- School of Life Sciences and Food Engineering, Hebei University of Engineering, Handan, China
The liver plays pivotal roles in immunologic responses, and correct hepatic adaptations in maternal immunology are required during pregnancy. In this review, we focus on anatomical and immunological maternal hepatic adaptations during pregnancy, including our recent reports in this area. Moreover, we summarize maternal pregnancy-associated liver diseases, including hyperemesis gravidarum; intrahepatic cholestasis of pregnancy; preeclampsia, specifically hemolysis, elevated liver enzymes, and low platelet count syndrome; and acute fatty liver of pregnancy. In addition, the latest information about the factors that regulate hepatic immunology during pregnancy are reviewed for the first time, including human chorionic gonadotropin, estrogen, progesterone, growth hormone, insulin like growth factor 1, oxytocin, adrenocorticotropic hormone, adrenal hormone, prolactin, melatonin and prostaglandins. In summary, the latest progress on maternal hepatic anatomy and immunological adaptations, maternal pregnancy-associated diseases and the factors that regulate hepatic immunology during pregnancy are discussed, which may be used to prevent embryo loss and abortion, as well as pregnancy-associated liver diseases.
1 Introduction
The liver contains macrophages (resident Kupffer cells, KCs), natural killer (NK) cells, natural killer T (NKT) cells, and reticuloendothelial cell networks that act as immune sentinels and effector cells to detect and capture circulating pathogens (1). There are physiological, hormonal and immunological adaptations in the mother during pregnancy, and the decidua and placenta form key immunological barriers to protect the fetus and promote its growth (2). However, the immunological responses at the receptive maternal-fetal interface are highly dynamic and not simply suppressed, and a tolerogenic maternal immune system is required for a successful pregnancy (3). Furthermore, peripheral maternal immune system components are dynamically modulated during normal pregnancy, and significant systemic immunological adaptation is necessary to avoid detrimental immune responses against the allogeneic fetus (4). As a frontline immune tissue, the liver can mount a rapid and robust immune response, and portal blood can transport a large number of foreign but harmless molecules (5). In addition, the liver works as a lymphoid organ to facilitate tolerance rather than immunoreactivity, which is essential for fetal immune tolerance (6).
Successful pregnancy is dependent on correct hepatic adaptations in maternal immunology. However, there has not been a review that focuses on maternal liver immunology during pregnancy. In this review, we first focus on our current understanding of anatomical and immunological maternal hepatic adaptations, as well as pregnancy-associated hepatic diseases. Then, we summarize the factors that regulate hepatic immunology during pregnancy. It is essential to avoid detrimental immune responses against the allogeneic fetus and prevent maternal hepatic diseases related to pregnancy.
2 Hepatic cellular anatomy
The microscopic architecture of the liver is similar in all mammals, although there are obvious differences in the hepatic lobation and gallbladder between rodents and humans. The internal structure of the liver consists of small hexagonal lobule that is the functional structural unit of the liver, and includes hepatocyte plates and a central vein (7). The terminal twigs of the portal vein, hepatic artery and bile duct surround the central vein at the portal field (8). In addition, the hepatic lobule contains the portal vein, hepatic artery, bile duct and hepatic sinusoid (Figure 1). The liver is composed of hepatocytes, biliary epithelial cells, stellate cells, KCs, and liver sinusoidal endothelial cells (LSECs) that cooperatively regulate hepatic function at multiple levels (7).
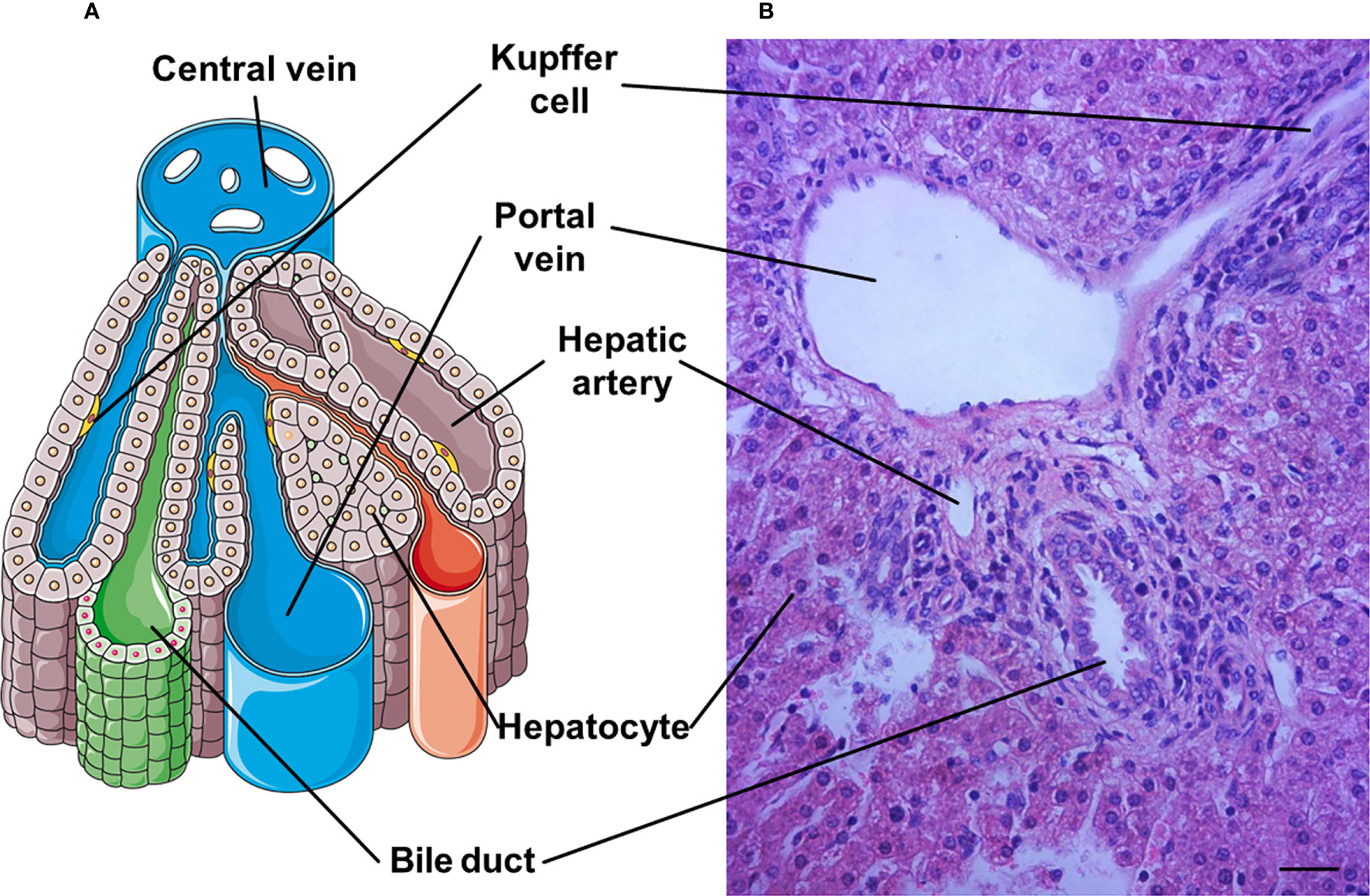
Figure 1 A representative hepatic lobule. (A) The representative diagram of a hepatic lobule. (B) Hepatic tissue stained by haematoxylin and eosin includes a representative histological image of hepatic lobule. A portal triad is a component of the hepatic lobule, consists of proper hepatic artery, hepatic portal vein, small bile duct and hepatic sinusiod. Branches of the portal vein and the hepatic artery merge upon entry into the liver lobule at the portal field for blood supply of the liver, and exits at the central vein. Bar = 50 µm.
3 Anatomical maternal hepatic adaptations
There is gestational hepatomegaly to meet the increasing metabolic demands during pregnancy. The size of the liver increases, and is proportional to the increase in body weight of pregnant rats. Furthermore, liver growth during pregnancy is related to maternal body weight gain, fetal number and hepatocyte hypertrophy, which is induced by circulating reproductive hormones in rodents (9). Moreover, the increase in liver size with pregnancy is regulated by the reproductive state and fetal growth during pregnancy in women (10). In addition, pregnancy-induced hepatomegaly is initiated following implantation, peaks at parturition and is associated with interleukin (IL)-6, tumor necrosis factor (TNF)-α, c-JUN and IL-1β, and the activation of hepatic signal transducer and activator of transcription (STAT) 3, β-catenin and epidermal growth factor receptor (11).
It has been reported that portal venous and total liver blood flows are significantly increased after 28 weeks gestation, but the hepatic arterial blood flow shows no significant changes during pregnancy in humans (12). Furthermore, the resistive index in the common hepatic artery decreases during the third trimester of pregnancy, and this decrease is related to systemic arterial vasodilatation during normal pregnancy (13). Moreover, the flow velocity of the portal vein is higher in the first trimester than in the second and third trimesters and can be used as an indicator of physiological alterations related to pregnancy (14). However, there may be more cytokines and growth factors related to anatomical maternal hepatic adaptations during pregnancy, and so further studies on this area may be needed.
4 The immunological structure of the liver
Two blood supplies in the liver, the hepatic artery and the portal vein, carry potential pathogens, microbe-derived molecules, nutrients, and old, oxidized, or damaged molecules for clearance (5). The small diameter of the sinusoids, minimal systemic venous pressure and perturbations in sinusoidal flow slow blood flow in the liver, which lengthens the time during which the liver can facilitate intimate interactions between resident immune cells and nonhematopoietic hepatic cells, and ultimately, the blood leaves the liver via the central hepatic veins (15).
In the liver, sinusoidal blood is actively scanned by a dense network of intravascular macrophages (such as KCs), a monolayer of LSECs, dendritic cells (DCs), hepatic stellate cells (HSCs), and liver-resident lymphocytes, including NKT cells, NK cells, and γδ-T cells, and these cells can activate and initiate the immune response through the production of cytokines and the recruitment of additional immune cells (16). The hepatic immunological structure mainly includes hepatocytes, HSCs, LSECs, KCs, DCs and lymphocytes, which are involved in the modulation of maternal immune responses (Figure 2).
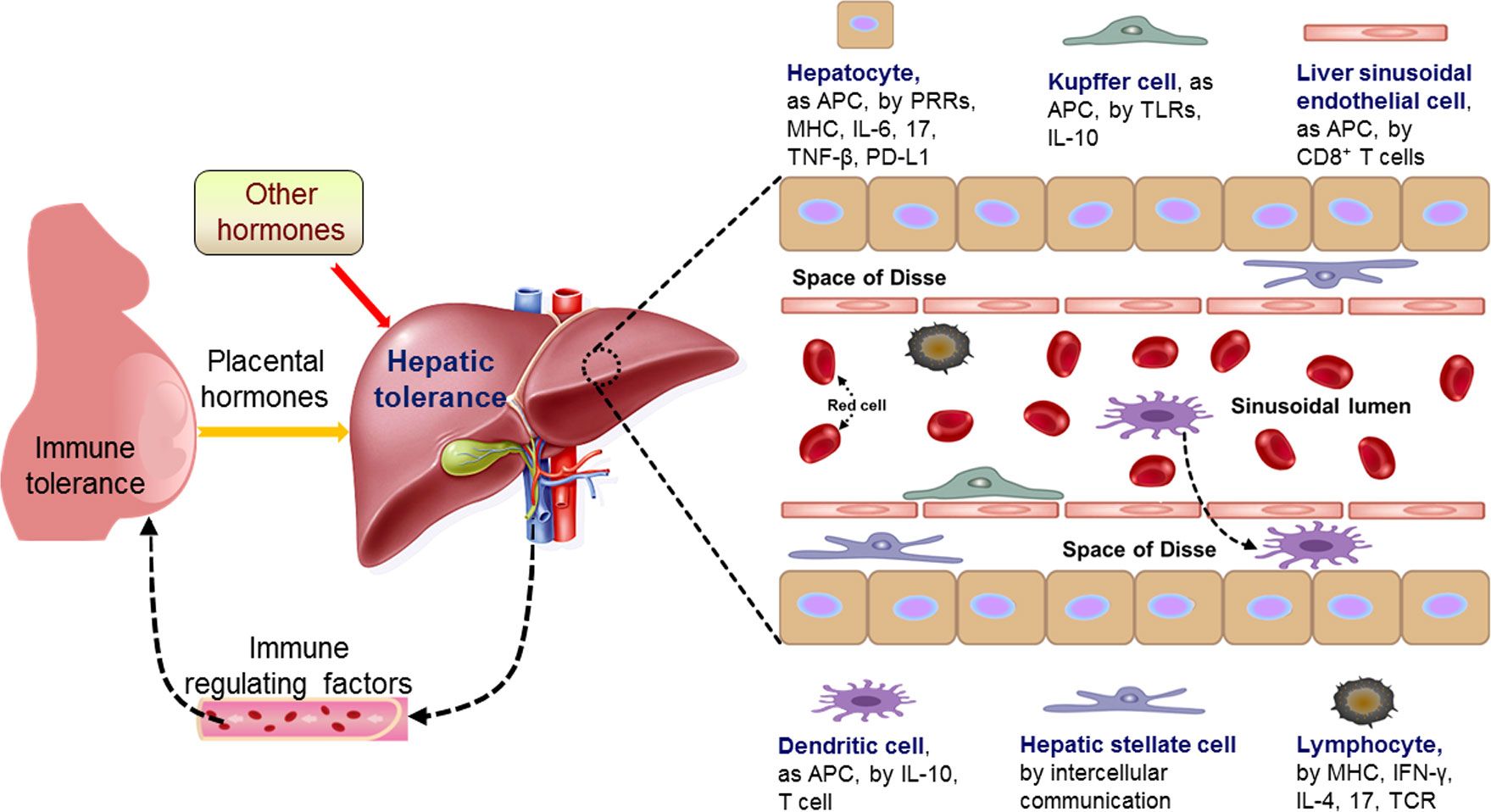
Figure 2 Pregnancy modulates maternal hepatic immune tolerance by placental hormones and other hormones. During pregnancy, the immune functions of maternal hepatic cells are modulated by placental hormones and other hormones, which lead to hepatic tolerance based on the recent advances. The changed immune regulating factors from the liver modulate maternal immune tolerance through blood circulation. Hepatocytes as antigen-presenting cells (APCs), are involved in regulating hepatic immune tolerance by modulation of pattern recognition receptor (PRRs), major histocompatibility complex (MHC), interleukin-6 (IL-6), IL-17, tumor necrosis factor (TNF)-β and programmed death ligand 1 (PD-L1), and kupffer cells, as APCs, are by regulation of toll-like receptors (TLRs) and IL-10. Furthermore, liver sinusoidal endothelial cells, as APCs, participate in hepatic immune tolerance by activation of CD8+ T cells and dendritic cells, as APCs, are implicated in hepatic immune tolerance by IL-10 and T cell. Moreover, hepatic stellate cells are by intercellular communication, and lymphocytes are by MHC, interferon-gamma (IFN-γ), IL-4, IL-17 and T cell receptor (TCR). Liver DCs can transverse the LSECs, enter the space of Disse.
4.1 Hepatocytes
The primary roles of hepatocytes are metabolism, protein production (including immune proteins), and toxin neutralization, and these cells express a wide variety of immune receptors (including pattern recognition receptor and major histocompatibility complex (MHC)) that are involved in hepatocyte-mediated immunity and the adaptive immune response (17, 18). Studies have shown that hepatocytes act as antigen-presenting cells (APCs) to activate naive CD4+ or CD8+ T cells (19, 20). In addition, proinflammatory hepatocytes are implicated in the recruitment of macrophages, and the recruited macrophages release cytokines (including IL-6, TNF-β, and IL-17) to induce the expression of inhibitory ligands, such as programmed death ligand 1 (PD-L1), in hepatocytes (21). Furthermore, hepatocytes produce a wide variety of innate immune proteins, including liver-enriched transcription factors and proinflammatory mediators, which modulate the expression of these innate immune proteins (22). Therefore, hepatocytes are implicated in the maternal adaptive immune response.
4.2 Kupffer cells
KCs are the largest population of liver-resident macrophages, and fill the sinusoidal lumens to scan for any foreign material in the blood. KCs express Toll-like receptors (TLRs), complement receptors, antibody receptors, and molecules that are involved in detecting, binding and internalizing pathogens, and activating KCs (1).
KCs can act as incompetent APCs, and suppress T-cell activation induced by other potent APCs, contributing to liver-mediated systemic immune tolerance (23). In addition, hepatic regulatory T cells (Tregs) and KCs prevent the establishment of the cytotoxic T lymphocyte response by inducing the secretion of the immunosuppressive cytokine IL-10 and create a local suppressive microenvironment in the liver (24). Moreover, KCs clear activated self cells, limit the potential of these cells to secrete inflammatory mediators, and participate in regulating inflammation (1). Therefore, KCs are involved in hepatic immunoregulation by producing immune-related cytokines and chemokines and cooperating with other innate immune cells.
4.3 Liver sinusoidal endothelial cells
LSECs form the wall of the hepatic sinusoids and play key roles in the maintenance of hepatic homeostasis and control of the hepatic immune response (25). LSECs have anti-inflammatory and anti-fibrogenic properties by inhibiting KC and HSC activation, and regulating intrahepatic vascular resistance and portal pressure (26). In addition, LSECs not only present endogenous antigens to CD8+ T cells but also efficiently function as true APCs in the cross-presentation of exogenous antigens. Moreover, LSECs can cross-present soluble exogenous antigens in the circulation to CD8+ T cells, leading to CD8+ T-cell tolerance rather than immunity (27). Therefore, LSECs act as APCs and modulate KC and HSC activation to participate in hepatic immunoregulation.
4.4 Liver dendritic cells
Liver DCs are derived from the bone marrow and are typically located around the central veins and portal tracts (16). DCs are the major APCs within all tissues of the body, and liver DCs can serve as a priming site for liver-infiltrating T cells in response to inflammation (28, 29). In addition, liver DCs internalize antigens derived from the blood, but DCs are poor APCs and promote tolerance rather than the activation of T cells under basal conditions (1). Furthermore, liver DCs mature during intrahepatic translocation from the portal circulation to the central vein (30), and liver DCs can transverse LSECs, enter the space of Disse and exit the liver via lymphatic drainage (31).
Liver DCs can be broadly divided into myeloid DCs (mDCs), plasmacytoid DCs (pDCs) and other DCs, and mDCs are further defined as CD11b+ and CD11c+ cells in mice (32). In contrast to mDCs and pDCs in the liver, CD11c+CD8+ DCs support robust T-cell activation, leading to the generation of T helper 1 (Th1)-type responses (29, 33). Furthermore, the suppression of immunosuppressive signals, such as the blockade of IL-10, can improve the development of DCs that enhance antigen presentation, costimulatory molecule expression, and T-cell activation in the liver (34). Therefore, liver DCs regulate hepatic immune tolerance by modulating T-cell activation depending on signals from the local environment.
4.5 Hepatic stellate cells
HSCs reside in the space of Disse, have an astral phenotype and play central roles in liver development, hepatocyte homeostasis, and the coordinated response of the liver to injury (35). There is intercellular communication via soluble mediators and cytokines/chemokines between HSCs and neighboring cell types, including hepatocytes, biliary epithelial cells, hepatic progenitor cells, KCs, bone marrow-derived macrophages, LSECs, infiltrating immune cells, and nerve cells (29). HSCs can be activated by many signaling molecules and convergent pathways that include fibrogenic, proliferative, and inflammatory cytokines, hedgehog signaling, metabolic reprogramming, cholesterol signaling, and oxidative stress. Furthermore, cytokines and growth factors secreted by hepatic immune cells are the most potent activating signals for HSCs, and activated HSCs express innate immune receptors related to TLRs and the complement pathway that are direct activators of HSCs (35). Therefore, hepatic immune cells secrete cytokines and growth factors that activate HSCs in a paracrine manner, which regulate hepatocyte homeostasis and the adaptive response.
4.6 Liver-resident lymphocytes
The liver contains both resident and transiting lymphocytes. There are multiple types of liver-resident lymphocytes, including memory CD8+ T cells, unconventional T cells (invariant NKT cells, mucosal-associated invariant T-cells, and γδT cells), NK cells and other innate lymphoid cells (36). CD8+ T cells are enriched in the liver, and the largest population of γδ T cells is in the liver. In addition, nearly half of lymphocytes in the liver express the T-cell receptor (TCR) (37). Furthermore, γδ T cells are distinct from conventional T-cells and can bind to several different foreign and self ligands in both MHC-dependent and MHC-independent manners, contributing to the immune response and immunopathology (38).
Liver NK cells express a restricted TCR repertoire and respond to lipid antigens to directly kill target cells via perforin and granzyme. In addition, NK cells can initiate both inflammatory and anti-inflammatory responses and produce a wide array of cytokines, including interferon-gamma (IFN-γ), IL-4 and IL-17 (39). There is an expanded population of liver-resident CD4+ T cells in patients with primary sclerosing cholangitis (PSC) compared to healthy individuals, and naive CD4+ T cells in PSC patients trend to develop into Th17 cells (40). Therefore, liver-resident lymphocytes produce cytokines that modulate the hepatic immune response and immunopathology, as well as systemic immune status.
5 Immunological adaptations in the maternal liver
In the liver, self and foreign antigens are presented by DCs, LSECs, KCs, HSCs and hepatocytes, which induce the expression of anti-inflammatory mediators and inhibitory cell surface ligands associated with T-cell activation, and contribute to systemic tolerance and the local immunosuppressive milieu (41). In addition, hepatic microenvironmental factors induce the expression of tolerogenic human leukocyte antigen G proteins in hepatic immune cells, hepatocytes and liver epithelial cells, which are associated with the tolerogenic properties of the liver (42). Furthermore, as a type-1 membrane glycoprotein, CD200 contains two immunoglobulin superfamily domains, is expressed in hepatic endothelial structures, and plays a pivotal role in the systemic immune tolerogenic effect of the liver (43). On the other hand, trophoblasts express CD200, which counter-regulates the Th1-type proinflammatory cytokine-inducible fibrinogen-related prothrombinase fgl2. However, fgl2 can terminate pregnancy via thrombin activation, and CD200-dependent downregulation of the Th1-type cytokine response is related to maternal hepatic tolerance during pregnancy (44). Moreover, acetaminophen-induced liver inflammation interferes with maternal immune and endocrine adaptation, which increases plasma alanine aminotransferase, decreases plasma progesterone, and morphologically alters the placenta (45).
There are increases in the expression of interferon-stimulated gene 15-kDa protein (ISG15) and myxovirus resistance protein 1 (Mx1) in the bovine liver during the late peri-implantation period (46). Our previous studies showed ISG15 and ISG15-conjugated proteins are upregulated in the ovine liver during early pregnancy. In addition, 2’,5’-oligoadenylate synthetase 1, Mx1, interferon-gamma-inducible protein 10 and STAT1 are increased in the maternal liver during early pregnancy (47).
Our previous studies showed that early pregnancy regulates the expression of Th cytokines in the ovine maternal liver, including IL-2, IFN-γ, TNF-β, IL-4, IL-5, IL-6, and IL-10 (48), and the TLR pathway is modulated in the ovine maternal liver during early pregnancy (49). The expression of nuclear factor kappaB (NF-κB) signaling in the ovine maternal liver, including NF-κB1 (p50), NF-κB2 (p52), RelA (p65), RelB and c-Rel, is modulated during early pregnancy in sheep (50). In addition, early pregnancy modulates the expression of the IkappaB (IκB) family, including B-cell leukemia-3 (BCL-3), IκBα, IκBβ, IκBϵ, IKKγ, IκBNS and IκBζ in the livers of ewes (51). Furthermore, the expression of complement components in the maternal liver, including C1q, C1r, C1s, C2, C4a, C5b and C9, is changed in ewes (52). Moreover, the expression of CD4 is improved, and nod-like receptor expression is regulated in the maternal liver during early pregnancy in sheep (53, 54). Therefore, pregnancy modulates the expression of many cytokines and signaling pathways, which contribute to systemic tolerance and the local immunosuppressive milieu in the liver (Figure 3). However, more studies are needed to confirm whether other cytokines and signaling pathways are associated with maternal hepatic adaptations during pregnancy.
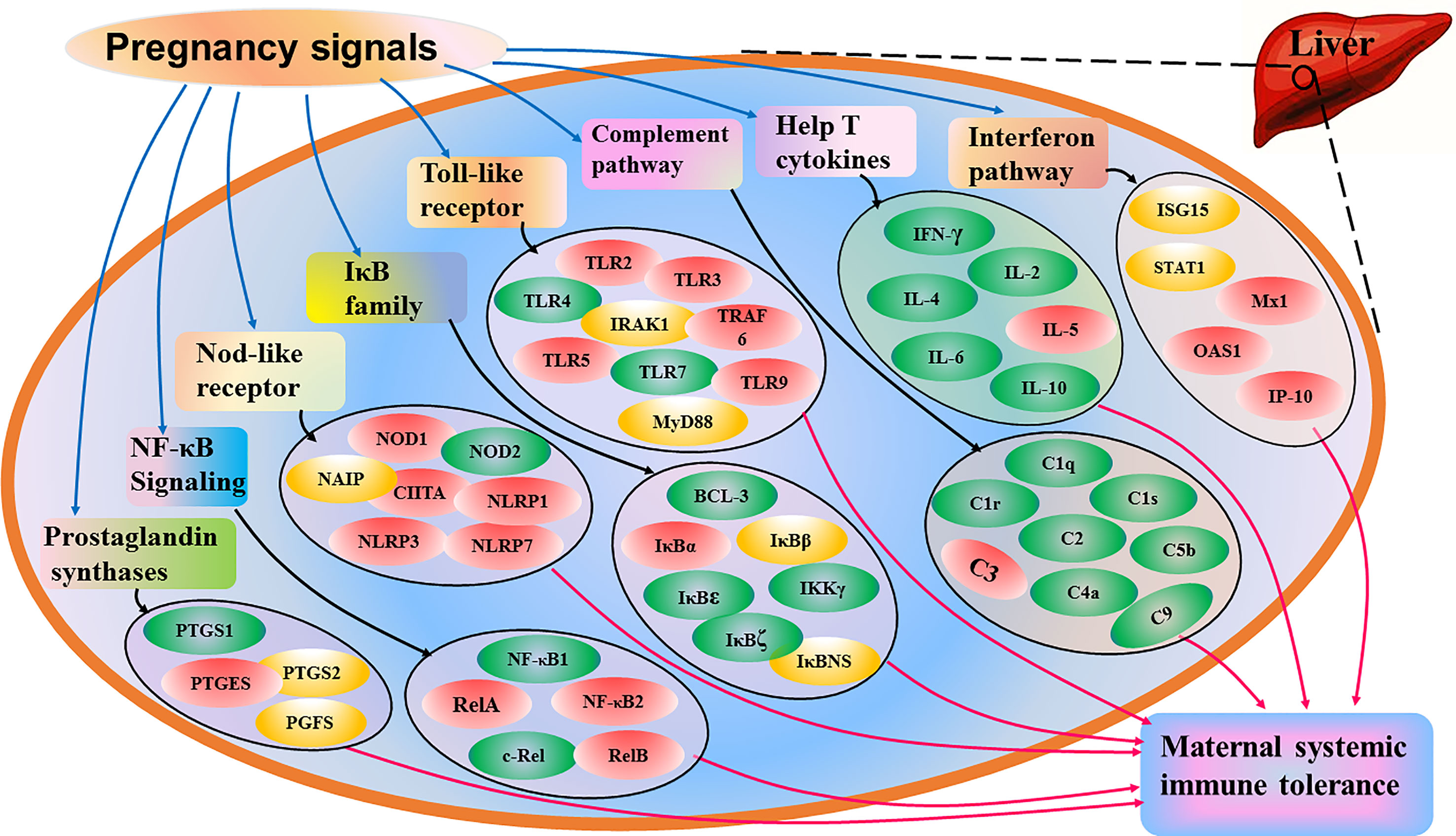
Figure 3 Sketch of immunological adaptations in maternal liver during pregnancy based on the recent advances. Pregnancy signals induce changed expression of interferon pathway, help T cytokines, complement pathway, toll-like receptor, IkappaB (IκB) family, nuclear factor kappaB (NF-κB) signaling, nod-like receptor and prostaglandins E synthase, which contributes to maternal systemic immune tolerance during pregnancy. Interferon pathway includes interferon-stimulated gene 15 kDa protein (ISG15), signal transducer and activator of transcription 1 (STAT1), myxovirusresistance protein 1 (Mx1), 2’,5’-oligoadenylate synthetase 1 (OAS1) and interferon-gamma-inducible protein 10 (IP-10), and help T cytokines include interleukin (IL)-2, interferon-gamma (IFN-γ), tumor necrosis factor (TNF)-β, IL-4, IL-5, IL-6 and IL-10. Complement pathway includes complement components C1q, C1r, C1s, C2, C3, C4, C5 and C9, and toll-like receptors (TLRs) include TLR2, TLR3, TLR4, TLR5, TLR7, TLR9, myeloid differentiation primary-response protein 88 (MyD88), tumor necrosis factor receptor associated factor 6 (TRAF6) and interleukin-1-receptor-associated kinase 1 (IRAK1). IkappaB (IκB) family includes B cell leukemia-3 (BCL-3), IκBα, IκBβ, IκBϵ, IKKγ, IκBζ and IκBNS, and nuclear factor kappaB (NF-κB) signaling includes NF-κB1, NF-κB2, RelA, RelB and c-Rel. Nucleotide-binding oligomerization domain (NOD) receptors (NLRs) NOD1, NOD2, major histocompatibility complex class II transactivator (CIITA), neuronal apoptosis inhibitor protein (NAIP), NLR family, pyrin domain-containing 1 (NLRP1), NLRP3 and NLRP7, and prostaglandin synthases include prostaglandin-endoperoxide synthase 1 (PTGS1), PTGS2, PGE synthase (PTGES) and PGF synthase (PGFS). Note: red, stimulators; green, negative regulators; yellow, changed.
6 Maternal pregnancy-associated liver diseases
There are four main pregnancy-associated liver diseases that include hyperemesis gravidarum (HG); intrahepatic cholestasis of pregnancy (ICP); liver disorders associated with preeclampsia, specifically hemolysis, elevated liver enzymes, and low platelet count syndrome (HELLP); and acute fatty liver of pregnancy (AFLP) (Figure 4). These liver diseases are closely associated with significant maternal and fetal/neonatal morbidity and mortality (55). Inflammation induced by environmental factors and general stress leads to defects in immune tolerance of the maternal liver. The local and systemic immune tolerance is related to liver resident nonconventional APCs, including LSECs, HSCs and hepatocytes (44).
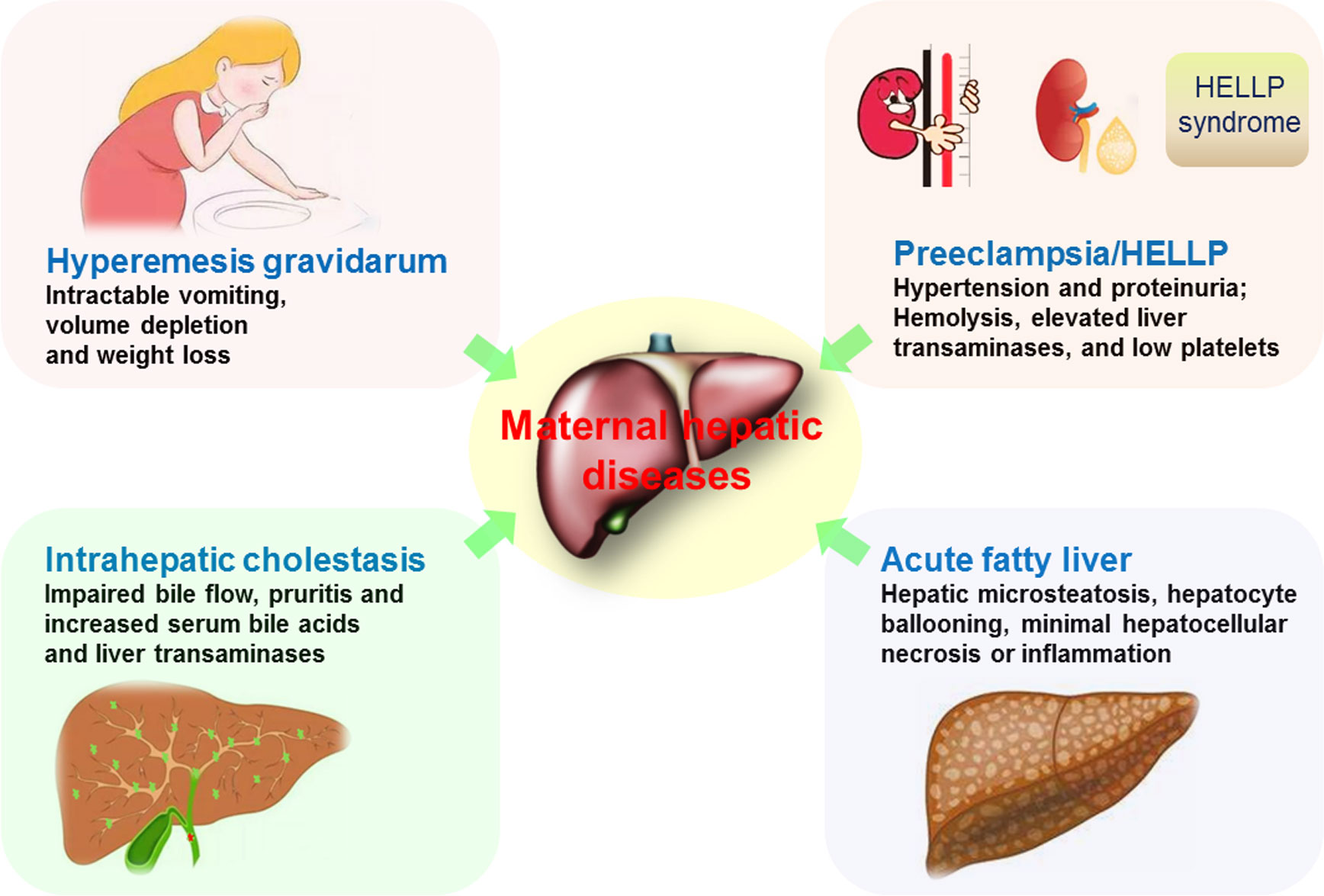
Figure 4 Maternal pregnancy-associated liver diseases. Hyperemesis gravidarum is described as intractable vomiting that leads to volume depletion and weight loss. Intrahepatic cholestasis has the impaired bile flow that results in pruritis and increases in serum bile acids and liver transaminases. Preeclampsia presents hypertension and proteinuria, and HELLP is associated with preeclampsia, characterized by hemolysis, elevated liver enzymes, and low platelet count syndrome. Acute fatty liver of pregnancy is characterized by hepatic microsteatosis, hepatocyte ballooning, minimal hepatocellular necrosis or inflammation.
6.1 Hyperemesis gravidarum
HG is described as intractable vomiting during pregnancy, results in volume depletion and weight loss, and starts at 4 or 5 weeks during the first trimester of pregnancy (56). HG has a multifactorial etiology, including molar pregnancy, multifetal pregnancy, hypoadrenalism, hyperthyroidism, cerebral malignancy, and gastrointestinal obstruction (55).
The causes of HG are related to hormones in maintaining pregnancy, and the level of human chorionic gonadotropin (hCG) correlates closely with HG during pregnancy (57). Ketonuria levels and inflammatory markers platelet-to-lymphocyte ratio, monocyte-to-lymphocyte ratio, and neutrophil-to-lymphocyte ratio are higher in pregnant patients with HG (58). Th1 and Th2 cytokines, estrogen, progesterone receptor, growth/differentiation factor 15 and its receptor, glial-derived neurotrophic factor family receptor alpha-like are closely related to HG (44, 56). Th cytokines, including IFN-γ, IL-2, IL-4, IL-6 and IL-10, decrease, but IL-5 increases in the maternal liver during early pregnancy in an animal model (48). Progesterone receptor expression level upregulates in the maternal liver during early pregnancy, which is associated with the modulation of maternal hepatic immune function in an animal model (59).
The treatment for HG includes pharmaceutical treatment and replenishing lost nutrients. Pharmaceutical treatment includes antiemetics plus correcting electrolytes, as well as intravenous fluids and considering admission if needed (44). The prevention includes nutritional support, such as vitamin replacement, and enteral tube feeding (55). Further study should focus on establishing underlying etiology for designing individualized management strategies, and long-term safety of new therapies for the mother.
6.2 Intrahepatic cholestasis of pregnancy
ICP is defined as impaired bile flow, the accumulation of bile constituents in the liver and blood, which causes pruritus and increases in serum bile acids and liver transaminases, and is also related to fetal distress, spontaneous and iatrogenic preterm birth, and stillbirth (60). ICP starts at the last trimester of pregnancy in the majority of patients, and also possibly onsets earlier (44).
The causes of ICP are related to mechanical obstruction and deficiency in cellular transporters. The familial cholestasis type 1, bile salt export pump and multidrug resistance p-glycoprotein 3, ABC transporter superfamily, vascular endothelial growth factor, estrogen, MHC class I molecule, vascular cell adhesion molecule, Th cytokines are closely associated with ICP (44, 61). In addition, peroxisomal acyl-CoA oxidase 1, L-palmitoylcarnitine, and glycocholic acid expression levels in placental and serum are significantly higher in third-trimester ICP patients (62).
The mainly treatment for ICP is with ursodeoxycholic acid (UDCA) that is a bile acid. UDCA treatment reduces spontaneous preterm birth in ICP, in particular in singleton pregnancies with maternal serum bile acid concentrations 40 μmol/L or more, but has no significant effect on the prevalence of stillbirth in women with ICP (63). Rifampicin combines with UDCA treatment reduces pruritus and serum bile acid concentration, and Rifampicin can improve cholestatic pruritus in nonpregnant individuals (55). Studies are needed for the future on improving pregnancy outcome and maternal pruritus using new therapies, and identifying women at risk of future hepatobiliary disease via genetic tests.
6.3 Preeclampsia/HELLP
The preeclampsia is definited as the presence of hypertension and proteinuria occurring after 20 weeks of gestation in a previously normotensive patient. Systemic manifestations of preeclampsia include low platelets or elevated liver enzymes, and preeclampsia also results in other maternal organ dysfunction and fetal growth restriction (64). HELLP syndrome is a pregnancy-associated liver disease, and termed as a severe complication of preeclampsia.
The causes of preeclampsia/HELLP are mainly related to abnormal placentation, trophoblast invasion and maternal-fetal interface (65), which result in increased release of inflammatory mediators into the maternal circulation, imbalance between pro- and anti-angiogenic factors, liver and vascular dysfunction, damaged endothelium, vasospasm, platelet aggregation, endothelial damage and hemolytic anemia (55). There are many cytokines that are related to preeclampsia/HELLP, including TNF-α, transforming growth factor-β, a disintegrin and metalloproteases with thrombospondin type 1 motif 12, IL-1 family, IL-17, IL-18-binding protein, IL-33, intercellular adhesion molecule-1, monocyte chemoattractant peptide 1, pentraxin 3, soluble vascular adhesion molecule 1, C reactive protein, soluble fms-like tyrosine kinase-1, vascular endothelial growth factor A, pregnancy-associated plasma protein A2, aspartate aminotransferase, alanine aminotransferase, Th cytokines, soluble Fas ligand, soluble CD40 ligand (44).
The mainly treatment for preeclampsia is with low dose prophylactic aspirin, MgSO4, oral calcium, vitamin D, melatonin, detoxification of reactive oxygen species, and treatment for HELLP with corticosteroid (44). It is reported that prophylactic low-dose aspirin can reduce the risk of preterm preeclampsia, but no drugs can influence disease progression except for delivery (66). Future researches needed include identification of new and refinement of biomarkers for predicting preeclampsia, and evaluation of hemostasis markers of acute liver injury for aiding the management of bleeding/thrombosis complications in HELLP.
6.4 Acute fatty liver of pregnancy
AFLP is definited as jaundice with rapidly progressive liver failure in the third trimester of pregnancy, and characterized by hepatic microsteatosis, hepatocyte ballooning, minimal hepatocellular necrosis or inflammation (55).
The causes of AFLP are associated with long-chain acyl-coenzyme A dehydrogenase deficiency, mitochondrial dysfunction and abnormal β-oxidation of fatty acids long-chain fatty acid (44). In addition, the maternal characteristics, including first pregnancy, multifetal pregnancy, male fetus, and low body mass index, are related to AFLP (55).
The mainly treatment for AFLP is with intensive care treatment, including plasma exchange, plasma perfusion, or a combination of both. Immediate delivery is also a choice in most cases, and severe cases may require liver transplantation (44). There are infectious complications in AFLP, so low dose antibiotics treatment is recommended (55). Further studies are needed in identification of biomarkers of at-risk pregnancies to minimize maternal and fetal complications, and delineation of risk factors, such as environmental risks, maternal conditions and family history.
7 Factors that regulate hepatic immunology
Maternal endocrine hormone profiles are modulated during pregnancy, and these hormones include steroid hormones, growth hormone (GH)/insulin-like growth factor 1 (IGF-1), prolactin, adrenocorticotropic hormone (ACTH), human chorionic gonadotropin (hCG), metanephrines, and aldosterone (67). In summary, the factors that regulate hepatic immunology include hCG, estrogen, progesterone, GH/IGF-1, prolactin, ACTH, aldosterone, adrenaline, melatonin, oxytocin and prostaglandins (PGs), which are implicated in the regulation of hepatic immunological adaptations during pregnancy (Figure 5).
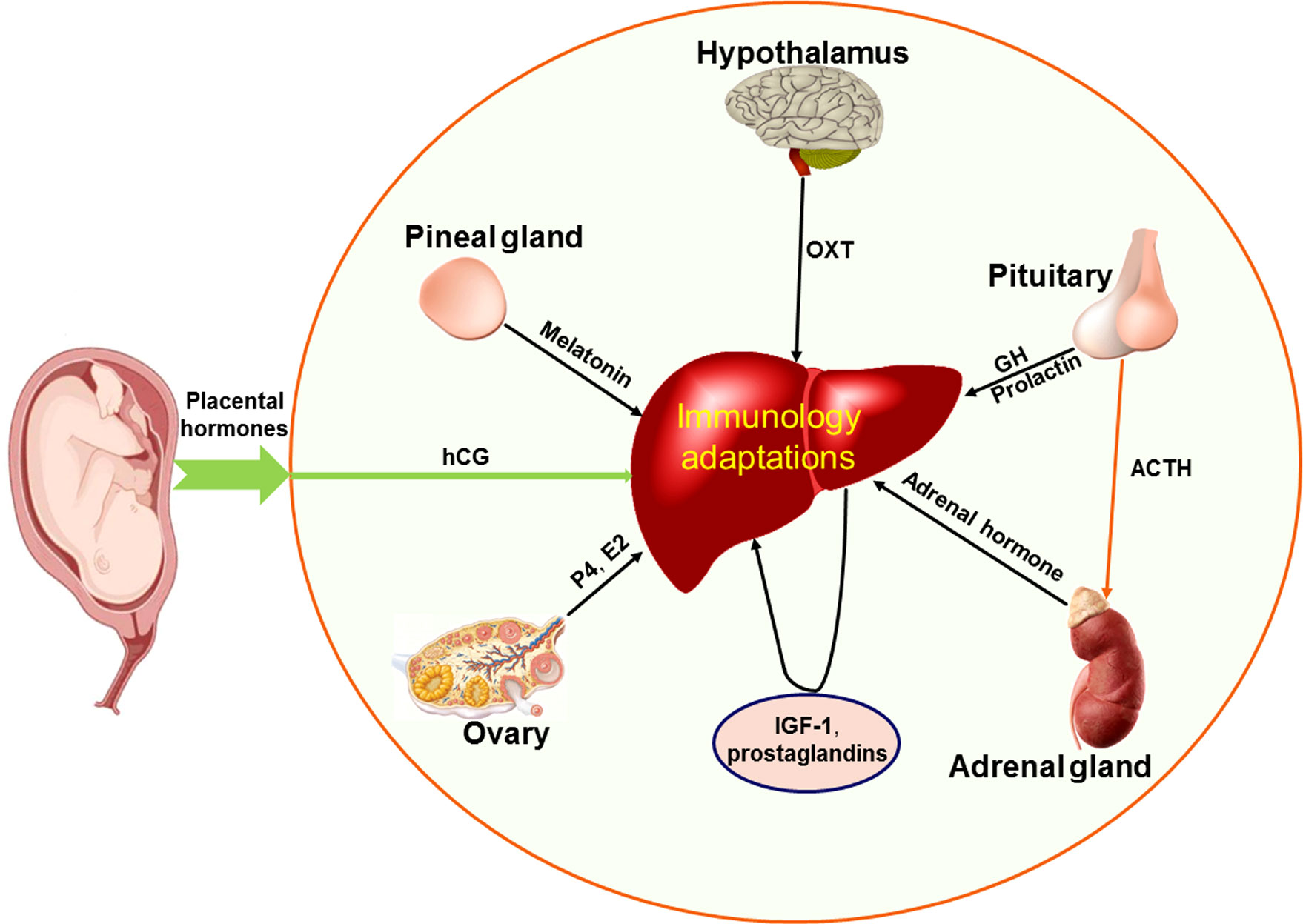
Figure 5 Factors that regulate maternal hepatic immunological adaptations during pregnancy based on the recent advances. During pregnancy, placental hormones (including human chorionic gonadotropin, hCG) have effects on the liver, ovaries, hypothalamus, pituitary gland, pineal gland and adrenal glands, which participate in regulating maternal hepatic immunological adaptations during pregnancy. Other hormones and hCG directly modulate the hepatic immunology. The other hormones include estrogen (E2) and progesterone (P4) mainly produced by ovaries, insulin like growth factor 1 (IGF-1) and prostaglandins produced by the liver in a paracrine manner, oxytocin (OXT) produced by hypothalamus, growth hormone (GH), adrenocorticotropic hormone (ACTH) and prolactin produced by pituitary gland, melatonin secreted by the pineal gland, and adrenal hormone mainly produced by adrenal glands.
7.1 Human chorionic gonadotropin
The placenta secretes hCG, which has a series of effects on the survival of the embryo during pregnancy (68). It has been reported that chorionic gonadotropin can induce maternal hepatocyte proliferation during early pregnancy in mice (69). Silent mating type information regulation 2 homolog 1 and forkhead box o3 axis in hepatocytes is regulated by hCG, which participates in immune suppression, and has therapeutic effects on inflammatory liver diseases (70). However, hCG is also closely related to HG during pregnancy (57). Therefore, the therapeutic effects of hCG on pregnancy-associated liver diseases need further study.
7.2 Estrogen
Serum estrogen levels increase during normal pregnancy (71), and estrogen stimulates hepatocyte proliferation in late pregnancy in mice (67). In addition, significant increases in estrogen increase CD4+ CD25+ Treg cells in peripheral blood to influence the immune function of pregnant women (72). Estrogen signaling is related to hepatic oxidative damage and the expression level of peroxisome proliferator-activated receptor-γ (PPARG) coactivator 1α (PGC1A) in the mouse liver, and these factors are involved in steatohepatitis (73). However, estrogen has protective effects on liver diseases, including nonalcoholic fatty liver disease (NAFLD) and nonalcoholic steatohepatitis (NASH) (74). Therefore, further investigations may focus on the effects of estrogen on liver health during normal pregnancy.
7.3 Progesterone
Serum progesterone levels are upregulated during normal pregnancy, and the immune system is modulated by circulating progesterone, which plays a key role in halothane-induced liver injury via immune-related responses (75). Progesterone is related with decreases in the inflammatory reactions, the activation of immune cells and the production of cytokines, which are essential for the successful pregnancy (76). Our recent study showed that progesterone receptor and progesterone-induced blocking factor were upregulated in the ovine liver during early pregnancy, which is involved in the regulation of maternal hepatic immune and other functions in an endocrine manner (59). Progesterone can increase hepatitis E virus (HEV) replication and results in severe HEV through activation of the nonclassical progesterone receptor membrane component 1/2 signaling pathway, rather than immunomodulation of HEV-induced interferon response (77). Vaginal progesterone levels are positively related to ICP, and progesterone metabolites are increased during pregnancy, which enhances the risk of ICP (78). Progesterone use is related to more severe lobular inflammation and influences NAFLD pathobiology (79). High levels of progesterone are favorable for pregnancy maintenance but are also the main cause of maternal pregnancy-associated liver diseases. Therefore, the effects of progesterone on liver health should be considered in future studies.
7.4 Growth hormone/insulin like-growth factor 1
GH produced by the pituitary gland regulates the production of IGF-1 by the liver, and its effects are directly mediated by the GH receptor (GHR). There is an inverse association between hepatic expression of the IGF system (IGF-1 and GHR) and certain cytokines (IL-1β, IL-18 and TNF-α) and acute-phase proteins (serum amyloid A and haptoglobin) in the liver, which are associated with hepatic immunomodulation in pigs (80). In addition, GH activates c-JUN to increase the induction of the regulator of calcineurin 1-4, which affects the activity of calcineurin in the rat liver and participates in T-cell activation and gluconeogenesis (81). Furthermore, IGF-1 inhibits the expression of TNF-α, IL-1β, and cytokine-induced neutrophil chemoattractant 1 and accelerates the degradation of inducible nitric oxide synthase caused by D-galactosamine and lipopolysaccharide to prevent liver injury through an NF-κB-independent pathway in rats (82). GH replacement therapy improves NAFLD/NASH, and IGF-I treatment can improve NASH and cirrhosis in animal models (83).
Hepatic GH-binding protein and GHR mRNA levels are upregulated during pregnancy (84), but the expression levels of hepatic GHR 1A and IGF-1 mRNA are downregulated at parturition in cows (85). In addition, the expression level of IGF binding protein 4 (IGFBP-4) mRNA is increased in the maternal liver, but the expression level of IGFBP-3 mRNA is decreased during pregnancy in rats (86). Therefore, GH and IGF-1 are involved in the regulation of maternal liver function during pregnancy and have therapeutic effects on liver diseases.
7.5 Prolactin
Prolactin is primarily synthesized in the anterior pituitary gland, as well as the central nervous system, the immune system, and the uterus (87). In addition, prolactin improves normal liver growth, survival, and regeneration in prolactin receptor (PRLR)-dependent and/or independent manners, which are associated with hepatic IL-6, a suppressor of cytokine signaling-3 in rodents (88). Furthermore, prolactin suppresses mitogen-activated protein kinase-dependent activation of c-Myc via PRLR to prevent tumor-promoting liver inflammation in mice (89).
Our recent study showed that early pregnancy inhibited the expression of prolactin and PRLR in the liver in ewes, and PRLR protein was located in hepatocytes, endothelial cells of the proper hepatic arteries and hepatic portal veins (90). PRLR is suppressed in mammary glandular tissue during periods of high progesterone levels and increases when the serum progesterone level drops in mice (78). Prolactin ameliorates hepatic steatosis via PRLR and fatty acid translocase, which can be used to improve NAFLD (91). Therefore, prolactin has therapeutic effects on liver diseases, but the use of prolactin in liver disease therapy during pregnancy should be done with caution.
7.6 ACTH, aldosterone and adrenaline
Adrenal glands mainly produce corticosteroid-, aldosterone-, cortisol- and adrenaline-type hormones. Aldosterone promotes caveolin 1-related selective autophagy and F-actin remodeling by activating the AMP-activated protein kinase (AMPK)-ULK1 pathway and inhibiting the NO-dependent pathway, which induces LSEC defenestration via aldosterone-induced oxidation (92). In addition, aldosterone induces the activation of primary mouse HSCs by promoting the expression and assembly of the NOD-like receptor (NLR) family, pyrin domain containing 3 inflammasome and liver fibrosis (93). Mineralocorticoid receptor (MR) is activated by aldosterone or glucocorticoids, as well as pathological milieu factors, and associated with the development of NAFLD and NASH (94). Treatment with eplerenone, an MR antagonist, inhibits the development of fibrosis and portal hypertension related complications in an animal model (95). However, effects of ACTH, aldosterone and adrenaline on the maternal liver during pregnancy should be investigated.
7.7 Melatonin
Melatonin is secreted by the pineal gland, and acts directly on the liver to elevate plasma glucose levels via melatonin receptor 1B in mouse hepatocytes in a dose-dependent manner (96). Prenatal glucocorticoid overexposure results in maternal hepatic steatosis, the downregulation of leptin and the upregulation of caspase 3 and TNF-α proteins levels, DNA methyltransferase activity and DNA methylation, and melatonin can rescue these adverse effects in the rat liver (97).
Serum melatonin levels are significantly lower in women with ICP than in healthy pregnant women (98). Our study showed that melatonin receptor 1A was upregulated in the maternal liver, but melatonin receptor 1B is downregulated during early pregnancy in sheep (54). Sato et al. showed melatonin administration not only had antioxidant effects, but also attenuated liver steatosis in NAFLD, increased functions and enzyme activity in mitochondria to inhibit lipid accumulation in the liver (99). Therefore, the use of melatonin in maternal pregnancy-associated liver disease therapy should be explored.
7.8 Oxytocin
Oxytocin is mainly produced by the hypothalamus, and oxytocin treatment modulates hepatic immune and inflammatory processes to protect against sepsis-induced oxidative damage (100). Oxytocin is synthesized by magnocellular neurons, and oxytocin secretion is upregulated by intracerebroventricular kisspeptin in late pregnancy (101). In addition, oxytocin treatment induces thermogenic gene expression, which ameliorates fatty liver in an animal model (102). Therefore, more studies may focus on the use of oxytocin to treat maternal pregnancy-associated liver disease.
7.9 Prostaglandins
PGs can suppress generation of the reactive oxygen species, modulate production of the inflammatory cytokines and cell adhesion molecules, which have protective effects on ischemia reperfusion-injured livers (103). Intraportal PGE1 infusion can protect extrahepatic cholestatic liver from ischemia reperfusion injury by reducing oxidative stress damage, intrahepatic neutrophil infiltration and hepatocyte apoptosis in rat model of extrahepatic cholestasis (104).
Mesenchymal stem cell (MSC) treatment enhances YAP and β-catenin expression with increasing PGE2 production in the ischemic livers, indicating that PGE2 is related to MSC-mediated immunotherapy of liver sterile inflammatory injury (105). PGE2 levels are higher throughout late pregnancy in mice, and high levels of PGE2 during late pregnancy protect fetuses from inflammatory damage related to IL-1β (106). In addition, prostaglandin-endoperoxide synthase 2, PGE synthase and PGF synthase levels are upregulated in the ovine maternal liver during early pregnancy, which are related to the hepatic immune adjustment during early pregnancy in sheep (107). Therefore, PGs could be used to treat liver disease, but more studies are needed to confirm this use during pregnancy.
8 Conclusion
Pregnancy changes the expression of many cytokines and signaling pathways (Figure 3), which are related to the maintenance of maternal liver homeostasis and immune tolerance, as well as pregnancy-associated diseases. Maternal immunological adaptations in the liver are necessary for successful pregnancy and are directly associated with avoiding embryo loss and abortion. In addition, the liver disorders in pregnancy may be mainly associated with hCG, Th1 and Th2 cytokines, estrogen and progesterone. In this article, the factors that regulate hepatic immunology are discussed, which may be used to avoid detrimental immune responses against the allogeneic fetus and prevent maternal hepatic diseases related to pregnancy. However, many mysteries still exist regarding the modulation of hepatic immune functions during pregnancy. Therefore, future studies are needed in these areas, which will bring new hopes for the prevention of embryo loss and abortion during pregnancy, as well as pregnancy-associated immunological diseases.
Author contributions
All authors contributed to the development of this review article and approved the submitted version. LY and YM searched the literature and drafted the manuscript. LY and YS conceived and designed the review. HF and LZ examined the literature and made the figures. LY made a critical revision of the review. All authors contributed to the article and approved the submitted version.
Funding
This work was supported by the grants from Hebei Postgraduate Innovation Ability Funding Project (CXZZSS2023125), Hebei Natural Science Foundation, China (C2021402019 and C2022402038), and Hebei Science and Technology Agency, China (22326602D).
Conflict of interest
The authors declare that the research was conducted in the absence of any commercial or financial relationships that could be construed as a potential conflict of interest.
Publisher’s note
All claims expressed in this article are solely those of the authors and do not necessarily represent those of their affiliated organizations, or those of the publisher, the editors and the reviewers. Any product that may be evaluated in this article, or claim that may be made by its manufacturer, is not guaranteed or endorsed by the publisher.
References
1. Jenne CN, Kubes P. Immune surveillance by the liver. Nat Immunol (2013) 14:996–1006. doi: 10.1038/ni.2691
2. Ander SE, Diamond MS, Coyne CB. Immune responses at the maternal-fetal interface. Sci Immunol (2019) 4:eaat6114. doi: 10.1126/sciimmunol.aat6114
3. Mor G, Aldo P, Alvero AB. The unique immunological and microbial aspects of pregnancy. Nat Rev Immunol (2017) 17:469–82. doi: 10.1038/nri.2017.64
4. Abu-Raya B, Michalski C, Sadarangani M, Lavoie PM. Maternal immunological adaptation during normal pregnancy. Front Immunol (2020) 11:575197. doi: 10.3389/fimmu.2020.575197
5. Kubes P, Jenne C. Immune responses in the liver. Annu Rev Immunol (2018) 36:247–77. doi: 10.1146/annurev-immunol-051116-052415
6. Bogdanos DP, Gao B, Gershwin ME. Liver immunology. Compr Physiol (2013) 3:567–98. doi: 10.1002/cphy.c120011
7. Trefts E, Gannon M, Wasserman DH. The liver. Curr Biol (2017) 27:R1147–R51. doi: 10.1016/j.cub.2017.09.019
8. Frevert U, Engelmann S, Zougbédé S, Stange J, Ng B, Matuschewski K, et al. Intravital observation of plasmodium berghei sporozoite infection of the liver. PLoS Biol (2005) 3:e192. doi: 10.1371/journal.pbio.0030192
9. Milona A, Owen BM, van Mil S, Dormann D, Mataki C, Boudjelal M, et al. The normal mechanisms of pregnancy-induced liver growth are not maintained in mice lacking the bile acid sensor Fxr. Am J Physiol Gastrointest Liver Physiol (2010) 298:G151–8. doi: 10.1152/ajpgi.00336.2009
10. Bartlett AQ, Vesco KK, Purnell JQ, Francisco M, Goddard E, Guan X, et al. Pregnancy and weaning regulate human maternal liver size and function. Proc Natl Acad Sci U S A (2021) 118:e2107269118. doi: 10.1073/pnas.2107269118
11. Dai G, Bustamante JJ, Zou Y, Myronovych A, Bao Q, Kumar S, et al. Maternal hepatic growth response to pregnancy in the mouse. Exp Biol Med (2011) 236:1322–32. doi: 10.1258/ebm.2011.011076
12. Nakai A, Sekiya I, Oya A, Koshino T, Araki T. Assessment of the hepatic arterial and portal venous blood flows during pregnancy with Doppler ultrasonography. Arch Gynecol Obstet (2002) 266:25–9. doi: 10.1007/pl00007495
13. Mandic-Markovic VD, Mikovic ZM, Djukic MK, Vasiljevic MD, Jankovic G. Doppler Parameters of the maternal hepatic artery blood flow in normal pregnancy: maternal hepatic artery blood flow in normal pregnancy. Eur J Obstet Gynecol Reprod Biol (2014) 181:275–9. doi: 10.1016/j.ejogrb.2014.08.010
14. Bozgeyik Z, Ozdemir H, Kocakoc E, Simsek M. Hepatic and portal venous Doppler waveforms and flow velocities in normal pregnancy. Med Sci Monit (2009) 15:CR624–7.
15. Robinson MW, Harmon C, O'Farrelly C. Liver immunology and its role in inflammation and homeostasis. Cell Mol Immunol (2016) 13:267–76. doi: 10.1038/cmi.2016.3
16. Racanelli V, Rehermann B. The liver as an immunological organ. Hepatology (2006) 43:S54–62. doi: 10.1002/hep.21060
17. Getachew A, Hussain M, Huang X, Li Y. Toll-like receptor 2 signaling in liver pathophysiology. Life Sci (2021) 284:119941. doi: 10.1016/j.lfs.2021.119941
18. Paul-Heng M, Leong M, Cunningham E, Bunker DLJ, Bremner K, Wang Z, et al. Direct recognition of hepatocyte-expressed MHC class I alloantigens is required for tolerance induction. JCI Insight (2018) 3:e97500. doi: 10.1172/jci.insight.97500
19. Bertolino P, Bowen DG, McCaughan GW, Fazekas de St Groth B. Antigen-specific primary activation of CD8+ T cells within the liver. J Immunol (2001) 166:5430–8. doi: 10.4049/jimmunol.166.9.5430
20. Bertolino P, McCaughan GW, Bowen DG. Role of primary intrahepatic T-cell activation in the 'liver tolerance effect'. Immunol Cell Biol (2002) 80:84–92. doi: 10.1046/j.0818-9641.2001.01048.x
21. Sun X, Wu J, Liu L, Chen Y, Tang Y, Liu S, et al. Transcriptional switch of hepatocytes initiates macrophage recruitment and T-cell suppression in endotoxemia. J Hepatol (2022) 77:436–52. doi: 10.1016/j.jhep.2022.02.028
22. Zhou Z, Xu MJ, Gao B. Hepatocytes: a key cell type for innate immunity. Cell Mol Immunol (2016) 13:301–15. doi: 10.1038/cmi.2015.97
23. You Q, Cheng L, Kedl RM, Ju C. Mechanism of T cell tolerance induction by murine hepatic Kupffer cells. Hepatology (2008) 48:978–90. doi: 10.1002/hep.22395
24. Breous E, Somanathan S, Vandenberghe LH, Wilson JM. Hepatic regulatory T cells and kupffer cells are crucial mediators of systemic T cell tolerance to antigens targeting murine liver. Hepatology (2009) 50:612–21. doi: 10.1002/hep.23043
25. Gracia-Sancho J, Caparrós E, Fernández-Iglesias A, Francés R. Role of liver sinusoidal endothelial cells in liver diseases. Nat Rev Gastroenterol Hepatol (2021) 18:411–31. doi: 10.1038/s41575-020-00411-3
26. Hammoutene A, Rautou PE. Role of liver sinusoidal endothelial cells in non-alcoholic fatty liver disease. J Hepatol (2019) 70:1278–91. doi: 10.1016/j.jhep.2019.02.012
27. Limmer A, Ohl J, Kurts C, Ljunggren HG, Reiss Y, Groettrup M, et al. Efficient presentation of exogenous antigen by liver endothelial cells to CD8+ T cells results in antigen-specific T-cell tolerance. Nat Med (2000) 6:1348–54. doi: 10.1038/82161
28. Eckert C, Klein N, Kornek M, Lukacs-Kornek V. The complex myeloid network of the liver with diverse functional capacity at steady state and in inflammation. Front Immunol (2015) 6:179. doi: 10.3389/fimmu.2015.00179
29. Heymann F, Tacke F. Immunology in the liver–from homeostasis to disease. Nat Rev Gastroenterol Hepatol (2016) 13:88–110. doi: 10.1038/nrgastro.2015.200
30. Sato T, Yamamoto H, Sasaki C, Wake K. Maturation of rat dendritic cells during intrahepatic translocation evaluated using monoclonal antibodies and electron microscopy. Cell Tissue Res (1998) 294:503–14. doi: 10.1007/s004410051201
31. Kudo S, Matsuno K, Ezaki T, Ogawa M. A novel migration pathway for rat dendritic cells from the blood: hepatic sinusoids-lymph translocation. J Exp Med (1997) 185:777–84. doi: 10.1084/jem.185.4.777
32. Crispe IN. Liver antigen-presenting cells. J Hepatol (2011) 54:357–65. doi: 10.1016/j.jhep.2010.10.005
33. Doherty DG. Immunity, tolerance and autoimmunity in the liver: a comprehensive review. J Autoimmun (2016) 66:60–75. doi: 10.1016/j.jaut.2015.08.020
34. Kingham TP, Chaudhry UI, Plitas G, Katz SC, Raab J, DeMatteo RP. Murine liver plasmacytoid dendritic cells become potent immunostimulatory cells after Flt-3 ligand expansion. Hepatology (2007) 45:445–54. doi: 10.1002/hep.21457
35. Carter JK, Friedman SL. Hepatic stellate cell-immune interactions in NASH. Front Endocrinol (2022) 13:867940. doi: 10.3389/fendo.2022.867940
36. Wang Y, Zhang C. The roles of liver-resident lymphocytes in liver diseases. Front Immunol (2019) 10:1582. doi: 10.3389/fimmu.2019.01582
37. Parker GA, Picut CA. Liver immunobiology. Toxicol Pathol (2005) 33:52–62. doi: 10.1080/01926230590522365
38. Vantourout P, Hayday A. Six-of-the-best: unique contributions of γδ T cells to immunology. Nat Rev Immunol (2013) 13:88–100. doi: 10.1038/nri3384
39. Brennan PJ, Brigl M, Brenner MB. Invariant natural killer T cells: an innate activation scheme linked to diverse effector functions. Nat Rev Immunol (2013) 13:101–17. doi: 10.1038/nri3369
40. Poch T, Krause J, Casar C, Liwinski T, Glau L, Kaufmann M, et al. Single-cell atlas of hepatic T cells reveals expansion of liver-resident naive-like CD4+ T cells in primary sclerosing cholangitis. J Hepatol (2021) 75:414–23. doi: 10.1016/j.jhep.2021.03.016
41. Tiegs G, Lohse AW. Immune tolerance: what is unique about the liver. J Autoimmun (2010) 34:1–6. doi: 10.1016/j.jaut.2009.08.008
42. Amiot L, Vu N, Samson M. Biology of the immunomodulatory molecule HLA-G in human liver diseases. J Hepatol (2015) 62:1430–7. doi: 10.1016/j.jhep.2015.03.007
43. Wright GJ, Jones M, Puklavec MJ, Brown MH, Barclay AN. The unusual distribution of the neuronal/lymphoid cell surface CD200 (OX2) glycoprotein is conserved in humans. Immunology (2001) 102:173–9. doi: 10.1046/j.1365-2567.2001.01163.x
44. Bremer L, Schramm C, Tiegs G. Immunology of hepatic diseases during pregnancy. Semin Immunopathol (2016) 38:669–85. doi: 10.1007/s00281-016-0573-1
45. Thiele K, Solano ME, Huber S, Flavell RA, Kessler T, Barikbin R, et al. Prenatal acetaminophen affects maternal immune and endocrine adaptation to pregnancy, induces placental damage, and impairs fetal development in mice. Am J Pathol (2015) 185:2805–18. doi: 10.1016/j.ajpath.2015.06.019
46. Meyerholz MM, Mense K, Knaack H, Sandra O, Schmicke M. Pregnancy-induced ISG-15 and MX-1 gene expression is detected in the liver of Holstein-Friesian heifers during late peri-implantation period. Reprod Domest Anim (2016) 51:175–7. doi: 10.1111/rda.12638
47. Yang L, Li N, Zhang L, Bai J, Zhao Z, Wang Y. Effects of early pregnancy on expression of interferon-stimulated gene 15, STAT1, OAS1, MX1, and IP-10 in ovine liver. Anim Sci J (2020) 91:e13378. doi: 10.1111/asj.13378
48. Yang L, Bai J, Zhao Z, Li N, Wang Y, Zhang L. Differential expression of T helper cytokines in the liver during early pregnancy in sheep. Anim Reprod (2019) 16:332–9. doi: 10.21451/1984-3143-AR2018-0141
49. Gao M, Cai C, Han X, Wang L, Zhang W, Zhang L, et al. The early stage of pregnancy modulates toll-like receptor signaling in the ovine liver. J Appl Anim Res (2021) 49:374–81. doi: 10.1080/09712119.2021.1990935
50. Fang S, Zhang T, Qiao H, Hao S, Zhang L, Yang L. Expression of nuclear factor kappa B components in the ovine maternal liver in early pregnancy periods. Anim Sci J (2022) 93:e13724. doi: 10.1111/asj.13724
51. Zhang L, Ren Y, Li Y, Meng Y, Fang H, Yang L. Regulation of nod-like receptor expression in the liver of ewes during early pregnancy. J Anim Feed Sci (2023) 32:267–79. doi: 10.22358/jafs/161622/2023
52. Feng P, Yang G, Zhang W, Zhang L, Wu J, Yang L. Early pregnancy regulates expression of complement components in ovine liver. Anim Sci J (2021) 92:e13660. doi: 10.1111/asj.13660
53. Cai C, Ren Y, Cao J, Fang S, Zhang L, Yang L. Expression of IkappaB family in the ovine liver during early pregnancy. Animals (2023) 13:1057. doi: 10.3390/ani13061057
54. Bai J, Zhang L, Zhao Z, Li N, Wang B, Yang L. Expression of melatonin receptors and CD4 in the ovine thymus, lymph node, spleen and liver during early pregnancy. Immunology (2020) 160:52–63. doi: 10.1111/imm.13180
55. Terrault NA, Williamson C. Pregnancy-associated liver diseases. Gastroenterology (2022) 163:97–117.e1. doi: 10.1053/j.gastro.2022.01.060
56. Liu C, Zhao G, Qiao D, Wang L, He Y, Zhao M, et al. Emerging progress in nausea and vomiting of pregnancy and hyperemesis gravidarum: challenges and opportunities. Front Med (2022) 8:809270. doi: 10.3389/fmed.2021.809270
57. Niebyl JR. Clinical practice. nausea and vomiting in pregnancy. N Engl J Med (2010) 363:1544–50. doi: 10.1056/NEJMcp1003896
58. Soysal C, Işıkalan MM, Bıyık İ, Erten Ö, İnce O. The relationship between inflammation markers and ketonuria in hyperemesis gravidarum. J Obstet Gynaecol Res (2021) 47:3078–83. doi: 10.1111/jog.14857
59. Zhang L, Zhuang C, Zhao Z, Li N, Bai J, Yang L. Effect of early pregnancy on the expression of progesterone receptor and progesterone-induced blocking factor 1 in ovine liver. Czech J Anim Sci (2019) 64:317–23. doi: 10.17221/21/2019-CJAS
60. Palmer KR, Xiaohua L, Mol BW. Management of intrahepatic cholestasis in pregnancy. Lancet (2019) 393:853–4. doi: 10.1016/S0140-6736(18)32323-7
61. Schneider G, Paus TC, Kullak-Ublick GA, Meier PJ, Wienker TF, Lang T, et al. Linkage between a new splicing site mutation in the MDR3 alias ABCB4 gene and intrahepatic cholestasis of pregnancy. Hepatology (2007) 45:150–8. doi: 10.1002/hep.21500
62. Dong R, Ye N, Zhao S, Wang G, Zhang Y, Wang T, et al. Studies on novel diagnostic and predictive biomarkers of intrahepatic cholestasis of pregnancy through metabolomics and proteomics. Front Immunol (2021) 12:733225. doi: 10.3389/fimmu.2021.733225
63. Ovadia C, Sajous J, Seed PT, Patel K, Williamson NJ, Attilakos G, et al. Ursodeoxycholic acid in intrahepatic cholestasis of pregnancy: a systematic review and individual participant data meta-analysis. Lancet Gastroenterol Hepatol (2021) 6:547–58. doi: 10.1016/S2468-1253(21)00074-1
64. Rana S, Lemoine E, Granger JP, Karumanchi SA. Preeclampsia: pathophysiology, challenges, and perspectives. Circ Res (2019) 124:1094–112. doi: 10.1161/CIRCRESAHA.118.313276
65. Alese MO, Moodley J, Naicker T. Preeclampsia and HELLP syndrome, the role of the liver. J Matern Fetal Neonatal Med (2021) 34:117–23. doi: 10.1080/14767058.2019.1572737
66. Chappell LC, Cluver CA, Kingdom J, Tong S. Pre-eclampsia. Lancet (2021) 398:341–54. doi: 10.1016/S0140-6736(20)32335-7
67. Morton A, Teasdale S. Physiological changes in pregnancy and their influence on the endocrine investigation. Clin Endocrinol (2022) 96:3–11. doi: 10.1111/cen.14624
68. Gridelet V, Perrier d'Hauterive S, Polese B, Foidart JM, Nisolle M, Geenen V. Human chorionic gonadotrophin: new pleiotropic functions for an "old" hormone during pregnancy. Front Immunol (2020) 11:343. doi: 10.3389/fimmu.2020.00343
69. Cho J, Tsugawa Y, Imai Y, Imai T. Chorionic gonadotropin stimulates maternal hepatocyte proliferation during pregnancy. Biochem Biophys Res Commun (2021) 579:110–5. doi: 10.1016/j.bbrc.2021.09.039
70. Steinmetz C, Kashyap A, Zhivkova N, Alizor H, Ernst I, Gottfried-Brand D, et al. Activation of silent mating type information regulation 2 homolog 1 by human chorionic gonadotropin exerts a therapeutic effect on hepatic injury and inflammation. Hepatology (2017) 65:2074–89. doi: 10.1002/hep.29072
71. Varas SM, Jahn GA. The expression of estrogen, prolactin, and progesterone receptors in mammary gland and liver of female rats during pregnancy and early postpartum: regulation by thyroid hormones. Endocr Res (2005) 31:357–70. doi: 10.1080/07435800500454528
72. Xiong YH, Yuan Z, He L. Effects of estrogen on CD4(+) CD25(+) regulatory T cell in peripheral blood during pregnancy. Asian Pac J Trop Med (2013) 6:748–52. doi: 10.1016/S1995-7645(13)60131-5
73. Besse-Patin A, Léveillé M, Oropeza D, Nguyen BN, Prat A, Estall JL. Estrogen signals through peroxisome proliferator-activated receptor-γ coactivator 1α to reduce oxidative damage associated with diet-induced fatty liver disease. Gastroenterology (2017) 152:243–56. doi: 10.1053/j.gastro.2016.09.017
74. Lee C, Kim J, Jung Y. Potential therapeutic application of estrogen in gender disparity of nonalcoholic fatty liver disease/nonalcoholic steatohepatitis. Cells (2019) 8:1259. doi: 10.3390/cells8101259
75. Toyoda Y, Miyashita T, Endo S, Tsuneyama K, Fukami T, Nakajima M, et al. Estradiol and progesterone modulate halothane-induced liver injury in mice. Toxicol Lett (2011) 204:17–24. doi: 10.1016/j.toxlet.2011.03.031
76. Raghupathy R, Szekeres-Bartho J. Progesterone: a unique hormone with immunomodulatory roles in pregnancy. Int J Mol Sci (2022) 23(3):1333. doi: 10.3390/ijms23031333
77. Sooryanarain H, Ahmed SA, Meng XJ. Progesterone-mediated enhancement of hepatitis E virus replication in human liver cells. mBio (2021) 12:e0143421. doi: 10.1128/mBio.01434-21
78. Xu L, Yuan Y, Che Z, Tan X, Wu B, Wang C, et al. The hepatoprotective and hepatotoxic roles of sex and sex-related hormones. Front Immunol (2022) 13:939631. doi: 10.3389/fimmu.2022.939631
79. Yang JD, Abdelmalek MF, Guy CD, Gill RM, Lavine JE, Yates K, et al. Nonalcoholic steatohepatitis clinical research network. patient sex, reproductive status, and synthetic hormone use associate with histologic severity of nonalcoholic steatohepatitis. Clin Gastroenterol Hepatol (2017) 15:127–131.e2. doi: 10.1016/j.cgh.2016.07.034
80. Slifierz MJ, Friendship R, de Lange CF, Slavic D, Grgic H, Farzan A. Immunomodulatory factors and infectious agents associated with the hepatic gene expression of the IGF system in nursery pigs. Animal (2014) 8:844–51. doi: 10.1017/S1751731114000275
81. Nakata T, Toyoshima Y, Yagi T, Katsumata H, Tokita R, Minami S. Growth hormone increases regulator of calcineurin 1-4 (Rcan1-4) mRNA through c-JUN in rat liver. PLoS One (2020) 15:e0235270. doi: 10.1371/journal.pone.0235270
82. Hijikawa T, Kaibori M, Uchida Y, Yamada M, Matsui K, Ozaki T, et al. Insulin-like growth factor 1 prevents liver injury through the inhibition of TNF-alpha and iNOS induction in d-galactosamine and LPS-treated rats. Shock (2008) 29:740–7. doi: 10.1097/shk.0b013e31815d0780
83. Takahashi Y. The role of growth hormone and insulin-like growth factor-I in the liver. Int J Mol Sci (2017) 18:1447. doi: 10.3390/ijms18071447
84. Ilkbahar YN, Southard JN, Talamantes F. Transcriptional upregulation of hepatic GH receptor and GH-binding protein expression during pregnancy in the mouse. J Mol Endocrinol (1999) 23:85–96. doi: 10.1677/jme.0.0230085
85. Radcliff RP, McCormack BL, Crooker BA, Lucy MC. Growth hormone (GH) binding and expression of GH receptor 1A mRNA in hepatic tissue of periparturient dairy cows. J Dairy Sci (2003) 86:3933–40. doi: 10.3168/jds.S0022-0302(03)74002-8
86. Rosato R, Lindenbergh-Kortleve D, Neck Jv, Drop S, Jahn G. Effect of chronic thyroxine treatment on IGF-I, IGF-II and IGF-binding protein expression in mammary gland and liver during pregnancy and early lactation in rats. Eur J Endocrinol (2002) 146:729–39. doi: 10.1530/eje.0.1460729
87. Freeman ME, Kanyicska B, Lerant A, Nagy G. Prolactin: structure, function, and regulation of secretion. Physiol Rev (2000) 80:1523–631. doi: 10.1152/physrev.2000.80.4.1523
88. Moreno-Carranza B, Goya-Arce M, Vega C, Adán N, Triebel J, López-Barrera F, et al. Prolactin promotes normal liver growth, survival, and regeneration in rodents: effects on hepatic IL-6, suppressor of cytokine signaling-3, and angiogenesis. Am J Physiol Regul Integr Comp Physiol (2013) 305:R720–6. doi: 10.1152/ajpregu.00282.2013
89. Hartwell HJ, Petrosky KY, Fox JG, Horseman ND, Rogers AB. Prolactin prevents hepatocellular carcinoma by restricting innate immune activation of c-myc in mice. Proc Natl Acad Sci U S A (2014) 111:11455–60. doi: 10.1073/pnas.1404267111
90. Feng P, Wu J, Ren Y, Zhang L, Cao J, Yang L. Early pregnancy regulates the expression of prolactin and its receptor in the thymus, the liver, the spleen and lymph nodes in sheep. Domest Anim Endocrinol (2022) 81:106731. doi: 10.1016/j.domaniend.2022.106731
91. Zhang P, Ge Z, Wang H, Feng W, Sun X, Chu X, et al. Prolactin improves hepatic steatosis via CD36 pathway. J Hepatol (2018) 68:1247–55. doi: 10.1016/j.jhep.2018.01.035
92. Luo X, Wang D, Luo X, Zhu X, Wang G, Ning Z, et al. Caveolin 1-related autophagy initiated by aldosterone-induced oxidation promotes liver sinusoidal endothelial cells defenestration. Redox Biol (2017) 13:508–21. doi: 10.1016/j.redox.2017.07.011
93. Li Y, Zhang Y, Chen T, Huang Y, Zhang Y, Geng S, et al. Role of aldosterone in the activation of primary mice hepatic stellate cell and liver fibrosis via NLRP3 inflammasome. J Gastroenterol Hepatol (2020) 35:1069–77. doi: 10.1111/jgh.14961
94. Schreier B, Zipprich A, Uhlenhaut H, Gekle M. Mineralocorticoid receptors in non-alcoholic fatty liver disease. Br J Pharmacol (2022) 179:3165–77. doi: 10.1111/bph.15784
95. Schreier B, Wolf A, Hammer S, Pohl S, Mildenberger S, Rabe S, et al. The selective mineralocorticoid receptor antagonist eplerenone prevents decompensation of the liver in cirrhosis. Br J Pharmacol (2018) 175:2956–67. doi: 10.1111/bph.14341
96. Poon AM, Choy EH, Pang SF. Modulation of blood glucose by melatonin: a direct action on melatonin receptors in mouse hepatocytes. Biol Signals Recept (2001) 10:367–79. doi: 10.1159/000046904
97. Tiao MM, Huang LT, Chen CJ, Sheen JM, Tain YL, Chen CC, et al. Melatonin in the regulation of liver steatosis following prenatal glucocorticoid exposure. BioMed Res Int (2014) 2014:942172. doi: 10.1155/2014/942172
98. Çelik S, Guve H, Çalışkan C, Çelik S. The role of melatonin, IL-8 and IL-10 in intrahepatic cholestasis of pregnancy. Z Geburtshilfe Neonatol (2021) 225:238–43. doi: 10.1055/a-1233-9084
99. Sato K, Meng F, Francis H, Wu N, Chen L, Kennedy L, et al. Melatonin and circadian rhythms in liver diseases: functional roles and potential therapies. J Pineal Res (2020) 68:e12639. doi: 10.1111/jpi.12639
100. Işeri SO, Sener G, Saglam B, Gedik N, Ercan F, Yegen BC. Oxytocin protects against sepsis-induced multiple organ damage: role of neutrophils. J Surg Res (2005) 126:73–81. doi: 10.1016/j.jss.2005.01.021
101. Abbasi M, Perkinson MR, Seymour AJ, Piet R, Campbell RE, Iremonger KJ, et al. Local kisspeptin excitation of rat oxytocin neurones in late pregnancy. J Physiol (2022) 600:1753–70. doi: 10.1113/JP282531
102. Yuan J, Zhang R, Wu R, Gu Y, Lu Y. The effects of oxytocin to rectify metabolic dysfunction in obese mice are associated with increased thermogenesis. Mol Cell Endocrinol (2020) 514:110903. doi: 10.1016/j.mce.2020.110903
103. Hossain MA, Wakabayashi H, Izuishi K, Okano K, Yachida S, Maeta H. The role of prostaglandins in liver ischemia-reperfusion injury. Curr Pharm Des (2006) 12:2935–51. doi: 10.2174/138161206777947678
104. Xu F, Liu X, Wang C, Dai C. Prostaglandin E1 preconditioning attenuates liver ischemia reperfusion injury in a rat model of extrahepatic cholestasis. BioMed Res Int (2018) 2018:3812424. doi: 10.1155/2018/3812424
105. Li C, Jin Y, Wei S, Sun Y, Jiang L, Zhu Q, et al. Hippo signaling controls NLR family pyrin domain containing 3 activation and governs immunoregulation of mesenchymal stem cells in mouse liver injury. Hepatology (2019) 70:1714–31. doi: 10.1002/hep.30700
106. Guo Q, Li J, Zhong L, Cai H, Wang H, Yan H, et al. Prostaglandin-E2 deficiency during late pregnancy and the associated increase in interleukin-1β derived from periaortic lymph nodes lead to abortion. Mol Hum Reprod (2019) 25:825–37. doi: 10.1093/molehr/gaz058
Keywords: hormone, immunology, liver, pregnancy, growth factor
Citation: Yang L, Meng Y, Shi Y, Fang H and Zhang L (2023) Maternal hepatic immunology during pregnancy. Front. Immunol. 14:1220323. doi: 10.3389/fimmu.2023.1220323
Received: 10 May 2023; Accepted: 20 June 2023;
Published: 30 June 2023.
Edited by:
Julia Szekeres-Bartho, University of Pécs, HungaryCopyright © 2023 Yang, Meng, Shi, Fang and Zhang. This is an open-access article distributed under the terms of the Creative Commons Attribution License (CC BY). The use, distribution or reproduction in other forums is permitted, provided the original author(s) and the copyright owner(s) are credited and that the original publication in this journal is cited, in accordance with accepted academic practice. No use, distribution or reproduction is permitted which does not comply with these terms.
*Correspondence: Ling Yang, eWFuZ2xpbmdAaGViZXUuZWR1LmNu