- 1Institute of Cell Biochemistry, Hannover Medical School, Hannover, Germany
- 2Kusuma School of Biological Sciences, Indian Institute of Technology Delhi, New Delhi, India
- 3Institute of Medical Microbiology and Hospital Epidemiology, Hannover Medical School, Hannover, Germany
- 4German Center for Infection Research (DZIF), Partner Site Hannover-Braunschweig, Hannover, Germany
Autophagy plays an important role in recognizing and protecting cells from invading intracellular pathogens such as Salmonella. In this work, we investigated the role of p38MAPK/MK2 in modulating the host cell susceptibility to Salmonella infection. Inhibition of p38MAPK or MK2 led to a significant increase of bacterial counts in Salmonella infected mouse embryonic fibroblasts (MEFs), as well as in MK2-deficient (Mk2-/-) cells. Furthermore, western blot analysis showed that Mk2-/- cells have lower level of LC3 lipidation, which is the indicator of general autophagy compared to Mk2-rescued cells. In Mk2-/- cells, we also observed lower activated TANK-binding kinase-1 phosphorylation on Ser172 and p62/SQTM1-Ser403 phosphorylation, which are important to promote the translocation of p62 to ubiquitinated microbes and required for efficient autophagy of bacteria. Furthermore, immunofluorescence analysis revealed reduced colocalization of Salmonella with LC3 and p62 in MEFs. Inhibition of autophagy with bafilomycin A1 showed increased bacterial counts in treated cells compared to control cell. Overall, these results indicate that p38MAPK/MK2-mediated protein phosphorylation modulates the host cell susceptibility to Salmonella infection by affecting the autophagy pathways.
1 Introduction
Autophagy is a highly conserved and tightly regulated process of intracellular protein degradation in eukaryotic cells that is activated by cellular stressors such as nutrient starvation, damaged organelles, misfolded proteins and proteotoxic aggregates, infections etc. (1, 2). In this mode of degradation, the cytoplasmic substrates are engulfed into double-membrane vesicles, called autophagosomes, which then fuse with lysosomes for eventual degradation. Autophagy is not only important in cell homeostasis, but is also crucial for the host to defend against intracellular pathogens (3). Selective autophagy, called xenophagy, represents a direct antimicrobial mechanism. It is mediated by ubiquitination and colocalization of pathogens with autophagy receptor proteins such as sequestosome 1(SQSTM1)/p62, nuclear dot protein 52 (NDP52/CALCOCO2) and optineurin (OPTN) (4, 5). Several studies have demonstrated diverse autophagy-evasion mechanisms by intracellular pathogens ranging from blocking of targeting to the autophagosomes, interfering with autophagosome-lysosome fusion to establish their niches inside the cells (5).
Salmonella enterica serovar Typhimurium (S. Typhimurium) is a Gram-negative and facultative intracellular bacterial pathogens that causes a variety of diseases from gastroenteritis to typhoid fever in humans and mice (6). S. Typhimurium uses its needle-like protein complex called type III secretion systems (T3SSs) encoded by Salmonella pathogenicity island 1 (SPI-1) and SPI-2 to deliver bacterial effectors to the host cells to manipulate host cell signaling, cytoskeletal and vesicular pathways (7). SPI-1 genes are important for bacterial invasion, whereas SPI-2 genes are required for intracellular bacterial survival (8). Upon invasion into mammalian cells, most Salmonella remain in a single membrane-bound compartment called the Salmonella-containing vacuole (SCV) that acquires late endosomal markers such as the lysosomal-associated membrane protein 1 (LAMP-1). However, some bacteria escape from the SCV and replicate rapidly in the host cell cytosol. A previous study showed that autophagy targets Salmonella found in damaged SCVs and in the cytosol for degradation and restricts Salmonella replication (9).
The p38MAPK pathway and its downstream signaling MAPKAP kinase 2 (MK2) are important for the induction of innate immunity and the regulation of inflammatory responses. They are involved in transcriptional and post-transcriptional regulation of cytokine expression (10–12). Our previous studies have shown that MK2-and MK2/3-deficient mice display an impaired inflammatory response against LPS-induced endotoxic shock and increased susceptibility to Listeria monocytogenes infection because of impaired production of TNF (13–15). Oral administration of S. Typhimurium activated the p38MAPK pathway in mice and inhibition of the p38MAPK pathway using SB203580, a specific reversible p38MAPK inhibitor, was shown to abrogate S. Typhimurium-triggered expression of the antimicrobial peptide cryptdin in Paneth cells in vivo (16). The cellular and molecular mechanism how p38MAPK/MK2 influence the survival of S. Typhimurium remains elusive. Here, we demonstrate that the p38MAPK/MK2 signaling axis regulates intracellular S. Typhimurium survival by modulating autophagy pathways in the host cells.
2 Materials and methods
2.1 Bacteria
S. Typhimurium SL1344 (S. Tm) WT (17), S. Tm ΔinvA, S. Tm ΔsopB, S. Tm ΔsopE/E2, S. Tm ΔavrA and S.Tm eGFP (pFPV25.1) (18) were grown overnight at 37°C with shaking in lysogeny broth (LB) supplemented with 100 μg/ml streptomycin, 100 μg/mL ampicillin, or 50 μg/mL kanamycin, when appropriate.
2.2 Cell lines
Mouse embryonic fibroblast (MEFs) WT, MK2 deficient cells transduced with retroviral constructs pMMP–IRES–GFP (vector), MK2 deficient cells transduced with retroviral constructs pMMP–MK2–IRES–GFP (MK2), MK2 deficient cells transduced with retroviral constructs pMMP–MK2KR–IRES–GFP (MK2-K79R), kinase-dead MK2 mutants in which lysine79 in catalytic subdomain I is replaced with arginine, MK2 deficient HEK293T cells (MK2-/-), MK2 sufficient HEK293T (MK2+/+), immortalized bone marrow derived macrophages (iBMDM) from MK2 deficient cells transduced with retroviral constructs pMMP–IRES–GFP (vector), MK2 deficient cells transduced with retroviral constructs pMMP–MK2–IRES–GFP (MK2). The generation of MEF cell line was done by cotransfection of primary MEFs with pSV40Tag encoding simian virus 40 large T-antigen and pREP8 plasmid (Invitrogen) in a 10:1 mixture. Colonies were selected with 3 mM histidinol (Sigma) (19). Immortalized murine macrophages (iBMDMs) were established from bone marrow-derived macrophages (BMDM) that were isolated from mice deficient for MK2 or from wild type mice by retroviral infection with a virus encoding both the v-Raf and the v-Myc oncogenes as described in detail previously (20). Bone marrow derived macrophages (BMDM) were isolated from Mk2+/+ and Mk2-/- mice were cultivated as described (21). All cells were cultured in DMEM GlutaMAX (DMEM-Life Technologies) supplemented with 10% (v/v) fetal bovine serum and 1x Penicillin Streptomycin.
2.3 Gentamicin protection assay
Cells as indicated were seeded in 24-well plates (105 cells/well) [for bacterial count and immunofluorescence analysis (IF)] or 6- well plates (4x105 cells/well) [for western blot (WB)] and incubated overnight in 5% CO2 at 37°C. The next day, culture medium was replaced with DMEM without Penicillin Streptomycin and indicated substance such as DMSO, the pan-p38 inhibitor BIRB-796 (BIRB, 1µM), the MK2 inhibitor PF-3644022 (PF22, 5µM), the autophagy inhibitor Bafilomycin A1 (BafA1, 20nM), the mTOR inhibitor Rapamycin (1µM), the TBK1 inhibitor BX795 (1µM), or TNF-α (20ng/mL) for at least one hour before infection. Cells were then infected with different Salmonella strains at MOI 10, 100, 500, 1000 (as indicated) for 1 hours. For IF and WB, cells were infected at MOI 100. Cells were washed 3 times with PBS and culture medium was replaced with medium supplemented with 100 μg/mL gentamicin (Sigma) and indicated substances as above for 1 hours to kill extracellular bacteria. For 2 hours post infection time point, cells were lysed in PBS containing 1% (v/v) Triton X-100 and 0.1% (v/v) sodium dodecyl sulfate (SDS) (for bacterial counts) or were fixed with 4% PFA (for IF) or lysed in with Laemmli loading buffer (for WB). The cell lysates were then serially diluted in PBS and plated on LB agar for colony-forming unit (CFU) count. For later time points, culture medium was replaced with a medium supplemented with 10 μg/mL gentamicin (Sigma).
2.4 Immunofluorescence
Fixed cells were washed 3 times with PBS, and permeabilized with Triton-X100 (0.25%) in PBS for 30 min at RT. Blocking was done using 4% bovine serum albumin (BSA) in PBS (blocking buffer) for 1 h at 4°C. Cells were then incubated with first antibodies in blocking buffer and incubated overnight at 4°C. The following first antibodies were used: LC3 (mbl international), p62 (Abcam), Salmonella O Antiserum Group B (BD Difco), Salmonella Typhimurium (Clone: B2363M, Meridian Life Science), LAMP-1 (eBioscience) and Anti-Ubiquitinylated proteins Antibody, clone FK2 (Sigma). After 3 times washing with PBS, secondary antibodies were added and incubated for 1h at RT. The following secondary antibodies were used: Alexa (Ax) 488- conjugated goat anti-mouse IgG, Ax488-conjugated goat anti-rabbit IgG, Ax555-conjugated goat anti-rabbit IgG, Ax568-conjugated goat anti-mouse IgG, Ax568-conjugated goat anti-rat IgG (Thermofisher Scientific). Phalloidin-iFluor 647 (1:1000) (Abcam) and DAPI (Invitrogen) were applied to visualize F-actin and nuclei, respectively. Images were obtained on a Cytation 1 (BioTek) at 20x magnification in 5-10 fields of view per samples using Gen 11 software (BioTek). Brightness and contrast were adjusted using ImageJ 1.53k software. Gen 11 software (BioTek) was used to quantify median fluorescence intensity (MFI) colocalization of LC3 and p62 with S.Tm eGFP and percentage of colocalization of LAMP-1 and ubiquitinated protein with Salmonella. GFP staining with size of 0.5 – 5 µm was defined as primary mask to identify the bacteria, then the mask was expanded for 1 µm. For p62 and LC3 colocalization with Salmonella, MFI of Ax555 (LC3) and Ax568 (p62) inside the primary mask were measured. The results are defined as the median of Ax555 or Ax568 fluorescence intensity colocalized with STm-GFP. For LAMP-1 and ubiquitin colocalization with Salmonella, MFI of Ax568 inside the primary mask were measure. The threshold was determined from highest MFI of the bacteria completely absence of LAMP-1 or ubiquitin staining. Furthermore, the percentage of LAMP-1 or ubiquitin positive staining were calculated and determined. The results are defined as percentage of LAMP-1 or ubiquitin positive staining to total bacteria.
2.5 Western blot
Cells were directly lysed by boiling in 2x SDS-PAGE loading buffer then separated by SDS-PAGE and transferred to polyvinylidene difluoride (PVDF) membrane. After blocking with 3% milk in PBS with 0.1% Tween 20, membranes were incubated with primary antibody in PBS with 3%BSA and 0.1% Tween 20. The following primary antibodies were used: LC3 (MBL International), p62 (Abcam), p-p62 Ser403 (MBL International), TBK1/NAK, p-TBK1/NAK Ser172, Atg5 (D5F5U), p-MK2 (Phospho-MAPKAPK-2) Thr222, MK2 (MAPKAPK-2) (Cell Signaling Technology), EF-2 (Santa Cruz Biotech), GAPDH (Abcam). Immunoreactive bands were visualized using appropriate secondary antibodies and enhanced chemiluminescence detection reagents. Images were obtained using the ImageQuant LAS 3000 system.
2.6 Statistical analysis
Data were analyzed using Prism V7.0d software (GraphPad). Statistical analysis was done using one-way analysis of variance (ANOVA) followed by Tukey’s multiple comparison test or T-Test as indicated. Graphs display the mean values ± SD, unless stated otherwise.
3 Results
3.1 p38MAPK/MK2 signaling restricts intracellular infection by Salmonella
To investigate the role and detailed mechanisms of p38MAPK/MK2 pathway in cellular response to Salmonella infection, we first performed the gentamicin protection assay for infection efficacy. Therefore, we infected murine embryonic fibroblast (MEFs) with S. Typhimurium for 1h, treated cells with gentamicin to inactivate extracellular bacteria, lysed cells and counted bacteria numbers at 2 and 6 hours post infection (p.i.). Interestingly, immunofluorescence analysis of individual cells infected with S. Typhimurium expressing GFP showed increased bacterial number after p38MAPK inhibitor BIRB796 (BIRB) and MAPKAPK2 (MK2) inhibitor PF-3644022 (PF22) treatment at 2h p.i. and 6h p.i. (Figures 1A, B). Furthermore, we observed increased S. Typhimurium counts in the presence of the BIRB and the PF22 (Figure 1C). To convincingly prove that MK2 contributes to S. Typhimurium control, we performed similar experiments in MK2-deficient MEFs. Consistent with the findings from pharmacological inhibition of p38/MK2 pathway, cells lacking MK2 showed higher intracellular counts of GFP-labelled bacteria compared to cells rescued with retroviral expression of MK2 (Figures 1D, E). Furthermore, MK2-deficient MEFs and MEFs expressing a kinase-dead MK2 mutant in which lysine 79 in the ATP-binding catalytic subdomain I is replaced with arginine (19) showed higher bacterial counts compared to wild type Mk2-rescued MEFs at 2 hours and 6 hours p.i. (Figure 1F). Interestingly, unlike at later time points, at 90 minutes p.i., MK2-deficient and wild-type Mk2-rescued MEFs showed similar bacterial counts (Supplementary Figure 1A). This indicates that there are similar levels of invasion of Salmonella in both cell lines, however the differences arise after cell entry.
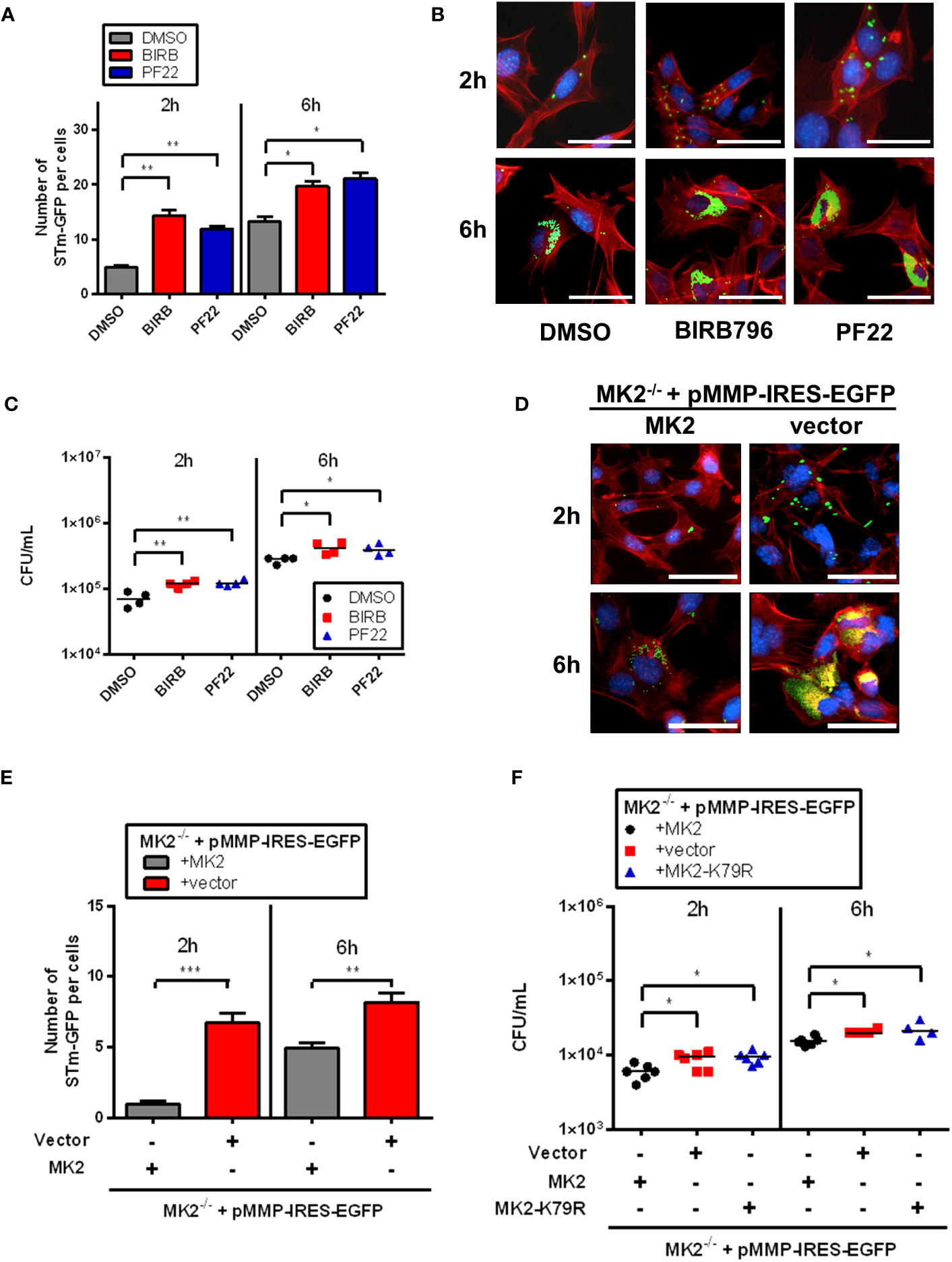
Figure 1 p38MAPK/MK2 signaling influence survival of intracellular S. Typhimurium. (A) Quantification and (B) immunofluorescence staining of MEF WT treated with DMSO (grey), BIRB (red) and PF22 (blue) were infected with STm-GFP (green), fixed at 2h and 6h p.i. Nuclei were stained with DAPI (blue). F-actin (red) were stained with Phalloidin-647. The results are defined as number of STm-GFP per cells. Original magnification: 200x. Scale bars = 100 μm. One-way ANOVA with Tukey’s multiple comparison test, *p<0.05, **p<0.01. (C) MEF WT treated with DMSO (black closed circle), BIRB (red closed square) and PF22 (blue closed triangle) were infected with S. Typhimurium (STm) WT at MOI 10 for 1 h, washed three times with PBS, then incubated for 1 hours in media supplemented with 100 μg/mL gentamicin. At 2h and 6h p.i, cells were lysed, serially diluted and plated on LB agar plates to quantify intracellular bacteria. The results are defined as colony forming unit (CFU)/mL. One-way ANOVA with Tukey’s multiple comparison test, *p<0.05, **p<0.01. (D) Immunofluorescence staining and (E) quantification of Mk2-rescued (MK2-grey) and Mk2-/- (red) MEFs were lentivirally transduced with vectors for empty control (vector) were infected with STm-GFP (green), fixed at 2h and 6h p.i. Nuclei were stained with DAPI (blue). F-actin (red) were stained with Phalloidin-647. The results are defined as number of STm-GFP per cells. Original magnification: 200x. Scale bars = 100 μm. Unpaired t-test. **p < 0.01, ***p < 0.001. (F) Mk2-rescued (black closed circle), Mk2-/- (red closed square) and MEFs expressing a kinase-dead MK2 mutant in which lysine 79 (Mk2K79R, blue closed triangle) were infected with S. Typhimurium WT at MOI 10 for 1 hour, washed three times with PBS, then incubated for 1 hours in media supplemented with 100 μg/mL gentamicin. At 2h and 6h p.i, the cells were lysed, collected, serially diluted and plated on LB agar plates to quantify intracellular bacteria. The results are defined as CFU/mL. One-way ANOVA with Tukey’s multiple comparison test, *p<0.05. Data points, means and SD of representative results of at least three independent experiments are depicted.
Macrophages are important hosts for intracellular Salmonella and hence we also verified our findings in primary bone-marrow-derived macrophages (BMDMs). We used immortalized bone marrow-derived macrophages (iBMDM) with a retroviral MK2 rescue and obtained similar results at 4 hours p.i (Supplementary Figure 1B). Furthermore, we generated and compared BMDMs from MK2-deficient and wild-type mice in similar assays and observed higher intracellular Salmonella counts in MK2-deficient BMDMs (Supplementary Figure 1C). Moreover, similar results were observed in MK2-deficient HEK293T cells compared to wild-type control cells (MK2 WT) at 2 hours p.i. (Supplementary Figure 1D). Thus, our findings show that p38MAPK/MK2-mediated protein phosphorylation modulates the host cell susceptibility to Salmonella infection in different relevant cell types.
3.2 MK2-deficient MEFs display a higher abundance of cytosolic Salmonella
After entry into the host cell, Salmonella resides within a modified phagosomal compartment termed the Salmonella-containing vacuole (SCV) that acquire late endosomal markers, such as LAMP-1. Most intracellular Salmonella remain and replicate in the SCV. However, a small percentage of bacteria can damage the SCV and escape into the host cytosol. Cytosolic Salmonella are rapidly recognized by the ubiquitin E3 ligases resulting in the formation of ubiquitin chain layer surrounding the bacteria, marked by autophagy adaptor molecules for later downstream autophagy activation to eliminate the bacteria. Meanwhile, Salmonella residing in damaged SCVs can also be targeted for autophagic elimination (9). To investigate whether p38MAPK/MK2 signaling affects subcellular localization of intracellular bacteria, we infected MEFs with S. Typhimurium-GFP and analyzed the samples by co-immunofluorescence microscopy using an anti-LAMP1 antibody. At 2 h p.i., a higher percentage of bacteria colocalized with LAMP-1 (SCV) in MK2 expressing MEFs (93.4%) compared to MK2-deficient MEFs (85.9%) (Figure 2A). We also observed a higher percentage of Salmonella colocalized with ubiquitin in MK2-/- MEFs (11.23%) suggesting that higher abundance of cytosolic Salmonella in Mk2-deficient MEFs compared to Mk2-rescued MEFs (2.33%) (Figure 2B).
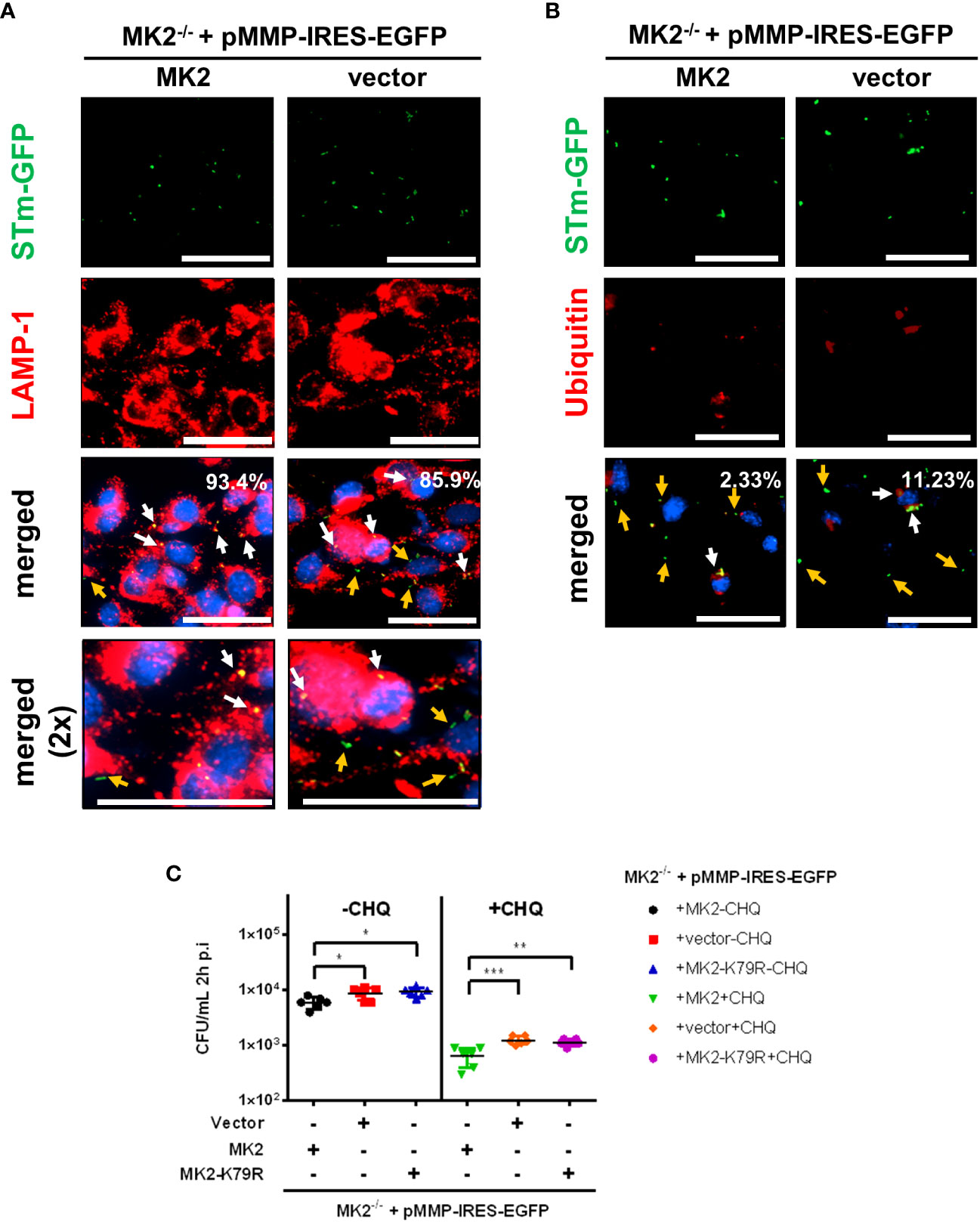
Figure 2 In cells lacking MK2, bacteria are abundance in host cytosol. Mk2-rescued (MK2) and Mk2-/- (vector) were infected with STm-GFP (green) at MOI 100. Infected cells were fixed at 2h p.i, stained with (A) anti-LAMP-1 and (B) anti-ubiquitin antibody followed by Ax568-labeled secondary antibody (red). Percent colocalization was determined by counting the number of bacteria with higher MFI from predetermined threshold to the total bacteria. White arrows showed colocalized STm, whereas orange arrows showed non-colocalized STm. Nuclei were stained with DAPI (blue). The experiment shown is representative of three independent experiments. (C) Mk2-rescued (MK2), Mk2-/- (vector) and Mk2K79R MEFs were infected with STm WT at MOI 10. Medium with gentamicin (100 μg/mL) and with (+CHQ) or without (-CHQ) 400 μM chloroquine were added 1 hour after infection. At 2h p.i, cells were washed with PBS and lysed. The results are defined as CFU/mL. One-way ANOVA with Tukey’s multiple comparison test, *p<0.05, **p<0.01, ***p < 0.001. The experiment shown is representative of three independent experiments.
Subsequently, we used a combination of chloroquine (CHQ) resistance assay and gentamicin protection assay to quantitatively determine cytosolic Salmonella counts. CHQ is a lysosomotropic agent that accumulates only within the SCV and leads to the killing of vacuolar bacteria by a still unknown mechanisms (22). MK2-deficient and MK2KR -rescued MEFs showed higher viable Salmonella counts compared to wild-type Mk2-rescued MEFs at 2 hours p.i. with or without CHQ treatment (Figure 2C), indicating that MEF cells lacking MK2 activity accumulate higher cytosolic Salmonella load.
3.3 The autophagic capture of Salmonella was affected by MK2 in MEFs
Autophagy, known for the degradation of cytosolic components in eukaryotic cells, plays an important role in recognizing and protecting cells from invading intracellular pathogens such as Salmonella. To understand the mechanisms of MK2 mediated control of susceptibility to Salmonella infection, we compared the levels of lipidated LC3 (LC3-II) levels between MK2-deficient and expressing MEFs infected with S. Typhimurium. We found that LC3-II levels after infection were lower in Mk2-/- MEFs compared to Mk2-rescued MEFs (Figure 3A). Non-lipidated LC3 (LC3-I) was also upregulated upon infection in both cell lines, with the MK2-rescued MEFs showing slightly increased levels. Mechanisms involved in this upregulation are unknown, but MAPK pathways have been previously reported to regulate LC3-I levels (23). p62 (also known as SQSTM1) is an autophagy receptor and substrate with multiple protein-protein interaction domains, including an ubiquitin-associated (UBA) domain for ubiquitinated cargo binding and an LC3 interaction region (LIR) for binding to LC3. Similar amount of SQSTM1/p62 were detected in the presence or absence of MK2 (Figure 3A). Interestingly, p62 phosphorylation at Ser403 was increased in Mk2-rescued MEFs at 2 and 4 h p.i and decreased again at 6 h p.i., even when compared to uninfected MEFs. In contrast, Mk2-/- MEFs showed lower p62 phosphorylation at Ser403 compared to Mk2-rescued MEFs at all-time points. In addition, no differences were detected in the levels of ATG5, a protein essential for the early steps in autophagosome formation (Figure 3A).
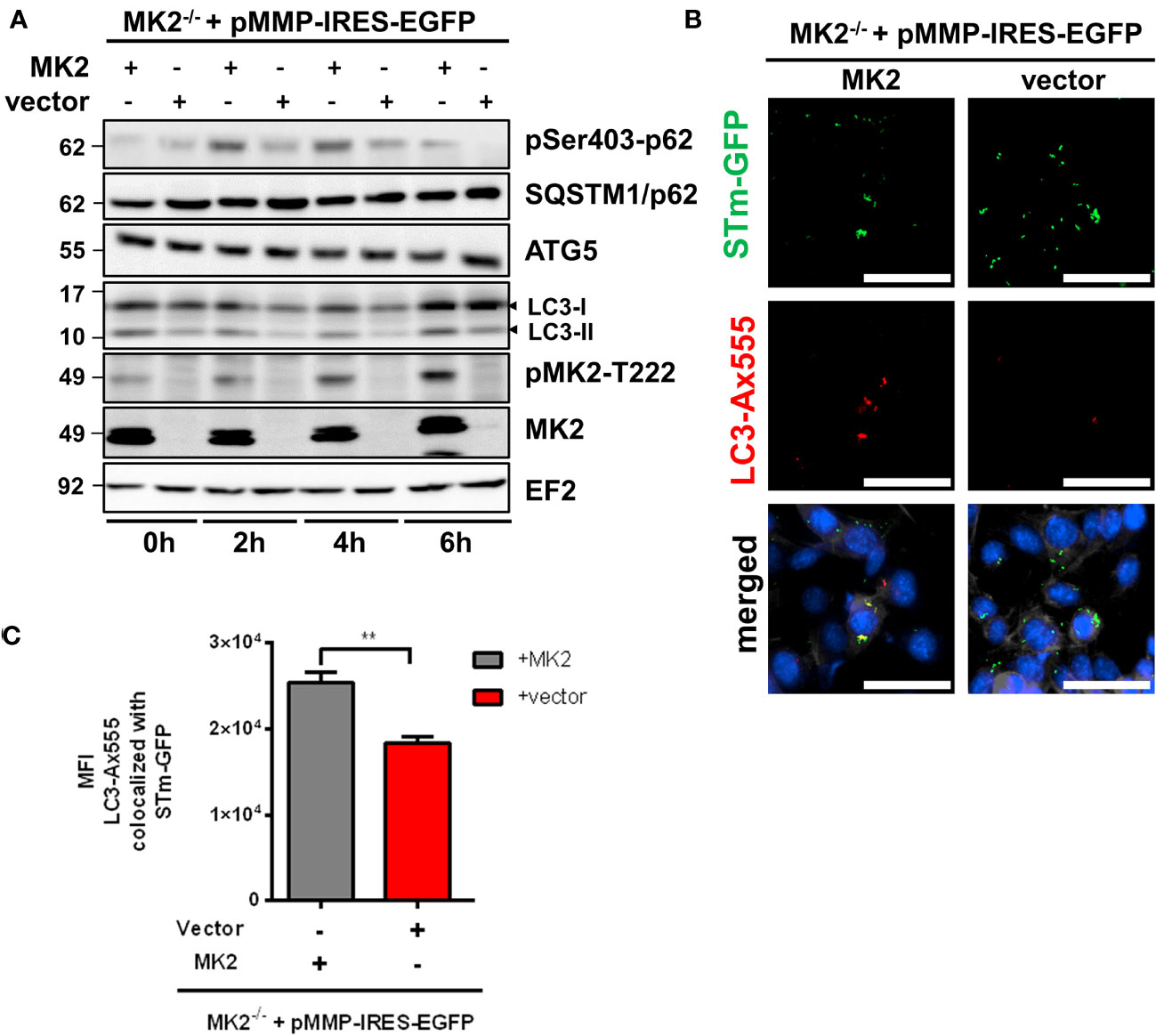
Figure 3 MK2 is required to activate and recruit the autophagy machinery to S. Typhimurium. (A) Mk2-rescued (MK2) and Mk2-/- (vector) were infected with STm WT at MOI 100. Western blot was performed to cells infected at 0, 2, 4 and 6h p.i,. Representative blots from three independent experiments are shown. (B) Mk2-rescued (MK2) and Mk2-/- (vector) were infected with STm-GFP (green) at MOI 100. Infected cells were fixed at 2h p.i, stained with LC3 antibody followed by Ax555-labeled secondary antibody (red). Nuclei were stained with DAPI (blue). F-actin (white) were stained with Phalloidin-647. Original magnification: 200x. Scale bars = 100 μm. (C) Quantification of LC3-Ax555 colocalized with STm-GFP. The results are defined as the median of Ax555 fluorescence intensity colocalized with STm-GFP. Unpaired t-test. **p < 0.01.
We then used immunofluorescence microscopy to specifically look at autophagic clearance of Salmonella. Co-immunofluorescence staining of MEFs infected with Salmonella expressing GFP showed reduced colocalization of Salmonella with LC3 in Mk2-/- MEFs in comparison to Mk2-rescued MEFs at 2 h p.i. (Figures 3B, C). Similar results were observed in the colocalization of Salmonella with the autophagy receptor p62/SQSTM1, known to be essential for efficient autophagy of bacteria (Supplementary Figures 2A, B). Inhibition of autophagy with bafilomycin A1 (BafA1) led to increased viable Salmonella counts in MK2-expressing MEFs, comparable to that in untreated MK2-deficient cells. This indicates that the positive role of MK2 in restricting Salmonella is probably via modulation of autophagy (Figure 4A). Consistently, there was no further increase in bacterial counts observed upon treating MK2-deficient cells with BafA1. Immunofluorescence analysis of individual cells infected with S. Typhimurium expressing GFP showed increased bacterial numbers after BafA1 treatment at 2h p.i. (Figures 4B, C). Furthermore, immunofluorescence staining also showed reduced colocalization of Salmonella with LC3 puncta in BafA1 treated cells (Figures 4C, D). Interestingly, activation of autophagy by treatment with the mTOR inhibitor rapamycin was sufficient to reduce the bacterial counts in MK2-deficient MEFs to levels comparable to that in MK2 expressing cells (Figure 4E). This clearly supports a model where p38/MK2 plays essential role in activating selective autophagy of bacterial cells.
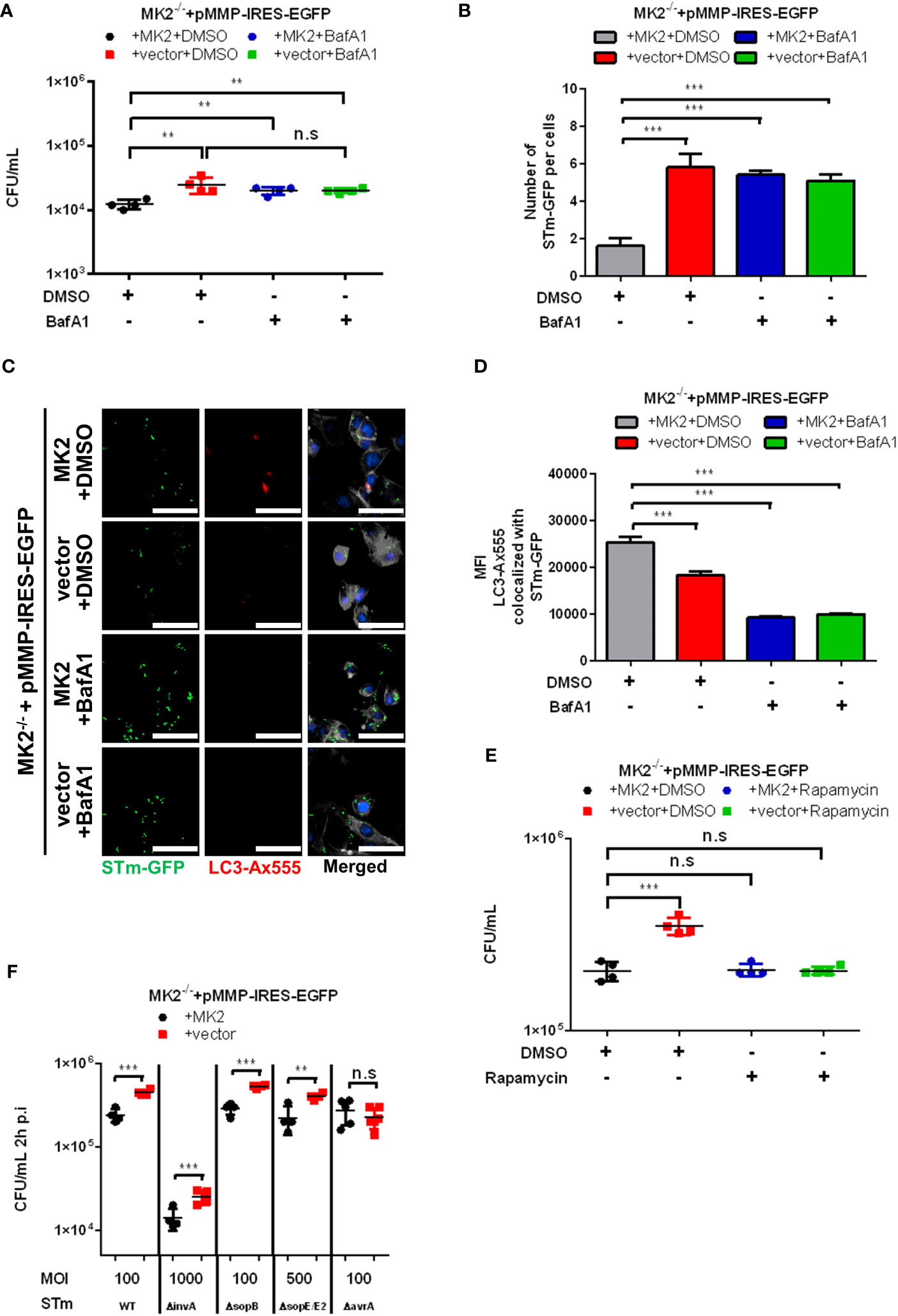
Figure 4 Autophagy activity is required to control intracellular S. Typhimurium. (A) Mk2-rescued (MK2) and Mk2-/- (vector) treated with DMSO or Bafilomycin A1 (BafA1) were infected with STm WT at MOI 10 for 1 hour, then washed with PBS. Medium with gentamicin (100 μg/mL) and with or without BafA1 were added. At 2h p.i, cells were washed with PBS and lysed. The results are defined as CFU/mL. One-way ANOVA with Tukey’s multiple comparison test, **p<0.01, n.s, not significant. The experiment shown is representative of three independent experiments. (B) Quantification and (C) immunofluorescence staining of Mk2-rescued (MK2) and Mk2-/- (vector) treated with DMSO or BafA1 were infected with STm-GFP (green) at MOI 100 for 1 hour. Infected cells were fixed at 2h p.i, stained with LC3 antibody followed by Ax568-labeled secondary antibody (red). Nuclei were stained with DAPI (blue). F-actin (white) were stained with Phalloidin-647. The results are defined as number of STm-GFP per cells. Original magnification: 200x. Scale bars = 100 μm. One-way ANOVA with Tukey’s multiple comparison test, ***p < 0.001. (D) Quantification of LC3-Ax555 colocalized with STm-GFP. The results are defined as the median of Ax555 fluorescence intensity colocalized with STm-GFP. One-way ANOVA with Tukey’s multiple comparison test, ***p < 0.001. The experiment shown is representative of three independent experiments. (E) Mk2-rescued (MK2) and Mk2-/- (vector) with DMSO or rapamycin were infected with STm WT at MOI 10 for 1 hour. Medium with gentamicin (100 μg/mL) and with or without rapamycin were added. At 2h p.i, cells were washed with PBS and lysed. The results are defined as CFU/mL. One-way ANOVA with Tukey’s multiple comparison test, n.s = not significant, ***p<0.001. The experiment shown is representative of three independent experiments. (F) Mk2-rescued (MK2) and Mk2-/- (vector) were infected with STm WT, STm ΔinvA, STm ΔsopB, STm ΔsopE/E2 and STm ΔavrA with stated MOI for 1 hour. Medium with gentamicin (100 μg/mL) were added. At 2h p.i, cells were washed with PBS and lysed. The results are defined as CFU/mL. Unpaired t-test. N.s, not significant, **p < 0.01, ***p < 0.001. The experiment shown is representative of three independent experiments.
It is known that Salmonella injects its effector proteins into the host cell cytosol by type III secretion system (T3SS) to avoid the autophagy pathway and for remodeling of the SCV for intracellular survival (24). The T3SS-1 translocated effectors play an important role to influence the host’s cellular functions to facilitate Salmonella invasion and mediate their persistence in the host cells (7). AvrA is one of the Salmonella effectors secreted by the SPI-1 T3SS and is known to inhibit the host c-Jun N-terminal kinase (JNK)/AP-1 and NF-κB signaling pathways (25) and to suppress autophagy by reducing Beclin-1 protein (26). Therefore, we then infected MEFs with S. Typhimurium ΔavrA and other SPI-1 mutants such as ΔinvA, ΔsopB, ΔsopE/ΔsopE2. MEFs infected with S. Typhimurium ΔavrA showed similar bacterial counts in both Mk2-/- and Mk2-rescued MEFs at 2h p.i. (Figure 4F) whereas, other SPI-1 mutants showed increased S. Typhimurium counts in Mk2-deficient MEFs at 2h p.i. compared to Mk2-rescued MEFs, similar to the S. Typhimurium WT strain infected MEFs (Figure 4F). These data support the idea that p38MAPK/MK2 signaling modulates the host cell susceptibility to Salmonella infection by affecting the autophagy pathways downstream to AvrA.
3.4 Exogenous TNF-α suppresses Salmonella counts in MK2-/- MEFs
It has been known for a long time that MK2 is essential for tumor necrosis factor-α (TNF) biosynthesis (13). Since a previous study also showed that TNF-stimulated p62-mediated autophagic activity restricted survival of Shigella and Listeria ActA mutant (27), we infected MEFs with S. Typhimurium after TNF treatment. Upon treatment with exogenous TNF, we observed decreased Salmonella counts in Mk2-/- MEFs in comparison to untreated Mk2-/- MEFs (Figure 5A). Immunofluorescence analysis in TNF treated S. Typhimurium infected Mk2-/- MEFs showed reduced bacterial number in comparison to untreated MEFs (Figures 5B, C). Hence, addition of exogenous TNF can stimulate cellular response, rescues MK2-deficiency and restricts the survival of S. Typhimurium.
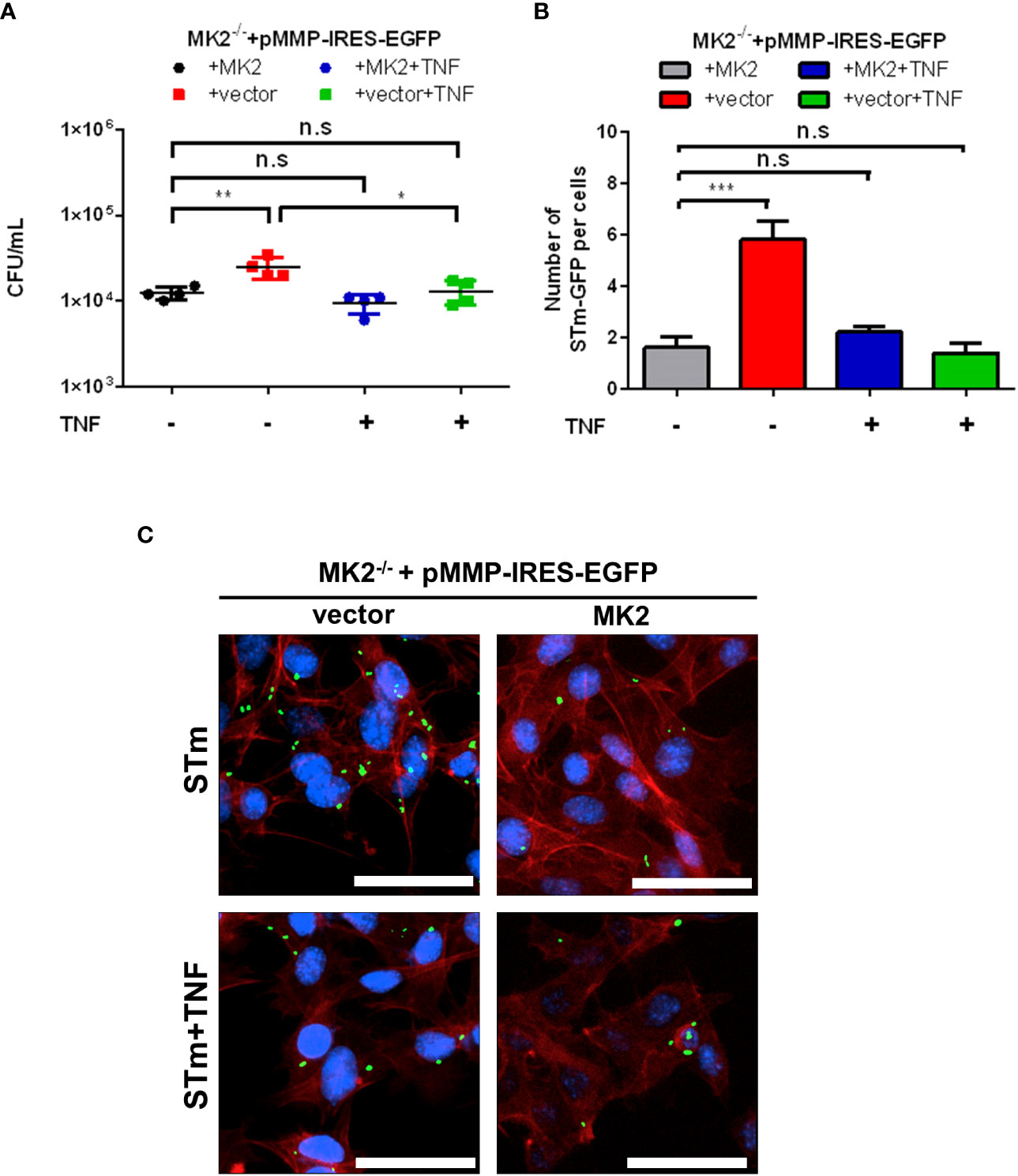
Figure 5 Exogenous TNF treatment influence intracellular S. Typhimurium survival in MEFs. (A) Mk2-rescued (MK2) and Mk2-/- (vector) treated with or without TNF were infected with STm WT at MOI 10 for 1 hour. Medium with gentamicin (100 μg/mL) and with or without TNF were added. At 2h p.i, cells were washed with PBS and lysed. The results are defined as CFU/mL. One-way ANOVA with Tukey’s multiple comparison test, n.s = not significant, *p<0.05, **p<0.01. The experiment shown is representative of three independent experiments. (B) Quantification and (C) immunofluorescence staining of Mk2-rescued (MK2) and MK2-/- (vector) treated with or without TNF were infected with STm-GFP (green) for 1 hour, fixed at 2h. Nuclei were stained with DAPI (blue). F-actin (red) were stained with Phalloidin-647. Original magnification: 200x. Scale bars = 100 μm. The results are defined as number of STm-GFP per cells. One-way ANOVA with Tukey’s multiple comparison test, n.s = not significant,***p<0.001. The experiment shown is representative of three independent experiments.
3.5 Reduced TBK1 activation by Salmonella infection of Mk2-/- MEFs
TANK-binding kinase-1 (TBK1), an integral component of Type I interferon induction by microbial infection, is both important for autophagic maturation by coordinating assembly and function of the autophagic machinery (28) and protection of vacuolar integrity during intracellular bacterial infection (29). Catalytically active TBK1 is recruited to cytosol-invading Salmonella and then induce anti-bacterial autophagy via the upstream autophagy regulator WIPI2 (30) and phosphorylation of the autophagic adaptor p62 at Ser-403 (31). Interestingly, IKKϵ and TBK1 are also activated in cells after stimulation with TNF (32). Since we detected reduced p62-S403 phosphorylation in Salmonella infected MK2-deficient cells (Figure 3A), we employed western blot to determine whether MK2 signaling affects TBK1 activation. Similar levels of TBK1 were observed in all time points for both genotypes. However, activated TBK1 (pS172-TBK1) was higher in Mk2-rescued MEFs after infection in comparison to Mk2-/- MEFs (Figure 6A). In addition, MK2-K79R MEFs showed a level of pS172-TBK1 similar to Mk2-rescued MEFs after infection indicating that a rescue of the p38MAPK level by expression of MK2 protein as described in Kotlyarov et al. (33) and Ronkina et al. (19) is sufficient for TBK1 activation (Supplementary Figure 3). Furthermore, treatment with the TBK1 inhibitor BX795 resulted in increased Salmonella counts in MK2 expressing MEFs (Figure 6B) indicating a role for TBK1 in restricting Salmonella infection. In agreement with this finding, immunofluorescence microscopy of BX795 treated S. Typhimurium infected MEFs showed increased bacterial number in comparison to untreated S. Typhimurium infected cells (Figures 6C, D). These data indicate that a p38MAPK/MK2-dependent activation of TBK1 during bacterial infection is essential to restrict Salmonella infection and there is a defect in TBK1-regulated bacterial clearance in Mk2-/- MEFs.
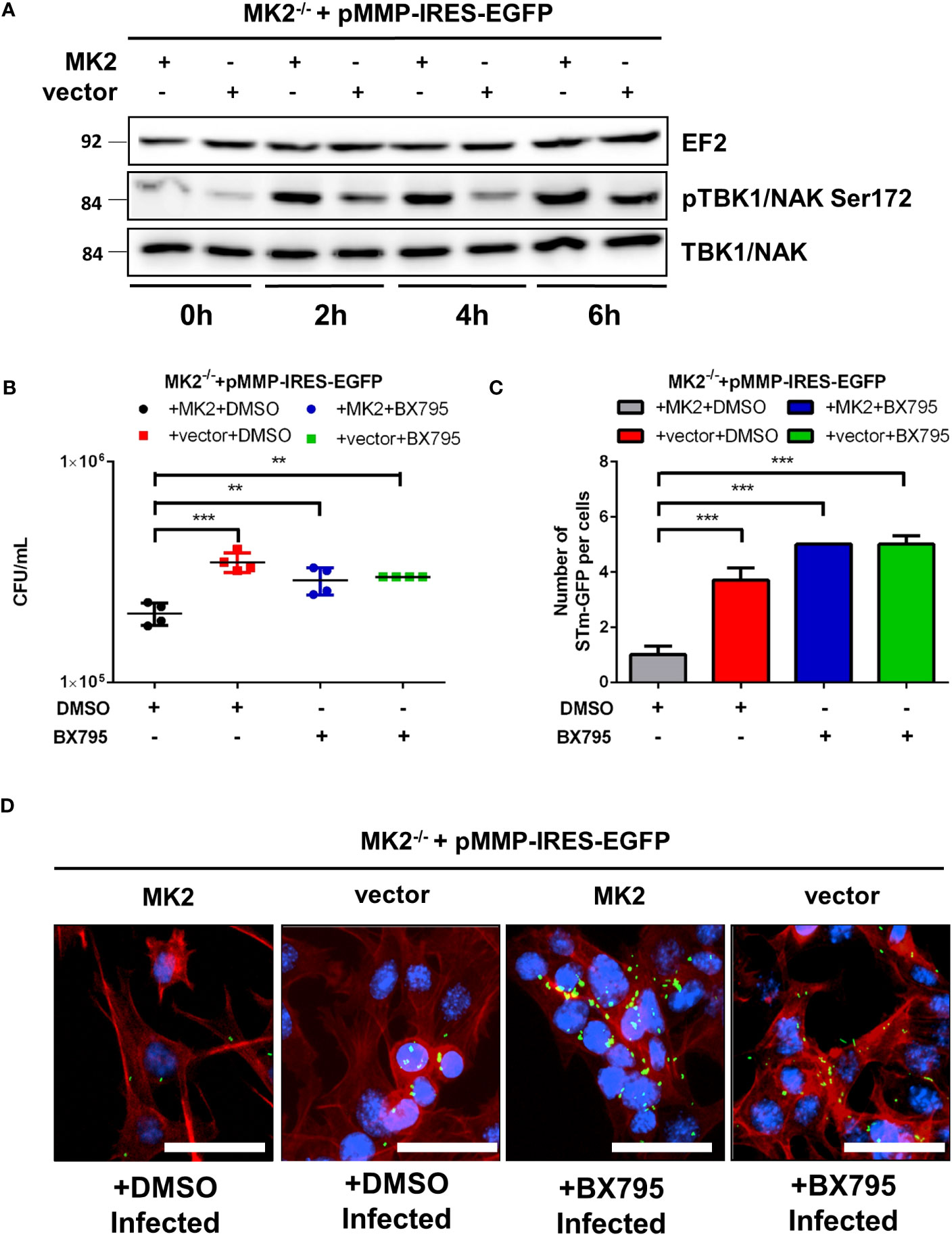
Figure 6 TBK1 activation is required to control intracellular S. Typhimurium. (A) Mk2-rescued (MK2) and Mk2-/- (vector) were infected with STm WT at MOI 100 for 1 hour. Western blot was performed to cells infected at 0, 2, 4 and 6h p.i,. Representative blots from three independent experiments are shown. (B) Mk2-rescued (MK2) and MK2-/- (vector) treated with DMSO or BX795 were infected with STm WT at MOI 10 for 1 hour. Medium with gentamicin (100 μg/mL) and BX795 were added. At 2h p.i, cells were washed with PBS and lysed. The results are defined as CFU/mL. One-way ANOVA with Tukey’s multiple comparison test, **p<0.01, ***p<0.001. The experiment shown is representative of three independent experiments. (C) Quantification and (D) immunofluorescence staining of Mk2-rescued (MK2) and Mk2-/- (vector) treated with DMSO or BX795 for 1 hour were infected with STm-GFP (green), fixed at 2h p.i. Nuclei were stained with DAPI (blue). F-actin (red) were stained with Phalloidin-647. Original magnification: 200x. Scale bars = 100 μm. The results are defined as number of STm-GFP per cells. One-way ANOVA with Tukey’s multiple comparison test, ***p<0.001. The experiment shown is representative of three independent experiments.
4 Discussion
The p38MAPK/MK2 pathway is crucial for innate immunity and the inflammatory response in vivo and in vitro. In this study, inhibition of p38MAPK/MK2 in wild type cells and the use of MK2-deficient cells in a model of early S. Typhimurium infection showed increased bacterial survival in MEFs, macrophages and HEK293T cells. In our previous study, MK2-deficient mice displayed higher susceptibility to L. monocytogenes infection due to impaired control of bacterial growth. Lower production of TNF and IFN-γ were observed in this infection model in vivo and in vitro. In addition, decreased phagocytosis of bacteria but normal oxidative burst activity were observed in Mk2-/- macrophages (14). We also demonstrated previously that p38MAPK/MK2 pathway repressed cell apoptosis via RIPK1 in an Yersinia enterocolitica infection model (34).
Upon invasion, Salmonella used their T3SSs to hijack host factors for their interests including the establishment of SCV and Salmonella-induced filaments (SIFs), which are important for their survival inside the host cells. However, some bacteria can penetrate and escape from the SCV and replicate rapidly in the host cell cytosol. From our study, microscopy and chloroquine resistance assays revealed that in the absence of MK2, a higher percentage of bacteria was detected in the host’s cytosol. It is known that upon exposure to the cytoplasm, Salmonella are quickly recognized by the ubiquitin E3-ligases of the host cells and ubiquitinated (35). Ubiquitinated bacteria then activate autophagic adaptors such as NDP52, OPTN, and p62, resulting the interaction with autophagic membrane anchoring protein LC3 that lead the bacteria to autophagosomes (27, 36). However, a different mechanism, in which Salmonella residing in damaged SCVs could also be targeted for autophagy by galectin 8, further adaptors and ubiquitin resulting in autophagic elimination, has also been described (37).
Autophagy as a part of innate immunity is an important mechanism to eliminate intracellular pathogens. Salmonella, Shigella, Listeria, Mycobacterium and other invasive bacterial pathogens have been known to induce autophagy (38). Losier et al. reported that adenosine monophosphate-activated protein kinase (AMPK), an upstream activator or the autophagy pathway, was stimulated upon detection of outer membrane vesicles from Salmonella. Upon AMPK activation, mammalian target of rapamycin complex 1 (mTORC1)-mediated repression of autophagy was relieved, positioning the cell for a rapid induction of autophagy against bacteria (39). In our current study, addition of mTOR inhibitor rapamycin showed reduced bacterial counts, which might result from induction of general autophagy. On the contrary, inhibition of autophagy with BafA1 treatment showed increased bacterial counts. Although p38MAPK/MK2/MK3 regulated autophagy by phosphorylation of Beclin-1 at Serine 90 upon starvation in HeLa cells has been described (40), it still remains open whether the regulation of autophagy by p38MAPK/MK2 upon Salmonella infection in MEFs follows the same mechanism.
In this study, we observed that in the absence of MK2, Salmonella infection leads to lower levels of LC3-II and serine 403 phosphorylated p62. Likewise, microscopy analysis showed lower colocalization of Salmonella with LC3 and p62 in Mk2-/- cells. During bacterial infection, phosphorylation of Serine 403 in the UBA domain of p62 by TBK-1 increases p62’s affinity for polyubiquitinated proteins and protein aggregates resulting in activation of the autophagic machinery and autophagic clearance (28, 41). Our data indicate that MK2 is upstream to this TBK1-dependent clearance process and that the level of activated TBK1 (pS172-TBK1) was higher in MK2 expressing MEFs after infection in comparison to MK2-deficient MEFs. TBK1 plays important role in many cellular processes such as innate immunity, inflammatory cytokine production, autophagy and cell death. It is activated by pattern-recognition receptors including Toll-like receptors and intracellular receptors including RIG-I, MDA5 or DAI. TBK1 is also important for the activation of type I interferon (IFN) (42). Focal adhesion kinase (FAK)-interacting protein of 200 kDa (FIP200), a component of the autophagy inducing the UNC-51-like autophagy activating kinase 1 (ULK1) complex, controls the TBK1 activation and p62 phosphorylation at Ser403 (31). The initiation of autophagy process is regulated by the ULK1 complex and the class III phosphatidylinositol 3-kinase (PtdIns3K) lipid kinase complex. Beclin 1 (BECN1), the regulatory component of this complex is a substrate of MK2 and a potential mediator of MK2-mediated control of TBK1 activation (40).
During autophagosome induction, TBK1 associates with gradient fractions consisting of the p62 and BECN1-PI3K complex component UVRAG (28). TBK1 activation is a prerequisite for recruitment of cytoplasmic Salmonella to autophagosomes (30). Salmonella AvrA has been shown to downregulate BECN1 protein levels to suppress autophagy (26). In the context of pathogen-induced autophagy, TBK1 phosphorylation was enhanced in BECN1 knockout cells upon Sendai virus infection, as BECN1 was shown to enhance autophagy and suppress TBK1 activation (43). Therefore, we hypothesize that sensing of Salmonella in the cytoplasm leads to TBK1 activation and downstream autophagosome assembly mediated by BECN1 and p62, while the bacteria attempt to inactivate this signaling by degrading BECN1 using AvrA-dependent mechanisms. The lack of MK2-dependence on bacterial survival in AvrA-deficient Salmonella indicate that BECN1 could be the target of MK2. TBK1 activation is downregulated and/or delayed in MK2-deficient cells. The complex modification and interactome pattern of BECN1, regulated by MK2-mediated phosphorylation (40) could regulate the balance between autophagy initiation and TBK1 activation. In addition, the MK2-K79R mutant could rescue the TBK1 activation-defect associated with MK2-deficiency. However, the catalytic inactive mutant could not rescue the bacterial susceptibility of MK2 KO cells (Figure 1F). It seems that the catalytic activity of MK2 is not necessary to activate TBK1, although its activity is important for eliminating Salmonella. Thus, this needs to be studied further and it should be taken into account that expression of both, active and catalytic dead MK2 is able to stabilize p38MAPK and restore p38 signaling (19, 33).
The transcription factor EB (TFEB) regulates various cellular responses including autophagy and the lysosomal pathway in response to different stress conditions, such as nutrient deprivation, organelle damage and pathogen invasion. Recently, it has been demonstrated that p38MAPK phosphorylates TFEB at serine 401, activates numerous immune genes and impairs the polarization of M0 into M1 inflammatory macrophages (44). Also, the localization of TFEB to lysosomes via mTOR interaction results in TFEB phosphorylation at serine 211. Subsequently, phosphorylated TFEB locates to the cytoplasm whereas the dephosphorylated TFEB translocates to the nucleus which then activates its target genes including autophagy and immune genes (45). Interestingly, the small molecule autophagy inducer acacetin can reduce intracellular Salmonella infection by inducing TFEB dephosphorylation, thereby increasing autolysosomal and lysosomal populations in the cells and restricting replication of intracellular Salmonella (46).
Some pathogens have evolved escape mechanisms to overcome autophagy that are important for their survival inside the cells (9, 38). The various SPI-1 effector proteins identified in Salmonella have different roles during Salmonella infection, such as alteration of host cytoskeleton, cell metabolism and regulation of host inflammatory response (47). Our current results revealed that SPI-1 mutants such as ΔinvA, ΔsopB, ΔsopE/ΔsopE2 showed increased S. Typhimurium counts in Mk2-/- MEFs that are similar to MEFs cells infected with S. Typhimurium WT. The S. Typhimurium invA is one of the invasion genes required for invasion of non-phagocytic cells (48). The Salmonella outer proteins (Sop)B, an inositol phosphatase that is also known as SigD, blocks inositol phosphate signaling pathways and induces Akt activation that inhibit apoptosis (49, 50). SopE and its homolog SopE2, a Rho GTPase exchange factor, activates Cdc42 and Rac1 that induces actin cytoskeleton rearrangements, membrane ruffling and promotes bacterial invasion (51, 52). Obviously, these genes play MK2-independent roles in Salmonella infection. Interestingly, only S. Typhimurium ΔavrA showed similar bacterial counts in Mk2-rescued and Mk2-/- MEFs at 2h p.i. The AvrA protein plays a critical role in suppressing activation of the NF-κB pathway, apoptosis via JNK pathway and autophagy by reducing Beclin-1 protein (26, 53, 54). These functions of AvrA overlap with MK2/3 functions and might explain the phenotype observed in our study.
Our experiments demonstrated that the addition of exogenous TNF showed reduced bacterial numbers in Mk2-/- MEFs in comparison with untreated Salmonella infected Mk2-/- MEFs. Since it is known that MK2 regulated biosynthesis of TNF and other cytokines at the post-transcriptional level and inactivation the mRNA-destabilizing and translation-inhibiting protein tristetraprolin (TTP) (13, 55), it is likely that addition of TNF could at least partially rescue early effects of MK2/3-deficiency. TNF has been shown to induce autophagy to eliminate intracellular pathogens, such as Toxoplasma gondii, Listeria and Shigella (27, 56). In Shigella infection, addition of exogenous TNF increased septin cage formation and entrapment of bacteria to be targeted by autophagy leading to inhibition of Shigella’s cell-to-cell spread (57).
However, the precise role of autophagy, TFEB regulation and TNF mediated mechanisms of host defense to limit bacterial dissemination still needs further investigation. In summary, we demonstrated here that p38MAPK/MK2 signaling is important to defend cells against intracellular Salmonella infection by affecting the autophagic pathways (Supplementary Figure 4). Especially, TBK activation seems the critical factor influenced by p38MAPK/MK2 signaling upon Salmonella infection.
Data availability statement
The raw data supporting the conclusions of this article will be made available by the authors, without undue reservation.
Ethics statement
Ethical approval was not required for the studies on humans in accordance with the local legislation and institutional requirements because only commercially available established cell lines were used. Ethical approval was not required for the studies on animals in accordance with the local legislation and institutional requirements because only commercially available established cell lines were used.
Author contributions
AS, GG, and AK conceived and designed the experiments. AS performed the experiments and analyzed the data. AS, MBM, GG, AK, and MG wrote and edited the paper. All authors contributed to the article and approved the submitted version.
Funding
This study was without external funding. It was supported by Insitute of Cell Biochemistry, Hannover Medical School, 30625 Hannover, Germany; Institute of Medical Microbiology and Hospital Epidemiology, Hannover Medical School and German Center for Infection Research (DZIF), Partner Site Hannover-Braunschweig, 30625 Hannover, Germany.
Acknowledgments
We would like to thank Natalia Ronkina, Chanchal Chauhan, Sarah Herr, Kathrin Laaß, Anneke Dörrie and Tatiana Yakovleva (Institute of Cell Biochemistry, Hannover Medical School) for their expert technical help performing experiments and discussion.
Conflict of interest
The authors declare that the research was conducted in the absence of any commercial or financial relationships that could be construed as a potential conflict of interest.
Publisher’s note
All claims expressed in this article are solely those of the authors and do not necessarily represent those of their affiliated organizations, or those of the publisher, the editors and the reviewers. Any product that may be evaluated in this article, or claim that may be made by its manufacturer, is not guaranteed or endorsed by the publisher.
Supplementary material
The Supplementary Material for this article can be found online at: https://www.frontiersin.org/articles/10.3389/fimmu.2023.1245443/full#supplementary-material
Supplementary Figure 1 | (A) MEFs Mk2-rescued (closed circle), Mk2-/- (closed square) were infected with S. Typhimurium WT at MOI 10 for 10 minutes or 30 minutes, washed three times with PBS, then incubated for 1 hours in media supplemented with 100 μg/mL gentamicin. At 70 minutes and 90 minutes p.i, the cells were lysed, collected, serially diluted and plated on LB agar plates to quantify intracellular bacteria. The results are defined as CFU/mL. Unpaired t-test, n.s = not significant. (B) iBMDM Mk2-rescued and Mk2-/- (vector) and (C) BMDM Mk-+/+ (closed circle), Mk2-/- (closed square) were infected with S. Typhimurium WT at MOI 10 for 30 minutes, washed three times with PBS, then incubated for 1 hours in media supplemented with 100 μg/mL gentamicin. At 1.5h and 4h p.i, the cells were lysed, collected, serially diluted and plated on LB agar plates to quantify intracellular bacteria. The results are defined as CFU/mL. Unpaired t-test, n.s = not significant, **p<0.01. (D) HEK293T cells WT (closed circle) and MK2-/- (closed square) were infected with S. Typhimurium WT at MOI 10 for 1 hour, washed three times with PBS, then incubated for 1 hours in media supplemented with 100 μg/mL gentamicin. At 2h and 6h p.i, the cells were lysed, collected, serially diluted and plated on LB agar plates to quantify intracellular bacteria. The results are defined as CFU/mL. Unpaired t-test, **p<0.01.
Supplementary Figure 2 | (A) Mk2-rescued (MK2) and Mk2-/- (vector) were infected with STm-GFP (green) at MOI 100. Infected cells were fixed at 2h p.i, stained with p62 antibody followed by Ax568-labeled secondary antibody (red). Nuclei were stained with DAPI (blue). F-actin (white) were stained with Phalloidin-647. Original magnification: 200x. Scale bars = 100 μm. (B) Quantification of LC3-Ax568 colocalized with STm-GFP. The results are defined as the median of Ax568 fluorescence intensity colocalized with STm-GFP. Unpaired t-test. ***p < 0.001.
Supplementary Figure 3 | MEFs expressing a kinase-dead MK2 mutant, in which lysine 79 was replaced by an arginine (Mk2K79R), were infected with STm WT at MOI 100 for 1 hour. Lysates from cells infected for 0, 2, 4 and 6h were separated by SDS-PAGE and TBK1/NAK expression and phosphorylation at S172 (TBK1/NAK Ser 172) were detected by western blot. Elongation factor 2 (EF2) serves as loading control.
Supplementary Figure 4 | A proposed model of regulation of intracellular S. Typhimurium (STm) survival by p38MAPK/MK2-dependent modulation of autophagy pathways in the host cells. (1) After entry into the host cells, Salmonella escape from SCV into the host cytosol. (2) p38MAPK/MK2 are activated by cellular stressors, such as inflammatory cytokines (e.g. IL-1 and TNF), oxidative stress, starvation or infection, and regulate the abundance of cytosolic Salmonella after infection. (3) TBK1 is activated and phosphorylates p62 at serine 403 in the UBA domain. (4) UBA domain phosphorylation enhances the activity of p62 and binds to ubiquitinated Salmonella that escaped to the cytosol. (5) In parallel, MK2 phosphorylates Beclin 1 that induces (6) phagophore nucleation, (7) autophagosome formation and (8) fusion of autophagosomes with lysosomes leads to degradation of the bacteria in autolysosomes. (9) AvrA Salmonella effector suppresses autophagy by inhibiting JNK signaling and reducing Beclin-1 protein level. P -phosphorylation; Ub – ubiquitinylation.
References
1. Levine B, Kroemer G. Autophagy in the pathogenesis of disease. Cell (2008) 132:27–42. doi: 10.1016/j.cell.2007.12.018
2. Kaur J, Debnath J. Autophagy at the crossroads of catabolism and anabolism. Nat Rev Mol Cell Biol (2015) 16:461–72. doi: 10.1038/nrm4024
3. Mizushima N, Yoshimori T, Levine B. Methods in mamMalian autophagy research. Cell (2010) 140:313–26. doi: 10.1016/j.cell.2010.01.028
4. Shahnazari S, Brumell JH. Mechanisms and consequences of bacterial targeting by the autophagy pathway. Curr Opin Microbiol (2011) 14:68–75. doi: 10.1016/j.mib.2010.11.001
5. Riebisch AK, Mühlen S, Beer YY, Schmitz I. Autophagy—A story of bacteria interfering with the host cell degradation machinery. Pathogens (2021) 10:110. doi: 10.3390/pathogens10020110
6. Gal-Mor O, Boyle EC, Grassl GA. Same species, different diseases: how and why typhoidal and non-typhoidal Salmonella enterica serovars differ. Front Microbiol (2014) 5:391. doi: 10.3389/fmicb.2014.00391
7. Hansen-Wester I, Hensel M. Salmonella pathogenicity islands encoding type III secretion systems. Microbes Infect (2001) 3:549–59. doi: 10.1016/S1286-4579(01)01411-3
8. Waterman SR, Holden DW. Functions and effectors of the Salmonella pathogenicity island 2 type III secretion system. Cell Microbiol (2003) 5:501–11. doi: 10.1046/j.1462-5822.2003.00294.x
9. Wu S, Shen Y, Zhang S, Xiao Y, Shi S. Salmonella interacts with autophagy to offense or defense. Front Microbiol (2020) 11:721. doi: 10.3389/fmicb.2020.00721
10. Gaestel M. MAPKAP kinases — MKs — two’s company, three’s a crowd. Nat Rev Mol Cell Biol (2006) 7:120–30. doi: 10.1038/nrm1834
11. Gaestel M, Kotlyarov A, Kracht M. Targeting innate immunity protein kinase signalling in inflammation. Nat Rev Drug Discov (2009) 8:480–99. doi: 10.1038/nrd2829
12. Ronkina N, Gaestel M. MAPK-activated protein kinases: servant or partner? Annu Rev Biochem (2022) 91:505–40. doi: 10.1146/annurev-biochem-081720-114505
13. Kotlyarov A, Neininger A, Schubert C, Eckert R, Birchmeier C, Volk H-D, et al. MAPKAP kinase 2 is essential for LPS-induced TNF-α biosynthesis. Nat Cell Biol (1999) 1:94–7. doi: 10.1038/10061
14. Lehner MD, Schwoebel F, Kotlyarov A, Leist M, Gaestel M, Hartung T. Mitogen-activated protein kinase-activated protein kinase 2-deficient mice show increased susceptibility to listeria monocytogenes infection. J Immunol (2002) 168:4667–73. doi: 10.4049/jimmunol.168.9.4667
15. Ronkina N, Kotlyarov A, Dittrich-Breiholz O, Kracht M, Hitti E, Milarski K, et al. The mitogen-activated protein kinase (MAPK)-activated protein kinases MK2 and MK3 cooperate in stimulation of tumor necrosis factor biosynthesis and stabilization of p38 MAPK. Mol Cell Biol (2007) 27:170–81. doi: 10.1128/MCB.01456-06
16. Salzman NH, Chou MM, de Jong H, Liu L, Porter EM, Paterson Y. Enteric salmonella infection inhibits paneth cell antimicrobial peptide expression. Infect Immun (2003) 71(3):1109–15. doi: 10.1128/IAI.71.3.1109-1115.2003
17. Hoiseth SK, Stocker BA. Aromatic-dependent Salmonella typhimurium are non-virulent and effective as live vaccines. Nature (1981) 291:238–9. doi: 10.1038/291238a0
18. Cormack BP, Valdivia RH, Falkow S. FACS-optimized mutants of the green fluorescent protein (GFP). Gene (1996) 173:33–8. doi: 10.1016/0378-1119(95)00685-0
19. Ronkina N, Menon MB, Schwermann J, Arthur JSC, Legault H, Telliez J-B, et al. Stress induced gene expression: a direct role for MAPKAP kinases in transcriptional activation of immediate early genes. Nucleic Acids Res (2011) 39:2503–18. doi: 10.1093/nar/gkq1178
20. Ehlting C, Ronkina N, Böhmer O, Albrecht U, Bode KA, Lang KS, et al. Distinct Functions of the Mitogen-activated Protein Kinase-activated Protein (MAPKAP) Kinases MK2 and MK3: MK2 MEDIATES LIPOPOLYSACCHARIDE-INDUCED SIGNAL TRANSDUCERS AND ACTIVATORS OF TRANSCRIPTION 3 (STAT3) ACTIVATION BY PREVENTING NEGATIVE REGULATORY EFFECTS OF MK3* *This work was supported by the Deutsche Forschungsgemeinschaft (Bonn), through Collaborative Research Centers 575 (to J. G. B. and D. H.), and 542 (to J. B.) and through Project Ga 453/11 (to M. G.). J Biol Chem (2011) 286:24113–24. doi: 10.1074/jbc.M111.235275
21. Menon MB, Yakovleva T, Ronkina N, Suwandi A, Odak I, Dhamija S, et al. Lyz2-cre-mediated genetic deletion of septin7 reveals a role of septins in macrophage cytokinesis and kras-driven tumorigenesis. Front Cell Dev Biol (2022) 9:795798. doi: 10.3389/fcell.2021.795798
22. Knodler LA, Nair V, Steele-Mortimer O. Quantitative assessment of cytosolic Salmonella in epithelial cells. PloS One (2014) 9:e84681. doi: 10.1371/journal.pone.0084681
23. Kim J-H, Hong S-K, Wu P-K, Richards AL, Jackson WT, Park J-I. Raf/MEK/ERK can regulate cellular levels of LC3B and SQSTM1/p62 at expression levels. Exp Cell Res (2014) 327:340–52. doi: 10.1016/j.yexcr.2014.08.001
24. Castanheira S, García-del Portillo F. Salmonella populations inside host cells. Front Cell Infect Microbiol (2017) 7:432. doi: 10.3389/fcimb.2017.00432
25. Wu H, Jones RM, Neish AS. The Salmonella effector AvrA mediates bacterial intracellular survival during infection in vivo. Cell Microbiol (2012) 14:28–39. doi: 10.1111/j.1462-5822.2011.01694.x
26. Jiao Y, Zhang Y, Lin Z, Lu R, Xia Y, Meng C, et al. Salmonella enteritidis effector avrA suppresses autophagy by reducing beclin-1 protein. Front Immunol (2020) 11:686. doi: 10.3389/fimmu.2020.00686
27. Mostowy S, Sancho-Shimizu V, Hamon MA, Simeone R, Brosch R, Johansen T, et al. p62 and NDP52 proteins target intracytosolic shigella and listeria to different autophagy pathways. J Biol Chem (2011) 286:26987–95. doi: 10.1074/jbc.M111.223610
28. Pilli M, Arko-Mensah J, Ponpuak M, Roberts E, Master S, Mandell MA, et al. TBK-1 promotes autophagy-mediated antimicrobial defense by controlling autophagosome maturation. Immunity (2012) 37:223–34. doi: 10.1016/j.immuni.2012.04.015
29. Radtke AL, Delbridge LM, Balachandran S, Barber GN, O’Riordan MX. TBK1 protects vacuolar integrity during intracellular bacterial infection. PloS Pathog (2007) 3:e29. doi: 10.1371/journal.ppat.0030029
30. Thurston TL, Boyle KB, Allen M, Ravenhill BJ, Karpiyevich M, Bloor S, et al. Recruitment of TBK1 to cytosol-invading Salmonella induces WIPI2-dependent antibacterial autophagy. EMBO J (2016) 35:1779–92. doi: 10.15252/embj.201694491
31. Schlütermann D, Berleth N, Deitersen J, Wallot-Hieke N, Friesen O, Wu W, et al. FIP200 controls the TBK1 activation threshold at SQSTM1/p62-positive condensates. Sci Rep (2021) 11:13863. doi: 10.1038/s41598-021-92408-4
32. Clark K, Plater L, Peggie M, Cohen P. Use of the pharmacological inhibitor BX795 to study the regulation and physiological roles of TBK1 and IκB kinase ϵ: A DISTINCT UPSTREAM KINASE MEDIATES SER-172 PHOSPHORYLATION AND ACTIVATION*. J Biol Chem (2009) 284:14136–46. doi: 10.1074/jbc.M109.000414
33. Kotlyarov A, Yannoni Y, Fritz S, Laaß K, Telliez J-B, Pitman D, et al. Distinct cellular functions of MK2. Mol Cell Biol (2002) 22:4827–35. doi: 10.1128/MCB.22.13.4827-4835.2002
34. Menon MB, Gropengießer J, Fischer J, Novikova L, Deuretzbacher A, Lafera J, et al. p38MAPK/MK2-dependent phosphorylation controls cytotoxic RIPK1 signalling in inflammation and infection. Nat Cell Biol (2017) 19:1248–59. doi: 10.1038/ncb3614
35. Wang L, Yan J, Niu H, Huang R, Wu S. Autophagy and ubiquitination in salmonella infection and the related inflammatory responses. Front Cell Infect Microbiol (2018) 8:78. doi: 10.3389/fcimb.2018.00078
36. Gomes LC, Dikic I. Autophagy in antimicrobial immunity. Mol Cell (2014) 54:224–33. doi: 10.1016/j.molcel.2014.03.009
37. Thurston TLM, Wandel MP, von Muhlinen N, Foeglein Á, Randow F. Galectin 8 targets damaged vesicles for autophagy to defend cells against bacterial invasion. Nature (2012) 482:414–8. doi: 10.1038/nature10744
38. Cemma M, Brumell JH. Interactions of pathogenic bacteria with autophagy systems. Curr Biol (2012) 22:R540–5. doi: 10.1016/j.cub.2012.06.001
39. Losier TT, Akuma M, McKee-Muir OC, LeBlond ND, Suk Y, Alsaadi RM, et al. AMPK Promotes Xenophagy through Priming of Autophagic Kinases upon Detection of Bacterial Outer Membrane Vesicles. Cell Rep (2019) 26:2150–2165.e5. doi: 10.1016/j.celrep.2019.01.062
40. Wei Y, An Z, Zou Z, Sumpter R Jr, Su M, Zang X, et al. The stress-responsive kinases MAPKAPK2/MAPKAPK3 activate starvation-induced autophagy through Beclin 1 phosphorylation. eLife (2015) 4:e05289. doi: 10.7554/eLife.05289
41. Matsumoto G, Wada K, Okuno M, Kurosawa M, Nukina N. Serine 403 phosphorylation of p62/SQSTM1 regulates selective autophagic clearance of ubiquitinated proteins. Mol Cell (2011) 44:279–89. doi: 10.1016/j.molcel.2011.07.039
42. Clément J-F, Meloche S, Servant MJ. The IKK-related kinases: from innate immunity to oncogenesis. Cell Res (2008) 18:889–99. doi: 10.1038/cr.2008.273
43. Jin S, Tian S, Chen Y, Zhang C, Xie W, Xia X, et al. USP19 modulates autophagy and antiviral immune responses by deubiquitinating Beclin-1. EMBO J (2016) 35:866–80. doi: 10.15252/embj.201593596
44. Martina JA, Jeong E, Puertollano R. p38 MAPK-dependent phosphorylation of TFEB promotes monocyte-to-macrophage differentiation. EMBO Rep (2023) 24:e55472. doi: 10.15252/embr.202255472
45. Roczniak-Ferguson A, Petit CS, Froehlich F, Qian S, Ky J, Angarola B, et al. The transcription factor TFEB links mTORC1 signaling to transcriptional control of lysosome homeostasis. Sci Signal (2012) 5:ra42–2. doi: 10.1126/scisignal.2002790
46. Ammanathan V, Mishra P, Chavalmane AK, Muthusamy S, Jadhav V, Siddamadappa C, et al. Restriction of intracellular Salmonella replication by restoring TFEB-mediated xenophagy. Autophagy (2020) 16:1584–97. doi: 10.1080/15548627.2019.1689770
47. Lou L, Zhang P, Piao R, Wang Y. Salmonella pathogenicity island 1 (SPI-1) and its complex regulatory network. Front Cell Infect Microbiol (2019) 9:270. doi: 10.3389/fcimb.2019.00270
48. Galan JE, Ginocchio C, Costeas P. Molecular and functional characterization of the Salmonella invasion gene invA: homology of InvA to members of a new protein family. J Bacteriol (1992) 174:4338–49. doi: 10.1128/jb.174.13.4338-4349.1992
49. Marcus SL, Wenk MR, Steele-Mortimer O, Finlay BB. A synaptojanin-homologous region of Salmonella typhimurium SigD is essential for inositol phosphatase activity and Akt activation. FEBS Lett (2001) 494:201–7. doi: 10.1016/S0014-5793(01)02356-0
50. Knodler LA, Finlay BB, Steele-Mortimer O. The salmonella effector protein sopB protects epithelial cells from apoptosis by sustained activation of akt*. J Biol Chem (2005) 280:9058–64. doi: 10.1074/jbc.M412588200
51. Hardt WD, Chen LM, Schuebel KE, Bustelo XR, Galán JES. typhimurium encodes an activator of Rho GTPases that induces membrane ruffling and nuclear responses in host cells. Cell (1998) 93:815–26. doi: 10.1016/S0092-8674(00)81442-7
52. Stender S, Friebel A, Linder S, Rohde M, Mirold S, Hardt W-D. Identification of SopE2 from Salmonella typhimurium, a conserved guanine nucleotide exchange factor for Cdc42 of the host cell. Mol Microbiol (2000) 36:1206–21. doi: 10.1046/j.1365-2958.2000.01933.x
53. Collier-Hyams LS, Zeng H, Sun J, Tomlinson AD, Bao ZQ, Chen H, et al. Cutting edge: Salmonella AvrA effector inhibits the key proinflammatory, anti-apoptotic NF-kappa B pathway. J Immunol (2002) 169:2846–50. doi: 10.4049/jimmunol.169.6.2846
54. Jones RM, Wu H, Wentworth C, Luo L, Collier-Hyams L, Neish AS. Salmonella avrA coordinates suppression of host immune and apoptotic defenses via JNK pathway blockade. Cell Host Microbe (2008) 3:233–44. doi: 10.1016/j.chom.2008.02.016
55. Ronkina N, Shushakova N, Tiedje C, Yakovleva T, Tollenaere MAX, Scott A, et al. The role of TTP phosphorylation in the regulation of inflammatory cytokine production by MK2/3. J Immunol (2019) 203:2291–300. doi: 10.4049/jimmunol.1801221
56. Subauste CS, Andrade RM, Wessendarp M. CD40-TRAF6 and autophagy-dependant anti-microbial activity in macrophages. Autophagy (2007) 3:245–8. doi: 10.4161/auto.3717
Keywords: p38MAPK, MK2, cell signaling, Salmonella, infection, autophagy, xenophagy, MEFs
Citation: Suwandi A, Menon MB, Kotlyarov A, Grassl GA and Gaestel M (2023) p38MAPK/MK2 signaling stimulates host cells autophagy pathways to restrict Salmonella infection. Front. Immunol. 14:1245443. doi: 10.3389/fimmu.2023.1245443
Received: 26 June 2023; Accepted: 28 August 2023;
Published: 12 September 2023.
Edited by:
Felix Ngosa Toka, Ross University School of Veterinary Medicine, Saint Kitts and NevisReviewed by:
Adriana Mantegazza, Thomas Jefferson University, United StatesAngel Nebreda, Institute for Research in Biomedicine, Spain
Copyright © 2023 Suwandi, Menon, Kotlyarov, Grassl and Gaestel. This is an open-access article distributed under the terms of the Creative Commons Attribution License (CC BY). The use, distribution or reproduction in other forums is permitted, provided the original author(s) and the copyright owner(s) are credited and that the original publication in this journal is cited, in accordance with accepted academic practice. No use, distribution or reproduction is permitted which does not comply with these terms.
*Correspondence: Abdulhadi Suwandi, c3V3YW5kaS5hYmR1bGhhZGlAbWgtaGFubm92ZXIuZGU=; Matthias Gaestel, Z2Flc3RlbC5tYXR0aGlhc0BtaC1oYW5ub3Zlci5kZQ==