- Department of Thyroid and Neck, Affiliated Cancer Hospital of Zhengzhou University, Henan Cancer Hospital, Zhengzhou, China
Protein post-translational modification (PTM) is a regulatory mechanism for protein activity modulation, localization, expression, and interactions with other cellular molecules. It involves the addition or removal of specific chemical groups on the amino acid residues of proteins. Its common forms include phosphorylation, ubiquitylation, methylation, and acetylation. Emerging research has highlighted lactylation, succinylation, and glycosylation. PTMs are involved in vital biological processes. The occurrence and development of diseases depends on protein abundance and is regulated by various PTMs. In addition, advancements in tumor immunotherapy have revealed that protein PTM is also involved in the proliferation, activation, and metabolic reprogramming of immune cells in tumor microenvironment. These PTMs play an important role in tumor immunotherapy. In this review, we comprehensively summarize the role of several types of PTMs in tumor immunotherapy. This review could provide new insights and future research directions for tumor immunotherapy.
1 Introduction
Tumor immunotherapy is a novel and effective treatment that overcomes tumor immune escape by activating or reversing immune cells with failed functions, thereby inhibiting or killing tumor cells (1). According to molecular mechanisms, it includes immune checkpoint inhibitors (ICIs), acceptance and commitment therapy (ACT), and monoclonal antibody therapy (2). ICIs can block the inhibitory effect of tumor cells on immune cells. In the 1990s, immunologists James P. Alison and Tasuku Honjo discovered ICIs, which marked the new era of tumor immunotherapy (3). In 2011, ipilimumab, a cytotoxic T lymphocyte antigen-4 antibody, was first used to treat melanoma (4). ACT suppresses tumors mainly by injecting specific immune cells targeting cancer cells into patients after being expanded and cultured in vitro (5). Anti-CD19 chimeric antigen receptor T-cell therapy (CAR-T) for B-cell lymphoma has been approved for clinical use (6). Monoclonal antibody therapy can inhibit tumors mainly by recruiting T cells to the tumor site and directly targeting tumor cells (7). Monoclonal antibodies are widely used in the field of tumor immunotherapy. Currently, the Food and Drug Administration has approved more than 100 monoclonal antibody products to enter the market (8). Immunotherapy can treat various solid and hematological tumors. New immunotherapy targets and corresponding immunotherapeutic drugs have been continuously discovered. Thus, treatment strategies for tumors have gradually shifted from inhibiting malignant proliferation and invasion of tumor cells to exploring the complex relationship between the tumor and the microenvironment around the tumor (9).
Post-translational modification (PTM) is a covalent modification of the side chains of amino acids in translated proteins. Under physiologic and pathologic conditions, it can expand the functional diversity of proteins by regulating protein folding, activity, stability, localization, signal transduction, and binding (10). Its main forms include ubiquitin, phosphorylation, methylation, acetylation, glycosylation, and succinylation (11). It is closely related to immune cell activation, signal regulation, immune response, and tumor metabolic reprogramming (12–14). It can affect the efficacy of immunotherapy directly or indirectly by regulating immune checkpoints or remodeling tumor immune microenvironment (15–17). In this review, we summarize potential mechanisms of several types of PTMs affecting cancer development and immunotherapy (Figure 1).
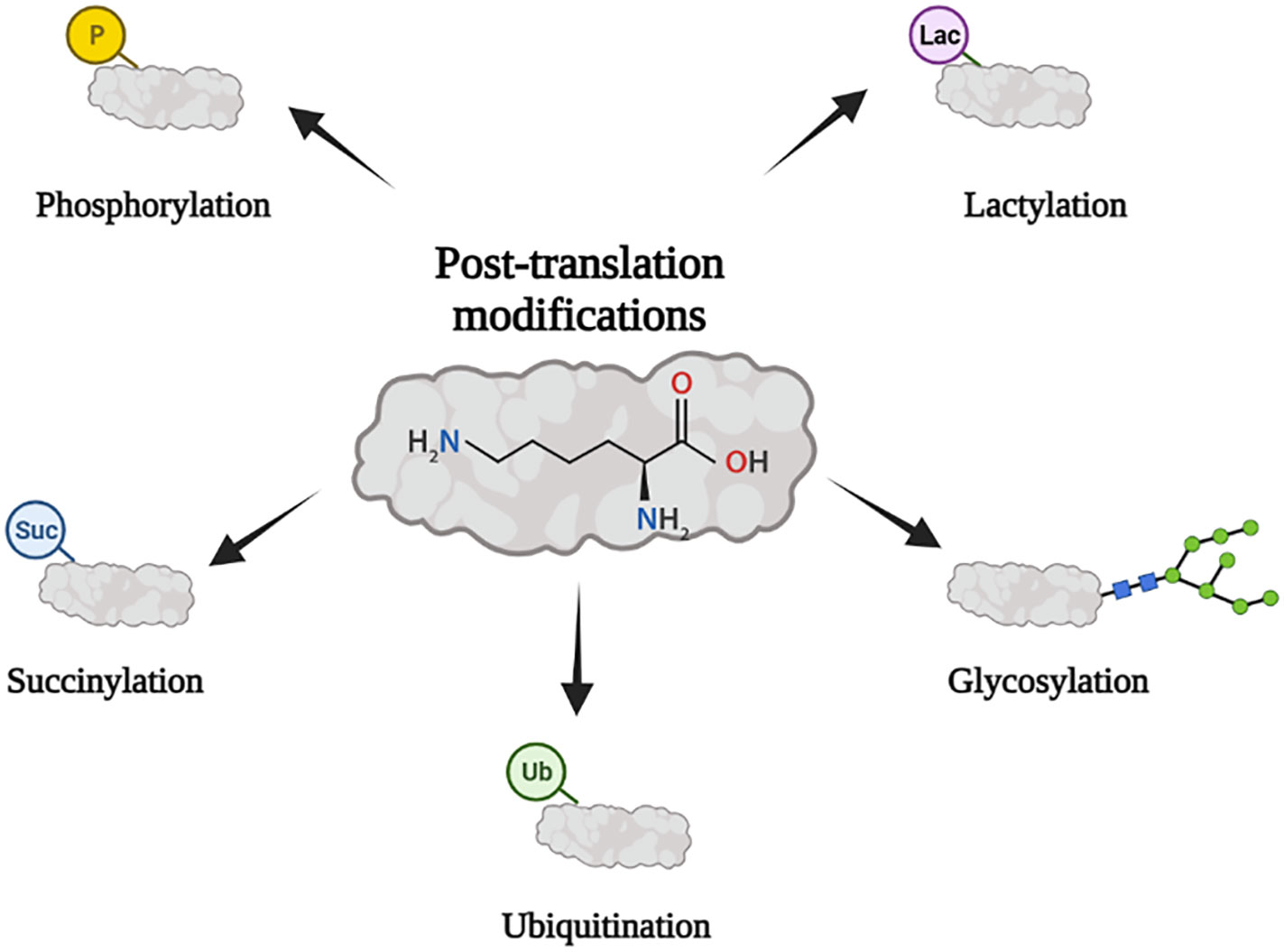
Figure 1 PTM is closely related to tumor immunity. Its main forms include ubiquitination, phosphorylation, glycosylation, succinylation, and lactylation.
2 Phosphorylation and tumor immunity
Phosphorylation is a classical and reversible PTM in which phosphate groups are covalently modified to amino acid residues after catalysis of protein kinases. It is the most common and essential PTM in eukaryotes. Approximately 30% of the proteins in mammals can be phosphorylated (18). Protein phosphorylation plays an important role in cell division, signal transduction, gene expression regulation, and protein interaction (19). Therefore, mutation of a protein phosphate site can lead to the occurrence and progression of cancer by inducing tumor cell proliferation, invasion, and metastasis and inhibiting apoptosis (18, 20). The activation or inhibition of mitogen-activated protein kinases, such as phosphoinositide 3-kinase (PI3K) and Akt kinase, and other signaling pathways is related to the phosphorylation and dephosphorylation of related proteins or enzymes in tumors, followed by regulation of the proliferation, differentiation, apoptosis, and migration of tumor cells (18).
The progression and inhibition of breast cancer are significantly related to the phosphorylation of upstream and downstream regulatory factors of nitric oxide (NO) (21). The growth and proliferation of breast cancer cells are partly induced by NO synthase, which maintains the phosphorylation of Akt and mitogen-activated protein kinase 1/2 (extracellular signal-regulated protein kinases 1 and 2 [ERK1/2]) (22, 23). However, a high NO concentration can induce apoptosis of breast cancer cells through dephosphorylation of Akt and ERK (24). Nuclear transcription factor kappa B-interacting long noncoding RNA (lncRNA) can inhibit breast cancer metastasis by blocking inhibitor of nuclear factor kappa B (NF-𝜅B) phosphorylation (25). Chao et al.’s study revealed that fructose-1,6-bisphosphatase 1 and 6‐phosphofructose‐2‐kinase/fructose‐2,6‐bisphosphatase 3 can promote breast cancer cell genesis, glycolysis, and paclitaxel resistance through phosphorylation by proviral insertion in murine lymphomas 2 (26, 27). The PI3K–Akt–mammalian target of rapamycin kinase pathway is abnormally activated in non-small cell lung cancer, and overexpression of phosphorylated Akt leads to tumor cell proliferation (28). Phosphorylation of S308 and S30 of cyclase-associated protein 1 can stimulate the proliferation, migration, and metastasis of lung cancer cells (29).
The search for immune checkpoints and ICIs is a research direction in the field of tumor immunotherapy (30). Considering the significant effect of phosphorylation on tumor characteristics, inhibitors targeting phosphokinases or phosphorylated molecules can be used as targets for tumor therapy (31). Phosphorylated transforming growth factor beta (TGF-β)-induced factor homeobox 2 (TGIF2) can induce epithelial–mesenchymal transition (EMT) and metastasis of lung adenocarcinoma, and p-TGIF2 is a potential therapeutic target for lung adenocarcinoma metastasis (32). The expression level of the CD274 molecule programmed cell death ligand-1 (PD-L1) in tumors is regulated in many aspects of translation and post-translation. Guo proved that hexokinase 2 can be used as a protein kinase to phosphorylate the Thr291 site of I𝜅Bα, thereby promoting combined protease u-calpain and I𝜅Bα and degrading I𝜅Bα, in turn promoting the entry of the NF-𝜅B transcription subunit into the nucleus and the PD-L1 expression, ultimately leading to the immune escape of the tumor (33). Combined hexokinase 2 inhibitor and PD-1 antibody in glioma can significantly improve the therapeutic effect of the PD-1 antibody. Li showed that epidermal growth factor receptor (EGFR) overexpression in tumors inhibited the phosphorylation of PD-L1 through glycogen synthase kinase-3β/α (GSK3β/α), which hindered the ubiquitination and improved the stability of PD-L1 (34). In contrast, the EGFR inhibitor osimertinib can interfere with the aforementioned process, induce ubiquitin, degrade PD-L1, and enhance the antitumor immune function of T cells (35, 36). Chen et al. found that interleukin (IL)-6 can phosphorylate PD-L1 by activating Janus kinase 1 (JAK1), in turn catalyzing PD-L1 glycosylation, enhancing its stability, and promoting tumor immune escape (37). In an animal model, the anti-IL-6 antibody combined with anti-T-cell immunoglobulin 3 induced the synergistic T-cell killing effect (37). Drugs targeting phosphokinases can be used as the focus of tumor immunotherapy. Thus, the potential application value of PTM in tumor immunotherapy has been sufficiently proven (Figure 2).
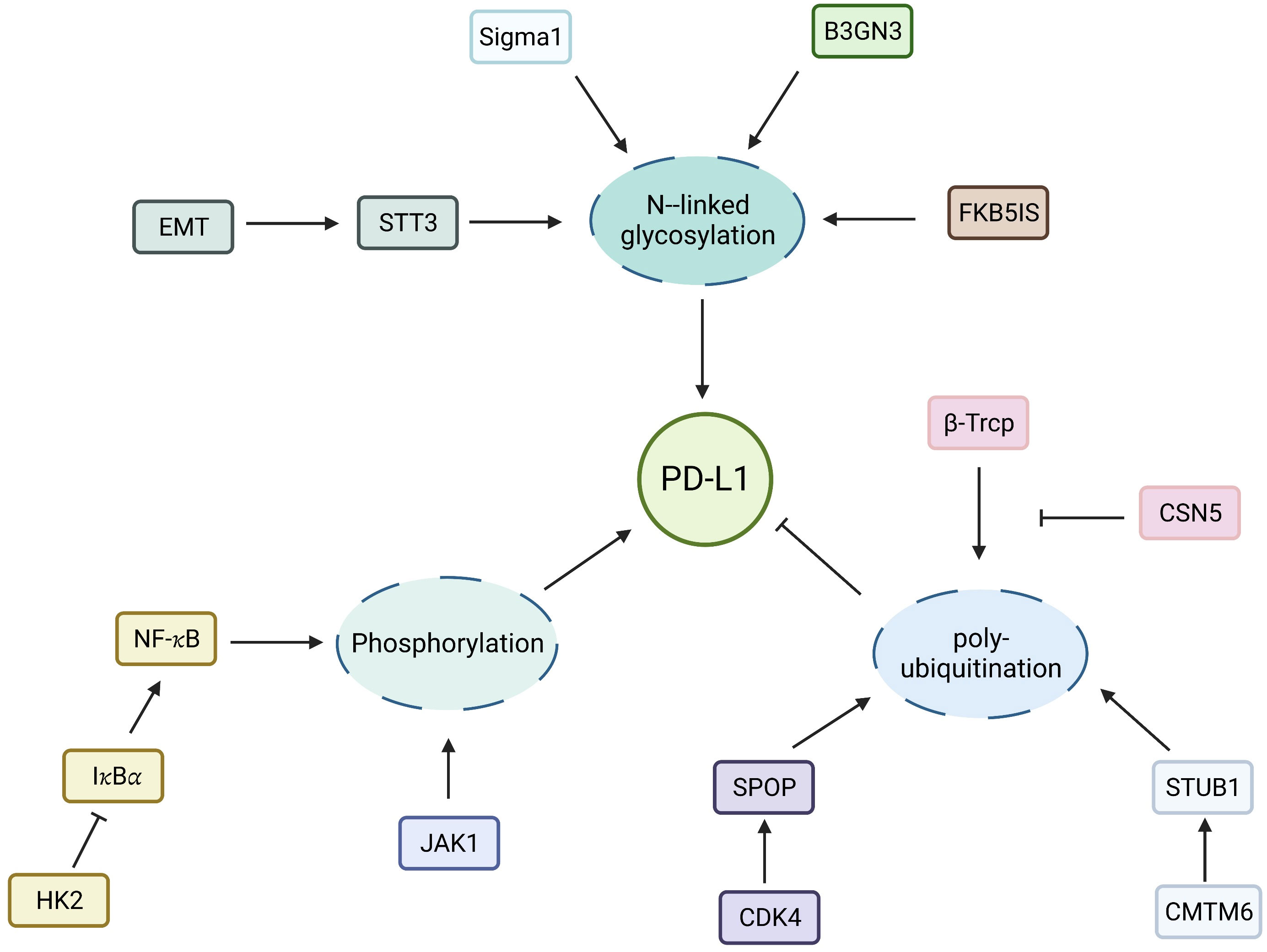
Figure 2 Regulation of PD-L1 by PTMs. Molecular regulation of PD-L1 N- linked glycosylation, phosphorylation and ubiquitination.
Some immune drugs related to phosphorylation have been developed and applied. MYCi975, a small-molecule compound, can increase the degradation of MYC by enhancing the phosphorylation of MYC on threonine-58, mediated by proteasome. In addition, it can upregulate PD-L1 and make tumors sensitive to PD1 immunotherapy (38). Simvastatin, a potential therapeutic drug for immunotherapy in colorectal cancer, inhibits the phosphorylation of YAP, mediated by the lncRNA SNHG29, and promotes antitumor immunity by inhibiting the PD-L1 expression (39). Ursodeoxycholic acid, a clinically approved compound, can enhance antitumor immunity by phosphorylating TGF-β at T282 along the Takeda G-protein-coupled receptor 5–cyclic adenosine monophosphate–protein kinase A axis and inhibiting the differentiation and activation of Treg cells in mice (40). Elesclomol was identified in the differential cytotoxicity screening of the internal tool compound library. It promoted YAP phosphorylation and inhibited its nuclear accumulation through the reactive oxygen species/large tumor suppressor kinase 1 kinase signaling pathway (41). In addition, the PD-L1 expression and signal transducer and activator of transcription 3 phosphorylation increased after the nintedanib therapy for lung cancer. Nintedanib combined with αPD-L1 can enhance the therapeutic response of ICIs, further activating the tumor immune microenvironment and showing remarkable antitumor effects (42) (Table 1).
Targeted phosphorylation performs therapeutic and predictive functions in tumor immunity. The immunohistochemistry of ERK1/2 phosphorylation can predict the overall survival rate of patients with independent recurrent glioblastoma after blocking PD-1 (43). Therefore, the development of related kits would help adjust the treatment plan of patients with PD-1 blockade.
3 Ubiquitylation and tumor immunity
In ubiquitination, ubiquitin molecules are covalently attached to specific residues of substrate proteins. Ubiquitination, a dynamic and reversible process, plays important roles in protein localization, metabolism, function, regulation, and degradation. Signal transduction proteins are regulated by PTM, and their ubiquitin level is second only to phosphorylation (44). Some ubiquitin-modified proteins change their function and location (45), while most are degraded by the ubiquitin–proteasome system (UPS) or lysosome degradation pathways, thus regulating various life activities, such as cell cycle, proliferation, apoptosis, differentiation, gene expression, transcriptional regulation, signal transduction, damage repair, inflammation, and immunity (46, 47). Currently, three ubiquitin enzymes are involved in ubiquitination modification: ubiquitin-activating enzyme (E1), ubiquitin-binding enzyme (E2), and ubiquitin ligase (E3). Ubiquitination is terminated by deubiquitinating proteins (DUBs). Among them, the E3 ubiquitin ligase can recognize the type of substrate protein; therefore, the specificity of the ubiquitin ligase is mainly realized by the ubiquitin ligase. The E3 ubiquitin ligase is believed to play an important role in tumor immunity (48, 49).
Casitas B-lineage lymphoma proto-oncogene b (CBL-b), as a E3 ubiquitin ligase, is an immune tolerance factor directly related to T-cell activation (50). Naramura’s study revealed that c-cbl-knockout T cells were more responsive to CD3 stimulation and promoted T-cell receptor beta variable 20/OR9-2 clearance on the cell surface, thereby inhibiting T-cell activation (51). Mutations in the ubiquitin-mediated protein degradation system can be involved in at least 10% of tumorigenesis and development (47, 52). The ubiquitin protein ligase E3 component n-recognin 5 (UBR5), an E3 ubiquitin ligase, is essential for the embryonic development of mammals (53). Elevated UBR5 expression is closely related to the survival and poor prognosis of patients with ovarian cancer (54). The E3 ubiquitin ligase regulates the activity and function of immune cells and plays an important role in regulating tumor cells and microenvironment (55).
UPS is the intracellular system responsible for protein degradation. Abnormal activation of the system accelerates the degradation of intracellular proteins. UPS can affect the survival of tumor cells by promoting the degradation of tumor-suppressor proteins, such as the tumor protein p53, or blocking the degradation of carcinogenic proteins. Song’s study proved that tumor-derived UBR5 plays a dual role in promoting tumorigenesis and affecting immune microenvironment. UBR5 can regulate tumor spheroid formation of ovarian cancer through the p53–β–catenin pathway and then enhance immunosuppression by recruiting tumor-associated macrophages (TAMs) (54). Considering the aforementioned mechanism, targeted UBR5 significantly inhibits tumor growth, eliminates the ability of ovarian cancer to resist conventional chemotherapy and immunotherapy, and significantly improves the effect of the standard treatment of ovarian cancer. In lung cancer, ubiquitin ligase interleukin 17 receptor B (CRL4) and WD repeat domain 4 promotes the progression of lung cancer through ubiquitin degradation of the promyelocytic leukemia protein. Therefore, targeted regulation of the E3 ubiquitin ligase or its substrate protein can provide new opportunities for tumor immunotherapy (56). Yu et al. showed that the ubiquitin-binding enzyme E2T (UBE2T) can promote the entry of β-catenin into the nucleus through ubiquitin degradation of the receptor for activated C kinase 1, thus promoting the occurrence and development of gastric cancer (57). The team further targeted the upstream ubiquitin-binding enzyme UBE2T to develop a small molecular inhibitor M435-1279 with low cytotoxicity that can inhibit the progression of gastric cancer in vivo and in vitro (Figure 3).
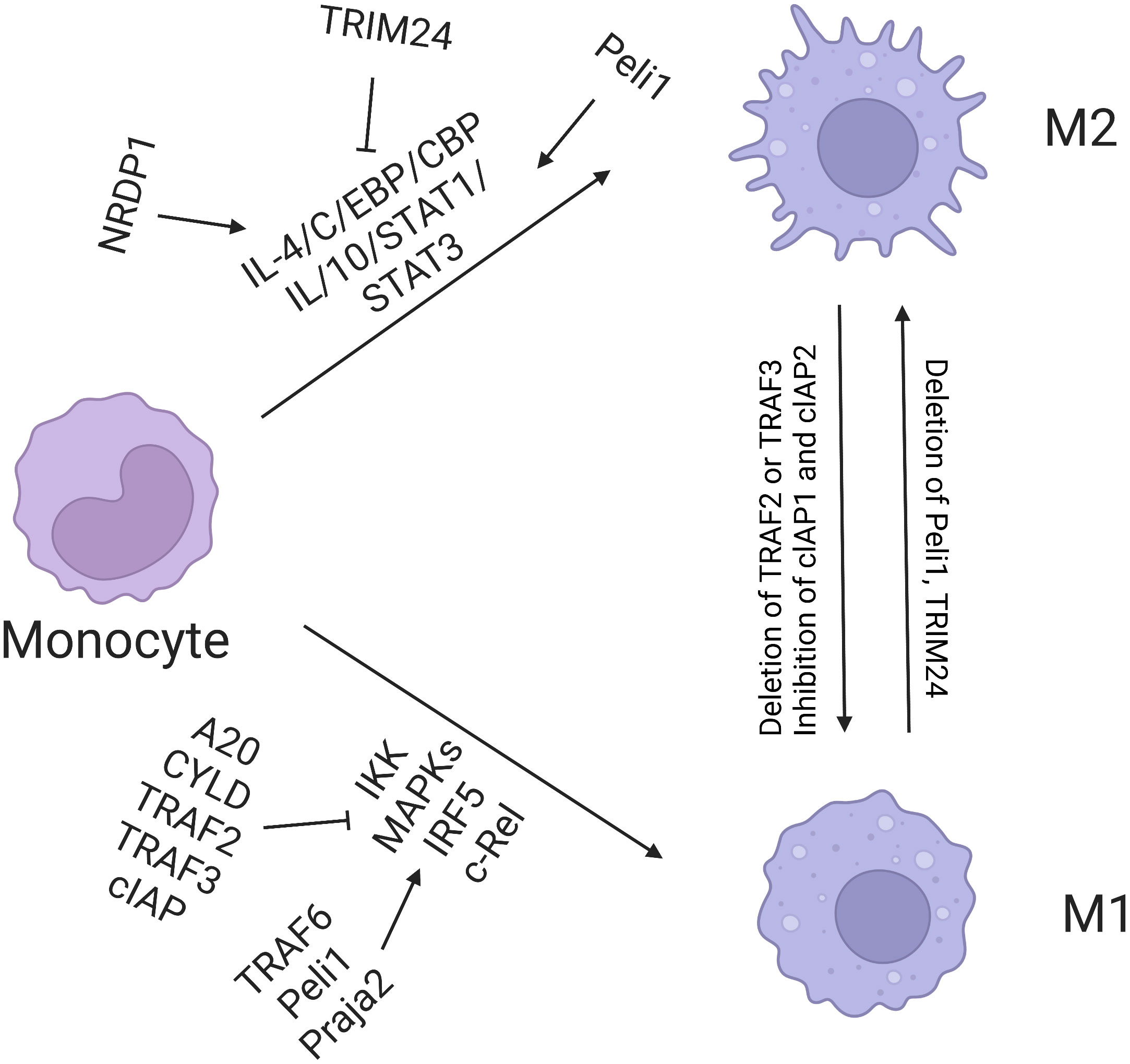
Figure 3 Regulation of macrophage polarization by ubiquitination. E3 ligases TRAF6, Peli1, Praja2, TRAF2, TRAF3, and cIAP promote M1 polarization, among which A20 and CYLD inhibit this process. Nrdp1 promotes the expression of M2 gene induced by IL-4 by mediating ubiquitination of K63 and activation of transcription factor C/EBP. TRIM24 inhibits M2 polarization by ubiquitination of acetyltransferase CBP.
Owing to its role in tumor immunity, ubiquitination would be helpful in clinical immunotherapy to find new E3s and DUBs for antitumor immunomodulation and clarify their functional mechanisms. Finding and developing specific inhibitors targeting the E3 ligase and DUBs are important for clinical applications of ubiquitination. In addition to developing inhibitors, adoptive cell therapy has a clinical application potential, such as knocking-out specific E3 and DUB to improve the therapeutic effect. This method is particularly attractive for adoptive T-cell and natural killer cell therapy based on the chimeric antigen receptor.
4 Succinylation and tumor immunity
Succinylation modification is a type of PTM that mainly occurs in lysine residues. Compared with methylation and acetylation modification, succinylation modification has a greater effect on the structure and function of proteins. Enzymes in cell metabolism, particularly mitochondrial metabolism, are widely regulated by succinylation modification. Currently, the regulatory enzyme system of succinylation (including transferase and de-modifier enzyme) and biological function have become hot research topics.
Lu et al. first discovered histone-succinylated transferase-lysine acetyltransferase 2A, which can use succinyl coenzyme A as a substrate to catalyze the succinylation of the histone H3K79 site, thus promoting the transcription of oncogenes, tumorigenesis, and cancer development (58). In addition, glutaminase was overexpressed in pancreatic ductal carcinoma. Compared with normal cells, the growth and survival of pancreatic ductal cancer cells depended more on glutamine metabolism. In addition, glutaminase was overexpressed in pancreatic ductal carcinoma. Compared with normal cells, the growth and survival of pancreatic ductal cancer cells depended more on glutamine metabolism. Succinylation modification occurred on the glutaminase protein. Succinylation at the K311 site of the glutaminase protein was directly mediated by succinyl coenzyme A, which promoted the conversion of glutaminase from the monomer to the tetramer form. As a result, catabolism of glutamine was enhanced (59).
Tumor immunotherapy is closely related to protein succinylation modification. Tumor immune metabolism in immune cell proliferation, differentiation, response, and outcome is a research frontier worldwide. Advancements in this research would aid our understanding of immune cell biology in theory and exhibit an application prospect in maintaining immune homeostasis and tumor immunotherapy. When activated by lipopolysaccharide, macrophages consume abundant glucose, enhance glycolysis, express M1 molecular markers, and produce abundant inflammatory factor IL-1β. The mechanism involves the accumulation of succinate, the intermediate product of glucose metabolism. Succinate promotes hypoxia inducible factor-1 (HIF-1α) to enhance the transcription of IL-1β, in which the activation of pyruvate kinase M2 (PKM2), a key enzyme in glycolysis, plays an important role. Yang et al. further discovered that PKM2 is desuccinylated by sirtuin-5 (60). In SIRT5-deficient cells, the succinylation level of PKM2 increased; PKM2 transformed from the tetramer to the dimer form; and pyruvate kinase activity decreased. Dimerized PKM2 enters the nucleus and cooperates with HIF-1α to bind to the promoter region of IL-1β, which significantly enhances the transcription of IL-1β and glycolysis of macrophages. These results suggest that SIRT5 regulates macrophage metabolism and plays an important role in the malignant transformation of colitis and even colitis cancer. Metabolic changes in tumor microenvironment significantly regulate tumor immune sensitivity, but the underlying mechanism remains unclear. Cheng et al. found that tumors deficient in fumarate hydratase (FH) showed functional CD8+ T-cell activation, expansion, and inhibition and enhanced malignant proliferation (61). Regarding the mechanism, FH depletion in tumor cells can accumulate fumarate in tumor interstitial fluid. Elevated fumarate levels can directly succinate ZAP70 at C96 and C102 sites and eliminate the activity of infiltrating CD8+ T cells, thus inhibiting the activation of CD8+ T cells and antitumor immune response in vitro and in vivo. In addition, removing fumarate by increasing the FH expression can significantly enhance the antitumor effect of anti-CD19 CAR T cells. Thus, these findings prove the role of fumarate in controlling TCR signal transduction and suggest that the accumulation of fumarate in tumor microenvironment is a metabolic disorder of the antitumor function of CD8+ T cells. Potentially, fumarate depletion may be an important strategy for tumor immunotherapy.
Immune-targeted drugs for succinylation have not been confirmed in the clinical treatment of cancer. They remain in the research stage in vivo and in vitro. Nevertheless, in a previous study, 90Y-labeled succinylated streptavidin significantly inhibited the growth of breast cancer in the pre-targeted radiotherapy group (p < 0.05) (62). In ovarian cancer cells, inhibition of dihydrothionyl succinyltransferase, a subunit of α-KGDC in the tricarboxylic acid cycle, reduced oxidative phosphorylation and the expression and function of immunosuppressant markers in myeloid cells (63). Lactb is a positive regulator of the NF-κB signal in dendritic cells, and succinylation of the lysine 288 residue is inhibited by Suclg2. Therefore, the development of succinylated immune-targeting drugs may be a research direction for immunotherapy.
5 Lactylation and tumor immunity
Lactic acid is a metabolite of cellular glycolysis. However, it has been considered a simple cellular energy substance and metabolite. Its regulatory role in biological function has been unknown. Lactic acid-mediated protein PTM lactylation plays a regulatory role in immune cells and cancer metabolism.
Regarding the tumor metabolism, Zhao et al. found that lactic acid accumulated during metabolism can be used as a precursor to induce lactylation of histone lysine and participate in the homeostasis regulation of M1 macrophages infected by bacteria (64). In addition to a study on the epigenetic regulation of histone lactic acid modification, Gao et al. drew a panoramic map of lactic acid modification in hepatocellular carcinoma for the first time. Lactylation occurs in histones and plays a global regulatory role in hepatocellular carcinoma by affecting widely distributed non-histone proteins. E1A-binding protein p300. Histone deacetylase causes the activation and deactivation of non-histone lactylation (65). Lu et al. found that the metabolite lactic acid affects the tumor microenvironment and promotes tumorigenesis by regulating the lactylation of M protein Lys72 in Treg cells and enhancing TGF-β signal transduction, which provides a new theoretical basis for cancer immunotherapy by targeting Treg cells (66). Zhao et al. found that activated macrophages play an important role in ulcerative colitis. Lactic acid can enhance histone H3K18 lactylation in macrophages, inhibit macrophage coking, and restore intestinal immune function (67). In addition, Zhang et al. found that lactic acid-mediated lactylation of PKM2 at the K62 site can enhance the pyruvate kinase activity of PKM2 to inhibit the Warburg effect and ultimately promote the transition of macrophages from the proinflammatory to the repair phenotype (68). The underexpression of sirtuin 3 in hepatocellular carcinoma promotes the lactylation of cyclin E2, which in turn promotes tumor progression, and sirtuin 3 is a potential therapeutic target for hepatocellular carcinoma (69).
The lactate score model can be used to predict tumor immune escape (70). A lactic acid-related model study on gastric cancer revealed numerous infiltrated immune cells (macrophages to the highest degree), characterized by an increased lactic acid score. ICIs showed a decreased response rate in gastric cancer with a high lactate score. Tumors with a high lactate fraction have high tumor immune dysfunction, implying higher risks of immune escape and dysfunction. These findings indicate that the lactate score can be used to predict malignant progression and immune evasion of gastric cancer. However, the application of lactic acid drugs in clinical tumor immunity remains under development.
6 Glycosylation and tumor immunity
Glycosylation is an important protein PTM in which O-linked N-acetylglucosamine (O-GlcNAcylation) refers to addition of monosaccharide modification to serine and/or threonine residues of protein in cells, which is the most common glycosylation form in eukaryotes (71). This modification is a highly dynamic modification method, which would change with the nutritional status in cells and extracellular stimuli. It widely occurs in intracellular proteins and regulates important biological processes, such as gene transcription, signal transduction, protein synthesis, and metabolic reprogramming.
As early as 1991, Crowley et al. discovered the effect of non-enzymatic glycosylation on the function of mesangial cells (72). Khidekel discovered a new strategy to monitor the glycosylation kinetics of O-GlcNAc using protein omics based on quantitative mass spectrometry (73). Fogel et al. found that site-specific N-glycosylation affects the structure and function of binding synaptic cell adhesion molecule interaction (74). In addition, some studies have summarized the current knowledge of immunoglobulin glycosylation and paid special attention to the research and vaccination for infectious diseases, considered to be a field with many interesting opportunities (75). Hu et al. analyzed 83 high-grade serous ovarian cancer and 23 non-tumor tissues prospectively with comprehensive protein omics and glycochemistry. Tyagi et al. comprehensively summarized the discovery of RNA glycosylation, conceptually understood its previous potential discovery and its biological consequences, and explained the dynamic impact of this modification on its molecular versatility, determining the immunological fate of cancer and the potential impact of glycosylation on cell interaction, signal transduction, immunomodulation, cancer escape, and proliferation (76). Shi et al. found that glucose metabolism in TAM was modified by enhancing O-GlcNAcylation, promoting tumor metastasis and chemotherapy resistance. They revealed that M2-like TAM is the immune cell subgroup with the strongest glucose uptake ability in tumor microenvironment and discovered the new function of O-linked N-acetylglucosamine transferase located in lysosomes (77). They clarified the significance of competitive uptake and utilization of glucose by TAM, particularly M2-like TAM, in shaping cell-specific tumor-promoting function, which provided a potential target for tumor treatment.
The star molecule of glycosylation in tumor immunotherapy is PD-L1. Glycosylation can stabilize PD-L1, which prevents PD-L1 from degradation of 26S proteasome mediated by GSK3β, thus enhancing its interaction with PD-1 on CD8+ T cells. In addition, the catalytic subunit of oligo-glycosyltransferase STT3 transfers the core glycan structure to PD-L1, which leads to EMT. Combined PD-L1 and PD-1 is also influenced by the glycosylation of PD-L1. In a study on EGF/EGFR signal transduction, PD-L1/PD-1 interaction requires β 1,3-N- acetylglucosamine transferase 3 (B3GNT3) to mediate the glycosylation of poly-N-acetyllactosamine on PD-L1 N192 and N200. The 4T1 cells lacking B3GNT3 expression grew in severe combined immunodeficiency mice, but not in immunocompetent BALB/c mice (78). Therefore, glycosylation targeting PD-L1 is a breakthrough in tumor immunotherapy.
7 Conclusions
PTMs are chemical changes that occur after protein synthesis that play a vital role in regulating protein function, stability, localization, and interactions. In addition to the types of PTMs summarized above, other PTMs play a vital role in tumor immunity. Currently, immune-related PTM drugs mostly include phosphorylation inhibitors. In addition, some PTM models can predict tumor immune evasion, such as the lactic acid score model. Immune-targeted drugs for succinylation have not been confirmed in the clinical treatment of cancer and remain in the research stage in vivo and in vitro. In addition to developing inhibitors, adoptive cell therapy carries clinical application potential, such as knocking-out specific PTM-related proteins to improve the therapeutic effect. This method is particularly attractive for adoptive T-cell and natural killer cell therapy based on the chimeric antigen receptor. This may be the direction of PTM immunotherapy in the future. In general, protein PTM is a regulator of tumor immunity. Its disorders affect various immune processes, including T-cell activation, immune checkpoint regulation, cytokine production, and immune cell interaction in the tumor microenvironment. Understanding its role in tumor immunity may provide insights for the development of new immunotherapies and targeted therapies for cancer.
Author contributions
Conceptualization: YL and HH. Formal analysis: YL. Investigation: HH. Resources: YL. Data curation: HH. Writing—original draft preparation: YL. Writing—review and editing: HH. Visualization: HH. Supervision: RZ. Project administration: RZ. Funding acquisition: YL. All authors have read and agreed to the published version of the manuscript.
Funding
This research is funded by the Henan Province Science and Technology Research Program Project (222102310612).
Acknowledgments
The authors would like to thank all the reviewers who participated in the review, as well as MJEditor (www.mjeditor.com) for providing English editing services during the preparation of this manuscript.
Conflict of interest
The authors declare that the research was conducted in the absence of any commercial or financial relationships that could be construed as a potential conflict of interest.
Publisher’s note
All claims expressed in this article are solely those of the authors and do not necessarily represent those of their affiliated organizations, or those of the publisher, the editors and the reviewers. Any product that may be evaluated in this article, or claim that may be made by its manufacturer, is not guaranteed or endorsed by the publisher.
References
1. Ando Y, Mariano C, Shen K. Engineered in vitro tumor models for cell-based immunotherapy. Acta Biomater (2021) 132:345–59.
2. Yakkala C, Denys A, Kandalaft L, Duran R. Cryoablation and immunotherapy of cancer. Curr Opin Biotechnol (2020) 65:60–4.
3. Friedrich MJ. Immunotherapy 2.0: improving the response to checkpoint inhibitors. JAMA (2019) 321:131–3.
4. Lesterhuis WJ, Haanen JB, Punt CJ. Cancer immunotherapy–revisited. Nat Rev Drug Discovery (2011) 10:591–600.
5. Du S, Dong J, Jin S, Zhang H, Zhang Y. Acceptance and Commitment Therapy for chronic pain on functioning: A systematic review of randomized controlled trials. Neurosci Biobehav Rev (2021) 131:59–76.
6. Huang R, Li X, He Y, Zhu W, Gao L, Liu Y, et al. Recent advances in CAR-T cell engineering. J Hematol Oncol (2020) 13:86.
8. Mullard A. FDA approves 100th monoclonal antibody product. Nat Rev Drug Discovery (2021) 20:491–5.
9. Wang F, Malnassy G and Qiu W. The epigenetic regulation of microenvironment in hepatocellular carcinoma. Front Oncol (2021) 11:653037.
10. Czuba LC, Hillgren KM, Swaan PW. Post-translational modifications of transporters. Pharmacol Ther (2018) 192:88–99.
11. Wang R, Wang G. Protein modification and autophagy activation. Adv Exp Med Biol (2019) 1206:237–59.
12. Fang Y, Xu X, Ding J, Yang L, Doan MT, Karmaus PWF, et al. Histone crotonylation promotes mesoendodermal commitment of human embryonic stem cells. Cell Stem Cell (2021) 28:748–763.e747.
13. Kim EJ, Liu P, Zhang S, Donahue K, Wang Y, Schehr JL, et al. BAF155 methylation drives metastasis by hijacking super-enhancers and subverting anti-tumor immunity. Nucleic Acids Res (2021) 49:12211–33.
14. Kukkula A, Ojala VK, Mendez LM, Sistonen L, Elenius K and Sundvall M. Therapeutic potential of targeting the SUMO pathway in cancer. Cancers (Basel) (2021) 13(17):4402.
15. Binnewies M, Roberts EW, Kersten K, Chan V, Fearon DF, Merad M, et al. Understanding the tumor immune microenvironment (TIME) for effective therapy. Nat Med (2018) 24:541–50.
16. Dai X, Gao Y, Wei W. Post-translational regulations of PD-L1 and PD-1: Mechanisms and opportunities for combined immunotherapy. Semin Cancer Biol (2022) 85:246–52.
17. Yamaguchi H, Hsu JM, Yang WH, Hung MC. Mechanisms regulating PD-L1 expression in cancers and associated opportunities for novel small-molecule therapeutics. Nat Rev Clin Oncol (2022) 19:287–305.
18. Liu X, Zhang Y, Wang Y, Yang M, Hong F, Yang S. Protein phosphorylation in cancer: role of nitric oxide signaling pathway. Biomolecules (2021) 11(7):1009.
19. Xia S, Zhai Y, Wang X, Fan Q, Dong X, Chen M, et al. Phosphorylation of polysaccharides: A review on the synthesis and bioactivities. Int J Biol Macromol (2021) 184:946–54.
20. Arrington JV, Hsu CC, Elder SG, Andy Tao W. Recent advances in phosphoproteomics and application to neurological diseases. Analyst (2017) 142:4373–87.
21. Mishra D, Patel V, Banerjee D. Nitric oxide and S-nitrosylation in cancers: emphasis on breast cancer. Breast Cancer (Auckl) (2020) 14:1178223419882688.
22. Jin YJ, Chennupati R, Li R, Liang G, Wang S, Iring A, et al. Protein kinase N2 mediates flow-induced endothelial NOS activation and vascular tone regulation. J Clin Invest (2021) 131(21):e145734.
23. Hong PP, Zhu XX, Yuan WJ, Niu GJ, Wang JX. Nitric oxide synthase regulates gut microbiota homeostasis by ERK-NF-κB pathway in shrimp. Front Immunol (2021) 12:778098.
24. Wink DA, Ridnour LA, Cheng R, Switzer CW, Glynn S, Ambs S. The oncogenic properties of the redox inflammatory protein inducible nitric oxide synthase in ER(-) breast cancer. Redox Biol (2015) 5:413.
25. Liu B, Sun L, Liu Q, Gong C, Yao Y, Lv X, et al. A cytoplasmic NF-kappaB interacting long noncoding RNA blocks IkappaB phosphorylation and suppresses breast cancer metastasis. Cancer Cell (2015) 27:370–81.
26. Lu C, Qiao P, Sun Y, Ren C, Yu Z. Positive regulation of PFKFB3 by PIM2 promotes glycolysis and paclitaxel resistance in breast cancer. Clin Transl Med (2021) 11:e400.
27. Lu C, Ren C, Yang T, Sun Y, Qiao P, Han X, et al. Fructose-1, 6-bisphosphatase 1 interacts with NF-kappaB p65 to regulate breast tumorigenesis via PIM2 induced phosphorylation. Theranostics (2020) 10:8606–18.
28. Yonesaka K, Tanizaki J, Maenishi O, Haratani K, Kawakami H, Tanaka K, et al. HER3 augmentation via blockade of EGFR/AKT signaling enhances anticancer activity of HER3-targeting patritumab deruxtecan in EGFR-mutated non-small cell lung cancer. Clin Cancer Res (2022) 28:390–403.
29. Zeng J, Li X, Liang L, Duan H, Xie S, Wang C. Phosphorylation of CAP1 regulates lung cancer proliferation, migration, and invasion. J Cancer Res Clin Oncol (2022) 148:137–53.
30. Morad G, Helmink BA, Sharma P, Wargo JA. Hallmarks of response, resistance, and toxicity to immune checkpoint blockade. Cell (2021) 184:5309–37.
31. Pan S, Chen R. Pathological implication of protein post-translational modifications in cancer. Mol Aspects Med (2022) 86:101097.
32. Du R, Wang C, Liu J, Wang K, Dai L, Shen W. Phosphorylation of TGIF2 represents a therapeutic target that drives EMT and metastasis of lung adenocarcinoma. BMC Cancer (2023) 23:52.
33. Guo D, Tong Y, Jiang X, Meng Y, Jiang H, Du L, et al. Aerobic glycolysis promotes tumor immune evasion by hexokinase2-mediated phosphorylation of IkappaBalpha. Cell Metab (2022) 34:1312–1324 e1316.
34. Li CW, Lim SO, Xia W, Lee HH, Chan LC, Kuo CW, et al. Glycosylation and stabilization of programmed death ligand-1 suppresses T-cell activity. Nat Commun (2016) 7:12632.
35. Qian G, Guo J, Vallega KA, Hu C, Chen Z, Deng Y, et al. Membrane-associated RING-CH 8 functions as a novel PD-L1 E3 ligase to mediate PD-L1 degradation induced by EGFR inhibitors. Mol Cancer Res (2021) 19:1622–34.
36. de Scordilli M, Michelotti A, Bertoli E, De Carlo E, Bearz A. Targeted therapy and immunotherapy in early-stage non-small cell lung cancer: current evidence and ongoing trials. Int J Mol Sci (2022) 23(13):7222.
37. Chan LC, Li CW, Xia W, Hsu JM, Lee HH, Cha JH, et al. IL-6/JAK1 pathway drives PD-L1 Y112 phosphorylation to promote cancer immune evasion. J Clin Invest (2019) 129:3324–38.
38. Han H, Jain AD, Truica MI, Izquierdo-Ferrer J, Anker JF, Lysy B, et al. Small-molecule MYC inhibitors suppress tumor growth and enhance immunotherapy. Cancer Cell (2019) 36:483–497.e415.
39. Ni W, Mo H, Liu Y, Xu Y, Qin C, Zhou Y, et al. Targeting cholesterol biosynthesis promotes anti-tumor immunity by inhibiting long noncoding RNA SNHG29-mediated YAP activation. Mol Ther (2021) 29:2995–3010.
40. Shen Y, Lu C, Song Z, Qiao C, Wang J, Chen J, et al. Ursodeoxycholic acid reduces antitumor immunosuppression by inducing CHIP-mediated TGF-β degradation. Nat Commun (2022) 13:3419.
41. Li Y, Yang J, Zhang Q, Xu S, Sun W, Ge S, et al. Copper ionophore elesclomol selectively targets GNAQ/11-mutant uveal melanoma. Oncogene (2022) 41:3539–53.
42. Tu J, Xu H, Ma L, Li C, Qin W, Chen X, et al. Nintedanib enhances the efficacy of PD-L1 blockade by upregulating MHC-I and PD-L1 expression in tumor cells. Theranostics (2022) 12:747–66.
43. Arrieta VA, Chen AX, Kane JR, Kang SJ, Kassab C, Dmello C, et al. ERK1/2 phosphorylation predicts survival following anti-PD-1 immunotherapy in recurrent glioblastoma. Nat Cancer (2021) 2:1372–86.
44. Hwang JT, Lee A, Kho C. Ubiquitin and ubiquitin-like proteins in cancer, neurodegenerative disorders, and heart diseases. Int J Mol Sci (2022) 23(9):5053.
45. Vaughan RM, Kupai A, Rothbart SB. Chromatin regulation through ubiquitin and ubiquitin-like histone modifications. Trends Biochem Sci (2021) 46:258–69.
46. Cruz Walma DA, Chen Z, Bullock AN, Yamada KM. Ubiquitin ligases: guardians of mamMalian development. Nat Rev Mol Cell Biol (2022) 23:350–67.
47. Dang F, Nie L, Wei W. Ubiquitin signaling in cell cycle control and tumorigenesis. Cell Death Differ (2021) 28:427–38.
48. Çetin G, Klafack S, Studencka-Turski M, Krüger E, Ebstein F. The ubiquitin-proteasome system in immune cells. Biomolecules (2021) 11(1):60.
49. Garcia-Sanchez JA, Ewbank JJ, Visvikis O. Ubiquitin-related processes and innate immunity in C. elegans Cell Mol Life Sci (2021) 78:4305–33.
50. Kumar J, Kumar R, Kumar Singh A, Tsakem EL, Kathania M, Riese MJ, et al. Deletion of Cbl-b inhibits CD8(+) T-cell exhaustion and promotes CAR T-cell function. J Immunother Cancer (2021) 9(1):e001688.
51. Naramura M, Jang IK, Kole H, Huang F, Haines D, Gu H. c-Cbl and Cbl-b regulate T cell responsiveness by promoting ligand-induced TCR down-modulation. Nat Immunol (2002) 3:1192–9.
52. Martinez-Jimenez F, Muinos F, Lopez-Arribillaga E, Lopez-Bigas N, Gonzalez-Perez A. Systematic analysis of alterations in the ubiquitin proteolysis system reveals its contribution to driver mutations in cancer. Nat Cancer (2020) 1:122–35.
53. Kim JG, Shin HC, Seo T, Nawale L, Han G, Kim BY, et al. Signaling pathways regulated by UBR box-containing E3 ligases. Int J Mol Sci (2021) 22(15):8323.
54. Song M, Yeku OO, Rafiq S, Purdon T, Dong X, Zhu L, et al. Tumor derived UBR5 promotes ovarian cancer growth and metastasis through inducing immunosuppressive macrophages. Nat Commun (2020) 11:6298.
55. Wu Y, Jiao H, Yue Y, He K, Jin Y, Zhang J, et al. Ubiquitin ligase E3 HUWE1/MULE targets transferrin receptor for degradation and suppresses ferroptosis in acute liver injury. Cell Death Differ (2022) 29:1705–18.
56. Wang YT, Chen J, Chang CW, Jen J, Huang TY, Chen CM, et al. Ubiquitination of tumor suppressor PML regulates prometastatic and immunosuppressive tumor microenvironment. J Clin Invest (2017) 127:2982–97.
57. Yu Z, Jiang X, Qin L, Deng H, Wang J, Ren W, et al. A novel UBE2T inhibitor suppresses Wnt/beta-catenin signaling hyperactivation and gastric cancer progression by blocking RACK1 ubiquitination. Oncogene (2021) 40:1027–42.
58. Wang Y, Guo YR, Liu K, Yin Z, Liu R, Xia Y, et al. KAT2A coupled with the alpha-KGDH complex acts as a histone H3 succinyltransferase. Nature (2017) 552:273–7.
59. Tong Y, Guo D, Lin SH, Liang J, Yang D, Ma C, et al. SUCLA2-coupled regulation of GLS succinylation and activity counteracts oxidative stress in tumor cells. Mol Cell (2021) 81:2303–2316.e2308.
60. Wang F, Wang K, Xu W, Zhao S, Ye D, Wang Y, et al. SIRT5 desuccinylates and activates pyruvate kinase M2 to block macrophage IL-1beta production and to prevent DSS-induced colitis in mice. Cell Rep (2017) 19:2331–44.
61. Cheng J, Yan J, Liu Y, Shi J, Wang H, Zhou H, et al. Cancer-cell-derived fumarate suppresses the anti-tumor capacity of CD8(+) T cells in the tumor microenvironment. Cell Metab (2023) 35(6):961–978.e10.
62. Gu W, Yudistiro R, Hanaoka H, Katsumata N, Tsushima Y. Potential of three-step pretargeting radioimmunotherapy using biotinylated bevacizumab and succinylated streptavidin in triple-negative breast cancer xenograft. Ann Nucl Med (2021) 35:514–22.
63. Udumula MP, Sakr S, Dar S, Alvero AB, Ali-Fehmi R, Abdulfatah E, et al. Ovarian cancer modulates the immunosuppressive function of CD11b(+)Gr1(+) myeloid cells via glutamine metabolism. Mol Metab (2021) 53:101272.
64. Zhang D, Tang Z, Huang H, Zhou G, Cui C, Weng Y, et al. Metabolic regulation of gene expression by histone lactylation. Nature (2019) 574:575–80.
65. Yang Z, Yan C, Ma J, Peng P, Ren X, Cai S, et al. Lactylome analysis suggests lactylation-dependent mechanisms of metabolic adaptation in hepatocellular carcinoma. Nat Metab (2023) 5:61–79.
66. Gu J, Zhou J, Chen Q, Xu X, Gao J, Li X, et al. Tumor metabolite lactate promotes tumorigenesis by modulating MOESIN lactylation and enhancing TGF-beta signaling in regulatory T cells. Cell Rep (2022) 39:110986.
67. Sun S, Xu X, Liang L, Wang X, Bai X, Zhu L, et al. Lactic Acid-Producing Probiotic Saccharomyces cerevisiae Attenuates Ulcerative Colitis via Suppressing Macrophage Pyroptosis and Modulating Gut Microbiota. Front Immunol (2021) 12:777665.
68. Wang J, Yang P, Yu T, Gao M, Liu D, Zhang J, et al. Lactylation of PKM2 suppresses inflammatory metabolic adaptation in pro-inflammatory macrophages. Int J Biol Sci (2022) 18:6210–25.
69. Jin J, Bai L, Wang D, Ding W, Cao Z, Yan P, et al. SIRT3-dependent delactylation of cyclin E2 prevents hepatocellular carcinoma growth. EMBO Rep (2023) 24:e56052.
70. Yang H, Zou X, Yang S, Zhang A, Li N, Ma Z. Identification of lactylation related model to predict prognostic, tumor infiltrating immunocytes and response of immunotherapy in gastric cancer. Front Immunol (2023) 14:1149989.
71. Magalhães A, Duarte HO, Reis CA. The role of O-glycosylation in human disease. Mol Aspects Med (2021) 79:100964.
72. Crowley ST, Brownlee M, Edelstein D, Satriano JA, Mori T, Singhal PC, et al. Effects of nonenzymatic glycosylation of mesangial matrix on proliferation of mesangial cells. Diabetes (1991) 40:540–7.
73. Khidekel N, Ficarro SB, Clark PM, Bryan MC, Swaney DL, Rexach JE, et al. Probing the dynamics of O-GlcNAc glycosylation in the brain using quantitative proteomics. Nat Chem Biol (2007) 3:339–48.
74. Fogel AI, Li Y, Giza J, Wang Q, Lam TT, Modis Y, et al. N-glycosylation at the SynCAM (synaptic cell adhesion molecule) immunoglobulin interface modulates synaptic adhesion. J Biol Chem (2010) 285:34864–74.
75. Alter G, Ottenhoff THM, Joosten SA. Antibody glycosylation in inflammation, disease and vaccination. Semin Immunol (2018) 39:102–10.
76. Tyagi W, Pandey V, Pokharel YR. Membrane linked RNA glycosylation as new trend to envision epi-transcriptome epoch. Cancer Gene Ther (2023) 30:641–6.
77. Shi Q, Shen Q, Liu Y, Shi Y, Huang W, Wang X, et al. Increased glucose metabolism in TAMs fuels O-GlcNAcylation of lysosomal Cathepsin B to promote cancer metastasis and chemoresistance. Cancer Cell (2022) 40:1207–1222 e1210.
Keywords: post-translational modification, tumor immunotherapy, phosphorylation, ubiquitylation, succinylation
Citation: Li Y, Zhang R and Hei H (2023) Advances in post-translational modifications of proteins and cancer immunotherapy. Front. Immunol. 14:1229397. doi: 10.3389/fimmu.2023.1229397
Received: 26 May 2023; Accepted: 21 July 2023;
Published: 22 August 2023.
Edited by:
Xiangpeng Dai, Jilin University, ChinaReviewed by:
Kai Xu, Huazhong University of Science and Technology, ChinaWei Su, The First Affiliated Hospital of Xinxiang Medical University, China
Jia Liu, Qingdao University, China
Copyright © 2023 Li, Zhang and Hei. This is an open-access article distributed under the terms of the Creative Commons Attribution License (CC BY). The use, distribution or reproduction in other forums is permitted, provided the original author(s) and the copyright owner(s) are credited and that the original publication in this journal is cited, in accordance with accepted academic practice. No use, distribution or reproduction is permitted which does not comply with these terms.
*Correspondence: Hu Hei, tigerblack@126.com
†These authors share first authorship