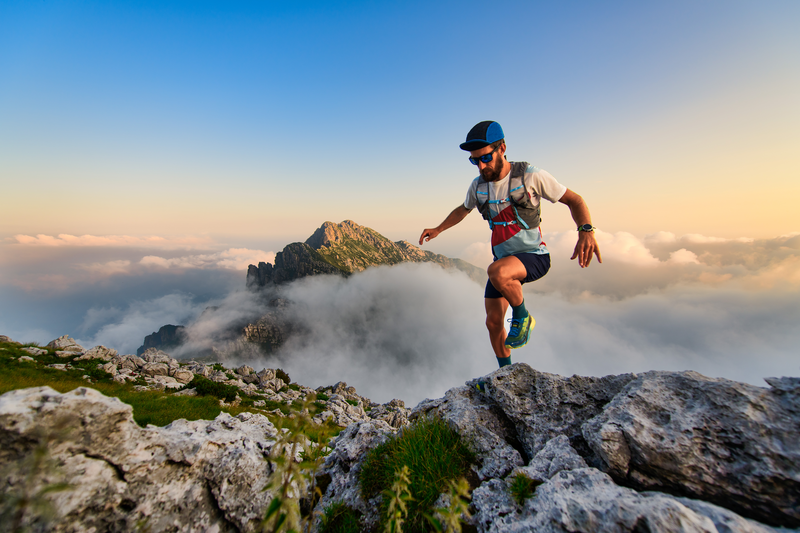
94% of researchers rate our articles as excellent or good
Learn more about the work of our research integrity team to safeguard the quality of each article we publish.
Find out more
ORIGINAL RESEARCH article
Front. Immunol. , 28 August 2023
Sec. Cancer Immunity and Immunotherapy
Volume 14 - 2023 | https://doi.org/10.3389/fimmu.2023.1239097
This article is part of the Research Topic Immunotherapy and Multimodality Therapy for Lung Cancer View all 27 articles
Introduction: There are limited data on the influence of different anti-cancer therapies on lymphocyte subpopulations and their relationships to survival of non-small cell lung cancer (NSCLC) patients. This study aimed to assess the effect of immunotherapy, chemotherapy, immunochemotherapy, adjuvant chemotherapy after surgery, and antibodies against Vascular Endothelial Growth Factors (VEGF) on B cell, T cell, and NK cell subpopulations, and the survival time of NSCLC patients.
Methods: A total of 32 consecutive NSCLC patients were recruited at Pulmonology Clinic, Leipzig from January 2018 to March 2020 and enrolled in this study. Immunophenotyping was done using a FACS Canto II flow cytometer (BD Biosciences) before the administration of the planned therapy and during therapy with up to 7 observational windows for each patient targeting 130 immunologic parameters.
Results: Absolute transitional B cells was significantly increased after immunotherapy (p = 0.032), immunochemotherapy (p = 0.030), and antibodies against VEGF (p = 0.024). Similarly, absolute counts and percentage of B cells were significantly increased after adjuvant chemotherapy (p = 0.023). However, absolute counts and percentage of transitional B cells are significantly decreased after chemotherapy (p = 0.001). Activated cytotoxic T cells were significantly increased after immunotherapy (p = 0.031) and immunochemotherapy (p = 0.030). The overall survival rate of NSCLC patients was 31%.
Conclusions: In conclusion, this study suggests that different types of anti-cancer therapies affect lymphocyte subpopulations of NSCLC patients. Further large-scale and multicentre studies are required to confirm our results and to evaluate the prognostic value of lymphocyte subpopulations.
Lung cancer is one of the most frequent malignancies with 2.2 million new cases a year and the most common cause of cancer death in the world (1). Non-small cell lung cancer (NSCLC) is about 80–85% of all lung cancer cases (2). Majority (> 55%) of NSCLC patients are diagnosed late at the advanced stages of the disease (3). Currently, the treatment options for NSCLC are systemic therapies such as chemotherapies, drugs targeting commonly mutated pathways in lung cancer, and immune checkpoint inhibitors, surgical resection of the primary tumor or metastatic lesion and radiation therapy (4). The management and therapy of patients with advanced NSCLC have changed markedly in the last few years. Early detection methods and therapeutic options have been improved tremendously. However, the overall survival rate of patients with advanced NSCLC did not improve much and is still dismal (5). The reported 5-year survival rate of NSCLC patients was 17.8%, which is one of the highest fatality rates in non-communicable diseases (6). Reliable markers of treatment response and survival outcomes are urgently needed to enable early adaptation of treatment strategies in advanced NSCLC patients. Tumorigenesis and programmed cell death ligand 1 (PD-L1) status became standard markers influencing therapy regimes (7). Expression of PD-L1 has shown a significant predictive role (8). Other biomarkers such as lung immune prognostic index (LIPI) and tumor mutation burden (TMB) have revealed inconsistent results (9). Several studies have also demonstrated that lung cancer patients have lower levels of CD4+/CD8+ ratio, CD4+T cells, NK cell levels, and higher regulatory T cells (Tregs) than healthy subjects (10, 11). Patient-related factors such as age and comorbidities also affect peripheral blood lymphocyte subpopulations and treatment outcomes of NSCLC patients (12). Anti-cancer therapies, tumor surgery and wound healing may also have an impact on the immunophenotyping of lymphocyte subpopulations. So far, there are limited data concerning changes or developmental shifts in the most relevant B cell, T cell, and NK cell subpopulations caused by different anti-cancer therapies. Understanding the potential changes in lymphocyte subpopulations during different therapies is crucial to understand the effect of different therapies on the immune system and to adapt effective therapy regimens for better management of NSCLC patients. Therefore, in this study, we investigated the influence of immunotherapy, chemotherapy, immunochemotherapy, adjuvant chemotherapy after surgery, and antibodies against Vascular Endothelial Growth Factors (VEGF) on B cell, T cell, and NK cell subpopulations. Additionally, the relationship between therapy regimens and survival was assessed.
A longitudinal cohort study was performed on 32 consecutive NSCLC patients who visited the Pulmonology Clinic, Leipzig from January 10, 2018 to March 3, 2020. Socio-demographic information such as age, sex, smoking status, and clinical information such as type of cancer and the stage of cancer were collected from patients using a standardized questionnaire. Active smokers were defined as patients actively smoking at the time of data collection or up to 6 months before the data collection. To be qualified as former smoker, it was required that the patient quit smoking more than 6 months prior to data collection. Immunophenotyping was done before the administration of the planned therapy and during therapy with up to 7 observational windows (3 to 4 weeks each window) in the laboratory of the Institute of Clinical Immunology, University Hospital Leipzig resulting in a total of 135 observations with 130 immunologic parameters assessed. The median follow-up period for the study participants included in this study was 1,220 days.
Ethical approval was made by the Medical Faculty of the University of Leipzig ethics committee (Ref. No: 201/18-ek). The socio-demographic information, clinical data, and venous blood were collected from NSCLC patients following the ethical considerations recommended by the Declaration of Helsinki of 1975, revised in 2013. Data identifying study participants were removed prior to analyses. Safeguards for appropriate and ethical use of data were in place. Informed consent was acquired from all NSCLC patients included in the study.
Patients were treated with one of the following five different treatment options: Immunotherapy (Nivolumab, Pembrolizumab, or Atezolizumab), Cytotoxic chemotherapy (Carboplatin + Nab-Paclitaxel, Carboplatin + Pemetrexed + Bevacizumab or Cisplatin + Pemetrexed + Bevacizumab), Immunochemotherapy (Carboplatin + Pemetrexed + Pembrolizumab, Carboplatin + Nab-Paclitaxel + Atezolizumab, Carboplatin + Nab-Paclitaxel + Pembrolizumab or Carboplatin + Nab-Paclitaxel + Bevacizumab + Atezolizumab), Adjuvant chemotherapy after tumor surgery (Cisplatin + Vinorelbin or Carboplatin + Vinorelbin) and Antibodies against VEGF (Bevacizumab).
Ethylenediaminetetraacetic acid (EDTA) venous blood was collected from each patient in the pulmonology clinic before the administration of the planned therapy at the time of treatment start and during observational windows. EDTA blood specimen was immediately brought to the laboratory of the Institute of Clinical Immunology for analysis of the cellular immune status. Blood samples were processed and analyzed within 3 hours of collection.
The staining and flow cytometry analysis of B cell, T cell, and NK cell subpopulations were performed according to previously published protocols (13, 14). In brief, whole blood sample was divided into 8 different panel tests to analyze the following specific cell populations: (i) general lymphocytes, (ii) B cell subsets, (iii) CD4 T cell subsets, (iv) CD8 T cell subsets, (v) regulatory T cells, (vi) recent thymic emigrants (RTEs), (vii) NK cell subsets, and (viii) NK cell activation markers. To each panel test, a 100 µl whole blood specimen was added and incubated with specific antibodies to the chosen cell subsets. The antibody mixes, cell markers, fluorochromes, IgG subtypes, antibody concentration, and clones used for every panel test are explained in Supplementary Table S1.
To decide the optimum dilution for each staining, each antibody for eight-color analysis panels was titrated separately and compared with isotype controls. Cell surface markers were stained for 15 minutes in the dark at room temperature and then erythrocytes were lysed by lysis buffer (BD Biosciences, Heidelberg, Germany) after incubation or 10 minutes. After centrifugation and washing with phosphate-buffered saline (PBS) (Biochrom, Berlin, Germany), cells were fixed with 200 µl PBS that contains 1% formaldehyde and ready for flow cytometry analysis.
The flowcytometry data acquisition was made using FACS Canto II (BD Biosciences) machine. The FACS Canto II is has three lasers: a 647 nm red laser, a 488 nm blue laser, and a 405 nm violet laser. During the analysis of all panel tests, automatic standard compensation was done, except for the B cell subsets panel, where the spectral overlap of the allophycocyanin (APC) toward peridinin-chlorophyll-protein (PerCP) was set to 12.66, to achieve optimal separation of memory and naive B cells. A total of 50,000 cell events were collected in the lymphocyte collection gate (CD45+ versus SSClow) for eight-color analysis, and the FACS DIVA (BD Biosciences) software version was used for data analysis 8. The absolute cell counts were determined using the relative flow cytometric cell counts measured by BD FACS Canto II Flow Cytometer set in relation to absolute cell counts analyzed by Sysmex XP-300 automated hematology analyzer (Sysmex Germany GmbH, Bornbarch, Germany) based on the standard protocol.
The general lymphocyte gating strategy gives an overview of changes in the lymphocyte subpopulations. B cells (CD19+), T cells (CD3+), and NK cells (CD16+/56+) as well as HLA-DR+CD38+ activated T cells were gated from the lymphocyte gate using specific markers. Moreover, NK-T cells (T cells co-expressing CD16 molecule) were separated by gating CD3 versus CD16/56. The CD8 versus CD4 plot was used to separate 4 different T cell subsets: CD4+ and CD8+ T cells, CD4-/CD8- double-negative and CD4+/CD8+ double-positive T cells (Figure 1).
Figure 1 General lymphocytes gating strategy. Based on lymphocyte separation (SSC vs. CD45) (A), T cells, B cells (B), NK cells, and NK-T cells (C) were detected. From CD3+T cells gating, T helper (CD4+) cells, cytotoxic T cells (CD8+), CD4 and CD8 double positive and double negative T cells (D) and T cells activation status by HLA-DR+CD38+ expression were determined (E).
B cells (CD19+) subsets were idetnified from the lymphocyte gate. The CD21 vs. CD27combination was used for the separation of immature, CD21low, and memory B cells. Double-negative immature B cells were used as a baseline for CD21+ or CD27+ B cells. Non-switched and switched memory B cells were separated from the CD27 gate by IgD versus IgM demarcation. The CD38low versus CD21low plot creates the gates for the activated CD21lowCD38low B cells and immature transitional B cells, which are specified to be CD21lowCD38+ B cells. IgM+ and CD38+ co-expressions were used for gating Transitional B cells. From this plot, plasma-blasts were separated using CD38+ expression and the absence of surface IgM. Additionally, CD27+CD138+ peripheral plasma cells were identified (Supplementary Figure S1(I)).
T cells were separated from other lymphocytes using CD3 staining. To analyze T cell subpoulations, a combination of CD45RO, CD45RA, and CCR7 markers was used to separate effector cells (CD45RA+CD45RO-CCR7-), naive (CD45RA+CD45RO-CCR7+), central memory (CD45RA-CD45RO+CCR7+) and effector memory (CD45RA-CD45RO+CCR7-) cells. Additionally, from the CD3+CD4+ T helper cell population, regulatory T cells were detected as CD25+CD127 low cells (Supplementary Figure S1(II)).
For NK cells subsets analysis, T cells were excluded by gating the CD3 negative cells. From the CD3 negative cells, CD16 and CD56 markers were used to separate mature NK cells (CD16+CD56dim) and CD56 bright NK cells. In the next step, the expression of NK cells activation markers such as Nkp30, Nkp44, Nkp46, and CD57 was analyzed on mature NK cells (Supplementary Table S1(III)).
Descriptive statistics were used to analyze demographic and clinical characteristics of patients before and after anti-cancer treatment. Treatment effects on the immunologic parameters compared to their baseline values were determined by applying linear mixed models (random intercept and random slope) with the statistical software suite R (version 4.2.0, R packages “lme4” and “lmerTest”) to consider multiple measurements per subject. Time points were assumed to be equidistant for this purpose. We further adjusted for age. Parameters were initially log- or logit-transformed if they were on an absolute or percentage scale, respectively. We corrected for multiple significance testing of changes in lymphocyte subpopulations after anti-cancer treatment by controlling the False Discovery Rate (FDR, Benjamini–Hochberg) for the 130 tests performed, so that FDR is less than or equal to 5%. In the following we denote the corrected p-values (according to FDR) as “q-values”. For survival analysis, the ratios of survivors to all included patients within each treatment option were calculated.
A total of 32 patients with NSCLC were enrolled in this study. Median age of patients included in this study was 68 years (range, 50–83 years), and 22 (68.7%) patients were ≥ 65 years old. The majority of study participants were male (N=24, 75%). The most frequent type of NSCLC, was squamous cell carcinoma in 16 (50%) of patients. Moreover, 20 (62.5%) patients had stage IV advanced NSCLC. Almost all patients 30 (96.9%) were current or former smokers. Immunochemotherapy was the most commonly administered therapy (N=12, 37.5%) followed by cytotoxic chemotherapy (N=8, 25%), adjuvant chemotherapy after surgery (N=7, 21.9%), and immunotherapy (N=4, 12.5%) (Table 1).
Table 1 Socio-demographic and clinical characteristics of study participants by treatment group (N = 32).
Absolute number of transitional B cells was significantly increased after therapy with immunotherapy (q=0.032), immunochemotherapy (q=0.030), and antibodies against VEGF (q=0.024). The transitional B cells percentage was significantly increased after immunotherapy (q=0.033). The percentage of B cells and absolute number of B cells were significantly increased after adjuvant chemotherapy (q=0.023). However, the percentage and absolute number of transition cells were significantly decreased after chemotherapy (q=0.001). Likewise, the absolute number of memory B cells and non-switched B cells were decreased significantly after adjuvant chemotherapy (q=0.023, and q=0.018, respectively) (Table 2, Figure 2).
Figure 2 Heatmap showing changes in lymphocyte subpopulations after treatment with different anti-cancer therapies and aging. Statistically significant changes in lymphocyte subpopulations are indicated by dark red and red color boxes (FDR = 0 - 1% and 1 - 5%, respectively).
After immunotherapy, a significant increase was observed in activated cytotoxic T cells (CD8/CD38) (q=0.050), activated cytotoxic T cells (HLA-DR+) (q=0.031), and activated cytotoxic (HLA-DR+CD38+) T cells (q=0.031). Similarly, after immunochemotherapy, a significant increase was revealed in activated cytotoxic T cells (CD8/CD38) (q<0.0001), activated cytotoxic T cells (HLA-DR+) (q=0.030), and activated cytotoxic (HLA-DR+CD38+) T cells (q=0.002). There was a trend that the percentage of cytotoxic T cells (CD8+) and T cells (CD3+) were increased after immunotherapy (q=0.057) and immunochemotherapy (q=0.080), respectively. Chemotherapy, adjuvant chemotherapy, and antibodies against VEGF therapies did not induce significant changes in numbers of T cell subpopulation and activation (Table 3, Supplementary Table S2, Figure 2).
In our study, none of the treatment options used had a statistically significant effect on NK cell subsets. However, an increased trend in the percentage of immature NK cells II (CD56+CD16-) after immunotherapy (q=0.057) and a decreased trend in the percentage of CD16+CD56 NK cells (cytolytic activity) after immunochemotherapy (q=0.059) was observed (Table 4; Figure 2).
Table 4 Changes in the NK cell subpopulations in NSCLC patients after different anti-cancer therapies.
In addition to lymphocytes, we assessed possible changes in monocytes and granulocytes after our therapy options. Percentages of monocytes significantly increased from 3.7% to 11.7% (q=0.033) after immunotherapy. Moreover, in the chemotaxis of granulocytes, the number of migrated granulocytes was significantly increased (q=0.023), while chemotaxis of granulocytes and percentages of active granulocytes (L-selectin) significantly decreased (q=0.018) after adjuvant chemotherapy. Other therapy options did not show significant changes in monocyte and granulocyte parameters (Supplementary Table S2).
Age showed significant association with CD4+ T-lymphocytes, naive (CD45RA+CCR7+) CD8+T cells, and CD4- recent thymus migrations cells (q<0.05). However, age was not significantly associated with lymphocyte subpopulations showing significant changes after anti-cancer therapies. These findings suggest that the observed changes in lymphocyte subpopulations after anti-cancer therapies are not confounded by age (Figure 2, Supplementary Figure S2).
Overall, 9/29 (31%) of the patients survived. For adjuvant chemotherapy we observed that 4/5 (80%) of patients survived. Patients with immunotherapy and immunochemotherapy achieved comparable survival with 2/7 (29%) and 2/9 (22%), respectively. Within chemotherapy 0/7 (0%) of patients survived in contrast to the patient who received therapy with antibodies against VEGF (1/1 (100%)).
This study was aimed to analyse the impact of different therapeutic regimens on the immune system of NSCLC patients investigating changes in B-, T-, and NK cell subsets during therapy.
The absolute numbers of transitional B cells were significantly increased after therapy with immunotherapy, immunochemotherapy, and antibodies against VEGF. Percentages of transitional B cells also significantly increased after immunotherapy. Similarly, absolute numbers and percentages of B cells were significantly increased after adjuvant chemotherapy. The observed upregulation of B cells and transitional B cells during these therapies suggest activation of immune defense. These effects of therapies on B cells also imply the potential use of these cells as a target for vaccination against lung cancer. In line with this, targeted strategies to enhance B cells by using stimulated B cell ligands have been applied to inhibit lung metastasis and tumor growth (15, 16).
B cells are important immune cells that support an effective antitumor immune response by their capability to present tumor antigens, activate cytotoxic T cell and T-lymphocytes, response, as well as their ability to generate cytokines and anti-tumor antibodies (17–19). In line with the findings of this study, previous studies suggest that B cell counts increase in tumors of patients responding to immunotherapy, and tumor-infiltrating B cells were associated with, tumor stage and longer survival in NSCLC (20, 21). Even though the importance of B cells in making anti-cancer immune responses was progressively unraveled in the last years, a better understanding about the role of different B cell subsets, particularly transitional B cells is still required in cancer patients (17).
Interestingly, absolute numbers and percentages of transitional B cells significantly decreased after chemotherapy. Similarly, the absolute number of memory B cells and non-class switched B cells significantly decreased after adjuvant chemotherapy. Down-regulation of B cells during chemotherapy suggests a potential suppression of immune defense due to cytotoxic side-effects on the bone marrow. A previous study also reported that chemotherapy reduced the absolute counts of B cells (22). Another study showed that chemotherapy reduced absolute counts of B cells followed by a slow increase but not reaching baseline levels. This suggests that the chemotherapy has a long-term effect on lymphocyte subsets (23).
In this study, we noticed a significant increase in activated cytotoxic T cells in patients treated with immunotherapy and immunochemotherapy. The immune system activation by immunotherapy and immunochemotherapy is a desired therapeutic effect. T lymphocytes are the most important inflammatory cells that infiltrate the tumor, exert a direct cytotoxic effect, or cause lysis of tumor by cytokine release (24–26). CD8+ T cells can also increase and differentiate into CD8+ T cells that can infiltrate tumors by peripheral blood migration and play an essential role in the direct killing of tumor cells (27). Our finding is in line with the reported increase of CD8+ T cells after immunotherapy in lung cancer patients (28). Another study also suggested that as part of a successful immunotherapy response, effector memory tumor antigen-specific cytotoxic T cells are increased (29). However, in contrast to our findings, other studies reported decreased proportion of CD8+ T cells after immunotherapy as compared to baseline values (30, 31).
The observed differences in cytotoxic T cell response after immunotherapy could be explained as follows. First, lung cancer patients treated with PD-1 inhibitors are characterized by expressing effector-like phenotypes (HLA-DR+, CD38+, Bcl-2lo), co-stimulatory molecules (CD28, CD27, ICOS), a highly specific subset of proliferating CD8+ T cells in the peripheral blood, and high levels of PD-1 (32). Second, different timings of blood sampling could contribute to the observed contradictory findings across studies. CD8+ T cell response induction by blocking the PD-1 pathway in the peripheral blood is transient and detected during the first four weeks after treatment start. Then these specific CD8+ T cells migrate to the tumor sites (32).
Although none of the therapies investigated in this study showed a significant effect on NK cells, a trend increase in the percentage of immature NK cells was observed after immunotherapy. A decrease in the percentage of CD16+CD56 NK cells was also observed after immunochemotherapy. A previous study demonstrated that the PD-L1 leads blockage of causes activation of NK cells and increases the direct antitumor effect of NK cells (33). Another study showed that in NSCLC patients, NK cell activity is a marker to predict the immunotherapy response (34). Drugs modulating the function and proliferative activity of NK cells have been developed to produce synergistic effect in combination with immunotherapy (35, 36).
In addition to lymphocyte subpopulations, we assessed the possible effects of anti-cancer therapies on monocytes and granulocytes. Percentage of monocytes showed a significant increase after immunotherapy. This could be interpreted as the effect of a T cell interaction and activation by immunotherapy as described in a previous study (37). In several tumor types, an increase in HLA-DR low monocytes has been reported (38). As already described for HLA-DR low monocytes in sepsis, these monocytic cells might decrease T cell function in patients with cancer (39). Moreover, the number of migrated granulocytes in this chemotaxis study was significantly increased, while the percentage of active granulocytes (L-selectin) decreased after adjuvant chemotherapy. This is possibly due to the consequence of high baseline activity, postoperative inflammation, and wound healing processes. Previous studies also reported that the number of neutrophils in tumor tissues and blood is correlated with poor patient outcomes and disease progression (40). Neutrophil count was associated with the percentage of HLA-DR low monocytes in patients with lung cancer managed by surgery of the primary tumor as an important subpopulation of the myeloid-derived suppressor cells (MDSC). Nevertheless, in the late tumor stages, this observation was not confirmed (41).
In the present study, NSCLC patients treated with adjuvant chemotherapy showed the highest survival of 80%. In contrast, patients treated with chemotherapy alone showed the lowest survival with 0%. This could be explained by the fact that adjuvant chemotherapy is usually applied for early-stage NSCLC; stage IIA, IIB, and IIIA NSCLC to kill remaining cancer cells after surgery (42). Adding immunotherapy to chemotherapy in a palliative setting increased observed survival to 22% compared to chemotherapy alone.
This is consistent with previous reports showing a significant increase in overall response rates, overall survival and progression-free survival in NSCLC patients treated with immunotherapy compared to chemotherapy (43). Five-year follow-up study showed a significantly increased median time of overall survival, which doubled from 13.4 months in chemotherapy to 26.3 months in immunotherapy; and a higher overall survival rate, 31.9% in the immunotherapy group compared to 16.3% in the chemotherapy group (44). Immunotherapy enhances the immune system that targets cancer cells and slows or stops the cancer cells metastasis and growth (45). Previous studies also showed that improved survival is correlated with a strong immune response to tumers. Moreover, higher numbers of CD8+ T cells and natural killer cells are linked with better patient survival (46, 47).
We repeated all the analyses twice, with further adjustment for tumor stage, and with further adjustment for tumor stage and exclusion of patients with adjuvant therapy to check that stage of tumor did not bias our initial analysis with adjustment for age only. Indeed, the results were comparable yielding no significant additional information.
This study has some limitations to be mentioned. Firstly, despite multiple measurements, due to the limited sample size our study might be underpowered to identify smaller changes in the lymphocyte subpopulations before and after treatment with different anti-cancer therapies. Likewise, survival analysis is limited by the small sample size. Secondly, this study was conducted at a single health care facility, therefore, selection bias cannot be fully excluded.
Since this study was the first one investigating such a large spectrum of parameters in cytomics, further research is needed to determine prognostic significance, further potential confounding factors and long-term survival.
The original contributions presented in the study are included in the article/Supplementary Material, further inquiries can be directed to the corresponding author/s.
The studies involving humans were approved by ethics committee of the University of Leipzig. The studies were conducted in accordance with the local legislation and institutional requirements. The participants provided their written informed consent to participate in this study.
Conceptualization, PG, CG, MS, US. Methodology, PG, CG, US. Formal analysis, AK, BT. Investigation, PG, AB. Data curation, PG, CG, AK, BT. Original draft preparation, BT. Review and editing, PG, CG, US, AK, BT, AB, MS. Project administration, PG, CG. All authors contributed to the article and approved the submitted version.
Funded by the Open Access Publishing Fund of Leipzig University supported by the German Research Foundation within the program Open Access Publication Funding. Markus Scholz was supported by “ChemoTox-AI”, a project financed by the German Federal Ministry of Education and Research (BMBF) within the Computational Life Science line of funding (grant number 031L0261).
The authors would like to acknowledge Katrin Bauer, Bettina Glatte, and Sabine Seiffert for their contribution to flow cytometry analysis in the institute of Clinical Immunology laboratory. We also would like to thank the staff members of the Pulmonology Clinic for their assistance in blood sample collection and timely delivery of blood samples to the institute of Clinical Immunology laboratory.
CG declare to have received honoraria for lectures and advisory boards from BMS, MSD, Roche, and Regeneron. MS received funding from Pfizer Inc. for a project not related to this research.
The remaining authors declare that the research was conducted in the absence of any commercial or financial relationships that could be construed as a potential conflict of interest.
All claims expressed in this article are solely those of the authors and do not necessarily represent those of their affiliated organizations, or those of the publisher, the editors and the reviewers. Any product that may be evaluated in this article, or claim that may be made by its manufacturer, is not guaranteed or endorsed by the publisher.
The Supplementary Material for this article can be found online at: https://www.frontiersin.org/articles/10.3389/fimmu.2023.1239097/full#supplementary-material
1. Ferlay J, Colombet M, Soerjomataram I, Parkin DM, Piñeros M, Znaor A, et al. Cancer statistics for the year 2020: An overview. Int J Cancer (2021) 149:778–89. doi: 10.1002/ijc.33588
2. Inamura K. Lung cancer: understanding its molecular pathology and the 2015 WHO classification. Front Oncol (2017) 7:193/full. doi: 10.3389/fonc.2017.00193/full
3. Siegel RL, Miller KD, Jemal A. Cancer statistics, 2019. CA Cancer J Clin (2019) 69:7–34. doi: 10.3322/caac.21551
4. Zappa C, Mousa SA. Non-small cell lung cancer: current treatment and future advances. Transl Lung Cancer Res (2016) 5:288–300. doi: 10.21037/tlcr.2016.06.07
5. Wang T, Nelson RA, Bogardus A, Grannis FW. Five-year lung cancer survival. Cancer (2010) 116:1518–25. doi: 10.1002/cncr.24871
6. Wong MCS, Lao XQ, Ho KF, Goggins WB, Tse SLA. Incidence and mortality of lung cancer: global trends and association with socioeconomic status. Sci Rep (2017) 7:14300. doi: 10.1038/s41598-017-14513-7
7. Ettinger DS, Wood DE, Aisner DL, Akerley W, Bauman JR, Bharat A, et al. Non–small cell lung cancer, version 3.2022, NCCN clinical practice guidelines in oncology. J Natl Compr Cancer Netw (2022) 20:497–530. doi: 10.6004/jnccn.2022.0025
8. Aguiar PN, De Mello RA, Hall P, Tadokoro H, Lima Lopes G. PD-L1 expression as a predictive biomarker in advanced non-small-cell lung cancer: updated survival data. Immunotherapy (2017) 9:499–506. doi: 10.2217/imt-2016-0150
9. Proto C, Ferrara R, Signorelli D, Lo Russo G, Galli G, Imbimbo M, et al. Choosing wisely first line immunotherapy in non-small cell lung cancer (NSCLC): what to add and what to leave out. Cancer Treat Rev (2019) 75:39–51. doi: 10.1016/j.ctrv.2019.03.004
10. Wei Z, Zhang W, Gao F, Wu Y, Zhang G, Liu Z, et al. Impact of lymphocyte subsets on chemotherapy efficacy and long-term survival of patients with advanced non-small-cell lung cancer. Zhongguo Yi Xue Ke Xue Yuan Xue Bao (2017) 39:371–6. doi: 10.3881/j.issn.1000-503X.2017.03.012
11. Qiu J, Che G, Liu F, Sha X, Ju S, Ma H, et al. The detection and clinical significance of peripheral regulatory CD4+CD25hiCD127low T cells in patients with non-small cell lung cancer. Clin Transl Oncol (2019) 21:1343–7. doi: 10.1007/s12094-019-02063-5
12. Wang S, Wong ML, Hamilton N, Ben DJ, TM J, Walter LC. Impact of age and comorbidity on non–small-cell lung cancer treatment in older veterans. J Clin Oncol (2012) 30:1447–55. doi: 10.1200/JCO.2011.39.5269
13. Boldt A, Borte S, Fricke S, Kentouche K, Emmrich F, Borte M, et al. Eight-color immunophenotyping of T-, B-, and NK cell subpopulations for characterization of chronic immunodeficiencies. Cytom Part B Clin Cytom (2014) 86:191–206. doi: 10.1002/cyto.b.21162
14. Maecker HT, McCoy JP, Nussenblatt R. Standardizing immunophenotyping for the human immunology project. Nat Rev Immunol (2012) 12:191–200. doi: 10.1038/nri3158
15. Gonzalez NK, Wennhold K, Balkow S, Kondo E, Bölck B, Weber T, et al. In vitro and in vivo imaging of initial B-T cell interactions in the setting of B cell based cancer immunotherapy. Oncoimmunology (2015) 4:e1038684. doi: 10.1080/2162402X.2015.1038684
16. Sorrentino R, Morello S, Forte G, Montinaro A, De VG, Luciano A, et al. B cells contribute to the antitumor activity of cpG-oligodeoxynucleotide in a mouse model of metastatic lung carcinoma. Am J Respir Crit Care Med (2011) 183:1369–79. doi: 10.1164/rccm.201010-1738OC
17. Largeot A, Pagano G, Gonder S, Moussay E, Paggetti J. The B-side of cancer immunity: the underrated tune. Cells (2019) 8. doi: 10.3390/cells8050449
18. Sharonov GV, Serebrovskaya EO, Yuzhakova DV, Britanova OV, Chudakov DM. B cells, plasma cells and antibody repertoires in the tumour microenvironment. Nat Rev Immunol (2020) 20:294–307. doi: 10.1038/s41577-019-0257-x
19. Kinker GS, Vitiello GAF, Ferreira WAS, Chaves AS, Cordeiro de Lima VC, Medina TDS. B cell orchestration of anti-tumor immune responses: A matter of cell localization and communication. Front Cell Dev Biol (2021) 9:678127/full. doi: 10.3389/fcell.2021.678127/full
20. Germain C, Gnjatic S, Tamzalit F, Knockaert S, Remark R, Goc J, et al. Presence of B cells in tertiary lymphoid structures is associated with a protective immunity in patients with lung cancer. Am J Respir Crit Care Med (2014) 189:832–44. doi: 10.1164/rccm.201309-1611OC
21. Wang SS, Liu W, Ly D, Xu H, Qu L, Zhang L. Tumor-infiltrating B cells: their role and application in anti-tumor immunity in lung cancer. Cell Mol Immunol (2019) 16:6–18. doi: 10.1038/s41423-018-0027-x
22. Spitzer MH, Carmi Y, Reticker-Flynn NE, Kwek SS, Madhireddy D, Martins MM, et al. Systemic immunity is required for effective cancer immunotherapy. Cell (2017) 168:487–502.e15. doi: 10.1016/j.cell.2016.12.022
23. Xia Y, Li W, Li Y, Liu Y, Ye S, Liu A, et al. The clinical value of the changes of peripheral lymphocyte subsets absolute counts in patients with non-small cell lung cancer. Transl Oncol (2020) 13:100849. doi: 10.1016/j.tranon.2020.100849
24. Aerts JG, Hegmans JP. Tumor-specific cytotoxic T cells are crucial for efficacy of immunomodulatory antibodies in patients with lung cancer. Cancer Res (2013) 73:2381–8. doi: 10.1158/0008-5472.CAN-12-3932
25. Kataki A, Scheid P, Piet M, Marie B, Martinet N, Martinet Y, et al. Tumor infiltrating lymphocytes and macrophages have a potential dual role in lung cancer by supporting both host-defense and tumor progression. J Lab Clin Med (2002) 140:320–8. doi: 10.1067/mlc.2002.128317
26. Martínez-Lostao L, Anel A, Pardo J. How do cytotoxic lymphocytes kill cancer cells? Clin Cancer Res (2015) 21:5047–56. doi: 10.1158/1078-0432.CCR-15-0685
27. Zhang N, Bevan MJ. CD8(+) T cells: foot soldiers of the immune system. Immunity (2011) 35:161–8. doi: 10.1016/j.immuni.2011.07.010
28. Saravia J, Chapman NM, Chi H. Helper T cell differentiation. Cell Mol Immunol (2019) 16:634–43. doi: 10.1038/s41423-019-0220-6
29. Principe N, Kidman J, Goh S, Tilsed CM, Fisher SA, Fear VS, et al. Tumor infiltrating effector memory antigen-specific CD8+ T cells predict response to immune checkpoint therapy. Front Immunol (2020) 11:584423/full. doi: 10.3389/fimmu.2020.584423/full
30. Yan Y, Wang X, Liu C, Jia J. Association of lymphocyte subsets with efficacy and prognosis of immune checkpoint inhibitor therapy in advanced non-small cell lung carcinoma: a retrospective study. BMC Pulm Med (2022) 22:166. doi: 10.1186/s12890-022-01951-x
31. Zhuo M, Chen H, Zhang T, Yang X, Zhong J, Wang Y, et al. The potential predictive value of circulating immune cell ratio and tumor marker in atezolizumab treated advanced non-small cell lung cancer patients. Cancer Biomarkers (2018) 22:467–76. doi: 10.3233/CBM-171089
32. Kamphorst AO, Pillai RN, Yang S, Nasti TH, Akondy RS, Wieland A, et al. Proliferation of PD-1+ CD8 T cells in peripheral blood after PD-1–targeted therapy in lung cancer patients. Proc Natl Acad Sci (2017) 114:4993–8. doi: 10.1073/pnas.1705327114
33. Kamata T, Suzuki A, Mise N, Ihara F, Takami M, Makita Y, et al. Blockade of programmed death-1/programmed death ligand pathway enhances the antitumor immunity of human invariant natural killer T cells. Cancer Immunol Immunother (2016) 65:1477–89. doi: 10.1007/s00262-016-1901-y
34. Choi MG, Kim YJ, Lee JC, Rho JK, Choi C. Efficacy of natural killer cell activity as a biomarker for predicting immunotherapy response in non-small cell lung cancer. Thorac Cancer (2020) 11:3337–45. doi: 10.1111/1759-7714.13677
35. Zingoni A, Fionda C, Borrelli C, Cippitelli M, Santoni A, Soriani A. Natural killer cell response to chemotherapy-stressed cancer cells: role in tumor immunosurveillance. Front Immunol (2017) 8:1194. doi: 10.3389/fimmu.2017.01194
36. Yang Y, Li L, Jiang Z, Wang B, Pan Z. Anlotinib optimizes anti-tumor innate immunity to potentiate the therapeutic effect of PD-1 blockade in lung cancer. Cancer Immunol Immunother (2020) 69:2523–32. doi: 10.1007/s00262-020-02641-5
37. Vermare A, Guérin MV, Peranzoni E, Bercovici N. Dynamic CD8+ T cell cooperation with macrophages and monocytes for successful cancer immunotherapy. Cancers (Basel) (2022) 14:3546. doi: 10.3390/cancers14143546
38. Greten TF, Manns MP, Korangy F. Myeloid derived suppressor cells in human diseases. Int Immunopharmacol (2011) 11:802–7. doi: 10.1016/j.intimp.2011.01.003
39. Döcke WD, Randow F, Syrbe U, Krausch D, Asadullah K, Reinke P, et al. Monocyte deactivation in septic patients: Restoration by IFN-γ treatment. Nat Med (1997) 3:678–81. doi: 10.1038/nm0697-678
40. Kasuga I, Makino S, Kiyokawa H, Katoh H, Ebihara Y, Ohyashiki K. Tumor-related leukocytosis is linked with poor prognosis in patients with lung carcinoma. Cancer (2001) 92:2399–405. doi: 10.1002/1097-0142(20011101)92:9<2399::AID-CNCR1588>3.0.CO;2-W
41. Riemann D, Cwikowski M, Turzer S, Giese T, Grallert M, Schütte W, et al. Blood immune cell biomarkers in lung cancer. Clin Exp Immunol (2019) 195:179–89. doi: 10.1111/cei.13219
42. Arriagada R, Cartmell-Davies J, Girling DJ, Le Chevalier T, Marsoni S, Parmar MKB, et al. Chemotherapy in non-small cell lung cancer: a meta-analysis using updated data on individual patients from 52 randomised clinical trials. Non-small Cell Lung Cancer Collaborative Group. BMJ (1995) 311:899–909. doi: 10.1136/bmj.311.7010.899
43. Reck M, Rodríguez-Abreu D, Robinson AG, Hui R, Csőszi T, Fülöp A, et al. Pembrolizumab versus chemotherapy for PD-L1–positive non–small-cell lung cancer. N Engl J Med (2016) 375:1823–33. doi: 10.1056/NEJMoa1606774
44. Reck M, Rodríguez-Abreu D, Robinson AG, Hui R, Csőszi T, Fülöp A, et al. Five-year outcomes with pembrolizumab versus chemotherapy for metastatic non–small-cell lung cancer with PD-L1 tumor proportion score ≥ 50%. J Clin Oncol (2021) 39:2339–49. doi: 10.1200/JCO.21.00174
45. Banchereau J, Steinman RM. Dendritic cells and the control of immunity. Nature (1998) 392:245–52. doi: 10.1038/32588
46. Zhuang X, Xia X, Wang C, Gao F, Shan N, Zhang L, et al. A high number of CD8+ T cells infiltrated in NSCLC tissues is associated with a favorable prognosis. Appl Immunohistochem Mol Morphol (2010) 18:24–8. doi: 10.1097/PAI.0b013e3181b6a741
Keywords: non-small cell lung cancer, B cells, T cells, NK cells, anti-cancer therapies
Citation: Gessner P, Tessema B, Scholz M, Sack U, Boldt A, Kühnapfel A and Gessner C (2023) The influence of anti-cancer therapies on lymphocyte subpopulations of lung cancer patients. Front. Immunol. 14:1239097. doi: 10.3389/fimmu.2023.1239097
Received: 12 June 2023; Accepted: 07 August 2023;
Published: 28 August 2023.
Edited by:
Yuan Wan, Binghamton University, United StatesCopyright © 2023 Gessner, Tessema, Scholz, Sack, Boldt, Kühnapfel and Gessner. This is an open-access article distributed under the terms of the Creative Commons Attribution License (CC BY). The use, distribution or reproduction in other forums is permitted, provided the original author(s) and the copyright owner(s) are credited and that the original publication in this journal is cited, in accordance with accepted academic practice. No use, distribution or reproduction is permitted which does not comply with these terms.
*Correspondence: Christian Gessner, Y2guZ2Vzc25lckBwbmV1bW9sb2dlLWxlaXB6aWcuZGU=
† These authors share first authorship
‡ These authors share senior authorship
Disclaimer: All claims expressed in this article are solely those of the authors and do not necessarily represent those of their affiliated organizations, or those of the publisher, the editors and the reviewers. Any product that may be evaluated in this article or claim that may be made by its manufacturer is not guaranteed or endorsed by the publisher.
Research integrity at Frontiers
Learn more about the work of our research integrity team to safeguard the quality of each article we publish.