- 1Department of Cancer and Inflammation Research, Institute of Molecular Medicine, University of Southern Denmark, Odense, ;Denmark
- 2Department of Oncology, Odense University Hospital, Odense, Denmark
- 3Academy of Geriatric Cancer Research (AgeCare), Odense University Hospital, Odense, Denmark
Introduction
Mammalian cells have evolved defense systems to detect and respond to viral infections by producing cytokines that activate and shape the antiviral immune response (1, 2). Recent studies have revealed that cancer cells can also activate antiviral signaling in response to therapy-induced DNA damage and demethylation (3–6). This activation initiates the production of type I interferon (IFN), which is crucial for a T-cell response against cancer cells (4–6) and supports anti-tumor immunity (7). However, it has become apparent that therapy-induced activation of antiviral signaling can also trigger interleukin-1 (IL-1)-driven antiviral responses, which may not be advantageous as IL-1 promotes the production of cytokines that directly enhance tumor growth or inhibit anti-tumor immunity through the recruitment of myeloid-derived suppressor cells (MDSCs) to the tumor microenvironment (TME) (8). Therefore, it is important to understand the interplay between IFN and IL-1 antiviral responses in cancer cells and how this may impact immune evasion and anti-cancer therapy.
IFN and IL-1 antiviral response pathways
The human body is constantly exposed to a wide range of pathogens, including viruses that can cause severe illnesses. To combat these pathogens, the immune system has evolved a complex network of defense mechanisms, including the antiviral response pathways (1, 2). The pathways are initiated by specialized receptors called pattern recognition receptors, which recognize viral components such as viral RNA or DNA. These receptors include surface molecules such as Toll-like and lectin receptors, as well as cytoplasmic receptors such as retinoic acid-inducible gene (RIG-I) and melanoma differentiation-associated gene 5 (MDA-5). The receptors are expressed in various cells, including epithelial and immune cells.
Activation of pattern recognition receptors triggers signaling cascades that converge into activation of IRF3 and IRF7 transcription factors and/or NF-κB pathways. This ultimately leads to the production of type I and III IFNs, which are cytokines that play a crucial role in the antiviral response (9, 10). Type I and III IFN bind to specific receptors on the surface of infected and neighboring cells, triggering a signaling pathway that results in the upregulation of a variety of antiviral effector molecules. The molecules can directly inhibit viral replication or induce apoptosis, thereby limiting the spread of the virus. In addition, the antiviral response leads to production of proinflammatory cytokines that support innate and adaptive immunity, including IL-1, which plays a crucial role in regulating the immune response to viral infections. Like IFNs, IL-1 contributes to the overall antiviral signaling by promoting local and systemic inflammation and activating immune cells (11). The expression of IL-1 is also mediated by IRF and NF-κB signaling pathways. Despite the important roles of both the IFN and IL-1 response pathways in the antiviral defense, the relationship between them remains poorly understood.
Diverging roles of IFN and IL-1 antiviral pathways in cancer
Intriguingly, cancer cells can also activate antiviral signaling in the absence of viral infection through two distinct mechanisms: (i) the release of fragmented dsDNA into the cytosol due to genomic instability or (ii) epigenetic activation and transcription of dsRNAs from endogenous retroviruses (ERVs) in the genome (3–6). These mechanisms occur spontaneously in cancer cells due to their genomic and epigenetic instability and are strongly triggered by standard anti-cancer therapies such as radiation therapy, chemotherapy, and hypomethylating agents (3–6, 12–15). Activation of antiviral signaling in cancer cells can induce the expression of type I IFNs (3–6, 12–15), which generally inhibit cancer cell growth and support the function of immune cells with anti-cancer effects, such as T cells and NK cells (7). This suggests a beneficial role of spontaneous or therapy-induced activation of antiviral signaling in cancer cells.
However, recent research challenges the notion that IFNs are the primary drivers of antiviral responses in cancer cells. Evidence suggests that in a substantial subset of melanoma, breast and ovarian cancers, IL-1 is a potent moderator of the antiviral response to treatment with hypomethylating agents (8). In contrast to IFNs, IL-1 is considered to have unfavorable effects on anti-tumor immunity (11), largely due to its ability to enhance the recruitment of immune repressive cells to the TME (11, 16–24). In agreement, IL-1-driven antiviral response in cancer cells promote the expression of multiple myeloid cell chemoattractant, which recruit MDSCs to tumors (8). MDSCs are known to restrict the activation, proliferation and functionality of T cells in the TME, leading to repression of anti-tumor immunity (25–29). Additionally, tumor infiltration of MDSCs is associated with tumor progression and poor response to various therapies, including chemotherapy, radiation, and immunotherapy, across multiple tumor types (30). Therefore, many tumors may evade immune control and anti-cancer treatment by rewiring antiviral pathways to substitute a lethal IFN-driven inflammatory response with an IL-1-driven response (Figure 1).
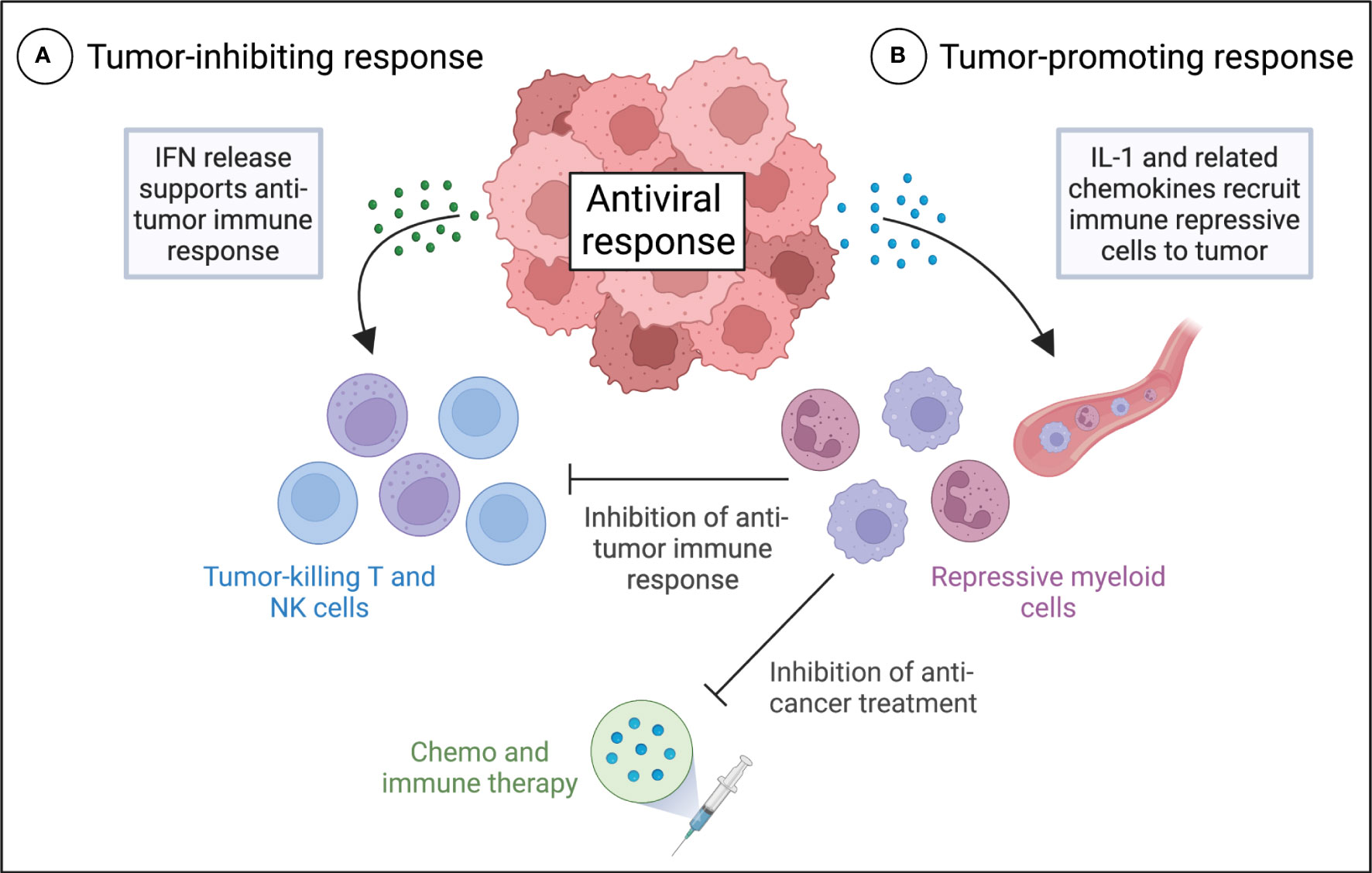
Figure 1 Different roles of IFN and IL-1 antiviral signaling in anti-tumor immunity and the therapeutic response of tumors. Proposed model for tumor inhibiting IFN-driven (A) and tumor promoting IL-1-driven (B) antiviral responses in cancer cells and their effects on the anti-tumor immune response and anti-cancer treatment. (A) Activation of IFN expression in tumors by the antiviral response results in autocrine and paracrine IFN signaling and production of a variety of antiviral effector molecules that support anti-tumor immunity. (B) Activation of IL-1 expression in tumors results in the production of multiple myeloid cell chemoattractant, which recruit MDSCs to the tumor microenvironment. MDSCs may restrict the activation, proliferation and functionality of T cells in the tumor microenvironment, leading to repression of anti-tumor immunity, and they may promote a poor response to various therapies, including chemotherapy, radiation, and immunotherapy.
Interaction between IFN and IL-1 antiviral pathways
There is growing evidence to suggest that the interplay between IL-1 and IFNs is crucial in maintaining a delicate balance in the innate inflammatory response. Studies have shown that both IFN-α and IFN-β can downregulate the transcription of IL-1α and IL-1β, as well as inhibit the processing of the inflammasome that produces bioactive IL-1 (31–33). Additionally, numerous studies have shown that type I IFNs induce the expression of IL-1RA, which is an antagonist of the IL-1 receptor (34–36). These effects have also been observed in patients receiving type I IFN therapy (37). Thus, it appears that type I and III IFNs are capable of suppressing IL-1 activity at multiple levels.
The IFN-mediated regulation of IL-1 activity can have opposing effects during pathogen infections, depending on the situation. In some cases, the inhibition of IL-1 activity by IFNs can impair the host ability to mount a robust immune response against the pathogen. However, in situations where excessive IL-1 activity could lead to immunopathology, IFN-mediated suppression of IL-1 can be beneficial in limiting tissue damage and inflammation. Although much less studied, available data also suggest that IL-1 potently antagonizes type I IFN responses by directly regulating both transcription and translation of IFN-β (33, 38) as well as attenuating IFN-α/β-induced STAT phosphorylation (39).
Although limited, the available data suggest that there is crosstalk between IFNs and IL-1 in cancer as well. Specifically, it has been demonstrated that activation of antiviral signaling by DNA methyltransferase inhibitor-mediated de-repression of ERVs induce the expression of IFN and IL-1 genes in a complex pattern, with some cancer cell lines expressing either one alone or both together (8). This suggest that IFN and IL-1 antiviral responses are not mutually exclusive, despite the negative crosstalk between these signaling pathways. Additionally, it was found that DNA methyltransferase inhibitor-mediated induction of IL-1 was strongly suppressed by the presence of type I and III IFN, whereas IL-1 did not inhibit IFN expression. These findings suggests that there is interplay between IFN and IL-1 in cancer.
Discussion
Antiviral signaling is increasingly recognized as a critical regulator of both tumor development and anti-tumor immunity. While much research has focused on the role of IFNs in this process (4, 5), recent evidence suggests that IL-1 may also play a significant role (8). Therefore, understanding the complex interplay between these two signaling pathways in tumors and their divergent roles in anti-tumor immunity is crucial.
For instance, it is well known that IFN signaling is often lost in cancer, but it is not known how this affects IL-1 activity and ultimately anti-tumor immunity. Therefore, studying the relationship between IFN and IL-1 in tumors will be essential to develop effective cancer therapies that activate antiviral signaling. It will also be important to comprehensively characterize the molecular differences that determine the relative activation IFN and IL-1 signaling in tumors. This understanding will provide profound biological insight into tumor biology and aid the identification of biomarkers for cancer patient stratification with respect to treatment with drugs that activate antiviral signaling, such as agents that induce DNA damage and demethylation.
Overall, elucidating the interplay between IFNs and IL-1 in cancer will have far-reaching implications for the development of novel cancer therapies and personalized medicine.
Author contributions
MG wrote the manuscript.
Funding
The study was supported by the Region of Southern Denmark, Pink Tribute, Einar Willumsens foundation, Carl and Ellen Hertzs foundation, Phycician Sofus Carl Emil Friis and spouse’s foundation, Neye foundation, Agnes and Poul Friis foundation, Emil C. Hertz and spouse’s foundation, Frimodt-Heineke foundation, Olga Doris Friis foundation, Dagmar Marshalls foundation, the Danish Cancer Society, the Novo Nordisk foundation, and the Danish Research Council for Independent Research. The funders were not involved in the study design, collection, analysis,interpretation of data, the writing of this article or the decision to submit it for publication.
Conflict of interest
The author declares that the research was conducted in the absence of any commercial or financial relationships that could be construed as a potential conflict of interest.
Publisher’s note
All claims expressed in this article are solely those of the authors and do not necessarily represent those of their affiliated organizations, or those of the publisher, the editors and the reviewers. Any product that may be evaluated in this article, or claim that may be made by its manufacturer, is not guaranteed or endorsed by the publisher.
References
1. Briard B, Place DE, Kanneganti TD. DNA Sensing in the innate immune response. Physiol (Bethesda) (2020) 35(2):112–24. doi: 10.1152/physiol.00022.2019
2. Musella M, Manic G, De Maria R, Vitale I, Sistigu A. Type-i-interferons in infection and cancer: unanticipated dynamics with therapeutic implications. Oncoimmunology (2017) 6(5):e1314424. doi: 10.1080/2162402X.2017.1314424
3. Bakhoum SF, Ngo B, Laughney AM, Cavallo JA, Murphy CJ, Ly P, et al. Chromosomal instability drives metastasis through a cytosolic DNA response. Nature (2018) 553(7689):467–72. doi: 10.1038/nature25432
4. Chiappinelli KB, Strissel PL, Desrichard A, Li H, Henke C, Akman B, et al. Inhibiting DNA methylation causes an interferon response in cancer via dsRNA including endogenous retroviruses. Cell (2017) 169(2):361. doi: 10.1016/j.cell.2017.03.036
5. Roulois D, Loo Yau H, Singhania R, Wang Y, Danesh A, Shen SY, et al. DNA-Demethylating agents target colorectal cancer cells by inducing viral mimicry by endogenous transcripts. Cell (2015) 162(5):961–73. doi: 10.1016/j.cell.2015.07.056
6. Feng X, Tubbs A, Zhang C, Tang M, Sridharan S, Wang C, et al. ATR inhibition potentiates ionizing radiation-induced interferon response via cytosolic nucleic acid-sensing pathways. EMBO J (2020) 39(14):e104036. doi: 10.15252/embj.2019104036
7. Zitvogel L, Galluzzi L, Kepp O, Smyth MJ, Kroemer G. Type I interferons in anticancer immunity. Nat Rev Immunol (2015) 15(7):405–14. doi: 10.1038/nri3845
8. Traynor S, Terp MG, Nielsen AY, Guldberg P, Jakobsen M, Pedersen PG, et al. DNA Methyltransferase inhibition promotes recruitment of myeloid-derived suppressor cells to the tumor microenvironment through induction of tumor cell-intrinsic interleukin-1. Cancer Lett (2023) 552:215982. doi: 10.1016/j.canlet.2022.215982
9. McNab F, Mayer-Barber K, Sher A, Wack A, O'Garra A. Type I interferons in infectious disease. Nat Rev Immunol (2015) 15(2):87–103. doi: 10.1038/nri3787
10. Parker BS, Rautela J, Hertzog PJ. Antitumour actions of interferons: implications for cancer therapy. Nat Rev Cancer (2016) 16(3):131–44. doi: 10.1038/nrc.2016.14
11. Apte RN, Voronov E. Is interleukin-1 a good or bad 'guy' in tumor immunobiology and immunotherapy? Immunol Rev (2008) 222:222–41. doi: 10.1111/j.1600-065X.2008.00615.x
12. Sistigu A, Yamazaki T, Vacchelli E, Chaba K, Enot DP, Adam J, et al. Cancer cell-autonomous contribution of type I interferon signaling to the efficacy of chemotherapy. Nat Med (2014) 20(11):1301–9. doi: 10.1038/nm.3708
13. Parkes EE, Walker SM, Taggart LE, McCabe N, Knight LA, Wilkinson R, et al. Activation of STING-dependent innate immune signaling by s-Phase-Specific DNA damage in breast cancer. J Natl Cancer Inst (2017) 109(1). doi: 10.1093/jnci/djw199
14. Hartlova A, Erttmann SF, Raffi FA, Schmalz AM, Resch U, Anugula S, et al. DNA Damage primes the type I interferon system via the cytosolic DNA sensor STING to promote anti-microbial innate immunity. Immunity (2015) 42(2):332–43. doi: 10.1016/j.immuni.2015.01.012
15. Mackenzie KJ, Carroll P, Martin CA, Murina O, Fluteau A, Simpson DJ, et al. cGAS surveillance of micronuclei links genome instability to innate immunity. Nature (2017) 548(7668):461–5. doi: 10.1038/nature23449
16. Bunt SK, Sinha P, Clements VK, Leips J, Ostrand-Rosenberg S. Inflammation induces myeloid-derived suppressor cells that facilitate tumor progression. J Immunol (2006) 176(1):284–90. doi: 10.4049/jimmunol.176.1.284
17. Kaplanov I, Carmi Y, Kornetsky R, Shemesh A, Shurin GV, Shurin MR, et al. Blocking IL-1beta reverses the immunosuppression in mouse breast cancer and synergizes with anti-PD-1 for tumor abrogation. Proc Natl Acad Sci U.S.A. (2019) 116(4):1361–9. doi: 10.1073/pnas.1812266115
18. Elkabets M, Ribeiro VS, Dinarello CA, Ostrand-Rosenberg S, Di Santo JP, Apte RN, et al. IL-1beta regulates a novel myeloid-derived suppressor cell subset that impairs NK cell development and function. Eur J Immunol (2010) 40(12):3347–57. doi: 10.1002/eji.201041037
19. Tannenbaum CS, Rayman PA, Pavicic PG, Kim JS, Wei W, Polefko A, et al. Mediators of inflammation-driven expansion, trafficking, and function of tumor-infiltrating MDSCs. Cancer Immunol Res (2019) 7(10):1687–99. doi: 10.1158/2326-6066.CIR-18-0578
20. Song X, Krelin Y, Dvorkin T, Bjorkdahl O, Segal S, Dinarello CA, et al. CD11b+/Gr-1+ immature myeloid cells mediate suppression of T cells in mice bearing tumors of IL-1beta-secreting cells. J Immunol (2005) 175(12):8200–8. doi: 10.4049/jimmunol.175.12.8200
21. Bunt SK, Yang L, Sinha P, Clements VK, Leips J, Ostrand-Rosenberg S. Reduced inflammation in the tumor microenvironment delays the accumulation of myeloid-derived suppressor cells and limits tumor progression. Cancer Res (2007) 67(20):10019–26. doi: 10.1158/0008-5472.CAN-07-2354
22. Aggen DH, Ager CR, Obradovic AZ, Chowdhury N, Ghasemzadeh A, Mao W, et al. Blocking IL1 beta promotes tumor regression and remodeling of the myeloid compartment in a renal cell carcinoma model: multidimensional analyses. Clin Cancer Res (2021) 27(2):608–21. doi: 10.1158/1078-0432.CCR-20-1610
23. Najjar YG, Rayman P, Jia X, Pavicic PG Jr., Rini BI, Tannenbaum C, et al. Myeloid-derived suppressor cell subset accumulation in renal cell carcinoma parenchyma is associated with intratumoral expression of IL1beta, IL8, CXCL5, and mip-1alpha. Clin Cancer Res (2017) 23(9):2346–55. doi: 10.1158/1078-0432.CCR-15-1823
24. Jiang H, Gebhardt C, Umansky L, Beckhove P, Schulze TJ, Utikal J, et al. Elevated chronic inflammatory factors and myeloid-derived suppressor cells indicate poor prognosis in advanced melanoma patients. Int J Cancer (2015) 136(10):2352–60. doi: 10.1002/ijc.29297
25. Kim IS, Gao Y, Welte T, Wang H, Liu J, Janghorban M, et al. Immuno-subtyping of breast cancer reveals distinct myeloid cell profiles and immunotherapy resistance mechanisms. Nat Cell Biol (2019) 21(9):1113–26. doi: 10.1038/s41556-019-0373-7
26. Albrengues J, Shields MA, Ng D, Park CG, Ambrico A, Poindexter ME, et al. Neutrophil extracellular traps produced during inflammation awaken dormant cancer cells in mice. Science (2018) 361(6409). doi: 10.1126/science.aao4227
27. Gonda K, Shibata M, Ohtake T, Matsumoto Y, Tachibana K, Abe N, et al. Myeloid-derived suppressor cells are increased and correlated with type 2 immune responses, malnutrition, inflammation, and poor prognosis in patients with breast cancer. Oncol Lett (2017) 14(2):1766–74. doi: 10.3892/ol.2017.6305
28. Jimenez-Cortegana C, Galassi C, Klapp V, Gabrilovich DI, Galluzzi L. Myeloid-derived suppressor cells and radiotherapy. Cancer Immunol Res (2022) 10(5):545–57. doi: 10.1158/2326-6066.CIR-21-1105
29. Takeuchi S, Baghdadi M, Tsuchikawa T, Wada H, Nakamura T, Abe H, et al. Chemotherapy-derived inflammatory responses accelerate the formation of immunosuppressive myeloid cells in the tissue microenvironment of human pancreatic cancer. Cancer Res (2015) 75(13):2629–40. doi: 10.1158/0008-5472.CAN-14-2921
30. Gjerstorff MF, Traynor S, Gammelgaard OL, Johansen S, Pedersen CB, Ditzel HJ, et al. PDX models: a versatile tool for studying the role of myeloid-derived suppressor cells in breast cancer. Cancers (Basel) (2022) 14(24). doi: 10.3390/cancers14246153
31. Novikov A, Cardone M, Thompson R, Shenderov K, Kirschman KD, Mayer-Barber KD, et al. Mycobacterium tuberculosis triggers host type I IFN signaling to regulate IL-1beta production in human macrophages. J Immunol (2011) 187(5):2540–7. doi: 10.4049/jimmunol.1100926
32. Mayer-Barber KD, Andrade BB, Barber DL, Hieny S, Feng CG, Caspar P, et al. Innate and adaptive interferons suppress IL-1alpha and IL-1beta production by distinct pulmonary myeloid subsets during mycobacterium tuberculosis infection. Immunity (2011) 35(6):1023–34. doi: 10.1016/j.immuni.2011.12.002
33. Mayer-Barber KD, Andrade BB, Oland SD, Amaral EP, Barber DL, Gonzales J, et al. Host-directed therapy of tuberculosis based on interleukin-1 and type I interferon crosstalk. Nature (2014) 511(7507):99–103. doi: 10.1038/nature13489
34. Huang Y, Blatt LM, Taylor MW. Type 1 interferon as an antiinflammatory agent: inhibition of lipopolysaccharide-induced interleukin-1 beta and induction of interleukin-1 receptor antagonist. J Interferon Cytokine Res (1995) 15(4):317–21. doi: 10.1089/jir.1995.15.317
35. Tilg H, Mier JW, Vogel W, Aulitzky WE, Wiedermann CJ, Vannier E, et al. Induction of circulating IL-1 receptor antagonist by IFN treatment. J Immunol (1993) 150(10):4687–92.
36. Coclet-Ninin J, Dayer JM, Burger D. Interferon-beta not only inhibits interleukin-1beta and tumor necrosis factor-alpha but stimulates interleukin-1 receptor antagonist production in human peripheral blood mononuclear cells. Eur Cytokine Netw (1997) 8(4):345–9.
37. Reznikov LL, Puren AJ, Fantuzzi G, Muhl H, Shapiro L, Yoon DY, et al. Spontaneous and inducible cytokine responses in healthy humans receiving a single dose of IFN-alpha2b: increased production of interleukin-1 receptor antagonist and suppression of IL-1-induced IL-8. J Interferon Cytokine Res (1998) 18(10):897–903. doi: 10.1089/jir.1998.18.897
38. Kohase M, Zhang YH, Lin JX, Yamazaki S, Sehgal PB, Vilcek J. Interleukin-1 can inhibit interferon-beta synthesis and its antiviral action: comparison with tumor necrosis factor. J Interferon Res (1988) 8(4):559–70. doi: 10.1089/jir.1988.8.559
Keywords: interleukin 1, interferon, tumor immunity, therapeutic resistance, myeloid-derived suppressor cells (MDSC)
Citation: Gjerstorff MF (2023) Crosstalk between interferon and interleukin-1 antiviral signaling in cancer cells: implications for immune evasion and therapeutic resistance. Front. Immunol. 14:1219870. doi: 10.3389/fimmu.2023.1219870
Received: 09 May 2023; Accepted: 29 May 2023;
Published: 08 June 2023.
Edited by:
Xiaowen Liu, Fudan University, ChinaReviewed by:
Martina Musella, Catholic University of the Sacred Heart, ItalyEleonora Aricò, National Institute of Health (ISS), Italy
Flávia Castro, Universidade do Porto, Portugal
Copyright © 2023 Gjerstorff. This is an open-access article distributed under the terms of the Creative Commons Attribution License (CC BY). The use, distribution or reproduction in other forums is permitted, provided the original author(s) and the copyright owner(s) are credited and that the original publication in this journal is cited, in accordance with accepted academic practice. No use, distribution or reproduction is permitted which does not comply with these terms.
*Correspondence: Morten Frier Gjerstorff, bWdqZXJzdG9yZmZAaGVhbHRoLnNkdS5kaw==