- 1Department of Life Science, College of Natural Sciences, Hanyang University, Seoul, Republic of Korea
- 2Department of Dermatology and Cutaneous Biology Research Institute, Gangnam Severance Hospital, Yonsei University College of Medicine, Seoul, Republic of Korea
- 3Department of Pediatrics, Stanford University School of Medicine, Stanford, CA, United States
- 4Department of Dermatology, Hanyang University College of Medicine, Seoul, Republic of Korea
- 5Hanyang Institute of Bioscience and Biotechnology, Hanyang University, Seoul, Republic of Korea
- 6Graduate School of Medical Science and Engineering, Korea Advanced Institute of Science and Technology (KAIST), Daejeon, Republic of Korea
- 7The Center for Viral Immunology, Korea Virus Research Institute, Institute for Basic Science (IBS), Daejeon, Republic of Korea
- 8Research Institute for Natural Sciences, Hanyang University, Seoul, Republic of Korea
- 9Research Institute for Convergence of Basic Sciences, Hanyang University, Seoul, Republic of Korea
Psoriasis is a chronic inflammatory skin disease characterized by hyperplasia of keratinocytes and immune cell infiltration. The IL-17-producing T cells play a key role in psoriasis pathogenesis, while regulatory T (Treg) cells are diminished during psoriatic inflammation. Current psoriasis treatments largely focus on IL-17 and IL-23, however, few studies have explored therapeutic drugs targeting an increase of Treg cells to control immune homeostasis. In this study, we investigated the effects of a cytotoxic T lymphocyte antigen-4 (CTLA-4) signaling peptide (dNP2-ctCTLA-4) in Th17, Tc17, γδ T cells, Treg cells in vitro and a mouse model of psoriasis. Treatment with dNP2-ctCTLA-4 peptide showed a significant reduction of psoriatic skin inflammation with increased Treg cell proportion and reduced IL-17 production by T cells, indicating a potential role in modulating psoriatic skin disease. We compared dNP2-ctCTLA-4 with CTLA-4-Ig and found that only dNP2-ctCTLA-4 ameliorated the psoriasis progression, with increased Treg cells and inhibited IL-17 production from γδ T cells. In vitro experiments using a T cell-antigen presenting cell co-culture system demonstrated the distinct mechanisms of dNP2-ctCTLA-4 compared to CTLA-4-Ig in the induction of Treg cells. These findings highlight the therapeutic potential of dNP2-ctCTLA-4 peptide in psoriasis by augmenting Treg/Teff ratio, offering a new approach to modulating the disease.
Introduction
Psoriasis is a chronic inflammatory skin disease that affects approximately 2-3% of adults worldwide (1). Clinical features of psoriasis present as erythematous plaques with silvery-scale (2). It is characterized by systemic inflammation, which is associated with comorbid diseases such as arthritis, inflammatory bowel diseases, and cardiovascular diseases (3). The pathogenesis of psoriasis is complex and involves the infiltration of various immune cells, such as T cells and dendritic cells, into the skin (4). The activation of IL-17-secreting T cells through the IL-23-IL-17A axis plays a crucial role in the exacerbation of inflammation. Pro-inflammatory cytokines such as TNF-α, IL-6, and IL-1β secreted from keratinocytes, as well as effector cytokines such as IL-12 and IL-23 secreted from dendritic cells, contribute to the activation of Th17 and Tc17 cells (5, 6). In addition, innate lymphoid cell 3 (ILC3) and γδ T cells are also involved in skin inflammation by secreting IL-17A cytokine (7–12).
Psoriasis treatment strategies are determined based on the severity of the patient (13–15). Topical steroids and/or calcipotriol are commonly used to treat mild cases. For moderate-to-severe patients, systemic therapy including cyclosporin A, methotrexate, and narrow-band ultraviolet B is administered, and more recently, biologics and small molecule drugs have been used to target specific molecules involved in inflammation (16). These drugs include secukinumab and ixekizumab, which target the IL-17A cytokine, and guselkumab and risankizumab, which simultaneously inhibit IL-23 by targeting the p19 molecule (2, 3, 17–19).
Regulatory T (Treg) cells play a crucial role in maintaining immune homeostasis and regulating inflammatory responses (20, 21). These cells can be divided into two subsets: Natural Treg cells that develop in the thymus and induced Treg cells that are induced in the periphery and express the transcription factor Forkhead box P3 (Foxp3), which confers immunosuppressive function (20). Treg cells suppress effector T cell responses by depleting IL-2 signaling through high CD25 (IL-2Rα) expression, regulating co-stimulatory signaling through constitutive expression of CTLA-4, and secreting suppressive cytokines such as IL-10 (22–24). Defects in the number or suppressive function of Treg cells have been implicated in autoimmune diseases, including type 1 diabetes, multiple sclerosis, systemic lupus erythematosus, and psoriasis (25–29). The conversion of Treg cells to IL-17A-secreting cells that have lost their suppressive function has been shown in several autoimmune diseases including psoriasis (30–32). In animal models of psoriasis, depletion of Treg cells results in increased disease severity (33–35), highlighting their crucial role in disease regulation. Despite this knowledge, there are currently no treatments available specifically targeting Treg cell augmentation in psoriasis. In this study, we investigated a synthesized peptide that combines the signaling domain of CTLA-4 with a cell-penetrating peptide (CPP) to promote the generation of Foxp3+ Treg cells during psoriatic skin inflammation. Our findings indicate that the dNP2-ctCTLA-4 peptide holds promise as a therapeutic agent for psoriasis by inducing endogenous Treg cells in vivo.
Results
Induction of Foxp3+ Treg cells by dNP2-ctCTLA-4 peptide
Previously we generated the recombinant protein and a synthetic peptide of the cytoplasmic domain of the cytotoxic T lymphocyte antigen-4 (CTLA-4) in conjugation with a CPP dNP2 (dNP2-ctCTLA-4) (36–38). This protein and peptide showed successful immune modulatory effects on T cell activation during autoimmune or allergic disease with an increase of Foxp3 expressing Treg cells (36, 38–40). Building on this knowledge, we aimed to investigate whether the dNP2-ctCTLA-4 could be effective in treating psoriasis with increased Treg cells. As both Th17 (CD4) and Tc17 (CD8) cells are important pathogenic cells in psoriasis, we examined the functional effects of the dNP2-ctCTLA-4 peptide on mouse CD4 and CD8 T cells in vitro. In our experiments, we cultured TCRαβ+CD4+CD25-CD62LhighCD44low naïve CD4 T cells under Treg condition (TGF-β, IL-2) with or without the peptide. The results demonstrated a significant increase in CD25+Foxp3+ Treg cells when the peptide was present (Figures 1A, B). Furthermore, when we cultured these naïve CD4 T cells under Th17+IL-2 conditions (TGF-β, IL-6, IL-1β, IL-23, IL-2), dNP2-ctCTLA-4 exhibited inhibitory effects on IL-17 production while simultaneously increasing Foxp3 expression (Figures 1C, D). Similar observations were made with TCRαβ+CD8+CD25-CD62LhighCD44low naïve CD8 T cells, which were differentiated under Treg condition (TGF-β, IL-2) in the presence of dNP2-ctCTLA-4. The peptide treatment led to a significant increase in CD25+Foxp3+ CD8 T cells (Figures 1E, F). Moreover, when naïve CD8 T cells were cultured under Tc17+IL-2 conditions (TGF-β, IL-6, IL-1β, IL-23, IL-2), the peptide demonstrated a reduction in IL-17 production while promoting Foxp3 expression (Figures 1G, H). To investigate whether dNP2-ctCTLA-4 can intrinsically increase TGF-β mediated Foxp3 expression, naïve CD8 T cells were stimulated with anti-CD3 and TGF-β in the presence or absence of dNP2-ctCTLA-4 peptide. The dNP2-ctCTLA-4 peptide reduced SMAD2 linker region phosphorylation and the amount of p-ERK. This result is consistent with our previous research confirmed in the splenocytes (38), suggesting that dNP2-ctCTLA-4 peptide intrinsically enhances TGF-β signaling in CD8 T cells, leading to an increase in Foxp3+ T cells (Figure 1I). In summary, our findings demonstrate that the dNP2-ctCTLA-4 peptide has the ability to induce Foxp3 expression in both CD4 and CD8 T cells under various stimulatory conditions. These results suggest the therapeutic potential of the peptide in modulating Th17/Tc17 responses associated with psoriasis.
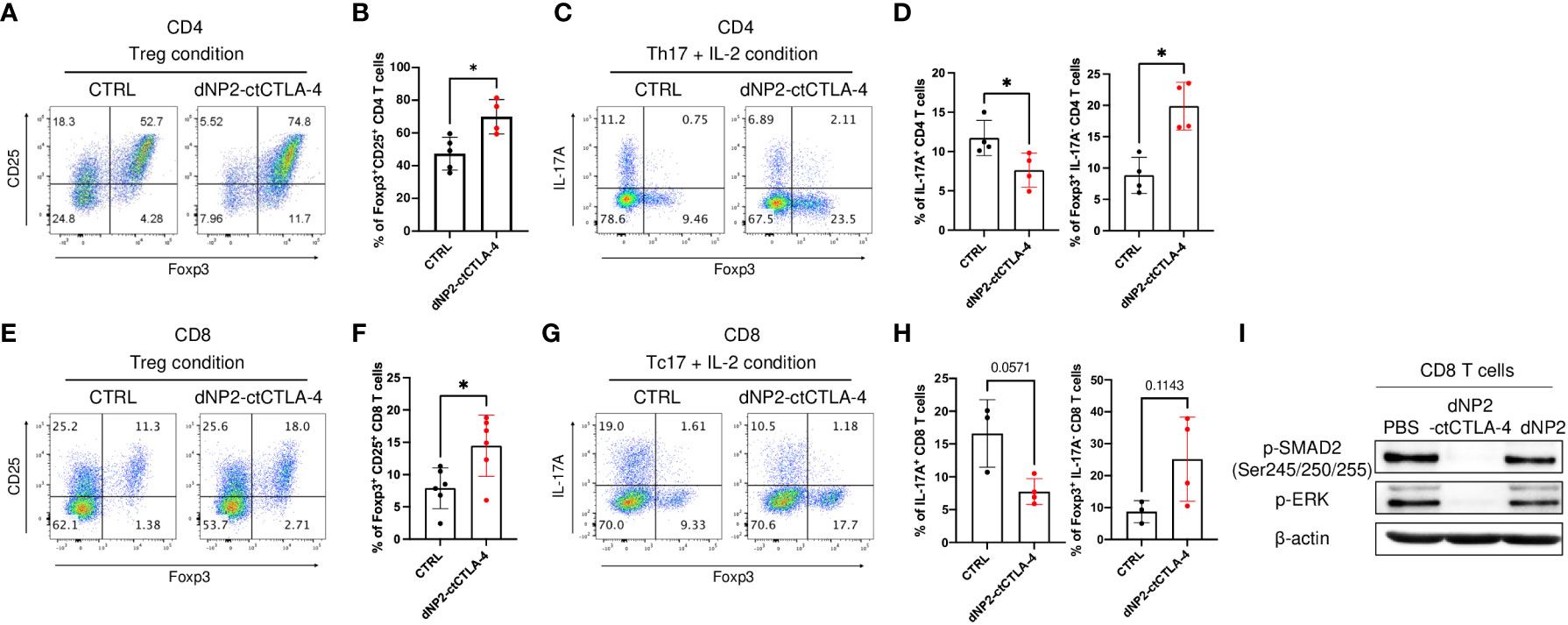
Figure 1 dNP2-ctCTLA-4 effectively induces Foxp3+ Treg cells in both CD4 and CD8 T cells. (A-D) Mouse naïve CD4 T cells were stimulated with anti-CD3/CD28 mAb in the presence or absence of dNP2-ctCTLA-4 for 3 or 4 days. Representative flow cytometric dot plot (A) and bar graphs (B) of expression of CD25 and Foxp3 under Treg differentiation condition (n=4~5). Representative flow cytometric dot plot (C) and bar graphs (D) of expression of IL-17A and Foxp3 under Th17 with IL-2 differentiation condition (n=4). (E-H) Mouse naïve CD8 T were stimulated with anti-CD3/CD28 mAb in the presence or absence of dNP2-ctCTLA-4 for 3 days. Representative flow cytometric dot plot (E) and bar graphs (F) of expression of CD25 and Foxp3 under Treg differentiation condition (n=6). Representative flow cytometric dot plot (G) and bar graphs (H) of expression of IL-17A and Foxp3 under Tc17 with IL-2 differentiation condition (n=3-4). (I) Mouse naïve CD8 T cells were stimulated with anti-CD3/CD28 mAb and TGF-β in the presence of dNP2-ctCTLA-4 or dNP2 peptide. The lysate was analyzed by immunoblotting of the p-SMAD2 linker region and p-ERK. Data are presented as mean ± S.D. Statistical significance was determined by the Mann-Whitney test. n.s. = nonsignificant, *p < 0.05.
Amelioration of psoriasis-like skin inflammation by dNP2-ctCTLA-4
To assess the potential of the dNP2-ctCTLA-4 peptide in inducing Treg cells and alleviating psoriatic skin inflammation, we conducted in vivo experiments using the well-established imiquimod (IMQ)-induced psoriasis mouse model (41), which closely resembles human psoriasis (42). The IMQ-induced psoriasis mouse model was established by daily application of IMQ to the back skin of mice until day-5. Concurrently, the mice received intraperitoneal administration of 100 μg of the dNP2-ctCTLA-4 peptide three times daily from day-1 to 5 (Figure 2A). Our findings demonstrated that the dNP2-ctCTLA-4 peptide significantly inhibited the progression of psoriasis (Figure 2B) and effectively improved the psoriasis area and severity index (PASI) score (Figure 2C). Histological analysis of dorsal tissue samples revealed a reduction in epidermal hyperplasia and immune cell infiltration (Figure 2D). Flow cytometry analysis of skin-draining lymph nodes (SDLN) showed a notable increased in the proportion of Foxp3+ CD4 T cells, accompanied by a reduction in IL-17A-producing γδ T cells (Figures 2E, F). Taken together, these results highlight the potential of the dNP2-ctCTLA-4 peptide to induce Foxp3+ Treg cells in vivo, consequently ameliorating psoriasis-like skin inflammation. This induction of Treg cells leads to a significantly increased Treg/Teff ratio (Figure 2G), underscoring the therapeutic efficacy of the dNP2-ctCTLA-4 peptide in the treatment of psoriatic skin inflammation.
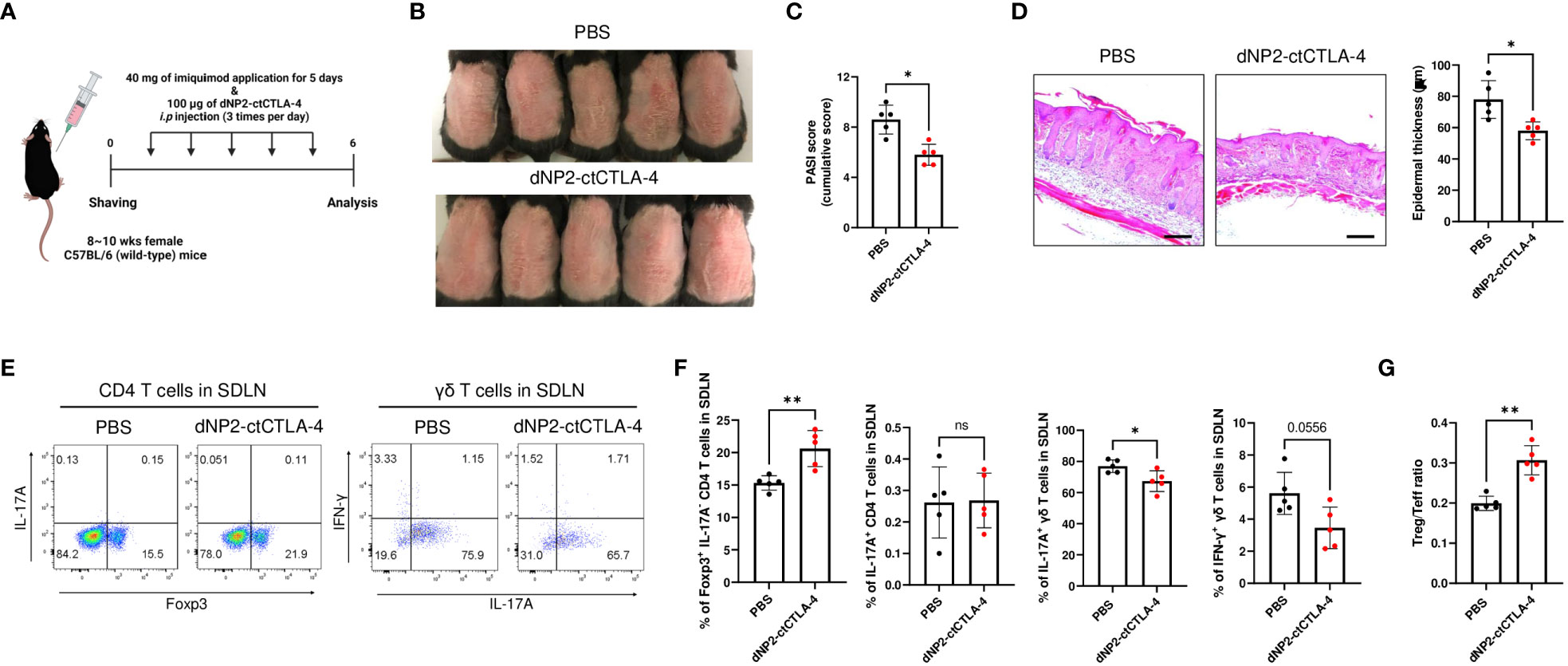
Figure 2 dNP2-ctCTLA-4 ameliorates psoriatic skin inflammation, accompanied by an increase in Foxp3+ CD4 T cells. (A) Experimental scheme of drug treatment in the imiquimod-induced psoriasis murine model. (B) Comparison of representative gross back skin phenotypes after the last IMQ application between the control and dNP2-ctCTLA-4 treated groups (n=5 per group). (C) Cumulative PASI scores (erythema, thickness, scaling). (D) Histological analysis of H&E-stained back skin samples (left, x100 magnification, scale bar = 200 μm) and epidermal thickness measured by ImageJ software (right). (E) Representative dot plots and (F) bar graphs of Foxp3, IL-17A, IFN-γ expression of isolated lymphocytes (CD4 T cells, γδ T cells) in SDLN. (G) The Treg/Teff ratio in SDLN. Data are presented as mean ± S.D. Statistical significance was determined by Mann–Whitney test. n.s., nonsignificant; *p < 0.05, **p < 0.01.
Suppression of psoriatic skin inflammation by dNP2-ctCTLA-4, but not CTLA-4-Ig
CTLA-4-Ig (Abatacept) is a US Food and Drug Administration (FDA)-approved drug primarily used for rheumatoid arthritis treatment. It consists of the extracellular domain of CTLA-4 fused with the Fc region of an immunoglobulin (43, 44). By binding to B7 molecules, CTLA-4-Ig effectively inhibits the co-stimulatory signal of T cells, thereby preventing their activation (45). In contrast, the dNP2-ctCTLA-4 peptide utilized in our study employs the cytoplasmic domain of CTLA-4 to modulate intracellular signaling and result in Foxp3-expressing Treg cells. Considering the distinct domains of CTLA-4 targeted by these two agents (Figure 3A), we hypothesized that they would exhibit different mechanisms of action in treating psoriasis. To evaluate this hypothesis, equal doses of CTLA-4-Ig and dNP2-ctCTLA-4 peptide were administered to mice with IMQ-induced psoriatic skin inflammation, following the same protocol as in the previous experiment (Figure 3B). As shown in the figure, CTLA-4-Ig did not effectively inhibit psoriatic skin inflammation, while the dNP2-ctCTLA-4 peptide significantly reduced psoriasis symptoms and PASI score (Figures 3C, D). Additionally, only the group treated with the dNP2-ctCTLA-4 peptide demonstrated a decrease in epidermal layer thickness and infiltration of inflammatory cells (Figure 3E). These compelling findings suggest that the dNP2-ctCTLA-4 peptide, which specifically targets the intracellular signaling events related to the cytoplasmic domain of CTLA-4, would be more effective in regulating psoriatic skin inflammation compared to CTLA-4-Ig. The differential mechanisms of action of these two agents provide further insights into their distinct therapeutic potential for managing psoriasis.
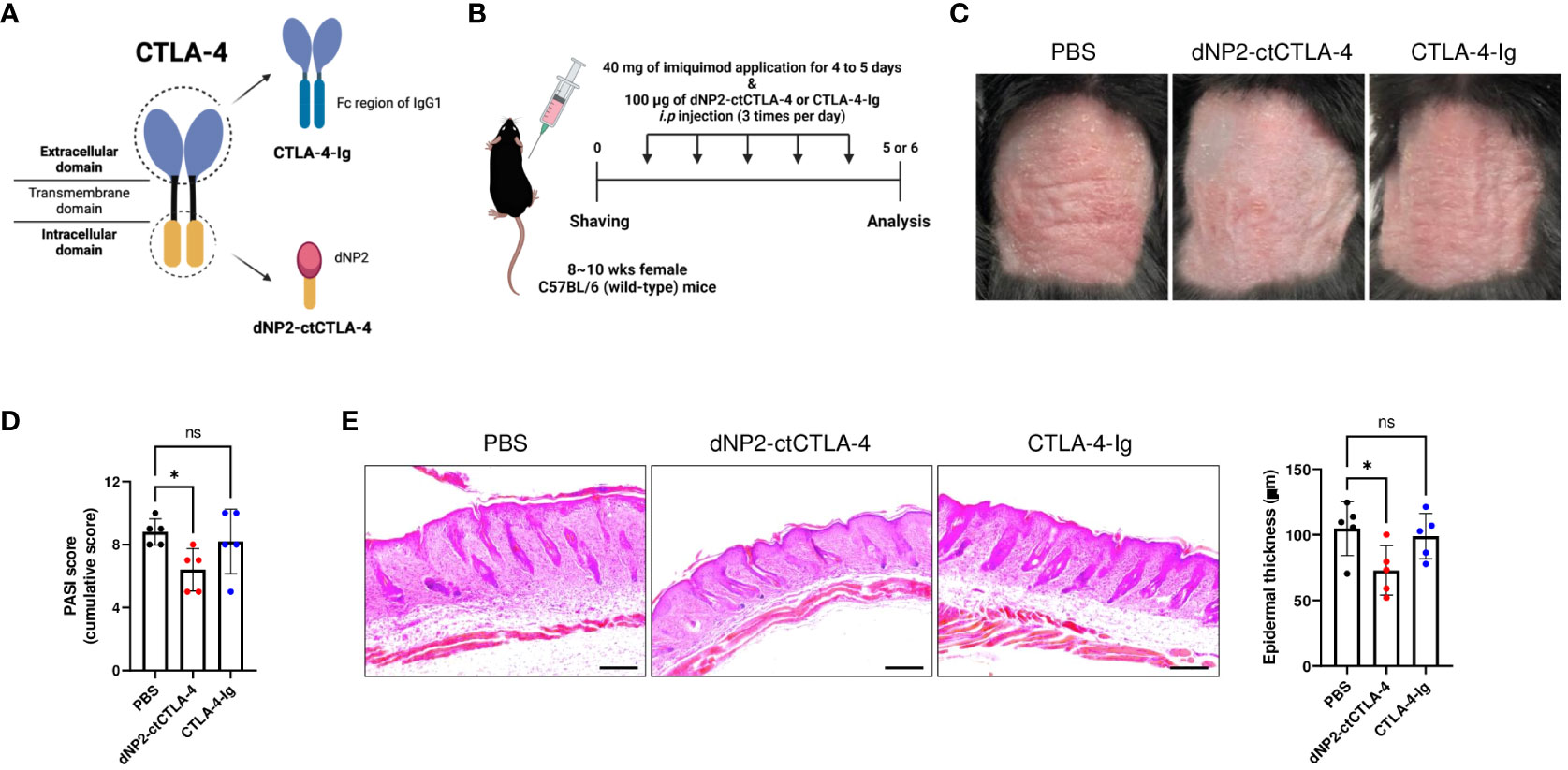
Figure 3 dNP2-ctCTLA-4 effectively improves psoriatic skin inflammation, while CTLA-4-Ig does not. (A) Schematic images of dNP2-ctCTLA-4 and CTLA-4-Ig utilizing each domain of CTLA-4. (B) Experimental scheme of drug treatment in the IMQ-induced psoriasis murine model. (C) Comparison of representative back skin phenotypes after last IMQ application between dNP2-ctCTLA4 and CTLA4-Ig treated group (n=5 per group). (D) Cumulative PASI scores (erythema, thickness, scaling). (E) Histological analysis of H&E-stained back skin samples (left, x100 magnification, scale bar = 200 μm) and epidermal thickness measured by ImageJ software (right). Data are presented as mean ± S.D. Statistical significance was determined by the Kruskal-Wallis test. n.s., nonsignificant; *p < 0.05.
Induction of CD25+ Foxp3+ Treg cells and suppression of IL-17A-producing effector T cells by dNP2-ctCTLA-4, but not CTLA-4-Ig
To investigate the impact of dNP2-ctCTLA-4 peptide and CTLA-4-Ig on lymphoid cells in psoriasis-like skin inflammation, we conducted a flow cytometry analysis. Intriguingly, the dNP2-ctCTLA-4 peptide significantly induced CD25+ Foxp3+ Treg cells, whereas CTLA-4-Ig could not in SDLN (Figures 4A, B). Neither treatment showed a statistically significant tendency to inhibit the production of IL-17A and IFN-γ in CD4 T cells (Figure 4B). Although the dNP2-ctCTLA-4 peptide resulted in a slightly reduced tendency in the proportion of IL-17A-producing γδ T cells (Figures 4C, D), it exhibited a significantly increased Treg/Teff ratio compared to CTLA-4-Ig, confirming its potential as an effective regulator of psoriatic skin inflammation (Figure 4E). Consistent with these findings, the dNP2-ctCTLA-4 peptide also demonstrated a significant reduction in the absolute cell number of infiltrated CD45+ lymphocytes into the skin tissue, as compared to control group (Figures 4F, G). However, it is worth noting that neither CTLA-4-Ig nor the dNP2-ctCTLA-4 peptide appeared to reduce the proportion of IL-17+ γδ T cells in the skin. Overall, this result suggests that CTLA-4-Ig might not be an effective molecule for controlling psoriatic skin inflammation, whereas the dNP2-ctCTLA-4 peptide could serve as a promising novel therapeutic option, as it exhibits a mechanism of increasing the Treg/Teff ratio.
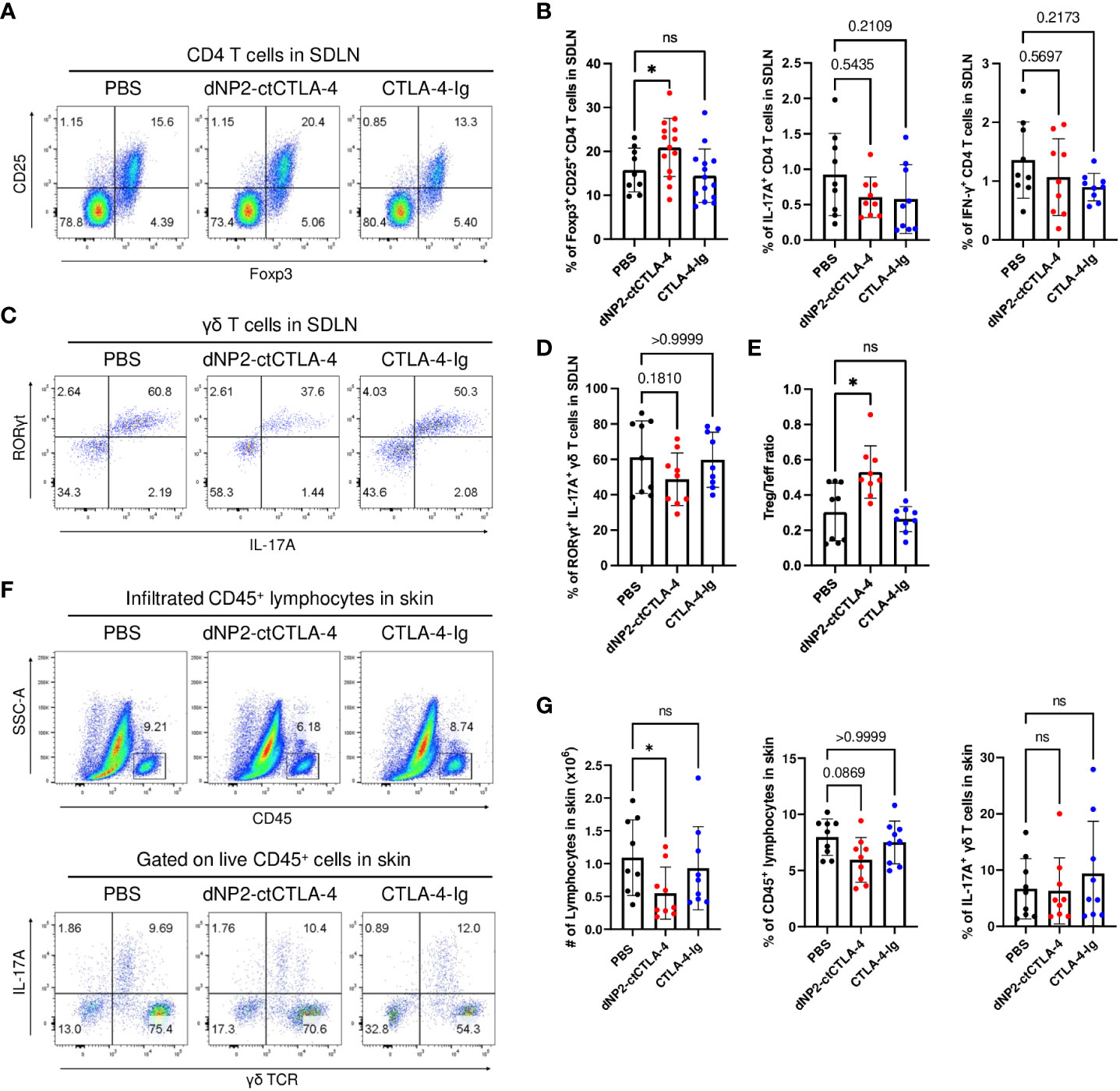
Figure 4 dNP2-ctCTLA-4 increases CD25+ Foxp3+ Treg cells in the SDLN of the mice, whereas CTLA-4-Ig does not. (A) Representative dot plots and (B) bar graphs of flow cytometric analysis in SDLN to identify CD25+ Foxp3+ CD4 T cells (n=9-14 per group), IL-17A+ CD4 T cells (n=9 per group) and IFN-γ+ CD4 T cells (n=9 per group). (C) Representative dot plots and (D) bar graphs of flow cytometric analysis in SDLN to identify IL-17A+ RORγt+ γδ T cells (n=9 per group). (E) The Treg-Teff ratio in SDLN (n=9 per group). (F) Representative dot plots and (G) bar graphs of flow cytometric analysis in the skin tissue to identify infiltrated lymphocytes, and IL-17A+ γδ T cells (n=9 per group). Data are presented as mean ± S.D. Statistical significance was determined by the Kruskal-Wallis test or Brown-Forsythe and Welch ANOVA tests. n.s., nonsignificant; *p < 0.05.
Distinct mechanisms of action by dNP2-ctCTLA-4 and CTLA-4-Ig during Th17 and Treg differentiation in T cell -antigen presenting cells (APC) coculture system
To gain further insights into the distinct mechanisms underlying T cell regulation by dNP2-ctCTLA-4 peptide and CTLA-4-Ig, we performed experiments utilizing FACS-sorted naïve CD4 T cells derived from 2D2 mice. These cells were stimulated with MOG35-55 peptide in the presence of APCs to induce Th17 and Treg cells, while being exposed to either the dNP2-ctCTLA-4 peptide or CTLA-4-Ig. Our findings revealed noteworthy disparities between the two agents. Specifically, the dNP2-ctCTLA-4 peptide demonstrated induction of Foxp3+ Treg cells under Th17 differentiation conditions (IL-6, TGF-β), which was not observed with CTLA-4-Ig (Figures 5A, B). Intriguingly, CTLA-4-Ig exhibited a tendency to inhibit IL-17+ CD4 T cells, a response not observed with the dNP2-ctCTLA-4 peptide. We further examined key functional molecules in Treg functions including CD25 and CD39. Notably, the dNP2-ctCTLA-4 peptide significantly upregulated both CD25 and CD39 expression in Foxp3+ Treg cells compared to CTLA-4-Ig (Figures 5C, D). Moreover, the dNP2-ctCTLA-4 peptide induced CD25+ Foxp3+ Treg cells under Treg conditions, while CTLA-4-Ig failed to elicit a similar response (Figures 5E, F). We observed an increase in the CD25high CTLA-4high population within Foxp3+ Treg cells under Treg conditions when treated with the dNP2-ctCTLA-4 peptide, but not with CTLA-4-Ig (Figures 5G, H). Notably, the expression level of CTLA-4 tended to be increased in Foxp3+ CD4 T cells by the dNP2-ctCTLA-4 peptide, whereas CTLA-4-Ig did not elicit a similar effect (Figures 5I, J). Collectively, these findings highlight that the dNP2-ctCTLA-4 peptide induces Foxp3+ Treg cells with enhanced Treg characteristics, while CTLA-4-Ig suppresses Th17 responses. Thus, our results suggest that the mechanisms underlying the actions of CTLA-4-Ig and the dNP2-ctCTLA-4 peptide are fundamentally different which provides a plausible explanation for the divergent outcomes observed in the psoriasis experiments.
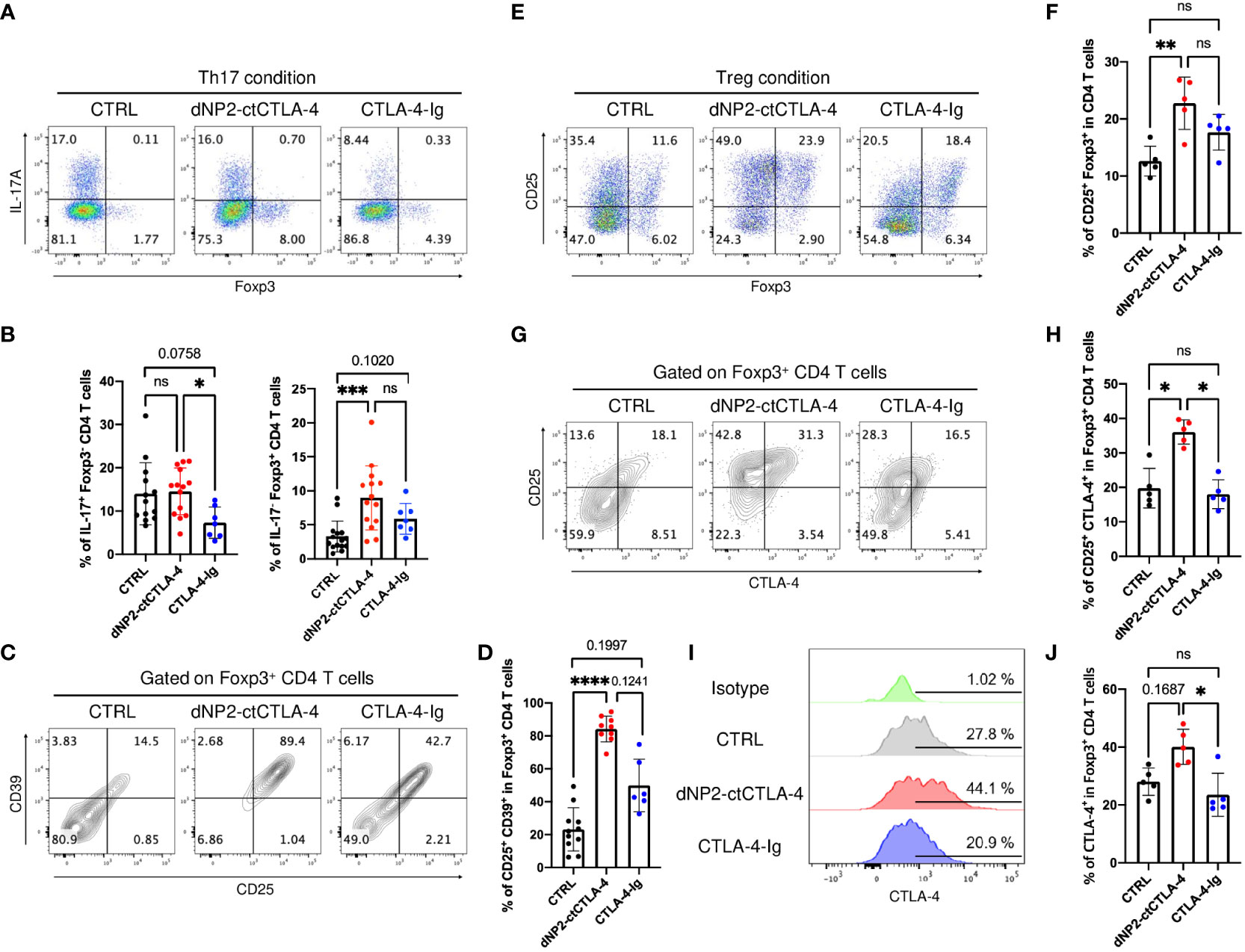
Figure 5 dNP2-ctCTLA-4 and CTLA-4-Ig exhibit distinct functional effects on T cell activation and differentiation. (A-D) Naïve CD4 T cells from 2D2 mice were stimulated with MOG35-55 peptide loaded by APCs in the presence of 3~5 μg/ml of dNP2-ctCTLA-4 or CTLA-4-Ig under Th17 condition. Representative flow cytometric dot plot (A) and bar graphs (B) of expression of IL-17 and Foxp3 under Th17 differentiation condition (n=6-14). Representative flow cytometric dot plot (C) and bar graphs (D) of Treg markers including CD25 and CD39 in Foxp3+ CD4 T cells (n=6-11). (E-J) Naïve CD4 T cells from 2D2 mice were stimulated with MOG35-55 peptide loaded by antigen-presenting cells in the presence of 3~5 μg/ml of dNP2-ctCTLA-4 or CTLA-4-Ig under Treg condition (n=5). Representative flow cytometric dot plot (E) and a bar graph (F) of Treg induction. Representative flow cytometric dot plot (G) and a bar graph (H) of Treg marker including CD25 and CTLA-4 in Foxp3+ CD4 T cells. Representative flow cytometric histogram (I) and bar graphs (J) of CTLA-4 expression in Foxp3+ CD4 T cells. Data are presented as mean ± S.D. Statistical significance was determined by the Kruskal-Wallis test. n.s., nonsignificant; *p < 0.05, **p<0.01, ***<0.001, ****<0.0001.
Discussion
In this study, we conducted a comprehensive investigation into the therapeutic potential of a dNP2-ctCTLA-4 peptide utilizing an IMQ-induced psoriasis-like skin inflammation mouse model (Figure 6). Our findings substantiated the effectiveness of dNP2-ctCTLA-4, which employs a CPP fused with the cytoplasmic domain of CTLA-4. The peptide demonstrated its remarkable efficacy in inducing Foxp3-expressing T cells in both CD4 and CD8 T cells by inhibiting the p-ERK and the p-SMAD2/3 linker region in vitro. In vivo studies further demonstrated its ability to effectively ameliorate the progression of psoriatic skin inflammation and augment the population of Foxp3+ CD4 T cells. Notably, the Treg cells induced by the dNP2-ctCTLA-4 peptide exhibited heightened expression of functional markers, such as CD25, CD39, and CTLA-4 suggesting the induction of potent suppressive Treg cells. In contrast, CTLA-4-Ig, which employs the extracellular domain of CTLA-4, displayed a propensity to reduce Treg cell populations in vitro and provided ineffective in inhibiting psoriasis progression. Our findings shed light on Treg induction as a novel strategy for mitigating psoriasis-like skin inflammation and underscore the potential of dNP2-ctCTLA-4 as a promising therapeutic agent for autoimmune diseases, including psoriasis.
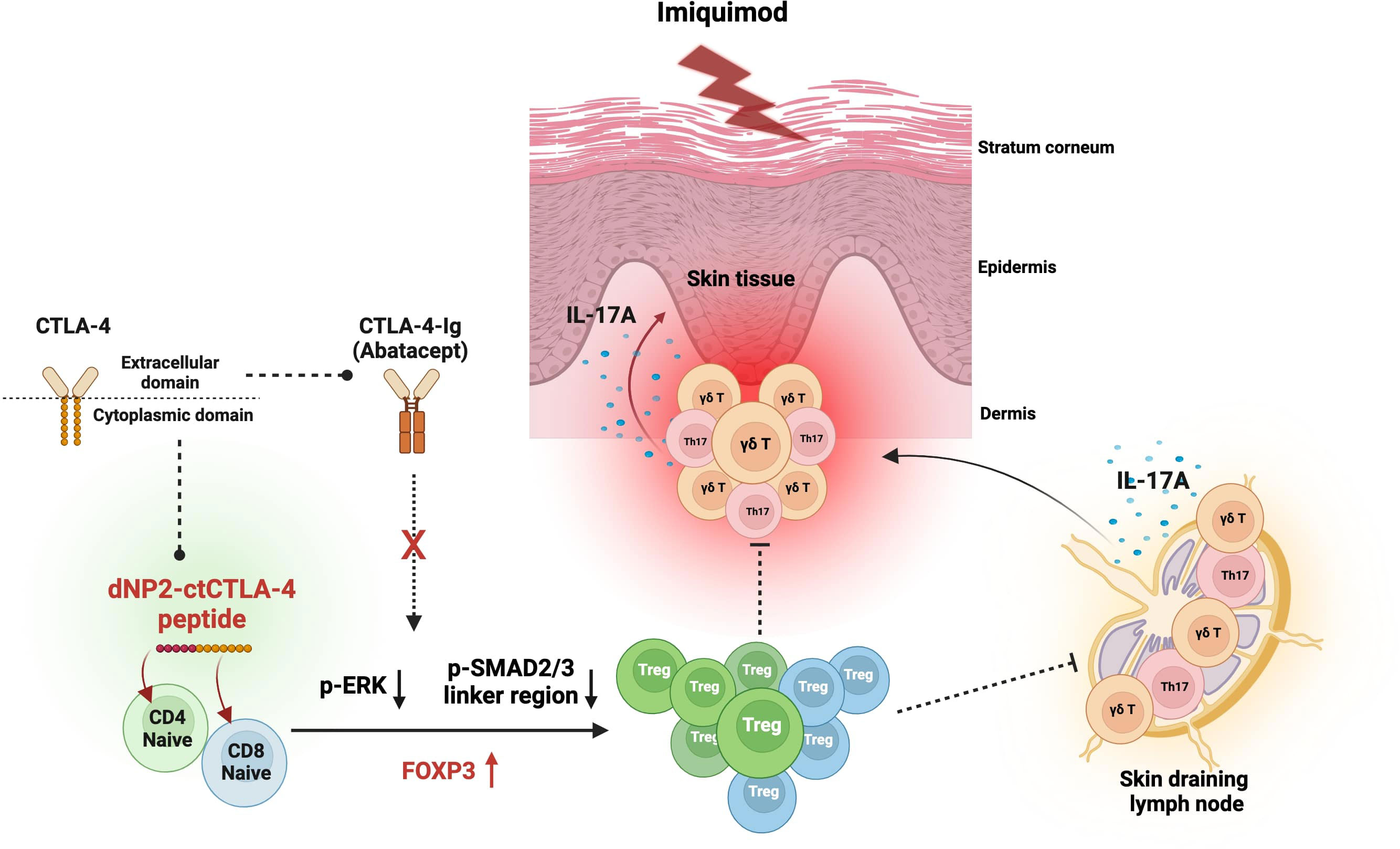
Figure 6 Graphical summary of the effects of dNP2-ctCTLA-4 on psoriatic skin inflammation. Diagram created with BioRender (BioRender.com). The dNP2-ctCTLA-4 peptide is composed of dNP2, a cell-penetrating peptide, and the cytoplasmic domain of CTLA-4. In contrast, CTLA-4-Ig (Abatacept) is a fusion protein that combines the Fc region of immunoglobulin IgG1 and the extracellular domain of CTLA-4. The dNP2-ctCTLA-4 peptide induces Foxp3 expression in both CD4 and CD8 T cells by inhibiting the p-ERK and p-SMAD2/3 linker regions. However, CTLA-4-Ig is incapable of inducing Foxp3+ Treg cells. The dNP2-ctCTLA-4 peptide can suppress IL-17A production in γδ T cells, Th17 and Tc17 cells while inducing Treg cells, thereby presumably alleviating psoriatic skin inflammation.
Psoriasis, a chronic inflammatory skin disease, is characterized by an immunological imbalance, resulting in the excessive secretion of IL-17A cytokine. This dysregulation occurs through the IL-23/IL-17A axis and involves multiple immune cell types. While IL-17-secreting αβ T-cells (Th17, Tc17) are the major contributor to pathogenesis in human psoriasis patients, γδ T cells are primarily responsible for inflammatory responses in mouse models (46–48). The γδ T cells expressing the Vγ4 and Vγ6 chain are found in the dermal layer of skin tissue (49–51) and known to secrete IL-17A in response to stimuli such as IL-1β and IL-23 (52, 53). They also migrate to inflamed areas by expressing C-C motif chemokine receptor 6 (CCR6) on their surface (54–56). Although there is evidence of Treg cells suppressing the activation of γδ T cells (57–59), the interplay between these cell types remains relatively unexplored. In this study, we demonstrated that dNP2-ctCTLA-4 peptide induces Treg cells in an inflammatory environment. It effectively suppresses IL-17A production by γδ T cells in SDLN, consequently reducing immune cell infiltration into the skin tissue. Furthermore, the dNP2-ctCTLA-4 peptide promotes the generation of Foxp3+ Treg cells while inhibiting IL-17A production during Th17 and Tc17 differentiation in vitro. In our previous report, we confirmed that the dNP2-ctCTLA-4 peptide can be delivered to T cells (36, 60) and inhibit T cell activation. We also demonstrated the peptide interferes with PKC-η to increase Treg cells, inhibiting progression of experimental autoimmune encephalomyelitis (EAE) (38). In the induced psoriasis model involving the ear, our findings once again demonstrated the efficacy of the dNP2-ctCTLA-4 peptide in effectively suppressing psoriatic skin inflammation (Supplementary Figures S1A, B in Supplementary Material). Notably, we observed a significant reduction in Il17a mRNA expression within the skin (Supplementary Figure S1C in Supplementary Material). Furthermore, we observed that the activation of γδ T cells, which are known to be activated by IL-1β and IL-23, could be effectively inhibited by the dNP2-ctCTLA-4 peptide (Supplementary Figures S1D, E in Supplementary Material). Therefore, we have expected that dNP2-ctCTLA-4 peptide could regulate both IL-17-secreting αβ T cells and IL-17A+ γδ T cells. These results provide compelling evidence that the dNP2-ctCTLA-4 peptide acts as a potent regulator of immune responses in the context of psoriatic skin inflammation. Its ability to attenuate the expression of IL-17A by Th17 and Tc17, a critical driver of psoriasis pathology, and inhibit the activation of γδ T cells further support its therapeutic potential in this disease.
CTLA-4 (CD152) is an immune checkpoint molecule that plays a crucial role in regulating T cell activity by competing with CD28 signaling to bind to the B7 molecules expressed on the surface of APCs (61–64). CTLA-4-Ig (Abatacept), a drug that combines the extracellular domain of CTLA-4 with the Fc region of an immunoglobulin, has been FDA-approved as a treatment for rheumatoid arthritis, active psoriatic arthritis. Our findings showed that only the dNP2-ctCTLA-4 peptide was effective in increasing Treg cells and Treg/Teff ratio, while CTLA-4-Ig did not show any therapeutic effect. In our T cell-APC coculture experiment, we observed that CTLA-4-Ig successfully suppressed antigen-specific T cell activation. However, when it came to alleviating psoriatic skin inflammation, CTLA-4-Ig did not yield the same results as the dNP2-ctCTLA-4 peptide. This discrepancy may be attributed to the fact that the IMQ-induced psoriasis model predominantly relies on the involvement of γδ T cells. Additionally, several studies have shown that CTLA-4-Ig reduces Treg cells (65, 66) and combination therapies involving rapamycin, which induces Treg cells, have been attempted to overcome this limitation (67). Clinically, CTLA-4-Ig has not been successful in treating psoriasis. Although CTLA-4-Ig was studied in a phase 1 clinical trial for patients with psoriasis vulgaris (NCT00306878, NCT0027722); the study was discontinued in 2011, and no results have been reported. While CTLA-4-Ig is used in patients with psoriatic arthritis, it only had a moderate impact on psoriasis symptoms (68). Moreover, it has been reported that CTLA-4-Ig failed to prevent psoriasis relapse after discontinuation of Ustekinumab treatment (69). Obviously, the cytoplasmic and extracellular domains of CTLA-4 play distinct roles in modulating inflammation, each operating through different mechanisms. The cytoplasmic domain of CTLA-4 encompasses various functional residues, including a crucial lysine residue that interacts with PKC-η protein (70). Our previous study demonstrated that the mutant form of the lysine residue of dNP2-ctCTLA-4 peptide failed to induce Foxp3+ Treg cells and ameliorate the progression of EAE disease, whereas the wild-type form of dNP2-ctCTLA-4 peptide effectively regulated EAE by inducing Treg cells (38). The dNP2-ctCTLA-4 peptide enhances TGF-β-Smad2/3 signaling, leading to increased Foxp3 expression in CD4 T cells through its binding with PKC-η protein. These findings highlight the importance of considering the differential mechanisms of action when designing therapies targeting CTLA-4 for the management of psoriasis.
Biologics have emerged as effective treatments for psoriasis, targeting specific cytokines, such as IL-17A and IL-23 (17, 71). However, despite their success, there are still some non-responders (72, 73) and currently available biologics do not offer a complete cure for psoriasis, often leading to relapse upon discontinuation. Treg cells play a critical role in maintaining immune tolerance and prevent autoimmune diseases. In a notable study, it was demonstrated that inducing Treg cells by topical application of short-chain fatty acids was shown to inhibit psoriatic skin inflammation reducing Il17a mRNA levels while increasing Foxp3 and Il10 mRNA levels in the skin (74). Moreover, the vitamin D analog, Maxacalcitol, significantly inhibits IMQ-induced psoriasiform dermatitis and reduces IL-17A mRNA expression in the skin, while also increasing Foxp3+ Treg cells (75). These findings support the notion that inducing Treg cells could serve as an effective treatment strategy for psoriasis, offering sustained therapeutic efficacy without recurrence after the cessation of therapy. Therefore, given the potential of Treg cells in regulating immune responses and the observed benefits of Treg cell induction in psoriasis, there is a promising opportunity to develop therapies that specifically target and modulate Treg cells, providing a novel approach for the treatment of psoriasis and other autoimmune conditions. Furthermore, the combination therapy of IL-17 or IL-23 blockade with Treg-inducing molecules such as dNP2-ctCTLA-4 peptide may hold therapeutic potential for more complete regulation of the disease.
Taken together, our findings suggest that the dNP2-ctCTLA-4 peptide holds promise as a novel therapeutic approach for psoriasis, offering the potential to modulate immune responses, suppress inflammation, and mitigate the pathogenic effects associated with controlling IL-17A producing Th17, Tc17, and γδ T cells via increasing Treg cells. Further research is warranted to fully elucidate the underlying mechanisms and explore the translational potential of the dNP2-ctCTLA-4 peptide as a targeted treatment for psoriasis.
Materials and methods
Mice
Female C57BL/6 mice aged 8 to 10 weeks were purchased from Daehan Biolink (DBL, Korea), while 2D2 TCR-transgenic mice were obtained from the Jackson Laboratory (Bar Harbor, ME, USA). The mice were housed and bred in a specific pathogen-free animal facility at Hanyang University under controlled conditions of constant temperature (21 ± 1°C), humidity (50 ± 5%), and a 12-hour light/dark cycle, and provided with regular chow and autoclaved water. All mouse experimental procedures were carried out in accordance with the guidelines of the Institutional Animal Care and Use Committees of Hanyang University (Animal Experiment Approval Number: 2022-0002A) and all mice studies were randomized in a blinded manner in psoriasis mouse models.
Imiquimod-induced psoriasis-like mouse model
To induce psoriasis, 8- to 10-week-old female C57BL/6 mice purchased from Daehan Biolink (DBL, Korea) were used. On Day 0, hair was removed from the dorsal skin using a clipper and hair removal cream. Starting from Day 1, 40 mg of 5% Aldara Cream (3M Pharmaceuticals, Leicestershire, United Kingdom) was topically applied to the dorsal skin for 4 to 5 consecutive days. Psoriasis was induced under deep anesthesia with zoletil (30 mg/kg; Virvac, Carros, France) and rompun (10 mg/kg; Bayer Korea, Ansan, Korea). During disease induction, 100 μg of either dNP2-ctCTLA-4 or CTLA-4-Ig were administered intraperitoneally three times a day. On day 5 or 6, mice were sacrificed and the severity of inflammation in each tissue was assessed using flow cytometry (FACS) or histological analysis with hematoxylin and eosin (H&E) staining. Skin lesion severity was evaluated using the PASI scoring criteria, which considers three signs of erythema, scaling, and thickening, with a scale ranging from 0 (no symptoms) to 4 (very marked). To investigate the effect on ears, we applied 20 mg of 5% Aldara cream daily for six days, and administered 100 μg of protein via intraperitoneal injection. We measured the thickness of the ears using a micrometer (Mitutoyo, Kawasaki, Japan) every day and analyzed the data on day 7.
Skin cell preparation
To acquire single cells, the fat was initially scraped off, and the dorsal skin tissue of the mouse was then separated into the epidermal and dermal layers at 37°C for 90 minutes using 2.4 U/ml of dispase II (04 942 078 001; Roche). After separation, the tissue was cut into small pieces and digested in DMEM media containing 1 mg/ml of collagenase D (11 088 866 001; Sigma-Aldrich), 0.2 mg/ml of DNase I (10 104 159 001; Sigma-Aldrich), and 2% FBS (S 001 01; Welgene) at 225 rpm for 1 hour at 37°C. The enzymatic reaction was stopped by adding 0.5 M ethylenediaminetetraacetic acid (EDTA), followed by filtering through a 40 µm cell strainer and washing with PBS. Single cells were finally obtained, and flow cytometry analysis was performed after antibody staining.
Flow cytometry
Cells isolated from each tissue were analyzed using flow cytometry. Cells were restimulated with Cell Stimulation Cocktail (00-4975-03; eBioscience) to determine intracellular cytokine levels for 4 h at 37°C. To exclude dead cells, cells were stained with the Zombie Aqua Fixable Viability Kit (423101; BioLegend) at room temperature for 10 min. After washing with PBS, the surface proteins were stained with specific monoclonal antibodies for 20 min at 4°C. After washing with PBS, cells were fixed and permeabilized using Foxp3/Transcription Factor Staining Buffer Set (00-5523-00; eBioscience) for 30 min at room temperature. Finally, intracellular cytokine proteins were stained with monoclonal antibodies for 30 min at room temperature. FACS Canto II or FACS Symphony A3 was used to acquire data and FlowJo software version 10.8.0 was used to analyze the data.
Histology
For histological analysis of mouse back skin samples, the tissues were fixed in 4% paraformaldehyde overnight at 4°C, followed by embedding in paraffin. Sections of the skin tissue blocks were prepared and stained with hematoxylin and eosin to reveal the cellular architecture. The resulting H&E-stained sections were then mounted with a cover glass using a mounting solution. Subsequently, the sections were observed under an inverted microscope (Leica DMi8, Leica Microsystems), and images were captured and analyzed using Image J software version 2.0.0
In vitro T cell differentiation
Naïve CD4 T cells (TCRαβ+CD4+CD25-CD62LhighCD44low) or naïve CD8 T cells (TCRαβ+CD8+CD25-CD62LhighCD44low) were isolated from splenocytes of 8- to 10-week-old C57BL/6 mice using a FACSAria Fusion (BD Biosciences). Purified naïve CD4 T cells were activated with plate-bound anti-CD3 (2 μg/ml; 553057; BD Pharmingen) and anti-CD28 (2 μg/ml; 553294; BD Pharmingen) antibodies and differentiated with the following cytokine cocktails in a 96-well plate for 3 or 4 days: 20 U/ml of IL-2 (212-12; Peprotech), 2 ng/ml of TGF-β (240-B-002; R&D Systems) for Treg differentiation; or 5 μg/ml of anti-IL-4 (11B11; 16-7041-85; eBioscience), 5 μg/ml of anti-IFN-γ (XMG1.2; 16-7311-85; eBioscience), 30 ng/ml of IL-6 (406-ML; R&D Systems), 2 ng/ml of TGF-β, 20 ng/ml of IL-1β (401-ML-010; R&D Systems), 20 ng/ml of IL-23 (1887-ML-101; R&D Systems), 20 U/ml of IL-2 for Th17 differentiation. Additionally, cells were co-treated with 2 μM of dNP2-ctCTLA-4. Purified naïve CD8 T cells were activated with plate-bound anti-CD3 (3 μg/ml) and anti-CD28 (3 μg/ml) antibodies and differentiated with the following cytokine cocktails in a 96-well plate for 3 days: 25 U/ml of IL-2, 0.5 ng/ml of TGF-β for Treg differentiation; or 5 μg/ml of anti-IL-4, 5 μg/ml of anti-IFN-γ, 30 ng/ml of IL-6, 2 ng/ml of TGF-β, 20 ng/ml of IL-1β, 20 ng/ml of IL-23, 20 U/ml of IL-2 for Tc17 differentiation. Additionally, cells were co-treated with 2 μM of dNP2-ctCTLA-4.
In vitro co-culture assay
Naïve CD4 T cells (CD4+TCR-Vβ11+CD25-CD62LhighCD44low) were isolated from splenocytes of 8- to 10-week-old 2D2 mice using the CD4 T Cell Isolation Kit (Miltenyi Biotec) according to the manufacturer’s instructions, followed by FACS sorting. The antigen-presenting cells were irradiated with a 3500 rad gamma ray and seeded with the sorted naïve CD4 T cells at a 5:1 ratio. T cells were activated using 40 μg/ml of MOG35-55 (AnyGen, Korea), and differentiated using the following cytokine cocktails in a 96-well flat bottom plate for 3 days: 5 μg/ml of anti-IL-4 (11B11; 16-7041-85; eBioscience), 5 μg/ml of anti-IFN-γ (XMG1.2; 16-7311-85; eBioscience), 30 ng/ml of IL-6 (406-ML; R&D Systems), 2 ng/ml of TGF-β (240-B-002; R&D Systems) for Th17 differentiation; or 5 ng/ml of TGF-β for Treg differentiation. Additionally, cells were co-treated with dNP2-ctCTLA-4 or CTLA-4-Ig.
Immunoblotting
Naïve CD8 T cells were isolated and stimulated with 5μg/ml of anti-CD3/CD28 mAb and 5 ng/ml of TGF-β at 37°C, 30 minutes. Afterward, the cells were washed with PBS and lysed with RIPA buffer (Cell Signaling, Beverly, MA) containing 1 μM of NaF and 1 μM of PMSF for 30 minutes on ice. Immunoblotting was performed on PVDF membranes (Bio-Rad) using the following primary antibodies: phospho-Smad2, phospho-ERK, and β-actin mouse mAb.
Recombinant protein purification and peptide synthesis
dNP2-conjugated recombinant proteins were purified as previously described (36). Briefly, dNP2-ctCTLA-4 was purified by Ni-NTA affinity chromatography (Qiagen, Chatsworth, CA, USA) and desalted using a PD-10 Sephadex G-25 column (GE Healthcare, Uppsala, Sweden). To obtain highly purified proteins, an additional ion-exchange protein purification step was performed using SP Sepharose High Performance (GE Healthcare, Uppsala, Sweden). For the peptide form of dNP2-ctCTLA-4 (from AnyGen, Korea), the peptide was synthesized by solid-phase peptide synthesis as previously described (38). The purity of the final peptide products was more than 95%.
RNA extraction and quantitative PCR
To extract RNA from ear tissue, we used TRI Reagent RT (Molecular Research Center, Inc.) following the manufacturer’s protocol. The extracted RNA was then quantified using a UV spectrophotometer (CellTAGen, Inc., Seoul, Korea). Subsequently, cDNA synthesis was performed using a ReverTra Ace qPCR RT Master Mix (Toyobo, Osaka, Japan). For quantitative RT-PCR analysis, we used iQ SYBR Green Supermix (Bio-Rad, Hercules, CA, USA) with a Bio-Rad CFX Connect real-time PCR detection system. To ensure accuracy, we normalized expression levels to CD45.
Antibodies
The following monoclonal antibodies were used for cell surface staining: anti-mouse CD45 (30-F11; BioLegend), anti-mouse TCRβ (H57-597; eBioscience), anti-mouse TCRγδ (eBioGL3 (GL-3, GL3); eBioscience), anti-mouse Vγ1 (2.11; BioLegend), anti-mouse CD4 (RM4-5; BioLegend), anti-mouse CD25 (PC61; BioLegend), anti-mouse CD27 (LG.3A10; BD Biosciences), anti-mouse CD39 (Duha59; BioLegend), anti-mouse CD44 (IM7; BioLegend), anti-mouse CD62L (MEL-14; eBioscience). The following antibodies were used for intracellular staining: anti-mouse Foxp3 (FJK-16s; eBioscience), anti-RORγt (Q31-415 378; BD Biosciences), anti-mouse IL-17A (eBio17B7; eBioscience), anti-mouse IFN-γ (XMG1.2; eBioscience), anti-mouse CTLA-4 (UC10-4B9; eBioscience).
Statistical analysis
Statistical analysis was conducted using GraphPad Prism version 7.0 (GraphPad Software, San Diego, CA, USA). Mann–Whitney test, Kruskal–Wallis test and Brown-Forsythe and Welch ANOVA tests were employed for data analysis. The data are presented as mean ± S.D. or ± S.E.M., and p ≤ 0.05 was considered statistically significant. Additional information regarding sample size and specific statistical analyses can be found in the corresponding figure legend.
Data availability statement
The original contributions presented in the study are included in the article/Supplementary Material. Further inquiries can be directed to the corresponding authors.
Ethics statement
The animal study was approved by Institutional Animal Care and Use Committees of Hanyang University. The study was conducted in accordance with the local legislation and institutional requirements.
Author contributions
J-MC designed and supervised the project. W-SL conducted and analyzed an experiment using a psoriasis mouse back skin model. K-HN designed comparative analysis study using dNP2-ctCTLA-4 and CTLA-4-Ig by an in vitro T cell-APC co-culture assay and also performed the Tc17 experiment. JHK and W-JK initiated this study by analyzing psoriasis mouse ear model experiment and in vitro γδ T cell experiments. G-RK provided support recombinant proteins and synthetic peptides and performed in vitro Th17, Treg differentiation, and immunoblotting experiment. JEK and E-CS provided valuable technical advice on the psoriasis mouse model and contributed insightful comments on the results. W-SL, K-HN, and G-RK prepared the original draft and J-MC revised the draft. All authors contributed to the article and approved the submitted version.
Funding
This research received funding through grants awarded to J-MC from the Basic Science Research Program (No. NRF-2019R1A2C3006155) and to G-RK from the Sejong Science Fellow (No. 2023-00210407) of the National Research Foundation, sponsored by the Korean government.
Acknowledgments
The author expresses gratitude to In Young Song for providing technical support in FACS sorting, which was conducted at the Industry-University Cooperation Foundation of Hanyang University (Seoul).
Conflict of interest
The authors declare that the research was conducted in the absence of any commercial or financial relationships that could be construed as a potential conflict of interest.
The reviewer JL declared a shared affiliation with the author JHK to the handling editor at the time of review.
Publisher’s note
All claims expressed in this article are solely those of the authors and do not necessarily represent those of their affiliated organizations, or those of the publisher, the editors and the reviewers. Any product that may be evaluated in this article, or claim that may be made by its manufacturer, is not guaranteed or endorsed by the publisher.
Supplementary material
The Supplementary Material for this article can be found online at: https://www.frontiersin.org/articles/10.3389/fimmu.2023.1233514/full#supplementary-material
References
1. Nestle FO, Kaplan DH, Barker J. Psoriasis. N Engl J Med (2009) 361(5):496–509. doi: 10.1056/NEJMra0804595
2. Griffiths CEM, Armstrong AW, Gudjonsson JE, Barker J. Psoriasis. Lancet (2021) 397(10281):1301–15. doi: 10.1016/S0140-6736(20)32549-6
3. Greb JE, Goldminz AM, Elder JT, Lebwohl MG, Gladman DD, Wu JJ, et al. Psoriasis. Nat Rev Dis Primers. (2016) 2:16082. doi: 10.1038/nrdp.2016.82
4. Lowes MA, Suarez-Farinas M, Krueger JG. Immunology of psoriasis. Annu Rev Immunol (2014) 32:227–55. doi: 10.1146/annurev-immunol-032713-120225
5. Boehncke WH, Schon MP. Psoriasis. Lancet (2015) 386(9997):983–94. doi: 10.1016/S0140-6736(14)61909-7
6. Di Cesare A, Di Meglio P, Nestle FO. The IL-23/Th17 axis in the immunopathogenesis of psoriasis. J Invest Dermatol (2009) 129(6):1339–50. doi: 10.1038/jid.2009.59
7. Chen YL, Hardman CS, Yadava K, Ogg G. Innate lymphocyte mechanisms in skin diseases. Annu Rev Immunol (2020) 38:171–202. doi: 10.1146/annurev-immunol-082919-093554
8. Polese B, Zhang H, Thurairajah B, King IL. Innate lymphocytes in psoriasis. Front Immunol (2020) 11:242. doi: 10.3389/fimmu.2020.00242
9. Laggner U, Di Meglio P, Perera GK, Hundhausen C, Lacy KE, Ali N, et al. Identification of a novel proinflammatory human skin-homing Vgamma9Vdelta2 T cell subset with a potential role in psoriasis. J Immunol (2011) 187(5):2783–93. doi: 10.4049/jimmunol.1100804
10. Pantelyushin S, Haak S, Ingold B, Kulig P, Heppner FL, Navarini AA, et al. Rorgammat+ innate lymphocytes and gammadelta T cells initiate psoriasiform plaque formation in mice. J Clin Invest. (2012) 122(6):2252–6. doi: 10.1172/JCI61862
11. Cua DJ, Tato CM. Innate IL-17-producing cells: the sentinels of the immune system. Nat Rev Immunol (2010) 10(7):479–89. doi: 10.1038/nri2800
12. Castillo-Gonzalez R, Cibrian D, Sanchez-Madrid F. Dissecting the complexity of gammadelta T-cell subsets in skin homeostasis, inflammation, and Malignancy. J Allergy Clin Immunol (2021) 147(6):2030–42. doi: 10.1016/j.jaci.2020.11.023
13. Yan BX, Chen XY, Ye LR, Chen JQ, Zheng M, Man XY. Cutaneous and systemic psoriasis: classifications and classification for the distinction. Front Med (Lausanne). (2021) 8:649408. doi: 10.3389/fmed.2021.649408
14. Armstrong AW, Read C. Pathophysiology, clinical presentation, and treatment of psoriasis: A review. JAMA (2020) 323(19):1945–60. doi: 10.1001/jama.2020.4006
17. Ghoreschi K, Balato A, Enerback C, Sabat R. Therapeutics targeting the IL-23 and IL-17 pathway in psoriasis. Lancet (2021) 397(10275):754–66. doi: 10.1016/S0140-6736(21)00184-7
18. Song A, Lee SE, Kim JH. Immunopathology and immunotherapy of inflammatory skin diseases. Immune Netw (2022) 22(1):e7. doi: 10.4110/in.2022.22.e7
19. Sbidian E, Chaimani A, Garcia-Doval I, Doney L, Dressler C, Hua C, et al. Systemic pharmacological treatments for chronic plaque psoriasis: a network meta-analysis. Cochrane Database Syst Rev (2022) 5(5):CD011535. doi: 10.1002/14651858.CD011535.pub5
20. Togashi Y, Shitara K, Nishikawa H. Regulatory T cells in cancer immunosuppression - implications for anticancer therapy. Nat Rev Clin Oncol (2019) 16(6):356–71. doi: 10.1038/s41571-019-0175-7
21. Sakaguchi S, Yamaguchi T, Nomura T, Ono M. Regulatory T cells and immune tolerance. Cell (2008) 133(5):775–87. doi: 10.1016/j.cell.2008.05.009
22. Vignali DA, Collison LW, Workman CJ. How regulatory T cells work. Nat Rev Immunol (2008) 8(7):523–32. doi: 10.1038/nri2343
23. Shevyrev D, Tereshchenko V. Treg heterogeneity, function, and homeostasis. Front Immunol (2019) 10:3100. doi: 10.3389/fimmu.2019.03100
24. Josefowicz SZ, Lu LF, Rudensky AY. Regulatory T cells: mechanisms of differentiation and function. Annu Rev Immunol (2012) 30:531–64. doi: 10.1146/annurev.immunol.25.022106.141623
25. Goodman WA, Levine AD, Massari JV, Sugiyama H, McCormick TS, Cooper KD. IL-6 signaling in psoriasis prevents immune suppression by regulatory T cells. J Immunol (2009) 183(5):3170–6. doi: 10.4049/jimmunol.0803721
26. Sugiyama H, Gyulai R, Toichi E, Garaczi E, Shimada S, Stevens SR, et al. Dysfunctional blood and target tissue CD4+CD25high regulatory T cells in psoriasis: mechanism underlying unrestrained pathogenic effector T cell proliferation. J Immunol (2005) 174(1):164–73. doi: 10.4049/jimmunol.174.1.164
27. Yang L, Li B, Dang E, Jin L, Fan X, Wang G. Impaired function of regulatory T cells in patients with psoriasis is mediated by phosphorylation of STAT3. J Dermatol Sci (2016) 2):85–92. doi: 10.1016/j.jdermsci.2015.11.007
28. Buckner JH. Mechanisms of impaired regulation by CD4(+)CD25(+)FOXP3(+) regulatory T cells in human autoimmune diseases. Nat Rev Immunol (2010) 10(12):849–59. doi: 10.1038/nri2889
29. Dominguez-Villar M, Hafler DA. Regulatory T cells in autoimmune disease. Nat Immunol (2018) 19(7):665–73. doi: 10.1038/s41590-018-0120-4
30. Bovenschen HJ, van de Kerkhof PC, van Erp PE, Woestenenk R, Joosten I, Koenen HJ. Foxp3+ regulatory T cells of psoriasis patients easily differentiate into IL-17A-producing cells and are found in lesional skin. J Invest Dermatol (2011) 131(9):1853–60. doi: 10.1038/jid.2011.139
31. Soler DC, McCormick TS. The dark side of regulatory T cells in psoriasis. J Invest Dermatol (2011) 131(9):1785–6. doi: 10.1038/jid.2011.200
32. Jung MK, Kwak JE, Shin EC. IL-17A-producing foxp3(+) regulatory T cells and human diseases. Immune Netw (2017) 17(5):276–86. doi: 10.4110/in.2017.17.5.276
33. Hartwig T, Zwicky P, Schreiner B, Yawalkar N, Cheng P, Navarini A, et al. Regulatory T cells restrain pathogenic T helper cells during skin inflammation. Cell Rep (201) 25(13):3564–3572.e4. doi: 10.1016/j.celrep.2018.12.012
34. Stockenhuber K, Hegazy AN, West NR, Ilott NE, Stockenhuber A, Bullers SJ, et al. Foxp3(+) T reg cells control psoriasiform inflammation by restraining an IFN-I-driven CD8(+) T cell response. J Exp Med (2018) 215(8):1987–98. doi: 10.1084/jem.20172094
35. Choi CW, Kim BR, Yang S, Kim Y, Kang JS, Youn SW. Regulatory T cells suppress skin inflammation in the imiquimod-induced psoriasis-like mouse model. J Dermatol Sci (2020) 98(3):199–202. doi: 10.1016/j.jdermsci.2020.04.008
36. Lim S, Kim WJ, Kim YH, Lee S, Koo JH, Lee JA, et al. dNP2 is a blood-brain barrier-permeable peptide enabling ctCTLA-4 protein delivery to ameliorate experimental autoimmune encephalomyelitis. Nat Commun (2015) 6:8244. doi: 10.1038/ncomms9244
37. Koo JH, Kim GR, Nam KH, Choi JM. Unleashing cell-penetrating peptide applications for immunotherapy. Trends Mol Med (2022) 28(6):482–96. doi: 10.1016/j.molmed.2022.03.010
38. Kim GR, Kim WJ, Lim S, Lee HG, Koo JH, Nam KH, et al. In vivo induction of regulatory T cells via CTLA-4 signaling peptide to control autoimmune encephalomyelitis and prevent disease relapse. Adv Sci (Weinh). (2021) 8(14):2004973. doi: 10.1002/advs.202004973
39. Lim S, Kirkiles-Smith NC, Pober JS, Bothwell ALM, Choi JM. Regulation of human T cell responses by dNP2-ctCTLA-4 inhibits human skin and microvessel graft rejection. Biomaterials (2018) 183:128–38. doi: 10.1016/j.biomaterials.2018.08.049
40. Lim S, Ho Sohn J, Koo JH, Park JW, Choi JM. dNP2-ctCTLA-4 inhibits German cockroach extract-induced allergic airway inflammation and hyper-responsiveness via inhibition of Th2 responses. Exp Mol Med (2017) 49(8):e362. doi: 10.1038/emm.2017.107
41. Kim WJ, Koo JH, Cho HJ, Lee JU, Kim JY, Lee HG, et al. Protein tyrosine phosphatase conjugated with a novel transdermal delivery peptide, astrotactin 1-derived peptide recombinant protein tyrosine phosphatase (AP-rPTP), alleviates both atopic dermatitis-like and psoriasis-like dermatitis. J Allergy Clin Immunol (2018) 141(1):137–51. doi: 10.1016/j.jaci.2017.04.007
42. van der Fits L, Mourits S, Voerman JS, Kant M, Boon L, Laman JD, et al. Imiquimod-induced psoriasis-like skin inflammation in mice is mediated via the IL-23/IL-17 axis. J Immunol (2009) 182(9):5836–45. doi: 10.4049/jimmunol.0802999
43. Bluestone JA, St Clair EW, Turka LA. CTLA4Ig: bridging the basic immunology with clinical application. Immunity (2006) 24(3):233–8. doi: 10.1016/j.immuni.2006.03.001
44. Ruderman EM, Pope RM. Drug Insight: abatacept for the treatment of rheumatoid arthritis. Nat Clin Pract Rheumatol (2006) 2(12):654–60. doi: 10.1038/ncprheum0345
45. Chambers CA, Kuhns MS, Egen JG, Allison JP. CTLA-4-mediated inhibition in regulation of T cell responses: mechanisms and manipulation in tumor immunotherapy. Annu Rev Immunol (2001) 19:565–94. doi: 10.1146/annurev.immunol.19.1.565
46. Kabashima K, Honda T, Ginhoux F, Egawa G. The immunological anatomy of the skin. Nat Rev Immunol (2019) 19(1):19–30. doi: 10.1038/s41577-018-0084-5
47. Dainichi T, Kitoh A, Otsuka A, Nakajima S, Nomura T, Kaplan DH, et al. The epithelial immune microenvironment (EIME) in atopic dermatitis and psoriasis. Nat Immunol (2018) 19(12):1286–98. doi: 10.1038/s41590-018-0256-2
48. Pasparakis M, Haase I, Nestle FO. Mechanisms regulating skin immunity and inflammation. Nat Rev Immunol (2014) 14(5):289–301. doi: 10.1038/nri3646
49. Cai Y, Shen X, Ding C, Qi C, Li K, Li X, et al. Pivotal role of dermal IL-17-producing gammadelta T cells in skin inflammation. Immunity (2011) 35(4):596–610. doi: 10.1016/j.immuni.2011.08.001
50. Hartwig T, Pantelyushin S, Croxford AL, Kulig P, Becher B. Dermal IL-17-producing gammadelta T cells establish long-lived memory in the skin. Eur J Immunol (2015) 45(11):3022–33. doi: 10.1002/eji.201545883
51. Kim JH, Choi YJ, Lee BH, Song MY, Ban CY, Kim J, et al. Programmed cell death ligand 1 alleviates psoriatic inflammation by suppressing IL-17A production from programmed cell death 1-high T cells. J Allergy Clin Immunol (2016) 137(5):1466–1476.e3. doi: 10.1016/j.jaci.2015.11.021
52. Cai Y, Xue F, Qin H, Chen X, Liu N, Fleming C, et al. Differential Roles of the mTOR-STAT3 Signaling in Dermal gammadelta T Cell Effector Function in Skin Inflammation. Cell Rep (2019) 27(10):3034–3048.e5. doi: 10.1016/j.celrep.2019.05.019
53. Sutton CE, Lalor SJ, Sweeney CM, Brereton CF, Lavelle EC, Mills KH. Interleukin-1 and IL-23 induce innate IL-17 production from gammadelta T cells, amplifying Th17 responses and autoimmunity. Immunity (2009) 31(2):331–41. doi: 10.1016/j.immuni.2009.08.001
54. Campbell JJ, Ebsworth K, Ertl LS, McMahon JP, Newland D, Wang Y, et al. IL-17-Secreting gammadelta T Cells Are Completely Dependent upon CCR6 for Homing to Inflamed Skin. J Immunol (2017) 199(9):3129–36. doi: 10.4049/jimmunol.1700826
55. Mabuchi T, Singh TP, Takekoshi T, Jia GF, Wu X, Kao MC, et al. CCR6 is required for epidermal trafficking of gammadelta-T cells in an IL-23-induced model of psoriasiform dermatitis. J Invest Dermatol (2013) 133(1):164–71. doi: 10.1038/jid.2012.260
56. Hedrick MN, Lonsdorf AS, Shirakawa AK, Richard Lee CC, Liao F, Singh SP, et al. CCR6 is required for IL-23-induced psoriasis-like inflammation in mice. J Clin Invest. (2009) 119(8):2317–29. doi: 10.1172/jci37378
57. Park SG, Mathur R, Long M, Hosh N, Hao L, Hayden MS, et al. T regulatory cells maintain intestinal homeostasis by suppressing gammadelta T cells. Immunity (2010) 33(5):791–803. doi: 10.1016/j.immuni.2010.10.014
58. Yurchenko E, Levings MK, Piccirillo CA. CD4+ Foxp3+ regulatory T cells suppress gammadelta T-cell effector functions in a model of T-cell-induced mucosal inflammation. Eur J Immunol (2011) 41(12):3455–66. doi: 10.1002/eji.201141814
59. Li L, Wu CY. CD4+ CD25+ Treg cells inhibit human memory gammadelta T cells to produce IFN-gamma in response to M tuberculosis antigen ESAT-6. Blood (2008) 111(12):5629–36. doi: 10.1182/blood-2008-02-139899
60. Lim S, Lee JA, Koo JH, Kang TG, Ha SJ, Choi JM. Cell type preference of a novel human derived cell-permeable peptide dNP2 and TAT in murine splenic immune cells. PloS One (2016) 11(5):e0155689. doi: 10.1371/journal.pone.0155689
61. Kim GR, Choi JM. Current understanding of cytotoxic T lymphocyte antigen-4 (CTLA-4) signaling in T-cell biology and disease therapy. Mol Cells (2022) 45(8):513–21. doi: 10.14348/molcells.2022.2056
62. Choi JM, Kim SH, Shin JH, Gibson T, Yoon BS, Lee DH, et al. Transduction of the cytoplasmic domain of CTLA-4 inhibits TcR-specific activation signals and prevents collagen-induced arthritis. Proc Natl Acad Sci U S A. (2008) 105(50):19875–80. doi: 10.1073/pnas.0805198105
63. Choi JM, Ahn MH, Chae WJ, Jung YG, Park JC, Song HM, et al. Intranasal delivery of the cytoplasmic domain of CTLA-4 using a novel protein transduction domain prevents allergic inflammation. Nat Med (2006) 12(5):574–9. doi: 10.1038/nm1385
64. Alegre ML, Frauwirth KA, Thompson CB. T-cell regulation by CD28 and CTLA-4. Nat Rev Immunol (2001) 1(3):220–8. doi: 10.1038/35105024
65. Glatigny S, Hollbacher B, Motley SJ, Tan C, Hundhausen C, Buckner JH, et al. Abatacept targets T follicular helper and regulatory T cells, disrupting molecular pathways that regulate their proliferation and maintenance. J Immunol (2019) 202(5):1373–82. doi: 10.4049/jimmunol.1801425
66. Riella LV, Liu T, Yang J, Chock S, Shimizu T, Mfarrej B, et al. Deleterious effect of CTLA4-Ig on a Treg-dependent transplant model. Am J Transplant. (2012) 12(4):846–55. doi: 10.1111/j.1600-6143.2011.03929.x
67. Kirk AD, Guasch A, Xu H, Cheeseman J, Mead SI, Ghali A, et al. Renal transplantation using belatacept without maintenance steroids or calcineurin inhibitors. Am J Transplant. (2014) 14(5):1142–51. doi: 10.1111/ajt.12712
68. Mease PJ, Gottlieb AB, van der Heijde D, FitzGerald O, Johnsen A, Nys M, et al. Efficacy and safety of abatacept, a T-cell modulator, in a randomised, double-blind, placebo-controlled, phase III study in psoriatic arthritis. Ann Rheum Dis (2017) 76(9):1550–8. doi: 10.1136/annrheumdis-2016-210724
69. Harris KM, Smilek DE, Byron M, Lim N, Barry WT, McNamara J, et al. Effect of costimulatory blockade with abatacept after ustekinumab withdrawal in patients with moderate to severe plaque psoriasis: the PAUSE randomized clinical trial. JAMA Dermatol (2021) 157(11):1306–15. doi: 10.1001/jamadermatol.2021.3492
70. Kong KF, Fu G, Zhang Y, Yokosuka T, Casas J, Canonigo-Balancio AJ, et al. Protein kinase C-eta controls CTLA-4-mediated regulatory T cell function. Nat Immunol (2014) 15(5):465–72. doi: 10.1038/ni.2866
71. Lynde CW, Poulin Y, Vender R, Bourcier M, Khalil S. Interleukin 17A: toward a new understanding of psoriasis pathogenesis. J Am Acad Dermatol (2014) 71(1):141–50. doi: 10.1016/j.jaad.2013.12.036
72. Lebwohl MG, Gordon KB, Gallo G, Zhang L, Paul C. Ixekizumab sustains high level of efficacy and favourable safety profile over 4 years in patients with moderate psoriasis: results from UNCOVER-3 study. J Eur Acad Dermatol Venereol. (2020) 34(2):301–9. doi: 10.1111/jdv.15921
73. Bartos S, Hill D, Feldman SR. Review of maintenance of response to psoriasis treatments. J Dermatolog Treat (2016) 27(4):293–7. doi: 10.1080/09546634.2016.1177158
74. Schwarz A, Philippsen R, Schwarz T. Induction of regulatory T cells and correction of cytokine disbalance by short-chain fatty acids: implications for psoriasis therapy. J Invest Dermatol (2021) 141(1):95–104.e2. doi: 10.1016/j.jid.2020.04.031
Keywords: psoriasis, dNP2-ctCTLA-4, CTLA-4-Ig, Treg cells, IL-17A
Citation: Lee W-S, Nam K-H, Kim JH, Kim W-J, Kim JE, Shin E-C, Kim G-R and Choi J-M (2023) Alleviating psoriatic skin inflammation through augmentation of Treg cells via CTLA-4 signaling peptide. Front. Immunol. 14:1233514. doi: 10.3389/fimmu.2023.1233514
Received: 02 June 2023; Accepted: 11 September 2023;
Published: 25 September 2023.
Edited by:
Eva Reali, University of Ferrara, ItalyReviewed by:
June-Yong Lee, Yonsei University, Republic of KoreaNaeun Lee, Catholic University of Korea, Republic of Korea
Yeonseok Chung, Seoul National University, Republic of Korea
Copyright © 2023 Lee, Nam, Kim, Kim, Kim, Shin, Kim and Choi. This is an open-access article distributed under the terms of the Creative Commons Attribution License (CC BY). The use, distribution or reproduction in other forums is permitted, provided the original author(s) and the copyright owner(s) are credited and that the original publication in this journal is cited, in accordance with accepted academic practice. No use, distribution or reproduction is permitted which does not comply with these terms.
*Correspondence: Gil-Ran Kim, Z29kcWhyMzA3OUBoYW55YW5nLmFjLmty; Je-Min Choi, amVtaW5jaG9pQGhhbnlhbmcuYWMua3I=
†These authors have contributed equally to this work and share first authorship