- 1Programa de Pós-Graduação em Medicina Tropical, Universidade do Estado do Amazonas (UEA), Manaus, Brazil
- 2Fundação de Medicina Tropical Heitor Vieira Dourado (FMT-HVD), Manaus, Brazil
- 3Programa de Pós-Graduação em Imunologia Básica e Aplicada, Instituto de Ciências Biológicas, Universidade Federal do Amazonas (UFAM), Manaus, Brazil
- 4Diretoria de Ensino e Pesquisa, Fundação Hospitalar de Hematologia e Hemoterapia do Amazonas (HEMOAM), Manaus, Brazil
- 5Instituto de Patologia Tropical e Saúde Pública, Universidade Federal de Goiás (UFG), Goiânia, Brazil
- 6Escola de Enfermagem de Manaus, Universidade Federal do Amazonas (UFAM), Manaus, Brazil
- 7Programa de Pós-Graduação em Ciências Aplicadas à Hematologia, Fundação Hospitalar de Hematologia e Hemoterapia do Amazonas (HEMOAM) Universidade do Estado do Amazonas (UEA), Manaus, Brazil
- 8Instituto Todos pela Saúde, São Paulo, São Paulo, Brazil
- 9Instituto Leônidas & Maria Deane/Fundação Oswaldo Cruz (ILMD/Fiocruz Amazônia), Manaus, Brazil
Background: The novel coronavirus disease 2019 (COVID-19) presents with complex pathophysiological effects in various organ systems. Following the COVID-19, there are shifts in biomarker and cytokine equilibrium associated with altered physiological processes arising from viral damage or aggressive immunological response. We hypothesized that high daily dose methylprednisolone improved the injury biomarkers and serum cytokine profiles in COVID-19 patients.
Methods: Injury biomarker and cytokine analysis was performed on 50 SARS-Cov-2 negative controls and 101 hospitalized severe COVID-19 patients: 49 methylprednisolone-treated (MP group) and 52 placebo-treated serum samples. Samples from the treated groups collected on days D1 (pre-treatment) all the groups, D7 (2 days after ending therapy) and D14 were analyzed. Luminex assay quantified the biomarkers HMGB1, FABP3, myoglobin, troponin I and NTproBNP. Immune mediators (CXCL8, CCL2, CXCL9, CXCL10, TNF, IFN-γ, IL-17A, IL-12p70, IL-10, IL-6, IL-4, IL-2, and IL-1β) were quantified using cytometric bead array.
Results: At pretreatment, the two treatment groups were comparable demographically. At pre-treatment (D1), injury biomarkers (HMGB1, TnI, myoglobin and FABP3) were distinctly elevated. At D7, HMGB1 was significantly higher in the MP group (p=0.0448) compared to the placebo group, while HMGB1 in the placebo group diminished significantly by D14 (p=0.0115). Compared to healthy control samples, several immune mediators (IL-17A, IL-6, IL-10, MIG, MCP-1, and IP-10) were considerably elevated at baseline (all p≤0.05). At D7, MIG and IP-10 of the MP-group were significantly lower than in the placebo-group (p=0.0431, p=0.0069, respectively). Longitudinally, IL-2 (MP-group) and IL-17A (placebo-group) had increased significantly by D14. In placebo group, IL-2 and IL-17A continuously increased, as IL-12p70, IL-10 and IP-10 steadily decreased during follow-up. The MP treated group had IL-2, IFN-γ, IL-17A and IL-12p70 progressively increase while IL-1β and IL-10 gradually decreased towards D14. Moderate to strong positive correlations between chemokines and cytokines were observed on D7 and D14.
Conclusion: These findings suggest MP treatment could ameliorate levels of myoglobin and FABP3, but appeared to have no impact on HMGB1, TnI and NTproBNP. In addition, methylprednisolone relieves the COVID-19 induced inflammatory response by diminishing MIG and IP-10 levels. Overall, corticosteroid (methylprednisolone) use in COVID-19 management influences the immunological molecule and injury biomarker profile in COVID-19 patients.
1 Introduction
The ongoing Coronavirus disease 2019 (COVID-19) pandemic has presented a major challenge to clinicians, medical scientists and patients as well as existing healthcare systems. This very contagious respiratory infection is associated with a large spectrum of symptoms, a myriad of sequelae, complications, and death.
The etiological agent of the COVID-19, that is the severe acute respiratory syndrome coronavirus 2 (SARS-CoV-2), attaches and enters target human cells via the human host’s angiotensin converting enzyme receptor 2 (ACE2) and neuropilin-1 (NRP1) cell receptors with the activation of the serine transmembrane protease 2 (TMPRSS2) host receptor as it enters the target cells. The target cells with these receptors are mostly found in the respiratory and gastrointestinal tract receptors (1, 2). Subsequently, the viral invasion causes a complex interplay of the immunological, inflammatory, and coagulative cascades (3–7). In addition to the organ tropism demonstrated by the virus, SARS-CoV-2 infection can influence the course of the disease by potentially aggravating other underlying conditions, of which the systemic responses to the viral infection may cause organ dysfunction in affected systems (3–5).
In majority of the cases, the clinical features of COVID-19 presented with acute respiratory distress syndrome (ARDS) that could quickly evolve into pneumonia, respiratory failure, and death. The ARDS is often accompanied by a rise in the levels of inflammatory cytokines such as IL-2, IL-7, IL-6, IL-10, IP-10, MCP1 and TNF-α (8, 9), referred to as the cytokine storm. At the same time elevated serum and plasma levels of cytokines like IL-6, IL-10, TNF-α and IL-12R have been associated with disease severity (1, 10). To attenuate the cytokine storm effects and manage the cytokine-induced injury, corticosteroids such as methylprednisolone have been administered as therapeutic intervention to COVID-19 patients (11–18). Borrowing from the methylprednisolone use in treating Severe Acute Respiratory Syndrome Coronavirus (SARS-CoV) and Middle East respiratory syndrome coronavirus (MERS-CoV) outbreaks (19–22), corticosteroids became one of the first-line therapeutic intervention against COVID-19.
Understanding the host-pathogen interaction in COVID-19 is vital for improved treatment and management of the disease. With this, biomarkers to disease progression, prognosis, and response to treatment are important. Studies on COVID-19 patients demonstrated changes in damage associated molecular patterns (DAMPs) including high mobility group box 1 (HMGB1) and surfactant protein A (SP-A) (23–25). Other biomarkers like myoglobin and troponin I have been positively correlated with significantly higher risks of severe disease and mortality among COVID-19 patients (26–29). Data from meta-analyses further highlight the prognostic value of the cardiac markers, both Brain natriuretic peptides (BNP), and N-terminal pro-B-type natriuretic peptide (NT-proBNP) with regard to mortality and disease severity in patients with COVID-19 (28, 30–34). Similarly, COVID-19 has been associated with increased levels neuronal injury serum markers like neurofilament light chain (NfL), neuron-specific enolase (35–37) while biomarkers like NfL, Glial fibrillary acidic protein, and tau were significantly increased in patients with fatal outcome (38). From these studies, elevated levels of these biomarkers, among others, are often indicative of unfavorable outcomes and other complications in COVID-19.
Some works report different biomarkers that related to the state of the affected organs and systems, information about the progression/improvement of the disease and the response to treatment (3, 39–44). Immunological, inflammatory, coagulation, hematological, cardiac, biochemical, damage-associated molecular patterns and abnormal laboratory markers are some of the informative biomarkers used in COVID-19 investigations (3). Understanding the profiles of biomarkers and cytokines in infection, as well as the changes that occur after administration of systemic corticosteroid treatment, is critical to assessing the benefits and/or risks of this treatment (3).
Monitoring levels of immunological and tissue injury biomarkers is important to understand the dynamics of corticosteroid therapeutic responses in patients with COVID-19, as well as their effects. These biomolecules may serve as prognostic factors and/or biomarkers of therapeutic success in patients with COVID-19. This work aimed to determine the effect of methylprednisolone on tissue injury biomarkers and cytokine profiles compared to placebo in patients with COVID-19.
2 Methods
This was an analytical study using samples and secondary data from retrospective cohort, patients from the MetCOVID clinical trial (45, 46). In our study, baseline serum cytokine and injury biomarker profiles of COVID-19 infected patients who completed the 5-day methylprednisolone (MP) treatment and, the longitudinal effect of the MP intervention at 14-day follow-up on the cytokine and biomarker changes was determined and compared to the placebo group. Patient demographic and clinical data were also targeted for analysis.
2.1 Ethics statement
The Fundação de Medicina Tropical Doutor Heitor Vieira Dourado Research Ethics Committee approved this study under certificate CAAE 46193821.5.0000.0005. This was a sub study of a larger clinical trial study approved under certificate number 30615920.2.0000.0005. Likewise, the Fundação Hospitalar de Hematologia e Hemoterapia do Amazonas (HEMOAM) Ethics Committee approved the negative control group (CAAE 56413316.9.0000.0009). All donors were submitted to a serological screening at HEMOAM, which is recommended by Brazilian Blood Donor Bank Authorities in order to monitor blood borne pathogens and includes serological analysis for the Hepatitis B and C virus, HIV, DENV, HTLV, Syphilis and Chagas Disease.
2.2 The participants
The participants used in this study were drawn from the MetCOVID study (Clinical Trials Identifier NCT04343729). The MetCOVID clinical trial was a double-blinded, randomized, placebo-controlled, phase IIb clinical trial that aimed to assess the efficacy of intravenous methylprednisolone sodium succinate (MP), compared to placebo treatment. The methylprednisolone sodium succinate treatment (0.5 mg/kg) was administered twice daily for 5 days in hospitalized patients with suspected COVID-19 infection, while the placebo arm of the study received saline solution twice daily for 5 days (45, 46).
For our study, we selected participants of either gender that had no prior use of corticosteroids before enrollment into the MetCOVID trial and had completed their 5-day treatment dose, in both the methylprednisolone and placebo groups. An additional negative control group (n=50) was included for comparison in the cytokine analysis. These control samples, collected only time, were collected from donors at the Fundação Hospitalar de Hematologia e Hemoterapia do Amazonas (HEMOAM), the principal hematological reference hospital in Manaus city, Amazonas, Brazil, prior to the outbreak of the COVID-19 pandemic. The control samples were thus COVID-19 negative and were analyzed only during the baseline (D1) comparison with the two treatment groups.
Subsequent information on patient demographics, clinical laboratory analyses (hematological and biochemical) were extracted from the REDCap electronic database. Hematological and biochemical tests at baseline were conducted as described by Jeronimo et al. (45).
2.3 Biological sample collection and processing
Venous blood was collected into vacutainer tubes containing clot activator on days D1 (pre-treatment), D7, and D14. Serum was collected and kept at -80°C until needed.
2.4 Quantification of biomarkers
Serum levels of NTproBNP, Troponin I, Myoglobin, FABP3, and HMGB1 were determined using commercially available bead-based immunoassay kits (MILLIPLEX MAP HCVD1MAG-67K, HCVD2MAG-67K, HNS2MAG-95K, and HCYP4MAG-64K kits; Merck KGaA). The assay was performed following the manufacturer’s instructions. Serum dilution was adapted to 1:4, 1:10 and 1:100 for HMGB1, FABP3 and myoglobin, respectively, to improve the assay range of target analytes. Samples, standards, and controls were run in duplicate with a coefficient of variance (CV) <20%. For readout and data acquisition, a MAGPIX® reader (Luminex Corporation) with xPONENT® software (version 4.3) was used, while Curve fitting was done using Belysa® Curve Fitting Software version 1.0.19 (Merck KGaA, Darmstadt, Germany).
2.5 Quantification of chemokine and cytokines
Soluble serum immunological mediators (chemokine and cytokine) including CXCL8, CCL2, CXCL9, CXCL10, TNF, IFN-γ, IL-17A, IL-12p70, IL-10, IL-6, IL-4, IL-2 and IL-1β were quantified using arrays of cytometric beads (Human Chemokine, Human Inflammatory Cytokine and Human Cytokine Th1/Th2/Th17 BD™ kits, BD Biosciences, San Diego, CA, USA), according to the manufacturer’s instructions. Data was acquired using the FACSCanto II and analyzed using the FCAP-Array software version 3.0.1 (BD Biosciences, San Jose, CA, USA). Data were reported in picograms per milliliter (pg/mL) concentrations, according to the standard curves provided in the kits.
2.6 Statistical analysis
Descriptive statistics was done for demographic, laboratory and clinical data. For qualitative variables, the chi-square test was performed. Statistical significance in the differences in quantitative variables was assessed using parametric (unpaired t-test) or non-parametric (unpaired Mann-Whitney test) tests, after initially being subjected to a Shapiro-Wilk test for normality. ANOVA test (normally distributed variables) and two-tailed Kruskal-Wallis test with Dunn’s post-test (non-parametric variables) were used in multi-group comparisons. These tests were also done for biomarker and cytokine assay data. Cytokine results were log-transformed before doing the comparative statistical analysis. In all cases, significance was considered at p < 0.05. Statistical analyses were performed using STATA version 15.0 and GraphPad Prism, version 9.0.1 (GraphPad Software, San Diego, CA, USA).
2.7 Correlation network analysis
Cytokine correlation networks were set up to assess associations in the MP and placebo groups on D1, D7, and D14.Correlations between quantitative levels of chemokines and cytokines were determined using Spearman’s correlation coefficient in GraphPad Prism, version 9.0.1 (GraphPad Software, San Diego, CA, USA). After performing a correlation matrix analysis between immunological molecules, a database was created using the Microsoft Excel program, where only significant correlations were considered for further network development using the open source Cytoscape software (version 3.9.1) (Cytoscape Consortium San Diego, CA, USA). Following the software’s recommendations and instructions, the chemokine and cytokine networks were constructed. The correlation index (r) was used to categorize the correlation strength as weak (r ≤ 0.35), moderate (r ≥ 0.36 to r ≤ 0.67) or strong (r ≥ 0.68), as proposed earlier (47, 48). The levels of statistical significance defined in both cases were p < 0.05.
3 Results
3.1 Characterizing the baseline clinical, epidemiological and laboratory data of the patients
Our study included 101 patients categorized into two groups, 49 from MP group and 52 from the Placebo group. The patients had a median age of 60 years, were overweight (BMI=28 kg/m2), mostly males (68.3%) and mostly of an admixed racial background (79.2%). Most participants (91%) reported having suffered other comorbidities, particularly hypertension (59.8%), obesity (37.0%) and diabetes (29.4%) respectively. Most of the participants having a Glasgow coma score of between 13 and 15, the patients had little to mild brain injuries. The laboratory findings of neutrophils, platelets, blood glucose, ALT, AST, D-dimer, and IL-6 were observed to be above the reference values. On the other hand, lymphocyte levels were below the reference values. Overall, there were no significant differences in the baseline characteristics between patients randomized into the MP and placebo groups (Table 1).
To compare the differences in the hematological and biochemical profile of the patients after the intervention, data from D7 were compared to that at D1 (Table 2). It was observed that there were significant differences between the D1 and D7 results for hemoglobin and hematocrit (p=0.0162 and p=0.0166, respectively) in the placebo group. Patients who received treatment with MP showed a significant drop in C-reactive protein (CRP) levels on D7 compared to D1 (p=0.042) (Table 2). Although deaths were reported in both groups at D7, the numbers were not significantly different (p=0.218 and p=> 0.999, in Placebo and MP, respectively).
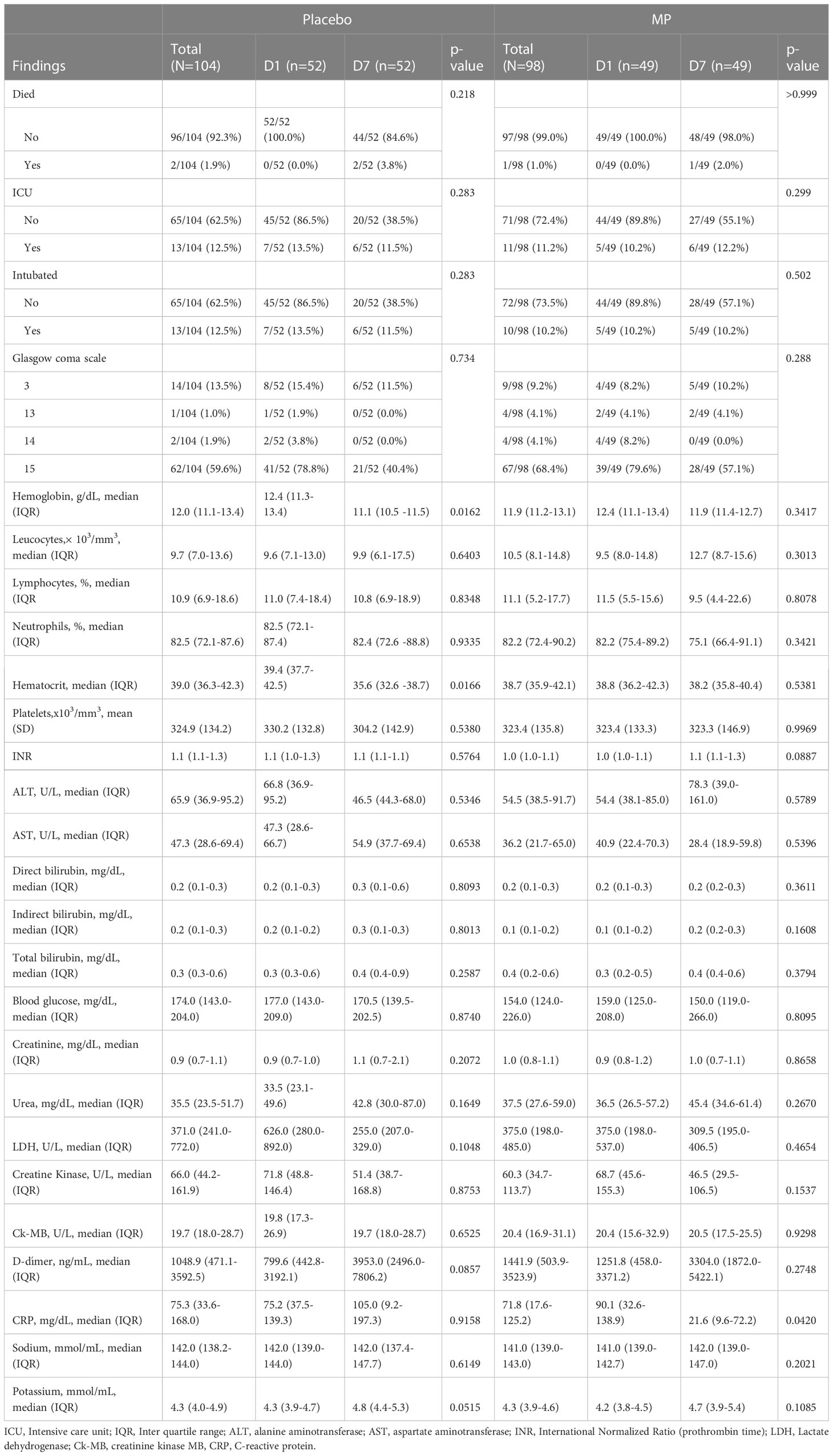
Table 2 Comparison between D1 (pre-treatment) and D7 (approximately 2 days post treatment) patient clinical and lab findings for each of the treatment groups.
When compared to the Placebo group, the MP group’s blood hemogram profile improved. However, these differences were not statistically significant (Table 3).
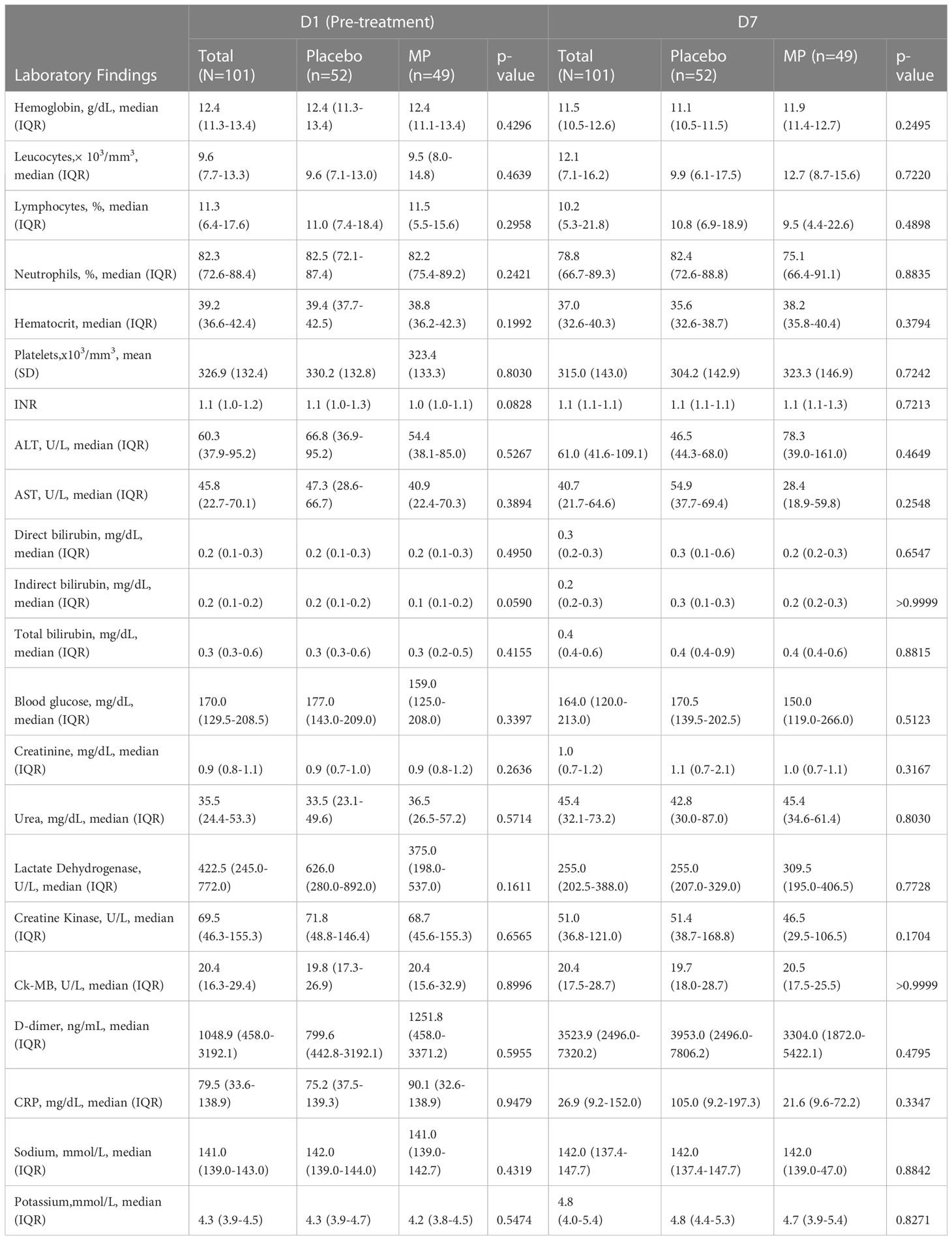
Table 3 A between-groups comparison of the lab hematological and biochemistry findings at D1 and D7.
3.2 Injury biomarker profile in the MP and placebo group
On admission, biomarker levels were comparable between the two groups, suggesting that the effect of COVID-19 infection on patients was similar. No significant differences were observed between the two groups at D1 vs. D7 and D14, except for HMGB1, which was significantly lower in the placebo group than MP group on D7 (p = 0.0447) (Table 4). Baseline and follow-up values of myoglobin, NTproBNP, troponin I, FABP were comparable between groups on different follow-up days (p>0.05).
A longitudinal analysis indicated that HMGB1 levels in the placebo group significantly decreased with time from a median of 205.99 ng/mL on D1 to 57.2 ng/mL on D14 (p=0.0115) (Table 5). In contrast, HMGB1 levels in the MP group were similar during follow-up (p = 0.1856). On the other hand, myoglobin in the placebo group had an increasing tendency from D1 to D14 (95.59 to 145.3 ng/ml), while in the MP group it decreased in the same period (128.77 to 82.62 ng/ml), but without significant difference (p>0.05). FABP3 levels in the placebo group seemed to stabilize between D1 and D7 before increasing on D14 (4486.49 to 6522 pg/mL) (p=0.4171), while in the MP group this biomarker steadily increased from D1 to D14 (5244.93 to 22003.67 pg/ml) (p=0.0509).
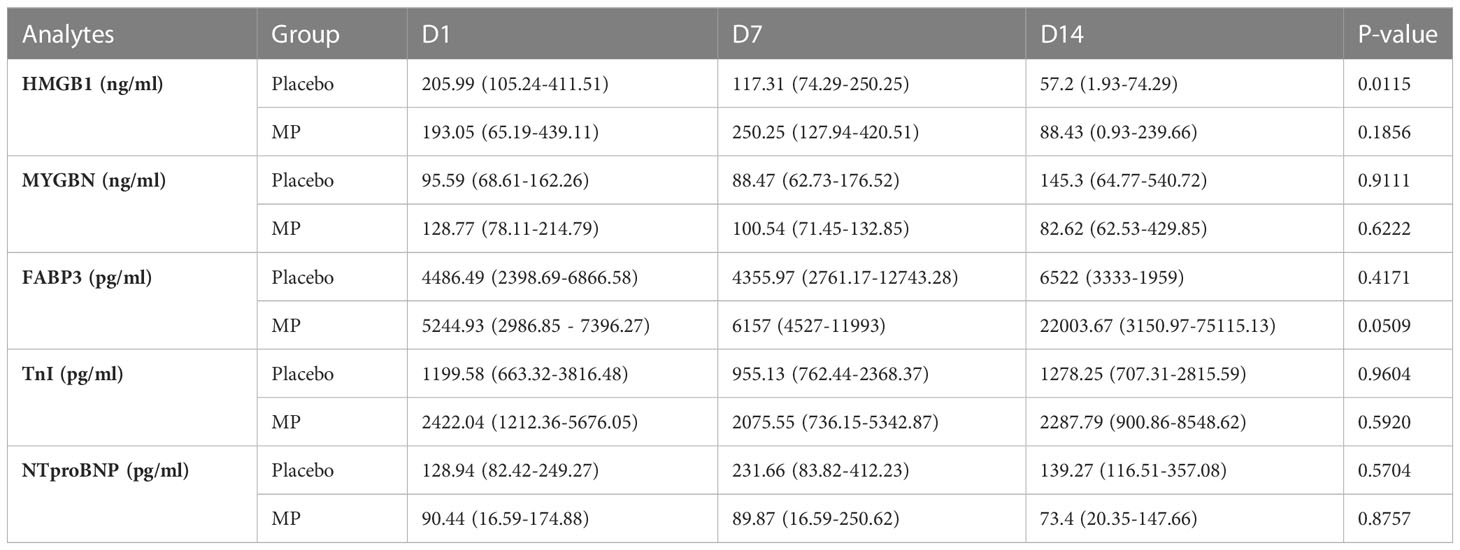
Table 5 Longitudinal evaluation of injury biomarkers in COVID-19 patients treated with MP and placebo over a 14-day period.
TnI levels in both groups were constant during follow-up. For NTproBNP, mean levels in the placebo group increased at D7 before falling at D14 (128.94 to 231.66 to 139.27 pg/ml at D14). In the MP group, NTproBNP remained constant on D1 and D7, approximately 90 pg/ml, before a slight drop on D14 to 73.4 pg/ml. Again, these observed changes were not significant (p>0.05) (Table 5).
3.3 The serum cytokine and chemokine of COVID-19 patients
Figure 1 shows the serum cytokine and chemokine profile of the COVID-19 patients at the D1 baseline. When compared to the control group at D1, levels of IL-10, IL-17A, IL-6, MIG/CXCL9, MCP-1/CCL2 and IP-10/CXCL10 were significantly elevated in the patients. A comparison between the MP and placebo groups at D7 revealed that the chemokines MIG/CXCL9 and IP-10/CXCL10 were significantly elevated in the Placebo group. No significant differences were observed in the serum immunological molecules at D14 (Figure 1_Supplementary Files).
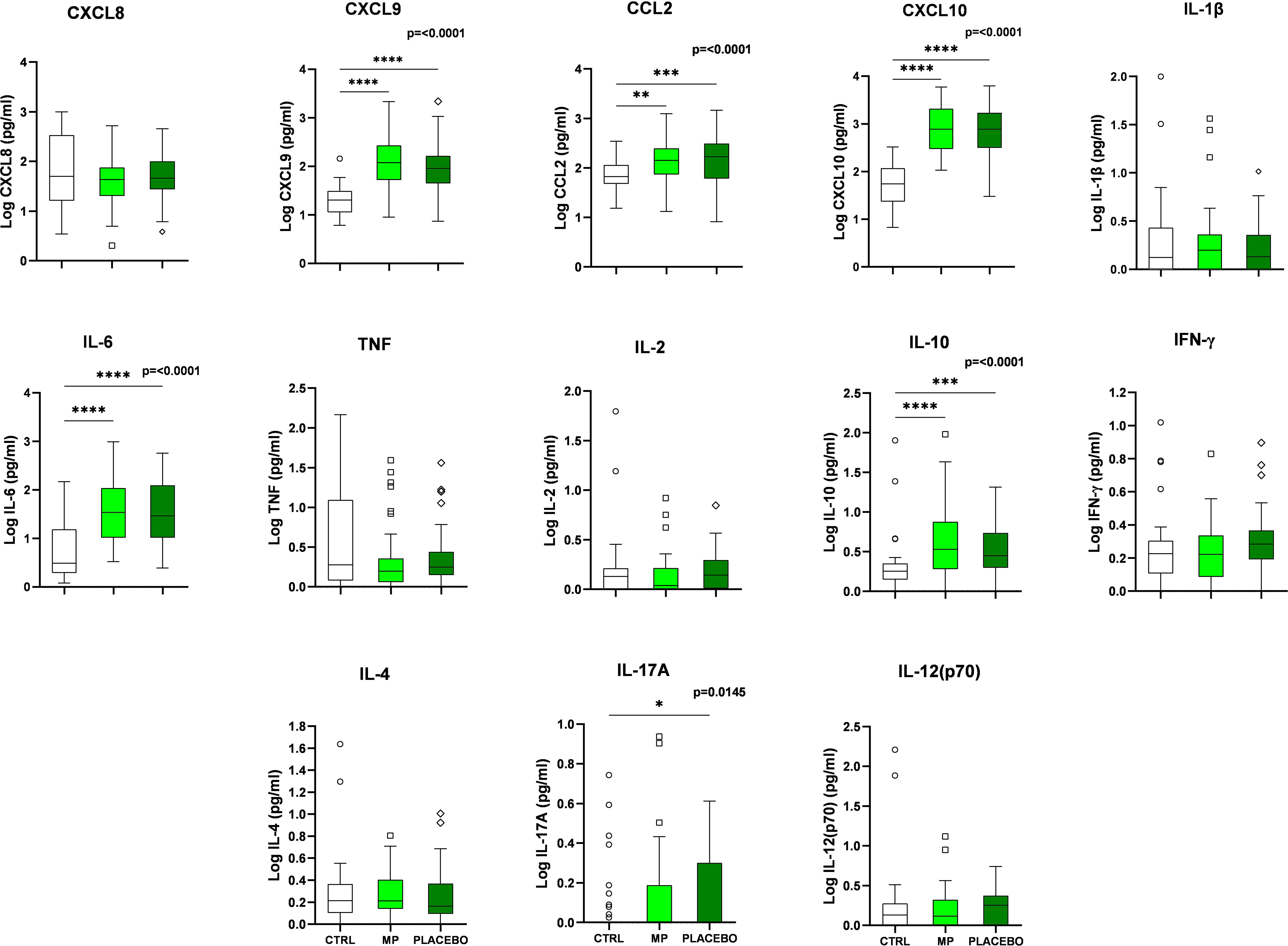
Figure 1 Serum cytokine and chemokine levels in COVID-19 infected patients compared to the healthy participants (negative controls) at baseline (D1). COVID-19 negative controls (CTRL), Methylprednisolone treated patients (MP), Placebo/normal saline treated patients (PLACEBO), Interleukin-2 (IL-2), Interleukin-4 (IL-4), Interleukin-8 (IL-8), Interleukin-1β (IL-1β), Interleukin-6 (IL-6), Interleukin-10 (IL-10), Interleukin-12p70 (IL-12p70), Interleukin-17A (IL-17A),Tumor Necrosis Factor (TNF), Interferon-γ (IFN-γ), Monokine-induced by Interferon-γ (MIG/CXCL9), Monocyte Chemoattractant Protein-1 (MCP-1/CCL2), and Interferon-γ-induced Protein-10 (IP-10/CXCL10). *p < 0.05, **p < 0.01, ***p < 0.001, and ****p < 0.0001.
When comparing the baseline cytokines/chemokines results (D1) of the controls and the MP group as seen in Figure 1, six immune mediators were significantly high. This number declined during the D7 analysis (Supplementary Figure 1). This decline occurred over time after the MP-intervention. Some of these immune molecules responded quickly, and others slowly, relative to the baseline levels.
3.4 Longitudinal profile of the immune modulators after treatment of COVID-19 patients
Figure 2 depicts the levels of cytokines and chemokines in the MP and placebo groups during follow-up. The MP group demonstrated a progressive increase in IL-2, IFN-γ, IL-17A and IL-12p70 concentrations towards D14, but only IL-2 had a significant increase by D14.The placebo group IL-2 and IL-17A levels followed the same progressive pattern (Figure 2), with only IL-17A increasing significantly by D14. On the other hand, concentrations of IL-1β, IL-10 and IP-10 (CXCL10) in MP group and IFN-γ, IL-12p70, IL-10, MCP-1 (CCL2), MIG (CXCL9) and IP-10 (CXCL10) in the placebo group decreased longitudinally (Figure 2).
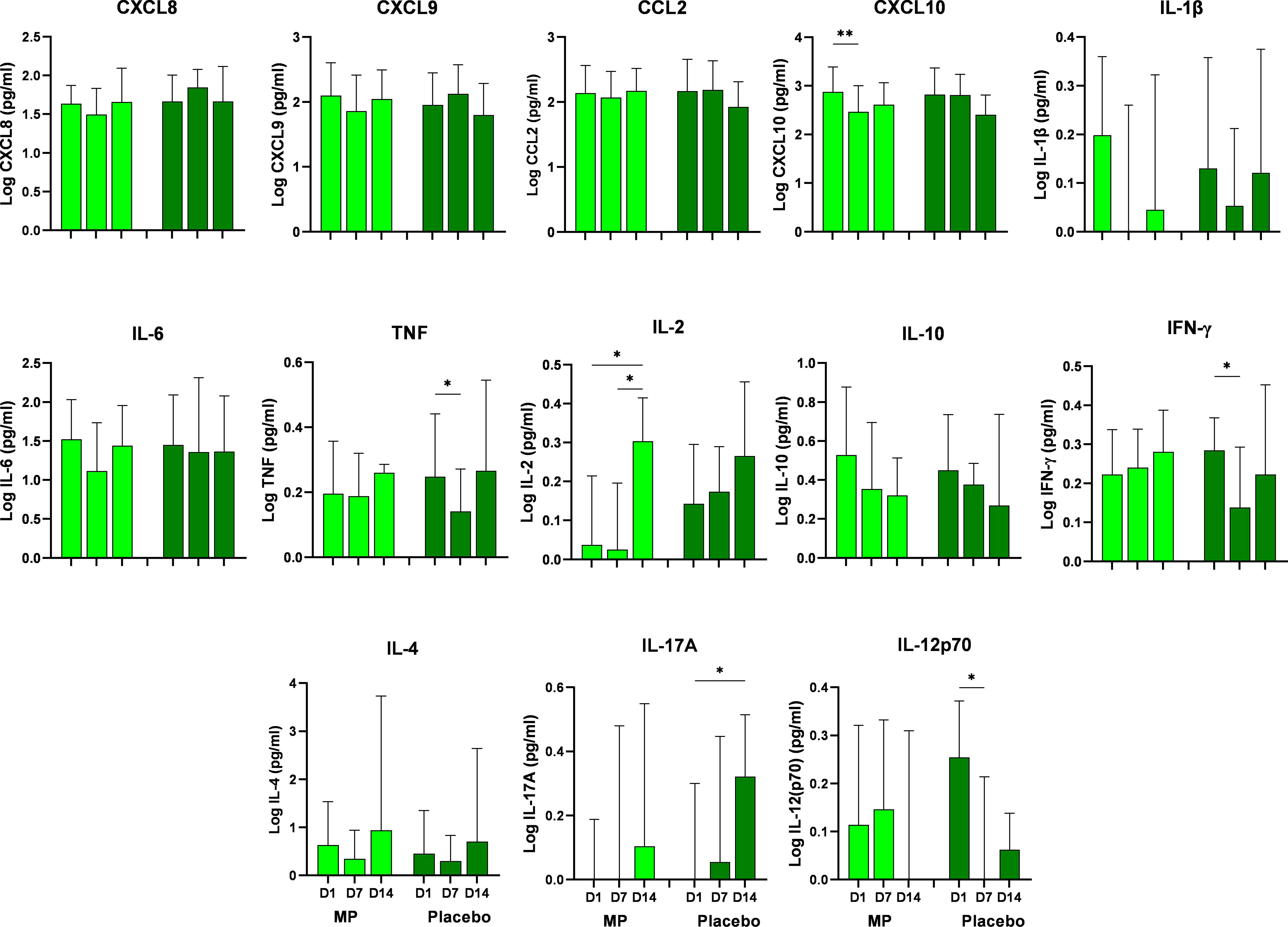
Figure 2 Serum concentrations of chemokines and cytokines in treated COVID-19 patients (MP-treated and Placebo groups) during the follow up (D1 to D14). Statistical difference between follow up times was considered significant when p < 0.05 (*). Statistical analysis was performed using the Kruskal-Wallis test followed by Dunn’s posttest or the Ordinary ANOVA test with Tukey’s post-test where necessary. Methylprednisolone treated patients (MP), Placebo/normal saline treated patients (Placebo), Interleukin-2 (IL-2), Interleukin-4 (IL-4), Interleukin-8 (IL-8), Interleukin-1β (IL-1β), Interleukin-6 (IL-6), Interleukin-10 (IL-10), Interleukin-12p70 (IL-12p70), Interleukin-17A (IL-17A),Tumor Necrosis Factor (TNF), Interferon-γ (IFN-γ), Monokine-induced by Interferon-γ (MIG/CXCL9), Monocyte Chemoattractant Protein-1 (MCP-1/CCL2), and Interferon-γ-induced Protein-10 (IP-10/CXCL10), D1- day 1, D7- day 7, D14 - day 14. *p < 0.05, **p < 0.01.
A longitudinal analysis of cytokine and chemokine levels from D1 to D14 showed significant increase in IL-2 (between D1and D14, and D7 and D14), and significant decrease between D1 and D7 in IP-10 (CXCL10) levels within the MP group. Significant changes were observed in the profile of IFN-γ, TNF and IL-17A within the Placebo group, mostly occurring between D1 and D7 (Figure 2). Specifically, these significant changes in the Placebo group were a decrease in IFN-γ, TNF, and IL-12p70 where all decreased by D7 and an increase in IL-17A by D14.
3.5 COVID-19 patients have complex biomarker networks with moderate to strong interactions in the circulating serum cytokines/chemokines of the MP-treated group
COVID-19 patients had an immunological marker interaction network different from the healthy non-COVID-19 controls, as shown in Figure 3. The control group showed strong correlations between TNF and IL-1β, CXCL8/IL-8 and IL-6, and CXCL8/IL-8 and TNF. On D1 (prior to intervention), weak levels of IL-10 regulation for cytokines and modest chemokine modulation by IL-10 response were detected in the placebo group, unlike the MP group, where strong positive correlations between IL-10 with IL-6 and IL-8/CXCL8 with IL-6 were observed. The two infected groups demonstrated numerous chemokine–cytokine (IL-10, IL-6 and IL-17A) correlations; the healthy controls had more of cytokine-cytokine relationships (IL-10/IL-6 with IL-1β, TNF, and IL-2).
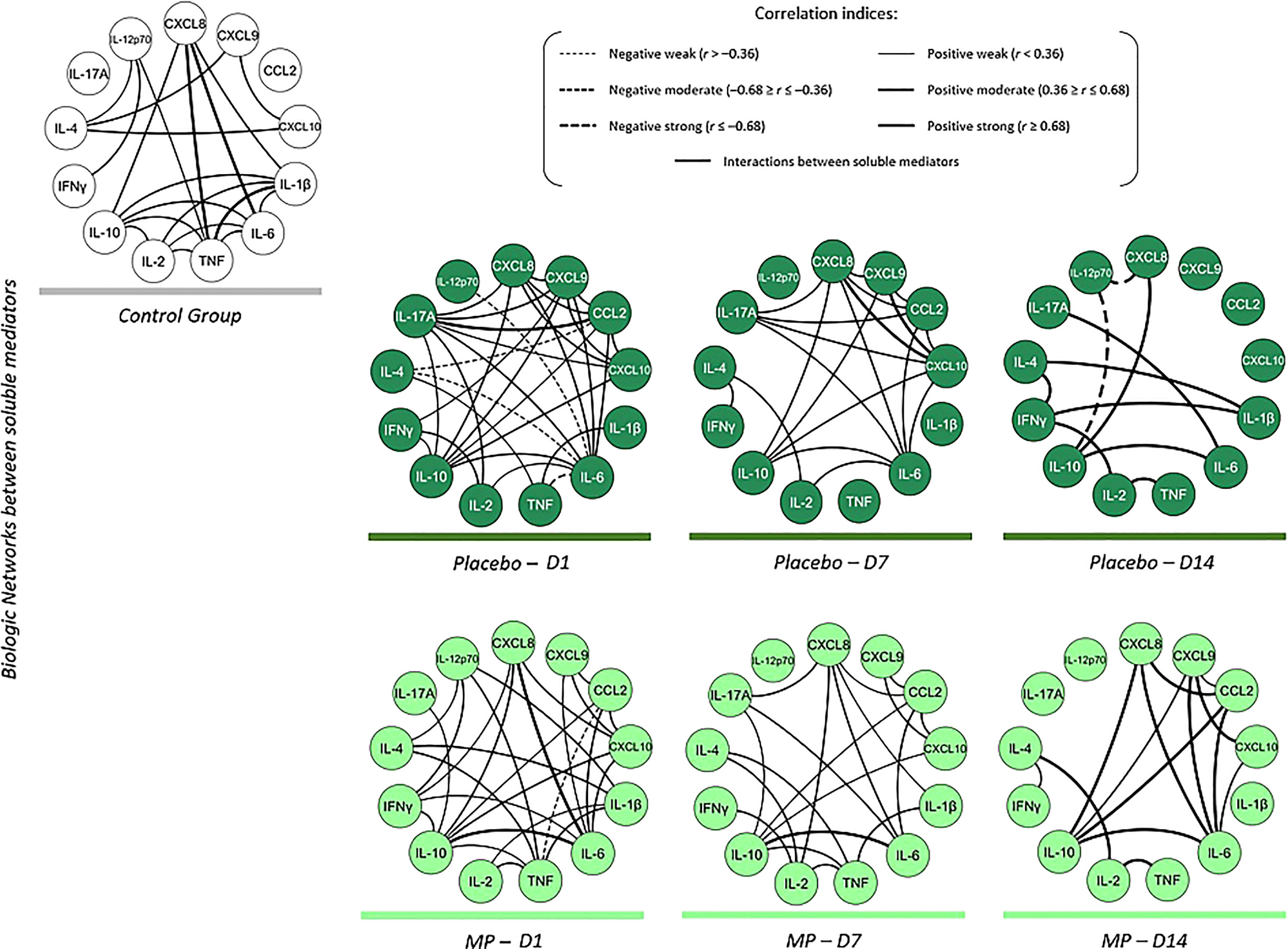
Figure 3 Immune mediator networks show the interactions between the groups in the follow-up of the study. Color codes were used to identify the different study groups as follows: Control group (), Placebo (
) and MP group (
). The different line sizes and types demonstrate the interrelationships between the chemokines and cytokines circulating in the peripheral blood from the different study groups. Dashed lines between molecules indicate a negative correlation, while solid lines indicate a positive correlation. The thickness of these indicates the strength of the correlation. The correlation index (r) used to categorize the strength of the correlation as weak (r ≤ 0.35), moderate (r≥0.36 to r ≤ 0.67) or strong (r≥0.68).
After the intervention, patients treated with MP showed moderately increased positive correlations of chemokines with other immune mediators during clinical follow-up (D7). A clear inflammatory response was revealed at D7 within the placebo treated patients. Of interest in the placebo group was the strong chemoattractant activities advanced by the strong correlations between IP-10/CXCL10 and IL-8/CXCL8, and by IP-10/CXCL10 with IL-8 and MIG/CXCL9.
Contrary to the observations on D1 and D7, there was predominantly strong positive correlation between cytokines and chemokines on D14 in both treatment groups. MP-treated group also presented few moderate chemokine-cytokine interactions. The D14 placebo group interactions were characterized by substantial correlations between pro-inflammatory and regulating immune molecules.
4 Discussion
The complex immunopathological response following SARS-CoV-2 infection is responsible for varied disease pathogenesis and clinical manifestations. This immunopathological response arises from activated immune responses against the SARS-CoV-2, and uncontrolled inflammatory responses characterized by marked pro-inflammatory cytokine release in patients with severe COVID-19, leading to lymphopenia, lymphocyte dysfunction, and granulocyte and monocyte abnormalities. Knowing the effect of corticosteroid intervention on the host’s immune responses and injury biomarkers during COVID-19 infection would allow for an early assessment of the benefit and/or risk of this treatment against immunity- or virus-induced pathology, and evidence of crosstalk between injury and inflammation biomolecules. Currently, the success of corticosteroid treatment varies on dose, treatment time, stage of disease and patient factors.
Baseline clinical results revealed similar observations, particularly lower lymphocyte counts, elevated neutrophil counts, high platelet counts, high dimer readings and impaired liver function. These observations corresponded similar observations among COVID-19 patients in China (49–51).
The use of methylprednisolone was associated with a significant reduction in CRP levels on day 7. Torres et al. also observed that patients who received corticosteroids showed differences in the systemic anti-inflammatory effect measured by CRP on day 3, with variations related to the presence or absence of lymphopenia (52).
A comparison of the D1 and D7 laboratory findings revealed no significant differences in the hematological and biochemistry parameters. However, we found significant decline in hemoglobin and hematocrit levels in the Placebo group, agreeing with findings from previous studies that analyzed the worsening of the disease (53–59). Comparing the changes in the groups after the corticosteroid treatment, they were not significantly different in the placebo group. This can be explained by time lost (in days) between onset of symptoms until inclusion, time to treatment and follow-up considering that clinical parameters vary with disease severity, patient’s immunity status, and viral characteristics like incubation time, replication phases, and duration of shedding (60), and all groups were of severe cases.
The High-mobility group box 1 (HMGB1) is a multifunctional nuclear protein actively secreted by necrotic cells in response to pathogens or endogenous inflammatory stimuli, and can be passively released by damaged lung parenchyma cells, as in the case of COVID-19 (42). Al-kuraishy et al. summarized that high levels of HMGB1 are synonymous with disease severity, development of cytokine storm (CS), acute lung injury and acute distress syndrome (ARDS) (61), and it is a potential prognostic factor for severe COVID-19 and mortality. HMGB1 levels in our cohort at D1 were significantly elevated. Sivakorn et al. showed that HMGB1 levels at ICU admission were higher in patients with COVID-19 than in healthy subjects (42). Taken together, these findings underscore the potential crucial role of HMGB1 as a biomarker for COVID-19 disease severity.
Despite the MP intervention, HMGB1 levels in treated patients were still elevated. Although there was a significant difference in HMGB1 levels in the Placebo group between D1 and D14. However, Gougeon et al. (62) and Rubartelli and Lotze (63) argue that DAMPs, such as HMGB1, once released are limited spatially and temporally until they get oxidized, essentially reducing inflammation by minimizing prolonged stimulation of its targets (62, 63). The high levels of circulating HMGB1 in our MP-treated group could arise from its potent action as an alarmin, in an attempt to promote an effective immune response against the pathogen (especially Th1) or that the damage still present. Although, the corticoid has the action of suppressing this response, it is not necessarily succeeding in regenerating tissue or reversing the damage, in which the alarmin is still “giving the warning”. The observation in our study is in line with claims that circulating levels of HMGB1 are elevated for prolonged periods in severe infections such as sepsis and COVID-19 (64) further confirming its role as a late mediator of systemic inflammation.
Several drugs, and peptide-based and small-molecule HMGB1-targeted therapeutics can act as specific HMGB1 antagonists, improving inflammation, ameliorating tissue injury and improving survival (65, 66). In COVID-19 for instance, dexamethasone can potentially modulate the HMGB1 signaling pathway in infected patients (67). This may explain the differential effect between different corticosteroid treatments, since unlike dexamethasone, MP or hydrocortisone cannot modulate the HMGB1-induced inflammatory response (68). The lack of effect of MP therapy on HMGB1 levels as seen in the present investigation may be explained by disparity in the capacity of various corticosteroids to modify HMGB1. These findings suggest that MP mode of action did not support or promote an immediate dissipation of HMGB1 production and its effects in patients with COVID-19.
Myoglobin is an iron and oxygen-binding protein that is important in the storage of oxygen in skeletal and cardiac muscles. Likewise, myoglobin has a prognostic value as a marker of myocardial injury in patients with COVID-19 (69). Our findings are in line with observations of myoglobin as an important COVID-19 biomarker. Yu et al. demonstrated that myoglobin was a predictive factor for in-hospital deaths from COVID-19. Corticosteroids can decrease inflammatory responses and reduce immune-mediated damage as seen in COVID-19 (70). Although not significantly, methylprednisolone intervention in our COVID-19 cohort improved serum creatine kinase and myoglobin levels.
Fatty acid binding protein 3 (FABP3), also known as Heart fatty acid binding protein (HFAB), is a highly specific myocardial injury serum biomarker (71). This protein reversibly and non-covalently binds long-chain fatty acids to facilitate intracellular cytoplasmic transport of fatty acids. Recent studies found that elevated levels of FABP3 were associated with severe COVID-19 (72). Our cohort demonstrated normal levels of FABP3 (ranging from 1.6 ng/mL to 19 ng/mL) in accordance with several cardiovascular disease studies (73–78). In addition to the treatment, the observed normality may have been influenced by the >36h delay from the onset of symptoms to when patients sought treatment. Although not significant, FABP3 levels in MP group on D14 were twice as high as in placebo-treated patients.
Troponin I and N-terminus of brain natriuretic peptide prohormone (NT-proBNP) are specific for myocardial injury and their increase is influenced by the severity of the disease. In our study, it was observed no significant difference in Troponin I (TnI) levels between placebo and MP groups on days 1, 7 and 14. Our results were also in agreement with the observations of Samprathi and Jayashree (3) who note that TnI and NT-proBNP are increased in patients with COVID-19 and serve as important cardiac biomarkers (3).
Another study reported that corticosteroids may protect patients with pneumonia against myocardial injury and poor cardiovascular outcomes (79). This study however used the serum high-sensitivity troponin (hs-cTnT) and not TnI as an indicator of myocardial injury, and patients received either methylprednisolone (20-80 mg/d), betamethasone (4-8 mg/d) or prednisone (25-50 mg/d) unlike the biomarker and MP dose used in our study.
Gao et al. observed that NT-proBNP was significantly higher in COVID-19 fatal cases compared with non-severe (80). Like TnI, high NT-proBNP is significantly indicative of poor prognosis among patients with COVID-19 suggesting that NT-proBNP is associated with the severity of the infection, leading to death. Other studies have confirmed similar observations regarding TnI and NT-proBNP and in-hospital mortality (28, 33, 51). NT-proBNP is secreted in response to increased myocardial wall stress, such as ischemia. Although NT-proBNP levels in MP group declined over time, it was not significant. Overall, NT-proBNP levels do not appear to be significantly influenced by MP treatment, perhaps due to the 1-week interval between assessments, but the biomarker is useful for assessing severity and predicting mortality.
Compared with controls, SARS-CoV-2 infection triggered an elevation in anti-inflammatory cytokine IL-10 and pro-inflammatory cytokine IL-6 and IL-17A. In addition, a significant elevation in the chemokine profile particularly in monocyte chemoattractant protein-1 (MCP-1), Th1 monocyte chemokines induced by interferon gamma (MIG) and IFN-γ-10 inducible protein (IP-10).These observations were similar to those from SARS patients (81), and COVID-19 patients (82–84). Additionally, some of the inflammatory cytokines and chemokines elevated in our study have been associated with the cytokine storm that cause acute lung injury and amplified inflammatory response that is deleterious to other organs (83, 85). Further, after the viral infection, these cytokines also stimulate pathways that result in immune cell differentiation, and the movement of leukocytes to infection sites further aggravating the inflammation and tissue injury (1, 86).
When compared to the placebo group, MP appeared to modify IL-8/CXCL8, IL-17A, IL-1β, IL-6, MIG/CXCL9, MCP-1/CCL2, and IP-10/CXCL10 levels by day 2 after end of therapy (D7). MP may inhibit the synthesis of NF-κB, which is responsible for the stimulation of expression of genes that code for inflammatory molecules like TNF, IL1, MCP-1/CCL2 and IL-8/CXCL8 (87). Studies have observed that the use of corticosteroids reduces TNF-alpha and IL-6 levels by reducing the systemic inflammatory response in patients hospitalized for CAP (88).
On D7, MIG/CXCL9 and IP-10/CXCL10 were significantly reduced in the MP group than the placebo. These cytokines are responsible for chemotaxis, differentiation, multiplication and tissue extravasation of leukocytes. They are strongly induced by IFN-γ (89), suggesting that the corticosteroid intervention interrupted the subsequent chemotactic effects influenced by IFN-γ in COVID-19. On a similar note, the significant difference in MIG and IP-10, as at D7 between the two groups could also be indicative of the difference in disease severity existing after the intervention and follow-up. As noted by Yang et al., although some cytokines were elevated in non-severe patients, they were also significantly lower than in severely ill COVID-19 patients (82).
Longitudinal data from the present study indicate that MP had a reducing effect on IL-2, IL-10 and IP-10. A longitudinal analysis of the cytokine profile after MP treatment showed a steady increase in IFN-γ and a corresponding decline in IL-10 between D1 and D14. This can be attributed to the short 5-day course of treatment and the short half-life of the drug (90). We postulate that the relatively high longitudinal levels of IFN-γ, IL-8, IL-6, MCP-1, MIG and IP-10 could be due to delayed viral clearance, disease severity (91) and possibly high SARS-CoV-2 viral load.
There was an observed difference in correlations between controls and pretreated COVID-19. In the control group, there was an active IL-10-IL-6 balance as well as correlation between IL-8/CXCL8, IL-1β, and TNF with other mediators. Compared with the control group, there was extensive cytokine/chemokine correlations centered on IL-17A, IL-10, IL-6, CCL2, CXCL8, CXCL9 and CXCL10 and fewer correlations with IL-1β in the COVID-19 patients at baseline. In severe cases, through inflammatory mediators like IFN-α, IL-1β, IL-6, CCL2, CCL5 and others, COVID-19 causes an inflammatory process that if uncontrolled evolves into lung tissue injuries, systemic inflammations and pathogenesis, and organ failure (1). The inflammatory profile and mediator profile of this disease may vary from factors such as patient immunodeficiency status, age, weight, gender, among others (1, 9, 92).
The corticoid treatment saw more correlations associated with IL-10, IL-2, TNF and IL-1β. Although all the associations here were mainly moderate positive, our analysis revealed strong correlation between IL-10 and IL-6 in MP treated, compared to CXCL10 with CXCL8 and CXCL9 in the placebo group. A proposed mechanism of action of these natural hormones and their synthetic analogs is to block the synthesis and secretion of cytokines, including TNF and IL-1, and other inflammatory mediators, such as prostaglandins, reactive oxygen species, and nitric oxide, produced by macrophages and other inflammatory cells (87).
Corticosteroids block the response of neutrophils to damage tissues and inhibit the chemotaxis of monocytes and neutrophils to sites of inflammation. Furthermore, these drugs block T-cell activation through inhibition of cytokine releases, thus decreasing levels of interleukin IL1, IL-2, IL-6, and TNF-α. Corticosteroids’ anti-inflammatory and immunosuppressive effects are dose- and time-dependent (70). By day 14, the MP treated patients exhibited strong correlations between IL-1, IL-6, CCL2, CXCL9, and CXCL8. On the contrary, patients receiving placebo therapy had the correlations only between IL-10, IL-6, and IL-1β and with none in the chemokines. Strong but negative correlations, IL-12p70 with IL-10 and CXCL8, were noted in the Placebo group.
In addition to drugs, several other factors, like host factors, can influence the production and correlation of human cytokines (93). The host factors can be genetic or non-genetic host factors like age, obesity and gender. Though our cohort was homogenous in age, sex and body mass index, we cannot rule out the potential influence cytokine gene variants. Hoffmann et al. report that there is a wide inter-ethnic variability in cytokine gene variants’ frequencies (IL-2, IL-6, IL-10, TNF, TGFB1, and IFN-γ) (94). These host genetic background is a critical influencer in COVID-19 outcome (95). A review study observed that variants in cytokine genes such as IL-1β, IL1R1, IL1RN, IL-6, IL-17A, FCGR2A, and TNF may be associated with disease susceptibility and/or severity, cytokine storm, and/or COVID-19 complications like thrombosis (96). Although we did not examine the pharmacogenomics of MP metabolism and presence of cytokine variants in our cohort, we cannot rule out their impact on the cytokine/chemokine profile. We also hypothesize that other than the MP treatment, that the injury biomarkers profile in our cohort may have influenced the observed mediator-mediator interrelationships.
Our data demonstrated that serum biomarkers HMGB1, myoglobin, FABP3 and troponin I were significantly altered during the initial phase of COVID-19. At D7, the MP had ameliorated levels of myoglobin and FABP3. Analysis of cytokine at pretreatment showed that the COVID-19 positive patients had significantly elevated levels of IL-17A, IL-6, IL-10, MIG, MCP-1 and IP-10. At D7, the MIG and IP-10 levels in MP group were significantly lower than placebo group. The intervention also lowered the IL-17A, IL-6, IL-1β, MCP-1, and IL-8 levels though not significantly. The MIG/CXCL9 induces chemotaxis, promote differentiation and multiplication of leukocytes, and cause tissue extravasation whereas the IP-10/CXCL10 as an inflammatory chemokine mediates immune responses through the activation and recruitment of leukocytes such as T cells, eosinophils, monocytes and NK cells (97). The observations at D7 are suggestive of how MP relieves the COVID-19 induced inflammatory response. Longitudinally, MP therapy influenced the IP-10 and IL-2 profiles, and decreased the IL-1β, and IL-10 levels. Conversely, IFN-γ levels steadily increased following this treatment. Interestingly IL-2 and IL-17A increased significantly in the placebo group, while IL-12p70, IL-10 and IP-10 decreased with time. Finally, unlike the 10-day dexamethasone study (16), the overall usage of MP for 5 days was not, in theory, effective in improving patient prognosis (45), despite the alterations in the cytokine profile.
This study had some limitations. The longitudinal decrease in sample size during injury-associated biomarker analysis and the lack of healthy controls restricted a full appreciation of biomarker dynamics. The sample size limitation subsequently affected our ability do a correlation network between the biomarker and cytokine profiles. The time interval between sample collection and analysis may have influenced the cytokine and biomarker levels detected considering that cytokines degrade during long time storage. In addition, considering that we used data extracted from patient records, there was incomplete collection or recording of full laboratory test data at D14 follow up. Despite these limitations, the results presented here help get an understanding the influence corticosteroid intervention has on the cytokine and biomarker profile of COVID-19 patients.
Conclusion
Overall, the use of high-daily dose methylprednisolone in severe COVID-19 management was beneficial to the immunological response, especially the pro-inflammatory cytokines, but limited on the injury biomarker profile. We theorize that the methylprednisolone effect the injury biomarker and cytokine profile could be dose-influenced because of the high daily dose used and treatment-time influenced considering the median 12 days delay from onset of symptoms to inclusion and start of the MP-treatment. We propose that more focused studies in future should explore influence of different doses, time to treatment, age, and sex postulates to evaluate how best to position and fortify methylprednisolone’s use in the management of inflammatory reactions associated with corona virus infections like SARS, MERS and COVID-19.
Data availability statement
The original contributions presented in the study are included in the article/Supplementary Material. Further inquiries can be directed to the corresponding author.
Ethics statement
The studies involving human participants were reviewed and approved by Fundação de Medicina Tropical Doutor Heitor Vieira Dourado. The patients/participants provided their written informed consent to participate in this study.
Author contributions
VM, AC, WM, and GM conceived the study concept and designed the experiments. VM, RN, CM, AL, and AC performed the experiments, and data acquisition. VM, AS, BS, and JN analyzed and interpreted the data, and did data visualization. VS advised on the data analysis and interpretation. VM, RN, and JN and wrote the manuscript. AA, AC, FV, ML, LG, VS, WM, and GM critically revised the manuscript and results. MB, AC, ML, WM, and GM provided administrative, technical, and material support and supervised the study. All authors contributed to the article and approved the submitted version.
Funding
Financial support was provided by grants from the Fundação de Amparo à Pesquisa do Estado do Amazonas (FAPEAM) under the Resolutions # 002/2008, 007/2018, 005/2019 and 005/2022, Conselho Nacional de Desenvolvimento Científico e Tecnológico (CNPq), and Coordenação de Aperfeiçoamento de Pessoal de Nível Superior scholarships (CAPES). The funders had no role in study design, data collection and analysis, decision to publish, or preparation of the manuscript.
Acknowledgments
VM, RN, AS, BS, and AL are recipients of the Fundação de Amparo à Pesquisa do Estado do Amazonas (FAPEAM) or CAPES scholarships. AC, WM, ML and GM are research fellows from Conselho Nacional de Desenvolvimento Científico e Tecnológico (CNPq). We give special thanks to the director and staff of Hospital e Pronto Socorro Delphina Rinaldi Abdel Aziz, IPCCB, FMT-HVD and HEMOAM as well as the many members of the MetCOVID Study Team for their outstanding support. We are also deeply grateful to Fabiane Bianca Albuquerque Barbosa, Nilberto Dias de Araújo and Fábio Magalhães-Gama for their technical support.
Conflict of interest
The authors declare that the research was conducted in the absence of any commercial or financial relationships that could be construed as a potential conflict of interest.
Publisher’s note
All claims expressed in this article are solely those of the authors and do not necessarily represent those of their affiliated organizations, or those of the publisher, the editors and the reviewers. Any product that may be evaluated in this article, or claim that may be made by its manufacturer, is not guaranteed or endorsed by the publisher.
Supplementary material
The Supplementary Material for this article can be found online at: https://www.frontiersin.org/articles/10.3389/fimmu.2023.1229611/full#supplementary-material
References
1. Oliveira DS, Medeiros NI, Gomes JAS. Immune response in COVID-19: What do we currently know? Microb Pathog (2020) 148:104484. doi: 10.1016/j.micpath.2020.104484
2. Hoffmann M, Kleine-Weber H, Schroeder S, Krüger N, Herrler T, Erichsen S, et al. SARS-CoV-2 cell entry depends on ACE2 and TMPRSS2 and is blocked by a clinically proven protease inhibitor. Cell (2020) 181(2):271–280.e8. doi: 10.1016/j.cell.2020.02.052
3. Samprathi M, Jayashree M. Biomarkers in COVID-19: an up-to-date review. Front Pediatr (2021) 8. doi: 10.3389/fped.2020.607647
4. Hajjar LA, Costa IBS da S, Rizk SI, Biselli B, Gomes BR, Bittar CS, et al. Intensive care management of patients with COVID-19: a practical approach. Ann Intensive Care (2021) 11(1):1–17. doi: 10.1186/s13613-021-00820-w
5. Daly JL, Simonetti B, Klein K, Chen KE, Williamson MK, Antón-Plágaro C, et al. Neuropilin-1 is a host factor for SARS-CoV-2 infection. Science (2020) 370(6518):861–5. doi: 10.1126/science.abd3072
6. Gavriatopoulou M, Korompoki E, Fotiou D, Ntanasis-Stathopoulos I, Psaltopoulou T, Kastritis E, et al. Organ-specific manifestations of COVID-19 infection. Clin Exp Med (2020) 20(4):493. doi: 10.1007/s10238-020-00648-x
7. Teixeira TA, Bernardes FS, Oliveira YC, Hsieh MK, Esteves SC, Duarte-Neto AN, et al. SARS-CoV-2 and Multi-Organ damage – What men’s health specialists should know about the COVID-19 pathophysiology. Int Braz J urol (2021) 47(3):637–46. doi: 10.1590/s1677-5538.ibju.2020.0872
8. Chen N, Zhou M, Dong X, Qu J, Gong F, Han Y, et al. Epidemiological and clinical characteristics of 99 cases of 2019 novel coronavirus pneumonia in Wuhan, China: a descriptive study. Lancet (2020) 395(10223):507–13. doi: 10.1016/S0140-6736(20)30211-7
9. Singhal T. A review of coronavirus disease-2019 (COVID-19). Indian J Pediatr (2020) 87(4):281–6. doi: 10.1007/s12098-020-03263-6
10. Long QX, Tang XJ, Shi QL, Li Q, Deng HJ, Yuan J, et al. Clinical and immunological assessment of asymptomatic SARS-CoV-2 infections. Nat Med (2020) 26(8):1200–4. doi: 10.1038/s41591-020-0965-6
11. Ye Z, Wang Y, Colunga-Lozano LE, Prasad M, Tangamornsuksan W, Rochwerg B, et al. Efficacy and safety of corticosteroids in COVID-19 based on evidence for COVID-19, other coronavirus infections, influenza, community-acquired pneumonia and acute respiratory distress syndrome: a systematic review and meta-analysis. CMAJ (2020) 192(27):E755–67. doi: 10.1503/cmaj.200645
12. Russell CD, Millar JE, Baillie JK. Clinical evidence does not support corticosteroid treatment for 2019-nCoV lung injury. Lancet (2020) 395:473–5. doi: 10.1016/S0140-6736(20)30317-2
13. Russell B, Moss C, George G, Santaolalla A, Cope A, Papa S, et al. Associations between immune-suppressive and stimulating drugs and novel COVID-19 - A systematic review of current evidence. Ecancermedicalscience (2020) 14:1022. doi: 10.3332/ecancer.2020.1022
14. Zhao JP, Hu Y, Du RH, Chen ZS, Jin Y, Zhou M, et al. [Expert consensus on the use of corticosteroid in patients with 2019-nCoV pneumonia]. Chinese journal of tuberculosis and respiratory diseases (2020) 43(3):183–4. doi: 10.3760/cma.j.issn.1001-0939.2020.03.008
15. Wu C, Chen X, Cai Y, Xia Jia’an, Zhou X, Xu S, et al. Risk factors associated with acute respiratory distress syndrome and death in patients with coronavirus disease 2019 pneumonia in Wuhan, China supplemental content. JAMA Intern Med (2020) 180(7):934–43. doi: 10.1001/jamainternmed.2020.0994
16. RECOVERY Collaborative Group. Dexamethasone in hospitalized patients with Covid-19. N Engl J Med (2021) 384(8):693–704. doi: 10.1056/NEJMoa2021436
17. Tu J, Mo X, Zhang X, Xun J, Chen X, Liu Y, et al. Effects of different corticosteroid therapy on severe COVID-19 patients: a meta-analysis of randomized controlled trials. Expert Rev Respir Med (2021) 16(1):1. doi: 10.1080/17476348.2021.1983429
18. National Health Commission of the People’s Republic of China. Diagnosis and treatment protocol for COVID-19 (Trial version 7). Chin Med J (2020) 133:1087–95. doi: 10.1097/CM9.0000000000000819
19. Crisan Dabija R, Antohe I, Trofor A, Antoniu SA. Corticosteroids in SARS-COV2 infection: certainties and uncertainties in clinical practice. Expert Rev Anti Infect Ther (2021) 19(12):1. doi: 10.1080/14787210.2021.1933437
20. Li H, Chen C, Hu F, Wang J, Zhao Q, Gale RP, et al. Impact of corticosteroid therapy on outcomes of persons with SARS-CoV-2, SARS-CoV, or MERS-CoV infection: a systematic review and meta-analysis. Leukemia (2020) 34(6):1503–11. doi: 10.1038/s41375-020-0848-3
21. Lee KH, Yoon S, Jeong GH, Kim JY, Han YJ, Hong SH, et al. Efficacy of corticosteroids in patients with SARS, MERS and COVID-19: A systematic review and meta-analysis. J Clin Med (2020) 9(8):1–17. doi: 10.3390/jcm9082392
22. Sung JJY, Wu A, Joynt GM, Yuen KY, Lee N, Chan PKS, et al. Severe acute respiratory syndrome: report of treatment and outcome after a major outbreak. Thorax (2004) 59(5):414–20. doi: 10.1136/thx.2003.014076
23. Fan X, Song JW, Wang SY, Cao WJ, Wang XW, Zhou MJ, et al. Changes of damage associated molecular patterns in COVID-19 patients. Infect Dis Immun (2021) 1(1):20–7. doi: 10.1097/01.ID9.0000733572.40970.6c
24. Vicentino ARR, Fraga-Junior V da S, Palazzo M, Tasmo NRA, Rodrigues DAS, Barroso SPC, et al. High mobility group box 1, ATP, lipid mediators, and tissue factor are elevated in COVID-19 patients: HMGB1 as a biomarker of worst prognosis. Clin Transl Sci (2023) 16(4):631. doi: 10.1111/cts.13475
25. Chen L, Long X, Xu Q, Tan J, Wang G, Cao Y, et al. Elevated serum levels of S100A8/A9 and HMGB1 at hospital admission are correlated with inferior clinical outcomes in COVID-19 patients. Cell Mol Immunol (2020) 17(9):992–4. doi: 10.1038/s41423-020-0492-x
26. Yu J-S, Chen R-D, Zeng L-C, Yang H-K, Li H. Myoglobin offers higher accuracy than other cardiac-specific biomarkers for the prognosis of COVID-19. Front Cardiovasc Med (2021) 8. doi: 10.3389/fcvm.2021.686328
27. Ma C, Tu D, Gu J, Xu Q, Hou P, Wu H, et al. The predictive value of myoglobin for COVID-19-related adverse outcomes: A systematic review and meta-analysis. Front Cardiovasc Med (2021) 8:757799. doi: 10.3389/fcvm.2021.757799
28. Stefanini GG, Chiarito M, Ferrante G, Cannata F, Azzolini E, Viggiani G, et al. Early detection of elevated cardiac biomarkers to optimise risk stratification in patients with COVID-19. Heart (2020) 106(19):1512–8. doi: 10.1136/heartjnl-2020-317322
29. Zeng J-H, Wu W-B, Qu J-X, Wang Y, Dong C-F, Luo Y-F, et al. Cardiac manifestations of COVID-19 in Shenzhen, China. Infection (2020) 48(6):861–70. doi: 10.1007/s15010-020-01473-w
30. Kaufmann CC, Ahmed A, Burger AL, Muthspiel M, Jäger B, Wojta J, et al. Biomarkers associated with cardiovascular disease in COVID-19. Cells (2022) 11(6):922. doi: 10.3390/cells11060922
31. Wungu CDK, Khaerunnisa S, Putri EAC, Hidayati HB, Qurnianingsih E, Lukitasari L, et al. Meta-analysis of cardiac markers for predictive factors on severity and mortality of COVID-19. Int J Infect Dis (2021) 105:551–9. doi: 10.1016/j.ijid.2021.03.008
32. Pranata R, Huang I, Lukito AA, Raharjo SB. Elevated N-terminal pro-brain natriuretic peptide is associated with increased mortality in patients with COVID-19: systematic review and meta-analysis. Postgrad Med J (2020) 96(1137):387–91. doi: 10.1136/postgradmedj-2020-137884
33. Caro-Codón J, Rey JR, Buño A, Iniesta AM, Rosillo SO, Castrejon-Castrejon S, et al. Characterization of NT-proBNP in a large cohort of COVID-19 patients. Eur J Heart Fail (2021) 23(3):456–464. doi: 10.1002/ejhf.2095
34. O’Donnell C, Ashland MD, Vasti EC, Lu Y, Chang AY, Wang P, et al. N-terminal pro-B-type natriuretic peptide as a biomarker for the severity and outcomes with COVID-19 in a nationwide hospitalized cohort. J Am Heart Assoc (2021) 10(24):e022913. doi: 10.1161/JAHA.121.022913
35. Ameres M, Brandstetter S, Toncheva AA, Kabesch M, Leppert D, Kuhle J, et al. Association of neuronal injury blood marker neurofilament light chain with mild-to-moderate COVID-19. J Neurol (2020) 267:3476–8. Springer Science and Business Media Deutschland GmbH. doi: 10.1007/s00415-020-10050-y
36. Sutter R, Hert L, De Marchis GM, Twerenbold R, Kappos L, Naegelin Y, et al. Serum Neurofilament Light Chain Levels in the Intensive Care Unit: Comparison between Severely Ill Patients with and without Coronavirus Disease 2019. Ann Neurol (2021) 89(3):610–6. doi: 10.1002/ana.26004
37. Cione E, Siniscalchi A, Gangemi P, Cosco L, Colosimo M, Longhini F, et al. Neuron-specific enolase serum levels in COVID-19 are related to the severity of lung injury. PloS One (2021) 16(5):e0251819. doi: 10.1371/journal.pone.0251819
38. De Lorenzo R, Loré NI, Finardi A, Mandelli A, Cirillo DM, Tresoldi C, et al. Blood neurofilament light chain and total tau levels at admission predict death in COVID-19 patients. J Neurol (2021) 1:1–7. doi: 10.1007/s00415-021-10595-6
39. Chen R, Huang Y, Quan J, Liu J, Wang H, Billiar TR, et al. HMGB1 as a potential biomarker and therapeutic target for severe COVID-19. Heliyon (2020) 6(12):e05672. doi: 10.1016/j.heliyon.2020.e05672
40. Abbasi B, Torres P, Ramos-Tuarez F, Dewaswala N, Abdallah A, Chen K, et al. Cardiac troponin-I and COVID-19: A prognostic tool for in-hospital mortality. Cardiol Res (2020) 11(6):398–404. doi: 10.14740/cr1159
41. Dekosky ST, Kochanek PM, Valadka AB, Clark RSB, Chou SHY, Au AK, et al. Blood biomarkers for detection of brain injury in COVID-19 patients. J Neurotrauma (2021) 38:1–43. Mary Ann Liebert Inc. doi: 10.1089/neu.2020.7332
42. Sivakorn C, Dechsanga J, Jamjumrus L, Boonnak K, Schultz MJ, Dondorp AM, et al. High mobility group box 1 and interleukin 6 at intensive care unit admission as biomarkers in critically ill COVID-19 patients. Am J Trop Med Hyg (2021) 105(1):73–80. doi: 10.4269/ajtmh.21-0165
43. Henry BM, De Oliveira MHS, Benoit S, Plebani M, Lippi G. Hematologic, biochemical and immune biomarker abnorMalities associated with severe illness and mortality in coronavirus disease 2019 (COVID-19): a meta-analysis. Clin Chem Lab Med (2020) 58(7):1021–8. doi: 10.1515/cclm-2020-0369
44. Chen R, Sang L, Jiang M, Yang Z, Jia N, Fu W, et al. Longitudinal hematologic and immunologic variations associated with the progression of COVID-19 patients in China. J Allergy Clin Immunol (2020) 146(1):89–100. doi: 10.1016/j.jaci.2020.05.003
45. Jeronimo CMP, Farias MEL, Val FFA, Sampaio VS, Alexandre MAA, Melo GC, et al. Methylprednisolone as Adjunctive Therapy for Patients Hospitalized With Coronavirus Disease 2019 (COVID-19; Metcovid): A Randomized, Double-blind, Phase IIb, Placebo-controlled Trial (2021).
46. Barros CMSS, Freire RS, Frota E, Rezende Santos AG, Farias MEL, Rodrigues MGA, et al. Short-course of methylprednisolone improves respiratory functional parameters after 120 days in hospitalized COVID-19 patients (Metcovid trial): A randomized clinical trial. Front Med (2021) 8:758405. doi: 10.3389/fmed.2021.758405
47. Taylor R. Interpretation of the correlation coefficient: A basic review. J Diagn Med Sonogr (1990) 6(1):35–9. doi: 10.1177/875647939000600106
48. Costa AG, Ramasawmy R, Val FFA, Ibiapina HNS, Oliveira AC, Tarragô AM, et al. Polymorphisms in TLRs influence circulating cytokines production in Plasmodium vivax malaria: TLR polymorphisms influence cytokine productions in malaria-vivax. Cytokine (2018) 110:374–80. doi: 10.1016/j.cyto.2018.04.008
49. Qin C, Zhou L, Hu Z, Zhang S, Yang S, Tao Y, et al. Dysregulation of immune response in patients with coronavirus 2019 (COVID-19) in Wuhan, China. Clin Infect Dis (2020) 71(15):762–8. doi: 10.1093/cid/ciaa248
50. Yao Y, Cao J, Wang Q, Shi Q, Liu K, Luo Z, et al. D-dimer as a biomarker for disease severity and mortality in COVID-19 patients: a case control study. J Intensive Care (2020) 8(1):1–11. doi: 10.1186/s40560-020-00466-z
51. Chen T, Wu D, Chen H, Yan W, Yang D, Chen G, et al. Clinical characteristics of 113 deceased patients with coronavirus disease 2019: retrospective study. BMJ (Clinical research ed.) (2020) 368:m1091. doi: 10.1136/bmj.m1091
52. Torres A, Ceccato A, Ferrer M, Gabarrus A, Sibila O, Cilloniz C, et al. Effect of corticosteroids on C-reactive protein in patients with severe community-acquired pneumonia and high inflammatory response: the effect of lymphopenia. J Clin Med (2019) 8(9):1461. doi: 10.3390/jcm8091461
53. Russo A, Tellone E, Barreca D, Ficarra S, Laganà G. Implication of COVID-19 on erythrocytes functionality: red blood cell biochemical implications and morpho-functional aspects. Int J Mol Sci (2022) 23(4):2171. doi: 10.3390/ijms23042171
54. Cavezzi A, Troiani E, Corrao S. COVID-19: hemoglobin, iron, and hypoxia beyond inflammation. A narrative review. Clin Pract (2020) 10(2):1271. doi: 10.4081/cp.2020.1271
55. Grau M, Ibershoff L, Zacher J, Bros J, Tomschi F, Diebold KF, et al. Even patients with mild COVID-19 symptoms after SARS-CoV-2 infection show prolonged altered red blood cell morphology and rheological parameters. J Cell Mol Med (2022) 26(10):3022–30. doi: 10.1111/jcmm.17320
56. Karimi Shahri M, Niazkar HR, Rad F. COVID-19 and hematology findings based on the current evidences: A puzzle with many missing pieces. Int J Lab Hematol (2021) 43(2):160–8. doi: 10.1111/ijlh.13412
57. Mao J, Dai R, Du RC, Zhu Y, Shui LP, Luo XH. Hematologic changes predict clinical outcome in recovered patients with COVID-19. Ann Hematol (2021) 100(3):675. doi: 10.1007/s00277-021-04426-x
58. Fouad SH, Allam MF, Taha SI, Okba AA, Hosny A, Moneer M, et al. Comparison of hemoglobin level and neutrophil to lymphocyte ratio as prognostic markers in patients with COVID-19. J Int Med Res (2021) 49(7). doi: 10.1177/03000605211030124
59. Lippi G, Mattiuzzi C. Hemoglobin value may be decreased in patients with severe coronavirus disease 2019. Hematol Transfus Cell Ther (2020) 42(2):116–7. doi: 10.1016/j.htct.2020.03.001
60. Li S, Hu Z, Song X. High-dose but not low-dose corticosteroids potentially delay viral shedding of patients with COVID-19. Clin Infect Dis (2020) 72(7):1297–8. doi: 10.1093/cid/ciaa829/5863128
61. Al-kuraishy HM, Al-Gareeb AI, Alkazmi L, Habotta OA, Batiha GES. High-mobility group box 1 (HMGB1) in COVID-19: extrapolation of dangerous liaisons. Inflammopharmacology (2022) 30(3):811–20. doi: 10.1007/s10787-022-00988-y
62. Gougeon ML, Melki MT, Sadi H. HMGB1, an alarmin promoting HIV dissemination and latency in dendritic cells. Cell Death Differ (2012) 19(1):96. doi: 10.1038/cdd.2011.134
63. Rubartelli A, Lotze MT. Inside, outside, upside down: damage-associated molecular-pattern molecules (DAMPs) and redox. Trends Immunol (2007) 28(10):429–36. doi: 10.1016/j.it.2007.08.004
64. Peltz ED, Moore EE, Eckels PC, Damle SS, Tsuruta Y, Johnson JL, et al. HMGB1 is markedly elevated within 6 hours of mechanical trauma in humans. Shock (2009) 32(1):17. doi: 10.1097/SHK.0b013e3181997173
65. Vanpatten S, Al-Abed Y. High mobility group box-1 (HMGb1): current wisdom and advancement as a potential drug target. J Med Chem (2018) 61(12):5093–107. doi: 10.1021/acs.jmedchem.7b01136
66. Ding X, Li S, Zhu L. Potential effects of HMGB1 on viral replication and virus infection-induced inflammatory responses: A promising therapeutic target for virus infection-induced inflammatory diseases. Cytokine Growth Factor Rev (2021) 62:54–61. doi: 10.1016/j.cytogfr.2021.08.003
67. Gerö D, Szoleczky P, Mó Dis K, Pribis JP, Al-Abed Y. Identification of pharmacological modulators of HMGB1-induced inflammatory response by cell-based screening. PloS One (2013) 8(6):e65994. doi: 10.1371/journal.pone.0065994
68. Annweiler C, Papon N, Sabatier J-M, Barré J. DAMPening severe COVID-19 with dexamethasone. Infect Disord-Drug Targets (2022) 22:11–2. doi: 10.2174/1871526521999210827142
69. Ma C, Tu D, Gu J, Xu Q, Hou P, Wu H, et al. The predictive value of myoglobin for COVID-19-related adverse outcomes: A systematic review and meta-analysis. Front Cardiovasc Med (2021) 8:1509. doi: 10.3389/fcvm.2021.757799
70. Antoon JW, Chakraborti C. Corticosteroids in the treatment of alcohol-induced rhabdomyolysis. Mayo Clin Proc (2011) 86(10):1005. doi: 10.4065/mcp.2011.0102
71. Hai-Long W, Xiao-Hua P, Jian-Jun Y, Xue-Mei T. The prognostic value of heart-type fatty acid binding protein in patients with acute coronary syndrome. J Coll Phys Surg Pakistan (2018) 28(1):56–61. doi: 10.29271/jcpsp.2018.01.56
72. Yin L, Mou H, Shao J, Zhu Y, Pang X, Yang J, et al. Correlation between Heart fatty acid binding protein and severe COVID-19: A case-control study. PloS One (2020) 15(4):e0231687. doi: 10.1371/journal.pone.0231687
73. Kleine AH, Glatz JFC, Van Nieuwenhoven FA, van der Vusse GJ. Release of heart fatty acid-binding protein into plasma after acute myocardial infarction in man. Mol Cell Biochem (1992) 116(1):155–62. doi: 10.1007/BF01270583
74. Trifonov IR, Katrukha AG, Iavelov IS, Averkov OV, Gratsianskiĭ NA. Diagnostic value of heart fatty-acid binding protein in early hospitalized patients with non ST elevation acute coronary syndrome. Kardiologiia (2003) 43(5):4–8.
75. Chan CPY, Sum KW, Cheung KY, Glatz JFC, Sanderson JE, Hempel A, et al. Development of a quantitative lateral-flow assay for rapid detection of fatty acid-binding protein. J Immunol Methods (2003) 279(1–2):91–100. doi: 10.1016/S0022-1759(03)00243-6
76. Ohkaru Y, Asayama K, Ishii H, Nishimura S, Sunahara N, Tanaka T, et al. Development of a sandwich enzyme-linked immunosorbent assay for the determination of human heart type fatty acid-binding protein in plasma and urine by using two different monoclonal antibodies specific for human heart fatty acid-binding protein. J Immunol Methods (1995) 178(1):99–111. doi: 10.1016/0022-1759(94)00248-U
77. Pagani F, Bonora R, Bonetti G, Panteghini M. Evaluation of a sandwich enzyme-linked immunosorbent assay for the measurement of serum heart fatty acid-binding protein. Ann Clin Biochem (2002) 39(Pt 4):404–5. doi: 10.1258/000456302760042173
78. Watanabe T, Ohkubo Y, Matsuoka H, Kimura H, Sakai Y, Ohkaru Y, et al. Development of a simple whole blood panel test for detection of human heart-type fatty acid-binding protein. Clin Biochem (2001) 34(4):257–63. doi: 10.1016/S0009-9120(01)00200-4
79. Cangemi R, Carnevale R, Cammisotto V, Nocella C, Bartimoccia S, Taliani G, et al. Corticosteroid use, myocardial injury and in-hospital cardiovascular events in patients with community-acquired pneumonia. Br J Clin Pharmacol (2022) 88(1):155–65. doi: 10.1111/bcp.14936
80. Gao L, Jiang D, Wen XS, Cheng XC, Sun M, He B, et al. Prognostic value of NT-proBNP in patients with severe COVID-19. Respir Res (2020) 21(1):1–7. doi: 10.1186/s12931-020-01352-w
81. Wong CK, Lam CWK, Wu AKL, Ip WK, Lee NLS, Chan IHS, et al. Plasma inflammatory cytokines and chemokines in severe acute respiratory syndrome. Clin Exp Immunol (2004) 136(1):95. doi: 10.1111/j.1365-2249.2004.02415.x
82. Yang L, Liu S, Liu J, Zhang Z, Wan X, Huang B, et al. COVID-19: immunopathogenesis and immunotherapeutics. Signal Transduct Target Ther (2020) 5(1):1–8. doi: 10.1038/s41392-020-00243-2
83. Akhmerov A, Marbán E. COVID-19 and the heart. Circ Res (2020) 126(10):1443–55. doi: 10.1161/CIRCRESAHA.120.317055
84. De Biasi S, Tartaro D, Gibellini L, Paolini A, Quong A, Petes C, et al. Endogenous control of inflammation characterizes pregnant women with asymptomatic or paucisymptomatic SARS-CoV-2 infection. Nat Commun (2021) 12(1):4677. doi: 10.1038/s41467-021-24940-w
85. Ponti G, Maccaferri M, Ruini C, Tomasi A, Ozben T. Biomarkers associated with COVID-19 disease progression. Crit Rev Clin Lab Sci (2020) 57(6):389–99:2020. doi: 10.1080/10408363.2020.1770685
86. Liu JM, Tan BH, Wu S, Gui Y, Suo J, Li YC. Evidence of central nervous system infection and neuroinvasive routes, as well as neurological involvement, in the lethality of SARS-CoV-2 infection. J Med Virol (2021) 93:1304–13. John Wiley and Sons Inc. doi: 10.1002/jmv.26570
87. Abbas AK, Lichtman AH, Pillai S. Cellular and Molecular Immunology (2021). Available at: https://www.elsevier.com/books/cellular-and-molecular-immunology/abbas/978-0-323-75748-5.
88. Ferrer M, Torres A, Martínez R, Ramírez P, Polverino E, Montull B, et al. Inhaled corticosteroids and systemic inflammatory response in community-acquired pneumonia: A prospective clinical study. Respirology (2014) 19(6):929–35. doi: 10.1111/resp.12324
89. Tokunaga R, Zhang W, Naseem M, Puccini A, Berger MD, Soni S, et al. CXCL9, CXCL10, CXCL11/CXCR3 axis for immune activation–a target for novel cancer therapy. Cancer Treat Rev. (2018) 63:40–7. doi: 10.1016/j.ctrv.2017.11.007
90. Kapugi M, Cunningham K. Corticosteroids. Orthop Nurs (2019) 38(5):340–1. doi: 10.1097/NOR.0000000000000595
91. Chen Y, Wang J, Liu C, Su L, Zhang D, Fan J, et al. IP-10 and MCP-1 as biomarkers associated with disease severity of COVID-19. Mol Med (2020) 26(1):1–12. doi: 10.1186/s10020-020-00230-x
92. Acosta-Ampudia Y, Monsalve DM, Rojas M, Rodríguez Y, Gallo JE, Salazar-Uribe JC, et al. COVID-19 convalescent plasma composition and immunological effects in severe patients. J Autoimmun (2021) 118:102598. doi: 10.1016/j.jaut.2021.102598
93. ter Horst R, Jaeger M, Smeekens SP, Oosting M, Swertz MA, Li Y, et al. Host and environmental factors influencing individual human cytokine responses. Cell (2016) 167(4):1111. doi: 10.1016/j.cell.2016.10.018
94. Hoffmann SC, Stanley EM, Cox ED, DiMercurio BS, Koziol DE, Harlan DM, et al. Ethnicity greatly influences cytokine gene polymorphism distribution. Am J Transplant Off J Am Soc Transplant Am Soc Transpl Surg (2002) 2(6):560–7. doi: 10.1034/j.1600-6143.2002.20611.x
95. Di Maria E, Latini A, Borgiani P, Novelli G. Genetic variants of the human host influencing the coronavirus-associated phenotypes (SARS, MERS and COVID-19): rapid systematic review and field synopsis. Hum Genomics (2020) 14(1):1–19. doi: 10.1186/s40246-020-00280-6
96. Fricke-Galindo I, Falfán-Valencia R. Genetics insight for COVID-19 susceptibility and severity: A review. Front Immunol (2021) 12. doi: 10.3389/fimmu.2021.622176
Keywords: SARS-CoV-2, methylprednisolone, biomarkers, injury, cytokine, immune mediators
Citation: Mwangi VI, Netto RLA, de Morais CEP, Silva AS, Silva BM, Lima AB, Neves JCF, Borba MGS, Val FFA, de Almeida ACG, Costa AG, Sampaio VS, Gardinassi LG, de Lacerda MVG, Monteiro WM and de Melo GC (2023) Temporal patterns of cytokine and injury biomarkers in hospitalized COVID-19 patients treated with methylprednisolone. Front. Immunol. 14:1229611. doi: 10.3389/fimmu.2023.1229611
Received: 26 May 2023; Accepted: 26 July 2023;
Published: 16 August 2023.
Edited by:
Soheil Ebrahimpour, Babol University of Medical Sciences, IranReviewed by:
Karen Ingrid Tasca, São Paulo State University, BrazilSamrad Mehrabi, Shiraz University of Medical Sciences, Iran
Copyright © 2023 Mwangi, Netto, de Morais, Silva, Silva, Lima, Neves, Borba, Val, de Almeida, Costa, Sampaio, Gardinassi, de Lacerda, Monteiro and de Melo. This is an open-access article distributed under the terms of the Creative Commons Attribution License (CC BY). The use, distribution or reproduction in other forums is permitted, provided the original author(s) and the copyright owner(s) are credited and that the original publication in this journal is cited, in accordance with accepted academic practice. No use, distribution or reproduction is permitted which does not comply with these terms.
*Correspondence: Gisely Cardoso de Melo, cardosogisely@gmail.com