- 1Center for Immunology, Masonic Cancer Center, Minneapolis, MN, United States
- 2University of Minnesota, Department of Laboratory Medicine and Pathology, Minneapolis, MN, United States
- 3Division of Hematology, Oncology and Transplantation, Department of Medicine, University of Minnesota, Minneapolis, MN, United States
CD4 T cells were initially described as helper cells that promote either the cellular immune response (Th1 cells) or the humoral immune response (Th2 cells). Since then, a plethora of functionally distinct helper and regulatory CD4 T cell subsets have been described. CD4 T cells with cytotoxic function were first described in the setting of viral infections and autoimmunity, and more recently in cancer and gut dysbiosis. Regulatory CD4 T cell subsets such as Tregs and T-regulatory type 1 (Tr1) cells have also been shown to have cytotoxic potential. Indeed, Tr1 cells have been shown to be important for maintenance of stem cell niches in the bone marrow and the gut. This review will provide an overview of cytotoxic CD4 T cell development, and discuss the role of inflammatory and Tr1-like cytotoxic CD4 T cells in maintenance of intestinal stem cells and in anti-cancer immune responses.
Historical overview
Naïve CD4 T cells upon activation differentiate into various specialized subsets that direct specific types of immune responses by producing specific cytokines. In 1986, Mossman and Coffman characterized two main populations of CD4 T cells in mice: IFNγ producing Th1 cells and IL4-producing Th2 cells (1). Subsequently, distinct subsets of CD4 T cells have been described including follicular-helper Tfh cells, IL17-producing Th17 cells, and FOXP3+ regulatory T cells (2–4) among others. Each CD4 T cell subset facilitates immune responses towards distinct types of pathogens through distinct mechanisms.
CD4 T cells have been traditionally associated with helper functions while CD8 T cells have been associated with cytotoxic functions. However, studies as early as the 1970s had identified CD4 T cells with cytotoxic activity in the context of allograft rejection (5–7). Even though subsequent studies identified CD4 T cell clones with cytotoxic potential in humans (8) and mice (9, 10), cytotoxic activity in CD4 T cells was considered an artifact of long-term in-vitro cultures. However, recent studies have identified antigen-specific cytotoxic CD4 T cells in patients with chronic viral infections such as CMV (11, 12), HIV (13, 14) and hepatitis (15), and in murine models of chronic viral infection (16). Cytotoxic CD4 T cells have also been shown to be induced in acute influenza infections (17, 18). While the earliest studies identified cytotoxic CD4 T cells in viral infection models, they have also been recently identified in anti-tumor immune responses and in chronic inflammatory responses during auto-immune/auto-inflammatory diseases (19, 20). These studies clearly indicate that cytotoxic CD4 T cells can be elicited under diverse acute and chronic inflammatory conditions in-vivo.
Although cytotoxic CD4 T cells were first thought to be a distinct CD4 T cell subset, they have now been shown to have features of various helper T cell subsets. Furthermore, inhibitory CD4 T cell subsets such as FOXP3+ Tregs and FOXP3- Tr1 cells have also been shown to have cytotoxic potential (21, 22). In this review, we summarize the key mechanisms by which CD4 T cells mediate cytotoxic functions, and propose 2 pathways that could regulate the differentiation of cytotoxic CD4 T cells. We also illuminate the role of cytotoxic CD4s in maintaining homeostasis in the gut mucosa and in immune responses against cancer. There will be a special focus on studies that have highlighted novel roles for Tr1-like cytotoxic CD4s with suppressive attributes in maintenance of intestinal stem cells and in cancers of the gut mucosa.
Identity and differentiation of cytotoxic CD4s
Mechanisms of cytotoxicity
The fusion of lysosomes to the membrane causes surface expression of the lysosomal proteins LAMP-1 (CD107a) and LAMP-2 (CD107b) - these are markers of degranulation and can be used to identify activated cytotoxic CD4 T cells upon in-vitro stimulation. More recently, another lysosomal granule protein NKG7 has been identified as a marker of degranulation of cytotoxic CD4 T cells (23). This has led to the development of a NKG7-Cre transgenic mouse, which when crossed to Rosa26- LoxP-STOP-LoxP fluorescent reporter mice can be used to identify cytotoxic CD4 T cells (23). Thus, cytotoxic CD4 T cells can be identified via a variety of markers.
Transcriptomic and proteomic data has shown that cytotoxic CD4s express various granzymes, including Granzyme A, B and K, as well as the cationic antibacterial protein granulysin (24, 25). GZMK and GZMA are also upregulated in Chimeric Antigen receptor (CAR) CD4 T cells, while GZMB and GZMM were enriched in CAR CD8 T cells in CLL (26). Thus, cytotoxic CD4 T cells can mediate killing through granules with cytotoxic function such as GZMB, but also through granules with both cytotoxic and non-cytotoxic functions such as GZMK. Interestingly GZMB+ and GZMK+ CD4s seem to be a part of distinct populations of cytotoxic CD4 T cells in human cancer patients (19). The differentiation pathway and lineage relationship between the GZMB+ and the GZMK+ cytotoxic CD4 T cells are still unknown.
Cytotoxic CD4 T cells are also capable of mediating killing via granule-independent mechanisms using death-inducing receptors. All helper T cell subsets have been shown to have some FasL-dependent cytolytic activity (27). In melanoma cells resistant to FAS-induced apoptosis, the death receptor TRAIL facilitated CD4 T cell-induced cancer cell death (28). The activating NK cell receptor NKG2D has been recently shown to be essential for cytotoxic CD4 T cell-mediated lysis of Tregs (29), thus raising the possibility that NKG2D+ CD4 T cells can mediate MHCII-independent “bystander” cytotoxicity. Signaling via SLAMF7, another NK cell receptor, has also been shown to promote cytolytic activity in CD4 T cells (24). Thus, cytotoxic CD4 T cells employ a number of mechanisms to mediate cell death.
Transcriptional regulation of cytotoxic molecules in CD4 T cells
Many key regulators of cytotoxic function in CD8 T cells have been shown to be critical regulators of cytotoxic function in CD4 T cells as well. The transcription factor EOMES has been shown to be a key regulator of cytotoxic CD4 T cells in mice and humans via the induction of perforin (30–32). However, other studies show that the transcription factor HOBIT, rather than EOMES, could be essential for expression of cytotoxic molecules in human CD4 T cells (33, 34). Apart from HOBIT, the IL2-dependent induction of the HOBIT homolog BLIMP1 was shown to be critical for induction of granzymes in tumor-specific CD4 T cells (35). Apart from IL2, the cytokine IL15 is also shown to promote the expression of granzyme B in CD4 T cells (35, 36). In CD8 T cells, IL15 is known to induce HOBIT but not BLIMP1 (37). Thus, induction of granzymes in CD4 T cells can be driven by IL2-induced BLIMP1 and IL15-induced HOBIT dependent mechanisms. In CD8 T cells, BLIMP1 is required for induction of Granzyme B in effector CD8 T cells while HOBIT was required for maintenance of Granzyme B in memory CD8 T cells (38). Whether such a temporal regulation occurs in cytotoxic CD4s is not known. While HOBIT and BLIMP1 are essential for induction of granzyme B in human CD4 T cells, they are not required for induction of perforin (39). RUNX3 is known to induce perforin in CD8 T cells (40). Thus, RUNX3 rather than EOMES could be important for inducing perforin in human CD4 T cells. To summarize, EOMES, RUNX3, BLIMP1 and HOBIT have been shown to be key transcription factors that are important for inducing cytotoxic function in CD4 T cells.
Runx3-dependent and Runx3-independent differentiation of cytotoxic CD4 T cells
While the above studies have defined transcription factors that regulate cytotoxic programs in CD4 T cells, the upstream signals that regulate cytotoxic CD4 T cell differentiation have not been as well explored. Based on the literature, there are 2 different pathways for the differentiation of cytotoxic CD4 T cells: a RUNX3-dependent pathway that relies on TCR signaling as the initiating event and a RUNX3-independent pathway that relies on signaling via the receptor CRTAM as the initiating event (summarized in Figure 1).
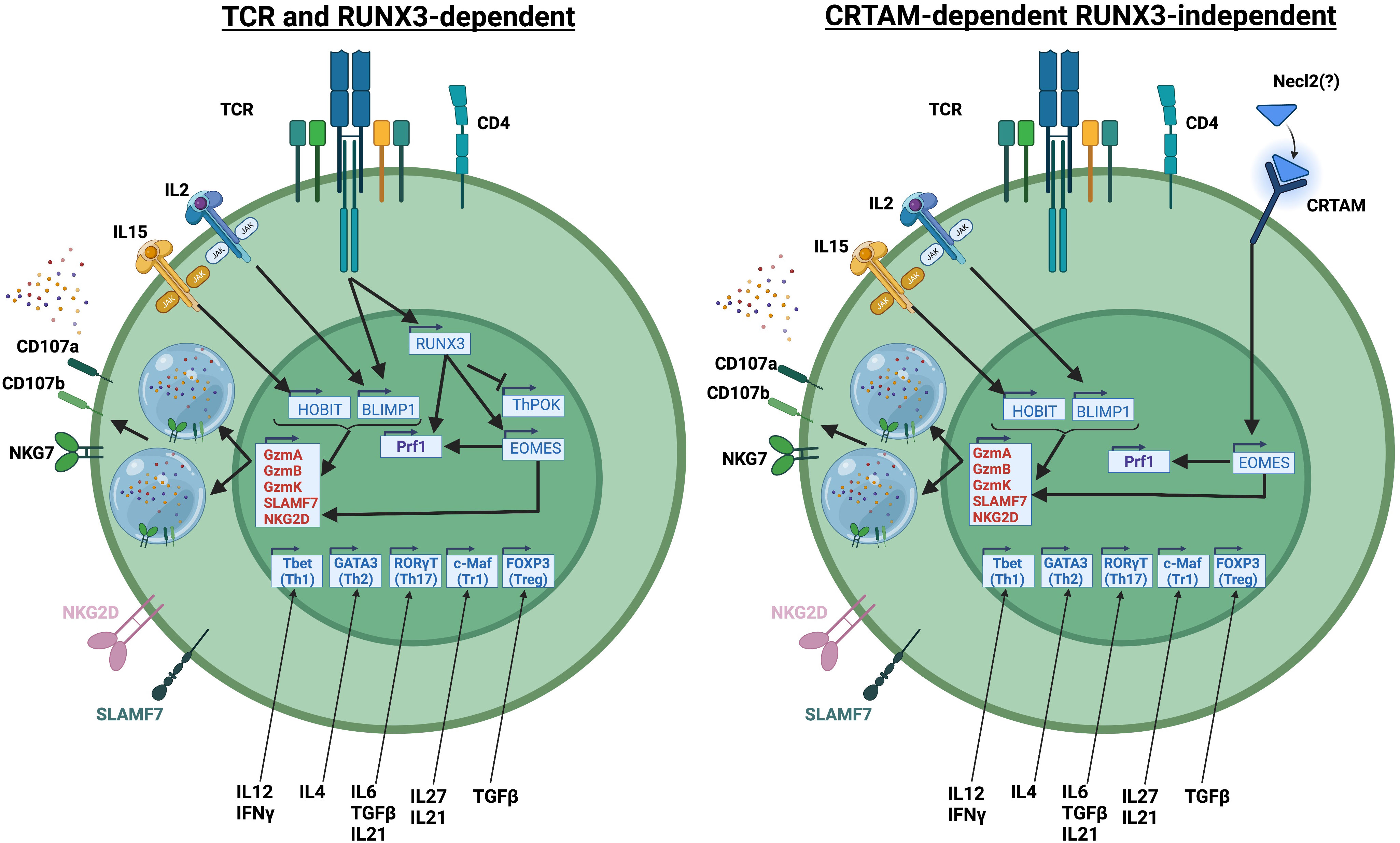
Figure 1 Representation of RUNX3-dependent and RUNX3-independent differentiation pathways of cytotoxic CD4 T cells. Created with BioRender.com.
The RUNX3-induced gene EOMES has a well-established role in inducing cytotoxic function in CD8 T cells. However, since THPOK maintains CD4 T cell identity by repressing CD8 T cell lineage genes (including Runx3), induction of Runx3 and Eomes in CD4 T cells would likely require downregulation of THPOK. Indeed, a recent study has shown that a significant population of CD4 T cells in mice have downregulated THPOK and upregulated RUNX3, and that these CD4 T cells eventually acquire cytotoxic function in a stepwise manner (41). However, another study showed that cytotoxic CD4 T cells in humans acquire cytotoxic function by upregulation of RUNX3 without repression of ThPOK (33). However, in that study, siRNA knockdown of THPOK showed that THPOK still negatively regulated the cytotoxic program in CD4 T cells. Whether cytotoxic CD4 T cells in humans can downregulate THPOK to improve their cytotoxic function is not known.
In mice, loss of THPOK and de-repression of RUNX3 were shown to be driven by chronic TCR signaling (41). In agreement with the findings from the mouse study, data from human patients has shown that Cytomegalovirus (CMV), a chronic viral infection, strongly expands cytotoxic CD4 T cells (33). CMV-specific cytotoxic CD4 T cells upregulate PD1, an indicator of active TCR signaling, and have reduced ability to produce the Th1 cytokines IFNγ and TNF (42). Interestingly however, the CMV-specific cytotoxic CD4 T cells do not express other exhaustion-associated markers such as TIM3 and LAG3 and are only minimally-responsive to PD1 blockade, suggesting that the TCR-induced cytotoxic phenotype is distinct from exhaustion (42).
In contrast to the RUNX3-dependent model, studies have shown that CRTAM, a molecule that regulates cytotoxic function in CD8 T cells and NK cells, can also induce cytotoxic CD4 T cells (43). Interestingly in this study, CRTAM directly regulates EOMES expression in a RUNX3-independent manner (43). The previously established roles of BLIMP1 and HOBIT in regulating the expression of granzymes and other cytolytic molecules would be compatible with both CRTAM-dependent and RUNX3-dependent models of cytotoxic CD4 T cell development. The factors downstream of RUNX3 or CRTAM that regulate cytotoxic CD4 T cell differentiation have not been well-described.
Although cytotoxic CD4 T cells are considered a distinct subset from helper CD4 T cells, cytotoxic CD4s can develop under different polarization conditions and have transcriptional signatures/features associated with the polarized CD4 T cell subset, including Th1, Th2, Th17, Tregs, and Tr1 cells (44–48). Whether or not the cytotoxic program can be induced in all helper T cell subsets, or whether cytotoxic CD4 T cells acquire different polarized states after differentiation is still unclear: while some studies show that cytotoxic CD4 T cell differentiation is TBET-dependent and occurs most efficiently under Th1-polarization conditions (33), other studies show that the regulation of the cytotoxic program occurs independent of TBET (35).
Definition of cytotoxic CD4 T cells: phenotype vs function
A major challenge in defining cytotoxic CD4 T cells is that CD4 T cells that express molecules associated with cytotoxic functions are not necessarily cytotoxic. Indeed, a population of GzmKhi GzmBlo CD8 T cells has been shown to have low cytotoxic potential – rather, these cells are key drivers of inflammation (49). Granzyme B has also been linked with non-cytotoxic functions, including regulating the polarization of Th0 and Th1 vs Th17 CD4 T cells (50). Perforin is important for the formation of pores to facilitate granzyme entry into the target cells. Thus, it is possible that expression of perforin, rather than granzymes, is a more reliable indicator of cytotoxic CD4 T cells. Indeed, deletion of perforin or inhibition of perforin activity has been shown to inhibit cytotoxic activity in CD4 T cells (24, 51). Furthermore memory CD4 T cells in humans express comparable granzyme B, but lower perforin, than memory CD8 T cells (52). This observation would fit with both the RUNX3-dependent and RUNX3-independent models of cytotoxic CD4 T cell differentiation. In both models, induction of EOMES and/or RUNX3, transcription factors that induce perforin, is a key step in the development of cytotoxic CD4 T cells.
As mentioned before, CD4 T cells can mediate cytotoxicity through death receptors such as FASL. However, all helper-T cell subsets have been shown to have some basal cytotoxic potential that is driven by the expression of the death receptor FasL (27). Thus, whether “cytotoxic” CD4 T cells expressing death receptors are a distinct subset from helper CD4 T cells is unclear. For this reason, perforin/granzyme expression and perforin-mediated killing remain the most reliable markers of cytotoxic CD4 T cells.
Cytotoxic CD4 T-cells at the gut mucosal surface
Introduction
Gut-associated lymphoid tissue (GALT) must maintain a fine balance between suppressive and pro-inflammatory states. This ensures tolerance towards complex commensal microbial communities while also eliciting a population of antigen-specific memory/effector populations poised for rapid response to pathogens. The GALT must do this while also maintaining barrier integrity and integrating signals that dictate the appropriate differentiation of immune subsets from multipotent progenitors. The complex topography of the GI tract influences the cross-talk between CD4 T cells and surrounding cells, which bidirectionally influences both epithelial and T-cell differentiation. Thus, CD4+ T-cells not only comprise a critical population of effector/helper cells, but additionally serve as a central nexus of GALT tissue signal integration.
Millions of crypt and villus structures comprise the epithelium of the gut. Under the epithelium exists the lamina propria and specialized lymph tissue known as Peyer’s patches. The gut epithelium itself is a single layer which is renewed by intestinal stem cells (ISC) every 3-7 days (53, 54). CD4+ T cells may be found in the lamina propria and Peyer’s patches, as well as the intestinal epithelium. Single-cell studies demonstrate that CD4+ T-cells are imprinted, during transition through the mesenteric lymph nodes, with transcriptional programs unique to either the lamina propria or the gut epithelium, implying differentiation occurs prior to tissue migration (55). Entry of CD4+ T-cells to the epithelium requires activation via interaction with antigen-presenting cells in peripheral sites, principally including Peyer’s patches and draining mesenteric lymph nodes (56–58). This results in upregulation of the homing receptor AlphaE/Beta7 (αE/β7). Migration to the epithelium coincides with an increase in cytotoxic potential. These intraepithelial αE/β7+ CD4s are poised to release effector cytokines IFNγ and TNF. FOXP3+ Tregs are restrained to the lamina propria and must lose FOXP3 in order to migrate into the epithelium (58). Thus, most CD4+ T-cells found in the epithelium are antigen-experienced long-lived resident effector/memory T-cells, poised for rapid activation and cytotoxicity (59).
Cytotoxic potential manifests in several transcriptionally diverse CD4+ T-cells of the gut, elicited under a variety of pathogenic challenges and disease states. These include phenotypically recognizable Th0, Th1, Th2, Th17, and Treg cell types (60). Expression of the cytotoxic markers Nkg7, FasL, and GzmB has been most closely associated with T-helper type 1 differentiation. Th1 cells are recognized by expression of IFNγ and the transcription factor TBET, but modern single-cell studies now challenge the concept that Th1 cells are a discrete population. scRNAseq analysis of murine colonic LP CD4 T cells instead revealed that CD4 T cells develop along a polarized transcriptional continuum (61). Transcriptional variation across single-cell clusters was determined more by the specific pathogen threat than the expression of cytokines or the master transcription factors TBET, GATA3 or RORγ. For example, while Salmonella classically elicits a phenotypic Th1 response, challenge with Salmonella led to the development of several clusters of effector CD4 T cells with varying expression levels of Th1-defining gene sets; Ifng and Tbx21 were diffusely expressed throughout each cluster. Furthermore, single progenitor cells could give rise to cells capable of expressing different canonical cytokines. Similar results were observed for cytotoxicity markers GzmB, FasL, and Nkg7. Caution should therefore be used when describing an association between cytotoxic markers and Th1 cells in the gut, given the broad transcriptional heterogeneity of T-helper subsets observed in this region.
Regardless of their specific co-existing helper functions or cytokine profiles, the development of cytotoxic CD4+ T-cells critically depends on the activity of the transcription factor THPOK. THPOK and RUNX transcription factors are critical for the induction of CD4 and CD8 T-cell differentiation during thymic development. DN thymocytes upregulate RUNX1 as cells transition to the DP stage (62). During subsequent MHCII-induced selection THPOK is up-regulated progressively as thymocytes down-regulate CD8 and become CD4+ SPs. RUNX3 and THPOK mutually antagonize each other during CD4 and CD8 T-cell thymic development, with CD4+ T-cell differentiation dependent on THPOK expression. In the periphery, CD4+ T-cells continue to express THPOK, which represses genes of the CD8 lineage including cytotoxic genes GzmB and Prf1 (63). A key observation was that mature CD4+ T-cells in the gut could downregulate THPOK in response to strong or chronic TCR activation, leading to re-expression of cytolytic machinery and generation of MHCII-restricted CD4 T-cells (41). THPOK downregulation is dependent on engagement of the TCR-MHCII and PD1/PDL1 axes (64). This likely leads to disruption of a positive-feedback auto-regulatory loop wherein THPOK expression is maintained via binding and repression of a silencer element (SilThpok). Disruption of THPOK expression is dependent on TBET-mediated re-expression of RUNX3, which binds to the SilThpok element (65). Thus, CD4+ T cell cytotoxic potential appears dependent on a TBET-RUNX3-THPOK silencing axis, leading to re-expression of canonical CD8+ cytolytic molecules.
Downregulation of THPOK induces expression of CD8αα, coincident with re-expression of GzmB and Prf1, leading to the development of double positive (CD4/CD8αα) cytotoxic intraepithelial lymphocytes. These CD4/8 DP IELs have significant anti-inflammatory properties, similar to FOXP3+ T-regulatory cells, and can in fact originate from either CD4+ conventional or FOXP3+ pTreg precursors (58, 66–69). This was shown by multiple groups applying lineage tracing using Rosa26-LoxP-STOP-tdTomato reporters, and single-cell techniques, that enable identification of ex-FOXP3+ cells (55, 70). Treg cells (Tomato+ FOXP3+) began in naïve states, then expanded into a Tomato+ FOXP3- subset (ex-Treg, or Treg-like) before differentiating into pre-intraepithelial lymphocyte (Pre-IEL) subsets, and finally a CD4+CD8αα+ intra-epithelial population. Treg-like cells had downregulated Foxp3 and acquired some markers of cytotoxicity (Nkg7, GzmB) and chemokine expression (Ccl5). CD4+ T-conventional (Tomato-) cells also transited through naïve and pre-IEL subsets with similar gene expression profiles, before also terminally differentiating into CD4+CD8αα+ cells. TCR diversity diminished as both Treg and Tconv populations differentiated, implying clonal selection. TCR clones were shared between populations comprising the Treg differentiation pathway, and between populations comprising the Tconv differentiation pathway, although not between populations of these two pathways, supporting the independence of the Treg and Tconv lineages. Functional experiments confirmed that TCR engagement by MHCII molecules expressed by epithelial cells was critical for differentiation of CD4 precursors into pre-IEL and CD4+CD8αα stages (64). These THPOK- CD4 T cells appear poised to exert either immunosuppressive or pro-inflammatory functions depending on surrounding inflammatory signals, and complement other suppressive populations. OT-II mice deficient in RUNX3 were unable to downregulate THPOK or develop CD4+ IELs; in response to OVA exposure, they developed colitis. This phenotype could be rescued by provision of THPOK-deficient OT-II CD4 T cells. IL15 exposure led to expression of the cytotoxic marker CD107 on OTII-specific THPOK- CD4+ T-cells, whereas it had no effect on THPOK+ CD4+ T-cells (41). Thus, the majority of cytotoxic CD4+ T-cells of the gut epithelium are CD4+CD8αα DP cells, which originated as either FOXP3+ pTregs or FOXP3- Tregs and developed via interactions with epithelial cells presenting antigens in an MHCII context. Their ultimate functionality depends on the cytokine milieu of the epithelium, with IL15 playing a principal role in cytotoxic capacity.
T-regulatory type 1 cells
In addition to FOXP3+ T regulatory cells and CD4+CD8αα DPs, a third class of potentially cytotoxic T-cells with regulatory potential has been described. T-regulatory type 1 (Tr1s) were first described as arising in a patient with severe combined immunodeficiency (SCID) who received mismatched allogeneic fetal liver and thymus transplants and developed stable mixed chimerism (71). Subsequent studies have demonstrated the importance of Tr1 cells at preventing allo-rejection in bone marrow and solid organ transplantation settings. Clinical efforts to utilize these properties have demonstrated the ability of Tr1 cell therapy to tolerize patients towards solid organ grafts from HLA-mismatched donors, potentially greatly expanding organ transplant candidacy, and preempting the need for lifelong immunosuppression in graft recipients. Tr1s are FOXP3- IL10-producing CD4 T cells with the potential to suppress immune responses to foreign- and self-antigens. Tr1s are not the only FOXP3- CD4+ cell type that can synthesize IL-10, and current studies do not fully address the lineage relationships between Tr1s and other IL-10-producing FOXP3- CD4 T cells. In settings of chronic infection, Th1 cells are known to switch to high production of both IFNγ and IL10 (72). However, Th1 production of IL10 may be transient, in contrast with the more sustained production observed by Tr1 cells. Tr1s produce relatively lower levels of Tbx21 and Egr2, but elevated levels of Gata3 as compared to IL10-producing Th1 cells, potentially hinting at separate developmental pathways and enabling their discrimination (73, 74). Phenotypically, Tr1s were proposed to be identifiable by CD49b and LAG3 positivity, in both humans and mice, although several reports of IL10-producing FOXP3- CD4 T cells did not evaluate them for these markers (75). Thus, for the purposes of this review, Tr1 cells are defined as antigen-experienced FOXP3- IL10+ CD4+ T-cells with well-demonstrated roles suppressing inflammation towards self-antigens, particularly in alloreactive settings.
Development and function of Tr1s
Tr1 cells originate from naïve CD4 T-cells in the periphery following chronic antigen stimulation in the presence of key cytokines including IL10 (76–79). IL10 appears sufficient but dispensable for Tr1 induction, and yet critical for maintenance of Tr1 suppressive function; Using dual-reporter Foxp3gfp/IL-10Thy-1.1 mice crossed to an IL10-deficient background, Maynard et al. showed that IL10 producing Tr1 cells continued to be found in normal frequencies in the epithelium and lamina propria of both the small and large intestine (80). However, IL10R-impaired CD4+ T-cells have deficiencies in IL10-secretion and are less capable of suppressing colitis (81). This demonstrates that IL10 can function in paracrine and/or autocrine manner to induce and sustain the maximal activity of Tr1 cells and is a key mediator of Tr1 suppressor activity. Other signals important for Tr1 differentiation include IL27 and TGFβ. An emerging model suggests that Tr1 induction requires (i) STAT1/3 activation via IL27 and/or IL10, (ii) aryl hydrocarbon receptor (AHR) or c-Maf upregulation, stimulated via TGFβ or ICOS-L binding, and (iii) TCR stimulation by cognate antigen presented in an MHCII context (reviewed in (82)). IL21 may also contribute, functioning in an autocrine manner to maintain Tr1 cells, as well as having pleiotropic immunomodulatory effects on several surrounding cell types. Overall, Tr1 induction and maintenance appears to depend on antigen stimulation, IL10 or IL27 exposure, and sustained autocrine stimulation via IL10 and/or IL21.
In addition to roles for AHR and c-MAF described above, recent evidence demonstrates the importance of the transcription factor EOMES at mediating Tr1 development, and in particular, at inducing cytotoxic potential among Tr1s [reviewed in (83)]. The majority of evidence to date has not clarified whether Tr1 cells are a distinct differentiation lineage, or simply a transient activation state. Recent evidence however posits that human EOMES+ Tr1s are a unique lineage of GZMK+ CD4+ T-cells (84). As described above, EOMES and TBET have a high degree of homology but have differential expression patterns; TBET is expressed in most CD4+ T-cells early during acute inflammation, and in particular under Th1-polarizing conditions. In contrast, EOMES induction seems to occur only after prolonged inflammation, e.g. >30 days in LCMV mouse models. EOMES, in concert with TBET, induces both Il10 expression and the cytotoxicity genes GzmB, Nkg7, Prf1; in human Tr1s GzmK is induced via direct binding of TBET and EOMES to its promoter. EOMES has been proposed as a possible lineage-defining factor for Tr1 cells, which is congruent with its capacity to block Foxp3 expression (32, 84–86). However, EOMES is not specific to Tr1-cells, and not all reported Tr1-like cells express EOMES, confounding efforts to perform lineage tracing based solely on this marker (75). Fate-mapping experiments, ideally using dual-reporter FOXP3/IL10 mice should conclusively reveal the origins of these cells under different contexts.
Tr1s expressing granzymes, perforin, and/or granulysin have been observed to mediate direct cytolysis of myeloid cells. Most studies of Tr1 cytotoxicity have focused on their capacity to induce death of myeloid APCs. Clinical-grade ex-vivo-expanded Tr1 cells with efficacy in suppressing Crohn’s disease were found to be cytotoxic towards myeloid cells over a timespan of several days (87). This effect was independent of perforin, but dependent on the activity of granzymes as well as granulysin, an antimicrobial peptide (88). Additionally, it was observed that IL10-producing CD4+ T-cells, expanded in vitro, were capable of killing APCs that presented islet autoantigens in an MHCII context, presumably preventing the development of islet autoantigen-specific T-effector cells in a linked-recognition mechanism (89). However, the majority of CD4+ T-cells examined were FOXP3+, suggesting that Tr1s were a minor contributor to this particular effect. A further nuance to many of these studies it that they rely on stimulation with anti-CD3 antibodies, which triggers expression of granzyme B in most T-cells (90). These caveats prompt the question of whether Tr1-mediated cytotoxicity has physiological relevance in unmanipulated in-vivo settings. EOMES+ Tr1 cells expressing granzymes and CD107a are overrepresented in patients with chronic lymphocytic leukemia (CLL) and B cell acute lymphoblastic leukemia (B-ALL) (91, 92). Cytotoxic CD4+ T-cells are also found in multiple solid tumor settings, but whether these are predominantly Tr1 cells is not established [reviewed in (19)]. FOXP3+ Tregs are also reportedly cytotoxic, in a perforin- and/or granzyme B-dependent manner (89, 93). Overall, it appears that Tr1s may induce direct cytotoxicity, particularly towards professional antigen-presenting cells, as part of their suppressor and anti-cancer functions. This raises the question as to whether they may also mediate direct cytotoxicity towards alternative antigen-presenting cells such as intestinal epithelial and stem cell populations, as discussed below.
Tolerogenic functions of T-regulatory type 1 cells vs Tregs in the gut
Recent studies have shed light on previously under-appreciated roles for FOXP3- Tr1 cells, vis-à-vis Foxp3+ Tregs, in maintaining gut homeostasis. Although both Tr1 and Treg cells play important roles in dampening inflammation and mitigating dysbiosis, subtle differences in their anatomic distributions may point to non-overlapping functions. In the lamina propria of mice, Tr1s comprise the major IL10 producing subset of the small intestine, while FOXP3+ Tregs are more common in the large intestine (94). Similarly, chronic stimulation of T-cells in IL10-reporter mice in vivo demonstrated predominant induction of Tr1 cells in the small intestine but FOXP3+ Tregs in the colon (95). In mouse models of bone marrow transplant EOMES+ Tr1 cells outnumber FOXP3+ Tregs in the lamina propria by about 4:1, and their presence is critical for the prevention of Graft-versus-host disease (86). Tr1 cells prevent colitis in adoptive transfer models, and pathogenic colitis-inducing Th17 cells are suppressed by both Foxp3+ and Foxp3- IL10-producing CD4+ T-cells (75, 77, 96). Studies of tissues from patients with Inflammatory Bowel Disease (IBD) found that an EOMES+ IL7R- CCR5+ Tr1 population is diminished in the inflamed intestinal lamina propria of patients with IBD, relative to matched healthy control tissue (84). Patients with Inflammatory Bowel Disease are known to have selective loss of IL10 secretion from Tr1 cells, while IL10 production from FOXP3+ Tregs appears unaffected, further emphasizing a specific importance of Tr1s to IBD (97). Importantly, adoptively transferred CD4+ FOXP3- cells are able to convert to FOXP3+ cells in recipient animals, but FOXP3+ cells were not observed to convert into FOXP3- cells, implying that Treg to Tr1 conversion is rare (80). TCR clonotype sharing has been observed between Tr1 and Th1 T cells but not FOXP3+ Tregs in colorectal cancer (98). Finally, peripherally-induced Tregs have a more defined role against foreign antigens, including the commensal bacteria found at higher concentrations in the large bowel, while Tr1s mediate activity against self-antigens. Thus, evidence to date suggests that Tr1 and Foxp3+ T-cells have distinct lineage origins, and rarely interconvert, but serve similar functional roles in distinct anatomic locations. Further research is needed to fully define non-redundant functions between these cell lineages.
Crosstalk between cytotoxic CD4 subsets and gut epithelial cells, and the potential for cancer initiation
Emerging evidence demonstrates that CD4+ T-cells influence the function and differentiation trajectories of intestinal stem cells (ISCs). ISCs reside in the crypt base and are surrounded by either Paneth cells in the small intestine or deep crypt secretory cells in the colon. They initially develop into progenitors called transient amplifying cells (TA), that proliferate and further differentiate into secretory cells (Paneth, goblet, enteroendocrine, and tuft) and absorptive cell types (M cells, and enterocytes). WNT, NOTCH, and BMP pathways converge to maintain the balance between self-renewal and progressive differentiation of ISCs. Reserve pools of slow-cycling cells reside just above the crypt base, and are able to reconstitute the ISC population after injury (53, 99–103). Additionally, lineage-committed progenitors and terminally differentiated cells may dedifferentiate into stem-like cells, thereby serving as extra “reserve” cells for ISC renewal (104). Overall, the gut epithelium is maintained via a complex milieu of progenitor cell types that can deviate from a hierarchical maturation pattern under conditions of inflammation or injury. At several steps in this process, perturbations in CD4+ T-cell-epithelial interactions may influence cancer initiation and progression.
CD4+ T-cells coordinate ISC differentiation fates that are appropriate to the type of pathogen encounter or inflammatory stimulus. This was showed elegantly by Biton et al, who used single-cell methods to determine that intestinal stem cells are comprised of at least 3 distinct subsets, ISC-I, ISC-II-, and ISC-III (105). Each ISC subset expressed MHCII molecules and presented antigens to CD4+ T-cells. Co-culture with different Th cell types skewed terminal differentiation towards different terminal states; for example, Th1 co-cultured ISCs differentiated towards Paneth and goblet cell lineages, while Th17 cells induced a TA cell fate. IL10 or co-culture with inducible IL10-producing Tregs led to ISC self-renewal and expansion, while co-culture with other Th cytokines downregulated self-renewal in favor of increased differentiation. The provision of IL10 by CD4+ T-cells in the ISC niche is reminiscent of recent independent findings of a similar phenomenon in the hematopoietic stem cell niche (106). It is intriguing to speculate that CD4 provision of IL10 may be a common phenomenon in all stem cell niches. IL10 provision in this niche may maladaptively contribute to early immune evasion by inhibiting the activity of cytotoxic T-cells that could otherwise remove mutation-harboring stem/progenitor cells. While this mechanism requires cognate recognition of MHCII expressing-ISCs, loss of MHCII expression from IECs/ISCs may also increase carcinogenesis. In one study, mice fed a high-fat diet developed alterations in commensal bacterial populations, leading to reduced IFNγ signaling and downregulation of immunomodulatory (H2-Aa,H2-Ab1,Ciita) and costimulatory molecules (Icosl,Sectm1a,Sectm1b) on ISCs/IECs (107). MHC class II-negative (MHC-II-) ISCs lacking the tumor suppressor APC, coupled with a high fat diet, exhibited greater tumor-initiating capacity than their MHC class II-positive (MHC-II+) counterparts. This suggests that surveillance of MHCII-expressing ISCs/IECs may be an important mechanism of cancer prevention, and suggests a possible mechanistic link between high-fat diets, shifts in commensal bacterial populations, and colon cancer initiation.
Further evidence supporting the importance of CD4+ T-cells in cancer initiation comes from a recent large atlas study of colonic epithelium (108). Mice in which the tumor suppressor APC gene was conditionally deleted in their ISCs (LGF5-CreERt2;ApcL/L) exhibited 2-fold greater tumorigenicity in an orthotopic, syngeneic colon transplantation assay. The greater rate of tumor incidence was not observed when transplanted into mice deficient in B- and T-cells, supporting the role of adaptive immune cells at preventing tumor initiation. In subsequent experiments, APC-mutated colon cancers were then initiated from either stem cells or differentiated cell precursors. Strikingly, despite identical mutational processes, these tumor types developed divergent immune microenvironments. Stem-cell-originating cancer cells co-localized with CD4+ T-cells expressing a high frequency of FOXP3+ cells, as well as upregulation of genes associated with exhaustion including Pdcd1, Ctla4, and Havcr2 (TIM3). GZMB+ T-cells were relatively infrequent. Mechanistically, Tregs may constrain cytotoxic CD4+ T-cell development in this context by sequestering excess IL2, thereby preventing BLIMP-1 and GZMB expression (35). In contrast to stem-cell originating cancer cells, tumor cells originating from differentiated cells expressed more robust antigen-presenting machinery and were enriched with a relatively high frequency of cytotoxic CD4+ and CD8+ T-cells. Differences in these mouse tumor models correlate with divergent human precancerous lesions – specifically, serrated polyps and conventional adenomas. Thus, the degree of infiltration by cytotoxic, IFNγ-rich T-cells in early cancer lesions reflects the inherent stemness and antigen-presenting capacity of the tumors cell of origin; these poised cytotoxic T-cells may be an independent variable, aside from tumor mutational burden, that affects clinical responsiveness to checkpoint blockade (109).
A final mechanism by which cytotoxic CD4+ T-cells contribute to tumorigenesis in the gut may be via chronic inflammatory signaling. Regulatory T-cell populations, including Tr1s, are depleted in inflammatory bowel disease (IBD), while inflammatory and cytotoxic CD4+ T-cells are relatively overrepresented (110). Inflammatory cytokines including IFNγ and TNF can damage the Paneth cell niche and T-cell-delivered IFNγ can delete ISCs via activation of JAK1/STAT1-mediated apoptosis (111–113). Recognition of ISCs/IECs by CD4+ T-cells can occur via MHCII-independent mechanisms. For example, in Crohn’s disease a unique population of CD4+ NKG2D+ lamina propria lymphocytes were found to functionally interact with epithelial cells via recognition of the stress protein MICA (114). Congruently, therapeutic blockade of αEβ7+ receptors, which is a promising clinical strategy in IBD, leads to greater efflux of cytotoxic/proinflammatory CD8+ and CD4+ T cells from the gut to the draining lymph nodes. This treatment does not affect T-regulatory cells, which express low levels of αEβ7+ (115). Additional studies are needed to determine whether strategies which diminish chronic inflammation in the gut lower the risk of colon cancer associated with IBD.
Role of cytotoxic CD4 T cells in immune responses against cancer
Importance of cytotoxic CD4 T cells in anti-tumor immune responses
A plethora of studies have described CD8 T cells as being the main T cell subset responsible for killing tumor cells and thus a critical component of anti-tumor immune responses. Within this framework, Th1-like helper CD4 T cells have been shown to be important for effective priming of anti-tumor CD8 T cells while CD4+ regulatory T cells have been shown to impede priming and effector function of anti-tumor CD8 T cells (116, 117). This is true both in the context of self/tumor-associated antigens, and mutation-derived neoantigens (116, 118). Indeed, a lower ratio of Tregs to non-Tregs has been associated with improved prognosis in some, but not all cancers (119). While CD4 T cells have been typically relegated to the ‘supporting cast’ of anti-tumor immune cells, studies in the last decade or so have shown that CD4 T cells with cytolytic properties are capable of being the main tumor-killing cell type in effective anti-tumor immune responses. The earliest evidence of this was studies by Jim Allison and Paul Antony showing that tumor-specific CD4 T cells transferred into lymphopenic hosts developed a cytotoxic phenotype upon tumor challenge (120, 121). These cytotoxic CD4 T cells were sufficient to kill tumor cells in a MHCII-dependent manner.
While the above studies were done in murine models of melanoma, this has also been shown in cancers of the mucosa such as mismatch repair sufficient colorectal cancer. This is a subtype of colorectal cancer that is associated with significantly worse outcomes than mismatch repair-deficient colorectal cancer. A recent study showed that GZMB+ cytotoxic CD4s that infiltrated into the center of the tumor were associated with improved prognosis in patients with MMR-sufficient colorectal cancer (122). Furthermore, the beneficial effects of neoadjuvant chemotherapy were associated with infiltration of cytotoxic CD4 T cells into the tumor center. Similar findings were also shown in patients with bladder cancer, where clonally expanded cytotoxic CD4 T cells were enriched in the tumor vs the surrounding healthy tissue and predicted response to anti-PD1 blockade (123). Interestingly in contrast to CD8 T cells, cytotoxic CD4 T cells were shown to be distinct subset from exhausted CD4 T cells. Apart from primary tumors, tumor-specific cytolytic CD4 T cells have been shown to be important for control of lung tumor metastases (124).
Cancer-associated cytotoxic CD4s share features with canonical T helper subsets
As mentioned before, cytotoxic CD4 T cells have been shown to have features of different helper T cell subsets - this has also been seen in cancer patients. The presence of cytotoxic CD4 T cells that have shared TCR clones with multiple helper T cell subsets suggests that the cytotoxic function in CD4 T cells is regulated independently of their helper function in cancer. Indeed, a recent study showed that IL2 and BLIMP1 induced cytotoxic function in tumor-specific CD4 T cells, and that this occurred independent of TBET-mediated induction of a Th1 polarization state (35). A recent study has shown that CD4 T cells specific for the cancer-testis antigen NY-ESO express genes related to multiple helper T cell subsets at levels comparable to conventional helper T cells (24). Furthermore, cytotoxic CD4 T cells from patients with breast cancer, liver cancer and head and neck cancer had significant clonal overlap with non-cytotoxic Th1, Th2 and Th17 CD4 T cells (24). Thus, cytotoxic CD4 T cells in cancer have attributes of other helper T cell subsets.
Pro-tumor and anti-tumor role of cytotoxic FOXP3− regulatory (Tr1) T cells in cancer
As mentioned before, cytotoxic CD4 T cells are capable of exhibiting attributes of various helper T cell subsets. However, immune-suppressive CD4 T cell subsets have also been shown to have cytotoxic function. These include FOXP3+ regulatory T cells (Tregs) and FOXP3− type-1 regulatory (Tr1) cells (47, 48). The role of Tregs, including their cytotoxic function, in anti-tumor immune responses has been extensively discussed in other reviews (125, 126). While FOXP3− type-1 regulatory (Tr1) cells have typically been associated with impairment of anti-tumor immune responses, recent studies have shown that Tr1 cells with cytotoxic function have context-dependent pro-tumor or anti-tumor roles (summarized in Figure 2). The most prominent studies highlighting the anti-tumor role for cytotoxic Tr1-like T cells comes from acute myeloid leukemia (AML), where cytotoxic Tr1 cells are known to efficiently kill myeloid leukemia cells while also suppressing Graft-Versus-Host Disease in bone marrow transplant patients (48, 127, 128). Interestingly although the Tr1-cells specifically killed AML blasts, this cytotoxicity was antigen-independent but MHC (HLA)-class I dependent (127). The cytotoxic activity of cytotoxic Tr1 cells against myeloid cells has also been extended to tumor-associated macrophages. Indeed in metastatic melanoma, cytotoxic GZMB+ Tr1 cells but not Tregs inhibit macrophage-mediated tumor growth via direct killing of the tumor-promoting macrophages (129). However, the cytotoxic Tr1-cells can also kill antigen-presenting cells that are critical for priming anti-tumor immune responses (130). Indeed, cytotoxic GZMK+ EOMES+ Tr1 cells have been associated with tumor progression in colorectal cancer, non-small-cell lung cancer, and in liver metastases (91, 98). Whether or not cytotoxic Tr1 cells equally target pro-tumor and anti-tumor myeloid cell subsets is still unclear. Additionally, the immune-suppressive impact of the Tr1-produced cytokine IL10 likely results in a net pro-tumor effect of the cytotoxic Tr1 cells (98). Thus, although cytotoxic Tr1-like cells have pro-tumor and anti-tumor roles, they likely have a net pro-tumor effect in the absence of immunotherapy.
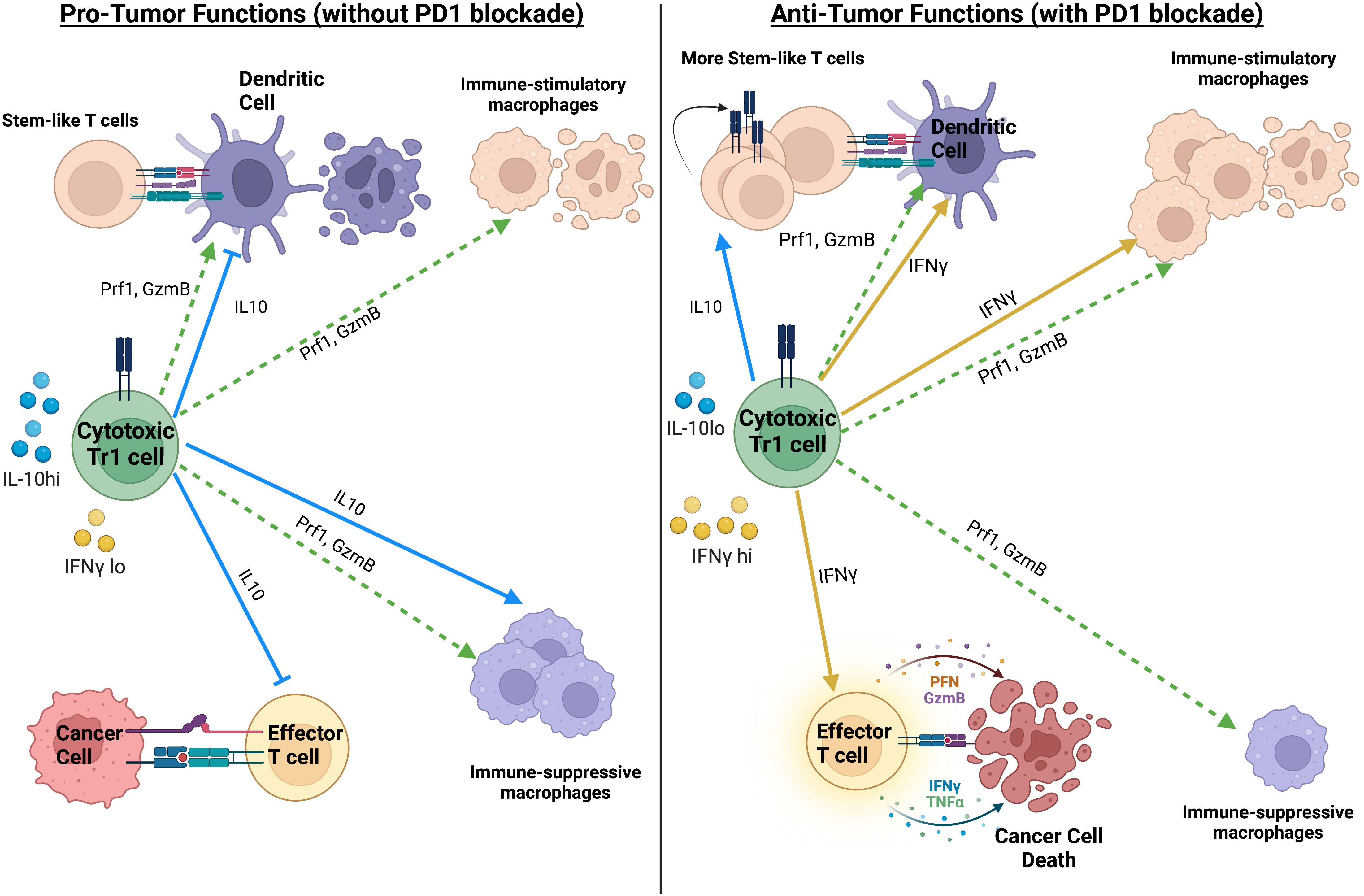
Figure 2 Summary of the pro-tumor and anti-tumor impact of Tr1-like cytotoxic CD4 T cells, in the absence or presence of PD1 blockade. The net impact of Tr1-like cytotoxic CD4 T cells on anti-tumor immunity likely depends on the ratio of IL10 to IFNγ. Created with BioRender.com.
Importance of cytotoxic CD4 T cells for response to checkpoint blockade therapy
Various studies have suggested a critical role for cytolytic CD4 T cells in response to PD1/PDL1 based checkpoint blockade therapies. In bladder cancer, a cytotoxic CD4 T cell gene signature strongly predicts response to atezolizumab (anti-PDL1) (131). The cytotoxic CD4 T cells from the bladder cancer patients directly killed matched tumor blasts in an MHCII-dependent manner. Furthermore, the association between cytotoxic CD4 T cells and response to anti-PDL1 therapy only held in patients with immune-inflamed tumors, not in immune excluded or desert tumors where the CD4 T cells would not be in contact with the tumor cells (131). These two observations suggest that direct killing of tumor cells by cytotoxic CD4 T cells was important for the efficacy of anti-PDL1 in bladder cancer. Similar observations have also been made in Hodgkin’s lymphoma and colorectal cancer in the context of PD1 blockade (98, 132, 133). Those studies suggest that cytotoxic CD4 T cells are important for killing tumor cells resistant to killing by CD8 T cells. However, the relative importance of helper vs cytotoxic functions of CD4 T cells in those contexts is still unclear. We addressed this question using a murine leukemia model, where we showed that leukemia elicited CD4 T cells with both a Th1-like helper and a cytotoxic phenotype (92). A combination of a targeted therapy and PDL1 blockade elicited clonal expansion of leukemia-specific helper-cytotoxic CD4 T cells, which resulted in a significant survival benefit relative to treatment with the targeted therapy or PDL1 blockade alone. Interestingly, the combination therapy had minimal impact on the expression of cytotoxic molecules in the leukemia specific CD4 T cells. Instead, molecules associated with improved helper function and immune cell recruitment such as CCL4 and CD40L were significantly increased in the therapy-expanded CD4 T cells, which was associated with an improved expansion of GZMB+ CD8 T cells. Depletion of CD4 and CD8 T cells also resulted in a loss of treatment efficacy, suggesting that cytotoxic CD8 T cells expanded with signals from helper-cytotoxic CD4 T cells were required for the treatment efficacy. However, CD4 T cell depletion resulted in unform lethality while CD8 T cell depletion had a less penetrant but still significant impact on survival. This suggested that although the helper function of CD4 T cells was the major driver of anti-leukemia immunity, the cytotoxic function of the CD4 T cells likely still played an important role in leukemia clearance (92).
Interestingly in colorectal cancer, cytotoxic CD4 T cells with a Tr1-like phenotype are positively correlated with response to PD1 blockade, which was in direct contrast to their negative association with survival in the absence of PD1 blockade (98). The Tr1-like cytotoxic CD4 T cells in colorectal cancer were suggested to differentiate from Th1-like cytotoxic CD4 T cells in the tumor (98). Thus, we hypothesize that the anti-tumor role of cytotoxic Tr1 cells in this context is driven by the ability of PD1 blockade to preferentially enhance production of Th1 cytokines such as IFNγ over IL10 in Tr1-like CD4 T cells from human cancer samples (134). We hypothesize that this change in the ratio of IFNγ to IL10 in the cytotoxic Tr1-like cells plays a key role in their anti-tumor role in the presence of PD1 blockade (see Figure 2). IL10 is known to drive macrophage polarization towards a pro-tumor regulatory M2-like phenotype while IFNγ is known to drive macrophage polarization towards a stimulatory anti-tumor M1-like phenotype (135). Furthermore, IL10 inhibits the maturation of dendric cells (136) while IFNγ promotes the expression of MHCII on dendritic cells (137). In limited quantities, IL10 has beneficial anti-tumor effects by promoting the maintenance of stem-like CD8 T cells (138). PD1 blockade has been shown to recruit stem-like CD8 T cells to tumors and draining lymph-nodes, where their maintenance is essential for durable anti-tumor immune responses (139–142). Thus, the cytotoxic CD4 T cells in colorectal cancer are skewed towards a Tr1-like suppressive phenotype in the absence of treatment, while PD1 blockade can convert the pro-tumor Tr1-like cytotoxic CD4 T cells towards a more anti-tumor Th1-like phenotype. The ratio of IL10 to IFNγ produced by the Tr1-like cytotoxic CD4 T cells could be a key determinant in whether Tr1-like cytotoxic CD4 T cells have a net pro-tumor or anti-tumor impact (summarized in Figure 2).
Cytotoxic CD4+ CAR T cells have improved persistence in cancer
Cytotoxic CD4 T cells have also shown to play key roles in long-term protection in the context of CAR T cell therapy. While CD8+ CAR T cells are highly efficient at killing target cells, they are more prone to activation-induced cell death that impacts their persistence. In contrast, CD4+ CAR T cells are less efficient at killing target cells but are less prone to activation-induced cell death (143). Indeed, CD4+ CAR T cells are shown to be more effective at persisting and in mediating protection against liquid and solid tumors in-vivo, in part due to their greater resistance to exhaustion vis-à-vis CD8+ CAR T cells (144, 145). Recent data from June and colleagues showed that CD4+ CAR T cells with a Tr1-like phenotype could be beneficial for long-term protection in-vivo (26). The authors tracked the proportion of CD4+ and CD8+ CAR T cells over time in patients with CLL. Although CD8+ CAR T cells dominated early on, the CD4+ CAR T cells eventually took over and were associated with long-term tumor control (26). A significant proportion of the CD4+ CAR T cells in the long-term survivors had a Tr1-like phenotype, including expression of IL10, EOMES and granzyme K (26). This suggests that a Tr1 phenotype is beneficial for persistence and long-term protection in the context of CAR T cell therapy. While CD4+ CAR T cells have been shown to persist longer, they have also been shown to induce a stronger cytokine release syndrome (146). Thus, the therapeutic utility of Tr1-like CD4+ CAR T cells in balancing tumor killing with mitigation of cytokine release syndrome would be worth exploring.
Conclusions and future directions
Cytotoxic potential, as implied by the expression of granzymes, perforin, FasL, and/or granulysin, manifests in alpha/beta CD4+ T-cells in a variety of anatomical sites and inflammatory states. Cytotoxic potential has been observed in all known CD4+ memory/effector subsets, including FOXP3+ T-regulatory cells, Tr1s, Th1, Th2, Th17, and among non-classical subsets. Regardless of the specific CD4+ T-cell subset involved, cytotoxicity typically requires TCR signaling via recognition of cognate peptide-MHCII, context-specific inflammatory cues, and upregulation of transcriptional programs closely shared with CD8+ cytotoxic lymphocytes. While no master transcription factor is singly responsible for inducing cytotoxicity, key roles have been identified for EOMES, BLIMP1, HOBIT, and RUNX3. Particularly in the mucosal epithelium of the gut, cytotoxicity is linked with downregulation of the critical CD4-identifying factor THPOK, and upregulation of CD8αα. The breadth of observed cytotoxic CD4+ T-cell activity suggests that CD4s that mediate cell killing have unique functions from CD8+ T-cells, NK cells, or other cytotoxic cell types. Alternatively, cytotoxic potential may sometimes occur as a secondary consequence of stimulation, but not result in direct cell killing. Recognition of cognate peptides presented in an MHCII context is mediated principally by CD4+ T-cells and represents a setting where cytotoxic CD4+ T-cells would be expected to have a unique advantage. Confirming this, cytotoxic CD4+ T-cells are now known to directly target immune cells that constitutively express MHCII molecules. Of greater importance are observations that non-immune cells upregulate MHCII in inflammatory settings, resulting in CD4-mediated cell killing of epithelial and mesenchymal targets. These findings complement others showing that cytotoxic CD4+ T cells play important roles in infection, autoimmunity, and in both hematologic and solid malignancies.
Several outstanding questions remain in the field. First, how does cytotoxicity delivered by CD4+ T-cells affect downstream immunity? For instance, what is the impact of CD4 T cell-mediated killing of APCs? Secondly, what are the ontogenies and distinct functions of FOXP3+ and FOXP3– regulatory T-cells that develop cytotoxic potential? Thirdly, what is the significance of cytotoxic CD4 T cells during ageing (147)? Is their increase simply a consequence of inflammaging; or do they have functional roles in controlling reactivated chronic viruses and/or removing senescent cells? Finally, what are the relative contributions of cytotoxic CD4s in anti-cancer immune therapies? The answers to these questions will illuminate the functional significance of this enigmatic CD4-T cell type.
Author contributions
The manuscript was written by HV and ST. MF edited and revised the manuscript before submission.
Conflict of interest
The authors declare that the research was conducted in the absence of any commercial or financial relationships that could be construed as a potential conflict of interest.
Publisher’s note
All claims expressed in this article are solely those of the authors and do not necessarily represent those of their affiliated organizations, or those of the publisher, the editors and the reviewers. Any product that may be evaluated in this article, or claim that may be made by its manufacturer, is not guaranteed or endorsed by the publisher.
References
1. Mosmann TR, Coffman RL. Th1 and Th2 cells: Different patterns of lymphokine secretion lead to different functional properties. Annu Rev Immunol (1989) 7(1):145–73. doi: 10.1146/annurev.iy.07.040189.001045
2. Crotty S. Follicular helper Cd4 T cells (Tfh). Annu Rev Immunol (2011) 29:621–63. doi: 10.1146/annurev-immunol-031210-101400
3. Steinman L. A brief history of T(H)17, the first major revision in the T(H)1/T(H)2 hypothesis of T cell-mediated tissue damage. Nat Med (2007) 13(2):139–45. doi: 10.1038/nm1551
4. Sakaguchi S. Regulatory T cells: History and perspective. Methods Mol Biol (Clifton NJ) (2011) 707:3–17. doi: 10.1007/978-1-61737-979-6_1
5. Wagner H, Götze D, Ptschelinzew L, Röllinghoff M. Induction of cytotoxic T lymphocytes against I-Region-Coded determinants: In vitro evidence for a third histocompatibility locus in the mouse. J Exp Med (1975) 142(6):1477–87. doi: 10.1084/jem.142.6.1477
6. Billings P, Burakoff S, Dorf ME, Benacerraf B. Cytotoxic T lymphocytes specific for I region determinants do not require interactions with h-2k or d gene products. J Exp Med (1977) 145(5):1387–92. doi: 10.1084/jem.145.5.1387
7. Billings P, Burakoff S, Dorf ME, Benacerraf B. Cytotoxic T lymphocytes induced against allogeneic I-region determinants react with ia molecules on trinitrophenyl-conjugated syngeneic target cells. J Exp Med (1977) 146(2):623–28. doi: 10.1084/jem.146.2.623
8. Feighery C, Stastny P. Hla-d region-associated determinants serve as targets for human cell-mediated lysis. J Exp Med (1979) 149(2):485–94. doi: 10.1084/jem.149.2.485
9. Lukacher AE, Morrison LA, Braciale VL, Malissen B, Braciale TJ. Expression of specific cytolytic activity by h-2i region-restricted, influenza virus-specific T lymphocyte clones. J Exp Med (1985) 162(1):171–87. doi: 10.1084/jem.162.1.171
10. Maimone MM, Morrison LA, Braciale VL, Braciale TJ. Features of target cell lysis by class I and class ii mhc-restricted cytolytic T lymphocytes. J Immunol (Baltimore Md 1950) (1986) 137(11):3639–43.
11. van Leeuwen EM, Remmerswaal EB, Heemskerk MH, ten Berge IJ, van Lier RA. Strong selection of virus-specific cytotoxic Cd4+ T-cell clones during primary human cytomegalovirus infection. Blood (2006) 108(9):3121–7. doi: 10.1182/blood-2006-03-006809
12. Jackson SE, Sedikides GX, Mason GM, Okecha G, Wills MR. Human cytomegalovirus (Hcmv)-specific Cd4+ T cells are polyfunctional and can respond to hcmv-infected dendritic cells in vitro. J Virol (2017) 91(6):e02128-16. doi: 10.1128/JVI.02128-16
13. Appay V, Zaunders JJ, Papagno L, Sutton J, Jaramillo A, Waters A, et al. Characterization of Cd4+ ctls ex vivo. J Immunol (Baltimore Md 1950) (2002) 168(11):5954–8. doi: 10.4049/jimmunol.168.11.5954
14. Johnson S, Eller M, Teigler JE, Maloveste SM, Schultz BT, Soghoian DZ, et al. Cooperativity of hiv-specific cytolytic Cd4 T cells and Cd8 T cells in control of hiv viremia. J Virol (2015) 89(15):7494–505. doi: 10.1128/JVI.00438-15
15. Aslan N, Yurdaydin C, Wiegand J, Greten T, Ciner A, Meyer MF, et al. Cytotoxic Cd4 T cells in viral hepatitis. J Viral Hepatitis (2006) 13(8):505–14. doi: 10.1111/j.1365-2893.2006.00723.x
16. Verma S, Weiskopf D, Gupta A, McDonald B, Peters B, Sette A, et al. Cytomegalovirus-specific Cd4 T cells are cytolytic and mediate vaccine protection. J Virol (2015) 90(2):650–8. doi: 10.1128/JVI.02123-15
17. Brown DM, Lee S, Garcia-Hernandez Mde L, Swain SL. Multifunctional Cd4 cells expressing gamma interferon and perforin mediate protection against lethal influenza virus infection. J Virol (2012) 86(12):6792–803. doi: 10.1128/JVI.07172-11
18. Hua L, Yao S, Pham D, Jiang L, Wright J, Sawant D, et al. Cytokine-dependent induction of Cd4+ T cells with cytotoxic potential during influenza virus infection. J Virol (2013) 87(21):11884–93. doi: 10.1128/JVI.01461-13
19. Oh DY, Fong L. Cytotoxic Cd4+ T cells in cancer: Expanding the immune effector toolbox. Immunity (2021) 54(12):2701–11. doi: 10.1016/j.immuni.2021.11.015
20. Park S, Anderson NL, Canaria DA, Olson MR. Granzyme-Producing Cd4 T Cells Cancer Autoimmune Disease. ImmunoHorizons (2021) 5(12):909–17. doi: 10.4049/immunohorizons.2100017
21. Loebbermann J, Thornton H, Durant L, Sparwasser T, Webster KE, Sprent J, et al. Regulatory T cells expressing granzyme b play a critical role in controlling lung inflammation during acute viral infection. Mucosal Immunol (2012) 5(2):161–72. doi: 10.1038/mi.2011.62
22. Gregori S, Goudy KS, Roncarolo MG. The cellular and molecular mechanisms of immuno-suppression by human type 1 regulatory T cells. Front Immunol (2012) 3:30. doi: 10.3389/fimmu.2012.00030
23. Ng SS, De Labastida Rivera F, Yan J, Corvino D, Das I, Zhang P, et al. The nk cell granule protein Nkg7 regulates cytotoxic granule exocytosis and inflammation. Nat Immunol (2020) 21(10):1205–18. doi: 10.1038/s41590-020-0758-6
24. Cachot A, Bilous M, Liu YC, Li X, Saillard M, Cenerenti M, et al. Tumor-specific cytolytic Cd4 T cells mediate immunity against human cancer. Sci Adv (2021) 7(9):eabe3348. doi: 10.1126/sciadv.abe3348
25. Hidalgo LG, Einecke G, Allanach K, Halloran PF. The transcriptome of human cytotoxic T cells: Similarities and disparities among allostimulated Cd4(+) ctl, Cd8(+) ctl and nk cells. Am J Transplant Off J Am Soc Transplant Am Soc Transplant Surgeons (2008) 8(3):627–36. doi: 10.1111/j.1600-6143.2007.02128.x
26. Melenhorst JJ, Chen GM, Wang M, Porter DL, Chen C, Collins MA, et al. Decade-long leukaemia remissions with persistence of Cd4+ car T cells. Nature (2022) 602(7897). doi: 10.1038/s41586-021-04390-6
27. Kotov DI, Kotov JA, Goldberg MF, Jenkins MK. Many Th cell subsets have fas ligand-dependent cytotoxic potential. J Immunol (Baltimore Md 1950) (2018) 200(6):2004–12. doi: 10.4049/jimmunol.1700420
28. Thomas WD, Hersey P. Tnf-related apoptosis-inducing ligand (Trail) induces apoptosis in fas ligand-resistant melanoma cells and mediates Cd4 T cell killing of target cells. J Immunol (Baltimore Md 1950) (1998) 161(5):2195–200.
29. Yang D, Tian Z, Zhang M, Yang W, Tang J, Wu Y, et al. Nkg2d+Cd4+ T cells kill regulatory T cells in a Nkg2d-Nkg2d ligand- dependent manner in systemic lupus erythematosus. Sci Rep (2017) 7(1):1288. doi: 10.1038/s41598-017-01379-y
30. Curran MA, Geiger TL, Montalvo W, Kim M, Reiner SL, Al-Shamkhani A, et al. Systemic 4-1bb activation induces a novel T cell phenotype driven by high expression of eomesodermin. J Exp Med (2013) 210(4):743–55. doi: 10.1084/jem.20121190
31. Qui HZ, Hagymasi AT, Bandyopadhyay S, St Rose MC, Ramanarasimhaiah R, Ménoret A, et al. Cd134 plus Cd137 dual costimulation induces eomesodermin in Cd4 T cells to program cytotoxic Th1 differentiation. J Immunol (Baltimore Md 1950) (2011) 187(7):3555–64. doi: 10.4049/jimmunol.1101244
32. Thelen B, Schipperges V, Knörlein P, Hummel JF, Arnold F, Kupferschmid L, et al. Eomes is sufficient to regulate il-10 expression and cytotoxic effector molecules in murine Cd4+ T cells. Front Immunol (2023) 14:1058267. doi: 10.3389/fimmu.2023.1058267
33. Serroukh Y, Gu-Trantien C, Hooshiar Kashani B, Defrance M, Vu Manh TP, Azouz A, et al. The transcription factors Runx3 and thpok cross-regulate acquisition of cytotoxic function by human Th1 lymphocytes. eLife (2018) 7:e30496. doi: 10.7554/eLife.30496
34. Oja AE, Vieira Braga FA, Remmerswaal EB, Kragten NA, Hertoghs KM, Zuo J, et al. The transcription factor hobit identifies human cytotoxic Cd4+ T cells. Front Immunol (2017) 8:325. doi: 10.3389/fimmu.2017.00325
35. Śledzińska A, Vila de Mucha M, Bergerhoff K, Hotblack A, Demane DF, Ghorani E, et al. Regulatory T cells restrain interleukin-2- and blimp-1-Dependent acquisition of cytotoxic function by Cd4+ T cells. Immunity (2020) 52(1):1–16. doi: 10.1016/j.immuni.2019.12.007
36. Broux B, Mizee MR, Vanheusden M, van der Pol S, van Horssen J, Van Wijmeersch B, et al. Il-15 amplifies the pathogenic properties of Cd4+Cd28- T cells in multiple sclerosis. J Immunol (Baltimore Md 1950) (2015) 194(5):2099–109. doi: 10.4049/jimmunol.1401547
37. Mackay LK, Minnich M, Kragten NA, Liao Y, Nota B, Seillet C, et al. Hobit and Blimp1 instruct a universal transcriptional program of tissue residency in lymphocytes. Sci (New York NY) (2016) 352(6284):459–63. doi: 10.1126/science.aad2035
38. Kragten NAM, Behr FM, Vieira Braga FA, Remmerswaal EBM, Wesselink TH, Oja AE, et al. Blimp-1 induces and hobit maintains the cytotoxic mediator granzyme b in Cd8 T cells. Eur J Immunol (2018) 48(10):1644–62. doi: 10.1002/eji.201847771
39. Zundler S, Becker E, Spocinska M, Slawik M, Parga-Vidal L, Stark R, et al. Hobit- and blimp-1-Driven Cd4+ tissue-resident memory T cells control chronic intestinal inflammation. Nat Immunol (2019) 20(3). doi: 10.1038/s41590-018-0298-5
40. Cruz-Guilloty F, Pipkin ME, Djuretic IM, Levanon D, Lotem J, Lichtenheld MG, et al. Runx3 and T-box proteins cooperate to establish the transcriptional program of effector ctls. J Exp Med (2009) 206(1):51–9. doi: 10.1084/jem.20081242
41. Mucida D, Husain MM, Muroi S, van Wijk F, Shinnakasu R, Naoe Y, et al. Transcriptional reprogramming of mature Cd4⁺ helper T cells generates distinct mhc class ii-restricted cytotoxic T lymphocytes. Nat Immunol (2013) 14(3):281–9. doi: 10.1038/ni.2523
42. Parry HM, Dowell AC, Zuo J, Verma K, Kinsella FAM, Begum J, et al. Pd-1 is imprinted on cytomegalovirus-specific Cd4+ T cells and attenuates Th1 cytokine production whilst maintaining cytotoxicity. PloS Pathog (2021) 17(3):e1009349. doi: 10.1371/journal.ppat.1009349
43. Takeuchi A, Badr Mel S, Miyauchi K, Ishihara C, Onishi R, Guo Z, et al. Crtam determines the Cd4+ cytotoxic T lymphocyte lineage. J Exp Med (2016) 213(1):123–38. doi: 10.1084/jem.20150519
44. Eshima K, Misawa K, Ohashi C, Iwabuchi K. Role of T-bet, the master regulator of Th1 cells, in the cytotoxicity of murine Cd4+ T cells. Microbiol Immunol (2018) 62(5):348–56. doi: 10.1111/1348-0421.12586
45. Lancki DW, Hsieh CS, Fitch FW. Mechanisms of lysis by cytotoxic T lymphocyte clones. lytic activity and gene expression in cloned antigen-specific Cd4+ and Cd8+ T lymphocytes. J Immunol (Baltimore Md 1950) (1991) 146(9):3242–9.
46. Hou L, Yuki K. Ccr6 and Cxcr6 identify the Th17 cells with cytotoxicity in experimental autoimmune encephalomyelitis. Front Immunol (2022) 13:819224. doi: 10.3389/fimmu.2022.819224
47. Bolivar-Wagers S, Larson JH, Jin S, Blazar BR. Cytolytic Cd4+ and Cd8+ regulatory T-cells and implications for developing immunotherapies to combat graft-Versus-Host disease. Front Immunol (2022) 13:864748. doi: 10.3389/fimmu.2022.864748
48. Chen PP, Cepika AM, Agarwal-Hashmi R, Saini G, Uyeda MJ, Louis DM, et al. Alloantigen-specific type 1 regulatory T cells suppress through ctla-4 and pd-1 pathways and persist long-term in patients. Sci Trans Med (2021) 13(617):eabf5264. doi: 10.1126/scitranslmed.abf5264
49. Jonsson AH, Zhang F, Dunlap G, Gomez-Rivas E, Watts GFM, Faust HJ, et al. Granzyme k+ Cd8 T cells form a core population in inflamed human tissue. Sci Trans Med (2022) 14(649). doi: 10.1126/scitranslmed.abo0686
50. Hoek KL, Greer MJ, McClanahan KG, Nazmi A, Piazuelo MB, Singh K, et al. Granzyme b prevents aberrant il-17 production and intestinal pathogenicity in Cd4+ T cells. Mucosal Immunol (2021) 14(5):1088–99. doi: 10.1038/s41385-021-00427-1
51. Fang M, Siciliano NA, Hersperger AR, Roscoe F, Hu A, Ma X, et al. Perforin-dependent Cd4+ T-cell cytotoxicity contributes to control a murine poxvirus infection. Proc Natl Acad Sci USA (2012) 109(25):9983–8. doi: 10.1073/pnas.1202143109
52. Lin L, Couturier J, Yu X, Medina MA, Kozinetz CA, Lewis DE. Granzyme b secretion by human memory Cd4 T cells is less strictly regulated compared to memory Cd8 T cells. BMC Immunol (2014) 15:36. doi: 10.1186/s12865-014-0036-1
53. Barker N, van Es JH, Kuipers J, Kujala P, van den Born M, Cozijnsen M, et al. Identification of stem cells in small intestine and colon by marker gene Lgr5. Nature (2007) 449(7165):1003–7. doi: 10.1038/nature06196
54. Barker N. Adult intestinal stem cells: Critical drivers of epithelial homeostasis and regeneration. Nat Rev Mol Cell Biol (2014) 15(1):19–33. doi: 10.1038/nrm3721
55. London M, Bilate AM, Castro TBR, Sujino T, Mucida. Stepwise Chromatin D. And transcriptional acquisition of an intraepithelial lymphocyte program. Nat Immunol (2021) 22(4):449–59. doi: 10.1038/s41590-021-00883-8
56. Cheroutre H, Lambolez F, Mucida D. The light and dark sides of intestinal intraepithelial lymphocytes. Nat Rev Immunol (2011) 11(7):445–56. doi: 10.1038/nri3007
57. Bandeira A, Mota-Santos T, Itohara S, Degermann S, Heusser C, Tonegawa S, et al. Localization of Gamma/Delta T cells to the intestinal epithelium is independent of normal microbial colonization. J Exp Med (1990) 172(1):239–44. doi: 10.1084/jem.172.1.239
58. Sujino T, London M, Hoytema van Konijnenburg DP, Rendon T, Buch T, Silva HM, et al. Tissue adaptation of regulatory and intraepithelial Cd4⁺ T cells controls gut inflammation. Sci (New York NY) (2016) 352(6293):1581–6. doi: 10.1126/science.aaf3892
59. Guy-Grand D, Malassis-Seris M, Briottet C, Vassalli P. Cytotoxic differentiation of mouse gut thymodependent and independent intraepithelial T lymphocytes is induced locally. correlation between functional assays, presence of perforin and granzyme transcripts, and cytoplasmic granules. J Exp Med (1991) 173(6):1549–52. doi: 10.1084/jem.173.6.1549
60. Takeuchi A, Saito T. Cd4 ctl, a cytotoxic subset of Cd4+ T cells, their differentiation and function. Front Immunol (2017) 8:194. doi: 10.3389/fimmu.2017.00194
61. Kiner E, Willie E, Vijaykumar B, Chowdhary K, Schmutz H, Chandler J, et al. Gut Cd4+ T cell phenotypes are a continuum molded by microbes, not by Th archetypes. Nat Immunol (2021) 22(2):216–28. doi: 10.1038/s41590-020-00836-7
62. Egawa T, Tillman RE, Naoe Y, Taniuchi I, Littman DR. The role of the runx transcription factors in thymocyte differentiation and in homeostasis of naive T cells. J Exp Med (2007) 204(8):1945–57. doi: 10.1084/jem.20070133
63. Wang L, Wildt KF, Castro E, Xiong Y, Feigenbaum L, Tessarollo L, et al. The zinc finger transcription factor Zbtb7b represses Cd8-lineage gene expression in peripheral Cd4+ T cells. Immunity (2008) 29(6):876–87. doi: 10.1016/j.immuni.2008.09.019
64. Moon S, Park Y, Hyeon S, Kim YM, Kim JH, Kim H, et al. Niche-specific mhc ii and pd-L1 regulate Cd4+Cd8αα+ intraepithelial lymphocyte differentiation. J Exp Med (2021) 218(4). doi: 10.1084/jem.20201665
65. Reis BS, Rogoz A, Costa-Pinto FA, Taniuchi I, Mucida D. Mutual expression of the transcription factors Runx3 and thpok regulates intestinal Cd4⁺ T cell immunity. Nat Immunol (2013) 14(3):271–80. doi: 10.1038/ni.2518
66. Naidoo BT. The cerebrospinal fluid in the healthy newborn infant. South Afr Med J = Suid-Afrikaanse tydskrif vir geneeskunde (1968) 42(35):933–5.
67. Brambilla G, Carlo P, Finollo R, Sciaba L. Dose-response curves for liver DNA fragmentation induced in rats by sixteen n-nitroso compounds as measured by viscometric and alkaline elution analyses. Cancer Res (1987) 47(13):3485–91.
68. Black SH. Preimplantation genetic diagnosis. Curr Opin Pediatr (1994) 6(6):712–6. doi: 10.1097/00008480-199412000-00018
69. Cao X, Cai SF, Fehniger TA, Song J, Collins LI, Piwnica-Worms DR, et al. Granzyme b and perforin are important for regulatory T cell-mediated suppression of tumor clearance. Immunity (2007) 27(4):635–46. doi: 10.1016/j.immuni.2007.08.014
70. Bilate AM, London M, Castro TBR, Mesin L, Bortolatto J, Kongthong S, et al. T Cell receptor is required for differentiation, but not maintenance, of intestinal Cd4+ intraepithelial lymphocytes. Immunity (2020) 53(5):1001–14.e20. doi: 10.1016/j.immuni.2020.09.003
71. Bacchetta R, Bigler M, Touraine JL, Parkman R, Tovo PA, Abrams J, et al. High levels of interleukin 10 production in vivo are associated with tolerance in scid patients transplanted with hla mismatched hematopoietic stem cells. J Exp Med (1994) 179(2):493–502. doi: 10.1084/jem.179.2.493
72. Jagannathan P, Eccles-James I, Bowen K, Nankya F, Auma A, Wamala S, et al. Ifnγ/Il-10 Co-producing cells dominate the Cd4 response to malaria in highly exposed children. PloS Pathog (2014) 10(1):e1003864. doi: 10.1371/journal.ppat.1003864
73. Gabrysová L, Nicolson KS, Streeter HB, Verhagen J, Sabatos-Peyton CA, Morgan DJ, et al. Negative feedback control of the autoimmune response through antigen-induced differentiation of il-10-Secreting Th1 cells. J Exp Med (2009) 206(8):1755–67. doi: 10.1084/jem.20082118
74. Uyeda MJ, Freeborn RA, Cieniewicz B, Romano R, Chen PP, Liu JM, et al. Bhlhe40 regulates il-10 and ifn- Γ production in T cells but does not interfere with human type 1 regulatory T cell differentiation. Front Immunol (2021) 12:683680. doi: 10.3389/fimmu.2021.683680
75. Gagliani N, Magnani CF, Huber S, Gianolini ME, Pala M, Licona-Limon P, et al. Coexpression of Cd49b and lag-3 identifies human and mouse T regulatory type 1 cells. Nat Med (2013) 19(6):739–46. doi: 10.1038/nm.3179
76. Freeborn RA, Strubbe S, Roncarolo MG. Type 1 regulatory T cell-mediated tolerance in health and disease. Front Immunol (2022) 13:1032575. doi: 10.3389/fimmu.2022.1032575
77. Groux H, O'Garra A, Bigler M, Rouleau M, Antonenko S, de Vries JE, et al. A Cd4+ T-cell subset inhibits antigen-specific T-cell responses and prevents colitis. Nature (1997) 389(6652):737–42. doi: 10.1038/39614
78. Levings MK, Gregori S, Tresoldi E, Cazzaniga S, Bonini C, Roncarolo MG. Differentiation of Tr1 cells by immature dendritic cells requires il-10 but not Cd25+Cd4+ tr cells. Blood (2005) 105(3):1162–9. doi: 10.1182/blood-2004-03-1211
79. Oida T, Zhang X, Goto M, Hachimura S, Totsuka M, Kaminogawa S, et al. Cd4+Cd25- T cells that express latency-associated peptide on the surface suppress Cd4+Cd45rbhigh-induced colitis by a tgf-Beta-Dependent mechanism. J Immunol (Baltimore Md 1950) (2003) 170(5):2516–22. doi: 10.4049/jimmunol.170.5.2516
80. Maynard CL, Harrington LE, Janowski KM, Oliver JR, Zindl CL, Rudensky AY, et al. Regulatory T cells expressing interleukin 10 develop from Foxp3+ and Foxp3- precursor cells in the absence of interleukin 10. Nat Immunol (2007) 8(9):931–41. doi: 10.1038/ni1504
81. Brockmann L, Gagliani N, Steglich B, Giannou AD, Kempski J, Pelczar P, et al. Il-10 receptor signaling is essential for Tr1 cell function in vivo. J Immunol (Baltimore Md 1950) (2017) 198(3):1130–41. doi: 10.4049/jimmunol.1601045
82. Matsuda M, Terada T, Kitatani K, Kawata R, Nabe T. Roles of type 1 regulatory T (Tr1) cells in allergen-specific immunotherapy. Front Allergy (2022) 3:981126. doi: 10.3389/falgy.2022.981126
83. Geginat J, Vasco C, Gruarin P, Bonnal R, Rossetti G, Silvestri Y, et al. Eomesodermin-expressing type 1 regulatory (Eomes+ Tr1)-like T cells: Basic biology and role in immune-mediated diseases. Eur J Immunol (2023) 53(5):e2149775. doi: 10.1002/eji.202149775
84. Gruarin P, Maglie S, De Simone M, Häringer B, Vasco C, Ranzani V, et al. Eomesodermin controls a unique differentiation program in human il-10 and ifn-Γ coproducing regulatory T cells. Eur J Immunol (2019) 49(1):96–111. doi: 10.1002/eji.201847722
85. Pearce EL, Mullen AC, Martins GA, Krawczyk CM, Hutchins AS, Zediak VP, et al. Control of effector Cd8+ T cell function by the transcription factor eomesodermin. Sci (New York NY) (2003) 302(5647):1041–3. doi: 10.1126/science.1090148
86. Zhang P, Lee JS, Gartlan KH, Schuster IS, Comerford I, Varelias A, et al. Eomesodermin promotes the development of type 1 regulatory T (Tr1) cells. Sci Immunol (2017) 2(10). doi: 10.1126/sciimmunol.aah7152
87. Guipouy D, Gertner-Dardenne J, Pfajfer L, German Y, Belmonte N, Dupré L. Granulysin- and granzyme-dependent elimination of myeloid cells by therapeutic ova-specific type 1 regulatory T cells. Int Immunol (2019) 31(4):239–50. doi: 10.1093/intimm/dxy083
88. Walch M, Dotiwala F, Mulik S, Thiery J, Kirchhausen T, Clayberger C, et al. Cytotoxic cells kill intracellular bacteria through granulysin-mediated delivery of granzymes. Cell (2014) 157(6):1309–23. doi: 10.1016/j.cell.2014.03.062
89. Tree TI, Lawson J, Edwards H, Skowera A, Arif S, Roep BO, et al. Naturally arising human Cd4 T-cells that recognize islet autoantigens and secrete interleukin-10 regulate proinflammatory T-cell responses Via linked suppression. Diabetes (2010) 59(6):1451–60. doi: 10.2337/db09-0503
90. Grossman WJ, Verbsky JW, Tollefsen BL, Kemper C, Atkinson JP, Ley TJ. Differential expression of granzymes a and b in human cytotoxic lymphocyte subsets and T regulatory cells. Blood (2004) 104(9):2840–8. doi: 10.1182/blood-2004-03-0859
91. Roessner PM, Llaó Cid L, Lupar E, Roider T, Bordas M, Schifflers C, et al. Eomes and il-10 regulate antitumor activity of T regulatory type 1 Cd4+ T cells in chronic lymphocytic leukemia. Leukemia (2021) 35(8):2311–24. doi: 10.1038/s41375-021-01136-1
92. Tracy SI, Venkatesh H, Hekim C, Heltemes-Harris LM, Knutson TP, Bachanova V, et al. Combining nilotinib and pd-L1 blockade reverses Cd4+ T-cell dysfunction and prevents relapse in acute b-cell leukemia. Blood (2022) 140(4):335–48. doi: 10.1182/blood.2021015341
93. Gondek DC, Lu LF, Quezada SA, Sakaguchi S, Noelle RJ. Cutting edge: Contact-mediated suppression by Cd4+Cd25+ regulatory cells involves a granzyme b-dependent, perforin-independent mechanism. J Immunol (Baltimore Md 1950) (2005) 174(4):1783–6. doi: 10.4049/jimmunol.174.4.1783
94. Denning TL, Norris BA, Medina-Contreras O, Manicassamy S, Geem D, Madan R, et al. Functional specializations of intestinal dendritic cell and macrophage subsets that control Th17 and regulatory T cell responses are dependent on the T Cell/Apc ratio, source of mouse strain, and regional localization. J Immunol (Baltimore Md 1950) (2011) 187(2):733–47. doi: 10.4049/jimmunol.1002701
95. Kamanaka M, Kim ST, Wan YY, Sutterwala FS, Lara-Tejero M, Galán JE, et al. Expression of interleukin-10 in intestinal lymphocytes detected by an interleukin-10 reporter knockin tiger mouse. Immunity (2006) 25(6):941–52. doi: 10.1016/j.immuni.2006.09.013
96. Huber S, Gagliani N, Esplugues E, O'Connor W, Huber FJ, Chaudhry A, et al. Th17 cells express interleukin-10 receptor and are controlled by Foxp3⁻ and Foxp3+ regulatory Cd4+ T cells in an interleukin-10-Dependent manner. Immunity (2011) 34(4):554–65. doi: 10.1016/j.immuni.2011.01.020
97. Alfen JS, Larghi P, Facciotti F, Gagliani N, Bosotti R, Paroni M, et al. Intestinal ifn-Γ-Producing type 1 regulatory T cells coexpress Ccr5 and programmed cell death protein 1 and downregulate il-10 in the inflamed guts of patients with inflammatory bowel disease. J Allergy Clin Immunol (2018) 142(5):1537–47.e8. doi: 10.1016/j.jaci.2017.12.984
98. Bonnal RJP, Rossetti G, Lugli E, De Simone M, Gruarin P, Brummelman J, et al. Clonally expanded eomes+ Tr1-like cells in primary and metastatic tumors are associated with disease progression. Nat Immunol (2021) 22(6):735–45. doi: 10.1038/s41590-021-00930-4
99. Sangiorgi E, Capecchi MR. Bmi1 is expressed in vivo in intestinal stem cells. Nat Genet (2008) 40(7):915–20. doi: 10.1038/ng.165
100. Montgomery RK, Carlone DL, Richmond CA, Farilla L, Kranendonk ME, Henderson DE, et al. Mouse telomerase reverse transcriptase (Mtert) expression marks slowly cycling intestinal stem cells. Proc Natl Acad Sci USA (2011) 108(1):179–84. doi: 10.1073/pnas.1013004108
101. Powell AE, Wang Y, Li Y, Poulin EJ, Means AL, Washington MK, et al. The pan-erbb negative regulator Lrig1 is an intestinal stem cell marker that functions as a tumor suppressor. Cell (2012) 149(1):146–58. doi: 10.1016/j.cell.2012.02.042
102. Carmon KS, Gong X, Lin Q, Thomas A, Liu Q. R-spondins function as ligands of the orphan receptors Lgr4 and Lgr5 to regulate Wnt/Beta-catenin signaling. Proc Natl Acad Sci USA (2011) 108(28):11452–7. doi: 10.1073/pnas.1106083108
103. de Lau W, Peng WC, Gros P, Clevers H. The r-Spondin/Lgr5/Rnf43 module: Regulator of wnt signal strength. Genes Dev (2014) 28(4):305–16. doi: 10.1101/gad.235473.113
104. Takeda N, Jain R, LeBoeuf MR, Wang Q, Lu MM, Epstein JA. Interconversion between intestinal stem cell populations in distinct niches. Sci (New York NY) (2011) 334(6061):1420–4. doi: 10.1126/science.1213214
105. Biton M, Haber AL, Rogel N, Burgin G, Beyaz S, Schnell A, et al. T Helper cell cytokines modulate intestinal stem cell renewal and differentiation. Cell (2018) 175(5):1307–20.e22. doi: 10.1016/j.cell.2018.10.008
106. Hernández-Malmierca P, Vonficht D, Schnell A, Uckelmann HJ, Bollhagen A, Mahmoud MAA, et al. Antigen presentation safeguards the integrity of the hematopoietic stem cell pool. Cell Stem Cell (2022) 29(5):760–75.e10. doi: 10.1016/j.stem.2022.04.007
107. Beyaz S, Chung C, Mou H, Bauer-Rowe KE, Xifaras ME, Ergin I, et al. Dietary suppression of mhc class ii expression in intestinal epithelial cells enhances intestinal tumorigenesis. Cell Stem Cell (2021) 28(11):1922–35.e5. doi: 10.1016/j.stem.2021.08.007
108. Chen B, Scurrah CR, McKinley ET, Simmons AJ, Ramirez-Solano MA, Zhu X, et al. Differential pre-malignant programs and microenvironment chart distinct paths to malignancy in human colorectal polyps. Cell (2021) 184(26):6262–80.e26. doi: 10.1016/j.cell.2021.11.031
109. DeStefano Shields CE, White JR, Chung L, Wenzel A, Hicks JL, Tam AJ, et al. Bacterial-driven inflammation and mutant braf expression combine to promote murine colon tumorigenesis that is sensitive to immune checkpoint therapy. Cancer Discov (2021) 11(7):1792–807. doi: 10.1158/2159-8290.CD-20-0770
110. Chen L, He Z, Reis BS, Gelles JD, Chipuk JE, Ting AT, et al. Ifn-Γ+ cytotoxic Cd4+ T lymphocytes are involved in the pathogenesis of colitis induced by il-23 and the food colorant red 40. Cell Mol Immunol (2022) 19(7):777–90. doi: 10.1038/s41423-022-00864-3
111. Günther C, Martini E, Wittkopf N, Amann K, Weigmann B, Neumann H, et al. Caspase-8 regulates tnf-α-Induced epithelial necroptosis and terminal ileitis. Nature (2011) 477(7364):335–9. doi: 10.1038/nature10400
112. Raetz M, Hwang SH, Wilhelm CL, Kirkland D, Benson A, Sturge CR, et al. Parasite-induced Th1 cells and intestinal dysbiosis cooperate in ifn-Γ-Dependent elimination of paneth cells. Nat Immunol (2013) 14(2):136–42. doi: 10.1038/ni.2508
113. Takashima S, Martin ML, Jansen SA, Fu Y, Bos J, Chandra D, et al. T Cell-derived interferon-Γ programs stem cell death in immune-mediated intestinal damage. Sci Immunol (2019) 4(42). doi: 10.1126/sciimmunol.aay8556
114. Allez M, Tieng V, Nakazawa A, Treton X, Pacault V, Dulphy N, et al. Cd4+Nkg2d+ T cells in crohn's disease mediate inflammatory and cytotoxic responses through mica interactions. Gastroenterology (2007) 132(7):2346–58. doi: 10.1053/j.gastro.2007.03.025
115. Dai B, Hackney JA, Ichikawa R, Nguyen A, Elstrott J, Orozco LD, et al. Dual targeting of lymphocyte homing and retention through α4β7 and αeβ7 inhibition in inflammatory bowel disease. Cell Rep Med (2021) 2(8):100381. doi: 10.1016/j.xcrm.2021.100381
116. Antony PA, Piccirillo CA, Akpinarli A, Finkelstein SE, Speiss PJ, Surman DR, et al. Cd8+ T cell immunity against a Tumor/Self-antigen is augmented by Cd4+ T helper cells and hindered by naturally occurring T regulatory cells. J Immunol (Baltimore Md 1950) (2005) 174(5):2591–601. doi: 10.4049/jimmunol.174.5.2591
117. Kreiter S, Vormehr M, van de Roemer N, Diken M, Löwer M, Diekmann J, et al. Mutant mhc class ii epitopes drive therapeutic immune responses to cancer. Nature (2015) 520(7549):692–6. doi: 10.1038/nature14426
118. Linnemann C, van Buuren MM, Bies L, Verdegaal EM, Schotte R, Calis JJ, et al. High-throughput epitope discovery reveals frequent recognition of neo-antigens by Cd4+ T cells in human melanoma. Nat Med (2015) 21(1):81–5. doi: 10.1038/nm.3773
119. Plitas G, Rudensky AY. Regulatory T cells in cancer (2020) 4:459–77. doi: 10.1146/annurev-cancerbio-030419-033428
120. Quezada SA, Simpson TR, Peggs KS, Merghoub T, Vider J, Fan X, et al. Tumor-reactive Cd4(+) T cells develop cytotoxic activity and eradicate Large established melanoma after transfer into lymphopenic hosts. J Exp Med (2010) 207(3):637–50. doi: 10.1084/jem.20091918
121. Xie Y, Akpinarli A, Maris C, Hipkiss EL, Lane M, Kwon EK, et al. Naive tumor-specific Cd4(+) T cells differentiated in vivo eradicate established melanoma. J Exp Med (2010) 207(3):651–67. doi: 10.1084/jem.20091921
122. Qi J, Liu X, Yan P, He S, Lin Y, Huang Z, et al. Analysis of immune landscape reveals prognostic significance of cytotoxic Cd4+ T cells in the central region of pmmr crc. Front Oncol (2021) 11:724232. doi: 10.3389/fonc.2021.724232
123. Oh DY, Kwek SS, Raju SS, Li T, McCarthy E, Chow E, et al. Intratumoral Cd4 + T cells mediate anti-tumor cytotoxicity in human bladder cancer. Cell (2020) 181(7):1612–25.e13. doi: 10.1016/j.cell.2020.05.017
124. Liu Q, Wang L, Lin H, Wang Z, Wu J, Guo J, et al. Tumor-specific Cd4+ T cells restrain established metastatic melanoma by developing into cytotoxic Cd4- T cells. Front Immunol (2022) 13:875718. doi: 10.3389/fimmu.2022.875718
125. Li C, Jiang P, Wei S, Xu X, Wang J. Regulatory T cells in tumor microenvironment: New mechanisms, potential therapeutic strategies and future prospects. Mol Cancer (2020) 19(1):116. doi: 10.1186/s12943-020-01234-1
126. González-Navajas JM, Fan DD, Yang S, Yang FM, Lozano-Ruiz B, Shen L, et al. The impact of tregs on the anticancer immunity and the efficacy of immune checkpoint inhibitor therapies. Front Immunol (2021) 12:625783(416). doi: 10.3389/fimmu.2021.625783
127. Locafaro G, Andolfi G, Russo F, Cesana L, Spinelli A, Camisa B, et al. Il-10-Engineered human Cd4(+) Tr1 cells eliminate myeloid leukemia in an hla class I-dependent mechanism. Mol Ther (2017) 25(10):2254–69. doi: 10.1016/j.ymthe.2017.06.029
128. Cieniewicz B, Uyeda MJ, Chen PP, Sayitoglu EC, Liu JM, Andolfi G, et al. Engineered type 1 regulatory T cells designed for clinical use kill primary pediatric acute myeloid leukemia cells. Haematologica (2021) 106(10):2588–97. doi: 10.3324/haematol.2020.263129
129. Yan H, Zhang P, Kong X, Hou X, Zhao L, Li T, et al. Primary Tr1 cells from metastatic melanoma eliminate tumor-promoting macrophages through granzyme b- and perforin-dependent mechanisms. Tumour Biol J Int Soc Oncodevelopmental Biol Med (2017) 39(4):1010428317697554. doi: 10.1177/1010428317697554
130. Magnani CF, Alberigo G, Bacchetta R, Serafini G, Andreani M, Roncarolo MG, et al. Killing of myeloid apcs Via hla class I, Cd2 and Cd226 defines a novel mechanism of suppression by human Tr1 cells. Eur J Immunol (2011) 41(6):1652–62. doi: 10.1002/eji.201041120
131. Oh DY, Kwek SS, Raju SS, Li T, McCarthy E, Chow E, et al. Intratumoral Cd4+ T cells mediate anti-tumor cytotoxicity in human bladder cancer. Cell (2020) 181(7):1612–25.e13. doi: 10.1016/j.cell.2020.05.017
132. Germano G, Lu S, Rospo G, Lamba S, Rousseau B, Fanelli S, et al. Cd4 T cell-dependent rejection of beta-2 microglobulin null mismatch repair-deficient tumors. Cancer Discov (2021) 11(7):1844–59. doi: 10.1158/2159-8290.CD-20-0987
133. Nagasaki J, Togashi Y, Sugawara T, Itami M, Yamauchi N, Yuda J, et al. The critical role of Cd4+ T cells in pd-1 blockade against mhc-Ii-Expressing tumors such as classic Hodgkin lymphoma. Blood Adv (2020) 4(17):4069–82. doi: 10.1182/bloodadvances.2020002098
134. Roberts A, Bentley L, Tang T, Stewart F, Pallini C, Juvvanapudi J, et al. Ex vivo modelling of pd-1/Pd-L1 immune checkpoint blockade under acute, chronic, and exhaustion-like conditions of T-cell stimulation. Sci Rep (2021) 11(1):4030. doi: 10.1038/s41598-021-83612-3
135. Chuang Y, Hung ME, Cangelose BK, Leonard JN. Regulation of the il-10-Driven macrophage phenotype under incoherent stimuli. Innate Immun (2016) 22(8):647–57. doi: 10.1177/1753425916668243
136. Mittal SK, Cho KJ, Ishido S, Roche PA. Interleukin 10 (Il-10)-Mediated immunosuppression: March-I induction regulates antigen presentation by macrophages but not dendritic cells. J Biol Chem (2015) 290(45):27158–67. doi: 10.1074/jbc.M115.682708
137. Akbar SM, Inaba K, Onji M. Upregulation of mhc class ii antigen on dendritic cells from hepatitis b virus transgenic mice by interferon-gamma: Abrogation of immune response defect to a T-Cell-Dependent antigen. Immunology (1996) 87(4):519–27. doi: 10.1046/j.1365-2567.1996.516576.x
138. Hanna BS, Llaó-Cid L, Iskar M, Roessner PM, Klett LC, Wong JKL, et al. Interleukin-10 receptor signaling promotes the maintenance of a pd-1int tcf-1+ Cd8+ T cell population that sustains anti-tumor immunity. Immunity (2021) 54(12):2825–41.e10. doi: 10.1016/j.immuni.2021.11.004
139. Beltra JC, Manne S, Abdel-Hakeem MS, Kurachi M, Giles JR, Chen Z, et al. Developmental relationships of four exhausted Cd8+ T cell subsets reveals underlying transcriptional and epigenetic landscape control mechanisms. Immunity (2020) 52(5):825–41.e8. doi: 10.1016/j.immuni.2020.04.014
140. Siddiqui I, Schaeuble K, Chennupati V, Fuertes Marraco SA, Calderon-Copete S, Pais Ferreira D, et al. Intratumoral Tcf1+Pd-1+Cd8+ T cells with stem-like properties promote tumor control in response to vaccination and checkpoint blockade immunotherapy. Immunity (2019) 50(1):195–211.e10. doi: 10.1016/j.immuni.2018.12.021
141. Connolly KA, Kuchroo M, Venkat A, Khatun A, Wang J, William I, et al. A reservoir of stem-like Cd8+ T cells in the tumor-draining lymph node preserves the ongoing antitumor immune response. Sci Immunol (2021) 6(64):eabg7836. doi: 10.1126/sciimmunol.abg7836
142. Jansen CS, Prokhnevska N, Master VA, Sanda MG, Carlisle JW, Bilen MA, et al. An intra-tumoral niche maintains and differentiates stem-like Cd8 T cells. Nature (2019) 576(7787):465–70. doi: 10.1038/s41586-019-1836-5
143. Liadi I, Singh H, Romain G, Roysam B, Cooper LJ, Varadarajan N. Defining potency of car+ T cells: Fast and furious or slow and steady. Oncoimmunology (2018) 8(10):e1051298. doi: 10.1080/2162402X.2015.1051298
144. Agarwal S, Hanauer JDS, Frank AM, Riechert V, Thalheimer FB, Buchholz CJ. In vivo generation of car T cells selectively in human Cd4+ lymphocytes. Mol Ther J Am Soc Gene Ther (2020) 28(8):1783–94. doi: 10.1016/j.ymthe.2020.05.005
145. Wang D, Aguilar B, Starr R, Alizadeh D, Brito A, Sarkissian A, et al. Glioblastoma-targeted Cd4+ car T cells mediate superior antitumor activity. JCI Insight (2018) 3(10). doi: 10.1172/jci.insight.99048
146. Bove C, Arcangeli S, Falcone L, Camisa B, Khoury REI, Greco B, et al. Cd4 car-T cells targeting Cd19 play a key role in exacerbating cytokine release syndrome, while maintaining long-term responses. J ImmunoTherapy Cancer (2023) 11(1):e005878. doi: 10.1136/jitc-2022-005878
Keywords: cytotoxic, Tr1 cell, tolerance, stem cell, immunotherapy
Citation: Venkatesh H, Tracy SI and Farrar MA (2023) Cytotoxic CD4 T cells in the mucosa and in cancer. Front. Immunol. 14:1233261. doi: 10.3389/fimmu.2023.1233261
Received: 01 June 2023; Accepted: 24 July 2023;
Published: 16 August 2023.
Edited by:
Colleen Jean Winstead, Epitopea Limited, United KingdomReviewed by:
Cecile Maire, University Medical Center Hamburg-Eppendorf, GermanyHiroshi Yano, Cornell University, United States
Copyright © 2023 Venkatesh, Tracy and Farrar. This is an open-access article distributed under the terms of the Creative Commons Attribution License (CC BY). The use, distribution or reproduction in other forums is permitted, provided the original author(s) and the copyright owner(s) are credited and that the original publication in this journal is cited, in accordance with accepted academic practice. No use, distribution or reproduction is permitted which does not comply with these terms.
*Correspondence: Sean I. Tracy, stracy@umn.edu; Michael A. Farrar, farra005@umn.edu