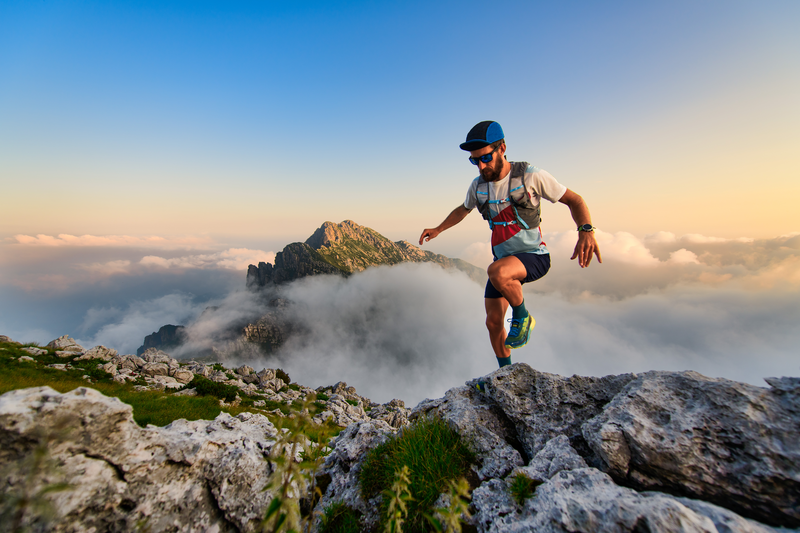
94% of researchers rate our articles as excellent or good
Learn more about the work of our research integrity team to safeguard the quality of each article we publish.
Find out more
STUDY PROTOCOL article
Front. Immunol. , 20 December 2023
Sec. Vaccines and Molecular Therapeutics
Volume 14 - 2023 | https://doi.org/10.3389/fimmu.2023.1212320
This article is part of the Research Topic Global Excellence in Translational Immunology: Asia and Australasia 2023 View all 7 articles
Maternal immunisation, a low cost and high efficacy intervention is recommended for its pathogen specific protection. Evidence suggests that maternal immunisation has another significant impact: reduction of preterm birth (PTB), the single greatest cause of childhood morbidity and mortality globally. Our overarching question is: how does maternal immunisation modify the immune system in pregnant women and/or their newborn to reduce adverse pregnancy outcomes and enhance the newborn infant’s capacity to protect itself from infectious diseases during early childhood? To answer this question we are conducting a multi-site, prospective observational cohort study collecting maternal and infant biological samples at defined time points during pregnancy and post-partum from nulliparous women. We aim to enrol 400 women and determine the immune trajectory in pregnancy and the impact of maternal immunisation (including influenza, pertussis and/or COVID-19 vaccines) on this trajectory. The results are expected to identify areas that can be targeted for future intervention studies.
Globally, preterm birth (PTB) remains one of the most significant causes of death and morbidity in childhood (1, 2). PTB is the single most important cause of death in children under five years of age, with one million children dying each year due to PTB-related complications (1). It is also an important cause of disability and major contributor to health care expenditure (3). Most PTB and stillbirths occur in low-income settings, with over 75% of cases occurring in Asia and sub-Saharan Africa, and morbidity and mortality rates of premature infants in these settings remain disproportionately high (1). Despite efforts to reduce PTB worldwide, rates of this adverse pregnancy outcome remain persistently elevated, independent of income setting (4, 5). Hence, there is an urgent need for more effective interventions to reduce PTB and its attendant neonatal and early childhood morbidity and mortality, particularly in low- and middle-income countries (LMICs).
Maternal vaccines are a well-established strategy used for prevention of pathogen-specific disease in mothers and infants through transplacental transfer of maternal antibodies. Routine tetanus toxoid-containing vaccination was the first routine immunisation program implemented for pregnant women (6). Beyond pathogen-specific benefits, some studies have also identified pathogen-agnostic or non-specific effects (NSE) of maternal vaccination protecting against several adverse birth outcomes, including PTB and stillbirth (7–11). Our group have previously published a systematic review of the safety of influenza vaccination in pregnancy (12). An unexpected finding of our review of the literature was an association between maternal immunisation and lower rates of PTB (12). However, this finding has not been consistent across other published studies. Nonetheless, we were intrigued by the possibility of immunisation being associated with reduced PTB and thus proceeded to explore this association in a large dataset of 270,851 women who gave birth in the Australian state of Victoria between Jan 2015 and Dec 2018 (11). We found that women receiving influenza vaccine and/or pertussis containing vaccine during pregnancy had a significantly lower risk of PTB than unvaccinated women (11). Further, the reduction in PTB we observed in association with maternal vaccination remained significant and consistent across four different influenza seasons (2015-2018) and across different times of the year (during and outside of influenza seasons) (11). The benefits therefore were unlikely related to preventing maternal infection or actual exposure to influenza virus. We have further confirmed this association when accounting for immortal time bias (13).
The term pathogen-agnostic or NSE of vaccination aims to capture protection against outcomes unrelated to prevention of the intended vaccine preventable disease (14). We will use the term NSE for the remainder of this article. These could theoretically be associated with any vaccine. A systematic review in 2016 sponsored by the World Health Organization concluded that the Bacille Calmette-Guerin (BCG) vaccine and measles vaccine were associated with mortality benefits greater than expected based on pathogen-specific effects alone (15). For example, although Mycobacterium tuberculosis is the intended target pathogen of BCG vaccines, BCG vaccination of newborns reduces risk for death from a wide range of infections unrelated to tuberculosis, including neonatal sepsis (16, 17). Mechanisms that have been postulated include changes in innate immunity, including a rapid increase in neutrophils (18).
Although the causes of PTB are not entirely understood, there are several risk factors and mechanisms that are known to be implicated in the process (19). Some studies suggest that in the case of preterm labour, multiple factors contribute to dysregulated inflammatory processes that result in early initiation of labour (19, 20). This trajectory, down an unfavourable path, may be underpinned by activity of the immune system- the so called “immune trajectory” in pregnancy. One hypothesis for the NSE of maternal immunisation on birth outcomes is that maternal vaccines may modulate the maternal and fetal immune trajectory such that these heightened inflammatory processes that contribute to PTB are reduced. Similar to the benefit of BCG on newborn host defence, maternal vaccination could for example activate innate immunity providing broad protection against pathogens, or enhance immune regulatory pathways, which together could reduce potentially harmful inflammatory responses during pregnancy (21, 22).
NSE of vaccination are increasingly being recognised for their health benefits in postnatal life. Yet, this has never been explored in prenatal life, i.e. during pregnancy. Our and others’ data indicate that maternal immunisation may increase immune resilience leading to longer gestation, and in turn, a healthy start to life. For the purpose of this study, we define “immune resilience” as the capacity to preserve and/or rapidly restore immune function that resist adverse outcomes and control other causes of inflammatory stress. Based on the above observations, and the clear need to understand mechanisms for other vaccines and interventions to tackle the substantial burden of adverse pregnancy outcomes, we designed the PREMITUR study.
The PREMITUR study hypothesises that maternal immunisation alters the immune trajectory in pregnant women in a way that leads to a reduction in adverse pregnancy outcomes. The specific aims of our study are:
Aim 1. To determine the immune trajectory of pregnancy that leads to term versus PTB.
Aim 2. To define the impact of maternal immunisation on the immune trajectory of pregnancy.
Aim 3. To delineate the impact of maternal immunisation on the immune trajectory of the infant.
Pregnancy induces rapid changes in systemic maternal immunity. In healthy term pregnancy these changes follow a healthy developmental trajectory (23–25), while the final common pathway leading to adverse pregnancy outcome appears to involve an altered immune trajectory (26). However, the immune trajectory of pregnancy in relation to adverse pregnancy outcomes has not been comprehensively captured via the powerful integration across the range of biological domains that we will conduct here (RNA, protein, metabolite, function – ‘multi-omics’). To our knowledge, there is only one recent publication that applied a similar integrated, multi-omic approach to PTB (27). While this published report clearly confirms feasibility and advantages of our systems biology approach, the previous study only assessed a single time point in a cross-sectional design, i.e. they did not attempt to determine the trajectory (27). And importantly, this published report did not take the impact of maternal immunisation into account. We have recently shown that integrating across the multi-omic space and conducting these assessments longitudinally over time in the same subject allows delineation of developmental trajectories that provide critical insight into complex biological processes (18, 28). Importantly, this integration across biology (multi-omics) and over time in the same subject allows for sound and rigorous statistical interrogation of group sample sizes with n ≤20 (18, 25).
Our group has also recently shown that one’s “immune baseline” can determine the outcomes of vaccination or infection (29, 30), and that for the immune trajectory of the newborn this ‘baseline’ is influenced by maternal immunity (31, 32). We have also shown that the ‘baseline’ in early life is not a stable steady state (as it is in adults), but a dynamic yet highly predictable (and predictive) trajectory (28). This suggests that impacting the mother’s immune trajectory in pregnancy through maternal immunisation may impact the immune trajectory of the infant. The concept of maternal immunity impacting immune ontogeny of her infant is already widely accepted for maternal antibodies influencing the infant’s antibody response to vaccination (33). But there is growing evidence that this mother-infant interaction goes far beyond adaptive immunity: i) maternal quadrivalent influenza vaccine (QIV) reduces acute airway infections in the infant beyond influenza (34) which may relate to vaccine-induced cytokine responses in the nasal mucosa of her infant (35); ii) maternal diphtheria, tetanus and acellular pertussis (dTpa) immunisation during pregnancy reduces the risk for acute airway infections beyond Bordetella pertussis in infants, which may relate to vaccine-induced cytokine transfer across the placenta (36). The theoretical underpinnings of our rationale for Aim 3 are thus supported by existing evidence. What is lacking is evaluation of the maternal-infant immune dyad in the context of maternal immunisation using the unbiased, systematic approach of systems biology proposed here. Our findings promise not only to unveil the mechanisms related to maternal immunisation but to identify pathways to target to improve the developmental trajectory and well-being of all children in general.
This is a prospective, observational cohort study.
There are four maternity services participating in this project- two private and two public. Together these services have over 25,000 births per year, of which half are primigravid women.
In this project we partner with both public and private providers of antenatal care. In Australia almost one quarter of births occur in the private sector although this group is rarely included in research. It is essential to consider the private sector to maximise the opportunities for translation of key findings and to ensure broad representation of women so findings can be generalizable.
Miracle Babies is Australia’s leading organisation supporting premature and sick newborns, their families and the hospitals that care for them. They are partnering on this project and will be providing input end-to-end for this study. This includes providing consumer representation on the project steering committee, design and review of the study protocol, review of the conduct and progress of the study, interpretation of the data and most importantly appropriate and sensitive dissemination of the findings.
A pregnant woman will be eligible for inclusion in the PREMITUR study if the following criteria are met
● Nulliparous
● Aged 18 to ≤45 years
● Gestation between 9 and 22 weeks at time of recruitment
● Willing and able to comply with all study requirements including timing and/or nature of required assessments and follow up of mother and baby to six weeks post-partum
A pregnant woman will be ineligible for inclusion in the PREMITUR if the following criteria apply:
● Multiparous
● Multiple pregnancy
● Risk factors for PTB e.g. previous cervical procedure such as cone biopsy
● Unable to provide consent
● Concomitant immunosuppressive medication likely to continue beyond first trimester
● Any other significant acute or chronic medical condition that in the opinion of the investigator may interfere with the interpretation of study results or place the participant at increased risk if they participate in the study.
● Unwilling and unable to comply with all study requirements including timing and/or nature of required assessments and follow up of mother and baby to six weeks post-partum (e.g. planning to relocate during pregnancy)
We aim to recruit 400 healthy nulliparous women over three years. We are restricting our recruitment to primigravid women.
Potential participants will be identified by one of two mechanisms. In the public system women will be identified from clinic lists as eligible by a research midwife who will alert the treating clinician. If deemed appropriate the treating clinician will introduce the study to the participant and seek their interest. If they are interested to receive further information then their details will be shared with the research midwife who will contact them, provide further information, answer questions and provide the participant information and consent form (PICF). Signed consent can be obtained either electronically or in paper form with a wet ink signature. A similar process is followed for women receiving private maternity care, although in this case identification of eligible women is via the private obstetrician (Figure 1).
Figure 1 Study Design (Created with BioRender.com).
Clinical data will be collected at baseline including established risk factors for PTB as well as past vaccination history. Pregnancy outcomes will be collected at birth. Venous blood (5mL) will be collected at each timepoint: 10-14 weeks, 20-22 weeks, 26-28 weeks, 34-36 weeks, and at birth (Figure 2).
Figure 2 Overview of study procedures (Created with BioRender.com).
We have chosen these time points to afford serial profiling while minimising burden and coinciding with routine pregnancy care appointments.
Specifically, blood will be collected for transcriptomic (RNASeq) as well as epigenetic (methylation) analysis and plasma harvested for proteomics (via Somalogic Inc.) and metabolomics (via Metabolon Inc.). Cell composition of this blood sample will also be assessed by flow cytometric analysis capturing most innate and adaptive immune subsets, from neutrophils, dendritic cells (DCs) and their subsets, to NK cells, monocytes, as well as most T cell subsets and B cell subsets. The Paxgene tube will be used for transcriptomics. As little as 55 microlitres of blood is required for proteomics and metabolomics, so 5mL per timepoint is sufficient for the proposed testing. Given the longitudinal nature of our study set up, samples will be batch randomized to reduce artifact, as well as corrected using ComBat batch to normalise across multiple analytical runs. Following distributional normalisation, we will apply the full power of longitudinal samples from the same participants, i.e., features will be normalised as change over time within each woman, using the WithinVariation function implemented in the R mixOmics package. With this, absolute values will be represented as relative change over time - i.e. biological trajectories. We will analyse multiomic signatures across women to define the immune trajectory of pregnancy. We will then contrast omic signatures across clinical groups (healthy term vs. PTB).
Assessment of the newborn infants’ response to maternal immunisation will focus on the nasal mucosa of the infants born to mothers enrolled in our study. Samples will be obtained on day of life 1 and at 6 weeks of age via a standardised, non-invasive and painless sampling procedure (Nasosorption), well-tolerated by newborn infants (and their parents) (37). These time points were chosen to coincide with infant health checks to reduce additional burden to parents. The neonatal nasal lining fluid absorption devices will be used to extract total proteins contrasting the infants’ developmental trajectory as a function of maternal immunisation status. The method of isolation of total proteins from the nasal lining fluids starts by thawing the devices on ice to preserve the integrity of the cells. Then, 300 ul of elution buffer (PBS (pH7.4) containing 0.05% (v/v) Tween20) is added. The synthetic absorptive matrix (SAM) is then removed from the nasosorption device using forceps by tearing it from the handle using sterile forceps. The SAM is then placed into a spinX column using a new pair of sterile forceps. After a centrifugation of the column and SAM at speed centrifugation(16,000 g) for 30 minutes at 4C, 260 ul of equate is reconstituted into a sterile tube and total proteins are quantified using Pierce BCA protein assay kit The protein identification and quantities will be assessed using Somascan, an untargeted Proteomics platform (Somalogic) capable of detecting more than 7000 proteins in less than 200ul of eluate (200ug/ml). In the testing assays performed by the study team, enough proteins were extracted from infant nasal lining devices and only a portion was used for proteomics analysis. The rest of the samples can be stored and used for targeted protein detection panels if interesting signals are detected from the initial untargeted analysis.
Our group and others have proven that when using this integrated multi-omic approach a sample size of 20 per group will suffice to identify robust (80% power) biological signatures related to pregnancy outcome with a two-sided significance criterion (alpha) of < 0.05. With this in mind, a sample size of 400 participants was chosen, assuming a background rate of PTB of 8%, it was estimated 32 women would delivery prematurely providing >20 per group.
We will compare the immune trajectory of all women with a preterm birth (estimated to be 8% of our total sample size) with a matched control group of women who deliver at term. We will not be performing multiomics on all participants recruited. To assess the immune trajectory in relation to pregnancy outcome longitudinally over time we will identify features both in the univariate and multivariate space. For univariate analysis, differentially expressed (DE) genes, proteins, or metabolites will be identified using DESeq2 v1.26. Correction for multiple comparisons will be done to control for false discoveries, filtering results based on a highly conservative p-value for significance (<0.001). Sparse Partial Least Squares - Discriminant Analysis (sPLS-DA) will be used to identify discriminatory features that co-vary with each other. Given the user-defined sparsity feature of sPLS-DA, and with the readout being classification accuracy, no p-value adjustment is necessary for this method. To assess the data in their biological context, i.e. integrated multi-omics, we will use two complementary integration strategies. NetworkAnalyst is based on known protein-protein interaction (PPI) networks and DIABLO, part of the mixOmics framework, a data-driven multi-omic integration approach. Given that DIABLO selects features that allow maximum class distinction (i.e. accurate classification of pregnancy outcome), this approach will also identify the earliest point during pregnancy that can accurately predict an adverse birth outcome. Finally, time course analyses that generate smoothing splines allow identification of groups of features that follow the same trajectory in a multivariate space. The DynOmics toolbox is an extension of the mixOmics toolbox used for multi-omic datasets built to identify these dynamic longitudinal changes in biological systems, while integrating multi-omic data.
We will compare the immune trajectory of 20 unvaccinated women with 20 women who received either one of the two vaccines recommended in pregnancy, and 20 vaccinated with both vaccines. Recruitment started prior to the availability of COVID-19 vaccines but continued during the rollout of COVID-19 vaccines. Data on any vaccination during pregnancy is captured and will be taken into account in the analysis. Based on our previous work, we expect ~10 women in our cohort to choose to not be vaccinated at all, and approximately 50 women to be vaccinated with either influenza or pertussis alone. If we are unable to recruit 10 controls who have received no vaccination we intend to use women who are vaccinated late in pregnancy as a control over the time periods they remain “unvaccinated”. We will employ the same blood processing and data analysis pipeline summarised for Aim 1.
Samples will be obtained on day of life 1 and 6 weeks of life (day of recommended first follow up) via our highly standardised, non-invasive thus non-painful sampling procedure (Nasosorption), well-tolerated by newborn infants (and their parents) as described. We will process and analyse these 120 samples as described above contrasting the infants’ immune trajectory as a function of maternal immunisation status.
Clinical Data will be collected across all study sites using REDCap, a secure electronic platform with built-in quality checks and access to the platform is limited to the study teams with clinical good practice up-to-date certificates, and unique credentials are required to log in.
To further ensure the quality of our data, we will be implementing quality assurance (QA) processes. These processes will be performed by the study team, and any discrepancies or issues that arise will be raised and resolved before sharing the data across the project analysis teams. Once the study is complete, the database will be locked, and data downloads will be restricted to read-only access.
In addition to clinical data, our study will also be collecting laboratory data from all study sites. MAESTRO (Multiomic Analytics, Experiments, and Sample Tracking Orchestrator) via RAN BioLinks, Ltd., a sample tracking platform will be used and access granted using specific credentials for each study team. MAESTRO is a modular platform that allows us to track and manage operational data in the laboratory, sample inventory, storage, and processing sites information as well as information about study samples, such as the collector, time, barcodes, volume, and any deviation from the protocol. It also enables easier integration with de-identified clinical data from REDCap in the future.
In addition to sample tracking, MAESTRO also includes a project management feature. This feature allows researchers to create centralized documentation, set goals, track milestones, manage team membership and roles attribution, and store up-to-date protocol documents. To ensure data quality, MAESTRO has a built-in a quality control layer. This layer automatically flags any inconsistencies or out-of-range data according to study-specific protocols and procedures.
To minimize the potential confounding effects of batch effects across different platforms, we will be aligning our sample batches for all “OMIC” platforms with the study block randomization. This approach ensures that we include study samples from the same participant across time points and representation of samples from different groups within each batch.
Each assay platform used in our study has predefined criteria for quality control of each experimental sample and assay. At a minimum, internal controls and standards will be run across all runs to ensure data quality.
Finally, to ensure confidentiality, all electronic data capture will be performed using secure, password-protected, encrypted devices. Paper records, including consent forms and medical records, will be stored in locked cabinets in secure locations with restricted access at the study sites. By taking these measures, we can ensure that our study meets the highest standards of data quality and confidentiality.
This trial was reviewed and approved by the Monash Health Human Research Ethics Committee (HREC). The trial is also registered on the Australian and New Zealand Clinical Trials Registry (ANZCTR). The trial will be conducted in compliance with local and National Health and Medical Research Council (NHMRC) guidelines. The outcomes of the clinical trial will be disseminated through local, national and international meetings and in peer-reviewed medical journals. Authorship will be allocated to final publication for study investigators and those providing substantive contributions to the design, conduct, interpretation, and reporting of the clinical trial.
In the ten minutes it has taken to read this manuscript, almost 300 more babies will have been born prematurely around the world. This global health problem needs urgent and innovative approaches. This project will capture for the first time the biological pathways responsible for protection against adverse pregnancy outcomes and prevention of illness in infants through increased immune resilience during pregnancy. This would confirm the place of maternal immunisation as part of a “three for one” deal including pathogen-specific protection for the mother and neonate, and non-specific immunological benefits leading to protection for the fetus. Finally, our work will empower women to base their choices around vaccination during pregnancy on evidence and insight. Specifically, our project not only has the potential to unlock mechanisms that underpin the beneficial effect of maternal immunisation, but provide immediate translational avenues, as all vaccines under study are already licensed and proven to be safe in pregnancy.
MG and TK conceived the concept for this study. MG, SC, JO, SK have contributed to study design and recruitment. TK, M-AD, RB-O and NA have contributed to methodology and planned analysis. All authors have contributed and reviewed the protocol and this manuscript. All authors contributed to the article and approved the submitted version.
This study is funded by the Australian Medical Research Future Fund (APP2007141). The funder has no role in the study design, data collection, data analyses, data interpretation, writing of the report or submitting of the report for publication.
The authors declare that the research was conducted in the absence of any commercial or financial relationships that could be construed as a potential conflict of interest.
All claims expressed in this article are solely those of the authors and do not necessarily represent those of their affiliated organizations, or those of the publisher, the editors and the reviewers. Any product that may be evaluated in this article, or claim that may be made by its manufacturer, is not guaranteed or endorsed by the publisher.
1. Chawanpaiboon S, Vogel JP, Moller A-B, Lumbiganon P, Petzold M, Hogan D, et al. Global, regional, and national estimates of levels of preterm birth in 2014: a systematic review and modelling analysis. Lancet Glob Health (2019) 7:e37–46. doi: 10.1016/S2214-109X(18)30451-0
2. United Nations. Levels and Trends in Child Mortality: 2020 Report. New York: United Nation’s Children’s Fund (2020).
3. Newnham JP, Schilling C, Petrou S, Morris JM, Wallace EM, Brown K, et al. The health and educational costs of preterm birth to 18 years of age in Australia. Aust N Z J Obstet Gynaecol (2022) 62:55–61. doi: 10.1111/ajo.13405
4. U.S 2021March of Dimes Report Card. Available at: https://www.marchofdimes.org/sites/default/files/2022-10/March-of-Dimes-2021-Full-Report-Card.pdf.
5. Australian Institute of Health and Welfare. Australia’s mothers and babies 2017- in brief. Canberra, Australia: Brief Canberra: AIHW (2019).
6. World Health Organization. Protecting all against tetanus: guide to sustaining maternal and neonatal tetanus elimination (MNTE) and broadening tetanus protection for all populations. Geneva: World Health Organization (2019).
7. Arriola CS, Vasconez N, Thompson MG, Olsen SJ, Moen AC, Bresee J, et al. Association of influenza vaccination during pregnancy with birth outcomes in Nicaragua. Vaccine (2017) 35:3056–63. doi: 10.1016/j.vaccine.2017.04.045
8. Olsen SJ, Mirza SA, Vonglokham P, Khanthamaly V, Chitry B, Pholsena V, et al. The effect of influenza vaccination on birth outcomes in a cohort of pregnant women in lao PDR, 2014-2015. Clin Infect Dis (2016) 63:487–94. doi: 10.1093/cid/ciw290
9. Regan AK, Moore HC, de Klerk N, Omer SB, Shellam G, Mak DB, et al. Seasonal trivalent influenza vaccination during pregnancy and the incidence of stillbirth: population-based retrospective cohort study. Clin Infect Dis (2016) 62:1221–7. doi: 10.1093/cid/ciw082
10. Legge A, Dodds L, MacDonald NE, Scott J, McNeil S. Rates and determinants of seasonal influenza vaccination in pregnancy and association with neonatal outcomes. CMAJ (2014) 186:E157–64. doi: 10.1503/cmaj.130499
11. Giles ML, Davey M-A, Wallace EM. Associations between maternal immunisation and reduced rates of preterm birth and stillbirth: A population based retrospective cohort study. Front Immunol (2021) 12(3194). doi: 10.3389/fimmu.2021.704254
12. Giles ML, Krishnaswamy S, Macartney K, Cheng A. The safety of inactivated influenza vaccines in pregnancy for birth outcomes: a systematic review. Hum Vaccin Immunother. (2019) 15(3):687–99. doi: 10.1080/21645515.2018.1540807
13. Gunatilaka A, Rolnik DL, Giles ML. Protective effect of maternal immunization on birth outcomes: A data linkage study. Int J Gynecol Obstet (2023) 00:1–7. doi: 10.1002/ijgo.14774
14. Aaby P, Benn CS, Flanagan KL, Klein SL, Kollmann TR, Lynn DJ, et al. The non-specific and sex-differential effects of vaccines. Nat Rev Immunol (2020) 20:464–70. doi: 10.1038/s41577-020-0338-x
15. Higgins JP, Soares-Weiser K, López-López JA, Kakourou A, Chaplin K, Christensen H, et al. Association of BCG, DTP and measles containing vaccines with childhood mortality: systematic review. BMJ (2016) 355:i5170. doi: 10.1136/bmj.i5170
16. Biering-Sørensen S, Aaby P, Lund N, Monteiro I, Jensen KJ, Eriksen HB, et al. Early BCG-Denmark and neonatal mortality among infants weighing <2500 g: A randomized controlled trial. Clin Infect Dis (2017) 65:1183–90. doi: 10.1093/cid/cix525
17. Aaby P, Roth A, Ravn H, Napirna BM, Rodrigues A, Lisse IM, et al. Randomized trial of BCG vaccination at birth to low-birth-weight children: Beneficial nonspecific effects in the neonatal period? J Infect Dis (2011) 204:245–52. doi: 10.1093/infdis/jir240
18. Brook B, Harbeson DJ, Shannon CP, Cai B, He D, Ben-Othman R, et al. BCG vaccination-induced emergency granulopoiesis provides rapid protection from neonatal sepsis. Sci Transl Med (2020) 12(542):eaax4517. doi: 10.1126/scitranslmed.aax4517
19. Goldenberg RL, Culhane JF, Iams JD, Romero R. Epidemiology and causes of preterm birth. Lancet (2008) 371(9606):75–84. doi: 10.1016/S0140-6736(08)60074-4
20. Romero R, Dey SK, Fisher SJ. Preterm labor: one syndrome, many causes. Science (2014) 345(6198):760–5. doi: 10.1126/science.1251816
21. Goodridge HS, Ahmed SS, Curtis N, Kollmann TR, Levy O, Netea MG, et al. Harnessing the beneficial heterologous effects of vaccination. Nat Rev Immunol (2016) 16(6):392–400. doi: 10.1038/nri.2016.43
22. Mantovani A, Netea MG. Trained innate immunity, epigenetics, and Covid-19. N Engl J Med (2020) 383(11):1078–80. doi: 10.1056/NEJMcibr2011679
23. Aghaeepour N, Ganio EA, Mcilwain D, Tsai AS, Tingle M, Van Gassen S, et al. An immune clock of human pregnancy. Sci Immunol (2017) 2(15):eaan2946. doi: 10.1126/sciimmunol.aan2946
24. Ghaemi MS, DiGiulio DB, Contrepois K, Callahan B, Ngo TTM, Lee-McMullen B, et al. Multiomics modeling of the immunome, transcriptome, microbiome, proteome and metabolome adaptations during human pregnancy. Bioinformatics (2019) 35(1):95–103. doi: 10.1093/bioinformatics/bty537
25. Aghaeepour N, Lehallier B, Baca Q, Ganio EA, Wong RJ, Ghaemi MS, et al. A proteomic clock of human pregnancy. Am J Obstet Gynecol. (2018) 218(3):347.e1–347.e14. doi: 10.1016/j.ajog.2017.12.208
26. Deshmukh H, Way SS. Immunological basis for recurrent fetal loss and pregnancy complications. Annu Rev Pathol (2019) 14:185–210. doi: 10.1146/annurev-pathmechdis-012418-012743
27. Jehan F, Sazawal S, Baqui AH, Nisar MI, Dhingra U, Khanam R, et al. Multiomics characterization of preterm birth in low- and middle-income countries. JAMA Netw Open (2020) 3(12):e2029655. doi: 10.1001/jamanetworkopen.2020.29655
28. Lee AH, Shannon CP, Amenyogbe N, Bennike TB, Diray-Arce J, Idoko OT, et al. Dynamic molecular changes during the first week of human life follow a robust developmental trajectory. Nat Commun (2019) 10(1):1092. doi: 10.1038/s41467-019-08794-x
29. Tsang JS, Dobaño C, VanDamme P, Moncunill G, Marchant A, Othman RB, et al. Improving vaccine-induced immunity: can baseline predict outcome? Trends Immunol (2020) 41(6):457–65. doi: 10.1016/j.it.2020.04.001
30. Shannon CP, Blimkie TM, Ben-Othman R, Gladish N, Amenyogbe N, Drissler S, et al. Multi-omic data integration allows baseline immune signatures to predict hepatitis B vaccine response in a small cohort. Front Immunol (2020) 11:578801. doi: 10.3389/fimmu.2020.578801
31. Kollmann TR, Marchant A, Way SS. Vaccination strategies to enhance immunity in neonates. Science (2020) 368(6491):612–5. doi: 10.1126/science.aaz9447
32. Marchant A, Amenyogbe N, Kollmann TR, Goetghebuer T. Maternal HIV infection alters antimicrobial immunity in exposed and uninfected infants. Pediatr Infect Dis J (2020) 39(5):e47–8. doi: 10.1097/INF.0000000000002614
33. Marchant A, Sadarangani M, Garand M, Dauby N, Verhasselt V, Pereira L, et al. Maternal immunisation: collaborating with mother nature. Lancet Infect Dis (2017) 17(7):e197–208. doi: 10.1016/S1473-3099(17)30229-3
34. Bischoff AL, Følsgaard NV, Vissing NH, Birch S, Brix S, Bisgaard H. Airway mucosal immune-suppression in neonates of mothers receiving A(H1N1)pnd09 vaccination during pregnancy. Pediatr Infect Dis J (2015) 34(1):84–90. doi: 10.1097/INF.0000000000000529
35. Habibi MS, Thwaites RS, Chang M, Jozwik A, Paras A, Kirsebom F, et al. Neutrophilic inflammation in the respiratory mucosa predisposes to RSV infection. Science (2020) 370(6513):eaba9301. doi: 10.1126/science.aba9301
36. Khodr ZG, Bukowinski AT, Gumbs GR, Conlin AMS. Tetanus, diphtheria, and acellular pertussis vaccination during pregnancy and reduced risk of infant acute respiratory infections. Vaccine (2017) 35(42):5603–10. doi: 10.1016/j.vaccine.2017.08.041
Keywords: pregnancy, vaccination, preterm birth, influenza, pertussis
Citation: Giles ML, Cole S, O’Bryan J, Krishnaswamy S, Ben-Othman R, Amenyogbe N, Davey M-A and Kollmann T (2023) The PRotective Effect of Maternal Immunisation on preTerm birth: characterising the Underlying mechanisms and Role in newborn immune function: the PREMITUR study protocol. Front. Immunol. 14:1212320. doi: 10.3389/fimmu.2023.1212320
Received: 18 May 2023; Accepted: 04 December 2023;
Published: 20 December 2023.
Edited by:
Adrian John Frederick Luty, Institut de Recherche Pour le Développement (IRD), FranceReviewed by:
Lovro Lamot, University of Zagreb, CroatiaCopyright © 2023 Giles, Cole, O’Bryan, Krishnaswamy, Ben-Othman, Amenyogbe, Davey and Kollmann. This is an open-access article distributed under the terms of the Creative Commons Attribution License (CC BY). The use, distribution or reproduction in other forums is permitted, provided the original author(s) and the copyright owner(s) are credited and that the original publication in this journal is cited, in accordance with accepted academic practice. No use, distribution or reproduction is permitted which does not comply with these terms.
*Correspondence: Michelle L. Giles, bWljaGVsbGUuZ2lsZXNAbW9uYXNoLmVkdQ==
†Present addresses: Rym Ben-Othman, RAN BioLinks Ltd, Richmond Hill, ON, Canada
Tobias Kollmann, Department of Microbiology and Immunology, Dalhousie University, Halifax, NS, Canada
Nelly Amenyogbe, Department of Microbiology and Immunology, Dalhousie University, Halifax, NS, Canada
Disclaimer: All claims expressed in this article are solely those of the authors and do not necessarily represent those of their affiliated organizations, or those of the publisher, the editors and the reviewers. Any product that may be evaluated in this article or claim that may be made by its manufacturer is not guaranteed or endorsed by the publisher.
Research integrity at Frontiers
Learn more about the work of our research integrity team to safeguard the quality of each article we publish.