- 1Department of Gastroenterology, Jinhua Wenrong Hospital, Jinhua, Zhejiang, China
- 2Department of Surgical Oncology, Hangzhou Cancer Hospital, Hangzhou, Zhejiang, China
- 3Department of Colorectal Surgery, Affiliated Jinhua Hospital, Zhejiang University School of Medicine, Jinhua, Zhejiang, China
- 4Department of Colorectal Surgery, the First Affiliated Hospital, Zhejiang University School of Medicine, Hangzhou, Zhejiang, China
As one of the main threats to human life (the fourth most dangerous and prevalent cancer), colorectal cancer affects many people yearly, decreases patients’ quality of life, and causes irreparable financial and social damages. In addition, this type of cancer can metastasize and involve the liver in advanced stages. However, current treatments can’t completely eradicate this disease. Chemotherapy and subsequent surgery can be mentioned among the current main treatments for this disease. Chemotherapy has many side effects, and regarding the treatment of this type of tumor, chemotherapy can lead to liver damage, such as steatohepatitis, steatosis, and sinus damage. These damages can eventually lead to liver failure and loss of its functions. Therefore, it seems that other treatments can be used in addition to chemotherapy to increase its efficiency and reduce its side effects. Biological therapies and immunotherapy are one of the leading suggestions for combined treatment. Antibodies (immune checkpoint blockers) and cell therapy (DC and CAR-T cells) are among the immune system-based treatments used to treat tumors. Immunotherapy targets various aspects of the tumor that may lead to 1) the recruitment of immune cells, 2) increasing the immunogenicity of tumor cells, and 3) leading to the elimination of inhibitory mechanisms established by the tumor. Therefore, immunotherapy can be used as a complementary treatment along with chemotherapy. This review will discuss different chemotherapy and immunotherapy methods for colorectal cancer. Then we will talk about the studies that have dealt with combined treatment.
1 Introduction
According to GLOBOCAN data, colorectal cancer (CRC) is the third most common cancer in men and the second in women. It is also known that its mortality is higher in men than in women (1). This type of cancer has the highest incidence rate in Europe, Australia, North America, and New Zealand (2). Instead, the incidence of this disease in Africa and South-Central Asia is the lowest among others (3). It seems that diet, environment, and genetics are the most influential factors in the susceptibility to this disease. Colorectal cancer (CRC) is divided into two groups based on DNA stability, mutation, and repair (4). The first type is characterized by DNA mismatch repair (dMMR), a high level of microsatellite instability (MSI-H), and reduced expression of beta 2 microglobulin (B2MG) (presence in the MHC-1 structure and contributing to its stability at the cell surface) (5). The second type has stable microsatellites (MSI-L) and is mismatch-repair-proficient (pMMR) (6). In addition, these two types of CRC differ in immune checkpoint ligands expression level, MSI-H tumors have high expression, and MSI-L type has low expression of immune checkpoint ligands (7, 8). Due to this characteristic, the two types of CRC respond differently to different immunotherapy treatments and knowing the type of colorectal cancer is essential for immunotherapy. As expected, cancers with instability in DNA experience fewer repairs and stably undergo mutations (9, 10). These mutations lead to the production of protein antigens (presented and new peptides on the surface of tumor cells) called neoantigens which have better immunogenicity than tumors with a lower mutation and show a high level of DNA repair (11).
Also, in another type of classification, four consensus molecular subtypes (CMSs) with a distinguishing feature are described: CMS1 (microsatellite instability immune, 14%), hypermutated, microsatellite unstable and robust immune activation; CMS2 (canonical, 37%), epithelial, marked WNT and MYC signaling activation; CMS3 (metabolic, 13%), epithelial and evident metabolic dysregulation; and CMS4 (mesenchymal, 23%), prominent TGF-β activation, stromal invasion, and angiogenesis. Samples with mixed features (13%) possibly represent a transition phenotype or intratumoral heterogeneity. So knowing the type of CRC is very important in choosing the proper treatment (12).
In addition, the results of studies have shown that chemotherapy as monotherapy cannot completely remove the tumor (13). Sometimes, even after surgery, the cancer recurs and can disrupt the patient’s life (14). Therefore, the use of simultaneous and combined treatments is suggested. One of the most important treatments that have received much attention today is tumor immunotherapy, which has shown promising results (15). Immunotherapy includes various treatments based on antibodies and T cell transfer; these are among the most critical cells used in CRC immunotherapy, and they are used in three ways, expanded without any change, TCR genetic manipulation, and CAR-T cell application (16). Also, dendritic cells (DCs) can be used to treat various tumors (17). Antibodies (Ab) used in the treatment of tumors target multiple pathways of tumor progression, including angiogenesis, tumor growth, metastasis, and immune suppression mechanisms, which have been very promising (18, 19). One group of the most important antibodies used in treating CRC (even in MSS type) is immune checkpoint blockers, which suppress the pathways developed by tumor cells to suppress the immune system (20, 21). In this article, we will first talk about approved chemotherapy and their combined uses. Then we will discuss the various available immunotherapies. Finally, we will discuss the talk about studies that use the combination of chemotherapy and immunotherapy for CRC treatment.
2 Colorectal cancer chemotherapy
Chemotherapy is one of the first treatment strategies after tumor diagnosis (22). However, this type of treatment should be personalized according to the patient’s tumor characteristics (23). Among the elements that should be checked include the general state of health, biology of the tumor (its aggressiveness), side effects of the chemotherapy regimen, left and right laterality and the primary location of the tumor, the drugs currently taken, other co-morbidities, and mutation status of important genes in colorectal cancer, including genes related to RAS and BRAF in tumor cells (24, 25). Mutations of RAS and BRAF genes can activate cell signaling pathways related to cell proliferation and differentiation (26). Investigating these types of mutations is very important because they can lead to resistance of cancer cells to treatment with EGFR inhibitors (27). Some chemotherapy drugs that the has approved include 5-fluorouracil, irinotecan, oxaliplatin, trifluoridine-tipiracil, and capecitabine (28, 29). These drugs exert their anti-tumor effects by different cells affecting growth pathways. However, using these drugs is not without harm to healthy cells, and they have different side effects, which we will discuss later.
2.1 Colorectal cancer treatment by 5-fluorouracil (5-FU)
5-FU (uracil analog) is an anti-metabolite drug (30) that replaces fluorine with hydrogen at the C5 position of uracil and ultimately leads to the formation of adenine-uracil/5-FU base pairs (31). 5-FU, after the entrance to a cell by the facilitated transport mechanism, converted intracellularly to several active metabolites, including 1) fluorouridine triphosphate (FUTP), 2) fluorodeoxyuridine triphosphate (FdUTP), and 3) fluorodeoxyuridine monophosphate (FdUMP) (31, 32). 5-FU normally exerts its antitumor effects (mediated by active metabolites) by three mechanisms. This drug can inhibit thymidylate synthase (TS) (33). This action disrupts intracellular deoxynucleotide pools required for DNA replication and cell proliferation (34). In addition, this drug can replace more than 40% of cellular RNA uracils, which can lead to the disruption of RNA synthesis (35). Also, this drug can be attached to cellular DNA after its anabolism inside the cell, leading to DNA fragmentation (36).
5-FU has been used orally or intravenously since 1990 (37, 38). However, due to the considerable variation in pharmacokinetics and unpredictable absorption, its oral use is not recommended and has been abandoned (39). The results of studies show that only 3% of 5-FU (prescribed dose) becomes toxic to cancer cells through anabolic actions (40). Although most of the administrated amounts of 5-FU are catabolized in the liver through the activity of the dihydropyridine dehydrogenase enzyme and turn into a non-toxic and inactive metabolite (41). Also, studies have shown that 20% of 5-FU prescribed through infusion is excreted through urine directly and without any change (42).
One of the drugs that can be used alongside 5-FU and increase its therapeutic efficiency is leucovorin (LV) (43, 44). The simultaneous use of these two drugs leads to an increase in the survival of patients, a decrease in side effects (chemoprotection), and an increase in the therapeutic potential of 5-FU (45). Side effects associated with the therapeutic use of 5-FU are divided into three categories. The first category is related to the effects of this drug on general conditions, including fatigue, mucositis, vomiting, diarrhea, nausea, fever, and stomatitis (46). The second category is its effects on immune system cells, blood cells, and other healthy cells, and it includes neutropenia, anemia, leukopenia, thrombocytopenia, skin rashes, and neuropathy (47). The third category comprises neurological abnormalities, including changes in cognitive function and cerebellar ataxia, which occur less often than the previous two categories (48). Cardiotoxicity (although its pathogenesis has not been completely determined) is one of the side effects of chemotherapy with 5-FU, which rarely happens, but can seriously affect the patient’s health (49).
Also, dihydropyrimidine dehydrogenase (DPYD), as a highly polymorphic gene, may be affected the treatment outcome in fluoropyrimidine-based treatments (50, 51). The product of this gene is the rate-limiting enzyme in fluoropyrimidine metabolism, or dihydropyrimidine dehydrogenase (DPD), whose function defects can lead to the accumulation of toxic metabolites from fluoropyrimidine (51). Investigations show that in patients with DPYD pathogenic variants receiving the standard dose of fluoropyrimidine chemotherapy, the risk of death due to treatment increases (52). Therefore, considering that the variants of this gene have been identified to a certain extent, it is recommended to investigate the variants of this gene before approving this type of drug. The results have shown that c.2194G>A is the most common polymorphism associated with DPYD, which is also associated with neutropenia (53). However, there is still no suitable single-nucleotide polymorphism (SNPs) panel to investigate DPYD variants, requiring more investigations.
The results of various studies show that this drug has multiple effects, including oxidative stress that causes myocardial damage, coronary artery spasm, ischemia caused by impaired oxygen transfer from red blood cells, and endothelial damage leading to thrombosis, and it causes cardiotoxicity and heart tissue damage (54). However, considering the therapeutic effects of 5-FU regardless of its side effects, this drug is being used in combination with other drugs in many clinical trials (Table 1).
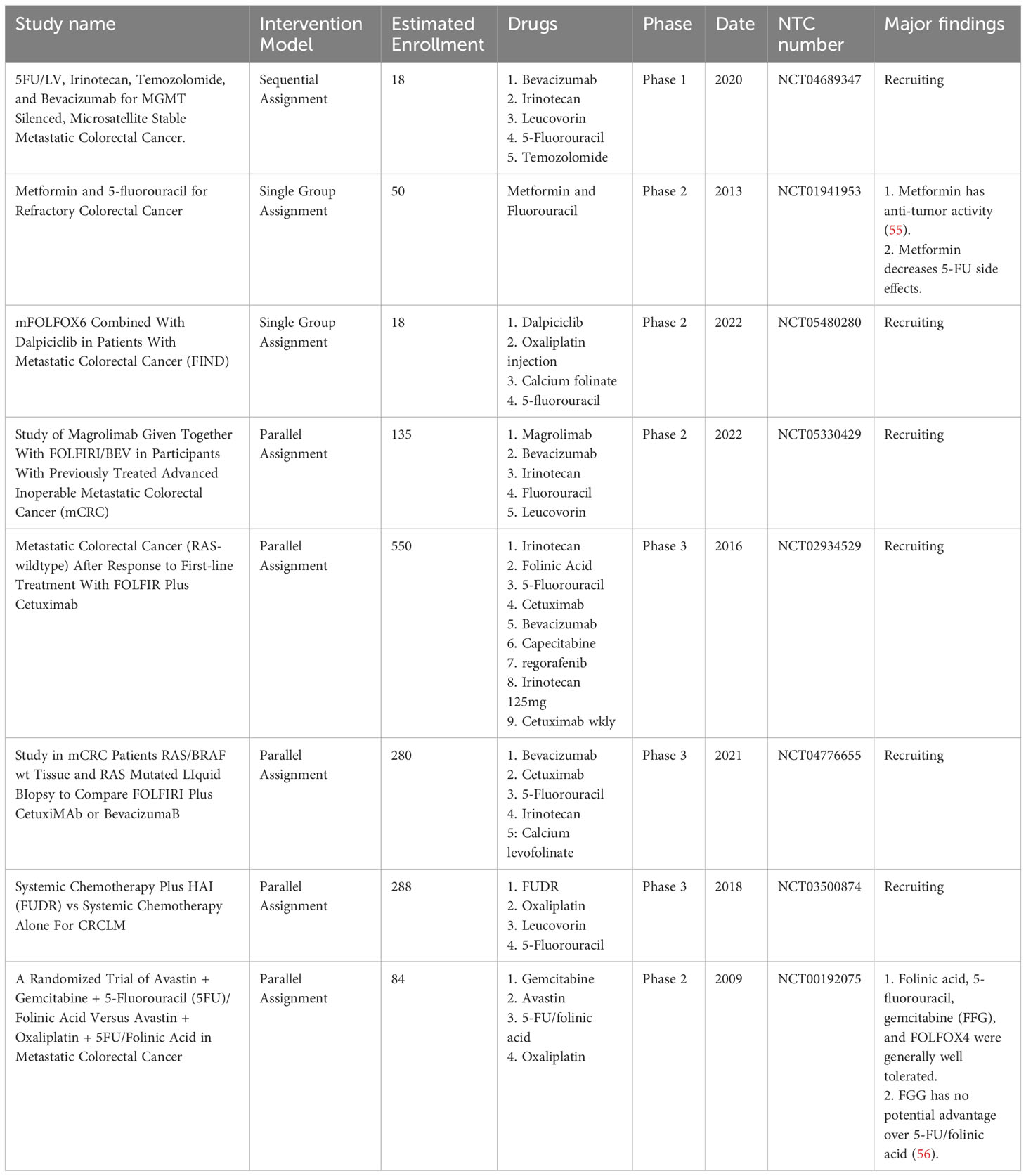
Table 1 Example of chemotherapy drugs application in combination with Fluorouracil in clinical trials.
2.2 Colorectal cancer treatment by capecitabine
Despite the success of using 5-FU in treating colorectal cancer, due to the short half-life of this drug (requiring multiple injections) and its rapid clearance from the body, researchers are looking for a way to redesign and use the therapeutic advantages of this drug (57). The results of researchers’ efforts in 2009 led to the discovery of capecitabine, a prodrug of 5-FU, which has advantages over 5-FU (58). Unlike 5-FU, this drug is oral, and after being absorbed through the patient’s digestive system, it is converted into 5-FU by successive enzymatic reactions, first in the liver and then in the tumor site (3 reactions) (58, 59). Thymidine phosphorylase (TP) is expressed in higher concentrations in neoplastic tissue (60, 61). This enzyme mediates capecitabine final conversion from 5’-deoxy-5-fluorouridine to 5-FU; therefore, the production of the active form of the drug is preferably done in tumor tissue (62, 63). Also, the results of studies have shown that the level of TP expression in tumor tissue is increased after exposure to radiotherapy and cytotoxic agents to help tumor eradication synergistically (64).
2.3 Colorectal cancer treatment by irinotecan (IRI)
IRI is a water-soluble semi-synthetic chemotherapy drug derived from camptothecin, which was approved for the treatment of lung cancer, cervical cancer, and ovarian cancer in Japan in 1994 (65, 66). This drug is also used to treat metastatic colorectal cancer (67). The results of clinical trials showed that the combination of IRI with 5-FU/LV can significantly increase the survival of patients compared to the 5-FU/LV receiving group (68). Also, this drug is used in combination with oxaliplatin and can help to improve patients’ conditions by inhibiting metastasis (69–71). Irinotecan has an acceptable tolerability profile, increases the duration of treatment, is not associated with cumulative toxicity in patients with metastatic CRC, and leads to improved patient survival and quality of life (QOL) (66). Various studies have shown that exposure to ethyl-10-hydroxy-camptothecin (SN38), the active metabolite of irinotecan, shows different results in different people and can lead to severe toxicity in patients receiving the drug (72). Also, the drug dosage should be strictly controlled in some patients, including patients with severe renal failure and patients with UDP-glucuronosyltransferase 1A1 (UGT1A1) polymorphism (73).
IRI exerts its antitumor function usually by inhibiting topoisomerase I (74). However, the results of studies show that this is not the only functional mechanism of IRI. Cells exposed to IRI experience extensive gene expression changes. SN38 interacts with the various vital proteins for the cell, including BCL-xL (75), which has an anti-apoptotic role, up-regulation of FAS (76), mouse double minute 2 homolog (MDM2) involved in TP53-mediated cell death (77), and activation of MAPK signaling pathway can lead to increased cancer cells apoptosis (78). The excretion and pharmacokinetics of IRI depend on several factors, such as dosage, liver function status, age, administration time, and gender (79).
One of the side effects of IRI application is neutropenia (more common in women than in men) in receiving patients (80). Several side effects with the use of this chemotherapy drug, including delayed and severe diarrhea, abdominal pain, steatohepatitis, metabolic changes in patients’ plasma (accumulation of acylcarnitines, nucleobases, and certain amino acids in plasma), similar cholinergic symptoms, oxidative stress in the liver and sweating they experience (81).
2.4 Colorectal cancer treatment by oxaliplatin (Ox)
Oxaliplatin is a platinum-derived drug used to treat metastatic colorectal cancer distributed throughout the body by binding to plasma proteins (82). About half of the Ox injected into patients is eliminated through urine, but its excretion in feces is insignificant (clearance unrelated to liver function) (83). Most of the side effects of this drug are related to the release of platinum-active species (84) and its binding to DNA sequences (usually to GA or GG), which prevents DNA repair and synthesis in healthy cells (85). Currently, Ox is not usually used alone in treating colorectal cancer, but this drug is combined with other chemotherapy and biological drugs (83). For example, in three randomized clinical trials, adding oxaliplatin to a regimen of leucovorin, capecitabine, and fluorouracil resulted in a 20% reduction in disease recurrence (86).
2.5 Colorectal cancer treatment by trifluridine-tipiracil
As it is clear from the name of the drug trifluridine/tipiracil, this drug consists of two parts. Trifluoridine is a thymidine-related nucleoid analog and replaces thymidine in DNA (87). This is while tipiracil strengthens the function of trifluridine by inhibiting the thymidine phosphorylase enzyme (88). Tipiracil leads to trifluridine replacement in DNA by preventing thymidine bases and ultimately prevents cell proliferation (87). The most common side effects associated with the use of this drug in metastatic colorectal cancer patients included neutropenia, anemia, thrombocytopenia, and leukopenia (89). Trifluoridine in this medicine, similar to what is seen in the therapeutic use of 5-FU, is converted into a monophosphorylated form by thymidine kinase (90). Still, unlike 5-FU, the monophosphorylated form of trifluorothymidine inhibits the activity of this enzyme by binding to the active site of the thymidylate synthase enzyme (90). It leads to cytotoxicity and non-production of thymidine by this enzyme. Subsequent phosphorylation by thymidine kinase produces trifluridine triphosphate, readily incorporated into the DNA of tumor cells (in place of thymidine bases), interferes with DNA function, and inhibits tumor growth (91). In clinical applications, this drug leads to the inhibition of tumor growth in a dose-dependent manner (92). The results of new studies show that this drug has high therapeutic efficiency, is very easy to use, and has fewer side effects than other chemotherapy drugs (93).
3 Colorectal cancer combinational chemotherapy
In many combination treatments related to colorectal cancer chemotherapy, 5-FU or capecitabine are usually the leading drugs. The results of the studies show that the therapeutic effects of capecitabine have more promising effects compared to the combined treatment of LV/5-FU (94, 95). However, chemotherapy regimens based on 5-FU have been designed over many years (96).
Among these treatments, we can mention FOLFOX, which includes folinic acid, 5-FU, and oxaliplatin (97, 98). The therapeutic efficacy of this combination is greater than the single use of oxaliplatin and 5-FU, but its primary mechanism is unclear (99). Today, this combination seems more effective in affecting the intestinal microbiota (100, 101). The 16S rRNA gene sequence analysis from new studies has shown that the abundance of Akkermansia muciniphila increases significantly in patients receiving the FOLFOX combination (102). Further studies on Akkermansia muciniphila showed that dipeptides containing branched-chain amino acids (BCAA) are one of the main factors in increasing the anticancer activity of the mentioned compound (102). The results of various studies have shown that CAPOX (folinic acid, capecitabine, oxaliplatin) and FOLFOX are the most effective regimens in treating advanced colon cancer (103), with the difference that in the CAPOX regimen, the infusion of 5 FU is replaced by an oral derivative of capecitabine (104).
FOLFIRI is one of the other combined chemotherapy options that consists of 400 mg/m2 5-FU (day one iv bolus), 600 mg/m2 5-FU (days 1 and 2 iv by ci), and 180 mg/m2 irinotecan (day 1 iv) and are repeated every two weeks (105, 106). Due to the low therapeutic efficiency of 5-FU (about 10 to 15%), combination treatments of chemotherapy drugs, including FOLFIRI, are used, which leads to an increase in efficiency of up to 45% (105). The results of new studies show that the treatment outcome can be predicted based on the profile of the immune system cells of a person with colon cancer, especially the Treg/TH ratio (107). So patients with a higher Treg/TH ratio respond better to FOLFIRI treatment than patients with a lower ratio (108). Also, a specific decrease in the population of regulatory T cells was observed in patients receiving FOLFIRI (109). Therefore, it seems that some patients’ high number of regulatory T cells does not have the immunological pressure to make the tumor more resistant. When these patients are faced with treatment, they respond to it in a better way (107).
The combination of capecitabine and irinotecan (CAPIRI) is another chemotherapy combination used less in studies than other combinations (110). However, the various results that have used chemotherapy compounds have acknowledged that these compounds cannot eradicate tumor cells. Additional treatments, including biological or immunological options, are needed in their cases. Table 2 summarizes some approved combined and single drug-based chemotherapy regimens.
4 Biological treatments for colorectal cancer
Many biological treatments are directly related to immunological treatments because the responsible for their function is an antibody or its derivatives; however, in this section, we will talk about drugs that can affect tumor biology. Currently, three biological agents, which are also considered immunological agents, include bevacizumab, an anti-VEGF-A antibody; cetuximab, an anti-EGF receptor antibody; and panitumumab, an anti-EGFR antibody, which has been approved for the first-line treatment of metastatic colorectal cancer (119). These antibodies prevent cancer cell proliferation by blocking growth receptors’ function. In CRC patients who have mutated RAS in association with BRAF V600E mutation, along with chemotherapy, bevacizumab is the only agent that can lead to increased treatment efficiency (120). In addition, panitumumab and other anti-EGFR antibody applications are limited to tumors with mutations in RAS (121). Therefore, knowing the tumor type and properties can help choose the appropriate treatment with biological agents.
In addition to approved biologic therapies, researchers are currently searching for new biologic drugs to treat CRC. One of the most critical aspects of various tumors is mesenchymal-epithelial transition (122). This phenomenon is facilitated by the binding of hepatocyte growth factor (HGF) to its tyrosine kinase receptor called c-MET (119, 123). Blocking this pathway is particularly important because of the significant role of mesenchymal-epithelial transition in metastasis (124). Therefore, the biological drugs under investigation are essential for this path. Onartuzumab, Tivantinib, Savolitinib, and Cabozantinib can be mentioned among these drugs (125). However, the use of biological treatments is not without side effects. For example, using bevacizumab may have serious side effects such as proteinuria, impaired wound healing, hypertension, arterial (but not venous) thromboembolic events, bowel perforation, bleeding, and leukoencephalopathy.
Due to the considerable overlap of biological treatments with immunological treatments, they are usually grouped together. However, because these treatments directly affect the biology of tumor cells and do not affect the immune system’s responses, they are known by this name.
5 Colorectal cancer immunotherapy
The results of previous studies have shown that increasing the penetration of T cells into the colorectal tumor can control tumor growth (126). After identifying the MHC-peptide complex and in the presence of co-stimulatory signals, T cells identify tumor cells and destroy them using different methods, including releasing granules (cytotoxic T lymphocytes) (127). In a constant challenge, tumor cells are established in the body and destroyed by the immune system’s cells (128). Tragedies begin when the immune cells cannot destroy malignant or neoplastic cells. An important point about the tumor is its microenvironment. As the cancer progresses, it evolves to suppress the immune system’s responses (129, 130). Different types of tumors use different mechanisms, but for example, they can reduce the expression of MHC-1 molecules (131), reduce the expression of co-stimulatory molecules (132), increase the expression of growth factors (133), and increase the expression of anti-inflammatory cytokines and inhibitory surface molecules (CTLA-4, TIM-3, PD-1, PD-L1, LAG-3, and A2AR) (134–136). In addition, tumor cells secrete extracellular vesicles (EVs) into the tumor environment, reaching the immune system cells and disrupting their functions (137). Therefore, even if tumor cells are destroyed by chemotherapy, there must be a competent immune system that cleans the dead cells and also eliminates the remaining tumor cells with its abilities.
For this reason, tumor immunotherapy’s importance has increased daily (Table 3). Tumor immunotherapy can be divided into several parts. Passive immunotherapy occurs through the transfer of antibodies (native or engineered) mRNA (151), and cytokines (152, 153), and active immunity, which is usually performed through transferring cells related to the immune system, including dendritic cells (DCs) (154) and T cells (155).
5.1 Colorectal cancer passive immunotherapy
MSI-H tumors usually experience infiltration of immune cells, including TCD4+ (TH1) cells and TCD8+ (156); however, the immune cells are functionally unresponsive. These cells (present in the tumor stroma) usually express immune checkpoints such as PD-1 and CTLA-4, which bind to ligands on the surface of tumor cells (such as PD-L1 and CD28) and suppress the function of immune cells, especially T cells (157). Also, as it was said, by reducing the expression of β2MG, these tumors express a low level of stable MHC-1 on the tumor cell surface and escape from being recognized by TCD8+ cells (158). However, MSI-L colorectal cancers experience less infiltration of immune cells into the tumor stroma and have low levels of PD-1 expression (immune cells) and PD-L1 expression (tumor cells) (159, 160). Therefore, it seems that antibodies called immune checkpoint blockers (ICb) based on tumor type can be used to a greater extent in treating MSI-H tumors than MSI-L tumors (157).
In addition to the importance of the above classification, there is another molecular classification (Consensus Molecular Subtype (CMS)) for colorectal cancer, and knowing these characteristics is important for choosing the type of immunotherapy (161). From a molecular point of view, colorectal tumors are divided into four groups. This division simultaneously considers tumor and immune cells’ main gene expression changes in the different environments mentioned. CMS1 is a group of CRC where many mutations are observed (161). They are synonymous with MSI-H type, and mutations associated with BRAF genes are frequently observed in these tumors, including about 1/7 of colorectal tumors (161). CMS2, also called the canonical type, comprises approximately 1/3 of CRC and is associated with mutations that activate the Wnt and Myc signaling pathways (12). CMS3 tumors are also called metabolic type and include 1/7 of colorectal tumors, which often have KRAS-related mutations and disrupt the metabolic pathways of cancer cells (12, 162). The last type and CMS4, also called mesenchymal type, constitute 1/4 of colorectal tumor cases and are associated with activation of the growth factor-β (TGF-β) pathway, increased stromal activation, angiogenesis, and inflammatory infiltration (12).
The importance of these classifications is that the tumor microenvironment of each of these classes is different and therefore requires different treatments (163). In terms of immunity, CMS2 and CMS3 tumors are called cold tumors, which are at a very low level in terms of immune system responses, and in fact, the immune response against these tumors is not well established (161, 164). However, CMS1 and CMS4 tumors are called hot tumors that have higher immune responses than the previous groups. But the noteworthy point is that CMS1 and CMS4 show different responses to treatments due to their features for the immunotherapy index (165). As mentioned before, the CMS1 group, which is similar to MSI-H, was diffuse immune tumor-infiltrating lymphocytes (TILs) rich in CD8+ and CD68+ macrophages (162), whereas CMS4 tumors differed with a different pattern of immune infiltration, including monocyte-derived cells, regulatory t cells (Treg), MDSCs, and TH17 cells (166). The main factor in immunosuppression in the CMS4 type is the production of TGF-β and its related mechanisms (167). A therapeutic strategy of combining selective TGFβ inhibitors with immune checkpoint blockers can reactivate a strong immune response in these models of colon cancer.
In addition to antibodies that play a role in inhibiting immune checkpoints, other antibodies, including antibodies against cytokines such as TGF-β, can reduce the suppression of tumor-related immune responses (168). Also, antibodies against specific tumor antigens can sometimes be used to increase the recognition of tumor cells by the innate immune system (169). In this model, antibody attachment to tumor cells can lead to the tumor cells’ apoptosis by mechanisms such as antibody-dependent cellular cytotoxicity (ADCC), performed by macrophages and NK cells, and complement-mediated cytotoxicity (CDC).
5.2 Colorectal cancer active immunotherapy
Active immunotherapy, which usually occurs by cell transfer, uses T cells and DCs for treatment. The use of T cells occurs in 3 ways. In the first case, tumor-infiltrated lymphocytes (TILs) are collected from the tumor site, expanded, and injected into the patient as an autograft (170). This action leads to an increase in the number of tumor-specific T cells. In the second type of t cell therapy, T cell receptors (TCRs) are changed using genetic engineering methods, and their ability to identify antigens related to tumor cells increases (171). Another approach that has attracted much attention is using T cells with chimeric antigen receptors (CAR-T cells) (16, 172). In this technology, the Scfv part of an antibody that identifies tumor-specific or associated antigens (TSA or TAA) and its combination with different intracellular domains involved in T cell activation signal transmission is usually used (173).
In some cases, the extracellular part of CAR consists of different parts of a receptor whose ligand is abundantly produced in tumor cells (174). However, the applications of CAR-T have various limitations, and it is a dynamic field in cancer treatment, which is still being researched (175). CAR-T cell-based treatment is often used in clinical trial studies (Table 4).
In the case of CRC, there are limitations in the use of CAR-T cells, which include the low chemotactic ability of these cells to migrate to the tumor site, the acidic environment resulting from the metabolism of tumor cells, induced hypoxia, and lack of nutrients (173, 178). In addition to these cases, the severe immunosuppressive microenvironment in CRC is also one of the treatment obstacles. Among these substances that suppress transplanted CAR-T cells are anti-inflammatory cytokines, anti-inflammatory cells including Tregs, MDSCs, and tumor-associated macrophages, as well as metabolites derived from tumor cells (such as kynurenine produced from tryptophan by IDO) (178, 179).
In the study conducted by Jie Xu et al., they produced a human epidermal growth factor receptor 2 (HER2) based CAR-T cell. This receptor also is expressed on the surface of many healthy cells. However, its expression level is higher in tumor cells such as ovarian, stomach, colorectal, breast, and lung cancer (180). This study showed that HER2-specific CAR-T could increase anti-tumor responses in the CRC mouse model and has a high therapeutic capacity (181).
In addition to T cells, under the influence of TME, DCs acquire different functional and phenotypic characteristics, which leads to their non-functionality in anti-tumor responses and even suppression of immune responses (182, 183). According to the characteristics of tumor microenvironment-infiltrated DCs (TIDCs), such as their maturation level and their interaction with other cells in the tumor environment, including TILs, they can have positive or negative effects on CRC prognosis (184, 185). In addition to TIDCs, in CRC patients, the number and function of circulating DCs are generally reduced, and the immature or progenitor phenotype is also associated with an increase (186, 187). In general, it can be said that a large population of TIDCs (checked using the S100 marker) is associated with a good prognosis and less metastasis in CRC (188, 189). It has also been shown that the higher number of mature TIDCs in CRC is associated with better disease prognosis and TH and CTL responses (as seen in MSI-CRC) (190). It has been shown that the therapeutic use of DCs expressing PD-L1 leads to an increase in the lymph infiltration of TCD8+ cells to the CRC site and is associated with increased patient survival (191). Both circulating DCs and TIDCs produce cytokines and anti-inflammatory factors, including VEGF, IL-10, and TGF-β, which ultimately suppress T-cell responses (184). Also, in these cells, the expression level of some genes, including genes related to COX-2 and HMGB1, increases and helps to suppress the immune system’s responses (185). The important point is that tumor cells affect DCs by producing various factors such as CCL2, CXCL5, CXCL1, and VEGF. Therefore, if dendritic cells are isolated from CRC patients and autologously transplanted to patients after expansion and differentiation, they can exert strong anti-immune responses against the tumor by stimulating T cells (192). Another approach is to use the stimulating factors of DC responses and the processing and presenting of their antigens by in situ administration of their stimulating molecules, such as CpG, FLT3L, TLR, and STING agonists (124, 193).
6 Colorectal cancer combination therapy
Considering the TME and the complex behavior of tumor cells in the face of different treatments, it seems that monotherapy cannot achieve the desired results. As mentioned earlier, researchers had reached this conclusion many years ago and therefore used the combination of different chemotherapy drugs along with radiotherapy and surgery. With the emergence and increase of treatments based on immunology and biology to prevent the many side effects of chemotherapy, these treatments became more desirable options. In this way, researchers are interested in using multiple immunotherapies with fewer side effects to reduce the dose of chemotherapy drugs and help eradicate tumor cells.
6.1 Cytokine combination with chemotherapy
In the meantime, many achievements have been made that show that immunotherapy increases the effectiveness of chemotherapy. Studies have shown the existence of interactions between 5-FU and IFN-α in increasing cytotoxicity for different cancers (194). In a study by Laurent et al., 5-FU and IFN-α were used to treat colorectal cancer (194). The results of this study show that IFN-α, in combination with 5-FU, increases the amount of DNA single-stranded and double-stranded breaks in colorectal cancer cells in vitro by modulation of converting enzymes for anticancer prodrugs (194). Other studies also showed that IFN-γ could increase the activity of enzymes related to 5-FU anabolism (TP and UP) and thus help to remove more tumor cells by increasing the active form of this drug (195). Although the experimental results were promising, the combined use of 5-FU and IFN-α in clinical studies could not significantly affect patients’ survival rate or tumor removal (196). For this reason, the combined use of this drug stopped.
6.2 Combination of immune checkpoint blockades and chemotherapy
According to the treatment experiences of immune checkpoint blockades with chemotherapy drugs, these drugs seem to increase immune system cells’ capacity for anti-tumor responses (197). For example, it has been shown that using 5-FU can lead to apoptosis of MDSCs in the TME, which leads to the removal of immune inhibition induced by these cells (198, 199). In another way, the use of oxaliplatin, which leads to the apoptosis of tumor cells (199), causes the appearance of various antigens and their removal by DCs for the pancreas to T cells and leads to an increase in tumor-specific responses (200, 201). In one study, an in vivo assay using an immune checkpoint blockade mouse colon cancer model showed that an antitumor response was induced in the combined use of oxaliplatin with immune checkpoint inhibitors and resulted in increased survival in this model (157). In addition, 5-FU and atezolizumab (202), a humanized antibody against PD-L1, were used in a clinical trial (202). To use this combination, patients are first treated with the FOLFOX chemotherapy combination; then, they are treated with 5-FU and atezolizumab (202). However, the results of this study showed that adding this therapeutic combination does not affect the progression-free survival (PFS) and overall survival (OS) of patients.
6.3 Combination of monoclonal antibodies with chemotherapy
Today, most patients with mCRC are treated with a first-line biologic agent, usually monoclonal antibodies against vascular endothelial growth factor (VEGF) or epidermal growth factor receptor (EGFR), depending on their RAS mutation status (203). As shown in Table 5, bevacizumab is a humanized anti-angiogenic antibody used to treat various tumors as a front line in combination with chemotherapy (208). The binding of this antibody to its ligand (VEGFR) leads to the suppression of new angiogenesis and normalization of blood vessels, which allows T cells to penetrate the tumor and activate them effectively (209, 210). According to those mentioned earlier, FOLFOXIRI and bevacizumab (targeting VEGF-A) in combination with atezolizumab (targeting PD-L1) were used in a clinical trial in the front line with chemotherapy, and the results obtained from it with the therapeutic use of bevacizumab and chemotherapy have been compared (211). The number of patients participating in this study was 218 patients, the results of which show that the PFS for patients receiving combined treatment (about 13 months) was higher compared to those receiving bevacizumab and chemotherapy alone (11.5 months) (p=0.07) (211). This change was not significant. Further studies have used FOLFOX combined with bevacizumab and nivolumab versus FOLFOX and bevacizumab for the treatment of CRC to investigate the effect of chemotherapy combined with immunotherapy in a clinical trial study (212). The primary endpoint of PFS was not met in this study. However, patients receiving FOLFOX combined with bevacizumab and nivolumab showed a higher PFS rate than patients receiving FOLFOX and bevacizumab after 12 months (212). Also, higher and more durable immune response rates were observed in the combined treatment compared to FOLFOX and bevacizumab, which indicates that the combined treatment of chemotherapy and tumor adenoma targeting with two antibodies that target different pathways has a higher therapeutic efficiency (Figure 1).
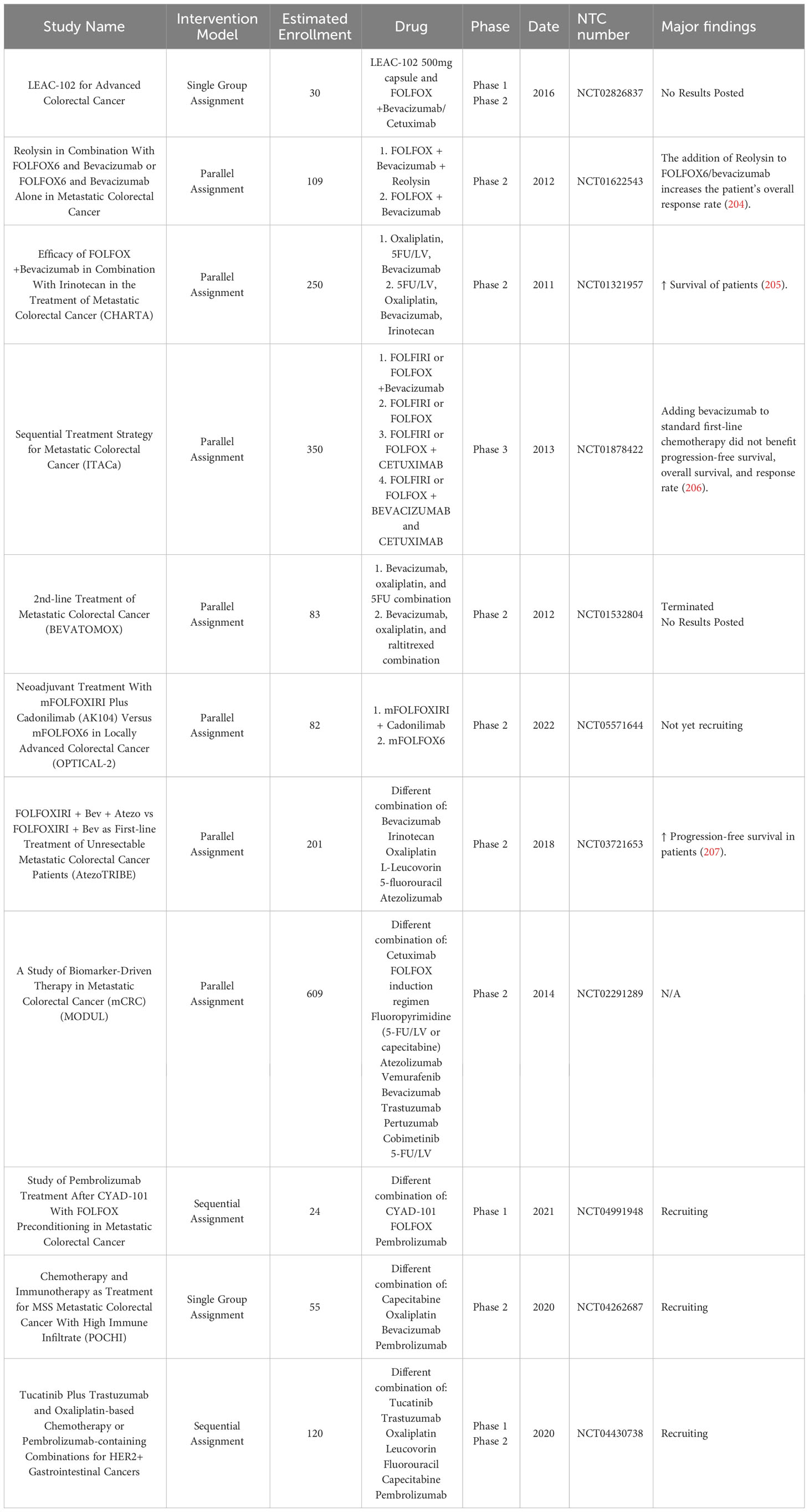
Table 5 Examples of Ab-based immunotherapy in combination with chemotherapy in clinical trials as novel therapeutics in colorectal cancer.
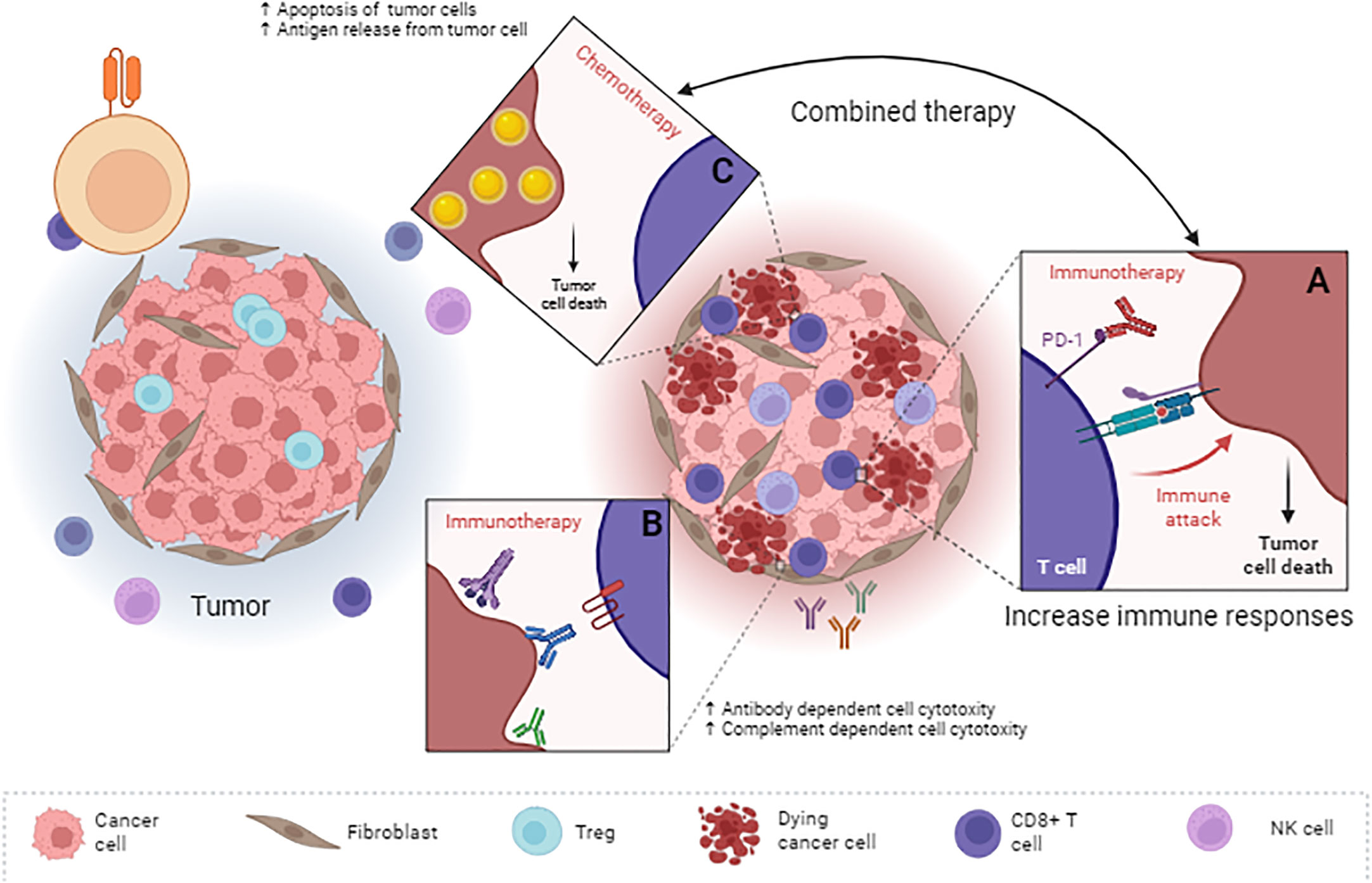
Figure 1 Combined use of chemotherapy and antibodies in the treatment of colorectal cancer. Antibodies can have different functions by targeting different tumor antigens. Some of them, such as immune checkpoint inhibitors (A) such as anti-PD-1 Ab and anti-CTLA-4 Ab, increase the response of immune cells, and some, by targeting a specific antigen (B), leading to the activation of ADCC or CDC. Chemotherapy can lead to apoptosis of tumor cells and the release of various types of antigens derived from tumor cells (C). On the one hand, the release of these antigens leads to an increase in the maturation of antigen-presenting cells. On the other hand, using immune checkpoint inhibitors leads to activating more immune system cells to deal with the tumor. In fact, these types of therapeutic compounds affect the tumor from different pathways and do not allow tumor recurrence after radiation or chemotherapy to the tumor cells. ADCC, Antibody-dependent cell cytotoxicity; CDC, Complement-dependent cell cytotoxicity.
Among other studies that have dealt with the combination of chemotherapy and immunotherapy, we can refer to the study of GOIRC-03-2018 in phase II, in which the combination of triple chemotherapy (FOLFOXIRI) with bevacizumab and nivolumab in patients with CRC containing mutations Noted in RAS/BRAF (213). The results of this study show that the combination of FOLFOXIRI with bevacizumab and nivolumab is safe and has shown promising results; that’s why this group has continued its work in phase 3, the results of which have not been published yet. Also, in another clinical trial, although the POCHI trial (NCT04262687) is currently investigating the combination of CAPOX and bevacizumab with pembrolizumab as first-line treatment in eligible patients with MSS mCRC who have a high immune infiltrate, and the results are expected to show goodness in patients (214).
Cetuximab, a chimeric antibody (mouse V and human FC), is an IgG1 antibody that can increase the elimination of tumor cells by increasing ADCC and the expression of MHC-2 molecules on the surface of DCs (215, 216). It has been approved as part of the treatment regimen of CRC patients in combination therapy. In this way, to investigate the effectiveness of cetuximab in combination treatment with chemotherapy, treatment with cetuximab in combination with FOLFOX was used (phase 2 clinical trial), and it has been shown that this combination has potential therapeutic effects in selected patients (217, 218). The results of phase 3 clinical trial (TAILOR study) related to the therapeutic use of the combination of cetuximab and FOLFOX show that all the relevant clinical objectives and endpoints have been met cetuximab in combination with FOLFOX is an effective standard care first-line treatment regimen approved for patients with mCRC (219). Also, a study that investigates the therapeutic efficacy of adding avelumab to FOLFOX and cetuximab shows that this addition does not have a specific adverse effect (220). However, adding avelumab to the above combination did not reach its first endpoint, and no significant improvement was observed in the patient’s condition.
6.4 Combination of CAR-T cells with chemotherapy
It seems that by modulating the phenotype and abundance of blood leukocytes, chemotherapy could facilitate the production of the most effective CAR-T cell products. Also, by increasing the activity of circulating CD8+ T lymphocytes and rebuilding the effective memory population, chemotherapy can strengthen immune system responses (221). However, chemotherapy’s effect on other immune system cells, such as neutrophils, is harmful and can sometimes lead to neutropenia. Also, the results of various studies show inconclusive data about the effect of chemotherapy on TCD4+ cells (Figure 2) (222). Interestingly, Bellone et al. found induced and spontaneous IFN-γ release by TCD8+ cells after each course of combined chemotherapy with cisplatin, gemcitabine, and 5-fluorouracil (up to 4 cycles) are unchanged in comparison with pre-treatment values, indicating preservation of effect function throughout the treatment course (221, 223, 224). Therefore, it seems that the use of chemotherapy before transferring the CAR-T cells can provide suitable conditions in the body for the activity of these cells. However, during our search in the literature until March 2023, no study simultaneously used chemotherapy drugs and CAR-T cells to treat colorectal cancer. We recommend that researchers also use this method to treat CRC because chemotherapy can increase the therapeutic efficiency of CAR-T cells based treatments.
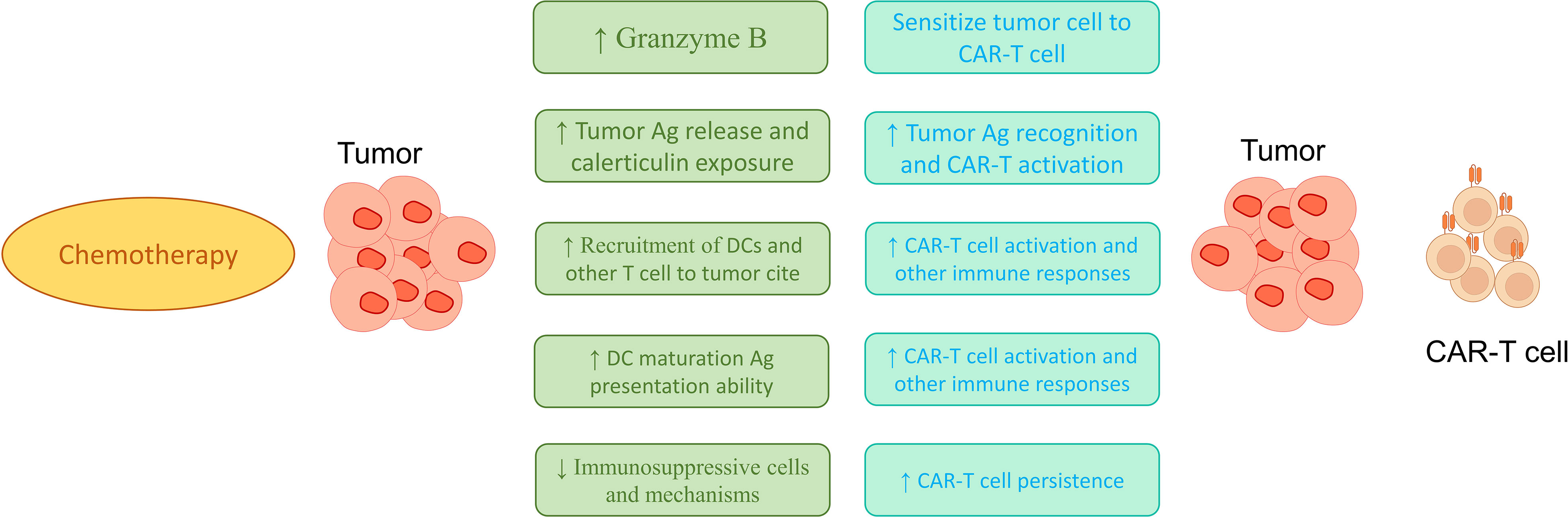
Figure 2 Possible mechanisms in increasing the effectiveness of treatments based on combinations of CAR-T cell and chemotherapy. As shown in the figure, the use of chemotherapy can increase the recruitment of immune cells, increase the maturation of dendritic cells, increase the activation of immune cells at the tumor site, and reduce the suppressive responses of immune cells to increase the potential therapeutic function of CAR-T cells.
7 Conclusion and future perspective
As mentioned in many articles and based on the opinion of scientists active in the field of tumor treatment, therapies based on a drug usually cannot overcome the complex TME created by tumor cells. Regarding chemotherapy, due to its side effects, the lower the dose of the drug, the better the patient’s health and the fewer side effects. As discussed throughout this review, tumors use different methods and pathways to overcome various checkpoints that inhibit tumor growth. Also, many studies have shown that, in many cases, the use of a single treatment can lead to tumor resistance to that treatment. For example, cancer cells can become resistant to chemotherapy drugs by expressing some membrane pumps and creating new strains of tumor cells resistant to the given treatment. Also, using antibodies against immune checkpoints (For example, anti-PD-1 Ab) can lead to compensatory expression of other immune checkpoints (LAG-3 expression) on the surface of tumor cells (225). Therefore, it seems that combinational treatments that simultaneously target several different mechanisms related to the growth and immunosuppression induced by the tumor can be of great help in the treatment of tumors. As we know, the primary system that ultimately leads to tumor eradication is the immune system. Therefore methods based on activating this system more and more specifically against tumor cells can help eradicate the tumor faster. One of the ways to get the proper treatment and, at the same time, get the appropriate treatment is to use immunotherapy along with chemotherapy. As discussed in Section 6, in many studies, the use of this combination has helped improve patients’ health. But it is worth noting that in some cases, no significant change in the improvement of the condition of patients was observed in the combined treatment group and single treatment with chemotherapy. The critical point about these treatments is that the combination of chemotherapy and the CAR-T cells in colorectal cancer was not observed in the literature. While theoretically and in clinical applications, this combination is highly effective in improving patients’ conditions in other tumors. It seems that the use of new compounds, such as small molecules, chemotherapy, and immunotherapy, can affect this tumor treatment. However, more studies are needed to investigate the efficacy and safety of combination treatments.
Author contributions
All authors contributed to the article and approved the submitted version.
Conflict of interest
The authors declare that the research was conducted in the absence of any commercial or financial relationships that could be construed as a potential conflict of interest.
Publisher’s note
All claims expressed in this article are solely those of the authors and do not necessarily represent those of their affiliated organizations, or those of the publisher, the editors and the reviewers. Any product that may be evaluated in this article, or claim that may be made by its manufacturer, is not guaranteed or endorsed by the publisher.
References
1. Macrae FA. Colorectal cancer: Epidemiology, risk factors, and protective factors. (2017) 2016. Available at: https://www.uptodate.com/contents/colorectal-cancer-epidemiology-risk-factors-and-protective-factors
2. Rawla P, Sunkara T, Barsouk A. Epidemiology of colorectal cancer: incidence, mortality, survival, and risk factors. Gastroenterol Rev/Przegląd Gastroenterologiczny (2019) 14(2):89–103. doi: 10.5114/pg.2018.81072
3. Bray F, Ferlay J, Soerjomataram I, Siegel RL, Torre LA, Jemal A. Global cancer statistics 2018: GLOBOCAN estimates of incidence and mortality worldwide for 36 cancers in 185 countries. CA: Cancer J Clin (2018) 68(6):394–424. doi: 10.3322/caac.21492
4. De Smedt L, Lemahieu J, Palmans S, Govaere O, Tousseyn T, Cutsem Van E, et al. Microsatellite instable vs stable colon carcinomas: analysis of tumour heterogeneity, inflammation and angiogenesis. Br J Cancer (2015) 113(3):500–9. doi: 10.1038/bjc.2015.213
5. Li K, Luo H, Huang L, Luo H, Zhu X. Microsatellite instability: a review of what the oncologist should know. Cancer Cell Int (2020) 20:1–13. doi: 10.1186/s12935-019-1091-8
6. Förster I, Brockmann M, Schildgen O, Schildgen V. Microsatellite instability testing in colorectal cancer using the QiaXcel advanced platform. BMC Cancer (2018) 18:1–6. doi: 10.1186/s12885-018-4400-z
7. Passardi A, Canale M, Valgiusti M, Ulivi P. Immune checkpoints as a target for colorectal cancer treatment. Int J Mol Sci (2017) 18(6):1324. doi: 10.3390/ijms18061324
8. Schrock A, Ouyang C, Sandhu J, Sokol E, Jin D, Ross J, et al. Tumor mutational burden is predictive of response to immune checkpoint inhibitors in MSI-high metastatic colorectal cancer. Ann Oncol (2019) 30(7):1096–103. doi: 10.1093/annonc/mdz134
9. Reilly NM, Novara L, Nicolantonio Di F, Bardelli A. Exploiting DNA repair defects in colorectal cancer. Mol Oncol (2019) 13(4):681–700. doi: 10.1002/1878-0261.12467
10. Liu B, Nicolaides NC, Markowitz S, Willson JK, Parsons RE, Jen J, et al. Mismatch repair gene defects in sporadic colorectal cancers with microsatellite instability. Nat Genet (1995) 9(1):48–55. doi: 10.1038/ng0195-48
11. Westdorp H, Fennemann FL, Weren RD, Bisseling TM, Ligtenberg MJ, Figdor CG, et al. Opportunities for immunotherapy in microsatellite instable colorectal cancer. Cancer Immunol Immunother (2016) 65:1249–59. doi: 10.1007/s00262-016-1832-7
12. Guinney J, Dienstmann R, Wang X, Reynies De A, Schlicker A, Soneson C, et al. The consensus molecular subtypes of colorectal cancer. Nat Med (2015) 21(11):1350–6. doi: 10.1038/nm.3967
13. Carrassa L, Damia G. DNA damage response inhibitors: Mechanisms and potential applications in cancer therapy. Cancer Treat Rev (2017) 60:139–51. doi: 10.1016/j.ctrv.2017.08.013
14. Tavare AN, Perry NJ, Benzonana L.L, Takata M, Ma D. Cancer recurrence after surgery: direct and indirect effects of anesthetic agents. Int J Cancer (2012) 130(6):1237–50. doi: 10.1002/ijc.26448
15. Boland PM, Ma WW. Immunotherapy for colorectal cancer. Cancers (2017) 9(5):50. doi: 10.3390/cancers9050050
16. Feng M, Zhao Z, Yang M, Ji J, Zhu D. T-cell-based immunotherapy in colorectal cancer. Cancer Lett (2021) 498:201–9. doi: 10.1016/j.canlet.2020.10.040
17. Aldahlawi AM, Abdullah ST. Dendritic cell−Based immunotherapies and their potential use in colorectal cancer immunotherapy. J Microsc Ultrastruct (2021) 10(3):107–13. doi: 10.4103/jmau.jmau_20_21
18. Sun X, Suo J, Yan J. Immunotherapy in human colorectal cancer: challenges and prospective. World J Gastroenterol (2016) 22(28):6362. doi: 10.3748/wjg.v22.i28.6362
19. Weiner LM, Murray JC, Shuptrine CW. Antibody-based immunotherapy of cancer. Cell (2012) 148(6):1081–4. doi: 10.1016/j.cell.2012.02.034
20. Singh PP, Sharma PK, Krishnan G, Lockhart AC. Immune checkpoints and immunotherapy for colorectal cancer. Gastroenterol Rep (2015) 3(4):289–97. doi: 10.1093/gastro/gov053
21. Ros J, Balconi F, Baraibar I, Gonzalez NS, Salva F, Tabernero J, et al. Advances in immune checkpoint inhibitor combination strategies for microsatellite stable colorectal cancer. Front Oncol (2023) 13. doi: 10.3389/fonc.2023.1112276
22. Gill S, Thomas R, Goldberg R. Colorectal cancer chemotherapy. Aliment Pharmacol Ther (2003) 18(7):683–92. doi: 10.1046/j.1365-2036.2003.01735.x
23. Kraus S, Nabiochtchikov I, Shapira S, Arber N. Recent advances in personalized colorectal cancer research. Cancer Lett (2014) 347(1):15–21. doi: 10.1016/j.canlet.2014.01.025
24. Leichsenring J, Koppelle A, Reinacher-Schick A. Colorectal cancer: personalized therapy. Gastrointest Tumors (2015) 1(4):209–20. doi: 10.1159/000380790
25. Li Z-N, Zhao L, Yu L.-F, Wei M-J. BRAF and KRAS mutations in metastatic colorectal cancer: Future perspectives for personalized therapy. Gastroenterol Rep (2020) 8(3):192–205. doi: 10.1093/gastro/goaa022
26. Gong J, Cho M, Fakih M. RAS and BRAF in metastatic colorectal cancer management. J Gastrointest Oncol (2016) 7(5):687. doi: 10.21037/jgo.2016.06.12
27. Yaeger R, Cercek A, EM, Reidy DL, Kemeny N, Wolinsky T, et al. Pilot Trial of Combined BRAF and EGFR Inhibition in BRAF-Mutant Metastatic Colorectal Cancer PatientsRAF plus EGFR Inhibition for BRAF-Mutant mCRC. Clin Cancer Res (2015) 21(6):1313–20. doi: 10.1158/1078-0432.CCR-14-2779
28. Eisterer W, Prager G. Chemotherapy, still an option in the twenty-first century in metastatic colorectal cancer? Cardiovasc Intervent Radiol (2019) 42:1213–20. doi: 10.1007/s00270-019-02278-7
29. Goyle S, Maraveyas A. Chemotherapy for colorectal cancer. Digest Surg (2006) 22(6):401–14. doi: 10.1159/000091441
30. Thomas D, Zalcberg J. 5-Fluorouracil: A pharmacological paradigm in the use of cytotoxics. Clin Exp Pharmacol Physiol (1998) 25(11):887–95. doi: 10.1111/j.1440-1681.1998.tb02339.x
31. Longley DB, Harkin DP, Johnston PG. 5-fluorouracil: mechanisms of action and clinical strategies. Nat Rev Cancer (2003) 3(5):330–8. doi: 10.1038/nrc1074
32. Ceilley RI. Mechanisms of action of topical 5-fluorouracil: review and implications for the treatment of dermatological disorders. J Dermatol Treat (2012) 23(2):83–9. doi: 10.3109/09546634.2010.507704
33. Pullarkat S, Stoehlmacher J, Ghaderi V, Xiong Y, Ingles S, Sherrod A, et al. Thymidylate synthase gene polymorphism determines response and toxicity of 5-FU chemotherapy. Pharmacogenom J (2001) 1(1):65–70. doi: 10.1038/sj.tpj.6500012
34. Kumar A, Singh AK, Singh H, Thareja S, Kumar P. Regulation of thymidylate synthase: an approach to overcome 5-FU resistance in colorectal cancer. Med Oncol (2023) 40(1):1–19. doi: 10.1007/s12032-022-01864-z
35. Hussain S. On a new proposed mechanism of 5-fluorouracil-mediated cytotoxicity. Trends Cancer (2020) 6(5):365–8. doi: 10.1016/j.trecan.2020.02.009
36. Blondy S, David V, Verdier M, Mathonnet M, Perraud A, Christou N. 5-Fluorouracil resistance mechanisms in colorectal cancer: From classical pathways to promising processes. Cancer Sci (2020) 111(9):3142–54. doi: 10.1111/cas.14532
37. Diasio RB, Harris BE. Clinical pharmacology of 5-fluorouracil. Clin Pharmacokinet (1989) 16:215–37. doi: 10.2165/00003088-198916040-00002
38. Parker WB, Cheng YC. Metabolism and mechanism of action of 5-fluorouracil. Pharmacol Ther (1990) 48(3):381–95. doi: 10.1016/0163-7258(90)90056-8
39. Milano G, Chamorey A-L. Clinical pharmacokinetics of 5-fluorouracil with consideration of chronopharmacokinetics. Chronobiol Int (2002) 19(1):177–89. doi: 10.1081/CBI-120002597
40. Miura K, Kinouchi M, Ishida K, Fujibuchi W, Naitoh T, Ogawa H, et al. 5-fu metabolism in cancer and orally-administrable 5-fu drugs. Cancers (2010) 2(3):1717–30. doi: 10.3390/cancers2031717
41. Vodenkova S, Buchler T, Cervena K, Veskrnova V, Vodicka P, Vymetalkova V. 5-fluorouracil and other fluoropyrimidines in colorectal cancer: Past, present and future. Pharmacol Ther (2020) 206:107447. doi: 10.1016/j.pharmthera.2019.107447
42. Mutharani B, Ranganathan P, Chen S-M. Temperature-reversible switched antineoplastic drug 5-fluorouracil electrochemical sensor based on adaptable thermo-sensitive microgel encapsulated PEDOT. Sens Actuators B: Chem (2020) 304:127361. doi: 10.1016/j.snb.2019.127361
43. Machover D. A comprehensive review of 5-fluorouracil and leucovorin in patients with metastatic colorectal carcinoma. Cancer: Interdiscip Int J Am Cancer Soc (1997) 80(7):1179–87. doi: 10.1002/(SICI)1097-0142(19971001)80:7<1179::AID-CNCR1>3.0.CO;2-G
44. Glimelius B, Stintzing S, Marshall J, and Yoshino T, Gramont A, et al. Metastatic colorectal cancer: Advances in the folate-fluoropyrimidine chemotherapy backbone. Cancer Treat Rev (2021) 98:102218. doi: 10.1016/j.ctrv.2021.102218
45. Hegde VS, Nagalli S. Leucovorin (StatPearls. StatPearls Publishing). (2020). http://www.ncbi.nlm.nih.gov/books/NBK553114/. Accessed May 17, 2022
46. Carlotto A, Hogsett VL, Maiorini EM, Razulis JG, Sonis ST, et al. The economic burden of toxicities associated with cancer treatment: review of the literature and analysis of nausea and vomiting, diarrhoea, oral mucositis and fatigue. Pharmacoeconomics (2013) 31:753–66. doi: 10.1007/s40273-013-0081-2
47. Louvet C, Andre T, Tigaud J, Gamelin E, Douillard J, Brunet R, et al. Phase II study of oxaliplatin, fluorouracil, and folinic acid in locally advanced or metastatic gastric cancer patients. J Clin Oncol (2002) 20(23):4543–8. doi: 10.1200/JCO.2002.02.021
48. Choi SM, Lee SH, Yang YS, Kim BC, Kim MK, Cho KH, et al. 5-fluorouracil-induced leukoencephalopathy in patients with breast cancer. J Korean Med Sci (2001) 16(3):328–34. doi: 10.3346/jkms.2001.16.3.328
49. Anaka M, Abdel-Rahman O. Managing 5FU cardiotoxicity in colorectal cancer treatment. Cancer Manage Res (2022) p:273–85. doi: 10.2147/CMAR.S273544
50. Del Re M, Cinieri S, Michelucci A, Salvadori S, Loupakis F, Schirripa M, et al. DPYD* 6 plays an important role in fluoropyrimidine toxicity in addition to DPYD* 2A and c. 2846A> T: a comprehensive analysis in 1254 patients. Pharmacogenom J (2019) 19(6):556–63. doi: 10.1038/s41397-019-0077-1
51. Innocenti F, Mills SC, Sanoff H, Ciccolini J, and Lenz H-J, Milano G, et al. All you need to know about DPYD genetic testing for patients treated with fluorouracil and capecitabine: A practitioner-friendly guide. JCO Oncol Pract (2020) 16(12):793–8. doi: 10.1200/OP.20.00553
52. Sharma BB, Rai K, Blunt H, Zhao W, Tosteson TD, Brooks GA, et al. Pathogenic DPYD variants and treatment-related mortality in patients receiving fluoropyrimidine chemotherapy: a systematic review and meta-analysis. Oncologist (2021) 26(12):1008–16. doi: 10.1002/onco.13967
53. Iachetta F, Bonelli C, Romagnani A, Zamponi R, Tofani L, Farnetti E, et al. The clinical relevance of multiple DPYD polymorphisms on patients candidate for fluoropyrimidine based-chemotherapy. An Italian case-control study. Br J Cancer (2019) 120(8):834–9. doi: 10.1038/s41416-019-0423-8
54. Polk A, Vistisen K, Vaage-Nilsen M, Nielsen DL. A systematic review of the pathophysiology of 5-fluorouracil-induced cardiotoxicity. BMC Pharmacol Toxicol (2014) 15(1):1–11. doi: 10.1186/2050-6511-15-47
55. MIranda VC, Faria L.D, Braghiroli MI.F.M, Jacobs M, Sabbaga J, Hoff PM, et al. A phase II trial of metformin and fluorouracil (MetFU) for patients (pts) with metastatic colorectal cancer (mCRC) refractory to standard treatment. Am Soc Clin Oncol (2014) 32:601. doi: 10.1200/jco.2014.32.3_suppl.601
56. Madajewicz S, Waterhouse DM, Ritch PS, Khan MQ, Higby DJ, Leichman CG, et al. Multicenter, randomized phase II trial of bevacizumab plus folinic acid, fluorouracil, gemcitabine (FFG) versus bevacizumab plus folinic acid, fluorouracil, oxaliplatin (FOLFOX4) as first-line therapy for patients with advanced colorectal cancer. Invest New Drugs (2012) 30:772–8. doi: 10.1007/s10637-010-9598-9
57. Hirsch BR, Zafar SY. Capecitabine in the management of colorectal cancer. Cancer Manage Res (2011) 3:79–89. doi: 10.2147/CMR.S11250
58. Walko CM, Lindley C. Capecitabine: a review. Clin Ther (2005) 27(1):23–44. doi: 10.1016/j.clinthera.2005.01.005
59. Etienne-Grimaldi M-C, Boyer J-C, Béroud C, Mbatchi L, Kuilenburg van A, Bobin-Dubigeon C, et al. New advances in DPYD genotype and risk of severe toxicity under capecitabine. PloS One (2017) 12(5):e0175998. doi: 10.1371/journal.pone.0175998
60. Takebayashi Y, Yamada K, Miyadera K, Sumizawa T, Furukawa T, Kinoshita F, et al. The activity and expression of thymidine phosphorylase in human solid tumours. Eur J Cancer (1996) 32(7):1227–32. doi: 10.1016/0959-8049(96)00061-5
61. Takayama T, Mugiya S, Sugiyama T, Aoki T, Furuse H, Liu H, et al. High levels of thymidine phosphorylase as an independent prognostic factor in renal cell carcinoma. Japanese J Clin Oncol (2006) 36(9):564–9. doi: 10.1093/jjco/hyl063
62. Meropol NJ, Gold PJ, Diasio RB, Andria M, Dhami M, Godfrey T, et al. Thymidine phosphorylase expression is associated with response to capecitabine plus irinotecan in patients with metastatic colorectal cancer. J Clin Oncol (2006) 24(25):4069–77. doi: 10.1200/JCO.2005.05.2084
63. Petrioli R, Bargagli G, Lazzi S, Pascucci A, Francini E, Bellan C, et al. Thymidine phosphorylase expression in metastatic sites is predictive for response in patients with colorectal cancer treated with continuous oral capecitabine and biweekly oxaliplatin. Anti-cancer Drugs (2010) 21(3):313–9. doi: 10.1097/CAD.0b013e328334d88a
64. Van Cutsem E, Cunningham D, Hoff PM, Maroun J. Thymidine phosphorylase (TP) activation: convenience through innovation. Oncologist (2001) 6(S4):1–2. doi: 10.1634/theoncologist.6-suppl_4-1
65. Tsuda H, Takatsuki K, Ohno R, Masaoka T, Okada K, Shirakawa S, et al. Treatment of adult T-cell leukaemia–lymphoma with irinotecan hydrochloride (CPT-11). Br J Cancer (1994) 70(4):771–4. doi: 10.1038/bjc.1994.394
66. Bailly C. Irinotecan: 25 years of cancer treatment. Pharmacol Res (2019) 148:104398. doi: 10.1016/j.phrs.2019.104398
67. Tejpar S, Peeters M, Humblet Y, Vermorken J, Hertogh De G, Roock De W, et al. Relationship of efficacy with KRAS status (wild type versus mutant) in patients with irinotecan-refractory metastatic colorectal cancer (mCRC), treated with irinotecan (q2w) and escalating doses of cetuximab (q1w): The EVEREST experience (preliminary data). J Clin Oncol (2008) 26(15_suppl):4001–1. doi: 10.1200/jco.2008.26.15_suppl.4001
68. Fuchs C, Mitchell EP, Hoff PM. Irinotecan in the treatment of colorectal cancer. Cancer Treat Rev (2006) 32(7):491–503. doi: 10.1016/j.ctrv.2006.07.001
69. Falcone A, Masi G, Brunetti I, Benedetti G, Bertetto O, Picone V, et al. The triplet combination of irinotecan, oxaliplatin and 5FU/LV (FOLFOXIRI) vs the doublet of irinotecan and 5FU/LV (FOLFIRI) as first-line treatment of metastatic colorectal cancer (MCRC): Results of a randomized phase III trial by the Gruppo Oncologico Nord Ovest (GONO). J Clin Oncol (2006) 24(18_suppl):3513–3. doi: 10.1200/jco.2006.24.18_suppl.3513
70. Ychou M, Conroy T, Seitz J, Gourgou S, Hua A, Mery-Mignard D, et al. An open phase I study assessing the feasibility of the triple combination: oxaliplatin plus irinotecan plus leucovorin/5-fluorouracil every 2 weeks in patients with advanced solid tumors. Ann Oncol (2003) 14(3):481–9. doi: 10.1093/annonc/mdg119
71. Zarate R, Rodriguez J, Bandres E, Patiño-García A, Ponz-Sarvise M, Viudez A, et al. Oxaliplatin, irinotecan and capecitabine as first-line therapy in metastatic colorectal cancer (mCRC): a dose-finding study and pharmacogenomic analysis. Br J Cancer (2010) 102(6):987–94. doi: 10.1038/sj.bjc.6605595
72. Fujita K-i, Kubota Y, and Ishida H, Sasaki Y. Irinotecan, a key chemotherapeutic drug for metastatic colorectal cancer. World J Gastroenterol (2015) 21(43):12234. doi: 10.3748/wjg.v21.i43.12234
73. Takano M, Sugiyama T. UGT1A1 polymorphisms in cancer: impact on irinotecan treatment. Pharmacogenom Personalized Med (2017) p:61–8. doi: 10.2147/PGPM.S108656
75. Lee B, Min JA, Nashed A, Lee S-O, Yoo JC, Chi S-W, et al. A novel mechanism of irinotecan targeting MDM2 and Bcl-xL. Biochem Biophys Res Commun (2019) 514(2):518–23. doi: 10.1016/j.bbrc.2019.04.009
76. Weekes J, Lam AK.-Y, Sebesan S, Ho Y-H. Irinotecan therapy and molecular targets in colorectal cancer: a systemic review. World J Gastroenterol: WJG (2009) 15(29):3597. doi: 10.3748/wjg.15.3597
77. Bartel F, Harris L.C, Wurl P, Taubert H. MDM2 and its splice variant messenger RNAs: expression in tumors and down-regulation using antisense oligonucleotides. Mol Cancer Res (2004) 2(1):29–35. doi: 10.1158/1541-7786.29.2.1
78. Petitprez A, Larsen AK. Irinotecan resistance is accompanied by upregulation of EGFR and Src signaling in human cancer models. Curr Pharm Design (2013) 19(5):958–64. doi: 10.2174/138161213804547204
79. Hahn RZ, Antunes MV, Verza SG, Perassolo MS, Suyenaga ES, Schwartsmann G, et al. Pharmacokinetic and pharmacogenetic markers of irinotecan toxicity. Curr Medicinal Chem (2019) 26(12):2085–107. doi: 10.2174/0929867325666180622141101
80. Riera P, Páez D. Elucidating the role of pharmacogenetics in irinotecan efficacy and adverse events in metastatic colorectal cancer patients. Expert Opin Drug Metab Toxicol (2021) 17(10):1157–63. doi: 10.1080/17425255.2021.1974397
81. Kciuk M, Marciniak B, Kontek R. Irinotecan—still an important player in cancer chemotherapy: a comprehensive overview. Int J Mol Sci (2020) 21(14):4919. doi: 10.3390/ijms21144919
82. Branca JJV, Carrino D, Gulisano M, Ghelardini C, Mannelli Cesare Di L, Pacini A. Oxaliplatin-induced neuropathy: Genetic and epigenetic profile to better understand how to ameliorate this side effect. Front Mol Biosci (2021) 8:643824. doi: 10.3389/fmolb.2021.643824
83. Comella P, Casaretti R, Sandomenico C, Avallone A, Franco L. Role of oxaliplatin in the treatment of colorectal cancer. Ther Clin Risk Manage (2009) p:229–38. doi: 10.2147/TCRM.S3583
84. Bratsos I, Jedner S, Gianferrara T, Alessio E. Ruthenium anticancer compounds: challenges and expectations. Chimia (2007) 61(11):692–2. doi: 10.2533/chimia.2007.692
85. Gabano E, Ravera M, Osella D. The drug targeting and delivery approach applied to Pt-antitumour complexes. A coordination point of view. Curr Medicinal Chem (2009) 16(34):4544–80. doi: 10.2174/092986709789760661
86. Huang W-K, Hsu H-C, Chang S-H, Chou W.-C, Chang P-H, Chiang S-F, et al. Real-world effectiveness of adjuvant oxaliplatin chemotherapy in stage III colon cancer: a controlled interrupted time series analysis. Front Pharmacol (2021) 12:693009. doi: 10.3389/fphar.2021.693009
87. Burness CB, Duggan ST. Trifluridine/tipiracil: a review in metastatic colorectal cancer. Drugs (2016) 76:1393–402. doi: 10.1007/s40265-016-0633-9
88. Petrelli F, Perego G, Ghidini A, Ghidini M, Borgonovo K, Scolari C, et al. A systematic review of salvage therapies in refractory metastatic colorectal cancer. Int J Colorectal Dis (2020) 35:783–94. doi: 10.1007/s00384-020-03571-5
89. White T. Metastatic colorectal cancer: Management with trifluridine/tipiracil. Number 2/April (2017) 21(2):E30–7. doi: 10.1188/17.CJON.E30-E37
90. Voutsadakis IA. Biomarkers of trifluridine-tipiracil efficacy. J Clin Med (2021) 10(23):5568. doi: 10.3390/jcm10235568
91. Roda D, Roselló S, Huerta M, Cervantes A. Trifluridine/tipiracil in earlier lines of chemotherapy for advanced colorectal cancer. Ann Oncol (2020) 31(9):1097–8. doi: 10.1016/j.annonc.2020.05.030
92. Matsuoka K, Nukatsuka M, and Kobunai T, Takechi T. Enhanced efficacy of trifluridine for ionizing radiation in human colorectal cancer cell lines. Cancer Res (2017) 77(13_Supplement):1055–5. doi: 10.1158/1538-7445.AM2017-1055
93. Kwakman JJ, Vink G, Vestjens J, Beerepoot L.V, Groot JW, Jansen RL, et al. Feasibility and effectiveness of trifluridine/tipiracil in metastatic colorectal cancer: real-life data from The Netherlands. Int J Clin Oncol (2018) 23:482–9. doi: 10.1007/s10147-017-1220-0
94. Rao S, Cunningham D, Price T, Hill M, Ross P, Tebbutt N, et al. Phase II study of capecitabine and mitomycin C as first-line treatment in patients with advanced colorectal cancer. Br J Cancer (2004) 91(5):839–43. doi: 10.1038/sj.bjc.6602039
95. Hénin E, You B, VanCutsem E, Hoff P, Cassidy J, Twelves C, et al. A dynamic model of hand-and-foot syndrome in patients receiving capecitabine. Clin Pharmacol Ther (2009) 85(4):418–25. doi: 10.1038/clpt.2008.220
96. Sandhu J, Lavingia V, Fakih M. Systemic treatment for metastatic colorectal cancer in the era of precision medicine. J Surg Oncol (2019) 119(5):564–82. doi: 10.1002/jso.25421
97. Chua W, Goldstein D, Lee C, Dhillon H, Michael M, Mitchell P, et al. Molecular markers of response and toxicity to FOLFOX chemotherapy in metastatic colorectal cancer. Br J Cancer (2009) 101(6):998–1004. doi: 10.1038/sj.bjc.6605239
98. Han SW, Lee HJ, Bae JM, Cho NY, Lee KH, Kim TY, et al. Methylation and microsatellite status and recurrence following adjuvant FOLFOX in colorectal cancer. Int J Cancer (2013) 132(9):2209–16. doi: 10.1002/ijc.27888
99. Akdeniz N, Kaplan MA, Uncu D, İnanç M, Kaya S, Dane F, et al. The comparison of FOLFOX regimens with different doses of 5-FU for the adjuvant treatment of colorectal cancer: a multicenter study. Int J Colorectal Dis (2021) 36:1311–9. doi: 10.1007/s00384-021-03888-9
100. Li J, Li J, Lyu N, Ma Y, Liu F, Feng Y, et al. Composition of fecal microbiota in low-set rectal cancer patients treated with FOLFOX. Ther Adv Chronic Dis (2020) 11:2040622320904293. doi: 10.1177/2040622320904293
101. Hou XY, Zhang P, Du HZ, Gao YQ, Sun RQ, Qin SY, et al. Prevotella contributes to individual response of FOLFOX in colon cancer. Clin Trans Med (2021) 11(9):e512. doi: 10.1002/ctm2.512
102. Hou X, Zhang P, Du HZ, Gao YQ, Sun RQ, Qin SY, et al. Akkermansia muciniphila potentiates the antitumor efficacy of FOLFOX in colon cancer. Front Pharmacol (2021) 12:725583. doi: 10.3389/fphar.2021.725583
103. Nagourney RA, Evans S, Tran PH, Nagourney AJ, Sugarbaker PH, et al. Colorectal cancer cells from patients treated with FOLFOX or CAPOX are resistant to oxaliplatin. Eur J Surg Oncol (2021) 47(4):738–42. doi: 10.1016/j.ejso.2020.09.017
104. Degirmencioglu S, Tanrıverdi O, Demiray AG, Senol H, Dogu GG, Yaren A. Retrospective comparison of efficacy and safety of CAPOX and FOLFOX regimens as adjuvant treatment in patients with stage III colon cancer. J Int Med Res (2019) 47(6):2507–15. doi: 10.1177/0300060519848258
105. Zaniboni A, Aitini E, Barni S, Ferrari D, Cascinu S, Catalano V, et al. FOLFIRI as second-line chemotherapy for advanced pancreatic cancer: a GISCAD multicenter phase II study. Cancer Chemother Pharmacol (2012) 69:1641–5. doi: 10.1007/s00280-012-1875-1
106. Lyskjaer I, Kronborg CS, Rasmussen MH, Sørensen BS, Demuth C, Rosenkilde M, et al. Correlation between early dynamics in circulating tumour DNA and outcome from FOLFIRI treatment in metastatic colorectal cancer. Sci Rep (2019) 9(1):1–10. doi: 10.1038/s41598-019-47708-1
107. Budithi A, Su S, Kirshtein A, Shahriyari L. Data driven mathematical model of FOLFIRI treatment for colon cancer. Cancers (2021) 13(11):2632. doi: 10.3390/cancers13112632
108. Xu T, Lu J, An H. The relative change in regulatory T cells/T helper lymphocytes ratio as parameter for prediction of therapy efficacy in metastatic colorectal cancer patients. Oncotarget (2017) 8(65):109079. doi: 10.18632/oncotarget.22606
109. Maeda K, Hazama S, Tokuno K, Kan S, Maeda Y, Watanabe Y. Impact of chemotherapy for colorectal cancer on regulatory T-cells and tumor immunity. Anticancer Res (2011) 31(12):4569–74.
110. Modest DP, Laubender RP, Stintzing S, Giessen C, Schulz C, Haas M, et al. Early tumor shrinkage in patients with metastatic colorectal cancer receiving first-line treatment with cetuximab combined with either CAPIRI or CAPOX: an analysis of the German AIO KRK 0104 trial. Acta Oncol (2013) 52(5):956–62. doi: 10.3109/0284186X.2012.752580
111. Lokich JJ, Ahlgren JD, Gullo JJ, and Philips JA, Fryer JG, et al. A prospective randomized comparison of continuous infusion fluorouracil with a conventional bolus schedule in metastatic colorectal carcinoma: a Mid-Atlantic Oncology Program Study. J Clin Oncol (1989) 7(4):425–32. doi: 10.1200/JCO.1989.7.4.425
112. Gustavsson B, Carlsson G, Machover D, Petrelli N, Roth A, Schmoll H-J, et al. A review of the evolution of systemic chemotherapy in the management of colorectal cancer. Clin Colorectal Cancer (2015) 14(1):1–10. doi: 10.1016/j.clcc.2014.11.002
113. Maindrault-Goebel F, Louvet C, Andre T, Carola E, Lotz J, Molitor J, et al. Oxaliplatin added to the simplified bimonthly leucovorin and 5-fluorouracil regimen as second-line therapy for metastatic colorectal cancer (FOLFOX6). Eur J Cancer (1999) 35(9):1338–42. doi: 10.1016/S0959-8049(99)00149-5
114. Andre T, Louvet C, Maindrault-Goebel F, Couteau C, Mabro M, Lotz J, et al. CPT-11 (irinotecan) addition to bimonthly, high-dose leucovorin and bolus and continuous-infusion 5-fluorouracil (FOLFIRI) for pretreated metastatic colorectal cancer. Eur J Cancer (1999) 35(9):1343–7. doi: 10.1016/S0959-8049(99)00150-1
115. Falcone A, Ricci S, Brunetti I, Pfanner E, Allegrini G, Barbara C, et al. Phase III trial of infusional fluorouracil, leucovorin, oxaliplatin, and irinotecan (FOLFOXIRI) compared with infusional fluorouracil, leucovorin, and irinotecan (FOLFIRI) as first-line treatment for metastatic colorectal cancer: the Gruppo Oncologico Nord Ovest. J Clin Oncol (2007) 25(13):1670–6. doi: 10.1200/JCO.2006.09.0928
116. Van Cutsem E, Twelves C, Cassidy J, Allman D, Bajetta E, Boyer M, et al. Oral capecitabine compared with intravenous fluorouracil plus leucovorin in patients with metastatic colorectal cancer: results of a large phase III study. J Clin Oncol (2001) 19(21):4097–106. doi: 10.1200/JCO.2001.19.21.4097
117. Cassidy J, Tabernero J, Twelves C, Brunet R, Butts C, Conroy T, et al. XELOX (capecitabine plus oxaliplatin): active first-line therapy for patients with metastatic colorectal cancer. J Clin Oncol (2004) 22(11):2084–91. doi: 10.1200/JCO.2004.11.069
118. Xu R-H, Muro K, Morita S, Iwasa S, Han SW, Wang W, et al. Modified XELIRI (capecitabine plus irinotecan) versus FOLFIRI (leucovorin, fluorouracil, and irinotecan), both either with or without bevacizumab, as second-line therapy for metastatic colorectal cancer (AXEPT): a multicentre, open-label, randomised, non-inferiority, phase 3 trial. Lancet Oncol (2018) 19(5):660–71. doi: 10.1016/S1470-2045(18)30140-2
119. Mahipal A, Grothey A. Role of biologics in first-line treatment of colorectal cancer. J Oncol Pract (2016) 12(12):1219–28. doi: 10.1200/JOP.2016.018382
120. Hiret S, Borg C, Bertaut A, Bouche O, Adenis A, Deplanque G, et al. Bevacizumab or cetuximab plus chemotherapy after progression with bevacizumab plus chemotherapy in patients with wtKRAS metastatic colorectal cancer: A randomized phase II study (Prodige 18–UNICANCER GI). Am Soc Clin Oncol (2016) 34:3514. doi: 10.1200/JCO.2016.34.15_suppl.3514
121. Cutsem EV, Lenz H-J, Kohne C, Heinemann V, Tejpar S, Melezínek I, et al. Fluorouracil, leucovorin, and irinotecan plus cetuximab treatment and RAS mutations in colorectal cancer. J Clin Oncol (2015) 33:692–700. doi: 10.1200/JCO.2014.59.4812
122. Wang Q, Yang S, Wang K, Sun S-Y. MET inhibitors for targeted therapy of EGFR TKI-resistant lung cancer. J Hematol Oncol (2019) 12(1):1–11. doi: 10.1186/s13045-019-0759-9
123. Lam BQ, Dai L, Qin Z. The role of HGF/c-MET signaling pathway in lymphoma. J Hematol Oncol (2016) 9(1):1–8. doi: 10.1186/s13045-016-0366-y
124. Wang Y, Johnson KC.C, and Gatti-Mays ME, Li Z. Emerging strategies in targeting tumor-resident myeloid cells for cancer immunotherapy. J Hematol Oncol (2022) 15(1):118. doi: 10.1186/s13045-022-01225-3
125. Xie Y-H, Chen Y-X, Fang J-Y. Comprehensive review of targeted therapy for colorectal cancer. Signal Transduct Target Ther (2020) 5(1):22. doi: 10.1038/s41392-020-0116-z
126. Schmitt M, Greten FR. The inflammatory pathogenesis of colorectal cancer. Nat Rev Immunol (2021) 21(10):653–67. doi: 10.1038/s41577-021-00534-x
127. Ganesh K, Stadler ZK, Cercek A, Mendelsohn RB, Shia J, Segal NH, et al. Immunotherapy in colorectal cancer: rationale, challenges and potential. Nat Rev Gastroenterol Hepatol (2019) 16(6):361–75. doi: 10.1038/s41575-019-0126-x
128. Bodduluri SR, Mathis S, Maturu P, Krishnan E, Satpathy SR, Chiltonb PM, et al. Mast cell–dependent CD8+ T-cell recruitment mediates immune surveillance of intestinal tumors in ApcMin/+ MiceMast cell mediated immune surveillance of intestinal cancer. Cancer Immunol Res (2018) 6(3):332–47. doi: 10.1158/2326-6066.CIR-17-0424
129. O’Sullivan D, Sanin DE, Pearce EJ, Pearce EL. Metabolic interventions in the immune response to cancer. Nat Rev Immunol (2019) 19(5):324–35. doi: 10.1038/s41577-019-0140-9
130. Grzywa TM, Sosnowska A, Matryba P, Rydzynska Z, Jasinski M, Nowisb D, et al. Myeloid cell-derived arginase in cancer immune response. Front Immunol (2020) 11:938. doi: 10.3389/fimmu.2020.00938
131. Dianat-Moghadam H, Mahari A, Salahlou R, Khalili M, and Azizi M, Sadeghzadeh H, et al. Immune evader cancer stem cells direct the perspective approaches to cancer immunotherapy. Stem Cell Res Ther (2022) 13(1):1–12. doi: 10.1186/s13287-022-02829-9
132. Marofi F, Achmad H, Bokov D, Abdelbasset WK, Alsadoon Z, Chupradit S, et al. Hurdles to breakthrough in CAR T cell therapy of solid tumors. Stem Cell Res Ther (2022) 13(1):1–19. doi: 10.1186/s13287-022-02819-x
133. Wu F, Yang J, Liu J, Wang Y, Mu J, Zeng Q, et al. Signaling pathways in cancer-associated fibroblasts and targeted therapy for cancer. Signal Transduct Target Ther (2021) 6(1):218. doi: 10.1038/s41392-021-00641-0
134. Kubli SP, Berger T, Araujo DV, Siu LL, Mak TW. Beyond immune checkpoint blockade: emerging immunological strategies. Nat Rev Drug Discovery (2021) 20(12):899–919. doi: 10.1038/s41573-021-00155-y
135. Shi A-P, Tang X-Y, Xiong Y-L, Zheng K-F, Liu K-F, Shi X-G, et al. Immune checkpoint LAG3 and its ligand FGL1 in cancer. Front Immunol (2022) 12:5962. doi: 10.3389/fimmu.2021.785091
136. Dermani FK, Samadi P, Rahmani G, Kohlan AK, Najafi R. PD-1/PD-L1 immune checkpoint: potential target for cancer therapy. J Cell Physiol (2019) 234(2):1313–25. doi: 10.1002/jcp.27172
137. Hazrati A, Soudi S, Malekpour K, Mahmoudi M, Rahimi A, Hashemi SM, et al. Immune cells-derived exosomes function as a double-edged sword: role in disease progression and their therapeutic applications. biomark Res (2022) 10(1):30. doi: 10.1186/s40364-022-00374-4
138. Koopman M, Antonini NF, Douma J, Wals J, Honkoop AH, Erdkamp FL, et al. Sequential versus combination chemotherapy with capecitabine, irinotecan, and oxaliplatin in advanced colorectal cancer (CAIRO): a phase III randomised controlled trial. Lancet (2007) 370(9582):135–42. doi: 10.1016/S0140-6736(07)61086-1
139. Ibrahim A, Hirschfeld S, Cohen MH, Griebel DJ, Williams GA, Pazdur R. FDA drug approval summaries: oxaliplatin. Oncologist (2004) 9(1):8–12. doi: 10.1634/theoncologist.9-1-8
140. Graham J, Muhsin M, Kirkpatrick P. Cetuximab. Nat Rev Drug Discovery (2004) 3(7):549–550. doi: 10.1038/nrd1445
141. Cohen MH, Chen H, Shord S, Fuchs C, He K, Zhao H, et al. Approval summary: cetuximab in combination with cisplatin or carboplatin and 5-fluorouracil for the first-line treatment of patients with recurrent locoregional or metastatic squamous cell head and neck cancer. Oncologist (2013) 18(4):460–6. doi: 10.1634/theoncologist.2012-0458
142. Cohen MH, Gootenberg J, Keegan P, Pazdur R. FDA drug approval summary: bevacizumab (Avastin®) plus carboplatin and paclitaxel as first-line treatment of advanced/metastatic recurrent nonsquamous non-small cell lung cancer. Oncologist (2007) 12(6):713–8. doi: 10.1634/theoncologist.12-6-713
143. Bekaii-Saab TS, Roda JM, Guenterberg KD, Ramaswamy B, Young DC, Ferketich AK, et al. A phase I trial of paclitaxel and trastuzumab in combination with interleukin-12 in patients with HER2/neu-expressing Malignancies. Mol Cancer Ther (2009) 8(11):2983–91. doi: 10.1158/1535-7163.MCT-09-0820
144. Ramanathan RK, Hwang JJ, Zamboni W.C, Sinicrope FA, Safran H, Wong MK, et al. Low overexpression of HER-2/Neu in advanced colorectal cancer limits the Usefulness of trastuzumab (Herceptin®) and irinotecan as therapy. A phase II trial. Cancer Invest (2004) 22(6):858–65. doi: 10.1081/CNV-200039645
145. Robert C, Schachter J, Long GV, Arance A, Grob JJ, Mortier L, et al. Pembrolizumab versus ipilimumab in advanced melanoma. New Engl J Med (2015) 372(26):2521–32. doi: 10.1056/NEJMoa1503093
146. Herbst RS, Baas P, Kim D-W, Felip E, Pérez-Gracia JL, Han J-Y, et al. Pembrolizumab versus docetaxel for previously treated, PD-L1-positive, advanced non-small-cell lung cancer (KEYNOTE-010): a randomised controlled trial. Lancet (2016) 387(10027):1540–50. doi: 10.1016/S0140-6736(15)01281-7
147. Le DT, Uram JN, Wang H, Bartlett BR, Kemberling H, Eyring AD, et al. PD-1 blockade in tumors with mismatch-repair deficiency. New Engl J Med (2015) 372(26):2509–20. doi: 10.1056/NEJMoa1500596
148. Hoos A, Ibrahim R, Korman A, Abdallah K, Berman D, Shahabi V, et al. Development of ipilimumab: contribution to a new paradigm for cancer immunotherapy. Semin Oncol (2010) 37:533–46. doi: 10.1053/j.seminoncol.2010.09.015
149. Hodi FS, O'day SJ, McDermott DF, Weber RW, Sosman JA, Haanen JB, et al. Improved survival with ipilimumab in patients with metastatic melanoma. New Engl J Med (2010) 363(8):711–23. doi: 10.1056/NEJMoa1003466
150. Wolchok JD, Kluger H, Callahan MK, Postow MA, Rizvi NA, Lesokhin AM, et al. Nivolumab plus ipilimumab in advanced melanoma. New Engl J Med (2013) 369(2):122–33. doi: 10.1056/NEJMoa1302369
151. Schlake T, Thess A, Thran M, Jordan I. mRNA as novel technology for passive immunotherapy. Cell Mol Life Sci (2019) 76:301–28. doi: 10.1007/s00018-018-2935-4
152. Arab A, Yazdian-Robati R, Behravan J. HER2-positive breast cancer immunotherapy: A focus on vaccine development. Archivum Immunol Therapiae Experiment (2020) 68:1–18. doi: 10.1007/s00005-019-00566-1
153. Akkın S, Varan G, Bilensoy E. A review on cancer immunotherapy and applications of nanotechnology to chemoimmunotherapy of different cancers. Molecules (2021) 26(11):3382. doi: 10.3390/molecules26113382
154. Mohsenzadegan M, Peng RW, Roudi R. Dendritic cell/cytokine-induced killer cell-based immunotherapy in lung cancer: what we know and future landscape. J Cell Physiol (2020) 235(1):74–86. doi: 10.1002/jcp.28977
155. Waldman AD, Fritz JM, Lenardo MJ. A guide to cancer immunotherapy: from T cell basic science to clinical practice. Nat Rev Immunol (2020) 20(11):651–68. doi: 10.1038/s41577-020-0306-5
156. Mulet-Margalef N, Linares J, Badia-Ramentol J, Jimeno M, Sanz Monte C, Manzano Mozo JL, et al. Challenges and therapeutic opportunities in the dMMR/MSI-H colorectal cancer landscape. Cancers (2023) 15(4):1022. doi: 10.3390/cancers15041022
157. Sahin IH, Akce M, Alese O, Shaib W, Lesinski GB, El-Rayes B, et al. Immune checkpoint inhibitors for the treatment of MSI-H/MMR-D colorectal cancer and a perspective on resistance mechanisms. Br J Cancer (2019) 121(10):809–18. doi: 10.1038/s41416-019-0599-y
158. Chen L, Jiang X, Li Y, Zhang Q, Li Q, Zhang X, et al. How to overcome tumor resistance to anti-PD-1/PD-L1 therapy by immunotherapy modifying the tumor microenvironment in MSS CRC. Clin Immunol (2022) 237:108962. doi: 10.1016/j.clim.2022.108962
159. Bai Z, Zhou Y, Ye Z, Xiong J, Lan H, Wang F, et al. Tumor-infiltrating lymphocytes in colorectal cancer: the fundamental indication and application on immunotherapy. Front Immunol (2022) 12:5926. doi: 10.3389/fimmu.2021.808964
160. Cui G. The mechanisms leading to distinct responses to PD-1/PD-L1 blockades in colorectal cancers with different MSI statuses. Front Oncol (2021) 11:573547. doi: 10.3389/fonc.2021.573547
161. Ciardiello D, Vitiello PP, Cardone C, Martini G, Troiani T, Martinelli E, et al. Immunotherapy of colorectal cancer: Challenges for therapeutic efficacy. Cancer Treat Rev (2019) 76:22–32. doi: 10.1016/j.ctrv.2019.04.003
162. Becht E, Reyniès A, Giraldo NA, Pilati C, Buttard B, Lacroix L, et al. Immune and stromal classification of colorectal cancer is associated with molecular subtypes and relevant for precision immunotherapyDistinct immune phenotypes of colorectal cancer molecular subtypes. Clin Cancer Res (2016) 22(16):4057–66. doi: 10.1158/1078-0432.CCR-15-2879
163. Wang W, Kandimalla R, Huang H, Zhu L, Li Y, Gao F, et al. Molecular subtyping of colorectal cancer: Recent progress, new challenges and emerging opportunities. Semin Cancer Biol (2019) 55:37–52. doi: 10.1016/j.semcancer.2018.05.002
164. Chowdhury S, Hofree M, Lin K, Maru D, Kopetz S, Shen JP. Implications of intratumor heterogeneity on consensus molecular subtype (CMS) in colorectal cancer. Cancers (2021) 13(19):4923. doi: 10.3390/cancers13194923
165. Källberg J, Harrison A, March V, Bērziņa S, Nemazanyy I, Kepp O, et al. Intratumor heterogeneity and cell secretome promote chemotherapy resistance and progression of colorectal cancer. Cell Death Dis (2023) 14(5):306.
166. Dienstmann R, Vermeulen L, Guinney J, Kopetz S, Tejpar S, Tabernero J, et al. Consensus molecular subtypes and the evolution of precision medicine in colorectal cancer. Nat Rev Cancer (2017) 17(2):79–92. doi: 10.1038/nrc.2016.126
167. Payandeh Z, Khalili S, Somi MH, Mard‐Soltani M, Baghbanzadeh A, Hajiasgharzadeh K, et al. PD-1/PD-L1-dependent immune response in colorectal cancer. J Cell Physiol (2020) 235(7-8):5461–75. doi: 10.1002/jcp.29494
168. Proctor M. TGF-β’s role in immune evasion of colorectal cancer and anti-TGF-β therapies. Microreviews Cell Mol Biol (2021) 8(4).:1–3
169. Sillo T, Beggs A, and Morton D, Middleton G. Mechanisms of immunogenicity in colorectal cancer. J Br Surg (2019) 106(10):1283–97. doi: 10.1002/bjs.11204
170. Zhou C, Cheng X, Tu S. Current status and future perspective of immune checkpoint inhibitors in colorectal cancer. Cancer Lett (2021) 521:119–29. doi: 10.1016/j.canlet.2021.07.023
171. Baulu E, Gardet C, Chuvin N, Depil S. TCR-engineered T cell therapy in solid tumors: State of the art and perspectives. Sci Adv (2023) 9(7):eadf3700. doi: 10.1126/sciadv.adf3700
172. Ray SK, Meshram Y, Mukherjee S. Cancer immunology and CAR-T cells: A turning point therapeutic approach in colorectal carcinoma with clinical insight. Curr Mol Med (2021) 21(3):221–36. doi: 10.2174/1566524020666200824103749
173. Li H, Yang C, Cheng H, Huang S, Zheng Y. CAR-T cells for Colorectal Cancer: Target-selection and strategies for improved activity and safety. J Cancer (2021) 12(6):1804. doi: 10.7150/jca.50509
174. Kosti P, Opzoomer JW, Larios-Martinez KI, Henley-Smith R, Scudamore CL, Okesola M, et al. Hypoxia-sensing CAR T cells provide safety and efficacy in treating solid tumors. Cell Rep Med (2021) 2(4):100227. doi: 10.1016/j.xcrm.2021.100227
175. Sterner RC, Sterner RM. CAR-T cell therapy: current limitations and potential strategies. Blood Cancer J (2021) 11(4):69. doi: 10.1038/s41408-021-00459-7
176. Katz SC, Moody AE, Guha P, Hardaway JC, Prince E, LaPorte J, et al. HITM-SURE: Hepatic immunotherapy for metastases phase Ib anti-CEA CAR-T study utilizing pressure enabled drug delivery. J ImmunoTher Cancer (2020) 8(2):e001097. doi: 10.1136/jitc-2020-001097
177. Wang Y, Chen M, Wu Z, Tong C, Dai H, Guo Y, et al. CD133-directed CAR T cells for advanced metastasis Malignancies: A phase I trial. Oncoimmunology (2018) 7(7):e1440169. doi: 10.1080/2162402X.2018.1440169
178. Wang H, Pan W. Challenges of chimeric antigen receptor-T/natural killer cell therapy in the treatment of solid tumors: focus on colorectal cancer and evaluation of combination therapies. Mol Cell Biochem (2022) 47:1–14. doi: 10.1007/s11010-022-04568-0
179. Liu Q, Li J, Zheng H, Yang S, Hua Y, Huang N, et al. Adoptive cellular immunotherapy for solid neoplasms beyond CAR-T. Mol Cancer (2023) 22(1):1–27. doi: 10.1186/s12943-023-01735-9
180. Tolmachev V, Orlova A, Sörensen J. The emerging role of radionuclide molecular imaging of HER2 expression in breast cancer. Semin Cancer Biol (2021) 72:185–97. doi: 10.1016/j.semcancer.2020.10.005
181. Xu J, Meng Q, Sun H, Zhang X, Yun J, Li B, et al. HER2-specific chimeric antigen receptor-T cells for targeted therapy of metastatic colorectal cancer. Cell Death Dis (2021) 12(12):1109. doi: 10.1038/s41419-021-04100-0
182. Bruni D, Angell HK, Galon J. The immune contexture and Immunoscore in cancer prognosis and therapeutic efficacy. Nat Rev Cancer (2020) 20(11):662–80. doi: 10.1038/s41568-020-0285-7
183. Yu I, Dakwar A, Takabe K. Immunotherapy: recent advances and its future as a neoadjuvant, adjuvant, and primary treatment in colorectal cancer. Cells (2023) 12(2):258. doi: 10.3390/cells12020258
184. Subtil B, Cambi A, Tauriello DV, Vries IJ.M. The therapeutic potential of tackling tumor-induced dendritic cell dysfunction in colorectal cancer. Front Immunol (2021) p:4048. doi: 10.3389/fimmu.2021.724883
185. Zhao L-p, Hu J-h, Hu D, Wang H-j, Huang C-g, Luo R-h, et al. Hyperprogression, a challenge of PD-1/PD-L1 inhibitors treatments: potential mechanisms and coping strategies. Biomed Pharmacother (2022) 150:112949. doi: 10.1016/j.biopha.2022.112949
186. Wang M, Zhang L, Chang W, Zhang Y. The crosstalk between the gut microbiota and tumor immunity: Implications for cancer progression and treatment outcomes. Front Immunol (2022) 13. doi: 10.3389/fimmu.2022.1096551
187. Inamura K. Gut microbiota contributes towards immunomodulation against cancer: new frontiers in precision cancer therapeutics. Semin Cancer Biol (2021) 70:11–23. doi: 10.1016/j.semcancer.2020.06.006
188. Gai X-D, Li C, Song Y, Lei Y-M, Yang B-X, et al. In situ analysis of FOXP3+ regulatory T cells and myeloid dendritic cells in human colorectal cancer tissue and tumor−draining lymph node. Biomed Rep (2013) 1(2):207–12. doi: 10.3892/br.2012.35
189. Nakayama Y, Inoue Y, Minagawa N, Katsuki T, Nagashima N, Onitsuka K, et al. Relationships between S-100 protein-positive cells and clinicopathological factors in patients with colorectal cancer. Anticancer Res (2003) 23(6):4423–6.
190. Bauer K, Michel S, Reuschenbach M, Nelius N, and Doeberitz Knebel von M, Kloor M, et al. Dendritic cell and macrophage infiltration in microsatellite-unstable and microsatellite-stable colorectal cancer. Familial Cancer (2011) 10:557–65. doi: 10.1007/s10689-011-9449-7
191. Miller TJ, Anyaegbu CC, Lee‐Pullen TF, Spalding L.J, Platell CF, McCoy MJ, et al. PD-L1+ dendritic cells in the tumor microenvironment correlate with good prognosis and CD8+ T cell infiltration in colon cancer. Cancer Sci (2021) 112(3):1173–83. doi: 10.1111/cas.14781
192. Kreidieh M, Mukherji D, Temraz S, Shamseddine A. Expanding the scope of immunotherapy in colorectal cancer: current clinical approaches and future directions. BioMed Res Int (2020) 2020. doi: 10.1155/2020/9037217
193. Chauhan DS, Dhasmana A, Laskar P, Prasad R, Jain NK, Srivastava R, et al. Nanotechnology synergized immunoengineering for cancer. Eur J Pharm Biopharm (2021) 163:72–101. doi: 10.1016/j.ejpb.2021.03.010
194. Laurent P, Tevaearai H, Eliason J, Givel J-C, Odartchenko N. Interferon alpha and 5′-deoxy-5-fluorouridine in colon cancer: effects as single agents and in combination on growth of xenograft tumours. Eur J Cancer (1994) 30(12):1859–65. doi: 10.1016/0959-8049(94)00341-2
195. Koshiji M, Adachi Y, Taketani S, Takeuchi K, Hioki K, Ikehara S, et al. Mechanisms underlying apoptosis induced by combination of 5-fluorouracil and interferon-γ. Biochem Biophys Res Commun (1997) 240(2):376–81. doi: 10.1006/bbrc.1997.7657
196. Matos AI, Carreira B, Peres C, Moura L.I, Conniot J, Fourniols T, et al. Nanotechnology is an important strategy for combinational innovative chemo-immunotherapies against colorectal cancer. J Controlled Release (2019) 307:108–38. doi: 10.1016/j.jconrel.2019.06.017
197. Lin W-Y, Wang S-S, Kang Y-N, Porpiglia AS, Chang Y, Huang C-H, et al. Do proton pump inhibitors affect the effectiveness of chemotherapy in colorectal cancer patients? A systematic review with meta-analysis. Front Pharmacol (2022) 13. doi: 10.3389/fphar.2022.1048980
198. Pilot T, Fratti A, Thinselin C, Perrichet A, Demontoux L, Limagne E, et al. Heat shock and HSP70 regulate 5-FU-mediated caspase-1 activation in myeloid-derived suppressor cells and tumor growth in mice. J Immunother Cancer (2020) 8(1):e000478. doi: 10.1136/jitc-2019-000478
199. Wang Z, Till B, Gao Q. Chemotherapeutic agent-mediated elimination of myeloid-derived suppressor cells. Oncoimmunology (2017) 6(7):e1331807. doi: 10.1080/2162402X.2017.1331807
200. Arango D, Wilson A, Shi Q, Corner G, Aranes M, Nicholas C, et al. Molecular mechanisms of action and prediction of response to oxaliplatin in colorectal cancer cells. Br J Cancer (2004) 91(11):1931–46. doi: 10.1038/sj.bjc.6602215
201. Cao P, Xia Y, He W, Zhang T, Hong L, Zheng P, et al. Enhancement of oxaliplatin-induced colon cancer cell apoptosis by alantolactone, a natural product inducer of ROS. Int J Biol Sci (2019) 15(8):1676. doi: 10.7150/ijbs.35265
202. Tabernero J, Grothey A, Arnold D, Gramont A, Ducreux M, P, et al. MODUL cohort 2: an adaptable, randomized, signal-seeking trial of fluoropyrimidine plus bevacizumab with or without atezolizumab maintenance therapy for BRAFwt metastatic colorectal cancer. ESMO Open (2022) 7(5):100559. doi: 10.1016/j.esmoop.2022.100559
203. Baraibar I, Mirallas O, Saoudi N, Ros J, Salvà F, Tabernero J, et al. Combined treatment with immunotherapy-based strategies for MSS metastatic colorectal cancer. Cancers (2021) 13(24):6311. doi: 10.3390/cancers13246311
204. Tang PA, Jonker DJ, Kennecke HF, Welch S, Cripps MC, Asmis TR, et al. IND. 210: A randomized phase 2 trial of FOLFOX6/bevacizumab with or without reovirus (Reo) in patients (pts) with metastatic colorectal cancer (mCRC). Am Soc Clin Oncol (2016) 34:3546. doi: 10.1200/JCO.2016.34.15_suppl.3546
205. Cremolini C, Antoniotti C, Stein A, Bendell J, Gruenberger T, Rossini D, et al. Individual patient data meta-analysis of FOLFOXIRI plus bevacizumab versus doublets plus bevacizumab as initial therapy of unresectable metastatic colorectal cancer. J Clin Oncol (2020) 38(28):3314–24. doi: 10.1200/JCO.20.01225
206. Passardi A, Nanni O, Tassinari D, Turci D, Cavanna L, Fontana A, et al. Effectiveness of bevacizumab added to standard chemotherapy in metastatic colorectal cancer: final results for first-line treatment from the ITACa randomized clinical trial. Ann Oncol (2015) 26(6):1201–7. doi: 10.1093/annonc/mdv130
207. Antoniotti C, Rossini D, Pietrantonio F, Catteau A, Salvatore L, Lonardi S, et al. Upfront FOLFOXIRI plus bevacizumab with or without atezolizumab in the treatment of patients with metastatic colorectal cancer (AtezoTRIBE): a multicentre, open-label, randomised, controlled, phase 2 trial. Lancet Oncol (2022) 23(7):876–87. doi: 10.1016/S1470-2045(22)00274-1
208. Terme M, Pernot S, Marcheteau E, Sandoval F, Benhamouda N, Colussi O, et al. VEGFA-VEGFR pathway blockade inhibits tumor-induced regulatory T-cell proliferation in colorectal CancerVEGF-A inhibition prevents tumor-induced treg proliferation. Cancer Res (2013) 73(2):539–49. doi: 10.1158/0008-5472.CAN-12-2325
209. Gavalas NG, Tsiatas M, Tsitsilonis O, Politi E, Ioannou K, Ziogas AC, et al. VEGF directly suppresses activation of T cells from ascites secondary to ovarian cancer via VEGF receptor type 2. Br J Cancer (2012) 107(11):1869–75. doi: 10.1038/bjc.2012.468
210. Limagne E, Euvrard R, Thibaudin M, Rébé C, Derangère V, Chevriaux A, et al. Accumulation of MDSC and Th17 cells in patients with metastatic colorectal cancer predicts the efficacy of a FOLFOX–bevacizumab drug treatment regimenMDSC and Th17 in FOLFOX–bevacizumab–treated mCRC patients. Cancer Res (2016) 76(18):5241–52. doi: 10.1158/0008-5472.CAN-15-3164
211. Cremolini C, Rossini D, Antoniotti C, Pietrantonio F, Lonardi S, Salvatore L, et al. LBA20 FOLFOXIRI plus bevacizumab (bev) plus atezolizumab (atezo) versus FOLFOXIRI plus bev as first-line treatment of unresectable metastatic colorectal cancer (mCRC) patients: Results of the phase II randomized AtezoTRIBE study by GONO. Ann Oncol (2021) 32:S1294–5. doi: 10.1016/j.annonc.2021.08.2094
212. Bordonaro R, Calvo A, Auriemma A, Hollebecque A, Rubovszky G, Saunders MP, et al. Trifluridine/tipiracil in combination with oxaliplatin and either bevacizumab or nivolumab in metastatic colorectal cancer: a dose-expansion, phase I study. ESMO Open (2021) 6(5):100270. doi: 10.1016/j.esmoop.2021.100270
213. Damato A, Bergamo F, Antonuzzo L, Nasti G, Pietrantonio F, Tonini G, et al. Phase II study of nivolumab in combination with FOLFOXIRI/bevacizumab as first-line treatment in patients with advanced colorectal cancer RAS/BRAF mutated (mut): NIVACOR trial. Am Soc Clin Oncol (2022). doi: 10.3389/fonc.2021.766500
214. Gallois C, Emile J-F, Kim S, Monterymard C, Gilabert M, Bez J, et al. Pembrolizumab with Capox Bevacizumab in patients with microsatellite stable metastatic colorectal cancer and a high immune infiltrate: The FFCD 1703-POCHI trial. Digest Liver Dis (2021) 53(10):1254–9. doi: 10.1016/j.dld.2021.06.009
215. Trotta AM, Ottaiano A, Romano C, Nasti G, Nappi A, Divitiis De C, et al. Prospective evaluation of cetuximab-mediated antibody-dependent cell cytotoxicity in metastatic colorectal cancer patients predicts treatment efficacyCetuximab-mediated ADCC in mCRC patients. Cancer Immunol Res (2016) 4(4):366–74. doi: 10.1158/2326-6066.CIR-15-0184
216. Wang L, Wei Y, Fang W, Lu C, Chen J, Cui G, et al. Cetuximab enhanced the cytotoxic activity of immune cells during treatment of colorectal cancer. Cell Physiol Biochem (2017) 44(3):1038–50. doi: 10.1159/000485404
217. Tabernero J, Cutsem Van E, Sastre J, Cervantes A, Laethem Van J-L, Humblet Y, et al. An international phase II study of cetuximab in combination with oxaliplatin/5-fluorouracil (5-FU)/folinic acid (FA)(FOLFOX-4) in the first-line treatment of patients with metastatic colorectal cancer (CRC) expressing epidermal growth factor receptor (EGFR). Preliminary results. J Clin Oncol (2004) 22(14_suppl):3512–2. doi: 10.1200/jco.2004.22.90140.3512
218. Stein A, Binder M, Bokemeyer C, Batran Al SE, Hinke A, Hegewisch-Becker S. Avelumab and cetuximab in combination with FOLFOX in patients with previously untreated metastatic colorectal cancer (MCRC): The phase II AVETUX-CRC trial (AIO KRK 0216). Am Soc Clin Oncol (2017). doi: 10.1200/JCO.2017.35.15_suppl.TPS3620
219. Qin S, Li J, Wang L, Xu J, Cheng Y, Bai Y, et al. Efficacy and tolerability of first-line cetuximab plus leucovorin, fluorouracil, and oxaliplatin (FOLFOX-4) versus FOLFOX-4 in patients with RAS wild-type metastatic colorectal cancer: the open-label, randomized, phase III TAILOR trial. J Clin Oncol (2018) 36(30):3031. doi: 10.1200/JCO.2018.78.3183
220. Stein A, Binder M, Goekkurt E, Lorenzen S, Riera-Knorrenschild J, Depenbusch R, et al. Avelumab and cetuximab in combination with FOLFOX in patients with previously untreated metastatic colorectal cancer (MCRC): Final results of the phase II AVETUX trial (AIO-KRK-0216). Am Soc Clin Oncol (2020). doi: 10.1200/JCO.2020.38.4_suppl.96
221. Truong NT, Gargett T, Brown MP, Ebert L.M. Effects of chemotherapy agents on circulating leukocyte populations: Potential implications for the success of CAR-T cell therapies. Cancers (2021) 13(9):2225. doi: 10.3390/cancers13092225
222. Verma R, Foster RE, Horgan K, Mounsey K, Nixon H, Smalle N, et al. Lymphocyte depletion and repopulation after chemotherapy for primary breast cancer. Breast Cancer Res (2016) 18(1):1–12. doi: 10.1186/s13058-015-0669-x
223. Wu X, Feng Q-M, Wang Y, Shi J, Ge H-L, Di W. The immunologic aspects in advanced ovarian cancer patients treated with paclitaxel and carboplatin chemotherapy. Cancer Immunol Immunother (2010) 59:279–91. doi: 10.1007/s00262-009-0749-9
224. Mackall CL, Fleisher TA, Brown MR, Andrich MP, Chen CC, Feuerstein IM, et al. Distinctions between CD8+ and CD4+ T-cell regenerative pathways result in prolonged T-cell subset imbalance after intensive chemotherapy. Blood J Am Soc Hematol (1997) 89(10):3700–7. doi: 10.1182/blood.V89.10.3700
Keywords: colorectal cancer, tumor treatment, chemotherapy, immunotherapy, cell therapy
Citation: He X, Lan H, Jin K and Liu F (2023) Can immunotherapy reinforce chemotherapy efficacy? a new perspective on colorectal cancer treatment. Front. Immunol. 14:1237764. doi: 10.3389/fimmu.2023.1237764
Received: 09 June 2023; Accepted: 25 August 2023;
Published: 18 September 2023.
Edited by:
Andrea Balla, San Raffaele Scientific Institute (IRCCS), ItalyReviewed by:
Elena De Mattia, Aviano Oncology Reference Center (IRCCS), ItalyHelmout Modjathedi, Kingston University, United Kingdom
Copyright © 2023 He, Lan, Jin and Liu. This is an open-access article distributed under the terms of the Creative Commons Attribution License (CC BY). The use, distribution or reproduction in other forums is permitted, provided the original author(s) and the copyright owner(s) are credited and that the original publication in this journal is cited, in accordance with accepted academic practice. No use, distribution or reproduction is permitted which does not comply with these terms.
*Correspondence: Ketao Jin, amlua2V0YW8yMDAxQHpqdS5lZHUuY24=; Fanlong Liu, ZmFubG9uZ19saXVAemp1LmVkdS5jbg==
†These authors have contributed equally to this work