- 1Animal Disease Research Unit, United States Department of Agricultural - Agricultural Research Service, Pullman, WA, United States
- 2Department of Veterinary Microbiology and Pathology, College of Veterinary Medicine, Washington State University, Pullman, WA, United States
Introduction: Live in vivo attenuated Babesia bovis vaccines produced by sequential passages in splenectomized calves have historically been used to control acute bovine babesiosis in endemic areas worldwide. However, several constraints prevent the widespread use of these vaccines, including the need for several splenectomized calves to produce vaccine batches, and potential inconsistent parasite attenuation, which contraindicates their use for highly Babesia-susceptible adult cattle. Thus, the use of vaccines based on well-defined in vitro culture attenuated B. bovis strains emerges as a more sustainable and efficient alternative. Previous work demonstrated that the culture attenuated strain Att-S74-T3Bo is non-tick transmissible and able to safely protect calves against needle challenge with a B. bovis virulent strain.
Methods and results: Herein we evaluated safety and efficacy of Att-S74-T3Bo in preventing acute babesiosis in adult (>1.5 year of age) cattle. Results demonstrated that Att-S74-T3Bo vaccination of adult animals (n=5) induced self-limiting signs of acute infection and protected the vaccinated animals against challenge with the homologous virulent B. bovis strain Vir-S74-T3Bo. Att-S74-T3Bo-vaccinated adult cattle developed significant (P<0.05) monocytosis, with concomitant neutropenia and CD4+ leukopenia, in peripheral blood early after vaccination. Also, vaccinated animals developed a specific signature of pro- and anti-inflammatory cytokine expression in peripheral blood and significant levels of IgM, total IgG, IgG1, and IgG2 against the B. bovis immunodominant antigen RAP-1 CT. Strikingly, none of the vaccinated animals showed any signs of acute babesiosis after challenge with Vir-S74-T3Bo. In contrast, control adult cattle (n=5) showed pathognomonic symptoms of acute babesiosis, and significant decrease (P<0.05) in lymphocytes, monocytes, and neutrophils, starting on day 7 post-challenge. All control animals developed severe acute disease and were euthanized on days 10 through 12 days post-challenge.
Discussion and conclusion: Evidence from this study indicates that Att-S74-T3Bo safely protects highly susceptible adult cattle against challenge with a homologous virulent strain of B. bovis. In conclusion, Att-S74-T3Bo may be considered as a potential efficient and sustainable attenuated candidate vaccine strain to control acute bovine babesiosis in highly susceptible adult cattle. Future studies should focus on increasing the number of animals vaccinated, duration of immunity, and efficacy of this attenuated strain against heterologous virulent parasite strains.
1 Introduction
Apicomplexan hemoparasites of the genus Babesia are the causative agents of babesiosis, a tickborne disease of vertebrates that has a detrimental impact on public and animal health (1, 2). Of particular concern is bovine babesiosis, also known as Texas cattle fever, considering the large number of cattle at-risk of developing the disease in tropical and sub-tropical areas of the world, and the devastating economic impact that it can have on animal protein production worldwide (2). The historical study performed by Smith and Kilbourne in the late 1800s not only defined bovine babesiosis as the first discovered arthropod-transmitted disease but also set the foundation for an intense and effective program to eradicate the Babesia-vector Rhipicephalus microplus ticks from the US and consequently eradication of the disease from the country in the early 1940s (3, 4). However, with current climate changes and consequential emergence and re-emergence of tick populations in the southern states of the US, along with the presence of exotic antelope species in the region and the large number of potential Babesia-infected cattle entering the US from Mexico, serious concerns have been raised regarding the re-introduction of bovine babesiosis into the country (5, 6). Should an outbreak of bovine babesiosis occur, the US cattle industry’s ability to produce animal protein would be significantly compromised with implication on food security.
Babesia bovis is the most virulent and one of the primary causative agents of bovine babesiosis; however, other Babesia species, such as Babesia bigemina, Babesia divergens, Babesia ovata, and Babesia major, have also been implicated in outbreaks among cattle herds worldwide (2, 7–9). Acute B. bovis infection in naïve animals is characterized by fever, anemia, drop in packed cell volume (PCV), anorexia, prostration, and high mortality. B. bovis acute infection can also result in neurological symptoms due to adhesion of infected red blood cells (iRBC) in the brain capillaries, a fatal condition that resembles severe cerebral malaria (10, 11). Animals that are able to survive the acute phase of the disease develop life-long chronic infection and become reservoirs for parasite acquisition and transmission by competent tick vectors. Currently available methods to minimize the impact of bovine babesiosis include the application of acaricides to control tick infestation, utilization of anti-Babesia drugs, and use of live in vivo attenuated vaccines. Unfortunately, none of these approaches are cost-effective and sustainable to control the disease in endemic areas and to prevent its introduction into at-risk regions. For instance, in vivo attenuated vaccines are relatively effective in protecting cattle against bovine babesiosis in endemic areas, but they present several constraints that preclude their wide-spread use. Among these limitations are: (a) the need of several splenectomized calves for vaccine preparation; (b) the potential risk of recombination between vaccine and field parasite strains during sexual reproduction inside tick vectors, which can result in the emergence of novel virulent daughter strains; and (c) insufficient parasite attenuation that prevents the vaccine use for highly Babesia-susceptible adult cattle (>1-year old) (12–14). Another limitation of the in vivo attenuated Babesia vaccines is the potential development of inadequate protection after vaccination, especially against heterologous parasite strains, which may cause outbreaks of acute bovine babesiosis in vaccinated herds of cattle (13, 15, 16). As a result of all these limitations, the use of live in vivo attenuated B. bovis vaccines is not currently licensed in the US. Pondering this scenario, and the current absence of subunit vaccines against B. bovis, or any other Babesia species, it becomes urgent to investigate effective and sustainable novel vaccines to prevent the devastating effects of acute bovine babesiosis.
Considering the need for developing control strategies against the disease, different factors, such as genetics, age, and immunological status, among others, are known to be implicated in determining the cattle’s susceptibility to acute B. bovis infection. It has been shown that European Bos taurus breeds are markedly more susceptible to the parasite than Bos indicus (2, 17). Also, it has been well-documented that young animals (<6 months of age) are more resistant to acute bovine babesiosis while adult cattle (>1 year of age) are significantly more susceptible (18–21). The causes for this age-related resistance against Babesia is not completely understood; however, it seems to be associated with early activation of innate immune mechanisms in calves rather than maternal immunity (18, 22). Interestingly, spleen removal results in recrudescence of the disease in cattle regardless of their age, and abrogation of the resistance in young animals, which indicates that splenic immunological mechanisms are involved in protection (23). In that context, the fact that live vaccines confer relatively solid protection and young cattle are resistant to B. bovis acute infection reveal that protective immunity can be achieved. Gaining more insight on the nature of the effective immunity mechanisms elicited in such protected animals may help develop efficient vaccines against bovine babesiosis.
It has been shown that long-term in vitro cultivation of Babesia can lead to attenuation, decreased population complexity, and selection of parasites that are no longer transmitted by competent ticks (24, 25). Considering that Babesia parasites are cultured in laboratories under standard protocols and controlled conditions using minimal animal products, the use of in vitro culture attenuated vaccine strains of B. bovis may emerge as a sustainable and more efficient alternative to in vivo attenuated strains for the control of acute bovine babesiosis. In a previous study, we demonstrated that infection with the in vitro culture attenuated B. bovis strain Att-S74-T3Bo safely protects young cattle against a lethal challenge with a homologous virulent strain, revealing novel immune correlates of protection against acute disease (26). In this work we expanded that knowledge and tested the hypotheses that: (a) Att-S74-T3Bo vaccination is safe for highly Babesia-susceptible adult cattle; and (b) that Att-S74-T3Bo-vaccinated animals are protected against challenge with a virulent homologous B. bovis strain.
2 Material and methods
2.1 Parasite strains, vaccination, and challenge
The culture attenuated B. bovis strain Att- S74-T3Bo, originated from the parental T2Bo strain (27), was used for vaccination of adult cattle in this study. This strain has been continuously maintained in culture for more than 10 years, under standard conditions, as previously described (28). Recently, we demonstrated that Att-S74-T3Bo is not tick transmissible, has low population complexity compared to its parental virulent strain, and has an attenuated phenotype in calves (24, 25). For vaccination, a total of five male Holstein adult animals (>1.5-year of age) were inoculated intravenously (IV) with Att-S74-T3Bo iRBC (106 iRBC/calf). Another group of five male Holstein cattle (>1.5-year of age) were inoculated IV with 106 normal RBC (nRBC)/calf as controls. After Att-S74-T3Bo vaccination, all animals were monitored daily for clinical signs of acute babesiosis, including fever, drop in PCV, presence of parasites in peripheral blood, anorexia, prostration, and neurological signs. At 30 days after Att-S74-T3Bo inoculation, vaccinated and control animals were challenged IV with one 1-ml stabilate containing 107 iRBC of the virulent homologous B. bovis strain Vir-S74-T3Bo. Previous studies have shown that a challenge dose of 107 iRBC of Vir-S74-T3Bo induces severe acute disease in calves with the onset of clinical signs starting approximately 9-10 days after infection (24, 26, 29, 30). After challenge, all animals were monitored daily for clinical signs of acute bovine babesiosis, as described above. Average results of temperature and PCV at several timepoints after vaccination and challenge in all experimental animals were compared by a two-tailed t test using GraphPad Prism software version 9 (GraphPad Software, San Diego, CA). A P value <0.05 was considered statistically significant.
2.2 Parasite load in peripheral blood
Parasite load in peripheral blood of animals after vaccination and challenge was measured by real-time quantitative PCR (qPCR), as previously described (31). Briefly, blood from experimental animals was collected via jugular venipuncture into Vacutainer® tubes containing ethylenediamine tetra acetic acid (EDTA) (BD Company, Franklin Lakes, NJ) at several timepoints after vaccination and challenge. Genomic DNA from whole blood was extracted using the QIAamp® DNA Blood Mini Kit (QIAGen, Valencia, CA) following the manufacture’s protocol. DNA was used for qPCR to amplify the single copy B. bovis msa-1 gene. For the msa-1 qPCR, specific primers (5’ gatgcgtttgcacatgctaag 3’ and 5’ cgggtacttcggtgctctca 3’) and probe (FAM 5’-cacgctcaagtaggaaattttgttaaacctgga-3’ TAMRA) were used, and reactions were performed under the following conditions: 95°C for 10 min, 40 cycles of 95°C for 30 sec and 55.8°C for 15 sec, and extension at 72°C for 1 min. A msa-1 standard curve was prepared with 100 to 107 plasmid copies, and all samples were run in technical triplicate, as described elsewhere (31). Results of parasite load in peripheral blood are presented as copies of B. bovis msa-1 gene per 100 μl of blood. Average results of parasite load in vaccinated and control animals were compared by a two-tailed t test and a P value <0.05 was considered statistically significant.
2.3 Complete blood count
Complete cell blood count was evaluated in all experimental animals at several timepoints after vaccination and challenge using the ProCyte One™ Hematology Analyzer (IDEXX Laboratories, Westbrook, ME). Briefly, peripheral blood from the experimental animals was collected in Vacutainer® tubes containing EDTA at several timepoints after vaccination and challenge. Blood samples were homogenized for 5 minutes, and the numbers of total leukocytes, lymphocytes, monocytes, neutrophils, and RBC were measured. Results of each leukocyte population are presented as 1,000 cells/μl of blood, and RBC are shown as 1,000,000 cells/μl of blood. Average results of cell count in all experimental animals were compared by a two-tailed t test, and a P value <0.05 was considered statistically significant.
2.4 Phenotypic analysis of peripheral blood mononuclear cells
Alterations in the phenotype percentage of peripheral blood mononuclear cells (PBMC) was examined by flow cytometric analysis, as previously described (26, 32). Peripheral blood from experimental animals was collected at several timepoints after infection in Vacutainer® tubes containing EDTA. PBMC were isolated using Histopaque®-1077 (Sigma, St. Louis, MO), as per a standard protocol. Cells were then labeled with monoclonal antibodies to surface markers, followed by secondary antibodies (Tables S1, S2), using standard flow cytometry protocols, as described elsewhere (26, 32, 33). Flow cytometric analysis was performed using the Guava® easyCyte™ flow cytometer (Luminex, Austin, TX), and data were acquired using the InCyte™ guavaSoft™ software version 3.1.1 (Luminex, Austin, TX). A single-color monoclonal antibody panel was used for phenotyping CD14+ monocytes, CD335+ NK cells, CD4+ T cells, CD8+ T cells, γδ T cells, and B cells in PBMC. A minimum of 20,000 events were collected for each cell population. After acquisition, data were analyzed using FCS Express™, version 6 (DeNovo™ Software, Pasadena, CA). Results are presented as percentage of target cell populations in PBMC. Average percentage of PBMC populations in experimental animals at several timepoints post-vaccination and -challenge were compared by a two-tailed t test. A P value <0.05 was considered statistically significant.
2.5 Humoral immune response
The presence of antibodies against the C-terminal segment of the B. bovis immunodominant antigen rhoptry associated protein 1 (RAP-1 CT) in serum from vaccinated and control animals was evaluated by indirect ELISA (iELISA), as previously described (34). Briefly, peripheral blood was collected in vacutainer with no anticoagulant, and serum samples were obtained by standard procedures. For iELISA, 96-well Immulon™ 2HB microtiter plates (Thermo Fisher Scientific, Waltham, MA) were coated overnight at 4°C with 50 µl of recombinant RAP-1 CT (2µg/ml). Plates were then washed three times using 200 µl blocking buffer (0.2% I-Block™ in 1xPBS with 0.1% Tween 20) and blocked with 300 µl of the same buffer for one hour at 30°C. After blocking, bovine serum samples were diluted 1/50 in blocking buffer, and 50 µl was added to triplicate individual wells. Plates were incubated for one hour at 30°C and then washed five times in 200 µl blocking buffer. After that, 50 µl of a 1/1000 dilution of anti-bovine IgM, total IgG, IgG1, or IgG2 peroxidase labeled secondary antibodies (SeraCare, Milford, MA) were added to each well, and plates were incubated for 45 minutes at 30°C. After incubation, plates were washed four times using 200 µl blocking buffer and two times with 200 µl 1xPBS with 0.1% Tween 20. Fifty-five µl of SureBlue™ TMB (SeraCare) was then added to each well and plates were incubated for 10 minutes. Reaction was stopped by adding 55 µl TMB stop solution (SeraCare), and absorbance was measured at 450 nm using an ELISA plate reader. Results are presented as normalized OD values, where the absorbance value at different time after vaccination and challenge of each individual animal was divided by the animal’s value obtained at day zero.
2.6 Cytokine ELISA
Concentration of bovine IL-1β, IL-4, IL-6, IL-8, IL-10, CXCL10, IFNγ, and TNFα in serum samples of vaccinated and challenged animals was evaluated by MILLIPLEX® Bovine Cytokine/Chemokine Magnetic Bead (Millipore-Sigma, Burlington, MA) following the manufacture’s protocol. Minimal detectable levels were as follows: 0.1 pg/ml IL-1β, 12.8 pg/ml IL-4, 2.6 pg/ml IL-6, 2.2 pg/ml IL-8, 0.96 pg/ml IL-10, 0.6 pg/ml CXCL10, 0.1pg/ml IFNγ, and 12.8 pg/ml TNFα. Standard curves and quality controls for each cytokine in the multiplex assay were prepared following the manufacture’s protocol. The LX-200™ instrument (Luminex, Austin, TX) was used for the multiplex analysis. Data acquisition was done by xPONENT® software (Luminex), and results were analyzed by Belysa® software (Millipore-Sigma). Levels of cytokine concentration in peripheral blood of all experimental animals were compared by the Mann-Whitney test, and a P value <0.05 was considered statistically significant.
3 Results
3.1 Vaccination with B. bovis Att-S74-T3Bo induces self-limiting acute infection in adult cattle
One major impediment for the use of live in vivo attenuated B. bovis vaccines in endemic areas is that adult cattle may develop symptoms of acute disease and die due to vaccination. Consequently, the currently available live vaccines are not recommended for adult animals. Therefore, our first step in this study was to evaluate the safety of Att-S74-T3Bo for highly susceptible adult cattle. Results showed that animals that received 106 iRBC of the attenuated parasite IV developed a mild but significant (P<0.05) increase of temperature at day 7 after vaccination compared to control non-vaccinated cattle (Figure 1A). Also, vaccinated animals presented a significant (P<0.05) drop in PCV compared to control animals from days 7 through 12 post-vaccination (Figure 1B). Increase of temperature and drop in PCV correlated with a significant (P<0.05) decrease of RBC in peripheral blood of vaccinated animals compared to controls from days 7 through 12 post-vaccination (Figure 1C). Data of parasite load in peripheral blood, determined by qPCR, indicated that Att-S74-T3Bo was able to infect and replicate in adult cattle. The first peak of parasite replication was observed at day 6 post-vaccination (Figure 1D), which preceded the increase of temperature and decrease of PCV, and consequent drop in RBC, on day 7 post-Att-S74-T3Bo inoculation. Importantly, all vaccinated animals recovered from the mild signs of acute infection without the administration of anti-pyretic treatment, anti-Babesia drug, or any additional veterinary intervention. Collectively, results demonstrated that Att-S74-T3Bo infected and caused self-limiting signs of acute disease with no evidence of neurological symptoms in the vaccinated animals. These features suggest that this in vitro culture attenuated B. bovis strain may be considered safe for administration into adult cattle.
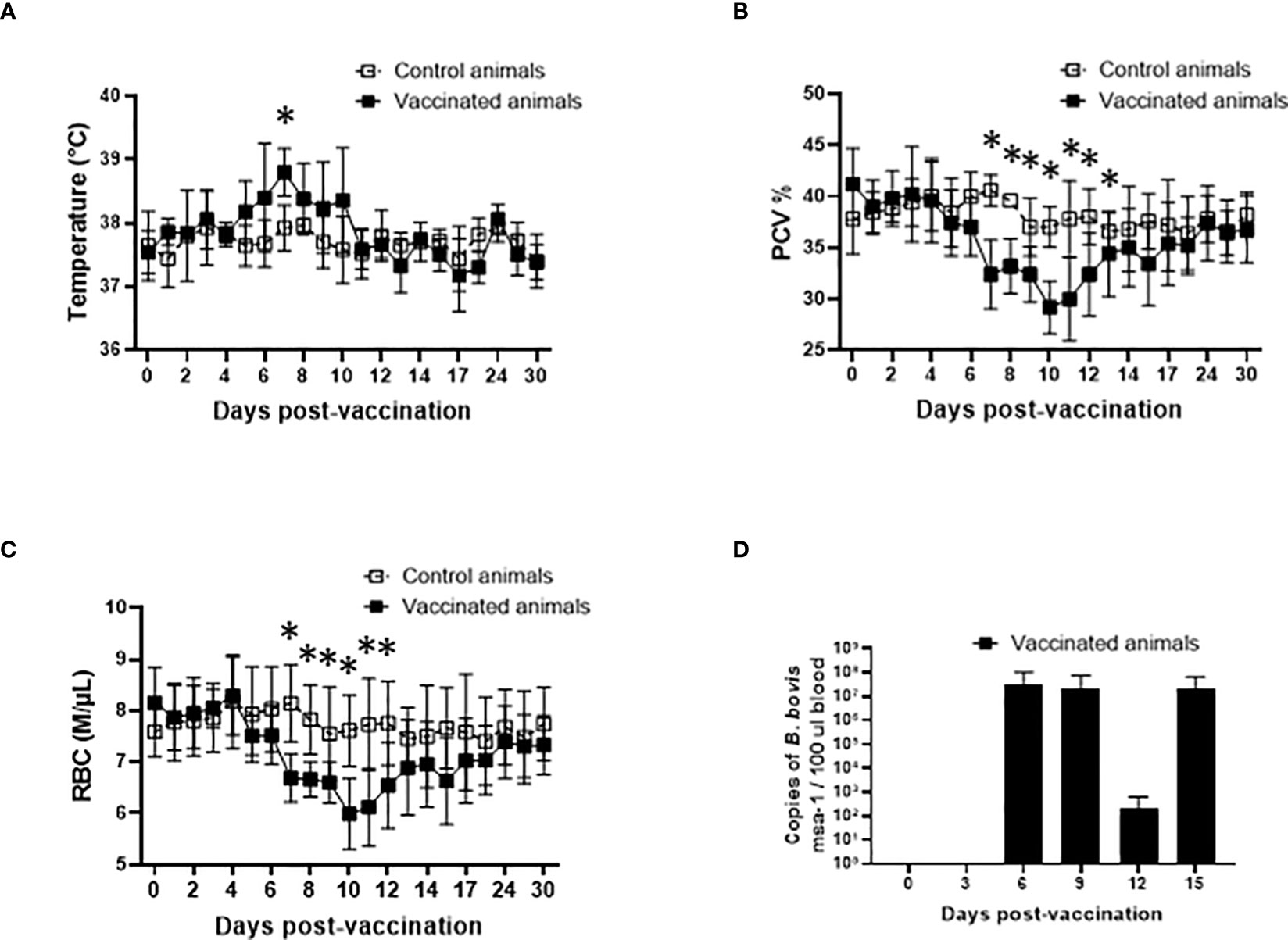
Figure 1 Temperature in Celsius degrees (A), percentage of packed cell volume (PCV) (B), absolute numbers of red blood cells (RBC), and parasite load in peripheral blood accessed by the copy numbers of the B. bovis msa-1 gene using real-time quantitative PCR (D) post-vaccination with the in vitro culture attenuated B. bovis strain Att-S74-T3Bo. Vaccinated animals (n=5). Control animals (n=5). * P<0.05.
3.2 Vaccination with B. bovis Att-S74-T3Bo protects adult cattle against challenge with homologous virulent parasite strain
After demonstrating that Att-S74-T3Bo vaccination did not induce severe acute disease in the experimental adult cattle, we evaluated the development of protection in the vaccinated animals. Data indicated that Att-S74-T3Bo-vaccinated cattle survived challenge with the homologous virulent B. bovis strain Vir-S74-T3Bo (Figure 2). Vaccinated animals were monitored for 30 days and showed no significant alterations in temperature, PCV, and RBC in peripheral blood after Vir-S74-T3Bo challenge (Figures 2A–C). In contrast, all control animals developed pathognomonic signs of acute bovine babesiosis, including significant (P<0.05) increase of temperature and decrease of PCV and RBC starting at day 8 and 9 post-challenge, respectively (Figures 2A–C). In addition, control animals showed inappetence and lethargy, starting around day 8 post-challenge. Results of parasite load in peripheral blood indicated replication of B. bovis in both vaccinated and control animals after challenge (Figure 2D). Interestingly, levels of parasite in peripheral blood were significantly (P<0.05) higher in vaccinated cattle than in control animals on days 9 and 11 after challenge. Control cattle were humanely euthanized on days 10 (n=2), 11 (n=1), and 12 (n=2) post-challenge due to the severity of clinical signs of acute disease (Figure 2E). Altogether, these results demonstrated that highly susceptible adult cattle vaccinated with Att-S74-T3Bo are protected against challenge with a virulent homologous parasite strain.
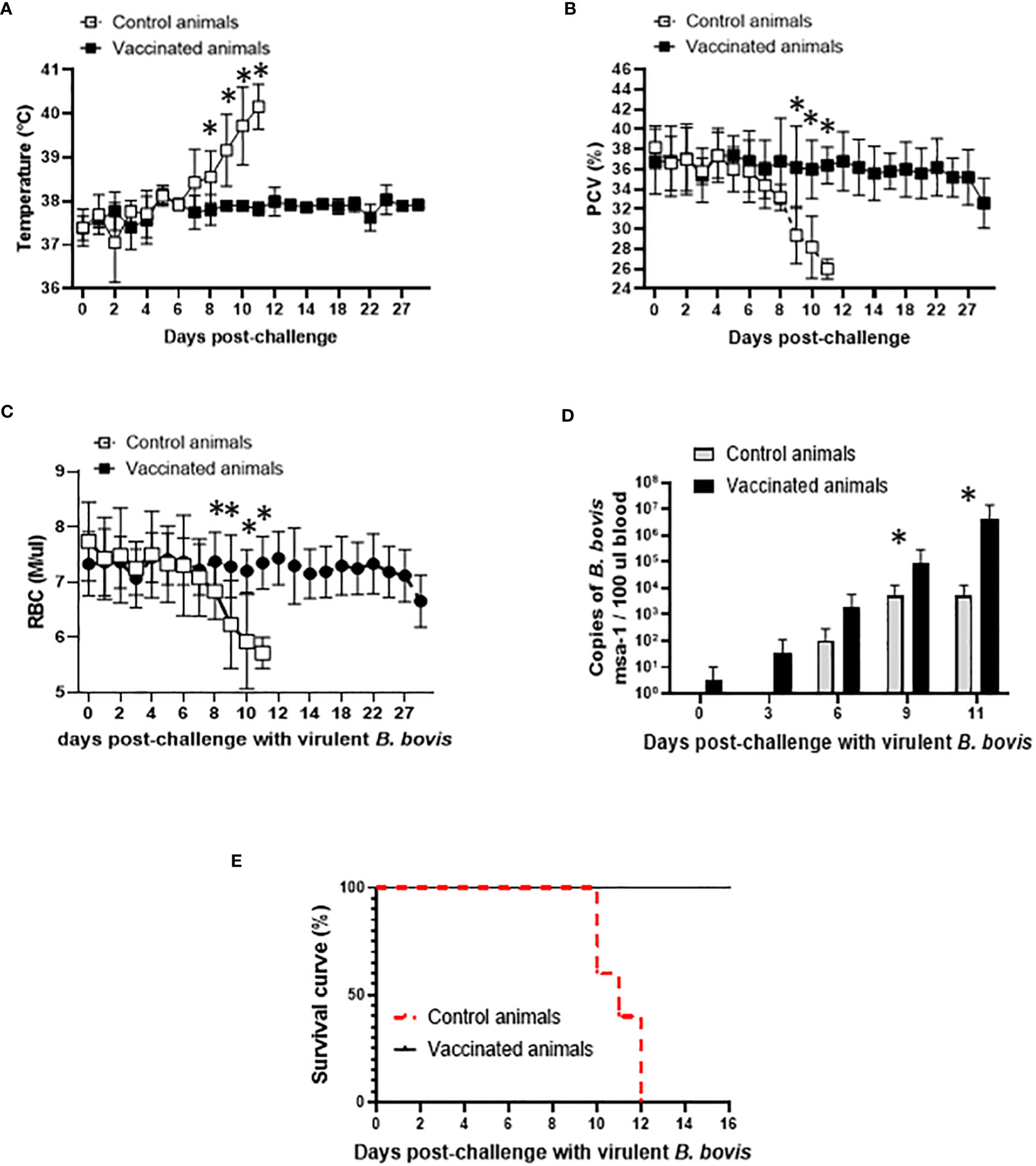
Figure 2 Temperature in Celsius degrees (A), percentage of packed cell volume (PCV) (B), absolute numbers of red blood cells (RBC) (C), parasite load in peripheral blood accessed by the copy numbers of the B. bovis msa-1 gene using real-time quantitative PCR (D), and Kaplan-Meier survival curve (E) after challenge with the virulent B. bovis strain Vir-S74-T3Bo. Vaccinated animals (n=5). Control animals (n=5). * P<0.05.
3.3 Att-S74-T3Bo-vaccinated adult cattle developed a specific protective profile of leukocytes in peripheral blood
The evidence that Att-S74-T3Bo vaccination protected adult cattle against challenge with Vir-S74-T3Bo led us to investigate alterations of leukocytes in peripheral blood from vaccinated protected animals in comparison to controls. As a first step in this investigation, peripheral blood of experimental animals was evaluated using a hematology analyzer to determine the alterations in the absolute numbers of total leukocytes, lymphocytes, monocytes, and neutrophils at different timepoints after vaccination and challenge (Figure 3). Results showed a significant (P<0.05) decrease of total leukocytes in Att-S74-T3Bo-vaccinated cattle on days 6 to 9 post-vaccination (Figure 3A). Even though no significant changes were observed in the levels of lymphocytes, vaccinated cattle showed a tendency toward lymphocytopenia between days 6 and 9 post-vaccination compared to controls (Figure 3A). In addition, Att-S74-T3Bo-vaccinated cattle showed a marked decrease (P<0.05) of neutrophils in peripheral blood from days 6 to 15 post-vaccination (Figure 3A). Interestingly, vaccinated animals developed a significant (P<0.05) monocytopenia at day 6 followed by marked monocytosis in peripheral blood, compared to controls, from day 11 to 17 post-vaccination (Figure 3A). Control animals showed significant (P<0.05) decrease of total leukocytes, lymphocytes, monocytes, and neutrophils in peripheral blood starting around day 7 to 8 post-challenge (Figure 3B). In contrast, no significant alterations in the absolute numbers of total leukocytes, lymphocytes, monocytes, and neutrophils were observed in peripheral blood of Att-S74-T3Bo-vaccinated cattle after challenge (Figure 3B). Next, we examined the profile of PBMC in experimental cattle after Att-S74-T3Bo vaccination and Vir-S74-T3Bo challenge (Figure 4). Gate strategy for the flow cytometric analysis to determine the percentage of CD14+ monocytes, CD335+ NK cells, CD4+ T cells, CD8+ T cells, γδ T cells, and B cells in PBMC of experimental animals is shown in the Supplemental Figure 1 (Figure S1). Results demonstrated a significant (P<0.05) decrease of CD4+ T cells in peripheral blood of cattle at day 6 post-vaccination compared to unvaccinated control animals (Figure 4A). Also, data demonstrated a significant (P<0.05) increase of the percentage of monocytes in peripheral of vaccinated cattle starting at day 12 post-Att-S74-T3Bo inoculation compared to controls (Figure 4A), confirming the results obtained from the whole blood analysis (Figure 3A). In addition, vaccinated animals presented a tendency toward decrease of CD8+ T cells in peripheral blood compared to controls at day 6 post-vaccination; however, no significant differences were observed (Figure 4A). No significant differences in the percentage of γδ T cells, NK cells, and B cells were observed in peripheral blood of vaccinated cattle in comparison to controls (Figure 4A). The percentage of CD4+ and CD8+ T cells decreased significantly (P<0.05) in peripheral blood of control animals, compared to vaccinated cattle, starting at days 9 and 10 post-Vir-S74-T3Bo challenge, respectively (Figure 4B). A significant (P<0.05) monocytosis in PBMC of control animals compared to vaccinated cattle was observed at day 11 post-challenge, by that time two control animals had to be euthanized due to the severity of the disease while the other three remaining cattle were showing severe signs of acute babesiosis. No significant differences in the percentage of γδ T cells, NK cells, and B cells in peripheral blood of vaccinated cattle compared to controls were observed after Vir-S74-T3Bo challenge (Figure 4B). Together, the results demonstrate the development of a specific pattern of alterations in leukocytes in peripheral blood of protected adult cattle vaccinated with Att-S74-T3Bo, which was mainly characterized by increase of monocytes with concomitant decrease of CD4+ T cells early after Att-S74-T3Bo vaccination.
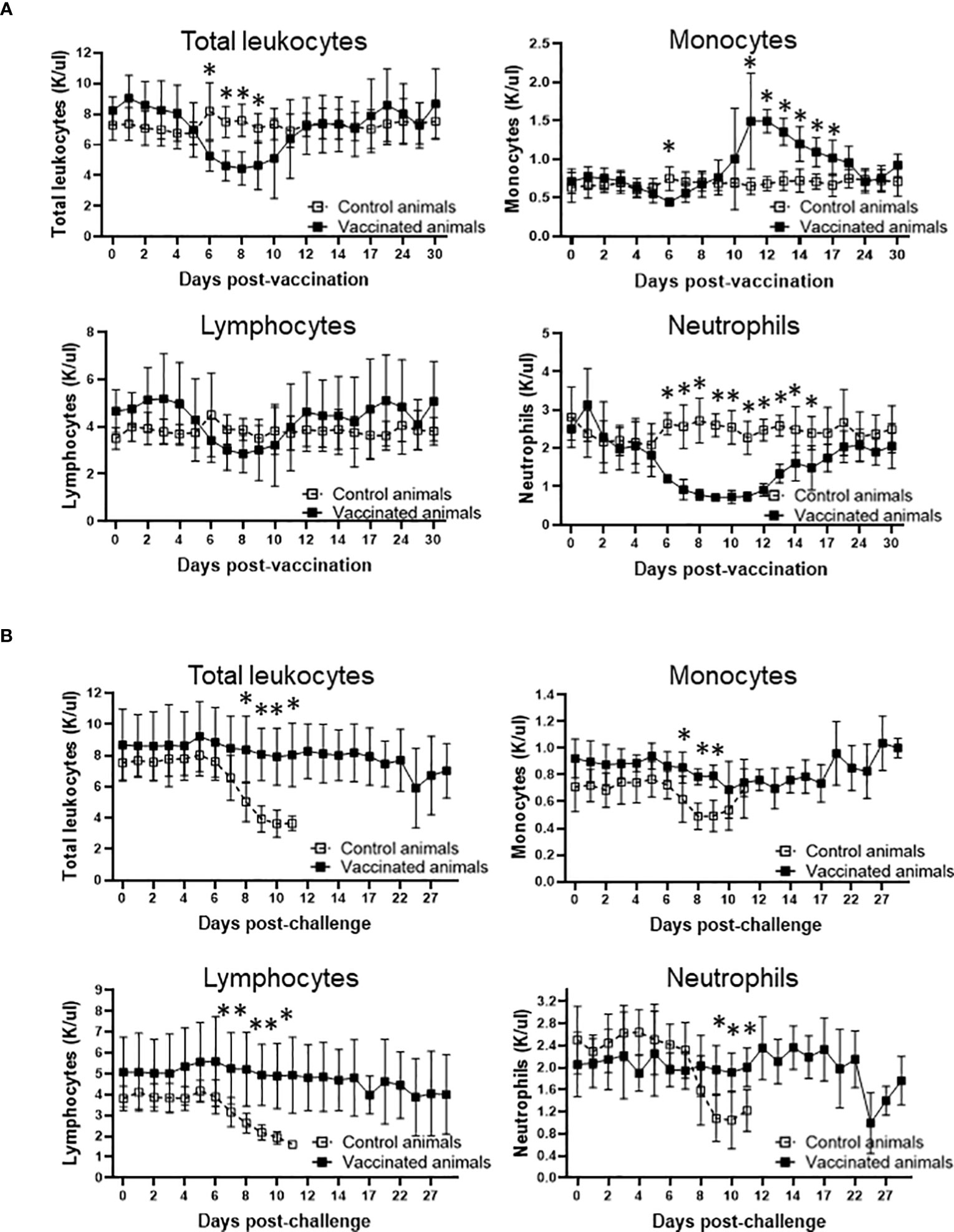
Figure 3 Profile of total leukocytes, lymphocytes, monocytes, and neutrophils in peripheral of vaccinated (n=5) and control (n=5) animals after vaccination with the in vitro culture attenuated B. bovis strain Att-S74-T3Bo (A) and challenge with the virulent B. bovis strain Vir-S74-T3Bo (B). Results are presented as average of 1,000 cells per µl of blood (K/µl) of experimental animals. * P<0.05.
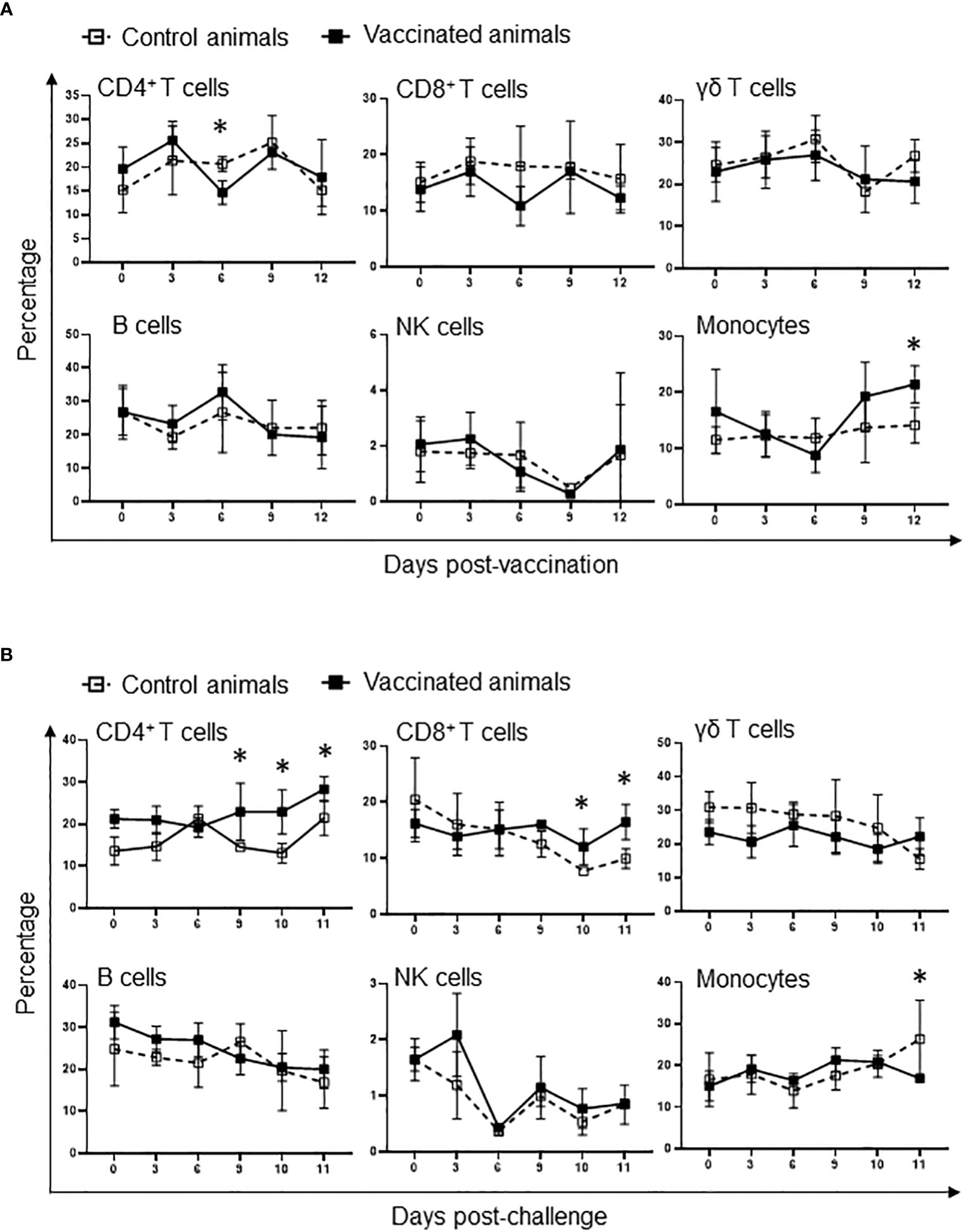
Figure 4 Flow cytometric analysis showing alterations of peripheral blood mononuclear cells (PBMC) after vaccination with the in vitro culture attenuated B. bovis strain Att-S74-T3Bo (A) and challenge with the virulent B. bovis strain Vir-S74-T3Bo (B). Results are presented as average of percentage of 30,000 cells of experimental animals. Vaccinated animals (n=5). Control animals (n=5). * P<0.05.
3.4 Protected Att-S74-T3Bo-vaccinated cattle developed early expression of pro- and anti-inflammatory cytokines in peripheral blood with significant humoral response to B. bovis RAP-1 CT
After demonstrating that Att-S74-T3Bo vaccinated adult cattle are protected against challenge with Vir-S74-T3Bo and that protected animals developed a signature pattern of leukocytes in peripheral blood, next we examined the immune responses of the experimental animals (Figure 5). We observed a significant increase in the level of IL-10 in peripheral blood of vaccinated cattle between days 6 and 9 post-Att-S74-T3Bo inoculation (Figure 5A). Similarly, IFNγ was significantly elevated in peripheral blood of vaccinated animals on days 6 and 9 post-vaccination (Figure 5A). In addition, no significant differences were detected in the levels of CXCL10, TNFα, IL-6, IL-8, and IL-4 after vaccination (Figure 5A). Control cattle showed significant (P<0.05) increase in CXCL10, IFNγ, and IL-10 at day 9 post-challenge, compared to Att-S74-T3Bo-vaccinated animals. In addtion, a significant increase in the concentration of IL-8 was observed in vaccinated animals at day 11 post-challenge (Figure 5B). No significant differences were detected in the level of TNFα, IL-6, and IL-4 in vaccinated and control animals after challenge (Figure 5B). All Att-S74-T3Bo-vaccinated cattle developed detectable levels of IgM against the immunodominant antigen B. bovis RAP-1 CT as early as 6 days post-inoculation (Figure 6A). Also, marked levels of IgG, IgG1, and IgG2 against RAP-1 CT were detected, starting at day 15 post-inoculation, in all five Att-S74-T3Bo-vaccinated animals (Figures 6B–D). Importantly, no boost effect was observed in the levels of IgM and IgG after challenging the vaccinated animals with the virulent B. bovis strain. Noteworthy, no levels of detectable IgM and IgG against RAP-1 CT were observed in the control animals that succumbed to acute disease on days 10 through 12 post-challenge (Figure 6). In summary, data showed that vaccinated, protected adult cattle developed a balance expression profile of pro- and anti-inflammatory cytokines in peripheral blood, as demonstrated by the levels of IL-10, CXCL10 and, IFNγ after Att-S74-T3Bo vaccination and challenge. Also, Att-S74-T3Bo vaccination induced markedly levels of antibodies that may play a role in protecting the animals from challenge with the virulent parasite strain. In addition, data showed that control animals developed a late cytokine response compared to cattle inoculated with attenuated parasites.
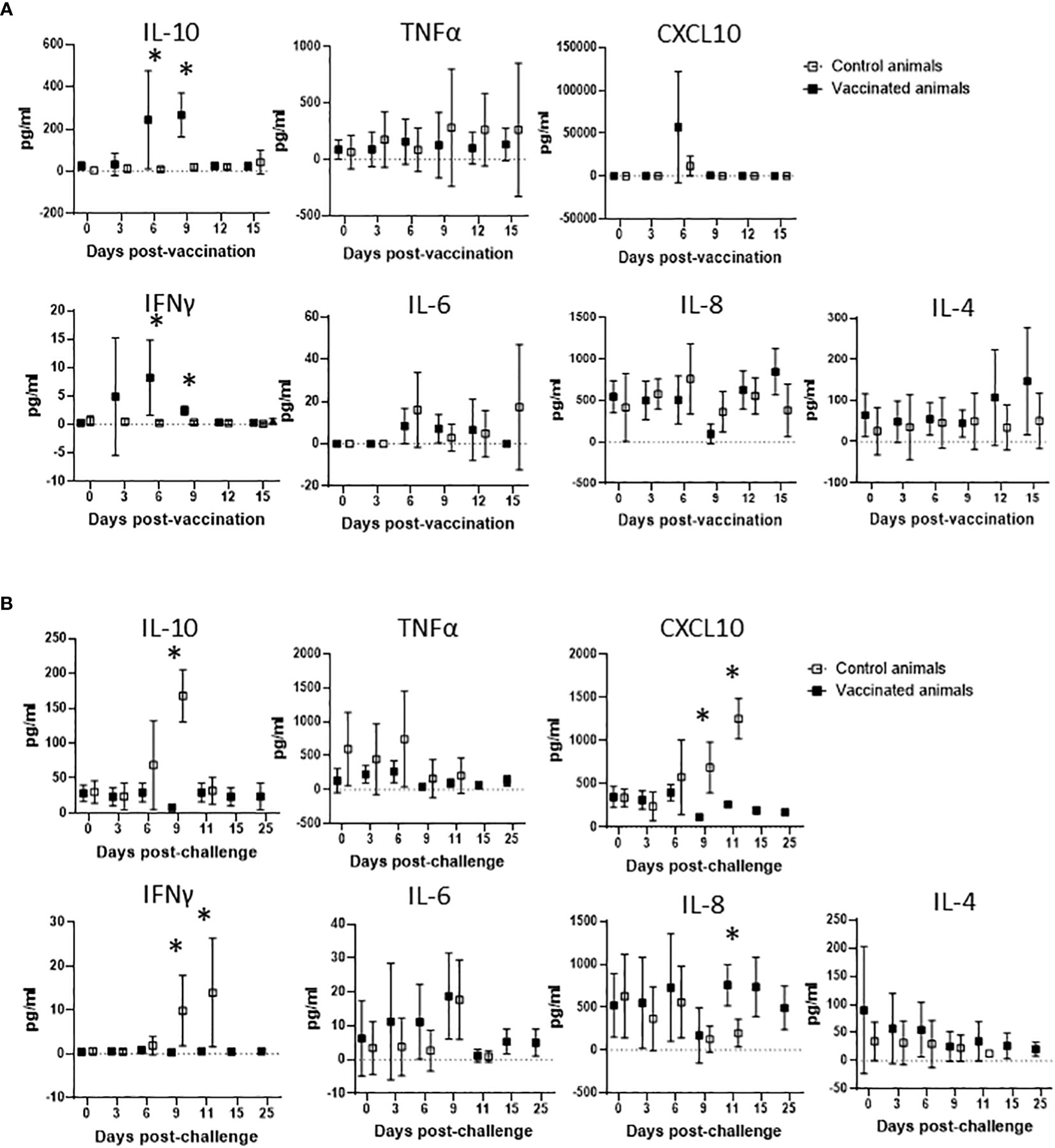
Figure 5 Expression profile of IL-10, TNFα, CXCL10, IFNγ, IL-6, IL-8, and IL-4 in peripheral blood of cattle vaccinated with the in vitro culture attenuated B. bovis strain Att-S74-T3Bo (A) following challenge with the virulent B. bovis strain Att-S74-T3Bo (B). Cytokine results are presented as means of picograms per ml of blood (pg/ml) of experimental animals. Vaccinate animals (n=5). Control animals (n=5). * P<0.05.
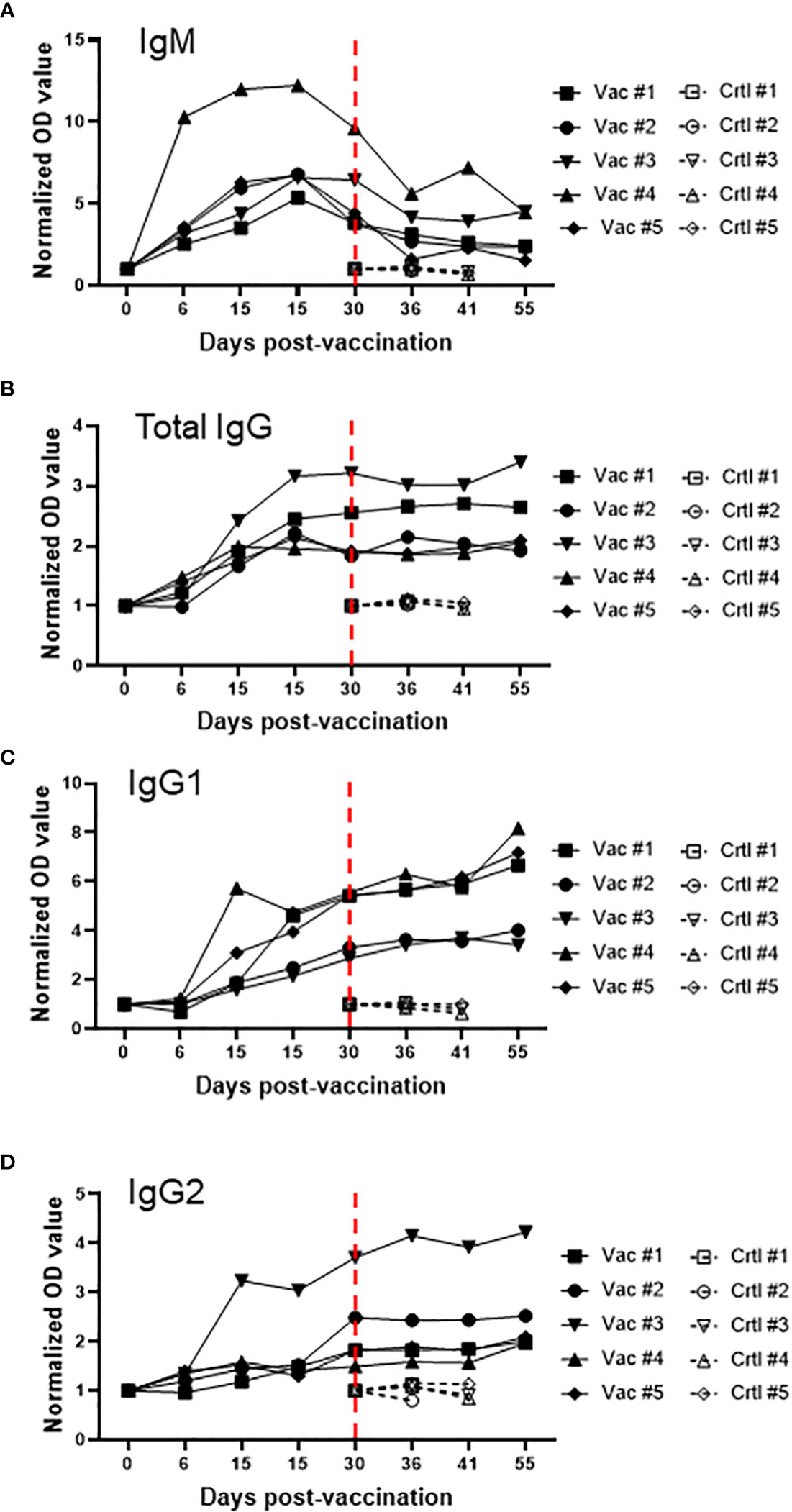
Figure 6 Levels of IgM (A), total IgG (B), IgG1 (C), and IgG2 (D) against the immunodominant antigen rhoptry associated protein 1 C-terminal (RAP-1 CT) after vaccination with the in vitro culture attenuated B. bovis strain Att-S74-T3Bo and challenge with the virulent B. bovis strain Vir-S74-T3Bo. Results are presented as normalized optical density of individual experimental animals. Animals vaccinated with Att-S74-T3Bo and challenged with Vir-S74-T3Bo (Vac). Control animals challenged with Vir-S74-T3Bo (Crtl). Dashed red lines indicate day zero of Vir-S74-T3Bo challenge.
4 Discussion
In this study we demonstrate that vaccination with the live in vitro culture attenuated B. bovis strain Att-S74-T3Bo is safe and protects adult cattle against acute bovine babesiosis. We also identified a pattern of leukocytes and cytokine expression in peripheral blood of protected animals, which increments the knowledge on correlates of protection against B. bovis acute infection found in a previous study (26). It has been well-documented that live in vivo attenuated Babesia vaccines generally protects cattle against acute bovine babesiosis (13). Despite this fact, these vaccines present several constraints that prevent their wide-spread use in cattle, especially in areas that are non-endemic for Babesia parasites, including the US. Among these limitations, at least two are a major concern. One is the need of a large number of animals for vaccine production, and the other is the risk of vaccinated cattle, especially adult animals (>1-year old), to develop acute disease due to vaccination, which prevents its use for highly susceptible adult cattle. In this study we started addressing these two concerns by using Att-S74-T3Bo, a previously defined in vitro culture attenuated strain of B. bovis (24–26). Despite of the reduced number of animals used in the present study, the data support the hypotheses that vaccination with Att-S74-T3Bo is safe for highly susceptible adult cattle and that it induces protection against challenge with a virulent homologous B. bovis strain. Results demonstrate that Att-S74-T3Bo-vaccinated cattle developed mild self-limiting acute infection, and that vaccination elicited a protective immune response against challenge with Vir-S74-T3Bo.
Currently available methodology to produce live in vivo attenuated B. bovis vaccines was developed in Australia more than 100 years ago (21). After that, this approach has been refined and slightly modified but in essence it consists of sequentially infecting several splenectomized calves with virulent B. bovis parasites. After approximately 20 cycles of infection/passages in individual splenectomized naïve calves, attenuate parasites are selected from the original population. Even though the genotypic and phenotypic differences between virulent and attenuated parasite populations after the passages in splenectomized calves remain unknown, the emerging attenuated parasites are then used as a live blood-based vaccine (2, 15, 16, 21). In this context, vaccination of cattle with live in vivo attenuated B. bovis parasites has been historically used as an approach to control acute babesiosis in endemic areas (35). However, field studies have reported failures of these vaccines with the emergence of outbreaks and development of acute disease in vaccinated herds of cattle. Outbreaks in vaccinated animals are associated with the presence of field isolates that are able to break through the vaccine immunity (36). On the other hand, attenuation through passages in splenectomized calves is both a biologically and logistically challenging process, and inadequate attenuation of vaccine strains is not uncommon. As a result, development of acute disease caused by vaccination may happen due to deficient selection of attenuated parasite populations during the passages in splenectomized calves or other factors, such as the breed and immunological status of the vaccinated animals, and the presence in the field of heterologous and/or homologous highly virulent strains. This is of particular concern considering the use of in vivo attenuated B. bovis vaccines for highly susceptible adult cattle. Consequently, these vaccines are only recommended for young (< 6 months of age) and not to adult animals (35). Another important point to be considered for the use of in vivo attenuated B. bovis vaccines is the possibility of recombination between vaccine and field strains of the parasite during sexual reproduction inside the tick vectors. Such situation could potentially produce new virulent parasite strains that may escape the vaccine immunity. Relevance of the present study relies on the fact of using the previously defined in vitro culture attenuated B. bovis strain Att-S74-T3Bo that safely protects highly susceptible adult cattle against challenge with a virulent homologous B. bovis strain. Collectively, this information adds to previous work showing that Att-S74-T3Bo has less population complexity compared to its parental strain and that it is not tick-transmissible (24, 25). Another major downside facing the wide-spread use of in vivo attenuated B. bovis vaccines is the difficulties in standardizing and large-scale producing parasite vaccine strains using splenectomized calves (16, 35). To address these concerns, alternative methods to attenuate Babesia parasites have been tested, including the use of chemical, radiation, and passages in in vitro culture (37–40). Here we show that a dose of 106 in vitro culture attenuated parasites is enough to safely protect adult cattle from acute disease caused by a homologous virulent B. bovis strain. Considering that the volume of B. bovis cultures can be easily expanded using standard laboratory protocols, promoting the increase in the percentage of parasitized erythrocytes, which can reach approximately 40%, it is predicted that no major issues are expected regarding standardization and large-scale production of this in vitro attenuated vaccine strain. Altogether, results presented by this study represent an important advance regarding the development of a sustainable, safe, and efficient live in vitro attenuated vaccine to control acute bovine babesiosis in adult cattle.
It was previously shown that Att-S74-T3Bo inoculation protects calves (<1 year of age) against challenge with a homologous virulent strain (26). Protected calves showed increase of monocytes with concomitant decrease of neutrophils and CD4+ T lymphocytes in peripheral blood on day 3 to 7 post-Att-S74-T3Bo inoculation. Here we demonstrated that Att-S74-T3Bo is also effective in protecting adult cattle and that the protected animals showed a similar pattern of PBMC alteration compared to young animals. However, in this study, the adult protected cattle developed a delayed monocytosis, neutropenia and CD4+ lymphopenia in peripheral blood compared to the previous observation in young animals (26). Interestingly, here we observed monocytopenia and the absence of monocytosis in control animals, which correlates with the onset of clinical signs of acute disease. Taken together, we infer that increased number of monocytes in peripheral blood after vaccination is a sine qua non event to elicit protection against acute B. bovis infection regardless the cattle age. Of note, it has also been shown that recruitment and activation of monocytes and macrophages are critical to control parasitemia of lethal and non-lethal Plasmodium infection (41, 42), which is consistent with the findings of this study. Monocytes have been recently categorized into subsets based on the expression of the surface markers CD14 and CD16 (32, 43, 44). Therefore, future studies are needed to investigate the kinetics of monocyte subsets in peripheral blood of protected cattle after Att-S74-T3Bo vaccination in comparison with control animals. Additional investigation is also necessary to determine the profile of cytokine expression in the monocyte subsets in protected cattle.
It has been demonstrated that development of a protective immune response against B. bovis involves a fine balance of early activation of innate immune mechanisms, and subsequent elicitation of adaptive humoral and cellular responses (26, 45, 46). Interestingly, the association between protection and development of a balance of pro-inflammatory and regulatory cytokines was also demonstrated in Plasmodium spp. and Theileria spp (47–49). Findings of this current study agree with this assumption by demonstrating that vaccinated protected adult animals showed early systemic expression of IL-10, a regulatory anti-inflammatory cytokine, and concomitant elevation of IFNγ, a pro-inflammatory cytokine. In contrast, control animals presented similar but delayed pattern of cytokines following challenge with a virulent B. bovis strain. Considering the elevated levels of IFNγ and CXCL10 in controls animals on day 9 post-challenge, we speculate that this untimely systemic production of pro-inflammatory cytokines may account for the severity of acute disease caused by the virulent strain in adult animals, as previously mentioned (18). It is also possible to consider that control animals experienced a transient immunosuppression with subsequent exacerbation of the inflammatory immune response after challenge, which may be in part responsible for the clinical signs of acute bovine babesiosis and death of adult animals. In this study, Att-S74-T3Bo-vaccinated cattle developed significant and early levels of IgM and IgG against the B. bovis immunodominant antigen RAP-1 CT, which is in agreement with previous observation (26). In contrast, control animals succumbed to acute disease around days 10 to 12 post-Vir-S74-T3Bo challenge without detectable levels of anti-RAP-1 CT antibodies. Therefore, it is plausible to assume that humoral immune response against B. bovis antigens may play a role in protecting the vaccinated animals after challenge. Collectively, results of blood cell alterations, cytokine expression in peripheral blood, and humoral immune response in vaccinated protected adult cattle increment the information on correlates of immune protection against B. bovis and may be relevant for vaccine development.
In conclusion, here we demonstrate that, under the present experimental conditions, Att-S74-T3Bo vaccination is safe for adult cattle and that vaccinated animals are protected against challenge with the homologous virulent strain Vir-S74-T3Bo. By using a sensitive method for cytokine detection and a systemic approach to investigate alterations of leukocytes in peripheral blood after vaccination and challenge, we identified markers for protection and disease progression in highly susceptible adult cattle. Considering this and previous studies, we are building a solid body of information on the Att-S74-T3Bo strain (24–26, 50). It is now known that this attenuated long-term cultured B. bovis strain presents a low population complexity compared to its parental strain (24), it is not tick-transmissible (25), and it protects calves against challenge with homologous virulent strain (26). Results from this study increment previous knowledge and provide additional evidence on the safety and efficacy of Att-S74-T3Bo in protecting adult cattle against acute bovine babesiosis. Future studies should determine the duration of the immunity induced by Att-S74-T3Bo vaccination and its efficacy against heterologous virulent strains of B. bovis and challenge with B. bovis-infected ticks.
Data availability statement
The original contributions presented in the study are included in the article/Supplementary Material. Further inquiries can be directed to the corresponding author.
Ethics statement
The animal study was reviewed and approved by Washington State University Institutional Animal Care and Use Committee.
Author contributions
Conception and design: RB, CS, MU. Collection and assembly of data: RB, JC-P, JL. Data analysis and interpretation: RB, JC-P, JL, CS, MU. Manuscript writing: All authors. Final approval of manuscript: All authors.
Funding
We acknowledge financial support from the USDA National Institute of Food and Agriculture (NIFA) (Award Number: 2020-67015-31809; Proposal Number: 2019-05375, Accession Number: 1022541), the International Development Research Center (IDRC) (Livestock Vaccine Innovation Fund (Grant 108525), funded by the Canadian Government and the Bill and Melinda Gates Foundation), and the United States Department of Agriculture (ARS-USDA CRIS 2090- 32000-040-00-D).
Acknowledgments
We would like to express our gratitude to Paul Lacy, Jinna Navas, Sarah Therrian, and Jamie Reed for their excellent technical assistance and animal care.
Conflict of interest
The authors declare that the research was conducted in the absence of any commercial or financial relationships that could be construed as a potential conflict of interest.
Publisher’s note
All claims expressed in this article are solely those of the authors and do not necessarily represent those of their affiliated organizations, or those of the publisher, the editors and the reviewers. Any product that may be evaluated in this article, or claim that may be made by its manufacturer, is not guaranteed or endorsed by the publisher.
Supplementary material
The Supplementary Material for this article can be found online at: https://www.frontiersin.org/articles/10.3389/fimmu.2023.1219913/full#supplementary-material
References
1. Krause PJ. Human babesiosis. Int J Parasitol (2019) 49(2):165–74. doi: 10.1016/j.ijpara.2018.11.007
2. Bock R, Jackson L, de Vos A, Jorgensen W. Babesiosis of cattle. Parasitology (2004) 129(Suppl):S247–69. doi: 10.1017/S0031182004005190
3. Smith TK FL. Investigations into the nature, causation, and prevention of Texas or southern cattle fever. Bull (United States Bureau Anim Industry) (1893) 1(1):301.
4. Graham OH, Hourrigan JL. Eradication programs for the arthropod parasites of livestock. J Med Entomol (1977) 13(6):629–58. doi: 10.1093/jmedent/13.6.629
5. Almazan C, Tipacamu GA, Rodriguez S, Mosqueda J, Perez de Leon A. Immunological control of ticks and tick-borne diseases that impact cattle health and production. Front Biosci (Landmark Ed) (2018) 23:1535–51. doi: 10.2741/4659
6. Esteve-Gasent MD, Rodríguez-Vivas RI, Medina RF, Ellis D, Schwartz A, Cortés Garcia B, et al. Research on integrated management for cattle fever ticks and bovine babesiosis in the United States and Mexico: Current status and opportunities for binational coordination. Pathog (Basel Switzerland) (2020) 9(11). doi: 10.3390/pathogens9110871
7. Ozubek S, Bastos RG, Alzan HF, Inci A, Aktas M, Suarez CE. Bovine babesiosis in Turkey: Impact, Current gaps, and opportunities for intervention. Pathog (Basel Switzerland) (2020) 9(12). doi: 10.3390/pathogens9121041
8. He L, Bastos RG, Sun Y, Hua G, Guan G, Zhao J, et al. Babesiosis as a potential threat for bovine production in China. Parasit Vectors (2021) 14(1):460. doi: 10.1186/s13071-021-04948-3
9. Schnittger L, Rodriguez AE, Florin-Christensen M, Morrison DA. Babesia: A world emerging. Infect Genet Evol (2012) 12(8):1788–809. doi: 10.1016/j.meegid.2012.07.004
10. Sondgeroth KS, McElwain TF, Allen AJ, Chen AV, Lau AO. Loss of neurovirulence is associated with reduction of cerebral capillary sequestration during acute Babesia bovis infection. Parasit Vectors (2013) 6(1):181. doi: 10.1186/1756-3305-6-181
11. Sondgeroth KS, McElwain TF, Ueti MW, Scoles GA, Reif KE, Lau AO. Tick passage results in enhanced attenuation of Babesia bovis. Infect Immun (2014) 82(10):4426–34. doi: 10.1128/IAI.02126-14
12. de Castro JJ. Sustainable tick and tickborne disease control in livestock improvement in developing countries. Vet Parasitol (1997) 71(2-3):77–97. doi: 10.1016/S0304-4017(97)00033-2
13. Bock RE, de Vos AJ. Immunity following use of Australian tick fever vaccine: A review of the evidence. Aust Vet J (2001) 79(12):832–9. doi: 10.1111/j.1751-0813.2001.tb10931.x
14. Rathinasamy V, Poole WA, Bastos RG, Suarez CE, Cooke BM. Babesiosis vaccines: Lessons learned, challenges ahead, and future glimpses. Trends Parasitol (2019) 35(8):622–35. doi: 10.1016/j.pt.2019.06.002
15. Callow LL, Dalgliesh RJ, de Vos AJ. Development of effective living vaccines against bovine babesiosis–the longest field trial? Int J Parasitol (1997) 27(7):747–67. doi: 10.1016/S0020-7519(97)00034-9
16. Callow LL, Mellors LT. A new vaccine for Babesia argentina infection prepared in splenectomised calves. Aust Vet J (1966) 42(12):464–5. doi: 10.1111/j.1751-0813.1966.tb14476.x
17. Daly GD, Hall TK. A note on the susceptibility of British and some zebu-type cattle to tick fever (Babesiosis). Aust Vet J (1955) 31:152.
18. Zintl A, Gray JS, Skerrett HE, Mulcahy G. Possible mechanisms underlying age-related resistance to bovine babesiosis. Parasite Immunol (2005) 27(4):115–20. doi: 10.1111/j.1365-3024.2005.00748.x
19. Trueman KF, Blight GW. The effect of age on resistance of cattle to Babesia bovis. Aust Vet J (1978) 54(6):301–5. doi: 10.1111/j.1751-0813.1978.tb02465.x
20. Howell JM, Ueti MW, Palmer GH, Scoles GA, Knowles DP. Persistently infected calves as reservoirs for acquisition and transovarial transmission of Babesia bovis by Rhipicephalus (Boophilus) microplus. J Clin Microbiol (2007) 45(10):3155–9. doi: 10.1128/JCM.00766-07
21. De Vos AJ, Bock RE. Vaccination against bovine babesiosis. Ann NY Acad Sci (2000) 916:540–5. doi: 10.1111/j.1749-6632.2000.tb05333.x
22. Goff WL, Johnson WC, Tuo W, Valdez RA, Parish SM, Barrington GM, et al. Age-related innate immune response in calves to Babesia bovis involves IL-12 induction and IL-10 modulation. Ann NY Acad Sci (2002) 969:164–8. doi: 10.1111/j.1749-6632.2002.tb04371.x
23. Goff WL, Bastos RG, Brown WC, Johnson WC, Schneider DA. The bovine spleen: interactions among splenic cell populations in the innate immunologic control of hemoparasitic infections. Vet Immunol Immunopathol (2010) 138(1-2):1–14. doi: 10.1016/j.vetimm.2010.07.006
24. Alzan HF, Bastos RG, Laughery JM, Scoles GA, Ueti MW, Johnson WC, et al. A Culture-Adapted strain of Babesia bovis has reduced subpopulation complexity and is unable to complete its natural life cycle in ticks. Front Cell Infect Microbiol (2022) 12:827347. doi: 10.3389/fcimb.2022.827347
25. Mazuz ML, Laughery JM, Lebovitz B, Yasur-Landau D, Rot A, Bastos RG, et al. Experimental Infection of calves with transfected attenuated Babesia bovis expressing the Rhipicephalus microplus Bm86 antigen and eGFP marker: Preliminary studies towards a dual anti-tick/Babesia vaccine. Pathog (Basel Switzerland) (2021) 10(2). doi: 10.3390/pathogens10020135
26. Bastos RG, Laughery JM, Ozubek S, Alzan HF, Taus NS, Ueti MW, et al. Identification of novel immune correlates of protection against acute bovine babesiosis by superinfecting cattle with in vitro culture attenuated and virulent Babesia bovis strains. Front Immunol (2022) 13:1045608. doi: 10.3389/fimmu.2022.1045608
27. Brayton KA, Lau AO, Herndon DR, Hannick L, Kappmeyer LS, Berens SJ, et al. Genome sequence of Babesia bovis and comparative analysis of apicomplexan hemoprotozoa. PloS Pathog (2007) 3(10):1401–13. doi: 10.1371/journal.ppat.0030148
28. Erp EE, Gravely SM, Smith RD, Ristic M, Osorno BM, Carson CA. Growth of Babesia bovis in bovine erythrocyte cultures. Am J Trop Med Hyg (1978) 27(5):1061–4. doi: 10.4269/ajtmh.1978.27.1061
29. Alzan HF, Bastos RG, Ueti MW, Laughery JM, Rathinasamy VA, Cooke BM, et al. Assessment of Babesia bovis 6cys A and 6cys B as components of transmission blocking vaccines for babesiosis. Parasit Vectors (2021) 14(1):210. doi: 10.1186/s13071-021-04712-7
30. Johnson WC, Hussein HE, Capelli-Peixoto J, Laughery JM, Taus NS, Suarez CE, et al. A Transfected Babesia bovis Parasite line expressing eGFP is able to complete the full life cycle of the parasite in mammalian and tick hosts. Pathog (Basel Switzerland) (2022) 11(6). doi: 10.3390/pathogens11060623
31. Bastos RG, Ueti MW, Guerrero FD, Knowles DP, Scoles GA. Silencing of a putative immunophilin gene in the cattle tick Rhipicephalus (Boophilus) microplus increases the infection rate of Babesia bovis in larval progeny. Parasit Vectors (2009) 2(1):57. doi: 10.1186/1756-3305-2-57
32. Bastos RG, Sears K, Dinkel KD, Knowles DP, Fry LM. Changes in the molecular and functional phenotype of bovine monocytes during T. parva infection. Infect Immun (2019) 87(12):e00703–19. doi: e00703–19
33. Bastos RG, Johnson WC, Brown WC, Goff WL. Differential response of splenic monocytes and DC from cattle to microbial stimulation with Mycobacterium bovis BCG and Babesia bovis merozoites. Vet Immunol Immunopathol (2007) 115(3-4):334–45. doi: 10.1016/j.vetimm.2006.11.001
34. Goff WL, McElwain TF, Suarez CE, Johnson WC, Brown WC, Norimine J, et al. Competitive enzyme-linked immunosorbent assay based on a rhoptry-associated protein 1 epitope specifically identifies Babesia bovis-infected cattle. Clin Diagn Lab Immunol (2003) 10(1):38–43. doi: 10.1128/CDLI.10.1.38-43.2003
35. de Waal DT, Combrink MP. Live vaccines against bovine babesiosis. Vet Parasitol (2006) 138(1-2):88–96. doi: 10.1016/j.vetpar.2006.01.042
36. Bock RE, de Vos AJ, Lew A, Kingston TG, Fraser IR. Studies on failure of T strain live Babesia bovis vaccine. Aust Vet J (1995) 72(8):296–300. doi: 10.1111/j.1751-0813.1995.tb03558.x
37. Yunker CE, Kuttler KL, Johnson LW. Attenuation of Babesia bovis by in vitro cultivation. Vet Parasitol (1987) 24(1-2):7–13. doi: 10.1016/0304-4017(87)90125-7
38. Al-Nazal HA, Cooper E, Ho MF, Eskandari S, Majam V, Giddam AK, et al. Pre-clinical evaluation of a whole-parasite vaccine to control human babesiosis. Cell Host Microbe (2021) 29(6):894–903.e5. doi: 10.1016/j.chom.2021.04.008
39. Rojas C, Figueroa JV, Alvarado A, Mejia P, Mosqueda JJ, Falcon A, et al. Bovine babesiosis live vaccine production: use of gamma irradiation on the substrate. Ann NY Acad Sci (2006) 1081:405–16. doi: 10.1196/annals.1373.059
40. Alvarez JA, Rojas C, Figueroa JV. An Overview of current knowledge on in vitro Babesia Cultivation for production of live attenuated vaccines for bovine babesiosis in Mexico. Front Vet Sci (2020) 7(364). doi: 10.3389/fvets.2020.00364
41. Brown IN, Dockrell HM, de Souza JB, Playfair JH. Blood-stage malaria in Biozzi high and low antibody responder mice: the importance of antibody and macrophages in immunity. Ann Immunol (Paris) (1984) 135c(2):231–40. doi: 10.1016/S0769-2625(84)81156-3
42. Chua CL, Brown G, Hamilton JA, Rogerson S, Boeuf P. Monocytes and macrophages in malaria: protection or pathology? Trends Parasitol (2013) 29(1):26–34. doi: 10.1016/j.pt.2012.10.002
43. Hussen J, Düvel A, Sandra O, Smith D, Sheldon IM, Zieger P, et al. Phenotypic and functional heterogeneity of bovine blood monocytes. PloS One (2013) 8:1–11. doi: 10.1371/journal.pone.0071502
44. Hussen J, Schuberth H-J. Heterogeneity of bovine peripheral blood monocytes. Front Immunol (2017) 8:1–9. doi: 10.3389/fimmu.2017.01875
45. Goff WL, Johnson WC, Horn RH, Barrington GM, Knowles DP. The innate immune response in calves to Boophilus microplus tick transmitted Babesia bovis involves type-1 cytokine induction and NK-like cells in the spleen. Parasite Immunol (2003) 25(4):185–8. doi: 10.1046/j.1365-3024.2003.00625.x
46. Brown WC, Norimine J, Knowles DP, Goff WL. Immune control of Babesia bovis infection. Vet Parasitol (2006) 138(1-2):75–87. doi: 10.1016/j.vetpar.2006.01.041
47. Claser C, De Souza JB, Thorburn SG, Grau GE, Riley EM, Rénia L, et al. Host resistance to plasmodium-induced acute immune pathology is regulated by interleukin-10 receptor signaling. Infect Immun (2017) 85(6). doi: 10.1128/IAI.00941-16
48. Frimpong A, Amponsah J, Adjokatseh AS, Agyemang D, Bentum-Ennin L, Ofori EA, et al. Asymptomatic malaria infection is maintained by a balanced pro- and anti-inflammatory response. Front Microbiol (2020) 11:559255. doi: 10.3389/fmicb.2020.559255
49. Hafalla JC, Claser C, Couper KN, Grau GE, Renia L, de Souza JB, et al. The CTLA-4 and PD-1/PD-L1 inhibitory pathways independently regulate host resistance to Plasmodium-induced acute immune pathology. PloS Pathog (2012) 8(2):e1002504. doi: 10.1371/journal.ppat.1002504
Keywords: bovine babesiosis, Babesia bovis, in vitro culture attenuated vaccines, Babesia immunity, Babesia vaccine
Citation: Bastos RG, Capelli-Peixoto J, Laughery JM, Suarez CE and Ueti MW (2023) Vaccination with an in vitro culture attenuated Babesia bovis strain safely protects highly susceptible adult cattle against acute bovine babesiosis. Front. Immunol. 14:1219913. doi: 10.3389/fimmu.2023.1219913
Received: 09 May 2023; Accepted: 07 July 2023;
Published: 31 July 2023.
Edited by:
Meiqing Shi, University of Maryland, College Park, United StatesReviewed by:
Perle Latre De Late, University of Missouri, United StatesAlba Marina Gimenez, University of São Paulo, Brazil
Copyright © 2023 Bastos, Capelli-Peixoto, Laughery, Suarez and Ueti. This is an open-access article distributed under the terms of the Creative Commons Attribution License (CC BY). The use, distribution or reproduction in other forums is permitted, provided the original author(s) and the copyright owner(s) are credited and that the original publication in this journal is cited, in accordance with accepted academic practice. No use, distribution or reproduction is permitted which does not comply with these terms.
*Correspondence: Reginaldo G. Bastos, reginaldo_bastos@wsu.edu