- 1Institute of Medical Sciences, University of Aberdeen, Aberdeen, United Kingdom
- 2Department of Ophthalmology, Beijing Hospital, National Center of Gerontology, Beijing, China
- 3Department of Ophthalmology and Visual Science, Graduate School of Medical and Dental Sciences, Tokyo Medical and Dental University, Tokyo, Japan
- 4Nuffield Department of Medicine, University of Oxford, Oxford, United Kingdom
- 5Eye Clinic, Aberdeen Royal Infirmary, Aberdeen, United Kingdom
Restoration of immunological tolerance to self antigens has been a major drive in understanding the mechanisms of, and developing new treatments for, autoimmune and autoinflammatory disease. Sessile dendritic cells (DC) are considered the main instruments underpinning immunological tolerance particularly the CD205+ (DEC205+) cDC1 subset in contrast to DCIR2+ cDC2 which mediate immunogenicity. Targeting DC using autoantigen peptide-antibody fusion proteins has been a well explored methodology for inducing tolerance. Here we show that subcutaneous (s.c.) inoculation of hen-egg lysozyme (HEL)-DEC205 Ig fusion prevents the development of spontaneous uveoretinitis (experimental autoimmune uveoretinitis, EAU) in a transgenic mouse model generated by crossing interphotoreceptor retinol binding protein (IRBP)-HEL (sTg HEL) with HEL specific TCR (sTg TCR) mice. Prolonged suppression of EAU required injections of HEL-DEC205 Ig once weekly, reflecting the half life of s.c. DC. Interestingly, HEL-DCIR2 Ig also had a suppressive effect on development of EAU but less so than DEC205 Ig while it had minimal effect on preventing the retinal atrophy associated with EAU. In addition, HEL-DEC205 Ig was only effective when administered s.c. rather than systemically and had no effect on EAU induced by adoptive transfer of HEL-activated T cells. These data demonstrate the importance of systemic (lymph node) rather than local (eye) antigen presentation in the development of EAU as well as suggest a potential therapeutic approach to controlling sight-threatening immune-mediated uveitis provided relevant antigen(s) can be identified.
Introduction
Uveitis is a major source of visual impairment accounting for up to 10% of all causes of blindness (1, 2). Infectious agents account for a significant proportion of cases but in a majority of patients no specific cause can be identified (3). Such cases are currently described as “undifferentiated” uveitis (4) although latent or persistent infection may be lurking undetected (5). Autoimmune/autoinflammatory processes are considered to play a role in many cases of undifferentiated uveitis, concepts based on experimental models of uveitis induced by retina-specific antigens (6). This has been supported by the generation of a transgenic T cell receptor (TCR) mouse model of spontaneous experimental autoimmune uveitis/uveoretinitis (EAU), in which ~30% of pathogenic T cells are specific for the retinal protein interphotoreceptor retinol binding protein (IRBP), underscoring a role for an autoimmune pathogenesis in this disease (7).
We have developed a model of spontaneous EAU, in which the neoantigen hen egg lysozyme (HEL) is expressed in the retina under control of the IRBP promoter (8). When single transgenic IRBP-HEL mice (sTg HEL mice) are crossed to mice expressing a transgenic T cell receptor (TCR) for HEL (sTg TCR mice), double transgenic mice (dTg IRBP : HEL TCR mice, hereafter termed dTg mice) spontaneously develop EAU with onset around post-natal day (P)20/21 and with 100% incidence. Inflammation progressively worsens to reach a peak at ~P30 and eventually leads to total retinal destruction, with phthisis bulbi (ocular shrinkage) occurring in some mice (9). The disease thus resembles the most severe forms of chronic, progressive undifferentiated uveitis in humans (10, 11).
Restoration of self-tolerance is the central therapeutic aim in autoimmune disease. In health, steady-state dendritic cells (DC) promote tolerance through a variety of mechanisms including deletion and anergy of autoreactive T cells but predominantly by maintaining the peripheral pool of T regulatory cells (Tregs) [reviewed in refs (12, 13)]. Since DC’s were shown to have specific cell surface molecules which reflected their functional state, attempts were made to modify the role of DC either for immunogenicity (DC vaccine) or for tolerance induction (14). Initially DEC205, a C-type lectin endocytic receptor, was considered to promote tolerance while delivery of antigens via a second molecule, DC inhibitory receptor 2 (DCIR2), was considered immunogenic and therefore more suitable to induce immune responses against pathogens and tumours. However, these distinctions became blurred as both molecules as well as other surface receptors, were found to have differential effects depending on the microenvironmental context.
Recent developments in DC biology and classification show that homeostatic immunological self-tolerance is sustained particularly by a subset of conventional DC (cDC1) which express CD11c, CXCR1 and DEC205 surface receptors [reviewed in ref (15)]. DEC205, has been shown to mediate tolerogenic properties of DC in the absence of micro-environmental challenge by acting as a scavenging receptor for apoptotic and necrotic cells (16, 17). However, DEC205+ DC may also participate in immunogenic/inflammatory responses indicating that the context of antigen uptake and presentation is important in determining the final immune outcome e.g. to foreign antigens (18).
DEC205 is one of a set of DC surface molecules including DCIR2, Langerin, CD11b, CD11c, CD47 and CD40 which have been used as fusion proteins to deliver antigens to DC. While most of these proteins have been delivered with aim of inducing enhanced immune responses in vaccination protocols e.g. against HIV and Leishmaniasis (19, 20), the use of DEC205 antibody, or short chain antibody fragments, fused to “self” antigens, in the absence of adjuvant or other proinflammatory stimuli, has been investigated for its potential to induce antigen-specific immunological tolerance for more than 15 years [reviewed in ref (21)]. Preclinical examples include models of diabetes (22), experimental autoimmune encephalomyelitis (23), experimental colitis (24), and arthritis (25). However, previous studies have indicated that inhibition using chimeric anti-DEC205 might not be sufficient to inhibit ongoing autoimmune responses (26) or chronic disease (27) (Table 1).
Reuter et al. (31) have specifically investigated the kinetics of antigen uptake using a range of antigen-antibody fusion proteins. They have shown that DEC205 is most efficiently targeted for antigen delivery in steady state cDC1, CD8α+DC. In contrast, in activated cDC1, CD8α+DC, and in mature cDC2, CD8α-DC where DEC 205 is upregulated, antigen-delivery is 50% less efficient (32). Thus the nature of DC and its surface receptor as well as the context in which it is targeted by an antigen-antibody fusion protein determine the immunological outcomes of tolerogenicity vs immunogenicity.
We have tested fusion proteins which combine HEL with antibodies to DC surface molecules, namely DEC205 (cDC1) and DCIR2 (cDC2) in dTg mice prior to the onset of EAU (P18). We show that weekly subcutaneous (s.c.) administration of the DEC205-HEL fusion protein completely abrogates development of EAU while DCIR2-HEL fusion protein is less effective but still significantly inhibitory. Both the inflammatory changes and the associated retinal degeneration (chorioretinal atrophy) are markedly reduced by treatment with DEC205-HEL fusion protein while DCIR2-HEL fusion protein is less effective in suppressing both inflammation and atrophy.
Materials and methods
Animals and EAU model
B10BR (H-2K) wild type and Tg mice were bred in established breeding colonies and housed in the Medical Research Facility, University of Aberdeen. Littermate male and female mice of different ages were used in the experiments. Generation of dTg mice was as described previously (8). sTg HEL mice expressing HEL antigen under the retina-specific IRBP promoter were crossed with sTg TCR (3A9) mice which have >70% HEL-specific peripheral CD4+ T cells. The dTg offspring spontaneously develop EAU at P20/21 with 100% incidence. Genotyping of the experimental mice was performed using standard in-house PCR procedures (9). Appropriate genotype and age matched mice were used in experiments as specified. All animal work was performed in accordance with guidelines of the Association for Research in Vision and Ophthalmology (ARVO) statement for the use of animals in ophthalmic and vision research and the regulations of the Animal License Act 2006 (amended 2019) (United Kingdom).
Preparation of fusion proteins
Plasmids containing the DNA constructs for DEC205-HEL, DCIR2-HEL, DEC205-OVA and DEC2O5-IgG isotype were obtained from R.M. Steinman and Michel Nussenzweig, Rockefeller University, NY, USA. Fusion proteins were prepared according to the protocol described previously (33). Briefly, both heavy chain and light chain antibody DNA was transfected into HEK293T cells with calcium-phosphate. Cells were grown in complete DMEM and incubated overnight until the next morning when cells were washed with PBS and cultured in complete DMEM for 4 days. Medium containing the hybrid antibodies was then spun down, filtered and kept on ice. Antibodies were purified on Protein G columns (Genescript, Piscataway, NJ, USA; cat. number L00209), and concentrated using Amicon Ultra-15 Centrifugal filter units (10K) (Millipore, UK; cat. number UFC9 010 008). The concentrations of purified antibodies were determined using Nanodrop, and Ig heavy chains fused to HEL peptide were verified by Western blotting before being injected into mice. Rabbit anti-mouse polyclonal antibodies were used for immunoblotting: anti-ovalbumin antibody (Abcam, UK; cat. number ab181688, 1:500 dilution), anti-lysozyme antibody (Rockland, CA, USA; cat number USA 200-401-072, 1:500 dilution) and secondary antibody Alexa Fluor 680 goat anti-rabbit IgG (H+L) (Invitrogen, UK 1:10000 dilution). The membranes were imaged with Odyssey Infrared Imaging System (Licor Biosciences, UK).
Treatment of dTg mice with fusion proteins
Fusion proteins (DEC205-HEL or DCIR2-HEL; 5µg) were inoculated into dTg mice s.c. into the nape of the neck either as a single dose on P18, or in 6 doses given in weekly intervals (P18, P25, P32, P39, P46, P53), or to assess treatment of ongoing disease in 5 doses (P25, P32, P39, P46, P53). Additional control groups included PBS injection, Ig isotype and DEC205-OVA fusion protein. The development and severity of uveitis was monitored using an otoscope-based fundus imaging system (see below) at the following time points: P21, P30, P45, and P60. For some experiments mice were inoculated with 7 doses of fusion protein (P18, P25, P32, P39, P46, P53, P60) and were examined at P50, P70, P85, P100 and P115.
Clinical evaluation of EAU
Mice were anaesthetised and fundi imaged using an otoscope-based fiber-optic light device as described previously (9). In brief, anaesthesia was induced with an intraperitoneal injection of a mixture of 40 mg/kg Vetalar® (Fort Dodge Animal Health Ltd., Southampton, UK) and 0.2 - 1.0 mg/kg Domitor® (Orion Pharma, Espoo, Finland) diluted in injectable water. Pupils were dilated with Minims 1% (w/v) tropicamide, and 2.5% (w/v) phenylephrine hydrochloride (both from Chauvin Pharmaceuticals Ltd, UK). Viscotears Carbomer 2 mg/g liquid gel (Alcon Eyecare UK Ltd., Camberley, UK) was used throughout the procedure as lubrication to prevent corneal drying and lens opacification. Images of the central and peripheral retina were taken. EAU severity was evaluated separately in terms of inflammation and chorioretinal atrophy using a scoring system as previously described (9, 34).
Histology
Mice (P60) were sacrificed and eyes removed immediately. Eyes were fixed in 2.5% (w/v) glutaraldehyde (Sigma, UK), embedded in resin and processed for standard hematoxylin and eosin (H&E) staining.
Flow cytometry
Single cell suspensions were prepared from lymph nodes and retinas for flow cytometry analysis. The eye-draining submandibular and skin-draining superficial cervical lymph nodes were collected for specific experiments. Lymph nodes were passed gently through a 70μm cell strainer. Intact, whole retinas were dissected from eye-cups after removing the anterior segment, lens and vitreous. Then retinas were digested in 1 ml PBS containing a final concentration of 10 μg/ml Liberase and 10 μg/ml DNase I (both from Roche, Mannheim, Germany) for 40 min at 37°C. The dissociated lymph node and retina cells were then washed and re-suspended in PBS containing 2% FBS. For the exclusion of dead cells, all samples were stained with Fixable Viability Dye eFluor455 according to manufacturer’s instruction (eBioscience, Hatfield, UK). The cells were then incubated with Fc block CD16/32 (clone 2.4G2) antibody for 10 min at 4°C, followed by surface staining of directly conjugated anti-CD4 (clone GK1.5) APC-Cy7 and anti-CD25 (clone PC61) PE antibodies (both from BD Biosciences, Oxford, UK) for 30 min at 4°C. For the staining of intracellular transcription factors, cells were incubated with Cytofix/Cytoperm (BD Biosciences, Oxford, UK) for 20 min at 4°C followed by staining with directly conjugated antibodies to FoxP3 (clone FJK16S) APC, T-bet (clone 4B10) PE-Cy7 (both from eBioscience, Hatfield, UK) and RORγt PerCp-Cy5.5 (clone Q31-378, BD Biosciences, Oxford, UK) for 30 min at 4°C. For intracellular cytokine staining, dissociated cells were first incubated in RPMI containing 10% FBS, 50ng/ml phorbol 12-myristate-13-acetate, 1μM ionomycin (both from Sigma-Aldrich, St. Louis, MO, USA) and monensin (BD GolgiStopTM, BD Biosciences, UK) for 5h at 5% CO2 and 37°C. The cells were then washed and stained with Fixable Viability Dye eFluor455 followed by incubation with Fc-receptor block CD16/32 and then surface staining with CD4 (clone GK1.5) APC-Cy7. Next, BD Cytofix/Cytoperm was used for fixation before staining of intracellular cytokines using directly conjugated anti-mouse IFNγ (clone XMG1.2) APC and IL-17A (clone TC11-18H10) PE (both from BD Biosciences, Oxford, UK). All flow cytometry experiments were acquired using a BD LSRII flow cytometer (BD Bioscience, UK) with collection of least 105 events for each sample. Data analysis was performed using FlowJo, LLC for Windows, version 10 (TreeStar Inc., Ashland, OR, USA).
Statistical analysis
Data are presented as mean ± SEM. N is indicated in the figure legends. Statistical analysis was performed using GraphPad Prism 9 (La Jolla, CA, USA). For parametric data, unpaired Student’s t-test was used to compare between two groups. For non-parametric data, differences between all groups were analysed using Kruskal-Wallis test and Mann-Whitney test was performed between specific groups of interest. Statistical significance was considered when p<0.05.
Results
Delivery of specific antigen via DEC 205 prevents spontaneous EAU in dTg mice
As indicated above, EAU in dTg mice develops spontaneously at eye opening (~P21) and progressively worsens to peak of inflammation (Grade 3-4) at P30 (Figures 1A, B). Chorioretinal atrophy accompanies the inflammation but develops more slowly (9, 35, 36). Severe inflammation persists until the entire retina is involved while progressively enlarging areas of chorioretinal atrophy reach a maximum (Grade 3-4) around P60 (Figure 1A). A single subcutaneous (s.c.) administration of DEC205-HEL fusion protein at P25 did not delay the progression of EAU compared to DCIR2-HEL, OVA-HEL or Ig isotype-HEL fusion proteins (data not shown). In contrast, repeat weekly doses (beginning P18) of DEC205-HEL by s.c. inoculation, effectively prevented development of EAU, both in terms of inflammation and chorioretinal atrophy through P60, leaving an essentially normal-appearing posterior segment of the eye compared to the isotype-HEL-treated control group (Figures 1A, B; p=0.0006 for inflammatory score and p=0.0092 for atrophy score at P60). Histological studies confirmed the anti-inflammatory effect of DEC205-HEL fusion protein: whereas PBS-treated dTg mice showed extensive retinal inflammatory cell infiltration and retinal thinning, with granuloma formation and loss of photoreceptors, dTg mice treated with DEC205-HEL fusion protein retained normal retinal morphology (Figure 1C). The isotype-HEL-treated control group also showed inflammatory and atrophic changes although the degree was markedly less severe than in PBS-treated group.
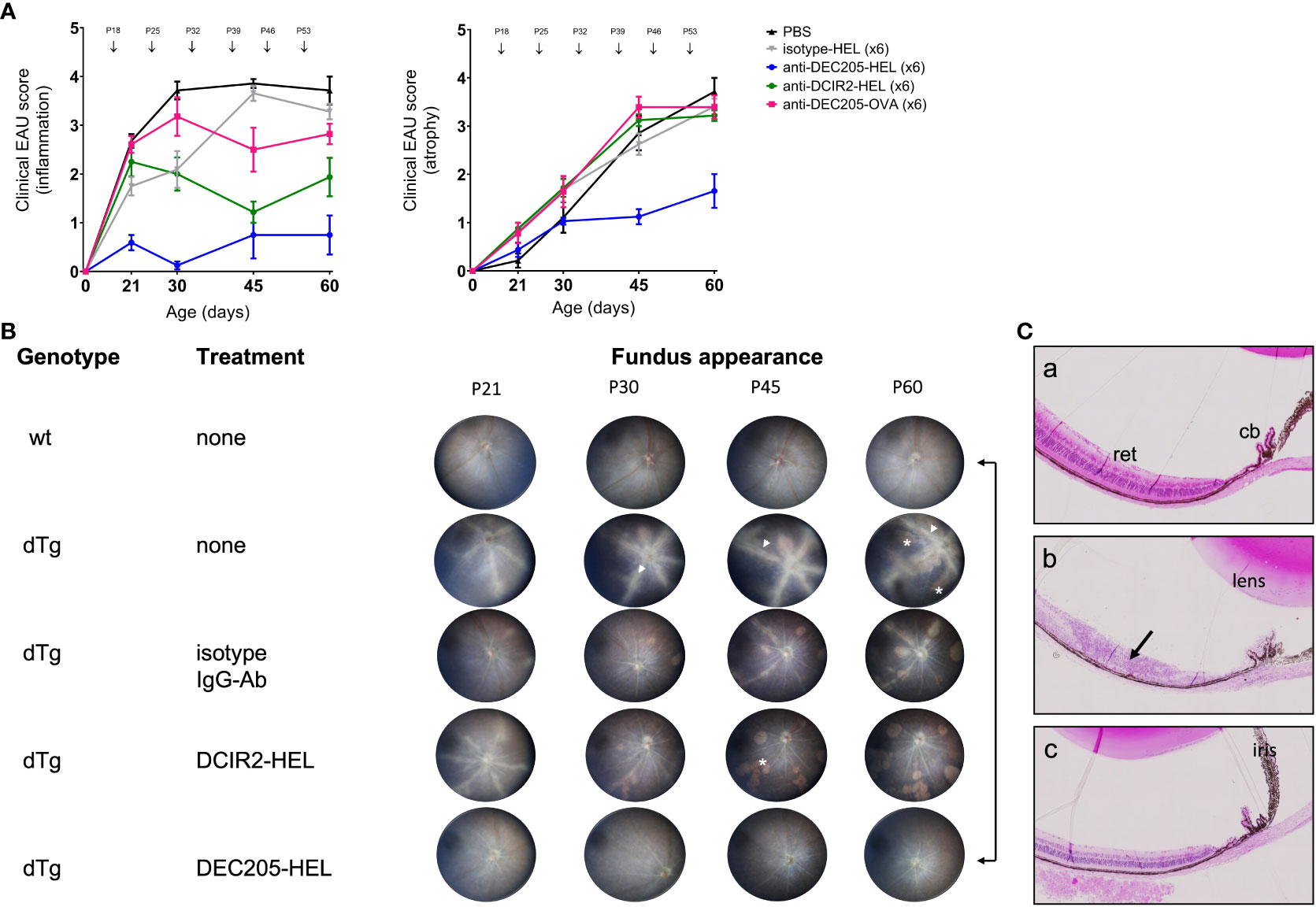
Figure 1 Prevention of Spontaneous Experimental Autoimmune Uveoretinitis (EAU) in HEL-IRBP Double Transgenic mice by Antibody-HEL fusion proteins. (A) Dtg HEL/TCR mice were treated with weekly s.c. injection of DEC205-HEL (), DCIR2-HEL (
), DEC205-OVA (
), isotype-HEL (
) and PBS (
) at postnatal (P) days indicated by arrows. Fundus images were acquired at P21, P30, P45, P60 and graded for inflammation and atrophy. Sample size: DEC205-HEL (N=8), DCIR2-HEL (N=8), DEC205-OVA (N=7), isotype-HEL (N=8) and PBS (N=7). Differences between groups were analysed using Mann-Whitney test. (B) Representative fundus images of healthy wild type mice, untreated dTg mice and dTg mice treated with weekly inoculation of fusion proteins as in Figure 1A and images taken at P21, P30, P45 and P60. Fundus images showing severe vasculitis (arrowhead), and atrophy (asterisk). (C) Histology of eyes at P30: (a) non-Tg normal mouse posterior segment; (b) dTg mouse showing severe retinal damage associated with extensive granulomatous inflammation (arrow); (c) dTg mouse treated x2 with DEC205-HEL fusion protein showing normal retinal morphology. cb: ciliary body; ret: retina.
Weekly injections of comparable doses of DCIR2-HEL fusion protein s.c. also suppressed inflammation but were less effective compared to DEC205-HEL and did not prevent chorioretinal atrophy when compared to isotype control-treated mice (Figure 1A; p=0.0036 for inflammatory score, p=0.4346 for atrophic score vs isotype control at P60; Supplementary Figure 1; Supplementary Tables 1A, B). Furthermore, delivery of HEL itself as an Ig isotype fusion protein had no suppressive effects on inflammation (p=0.0834 for Ig isotype fusion protein-treated mice vs PBS-treated mice). Delivery of an irrelevant antigen, OVA, fused to DEC205 antibody had no significant effects on the progression of disease (p=0.1192 for DEC205-OVA treated group vs isotype control) and also had no effect on chorioretinal atrophy which progressed inexorably (p= 0.9525 for DEC-205-OVA vs isotype control).
The DC inhibitory receptor DCIR2 identified by the 33D1 antibody used here is expressed by a subset of tolerogenic splenic DC’s (37) as well as immunogenic cDC2, CXCR1-ve DC (38). Accordingly, we explored whether delivery of this antibody intraperitoneally (i.p.) to target the spleen might enhance its immunosuppressive effect. However, s.c. delivery of DCIR2-HEL fusion protein was more effective in suppressing spontaneous uveitis than i.p. delivery (Figure 2: P60 inflammatory score s.c. vs isotype control p= 0.0036; P60 inflammatory score i.p. vs isotype control p= 0.5306). Neither route of administration of DCIR2 fusion protein affected progression of chorioretinal atrophy (P60 atrophic score s.c. vs isotype control p= 0.4346; P60 atrophic score i.p. vs isotype control p= 0.9390).
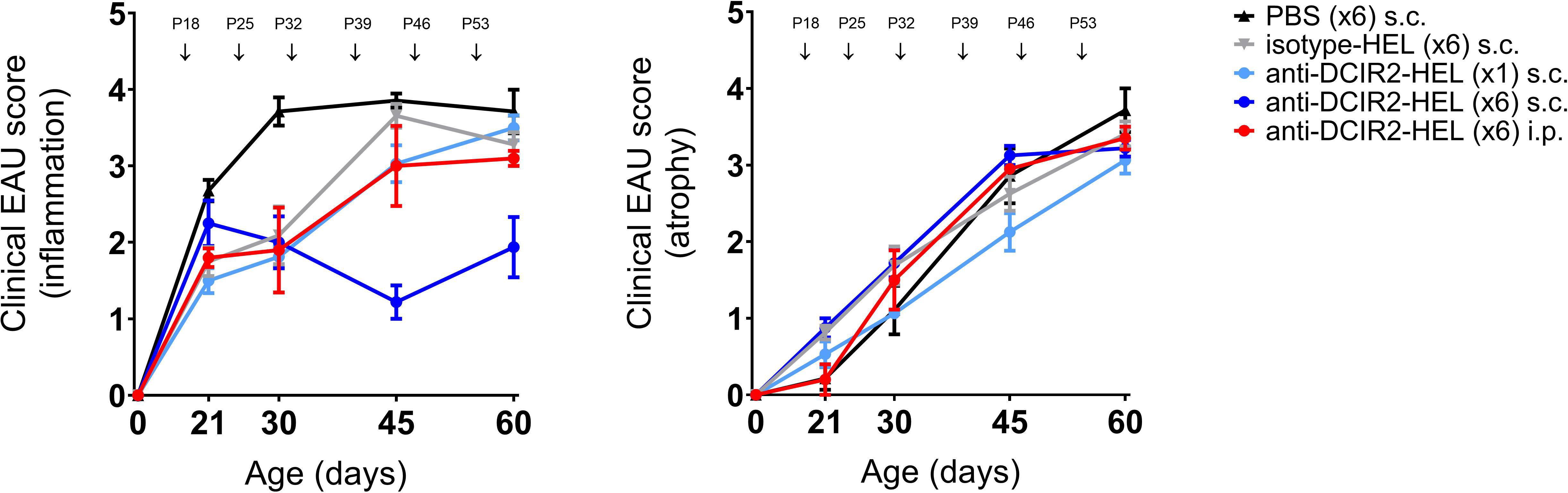
Figure 2 Prevention of spontaneous EAU by s.c. inoculation of DCIR2-HEL fusion protein but not with i.p. administration. Inflammation and atrophy scores of dTg mice treated s.c. with PBS (), IgG isotype-HEL (
), DCIR2-HEL (
) at P18, P25, P32,P39,P46, P53 or with a single s.c. inoculation of DCIR2-HEL (
) at P18 compared to i.p. administration of DCIR2-HEL fusion protein (
) at P18, P25, P32, P39, P46, P53. Weekly administration of DCIR2-HEL s.c. was significantly more efficient in prevention of inflammation compared to i.p delivery (p=0.0167). Sample size: DCIR2-HEL x6 s.c. (N=8), DCIR2-HEL x1 s.c. (N=8), DCIR2-HEL x6 i.p. (N=5), isotype-HEL (N=8) and PBS (N=7). Differences between groups were analysed using Mann-Whitney test.
Administration of weekly doses of DEC205-HEL and DCIR2-HEL fusion proteins, beginning after disease onset (P25), arrested any further development of inflammation (p=0.0061 for DEC205-HEL vs PBS at P60; p=0.0141 for DCIR2-HEL vs PBS at P60) but was only minimally effective in preventing progressive development of chorioretinal atrophy (p=0.0128 for DEC205-HEL vs PBS at P60; p=0.0042 for DCIR2-HEL vs PBS at P60) (Figure 3). In addition, cessation of DEC205-HEL treatment after P60 was accompanied by a recurrence of inflammation, but not to the same degree as in PBS-treated control mice (Figure 4: p=0.0038 for both inflammatory and atrophic score of P115 DEC205-HEL vs P60 PBS; Supplementary Table 3). Furthermore, signs of chorioretinal atrophy began to appear once treatment ceased (Figure 4).
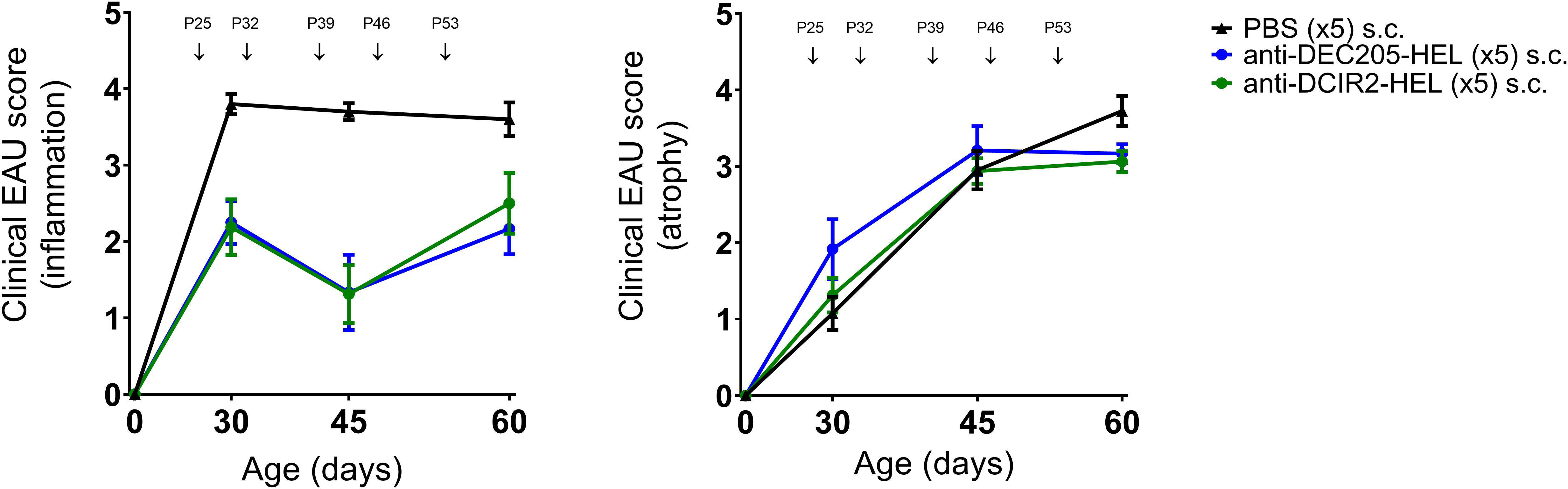
Figure 3 DEC205-HEL and DCIR2-HEL fusion proteins arrest progression of ongoing inflammation but not chorioretinal atrophy. dTg HEL/TCR mice were treated after onset of EAU with weekly s.c. PBS (), DEC205-HEL (
) or DCIR2-HEL (
) fusion proteins at P25, P32, P39, P46, and P53. Fundoscopy was performed at P30, P45, and P60 for evaluation of EAU progression. Differences between groups were analysed using Mann-Whitney test. DEC205-HEL and DCIR2-HEL fusion protein prevented progression of retinal inflammation (p=0.0061 and p=0.0141 respectively) but despite reaching significantly reduced score in comparison to PBS injected group did not prevent development of atrophy (p=0.0128 and p=0.0042 respectively). Sample size: DEC205-HEL (N=6), DCIR2-HEL (N=8), and PBS (N=10).
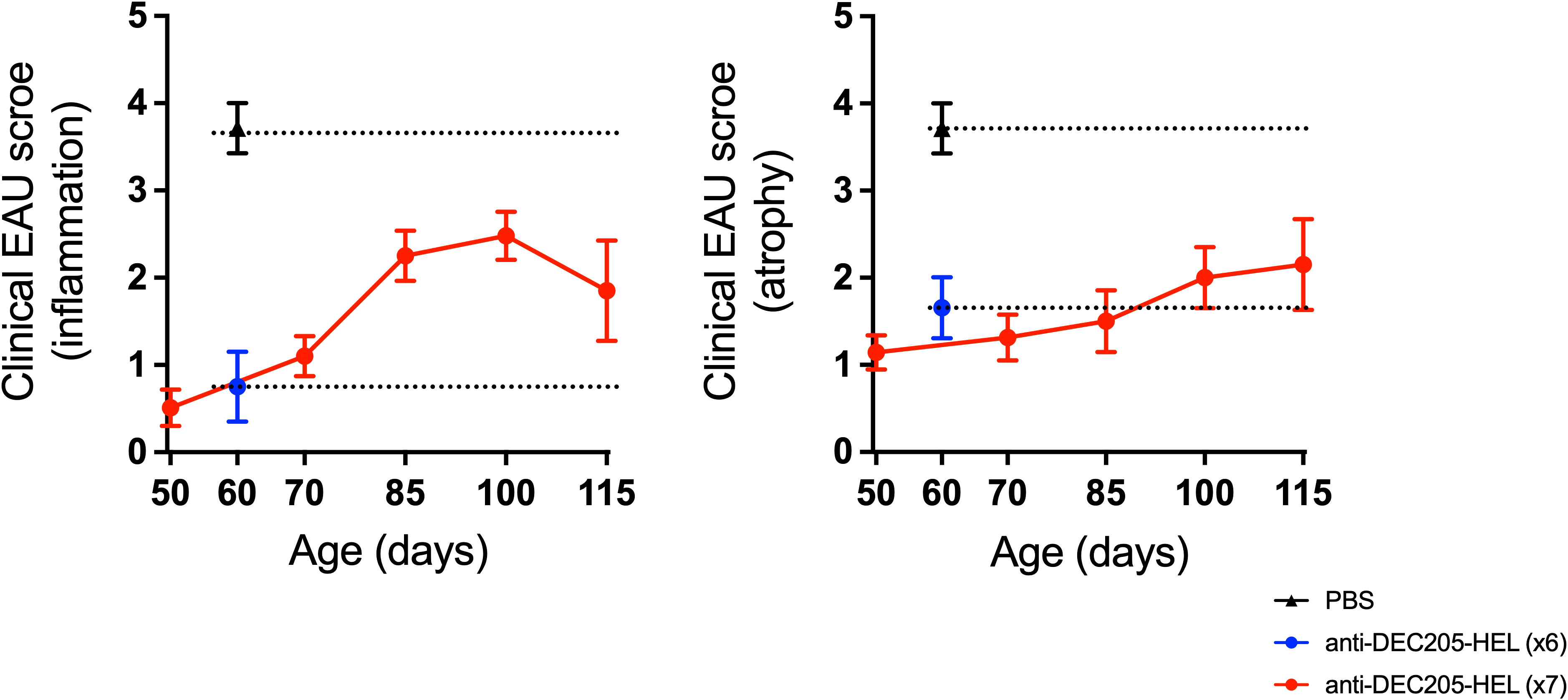
Figure 4 Development of EAU recommenced after cessation of DEC205-HEL fusion protein treatment but was less severe. dTg HEL/TCR mice received repeated weekly treatment of x7 DEC205-HEL fusion protein () via s.c. injection at P18, P25, P32, P39, P46, P53 and P60. Fundoscopy was performed at P50, P70, P85, P100 and P115 for evaluation of retinal inflammation and atrophy. Mice which received weekly treatment of PBS (
) reached grade 4 EAU by P60 (data for P60 time point included for reference). DEC205-HEL treated mice had minimal signs of EAU at P60 (
) but after stopping treatment beyond P60 began to develop signs of EAU (both inflammation plus atrophy) but until P115 did not reach the level of severity of the PBS treated mice (p=0.0038 for both inflammatory and atrophy). Sample size: P115 DEC205-HEL (N=5), P60 PBS (N=7), P60 DEC205-HEL (N=8). Differences between groups were analysed using Mann-Whitney U test.
Treatment with DEC 205-HEL fusion protein does not prevent EAU induced by adoptive transfer of HEL-activated CD4+ Tg T cells
We have previously shown that CD4+ HEL TCR (3A9) T cells, but not CD3 double negative (DN) cells, when adoptively transferred to sTg HEL mice leads to EAU 5d post transfer (9). HEL TCR specific T cells require activation with HEL protein in vitro in order to induce EAU by adoptive transfer while non-specific activation with anti-CD3/CD28 antibodies is ineffective (9). In addition, disease severity is less than in spontaneous EAU and is both cell dose-dependent and self-limited.
We wished to determine whether treatment with DEC205-HEL fusion protein would prevent EAU induced by adoptive transfer of in vitro HEL-activated T cells. sTg HEL mice were treated s.c. with DEC205-HEL fusion protein or PBS followed 1 day later with i.v. adoptive transfer of 5.0 x 106 HEL TCR (3A9) cells which had been incubated with 1μM HEL protein for 3 days in vitro. EAU developed beginning at day 5 post transfer and with 100% incidence by day 7. There was no difference in disease incidence or severity between DEC205-HEL treated mice and PBS treated control mice (Figure 5: p=0.4056 and p=0.6550 for DEC205-HEL vs PBS at day 5 and 7 post adoptive transfer respectively).
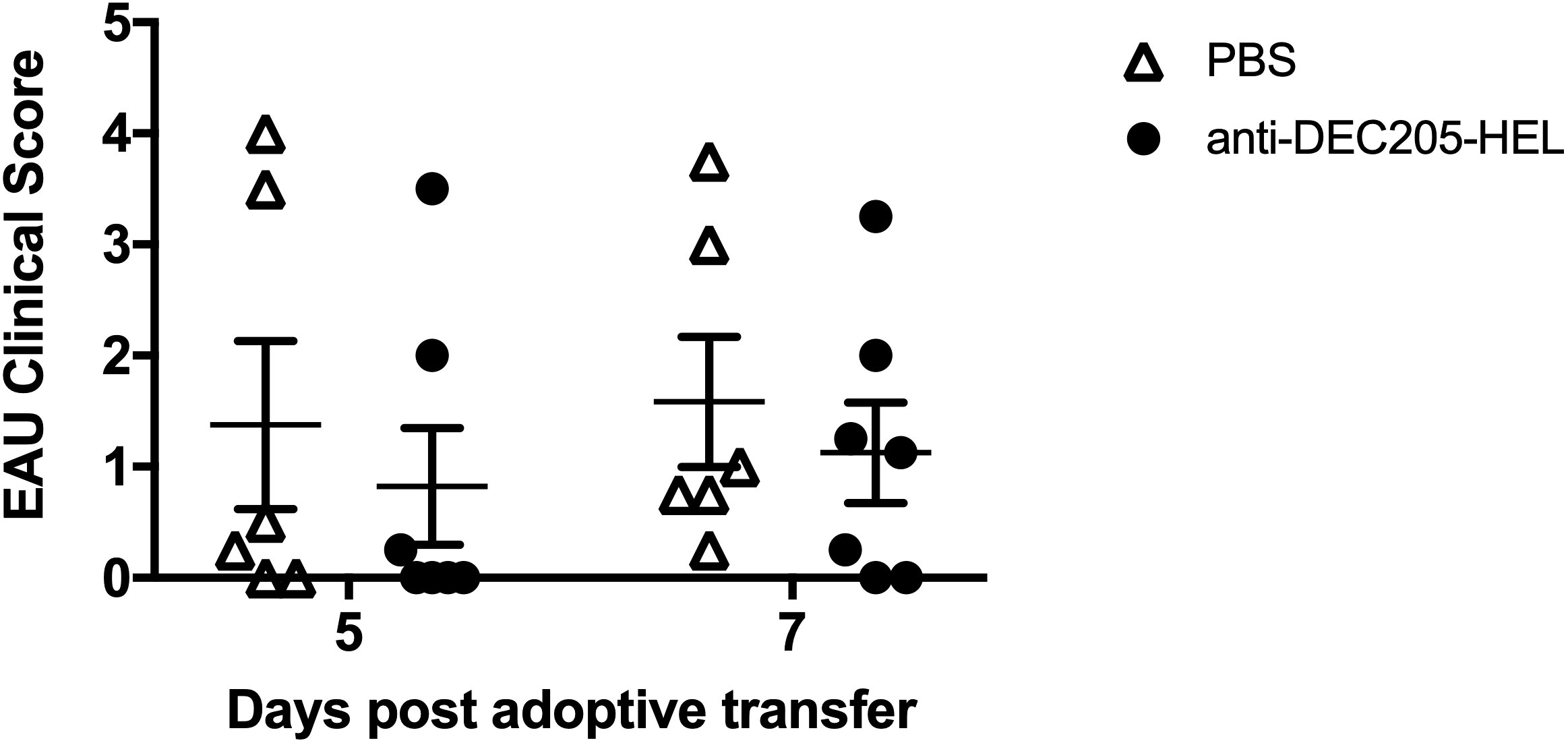
Figure 5 DEC 205-HEL s.c. fusion protein fails to prevent EAU induced by i.v. adoptive transfer of HEL-activated sTg TCR (3A9) lymphocytes to stg HEL mice. Lymph node and spleen cells were processed from sTg TCR (3A9) mice and cultured at 1:1 ratio with HEL protein (1μM) for 72h. sTg HEL mice were treated with s.c. injection of PBS or DEC205-HEL fusion protein at P21, 24h prior to adoptive transfer of 5 x 106 cultured cells via tail vein injection at P22. Fundus images of sTg HEL mice were taken at 5 days (P27) and 7 days (P29) post adoptive transfer for evaluation of EAU severity. There was no difference in EAU inflammatory scores between mice which received PBS and DEC205-HEL fusion protein (p=0.4056 and p=0.6550 for day 5 and 7 post adoptive transfer respectively). Sample size: DEC205-HEL (N=7), PBS (N=6). Differences between groups were analysed using Mann Whitney U test.
Treatment of dTg mice with DEC205-HEL fusion protein prevents infiltration of retinal IFNγ-producing cells and expansion of CD4+FoxP3+ Tregs in the eye-draining lymph node
We next explored the effect of DEC205-HEL fusion protein on lymphocyte populations in the skin- and eye-draining lymph nodes and in the retina in dTg mice as they developed spontaneous EAU. We have previously shown that dTg mice are profoundly lymphopenic at the onset of EAU (P21) which lessens as the disease progresses but persists for the course of the disease. We have also shown that both IL-17+ and INFγ+ CD4 T cells as well as CD3+ double negative (DN) T cells participate in the development of EAU, the latter population being more prominent in the initial stages of disease (9). Importantly, we have previously shown in mice that the submandibular lymph node (SMLN) is the eye-draining lymph node while the superficial cervical lymph node (SCLN) drains the periocular tissues and cervical skin (39, 40). We used this information to tease out changes in lymphocyte populations in these various sites.
We chose one time point (P26) when there would be significant suppression of spontaneous EAU after two doses (P18 and P25) of DEC205-HEL fusion protein (Figure 1A). We observed that there was no difference in the absolute numbers of IL-17+ or IFNγ+ CD4+ or DN T cells in either the eye-draining or the skin-draining lymph node Figure 6A; Supplementary Figure 3). In the retina, DEC205-HEL selectively reduced both the CD4+IFNγ+ and the DN IFNγ+ T cell populations, but had no effect on either IL-17+ T cell populations, despite this population being the first cells to infiltrate the retina in this model of EAU (9) (Figure 6B). However, there was a significant reduction in DN CD25+FoxP3- T cells in the retina, most likely a reflection of the overall markedly suppressed inflammation induced by DEC205-HEL (Figure 6B). Interestingly, there was also a significant reduction in CD4+CD25+FoxP3+ T cells in the eye-draining SMLN but not in the skin-draining SCLN although the lack of effect appeared attributable to a single outlier (Figure 6A).
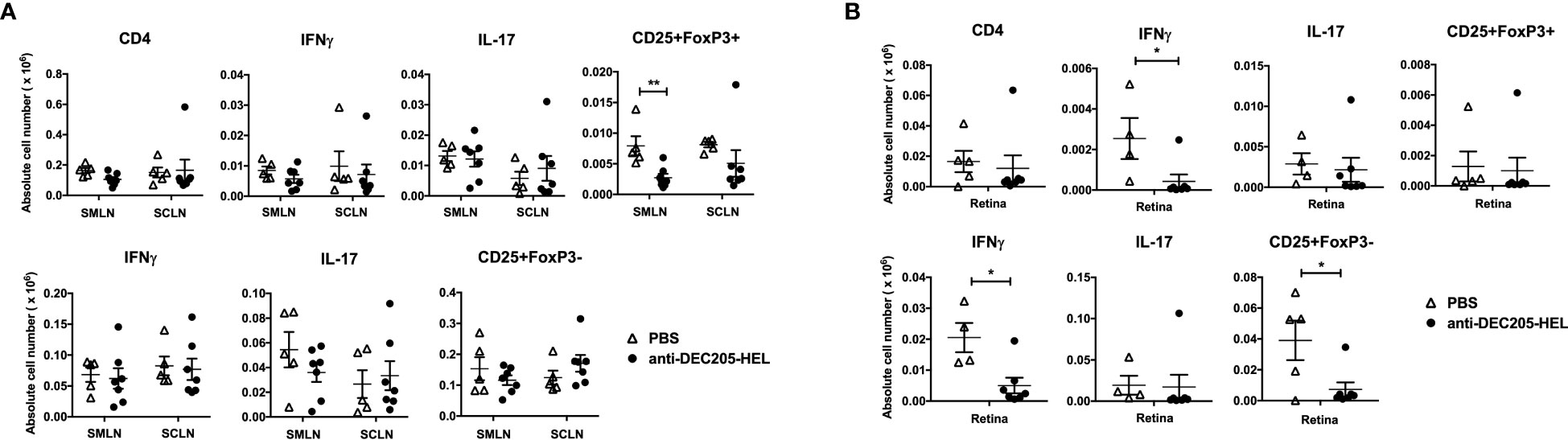
Figure 6 DEC205 fusion protein reduces INFγ+ but not IL-17+ effector cells in the retina. dTg mice were treated with s.c. injection of DEC205-HEL fusion protein or PBS at P18 and P25 before harvest of the eye draining submandibular lymph node (SMLN), skin draining superficial cervical lymph node (SCLN) and retina at P26. Tissues were processed for cell surface staining of CD4 and CD25, and intracellular staining of IFNγ, IL-17 and FoxP3. (A) Lymph node cells and (B) retina cells were analysed separately for CD4+ (upper panel) and CD4- DN cells (lower panel). (A) DEC205-HEL fusion protein treated dTg mice had fewer CD4+CD25+FoxP3+ cells in the SMLN (p=0.0057) and no difference was observed in the CD4-DN cells in the DLN between DEC205-HEL fusion protein and PBS treated dTg mice. (B) In the retina of DEC205-HEL fusion protein treated mice, both CD4+ and CD4-DN IFNγ+ were markedly reduced as well as CD4DNCD25+FoxP3- cells (p=0.0369, p=0.0107 and p=0.0237 respectively) Sample size: DEC205-HEL (N=7), PBS (N=at least 4 per group). Data were analysed using student’s t-test, with *p<0.05, **p<0.01 respectively.
We further examined the lymphocyte population in the skin-draining SCLN, the site for trafficking steady state DC targeted by inoculations of DEC205-HEL in the cervical skin (Figure 7A). Treatment of sTg TCR (3A9) mice with two doses of DEC205-HEL fusion protein (administered s.c. on day 0 and day 7) induced a significantly greater yield in the total number of cells within SCLN on day 8 of the experiment (Figure 7B). We then cultured cells isolated from the SCLN for 72h in the presence of HEL protein. Flow cytometric analysis revealed that in vivo exposure to DEC205-HEL fusion protein selectively led to a significant reduction in the percentage of the CD4+ T cell populations after three days of culture in the presence of HEL, and affected both the Th1 (T-bet) and the Th17 (RORγt) expression amongst CD4+ cells (Figure 7C).
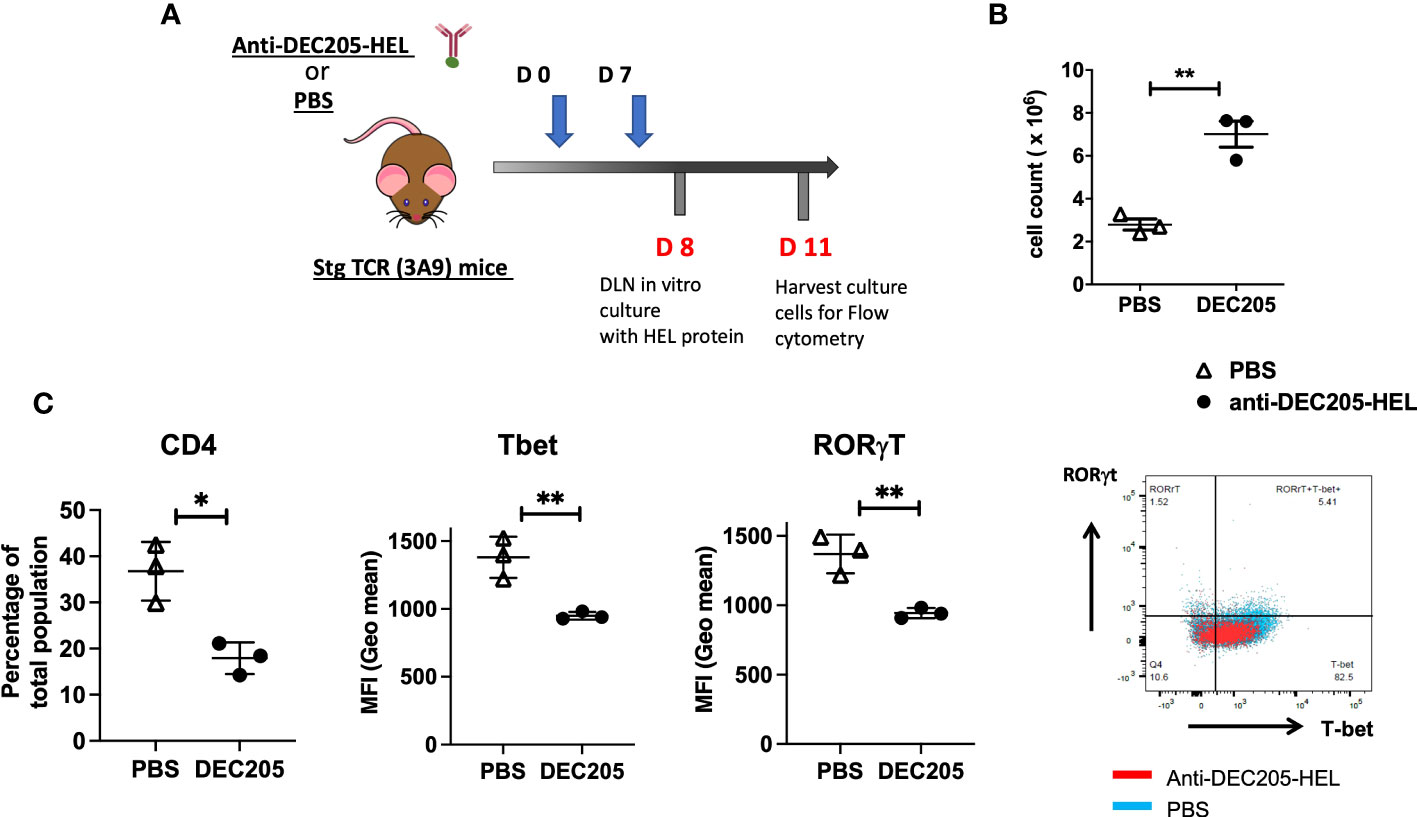
Figure 7 Lymphocytes from sTg DEC205-HEL fusion protein treated mice show reduced T cell activation in response to HEL specific antigen in vitro. (A) Experimental design: Adult (4-6 weeks) sTg TCR (3A9) mice were inoculated with DEC205-HEL fusion protein or PBS s.c. in the neck at day 0 and day 7 of experiment. The skin draining SCLN were harvested at day 8 cultured in vitro with 1μM HEL protein for 72h and harvested for flow cytometry of cell surface CD4 and intracellular T-bet and RORγt. (B) Absolute cell count of SCLN cells: a significantly greater yield was obtained from DEC205-HEL-treated mice compared to PBS control treated mice at day 8 post inoculation. (C) After stimulation in vitro with HEL protein, the percentage of CD4+T cells was markedly reduced, affecting both T-bet and RORγt expression levels. N=3 mice with in vitro cultured cells plated in triplicates and average calculated for each mouse. Data were analysed using student’s t-test, with *p<0.05, **p<0.01 respectively.
Discussion
The HEL : TCR dTg model of EAU is a CD4+ T cell mediated disease which develops spontaneously on photoreceptor maturation (~P20/21), and is driven by dysregulation in Teff/Treg cell balance occasioned by profound neonatal lymphopenia (9). Expansion into the lymphocyte space in lymphopenia is known to increase the risk of activating autoreactive T cells (41). The clinical signs in this model have many features resembling idiopathic uncontrolled intraocular inflammation (4) and can be prevented by adoptive transfer of antigen-experienced Tregs (9).
An alternative approach to adoptive Treg transfer in the treatment of inflammatory and autoimmune disease is targeted delivery of specific antigen to steady state DC using antibodies to cell surface proteins fused to the antigen in question. A range of antibodies has been used, targeting both cDC1 and cDC2 with different intended outcomes (17, 18, 21). Delivery of fusion proteins in the context of inflammation (e.g. with an adjuvant), promotes immunity and has been applied to promoting protective immunity against infections and cancer (20, 42–52). In contrast, targeting steady state DC in the absence of additional activation has been used to restore tolerance in autoimmune disease (23, 28, 29, 47, 53, 54).
Different DC surface proteins evoke different responses. For instance, targeting DEC205+ cDC1 in the absence of adjuvant is considered a useful strategy for promoting tolerance, while targeting DCIR2+ cDC2 may also promote tolerance but is more aligned with promoting immunity (30). Previous studies showed that differential expression of DEC205 and DCIR2 on subsets of DC influenced the outcome of antigen targeting, while monocytic DC (moDC) were significantly less effective in antigen uptake (14, 55). However, the specificity of the target antibody for particular DC subsets is not absolute and the expression of different surface molecules is context-dependent (17, 18).
Data in this report addresses antigen delivery via DC cell surface receptors using specific chimeric antibody fused to HEL in the model of HEL-antigen specific spontaneous EAU described here. Targeting antigen to DEC205 was most effective in preventing development of both the inflammatory disease and the associated chorioretinal atrophy but required early and regular weekly administration. Targeting antigen to DCIR2 was also effective in reducing/preventing inflammation but significantly less so, and was also less effective in preventing chorioretinal atrophy in this model. There was also a small suppressive effect of HEL-fused to IgG but not of irrelevant antigen (OVA) fused to DEC205 antibody, suggesting that at some level targeting proteins to surface receptors on steady state DC using chimeric antibody fusion proteins in the absence of inflammatory stimuli may variably modulate DC function partially by acting via inhibitory Fc receptors.
However, the defining data in this work is the very potent effect of DEC205-HEL fusion protein on suppressing spontaneous EAU. The site of Teff cell activation which initiates disease in this model is unclear but potential sites include the retina, the thymus and the eye-draining lymph node (SMLN) where HEL antigen has been detected in sTg mice (8, 56). Our data show that s.c. cervical skin delivery of DEC205-HEL leads to a marked reduction in both responsiveness of CD4+ T cells and expression of T-bet and RORγt after in vitro stimulation with HEL, with selective reduction of CD4+IFNγ+ and DN-IFNγ+ T cells in the retina in vivo. This correlated with a reduction in activated CD25+FoxP3- presumed effector T cells in the retina. Inhibition of CD4+IFNγ+ T cell activity would lead to disease inhibition since, as indicated above, this model is CD4 Th1 type mediated. Interestingly, there was little evidence of induction of Tregs in this model; indeed, there was an apparent reduction of CD4+Foxp3+ cells in the eye-draining SMLN (Figure 6). However, this was probably a reflection of the marked reduction in inflammation (EAU) induced by inhibition of Th1 Teff cells (Figure 7). Inflammation in this model is characterised by a significant expansion of Tregs in the retina and the eye-draining lymph node (9) and so the absence of inflammation would be accompanied by an apparent concomitant reduction in Tregs. Interestingly, there was no change in Treg populations in the skin draining SCLN, the site of drainage for migratory DEC205+ DCs and selective reduction in CD4+ T cell responsiveness to HEL protein following inoculation of DEC205-HEL (Figure 7C). This supports a mechanism of action for DEC205-HEL in this model involving inhibition of Th1 Teff cells rather than induction of Tregs. The mechanistic differences between this model and others in which DEC205-antigen fusion proteins mediate their tolerising effect by inducing Tregs, likely relate to the fact that inflammation in this dTg model is lymphopenia-driven in which there is a relative lack of Tregs from the onset of disease (9). An essential role for tolerising DC at the stage of antigen uptake and processing via DEC205-HEL treatment in this model, however, is underscored by the lack of effect of DEC205-HEL in the adoptive transfer model of the disease (Figure 5) in which T cell activation is induced by HEL in vitro prior to transfer. Furthermore, the results suggest that DC targeting by this method may be ineffective against fully activated T cells. These data conflict with those in an adoptive transfer model of acute colitis (24). However, in the colitis model, Treg, which are a prominent component of the colonic T cell population, appeared to play the greater part in disease control unlike the current model.
It is interesting to speculate on the site of action of DEC205-HEL fusion protein. Inoculation of the fusion protein into the skin will target skin-resident cDC1 as well as DC in the skin-draining SCLN. We have also observed that some soluble DEC205-HEL is likely to enter the circulation via lymph-to-blood transport in DLNs and reach other tissues such as the spleen (data not shown). However, targeting of antigen using DC surface receptor antibody-fusion proteins administered subcutaneously has confirmed local skin cDCs as the site where uptake of antigen for both tolerogenesis (21) and immunogenic vaccination (57) occurs. The life span of such DC as they traffic to the DLN to exert an effect on Teff cells appears to be ~7 days since weekly administration of DEC205-HEL fusion protein which was required to sustain a suppressive effect (Figure 1A); moreover, the effect became significantly reduced within days of stopping treatment (Figure 4). These data fit well with what is known about the life-span of DC in vivo but do not shed light on the site of Teff suppression by DEC205-HEL-treated DC.
Finally, the variable effect of DEC205-HEL and DCIR2-HEL on inflammation and chorioretinal atrophy is noteworthy. Inflammation-associated retinal damage presented as increasingly large areas of atrophy (9), expanding from a perivascular location in the control mice and partially in the DCIR2-HEL treated mice who, unlike the DEC205-HEL-treated mice, always had some degree of inflammation (Figure 1). In contrast, when inflammation was completely suppressed as in DEC205-HEL-treated mice the progressive development of atrophic changes suggested they were the result of a residual low grade, albeit ongoing inflammatory response. The role of inflammation in retinal degeneration is now widely recognised.
Overall, the data presented here demonstrate the value of DC targeting in a model of spontaneous autoimmune disease as a means to investigate immunological mechanisms of DC function in restoring immunological tolerance. As a treatment for autoimmune disease, DC targeting may also have value, provided relevant autoantigens can be identified. Currently, clinical trials evaluating tolerogenic DC for the treatment of autoimmune disease and prevention of graft rejection have mainly utilised peripheral blood monocyte’s, cultured in the presence of GM-CSF and IL-4 [reviewed in ref (58)]. However, harvesting rare circulating DC is a laborious and intense process and the possibility of directly targeting cDC1 (and 2) using fusion protein technology for delivery of antigens might be one way forward.
Data availability statement
The raw data supporting the conclusions of this article will be made available by the authors, without undue reservation.
Ethics statement
The animal study was approved by University of Aberdeen, Ethical Review Committee, University of Aberdeen, King’s College, Aberdeen AB24 3FX, United Kingdom. The study was conducted in accordance with the local legislation and institutional requirements.
Author contributions
IPK, TY, RF, KK and LK performed the experiments. RJC generated the transgenic mice and critiqued the paper. CM-G prepared the fusion protein constructs and critiqued the paper. TY, LK, and JVF designed the study. All authors contributed to the article and approved the submitted version.
Funding
This work was funded by Fight for Sight, The Eye Charity (CSO project grant award: 3031-3032). The Development Trust of the University of Aberdeen (Saving Sight in Grampian) (Grant No. RG-12663 and RG-14251).
Acknowledgments
We are grateful to Michel Nussenzweig and Ralph Steinman, of the Rockefeller University, New York, USA for providing DNA constructs for the fusion proteins used in this study and to Mariapia Degli-Esposti and Matt Wikstrom, University of Western Australia, Perth, Australia for discussions relating to the model system described in this work.
Conflict of interest
The authors declare that the research was conducted in the absence of any commercial or financial relationships that could be construed as a potential conflict of interest.
Publisher’s note
All claims expressed in this article are solely those of the authors and do not necessarily represent those of their affiliated organizations, or those of the publisher, the editors and the reviewers. Any product that may be evaluated in this article, or claim that may be made by its manufacturer, is not guaranteed or endorsed by the publisher.
Supplementary material
The Supplementary Material for this article can be found online at: https://www.frontiersin.org/articles/10.3389/fimmu.2023.1227633/full#supplementary-material
References
1. Jabs DA. Epidemiology of uveitis. Ophthalmic Epidemiol (2008) 15(5):283–4. doi: 10.1080/09286580802478724
2. Williams GJ, Brannan S, Forrester JV, Gavin MP, Paterson-Brown SP, Purdie AT, et al. The prevalence of sight-threatening uveitis in Scotland. Br J Ophthalmol (2007) 91(1):33–6. doi: 10.1136/bjo.2006.101386
3. Forrester JV, Kuffova L, Dick AD. Autoimmunity, autoinflammation and infection in uveitis. Am J Ophthalmol (2018) 189:77–85. doi: 10.1016/j.ajo.2018.02.019
4. Jabs DA, Nussenblatt RB, Rosenbaum JT, Standardization of Uveitis Nomenclature Working G. Standardization of uveitis nomenclature for reporting clinical data. Results of the First International Workshop. Am J Ophthalmol (2005) 140(3):509–16. doi: 10.1016/j.ajo.2005.03.057
5. Forrester JV, McMenamin PG, Dando SJ. CNS infection and immune privilege. Nat Rev Neurosci (2018) 19(11):655–71. doi: 10.1038/s41583-018-0070-8
6. Klaska IP, Forrester JV. Mouse models of autoimmune uveitis. Curr Pharm Des (2015) 21(18):2453–67. doi: 10.2174/1381612821666150316122928
7. Horai R, Silver PB, Chen J, Agarwal RK, Chong WP, Jittayasothorn Y, et al. Breakdown of immune privilege and spontaneous autoimmunity in mice expressing a transgenic T cell receptor specific for a retinal autoantigen. J Autoimmunity (2013) 44:21–33. doi: 10.1016/j.jaut.2013.06.003
8. Lambe T, Leung JC, Ferry H, Bouriez-Jones T, Makinen K, Crockford TL, et al. Limited peripheral T cell anergy predisposes to retinal autoimmunity. J Immunol (2007) 178(7):4276–83. doi: 10.4049/jimmunol.178.7.4276
9. Liu YH, Molzer C, Makinen K, Kamoi K, Corbett CLC, Klaska IP, et al. Treatment with foxP3+ Antigen-experienced T regulatory cells arrests progressive retinal damage in a spontaneous model of uveitis. Front Immunol (2020) 11:2071. doi: 10.3389/fimmu.2020.02071
10. Atmaca LS, Batioglu F, Atmaca Sonmez P. A long-term follow-up of Eales’ disease. Ocul Immunol Inflamm (2002) 10(3):213–21. doi: 10.1076/ocii.10.3.213.15598
11. Tan LT, Isa H, Lightman S, Taylor SR. Prevalence and causes of phthisis bulbi in a uveitis clinic. Acta Ophthalmol (2012) 90(5):e417–8. doi: 10.1111/j.1755-3768.2011.02319.x
12. Kitagawa Y, Sakaguchi S. Molecular control of regulatory T cell development and function. Curr Opin Immunol (2017) 49:64–70. doi: 10.1016/j.coi.2017.10.002
13. Steinman RM. Decisions about dendritic cells: past, present, and future. Annu Rev Immunol (2012) 30:1–22. doi: 10.1146/annurev-immunol-100311-102839
14. Dudziak D, Kamphorst AO, Heidkamp GF, Buchholz VR, Trumpfheller C, Yamazaki S, et al. Differential antigen processing by dendritic cell subsets in vivo. Science (2007) 315(5808):107–11. doi: 10.1126/science.1136080
15. Yin X, Chen S, Eisenbarth SC. Dendritic cell regulation of T helper cells. Annu Rev Immunol (2021) 39:759–90. doi: 10.1146/annurev-immunol-101819-025146
16. Iberg CA, Bourque J, Fallahee I, Son S, Hawiger D. TNF-alpha sculpts a maturation process in vivo by pruning tolerogenic dendritic cells. Cell Rep (2022) 39(2):110657. doi: 10.1016/j.celrep.2022.110657
17. Bourque J, Hawiger D. Variegated outcomes of T cell activation by dendritic cells in the steady state. J Immunol (2022) 208(3):539–47. doi: 10.4049/jimmunol.2100932
18. Bourque J, Hawiger D. Applications of antibody-based antigen delivery targeted to dendritic cells in vivo. Antibodies (Basel) (2022) 11(1):8. doi: 10.3390/antib11010008
19. Ngu LN, Nji NN, Ambada GE, Sagnia B, Sake CN, TChadji JC, et al. In vivo targeting of protein antigens to dendritic cells using anti-DEC-205 single chain antibody improves HIV Gag specific CD4+ T cell responses protecting from airway challenge with recombinant vaccinia-gag virus. Immun Inflammation Dis (2017) 7(2):55–67. doi: 10.1002/iid3.151
20. Matos I, Mizenina O, Lubkin A, Steinman RM, Idoyaga J. Targeting Leishmania major antigens to dendritic cells in vivo induces protective immunity. PloS One (2013) 8(6):e67453. doi: 10.1371/journal.pone.0067453
21. Castenmiller C, Keumatio-Doungtsop BC, van Ree R, de Jong EC, van Kooyk Y. Tolerogenic immunotherapy: targeting DC surface receptors to induce antigen-specific tolerance. Front Immunol (2021) 12:643240. doi: 10.3389/fimmu.2021.643240
22. Price JD, Hotta-Iwamura C, Zhao Y, Beauchamp NM, Tarbell KV. DCIR2+ cDC2 DCs and zbtb32 restore CD4+ T-cell tolerance and inhibit diabetes. Diabetes (2015) 64(10):3521–31. doi: 10.2337/db14-1880
23. Ring S, Maas M, Nettelbeck DM, Enk AH, Mahnke K. Targeting of autoantigens to DEC205(+) dendritic cells in vivo suppresses experimental allergic encephalomyelitis in mice. J Immunol (2013) 191(6):2938–47. doi: 10.4049/jimmunol.1202592
24. Wadwa M, Klopfleisch R, Buer J, Westendorf AM. Targeting antigens to dec-205 on dendritic cells induces immune protection in experimental colitis in mice. Eur J Microbiol Immunol (Bp) (2016) 6(1):1–8. doi: 10.1556/1886.2015.00048
25. Spiering R, Margry B, Keijzer C, Petzold C, Hoek A, Wagenaar-Hilbers J, et al. DEC205+ Dendritic cell-targeted tolerogenic vaccination promotes immune tolerance in experimental autoimmune arthritis. J Immunol (2015) 194(10):4804–13. doi: 10.4049/jimmunol.1400986
26. Petzold C, Schallenberg S, Stern JN, Kretschmer K. Targeted antigen delivery to DEC-205(+) dendritic cells for tolerogenic vaccination. Rev Diabetic Studies: RDS (2012) 9(4):305–18. doi: 10.1900/RDS.2012.9.305
27. Price JD, Beauchamp NM, Rahir G, Zhao Y, Rieger CC, Lau-Kilby AW, et al. CD8+ dendritic cell-mediated tolerance of autoreactive CD4+ T cells is deficient in NOD mice and can be corrected by blocking CD40L. J Leukocyte Biol (2014) 95(2):325–36. doi: 10.1189/jlb.0113013
28. Cao L, Chang H, Shi X, Peng C, He Y. Keratin mediates the recognition of apoptotic and necrotic cells through dendritic cell receptor DEC205/CD205. Proc Natl Acad Sci United States America (2016) 113(47):13438–43. doi: 10.1073/pnas.1609331113
29. Cao L, Shi X, Chang H, Zhang Q, He Y. pH-Dependent recognition of apoptotic and necrotic cells by the human dendritic cell receptor DEC205. Proc Natl Acad Sci United States America (2015) 112(23):7237–42. doi: 10.1073/pnas.1505924112
30. Tabansky I, Keskin DB, Watts D, Petzold C, Funaro M, Sands W, et al. Targeting DEC-205(-)DCIR2(+) dendritic cells promotes immunological tolerance in proteolipid protein-induced experimental autoimmune encephalomyelitis. Mol Med (2018) 24(1):17. doi: 10.1186/s10020-018-0017-6
31. Reuter A, Panozza SE, Macri C, Dumont C, Li J, Liu H, et al. Criteria for dendritic cell receptor selection for efficient antibody-targeted vaccination. J Immunol (2015) 194(6):2696–705. doi: 10.4049/jimmunol.1402535
32. Jones A, Bourque J, Kuehm L, Opejin A, Teague RM, Gross C, et al. Immunomodulatory functions of BTLA and HVEM govern induction of extrathymic regulatory T cells and tolerance by dendritic cells. Immunity (2016) 45(5):1066–77. doi: 10.1016/j.immuni.2016.10.008
33. Hawiger D, Inaba K, Dorsett Y, Guo M, Mahnke K, Rivera M, et al. Dendritic cells induce peripheral T cell unresponsiveness under steady state conditions in vivo. J Exp Med (2001) 194(6):769–79. doi: 10.1084/jem.194.6.769
34. Xu H, Koch P, Chen M, Lau A, Reid DM, Forrester JV. A clinical grading system for retinal inflammation in the chronic model of experimental autoimmune uveoretinitis using digital fundus images. Exp Eye Res (2008) 87(4):319–26. doi: 10.1016/j.exer.2008.06.012
35. Liu YH, Molzer C, Milne GC, Kuffova L, Forrester JV. Transmission Electron Microscopy Data on drusen-like deposits in the retinal degeneration sTg-IRBP: HEL mouse model. Data Brief (2019) 22:140–4. doi: 10.1016/j.dib.2018.12.007
36. Liu YH, Corbett C, Klaska IP, Makinen K, Nickerson JM, Cornall RJ, et al. Partial retinal photoreceptor loss in a transgenic mouse model associated with reduced levels of interphotoreceptor retinol binding protein (IRBP, RBP3). Exp Eye Res (2018) 172:54–65. doi: 10.1016/j.exer.2018.03.020
37. Stolley JM, Campbell DJ. A 33D1+ Dendritic cell/autoreactive CD4+ T cell circuit maintains IL-2-dependent regulatory T cells in the spleen. J Immunol (2016) 197(7):2635–45. doi: 10.4049/jimmunol.1600974
38. Calabro S, Liu D, Gallman A, Nascimento MS, Yu Z, Zhang TT, et al. Differential intrasplenic migration of dendritic cell subsets tailors adaptive immunity. Cell Rep (2016) 16(9):2472–85. doi: 10.1016/j.celrep.2016.07.076
39. Plskova J, Holan V, Filipec M, Forrester JV. Lymph node removal enhances corneal graft survival in mice at high risk of rejection. BMC Ophthalmol (2004) 4:3. doi: 10.1186/1471-2415-4-3
40. Plskova J, Duncan L, Holan V, Filipec M, Kraal G, Forrester JV. The immune response to corneal allograft requires a site-specific draining lymph node. Transplantation (2002) 73(2):210–5. doi: 10.1097/00007890-200201270-00010
41. Calzascia T, Pellegrini M, Lin A, Garza KM, Elford AR, Shahinian A, et al. CD4 T cells, lymphopenia, and IL-7 in a multistep pathway to autoimmunity. Proc Natl Acad Sci United States America (2008) 105(8):2999–3004. doi: 10.1073/pnas.0712135105
42. Ahmed EH, Brooks E, Sloan S, Schlotter S, Jeney F, Hale C, et al. Targeted delivery of BZLF1 to DEC205 drives EBV-protective immunity in a spontaneous model of EBV-driven lymphoproliferative disease. Vaccines (Basel) (2021) 9(6):555. doi: 10.3390/vaccines9060555
43. Apostolico JS, Lunardelli VA, Yamamoto MM, Souza HF, Cunha-Neto E, Boscardin SB, et al. Dendritic cell targeting effectively boosts T cell responses elicited by an HIV multiepitope DNA vaccine. Front Immunol (2017) 8:101. doi: 10.3389/fimmu.2017.00101
44. Flynn BJ, Kastenmuller K, Wille-Reece U, Tomaras GD, Alam M, Lindsay RW, et al. Immunization with HIV Gag targeted to dendritic cells followed by recombinant New York vaccinia virus induces robust T-cell immunity in nonhuman primates. Proc Natl Acad Sci United States America (2011) 108(17):7131–6. doi: 10.1073/pnas.1103869108
45. Henriques HR, Rampazo EV, Goncalves AJ, Vicentin EC, Amorim JH, Panatieri RH, et al. Targeting the non-structural protein 1 from dengue virus to a dendritic cell population confers protective immunity to lethal virus challenge. PloS Negl Trop Dis (2013) 7(7):e2330. doi: 10.1371/journal.pntd.0002330
46. Idoyaga J, Lubkin A, Fiorese C, Lahoud MH, Caminschi I, Huang Y, et al. Comparable T helper 1 (Th1) and CD8 T-cell immunity by targeting HIV gag p24 to CD8 dendritic cells within antibodies to Langerin, DEC205, and Clec9A. Proc Natl Acad Sci United States America (2011) 108(6):2384–9. doi: 10.1073/pnas.1019547108
47. Lehmann CH, Heger L, Heidkamp GF, Baranska A, Luhr JJ, Hoffmann A, et al. Direct delivery of antigens to dendritic cells via antibodies specific for endocytic receptors as a promising strategy for future therapies. Vaccines (Basel) (2016) 4(2):8. doi: 10.3390/vaccines4020008
48. Matos I, Mizenina O, Lubkin A, Steinman RM, Idoyaga J. Targeting leishmania major antigens to dendritic cells in vivo induces protective immunity. PloS One (2013) 8(6):e67453. doi: 10.1371/journal.pone.0067453
49. Neubert K, Lehmann CH, Heger L, Baranska A, Staedtler AM, Buchholz VR, et al. Antigen delivery to CD11c+CD8- dendritic cells induces protective immune responses against experimental melanoma in mice in vivo. J Immunol (2014) 192(12):5830–8. doi: 10.4049/jimmunol.1300975
50. Pereira LR, Vicentin ECM, Pereira SA, Maeda D, Alves R, Andreata-Santos R, et al. Intradermal delivery of dendritic cell-targeting chimeric mAbs genetically fused to type 2 dengue virus nonstructural protein 1. Vaccines (Basel) (2020) 8(4). doi: 10.3390/vaccines8040565
51. Rampazo EV, Amorim KN, Yamamoto MM, Panatieri RH, Rodrigues MM, Boscardin SB. Antigen targeting to dendritic cells allows the identification of a CD4 T-cell epitope within an immunodominant Trypanosoma cruzi antigen. PloS One (2015) 10(2):e0117778. doi: 10.1371/journal.pone.0117778
52. Silva-Sanchez A, Meza-Perez S, Flores-Langarica A, Donis-Maturano L, Estrada-Garcia I, Calderon-Amador J, et al. ESAT-6 Targeting to DEC205+ Antigen Presenting Cells Induces Specific-T Cell Responses against ESAT-6 and Reduces Pulmonary Infection with Virulent Mycobacterium tuberculosis. PloS One (2015) 10(4):e0124828. doi: 10.1371/journal.pone.0124828
53. Daniel C, Ploegh H, von Boehmer H. Antigen-specific induction of regulatory T cells in vivo and in vitro. Methods Mol Biol (2011) 707:173–85. doi: 10.1007/978-1-61737-979-6_11
54. Mahnke K, Ring S, Enk AH. Antibody targeting of “Steady-state” Dendritic cells induces tolerance mediated by regulatory T cells. Front Immunol (2016) 7:63. doi: 10.3389/fimmu.2016.00063
55. Kamphorst AO, Guermonprez P, Dudziak D, Nussenzweig MC. Route of antigen uptake differentially impacts presentation by dendritic cells and activated monocytes. J Immunol (2010) 185(6):3426–35. doi: 10.4049/jimmunol.1001205
56. Voigt V, Wikstrom ME, Kezic JM, Schuster IS, Fleming P, Makinen K, et al. Ocular antigen does not cause disease unless presented in the context of inflammation. Sci Rep (2017) 7(1):14226. doi: 10.1038/s41598-017-14618-z
57. Pastor Y, Ghazzaui N, Hammoudi A, Centlivre M, Cardinaud S, Levy Y. Refining the DC-targeting vaccination for preventing emerging infectious diseases. Front Immunol (2022) 13:949779. doi: 10.3389/fimmu.2022.949779
Keywords: DEC205, dendritic cells, fusion protein, autoimmune, uveitis, hen egg lysozyme
Citation: Klaska IP, Yu T, Fordyce R, Kamoi K, Cornall RJ, Martin-Granados C, Kuffova L and Forrester JV (2023) Targeted delivery of autoantigen to dendritic cells prevents development of spontaneous uveitis. Front. Immunol. 14:1227633. doi: 10.3389/fimmu.2023.1227633
Received: 23 May 2023; Accepted: 14 August 2023;
Published: 01 September 2023.
Edited by:
Andrew W. Taylor, Boston University, United StatesReviewed by:
Margaret Chang, Boston Children’s Hospital and Harvard Medical School, United StatesTanima Bose, Ludwig Maximilian University of Munich, Germany
Copyright © 2023 Klaska, Yu, Fordyce, Kamoi, Cornall, Martin-Granados, Kuffova and Forrester. This is an open-access article distributed under the terms of the Creative Commons Attribution License (CC BY). The use, distribution or reproduction in other forums is permitted, provided the original author(s) and the copyright owner(s) are credited and that the original publication in this journal is cited, in accordance with accepted academic practice. No use, distribution or reproduction is permitted which does not comply with these terms.
*Correspondence: John V. Forrester, j.forrester@abdn.ac.uk
†These authors have contributed equally to this work