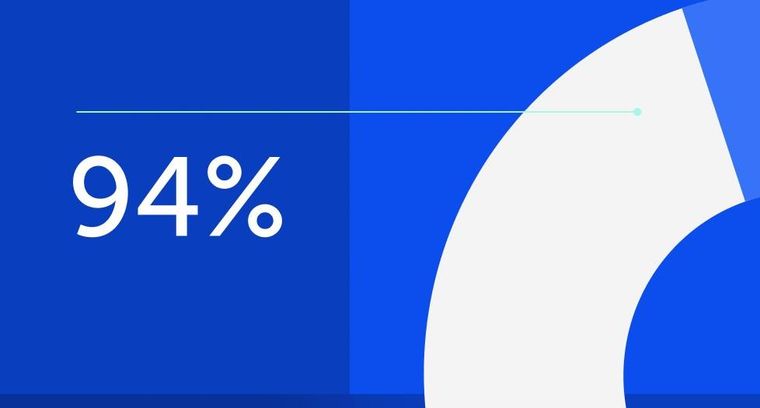
94% of researchers rate our articles as excellent or good
Learn more about the work of our research integrity team to safeguard the quality of each article we publish.
Find out more
MINI REVIEW article
Front. Immunol., 01 September 2023
Sec. Inflammation
Volume 14 - 2023 | https://doi.org/10.3389/fimmu.2023.1244586
This article is part of the Research TopicCellular and Systemic Interplay of Metabolism and Inflammation in the Pathogenesis of Lung DiseasesView all 11 articles
Adiponectin is an insulin sensitizing hormone that also plays a role in the regulation of inflammation. Although adiponectin can exert pro-inflammatory effects, more studies have reported anti-inflammatory effects, even in non-adipose tissues such as the lung. Obesity is considered an inflammatory disease, is a risk factor for lung diseases, and is associated with decreased levels of plasma adiponectin. The results of recent studies have suggested that adiponectin exerts anti-inflammatory activity in chronic obstructive pulmonary disease, asthma and invasive fungal infection. The signaling receptors of adiponectin, AdipoR1 and AdipoR2, are expressed by epithelial cells, endothelial cells, and immune cells in the lung. In this mini-review, we discuss the anti-inflammatory mechanisms of adiponectin in lung cells and tissues.
Adiponectin (APN) is a protein abundantly produced by adipocytes. It is present at high concentrations in plasma and regulates glucose levels, lipid metabolism, and insulin sensitivity. Plasma APN levels are relatively high in lean and healthy individuals. Decreased circulating levels of APN in obese individuals could enhance the risk of insulin resistance, type 2 diabetes, and cardiovascular disease (1). APN has also been reported to be produced by lymphocytes, skeletal muscle cells, cardiomyocytes, osteoblasts, and liver cells (2–5).
APN binds to signaling adiponectin receptors AdipoR1 and AdipoR2 (6) and the non-signaling receptor T-cadherin (7). While AdipoR1 binds to the globular form of APN, AdipoR2 preferentially binds to the high-molecular weight (HMW) form (8). The HMW isoform is consist of several linked hexamers and trimers (9). Interestingly, females have higher circulating levels of total APN with higher proportions of HMW isoform compares to males (10–12). Unlike the expression of APN, there are no sex differences in the expression of AdipoRs in adipose tissue (13).
Obesity is associated with a higher incidence of various diseases, including pulmonary disorders, such as asthma, chronic obstructive pulmonary disease, and pulmonary hypertension, and is also associated with decreased plasma adiponectin (14–18). Obesity-associated dysregulation of immune responses, inflammatory vigor, and adipose tissue immune cell infiltration are also believed to contribute to infectious disease pathogenesis (19).
According to the World Health Organization (WHO), the two most common chronic lung diseases are COPD and asthma, causing restricted airflow and breathing problem. APN has been implicated to play a role in the pathophysiology of COPD. Many studies revealed decreased serum APN in COPD patients (20). With a steadily increasing population of immunocompromised patients, disease caused by fungal infections remain a great threat to public health. Among lung fungal pathogens, such as Aspergillus, Cryptococcus, and Pneumocystis, Aspergillosis (approximately 57%) by Aspergillus spp. was most common (21). While the APN roles have been more thoroughly investigated in COPD and asthma, fewer studies have implicated in APN lung diseases. APN is required for metabolism and has a regulatory role in inflammation, primarily exerting anti-inflammatory effects. Here, we specifically review published studies that examine the anti-inflammatory activity of APN in inflammatory pulmonary diseases and cells of the lung.
Chronic Obstructive Pulmonary Disease (COPD) is a globally increasing cause of mortality with increasing prevalence over the past 20 years (22). Population aging, smoking and exposure to air pollution are leading risk factors for COPD (22–24). COPD patients showed increased numbers of lung inflammatory cells such as neutrophils, macrophages, and CD8+ T cells, with increased production of chemokines and cytokines (25). A mouse model of COPD with tobacco smoke exposure showed increased APN production in bronchoalveolar lavage fluid (BALF) and APN gene expression by airway epithelial cells (26), suggesting that APN has the potential to modulate the inflammatory response in COPD. Another study used an elastase-induced emphysema model to identify the possible role of APN in the pathogenesis of COPD (27). Elastase-induced emphysema is associated with reduced APN concentration. APN-deficient (APN KO) mice show a progressive COPD-like phenotype characterized by progressive emphysema, increased endothelial apoptosis and increased TNF-α activity. APN is associated with inflammation in COPD and is positively correlated with as the neutrophil-recruiting chemokine IL-8 (26). APN can therefore be a biomarker for disease severity and progression in patients of COPD (28).
Asthma is a chronic inflammatory disorder that affects airways in the lungs narrowed and swollen by inflammation and blocked by excess mucus. The most common asthma triggers include air pollen, dust products, animal dander, tobacco smoke and a wide range of fungi (29). In an obesity-related asthma mouse model used by Zhu et al., APN level in serum and BALF as well as adiponectin receptor (AdipoR) mRNA expression in lung were decreased and exogenous APN treatment increased both the APN level and AdipoR expression (30). In a mouse model of asthma by Medoff et al., ovalbumin (OVA)-sensitized and challenged APN KO mice exhibited increased pulmonary vascular remodeling, eosinophilic inflammation, and inflammatory chemokine gene expression compared to control mice (31). Furthermore, Obesity is associated with low-grade inflammation and enhances chronic inflammation in the airways of asthmatic obese patients. Expression of AdipoR2 and T-cadherin genes in bronchial epithelial cells was higher among obese patients with asthma than obese controls (32). Obesity in mice was associated with increased BALF macrophages, neutrophils and eosinophils, and increased Th2 cytokine production, including IL-13 and IL-5. Administration of exogenous APN reduced inflammation in obese mice, suggesting a therapeutic potential for the adiponectin pathway (33), and an APN receptor agonist reduced IL-4, IL-17, IL-23, and TNF-α in an OVA/lipopolysaccharide (LPS)-induced obese asthmatic model (34). In summary, APN treatment alleviated pathological changes in lung with reduction of eosinophils, total cell numbers in BALF, the eosinophil-attracting chemokine eotaxin and myeloperoxidase levels, which suggests that APN regulates cell migration into the airway and clearance of pulmonary inflammation in obesity-related asthma (30, 31).
In obese asthmatics, low APN was found more frequently than high (35) and non-obese individuals had higher levels of serum APN compared to obese (36). However, two other studies found no correlation between APN and obesity (37, 38). The differences in results of these studies might be related to demographic differences in age, gender, race, and disease severity. Although clinical data depend mainly on the serum APN, results from animal models and cell cultures demonstrate a potential benefit of APN pathway-stimulating therapy in asthmatics (35).
In invasive aspergillosis patients, excessive inflammation is associated with increased mortality (39). However, the effects of APN on anti-fungal immune responses in the lung remains unclear.
Fungal spores are easily aerosolized and inhaled (40). Different manifestations of Aspergillus infection include allergic bronchopulmonary aspergillosis, chronic pulmonary aspergillosis, and invasive aspergillosis. Allergic bronchopulmonary aspergillosis is considered poorly controlled asthma, while invasive aspergillosis occurs in immunocompromised patients such as those with COPD, solid organ or bone marrow transplant recipients, intensive care unit patients, and patients with severe viral infection (HIV, influenza A virus, COVID-19) (41–45). Invasive aspergillosis has an extremely high mortality rate (50-90%) in immunocompromised patients (46). Aspergillus fumigatus is most prevalent and the major cause of aspergillosis (47). APN KO mice with invasive aspergillosis exhibit increased disease pathology including decreased survival rate, increased fungal burden in the lung, increased cytokine production (IL-6 and TNF-α), and increased eosinophil recruitment (48). Aspiration of Aspergillus fumigatus conidia or chitin, one of the fungal cell wall components induced increased eosinophil recruitment in APN KO mice compared to wild-type controls (48, 49), and recombinant murine APN inhibited chitin-mediated eosinophil recruitment (49). Thus, it is likely that APN inhibits excessive inflammation in invasive aspergillosis, either directly or indirectly by enhancing antifungal immunity.
The roles of adiponectin in COPD, asthma, and pulmonary aspergillosis is summarized in Figure 1. Recent studies suggest a role for APN in regulating the inflammatory response in aspergillosis, COPD and asthma in either animal models or human patients (28, 35, 38). However, there are limited studies examining the role of APN during fungal infection in murine lungs (48, 49). Moreover, the number of the immunocompromised individuals is rapidly increasing, due to increased use of immunosuppressive therapies. Despite immune suppression, aspergillosis patients can succumb to an uncontrolled inflammatory response. Moreover, COPD and asthma patients showed increased hypersensitivity to Aspergillus fumigatus (29, 50, 51). More research is need to further unravel the anti-inflammatory mechanisms of the APN pathway in lung disease, especially in the context of fungal infection.
Figure 1 Adiponectin effect on lung disease. Obesity and aging are associated with loss of muscle mass, insulin resistance, and features of metabolic syndromes. Adiponectin (APN) activity is linked to metabolism and inhibition of inflammation. Decreases/deficiencies in plasma APN, even due to obesity, may contribute to adipose tissue inflammation, induction of asthma and TNF-α production in adipose and non-adipose tissues such as the lung. APN regulates inflammation in chronic pulmonary disease (COPD), asthma, fungal, bacterial, and viral infection. Adiponectin deficiency in COPD, aspergillosis, viral infection or tuberculosis resulted in increased expression of the proinflammatory cytokines TNF-α and IL-6, further suggesting that APN functions exerts anti-inflammatory activity. However, the effects of APN or AdipoRon on viral infection remain unknown. Treatment with APN or its agonist affects cytokine production; a decrease of IL-13 and IL-5 in asthma model and an increase of IL-8 in COPD, and increased IL-4, 1L-17, IL-23, and TNF-α in asthma. APN treatment also inhibited lung eosinophil recruitment in response to chitin.
Tuberculosis is a bacterial infectious disease that most often affects the lungs, which is caused by Mycobacterium tuberculosis. One-third of the world population is infected with this pathogen. Infection of M. tuberculosis alters adipose tissue morphology and contributes to an acute loss of body fat, which worsens pulmonary pathology. Comparing the lungs of M. tuberculosis-infected fat-ablated mice to infected fat-unablated mice, the levels of cytokines, such as TNF-α, IFN-γ, CD68, IL-12, and IL-10 were increased (52). APN may promote a reduction of TNF-α in the lungs in M. tuberculosis-infected mice (52). In acute lung injury model, APN attenuates LPS-induced lung injury in acute lung injury model (53). These reports suggest that APN regulates pulmonary pathology during bacterial infection.
Epidemiological evidence suggests an association between obesity and increased susceptibility to viral pneumonias associated with influenza, SARS-CoV-2, and COVID-19 cases (19, 54, 55). APN level is reduced in the patients infected with influenza A virus and COVID-19 with respiratory failure (56–58). Overexpression of IL-6, a key adipocyte-secreted inflammatory mediator, is an important risk factor worsening outcomes in influenza virus infection (59). In COVID-19, APN is generated by lymphocytes and downregulates the bone marrow production of granulocytes, with the activation of regulatory T cells (60). While the levels of cytokines in lung in COVID-19 patients have not been analyzed, the plasma levels of IL-6, TNF-α, and IL-10 were highly increased in severe COVID-19 patients with comparable levels to non-COVID-19 patients (61). So far, no study evaluated the effect of APN or AdipoRon on SARS-CoV-2 infection (62). These studies suggest that the APN pathway could be manipulated to provide protection against detrimental inflammation in response to viral infection.
APN and AdipoRs are expressed in the lung, suggesting an important role for the APN pathway in lung biological processes (63). Alveolar macrophages from APN KO mice released higher matrix metalloproteinases and TNF-α, which was suppressed by APN pretreatment (63). In humans, APN release from lung explants is negatively correlated with body mass index (64). Lung macrophages express both of the signaling APN receptors AdipoR1 and AdipoR2 (64). Lung macrophages treated with APN or its AdipoR1/R2 agonist AdipoRon resulted in decreased LPS- and poly (I:C)-induced production of TNF-α, IL-6, CXCL1 and CXCL8 (64). In the murine macrophage-like cell line RAW264, the globular domain of APN binds to the AdipoR1 and inhibits the TLR-induced NF-κB activity (65).
In lean mice, lung regulatory T cells (CD3+ CD4+ Foxp3+ cells) increased AdipoR1 expression, while obese mice with allergic inflammation had reduced AdipoR1 expression in lung regulatory T cells and increased expression in BALF eosinophils (66). Thus, adiponectin exerts anti-inflammatory effects on lung cells, likely due to signaling through adiponectin receptors.
Idiopathic pulmonary fibrosis is a common pulmonary disease, with high mortality, especially in older people. The idiopathic pulmonary fibrosis mouse model of bleomycin challenge induced a remarkable collagen fiber accumulation with extensive alveolar damage. Furthermore, APN treatment attenuated bleomycin-induced histopathology and inhibits TGF-β1-induced fibrosis in human lung fibroblasts with decreased TNF-α, IL-6, IL-1β, and IL-18 expression (67). Paraquat is an herbicide used worldwide and exposure to paraquat may cause acute injury and fibrosis in humans (68). In a pulmonary fibrosis model induced by paraquat, APN attenuated the fibrosis with decreased TGF-β1 and α-smooth muscle actin by regulating the NF-κB pathway (69). Human lung fibroblasts also express both AdipoR1 and AdipoR2, and APN treatment increased fibroblast expression of AdipoR1 but not AdipoR2. Knockdown of AdipoR1 using siRNA reversed the APN-mediated protective effect against paraquat-mediated fibrosis, demonstrating the importance of the APN-AdipoR1 pathway in fibroblasts for protection against pulmonary fibrosis (69).
In contrast, airway epithelial cells express significant levels of APN and AdipoR1, but not AdipoR2 in COPD patients and cultures of human lung epithelial cells (26). APN attenuated A549 cellular apoptosis and ameliorated cytotoxic effects induced by TNF-α and IL-1β by inhibiting NF-κB transactivation through AdipoR1. APN increased mRNA expression of the anti-inflammatory cytokine IL-10 in lung epithelial A549 cells (70). In summary, these studies provide evidence for a direct effect of APN on proliferation and inflammation of A549 epithelial cells with a protective role of APN in lung cells.
Hallmark features of acute lung injury include immune and endothelial cell activation and loss of vascular integrity (71). When acute lung injury was induced in control and APN KO mice by administration of LPS, APN KO mice appeared more ill with increased BALF protein concentration, increased production of TNF-α and IL-6, and decreased level of IL-10 in lung homogenates (53). Compared to the control mice with LPS injection, increased expression of IL-6, E-selectin, and Nox2 were detected in endothelial cells of APN KO mice (33). APN localizes to pulmonary vascular endothelium and APN deficiency leads to an age-dependent inflammatory vascular phenotype (72). APN deficiency also impairs mitochondrial function, promotes endothelial cell activation, and increases the susceptibility to LPS-induced acute lung injury (73). These finding suggests that parenchymal cells of the lung may play a role in the protective actions of APN.
In obesity-related asthma mice, APN treatment relieved inflammation and improved AMPK activity with a decrease in iNOS, Bcl-2, and NF-κB levels in lung (30). Treatment of Compound C, the AMPK inhibitor, reverses the effects of APN (30). It has been reported that APN directly binds to AdipoR1 and mediates signaling by activating AMPK.
M. tuberculosis infection increases the levels of both PPAR-γ and PPAR-α, key regulators of adipogenesis and lipid oxidation in the lungs (52). In LPS-induced acute lung injury model, NF-κB activation is APN is increased in APN KO mice (53).
In healthy mice, APN treatment induces the activation of p38 MAPK in Helios− regulatory T cells and upregulates the expression of AdipoR1 (74). In human epithelial cell line A549, activation of the APN-AdipoR1 pathway reduces cytotoxic effects inhibiting NF-κB activation and cytokine gene expression through ERK1/2 and AKT (70).
There is increasing data suggesting that APN exerts an anti-inflammatory effect in the lung. Lung cells, including immune cells, epithelial cells, and endothelial cells, express AdipoRs, indicating they also have signaling ability upon binding to APN or an AdipoR agonist such as AdipoRon. Although both pro- and anti-inflammatory properties have been reported, anti-inflammatory function of APN was mainly observed in lung cells (Table 1). APN can trigger the activation of AMPK, PPAR, ERK, and AKT through its receptors. It is well known that APN improves different lung diseases; enhancing the signaling might prove a therapeutic target. However, further research will clarify the roles and mechanism of APN pathway-induced protection in lung diseases, including fungal, bacterial, and viral infection, which could result in novel therapies that protect against infection, excessive inflammation, and other lung pathologies.
J-YL and ST wrote the manuscript. J-YL prepared illustrations. J-YL and ST revised the manuscript. All authors contributed to the article and approved the submitted version.
The research was funded by National Institutes of Health/National Institute of Allergy and Infectious Diseases [R21 AI163574] (ST).
Figures were created with BioRender.com.
The authors declare that the research was conducted in the absence of any commercial or financial relationships that could be construed as a potential conflict of interest.
All claims expressed in this article are solely those of the authors and do not necessarily represent those of their affiliated organizations, or those of the publisher, the editors and the reviewers. Any product that may be evaluated in this article, or claim that may be made by its manufacturer, is not guaranteed or endorsed by the publisher.
1. Li S, Shin HJ, Ding EL, Van Dam RM. Adiponectin levels and risk of type 2 diabetes: a systemic review and meta-analysis. JAMA (2009) 302:179–88. doi: 10.1001/jama.2009.976
2. Ma H, Gomez V, Lu L, Yang X, Wu X, Xiao SY. Expression of adiponectin and its receptors in livers of morbidly obese patients with non-alcoholic fatty liver disease. J Gastroenterol Hepatol (2009) 24:233–7. doi: 10.1111/J.1440-1746.2008.05548.X
3. Crawford LJA, Peake R, Price S, Morris TCM, Irvine AE. Adiponectin is produced by lymphocytes and is a negative regulator of granulopoiesis. J Leukoc Biol (2010) 88:807–11. doi: 10.1189/JLB.1109723
4. Berner HS, Lyngstadaas SP, Spahr A, Monjo M, Thommesen L, Drevon CA, et al. Adiponectin and its receptors are expressed in bone-forming cells. Bone (2004) 35:842–9. doi: 10.1016/J.BONE.2004.06.008
5. Delaigle AM, Jonas JC, Bauche IB, Cornu O, Brichard SM. Induction of adiponectin in skeletal muscle by inflammatory cytokines: In vivo and in vitro studies. Endocrinology (2004) 145:5589–97. doi: 10.1210/en.2004-0503
6. Yamauchi T, Kamon J, Ito Y, Yokomizo T, Kita S, Sugiyama T, et al. Cloning of adiponectin receptors that mediate antidiabetic metabolic effects. Nature (2003) 423:762–9. doi: 10.1038/nature01705
7. Hug C, Wang J, Ahmad NS, Bogan JS, Tsao TS, Lodish HF. T-cadherin is a receptor for hexameric and high-molecular-weight forms of Acrp30/adiponectin. Proc Natl Acad Sci U S A (2004) 101:10308–13. doi: 10.1073/PNAS.0403382101
8. Diep Nguyen T. Adiponectin: Role in physiology and pathophysiology. Int J Prev Med (2020) 11:136. doi: 10.4103/ijpvm.ijpvm_193_20
9. Garcia P, Sood A. Adiponectin in pulmonary disease and critically ill patients. Curr Med Chem (2012) 19:5493–500. doi: 10.2174/092986712803833263
10. Peake PW, Kriketos AD, Campbell LV, Shen Y, Charlesworth JA. The metabolism of isoforms of human adiponectin: studies in human subjects and in experimental animals. Eur J Endocrinol (2005) 153:409–17. doi: 10.1530/EJE.1.01978
11. Eglit T, Lember M, Ringmets I, Rajasalu T. Gender differences in serum high-molecular-weight adiponectin levels in metabolic syndrome. Eur J Endocrinol (2013) 168:385–91. doi: 10.1530/EJE-12-0688
12. Rudnicki M, Abdifarkosh G, Rezvan O, Nwadozi E, Roudier E, Haas TL. Female mice have higher angiogenesis in perigonadal adipose tissue than males in response to high-fat diet. Front Physiol (2018) 23:1452. doi: 10.3389/fphys.2018.01452
13. Rasmussen MS, Lihn AS, Pedersen SB, Bruun JM, Rasmussen M, Richelsen B. Adiponectin receptors in human adipose tissue: effects of obesity, weight loss, and fat depots. Obesity (2006) 14:28–35. doi: 10.1038/OBY.2006.5
14. Peters U, Dixon AE, Forno E. Obesity and asthma. J Allergy Clin Immunol (2018) 141:1169–79. doi: 10.1016/j.jaci.2018.02.004
15. Dixon AE, Peters U. The effect of obesity on lung function. Expert Rev Respir Med (2018) 12:755–67. doi: 10.1080/17476348.2018.1506331
16. Verberne LDM, Leemrijse CJ, Swinkels ICS, Van Dijk CE, De Bakker DH, Nielen MMJ. Overweight in patients with chronic obstructive pulmonary disease needs more attention: a cross-sectional study in general practice. NPJ Prim Care Respir Med (2017) 27:63. doi: 10.1038/S41533-017-0065-3
17. Medoff BD. Fat, fire and muscle - The role of adiponectin in pulmonary vascular inflammation and remodeling. Pulm Pharmacol Ther (2013) 26:420–6. doi: 10.1016/j.pupt.2012.06.006
18. Adamczak M, Wicek A, Funahashi T, Chudek J, Kokot F, Matsuzawa Y. Decreased plasma adiponectin concentration in patients with essential hypertension. Am J Hypertens (2003) 16:72–5. doi: 10.1016/s0895-7061(02)03197-7
19. Alarcon PC, Damen MSMA, Madan R, Deepe GS, Spearman P, Way SS, et al. Adipocyte inflammation and pathogenesis of viral pneumonias: an overlooked contribution. Mucosal Immunol (2021) 14:1224–34. doi: 10.1038/s41385-021-00404-8
20. Lin YH, Jiang TX, Hu SX, Shi YH. Association between serum adiponectin concentrations and chronic obstructive pulmonary disease: a meta-analysis. Biosci Rep (2020) 40:BSR20192234. doi: 10.1042/BSR20192234
21. Li Z, Li Y, Chen Y, Li J, Li S, Li C, et al. Trends of pulmonary fungal infections from 2013 to 2019: an AI-based real-world observational study in Guangzhou, China. Emerg Microbes Infect (2021) 10:450. doi: 10.1080/22221751.2021.1894902
22. Rodrigues S de O, da Cunha CMC, Soares GMV, Silva PL, Silva AR, Gonçalves-De-albuquerque CF. Mechanisms, pathophysiology and currently proposed treatments of chronic obstructive pulmonary disease. Pharmaceuticals (2021) 14:979. doi: 10.3390/PH14100979
23. Adeloye D, Song P, Zhu Y, Campbell H, Sheikh A, Rudan I. Global, regional, and national prevalence of, and risk factors for, chronic obstructive pulmonary disease (COPD) in 2019: a systematic review and modelling analysis. Lancet Respir Med (2022) 10:447–58. doi: 10.1016/S2213-2600(21)00511-7
24. Christenson SA, Smith BM, Bafadhel M, Putcha N. Chronic obstructive pulmonary disease. Lancet (2022) 399:2227–42. doi: 10.1016/S0140-6736(22)00470-6
25. Barnes PJ, Shapiro SD, Pauwels RA. Chronic obstructive pulmonary disease: molecular and cellular mechanisms. Eur Respir J (2003) 22:672–88. doi: 10.1183/09031936.03.00040703
26. Miller M, Cho JY, Pham A, Ramsdell J, Broide DH. Adiponectin and functional adiponectin receptor 1 are expressed by airway epithelial cells in chronic obstructive pulmonary disease. J Immunol (2009) 182:684–91. doi: 10.4049/JIMMUNOL.182.1.684
27. Nakanishi K, Takeda Y, Tetsumoto S, Iwasaki T, Tsujino K, Kuhara H, et al. Involvement of endothelial apoptosis underlying chronic obstructive pulmonary disease-like phenotype in adiponectin-null mice: Implications for therapy. Am J Respir Crit Care Med (2011) 183:1164–75. doi: 10.1164/rccm.201007-1091OC
28. Jaswal S, Saini V, Kaur J, Gupta S, Kaur H, Garg K. Association of adiponectin with lung function impairment and disease severity in chronic obstructive pulmonary disease. Int J Appl Basic Med Res (2018) 8:14–8. doi: 10.4103/IJABMR.IJABMR_65_17
29. Namvar S, Labram B, Rowley J, Herrick S. Aspergillus fumigatus—Host interactions mediating airway wall remodelling in asthma. J Fungi (Basel) (2022) 8:159. doi: 10.3390/jof8020159
30. Zhu L, Chen X, Chong L, Kong L, Wen S, Zhang H, et al. Adiponectin alleviates exacerbation of airway inflammation and oxidative stress in obesity-related asthma mice partly through AMPK signaling pathway. Int Immunopharmacol (2019) 67:396–407. doi: 10.1016/j.intimp.2018.12.030
31. Medoff BD, Okamoto Y, Leyton P, Weng M, Sandall BP, Raher MJ, et al. Adiponectin deficiency increases allergic airway inflammation and pulmonary vascular remodeling. Am J Respir Cell Mol Biol (2009) 41:397–406. doi: 10.1165/RCMB.2008-0415OC
32. Sideleva O, Suratt BT, Black KE, Tharp WG, Pratley RE, Forgione P, et al. Obesity and asthma: an inflammatory disease of adipose tissue not the airway. Am J Respir Crit Care Med (2012) 186:598–605. doi: 10.1164/RCCM.201203-0573OC
33. Shore SA, Terry RD, Flynt L, Xu A, Hug C. Adiponectin attenuates allergen-induced airway inflammation and hyperresponsiveness in mice. J Allergy Clin Immunol (2006) 118:389–95. doi: 10.1016/j.jaci.2006.04.021
34. Elaidy SM, Essawy SS, Hussain MA, El-Kherbetawy MK, Hamed ER. Modulation of the IL-23/IL-17 axis by fenofibrate ameliorates the ovalbumin/lipopolysaccharide-induced airway inflammation and bronchial asthma in rats. Naunyn Schmiedebergs Arch Pharmacol (2018) 391:309–21. doi: 10.1007/S00210-017-1459-Z
35. Otelea MR, Arghir OC, Zugravu C, Rascu A. Adiponectin and asthma: Knowns, unknowns and controversies. Int J Mol Sci (2021) 22:8971. doi: 10.3390/ijms22168971
36. Ozde C, Dogru M, Erdogan F, Ipek IO, Ozde S, Karakaya O. The relationship between adiponectin levels and epicardial adipose tissue thickness in non-obese children with asthma. Asian Pac J Allergy Immunol (2015) 33:289–95. doi: 10.12932/AP0599.33.4.2015
37. Zhang X, Zheng J, Zhang L, Liu Y, Chen GP, Zhang HP, et al. Systemic inflammation mediates the detrimental effects of obesity on asthma control. Allergy Asthma Proc (2018) 39:43–50. doi: 10.2500/AAP.2018.39.4096
38. Nasiri Kalmarzi R, Ataee P, Mansori M, Moradi G, Ahmadi S, Kaviani Z, et al. Serum levels of adiponectin and leptin in asthmatic patients and its relation with asthma severity, lung function and BMI. Allergol Immunopathol (Madr) (2017) 45:258–64. doi: 10.1016/J.ALLER.2016.09.004
39. Camargo JF, Husain S. Immune correlates of protection in human invasive aspergillosis. Clin Infect Dis (2014) 59:569–77. doi: 10.1093/CID/CIU337
40. Richardson M, Bowyer P, Sabino R. The human lung and Aspergillus: You are what you breathe in? Med Mycol (2019) 57:S145–54. doi: 10.1093/mmy/myy149
41. Koehler P, Cornely OA, Böttiger BW, Dusse F, Eichenauer DA, Fuchs F, et al. COVID-19 associated pulmonary aspergillosis. Mycoses (2020) 63:528–34. doi: 10.1111/myc.13096
42. Arastehfar A, Carvalho A, van de Veerdonk FL, Jenks JD, Koehler P, Krause R, et al. COVID-19 associated pulmonary aspergillosis (CAPA)—from immunology to treatment. J Fungi (Basel) (2020) 6:91. doi: 10.3390/jof6020091
43. Mardani M, Hakamifard A. COVID-19 and immunocompromised conditions: Ongoing challenging issue. Eur J Inflammation (2021) 19:1–3. doi: 10.1177/20587392211016114
44. Denning DW, Morgan EF. Quantifying deaths from aspergillosis in HIV positive people. J Fungi (Basel) (2022) 8:1131. doi: 10.3390/jof8111131
45. Koehler P, Bassetti M, Kochanek M, Shimabukuro-Vornhagen A, Cornely OA. Intensive care management of influenza-associated pulmonary aspergillosis. Clin Microbiol Infect (2019) 25:1501–9. doi: 10.1016/j.cmi.2019.04.031
46. Inoue K, Muramatsu K, Nishimura T, Fujino Y, Matsuda S, Fushimi K, et al. Association between early diagnosis of and inpatient mortality from invasive pulmonary aspergillosis among patients without immunocompromised host factors: a nationwide observational study. Int J Infect Dis (2022) 122:279–84. doi: 10.1016/j.ijid.2022.05.048
47. Dagenais TRTT, Keller NP. Pathogenesis of Aspergillus fumigatus in invasive aspergillosis. Clin Microbiol Rev (2009) 22:447–65. doi: 10.1128/CMR.00055-08
48. Amarsaikhan N, Tsoggerel A, Hug C, Templeton SP. The metabolic cytokine adiponectin inhibits inflammatory lung pathology in invasive aspergillosis. J Immunol (2019) 203:956–63. doi: 10.4049/JIMMUNOL.1900174
49. Amarsaikhan N, Stolz DJ, Wilcox A, Sands EM, Tsoggerel A, Gravely H, et al. Reciprocal inhibition of adiponectin and innate lung immune responses to chitin and. Aspergillus fumigatus Front Immunol (2019) 10:1057. doi: 10.3389/FIMMU.2019.01057
50. Redes JL, Basu T, Ram-Mohan S, Ghosh CC, Chan EC, Sek AC, et al. Aspergillus fumigatus-secreted alkaline protease 1 mediates airways hyperresponsiveness in severe asthma. Immunohorizons (2019) 3:368–77. doi: 10.4049/immunohorizons.1900046
51. Bafadhel M, McKenna S, Agbetile J, Fairs A, Desai D, Mistry V, et al. Aspergillus fumigatus during stable state and exacerbations of COPD. Eur Respir J (2014) 43:64–71. doi: 10.1183/09031936.00162912
52. Ayyappan JP, Ganapathi U, Lizardo K, Vinnard C, Subbian S, Perlin DS, et al. Adipose tissue regulates pulmonary pathology during TB Infection. mBio (2019) 16:e027771–18. doi: 10.1128/mBio.02771-18
53. Konter JM, Parker JL, Baez E, Li SZ, Ranscht B, Denzel M, et al. Adiponectin attenuates lipopolysaccharide-induced acute lung injury through suppression of endothelial cell activation. J Immunol (2012) 188:854–63. doi: 10.4049/JIMMUNOL.1100426
54. Lighter J, Phillips M, Hochman S, Sterling S, Johnson D, Francois F, et al. Obesity in patients younger than 60 years is a risk factor for COVID-19 hospital admission. Clin Infect Dis (2020) 71:896–7. doi: 10.1093/CID/CIAA415
55. Popkin BM, Du S, Green WD, Beck MA, Algaith T, Herbst CH, et al. Individuals with obesity and COVID-19: A global perspective on the epidemiology and biological relationships. Obes Rev (2020) 21:e13128. doi: 10.1111/OBR.13128
56. Tsatsanis C, Margioris AN, Kontoyiannis DP. Association between H1N1 infection severity and obesity-adiponectin as a potential etiologic factor. J Infect Dis (2010) 202:459–60. doi: 10.1086/653842
57. Kearns SM, Ahern KW, Patrie JT, Horton WB, Harris TE, Kadl A. Reduced adiponectin levels in patients with COVID-19 acute respiratory failure: A case-control study. Physiol Rep (2021) 9:e14843. doi: 10.14814/PHY2.14843
58. Perrotta F, Scialò F, Mallardo M, Signoriello G, D’Agnano V, Bianco A, et al. Adiponectin, leptin, and resistin are dysregulated in patients infected by SARS-CoV-2. Int J Mol Sci (2023) 24:1131. doi: 10.3390/ijms24021131
59. Yu X, Zhang X, Zhao B, Wang J, Zhu Z, Teng Z, et al. Intensive cytokine induction in pandemic H1N1 influenza virus infection accompanied by robust production of IL-10 and IL-6. PLoS One (2011) 6:e28680. doi: 10.1371/JOURNAL.PONE.0028680
60. Crawford LJA, Peake R, Price S, Morris TCM, Irvine AE. Adiponectin is produced by lymphocytes and is a negative regulator of granulopoiesis. J Leukoc Biol (2010) 88:807–11. doi: 10.1189/JLB.1109723
61. Flikweert AW, Kobold ACM, van der Sar-van der Brugge S, Heeringa P, Rodenhuis-Zybert IA, Bijzet J, et al. Circulating adipokine levels and COVID-19 severity in hospitalized patients. Int J Obes (2023) 47:126–37. doi: 10.1038/s41366-022-01246-5
62. Al-Kuraishy HM, Al-Gareeb AI, Bungau SG, Radu AF, Batiha GES. The potential molecular implications of adiponectin in the evolution of SARS-CoV-2: Inbuilt tendency. J King Saud Univ Sci (2022) 34:102347. doi: 10.1016/j.jksus.2022.102347
63. Summer R, Little FF, Ouchi N, Takemura Y, Aprahamian T, Dwyer D, et al. Alveolar macrophage activation and an emphysema-like phenotype in adiponectin-deficient mice. Am J Physiol Lung Cell Mol Physiol (2008) 294:L1035–42. doi: 10.1152/AJPLUNG.00397.2007
64. Salvator H, Grassin-Delyle S, Brollo M, Couderc LJ, Abrial C, Victoni T, et al. Adiponectin inhibits the production of TNF-α, IL-6 and chemokines by human lung macrophages. Front Pharmacol (2021) 12:718929. doi: 10.3389/FPHAR.2021.718929
65. Yamaguchi N, Argueta JGM, Masuhiro Y, Kagishita M, Nonaka K, Saito T, et al. Adiponectin inhibits Toll-like receptor family-induced signaling. FEBS Lett (2005) 579:6821–6. doi: 10.1016/J.FEBSLET.2005.11.019
66. Ramos-Ramírez P, Malmhäll C, Johansson K, Adner M, Lötvall J, Bossios A. Lung regulatory t cells express adiponectin receptor 1: Modulation by obesity and airway allergic inflammation. Int J Mol Sci (2020) 21:8990. doi: 10.3390/ijms21238990
67. Wang X, Yang J, Wu L, Tong C, Zhu Y, Cai W, et al. Adiponectin inhibits the activation of lung fibroblasts and pulmonary fibrosis by regulating the nuclear factor kappa B (NF-κB) pathway. Bioengineered (2022) 13:10098–110. doi: 10.1080/21655979.2022.2063652
68. Dinis-Oliveira RJ, Duarte JA, Sánchez-Navarro A, Remião F, Bastos ML, Carvalho F. Paraquat poisonings: Mechanisms of lung toxicity, clinical features, and treatment. Crit Rev Toxicol (2008) 38:13–71. doi: 10.1080/10408440701669959
69. Yao R, Cao Y, He YR, Lau WB, Zeng Z, Liang ZA. Adiponectin attenuates lung fibroblasts activation and pulmonary fibrosis induced by paraquat. PLoS One (2015) 10:e0125169. doi: 10.1371/JOURNAL.PONE.0125169
70. Nigro E, Scudiero O, Sarnataro D, Mazzarella G, Sofia M, Bianco A, et al. Adiponectin affects lung epithelial A549 cell viability counteracting TNFa and IL-1ß toxicity through AdipoR1. Int J Biochem Cell Biol (2013) 45:1145–53. doi: 10.1016/j.biocel.2013.03.003
71. Orfanos SE, Mavrommati I, Korovesi I, Roussos C. Pulmonary endothelium in acute lung injury: From basic science to the critically ill. Intensive Care Med (2004) 30:1702–14. doi: 10.1007/S00134-004-2370-X
72. Summer R, Fiack CA, Ikeda Y, Sato K, Dwyer D, Ouchi N, et al. Adiponectin deficiency: A model of pulmonary hypertension associated with pulmonary vascular disease. Am J Physiol Lung Cell Mol Physiol (2009) 297:432–8. doi: 10.1152/AJPLUNG.90599.2008
73. Shah D, Torres C, Bhandari V. Adiponectin deficiency induces mitochondrial dysfunction and promotes endothelial activation and pulmonary vascular injury. FASEB J (2019) 33:13617–31. doi: 10.1096/fj.201901123R
Keywords: adiponectin, lung immune response, adiponectin receptors, COPD, asthma, inflammatory pulmonary disease, infectious lung disease, obesity
Citation: Lim J-Y and Templeton SP (2023) Regulation of lung inflammation by adiponectin. Front. Immunol. 14:1244586. doi: 10.3389/fimmu.2023.1244586
Received: 22 June 2023; Accepted: 21 August 2023;
Published: 01 September 2023.
Edited by:
Slaven Crnkovic, Medical University of Graz, AustriaReviewed by:
Cassiano Felippe Gonçalves-de-Albuquerque, Rio de Janeiro State Federal University, BrazilCopyright © 2023 Lim and Templeton. This is an open-access article distributed under the terms of the Creative Commons Attribution License (CC BY). The use, distribution or reproduction in other forums is permitted, provided the original author(s) and the copyright owner(s) are credited and that the original publication in this journal is cited, in accordance with accepted academic practice. No use, distribution or reproduction is permitted which does not comply with these terms.
*Correspondence: Steven P. Templeton, c3B0ZW1wbGVAaXVwdWkuZWR1
Disclaimer: All claims expressed in this article are solely those of the authors and do not necessarily represent those of their affiliated organizations, or those of the publisher, the editors and the reviewers. Any product that may be evaluated in this article or claim that may be made by its manufacturer is not guaranteed or endorsed by the publisher.
Research integrity at Frontiers
Learn more about the work of our research integrity team to safeguard the quality of each article we publish.