, Maziarz K, Ermert D, Gulati S, Zheng B, et al. C4BP-IgM protein as a therapeutic approach to treat Neisseria gonorrhoeae infections. JCI Insight (2019) 4(23):131886. doi: 10.1172/jci.insight.131886
30. Ram S, Cullinane M, Blom AM, Gulati S, McQuillen DP, Monks BG, et al. Binding of C4b-binding protein to porin: a molecular mechanism of serum resistance of Neisseria gonorrhoeae. J Exp Med (2001) 193(3):281–95. doi: 10.1084/jem.193.3.281
31. Ram S, McQuillen DP, Gulati S, Elkins C, Pangburn MK, Rice PA. Binding of complement factor H to loop 5 of porin protein 1A: a molecular mechanism of serum resistance of nonsialylated Neisseria gonorrhoeae. J Exp Med (1998) 188(4):671–80. doi: 10.1084/jem.188.4.671
32. Madico G, Ngampasutadol J, Gulati S, Vogel U, Rice PA, Ram S. Factor H binding and function in sialylated pathogenic neisseriae is influenced by gonococcal, but not meningococcal, porin. J Immunol (2007) 178(7):4489–97. doi: 10.4049/jimmunol.178.7.4489
33. Duensing TD, Putten JP. Vitronectin binds to the gonococcal adhesin OpaA through a glycosaminoglycan molecular bridge. Biochem J (1998) 334(Pt 1):133–9. doi: 10.1042/bj3340133
34. Cole JG, Fulcher NB, Jerse AE. Opacity proteins increase Neisseria gonorrhoeae fitness in the female genital tract due to a factor under ovarian control. Infect Immun (2010) 78(4):1629–41. doi: 10.1128/IAI.00996-09
35. Ward ME, Watt PJ, Glynn AA. Gonococci in urethral exudates possess a virulence factor lost on subculture. Nature (1970) 227(5256):382–4. doi: 10.1038/227382a0
36. Parsons NJ, Curry A, Fox AJ, Jones DM, Cole JA, Smith H. The serum resistance of gonococci in the majority of urethral exudates is due to sialylated lipopolysaccharide seen as a surface coat. FEMS Microbiol Lett (1992) 69(3):295–9. doi: 10.1111/j.1574-6968.1992.tb05169.x
37. Elkins C, Carbonetti NH, Varela VA, Stirewalt D, Klapper DG, Sparling PF. Antibodies to N-terminal peptides of gonococcal porin are bactericidal when gonococcal lipopolysaccharide is not sialylated. Mol Microbiol (1992) 6(18):2617–28. doi: 10.1111/j.1365-2958.1992.tb01439.x
38. Gulati S, Schoenhofen IC, Whitfield DM, Cox AD, Li J, St Michael F, et al. Utilizing CMP-sialic acid analogs to unravel neisseria gonorrhoeae lipooligosaccharide-mediated complement resistance and design novel therapeutics. PloS Pathog (2015) 11(12):e1005290. doi: 10.1371/journal.ppat.1005290
39. Ram S, Lewis LA, Rice PA. Infections of people with complement deficiencies and patients who have undergone splenectomy. Clin Microbiol Rev (2010) 23(4):740–80. doi: 10.1128/CMR.00048-09
40. Lewis LA, Ram S. Complement interactions with the pathogenic Neisseriae: clinical features, deficiency states, and evasion mechanisms. FEBS Lett (2020) 594(16):2670–94. doi: 10.1002/1873-3468.13760
41. Borrow R, Taha MK, Giuliani MM, Pizza M, Banzhoff A, Bekkat-Berkani R. Methods to evaluate serogroup B meningococcal vaccines: From predictions to real-world evidence. J Infect (2020) 81(6):862–72. doi: 10.1016/j.jinf.2020.07.034
42. Joiner KA, Scales R, Warren KA, Frank MM, Rice PA. Mechanism of action of blocking immunoglobulin G for Neisseria gonorrhoeae. J Clin Invest (1985) 76(5):1765–72. doi: 10.1172/JCI112167
43. Rice PA, Vayo HE, Tam MR, Blake MS. Immunoglobulin G antibodies directed against protein III block killing of serum-resistant Neisseria gonorrhoeae by immune serum. J Exp Med (1986) 164(5):1735–48. doi: 10.1084/jem.164.5.1735
44. Plummer FA, Chubb H, Simonsen JN, Bosire M, Slaney L, Maclean I, et al. Antibody to Rmp (outer membrane protein 3) increases susceptibility to gonococcal infection. J Clin Invest (1993) 91(1):339–43. doi: 10.1172/JCI116190
45. Li G, Xie R, Zhu X, Mao Y, Liu S, Jiao H, et al. Antibodies with higher bactericidal activity induced by a Neisseria gonorrhoeae Rmp deletion mutant strain. PloS One (2014) 9(3):e90525. doi: 10.1371/journal.pone.0090525
46. Gulati S, Mu X, Zheng B, Reed GW, Ram S, Rice PA. Antibody to reduction modifiable protein increases the bacterial burden and the duration of gonococcal infection in a mouse model. J Infect Dis (2015) 212(2):311–5. doi: 10.1093/infdis/jiv024
47. Criss AK, Seifert HS. A bacterial siren song: intimate interactions between Neisseria and neutrophils. Nat Rev Microbiol (2012) 10(3):178–90. doi: 10.1038/nrmicro2713
48. Palmer A, Criss AK. Gonococcal defenses against antimicrobial activities of neutrophils. Trends Microbiol (2018) 26(12):1022–34. doi: 10.1016/j.tim.2018.07.003
49. Thongthai C, Sawyer WD. Studies on the virulence of Neisseria gonorrhoeae. I. Relation of colonial morphology and resistance to phagocytosis by polymorphonuclear leukocytes. Infect Immun (1973) 7(3):373–9. doi: 10.1128/iai.7.3.373-379.1973
50. Witt K, Veale DR, Smith H. Resistance of Neisseria gonorrhoeae to ingestion and digestion by phagocytes of human buffy coat. J Med Microbiol (1976) 9(1):1–12. doi: 10.1099/00222615-9-1-1
51. Veale DR, Goldner M, Penn CW, Ward J, Smith H. The intracellular survival and growth of gonococci in human phagocytes. J Gen Microbiol (1979) 113(2):383–93. doi: 10.1099/00221287-113-2-383
52. Ross SC, Densen P. Opsonophagocytosis of Neisseria gonorrhoeae: interaction of local and disseminated isolates with complement and neutrophils. J Infect Dis (1985) 151(1):33–41. doi: 10.1093/infdis/151.1.33
53. Sarantis H, Gray-Owen SD. Defining the roles of human carcinoembryonic antigen-related cellular adhesion molecules during neutrophil responses to Neisseria gonorrhoeae. Infect Immun (2012) 80(1):345–58. doi: 10.1128/IAI.05702-11
54. Sintsova A, Sarantis H, Islam EA, Sun CX, Amin M, Chan CH, et al. Global analysis of neutrophil responses to Neisseria gonorrhoeae reveals a self-propagating inflammatory program. PloS Pathog (2014) 10(9):e1004341. doi: 10.1371/journal.ppat.1004341
55. Söderholm N, Vielfort K, Hultenby K, Aro H. Pathogenic Neisseria hitchhike on the uropod of human neutrophils. PloS One (2011) 6(9):e24353. doi: 10.1371/journal.pone.0024353
56. Ragland SA, Schaub RE, Hackett KT, Dillard JP, Criss AK. Two lytic transglycosylases in Neisseria gonorrhoeae impart resistance to killing by lysozyme and human neutrophils. Cell Microbiol (2017) 19(3). doi: 10.1111/cmi.12662
57. Handing JW, Criss AK. The lipooligosaccharide-modifying enzyme LptA enhances gonococcal defence against human neutrophils. Cell Microbiol (2015) 17(6):910–21. doi: 10.1111/cmi.12411
58. Shafer WM, Qu X, Waring AJ, Lehrer RI. Modulation of Neisseria gonorrhoeae susceptibility to vertebrate antibacterial peptides due to a member of the resistance/nodulation/division efflux pump family. Proc Natl Acad Sci USA (1998) 95(4):1829–33. doi: 10.1073/pnas.95.4.1829
59. Handing JW, Ragland SA, Bharathan UV, Criss AK. The mtrCDE efflux pump contributes to survival of neisseria gonorrhoeae from human neutrophils and their antimicrobial components. Front Microbiol (2018) 9:2688. doi: 10.3389/fmicb.2018.02688
60. Ragland SA, Humbert MV, Christodoulides M, Criss AK. Neisseria gonorrhoeae employs two protein inhibitors to evade killing by human lysozyme. PloS Pathog (2018) 14(7):e1007080. doi: 10.1371/journal.ppat.1007080
61. Juneau RA, Stevens JS, Apicella MA, Criss AK. A thermonuclease of Neisseria gonorrhoeae enhances bacterial escape from killing by neutrophil extracellular traps. J Infect Dis (2015) 212(2):316–24. doi: 10.1093/infdis/jiv031
62. Johnson MB, Criss AK. Neisseria gonorrhoeae phagosomes delay fusion with primary granules to enhance bacterial survival inside human neutrophils. Cell Microbiol (2013) 15(8):1323–40. doi: 10.1111/cmi.12117
63. Werner LM, Alcott A, Mohlin F, Ray JC, Belcher Dufrisne M, Smirnov A, et al. Neisseria gonorrhoeae co-opts C4b-binding protein to enhance complement-independent survival from neutrophils. PloS Pathog (2023) 19(3):e1011055. doi: 10.1371/journal.ppat.1011055
64. Smirnov A, Daily KP, Gray MC, Ragland SA, Werner LM, Brittany Johnson M, et al. Phagocytosis via complement receptor 3 enables microbes to evade killing by neutrophils. J Leukoc Biol (2023) 114(1):1–20. doi: 10.1093/jleuko/qiad028
65. Château A, Seifert HS. Neisseria gonorrhoeae survives within and modulates apoptosis and inflammatory cytokine production of human macrophages. Cell Microbiol (2016) 18(4):546–60. doi: 10.1111/cmi.12529
66. Mosleh IM, Huber LA, Steinlein P, Pasquali C, Günther D, Meyer TF. Neisseria gonorrhoeae porin modulates phagosome maturation. J Biol Chem (1998) 273(52):35332–8. doi: 10.1074/jbc.273.52.35332
67. Ortiz MC, Lefimil C, Rodas PI, Vernal R, Lopez M, Acuña-Castillo C, et al. Neisseria gonorrhoeae modulates immunity by polarizing human macrophages to a M2 profile. PloS One (2015) 10(6):e0130713. doi: 10.1371/journal.pone.0130713
68. Escobar A, Rodas PI, Acuña-Castillo C. Macrophage-neisseria gonorrhoeae interactions: A better understanding of pathogen mechanisms of immunomodulation. Front Immunol (2018) 9:3044. doi: 10.3389/fimmu.2018.03044
69. Jerse AE. Experimental gonococcal genital tract infection and opacity protein expression in estradiol-treated mice. Infect Immun (1999) 67(11):5699–708. doi: 10.1128/IAI.67.11.5699-5708.1999
70. Song W, Condron S, Mocca BT, Veit SJ, Hill D, Abbas A, et al. Local and humoral immune responses against primary and repeat Neisseria gonorrhoeae genital tract infections of 17beta-estradiol-treated mice. Vaccine (2008) 26(45):5741–51. doi: 10.1016/j.vaccine.2008.08.020
71. Feinen B, Jerse AE, Gaffen SL, Russell MW. Critical role of Th17 responses in a murine model of Neisseria gonorrhoeae genital infection. Mucosal Immunol (2010) 3(3):312–21. doi: 10.1038/mi.2009.139
72. Liu Y, Russell MW. Diversion of the immune response to Neisseria gonorrhoeae from Th17 to Th1/Th2 by treatment with anti-transforming growth factor β antibody generates immunological memory and protective immunity. mBio (2011) 2(3):e00095–11. doi: 10.1128/mBio.00095-11
73. Liu Y, Islam EA, Jarvis GA, Gray-Owen SD, Russell MW. Neisseria gonorrhoeae selectively suppresses the development of Th1 and Th2 cells, and enhances Th17 cell responses, through TGF-β-dependent mechanisms. Mucosal Immunol (2012) 5(3):320–31. doi: 10.1038/mi.2012.12
74. Liu Y, Liu W, Russell MW. Suppression of host adaptive immune responses by Neisseria gonorrhoeae: role of interleukin 10 and type 1 regulatory T cells. Mucosal Immunol (2014) 7(1):165–76. doi: 10.1038/mi.2013.36
75. Liu Y, Egilmez NK, Russell MW. Enhancement of adaptive immunity to Neisseria gonorrhoeae by local intravaginal administration of microencapsulated interleukin 12. J Infect Dis (2013) 208(11):1821–9. doi: 10.1093/infdis/jit354
76. Liu Y, Perez J, Hammer LA, Gallagher HC, De Jesus M, Egilmez NK, et al. Intravaginal Administration of Interleukin 12 during Genital Gonococcal Infection in Mice Induces Immunity to Heterologous Strains of Neisseria gonorrhoeae. mSphere (2018) 3(1):e00421-17. doi: 10.1128/mSphere.00421-17
77. Liu Y, Hammer LA, Daamen J, Stork M, Egilmez NK, Russell MW. Microencapsulated IL-12 drives genital tract immune responses to intranasal gonococcal outer membrane vesicle vaccine and induces resistance to vaginal infection with diverse strains of neisseria gonorrhoeae. mSphere (2022) 8(1):e0038822. doi: 10.1128/msphere.00388-22
78. Gulati S, Zheng B, Reed GW, Su X, Cox AD, St Michael F, et al. Immunization against a saccharide epitope accelerates clearance of experimental gonococcal infection. PloS Pathog (2013) 9(8):e1003559. doi: 10.1371/journal.ppat.1003559
79. Gulati S, Beurskens FJ, de Kreuk BJ, Roza M, Zheng B, DeOliveira RB, et al. Complement alone drives efficacy of a chimeric antigonococcal monoclonal antibody. PloS Biol (2019) 17(6):e3000323. doi: 10.1371/journal.pbio.3000323
80. Kasper DL, Rice PA, McCormick WM. Bactericidal antibody in genital infection due to Neisseria gonorrhoeae. J Infect Dis (1977) 135(2):243–51. doi: 10.1093/infdis/135.2.243
81. Hook EW, Olsen DA, Buchanan TM. Analysis of the antigen specificity of the human serum immunoglobulin G immune response to complicated gonococcal infection. Infect Immun (1984) 43(2):706–9. doi: 10.1128/iai.43.2.706-709.1984
82. Buchanan TM, Eschenbach DA, Knapp JS, Holmes KK. Gonococcal salpingitis is less likely to recur with Neisseria gonorrhoeae of the same principal outer membrane protein antigenic type. Am J Obstet Gynecol (1980) 138(7 Pt 2):978–80. doi: 10.1016/0002-9378(80)91091-1
83. Plummer FA, Simonsen JN, Chubb H, Slaney L, Kimata J, Bosire M, et al. Epidemiologic evidence for the development of serovar-specific immunity after gonococcal infection. J Clin Invest (1989) 83(5):1472–6. doi: 10.1172/JCI114040
84. Hedges SR, Mayo MS, Mestecky J, Hook EW, Russell MW. Limited local and systemic antibody responses to Neisseria gonorrhoeae during uncomplicated genital infections. Infect Immun (1999) 67(8):3937–46. doi: 10.1128/IAI.67.8.3937-3946.1999
85. Hedges SR, Sibley DA, Mayo MS, Hook EW, Russell MW. Cytokine and antibody responses in women infected with Neisseria gonorrhoeae: effects of concomitant infections. J Infect Dis (1998) 178(3):742–51. doi: 10.1086/515372
86. Gagliardi MC, Starnino S, Teloni R, Mariotti S, Dal Conte I, Di Carlo A, et al. Circulating levels of interleukin-17A and interleukin-23 are increased in patients with gonococcal infection. FEMS Immunol Med Microbiol (2011) 61(1):129–32. doi: 10.1111/j.1574-695X.2010.00759.x
87. Masson L, Salkinder AL, Olivier AJ, McKinnon LR, Gamieldien H, Mlisana K, et al. Relationship between female genital tract infections, mucosal interleukin-17 production and local T helper type 17 cells. Immunology (2015) 146(4):557–67. doi: 10.1111/imm.12527
88. Mensforth S, Ayinde OC, Ross J. Spontaneous clearance of genital and extragenital Neisseria gonorrhoeae: data from GToG. Sex Transm Infect (2020) 96(8):556–61. doi: 10.1136/sextrans-2020-054500
89. Edwards JL, Apicella MA. The molecular mechanisms used by Neisseria gonorrhoeae to initiate infection differ between men and women. Clin Microbiol Rev (2004) 17(4):965–81. doi: 10.1128/CMR.17.4.965-981.2004
90. Gulati S, Rice PA, Ram S. Complement-dependent serum bactericidal assays for neisseria gonorrhoeae. Methods Mol Biol (2019) 1997:267–80. doi: 10.1007/978-1-4939-9496-0_16
91. Hobbs MM, Duncan JA. Experimental human infection with neisseria gonorrhoeae. Methods Mol Biol (2019) 1997:431–52. doi: 10.1007/978-1-4939-9496-0_25
92. Seifert HS, Wright CJ, Jerse AE, Cohen MS, Cannon JG. Multiple gonococcal pilin antigenic variants are produced during experimental human infections. J Clin Invest (1994) 93(6):2744–9. doi: 10.1172/JCI117290
93. Jerse AE, Cohen MS, Drown PM, Whicker LG, Isbey SF, Seifert HS, et al. Multiple gonococcal opacity proteins are expressed during experimental urethral infection in the male. J Exp Med (1994) 179(3):911–20. doi: 10.1084/jem.179.3.911
94. Schneider H, Griffiss JM, Boslego JW, Hitchcock PJ, Zahos KM, Apicella MA. Expression of paragloboside-like lipooligosaccharides may be a necessary component of gonococcal pathogenesis in men. J Exp Med (1991) 174(6):1601–5. doi: 10.1084/jem.174.6.1601
95. Schneider H, Schmidt KA, Skillman DR, Van De Verg L, Warren RL, Wylie HJ, et al. Sialylation lessens the infectivity of Neisseria gonorrhoeae MS11mkC. J Infect Dis (1996) 173(6):1422–7. doi: 10.1093/infdis/173.6.1422
96. Hobbs MM, Anderson JE, Balthazar JT, Kandler JL, Carlson RW, Ganguly J, et al. Lipid A's structure mediates Neisseria gonorrhoeae fitness during experimental infection of mice and men. mBio (2013) 4(6):e00892–13. doi: 10.1128/mBio.00892-13
97. Johannsen DB, Johnston DM, Koymen HO, Cohen MS, Cannon JG. A Neisseria gonorrhoeae immunoglobulin A1 protease mutant is infectious in the human challenge model of urethral infection. Infect Immun (1999) 67(6):3009–13. doi: 10.1128/IAI.67.6.3009-3013.1999
98. Cohen IR, Kellogg DS, Norins LC. Serum antibody response in experimental human gonorrhoea. Immunoglobulins G, A and M. Br J Vener Dis (1969) 45(4):325–7. doi: 10.1136/sti.45.4.325
99. Kellogg DS, Cohen IR, Norins LC, Schroeter AL, Reising G. Neisseria gonorrhoeae. II. Colonial variation and pathogenicity during 35 months in vitro. J Bacteriol (1968) 96(3):596–605. doi: 10.1128/jb.96.3.596-605.1968
100. Ramsey KH, Schneider H, Cross AS, Boslego JW, Hoover DL, Staley TL, et al. Inflammatory cytokines produced in response to experimental human gonorrhea. J Infect Dis (1995) 172(1):186–91. doi: 10.1093/infdis/172.1.186
101. Schmidt KA, Schneider H, Lindstrom JA, Boslego JW, Warren RA, Van de Verg L, et al. Experimental gonococcal urethritis and reinfection with homologous gonococci in male volunteers. Sex Transm Dis (2001) 28(10):555–64. doi: 10.1097/00007435-200110000-00001
102. Greenberg L, Diena BB, Ashton FA, Wallace R, Kenny CP, Znamirowski R, et al. Gonococcal vaccine studies in Inuvik. Can J Public Health (1974) 65(1):29–33.
104. Paynter J, Goodyear-Smith F, Morgan J, Saxton P, Black S, Petousis-Harris H. Effectiveness of a group B outer membrane vesicle meningococcal vaccine in preventing hospitalization from Gonorrhea in New Zealand: A retrospective cohort study. Vaccines (Basel) (2019) 7(1), 5. doi: 10.3390/vaccines7010005
105. Petousis-Harris H, Radcliff FJ. Exploitation of Neisseria meningitidis Group B OMV Vaccines Against N. gonorrhoeae to Inform the Development and Deployment of Effective Gonorrhea Vaccines. Front Immunol (2019) 10:683. doi: 10.3389/fimmu.2019.00683
106. Bruxvoort KJ, Lewnard JA, Chen LH, Tseng HF, Chang J, Marrazzo J, et al. Prevention of Neisseria gonorrhoeae with meningococcal B vaccine: a matched cohort study in Southern California. Clin Infect Dis (2022) 76(3):e1341–e9. doi: 10.1093/cid/ciac436
107. Raccagni AR, Galli L, Spagnuolo V, Bruzzesi E, Muccini C, Bossolasco S, et al. Meningococcus B vaccination effectiveness against neisseria gonorrhoeae infection in people living with HIV: A case-control study. Sex Transm Dis (2023) 50(5):247–51. doi: 10.1097/OLQ.0000000000001771
108. Craig AP, Gray RT, Edwards JL, Apicella MA, Jennings MP, Wilson DP, et al. The potential impact of vaccination on the prevalence of gonorrhea. Vaccine (2015) 33(36):4520–5. doi: 10.1016/j.vaccine.2015.07.015
109. Hui BB, Padeniya TN, Rebuli N, Gray RT, Wood JG, Donovan B, et al. A gonococcal vaccine has the potential to rapidly reduce the incidence of neisseria gonorrhoeae infection among urban men who have sex with men. J Infect Dis (2022) 225(6):983–93. doi: 10.1093/infdis/jiab581
110. Whittles LK, Didelot X, White PJ. Public health impact and cost-effectiveness of gonorrhoea vaccination: an integrated transmission-dynamic health-economic modelling analysis. Lancet Infect Dis (2022) 22(7):1030–41. doi: 10.1016/S1473-3099(21)00744-1
111. Looker KJ, Booton R, Begum N, Beck E, Shen J, Turner KME, et al. The potential public health impact of adolescent 4CMenB vaccination on Neisseria gonorrhoeae infection in England: a modelling study. BMC Public Health (2023) 23(1):1. doi: 10.1186/s12889-022-14670-z
112. Semchenko EA, Tan A, Borrow R, Seib KL. The serogroup B meningococcal vaccine bexsero elicits antibodies to neisseria gonorrhoeae. Clin Infect Dis (2019) 69(7):1101–11. doi: 10.1093/cid/ciy1061
113. Leduc I, Connolly KL, Begum A, Underwood K, Darnell S, Shafer WM, et al. The serogroup B meningococcal outer membrane vesicle-based vaccine 4CMenB induces cross-species protection against Neisseria gonorrhoeae. PloS Pathog (2020) 16(12):e1008602. doi: 10.1101/2020.05.13.094177
114. Thng C, Semchenko EA, Hughes I, O'Sullivan M, Seib KL. An open-label randomised controlled trial evaluating the efficacy of a meningococcal serogroup B (4CMenB) vaccine on Neisseria gonorrhoeae infection in gay and bisexual men: the MenGO study protocol. BMC Public Health (2023) 23(1):607. doi: 10.1186/s12889-023-15516-y
115. Matthias KA, Connolly KL, Begum AA, Jerse AE, Macintyre AN, Sempowski GD, et al. Meningococcal detoxified outer membrane vesicle vaccines enhance gonococcal clearance in a murine infection model. J Infect Dis (2022) 225(4):650–60. doi: 10.1093/infdis/jiab450
116. Zielke RA, Wierzbicki IH, Baarda BI, Gafken PR, Soge OO, Holmes KK, et al. Proteomics-driven antigen discovery for development of vaccines against gonorrhea. Mol Cell Proteomics (2016) 15(7):2338–55. doi: 10.1074/mcp.M116.058800
117. El-Rami FE, Zielke RA, Wi T, Sikora AE, Unemo M. Quantitative proteomics of the 2016 WHO. Mol Cell Proteomics (2019) 18(1):127–50. doi: 10.1074/mcp.RA118.001125
118. Baarda BI, Zielke RA, Holm AK, Sikora AE. Comprehensive bioinformatic assessments of the variability of neisseria gonorrhoeae vaccine candidates. mSphere (2021) 6(1):e00977-20. doi: 10.1128/mSphere.00977-20
119. Zhu T, McClure R, Harrison OB, Genco C, Massari P. Integrated Bioinformatic Analyses and Immune Characterization of New Neisseria gonorrhoeae Vaccine Antigens Expressed during Natural Mucosal Infection. Vaccines (Basel) (2019) 7(4), 153. doi: 10.3390/vaccines7040153
120. Sikora AE, Gomez C, Le Van A, Baarda BI, Darnell S, Martinez FG, et al. A novel gonorrhea vaccine composed of MetQ lipoprotein formulated with CpG shortens experimental murine infection. Vaccine (2020) 38(51):8175–84. doi: 10.1016/j.vaccine.2020.10.077
121. Fegan JE, Calmettes C, Islam EA, Ahn SK, Chaudhuri S, Yu RH, et al. Utility of hybrid transferrin binding protein antigens for protection against pathogenic neisseria species. Front Immunol (2019) 10:247. doi: 10.3389/fimmu.2019.00247
Keywords: immunology, gonococcus, Neisseria, STI, vaccines, gonorrhea
Citation: Belcher T, Rollier CS, Dold C, Ross JDC and MacLennan CA (2023) Immune responses to Neisseria gonorrhoeae and implications for vaccine development. Front. Immunol. 14:1248613. doi: 10.3389/fimmu.2023.1248613
Received: 27 June 2023; Accepted: 26 July 2023;
Published: 17 August 2023.
Edited by:
Laurel L. Lenz, University of Colorado Anschutz Medical Campus, United StatesReviewed by:
Michael W. Russell, University at Buffalo, United StatesAlison Criss, University of Virginia, United States
Copyright © 2023 Belcher, Rollier, Dold, Ross and MacLennan. This is an open-access article distributed under the terms of the Creative Commons Attribution License (CC BY). The use, distribution or reproduction in other forums is permitted, provided the original author(s) and the copyright owner(s) are credited and that the original publication in this journal is cited, in accordance with accepted academic practice. No use, distribution or reproduction is permitted which does not comply with these terms.
*Correspondence: Thomas Belcher, thomas.belcher@ndm.ox.ac.uk