- 1Instituto Sírio-Libanês de Ensino e Pesquisa, Hospital Sírio-Libanês, São Paulo, Brazil
- 2Faculdade Israelita de Ciências da Saúde Albert Einstein, Hospital Israelita Albert Einstein, São Paulo, Brazil
- 3Departamento de Imunologia, Instituto de Ciências Biomédicas, Universidade de São Paulo, São Paulo, Brazil
- 4Departamento de Oncologia Clínica e Experimental, Escola Paulista de Medicina (UNIFESP), São Paulo, Brazil
Regular and moderate exercise is being used for therapeutic purposes in treating several diseases, including cancer, cardiovascular diseases, arthritis, and even chronic kidney diseases (CKDs). Conversely, extenuating physical exercise has long been pointed out as one of the sources of acute kidney injury (AKI) due to its severe impact on the body’s physiology. AKI development is associated with increased tubular necrosis, which initiates a cascade of inflammatory responses. The latter involves cytokine production, immune cell (macrophages, lymphocytes, and neutrophils, among others) activation, and increased oxidative stress. AKI can induce prolonged fibrosis stimulation, leading to CKD development. The need for therapeutic alternative treatments for AKI is still a relevant issue. In this context arises the question as to whether moderate, not extenuating, exercise could, on some level, prevent AKI. Several studies have shown that moderate exercise can help reduce tissue damage and increase the functional recovery of the kidneys after an acute injury. In particular, the immune system can be modulated by exercise, leading to a better recovery from different pathologies. In this review, we aimed to explore the role of exercise not as a trigger of AKI, but as a modulator of the inflammatory/immune system in the prevention or recovery from AKI in different scenarios. In AKI induced by ischemia and reperfusion, sepsis, diabetes, antibiotics, or chemotherapy, regular and/or moderate exercise could modulate the immune system toward a more regulatory immune response, presenting, in general, an anti-inflammatory profile. Exercise was shown to diminish oxidative stress, inflammatory markers (caspase-3, lactate dehydrogenase, and nitric oxide), inflammatory cytokines (interleukin (IL)-1b, IL-6, IL-8, and tumor necrosis factor-α (TNF-α)), modulate lymphocytes to an immune suppressive phenotype, and decrease tumor necrosis factor-β (TGF-β), a cytokine associated with fibrosis development. Thus, it creates an AKI recovery environment with less tissue damage, hypoxia, apoptosis, or fibrosis. In conclusion, the practice of regular moderate physical exercise has an impact on the immune system, favoring a regulatory and anti-inflammatory profile that prevents the occurrence of AKI and/or assists in the recovery from AKI. Moderate exercise should be considered for patients with AKI as a complementary therapy.
Introduction
Acute kidney injury (AKI) is characterized by a sudden reduced glomerular filtration rate and, therefore, decreased renal function, with renal structural damage. In addition, AKI can lead to more severe diseases such as cardiovascular events and the development of chronic kidney disease (CKD) (1–4). AKI is common in all countries and has an increased risk of death at any stage. It occurs in 8%–32% of patients admitted to hospital and affects more than 50% of patients in intensive care units (ICUs) (1, 5). Although there is a high prevalence in ICU patients, AKI can also affect patients without a critical illness (6–8). The mechanisms that cause AKI are related to poor oxygen and nutrient delivery to the nephrons, an imbalance in reactive oxygen species (ROS) or nitric oxide (NO) production, and an increased energy demand. This leads to cell death through necrosis or apoptosis, or both, triggering a cascade of inflammatory response and renal damage and dysfunction (1, 9).
Physical exercise is a subcategory of physical activity that is planned and done with regularity to improve or maintain health and fitness (10–12). There are several ways to measure the intensity of exercise: training heart rate (THR), metabolic equivalent (MET—compares oxygen consumption during rest and during activity), and a certain percentage of the VO2max, which is the maximum oxygen consumption/uptake of an individual (11–13). Exercises are deemed to be extenuating if they cause each individual’s heart rate to reach 80% of its maximum level or if they cause them to consume ≥65% of their VO2 max over the entire exercise session (14, 15). Moderate exercise is typically defined as when an individual reaches 46%–63% of their VO2 max, attains a MET score of 3–5.9, or reaches 40%–60% of their THR (4, 13). With regard to the intensity of exercise, the studies included in this review followed those concepts of extenuating and moderate exercises, and most of them were based on the VO2 max percentage criteria. For exercise regularity, we used the same criteria as the American College of Sports Medicine Guidelines, in which exercises are considered regular when they are done three or more times a week for at least 4 weeks (10).
It is well known that extenuating exercise increases the risk of AKI (14–22). Strenuous exercise, in which study subjects had to run at their verified speed to elicit 65% VO2 max on a −10% gradient treadmill, led to dehydration and muscle damage. In addition, most of the time these exercises were carried out in a hot environment, and this combination appeared to exacerbate the risk of AKI (23). Exercise-induced AKI is characterized by muscle soreness, cramps or muscular stiffness, headaches, nausea, vomiting, and often changes in urine color. These symptoms are likely to be related to ischemic renal failure or kidney injury because of rhabdomyolysis (17, 24).
The immune system plays a major role in the pathophysiology of AKI and CKD (25, 26). In AKI, there is much necrotic cell death, damage-associated molecular patterns (DAMPs), monocytes, M1 macrophages, and proinflammatory cytokines, specifically interleukin (IL)-6, IL-8, and tumor necrosis factor-α (TNF-α) (26, 27). In addition, activated T cells contribute to nephrotoxicity, whereas B cells do not have a defined role in AKI pathogenesis and maintenance. This hyper-activated inflammatory response leads to more necrosis, which enhances inflammation, creating a vicious cycle of inflammation and renal tissue damage. In CKD, macrophages have an important role, producing IL-1β, TNF-α, and IL-6, which contribute to renal inflammation. Those cells also produce tumor necrosis factor-β (TGF-β) and promote extracellular matrix deposition, leading to renal fibrosis. T cells contribute to tubulointerstitial fibrosis by inducing tubular cell death and producing interferon-γ (IFN-γ) and TNF-α. B cells can have an important role in CKD pathogenesis depending on the cause of the disease; for example, if it is autoimmune, B cells can be the source of autoimmune antibodies that cause glomerulonephritis (25).
Physical exercise is closely related to our immune system. Exercise and muscle fatigue can cause the production of reactive oxygen species (ROS), induce adenosine triphosphate (ATP) depletion, and trigger an inflammatory reaction, leading to an increased number of T-lymphocytes after exercise (28). On the other hand, there is growing evidence that physical exercise also has an anti-inflammatory effect on the human body (4). Excess visceral adipose tissue causes a systemic inflammatory environment through the production of adipokines, including TNF-α and IL-6 (29, 30). In addition, obesity predisposes t-lymphocyte and macrophage infiltration in the visceral adipose tissue, which maintains the inflammatory state (29). Therefore, exercising acts as an anti-inflammatory stimulus by reducing the adipose tissue, and a randomized control trial showed that the bigger the adipose tissue loss, the greater the anti-inflammatory effect of exercise (31). That trial was done under a training protocol with aerobic exercises for 12 weeks, with intensities ranging from 50% to 85% VO2 max, and also showed that contracting skeletal muscles produces anti-inflammatory myokines, such as IL-6, IL-15, and irisin (31). IL-6 myokine results in anti-inflammatory effects that are different from what would usually be expected. It increases the circulation of IL-10 and IL-1ra and inhibits lipopolysaccharide (LPS)-induced TNF-α (32). Last, but not least, Stewart et al. showed that regular exercise over 12 weeks reduced the TLR4 expression in CD14+ cells, thereby contributing to a less active inflammatory environment (33).
In this review, we have focused on the effects of regular aerobic exercise on immune system regulation and the therapeutic potential of physical exercise in treating AKI induced by different triggers (hypoperfusion/ischemia and reperfusion, sepsis, diabetes, antibiotic use, chemotherapy, etc.) as shown in Figure 1.
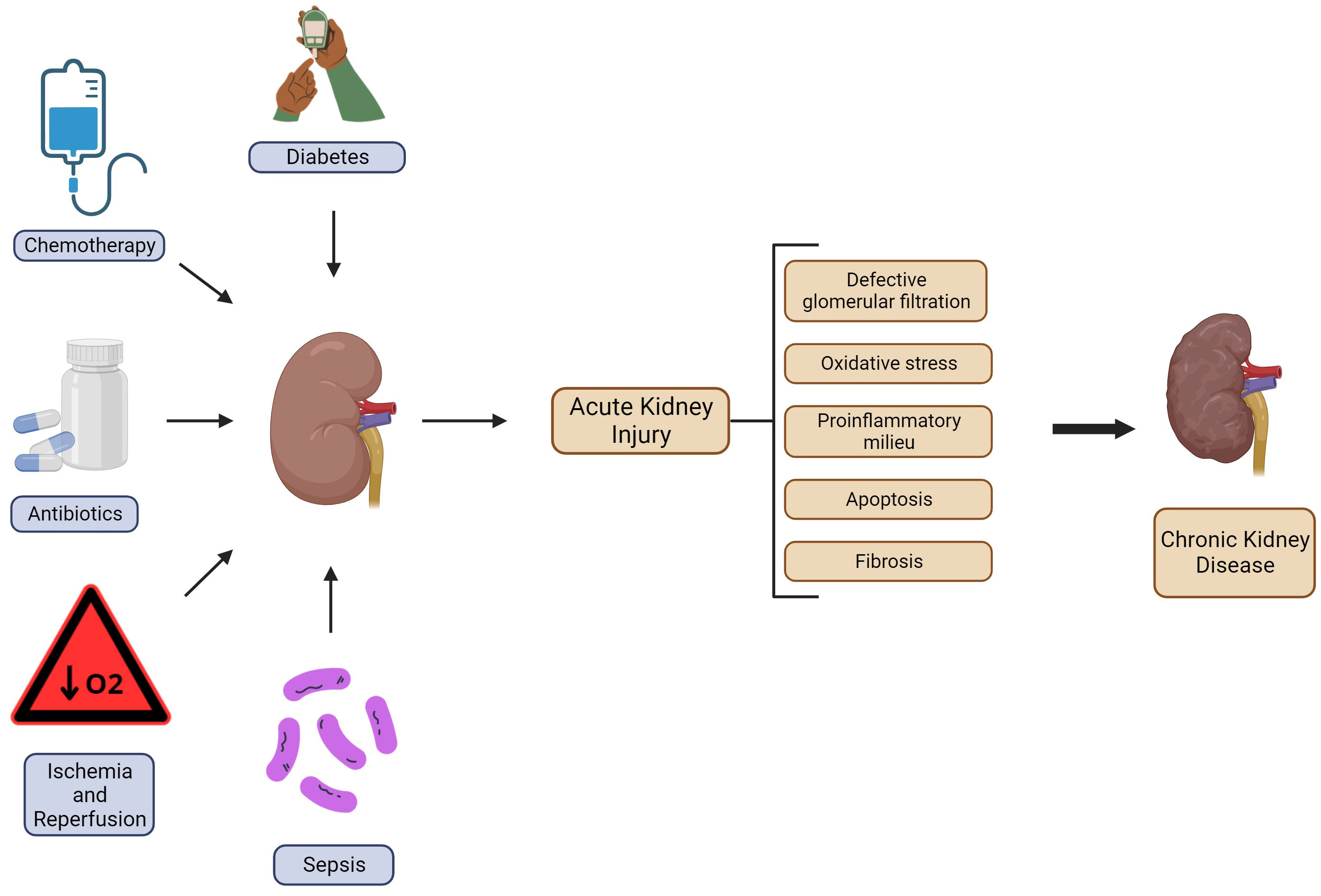
Figure 1 Schematic representation of AKI triggers and the mechanisms associated with CKD progression. Several situations can lead to AKI; the most common are diabetes, chemotherapy, use of antibiotics, ischemia and reperfusion, and sepsis. Once there is the onset of this pathologic condition, it can cause functional depletion, histologic damage, and a shift in the inflammatory response toward a proinflammatory milieu. In more severe cases it can progress to the development of CKD. Created with BioRender.com.
Ischemia and reperfusion
Renal hypoperfusion contributes to AKI development (3) and is extensively studied because of its contribution to the understanding of ischemia and reperfusion (IR) during kidney transplantation. A systematic review evaluating kidney transplant recipients, with structured training protocols consisting of exercises three times a week for 3–6 months, showed improvements in cardiorespiratory fitness and muscle strength (34). It has also been shown that cardiovascular reserves are relevant survival predictors for CKD and kidney transplantation (35). Based on these data, we assembled some studies that sought the effects of exercise on renal function in different hypoperfusion conditions.
Kidney IR is a widely utilized model based on clamping either one or both kidneys for a short period of time, which comprises the ischemia, and then removing the clamps to allow reperfusion. This process is known to cause great local inflammation that can affect the engraftment (36). In the Lima et al. (2019) (37) study, before IR induction, Wistar rats were subjected to 4 weeks of training five times a week, with progressive intensity and duration, not achieving exhaustion, and this group was named exercise plus ischemic/reperfusion (EX+IR). EX+IR rats presented with lower levels of serum creatinine, blood urea nitrogen (BUN), and proteinuria after 48 h of reperfusion than sedentary rats. The EX+IR group also had less renal tissue injury, probably due to the controlled cell death observed by caspase-3 expression (37). Similar results were observed in the Lee et al. (2010) study, in which rats underwent exercise training (running for 30 min a day, five times a week, for 4 weeks), and were then subjected to hemorrhagic shock (EHS). In addition to having a lower increase in BUN and serum creatinine levels, rats that went through the exercise program had higher survival rates and lower levels of the inflammatory marker lactate dehydrogenase (LDH) than rats that did not (38). These results show that preconditioning regular aerobic exercise is efficient in attenuating AKI outcomes.
Another instability of hemodynamic hypoperfusion that can lead to AKI is myocardial infarction, known as the “cardio-renal syndrome” (6). A myocardial infarction experimental model was performed in Wistar rats with a permanent left anterior descending artery ligation. Four weeks after myocardial infarction, the rats were randomly distributed into exercise-based or sedentary-based intervention groups, with and without L-arginine supplementation (39). The exercise protocol consisted of running on a treadmill at a speed of 10–17 meters per minute, for 20–50 min a day for 10 weeks. L-arginine has been used as a precursor of nitric oxide (NO), which has physiological properties such as vasodilatation and the reduction of oxidative stress (40). No significant difference was found in serum creatinine measurements among groups, although BUN levels were lower in the exercise group, with no difference from those in the exercise group that received supplementation. After 10 weeks, training with and without L-arginine supplementation decreased lipid peroxidation in myocardial infarction rats, leading to less kidney oxidative stress. This indicates that exercise after cardio-renal syndrome could ameliorate kidney function by diminishing inflammatory innate immunity (39).
Sepsis
Sepsis is a severe inflammatory disorder with high mortality and poor outcomes, causing end-organ dysfunction; it is a recurrent complication of AKI that raises mortality (6, 41). It has been shown that moderate and intense exercise can modulate neutrophils by improving the function of chemotaxis, phagocytosis, and microbicide (42).
In an animal sepsis model, after 4 weeks of treadmill training, rats received intravenously endotoxin (LPS from Escherichia coli). The training consisted of running on a treadmill at a speed of 12 meters per minute (m/min) for 15 min daily in the first week, 15 m/min for 30 min in the second week, 18m/min for 45 min in the third week, and 21 m/min for 60 min in the fourth week. In the acute phase, the trained group had increased neutrophils, and after 72 h the number of circulating lymphocytes was higher than in the sedentary group. The trained group also demonstrated attenuated septic responses and associated alterations due to endotoxin administration, and a reduced incidence of septic shock. They also exhibited less organ dysfunction measured by diminished levels of BUN and serum creatinine (kidney function biomarkers), creatine phosphokinase, LDH, aspartate aminotransferase, and alanine aminotransferase (liver damage biomarkers), and amylase and lipase (pancreas function biomarkers). The histopathological analysis also showed that endotoxin-induced organ damage was abrogated in trained rats. Exercise also attenuated the endotoxin-induced release of NO, free radicals, and proinflammatory cytokines such as TNF-α and IL-1β.
Mice subjected to the cecal ligation and puncture (CLP) sepsis model and that had previously trained on a treadmill at a speed of 8–12 m/min for 30 min three times a week for 8 weeks, showed less organ injury, fewer cell infiltration in the lungs, and increased survival rates. The trained animals presented with lower levels of the proinflammatory cytokines IL-1β, IL-6, and IL-8 in the peritoneal fluid and reduced IL-8 levels in the plasma. They also showed higher levels of the anti-inflammatory cytokine IL-10 in the peritoneal fluid and plasma, which demonstrates an attenuated inflammatory response (43).
In a severe polymicrobial sepsis model (fecal-induced peritonitis), mice were trained on a treadmill for 5 days a week for 6 weeks, with a stepwise adjustment of the duration and intensity of exercise from the first until the sixth week. Signs of morbidity were reduced and there was an increased survival rate (20% of the trained group survived while all the untrained animals died). In the acute phase post sepsis induction (6 h), trained mice demonstrated significantly lower concentrations of IL-6 and IL-10, with no difference in bacterial clearance between groups. After 24 hours IL-6 levels remained lower and increased bacterial clearance in all tissues was observed as being more significant in the lungs and blood. Moreover, regular exercise reduced signs of sepsis-associated AKI, with less tubular damage and twofold lower BUN levels (44). Trained mice also exhibited increased arginine and lysophosphatidylcholines plasma levels, metabolites that are likely to contribute to an immunomodulatory response that is beneficial for the host.
A major problem in sepsis is the phenomenon of a cytokine storm (45), which causes an amplified dysfunction response and depends on several factors, including individual genetics. Recent studies regarding COVID-19 sepsis and cytokine storms have helped to elucidate the relationship between this phenomenon and kidney injury, once the host immune response plays a major role in disease severity (46). Those studies have shown that cytokine-release syndrome causes tubular damage and endothelial dysfunction in kidney tissue, with IL-6 and IFNγ being the most relevant cytokines (47, 48). Those proinflammatory cytokines have their gene expression induced by the NF-kB pathway (47). In addition, IFNγ produced by natural killer cells in the tubulointerstitial tissue was demonstrated to be related to renal fibrosis and CKD progression (49). Furthermore, the systemic inflammation caused by a cytokine storm leads to septic shock, reducing cardiac output, and inducing pre-renal AKI (48). In addition, physical exercise in COVID-19 patients was shown to decrease inflammation, downregulating the mTOR and NF-kB pathways and possibly contributing to the prevention of severe outcomes (50). Thus, regular exercise has the potential to, at least partially, prevent cytokine storms by presenting a less exacerbated immune response against pathogen-associated molecular patterns (PAMPs) and DAMPs.
All of the abovementioned studies were conducted under aerobic exercise protocols, which are structured activities designed to increase cardiovascular and respiratory functions (10). Hence, aerobic physical exercise seems to protect against sepsis-associated organ injuries and reduce fatal outcomes and proinflammatory responses. These effects occur possibly due to the modulation of the immune system and the adaptation of kidney tissue, leading to a more efficient and balanced immune response. Other types of exercise, such as strength, mobility, and balance training, were not evaluated; therefore, further investigation is required to determine their contribution to sepsis-associated organ injuries.
Diabetes
It is well known that diabetes mellitus (DM) is associated with important clinical complications such as nephropathy, which is the most harmful chronic complication and increases the risk of CKD development (51, 52). Regular exercise training is highly recommended to patients with chronic diseases such as DM or hypertension, because of the benefits in preventing and managing the disease and better control of the symptoms, which has an impact on the disease’s outcome (53).
It has been shown in rats that aerobic exercise performed prior to DM induction improved metabolic control by reducing weight and controlling glycemic levels. The exercise protocol consisted of running on a treadmill 5 days a week for 4 weeks, for 20 min in the first week and increasing up to 1h by the end of the fourth week. The exercise also ameliorated renal function by increasing the glomerular filtration rate and decreasing proteinuria. The aerobic training attenuated structural changes, with fewer tubular and glomerular injuries as measured by the tubulointerstitial lesions score and glomerulosclerosis index. The trained group also presented less expansion of the extracellular matrix, with a decreased expression of TGF-β, and attenuated the accumulation of fibronectin and collagen IV. The same result was maintained, or even improved, with continuous exercise for 8 weeks after DM induction (54).
Similar results have been seen in a mouse model of moderate aerobic exercise training for 6 weeks, with 1 h of running on a treadmill per day at a speed of 12 m/min, 6 days/week, and after diabetes-induced renal injury. The exercise group achieved better glycemic control and decreased proteinuria and collagen IV levels. Furthermore, it was observed that aerobic exercise training mitigates mitochondrial dysfunction, with decreased superoxide production and improved membrane potential and ATP production. Tang et al. also found that aerobic exercise increased the renal expression of the immune modulator complex Sirtuin (Sirt)1/Peroxisome proliferator-activated receptor-gamma coactivator (PGC)-1α and this was correlated to a protective effect on kidney injury (55).
Another study conducted 8 weeks of training after DM induction, in which rats were subjected to exercise training that consisted of 60 min per day of running on a treadmill at 16 m/min, 5 days a week. It showed that exercise could improve renal function and reduce levels of albuminuria, lipid peroxidation, and thiobarbituric acid-reactive substances. These results suggest a decreased level of oxidative stress, showing an ameliorated urine excretion of NO and an absence of renal lesions (56). NO is synthesized in the tubular endothelium, over other tissues, and it is responsible for hemodynamic regulation, tubular function, vascularization control, and redox balance by increasing antioxidant products (57, 58).
Overall, exercise was shown to have renoprotective effects, preventing renal dysfunction and structural changes through immune regulation in diabetic-induced AKI.
Antibiotics
Gentamicin (Garamycin) is a widely used antibiotic in the treatment of severe Gram-negative bacterial infections. Unfortunately, it also causes a reduction in the glomerular filtration rate, increases the likelihood of proximal tubular necrosis, and induces oxidative stress in renal tissue, which is characterized by nephrotoxicity and usually leads to AKI (8, 59). Gentamicin is an aminoglycoside and the proximal tubule cells of the renal cortex have the ability to concentrate aminoglycosides several times more than plasma levels. Once gentamicin gets inside the cell it causes oxidative stress by acting on mitochondria and damaging cell membrane by phospholipidosis, leading to kidney injury (59, 60).
A study conducted in 2011 showed that rats treated with gentamicin for 10 days presented with decreased renal function and tissue injury, elevated NO levels in serum, diminished NO levels in urine, and no signs of expression of inducible nitric oxide synthase in the kidneys. All parameters were restored after 30 days without gentamicin (61). A similar study showed that 30 days of moderate running on a treadmill after 10 days of gentamicin treatment recovered NO urinary excretion. The rats ran at 16 m/min for 60 min a day, 5 days a week, for 30 days. The authors also evaluated another inflammatory component, ROS, which was measured through the presence of their byproduct, thiobarbituric acid-reactive substances. After the exercise, those substances were decreased in plasma, urine, and renal tissue, and there was increased production of the antioxidant defenses of the kidney catalase and glutathione. Gentamicin treatment elevated the pro-fibrotic cytokine TGF-β production and the lymphomononuclear infiltration in the renal tissue, which was restored to steady-state levels with exercise (62).
Taurine is an amino acid-like compound commonly found in mammalian tissues and is known to have a role in cellular plasma membrane stabilization and osmoregulation and have antioxidant effects (63). The use of taurine supplementation was shown to soothe kidney damage caused by gentamicin resulting in better renal function, more antioxidant agent activity, less hypoxia, and fewer histologic changes (64, 65). It has also been demonstrated that exercise increases taurine plasma concentrations (66–68) and decreases thiobarbituric acid-reactive substances (69). Because taurine is an essential amino acid found in various animal tissues in elevated concentrations, one could argue that the mechanism involved in gentamicin-nephrotoxicity reduction after exercise could be a result of the rise in taurine. Although further studies are required, these data suggest that moderate and regular exercise could improve the process of gentamicin-induced AKI recovery.
Chemotherapy
Chemotherapy is one of the oldest forms of cancer treatment, and it remains the most used option. Exercise has been proposed as a therapy to ameliorate the physical and psychological side effects of chemotherapy. Nephrotoxicity is one notable side effect associated with the use of chemotherapies, such as cisplatin or doxorubicin. Unfortunately, there is no efficient therapy to reduce cisplatin-induced nephrotoxicity (42, 70, 71).
A study with rats consisted of 30 min of continuous running on a treadmill, 5 days a week for 4 weeks prior to an intraperitoneal injection of cisplatin (5 mg/kg) or saline, in comparison to a sedentary-cisplatin or control group. Previous training ameliorated renal function and resulted in fewer tubulointerstitial lesions, less inflammation (as evidenced by decreased macrophage infiltration), and diminished IL-1β, as well as decreased urinary excretion of MCP-1 and TGF-β. Increased NO levels in renal tissue and phosphorylation of endothelial NO synthase (eNOS) were observed, contributing to renoprotection, which possibly indicates a preserved endothelial function (72).
Similar results were described by our group using mice that underwent exercise training for 6 weeks running on a treadmill 5 days a week, with the time spent training each day increasing from 30 min to 60 min each week. Exercise attenuated renal dysfunction and inflammation, with reduced cell death and increased expressions of IL-6 and heme oxygenase (HO-1) in the kidneys (73). HO-1 is an enzyme that catalyzes heme degradation. It is known to be a regulator of the inflammatory response as an antioxidant agent and is associated with cytoprotection in AKI and other renal diseases (74, 75).
We also investigated the role of adaptive immune cells in physical exercise AKI protection. Corroborating our previous data, less damage and fewer inflammatory profiles were seen. The training mouse group (submitted to the same training protocol used for Miyagi et al. (2014)) showed less renal dysfunction (serum creatinine levels) and less tissue damage, as shown by decreased KIM-1 expressions. The study suggests a different modulation of T cells in the kidneys after exercise, showing fewer CD4+ T cells with less activation (CD4+CD25+ and CD4+CD69+ decreased populations), but also a decrease in regulatory T cells (Treg CD4+FOXP3), which is believed to be a reflex of less T helper recruitment in general. Similarly, IL-10 and TNF-α production by CD4+ T cells in kidney lymph nodes were restored to basal levels in the exercise group when compared with the cisplatin-sedentary group (76).
Cardoso et al. (77) demonstrated that rats that regularly exercised on a voluntary running wheel for 2 months after doxorubicin-induced AKI showed less damage and better tissue recovery. They had fewer renal injuries, inflammatory responses, and collagen deposition in tubules, and milder thickening of the Bowman’s capsule. Although normal restorations could not be verified, it is evident that the attenuation of kidney damage after exercise likely improves kidney function. These results suggest that aerobic exercise is able to result in a less pronounced inflammatory profile by producing a lower amount of cytokines, leading to decreased kidney injuries (76), and influencing both innate and adaptive immune responses.
Other interesting data are that paclitaxel, a chemotherapic, has been associated with the amelioration of sepsis-induced AKI (78). The use of this chemotherapic in low doses seems to diminish fibrosis and modify miRNA expression patterns in kidney disease models in rats (79, 80). In this context, low doses of paclitaxel effects and immune modulation may have a synergic action with aerobic exercise effects. It is also interesting that the deacetylation of p53 has alleviated sepsis-induced AKI in an animal model (81) and that p53 plays an important role in AKI pathogenesis and repair (82). These data support the idea that cancer and AKI may have an intimate relationship, inclusive in their treatments, that is not yet well studied.
Collectively, these studies indicate that regular exercise might diminish AKI through downregulation of innate and adaptive immune effector responses.
Discussion
It is unquestionable that physical activity improves health, and it is positively correlated to the prevention and management of chronic diseases and the improvement of cardiologic and metabolic outcomes. It can also increase the expression of various growth factors and neurotransmitters in the brain, resulting in improvements in cognition, neurogenesis, and preventing different psychological diseases. Furthermore, exercise modulates the immune system, modifying its interaction with all tissues. Conversely, it is expected that a variety of responses among molecules, cell types, tissue, and organs stimulated during various types of exercise diverge in intensity, duration, and individual personal characteristics (83–85). AKI is an acute disease with the potential for late complications leading to CKD development with dialysis dependence (6), and it can affect transplant engraftment. The prevention and treatment of AKI still require major attention and further studies.
During exercise, our kidneys work to maintain adequate fluid and electrolyte homeostasis. In extenuating high-intensity exercise, blood redistribution to active body parts occurs, causing a decrease in renal perfusion and the filtration ratio (14, 86). On the other hand, moderate physical activity is also correlated with better kidney function and less kidney damage (87) and there are few solid studies describing the benefits of exercise in mitigating or even preventing AKI.
Tissue repair is mostly conducted by inflammation and fibrosis with collagen disposition. However, when this process occurs it may result in cell and tissue malfunction, and eventually culminate in CKD (88).
Moderate exercise has a positive impact on the immune system (42, 89). Physical exercise was able to modulate immune cells to a regulatory phenotype, associated with an increase in anti-inflammatory cytokines, regulating cytokine balance, such as TNF-α and IL-10 levels, as well as increases in IL-6 levels, and decreases of reactive oxygen and nitrogen species. This environment is less conducive to apoptosis and fibrotic persistence, therefore preserving glomerular filtration, preventing AKI, and diminishing the risk of CKD (4), as shown in the schematic in Figure 2.
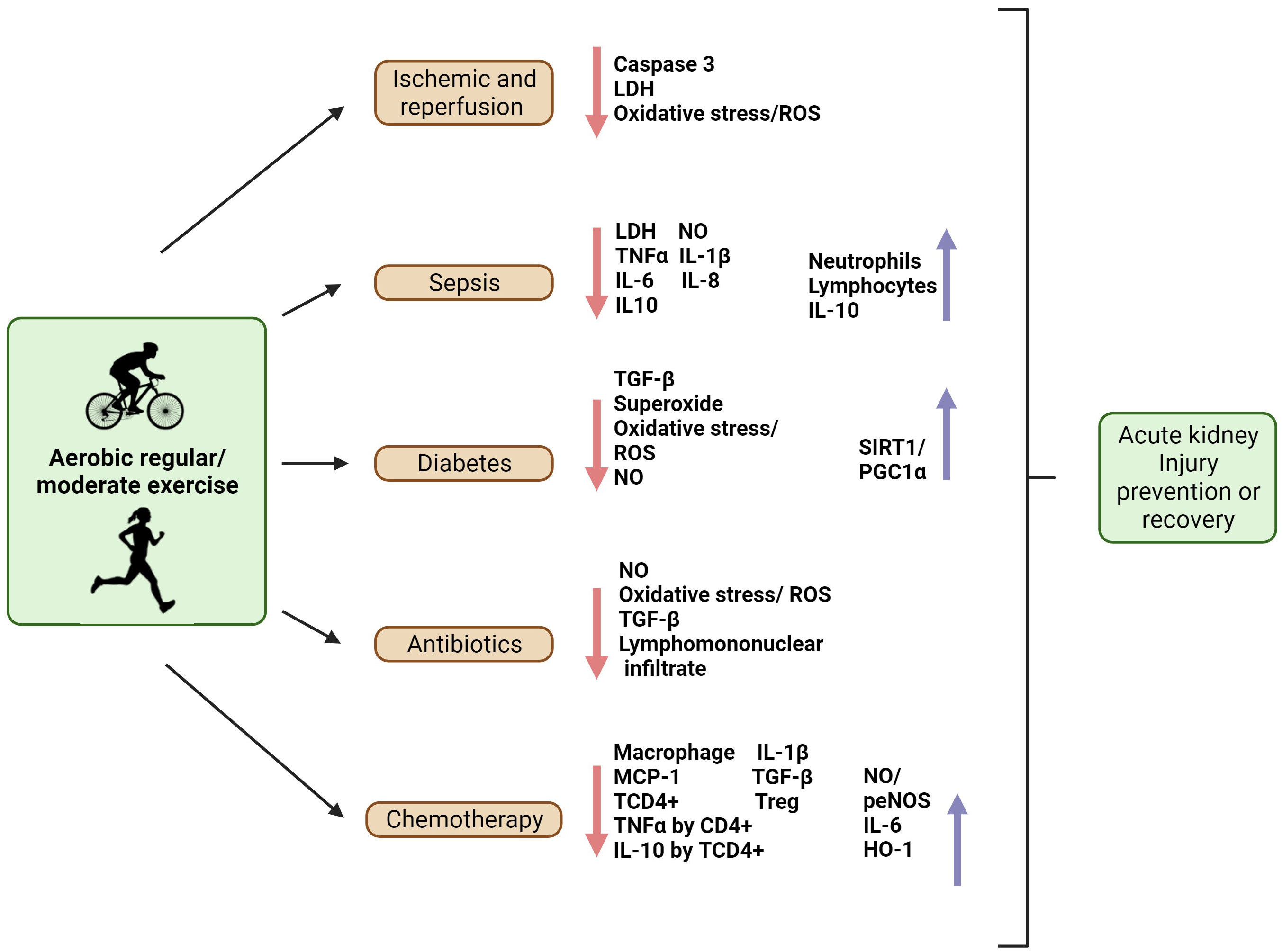
Figure 2 Aerobic regular/moderate exercise as an immunomodulator to prevent AKI or aid in the recovery of the kidney from AKI. In ischemia and reperfusion, exercise was shown to diminish caspase 3, oxidative stress (ROS), and LDH levels, providing a better recovery or preventing lesions in these situations. In the sepsis model, exercise promoted the recovery of neutrophils and lymphocytes, and decreased inflammatory markers such as LDH, NO, TNFα, and IL-1β, IL-6, and IL-8 levels; it might also modulate IL-10 levels. In diabetes, physical exercise prevents kidney injury by decreasing TGF-β, superoxide, oxidative stress, ROS, NO, and increasing Sirtuin (SIRT)1/Peroxisome proliferator-activated receptor-gamma coactivator (PGC)-1α. Exercise can prevent AKI induced by antibiotics by decreasing NO, ROS, TGF- β, and leukocyte infiltration. In chemotherapy-induced AKI, exercise can prevent kidney injury by decreasing macrophage, monocyte chemoattractant protein-1 (MCP-1), IL-1β, TGF-β, CD4+ T cells (TCD4+), regulatory T cells (Treg), TNF-α, and IL-10 levels produced by TCD4+, and inducing the production of NO, phosphorylation of endothelial NO synthase (p-eNOS), heme oxygenase (HO-)1, and IL-6. Created with BioRender.com.
These findings support the prescription of moderate and regular aerobic exercise for patients who are at risk of developing AKI and also for patients who have already been diagnosed with AKI. As physical exercise is a simple and low-cost intervention, this could be broadly applied at all levels of the health care system, producing great results by improving patients’ health and diminishing the burden of the disease.
Conclusion
In conclusion, regular practice of moderate physical exercise has an impact on the immune system, favoring a regulatory and anti-inflammatory profile, which prevents the development of and aids recovery from AKI. Protection from AKI induced by several triggers minimizes the risk of CKD. Under supervision, physical exercise has great potential for preventing AKI or treating patients with AKI.
Author contributions
AC-N and LB-A have contributed equally to this study. LB-A, WP, NC, and MA designed the review. AC-N, LB-A, EB-J, and MA wrote the text. AC-N, WP, NC, and MA revised the text and added intellectual content. AC-N, LB-A, EB-J, WP, and MA proofread the review. MA is the corresponding author. All authors contributed to the article and approved the submitted version.
Funding
This work was funded by Fundação de Amparo à Pesquisa do Estado de São Paulo (FAPESP) as grant (2017/05264-7) and fellowship (2018/20815-2 and, 2020/16491-7).
Conflict of interest
The authors declare that the research was conducted in the absence of any commercial or financial relationships that could be construed as a potential conflict of interest.
Publisher’s note
All claims expressed in this article are solely those of the authors and do not necessarily represent those of their affiliated organizations, or those of the publisher, the editors and the reviewers. Any product that may be evaluated in this article, or claim that may be made by its manufacturer, is not guaranteed or endorsed by the publisher.
References
1. Ronco C, Bellomo R, Kellum JA. Acute kidney injury. Lancet (2019) 394(10212):1949–64. doi: 10.1016/S0140-6736(19)32563-2
2. Chawla LS, Eggers PW, Star RA, Kimmel PL. Acute kidney injury and chronic kidney disease as interconnected syndromes. N Engl J Med (2014) 371(1):58–66. doi: 10.1056/NEJMra1214243
3. Kellum JA, Prowle JR. Paradigms of acute kidney injury in the intensive care setting. Nat Rev Nephrol (2018) 14(4):217–30. doi: 10.1038/nrneph.2017.184
4. Scheffer DDL, Latini A. Exercise-induced immune system response: Anti-inflammatory status on peripheral and central organs. Biochim Biophys Acta Mol Basis Dis (2020) 1866(10):165823. doi: 10.1016/j.bbadis.2020.165823
5. Hoste EAJ, Kellum JA, Selby NM, Zarbock A, Palevsky PM, Bagshaw SM, et al. Global epidemiology and outcomes of acute kidney injury. Nat Rev Nephrol (2018) 14(10):607–25. doi: 10.1038/s41581-018-0052-0
6. Makris K, Spanou L. Acute kidney injury: Definition, pathophysiology and clinical phenotypes. Clin Biochem Rev (2016) 37(2):85–98.
7. Watanabe T. [Prospect for the 21st century: the kidney]. Nihon Naika Gakkai Zasshi (2002) 91(12):3343–6.
8. Khalili H, Bairami S, Kargar M. Antibiotics induced acute kidney injury: incidence, risk factors, onset time and outcome. Acta Med Iran (2013) 51(12):871–8.
9. Togel F, Westenfelder C. Recent advances in the understanding of acute kidney injury. F1000Prime Rep (2014) 6:83. doi: 10.12703/P6-83
10. Pescatello LS, A.C.o.S. Medicine. ACSM's Guidelines for Exercise Testing and Prescription. Philadelphia, PA, USA: Wolters Kluwer Health (2014).
11. Hawley JA, Hargreaves M, Joyner MJ, Zierath JR. Integrative biology of exercise. Cell (2014) 159(4):738–49. doi: 10.1016/j.cell.2014.10.029
12. Garber CE, Blissmer B, Deschenes MR, Franklin BA, Lamonte MJ, Lee IM, et al. American College of Sports Medicine position stand. Quantity and quality of exercise for developing and maintaining cardiorespiratory, musculoskeletal, and neuromotor fitness in apparently healthy adults: guidance for prescribing exercise. Med Sci Sports Exerc (2011) 43(7):1334–59. doi: 10.1249/MSS.0b013e318213fefb
13. Kenney WL, Wilmore J, Costill D. Physiology of Sport and Exercise. 6th Edition. Champaign, IL, USA: Human Kinetics (2015).
14. Bongers CCWG, Alsady M, Nijenhuis T, Tulp ADM, Eijsvogels TMH, Deen PMT, et al. Impact of acute versus prolonged exercise and dehydration on kidney function and injury. Physiol Rep (2018) 6(11):e13734. doi: 10.14814/phy2.13734
15. Wu GL, Chen YS, Huang XD, Zhang LX. Exhaustive swimming exercise related kidney injury in rats - protective effects of acetylbritannilactone. Int J Sports Med (2012) 33(1):1–7. doi: 10.1055/s-0031-1284397
16. Lecina M, Castellar-Otín C, López-Laval I, Carrasco Páez L, Pradas F. Acute kidney injury and hyponatremia in ultra-trail racing: A systematic review. Medicina (Kaunas) (2022) 58(5):1–18. doi: 10.3390/medicina58050569
17. Kim J, Lee J, Kim S, Ryu HY, Cha KS, Sung DJ. Exercise-induced rhabdomyolysis mechanisms and prevention: A literature review. J Sport Health Sci (2016) 5(3):324–33. doi: 10.1016/j.jshs.2015.01.012
18. Spada TC, Silva JMRD, Francisco LS, Marçal LJ, Antonangelo L, Zanetta DMT, et al. High intensity resistance training causes muscle damage and increases biomarkers of acute kidney injury in healthy individuals. PloS One (2018) 13(11):e0205791. doi: 10.1371/journal.pone.0205791
19. Juett LA, James LJ, Mears SA. Effects of exercise on acute kidney injury biomarkers and the potential influence of fluid intake. Ann Nutr Metab (2020) 76 Suppl 1:53–9. doi: 10.1159/000515022
20. Kao WF, Hou SK, Chiu YH, Chou SL, Kuo FC, Wang SH, et al. Effects of 100-km ultramarathon on acute kidney injury. Clin J Sport Med (2015) 25(1):49–54. doi: 10.1097/JSM.0000000000000116
21. Mansour SG, Verma G, Pata RW, Martin TG, Perazella MA, Parikh CR. Kidney injury and repair biomarkers in marathon runners. Am J Kidney Dis (2017) 70(2):252–61. doi: 10.1053/j.ajkd.2017.01.045
22. Lipman GS, Krabak BJ, Waite BL, Logan SB, Menon A, Chan GK. A prospective cohort study of acute kidney injury in multi-stage ultramarathon runners: the Biochemistry in Endurance Runner Study (BIERS). Res Sports Med (2014) 22(2):185–92. doi: 10.1080/15438627.2014.881824
23. Junglee NA, Di Felice U, Dolci A, Fortes MB, Jibani MM, Lemmey AB, et al. Exercising in a hot environment with muscle damage: effects on acute kidney injury biomarkers and kidney function. Am J Physiol Renal Physiol (2013) 305(6):F813–20. doi: 10.1152/ajprenal.00091.2013
24. Kaito H, Ishimori S, Nozu K, Shima Y, Nakanishi K, Yoshikawa N, et al. Molecular background of urate transporter genes in patients with exercise-induced acute kidney injury. Am J Nephrol (2013) 38(4):316–20. doi: 10.1159/000355430
25. Qu L, Jiao B. The interplay between immune and metabolic pathways in kidney disease. Cells (2023) 12(12):1–17. doi: 10.3390/cells12121584
26. Singbartl K, Formeck CL, Kellum JA. Kidney-immune system crosstalk in AKI. Semin Nephrol (2019) 39(1):96–106. doi: 10.1016/j.semnephrol.2018.10.007
27. Sato Y, Yanagita M. Immune cells and inflammation in AKI to CKD progression. Am J Physiol Renal Physiol (2018) 315(6):F1501–12. doi: 10.1152/ajprenal.00195.2018
28. Finsterer J. Biomarkers of peripheral muscle fatigue during exercise. BMC Musculoskelet Disord (2012) 13:218. doi: 10.1186/1471-2474-13-218
29. Gleeson M, Bishop NC, Stensel DJ, Lindley MR, Mastana SS, Nimmo MA. The anti-inflammatory effects of exercise: mechanisms and implications for the prevention and treatment of disease. Nat Rev Immunol (2011) 11(9):607–15. doi: 10.1038/nri3041
30. Yudkin JS. Inflammation, obesity, and the metabolic syndrome. Horm Metab Res (2007) 39(10):707–9. doi: 10.1055/s-2007-985898
31. Wedell-Neergaard AS, Lang Lehrskov L, Christensen RH, Legaard GE, Dorph E, Larsen MK, et al. Exercise-induced changes in visceral adipose tissue mass are regulated by IL-6 signaling: A randomized controlled trial. Cell Metab (2019) 29(4):844–855 e3. doi: 10.1016/j.cmet.2018.12.007
32. Mathur N, Pedersen BK. Exercise as a mean to control low-grade systemic inflammation. Mediators Inflammation (2008) 2008:109502. doi: 10.1155/2008/109502
33. Stewart LK, Flynn MG, Campbell WW, Craig BA, Robinson JP, McFarlin BK, et al. Influence of exercise training and age on CD14+ cell-surface expression of toll-like receptor 2 and 4. Brain Behav Immun (2005) 19(5):389–97. doi: 10.1016/j.bbi.2005.04.003
34. Calella P, Hernández-Sánchez S, Garofalo C, Ruiz JR, Carrero JJ, Bellizzi V. Exercise training in kidney transplant recipients: a systematic review. J Nephrol (2019) 32(4):567–79. doi: 10.1007/s40620-019-00583-5
35. Ting SM, Iqbal H, Kanji H, Hamborg T, Aldridge N, Krishnan N, et al. Functional cardiovascular reserve predicts survival pre-kidney and post-kidney transplantation. J Am Soc Nephrol (2014) 25(1):187–95. doi: 10.1681/ASN.2013040348
36. Faucher Q, Alarcan H, Marquet P, Barin-Le Guellec C. Effects of ischemia-reperfusion on tubular cell membrane transporters and consequences in kidney transplantation. J Clin Med (2020) 9(8):1–29. doi: 10.3390/jcm9082610
37. de Lima WV, Visona I, Schor N, Almeida WS. Preconditioning by aerobic exercise reduces acute ischemic renal injury in rats. Physiol Rep (2019) 7(14):e14176. doi: 10.14814/phy2.14176
38. Lee CJ, Peng TC, Hsu BG, Harn HJ, Chao YF, Lee RP. Lack of exercise decreases survival and increases organ damage after hemorrhagic shock in rats. Biol Res Nurs (2010) 12(2):198–205. doi: 10.1177/1099800409353935
39. Ranjbar K, Nazem F, Sabrinezhad R, Nazari A. Aerobic training and L-arginine supplement attenuates myocardial infarction-induced kidney and liver injury in rats via reduced oxidative stress. Indian Heart J (2018) 70(4):538–43. doi: 10.1016/j.ihj.2017.08.011
40. Khalaf D, Krüger M, Wehland M, Infanger M, Grimm D. The Effects of Oral l-Arginine and l-Citrulline Supplementation on Blood Pressure. Nutrients (2019) 11(7):1–14. doi: 10.3390/nu11071679
41. Wu Y, Guo X, Peng Y, Fang Z, Zhang X. Roles and molecular mechanisms of physical exercise in sepsis treatment. Front Physiol (2022) 13:879430. doi: 10.3389/fphys.2022.879430
42. Giraldo E, Garcia JJ, Hinchado MD, Ortega E. Exercise intensity-dependent changes in the inflammatory response in sedentary women: role of neuroendocrine parameters in the neutrophil phagocytic process and the pro-/anti-inflammatory cytokine balance. Neuroimmunomodulation (2009) 16(4):237–44. doi: 10.1159/000212384
43. de Araújo CC, Silva JD, Samary CS, Guimarães IH, Marques PS, Oliveira GP, et al. Regular and moderate exercise before experimental sepsis reduces the risk of lung and distal organ injury. J Appl Physiol (1985) (2012) 112(7):1206–14. doi: 10.1152/japplphysiol.01061.2011
44. Sossdorf M, Fischer J, Meyer S, Dahlke K, Wissuwa B, Seidel C, et al. Physical exercise induces specific adaptations resulting in reduced organ injury and mortality during severe polymicrobial sepsis. Crit Care Med (2013) 41(10):e246–55. doi: 10.1097/CCM.0b013e31828a2ae3
45. Chousterman BG, Swirski FK, Weber GF. Cytokine storm and sepsis disease pathogenesis. Semin Immunopathol (2017) 39(5):517–28. doi: 10.1007/s00281-017-0639-8
46. Tay MZ, Poh CM, Rénia L, MacAry PA, Ng LFP. The trinity of COVID-19: immunity, inflammation and intervention. Nat Rev Immunol (2020) 20(6):363–74. doi: 10.1038/s41577-020-0311-8
47. Stasi A, Castellano G, Ranieri E, Infante B, Stallone G, Gesualdo L, et al. SARS-coV-2 and viral sepsis: immune dysfunction and implications in kidney failure. J Clin Med (2020) 9(12):1–23. doi: 10.3390/jcm9124057
48. Ahmadian E, Hosseiniyan Khatibi SM, Razi Soofiyani S, Abediazar S, Shoja MM, Ardalan M, et al. Covid-19 and kidney injury: Pathophysiology and molecular mechanisms. Rev Med Virol (2021) 31(3):e2176. doi: 10.1002/rmv.2176
49. Law BMP, Wilkinson R, Wang X, Kildey K, Lindner M, Rist MJ, et al. Interferon-gamma production by tubulointerstitial human CD56(bright) natural killer cells contributes to renal fibrosis and chronic kidney disease progression. Kidney Int (2017) 92(1):79–88. doi: 10.1016/j.kint.2017.02.006
50. Alves HR, Lomba GSB, Gonçalves-de-Albuquerque CF, Burth P. Irisin, exercise, and COVID-19. Front Endocrinol (Lausanne) (2022) 13:879066. doi: 10.3389/fendo.2022.879066
51. Pacilli A, Viazzi F, Fioretto P, Giorda C, Ceriello A, Genovese S, et al. Epidemiology of diabetic kidney disease in adult patients with type 1 diabetes in Italy: The AMD-Annals initiative. Diabetes Metab Res Rev (2017) 33(4):e2873. doi: 10.1002/dmrr.2873
52. Park CW. Diabetic kidney disease: from epidemiology to clinical perspectives. Diabetes Metab J (2014) 38(4):252–60. doi: 10.4093/dmj.2014.38.4.252
53. Harjutsalo V, Groop PH. Epidemiology and risk factors for diabetic kidney disease. Adv Chronic Kidney Dis (2014) 21(3):260–6. doi: 10.1053/j.ackd.2014.03.009
54. Amaral LS, Silva FA, Correia VB, Andrade CE, Dutra BA, Oliveira MV, et al. Beneficial effects of previous exercise training on renal changes in streptozotocin-induced diabetic female rats. Exp Biol Med (Maywood) (2016) 241(4):437–45. doi: 10.1177/1535370215609696
55. Tang LX, Wang B, Wu ZK. Aerobic exercise training alleviates renal injury by interfering with mitochondrial function in type-1 diabetic mice. Med Sci Monit (2018) 24:9081–9. doi: 10.12659/MSM.912877
56. Rodrigues AM, Bergamaschi CT, Araújo RC, Mouro MG, Rosa TS, Higa EM. Effects of training and nitric oxide on diabetic nephropathy progression in type I diabetic rats. Exp Biol Med (Maywood) (2011) 236(10):1180–7. doi: 10.1258/ebm.2011.011005
57. Stuart-Smith K. Demystified. Nitric oxide. Mol Pathol (2002) 55(6):360–6. doi: 10.1136/mp.55.6.360
58. Trepels T, Zeiher AM, Fichtlscherer S. The endothelium and inflammation. Endothelium (2006) 13(6):423–9. doi: 10.1080/10623320601061862
59. Ali BH, Za'abi M, Blunden G, Nemmar A. Experimental gentamicin nephrotoxicity and agents that modify it: a mini-review of recent research. Basic Clin Pharmacol Toxicol (2011) 109(4):225–32. doi: 10.1111/j.1742-7843.2011.00728.x
60. Quiros Y, Vicente-Vicente L, Morales AI, López-Novoa JM, López-Hernández FJ. An integrative overview on the mechanisms underlying the renal tubular cytotoxicity of gentamicin. Toxicol Sci (2011) 119(2):245–56. doi: 10.1093/toxsci/kfq267
61. Christo JS, Rodrigues AM, Mouro MG, Cenedeze MA, Simões Mde J, Schor N, et al. Nitric oxide (NO) is associated with gentamicin (GENTA) nephrotoxicity and the renal function recovery after suspension of GENTA treatment in rats. Nitric Oxide (2011) 24(2):77–83. doi: 10.1016/j.niox.2010.12.001
62. Oliveira CS, Rodrigues AM, Nogueira GB, Nascimento MA, Punaro GR, Higa EM. Moderate aerobic exercise on the recovery phase of gentamicin-induced acute kidney injury in rats. Life Sci (2017) 169:37–42. doi: 10.1016/j.lfs.2016.10.028
63. Seidel U, Huebbe P, Rimbach G. Taurine: A regulator of cellular redox homeostasis and skeletal muscle function. Mol Nutr Food Res (2019) 63(16):e1800569. doi: 10.1002/mnfr.201800569
64. Erdem A, Gündoğan NU, Usubütün A, Kilinç K, Erdem SR, Kara A, et al. The protective effect of taurine against gentamicin-induced acute tubular necrosis in rats. Nephrol Dial Transplant (2000) 15(8):1175–82. doi: 10.1093/ndt/15.8.1175
65. Miyazaki T, Bouscarel B, Ikegami T, Honda A, Matsuzaki YT. The protective effect of taurine against hepatic damage in a model of liver disease and hepatic stellate cells. Adv Exp Med Biol (2009) 643:293–303. doi: 10.1007/978-0-387-75681-3_30
66. Miyazaki T, Ishikura K, Honda A, Ra SG, Komine S, Miyamoto Y, et al. Increased N-acetyltaurine in serum and urine after endurance exercise in human. Adv Exp Med Biol (2015) 803:53–62. doi: 10.1007/978-3-319-15126-7_5
67. Miyazaki T, Nakamura Y, Ebina K, Mizushima T, Ra SG, Ishikura K, et al. Increased N-acetyltaurine in the skeletal muscle after endurance exercise in rat. Adv Exp Med Biol (2017) 975 Pt 1:403–11. doi: 10.1007/978-94-024-1079-2_33
68. Carru C, Da Boit M, Paliogiannis P, Zinellu A, Sotgia S, Sibson R, et al. Markers of oxidative stress, skeletal muscle mass and function, and their responses to resistance exercise training in older adults. Exp Gerontol (2018) 103:101–6. doi: 10.1016/j.exger.2017.12.024
69. Zhang M, Izumi I, Kagamimori S, Sokejima S, Yamagami T, Liu Z, et al. Role of taurine supplementation to prevent exercise-induced oxidative stress in healthy young men. Amino Acids (2004) 26(2):203–7. doi: 10.1007/s00726-003-0002-3
70. Schwartz AL, Mori M, Gao R, Nail LM, King ME. Exercise reduces daily fatigue in women with breast cancer receiving chemotherapy. Med Sci Sports Exerc (2001) 33(5):718–23. doi: 10.1097/00005768-200105000-00006
71. Smuder AJ. Exercise stimulates beneficial adaptations to diminish doxorubicin-induced cellular toxicity. Am J Physiol Regul Integr Comp Physiol (2019) 317(5):R662–72. doi: 10.1152/ajpregu.00161.2019
72. Francescato HDC, Almeida LF, Reis NG, Faleiros CM, Papoti M, Costa RS, et al. Previous exercise effects in cisplatin-induced renal lesions in rats. Kidney Blood Press Res (2018) 43(2):582–93. doi: 10.1159/000488964
73. Miyagi MY, Seelaender M, Castoldi A, de Almeida DC, Bacurau AV, Andrade-Oliveira V, et al. Long-term aerobic exercise protects against cisplatin-induced nephrotoxicity by modulating the expression of IL-6 and HO-1. PloS One (2014) 9(10):e108543. doi: 10.1371/journal.pone.0108543
74. Lever JM, Boddu R, George JF, Agarwal A. Heme oxygenase-1 in kidney health and disease. Antioxid Redox Signal (2016) 25(3):165–83. doi: 10.1089/ars.2016.6659
75. Correa-Costa M, Amano MT, Camara NO. Cytoprotection behind heme oxygenase-1 in renal diseases. World J Nephrol (2012) 1(1):4–11. doi: 10.5527/wjn.v1.i1.4
76. Miyagi MYS, Latancia MT, Testagrossa LA, Andrade-Oliveira V, Pereira WO, Hiyane MI, et al. Physical exercise contributes to cisplatin-induced nephrotoxicity protection with decreased CD4+ T cells activation. Mol Immunol (2018) 101:507–13. doi: 10.1016/j.molimm.2018.08.014
77. Cardoso DF, Coriolano HA, Duarte JA. Regular voluntary running has favorable histological effects on doxorubicin-induced kidney toxicity in Wistar rats. Cell Tissue Res (2018) 374(1):177–87. doi: 10.1007/s00441-018-2840-z
78. Xu L, Hu G, Xing P, Zhou M, Wang D. Paclitaxel alleviates the sepsis-induced acute kidney injury via lnc-MALAT1/miR-370-3p/HMGB1 axis. Life Sci (2020) 262:118505. doi: 10.1016/j.lfs.2020.118505
79. Zhang D, Sun L, Xian W, Liu F, Ling G, Xiao L, et al. Low-dose paclitaxel ameliorates renal fibrosis in rat UUO model by inhibition of TGF-beta/Smad activity. Lab Invest (2010) 90(3):436–47. doi: 10.1038/labinvest.2009.149
80. Sun L, Zhang D, Liu F, Xiang X, Ling G, Xiao L, et al. Low-dose paclitaxel ameliorates fibrosis in the remnant kidney model by down-regulating miR-192. J Pathol (2011) 225(3):364–77. doi: 10.1002/path.2961
81. Sun M, Li J, Mao L, Wu J, Deng Z, He M, et al. p53 deacetylation alleviates sepsis-induced acute kidney injury by promoting autophagy. Front Immunol (2021) 12:685523. doi: 10.3389/fimmu.2021.685523
82. Tang C, Ma Z, Zhu J, Liu Z, Liu Y, Liu Y, et al. P53 in kidney injury and repair: Mechanism and therapeutic potentials. Pharmacol Ther (2019) 195:5–12. doi: 10.1016/j.pharmthera.2018.10.013
83. Ruegsegger GN, Booth FW. Health benefits of exercise. Cold Spring Harb Perspect Med (2018) 8(7):1–15. doi: 10.1101/cshperspect.a029694
84. Chen FT, Hopman RJ, Huang CJ, Chu CH, Hillman CH, Hung TM, et al. The effect of exercise training on brain structure and function in older adults: A systematic review based on evidence from randomized control trials. J Clin Med (2020) 9(4):1–23. doi: 10.3390/jcm9040914
85. Pescatello LS, MacDonald HV, Lamberti L, Johnson BT. Exercise for hypertension: A prescription update integrating existing recommendations with emerging research. Curr Hypertens Rep (2015) 17(11):87. doi: 10.1007/s11906-015-0600-y
86. Bongers CCWG, Alsady M, Nijenhuis T, Hartman YAW, Eijsvogels TMH, Deen PMT, et al. Impact of acute versus repetitive moderate intensity endurance exercise on kidney injury markers. Physiol Rep (2017) 5(24):e13544. doi: 10.14814/phy2.13544
87. Martens RJH, van der Berg JD, Stehouwer CDA, Henry RMA, Bosma H, Dagnelie PC, et al. Amount and pattern of physical activity and sedentary behavior are associated with kidney function and kidney damage: The Maastricht Study. PloS One (2018) 13(4):e0195306. doi: 10.1371/journal.pone.0195306
88. Forni LG, Darmon M, Ostermann M, Oudemans-van Straaten HM, Pettilä V, Prowle JR, et al. Renal recovery after acute kidney injury. Intensive Care Med (2017) 43(6):855–66. doi: 10.1007/s00134-017-4809-x
Keywords: moderate exercise, immune system, cytokines, AKI -acute kidney injury, CKD - chronic kidney disease, regular exercise
Citation: Costanti-Nascimento AC, Brelaz-Abreu L, Bragança-Jardim E, Pereira WdO, Camara NOS and Amano MT (2023) Physical exercise as a friend not a foe in acute kidney diseases through immune system modulation. Front. Immunol. 14:1212163. doi: 10.3389/fimmu.2023.1212163
Received: 25 April 2023; Accepted: 26 September 2023;
Published: 20 October 2023.
Edited by:
Eduardo Zapaterra Campos, Federal University of Pernambuco, BrazilReviewed by:
Gabriel Rufino Estrela, National Institute of Diabetes and Digestive and Kidney Diseases (NIH), United StatesTayrine Ordonio Filgueira, Federal University of Pernambuco, Brazil
Copyright © 2023 Costanti-Nascimento, Brelaz-Abreu, Bragança-Jardim, Pereira, Camara and Amano. This is an open-access article distributed under the terms of the Creative Commons Attribution License (CC BY). The use, distribution or reproduction in other forums is permitted, provided the original author(s) and the copyright owner(s) are credited and that the original publication in this journal is cited, in accordance with accepted academic practice. No use, distribution or reproduction is permitted which does not comply with these terms.
*Correspondence: Mariane Tami Amano, bXRhbWFub0Btb2Noc2wub3JnLmJy
†These authors have contributed equally to this work