- 1Department of Gynecology, Cancer Hospital of Dalian University of Technology (Liaoning Cancer Hospital & Institute), Shenyang, Liaoning, China
- 2Department of Clinical Epidemiology, Shengjing Hospital of China Medical University, Shenyang, Liaoning, China
- 3Department of General Surgery, Shengjing Hospital of China Medical University, Shenyang, Liaoning, China
- 4Department of Oncology, Shengjing Hospital of China Medical University, Shenyang, Liaoning, China
- 5Medical Oncology Department of Gastrointestinal Cancer, Cancer Hospital of Dalian University of Technology (Liaoning Cancer Hospital & Institute), Shenyang, Liaoning, China
Cancer immunotherapy has exhibited promising antitumor effects in various tumors. Infiltrated regulatory T cells (Tregs) in the tumor microenvironment (TME) restrict protective immune surveillance, impede effective antitumor immune responses, and contribute to the formation of an immunosuppressive microenvironment. Selective depletion or functional attenuation of tumor-infiltrating Tregs, while eliciting effective T-cell responses, represents a potential approach for anti-tumor immunity. Furthermore, it does not disrupt the Treg-dependent immune homeostasis in healthy organs and does not induce autoimmunity. Yet, the shared cell surface molecules and signaling pathways between Tregs and multiple immune cell types pose challenges in this process. Noncoding RNAs (ncRNAs), including microRNAs (miRNAs) and long noncoding RNAs (lncRNAs), regulate both cancer and immune cells and thus can potentially improve antitumor responses. Here, we review recent advances in research of tumor-infiltrating Tregs, with a focus on the functional roles of immune checkpoint and inhibitory Tregs receptors and the regulatory mechanisms of ncRNAs in Treg plasticity and functionality.
1 Introduction
Immunotherapy for cancer has become the most promising approach in recent years (1). Therapeutic drugs and cellular therapies derived from immunotherapy have extended the lives of patients with advanced cancer. Among them, immune checkpoint inhibitors (ICIs), also known as co-inhibitory receptors (CIRs), are the most well-known treatment strategy. The expression of ICIs in T cells increases upon activation, forcing the anti-tumor T cells to retreat into a dormant or exhausted non-active state. Antibodies antagonizing ICIs are used to revive T cells and maintain their anti-tumor response (2). So far, immune checkpoint blockade (ICB) antibodies have shown success in some cancers. However, only partial responses or no response have been observed in 60%–70% of patients undergoing this therapy. Additionally, some patients experience immune-related adverse events (irAEs), including autoimmune diseases or immunopathological disorders (3). Therefore, the critical focus of immunotherapy is to identify the reasons behind the shortcomings of ICB treatment and explore more effective anti-tumor immune therapeutic approaches. It will help patients avoid excessive exposure to irAEs and achieve more positive treatment outcomes.
Regulatory T cells (Tregs) are an integral part of the immune system because they contribute primarily to maintaining collective immune system homeostasis and tolerance (4). Tregs inhibit the activation and differentiation of CD4+ helper T and CD8+ cytotoxic T cells, thereby inducing responses to autologous and tumor-expressed antigens (5, 6). The discovery of suppressor T cells was aided by identifying high and low expression of IL-2 receptor α subunit (CD25) and IL-7 receptor α subunit (CD127) respectively in both humans and mice. The further discovery of forkhead/winged helix transcription factor (Foxp3) expression in Tregs established the CD4+CD25+Foxp3+ classical combination marker (7–9). Adjusted expression of Foxp3 – via either a genetic or epigenetic route – significantly affect the immunosuppression of Tregs (10, 11). In the tumor microenvironment (TME), Tregs are majorly immunosuppressive, antagonize anti-tumor immunity and inhibit functions of other immune effector cells, and this aids tumor immune escape (12, 13). Interactions among Tregs, the TME and adjacent cells are critical for maintaining Tregs stability and plasticity. These interactions synergistically influenced the function and number of Tregs through inflammation, cytokine secretion, metabolic changes and transcriptional regulation (14–16). Notably, the infiltration of Tregs into the TME is closely related to tumor progression and poor prognoses (17, 18). However, animal studies have indicated that systemic depletion of Tregs can enhance anti-tumor immunity but can also lead to various autoimmune diseases (19, 20). Therefore, selectively depleting tumor-infiltrating Tregs within the TME without affecting Tregs in healthy tissues can elicit anti-tumor immunity without inducing detrimental autoimmunity. The effective identification of tumor-infiltrating Tregs and a clear understanding of the transition from healthy Tregs to tumor-infiltrating Tregs based on Tregs plasticity represent a promising direction for cancer immunotherapy.
Noncoding RNA (ncRNAs) do not translate proteins (21, 22) but instead act as “regulators” of cellular functions, including molecular signaling pathways in malignant tumors (23, 24). Among them, microRNA (miRNA), long non-coding RNA (lncRNA) and circular RNA (circRNA) are most important to current cancer research (23, 25, 26). They are widely involved in various malignant phenotypes of cancer. LncRNAs exhibit specific expression patterns across various immune cell types, ranging from hematopoietic stem cells (HSCs) to innate and adaptive immune cells in humans and mice. The expression of specific lncRNAs in human and murine immune cell types suggests their evolutionary conservation (27). Immune-specific ncRNAs exert their effects on hematopoietic differentiation through various mechanisms, including acting as ncRNA/protein decoys and functioning as protein scaffolds, transporters, and recruiters in the nucleus and cytoplasm (28). Notably, most immune-specific ncRNAs appear to recruit protein complexes to specific genomic loci, thereby regulating target gene expression at the epigenetic and transcriptional levels and consequently modulating immune cell activity and differentiation in the nucleus.
In this review, we summarize advancements in research of tumor-associated Tregs and highlight relevant interactions between ncRNA and Tregs. On the other hand, we discuss the potential effects of ICB antibodies on Treg-mediated immune suppression in the context of anti-tumor immunity and summarize the role of ncRNAs in this process. A better understanding of ncRNA-mediated tumor immune regulation, especially their role in the regulation of Tregs function, engenders possible insights into cancer immunotherapy.
2 The classifications and plasticity of Tregs
Tregs are sub-classified using four criteria, which are governed by plasticity and functional complexity. First, based on their origin, Tregs are divided into thymus Tregs (tTregs) and peripheral Tregs (pTregs) (29). In relation to ncRNA regulation, CD69/miR-155/SOCS-1 axis is a non-redundant key regulator involved in Tregs development and homeostasis (30). Intrathymic miR-181a/b-1 controlled Tregs cell formation by expressing an adequate signaling threshold. The miR-181a/b-1-deficient Treg showed elevated suppressive capacity and was inversely correlated with CTLA-4 protein levels in thymus and peripheral Tregs (31).
Second, based on their degree of activation, Tregs are divided into central Tregs (cTregs) and effector Tregs (eTregs) (32). CTregs, also known as resting or naive Tregs, are the major constituents of peripheral and secondary lymphoid Tregs. CTregs that express CD62L, CCR7 and are phenotypically similar to traditional naive T cells, are important for circulation in lymphoid organs (33). On the other hand, eTregs are mainly found in tissues and organs, with only a small fraction found in secondary lymphoid organs (34). eTregs are antigen-activated Tregs that highly express CD44, ICOS, and KLRG1 and other molecular and tissue-localization-related chemokine receptors, lack CD62L and CCR7 expressions, and can directly contribute to immunosuppression (35). miR-155 targets and reduces CD62L expression in Tregs (36). It is a critical regulatory factor in pregnancy immune adaptation, promoting Treg expansion to achieve pregnancy tolerance and prevent fetal loss (37). miR-744/CD134 mitigates immune rejection by regulating the expression of CD62L and Ki67 (38).
Third, based on their biological characteristics, Tregs are divided into natural Tregs (nTregs) and inducible Tregs (iTregs) (39). nTregs mature in the thymus, maintain immune tolerance, and control inflammatory responses by exerting inhibitory functions through cell-to-cell communication (39, 40). nTregs are generated from CD25+ Tregs and Foxp3lo Tregs progenitors through the acquisition of negative and positive selection programs, respectively, with distinct TCR (T-cell receptor) repertoires and transcriptomes (41). In contrast, iTregs are closely related to cancers, such as intratumoral iTregs that act in a tumor antigen-selective manner. They are activated and expanded in the TME when their TCRs specifically respond to autologous tumors and mutated neoantigens (42). Furthermore, the TCR repertoire of intratumoral iTregs significantly overlapped with circulating Tregs and was also able to exhibit specific responses to autologous tumors and mutated neoantigens. This suggests that TCRs derived from tumor antigen-specific Tregs are present in circulation and the TME, as both were sources of tumor-specific TCRs (42).
Fourth, Tregs have been classified based on cell surface markers. These include Th1-like Tregs (T-bet+ IFNγ+- Foxp3+), Th2-like Tregs (Gata3+ IRF4+ IL4+ Foxp3+) and Th17-like Tregs (IL-17+ RORγt+ Foxp3+). Th1-like Tregs are characterized by the expression of T-bet and CXCR3 (43) and inducing the transformation of each other subtype to Th1 is a potential therapeutic approach. Th2-like Tregs mainly express Gata3 and IRF4 and tend to secrete IL-4 and IL-13 (44), are potent immunosuppressors and promote activation through autocrine IL-2. They are found more in tissues than in circulation and ably migrate in response to chemokines in the TME (45). Th17-like Tregs expressed RORγt and shared some phenotypic and functional characteristics with conventional Th17 cells, such as expressing high levels of CCR4 and CCR6 and low levels of CXCR3. However, Th17-like Tregs expressed only low levels of CD161 and were mostly unable to secrete IL-22 and TNF-α but produced IL-17, thereby retaining their inhibitory function (46, 47). miR-17 modulates Tregs function by targeting co-regulators of the Foxp3 transcription factor (48). Furthermore, Foxp3 plays a dual role in controlling the dependency on IL-2 in Tregs. On the one hand, it inhibits IL-2 transcription; on the other hand, it promotes the expression of CD25 (IL-2Ra) (49, 50). Reprogramming T cell-derived extracellular vesicles through IL-2 surface engineering can induce effective anti-cancer effects through miRNA delivery (51).
In summary, Tregs differentiated into subclasses under various stimuli, and there was mutual transformation among the subclasses. Although this demonstrated the plasticity of Tregs, much of this plasticity remains unanswered. Does plasticity depend on the initial heterogeneity of Treg? Is there an inevitability of specific subsets of Treg with specific effects? Tumor-infiltrating Tregs may arise from circulation and tissue residency and be induced by the TME. Distinguishing their phenotypic and functional characteristics from Tregs in healthy tissues based on plasticity and stability is crucial for the development of immunotherapies that target Tregs.
3 The ncRNA associated regulatory mechanisms of Tregs in cancer
In most cancers, Tregs are in higher proportions in tumor than normal tissues and infiltrate tumor tissues earlier than effector T cells. Indeed, a high ratio of Treg to CD8+ T cells indicated a poor prognosis (52). Furthermore, the abnormal differentiation and distribution of Tregs in cancer patients are affected by altered genetic information, abnormal molecular expression and reprogramming of cellular metabolism (53). These factors either drive Tregs enrichment in the TME, resulting in an immunosuppressive microenvironment, or aid peripheral Tregs in their roles in the formation of pre-metastatic niches (54).
Helios gene promoted the preferential differentiation of CD4+ naive T cells into Tregs (55). Intratumoral Helios- deficient Tregs acquired effector T cell function and induced immune responses by expressing effector cytokines (56). In malignant pleural effusion (MPE) of Non-Small Cell Lung Cancer (NSCLC) patients, decreased miR-4772-3p levels relieved the repression of Helios, thereby enhancing the activity of Tregs (57). Widespread miR-146a in Tregs regulated IFNγ-dependent immune responses by targeting STAT1 (58). Similarly, the loss of miR-17-92 in CD4+ T led to tumor immune evasion (59). Expression profiling of miRNAs revealed that miRNAs modulated the biological characteristics of Tregs by acting on target genes such as FOXP3, CTLA-4 and GARP (59, 60). The expression of miR-21 affects the balance of Th17/Tregs in GC patients (61). Among them, the Foxp3+ Tregs subset has been a focal point of research in recent years. Peripheral Tregs can be further classified into three subtypes based on the expression of CD25 and Foxp3: Fr. I, naive or resting Tregs with the CD45RA+CD25loFoxp3lo phenotype; Fr. II, Fr. I differentiates into CD45RA+ CD25hiFoxp3hi effector Tregs following antigen stimulation; Fr. III, a subset of CD45RA+CD25loFoxp3loCD4+ T cells that produce pro-inflammatory cytokines but exhibit minimal suppressive activity (62, 63). The enrichment of Fr. III subtype in cancer tissues is associated with a more favorable prognosis compared to the Fr. II subtype (63). NF-κB-mediated miR-34a disrupts the equilibrium Treg/Th17 balance by directly targeting Foxp3 (64). ADAR1 enhanced Treg cell function via modulation of the miR-21b/Foxp3 axis (65).
The immunosuppressive function of Tregs is dependent upon high intracellular cAMP concentrations. One of the major metabolic pathways supporting Tregs survival and function was an altered lipid metabolism (66). In relation to ncRNA regulation, miR-142-5p inhibited the expression of cAMP-hydrolyzing enzyme phosphodiesterase-3b (Pde3b) at the post-transcriptional level to modulate immune metabolism, thereby controlling the function of Tregs (67). Furthermore, given the predominance of intratumoral Tregs in glucose uptake, both glycolysis and oxidative phosphorylation contributed to fatty acid synthesis and thus promoted Tregs expansion (68). Glycolytically produced lactate increased Foxp3+ Tregs expression by activating the NF-κB pathway and promoted prostate cancer (PC) invasion through miR-21/TLR8 (69). Under starvation conditions, Foxp3 expression in human Peripheral Blood Mononuclear Cells (PBMCs) was inversely correlated with the expression of miR-31 and miR-155, which may also be potential metabolic-related immunomodulatory tools (67).
Relatedly, lncRNAs were involved in the regulation of Tregs function at the molecular level in breast cancer, the lncRNA SNHG1 competitively bound to miR-448 and reduced expression of IDO, thus inhibited Tregs differentiation, and this hindered immune escape (70). Generally, highly expressed IDO in the TME led to a decrease in tryptophan and accumulation of kynurenine, inhibiting T cell activation and inducing the production of Tregs (71). In vitro and in vivo assays confirmed that lncRNA Flatr in T cells directly participated in the transcription of Foxp3 as lncRNA Flatr-deficient mice showed delayed induction of Tregs (72). Besides, high expression of ZC3H12D in Tregs and NSCLC influenced patient prognosis through the ZC3H12D-hsa-miR-4443-ENST00000630242 axis (73). The highly-expressed membrane and cytoplasmic-localized lnc-INSR aided Tregs formation of an immunosuppressive microenvironment by inducing aberrant activation of the PI3K/AKT pathway in childhood acute T lymphoblastic leukemia (74). Overexpressed LINC00301 in NSCLC tissue targeted TGF-β and this increased Tregs therein (75).
Similarly, miRNAs were important regulators as overexpressed miR-216a was associated with decreased overall survival in CRC, as shown via multiomics analysis, and Tregs that had infiltrated the TME were involved in the regulation of miR-216a functions (76). MiR-520b that was overexpressed in breast cancer (BC) tissues augmented activation of Tregs in TME and induced M2-type polarization of macrophages (77). Persistent HBV in hepatocellular carcinoma (HCC) tissue maintained TGF-β activity, which repressed miR-34a expression, which in turn increased both CCL22 and recruitment of Tregs (78). MiR-26a inhibited the HCC-induced effect of diethylnitrosamine (DEN) by reducing the abundance of Tregs (79). MiR-28 was involved in PD1+Foxp3+expression and influenced the exhaustion of TIM3+Foxp3+Tregs in vitro (80). The GATA3/miR-125a-5p/IL-6R axis explicated how Treg cells responded to inflammatory IL-6-rich conditions (81). Relatedly, MiR-124/STAT3 played a similar role in Tregs of glioma as exposure of T cells of glioblastoma patients to miR-124 reactivated the immune response (82). Mesenchymal stem cells modulated the CRC-TME immunocompetence via miR-150 and miR-7 (83). MiR-34 was downregulated in Tp53-mutated secondary adult acute myeloid leukemia (sAML), resulting in increased PD-1 expression and Treg enrichment (84) (Figure 1).
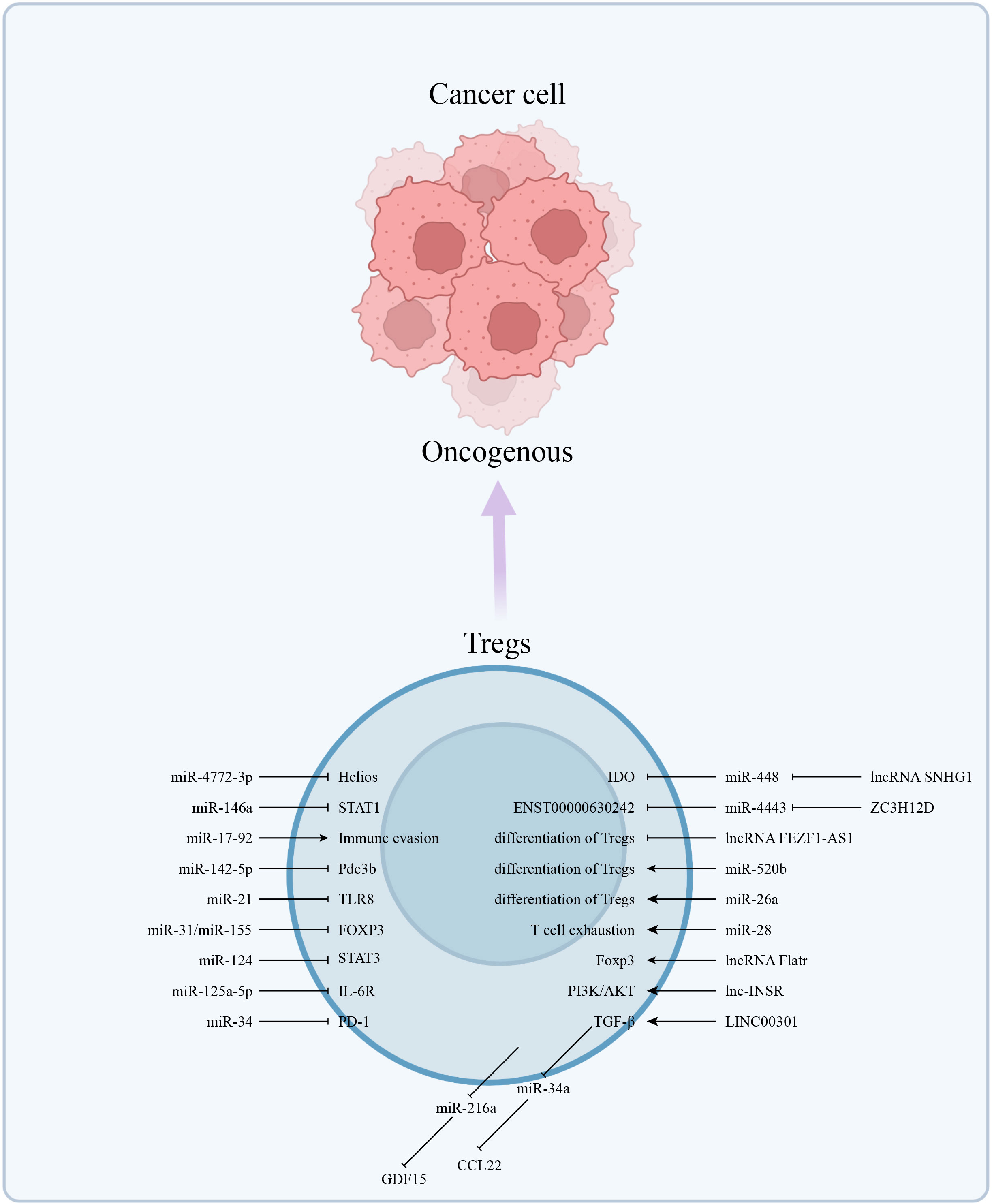
Figure 1 The abnormally expressed ncRNAs in Tregs affect the function of Tregs in multiple dimensions, such as affecting the differentiation of Tregs, the expression of ICIs, and the release of cytokines. Thereby establishing an immunosuppressive microenvironment and promoting the occurrence and development of cancer.
4 The ncRNA and Tregs cell-based communication in TME
4.1 Tregs and cancer cells in TME
Signals such as tumor-derived cytokines, exosomes in the TME and tumor antigens work to additionally induce the transformation of T cells into Tregs (85–88). For example, tumor-derived cytokines such as IL-2 and TGF-β are representative molecules that induced Foxp3+ Tregs (89, 90) and more on this was summarized by Tuzlak S et al. (91). Furthermore, tumor-derived exosomes, because of their diverse contents, induce Tregs via a more complex mechanism (92). First, there exist exosome-lncRNAs that induce Tregs at various levels. For example, CD73+γδT1 cells are the predominant Treg subset in breast cancer (BC). Their induction is triggered when lncRNA SNHG16 carried by BC cell-derived exosomes acts as a competing endogenous RNA (ceRNA) by sponging miR-16-5p, leading to the liberation of the target SMAD5 gene, thereby promoting the expression of CD73 and inducing T cell differentiation into Tregs (93). Another example is lncRNA RP11-323N12.5 that was overexpressed in gastric cancer (GC) and thus activated the Hippo signaling pathway in T cells and induced the differentiation of Tregs through exosome-carrier (94). Likewise, RP11-357H14.17, which was overexpressed in GC, is possibly involved in the differentiation of Tregs (95). One can hypothesize that exosomal miRNAs possibly play a similar role, and this is supported by the uniqueness of miRNAs in Tregs exosomes due to the enrichment of miR-146a-5p, miR-150-5p and miR-21-5p, and depletion of miR-106a-5p, miR-155- 5p and miR-19a-3p (84). Further, colorectal cancers (CRC) secreted miR-208b-containing exosomes by targeting PDCD4 to promote Tregs proliferation and reduce CRC sensitivity to oxaliplatin-based chemotherapy (96). The miR-124-3p-enriched exosomes significantly inhibited CRC growth, reduced Tregs infiltration into the TME, and prolonged the median survival time of tumor-bearing mice (97). Non-Small Cell Lung Cancer (NSCLC) and CRC-derived miR-214 were delivered into T cells via microvesicles (MVs), which subsequently downregulated phosphatases and PTEN and promoted Tregs expansion. The miR-214-induced Tregs promoted tumor growth through IL-10. Pertinently, the anti-miR-214 antisense oligonucleotides (ASOs) effectively blocked Tregs expansion and limited tumor growth in tumor-bearing mice (98). Relatedly, nasopharyngeal carcinoma (NPC) exosomal miR-243 targeted FGF11 to inhibit T cell proliferation and induce Tregs to not only differentiate, but also impair T cell function (99). Lastly, miR-10a-loaded exosomes resulted in increased expression levels of RORγt and Foxp3 in T cells that promoted Tregs differentiation (100).
4.2 Tregs and other immune cells in TME
Differentiated Tregs suppressed the antitumor immunity of effector T cells, NK cells, macrophages and DCs through multiple mechanisms, and functioned synergistically with MDSCs through crosstalk (101). Generally, Tregs inhibit antitumor immune functions of DCs cells by secreting inhibitory cytokines (IL-10, TGF-β and IL-35) (102, 103). In addition, CTLA-4 and LAG3 on the surface of Tregs combined with CD80/CD86 and MHC II, respectively, on the surface of DCs to induce immune tolerance of DCs (104, 105) suggesting that cell-to-cell transfer of ncRNAs via exosomes might be a novel mechanism by which Tregs regulated DCs function. Indeed miR-150-5p and miR-142-3p, upon entry into DCs, promoted an increase of IL-10 and a decrease of IL-6, which suppressed immune response in tissues (106). DCs cells influence the differentiation of Tregs as TGF-β selectively increased the expression of miR-27a in DCs through transcription factor SP1, and this hindered DC-mediated Th1 and Th17 cell differentiation but promoted Tregs differentiation (107). Akin to this was that overexpressed CTLA-4 in RORγt-deficient Treg that were isolated from tumors, increased Foxp3 expression in DCs cells (108). Exosomal miR-29a-3p and miR-21-5p released by macrophages in the epithelial ovarian cancer (EOC) microenvironment synergistically inhibited STAT3, resulting in an imbalanced Treg/Th17 ratio, which created an immunosuppressive microenvironment (109). Conversely, miR-15a/16-1 alleviated immunosuppression in HCC by disrupting CCL22-mediated communication between Kupffer cells and Tregs (110). The positive feedback loop formed between MDSCs and Tregs contributes to the formation of the immunosuppressive microenvironment. Tumor-induced MDSCs promoted the proliferation of Tregs in both a TGF-β-dependent and highly expressed CD73 manner, enhancing immunosuppressive effects (111, 112). Then, Tregs enhanced the expansion and suppressive functions of MDSCs by promoting the secretion of TGF-β and IL-35 (113, 114). MDSCs- and Tregs-associated miRNAs have been identified in acute lymphoblastic leukemia (115). Of note is that BMSCs-derived exosomal miR-23b-3p maintained Th17/Treg balance, by suppressing the PI3K/AKT/NF-κB signaling pathway (116) (Figure 2).
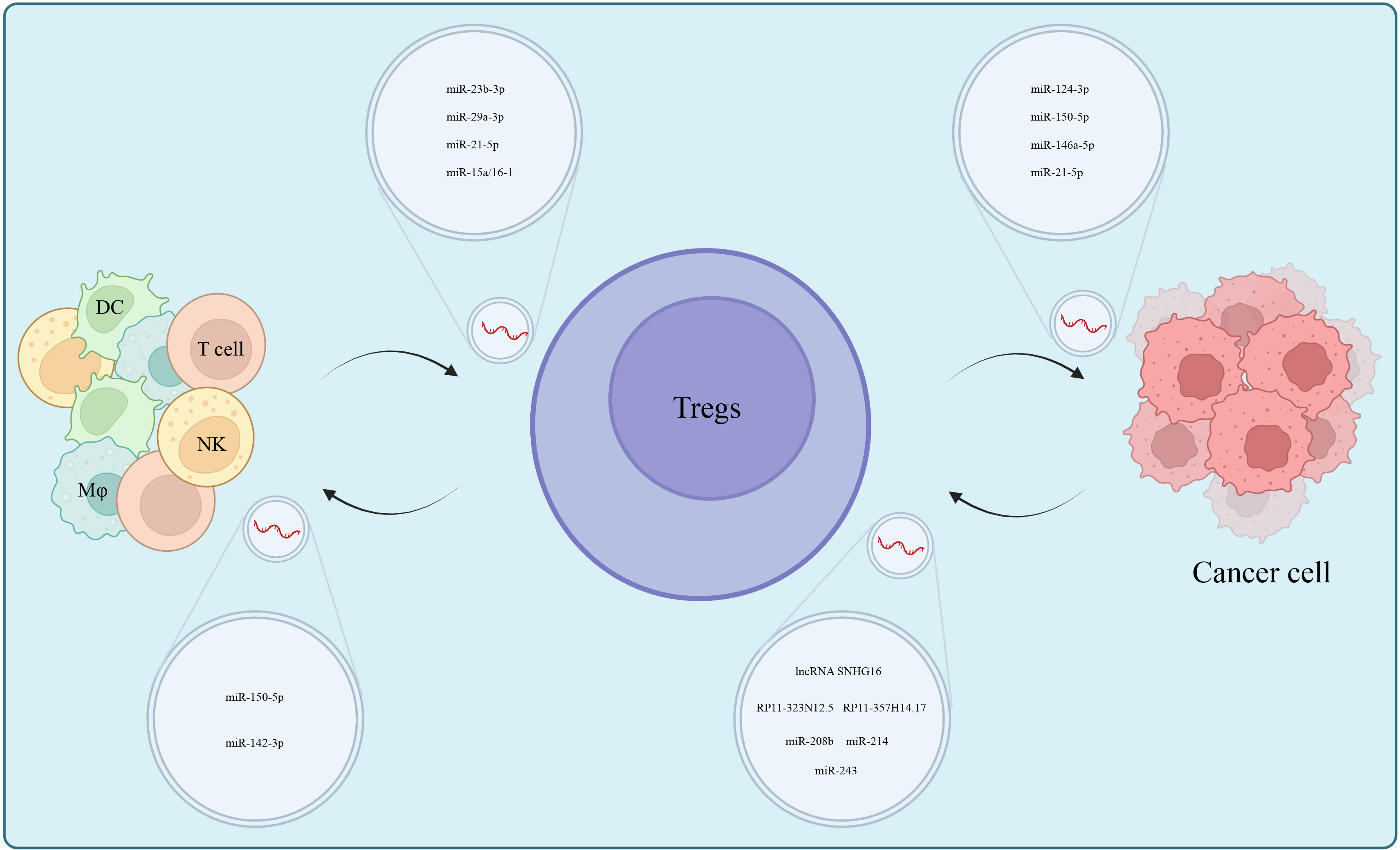
Figure 2 Exosomal ncRNAs mediate cell-to-cell interactions which centered on Tregs in the TME, participate in tumorigenesis and form an immunosuppressive microenvironment.
4.3 Tregs in circulation
NcRNAs in circulation are involved in the regulation of Tregs. MiR-21 expression was significantly elevated in the serum of diffuse large B-cell lymphoma (DLBCL) patients, and it promoted inducible co-stimulator (ICOS) expression on Tregs, thereby assisting the COS ligand (ICOSL) on endothelial cells to crosstalk with Tregs (117). The upregulated miR-21 was also associated with the different subsets of Th cells in esophageal squamous cell carcinoma (ESCC) (118). Overexpressed miR-182 in peripheral blood mononuclear cells (PBMCs) and serum from BC patients increased the expression of FOXP3, TGF-β and IL-17 in T cells and induced T cell differentiation into Tregs (119). Similarly, the upregulated linc-POU3F3 in PBMCs recruited TGF-β, increased the phosphorylation level of SMAD2/3, and ultimately promoted the distribution of Tregs among peripheral blood T cells of GC patients (120). MiR-27b-3p, miR-340-5p and miR-330-3p negatively regulated TGF-β and IL-10 in CD8+ T cells, limiting their differentiation into Tregs (121). Overexpression of miR-155 in peripheral blood and tissues of cervical cancer patients (CC) inhibited the expression of the target SOSC1 gene and induced an imbalanced Th17/Treg ratio (122). MiR-141 targeted CXCL1/CXCR2, which reduced Tregs recruitment in MPEs of NSCLC (123). MiR-568 mimiced its target NFAT5, thus suppressing Tregs cell activation and reducing Treg-derived IL-2 production (124). On the other hand, the differentiated Tregs regulated, via positive feedbacks, tumor development and immune evasion. Lnc-EGFR was overexpressed in Tregs and promoted HCC growth in an EGFR-dependent manner (125).
Many questions on circulating ncRNAs remain unanswered. Are they delivered in the form of exosomes or microvesicles, or are they purely independent RNA molecules? If they are free circulating molecules, how do they overcome blood flow shear stresses and avoid enzymatic degradation? Whatever the case, this long-distance signaling is the basis for the formation of pre-metastatic niches and molecular targets in liquid biopsies.
4.4 The role of immune-related ncRNA in prognostic prediction models for cancer
Given the broad regulatory roles of ncRNAs in the immunosuppressive microenvironment, their aberrant expression is possibly related to tumor prognosis. Indeed, the predictive models based on immune-related ncRNA expression contributed to evaluating the prognosis of head and neck squamous cell carcinoma (HNSCC) (126), GC (127), pancreatic cancer (128) and hepatocellular carcinoma (HCC) (129). MiR-146a expression in PBMCs was not only negatively correlated with Tregs but was also a marker of NSCLC liquid biopsy (130). The expression of miR-21 was associated with the inhibition of CD8+ T and was a potential diagnostic and prognostic marker for ESCC (131). The combined expression of miR-101, miR-548b, miR-554, and miR-1202 was a prognostic marker and potential therapeutic target for PCNSL (132). FGD5-AS1 promotes apoptosis of CD8+ T by influencing the expression of PD-L1 in NSCLC cells and was associated with poor prognosis of patients (133). The exosome circUHRF1 secreted by HCC cells contributes to immunosuppressive by inducing NK cell dysfunction and leading to adverse clinical outcomes (134).
5 Tregs-related tumor immunotherapy strategies
The TME has an elevated ratio of Tregs to effector T cells, which effectively suppresses autologous antitumor immune responses (Figure 3). Thus, reducing the infiltration of Tregs into the TME reverses this immunosuppression (135). This can be attained through either depletion of Tregs or reducing recruitment into the TME. Other ways include taking advantage of the plasticity of Tregs to transform them into an anti-tumor phenotype and, finally, applying ICIs therapy to change the biological behavior of Tregs.
5.1 Depletion and reduced recruitment of Tregs
Blockades of Tregs by CD25 restored IFN-γ production in CD8+ T cells and improved the efficacy of anti-VEGF therapy, which extended relapse-free survival durations in glioblastoma patients (136). Blocking IL-2 signaling —the use of anti-IL-2/anti-IL-2R to do so improved immune responses— impaired Tregs activity. Indeed, treatments with IL-2 mutant proteins reduced the number of Tregs and inhibited tumor growth (137). In addition, miR-142-3p in Tregs mediated cyclophosphamide (CY) depletion of Tregs by both targeting CD39 and altering intracellular ATP levels (138). Exogenous supplementation of miR-200c combined with the B16F10/GPI-IL-21 vaccine reduced Tregs recruitment, activated antitumor immunity and reduced melanoma metastasis (139). While depletion of Tregs appears to be a plausible therapeutic direction, Tregs that infiltrated TMEs lacked unique identifiers, thus creating a risk of clearing non-target Tregs, which may cause unexpected physiological damage. More insights are thus needed to make such treatments clinically feasible. Another promising therapeutic strategy is blocking the migration of Tregs into the TME by restricting intercellular communication that is based on CCR4 (140), CCL20 (141), CCL3-CCR1/CCR5 and CXCL12-CXCR4 (142). Relatedly, radiotherapy inhibited the specific recruitment of Tregs in Lewis lung cancer by upregulating miR-545 (143). A worthwhile research focus is the application of ncRNA for similar therapeutics.
5.2 Predisposing Tregs to an antitumor immunophenotype
This can be attained through first, curbing the transformation of CD4+T to Tregs —miR-17-92 (59, 144) is involved in the differentiation of CD4+ into Tregs— and second, inducing the transformation of Tregs to Th1 type —Th1-like differentiation was mediated by miR-27a (107). In addition, ncRNAs function as transit points in the drug-mediated transformation of Tregs. Shenmai injection inhibited the differentiation of CD4+ T cells into Tregs through the miR-103/GPER1 axis, thereby improving postoperative immune function in patients with papillary thyroid carcinoma (PTC) after 131 I radiotherapy (145). Ganoderma lucidum polysaccharides (GLPS) increased miR-125b expression, which then repressed Notch1 and Foxp3, restoring T cell function and limiting HCC growth (146). It is worth emphasizing that the oncogenic and tumor suppressor function of the Th17 subtype of Tregs in the TME is currently not fully understood. This together with the concomitant complex molecular regulation is a worthwhile research topic.
5.3 The role of ICIs in Tregs in cancer
Altered expression of ICIs underlies cancer evasion from immune surveillance (147), and immunotherapies that targeted CTLA-4 and PD-1 were effective against a variety of cancers (148). Indeed, targeting CTLA-4, TIGIT, PD-1, GITR and other co-inhibitory receptors to limit the function of Tregs possibly is an effective cancer treatment (149). Although tumor-infiltrating Tregs are functionally conserved, and tumor-infiltrating effector T cells are dysfunctional, co-suppressor receptors do not have opposing effects on Tregs and effector T cells. Much remains to be clarified on the effects of either checkpoint inhibition or stimulation not only on Tregs stability and function but also on Tregs and effector T cell activity and ratio (Figure 4).
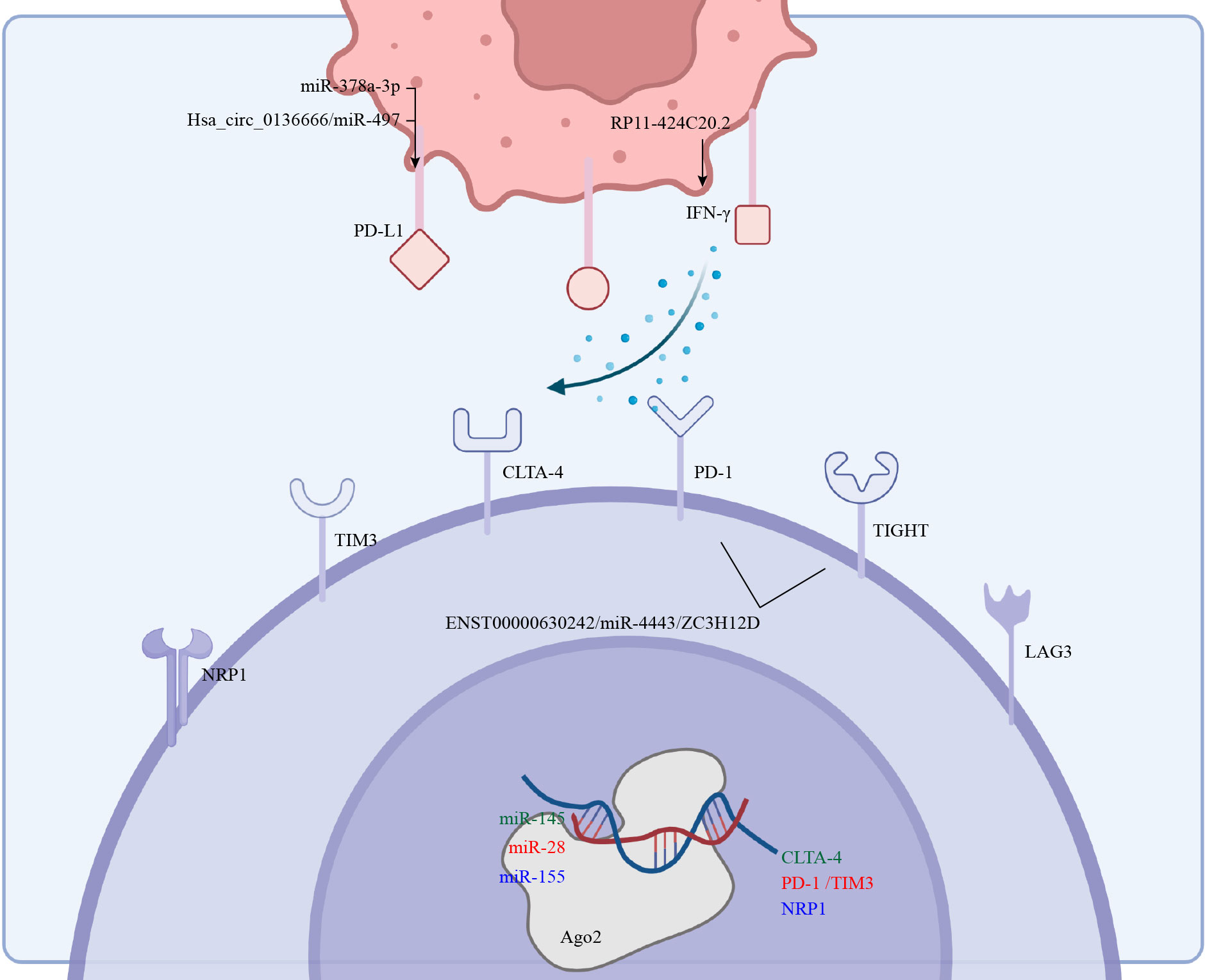
Figure 4 The ncRNAs in cancer cells and Tregs affect the expression of ICIs and ligands which induce immunotherapy resistance.
5.3.1 CTLA-4
CTLA-4 was the first identified immune checkpoint of Tregs that inhibited functions of CD4+ and CD8+ cells (150), and part of this inhibition was achieved through high-affinity binding to CD80 and CD86 (151). Abnormally expressed ncRNAs in Tregs were also an important factor in regulating CTLA-4 expression (108), as miR-145 negatively regulated the expression of CTLA-4 by binding to its 3’-UTR in Tregs (59). Thus, anti-CTLA-4 therapy could enhance the functions of effector T cells, only after surmounting these two challenges. First, anti-CTLA-4 therapies are yet to achieve the same effects as the current benchmark, anti-PD-1 therapies. Indeed, although anti-CTLA-4 mAbs were effective in depleting Foxp3+ Tregs in mouse tumors, similar success has not been attained in human tumors (151) because anti-CTLA-4 mAbs depleted Tregs in an Fc-dependent mechanism. Thus, antibodies with improved FcγR binding profiles were able to induce better intratumoral Tregs depletion and increased CD8+/Tregs ratio (152). This is possibly due to high antibody-dependent cell-mediated cytotoxicity (ADCC) and cellular phagocytosis (ADCP) (153, 154). On the other hand, treatment with anti-CTLA-4 mAb combined with IL15/IL15Rα complex depleted Tregs, which might have been related to the activation of NK cells (155). Yet even low-doses of anti-CTLA-4 combined with anti-PD-1 therapy led to immunotherapy-related adverse effects (irAEs) (156). Fortunately, recent results showed that the introduction of a tyrosine-to-histidine mutation in the polypeptide chain improved the pH sensitivity of anti-CTLA-4 mAb, thereby avoiding CTLA-4 downregulation and effectively depleting intratumoral Tregs, which then reduced occurrences of irAEs (157). Thus, either TLA-4 and OX40 bispecific antibodies or EZH2-based anti-CTLA-4 therapy are putative next-generation immunotherapies (158). Lastly, it was suggested that the expression of CTLA-4 maintains the balance between effector T cells and Tregs and adjusting its expression through RNA-related technologies was a therapy likely to destroy the immunosuppressive TME (159). The RP11-424C20.2/UHRF1 axis in HCC and thymoma affects CLTA-4 expression in an IFN-γ-dependent manner (160).
5.3.2 PD-1/PD-L1
Through binding with its ligand (PD-L1 or PD-L2) on the surface of Tregs, PD-1 restricted activation of the PI3K/AKT/mTOR pathway by dephosphorylating CD28 (161, 162). Further, exogenous TGF-β and PD-L1 induced T cells to differentiate into Tregs (163). No wonder anti-PD-1/PD-L1 therapy is patently the most effective current anti-tumor immunotherapy in clinical practice (164). In terms of ncRNA regulation, miR-378a-3p affected Tregs differentiation by directly regulating PD-L1 in HCC (165). Similarly, aberrantly activated Hsa_circ_0136666/miR-497/PD-L1 axis in CRC regulated Treg-mediated immune escape in a similar manner (166).
5.3.3 TIGIT
TIGIT competes with CD155 on the cell surface, and this influences the phenotypic variation of Tregs (167). TIGIT is transcriptionally regulated by FOXP3 and is therefore regarded as an identifier of pure and stable Tregs (168, 169). TIGIT+ Tregs were more effective at suppressing TH1 and TH17 cell responses than TIGIT- Tregs. Functionally, TIGIT works by inhibiting the PI3K–AKT pathway (170). Notably, the enrichment of TIGIT+ Tregs has been detected in various cancer groups (171, 172). In terms of ncRNA regulation, ENST00000630242 (lncRNA) was involved in the expression of TIGIT in NSCLC through a ceRNA mechanism (73).
5.3.4 LAG3
LAG3 is a co-inhibitory receptor that acts both intracellularly and extracellularly in Tregs cells. It is required for Tregs cell-mediated suppression of effector T cell proliferation (173, 174). On the other hand, LAG3 is highly expressed on IFNγ+FOXP3+ TH1-like Tregs and may be a potential marker of TH1-like Tregs. Similarly, the prevalence of LAG3+Treg in peripheral blood was higher in cancer patients than in healthy volunteers, but altogether lower than in the TME (175, 176). However, like CTLA-4, LAG3 enabled Tregs proliferation and limited Treg accumulation at sites of inflammation (104, 177). Moreover, the expression of LAG3 was proportional to that of CD25, which counteracted both activation of Treg cells and specific upregulation of LAG3-LAG3 functions in Treg are complex (177).
5.3.5 TIM3
TIM3 is not only highly expressed in tissue-Tregs but also often co-expressed with other inhibitory receptors (178). Infiltration of TIM3+Tregs into the TME of various cancers preceded that of the peripheral blood (179). Furthermore, TIM3+Tregs were a resident Tregs subset in colon cancer (180). In relation to ncRNA regulation, overexpressed miR-28 in melanoma-bearing mice inhibited the expression of PD-1 and TIM3 on Tregs and induced the depletion of TIM3+ Foxp3+ Tregs (80).
5.3.6 NRP1
NRP1 expressed by Tregs was an important molecule in the discrimination between thymus- and peripherally-derived Tregs (181). It was specifically expressed in immunosuppressive environments such as cancers and was a potential VEGF receptor (182). The expression of NRP1 promoted not only interactions between Tregs and DCs but also peripheral immune tolerance (183, 184). In relation to ncRNA regulation, deficiency of miR-155 impeded NRP1-mediated immune tolerance (185).
6 Conclusions and perspectives
Tregs are a key mediator of immune self-tolerance, which in turn facilitates autoimmunity and tumor immunosuppression. Moreover, Tregs are a highly plastic and heterogeneous cell population. Their immunosuppressive effects are a major obstacle to effective antitumor immunity. Thus, augmenting traditional cancer treatment methods with therapies that target these immunosuppressive effects can create effective anti-tumor effects that are especially relevant in the field of immunotherapy. The complex functions of intratumoral Tregs are affected by both exogenous and endogenous factors. Important endogenous factors are abnormal transcription and reprogrammed metabolism, whereas important exogenous factors are cytokines, chemokines, exosome contents, and metabolites in TME. Various ncRNAs patently play powerful roles in inducing the differentiation of Tregs and regulating the expression of ICIs. Thus, a possible strategy for improving cancer treatments is using immunotherapies that target ncRNA. Indeed, clinical trials of ncRNA-focused tumor therapies are ongoing (NCT03830619, NCT04269746, NCT04767750, NCT03057171), yet not one study has validated ncRNAs as targets for regulating Tregs in cancer immunotherapy. Exacerbating this dearth of research on ncRNAs-targeting therapies is the few studies on ncRNAs in Tregs —even among these few studies, the majority are on autoimmune diseases when the most impactful focal areas for cancer research would be to not only understand the role of ncRNAs in tumor-infiltrating Tregs and but also construct corresponding regulatory networks.
Tumor immunotherapy that targets Tregs remains both promising and challenging. For example, Tregs-related therapy has shifted from “elimination” to “inducing the functional differentiation of Tregs towards Th1-like Tregs”. Thus, exploring targets in this new direction, especially those related to the regulatory roles of ncRNAs, is a worthwhile research focus. A role of ICIs is Tregs markers and triggers for Treg-mediated inhibitory effects. Thus, it is also worthwhile to explicate how ncRNAs regulate these effector molecules.
In conclusion, the elaborate molecular mechanisms of how ncRNAs affect the differentiation and regulation of Tregs in various cancers remain enigmatic. Further functional studies on Tregs will not only aid our understanding of the role of ncRNAs in cancer immune responses and tumor immunotherapy but also develop Tregs as cancer immunotherapy targets.
Author contributions
YM wrote this manuscript. HP designed the research. HW gathered information and performed literature retrieval. YL draw the figures. XX performed the proofreading and revise the manuscript. All authors contributed to the article and approved the submitted version.
Funding
The Fundamental Research Funds for the Central Universities (LD202104), CSCO (Y-HR2019-0337).
Conflict of interest
The authors declare that the research was conducted in the absence of any commercial or financial relationships that could be construed as a potential conflict of interest.
Publisher’s note
All claims expressed in this article are solely those of the authors and do not necessarily represent those of their affiliated organizations, or those of the publisher, the editors and the reviewers. Any product that may be evaluated in this article, or claim that may be made by its manufacturer, is not guaranteed or endorsed by the publisher.
References
1. Märkl F, Huynh D, Endres S, Kobold S. Utilizing chemokines in cancer immunotherapy. Trends Cancer (2022) 8:670–82. doi: 10.1016/j.trecan.2022.04.001
2. Ribas A, Wolchok JD. Cancer immunotherapy using checkpoint blockade. Science (2018) 359:1350–5. doi: 10.1126/science.aar4060
3. Geisler AN, Phillips GS, Barrios DM, Wu J, Leung DYM, Moy AP, et al. Immune checkpoint inhibitor-related dermatologic adverse events. J Am Acad Dermatol (2020) 83:1255–68. doi: 10.1016/j.jaad.2020.03.132
4. Lucca LE, Dominguez-Villar M. Modulation of regulatory T cell function and stability by co-inhibitory receptors. Nat Rev Immunol (2020) 20:680–93. doi: 10.1038/s41577-020-0296-3
5. Li MO, Rudensky AY. T cell receptor signalling in the control of regulatory T cell differentiation and function. Nat Rev Immunol (2016) 16:220–33. doi: 10.1038/nri.2016.26
6. Van Der Veeken J, Gonzalez AJ, Cho H, Arvey A, Hemmers S, Leslie CS, et al. Memory of inflammation in regulatory T cells. Cell (2016) 166:977–90. doi: 10.1016/j.cell.2016.07.006
7. Hori S, Nomura T, Sakaguchi S. Control of regulatory T cell development by the transcription factor Foxp3. Science (2003) 299:1057–61. doi: 10.1126/science.1079490
8. Liu W, Putnam AL, Xu-Yu Z, Szot GL, Lee MR, Zhu S, et al. CD127 expression inversely correlates with FoxP3 and suppressive function of human CD4+ T reg cells. J Exp Med (2006) 203:1701–11. doi: 10.1084/jem.20060772
9. Sakaguchi S, Miyara M, Costantino CM, Hafler DA. FOXP3+ regulatory T cells in the human immune system. Nat Rev Immunol (2010) 10:490–500. doi: 10.1038/nri2785
10. Lu L, Barbi J, Pan F. The regulation of immune tolerance by FOXP3. Nat Rev Immunol (2017) 17:703–17. doi: 10.1038/nri.2017.75
11. Tanaka A, Sakaguchi S. Regulatory T cells in cancer immunotherapy. Cell Res (2017) 27:109–18. doi: 10.1038/cr.2016.151
12. Campbell C, Rudensky A. Roles of regulatory T cells in tissue pathophysiology and metabolism. Cell Metab (2020) 31:18–25. doi: 10.1016/j.cmet.2019.09.010
13. Sakaguchi S, Mikami N, Wing JB, Tanaka A, Ichiyama K, Ohkura N. Regulatory T cells and human disease. Annu Rev Immunol (2020) 38:541–66. doi: 10.1146/annurev-immunol-042718-041717
14. Proto JD, Doran AC, Gusarova G, Yurdagul A Jr., Sozen E, Subramanian M, et al. Regulatory T cells promote macrophage efferocytosis during inflammation resolution. Immunity (2018) 49:666–677.e666. doi: 10.1016/j.immuni.2018.07.015
15. Shi H, Chi H. Metabolic control of treg cell stability, plasticity, and tissue-specific heterogeneity. Front Immunol (2019) 10:2716. doi: 10.3389/fimmu.2019.02716
16. Wang K, Fu W. Transcriptional regulation of Treg homeostasis and functional specification. Cell Mol Life Sci (2020) 77:4269–87. doi: 10.1007/s00018-020-03534-7
17. Ye L, Zhang T, Kang Z, Guo G, Sun Y, Lin K, et al. Tumor-infiltrating immune cells act as a marker for prognosis in colorectal cancer. Front Immunol (2019) 10:2368. doi: 10.3389/fimmu.2019.02368
18. Landwehr LS, Altieri B, Schreiner J, Sbiera I, Weigand I, Kroiss M, et al. Interplay between glucocorticoids and tumor-infiltrating lymphocytes on the prognosis of adrenocortical carcinoma. J Immunother Cancer (2020) 8:e000469. doi: 10.1136/jitc-2019-000469
19. Shimizu J, Yamazaki S, Sakaguchi S. Induction of tumor immunity by removing CD25+CD4+ T cells: a common basis between tumor immunity and autoimmunity. J Immunol (1999) 163:5211–8. doi: 10.4049/jimmunol.163.10.5211
20. Sakurai Y, Usui Y, Hattori T, Takeuchi M, Takayama K, Karasawa Y, et al. Programmed cell death-1 pathway deficiency enhances autoimmunity leading to dacryoadenitis of mice. Am J Pathol (2021) 191:1077–93. doi: 10.1016/j.ajpath.2021.02.014
21. Djebali S, Davis CA, Merkel A, Dobin A, Lassmann T, Mortazavi A, et al. Landscape of transcription in human cells. Nature (2012) 489:101–8. doi: 10.1038/nature11233
22. Anastasiadou E, Jacob LS, Slack FJ. Non-coding RNA networks in cancer. Nat Rev Cancer (2018) 18:5–18. doi: 10.1038/nrc.2017.99
23. Esteller M, Pandolfi PP. The epitranscriptome of noncoding RNAs in cancer. Cancer Discovery (2017) 7:359–68. doi: 10.1158/2159-8290.CD-16-1292
24. Slack FJ, Chinnaiyan AM. The role of non-coding RNAs in oncology. Cell (2019) 179:1033–55. doi: 10.1016/j.cell.2019.10.017
25. Rupaimoole R, Slack FJ. MicroRNA therapeutics: towards a new era for the management of cancer and other diseases. Nat Rev Drug Discovery (2017) 16:203–22. doi: 10.1038/nrd.2016.246
26. Chen LL. The expanding regulatory mechanisms and cellular functions of circular RNAs. Nat Rev Mol Cell Biol (2020) 21:475–90. doi: 10.1038/s41580-020-0243-y
27. Zhou J, Xu J, Zhang L, Liu S, Ma Y, Wen X, et al. Combined single-cell profiling of lncRNAs and functional screening reveals that H19 is pivotal for embryonic hematopoietic stem cell development. Cell Stem Cell (2019) 24:285–298.e285. doi: 10.1016/j.stem.2018.11.023
28. Nojima T, Proudfoot NJ. Mechanisms of lncRNA biogenesis as revealed by nascent transcriptomics. Nat Rev Mol Cell Biol (2022) 23:389–406. doi: 10.1038/s41580-021-00447-6
29. Shevach EM, Thornton AM. tTregs, pTregs, and iTregs: similarities and differences. Immunol Rev (2014) 259:88–102. doi: 10.1111/imr.12160
30. Sánchez-Díaz R, Blanco-Dominguez R, Lasarte S, Tsilingiri K, Martín-Gayo E, Linillos-Pradillo B, et al. Thymus-derived regulatory T cell development is regulated by C-type lectin-mediated BIC/microRNA 155 expression. Mol Cell Biol (2017) 37:e00341-16. doi: 10.1128/MCB.00341-16
31. Łyszkiewicz M, Winter SJ, Witzlau K, Föhse L, Brownlie R, Puchałka J, et al. miR-181a/b-1 controls thymic selection of Treg cells and tunes their suppressive capacity. PloS Biol (2019) 17:e2006716. doi: 10.1371/journal.pbio.2006716
32. Smigiel KS, Richards E, Srivastava S, Thomas KR, Dudda JC, Klonowski KD, et al. CCR7 provides localized access to IL-2 and defines homeostatically distinct regulatory T cell subsets. J Exp Med (2014) 211:121–36. doi: 10.1084/jem.20131142
33. Hoffmann P, Eder R, Boeld TJ, Doser K, Piseshka B, Andreesen R, et al. Only the CD45RA+ subpopulation of CD4+CD25high T cells gives rise to homogeneous regulatory T-cell lines upon in vitro expansion. Blood (2006) 108:4260–7. doi: 10.1182/blood-2006-06-027409
34. Levine AG, Arvey A, Jin W, Rudensky AY. Continuous requirement for the TCR in regulatory T cell function. Nat Immunol (2014) 15:1070–8. doi: 10.1038/ni.3004
35. Pere H, Montier Y, Bayry J, Quintin-Colonna F, Merillon N, Dransart E, et al. A CCR4 antagonist combined with vaccines induces antigen-specific CD8+ T cells and tumor immunity against self antigens. Blood (2011) 118:4853–62. doi: 10.1182/blood-2011-01-329656
36. Divekar AA, Dubey S, Gangalum PR, Singh RR. Dicer insufficiency and microRNA-155 overexpression in lupus regulatory T cells: an apparent paradox in the setting of an inflammatory milieu. J Immunol (2011) 186:924–30. doi: 10.4049/jimmunol.1002218
37. Schjenken JE, Moldenhauer LM, Zhang B, Care AS, Groome HM, Chan HY, et al. MicroRNA miR-155 is required for expansion of regulatory T cells to mediate robust pregnancy tolerance in mice. Mucosal Immunol (2020) 13:609–25. doi: 10.1038/s41385-020-0255-0
38. Zhou B, Mei F, Wu C, Liu Z, Xu H, Cui Y. Effect of miR-744 on ameliorating heart allograft rejection in BALB/c mice via regulation of TNFRSF4 expression in regulatory T cells. Transplant Proc (2020) 52:398–405. doi: 10.1016/j.transproceed.2019.10.014
39. Wing JB, Tanaka A, Sakaguchi S. Human FOXP3(+) regulatory T cell heterogeneity and function in autoimmunity and cancer. Immunity (2019) 50:302–16. doi: 10.1016/j.immuni.2019.01.020
40. Grinberg-Bleyer Y, Oh H, Desrichard A, Bhatt DM, Caron R, Chan TA, et al. NF-κB c-rel is crucial for the regulatory T cell immune checkpoint in cancer. Cell (2017) 170:1096–1108.e1013. doi: 10.1016/j.cell.2017.08.004
41. Owen DL, Mahmud SA, Sjaastad LE, Williams JB, Spanier JA, Simeonov DR, et al. Thymic regulatory T cells arise via two distinct developmental programs. Nat Immunol (2019) 20:195–205. doi: 10.1038/s41590-018-0289-6
42. Ahmadzadeh M, Pasetto A, Jia L, DeNiger DC, Stevanović S, Robbins PF, et al. Tumor-infiltrating human CD4(+) regulatory T cells display a distinct TCR repertoire and exhibit tumor and neoantigen reactivity. Sci Immunol (2019) 4:eaao4310. doi: 10.1126/sciimmunol.aao4310
43. Di Pilato M, Kim EY, Cadilha BL, Prüßmann JN, Nasrallah MN, Seruggia D, et al. Targeting the CBM complex causes T(reg) cells to prime tumours for immune checkpoint therapy. Nature (2019) 570:112–6. doi: 10.1038/s41586-019-1215-2
44. Noval Rivas M, Burton OT, Wise P, Charbonnier LM, Georgiev P, Oettgen HC, et al. Regulatory T cell reprogramming toward a Th2-cell-like lineage impairs oral tolerance and promotes food allergy. Immunity (2015) 42:512–23. doi: 10.1016/j.immuni.2015.02.004
45. Halim L, ROmano M, Mcgregor R, Correa I, Pavlidis P, Grageda N, et al. An atlas of human regulatory T helper-like cells reveals features of th2-like tregs that support a tumorigenic environment. Cell Rep (2017) 20:757–70. doi: 10.1016/j.celrep.2017.06.079
46. Zhou L, Lopes JE, Chong MM, Ivanov Ii, Min R, Victora GD, et al. TGF-beta-induced Foxp3 inhibits T(H)17 cell differentiation by antagonizing RORgammat function. Nature (2008) 453:236–40. doi: 10.1158/2326-6066.CIR-17-0479
47. Ayyoub M, Deknuydt F, Raimbaud I, Dousset C, Leveque L, Bioley G, et al. Human memory FOXP3+ Tregs secrete IL-17 ex vivo and constitutively express the T(H)17 lineage-specific transcription factor RORgamma t. Proc Natl Acad Sci U.S.A. (2009) 106:8635–40. doi: 10.1073/pnas.0900621106
48. Yang HY, Barbi J, Wu CY, Zheng Y, Vignali PD, Wu X, et al. MicroRNA-17 modulates regulatory T cell function by targeting co-regulators of the foxp3 transcription factor. Immunity (2016) 45:83–93. doi: 10.1016/j.immuni.2016.06.022
49. Schubert LA, Jeffery E, Zhang Y, Ramsdell F, Ziegler SF. Scurfin (FOXP3) acts as a repressor of transcription and regulates T cell activation. J Biol Chem (2001) 276:37672–9. doi: 10.1074/jbc.M104521200
50. Ohkura N, Hamaguchi M, Morikawa H, Sugimura K, Tanaka A, Ito Y, et al. T cell receptor stimulation-induced epigenetic changes and Foxp3 expression are independent and complementary events required for Treg cell development. Immunity (2012) 37:785–99. doi: 10.1016/j.immuni.2012.09.010
51. Jung D, Shin S, Kang SM, Jung I, Ryu S, Noh S, et al. Reprogramming of T cell-derived small extracellular vesicles using IL2 surface engineering induces potent anti-cancer effects through miRNA delivery. J Extracell Vesicles (2022) 11:e12287. doi: 10.1002/jev2.12287
52. Darrasse-Jèze G, Bergot AS, Durgeau A, Billiard F, Salomon BL, Cohen JL, et al. Tumor emergence is sensed by self-specific CD44hi memory Tregs that create a dominant tolerogenic environment for tumors in mice. J Clin Invest (2009) 119:2648–62. doi: 10.1172/JCI36628
53. Ferreira LMR, Muller YD, Bluestone JA, Tang Q. Next-generation regulatory T cell therapy. Nat Rev Drug Discov (2019) 18:749–69. doi: 10.1038/s41573-019-0041-4
54. Dai E, Zhu Z, Wahed S, Qu Z, Storkus WJ, Guo ZS. Epigenetic modulation of antitumor immunity for improved cancer immunotherapy. Mol Cancer (2021) 20:171. doi: 10.1186/s12943-021-01464-x
55. Ng MSF, Roth TL, Mendoza VF, Marson A, Burt TD. Helios enhances the preferential differentiation of human fetal CD4(+) naïve T cells into regulatory T cells. Sci Immunol (2019) 4:eaav5947. doi: 10.1126/sciimmunol.aav5947
56. Yates K, Bi K, Haining WN, Cantor H, Kim HJ. Comparative transcriptome analysis reveals distinct genetic modules associated with Helios expression in intratumoral regulatory T cells. Proc Natl Acad Sci U.S.A. (2018) 115:2162–7. doi: 10.1073/pnas.1720447115
57. Yu WQ, Ji NF, Gu CJ, Sun ZX, Wang ZX, Chen ZQ, et al. Downregulation of miR-4772-3p promotes enhanced regulatory T cell capacity in Malignant pleural effusion by elevating Helios levels. Chin Med J (Engl) (2019) 132:2705–15. doi: 10.1097/CM9.0000000000000517
58. Lu LF, Boldin MP, Chaudhry A, Lin LL, Taganov KD, Hanada T, et al. Function of miR-146a in controlling Treg cell-mediated regulation of Th1 responses. Cell (2010) 142:914–29. doi: 10.1016/j.cell.2010.08.012
59. Fayyad-Kazan H, Rouas R, Fayyad-Kazan M, Badran R, El Zein N, Lewalle P, et al. MicroRNA profile of circulating CD4-positive regulatory T cells in human adults and impact of differentially expressed microRNAs on expression of two genes essential to their function. J Biol Chem (2012) 287:9910–22. doi: 10.1074/jbc.M111.337154
60. Jebbawi F, Fayyad-Kazan H, Merimi M, Lewalle P, Verougstraete JC, Leo O, et al. A microRNA profile of human CD8(+) regulatory T cells and characterization of the effects of microRNAs on Treg cell-associated genes. J Transl Med (2014) 12:218. doi: 10.1186/s12967-014-0218-x
61. Zheng X, Dong L, Wang K, Zou H, Zhao S, Wang Y, et al. MiR-21 participates in the PD-1/PD-L1 pathway-mediated imbalance of th17/treg cells in patients after gastric cancer resection. Ann Surg Oncol (2019) 26:884–93. doi: 10.1245/s10434-018-07117-6
62. Miyara M, Yoshioka Y, Kitoh A, Shima T, Wing K, Niwa A, et al. Functional delineation and differentiation dynamics of human CD4+ T cells expressing the FoxP3 transcription factor. Immunity (2009) 30:899–911. doi: 10.1016/j.immuni.2009.03.019
63. Saito T, Nishikawa H, Wada H, Nagano Y, Sugiyama D, Atarashi K, et al. Two FOXP3(+)CD4(+) T cell subpopulations distinctly control the prognosis of colorectal cancers. Nat Med (2016) 22:679–84. doi: 10.1038/nm.4086
64. Xie M, Wang J, Gong W, Xu H, Pan X, Chen Y, et al. NF-κB-driven miR-34a impairs Treg/Th17 balance via targeting Foxp3. J Autoimmun (2019) 102:96–113. doi: 10.1016/j.jaut.2019.04.018
65. Zhao Y, Zheng X, Li M, Zhao J, Wang X, Zhu H. ADAR1 improved Treg cell function through the miR-21b/Foxp3 axis and inhibits the progression of acute graft-versus-host disease after allogeneic hematopoietic stem cell transplantation. Int Immunopharmacol (2023) 115:109620. doi: 10.1016/j.intimp.2022.109620
66. Field CS, Baixauli F, Kyle RL, Puleston DJ, Cameron AM, Sanin DE, et al. Mitochondrial integrity regulated by lipid metabolism is a cell-intrinsic checkpoint for treg suppressive function. Cell Metab (2020) 31:422–437.e425. doi: 10.1016/j.cmet.2019.11.021
67. Anandagoda N, Willis JC, Hertweck A, Roberts LB, Jackson I, Gökmen MR, et al. microRNA-142-mediated repression of phosphodiesterase 3B critically regulates peripheral immune tolerance. J Clin Invest (2019) 129:1257–71. doi: 10.1172/JCI124725
68. Pacella I, Procaccini C, Focaccetti C, Miacci S, Timperi E, Faicchia D, et al. Fatty acid metabolism complements glycolysis in the selective regulatory T cell expansion during tumor growth. Proc Natl Acad Sci U.S.A. (2018) 115:E6546–e6555. doi: 10.1073/pnas.1720113115
69. Comito G, Iscaro A, Bacci M, Morandi A, Ippolito L, Parri M, et al. Lactate modulates CD4(+) T-cell polarization and induces an immunosuppressive environment, which sustains prostate carcinoma progression via TLR8/miR21 axis. Oncogene (2019) 38:3681–95. doi: 10.1038/s41388-019-0688-7
70. Pei X, Wang X, Li H. LncRNA SNHG1 regulates the differentiation of Treg cells and affects the immune escape of breast cancer via regulating miR-448/IDO. Int J Biol Macromol (2018) 118:24–30. doi: 10.1016/j.ijbiomac.2018.06.033
71. Huang B, Pan PY, Li Q, Sato AI, Levy DE, Bromberg J, et al. Gr-1+CD115+ immature myeloid suppressor cells mediate the development of tumor-induced T regulatory cells and T-cell anergy in tumor-bearing host. Cancer Res (2006) 66:1123–31. doi: 10.1158/0008-5472.CAN-05-1299
72. Brajic A, Franckaert D, Burton O, Bornschein S, Calvanese AL, Demeyer S, et al. The long non-coding RNA flatr anticipates foxp3 expression in regulatory T cells. Front Immunol (2018) 9:1989. doi: 10.3389/fimmu.2018.01989
73. Chen W, Guo Z, Wu J, Lin G, Chen S, Lin Q, et al. Identification of a ZC3H12D-regulated competing endogenous RNA network for prognosis of lung adenocarcinoma at single-cell level. BMC Cancer (2022) 22:115. doi: 10.1186/s12885-021-08992-1
74. Wang Y, Yang X, Sun X, Rong L, Kang M, Wu P, et al. Bone marrow infiltrated Lnc-INSR induced suppressive immune microenvironment in pediatric acute lymphoblastic leukemia. Cell Death Dis (2018) 9:1043. doi: 10.1038/s41419-018-1078-8
75. Sun CC, Zhu W, Li SJ, Hu W, Zhang J, Zhuo Y, et al. FOXC1-mediated LINC00301 facilitates tumor progression and triggers an immune-suppressing microenvironment in non-small cell lung cancer by regulating the HIF1α pathway. Genome Med (2020) 12:77. doi: 10.1186/s13073-020-00773-y
76. Tung CB, Li CY, Lin HY. Multi-omics reveal the immunological role and the theragnostic value of miR-216a/GDF15 axis in human colon adenocarcinoma. Int J Mol Sci (2021) 22:13636. doi: 10.3390/ijms222413636
77. Zhu Q, Yuan J, He Y, Hu Y. The effect of miR-520b on macrophage polarization and T cell immunity by targeting PTEN in breast cancer. J Oncol (2021) 2021:5170496. doi: 10.1155/2021/5170496
78. Yang P, Li QJ, Feng Y, Zhang Y, Markowitz GJ, Ning S, et al. TGF-β-miR-34a-CCL22 signaling-induced Treg cell recruitment promotes venous metastases of HBV-positive hepatocellular carcinoma. Cancer Cell (2012) 22:291–303. doi: 10.1016/j.ccr.2012.07.023
79. Badr AM, El-Ahwany E, Goda L, Nagy F, Helal N, El Deeb S. MicroRNA-26a systemic administration attenuates tumor formation in hepatocellular carcinoma mouse model. Pak J Pharm Sci (2021) 34:925–32.
80. Li Q, Johnston N, Zheng X, Wang H, Zhang X, Gao D, et al. miR-28 modulates exhaustive differentiation of T cells through silencing programmed cell death-1 and regulating cytokine secretion. Oncotarget (2016) 7:53735–50. doi: 10.18632/oncotarget.10731
81. Li D, Kong C, Tsun A, Chen C, Song H, Shi G, et al. MiR-125a-5p decreases the sensitivity of treg cells toward IL-6-mediated conversion by inhibiting IL-6R and STAT3 expression. Sci Rep (2015) 5:14615. doi: 10.1038/srep14615
82. Wei J, Wang F, Kong LY, Xu S, Doucette T, Ferguson SD, et al. miR-124 inhibits STAT3 signaling to enhance T cell-mediated immune clearance of glioma. Cancer Res (2013) 73:3913–26. doi: 10.1158/0008-5472.CAN-12-4318
83. François S, Usunier B, Forgue-Lafitte ME, L'homme B, Benderitter M, Douay L, et al. Mesenchymal stem cell administration attenuates colon cancer progression by modulating the immune component within the colorectal tumor microenvironment. Stem Cells Transl Med (2019) 8:285–300. doi: 10.1002/sctm.18-0117
84. Torri A, Carpi D, Bulgheroni E, Crosti MC, Moro M, Gruarin P, et al. Extracellular microRNA signature of human helper T cell subsets in health and autoimmunity. J Biol Chem (2017) 292:2903–15. doi: 10.1074/jbc.M116.769893
85. Downs-Canner S, Berkey S, Delgoffe GM, Edwards RP, Curiel T, Odunsi K, et al. Suppressive IL-17A(+)Foxp3(+) and ex-Th17 IL-17A(neg)Foxp3(+) T(reg) cells are a source of tumour-associated T(reg) cells. Nat Commun (2017) 8:14649. doi: 10.1038/ncomms14649
86. Anel A, Gallego-Lleyda A, De Miguel D, Naval J, Martínez-Lostao L. Role of exosomes in the regulation of T-cell mediated immune responses and in autoimmune disease. Cells (2019) 8:154. doi: 10.3390/cells8020154
87. Wang L, Simons DL, Lu X, Tu TY, Solomon S, Wang R, et al. Connecting blood and intratumoral T(reg) cell activity in predicting future relapse in breast cancer. Nat Immunol (2019) 20:1220–30. doi: 10.1038/s41590-019-0429-7
88. Lim SA, Wei J, Nguyen TM, Shi H, Su W, Palacios G, et al. Lipid signalling enforces functional specialization of T(reg) cells in tumours. Nature (2021) 591:306–11. doi: 10.1038/s41586-021-03235-6
89. Konkel JE, Zhang D, Zanvit P, Chia C, Zangarle-Murray T, Jin W, et al. Transforming growth factor-β Signaling in regulatory T cells controls T helper-17 cells and tissue-specific immune responses. Immunity (2017) 46:660–74. doi: 10.1016/j.immuni.2017.03.015
90. Bansal SS, Ismahil MA, Goel M, Zhou G, Rokosh G, Hamid T, et al. Dysfunctional and proinflammatory regulatory T-lymphocytes are essential for adverse cardiac remodeling in ischemic cardiomyopathy. Circulation (2019) 139:206–21. doi: 10.1161/CIRCULATIONAHA.118.036065
91. Tuzlak S, Dejean AS, Iannacone M, Quintana FJ, Waisman A, Ginhoux F, et al. Repositioning T(H) cell polarization from single cytokines to complex help. Nat Immunol (2021) 22:1210–7. doi: 10.1038/s41590-021-01009-w
92. Chatila TA, Williams CB. Regulatory T cells: exosomes deliver tolerance. Immunity (2014) 41:3–5. doi: 10.1016/j.immuni.2014.07.001
93. Ni C, Fang QQ, Chen WZ, Jiang JX, Jiang Z, Ye J, et al. Breast cancer-derived exosomes transmit lncRNA SNHG16 to induce CD73+γδ1 Treg cells. Signal Transduct Target Ther (2020) 5:41. doi: 10.1038/s41392-020-0129-7
94. Wang J, Huang F, Shi Y, Zhang Q, Xu S, Yao Y, et al. RP11-323N12.5 promotes the Malignancy and immunosuppression of human gastric cancer by increasing YAP1 transcription. Gastric Cancer (2021) 24:85–102. doi: 10.1007/s10120-020-01099-9
95. Xiaoli T, Wenting W, Meixiang Z, Chunlei Z, Chengxia H. Long noncoding RNA RP11-357H14.17 plays an oncogene role in gastric cancer by activating ATF2 signaling and enhancing treg cells. BioMed Res Int (2021) 2021:6635936. doi: 10.1155/2021/6635936
96. Ning T, Li J, He Y, Zhang H, Wang X, Deng T, et al. Exosomal miR-208b related with oxaliplatin resistance promotes Treg expansion in colorectal cancer. Mol Ther (2021) 29:2723–36. doi: 10.1016/j.ymthe.2021.04.028
97. Rezaei R, Baghaei K, Hashemi SM, Zali MR, Ghanbarian H, Amani D. Tumor-derived exosomes enriched by miRNA-124 promote anti-tumor immune response in CT-26 tumor-bearing mice. Front Med (Lausanne) (2021) 8:619939. doi: 10.3389/fmed.2021.619939
98. Yin Y, Cai X, Chen X, Liang H, Zhang Y, Li J, et al. Tumor-secreted miR-214 induces regulatory T cells: a major link between immune evasion and tumor growth. Cell Res (2014) 24:1164–80. doi: 10.1038/cr.2014.121
99. Ye SB, Zhang H, Cai TT, Liu YN, Ni JJ, He J, et al. Exosomal miR-24-3p impedes T-cell function by targeting FGF11 and serves as a potential prognostic biomarker for nasopharyngeal carcinoma. J Pathol (2016) 240:329–40. doi: 10.1002/path.4781
100. Bolandi Z, Mokhberian N, Eftekhary M, Sharifi K, Soudi S, Ghanbarian H, et al. Adipose derived mesenchymal stem cell exosomes loaded with miR-10a promote the differentiation of Th17 and Treg from naive CD4(+) T cell. Life Sci (2020) 259:118218. doi: 10.1016/j.lfs.2020.118218
101. Li C, Jiang P, Wei S, Xu X, Wang J. Regulatory T cells in tumor microenvironment: new mechanisms, potential therapeutic strategies and future prospects. Mol Cancer (2020) 19:116. doi: 10.1186/s12943-020-01234-1
102. Budhu S, Schaer DA, Li Y, Toledo-Crow R, Panageas K, Yang X, et al. Blockade of surface-bound TGF-β on regulatory T cells abrogates suppression of effector T cell function in the tumor microenvironment. Sci Signal (2017) 10:eaak9702. doi: 10.1126/scisignal.aak9702
103. Toomer KH, Malek TR. Cytokine signaling in the development and homeostasis of regulatory T cells. Cold Spring Harb Perspect Biol (2018) 10:a028597. doi: 10.1101/cshperspect.a028597
104. Liang B, Workman C, Lee J, Chew C, Dale BM, Colonna L, et al. Regulatory T cells inhibit dendritic cells by lymphocyte activation gene-3 engagement of MHC class II. J Immunol (2008) 180:5916–26. doi: 10.4049/jimmunol.180.9.5916
105. Bolton HA, Zhu E, Terry AM, Guy TV, Koh WP, Tan SY, et al. Selective Treg reconstitution during lymphopenia norMalizes DC costimulation and prevents graft-versus-host disease. J Clin Invest (2015) 125:3627–41. doi: 10.1172/JCI76031
106. Tung SL, Boardman DA, Sen M, Letizia M, Peng Q, Cianci N, et al. Regulatory T cell-derived extracellular vesicles modify dendritic cell function. Sci Rep (2018) 8:6065. doi: 10.1038/s41598-018-24531-8
107. Min S, Li L, Zhang M, Zhang Y, Liang X, Xie Y, et al. TGF-β-associated miR-27a inhibits dendritic cell-mediated differentiation of Th1 and Th17 cells by TAB3, p38 MAPK, MAP2K4 and MAP2K7. Genes Immun (2012) 13:621–31. doi: 10.1038/gene.2012.45
108. Rizzo A, Di Giovangiulio M, Stolfi C, Franzè E, Fehling HJ, Carsetti R, et al. RORγt-expressing tregs drive the growth of colitis-associated colorectal cancer by controlling IL6 in dendritic cells. Cancer Immunol Res (2018) 6:1082–92. doi: 10.1158/2326-6066.CIR-17-0698
109. Zhou J, Li X, Wu X, Zhang T, Zhu Q, Wang X, et al. Exosomes released from tumor-associated macrophages transfer miRNAs that induce a treg/th17 cell imbalance in epithelial ovarian cancer. Cancer Immunol Res (2018) 6:1578–92. doi: 10.1053/j.gastro.2021.10.015
110. Liu N, Chang CW, Steer CJ, Wang XW, Song G. MicroRNA-15a/16-1 prevents hepatocellular carcinoma by disrupting the communication between kupffer cells and regulatory T cells. Gastroenterology (2022) 162:575–89. doi: 10.1084/jem.20050463
111. Ghiringhelli F, Puig PE, Roux S, Parcellier A, Schmitt E, Solary E, et al. Tumor cells convert immature myeloid dendritic cells into TGF-beta-secreting cells inducing CD4+CD25+ regulatory T cell proliferation. J Exp Med (2005) 202:919–29. doi: 10.3389/fimmu.2012.00190
112. Ohta A, Kini R, Ohta A, Subramanian M, Madasu M, Sitkovsky M. The development and immunosuppressive functions of CD4(+) CD25(+) FoxP3(+) regulatory T cells are under influence of the adenosine-A2A adenosine receptor pathway. Front Immunol (2012) 3:190. doi: 10.4049/jimmunol.0902739
113. Kochetkova I, Golden S, Holderness K, Callis G, Pascual DW. IL-35 stimulation of CD39+ regulatory T cells confers protection against collagen II-induced arthritis via the production of IL-10. J Immunol (2010) 184:7144–53. doi: 10.4161/onci.21176
114. Fujimura T, Kambayashi Y, Aiba S. Crosstalk between regulatory T cells (Tregs) and myeloid derived suppressor cells (MDSCs) during melanoma growth. Oncoimmunology (2012) 1:1433–4. doi: 10.1016/j.humimm.2020.10.009
115. Labib Salem M, Zidan AA, Ezz El-Din El-Naggar R, Attia Saad M, El-Shanshory M, Bakry U, et al. Myeloid-derived suppressor cells and regulatory T cells share common immunoregulatory pathways-related microRNAs that are dysregulated by acute lymphoblastic leukemia and chemotherapy. Hum Immunol (2021) 82:36–45. doi: 10.1016/j.brainresbull.2020.09.003
116. Sun X, Zheng X, Zhang X, Zhang Y, Luo G. Exosomal microRNA-23b-3p from bone marrow mesenchymal stem cells maintains T helper/Treg balance by downregulating the PI3k/Akt/NF-κB signaling pathway in intracranial aneurysm. Brain Res Bull (2020) 165:305–15. doi: 10.1186/s13046-017-0551-z
117. Zheng Z, Xu PP, Wang L, Zhao HJ, Weng XQ, Zhong HJ, et al. MiR21 sensitized B-lymphoma cells to ABT-199 via ICOS/ICOSL-mediated interaction of Treg cells with endothelial cells. J Exp Clin Cancer Res (2017) 36:82. doi: 10.1007/s12026-019-09079-7
118. Samiei H, Sadighi-Moghaddam B, Mohammadi S, Gharavi A, Abdolmaleki S, Khosravi A, et al. Dysregulation of helper T lymphocytes in esophageal squamous cell carcinoma (ESCC) patients is highly associated with aberrant production of miR-21. Immunol Res (2019) 67:212–22. doi: 10.1111/cas.14764
119. Soheilifar MH, Vaseghi H, Seif F, Ariana M, Ghorbanifar S, Habibi N, et al. Concomitant overexpression of mir-182-5p and mir-182-3p raises the possibility of IL-17-producing Treg formation in breast cancer by targeting CD3d, ITK, FOXO1, and NFATs: A meta-analysis and experimental study. Cancer Sci (2021) 112:589–603. doi: 10.1111/cas.14764
120. Xiong G, Yang L, Chen Y, Fan Z. Linc-POU3F3 promotes cell proliferation in gastric cancer via increasing T-reg distribution. Am J Transl Res (2015) 7:2262–9. doi: 10.1002/jcp.28367
121. Rouas R, Merimi M, Najar M, El Zein N, Fayyad-Kazan M, Berehab M, et al. Human CD8(+) CD25 (+) CD127 (low) regulatory T cells: microRNA signature and impact on TGF-β and IL-10 expression. J Cell Physiol (2019) 234:17459–72. doi: 10.1002/jcp.28367
122. Zhang Y, Wang ZC, Zhang ZS, Chen F. MicroRNA-155 regulates cervical cancer via inducing Th17/Treg imbalance. Eur Rev Med Pharmacol Sci (2018) 22:3719–26. doi: 10.1158/1535-7163.MCT-14-0448
123. Lv M, Xu Y, Tang R, Ren J, Shen S, Chen Y, et al. miR141-CXCL1-CXCR2 signaling-induced Treg recruitment regulates metastases and survival of non-small cell lung cancer. Mol Cancer Ther (2014) 13:3152–62. doi: 10.1093/intimm/dxt065
124. Li W, Kong LB, Li JT, Guo ZY, Xue Q, Yang T, et al. MiR-568 inhibits the activation and function of CD4+ T cells and Treg cells by targeting NFAT5. Int Immunol (2014) 26:269–81. doi: 10.1038/ncomms15129
125. Jiang R, Tang J, Chen Y, Deng L, Ji J, Xie Y, et al. The long noncoding RNA lnc-EGFR stimulates T-regulatory cells differentiation thus promoting hepatocellular carcinoma immune evasion. Nat Commun (2017) 8:15129. doi: 10.3389/fimmu.2021.782216
126. Sun Q, Li Y, Yang X, Wu X, Liu Z, Mou Y, et al. Identification and validation of 17-lncRNA related to regulatory T cell heterogeneity as a prognostic signature for head and neck squamous cell carcinoma. Front Immunol (2021) 12:782216. doi: 10.3389/fonc.2021.666064
127. Ma E, Hou S, Wang Y, Xu X, Wang Z, Zhao J. Identification and validation of an immune-related lncRNA signature to facilitate survival prediction in gastric cancer. Front Oncol (2021) 11:666064. doi: 10.3389/fcell.2021.748442
128. Yu X, Dong P, Yan Y, Liu F, Wang H, Lv Y, et al. Identification of N6-methyladenosine-associated long non-coding RNAs for immunotherapeutic response and prognosis in patients with pancreatic cancer. Front Cell Dev Biol (2021) 9:748442. doi: 10.1155/2021/9798231
129. Yuan M, Wang Y, Sun Q, Liu S, Xian S, Dai F, et al. Identification of a nine immune-related lncRNA signature as a novel diagnostic biomarker for hepatocellular carcinoma. BioMed Res Int (2021) 2021:9798231. doi: 10.3389/fonc.2021.715677
130. Dezfuli NK, Alipoor SD, Dalil Roofchayee N, Seyfi S, Salimi B, Adcock IM, et al. Evaluation Expression of miR-146a and miR-155 in Non-Small-Cell Lung Cancer Patients. Front Oncol (2021) 11:715677. doi: 10.1002/jcla.24125
131. Samiei H, Ajam F, Gharavi A, Abdolmaleki S, Kokhaei P, Mohammadi S, et al. Simultaneous disruption of circulating miR-21 and cytotoxic T lymphocytes (CTLs): Prospective diagnostic and prognostic markers for esophageal squamous cell carcinoma (ESCC). J Clin Lab Anal (2022) 36:e24125. doi: 10.1371/journal.pone.0229577
132. Takashima Y, Kawaguchi A, Iwadate Y, Hondoh H, Fukai J, Kajiwara K, et al. miR-101, miR-548b, miR-554, and miR-1202 are reliable prognosis predictors of the miRNAs associated with cancer immunity in primary central nervous system lymphoma. PloS One (2020) 15:e0229577. doi: 10.1016/j.cbi.2021.109652
133. Xia Y, Wang WC, Shen WH, Xu K, Hu YY, Han GH, et al. Thalidomide suppresses angiogenesis and immune evasion via lncRNA FGD5-AS1/miR-454-3p/ZEB1 axis-mediated VEGFA expression and PD-1/PD-L1 checkpoint in NSCLC. Chem Biol Interact (2021) 349:109652. doi: 10.1186/s12943-020-01222-5
134. Zhang PF, Gao C, Huang XY, Lu JC, Guo XJ, Shi GM, et al. Cancer cell-derived exosomal circUHRF1 induces natural killer cell exhaustion and may cause resistance to anti-PD1 therapy in hepatocellular carcinoma. Mol Cancer (2020) 19:110. doi: 10.1158/2326-6066.CIR-18-0419
135. Ahmetlić F, Riedel T, Hömberg N, Bauer V, Trautwein N, Geishauser A, et al. Regulatory T cells in an endogenous mouse lymphoma recognize specific antigen peptides and contribute to immune escape. Cancer Immunol Res (2019) 7:600–8. doi: 10.1158/0008-5472.CAN-19-1577
136. Long Y, Tao H, Karachi A, Grippin AJ, Jin L, Chang YE, et al. Dysregulation of glutamate transport enhances treg function that promotes VEGF blockade resistance in glioblastoma. Cancer Res (2020) 80:499–509. doi: 10.4049/jimmunol.1700433
137. Carmenate T, Ortíz Y, Enamorado M, García-Martínez K, Avellanet J, Moreno E, et al. Blocking IL-2 signal in vivo with an IL-2 antagonist reduces tumor growth through the control of regulatory T cells. J Immunol (2018) 200:3475–84. doi: 10.1158/0008-5472.CAN-10-0283
138. Zhao J, Cao Y, Lei Z, Yang Z, Zhang B, Huang B. Selective depletion of CD4+CD25+Foxp3+ regulatory T cells by low-dose cyclophosphamide is explained by reduced intracellular ATP levels. Cancer Res (2010) 70:4850–8. doi: 10.1016/j.biopha.2016.03.013
139. Wang X, Zhao F, Shi F, He X, Pan M, Wu D, et al. Reinforcing B16F10/GPI-IL-21 vaccine efficacy against melanoma by injecting mice with shZEB1 plasmid or miR200c agomir. BioMed Pharmacother (2016) 80:136–44. doi: 10.1158/0008-5472.CAN-18-0365
140. Shabaneh TB, Molodtsov AK, Steinberg SM, Zhang P, Torres GM, Mohamed GA, et al. Oncogenic BRAF(V600E) governs regulatory T-cell recruitment during melanoma tumorigenesis. Cancer Res (2018) 78:5038–49. doi: 10.1186/s40425-019-0701-2
141. Wang D, Yang L, Yu W, Wu Q, Lian J, Li F, et al. Colorectal cancer cell-derived CCL20 recruits regulatory T cells to promote chemoresistance via FOXO1/CEBPB/NF-κB signaling. J Immunother Cancer (2019) 7:215. doi: 10.1016/j.canlet.2019.10.032
142. Wang R, Feng W, Wang H, Wang L, Yang X, Yang F, et al. Blocking migration of regulatory T cells to leukemic hematopoietic microenvironment delays disease progression in mouse leukemia model. Cancer Lett (2020) 469:151–61. doi: 10.1016/j.canlet.2019.10.032
143. Liao C, Xiao W, Zhu N, Liu Z, Yang J, Wang Y, et al. Radiotherapy suppressed tumor-specific recruitment of regulator T cells via up-regulating microR-545 in Lewis lung carcinoma cells. Int J Clin Exp Pathol (2015) 8:2535–44. doi: 10.4049/jimmunol.1203567
144. De Kouchkovsky D, Esensten JH, Rosenthal WL, Morar MM, Bluestone JA, Jeker LT. microRNA-17-92 regulates IL-10 production by regulatory T cells and control of experimental autoimmune encephalomyelitis. J Immunol (2013) 191:1594–605. doi: 10.1002/ddr.21459
145. Fang T, Li J, Wu X. Shenmai injection improves the postoperative immune function of papillary thyroid carcinoma patients by inhibiting differentiation into Treg cells via miR-103/GPER1 axis. Drug Dev Res (2018) 79:324–31. doi: 10.1186/s12967-015-0465-5
146. Li A, Shuai X, Jia Z, Li H, Liang X, Su D, et al. Ganoderma lucidum polysaccharide extract inhibits hepatocellular carcinoma growth by downregulating regulatory T cells accumulation and function by inducing microRNA-125b. J Transl Med (2015) 13:100. doi: 10.1186/s13073-019-0620-3
147. Shrestha R, Nabavi N, Lin YY, Mo F, Anderson S, Volik S, et al. BAP1 haploinsufficiency predicts a distinct immunogenic class of Malignant peritoneal mesothelioma. Genome Med (2019) 11:8. doi: 10.1001/jamaoncol.2019.2244
148. Uldrick TS, Gonçalves PH, Abdul-Hay M, Claeys AJ, Emu B, Ernstoff MS, et al. Assessment of the safety of pembrolizumab in patients with HIV and advanced cancer-A phase 1 study. JAMA Oncol (2019) 5:1332–9. doi: 10.1186/s40425-019-0785-8
149. Kim HR, Park HJ, Son J, Lee JG, Chung KY, Cho NH, et al. Tumor microenvironment dictates regulatory T cell phenotype: Upregulated immune checkpoints reinforce suppressive function. J Immunother Cancer (2019) 7:339. doi: 10.1182/blood-2017-06-741033
150. Rowshanravan B, Halliday N, Sansom DM. CTLA-4: a moving target in immunotherapy. Blood (2018) 131:58–67. doi: 10.1182/blood-2017-06-741033
151. Tekguc M, Wing JB, Osaki M, Long J, Sakaguchi S. Treg-expressed CTLA-4 depletes CD80/CD86 by trogocytosis, releasing free PD-L1 on antigen-presenting cells. Proc Natl Acad Sci U.S.A. (2021) 118:e2023739118. doi: 10.1016/j.ccell.2018.02.010
152. Arce Vargas F, Furness AJS, Litchfield K, Joshi K, Rosenthal R, Ghorani E, et al. Fc effector function contributes to the activity of human anti-CTLA-4 antibodies. Cancer Cell (2018) 33:649–663.e644. doi: 10.1080/2162402X.2017.1387705
153. Cari L, Nocentini G, Migliorati G, Riccardi C. Potential effect of tumor-specific Treg-targeted antibodies in the treatment of human cancers: A bioinformatics analysis. Oncoimmunology (2018) 7:e1387705. doi: 10.1080/2162402X.2017.1387705
154. Ha D, Tanaka A, Kibayashi T, Tanemura A, Sugiyama D, Wing JB, et al. Differential control of human Treg and effector T cells in tumor immunity by Fc-engineered anti-CTLA-4 antibody. Proc Natl Acad Sci U.S.A. (2019) 116:609–18. doi: 10.1158/2326-6066.CIR-18-0386
155. Sanseviero E, O'brien EM, Karras JR, Shabaneh TB, Aksoy BA, Xu W, et al. Anti-CTLA-4 activates intratumoral NK cells and combined with IL15/IL15Rα Complexes enhances tumor control. Cancer Immunol Res (2019) 7:1371–80. doi: 10.1056/NEJMoa1910836
156. Larkin J, Chiarion-Sileni V, Gonzalez R, Grob JJ, Rutkowski P, Lao CD, et al. Five-year survival with combined nivolumab and ipilimumab in advanced melanoma. N Engl J Med (2019) 381:1535–46. doi: 10.1038/s41422-019-0184-1
157. Zhang Y, Du X, Liu M, Tang F, Zhang P, Ai C, et al. Hijacking antibody-induced CTLA-4 lysosomal degradation for safer and more effective cancer immunotherapy. Cell Res (2019) 29:609–27. doi: 10.1186/s40425-019-0570-8
158. Kvarnhammar AM, Veitonmäki N, Hägerbrand K, Dahlman A, Smith KE, Fritzell S, et al. The CTLA-4 x OX40 bispecific antibody ATOR-1015 induces anti-tumor effects through tumor-directed immune activation. J Immunother Cancer (2019) 7:103. doi: 10.1002/eji.201242590
159. Miska J, Bas E, Devarajan P, Chen Z. Autoimmunity-mediated antitumor immunity: tumor as an immunoprivileged self. Eur J Immunol (2012) 42:2584–96. doi: 10.18632/aging.102197
160. Yang J, Zhang Y, Song H. A disparate role of RP11-424C20.2/UHRF1 axis through control of tumor immune escape in liver hepatocellular carcinoma and thymoma. Aging (Albany NY) (2019) 11:6422–39. doi: 10.1126/science.aaf1292
161. Hui E, Cheung J, Zhu J, Su X, Taylor MJ, Wallweber HA, et al. T cell costimulatory receptor CD28 is a primary target for PD-1-mediated inhibition. Science (2017) 355:1428–33. doi: 10.1016/j.immuni.2019.01.001
162. Miragaia RJ, Gomes T, Chomka A, Jardine L, Riedel A, Hegazy AN, et al. Single-cell transcriptomics of regulatory T cells reveals trajectories of tissue adaptation. Immunity (2019) 50:493–504.e497. doi: 10.1038/ni.3868
163. Maj T, Wang W, Crespo J, Zhang H, Wang W, Wei S, et al. Oxidative stress controls regulatory T cell apoptosis and suppressor activity and PD-L1-blockade resistance in tumor. Nat Immunol (2017) 18:1332–41. doi: 10.1038/s41571-021-00473-5
164. Doroshow DB, Bhalla S, Beasley MB, Sholl LM, Kerr KM, Gnjatic S, et al. PD-L1 as a biomarker of response to immune-checkpoint inhibitors. Nat Rev Clin Oncol (2021) 18:345–62. doi: 10.1080/21655979.2022.2031408
165. Li Y, Zhou T, Cheng X, Li D, Zhao M, Zheng WV. microRNA-378a-3p regulates the progression of hepatocellular carcinoma by regulating PD-L1 and STAT3. Bioengineered (2022) 13:4730–43. doi: 10.1016/j.cellsig.2021.110095
166. Xu YJ, Zhao JM, Gao C, Ni XF, Wang W, Hu WW, et al. Hsa_circ_0136666 activates Treg-mediated immune escape of colorectal cancer via miR-497/PD-L1 pathway. Cell Signal (2021) 86:110095. doi: 10.1038/ni.1674
167. Yu X, Harden K, Gonzalez LC, Francesco M, Chiang E, Irving B, et al. The surface protein TIGIT suppresses T cell activation by promoting the generation of mature immunoregulatory dendritic cells. Nat Immunol (2009) 10:48–57. doi: 10.4049/jimmunol.1401803
168. Bin Dhuban K, D'hennezel E, Nashi E, Bar-Or A, Rieder S, Shevach EM, et al. Coexpression of TIGIT and FCRL3 identifies Helios+ human memory regulatory T cells. J Immunol (2015) 194:3687–96. doi: 10.4049/jimmunol.1402381
169. Fuhrman CA, Yeh WI, Seay HR, Saikumar Lakshmi P, Chopra G, Zhang L, et al. Divergent phenotypes of human regulatory T cells expressing the receptors TIGIT and CD226. J Immunol (2015) 195:145–55. doi: 10.4049/jimmunol.1103627
170. Lozano E, Dominguez-Villar M, Kuchroo V, Hafler DA. The TIGIT/CD226 axis regulates human T cell function. J Immunol (2012) 188:3869–75. doi: 10.1016/j.immuni.2016.10.021
171. De Simone M, Arrigoni A, Rossetti G, Gruarin P, Ranzani V, Politano C, et al. Transcriptional landscape of human tissue lymphocytes unveils uniqueness of tumor-infiltrating T regulatory cells. Immunity (2016) 45:1135–47. doi: 10.1016/j.cell.2017.05.035
172. Zheng C, Zheng L, Yoo JK, Guo H, Zhang Y, Guo X, et al. Landscape of infiltrating T cells in liver cancer revealed by single-cell sequencing. Cell (2017) 169:1342–1356.e1316. doi: 10.1016/j.immuni.2004.08.010
173. Huang CT, Workman CJ, Flies D, Pan X, Marson AL, Zhou G, et al. Role of LAG-3 in regulatory T cells. Immunity (2004) 21:503–13. doi: 10.1073/pnas.1810580115
174. Magnuson AM, Kiner E, Ergun A, Park JS, Asinovski N, Ortiz-Lopez A, et al. Identification and validation of a tumor-infiltrating Treg transcriptional signature conserved across species and tumor types. Proc Natl Acad Sci U.S.A. (2018) 115:E10672–e10681. doi: 10.1126/science.1176077
175. Pan F, Yu H, Dang EV, Barbi J, Pan X, Grosso JF, et al. Eos mediates Foxp3-dependent gene silencing in CD4+ regulatory T cells. Science (2009) 325:1142–6. doi: 10.1016/j.immuni.2013.01.013
176. Sharma MD, Huang L, Choi JH, Lee EJ, Wilson JM, Lemos H, et al. An inherently bifunctional subset of Foxp3+ T helper cells is controlled by the transcription factor eos. Immunity (2013) 38:998–1012. doi: 10.4049/jimmunol.0903879
177. Camisaschi C, Casati C, Rini F, Perego M, De Filippo A, Triebel F, et al. LAG-3 expression defines a subset of CD4(+)CD25(high)Foxp3(+) regulatory T cells that are expanded at tumor sites. J Immunol (2010) 184:6545–51. doi: 10.1038/s41577-019-0224-6
178. Wolf Y, Anderson AC, Kuchroo VK. TIM3 comes of age as an inhibitory receptor. Nat Rev Immunol (2020) 20:173–85. doi: 10.1371/journal.pone.0058006
179. Yan J, Zhang Y, Zhang JP, Liang J, Li L, Zheng L. Tim-3 expression defines regulatory T cells in human tumors. PloS One (2013) 8:e58006. doi: 10.4161/onci.23849
180. Sakuishi K, Ngiow SF, Sullivan JM, Teng MW, Kuchroo VK, Smyth MJ, et al. TIM3(+)FOXP3(+) regulatory T cells are tissue-specific promoters of T-cell dysfunction in cancer. Oncoimmunology (2013) 2:e23849. doi: 10.3389/fimmu.2017.01228
181. Roy S, Bag AK, Singh RK, Talmadge JE, Batra SK, Datta K. Multifaceted role of neuropilins in the immune system: potential targets for immunotherapy. Front Immunol (2017) 8:1228. doi: 10.1002/ijc.28135
182. Piechnik A, Dmoszynska A, Omiotek M, Mlak R, Kowal M, Stilgenbauer S, et al. The VEGF receptor, neuropilin-1, represents a promising novel target for chronic lymphocytic leukemia patients. Int J Cancer (2013) 133:1489–96. doi: 10.1007/s00262-008-0646-7
183. Battaglia A, Buzzonetti A, Baranello C, Ferrandina G, Martinelli E, Fanfani F, et al. Metastatic tumour cells favour the generation of a tolerogenic milieu in tumour draining lymph node in patients with early cervical cancer. Cancer Immunol Immunother (2009) 58:1363–73. doi: 10.1517/14728222.2014.977784
184. Chaudhary B, Elkord E. Novel expression of Neuropilin 1 on human tumor-infiltrating lymphocytes in colorectal cancer liver metastases. Expert Opin Ther Targets (2015) 19:147–61. doi: 10.1038/s41598-021-95213-1
Keywords: Tregs, miRNA, lncRNA, immunotherapy, cancer
Citation: Ma Y, Xu X, Wang H, Liu Y and Piao H (2023) Non-coding RNA in tumor-infiltrating regulatory T cells formation and associated immunotherapy. Front. Immunol. 14:1228331. doi: 10.3389/fimmu.2023.1228331
Received: 24 May 2023; Accepted: 28 July 2023;
Published: 21 August 2023.
Edited by:
Gary S. Stein, University of Vermont, United StatesReviewed by:
Chuan Lan, the Affiliated Hospital of North Sichuan Medical College, ChinaCheng Fang, Sun Yat-sen University Cancer Center (SYSUCC), China
Luke Bu, National Institutes of Biomedical Innovation, Health and Nutrition, Japan
Copyright © 2023 Ma, Xu, Wang, Liu and Piao. This is an open-access article distributed under the terms of the Creative Commons Attribution License (CC BY). The use, distribution or reproduction in other forums is permitted, provided the original author(s) and the copyright owner(s) are credited and that the original publication in this journal is cited, in accordance with accepted academic practice. No use, distribution or reproduction is permitted which does not comply with these terms.
*Correspondence: Haiyan Piao, cGlhb2hhaXlhbjE5ODFAMTYzLmNvbQ==
†These authors have contributed equally to this work
‡ORCID: Yue Ma, orcid.org/0000-0001-8115-5204
Yang Liu, orcid.org/0000-0002-2129-9086
Haiyan Piao, orcid.org/0000-0002-3039-5758