- 1Institute of Integrated Traditional Chinese and Western Medicine, Tongji Hospital, Tongji Medical College, Huazhong University of Science and Technology, Wuhan, China
- 2Department of Integrated Traditional Chinese and Western Medicine, Tongji Hospital, Tongji Medical College, Huazhong University of Science and Technology, Wuhan, China
The incidence of human herpesvirus (HHVs) is gradually increasing and has affected a wide range of population. HHVs can result in serious consequences such as tumors, neonatal malformations, sexually transmitted diseases, as well as pose an immense threat to the human health. The cGAS-STING pathway is one of the innate immune pattern-recognition receptors discovered recently. This article discusses the role of the cGAS-STING pathway in human diseases, especially in human herpesvirus infections, as well as highlights how these viruses act on this pathway to evade the host immunity. Moreover, the author provides a comprehensive overview of modulators of the cGAS-STING pathway. By focusing on the small molecule compounds based on the cGAS-STING pathway, novel targets and concepts have been proposed for the development of antiviral drugs and vaccines, while also providing a reference for the investigation of disease models related to the cGAS-STING pathway. HHV is a double-stranded DNA virus that can trigger the activation of intracellular DNA sensor cGAS, after which the host cells initiate a cascade of reactions that culminate in the secretion of type I interferon to restrict the viral replication. Meanwhile, the viral protein can interact with various molecules in the cGAS-STING pathway. Viruses can evade immune surveillance and maintain their replication by inhibiting the enzyme activity of cGAS and reducing the phosphorylation levels of STING, TBK1 and IRF3 and suppressing the interferon gene activation. Activators and inhibitors of the cGAS-STING pathway have yielded numerous promising research findings in vitro and in vivo pertaining to cGAS/STING-related disease models. However, there remains a dearth of small molecule modulators that have been successfully translated into clinical applications, which serves as a hurdle to be overcome in the future.
1 Introduction
From ancient times to the present, the interaction between virus and host has never stopped. In the face of a viral invasion, the human immune system has evolved so that it can defend the human body against several diseases; nevertheless, in response to the strong immune defense system, viruses have also ingeniously evolved several strategies to evade immune surveillance and antiviral immune responses. There are nine types of human herpesvirus (HHV), these viruses can cause different diseases, including genital herpes, neonatal encephalitis, varicella, herpes zoster, roseola infantum, Kaposi’s sarcoma, infectious mononucleosis, and Hodgkin’s lymphoma. HHV can affect individuals of all age groups, from newborns to the elderly. Furthermore, it can cause serious consequences such as cancer or birth defects. HHV has already led to serious health problems worldwide.
At present, the primary antiviral drugs are nucleoside analogs that mainly comprise acyclovir and glucocorticoids. However, in clinical settings, no effective vaccines are available for many types of HHV (1). Therefore, there is an urgent need to discover new antiviral targets and develop new antiviral strategies.
The body’s first line of defense against pathogen invasion is the natural immune system. There is no doubt that it plays a crucial role in antiviral immunity. Many pattern recognition receptors (PRRs) are important components of this barrier; they recognize pathogen-associated molecular patterns and activate a sequence of signaling pathways in the body to generate a natural immune response.
Cyclic GMP – AMP synthase (cGAS) is a newly discovered intracellular nucleic acid receptor (2). It can detect the host’s double-stranded DNA produced as a result of body damage or foreign double-stranded DNA. Recent studies have reported that cGAS can detect genetic material produced by bacteria, thereby initiating a chain of immune responses.
Stimulator of interferon genes (STING) is a molecule that connected to cGAS downstream. Many studies have reported the role of the cGAS-STING pathway in various systemic diseases. The cGAS-STING pathway plays a role in the immune responses to various conditions, including respiratory system diseases, circulatory system diseases, digestive system diseases, nervous system diseases, viral infection, autoimmune diseases, tumorigenesis, and aging.
In the beginning, most researchers either focused on the interaction between one kind of virus or one disease and the cGAS-STING pathway or on the regulatory effects of a molecule on the cGAS-STING pathway. Besides, the cGAS-STING pathway is rarely involved in the immune escape of viruses.
In present study, we emphasize the role of the cGAS-STING pathway in various systemic diseases by briefly summarizing its role in diseases occurrence, and emphatically discussing the research progress on the cGAS-STING pathway in anti-human herpesvirus infections. Furthermore, we present some regulatory molecules for cGAS and STING. Our study provides new ideas for identifying more effective and comprehensive antiviral targets and developing new antiviral drugs and vaccines. It also provides a research basis for discovering effective therapeutic drugs for diseases associated with the cGAS-STING pathway in clinical settings.
2 cGAS-STING pathway
cGAS is a cytoplasmic DNA sensor that is present in an autoinhibited state when it is not bound to DNA (3). When exogenous or endogenous DNA is detected, cGAS binds to it in a DNA sequence-independent manner (4). Activated cGAS undergoes a conformational change to form a dimer, which is warranted for cGAS activation (5). Active cGAS dimers can promote the synthesis of ATP and GTP loops, secondary the second messenger known as cyclic GMP-AMP (cGAMP) (6). In turn, cGAMP binds to STING on the endoplasmic reticulum (ER) to form dimers, tetramers, and higher-order oligomers. Activated STING is transferred from the ER to the Golgi apparatus. During this process, STING recruits TANK-binding kinase 1 (TBK1). Phosphorylated TBK1, in turn, activates interferon regulatory factor 3 (IRF3). Dimerized IRF3 can trigger the production of type-I interferon (IFN) (7). In addition, STING activates IKK kinase, which phosphorylates the IκB family of inhibitors of nuclear factor-kappa B (NF-κB). The phosphorylated IκB protein is degraded via the ubiquitin-proteasome pathway; at this point, NF-κB enters the nucleus and functions together with IFN regulatory factors such as IRF3 to induce the expression of IFNs and inflammatory cytokines such as tumor necrosis factor (TNF), interleukin (IL)-1β, and IL-6 (3) (Figure 1).
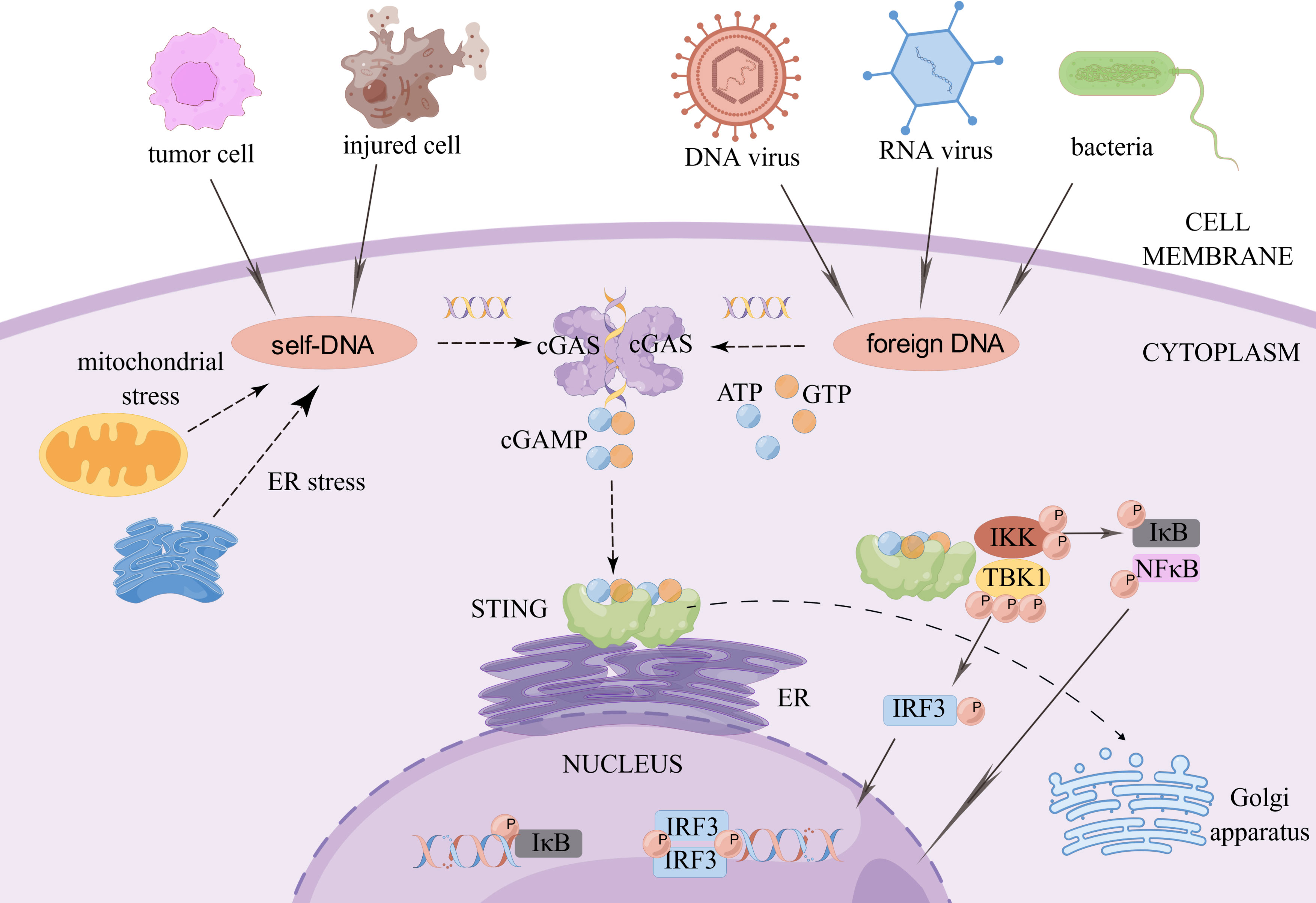
Figure 1 The cGAS-STING signaling pathway. When exogenous pathogen DNA invades, or when the organism experiences cellular damage resulting in leakage of self DNA, the intracytoplasmic DNA receptor cGAS is activated. Activated cGAS undergoes a conformational change and forms a dimer, which promotes the binding of ATP and GTP to generate cGAMP. cGAMP in turn binds to STING on the ER, which subsequently recruits TBK1. Phosphorylated TBK1 in turn activates IRF3. STING also activates IKK kinase, which phosphorylates the IκB family. The degradation of phosphorylated IκB proteins allows NF-κB to enter the nucleus and work with interferon regulatory factors such as IRF3 to induce the expression of interferons and inflammatory cytokines such as TNF, IL-1β and IL-6. Created by Figdraw. Export ID: RYIOTb98b8.
3 Function of the cGAS-STING pathway in diseases
In recent years, researches on the cGAS-STING pathway have focused on the role of this pathway in various aspects such as tumor immunity, viral infection, inflammation, and aging.
The activation of the cGAS-STING pathway in cancer cells triggers the autocrine and paracrine secretion of type I IFN. The infiltration rate of immune cells and the expression profile of immune-related genes are increased (8), augmenting the activation and infiltration of CD8+ T cells (9, 10). Moreover, the antigenicity of cancer cells is increased, increasing the possibility of being recognized and killed by cytotoxic T cells; this results in tumor growth inhibition in vivo (11, 12). In addition, mutant P53 can inhibit the cGAS-STING pathway, leading to tumors escaping the immune system (13).
Besides, the double-stranded DNA from exogenous pathogens and mitochondrial DNA produced by damaged organisms can activate the cGAS-STING signaling pathway to induce a variety of inflammatory responses (14), leading to tissue fibrosis.
In atherosclerosis, diabetic cardiomyopathy, and myocardial infarction models, the cGAS-STING pathway is activated and downstream molecules are expressed. However, STING inhibitors can block fibrosis and apoptosis in cardiomyocytes, protecting myocardial function and delaying heart failure progression (14–17).
Activation of the cGAS-STING signaling pathway is also involved in the development of inflammatory injury and fibrosis in the liver, lungs, intestine, and central nervous system. On the other hand, inhibition of the cGAS-STING pathway decreases the occurrence of inflammation, and organ damage and improves these pathologies (18–26).
Increasing evidence suggests that the cGAS-STING signaling pathway plays a key pathogenic role in autoimmune diseases such as systemic lupus erythematosus (SLE), rheumatoid arthritis (RA), Aicardi-Goutières syndrome (AGS), and STING-associated vasculopathy with onset in infancy (SAVI). It was found that the expression of the IFN-inducible gene IFIT3 and downstream IFNβ were increased in patients with SLE compared with healthy controls; furthermore, it was positively correlated with the activity of the cGAS-STING pathway (27). In addition, IFN-stimulated genes (ISGs) are abnormally elevated in patients with AGS (28).
In a mouse model of inflammatory arthritis, cGAS deficiency blocked the IFN response and decreased inflammatory cell infiltration and joint swelling (29). Deletion of cGAS or STING can saved DNase II-/- mice from fatal inflammatory diseases caused by DNA clearance defects (30) (Table 1).
The cGAS-STING signaling pathway inhibits a variety of viral infections. During viral infections, viral replication induces the responses of inflammatory factors and ISGs, and the cGAS-STING pathway is activated, subsequently inhibiting the progression of viral infection. However, deletion or silencing of cGAS or STING genes results in reduced production of type I IFN and enhanced viral infection, thus suggested that the host cGAS-STING signaling pathway plays an important role in limiting viral replication (31–34).
The recruitment, activation, and signaling of each molecule of the cGAS-STING pathway are inextricably linked to the role of this pathway. A study has observed that cGAS is not only present in the cytoplasm but also in the nucleus and that nuclear cGAS plays a role in innate immunity against viral infections by regulating histone arginine modification (35). The deubiquitination of STING promotes its stability as well as the expression of type I IFN and pro-inflammatory factors after DNA viral infections (36). Furthermore, ubiquitin-regulated X structural domain protein promotes the ubiquitination, dimerization, and transport of STING and positively regulates STING signaling and subsequent TBK1 recruitment and phosphorylation to promote antiviral immune responses (37). The recruitment of TBK1 to STING is important for both IRF3 and NF-κB activation; furthermore, the resulting type I IFN -mediated independent immune defense against viral infections is essential (38).
The interaction between virus and host is exceptional because viral infection leads to the activation of the host cell’s natural immunity against the virus, which has evolved multiple strategies to escape the host’s antiviral immunity and establish latent infection. Various viral proteins can function as inhibitors of intracellular DNA receptors and antagonize the antiviral responses of the body. Furthermore, they can interact with various molecules of the cGAS-STING pathway, achieving immune escape by inhibiting the enzymatic activity of cGAS; reducing the phosphorylation levels of STING, TBK1, and IRF3; and inhibiting the activation of the IFN gene so as to maintain viral replication. Immune escape of the virus not only occurs during initial infection but also persists during the latent and recurrence periods of the virus, with the interaction between the strengthening of the body’s immune system and virus evolution constantly occurring (Figure 2).
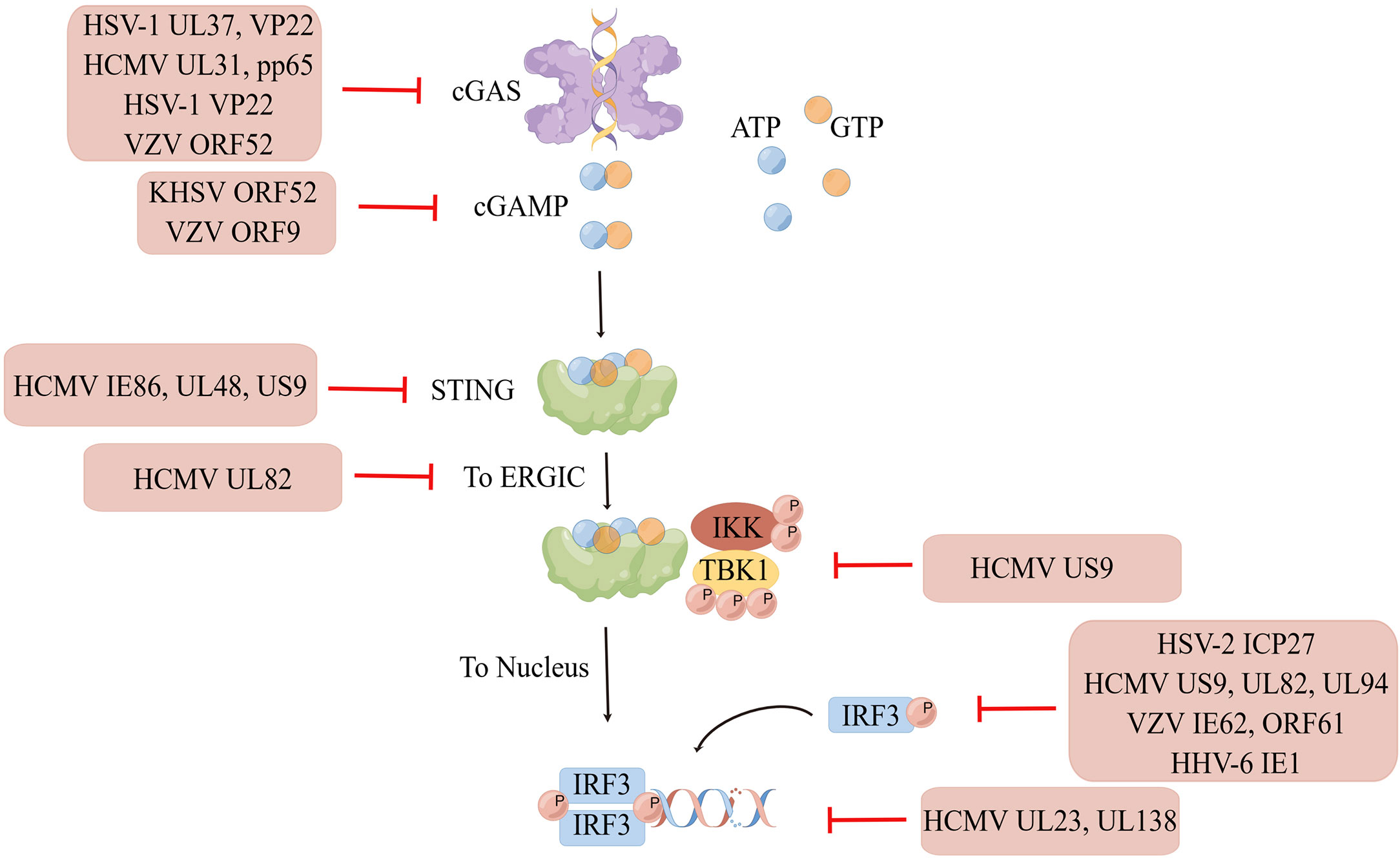
Figure 2 Immune escape of HHVs. Human herpesviruses can evade immune surveillance and maintain their replication by inhibiting the enzyme activity of cGAS, reducing the phosphorylation level of STING, TBK1, IRF3, and suppressing interferon gene activation. Created by Figdraw. Export ID: STPWPba3de.
4 Herpesvirus
Herpesviruses are a class of double-stranded DNA viruses that comprising a four-layered structure: an envelope, a tegument, a capsid, and a DNA core. They are one of the largest known viruses to date, with a diameter of approximately 200 nm. This virus class is divided into three subtypes: alpha, beta, and gamma, with highly prevalence worldwide. At present, nine types of herpesviruses can infect humans: herpes simplex virus-1 (HSV-1), herpes simplex virus-2(HSV-2),varicella-zoster virus(VZV), Epstein-Barr virus(EBV), human cytomegalovirus (HCMV), HHV-6A, HHV-6B, HHHV-7, and Kaposi’s sarcoma-associated herpesvirus(KSHV).Herpesvirus particles comprise a double-stranded DNA core and an icosahedral capsid surrounded by an unstructured proteinaceous matrix called the envelope. In turn, the envelope is surrounded by a lipid bilayer studded with branched glycoproteins (39).
HSV-1 primarily leads to cold sores, and neonatal herpes simplex encephalitis via vertical transmission from the mother to the child. On the other hand, HSV-2 primarily leads to genital herpes that manifests as clusters or scattered small blisters around the external genitalia or anus, which rupture after 2-4 days to form erosions or ulcers and are painful. After the initial genital herpes subsides, the remaining virus will be dormant for a long time; when the body’s resistance is decreased or provoking factors are encountered, the condition can relapse, with the recurrence symptoms being generally milder than the symptom of the initial infection.
Humans are the only natural host of VZV, and the skin is the main target organ of this virus. Varicella is more common among infants and preschool children and manifests as fever and patches of erythematous maculopapular rashes, herpes, and crusts on the skin and mucous membranes. The disease is self-limited and does not leave any scars; furthermore, lifelong immunity is achieved after illness. Herpes zoster is a common disease in adults, the elderly, or individuals with immunodeficiency and immunosuppression. It is caused by the activation of latent viruses. In the early stage, there is an abnormal feeling on the local skin such as itching or pain, followed by red rashes and herpes along the nerve distribution of the trunk.
EBV, also called HHV-4, is the causative agent of infectious mononucleosis and is strongly associated with the development of Burkitt lymphoma, nasopharyngeal cancer, and childhood lymphoma.
The clinical manifestations include pharyngitis, fever, and enlarged lymph nodes. HCMV, also called HHV-5, mostly exists in the form of latent infection. The general population is susceptible to HCMV, and can be repeatedly infected. Most HCMV infections are asymptomatic; however, HCMV can cause visceral diseases in some patients as well as infectious mononucleosis. Furthermore, HCMV causes neonatal infection via vertical transmission from the mother to the child, and congenital HCMV infections can be teratogenic in newborns. HHV-6 is more likely to infect patients with immunodeficiency. The clinical features of HHV-6 infection in adults are acute fever, upper respiratory tract symptoms, mononucleosis and rash. The rash is mostly in the form of macules, can be fused into pieces, and even develop into diffuse erythema; when the infection subsides, desquamation is observed.
On the other hand, HHV-6 infection in neonates is generally asymptomatic Only in severe cases, it may result in an infantile rash. In addition, HHV-7 infection is common in children. It can cause exanthema subitum, febrile seizures, or neurological complications. KSHV also called HHV-8 is closely related to the occurrence of Kaposi’s sarcoma in patients with AIDS.
Classic Kaposi’s sarcoma is characterized by purple, reddish-blue, or dark brown maculopapular rashes, plaques, and nodules, with ulcer formation in some cases. It is particularly common at the extremities and may be accompanied by lymphedema. HHV can be latent in the ganglia for a long time after the initial infection and can relapse when human immunity is weakened. To date, no effective vaccines against HHV have been developed (Table 2).
5 cGAS-STING pathway and HHV infections
5.1 cGAS-STING pathway and HSV infections
HSV-1 infection triggers the cGAS-STING and Toll-like receptor 3 pathways. Both these pathways are essential to attenuate viral replication (40). Some researchers have hypothesized that in HSV-1 infection, STING binds to NLR family pyrin domain containing 3 via two pathways to promote inflammasome activation and that the cGAS-STING-NLRP3 signaling pathway is crucial for the host to resist HSV-1 infection (41). When the cGAS-STING pathway is inhibited because of various reason or when type I IFN signaling is impaired, the innate antiviral immune response is also inhibited; as a result, HSV replication is enhanced, and the host is more resistant to this virus (42–44). Compared with adults, neonates with HSV infection, neonatal cord blood mononuclear cells and peripheral blood mononuclear cells displayed significantly decreased cGAS expression at the mRNA and protein levels. Furthermore, the production of cGAMP, a secondary messenger, and activation of the transcription factor IRF3 were markedly decreased, possibly leading to the high susceptibility of neonates to DNA viral infections (45).
HSV-1 can induce cytokine responses and apoptosis (46). Studies on clinical cases of herpes simplex encephalitis, mouse models, and primary cell cultures have reported that when microglia are infected with HSV-1, a low viral load can induce type I IFN response, whereas a high viral load can induce cGAS-STING pathway-dependent apoptosis (47). Moreover, in a mouse model of genital herpes, specific activation of the STING pathway in the vagina causes activation of the IFN system, limiting inflammatory responses to control HSV-2 infection in vivo (48).
HSV is a DNA virus that has developed multiple strategies to evade host immune responses. Some HSV proteins can act on cGAS to achieve immune escape. For example, the HSV-1 surface protein UL37 can deamidate cGAS in human and mice. Deamidation impairs the ability of cGAS to catalyze cGAMP synthesis. HSV-1 with a deamidase deficiency in UL37 induces a potent antiviral response (49). Moreover, VP22, an HSV-1 body tegument protein, can interact with cGAS to inhibit its enzymatic activity and help continuously evade the innate antiviral response of the host (50). In addition, VP22 can effectively disrupt pre-formed cGAS DNA cohesion in cells, and ORF9, a tegument protein of VZV, acts similarly to inhibit cGAS-STING phase separation (51).
VP1-2, a deubiquitinating enzyme (DUB) of HSV-1 can act on STING, TBK1, IRF3 and other pathway molecules. DUB can decrease the phosphorylation levels of TBK1 and IRF3 by inhibiting the ubiquitination of STING, thereby realizing the immune escape of HSV in the brain. Human or mouse microglia infected with HSV1with a DUB-active mutant of VP1-2 protein can increase the expression of IFNs and decrease viral replication in the brain (52). Moreover, ICP27, an immediate-early protein of HSV-2, can directly bind to IRF3 and inhibit its phosphorylation and nuclear translocation (53).
The surface proteins of HSV-1 are effective IFN antagonists. For example, HSV-1 US3 functions against the antiviral immunity of the host by targeting the activation of β-catenin in the cGAS-STING-pathway (54). Furthermore, VP22 can downregulate IFN-γ promoter activation and IFN-γ production as well as inhibit the expression of IFN and its downstream antiviral genes (50). Taken together, VP22 plays a role in inhibiting the cGAS - STING-mediated antiviral innate immune signaling pathway. In addition, HSV-2 immediate early protein ICP27 inhibited the activation of IFN-β promoter and the production of IFN-βat the mRNA and protein levels (53). HSV-2 glycoprotein E and glycoprotein C act synergistically to protect the virus from antibody- and complement-mediated neutralization (55).
5.2 cGAS-STING pathway and HCMV infections
STING et al. PRRs pathway can recognize CMV and generate type I IFN-based immune defense to resist infection (56). In CMV-infected cell and animal models, STING is needed to initiate first-stage type I IFN production and inhibit CMV replication (57). Studies have reported that primary human endothelial cells produce a strong type I IFN response to HCMV invasion; this depends on cGAS, STING, and IRF3 signaling. Furthermore, HCMV can stimulate primary human monocyte-derived macrophages and dendritic cells and trigger type I IFN production in a cGAS-dependent cGAMP formation way. In addition, IFN-γ-inducible protein 16(IFI16) recognizes the herpesvirus genome and induces inflammasome production and IFN-β responses. As a restriction factor of viral lytic replication, IFI16 inhibits viral DNA replication, particularly HCMV replication (58, 59). Studies have reported that monocyte-derived cells without cGAS exhibit an impaired type I IFN response (60, 61).
In addition, CMV can evade the antiviral immunity of the body at multiple stages. First, upstream of the pathway, HCMV UL31 protein, cGAS inhibitor, can directly interact with cGAS, inhibit the enzymatic function of cGAS, and decrease cGAMP production. Furthermore, the overexpression of UL31 can significantly decrease the antiviral responses, whereas UL31 knockdown can upregulate the expression of type I IFN and downstream antiviral genes. In addition, wild-type HCMVs can replicate more efficiently than UL31-deficient HCMVs (62). PP65, a tegument protein of HCMV selectively binds to cGAS and prevents its interaction with STING, inactivating signaling via the cGAS-STING-IRF3 axis and inhibiting IFN-β production (63). Infection with the PP65-deficient mutant virus can reduce stronger IFN responses and proinflammatory chemokines (64).
HCMV can interact with STING and its downstream molecules. For example, the IE86 protein of HCMV can promote the proteasome-dependent degradation of STING. Furthermore, UL122, encoding the IE86 protein, can strongly inhibit STING-induced IFN-β promoter activation (65). UL82, a tegument protein of HCMV, can function as a negative regulator of STING-dependent antiviral responses and inhibit the transport of STING from the ER to perinuclear microsomes. Furthermore, it can inhibit the recruitment of TBK1 and IRF3 to the STING complex. Wild-type HCMV exhibits better replication efficiency than UL82-deficient mutants (66). Moreover, pUL48, a tegument protein of HCMV, can encode DUB for the deubiquitination of TRAF6, TRAF3, IRAK1, IRF7 and STING, thereby inhibiting type I IFN responses, obtaining tumor precursor function, and promoting tumor formation (67). HCMV US9 can disrupt STING oligomerization and STING and tbk1 binding via competitive interactions. Furthermore, it can inhibit the nuclear translocation of IRF3 and its cytosolic domain to inhibit IRF3 activation (68).
PUL83, a tegument protein of HCMV, can interact with IFI16 to stimulate the immediate early promoter of the virus and inhibit IFN signaling, thereby inhibiting the expression of antiviral genes in infected cells and achieving immune escape (64, 69, 70). Ul23, a tegument protein of HCMV, can significantly decrease the expression of ISGs and the activity of the promoter of the response elements of ISGs during HCMV infection. UL23 is a key factor in the negative regulation of type I IFN-mediated immune responses (71). HCMV inhibits the cGAS-STING-TBK1 pathway and decreases IFN-β mRNA accumulation via its latent associated protein UL138 (72).
UL94, an epidermal protein of HCMV, inhibits cGAS-STING-mediated antiviral responses, interacts with IRF3-activated mediator STING, disrupts STING dimerization and translocation, and hinders the recruitment of TBK1 to the STING signalosome. Ectopic expression of UL94 can impair cytoplasmic double-stranded DNA and DNA virus-induced type I IFN induction and enhance viral replication. In contrast, UL94 deficiency can enhance HCMV-induced transcription of type I IFN and downstream antiviral effectors and impair viral replication (73).
5.3 cGAS-STING pathway and KSHV infections
A study have reported that mitochondrial DNA on the surface of the extracellular vesicles (EVs) of KSHV is it’s a causative factor (74). KSHV-infected cells induce ISG responses. ISGs and IRF-activating genes were significantly activated in the EVs of human endothelial cells treated with KSHV EVs. This suggests that the cGAS-STING pathway is associated with KSHV EV-mediated expression of ISGs (74). In addition, some researchers have suggested the importance of STING in limiting bystander cell transmission than in inhibiting viral replication when endotheliocytes are infected with KSHV (75). Study have reported that new KSHV and HSV-1 infections and latent KSHV and EBV infections can induce the interaction of the H2B-IFI16-BRCA1 complex with intracellular cGAS and STING, leading to the phosphorylation of TBK1 and IRF3, nuclear translocation of IRF3, and production of IFN-γ (76).
The cGAS-dependent response induced by KSHV infection can limit infection, whereas KSHV also exhibits unique mechanisms to antagonize host cGAS sensing (77). ORF52 is a tegument protein abundantly present in extracellular viral particles. Studies have shown that KSHV cGAS inhibitors (KicGAS) encoded by ORF52 inhibit DNA-induced phase separation and cGAS activation, thereby inhibiting cGAS enzymatic activity. KicGAS optimizes the production of infectious virus particles in addition to immune evasion. The loss of ORF52 reduces virion production and causes infectious defects in the virus, with the concomitant enhancement of cGAS signaling (77–79). Viral IFN regulatory factor 1(vIRF1), inhibits STING phosphorylation and activation by preventing the STING and TBK1interaction, thus inhibiting the DNA-sensing pathway. Virf1-expressing cells can inhibit IFN-β production after infection with pathogenic DNA. In summary, gamma herpesviruses encode inhibitors to block cGAS-STING-mediated antiviral immunity. The regulation of this pathway is important for the transmission and lifelong persistence of herpesviruses in the population (80).
The cGAS-STING pathway is activated during KSHV primary infection, as well as plays an important role in reactivating KSHV latency. Latency-associated nuclear antigen (LANA) in KSHV is mainly localized in the nucleus of latently infected cells and is essential for maintaining and replicating of latently infected viral DNA. The cytosolic isoforms of LANA act as antagonists of the cytosolic DNA sensor cGAS. LANA and its KSHV isoform inhibit TBK1 and IRF3 phosphorylation and cGAS-STING dependent IFN production by directly interacting with cGAS during virus latency reactivation. This eventually antagonizes the restriction of KSHV replication and counteracts innate immune responses, thus promoting virus reactivation in cells (81, 82).
5.4 cGAS-STING pathway and VZV infections
Type I IFN induction during VZV infection depends on the cGAS/STING DNA sensing pathway, and VZV recognition by cGAS limits its replication (83). STING mediated the host defense of dermal cells against VZV infection. STING inhibition by small interfering RNA- or short hairpin RNA-mediated gene disruption increased viral replication, reduced IRF3 phosphorylation, and induced IFN and proinflammatory cytokines. The pretreatment with STING agonists reduced VZV glycoprotein E levels and viral replication. Moreover, increased IFN-λ secretion in the STING-dependent pathway was observed after VZV infection (84). A study showed that transcripts of STING-encoding genes were selectively concentrated in exosomes secreted by VZV-infected lymphocytes (85). Reduced STING expression increased viral replication in primary fibroblasts, whereas STING overexpression inhibited VZV plaque formation (86).
Some VZV proteins are powerful antagonists of the IFN signaling pathway. The VZV immediate early proteinORF61 eliminates the natural immune response by degrading activated IRF3 and downregulating the IRF3-mediated IFN-β pathway (87). In addition, the VZV immediate early protein IE62 induces VZV gene expression upon VZV entry into cells and inhibits IFN-dependent antiviral defense. IE62 blocks TBK1-mediated IFN-β secretion and IRF3 phosphorylation (88). The VZV tegument protein ORF9 inhibits cGAMP production by functioning as a cGAS antagonist. Virus-expressed ORF9 binds to endogenous cGAS, resulting in an attenuated response of type I IFN (83).
5.5 cGAS-STING pathway and other HHVs
The levels of STING and programmed cell death ligand 1 (PD-L1) were significantly higher in EBV-associated gastric cancer than in non-EBV-associated gastric cancer. STING levels in EBV-associated gastric cancer were positively correlated with PD-L1 levels (89). C-176, a STING inhibitor, inhibits the EBV-induced transformation of peripheral blood mononuclear cells. Furthermore, C-176 treatment inhibited tumor formation and prolonged survival in a mouse model of EBV-associated lymphoid tissue proliferative disease (90). In human airway epithelial cells, HHV type IV EBV induces tripartite motif-containing protein 29 to suppress innate immune activation, leading to persistent DNA viral infection (91). The immediate early protein IE1 of human HHV type 6 is one of the first viral proteins synthesized upon virus entry and is a potent inhibitor of IFN-β gene expression. A study showed that in the presence of IE1, IRF3 did not efficiently bind to the IFN-β promoter sequence, and the dimerization and nuclear translocation of IRF3 decreased in the IE1-expressing cells (92). Study has found that the STING/STAT6 pathway was upregulated in HHV-6A-infected natural killer (NK) cells. NK cells infected with HHV-6B and HHV-7 showed significantly increased chemokine C-C motif ligand (CCL) 3, IFN-α, TNF-α, IL-8, and IFN-γ levels and slightly increased IL-4 and CCL4levels. HHV-6A-infected NK cells showed significantly increased IL-4 and IL-13 levels and slightly increased IL-10, TNF-α, IFN-α, and IFN-γ levels (93) (Table 3).
6 Modulators of the cGAS-STING pathway
Various disease models, including autoimmune diseases, inflammation, organ damage and fibrosis, tumor occurrence and treatment, and viral infection, have been used in studies on modulators related to the cGAS-STING pathway. With the help of computer simulations or high-throughput sequencing, various compounds that act on this pathway have been artificially synthesize based on the spatial structure of cGAS, STING, or their ligands.
Modulators of the cGAS-STING pathway were first developed for tumor therapy, and STING agonists have been used in combination with other drugs to inhibit tumor growth. For example, the combination of cGAMP, a STING activator, with saponin adjuvants can increase the effectiveness of influenza vaccines in elderly hosts without additional doses or additional vaccinations (94). The combination of cGAMP and celecoxib, the cyclooxygenase 2 inhibitor, induces local and systemic antitumor immunity, thereby inhibiting tumor growth (95). The combination of STING agonists and eribulin, which is used to treat triple-negative breast cancer, increased the levels of the immunogenic cytokine IFNβ (96). The combination of STING agonists and atezolizumab inhibits breast cancer 4T1 cell growth in mic by increasing the levels of TNF-α, IFN-β, IL-10, and IFN-γ in surrounding blood and tumor masses (P < 0.01). In a tumor-bearing mouse model, these two drugs increased CD8+ cytotoxic T cells and reduced FOXP3+ T regulatory cells (97).
The systemic administration of STING agonists raises some safety concerns, and intratumoral injections are limited by tumor accessibility. Some researchers have combined STING agonists with antibodies targeting tumor cells via a cleavage linker to obtain antibody-drug conjugates (ADCs). ADCs exert strong anti-tumor effects and show good tolerance for systemic administration. STING ADCs promote multiple aspects of innate and adaptive anti-tumor immune responses, including the activation of dendritic cells, T cells, NK cells, NK T cells and the promotion of M2 to M1 polarization of tumor-associated macrophages (98). Some researchers have combined STING agonists with capsid-like hollow polymer nanoparticles. These nanoparticles are morphologically similar to natural virions and can facilitate co-presentation of antigens and STING agonists to increase immune responses (99).
Similarly, to overcome limitations associated with drug delivery in the body, researchers have designed nanoparticles for intratumoral injection. These nanoparticles are endowed with deoxyribonuclease resistance, which increases cellular uptake and promotes the escape of IFN-stimulated DNA endosomal into the cytoplasm, eventually mediating the production of pro-inflammatory cytokines by strongly activating the STING pathway of cGAS (100). The binding of STING agonists to these nanoparticles reduced reduce tumor burden by > 50% - 80% and significantly increased median survival in melanoma (YUMM1.7) and malignant breast adenoma (E0771) models (101). In the B16-f10 mouse melanoma lung metastasis model, lipid nanoparticle therapy containing a STING agonist improved anti-PD-1 resistance in the mice (102). Mn2+ is a cGAS-STING agonist that can significantly enhance anti-tumor immunity. Researchers introduced MnOx into the surface of Mn2+-loaded Pb nanoparticles to construct Mn2+ -rich photonic nanodrugs (MnPB-MnOx). All components of MnPB-MnOx were biocompatible and biodegradable, making cGAS-STING more available for activation. The local treatment of mice carrying 4T1 breast cancer cells in situ showed that MnPB-MnOx elicited a systemic response to suppress distal tumors (103).
STING agonists play an important role as antivirals. A synthetic analog of 3’,3’-c-di(2’F,2’dAMP), 2’,3’-cyclic GMP-AMP, and its precursor pivaloyloxymethyl exert strong anti-hepatitis B virus (HBV) effects in primary human hepatocytes regardless of HBV genotype (104). Zhang X et al. reported that the human STING (h-STING) agonist 6-bromo-N-(naphthalen-1-yl) benzo[d][1,3]dioxole-5-carboxamide can induce a broad-spectrum initial antiviral immunity. The treatment of primary human fibroblasts with this agonist-induced an antiviral state that inhibited several flavivirus infections (105). Naturally, STING can be activated by circular dinucleotides (CDNs) such as cGAMP. A synthetic CDN, ADU-S100, has been reported to be effective in activating STING and is being evaluated for the treatment of cancer in clinical trials. ADU-S100 is mostly injected intratumorally in animal experiments. The intraperitoneal injection of STING agonists can inhibit abnormal angiogenesis of tumors and can increase pericyte coverage. ADU-S100can normalize tumor vascularity and induce the formation of tertiary lymphoid structure in a tumor microenvironment (106), thereby promoting activated CD8+ T-cell infiltrate peritoneal tumor nodules (107).
The STING agonist 5,6-dimethylfuranone-4-acetic acid (DMXAA) was originally developed by the Auckland Cancer Society Research Centre as an anti-cancer drug and later discovered to be a mouse STING(m-STING) molecular-specific agonist. In vivo studies have shown that DMXAA can reduce the size of tumor blood vessels and increase the levels of tumor antigens (108). By performing single-cell RNA sequencing, researchers demonstrated that DMXAA could generate a chemokine environment to promote the recruitment of chimeric antigen receptor (CAR) T cells, thereby promoting the transport and persistence of CAR T cells (109). The antiviral action of DMXAA is crucial; DMXAA induces the STING signaling activation of macrophages in chronic HBV mice and inhibits the transcription of covalently closed circular DNA of HBV and its replication by epigenetic modification of hepatocytes (110). In addition, DMXAA administration to HSV-1-infected mice can reduce the viral load of their peripheral and central nervous systems, thereby increasing mouse survival (111). The cGAS-STING pathway promotes the development of inflammatory diseases, and DMXAA aggravates the severity of these diseases. A study showed that chronic exposure to DMXAA led to hepatic steatosis and inflammation in wild-type mice, but not in STING-deficient mice (112). In an acute pancreatitis model, DMXAA administration produced more severe symptoms in test mice than in control mice. Activated STING could sense acinar cell death by detecting DNA in acinar cells, and thus activated signaling pathways that promote inflammation (113). In cecal ligation perforation-induced sepsis mice, mice treated with the STING agonist DMXAA showed more intestinal cell apoptosis and severe systemic inflammatory responses than STING knockout mice (114).
Ramanjulu et al. developed a binding strategy to synergize the action of two symmetry-based amidobenzimidazole (ABZI) compounds. They developed ABZIs (diABZIs) with more powerful cell functions, strong binding capacity to STING (115), and more effectivity than that possessed by CDNs (116). diABZIs can stimulate h-STING molecules, induce type I IFN production, strengthen antiviral immunity, and exert inhibitory effects on various viral infections. Studies have shown that diABZIs exhibit potent antiviral activities against the human coronaviruses HCoV-229E and HCoV-OC43 via the IFN pathway and can prevent SARS-CoV-2 infection (117). Moreover, treatment with a low dose if diABZI (0.1 μM) effectively reduced the SARS-CoV-2 viral load on the surface of human small airway epithelial cells. After diABZI treatment, viral RNA levels measured by quantitative reverse transcription polymerase chain reaction were approximately 1000-fold (118). Furthermore, diABZI prevented epithelial damage in the reconstituted primary human bronchial airway epithelial acute lung injury (ALI) system (117). In k18-ace2 transgenic mice infected with SARS-CoV-2, the nasal administration of diABZI-4 before or after viral interference completely prevented the onset of severe respiratory diseases (119). The intravenous injection of diABZI into immunocompetent mice containing syngeneic colon tumors elicited strong antitumor activity and resulted in complete and durable tumor regression (115).
STING inhibitors can inhibit the activation of STING in vivo, thereby reducing organ damage and inflammatory responses.
Injecting the STING inhibitor C-176 in a diabetic cardiomyopathy mouse model significantly blocked inflammation and apoptosis in cardiomyocytes (14). C-176 administered mice effectively mitigated lung inflammation and fibrosis induced by graphitized multi-walled carbon nanotubes (23). Pretreatment with C-176 can prevent the nuclear displacement of NF-κB p65 and p-IRF3, thereby mitigating kidney damage in mice sensitized by trichloroethylene (120). The pharmacological blockade of STING with C-176 improves atherosclerotic formation in APOE-/- mice (121). Pretreating mice with acute lung injury with C-176 can reduce the production of inflammatory cytokines, including TNF-α, IL-6, IL-12, and IL-1β, and inhibit the gene expression of chemokines and adhesion molecule vascular cell adhesion protein-1 in lung tissues, thereby reducing lipopolysaccharide-induced mouse ALI (122). Researchers have developed spherical polyethyleneimine-coated mesoporous polydopamine nanoparticles loaded with C-176 (PEI-PDA@C-176 NPs) for RA treatment. The intra-articular injection of PEI-PDA@C-176 NPs effectively reduced dsDNA-induced arthritis and collagen-induced arthritis in a mouse model (123).
H-151, another novel STING inhibitor, targets STING molecules in humans and mice and protects various organs from damage. In a mouse model of intestinal ischemia-reperfusion(I/R) injury, H-151 treatment decreased p-IRF3, serum tissue damage marker (lactate dehydrogenase and aspartate aminotransferase), and cytokine (IL-1β and IL-6) levels, as well as reduced ischemia-reperfusion-induced intestinal and lung injuries and inflammation (124). In cisplatin-induced kidney injury mice, H151 treatment significantly improved the kidney injury; tubular cell apoptosis and tubular damage markers were effectively weakened, whereas renal function kidney morphology and renal inflammation were significantly improved. Cisplatin-induced mitochondrial damage and mitochondrial gene expression were reversed, mitochondrial morphology was improved, and mitochondrial DNA content was restored (125). In animal experiments, on the 28th day after myocardial infarction modeling in mice, the H-151 treatment exerted a significant inhibitory effect on the cGAS-STING-IRF3 pathway and inflammatory responses, especially the type I IFN response. Moreover, H-151 treatment reduced the apoptosis of adult cardiomyocytes and fibrosis of cardiac fibroblasts in vivo, thus protecting myocardial functions (15). Similarly, some researchers showed that three weeks after myocardial infarction in mice, theH-151 inhibited STING to reduce infarct dilation and scarring. Left ventricular systolic function increased to near-normal levels, and myocardial hypertrophy was reduced after H-151 treatment (16).
The cGAS inhibitor RU.521 attenuated the clinical signs of colitis in wild-type mice and decreased cGAMP and STING levels and TBK1 and IRF3 phosphorylation in colonic tissues (126). In pulmonary ventilation I/R rat models, cGAS-STING pathway inhibition attenuated ER stress, thereby reducing lung injuries and promoting lung functions (127). In a mouse model of sepsis, the cGAS inhibitor RU.521significantly increased cardiac functions and greatly reduced inflammatory responses, oxidative stress, and apoptosis in septic mouse hearts (128). In a mouse model of cerebral venous sinus thrombosis (CVST), RU.521 treatment reduced the levels of 2’3’-cGAMP, STING, and downstream inflammatory cytokines. It also reduced oxidative stress, decreased the number of microglia and neutrophils, improved neuronal apoptosis, and reduced neurological deficits caused by CVST (129).
In recent years, a series of compounds targeting the cGAS-STING pathway have been synthesized by different means.
Some of them are small molecule cyclic urea activators that can efficiently activate different STING molecules in humans (130), whereas some compounds are CDNs that can induce IFN secretion at higher levels (131). Furthermore, some of these compounds are thieno [2,3-d]imidazole derivatives that can induce tumor regression in mice without inducing weight loss as noval STING agonists (132), and some are oral STING agonists that can induce long-lasting antitumor immunity when combined with other drugs to overcome immunotherapy resistance synergistically (133). Moreover, some of these compounds are new covalent cGAS inhibitors that exert better inhibitory effects and selectivity than exerted by RU.521 (134), and some are new small-molecule human cGAS inhibitors with high binding affinity and cellular activity in vitro (135).
These approaches provide a basis for future studies to develop more efficient modulators for the cGAS-STING pathway and establish ideas for the clinical development of new therapeutic agents (Table 4).
7 Discussion
The summary of studies results in recent years showed that the cGAS-STING pathway played different roles in different disease models. We should look at its role dialectically.
In inflammatory and autoimmune diseases, the cGAS-STING pathway promotes inflammatory molecule secretion and recruitment, induces cell apoptosis, and aggravates the occurrence of fibrosis. However, in tumor development and antiviral infections, the cGAS-STING pathway plays a role in preventing disease development. It can promote CD8+ T-cell infiltration, change the tumor microenvironment, and inhibit tumor growth (97, 107). For antivirals, the activation of the cGAS-STING pathway increases the type-I-IFN-dominated antiviral responses (7).
HHV infection involves diverse susceptible people and disease spectra. HHV can cause neonatal infections, tumors, sexually transmitted diseases, and AIDS; thus, it is destructive to human health. Hence, attention should be paid to HHV infections and effective drugs should be promptly identified against the viral infections.
The cGAS-STING pathway exerts antiviral effects by inducing type I IFN production, providing a basis for treating HHV infection. However, HHVs also inhibit the cGAS-STING pathway via various viral proteins to escape immune surveillance. Viral proteins can interfere with various steps of the pathway, including inhibiting cGAS enzyme activity, reducing cGAMP secretion, disrupting cGAS-DNA binding, deubiquitinating STING, blocking STING intracellular transfer, inhibiting the recruitment of IRF3 and TBK1, inhibiting IRF3 phosphorylation and nuclear transport processes, and inhibiting IFN gene transcription. Thus, inhibiting viral replication may be possible by mutating these viral proteins. These findings provide insights for developing new antiviral agents.
In this review, we have summarized the role of the cGAS-STING pathway in different diseases, especially in anti- HHV infections, to bridge the gap in the interaction mechanism between some HHVs and the cGAS-STING pathway. We hope that this review provides a basis for future antiviral research as well as provides information on new targets and mechanisms to develop clinical drugs and vaccines.
However, the application of the cGAS STING pathway in diseases involves many aspects, and the discussion in the present review may not be detailed enough. EBV, HHV-6, HHV-7, and HHV-8 cause asymptomatic infections in the early clinical stage, and in some patients, symptoms only appear when the infections lead to serious complications or comorbidities. Thus, some gaps in the interaction between these viruses and the cGAS-sting pathway, which needs to be addressed and explored in future studies.
In recent years, agonists or inhibitors for the cGAS-STING pathway have also been actively developed. Small-molecule modulators with high efficacy and absorption rates and low side effects need to be developed. cGAS-STING pathway modulators have been studied intensively from the perspective of anti-tumor therapy. Many researchers have loaded STING agonists with nanoparticle, either by intratumoral injection or in combination with other drugs, to overcome limitations such as antitumor drug resistance and drug transportation in the body.
Besides, antiviral studies have shown that cGAS-STING pathway modulators exert good effects in vivo and in vitro; however, a few drugs are supported by clinical trial data (118, 136, 137). Many modulators have played an essential role theoretically in vitro and in vivo; however, owing to the specificity of human and mouse STING, as well as the metabolism and absorption of unknown drugs in the human body, cGAS-STING pathway modulators that can be used in clinical practice are yet to be explored. Therefore, future studies should be directed toward exploring such modulators.
Author contributions
XJ and CH put forward the idea of this study. XJ prepared the initial manuscript, figures, and tables. XJ, CH, and ZC contributed to revising the final version. All authors contributed to the article and approved the submitted version.
Funding
This work was supported by the National Natural Science Foundation of China (No. 82004199).
Conflict of interest
The authors declare that the research was conducted in the absence of any commercial or financial relationships that could be construed as a potential conflict of interest.
Publisher’s note
All claims expressed in this article are solely those of the authors and do not necessarily represent those of their affiliated organizations, or those of the publisher, the editors and the reviewers. Any product that may be evaluated in this article, or claim that may be made by its manufacturer, is not guaranteed or endorsed by the publisher.
References
1. Gershon AA, Gershon MD, Shapiro ED. Live attenuated varicella vaccine: Prevention of varicella and of zoster. J Infect Dis (2021) 224(12 Suppl 2):S387–S97. doi: 10.1093/infdis/jiaa573
2. Wu J, Sun L, Chen X, Du F, Shi H, Chen C, et al. Cyclic gmp-amp is an endogenous second messenger in innate immune signaling by cytosolic DNA. Sci (New York NY) (2013) 339(6121):826–30. doi: 10.1126/science.1229963
3. Chen Q, Sun L, Chen ZJ. Regulation and function of the cgas-sting pathway of cytosolic DNA sensing. Nat Immunol (2016) 17(10):1142–9. doi: 10.1038/ni.3558
4. Zhang X, Wu J, Du F, Xu H, Sun L, Chen Z, et al. The cytosolic DNA sensor cgas forms an oligomeric complex with DNA and undergoes switch-like conformational changes in the activation loop. Cell Rep (2014) 6(3):421–30. doi: 10.1016/j.celrep.2014.01.003
5. Hopfner K-P, Hornung V. Molecular mechanisms and cellular functions of cgas-sting signalling. Nat Rev Mol Cell Biol (2020) 21(9):501–21. doi: 10.1038/s41580-020-0244-x
6. Galluzzi L, Vanpouille-Box C, Bakhoum SF, Demaria S. Snapshot: Cgas-sting signaling. Cell (2018) 173(1):276.e1. doi: 10.1016/j.cell.2018.03.015
7. Srikanth S, Woo JS, Wu B, El-Sherbiny YM, Leung J, Chupradit K, et al. The ca2+ Sensor stim1 regulates the type I interferon response by retaining the signaling adaptor sting at the endoplasmic reticulum. Nat Immunol (2019) 20(2):152–+. doi: 10.1038/s41590-018-0287-8
8. Zhu C, Li J, Yao M, Fang C. Potential for treatment benefit of sting agonists plus immune checkpoint inhibitors in oral squamous cell carcinoma. BMC Oral Health (2021) 21(1):506. doi: 10.1186/s12903-021-01813-8
9. Cen D, Ge Q, Xie C, Zheng Q, Guo J, Zhang Y, et al. Zns@Bsa nanoclusters potentiate efficacy of cancer immunotherapy. Advanced Materials (2021) 33(49):e2104037. doi: 10.1002/adma.202104037
10. Li Q, Chen Q, Yang X, Zhang Y, Lv L, Zhang Z, et al. Cocktail strategy based on a dual function nanoparticle and immune activator for effective tumor suppressive. J Nanobiotechnology (2022) 20(1):84. doi: 10.1186/s12951-022-01241-y
11. Wang H, Xia L, Yao C-C, Dong H, Yang Y, Li C, et al. Nlrp4 negatively regulates type I interferon response and influences the outcome in anti-programmed cell death protein (Pd)-1/pd-ligand 1 therapy. Cancer Sci (2022) 113(3):838–51. doi: 10.1111/cas.15243
12. Falahat R, Berglund A, Putney RM, Perez-Villarroel P, Aoyama S, Pilon-Thomas S, et al. Epigenetic reprogramming of tumor cell-intrinsic sting function sculpts antigenicity and T cell recognition of melanoma. Proc Natl Acad Sci United States America (2021) 118(15):e2013598118. doi: 10.1073/pnas.2013598118
13. Ghosh M, Saha S, Bettke J, Nagar R, Parrales A, Iwakuma T, et al. Mutant P53 aids cancer cells in evading lethal innate immune responses. Cancer Discovery (2021) 11(5):OF14–OF. doi: 10.1158/2159-8290.Cd-rw2021-022
14. Ma XM, Geng K, Law BY-K, Wang P, Pu YL, Chen Q, et al. Lipotoxicity-induced mtdna release promotes diabetic cardiomyopathy by activating the cgas-sting pathway in obesity-related diabetes. Cell Biol Toxicol (2022) 39(1):277–99. doi: 10.1007/s10565-021-09692-z
15. Hu S, Gao Y, Gao R, Wang Y, Qu Y, Je Y, et al. The selective sting inhibitor H-151 preserves myocardial function and ameliorates cardiac fibrosis in murine myocardial infarction. Int Immunopharmacol (2022) 107:108658. doi: 10.1016/j.intimp.2022.108658
16. Rech L, Abdellatif M, Stangl V, Mabotuwana N, Hardy S, Rainer PP, et al. Small molecule sting inhibition improves myocardial infarction remodeling. Life Sci (2022) 291:120263. doi: 10.1016/j.lfs.2021.120263
17. Pallares J, Torreguitart N, Arque G, Portero-Otin M, Purroy F. Human atheromatous plaques expressed sensing adaptor sting, a potential role in vascular inflammation pathogenesis. Thromb Haemostasis (2022) 122(09):1621–3. doi: 10.1055/a-1772-1192
18. Aguado J, Chaggar HK, Gomez-Inclan C, Shaker MR, Leeson HC, Mackay-Sim A, et al. Inhibition of the cgas-sting pathway ameliorates the premature senescence hallmarks of ataxia-telangiectasia brain organoids. Aging Cell (2021) 20(9):e13468. doi: 10.1111/acel.13468
19. Zhao Y, Liu B, Xu L, Yu S, Fu J, Wang J, et al. Ros-induced mtdna release: The emerging messenger for communication between neurons and innate immune cells during neurodegenerative disorder progression. Antioxidants (2021) 10(12):1917. doi: 10.3390/antiox10121917
20. Kwon O-C, Song J-J, Yang Y, Kim S-H, Kim JY, Seok M-J, et al. Sgk1 inhibition in glia ameliorates pathologies and symptoms in parkinson disease animal models. EMBO Mol Med (2021) 13(4):e13076. doi: 10.15252/emmm.202013076
21. Barrett JP, Knoblach SM, Bhattacharya S, Gordish-Dressman H, Stoica BA, Loane DJ. Traumatic brain injury induces cgas activation and type I interferon signaling in aged mice. Front Immunol (2021) 12:710608. doi: 10.3389/fimmu.2021.710608
22. Chen C, Zhang Y, Tao M, Zhao X, Feng Q, Fei X, et al. Atrial natriuretic peptide attenuates colitis via inhibition of the cgas-sting pathway in colonic epithelial cells. Int J Biol Sci (2022) 18(4):1737–54. doi: 10.7150/ijbs.67356
23. Han B, Wang X, Wu P, Jiang H, Yang Q, Li S, et al. Pulmonary inflammatory and fibrogenic response induced by graphitized multi-walled carbon nanotube involved in cgas-sting signaling pathway. J Hazardous Materials (2021) 417:125984. doi: 10.1016/j.jhazmat.2021.125984
24. Liang L, Shen Y, Hu Y, Liu H, Cao J. Cgas exacerbates schistosoma japonicum infection in a sting-type I ifn-dependent and independent manner. PloS Pathog (2022) 18(2):e1010233. doi: 10.1371/journal.ppat.1010233
25. Liu Z, Wang M, Wang X, Bu Q, Wang Q, Su W, et al. Xbp1 deficiency promotes hepatocyte pyroptosis by impairing mitophagy to activate mtdna-cgas-sting signaling in macrophages during acute liver injury. Redox Biol (2022) 52:102305. doi: 10.1016/j.redox.2022.102305
26. Larrick JW, Mendelsohn AR. Modulation of cgas-sting pathway by nicotinamide riboside in alzheimer's disease. Rejuvenation Res (2021) 24(5):397–402. doi: 10.1089/rej.2021.0062
27. Wang J, Dai M, Cui Y, Hou G, Deng J, Gao X, et al. Association of abnormal elevations in ifit3 with overactive cyclic gmp-amp synthase/stimulator of interferon genes signaling in human systemic lupus erythematosus monocytes. Arthritis Rheumatol (2018) 70(12):2036–45. doi: 10.1002/art.40576
28. Mackenzie KJ, Carroll P, Lettice L, Tarnauskaitė Ž, Reddy K, Dix F, et al. Ribonuclease H2 mutations induce a cgas/sting-dependent innate immune response. EMBO J (2016) 35(8):831–44. doi: 10.15252/embj.201593339
29. Willemsen J, Neuhoff M-T, Hoyler T, Noir E, Tessier C, Sarret S, et al. Tnf leads to mtdna release and cgas/sting-dependent interferon responses that support inflammatory arthritis. Cell Rep (2021) 37(6):109977. doi: 10.1016/j.celrep.2021.109977
30. Li T, Yum S, Li M, Chen X, Zuo X, Chen ZJ. Tbk1 recruitment to sting mediates autoinflammatory arthritis caused by defective DNA clearance. J Exp Med (2022) 219(1):e20211539. doi: 10.1084/jem.20211539
31. Iampietro M, Dumont C, Mathieu C, Spanier J, Robert J, Charpenay A, et al. Activation of cgas/sting pathway upon paramyxovirus infection. Iscience (2021) 24(6):102519. doi: 10.1016/j.isci.2021.102519
32. Xu Y, Zhang Y, Sun S, Luo J, Jiang S, Zhang J, et al. The innate immune DNA sensing cgas-sting signaling pathway mediates anti-prrsv function. Viruses-Basel (2021) 13(9):1829. doi: 10.3390/v13091829
33. Sharma KB, Chhabra S, Aggarwal S, Tripathi A, Banerjee A, Yadav AK, et al. Proteomic landscape of Japanese encephalitis virus-infected fibroblasts. J Gen Virol (2021) 102(9). doi: 10.1099/jgv.0.001657
34. Krump NA, Wang R, Liu W, Yang JF, Ma T, You J. Merkel cell polyomavirus infection induces an antiviral innate immune response in human dermal fibroblasts. J Virol (2021) 95(13):e0221120. doi: 10.1128/jvi.02211-20
35. Cui S, Yu Q, Chu L, Cui Y, Ding M, Wang Q, et al. Nuclear cgas functions non-canonically to enhance antiviral immunity via recruiting methyltransferase prmt5. Cell Rep (2020) 33(10):108490. doi: 10.1016/j.celrep.2020.108490
36. Zhang M, Zhang M-X, Zhang Q, Zhu G-F, Yuan L, Zhang D-E, et al. Usp18 recruits usp20 to promote innate antiviral response through deubiquitinating sting/mita. Cell Res (2016) 26(12):1302–19. doi: 10.1038/cr.2016.125
37. Yang L, Wang L, Ketkar H, Ma J, Yang G, Cui S, et al. Ubxn3b positively regulates sting-mediated antiviral immune responses. Nat Commun (2018) 9:2329. doi: 10.1038/s41467-018-04759-8
38. Yum S, Li M, Fang Y, Chen ZJ. Tbk1 recruitment to sting activates both irf3 and nf-kappa B that mediate immune defense against tumors and viral infections. Proc Natl Acad Sci United States America (2021) 118(14):e2100225118. doi: 10.1073/pnas.2100225118
39. Kukhanova MK, Korovina AN, Kochetkov SN. Human herpes simplex virus: Life cycle and development of inhibitors. Biochemistry-Moscow (2014) 79(13):1635–52. doi: 10.1134/s0006297914130124
40. Latif MB, Raja R, Kessler PM, Sen GC. Relative contributions of the cgas-sting and tlr3 signaling pathways to attenuation of herpes simplex virus 1 replication. J Virol (2020) 94(6):e01717–19. doi: 10.1128/jvi.01717-19
41. Wang W, Hu D, Wu C, Feng Y, Li A, Liu W, et al. Sting promotes nlrp3 localization in er and facilitates nlrp3 deubiquitination to activate the inflammasome upon hsv-1 infection. PloS Pathog (2020) 16(3):e1008335. doi: 10.1371/journal.ppat.1008335
42. Jia M, Qin D, Zhao C, Chai L, Yu Z, Wang W, et al. Redox homeostasis maintained by gpx4 facilitates sting activation. Nat Immunol (2020) 21(7):727–+. doi: 10.1038/s41590-020-0699-0
43. Chu L, Li C, Li Y, Yu Q, Yu H, Li C, et al. Perillaldehyde inhibition of cgas reduces dsdna-induced interferon response. Front Immunol (2021) 12:655637. doi: 10.3389/fimmu.2021.655637
44. Guo Y, Jiang F, Kong L, Wu H, Zhang H, Chen X, et al. Otud5 promotes innate antiviral and antitumor immunity through deubiquitinating and stabilizing sting. Cell Mol Immunol (2021) 18(8):1945–55. doi: 10.1038/s41423-020-00531-5
45. Wang Z-S, Y-l L, Mi N, Duan D-Y. Intracellular DNA sensing pathway of cgas-cgamp is decreased in human newborns and young children. Mol Immunol (2017) 87:76–85. doi: 10.1016/j.molimm.2017.04.007
46. Lum KK, Song B, Federspiel JD, Diner BA, Howard T, Cristea IM. Interactome and proteome dynamics uncover immune modulatory associations of the pathogen sensing factor cgas. Cell Syst (2018) 7(6):627–+. doi: 10.1016/j.cels.2018.10.010
47. Reinert LS, Rashidi AS, Tran DN, Katzilieris-Petras G, Hvidt AK, Gohr M, et al. Brain immune cells undergo cgas/sting-dependent apoptosis during herpes simplex virus type 1 infection to limit type iifn production. J Clin Invest (2021) 131(1):e136824. doi: 10.1172/jci136824
48. Skouboe MK, Knudsen A, Reinert LS, Boularan C, Lioux T, Perouzel E, et al. Sting Agonists Enable Antiviral Cross-Talk between Human Cells and Confer Protection against Genital Herpes in Mice. PloS Pathog (2018) 14(4):e1006976. doi: 10.1371/journal.ppat.1006976
49. Zhang J, Zhao J, Xu S, Li J, He S, Zeng Y, et al. Species-specific deamidation of cgas by herpes simplex virus ul37 protein facilitates viral replication. Cell Host Microbe (2018) 24(2):234–+. doi: 10.1016/j.chom.2018.07.004
50. Huang J, You H, Su C, Li Y, Chen S, Zheng C. Herpes simplex virus 1 tegument protein vp22 abrogates cgas/sting-mediated antiviral innate immunity. J Virol (2018) 92(15):e00841–18. doi: 10.1128/jvi.00841-18
51. Xu G, Liu C, Zhou S, Li Q, Feng Y, Sun P, et al. Viral tegument proteins restrict cgas-DNA phase separation to mediate immune evasion. Mol Cell (2021) 81(13):2823–+. doi: 10.1016/j.molcel.2021.05.002
52. Bodda C, Reinert LS, Fruhwurth S, Richardo T, Sun C, Zhang B-C, et al. Hsv1 vp1-2 deubiquitinates sting to block type I interferon expression and promote brain infection. J Exp Med (2020) 217(7):e20191422. doi: 10.1084/jem.20191422
53. Guan X, Zhang M, Fu M, Luo S, Hu Q. Herpes simplex virus type 2 immediate early protein icp27 inhibits ifn-beta production in mucosal epithelial cells by antagonizing irf3 activation. Front Immunol (2019) 10:290. doi: 10.3389/fimmu.2019.00290
54. You H, Lin Y, Lin F, Yang M, Li J, Zhang R, et al. Beta-catenin is required for the cgas/sting signaling pathway but antagonized by the herpes simplex virus 1 us3 protein. J Virol (2020) 94(5):e01847–19. doi: 10.1128/jvi.01847-19
55. Awasthi S, Lubinski JM, Shaw CE, Barrett SM, Cai M, Wang F, et al. Immunization with a vaccine combining herpes simplex virus 2 (Hsv-2) glycoprotein C (Gc) and gd subunits improves the protection of dorsal root ganglia in mice and reduces the frequency of recurrent vaginal shedding of hsv-2 DNA in Guinea pigs compared to immunization with gd alone. J Virol (2011) 85(20):10472–86. doi: 10.1128/jvi.00849-11
56. Piersma SJ, Poursine-Laurent J, Yang L, Barber GN, Parikh BA, Yokoyama WM. Virus infection is controlled by hematopoietic and stromal cell sensing of murine cytomegalovirus through sting. Elife (2020) 9:e56882. doi: 10.7554/eLife.56882
57. Lio C-WJ, McDonald B, Takahashi M, Dhanwani R, Sharma N, Huang J, et al. Cgas-sting signaling regulates initial innate control of cytomegalovirus infection. J Virol (2016) 90(17):7789–97. doi: 10.1128/jvi.01040-16
58. Roy A, Dutta D, Iqbal J, Pisano G, Gjyshi O, Ansari MA, et al. Nuclear innate immune DNA sensor ifi16 is degraded during lytic reactivation of kaposi's sarcoma-associated herpesvirus (Kshv): Role of ifi16 in maintenance of kshv latency. J Virol (2016) 90(19):8822–41. doi: 10.1128/jvi.01003-16
59. Gariano GR, Dell'Oste V, Bronzini M, Gatti D, Luganini A, De Andrea M, et al. The intracellular DNA sensor ifi16 gene acts as restriction factor for human cytomegalovirus replication. PloS Pathog (2012) 8(1):e1002498. doi: 10.1371/journal.ppat.1002498
60. Paijo J, Doering M, Spanier J, Grabski E, Nooruzzaman M, Schmidt T, et al. Cgas senses human cytomegalovirus and induces type I interferon responses in human monocyte-derived cells. PloS Pathog (2016) 12(4):e1005546. doi: 10.1371/journal.ppat.1005546
61. Paijo J, Doering M, Spanier J, Grabski E, Garbe A, Messerle M, et al. Hcmv infected human monocyte-derived macrophages and dc produce type I ifn in a cgas-dependent manner. Cytokine (2015) 76(1):109–. doi: 10.1016/j.cyto.2015.08.243
62. Huang Z-F, Zou H-M, Liao B-W, Zhang H-Y, Yang Y, Fu Y-Z, et al. Human cytomegalovirus protein ul31 inhibits DNA sensing of cgas to mediate immune evasion. Cell Host Microbe (2018) 24(1):69–+. doi: 10.1016/j.chom.2018.05.007
63. Biolatti M, Dell'Oste V, Pautasso S, Gugliesi F, von Einem J, Krapp C, et al. Human cytomegalovirus tegument protein pp65 (Pul83) dampens type I interferon production by inactivating the DNA sensor cgas without affecting sting. J Virol (2018) 92(6):e01774–17. doi: 10.1128/jvi.01774-17
64. Browne EP, Shenk T. Human cytomegalovirus ul83-coded pp65 virion protein inhibits antiviral gene expression in infected cells. Proc Natl Acad Sci United States America (2003) 100(20):11439–44. doi: 10.1073/pnas.1534570100
65. Kim J-E, Kim Y-E, Stinski MF, Ahn J-H, Song Y-J. Human cytomegalovirus ie2 86 kda protein induces sting degradation and inhibits cgamp-mediated ifn-beta induction (Vol 8, pg 1854, 2017). Front Microbiol (2018) 9:350. doi: 10.3389/fmicb.2018.00350
66. Fu Y-Z, Su S, Gao Y-Q, Wang P-P, Huang Z-F, Hu M-M, et al. Human cytomegalovirus tegument protein ul82 inhibits sting-mediated signaling to evade antiviral immunity. Cell Host Microbe (2017) 21(2):231–43. doi: 10.1016/j.chom.2017.01.001
67. Kumari P, Saha I, Narayanan A, Narayanan S, Takaoka A, Kumar NS, et al. Essential role of hcmv deubiquitinase in promoting oncogenesis by targeting anti-viral innate immune signaling pathways. Cell Death Dis (2017) 8:e3078. doi: 10.1038/cddis.2017.461
68. Choi HJ, Park A, Kang S, Lee E, Lee TA, Ra EA, et al. Human cytomegalovirus-encoded us9 targets mavs and sting signaling to evade type I interferon immune responses. Nat Commun (2018) 9:125. doi: 10.1038/s41467-017-02624-8
69. Li T, Chen J, Cristea IM. Human cytomegalovirus tegument protein pul83 inhibits ifi16-mediated DNA sensing for immune evasion. Cell Host Microbe (2013) 14(5):591–9. doi: 10.1016/j.chom.2013.10.007
70. Cristea IM, Moorman NJ, Terhune SS, Cuevas CD, O'Keefe ES, Rout MP, et al. Human cytomegalovirus pul83 stimulates activity of the viral immediate-early promoter through its interaction with the cellular ifi16 protein. J Virol (2010) 84(15):7803–14. doi: 10.1128/jvi.00139-10
71. Feng L, Li W, Wu X, Li X, Yang X, Ran Y, et al. Human cytomegalovirus ul23 attenuates signal transducer and activator of transcription 1 phosphorylation and type I interferon response. Front Microbiol (2021) 12:692515. doi: 10.3389/fmicb.2021.692515
72. Albright ER, Mickelson CK, Kalejta RF. Human cytomegalovirus ul138 protein inhibits the sting pathway and reduces interferon beta mrna accumulation during lytic and latent infections. Mbio (2021) 12(6):e0226721. doi: 10.1128/mBio.02267-21
73. Zou H-M, Huang Z-F, Yang Y, Luo W-W, Wang S-Y, Luo M-H, et al. Human cytomegalovirus protein ul94 targets mita to evade the antiviral immune response. J Virol (2020) 94(12):e00022–20. doi: 10.1128/JVI.00022-20
74. Jeon H, Lee J, Lee S, Kang S-K, Park SJ, Yoo S-M, et al. Extracellular vesicles from kshv-infected cells stimulate antiviral immune response through mitochondrial DNA. Front In Immunol (2019) 10:876. doi: 10.3389/fimmu.2019.00876
75. Vogt D, Zaver S, Ranjan A, DiMaio T, Gounder AP, Smith JG, et al. Sting is dispensable during kshv infection of primary endothelial cells. Virology (2020) 540:150–9. doi: 10.1016/j.virol.2019.11.012
76. Iqbal J, Ansari MA, Kumar B, Dutta D, Roy A, Chikoti L, et al. Histone H2b-ifi16 recognition of nuclear herpesviral genome induces cytoplasmic interferon-beta responses. PloS Pathog (2016) 12(10):e1005967. doi: 10.1371/journal.ppat.1005967
77. Li W, Avey D, Fu B, J-j Wu, Ma S, Liu X, et al. Kaposi's sarcoma-associated herpesvirus inhibitor of cgas (Kicgas), encoded by orf52, is an abundant tegument protein and is required for production of infectious progeny viruses. J Virol (2016) 90(11):5329–42. doi: 10.1128/jvi.02675-15
78. Wu J-J, Li W, Shao Y, Avey D, Fu B, Gillen J, et al. Inhibition of cgas DNA sensing by a herpesvirus virion protein. Cell Host Microbe (2015) 18(3):333–44. doi: 10.1016/j.chom.2015.07.015
79. Bhowmik D, Du M, Tian Y, Ma S, Wu J, Chen Z, et al. Cooperative DNA binding mediated by kicgas/orf52 oligomerization allows inhibition of DNA-induced phase separation and activation of cgas. Nucleic Acids Res (2021) 49(16):9389–403. doi: 10.1093/nar/gkab689
80. Ma Z, Jacobs SR, West JA, Stopford C, Zhang Z, Davis Z, et al. Modulation of the cgas-sting DNA sensing pathway by gammaherpesviruses. Proc Natl Acad Sci United States America (2015) 112(31):E4306–E15. doi: 10.1073/pnas.1503831112
81. Zhang G, Chan B, Samarina N, Abere B, Weidner-Glunde M, Buch A, et al. Cytoplasmic isoforms of kaposi sarcoma herpesvirus lana recruit and antagonize the innate immune DNA sensor cgas. Proc Natl Acad Sci United States America (2016) 113(8):E1034–E43. doi: 10.1073/pnas.1516812113
82. Mariggiò G, Koch S, Zhang G, Weidner-Glunde M, Rückert J, Kati S, et al. Kaposi sarcoma herpesvirus (Kshv) latency-associated nuclear antigen (Lana) recruits components of the mrn (Mre11-rad50-nbs1) repair complex to modulate an innate immune signaling pathway and viral latency. PloS Pathog (2017) 13(4):e1006335. doi: 10.1371/journal.ppat.1006335
83. Hertzog J, Zhou W, Fowler G, Rigby RE, Bridgeman A, Blest HT, et al. Varicella-zoster virus orf9 is an antagonist of the DNA sensor cgas. EMBO J (2022) 41(14):e109217. doi: 10.15252/embj.2021109217
84. Kim J-A, Park S-K, Seo S-W, Lee C-H, Shin OS. Sting is involved in antiviral immune response against vzv infection via the induction of type I and iii ifn pathways. J Invest Dermatol (2017) 137(10):2101–9. doi: 10.1016/j.jid.2017.03.041
85. Gershon M, Gershon A. Varicella-zoster virus and the enteric nervous system. J Infect Dis (2018) 218:S113–S9. doi: 10.1093/infdis/jiy407
86. Kim J-A, Park S-K, Kumar M, Lee C-H, Shin OS. Insights into the role of immunosenescence during varicella zoster virus infection (Shingles) in the aging cell model. Oncotarget (2015) 6(34):35324–43. doi: 10.18632/oncotarget.6117
87. Zhu H, Zheng C, Xing J, Wang S, Li S, Lin R, et al. Varicella-zoster virus immediate-early protein orf61 abrogates the irf3-mediated innate immune response through degradation of activated irf3. J Virol (2011) 85(21):11079–89. doi: 10.1128/JVI.05098-11
88. Sen N, Sommer M, Che X, White K, Ruyechan WT, Arvin AM. Varicella-Zoster Virus Immediate-Early Protein 62 Blocks Interferon Regulatory Factor 3 (Irf3) Phosphorylation at Key Serine Residues: A Novel Mechanism of Irf3 Inhibition among Herpesviruses. J Virol (2010) 84(18):9240–53. doi: 10.1128/jvi.01147-10
89. Sun Q, Fu Y, Chen X, Li L, Wu H, Liu Y, et al. Prognostic perspectives of sting and pd-L1 expression and correlation with the prognosis of epstein-barr virus-associated gastric cancers. Gut Liver (2022) 16(6):875–91. doi: 10.5009/gnl210359
90. Miyagi S, Watanabe T, Hara Y, Arata M, Uddin MK, Mantoku K, et al. A sting inhibitor suppresses ebv-induced B cell transformation and lymphomagenesis. Cancer Sci (2021) 112(12):5088–99. doi: 10.1111/cas.15152
91. Xing J, Zhang A, Zhang H, Wang J, Li XC, Zeng M-S, et al. Trim29 promotes DNA virus infections by inhibiting innate immune response. Nat Commun (2017) 8:945. doi: 10.1038/s41467-017-00101-w
92. Jaworska J, Gravel A, Fink K, Grandvaux N, Flamand L. Inhibition of transcription of the beta interferon gene by the human herpesvirus 6 immediate-early 1 protein. J Virol (2007) 81(11):5737–48. doi: 10.1128/JVI.02443-06
93. Bortolotti D, Gentili V, Caselli E, Sicolo M, Soffritti I, D'Accolti M, et al. DNA sensors' Signaling in nk cells during hhv-6a, hhv-6b and hhv-7 infection. Front Microbiol (2020) 11:226. doi: 10.3389/fmicb.2020.00226
94. Vassilieva EV, Taylor DW, Compans RW. Combination of sting pathway agonist with saponin is an effective adjuvant in immunosenescent mice. Front In Immunol (2019) 10:3006. doi: 10.3389/fimmu.2019.03006
95. Kosaka A, Yajima Y, Yasuda S, Komatsuda H, Nagato T, Oikawa K, et al. Celecoxib promotes the efficacy of sting-targeted therapy by increasing antitumor cd8+ T-cell functions via modulating glucose metabolism of cd11b+ Ly6g+ Cells. Int J Cancer (2023) 152(8):1685–97. doi: 10.1002/ijc.34394
96. Takahashi-Ruiz L, Fermaintt CS, Wilkinson NJ, Chan PYW, Mooberry SL, Risinger AL. The microtubule destabilizer eribulin synergizes with sting agonists to promote antitumor efficacy in triple-negative breast cancer models. Cancers (Basel) (2022) 14(23):5962. doi: 10.3390/cancers14235962
97. Yin M, Hu J, Yuan Z, Luo G, Yao J, Wang R, et al. Sting agonist enhances the efficacy of programmed death-ligand 1 monoclonal antibody in breast cancer immunotherapy by activating the interferon-Β Signalling pathway. Cell Cycle (2022) 21(8):767–79. doi: 10.1080/15384101.2022.2029996
98. Wu Y-T, Fang Y, Wei Q, Shi H, Tan H, Deng Y, et al. Tumor-targeted delivery of a sting agonist improvescancer immunotherapy. Proc Natl Acad Sci United States America (2022) 119(49):e2214278119. doi: 10.1073/pnas.2214278119
99. Lin LC-W, Huang C-Y, Yao B-Y, Lin J-C, Agrawal A, Algaissi A, et al. Viromimetic sting agonist-loaded hollow polymeric nanoparticles for safe and effective vaccination against middle east respiratory syndrome coronavirus. Adv Funct Mater (2019) 29(28):1807616. doi: 10.1002/adfm.201807616
100. Garland KM, Rosch JC, Carson CS, Wang-Bishop L, Hanna A, Sevimli S, et al. Pharmacological activation of cgas for cancer immunotherapy. Front In Immunol (2021) 12:753472. doi: 10.3389/fimmu.2021.753472
101. Wehbe M, Wang-Bishop L, Becker KW, Shae D, Baljon JJ, He X, et al. Nanoparticle delivery improves the pharmacokinetic properties of cyclic dinucleotide sting agonists to open a therapeutic window for intravenous administration. J Control Release (2021) 330:1118–29. doi: 10.1016/j.jconrel.2020.11.017
102. Nakamura T, Sato T, Endo R, Sasaki S, Takahashi N, Sato Y, et al. Sting agonist loaded lipid nanoparticles overcome anti-pd-1 resistance in melanoma lung metastasis via nk cell activation. J For Immunotherapy Cancer (2021) 9(7):e002852. doi: 10.1136/jitc-2021-002852
103. Zheng Y, Chen J, Song X-R, Chang M-Q, Feng W, Huang H, et al. Manganese-enriched photonic/catalytic nanomedicine augments synergistic anti-tnbc photothermal/nanocatalytic/immuno-therapy via activating cgas-sting pathway. Biomaterials (2023) 293:121988. doi: 10.1016/j.biomaterials.2022.121988
104. Pimkova Polidarova M, Vanekova L, Brehova P, Dejmek M, Vavrina Z, Birkus G, et al. Synthetic stimulator of interferon genes (Sting) agonists induce a cytokine-mediated anti-hepatitis B virus response in nonparenchymal liver cells. ACS Infect Dis (2023) 9(1):23–32. doi: 10.1021/acsinfecdis.2c00424
105. Zhang X, Liu B, Tang L, Su Q, Hwang N, Sehgal M, et al. Discovery and mechanistic study of a novel human-stimulator-of-interferon-genes agonist. ACS Infect Dis (2019) 5(7):1139–49. doi: 10.1021/acsinfecdis.9b00010
106. Chelvanambi M, Fecek RJ, Taylor JL, Storkus WJ. Sting agonist-based treatment promotes vascular norMalization and tertiary lymphoid structure formation in the therapeutic melanoma microenvironment. J For Immunotherapy Cancer (2021) 9(2):e001906. doi: 10.1136/jitc-2020-001906
107. Lee SJ, Yang H, Kim WR, Lee YS, Lee WS, Kong SJ, et al. Sting activation norMalizes the intraperitoneal vascular-immune microenvironment and suppresses peritoneal carcinomatosis of colon cancer. J For Immunotherapy Cancer (2021) 9(6):e002195. doi: 10.1136/jitc-2020-002195
108. Graham PT, Nowak AK, Cornwall SMJ, Larma I, Nelson DJ. The sting agonist, dmxaa, reduces tumor vessels and enhances mesothelioma tumor antigen presentation yet blunts cytotoxic T cell function in a murine model. Front In Immunol (2022) 13:969678. doi: 10.3389/fimmu.2022.969678
109. Xu N, Palmer DC, Robeson AC, Shou P, Bommiasamy H, Laurie SJ, et al. Sting agonist promotes car T cell trafficking and persistence in breast cancer. J Exp Med (2021) 218(2):e20200844. doi: 10.1084/jem.20200844
110. Li Y, He M, Wang Z, Duan Z, Guo Z, Wang Z, et al. Sting signaling activation inhibits hbv replication and attenuates the severity of liver injury and hbv-induced fibrosis. Cell Mol Immunol (2022) 19(1):92–107. doi: 10.1038/s41423-021-00801-w
111. Cerón S, North BJ, Taylor SA, Leib DA. The sting agonist 5,6-dimethylxanthenone-4-acetic acid (Dmxaa) stimulates an antiviral state and protects mice against herpes simplex virus-induced neurological disease. Virology (2019) 529:23–8. doi: 10.1016/j.virol.2019.01.006
112. Yu Y, Liu Y, An W, Song J, Zhang Y, Zhao X. Sting-mediated inflammation in kupffer cells contributes to progression of nonalcoholic steatohepatitis. J Clin Invest (2019) 129(2):546–55. doi: 10.1172/JCI121842
113. Zhao Q, Wei Y, Pandol SJ, Li L, Habtezion A. Sting signaling promotes inflammation in experimental acute pancreatitis. Gastroenterology (2018) 154(6):1822–35.e2. doi: 10.1053/j.gastro.2018.01.065
114. Hu Q, Ren H, Li G, Wang D, Zhou Q, Wu J, et al. Sting-mediated intestinal barrier dysfunction contributes to lethal sepsis. EBioMedicine (2019) 41:497–508. doi: 10.1016/j.ebiom.2019.02.055
115. Ramanjulu JM, Pesiridis GS, Yang J, Concha N, Singhaus R, Zhang S-Y, et al. Design of amidobenzimidazole sting receptor agonists with systemic activity. Nature (2018) 564(7736):439–43. doi: 10.1038/s41586-018-0705-y
116. Zhu Q, Hu H, Liu H, Shen H, Yan Z, Gao L. A synthetic sting agonist inhibits the replication of human parainfluenza virus 3 and rhinovirus 16 through distinct mechanisms. Antiviral Res (2020) 183:104933. doi: 10.1016/j.antiviral.2020.104933
117. Zhu Q, Zhang Y, Wang L, Yao X, Wu D, Cheng J, et al. Inhibition of coronavirus infection by a synthetic sting agonist in primary human airway system. Antiviral Res (2021) 187:105015. doi: 10.1016/j.antiviral.2021.105015
118. Li M, Ferretti M, Ying B, Descamps H, Lee E, Dittmar M, et al. Pharmacological activation of sting blocks sars-cov-2 infection. Sci Immunol (2021) 6(59):eabi9007. doi: 10.1126/sciimmunol.abi9007
119. Humphries F, Shmuel-Galia L, Jiang Z, Wilson R, Landis P, Ng S-L, et al. A diamidobenzimidazole sting agonist protects against sars-cov-2 infection. Sci Immunol (2021) 6(59):eabi9002. doi: 10.1126/sciimmunol.abi9002
120. Ma J, Liu Z, Zhou S, Chen M, Gao L, Zuo X, et al. Renal tubular in tce-sensitization-induced immune kidney injury: Role of mitochondrial DNA in activating the cgas-sting signaling pathway. Int Immunopharmacol (2022) 113(Pt B):109432. doi: 10.1016/j.intimp.2022.109432
121. Pham PT, Fukuda D, Nishimoto S, Kim-Kaneyama J-R, Lei X-F, Takahashi Y, et al. Sting, a cytosolic DNA sensor, plays a critical role in atherogenesis: A link between innate immunity and chronic inflammation caused by lifestyle-related diseases. Eur Heart J (2021) 42(42):4336–48. doi: 10.1093/eurheartj/ehab249
122. Wu B, Xu M-M, Fan C, Feng C-L, Lu Q-K, Lu H-M, et al. Sting inhibitor ameliorates lps-induced ali by preventing vascular endothelial cells-mediated immune cells chemotaxis and adhesion. Acta Pharmacol Sin (2022) 43(8):2055–66. doi: 10.1038/s41401-021-00813-2
123. Shen H, Jin L, Zheng Q, Ye Z, Cheng L, Wu Y, et al. Synergistically targeting synovium sting pathway for rheumatoid arthritis treatment. Bioact Mater (2023) 24:37–53. doi: 10.1016/j.bioactmat.2022.12.001
124. Kobritz M, Borjas T, Patel V, Coppa G, Aziz M, Wang P. H151, a small molecule inhibitor of sting as a novel therapeutic in intestinal ischemia-reperfusion injury. Shock (2022) 58(3):241–50. doi: 10.1097/SHK.0000000000001968
125. Gong W, Lu L, Zhou Y, Liu J, Ma H, Fu L, et al. The novel sting antagonist H151 ameliorates cisplatin-induced acute kidney injury and mitochondrial dysfunction. Am J Physiol Renal Physiol (2021) 320(4):F608–F16. doi: 10.1152/ajprenal.00554.2020
126. Ma C, Yang D, Wang B, Wu C, Wu Y, Li S, et al. Gasdermin D in macrophages restrains colitis by controlling cgas-mediated inflammation. Sci Adv (2020) 6(21):eaaz6717. doi: 10.1126/sciadv.aaz6717
127. Huang R, Shi Q, Zhang S, Lin H, Han C, Qian X, et al. Inhibition of the cgas-sting pathway attenuates lung ischemia/reperfusion injury via regulating endoplasmic reticulum stress in alveolar epithelial type ii cells of rats. J Inflammation Res (2022) 15:5103–19. doi: 10.2147/JIR.S365970
128. Xu Q, Xiong H, Zhu W, Liu Y, Du Y. Small molecule inhibition of cyclic gmp-amp synthase ameliorates sepsis-induced cardiac dysfunction in mice. Life Sci (2020) 260:118315. doi: 10.1016/j.lfs.2020.118315
129. Ding R, Li H, Liu Y, Ou W, Zhang X, Chai H, et al. Activating cgas-sting axis contributes to neuroinflammation in cvst mouse model and induces inflammasome activation and microglia pyroptosis. J Neuroinflamm (2022) 19(1):137. doi: 10.1186/s12974-022-02511-0
130. Basu S, Middya S, Banerjee M, Ghosh R, Pryde DC, Yadav DB, et al. The discovery of potent small molecule cyclic urea activators of sting. Eur J Med Chem (2022) 229:114087. doi: 10.1016/j.ejmech.2021.114087
131. Vavřina Z, Perlíková P, Milisavljević N, Chevrier F, Smola M, Smith J, et al. Design, synthesis, and biochemical and biological evaluation of novel 7-deazapurine cyclic dinucleotide analogues as sting receptor agonists. J Med Chem (2022) 65(20):14082–103. doi: 10.1021/acs.jmedchem.2c01305
132. Niu J, Bai H, Li Z, Gao Y, Zhang Y, Wang X, et al. Discovery of novel thieno[2,3-D]Imidazole derivatives as agonists of human sting for antitumor immunotherapy using systemic administration. Eur J Med Chem (2022) 238:114482. doi: 10.1016/j.ejmech.2022.114482
133. Pan B-S, Perera SA, Piesvaux JA, Presland JP, Schroeder GK, Cumming JN, et al. An orally available non-nucleotide sting agonist with antitumor activity. Sci (New York NY) (2020) 369(6506):eaba6098. doi: 10.1126/science.aba6098
134. Song J, Yang R-R, Chang J, Liu Y-D, Lu C-H, Chen L-F, et al. Discovery and characterization of a novel cgas covalent inhibitor for the treatment of inflammatory bowel disease. Acta Pharmacol Sin (2022) 44(4):791–800. doi: 10.1038/s41401-022-01002-5
135. Padilla-Salinas R, Sun L, Anderson R, Yang X, Zhang S, Chen ZJ, et al. Discovery of small-molecule cyclic gmp-amp synthase inhibitors. J Org Chem (2020) 85(3):1579–600. doi: 10.1021/acs.joc.9b02666
136. Ding C, Song Z, Shen A, Chen T, Zhang A. Small molecules targeting the innate immune cgas−Sting−Tbk1 signaling pathway. Acta Pharm Sin B (2020) 10(12):2272–98. doi: 10.1016/j.apsb.2020.03.001
Keywords: cGAS, STING, human herpesvirus, innate immunity, immune escape
Citation: Jin X, Wang W, Zhao X, Jiang W, Shao Q, Chen Z and Huang C (2023) The battle between the innate immune cGAS-STING signaling pathway and human herpesvirus infection. Front. Immunol. 14:1235590. doi: 10.3389/fimmu.2023.1235590
Received: 06 June 2023; Accepted: 21 July 2023;
Published: 02 August 2023.
Edited by:
Pei-Hui Wang, Shandong University, ChinaReviewed by:
Daniel Prantner, University of Maryland, United StatesCong Huang, Peking University, China
Copyright © 2023 Jin, Wang, Zhao, Jiang, Shao, Chen and Huang. This is an open-access article distributed under the terms of the Creative Commons Attribution License (CC BY). The use, distribution or reproduction in other forums is permitted, provided the original author(s) and the copyright owner(s) are credited and that the original publication in this journal is cited, in accordance with accepted academic practice. No use, distribution or reproduction is permitted which does not comply with these terms.
*Correspondence: Zhuo Chen, chenz@tjh.tjmu.edu.cn; Cong Huang, huangcong91@tjh.tjmu.edu.cn