- 1Reproductive Medicine Center, Department of Obstetrics and Gynecology, The First Affiliated Hospital of Anhui Medical University, Hefei, China
- 2National Health Commission (NHC) Key Laboratory of Study on Abnormal Gametes and Reproductive Tract, Anhui Medical University, Hefei, China
- 3Key Laboratory of Population Health Across Life Cycle, Anhui Medical University, Hefei, Anhui, China
- 4Anhui Key Laboratory of Reproductive Health and Genetics, Anhui Medical University, Hefei, Anhui, China
- 5Biopreservation and Artificial Organs, Anhui Provincial Engineering Research Center, Anhui Medical University, Hefei, Anhui, China
HMGB1 that belongs to the High Mobility Group-box superfamily, is a nonhistone chromatin associated transcription factor. It is present in the nucleus of eukaryotes and can be actively secreted or passively released by kinds of cells. HMGB1 is important for maintaining DNA structure by binding to DNA and histones, protecting it from damage. It also regulates the interaction between histones and DNA, affecting chromatin packaging, and can influence gene expression by promoting nucleosome sliding. And as a DAMP, HMGB1 binding to RAGE and TLRs activates NF-κB, which triggers the expression of downstream genes like IL-18, IL-1β, and TNF-α. HMGB1 is known to be involved in numerous physiological and pathological processes. Recent studies have demonstrated the significance of HMGB1 as DAMPs in the female reproductive system. These findings have shed light on the potential role of HMGB1 in the pathogenesis of diseases in female reproductive system and the possibilities of HMGB1-targeted therapies for treating them. Such therapies can help reduce inflammation and metabolic dysfunction and alleviate the symptoms of reproductive system diseases. Overall, the identification of HMGB1 as a key player in disease of the female reproductive system represents a significant breakthrough in our understanding of these conditions and presents exciting opportunities for the development of novel therapies.
1 Introduction
High-mobility group proteins (HMG) were first extracted and identified in the bovine thymus in 1973 and named for their high mobility in gel electrophoresis (1). Based on their functional sequence motif characteristics, HMG proteins are divided into three superfamilies: HMGB, HMGA, and HMGN (2). As the most abundant protein among all HMG family members, HMGB1 is a multifunctional protein that plays a crucial role in various cellular processes (3). As a DNA-binding nuclear factor, it regulates the transcriptional activity of genes, controls DNA replication and repair, and facilitates telomere maintenance and nucleosome assembly (4). In addition to its intracellular functions, HMGB1 can be transferred into the extracellular environment (5). the released or secreted HMGB1 functions as a damage-associated molecular pattern (DAMPs) that can interact with pattern recognition receptors (PRRs), such as receptors for advanced glycation end-products (RAGE) and toll-like receptors (TLRs) (6). Under normal conditions, HMGB1 can promote cell proliferation and differentiation, promote inflammatory reaction and immune response, participate in tissue repair and regeneration, and regulate gene expression and transcription (7–10). In pathological conditions, HMGB1 plays a crucial role in amplifying the inflammatory response and driving the pathogenesis of many diseases. For instance, HMGB1 has been implicated in several malignancies, including breast, lung, and colorectal cancers (11). Overall, these findings highlight the importance of HMGB1 in multiple cellular processes and suggest that it may be a potential therapeutic target for various diseases.
Pregnancy is a complex physiological process. During pregnancy, the maternal immune system is constantly changing in response to fetal development and environmental signals. Inflammation is a critical mechanism in the establishment of pregnancy, the initiation of labor, and the development of many pregnancy complications (12, 13). Normal pregnancy has three distinct immunological phases that are characterized by distinct biological processes. The first trimester of pregnancy is a pro-inflammatory phase. Early pregnancy includes events such as decidualization, implantation, trophoblast development, and placental growth. At this stage, the blastocyst breaks through the uterus lining and invades the endometrial tissue (14). Therefore, an inflammatory environment is necessary for repairing the uterine epithelium and removing cellular debris. In the second trimester of pregnancy, the fetus grows rapidly while the mother, placenta, and fetus work together to induce an anti-inflammatory state. During the final trimester of pregnancy, the baby’s organs fully develop and the mother’s body prepares for birth. The uterus contracts due to a pro-inflammatory environment in this stage, which helps with the expulsion of the baby and placenta (15). Whether before or at any stage of pregnancy, immune disorders can have a significant impact on pregnancy outcomes.
Over the last decade, extensive studies have demonstrated that HMGB1 plays a vital role throughout pregnancy and is involved in various diseases of the female reproductive system (Figure 1). HMGB1 promotes uterine decidualization and embryo implantation, and embryonic development in the first trimester (16–19), and cervical ripening and delivery in the third trimester (20). However, high level of HMGB1 levels may result in female reproductive disorders, including recurrent spontaneous abortion (RSA) (21), gestational diabetes mellitus (GDM) (22), preterm birth (PTB) (23), preeclampsia (PE) (24), polycystic ovary syndrome (PCOS) (25), and endometriosis (26). Further research is needed to determine the precise role of HMGB1 in normal pregnancy and the development of reproductive disorders. HMGB1 may serve as a valuable biomarker for the early prediction of these diseases and provide new ideas for their prevention and treatment. This review provides an overview of the function of HMGB1 and explores available HMGB1 inhibitors in the female reproductive system.
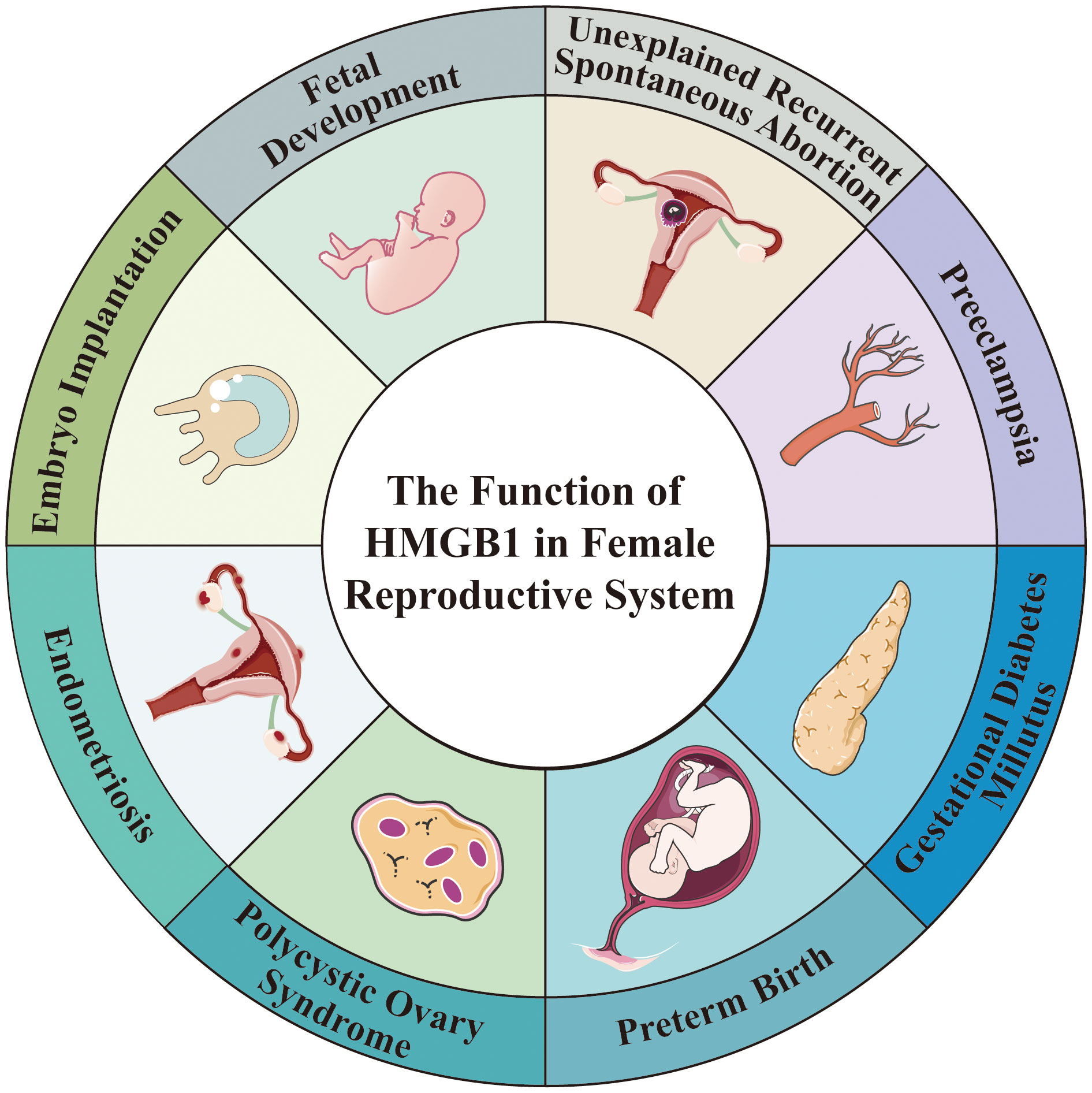
Figure 1 The Function of HMGB1 in Female Reproductive System. HMGB1 is involved in fetal development and embryo implantation, as well as a variety of diseases in female reproductive system, such as preeclampsia, preterm birth, unexplained recurrent spontaneous abortion, gestational diabetes millutus, polycystic ovary syndrome, endometriosis.
2 The overview of HMGB1 as a DAMP
HMGB1 is an evolutionarily highly conserved nuclear non-histone DNA binding protein that was discovered in 1973. The structure of HMGB1 is shown in Figure 2, and consists of two consecutive positively charged DNA binding domains, called HMG A box (9-79 amino acids) and B box (89-162 amino acids), a highly negatively charged C-terminal tail (186–215 amino acids) and a short but functionally significant N-terminal region (27).
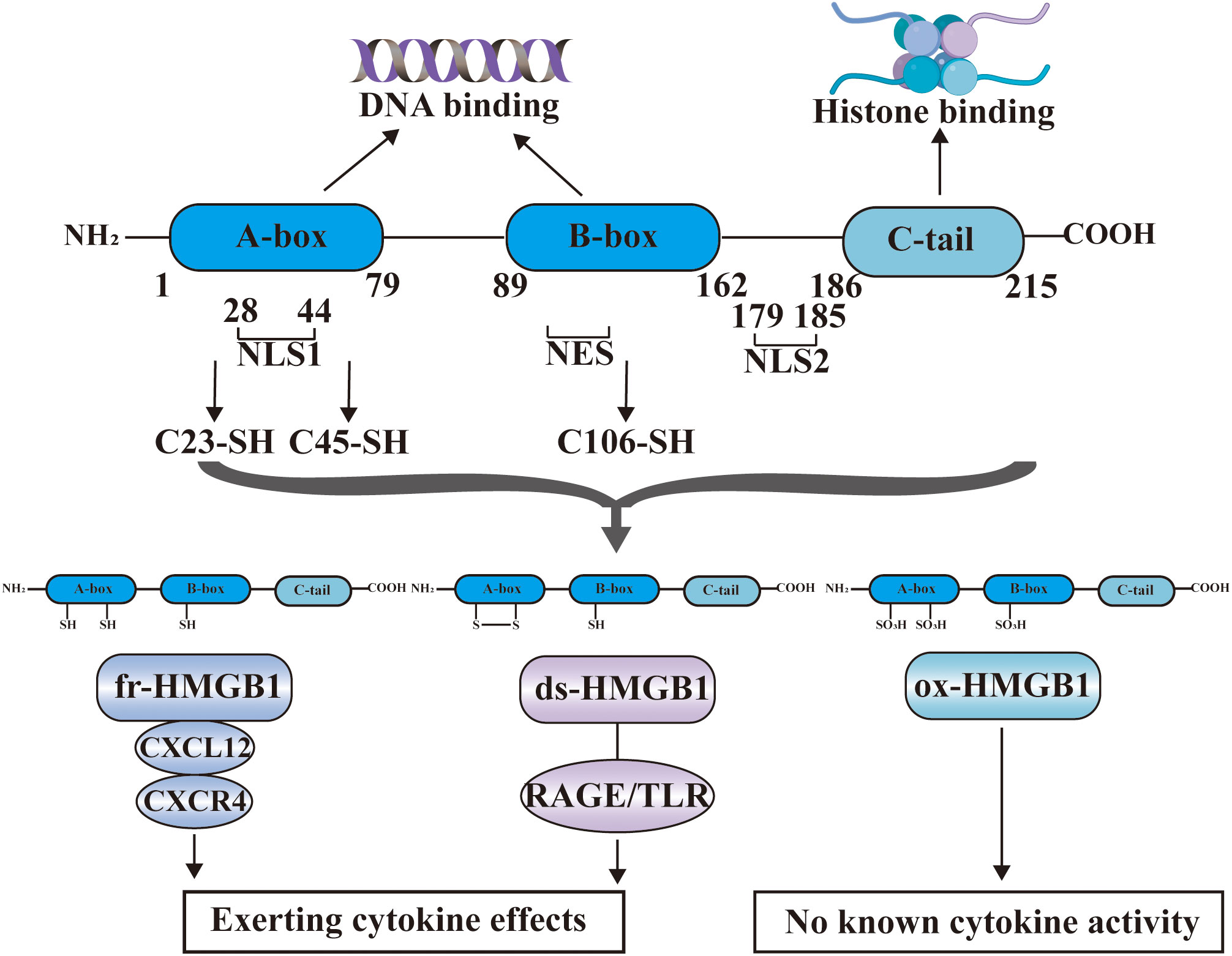
Figure 2 The structure of HMGB1. HMGB1 consists of HMG A box (9-79 amino acids), B box (89-162 amino acids), a highly negatively charged C-terminal tail (186-215 amino acids) and a short N-terminal region. The A and B boxes in the nucleus can bind DNA, and the C-terminal region can bind histones. HMGB1 has two nuclear localization signal regions (NLS1 and NLS2) and one nuclear export signal (NES). HMGB1 has three cysteine residues at amino acids C23, C45, and C106. C23 and C45 are located in A box, while C106 is located in B box. The redox state of these three cysteines determines that HMGB1 has three redox forms: (1) Fully reduced HMGB1 (fr-HMGB1) can bind to CXCL12 and then stimulate leukocyte recruitment through the CXCR4 receptor. (2) Partially reduced HMGB1 called disulfide bond HMGB1 (ds-HMGB1) can trigger inflammatory responses via receptors including RAGE and TLRs. (3) Completely oxidized HMGB1 (ox-HMGB1) has no known cytokine activity.
In the nucleus, the A and B boxes bind to DNA, whereas the C-terminal region binds to histones. HMGB1 binds to DNA with low affinity. As a shuttle protein translocating between the nucleus and cytoplasm, HMGB1 has two nuclear localization signal regions (NLS1 and NLS2) and one nuclear export signal (NES) (28). HMGB1 is the most mobile protein in the nucleus, and can pass through the nucleus and enter the cytoplasm within 1-2 seconds (3, 29). HMGB1 is transferred into the extracellular environment through two different mechanisms: (1) Active secretion: Immune cells and some other cells like epithelial cells and endothelial cells can actively secrete HMGB1 when they are stimulated by various factors (30–33). These factors include lipopolysaccharide (LPS), pathogen infections, and endogenous host stimuli (34, 35). This active release of HMGB1 can occur through two models. In one model, the cells with stimuli directly secrete HMGB1 into the extracellular space (36). In the other model, HMGB1 is packaged into intracellular vesicles, such as exosome. These vesicles then fuse with the cell membrane, leading to the release of HMGB1 outside the cell (37). (2) Passive release: HMGB1 can also be passively released from various types of cell death in response to different stimuli or damages. These types of cell death include necrosis, necroptosis, apoptosis, autophagy-dependent cell death, NETosis, pyroptosis, PANoptosis and ferroptosis (38–44). Extracellularly, HMGB1 participates in inflammatory response as DAMPs, thereby, playing a role in the occurrence and development of various physiological processes and diseases (45). The B box of HMGB1 is a functional domain that recognizes pattern recognition receptors (PRRs) and can induce macrophages to secrete proinflammatory cytokines (6). In contrast, A box can antagonize the activity of cytokines, and A box alone can act as a competitive antagonist of HMGB1 and inhibit the activity of HMGB1 (46).
HMGB1 contains three cysteine residues at amino acids C23, C45, and C106 (47). C23 and C45 are located in A box, where a disulfide bond can be formed between them, and C106 is located in B box (48). The redox state of these three cysteines determines whether HMGB1 has three redox forms that can regulate the extracellular activity of HMGB1 (47). Fully reduced HMGB1 (fr-HMGB1) has three conserved cysteines containing thiol groups that can form a complex with other chemokines, such as CXCL12 and stimulate leukocyte recruitment through the CXCR4 receptor (49). Partially reduced HMGB1 called disulfide bond HMGB1 (ds-HMGB1), has a disulfide bond between C23 and C45, which can trigger inflammatory responses via TLR2/4 or other receptors such as RAGE and TLR9 (50, 51). Completely oxidized HMGB1 (ox-HMGB1) has no immunological competence because it cannot activate macrophages or dendritic cells (52–54). In addition, HMGB1 is also subject to various types of post-translational modifications (PTMs), including acetylation, methylation, phosphorylation, N-glycosylation, phosphorylation, and ADP-ribosylation, which determine their subcellular localization and biological activity (27).
HMGB1 released outside cells binds to cell surface receptors on target cells as a DAMP (6). To date, a large number of studies have reported the related receptors of HMGB1, including toll-like receptors (TLR) 2-4/7/9, the receptor for advanced glycation end products (RAGE), integrin, cluster of differentiation 24 (CD24), T-cell immunoglobulin and mucin-3 (TIM3) and C-X-C chemokine receptor type 4 (CXCR4) (49, 55–57). So far, TLR4 and RAGE have been extensively studied and proven to be specific HMGB1 receptors in a large number of studies.HMGB1 combined with RAGE or TLR4 can activate a variety of signaling pathways, including the mitogen-activated protein kinase (MAPK) signaling pathway to mediate the occurrence of immune reactions such as autophagy (58), pyroptosis (59) and apoptosis (60) or the amplification of inflammation, thus playing a crucial role in diabetes, epilepsy, tumor and other diseases (3). Similarly, HMGB1 also plays a role in normal pregnancy and disease in female reproductive system by interacting with its receptors.
3 The role of HMGB1 in normal pregnancy
3.1 HMGB1 facilitates embryo implantation
Embryo implantation occurs when a fertilized egg attaches to the uterine lining and begins to grow (61). Various factors, including hormones, growth factors, and cytokines regulate the process of embryo implantation, enabling the embryo to connect with the maternal blood supply and receive the nutrients and oxygen required for grow (62). Successful implantation requires the synchronization of blastocysts with implantation ability and the uterus in a receptive state (63). Implantation failure is an important factor leading to pregnancy failure. There are three main reasons for implantation failure: insufficient uterine receptivity, problems with the embryo itself, and systemic causes (63).
In rodents, specific changes occur in the uterus during the pre-implantation period. These changes are divided into three stages: prereceptive, receptive, and nonreceptive stages, corresponding to gestation day (GD) 2&3, GD4, and GD5 in mice. On GD3, the uterus is not yet ready for implantation due to low progesterone(P4) levels. On GD4, with increased progesterone and estrogen secretion, the uterus becomes acceptable and blastocysts can successfully implant. On GD5, the uterus becomes nonreceptive again (64, 65). To examine the spatiotemporal expression of Hmgb1 in uterus during the pre-implantation period, Aikawa S et al. performed in situ hybridization using digoxigenin. On GD1-3, Hmgb1 expression is predominantly localized in epithelial cells with some stromal cell localization on GD3. In stroma cells, Hmgb1 signals are primarily observed on GD4&5. Western blotting results show that HMGB1 protein levels decrease with pregnancy progression as assessed on GD4,8&16. The specific spatial and temporal expression of Hmgb1 at this stage suggests that it plays an essential role in the pre-implantation period (17). Besides, HMGB1, estrogen, and progesterone show regular spatial and temporal expression characteristics during implantation period (17). Progesterone signaling through progesterone receptor (PR) regulates decidualization and endometrial homeostasis by promoting the thickening of the endometrium, inhibiting uterine contraction and regulating the immune system (66–68). Nuclear HMGB1 contributes to successful blastocyst implantation by interregulating with the P4-PR signaling pathway (17, 69). It has been observed that when the Hmgb1 gene is knockout, the levels of the P4-responsive gene Hoxa10 in the stromal cells are significantly decreased, thus resulting in reduced efficiency of P4-PR signal transduction (17). This highlights the vital role HMGB1 plays in regulating the P4-PR signaling pathway, which is crucial for successful blastocyst implantation. Additionally, it has been noted that HMGB1 influences the proliferation and differentiation of uterine stromal cells by targeting various proteins such as bone morphogenetic protein (Bmp2), kruppel-like factor 5 (Klf5), cAMP, as well as the G1 phase cell cycle regulator Cyclin D3 (Ccnd3) (16). This emphasizes the multifaceted role nuclear HMGB1 plays in regulating various aspects of blastocyst implantation and signifies its importance in the reproductive process.
Decidualization is the process by which endometrial stromal cells differentiating into decidual stromal cells during early pregnancy, and is accompanied by changes in the immune microenvironment at the maternal-fetal interface. Decidualization plays an extremely important role in embryo implantation, pregnancy establishment and maintenance, and delivery initiation (70). The expression of HMGB1 plays a crucial role in this process. The reduction in HMGB1 expression leads to a cascade of events that inhibits the expression of Bmp2, resulting in the down-regulation of prolactin family 8, subfamily a, member 2 (Prl8a2), an important marker produced by decidual cells. Consequently, uterine stromal cells cannot perform the necessary decidual response, which can ultimately affect further embryonic development (16). In addition to its role in uterine decidualization, HMGB1 also aids in blastocyst implantation by limiting macrophage accumulation and attenuating inflammatory responses in the endometrium. Hmgb1-deficient mice have been found to have higher pre-implantation levels of Ccl2 and Csf1, both of which are involved in priming macrophages (17). This ultimately leads to an increase in pre-implantation macrophage accumulation and inflammatory responses. However, it is important to note that the role of HMGB1 in blastocyst implantation is complex, as the elevated expression of HMGB1 in endometrial epithelial cells can decreases the adhesion ability of epithelial cells, thus affecting blastocyst implantation, which is one of the pathogenic mechanisms of recurrent implantation failure (71). Therefore, the precise regulation of HMGB1 expression is critical for successful embryo implantation.
3.2 HMGB1 contributes to fetal development: since most of information is related to fetal tissue development
The role of HMGB1 in fetal development is multifaceted and occurs at various stages of gestation. Both endogenous and exogenous HMGB1 affect normal early embryonic development (72). Through its ability to regulate mitochondrial autophagy in the cytoplasm, HMGB1 facilitates cell proliferation and differentiation, thereby aiding in embryo development (73). Interestingly, HMGB1 is found in mouse oocytes and embryos, and its gene expression potentially plays a role in controlling apoptosis during preimplantation via the p53 signaling pathway (72). Bagherpoor et al. found the downregulation of HMGB1 in undifferentiated pluripotent human embryonic stem cells (hESCs) did not affect cell pluripotency. However, in differentiated hESCs, the downregulation of HMGB1 results in decreased telomerase activity, reduced cell proliferation, increased apoptosis, and decreased differentiation to the neural ectodermal lineage (74). Overall, HMGB1 is essential for embryonic development and supports cell growth and differentiation. In addition, HMGB1 protects preimplantation embryonic development by decreasing the expression of Bak and Casp3 genes involved in apoptosis (72, 75).
HMGB1 also promotes the development of the nervous system (19). It has been reported that HMGB1 knockdown in zebrafish embryos leads to forebrain developmental defects in the forebrain due to increased Wnt/β-catenin expression (76). Consistent with these findings, another study showed that HMGB1-dependent CXCL12/CXCR4 signaling pathway is present in the developing mammalian central nervous system (CNS). In addition, a significant reduction in receptor RAGE in the CNS of HMGB1 knockdown mice was accompanied by Wnt/β-catenin overexpression, leading to the downregulation of several developmental factors. These factors include several neurogenesis factors (e.g. Ascl1, Neurod1, Sox2, Tbr2 and Bcl2), developmental factors (e.g. Pax6, Shh, Foxg1 and Emx2) and differentiation factors (BMP2, BMP4 and Tgf1). The decreased expression of these factors leads to increased neuronal apoptosis and decreased proliferation (77). These findings demonstrated that HMGB1 is a key regulator of embryonic brain development.
Moreover, HMGB1 is a crucial factor in the growth and development of bone tissues and limbs. HMGB1 accumulates in the hypertrophic chondrocytes on the growth plate and is then transferred from the cell nucleus to the cytoplasm. During the early stages of cartilage maturation, it is secreted. By acting as a chemotactic factor, it attracts osteoclasts, osteoblasts, and endothelial cells to invade the primary ossification center, thereby promoting intramembranous ossification within the cartilage (78). This promotes the formation of new bone tissue and contributes to the proper development of limbs.
Furthermore, HMGB1 exerts pro-angiogenic effects by inducing MAPK/ERK1/2 activation, cell proliferation, and chemotaxis in endothelial cells from different sources (79, 80). To prove this conclusion, Mitola et al. demonstrated this conclusion by implanting HMGB1-loaded alginate beads on chick chorioallantoic membranes at a developmental stage of 11 days (80). However, the current conclusion lacks evidence in rodent and human embryonic development. Interestingly, the expression of HMGB1 and the receptor RAGE was also observed in dental cell types in the late embryonic stage of rats, indicating that HMGB1 may also promote tooth development (81). In general, current research suggests that HMGB1 plays a crucial role in embryonic development at various stages and in successful pregnancy. However, more research is required to fully understand the complex interactions between HMGB1 and other factors that contribute to successful early life development.
4 The role of HMGB1 in female reproductive system diseases
In this section, we will discuss the role of HMGB1 in various conditions that influence female reproductive health by spanning both gynecological disorders and pregnancy-related complications. We aim to present a comprehensive landscape of HMGB1’s role across a timeline that begins prior to conception with issues affecting fertility, such as polycystic ovary syndrome and endometriosis, and extends through varying stages of pregnancy, encapsulating pregnancy complications like unexplained recurrent spontaneous abortion, preeclampsia, gestational diabetes mellitus, and preterm birth. We hope a chronological approach aids in building a comprehensive understanding of the omnipresence of HMGB1’s role throughout the female reproductive journey.
4.1 Polycystic ovary syndrome
Polycystic ovary syndrome (PCOS) is a common condition affecting the female reproductive system. It can cause infertility and complications during pregnancy, and affects the physical and mental health of 4%-20% of women of reproductive age globally (82, 83). The main clinical manifestations of PCOS include irregular menstruation (hypomenorrhea or amenorrhea), androgen excess, and multiple ovarian cysts, often accompanied by insulin resistance (IR), obesity, type 2 diabetes, and cardiovascular disease (83, 84). It has also been suggested that inflammation plays an important role in the pathogenesis of PCOS (85). As a molecule associated with several inflammatory diseases, HMGB1 is elevated in both the peripheral blood and follicular fluid of women with PCOS, particularly those with insulin resistance, compared with non-PCOS women (25, 86–90). During the development and maturation of granulosa cells (GC), HMGB1 interacting with TLRs, and may be involved in ovarian innate immunity and ovarian follicle maturation regulated by follicle-stimulating hormone (FSH) (91). However, excess extracellular HMGB1promotes autophagy of granulosa cells (86). Research has shown that inhibition of HMGB1 or the TLR4/NF-κB signaling pathway can improve inflammatory PCOS with insulin resistance (25). Further research is required to understand the mechanisms underlying the relationship between inflammation and PCOS.
4.2 Endometriosis
Endometriosis refers to the growth of endometrium-like tissue outside the uterine cavity, such as in the ovaries and the peritoneum (92). Approximately 10% of women of the reproductive age are affected by endometriosis (93). Endometriosis can cause a range of symptoms, including dysmenorrhea, deep pelvic pain, and infertility (94, 95). Although the underlying cause of endometriosis is not fully understood, but inflammation is believed to play a major role in its development (96). Researchers have found that HMGB1 is present in the endometrial cells of women with endometriosis, compared to those who do not (97). HMGB-1 may contribute to the development of endometriosis in part by regulating the inflammatory response and autophagy (97). HMGB1 expression is significantly increased during the secretory phase of the menstrual cycle (31). The extracellular secretion of HMGB1 appears to enhance the proliferation of endometrial stromal cells, contributing to the development of inflammatory responses in the endometrium (98). This effect can be inhibited by TLR4 antagonists and NF-κB inhibitors, suggesting that the HMGB1-TLR4-NF-κB pathway is involved in the development of aseptic inflammation in endometrial tissue (98). These findings were supported by subsequent studies confirming the role of HMGB1 in the pathogenesis of endometriosis (99, 100). The study conducted by Cao et al. not only localized HMGB1 in endometriosis patients but also found that its circulating levels were higher in these patients than in women without the disease, indicating its potential as a biomarker for detecting endometriosis (101). The researchers further examined the relationship between HMGB1 and glycolysis-related indicators pyruvate kinase M2 (PKM2) and hexokinase 2 (HK2). They discovered a positive correlation between their levels with HMGB1 expression, suggesting that HMGB1 may play a role in the pathogenesis of endometriosis by affecting glycolysis (26). In addition, the effects of HMGB1 on endometriosis may be linked to pyroptosis (102). However, it was also observed that HMGB1 was not significantly upregulated in patients with severe endometriosis (103). Therefore, more research is necessary to determine the viability of HMGB1 as a biomarker for endometriosis.
4.3 Unexplained recurrent spontaneous abortion
Recurrent spontaneous abortion (RSA) is a common pregnancy complication of two or more failed clinical pregnancies (104). This complication involves multiple factors, such as chromosomal abnormalities, age, antiphospholipid syndrome, uterine malformations, thrombosis, hormonal or metabolic disorders, infections, autoimmunity, sperm quality, and lifestyle issues (105). Despite extensive studies, no apparent causative factor exists for unexplained recurrent spontaneous abortion (URSA) in 50% to 75% of the patients diagnosed with RSA (106). Recent research has indicated that underlying immunological alterations may contribute to the development of URSA. In addition, studies have shown that abnormal expression of HMGB1 may be closely associated with the development of URSA.
Jin et al. found that individuals with HMGB1 rs2249825C/G polymorphism had a higher risk of RSA and also experience higher expression of HMGB1 in the chorionic villi. This indicates a strong association between the two factors (107). Patients with URSA have higher levels of serum HMGB1 compared to normal pregnant women (21, 108, 109), which is also observed in patients with PE. In patients with URSA, the maternal-fetal interface is disorganized with cell arrangement and nuclear rupture issues. There were more infiltrating cells in the chorionic villi and decidua than in normal pregnancies (21, 108, 109). The co-localization of HMGB1 with CD45 and Vimentin, but not CK7, suggests that increased HMGB1 at the maternal-fetal interface is likely due to immune cell secretion and passive release from ecdysteroid stromal cells following necrosis, rather than chorionic epithelial cells (108). Further studies have shown that the immune cells that secrete HMGB1 are predominantly macrophages (21). In both mouse models and human decidua tissues, the receptors RAGE, TLR2, and TLR4 were upregulated in the decidua of the URSA group, along with elevated expression of HMGB1. As a result, the expression levels of the inflammasome NLRP-3 and the pyroptosis-related proteins caspase-1 and GSDMD were also increased. These findings suggest that the role of HMGB1 goes beyond merely amplifying the inflammatory response, and it plays a significant role in the pathogenesis of URSA by inducing pyroptosis. Therefore, HMGB1 amplifies the inflammatory response not only by interacting with RAGE, TLR2, and TLR4 receptors, thereby activating the NF-κB signaling pathway, but also by inducing pyroptosis during the pathogenesis of URSA (21).
Furthermore, the use of HTR8/SVneo cells induced with lipopolysaccharide (LPS) as an in vitro model provides an opportunity for researchers to investigate the impact of inflammation on the uteroplacental interface. The experiment conducted by Zhou et al. revealed that the exposure to LPS has a significant impact on the ability of HTR8/SVneo cells to grow and move, which are essential functions for a healthy pregnancy. However, HMGB1 knockdown restores these two abilities and blocks the elevated expression of Beclin1 and LC3 in HTR-8/SVneo cells after LPS induction (110). This suggests that HMGB1 may also be involved in the development of miscarriage through the induction of autophagy. In summary, an unusual increase in HMGB1 expression in the maternal-fetal interface and circulatory system can affectseveral crucial processes in pregnancy, ultimately increasing the likelihood of miscarriage. To decrease the occurrence of URSA and improve the birth rate in women of childbearing age, it is crucial to conduct further studies on the precise mechanism by which HMGB1 mediates URSA.
4.4 Preeclampsia
Preeclampsia (PE) is a common characteristic complication of pregnancy, which complicates 2% to 4% of pregnancies globally and causes approximately 46,000 maternal deaths and 500,000 fetal and new-born deaths annually (111). It usually occurs after 20 weeks of gestation and often presents with hypertension, proteinuria, thrombocytopenia, liver and kidney function damage, headaches and other symptoms (111, 112). To date, the etiology and pathogenesis of PE have not been fully elucidated. According to the current research, the main factors involved in the pathogenesis of PE include insufficient recasting the uterine spiral arterioles, disturbance of immune regulation, excessive activation of inflammation, damage of vascular endothelium and genetic factors (111).
Numerous studies have demonstrated that women with PE have elevated levels of HMGB1 in multiple tissues, including plasma, placenta, trophoblast tissue, fetal membrane, and decidua (113–115), particularly those with severe or early-onset PE (116, 117). HMGB1 likely contributes to the pathogenesis of PE through several signaling pathways. For instance, it has been discovered that the levels of HMGB1 are significantly higher in the micro- and nano-vesicles of PE placental explants, which cause endothelial cell activation (118). Additionally, hypoxia-induced high levels of HMGB1 secretion in trophoblast tissue can lead to increased endothelial cell permeability through the TRL4/caveolin-1 (Cav-1) pathway, which may be a key factor in the clinical manifestations of PE hyperalbuminuria and systemic edema (114). Besides, elevated plasma HMGB1 stimulates group 3 innate lymphoid cells (ILC3) differentiation and increases IL-17 production (119). Moreover, in PE patients, the binding of HMGB1 to RAGE enhances the NF-κB signaling pathway, which leads to the elevation of pro-inflammatory cytokines such as IL-6 and TNF-α (120–122). It is known that IL-6 may be involved in the overexpression of placental sFlt-1 (123), and TNF-α can reduce the level of endothelial nitric oxide synthase (124), and the combination of these two causes excessive inflammatory reaction and vascular dysfunction, increased circulating endothelial particles and thrombophilia in the PE mother. Therefore, it can be inferred that HMGB1 is an important mediator for the promotion of PE generation.
Through RAGE-NF-κB-IL-6/CCL2 signaling pathway, HMGB1 has been shown to have a critical role in stimulating adipocytes, leading to the further development of inflammation in pregnant women with PE (125). According to Tangerås et al., the syncytial layer is the most important HMGB1-TLR4 activation site, and elevated HMGB1 in the syncytial layer of patients with PE induces TLR4-dependent IL-8 release through the inflammatory isoform of HMGB1 in placental explants and trophoblasts (126). This plays a significant role in the occurrence of local placental inflammation. In addition, hypoxic trophoblast HMGB1 can also induce human umbilical vein endothelial cells (HUVEC) to produce cytotoxicity and leukocyte arrest, as well as higher expression of cell adhesion molecules (VCAM-1 and ICAM-1), thereby causing cell damage (127). These findings suggest that HMGB1 plays a vital role in the development of PE, and that it is possible to prevent and treating PE through anti-HMGB1 therapy. Further research in this area is necessary to fully understand the role of HMGB1 in PE and its potential as a therapeutic target.
4.5 Gestational diabetes mellitus
Gestational diabetes mellitus(GDM) is the most common gestational metabolic disease, which refers to diabetes mellitus with normal glucose metabolism before pregnancy and develops only during pregnancy (128). GDM is a significantly harmful disease to both the mother and fetus. It is associated with a high risk of many adverse pregnancy outcomes, such as pre-eclampsia (PE), infection, fetal growth restriction, giant fetus, miscarriage, and postpartum type 2 diabetes (128, 129). Exploring the pathogenesis and treatment of GDM has always had an important place in obstetrics and gynecological research.
Using a cross-sectional comparison of the plasma levels of HMGB1 in 75 pregnant women with positive glucose tolerance tests and 48 pregnant women with negative glucose tolerance tests, Giacobbe et al. found that circulating levels of HMGB1 were higher in patients with GDM than in women with normal pregnancies (130). However, another study that compared plasma HMGB1 levels in GDM patients and normal pregnant women did not support this finding. No significant correlation were observed between HMGB1 levels and GDM. Surprisingly, another study found that maternal age was significantly associated with HMGB1 in patients with GDM but not in the normal pregnancy group (131). Thus, maternal age, a common risk factor for GDM, may influence the incidence of GDM by interacting with HMGB1. Similarly, Santangelo et al. also discovered no significant difference in the plasma HMGB1 levels between pregnant women with GDM and those with normal glucose tolerance. However, they observed an increase in the expression of HMGB1 protein expression in the fetal membrane tissue of patients with GDM. It is associated with high expression of VPAC2 (a VIP receptor) and RAGE receptors in the omental adipose tissue (22). The interaction between HMGB1 and RAGE has been previously shown to result in the secretion of inflammatory cytokines (132), suggesting that the increased expression of HMGB1 in patients with GDM may contribute to the chronic inflammatory state, which is relevant to obesity and insulin resistance. Further, studies are still needed to explore the relationship between HMGB1 and the pathogenesis of GDM.
4.6 Preterm birth
Preterm birth(PTB) is a term used to describe delivery that occurs before the 37th week of gestation (133). The global preterm birth rate is about 10% (134, 135). Approximately 70% of preterm birth are spontaneous, while 30% are related to maternal and/or fetal conditions (136, 137). Preterm premature rupture of membrane (pPROM) refers to the rupture of the amniotic sac (membranes) before 37 weeks’ gestation, which contributes to 30-40% of all preterm birth (137). Studies have shown that intra-amniotic infection and/or inflammation have a significant causal relationship with preterm birth (138, 139). In recent years, several studies have demonstrated elevated levels of HMGB1 in the amniotic fluid and plasma of patients with preterm birth compared to those with normal pregnancies (138, 140–145). HMGB1 induces preterm birth in mouse models (146). These findings suggest that HMGB1 may serve as a new non-invasive biomarker for PTB diagnosis.
In normal pregnancies, HMGB1 levels in the amniotic fluid (AF) are not regulated by gestational age (GA) and are higher at delivery than before delivery (140). HMGB1 promotes immune activation at the fetal-maternal interface, thereby facilitating delivery. Elevated levels of HMGB1 have been observed in the serum of mothers with chorioamnionitis-associated preterm birth (147). Intra-amniotic inflammation induces upregulation of HMGB1 expression and release of HMGB1 in the amnion through inhibition of miR-548 and miR-199a-3p (148, 149). In aseptic inflammation-associated preterm delivery, it was found that HMGB1 was released into the amniotic fluid after undergoing acetylation modification. Additionally, RAGE and TLR2/4 showed a dose-dependent increase in response to HMGB1, ultimately activating the p38MAPK signaling pathway. As a result, the expression of pro-inflammatory cytokines such as IL-1β, IL-6, IL-8, and TNF-α was significantly elevated, promoting inflammation unrelated to infection but somewhat related to aging (142). Previous research has shown that HMGB1 can promote inflammation in the chorioamnion by increasing the mRNA expression and protein concentration of NLRP3 and NOD2 while at the same time mediating the release of mature IL-1β and IL-6 by activating caspase-1 (146). Both IL-1β and IL-6 have been found to play crucial roles in the development of inflammation and can ultimately lead to preterm birth and delivery (150–152).
Furthermore, research has demonstrated that HMGB1 plays a crucial role in promoting the proliferation and activation of iNKT cells during metaphase. Once activated, these iNKT cells act by secrete a range of cytokines and lysis granules, such as IFN-γ, perforin and granzyme B, which may cause damage to both fetal and maternal tissues. As a result, the release of HMGB1 is more enhanced, leading to further inflammation and the promotion of PTB development (153). In addition, one study found that the mRNA expression and protein levels of several molecules, including HMGB1, RAGE, NF-κB/p65, matrix metalloproteinase (MMP)-9, and MMP-2, were significantly increased in the HMGB1-RAGE pathway in pregnant women who had experienced pPROM compared with those who had experienced normal full-term pregnancies. This suggests that the nucleoplasmic translocation of HMGB1 in pPROM placentas may cause it to bind to its receptor RAGE, which then stimulates the activity of NF-κB/p65. The activation of NF-κB/p65 triggers the release of MMP-9 and MMP-2. Therefore, HMGB1 is implicated in the progression of pPROM (154). According to the above researches, a significant relationship exists between HMGB1 and PTB pathogenesis. The prevention and treatment of PTB by targeting anti-HMGB1 has gradually become more encouraging.
The function of HMGB1 in the female reproductive system is twofold. It has been discovered to play a crucial role in the development of various conditions such as preeclampsia, preterm birth, gestational diabetes mellitus, unexplained recurrent spontaneous abortion, polycystic ovary syndrome, and endometriosis. With such a broad range of connections, HMGB1 may act as a biomarker for anticipating the beginning of these ailments. The early detection of HMGB1 levels can help prevent the onset of these health conditions in women through anti-HMGB1 treatment.
5 HMGB1-targeted therapeutic agents
5.1 Glycyrrhizin
Glycyrrhizin (GL) is a natural compound that is commonly found in large quantities in the roots and rhizomes of Glycyrrhiza glabra (155). It is a triterpene diol conjugate (156). Specifically, it has been discovered that GL forms a direct bond with HMGB1. This occurs when the GL interacts with two shallow concave surfaces that are created by the two arms of the two HMG boxes (157). GL can inhibit HMGB1-TLR4-NF-kB mRNA expression level and has physical interaction with HMGB1 and TLR4 observed in molecular docking (158). This makes it a valuable tool for the treatment of various diseases associated with HMGB1. Initially, GL was used primarily for the treatment of chronic hepatitis. Still, it is becoming increasingly significant in treating various other diseases such as oncology, lung diseases, cardiovascular diseases, sepsis, and more (159–165). Moreover, GL has shown great potential in treating pregnancy-related complications, making them a valuable tool for maternal and fetal health.
In patients with PE, the placenta is defective and trophoblast cells are hypoxic (166), which leads to increased secretion of HMGB1 by hypoxic trophoblastic tissues. The secretion of HMGB1 induces endothelial cell hyperpermeability via the TLR4/caveolin-1 pathway. Consequently, this leads to the development of generalized edema and hyperuria in patients with preeclampsia (114). However, according to Jiang et al., the concentration of 200 mg/ml of GL was found to reduce the permeability of hypoxic JEG-3-CM-induced human umbilical vein endothelial cells (HUVEC) by 89.8% (114). This finding suggests that GL can reduce endothelial cell permeability in patients with PE.
GL has a potent anti-inflammatory effect and can be used to treat pregnancy-related issues. Excessive glucose can cause inflammation in trophoblast cells, leading to increased HMGB1 expression. HMGB1 interacts with TLR4, resulting in the secretion of IL-6 and IL-8, which slows down trophoblast migration. However, GL can reverse the above effect caused by excessive glucose (167). Furthermore, in rodent models of PE, oral GL reduces HMGB1 and inflammatory factors including IL-1 and IL-6, both in serum and in the placenta (121). These results illustrate GL reduces inflammation in pregnancy complications by suppressing HMGB1 release and bioactivity. GL can also block the process of HMGB1-mediated senescence activation through the p38MAPK pathway in fetal membranes and placenta of PTB (142). This is an important finding as it indicates that GL may play a role in preventing preterm birth. Further studies have been conducted on the safety of GL administration during pregnancy. One such study involved the oral gavage of GD10-19 in pregnant rats, which showed no adverse effects on blood pressure and proteinuria (121). This indicates that GL is safe for use during pregnancy, at least in rats. Further research is necessary before recommending its use in other animals or humans.
5.2 Recombinant thrombomodulin
Thrombomodulin (TM), also referred to as CD141, is a glycoprotein found on the surface of endothelial cells (168, 169). It has a lectin-like domain that can bind to and neutralize HMGB1 (170–172). Recently, recombinant thrombomodulin (rTM), a commercial form of TM, was found to have anti-inflammatory effects and to improve body function by inhibiting HMGB1 in various diseases (173).
TM is highly expressed in placental trophoblast cells during normal pregnancies (174), and reduced TM expression has been observed in women with placental defects such as PE and miscarriage (175). It has been hypothesized that the TM plays a role in maintaining placental function. Indeed, was discovered that treating a mouse model of recurrent miscarriage induced by angiotensin II (Ang II)-related PE with rTM significantly inhibited multiple pathways mediated by HMGB1. rTM leads to decreased adverse pregnancy outcomes (176).
In PE, effect of elevated HMGB1 binding to its receptors is the activation of the HIF-regulated hypoxic stress response through NF-κB transcriptional upregulation and HIF-1α expression. It also inhibited placental angiogenesis by reducing placental growth factor (PlGF) production and enhancing sFlt-1 expression (120). In mice with pathological pregnancies, the use of rTM hindered the rise of HMGB1 levels in the blood and the production of pro-inflammatory cytokines such as IL-6 and TNF-α in the placenta. Additionally, it decreased the accumulation of HIF-1α protein, increased PlGF expression in the placenta, and reduced the buildup of fibrinogen in the placental vagus region (120). Therefore, rTM ultimately improved the fetal resorption rate and fetal growth restriction in the recurrent miscarriage model and reduced symptoms such as hypertension and proteinuria in the PE model (120, 176, 177). Moreover, rTM is too large to cross the placenta and affect the fetus (177), making it a promising drug for treating pregnancy complications such as pre-eclampsia, recurrent miscarriage, and fetal growth restriction.
5.3 Low molecular weight heparin
Low molecular weight heparin (LMWH) is a commonly used anticoagulant for treating pregnancy complications such as recurrent miscarriage, pre-eclampsia, and fetal growth restriction (153, 178). Although there is no evidence for the beneficial effects of heparin in reducing adverse neonatal outcomes (179). This helps to avoid adverse pregnancy outcomes (180, 181). Recent studies have shown that LMWH can bind to HMGB1, resulting in a reduced affinity of HMGB1 for RAGE. This effect may play a role in protecting the placental function and improving pregnancy (182). According to Zenerino et al., after 48 hours of LMWH treatment, the levels of HMGB1, RAGE, IL-6, and TNF-α were found to be reduced in a physiological placental villous explant model (178). This finding suggests that LMWH regulates the HMGB1/RAGE pro-inflammatory axis in human placenta. Moreover, LMWH has been discovered to hinder the growth of iNKT cells when co-cultured with APC in the presence of HMGB1 (178). This finding implies that LMWH may be effective in preventing preterm births without acute chorioamnionitis. In a mouse model of LPS-induced PE, LMWH enhanced pregnancy outcomes by reducing iron-regulator expression and boosting iron absorption (153). Further investigations are required to determine how heparin improves pregnancy rates. This is because heparin binds to both HMGB1 and RAGE (183), making it unclear which molecule is responsible for the inhibition of the HMGB1-RAGE axis. In addition, it is important to be cautious when using LMWH during severe PE as it can stimulate the placental expression and release of sFLT-1 (184).
5.4 Other agents
The following drugs can also target HMGB1 to provide new approaches for the treatment of pregnancy-related complications (Table 1; Supplementary Table 1). Aspirin has potential therapeutic benefits in treating pregnancy complications such as recurrent abortions (189). Salicylic acid (SA) has been found to have binding sites on HMGB1 in a specific domain through nuclear magnetic resonance (NMR) spectroscopy studies (185). Acetylsalicylic acid, commonly known as aspirin, is thought to work by inhibiting HMGB1 through its binding with SA (185). Aspirin has been shown to reduce expression levels of HMGB1 in both decidua tissue and peripheral blood in the mouse model of recurrent miscarriage, as well as decrease expression of receptors RAGE, TLR2 and TLR4 (21, 109). Magnesium sulfate, as a first-line drug for PE treatment, can inhibit vascular endothelial cell (VEC) apoptosis via the miR218-5p/HMGB1 axis (186). MiR-218-5p has the potential to bind to the 3’-UTR of HMGB1 and can negatively regulate the expression of HMGB1 (190). Compared with the rats in the normal group, miR-218-5p expression decreased in the placental tissues and VECs of the rats in the PE, while HMGB1 increased. Magnesium sulfate can reverse the changes and thus play a role in treating PE (186). In addition, studies have shown that Epigallocatechin gallate (EGCG) can reduce the expression of HMGB1 in hypoxic trophoblast cells in a dose-dependent manner. This helps to improve the cells’ angiogenic state and reduce endothelial dysfunction for treating PE (187). A water-soluble derivative of tanshinone IIA called sodium tanshinone IIA sulfonate (STS) has been discovered to inhibit the expression and release of HMGB1 in the hypoxic trophoblast. STS can be absorbed by the intestine and is capable of reversing HMGB1-induced cytotoxicity, leukocyte arrest, as well as the high expression of cell adhesion molecules including VCAM-1 and ICAM-1, which implies STS has the potential to treat PE (127). Researchers have discovered that Clarithromycin can extend gestation in mice with aseptic intra-amniotic inflammation induced by HMGB1. This results in a reduction of preterm birth rates and helps improve neonatal survival (188). Besides, there is a study showing that betamethasone treatment may also prevent preterm birth caused by HMGB1 (139).
6 Conclusions and perspectives
This review emphasizes the multiple roles of HMGB1 playing in female reproductive system and some potential HMGB1-targeted therapies (Figure 3). Under physiological conditions, HMGB1 is essential for the critical stages of pregnancy, such as uterine decidualization, embryo implantation, and early fetal development. HMGB1 also participates in the pathogenesis of diseases in female reproductive system as a DAMP. Therefore, HMGB1-targeted drugs may offer new ways to prevent or treat these diseases. However, more studies are needed to clarify the following questions (1): The precise mechanism by which HMGB1 plays roles in female reproductive system: Although there has been much research to explore the function of HMGB1, it’s necessary to further figure out every node associated with HMGB1and their connection in female reproductive system. (2) The specific mechanism of HMGB1-targeted therapies in female reproductive system: In this review, we refers to some kinds of promising HMGB1-targeted drugs including glycyrrhizin, recombinant thrombomodulin, low molecular weight heparin, aspirin and so on. But many issues related to them are still unclear. What are the binding targets of these drugs to HMGB1? How do they directly or indirectly regulate the signaling pathway where HMGB1 is located? Will they also target other signaling pathways? What are their administration methods, precautions, and adverse reactions? Is there any new HMGB1 Targeted therapy?
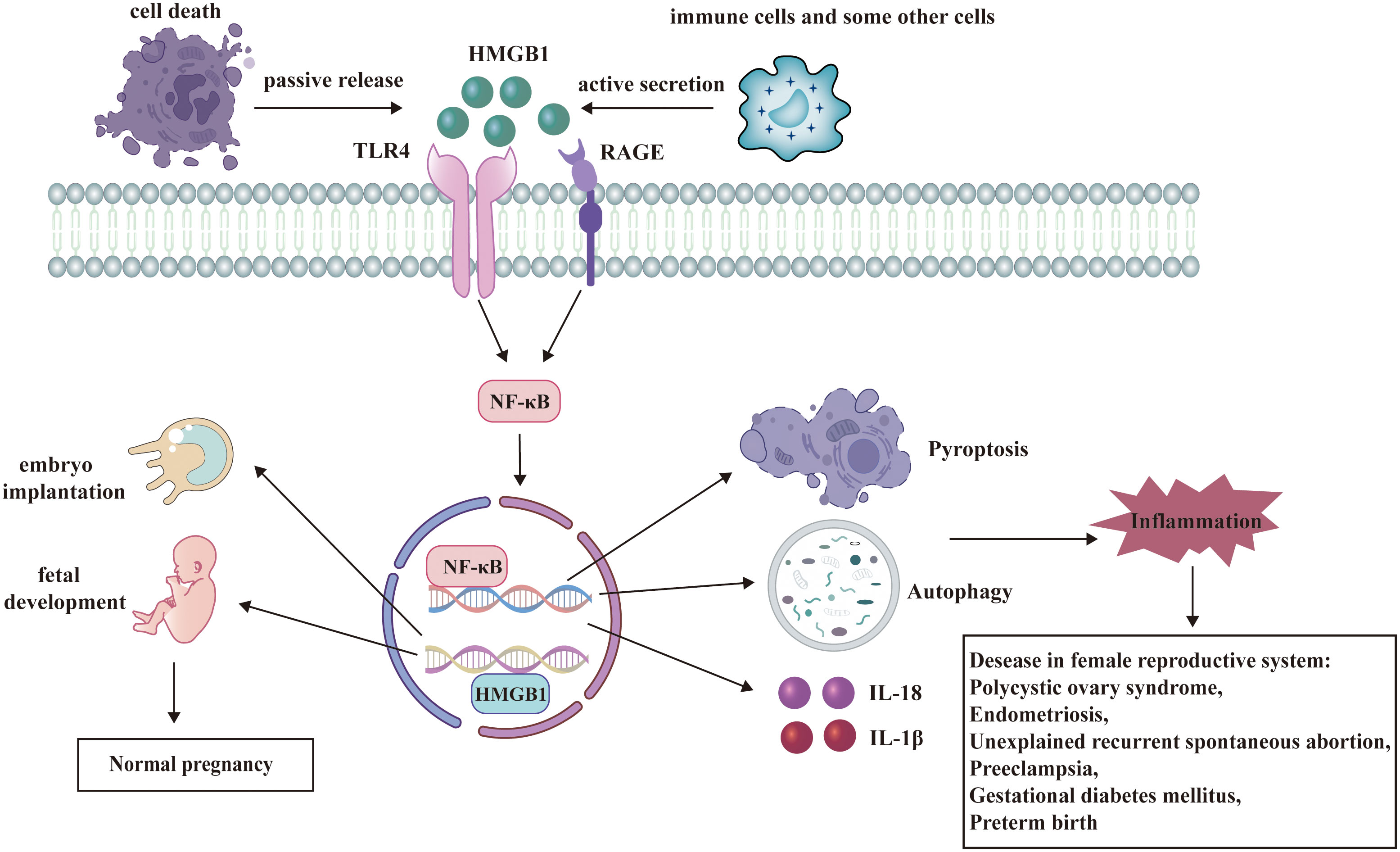
Figure 3 HMGB1 has a dual role in female reproductive system. HMGB1 participates in the regulation of many signaling pathways and plays significant roles in female reproductive system. Under physiological conditions, HMGB1 facilitates embryo implantation and fetal development. As a DAMP, HMGB1 binds to its receptors and activates several pathways such as autophagy, pyroptosis, and release of inflammatory cytokines. These processes contribute to the pathogenesis of diseases in female reproductive system.
Author contributions
YR, ZZ, YC and HZ designed the study and edited the final text. YR, ZD, XH and QZ collected the data from publications, developed the database and wrote the manuscript. YR prepared the figures. YR prepared the tables. BC, PZ, ZW, ZZ, YC and HZ contributed to the manuscript revision and critical discussion. All authors contributed to the article and approved the submitted version.
Funding
This research was supported by the National Natural Sciences Foundation of China (No. 32000642), the Young and Middle-aged Doctors in Reproductive Medicine Research-Basic Research Projects (No. BJHPA-2022-SHZHYXZHQNYJ-JICH-003), the Nonprofit Central Research Institute Fund of Chinese Academy of Medical Sciences (No. 2019PT310002), the Research Fund of Anhui Institute of translational medicine (2021zhyx-B16).
Acknowledgments
This work was supported by the Reproductive Center of the First Affiliated Hospital of Anhui Medical University.
Conflict of interest
The authors declare that the research was conducted in the absence of any commercial or financial relationships that could be construed as a potential conflict of interest.
Publisher’s note
All claims expressed in this article are solely those of the authors and do not necessarily represent those of their affiliated organizations, or those of the publisher, the editors and the reviewers. Any product that may be evaluated in this article, or claim that may be made by its manufacturer, is not guaranteed or endorsed by the publisher.
Supplementary material
The Supplementary Material for this article can be found online at: https://www.frontiersin.org/articles/10.3389/fimmu.2023.1238785/full#supplementary-material
References
1. Goodwin GH, Sanders C, Johns EW. A new group of chromatin-associated proteins with a high content of acidic and basic amino acids. Eur J Biochem (1973) 38(1):14–9. doi: 10.1111/j.1432-1033.1973.tb03026.x
2. Bustin M. Revised nomenclature for high mobility group (Hmg) chromosomal proteins. Trends Biochem Sci (2001) 26(3):152–3. doi: 10.1016/S0968-0004(00)01777-1
3. Kang R, Chen R, Zhang Q, Hou W, Wu S, Cao L, et al. Hmgb1 in health and disease. Mol Aspects Med (2014) 40:1–116. doi: 10.1016/j.mam.2014.05.001
4. Martinotti S, Patrone M, Ranzato E. Emerging roles for hmgb I protein in immunity, inflammation, and cancer. Immunotargets Ther (2015) 4:101–9. doi: 10.2147/ITT.S58064
5. Wang S, Zhang Y. Hmgb1 in inflammation and cancer. J Hematol Oncol (2020) 13(1):116. doi: 10.1186/s13045-020-00950-x
6. Yuan S, Liu Z, Xu Z, Liu J, Zhang J. High mobility group box 1 (Hmgb1): A pivotal regulator of hematopoietic Malignancies. J Hematol Oncol (2020) 13(1):91. doi: 10.1186/s13045-020-00920-3
7. Ding M, Gao Y, Gu Y, Song D, Wang J, Ni J, et al. Hmgb1 can activate cartilage progenitor cells in response to cartilage injury through the cxcl12/cxcr4 pathway. Endokrynol Pol (2023) 74(1):74–82. doi: 10.5603/EP.a2022.0084
8. Chen Y, Yu C, Jiang S, Sun L. Japanese flounder hmgb1: A damp molecule that promotes antimicrobial immunity by interacting with immune cells and bacterial pathogen. Genes (Basel) (2022) 13(9):1509. doi: 10.3390/genes13091509
9. Chang Q, Fujio M, Tsuboi M, Bian H, Wakasugi M, Hibi H. High-mobility group box 1 accelerates distraction osteogenesis healing via the recruitment of endogenous stem/progenitor cells. Cytotherapy (2023) 25(9):946–955. doi: 10.1016/j.jcyt.2023.05.013
10. He H, Wang X, Chen J, Sun L, Sun H, Xie K. High-mobility group box 1 (Hmgb1) promotes angiogenesis and tumor migration by regulating hypoxia-inducible factor 1 (Hif-1α) expression via the phosphatidylinositol 3-kinase (Pi3k)/akt signaling pathway in breast cancer cells. Med Sci Monitor Int Med J Exp Clin Res (2019) 25:2352–60. doi: 10.12659/MSM.915690
11. Wu L, Yang L. The function and mechanism of hmgb1 in lung cancer and its potential therapeutic implications. Oncol Lett (2018) 15(5):6799–805. doi: 10.3892/ol.2018.8215
12. Menon R. Fetal Inflammatory Response at the Fetomaternal Interface: A Requirement for Labor at Term and Preterm. Immunol Rev (2022) 308(1):149–67. doi: 10.1111/imr.13075
13. Nadeau-Vallee M, Obari D, Palacios J, Brien ME, Duval C, Chemtob S, et al. Sterile inflammation and pregnancy complications: A review. Reproduction (2016) 152(6):R277–R92. doi: 10.1530/REP-16-0453
14. Mikhailova V, Grebenkina P, Khokhlova E, Davydova A, Salloum Z, Tyshchuk E, et al. Pro- and anti-inflammatory cytokines in the context of nk cell-trophoblast interactions. Int J Mol Sci (2022) 23(4):2387. doi: 10.3390/ijms23042387
15. Mor G, Cardenas I. The immune system in pregnancy: A unique complexity. Am J Reprod Immunol (New York NY 1989) (2010) 63(6):425–33. doi: 10.1111/j.1600-0897.2010.00836.x
16. Wang K, Yang ZQ, Yu HF, Wang YS, Guo B, Yue ZP. High mobility group box 1 regulates uterine decidualization through bone morphogenetic protein 2 and plays a role in kruppel-like factor 5-induced stromal differentiation. Cell Physiol Biochem (2018) 48(6):2399–408. doi: 10.1159/000492655
17. Aikawa S, Deng W, Liang X, Yuan J, Bartos A, Sun X, et al. Uterine deficiency of high-mobility group box-1 (Hmgb1) protein causes implantation defects and adverse pregnancy outcomes. Cell Death Differ (2020) 27(5):1489–504. doi: 10.1038/s41418-019-0429-z
18. Itou J, Taniguchi N, Oishi I, Kawakami H, Lotz M, Kawakami Y. Hmgb factors are required for posterior digit development through integrating signaling pathway activities. Dev Dyn (2011) 240(5):1151–62. doi: 10.1002/dvdy.22598
19. Yu L, Guan YJ, Gao Y, Wang X. Rpl30 and hmgb1 are required for neurulation in golden hamster. Int J Neurosci (2009) 119(8):1076–90. doi: 10.1080/00207450802330504
20. D'Angelo G, Marseglia L, Granese R, Di Benedetto A, Giacobbe A, Impellizzeri P, et al. Different concentration of human cord blood hmgb1 according to delivery and labor: A pilot study. Cytokine (2018) 108:53–6. doi: 10.1016/j.cyto.2018.03.019
21. Zhu D, Zou H, Liu J, Wang J, Ma C, Yin J, et al. Inhibition of hmgb1 ameliorates the maternal-fetal interface destruction in unexplained recurrent spontaneous abortion by suppressing pyroptosis activation. Front Immunol (2021) 12:782792. doi: 10.3389/fimmu.2021.782792
22. Santangelo C, Filardi T, Perrone G, Mariani M, Mari E, Scazzocchio B, et al. Cross-talk between fetal membranes and visceral adipose tissue involves hmgb1-rage and vip-vpac2 pathways in human gestational diabetes mellitus. Acta Diabetol (2019) 56(6):681–9. doi: 10.1007/s00592-019-01304-x
23. Radnaa E, Richardson LS, Sheller-Miller S, Baljinnyam T, de Castro Silva M, Kumar Kammala A, et al. Extracellular vesicle mediated feto-maternal hmgb1 signaling induces preterm birth. Lab Chip (2021) 21(10):1956–73. doi: 10.1039/d0lc01323d
24. Hu Y, Yan R, Zhang C, Zhou Z, Liu M, Wang C, et al. High-mobility group box 1 from hypoxic trophoblasts promotes endothelial microparticle production and thrombophilia in preeclampsia. Arterioscler Thromb Vasc Biol (2018) 38(6):1381–91. doi: 10.1161/atvbaha.118.310940
25. Yang Y, Yang L, Qi C, Hu G, Wang L, Sun Z, et al. Cryptotanshinone alleviates polycystic ovary syndrome in rats by regulating the hmgb1/tlr4/nf−Κb signaling pathway. Mol Med Rep (2020) 22(5):3851–61. doi: 10.3892/mmr.2020.11469
26. Sun Y, Wang Q, Wang M, Sun F, Qiao P, Jiang A, et al. Chip induces ubiquitination and degradation of hmgb1 to regulate glycolysis in ovarian endometriosis. Cell Mol Life Sci CMLS (2022) 80(1):13. doi: 10.1007/s00018-022-04637-z
27. Chen R, Kang R, Tang D. The mechanism of hmgb1 secretion and release. Exp Mol Med (2022) 54(2):91–102. doi: 10.1038/s12276-022-00736-w
28. Kwak MS, Kim HS, Lee B, Kim YH, Son M, Shin J-S. Immunological significance of hmgb1 post-translational modification and redox biology. Front In Immunol (2020) 11:1189. doi: 10.3389/fimmu.2020.01189
29. Sapojnikova N, Maman J, Myers FA, Thorne AW, Vorobyev VI, Crane-Robinson C. Biochemical observation of the rapid mobility of nuclear hmgb1. Biochim Et Biophys Acta (2005) 1729(1):57–63. doi: 10.1016/j.bbaexp.2005.03.002
30. Volmari A, Foelsch K, Zierz E, Yan K, Qi M, Bartels K, et al. Leukocyte-derived high-mobility group box 1 governs hepatic immune responses to listeria monocytogenes. Hepatol Commun (2021) 5(12):2104–20. doi: 10.1002/hep4.1777
31. Li Y, Chen S-T, He Y-Y, Li B, Yang C, Yang Z-S, et al. The regulation and function of acetylated high-mobility group box 1 during implantation and decidualization. Front In Immunol (2023) 14:1024706. doi: 10.3389/fimmu.2023.1024706
32. Zhao Z, Li G, Wang Y, Li Y, Xu H, Liu W, et al. Cytoplasmic hmgb1 induces renal tubular ferroptosis after ischemia/reperfusion. Int Immunopharmacol (2023) 116:109757. doi: 10.1016/j.intimp.2023.109757
33. Gao S, Liu K, Ku W, Wang D, Wake H, Qiao H, et al. Histamine induced high mobility group box-1 release from vascular endothelial cells through H1 receptor. Front In Immunol (2022) 13:930683. doi: 10.3389/fimmu.2022.930683
34. Yu F, Tian W, Dong J. Anagliptin prevents lipopolysaccharide (Lps)- induced inflammation and activation of macrophages. Int Immunopharmacol (2022) 104:108514. doi: 10.1016/j.intimp.2021.108514
35. Chaudhary N, Srivastava S, Dave U, Ojha A, Guchhait P, Chandele A, et al. High-mobility group box 1 protein promotes dengue virus replication by interacting with untranslated regions of viral genome. Virus Res (2022) 309:198668. doi: 10.1016/j.virusres.2021.198668
36. Min HJ, Kim JH, Yoo JE, Oh JH, Kim KS, Yoon JH, et al. Ros-dependent hmgb1 secretion upregulates il-8 in upper airway epithelial cells under hypoxic condition. Mucosal Immunol (2017) 10(3):685–94. doi: 10.1038/mi.2016.82
37. Zhao Y, Zhang J, Lu H, Mao Y, Qin J, Wang Y, et al. Cardiopulmonary bypass-derived plasma exosomal hmgb1 contributes to alveolar epithelial cell necroptosis via mtdna/cgas/sting pathway. Shock (Augusta Ga) (2022) 58(6):534–41. doi: 10.1097/SHK.0000000000002006
38. Zhao ZB, Marschner JA, Iwakura T, Li C, Motrapu M, Kuang M, et al. Tubular epithelial cell hmgb1 promotes aki-ckd transition by sensitizing cycling tubular cells to oxidative stress: A rationale for targeting hmgb1 during aki recovery. J Am Soc Nephrol (2023) 34(3):394–411. doi: 10.1681/ASN.0000000000000024
39. Nwikue G, Olsson-Brown A, Aboheimed N, Yip V, Jolly C, Luchian A, et al. Tnf-Α Induced extracellular release of keratinocyte high-mobility group box 1 in stevens-johnson syndrome/toxic epidermal necrolysis: Biomarker and putative mechanism of pathogenesis. J Dermatol (2023) 00:1–11. doi: 10.1111/1346-8138.16847
40. Ren Y, Cao L, Wang L, Zheng S, Zhang Q, Guo X, et al. Autophagic secretion of hmgb1 from cancer-associated fibroblasts promotes metastatic potential of non-small cell lung cancer cells via nfκb signaling. Cell Death Dis (2021) 12(10):858. doi: 10.1038/s41419-021-04150-4
41. Sabbione F, Keitelman IA, Iula L, Ferrero M, Giordano MN, Baldi P, et al. Neutrophil extracellular traps stimulate proinflammatory responses in human airway epithelial cells. J Innate Immun (2017) 9(4):387–402. doi: 10.1159/000460293
42. Volchuk A, Ye A, Chi L, Steinberg BE, Goldenberg NM. Indirect regulation of hmgb1 release by gasdermin D. Nat Commun (2020) 11(1):4561. doi: 10.1038/s41467-020-18443-3
43. Kwak MS, Choi S, Kim J, Lee H, Park IH, Oh J, et al. Sars-Cov-2 infection induces hmgb1 secretion through post-translational modification and panoptosis. Immune Netw (2023) 23(3):e26. doi: 10.4110/in.2023.23.e26
44. Zhang D, Wu C, Ba D, Wang N, Wang Y, Li X, et al. Ferroptosis contribute to neonicotinoid imidacloprid-evoked pyroptosis by activating the hmgb1-rage/tlr4-nf-Κb signaling pathway. Ecotoxicology Environ Saf (2023) 253:114655. doi: 10.1016/j.ecoenv.2023.114655
45. Hayase N, Doi K, Hiruma T, Inokuchi R, Hamasaki Y, Noiri E, et al. Damage-associated molecular patterns in intensive care unit patients with acute liver injuries: A prospective cohort study. Med (Baltimore) (2018) 97(41):e12780. doi: 10.1097/md.0000000000012780
46. Gong W, Zheng Y, Chao F, Li Y, Xu Z, Huang G, et al. The anti-inflammatory activity of hmgb1 a box is enhanced when fused with C-terminal acidic tail. J BioMed Biotechnol (2010) 2010:915234. doi: 10.1155/2010/915234
47. Yang H, Lundbäck P, Ottosson L, Erlandsson-Harris H, Venereau E, Bianchi ME, et al. Redox modifications of cysteine residues regulate the cytokine activity of hmgb1. Mol Med (Cambridge Mass) (2021) 27(1):58. doi: 10.1186/s10020-021-00307-1
48. Deng M, Scott MJ, Fan J, Billiar TR. Location is the key to function: Hmgb1 in sepsis and trauma-induced inflammation. J Leukocyte Biol (2019) 106(1):161–9. doi: 10.1002/JLB.3MIR1218-497R
49. Fassi EMA, Sgrignani J, D'Agostino G, CecChinato V, Garofalo M, Grazioso G, et al. Oxidation state dependent conformational changes of hmgb1 regulate the formation of the cxcl12/hmgb1 heterocomplex. Comput Struct Biotechnol J (2019) 17:886–94. doi: 10.1016/j.csbj.2019.06.020
50. Yang H, Wang H, Ju Z, Ragab AA, Lundbäck P, Long W, et al. Md-2 is required for disulfide hmgb1-dependent tlr4 signaling. J Exp Med (2015) 212(1):5–14. doi: 10.1084/jem.20141318
51. Venereau E, Schiraldi M, Uguccioni M, Bianchi ME. Hmgb1 and leukocyte migration during trauma and sterile inflammation. Mol Immunol (2013) 55(1):76–82. doi: 10.1016/j.molimm.2012.10.037
52. Venereau E, Casalgrandi M, Schiraldi M, Antoine DJ, Cattaneo A, De Marchis F, et al. Mutually exclusive redox forms of hmgb1 promote cell recruitment or proinflammatory cytokine release. J Exp Med (2012) 209(9):1519–28. doi: 10.1084/jem.20120189
53. Yang H, Hreggvidsdottir HS, Palmblad K, Wang H, Ochani M, Li J, et al. A critical cysteine is required for hmgb1 binding to toll-like receptor 4 and activation of macrophage cytokine release. Proc Natl Acad Sci United States America (2010) 107(26):11942–7. doi: 10.1073/pnas.1003893107
54. Kazama H, Ricci J-E, Herndon JM, Hoppe G, Green DR, Ferguson TA. Induction of immunological tolerance by apoptotic cells requires caspase-dependent oxidation of high-mobility group box-1 protein. Immunity (2008) 29(1):21–32. doi: 10.1016/j.immuni.2008.05.013
55. Techarang T, Jariyapong P, Viriyavejakul P, Punsawad C. High mobility group box-1 (Hmgb-1) and its receptors in the pathogenesis of malaria-associated acute lung injury/acute respiratory distress syndrome in a mouse model. Heliyon (2021) 7(12):e08589. doi: 10.1016/j.heliyon.2021.e08589
56. Chen G-Y, Tang J, Zheng P, Liu Y. Cd24 and siglec-10 selectively repress tissue damage-induced immune responses. Sci (New York NY) (2009) 323(5922):1722–5. doi: 10.1126/science.1168988
57. Chiba S, Baghdadi M, Akiba H, Yoshiyama H, Kinoshita I, Dosaka-Akita H, et al. Tumor-Infiltrating Dcs Suppress Nucleic Acid-Mediated Innate Immune Responses through Interactions between the Receptor Tim-3 and the Alarmin Hmgb1. Nat Immunol (2012) 13(9):832–42. doi: 10.1038/ni.2376
58. Zhang W, Song J, Zhang Y, Ma Y, Yang J, He G, et al. Intermittent high glucose-induced oxidative stress modulates retinal pigmented epithelial cell autophagy and promotes cell survival via increased hmgb1. BMC Ophthalmol (2018) 18(1):192. doi: 10.1186/s12886-018-0864-5
59. Liu L, Wang N, Kalionis B, Xia S, He Q. Hmgb1 plays an important role in pyroptosis induced blood brain barrier breakdown in diabetes-associated cognitive decline. J Neuroimmunology (2022) 362:577763. doi: 10.1016/j.jneuroim.2021.577763
60. Liu C, She Y, Huang J, Liu Y, Li W, Zhang C, et al. Hmgb1-nlrp3-P2x7r pathway participates in pm-induced hippocampal neuron impairment by regulating microglia activation. Ecotoxicology Environ Saf (2022) 239:113664. doi: 10.1016/j.ecoenv.2022.113664
61. Huang C-C, Hsueh Y-W, Chang C-W, Hsu H-C, Yang T-C, Lin W-C, et al. Establishment of the fetal-maternal interface: Developmental events in human implantation and placentation. Front In Cell Dev Biol (2023) 11:1200330. doi: 10.3389/fcell.2023.1200330
62. Park JY, Mani S, Clair G, Olson HM, Paurus VL, Ansong CK, et al. A microphysiological model of human trophoblast invasion during implantation. Nat Commun (2022) 13(1):1252. doi: 10.1038/s41467-022-28663-4
63. Cai Y, Li N, Li H. Combining endometrial assembloids and blastoids to delineate the molecular roadmap of implantation. Stem Cell Rev Rep (2023) 19(5):1268–1282. doi: 10.1007/s12015-023-10527-z
64. Wang H, Dey SK. Roadmap to embryo implantation: Clues from mouse models. Nat Rev Genet (2006) 7(3):185–99. doi: 10.1038/nrg1808
65. Yoshinaga K. A sequence of events in the uterus prior to implantation in the mouse. J Assisted Reprod Genet (2013) 30(8):1017–22. doi: 10.1007/s10815-013-0093-z
66. Chi R-PA, Wang T, Adams N, Wu S-P, Young SL, Spencer TE, et al. Human endometrial transcriptome and progesterone receptor cistrome reveal important pathways and epithelial regulators. J Clin Endocrinol Metab (2020) 105(4):e1419–e39. doi: 10.1210/clinem/dgz117
67. Jin Z, Shi H, Lu M, Bu Z, Huo M, Zhang Y. Endometrial thickness changes after progesterone administration do not affect the pregnancy outcomes of frozen-thawed euploid blastocyst transfer: A retrospective cohort study. Fertility Sterility (2021) 116(6):1502–12. doi: 10.1016/j.fertnstert.2021.08.008
68. Putnam CD, Brann DW, Kolbeck RC, Mahesh VB. Inhibition of uterine contractility by progesterone and progesterone metabolites: Mediation by progesterone and gamma amino butyric acida receptor systems. Biol Reprod (1991) 45(2):266–72. doi: 10.1095/biolreprod45.2.266
69. Zicari A, Centonze C, Realacci M, Buchetti B, Pietropolli A, Ticconi C. Estradiol 17-beta and progesterone modulate inducible nitric oxide synthase and high mobility group box 1 expression in human endometrium. Reprod Sci (Thousand Oaks Calif) (2008) 15(6):559–66. doi: 10.1177/1933719107312560
70. Gellersen B, Brosens JJ. Cyclic decidualization of the human endometrium in reproductive health and failure. Endocrine Rev (2014) 35(6):851–905. doi: 10.1210/er.2014-1045
71. Han M, Cao Y, Zhou W, Zhou M, Zhou X, Zhang D, et al. Increased expression of hmgb1 in the implantation phase endometrium is related to recurrent implantation failure. Mol Biol Rep (2022) 49(3):1701–10. doi: 10.1007/s11033-021-06979-6
72. Cui X-S, Shen X-H, Kim N-H. High mobility group box 1 (Hmgb1) is implicated in preimplantation embryo development in the mouse. Mol Reprod Dev (2008) 75(8):1290–9. doi: 10.1002/mrd.20694
73. Tang D, Kang R, Livesey KM, Kroemer G, Billiar TR, Van Houten B, et al. High-mobility group box 1 is essential for mitochondrial quality control. Cell Metab (2011) 13(6):701–11. doi: 10.1016/j.cmet.2011.04.008
74. Bagherpoor AJ, Dolezalova D, Barta T, Kučírek M, Sani SA, Ešner M, et al. Properties of human embryonic stem cells and their differentiated derivatives depend on nonhistone DNA-binding hmgb1 and hmgb2 proteins. Stem Cells Dev (2017) 26(5):328–40. doi: 10.1089/scd.2016.0274
75. Shen X-H, Jin Y-X, Ko Y-G, Chung H-J, Cui X-S, Kim N-H. High mobility group box 1 (Hmgb1) enhances porcine parthenotes developing in vitro in the absence of bsa. Theriogenology (2006) 66(9):2077–83. doi: 10.1016/j.theriogenology.2006.05.019
76. Zhao X, Kuja-Panula J, Rouhiainen A, Y-c C, Panula P, Rauvala H. High mobility group box-1 (Hmgb1; amphoterin) is required for zebrafish brain development. J Biol Chem (2011) 286(26):23200–13. doi: 10.1074/jbc.M111.223834
77. Zhao X, Rouhiainen A, Li Z, Guo S, Rauvala H. Regulation of neurogenesis in mouse brain by hmgb1. Cells (2020) 9(7):1714. doi: 10.3390/cells9071714
78. Taniguchi N, Yoshida K, Ito T, Tsuda M, Mishima Y, Furumatsu T, et al. Stage-specific secretion of hmgb1 in cartilage regulates endochondral ossification. Mol Cell Biol (2007) 27(16):5650–63. doi: 10.1128/MCB.00130-07
79. Palumbo R, Sampaolesi M, De Marchis F, Tonlorenzi R, Colombetti S, Mondino A, et al. Extracellular hmgb1, a signal of tissue damage, induces mesoangioblast migration and proliferation. J Cell Biol (2004) 164(3):441–9. doi: 10.1083/jcb.200304135
80. Mitola S, Belleri M, Urbinati C, Coltrini D, Sparatore B, Pedrazzi M, et al. Cutting edge: Extracellular high mobility group box-1 protein is a proangiogenic cytokine. J Immunol (Baltimore Md 1950) (2006) 176(1):12–5. doi: 10.4049/jimmunol.176.1.12
81. Sugars R, Karlström E, Christersson C, Olsson ML, Wendel M, Fried K. Expression of hmgb1 during tooth development. Cell Tissue Res (2007) 327(3):511–9. doi: 10.1007/s00441-006-0293-2
82. Singh R, Kaur S, Yadav S, Bhatia S. Gonadotropins as pharmacological agents in assisted reproductive technology and polycystic ovary syndrome. Trends Endocrinol Metab (2023) 34(4):194–215. doi: 10.1016/j.tem.2023.02.002
83. Mu L, Ye Z, Hu J, Zhang Y, Chen K, Sun H, et al. Ppm1k-regulated impaired catabolism of branched-chain amino acids orchestrates polycystic ovary syndrome. EBioMedicine (2023) 89:104492. doi: 10.1016/j.ebiom.2023.104492
84. Shrivastava S, Conigliaro RL. Polycystic ovarian syndrome. Med Clin North Am (2023) 107(2):227–34. doi: 10.1016/j.mcna.2022.10.004
85. Patel S. Polycystic ovary syndrome (Pcos), an inflammatory, systemic, lifestyle endocrinopathy. J Steroid Biochem Mol Biol (2018) 182:27–36. doi: 10.1016/j.jsbmb.2018.04.008
86. Kumariya S, Ubba V, Jha RK, Gayen JR. Autophagy in ovary and polycystic ovary syndrome: Role, dispute and future perspective. Autophagy (2021) 17(10):2706–33. doi: 10.1080/15548627.2021.1938914
87. Zhang C, Hu J, Wang W, Sun Y, Sun K. Hmgb1-induced aberrant autophagy contributes to insulin resistance in granulosa cells in pcos. FASEB J (2020) 34(7):9563–74. doi: 10.1096/fj.202000605RR
88. Cirillo F, Catellani C, Lazzeroni P, Sartori C, Tridenti G, Vezzani C, et al. Hmgb1 is increased in adolescents with polycystic ovary syndrome (Pcos) and decreases after treatment with myo-inositol (Myo) in combination with alpha-lipoic acid (Ala). Gynecological Endocrinol (2020) 36(7):588–93. doi: 10.1080/09513590.2020.1725967
89. Cirillo F, Catellani C, Sartori C, Lazzeroni P, Morini D, Nicoli A, et al. Cftr and foxo1 gene expression are reduced and high mobility group box 1 (Hmgb1) is increased in the ovaries and serum of women with polycystic ovarian syndrome. Gynecological Endocrinol (2019) 35(10):842–6. doi: 10.1080/09513590.2019.1599349
90. Wang H-H, Lin M, Xiang G-d. Serum hmgb1 levels and its association with endothelial dysfunction in patients with polycystic ovary syndrome. Physiol Res (2018) 67(6):911–9. doi: 10.33549/physiolres.933831
91. Xie Y, Zhang K, Zhang K, Zhang J, Wang L, Wang X, et al. Toll-like receptors and high mobility group box 1 in granulosa cells during bovine follicle maturation. J Cell Physiol (2020) 235(4):3447–62. doi: 10.1002/jcp.29234
92. Chen S, Liu Y, Zhong Z, Wei C, Liu Y, Zhu X. Peritoneal immune microenvironment of endometriosis: Role and therapeutic perspectives. Front In Immunol (2023) 14:1134663. doi: 10.3389/fimmu.2023.1134663
93. Shafrir AL, Farland LV, Shah DK, Harris HR, Kvaskoff M, Zondervan K, et al. Risk for and consequences of endometriosis: A critical epidemiologic review. Best Pract Res Clin Obstet Gynaecol (2018) 51:1–15. doi: 10.1016/j.bpobgyn.2018.06.001
94. Vercellini P, Viganò P, Bandini V, Buggio L, Berlanda N, Somigliana E. Association of endometriosis and adenomyosis with pregnancy and infertility. Fertility Sterility (2023) 119(5):727–740. doi: 10.1016/j.fertnstert.2023.03.018
95. Allaire C, Bedaiwy MA, Yong PJ. Diagnosis and management of endometriosis. CMAJ (2023) 195(10):E363–E71. doi: 10.1503/cmaj.220637
96. Lamceva J, Uljanovs R, Strumfa I. The main theories on the pathogenesis of endometriosis. Int J Mol Sci (2023) 24(5):4254. doi: 10.3390/ijms24054254
97. Huang J, Chen X, Lv Y. Hmgb1 mediated inflammation and autophagy contribute to endometriosis. Front Endocrinol (Lausanne) (2021) 12:616696. doi: 10.3389/fendo.2021.616696
98. Yun BH, Chon SJ, Choi YS, Cho S, Lee BS, Seo SK. Pathophysiology of endometriosis: role of high mobility group box-1 and toll-like receptor 4 developing inflammation in endometrium. PloS One (2016) 11(2):e0148165. doi: 10.1371/journal.pone.0148165
99. Li Y, Lv X, Jiang M, Jin Z. Sitagliptin ameliorates hypoxia-induced damages in endometrial stromal cells: An implication in endometriosis. Bioengineered (2022) 13(1):800–9. doi: 10.1080/21655979.2021.2012950
100. Yun BH, Kim S, Chon SJ, Kim GH, Choi YS, Cho S, et al. High mobility group box-1 promotes inflammation in endometriotic stromal cells through toll-like receptor 4/nuclear factor-kappa B. Am J Trans Res (2021) 13(3):1400–10.
101. Cao Y, Liu X, Guo S-W. Plasma high mobility group box 1 (Hmgb1), osteopontin (Opn), and hyaluronic acid (Ha) as admissible biomarkers for endometriosis. Sci Rep (2019) 9(1):9272. doi: 10.1038/s41598-019-45785-w
102. Huang Y, Li R, Hu R, Yao J, Yang Y. Peg2-induced pyroptosis regulates the expression of hmgb1 and promotes hem15a migration in endometriosis. Int J Mol Sci (2022) 23(19):11707. doi: 10.3390/ijms231911707
103. Jaeger-Lansky A, Schmidthaler K, Kuessel L, Gstöttner M, Waidhofer-Söllner P, Zlabinger GJ, et al. Local and systemic levels of cytokines and danger signals in endometriosis-affected women. J Reprod Immunol (2018) 130:7–10. doi: 10.1016/j.jri.2018.07.006
104. Practice Committee of the American Society for Reproductive Medicine . Definitions of infertility and recurrent pregnancy loss: A committee opinion. Fertility Sterility (2020) 113(3):533–5. doi: 10.1016/j.fertnstert.2019.11.025
105. Practice Committee of the American Society for Reproductive Medicine . Evaluation and treatment of recurrent pregnancy loss: A committee opinion. Fertility Sterility (2012) 98(5):1103–11. doi: 10.1016/j.fertnstert.2012.06.048
106. Dimitriadis E, Menkhorst E, Saito S, Kutteh WH, Brosens JJ. Recurrent pregnancy loss. Nat Rev Dis Primers (2020) 6(1):98. doi: 10.1038/s41572-020-00228-z
107. Jin H, Wu J, Yang Q, Cai Y, He W, Liu C. High mobility group box 1 protein polymorphism affects susceptibility to recurrent pregnancy loss by up-regulating gene expression in chorionic villi. J Assist Reprod Genet (2015) 32(7):1123–8. doi: 10.1007/s10815-015-0493-3
108. Wang J, Zhu D, Yin J, Ma C, Peng X, Zou H, et al. Upregulated hmgb1 levels in maternal-fetal interface of patients with unexplained recurrent spontaneous abortion from different sources. J Matern Fetal Neonatal Med (2021) 35(25):6542–6549. doi: 10.1080/14767058.2021.1918084
109. Zou H, Yin J, Zhang Z, Xiang H, Wang J, Zhu D, et al. Destruction in maternal-fetal interface of ursa patients via the increase of the hmgb1-rage/tlr2/tlr4-nf-kappab signaling pathway. Life Sci (2020) 250:117543. doi: 10.1016/j.lfs.2020.117543
110. Zhou F, Wang Y, Tan Y, Wu C, Chen Y. Hmgb1 regulates lipopolysaccharide-induced cellular dysfunction in htr8/svneo cells: Implications for the role of hmgb1 in unexplained spontaneous miscarriage. Placenta (2021) 112:16–22. doi: 10.1016/j.placenta.2021.06.012
111. Magee LA, Nicolaides KH, von Dadelszen P. Preeclampsia. N Engl J Med (2022) 386(19):1817–32. doi: 10.1056/NEJMra2109523
112. Kahramanoglu Ö, Schiattarella A, Demirci O, Sisti G, Ammaturo FP, Trotta C, et al. Preeclampsia: State of art and future perspectives. A special focus on possible preventions. J Obstet Gynaecol (2022) 42(5):766–77. doi: 10.1080/01443615.2022.2048810
113. Holmlund U, Wähämaa H, Bachmayer N, Bremme K, Sverremark-Ekström E, Palmblad K. The novel inflammatory cytokine high mobility group box protein 1 (Hmgb1) is expressed by human term placenta. Immunology (2007) 122(3):430–7. doi: 10.1111/j.1365-2567.2007.02662.x
114. Jiang R, Cai J, Zhu Z, Chen D, Wang J, Wang Q, et al. Hypoxic trophoblast hmgb1 induces endothelial cell hyperpermeability via the trl-4/caveolin-1 pathway. J Immunol (Baltimore Md 1950) (2014) 193(10):5000–12. doi: 10.4049/jimmunol.1303445
115. Pradervand PA, Clerc S, Frantz J, Rotaru C, Bardy D, Waeber B, et al. High mobility group box 1 protein (Hmgb-1): A pathogenic role in preeclampsia? Placenta (2014) 35(9):784–6. doi: 10.1016/j.placenta.2014.06.370
116. Naruse K, Sado T, Noguchi T, Tsunemi T, Yoshida S, Akasaka J, et al. Peripheral rage (Receptor for advanced glycation endproducts)-ligands in normal pregnancy and preeclampsia: Novel markers of inflammatory response. J Reprod Immunol (2012) 93(2):69–74. doi: 10.1016/j.jri.2011.12.003
117. CW I, Romão-Veiga M, Matias ML, Fioratti EG, Peraçoli JC, Borges VT, et al. Increased expression of nlrp3 inflammasome in placentas from pregnant women with severe preeclampsia. J Reprod Immunol (2017) 123:40–7. doi: 10.1016/j.jri.2017.09.002
118. Xiao X, Xiao F, Zhao M, Tong M, Wise MR, Stone PR, et al. Treating normal early gestation placentae with preeclamptic sera produces extracellular micro and nano vesicles that activate endothelial cells. J Reprod Immunol (2017) 120:34–41. doi: 10.1016/j.jri.2017.04.004
119. Barnie PA, Lin X, Liu Y, Xu H, Su Z. Il-17 producing innate lymphoid cells 3 (Ilc3) but not th17 cells might be the potential danger factor for preeclampsia and other pregnancy associated diseases. Int J Clin Exp Pathol (2015) 8(9):11100–7.
120. Oda H, Nagamatsu T, Schust DJ, Cabral H, Miyazaki T, Iriyama T, et al. Recombinant thrombomodulin attenuates preeclamptic symptoms by inhibiting high-mobility group box 1 in mice. Endocrinology (2021) 162(4):bqaa248. doi: 10.1210/endocr/bqaa248
121. Liu F, Yang X, Xing J, Han K, Sun Y. Glycyrrhizin potentially suppresses the inflammatory response in preeclampsia rat model. Pregnancy Hypertens (2021) 23:34–40. doi: 10.1016/j.preghy.2020.10.007
122. Jabalie G, Ahmadi M, Koushaeian L, Eghbal-Fard S, Mehdizadeh A, Kamrani A, et al. Metabolic syndrome mediates proinflammatory responses of inflammatory cells in preeclampsia. Am J Reprod Immunol (2019) 81(3):e13086. doi: 10.1111/aji.13086
123. Zhou CC, Irani RA, Dai Y, Blackwell SC, Hicks MJ, Ramin SM, et al. Autoantibody-mediated il-6-dependent endothelin-1 elevation underlies pathogenesis in a mouse model of preeclampsia. J Immunol (Baltimore Md 1950) (2011) 186(10):6024–34. doi: 10.4049/jimmunol.1004026
124. Kim J, Lee K-S, Kim J-H, Lee D-K, Park M, Choi S, et al. Aspirin prevents tnf-Α-induced endothelial cell dysfunction by regulating the nf-Κb-dependent mir-155/enos pathway: Role of a mir-155/enos axis in preeclampsia. Free Radic Biol Med (2017) 104:185–98. doi: 10.1016/j.freeradbiomed.2017.01.010
125. Akasaka J, Naruse K, Sado T, Uchiyama T, Makino M, Yamauchi A, et al. Involvement of receptor for advanced glycation endproducts in hypertensive disorders of pregnancy. Int J Mol Sci (2019) 20(21):5462. doi: 10.3390/ijms20215462
126. Tangerås LH, Silva GB, Stødle GS, Gierman LM, Skei B, Collett K, et al. Placental inflammation by hmgb1 activation of tlr4 at the syncytium. Placenta (2018) 72-73:53–61. doi: 10.1016/j.placenta.2018.10.011
127. Zhao M, Feng Y, Xiao J, Liang J, Yin Y, Chen D. Sodium tanshinone iia sulfonate prevents hypoxic trophoblast-induced endothelial cell dysfunction via targeting hmgb1 release. J Biochem Mol Toxicol (2017) 31(7):21903. doi: 10.1002/jbt.21903
128. McIntyre HD, Catalano P, Zhang C, Desoye G, Mathiesen ER, Damm P. Gestational diabetes mellitus. Nat Rev Dis Primers (2019) 5(1):47. doi: 10.1038/s41572-019-0098-8
129. Iams JD. Clinical practice. Prevention of preterm parturition. N Engl J Med (2014) 370(3):254–61. doi: 10.1056/NEJMcp1103640
130. Giacobbe A, Granese R, Grasso R, Salpietro V, Corrado F, Giorgianni G, et al. Association between maternal serum high mobility group box 1 levels and pregnancy complicated by gestational diabetes mellitus. Nutr Metab Cardiovasc Dis (2016) 26(5):414–8. doi: 10.1016/j.numecd.2016.02.007
131. Hill AV, Menon R, Perez-Patron M, Carrillo G, Xu X, Taylor BD. High-mobility group box 1 at the time of parturition in women with gestational diabetes mellitus. Am J Reprod Immunol (2019) 82(5):e13175. doi: 10.1111/aji.13175
132. Nativel B, Marimoutou M, Thon-Hon VG, Gunasekaran MK, Andries J, Stanislas G, et al. Soluble hmgb1 is a novel adipokine stimulating il-6 secretion through rage receptor in sw872 preadipocyte cell line: Contribution to chronic inflammation in fat tissue. PloS One (2013) 8(9):e76039. doi: 10.1371/journal.pone.0076039
133. Casirati A, Somaschini A, Perrone M, Vandoni G, Sebastiani F, Montagna E, et al. Preterm birth and metabolic implications on later life: A narrative review focused on body composition. Front Nutr (2022) 9:978271. doi: 10.3389/fnut.2022.978271
134. Wise J. Un warns of preterm birth rates flatlining in every region. BMJ (Clinical Res ed) (2023) 381:1054. doi: 10.1136/bmj.p1054
135. Chawanpaiboon S, Vogel JP, Moller A-B, Lumbiganon P, Petzold M, Hogan D, et al. Global, regional, and national estimates of levels of preterm birth in 2014: A systematic review and modelling analysis. Lancet Glob Health (2019) 7(1):e37–46. doi: 10.1016/S2214-109X(18)30451-0
136. Boeckel S, Hrabalkova L, Baker TL, MacPherson H, Frew L, Boyle AK, et al. Cathelicidins and the onset of labour. Sci Rep (2019) 9(1):7356. doi: 10.1038/s41598-019-43766-7
137. Goldenberg RL, Culhane JF, Iams JD, Romero R. Epidemiology and causes of preterm birth. Lancet (2008) 371(9606):75–84. doi: 10.1016/S0140-6736(08)60074-4
138. Splichalova A, Slavikova V, Splichalova Z, Splichal I. Preterm life in sterile conditions: A study on preterm, germ-free piglets. Front In Immunol (2018) 9:220. doi: 10.3389/fimmu.2018.00220
139. Galaz J, Romero R, Arenas-Hernandez M, Panaitescu B, Para R, Gomez-Lopez N. Betamethasone as a potential treatment for preterm birth associated with sterile intra-amniotic inflammation: A murine study. J Perinatal Med (2021) 49(7):897–906. doi: 10.1515/jpm-2021-0049
140. Baumbusch MA, Buhimschi CS, Oliver EA, Zhao G, Thung S, Rood K, et al. High mobility group-box 1 (Hmgb1) levels are increased in amniotic fluid of women with intra-amniotic inflammation-determined preterm birth, and the source may be the damaged fetal membranes. Cytokine (2016) 81:82–7. doi: 10.1016/j.cyto.2016.02.013
141. Girard S, Heazell AEP, Derricott H, Allan SM, Sibley CP, Abrahams VM, et al. Circulating cytokines and alarmins associated with placental inflammation in high-risk pregnancies. Am J Reprod Immunol (New York NY 1989) (2014) 72(4):422–34. doi: 10.1111/aji.12274
142. Bredeson S, Papaconstantinou J, Deford JH, Kechichian T, Syed TA, Saade GR, et al. Hmgb1 promotes a P38mapk associated non-infectious inflammatory response pathway in human fetal membranes. PloS One (2014) 9(12):e113799. doi: 10.1371/journal.pone.0113799
143. Romero R, Chaiworapongsa T, Savasan ZA, Hussein Y, Dong Z, Kusanovic JP, et al. Clinical chorioamnionitis is characterized by changes in the expression of the alarmin hmgb1 and one of its receptors, srage. J Matern Fetal Neonatal Med (2012) 25(6):558–67. doi: 10.3109/14767058.2011.599083
144. Dubicke A, Fransson E, Centini G, Andersson E, Byström B, Malmström A, et al. Pro-inflammatory and anti-inflammatory cytokines in human preterm and term cervical ripening. J Reprod Immunol (2010) 84(2):176–85. doi: 10.1016/j.jri.2009.12.004
145. Dubicke A, Andersson P, Fransson E, Andersson E, Sioutas A, Malmström A, et al. High-mobility group box protein 1 and its signaling receptors in human preterm and term cervix. J Reprod Immunol (2010) 84(1):86–94. doi: 10.1016/j.jri.2009.09.010
146. Plazyo O, Romero R, Unkel R, Balancio A, Mial TN, Xu Y, et al. Hmgb1 induces an inflammatory response in the chorioamniotic membranes that is partially mediated by the inflammasome. Biol Reprod (2016) 95(6):130. doi: 10.1095/biolreprod.116.144139
147. Qiu XY, Sun L, Han XL, Chang Y, Cheng L, Yin LR. Alarmin high mobility group box-1 in maternal serum as a potential biomarker of chorioamnionitis-associated preterm birth. Gynecol Endocrinol (2017) 33(2):128–31. doi: 10.1080/09513590.2016.1214260
148. Peng J, Jiang J, Wang H, Feng X, Dong X. Mir−199a−3p suppresses cervical epithelial cell inflammation by inhibiting the hmgb1/tlr4/nf−Κb pathway in preterm birth. Mol Med Rep (2020) 22(2):926–38. doi: 10.3892/mmr.2020.11184
149. Son GH, Kim Y, Lee JJ, Lee KY, Ham H, Song JE, et al. Microrna-548 regulates high mobility group box 1 expression in patients with preterm birth and chorioamnionitis. Sci Rep (2019) 9(1):19746. doi: 10.1038/s41598-019-56327-9
150. Romero R, Parvizi ST, Oyarzun E, Mazor M, Wu YK, Avila C, et al. Amniotic fluid interleukin-1 in spontaneous labor at term. J Reprod Med (1990) 35(3):235–8.
151. Romero R, Mazor M, Brandt F, Sepulveda W, Avila C, Cotton DB, et al. Interleukin-1 alpha and interleukin-1 beta in preterm and term human parturition. Am J Reprod Immunol (New York NY 1989) (1992) 27(3-4):117–23. doi: 10.1111/j.1600-0897.1992.tb00737.x
152. Romero R, MIranda J, Chaiworapongsa T, Korzeniewski SJ, Chaemsaithong P, Gotsch F, et al. Prevalence and clinical significance of sterile intra-amniotic inflammation in patients with preterm labor and intact membranes. Am J Reprod Immunol (New York NY 1989) (2014) 72(5):458–74. doi: 10.1111/aji.12296
153. Kato M, Negishi Y, Shima Y, Kuwabara Y, Morita R, Takeshita T. Inappropriate activation of invariant natural killer T cells and antigen-presenting cells with the elevation of hmgb1 in preterm births without acute chorioamnionitis. Am J Reprod Immunol (2021) 85(1):e13330. doi: 10.1111/aji.13330
154. Yan H, Zhu L, Zhang Z, Li H, Li P, Wang Y, et al. Hmgb1-rage signaling pathway in pprom. Taiwanese J Obstetrics Gynecology (2018) 57(2):211–6. doi: 10.1016/j.tjog.2018.02.008
155. Wahab S, Annadurai S, Abullais SS, Das G, Ahmad W, Ahmad MF, et al. Glycyrrhiza glabra (Licorice): A comprehensive review on its phytochemistry, biological activities, clinical evidence and toxicology. Plants (Basel) (2021) 10(12):2751. doi: 10.3390/plants10122751
156. Luo P, Liu D, Li J. Pharmacological perspective: Glycyrrhizin may be an efficacious therapeutic agent for covid-19. Int J Antimicrob Agents (2020) 55(6):105995. doi: 10.1016/j.ijantimicag.2020.105995
157. Mollica L, De Marchis F, Spitaleri A, Dallacosta C, Pennacchini D, Zamai M, et al. Glycyrrhizin binds to high-mobility group box 1 protein and inhibits its cytokine activities. Chem Biol (2007) 14(4):431–41. doi: 10.1016/j.chembiol.2007.03.007
158. Paudel YN, Khan SU, Othman I, Shaikh MF. Naturally occurring hmgb1 inhibitor, glycyrrhizin, modulates chronic seizures-induced memory dysfunction in zebrafish model. ACS Chem Neurosci (2021) 12(18):3288–302. doi: 10.1021/acschemneuro.0c00825
159. Zhu K, Zhu X, Liu S, Yu J, Wu S, Hei M. Glycyrrhizin attenuates hypoxic-ischemic brain damage by inhibiting ferroptosis and neuroinflammation in neonatal rats via the hmgb1/gpx4 pathway. Oxid Med Cell Longev (2022) 2022:8438528. doi: 10.1155/2022/8438528
160. Wu Z, Wang Z, Xie Z, Zhu H, Li C, Xie S, et al. Glycyrrhizic Acid Attenuates the Inflammatory Response after Spinal Cord Injury by Inhibiting High Mobility Group Box-1 Protein through the P38/Jun N-Terminal Kinase Signaling Pathway. World Neurosurg (2022) 158:e856–e64. doi: 10.1016/j.wneu.2021.11.085
161. Wang Z, Yang X, Wang X, Liang F, Tang Y. Glycyrrhizin attenuates caspase-11-dependent immune responses and coagulopathy by targeting high mobility group box 1. Int Immunopharmacol (2022) 107:108713. doi: 10.1016/j.intimp.2022.108713
162. Niu Z, Lin J, Hao C, Xu X, Wang C, Dai K, et al. Glycyrrhizic Acid Attenuates Pulmonary Fibrosis of Silicosis by Inhibiting the Interaction between Hmgb1 and Brg1 through Pi3k/Akt/Mtor Pathway. Int J Environ Res Public Health (2022) 19(14):8743. doi: 10.3390/ijerph19148743
163. Jain R, Hussein MA, Pierce S, Martens C, Shahagadkar P, Munirathinam G. Oncopreventive and oncotherapeutic potential of licorice triterpenoid compound glycyrrhizin and its derivatives: molecular insights. Pharmacol Res (2022) 178:106138. doi: 10.1016/j.phrs.2022.106138
164. Yuan Y, Li B, Peng W, Xu Z. Protective effect of glycyrrhizin on coronary microembolization-induced myocardial dysfunction in rats. Pharmacol Res Perspect (2021) 9(1):e00714. doi: 10.1002/prp2.714
165. Saïdi H, Melki MT, Gougeon ML. Hmgb1-dependent triggering of hiv-1 replication and persistence in dendritic cells as a consequence of nk-dc cross-talk. PloS One (2008) 3(10):e3601. doi: 10.1371/journal.pone.0003601
166. Cheng S, Huang Z, Banerjee S, Jash S, Buxbaum JN, Sharma S. Evidence from human placenta, endoplasmic reticulum-stressed trophoblasts, and transgenic mice links transthyretin proteinopathy to preeclampsia. Hypertension (2022) 79(8):1738–54. doi: 10.1161/HYPERTENSIONAHA.121.18916
167. Heim KR, Mulla MJ, Potter JA, Han CS, Guller S, Abrahams VM. Excess glucose induce trophoblast inflammation and limit cell migration through hmgb1 activation of toll-like receptor 4. Am J Reprod Immunol (2018) 80(5):e13044. doi: 10.1111/aji.13044
168. Boron M, Hauzer-Martin T, Keil J, Sun X-L. Circulating thrombomodulin: release mechanisms, measurements, and levels in diseases and medical procedures. TH Open (2022) 6(3):e194–212. doi: 10.1055/a-1801-2055
169. Conway EM. Thrombomodulin and its role in inflammation. Semin Immunopathol (2012) 34(1):107–25. doi: 10.1007/s00281-011-0282-8
170. Li Y-H, Kuo C-H, Shi G-Y, Wu H-L. The role of thrombomodulin lectin-like domain in inflammation. J Biomed Sci (2012) 19(1):34. doi: 10.1186/1423-0127-19-34
171. Herzog C, Lorenz A, Gillmann H-J, Chowdhury A, Larmann J, Harendza T, et al. Thrombomodulin's lectin-like domain reduces myocardial damage by interfering with hmgb1-mediated tlr2 signalling. Cardiovasc Res (2014) 101(3):400–10. doi: 10.1093/cvr/cvt275
172. Ito T, Kawahara K, Okamoto K, Yamada S, Yasuda M, Imaizumi H, et al. Proteolytic cleavage of high mobility group box 1 protein by thrombin-thrombomodulin complexes. Arterioscler Thromb Vasc Biol (2008) 28(10):1825–30. doi: 10.1161/atvbaha.107.150631
173. Watanabe-Kusunoki K, Nakazawa D, Ishizu A, Atsumi T. Thrombomodulin as a physiological modulator of intravascular injury. Front In Immunol (2020) 11:575890. doi: 10.3389/fimmu.2020.575890
174. Oda H, Nagamatsu T, Cabral H, Miyazaki T, Iriyama T, Kawana K, et al. Thrombomodulin promotes placental function by up-regulating placental growth factor via inhibition of high-mobility-group box 1 and hypoxia-inducible factor 1α. Placenta (2021) 111:1–9. doi: 10.1016/j.placenta.2021.06.002
175. Kohli S, Singh KK, Gupta A, Markmeyer P, Lochmann F, Gupta D, et al. Placental thromboinflammation impairs embryonic survival by reducing placental thrombomodulin expression. Blood (2021) 137(7):977–82. doi: 10.1182/blood.2020005225
176. Sano T, Terai Y, Daimon A, Nunode M, Nagayasu Y, Okamoto A, et al. Recombinant human soluble thrombomodulin as an anticoagulation therapy improves recurrent miscarriage and fetal growth restriction due to placental insufficiency - the leading cause of preeclampsia. Placenta (2018) 65:1–6. doi: 10.1016/j.placenta.2018.03.006
177. Oda H, Nagamatsu T, Osuga Y. Thrombomodulin and pregnancy in the limelight: Insights into the therapeutic aspect of thrombomodulin in pregnancy complications. J Thromb Haemost (2022) 20(5):1040–55. doi: 10.1111/jth.15680
178. Zenerino C, Nuzzo AM, Giuffrida D, Biolcati M, Zicari A, Todros T, et al. The hmgb1/rage pro-inflammatory axis in the human placenta: Modulating effect of low molecular weight heparin. Molecules (2017) 22(11):1997. doi: 10.3390/molecules22111997
179. Mazarico E, Molinet-Coll C, Martinez-Portilla RJ, Figueras F. Heparin therapy in placental insufficiency: Systematic review and meta-analysis. Acta Obstet Gynecol Scand (2020) 99(2):167–74. doi: 10.1111/aogs.13730
180. Guerby P, Fillion A, O'Connor S, Bujold E. Heparin for preventing adverse obstetrical outcomes in pregnant women with antiphospholipid syndrome, a systematic review and meta-analysis. J Gynecology Obstetrics Hum Reprod (2021) 50(2):101974. doi: 10.1016/j.jogoh.2020.101974
181. Zheng L, Xia B, Yuan Y, Wang Y, Wang Y. Low-molecular-weight heparin in addition to low-dose aspirin for preventing preeclampsia and its complications: A systematic review and meta-analysis. Front Cardiovasc Med (2022) 9:1073148. doi: 10.3389/fcvm.2022.1073148
182. Ling Y, Yang Z-Y, Yin T, Li L, Yuan W-W, Wu H-S, et al. Heparin changes the conformation of high-mobility group protein 1 and decreases its affinity toward receptor for advanced glycation endproducts in vitro. Int Immunopharmacol (2011) 11(2):187–93. doi: 10.1016/j.intimp.2010.11.014
183. Liu R, Mori S, Wake H, Zhang J, Liu K, Izushi Y, et al. Establishment of in vitro binding assay of high mobility group box-1 and S100a12 to receptor for advanced glycation endproducts: Heparin's effect on binding. Acta Med Okayama (2009) 63(4):203–11. doi: 10.18926/AMO/31812
184. Yinon Y, Ben Meir E, Margolis L, Lipitz S, Schiff E, Mazaki-Tovi S, et al. Low molecular weight heparin therapy during pregnancy is associated with elevated circulatory levels of placental growth factor. Placenta (2015) 36(2):121–4. doi: 10.1016/j.placenta.2014.12.008
185. Choi HW, Tian M, Song F, Venereau E, Preti A, Park S-W, et al. Aspirin's active metabolite salicylic acid targets high mobility group box 1 to modulate inflammatory responses. Mol Med (Cambridge Mass) (2015) 21(1):526–35. doi: 10.2119/molmed.2015.00148
186. Zheng J, Tian M, Liu L, Jia X, Sun M, Lai Y. Magnesium sulfate reduces vascular endothelial cell apoptosis in rats with preeclampsia via the mir-218-5p/hmgb1 pathway. Clin Exp Hypertension (New York NY 1993) (2022) 44(2):159–66. doi: 10.1080/10641963.2021.2013492
187. Zhong M, Peng J, Xiang L, Yang X, Wang X, Zhu Y. Epigallocatechin gallate (Egcg) improves anti-angiogenic state, cell viability, and hypoxia-induced endothelial dysfunction by downregulating high mobility group box 1 (Hmgb1) in preeclampsia. Med Sci Monit (2020) 26:e926924. doi: 10.12659/msm.926924
188. Galaz J, Romero R, Arenas-Hernandez M, Farias-Jofre M, Motomura K, Liu Z, et al. Clarithromycin prevents preterm birth and neonatal mortality by dampening alarmin-induced maternal-fetal inflammation in mice. BMC Pregnancy Childbirth (2022) 22(1):503. doi: 10.1186/s12884-022-04764-2
189. Xu X, Wang J, Zhu D, Yin J, Liu J, Wu X, et al. Low-dose aspirin protects unexplained recurrent spontaneous abortion via downregulation of hmgb1 inflammation activation. Front Endocrinol (Lausanne) (2022) 13:914030. doi: 10.3389/fendo.2022.914030
Keywords: HMGB1, pregnancy complications, female reproductive system diseases, inflammation, anti-HMGB1
Citation: Ren Y, Zhu D, Han X, Zhang Q, Chen B, Zhou P, Wei Z, Zhang Z, Cao Y and Zou H (2023) HMGB1: a double-edged sword and therapeutic target in the female reproductive system. Front. Immunol. 14:1238785. doi: 10.3389/fimmu.2023.1238785
Received: 12 June 2023; Accepted: 03 August 2023;
Published: 18 August 2023.
Edited by:
Myoungsun Son, Feinstein Institute for Medical Research, United StatesReviewed by:
Huan Yang, Feinstein Institute for Medical Research, United StatesClaire Enid Kendal-Wright, Chaminade University of Honolulu, United States
Ozlem Guzeloglu-Kayisli, University of South Florida, United States
Copyright © 2023 Ren, Zhu, Han, Zhang, Chen, Zhou, Wei, Zhang, Cao and Zou. This is an open-access article distributed under the terms of the Creative Commons Attribution License (CC BY). The use, distribution or reproduction in other forums is permitted, provided the original author(s) and the copyright owner(s) are credited and that the original publication in this journal is cited, in accordance with accepted academic practice. No use, distribution or reproduction is permitted which does not comply with these terms.
*Correspondence: Huijuan Zou, hienjoyshine@aliyun.com; Yunxia Cao, caoyunxia6@126.com; Zhiguo Zhang, zzg_100@163.com