- 1Division of Rheumatology, Department of Medicine, Mayo Clinic, Rochester, MN, United States
- 2Department of Urology, Tianjin Institute of Urology, The Second Hospital of Tianjin Medical University, Tianjin, China
- 3Department of Quantitative Health Sciences, Mayo Clinic, Rochester, MN, United States
- 4Department of Cancer Biology, Mayo Clinic, Jacksonville, FL, United States
- 5Department of Immunology, Mayo Clinic, Jacksonville, FL, United States
- 6Department of Immunology, Mayo Clinic, Rochester, MN, United States
Introduction: The longitudinal responses towards multiple doses of COVID-19 mRNA vaccines in patients with systemic autoimmune diseases remain incompletely understood. While observational studies suggested the safety of COVID-19 mRNA vaccines in rheumatic disease patients, laboratory evidence is lacking.
Methods: Here we evaluated seroreactivity, clinical manifestions, and multiple disease biomarkers after 2 or 3 doses of COVID-19 mRNA vaccines in a cohort of patients with rheumatic diseases.
Results: Most patients generated high SARS-CoV-2 spike-specific neutralizing antibodies comparable to those in healthy controls after 2 doses of mRNA vaccines. The antibody level declined over time but recovered after the third dose of the vaccine. Patients with systemic lupus erythematosus (SLE) or psoriatic arthritis (PsA) remained without significant flares post-vaccination. The changes in anti-dsDNA antibody concentration and expression of type I interferon (IFN) signature genes were highly variable but did not show consistent or significant increases. Frequency of double negative 2 (DN2) B cells remained largely stable.
Discussion: Our data provide experimental evidences indicating the efficacy and safety of repeated COVID-19 mRNA vaccination in rheumatic disease patients.
Introduction
The coronavirus disease 2019 (COVID-19) messenger RNA (mRNA) vaccines have achieved remarkable success in fighting the COVID-19 pandemic caused by SARS-CoV-2. Because patients receiving immunosuppressive medicines were excluded in initial clinical trials and were less represented in many subsequent longitudinal studies, our understanding of the longitudinal immune responses towards COVID-19 mRNA vaccines in these patients remains incompletely understood. Furthermore, although several studies have indicated that COVID-19 mRNA vaccines usually do not exacerbate existing systemic autoimmunity, the current data are mostly observational, often over a short period (e.g., a few days after 2nd dose of vaccine) and may not account for the potential latent time for disease flare (1–8). Because changes in many serological, cellular, and molecular markers can precede disease onset, we reason that these biomarkers may provide a better indication of whether COVID-19 mRNA vaccines have any impact on disease activity. One consideration is that overactivation of type I interferon (IFN) has been associated with multiple rheumatic diseases, including systemic lupus erythematosus (SLE) and Sjögren’s syndrome. mRNA vaccines are known to provoke type I IFN signaling (9). Thus, it is plausible that repeated mRNA vaccination might lead to disease exacerbation partly due to type I IFN activation. To address this question, we evaluated disease activity, immune responses, cellular compositions, autoantibodies, and type I IFN signatures in samples from a cohort of rheumatic disease patients collected over 2-3 doses of COVID-19 mRNA vaccines.
Patients and methods
Study population and sample collection/storage
Patients were prospectively recruited from the Division of Rheumatology at Mayo Clinic Rochester in 2021. The study was approved by the Institutional Review Board of Mayo Clinic Rochester (IRB 21-000501). Written informed consent was obtained from all participating patients. All patients received at least two doses of COVID-19 mRNA vaccines (either Pfizer or Moderna mRNA vaccines). Among them, 13 received three doses of COVID-19 mRNA vaccination. Sex and age matched healthy controls’ plasma was collected by one month (n = 20) and six months (n = 5) after their 2nd dose vaccination at Mayo Clinic Rochester or Florida. Unvaccinated healthy control plasma was collected before the COVID-19 pandemic (n = 7). Throughout our study, Visit 1 (V1) represents “before the 1st dose of COVID-19 mRNA vaccination”; Visit 2 (V2) represents “1 month after the 2nd dose vaccination”; Visit 3 (V3) represents “4 months after the 2nd dose vaccination”; and Visit 4 (V4) represents “1 month after the 3rd dose of vaccination”; these times correspond to the collection of patients’ whole blood samples. Patient demographics, disease diagnosis and medication were detailed in Supplementary Table 1. Whole blood samples were preserved in DNA/RNA Shield (Zymo Research) and stored at -80°C. Peripheral blood mononuclear cells (PBMC) were isolated using Ficoll density gradient centrifugation and were resuspended in freezing medium. Subsequently, PBMC were cryopreserved in liquid nitrogen until use.
Enzyme-linked immunosorbent assay for recombinant SARS-CoV-2 spike protein
Ninety-six-well plates were coated with 1 μg/mL SARS-CoV-2 (2019-nCoV) Spike S1-His Recombinant Protein (Sino Biological) in PBS and incubated overnight at 4°C. Human plasma samples were heat-inactivated at 56°C for 1 hour. Plates were blocked with 3% Blotting-Grade Blocker (BIO-RAD) in 0.05% PBST for 1 hour at 37°C. Plasma samples were serially diluted in three-fold with 1% Blotting-Grade Blocker (BIO-RAD) in 0.05% PBS-T (dilution buffer), starting at 1:450 to 1:109350 dilution. SARS-CoV-2 Spike Protein (CR3022) Human IgG1 mAb (Cell Signaling) was included on each plate as Internal control to convert OD values into antibody concentrations. Diluted plasma samples were added to each well and incubated for 2 hours at room temperature using a plate mixer. Samples were run in duplicate. In each plate, convalescent plasma diluted samples were included as positive controls; unvaccinated healthy control diluted plasma samples were included as the negative control. Wells only added with dilution buffer were set in each plate as background readout. Detection relied on an enzyme-labeled secondary antibody, 1:10,000 diluted Goat Anti-Human IgG ads-HRP (Southern Biotech), or 1:1000 diluted Goat Anti-Human IgA ads-HRP (Southern Biotech) was added to each well for 1 hour at room temperature using a plate mixer. After incubation, TMB One Component HRP Microwell Substrate (Surmodics) was added to each well, and the reaction was terminated by 2M H2SO4. The absorbances were detected using a microplate reader at an optical density (OD) of 450 nm and 620 nm. The IgG original concentration and IgA half maximum dilution of the plasma samples were calculated and generated by GraphPad Prism 9.
SARS-CoV-2 surrogate neutralization assay
To detect SARS-CoV-2 total neutralizing antibodies and to identify individuals with an adaptive immune response to SARS-CoV-2, we used cPass SARS-CoV-2 Neutralization Antibody Detection Kit (GenScript) as a surrogate neutralization assay, which measures the ability of plasma to block the interaction between the ACE2 receptor protein and the receptor binding domain (RBD) of the viral spike protein. Convalescent plasma, healthy control plasma samples, and all 13 patients who have Visit 4 timepoint plasma samples were tested according to the manufacturer’s protocol. Human plasma samples were heat-inactivated at 56°C for 1 hour. Test plasma samples, positive and negative controls were diluted by sample dilution buffer with a ratio of 1:10. All plasma samples and controls were tested in duplicate. After reading the absorbance in a microtiter plate reader at 450 nm, the human plasma samples can be separated as a positive result and a negative result. Following the selection, the human plasma samples with a positive result were serially diluted in three-fold with sample dilution buffer, starting at 1:20 to 1:4860 dilution. The diluted plasma samples were tested with the same kit to obtain the titer measurement readout. The half-maximum dilution of the plasma samples with a positive result was calculated and generated by GraphPad Prism 9.
Anti-double stranded DNA ELISA assay
To measure the specific IgG autoantibodies against dsDNA in human plasma samples, we used QUANTA Lite dsDNA SC ELISA (Inova Diagnostics) to test patients’ samples according to the manufacturer’s protocols. Human plasma samples were diluted by the sample dilution buffer with a ratio of 1:100, as recommended. The calibrator samples, positive, negative, and single-stranded controls, were involved in each plate. Read the optical density (OD) of each well at 450nm on a microplate reader. The original concentration of the plasma samples was calculated by ratio.
Whole blood RNA isolation and real-time PCR
Whole blood RNA was isolated using Quick-RNA Whole Blood (Zymo Research) according to the manufacturer’s instructions and protocols. RNA concentration was measured by spectrophotometry, and the sample’s purity was evaluated according to 260/280 nm ratio. And cDNA was generated from RNA through PrimeScript RT Master Mix (TaKaRa). Then, cDNA was amplified by Real-Time PCR (Applied Biosystems) using Applied ABI PowerUp™ SYBR™ Green Master Mix (Life Technologies) and specific primers for the interest genes (MX1, IFIT1, IFI44). All reactions were run in duplicate under the same thermal cycling conditions as follows: 95°C for 10 min (polymerase activation) followed by 40 cycles at 95°C for 30s, 52-60°C for 30s, and 72°C for 30s. The results were quantified using the comparative (2-ΔΔCt) method. The Ct value of the target gene was normalized to the Ct value of the housekeeping gene, β-actin. Each patient’s data were reported as fold increase of the target gene mRNA level compared to his/her Visit 1 sample. The primer sequences are listed in Supplementary Table 2.
Flow cytometry
Cryopreserved PBMCs were thawed and stained with antibodies or viability dyes listed in Supplementary Table 3. Samples were acquired on a 4-laser Attune NxT flow cytometer (ThermoFisher Scientific). Up to 1.5 × 106 PBMCs were acquired for each sample. All samples were acquired with consistent settings on the same day. Data were analyzed with FlowJo 10.8.1 (Becton Dickinson).
Disease activity assessment
Medical charts were retrospectively reviewed to assess disease activity at the closest time before the COVID-19 mRNA vaccination and at the last visit (visit 3 or 4). For patients with SLE, disease activity was measured using the SLE disease activity index (SLEDAI-2k) (10) and a change in the modified lupus low disease activity status (mLLDAS) was considered a flare (11). For patients with psoriatic arthritis (PsA), we considered the increase in at least one joint for both tender and swollen joints counts (0–28), the onset of new extra-articular manifestations, or the increase in therapy to define if a patient presented a flare of the disease during that visits. Due to the small number of patients with other diagnosis, we didn’t pursue to assess their disease activity status.
Statistical analysis
Descriptive statistics were used to summarize the patients’ characteristics. Continuous variables were compared using the unpaired and paired Student T-test. P-values < 0.05 were considered statistically significant. The statistical analysis was performed using the GraphPad Prism 9 software (GraphPad).
Results
Robust humoral immune response to COVID-19 mRNA vaccine in rheumatic patients
Although recent literature indicates that some patients with rheumatic diseases had modestly reduced humoral immune response following two doses of COVID-19 mRNA vaccination, there was a considerable variation (2, 12). We first sought to determine if our cohort of rheumatic patients had good immune responses toward the COVID-19 mRNA vaccines (Moderna/Pfizer). To this end, we longitudinally measured human IgG and IgA antibodies against the SARS-CoV-2 spike protein S1 subunit at different time points before and after vaccination.
We separated our cohort of rheumatic disease patients into three groups: patients diagnosed with SLE, Sjogren’s, and idiopathic inflammatory myopathies, which are considered systemic autoimmune diseases, patients diagnosed with PsA and ankylosing spondylitis, which are considered inflammatory arthritides, and patients treated with rituximab and belimumab, two patients diagnosed with SLE and the other with Sjogren’s. Rituximab and belimumab-treated patients were separated because both directly target B cells and rituximab is known to have significantly negative effects on the humoral immune response to SARS-CoV-2 mRNA vaccines (2, 13–15).
Our ELISA results showed that anti-SARS-CoV-2 spike S1 IgG levels increased from barely detectable pre-vaccination to an equivalent level as those in vaccinated healthy controls (HC) at V2 (1 month after 2nd dose). Then they declined at V3 (4 months after 2nd dose). At V4 (after the 3rd dose), the IgG levels recovered to a similar level compared to those at V2 (Figures 1A, D, E). The exceptions are those receiving rituximab or belimumab whose anti-SARS-CoV-2 spike S1 IgG levels remained undetectable throughout all visits, consistent with previous reports (13, 15–17) (Figure 1A). IgA provides vital immune protection, especially in the mucosal sites. The dynamics of anti-SARS-CoV-2 spike S1 IgA titers broadly resembled those of anti-SARS-CoV-2 spike S1 IgG, except that the two rituximab-treated patients unexpectedly mounted measurable levels of S1 specific IgA at V4 (Figure 1B and Supplementary Figure 1A). The reduction of IgG and IgA levels at V3 compared to V2, and the recoveries at V4 compared to V3, were mostly statistically significant (Figure 1D and Supplementary Figure 1A). Furthermore, we evaluated the serum neutralizing ability using a surrogate angiotensin converting-enzyme 2 (ACE2) binding assay, which measures the ability of antibodies in plasma to block the interaction between the human angiotensin-converting enzyme-2 (hACE2) receptor protein and the receptor binding domain (RBD) of the viral spike protein. The data revealed similar dynamics as in the serum IgG and IgA titer results. The patient V2 samples showed a robust ability to block ACE2 binding, which declined at V3 and recovered at V4. We did not detect any neutralizing ability in the samples from the two rituximab-treated patients, suggesting that the measurable anti-SARS-CoV-2 S1 IgA in these two patients did not have the neutralizing capability (Figure 1C). Statistical analyses showed that patients with rheumatic diseases (excluding the rituximab/belimumab-treated patients) generated anti-SARS-CoV-2 spike S1 IgG and IgA one month after 2nd dose at a level comparable to healthy control groups (Figure 1E and Supplementary Figure 1B). While the antibodies waned at V3, they recovered to a similar (IgG) or slightly higher level (IgA) as in V2 after the 3rd dose (Figure 1D and Supplementary Figure 1A). There was no significant difference between the systemic autoimmune disease group and the inflammatory arthritis group at each visit (Supplementary Figure 2). Together, these data showed that patients with rheumatic diseases could have a robust humoral immune response towards COVID-19 mRNA vaccines after two doses, followed by a gradual decline and a strong recovery of anti-SARS-CoV-2 S1 IgG and IgA titers after a 3rd dose. Patients treated with rituximab or belimumab might have negligible SARS-CoV-2 specific IgG titers in all visits and some measurable level of IgA after the 2nd and 3rd dose of vaccination. However, these IgA might not confer neutralization capability.
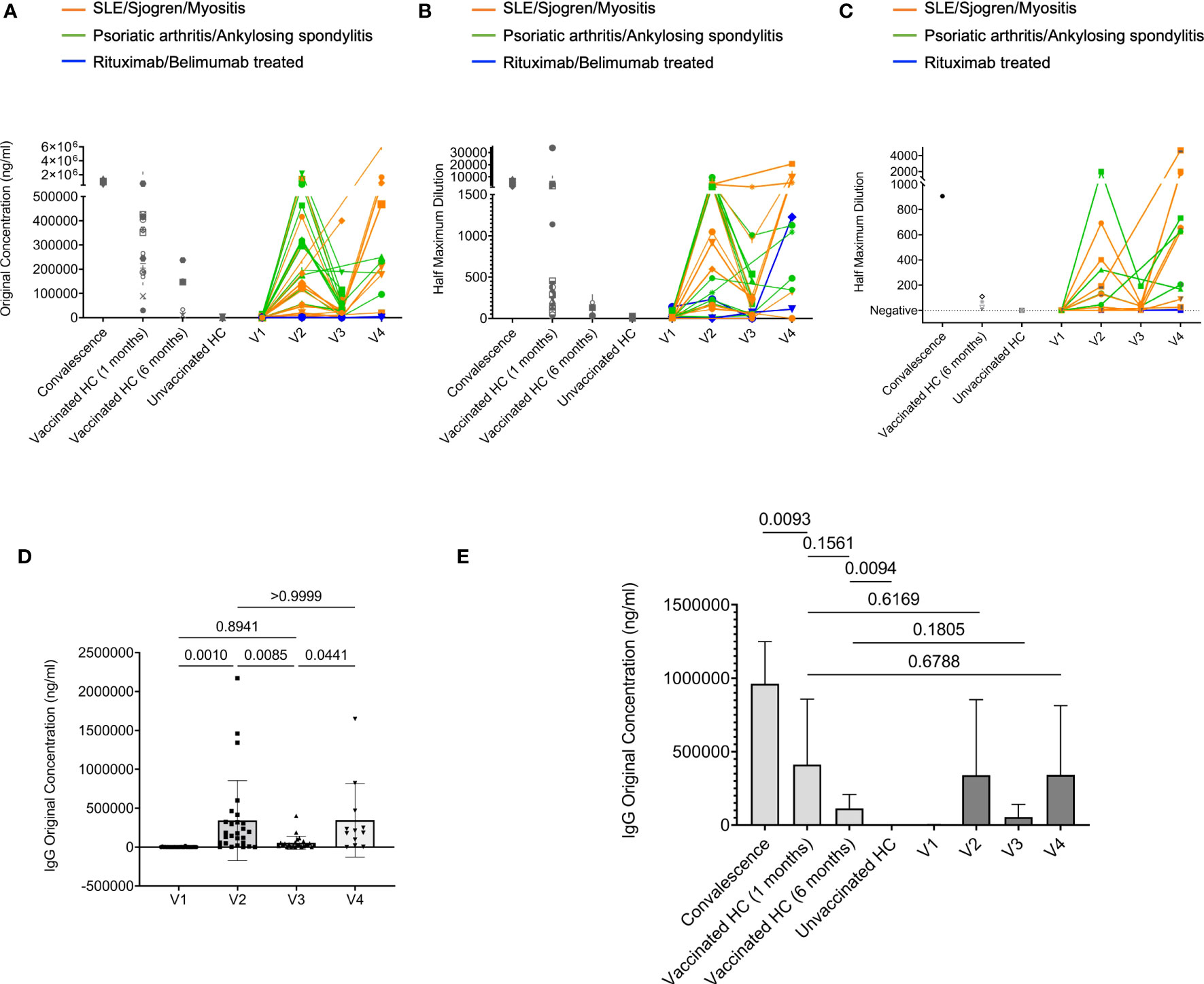
Figure 1 Humoral response to COVID-19 mRNA vaccine in rheumatic disease patients. Throughout, orange lines represent patients confirmed with SLE, Sjogren, and Myositis. Green lines represent patients confirmed with Psoriatic arthritis and Ankylosing spondylitis. Blue lines represent two patients treated with rituximab confirmed with SLE and Sjogren, respectively. And one patient treated with belimumab confirmed with SLE. (A), Concentration of human IgG against the SARS-CoV-2 spike S1 protein was obtained by ELISA. Orange subgroup (n = 13). Green subgroup (n = 12). Blue subgroup (n = 3). Healthy controls (HC) post-vaccinated 1-month (n = 20). HC post-vaccinated 6-month (n = 5). Unvaccinated healthy controls (HC) (n = 7). (B), Quantification of human IgA titers against the SARS-CoV-2 spike S1 protein was obtained by ELISA. (C), The ability of serum antibodies to block SARS-CoV-2 binding to human angiotensin-converting enzyme-2 (hACE2) receptor measured by surrogate neutralization. (D), Comparisons of IgG concentration in rheumatic disease patients’ plasma samples between different visits. (E), Comparisons of IgG concentration among indicated groups. P values were derived from paired t-tests (D) and unpaired t-tests (E).
Repeated SARS-CoV-2 vaccinations do not have a significant impact on disease activity and multiple disease biomarkers
When we assessed the change in clinical status among patients with SLE, three out of twelve showed a modest increase in SLEDAI scores at their last visit compared to before vaccination (Supplementary Table 4), but only for two patients this represented a flare (change from their mLLDAS) manifested as new joint inflammation (both at V3; Figure 2). The majority of the patients with SLE (83%, 10/12) remained stable or even improved their disease activity. Among patients with PsA, only one out of ten presented a flare of the disease at the last visit, and this patient was active since before COVID-19 vaccination.
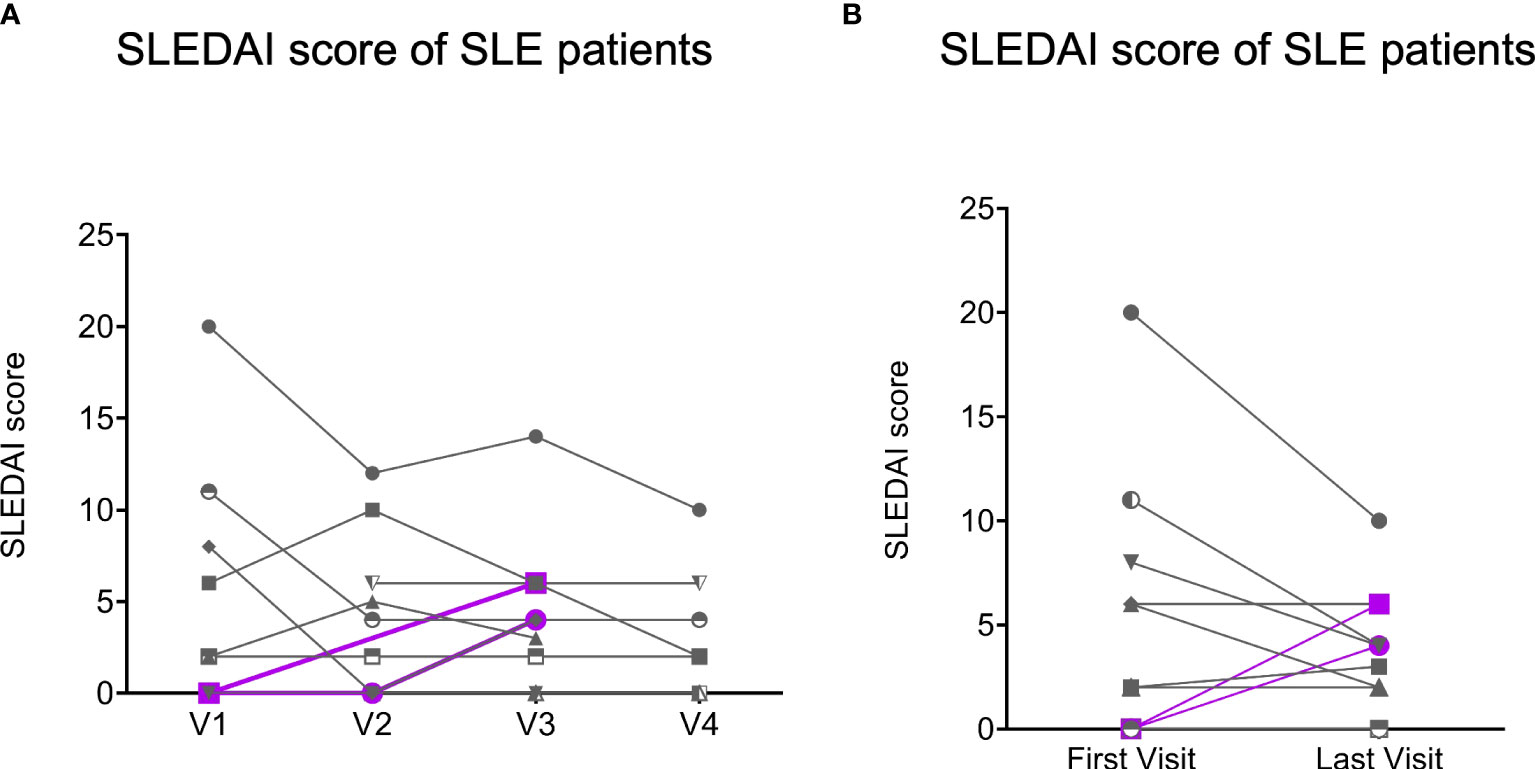
Figure 2 The Systemic Erythematosus Disease Activity Index (SLEDAI) scores of SLE patients (n = 12). Gray lines represent the SLE patients (n = 10) with decreasing or stable SLEDAI scores (no flare), and purple lines represent the SLE patients (n = 2) with increasing SLEDAI scores (flare) at the last visits compare to their first visits. (A), SLEDAI scores of SLE patients (n = 12) with all visits data. (B), SLEDAI scores of SLE patients (n = 12) was obtained at their first visits and last visits.
Because many molecular and cellular phenotypes can long precede autoimmune disease onset or flare, we decided to evaluate several key biomarkers associated with systemic rheumatic diseases. These included anti-dsDNA concentration, expression of type I IFN signature genes, and the frequency of IgD and CD27 ‘double-negative’ (DN) B cells lacking CD21 expression but expressing CD11c (DN2 cells), which expanded in patients with active SLE and correlated with increased morbidity in COVID-19 patients (18, 19). Plasma anti-dsDNA concentration was measured with ELISA. Samples from vaccinated healthy donors were included as controls. We verified that many SLE patients, but not healthy controls, had relatively high level of anti-dsDNA autoantibodies (Supplementary Figure 3). When we compared anti-dsDNA levels between V1 and each subsequent visit, we did not observe a consistent increase of anti-dsDNA in any comparison (Figure 3). Thus, 2 or 3 doses of COVID-19 mRNA vaccines may not substantially affect anti-dsDNA antibody levels in SLE patients.
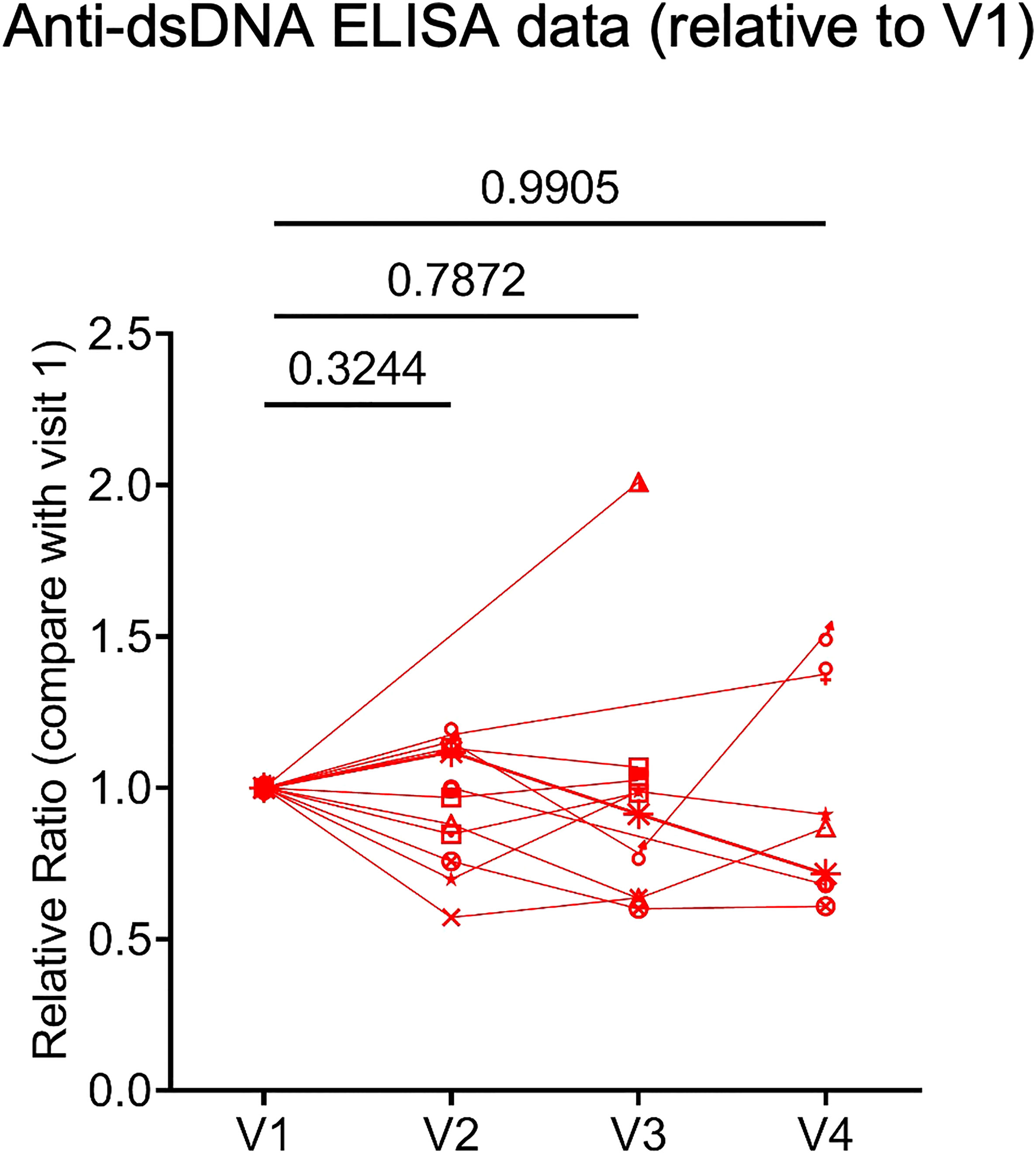
Figure 3 Human plasma anti-double stranded deoxyribonucleic acid (anti-dsDNA) level. Concentration of anti-dsDNA in SLE patients (n = 12) plasma samples relative to those in V1. Concentration of anti-dsDNA was obtained by ELISA. P values were calculated with paired parametric t-test.
Increased type I IFN signature is frequently associated with systemic autoimmunity. We examined the mRNA expression of three type I IFN signature genes (MX1, IFIT1, and IFI44) (20) in whole blood samples by real-time PCR. We observed a trend of increased IFI44 and IFIT1 expression at V2 relative to V1. At V3 and V4, none of the three genes showed any trend of increased expression compared to V1. In fact, the expression of IFI44 at V4 was significantly reduced compared to V1 (Figure 4). Therefore, 2 or 3 doses of COVID-19 mRNA vaccines may not consistently increase the expression of type I IFN signature genes.
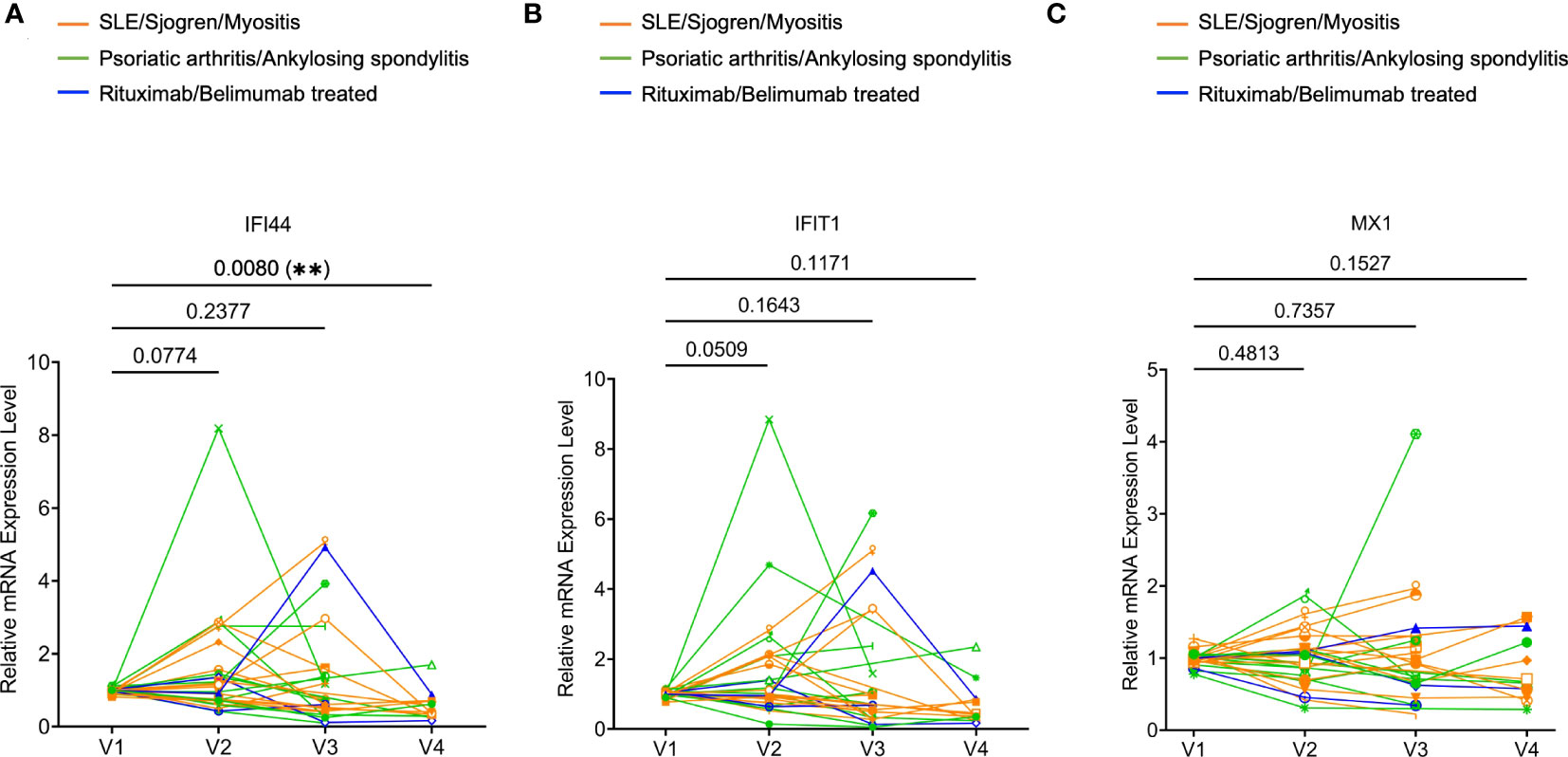
Figure 4 Expression of type I interferon (IFN) signature genes in rheumatic disease patients’ whole blood following SARS-CoV-2 mRNA vaccination. Orange subgroup (n = 13). Green subgroup (n = 12). Blue subgroup (n = 3). Relative mRNA expression of IFI44 (A), IFIT1 (B), and MX1 (C) were measured by RT-qPCR. P values were derived from a paired parametric t-test to show comparisons between indicated groups. **P < 0.01.
Finally, we sought to evaluate the cellular changes following COVID-19 mRNA vaccination using flow cytometry (see Supplementary Figure 4 for gating strategy). For non-rituximab/belimumab-treated patients, the frequency of CD19+ B cells showed a general trend of slight reduction relative to V1 (reached statistical significance only at V3). The two patients who were treated with rituximab had CD19+ frequencies below 0.02% at every visit, confirming the efficient B cell depletion (Supplementary Figure 5A). Within B cells, CD27+CD38+ plasmablasts remained stable throughout all visits (Supplementary Figure 5B). The two rituximab treated patients showed highly increased transitional B cell frequencies at V2 or V3 (Supplementary Figure 5C). However, due to the extremely low CD19+ frequency, the biological impact of such changes was likely limited. Extrafollicular DN2 B cells (CD19+IgD–CD27–CD11c+CD21–) are associated with systemic autoimmunity. We found that patients had widely variable DN2 B cell frequencies, and they did not show any significant alterations over the course of the study (Figure 5). Moreover, examination of T cells showed that total CD3+ T cell frequency, but not CD4+ or CD8+ T cell frequencies, slightly increased over the course of vaccination (Supplementary Figure 5D-F). TCR activated conventional CD4+ T cells (CD4+CD25–CD127+HLADR+CD38+) and the specialized B cell-interacting follicular helper T cells (Tfh)-like cells (CD4+CD45RA–CXCR5+) remained stable (Supplementary Figure 5G, H). Thus, overall lymphocyte homeostasis, including autoimmunity-associated extrafollicular DN2 B cells, may not be substantially altered by COVID-19 mRNA vaccination in rheumatic disease patients.
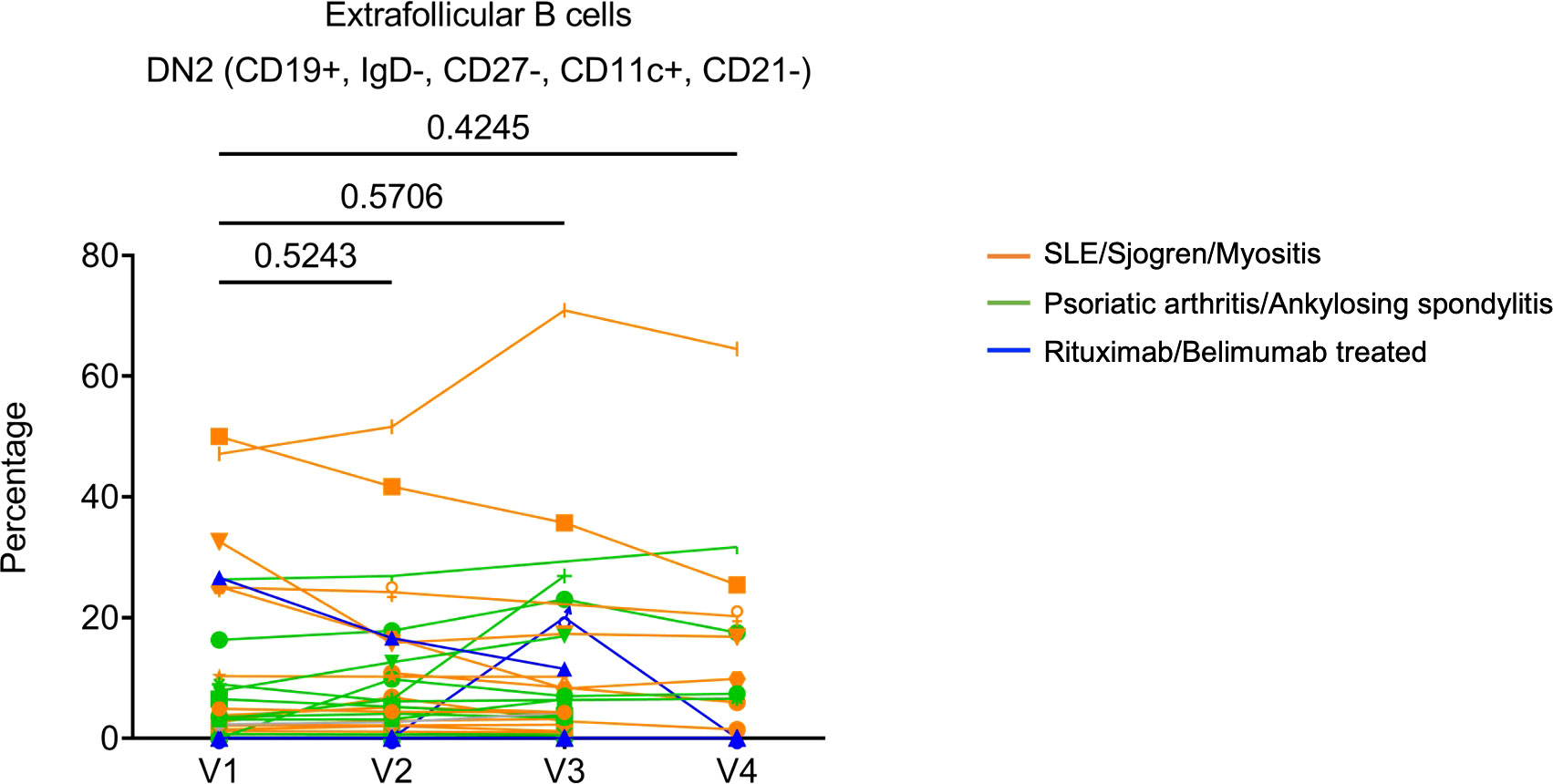
Figure 5 The frequencies of Extrafollicular DN2 B cells (CD19+IgD–CD27–CD11c+CD21–) following SARS-CoV-2 mRNA vaccination in patients’ PBMC. Orange subgroup (n = 13). Green subgroup (n = 12). Blue subgroup (n = 3). P values were generated by paired parametric t-test.
Discussion
Our study shows that following two doses of COVID-19 mRNA vaccines, most of the patients with rheumatic diseases elicit robust humoral immunity. The anti-SARS-CoV-2 antibody titers and neutralization ability declined over time after 2nd dose of the vaccine but mostly recovered after a 3rd dose of the COVID-19 mRNA vaccine. Previous studies have mostly focused on immune responses after two doses of COVID-19 vaccines, most of which reported a slightly reduced antibody response (1, 12). If the patients were treated with rituximab or belimumab, their humoral response to COVID-19 mRNA vaccines was severely curtailed or even abolished (13, 15–17). Our data are consistent with the detrimental effects of rituximab and belimumab on the humoral response to COVID-19 mRNA vaccines. The impact of belimumab on humoral immune response to SARS-CoV2 remains controversial (21, 22). Further investigation is warranted. On the other hand, we did not observe a substantial reduction of anti-SARS-CoV-2 immunoglobulin in patients not treated with rituximab or belimumab compared to healthy controls. Moreover, our data show that most patients can mount a vigorous antibody response after a 3rd vaccination, which restores the antibody level to a similar or even higher level to the one observed one month after 2nd dose. Thus, our results support that COVID-19 mRNA vaccines are highly immunogenic in patients with rheumatic diseases and the importance of booster vaccination.
One of the outstanding questions is the safety of COVID-19 mRNA vaccines in rheumatic disease patients. Autoimmune responses can be triggered by immune challenges, including vaccination, evidenced by rare myocarditis following COVID-19 mRNA vaccination in young adults (23). Thus, we did not observe a widespread increase in disease activity of patients with SLE or PsA after COVID-19 mRNA vaccination.
We also provide the first molecular and cellular evidence supporting the safety of long-term use of the COVID-19 mRNA vaccine in systemic rheumatic disease patients. While clinical observations support the safety of COVID-19 mRNA vaccines in rheumatic disease patients, anecdotal reports indicate a possible link between COVID-19 mRNA vaccines and disease flare (24). Moreover, previous literature has demonstrated that COVID-19 mRNA vaccines can stimulate serum type I IFN production, which is critical for optimal cellular immune response to the vaccine (9). On the other hand, there is evidence implicated that high mRNA transcripts of genes regulated by type I IFN, also known as type I IFN signatures, are correlated with the disease activity and the pathogenesis of several systemic autoimmune diseases, including SLE, Sjogren’s syndrome, rheumatoid arthritis and myositis (25). Therefore, we assessed the potential impacts of 2 or 3 doses of COVID-19 mRNA vaccines on disease activity and various disease activity biomarkers, including anti-dsDNA antibody level, expression of type I IFN signature genes, and cellular components. Reassuringly, the data showed that neither clinical disease activity (measured by SLEDAI in lupus patients) nor biomarkers indicate increased disease exacerbation following three doses of COVID-19 mRNA vaccines. Our findings corroborate the current clinical observations that COVID-19 mRNA vaccines are generally safe for rheumatic disease patients (4). Furthermore, at the time of preparing this manuscript, people in the US are getting updated bivalent COVID-19 mRNA vaccines as a 4th dose. Our data suggest that such repeat mRNA vaccination might not increase the risk of disease exacerbation.
The major limitation of our study is the small cohort size, which precludes any definitive conclusions. Research with a larger cohort is warranted to verify our observations. Nevertheless, our study provides an initial empirical and experimental evidence indicating the efficacy and safety of COVID-19 mRNA vaccines and supporting their wide usage in patients with systemic autoimmunity.
Data availability statement
The raw data supporting the conclusions of this article will be made available by the authors, without undue reservation.
Ethics statement
The studies involving human participants were reviewed and approved by The Institutional Review Board of Mayo Clinic Rochester. The patients/participants provided their written informed consent to participate in this study.
Author contributions
Study conception and design. ZA, KW, CW, UT, AD-G, HZ. Acquisition of data. ZA, XZ, YL, JJ, KM-F, JS, SC, KK, GF-P, AS-R. Analysis and interpretation of data. ZA, GF-P, XZ, CC, AD-G, HZ.
Funding
Supported by the National Institute of Arthritis and Musculoskeletal and Skin Disease, NIH (grant AR-077518), the National Institute of Allergy and Infectious Diseases, NIH (grant AI-162678) and Mayo Foundation for Medical Education and Research
Acknowledgments
The authors would like to thank the patients who participated in the study.
Conflict of interest
The authors declare that the research was conducted in the absence of any commercial or financial relationships that could be construed as a potential conflict of interest.
Publisher’s note
All claims expressed in this article are solely those of the authors and do not necessarily represent those of their affiliated organizations, or those of the publisher, the editors and the reviewers. Any product that may be evaluated in this article, or claim that may be made by its manufacturer, is not guaranteed or endorsed by the publisher.
Supplementary material
The Supplementary Material for this article can be found online at: https://www.frontiersin.org/articles/10.3389/fimmu.2023.1224702/full#supplementary-material
Supplementary Figure 1 | Statistical analyses of antibody titer data between visits. (A), Comparisons of IgA titers half maximum dilution in rheumatic disease patients’ plasma samples between different visits. (B), Comparisons of IgA titers half maximum dilution among indicated groups. P values were derived from paired t-tests (A) and unpaired t-tests (B).
Supplementary Figure 2 | Statistical analyses of antibody titer between disease groups at different visits. (A-C), Comparisons of anti-SARS-CoV-2 S1 protein IgG concentration between HC (gray), SLE/Sjogren/Myostitis (orange), and Psoriatic arthritis/Ankylosing spondylitis (green) at V2 (A), V3 (B) and V4 (C). (D-F), Comparisons of anti-SARS-CoV-2 S1 protein IgA titers between HC (gray), SLE/Sjogren/Myostitis (orange), and Psoriatic arthritis/Ankylosing spondylitis (green) at V2 (D), V3 (E) and V4 (F). P values were calculated by unpaired parametric t-test.
Supplementary Figure 3 | Human plasma anti-double stranded deoxyribonucleic acid (anti-dsDNA) level. Concentration of anti-dsDNA in human plasma samples was obtained by ELISA. SLE patients (Red, n = 12). Healthy controls post-vaccinated six months (Black, n = 5).
Supplementary Figure 4 | Representative flow cytometry plots of the gating strategies. (A), Gating strategies for T cell subsets. (B), Gating strategies for B cell subsets.
Supplementary Figure 5 | Summaries of the immune cell subset frequencies in rheumatic disease patients’ PBMC samples following SARS-CoV-2 mRNA vaccination. (A, B) cells (CD19+). (B), Antibody secreting cells (ASC, CD19+CD27+CD38+). (C), Transitional B cells (CD19+CD27-CD38+CD24+). (D), T cells (CD3+). (E), CD4+ T cells (CD3+CD4+). (F), CD8+ T cells (CD3+CD8+). (G), TCR activated conventional CD4+ T cells (CD4+CD25–CD127+HLADR+CD38+). (H), Circulating follicular helper T cell (Tfh)-like cells (CD3+CD4+CD45RA–CXCR5+). P values were calculated by paired parametric t-test. Orange subgroup (n = 13). Green subgroup (n = 12). Blue subgroup (n = 3).
References
1. Geisen UM, Berner DK, Tran F, Sumbul M, Vullriede L, Ciripoi M, et al. Immunogenicity and safety of anti-SARS-CoV-2 mRNA vaccines in patients with chronic inflammatory conditions and immunosuppressive therapy in a monocentric cohort. Ann Rheum Dis (2021) 80(10):1306–11. doi: 10.1136/annrheumdis-2021-220272
2. Izmirly PM, Kim MY, Samanovic M, Fernandez-Ruiz R, Ohana S, Deonaraine KK, et al. Evaluation of immune response and disease status in systemic lupus erythematosus patients following SARS-coV-2 vaccination. Arthritis Rheumatol (2022) 74(2):284–94. doi: 10.1002/art.41937
3. Syversen SW, Jyssum I, Tveter AT, Sexton J, Christensen IE, Tran TT, et al. Immunogenicity and safety of a three-dose SARS-CoV-2 vaccination strategy in patients with immune-mediated inflammatory diseases on immunosuppressive therapy. RMD Open (2022) 8(2):e002417. doi: 10.1136/rmdopen-2022-002417
4. Machado PM, Lawson-Tovey S, Strangfeld A, Mateus EF, Hyrich KL, Gossec L, et al. Safety of vaccination against SARS-CoV-2 in people with rheumatic and musculoskeletal diseases: results from the EULAR Coronavirus Vaccine (COVAX) physician-reported registry. Ann Rheum Dis (2022) 81(5):695–709. doi: 10.1136/annrheumdis-2021-221490
5. Larsen ES, Nilsson AC, Moller S, Voss AB, Johansen IS. Immunogenicity and risk of disease flare after a three-dose regimen with SARS-CoV-2 vaccination in patients with systemic lupus erythematosus: results from the prospective cohort study COVAC-SLE. Clin Exp Rheumatol (2022) 41(3):676–84. doi: 10.55563/clinexprheumatol/b8a6zb
6. Kartnig F, Mrak D, Simader E, Tobudic S, Radner H, Mandl P, et al. Safety and immunogenicity of a third COVID-19 vaccination in patients with immune-mediated inflammatory diseases compared with healthy controls. Ann Rheum Dis (2023) 82(2):292–300. doi: 10.1136/ard-2022-222682
7. Picchianti Diamanti A, Navarra A, Cuzzi G, Aiello A, Salemi S, Di Rosa R, et al. The third dose of BNT162b2 COVID-19 vaccine does not "Boost" Disease flares and adverse events in patients with rheumatoid arthritis. Biomedicines (2023) 11(3):687. doi: 10.3390/biomedicines11030687
8. Syversen SW, Jyssum I, Tveter AT, Tran TT, Sexton J, Provan SA, et al. Immunogenicity and safety of standard and third-dose SARS-coV-2 vaccination in patients receiving immunosuppressive therapy. Arthritis Rheumatol (2022) 74(8):1321–32. doi: 10.1002/art.42153
9. Li C, Lee A, Grigoryan L, Arunachalam PS, Scott MKD, Trisal M, et al. Mechanisms of innate and adaptive immunity to the Pfizer-BioNTech BNT162b2 vaccine. Nat Immunol (2022) 23(4):543–55. doi: 10.1038/s41590-022-01163-9
10. Gladman DD, Ibanez D, Urowitz MB. Systemic lupus erythematosus disease activity index 2000. J Rheumatol (2002) 29(2):288–91.
11. Ugarte-Gil MF, Hanly J, Urowitz M, Gordon C, Bae SC, Romero-Diaz J, et al. Remission and low disease activity (LDA) prevent damage accrual in patients with systemic lupus erythematosus: results from the Systemic Lupus International Collaborating Clinics (SLICC) inception cohort. Ann Rheum Dis (2022) 81(11):1541–8. doi: 10.1136/ard-2022-222487
12. Deepak P, Kim W, Paley MA, Yang M, Carvidi AB, Demissie EG, et al. Effect of immunosuppression on the immunogenicity of mRNA vaccines to SARS-coV-2 : A prospective cohort study. Ann Intern Med (2021) 174(11):1572–85. doi: 10.7326/M21-1757
13. Marty PK, Van Keulen VP, Erskine CL, Shah M, Hummel A, Stachowitz M, et al. Antigen specific humoral and cellular immunity following SARS-coV-2 vaccination in ANCA-associated vasculitis patients receiving B-cell depleting therapy. Front Immunol (2022) 13:834981. doi: 10.3389/fimmu.2022.834981
14. Raptis CE, Berger CT, Ciurea A, Andrey DO, Polysopoulos C, Lescuyer P, et al. Type of mRNA COVID-19 vaccine and immunomodulatory treatment influence humoral immunogenicity in patients with inflammatory rheumatic diseases. Front Immunol (2022) 13:1016927. doi: 10.3389/fimmu.2022.1016927
15. Mrak D, Tobudic S, Koblischke M, Graninger M, Radner H, Sieghart D, et al. SARS-CoV-2 vaccination in rituximab-treated patients: B cells promote humoral immune responses in the presence of T-cell-mediated immunity. Ann Rheum Dis (2021) 80(10):1345–50. doi: 10.1136/annrheumdis-2021-220781
16. Felten R, Gallais F, Schleiss C, Chatelus E, Javier RM, Pijnenburg L, et al. Cellular and humoral immunity after the third dose of SARS-CoV-2 vaccine in patients treated with rituximab. Lancet Rheumatol (2022) 4(1):e13–e6. doi: 10.1016/S2665-9913(21)00351-9
17. Jyssum I, Kared H, Tran TT, Tveter AT, Provan SA, Sexton J, et al. Humoral and cellular immune responses to two and three doses of SARS-CoV-2 vaccines in rituximab-treated patients with rheumatoid arthritis: a prospective, cohort study. Lancet Rheumatol (2022) 4(3):e177–e87. doi: 10.1016/S2665-9913(21)00394-5
18. Jenks SA, Cashman KS, Zumaquero E, Marigorta UM, Patel AV, Wang X, et al. Distinct effector B cells induced by unregulated toll-like receptor 7 contribute to pathogenic responses in systemic lupus erythematosus. Immunity (2018) 49(4):725–39 e6. doi: 10.1016/j.immuni.2018.08.015
19. Woodruff MC, Ramonell RP, Nguyen DC, Cashman KS, Saini AS, Haddad NS, et al. Extrafollicular B cell responses correlate with neutralizing antibodies and morbidity in COVID-19. Nat Immunol (2020) 21(12):1506–16. doi: 10.1038/s41590-020-00814-z
20. Palli E, Kravvariti E, Tektonidou MG. Type I interferon signature in primary antiphospholipid syndrome: clinical and laboratory associations. Front Immunol (2019) 10:487. doi: 10.3389/fimmu.2019.00487
21. Boedecker-Lips SC, Classen P, Kraus D, Weinmann-Menke J. Belimumab is not associated with COVID-19 mRNA vaccination failure in systemic lupus erythematosus. Rheumatol (Oxford) (2023) 62(3):e34–e5. doi: 10.1093/rheumatology/keac459
22. Sieiro Santos C, Calleja Antolin S, Moriano Morales C, Garcia Herrero J, Diez Alvarez E, Ramos Ortega F, et al. Immune responses to mRNA vaccines against SARS-CoV-2 in patients with immune-mediated inflammatory rheumatic diseases. RMD Open (2022) 8(1):e001898. doi: 10.1136/rmdopen-2021-001898
23. Oster ME, Shay DK, Su JR, Gee J, Creech CB, Broder KR, et al. Myocarditis cases reported after mRNA-based COVID-19 vaccination in the US from december 2020 to august 2021. JAMA (2022) 327(4):331–40. doi: 10.1001/jama.2021.24110
24. Xie Y, Liu Y, Liu Y. The flare of rheumatic disease after SARS-coV-2 vaccination: A review. Front Immunol (2022) 13:919979. doi: 10.3389/fimmu.2022.919979
Keywords: COVID-19 mRNA vaccine, systemic lupus erythematosus, Sjögren’s syndrome, psoriatic arthritis, SARS-CoV-2
Citation: An Z, Figueroa-Parra G, Zhou X, Li Y, Jaquith J, McCarthy-Fruin K, Sletten J, Warrington KJ, Weyand C, Crowson CS, Chumsri S, Knutson KL, Sanchez-Rodriguez A, Thanarajasingam U, Duarte-García A and Zeng H (2023) Immune responses and disease biomarker long-term changes following COVID-19 mRNA vaccination in a cohort of rheumatic disease patients. Front. Immunol. 14:1224702. doi: 10.3389/fimmu.2023.1224702
Received: 18 May 2023; Accepted: 17 July 2023;
Published: 31 July 2023.
Edited by:
Andras Perl, Upstate Medical University, United StatesReviewed by:
Robin Arcani, Assistance Publique Hôpitaux de Marseille, FranceMaria Manuela Rosado, Sapienza University of Rome, Italy
Copyright © 2023 An, Figueroa-Parra, Zhou, Li, Jaquith, McCarthy-Fruin, Sletten, Warrington, Weyand, Crowson, Chumsri, Knutson, Sanchez-Rodriguez, Thanarajasingam, Duarte-García and Zeng. This is an open-access article distributed under the terms of the Creative Commons Attribution License (CC BY). The use, distribution or reproduction in other forums is permitted, provided the original author(s) and the copyright owner(s) are credited and that the original publication in this journal is cited, in accordance with accepted academic practice. No use, distribution or reproduction is permitted which does not comply with these terms.
*Correspondence: Hu Zeng, zeng.hu1@mayo.edu
†These authors have contributed equally to this work and share first authorship