- 1Institute of Cardiovascular Science, Translational Medicine Institute, Xi’an Jiaotong University Health Science Center, Xi’an, Shaanxi, China
- 2Department of Cardiology, The Second Affiliated Hospital of Xi’an Jiaotong University, Xi’an, Shaanxi, China
- 3Department of Vascular Surgery, The Second Hospital of Tianjin Medical University, Tianjin, China
- 4Department of Surgery, Stanford University School of Medicine, Stanford, CA, United States
- 5Key Laboratory of Environment and Genes Related to Diseases, Ministry of Education, Xi’an, Shaanxi, China
Background: C-reactive protein (CRP) levels are elevated in patients with abdominal aortic aneurysms (AAA). However, it has not been investigated whether CRP contributes to AAA pathogenesis.
Methods: CRP deficient and wild type (WT) male mice were subjected to AAA induction via transient intra-aortic infusion of porcine pancreatic elastase. AAAs were monitored by in situ measurements of maximal infrarenal aortic external diameters immediately prior to and 14 days following elastase infusion. Key AAA pathologies were assessed by histochemical and immunohistochemical staining procedures. The influence of CRP deficiency on macrophage activation was evaluated in peritoneal macrophages in vitro.
Results: CRP protein levels were higher in aneurysmal than that in non-aneurysmal aortas. Aneurysmal aortic dilation was markedly suppressed in CRP deficient (aortic diameter: 1.08 ± 0.11 mm) as compared to WT (1.21 ± 0.08 mm) mice on day 14 after elastase infusion. More medial elastin was retained in CRP deficient than in WT elastase-infused mice. Macrophage accumulation was significantly less in aneurysmal aorta from CRP deficient than that from WT mice. Matrix metalloproteinase 2 expression was also attenuated in CRP deficient as compared to WT aneurysmal aortas. CRP deficiency had no recognizable influence on medial smooth muscle loss, lymphocyte accumulation, aneurysmal angiogenesis, and matrix metalloproteinase 9 expression. In in vitro assays, mRNA levels for tumor necrosis factor α and cyclooxygenase 2 were reduced in lipopolysaccharide activated peritoneal macrophages from CRP deficient as compared to wild type mice.
Conclusion: CRP deficiency suppressed experimental AAAs by attenuating aneurysmal elastin destruction, macrophage accumulation and matrix metalloproteinase 2 expression.
Introduction
Abdominal aortic aneurysms (AAA) are local dilation of aortic segments particularly infrarenal aorta resulted from media destruction and diagnosed when the diameter exceeds 50% of adjacent aortic diameter (1). AAAs progress asymptomatically but fatal upon premature rupture with an estimated annual death of 100,000 worldwide (1–3). While poorly defined, inflammation is crucial in AAA pathogenesis (1, 4).
C-reactive protein (CRP) is an acute inflammatory protein and increases dramatically in response to almost tissue injury, infection and inflammation, thus being used as an inflammatory marker in clinic (5–9). CRP binds phosphorylated choline of pneumococcal C-polysaccharides, activates classical complement pathway or interacts with the Fc gammaR1/2 (FcγR1/2) receptor, altogether promoting the clearance of pathogens and cellular debris (10). CRP also modulates the function of immune cells including macrophages and lymphocytes (11–15). Thus, CRP is important for both host defense and human disease pathogenesis by regulating innate and adaptive immunity.
In clinical AAAs, it has been reported that the CRP levels were positively associated with aneurysm diameter (16–20). CRP was also highly expressed in aneurysmal as compared to non-aneurysmal aortas (21). Additionally, serum CRP levels have been used for helping AAA diagnosis as well as predicting clinical outcomes following AAA repair (22, 23). However, it has not been investigated whether CRP mediates AAA pathogenesis. Therefore, this study assessed the influence of CRP deficiency on experimental AAA formation and progression in the intra-aortic elastase infusion-induced AAA model.
Materials and methods
Mice
CRP deficient mice were previously generated using CRISPR/Cas9 and homologous recombination technology to knock-in a STOP cassette at the ATG site of the CRP gene on C57BL/6 genetic background) at Shanghai Biomodel Organism Science & Technology Development Co., Ltd (Shanghai, China) (24). CRP deficient and C57BL/6 wild type (WT) mice were used for all experiments. The use and care of animals in this study were approved by the Laboratory Animal Management Committee of Xi’an Jiaotong University, Xi’an, China (No. 2022-623).
Identification of CRP deficient mice
Genomic DNA was extracted from tail tips of less than 3 weeks old mice. Genotyping was conducted using the polymerase chain reaction (PCR) assay and gene-specific primer set. PCR primers were sense primer P1, (GCAGTTGGCCAGGGAAAGTT) and antisense primer P2 (CATGATCAGAAGGCACCAGAGTAG) for WT allele (PCR product size: 552 base pairs) and sense primer P1 and antisense primer P3 (CCTCGCCGGACACGCTGAA) for targeted allele (PCR product size: 637 base pairs), respectively. Quantitative real-time reverse transcription- polymerase chain reaction (qRT-PCR) was assessed CRP mRNA levels in liver tissues using the primers (Table 1). Western blotting analysis was utilized to determine CRP protein expression. Antibodies for Western blotting were a goat anti-mouse CRP polyclonal antibody (1:4000, Cat#: BAF1829, R&D Systems, Inc, Minneapolis, MN, USA), a rabbit anti-mouse β-actin polyclonal antibody (1:10000, Cat#: bs-0061R, Bioss Technology, Beijing, China), and an horseradish peroxidase-conjugated donkey anti-goat polyclonal antibody (1:5000, Cat#: EK030, Zhuangzhi Biological Technology, Xi’an, China) or goat anti-rabbit polyclonal antibody (1:10000, Cat#: bs-40295G, Bioss Technology) (25).
AAA creation in mice
Male CRP-deficient and WT control mice at 9 weeks of age were used for experiments. AAAs were induced in infrarenal aorta under sterile condition using porcine pancreatic elastase (PPE) infusion method as previously described (26–28). Briefly, mice were anesthetized by 2% isoflurane inhalation, and a laparotomy was created to expose the infrarenal aorta. Mice were infused with PPE solution for 5 minutes (1.5 units/mL, Cat#: E-1250, Sigma-Aldrich, St Louis, MO, USA) through the aortotomy in temporarily controlled infrarenal aortic segment using a pressure pump (29). Thereafter, aortotomy and laparotomy were sequentially closed using 10-0 and 6-0 silk sutures, respectively. Mice were recovered, housed in individual cages, and monitored daily for morbidity and mortality.
Measurements of aneurysmal aortic diameters
External infrarenal aortic diameters were measured in situ using a digital microscope. Briefly, infrarenal aortic segment was photographed immediately prior to (baseline) and 14 days following PPE. Maximal external infrarenal aortic diameters were determined using Motic Image Plus 3.0 ML software (Motic Electric Group Co., Ltd, Xiamen, China). An AAA was defined as a more than 50% increase in external diameter over the baseline level (29).
Immunostaining of CRP in experimental aneurysmal aorta
Mice were euthanized by carbon dioxide inhalation 14 days following PPE infusion. Aortas were harvested, embedded in optical cutting temperature compound, sectioned (6 μm) and fixed with cold acetone. Infrarenal aortas from naïve WT mice were processed identically and served as non-aneurysmal controls. Frozen sections were stained with hematoxylin and eosin (H&E) and a standard streptavidin peroxidase immunohistochemical method for the assessment of morphological alterations and CRP protein expression, respectively. Reagents for CRP tissue immunostaining were a rabbit anti-mouse CRP polyclonal antibody (1:200, Cat#: bs-0155R) and normal rabbit IgG (1:200, Cat#: bs-0295P) from Bioss Technology. Other reagents were biotinylated goat anti-rabbit IgG antibody (1:400, Cat#: BA-1000) and AEC substrate kit (Cat#: SK-4200, Vector Laboratories, Inc, Newark, CA, USA) and streptavidin-peroxidase conjugate (1:200, Cat#: 016-030-084, Jackson ImmunoResearch, West Grove, PA, USA).
Histological analysis of medial elastin degradation and smooth muscle cell depletion in aneurysmal aortas
H&E and Elastic van Gieson (EVG) staining were performed frozen aortic sections to assess general morphological changes and elastin contents, respectively. To assess SMC retention, frozen aortic sections were sequentially stained with a goat anti-SMC α-actin polyclonal antibody (1:200, Cat#: NB300-978, Novus Biologicals, Centennial, CO, USA), a biotinylated rabbit anti-goat IgG antibody (1:400, Cat#: BA-5000, Vector Laboratories, Inc) and streptavidin-peroxidase conjugate (1:200, Cat#: 016-030-084, Jackson ImmunoResearch), and staining was visualized using the AEC substrate kit (Vector Laboratories, Inc). Elastin fragmentation and SMC loss were scored as the grade I (mild) to IV (severe) using a histological grading system as reported previously (26–31).
Immunohistochemical staining for leukocytes, angiogenesis and matrix metalloproteinases in aneurysmal aortas
A standard biotin-streptavidin-peroxidase method was used for all immunohistochemical staining. Reagents used in this study were monoclonal antibodies against CD68 (macrophages, 1:200, clone #: FA-11, Cat#: 137002), CD4 (CD4+ T cells, 1:200, clone #: GK1.5, Cat#: 100402), CD8 (CD8+ T cells, 1:200, clone #: 53-6.7, Cat#: 100702), B220 (B cells, 1:200, clone #: RA3-6B2, Cat#: 103202) and CD31 (1:200, clone#: 390, Cat#: 102402) (all above mentioned primary antibodies from Biolegend Inc, San Diego, CA, USA), goat anti-mouse polyclonal antibodies against MMP2 (1:200, Cat#: AF1488, R&D Systems) and MMP9 (1:200, Cat#: AF909, R&D Systems), a biotinylated goat anti-rat secondary antibody (1:400, Cat#: BA-9400, Vector Laboratories, Inc) or rabbit anti-goat IgG secondary antibody (1:400, Cat#: BA-5000, Vector Laboratories, Inc), and streptavidin-peroxidase conjugate (1:200, Cat#: 016-030-084, Jackson ImmunoResearch) (27–32). Macrophage accumulation was scored as the grade I to IV, and the densities of CD4+ T cells, CD8+ T cells, B220+ B cells and angiogenesis were quantified as the number of positively stained cells or neovessels per aortic cross section (ACS) (30). MMP expression levels were quantified as a positively stained area percentage of aortic wall using WinRood 6.5 image software, Mitani Co. Ltd., Tokyo, Japan.
Measurements of macrophage activation in vitro
Primary macrophages were isolated from 8 weeks old WT and CRP deficient mice 3 days following intraperitoneal injection of 3% thioglycolate and suspended in RPMI-1640 medium containing 10% fetal bovine serum and 1% penicillin/streptomycin. Following 6 hours activation with lipopolysaccharide (LPS, 50 ng/mL, Cat#: L2630, Sigma-Aldrich) or incubation with vehicle at 37°C, 5% CO2 for 6 h, cells were harvested. For interleukin-4 (IL-4) stimulated experiment, macrophages were treated with IL-4 (10 ng/mL; Cat#: 214-14, PeproTech, Inc. Cranbury, NJ, USA) or vehicle for 16 hours. Total RNA was extracted with RNAiso Plus (Cat#: 9109), and complementary DNA was synthesized PrimeScript RT kit (Cat#: RR036A) (all from Takara Bio Inc, Kusatsu, Shiga, Japan) and amplified using RealStar Green Power mix (Cat#: A311-10; GenStar, Beijing, China) and gene-specific primers (Table 1). mRNA levels were quantitated as fold changes in relative to vehicle-treated macrophages.
Statistical analysis
All statistical analyses were performed using the GraphPad software Version 9.0 (Boston, MA, USA). Data on continuous variables were presented as either mean ± standard deviation if normally distributed, and statistical significance was tested using Student’s t-test, or one, two–way ANOVA followed by two group comparison tests. Otherwise, data were given as median and interquartile range, and statistical significance was determined using nonparametric Mann-Whitney test. Statistical significance level was set at p<0.05.
Results
CRP protein is increased in experimental aneurysmal aortas
To examine whether CRP protein expression is altered in aneurysmal aortas, we stained aortic frozen sections from aneurysmal (PPE-infused) and non-aneurysmal WT mice. As depicted in Figure 1, rare or no positive staining was not seen in non-aneurysmal aorta. In contrast, an intense and diffusion CRP staining was noted in aneurysmal aortas (Figure 1). In serial sections, CRP staining was coincident with inflammatory cell accumulation in aneurysmal aortas.
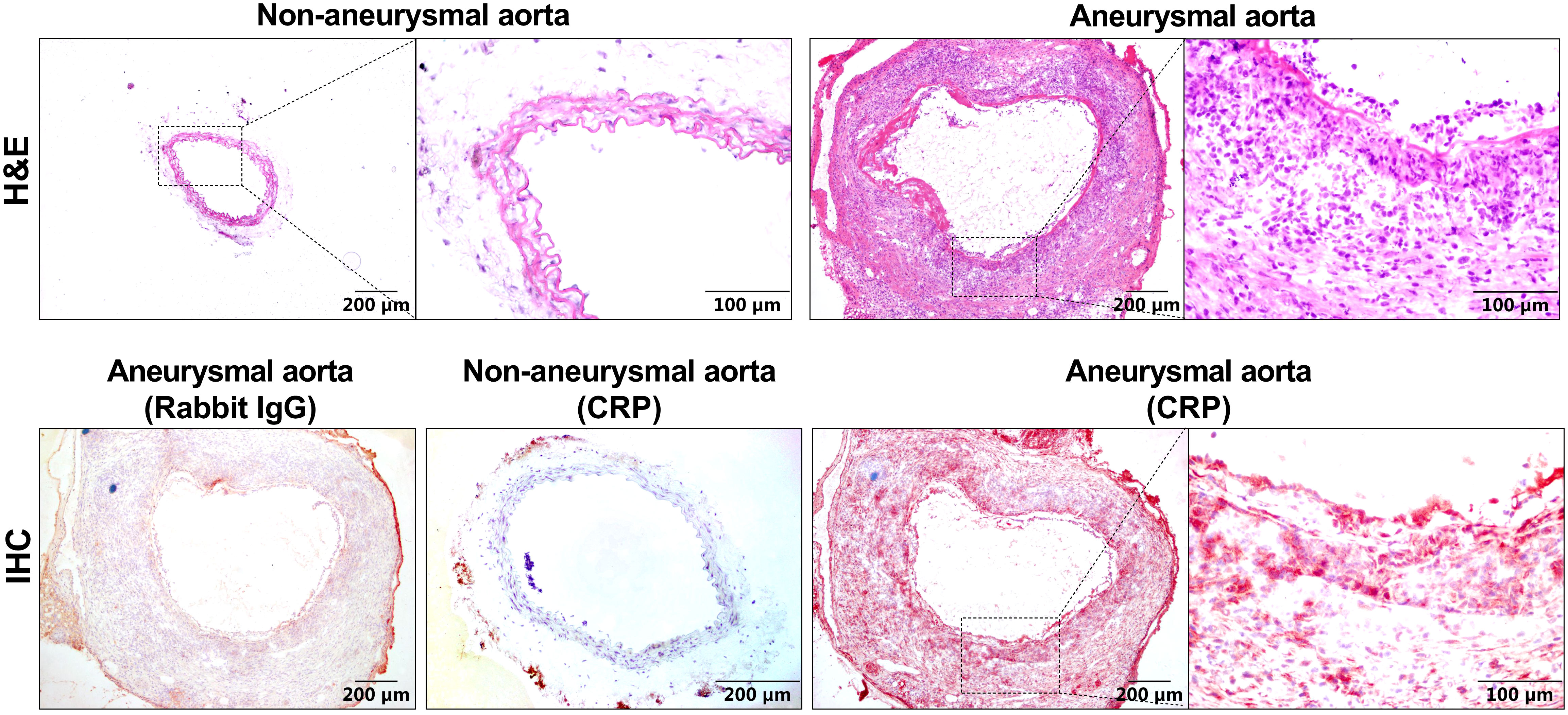
Figure 1 CRP expression is elevated in experimental aneurysmal aortas. H&E staining or CRP immunostaining using a rabbit anti-mouse CRP antibody or normal rabbit IgG as the negative control were performed on frozen sections from non-aneurysmal and aneurysmal aortas.
CRP deficiency mitigates experimental aneurysmal aortic dilation
To clarify the effect of CRP in experimental AAAs, previously generated CRP-deficient (CRP-/-) mice were used in this study (24). The homozygotes of CRP-deficient mice were screened by PCR genotyping and Western blotting (Figure 2A). Luminal PPE infusion in infrarenal aorta was performed to induce AAAs in both WT and CRP-/- mice. Fourteen days following PPE infusion, aortic dilation, as measured by external aortic diameter, was seen in both WT and CRP-/- mice as compared to the baseline level. However, maximal external aortic diameter was significantly smaller in CRP-/- (1.08 ± 0.11 mm) than that in WT (1.21 ± 0.08 mm) mice on day 14 after PPE infusion (Figures 2B, C). When subtracting aortic dilation due to the pressed infusion (approximately 0.8 mm), CRP deficiency per se led to a 32% reduction in aneurysmal enlargement (Figure 2C). Even considering the influence of baseline aortic diameter, a remarkable reduction in external aortic diameter was observed in CRP-/- as compared to WT mice 14 days following PPE infusion (Figure 2D). Thus, CRP may in part mediate experimental aneurysmal expansion.
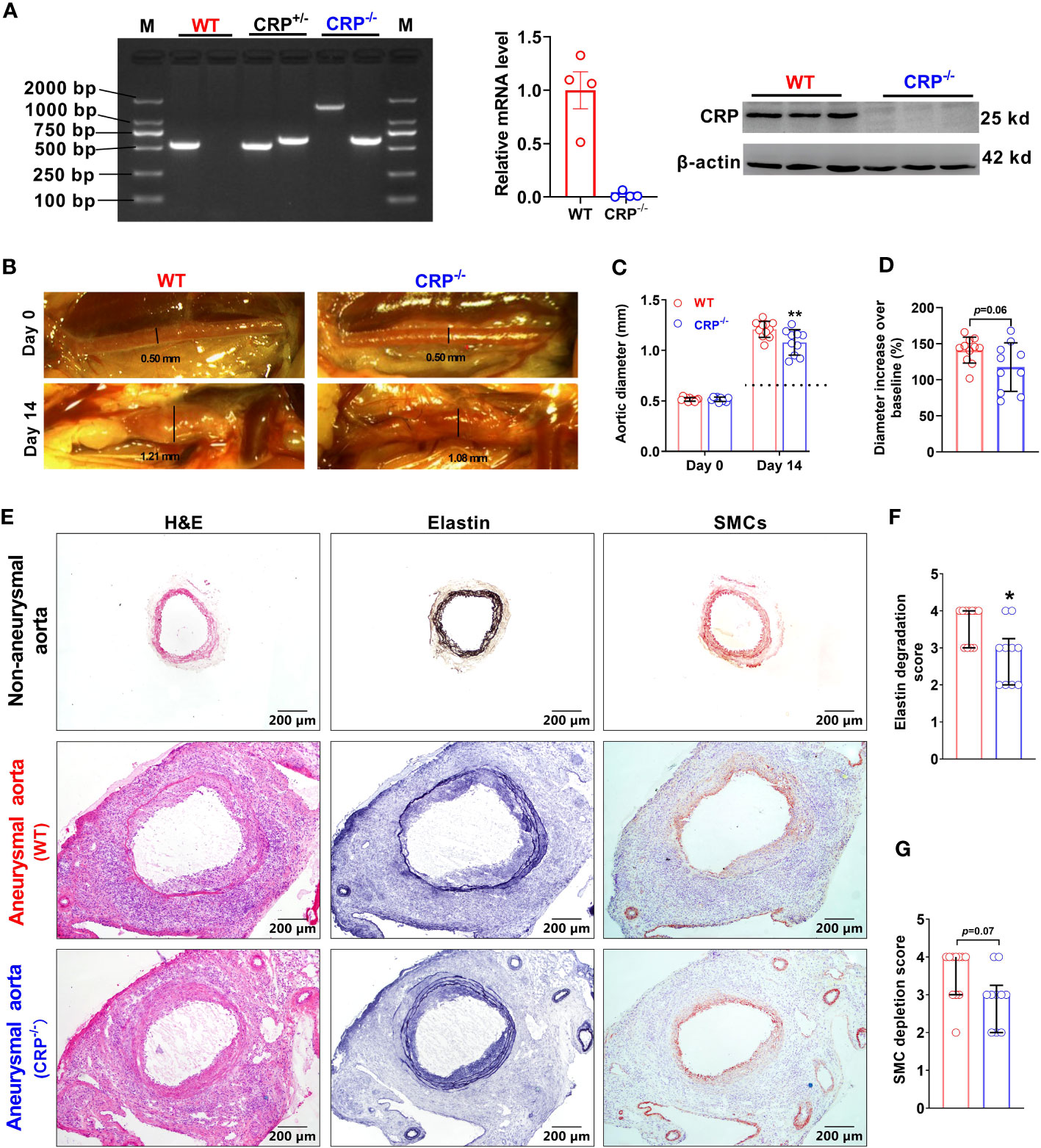
Figure 2 CRP deficiency suppresses experimental aneurysmal dilation. (A): Phenotype identification of CRP deficient mice. Phenotyping for CRP homozygotes (CRP deficient mice: CRP-/-), CRP heterozygotes (CRP+/-) and wild type (WT) mice using PCR assay. CRP expression levels in the livers of CRP-/- and WT mice were determined via qRT-PCR and Western blotting analyses. (B): Representative photographic images for infrarenal aortas of wild type and CRP-/- mice prior to and 14 days after the elastase infusion. AAAs were induced in male CRP-/- and its wild type control mice using intra-aortic infusion of PPE. Influence on AAAs were assessed via in situ measurements of maximal infrarenal aortic diameters. Dotted line indicates aortic expansion even after PPE solvent (PBS) pressed infusion (about 0.8 mm in our lab) (C, D): Maximal infrarenal aortic external diameters presented as absolute diameter on days 0 (baseline) and 14 after PPE infusion (C) or the percentage of diameters over baseline (D). n=10-11 mice per group. Two-ANOVA followed by two group comparison, **p<0.01 compared to wild type mice at same timepoint (C). Student t-test, p=0.06 compared to wild type mice (D). (E): Representative aortic images for H&E (left panels), elastin via Elastic Van Gieson (middle panels), and SMCs via an anti-SMC α antibody immunostaining (right panels) from non-aneurysmal and aneurysmal (wild type and CRP-/-) mice. (F, G): Quantification of medial elastin degradation (F) and SMC depletion (G) scores (media and interquartile) of wild type and CRP-/- aneurysmal aortas. Nonparametric Mann-Whitney test, *p<0.05 compared to wild type mice.
CRP deficiency attenuates medial elastin degradation and SMC loss
In EVG staining for medial elastin, more medial elastin was maintained in CRP-/- [score as 3 (2–3) (median with interquartile range)] as compared to WT [score as 4 (3–4)] mice (Figures 2E, F, p=0.019). Similarly, substantial retention of SMCs was noted in CRP-/- [score as 3 (2–3)] as compared to WT [score as 4 (3–4)]. However, the difference in SMC grades did not reach statistical significant level (Figures 2E, G) probably due to insufficient sample size. Thus, experimental AAA suppression mediated by CRP deficiency is associated with marked preservation of medial elastin.
CRP deficiency reduces macrophages accumulation in aneurysmal aorta
Leukocytes contribute to experimental AAA pathogenesis (29, 33). In non-aneurysmal aorta, few leukocytes, almost CD68+ macrophages in aortic adventitia, were stained positively with subset-specific mAbs (Figure 3A). However, leukocytes infiltrated intensely throughout aortic wall in aneurysmal aortas (Figure 3A). CD68+ macrophages accumulated significantly less in CRP-/- mice [score as 3 (2–4) (median with interquartile range)] than WT mice did [score as 4 (3–4)] (p=0.025) (Figures 3A, B). While CD4+ T cells, CD8+ T cells and B220+ B cells also accumulated in CRP-/- and WT mice, no significant difference in either subset was seen between two mouse strains (Figures 3A, C–E).
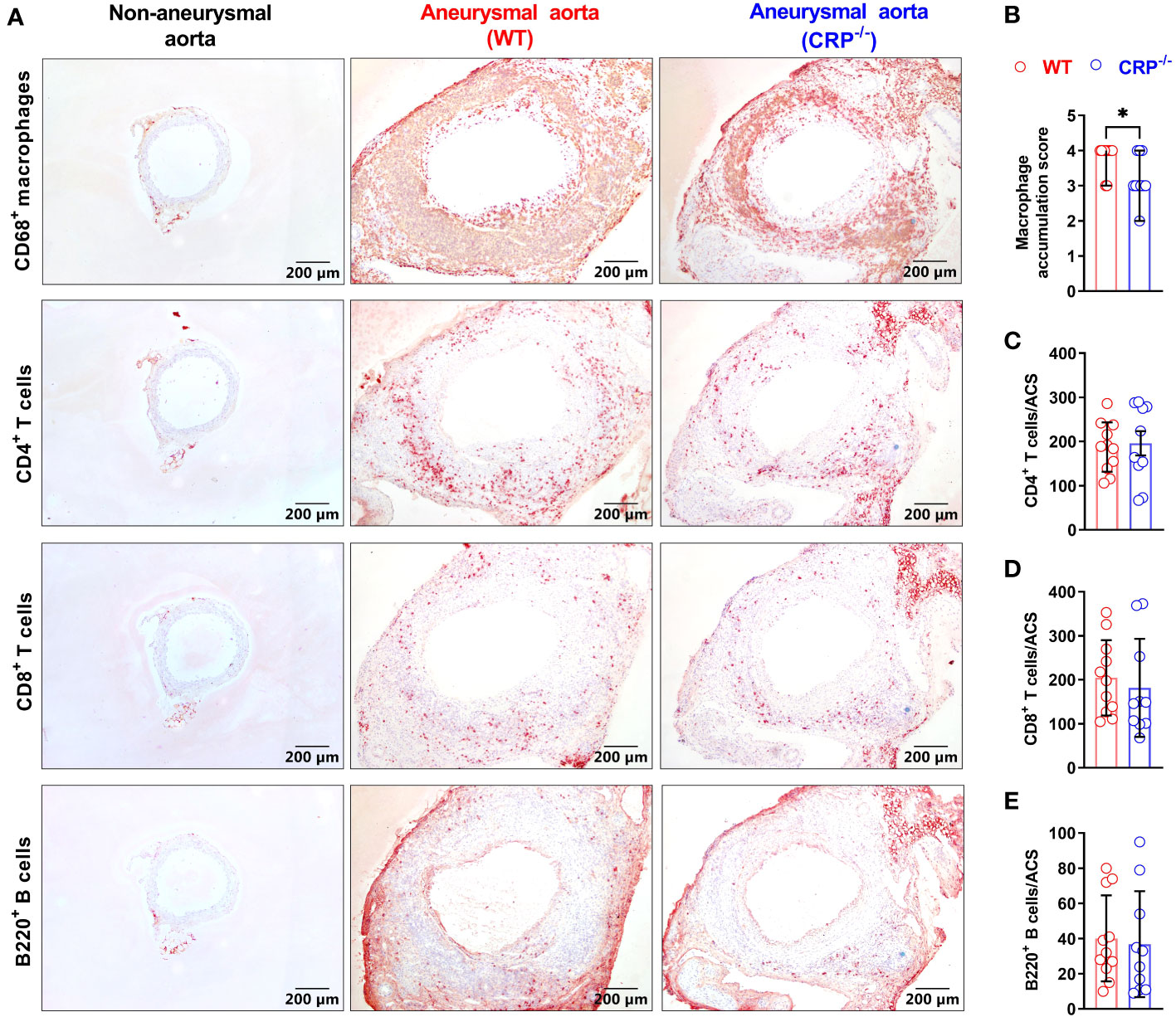
Figure 3 CRP deficiency alters aortic leukocyte accumulation. (A): Representative aortic immunostaining images for CD68+ macrophages, CD4+ T cells, CD8+ T cells and B220+ B cells from non-aneurysmal normal aorta (left panels) and aneurysmal (wild type: middle panels; CRP-/- mice: right panels). (B): Quantification of macrophage accumulation scores (media and interquartile) in WT and CRP-/- aneurysmal aorta. N=10-11 mice per group, Non-parametric Mann-Whitney, *P<0.01 compared to WT mice. (C–E): Quantification of different lymphocyte subsets of aortic leukocytes [mean ± standard deviation for positive cells/aortic cross-section (ACS)] from the aneurysmal aortas of WT and CRP-/- mice. No statistical difference in all lymphocyte subsets between two mouse strains.
CRP deficiency diminishes MMP2 expression in aneurysmal aorta
Both MMP2 and MMP9 are important mediators of AAA pathogenesis (34). In our immunohistochemical staining, MMP2 expression was reduced in PPE-infused CRP-/- [expressed as positive staining area ratio (%): 49.51 ± 13.77] as compared to WT (29.55 ± 9.98) mice (Figure 4A), with a 40% reduction in CRP-/- mice (Figure 4B). However, there was no significant difference in MMP9 expression between PPE-infused CRP-/- and WT mice (Figures 4A, C).
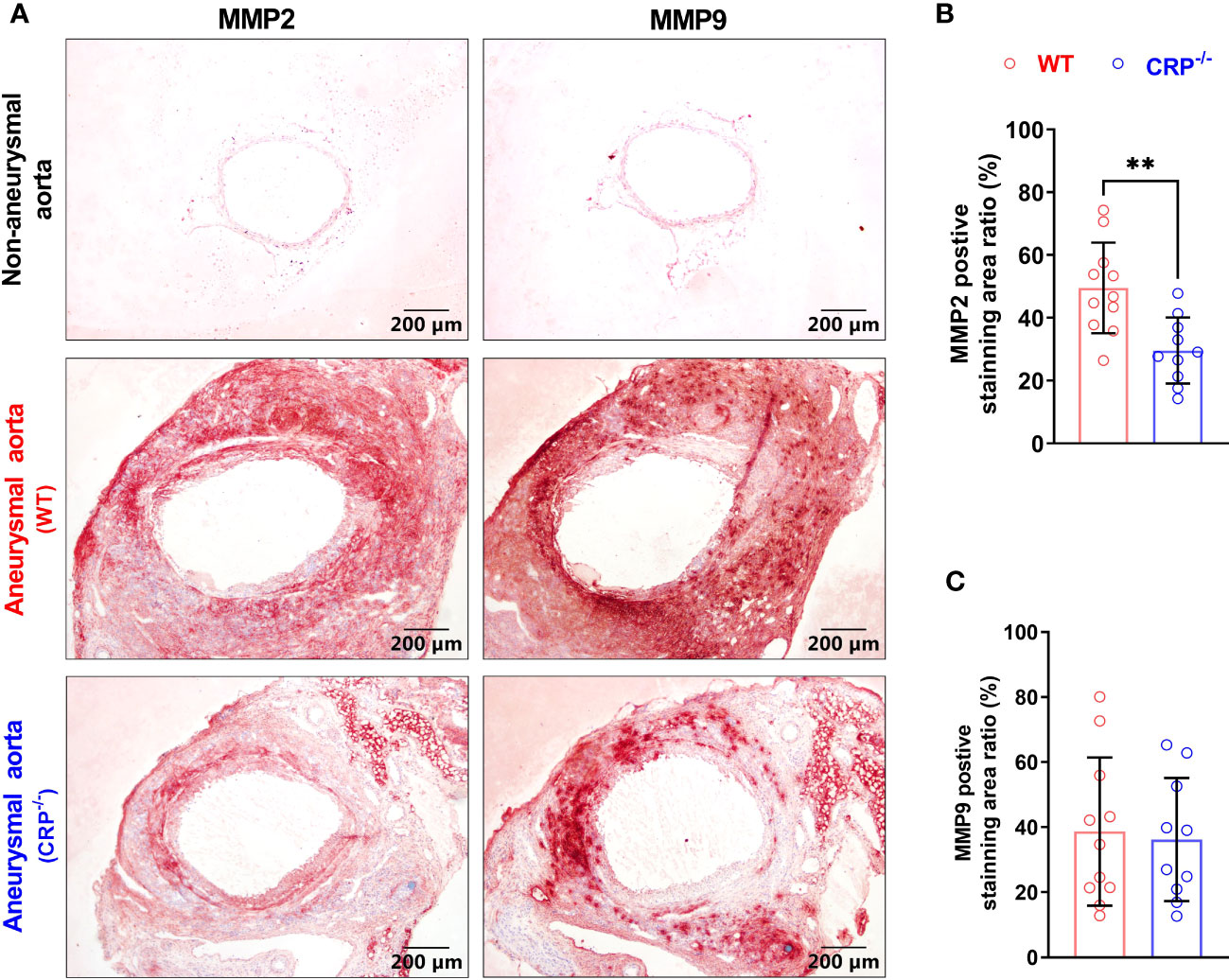
Figure 4 CRP deficiency reduces aneurysmal aortic MMP2 expression. (A): Representative immunostaining images for MMP2 and MMP9 in non-aneurysmal normal or aneurysmal (WT and CRP-/-) aortas. (B, C): Quantification of MMP2 and MMP9 expression (mean ± standard for the percentage of positively stained area in total aortic cross section area) in wild type and CRP-/- aneurysmal aortas. Student’s t tests, n=10-11 mice per group, **p<0.01 compared to wild type mice.
CRP deficiency has no recognizable impact on angiogenesis in aneurysmal aorta
Angiogenesis, an additional key AAA pathology, also contributes to the progression of AAAs (35, 36). Angiogenesis, as determined by the density of CD31+ neovessels, was not differentiated between CRP-/- (50.82 ± 13.40/ACS) and WT (43.90 ± 12.23/ACS) mice (Figure 5). Thus, neoangiogenesis may have no or limited contribution to the suppression of experimental AAAs by CRP deficiency.
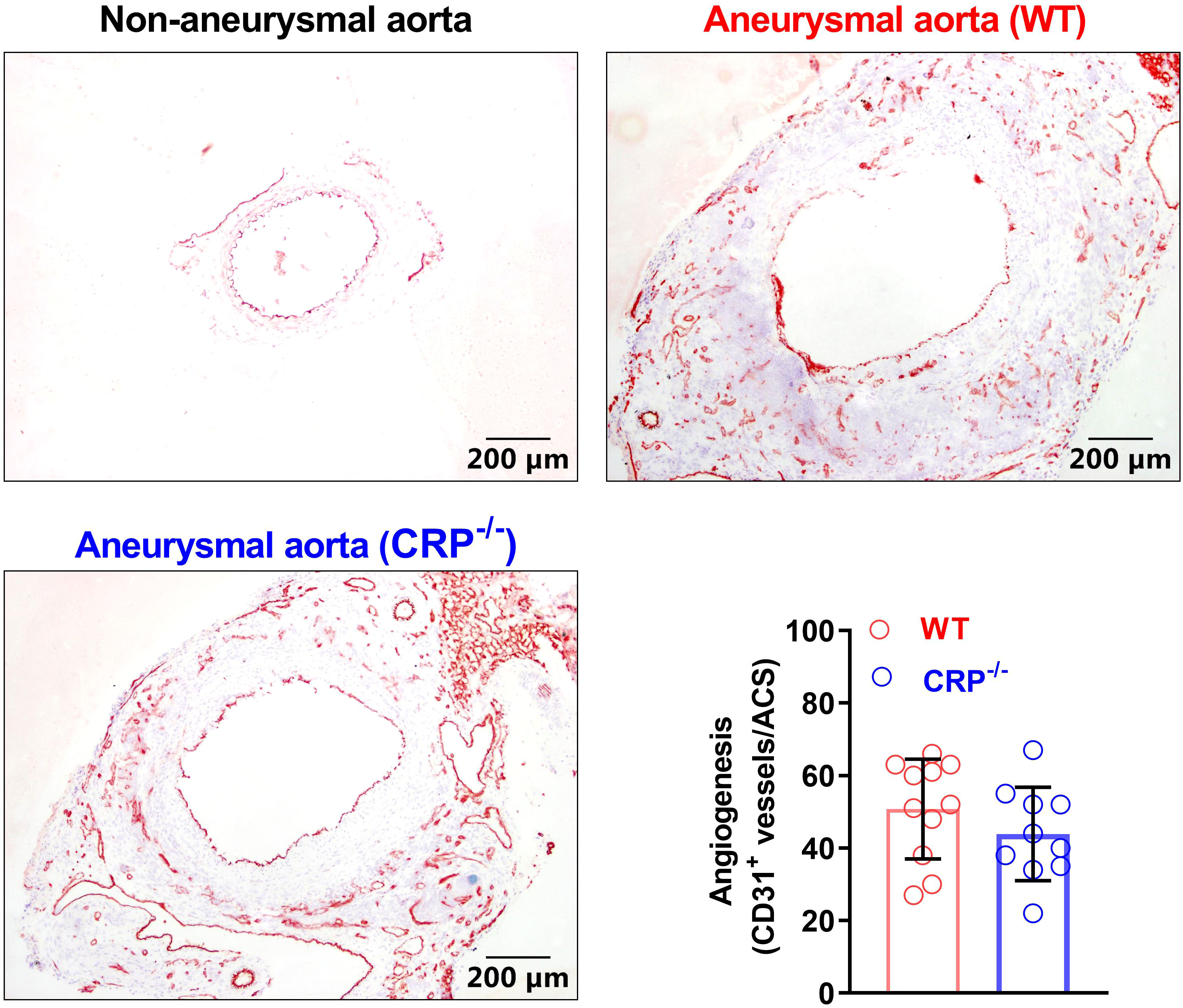
Figure 5 CRP deficiency has no remarkable impact on angiogenesis in aneurysmal aortas. Frozen sections were prepared from the aortas of non-aneurysmal normal (upper left) and aneurysmal (wild type: upper right; CRP-/-: lower left) mice (n=10-11 mice per group) and stained with an anti-CD31 antibody to assess aneurysmal angiogenesis. Lower right panel: angiogenesis in wild and CRP-/- aneurysmal mouse aortas was quantitated as the number of CD31-positive neovessels per ACS. Data are mean ± standard deviation. Student’s t tests, no significant difference between two groups.
CRP deficiency limits classic macrophage activation in vitro
Classic macrophage activation (conventionally known as proinflammatory M1 macrophage activation/polarization) promotes AAA formation and progression (33, 37). In classical activated macrophage by LPS, the expression levels of mRNA for TNF-α and COX-2, but not IL-1β, IL-6 (M1 marker genes) were significantly reduced in CRP deficient as compared to WT macrophages (Figure 6). However, no significant influences were found for the expression levels of all alternative activation macrophage markers (conventionally knowns as anti-inflammatory M2 macrophages) after activated by IL-4 (Supplementary Figure 1).
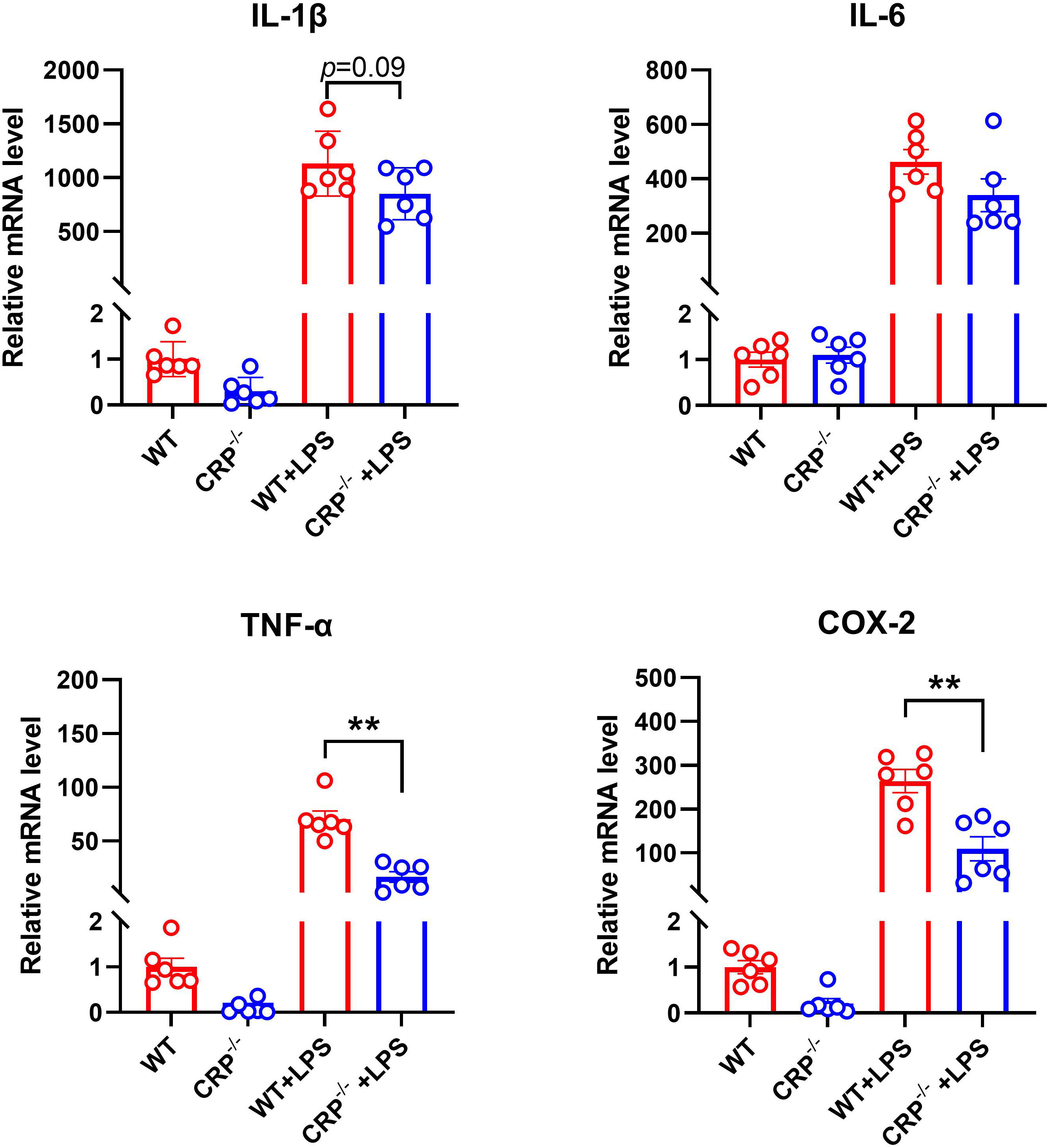
Figure 6 CRP deficiency impairs classic proinflammatory macrophage activation/polarization. Peritoneal macrophages from non-aneurysmal wild type (WT) and CRP-/- mice underwent the classic activation (conventionally known as M1 macrophages) in the presence of LPS (50 ng/ml) or vehicle alone for 6 hours. The mRNA expression levels of indicated classic activation macrophage marker genes were detected by using qRT-PCR. mRNA levels are presented as mean ± standard deviation fold changes relative to vehicle-treated WT macrophages. Two-way ANOVA followed two group comparison, **p<0.01 between two groups, n=6 biological repeats for each group.
Discussion
Although the detailed mechanism of AAA pathogenesis is still unclear, the involvement of inflammation in the formation and progression of AAAs has become a consensus in this field (38). CRP, as one of the important inflammatory acute phase proteins, is involved in the development of many cardiovascular diseases (39–45). In this study, we found increased expression of CRP protein in experimental AAAs. CRP deficiency inhibited experimental AAA enlargement in the PPE infusion AAA model. Histologically, the suppression of experimental AAAs by CRP deficiency was associated with the attenuation of medial elastin destruction, aneurysmal wall macrophage accumulation and MMP2 expression. Additionally, CRP deficiency partially inhibited proinflammatory macrophage activation/polarization. Thus, our study indicated that CRP may in part mediate AAA pathogenesis.
In previous studies, CRP has been shown to regulate phenotypic differentiation and activity of macrophages (46–48). In patients with certain cardiovascular diseases, CRP expression levels was positively correlated with M1 macrophage activation (48). This was consistent with our findings that CRP deficiency downregulated the expression levels of M1 macrophages marker genes. Nuclear factor kappa B (NF-κB) regulates proinflammatory macrophages polarization as well as CRP activity, thus reduced M1 macrophage polarization due to CRP deficiency may potentially associate with altered NF-κB signaling activity (42, 47, 49, 50). Additionally, reduced M1 macrophage activation in CRP deficient macrophages may attenuate the expression of proaneurysmal mediators including cytokines and MMPs consequently leading to experimental AAA inhibition.
We found that CRP expression was elevated in experimental AAA lesion. While we have no data showing the source for increased CRP, this may result from locally and/or systemically increased CRP as reported in other pathological condition (51, 52). Macrophages have been reported to be the main cellular source for non-liver derived CRP (53). CRP interacts with macrophage FcγR1/2 or lectin-like oxidized LDL receptor 1 (LOX-1) and bind to Oxidized low-density lipoprotein (Ox-LDL), thus regulating macrophage functional activity through multiple pathways and mediating vascular inflammatory diseases (10, 54–57). Alternatively, the interaction of CRP with LOX-1 and Ox-LDL also enhances CRP expression in endothelial cells, which further promote vascular inflammation (58). Therefore, the potential modulation of macrophage activity by CRP may partially contribute to experimental AAA pathogenesis in the PPE AAA model.
Although the loss of endogenous CRP reduced experimental AAAs, the inhibition was not strong as demonstrated for other agents including metformin (59–61). Therefore, we need more experimental evidence to validate whether CRP can be a therapeutic target for AAA disease. In summary, this study demonstrates a partial role of CRP in experimental AAA pathogenesis of AAAs using CRP deficient mice.
Data availability statement
The data presented in the study are included in the article/Supplementary Material. Further inquiries can be directed to the corresponding author.
Ethics statement
The animal study was approved by Laboratory Animal Management Committee of Xi’an Jiaotong University. The study was conducted in accordance with the local legislation and institutional requirements.
Author contributions
SZ, YW, EL, and BX designed this study; YF, HL, KL, SZ, JD, PW, ML, HW, XH, HH, RW, LB, WW, and YL collected and analyzed data, as well as drafted the manuscript; YF, HL, KL, PW, ML, HW, XH, and HH performed experiments; YW, NA, BX, and SZ critically revised manuscript. All authors contributed to the article and approved the submitted version.
Funding
This work was partly supported by the Natural Science Project of Shaanxi Province (2023-CX-PT-17 to SZ, 2022PT-41 to CX), Natural Science Project of Xi’an Jiaotong University (YXJLRH2022073 to SZ and JD) and Project of Key Laboratory of Medical Large Animal Models of Guangdong Province (Klmlam 202204 to SZ).
Conflict of interest
The authors declare that the research was conducted in the absence of any commercial or financial relationships that could be construed as a potential conflict of interest.
Publisher’s note
All claims expressed in this article are solely those of the authors and do not necessarily represent those of their affiliated organizations, or those of the publisher, the editors and the reviewers. Any product that may be evaluated in this article, or claim that may be made by its manufacturer, is not guaranteed or endorsed by the publisher.
Supplementary material
The Supplementary Material for this article can be found online at: https://www.frontiersin.org/articles/10.3389/fimmu.2023.1233807/full#supplementary-material
Supplementary Figure 1 | The effect of CRP deficiency in IL-4 induced M2 polarization in cultured macrophages. Peritoneal macrophages from non- WT and CRP-/- mice underwent the alternative activation (conventionally known as M2 polarization) in the presence of IL-4 (10 ng/ml) or vehicle alone. The mRNA expression levels of indicated M2 macrophage marker genes were measured by using qRT-PCR. Two-way ANOVA followed two group comparison, no significant influence of CRP deficiency on either M2 maker mRNA levels, n=3 biological repeats for each group.
References
1. Sakalihasan N, Michel JB, Katsargyris A, Kuivaniemi H, Defraigne JO, Nchimi A, et al. Abdominal aortic aneurysms. Nat Rev Dis Primers (2018) 4(1):34. doi: 10.1038/s41572-018-0030-7
2. Sampson UK, Norman PE, Fowkes FG, Aboyans V, Yanna S, Harrell FE Jr., et al. Global and regional burden of aortic dissection and aneurysms: Mortality trends in 21 world regions, 1990 to 2010. Glob Heart (2014) 9(1):171–80 e10. doi: 10.1016/j.gheart.2013.12.010
3. Singh K, Bonaa KH, Jacobsen BK, Bjork L, Solberg S. Prevalence of and risk factors for abdominal aortic aneurysms in a population-based study : The tromso study. Am J Epidemiol (2001) 154(3):236–44. doi: 10.1093/aje/154.3.236
4. Jagadesham VP, Scott DJ, Carding SR. Abdominal aortic aneurysms: An autoimmune disease? Trends Mol Med (2008) 14(12):522–9. doi: 10.1016/j.molmed.2008.09.008
5. Pepys MB, Hirschfield GM. C-reactive protein: A critical update. J Clin Invest (2003) 111(12):1805–12. doi: 10.1172/JCI18921
6. Shine B, de Beer FC, Pepys MB. Solid phase radioimmunoassays for human C-reactive protein. Clin Chim Acta (1981) 117(1):13–23. doi: 10.1016/0009-8981(81)90005-x
7. Bottazzi B, Doni A, Garlanda C, Mantovani A. An integrated view of humoral innate immunity: Pentraxins as a paradigm. Annu Rev Immunol (2010) 28:157–83. doi: 10.1146/annurev-immunol-030409-101305
8. Sproston NR, Ashworth JJ. Role of C-reactive protein at sites of inflammation and infection. Front Immunol (2018) 9:754. doi: 10.3389/fimmu.2018.00754
9. Thompson D, Pepys MB, Wood SP. The physiological structure of human C-reactive protein and its complex with phosphocholine. Structure (1999) 7(2):169–77. doi: 10.1016/S0969-2126(99)80023-9
10. Marnell LL, Mold C, Volzer MA, Burlingame RW, Du Clos TW. C-reactive protein binds to fc gamma ri in transfected cos cells. J Immunol (1995) 155(4):2185–93. doi: 10.4049/jimmunol.155.4.2185
11. Devaraj S, Yun JM, Duncan-Staley C, Jialal I. C-reactive protein induces M-csf release and macrophage proliferation. J Leukoc Biol (2009) 85(2):262–7. doi: 10.1189/jlb.0808458
12. Cai SY, Nie L, Chen J. C-reactive protein/serum amyloid P promotes pro-inflammatory function and induces M1-type polarization of monocytes/macrophages in mudskipper, boleophthalmus pectinirostris. Fish Shellfish Immunol (2019) 94:318–26. doi: 10.1016/j.fsi.2019.09.021
13. Zhang L, Liu SH, Wright TT, Shen ZY, Li HY, Zhu W, et al. C-reactive protein directly suppresses th1 cell differentiation and alleviates experimental autoimmune encephalomyelitis. J Immunol (2015) 194(11):5243–52. doi: 10.4049/jimmunol.1402909
14. Kim Y, Ryu J, Ryu MS, Lim S, Han KO, Lim IK, et al. C-reactive protein induces G2/M phase cell cycle arrest and apoptosis in monocytes through the upregulation of B-cell translocation gene 2 expression. FEBS Lett (2014) 588(4):625–31. doi: 10.1016/j.febslet.2014.01.008
15. Whisler RL, Newhouse YG, Mortensen RF. C-reactive protein- (Crp) mediated modulation of human B cell colony development. J Immunol (1983) 130(1):248–53. doi: 10.4049/jimmunol.130.1.248
16. Shangwei Z, Yingqi W, Jiang X, Zhongyin W, Juan J, Dafang C, et al. Serum high-sensitive C-reactive protein level and crp genetic polymorphisms are associated with abdominal aortic aneurysm. Ann Vasc Surg (2017) 45:186–92. doi: 10.1016/j.avsg.2017.05.024
17. Stather PW, Sidloff DA, Dattani N, Gokani VJ, Choke E, Sayers RD, et al. Meta-analysis and meta-regression analysis of biomarkers for abdominal aortic aneurysm. Br J Surg (2014) 101(11):1358–72. doi: 10.1002/bjs.9593
18. Cersit S, Ocal L, Keskin M, Gursoy MO, Kalcik M, Bayam E, et al. Association of C-reactive protein-to-albumin ratio with the presence and progression of abdominal aortic aneurysm. Angiology (2021) 72(2):153–8. doi: 10.1177/0003319720954084
19. Qin Y, Yang Y, Liu R, Cao X, Liu O, Liu J, et al. Combined cathepsin S and hs-crp predicting inflammation of abdominal aortic aneurysm. Clin Biochem (2013) 46(12):1026–9. doi: 10.1016/j.clinbiochem.2013.05.065
20. Badger SA, Soong CV, O'Donnell ME, Mercer C, Young IS, Hughes AE. C-reactive protein (Crp) elevation in patients with abdominal aortic aneurysm is independent of the most important crp genetic polymorphism. J Vasc Surg (2009) 49(1):178–84. doi: 10.1016/j.jvs.2008.07.081
21. Kim EN, Yu J, Lim JS, Jeong H, Kim CJ, Choi JS, et al. Crp immunodeposition and proteomic analysis in abdominal aortic aneurysm. PLoS One (2021) 16(8):e0245361. doi: 10.1371/journal.pone.0245361
22. De Haro J, Bleda S, Acin F. C-reactive protein predicts aortic aneurysmal disease progression after endovascular repair. Int J Cardiol (2016) 202:701–6. doi: 10.1016/j.ijcard.2015.09.122
23. Astrom Malm I, De Basso R, Blomstrand P, Wagsater D. Association of il-10 and crp with pulse wave velocity in patients with abdominal aortic aneurysm. J Clin Med (2022) 11(5):1182. doi: 10.3390/jcm11051182
24. Li HY, Tang ZM, Wang Z, Lv JM, Liu XL, Liang YL, et al. C-reactive protein protects against acetaminophen-induced liver injury by preventing complement overactivation. Cell Mol Gastroenterol Hepatol (2022) 13(1):289–307. doi: 10.1016/j.jcmgh.2021.09.003
25. Chen J, Luo H, Tao M, Liu Z, Wang G. Quantitation of nucleoprotein complexes by uv absorbance and bradford assay. Biophys Rep (2021) 7(6):429–36. doi: 10.52601/bpr.2021.210028
26. Liu H, Tian K, Xia C, Wei P, Xu B, Fu W, et al. Kunming mouse strain is less susceptible to elastase-induced abdominal aortic aneurysms. Anim Model Exp Med (2022) 5(1):72–80. doi: 10.1002/ame2.12197
27. Fu W, Liu H, Wei P, Xia C, Yu Q, Tian K, et al. Genetic deficiency of protein inhibitor of activated stat3 suppresses experimental abdominal aortic aneurysms. Front Cardiovasc Med (2023) 10:1092555. doi: 10.3389/fcvm.2023.1092555
28. Liu H, Wei P, Fu W, Xia C, Li Y, Tian K, et al. Dapagliflozin ameliorates the formation and progression of experimental abdominal aortic aneurysms by reducing aortic inflammation in mice. Oxid Med Cell Longev (2022) 2022:8502059. doi: 10.1155/2022/8502059
29. Tian K, Xia C, Liu H, Xu B, Wei P, Fu W, et al. Temporal and quantitative analysis of aortic immunopathologies in elastase-induced mouse abdominal aortic aneurysms. J Immunol Res (2021) 2021:6297332. doi: 10.1155/2021/6297332
30. Ikezoe T, Shoji T, Guo J, Shen F, Lu HS, Daugherty A, et al. No effect of hypercholesterolemia on elastase-induced experimental abdominal aortic aneurysm progression. Biomolecules (2021) 11(10):1434. doi: 10.3390/biom11101434
31. Xu B, Iida Y, Glover KJ, Ge Y, Wang Y, Xuan H, et al. Inhibition of vegf (Vascular endothelial growth factor)-a or its receptor activity suppresses experimental aneurysm progression in the aortic elastase infusion model. Arterioscler Thromb Vasc Biol (2019) 39(8):1652–66. doi: 10.1161/ATVBAHA.119.312497
32. Tanaka H, Xu B, Xuan H, Ge Y, Wang Y, Li Y, et al. Recombinant interleukin-19 suppresses the formation and progression of experimental abdominal aortic aneurysms. J Am Heart Assoc (2021) 10(17):e022207. doi: 10.1161/JAHA.121.022207
33. Raffort J, Lareyre F, Clement M, Hassen-Khodja R, Chinetti G, Mallat Z. Monocytes and macrophages in abdominal aortic aneurysm. Nat Rev Cardiol (2017) 14(8):457–71. doi: 10.1038/nrcardio.2017.52
34. Longo GM, Xiong W, Greiner TC, Zhao Y, Fiotti N, Baxter BT. Matrix metalloproteinases 2 and 9 work in concert to produce aortic aneurysms. J Clin Invest (2002) 110(5):625–32. doi: 10.1172/JCI15334
35. Lukasiewicz A, Reszec J, Kowalewski R, Chyczewski L, Lebkowska U. Assessment of inflammatory infiltration and angiogenesis in the thrombus and the wall of abdominal aortic aneurysms on the basis of histological parameters and computed tomography angiography study. Folia Histochem Cytobiol (2012) 50(4):547–53. doi: 10.5603/20323
36. Thompson MM, Jones L, Nasim A, Sayers RD, Bell PR. Angiogenesis in abdominal aortic aneurysms. Eur J Vasc Endovasc Surg (1996) 11(4):464–9. doi: 10.1016/s1078-5884(96)80183-3
37. Song H, Yang Y, Sun Y, Wei G, Zheng H, Chen Y, et al. Circular rna cdyl promotes abdominal aortic aneurysm formation by inducing M1 macrophage polarization and M1-type inflammation. Mol Ther (2022) 30(2):915–31. doi: 10.1016/j.ymthe.2021.09.017
38. Shi J, Guo J, Li Z, Xu B, Miyata M. Importance of nlrp3 inflammasome in abdominal aortic aneurysms. J Atheroscler Thromb (2021) 28(5):454–66. doi: 10.5551/jat.RV17048
39. Fu Y, Wu Y, Liu E. C-reactive protein and cardiovascular disease: From animal studies to the clinic (Review). Exp Ther Med (2020) 20(2):1211–9. doi: 10.3892/etm.2020.8840
40. Ji SR, Zhang SH, Chang Y, Li HY, Wang MY, Lv JM, et al. C-reactive protein: The most familiar stranger. J Immunol (2023) 210(6):699–707. doi: 10.4049/jimmunol.2200831
41. Razavi E, Ramezani A, Kazemi A, Attar A. Effect of treatment with colchicine after acute coronary syndrome on major cardiovascular events: A systematic review and meta-analysis of clinical trials. Cardiovasc Ther (2022) 2022:8317011. doi: 10.1155/2022/8317011
42. Agrawal A, Cha-Molstad H, Samols D, Kushner I. Overexpressed nuclear factor-kappab can participate in endogenous C-reactive protein induction, and enhances the effects of C/ebpbeta and signal transducer and activator of transcription-3. Immunology (2003) 108(4):539–47. doi: 10.1046/j.1365-2567.2003.01608.x
43. Romero-Vazquez S, Adan A, Figueras-Roca M, Llorenc V, Slevin M, Vilahur G, et al. Activation of C-reactive protein proinflammatory phenotype in the blood retinal barrier in vitro: Implications for age-related macular degeneration. Aging (Albany NY) (2020) 12(14):13905–23. doi: 10.18632/aging.103655
44. Slevin M, Garcia-Lara E, Capitanescu B, Sanfeliu C, Zeinolabediny Y, AlBaradie R, et al. Monomeric C-reactive protein aggravates secondary degeneration after intracerebral haemorrhagic stroke and may function as a sensor for systemic inflammation. J Clin Med (2020) 9(9):3053. doi: 10.3390/jcm9093053
45. Ruiz-Fernandez C, Gonzalez-Rodriguez M, Francisco V, Rajab IM, Gomez R, Conde J, et al. Monomeric C reactive protein (Mcrp) regulates inflammatory responses in human and mouse chondrocytes. Lab Invest (2021) 101(12):1550–60. doi: 10.1038/s41374-021-00584-8
46. Kaplan M, Shur A, Tendler Y. M1 macrophages but not M2 macrophages are characterized by upregulation of crp expression via activation of nfkappab: A possible role for ox-ldl in macrophage polarization. Inflammation (2018) 41(4):1477–87. doi: 10.1007/s10753-018-0793-8
47. Devaraj S, Jialal I. C-reactive protein polarizes human macrophages to an M1 phenotype and inhibits transformation to the M2 phenotype. Arterioscler Thromb Vasc Biol (2011) 31(6):1397–402. doi: 10.1161/ATVBAHA.111.225508
48. Bonaventura A, Mach F, Roth A, Lenglet S, Burger F, Brandt KJ, et al. Intraplaque expression of C-reactive protein predicts cardiovascular events in patients with severe atherosclerotic carotid artery stenosis. Mediators Inflammation (2016) 2016:9153673. doi: 10.1155/2016/9153673
49. Yang X, Hu W, Zhang Q, Wang Y, Sun L. Puerarin inhibits C-reactive protein expression via suppression of nuclear factor kappab activation in lipopolysaccharide-induced peripheral blood mononuclear cells of patients with stable angina pectoris. Basic Clin Pharmacol Toxicol (2010) 107(2):637–42. doi: 10.1111/j.1742-7843.2010.00548.x
50. Voleti B, Agrawal A. Regulation of basal and induced expression of C-reactive protein through an overlapping element for oct-1 and nf-kappab on the proximal promoter. J Immunol (2005) 175(5):3386–90. doi: 10.4049/jimmunol.175.5.3386
51. Jabs WJ, Theissing E, Nitschke M, Bechtel JF, Duchrow M, Mohamed S, et al. Local generation of C-reactive protein in diseased coronary artery venous bypass grafts and normal vascular tissue. Circulation (2003) 108(12):1428–31. doi: 10.1161/01.CIR.0000092184.43176.91
52. Torzewski J, Torzewski M, Bowyer DE, Frohlich M, Koenig W, Waltenberger J, et al. C-reactive protein frequently colocalizes with the terminal complement complex in the intima of early atherosclerotic lesions of human coronary arteries. Arterioscler Thromb Vasc Biol (1998) 18(9):1386–92. doi: 10.1161/01.atv.18.9.1386
53. Ciubotaru I, Potempa LA, Wander RC. Production of modified C-reactive protein in U937-derived macrophages. Exp Biol Med (Maywood) (2005) 230(10):762–70. doi: 10.1177/153537020523001010
54. Meuwissen M, van der Wal AC, Niessen HW, Koch KT, de Winter RJ, van der Loos CM, et al. Colocalisation of intraplaque C reactive protein, complement, oxidised low density lipoprotein, and macrophages in stable and unstable angina and acute myocardial infarction. J Clin Pathol (2006) 59(2):196–201. doi: 10.1136/jcp.2005.027235
55. Bharadwaj D, Stein MP, Volzer M, Mold C, Du Clos TW. The major receptor for C-reactive protein on leukocytes is fcgamma receptor ii. J Exp Med (1999) 190(4):585–90. doi: 10.1084/jem.190.4.585
56. Fujita Y, Kakino A, Harada-Shiba M, Sato Y, Otsui K, Yoshimoto R, et al. C-reactive protein uptake by macrophage cell line via class-a scavenger receptor. Clin Chem (2010) 56(3):478–81. doi: 10.1373/clinchem.2009.140202
57. Bournazos S, Gupta A, Ravetch JV. The role of igg fc receptors in antibody-dependent enhancement. Nat Rev Immunol (2020) 20(10):633–43. doi: 10.1038/s41577-020-00410-0
58. Stancel N, Chen CC, Ke LY, Chu CS, Lu J, Sawamura T, et al. Interplay between crp, atherogenic ldl, and lox-1 and its potential role in the pathogenesis of atherosclerosis. Clin Chem (2016) 62(2):320–7. doi: 10.1373/clinchem.2015.243923
59. Itoga NK, Rothenberg KA, Suarez P, Ho TV, Mell MW, Xu B, et al. Metformin prescription status and abdominal aortic aneurysm disease progression in the U.S. Veteran population. J Vasc Surg (2019) 69(3):710–6 e3. doi: 10.1016/j.jvs.2018.06.194
60. Fujimura N, Xiong J, Kettler EB, Xuan H, Glover KJ, Mell MW, et al. Metformin treatment status and abdominal aortic aneurysm disease progression. J Vasc Surg (2016) 64(1):46–54 e8. doi: 10.1016/j.jvs.2016.02.020
Keywords: abdominal aortic aneurysms, C-reactive protein, macrophages, matrix metalloproteinase 2, inflammation
Citation: Fu Y, Liu H, Li K, Wei P, Alam N, Deng J, Li M, Wu H, He X, Hou H, Xia C, Wang R, Wang W, Bai L, Xu B, Li Y, Wu Y, Liu E and Zhao S (2023) C-reactive protein deficiency ameliorates experimental abdominal aortic aneurysms. Front. Immunol. 14:1233807. doi: 10.3389/fimmu.2023.1233807
Received: 02 June 2023; Accepted: 25 August 2023;
Published: 11 September 2023.
Edited by:
Alok Agrawal, East Tennessee State University, United StatesReviewed by:
Karlheinz Peter, Baker Heart and Diabetes Institute, AustraliaShang-Rong Ji, Lanzhou University, China
Copyright © 2023 Fu, Liu, Li, Wei, Alam, Deng, Li, Wu, He, Hou, Xia, Wang, Wang, Bai, Xu, Li, Wu, Liu and Zhao. This is an open-access article distributed under the terms of the Creative Commons Attribution License (CC BY). The use, distribution or reproduction in other forums is permitted, provided the original author(s) and the copyright owner(s) are credited and that the original publication in this journal is cited, in accordance with accepted academic practice. No use, distribution or reproduction is permitted which does not comply with these terms.
*Correspondence: Sihai Zhao, c2loYWl6aGFvQHhqdHUuZWR1LmNu; Enqi Liu, bGl1ZW5xaUB4anR1LmVkdS5jbg==
†These authors share first authorship