- 1General Intensive Care Unit, Hadassah-Hebrew University Medical Center, Jerusalem, Israel
- 2Medical Intensive Care Unit, Hadassah-Hebrew University Medical Center, Jerusalem, Israel
- 3Department of Emergency Medicine, Hadassah-Hebrew University Medical Center and Hebrew University-Hadassah Faculty of Medicine, Jerusalem, Israel
- 4Infectious Diseases Division, Barzilai Medical Center, Ashkelon, Israel
- 5Ziv Medical Center and Azrieli Faculty of Medicine, Bar Ilan University, Safed, Israel
- 6Enlivex Therapeutics Ltd., Ness Ziona, Israel
- 7Department of Medicine, Hadassah-Hebrew University Medical Center, Jerusalem, Israel
- 8The Institute of Rheumatology-Immunology-Rheumatology, The Wohl Institute for Translational Medicine, Hadassah-Hebrew University Medical Center and Hebrew University-Hadassah Faculty of Medicine, Jerusalem, Israel
Background: Hyper-inflammatory immune response, a hallmark of severe COVID-19, is associated with increased mortality. Acute respiratory distress syndrome (ARDS) is a common manifestation. We undertook two phase I/II studies in five and then 16 subjects with severe/critical COVID-19 to assess the safety and preliminary efficacy of apoptotic cells (Allocetra™-OTS, Enlivex Therapeutics), a cellular immunomodulatory therapy that reprograms macrophages to reduce hyper-inflammatory response severity.
Methods: Eligible patients presenting to the Emergency Room with severe COVID-19 and respiratory dysfunction received one intravenous administration of Allocetra™-OTS and were monitored for adverse events (AEs) for 28 days. The primary aim was to determine the safety profile of treatment; secondary aims were recovery from ARDS, intensive care unit (ICU) and hospital length-of-stay, and mortality. Immune modulator markers were measured to elucidate the mechanism of action of Allocetra™-OTS.
Results: 21 patients with severe-critical COVID-19 of Gamma, Alpha and Delta variants, were treated with a single dose of apoptotic cells. 19/21 patients had mild-to-severe ARDS at presentation. Median age was 53 years, 16/21 were males, 16/21 were overweight/obese. No serious related adverse events (SAEs) were reported. All 21 study subjects survived to day 28 (end of study); 19/21 recovered completely. Comparable mortality rates at the hospital were 3.8%−8.9% for age- and gender-matched patients, and 39%−55% for critical patients. Recovering patients exhibited rapid ARDS resolution and parallel resolution of inflammation markers and elevated cytokines/chemokines.
Conclusion: In patients with severe/critical COVID-19 associated with ARDS, Allocetra™-OTS was safe, well-tolerated, and showed promising results for resolution of respiratory failure and inflammation.
Trial registration: https://clinicaltrials.gov/ct2/show/study/NCT04513470, https://clinicaltrials.gov/ct2/show/study/NCT04590053, Identifiers NCT04513470, NCT04590053.
1 Introduction
Severe acute respiratory syndrome coronavirus disease 2019 caused a global pandemic of coronavirus disease 2019 (COVID-19). Worldwide, the case fatality rate (CFR) of COVID–19 ranged between ~4% during the wild-type/alpha/delta virus infection period before the availability of vaccines, and ~1% during the dominance period of the Omicron SARS-COV-2 variants (1). During the wild-type/alpha/delta virus infection period, COVID-19 also resulted in complications among hospitalized patients, resulting in up to 15–25% of in-patients being admitted to the intensive care unit (ICU) (2–4). Acute respiratory distress syndrome (ARDS) is a severe pulmonary condition and one of the major complications of COVID-19 (5, 6). The pathogenesis of ARDS involves diverse immune cells of both the innate and adaptive immune systems that mediate the propagation of lung injury triggered by an insult such as severe viral infection. The resultant lung infiltrates and hypoxemia may be life-threatening. The immune response in COVID-19, which may result in ARDS, involves the unbalanced secretion of various inflammatory cytokines and chemokines, including TNF-α, IL-1β, IFN-γ, IL-6, IL-10, IL–1RA, IP–10, MCP–1, and IL-8 (CXCL8). These cytokines and chemokines were found to be independent and significant predictors of disease severity and death (7–10). Yang et al. showed that 81% of patients lost to COVID-19 also had ARDS (11). Moreover, a meta-analysis of 45 studies across 17 countries showed that more than 75% of COVID–19 patients admitted to the ICU developed ARDS (12).
This vigorous host response to infection, sometimes termed “cytokine storm,” is due to an immune system gone awry and an inflammatory response flaring out of control. This term has captured the attention of the public and the scientific community alike and is increasingly being used in both popular media and scientific literature. Indeed, recent publications have indicated that many of the complications of COVID-19 are related to the cytokine storm (13, 14).
In a previous clinical study using apoptotic cells (Allocetra-OTS, Enlivex Therapeutics, Israel) for immune modulation (ClinicalTrials.gov Identifier: NCT03925857), we observed that administration of Allocetra-OTS to patients with sepsis was safe and had a significant immuno-modulating effect, leading to resolution of the cytokine storm. All patients were alive at the end of the study. Based on comparisons with mortality score prediction and historical matched-controls, and the resolution of organ dysfunction compared to matched historical controls, a clear significant beneficial effect was seen (15).
In patients with severe COVID-19 there may be an underlying immunological mechanism of action, comparable to a mechanism we recently showed in sepsis (15), which is a hyper-inflammatory pathway associated with increased mortality. Therefore, two studies of five and 16 subjects (NCT04513470 and NCT04590053, respectively) were designed to determine the safety and efficacy of treatment with Allocetra-OTS in patients with severe/critical COVID-19.
2 Materials and methods
2.1 Study approval
The studies were approved by the local ethics committee and the Israeli Ministry of Health (Approval no. HMO–0204–20, and HMO–0770–20) and were performed according to institutional guidelines. Written informed consent was obtained in accordance with the Declaration of Helsinki from all study subjects and apoptotic cell donors.
2.2 Study design
The primary aim of these phase I/II studies (ClinicalTrials.gov Identifiers: NCT04513470 and NCT04590053, respectively) was to determine the safety profile of apoptotic cell infusion in subjects presenting to the Emergency Room (ER) with severe COVID-19 and respiratory dysfunction. Eligible patients with severe COVID-19 received treatment with Allocetra-OTS (Enlivex Therapeutics Ltd., Israel) apoptotic cells administered IV, followed by monitoring for adverse events (AEs) for 28 days. The secondary aims were to measure recovery from ARDS, intensive care unit (ICU) and hospital length-of-stays, and mortality.
Patients included in these studies were adult males and females aged 18–85 years, diagnosed with COVID-19, as defined below:
● Laboratory confirmation of SARS-COV2 infection by reverse-transcription polymerase chain reaction (RT-PCR) from any diagnostic sampling source.
● Patients classified as severe or critical according to National Institute of Health (NIH) severity classification (16).
● Illness with at least one of the following findings: (a) radiographic lung infiltrates, (b) arterial oxygen saturation (SpO2) ≤ 94% on room air, (c) requiring supplemental oxygen, with a partial pressure to fractional inspired concentration of oxygen (P/F) ratio of ≤350, ≥150.
● Exclusion criteria included: pregnancy, lactation, and woman of childbearing age who were not willing to use acceptable contraceptive measures for the entire study duration; other organ failures requiring organ support (not including mechanical ventilation), including kidney disease at stage 4 or requiring dialysis (i.e. estimated glomerular filtration rate (eGFR) < 30); malignancy; other serious systemic disease; psychosis; participation in other clinical trials or treatment with any experimental agents that may contradict this trial (e.g. biologics); co-infection with human immunodeficiency virus (HIV) or tuberculosis; known immuno-compromised state or medications known to be immunosuppressive; intubation (due to inability to sign an informed consent); partial arterial oxygen fraction (PaO2) to fractional inspired oxygen fraction (FiO2) (P/F) ratio or oxygen saturation to fractional inspired oxygen concentration (S/F) ratio <150 or change in status of eligibility manifested by a rapid decline in P/F ratio between eligibility status and actual drug delivery.
The National Early Warning Score 2 (NEWS2) (17) and 7-point ordinal scores were measured at enrollment and at each study time point. We also obtained blood samples for exploratory biological tests, including pro- and anti-inflammatory cytokines, chemokines, and growth factor levels in the serum.
Allocetra-OTS was administered as a single dose of 140×106 cells/kg or as a fixed dose of 109/patient, on day +1 (day 0 was time of diagnosis in the ER), following initiation of available standard-of-care (SOC) treatment for COVID-19, including remdesivir (Verklury, Gilead Sciences, Foster City, CA, USA), dexamethasone, and prophylactic enoxaparin. Interim safety analyses were performed by the Data and Safety Monitoring Board (DSMB) after 3 and 5 patients.
2.3 Alloctera™-OTS preparation
Enlivex Therapeutics Ltd. developed Allocetra-OTS based on the known activity of naturally occurring apoptotic cells to induce a pro-homeostatic state for both macrophages and dendritic cells (DCs) (18–22), contributing to the maintenance of peripheral homeostasis of almost all immune-triggered mechanisms in sepsis. Allocetra-OTS is composed of frozen non-matched human leukocyte antigen (HLA) mononuclear enriched leukocytes derived from a single donor via leukapheresis (Cell-Generation, Ltd. Hadassah Bio Park, Jerusalem, Israel), followed by freezing and preserving in liquid nitrogen (LN2). Following controlled thawing, cells are incubated with corticosteroids and washed to achieve an early apoptotic state. The cells are then washed again, eliminating both corticosteroid residues as well as material that may have been secreted by apoptotic cells. Once the cells are in an early apoptotic state, they are further Gamma or X-ray irradiated at 4000cGy to prevent T cell proliferation of potentially viable cells and therefore avoid the possible development of an acute graft-versus-host (GvHD)-like response and preserved at 2–8°C. Cell populations contain at least 40% early apoptotic cells in the form of a liquid suspension that is administered intravenously (IV). Following irradiation, the product is stable for up to 96 hours and kept at 2–8°C to avoid progression of apoptosis. All blood donors of Allocetra-OTS are tested for bacterial and virus infections as required for blood and plasma donation. The final product is retested for infection using pre-release Gram-stain and post-release sterility culture.
2.4 Potency assay
PBMC-derived monocytes/macrophages were co-cultured with Allocetra-OTS at ratios of 1:16, 1:32 and 1:64 for 1 hour. Following incubation, co-cultures were exposed to LPS and incubated for an additional 3 hours and tested by flow-cytometry for HLA-DR expression. Untreated monocytes/macrophages were used to determine basal expression levels. Monocytes/macrophages stimulated with LPS alone (without pre-incubation with Allocetra-OTS) were used as positive controls to determine upregulated expression levels in maturation response that was significantly inhibited upon adding Allocetra-OTS. This assay was based on an assay used in a previous clinical study (21) where maturation of dendritic cells was used for ApoCell potency effect.
2.5 Luminex® cytokine/chemokine analysis
Serum cytokine/chemokine measurements were performed as previously described (15) using the Luminex MAGPIX system (Luminex Corp, Texas, USA) and analyzed with Milliplex analysis software (Millipore MA, USA).
2.6 ELISA analysis
The following cytokines/chemokines were measured by sandwich ELISA kits: IL-18 (R&D), MCP-3 (R&D), TNFR1 (R&D).
2.7 Statistics
Comparisons between treated COVID-19 patients and healthy individuals were done using the non-parametric Mann-Whitney test. Comparisons between blood counts/cytokine levels from day 1 to day 28 for each patient were analyzed using the Wilcoxon matched-pairs signed-rank test. Means, standard deviations, medians, interquartile ranges, and ranges in each treatment group were presented, as appropriate. Statistical analysis was performed using GraphPad (Prism, San Diego, CA, USA).
3 Results
Following confirmation of the safety of the study in the first five patients, a follow-up study with an additional 16 patients, using the same protocol (apart from dose that was calculated 70x106/kg in the first five patients and a fixed dose of 109 cells per patient in the rest of the patients) was carried out. The results of the two studies together are presented here, as the same protocol was used in both.
A total of 25 patients were recruited to two consecutive studies (NCT04513470 and NCT04590053); 21/25 patients were included (median age of 53 years, 16 males (76.2%)). Patient 04 (Study NCT04513470) withdrew, patients 01-006 and 01-011 withdrew their consent, and Patient 01-010 was disqualified after recruitment due to late screen failure (Study NCT04590053). All patients received the standard of care (SOC) for COVID-19, including remdesivir (17/21, 81%), dexamethasone (21/21, 100%), and prophylactic enoxaparin (21/21, 100%). The data safety management board (DSMB) met following inclusion of 3/5 patients in the first study for a safety review and approval to continue the study, and for final review after 21 patients were enrolled. Allocetra-OTS infusion in the first five patients met the protocol for defined safety criteria at day 14 and the study proceeded to the second round of patient recruitment at the same dose, which also met the protocol-defined criteria for safety.
Patient characteristics are presented in Table 1.
3.1 Clinical course
Of the 21 patients, 11 were classified as having “severe” COVID-19 and 10 as “critical” COVID-19 based on the National Institute of Health (NIH) severity classification (23). Additionally, 19/21 patients had mild-to-severe (ARDS) upon hospitalization, with an initial median 7-point severity scale of 4 (range 3–4) and median NEWS2 score of 5 (range 2–8). All patients were alive at completion of 28 days of follow-up, and 19/21 (90.5%) had completely recovered. Polymerase chain reaction (PCR) testing was negative at discharge for 12/15 patients who were tested. A summary of clinical characterizations of patients included in the study is presented in Table 2.
3.2 Safety parameters
Safety was evaluated by monitoring for serious adverse events (SAEs) and adverse events (AEs). All patients survived 28 days of follow-up. Three unrelated SAEs were documented in two patients who required mechanical ventilation. No AEs related to investigational product administration were reported in 21 patients, with one AE possibly related to the investigational product administration in these studies (shivering following investigational product administration) (Table 3).
3.3 Preliminary efficacy results
3.3.1 Morbidity and mortality
All 21 patients from both studies were alive on day 28; 19/21 had been discharged healthy from the hospital and 2/21 were in ICU on day 28. On the 7-point ordinal severity scale, the initial median score was 4 (range 3–4) and the initial median NEWS2 score was 5 (range 2–8). A complete clinical recovery and hospital discharge were achieved for 19/21 patients, as reflected by the normal ordinal score (achieved by a median 7 days) and NEWS2 score (achieved by a median of 6 days) at hospital discharge.
3.3.2 Recovery from ARDS
Prevention of respiratory deterioration associated with COVID-19, particularly ARDS, was a preliminary efficacy parameter tested in this study. Out of 21 patients, there were 19 patients with ARDS, of whom 17/19 (89.5%) completely recovered. Specifically, the recovered patients reached a P/F ratio of more than 315mmHg (indicative of recovery from ARDS), after a median time of 6 days (range 2–14).
Despite the severe and critical clinical conditions and ARDS in 19/21 patients, there were no mortalities within 28 days. At the same time and for the same variants (gamma, alpha, and delta) mortality at Hadassah Medical Center was 5.1% and 8.9%, for all patients aged 50–60 and 60–70, respectively. For females, the mortality rates were 3.8% and 4.4.% for females aged 50−60 and 60−70, respectively. The corresponding mortality rates among males were higher, 6.0% and 12.1% for male patients aged 50–60 and 60–70, respectively (Figure 1A). The mortality rates among critical COVID–19 patients (10/21 of the patients in this study, all males) hospitalized in Hadassah Medical Center during that period were even higher: 39.3% and 47.8% for critical patients aged 50–60 and 60–70, respectively; 55.6% and 40.0% for females ages 50–60 and 60–70, respectively; and 31.6% and 51.6% for males ages 50–60 and 60–70, respectively (Figure 1B).
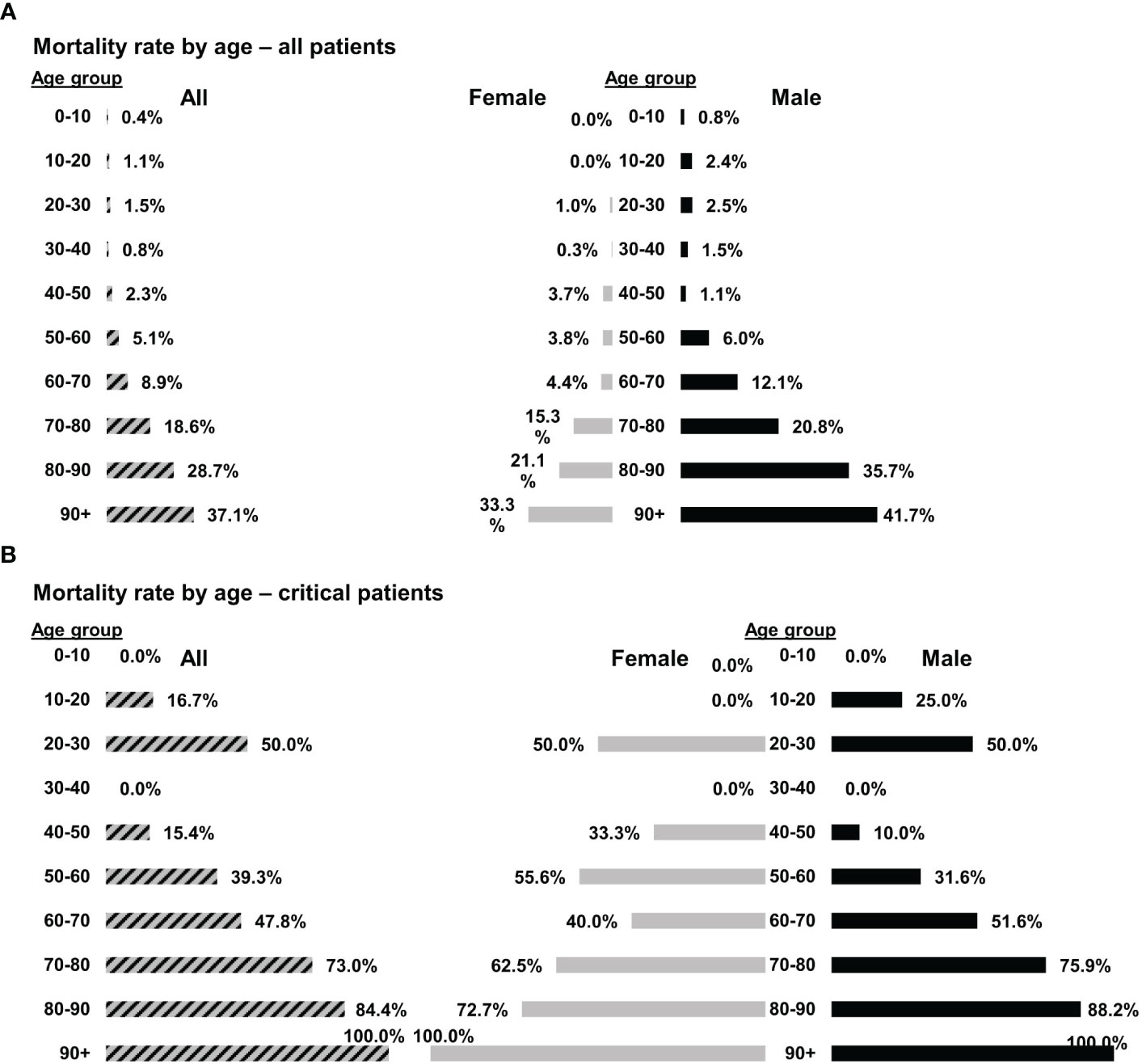
Figure 1 Mortality rates of COVID-19 patients at Hadassah Medical Center. The mortality rates of (A) all COVID-19 patients (n = 4,177) or (B) only critical COVID-19 patients (n = 197) that were hospitalized at the Hadassah Medical Center during the time of the studies.
3.3.3 Length of stay in ICU and hospital
Since most of the patients had recovered from ARDS and COVID-19, and no mortality occurred, we were next interested to verify that these promising results were expressed in the duration of hospital stay.
Median total hospitalization after investigational product administration was 6 days (range 2–28) for all the patients (10 critical, 11 severe patients), and the average was 7.6 ± 7 days. The average time for discharge from the hospital after investigational product administration for the 19/21 patients who were discharged was 5.5 ± 2.4 days (range 2–12, median 6 days). Only 2/21 patients (01-012 and 03-001, both critical at admission) were admitted to the ICU, where they remained until the end of the study.
3.3.4 Laboratory results
Laboratory evaluation included blood and serum levels, as detailed below. Complete blood count (CBC), acute phase and cardiac markers, and liver markers and enzymes of all 21 patients were documented. Figures 2–4 and Tables S1–S3 show their levels during the study period. White blood cell (WBC) counts were within normal limits throughout the study in 18/21 patients, and indeed were mildly decreased by 23% (IQR 8-35%) (Figure 2A). Neutrophil counts were above normal on admission in 7/21 patients (Figure 2B), and 10/21 patients presented to the ICU with an increased neutrophil percentage (Figure 2C). Both neutrophil count and percentage decreased significantly during the follow-up period, with median decreases of 45% and 29%, respectively between days 1 and 28 (p=0.0334 and p<0.0001 for neutrophil count and percentage, respectively, Wilcoxon matched-pairs test).
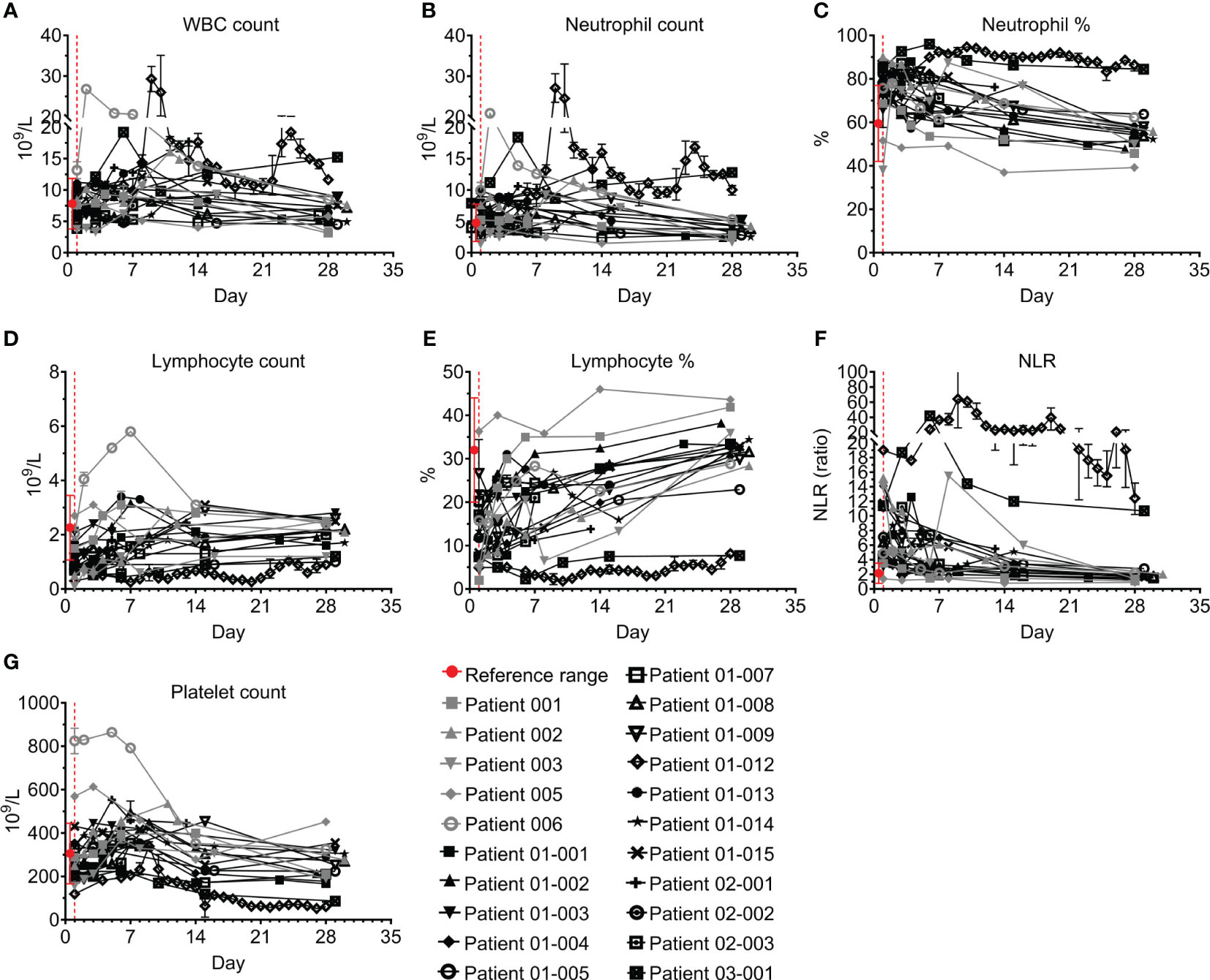
Figure 2 Blood counts of COVID-19 patients treated with Allocetra-OTS. Severe/critical COVID-19 patients from studies NCT04513470 (gray symbols) and NCT04590053 (black symbols) were treated with Allocetra-OTS on day 1 (red dotted line); blood samples were analyzed for (A) white blood cells (WBCs), (B, C) neutrophils, (D, E) lymphocytes, (F) neutrophil/lymphocyte ratio (NLR), and (G) platelet counts at the indicated timepoints. The first blood sampling for each patient was taken before IV administration of Allocetra-OTS. Data are shown as average ± range.
Most patients presented with lymphopenia; lymphocyte counts were below normal levels in 14/21 (Figure 2D) and 20/21 had a lymphocyte percentage below the normal range (Figure 2E). All patients except for patients 01-012 and 03-001 had a gradual increase in lymphocyte numbers and percentages, which recovered to normal levels between days 1 and 28 with median increases of 1.9- and 2.1-fold, respectively (p<0.0001, Wilcoxon matched-pairs test). In accordance with the slight neutrophilia and lymphopenia in most of the patients, 18/21 patients had a hypernormal neutrophil-to-lymphocyte ratio (NLR) upon admission to the ICU (median of 6.4, IQR: 4.5–11.3, compared to a normal range of 0.78–3.53). Except for patients 01-012 and 03-001, who had severe lymphopenia and a high NLR index throughout the study, the NLR of all the patients was significantly decreased following treatment (median decrease of 75%, IQR:29–74%) and reached normal levels by day 28 (Figure 2F; p<0.0001, Wilcoxon matched-pairs test). 17/21 patients had normal platelet counts during follow-up; two patients (005 and 006) had high platelet counts on admission followed by a decrease to normal levels; and patients 01-012 and 03-001 had unresolved severe thrombocytopenia (median platelet count <100×109/L) (Figure 2G).
All patients had elevated C-reactive protein (CRP) levels and 18/21 had elevated ferritin levels; however, these acute phase markers significantly declined in parallel with resolution of inflammation (Figures 3A, B; p<0.0001, Wilcoxon matched-pairs test). CRP levels remained above the normal range in 10/18 patients who were tested on Day 28, especially in patients 01-012 and 03-001. Ferritin levels were within the normal range by day 28 in 14/17 patients who were tested. Most patients had normal or mildly elevated D-dimer levels, which either decreased or remained unchanged during the study. Patients 01-014, 01-012, and 03-001 had an initial increase of D-dimer. This was followed by a decrease in patient 01-14, while patients 01-012 and 03-001 maintained hyper-normal levels of D-dimer throughout the hospitalization period (Figure 3C). 15/19 of the tested patients had normal creatine phosphokinase (CPK) levels throughout the study; patients 01-002, 01-003, 01-008, and 02-002 initially had higher than normal CPK levels, which were gradually decreased to normal, while Patient 01-012 started with normal CPK levels and gradually developed elevated CPK (Figure 3D). Patient 01-012 also had sustained high creatinine levels, while all the other patients had normal creatinine levels (Figure 3E). As shown in Figure 4, most patients had abnormal levels of liver enzymes and metabolites, suggesting aberrant liver function; aspartate transaminase (AST) levels upon admission were high in 13/20 of the tested patients with a gradual decline to the normal range by day 7 and significantly decreased AST on day 28 compared to day 1 (Figure 4A, p=0.0434, Wilcoxon matched-pairs test). The trend of alanine transaminase (ALT) was similar to AST, with 9/19 of the tested patients having abnormally high ALT on admission followed by decreasing ALT up to day 28 in most of these patients (Figure 4B, p=0.0407, Wilcoxon matched-pairs test). ALP and bilirubin were within the normal range in almost all patients (Figures 4C, D). Lactate dehydrogenase (LDH) was high in 19/20 of the patients upon admission, and significantly decreased towards normal levels by day 14 up to day 28 in all the patients except for patient 01-012, whose LDH levels were increased by 2.3-fold (Figure 4E, p=0.0038, Wilcoxon matched-pairs test).
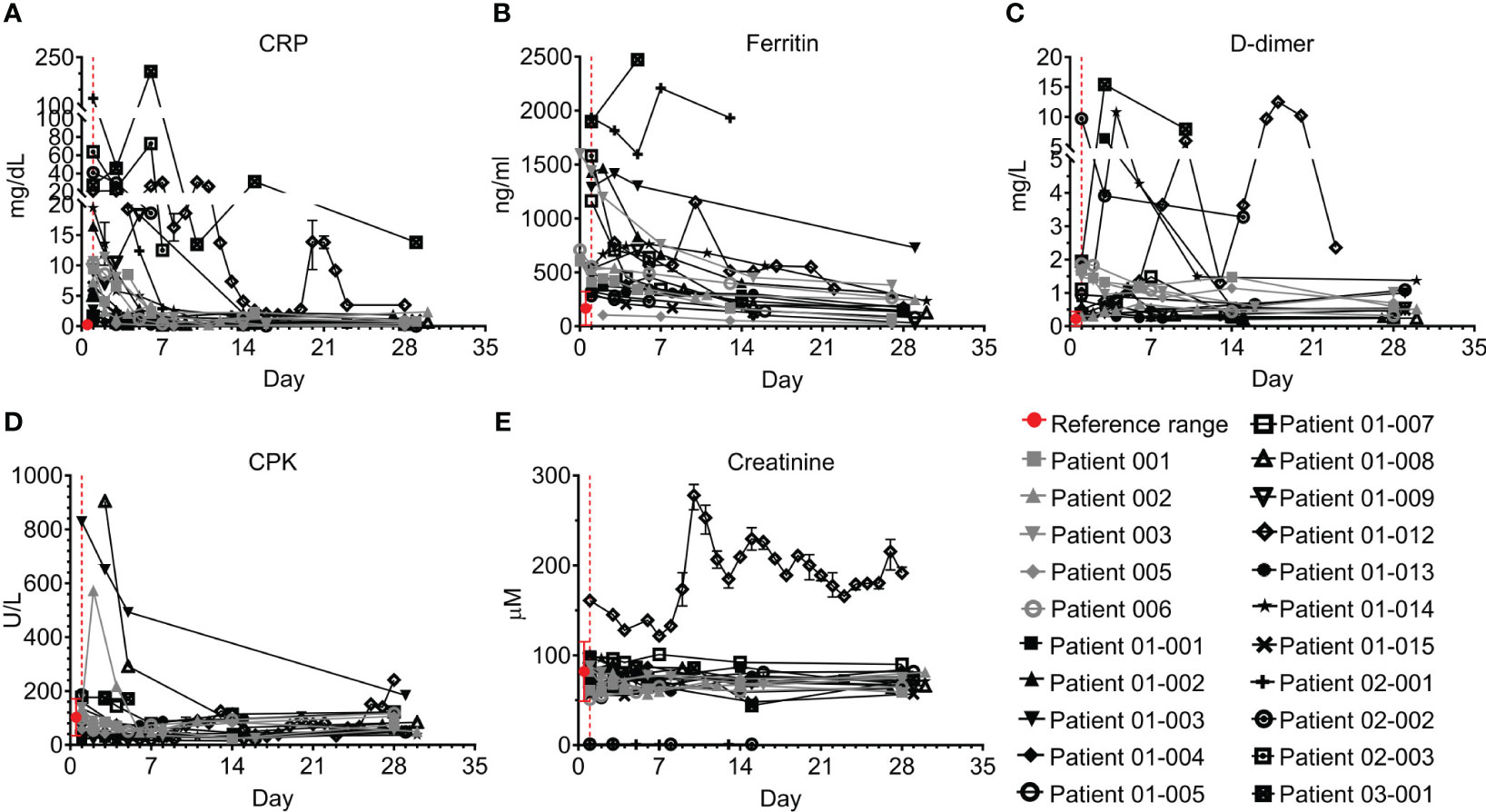
Figure 3 Acute phase and cardiac markers of COVID-19 patients treated with Allocetra-OTS. Severe/critical COVID-19 patients from studies NCT04513470 (gray symbols) and NCT04590053 (black symbols) were treated with Allocetra-OTS on day 1 (red dotted line). Blood samples were analyzed for (A) CRP, (B) ferritin, (C) D-dimer, (D) CPK, and (E) creatinine at the indicated timepoints. The first blood sampling for each patient was taken before IV administration of Allocetra-OTS. Data are shown as average ± range.
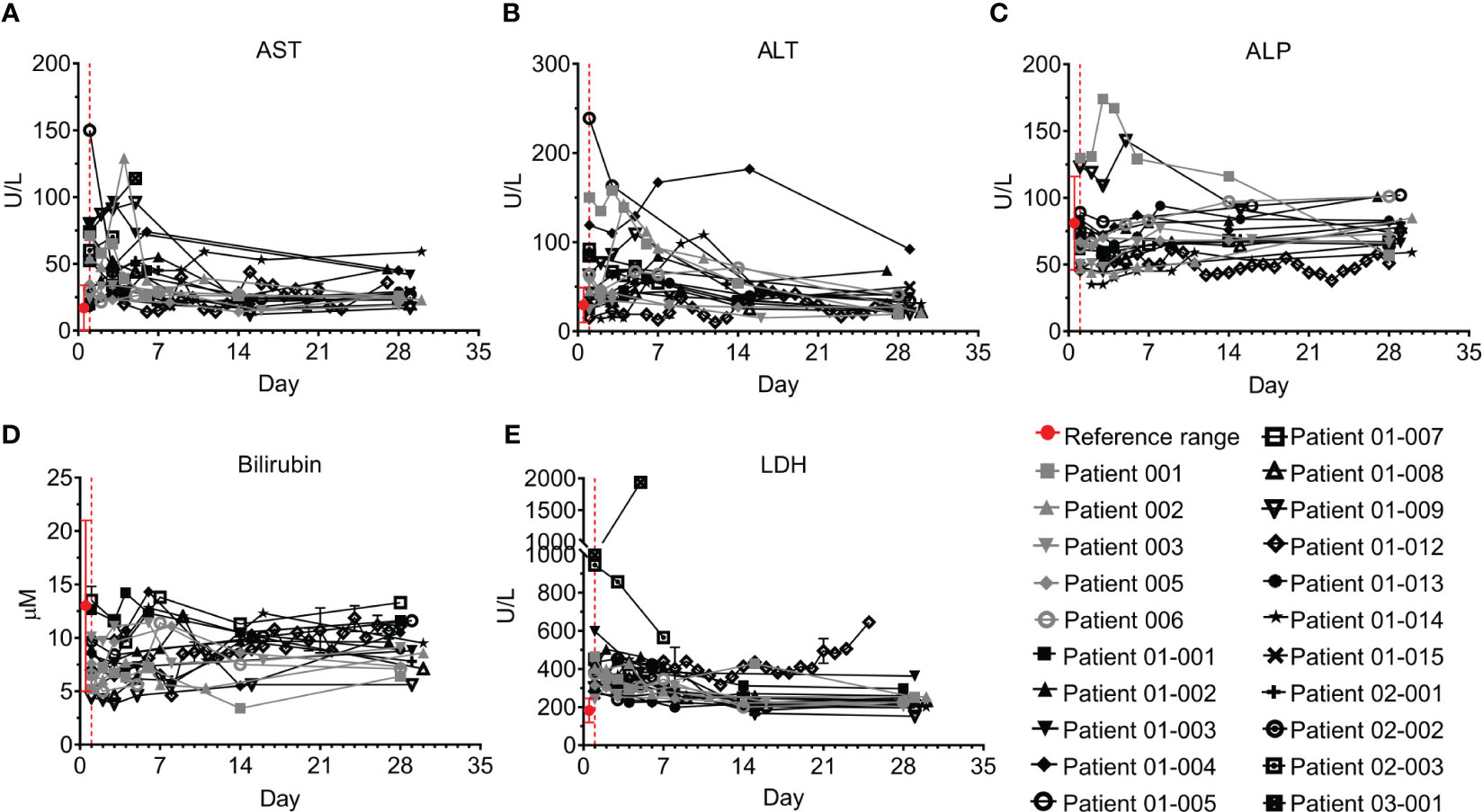
Figure 4 Liver markers and enzymes of COVID-19 patients treated with Allocetra-OTS. Severe/critical COVID-19 patients from studies NCT04513470 (gray symbols) and NCT04590053 (black symbols) were treated with Allocetra-OTS on day 1 (red dotted line). Blood samples were analyzed for (A) AST, (B) ALT, (C) alkaline phosphatase (ALP), (D) total bilirubin, and (E) LDH at the indicated timepoints. The first blood sampling for each patient was taken before IV administration of Allocetra-OTS. Data are shown as average ± range.
Of note, patients 01-012 and 03-001, who had markedly elevated neutrophils and CRP, did not improve as the others had and required treatment on mechanical ventilation and extracorporeal membrane oxygenation (ECMO).
Overall, for most patients, lymphocyte counts improved, and levels of acute phase reactants, CRP, ferritin, and D-dimer, decreased following treatment with Allocetra-OTS, correlating with amelioration of their clinical status. Most patients had mild elevation of liver enzymes before Allocetra-OTS administration that then resolved by day 28.
3.4 Exploratory endpoints, effect of Allocetra-OTS on cytokines, chemokines, and growth factors
3.4.1 Pro-inflammatory cytokines
Eight pro-inflammatory cytokines were tested, including TNF-α, IL-1β, IL-2, IL-6, IL-18, and interferons α, β, and γ (Figure 5; Table S4).
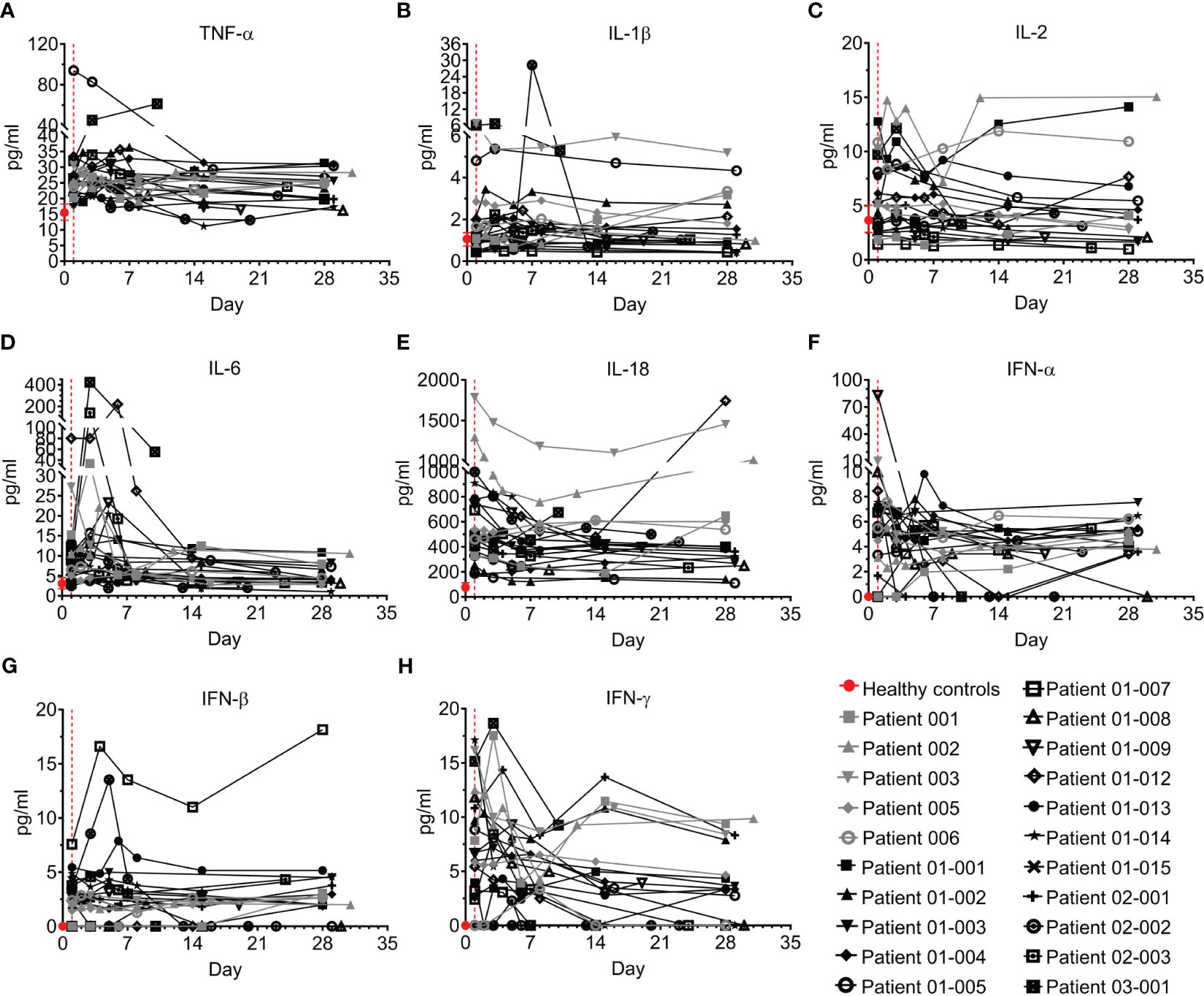
Figure 5 Pro-inflammatory cytokines of COVID-19 patients treated with Allocetra-OTS. Severely/critically ill COVID-19 patients from studies NCT04513470 (gray symbols) and NCT04590053 (black symbols) were treated with Allocetra-OTS on day 1 (red dotted line). Serum samples were analyzed for (A) TNF-α, (B) IL-1β, (C) IL-2, (D) IL-6, (E) IL-18, (F) IFN-α, (G) IFN-β, and (H) IFN-γ cytokine concentration at the indicated timepoints. The first blood sampling for each patient was taken before IV administration of Allocetra-OTS. Data are shown as average ± range.
TNF-α has previously been reported to be elevated in the serum and plasma of COVID–19 patients compared to healthy individuals (24–26). Accordingly, most of the patients in this study had elevated TNF-α upon admission to the hospital, with median of 25.2 pg/ml (IQR: 21.9–27.2) versus a median level of 14.6 pg/ml (IQR: 13.1–18.2) in healthy individuals (p=0·0002, Mann-Whitney test). Although TNF-α levels in 10/21 of the patients (47.6%) decreased following treatment, in four patients (19%) the level was unchanged, and 6/21 patients (28.6%) had mild increases of TNF–α by day 28. Patient 03-001 had an unusual 2-fold elevation of TNF-α (Figure 5A).
Unlike TNF-α, the levels of IL-1β and IL-2 were not significantly different than those of healthy individuals (Figures 5B, C). As reported in other studies (7, 8, 27–30), IL-6 levels of the majority of the COVID–19 patients in the present study (18/21, 85.7%) were significantly higher than healthy individuals (median of 9 pg/ml and 3 pg/ml in COVID–19 patients and healthy individuals, respectively; p=0·0018, Mann-Whitney test). Following treatment, IL-6 levels had rapidly and significantly decreased by day 7 and reached normal levels at day 28 (median concentration 4.1 pg/ml, p<0.0001, Wilcoxon matched-pairs test), with a median decrease of 35% (IQR: 18%–56%). Patients 01-012, 02-003 and 03-001 had an initial increase of IL-6 until days 3–7, followed by a delayed decrease (Figure 5D). IL-18 was also significantly higher than normal in the COVID–19 patients (median of 490 pg/ml and 69 pg/ml in COVID–19 patients and healthy individuals, respectively; p<0.0001, Mann-Whitney test). Although 14/21 patients (66.6%) had decreased IL-18 levels following treatment (median decrease of 29% at day 28 vs day 1), the IL-18 of six patients (28.6%) was increased, including patients 001, 005, 006, 01-012, and 03-001 (Figure 5E). Serum concentrations of IFN-α and IFN-β were low and remained unchanged in most patients, except for patient 01-007, who had an aberrant increase in IFN-β (Figures 5F, G).
Unlike IFN-α and IFN-β, levels of IFN-γ in COVID–19 patients upon admission to the ICU was significantly higher than normal (median of 6.7 pg/ml and 0 pg/ml in COVID–19 patients and healthy individuals, respectively, p=0.0006, Mann-Whitney test). By day 28, most patients (15/21, 71.4%) had significantly decreased IFN-γ with resolution of the disease (Figure 5H, p=0.0003, Wilcoxon matched-pairs test).
3.4.2 Anti-inflammatory cytokines
Interestingly, anti-inflammatory cytokines are also upregulated in COVID–19 (30, 31) in parallel with pro-inflammatory cytokines. Five anti-inflammatory cytokines were tested, including IL-10, IL-1Ra, IL-2Rα, TNFR-1, and IL-4 (Figure 6; Table S5). IL-10 was previously found to be elevated in COVID-19 patients and to be correlated with disease severity (29, 32, 33). Indeed, in the present study, IL-10 levels of COVID–19 patients on day 1 were 6.2-fold higher than levels in healthy individuals (median of 5.5 pg/ml and 0.9 pg/ml in COVID–19 patients and healthy individuals, respectively, p<0.0001, Mann-Whitney test). This was followed by rapid and substantial decline of IL-10 to normal levels in almost all the patients on days 14-to-28 (Figure 6A, p<0.0001, Wilcoxon matched-pairs test).
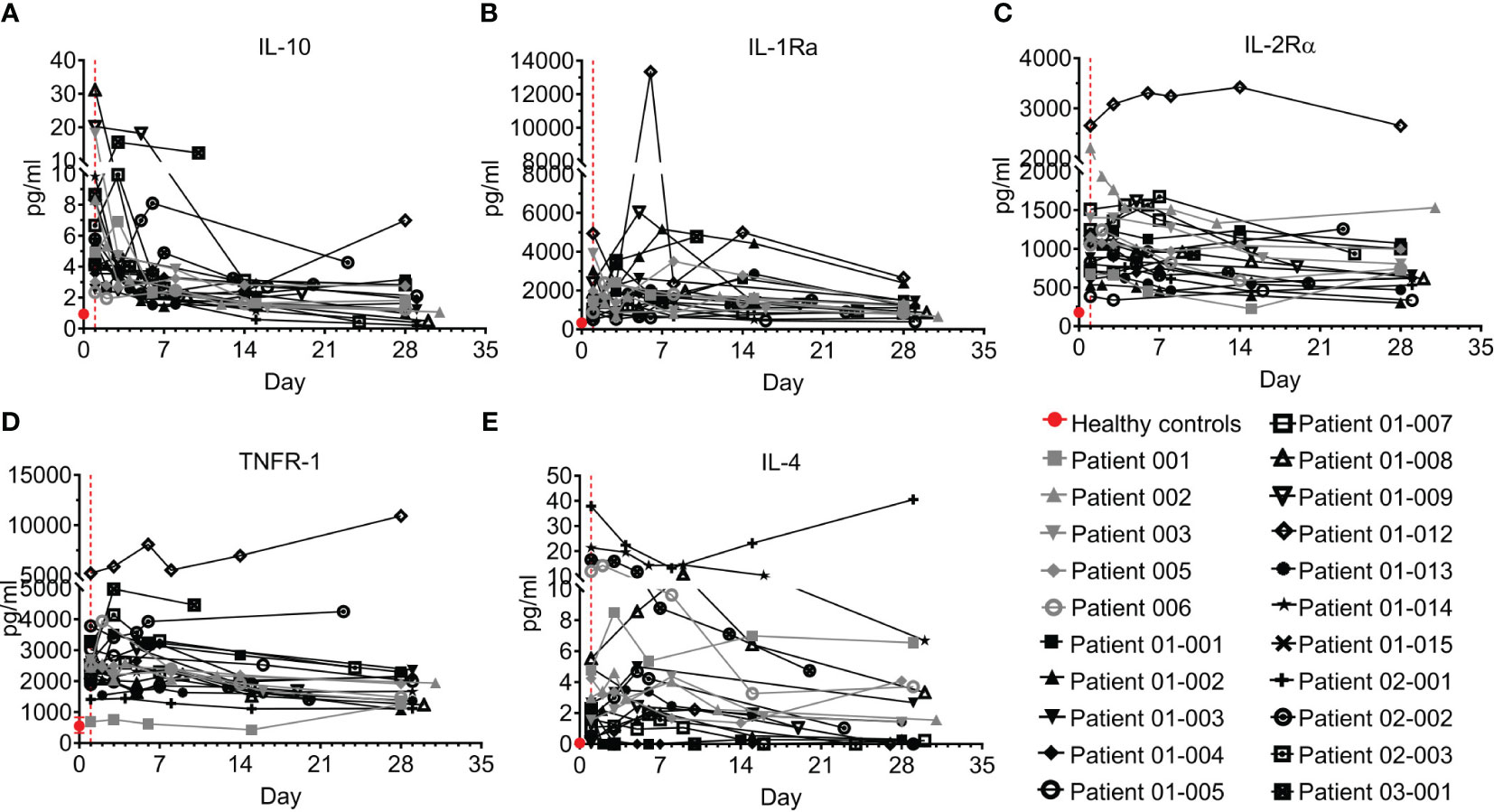
Figure 6 Anti-inflammatory cytokines of COVID-19 patients treated with Allocetra-OTS. Severe/critical COVID-19 patients from studies NCT04513470 (gray symbols) and NCT04590053 (black symbols) were treated with Allocetra-OTS on day 1 (red dotted line). Serum samples were analyzed for (A) IL-10, (B) IL-1Ra, (C) IL-2Rα, (D) TNFR-1, and (E) IL-4 cytokine concentration at the indicated timepoints. The first blood sampling for each patient was taken before IV administration of Allocetra-OTS. Data are shown as average ± range.
IL-1Ra, IL-2Rα, and TNFR-1 followed a similar trend. The majority of COVID–19 patients who presented with high levels of anti-inflammatory cytokines had a gradual decrease in those cytokines with resolution of the disease (Figures 6B–D). Unlike the majority of COVID–19 patients, patients 01-012 and p 03-001 had increasing and sustained high levels of IL-10, IL-1Ra, IL-2Rα, and TNFR-1. The pattern of IL-4 was slightly different from the other anti-inflammatory cytokines; while 20/21 patients (95%) presented with higher-than-normal IL-4 levels, most patients had decreased IL-4 levels during the follow-up period, except for patient 02-001, who manifested high levels of IL-4 throughout the study (Figure 6E).
Overall, the trend of the anti-inflammatory cytokines resembled that of the pro-inflammatory cytokines. Most patients had elevated IL-10, IL-1Ra, IL-2Rα, and TNFR-1, which gradually decreased as COVID-19 resolved.
3.4.3 Chemokines
Chemokines play pivotal roles in regulating the migration and infiltration of monocytes/macrophages and neutrophils to sites of inflammation and have been reported to be involved in COVID–19 (9, 34–36). Six chemokines were tested here, including monocyte chemoattractant protein 1 (MCP-1), macrophage inflammatory protein-1 alpha (MIP-1α), interferon gamma-induced protein 10 (IP-10), IL-8, MCP-3, and monokine induced by Gamma interferon (MIG, CXCL9) (Figure 7; Table S6). Overall, levels in most patients screened with MCP-1, MIP-1α, IL-8, and MCP-3 were above the concentration range of healthy individuals; however, IP-10 and MIG were markedly elevated in most patients upon admission to the ICU and then rapidly declined with the resolution of COVID–19. The levels of IP-10 at the end of the follow-up period remained high in patients 01-012 and 03-001, who also maintained high levels of IL-8 and MIG.
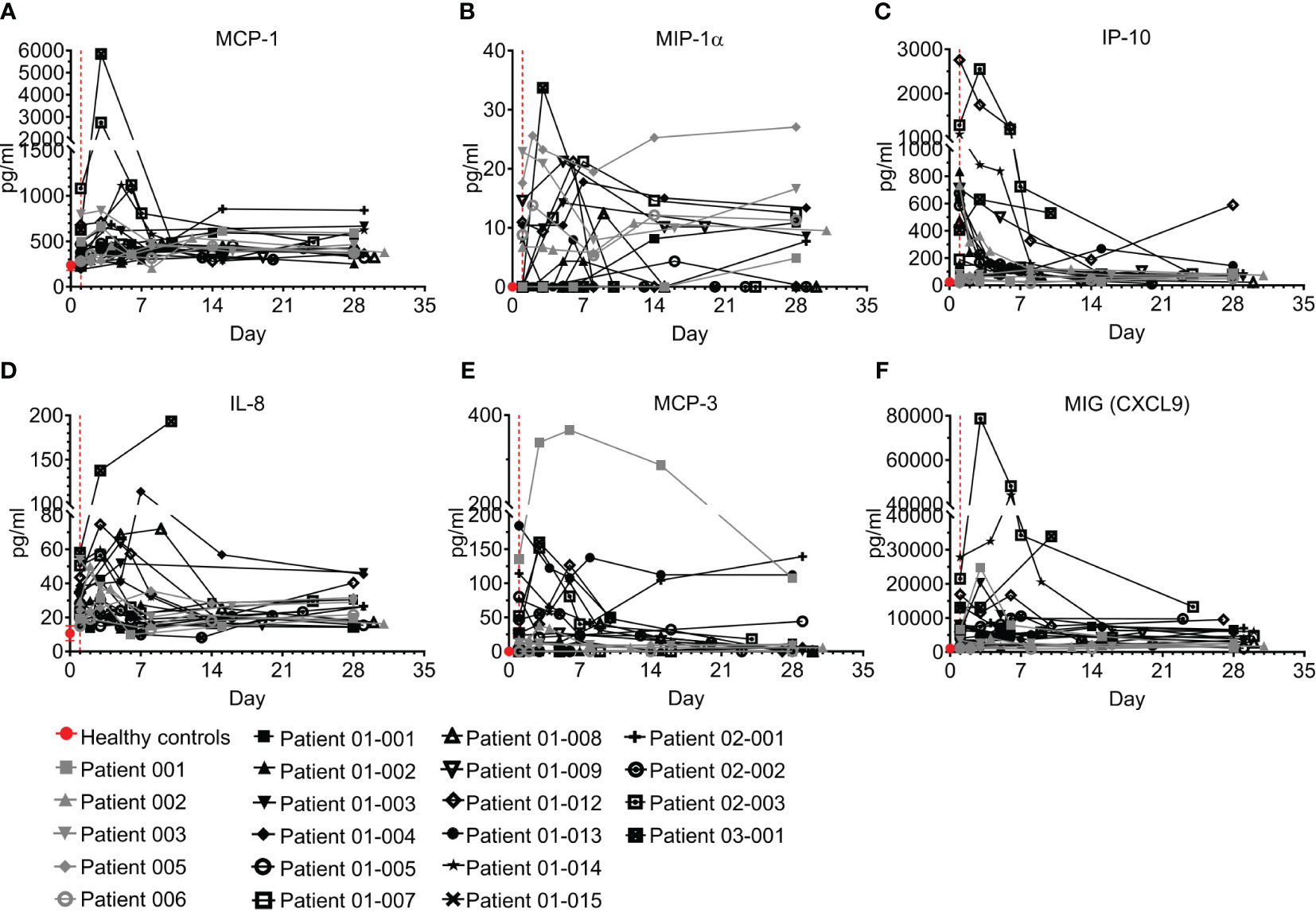
Figure 7 Chemokines of COVID-19 patients treated with Allocetra-OTS. Severe/critical COVID-19 patients from studies NCT04513470 (gray symbols) and NCT04590053 (black symbols) were treated with Allocetra-OTS on day 1 (red dotted line). Serum samples were analyzed for (A) MCP-1, (B) MIP-1α, (C) IP-10, (D) IL-8, (E) MCP-3, and (F) MIG (CXCL9) chemokine concentration at the indicated timepoints. The first blood sampling for each patient was taken before IV administration of Allocetra-OTS. Data are shown as average ± range.
3.4.4 Hematopoietic growth factors (HGFs)
An HGF is a relatively stable, secreted, or membrane-bound glycoprotein that causes immune cells to mature and/or proliferate, and also has profound effects on cell functions and behaviors. HGFs were shown to be upregulated in COVID–19 and were associated with disease severity and outcome (26, 37–39). Three growth factors were tested in the study and are presented here, including G-CSF, IL-7, and GM-CSF (Figure 8; Table S7). While GM-CSF was mostly undetected in both in healthy individuals and COVID–19 patients, ICU admission levels of G-CSF and IL-7 were significantly higher in COVID–19 patients than in healthy individuals (Figures 8A, B; p=0.0002 and p<0.0001 for G-CSF and IL-7, respectively). Following treatment, both HGFs were significantly decreased by day 7 and at day 28 were within or slightly above the normal range (Figures 8A, B; p=0.0446 and p<0.0001, for G-CSF and IL-7, respectively, Wilcoxon matched-pairs test). Overall, the trend of G-CSF and IL-7 HGFs resembled that of most of the cytokines and chemokines measured.
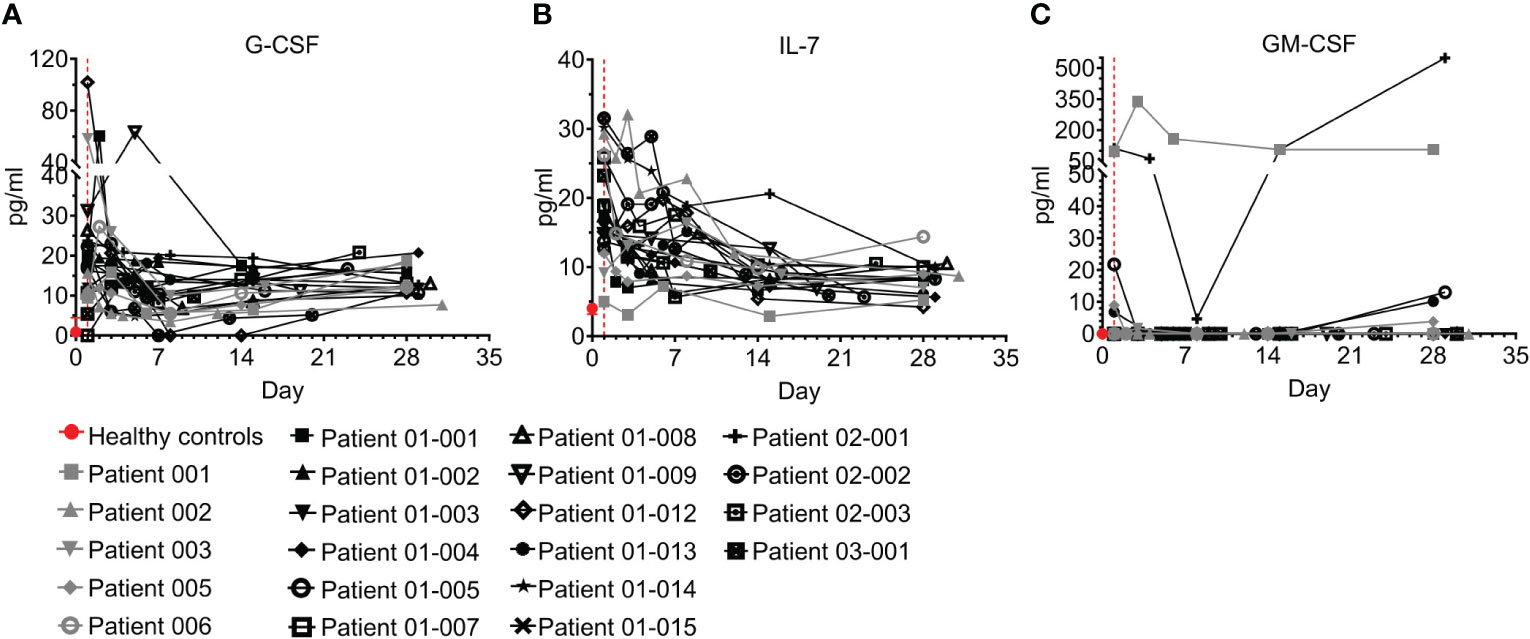
Figure 8 Hematopoietic growth factors (HGFs) of COVID-19 patients treated with Allocetra-OTS. Severe/critical COVID-19 patients from studies NCT04513470 (gray symbols) and NCT04590053 (black symbols) were treated with Allocetra-OTS on day 1 (red dotted line). Serum samples were analyzed for (A) G-CSF, (B) IL-7, and (C) GM-CSF HGF concentration at the indicated timepoints. The first blood sampling for each patient was taken before IV administration of Allocetra-OTS. Data are shown as average ± range.
3.5 Summary
In summary, most patients had elevated pro-, and anti-inflammatory cytokines, chemokines, and HGFs upon entering the study. Following treatment with Allocetra–OTS, most of these inflammatory immune-modulators, including IL-6, IFN-γ, IL-10, TNFR1, IP-10, MCP-3, and IL-7, gradually decreased to normal levels with resolution of COVID-19.
4 Discussion
Apoptotic cells (Allocetra–OTS) were given to 21 COVID–19 patients (earlier strains; G, alpha and delta), 11 with severe and 10 with critical illness. 19/21 patients had significant ARDS and all patients received supplemental oxygen via high-flow nasal cannula. Overall, the treatment with Allocetra-OTS was safe. There were only three serious adverse events (SAEs) and all were documented as “unrelated” to investigational product. Allocetra-OTS was also well tolerated when given in conjunction with standard therapy (remdesivir, enoxaparin, and dexamethasone). 19/21 of subjects showed early recovery, with 5.5 days on average until discharge; only 2/21 patients were still hospitalized by day 28 (end of study). 19 patients had mild-to-severe ARDS, and 17/19 (89.5%) completely recovered within a few days. For comparison, at the same time and for the same variants (G, alpha and delta), the mortality rates among critical COVID–19 patients (10/21 of the patients in this study) that were hospitalized in Hadassah Medical Center at that time period were much higher; 39.3% and 47.8%, for critical patients aged 50–60 and 60–70 years, respectively; females 55.6% and 40.0%, for ages 50–60 and 60–70, respectively; and males 31.6% and 51.6% for ages 50–60 and 60–70, respectively (Figure 1B). Although there was no control group in this study, the concomitant mortality rates with these variants, gender, severity, and ARDS in our institution were between 15%−40%.
Similar to patients with other severe inflammatory conditions, such as sepsis (11, 40, 41), most of the COVID–19 patients in this study were admitted to the ICU with high levels of CRP, lymphopenia, an elevated neutrophil count, and high NLR. Cytokine/chemokine inflammatory markers resolved following treatment with Allocetra–OTS, a pattern similar to the trends that we observed after treatment of patients with mild-to-moderate sepsis with Allocetra-OTS (15).
Immunological dysregulation that is manifested by a cytokine storm has emerged as a hallmark of COVID–19-related ARDS (8, 9). Accordingly, chemokines and pro- as well as anti-inflammatory cytokines were present in high concentrations in the serum of the COVID-19 patients in this study. Following treatment with Allocetra-OTS there was rapid reduction of TNF-α and IL-6, both independent predictors of disease severity and mortality (25, 29). The resolution of cytokine storm was also demonstrated by a substantial decrease in the levels of IFN-γ, IL-10, TNFR-1, and IP-10, all of which are involved in the imbalanced and harmful immune-response typical of severe COVID-19 patients (29, 32, 35, 38). Of note, only 2/21 patients did not experience resolution of the cytokine storm; these two patients were critical patients with a NEWS2 score higher than 7 at admission. Both were hospitalized in the intensive care unit (ICU) and progressed to mechanical ventilation and then extracorporeal membrane oxygenation (ECMO) within a week from the beginning of the study. This observation may suggest that recovery is very much related to the resolution of cytokine storms.
In this study, patterns seen with a resolution of the cytokine storm in patients with severe COVID-19 following treatment with Allocetra-OTS shared some striking similarities with patterns in a previous Phase 1b study that we conducted to determine the safety profile of Allocetra-OTS treatment in patients with mild-moderate sepsis (15). Similarities included resolution of lymphopenia and neutrophilia and a rapid decrease in the serum concentrations of CRP, TNF-α, IL-6, IFN-γ, IL-10, TNFR-1, and IP-10 following treatment with Allocetra-OTS.
Elevations in these common disease markers and their resolution following treatment with Allocetra-OTS suggests two main insights: (i) severe COVID–19 and sepsis possibly share common pathogenesis of cytokine triggering mechanisms; (ii) these mechanisms may involve activated macrophages, which are major initiators and inducers of cytokine storms (35) and which are the target of Allocetra-OTS apoptotic cells. Indeed, in both sepsis and severe COVID-19, pathogen-related signals trigger the activation of tissue macrophages, which in turn induce an initial hyper-inflammatory response characterized by the release of pro-inflammatory cytokines like TNF and IL-6 (42–45). This includes the release of chemokines and the subsequent recruitment and activation of the adaptive immune system. This prolonged activation also involves the secretion of anti-inflammatory cytokines such as IL-10, which can lead to terminal immune-suppression (43, 46).
Therefore, Allocetra-OTS may offer a holistic therapeutic approach for those disease processes that involve a severely imbalanced immune-response that cannot be resolved using monotherapies.
These results suggest that immune modulation of cytokine storm with Allocetra may offer a mode of treatment for critically ill patients with ARDS; however, to verify that a controlled study is needed.
Data availability statement
The raw data supporting the conclusions of this article will be made available by the authors, without undue reservation.
Ethics statement
The studies involving human participants were reviewed and approved by the local ethics committee and the Israeli Ministry of Health. The patients/participants provided their written informed consent to participate in this study.
Author contributions
PH designed the research studies, treated the patients, and wrote the manuscript. AA designed the research studies, and wrote the manuscript. AhN and SM treated the patients and wrote the manuscript. KAJ, NM and AkN treated the patients. LB designed the research studies and treated the patients. OH helped in the design of the study. YS designed the research studies, conducted the experiments, acquired and analyzed the data, and provided reagents. BR acquired and analyzed the data, and wrote the manuscript. DM designed the research studies, acquired and analyzed the data, and wrote the manuscript. All authors contributed to the article and approved the submitted version.
Funding
The authors declare that this study received funding from Enlivex Therapeutics Ltd. The funder was not involved in the study design, collection, analysis, interpretation of data, the writing of this article or the decision to submit it for publication.
Conflict of interest
PH received honoraria from Enlivex Ltd as a consultant. DM is the founder and the Chief Scientific Officer of Enlivex Therapeutics Ltd. YS, BR, LB and OH are employed by Enlivex Therapeutics Ltd.
The remaining authors declare that the research was conducted in the absence of any commercial or financial relationships that could be construed as a potential conflict of interest.
Publisher’s note
All claims expressed in this article are solely those of the authors and do not necessarily represent those of their affiliated organizations, or those of the publisher, the editors and the reviewers. Any product that may be evaluated in this article, or claim that may be made by its manufacturer, is not guaranteed or endorsed by the publisher.
Supplementary material
The Supplementary Material for this article can be found online at: https://www.frontiersin.org/articles/10.3389/fimmu.2023.1242551/full#supplementary-material
References
1. Mathieu E, Ritchie H, Rodés-Guirao L, Appel C, Giattino G, Hasell J, et al. Coronovirus Pandemic (COVID-19) (2020). Available at: https://ourworldindata.org/coronavirus.
2. Group ICC, Kartsonaki C. Characteristics and outcomes of an international cohort of 400,000 hospitalised patients with Covid-19. medRxiv (2021).
3. Carbonell R, Urgeles S, Rodriguez A, Bodi M, Martin-Loeches I, Sole-Violan J, et al. Mortality comparison between the first and second/third waves among 3,795 critical COVID-19 patients with pneumonia admitted to the ICU: A multicentre retrospective cohort study. Lancet Reg Health Eur (2021) 11:100243. doi: 10.1016/j.lanepe.2021.100243
4. Dongelmans DA, Termorshuizen F, Brinkman S, Bakhshi-Raiez F, Arbous MS, de Lange DW, et al. Characteristics and outcome of COVID-19 patients admitted to the ICU: a nationwide cohort study on the comparison between the first and the consecutive upsurges of the second wave of the COVID-19 pandemic in the Netherlands. Ann Inten Care (2022) 12(1):5. doi: 10.1186/s13613-021-00978-3
5. Fan E, Beitler JR, Laurent L, Calfee CS, Ferguson ND, Slutsky AS, et al. COVID-19-associated acute respiratory distress syndrome: is a different approach to management warranted? Lancet Respir Med (2020) 8(8):816–21. doi: 10.1016/S2213-2600(20)30304-0
6. Juanes-Velasco P, Landeira-Vinuela A, Garcia-Vaquero ML, Lecrevisse Q, Herrero R, Ferruelo A, et al. SARS-CoV-2 infection triggers auto-immune response in ARDS. Front Immunol (2022) 13:732197. doi: 10.3389/fimmu.2022.732197
7. Del Valle DM, Kim-Schulze S, Huang HH, Beckmann ND, Nirenberg S, Wang B, et al. An inflammatory cytokine signature predicts COVID-19 severity and survival. Nat Med (2020) 26(10):1636–43. doi: 10.1038/s41591-020-1051-9
8. Ronit A, Berg RMG, Bay JT, Haugaard AK, Ahlstrom MG, Burgdorf KS, et al. Compartmental immunophenotyping in COVID-19 ARDS: A case series. J Allergy Clin Immunol (2021) 147(1):81–91. doi: 10.1016/j.jaci.2020.09.009
9. Chen Y, Wang J, Liu C, Su L, Zhang D, Fan J, et al. IP-10 and MCP-1 as biomarkers associated with disease severity of COVID-19. Molec Med (2020) 26(1):97. doi: 10.1186/s10020-020-00230-x
10. Spadaro S, Park M, Turrini C, Tunstall T, Thwaites R, Mauri T, et al. Biomarkers for acute respiratory distress syndrome and prospects for personalised medicine. J Inflamm (2019) 16(1):1. doi: 10.1186/s12950-018-0202-y
11. Yang JM, Koh HY, Moon SY, Yoo IK, Ha EK, You S, et al. Allergic disorders and susceptibility to and severity of COVID-19: A nationwide cohort study. J Allergy Clin Immunol (2020) 146(4):790–8. doi: 10.1016/j.jaci.2020.08.008
12. Tan E, Song J, Deane AM, Plummer MP. Global impact of coronavirus disease 2019 infection requiring admission to the ICU: a systematic review and meta-analysis. Chest (2021) 159(2):524–36. doi: 10.1016/j.chest.2020.10.014
13. Huang R, Xia J, Chen Y, Shan C, Wu C. A family cluster of SARS-CoV-2 infection involving 11 patients in Nanjing, China. Lancet Infect Dis (2020) 20(5):534–5. doi: 10.1016/S1473-3099(20)30147-X
14. Mehta P, McAuley DF, Brown M, Sanchez E, Tattersall RS, Manson JJ, et al. COVID-19: consider cytokine storm syndromes and immunosuppression. Lancet (2020) 395(10229):1033–4. doi: 10.1016/S0140-6736(20)30628-0
15. van Heerden PV, Abutbul A, Sviri S, Zlotnick E, Nama A, Zimro S, et al. Apoptotic cells for therapeutic use in cytokine storm associated with sepsis- a phase Ib clinical trial. Front Immunol (2021) 12:718191. doi: 10.3389/fimmu.2021.718191
16. Coronovirus Disease 2019 (COVID-19) Treatment Guidelines (2023). Available at: https://www.covid19treatmentguidelines.nih.gov/.
17. National Early Warning Score (NEWS2): Standardixing the Assessment of Acute Illness Severity in the NHS (2017). Available at: https://www.rcplondon.ac.uk/projects/outputs/national-early-warning-score-news-2.
18. Trahtemberg U, Mevorach D. Apoptotic cells induced signaling for immune homeostasis in macrophages and dendritic cells. Front Immunol (2017) 8:1356. doi: 10.3389/fimmu.2017.01356
19. Moon BH, Park SK, Jang DK, Jang KS, Kim JT, Han YM. Use of APACHE II and SAPS II to predict mortality for hemorrhagic and ischemic stroke patients. J Clin Neurosci (2015) 22(1):111–5. doi: 10.1016/j.jocn.2014.05.031
20. Minne L, Abu-Hanna A, de Jonge E. Evaluation of SOFA-based models for predicting mortality in the ICU: A systematic review. Crit Care (2008) 12(6):R161. doi: 10.1186/cc7160
21. Mevorach D, Zuckerman T, Reiner I, Shimoni A, Samuel S, Nagler A, et al. Single infusion of donor mononuclear early apoptotic cells as prophylaxis for graft-versus-host disease in myeloablative HLA-matched allogeneic bone marrow transplantation: a phase I/IIa clinical trial. Biol Blood Marrow Transplant (2014) 20(1):58–65. doi: 10.1016/j.bbmt.2013.10.010
22. Knaus WA, Draper EA, Wagner DP, Zimmerman JE. APACHE II: a severity of disease classification system. Crit Care Med (1985) 13(10):818–29. doi: 10.1097/00003246-198510000-00009
23. Clinical Spectrum of SARS-CoV-2 Infection (2023). Available at: https://www.covid19treatmentguidelines.nih.gov/overview/clinical-spectrum/.
24. Qin C, Zhou L, Hu Z, Zhang S, Yang S, Tao Y, et al. Dysregulation of immune response in patients with coronavirus 2019 (COVID-19) in Wuhan, China. Clin Infect Dis (2020) 71(15):762–8. doi: 10.1093/cid/ciaa248
25. Mortaz E, Tabarsi P, Jamaati H, Dalil Roofchayee N, Dezfuli NK, Hashemian SM, et al. Increased serum levels of soluble TNF-α receptor is associated with ICU mortality in COVID-19 patients. Front Immunol (2021), 12. doi: 10.3389/fimmu.2021.592727
26. Huang C, Wang Y, Li X, Ren L, Zhao J, Hu Y, et al. Clinical features of patients infected with 2019 novel coronavirus in Wuhan, China. Lancet (2020) 395(10223):497–506. doi: 10.1016/S0140-6736(20)30183-5
27. Santa Cruz A, Mendes-Frias A, Oliveira AI, Dias L, Matos AR, Carvalho A, et al. Interleukin-6 is a biomarker for the development of fatal severe acute respiratory syndrome coronavirus 2 pneumonia. Front Immunol (2021) 12:613422. doi: 10.3389/fimmu.2021.613422
28. Liu T, Zhang J, Yang Y, Ma H, Li Z, Zhang J, et al. The role of interleukin-6 in monitoring severe case of coronavirus disease 2019. EMBO Mol Med (2020) 12(7):e12421. doi: 10.15252/emmm.202012421
29. Han H, Ma Q, Li C, Liu R, Zhao L, Wang W, et al. Profiling serum cytokines in COVID-19 patients reveals IL-6 and IL-10 are disease severity predictors. Emerg Microbes Infect (2020) 9(1):1123–30. doi: 10.1080/22221751.2020.1770129
30. Liu QQ, Cheng A, Wang Y, Li H, Hu L, Zhao X, et al. Cytokines and their relationship with the severity and prognosis of coronavirus disease 2019 (COVID-19): a retrospective cohort study. BMJ Open (2020) 10(11):e041471. doi: 10.1136/bmjopen-2020-041471
31. Islam H, Chamberlain TC, Mui AL, Little JP. Elevated interleukin-10 levels in COVID-19: potentiation of pro-inflammatory responses or impaired anti-iflammatory sction? Front Immunol (2021) 12:677008. doi: 10.3389/fimmu.2021.677008
32. Dhar SK, Vishnupriyan K, Damodar S, Gujar S, Das M. IL-6 and IL-10 as predictors of disease severity in COVID-19 patients: results from meta-analysis and regression. Heliyon (2021) 7(2):e06155. doi: 10.1016/j.heliyon.2021.e06155
33. Jafrin S, Aziz MA, Islam MS. Elevated levels of pleiotropic interleukin-6 (IL-6) and interleukin-10 (IL-10) are critically involved with the severity and mortality of COVID-19: an updated longitudinal meta-analysis and systematic review on 147 studies. biomark Insights (2022) 17. doi: 10.37766/inplasy2022.4.0046
34. Bülow Anderberg S, Luther T, Berglund M, Larsson R, Rubertsson S, Lipcsey M, et al. Increased levels of plasma cytokines and correlations to organ failure and 30-day mortality in critically ill Covid-19 patients. Cytokine (2021) 138:155389. doi: 10.1016/j.cyto.2020.155389
35. Lev S, Gottesman T, Sahaf Levin G, Lederfein D, Berkov E, Diker D, et al. Observational cohort study of IP-10’s potential as a biomarker to aid in inflammation regulation within a clinical decision support protocol for patients with severe COVID-19. PloS One (2021) 16(1):e0245296. doi: 10.1371/journal.pone.0245296
36. Wang J, Yang X, Li Y, Huang J-A, Jiang J, Su N. Specific cytokines in the inflammatory cytokine storm of patients with COVID-19-associated acute respiratory distress syndrome and extrapulmonary multiple-organ dysfunction. Virol J (2021) 18(1):117. doi: 10.1186/s12985-021-01588-y
37. Hue S, Beldi-Ferchiou A, Bendib I, Surenaud M, Fourati S, Frapard T, et al. Uncontrolled innate and impaired adaptive immune responses in patients with COVID-19 acute respiratory distress syndrome. Amer J Resp Crit Care Med (2020) 202(11):1509–19. doi: 10.1164/rccm.202005-1885OC
38. Wang G-L, Gao H-X, Wang Y-L, Wei X, Liu Y-Z, Lu J-H, et al. Serum IP-10 and IL-7 levels are associated with disease severity of coronavirus disease 2019. Cytokine (2021) 142:155500. doi: 10.1016/j.cyto.2021.155500
39. Zhou Y, Fu B, Zheng X, Wang D, Zhao C, Qi Y, et al. Pathogenic T-cells and inflammatory monocytes incite inflammatory storms in severe COVID-19 patients. Nat Sci Rev (2020) 7(6):998–1002. doi: 10.1093/nsr/nwaa041
40. Liu S, Wang X, She F, Zhang W, Liu H, Zhao X. Effects of neutrophil-to-lymphocyte ratio combined with interleukin-6 in predicting 28-day mortality in patients with sepsis. Front Immunol (2021) 12:639735. doi: 10.3389/fimmu.2021.639735
41. Li X, Xu Z, Pang X, Huang Y, Yang B, Yang Y, et al. Interleukin-10/lymphocyte ratio predicts mortality in severe septic patients. PloS One (2017) 12(6):e0179050. doi: 10.1371/journal.pone.0179050
42. Arango Duque G, Descoteaux A. Macrophage cytokines: involvement in immunity and infectious diseases. Front Immunol (2014) 5:491. doi: 10.3389/fimmu.2014.00491
43. Gallo CG, Fiorino S, Posabella G, Antonacci D, Tropeano A, Pausini E, et al. COVID-19, what could sepsis, severe acute pancreatitis, gender differences, and aging teach us? Cytokine (2021) 148:155628. doi: 10.1016/j.cyto.2021.155628
44. Diamond MS, Kanneganti T-D. Innate immunity: the first line of defense against SARS-CoV-2. Nat Immunol (2022) 23(2):165–76. doi: 10.1038/s41590-021-01091-0
45. Zhang Y-Y, Ning B-T. Signaling pathways and intervention therapies in sepsis. Signal Transduction Targeted Ther (2021) 6(1):407. doi: 10.1038/s41392-021-00471-0
Keywords: apoptotic cells, cytokine storm, ARDS, macrophage reprogramming, COVID-19
Citation: van Heerden PV, Abutbul A, Naama A, Maayan S, Makram N, Nachshon A, abu Jabal K, Hershkovitz O, Binder L, Shabat Y, Reicher B and Mevorach D (2023) Apoptotic cells for treatment of acute respiratory distress syndrome associated with COVID-19. Front. Immunol. 14:1242551. doi: 10.3389/fimmu.2023.1242551
Received: 19 June 2023; Accepted: 17 July 2023;
Published: 02 August 2023.
Edited by:
Amiram Ariel, University of Haifa, IsraelReviewed by:
Amira Othman, Queen’s University, CanadaThierry Mp Gauthier, National Institutes of Health (NIH), United States
Copyright © 2023 van Heerden, Abutbul, Naama, Maayan, Makram, Nachshon, abu Jabal, Hershkovitz, Binder, Shabat, Reicher and Mevorach. This is an open-access article distributed under the terms of the Creative Commons Attribution License (CC BY). The use, distribution or reproduction in other forums is permitted, provided the original author(s) and the copyright owner(s) are credited and that the original publication in this journal is cited, in accordance with accepted academic practice. No use, distribution or reproduction is permitted which does not comply with these terms.
*Correspondence: Dror Mevorach, bWV2b3JhY2hkQGhhZGFzc2FoLm9yZy5pbA==