- 1The First Dongguan Affiliated Hospital of Guangdong Medical University, Dongguan, Guangdong, China
- 2Department of Pathophysiology, Guangdong Medical University, Dongguan, Guangdong, China
- 3School of Pharmaceutical Sciences, Guangdong Medical University, Dongguan, China
- 4The Second Clinical Medical College of Guangdong Medical University, Dongguan, China
- 5School of Medical Technology, Guangdong Medical University, Dongguan, China
- 6Biomedical Innovation Center, Guangdong Medical University, Dongguan, China
- 7Institute of Marine Medicine, Guangdong Medical University, Zhanjiang, China
There is growing evidence that mesenchymal stem cell-derived extracellular vesicles and exosomes can significantly improve the curative effect of oxidative stress-related diseases. Mesenchymal stem cell extracellular vesicles and exosomes (MSC-EVs and MSC-Exos) are rich in bioactive molecules and have many biological regulatory functions. In this review, we describe how MSC-EVs and MSC-Exos reduce the related markers of oxidative stress and inflammation in various systemic diseases, and the molecular mechanism of MSC-EVs and MSC-Exos in treating apoptosis and vascular injury induced by oxidative stress. The results of a large number of experimental studies have shown that both local and systemic administration can effectively inhibit the oxidative stress response in diseases and promote the survival and regeneration of damaged parenchymal cells. The mRNA and miRNAs in MSC-EVs and MSC-Exos are the most important bioactive molecules in disease treatment, which can inhibit the apoptosis, necrosis and oxidative stress of lung, heart, kidney, liver, bone, skin and other cells, and promote their survive and regenerate.
1 Introduction
Mesenchymal stem cells belong to a heterogeneous cell population of stromal cells. They proliferate and differentiate in vitro in the form of plastic adherent cells. They can be isolated from many human tissues and differentiate into mesoderm and endoderm (1), neuroectodermal cells (2) and other embryonic lineage cells (3). Mesenchymal stem cells have been proven to have a variety of biological functions. They can interact with cells in the immune system for immune regulation, inhibit tumor necrosis factor (TNF), upregulate IL-10 (4), reduce inflammation, inhibit respiratory burst and Activate the spontaneous apoptosis of neutrophils, etc. (5). Although mesenchymal stem cells have many applications in the field of life sciences, their effectiveness is restricted by many factors. For example, the phenomenon of immune rejection of allogeneic mesenchymal stem cells (6), low persistence of curative effect of limited infusion of mesenchymal stem cells, deprivation of nutrients and growth factors and limitation of oxygen transport during mesenchymal stem cell transplantation (7), Passaged late mesenchymal stem cells trigger immediate menstrual blood-mediated inflammatory response (IBMIR) and form blood activation markers, etc. (8). In recent years, due to the vigorous development of research on exosomes derived from mesenchymal stem cells (MSC-Exos), it has been found that exosomes can avoid some of the shortcomings of mesenchymal stem cells in the treatment of various diseases.
Exosomes are spherical particles with a diameter of about 40 ~ 150 nm, which are released after the fusion of vesicles and cell membrane (9). It has lipid bilayer membrane structure, which is produced in cell culture or body fluid supernatant, such as blood, saliva, urine, breast milk, cerebrospinal fluid, bile and lymph, and can secrete exosomes (10–12). Compared with plasma membrane, exosomal membrane is harder and more stable in external environment. Exosomes carry a lot of genetic material similar to stem cells, including microRNAs (miRNAs) and mRNAs (13). In addition, exosomes contain a specific family of proteins such as heat shock protein integrins and tetrathione involved in membrane transport and fusion (14–16). It is worth noting that exosomes-based cell origin exosomes also play a unique role in cell communication (17). MSC-Exos has become the preferred treatment for many diseases and is a safe and effective stem cell-free replacement therapy (18). Exosomes are more stable and modifiable than mesenchymal stem cells and have no risk of tumor formation Due to the nanometer size and lipid bilayer structure of exosomes, exosomes can easily cross the biological barrier and enter the target organs (19). The therapeutic effect of MSC-Exos has been confirmed in a variety of diseases, including lung injury, myocardial injury, kidney injury, nerve injury, skin injury and aging (20).
When a living cell is damaged by free radicals or non-free radicals, it will obtain electrons from its molecules, which will produce a chain reaction and eventually lead to the damage of cell structure. Among these molecules, molecules from ROS (reactive oxygen species) have major biological effects, and the concept of oxidative stress is derived from this (21). Oxidative stress injury refers to the condition that oxygen and oxygen-derived free radicals exceed the natural antioxidant defense capacity of cells (22). The aggravation and prolongation of symptoms caused by oxidative stress is always a major problem in various common clinical diseases. The antioxidant activity of MSC-Exos and MSC-EVs has its unique advantages in inhibiting oxidative damage and alleviating inflammatory reaction MSC-Exos and MSC-EVs can increase calcium inflow, reduce the concentration of pro-inflammatory factors and reduce the production of ROS (23). Exosomal therapy has a looser regulatory approach than cell therapy and is considered as a “biological drug” with broad development prospects (24). In recent years, the research on exosomes is very active, and the articles on exosomes in the treatment of oxidative stress have accumulated a certain amount. This paper mainly reviews the mechanism, advantages, disadvantages and prospects of MSC-Exos and MSC-EVs derived from mesenchymal stem cells in the treatment of oxidative stress-related diseases in various systems. Based on previous studies, it details the related mechanisms, looks for the intersection of research directions, and deeply discusses the future research directions in this field (Figure 1).
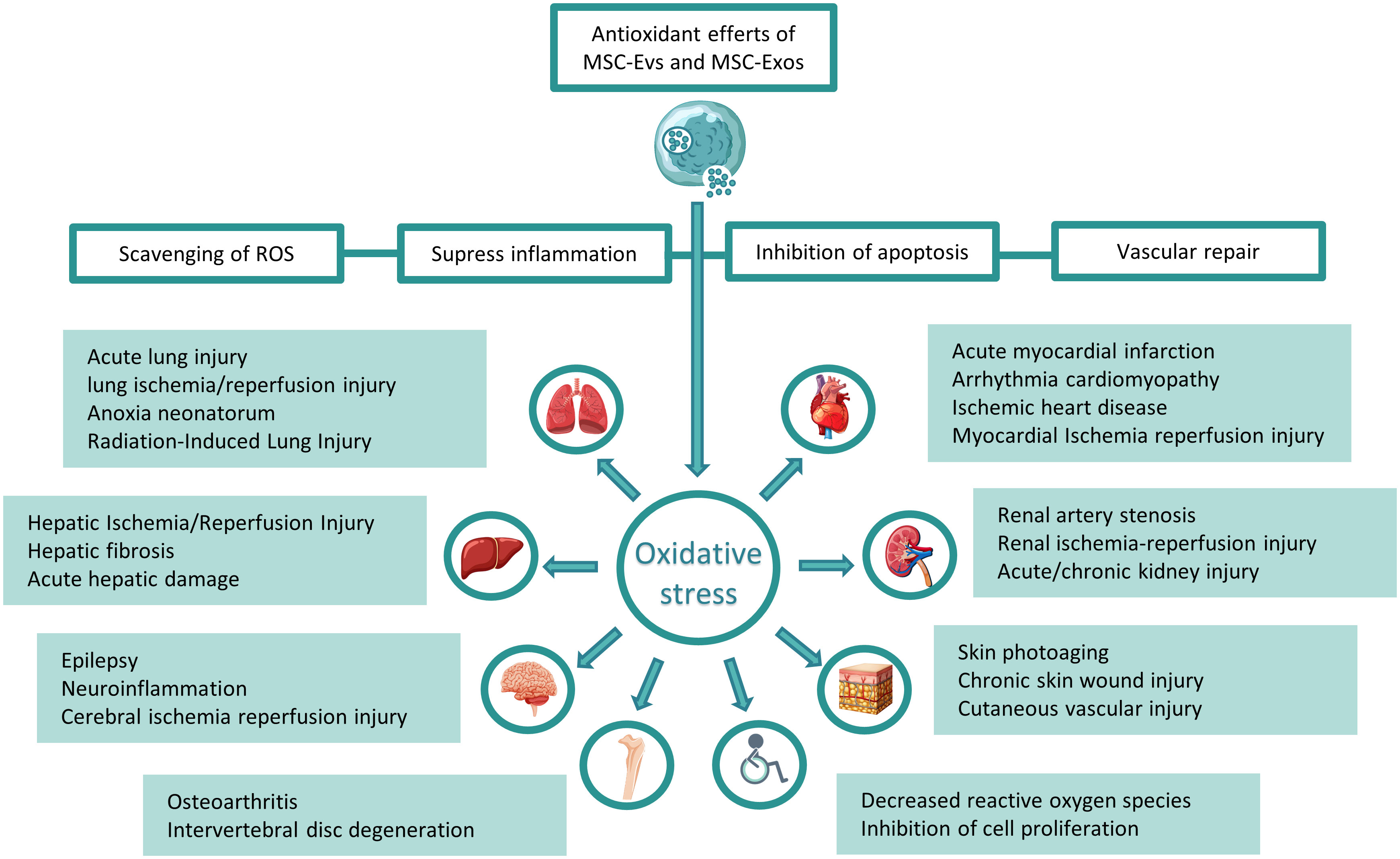
Figure 1 Treatment of related systemic diseases caused by oxidative stress with mesenchymal stem cell exosomes and vesicles: Mesenchymal stem cell-derived exosomes and exosomes can decrease ROS, inhibit inflammation, reduce cell apoptosis, and enhance angiogenesis, thereby treating respiratory, circulatory, and digestive systems, nervous system, motor system and other system diseases caused by oxidative stress.
2 Respiratory diseases
Therapies based on MSC-EVs or MSC-Exos have great application prospect in the treatment of oxidative stress-related lung injury. For example, acute lung injury (ALI), neonatal hypoxia-ischemia-reperfusion COVID-19 radiation lung injury, etc.
The main characteristic of ALI progression is oxidative stress response (25). The accumulation of excess pro-inflammatory factors can lead to the occurrence of oxidative stress in lung tissue, which then leads to the occurrence of ALI. At present, many drugs in the market cannot pass through the lung blood-air barrier, which leads to low curative effect, which is the main problem in treating ALI. Studies have shown that MSC-EVs can cross the blood-air barrier and other biological barriers in the lungs to enhance the therapeutic effect (26). Hyperoxia-induced lung injury can lead to the imbalance of oxidative-antioxidant system in vivo and lead to oxidative stress injury. The negative effects of ischemia-reperfusion are caused by the induction of inflammation and oxidative stress and the damage of cell energy metabolism, which leads to a series of harmful biological events from ion homeostasis failure to cell death (27). In severe acute respiratory syndrome (SARS) caused by SARS-associated coronavirus (SARS-CoV), the immune response to virus infection and cytokine storm play a key role in the severity of the disease (28). Cytokine storm will lead to the development of oxidative stress due to ROS produced by immune cells (29). Complex pathophysiological mechanisms indicate that severe COVID-19 is more suitable for multi-effect drug therapy than single target drug (30). Therefore, it is an innovative method to use MSC-EVs or MSC-Exos combined with COVID-19 clinical drugs.
Determining the effective therapeutic components carried by exosomes is the basis for explaining the therapeutic mechanism, including effective secretory proteins and microRNA (miRNA), etc. (31) At the same time, it is also important to further understand the therapeutic mechanism of exosomes in the lung, including antioxidant and anti-inflammatory treatment of target cells or injured tissues. miRNA is a small non-coding RNA molecule with 19-25 nucleotides that play various roles in physiology and disease progression (32). MSC-EVs have been shown to promote the repair of lung injury by delivering miR125amiR-181b and miR-126 in the field of ALI (25). Both inhalation and tail vein injection of MSC-EVs decreased the levels of pro-inflammatory cytokines IL-1 β MCP-1IL-1 α TNF α and IL-12 and increased the levels of anti-inflammatory cytokines IL-10. HE staining showed that MSC-EVs could improve pulmonary morphological changes such as alveolar wall thickening, alveolar septum congestion and inflammatory infiltration after lipopolysaccharide (LPS) stimulation (33). In addition, NF-kB is a key transcription factor, which has been proved to be a key signal factor for regulating pro-inflammatory cytokines in sepsis-induced ALI (25). The increase of pathological score showed that MSC-EVs treatment reversed the changes related to inflammatory infiltration. These studies suggest that MSC-EVs and MSC-Exos can improve the damage and pathological changes of oxidative stress-related lung diseases in the early stage and are excellent new therapeutic drugs.
2.1 MSC-EVs and MSC-Exos improve the related indexes of pulmonary oxidative stress
The antioxidant effects of MSC-EVs and MSC-Exos are closely related to their secreted proteins and microRNA. In exosomes-treated rat alveolar macrophages (NR8383 cells), the activity of SOD and GSH increased and the content of MDA decreased, and the effect was more obvious when the exosomes overexpressed miR-22-3p However, inhibiting FZD6 in exosomes inhibited SOD and GSH activities and increased MDA content, which indicated that miR-22-3p improved the antioxidant activity of cells through FZD6 (32). After adding MSC-EVs to raw 264.7 cells, the expression of Nrf2HO-1HMGB1 and other oxidative regulators decreased, and the number of 8-OHdG positive cells decreased. After knocking out Nrf2 in MSC-EVs and adding MSC-EVs again in raw 264.7 cells stimulated by LPS, the expression of HO-1IL-6 was up-regulated, and the expression of Nrf2Keap-1TNF-α did not change. These results indicate that knockout of Nrf2 in MSC-EVs can weaken the anti-inflammatory and antioxidant activities of EVs (33)
Transcriptome sequencing of mouse ALI model treated with MSC-EVs for 4 days showed that many genes related to immune regulation and oxidative stress were less different than those of ALI mice (34). In addition, a series of genes such as TLR4,Arg-1 and HMOX1 have been shown to be involved in immune regulation and antioxidant activity HO-1 is an antioxidant enzyme that can inhibit apoptosis, inflammation and cell proliferation to reduce cell death in ALI animal models by inducing several detoxification enzymes and antioxidant proteins (25). In summary, MSC-EVs plays an important therapeutic role in immune regulation and reduction of oxidative stress in ALI mouse model (33).
2.2 MSC-EVs and MSC-Exos in the treatment of lung cell injury and apoptosis induced by oxidative stress
MSC-EVs and MSC-Exos have been proved by many studies to alleviate lung pathological injury and apoptosis.
In vitro, the cell viability of NR8383 cells decreased and apoptosis was promoted after LPS treatment, while miR-22-3p up-regulated MSC-EVs increased cell viability and inhibited cell apoptosis FZD6 belongs to the “curl” gene family and is highly expressed in both adult and fetal lung tissues (35). Up-regulation of miR-22-3p or down-regulation of FZD6 in MSC-Exos can improve cell viability and inhibit apoptosis (32). In addition, up-regulation of miR-30b-3p of MSC-Exos in LPS-treated mouse lung epithelial cells (MLE-12 cells) also showed increased cell proliferation and inhibition of apoptosis (36).
In vivo experiment, the acute lung injury induced by sepsis in mice was basically normal after MSCs-EVs treatment, and the blood barrier of type I alveolar cells and endothelial cells were only slightly swollen. After exosomes treatment, the edema and bleeding of mice were alleviated (25). In the survival analysis, Kaplan-Meier survival curve showed that the survival rate of mice with cecal ligation and puncture (CLP) treated with MSCs-EVs was significantly higher (25). MiR-21-5p carried by MSC-Exos is a kind of cancer-promoting miRNA, which can effectively resist apoptosis. Ji Wei Li et al. treated mouse bone marrow-derived MSCs with hypoxia or miR-21-5p antagomir respectively to increase or decrease miR-21-5p concentration in MSC-Exos. It was found that MSC-Exos treatment weakened mouse lung ischemia-reperfusion injury in a miR-21-5p-dependent manner, effectively reduced oxidative stress-induced apoptosis and partially reduced hypoxia/reoxygenation-induced pro-inflammatory “M1” polarization of alveolar macrophages (37).
EVs can release glycosaminoglycan serum hyaluronic acid (HA) and improve energy metabolism of lung cells exposed to ischemia-reperfusion. This is particularly important in protecting against ischemic injury because HA is essential for maintaining the integrity of lung epithelial cells and inducing tissue healing and regeneration HA released by mesenchymal stem cells can trap immune cells in extracellular matrix (38–40) and prevent leukocytes from adhering to activated endothelial cells (40, 41). The impaired energy metabolism after ischemia-reperfusion injury is the main reason for the failure of ion homeostasis, which leads to cell swelling and apoptosis/autophagy activation. The treatment of MSC-EVs can restore ATP to baseline level and promote leukocyte homing in perfusion lung injury (27). These results suggest that MSC-EVs and MSC-Exos can enhance the inhibition of cell regeneration and apoptosis in oxidative stress-induced lung injury, especially miRNA in exosomes.
2.3 MSC-EVs and MSC-Exos in the treatment of lung inflammatory injury induced by oxidative stress
MSC-EVs and MSC-Exos play a significant biological role in anti-inflammation. ROS signal plays an important role in the occurrence and development of inflammatory injury. Excessive ROS promotes cell injury and death when the production and elimination of ROS are unbalanced (42). MSC-EVs and MSC-Exos can regulate and attenuate the inflammatory injury induced by oxidative stress in the lungs.
The expression of CD86 in mouse mononuclear macrophages was increased by LPS stimulation in vitro. After administration of MSC-EVs, the expression of CD86 decreased significantly, and the expression of Arg-1 increased, which indicated that macrophages polarized towards M2 anti-inflammatory direction (33).
In vivo MSC-EVs significantly reduced the levels of pro-inflammatory cytokines including TNF-alpha IL-1bIL-6MPO and significantly increased IL-10 levels and resulted in neutropenia in alveolar lavage fluid in a sepsis-induced acute lung injury model in mice. The phosphorylation activation of MAPK pathway is thought to play an important role in the pathogenesis of ALI and administration of MSC-EVs significantly inhibits their phosphorylation. Treatment with MSC-EVs decreased the phosphorylation level of NF-kB p65 and inhibited the increase of NF-kB p65 degradation. In addition, TLR4 also plays an important role in the regulation of inflammation and signal transduction. The decreased expression of TLR4 and its downstream signals (IL-1 β IL-6 and NF-kabb-p65) after MSC-EVs treatment also indicates that MSC-EVs can alleviate inflammation stimulated by LPS (33). These phenomena suggest that MSC-EVs play an important role in inhibiting inflammatory markers related to oxidative stress in the lungs.
2.4 MSC-EVs and MSC-Exos in the treatment of pulmonary vascular injury induced by oxidative stress
Vascular endothelial growth factor (VEGF) plays an important role in promoting angiogenesis and enhancing vascular permeability. Tracheal transplantation of MSC-EVs in rat lung tissue can significantly improve hyperoxia-induced angiogenesis damage and reduce the number of apoptotic cells in lung tissue (43).
Pericytes are cells that surround capillaries and veins throughout the body. Franco et al. (44) reported that pericytes can protect vascular endothelial cells from cytotoxicity. Covering vascular endothelial cells with pericyte prevents EVs from being phagocysed by vascular endothelial cells. Pericyte-dependent survival signals are forced by paracrine and autocrine circulation involving VEGF-A expression. In addition, some studies have shown that the expression of VEGF in differentiated pericyte enhances the survival of endothelial cells and the stability of microvessels (45). In general, these findings suggest that the improvement of angiogenesis of MSC-EVs is not mediated directly by phagocytosis of vascular endothelial cells, but indirectly induced by phagocytosis of pericyte by EVs. These results indicate that VEGF protein and mRNA carried by EVS are very important for angiogenesis, and the specific mechanism needs further study (43).
In conclusion, MSC-EVs or MSC-Exos play a positive role in various diseases of respiratory system. Firstly, exosomal proteins and miRNA have powerful functions, which can reach lung injury tissues through their targeting and homing properties to treat oxidative stress, inflammation and vascular injury (46). Secondly, exosomes can be used as carriers to encapsulate drugs across the air-blood barrier and alveolar epithelial-endothelial barrier to reach some places that drugs cannot reach. Finally, exosomes also show potential in disease diagnosis. Exosomes released by infected cells carry many pathogen-derived molecules and can be used as biomarkers of specific infectious factors. In addition to the frontier research directions such as exosomes and miRNAs, exosomes and autophagy, we believe that the combination of exosomes of infected cells and omics techniques to find biomarkers of various lung diseases may be the future research direction (47).
3 Diseases of circulatory system
It is well known that cytokines secreted by MSCs can enhance cardiomyocyte proliferation, reduce cardiomyocyte apoptosis, improve the microenvironment of damaged sites and repair damaged tissues (48).
3.1 MSC-EVs and MSC-Exos improve the related indexes of oxidative stress in circulatory system
Exosomes extracted from mesenchymal stem cells can improve myocardial dysfunction after hypoxia and myocardial infarction. Studies have shown that miR-182-5p carried by MSC-exos can treat myocardial ischemia-reperfusion (I/R) injury. MiR-199a-3p and miR-214 are similar to miR-182-5p in the response to I/R in rat myocardial cells, and both of them can improve the survival rate of myocardial cells and thus treat myocardial ischemia-reperfusion injury (49). At the same time, after hypoxia-induced injury, a low expression level of miR-182-5p was also observed in rat cardiomyocytes. Exosomal miR-182-5p engrafted NLRP3 inflammasome activation and cellular oxidative stress, thereby alleviating myocardial ischemia-reperfusion injury NLRP3 plays a key role in many diseases and can be activated by many types of agonists or risks. miRNA (miR-223) can bind to the 3 ‘UTR of NLRP3 to inhibit the inflammatory response caused by NLRP3 (50). On the other hand, overexpression of miR-182-5p downregulates Toll-like receptor 4 (TLR4), inactivates proinflammatory cytokines, TNF-α, and IL-6, thereby ameliorating liver I/R injury. In addition, miR-182-5p can also down-regulate TLR4 and inhibit ROS production to treat oxidative stress injury and apoptosis induced by atherosclerosis (51).
O’Brien CG et al. participated in the Seneca trial sponsored by the National Institutes of Health/National Heart Lung and Blood Institute. Peripheral blood mononuclear cells were successfully induced into induced pluripotent stem cells (IPSCs) and then differentiated into cardiomyocytes (ICMs). Using these AIC patient-specific MSCs, it was demonstrated that specific MSCs successfully reactivated iCMs after doxorubicin (DOX) injury. This effect is due to the active mitochondria carried by MSC-EVs (51).
Previous studies have shown that the re-expression of lncRNA alpha-2-macroglobulin antisense RNA 1 (Lnc RNA A2M-AS1) and Lnc RNA A2M-AS1 in myocardial infarction can significantly attenuate the hypoxia/resuscitation effect through the expression of inflammatory factor receptor IL1R2 (52). Oxygen (H/R)-induced cardiomyocyte apoptosis suggested that Lnc RNA A2M-AS1 may be involved in myocardial I/R injury.
AC16 cells were pretreated with exosomes and treated with ischemia-reperfusion. Cell proliferation was detected by CCK-8 assay, which showed that hMSCs-Exos treatment could reverse the decline in cell viability caused by ischemia reperfusion (53). Furthermore, H/R resulted in the apoptotic rate of AC16 cells, accompanied by downregulation of Bcl-2 protein levels and upregulation of Bax and Caspase-3 protein levels, which was attenuated in hMSCs-Exos treatment. hMSCs-Exos treated the content of LDH and MDA in H/R AC16 cells, but increased the content of SOD, thereby inhibiting oxidative stress. These results suggest that exosomes isolated from hMSCs can protect cardiomyocytes from H/R injury (54). It was further found that the level of Lnc RNA A2M-AS1-Exos was upregulated in AC16 cells cultured with hMSCs-Exos, alleviating H/R-induced apoptosis and oxidative stress in cardiomyocytes (54). Sánchez-Sánchez R et al. found that miR-4732-3p can induce apoptosis, ROS level and LDH activity in neonatal rat cardiomyocytes after OGD (glucose and oxygen deprivation model), prevent fibroblast migration and myofibroblast differentiation, induce in vitro and in vivo Angiogenesis. Intramyocardial injection of miR-4732-3p in exosomes in infarcted nude rats confers cardioprotection through functional and morphometric studies (55).
3.2 MSC-EVs and MSC-Exos in the treatment of circulatory vascular injury induced by oxidative stress
In recent years, a large number of evidences have shown that exosomes have protective effect in ischemic heart, which can alleviate myocardial I/R injury, promote cardiac regeneration and angiogenesis and inhibit fibrosis (56, 57). It has been proved that MSC-Exos overexpressing miR-486-5p can restore cardiac function after myocardial infarction in mice and non-human primates (58). Delivery of MSC-EVs containing miR-1505p in I/R rat model also alleviates poor myocardial remodeling (59). MSC-EVs-derived miR-21a5p induces cardiac protection in mice after I/R injury (60). adenovirus-transmitted miR-148a prevents ventricular remodeling in pressure overloaded mice (61). In mechanism, miR-210 was found to enhance myocardial vascularization in AMI rat model after myocardial transduction by up-regulating the expression of hepatocyte growth factor (62). Nevertheless, some miRNA in MSC-EVs may still be harmful to heart function, and the strategy of eliminating specific packaged miRNA molecules has been proved to improve the anti-apoptosis and angiogenesis of EVs (63).
Overexpression of Notch1 intracellular domain (N1ICD) in MSC-EVs can prevent apoptosis of CMS (cardiomyocytes) and promote cardiac angiogenesis under oxidative stress and ischemia injury. Notch plays an important role in cardiac repair after myocardial injury, Overexpressed MSC-EVs of N1ICD have good curative effect on angiogenesis of ischemic myocardium, proliferation of CMS (myocardial cells), improvement of cardiac function and fibrosis, Notch1 is strong for heart (64).
3.3 MSC-EVs and MSC-Exos in the treatment of cardiomyocyte apoptosis induced by oxidative stress
Mesenchymal stem cells can secrete a large number of soluble factors to promote myocardial cell proliferation, reduce apoptosis, improve ischemic microenvironment and mobilize endogenous cardiac stem cells by paracrine. However, studies have shown that although exogenous mesenchymal stem cells prefer to homing to myocardial ischemia sites, their survival rate in infarct areas is low (65). MSCs-Exos mediates cell-to-cell communication through horizontal transfer of bioactive RNA molecules and proteins (66). It is important that exosomes have good stability without the risk of chromosome loss and poor immune responses are rare (48). Therefore, MSCs-Exos is considered as an ideal drug delivery carrier and has great prospects in the treatment of oxidative stress-induced cardiomyocyte apoptosis.
3.4 MSC-EVs, MSC-Exos and autophagy of myocardial cells
Autophagy is involved in regulating the metabolic balance between synthesis, degradation and reuse of cellular substances. Both autophagy defect and overactivation will cause damage to homeostasis to some extent (67). Bafecycin A1 (Baf-A1) inhibited autophagy, and observed the effect of exosomes on autophagy. Compared with pure H2O2-induced autophagy, the expression of Beclin-1 and LC3B-II in exosomes + H2O2-treated cells increased and the expression of P62 decreased, while the protein levels of LC3B-IIBeclin-1 and P62 in H2O2 + exosomes + Baf-A1-treated cells were higher than those in H2O2 + exosomes and H2O2-treated cells. Therefore, autophagy induced by MSCs-Exos may be an important mechanism of cell protection after H2O2 (48). Autophagy is known to be associated with many pathways involving MAPK/mTOR and Akt/mTOR. Enhanced autophagy after hypoxia or ischemia injury has myocardial protection. Excessive ROS production in post-ischemia reperfusion stage can lead to autophagy. Matsui et al. reported that activation of MAPK pathway can induce autophagy, but activation of Beclin-1 pathway can induce autophagy, which may lead to cell death during reperfusion after ischemia (30). Therefore, ROS-induced autophagy is multifaceted (68).
At present, the research on the application of MSC-EVs and MSC-Exos in circulatory system diseases mainly focuses on microRNA. Numerous studies have found which microRNA in exosomes has a therapeutic effect on circulatory system diseases through sequencing technology. For example, miR-182-5p of MSC-Exos can improve myocardial I/R injury through the expression of GSDMD (51), miR-21a5p derived from MSC-EVs induced cardioprotection in mice after I/R injury (60), etc. Exosomes are natural carriers of bioactive molecules, and using them as carriers of drugs or siRNA to control gene expression and accelerate disease recovery may be a research hotspot in the future of exosomes in the circulatory system (69).
4 Diseases of digestive system
Oxidative stress is the main pathogenic phenomenon peculiar to liver diseases, which may lead to common liver diseases (70). Under normal circumstances, hepatocytes can balance the advantages and disadvantages of oxidative stress, control the level of oxidative stress within a reasonable range, and have the ability to regulate the balance of oxidants and antioxidants. However, due to the damage caused by toxins, there will be an imbalance between these particles. Oxidative stress is caused by mitochondrial dysfunction of hepatocytes, which leads to ROS production. This will not only induce irreversible changes in lipid protein and DNA content, but also regulate the pathway of controlling normal biological function (71). Studies have shown that MSCs-Exo can produce beneficial effects in animal models of various liver diseases, including liver injury, liver fibrosis, ischemia-reperfusion and so on.
4.1 MSC-EVs and MSC-Exos improve the related indexes of oxidative stress in liver injury
MSC-Exos can be used as an antioxidant to oxidative stress in mice with liver injury. In vitro, Hiroaki Haga et al. evaluated the effects of MSC-EVs on ROS production and NF-kB activity in normal mouse stem cells induced by H2O2 ROS activity was observed after 1 hour of H2O2. This activity was significant after 24 hours pre-incubation with MSC-EVs. The same NF-kB activity was also detected by MSC-EVs. Therefore, the results show that MSC-EVs can regulate the response to oxidative stress in liver IRI (72). GPX1 is an antioxidant that can induce oxidative stress injury induced by H2O2 to promote cell survival. When GPX1 in hucMSC-Exos is knocked out, the antioxidant activity of exosomes indicates that GPX1 is an important factor in hucMSC-Exos-mediated antioxidant activity and liver protection. Inducing increased GPX1 activity can induce liver injury and activate mitochondrial apoptosis pathway in hepatocytes (70). In vivo, hucMSC-Exos significantly inhibited the activation of oxidative stress products 8-OHdG and SOX9 in CCl4-induced liver tumor model. In CCl4-induced acute liver injury model, 8-OHdG also had more obvious antioxidant and liver protection effects than biphenyl ester (DDB) treatment, and hucMSC-Exos was a more effective antioxidant than DDB (73).
4.2 MSC-EVs and MSC-Exos in the treatment of liver inflammatory injury induced by oxidative stress
Macrophages and Kupffer cells are involved in regulating liver inflammation and hepatocyte death in liver. Studies have shown that exosomes can promote disease recovery by expressing inflammatory factors (72). MSC-EVs can reduce ROS production and overexpression of inflammatory cytokines such as IL-6 and IL-1B due to activation of the NF-kB signaling pathway. In addition, MSC-EVs can inhibit inflammation in liver through NLRP12, which is a negative regulator of inflammatory activity in vitro immune system and others, and plays a role through attenuated NF-kB (72).
4.3 MSC-EVs and MSC-Exos in the treatment of hepatocyte apoptosis induced by oxidative stress
The results of clinical studies show that HUCMSCs transplantation can improve the blood supply of hepatocyte extensive necrosis and other clinical symptoms in decompensated cirrhosis (70). The levels of ALT and AST, the markers of hepatocyte injury, and the levels of Caspase-3 and Bcl-2, the activity of Bax and the anti-apoptosis protein increased after treatment with hucMSC-Exos. These results suggest that hucMSC-Exos treatment can improve liver I/R injury (74). In acetaminophen (APAP) and hydrogen peroxide (HP) induced hepatocyte injury models treated with hucMSC-Exos, cell activity increased, necrosis and apoptosis decreased, LDH activity decreased and ROS decreased. In CCl4-induced acute liver injury cells treated with hucMSC-Exos, Bax and activated caspase 3 expressed TUNEL positive cells can inhibit hepatocyte degeneration and hepatic lobules at the same time, and even partially save the life of mice in acute liver injury model. It is confirmed that hucMSC-Exos can induce acute extensive liver injury induced by CCl4 (73). ERK1/2 phosphorylation and Bcl-2 expression induced by glutathione peroxidase 1 (GPX1) hucMSC-Exos in mice. The phosphorylation of I-Kappa-B Kinase b (IKKb) and NF-kB was inhibited 24 hours after hucMSC-Exos treatment. The expression of Casp-9 and Casp-3 was inhibited HucMSC-Exos inhibited the I-Kappa-B Kinase b (IKKb) NF-kBcaspase 3 pathway and pNF-kB nuclear translocation in CCl4-injured hepatocytes in a dose-dependent manner and induced Bcl-2 expression and ERK1/2 phosphorylation to reverse oxidative stress-induced apoptosis (70).
4.4 MSC-EVs and MSC-Exos regulate iron death in the treatment of liver injury
Iron death is a regulatory cell death caused by lipid peroxidation. Iron death is very important for preventing various liver diseases, including liver fibrosis. HSCs iron prolapse has become the target of inhibiting liver fibrosis (75). Benzyl chloride 1 (BECN1) is the key regulator of iron sag (76). It was found that BECN1 enriched down-regulated GPX4 in both MSCs-EVs and MSC-Exos, which contributed to iron sagging, which was necessary to activate HSCs (77). An increase in BECN1 was detected after MSC-Exos treatment, and a decrease in GPX4 and alpha-SMA (HSCs-activated markers) was also found in fibrotic mouse livers and collagen deposition. Therefore, MSC-Exos containing BECN1 can induce BECN1/GPX4-mediated iron prolapse and activation of HSCs in mouse fibrotic liver. When BECN1 was knocked out, ROS expressed by GPX4 produced mitochondrial membrane potential. Therefore, BECN1 can induce iron poisoning by down-regulating GPX4. BECN1 overexpression can produce ROS and decrease mitochondrial membrane potential in vivo experiments also showed the same results (77).
In the current report, MSCs-EVs and MSC-Exos were not only able to down-regulate inflammatory factors and oxidative stress indicators in diseases of digestive system, but exosomes were also found to regulate iron death in liver fibrosis. Iron death, a novel type of programmed cell death, occurs in a wide range of injured cells, and there are numerous research points that can be explored, which deserve to be deeply investigated.
5 Urinary system disease
Combination of MSC-EVs and MSC-Exos transport with renal artery revascularization can improve renal function and structure and narrow oxidative stress, apoptosis, fibrosis and microvascular remodeling (78, 79). Renal artery stenosis (RAS) is very common in patients with chronic kidney disease. RAS patients are prone to renovascular hypertension and progress to end-stage renal disease (80). MSC-EVs have been shown to alleviate renal inflammation and microvascular damage and improve hemodynamics and function beyond stenosis in porcine RAS (81, 82). This suggests that MSC-EVs are effective in preserving stenosis.
5.1 MSC-EVs and MSC-Exos regulate renal oxidative stress-related diseases through mitochondria
Mitochondria regulate many functions of renal cells, including redox state, survival, proliferation and death. The damage of mitochondrial structure and function is often accompanied by oxidative stress, which is mainly due to the production of superoxide (83) and H202 by complex I and III, which damages several components of mitochondria and forms a vicious circle of mitochondrial damage and oxidative stress. Mitosis can improve the revascularization results of experimental (84) and clinical RAS and protect renal function. Studies have shown that MSC-EVs and MSC-Exos play an important filamentous protective role in stenotic kidney, which improves mitochondrial density and mitochondrial swelling (85).
The important role of mitochondria in mammalian cells is to produce ATP through OXPHOS (86). Loss of a nuclear-encoded mitochondrial protein, TFAM, causes mtDNA depletion and OXPHOS (87, 88). After treatment of HK-2 with H202, the basal respiration rate, the maximum respiration rate, the ATP production respiration rate and the standby respiration capacity level all made MSC-EVs reverse these phenomena. Therefore, MSC-EVs can function as mitochondria (89).
Faisal A Alzahrani and others found that MSC-Exos significantly decreased the levels of MDA,HIF-1 α, mRNA and NADPH oxidase 2 (NOX2) protein and increased the levels of three antioxidant enzymes and HO-1 mRNA. The changes of oxidative stress or antioxidant related parameters were more prominent after ischemia. This indicates that MSC-Exos can play a role by inducing antioxidant activity and inhibiting oxidative stress in injured kidney tissue (90). Pallavi Bhargava et al. (86) used renal I/R injury model to evaluate the therapeutic effect of MSC-EVs. The results showed that the rate of renal tubular necrosis, the expression of KIM-1 and the number of apoptotic cells in I/R mice, And I/R mice showed higher levels of renal cytokines mRNAs (IL-6,IL-1 β and ICAM1) and serum cytokines (TNF-α and TWEAK) (91, 92). MSC-EVs treatment can reverse the levels of these cytokines.
5.2 MSC-EVs and MSC-Exos regulate related indexes of kidney oxidative stress
It is well known that most of the beneficial effects of MSCs are mediated by their exosomes containing miRNA, mRNA and LncRNA. These exosomal RNA are responsible for intercellular communication through which RNA-based information is transmitted to recipient cells (93). Interestingly, when exosomes were pretreated with RNase, the ameliorative effect of exosomes on renal ischemia-reperfusion injury was eliminated, which means that exosomal RNA has an ameliorative effect on oxidative stress in renal injury (94). In addition, Rafael S Lindoso et al. (95) reported that the ameliorative effect of MSCs-Exos on Renal ischemia-reperfusion injury and metabolic syndrome is related to the expression of some miRNA involved in apoptosis and hypoxia, which means that exosomes can improve their effects by post-transcriptional targeting of some genes in cells by exosomal miRNA Melatonin (Mel) preconditioning may induce MSCs to produce exosomes with higher expression of RNA vectors, which induce renal repair by inhibiting certain molecules involved in oxidative stress cell apoptosis and inflammation (90).
5.3 MSC-EVs and MSC-Exos in the treatment of apoptosis induced by oxidative stress
Renal ischemia-reperfusion injury may accelerate the development of chronic kidney (CKD). Renal ischemia-reperfusion injury releases free radicals and mitochondrial function induces apoptosis and inflammation (96, 97). The degree of renal function was evaluated by detecting BUN in plasma and creatinine in serum BUN and creatinine levels decreased sharply after 4 weeks of renal ischemia-reperfusion injury, but they were still higher than normal renal tissue. These elevated levels improved significantly after MSC-Exos preconditioning and creatinine levels returned to normal at 4 weeks. Therefore, the renal dysfunction after renal ischemia-reperfusion injury is relieved after MSC-Exos treatment, which means that MSC-Exos has broad prospects in the treatment of CKD (90). Exosomes can improve renal ischemia-reperfusion injury by interfering with apoptosis of renal cells. This inhibition of apoptosis can be evaluated by measuring the activity of caspase-3, the final marker of apoptosis. qPCR results showed that apoptosis markers Bax,PARP1 and caspase-3 were up-regulated and anti-apoptosis marker. Bcl2 was down-regulated in renal ischemia-reperfusion injury rats Bax,PARP1 and caspase-3 mRNA levels, and Bcl2 mRNA levels were significantly increased after treatment with MSC-Exos. These results suggest that the ameliorative effect of MSC-Exos on renal ischemia-reperfusion injury is related to the inhibition of apoptosis in damaged kidneys (90). In addition, MSC-Exos had similar anti-apoptosis effects on ischemia-reperfusion injury (98, 99) cisplatin-induced acute kidney injury (100, 101) and glycerol-induced acute kidney injury (102). This anti-apoptosis effect is also related to enhanced proliferation of renal tubular epithelial cells and improved renal function and structure (90).
5.4 MSC-EVs and MSC-Exos in the treatment of vascular injury induced by oxidative stress
Transcription factor Sox9 plays a key role in renal development, and its dysfunction can lead to severe renal dysplasia (103). It is reported that the improvement of AKI by MSC-Exos is mediated by up-regulating the expression of Sox9 in renal tubular cells (104). Injection of MSC-Exos in renal ischemia-reperfusion injury rats induced the expression of various angiogenic factors, resulting in the improvement of renal function (94, 105), suggesting that these angiogenic factors may be involved in exosomal-induced renal repair. Studies reveal the important role of bFGF, HGF and Sox9 in renal tubular regeneration after renal ischemia-reperfusion injury (106). The expression of these regeneration markers was also induced after treatment with MSC-Exos (104).
MSC-EVs and MSC-Exos have a dual role including regeneration and reduction of inflammation and oxidative stress in urinary tract diseases. It is rich in growth factors that can provide nutrients. At the same time, in vitro, mesenchymal stem cells can transfer many cytokines with anti-apoptosis, anti-inflammatory angiogenesis and immunomodulation properties into conditioned medium to promote the recovery of model animal diseases effectively. However, the safety and ethics of this therapy should be further explored and corrected in order to try to apply it to clinical practice (107).
6 Diseases of nervous system
Stem cell therapy shows great prospect in nervous system diseases. However, stem cell therapy is limited by its safety ethics or national legislation. Many evidences show that MSC-Exos and MSC-EVs are more effective than their parent cells in the treatment and recovery of nervous system diseases (108). EVs have many biological characteristics, including crossing the blood-brain barrier and the ability to resist freezing and thawing, which is beneficial for EVs to play a therapeutic role in nervous system defects (109). EV carries a variety of complex RNA and protein. EV has a good ability to regulate oxidative stress pathophysiology and immune response in nervous system diseases. Especially, the ability of miRNA transfer to target cells mediated by EVs plays a key role in antioxidant activity (110) and MSCs pretreated with H2O2 have better antioxidant activity (111). Studies have shown that EVs contain a series of up-regulated antioxidants miRNA, such as miR-215-5p,miR-424-5p,miR-31-3p,miR-193b-3p and miR-200b-3p. This indicates that exosomal miRNA plays an important role in antioxidant stress (112).
6.1 MSC-EVs and MSC-Exos improve the indicators related to oxidative stress in the nervous system
Seizures have a disproportionate impact on patients with traumatic brain injury and stroke (113). ROS overproduction caused by oxidative stress is an important pathophysiological mechanism in human epilepsy. Oxidative stress in epilepsy-causing hippocampal neurons can lead to neuronal apoptosis, cell loss and mitochondrial function. Therefore, targeting the changes in the pathophysiology of oxidative stress in the hippocampus has a significant improvement effect on the disease (114). Electrophysiological disturbances are often manifested in neuronal function (115), depolarization responses lead to spontaneous firing and AP numbers, and input resistance can lead to impaired excitability of neuronal cells (116), especially CA1 pyramidal neurons, in Oxidative stress is particularly vulnerable during epilepsy. Improvement of neuronal membrane excitability after EVs treatment, suggesting the ability of MSC-EVs to restore hippocampal electrophysiology in cellular and animal models.
SAMPs are involved in oxidative responses and cellular homeostasis, including iNOS, HMGB1, HO-1, and Nrf2 (117). MSC-EVs treatment resulted in improvement of SAMPs, suggesting that MSC-EVs have excellent therapeutic effect on H2O2-induced oxidative neuronal injury. Furthermore, There are two types of glutamate receptors that control oxidative stress levels through mediated signal transduction. Glut 1 is also strongly associated with the proliferation and death of neurons after the cell is stimulated (117). Reversal of the expression of SAMPs, AMPA, and Glut1 after EVs treatment indicated that MSC-EVs attenuated seizure-induced oxidative stress in the mouse hippocampus.
Nrf2 is closely related to antioxidant, and the antioxidant effect of Nrf2 enables it to play a neuroprotective role in epilepsy and other neurological diseases through targeted therapy. The function of NRF2 is closely related to KEAP1, which plays a coordinated role in the expression of several target genes, such as NADPH quinone oxidoreductase 1(NQO1) and heme oxygenase 1(HO-1), which encode antioxidant mediators and have protective effects against hippocampal neuronal damage caused by seizures (118). MSC-EVs are rich in antioxidant miRNAs, and knockdown of Nrf2 abolished the antioxidant capacity of MSC-EVs against epilepsy-induced hippocampal injury, suggesting that the Nrf2 defense system is involved in the antioxidant effect of MSC-EVs in epilepsy (112).
Qiang Luo et al. pretreated hippocampal neurons with 10 μg MSC-EVs, and then with 100 μM H2O2, MSC-EVs pretreated the activities of FRAP, CAT, SOD and GSH-PX. Furthermore, ROS production in hippocampal neurons of H2O2 was assessed by flow cytometry, and a significant rate of ROS production was detected in the group pretreated with MSC-EVs. Experiments showed that H2O2 led to increased expression of 8-OHdG (DNA damage marker), 4-HNE (lipid peroxidation marker) and DT (protein oxidation marker), and EVs treatment significantly enhanced the expression of these markers, and immunofluorescence staining confirmed that MSC-EVs can process and repair DNA damage. These results suggest that MSC-EVs have a strong antioxidant capacity in hippocampal neurons in response to H2O2 (112).
Through miRNA sequencing technology, find and identify substances that may have antioxidant potential in MSC-EVs. Comparing the differentially expressed miRNAs between MSC-EVs and H2O2-derived MSCs-EVs, many miRNAs were found to be upregulated in H2O2-derived MSCs-EVs, such as miR-215-5p, miR-424-5p, miR-31-3p, miR- 193b-3p and miR-200b-3p (119). GO classification showed that exosomal miRNA target genes were closely related to antioxidant active molecules. miRNA transfection revealed that miRNA inhibitors reduced oxidative stress-induced 8-OHdG concentrations in hippocampal neurons. This suggests that these miRNAs in MSC-EVs exert an antioxidant effect on H2O2 in hippocampal neurons (112).
Calcium, as an intracellular messenger, is ubiquitous in oxidative stress (120). mitochondria are important organelles in the control of calcium homeostasis (121). MSC-EVs can restore seizure-induced hippocampal neuronal morphological changes and mitochondrial function. The expression of TOM20, FIS1, and COXIV after EVs treatment indicated that MSC-EVs could restore mitochondrial/fusion and respiratory chains, so calcium and mitochondrial changes are critical for maintaining the stability of neuronal function (122). These results indicated that MSC-EVs improved calcium transients and mitochondrial function in primary cultures in H2O2.
The MWM test, which measures cognitive ability, found that MSC-EVs-treated seizure mice had shorter escape latencies. These data suggest that MSC-EVs treatment promotes functional reconstitution of hippocampal neurons during epileptic chronic seizures (123). Qiang Luo et al. pretreated hippocampal neurons with MSC-EVs to induce the uptake of nanoparticles, and then used H2O2 cells to induce oxidative stress. The results showed that the activities of various antioxidant enzymes decreased and excessive ROS production (124). It is evident that MSC-EVs have a significant antioxidant effect on seizure-induced neuronal damage by reversing H2O2-induced oxidative stress (112).
6.2 MSC-EVs and MSC-Exos treat inflammatory injury of nervous system induced by oxidative stress
Astrocytes play an important role in the formation of blood-brain barrier. They can produce and express neurotransmitters and some neurotransmitter receptors In addition, astrocytes can biotransform exogenous compounds and help regulate ionization around neurons. Studies have shown that astrocytes can be activated by inflammation or ROS (125). Sexual astrocyte activation can enable mitochondrial function (126). There is an extensive interaction between Nrf2 and NF-κB, which has been shown to be involved in the regulation of transcriptional, anti-oxidative, and anti-inflammatory pathways, regulation of NRF2 and NF-κB signaling pathway can reduce some inflammatory factors and improve the function of astrocytes (127).
MSC-Exos has a good therapeutic effect on inflammatory astrocytes and can improve a series of diseases caused by inflammatory astrocytes (128). MSC-Exos can enhance the cytotoxicity of astrocytes induced by LPS; The markers of reactive astrocyte proliferation, such as GFAP,C3,CD81 and Ki67, increased significantly after MSC-Exos treatment of inflammatory astrocytes. TNF α and IL-1 β in culture medium decreased after MSC-Exos treatment of LPS-induced astrocytes. These results indicate that MSC-Exos has a good effect on inhibiting LPS-induced decrease of cytotoxic astrocyte proliferation and inflammatory response (127).
Panpan Xian et al. used calcium imaging to study calcium changes in primary cultures of astrocytes. The data revealed that different groups of astrocytes had different fluorescent properties. MSC-Exos treatment of LPS-induced hippocampal astrocytes significantly enhanced their Ca2+ influx, and LPS resulted in faster changes in response rise time and decay time. It is worth noting that MSC-Exos has a significant therapeutic effect on astrocyte Ca2+ oscillation rate and mitochondrial dysfunction in patients with LPS (129).
In conclusion, mesenchymal stem cell exosomes can reduce inflammation and oxidative stress in nervous system diseases. Exosomes are closely related to the pathogenesis of central nervous system diseases, so exosomes have the potential to become unique biomarkers of nervous system diseases. In addition, exosomes can cross the blood-brain barrier, making them a new candidate for drug carriers for nervous system diseases.
7 Skin tissue trauma and repair
With the development of regenerative medicine, autologous mesenchymal stem cells can be cultured in vitro and then injected into vivo to promote the regeneration and repair of damaged tissues (130). More and more evidences show that exosomes have excellent therapeutic effects in various disease models besides stem cells. Exogenous skin aging is usually caused by various chronic injuries such as drinking, smoking and ultraviolet radiation. Cause skin aging based on the important role of ROS in photoaging. Protect skin from photoaging by producing ROS or inducing antioxidant defense (131, 132). MSC-Exos can inhibit oxidative damage of H2O2 keratinocytes, improve antioxidant activity, reduce oxidative reactivity and improve abnormal calcium and mitochondrial changes induced by oxidative stress. Subcutaneous injection of MSC-Exos can alleviate ultraviolet-induced skin tissue damage and inflammatory reaction in mice, inhibit cell proliferation and collagen deposition in skin of mice irradiated by ultraviolet radiation, alleviate oxidative damage of mice irradiated by ultraviolet radiation, improve antioxidant activity and alleviate oxidative reaction in mice. All in all, exosomes can promote wound healing and recovery through oxidative stress in various ways in the treatment of skin injury and photoaging (23, 132).
7.1 MSC-EVs and MSC-Exos in the treatment of photoaging injury induced by oxidative stress
It is well known that DNA damage is a marker of oxidative stress. Skin cells will produce ROS and DNA damage after oxidation (133). The results showed that MSC-Exos treatment could prevent ROS formation and DNA damage induced by oxidative stress in mouse keratinocytes.
R. S. Stern demonstrated that both MSC-EVs and Fb-EVs could proliferate cells and prevent cell cycle arrest induced by UVB and intracellular ROS levels induced by UVB radiation. In addition, the expression of MMP-1, the expression of Col-1, the expression of MSC-EVs and the enhancement of antioxidant activity of Fb-EVs in senescent cells after EVs treatment may be related to the up-regulation of GPX-1 gene expression (134, 135). Oxidative stress can lead to inflammation and inflammation can also induce subsequent oxidative damage (136). S. Candel et al. observed that MSC-Exos injection induced the expression of pro-inflammatory cytokines (TNF α IL-1 β and IL-6) in mouse skin after ultraviolet irradiation, which means that MSC-Exos alleviated inflammatory reaction and oxidative damage in mice exposed to ultraviolet irradiation (137).
MSC-EVs can transfer MSC-EVs through GPX-1 protein to achieve antioxidant effect. Higher levels of GPX-1 can be observed in EVs treated cells (70, 138). To elucidate the mechanism of EVs-dependent ROS, the expression of antioxidant protein GPX-1 after EVs treatment, but the expression of SOD1, SOD2 and catalase was not (139).
Keratinocytes are the main constituent cells of the epidermis and form an important skin barrier to prevent damage caused by ultraviolet radiation, water loss, pathogens, fungi and viruses (140). Studies have shown that Nrf2 is very important in regulating cell homeostasis, including antioxidant proteins, detoxification enzymes, drug transporters and many cytoprotective proteins (141). Regulation of Nrf2 pathway of keratinocytes to external oxidation is a promising treatment strategy for skin photoaging injury. The results of M. Schafer et al. showed that the recovery effect of MSC-Exos after oxidation was related to the down-regulation of Nrf2. By knocking down Nrf2, we can explore the detailed mechanism of MSC-Exos activity on oxidative keratinocyte reactivity (141). The results of Wang T et al. Show that MSC-EXOS can improve oxidative stress in both in vivo and in vitro experiments. Exosomes can improve oxidative stress injury of H2O2 pretreated keratinocytes at cellular level. Exosomes at animal level can improve DNA damage and mitochondrial changes of mouse skin after ultraviolet radiation. Exosomal therapy enhances the antioxidant capacity of skin as shown by iron ion antioxidant capacity and enhances the activity of glutathione POD or superoxide dismutase in cell and skin damage induced by oxidative stress. Therefore, MSC-Exos may be used as a potential skin nano-therapeutic agent to treat skin diseases or disorders caused by oxidative stress (23).
7.2 MSC-EVs and MSC-Exos in the treatment of chronic wound injury induced by oxidative stress
MSC-EVs not only has a broad prospect in the study of skin photoaging, but also has a good therapeutic effect on chronic skin injury. Diabetic ulcer is a chronic trauma characterized by an inflammatory state of hypoxia and undernutrition caused by elevated blood glucose levels followed by an elevated hypoxia of oxidative stress leading to the death of fibroblasts and other skin cell types (142, 143). Parvaiz A Shiekh et al. pretreated HDFs with EVs for 6 hours and then put them in hyperglycemia in order to study the cell survival of HDFs (fibroblasts) under hyperglycemia (143). All EVs were able to protect HDFs from cytotoxic hyperglycemia at least 24 hours after treatment until at least 72 hours after determination. Interestingly, the results are even better than those of the positive control group-complete medium. They found that both AT-MSCs and HF-MSCs can oxidative stress HDFs metabolism and activity under hyperglycemia (144).
7.3 MSC-EVs and MSC-Exos in the treatment of skin vascular injury induced by oxidative stress
The biology of angiogenesis includes the proliferation and migration of endothelial cells and angiogenesis. New blood vessels can supply oxygen and nutrition to the wound site, so the formation of new blood vessels can determine the effect of chronic wound healing (145, 146). Many studies have shown that inflammatory reaction of oxidative stress tissue leads to stagnation of wound healing. In addition, limited vascular function and angiogenesis will cause hypoxia in chronic injuries and lead to prolonged wound healing (147). Xiao X et al. made wound models and performed healing operations on 8-week-old and 64-week-old mice. The results showed that the healing ability of old mice was weaker than that of young mice. Quantitative measurements showed that MSC-EVs had a high level of reepithelization and a low level of scar formation. The quantitative analysis of neovascularization density confirmed the beneficial effect of MSC-EVs on wound vascular reconstruction (148).
MSC-EVs play an important role in regulating functional recovery and treating wound healing. MiR-146a and Src play an important role in promoting angiogenesis and wound healing, thus dephosphorylating Src (148). Next-generation mRNA sequencing and proteomics showed that EVs contain many angiogenic genes and proteins including growth factors, nuclear receptors, adhesion molecules, protease inhibitors, matrix protein transcription factors and other factors involved in angiogenesis, suggesting that MSC-EVs have important angiogenic potential (149).
7.4 MSC-EVs and MSC-Exos for skin aging induced by oxidative stress
The pursuit of eternal youth to resist aging is the lifelong pursuit of human beings. Using MSC-EVs to reduce oxidative stress to achieve anti-aging effects, this research direction has become the focus of many scholars, and many research results have been achieved. H2O2 reduced the expression of skin moisture-related mRNAs (aquaporin-1 and aquaporin-3) and hyaluronic acid, while MSC-Exos reversed these effects, and H2O2-induced cellular senescence was also reproduced in fibroblasts. Matsuoka T et al. found that over time, the downregulation of SIRT1 leads to the acetylation expression of p53, thereby inducing the expression of p21, a downstream molecule of p53, delaying the cell cycle and leading to cell senescence. MSC-Exos enhanced these transduction systems, effectively blocking the increase in intracellular β-galactosidase activity and the accumulation of ROS (150).
The anti-aging effect of MSC-EVs and its mechanism are still unclear, especially the effect on endothelial cell (EC) senescence. Xiao X et al. investigated the in vitro effects of MSC-EVs on oxidative stress-induced aging of human umbilical vein endothelial cells (HUVEC), as well as the in vivo effects on natural aging and diabetic mouse wound healing models (151). In addition, they investigated its molecular mechanism using miRNA sequencing and phosphokinase antibody arrays. It is suggested that MSC-EVs can be used as nanotherapeutics through the miR-146/Src pathway. In senescent HUVECs, MSC-EVs treatment prevented senescence-induced functions and promoted angiogenesis, cell migration and proliferation ability, mitochondrial function, and ROS levels (148).
Src kinase family is inextricably linked with aging. Senescence can activate the Src kinase family, which leads to oxidative stress, lipid peroxidation and DNA strand breakage, and finally leads to fatal damage to cells. Studies have shown that Src family inhibitors (PP2) can completely block H2O2-induced ECS aging. MSC-Exos has the same inhibitory effect as PP2. MSC-Exos can prevent senescence by inhibiting the activation of Src (152).
Studies by Zou et al. have shown that H2O2 can induce senescence in HUVEC and human aortic myocytes by up-regulating the alternative splicing body, Oct4A (153). H2O2-induced EC senescence induces a DNA damage response that activates p53 and p16, two important cell cycle regulatory pathways (154). High glucose can induce the senescence of HUVECs through the expression level of mitochondrial sirtuin (SIRT3), the expression of SA-gal, and the tube-forming ability of HUVECs (155). Oxidative stress is involved in the pathogenesis of diabetic vascular abnormalities, inducing premature senescence through DNA damage, and streptozotocin (STZ)-induced diabetes can induce senescence in ECs (156).
The miRNAs carried by MSC-EXOS has a significant effect on the treatment of cell senescence and the promotion of angiogenesis. Studies have shown that four miRNAs, miR-146a-5pmiR-34b-3pmiR-28-3p and miR-412-5p, play a promoting role in the treatment of aging ECs (157). High expression of miR-146a in MSC-EVS, when miR-146a inhibitor was used, the effect of MSC-EVS on aging disappeared. In addition, Xiao X et al. found that miR-146a can inhibit Src phosphorylation and its downstream target cavelin-1, thus inhibiting aging (148).
A large body of evidence shows that ROS can induce or accelerate ECs senescence at multiple subcellular levels. In cultured senescent HUVECs, MSC-EVs treatment prevented oxidative stress-induced ROS formation and DNA damage. Mitochondria are not the main source of ATP in ECs, but as ROS organelles, play an important role in the response of cells to pairs (158) and maintain the homeostasis of ECs (158). Experiments showed that aging-induced mitochondrial function of HUVECs could be improved by MSC-EVs treatment. Taken together, MSC-EVs have a comprehensive rescue effect on aging-induced endothelial cell function.
MSC-EVs and MSC-Exos have excellent therapeutic effects in both acute and chronic skin injuries. Exosomes can improve various functions caused by skin aging by inflammation and oxidative stress in skin injury. In recent reports, some scholars began to explore the effects of MSC-EVs and MSC-Exos on iron death and copper death induced by skin injury, which may be a research hotspot in the treatment of skin injury with exosomes in the future.
8 Diseases of motor system
ROS is involved in regulating many chondrocyte activities such as cell proliferation and matrix remodeling (159). Osteoarthritis (OA) is a joint disease characterized by cartilage degeneration and low-grade synovitis In damaged joints, chondrocyte homeostasis is gradually increased with the gradual increase of oxidative stress (160). MSC-EVs treatment of OA joint cells can down-regulate inflammatory factors and increase the synthesis of extracellular matrix of chondrocytes (160). Low levels of ROS play an indelible role in various physiological processes, but the formation of ROS can lead to tissue damage. The endogenous mechanism of MSC-EVs is activated by oxidative stress and can offset the influence of ROS. Therefore, oxidative stress induces both antioxidant reaction and autophagy, which leads to excessive production of active substances and oxidative damage to macromolecules (21).
Bone marrow mesenchymal stem cells are exposed to radiation, which will affect their survival and differentiation potential and lead to bone loss (161). Radiation can cause DNA damage on bone marrow mesenchymal stem cells, chromosome aberration, reactive oxygen species and cell senescence, which hinder the proliferation ability of bone marrow mesenchymal cells (162). In addition, radiation has a great influence on the differentiation of bone marrow mesenchymal stem cells, which will lead to the first choice of bone marrow mesenchymal stem cells to differentiate into adipocytes instead of osteoblasts, and finally lead to fat accumulation (163). Recently, some scholars have shown that BMSC-Exos can treat the effect of radiation on the differentiation of bone marrow mesenchymal stem cells. Liu et al. (164) transplantation of BMSC-Exos to save osteoporotic phenotype of recipient bone marrow mesenchymal cells improves bone through epigenetic regulation. In addition, Liu et al. (165) found that BMSC-Exos transplantation can prevent femoral head necrosis. The main preventive mechanism is to promote angiogenesis and prevent bone loss (166).
8.1 MSC-EVs and MSC-Exos regulate oxidative stress-related indicators in bone
It is known that 4-hydroxynonenal (HNE), a product of lipid peroxidation, can form a variety of protein complexes, which affect the activity and physiological function of OA chondrocytes. It is found that EVs treatment of OA chondrocytes can significantly form HNE complexes (160). Members of the POD (Prdx) family participate in the fight against ROS-induced cartilage injury. The exact mechanism of antioxidant protection of Prdx6 has not been clarified. Some scholars suggest that it may be directly scavenging low molecular weight peroxides and phospholipid peroxides. The protein expressed the activities of POD phospholipase A2 and lysophosphatidylcholine acyltransferase, which participated in the repair of cell membrane (167).
Mesenchymal stem cell injury is an important pathological mechanism of radiation-induced bone loss. Radiation can cause bone marrow mesenchymal stem cells to produce reactive oxygen species. Excessive ROS can lead to DNA damage (163, 168). Therefore, it is extremely important to treat radiation-induced bone loss and remove reactive oxygen species and DNA damage. Studies have shown that MSCs-Exos has excellent effects on oxidative stress and alleviating DNA damage. After irradiation, the kinds of reactive oxygen species will cause cell damage. DCF fluorescence in BM-MSCs is significant after exosomal treatment. The results of Western Blot also showed the expression of antioxidant proteins after co-incubation with exosomes. These results indicate that exudate can enhance the antioxidant capacity of BM-MSC after irradiation (166).
ATF6 is the target gene of miR-31-5P. When miR-31-5P is elevated, ATF6 does not promote endoplasmic reticulum stress of endothelial progenitor cells, which leads to apoptosis and calcification of endothelial progenitor cells. Recently, it has been reported that oxidative stress induces endoplasmic reticulum stress of endothelial progenitor cells (169). MSC-Exos down-regulates the expression of ATF6,CHOP,XBP1 and GRP78, suggesting that MSC-Exos has protective effect on endothelial endoplasmic reticulum stress induced by oxidative stress (170).
In the H2O2-induced NP cell injury model, Western blot showed that the levels of apoptotic proteins such as caspase-9 and caspase-3 were significant after H2O2 treatment, however, these were inhibited by exosome pretreatment and positive cells stained by TUNEL Significantly (17). Daisuke Sakai et al. used a microscope to observe the status of NP and annulus fibrosus, and a histological grading system to evaluate disc degeneration (171), and found slight changes in NP organization after exosome treatment. Using both X-ray and MRI examinations, exosome-treated disc height decreased more slowly at 2, 4, and 8 weeks, and IVDD treated with exosomes showed significantly higher intensity, suggesting that exosomes can delay Progression of IVDD (17).
8.2 MSC-EVs and MSC-Exos in the treatment of bone inflammation induced by oxidative stress
A great deal of evidence shows that inflammatory mediators can induce oxidative stress, which leads to the decrease of chondrocyte viability and the change of chondrocyte function (160). Proinflammatory cytokines produced by different joint cells can promote cartilage degradation. IL-1β stimulates OA chondrocytes to produce inflammatory cytokines. MSCs-EVs, which can exert anti-inflammatory and anti-catabolic effects. IL-6 and different cytokines can induce collagenase to produce cartilage degradation (160) and inhibit the expression of type II collagen (172). EVs can release IL-6. MSCs-EVs also demonstrated the release of MMP-13, a major collagenase that degrades type II collagen and promotes the development of OA chondrocytes into a state of like differentiation (173). Therefore, EVs can not only produce inflammatory mediators, but also control the consequences of mediator activation of cells.
NLRP3/IL-1 β plays a key role in inflammation through TXNIP activation (174). In H2O2-treated NP cells, H2O2 was significantly associated with inflammatory activation of the genes IL-1 β,TXNIP and NLRP3, which were inhibited by exosomal preconditioning. Western Blot and cellular immunity also showed the same results indicating that exosomes attenuated H2O2-induced activation of TXNIP-NLRP3 inflammatory corpuscles (17).
8.3 MSC-EVs and MSC-Exos down-regulate bone oxidative stress injury through mitochondria
Exosomes have obvious protective effect on the deterioration of mitochondria, exosomes can down-regulate ROS level and decrease apoptosis of NP cells, which can be seen from the low expression of caspase-3 and caspase-9. In addition, exosomes successfully expressed NLRP3 and TXNIP, thus inhibiting the decomposition of IL-1 β (17). Although systemic delivery of exosomes is generally considered to be the simplest, biological distribution indicates accumulation in the liver, spleen and lungs (175). Particularly when the avascular nature of IVDD is considered, local delivery of the subplate region is considered to be a good alternative. Previous evidence in vivo and in vitro indicates that oxidation products are widely present in IVDD (176). Cardiovascular calcification induced by oxidative stress products (177) and apoptosis and calcification of endothelial progenitor cells. These studies suggest that oxidative stress is a common pathological condition for apoptosis and calcification, including endothelial progenitor cells (178).
TEM showed that exosomal treatment alleviated mitochondrial morphological abnormalities and mitochondrial cristae breakage and disappearance induced by H2O2. Exosomal pretreatment also inhibited the production of mitochondrial ROS in NP cells induced by H2O2 (17). Proteomics MSC-Exos showed that 10.7% of exosomal proteins originated from mitochondria and 14.3% of exosomal proteins participated in ATP binding. In addition, 3.8% of exosomal proteins were involved in metabolism. It is important that MSC-Exos are enriched in different parts of mitochondria, including mitochondrial inner membrane, envelope, matrix, outer membrane and nucleoids. All in all, these data suggest that MSC-Exos may provide NP cells with mitochondrial proteins. Damaged mitochondria can be recovered by this treatment (17).
The progression of degenerative NP cells is accompanied by matrix degeneration caused by inflammation (179, 180). In order to study whether MSC-Exos can enhance mitochondrial biogenesis, Pengfei Chen et al. discovered that 10.3% of exosomes were derived from mitochondria through proteomics. Molecular function showed that 15.4% of exosomal proteins were involved in ATP binding. The enrichment of GO pathway also showed that mitochondrial fragments including mitochondrial inner membrane, envelope, matrix, space outer membrane and nucleoid were enriched in MSC-Exos (181).
Mitochondrial injury induced by mitochondrial electron transport chain complex I inhibitor reveals the chondroprotective mechanism of exosomes and whether it is inhibited by mitochondrial function. The exosomes restored the normal appearance of mitochondria in the chondrocytes (182). Chondrocytes treated with exosomes also showed mitochondrial and mtDNA content. In addition, rotenone treatment significantly inhibited the production of mitochondrial ROS in chondrocytes, which was significantly inhibited by exon treatment. The intracellular ATP level of exosomes-treated chondrocytes was 21% higher than that of rotenone-treated chondrocytes in function. These results indicate that MSC-Exos provide mitochondrial proteins to chondrocytes and thus restore damaged mitochondria (181).
The targeting and homing ability of exosomes is particularly important for their treatment in the locomotor system. Exosome hydrogel scaffolds are able to penetrate deeper into the wound and connect to the injury site when treating injuries in the locomotor system, and exosomes are homed to the peri-wound area for a more effective therapeutic effect. The combination of exosomes and materials and translation to clinical applications may be a hotspot for future research (183).
9 Senescence
Aging has been a topic of great concern to human beings. Aging is accompanied by the accumulation of aging cells, which changes the communication between cells and damages the homeostasis of tissues and the regeneration potential of organs (184). Recently, MSC-EVs have proved to be more effective and challenging than current stem cell-based treatments. Extracellular vesicles contain cell-specific proteins, lipids and nucleic acids, which may be released and absorbed by all types of cells to induce functional changes through horizontal transfer of their cargo. Non-aging mesenchymal stem cells cultured in low physiological oxygen tension (3%) to premature aging mesenchymal stem cells. Extracellular vesicles cultured in high oxygen (the usual oxygen culture condition is 21%) have many beneficial characteristics (185). Free radical theory holds that oxidative stress induced cell damage is one of the main causes of various senile diseases, which changes the biological structure and function (186). In particular, the cells treated with H2O2 will produce a large amount of reactive oxygen species (ROS), and the excessive accumulation of ROS will damage the macromolecular function and membrane system of cells, which will irreversibly damage various senile diseases and senescence (187, 188).
9.1 MSC-EVs and MSC-Exos inhibit senescence through ROS
Aging induces many cell disorders. The regeneration of mesenchymal stem cells in old age is a hot spot in regenerative medicine research in recent years. MSC-EVs have become a new tool for stem cell regeneration because of their systemic effect and safe gene transfer ability (189). In the present study, the role of aging-related ROS in the function and regeneration of mesenchymal stem cells in infant EVs was studied. The data clearly showed that the elderly MSCs showed down-regulation of SOD1 and SOD3, which led to ROS elevation and down-regulation of MEK/ERK pathway, which was related to the impaired ability of MSCs to necrotic area in flap model. In addition, edaravone or co-overexpression of SOD1 and SOD3 can save ROS increase and cell senescence of mesenchymal stem cells in old age, thus improving their function (190). It is worth noting that EVs derived from infant bone marrow mesenchymal stem cells in type 1 and type 2 diabetic mice can revitalize elderly bone marrow mesenchymal stem cells by inhibiting ROS production and accelerating cell aging to promote proliferation and in vivo function (191).
EVs are a powerful tool that not only inhibits ROS production, but also restores altered intercellular communication, improves stem cell function and stem cell quality, and thus delays stem cell failure in aging. It has been shown that treatment of senescent MSCs with non-senescent MSC-EVs can induce glycolytic oxidative phosphorylation of SA-β-galactosidase activity and over-expression of pluripotent factors (OCT4, SOX2, KLF4 and cMYC or OSKM). In addition, the cargo of these EVs induces up-regulation of miR-302b and HIF-1 α levels in target cells. It is concluded that miR-302b triggers the up-regulation of HIF-1 α and activates different pathways to delay premature senescence, improve stem and transform energy metabolism into glycolysis (192).
9.2 MSC-EVs and MSC-Exos cell proliferation inhibits senescence
miR-302b can proliferate cells and protect cells from oxidant-induced death of human mesenchymal stem cells (193). Kim, J. Y et al. studied ROS levels and cell death of human dental pulp stem cells (hDPSCs) after EVs treatment. It was found that the cells cultured under physiological hypoxia showed ROS and apoptosis levels compared with those cultured under 21% oxygen. Interestingly, no changes in ROS levels were observed after treatment, and cell cycles parallel to these observations showed no difference in G0/G1 phase, S phase and G2/M phase after EVs treatment. Cells cultured at 3% O2 showed higher levels in G0/G1 phase, S phase and G2/M phase, which indicated the overall proliferation of cells (191). Studies have shown that EVs can promote the function of aged AT-MSCs by inducing proliferation and up-regulating the expression of damaged cytokines, thus promoting the necrotic area of aged AT-MSCs in type 1 and type 2 diabetic mice. EVs significantly increased the proliferation of AT-MSCs in the elderly and increased the number of β-gal positive AT-MSCs in the elderly. Due to the up-regulation of SOD1 and SOD3 protein expression, the accumulation of ROS in aged AT-MSCs cells was induced by the addition of EVs. In addition, the addition of EVs up-regulated the expression of wound healing related cytokines (SDF-1VEGFAng1 and Flk1) in the elderly AT-MSCs. Transplantation studies show that the ability of aged AT-MSCs to show obvious necrotic area of flap mice after adding EVs is similar to that of infant AT-MSCs (191). Impaired expression of growth factors responsible for homing (SDF1) and angiogenesis (VEGF, Ang1, bFGF) was observed in elderly AT-MSCs. These growth factors are involved in regulating the function of EC and endothelial precursor cells (EPC) (191).
10 Immune
Studies have shown that MSC-EVs and MSC-Exos play both immune activation and immunosuppressive functions in cancer. The immune activation of exosomes mainly depends on the antigen presentation of exosomes, and the immunosuppression of exosomes mainly depends on the ligand protein and miRNA carried by exosomes (194). It has been reported that the existence of exosomes can provide several different mediators for cancer cells to form tumorigenic microorganisms, which belongs to the immunosuppressive effect of exosomes in cancer (195, 196). Many scholars have taken this as the breakthrough point to study cancer treatment. For example, Giovanna Andreola reported that FasL-positive exosomes released by melanoma cells can induce apoptosis of FasL-mediated Jurkat T lymphocytes (197). Phenotypically similar pro-apoptotic exosomes in the plasma of cancer patients indicate that these exosomes have a potential role in regulating host immunity and that they may become prognostic markers (198). Exosomes not only play an immunosuppressive role, but also play an immune activation role through other mechanisms. For example, exosomes released by mycobacterium-infected macrophages contain components that promote activation of adjacent uninfected macrophages (199) and many other exosomes-mediated to promote immune responses during infection with different types of microorganisms (200). In addition, synovial fibroblasts from patients with rheumatoid arthritis have been shown to release exosomes containing membrane-bound TNF-alpha that inhibit activation-induced cell death in CD4 T cells (201). Many of these studies suggest that exosomes may operate simultaneously in congenital and adaptive immune activation (202, 203) (Figure 2).
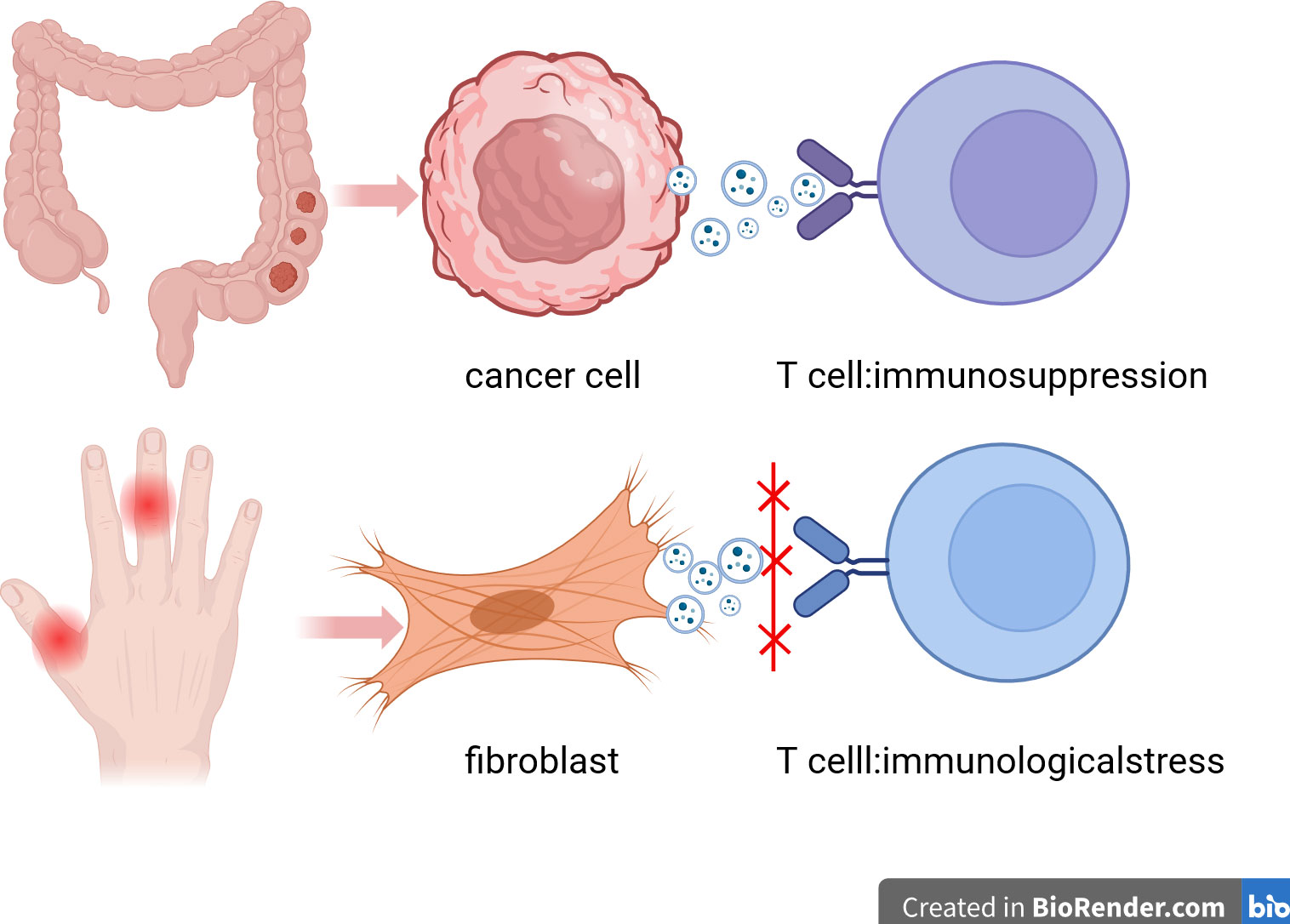
Figure 2 Immunosuppression and immune activation of exosomes. This figure describes the immunosuppressive effect of exosomes in cancer and the activation of immune response in synovial fibroblasts of patients with rheumatoid arthritis. Exosomes can help tumor cells escape killing by using human immunosuppressive mechanism. Exosomes can also be used as blockers of immune checkpoints to activate the immune response of T cells to kill tumor cells .
11 Conclusions and future directions
In conclusion, MSC-EVs and MSC-Exos are closely related to the regulation of oxidative stress injury in many systemic diseases. It has been fully verified in both cell model (Table 1) and animal model (Table 2). Oxidative stress can cause inflammatory factors to damage mitochondrial function, inhibit cell proliferation and enhance cell apoptosis. Inhibition of oxidative stress injury is of great significance to the treatment of various systemic diseases (233).
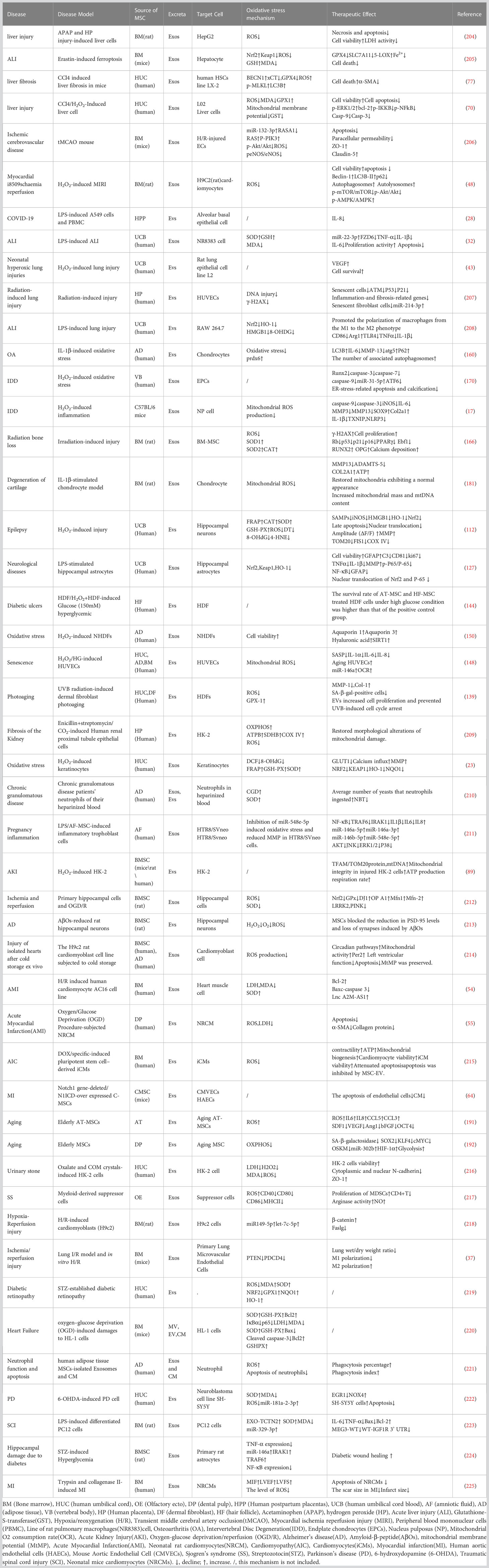
Table 1 Cell model: Summary of the mechanism of MSC-EVs and MSC-Exos in the treatment of oxidative stress-related diseases.
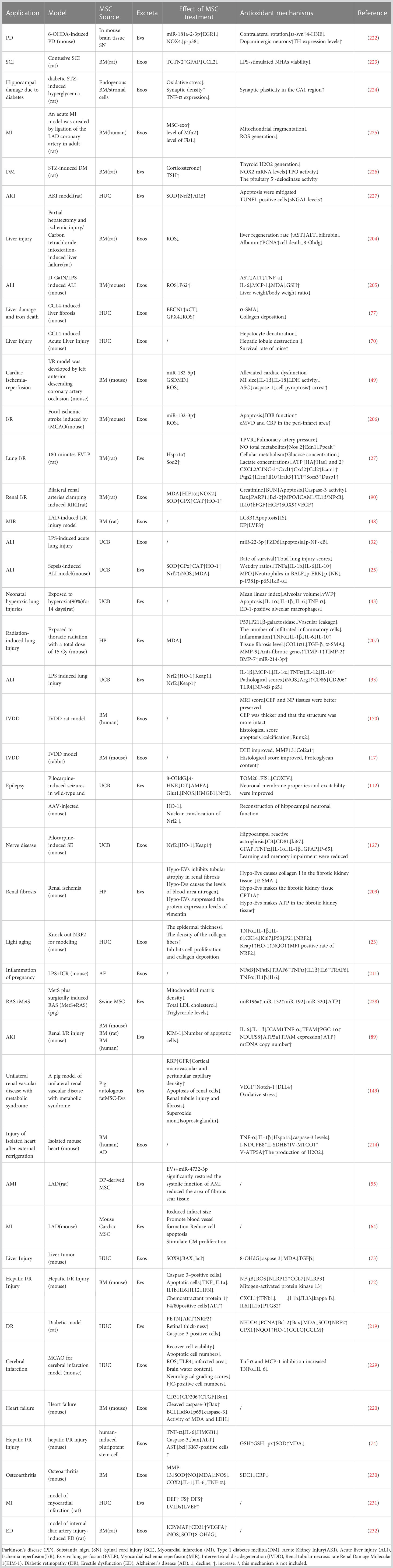
Table 2 Animal model: Summary of the mechanism of MSC-EVs and MSC-Exos in the treatment of oxidative stress-related diseases.
In the study of the mechanism of stem cell exosomes, iron death, copper death, autophagy and proteomics may be the hot spots in the future. In the application research of stem cell exosomes, hydrogel, collagen and other materials combined with exosomes to form composite scaffolds have been carried out by many scholars in cell experiments and animal experiments, but its clinical transformation has not yet been realized. Verifying its human safety and ethical rationality is the direction that needs to be worked hard now.
Regulatory proteins and miRNA in MSC-EVs and MSC-Exos are the core of treatment. However, the same protein and miRNA seem to show different results in different studies (234). At present, no research has shown the reason for this result. Potential effects of stem cell origin, such as the age of stem cell donors, may be related to the production of oxidative stress and inflammatory mediators, which may affect their immunomodulatory function (235). It may also be caused by sex, for example, female MSCs cause greater immunomodulatory effect than male MSCs (236). For example, some studies have not clarified the effective proteins in MSC-EVs and MSC-Exos. Although researchers have used proteomic databases to show which antioxidant proteins MSC-EVs and MSC-Exos contain, further work is needed to isolate and accurately identify these proteins (237). In addition, only one dose was used in some studies, and it is necessary to explore the possible dose-dependent protective effects of MSC-EVs and MSC-Exos (17).
At present, many functions of MSC-EVs and MSC-Exos have been discovered one after another, but these studies also have some limitations, MSC-EVs and MSC-Exos may have different inhibitory effects in different cell species and animal species (16). In addition, the differences in the isolation and application steps of EVs in different studies may lead to different effects of the same regulatory protein and miRNA. In order to ensure the repeatability of the effects of MSC-EVs and MSC-Exos, it is necessary to explore and formulate a protocol to control all the steps of their isolation and application (160). Therefore, MSC-EVs and MSC-Exos in the treatment of oxidative stress injury of various systems need to use models closer to human pathology for better clinical use (235).
Author contributions
All authors contributed to the conception and design of the review central idea. WZ wrote the first draft of the manuscript; YX and TW prepared the tables and retrieved literature; XP and YZ corrected the drafts of the paper. All authors contributed to the article and approved the submitted version.
Funding
Our work described in the present manuscript is supported by research grants from Guangdong Provincial Natural Science Foundation, Guangdong, China, No.2021A1515012437 and Guangdong Provincial Scientific Research Project, Guangdong Provincial Department of Education, No. 2021KTSCX040, Guangdong, China.
Acknowledgments
We thank all the authors who participated in the research on exosomes in the treatment of oxidative stress-related diseases, and thank the editors and reviewers for their valuable opinions and suggestions to improve the paper.
Conflict of interest
The authors declare that the research was conducted in the absence of any commercial or financial relationships that could be construed as a potential conflict of interest.
Publisher’s note
All claims expressed in this article are solely those of the authors and do not necessarily represent those of their affiliated organizations, or those of the publisher, the editors and the reviewers. Any product that may be evaluated in this article, or claim that may be made by its manufacturer, is not guaranteed or endorsed by the publisher.
References
1. Petersen BE, Bowen WC, Patrene KD, Mars WM, Sullivan AK, Murase N, et al. Bone marrow as a potential source of hepatic oval cells. Science (1999) 284:1168–70. doi: 10.1126/science.284.5417.1168
2. Pittenger MF, Mackay AM, Beck SC, Jaiswal RK, Douglas R, Mosca JD, et al. Multilineage potential of adult human mesenchymal stem cells. Science (1999) 284:143–7. doi: 10.1126/science.284.5411.143
3. Kopen GC, Prockop DJ, Phinney DG. Marrow stromal cells migrate throughout forebrain and cerebellum, and they differentiate into astrocytes after injection into neonatal mouse brains. Proc Natl Acad Sci U.S.A. (1999) 96:10711–6. doi: 10.1073/pnas.96.19.10711
4. Aggarwal S, Pittenger MF. Human mesenchymal stem cells modulate allogeneic immune cell responses. Blood (2005) 105:1815–22. doi: 10.1182/blood-2004-04-1559
5. Raffaghello L, Bianchi G, Bertolotto M, Montecucco F, Busca A, Dallegri F, et al. Human mesenchymal stem cells inhibit neutrophil apoptosis: a model for neutrophil preservation in the bone marrow niche. Stem Cells (2008) 26:151–62. doi: 10.1634/stemcells.2007-0416
6. Ankrum J, Karp JM. Mesenchymal stem cell therapy: Two steps forward, one step back. Trends Mol Med (2010) 16:203–9. doi: 10.1016/j.molmed.2010.02.005
7. Muschler GF, Nakamoto C, Griffith LG. Engineering principles of clinical cell-based tissue engineering. J Bone Joint Surg Am (2004) 86:1541–58. doi: 10.2106/00004623-200407000-00029
8. Moll G, Rasmusson-Duprez I, Von Bahr L, Connolly-Andersen AM, Elgue G, Funke L, et al. Are therapeutic human mesenchymal stromal cells compatible with human blood? Stem Cells (2012) 30:1565–74. doi: 10.1002/stem.1111
9. Mathivanan S, Fahner CJ, Reid GE, Simpson RJ. ExoCarta 2012: database of exosomal proteins, RNA and lipids. Nucleic Acids Res (2012) 40:D1241–4. doi: 10.1093/nar/gkr828
10. Caplan AI, Correa D. The MSC: an injury drugstore. Cell Stem Cell (2011) 9:11–5. doi: 10.1016/j.stem.2011.06.008
11. Morrison TJ, Jackson MV, Cunningham EK, Kissenpfennig A, Mcauley DF, O’kane CM, et al. Mesenchymal stromal cells modulate macrophages in clinically relevant lung injury models by extracellular vesicle mitochondrial transfer. Am J Respir Crit Care Med (2017) 196:1275–86. doi: 10.1164/rccm.201701-0170OC
12. Ogisu K, Fujio M, Tsuchiya S, Tsuboi M, Qi C, Toyama N, et al. Conditioned media from mesenchymal stromal cells and periodontal ligament fibroblasts under cyclic stretch stimulation promote bone healing in mouse calvarial defects. Cytotherapy (2020) 22:543–51. doi: 10.1016/j.jcyt.2020.05.008
13. Zhang Z, Mi T, Jin L, Li M, Zhanghuang C, Wang J, et al. Comprehensive proteomic analysis of exosome mimetic vesicles and exosomes derived from human umbilical cord mesenchymal stem cells. Stem Cell Res Ther (2022) 13:312. doi: 10.1186/s13287-022-03008-6
14. Subra C, Laulagnier K, Perret B, Record M. Exosome lipidomics unravels lipid sorting at the level of multivesicular bodies. Biochimie (2007) 89:205–12. doi: 10.1016/j.biochi.2006.10.014
15. Simons M, Raposo G. Exosomes–vesicular carriers for intercellular communication. Curr Opin Cell Biol (2009) 21:575–81. doi: 10.1016/j.ceb.2009.03.007
16. Lee Y, El Andaloussi S, Wood MJ. Exosomes and microvesicles: extracellular vesicles for genetic information transfer and gene therapy. Hum Mol Genet (2012) 21:R125–34. doi: 10.1093/hmg/dds317
17. Xia C, Zeng Z, Fang B, Tao M, Gu C, Zheng L, et al. Mesenchymal stem cell-derived exosomes ameliorate intervertebral disc degeneration via anti-oxidant and anti-inflammatory effects. Free Radical Biol Med (2019) 143:1–15. doi: 10.1016/j.freeradbiomed.2019.07.026
18. Wang ZG, He ZY, Liang S, Yang Q, Cheng P, Chen AM. Comprehensive proteomic analysis of exosomes derived from human bone marrow, adipose tissue, and umbilical cord mesenchymal stem cells. Stem Cell Res Ther (2020) 11:511. doi: 10.1186/s13287-020-02032-8
19. Ma M, Li B, Zhang M, Zhou L, Yang F, Ma F, et al. Therapeutic effects of mesenchymal stem cell-derived exosomes on retinal detachment. Exp Eye Res (2020) 191:107899. doi: 10.1016/j.exer.2019.107899
20. Ebrahim N, Ahmed IA, Hussien NI, Dessouky AA, Farid AS, Elshazly AM, et al. Mesenchymal Stem Cell-Derived Exosomes Ameliorated Diabetic Nephropathy by Autophagy Induction through the mTOR Signaling Pathway. Cells (2018) 7(12):226. doi: 10.20944/preprints201809.0153.v1
21. Filomeni G, De Zio D, Cecconi F. Oxidative stress and autophagy: the clash between damage and metabolic needs. Cell Death Differ (2015) 22:377–88. doi: 10.1038/cdd.2014.150
22. Bisht S, Faiq M, Tolahunase M, Dada R. Oxidative stress and male infertility. Nat Rev Urol (2017) 14:470–85. doi: 10.1038/nrurol.2017.69
23. Wang T, Jian Z, Baskys A, Yang J, Li J, Guo H, et al. MSC-derived exosomes protect against oxidative stress-induced skin injury via adaptive regulation of the NRF2 defense system. Biomaterials (2020) 257:120264. doi: 10.1016/j.biomaterials.2020.120264
24. Silva AKA, Morille M, Piffoux M, Arumugam S, Mauduit P, Larghero J, et al. Development of extracellular vesicle-based medicinal products: A position paper of the group “Extracellular Vesicle translatiOn to clinicaL perspectiVEs - EVOLVE France”. Adv Drug Delivery Rev (2021) 179:114001. doi: 10.1016/j.addr.2021.114001
25. Chen J, Li C, Liang Z, Li C, Li Y, Zhao Z, et al. Human mesenchymal stromal cells small extracellular vesicles attenuate sepsis-induced acute lung injury in a mouse model: the role of oxidative stress and the mitogen-activated protein kinase/nuclear factor kappa B pathway. Cytotherapy (2021) 23:918–30. doi: 10.1016/j.jcyt.2021.05.009
26. Qiao Q, Liu X, Yang T, Cui K, Kong L, Yang C, et al. Nanomedicine for acute respiratory distress syndrome: The latest application, targeting strategy, and rational design. Acta Pharm Sinica. B (2021) 11:3060–91. doi: 10.1016/j.apsb.2021.04.023
27. Lonati C, Bassani GA, Brambilla D, Leonardi P, Carlin A, Maggioni M, et al. Mesenchymal stem cell-derived extracellular vesicles improve the molecular phenotype of isolated rat lungs during ischemia/reperfusion injury. J Heart Lung Transplant (2019) 38:1306–16. doi: 10.1016/j.healun.2019.08.016
28. Shevtsova Y, Goryunov K, Babenko V, Pevzner I, Vtorushina V, Inviyaeva E, et al. Development of an in vitro model of SARS-coV-induced acute lung injury for studying new therapeutic approaches. Antioxidants (Basel Switzerland) (2022) 11(10):1910. doi: 10.3390/antiox11101910
29. Vardakas P, Skaperda Z, Tekos F, Kouretas D. ROS and COVID. Antioxidants (Basel) (2022) 11(2):339. doi: 10.3390/antiox11020339
30. Sengupta V, Sengupta S, Lazo A, Woods P, Nolan A, Bremer N. Exosomes derived from bone marrow mesenchymal stem cells as treatment for severe COVID-19. Stem Cells Dev (2020) 29:747–54. doi: 10.1089/scd.2020.0080
31. Risbud MV, Shapiro IM. Role of cytokines in intervertebral disc degeneration: pain and disc content. Nat Rev Rheumatol (2014) 10:44–56. doi: 10.1038/nrrheum.2013.160
32. Zheng Y, Liu J, Chen P, Lin L, Luo Y, Ma X, et al. Exosomal miR-22-3p from human umbilical cord blood-derived mesenchymal stem cells protects against lipopolysaccharid-induced acute lung injury. Life Sci (2021) 269:119004. doi: 10.1016/j.lfs.2020.119004
33. Zhao R, Wang L, Wang T, Xian P, Wang H, Long Q. Inhalation of MSC-EVs is a noninvasive strategy for ameliorating acute lung injury. J Control Release (2022) 345:214–30. doi: 10.1016/j.jconrel.2022.03.025
34. Sasaki H, Takayama K, Matsushita T, Ishida K, Kubo S, Matsumoto T, et al. Autophagy modulates osteoarthritis-related gene expression in human chondrocytes. Arthritis Rheum (2012) 64:1920–8. doi: 10.1002/art.34323
35. Piga R, Van Dartel D, Bunschoten A, Van Der Stelt I, Keijer J. Role of Frizzled6 in the molecular mechanism of beta-carotene action in the lung. Toxicology (2014) 320:67–73. doi: 10.1016/j.tox.2014.03.002
36. Yi X, Wei X, Lv H, An Y, Li L, Lu P, et al. Exosomes derived from microRNA-30b-3p-overexpressing mesenchymal stem cells protect against lipopolysaccharide-induced acute lung injury by inhibiting SAA3. Exp Cell Res (2019) 383:111454. doi: 10.1016/j.yexcr.2019.05.035
37. Li JW, Wei L, Han Z, Chen Z. Mesenchymal stromal cells-derived exosomes alleviate ischemia/reperfusion injury in mouse lung by transporting anti-apoptotic miR-21-5p. Eur J Pharmacol (2019) 852:68–76. doi: 10.1016/j.ejphar.2019.01.022
38. Coulson-Thomas VJ, Gesteira TF, Hascall V, Kao W. Umbilical cord mesenchymal stem cells suppress host rejection: the role of the glycocalyx. J Biol Chem (2014) 289:23465–81. doi: 10.1074/jbc.M114.557447
39. Kota DJ, Dicarlo B, Hetz RA, Smith P, Cox CS Jr., Olson SD. Differential MSC activation leads to distinct mononuclear leukocyte binding mechanisms. Sci Rep (2014) 4:4565. doi: 10.1038/srep04565
40. Kota DJ, Prabhakara KS, Cox CS, Olson SD. MSCs and hyaluronan: sticking together for new therapeutic potential? Int J Biochem Cell Biol (2014) 55:1–10. doi: 10.1016/j.biocel.2014.07.022
41. Day AJ, De La Motte CA. Hyaluronan cross-linking: a protective mechanism in inflammation? Trends Immunol (2005) 26:637–43. doi: 10.1016/j.it.2005.09.009
42. Kellner M, NooNepalle S, Lu Q, Srivastava A, Zemskov E, Black SM. ROS signaling in the pathogenesis of Acute Lung Injury (ALI) and Acute Respiratory Distress Syndrome (ARDS). Adv Exp Med Biol (2017) 967:105–37. doi: 10.1007/978-3-319-63245-2_8
43. Ahn SY, Park WS, Kim YE, Sung DK, Sung SI, Ahn JY, et al. Vascular endothelial growth factor mediates the therapeutic efficacy of mesenchymal stem cell-derived extracellular vesicles against neonatal hyperoxic lung injury. Exp Mol Med (2018) 50:1–12. doi: 10.1038/s12276-018-0055-8
44. Franco M, Roswall P, Cortez E, Hanahan D, Pietras K. Pericytes promote endothelial cell survival through induction of autocrine VEGF-A signaling and Bcl-w expression. Blood (2011) 118:2906–17. doi: 10.1182/blood-2011-01-331694
45. Geevarghese A, Herman IM. Pericyte-endothelial crosstalk: implications and opportunities for advanced cellular therapies. Transl Res (2014) 163:296–306. doi: 10.1016/j.trsl.2014.01.011
46. Tang P, Gu JM, Xie ZA, Gu Y, Jie ZW, Huang KM, et al. Honokiol alleviates the degeneration of intervertebral disc via suppressing the activation of TXNIP-NLRP3 inflammasome signal pathway. Free Radic Biol Med (2018) 120:368–79. doi: 10.1016/j.freeradbiomed.2018.04.008
47. Vejpongsa P, Yeh ET. Topoisomerase 2β: a promising molecular target for primary prevention of anthracycline-induced cardiotoxicity. Clin Pharmacol Ther (2014) 95:45–52. doi: 10.1038/clpt.2013.201
48. Liu L, Jin X, Hu CF, Li R, Zhou Z, Shen CX. Exosomes derived from mesenchymal stem cells rescue myocardial ischaemia/reperfusion injury by inducing cardiomyocyte autophagy via AMPK and akt pathways. Cell Physiol Biochem (2017) 43:52–68. doi: 10.1159/000480317
49. Yue R, Lu S, Luo Y, Zeng J, Liang H, Qin D, et al. Mesenchymal stem cell-derived exosomal microRNA-182-5p alleviates myocardial ischemia/reperfusion injury by targeting GSDMD in mice. Cell Death Discovery (2022) 8:202. doi: 10.1038/s41420-022-00909-6
50. Bauernfeind F, Rieger A, Schildberg FA, Knolle PA, Schmid-Burgk JL, Hornung V. NLRP3 inflammasome activity is negatively controlled by miR-223. J Immunol (2012) 189:4175–81. doi: 10.4049/jimmunol.1201516
51. Qin SB, Peng DY, Lu JM, Ke ZP. MiR-182-5p inhibited oxidative stress and apoptosis triggered by oxidized low-density lipoprotein via targeting toll-like receptor 4. J Cell Physiol (2018) 233:6630–7. doi: 10.1002/jcp.26389
52. Song XL, Zhang FF, Wang WJ, Li XN, Dang Y, Li YX, et al. LncRNA A2M-AS1 lessens the injury of cardiomyocytes caused by hypoxia and reoxygenation via regulating IL1R2. Genes Genomics (2020) 42:1431–41. doi: 10.1007/s13258-020-01007-6
53. Zhang B, Xu L, Zhuo N, Shen J. Resveratrol protects against mitochondrial dysfunction through autophagy activation in human nucleus pulposus cells. Biochem Biophys Res Commun (2017) 493:373–81. doi: 10.1016/j.bbrc.2017.09.015
54. Yu H, Pan Y, Dai M, Wang X, Chen H. Mesenchymal stem cell-originated exosomal Lnc A2M-AS1 alleviates hypoxia/reperfusion-induced apoptosis and oxidative stress in cardiomyocytes. Cardiovasc Drugs Ther (2022). doi: 10.1007/s10557-022-07339-7
55. Sánchez-Sánchez R, Gómez-Ferrer M, Reinal I, Buigues M, Villanueva-bádenas E, Ontoria-oviedo I, et al. miR-4732-3p in extracellular vesicles from mesenchymal stromal cells is cardioprotective during myocardial ischemia. Front Cell Dev Biol (2021) 9:734143. doi: 10.3389/fcell.2021.734143
56. Chen GH, Xu J, Yang YJ. Exosomes: promising sacks for treating ischemic heart disease? Am J Physiol Heart Circ Physiol (2017) 313:H508–h523. doi: 10.1152/ajpheart.00213.2017
57. Zhou H, Wang B, Yang Y, Jia Q, Qi Z, Zhang A, et al. Exosomes in ischemic heart disease: novel carriers for bioinformation. BioMed Pharmacother (2019) 120:109451. doi: 10.1016/j.biopha.2019.109451
58. Li Q, Xu Y, Lv K, Wang Y, Zhong Z, Xiao C, et al. Small extracellular vesicles containing miR-486-5p promote angiogenesis after myocardial infarction in mice and nonhuman primates. Sci Transl Med (2021) 13(584):eabb0202. doi: 10.1126/scitranslmed.abb0202
59. Ou H, Teng H, Qin Y, Luo X, Yang P, Zhang W, et al. Extracellular vesicles derived from microRNA-150-5p-overexpressing mesenchymal stem cells protect rat hearts against ischemia/reperfusion. Aging (Albany NY) (2020) 12:12669–83. doi: 10.18632/aging.102792
60. Luther KM, Haar L, Mcguinness M, Wang Y, Lynch Iv TL, Phan A, et al. Exosomal miR-21a-5p mediates cardioprotection by mesenchymal stem cells. J Mol Cell Cardiol (2018) 119:125–37. doi: 10.1016/j.yjmcc.2018.04.012
61. Raso A, Dirkx E, Philippen LE, Fernandez-Celis A, De Majo F, Sampaio-Pinto V, et al. Therapeutic delivery of miR-148a suppresses ventricular dilation in heart failure. Mol Ther (2019) 27:584–99. doi: 10.1016/j.ymthe.2018.11.011
62. Fan ZG, Qu XL, Chu P, Gao YL, Gao XF, Chen SL, et al. MicroRNA-210 promotes angiogenesis in acute myocardial infarction. Mol Med Rep (2018) 17:5658–65. doi: 10.3892/mmr.2018.8620
63. Zhang LL, Xiong YY, Yang YJ. The vital roles of mesenchymal stem cells and the derived extracellular vesicles in promoting angiogenesis after acute myocardial infarction. Stem Cells Dev (2021) 30:561–77. doi: 10.1089/scd.2021.0006
64. Xuan W, Khan M, Ashraf M. Extracellular vesicles from notch activated cardiac mesenchymal stem cells promote myocyte proliferation and neovasculogenesis. Front Cell Dev Biol (2020) 8:11. doi: 10.3389/fcell.2020.00011
65. Martin-Rendon E, Sweeney D, Lu F, Girdlestone J, Navarrete C, Watt SM. 5-Azacytidine-treated human mesenchymal stem/progenitor cells derived from umbilical cord, cord blood and bone marrow do not generate cardiomyocytes in vitro at high frequencies. Vox Sang (2008) 95:137–48. doi: 10.1111/j.1423-0410.2008.01076.x
66. Lugea A, Waldron RT. Exosome-mediated intercellular communication between stellate cells and cancer cells in pancreatic ductal adenocarcinoma. Pancreas (2017) 46:1–4. doi: 10.1097/MPA.0000000000000686
67. Bélanger M, Rodrigues PH, Dunn W. A., JR., Progulske-Fox A. Autophagy: a highway for Porphyromonas gingivalis in endothelial cells. Autophagy (2006) 2:165–70. doi: 10.4161/auto.2828
68. Matsui Y, Takagi H, Qu X, Abdellatif M, Sakoda H, Asano T, et al. Distinct roles of autophagy in the heart during ischemia and reperfusion: roles of AMP-activated protein kinase and Beclin 1 in mediating autophagy. Circ Res (2007) 100:914–22. doi: 10.1161/01.RES.0000261924.76669.36
69. Lin B, Yang J, Song Y, Dang G, Feng J. Exosomes and atherogenesis. Front Cardiovasc Med (2021) 8:738031. doi: 10.3389/fcvm.2021.738031
70. Yan Y, Jiang W, Tan Y, Zou S, Zhang H, Mao F, et al. hucMSC exosome-derived GPX1 is required for the recovery of hepatic oxidant injury. Mol therapy: J Am Soc Gene Ther (2017) 25:465–79. doi: 10.1016/j.ymthe.2016.11.019
71. Peralta C, Jiménez-Castro MB, Gracia-Sancho J. Hepatic ischemia and reperfusion injury: effects on the liver sinusoidal milieu. J Hepatol (2013) 59:1094–106. doi: 10.1016/j.jhep.2013.06.017
72. Haga H, Yan IK, Borrelli DA, Matsuda A, Parasramka M, Shukla N, et al. Extracellular vesicles from bone marrow-derived mesenchymal stem cells protect against murine hepatic ischemia/reperfusion injury. Liver Transpl (2017) 23:791–803. doi: 10.1002/lt.24770
73. Jiang W, Tan Y, Cai M, Zhao T, Mao F, Zhang X, et al. Human umbilical cord MSC-derived exosomes suppress the development of CCl(4)-induced liver injury through antioxidant effect. Stem Cells Int (2018) 2018:6079642. doi: 10.1155/2018/6079642
74. Nong K, Wang W, Niu X, Hu B, Ma C, Bai Y, et al. Hepatoprotective effect of exosomes from human-induced pluripotent stem cell-derived mesenchymal stromal cells against hepatic ischemia-reperfusion injury in rats. Cytotherapy (2016) 18:1548–59. doi: 10.1016/j.jcyt.2016.08.002
75. Sui M, Jiang X, Chen J, Yang H, Zhu Y. Magnesium isoglycyrrhizinate ameliorates liver fibrosis and hepatic stellate cell activation by regulating ferroptosis signaling pathway. BioMed Pharmacother (2018) 106:125–33. doi: 10.1016/j.biopha.2018.06.060
76. Kang R, Zhu S, Zeh HJ, Klionsky DJ, Tang D. BECN1 is a new driver of ferroptosis. Autophagy (2018) 14:2173–5. doi: 10.1080/15548627.2018.1513758
77. Tan Y, Huang Y, Mei R, Mao F, Yang D, Liu J, et al. HucMSC-derived exosomes delivered BECN1 induces ferroptosis of hepatic stellate cells via regulating the xCT/GPX4 axis. Cell Death Dis (2022) 13:319. doi: 10.1038/s41419-022-04764-2
78. Eirin A, Zhu XY, Krier JD, Tang H, Jordan KL, Grande JP, et al. Adipose tissue-derived mesenchymal stem cells improve revascularization outcomes to restore renal function in swine atherosclerotic renal artery stenosis. Stem Cells (2012) 30:1030–41. doi: 10.1002/stem.1047
79. Ebrahimi B, Eirin A, Li Z, Zhu XY, Zhang X, Lerman A, et al. Mesenchymal stem cells improve medullary inflammation and fibrosis after revascularization of swine atherosclerotic renal artery stenosis. PLoS One (2013) 8:e67474. doi: 10.1371/journal.pone.0067474
80. Textor SC, Lerman LO. Paradigm shifts in atherosclerotic renovascular disease: where are we now? J Am Soc Nephrol (2015) 26:2074–80. doi: 10.1681/ASN.2014121274
81. Eirin A, Zhu XY, Ebrahimi B, Krier JD, Riester SM, Van Wijnen AJ, et al. Intrarenal delivery of mesenchymal stem cells and endothelial progenitor cells attenuates hypertensive cardiomyopathy in experimental renovascular hypertension. Cell Transplant (2015) 24:2041–53. doi: 10.3727/096368914X685582
82. Eirin A, Zhu XY, Puranik AS, Tang H, Mcgurren KA, Van Wijnen AJ, et al. Mesenchymal stem cell-derived extracellular vesicles attenuate kidney inflammation. Kidney Int (2017) 92:114–24. doi: 10.1016/j.kint.2016.12.023
83. Murphy MP. Mitochondrial dysfunction indirectly elevates ROS production by the endoplasmic reticulum. Cell Metab (2013) 18:145–6. doi: 10.1016/j.cmet.2013.07.006
84. Eirin A, Li Z, Zhang X, Krier JD, Woollard JR, Zhu XY, et al. A mitochondrial permeability transition pore inhibitor improves renal outcomes after revascularization in experimental atherosclerotic renal artery stenosis. Hypertension (2012) 60:1242–9. doi: 10.1161/HYPERTENSIONAHA.112.199919
85. Kaasik A, Safiulina D, Zharkovsky A, Veksler V. Regulation of mitochondrial matrix volume. Am J Physiol Cell Physiol (2007) 292:C157–63. doi: 10.1152/ajpcell.00272.2006
86. Bhargava P, Schnellmann RG. Mitochondrial energetics in the kidney. Nat Rev Nephrol (2017) 13:629–46. doi: 10.1038/nrneph.2017.107
87. Kang D, Kim SH, Hamasaki N. Mitochondrial transcription factor A (TFAM): roles in maintenance of mtDNA and cellular functions. Mitochondrion (2007) 7:39–44. doi: 10.1016/j.mito.2006.11.017
88. Kunkel GH, Chaturvedi P, Tyagi SC. Mitochondrial pathways to cardiac recovery: TFAM. Heart Fail Rev (2016) 21:499–517. doi: 10.1007/s10741-016-9561-8
89. Zhao M, Liu S, Wang C, Wang Y, Wan M, Liu F, et al. Mesenchymal stem cell-derived extracellular vesicles attenuate mitochondrial damage and inflammation by stabilizing mitochondrial DNA. ACS nano (2021) 15:1519–38. doi: 10.1021/acsnano.0c08947
90. Alzahrani FA. Melatonin improves therapeutic potential of mesenchymal stem cells-derived exosomes against renal ischemia-reperfusion injury in rats. Am J Transl Res (2019) 11:2887–907.
91. Stallons LJ, Whitaker RM, Schnellmann RG. Suppressed mitochondrial biogenesis in folic acid-induced acute kidney injury and early fibrosis. Toxicol Lett (2014) 224:326–32. doi: 10.1016/j.toxlet.2013.11.014
92. West AP, Khoury-Hanold W, Staron M, Tal MC, Pineda CM, Lang SM, et al. Mitochondrial DNA stress primes the antiviral innate immune response. Nature (2015) 520:553–7. doi: 10.1038/nature14156
93. Quesenberry PJ, Dooner MS, Aliotta JM. Stem cell plasticity revisited: the continuum marrow model and phenotypic changes mediated by microvesicles. Exp Hematol (2010) 38:581–92. doi: 10.1016/j.exphem.2010.03.021
94. Zou X, Gu D, Xing X, Cheng Z, Gong D, Zhang G, et al. Human mesenchymal stromal cell-derived extracellular vesicles alleviate renal ischemic reperfusion injury and enhance angiogenesis in rats. Am J Transl Res (2016) 8:4289–99.
95. Lindoso RS, Collino F, Bruno S, Araujo DS, Sant’anna JF, Tetta C, et al. Extracellular vesicles released from mesenchymal stromal cells modulate miRNA in renal tubular cells and inhibit ATP depletion injury. Stem Cells Dev (2014) 23:1809–19. doi: 10.1089/scd.2013.0618
96. Williams P, Lopez H, Britt D, Chan C, Ezrin A, Hottendorf R. Characterization of renal ischemia-reperfusion injury in rats. J Pharmacol Toxicol Methods (1997) 37:1–7. doi: 10.1016/S1056-8719(96)00141-4
97. Zager RA, Johnson AC, Lund S. Uremia impacts renal inflammatory cytokine gene expression in the setting of experimental acute kidney injury. Am J Physiol Renal Physiol (2009) 297:F961–70. doi: 10.1152/ajprenal.00381.2009
98. Gatti S, Bruno S, Deregibus MC, Sordi A, Cantaluppi V, Tetta C, et al. Microvesicles derived from human adult mesenchymal stem cells protect against ischaemia-reperfusion-induced acute and chronic kidney injury. Nephrol Dial Transplant (2011) 26:1474–83. doi: 10.1093/ndt/gfr015
99. Zou X, Zhang G, Cheng Z, Yin D, Du T, Ju G, et al. Microvesicles derived from human Wharton’s Jelly mesenchymal stromal cells ameliorate renal ischemia-reperfusion injury in rats by suppressing CX3CL1. Stem Cell Res Ther (2014) 5:40. doi: 10.1186/scrt428
100. Bruno S, Grange C, Collino F, Deregibus MC, Cantaluppi V, Biancone L, et al. Microvesicles derived from mesenchymal stem cells enhance survival in a lethal model of acute kidney injury. PloS One (2012) 7:e33115. doi: 10.1371/journal.pone.0033115
101. Zhou Y, Xu H, Xu W, Wang B, Wu H, Tao Y, et al. Exosomes released by human umbilical cord mesenchymal stem cells protect against cisplatin-induced renal oxidative stress and apoptosis in vivo and in vitro. Stem Cell Res Ther (2013) 4:34. doi: 10.1186/scrt194
102. Bruno S, Tapparo M, Collino F, Chiabotto G, Deregibus MC, Soares Lindoso R, et al. Renal regenerative potential of different extracellular vesicle populations derived from bone marrow mesenchymal stromal cells. Tissue Eng Part A (2017) 23:1262–73. doi: 10.1089/ten.tea.2017.0069
103. Kumar S, Liu J, Pang P, Krautzberger AM, Reginensi A, Akiyama H, et al. Sox9 activation highlights a cellular pathway of renal repair in the acutely injured MamMalian kidney. Cell Rep (2015) 12:1325–38. doi: 10.1016/j.celrep.2015.07.034
104. Zhu F, Chong Lee Shin OLS, Pei G, Hu Z, Yang J, Zhu H, et al. Adipose-derived mesenchymal stem cells employed exosomes to attenuate AKI-CKD transition through tubular epithelial cell dependent Sox9 activation. Oncotarget (2017) 8:70707–26. doi: 10.18632/oncotarget.19979
105. Choi HY, Moon SJ, Ratliff BB, Ahn SH, Jung A, Lee M, et al. Microparticles from kidney-derived mesenchymal stem cells act as carriers of proangiogenic signals and contribute to recovery from acute kidney injury. PloS One (2014) 9:e87853. doi: 10.1371/journal.pone.0087853
106. Little MH, Kairath P. Does renal repair recapitulate kidney development? J Am Soc Nephrol (2017) 28:34–46. doi: 10.1681/ASN.2016070748
107. Hong C, Seo H, Kwak M, Jeon J, Jang J, Jeong EM, et al. Increased TRPC5 glutathionylation contributes to striatal neuron loss in Huntington’s disease. Brain (2015) 138:3030–47. doi: 10.1093/brain/awv188
108. Zhang ZG, Buller B, Chopp M. Exosomes - beyond stem cells for restorative therapy in stroke and neurological injury. Nat Rev Neurol (2019) 15:193–203. doi: 10.1038/s41582-018-0126-4
109. Holm MM, Kaiser J, Schwab ME. Extracellular vesicles: multimodal envoys in neural maintenance and repair. Trends Neurosci (2018) 41:360–72. doi: 10.1016/j.tins.2018.03.006
110. Santulli G. Exosomal microRNA: The revolutionary endogenous Innerspace nanotechnology. Sci Transl Med (2018) 10(467):eaav9141. doi: 10.1126/scitranslmed.aav9141
111. Garrido-Pascual P, Alonso-Varona A, Castro B, Burón M, Palomares T. H(2)O(2)-preconditioned human adipose-derived stem cells (HC016) increase their resistance to oxidative stress by overexpressing Nrf2 and bioenergetic adaptation. Stem Cell Res Ther (2020) 11:335. doi: 10.1186/s13287-020-01851-z
112. Luo Q, Xian P, Wang T, Wu S, Sun T, Wang W, et al. Antioxidant activity of mesenchymal stem cell-derived extracellular vesicles restores hippocampal neurons following seizure damage. Theranostics (2021) 11:5986–6005. doi: 10.7150/thno.58632
113. Thijs RD, Surges R, O’brien TJ, Sander JW. Epilepsy in adults. Lancet (2019) 393:689–701. doi: 10.1016/S0140-6736(18)32596-0
114. Pauletti A, Terrone G, Shekh-Ahmad T, Salamone A, Ravizza T, Rizzi M, et al. Targeting oxidative stress improves disease outcomes in a rat model of acquired epilepsy. Brain (2019) 142:e39. doi: 10.1093/brain/awz130
115. Zhu X, Shen K, Bai Y, Zhang A, Xia Z, Chao J, et al. NADPH oxidase activation is required for pentylenetetrazole kindling-induced hippocampal autophagy. Free Radic Biol Med (2016) 94:230–42. doi: 10.1016/j.freeradbiomed.2016.03.004
116. Ordemann GJ, Apgar CJ, Brager DH. D-type potassium channels norMalize action potential firing between dorsal and ventral CA1 neurons of the mouse hippocampus. J Neurophysiol (2019) 121:983–95. doi: 10.1152/jn.00737.2018
117. Hirao H, Dery KJ, Kageyama S, Nakamura K, Kupiec-Weglinski JW. Heme Oxygenase-1 in liver transplant ischemia-reperfusion injury: From bench-to-bedside. Free Radic Biol Med (2020) 157:75–82. doi: 10.1016/j.freeradbiomed.2020.02.012
118. Devinsky O, Vezzani A, O’brien TJ, Jette N, Scheffer IE, De Curtis M, et al. Epilepsy. Nat Rev Dis Primers (2018) 4:18024. doi: 10.1038/nrdp.2018.24
119. Haut SR, Velísková J, Moshé SL. Susceptibility of immature and adult brains to seizure effects. Lancet Neurol (2004) 3:608–17. doi: 10.1016/S1474-4422(04)00881-6
120. Berridge MJ, Bootman MD, Roderick HL. Calcium signalling: dynamics, homeostasis and remodelling. Nat Rev Mol Cell Biol (2003) 4:517–29. doi: 10.1038/nrm1155
121. Rahman S. Mitochondrial diseases and status epilepticus. Epilepsia (2018) 59 Suppl 2:70–7. doi: 10.1111/epi.14485
122. Burté F, Carelli V, Chinnery PF, Yu-Wai-Man P. Disturbed mitochondrial dynamics and neurodegenerative disorders. Nat Rev Neurol (2015) 11:11–24. doi: 10.1038/nrneurol.2014.228
123. Hausenloy DJ, Yellon DM. Myocardial ischemia-reperfusion injury: a neglected therapeutic target. J Clin Invest (2013) 123:92–100. doi: 10.1172/JCI62874
124. Jiang T, Sun Q, Chen S. Oxidative stress: A major pathogenesis and potential therapeutic target of antioxidative agents in Parkinson’s disease and Alzheimer’s disease. Prog Neurobiol (2016) 147:1–19. doi: 10.1016/j.pneurobio.2016.07.005
125. Verkhratsky A, Steardo L, Parpura V, Montana V. Translational potential of astrocytes in brain disorders. Prog Neurobiol (2016) 144:188–205. doi: 10.1016/j.pneurobio.2015.09.003
126. Pekny M, Pekna M, Messing A, Steinhäuser C, Lee JM, Parpura V, et al. Astrocytes: a central element in neurological diseases. Acta Neuropathol (2016) 131:323–45. doi: 10.1007/s00401-015-1513-1
127. Xian P, Hei Y, Wang R, Wang T, Yang J, Li J, et al. Mesenchymal stem cell-derived exosomes as a nanotherapeutic agent for amelioration of inflammation-induced astrocyte alterations in mice. Theranostics (2019) 9:5956–75. doi: 10.7150/thno.33872
128. Long Q, Upadhya D, Hattiangady B, Kim DK, An SY, Shuai B, et al. Intranasal MSC-derived A1-exosomes ease inflammation, and prevent abnormal neurogenesis and memory dysfunction after status epilepticus. Proc Natl Acad Sci U.S.A. (2017) 114:E3536–e3545. doi: 10.1073/pnas.1703920114
129. Levy S. Function of the tetraspanin molecule CD81 in B and T cells. Immunol Res (2014) 58:179–85. doi: 10.1007/s12026-014-8490-7
130. Kraitchman DL, Tatsumi M, Gilson WD, Ishimori T, Kedziorek D, Walczak P, et al. Dynamic imaging of allogeneic mesenchymal stem cells trafficking to myocardial infarction. Circulation (2005) 112:1451–61. doi: 10.1161/CIRCULATIONAHA.105.537480
131. Kammeyer A, Luiten RM. Oxidation events and skin aging. Ageing Res Rev (2015) 21:16–29. doi: 10.1016/j.arr.2015.01.001
132. Poon F, Kang S, Chien AL. Mechanisms and treatments of photoaging. Photodermatol Photoimmunol Photomed (2015) 31:65–74. doi: 10.1111/phpp.12145
133. Kuehne A, Emmert H, Soehle J, Winnefeld M, Fischer F, Wenck H, et al. Acute activation of oxidative pentose phosphate pathway as first-line response to oxidative stress in human skin cells. Mol Cell (2015) 59:359–71. doi: 10.1016/j.molcel.2015.06.017
134. Abdel-Malek ZA, Kadekaro AL, Swope VB. Stepping up melanocytes to the challenge of UV exposure. Pigment Cell Melanoma Res (2010) 23:171–86. doi: 10.1111/j.1755-148X.2010.00679.x
135. Wang SQ, Balagula Y, Osterwalder U. Photoprotection: a review of the current and future technologies. Dermatol Ther (2010) 23:31–47. doi: 10.1111/j.1529-8019.2009.01289.x
136. Rodgers K, Jadhav SS. The application of mesenchymal stem cells to treat thermal and radiation burns. Adv Drug Delivery Rev (2018) 123:75–81. doi: 10.1016/j.addr.2017.10.003
137. Velarde MC, Demaria M, Melov S, Campisi J. Pleiotropic age-dependent effects of mitochondrial dysfunction on epidermal stem cells. Proc Natl Acad Sci U.S.A. (2015) 112:10407–12.
138. Zhang B, Shen L, Shi H, Pan Z, Wu L, Yan Y, et al. Exosomes from human umbilical cord mesenchymal stem cells: identification, purification, and biological characteristics. Stem Cells Int (2016) 2016:1929536. doi: 10.1155/2016/1929536
139. Deng M, Yu T, Li D, Wang X, Zhou G, Liu W, et al. Human umbilical cord mesenchymal stem cell-derived and dermal fibroblast-derived extracellular vesicles protect dermal fibroblasts from ultraviolet radiation-induced photoaging in vitro. Photochemical photobiological sciences: Off J Eur Photochem Assoc Eur Soc Photobiol (2020) 19:406–14. doi: 10.1039/c9pp00421a
140. Zhang Z, Zi Z, Lee EE, Zhao J, Contreras DC, South AP, et al. Differential glucose requirement in skin homeostasis and injury identifies a therapeutic target for psoriasis. Nat Med (2018) 24:617–27. doi: 10.1038/s41591-018-0003-0
141. Schäfer M, Farwanah H, Willrodt AH, Huebner AJ, Sandhoff K, Roop D, et al. Nrf2 links epidermal barrier function with antioxidant defense. EMBO Mol Med (2012) 4:364–79. doi: 10.1002/emmm.201200219
142. Nunan R, Harding KG, Martin P. Clinical challenges of chronic wounds: searching for an optimal animal model to recapitulate their complexity. Dis Model Mech (2014) 7:1205–13. doi: 10.1242/dmm.016782
143. Shiekh PA, Singh A, Kumar A. Exosome laden oxygen releasing antioxidant and antibacterial cryogel wound dressing OxOBand alleviate diabetic and infectious wound healing. Biomaterials (2020) 249:120020. doi: 10.1016/j.biomaterials.2020.120020
144. Las Heras K, Royo F, Garcia-Vallicrosa C, Igartua M, Santos-Vizcaino E, Falcon-Perez J, et al. Extracellular vesicles from hair follicle-derived mesenchymal stromal cells: isolation, characterization and therapeutic potential for chronic wound healing. Stem Cell Res Ther (2022) 13:147. doi: 10.1186/s13287-022-02824-0
145. Martin P. Wound healing–aiming for perfect skin regeneration. Science (1997) 276:75–81. doi: 10.1126/science.276.5309.75
146. Las Heras K, Igartua M, Santos-Vizcaino E, Hernandez RM. Chronic wounds: Current status, available strategies and emerging therapeutic solutions. J Control Release (2020) 328:532–50. doi: 10.1016/j.jconrel.2020.09.039
147. Schäfer M, Werner S. Oxidative stress in normal and impaired wound repair. Pharmacol Res (2008) 58:165–71. doi: 10.1016/j.phrs.2008.06.004
148. Xiao X, Xu M, Yu H, Wang L, Li X, Rak J, et al. Mesenchymal stem cell-derived small extracellular vesicles mitigate oxidative stress-induced senescence in endothelial cells via regulation of miR-146a/Src. Signal transduction targeted Ther (2021) 6:354. doi: 10.1038/s41392-021-00765-3
149. Eirin A, Zhu X, Jonnada S, Lerman A, Van Wijnen A, Lerman L. Mesenchymal stem cell-derived extracellular vesicles improve the renal microvasculature in metabolic renovascular disease in swine. Cell Transplant (2018) 27:1080–95. doi: 10.1177/0963689718780942
150. Matsuoka T, Takanashi K, Dan K, Yamamoto K, Tomobe K, Shinozuka T. Effects of mesenchymal stem cell-derived exosomes on oxidative stress responses in skin cells. Mol Biol Rep (2021) 48:4527–35. doi: 10.1007/s11033-021-06473-z
151. Fornaro A, Olivotto I, Rigacci L, Ciaccheri M, Tomberli B, Ferrantini C, et al. Comparison of long-term outcome in anthracycline-related versus idiopathic dilated cardiomyopathy: a single centre experience. Eur J Heart Fail (2018) 20:898–906. doi: 10.1002/ejhf.1049
152. Haendeler J, Hoffmann J, Diehl JF, Vasa M, Spyridopoulos I, Zeiher AM, et al. Antioxidants inhibit nuclear export of telomerase reverse transcriptase and delay replicative senescence of endothelial cells. Circ Res (2004) 94:768–75. doi: 10.1161/01.RES.0000121104.05977.F3
153. Han YM, Bedarida T, Ding Y, Somba BK, Lu Q, Wang Q, et al. β-Hydroxybutyrate Prevents Vascular Senescence through hnRNP A1-Mediated Upregulation of Oct4. Mol Cell (2018) 71:1064–1078.e5. doi: 10.1016/j.molcel.2018.07.036
154. Shakeri H, Gevaert AB, Schrijvers DM, De Meyer GRY, De Keulenaer GW, Guns PDF, et al. Neuregulin-1 attenuates stress-induced vascular senescence. Cardiovasc Res (2018) 114:1041–51. doi: 10.1093/cvr/cvy059
155. Chen T, Ma C, Fan G, Liu H, Lin X, Li J, et al. SIRT3 protects endothelial cells from high glucose-induced senescence and dysfunction via the p53 pathway. Life Sci (2021) 264:118724. doi: 10.1016/j.lfs.2020.118724
156. Rossini AA, Like AA, Chick WL, Appel MC, Cahill GF Jr. Studies of streptozotocin-induced insulitis and diabetes. Proc Natl Acad Sci U.S.A. (1977) 74:2485–9.
157. Fang SB, Zhang HY, Wang C, He BX, Liu XQ, Meng XC, et al. Small extracellular vesicles derived from human mesenchymal stromal cells prevent group 2 innate lymphoid cell-dominant allergic airway inflammation through delivery of miR-146a-5p. J Extracell Vesicles (2020) 9:1723260. doi: 10.1080/20013078.2020.1723260
158. Erusalimsky JD. Vascular endothelial senescence: from mechanisms to pathophysiology. J Appl Physiol (1985) (2009) 106:326–32. doi: 10.1152/japplphysiol.91353.2008
159. Ahmad R, Sylvester J, Ahmad M, Zafarullah M. Involvement of H-Ras and reactive oxygen species in proinflammatory cytokine-induced matrix metalloproteinase-13 expression in human articular chondrocytes. Arch Biochem Biophys (2011) 507:350–5. doi: 10.1016/j.abb.2010.12.032
160. Guillén M, Tofiño-Vian M, Silvestre A, Castejón M, Alcaraz M. Role of peroxiredoxin 6 in the chondroprotective effects of microvesicles from human adipose tissue-derived mesenchymal stem cells. J orthopaedic translation (2021) 30:61–9. doi: 10.1016/j.jot.2021.08.003
161. Lo WJ, Lin CL, Chang YC, Bai LY, Lin CY, Liang JA, et al. Total body irradiation tremendously impair the proliferation, differentiation and chromosomal integrity of bone marrow-derived mesenchymal stromal stem cells. Ann Hematol (2018) 97:697–707. doi: 10.1007/s00277-018-3231-y
162. Alessio N, Del Gaudio S, Capasso S, Di Bernardo G, Cappabianca S, Cipollaro M, et al. Low dose radiation induced senescence of human mesenchymal stromal cells and impaired the autophagy process. Oncotarget (2015) 6:8155–66. doi: 10.18632/oncotarget.2692
163. Zou Q, Hong W, Zhou Y, Ding Q, Wang J, Jin W, et al. Bone marrow stem cell dysfunction in radiation-induced abscopal bone loss. J Orthop Surg Res (2016) 11:3. doi: 10.1186/s13018-015-0339-9
164. Liu S, Liu D, Chen C, Hamamura K, Moshaverinia A, Yang R, et al. MSC transplantation improves osteopenia via epigenetic regulation of notch signaling in lupus. Cell Metab (2015) 22:606–18. doi: 10.1016/j.cmet.2015.08.018
165. Liu X, Li Q, Niu X, Hu B, Chen S, Song W, et al. Exosomes secreted from human-induced pluripotent stem cell-derived mesenchymal stem cells prevent osteonecrosis of the femoral head by promoting angiogenesis. Int J Biol Sci (2017) 13:232–44. doi: 10.7150/ijbs.16951
166. Zuo R, Liu M, Wang Y, Li J, Wang W, Wu J, et al. BM-MSC-derived exosomes alleviate radiation-induced bone loss by restoring the function of recipient BM-MSCs and activating Wnt/β-catenin signaling. Stem Cell Res Ther (2019) 10:30. doi: 10.1186/s13287-018-1121-9
167. Fisher AB. Peroxiredoxin 6 in the repair of peroxidized cell membranes and cell signaling. Arch Biochem Biophys (2017) 617:68–83. doi: 10.1016/j.abb.2016.12.003
168. Hou J, Han ZP, Jing YY, Yang X, Zhang SS, Sun K, et al. Autophagy prevents irradiation injury and maintains stemness through decreasing ROS generation in mesenchymal stem cells. Cell Death Dis (2013) 4:e844. doi: 10.1038/cddis.2013.338
169. Zhao CQ, Zhang YH, Jiang SD, Jiang LS, Dai LY. Both endoplasmic reticulum and mitochondria are involved in disc cell apoptosis and intervertebral disc degeneration in rats. Age (Dordr) (2010) 32:161–77. doi: 10.1007/s11357-009-9121-4
170. Xie L, Chen Z, Liu M, Huang W, Zou F, Ma X, et al. MSC-Derived Exosomes Protect Vertebral Endplate Chondrocytes against Apoptosis and Calcification via the miR-31-5p/ATF6 Axis. Mol Ther Nucleic Acids (2020) 22:601–14. doi: 10.1016/j.omtn.2020.09.026
171. Sakai D, Mochida J, Iwashina T, Hiyama A, Omi H, Imai M, et al. Regenerative effects of transplanting mesenchymal stem cells embedded in atelocollagen to the degenerated intervertebral disc. Biomaterials (2006) 27:335–45. doi: 10.1016/j.biomaterials.2005.06.038
172. Porée B, Kypriotou M, Chadjichristos C, Beauchef G, Renard E, Legendre F, et al. Interleukin-6 (IL-6) and/or soluble IL-6 receptor down-regulation of human type II collagen gene expression in articular chondrocytes requires a decrease of Sp1.Sp3 ratio and of the binding activity of both factors to the COL2A1 promoter. J Biol Chem (2008) 283:4850–65. doi: 10.1074/jbc.M706387200
173. Goldring MB, Otero M, Plumb DA, Dragomir C, Favero M, El Hachem K, et al. Roles of inflammatory and anabolic cytokines in cartilage metabolism: signals and multiple effectors converge upon MMP-13 regulation in osteoarthritis. Eur Cell Mater (2011) 21:202–20. doi: 10.22203/eCM.v021a16
174. Han Y, Xu X, Tang C, Gao P, Chen X, Xiong X, et al. Reactive oxygen species promote tubular injury in diabetic nephropathy: The role of the mitochondrial ros-txnip-nlrp3 biological axis. Redox Biol (2018) 16:32–46. doi: 10.1016/j.redox.2018.02.013
175. Piazza N, Dehghani M, Gaborski TR, Wuertz-Kozak K. Therapeutic potential of extracellular vesicles in degenerative diseases of the intervertebral disc. Front Bioeng Biotechnol (2020) 8:311. doi: 10.3389/fbioe.2020.00311
176. Su T, Xiao Y, Xiao Y, Guo Q, Li C, Huang Y, et al. Bone marrow mesenchymal stem cells-derived exosomal miR-29b-3p regulates aging-associated insulin resistance. ACS Nano (2019) 13:2450–62. doi: 10.1021/acsnano.8b09375
177. Aghagolzadeh P, Radpour R, Bachtler M, Van Goor H, Smith ER, Lister A, et al. Hydrogen sulfide attenuates calcification of vascular smooth muscle cells via KEAP1/NRF2/NQO1 activation. Atherosclerosis (2017) 265:78–86. doi: 10.1016/j.atherosclerosis.2017.08.012
178. Ageta H, Tsuchida K. Post-translational modification and protein sorting to small extracellular vesicles including exosomes by ubiquitin and UBLs. Cell Mol Life Sci (2019) 76:4829–48. doi: 10.1007/s00018-019-03246-7
179. Pockert AJ, Richardson SM, Le Maitre CL, Lyon M, Deakin JA, Buttle DJ, et al. Modified expression of the ADAMTS enzymes and tissue inhibitor of metalloproteinases 3 during human intervertebral disc degeneration. Arthritis Rheum (2009) 60:482–91. doi: 10.1002/art.24291
180. Tian Y, Yuan W, Fujita N, Wang J, Wang H, Shapiro IM, et al. Inflammatory cytokines associated with degenerative disc disease control aggrecanase-1 (ADAMTS-4) expression in nucleus pulposus cells through MAPK and NF-κB. Am J Pathol (2013) 182:2310–21. doi: 10.1016/j.ajpath.2013.02.037
181. Chen P, Zheng L, Wang Y, Tao M, Xie Z, Xia C, et al. Desktop-stereolithography 3D printing of a radially oriented extracellular matrix/mesenchymal stem cell exosome bioink for osteochondral defect regeneration. Theranostics (2019) 9:2439–59. doi: 10.7150/thno.31017
182. Aghajani Nargesi A, Lerman LO, Eirin A. Mesenchymal stem cell-derived extracellular vesicles for kidney repair: current status and looming challenges. Stem Cell Res Ther (2017) 8:273. doi: 10.1186/s13287-017-0727-7
183. Bigarella CL, Liang R, Ghaffari S. Stem cells and the impact of ROS signaling. Development (2014) 141:4206–18. doi: 10.1242/dev.107086
184. Davalli P, Mitic T, Caporali A, Lauriola A, D’arca D. ROS, cell senescence, and novel molecular mechanisms in aging and age-related diseases. Oxid Med Cell Longev (2016) 2016:3565127. doi: 10.1155/2016/3565127
185. Deretic V, Saitoh T, Akira S. Autophagy in infection, inflammation and immunity. Nat Rev Immunol (2013) 13:722–37. doi: 10.1038/nri3532
186. Harman D. Free radical theory of aging: an update: increasing the functional life span. Ann N Y Acad Sci (2006) 1067:10–21. doi: 10.1196/annals.1354.003
187. Kawanishi S, Hiraku Y, Oikawa S. Mechanism of guanine-specific DNA damage by oxidative stress and its role in carcinogenesis and aging. Mutat Res (2001) 488:65–76. doi: 10.1016/S1383-5742(00)00059-4
188. Saxena S, Vekaria H, Sullivan PG, Seifert AW. Connective tissue fibroblasts from highly regenerative mammals are refractory to ROS-induced cellular senescence. Nat Commun (2019) 10:4400. doi: 10.1038/s41467-019-12398-w
189. Dixon SJ, Lemberg KM, Lamprecht MR, Skouta R, Zaitsev EM, Gleason CE, et al. Ferroptosis: an iron-dependent form of nonapoptotic cell death. Cell (2012) 149:1060–72. doi: 10.1016/j.cell.2012.03.042
190. Feng C, Yang M, Lan M, Liu C, Zhang Y, Huang B, et al. ROS: crucial intermediators in the pathogenesis of intervertebral disc degeneration. Oxid Med Cell Longev (2017) 2017:5601593. doi: 10.1155/2017/5601593
191. Khanh VC, Yamashita T, Ohneda K, Tokunaga C, Kato H, Osaka M, et al. Rejuvenation of mesenchymal stem cells by extracellular vesicles inhibits the elevation of reactive oxygen species. Sci Rep (2020) 10:17315. doi: 10.1038/s41598-020-74444-8
192. Mas-Bargues C, Sanz-Ros J, Román-Domínguez A, Gimeno-Mallench L, Inglés M, Viña J, et al. Extracellular vesicles from healthy cells improves cell function and stemness in premature senescent stem cells by miR-302b and HIF-1α Activation. Biomolecules (2020) 10(6):957. doi: 10.3390/biom10060957
193. Kim JY, Shin KK, Lee AL, Kim YS, Park HJ, Park YK, et al. MicroRNA-302 induces proliferation and inhibits oxidant-induced cell death in human adipose tissue-derived mesenchymal stem cells. Cell Death Dis (2014) 5:e1385. doi: 10.1038/cddis.2014.344
194. Zhang L, Yu D. Exosomes in cancer development, metastasis, and immunity. Biochim Biophys Acta Rev Cancer (2019) 1871:455–68. doi: 10.1016/j.bbcan.2019.04.004
195. Wieckowski EU, Visus C, Szajnik M, Szczepanski MJ, Storkus WJ, Whiteside TL. Tumor-derived microvesicles promote regulatory T cell expansion and induce apoptosis in tumor-reactive activated CD8+ T lymphocytes. J Immunol (2009) 183:3720–30. doi: 10.4049/jimmunol.0900970
196. Szajnik M, Czystowska M, Szczepanski MJ, Mandapathil M, Whiteside TL. Tumor-derived microvesicles induce, expand and up-regulate biological activities of human regulatory T cells (Treg). PloS One (2010) 5:e11469. doi: 10.1371/journal.pone.0011469
197. Andreola G, Rivoltini L, Castelli C, Huber V, Perego P, Deho P, et al. Induction of lymphocyte apoptosis by tumor cell secretion of FasL-bearing microvesicles. J Exp Med (2002) 195:1303–16. doi: 10.1084/jem.20011624
198. Huber V, Fais S, Iero M, Lugini L, Canese P, Squarcina P, et al. Human colorectal cancer cells induce T-cell death through release of proapoptotic microvesicles: role in immune escape. Gastroenterology (2005) 128:1796–804. doi: 10.1053/j.gastro.2005.03.045
199. Bhatnagar S, Schorey JS. Exosomes released from infected macrophages contain Mycobacterium avium glycopeptidolipids and are proinflammatory. J Biol Chem (2007) 282:25779–89. doi: 10.1074/jbc.M702277200
200. Schorey JS, Harding CV. Extracellular vesicles and infectious diseases: new complexity to an old story. J Clin Invest (2016) 126:1181–9. doi: 10.1172/JCI81132
201. Zhang HG, Liu C, Su K, Yu S, Zhang L, Zhang S, et al. A membrane form of TNF-alpha presented by exosomes delays T cell activation-induced cell death. J Immunol (2006) 176:7385–93. doi: 10.4049/jimmunol.176.12.7385
202. Chaput N, Flament C, Viaud S, Taieb J, Roux S, Spatz A, et al. Dendritic cell derived-exosomes: biology and clinical implementations. J Leukoc Biol (2006) 80:471–8. doi: 10.1189/jlb.0206094
203. Barros FM, Carneiro F, MaChado JC, Melo SA. Exosomes and immune response in cancer: friends or foes? Front Immunol (2018) 9:730. doi: 10.3389/fimmu.2018.00730
204. Damania A, Jaiman D, Teotia AK, Kumar A. Mesenchymal stromal cell-derived exosome-rich fractionated secretome confers a hepatoprotective effect in liver injury. Stem Cell Res Ther (2018) 9:31. doi: 10.1186/s13287-017-0752-6
205. Zhao S, Huang M, Yan L, Zhang H, Shi C, Liu J, et al. Exosomes Derived from Baicalin-Pretreated Mesenchymal Stem Cells Alleviate Hepatocyte Ferroptosis after Acute Liver Injury via the Keap1-NRF2 Pathway. Oxid Med Cell Longev (2022) 2022:8287227. doi: 10.1155/2022/8287227
206. Pan Q, Kuang X, Cai S, Wang X, Du D, Wang J, et al. miR-132-3p priming enhances the effects of mesenchymal stromal cell-derived exosomes on ameliorating brain ischemic injury. Stem Cell Res Ther (2020) 11:260. doi: 10.1186/s13287-020-01761-0
207. Lei X, He N, Zhu L, Zhou M, Zhang K, Wang C, et al. Mesenchymal Stem Cell-Derived Extracellular Vesicles Attenuate Radiation-Induced Lung Injury via miRNA-214-3p. Antioxid Redox Signal (2021) 35:849–62. doi: 10.1089/ars.2019.7965
208. Xu N, Shao Y, Ye K, Qu Y, Memet O, He D, et al. Mesenchymal stem cell-derived exosomes attenuate phosgene-induced acute lung injury in rats. Inhal Toxicol (2019) 31:52–60. doi: 10.1080/08958378.2019.1597220
209. Gao Z, Zhang C, Peng F, Chen Q, Zhao Y, Chen L, et al. Hypoxic mesenchymal stem cell-derived extracellular vesicles ameliorate renal fibrosis after ischemia-reperfusion injure by restoring CPT1A mediated fatty acid oxidation. Stem Cell Res Ther (2022) 13:191. doi: 10.1186/s13287-022-02861-9
210. Taghavi-Farahabadi M, Mahmoudi M, Mahdaviani SA, Baghaei K, Rayzan E, Hashemi SM, et al. Improving the function of neutrophils from chronic granulomatous disease patients using mesenchymal stem cells’ exosomes. Hum Immunol (2020) 81:614–24. doi: 10.1016/j.humimm.2020.05.009
211. Yang C, Lim W, Park J, Park S, You S, Song G. Anti-inflammatory effects of mesenchymal stem cell-derived exosomal microRNA-146a-5p and microRNA-548e-5p on human trophoblast cells. Mol Hum Reprod (2019) 25:755–71. doi: 10.1093/molehr/gaz054
212. Guo XF, Gu SS, Wang J, Sun H, Zhang YJ, Yu PF, et al. Protective effect of mesenchymal stem cell-derived exosomal treatment of hippocampal neurons against oxygen-glucose deprivation/reperfusion-induced injury. World J Emerg Med (2022) 13:46–53. doi: 10.5847/wjem.j.1920-8642.2022.015
213. De Godoy MA, Saraiva LM, De Carvalho LRP, Vasconcelos-Dos-Santos A, Beiral HJV, Ramos AB, et al. Mesenchymal stem cells and cell-derived extracellular vesicles protect hippocampal neurons from oxidative stress and synapse damage induced by amyloid-β oligomers. J Biol Chem (2018) 293:1957–75. doi: 10.1074/jbc.M117.807180
214. Scott SR, March KL, Wang IW, Singh K, Liu J, Turrentine M, et al. Bone marrow- or adipose-mesenchymal stromal cell secretome preserves myocardial transcriptome profile and ameliorates cardiac damage following ex vivo cold storage. J Mol Cell Cardiol (2022) 164:1–12. doi: 10.1016/j.yjmcc.2021.11.002
215. O’Brien CG, Ozen MO, Ikeda G, Vaskova E, Jung JH, Bayardo N, et al. Mitochondria-rich extracellular vesicles rescue patient-specific cardiomyocytes from doxorubicin injury: insights into the SENECA trial. JACC CardioOncol (2021) 3:428–40. doi: 10.1016/j.jaccao.2021.05.006
216. Li D, Zhang D, Tang B, Zhou Y, Guo W, Kang Q, et al. Exosomes from human umbilical cord mesenchymal stem cells reduce damage from oxidative stress and the epithelial-mesenchymal transition in renal epithelial cells exposed to oxalate and calcium oxalate monohydrate. Stem Cells Int (2019) 2019:6935806. doi: 10.1155/2019/6935806
217. Rui K, Hong Y, Zhu Q, Shi X, Xiao F, Fu H, et al. Olfactory ecto-mesenchymal stem cell-derived exosomes ameliorate murine Sjögren’s syndrome by modulating the function of myeloid-derived suppressor cells. Cell Mol Immunol (2021) 18:440–51. doi: 10.1038/s41423-020-00587-3
218. Zou L, Ma X, Wu B, Chen Y, Xie D, Peng C. Protective effect of bone marrow mesenchymal stem cell-derived exosomes on cardiomyoblast hypoxia-reperfusion injury through the miR-149/let-7c/Faslg axis. Free Radic Res (2020) 54:722–31. doi: 10.1080/10715762.2020.1837793
219. Sun F, Sun Y, Zhu J, Wang X, Ji C, Zhang J, et al. Mesenchymal stem cells-derived small extracellular vesicles alleviate diabetic retinopathy by delivering NEDD4. Stem Cell Res Ther (2022) 13:293. doi: 10.1186/s13287-022-02983-0
220. Yan F, Cui W, Chen Z. Mesenchymal stem cell-derived exosome-loaded microRNA-129-5p inhibits TRAF3 expression to alleviate apoptosis and oxidative stress in heart failure. Cardiovasc Toxicol (2022) 22:631–45. doi: 10.1007/s12012-022-09743-9
221. Mahmoudi M, Taghavi-Farahabadi M, Rezaei N, Hashemi SM. Comparison of the effects of adipose tissue mesenchymal stromal cell-derived exosomes with conditioned media on neutrophil function and apoptosis. Int Immunopharmacol (2019) 74:105689. doi: 10.1016/j.intimp.2019.105689
222. Ma J, Shi X, Li M, Chen S, Gu Q, Zheng J, et al. MicroRNA-181a-2-3p shuttled by mesenchymal stem cell-secreted extracellular vesicles inhibits oxidative stress in Parkinson’s disease by inhibiting EGR1 and NOX4. Cell Death Discovery (2022) 8:33. doi: 10.1038/s41420-022-00823-x
223. Liu J, Lin M, Qiao F, Zhang C. Exosomes Derived from lncRNA TCTN2-Modified Mesenchymal Stem Cells Improve Spinal Cord Injury by miR-329-3p/IGF1R Axis. J Mol Neurosci (2022) 72:482–95. doi: 10.1007/s12031-021-01914-7
224. Kubota K, Nakano M, Kobayashi E, Mizue Y, Chikenji T, Otani M, et al. An enriched environment prevents diabetes-induced cognitive impairment in rats by enhancing exosomal miR-146a secretion from endogenous bone marrow-derived mesenchymal stem cells. PloS One (2018) 13:e0204252. doi: 10.1371/journal.pone.0204252
225. Liu X, Li X, Zhu W, Zhang Y, Hong Y, Liang X, et al. Exosomes from mesenchymal stem cells overexpressing MIF enhance myocardial repair. J Cell Physiol (2020) 235:8010–22. doi: 10.1002/jcp.29456
226. Da Silva D, De Freitas ML, Cahil GM, De São José VS, Neto FM, Cardoso RC, et al. Influence of stem cell therapy on thyroid function and reactive oxygen species production in diabetic rats. Horm Metab Res (2018) 50:331–9. doi: 10.1055/a-0588-7944
227. Zhang G, Zou X, Huang Y, Wang F, Miao S, Liu G, et al. Mesenchymal stromal cell-derived extracellular vesicles protect against acute kidney injury through anti-oxidation by enhancing Nrf2/ARE activation in rats. Kidney Blood Press Res (2016) 41:119–28. doi: 10.1159/000443413
228. Farahani RA, Zhu XY, Tang H, Jordan KL, Lerman A, Lerman LO, et al. Metabolic syndrome alters the cargo of mitochondria-related microRNAs in swine mesenchymal stem cell-derived extracellular vesicles, impairing their capacity to repair the stenotic kidney. Stem Cells Int (2020) 2020:8845635. doi: 10.1155/2020/8845635
229. Cai G, Cai G, Zhou H, Zhuang Z, Liu K, Pei S, et al. Mesenchymal stem cell-derived exosome miR-542-3p suppresses inflammation and prevents cerebral infarction. Stem Cell Res Ther (2021) 12:2. doi: 10.1186/s13287-020-02030-w
230. Jin Z, Ren J, Qi S. Exosomal miR-9-5p secreted by bone marrow-derived mesenchymal stem cells alleviates osteoarthritis by inhibiting syndecan-1. Cell Tissue Res (2020) 381:99–114. doi: 10.1007/s00441-020-03193-x
231. Firoozi S, Pahlavan S, Ghanian MH, Rabbani S, Barekat M, Nazari A, et al. Mesenchymal stem cell-derived extracellular vesicles alone or in conjunction with a SDKP-conjugated self-assembling peptide improve a rat model of myocardial infarction. Biochem Biophys Res Commun (2020) 524:903–9. doi: 10.1016/j.bbrc.2020.02.009
232. Liu Y, Zhao S, Luo L, Wang J, Zhu Z, Xiang Q, et al. Mesenchymal stem cell-derived exosomes ameliorate erection by reducing oxidative stress damage of corpus cavernosum in a rat model of artery injury. J Cell Mol Med (2019) 23:7462–73. doi: 10.1111/jcmm.14615
233. Lamkanfi M, Dixit VM. Mechanisms and functions of inflammasomes. Cell (2014) 157:1013–22. doi: 10.1016/j.cell.2014.04.007
234. Milkovic L, Cipak Gasparovic A, Cindric M, Mouthuy PA, Zarkovic N. Short overview of ROS as cell function regulators and their implications in therapy concepts. Cells (2019) 8(8):793. doi: 10.3390/cells8080793
235. Boulestreau J, Maumus M, Rozier P, Jorgensen C, Noël D. Mesenchymal stem cell derived extracellular vesicles in aging. Front Cell Dev Biol (2020) 8:107. doi: 10.3389/fcell.2020.00107
236. Mckinnirey F, Herbert B, Vesey G, Mccracken S. Immune modulation via adipose derived Mesenchymal Stem cells is driven by donor sex in vitro. Sci Rep (2021) 11:12454. doi: 10.1038/s41598-021-91870-4
Keywords: mesenchymal stem cells, extracellular vesicles, exosomes, oxidative stress, inflammation, cell proliferation
Citation: Zhang W, Wang T, Xue Y, Zhan B, Lai Z, Huang W, Peng X and Zhou Y (2023) Research progress of extracellular vesicles and exosomes derived from mesenchymal stem cells in the treatment of oxidative stress-related diseases. Front. Immunol. 14:1238789. doi: 10.3389/fimmu.2023.1238789
Received: 12 June 2023; Accepted: 24 July 2023;
Published: 14 August 2023.
Edited by:
Chao Yang, Zhejiang Ocean University, ChinaReviewed by:
Yimei Feng, Xinqiao Hospital, ChinaYan Wang, Guangdong Pharmaceutical University, China
Yujie Shi, Peking University, China
Copyright © 2023 Zhang, Wang, Xue, Zhan, Lai, Huang, Peng and Zhou. This is an open-access article distributed under the terms of the Creative Commons Attribution License (CC BY). The use, distribution or reproduction in other forums is permitted, provided the original author(s) and the copyright owner(s) are credited and that the original publication in this journal is cited, in accordance with accepted academic practice. No use, distribution or reproduction is permitted which does not comply with these terms.
*Correspondence: Yanfang Zhou, eWZ6aG91QGdkbXUuZWR1LmNu; Xinsheng Peng, eHNocGVuZ0BnZG11LmVkdS5jbg==